- 1IPMA-DivAv - Portuguese Institute for the Sea and Atmosphere, Division of Aquaculture Upgrading and Bioprospection, Algés, Portugal
- 2CIIMAR - Interdisciplinary Centre of Marine and Environmental Research, University of Porto, Matosinhos, Portugal
- 3UCIBIO - Applied Molecular Biosciences Unit, NOVA School of Science and Technology, Department of Chemistry, NOVA University of Lisbon, Caparica, Portugal
- 4MARE – Marine and Environmental Sciences Center & ARNET - Aquatic Research Network, Faculty of Sciences, University of Lisbon, Lisbon, Portugal
- 5IPMA-EPPO - Portuguese Institute for the Sea and Atmosphere, Aquaculture Research Station, Olhão, Portugal
- 6Associate Laboratory i4HB - Institute for Health and Bioeconomy, NOVA School of Science and Technology, NOVA University of Lisbon, Caparica, Portugal
The increasing demand for healthy aquatic products has contributed to the rapid growth of aquaculture. Despite the many progresses, over the years, in aquaculture production, animal welfare and reduction of the stress induced by captivity remain a challenge in this sector, and overcoming it is fundamental to improve animal growth performance and resilience to environmental threats (e.g., disease outbreaks and/or climate change impacts). To address this challenge, aquafeeds biofortified with natural ingredients with functional properties, such as macroalgae, have emerged as a promising strategy to enhance fish immunity. The present study aimed at evaluating the potential of Laminaria digitata to improve juvenile gilthead seabream (Sparus aurata) physiological status and resilience, through the modulation of immunomodulatory and antioxidant responses. To explore this hypothesis, four independent treatments were carried out in which fish were fed with different experimental aquafeeds: control feed and biofortified feeds with 1.5%, 3% and 6% of whole L. digitata (incorporated as a dried and powdered ingredient). Fish (n=9) were collected from each treatment upon 30 and 60 days of biofortification in order to assess animal growth and condition indexes, haematological parameters, innate humoral parameters (antiprotease (AP) activity, peroxidase (POD) activity and immunoglobulin M (IgM)) and oxidative stress biomarkers (lipid peroxidation (LPO), catalase (CAT) activity, glutathione S-transferase (GST) activity, superoxide dismutase (SOD) activity and total antioxidant capacity (T-AOC)). Significant differences (α = 0.05) between treatments in biomarker responses were tested through a nested-design ANOVA, followed by Tukey HSD multiple comparisons. Pearson correlations between the different endpoints were also explored. Results indicate that a dietary inclusion of 1.5% L. digitata is the most effective biofortification dose, significantly improving antioxidant responses of S. aurata, by increasing levels of CAT, GST and T-AOC, along with the reduction in LPO levels. Additionally, the inclusion of 1.5% L. digitata enhances immunity by increasing the levels of IgM and POD, while maintaining the growth performance and condition indices of the fish. These findings underscore the potential utilization of L. digitata as a functional feed ingredient in aquaculture with relevant immune and antioxidant attributes.
1 Introduction
Aquaculture is one of the fastest growing food sectors worldwide, driven by the increasing demand for healthy and nutritious aquatic products (FAO, 2022; Verdegem et al., 2023). Despite significant investments in technological advances aimed at optimizing the production and value of farmed seafood, nowadays, aquaculture expansion continues to be defied by several environmental threats that hamper farmed animals’ welfare, growth performance, quality, and survival. Among these threats, disease outbreaks are a primary concern for the sector, causing substantial animal and economical losses (Cain, 2022). In many instances, disease outbreaks in aquaculture facilities are not the result of an isolated factor (exposure to a specific pathogen), but rather a combination of multiple variables, such as the occurrence of co-infections (viral, bacterial, parasitic or fungal infections), exposure to abiotic conditions outside the species’ optimal range, and inappropriate nutrition, leading to a compromised immune system and increased formation of free radicals responsible for cell oxidation and damage (Yarahmadi et al., 2016; FAO, 2018; Cain, 2022). This complex interplay between the various stressors at stake and their effects emphasizes the urgent need to develop strategies to improve the welfare and resilience of aquaculture animals to environmental stressors.
Finding eco-friendly and cost-efficient ways of enhancing farmed animals’ immune system and antioxidant responses has now become more important than ever, as disease outbreaks are expected to become more frequent and severe in the future due to the foreseen changes of the climate, resulting in even greater losses for the aquaculture sector (FAO, 2022). It is widely acknowledged that the overall performance and disease resistance of fish are highly reliant on proper nutrition (Oliva-Teles, 2012). In this sense, an increasingly popular and promising strategy that is gaining attention in the aquaculture sector involves the use of functional feeds enriched with natural immunostimulant ingredients. The purpose of these feeds is not only covering the specific nutritional requirements of farmed fish species, but also to promote animal well-being and optimal functionality by enhancing their immune status (Passos et al., 2021). Seaweeds play a crucial role in this approach, as they are natural products with great potential as aquafeed ingredients, being rich in nutrients and biological active compounds, specifically polysaccharides (e.g., alginates, carrageenans, agar, fucoidan, β-glucan and mannitol), polyphenols (e.g., phenolic acids, phlorotannins, flavonoids, and halogenated derivatives) and carotenoids (e.g., fucoxanthin and β-carotene) (Holdt and Kraan, 2011; Costa et al., 2021). These bioactive compounds have demonstrated anti-inflammatory, immunomodulatory, antioxidant and antibacterial properties, making seaweeds valuable allies against fish pathogens and promoting overall fish health (Morais et al., 2020; Silva et al., 2020; Cai et al., 2021). Previous studies have reported that incorporating a wide range of seaweed species into aquafeeds yields promising health and immune outcomes for different aquatic species (Peixoto et al., 2019; Shi et al., 2019; Passos et al., 2021; Thépot et al., 2021; Thépot et al., 2022). However, while a fair amount of information on red and green macroalgae species’ nutritive and functional potential is already available, literature on brown macroalgae is still scarce. Laminaria digitata, a brown seaweed belonging to the Laminaria genus (Laminariaceae, Ochrophyta) is a native Irish species that is globally harvested and cultured (Purcell-Meyerink et al., 2021). The use of this species has had diverse applications in the food, pharmaceutical, bioenergy and animal sectors (Purcell-Meyerink et al., 2021), and recent studies have investigated its potential as a feed ingredient for several terrestrial farmed animal species, including chickens (Costa et al., 2022), pigs (Ribeiro et al., 2022; Ribeiro et al., 2023) and ruminants (Belanche et al., 2016; De La Moneda et al., 2019). Within the aquaculture context, only a very limited number of studies had demonstrated the positive impact of L. digitata in marine aquafeeds (Ribeiro et al., 2015; Ferreira et al., 2022b), specifically regarding its bioactive properties and their effects on immune parameters (Cipriano-Maack et al., 2016; Palstra et al., 2018). Hence, more research is needed to fully understand the potential of L. digitata as functional feed ingredient and validate its use as an efficient non-chemically based approach to mitigate disease outbreaks in aquaculture. To this purpose, defining the effective doses of seaweeds’ inclusion in aquafeeds (i.e., the dose that can trigger an immune and/or overall health improvement, while maintaining optimal feed digestibility and animal growth) is a crucial and rather challenging step, especially in the rearing of carnivorous marine species (e.g., gilthead seabream, Sparus aurata) that have particular nutritional requirements (FAO, 2023).
The main objective of the present study was to assess the functional potential of L. digitata (at different inclusion levels) as an effective approach to improve the immune (antiprotease activity, peroxidase activity and immunoglobulin M), antioxidant responses (lipid peroxidation, catalase activity, glutathione S-transferase activity, superoxide dismutase activity and total antioxidant capacity) and overall physiological well-being of farmed marine fish (Sparus aurata). The results herein presented are expected to highlight the potential of L. digitata as a promising functional feed ingredient whose use may contribute to the implementation of smarter, tailor-made and more environmentally-friendly aquaculture practices.
2 Materials and methods
2.1 Ethical statement
The trial was performed by researchers certified in animal experimentation (EU functions A and B), and the animal handling and sampling procedures were conducted in compliance with the ARRIVE guidelines (Animal research: reporting of in vivo experiments) and ethics for the care and use of animals, following the recommendations of the Federation of European Laboratory Animal Science Associations (FELASA) and Portuguese legislation for Laboratory Animal Science (EU Directive 2010/63; Decreto-Lei n° 113/2013). The study was approved by IPMA’s Animal Welfare and Ethics Body (ORBEA), overseen by the National Authority for the use of live animals, also known as the Directorate-General for Food and Veterinary (DGAV).
2.2 Seaweed collection and experimental diets
Brown macroalga, Laminaria digitata, were harvested by boat from the Parc Marin d’Iroise, a special area of conservation off the West coast of Brittany, France. The harvesters use the “Scoubidou” technology (Pérez and Barbaroux, 1997) to gather the biomass between 5-10 m deep which is landed in Lannildut and transported by truck 15 km away to Algaia’s factory in Lannilis. At the industrial facility, the biomass was selected, milled, and dried using a bench-top fluid-bed technology (TG200, Retsch, Germany). Dried samples were stored, packed, and sent to a company specialised in fish feed production (SPAROS Lda, Olhão, Portugal), which manufactured four diets with similar nutritional composition, considering the nutritional needs of juvenile gilthead seabream (Sparus aurata) (detailed feed composition can be consulted in Table 1). All diets were formulated with the same feed ingredients, differing only in the percentage of powdered dry macroalga inclusion in detriment of wheat meal: i) one commercial control diet without seaweed supplementation (0%, CTR); ii) supplemented with 1.5% L. digitata (1.5-LD; low; corresponding the most economically viable alternative); iii) supplemented with 3% L. digitata (3-LD; average); and iv) supplemented with 6% L. digitata (6-LD; high; though, less economically viable alternative).
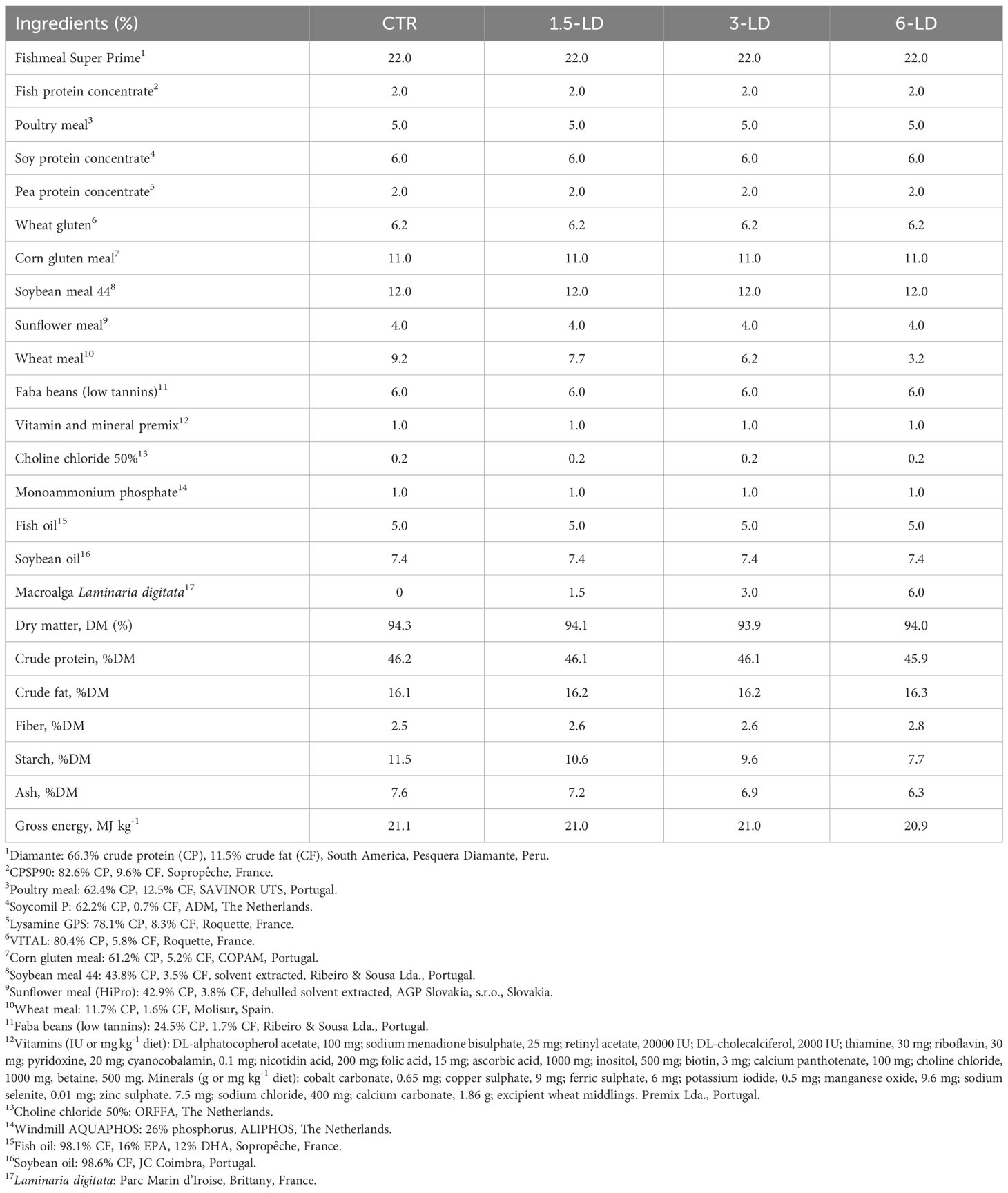
Table 1 Ingredient composition (%) and proximate analysis (%DM) of the experimental diets (CTR, 1.5-LD, 3-LD, and 6-LD) used to feed Sparus aurata juveniles.
2.3 Experimental trial and fish sampling
Sparus aurata specimens were reared until the juvenile stage (8.05 ± 0.60 g total weight; 8.2 ± 0.1 cm total length; mean ± standard deviation, n=120) at the Aquaculture Research Station of the Portuguese Institute for the Sea and Atmosphere (EPPO-IPMA, Olhão, Portugal) using routine hatchery conditions, and transported to IPMA’s Live Marine Organisms Bioterium (LABVIVOS) in Algés (Portugal). After a 3-week quarantine period, fish were transplanted to the experimental systems, being randomly distributed in 12 rectangular shaped glass tanks (50 L each, total volume), within independent recirculating aquaculture systems (RAS). The experimental setup used is schematized in Figure 1 and comprised four treatments: i) Control treatment (commercial diet without seaweed supplementation); ii) 1.5% Laminaria digitata (feed with 1.5% inclusion of L. digitata); iii) 3% Laminaria digitata (feed with 3% inclusion of L. digitata); and iv) 6% Laminaria digitata (feed with 6% inclusion of L. digitata).
To maintain seawater quality and abiotic parameters at the adequate levels, each system was equipped with: i) protein skimmers (V2Skim Pro Protein Skimmer 450, TMC Iberia, Portugal) to remove excessive organic compounds in the water; ii) ultra-violet (UV) disinfection (VE2cton 200, TMC Iberia, Portugal); iii) physical filtration composed of a filter bag (400 micron; TMC Iberia, Portugal), filter sponge and glass wool; iv) biological filtration (V2Bio 1000 Fluidised Sand Bed Filter and Bio Balls 1.5” Aquarium Pond Filter); v) automatic seawater refrigeration systems (Foshan WEINUO Refrigeration Equipment Co., Ltd, China) and submerged digital thermostats (300W, V2Therm Digital Heaters, TMC Iberia, Portugal) to control seawater temperature; and vi) submerged air stones displayed in each tank to control dissolved oxygen. Furthermore, fish faeces were removed daily in each incubation tank, and a 25% seawater renewal was performed. The system temperature was maintained at 20.0 ± 0.5°C and dissolved oxygen was kept at 7.2 ± 0.2 mg L-1, whereas salinity and pH were 35.0 ± 0.5‰ and 8.0 ± 0.1 units, respectively. Throughout the experiment we adhered to a 12h light/12h dark photoperiod regime. Water quality parameters such as ammonia (NH3/NH4), nitrite (NO2-) and nitrate (NO3-) levels were measured on a weekly basis using colorimetric tests (Tropic Marin, USA) and were kept below detectable levels, except for nitrates which were kept < 50 mg L-1. Temperature was measured daily with a portable precision thermometer (TFX 430, Ebro Electronic, Germany), while the remaining seawater abiotic parameters (salinity, dissolved oxygen and pH) were monitored using a multi-parameter measuring instrument (Multi 3420 SET G, WTW, Germany), connected to an IDS (intelligent, digital sensor) digital conductivity cell (TetraCon® 925, WTW, Germany), an optical IDS DO sensor (FDO® 925, WTW, Germany) and an IDS pH-electrode (SenTix® 940, WTW, Germany). The seawater abiotic parameters were adjusted whenever necessary and remained stable throughout the experiment. Prior to initiating the trial, fish were allowed to acclimate to the experimental tanks for a week. During this period (and the quarantine), all animals were hand-fed twice a day with the commercial control diet (2% average body weight, bw). Once acclimated to experimental conditions, the feeding trial was initiated by hand-feeding fish with different experimental feeds (CTR, 1.5%, 3% or 6%) according to the treatment. No mortality occurred during the 60 days of trial. After 30 days (T30) and 60 days (T60) of feeding trial, nine fish per treatment (i.e. three fish from each replicate tank) were randomly collected and anaesthetized by immersion for 10 minutes in an overdosed tricaine methanesulfonate solution (2 g L-1 of MS-222, Acros Organics, Belgium), buffered with sodium bicarbonate (NaHCO3, Sigma-Aldrich, USA), using a ratio of 1:2 of MS-222:Sodium bicarbonate to reduce fish stress. Once the anaesthetic took effect, fish were weighted (g, total weight, TW) on an analytical scale (EMS 300-3, Kern & Sohn, Germany), measured (cm; total length, TL) and peripheral blood was collected from the caudal vein using heparinized syringes. A fraction of fish blood was used to perform haematological analyses, while the remaining blood was transferred into a microtube (1.5 mL) with 20 μL of heparin (3000 U mL-1 in saline solution 0.9% NaCl, Sigma-Aldrich, USA), centrifuged at 10,000 ×g and 4°C for 10 minutes (Fisher Scientific AccuSpin Micro 17R Centrifuge, Germany) to isolate plasma, and then stored at -80°C until assayed for innate humoral parameters. The fish was subsequently dissected, and the spleen was collected, weighed and homogenized in ice-cold conditions with 1.0 mL of phosphate buffered saline pH 7.4 (PBS; 137 mM NaCl, 2.7 mM KCl, 10 mM Na2HPO4, 1.8 mM KH2PO4; reagents from Sigma-Aldrich, USA), using an Ultra-Turrax® device (T25 digital, Ika, Germany). Homogenates were centrifuged in 1.5 mL microtubes at 10,000 ×g and 4°C for 10 min, and the supernatants were transferred to new microtubes, immediately frozen and kept at -80°C until further analyses.
2.4 Fish growth and body condition indexes
According to Ricker (1975), Fulton’s condition index (K) was calculated directly from the biometric data to determine the condition of the fish:
where TW is the fish total weight (g), and TL is the total length (cm).
To determine the condition of the spleen and assess its relative size compared to the total body weight of the fish, the splenosomatic index (SSI) was calculated using the following equation:
where TW is the fish total weight (g).
The weight gain rate (WGR) was calculated using the following formula:
The specific growth rate (SGR) was determined to describe the growth rate of fish, based on the natural logarithm of body weight, using the final body weight and the initial mean body weight and the number of days in the experiment:
The survival ratio (SR) was calculated using the formula:
Feed conversion ratio (FCR) was measured based on the amount of feed fed and the fish weight gain during a period, with lower FCR values indicating higher efficiency:
2.5 Haematological parameters
A fraction of the peripheral blood was used immediately after collection to perform the trypan blue exclusion test for cell viability (Strober, 2001).
Briefly, a 0.4% solution of trypan blue (Sigma-Aldrich, USA) was prepared in PBS pH 7.2 (137 mM NaCl, 2.7 mM KCl, 10 mM Na2HPO4, 1.8 mM KH2PO4; reagents from Sigma-Aldrich, USA) and then 500 µL of diluted blood samples (1:50 in PBS) were added to 100 μL trypan blue in 1.5 mL microtubes. The mixed sample was incubated (≈ 3 minutes), loaded on a Neubauer chamber (Improved Neubauer Hausser Bright-Line, USA) and analysed using an optical microscope (LEICA DM2500, Germany) (three observations per treatment; the average of the three measurements was used in statistical analysis).
Fish blood smears were also performed on precleaned glass microscopy slides (n= 3 individuals per treatment, i.e., one smear per individual; VWR, USA) by placing a drop of blood that was spread by capillarity. The smears were allowed to air-dry overnight at room temperature, and then fixed with methanol (95%) (Sigma-Aldrich, USA) for 15 s (Kaplow and Ladd, 1965). After 24 h, the fixed blood smears were stained with the ready-to-use Hemacolor staining reagent (Hemacolor® Rapid staining of blood smear, Sigma-Aldrich), according to manufacturer instructions and mounted with Entellan® (Sigma-Aldrich, USA) medium. Then, slides were examined using an optical microscope (LEICA DM2500, Germany) and a minimum of 500 cells per slide were examined. Normal blood cells (erythrocytes and leukocytes) and erythrocytes nuclear abnormalities (ENAs) were counted based on criteria outlined by Carrasco et al. (1990).
2.6 Biochemical analyses
For biochemical biomarkers, all samples were analysed, at least, in triplicate, using 96-well microplates (Greiner Bio-one, Austria) and a Multiskan GO 1510 microplate reader (ThermoFisher Scientific, USA). All reagents and standards were of pro analysis (or superior) grade. The procedures are extensively described in the Supplementary Materials (see Supplementary Table S1).
2.6.1 Innate humoral parameters
Plasma samples were used to assess the following humoral immunological parameters: antiprotease (AP) activity, peroxidase (POD) activity and immunoglobulin M (IgM).
2.6.1.1 Antiprotease activity
Total antiprotease activity was determined following a method developed by Ellis (1990), modified by Hanif et al. (2004), with minor adaptations described in detail by Espinosa-Ruiz et al. (2021). This approach is based on the ability of plasma antiproteases to inhibit trypsin activity. The absorbance was measured at 450 nm and the inhibitory capacity of antiprotease was expressed as percentage trypsin inhibition, using the positive control as described by Zuo and Woo (1997).
2.6.1.2 Peroxidase activity
Peroxidase activity was assessed according to Quade and Roth (1997), with minor modifications described in detail by Espinosa-Ruiz et al. (2021). Peroxidase activity was determined by oxidation of 3,3′,5,5′ -Tetramethylbenzidine (TMB), with the absorbance measured at 450 nm. Peroxidase activity (units mL-1 plasma) was calculated by defining one unit of peroxidase as the amount required to produce an absorbance change of 1 OD (optical density).
2.6.1.3 Immunoglobulin M
Plasma immunoglobulin M was measured by an indirect Enzyme-Linked Immunosorbent Assay (ELISA) based on the protocol of Cuesta and Meseguer (2004), with some modifications described in detail by Espinosa-Ruiz et al. (2021). The absorbance was read at 450 nm and IgM concentrations (mg mL-1) were quantified based on a calibration curve ranging from 0 to 200 µg mL-1, performed with a positive control standard (IgM from human serum, Sigma-Aldrich, USA). Negative controls consisted of samples with no plasma or without primary antibody, whose absorbance values were subtracted for each sample value.
2.6.2 Oxidative stress
Spleen samples were used to determine oxidative stress biomarkers: lipid peroxidation (LPO), catalase (CAT) activity, glutathione S-transferase (GST) activity, superoxide dismutase (SOD) activity and total antioxidant capacity (T-AOC). Results (except for SOD, % inhibition) were normalized according to samples protein content (i.e., expressed in mg of total protein) determined through the Bradford (1976) method.
2.6.2.1 Lipid peroxidation
Lipid peroxidation was determined using a 96-well microplates protocol adapted from the thiobarbituric acid reactive substances (TBARS) method of Uchiyama and Mihara (1978). LPO was determined through the quantification of specific end-product of the lipid oxidative degradation process, i.e., malondialdehyde (MDA). MDA concentrations were quantified based on an eight-point calibration curve ranging from 0 to 0.3 mM TBARS, performed with malondialdehyde bis-(dimethyl acetal) standards (MDA; Merck, Germany). The absorbance was measured at 532 nm and the results were expressed in relation to total protein content, in nmol mg-1 protein.
2.6.2.2 Catalase activity
Catalase activity (EC 1.11.1.6) was determined following the method of Johansson and Borg (1988), adapted to 96-well microplates, as described by Maulvault et al. (2017). A calibration curve was built using formaldehyde standards, with concentrations ranging from 0 to 75 μM of formaldehyde (Sigma-Aldrich, USA). Absorbance was measured at 540 nm and results were expressed in terms of total protein content (nmol min-1 mg-1 protein). The formaldehyde concentration of the samples was first calculated using the equation derived from the linear regression of the standard curve. Catalase activity was then calculated, considering that one unit of catalase is defined as the amount of enzyme that produces 1.0 nmol of formaldehyde per minute at 25°C.
2.6.2.3 Glutathione S-transferase activity
Glutathione S-transferase activity (EC 2.5.1.18) was determined according to the method of Habig et al. (1974), and adapted to 96-well microplates, described by Maulvault et al. (2017). This method uses 1-Chloro-2,4-dinitrobenzene (CDNB; Sigma-Aldrich, Germany) as substrate and is based on the enzyme’s ability to conjugate xenobiotics into less toxic conjugates, that is the conjugation of glutathione (GSH) thiol group with CDNB (1-chloro-2,4-dinitrobenzene) substrate. The formation of this conjugate is proportional to the increase of GST activity and is followed spectrophotometrically every minute. Absorbance was read at 340 nm every minute for 6 minutes and the results were expressed relative to the total protein content of the samples as nmol min-1 mg-1 protein.
2.6.2.4 Superoxide dismutase activity
Superoxide dismutase activity (EC 1.15.1.1) was determined following the methodology described by Sun et al. (1988), adapted to 96-well microplates, and used by Maulvault et al. (2017). This method uses Nitro Blue Tetrazolium (NBT) and Xanthine Oxidase (XOD) as substrate. The absorbance was recorded at 560 nm every minute for 5 min, and then read at 10 and 15 min. The results were presented as the percentage of enzyme inhibition.
2.6.2.5 Total antioxidant capacity
Total antioxidant capacity was determined according to the method described by Kambayashi et al. (2009). T-AOC was calculated from a seven-point calibration curve, based on a series of Trolox standards, ranging from 0 to 0.33 mM, performed with (±)-6-Hydroxy-2,5,7,8-tetramethylchromane-2-carboxylic acid (Trolox, Sigma-Aldrich, USA). The absorbance was measured at 410 nm and the results were expressed in relation to total protein content, in nmol mg-1 protein.
2.7 Data analysis
Significant differences between treatments in growth parameters, body condition indexes , haematology results and biomarker levels were assessed using a one-way nested-ANOVA analysis (with time, T30 and T60, nested in treatment). Data were Log transformed, whenever necessary, to comply with the assumptions of normality (Kolmogorov–Smirnov’s test) and homogeneity of variances (Levene’s test) required for this analysis. Subsequently, the post-hoc Tukey HSD test was carried out to identify significant differences. Significant correlations between biomarker levels and animal condition indexes (K and SSI), as well as T-AOC and LPO between the other oxidative stress biomarkers (CAT, SOD and GST), were determined by means of Pearson’s correlation analysis. Statistical analyses were performed at a significance level of 0.05, using STATISTICA™ software (Version 7.0, StatSoft Inc., USA).
3 Results
3.1 Fish growth and body condition indexes
Growth performance and body condition indexes are presented in Table 2. Upon 30 and 60 days of feeding trial (T30 and T60), no significant differences were observed among treatments (p > 0.05). As for the effect of feeding duration (i.e., T30 versus T60, for the same treatment), a significant increase in total weight (TW), total length (TL) and weight gain rate (WGR) parameters was observed in the control treatment (↑26.9% TW; ↑7.6% TL; ↑34.6% WGR) and in the treatments with 3% L. digitata (↑23.3% TW; ↑8.0% TL; ↑43.0% WGR) and 6% L. digitata (↑21.9% TW; ↑6.8% TL; ↑45.1% WGR).
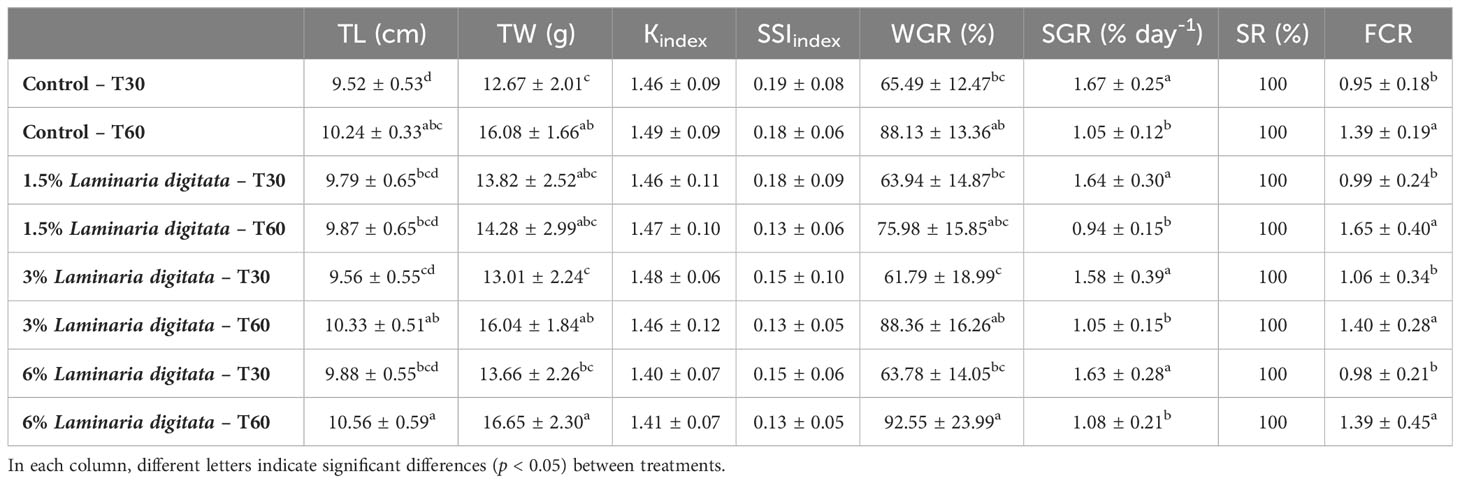
Table 2 Total length (TL; cm) and weight (TW; g), Fulton’s condition index (K), splenosomatic index (SSI), weight gain rate (WGR), specific growth rate (SGR), survival ratio (SR) and feed conversion ratio (FCR) in Sparus aurata after 30 days (T30) and 60 days (T60) of feeding trial (mean ± SD; n=9).
The specific growth rate (SGR) was significantly higher during the first 30 days of the feeding trial (p < 0.05) in all treatments, while the feed conversion rate (FCR) was significantly lower (p < 0.05; Table 2).
Fulton’s condition index (K), splenosomatic index (SSI) and survival ratio (SR) were not significantly affected by treatment or time (p > 0.05; Table 2).
A strong positive correlation was found between fish total weight and length (r = 0.941; p < 0.001), yet no significant correlations were found between K and the splenosomatic index (SSI, r = 0.096; p > 0.05; Table 3).
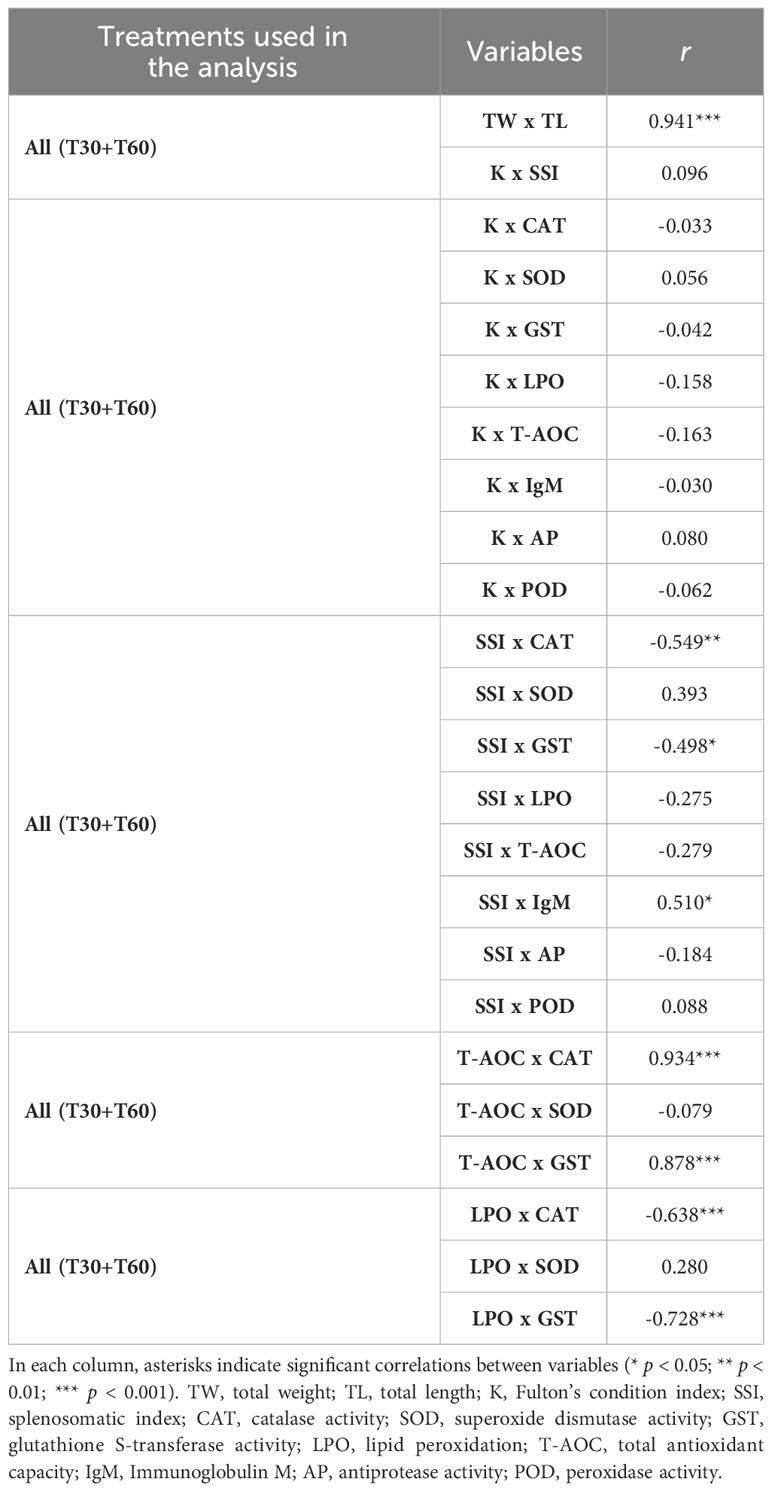
Table 3 Pearson correlation coefficients (r) between animal growth, condition indexes and biomarker responses.
3.2 Haematological profile
The haematological profile was evaluated in juvenile Sparus aurata fed for 30 and 60 days with aquafeeds supplemented with different levels of L. digitata. The results indicated that the experimental diets did not significantly affect the percentages of erythrocytes, leucocytes, viable erythrocytes, and ENAs at both T30 and T60 sampling dates (see Table 4; p > 0.05).
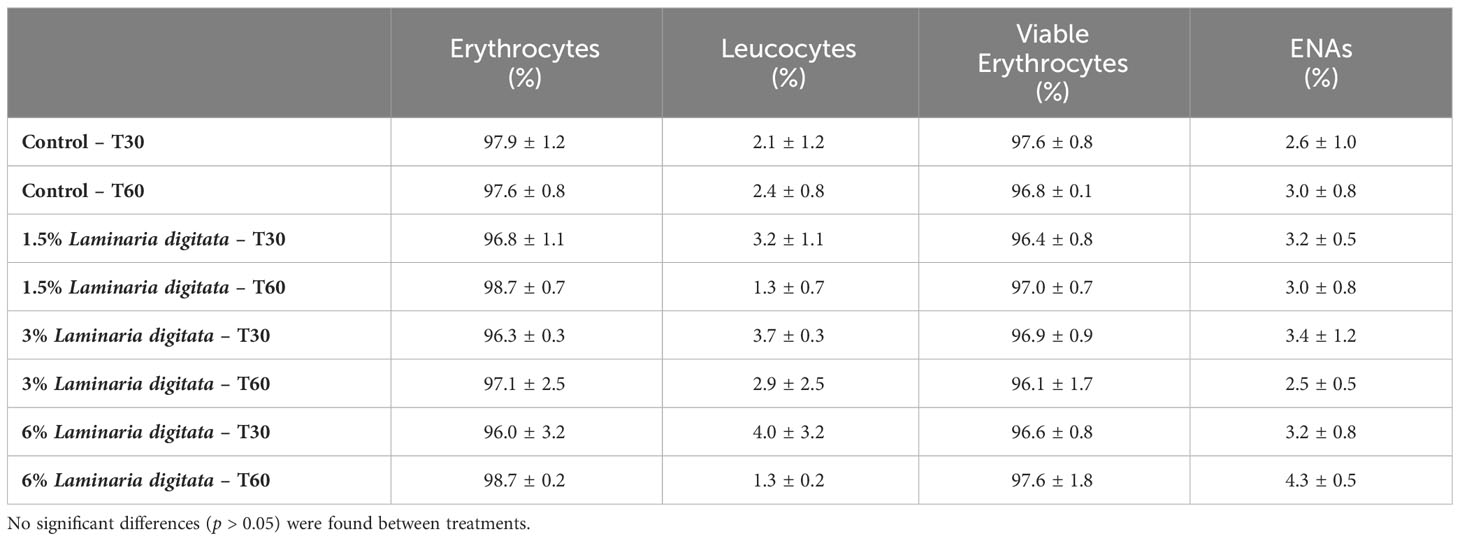
Table 4 Percentage of erythrocytes and leukocytes in relation to total cell counts, as well as percentage of viable erythrocytes and erythrocytes nuclear abnormalities (ENAs) in relation to total erythrocyte counts (mean ± SD; n=3). .
Leucocyte percentages across the different treatments consistently remained below 4.0% of the total cell counts, whereas the erythrocyte percentages were consistently high, accounting for over 96.0% of total cells (Table 4). Moreover, out of these erythrocytes, more than 96.1% were considered viable, and the occurrence of nuclear abnormalities was minimal, with percentages ranging between 2.5 and 4.3% (Table 4).
3.3 Immune humoral parameters
Fish immune humoral responses upon 30 and 60 days of feeding trial are presented in Figure 2. The duration of feeding (30 or 60 days) had no significant impact on the immune parameters of fish fed with the control feed (p > 0.05), indicating that the immune response of the control treatment remained consistent regardless of the feeding duration. The same trend was observed in fish fed with the experimental feeds containing 3% (3-LD) and 6% (6-LD) of L. digitata. However, fish fed with the feed containing 1.5% of macroalga (1.5-LD) exhibited a different pattern, i.e., a reduction in IgM content (↓35%; Figure 2A) and POD activity (↓41%; Figure 2C) between 30 and 60 days. Nevertheless, the IgM content and POD activity were significantly higher in fish fed with 1.5% of L. digitata (1.5-LD) compared to the remaining treatments. In terms of IgM, the 3-LD and 6-LD feeds did not differ significantly from the CTR, while for POD, the 3-LD feed was significantly higher than the CTR, but lower than the 1.5-LD treatment. Notably, antiprotease activity did not show significant differences between the experimental feeds with L. digitata inclusion and the respective commercial feed, with only a slight difference observed between 6-LD-T30 and 1.5-LD-T60 (Figure 2B).
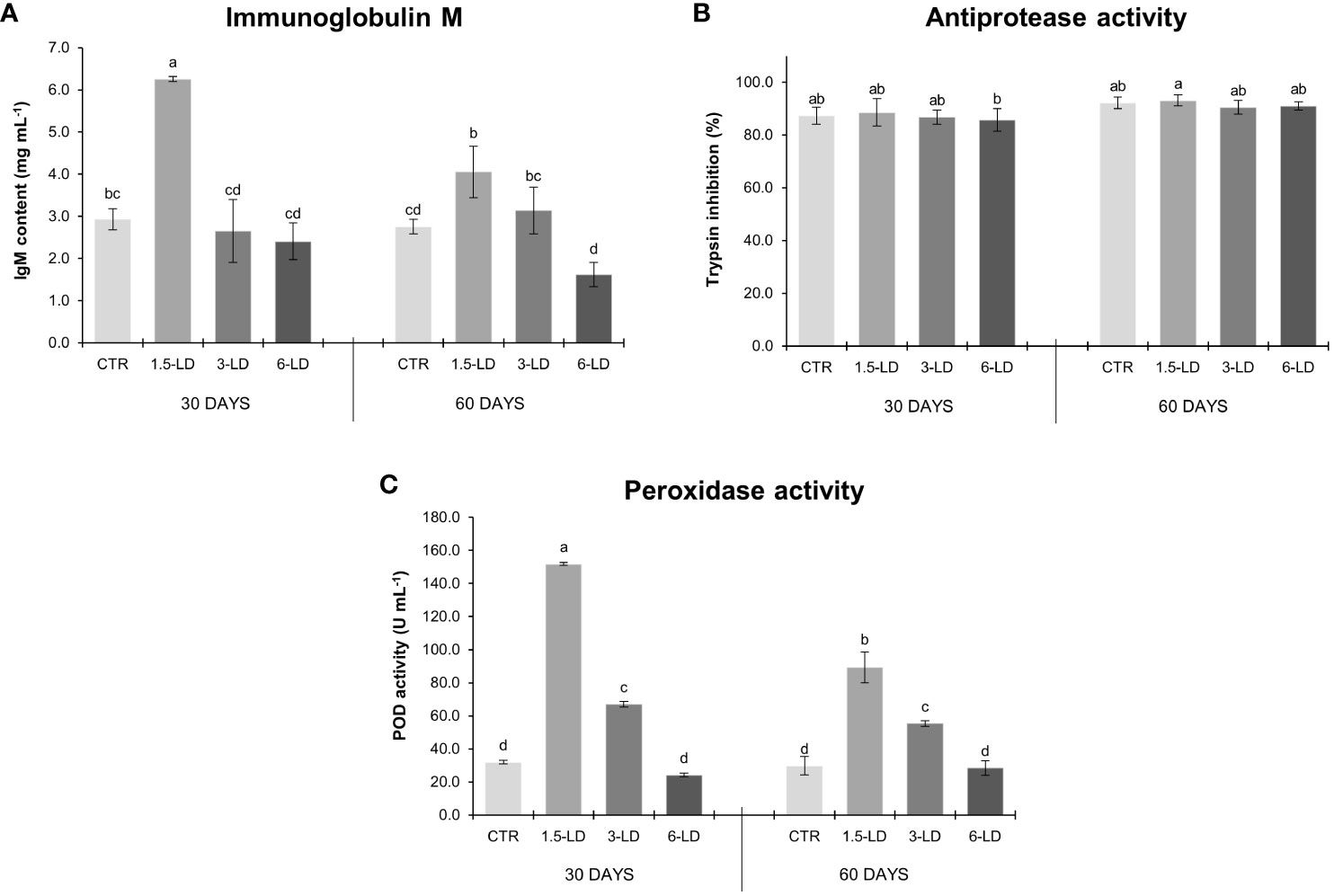
Figure 2 Plasma immune parameters of gilthead seabream juveniles after 30 and 60 days of feeding trial: immunoglobulin M (A, mg mL-1), antiprotease activity (B, expressed in terms of % trypsin inhibition) and peroxidase activity (C, U mL-1) (mean ± SD; n=6). Different lowercase letters indicate significant differences between dietary treatments in both sampling dates (p < 0.05). CTR, control treatment; 1.5-LD, feed with 1.5% inclusion of L. digitata; 3-LD, feed with 3% inclusion of L. digitata; 6-LD, feed with 6% inclusion of L. digitata; IgM, immunoglobulin M; POD, peroxidase.
No significant correlations were found between K and the immune humoral parameters (IgM, AP, and POD; p > 0.05; Table 3). However, there was a positive and significant correlation between SSI and IgM (r = 0.510 and p < 0.05; Table 3).
3.4 Oxidative stress biomarkers
Fish antioxidant responses upon 30 and 60 days of feeding trial are presented in Figure 3. The analysed biomarkers (LPO, CAT, GST, SOD, and T-AOC) showed no significant differences between CTR-T30 and CTR-T60, indicating that the duration of the experiment (30 or 60 days) had no impact on the oxidative stress response of animals from the control treatment. An identical trend was observed in the remain treatments, except in fish supplemented with 1.5% of L. digitata (1.5-LD), where a slight reduction in the activity of CAT (↓24%; Figure 3B) and T-AOC (↓24%; Figure 3E) was observed between 30 and 60 days. Regarding the effects of treatments, all feeds with L. digitata inclusion showed higher CAT activity than the CTR treatment, i.e., a 6.8-fold, 2.1-fold and 2.5-fold increase at T30, and 9.3-fold, 3.5-fold and 5.5-fold increase at T60, in 1.5-LD, 3-LD and 6-LD treatments, respectively (Figure 3B). In the case of T-AOC, only fish fed with 1.5% and 6% of L. digitata showed a significant increase in relation to the CTR treatment (~3.0-fold in 1.5-LD and 1.8-fold in 6-LD treatments) regardless of trial duration (Figure 3E). Regarding LPO, only fish fed with 1.5% macroalga revealed significant differences from the CTR treatment (~0.7-fold decrease at T30 and T60; Figure 3A). GST had the highest activity in treatment 1.5-LD, with a 4.8-fold increase over the CTR treatment at T30 and 11.6-fold increase at T60 (Figure 3C). Fish fed with 6% of L. digitata also differed from those of the CTR treatment, but to a lower extent, revealing a 2.1-fold increase at T30 and 6.0-fold increase at T60 (Figure 3C). GST levels in fish from treatment 3-LD also significantly differed from those of CTR fish at T60, evidencing a 3.2-fold increase (Figure 3C). SOD activity did not differ significantly between treatments or feeding durations (Figure 3D).
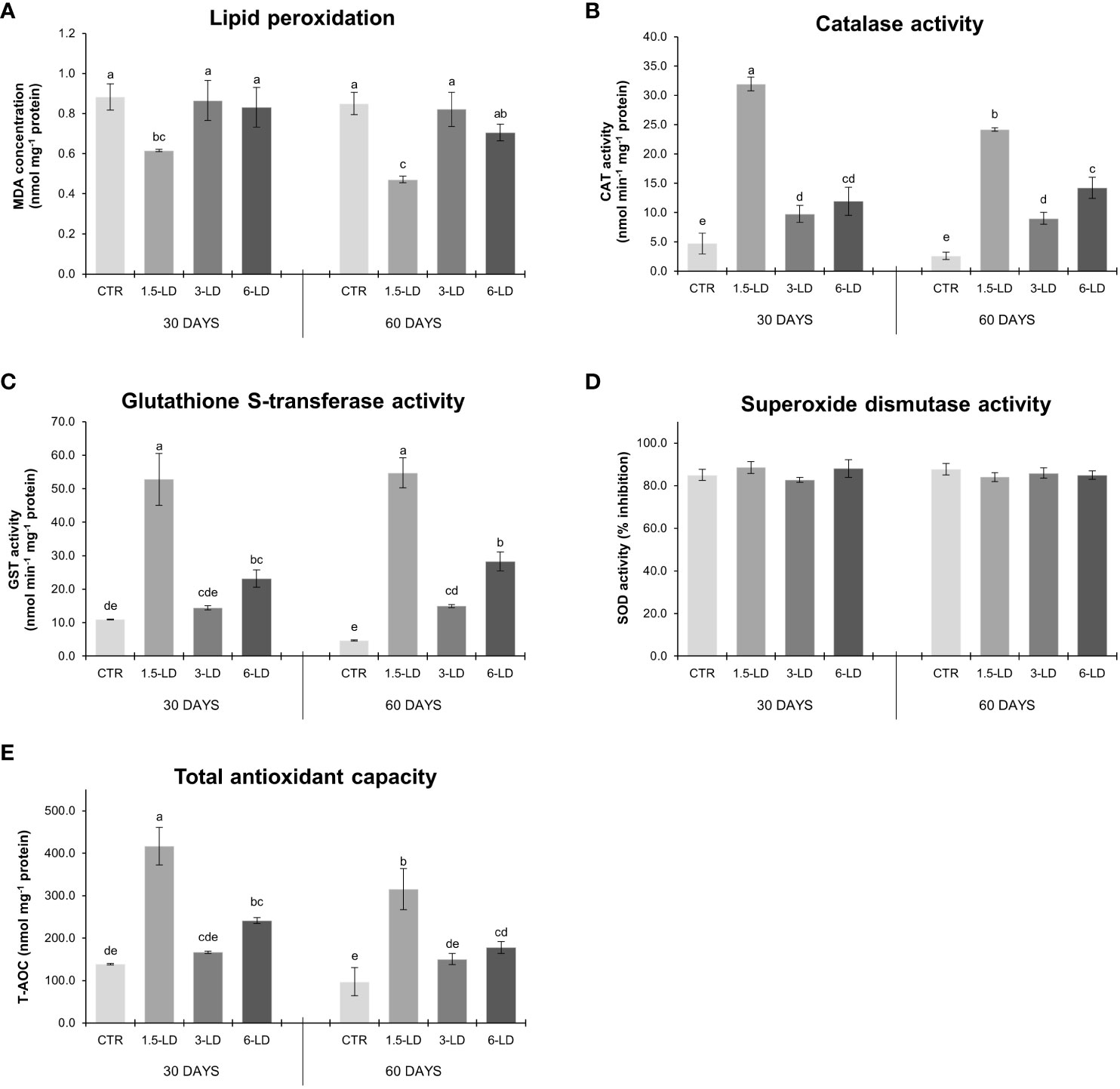
Figure 3 Oxidative stress biomarkers in the spleen of gilthead seabream juveniles after 30 and 60 days of feeding trial: lipid peroxidation (A, expressed as MDA concentration, nmol mg-1 protein), catalase activity (B, nmol min-1 mg-1 protein), glutathione S-transferase activity (C, nmol min-1 mg-1 protein), superoxide dismutase activity (D, % inhibition) and total antioxidant capacity (E, nmol mg-1 protein) (mean ± SD; n=6). Different lowercase letters indicate significant differences between dietary treatments in both sampling dates (p < 0.05). CTR, control treatment; 1.5-LD, feed with 1.5% inclusion of L. digitata; 3-LD, feed with 3% inclusion of L. digitata; 6-LD, feed with 6% inclusion of L. digitata; MDA, malondialdehyde; CAT, catalase; GST, glutathione S-transferase; SOD, superoxide dismutase; T-AOC, total antioxidant capacity.
No significant correlations were found between K and oxidative stress biomarkers (CAT, SOD, GST, LPO and T-AOC) (Table 3). However, SSI was significantly and negatively correlated with CAT (r = -0.549 and p < 0.01) and GST (r = -0.498 and p< 0.05) (Table 3). CAT and GST activities showed a very strong positive correlation with T-AOC (r = 0.934 and p < 0.001, and r = 0.878 and p < 0.001, respectively), as well as a significant negative correlation with LPO levels (r = -0.638 and p < 0.001, and r = -0.728 and p < 0.001, respectively; Table 3).
4 Discussion
The incorporation of novel and sustainably produced ingredients in aquafeed formulations has become one of the focal points in modern aquaculture research (FAO, 2022). Among these promising alternatives, macroalgae have emerged as noteworthy candidates, acquiring significant attention as potential feed ingredients to improve fish health due to their anti-inflammatory, antioxidant, and antimicrobial properties (Morais et al., 2020; Silva et al., 2020; Cai et al., 2021).
In the present study, we explored the effects of incorporating a brown macroalga, Laminaria digitata, as a functional ingredient in aquafeeds to bolster immunity and antioxidant responses in juvenile Sparus aurata. The results herein presented suggest that, overall, the inclusion of this seaweed in aquafeeds yields a positive effect on fish health.
Animal condition indexes serve as key indicators of the overall welfare, which is shaped by a complex interaction of factors such as feeding conditions, parasitic infections, physiological aspects, as well as physical and biological environment (Le Cren, 1951). Animals that remain in good condition are more likely to possess a robust and effective immune system, capable of defending themselves against pathogens and maintaining overall well-being (Zaccone, 2009). In our study, as the factor under analysis was the type of diet, we can state that the inclusion of L. digitata in the aquafeed formulation at the tested levels (1.5%, 3%, and 6%) did not compromise fish welfare. In other words, despite the differences observed throughout time, which are most likely related with the expected fish growth, animal condition (assessed through K and SSI indexes) remained consistently stable across all treatments. The maintenance of a consistent and appropriate (i.e., high K, WGR and SGR values) growth across all treatments suggests that the dietary inclusion of L. digitata did not hinder nutrient utilization or led to any adverse effects on the S. aurata’s metabolism, indicating that the seaweed was efficiently digested and its nutrients available to the fish. The fact that the FCR was consistently lower during the first 30 days of feeding trial shows that the fish were able to grow with a relatively lower amount of feed during this period, indicating a higher efficiency in converting feed into growth. Furthermore, this was also reflected in higher SGR values. The results of this study align with previous research that has demonstrated the potential of several species of brown macroalgae as a valuable eco-innovative aquafeed ingredient to enhance fish health and growth (Pham et al., 2006; Ribeiro et al., 2015; Kamunde et al., 2019; Shi et al., 2019; Negm et al., 2021; Maghawri et al., 2023).
The haematological profile evaluation of S. aurata indicated that the experimental feeds supplemented with L. digitata did not affect the type and number of blood cells produced by juvenile fish. In addition, the absence of significant alterations in the number of viable erythrocytes and/or erythrocyte nuclear abnormalities (ENAs) indicates that aquafeeds biofortified with L. digitata up to 6% did not have cytotoxic and/or genotoxic potential, a result also aligned with the high K values observed in all treatments. The consistent leucocyte percentages below 4.0% of the total cell counts across all treatments indicate that there were no signs of inflammation or acute immune responses in the fish. Leucocytes play a crucial role in the fish’s immune response. They are responsible for identifying and fighting off pathogens, such as bacteria and viruses, and play a role in inflammatory responses (Ellis, 1977; Fánge, 1992). An increase in leucocyte percentages could be an indication of an active immune response, usually in response to an infection, inflammation, or a stressful condition (Seibel et al., 2021). In the context of the present study, the low leucocyte percentages suggest that the fish’s immune system remained stable and indicate the absence of exposure to infectious agents or other stressors that could trigger an immune response. Furthermore, the high erythrocyte percentages observed in all treatments, accounting for over 96.0% of total cells, strongly suggest that the fish had a stable and efficient oxygen-carrying capacity, which is essential for overall metabolic activities (Nikinmaa et al., 2019; Esmaeili, 2021). Additionally, the finding that more than 96.1% of erythrocytes were viable, with a minimal percentage of nuclear abnormalities, indicates that the fish were in good haematological health and well-adapted to the experimental conditions (Witeska et al., 2022). The findings from our study are consistent with those of Passos et al. (2021), who explored the effects of diets supplemented with Gracilaria gracilis in Sparus aurata and found no significant differences in erythrocyte and leukocyte counts between the supplemented feeds and the control treatment. However, other studies have shown contrasting results, highlighting the significant impact of seaweed supplementation in aquafeeds on the haematological profile of fish. For example, Maghawri et al. (2023) incorporated brown algae Padina pavonica as a dietary supplement in Oreochromis niloticus, while Quezada-Rodríguez and Fajer-Ávila (2016) utilized extracts from the green alga Ulva clathrata for the same fish species. Additionally, Sattanathan et al. (2020) investigated the effects of dietary inclusion of Chaetomorpha aerea (Chlorophyta) in Labeo rohita against post-challenges of Aeromonas hydrophila, and all these three studies observed an increase in the percentage of erythrocytes and leucocytes compared to the control. Their findings suggest a protective response during times of stress, as dietary changes may trigger physiological responses, activating the immune system as a precautionary measure to maintain homeostasis and defend against potential stressors or pathogenic challenges associated with the new feeds. However, given the complexity of dietary interactions and the variability in outcomes observed across different fish species, types of seaweed, and experimental conditions, further research is needed to fully elucidate the underlying mechanisms responsible for these effects.
The analysis of immune humoral parameters (IgM, AP, and POD) among the different dietary treatments provided valuable insights into the potential immunomodulatory effects of L. digitata in S. aurata juveniles. Immunoglobulin M (IgM) is an essential antibody involved in both adaptive and innate immune responses, which recognises and neutralises invading pathogens, thereby enhancing fish ability to combat infections (Mashoof and Criscitiello, 2016). Additionally, POD are enzymes with an important microbicidal role, effectively utilizing hydrogen peroxide (H2O2) as a co-substrate to generate highly reactive species. These reactive species play a vital role in phagocytic activities, as they are responsible for damaging and killing pathogens and microbial invaders (Koeth et al., 2013; Guardiola et al., 2014). Meanwhile, AP plays a crucial role in neutralizing pathogen proteases by utilizing various protease inhibitors such as α1-antitrypsin (AAT) and α2-macroglobulin (α2M), which possess anti-enzyme activity. By controlling the activity of proteases, AP helps to maintain tissue integrity, preventing excessive tissue damage, and ensuring effective immune processes without harming surrounding tissues (Priyadarshini et al., 2017). Together, these three immune components contribute significantly to the fish’s defence against infections, promoting overall health and well-being.
In our study, a remarkable increase in IgM content and POD activity was observed in the fish that consumed the feed containing 1.5% L. digitata (1.5-LD). These results suggest that the inclusion of 1.5% L. digitata in the feed positively influenced the fish’s immune responses, enhancing their ability to combat pathogens and strengthening their immune defence mechanisms. Our findings are in line with previous studies that have established immunomodulatory roles of various macroalgae components, their impact on immunity parameters, and their contribution to maintaining overall health in different species (Peixoto et al., 2016; Hoseinifar et al., 2018; Shi et al., 2019; Negm et al., 2021). The inclusion of 3% L. digitata in aquafeeds only showed a significant increase in POD activity compared to the control, although with lower values than the 1.5-LD treatment. This suggests that a 3% inclusion of L. digitata also potentially contributed to an improved defence against pathogens, but never exceeding the effect observed with the 1.5-LD treatment. Conversely, the absence of significant differences in immune parameters (IgM, AP and POD) between the fish fed with 6% of L. digitata and those fed with a non-biofortified aquafeed (CTR treatment) indicates that macroalga inclusion levels above 3% do not lead to immunomodulatory improvements in juvenile S. aurata. These specific results contrast with those of Ferreira et al. (2022a), who observed a more pronounced immune response, in a dose-dependent manner, when using a blend of microalgae (Nannochloropsis oceanica - Eustigmatophyceae and Chlorella vulgaris - Chlorophyta) and macroalgae (Gracilaria gracilis - Rhodophyta and Ulva rigida - Chlorophyta) in European seabass (Dicentrarchus labrax). Some potential explanations for our observation include:
i) Dose-response relationship: The immune response may follow a dose-response relationship, where the effects are proportional to the dose or concentration of L. digitata. Fish immune response may reach a saturation point or threshold beyond which further immunomodulatory benefits are not achieved. This means that the immunomodulatory effects were already maximized at the 1.5% inclusion of L. digitata, and increasing the percentage of algae inclusion will not result in additional significant improvements in the immune responses;
ii) Antagonistic effect: It is possible that higher levels of L. digitata may negatively interfere with the immune response, possibly due to the presence of other compounds in the seaweed that can negatively affect fish immune function or result in other adverse physiological effects. For instance, brown seaweeds in general, and L. digitata in particular, are known to bioaccumulate high levels of metals (e.g., cadmium (Cd) and copper (Cu)) and other chemical contaminants (e.g., iodine (I) and inorganic arsenic (iAs) (Maulvault et al., 2015; Ratcliff et al., 2015; Anacleto et al., 2017; Ronan et al., 2017; Barbosa et al., 2020), with toxicological attributes to both humans and animals when exposure occurs beyond tolerable levels (Laverock et al., 1995; Garai et al., 2021). Hence, the less beneficial outcomes observed at higher inclusion percentages of L. digitata may be related to an increased presence of compounds with potentially negative effects (e.g., toxic metals) that, when present above certain concentrations, may play an antagonistic role upon those with positive immunostimulant and antioxidant properties (e.g., phenolic compounds, polysaccharides, and carotenoids). Considering that the present data, by itself, only allows for speculations (i.e., there is no information on the levels of potentially bioactive or toxic compounds in the experimental aquafeeds used in the trial), future studies should undertake a broad biochemical characterization and identification of relevant compounds in raw materials (i.e., dried macroalgae) that may be used as alternative ingredients in aquafeeds.
Regarding AP activity, overall, the inclusion of L. digitata in the feeds did not have a significant impact on the regulation of proteases activity in juvenile fish. Hence, the results obtained in this specific biomarker contrast somewhat the trends observed in the other studied immunity parameters and, thus, do not clearly point out to a lower or higher susceptibility to disease in fish biofortified with L. digitata. Yet, considering that the main role of these enzymes is to neutralize proteases produced by bacteria, viruses, fungi and protozoans, future studies focused on exploring the immunostimulatory potential of L. digitata should, perhaps, include pathogen challenge tests in the experimental design, as such an approach would likely yield more evident responses.
Another notable finding is that, between T30 and T60, immune parameters were stable in CTR, 3-LD, and 6-LD treatments, but a decrease in IgM content and POD activity was observed in the 1.5-LD treatment. This significant decrease is an intriguing finding that warrants further investigation, since several possible explanations exist for this pattern. An adaptive response of the S. aurata immune system to continuous feeding of diets containing L. digitata is one possibility. Initially, the inclusion of macroalga may have stimulated a more robust immune response; however, over time, the immune system may have adapted to the constant presence of the dietary component, leading to a reduction in IgM content and POD activity. Another potential influencing factor could have been changes in diet composition during the experimental period, as it is well-established that some ingredients, including bioactive compounds and nutrients, can undergo degradation or loss over time (Filipe et al., 2023). Therefore, it is premature to conclude that 30 days is inherently better than 60 days based only on this finding. To draw robust conclusions, further investigations are needed to explore the underlying mechanisms and the long-term effects of L. digitata inclusion on the immune response of juvenile S. aurata.
The interaction between the immune response and its antioxidant defence mechanisms is crucial for maintaining a healthy and functional immune system in fish. Spleen is the major secondary lymphoid organ in fish, thus, hosting a wide range of immunological (including the filtration of blood-borne pathogens and antigens), hematopoietic and red blood cell clearance mechanisms (Lewis et al., 2019; Mokhtar et al., 2023). Additionally, oxidative stress biomarkers are important indicators of physiological status and overall condition at the organ and whole-body levels.
In the present study, the activity of three antioxidant enzymes, namely superoxide dismutase (SOD), catalase (CAT), and glutathione S-transferase (GST), along with two essential antioxidant parameters, total antioxidant capacity (T-AOC) and lipid peroxidation (LPO), were assessed in fish spleen. These antioxidant enzymes play a major role in antioxidant mechanisms, protecting the cells from oxidative damage: SOD is the first line of defence against reactive oxygen species (ROS), catalysing the conversion of superoxide radicals (O2-) into hydrogen peroxide (H2O2) and oxygen (O2) (Lesser, 2006); then, CAT is responsible to neutralize hydrogen peroxide (H2O2) into less harmful compounds, namely water (H2O) and oxygen (O2) (Lesser, 2006); and GST catalyse the conjugation of glutathione (GSH) with several toxic compounds, including ROS that escape from the first line of defence (Domingues et al., 2010). This mechanism makes the conjugation products less toxic and more water-soluble, facilitating their excretion. LPO and T-AOC are also essential indicators of antioxidant responses, since LPO represents a complex chain reaction causing cellular damage through oxidative degradation of lipids (Gutteridge and Halliwell, 1990), while T-AOC reflects the organism’s overall ability to combat oxidative stress and neutralize ROS (Silvestrini et al., 2023).
In our study, the feed with 1.5% inclusion of L. digitata (1.5-LD) also emerged as the most effective in promoting antioxidant responses in juvenile S. aurata. The increase in CAT and GST activities, as well as T-AOC, alongside the corresponding decrease in LPO levels observed in 1.5-LD treatment indicates that L. digitata had a considerable impact on fish antioxidant defence mechanisms. Our results are consistent with previous studies reporting that seaweed supplementation modulates antioxidant status and oxidative stress in fish (Kamunde et al., 2019; Shi et al., 2019; Negm et al., 2021). The abundant presence of bioactive compounds in L. digitata provides a plausible explanation for the increased activity of antioxidant enzymes, since it is already known that this macroalga is rich in polyphenols (phenolic acids, phlorotannins, flavonoids, and halogenated derivatives), carotenoids (e.g., fucoxanthin and β-carotene) and polysaccharides (e.g., alginates, fucoidan, laminarin and mannitol) (Holdt and Kraan, 2011; Schiener et al., 2014; Bonfim-Mendonca et al., 2017; Vissers et al., 2017; Fernando et al., 2021). These compounds have demonstrated their remarkable ability to neutralize free radicals and reduce oxidative stress within cells, thus displaying antioxidant and anti-inflammatory properties. When incorporated into the fish diet, these antioxidants may have increased the activity of CAT and GST enzymes and raised the organism’s total antioxidant capacity (T-AOC). Consequently, this increased cellular protection against oxidative damage led to a decrease in LPO levels.
The inclusion of 3% and 6% of L. digitata also increased CAT activity (both percentages of inclusion), GST activity and T-AOC (only 6%). However, the magnitude of the increase in these biomarker activities was consistently lower for the higher inclusion levels of L. digitata. This pattern aligns with the findings observed in the immune responses (IgM and POD), indicating that increasing the macroalga concentration in the feeds does not add any benefit from the immune, antioxidant and overall animal condition points of view.
SOD activity showed no significant differences between the experimental feeds and the CTR treatment, meaning that the inclusion of L. digitata in the feeds did not have a substantial impact on this enzyme. This observation highlights the complexity of the fish’s antioxidant response, which involves multiple enzymes and pathways working in harmony to maintain redox balance and protect against oxidative stress.
In line with the pattern observed in IgM content and POD activity (immune responses), CAT activity and T-AOC showed a decrease between 30 and 60 days with the 1.5-LD feed. This decrease can be explained, once again, by the adaptation of the organism to the functional feed, as the fish adjusted to the new diet, leading to reduced antioxidant response needs. Alternatively, the effects of L. digitata bioactive compounds on CAT activity and T-AOC might be more pronounced at fish early life stages, decreasing over time due to metabolism or other regulatory mechanisms. This decrease may indicate that the fish’s redox balance has been achieved and maintained at a healthy level after the initial adaptation phase, suggesting that the antioxidants provided by the 1.5% L. digitata feed are sufficient to preserve redox homeostasis and protect cells against oxidative damage. Additionally, genetic regulation can also play a role in stabilizing antioxidant enzyme activity over time, ensuring overall antioxidant response homeostasis and adaptability to dietary changes for maintaining cellular health (Martin and Król, 2017; Mierziak et al., 2021).
The immune and antioxidant responses in organisms are highly complex and influenced by a wide array of factors. These factors include various aspects, such as diet composition, prevailing environmental conditions, exposure to pathogens, physiological changes, among others. Although this study focused specifically on S. aurata under optimal experimental conditions, it is essential to recognize that different fish species or varying environmental factors could yield different responses to the inclusion of L. digitata in aquafeeds.
The valuable findings from this study constitute a substantial contribution to the scientific understanding of fish health and underscore the potential utilization of L. digitata as a functional alternative feed ingredient in aquaculture. Further research on the interactions between dietary components, fish physiology, and immune-antioxidant responses will undoubtedly open new windows of opportunity in fish nutrition, contributing to improve animal welfare, quality and resilience to environmental stressors (e.g. pathogens, pollutants, climate change) that may challenge the survival of farmed animals and the economic viability of the sector.
5 Conclusions
This study highlights the promising potential of Laminaria digitata as a functional and eco-innovative aquafeed ingredient to improve immunity and antioxidant responses in juvenile Sparus aurata.
The results indicate that a dietary inclusion of 1.5% L. digitata is the most cost-effective inclusion percentage, significantly improving the antioxidant responses of S. aurata, through increased levels of CAT, GST and T-AOC, as well as reduced LPO levels. Additionally, the inclusion of 1.5% L. digitata showed to enhance immunity by inducing IgM synthesis and POD activity, while maintaining the growth performance and condition indexes of the fish.
Although aquafeeds biofortified with higher incorporation percentages of L. digitata (3% and 6%) showed no cytotoxic and/or genotoxic potential, the lack of consistent and clear beneficial effects in fish fed with these doses of macroalga suggests that future studies should prioritise inclusion percentages below 3%. Indeed, in this study it seemed evident that “less is more” when it comes to incorporating L. digitata in aquafeeds, as this approach appears to be more advantageous in terms of achieving optimal animal health status and considering cost-effectiveness.
Data availability statement
The original contributions presented in the study are included in the article/Supplementary Material. Further inquiries can be directed to the corresponding author.
Ethics statement
The animal study was approved by IPMA’s Animal Welfare and Ethics Body (ORBEA). The study was conducted in accordance with the local legislation and institutional requirements.
Author contributions
IM: Data curation, Formal analysis, Investigation, Methodology, Writing – original draft, Writing – review & editing. MD: Investigation, Writing – review & editing. AG: Investigation, Writing – review & editing. PP-F: Resources, Writing – review & editing. MD: Supervision, Writing – review & editing. AM: Resources, Supervision, Writing – review & editing. ALM: Conceptualization, Formal analysis, Funding acquisition, Investigation, Methodology, Project administration, Resources, Supervision, Writing – review & editing.
Funding
The author(s) declare financial support was received for the research, authorship, and/or publication of this article. This work was supported by Fundação Portuguesa para a Ciência e Tecnologia (FCT I.P.), under the framework of the project Aqua-CLIMADAPT (PTDC/CTA-AMB/0592/2021, https://doi.org/10.54499/PTDC/CTA-AMB/0592/2021) and the “Applied Molecular Biosciences Unit” (UCIBIO) which is financed by national funds from FCT/MCTES (UIDB/04378/2020), the project LA/P/0140/2020 of the Associate Laboratory Institute for Health and Bioeconomy—i4HB. IM also acknowledges FCT I.P. for the PhD grant (2020.04413.BD).
Acknowledgments
The authors acknowledge the team from the Aquaculture Research Station of Olhão (EPPO – IPMA I.P.) for the technical support provided during animal rearing and transplantation, as well as Leonardo Mata from GOPSIS Lda. for supplying the macroalga Laminaria digitata and Jorge Dias from SPAROS Lda. for supplying the experimental feeds.
Conflict of interest
The authors declare that the research was conducted in the absence of any commercial or financial relationships that could be construed as a potential conflict of interest.
The author(s) declared that they were an editorial board member of Frontiers, at the time of submission.
Publisher’s note
All claims expressed in this article are solely those of the authors and do not necessarily represent those of their affiliated organizations, or those of the publisher, the editors and the reviewers. Any product that may be evaluated in this article, or claim that may be made by its manufacturer, is not guaranteed or endorsed by the publisher.
Supplementary material
The Supplementary Material for this article can be found online at: https://www.frontiersin.org/articles/10.3389/fmars.2024.1325244/full#supplementary-material
References
Anacleto P., Van Den Heuvel F. H., Oliveira C., Rasmussen R. R., Fernandes J. O., Sloth J. J., et al. (2017). Exploration of the phycoremediation potential of Laminaria digitata towards diflubenzuron, lindane, copper and cadmium in a multitrophic pilot-scale experiment. Food Chem. Toxicol. 104, 95–108. doi: 10.1016/j.fct.2017.01.030
Barbosa V., Maulvault A. L., Anacleto P., Santos M., Mai M., Oliveira H., et al. (2020). Enriched feeds with iodine and selenium from natural and sustainable sources to modulate farmed gilthead seabream (Sparus aurata) and common carp (Cyprinus carpio) fillets elemental nutritional value. Food Chem. Toxicol. 140, 111330. doi: 10.1016/j.fct.2020.111330
Belanche A., Jones E., Parveen I., Newbold C. J. (2016). A Metagenomics Approach to Evaluate the Impact of Dietary Supplementation with Ascophyllum nodosum or Laminaria digitata on Rumen Function in Rusitec Fermenters. Front. Microbiol. 7. doi: 10.3389/fmicb.2016.00299
Bonfim-Mendonca P., Capoci I. R. G., Tobaldini-Valerio F., Negri M., Svidzinski T. I. E. (2017). Overview of β-glucans from Laminaria spp.: immunomodulation properties and applications on biologic models. Int. J. Mol. Sci. 18 (9), 1629. doi: 10.3390/ijms18091629
Bradford M. M. (1976). A rapid and sensitive method for the quantitation of microgram quantities of protein utilizing the principle of protein-dye binding. Anal. Biochem. 72 (1–2), 248–254. doi: 10.1016/0003-2697(76)90527-3
Cai J., Lovatelli A., Aguilar-Manjarrez J., Cornish L., Dabbadie L., Desrochers A., et al. (2021). “Seaweeds and microalgae: an overview for unlocking their potential in global aquaculture development,” in FAO Fisheries and Aquaculture Circular No. 1229 (Rome: FAO). doi: 10.4060/cb5670en
Cain K. D. (2022). The many challenges of disease management in aquaculture. J. World Aquaculture Soc. 53 (6), 1080–1083. doi: 10.1111/jwas.12936
Carrasco K. R., Tilbury K. L., Myers M. G. (1990). Assessment of the Piscine Micronucleus Test as an in situ biological indicator of Chemical Contaminant Effects. Can. J. Fisheries Aquat. Sci. 47 (11), 2123–2136. doi: 10.1139/f90-237
Cipriano-Maack A. N., Wood C. T., Culloty S. C. (2016). Immunostimulatory effect of diet (Laminaria digitata and Mytilus edulis) in the edible sea urchin, Paracentrotus lividus. Aquaculture Int. 25 (1), 341–354. doi: 10.1007/s10499-016-0032-y
Costa M., Cardoso C., Afonso C., Bandarra N. M., Prates J. (2021). Current knowledge and future perspectives of the use of seaweeds for livestock production and meat quality: a systematic review. J. Anim. Physiol. Anim. Nutr. 105 (6), 1075–1102. doi: 10.1111/jpn.13509
Costa M., Lopes P., Assunção J. P., Alfaia C. M., Coelho D., Mourato M. P., et al. (2022). Combined effects of dietary Laminaria digitata with alginate lyase on plasma metabolites and hepatic lipid, pigment and mineral composition of broilers. BMC Vet. Res. 18 (1). doi: 10.1186/s12917-022-03250-3
Cuesta A., Meseguer J. (2004). Total serum immunoglobulin M levels are affected by immunomodulators in seabream (Sparus aurata L.). Vet. Immunol. Immunopathol. 101 (3–4), 203–210. doi: 10.1016/j.vetimm.2004.04.021
De La Moneda A., Carro M. J., Weisbjerg M. R., Roleda M. Y., Lind V., Novoa-Garrido M., et al. (2019). Variability and potential of seaweeds as ingredients of ruminant diets: an in vitro study. Animals 9 (10), 851. doi: 10.3390/ani9100851
Domingues I., Agra A. R., Monaghan K. A., Soares A. M., Nogueira A. J. (2010). Cholinesterase and glutathione-S-transferase activities in freshwater invertebrates as biomarkers to assess pesticide contamination. Environ. Toxicol. Chem. 29 (1), 5–18. doi: 10.1002/etc.23
Ellis A. (1977). The leucocytes of fish: A review. J. Fish Biol. 11 (5), 453–491. doi: 10.1111/j.1095-8649.1977.tb04140.x
Ellis A. E. (1990). “Lysozyme assays,” in Techniques in Fish Immunology. Eds. Stolen J. S., Fletcher T. C., Anderson D. P., Roberson B. S., Van Muiswinkel W. B. (Fair Haven: SOS Publications), 101–113.
Esmaeili M. (2021). Blood Performance: a new formula for fish growth and health. Biology 10 (12), 1236. doi: 10.3390/biology10121236
Espinosa-Ruiz C., Manuguerra S., Morghese M., Beltrán J. M. G., Esteban M.Á., Giuga M., et al. (2021). Immunity and inflammatory responses in gilthead sea bream (Sparus aurata L.) exposed to sub-lethal mixture of carbamazepine, cadmium chloride and polybrominated diphenyl ether. Fish Shellfish Immunol. 111, 25–35. doi: 10.1016/j.fsi.2020.12.013
Fánge R. (1992). “Fish blood cells,” in Fish Physiology. Eds. Hoar W. S., Randall D. J., Farrell A. P. (London: Academic Press Inc.) 12 (Part B), 1–54. doi: 10.1016/s1546-5098(08)60008-4
FAO (2018). Impacts of climate change on fisheries and aquaculture: synthesis of current knowledge, adaptation and mitigation options. FAO Fisheries and Aquaculture Technical Paper No. 627 (Rome: FAO), 628 pp.
FAO (2022). The State of World Fisheries and Aquaculture 2022. Towards Blue Transformation (Rome: FAO). doi: 10.4060/cc0461en
FAO (2023)Fisheries and aquaculture software. In: FishStatJ-Software for Fishery Statistical Time Series (Rome: FAO Fisheries and Aquaculture Department). Available at: https://www.fao.org/fishery/en/topic/166235?lang=en (Accessed July 2, 2023).
Fernando I. P. S., Lee W., Ahn G. (2021). Marine algal flavonoids and phlorotannins; an intriguing frontier of biofunctional secondary metabolites. Crit. Rev. Biotechnol. 42 (1), 23–45. doi: 10.1080/07388551.2021.1922351
Ferreira M., MaChado M. G. C., Mota C. S., Abreu H., Silva J., Maia M. R., et al. (2022a). Micro- and macroalgae blend modulates the mucosal and systemic immune responses of European seabass (Dicentrarchus labrax) upon infection with Tenacibaculum maritimum. Aquaculture 566, 739222. doi: 10.1016/j.aquaculture.2022.739222
Ferreira M., Ribeiro P., Ribeiro L., Barata M., Domingues V. F., Sousa S., et al. (2022b). Biofortified diets containing algae and selenised yeast: effects on growth performance, nutrient utilization, and tissue composition of gilthead seabream (Sparus aurata). Front. Physiol. 12. doi: 10.3389/fphys.2021.812884
Filipe D., Gonçalves M. P., Fernandes H., Oliva-Teles A., Peres H., Belo I., et al. (2023). Shelf-life performance of fish feed supplemented with bioactive extracts from fermented olive mill and winery by-products. Foods 12 (2), 305. doi: 10.3390/foods12020305
Garai P., Banerjee P., Mondal P. K., Ch N., Saha R. (2021). Effect of heavy metals on fishes: toxicity and bioaccumulation. J. Clin. Toxicol., 11 (18), 1–10. doi: 10.35248/2161-0495.21.s18.001
Guardiola F. A., Cuesta A., Arizcun M., Meseguer J., Esteban M.Á. (2014). Comparative skin mucus and serum humoral defence mechanisms in the teleost gilthead seabream (Sparus aurata). Fish Shellfish Immunol. 36 (2), 545–551. doi: 10.1016/j.fsi.2014.01.001
Gutteridge J. M., Halliwell B. (1990). The measurement and mechanism of lipid peroxidation in biological systems. Trends Biochem. Sci. 15 (4), 129–135. doi: 10.1016/0968-0004(90)90206-q
Habig W. H., Pabst M. J., Jakoby W. B. (1974). Glutathione S-transferases. J. Biol. Chem. 249 (22), 7130–7139. doi: 10.1016/s0021-9258(19)42083-8
Hanif A., Bakopoulos V., Dimitriadis G. J. (2004). Maternal transfer of humoral specific and non-specific immune parameters to sea bream (Sparus aurata) larvae. Fish Shellfish Immunol. 17 (5), 411–435. doi: 10.1016/j.fsi.2004.04.013
Holdt S. L., Kraan S. (2011). Bioactive compounds in seaweed: functional food applications and legislation. J. Appl. Phycol. 23 (3), 543–597. doi: 10.1007/s10811-010-9632-5
Hoseinifar S. H., Yousefi S., Capillo G., Paknejad H., Khalili M., Tabarraei A., et al. (2018). Mucosal immune parameters, immune and antioxidant defence related genes expression and growth performance of zebrafish (Danio rerio) fed on Gracilaria gracilis powder. Fish Shellfish Immunol. 83, 232–237. doi: 10.1016/j.fsi.2018.09.046
Johansson L., Borg L. E. (1988). A spectrophotometric method for determination of catalase activity in small tissue samples. Anal. Biochem. 174 (1), 331–336. doi: 10.1016/0003-2697(88)90554-4
Kambayashi Y., Binh N. T., Asakura H., Hibino Y., Hitomi Y., Nakamura H., et al. (2009). Efficient assay for total antioxidant capacity in human plasma using a 96-well microplate. J. Clin. Biochem. Nutr. 44 (1), 46–51. doi: 10.3164/jcbn.08-162
Kamunde C., Sappal R., Melegy T. M. (2019). Brown seaweed (AquaArom) supplementation increases food intake and improves growth, antioxidant status and resistance to temperature stress in Atlantic salmon, Salmo salar. PloS One 14 (7), e0219792. doi: 10.1371/journal.pone.0219792
Kaplow L. S., Ladd C. (1965). Brief report: Simplified myeloperoxidase stain using benzidine dihydrochloride. Blood 26 (2), 215–219. doi: 10.1182/blood.v26.2.215.215
Koeth R. A., Haselden V., Tang W. H. W. (2013). “Myeloperoxidase in cardiovascular disease,” in Advances in Clinical Chemistry. Ed. Makowski G. S. (USA: Academic Press, Elsevier) 62, 1–32. doi: 10.1016/b978-0-12-800096-0.00001-9
Laverock M., Stephenson M., Macdonald C. (1995). Toxicity of iodine, iodide, and iodate to Daphnia magna and rainbow trout (Oncorhynchus mykiss). Arch. Environ. Contamination Toxicol. 29 (3), 344–350. doi: 10.1007/bf00212499
Le Cren E. D. (1951). The length-weight relationship and seasonal cycle in gonad weight and condition in the perch (Perca fluviatilis). J. Anim. Ecol. 20 (2), 201. doi: 10.2307/1540
Lesser M. P. (2006). Oxidative stress in marine environments: Biochemistry and Physiological Ecology. Annu. Rev. Physiol. 68 (1), 253–278. doi: 10.1146/annurev.physiol.68.040104.110001
Lewis S. M., Williams A., Eisenbarth S. C. (2019). Structure and function of the immune system in the spleen. Sci. Immunol. 4 (33). doi: 10.1126/sciimmunol.aau6085
Maghawri A., Marzouk S. S., El-Din H. M. E., Nashaat M. (2023). Effect of brown algae Padina pavonica as a dietary supplement on growth performance and health status of cultured Oreochromis niloticus. Egyptian J. Aquat. Res. 49 (3), 379–385. doi: 10.1016/j.ejar.2023.03.001
Martin S., Król E. (2017). Nutrigenomics and immune function in fish: new insights from omics technologies. Dev. Comp. Immunol. 75, 86–98. doi: 10.1016/j.dci.2017.02.024
Mashoof S., Criscitiello M. F. (2016). Fish immunoglobulins. Biology 5 (4), 45. doi: 10.3390/biology5040045
Maulvault A. L., Barbosa V., Alves R. J., Custódio A. L., Cunha S. C., Repolho T., et al. (2017). Ecophysiological responses of juvenile seabass (Dicentrarchus labrax) exposed to increased temperature and dietary methylmercury. Sci. Total Environ. 586, 551–558. doi: 10.1016/j.scitotenv.2017.02.016
Maulvault A. L., Cunha S. C., Barbosa V., Sloth J. J., Rasmussen R. R., Tediosi A., et al. (2015). Toxic elements and speciation in seafood samples from different contaminated sites in Europe. Environ. Res. 143, 72–81. doi: 10.1016/j.envres.2015.09.016
Mierziak J., Kostyn K., Boba A., Czemplik M., Kulma A., Wojtasik W. (2021). Influence of the bioactive diet components on the gene expression regulation. Nutrients 13 (11), 3673. doi: 10.3390/nu13113673
Mokhtar D. M., Zaccone G., Alesci A., Kuciel M., Hussein M. T., Sayed R. K. A. (2023). Main components of fish immunity: An overview of the fish immune system. Fishes 8 (2), 93. doi: 10.3390/fishes8020093
Morais T., Inácio A. R., Coutinho T., Ministro M., Cotas J., Pereira L., et al. (2020). Seaweed potential in the animal feed: A review. J. Mar. Sci. Eng. 8 (8), 559. doi: 10.3390/jmse8080559
Negm S. S., Ismael N. E., Ahmed A. I., Asely A. M. E., Naiel M. (2021). The efficiency of dietary Sargassum aquifolium on the performance, innate immune responses, antioxidant activity, and intestinal microbiota of Nile Tilapia (Oreochromis niloticus) raised at high stocking density. J. Appl. Phycol. 33 (6), 4067–4082. doi: 10.1007/s10811-021-02572-3
Nikinmaa M., Berenbrink M., Brauner C. J. (2019). Regulation of erythrocyte function: Multiple evolutionary solutions for respiratory gas transport and its regulation in fish. Acta Physiologica 227 (2). doi: 10.1111/apha.13299
Oliva-Teles A. (2012). Nutrition and health of aquaculture fish. J. Fish Dis. 35 (2), 83–108. doi: 10.1111/j.1365-2761.2011.01333.x
Palstra A. P., Kals J., Garcia A. B., Dirks R. P., Poelman M. (2018). Immunomodulatory effects of dietary seaweeds in LPS challenged Atlantic salmon Salmo salar as determined by deep RNA sequencing of the head kidney transcriptome. Front. Physiol. 9. doi: 10.3389/fphys.2018.00625
Passos R. P., Correia A. M. H., Ferreira I., De Souza M. A., Pires D., Gomes E., et al. (2021). Effect on health status and pathogen resistance of gilthead seabream (Sparus aurata) fed with diets supplemented with Gracilaria gracilis. Aquaculture 531, 735888. doi: 10.1016/j.aquaculture.2020.735888
Peixoto M. G. M., Ferraz R. R. N., Magnoni L. J., Pereira R. N., Gonçalves J., Calduch-Giner J. A., et al. (2019). Protective effects of seaweed supplemented diet on antioxidant and immune responses in European seabass (Dicentrarchus labrax) subjected to bacterial infection. Sci. Rep. 9 (1). doi: 10.1038/s41598-019-52693-6
Peixoto M. J., Salas-Leiton E., Pereira L. F., De Queiroz A. C., Magalhães F., Pereira R., et al. (2016). Role of dietary seaweed supplementation on growth performance, digestive capacity and immune and stress responsiveness in European seabass (Dicentrarchus labrax). Aquaculture Rep. 3, 189–197. doi: 10.1016/j.aqrep.2016.03.005
Pérez R., Barbaroux O. (1997). Ces algues qui nous entourent: conception actuelle, rôle dans la biosphère, utilisations, culture (Versailles, France: Edition Quae), 272.
Pham M. A., Lee K., Lee B., Lim S., Kim S., Lee Y., et al. (2006). Effects of Dietary Hizikia fusiformis on Growth and Immune Responses in Juvenile Olive Flounder (Paralichthys olivaceus). Asian-australasian J. Anim. Sci. 19 (12), 1769–1775. doi: 10.5713/ajas.2006.1769
Priyadarshini S. K., Subramani P. A., Michael R. D. (2017). Modulation of the innate immune responses in the striped snakehead murrel, Channa striata upon experimental infection with live and heat killed Aeromonas hydrophila. Open Vet. J. 7 (2), 157. doi: 10.4314/ovj.v7i2.13
Purcell-Meyerink D., Packer M. A., Wheeler T. M., Hayes M. (2021). Aquaculture Production of the Brown Seaweeds Laminaria digitata and Macrocystis pyrifera: Applications in Food and Pharmaceuticals. Molecules 26 (5), 1306. doi: 10.3390/molecules26051306
Quade M. J., Roth J. A. (1997). A rapid, direct assay to measure degranulation of bovine neutrophil primary granules. Vet. Immunol. Immunopathol. 58 (3–4), 239–248. doi: 10.1016/s0165-2427(97)00048-2
Quezada-Rodríguez P., Fajer-Ávila E. J. (2016). The dietary effect of ulvan from Ulva clathrata on hematological-immunological parameters and growth of tilapia (Oreochromis niloticus). J. Appl. Phycol. 29 (1), 423–431. doi: 10.1007/s10811-016-0903-7
Ratcliff J. J., Wan A. H. L., Edwards M. D., Soler-Vila A., Johnson M. P., Abreu H., et al. (2015). Metal content of kelp (Laminaria digitata) co-cultivated with Atlantic salmon in an Integrated Multi-Trophic Aquaculture system. Aquaculture 450, 234–243. doi: 10.1016/j.aquaculture.2015.07.032
Ribeiro D. S., Alfaia C. M., Medina-Pestana J. O., Carvalho D., Costa M., Martins C. F., et al. (2022). Influence of feeding weaned piglets with Laminaria digitata on the quality and nutritional value of meat. Foods 11 (7), 1024. doi: 10.3390/foods11071024
Ribeiro A. R., Gonçalves A., Colen R., Nunes M. L., Dinis M. T., Dias J. (2015). Dietary macroalgae is a natural and effective tool to fortify gilthead seabream fillets with iodine: Effects on growth, sensory quality and nutritional value. Aquaculture 437, 51–59. doi: 10.1016/j.aquaculture.2014.11.028
Ribeiro D. M., Pinto R. M. A., Lopes P. A., Pestana J. M., Alfaia C. M., Costa M. M., et al. (2023). Effect of Laminaria digitata dietary inclusion and CAZyme supplementation on blood cells, serum metabolites and hepatic lipids and minerals of weaned piglets. Sci. Rep. 13 (1). doi: 10.1038/s41598-023-33835-3
Ricker W. E. (1975). Computation and interpretation of biological statistics of fish populations (Canada: Bulletin of the Fisheries Board of Canada), 191.
Ronan J., Stengel D. B., Raab A., Feldmann J., O’Hea L., Bralatei E., et al. (2017). High proportions of inorganic arsenic in Laminaria digitata but not in Ascophyllum nodosum samples from Ireland. Chemosphere 186, 17–23. doi: 10.1016/j.chemosphere.2017.07.076
Sattanathan G., Thanikaivelan P., Padmapriya S., Anand A. V., Park S., Kim I. H., et al. (2020). Influences of dietary inclusion of algae Chaetomorpha aerea enhanced growth performance, immunity, haematological response and disease resistance of Labeo rohita challenged with Aeromonas hydrophila. Aquaculture Rep. 17, 100353. doi: 10.1016/j.aqrep.2020.100353
Schiener P., Black K. D., Stanley M. S., Green D. H. (2014). The seasonal variation in the chemical composition of the kelp species Laminaria digitata, Laminaria hyperborea, Saccharina latissima and Alaria esculenta. J. Appl. Phycol. 27 (1), 363–373. doi: 10.1007/s10811-014-0327-1
Seibel H., Baßmann B., Rebl A. (2021). Blood will Tell: What hematological analyses can reveal about fish welfare. Front. Vet. Sci. 8. doi: 10.3389/fvets.2021.616955
Shi Q., Hua R., Hao M., Zhu D., Aweya J. J., Li S., et al. (2019). Effects of dietary Sargassum horneri on growth performance, serum biochemical parameters, hepatic antioxidant status, and immune responses of juvenile black sea bream Acanthopagrus schlegelii. J. Appl. Phycol. 31 (3), 2103–2113. doi: 10.1007/s10811-018-1719-4
Silva A. F., Da Silva S. M., Carpena M., Garcia-Oliveira P., Simal-Gandara J., Morais S., et al. (2020). Macroalgae as a source of valuable antimicrobial compounds: extraction and applications. Antibiotics 9 (10), 642. doi: 10.3390/antibiotics9100642
Silvestrini A., Meucci E., Ricerca B. M., Mancini A. (2023). Total Antioxidant Capacity: biochemical aspects and clinical significance. Int. J. Mol. Sci. 24 (13), 10978. doi: 10.3390/ijms241310978
Strober W. (2001). Trypan blue exclusion test of cell viability. Curr. Protoc. Immunol. doi: 10.1002/0471142735.ima03bs21. Appendix 3B.
Sun Y., Oberley L. W., Li Y. (1988). A simple method for clinical assay of superoxide dismutase. Clin. Chem. 34 (3), 497–500. doi: 10.1093/clinchem/34.3.497
Thépot V., Campbell A. H., Paul N. A., Rimmer M. A. (2021). Seaweed dietary supplements enhance the innate immune response of the mottled rabbitfish, Siganus fuscescens. Fish Shellfish Immunol. 113, 176–184. doi: 10.1016/j.fsi.2021.03.018
Thépot V., Campbell A. H., Rimmer M. A., Jelocnik M., Johnston C. I., Evans B., et al. (2022). Dietary inclusion of the red seaweed Asparagopsis taxiformis boosts production, stimulates immune response and modulates gut microbiota in Atlantic salmon, Salmo salar. Aquaculture 546, 737286. doi: 10.1016/j.aquaculture.2021.737286
Uchiyama M., Mihara M. (1978). Determination of malonaldehyde precursor in tissues by thiobarbituric acid test. Anal. Biochem. 86 (1), 271–278. doi: 10.1016/0003-2697(78)90342-1
Verdegem M. C., Buschmann A. H., Latt U. W., Dalsgaard A. J. T., Lovatelli A. (2023). The contribution of aquaculture systems to global aquaculture production. J. World Aquaculture Soc. 54 (2), 206–250. doi: 10.1111/jwas.12963
Vissers A. M., Caligiani A., Sforza S., Vincken J., Gruppen H. (2017). Phlorotannin composition of Laminaria digitata. Phytochem. Anal. 28 (6), 487–495. doi: 10.1002/pca.2697
Witeska M., Kondera E., Ługowska K., Bojarski B. (2022). Hematological methods in fish – Not only for beginners. Aquaculture 547, 737498. doi: 10.1016/j.aquaculture.2021.737498
Yarahmadi P., Miandare H. K., Fayaz S., Caipang C. M. A. (2016). Increased stocking density causes changes in expression of selected stress- and immune-related genes, humoral innate immune parameters and stress responses of rainbow trout (Oncorhynchus mykiss). Fish Shellfish Immunol. 48, 43–53. doi: 10.1016/j.fsi.2015.11.007
Zaccone G. (2009). Fish Defenses Vol. 2: Pathogens, Parasites and Predators (Boca Raton: CRC Press). doi: 10.1201/9780429187285
Zuo X., Woo P. T. K. (1997). Natural anti-proteases in rainbow trout, Oncorhynchus mykiss and brook charr, Salvelinus fontinalis and the in vitro neutralization of fish α2-macroglobulin by the metalloprotease from the pathogenic hemoflagellate, Cryptobia salmositica. Parasitology 114 (4), 375–382. doi: 10.1017/S0031182096008578
Keywords: aquaculture, functional feeds, seaweeds, animal welfare, immune responses, antioxidant responses
Citation: Marmelo I, Dias M, Grade A, Pousão-Ferreira P, Diniz MS, Marques A and Maulvault AL (2024) Immunomodulatory and antioxidant effects of functional aquafeeds biofortified with whole Laminaria digitata in juvenile gilthead seabream (Sparus aurata). Front. Mar. Sci. 11:1325244. doi: 10.3389/fmars.2024.1325244
Received: 20 October 2023; Accepted: 11 January 2024;
Published: 30 January 2024.
Edited by:
Amina Zuberi, Quaid-i-Azam University, PakistanReviewed by:
Leonel Pereira, University of Coimbra, PortugalSevdan Yilmaz, Çanakkale Onsekiz Mart University, Türkiye
Copyright © 2024 Marmelo, Dias, Grade, Pousão-Ferreira, Diniz, Marques and Maulvault. This is an open-access article distributed under the terms of the Creative Commons Attribution License (CC BY). The use, distribution or reproduction in other forums is permitted, provided the original author(s) and the copyright owner(s) are credited and that the original publication in this journal is cited, in accordance with accepted academic practice. No use, distribution or reproduction is permitted which does not comply with these terms.
*Correspondence: Isa Marmelo, aXNhLm1hcm1lbG9AaXBtYS5wdA==; aXNhbW1hcm1lbG9AZ21haWwuY29t