- 1Hopkins Marine Station, Oceans Department, Stanford University, Pacific Grove, CA, United States
- 2Stanford Center for Ocean Solutions, Stanford University, Pacific Grove, CA, United States
- 3Comunidad y Biodiversidad A. C., Guaymas, Sonora, Mexico
- 4Departamento de Conservación de la Biodiversidad, El Colegio de la Frontera Sur (ECOSUR), San Cristóbal de las Casas, Chiapas, Mexico
- 5Facultad de Ciencias Marinas, Universidad Autónoma de Baja California, Ensenada, Mexico
- 6Moffat & Nichol, Long Beach, CA, United States
- 7Facultad de Economia, Universidad de Los Andes, Bogota, Colombia
- 8Department of Economics, University of Massachusetts, Amherst, MA, United States
- 9Sociedad Cooperativa de Producción Pesquera Buzos y Pescadores, Baja California Sur, Mexico
- 10Center for Oceans, Conservation International, Honolulu, HI, United States
- 11Coastal Science and Policy, University of California Santa Cruz, Santa Cruz, CA, United States
- 12Institute of Marine Sciences, University of California Santa Cruz, Santa Cruz, CA, United States
- 13Reef Check of California, Santa Cruz, CA, United States
- 14Marine Stewardship Council, London, United Kingdom
- 15California Academy of Science, Center for Biodiversity and Community Science, San Francisco, CA, United States
- 16Department of Human Ecology, Rutgers University, School of Environmental and Biological Science, New Brunswick, NJ, United States
- 17Department of Civil and Environmental Engineering and Oceans Department, Stanford University, Stanford, CA, United States
- 18Cooperative Institute for Marine and Atmospheric Studies, University of Miami, Miami, FL, United States
- 19School of Environmental, Civil, Agricultural and Mechanical Engineering, University of Georgia, Athens, GA, United States
Coastal ecosystems and human communities are threatened worldwide by climate change, and shocks from social, market and political change. There is an urgent global need to promote resilient food production and livelihoods in the face of these shocks. Small-scale fisheries (SSF) in rural settings can be particularly vulnerable as they frequently lack the resources, rights and infrastructure to respond to shocks originating outside the focal systems. We examined ecological and social outcomes of environmental extremes in a SSF socio-ecological system (SES) by using long-term oceanographic (between 2010-2019) and ecological (2006-2018) data tracking change in a kelp forest ecosystem of Baja California, Mexico, and concurrent documentation of proactive and reactive actions of a fishing community organized in a cooperative. Results indicate a complex landscape of ‘winners’ and ‘losers’ among species and fisheries exposed to unprecedented environmental extremes, including marine heat waves and prolonged hypoxia, and a suite of adaptive actions by the local fishing cooperative, and others in the region, that have helped confront these rapid and drastic changes. Cooperatives have established voluntary marine reserves to promote recovery of affected populations and have invested in diversification of activities enabled by access rights, collective decision-making, and participatory science programs. Results indicate that local actions can support social and ecological resilience in the face of shocks, and that enabling locally-driven adaptation pathways is critical to resilience. This case study highlights the crucial importance of strengthening and supporting rights, governance, capacity, flexibility, learning, and agency for coastal communities to respond to change and sustain their livelihoods and ecosystems in the long run.
Introduction
Coastal marine ecosystems around the world and the human communities and economic sectors that depend on them are taking the brunt of an increasingly variable climate, characterized by increasing frequency and intensity of heat waves, El Niño-Southern Oscillation (ENSO), storms, hypoxia, and ocean acidification (Doney et al., 2009; Alheit and Bakun, 2010; Hoegh-Guldberg and Bruno, 2010; Knutson et al., 2010; Sydeman et al., 2013; Breitburg et al., 2018; Laffoley and Baxter, 2019; Arafeh-Dalmau et al., 2020). Documented ecological and socioeconomic impacts of climate and oceanographic variability include changes in ocean productivity, food web dynamics, abundance of habitat-forming species, species distributions, a greater incidence of disease, collapse and geographic shifts of fisheries, and negative impacts on coastal communities and their economies (Hoegh-Guldberg and Bruno, 2010; Cheung et al., 2011).
Faced with complex and multifaceted interactions and impacts of climate change and other global shocks on coastal social-ecological systems (SES), such as the COVID-19 global pandemic, it is critically important that we improve our understanding of how coastal ecosystems and people respond. This is of particular importance for small-scale fisheries (SSF). SSF and their marine resources constitute major examples of coastal SES. SSF employ over 90% of fishers and fish workers worldwide, nearly half of which are women (Kelleher, 2012; FAO, 2020; Virdin et al., 2023). They contribute nearly half of fisheries catch globally, two thirds of aquatic food for human consumption (Berkes, 2001; Kelleher, 2012; FAO, 2020), and are crucially important to global food security, nutrition and livelihoods for communities worldwide (Smith et al., 2011; Teh and Sumaila, 2013; Short et al., 2021). SSF can be particularly vulnerable to climatic impacts and other shocks, as they are often characterized by low mobility, limited resources to manage fisheries, and limited options for alternative livelihoods (Berkes, 2001). For example, when they are spatially confined, SSF are less able than larger-scale fisheries to adapt to environmental change by moving to other fishing grounds, though local adaptation can occur through a suite of behaviors and responses that can vary between and within SES (Blythe et al., 2014; Ojea et al., 2020). Because of the overwhelming biological, social, economic, health and cultural importance of SSF and their marine resources, understanding the factors and feedbacks influencing their resilience to environmental variability and other shocks is a key priority.
We therefore ask how ecological responses affect local livelihoods and economies, e.g., through changes in fisheries productivity; how human responses affect local ecosystems, e.g., through changes in fishing behavior; and what are the possible options and strategies for mitigating and adapting to change. We examine resilience and adaptive capacity in a small-scale fisheries SES in the face of extreme environmental variability and uncertainty. By resilience we mean the ability of a social-ecological system to absorb disturbance while retaining function and provision of ecosystem services (Holling, 1973; Folke et al., 2004; Gunderson et al., 2015). It is dependent on characteristics of the ecosystems (Levin and Lubchenco, 2008; Palumbi et al., 2008) as well as the adaptive capacity of the social component, shown in how resource users anticipate, respond to, and recover from change (Adger and Vincent, 2005; Smit and Wandel, 2006; Walker and Salt, 2006; Ostrom, 2009; Cinner et al., 2012). Adaptive capacity describes the ability of human communities to influence their own resilience and the resilience of the ecosystem on which they depend (Walker et al., 2004; Nelson et al., 2007; Defeo et al., 2016).
Climate extremes are illustrative of a suite of potential shocks that impact coastal SES worldwide, associated with, e.g., environmental, market, social and political change. The framework is social-ecological, with a focus on both the social and the ecological, and our approach is participatory, with fishers, community members, and fisheries managers actively contributing to the design (in multi-stakeholder workshops) and conduction (through participation in data collection in the field) of the research, and fishing cooperative members co-authors in this paper. We use long-term nearshore oceanographic and ecological monitoring and concurrent documentation of proactive and reactive actions by the fishing cooperative of Isla Natividad, in Baja California, Mexico, as a case study. This is an upwelling ecosystem characterized by high productivity of kelp forests and associated species of commercial interest, including abalone, lobster, and finfish. In particular, we asked: (1) What are the responses of the kelp forest assemblage to environmental variability and extremes? (2) How have environmental variability and ecological responses affected local fisheries? (3) What proactive and reactive actions have been taken by the fishing community to adapt to change? A better understanding of how coastal SES respond to shocks associated with environmental variability and extremes offers insights into their adaptive capacity to climate, social, political and economic change and highlights possible strategies for supporting resilience. Thus, a key goal is not only to assess risks associated with climate change and extremes, but also to identify possible solutions to mitigate their detrimental effects and promote resilient human-natural coastal systems.
Although local actions cannot directly mitigate the effects of events like hypoxia or an extreme ENSO condition, which are influenced by larger scale processes, human-natural feedbacks can play a large role in determining the resilience of the local socio-ecological system to such events. In the context of SSF, adaptive capacity has been understood and measured in terms of social organization, human agency, flexibility, people’s capacity to learn, access to material assets, and socio-cognitive constructs (Cinner and Barnes, 2019). As a latent quality, adaptive capacity can materialize into adaptations contingent on a diverse set of socio-cultural factors such as risk perception and access to information (Burch and Robinson, 2007). For example, if a community perceives low risk associated with environmental change or lacks access to adequate information on the potential impacts of environment change, they are less likely to mobilize an adaptation strategy. Moreover, a culture of cooperation may provide the capacity to act on available information (e.g., Sánchez-Bajo and Roelants, 2011). The identification of these system-level characteristics is relevant to both advancing the scientific knowledge and theory of coupled systems dynamics, and to guiding their management, particularly under high risk and uncertainty from environmental change and other shocks. In particular, there is an urgent and critical need to inform adaptation pathways (e.g. Wise et al., 2014) in coastal SES in the face of global change.
Depending on their characteristics and behaviors, ecosystems and local communities might exhibit different responses to the impacts of environmental extremes and other changes (Figure 1). The responses of local ecosystems and the communities that depend on them can result in more or less resilient SES, from different ecological and social scenarios. These alternative scenarios were drawn based on review of the literature on SES resilience and adaptive capacity (e.g. Steneck et al., 2011; Cinner et al., 2012; Defeo et al., 2016, and references above) and extensive vetting within our co-authors group, in an iterative process carried out through multiple workshops. Our primary focus is on environmental variability and extremes, but similar considerations apply to other perturbations from social, market or political change.
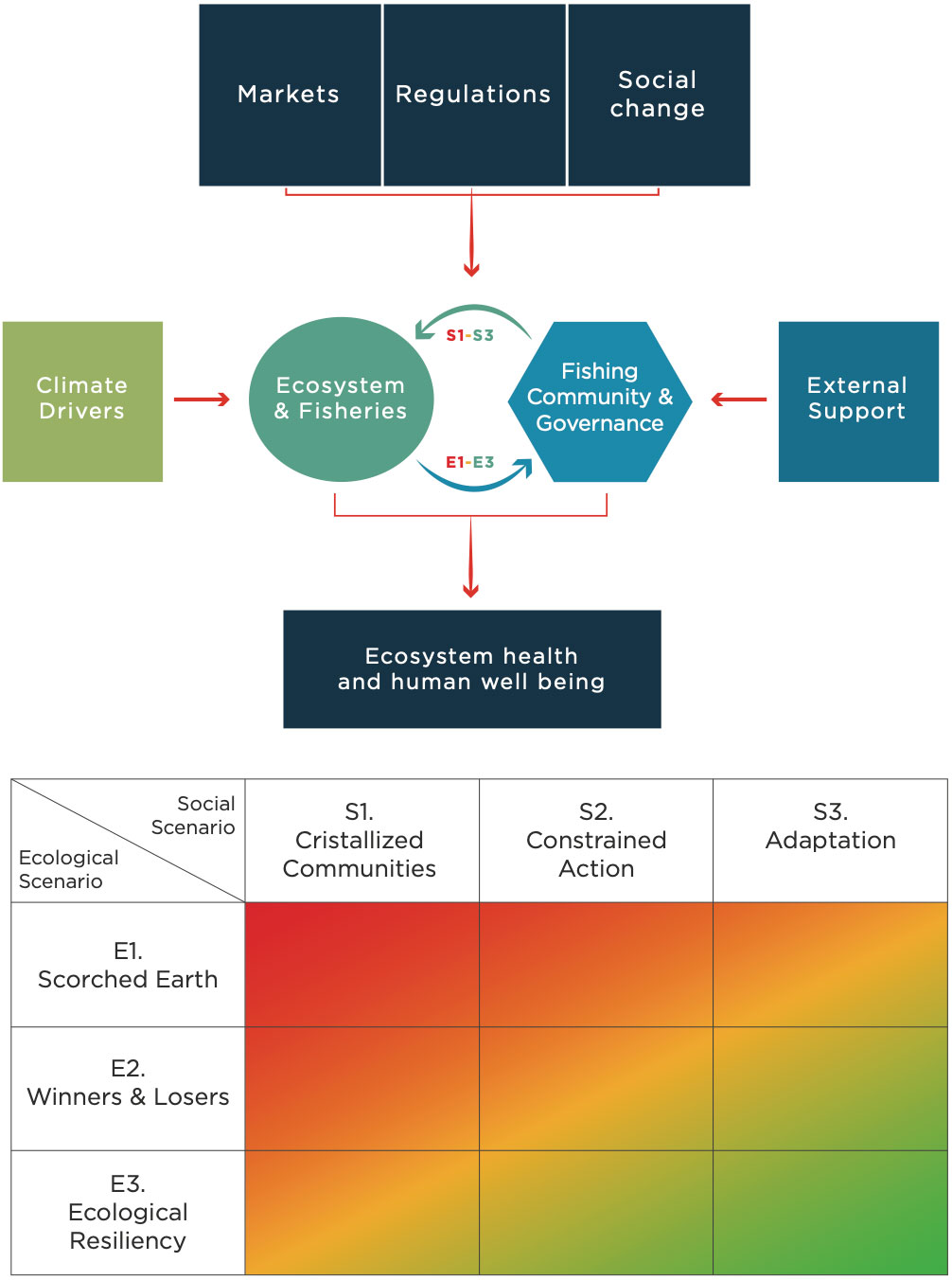
Figure 1 Conceptual framework and possible ecological and social scenarios. Depending on their characteristics and behaviors, as well as support from governments, academic institutions, or CSOs, ecosystems and local communities might exhibit different responses to the impacts of environmental change or other perturbations from social, market or political change. The responses of local ecosystems and the communities that depend on them can result in more or less resilient SES, from different ecological and social scenarios.
We propose three SES scenarios regarding possible ecosystem responses to coastal oceanographic variability (Figure 1).
Scorched earth scenario (E1)
Increases in the severity of oceanographic variability impacts multiple species and ecological processes, resulting in fisheries collapse and less diverse ecosystems, with likely negative social outcomes on all current and most possible future uses of the coastal marine environment. Under this scenario, ensuring the persistence and recovery of contemporary fisheries may be beyond current management tools.
Winners and losers scenario (E2)
Some species have low vulnerability or even thrive under changed conditions because they are not directly affected by associated stressors or are highly mobile and can behaviorally avoid adverse conditions. In contrast, more vulnerable species are negatively impacted. Differential vulnerabilities result in negative impacts on some fisheries, however there is still potential to support future alternative fisheries and activities. In addition, the persistence and recovery of impacted fisheries may be promoted through appropriate management actions.
Ecological resiliency scenario (E3)
Highly variable species responses, high redundancy within coastal marine ecosystems and fisheries, coupled with effective conservation and management actions, maintain ecosystem function and viable fisheries in the face of climate variability. Human communities can switch to a portfolio of economic alternatives that compensates for their losses. There might be short-term costs for fishers to adapt to such a portfolio, which are compensated in the long term.
There are at least three corresponding SES scenarios for social responses to ecosystem change or other external shocks:
Crystallized communities scenario (S1)
Coastal communities, and the governments that regulate their fisheries access rights, management options, strategies and scientific capacity available to communities, may be unwilling or unable to invest in conservation or adaptation strategies.
This may be due to a lack of tenure rights to marine resources that prevents the development of alternative uses of coastal ecosystems, a lack of resources and infrastructure for adaptation, exceedingly high and immediate opportunity costs, out-migration due to diminishing opportunities, distrust or disinterest in science, government or civil society organizations. All these may affect the supply of labor for fishing, a view that resource declines are part of a natural cycle and unlikely to be addressed through local action, or a lack of access to information, low-risk perception, or other socio-cultural factors. Also, local political or economic elites and organized crime may be interested in maintaining the status quo.
Constrained action scenario (S2)
Coastal communities are willing to invest in actions aimed at adapting to change but face institutional, social, cultural or economic constraints that limit or delay action. A lack of rights or other regulations does not allow them to diversify their economic portfolio, or they do not have the adequate resources or agency to mobilize these rights. Fishers that rely on vulnerable species suffer a larger economic loss, enhancing the inequalities across fishers groups.
Adaptation scenario (S3)
Coastal communities and government partners in co-management are willing and able to devise and implement adaptation strategies, enabled by social organization, capacity to learn, flexibility to change among strategies, secure rights and other assets, human agency, and socio-cognitive constructs (McCay et al., 2014; Cinner and Barnes, 2019). The institutional environment of norms, regulations and markets allows them to engage in such adaptation strategies.
Different combinations of the possible ecological and social responses to environmental change or other shocks will result in different outcomes for SES (Figure 1), that might range from becoming locked in a social-ecological trap (Holling, 2001; Cinner, 2011; Steneck et al., 2011) to exhibiting high resilience and adaptive capacity (Koontz et al., 2015). For instance, in the lack of tenure rights and cooperative solutions, uncontrolled fishing effort leads to the overexploitation and, in some instances, the collapse of fisheries (as in scenarios E1). This reduces income and, as a consequence, the capacity to accumulate organizational and economic capital (Gordon, 1954; Schaefer, 1957). Lack of capital and capacity prevents the community from adopting solutions aimed at diversifying activities or temporarily reducing harvesting to allow for fishery recovery (as in scenarios S1), in a reinforcing cycle between poverty and overexploitation of renewable resources. Therefore, each combination of social-ecological scenarios will feedback into each other and either amplify a positive feedback loop, or weaken it, resulting in a possible shift to alternate scenarios. Climate variability can also shift an ecosystem from one scenario to another, with shifts more likely in those scenarios that are less stable (weakening feedback loop; Figure 1).
Methods
Study site and system
Oceanographic and ecological participatory research was conducted between 2006-2018 in Isla Natividad, Baja California Sur, Mexico (Figure 2). The island, which is 8 km from the mainland and is within the Vizcaino Biosphere Reserve, has 500 inhabitants, 84 belonging to the fishing cooperative Buzos y Pescadores de la Baja California at the time of this study. The history and governance of this and related cooperatives are described in detail in McCay et al. (2014). Nearshore fisheries along the coast of the Baja California Peninsula are organized into local fishing cooperatives that, starting in the 1930s, were granted exclusive fishing rights on local stocks (concessions), including abalone, lobster and other benthic resources. The study zone and its fisheries have experienced various major shocks in the past, including severe ENSO effects combined with overfishing and illegal fishing of key resources in the 1980s, which greatly reduced the productivity of fisheries such as abalone and impacted the SES. Following that period, co-management was introduced and new territory-based access rights for community-based cooperatives were defined. In exchange for exclusive fishing zones, cooperative members are legally required to work collectively, pay dues, and assist governmental authorities in monitoring and enforcing their concessions (Young, 2001; McCay et al., 2014). Since 1992, cooperatives have been required to commit to sustainable management before being granted concessions, valid for up to 20 years. Management plans are worked out cooperatively with government agencies and include quotas, minimum legal sizes, zoning, seasonal closures, fishing gear restrictions, effort control, and continued monitoring of exploited populations (Hernandez and Kempton, 2003; Ponce-Díaz et al., 2009; McCay et al., 2014). The federation of the 11 cooperatives of the Vizcaino region (FEDECOOP), to which the cooperative Buzos y Pescadores belongs, was the first small-scale fishery from a developing country to receive, in 2004, an eco-certification from the Marine Stewardship Council for their red spiny lobster (Panulirus interruptus) fishery (Pérez-Ramírez et al., 2016). The cooperatives also rely on other fisheries, including several finfish species for which they do not hold exclusive rights and have not historically developed and enforced management plans (Shester and Micheli, 2011; Micheli et al., 2014a; Micheli et al., 2014b).
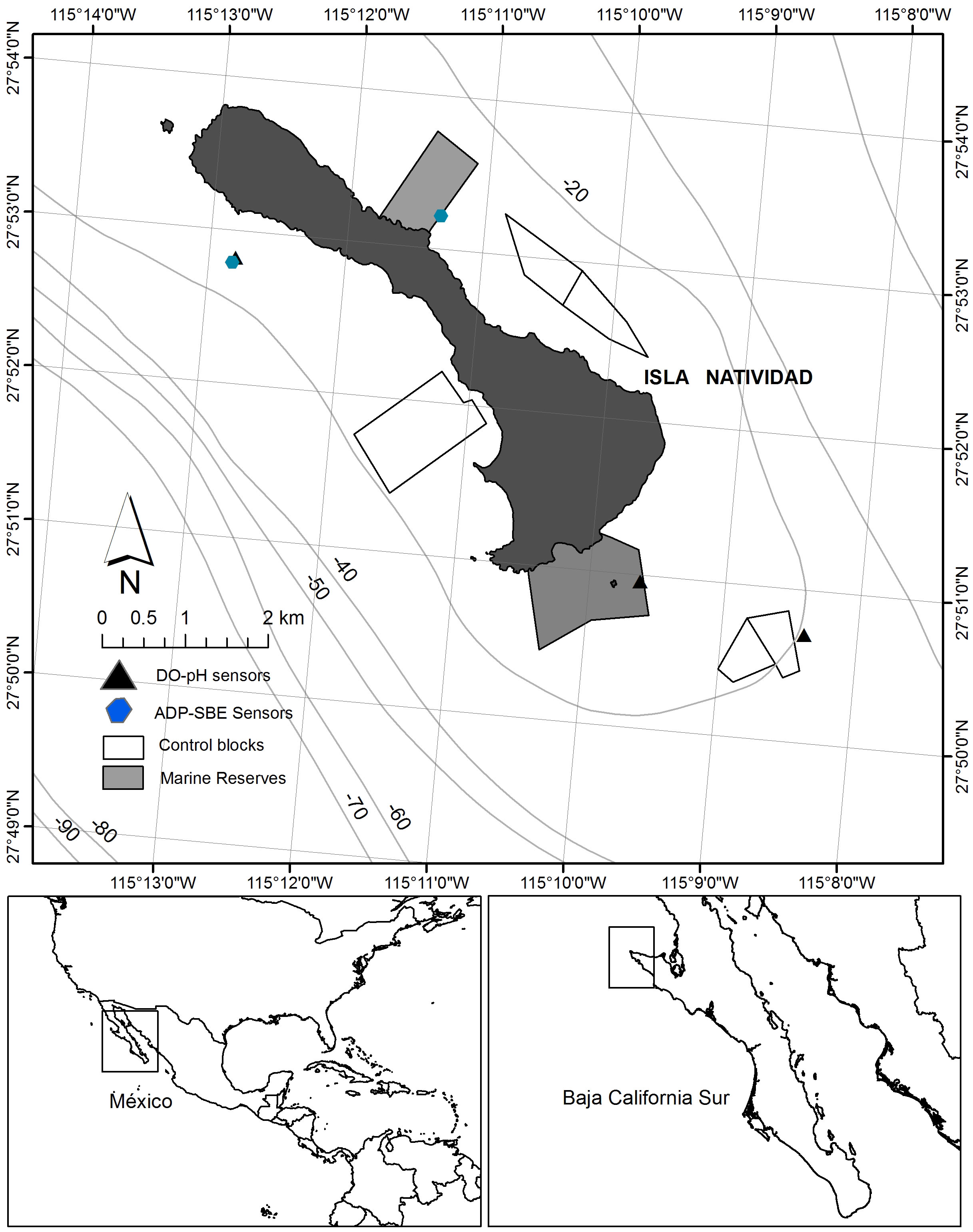
Figure 2 Location of Isla Natividad, Baja California Sur, Mexico. The top panel shows the location and boundaries of the two marine reserves established and enforced by the local community in 2006, and the three reference fished areas monitored yearly, between 2006-2018. The black triangle indicates the location of the nearshore oceanographic mooring. Coordinates (lat and long) indicate the exact location of Isla Natividad and study sites around it. The bottom right panel shows the location of the Baja California Peninsula (within the rectangle), on the southwestern side of the North American Continent. The Bottom left panel shows the location of Isla Natividad (within the rectangle), on the Pacific Ocean side of the Baja California Peninsula.
The coastal marine ecosystems along the Pacific coast of Baja California have extremely high levels of productivity and biological diversity associated with upwelling and biogeographic transitions (Arriaga, 1998). These upwelling ecosystems are influenced by basin-wide variation in climate and oceanographic conditions associated with the California Current, ENSO, the Pacific Decadal Oscillation (PDO), the North Pacific Gyre Oscillation (NPGO), and the warm-water anomaly of 2013/2015, referred to also as “the blob” (Cavole et al., 2016; Di Lorenzo and Mantua, 2016). These climatic cycles can result in large fluctuations in ocean temperature and upwelling intensity (Mann and Lazier, 1996), and can influence primary production and recruitment of fish and invertebrates, with significant impacts on fishery production and on responses of target species to fishing pressure (Barber and Chavez, 1983; Barber and Chavez, 1986; Chavez et al., 2003; Cheung et al., 2010; Carr and Reed, 2016). In addition to more variable physical conditions, recent studies have highlighted a suite of chemical changes associated with climate change, including ocean acidification and deoxygenation (Chan et al., 2008; Feely et al., 2008). In particular, severe hypoxic conditions have been observed off the coast of Oregon, USA since 2002, and subsequently at other locations within the California Current (Chan et al., 2008; Micheli et al., 2012; Booth et al., 2014). Documentation of climate-driven hypoxia on continental shelves and coastal areas (Chan et al., 2008; Micheli et al., 2012; Booth et al., 2014; Breitburg et al., 2018) and the observed acidification trends (Feely et al., 2008; Wootton et al., 2008; Doney, 2010) suggest that hypoxia and acidification will strongly affect the structure, function and provision of important services by coastal marine ecosystems.
Despite the productive and diverse marine communities and successful fisheries found within the Vizcaino region, some key resources (e.g., the economically valuable pink and green abalone, Haliotis corrugata and H. fulgens) have undergone drastic decline compared to historical levels due to the combined impacts of overfishing and severe ENSO events in the early 1980s, 1990s and in 1997/98 (Shepherd et al., 1998; Morales-Bojorquez et al., 2008). In February 2006 the cooperative of Isla Natividad voluntarily and by democratic vote set aside 8% of their abalone fishing ground in two no-take, fully protected marine reserves with the primary goal of recovering depleted abalone populations, while continuing to fish in the rest of the fishing ground. In addition to establishment of the voluntary reserve, the cooperative initiated a community-based monitoring program of the main fisheries’ target species and other kelp forest taxa. These two well-enforced reserves and the monitoring program have provided an unprecedented opportunity to tease apart the relative influences of fishing and environmental variability on harvested populations and whole ecosystems. Following Isla Natividad, four additional cooperatives have subsequently established marine reserves. Here we focus on Isla Natividad because it has the longest biophysical monitoring time series.
Coastal monitoring at Isla Natividad, Baja California Sur, Mexico
Ecological monitoring
To understand how the coastal ecosystems of the Pacific coast of Baja California might respond to climatic and oceanographic variability, in the presence and in the absence of fishing, we conducted ecological and oceanographic monitoring of commercial and non-commercial species of algae, fish and invertebrates within and outside the two marine reserves beginning in July 2006, shortly after the reserves were established. Participatory (community-based) monitoring was designed and conducted jointly by cooperative members, along with Civil Society Organizations (CSOs) and academic scientists (Micheli et al., 2012; Fulton et al., 2019). All divers were re-trained yearly by CSO personnel (Reef Check of California and Comunidad y Biodiversidad, A.C.) to ensure consistency, and all data were carefully checked by CSO and academic scientists. The annual ecological surveys consisted of visual census of replicate 30m long, 2m wide transects, haphazardly placed on rocky reef habitat, conducted using SCUBA between depths of 5-20m in three controls, fished sites and two marine reserves at Isla Natividad (late July - early August) between 2006-2018. Eleven to 30 transects per site were surveyed every year (avg=22.1, total=1367 transects). Divers recorded commercially and ecologically important fish species observed within a 2-m wide by 2-m high window along the 30-m benthic transect (120m3), and mobile macro-invertebrates and kelp (numbers of individuals and stipes of the giant kelp, Macrocystis pyrifera, and the southern sea palm, Eisenia arborea) within the 30x2-m belt transects (60m2). A point-contact method was used to characterize the habitat, quantifying percent cover of benthic algae and sessile invertebrates. The presence of different benthic groups (e.g., the benthic commercially harvested red alga Gelidium robustum) was recorded at 20-cm intervals along the 30-m transects.
Here we report results for the main benthic invertebrates and algae for which the cooperative holds exclusive access rights: the pink and green abalone; the spiny lobster; the sea cucumber Parastichopus parvimensis; the wavy turban snail, Megastraea undosa; and the red alga Gelidium sp. For kelps, which form the productive marine forests on which these fisheries depend, and their main consumers, we analyzed both harvested species (the giant kelp; and the red sea urchin Mesocentrotus franciscanus), and kelp (Eisenia arborea), sea urchin (the black and purple sea urchins, Centrostephanus coronatus and Strongylocentrotus purpuratus), and mollusks (the keyhole limpet Megathura crenulata) that are not currently harvested, but play important ecological roles as foundation species and key grazers and could support future fisheries. Finally, we report results for two abundant kelp-associated finfish species that are fished locally (the sheephead, Semychossiphus pulcher, and the kelp bass, Paralabrax clathratus), but for which the cooperative does not hold exclusive access rights (restricted to invertebrates and algae). Several finfish species (yellowtail, whitefish, sheephead, and kelp bass) are caught for local consumption and sale in national markets. Finfish prices are low (US$1-10/kg) and the contribution to the local economy has historically been limited for this fishing cooperative. The cooperative keeps records for the catch of all commercial species, which were included in these analyses as well.
Oceanographic monitoring
Following abalone and sea urchin mass mortalities in 2009 (Micheli et al., 2012), we initiated coastal oceanographic monitoring, beginning in May 2010. Aanderaa Dissolved oxygen (DO) and temperature (T) optodes (Aanderaa 3830) and pH sensors (SeaFET) were deployed at 14 m depth, attached to large cement moorings. Due to differences in battery capacities, the DO-T sensors were programmed to sample every 15 minutes while the pH sensors were programmed to sample at hourly intervals. Discrete water samples were collected at the beginning and at the end of each SeaFET deployment for analyses of water carbonate chemistry (salinity, DIC, and TA) and pH data calibration (Martz et al., 2003; Fulton et al., 2019). The DO-T sensors were factory-calibrated by the manufacturing company (Aanderaa Data Instruments). Beginning in 2013, the DO-T sensors were replaced with Sea-Bird 37 CTDOs (Sea-Bird Scientific) and MiniDOT (Precision Measurement Engineering, Inc.) dissolved oxygen and temperature sensors (both programmed to log data at 10-min intervals). Instruments were retrieved and cross-calibrated at 6-month intervals. Seawater dissolved oxygen concentration and temperature were monitored continuously between 2010-2019, whereas pH was monitored for intermittent time periods (Supplementary Figure S2).
Statistical analyses
Temporal trends of fish, invertebrate and macroalgal abundance (through the 13 years of monitoring), and effects of protection through no-take reserve establishment were examined using generalized linear mixed models (GLMMs) (e.g. Stroup, 2012) constructed with the ‘glmmTMB’ package in R v.3.4.3. Models included protection (2 levels: no take marine reserves and fished reference sites), year [2006-2018], an interaction term between year and protection (testing for the effect of protection in reserves), and depth as fixed effects, with sampling sites included as random intercepts to account for inter-site variability in baseline abundance. Because count data were over-dispersed and not normally distributed, we applied a negative binomial (NB) distribution with a log link function. We also compared models with zero-inflated Poisson, and binomial models for percent cover data (for the red alga Gelidium sp.). We selected models using AIC and by reviewing the fit of predicted values. Since all species were fit best by NB, here we report only results of these models. GLMM coefficients and confidence intervals testing for significant (at alpha=0.05) effects of protection in no-take reserves, time (year), their interaction, and water depth are reported in Supplementary Figure S4.
Social science methods to understand impacts of and responses to oceanographic, ecological and market changes
The authors of this study comprise diverse positionalities and backgrounds with representation from Mexican and non-Mexican academic and practitioner institutions and members of the Baja-based fishing cooperatives. They bring extensive collective experience working in and with the cooperatives, fisheries, and communities involved in this research spanning decades. The social and economic component of this longitudinal research relied upon multiple social research techniques to inform our understanding of community impacts of and responses to change (i.e. Ponce-Díaz et al., 2009; Shester and Micheli, 2011; McCay et al., 2014; Finkbeiner, 2015; Finkbeiner et al., 2018). These include fisheries catch surveys conducted on small boats together with fishers; participant observation and survey research among fishers and their families; and formal and informal interviews and interactions with cooperative leadership and staff, co-management leaders and staff of the Federation, and Mexican national scientists whose work informed fisheries modeling and quota setting with the coops. Our goal was to use multiple and triangulated methods to develop a coherent narrative of the history of events connected with environmental and market change.
The general understandings of the culture and history of resilience and adaptation within the cooperative-based fisheries that are core to this analysis come in part from a formal social-ecological survey focused on responses to system shocks and ecological changes such as past ENSO events (McCay et al., 2014). We conducted a paired social-ecological survey with the spouses/households of those fishers to understand local adaptations from a holistic perspective of fishers and divers as well as their families who adapted to changing ecological and economic resources. The social-ecological survey used a randomized 10% sample of fishers/households belonging to four fishing cooperatives on the mainland of the Vizcaino peninsula, plus the cooperative that runs the seafood processing plant on the peninsula. The findings from these surveys found agreement with findings from interviews conducted with leaders and key informant fishers of the Isla Natividad cooperative who were not part of the random sample.
The team also conducted formal and informal interviews with key informants positioned throughout the local/regional seafood supply chain to contextualize the survey responses. We specifically documented the actions taken by the Isla Natividad fishing cooperative as well as the other FEDECOOP cooperatives in response to environmental change. We conducted oral history interviews with elders and other key informants in all the communities and cooperatives of the federation, and unstructured interviews with administrative and technical staff throughout the study period, again focusing on responses to ecological and market change.
Participant observation was also central to our understanding of how the cooperatives responded to change. In particular, we participated in the biannual Isla Natividad cooperative general assemblies (Supplementary Figure S1) between 2005 and 2018, as well as additional assemblies and workshops, held in the communities where the cooperatives operate and in Ensenada, Baja California, where the central offices of the cooperatives are located. These meetings convening cooperative members and leaders allowed discussion of challenges, and documentation and vetting of adaptive measures, such as the establishment and assessment of marine reserves, fisheries improvement projects, aquaculture and mariculture, topics and proposals which emerged from co-production of knowledge and co-management arrangements with government and research scientists.
Finally, field economic experiments (common pool resource games) and concurrent structured interviews were conducted during the study period with six fishing cooperatives and 180 fishers inside and outside of the FEDECOOP structure (Finkbeiner et al., 2018). The objective of this study was to understand how increasing environmental and social uncertainty impacts fishing behavior and the underlying mechanisms that mediate the use of different strategies. The common pool resource game was structured after the exact biological, social and institutional dynamics of the Baja abalone fishery with real economic incentives. The concurrent structured interview administered with all 180 fisher participants sought to understand fisher risk attitudes towards different environmental and social sources of uncertainty and to contextualize the results of the experiments thus increasing internal validity.
Analysis of adaptive responses proceeded iteratively. Observations and interview results were initially assessed through iterative discussions across the co-author group, which includes cooperative members. Any gaps identified were filled through additional conversation and clarification with fishing cooperatives members and directors as well as others involved, especially in the co-management arrangements with state and federal government agencies. We then adopted a qualitative, empirically grounded approach to characterizing the community’s responses to changes and shocks and to link these responses to the alternative a priori scenarios we present above.
Because we focused mainly on the cooperatives, which were partners in the research projects, we acknowledge that we may have missed all the voices within the communities, nor can we thereby depict the full spectrum of adaptive strategies used by households and individuals, particularly the most vulnerable. We reiterate that results must be interpreted with the caveat that diverse community members experience social, ecological and economic shifts differently, depending on a variety of factors. Nonetheless, overall and for the purposes of this study, our thorough and multi-method ethnographic and quantitative study of fishers with rights to participate in the concession fisheries and their families produced a coherent and well-substantiated history of responses to environmental and market changes over time. We also observed a collective understanding and retelling of those events within the fisheries and communities, a cultural dimension of adaptation and resilience.
Results
Extreme oceanographic variability during 2010-2019
In situ coastal oceanographic monitoring conducted at Isla Natividad beginning in 2010 revealed high local year-to-year variability in water temperature, dissolved oxygen (DO) concentrations and pH (Figure 3; Supplementary Figure S2). As observed throughout the California Current and other upwelling ecosystems (Nam et al., 2011; Booth et al., 2012; Booth et al., 2014), short pulses (up to 12 hours) of low DO-low pH-low T occurred during the upwelling season in each year (Figure 3). In addition, prolonged (up to 2 weeks) low-oxygen conditions (sublethal levels DO 4.6 mg/L, and lethal to most nearshore animal species at DO 2 mg/L; Vaquer-Sunyer and Duarte, 2008) were documented in summer 2010, 2011, 2012, 2013, 2014 and 2018, while extreme warming occurred in late summer-early fall of 2012, 2014, 2015 and 2016. In 2010, the maximum and average water temperatures at 14 m depth were 17.1C and 14.4C, respectively, but reached 26.7-27.3C (max) and 17.3-18.9C (average) during warm years, including the marine heat wave of 2014-16 (Figure 3). Extended periods of low DO occurred in spring and summer of 2010 (up to 325.5 hours consecutively at or below DO 4.6 mg/L, avg. = 35.4). Low DO events also occurred in summer 2011, 2012, 2013, 2014 and 2018, though shorter in durations (up to 240.2 consecutive hours in 2011, 177.4 in 2012, 214.8 in 2013, 215 in 2014, and 128.7.in 2018 Figure 3). Low DO conditions were associated with low pH, with values as low as 7.46 recorded in 2012, and 7.5-7.63 in 2010 and 2011 (Supplementary Figure S2).
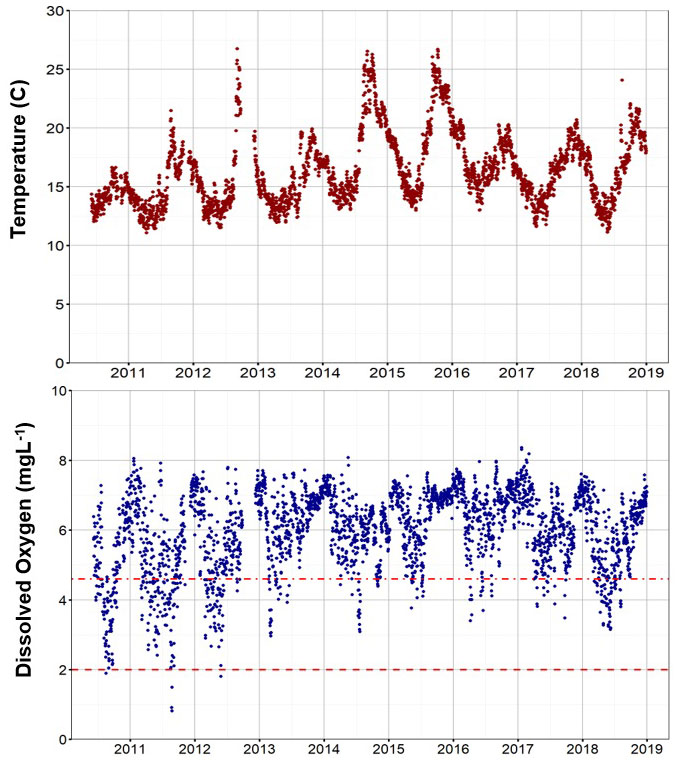
Figure 3 Daily average water temperature and DO concentrations at 14 m depth between 2010 and 2019. The dashed lines mark the 4.6 and 2 mg/L DO thresholds reported to be associated with sublethal and lethal effects, respectively, in multiple marine species tested in the laboratory (from Vaquer-Sunyer and Duarte, 2008).
Ecological responses to oceanographic variability
The 13-year monitoring (2006-2018) revealed an E2 scenario with Winner and Losers (Figure 1). Finfish species, the macroalgae Eisenia arborea and Gelidium sp. and the black sea urchin Centrostephanus coronatus showed no significant change or significantly increased in abundance through time (Figure 4 and Supplementary Figures S3-4). The abundances of these species were unaffected by hypoxia and heat waves (Figure 4 and Supplementary Figures S3-4), possibly due to fish mobility and capacity to move away from adverse local conditions, and high tolerance for these stressors in the sedentary species.
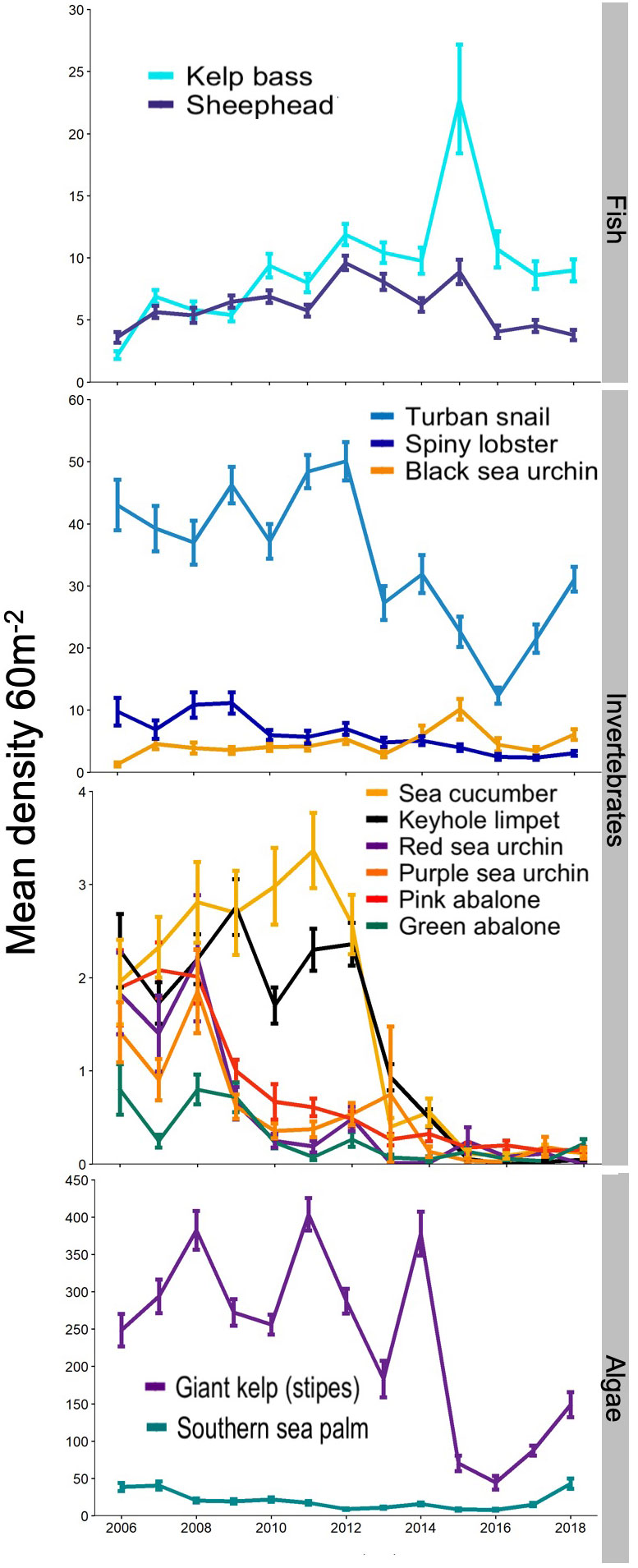
Figure 4 Abundance of algae, invertebrates, and fish from 2006-2018. Abundances within reserves (2 sites) and at fished sites (3 sites) are combined. Values are average densities estimated from 30x2 m transects (11-30 transects per site in each year, + 1 SE).
All benthic invertebrate species for which the cooperatives of Baja California hold exclusive rights exhibited significant decline (Figure 4 and Supplementary Figures S3-4), with signs of recovery after the extreme ENSO of 2016 for some species (spiny lobster, green abalone and wavy turban snail; Figure 4). Major declines were evident for pink abalone and red sea urchins starting in 2009, green abalone starting in 2010, and sea cucumbers and turban snail starting in 2013 (Figure 4). Spiny lobster also exhibited significant decline in abundance, based on visual census, although less drastically than sedentary benthic invertebrates (Figure 4 and Supplementary Figure S3-4). Green abalone, turban snail and sea cucumber showed a significant interaction between protection and year, indicative of a significant positive effect of the no-take reserves (Supplementary Figures S3-4). Turban snail, sea cucumber and green abalone abundance also began increasing in 2016-2017, after the marine heat wave, driven by increasing abundance in the reserves.
Invertebrate species for which the cooperative does not hold concession rights or for which there are currently no markets varied in their temporal trends (Figure 4), and, not surprisingly, without significant differences between fished and protected sites as these species were not harvested at any of the sites (Supplementary Figures S3-4). The purple sea urchin (Strongylocentrotus purpuratus) declined significantly in 2009, in contrast with the black sea urchin (Centrostephanus coronatus), which is considered a warm water species and, as reported above, has increased in abundance during the 13 years of monitoring. Similarly to turban snail and sea cucumber, the keyhole limpet Megathura crenulata decreased in abundance starting in 2013, following the first warming event in 2012.
Macroalgae showed high interannual variability, recovered rapidly from decline associated with heat waves and were unaffected by hypoxia. The giant kelp, Macrocystis pyrifera, that is harvested for agar production and as feed for abalone aquaculture, declined significantly following the extreme warming events in 2012, 2014 and 2015 (Figure 4 and Supplementary Figures S3-4). Kelp frond density were reduced significantly, and the entire kelp canopy was lost by early fall. However, giant kelp recovered over the following months and canopies were again present by spring of the following year, though densities in 2013 were lower than in previous years and the recovery observed in spring 2015 was reversed in summer 2015, likely due to high temperatures associated with the strong 2015/16 ENSO (Figure 4). The commercially harvested red alga Gelidium robustum does not exhibit significant change in percent cover over the 13-year monitoring period (Supplementary Figures. S3-4). Finally, the subcanopy kelp species, Eisenia arborea showed a steady decline throughout the monitoring period with a slight increase after 2013 and a strong increase in 2017 (Figure 4), and no significant change overall (Supplementary Figure S4).
Fishing cooperative impacts of and responses to oceanographic, ecological and market changes
Starting in 2009, catches of several invertebrate species have declined, coinciding with mass mortalities (Figure 5). Commercial exploitation of the high-value green and pink abalones, that are sold for up to US$100-120/kg, was voluntarily halted by the cooperative between 2011 and 2016. The fishery reopened in 2017 with conservative quotas (Smith et al., 2022). The red sea urchin has not been commercially exploited since 2008 (Figure 5). After the decline of abalone populations and the voluntary closure of these high-value fisheries, the cooperative focused their effort on other more stable species such as the lobster, turban snail, sea cucumber and red algae (Figure 5). However, starting with the local warming event of 2012, turban snail and sea cucumber have also declined, and catches are reduced for these species as well (Figure 5). Catches of red algae increased between 2014 and 2016 but have declined after 2016. The value of red algae is low (US$0.9-1.2/kg) and, while important in providing employment in harvesting and processing, this fishery provides limited income for the cooperatives. Nevertheless, during COVID-19, it was the only open fishery due to its use for medical reasons (Lopez-Ercilla et al., 2021). Lobster catches have remained high throughout the study period, including record years in 2012, 2013 and 2014 (Figure 5), and increased value of lobster (to US$45-65/kg) partly compensated for the loss of income from the decline and closure of other fisheries, with lobster accounting for up to 90% of income from fishing. It is important to highlight, however, that a diverse portfolio of fisheries and processing activities providing employment and livelihood is a key social benefit, beyond total economic value.
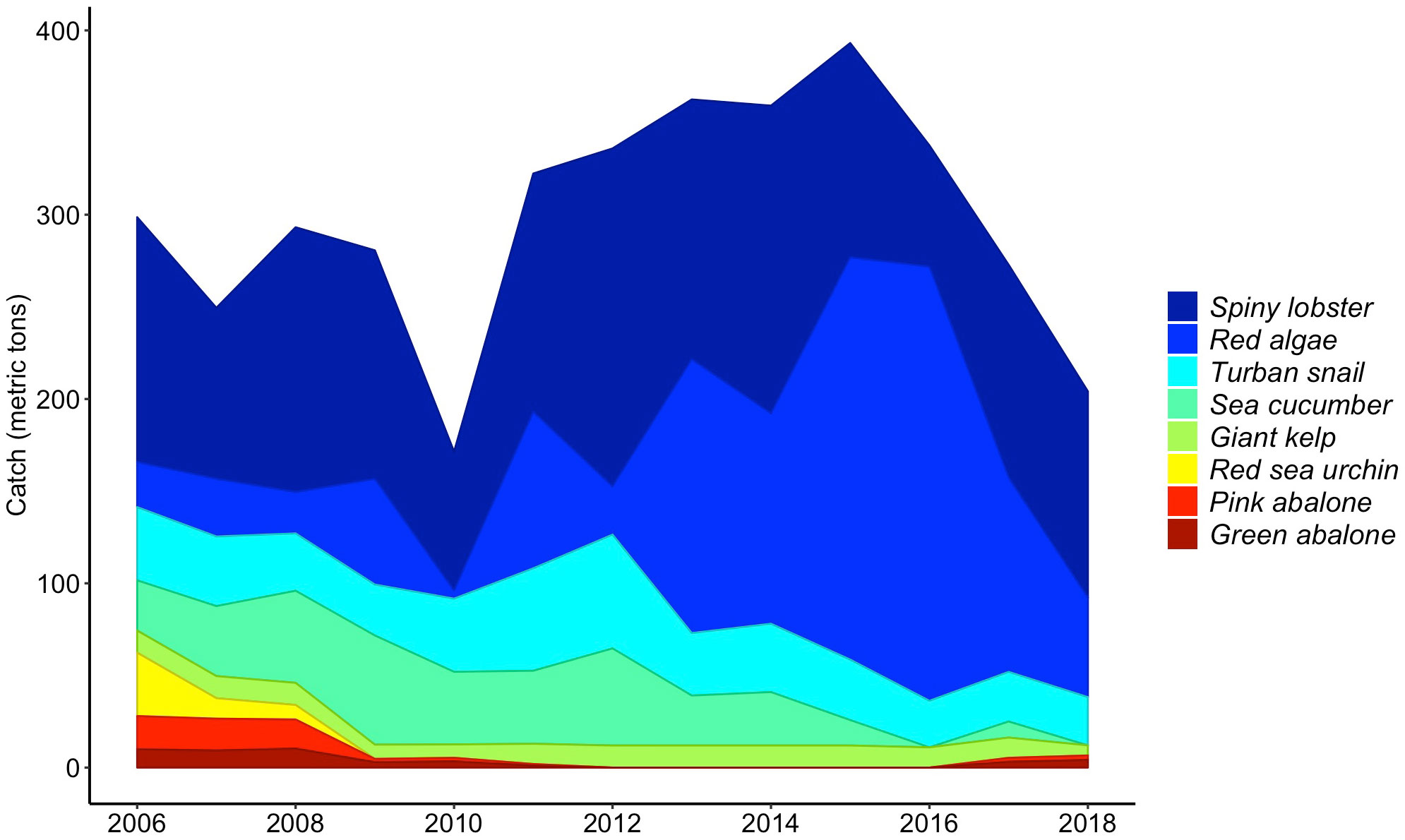
Figure 5 Time series of catch (in metric tons) from 2006-2018 for the species for which the cooperative holds exclusive access rights.
Analysis of management actions and other initiatives on Isla Natividad, as well as in the broader region, in response to environmental change and uncertainty has shed light on the adaptation strategies that have been implemented (Figure 6). Adaptive behaviors have been both reactive (e.g., fishery closure after mass mortality, Figure 6) and proactive in scope (such as establishment of voluntary no-take marine reserves, and development of aquaculture, mariculture, and eco-tourism; Figure 6), in an attempt to preempt future environmental and related socio-economic crises and respond to unprecedented or novel environmental change. The cooperatives of Baja California have long sought ways to diversify, being aware of the risks of high dependence on few species, and in this sense have been proactive. However, actions toward diversification have depended on specific events that call for corrective action, like investing more in fin-fishing and harvesting of algae when the abalone fishery is closed (Figure 6).
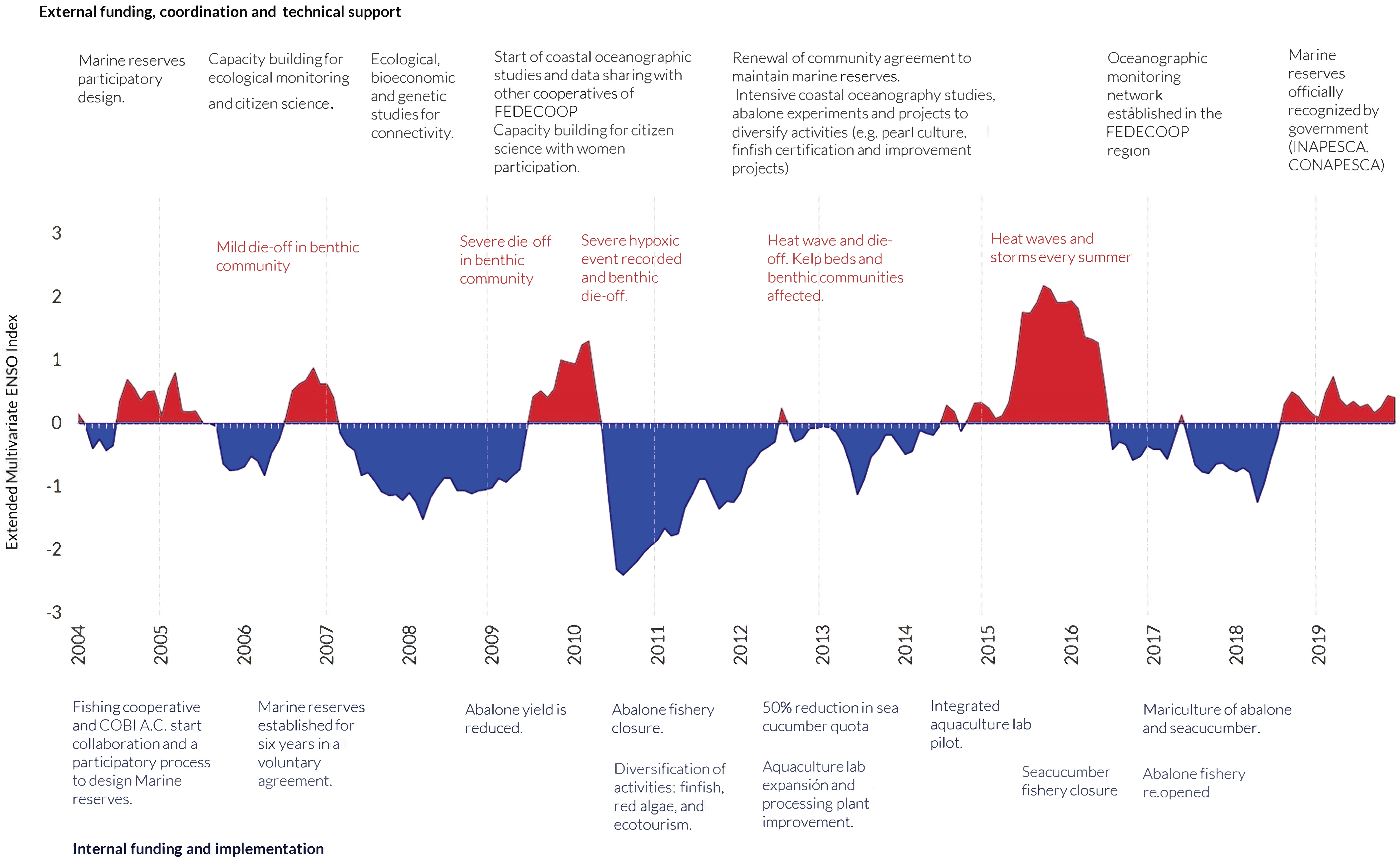
Figure 6 Major oceanographic and ecological changes, and adaptive actions taken by the local community to confront fisheries declines.
Perhaps the best example of how the Isla Natividad cooperative has proactively demonstrated strong fisheries management response and adaptive behavior is the voluntary and democratic declaration, in 2006, of their self-enforced no-take marine reserves. This was not the first attempt by Isla Natividad to close fishing areas: as early as 1989, the Isla Natividad cooperative decided to establish a voluntary closure of selected areas for abalone recovery, that was re-opened to fishing three years later. The abalone mass mortality event of 2009/2010 demonstrated the importance of the voluntary marine reserves established three years earlier. While the reserves could not mitigate the effects of the mass mortalities associated with oceanographic changes, they increased the capacity of the SES to recover by protecting large abalone with higher reproductive potential (Micheli et al., 2012), resulting in population recovery and re-opening of the fishery in 2017 (Smith et al., 2022).
A possible adaptation strategy and management response to environmental change is the diversification of the fishing portfolio and economic opportunities. In 2011 when the cooperative decided to temporarily close all abalone fishing to allow the population to recover, they relied on the harvest of snail, sea cucumber, and finfish, expanded their aquaculture hatchery and lab, upgraded their processing plant and cannery on the island, and built cabins for ecotourism. In 2012/2013 when the community experienced devastating tropical storms, record high temperatures, and complete kelp forest loss, adaptation strategies included pearl mariculture pilot studies, market research in their yellowtail fishery, development of an ecotourism business plan, and expansion of oceanographic and ecological monitoring. In 2014/2015, during a marine heat wave, the island was also hit by Hurricane Simon and other tropical storms, and kelp forest recovery was further inhibited. The community responded by ramping up efforts in seaweed harvesting, finfish fishing, and abalone and sea cucumber polyculture (Bauer et al., 2019). During this time, they also submitted a proposal to the federal government fisheries agency INAPESCA (Instituto Nacional de Pesca) to consider the formal designation of their voluntary marine reserves as Fisheries Refuges (Refugios Pesqueros), which was approved in 2018 (DOF, 2018) for five years. Abalone fisheries were re-started in 2017 and continue to date. The cooperative has been voluntarily fishing only approximately half of the quota established by the government fisheries agency, to allow for recovery and avoid overexploitation while population densities are still low. Investments have also continued into further diversifying production. These include experimental at-sea abalone mariculture and fisheries improvement projects aimed at increasing the value of yellowtail and ocean whitefish, conducted in partnership with CSOs.
Cooperatives have also been increasingly committing to and investing in monitoring and participating in research. For example, in 2017, ten cooperatives along the Pacific coast of Baja California, including in Isla Natividad, established a nearshore oceanographic monitoring network using low cost oceanographic sensors (Low et al., 2021), and several cooperatives are actively participating in oceanographic, genetic, and ecological studies through collaboration with academic institutions and local CSOs (e.g. Micheli et al., 2012; Munguia-Vega et al., 2015; Boch et al., 2018; Woodson et al., 2019; Fulton et al., 2019; Smith et al., 2022).
Discussion
Environmental extremes from marine heat waves, storms, escalating hypoxia and acidification are affecting marine ecosystems and coastal communities worldwide. This case study documents oceanographic, ecological and human responses to regional events (e.g. marine heat waves and extreme hypoxia) that have affected the Pacific basin. During the study period, nearshore ecosystems and fisheries of the southern California Current have been affected by both extreme hypoxia and marine heat waves (Micheli et al., 2012; Boch et al., 2018; Woodson et al., 2019; Arafeh-Dalmau et al., 2019; Beas-Luna et al., 2020).
Between 2009-2016 this region experienced a series of devastating tropical storms and hurricanes (including hurricane Simon in 2014), as well record high temperatures (e.g., during a marine heat wave in 2014/16). Long-term in situ oceanographic monitoring documented local warming events that preceded the region-wide marine heat wave of 2014-16 (in summer 2012) and affected the Pacific coast of Baja California Sur, but not northern Baja (Woodson et al., 2019). In situ measurements further documented extreme and prolonged local hypoxic and acidic conditions that exceed what was previously reported in the California current region (e.g., Booth et al., 2012; Frieder et al., 2012; Booth et al., 2014). These extreme conditions caused significant ecological shifts, including loss of kelp canopy, invertebrate mass mortalities, and shifts in community structure (Micheli et al., 2012; Arafeh-Dalmau et al., 2020; Beas-Luna et al., 2020). However, not all species were negatively impacted, and abundance and catch trends varied among species (Figures 4, 5).
Simultaneous observations of the bio-physical and human responses of this coastal SES reveals a convergence to ecological ‘winners and losers’ and fishing community ‘adapters’, in some cases within financial and capacity constraints (Figure 1), in response to extreme environmental shocks. Vulnerability and resilience vary across resources and ecosystem components, and the fishing community has undertaken a suite of proactive and reactive adaptive responses, enabled by secure tenure rights, financial assets, flexibility and the ability to diversify, social organizations that support collective action and effective leadership, learning from past exposure and disturbance, human agency, and external support by and collaboration with CSOs and researchers (McCay et al., 2014; Finkbeiner, 2015; Finkbeiner et al., 2018; Cinner and Barnes, 2019). Thus, our case study appears to combine E2 and S3, whereby the differential vulnerabilities of major fish stocks keep open possibilities for adaptive measures on the part of the fishing communities that enhance both ecological and social resilience. Ecological “winners and losers” help the whole system meet basic functions, continuing to provide livelihoods for fishery-dependent communities and opportunities for ecological conservation and restoration.
Faced with declining catches or sudden environmental shifts, fishers may react in several ways, each with different potentially short- and long-term consequences for ecosystems and livelihoods. Regardless of the specific action, risk can be reduced by making management more robust to uncertainty, such as through monitoring and using adaptive management that reduces uncertainty by promoting learning (Charles, 2007; Mumford et al., 2009), strengthening leadership and local institutions, providing adequate access to resources and rights and by explicitly articulating the potential risks, trade-offs and potential synergies of different adaptive actions (Gutiérrez et al., 2011; Finkbeiner, 2015; Finkbeiner et al., 2017).
In Isla Natividad and other fishing cooperatives of the Pacifico Norte, adaptation strategies for responding to extreme climatic influences on fisheries and mariculture have included suspending fishing activities after the occurrence of a natural disaster to help recover fishery resources and ecosystems (Smith et al., 2022), temporarily transferring target species and mariculture structures away from high-risk areas and to local refugia (Woodson et al., 2019) and implementing monitoring programs to track the recovery of marine resources and ecosystems (Fulton et al., 2019). Additional strategies could include switching fisheries and mariculture target species to reduce sensitivity to extreme events, using climate-proof technologies in aquaculture such as closed recirculating systems, and establishing an early warning system to assist decision makers in performing time-adaptive measures.
Previous research in the region has also demonstrated the importance of diversification (of catch and livelihood portfolios) as a strategy for minimizing inter-annual loss in income during periods of low productivity in the marine ecosystems (during El Niño years) and during periods of high market volatility (Finkbeiner, 2015). Under escalating environmental variability and other risks, strengthening and expanding coastal communities access rights and institutional capacity to enable continued diversification, particularly for production and markets targeting Anthropocene “winners” (e.g. species with broad environmental tolerance, high growth rates and short generation times) could be key strategies for supporting adaptation.
Enabling conditions and barriers to adaptation
Globally, the willingness of stakeholders to take action and invest in these different possible responses to change is influenced by a suite of complex drivers and interactions, including the perception of risk and of the anticipated costs and benefits of these initiatives and responses (Lynham, 2017). Therefore, the actual implementation of adaptive measures has faced numerous barriers, often relating to inadequate governance and institutional structures (Bennett et al., 2014; Eisenack et al., 2014) and lack of access to or inequitable distribution of financial resources (Bennett et al., 2014; Eisenack et al., 2014). Barriers also include lack of access to relevant information for adaptation (Bennett et al., 2014; Eisenack et al., 2014; Nunn et al., 2014), social and cultural norms that prevent adoption of viable adaptation options (Eisenack et al., 2014; Nunn et al., 2014), and conflicting and competing values (Wolf et al., 2013; Nunn et al., 2014), as well as the severity of extreme events and processes, such as reproductive failure at low densities, that prevent stock recovery (Aalto et al., 2019), and other factors that undermine resilience, such as loss of biodiversity, habitat degradation, pollution, invasive species, and pathogens (Holbrook and Johnson, 2014).
The case of Isla Natividad and its confederates in the region appears exceptional. However, every case study is exceptional in some way, and understanding the context and specific history of what makes it exceptional can be important. Critical to this story of environmental change, social learning and adaptation is the evolution of trust and cooperation between fishers in the region, the Mexican government, civil society organizations, and local and external scientists over the course of decades, commencing in the time of resource crisis (Ponce-Díaz et al., 2009).
Similar trajectories have been described for other regions in Latin America (Castilla et al., 1998; Gelcich et al., 2010; Defeo et al., 2016). For example, self-organization and social cohesion have been key drivers for the success of this and other co-management systems (Defeo and Castilla, 2005; McCay et al., 2014). Similar to the case of Isla Natividad, in the lobster cooperative of Punta Allen, Mexico, geographic isolation has promoted a strong collective organization, and an ability to develop and self-enforce rules with minimal government intervention. As a result, the Punta Allen lobster fishery was more resilient to unexpected shocks, such as Hurricane Gilbert in 1988, than other fisheries in the Yucatan region (Castilla and Defeo, 2001; Defeo et al., 2016).
After the El Niño event of 1982/1983, Mexico’s abalone populations were in dire shape and the commercial fishery was on the verge of collapse. At this critical junction, fishers from the region agreed to partake in co-management of the resource as well as in scientific research with government officials and external scientists – a responsibility that they have upheld to this day. Resulting from this process, strong social capital has emerged over time, both within the cooperatives and beyond, with government, CSOs and scientists. The 1997/1998 El Niño and Hurricane Nora provided another opportunity for the cooperatives to learn from severe environmental change and the importance of preemptively safeguarding resources. Understanding their role as active agents capable of buffering the effects of environmental change generated at higher scales is indicative of high levels of perceived human agency among fishers in the region. This history explains, in part, why adaptive capacity has materialized into the adaptive behaviors and action exemplified by the Isla Natividad case study; perceived risk from environmental change is high in this and other cooperatives that have experience with previous crises, and the cooperative has access to high quality information and data about the ecological impacts of environmental change based on their long history of working side by side with scientists.
Behavioral economic experiments and interviews with fishers from six cooperatives in this region, including Isla Natividad, have revealed that, when faced with increasing environmental and social uncertainties (i.e., poaching), fishers voluntarily reduce their fishing effort to compensate for potential losses associated with mass mortality events or illegal take, corroborating a strong sense of human agency (Finkbeiner et al., 2018). These economic experiments also indicated that participants from cooperatives that managed a more diverse portfolio of species, had mechanisms for social learning and communication, and that had stronger internal self-governance schemes behaved in the games with more restraint when faced with the shocks. Such behavioral studies are key to understanding the conditions and opportunities for moving the SES towards greater resilience and adaptive capacity (scenarios S3 and E3; Figure 1).
The Isla Natividad community’s ability to mobilize adaptive strategies and management responses during difficult times is contingent on their material assets and strong rights to access and manage environmental resources (McCay et al., 2014; Short et al., 2021). However, their ability to respond to environmental crises may become more constrained over time with the progression of climate change. For example, the fact that their fishery has become more reliant on lobster - which is almost entirely dependent on an export market - as other species lose under more severe climate change scenarios makes the cooperative much more vulnerable to economic catastrophes, as in the case of the first year of the COVID-19 pandemic. In this scenario, their ability to invest in other resources or diversify would become severely compromised (scenario S2; Figure 1).
Recent signs of recovery of the mollusk fisheries, particularly the valuable abalone fishery (Smith et al., 2022), and development of commercial finfish fisheries and mariculture suggest that conservation and diversification investments are at least partly contributing to avoiding this possible social-ecological trap. This resilience is exemplified by the COVID-19 crisis, that led to the closure of foreign markets and therefore the lobster fisheries, that are primarily exported live, with significant economic losses for the cooperatives of this region (Knight et al., 2020). Because abalone are canned, fish can be frozen, and Gelidium dried before export, these fisheries have continued, thereby maintaining employment and securing income, once markets reopened.
Surveys of 397 (24% women) fishers and fish workers across Mexico conducted from April to December 2020 have revealed that, overall, 58% of respondents reported they had taken actions to address the pandemic impacts – such as changing the way they sell their products, finding alternative markets, and activating the fishing organizations’ emergency funds - with the greatest fraction in Baja California, followed by Campeche and Quintana Roo (Lopez-Ercilla et al., 2021). The 42% of respondents in these surveys who reported they were unable to adapt, and the many cooperatives and communities throughout the region that faced major hardship in the pandemic provide counterfactuals for this case study and highlight how lacking the conditions that have enabled resilience in Isla Natividad and other systems constrain adaptation (scenario S1, Figure 1). The large fraction of SSF actors across Mexico, and around the world, that were unable to take adaptive actions in response to the market shock from the global pandemic further highlight the urgent need of expanding investment in social and human capital, technology, and local and national markets as key to resilience in the face of major climate and global market shocks (Short et al., 2021).
Marine reserves can provide an ecological safety net for communities’ income by enhancing greater resilience to environmental shocks (Micheli et al., 2012; Roberts et al., 2017; Aalto et al., 2019; Smith et al., 2022). However, ultimately, the capacity to adapt to these shocks will depend on the collective action capabilities of these groups to make decisions that require coordination and self-governance to avoid pushing the ecological decline further when facing these impacts. The resilience of the ecosystem has to be complemented by the resilience of the organization and the capacity of the fishers to adapt. For example, in the case reported here, by closing fisheries showing signs of decline and by diversifying income sources (Figure 6).
In contrast, open access fisheries, or fisheries vulnerable to poaching because of the absence of property rights (de jure open access) or ineffective enforcement and non-compliance (de facto open access), tend to overexploit their resources which, ultimately, reduces incomes and, in turn, limits capacity to accumulate capital. This dissipates existing capital and trust in the regulatory institutions. Other fisheries in the Baja California peninsula show that this is the case (Finkbeiner, 2015; Frawley et al., 2019): when financial and organizational capital and trust are lacking for contingent, historical reasons, programs that support local fisheries by increasing their economic capacity might be necessary to help fishing communities escape or avoid such social-ecological traps (E1-S1, Figure 1).
Whereas some cooperatives, like Buzos y Pescadores in Isla Natividad, have been proactively steering changes to adapt to climate change and absorb environmental shocks, other cooperatives in the Vizcaino peninsula struggled to support their members and to explore adaptive strategies in the face of the same exogenous challenges (Finkbeiner, 2015; Valdez-Rojas et al., 2022). Several circumstances may favor or hinder adaptive actions. For instance, geographic isolation of the Isla Natividad fishery is a condition that enhances its adaptive capacity insofar as it depends on exclusive access: exclusive access and quota systems are much more likely to be enforced in isolated fisheries than in fisheries where proximity to other communities makes poaching and free riding harder to control (McCay et al., 2014). Illegal fishing, in particular, is not a major issue in Isla Natividad, but it is a key challenge in SSF worldwide (e.g. Oyanedel et al., 2017).
Another key factor conducive to high adaptive capacity is the accumulated financial, knowledge, organizational and social capital. The high market value of lobster and abalone have contributed to a situation of low levels of poverty for many fishing cooperatives in this region (McCay et al., 2014). Poverty dramatically reduces capacity for adaptation: in conditions of poverty, fishing cooperatives lack the economic resources, access to knowledge, technical means and institutional infrastructure to diversify and invest in new activities, enforce quota limits and fishing closures, and incur short-term costs associated with the establishment of no-take zones. Moreover, they might lack the sense of trust in the institutions they are part of, and the willingness to engage in collective actions to protect and avoid overexploiting the natural resources they depend on.
Conclusions
In conclusion, this case study exemplifies how a fishing cooperative has been able to confront, adapt and overcome extreme environmental change and uncertainty. Over the course of this study period (2006-2018), the Isla Natividad fishing cooperative has implemented numerous adaptive strategies to address marine heatwaves, hypoxia events, tropical storms, kelp forest loss and the decline or mass mortality of important species, such as abalone.
These adaptive strategies demonstrate strength in each of the six domains of adaptive capacity (Cinner and Barnes, 2019). Through their ability to diversify into other species (even if lower value such as algae) and into other livelihood opportunities such as mariculture, aquaculture, and tourism, they show a high degree of flexibility. Importantly, flexibility is contingent on the mobilization of assets such as resources and rights. At critical times, the cooperative has been able to mobilize and invest their assets into alternative species and livelihoods, contingent on their strong property rights. The cooperative has also demonstrated a strong commitment to learning, indicated by their engagement in monitoring local oceanographic and ecological conditions with external researchers, experimental fisheries and market research. As adaptive capacity is a latent quality, human agency is absolutely critical for mobilizing both reactive and proactive adaptive strategies. The cooperative has shown strong agency through their mobilization of both reactive and proactive strategies such as through the reactive establishment of fishery closures in times of crisis and decline. Similarly, the cooperative has exemplified very practical risk attitudes and a resilience mindset (Mason et al., 2022) as demonstrated through the proactive, voluntary and democratic establishment of fully-protected marine reserves. And finally, social organization underpins all adaptive strategies mobilized by this cooperative, as shown by strong collective action, but specifically as demonstrated through cooperative decisions to enforce internal rules on quota reduction.
We have also alluded to the importance of context and history, particularly in response to major environmental events, which in this case appears to have led to enabling narratives and cultural understandings, and a high level of perceived human agency (Finkbeiner et al., 2018). This specificity limits generalizing from this case study but does not diminish the broader finding that coastal communities can take strong initiatives to respond adaptively to change and sustain their livelihoods and ecosystems. Within the constraints of specific contexts and histories, similar enabling conditions and processes hold great potential for supporting food security and livelihoods in coastal settings worldwide (e.g. Short et al., 2021).
Data availability statement
The raw data supporting the conclusions of this article will be made available by the authors, without undue reservation.
Ethics statement
The studies involving humans were approved by Stanford University, IRB, Panel on Non-medical Human Subjects research. The studies were conducted in accordance with the local legislation and institutional requirements. The participants provided their written informed consent to participate in this study.
Author contributions
FM: Conceptualization, Data curation, Formal analysis, Funding acquisition, Investigation, Methodology, Project administration, Resources, Supervision, Validation, Writing – original draft, Writing – review & editing. AS-A: Conceptualization, Funding acquisition, Investigation, Project administration, Resources, Supervision, Writing – review & editing. EA: Investigation, Writing – review & editing. RB-L: Investigation, Visualization, Writing – review & editing. CB: Conceptualization, Data curation, Formal analysis, Investigation, Methodology, Visualization, Writing – review & editing. JC: Conceptualization, Investigation, Methodology, Writing – review & editing. GDL: Conceptualization, Funding acquisition, Investigation, Methodology, Writing – review & editing. ED: Investigation, Resources, Writing – review & editing. AE-M: Conceptualization, Investigation, Resources, Writing – review & editing. EF: Conceptualization, Investigation, Writing – review & editing. JF: Investigation, Resources, Writing – review & editing. SF: Investigation, Resources, Writing – review & editing. AH: Conceptualization, Data curation, Investigation, Methodology, Project administration, Resources, Supervision, Writing – review & editing. AL: Conceptualization, Investigation, Project administration, Resources, Writing – review & editing. NL: Data curation, Investigation, Validation, Writing – review & editing. RM: Conceptualization, Data curation, Investigation, Resources, Writing – review & editing. BM: Conceptualization, Funding acquisition, Investigation, Methodology, Writing – review & editing. SM: Conceptualization, Investigation, Methodology, Resources, Writing – review & editing. MP: Conceptualization, Investigation, Project administration, Writing – review & editing. AR: Conceptualization, Data curation, Investigation, Project administration, Resources, Writing – review & editing. AS: Data curation, Formal analysis, Investigation, Methodology, Project administration, Validation, Visualization, Writing – review & editing. JT: Conceptualization, Funding acquisition, Investigation, Project administration, Resources, Supervision, Writing – review & editing. LV-V: Conceptualization, Investigation, Methodology, Project administration, Visualization, Writing – review & editing. CBW: Conceptualization, Data curation, Formal analysis, Funding acquisition, Investigation, Methodology, Resources, Validation, Visualization, Writing – review & editing.
Funding
The author(s) declare financial support was received for the research, authorship, and/or publication of this article. This work was supported by grants from The Walton Family Foundation, Packard Foundation, Marisla Foundation, Sander Family Foundation, and the US National Science Foundation (grants DEB121244, BioOce 1736830 and DISES 2108566).
Acknowledgments
We thank Wendy Weisman, Grant Murray, and Saudiel Ramirez-Sanchez for their contributions to the social surveys and J. C. Castilla for invaluable feedback on the manuscript. We are grateful to the members of the cooperative Buzos y Pescadores and the Isla Natividad community, the staff and directors of the Federacion Regional de Sociedades Cooperativas de la Industria Pesquera Baja California, F.C.L. (FEDECOOP), and the Reserva de la Biosfera El Vizcaino CONANP staff for their participation and support.
Conflict of interest
The authors declare that the research was conducted in the absence of any commercial or financial relationships that could be construed as a potential conflict of interest.
Publisher’s note
All claims expressed in this article are solely those of the authors and do not necessarily represent those of their affiliated organizations, or those of the publisher, the editors and the reviewers. Any product that may be evaluated in this article, or claim that may be made by its manufacturer, is not guaranteed or endorsed by the publisher.
Supplementary material
The Supplementary Material for this article can be found online at: https://www.frontiersin.org/articles/10.3389/fmars.2024.1322108/full#supplementary-material
References
Aalto E. A., Micheli F., Boch C. A., Espinosa Montes J. A., Woodson C. B., De Leo G. A. (2019). Catastrophic mortality, Allee effects, and marine protected areas. Am. Nat. 193, 391–408. doi: 10.1086/701781
Adger W. N., Vincent K. (2005). Uncertainty in adaptive capacity. Comptes Rendus Geosci. 337, 399–410. doi: 10.1016/j.crte.2004.11.004
Alheit J., Bakun A. (2010). Population synchronies within and between ocean basins: Apparent teleconnections and implications as to physical-biological linkage mechanisms. J. Mar. Systems 79, 267–285. doi: 10.1016/j.jmarsys.2008.11.029
Arafeh-Dalmau N., Montaño-Moctezuma G., Martinez J. A., Beas-Luna R., Schoeman D. S., Torres Moye G. (2019). Extreme marine heatwaves alter kelp forest community near its equatorward distribution limit. Front. Mar. Sci. 6, 499. doi: 10.3389/fmars.2019.00499
Arafeh-Dalmau N., Schoeman D. S., Montaño-Moctezuma G., Micheli F., Rogers-Bennett L., Olguin-Jacobson C., et al. (2020). Marine heatwaves threaten kelp forests. Sci. Lett. 367, 635.
Arriaga C. L. (1998). Regiones marinas prioritarias de México (México: Comisión Nacional para el Conocimiento y uso de la Biodiversidad).
Barber R. T., Chavez E. P. (1983). Biological consequences of el niño. Science 222, 1203–1210. doi: 10.1126/science.222.4629.1203
Barber R. T., Chavez E. P. (1986). Ocean variability in relation to living resources during the 1982/83 El Niño. Nature 319, 279–285. doi: 10.1038/319279a0
Bauer J., Vazquez-Vera L., Espinoza A., Lorda J., Beas R., Vela C., et al. (2019). Multi-trophic aquaculture of green abalone Haliotis fulgens and warty sea cucumber Parastichopus parvimensis enhances production. J. Shellfish Res. 38 (2), 455–461. doi: 10.2983/035.038.0229
Beas-Luna R., Micheli F., Woodson C. B., Carr M., Malone D., Torre J., et al. (2020). Geographic variation in responses of kelp forest communities of the California Current to recent climatic changes. Global Change Biol. 26, 6457–6473. doi: 10.1111/gcb.15273
Bennett N. J., Dearden P., Murray G., Kadfak A. (2014). The capacity to adapt?: communities in a changing climate, environment, and economy on the northern Andaman coast of Thailand. Ecol. Soc. 19 (2), 5. doi: 10.5751/ES-06315-190205
Berkes F. (2001). Managing small-scale fisheries: alternative directions and methods (Ottawa, Canada: IDRC). 320pp.
Blythe J. L., Murray G., Flaherty M. (2014). Strengthening threatened communities through adaptation: insights from coastal Mozambique. Ecol. Soc. 19 (2). doi: 10.5751/ES-06408-190206
Boch C. A., Micheli F., Al Najjar M. A., Monismith S. G., Beers J. M., Bonilla J. C., et al. (2018). Local oceanographic variability influences the performance of juvenile abalone under climate change. Sci. Rep. 8, 5501. doi: 10.1038/s41598-018-23746-z
Booth J. A. T., McPhee-Shaw E. E., Chua P., Kingsley E., Denny M., Phillips R., et al. (2012). Natural intrusions of hypoxic, low pH water into nearshore marine environments on the California coast. Continental Shelf Res. 45, 108–115. doi: 10.1016/j.csr.2012.06.009
Booth J. A. T., Woodson C. B., Sutula M., Micheli F., Weisberg S. B., Bograd S. J., et al. (2014). Patterns and potential drivers of declining oxygen content along the southern California coast. Limnol. Oceanogr. 59, 1–14. doi: 10.4319/lo.2014.59.4.1127
Breitburg D., Levin L. A., Oschlies A., Gregoire M., Chavez F. P., Conley D. J., et al. (2018). Declining oxygen in the global ocean and coastal waters. Science 359 (6371), eaam7240. doi: 10.1126/science.aam7240
Burch S., Robinson J. (2007). A framework for explaining the links between capacity and action in response to global climate change. Climate Policy 7 (4), 304–316. doi: 10.1080/14693062.2007.9685658
Carr M. H., Reed D. C. (2016). “Chapter 17: shallow rocky reefs and kelp forests,” in Ecosystems of California. Eds. Mooney H., Zavaleta E. (Oakland, California: University of California).
Castilla J. C., Defeo O. (2001). Latin-American benthic shellfisheries: emphasis on co-management and experimental practices. Rev. Fish Biol. Fisheries 11, 1–30. doi: 10.1023/A:1014235924952
Castilla J. C., Manriquez P. H., Alvarado J. L., Rosson A., Pino C., Espoz C., et al. (1998). Artisanal Caletas: As units of production and co-managers of benthic invertebrates in Chile. Can. J. Fisheries Aquat. Sci. 125, 407–413.
Cavole L. M., Demko A. M., Diner R. E., Giddings A., Koester I., Pagniello C. M. L. S., et al. (2016). Biological impacts of the 2013–2015 warm-water anomaly in the Northeast Pacific: Winners, losers, and the future. Oceanography 29, 273–285. doi: 10.5670/oceanog.2016.32
Chan F., Barth J. A., Lubchenco J., Kirincich A., Weeks H., Peterson W. T., et al. (2008). Emergence of anoxia in the California Current large marine ecosystem. Science 319 (5865), 920. doi: 10.1126/science.1149016
Charles A. (2007). “Adaptive co-management for resilient resource systems: some ingredients and the implications of their absence,” in Adaptive co-management: Collaboration, learning, and multi-level governance. Eds. Artmitage D., Berkes F., Doubleday N. (Vancouver, Canada: UBC Press).
Chavez F. P., Ryan J., Lluch-Cota S. E., Ñiquen C M. (2003). From anchovies to sardines and back: multidecadal change in the Pacific Ocean. Science 299, 217–221. doi: 10.1126/science.1075880
Cheung W. W. L., Lam V. W. Y., Sarmiento J. L., Kearney K., Watson R., Zeller D., et al. (2010). Large-scale redistribution of maximum fisheries catch potential in the global ocean under climate change. Global Change Biol. 16, 24–35. doi: 10.1111/j.1365-2486.2009.01995.x
Cheung W. W. L., Meeuwig J. J., Lam V. W. Y. (2011). “Ecosystem-based fisheries management in the face of climate change,” in Ecosystem approaches to fisheries: A global perspective. Eds. Christensen V., Maclean J. (New York, NY: Cambridge University Press), 171–188.
Cinner J. E. (2011). Social-ecological traps in reef fisheries. Global Environ. Change 21, 835–839. doi: 10.1016/j.gloenvcha.2011.04.012
Cinner J. E., Barnes M. L. (2019). Social dimensions of resilience in social-ecological systems. One Earth 1 (1), 51–56. doi: 10.1016/j.oneear.2019.08.003
Cinner J. E., McClanahan T. R., MacNeil M. A., Graham N. A. J., Daw T. M., Mukminin A., et al. (2012). Comanagement of coral reef social-ecological systems. PNAS 109 (14), 5219–5222. doi: 10.1073/pnas.1121215109
Defeo O., Castilla J. C. (2005). More than one bag for the world fishery crisis and keys for co-management successes in selected artisanal Latin American shellfisheries. Rev. Fish Biol. Fisheries 15, 265–283. doi: 10.1007/s11160-005-4865-0
Defeo O., Castrejon M., Perez-Castaneda R., Castilla J.-C., Gutierrez N. L., Essington T., et al. (2016). Co-management in Latin American small-scale shellfisheries: assessment from long-term case studies. Fish Fisheries 17, 176–192. doi: 10.1111/faf.12101
Diario Oficial de la Federación (DOF) (2018). Acuerdo por el que se establecen sos zonas de refugio pesquero en Isla Natividad (Mexico: BCS).
Di Lorenzo E., Mantua N. (2016). Multi-year persistence of the 2014/15 North Pacific marine heatwave. Nat. Climate Change 6, 1042–1047. doi: 10.1038/nclimate3082
Doney S. C. (2010). The growing human footprint on coastal and open-ocean biogeochemistry. Science 328, 1512–11516. doi: 10.1126/science.1185198
Doney S. C., Fabry V., Feely R., Kleypas J. (2009). Ocean acidification: the other CO2 problem. Mar. Sci. 1, 169–192. doi: 10.1146/annurev.marine.010908.163834
Eisenack K., Moser S. C., Hoffman E., Klein R. J. T., Oberlack C., Pechan A., et al. (2014). Explaining and overcoming barriers to climate change adaptation. Nat. Climate Change 4, 867–872. doi: 10.1038/nclimate2350
FAO. (2020). “The state of world fisheries and aquaculture 2020,” in Sustainability in action. Rome, Italy. doi: 10.4060/ca9229en
Feely R. A., Sabine C. L., Hernandez-Ayon J. M., Ianson D., Hales B. (2008). Evidence for upwelling of corrosive “acidified” water onto the continental shelf. Science 320, 1490–1492. doi: 10.1126/science.1155676
Finkbeiner E. M. (2015). The role of diversification in dynamic small-scale fisheries: lessons from Baja California Sur, Mexico. Global Environ. Change 32, 139–152. doi: 10.1016/j.gloenvcha.2015.03.009
Finkbeiner E., Micheli F., Bennett N. J., Ayers A. L., Le Cornu E., Doerr A. N. (2017). Examining trade-offs in climate change responses in the context of Pacific Island fisheries. Mar. Policy 88, 359–364. doi: 10.1016/j.marpol.2017.09.032
Finkbeiner E., Micheli F., Saenz-Arroyo A., Vazquez-Vera L., Perafan C. A., Cardenas J. C. (2018). Local response to global uncertainty: insights from experimental economics. Global Environ. Change 48, 151–157. doi: 10.1016/j.gloenvcha.2017.11.010
Folke C., Carpenter S., Walker B., Scheffer M., Elmqvist T., Gunderson L., et al. (2004). Regime shifts, resilience, and biodiversity in ecosystem management. Annu. Rev. Ecol. Syst. 35, 557–581. doi: 10.1146/annurev.ecolsys.35.021103.105711
Frawley T., Finkbeiner E., Crowder L. (2019). Environmental and institutional degradation in the globalized economy: lessons from small-scale fisheries in the Gulf of California. Ecol. Soc. 24 (1), 7. doi: 10.5751/ES-10693-240107
Frieder C. A., Nam S. H., Martz T. R., Levin L. A. (2012). High temporal and spatial variability of dissolved oxygen and pH in a nearshore California kelp forest. Biogeosciences 9, 3917–3930. doi: 10.5194/bg-9-3917-2012
Fulton S., Hernandez-Velasco A., Suarez-Castillo A., Fernandez-Rivera Melo F., Rojo M., Saenz-Arroyo A., et al. (2019). “From fishing fish to fishing data: the role of artisanal fishers in conservation and resource management in Mexico,” in Viability and sustainability of small-scale fisheries in latin America and The Caribbean. Eds. Salas S., Barragán-Paladines M. J., Chuenpagdee R. (Basel, Switzerland: Springer International Publishing), 151–175.
Gelcich S., Hughes T. P., Olsson P., Folke C., Defeo O., Fernandez M., et al. (2010). Navigating transformations in governance of Chilean marine coastal resources. PNAS 107, 16751–17060. doi: 10.1073/pnas.1012021107
Gordon H. S. (1954). The economic theory of a common property resource: the fishery. J. Political Economy 62 (2), 124–142. doi: 10.1086/257497
Gunderson A. R., Armstrong E. J., Stillman J. H. (2015). Multiple stressors in a changing world: the need for an improved perspective on physiological responses to the dynamic marine environment. Annu. Rev. Mar. Sci. 13 (41), 1–22.
Gutiérrez N., Hilborn R., Defeo O. (2011). Leadership, social capital and incentives promote successful fisheries. Nature 470, 386–389. doi: 10.1038/nature09689
Hernandez A., Kempton W. (2003). Changes in fisheries management in Mexico: Effects of increasing scientific input and public participation. Ocean Coast. Manage. 46, 507–526. doi: 10.1016/S0964-5691(03)00032-2
Hoegh-Guldberg O., Bruno J. F. (2010). The impact of climate change on the world’s marine ecosystems. Science 328, 1523–1528. doi: 10.1126/science.1189930
Holbrook N. J., Johnson J. E. (2014). Climate change impacts and adaptation of commercial marine fisheries in Australia: a review of the science. Climatic Change 124, 703–715. doi: 10.1007/s10584-014-1110-7
Holling C. S. (1973). Resilience and stability of ecological systems. Annu. Rev. Ecol. Syst. 4, 1–23. doi: 10.1146/annurev.es.04.110173.000245
Holling C. S. (2001). Understanding the complexity of economic, ecological, and social systems. Ecosystems 4, 90–405. doi: 10.1007/s10021-001-0101-5
Kelleher K. (2012). Hidden harvest: the global contribution of capture fisheries. Report no. 66469-GLB (Washington, DC: The World Bank).
Knight C., Burnham T. L. U., Mansfield E. J., Crowder L. B., Micheli F. (2020). Pandemic reveals vulnerability of small-scale fisheries to global market systems. Lancet Planetary Health 4 (6), e219. doi: 10.1016/S2542-5196(20)30128-5
Knutson T. R., McBride J. L., Chan J., Emmanuel K., Holland G., Landsea C., et al. (2010). Tropical cyclones and climate change. Nat. Geosci. 3, 157–163. doi: 10.1038/ngeo779
Koontz T. M., Gupta D., Mudliar P., Ranjan P. (2015). Adaptive institutions in social-ecological systems governance: A synthesis framework. Environ. Sci. Policy 53, 1–13. doi: 10.1016/j.envsci.2015.01.003
Laffoley D., Baxter J. M. (2019). Ocean deoxygenation: Everyone’s problem - Causes, impacts, consequences and solutions. Full report (Gland, Switzerland: IUCN). 580pp.
Levin S. A., Lubchenco J. (2008). Resilience, robustness, and marine ecosystem-based management. BioScience 58, 27–32. doi: 10.1641/B580107
Lopez-Ercilla I., Espinosa-Romero M. J., Fernandez Rivera-Melo F. J., Fulton S., Fernandez R., Torre J., et al. (2021). The voice of Mexican small-scale fishers in times of COVID-19: Impacts, responses, and digital divide. Mar. Policy 131, 104606. doi: 10.1016/j.marpol.2021.104606
Low N. H. N., Micheli F., Aguilar J. D., Romero Arce D., Boch C. A., Bracamontes M.Á., et al. (2021). Variable exposure to coastal hypoxia across the southern California Current System. Sci. Rep. 11, 10929. doi: 10.1038/s41598-021-89928-4
Lynham J. (2017). Identifying peer effects using gold rushers. Land Economics 93, 527–548. doi: 10.3368/le.93.3.527
Martz T. R., Carr J. J., French C. R., Degrandpre M. D. (2003). A submersible autonomous sensor for spectrophotometric pH measurements of natural waters. Ann. Chem. 75, 1844–1850. doi: 10.1021/ac020568l
Mason J. G., Eurich J. G., Lau J. D., Battista W., Free C. M., Mills K. E., et al. (2022). Attributes of climate resilience in fisheries: From theory to practice. Fish Fisheries 23 (3), 522–544. doi: 10.1111/faf.12630
McCay B. J., Micheli F., Ponce-Díaz G., Murray G., Shester G., Ramirez-Sanchez S., et al. (2014). Cooperatives, concessions, and co-management on the Pacific coast of Mexico. Mar. Policy 44, 49–59. doi: 10.1016/j.marpol.2013.08.001
Micheli F., De Leo G., Butner C., Martone R. G., Shester G. (2014a). A risk-based framework for assessing the cumulative impact of multiple fisheries. Biol. Conserv. 176, 224–235. doi: 10.1016/j.biocon.2014.05.031
Micheli F., De Leo G., Shester G. G., Martone R. G., Lluch-Cota S. E., Butner C., et al. (2014b). A system-wide approach to supporting improvement in seafood production practices and outcomes. Front. Ecol. Environ. 12, 297–305. doi: 10.1890/110257
Micheli F., Saenz-Arroyo A., Greenley A., Vazquez L., Espinoza Montes J. A., Rossetto M., et al. (2012). Evidence that marine reserves enhance resilience to climatic impacts. PloS One 7, e40832. doi: 10.1371/journal.pone.0040832
Morales-Bojorquez E., Mucino-Diaz M. O., Velez-Barajas J. A. (2008). Analysis of the decline of the abalone fishery (Haliotis fulgens and H. corrugata) along the west central coast of the Baja California peninsula, Mexico. J. Shellfish Res. 27, 865–870. doi: 10.2983/0730-8000(2008)27[865:AOTDOT]2.0.CO;2
Mumford J. D., Leach A. W., Levontin P., Kell L. T. (2009). Insurance mechanisms to mediate economic risks in marine fisheries. ICES J. Mar. Sci. 66, 950–959. doi: 10.1093/icesjms/fsp100
Munguia-Vega A., Sáenz-Arroyo A., Greenley A. P., Espinoza Montes J. A., Palumbi S., Rossetto M., et al. (2015). Marine reserves help preserving genetic diversity after impacts derived from climate variability: lessons from the pink abalone in Baja California. Global Ecol. Conserv. 4, 264–276. doi: 10.1016/j.gecco.2015.07.005
Nam S., Kim H. J., Send U. (2011). Amplification of hypoxic and acidic events by la Niña conditions on the continental shelf off California. Geophys. Res. Lett. 38, 1–5. doi: 10.1029/2011GL049549
Nelson D. R., Adger W. N., Brown K. (2007). Adaptation to environmental change: contributions of a resilience framework. Annual Rev. Environ. Resour. 32, 395–419. doi: 10.1146/annurev.energy.32.051807.090348
Nunn P. D., Aalbersberg W., Lata S., Gwilliam M. (2014). Beyond the core: community governance for climate-change adaptation in peripheral parts of Pacific Island Countries. Regional Environ. Change 14, 221–235. doi: 10.1007/s10113-013-0486-7
Ojea E., Lester S. E., Salgueiro-Otero D. (2020). Adaptation of fishing communities to climate-driven shifts in target species. One Earth 2, 544–556. doi: 10.1016/j.oneear.2020.05.012
Ostrom E. (2009). A general framework for analyzing sustainability of social-ecological systems. Science 325, 419–422. doi: 10.1126/science.1172133
Oyanedel R., Keim A., Castilla J. C., Gelcich S. (2017). Illegal fishing and territorial user rights in Chile. Conserv. Biol. 32, 619–627. doi: 10.1111/cobi.13048
Palumbi S. R., Mcleod K. L., Grunbaum D. (2008). Ecosystems in action: Lessons from marine ecology about recovery, resistance, and reversibility. Bioscience 58, 33–42. doi: 10.1641/B580108
Pérez-Ramírez M., Castrejón M., Gutiérrez N. L., Defeo O. (2016). The Marine Stewardship Council certification in Latin America and the Caribbean: A review of experiences, potentials and pitfalls. Fisheries Res. 182, 50–58. doi: 10.1016/j.fishres.2015.11.007
Ponce-Díaz G., Weisman W., McCay B. (2009). Co-responsabilidad y participación en el manejo de pesquerías en México: lecciones de Baja California Sur. Pesca y Conservación 1, 1–9.
Roberts C. M., O’Leary B. C., McCauley D. J., Cury P. M., Duarte C. M., Lubchenco J., et al. (2017). Marine reserves can mitigate and promote adaptation to climate change. PNAS 114 (24), 6167–6175. doi: 10.1073/pnas.1701262114
Sánchez-Bajo C., Roelants B. (2011). Capital and the debt trap: Learning from cooperatives in the global crisis (New York, NY: Palgrave Macmillan).
Schaefer M. B. (1957). Some considerations of population dynamics and economics in relation to the management of marine fishes. J. Fisheries Res. Board Canada 14, 669–681. doi: 10.1139/f57-025
Shepherd S. A., Turrubiates-Morales J. R., Hall K. (1998). Decline of the abalone fishery at La Natividad, México: overfishing or climate change? J. Shellfish Res. 17, 839–846.
Shester G., Micheli F. (2011). Conservation challenges for small-scale fisheries: Bycatch and habitat impacts of traps and gillnets. Biol. Conserv. 144, 673–1681. doi: 10.1016/j.biocon.2011.02.023
Short R. E., Gelcich S., Little D. C., Micheli F., Allison E. H., Basurto X., et al. (2021). Harnessing diversity of small-scale actors key to aquatic food futures. Nat. Food 2, 733–741. doi: 10.1038/s43016-021-00363-0
Smit B., Wandel J. (2006). Adaptation, adaptive capacity and vulnerability. Global Environ. Change 16, 82–292. doi: 10.1016/j.gloenvcha.2006.03.008
Smith A., Aguilar J. D., Boch C. A., De Leo G., Hernandez-Velasco A., Houck S., et al. (2022). Rapid recovery of depleted abalone in Isla Natividad, Baja California, Mexico. Ecosphere. doi: 10.1002/ecs2.4002
Smith M. D., Roheim C. A., Crowder L. B., Halpern B. S., Turnipseed M., Anderson J. L., et al. (2011). Sustainability and global seafood. Science 327, 784–786. doi: 10.1126/science.1185345
Steneck R. S., Hughes T. P., Cinner J. E., Adger W. N., Arnold S. N., Berkes F., et al. (2011). Creation of a gilded trap by the high economic value of the Maine lobster fishery. Conserv. Biol. 25, 904–912. doi: 10.1111/j.1523-1739.2011.01717.x
Sydeman W. J., Santora J. A., Thompson S. A., Marinovic B., Di Lorenzo E. (2013). Increasing variance in North Pacific climate relates to unprecedented ecosystem variability off California. Global Change Biol. 19, 1662–1675. doi: 10.1111/gcb.12165
Teh L. C. L., Sumaila U. R. (2013). Contribution of marine fisheries to worldwide employment. Fish Fisheries 14, 77–88. doi: 10.1111/j.1467-2979.2011.00450.x
Valdez-Rojas C., Beas-Luna R., Lorda J., Zepeda-Domınguez J. A., Montaño-Moctezuma G., Medellín-Ortíz A., et al. (2022). Using a social-ecological systems perspective to identify context-specific actions to build resilience in small-scale fisheries in Mexico. Front. Mar. Sci. 9, 904859. doi: 10.3389/fmars.2022.904859
Vaquer-Sunyer R., Duarte C. M. (2008). Thresholds of hypoxia for marine biodiversity. Proc. Natl. Acad. Sci. 105, 15452–15457. doi: 10.1073/pnas.0803833105
Virdin J., Basurto X., Nico G., Harper S., Mancha-Cisneros M. M., Vannuccini S., et al. (2023). Fishing for subsistence constitutes a livelihood safety net for populations dependent on aquatic foods around the world. Nat. Food. doi: 10.1038/s43016-023-00844-4
Walker B., Holling C. S., Carpenter S. R., Kinzig A. (2004). Resilience, adaptability and transformability in social–ecological systems. Ecol. Soc. 9 (2), 5. doi: 10.5751/ES-00650-090205
Walker B. H., Salt D. (2006). Resilience thinking: sustaining ecosystems and people in a changing world (Washington DC: Island Press).
Wise R. M., Fazey I., Stafford Smith M., Park S. E., Eakin H. C., Archer Van Garderen E. R. M., et al. (2014). Reconceptualising adaptation to climate change as part of pathways of change and response. Global Environ. Change 28, 325–336. doi: 10.1016/j.gloenvcha.2013.12.002
Wolf J., Allice I., Bell T. (2013). Values, climate change, and implications for adaptation: Evidence from two communities in Labrador, Canada. Global Environ. Change 23 (2), 548–562. doi: 10.1016/j.gloenvcha.2012.11.007
Woodson C. B., Micheli F., Boch C., Al-Najjar M., Espinoza A., Hernandez A., et al. (2019). Harnessing marine microclimates for climate change adaptation and marine conservation. Conserv. Lett. 12, e12609. doi: 10.1111/conl.12609
Wootton J. T., Pfister C. A., Forester J. D. (2008). Dynamic patterns and ecological impacts of declining ocean pH in a high-resolution multi-year dataset. Proc. Natl. Acad. Sci. 105, 18848–18853. doi: 10.1073/pnas.0810079105
Keywords: adaptive capacity, climate adaptation, co-management, fisheries sustainability, resilience, small-scale fisheries, social-ecological systems
Citation: Micheli F, Saenz-Arroyo A, Aalto E, Beas-Luna R, Boch CA, Cardenas JC, De Leo GA, Diaz E, Espinoza-Montes A, Finkbeiner E, Freiwald J, Fulton S, Hernández A, Lejbowicz A, Low NHN, Martinez R, McCay B, Monismith S, Precoma-de la Mora M, Romero A, Smith A, Torre J, Vazquez-Vera L and Woodson CB (2024) Social-ecological vulnerability to environmental extremes and adaptation pathways in small-scale fisheries of the southern California Current. Front. Mar. Sci. 11:1322108. doi: 10.3389/fmars.2024.1322108
Received: 15 October 2023; Accepted: 15 January 2024;
Published: 08 February 2024.
Edited by:
Wei-Bo Chen, National Science and Technology Center for Disaster Reduction(NCDR), TaiwanReviewed by:
Michele L. Barnes, James Cook University, AustraliaMuhammad Nur Arkham, Politeknik Kelautan dan Perikanan Dumai, Indonesia
Lida Teneva, Independent Researcher, Sacramento, CA, United States
Copyright © 2024 Micheli, Saenz-Arroyo, Aalto, Beas-Luna, Boch, Cardenas, De Leo, Diaz, Espinoza-Montes, Finkbeiner, Freiwald, Fulton, Hernández, Lejbowicz, Low, Martinez, McCay, Monismith, Precoma-de la Mora, Romero, Smith, Torre, Vazquez-Vera and Woodson. This is an open-access article distributed under the terms of the Creative Commons Attribution License (CC BY). The use, distribution or reproduction in other forums is permitted, provided the original author(s) and the copyright owner(s) are credited and that the original publication in this journal is cited, in accordance with accepted academic practice. No use, distribution or reproduction is permitted which does not comply with these terms.
*Correspondence: Fiorenza Micheli, TWljaGVsaUBzdGFuZm9yZC5lZHU=