- National Research Council – Institute for Marine Biological Resources and Biotechnology (CNR-IRBIM), Largo Fiera della Pesca, Ancona, Italy
Krill is a fundamental resource in the pelagic food web of the Ross Sea, constituting an important link between primary production and top predators. A series of Italian research voyages to the Ross Sea from 1994 to 2016 have contributed to our understanding of the dynamics of krill populations inhabiting the Ross Sea. Only the surveys in 1994 and 2004 reported information on krill’s predators through visual census data, and 2004 data were not object of publication until now. Analyzing Euphausia superba and Euphausia crystallorophias abundance spatial distribution in the study area in relation to the distribution of its key natural predators have shown a significant relationship between the spatial distribution of minke whales’ abundance and the density of E. superba biomass, indicating a classical predator-prey interaction. Moreover, krill biomass density data in the water column were analyzed together with the main environmental data from CTD samplings. The analysis of krill density data in relation to environmental factors throughout the water column revealed a significant relation between E. superba abundance and salinity, a result that may be linked to the presence of ice melting effects improving environment productivity conditions.
1 Introduction
The knowledge of krill role in the pelagic food webs in Antarctic and sub-Antarctic waters has been greatly enhanced through extensive research efforts focused on these crustaceans in such environments (Cavan et al., 2019; Testa et al., 2022). The dynamics of these animals could be influenced by many biotic and abiotic factors. Among the main biotic factors, we could mention prey availability, predators’ presence, and competitors for food (Meyer et al., 2020), whereas, among the main abiotic factors, we could consider ice cover, water temperature, salinity, dissolved oxygen and water circulation (Leonori et al., 2017; Veytia et al., 2020; De Felice et al., 2022).
Many species rely on krill as a main food source in Antarctic waters. Focusing on the top predators of the pelagic food chain, penguins (Watters et al., 2020), seals (Melbourne-Thomas, 2020) and whales (Konishi et al., 2014; Smetacek, 2021), in particular, show a huge percentage of their diet as consisting in these small crustaceans (Trathan and Hill, 2016). One study, based on isotope analysis, dealing with penguins’ diet in the Ross Sea (Jafari et al., 2021) has shown that the relative krill and fish consumption by Adélie penguins (Pygoscelis adeliae) and emperor penguins (Aptenodites forsteri) changed in relation to the prey availability, in function of seasonal sea ice dynamics and penguin life cycle phases. Dietary variability of Adélie penguin was already known, but not in emperor penguin. In contrast, other authors (Hong et al., 2021), from isotope analysis, found that emperor penguins in the Ross Sea maintain preference for the typology of prey (Antarctic silverfish), while Adélie penguin chicks at Cape Hallett mostly fed on Antarctic krill (Euphausia superba), since this krill species is particularly abundant there, but Adélie penguin chicks at Inexpressible Island, located near Terra Nova Bay, mainly fed on both Antarctic silverfish and ice krill (Euphausia crystallorophias), demonstrating a regional characterization of the diet and an adaptation to prey availability. In any case krill reveals to be one of the preferred preys, at least for Adélie penguin.
Seals are very important krill consumers. Crabeater seals prefer to breed close to krill and are sensible to the conditions of seasonal sea for breeding success. Weddell seals are less dependent on krill as a food source and remain connected to the stable ice and to the preys that can be found there (Wege et al., 2021). Trathan et al. (2022) found a positive response relationship between predator offspring mass (Antarctic fur seals, gentoo penguins and macaroni penguins) and the spatial distribution of krill, measuring the patchiness in krill distribution, while they found little relation between predator performance and krill density levels at South Georgia. This result evidence the importance of the information derived from krill spatial distribution when studying krill predators’ diet and breeding success.
Marine mammals often play a fundamental role concerning krill predation. Miller et al. (2019) have shown that blue whales prefer to concentrate where Antarctic krill swarms are bigger and shallower in order to maximize the energy intake respect to the predation effort. The spatial distribution of minke whales (Balaenoptera bonaerensis) in the Ross Sea was studied together with the distribution of Antarctic and ice krill using Generalized Additive Models (GAMs); the results showed that the distribution of these whales was influenced by many factors, such as longitude, distance from shelf break, oceanographic conditions, and densities of both krill species.
Following the examples given above, it could be expected that the spatial distributions of these top predators would overlap, at least partially, with those of the krill species, especially Euphausia superba, in the Ross Sea.
For similar reasons, krill preys, mainly represented by phytoplankton species and at a minor level by mesozooplankton species (Hellessey et al., 2020; De Felice et al., 2023), could influence abundance level and spatial distribution of Euphausiids in Antarctica. Different krill species are competitors for food, a fact evidenced by their similar feeding appendages and their diet (Haberman et al., 2003). It is also important to note, at least in the Ross Sea, that the cores of the two most relevant krill populations, E. superba and E. crystallorophias, tend to separate spatially, passing from austral spring to austral summer (Azzali et al., 2006; Leonori et al., 2017). The abovementioned behavior represents a potential strategy for reducing the risk of starvation resulting from competition for food resources.
The main aim of this work was to investigate potential correlations between the spatial distribution of krill biomass density and the distribution of krill predators in January 2004, having the opportunity of available data on krill predators. The prevalent localization of krill in the water column and the possible influence on the krill density of environmental variables along the water column were also studied, benefitting from in situ CTD data acquired during the acoustic survey.
2 Materials and methods
2.1 Study area, target species and sampling procedures
Acoustic and biological data concerning E. superba and E. crystallorophias came from an acoustic survey conducted in the western sector of the Ross Sea (Antarctica) and the adjacent Southern Ocean in January 2004. The study was conducted within the framework of the 19th Italian National Program for Research in Antarctica (PNRA) Expedition aboard the research vessel “Italica”. The area boundary coordinates were 69° and 76° S latitude and 165° E and 175° W longitude. The acoustic survey targeting krill was carried out from 28/12/2003 to 23/01/2004, synoptically with the census on krill predators. Both the acoustic and the visual surveys were taken 24 hours a day. The acoustic monitoring followed CCAMLR guidelines and focused on the top 200 m of the water column, the stratum with the core of krill abundance in the study area, as confirmed by the scarce krill abundance level found in the stratum between 200 and 300 meters during the following 2014 survey (Leonori et al., 2017). Krill swarms were monitored acoustically at three frequencies (38, 120, and 200 kHz), in order to separate krill echoes from non-target signals and separate the two krill species between them. Krill aggregations were identified in echograms on the base of the experience from the previous surveys and relying on frequency comparison methodology as described in Azzali et al. (2004a) to discriminate in the categories “E. superba”, “E. crystallorophias” and “other”. Periodical trawl hauls were carried out to obtain ground truth information. Bottom depth ranged from about 300 to 3500 m; the highest depths were in the northern area at the border between the Ross Sea and the Southern Ocean. Specimens were collected by means of the HPRI-1000 plankton net (mesh size 1 mm), which was designed by CNR-IRBIM (Ancona, Italy); haul position was decided on the basis of the observation of krill swarms during the acoustic survey (Azzali et al., 2004b; De Felice et al., 2023). The sampling net was equipped with SIMRAD ITI system that allowed, by acoustic wireless connection, to know information about the catch stratum, such as temperature, net depth and vertical opening. Oceanographic sampling was carried out by means of CTD probe Sea Bird Electronics SBE 911 plus.
For what concerns biomass estimation procedure, the density contrast (g) and sound speed contrast (h) coefficients applied for the two species were derived from Foote et al. (1990); the average tilt angle was hypothesized to be 15°. The fluid sphere model was applied for biomass calculation (Simmonds and MacLennan, 2005) in all the acoustic surveys carried out in the Ross Sea since 1989 by the CNR Institute of Ancona. The fluid sphere model has been improved in time (Anderson, 1950; Johnson, 1977; Stanton et al., 1993; Macaulay, 1994) and represents a valid tool for krill biomass evaluation. Hewitt et al. (2004) length-weight relationship was used for conversion from numbers to biomass. Biomass estimates were expressed as mean krill density per elementary statistical sampling rectangle (Simmonds and MacLennan, 2005). The ESSR method has been employed in all previous surveys, because it is suitable for acoustic monitoring of large areas. Western Ross Sea was subdivided into rectangular grid cells, spaced at intervals of 1° in longitude, but variable in latitude, according to the Earth’s curvature variation at the poles. All rectangles had an area of 600 nm2; the origin of the grid (rectangle A0) was set at coordinates 64° 25.1′ S 164°30.0′ E. Numbers and letters (in alphabetical order) proceed from the coast to offshore and southwards. Species abundance and biomass in each rectangle were estimated from the average NASC value of the Elementary Sampling Distance Units (ESDUs) laying within the rectangle.
2.2 Study of the relationships between krill, its predators and environmental parameters
Up to now, several acoustic surveys were conducted in the western Ross Sea during Italian expeditions to Antarctica (De Felice et al., 2022), but synoptic information on krill predators was often lacking. Apart from the survey held in January 2004 (Azzali et al., 2004b), only the two surveys carried out in 1994 had this information that was reported in Saino and Guglielmo (2000); however, krill dataset was not studied in relation with its predator dataset at that time, but data were analyzed and reported separately.
In the present paper krill distribution was studied both as spatial distribution over the whole study area in relation with the main krill predators and also along the water column at selected haul positions in relation with environmental parameters.
Consequently, as a first approach, the spatial distribution of krill biomass density within the study area was analyzed using multiple regression analysis (DISTLM), together with estimates of abundance of the main krill natural predators derived from visual census (strip transect methodology) conducted during the survey.
Visual census on krill predators took place from a dedicated platform located at 14 meters (level of the ship’s bridge) on the right side of the ship. Individuals spotted on that side within a strip of varying width, dependent on visibility and weather conditions, were considered. The census of krill predators was achieved with both traditional and digital binoculars; the latter allowed to take photos of the observed animals and store them, with relative time and geographical position, in the acoustic database. This allowed a more accurate recognition of the animals and facilitated the studying of the interaction between krill and its predators. Density values were calculated taking into account the number of individuals sighted for each species within each statistical rectangle (as defined for the estimation of krill biomass) and the total observed area (number of miles with sighting activity multiplied by the strip’s width). Four predator species were selected based on their dietary dependence on krill and their average krill consumption (minke whale) or the high density of these predators, as determined from visual census (crabeater seal, emperor penguin and Adélie penguin). Marine birds, as skuas and petrels, were not considered in this analysis, even if petrel species in particular presented very high densities, since they can predate krill only at surface and the relative data collected by means of visual census could contain more bias, since these animals usually follow the vessel, with the risk of multiple counts for the same specimen. In general, krill predators’ species identified in the study area were the same as reported in Saino and Guglielmo (2000) and are comparable to what found by Double et al. (2015) and Naganobu et al. (2006) for the portion of the area that is common with the data presented in this work. Limiting to penguins, seals and marine mammals, the species that were observed in decent numbers were respectively: Adélie penguin, emperor penguin, crabeater seal, Weddell seal, minke whale and killer whale. No humpback whales were observed and there was only one sighting of blue whale, but not during official visual census monitoring. The aforementioned data was averaged within the same statistical rectangles to prepare them for subsequent analysis with DISTLM, using the best “AIC (Akaike Information Criterion)” as selection criterion. Data were log (x+1) normalized and were formatted for processing using GIS software.
In second instance, acoustic data was matched with CTD data corresponding to the fishing stations in the core abundance areas for the two krill populations, that corresponded respectively with their highest catches with the trawl (Figure 1). Hauls nos. 1, 27, 28 and 30 were selected for E. superba, while hauls nos. 7, 8, 10, 13, 17 were selected for E. crystallorophias. The density of krill (Nautical Area Scattering Coefficient in m2/nm2) measured at the frequency of 120 kHz, which is the reference for biomass calculation, was obtained by layers of 20 meters stretching from 15 up to 195 meters (Azzali et al., 2004a; Leonori et al., 2017). The above data was then analyzed by means of DISTLM, using the best “AIC” as selection criterion. Temperature data were log (x+2) normalized, because values could be below -1°C in some cases, while the other variables were log (x+1) normalized.
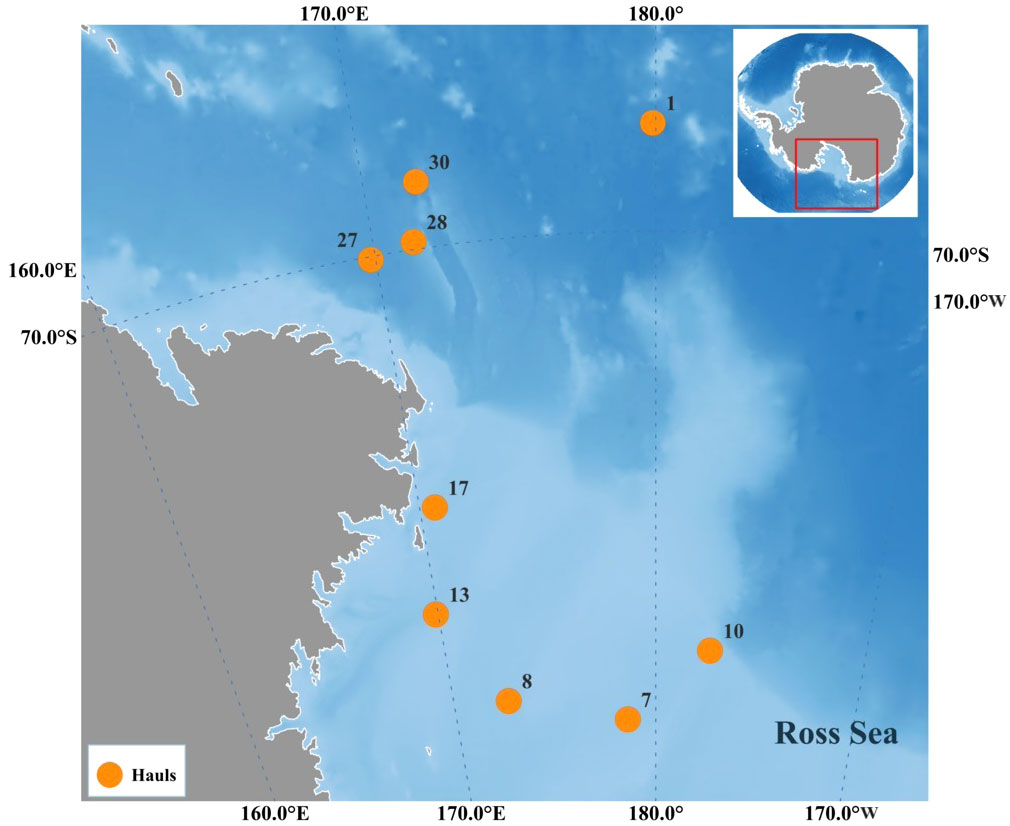
Figure 1 Geographic positions of the pelagic hauls with the highest catch levels of E. superba and E. crystallorophias.
Figure 2 shows vertical profiles of fluorescence (as proxy for phytoplankton) and krill density, after normalizing the data for hauls where the fluorescence parameter was available. This was done to visually check any potential correspondence in abundance peaks, which could indicate predator-prey interaction.
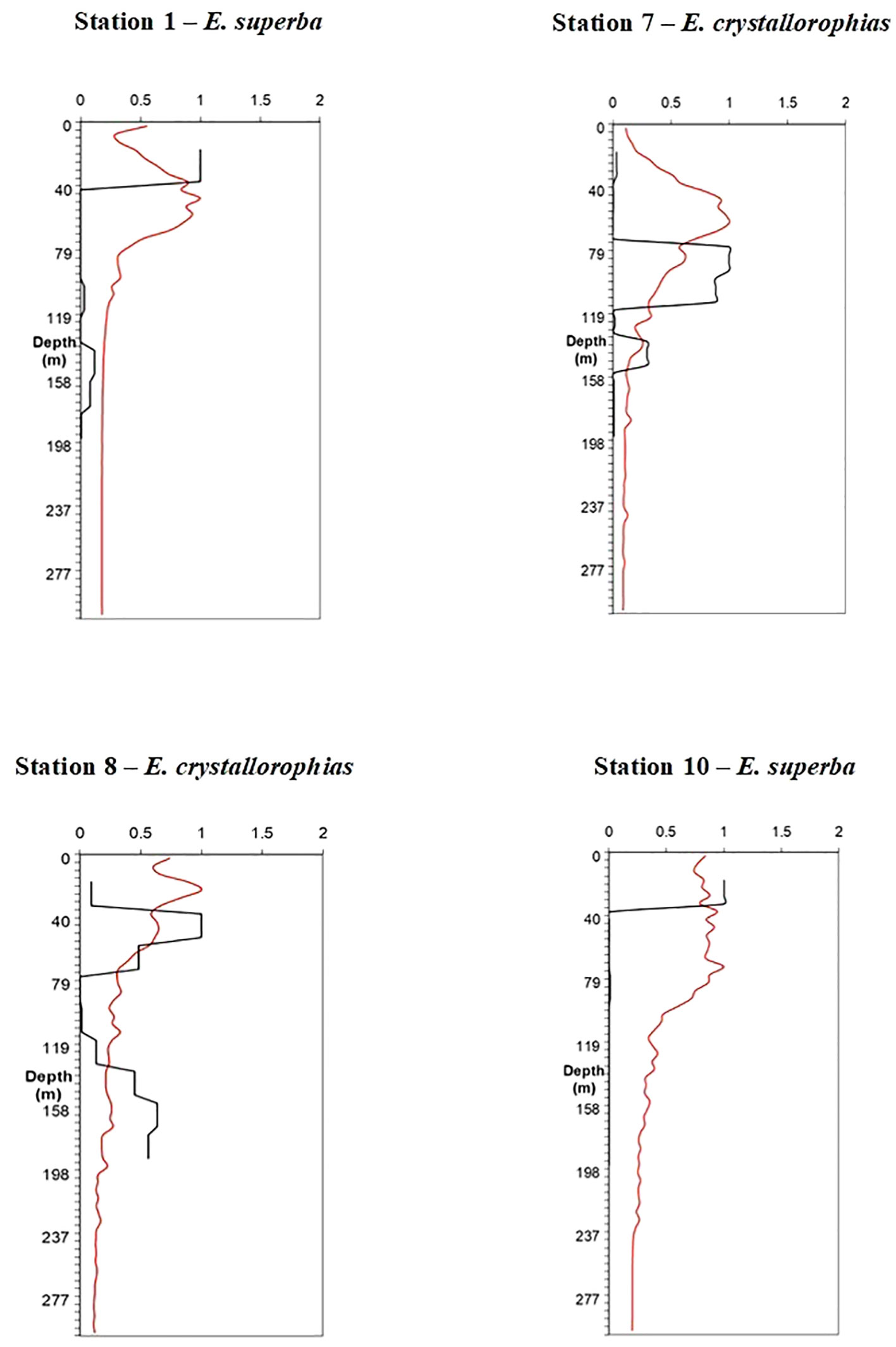
Figure 2 Vertical profiles of krill density compared with fluorescence for hauls 1, 7, 8 and 10 where the fluorimeter was operative. In black krill density profile, in red fluorescence.
3 Results
3.1 Spatial distribution of krill in relation to the density of their main natural predators
Krill biomass density data from the two species was studied by DISTLM analysis against abundance of the main natural predators. Maps depicting the spatial distribution of E. superba, E. crystallorophias and of natural krill predators are reported in Figure 3. The results of this analysis are shown in Tables 1 and 2; the only statistically significant relationship observed was between the density of minke whales and the biomass density of E. superba with a best estimate for AIC criterion being 235.49 for the model considering only minke whale density as explanatory variable.
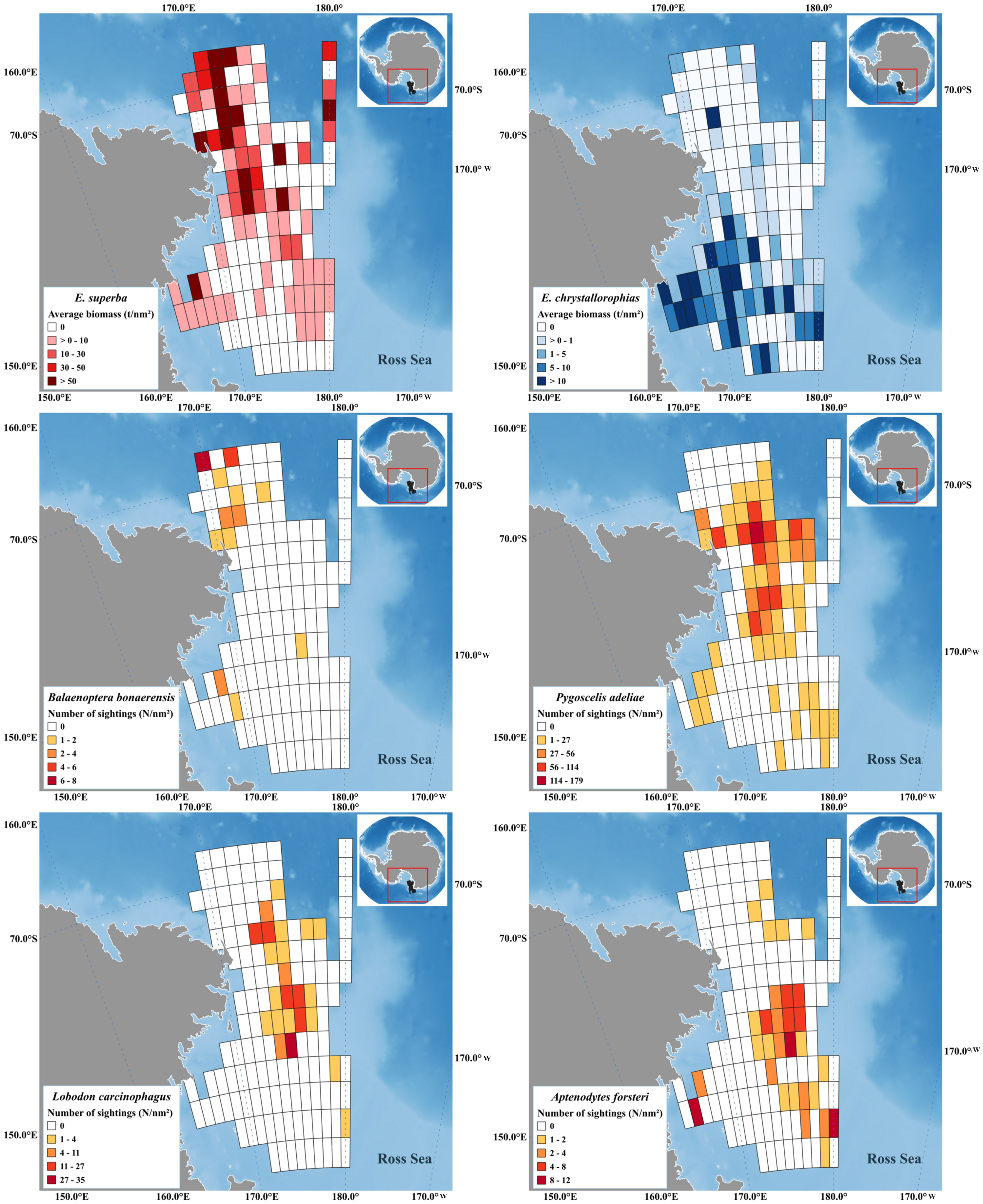
Figure 3 Spatial distribution of E. superba and E. crystallorophias density (above) in comparison with the spatial distribution of krill’s natural predators in January 2004 (below) along the grid of statistical rectangles in use for the estimation of krill biomass.
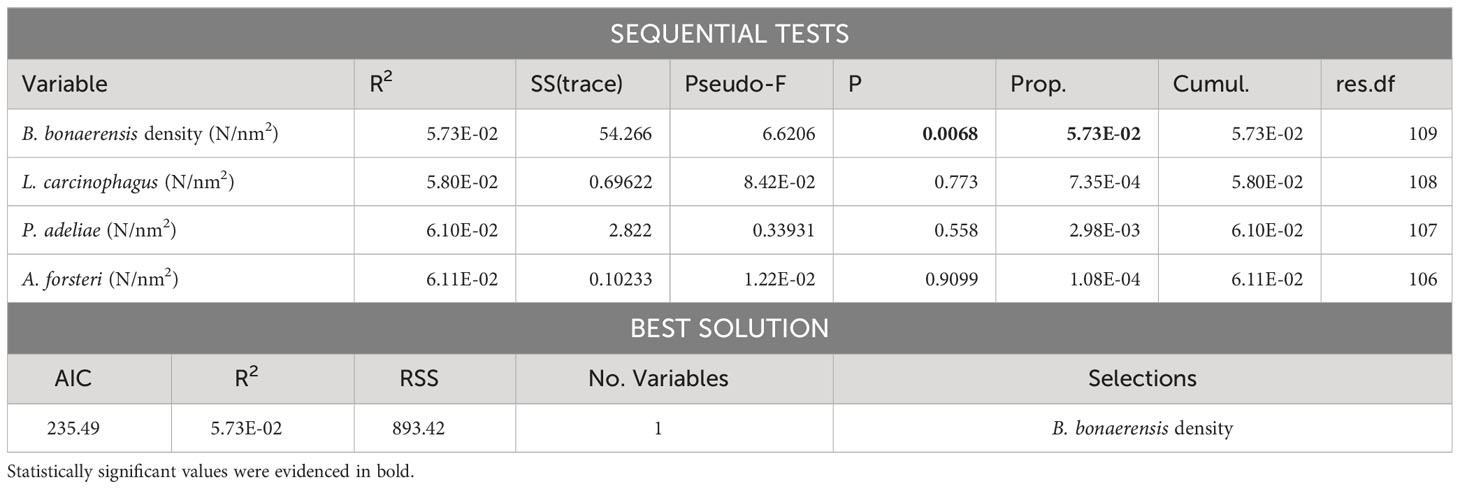
Table 1 Results of DISTLM models run on E. superba density values vs. the abundance of its main predators.
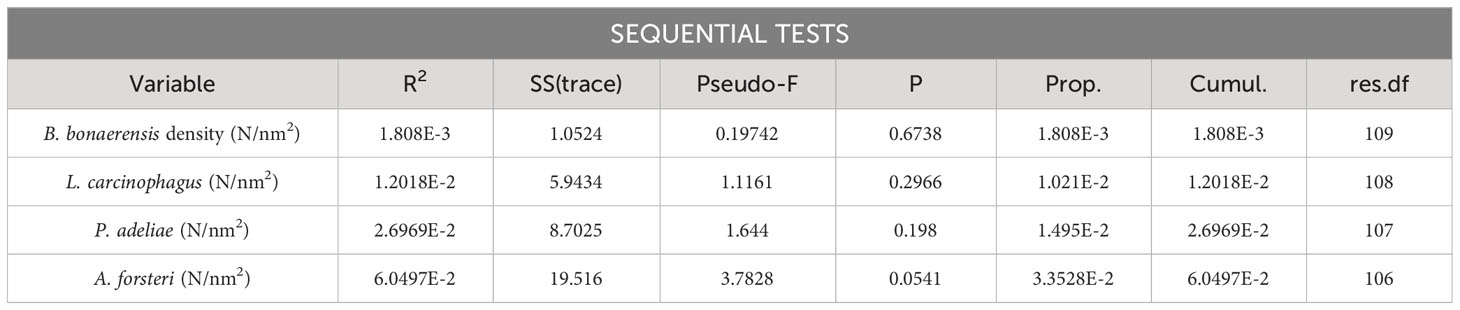
Table 2 Results of DISTLM models run on E. crystallorophias density values vs. the abundance of its main predators.
3.2 Krill density in relation to environmental parameters
Table 3 shows the results of the multivariate multiple regression applied to krill density data and CTD data recorded throughout the water column. The main environmental parameters measured by CTD probe were averaged within the corresponding depth ranges as it was done for krill density. The analysis was carried out considering one krill species at a time versus all the environmental parameters (temperature, salinity and water density) except for fluorescence, as the fluorimeter broke after the first 10 stations.
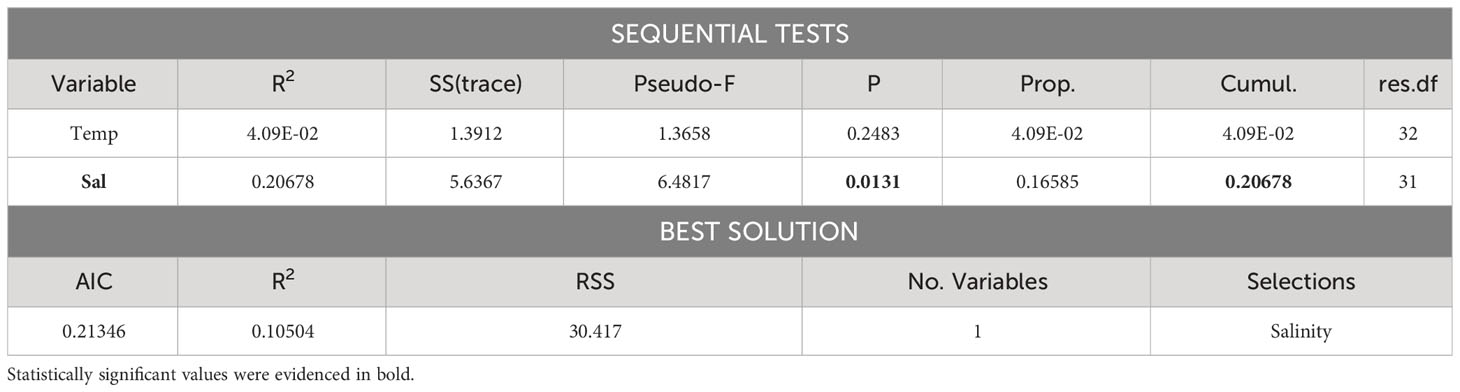
Table 3 Results of multivariate multiple regression on Antarctic krill density data and environmental parameters measured by CTD probe for layers of 20 meters between 15 and 195 meters.
Due to autocorrelation issues between water density and salinity, water density was discarded from the analysis.
Salinity exhibited a highly significant correlation with E. superba density, with AIC being -1.8898 in the best model considering only this correlation.
Examining in detail Table 4, which provides insights into the vertical distribution of krill in the water column in relation with fluorescence peaks, as proxy of phytoplankton, it becomes evident that E. superba in proximity of the considered stations tended to be located within the first 40 meters, apart from station 30 where it was abundant up to about 60 meters. On the contrary, E. crystallorophias was generally located in deeper strata, specifically within depth intervals of 80-120 meters at station 7, 40-80 meters and 140-200 meters at station 8, 60-120 meters at station 13, and finally within 100 meters at station 17. The overall vertical distribution bands for the two species were not significantly different, as the trend showed that E. superba predominantly occupied the upper meters of the water column while E. crystallorophias presented peaks of abundance below 40-60 meters. This suggests circadian movements along the water column, potentially associated with the time of day.
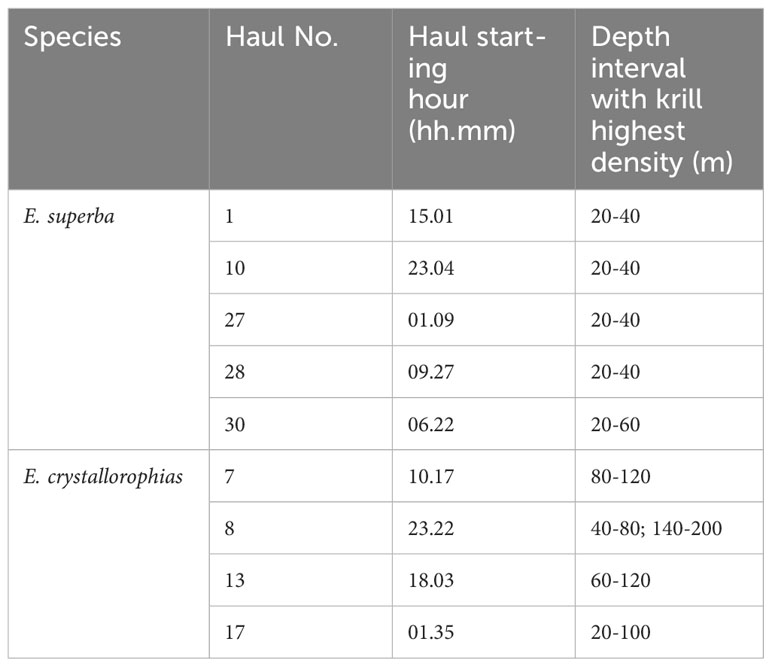
Table 4 Depth intervals in which E. superba and E. crystallorophias swarms occurred in concomitance with the fishing hauls selected for the analyses and local starting time of trawling operations.
4 Discussion
Multiple regression analysis applied to krill biomass data and krill predators’ abundance data showed a significant relationship for Antarctic krill only in the case of Balaenoptera bonaerensis. This result is in agreement with the literature that identifies in particular Antarctic krill as preferred prey for the numerous specimens of B. bonaerensis present in the Ross Sea and surrounding areas (Tamura and Konishi, 2009; Murase et al., 2013; Ishikawa et al., 2022). It suggests that minke whales tend to reside in areas with a high abundance of E. superba for feeding purposes. Several other species of marine mammals have krill as their main prey, for example humpback whale (Pallin et al., 2023), blue whale (Miller et al., 2019) and other baleen whales (Savoca et al., 2021) and in certain cases it was demonstrated that their migration seems to be well coordinated with environmental conditions and krill availability (Szesciorka et al., 2020). Our study area involves the western Ross Sea and the portion of the Southern Ocean bordering with it, extending from 69° to 76° S in latitude and from 165° E to 175° W in longitude. Considering the results by Double et al. (2015), going from 65° to 70° S of latitude we have sightings of humpback whales, blue whales, fin whales and minke whales, but south of 70° S the only marine mammals that were identified were minke whales and killer whales. These results are quite consistent with our study, confirming the predominant presence of B. bonaerensis and Orcinus orca in the Ross Sea among marine mammals, and, for the first species, its strong dependence on krill as a food source. It is highly probable that minke whales concentrate in the area north of Cape Adare, because they can find big E. superba swarms that can give a high energy level with limited efforts, a mechanism similar to what described by Miller et al. (2019) for Balaenoptera musculus. Minke whale exhibits also a marked preference for E. superba respect to E. crystallorophias; this fact is reflected by the difference in abundance of B. bonaerensis comparing the core of its distribution (and core for Antarctic krill) in the northern part of the study area, respect to the core of the distribution of ice krill in the central coastal part of the Ross Sea, where minke whales are present, but in much lower density. The spatial distribution of E. superba and E. crystallorophias in January 2004 reflects quite well what has been observed for all the years in which the acoustic survey was conducted in December-January (Davis et al., 2017; Leonori et al., 2017; De Felice et al., 2022) with Antarctic krill concentrated along the northwestern shelf break and ice krill mainly found in the central part. This could be a good condition for krill to avoid competition for food, and predators highly specialized with a diet based prevalently on krill, adapt to this distribution.
An exception in our dataset was constituted by crabeater seal (Lobodon carcinophagus) that has a relatively high dependence on krill as a food source (Nachtsheim et al., 2017; Bengtson and Stewart, 2018), but did not show a significant relation with krill biomass spatial distribution. A possible explanation could be that crabeater seals are observed and registered easily while they are resting on ice sheets but are less visible when in the water actively feeding on krill. Almost all the sightings of this species were relative to individuals resting on ice and their spatial distribution (Figure 3), resulting from our study, confirms that they are found prevalently on floating sea ice during austral summer, quite far from the coast, in contrast to Weddell seals that are mainly found on stable ice along the coast (Wege et al., 2021). Another possible reason for the absence of a correlation between the spatial distribution of krill and crabeater seals in western Ross Sea could be a switch in the diet composition by these animals in years when krill biomass is not particularly abundant, such as the year considered in this work (Leonori et al., 2017). It is not known how adaptable these seals could be for the diet, being considered highly specialists in feeding krill, but they have shown a shift in the diet towards other prey in the Antarctic Peninsula when krill biomass was at low levels (Hückstädt et al., 2012).
The situation for what concerns dietary preferences in penguins living in Antarctica is varied, in the sense that different results were obtained in different researches. Emperor penguin (A. forsteri) seems to be less dependent on krill as a food source (Putz, 1995; Gales et al., 1990; Zimmer et al., 2007), showing a wider range of possible preys also depending on the study area. When comparing emperor penguin and Adélie penguin, basing on stable isotope analysis (Hong et al., 2021), it was found that emperor penguins in the Ross Sea maintain preference for the typology of prey (Antarctic silverfish), while Adélie penguin chicks at Cape Hallett versus Terra Nova Bay presented a regional characterization of the diet and an adaptation to prey availability. By contrast, another study, based on isotope analysis, dealing with penguins’ diet in the Ross Sea (Jafari et al., 2021) has shown that the relative krill and fish consumption by Adélie penguins (Pygoscelis adeliae) and emperor penguins (Aptenodites forsteri) changed in relation to the prey availability, in function of seasonal sea ice dynamics and penguin life cycle phases. Dietary variability of Adélie penguin is generally confirmed by the various studies, but it is not always the case for emperor penguin. However, krill reveals to be one of the preferred prey, at least for Adélie penguin. In conclusion, it is not surprising that we have not found any relation between krill and emperor penguin, but it could be expected to find it with Adélie penguin; a possible reason for not finding any relation could be that we monitored these penguins during phases of active movement to or from feeding areas. Jafari et al. (2021) identified in spring and summer an active feeding period for P. adeliae, but we should take into account that duration of foraging trips by these animals could be very different within Antarctic areas (Juáres et al., 2016; Olmastroni et al., 2020).
As regards krill vertical distribution along the water column, the results have evidenced that the highest densities of the two krill species under investigation were mainly located within the first 100 meters, with occasional exceptions for E. crystallorophias, which in some cases was found even deeper. These results seem to be in line with those of other authors for the summer season, particularly in the case of E. superba (Pauly et al., 2000; Amakasu et al., 2011). However, it has been suggested that E. superba swarms make more than one vertical migration per day (Swadling, 2006). Probably, we could not detect migrations from the surface to deeper waters and back for E. superba due to the limited number of hauls considered, covering the daily time span only partially. However, it was possible to identify these movements for E. crystallorophias. In the initial stations, where the fluorescence data were available, a certain correspondence could be observed between the fluorescence peak and the peak of krill density when E. superba predominated (stations 1 and 10). By contrast, when E. crystallorophias prevailed (stations 7 and 8), the krill peak density was slightly deeper than that of fluorescence.
For what concerns the possible relations between krill and environmental parameters along the water column, the only significant one was found between Antarctic krill and salinity. This relation seems to be a similar result to the inverse correlation found by Leonori et al. (2017) between E. superba biomass and salinity in the water column. A possible interpretation for this relation is that a decrease in salinity could be associated with ice melting conditions that often mean higher availability of food freed from entrapping ice (Nicol, 2006; Murase et al., 2013) and consequently an increase in prey availability for krill, once again confirming the importance of the trophic factor for these animals. This result could also be connected to the buoyancy of krill. Krill is capable to swim changing stratum in water column quite well, as also evidenced by the data from this paper, but in certain condition, such as when their stomach is full and they rest, they are transported passively downwards (Tarling and Thorpe, 2017) and the extent of this transport could depend on salinity.
5 Conclusion
The analyses presented in this paper focused on the interactions between krill and its predators. The spatial distribution of E. superba and E. crystallorophias was studied together with four predator species; the results evidenced a relation between the abundance of B. bonaerensis and the biomass density of E. superba, confirming the high dependence of minke whales on krill as a prey. Krill density, particularly that of E. superba, showed a significant correlation only with salinity throughout the water column, a result that could be linked to local increases in productivity due to ice melting, but also to the regulation of water density of krill sinking, when resting, in relation to the buoyancy of these animals.
Data availability statement
The raw data supporting the conclusions of this article will be made available by the authors, upon pertinent request.
Ethics statement
The manuscript presents research on animals that do not require ethical approval for their study.
Author contributions
AD: Conceptualization, Writing – review & editing, Data curation, Formal Analysis, Methodology, Writing – original draft, Validation. IB: Data curation, Writing – review & editing, Validation. IC: Data curation, Writing – review & editing, Validation, Visualization. GC: Data curation, Writing – review & editing, Validation. IL: Conceptualization, Formal Analysis, Methodology, Supervision, Writing – review & editing, Data curation.
Funding
The author(s) declare financial support was received for the research, authorship, and/or publication of this article. Authors are grateful to the Italian National Antarctic Scientific Commission (CSNA) and the Italian National Research Program in Antarctica (PNRA) for financial support: research project 2002/8.4 (KEP).
Acknowledgments
The authors would like to thank the Italian National Research Program in Antarctica (PNRA). Special thanks go to Dr. Massimo Azzali for inspiring this work. We also wish to express our gratitude to the crew of R/V Italica and all the researchers involved for their assistance in conducting the scientific surveys.
Conflict of interest
The authors declare that the research was conducted in the absence of any commercial or financial relationships that could be construed as a potential conflict of interest.
Publisher’s note
All claims expressed in this article are solely those of the authors and do not necessarily represent those of their affiliated organizations, or those of the publisher, the editors and the reviewers. Any product that may be evaluated in this article, or claim that may be made by its manufacturer, is not guaranteed or endorsed by the publisher.
References
Amakasu K., Ono A., Hirano D., Moteki M., Ishimaru T. (2011). Distribution and density of Antarctic krill (Euphausia superba) and ice krill (E. crystallorophias) off Adelie Land in austral summer 2008 estimated by acoustical methods. Pol. Sci. 5, 187–194. doi: 10.1016/j.polar.2011.04.002
Azzali M., Leonori I., De Felice A., Russo A. (2006). Spatial–temporal relationships between two euphausiid species in the Ross Sea. Chem. Ecol. 22, S219–S233. doi: 10.1080/02757540600670836
Azzali M., Leonori I., Lanciani G. (2004a). A hybrid approach to acoustic classification and length estimation of krill. CCAMLR Sci. 11, 33–58.
Azzali M., Russo A., Sala A., De Felice A., Catalano B. (2004b). Preliminary results of a survey on krill, environment and predators in CCAMLR Division 88.1 carried out in December 2003 and in January 2004 (Project 8.4). CCAMLR WG-EMM-04/71 (Hobart, Australia: CCAMLR), 23 p.
Bengtson J. L., Stewart B. S. (2018). “Crabeater seal: lobodon carcinophaga,” in Encyclopedia of marine mammals, 3rd ed. Eds. Würsig B., Thewissen J. G. M., Kovacs K. M. (Academic Press), 230–232, ISBN: 9780128043271. doi: 10.1016/B978-0-12-804327-1.00098-4
Cavan E. L., Belcher A., Atkinson A., Hill S. L., Kawaguchi S., McCormack S., et al. (2019). The importance of Antarctic krill in biogeochemical cycles. Nat. Commun. 10, 4742. doi: 10.1038/s41467-019-12668-7
Davis L. B., Hofmann E. E., Klinck J. M., Piñones A., Dinniman M. S. (2017). Distributions of krill and Antarctic silverfish and correlations with environmental variables in the western Ross Sea, Antarctica. Mar. Ecol. Prog. Ser. 584, 45–65. doi: 10.3354/meps12347
De Felice A., Biagiotti I., Canduci G., Costantini I., Malavolti S., Giuliani G., et al. (2022). Is it the same every summer for the euphausiids of the ross sea? Divers. 14, 433. doi: 10.3390/d14060433
De Felice A., Manini E., Biagiotti I., Leonori I. (2023). Bioenergetics of Euphausia superba and Euphausia crystallorophias in the Ross Sea. Divers. 15, 480. doi: 10.3390/d15040480
Double M. C., Miller B. S., Leaper R., Olson P., Cox M. J., Miller E., et al. (2015). Cruise report on blue whale research from the NZ/Aus Antarctic Ecosystems Voyage 2015 of the Southern Ocean Research Partnership (International Whaling Commission).
Foote K. G., Everson I., Watkins J. L., Bone D. G. (1990). Target strengths of antarctic krill (Euphausia superba) at 38 and 120 kHz. J. Acoust. Soc Am. 87, 16–24.
Gales N. J., Klages N. T. W., Williams R., Woehler E. J. (1990). The diet of the emperor penguin, Aptenodytes forsteri, in Amanda Bay, Princess Elizabeth Land, Antarctica. Ant. Sci. 2, 23–28. doi: 10.1017/S0954102090000037
Haberman K. L., Ross R. M., Quetin L. B. (2003). Diet of the Antarctic krill (Euphausia superba Dana): II. Selective grazing in mixed phytoplankton assemblages. J. Exp. Mar. Biol. Ecol. 283, 97–113.
Hellessey N., Johnson R., Ericson J. A., Nichols P. D., Kawaguchi S., Nicol S., et al. (2020). Antarctic krill lipid and fatty acid content variability is associated to satellite derived chlorophyll a and sea surface temperatures. Sci. Rep. 10, 6060.
Hewitt R. P., Watkins J., Naganobu N., Sushin V., Brierley A. S., Demer D., et al. (2004). Biomass of antarctic krill in the scotia sea in January/February 2000 and its use in revising an estimate of precautionary yield. Deep-Sea Res. II 51, 1215–1236. doi: 10.1016/j.dsr2.2004.06.011
Hong S.-Y., Gal J.-K., Lee B.-Y., Son W.-J., Jung J.-W., La H.-S., et al. (2021). Regional differences in the diets of adélie and emperor penguins in the ross sea, Antarctica. Anim. 11, 2681. doi: 10.3390/ani11092681
Hückstädt L. A., Burns J. M., Koch P. L., McDonald B. I., Crocker D. E., Costa D. P. (2012). Diet of a specialist in a changing environment: the crabeater seal along the western Antarctic Peninsula. Mar. Ecol. Prog. Ser. 455, 287–301. doi: 10.3354/meps09601
Ishikawa H., Otsuki M., Tamura T., Konishi K., Bando T., Ishizuka M., et al. (2022). Foraging ecology of mature male Antarctic minke whales (Balaenoptera bonaerensis) revealed by stable isotope analysis of baleen plates. Pol. Sci. 31, 100785. doi: 10.1016/j.polar.2021.100785
Jafari V., Maccapan D., Careddu G., Sporta Caputi S., Calizza E., Rossi L., et al. (2021). Spatial and temporal diet variability of Adélie (Pygoscelis adeliae) and Emperor (Aptenodytes forsteri) Penguin: a multi tissue stable isotope analysis. Pol. Biol. 44, 1869–1881. doi: 10.1007/s00300-021-02925-1
Johnson R. K. (1977). Sound scattering from a fluid sphere revisited. J. Acoust. Soc Am. 61, 375–377.
Juáres M. A., Santos M., Mennucci J. A., Coria N. R., Mariano−Jelicich R. (2016). Diet composition and foraging habitats of Adélie and gentoo penguins in three different stages of their annual cycle. Mar. Biol. 163, 105. doi: 10.1007/s00227-016-2886-y
Konishi K., Hakamada T., Kiwada H., Kitakado T., Walløe L. (2014). Decrease in stomach contents in the Antarctic minke whale (Balaenoptera bonaerensis) in the Southern Ocean. Pol. Biol. 37, 205–215. doi: 10.1007/s00300-013-1424-3
Leonori I., De Felice A., Canduci G., Costantini I., Biagiotti I., Giuliani G., et al. (2017). Krill distribution in relation to environmental parameters in mesoscale structures in the Ross Sea. J. Mar. Syst. 166, 159–171. doi: 10.1016/j.jmarsys.2016.11.003
Macaulay M. C. (1994). A generalized target strength model for euphausiids, with application to other zooplankton. J. Acoust. Soc Am. 95, 2452–2466.
Melbourne-Thomas J. (2020). Climate shifts for krill predators. Nat. Clim. Change 10, 390–391. doi: 10.1038/s41558-020-0756-6
Meyer B., Atkinson A., Bernard K. S., Brierley A. S., Driscoll R., Hill S. L., et al. (2020). Successful ecosystem-based management of Antarctic krill should address uncertainties in krill recruitment, behaviour and ecological adaptation. Commun. Earth Env. 1, 28. doi: 10.1038/s43247-020-00026-1
Miller E. J., Potts J. M., Cox M. J., Miller B. S., Calderan S., Leaper R., et al. (2019). The characteristics of krill swarms in relation to aggregating Antarctic blue whales. Sci. Rep. 9, 16487. doi: 10.1038/s41598-019-52792-4
Murase H., Kitakado T., Hakamada T., Matsuoka K., Nishiwaki S., Naganobu M. (2013). Spatial distribution of Antarctic minke whales (Balaenoptera bonaerensis) in relation to spatial distributions of krill in the Ross Sea, Antarctica. Fish. Ocean. 22, 154–173. doi: 10.1111/fog.12011
Nachtsheim D. A., Jerosch K., Hagen W., Plotz J., Bornemann H. (2017). Habitat modelling of crabeater seals (Lobodon carcinophaga) in the Weddell Sea using the multivariate approach Maxent. Pol. Biol. 40, 961–976. doi: 10.1007/s00300-016-2020-0
Naganobu M., Nishiwaki S., Yasuma H., Matsukura R., Takao Y., Taki K., et al. (2006). Interactions between oceanography, krill and baleen whales in the Ross Sea and Adjacent Waters: An overview of Kaiyo Maru-JARPA joint survey in 2004/05. Paper SC/D06/J23 presented to the JARPA Review Meeting, December 2006 (unpublished). 33pp.
Nicol S. (2006). Krill, currents, and sea ice: Euphausia superba and its changing environment. Biosci. 56, 111–120. doi: 10.1641/0006-3568(2006)056[0111:KCASIE]2.0.CO;2
Olmastroni S., Fattorini N., Pezzo F., Focardi S. (2020). Gone fishing: Adélie penguin site-specific foraging tactics and breeding performance. Ant. Sci. 32, 199–209. doi: 10.1017/S0954102020000085
Pallin L. J., Kellar N. M., Steel D., Botero-Acosta N., Scott Baker C., Conroy J. A., et al. (2023). A surplus no more? variation in krill availability impacts reproductive rates of antarctic baleen whales. Glob. Ch. Biol. 29, 2108–2121. doi: 10.1111/gcb.16559
Pauly T., Nicol S., Higginbottom I., Hosie G., Kitchener J. (2000). Distribution and abundance of antarctic krill (Euphausia superba) off east antarctica (80-150°E) during the austral summer of 1995/1996. Deep-Sea Res. II 47, 2465–2488.
Putz K. (1995). The post-moult diet of Emperor Penguins (Aptenodytes forsteri) in the eastern Weddell Sea, Antarctica. Pol. Biol. 15, 457 463.
Saino N., Guglielmo L. (2000). “ROSSMIZE expedition: Distribution and biomass of birds and mammals in the western ross sea,” in Ross sea ecology. Ed. Faranda F. M., et al (Heidelberg: Springer-Verlag Berlin).
Savoca M. S., Czapanskiy M. F., Kahane-Rapport S. R., Gough W. T., Fahlbusch J. A., Bierlich K. C., et al. (2021). Baleen whale prey consumption based on high-resolution foraging measurements. Nat. 599, 85–90. doi: 10.1038/s41586-021-03991-5
Stanton T. K., Clay C. S., Chu D. (1993). Ray representation of sound scattering by weakly scattering deformed fluid cylinders: Simple physics and application to zooplankton. J. Acoust. Soc Am. 94, 3452–3454.
Swadling K. M. (2006). Krill migration: up and down all night. Curr. Biol. 16, R173–R175. doi: 10.1016/j.cub.2006.02.044
Szesciorka A. R., Ballance L. T., Širović A., Rice A., Ohman M. D., Hildebrand J. A., et al. (2020). Timing is everything: Drivers of interannual variability in blue whale migration. Sci. Rep. 10, 7710. doi: 10.1038/s41598-020-64855-y
Tamura T., Konishi K. (2009). Feeding habits and prey consumption of Antarctic minke whale (Balaenoptera bonaerensis) in the Southern Ocean. J. Northw. Atl. Fish Sci. 42, 13–25. doi: 10.2960/J.v42.m652
Tarling G. A., Thorpe S. E. (2017). Oceanic swarms of Antarctic krill perform satiation sinking. Proc. R. Soc B 284, 20172015. doi: 10.1098/rspb.2017.2015
Testa G., Neira S., Giesecke R., Pinones A. (2022). Projecting environmental and krill fishery impacts on the Antarctic Peninsula food web in 2100. Prog. Ocean. 206, 102862. doi: 10.1016/j.pocean.2022.102862
Trathan P. N., Fielding S., Warwick-Evans V., Freer J., Perry F. (2022). Seabird and seal responses to the physical environment and to spatio-temporal variation in the distribution and abundance of Antarctic krill at South Georgia, with implications for local fisheries management. ICES J. Mar. Sci. 79, 2373–2388. doi: 10.1093/icesjms/fsac168
Trathan P. N., Hill S. L. (2016). “The importance of krill predation in the southern ocean,” in Biology and ecology of Antarctic krill. Advances in polar ecology. Ed. Siegel V. (Springer, Cham). doi: 10.1007/978-3-319-29279-3_9
Veytia D., Corney S., Meiners K. M., Kawaguchi S., Murphy E. J., Bestley S. (2020). Circumpolar projections of Antarctic krill growth potential. Nat. Clim. Change 10, 568–575. doi: 10.1038/s41558-020-0758-4
Watters G. M., Hinke J. T., Reiss C. S. (2020). Long-term observations from Antarctica demonstrate that mismatched scales of fsheries management and predator-prey interaction lead to erroneous conclusions about precaution. Sci. Rep. 10, 2314. doi: 10.1038/s41598-020-59223-9
Wege M., Salas L., LaRue M. (2021). Ice matters: Life-history strategies of two Antarctic seals dictate climate change eventualities in the Weddell Sea. Glob. Change Biol. 27, 6252–6262. doi: 10.1111/gcb.15828
Keywords: krill, pelagic ecosystem, krill predators, Ross Sea, environment
Citation: De Felice A, Biagiotti I, Costantini I, Canduci G and Leonori I (2024) Interactions between krill and its predators in the western Ross Sea. Front. Mar. Sci. 11:1302498. doi: 10.3389/fmars.2024.1302498
Received: 26 September 2023; Accepted: 21 February 2024;
Published: 07 March 2024.
Edited by:
Antonia Granata, University of Messina, ItalyReviewed by:
Won Young Lee, Korea Polar Research Institute, Republic of KoreaRichard O’Driscoll, National Institute of Water and Atmospheric Research (NIWA), New Zealand
Copyright © 2024 De Felice, Biagiotti, Costantini, Canduci and Leonori. This is an open-access article distributed under the terms of the Creative Commons Attribution License (CC BY). The use, distribution or reproduction in other forums is permitted, provided the original author(s) and the copyright owner(s) are credited and that the original publication in this journal is cited, in accordance with accepted academic practice. No use, distribution or reproduction is permitted which does not comply with these terms.
*Correspondence: Iole Leonori, aW9sZS5sZW9ub3JpQGNuci5pdA==