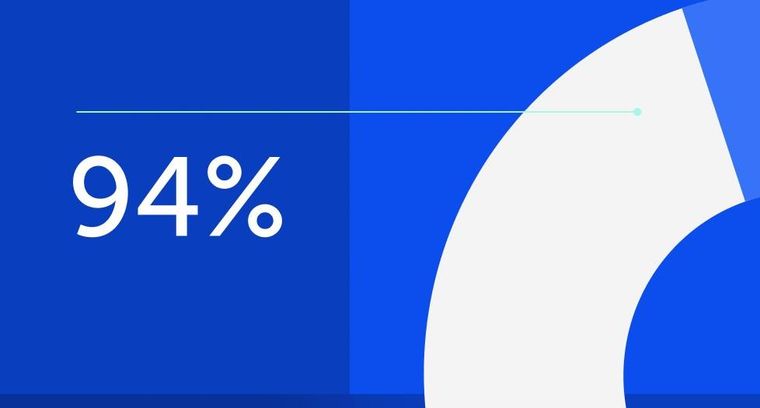
94% of researchers rate our articles as excellent or good
Learn more about the work of our research integrity team to safeguard the quality of each article we publish.
Find out more
ORIGINAL RESEARCH article
Front. Mar. Sci., 05 February 2024
Sec. Marine Fisheries, Aquaculture and Living Resources
Volume 11 - 2024 | https://doi.org/10.3389/fmars.2024.1295196
This article is part of the Research TopicSea Cucumbers: The Sustainability of Emergent and Historical ResourcesView all 12 articles
Sea cucumbers, integral components of benthic ecosystems, have become subjects of scientific scrutiny owing to their intricate morphology and ecological importance. Due to increasing demand, several species of these echinoderms have become overexploited. As a consequence, NE-Atlantic species became new targets for the international markets. There is a vital need for comprehensive biological data to establish and enhance holothurian fisheries management. In the absence of such data, there is a risk of ineffective fisheries regulations, particularly for susceptible commercial species in the NE-Atlantic, which could lead to overexploitation. Establishing effective fisheries regulations requires a foundation of fundamental biological information, such as growth rates from target populations. This work aims to determine the growth parameters for three commercial sea cucumber species from the NE-Atlantic, in a marine protected area, Holothuria mammata, H. forskali and H. arguinensis. This presents a challenge, as sea cucumbers lack significant calcified structures for age determination, and assessing size is complicated because of their body size plasticity. Thus, a von Bertalanffy model was fitted to length-frequency data using the ELEFAN method with the simulated annealing procedure. Underwater length measurements of the three sea cucumber species, in a relaxed state, were systematically recorded over a span of 18 months, with measurements taken every 1.5 months. The results provide the growth parameters of each species. Models considering seasonal growth were better fits to the data. The three species had different growth rates and periods of no growth coincided with the reproductive season. Mortality was lower for the species growing slower. This is crucial information to support decision-making processes regarding stock management, such as setting limits to fisheries considering stock condition associated with environmental variability.
The ecological role of sea cucumbers has long been described as vital for marine ecosystems. Through their feeding behaviour, holothurians help recycle nutrients, stimulate microalgae growth, mix the upper sediment layers and improve physicochemical processes of benthic habitats (Uthicke, 1999; MacTavish et al., 2012; Purcell et al., 2016). However, the high economic value of sea cucumbers driven by an ever-increasing demand, has led to an overexploitation of several sea cucumber species by the majority of fisheries worldwide (Purcell et al., 2013; González-Wangüemert et al., 2016). As a result of local stock depletion and closure of some regional and national fisheries (Purcell, 2014), the exploitation of natural stocks expanded to other areas endangering additional species (Purcell et al., 2013; Eriksson and Clarke, 2015). In the North-eastern Atlantic and the Mediterranean Sea, several sea cucumber species, new to the international trade markets, are now targeted for directed fisheries (González-Wangüemert et al., 2018; Vafidis and Antoniadou, 2023). The absence of time-series data on landings, biometry, and the density of natural holothurian stocks presents a significant obstacle to assess the population’s health. However, in some regions, a decrease in density, abundance, genetic diversity, loss of the largest size classes and even local stock depletion are evidence of overexploitation due to fishing pressure (some occurring illegally; González-Wangüemert et al., 2014; González-Wangüemert et al., 2018). Holothuria (Holothuria) mammata Grube, 1840 and Holothuria (Holothuria) arguinensis Koehler and Vaney 1906 are commercially relevant and targeted species in the in the NE-Atlantic and Mediterranean (González-Wangüemert et al., 2014; González-Wangüemert et al., 2018; Dereli and Aydın, 2021; Vafidis and Antoniadou, 2023), while Holothuria (Panningothuria) forskali Delle Chiaje, 1824 has seen an increasing interest (González-Wangüemert et al., 2016) with values going for over $ 100 USD per dry kg on the market (Sousa et al., 2020). Illegal captures of sea cucumbers, mainly H. arguinensis and H. mammata, have increased for the past years with populations showing local signs of overexploitation (González-Wangüemert et al., 2018).
For these species, there is a lack of knowledge on their ecology and biology which could ultimately lead to misguided or unsound decision-making (Lovatelli et al., 2004; Purcell et al., 2010). Individual growth is hard to estimate since sea cucumbers’ length and weight vary and are easily influenced by manipulation, due to physiological responses to environmental stimuli (e.g. muscle contraction and release of viscera or water). Length measurements should be standardized to avoid measurement errors and this is more easily achieved either by measuring individuals underwater, avoiding any contact, or by taking post-mortem measurements (Azevedo e Silva et al., 2021). Individual recognition techniques such as tagging, are usually shed by holothurians (Conand, 1981; Shelley, 1982) and have only been relatively successful in suspension feeders (Gianasi et al., 2015). Determining growth through the identification of annual growth rings in calcified structures has recently been described for sea cucumbers and this method could facilitate the determination of growth rates for holothuroids (Sun et al., 2019).
Generally, modelling approaches have provided successful growth estimations, through growth parameters, for sea cucumber populations. Understanding growth parameters is crucial not only for the preservation and control of a particular species, but also for mitigating the impact of fishing on natural populations. This knowledge enables us to establish fishery regulations grounded in biological information. A von Bertalanffy model applied with the ELEFAN_SA (simulated annealing optimization) procedure allows for an unconstrained search of parameters within the multi-dimensional space. This is an advantage since there is no need for an a priori fixing of parameters during search routines (Mildenberger et al., 2017), which could lead to biased results. Still, ELEFAN requires users to provide search conditions for each parameter, as well as length-class size and the number of length-class for calculation of the moving average (Zhou et al., 2022). This procedure is an analysis toolbox compiling single-species stock assessment methods specifically designed for data-limited fisheries analysis using length-frequency data (Mildenberger et al., 2017), which is an advantage.
Growth parameters of a population allow us to understand its populational dynamics, which will fundamentally lead to a better management of natural stocks. Estimating growth parameters is an important step that, not only guarantees correct assumptions for stock management, but also leads to a better understanding of the species ecology and biology. The aim of this study was to determine the growth parameters and natural mortality through length-frequency data for the three commercial NE-Atlantic sea cucumbers, H. forskali, H. mammata and H. arguinensis, in a rocky reef habitat, filling this knowledge gap. This will allow us to understand these species different growth strategies, providing crucial information that will support decision-making policies regarding stock management and the conservation of these ecologically important species.
The study was focused on a NE-Atlantic coastal area in the southwest of Portugal, within a marine protected area (MPA), the Arrábida Marine Park (38°26’50.4”N; 9°01’58.7”W; Figure 1), with regulation that bans most fishing activities (none of which susceptible of capturing sea cucumbers) and restricts human presence. Most places are in fact inaccessible unless with maritime transportation, which is also forbidden in some areas. Although fisheries are mostly banned in this MPA and local marine authorities display a regular surveillance for illegal activities, sea cucumbers are not yet a fishing target in this region. So, at the moment, these are unexploited populations. Different habitats compose this MPA, but it is mostly dominated by rocky reefs that end in a sandy floor (Gonçalves et al., 2002; Félix et al., 2021). For these reasons, this area allows the study of sea cucumbers’ population parameters in the most pristine-like conditions possible in the Portuguese coastline.
Starting in February 2018 and until March 2019, a visual census sampling was done every one and a half months, in 30 m x 3 m transects, to record length measurements (to the nearest 0.1mm) of the three local sea cucumber species with commercial interest (H. forskali, H. mammata and H. arguinensis). Sampling was done through scuba diving, in depths ranging from two to ten meters, depending on the depth of the sampling site. Since at least H. arguinensis and H. mammata have displacement behaviours between adjacent habitats (Félix et al., 2021; Azevedo e Silva et al., 2023), sampling was conducted considering full heterogeneity of habitats, in order to ensure the distribution of the species in the region was covered and to guarantee the growth functions would be adequate for the population of the three species. Length of sea cucumbers is variable and easily influenced by manipulation, so length measurements were carefully done underwater with a measuring tape by a team of two divers, where one measures the sea cucumber and the other registers the species and length. The diver measuring length remained the same, ensuring there were no errors attributable to user input. The measurements were carried out in sea cucumbers in a relaxed state, avoiding any handling related stress and following the proposed standardized methodology for comparability (Azevedo e Silva et al., 2021).
All length data obtained from sampling were grouped in different size classes according to the species analysed. H. arguinensis was grouped in three cm classes, H. mammata in two cm classes and H. forskali was grouped in one cm classes. While smaller bin sizes can provide more information, the sample size for H. arguinensis was only 177, resulting in modes with only 1 individual in several months when the bin size was two cm. Therefore, to avoid fitting the model to several modes with only 1 individual, a larger bin size was selected.
All analyses were conducted using the statistical computing software “R” (version 3.5.1). Non-seasonal and seasonal von Bertalanffy Growth Models (VBGF) were fitted to the restructured length frequency data with the ELEFAN (Electronic Length Frequency Analysis) procedure (Pauly, 1987) from the TropFishR package (Mildenberger et al., 2017). Data is restructured through a process that emphasizes the cohorts through peaks and troughs in the data set, calculating a count score for each length bin based on count deviations as compared to the selected Moving Average - MA (Pauly et al., 1992).
The non-seasonal VBGF (Equation 1) is described by the following function (Von Bertalanffy, 1938):
where: Lt is length at age; L∞ is the asymptotic length or the mean length that the species of a given population would reach if they were to grow forever; K is the rate at which asymptotic size is approached; t0 is the age at which length is zero. Instead of the parameter t0, ELEFAN uses a yearly repeating temporal starting point for each cohort’s growth curve (time of the year when length of cohort equals zero), which TropFishR returns as “anchor time” (tanchor). This value ranges between 0 and 1.
The seasonal VBGF (2) was modelled with a Hoening function (Somers, 1988), described by the following:
where, the new parameter C is the relative amplitude of the seasonal oscillations and ts is the phase of the seasonal oscillations denoting the time of year corresponding to the start of the convex segment of sinusoidal oscillation (when growth turns positive). The Hoening function includes seasonality in growth, determining the amplitude of the effect of the season in growth and the beginning of the seasonal oscillation (Pauly et al., 1992).
The time of the year when the growth rate is slowest, known as the winter point (WP), was calculated according to Equation 3:
Growth performance (Equation 4) comparisons were made using the growth performance index (Φ’) described by the following function (Pauly and Munro, 1984):
The R package TropFishR (Mildenberger et al., 2017) performs the restructuring procedure within the ELEFAN functions. The VBGF models were fitted with the “ELEFAN_SA” optimization procedure, a method based on simulated annealing that offers significant improvements to the estimation of the VBGF parameters while also searching over all parameters simultaneously (Mildenberger et al., 2017; Taylor and Mildenberger, 2017). The algorithm searches for the best fit through a series of iterations (consecutive curve fitting attempts) and allows for unconstrained search for optimum combinations of growth parameters, including seasonality (Schwamborn et al., 2019).
Moving Average (MA) was set based on three different scenarios for both H. forskali and H. mammata. First scenario considers a general rule of thumb where an MA setting that approximates the number of bins spanning the width of the smallest mode, which should reflect the youngest cohort following recruitment to the fishery (Taylor and Mildenberger, 2017). Second setting is the value immediately bellow. The third setting was the value immediately above. For H. arguinensis, since in the first scenario MA = 3, the other two scenarios were adapted to MA = 5 and MA = 7. Different scenarios were tested since the MA settings can significantly affect the scoring of the growth curve (Taylor and Mildenberger, 2017). The remaining settings for the functions were chosen, ensuring the widest possible search for overall maximum and a large number of iterations (SA temp = 6e5;maxit = 1000; SA_time =2 min), to ensure full convergence of iterations towards one best fit value (Schwamborn et al., 2018; Schwamborn et al., 2019). The search space used during fit optimization was constant for all species with tanchor = 0-1, K = 0.1-2, C = 0-1 and ts = 0-1, varying only in the L∞ parameter, where the search space was defined by the bounds Lmax * 0.8 and Lmax * 1.2 (where Lmax is the maximum length registered for the species throughout the entire sampling period). Then local maxima were analysed in order to overcome the possibility of the curve fitting algorithms being captured by local maxima (Schwamborn et al., 2019). Fitting scores (Rn) were recorded for comparison, estimated according to Equation 5:
where the Estimated Sum of Peaks (ESP) is the sum of peak values crossed by the growth curves, with the caveat that positively crossed bins are only counted once, while negatively crossed bins are counted every time they are encountered (Pauly, 1985). The Available Sum of Peaks (ASP) is the sum of all positive peaks, which represents a maximum possible score (if negative bins are crossed).
Natural mortality (M) was estimated with the two VBGF parameter (K, L∞) based on the method from Then et al. (2015) [33] with the TropFishR package (Mildenberger et al., 2017). Age-at-length was back calculated from length using the seasonal estimated VBGF function, according to Equation 2 (Ramírez-González et al., 2020) and finally, mean monthly growth rate was calculated as averages over the previous 12 months for age groups (Wilhelm et al., 2017; Ahmed et al., 2020).
A total of 2019 H. mammata, 3590 H. forskali and 177 H. arguinensis were sampled between February 2018 and March 2019, all included in the VBGF models. The three species presented unimodal length distribution where the total length ranged from 11.9 to 51 cm for Holothuria mammata, 7 to 33 cm for Holothuria forskali and 8 to 49 cm for Holothuria arguinensis (Figure 2).
Figure 2 Length class distribution of Holothuria mammata, Holothuria forskali and Holothuria arguinensis sampled in the Arrábida coastline, SW of Portugal from February 2018 to March 2019.
The different Moving Averages resulted in different model fitting scores (Rn) for the three species, where models selected were the ones with the highest fit and reasonable parameters (Table 1). Non-seasonal models presented worse fits to the data than seasonal models and this difference was more evident for Holothuria forskali.
Table 1 Parameters for the seasonal VBGF models fitted with the simulated annealing optimization procedure.
Among the models the one with a MA width of 5 bins had the highest Rn for H. mammata and H. arguinensis, while for H. forskali, the MA that generated the best fit was 9. H. forskali revealed strong seasonal oscillation effect (C = 0.994) while this factor was lower for H. mammata and H. arguinensis (0.5999 and 0.7790, respectively; Table 1). The natural mortality rate (M) was higher for H. forskali.
Both H. mammata and H. arguinensis models show a good overall fit as reflected by the intersection of growth curves with positively scored bins (Figure 3) and the higher Rn scores, while for H. forskali this is only evident for younger cohorts.
Figure 3 Age cohorts (dashed lines) estimated by the VBGF models run with the optimized simulated annealing ELEFAN procedure, fitted to the length-frequency data of Holothuria mammata, Holothuria arguinensis and Holothuria forskali. Black and white bars represent positively and negatively scored bins, respectively. Moving average settings considered were 5 for H. mammata and H. arguinensis and 9 for H. forskali.
The Winter point (WP) was 0.6 (July) for H. mammata, 0.17 (February) for H. forskali and 0.35 for H. arguinensis (April; Figure 4). Growth coefficient (K) was the lowest for H. mammata and higher for H. forskali and H. arguinensis and this is notable on the monthly mean growth rate, where H. forskali reaches asymptotic length faster.
Figure 4 Length-at-age for seasonally oscillating Von Bertalanffy growth curve depicting Winter Point (WP) and Monthly mean (12 months) growth rate for Holothuria mammata, Holothuria forskali and Holothuria arguinensis.
The studied region allowed the study of unexploited sea cucumber populations, which proved advantageous in accurately estimating natural growth parameters. A common occurrence in natural populations under severe fishing pressure is the absence of large individuals (Pauly et al., 1998). This leads to an underestimation of L∞ (Schwamborn et al., 2018) and an overestimation of K, due to the known interaction between the growth constant K and L∞. Consequently, an overestimation of K leads to an underestimation of a population’s ability to recover from fishing pressure (Schwamborn et al., 2019). The growth parameters provided by this study are therefore considered to be essential for these species since they are closer to a pristine condition.
To the best of our knowledge there are no reported growth parameters for H. mammata. The population of H. mammata in Arrábida’s coast is the healthiest found up to this moment (Félix et al., 2021), showing the largest individuals and the highest densities so far. It also has unimodal size-class distributions (Félix et al., 2021), as opposed to what has been reported by other studies (González-Wangüemert et al., 2014; Aydin and Erkan, 2015; González-Wangüemert et al., 2018). This is a reflection of good environmental conditions, successful recruitment and an unexploited population (Félix et al., 2021). Mortality rate of H. mammata was the lowest between the three species from this study. However, its growth rate is also the lowest. H. mammata is usually present in rocky reefs, hiding in crevices, and they either need to move to sandy patches to feed or they present diel feeding behaviour (Félix et al., 2021). These behaviours are size dependent and despite increasing survival, which is an adaptation against predation or disturbances from hydrodynamics, they represent a limitation to feeding opportunities and consequently, to the growth rate. This is an indication of a trade-off between growth and survival, as suggested for temperate species (Morgan, 2012), highlighting that while this species exhibits a lower mortality rate, representative of enhanced survival mechanisms, its growth rate is comparatively lower. The trade-off concept suggests that the allocation of resources to enhance survival, such as increased adaptations against predation or disturbances from hydrodynamics, may come at a cost to growth opportunities.
Holothuria forskali is an understudied species with few studies focusing on its biology and ecology (e.g. Tuwo and Conand, 1992; Santos et al., 2017; David et al., 2020; Azevedo e Silva et al., 2021; Ballesteros et al., 2021; Félix et al., 2024). To the best of our knowledge, this is the first study to report growth parameters for this species. H. forskali is the species with the highest growth rate between the three species, indicating that its asymptotic length is achieved faster. Considering this is the only species of the three with a defensive mechanism against predators (Cuvierian tubules; Muller et al., 1970; Azevedo e Silva et al., 2021), our findings indicate an evolutionary strategy in H. forskali, suggesting accelerated growth during the early stages: Given the reduced predation risk of this species, this growth pattern is inferred as an adaptive response to mitigate higher environmental stress conditions, since larger individuals would be more likely to remain stable in the substrate (Olaya-Restrepo et al., 2018). In fact, H. forskali is the more resilient species of all three to environmental stressors like hydrodynamics (Félix et al., 2024). However, it should be noted that K is relative to L∞ so a higher K does not necessarily mean that it is growing faster in absolute terms. In fact, according to the monthly growth rates, H. forskali and H. arguinensis seem to grow at around the same rate for the first year, after which H. forskali’s growth declines abruptly. Apart from having the highest K between the three species of this study, mortality rate of H. forskali is also the highest. In temperate coastal regions, where food may be seasonally abundant, the trade-off between achieving fast growth and reducing mortality explains a higher mortality in faster growing sea cucumbers (Hammond, 1982; Sonnenholzner, 2003; Morgan, 2012). Mortality could be due to predation of lower size class individuals or their inability to deal with physical stressors. A faster growth rate could be considered as an adaptive advantage since faster growth may have positive implications on mortality for some species (Ahmed et al., 2020). The models fit particularly well for the younger cohorts, however, this is not evident for older cohorts. The sample size is big enough to be considered representative, but it could be difficult to distinguish between the older, bigger cohorts. Since H. forskali eviscerates the Cuvierian tubules as a defensive mechanism, regenerating them comes at an energy cost (Vandenspiegel et al., 2000). The release of Cuvierian tubules could be a relevant factor contributing to the variability in growth rates. It can also be hypothesized that, while regenerating the Cuvierian tubules, this species may become more vulnerable to predation. Furthermore, in this region, H. forskali, shows evidence of two consecutive yearly reproductive peaks, which may have an added impact on somatic growth (unpublished data from the project at seacucumber.eu/en/projecto).
H. arguinensis presented a higher growth rate (K = 0.35 and L∞ = 53.03) than what was already reported for the Atlantic coast of Morocco (K = 0.17 and L∞ = 25.96; 0.29 and L∞ = 25.44; Haddi et al., 2021), but revealed a much slower growth rate than the populations at Ria Formosa, south of Portugal (K = 0.88 and L∞ = 69.90; Olaya-Restrepo et al., 2018). It is noteworthy, however, that the study for the Atlantic coast of Morocco used a recalculated length, based on both length and width, and this should be borne in mind when making a comparison. In the present study area, this species occurs exclusively on subtidal habitats. Nutrient rich subtidal environments provide more stable conditions for sea cucumber populations (Félix et al., 2021), particularly for H. arguinensis (Azevedo e Silva et al., 2023), but nutrient increases are seasonal and very dependent on upwelling events (Wooster et al., 1976). Moreover, faster growth rates in sea cucumbers under high stress conditions and in disturbed environments may help reducing mortality in younger holothurians (Morgan, 2012), so this could be considered as an adaptative advantage. In fact, the growth rate reported in that study (Olaya-Restrepo et al., 2018) is among the highest reported for sea cucumbers, even when considering tropical sea cucumbers and this could be linked to the high disturbance of the habitats coupled with the productivity of that ecosystem (Brito et al., 2009; Olaya-Restrepo et al., 2018). Nevertheless, the high fishing pressure in Ria Formosa showed already a significant reduction of the larger size classes (González-Wangüemert et al., 2018), which may have an influence in the estimation of the growth rate, increasing it. The combination of all factors may add up to justify the unusually high growth rate of H. arguinensis in Ria Formosa.
Assuming high growth rates and its advantages on the sustainability of the stocks can lead to neglecting a conservative management of the species and to a depletion of the stocks. This oversight could potentially lead to a slow, decade-long recovery, as pointed out for Holothuria nobilis (Uthicke et al., 2004). Slower growth rates have fundamental implications on the health of populations, primarily under high fishing pressure. It is important to ensure a highly conservative management for this species, guaranteeing an efficient monitoring of the populations. Even though Ria Formosa is a protected area, easy access to sea cucumbers facilitates illegal harvesting and implementation of restrictive measures should be reinforced.
Seasonal VBGF functions revealed a better fit to the data for the three species than non-seasonal VBGF functions. These results confirm seasonality in the growth of H. mammata, H. forskali and H. arguinensis, with seasonality being more evident in H. forskali species. In fact, H. forskali reveals a period of no growth during winter (February), while H. mammata and H. arguinensis have slower growth during early summer (June-July) and spring (April), respectively. Seasonal growth has been described for most temperate sea cucumber species (Morgan, 2012; Olaya-Restrepo et al., 2018), where annual cycles may limit food availability, drive reproductive behaviour and increase metabolism, leading to a fluctuation of body weight (Morgan, 2012) and consequently, body length (Azevedo e Silva et al., 2021). Indeed, H. forskali’s period of no growth coincides with the reported reproductive period for this species (Tuwo and Conand, 1992; Santos et al., 2017; unpublished data from the project at seacucumber.eu/en/projecto) and H. mammata and H. arguinensis’ slower growth periods also coincide with their reported reproductive periods (Marquet et al., 2017; Venâncio et al., 2022). The energy input into reproduction during breeding season (Poot-Salazar et al., 2014; Olaya-Restrepo et al., 2018) is consistent with our results that reveal growth during this period is slower or halted. Moreover, considering that sea cucumbers invest more energy into reproduction than growth (Ramírez-González et al., 2020), it should also be highlighted that most relative growth of the three species occurs during somatic growth, when sea cucumbers are not yet adult or ready to reproduce.
In this region, the productivity increases in the summer (Wooster et al., 1976; Costa et al., 2013), which coincides with the period when H. forskali’s growth increases. H. forskali presents a more selective feeding behaviour than the other two species, foraging on hard surfaces and selectively choosing food items, mostly composed of algae (Azevedo e Silva et al., 2021). Therefore, H. forskali may be more susceptible to seasonality that influences food quantity and quality. Temperature has been known to be a factor regulating the reproductive cycle of holothurians (Ahmed et al., 2020) and increase metabolism. In the study area, the temperature began increasing in January, peaking in October, but being generally higher during summer months (Félix et al., 2021; Venâncio et al., 2022). A higher temperature only seems to impact positively the growth of H. forskali as it likely increases its metabolism and positively affects food supply. On the other hand, it seems to negatively affect the growth of H. arguinensis and H. mammata. Since temperature seems to offset reproductive events for both these species (Marquet et al., 2017; Venâncio et al., 2022) this relationship between growth and temperature is explained by an allocation of resources into reproduction, a phenomenon described for sea cucumbers (Ru et al., 2018; Ahmed et al., 2020).
In the future, validating length-at-age model results with age determined from growth rings (Sun et al., 2019) will lead to more confidence in the models and more robust results. Growth studies provide valuable information that can help implement and improve management measures. However, studied populations should be monitored since a change in growth parameters could be the result of a change in the health of a population. The implications that incorrect assumptions can have on models are greatly enhanced by management decisions based on inflated growth estimates. The population traits of the species in the present study, in conjunction with the important ecological role of sea cucumbers, emphasize the need for conservative regulatory measures.
The raw data supporting the conclusions of this article will be made available by the authors, without undue reservation.
Ethical approval was not required for the study involving animals in accordance with the local legislation and institutional requirements because there was no impact on the animals studied. Sea cucumbers were measured with a tape underwater without handling. All permits required were obtained from the national entity.
FA: Conceptualization, Data curation, Formal analysis, Funding acquisition, Investigation, Methodology, Resources, Software, Visualization, Writing – original draft, Writing – review & editing. AB: Formal analysis, Funding acquisition, Project administration, Supervision, Visualization, Writing – review & editing. TS: Investigation, Methodology, Resources, Validation, Writing – review & editing. AP: Formal analysis, Funding acquisition, Investigation, Resources, Supervision, Validation, Visualization, Writing – review & editing. JS: Formal analysis, Investigation, Methodology, Validation, Visualization, Writing – review & editing. EV: Investigation, Methodology, Visualization, Writing – review & editing. PF: Conceptualization, Data curation, Formal analysis, Investigation, Methodology, Project administration, Resources, Supervision, Validation, Visualization, Writing – review & editing.
The author(s) declare financial support was received for the research, authorship, and/or publication of this article. This work was funded by the Operational Program Mar2020 MAR-02.01.01-FEAMP-0052 “Newcumber - Avanços para o cultivo sustentável de pepinos do mar”. This study had the support of Fundação para a Ciência e a Tecnologia (FCT) through projects UIDB/04292/2020 (https://doi.org/10.54499/UIDB/04292/2020) and UIDP/04292/2020 (https://doi.org/10.54499/UIDP/04292/2020) awarded to MARE and through project LA/P/0069/2020 (https://doi.org/10.54499/LA/P/0069/2020) granted to the Associate Laboratory ARNET. It also had the support of AB with the Scientific Stimulus Programme – CEECIND/00095/2017, of AP under the Scientific Employment Stimulus - Institutional Call - CEECINST/00051/2018 and FA and JS through the individual research grants (SFRH/BD/09563/2020 and SFRH/BDANA/02949/2023).
The authors wish to thank ICNF and H2O Lisbon Dive Club for the field assistance and all the volunteers who assisted with our work: Rui, Joshua, Hugo, João Francisco, Mariana, Matilde and Daniel.
The authors declare that the research was conducted in the absence of any commercial or financial relationships that could be construed as a potential conflict of interest.
All claims expressed in this article are solely those of the authors and do not necessarily represent those of their affiliated organizations, or those of the publisher, the editors and the reviewers. Any product that may be evaluated in this article, or claim that may be made by its manufacturer, is not guaranteed or endorsed by the publisher.
Ahmed Q., Bilgin S., Ali Q. M. (2020). Comparison of electronic length frequency analysis (ELEFAN) for estimation of growth parameters for lollyfish, Holothuria (Holothuria) atra and sand sea cucumber, Holothuria (Thymiosycia) arenicola (Holothuroidea: Echinodermata) in the north Arabian Sea. Mar. Sci. Technol. Bull. 9 (2), 145–158. doi: 10.33714/masteb.744301
Aydin M., Erkan S. (2015). Identification and some biological characteristics of commercial sea cucumber in the Turkey coast waters. Int. J. Fisheries Aquat. Stud. 3 (1), 260–265. Available at: https://www.fisheriesjournal.com/vol3issue1/Pdf/3-1-50.1.pdf.
Azevedo e Silva F., Brito A. C., Pombo A., Simões T., Marques T. A., Rocha C., et al. (2023). Spatiotemporal distribution patterns of the sea cucumber holothuria arguinensis on a rocky-reef coast (Northeast atlantic). Estuaries Coasts 46 (4), 1035–1045. doi: 10.1007/s12237-023-01201-1
Azevedo e Silva F., Brito A. C., Simões T., Pombo A., Marques T. A., Rocha C., et al. (2021). Allometric relationships to assess ontogenetic adaptative changes in three NE Atlantic commercial sea cucumbers (Echinodermata, Holothuroidea). Aquat. Ecol. 55, 711–720. doi: 10.1007/s10452-021-09856-3
Ballesteros T., Tubío A., Rodríguez R., Hernández A., Costas D., Troncoso J. (2021). Reproductive cycle of the sea cucumber Holothuria forskali (Holothuriida: Holothuriidae) in the Ría de Vigo (NW of Spain). Rev. Biol. Trop. 69, 101–117. doi: 10.15517/rbt.v69iSuppl.1.46331
Brito A., Newton A., Tett P., Fernandes T. F. (2009). Temporal and spatial variability of microphytobenthos in a shallow lagoon: Ria Formosa (Portugal). Estuarine Coast. Shelf Sci. 83 (1), 67–76. doi: 10.1016/j.ecss.2009.03.023
Conand C. (1981). Sexual cycle of three commercially important holothurian species (Echinodermata) from the lagoon of New Caledonia. Bull. Mar. Sci. 31 (3), 523–543. Available at: https://www.documentation.ird.fr/hor/fdi:02211.
Costa B. H. E., Erzini K., Caselle J. E., Folhas H., Gonçalves E. J. (2013). Reserve effect within a temperate marine protected area in the north-eastern Atlantic (Arrábida Marine Park, Portugal). Mar. Ecol. Prog. Ser. 481, 11–24. doi: 10.3354/meps10204
David F., Hubas C., Laguerre H., Badou A., Herault G., Bordelet T., et al. (2020). Food sources, digestive efficiency and resource allocation in the sea cucumber Holothuria forskali (Echinodermata: Holothuroidea): Insights from pigments and fatty acids. Aquaculture Nutr. 26 (5), 1568–1583. doi: 10.1111/anu.13103
Dereli H., Aydın M. (2021). Sea cucumber fishery in Turkey: Management regulations and their efficiency. Regional Stud. Mar. Sci. 41, 101551. doi: 10.1016/j.rsma.2020.101551
Eriksson H., Clarke S. (2015). Chinese market responses to overexploitation of sharks and sea cucumbers. Biol. Conserv. 184, 163–173. doi: 10.1016/j.biocon.2015.01.018
Félix P. M., Azevedo e Silva F., Simões T., Pombo A., Marques T. A., Rocha C., et al (2024). Modelling the habitat preferences of the NE-Atlantic sea cucumber Holothuria forskali: demographics and abundance. Ecol. Informatics. 80, 102476. doi: 10.1016/j.ecoinf.2024.102476
Félix P. M., Pombo A., Azevedo e Silva F., Simões T., Marques T. A., Melo R., et al. (2021). Modelling the distribution of a commercial NE-atlantic sea cucumber, holothuria mammata: demographic and abundance spatio-temporal patterns. Front. Mar. Sci. 8. doi: 10.3389/fmars.2021.675330
Gianasi B. L., Verkaik K., Hamel J. F., Mercier A. (2015). Novel use of PIT tags in sea cucumbers: Promising results with the commercial species Cucumaria frondosa. PloS One 10 (5), e0127884. doi: 10.1371/journal.pone.0127884
Gonçalves E. J., Henriques M., Almada V. C. (2002). Use of a temperate reef-fish community to identify priorities in the establishment of a marine protected area. In: Beumer J. P., Grant A., Smith D. C. (Eds). Aquatic Protected Areas: What Works Best And How Do We Know? Proceedings of the World Congress on Aquatic Protected Areas, Cairns, Australia – August 2002, pp. 261–272.
González-Wangüemert M., Aydin M., Conand C. (2014). Assessment of sea cucumber populations from the Aegean Sea (Turkey): First insights to sustainable management of new fisheries. Ocean Coast. Manage. 10 (1), 21–39. doi: 10.1016/j.ocecoaman.2014.02.014
González-Wangüemert M., Domínguez-Godino J. A., Cánovas F. (2018). The fast development of sea cucumber fisheries in the Mediterranean and NE Atlantic waters: From a new marine resource to its over-exploitation. Ocean Coast. Manage. 151, 165–177. doi: 10.1016/j.ocecoaman.2017.10.002
González-Wangüemert M., Valente S., Henriques F., Domínguez-Godino J. A., Serrão E. A. (2016). Setting preliminary biometric baselines for new target sea cucumbers species of the NE Atlantic and Mediterranean fisheries. Fisheries Res. 179, 57–66. doi: 10.1016/j.fishres.2016.02.008
Haddi I., Benzha F., Maanan M., Siddique S., Rhinane H., Charouki N., et al. (2021). Population index and growth estimation based on SLW index of the sea cucumber (Holothuria arguinensis Koehler & Vaney 1906) in the moroccan waters of the atlantic coast. Thalassas: Int. J. Mar. Sci. 38, 535–551. doi: 10.1007/s41208-021-00362-9
Hammond L. S. (1982). Patterns of feeding and activity in deposit-feeding holothurians and echinoids ( Echinodermata) from a shallow back-reef lagoon, Discovery Bay, Jamaica. Bull. Mar. Sci. 32 (2), 549–571.
Lovatelli A., Conand C., Purcell S., Uthicke S., Hamel J.-F., Mercier A. (2004). “Advances in sea cucumber aquaculture and management,” in FAO Fisheries Technical Paper No. 463 (Rome: FAO).
MacTavish T., Stenton-Dozey J., Vopel K., Savage C. (2012). Deposit-feeding sea cucumbers enhance mineralization and nutrient cycling in organically-enriched coastal sediments. PloS One 7 (11), e50031. doi: 10.1371/journal.pone.0050031
Marquet N., Conand C., Power D. M., Canário A. V. M., González-Wangüemert M. (2017). Sea cucumbers, Holothuria arguinensis and H. mammata, from the southern Iberian Peninsula: Variation in reproductive activity between populations from different habitats. Fisheries Res. 191, 120–130. doi: 10.1016/j.fishres.2017.03.007
Mildenberger T. K., Taylor M. H., Wolff M. (2017). TropFishR: an R package for fisheries analysis with length-frequency data. Methods Ecol. Evol. 8 (11), 1520–1527. doi: 10.1111/2041-210X.12791
Morgan A. D. (2012). Use of a growth model to estimate size at age in the temperate sea cucumber Australostichopus mollis. SPC Beche-de-Mer Bull. 32, 24–32.
Muller W. E. G., Zahn R. K., Schmid K. (1970). Morphology and function of cuvier organs of holothuria-forskali delle chiaje (echinodermata, holothuroidea, aspidochirota, holothuriidae). Z. Fur Wissenschaftliche Zoologie 181 (3–4), 219.
Olaya-Restrepo J., Erzini K., González-Wangüemert M. (2018). Estimation of growth parameters for the exploited sea cucumber holothuria arguinensis from south Portugal. Fishery Bull. 116 (1), 1–8. doi: 10.7755/FB.116.1.1
Pauly D. (1985). On improving operation and use of the elefan programs. part 1, avoiding" drift" of K towards low values. Fishbyte 3 (3), 13–14.
Pauly D. (1987). A review of the ELEFAN system for analysis of length-frequency data in fish and aquatic invertebrates. ICLARM Conf. Proc. 13 (232), 7–34.
Pauly D., Christensen V., Dalsgaard J., Froese R., Torres F. Jr. (1998). Fishing down marine food webs. Science 279 (5352), 860–863. doi: 10.1126/science.279.5352.860
Pauly D., Munro J. L. (1984). Once more on the comparison of growth in fish and invertebrates. Fishbyte 2 (1), 1–21.
Pauly D., Soriano-Bartz M., Mor Eauc J., Jarre-Teichmann A. (1992). А new model accounting for seasonal cessation of growth in fishes. Mar. Freshw. Res. 43 (5), 1151–1156. doi: 10.1071/MF9921151
Poot-Salazar A., Hernández-Flores Á., Ardisson P. L. (2014). Use of the SLW index to calculate growth function in the sea cucumber Isostichopus badionotus. Sci. Rep. 4(1), 5151. doi: 10.1038/srep05151
Purcell S. W. (2014). Value, market preferences and trade of beche-de-mer from pacific island sea cucumbers. PloS One 9, 1–8. doi: 10.1371/journal.pone.0095075
Purcell S. W., Conand C., Uthicke S., Byrne M. (2016). Ecological roles of exploited sea cucumbers. Oceanogr. Mar. Biol. 54, 367–386. doi: 10.1201/9781315368597-8
Purcell S. W., Lovatelli A., Vasconcellos M., Ye Y. (2010). “Managing sea cucumber fisheries with an ecosystem approach,” in FAO. Fisheries technical paper (Rome: FAO), vol. 520, 157. Available at: http://www.fao.org/docrep/012/i1384e/i1384e00.htm.
Purcell S. W., Mercier A., Conand C., Hamel J. F., Toral-Granda M. V., Lovatelli A., et al. (2013). Sea cucumber fisheries: Global analysis of stocks, management measures and drivers of overfishing. Fish Fisheries 14 (1), 34–59. doi: 10.1111/j.1467-2979.2011.00443.x
Ramírez-González J., Moity N., Andrade-Vera S., Mackliff H. R. (2020). Estimation of age and growth and mortality parameters of the sea cucumber Isostichopus fuscus (Ludwig 1875) and implications for the management of its fishery in the Galapagos Marine Reserve. Aquaculture Fisheries 5 (5), 245–252. doi: 10.1016/j.aaf.2020.01.002
Ru X., Zhang L., Liu S., Sun J., Yang H. (2018). Energy budget adjustment of sea cucumber Apostichopus japonicus during breeding period. Aquaculture Res. 49 (4), 1657–1663. doi: 10.1111/are.13621
Santos R., Dias S., Tecelão C., Pedrosa R., Pombo A. (2017). Reproductive biological characteristics and fatty acid profile of Holothuria mammata (Grube 1840). SPC Beche-de-Mer Inf. Bull. 37, 58–64.
Schwamborn R., Mildenberger T. K., Taylor M. H. (2018). fishboot: Bootstrap-based methods for the study of fish stocks and aquatic populations. (Vienna, Austria: R Foundation for Statistical Computing).
Schwamborn R., Mildenberger T. K., Taylor M. H. (2019). Assessing sources of uncertainty in length-based estimates of body growth in populations of fishes and macroinvertebrates with bootstrapped ELEFAN. Ecol. Model. 393, 37–51. doi: 10.1016/j.ecolmodel.2018.12.001
Shelley C. C. (1982). Aspects of the distribution, reproduction, growth and’fishery’potential of holothurians (beche-de-mer) in the papuan coastal lagoon (Papua New Guinea: University of Papua New Guinea).
Sonnenholzner J. (2003). Seasonal variation in the food composition of Holothuria theeli (Holothuroidea: Aspidochirotida) with observations on density and distribution patterns at the central coast of Ecuador. Bull. Mar. Sci. 73 (3), 527–543.
Sousa J., Félix P. M., Brito A. C., Venâncio E., Azevedo e Silva F., Simões T., et al. (2020). The effects of stocking density on physiological traits in Holothuria forskali broodstock. Aquaculture Res. 52(4), 1–13. doi: 10.1111/are.15007
Sun J., Hamel J.-F., Gianasi B. L., Mercier A. (2019). Age determination in echinoderms: first evidence of annual growth rings in holothuroids. Proc. R. Soc. B 286 (1906), 20190858. doi: 10.1098/rspb.2019.0858
Taylor M. H., Mildenberger T. K. (2017). Extending electronic length frequency analysis in R. Fisheries Manage. Ecol. 24 (4), 330–338. doi: 10.1111/fme.12232
Then A. Y., Hoenig J. M., Hall N. G., Hewitt D. A., Jardim H. (2015). Evaluating the predictive performance of empirical estimators of natural mortality rate using information on over 200 fish species. ICES J. Mar. Sci. 72 (1), 82–92. doi: 10.1093/icesjms/fsu136
Tuwo A., Conand C. (1992). Reproductive biology of the holothurian Holothuria forskali (Echinodermata). J. Mar. Biol. Assoc. United Kingdom 72 (4), 745–758. doi: 10.1017/S0025315400060021
Uthicke S. (1999). Sediment bioturbation and impact of feeding activity of Holothuria (Halodeima) atra and Stichopus chloronotus, two sediment feeding holothurians, at Lizard Island, great barrier reef. Bull. Mar. Sci. 64 (1), 129–141.
Uthicke S., Welch D., Benzie J. A. H. (2004). Slow growth and lack of recovery in overfished holothurians on the Great Barrier Reef: Evidence from DNA fingerprints and repeated large-scale surveys. Conserv. Biol. 18 (5), 1395–1404. doi: 10.1111/j.1523-1739.2004.00309.x
Vafidis D., Antoniadou C. (2023). Holothurian fisheries in the hellenic seas: seeking for sustainability. Sustainability (Switzerland) 15 (12), 9799. doi: 10.3390/su15129799
Vandenspiegel D., Jangoux M., Flammang P. (2000). Maintaining the line of defense: Regeneration of Cuvierian tubules in the sea cucumber Holothuria forskali (Echinodermata, Holothuroidea). Biol. Bull. 198 (1), 34–49. doi: 10.2307/1542802
Venâncio E., Félix P. M., Brito A. C., Silva F. A. E., Simões T., Sousa J., et al. (2022). Reproductive biology of the sea cucumber holothuria mammata (Echinodermata: holothuroidea). Biology 11 (5), 622. doi: 10.3390/biology11050622
Von Bertalanffy L. (1938). A quantitative theory of organic growth (inquiries on growth laws. II). Hum. Biol. 10 (2), 181–213.
Wilhelm M. R., Moloney C. L., Paulus S., Roux J. P. (2017). Fast growth inferred for northern Benguela shallow-water hake Merluccius capensis using annual survey-and monthly commercial length-frequency distributions. Fisheries Res. 193, 7–14. doi: 10.1016/j.fishres.2017.03.001
Wooster W. S., Bakun A., McLain D. R. (1976). Seasonal upwelling cycle along the eastern boundary of the North Atlantic. J. Mar. Res. 34 (2), 131–141.
Keywords: Holothuroidea, ELEFAN von Bertalanffy, seasonal growth model, length frequency, age at length
Citation: Azevedo e Silva F, Brito AC, Simões T, Pombo A, Sousa J, Venâncio E and Félix PM (2024) Estimating age and growth parameters for three commercial NE-Atlantic sea cucumbers, Holothuria mammata, H. forskali and H. arguinensis, in a marine protected area. Front. Mar. Sci. 11:1295196. doi: 10.3389/fmars.2024.1295196
Received: 15 September 2023; Accepted: 18 January 2024;
Published: 05 February 2024.
Edited by:
Amalia Pérez-Jiménez, University of Granada, SpainReviewed by:
Chryssanthi Antoniadou, Aristotle University of Thessaloniki, GreeceCopyright © 2024 Azevedo e Silva, Brito, Simões, Pombo, Sousa, Venâncio and Félix. This is an open-access article distributed under the terms of the Creative Commons Attribution License (CC BY). The use, distribution or reproduction in other forums is permitted, provided the original author(s) and the copyright owner(s) are credited and that the original publication in this journal is cited, in accordance with accepted academic practice. No use, distribution or reproduction is permitted which does not comply with these terms.
*Correspondence: Francisco Azevedo e Silva, ZmhzaWx2YUBmYy51bC5wdA==
Disclaimer: All claims expressed in this article are solely those of the authors and do not necessarily represent those of their affiliated organizations, or those of the publisher, the editors and the reviewers. Any product that may be evaluated in this article or claim that may be made by its manufacturer is not guaranteed or endorsed by the publisher.
Research integrity at Frontiers
Learn more about the work of our research integrity team to safeguard the quality of each article we publish.