- 1School of Food and Pharmacy, Zhejiang Ocean University, Zhoushan, China
- 2Key Lab of Mariculture and Enhancement of Zhejiang Province, Zhejiang Marine Fisheries Research Institute, Zhoushan, China
- 3Laboratory of Fish Disease, Wenzhou Fisheries Technology Extension Service, Wenzhou, China
Tiamulin (TML) has been studied and analyzed in pigs, cattle, chickens, ducks, and other domestic animals, however, its metabolic state in fish has not been well explored. This study investigated TML metabolism in Crucian carp (Carassius carassius). After intraperitoneal injection of TML into Crucian carp, ultra-high performance liquid chromatography with quadrupole and time-of-flight mass spectrometry (UPLC/Q-TOF MS) analysis, was conducted to identify TML metabolites. The UPLC/Q-TOF MS analysis and the relative molecular mass of the metabolites obtained from related literature identified five metabolites in Crucian carp. These metabolites were M1 (510.2908, C28H48NO5S+), M2 (510.2908, C28H48NO5S+), M3 (466.2750, C26H44NO4S+), M4 (482.2663, C26H44NO5S+), and M5 (482.2663, C26H44NO5S+). The enrichment and metabolism of TML and its metabolites in Crucian carp were investigated using the drug bath method combined with ultra-high performance liquid chromatography-tandem mass spectrometry (UPLC-MS/MS). TML exhibited an overall trend of an initial increase followed by a decrease. Moreover, the drug enrichment rate was fast and reached saturation after two days. The bioconcentration factor of TML in Crucian carp was 3.01. However, the drug had a slow elimination rate, with its complete metabolism occurring after 20 days.
1 Introduction
Tiamulin (TML) is a semi-synthetic derivative of pleuromutilin, a naturally occurring diterpene antibiotic produced by the fungi Pleurotus mutilus and Clitopilus(Witkamp et al., 1996; Liu et al., 2021). It was discovered by Frederick Kavanagh in 1951 in the USA and was widely used after the 1960s(Kavanagh et al., 1951). TML exhibits higher in vitro and in vivo antimicrobial activities against gram-positive and gram-negative microorganisms(Hanson et al., 2002; Goethe et al., 2019). Therefore, it was mainly used as a prophylaxis for dysentery, pneumonia and, mycoplasmal infections in pigs and poultry(Cromwell and Stahly, 1985; Borak et al., 2020). TML has also been used as a growth promoter to enhance growth in animal feed(Schuhmacher et al., 2006). However, its misuse may cause residues in edible animal tissues, the residues of TML may cause other bacteria in the human body to mutate into drug-resistant bacteria, affecting the efficacy of antimicrobial agents. The emergence of these resistance genes in animals poses a potential threat to human medicine and increases the difficulty of prevention and treatment of human diseases(Wang et al., 2004; Duijkeren et al., 2009). Thus, the maximum residue limits (MRLs) of TML in edible tissues (100 μg kg−1 for muscle and 500 μg kg−1 for liver, respectively) have been established by the European Commission (EC), United States, and China.
Being an effective diterpene antibiotic, TML has recently gained attention in aquaculture. Several studies(Bosse and Post, 2010; Irgang and Avendaño-Herrera, 2022) showed that TML could prevent Tenacibaculosis caused by Tenacibaculum dicentrarchi in salmon and Yersinia rucheri in rainbow trout. Moreover, TML could also promote the growth of aquatic animals reducing their susceptibility to diseases. However, there are concerns about the pharmacokinetics and distribution of TML and its metabolites in pigs(Cambeiro-Pérez et al., 2020), cattle(Kriukova et al., 2020), chickens(Garmyn et al., 2017), and other livestock. There are few TML pharmacokinetics(Liu et al., 2021) and residue depletion studies(Cuiyv et al., 2021) in fish. A recent report(Sun et al., 2017) showed significant metabolic differences in TML in various farm animals, which aroused our interest in TML metabolites in fish. The types and distribution of TML metabolites in fish are still unknown, and whether they are consistent with those in terrestrial animals needs further studies.
Yang et al. (Yang et al., 2019) and Fu et al. (Fu et al., 2020) posit that Crucian carp (C. carassius) is one of the most significant freshwater fish species in China and serves as an excellent animal model for various toxicological studies. In this study, we first established a quantitative ultraperformance liquid chromatography-tandem mass spectrometry (UPLC-MS/MS) method for determining the TML and identified the TML metabolites using the quadrupole time-of-flight (Q-TOF) method. We further evaluated the bioconcentration, elimination and metabolism patterns of TML in fish using Crucian carp as the animal model. The results provide basic information for the food safety assessment of TML, and for appropriate TML usage in aquaculture.
2 Materials and methods
2.1 Chemicals and reagents
The Tiamulin and Tiamulin-13C4 Fumarate (internal standard, IS) were purchased from Sigma Company (USA). Chromatographically pure-grade methanol, acetonitrile, n-hexane, and ethyl acetate were supplied by Merck Company (Darmstadt, Germany). Formic acid and ammonium acetate (all chromatographic pure) were obtained from Sigma Company (USA). Ultrapure water was obtained from a Milli-Q Water Purification System (Millipore, Bedford, USA). Other chemicals were of analytical grade.
2.2 Animals
A total of 120 Crucian carps with a wet weight of 195 ± 20 g and a body length of 19 ± 1 cm were purchased from Lincheng Market (Zhoushan, China). The samples were analyzed by UPLC-MS/MS to ensure that they were free of TML residues. Dechlorinated tap water was subjected to 72 h of aeration and was used as experimental water. The water temperature was 26 ± 1°C and was kept in glass tanks under a 14/10 h light/dark cycle. The water was supplied with commercial fish food and changed once a day. The fish were reared under laboratory conditions for more than two weeks and were ensured to exhibit normal activity, good health, and disease-free during the rearing period, with natural mortality of less than 5%. All animal experiments were approved by the Animal Welfare Committee of Zhejiang Marine Fisheries Research Institute (Zhoushan, Zhejiang, China) (ID Number:2023-JG-01).
2.3 Instrumental conditions
UPLC-Q-TOF/MS conditions: TML and its metabolites were detected using an ACQUITY UPLC system (Waters Co., USA) coupled with a hybrid Q-TOF/MS (Waters, UK). TML metabolites were separated using the ACQUITY ultraperformance liquid chromatography system with an Acquity UPLC BEH C18 column (50 mm×2.1 mm, 1.7 μm particle size) (Waters, USA) at 40°C. The mobile phase (water containing 0.05% v/v formic acid and ammonium acetate 5 mmol L-1 (solvent A) and acetonitrile (solvent B) was pumped at 0.3 mL min-1. The gradient elution program was run as follows: 0-0.5 min, 20% solvent B; 0.5-1.5 min, 20-60% solvent B; 1.5-6.0 min, 60% solvent B; 6.0-7.0 min, 60-20% solvent B; 7.0-8.0 min, 20% solvent B (injection volume; 10 μL). The analyte was detected by MSe using electrospray (ESI) positive ion mode. LockSpray solution: leucine enkephalin (positive ion m/z 556.2771); Typical source conditions for maximum intensity of precursor ions were as follows: capillary voltage, 3.5 kV; source temperature, 119°C; desolvation temperature, 385°C; cone gas (N2) flow rate, 50 L h-1; desolvation gas (N2) flow rate, 600 L h-1. Data were acquired from 100 to 700 Da. Low energy data were acquired at a collision energy of 5 eV and high energy data using a ramped collision energy of 10−45 eV. Data processing was carried out using Metabolynx software (version 4.1).
UPLC-MS/MS conditions: Quantification of TML was performed on an Acquity UPLC unit coupled with a Quattro Premier XE mass spectrometer equipped with an electrospray ionization (ESI) source (Waters Co.). Chromatographic separation was achieved using a Waters BEH C18 UPLC column (2.1 mm 50 mm, 1.7 μm) at 40 °C. The mobile phase (water containing 0.05% v/v formic acid and ammonium acetate 5 mmol L-1 (solvent A) and acetonitrile (solvent B) was pumped at 0.3 mL min-1. The gradient elution program was run as follows: 0-0.5 min, 20% solvent B; 0.5-1.5 min, 20-30% solvent B; 1.5-4.0 min, 30-40% solvent B; 4.0-4.5 min, 40-20% solvent B; 4.5-5.0 min, 20% solvent B (injection volume; 10 μL). TML was quantified using multiple reaction monitoring (MRM) in the positive ESI mode and the optimized MS parameters were set as follows: capillary voltage, 3.5 kV; source temperature, 119°C; desolvation temperature, 385°C; cone gas (N2) flow rate, 50 L h-1; desolvation gas (N2) flow rate, 55 L h-1. Data acquisition and processing were operated through Masslynx V4.1 workstation. Individual cone and collision energy voltages, as well as multiple reaction monitoring mass transitions are summarized in Table 1.
2.4 Preparation of standard solutions and samples
Tiamulin stock solution (100 μg mL-1) was prepared in methanol. The stock solution was diluted in acetonitrile to prepare the working solutions which were further diluted in blank plasma to prepare the calibration standards at concentrations of 5, 20, 50, 200, 500, and 1000 ng mL-1. Each standard was spiked with the IS at 20 μg mL-1 concentration.
Weighed 2 ± 0.01 g of the homogeneous tissue samples into a 50 mL centrifuge tube and added 50 μL of 100 ng/mL IS and 10 mL of 2% formic acid-acetonitrile. The mixture was vortexed for 30 s and ultrasonically extracted for 10 min. The resulting solution was centrifuged at 4200 g for 10 min, and the supernatant was transferred into a 15 mL centrifuge tube for purification.
The Oasis MCX (3 mL, 60 mg) solid phase extraction column was activated with 3 mL of methanol and balanced with 2% formic acid water. The supernatant (10 mL) was then passed through the solid phase extraction column at a flow rate of 1-2 drops per second. After sample loading, the solid phase extraction column was eluted with 3 mL of 2% formic acid water, followed by the same volumes of methanol and n-hexane, and the residual liquid in the column was dried. The effluent was discarded, and the eluent was collected in a 15 mL centrifuge tube at a flow rate of 1 drop per second with 4 mL of 5% ammonia-methanol solution. The eluent was dried with nitrogen at 50°C in a water bath, after which 1.0 mL of 5 mmol L-1 ammonium acetate-acetonitrile (v/v=4:1) solution containing 0.05% formic acid was added. Finally, the eluent was filtered through a 0.22 μm organic phase filter membrane for further analysis.
2.5 Optimization of tiamulin extraction conditions
TML extraction conditions were investigated to achieve the best extraction in the shortest time possible. Four different solvents (2% formic acid-acetonitrile, acetonitrile, methanol and ethyl acetate) and two types of extraction columns (Oasis MCX SPE and Oasis HLB SPE columns) were used to extract the samples to be tested. Thereafter, the high and low recoveries of TML were compared to determine the solvent with an ideal extraction effect and the column with a good purification effect.
2.6 Method validation
The performance of the established method was assessed with reference to the validation for the bioanalytical methods of the US Food and Drug Administration(FDA) (U.S. Food and Drug Administration, 2018). The validation parameters included calibration curves, accuracy, recovery, precision, dilution accuracy, limits of detection (LODs) and limit of quantification (LOQs).
2.7 Pharmacokinetics
2.7.1 Tissue distribution of tiamulin in Crucian carp
Sixty Crucian carps were randomly selected and set as the TML treatment group. Four plastic tanks were set for treatment, each containing 40 L of water and 15 organisms. Two parallel control groups were set up. We intraperitoneally injected 1 mL of 10 μg mL-1 TML into the Crucian carp in the treatment tanks, and the blank control groups were injected with the same dose of saline. Three fish were randomly collected from each group at 0.1, 0.5, 1, 4, 12, 24, 72 and 96 h after treatment administration. Each individual fish was randomly selected from separate glass tanks as a replicate. The collected fish were used for heart, liver, spleen, kidney, skin, gallbladder, gill, intestine, gonad, muscle, and plasma sampling using anatomical tools. The sampled tissues were weighed and stored at -20°C for the UPLC/Q-TOF MS analysis.
2.7.2 Enrichment and metabolism of tiamulin in Crucian carp
The drug treatment concentration for the pre-experiment was 600 ng mL-1. The experiment was conducted in three glass tanks, each containing 20 Crucian carps in 10 L of liquor. Two parallel control groups were also set up. Unlike the drug bath treatment groups, the blank control groups were not treated with drugs. The water was changed daily, followed by drug administration The drug bath exposure treatment was conducted for 7 days, after which the drug exposure treatment and the blank control groups were reared in clean water for a two-week purification. The water was changed once a day. The sampling time points were set to 1, 2, 3, 5, 7, 8, 9, 11, 14, and 21 days after the treatment. A fish was collected from each tank and washed with tap water after dechlorination, and the excess water on the fish surface was bolt-dried with absorbent paper, followed by weighing the fish. For the drug exposure treatment, 1 mL of the treatment water was collected at 1, 2, 3, 5, 7, 8, 9, 11, 14, and 21 days before administration, and frozen at -20°C for subsequent concentration analysis. Each individual fish was randomly selected from separate glass tanks as a replicate, and subsequently the whole fish of crucian carp fish were homogenized for experimental processing.
The bioconcentration factor (BCF) refers to the ratio of the compound concentration in the organism to that dissolved in water. The formula was BCF=Cf/Cw, where, when the bioaccumulation reaches equilibrium. Cf is the TML concentration in fish, and Cw represents the TML concentration in water. The half-life (T1/2) of the drug reflects its metabolic status and is calculated by the formula: T1/2 = 0.693/k; k=-(InC0-InC)/t, where T1/2 is the half-life of the drug; k is the elimination rate constant; C0 and C are the drug concentrations of the drug elimination curve; t is the time difference corresponding to the two concentrations.
2.8 Data analysis
Results are presented as mean ± st.dev. The levels of significance were set at P< 0.05. The SPSS version 25.0 (International Business Machines Corporation, Armonk, NY, USA) was used for the statistical analysis.
3 Results and discussion
3.1 Method validation
The standard calibration curve of the TML had a good linear correlation over the range of 5-1000 ng mL-1 with the weighted R2 of 0.9964, indicating that the method had a high selectivity with no interferences. The regression equation was: y=2.87543x+5.00915. Moreover, sample concentrations of S/N=3 and S/N=10 were selected as the LOD and LOQ of the method, respectively. The results showed that the LOD and LOQ of TML were 0.03 μg kg-1 and 0.1 μg kg-1, respectively. The recoveries of TML in the muscle, liver and intestine ranged from 80.35 to 108.24% (Table 2). Thus, the method had a high accuracy and good reproducibility for TML quantification in the muscle and liver tissues of Crucian carp (Cuiyv et al., 2021).
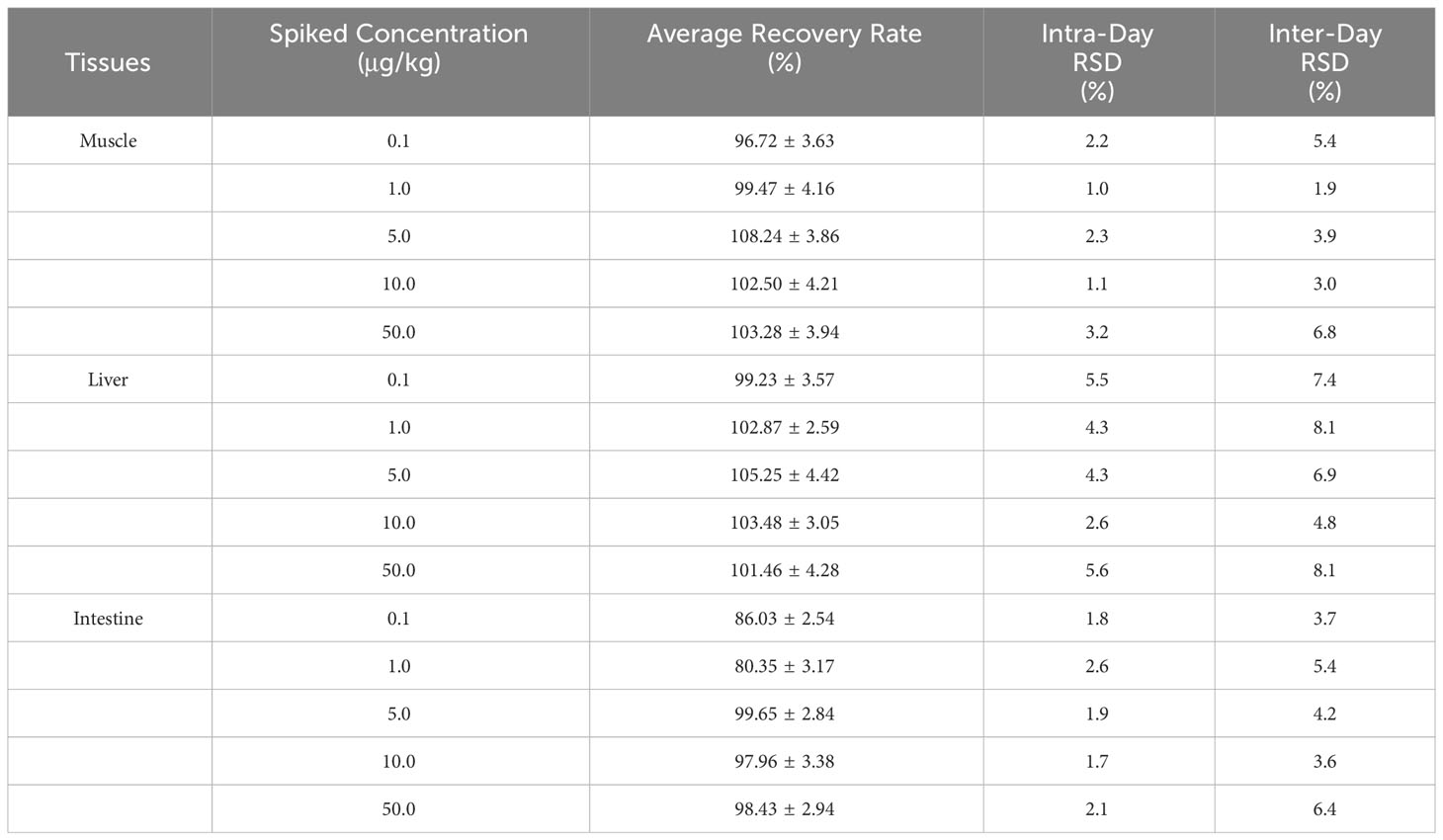
Table 2 Intra-day/inter-day precision, extraction recovery of TML in Crucian carp muscle, liver, and intestine (n=3).
Tiamulin can be quantified based on the calibration curve of a commercial standard using the UPLC method. However, without a standard, the metabolite quantity can be calculated using the ratio of the metabolite peak area to the wet weight of the sampled tissue. This method (Zalko et al., 2006) has been widely used to analyze metabolites with similar ionization efficiency in mass-spectrometry. Therefore, we established and validated the method by adding TML standard solutions to blank samples, which can be used to quantify TML and assess its metabolite conversion via the semi-quantitative relative abundance (Tan et al., 2020).
To detect TML and its metabolites in biological samples accurately, we performed sample pretreatment to remove interferences. In this study, we established a modified method using different extraction columns and extraction solvents to improve extraction efficiency. TML recovery results are shown in Figure 1. TML recovery using 2% formic acid-acetonitrile, acetonitrile and ethyl acetate was higher, but the average recovery by acetonitrile extraction using the Oasis MCX SPE column was much higher (more than 100%). This indicated that the recovery was influenced by matrix effects. The recoveries of both columns using methanol were very low and did not meet the requirements. When the Oasis HLB SPE column was used for the extraction, pigment deposition occurred, and the color gradually deepened with the increased loading volume, eventually leading to the blockage of the solid phase extraction column and causing losses. In summary, the samples were ultimately extracted with 2% formic acid-acetonitrile, processed by ultrasonic centrifugation, and purified by passing through the Oasis MCX SPE column (Yin et al., 2016).
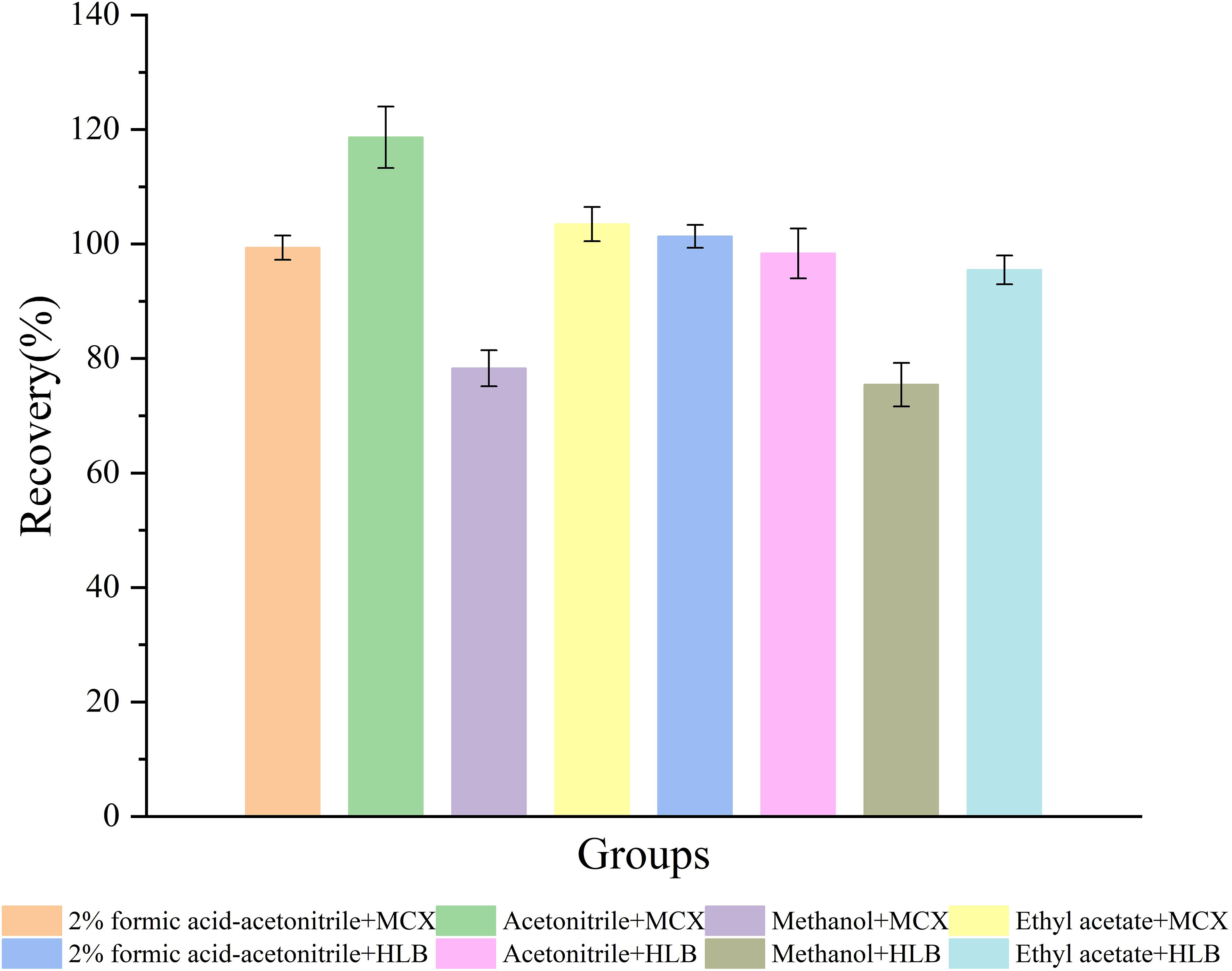
Figure 1 Recoveries of TML by different extraction columns (Oasis MCX SPE and Oasis HLB SPE columns) and extraction solvents (2% formic acid-acetonitrile, acetonitrile, methanol, and ethyl acetate) in fish samples spiked at 50 ng mL-1.
Ciucă et al. (Ciucă et al., 2023) established a high-performance liquid chromatography-tandem mass spectrometry (HPLC-MS/MS) method for analyzing TML residues in pig, rabbit and poultry tissues. The recoveries of samples with 0.5, 1 and 1.5 maximum residue limits exceeded 75.0%, and the coefficient of variation was less than 15%. Moreover, You et al. (You et al., 2021) proposed a label-free electrochemical immunosensor for sensitively detecting TML in pork. TML concentration showed a good linear relationship in the range of 0.05-100 ng mL-1, and its detection limit was 0.04 ng mL-1, with high accuracy. However, the recovery and sensitivity of our method were higher than those of these two methods and thus provides an effective methodology for TML detection in fish.
3.2 Metabolism of tiamulin in Crucian carp
Various tissues were isolated from Crucian carp after the intraperitoneal injection administration. The analysis was performed using high-performance liquid chromatography with quadrupole time-of-flight mass spectrometry (UPLC/Q-TOF MS). Five TML metabolites were identified in Crucian carp tissues based on their relative molecular masses and relevant literature information from MS/MS data. These metabolites included M1 (510.2908, C28H48NO5S+), M2 (510.2908, C28H48NO5S+), M3 (466.2750, C26H44NO4S+), M4 (482.2663, C26H44NO5S+), and M5 (482.2663, C26H44NO5S+). The secondary scanning mass spectra of these five metabolites and their MS/MS fragment ion analysis are shown in Figures 2A–C.
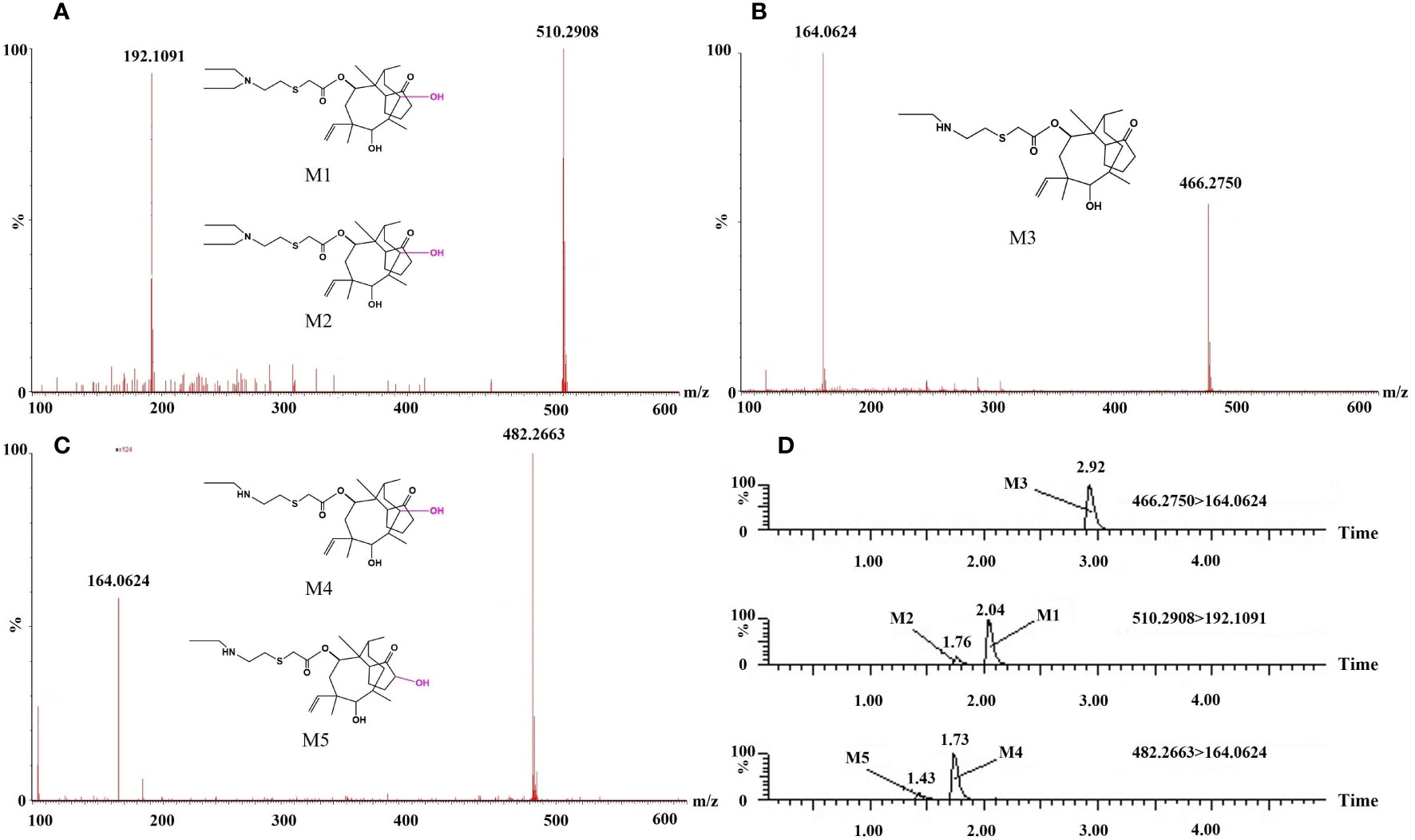
Figure 2 Mass spectra (A–C) and chromatograms (D) of the five tiamulin metabolites in Crucian carp tissues.
Sun et al. (Sun et al., 2017) used UPLC/Q-TOF MS for in vitro and in vivo identification of TML metabolites in different farm animals such as rats, chickens, pigs, goats and dairy cows. A total of 26 tiamulin metabolites were identified in vitro and in vivo. However, we only detected five TML metabolites in Crucian carp, showing the differences in the amount of TML metabolites among species.
Kolanczyk et al. (Kolanczyk et al., 2018) suggested that mammals generally exhibit a greater abundance of drug metabolites compared to fish. Figure 2 shows the chromatograms of the five TML metabolites in Crucian carp tissues. The m/z values of M1 and M2 were both 510.2908, with an error of 1.1-3.8 ppm between the calculated and measured values, indicating that the metabolites were products of hydroxylation or partial oxidation of nitrogen and sulfur. The retention times of the metabolites on the chromatograms differed due to their different reaction sites. The elemental composition of M3 was C26H44NO4S+ (m/z 466.2750), and compared with TML, M3 decreased by one ethyl group (C2H4), suggesting that M3 may be a de ethyl TML. M4 and M5 contained C26H44NO5S+ with m/z values of 482.2663, 16 Da higher than M3. This indicated that these metabolites were hydroxylated or oxidized products of M3.
3.3 Tissue-specific distribution
The tissue-specific distribution of TML in crucian carp are shown in Figure 3. TML distribution in the collected tissues (heart, liver, spleen, kidney, skin, gallbladder, gill, intestine, gonads, muscle, and plasma) was significantly different. The highest TML concentrations were found in the gills at 0.5 h but were not detected in the skin and gall bladder. TML concentration showed an initial increase, followed by a decreasing trend in all tissues except the heart (Yang et al., 2022).
Shang et al. (Shang et al., 2010) found that 90% of the orally administered TML was absorbed in the intestinal canal of pigs within a very short time, and the peak blood concentration could be reached at 2-4 h; however, the highest liver content was observed after absorption. In contrast, in this study, the intraperitoneally administered TML was distributed to the heart with sufficient oxygen supply in a short period, and the highest concentration of the drug was reached at 0.5 h in the gills. We infer that some drugs are enriched in the gills through blood circulation, which are important metabolic tissues for these drugs. Some studies also support our viewpoint. Ling et al. (Ling et al., 2016) found certain residues of the sanguinarine in the gills of common carp during the pharmacokinetic evaluation experiment of intraperitoneal injection of sanguinarine.
3.4 Enrichment and metabolism of tiamulin in Crucian carp
As shown in Figure 4A, TML was rapidly incorporated into the fish body in the first three days of the drug bath treatment, and the drug concentration increased rapidly to a peak of 1927.42 ng g-1. After the third day, TML was saturated, and its concentration remained the same in Crucian carp, possibly because in an equilibrium state, complete saturation occurs quickly at higher exposure concentrations. However, after the 7th day (the end of the drug bath treatment), the TML concentration exhibited a significant downward trend. On the 8th day, the drug concentration decreased to 1323.68 ng g-1 and continued to decrease gradually on the subsequent days, reaching 55.33 ng g-1 on the 21st day, thus indicating the drug had been metabolized.
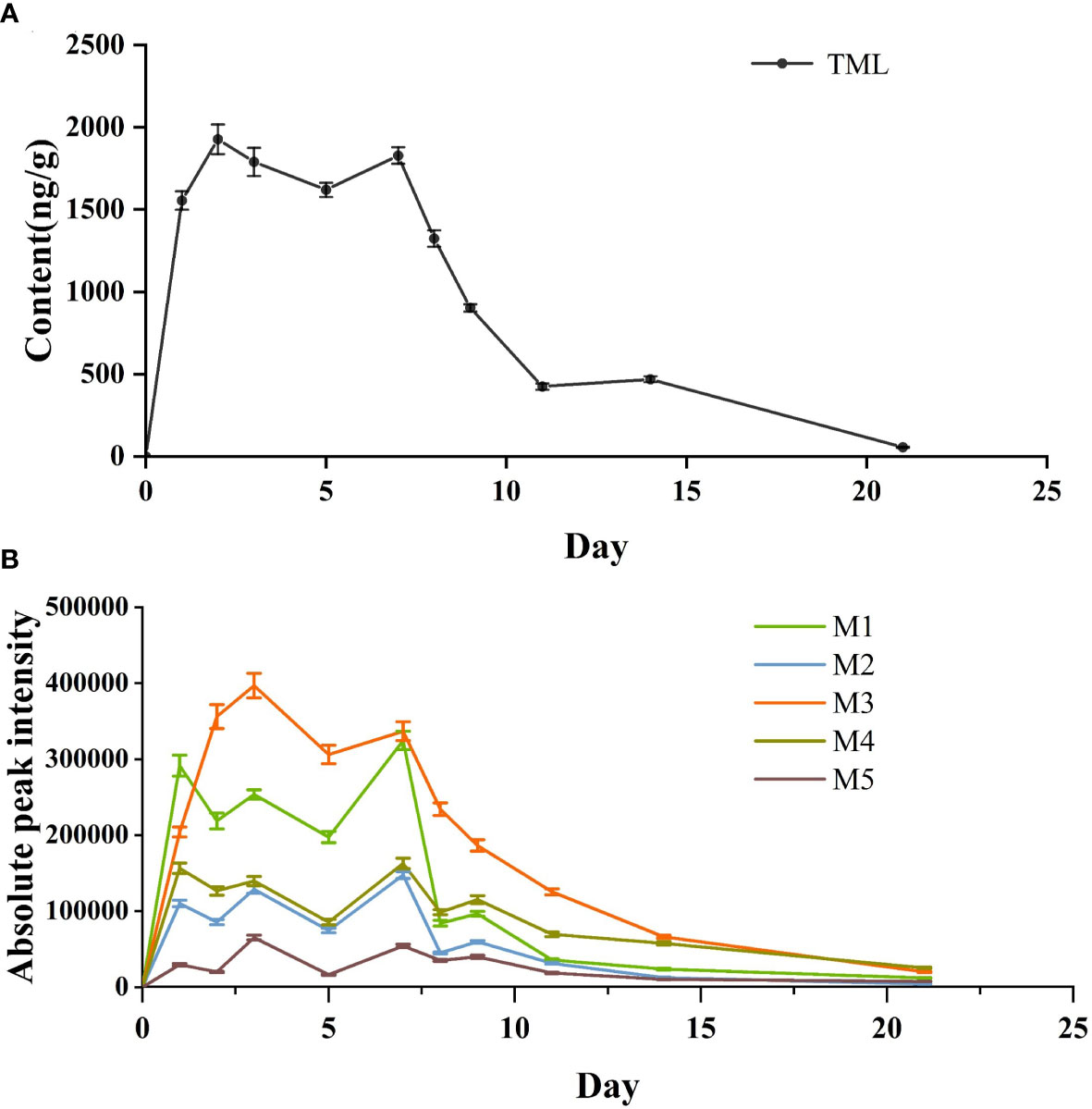
Figure 4 Concentration-time curves of TML (A) and peak areas of extraction chromatograms of TML metabolites (B) detected in in vivo of Crucian carp..
This was similar to fenoxaprop-ethyl enrichment and metabolism in zebrafish (Xu et al., 2019). In the accumulation stage, the drug concentration in the fish showed a positive correlation with time. When the drug reaches a certain concentration in the body, it rapidly begins to enter the elimination stage until it is completely metabolized. The metabolic trends of the five metabolites were consistent with those of TML. As shown in Figure 4B, the two metabolites with the highest peak areas were M1 and M3. The overall change trend of the five metabolites also involved an initial increase followed by a decrease, reflecting the absorption and release process of tiamulin in Crucian carp (Liu et al., 2014).
The bioconcentration factor (BCF) determines the enrichment and accumulation trend of organic compounds in organisms. The BCF of TML in Crucian carp was 3.01, much lower than that of most heavy metals in fish. Xu et al. (Xu et al., 2020) determined the accumulation of Cr, As, Cd, Pb, Zn, Cu, Ni, and Hg in eight types of fish obtained from six caves. The results showed that the BCFs of these metals were all greater than 5000. Furthermore, Cui et al. (Cui et al., 2023) studied the enrichment and metabolism of organophosphorus pesticide malathion and its metabolites in zebrafish. The BCF of zebrafish was 12.9 after 15 days of exposure to the pesticide. These differences in the BCFs may be related to drug solubility in water or its metabolism degree in fish. The water solubility of TML is better than that of malathion, resulting in higher drug concentrations in water but with a lower BCF.
The half-life (T1/2) of the drug reflects its metabolic status in the case of the TML tested in the Crucian carp was of 3.74 d. Peng et al. (Peng et al., 2021) found that the half-life of the nonylphenol in zebrafish was 1.75-2.45 d. Xu et al. (Xu et al., 2019) found that the half-life of the fenoxaprop-ethyl in zebrafish was 0.92-1.72 d. The metabolism of TML was slower in crucian carp compared to other compounds. And the tissue-specific distribution experiment showed that the TML was eliminated within 24 h of the administration but was not completely eliminated by the second week of purification experiment. This indicates that the same drug could exhibit different half-lives under different administration methods or dose conditions.
4 Conclusion
This study developed a preliminary method for detecting TML, a truncated pleuromutilin antibiotic, in fish tissues. The extraction conditions of TML were evaluated and optimized using four extraction solvents and two extraction columns to determine the suitable method. TML was injected intraperitoneally into Crucian carp, and five TML metabolites were identified from Crucian carp tissues using the UPLC/Q-TOF MS technique based on related literature. The drug bath treatment was used to determine the enrichment and release pattern of TML in Crucian carp. The enrichment efficiency of TML was higher on the first day, and reached saturation on the second day. TML had a slow metabolic rate with its complete metabolism occurring after 20 days. Based on these results, we provide a reference for the TML absorption and metabolism mechanism in fish. However, further in-depth research is needed to explore the application prospect of TML in fish biology.
Data availability statement
The original contributions presented in the study are included in the article/Supplementary Material. Further inquiries can be directed to the corresponding author.
Ethics statement
The animal study was approved by Institutional Animals Care and Use Committee of Zhejiang Marine Fisheries Research Institute, Zhoushan, China, under permit No.2023-JG-01. The study was conducted in accordance with the local legislation and institutional requirements.
Author contributions
QW: Data curation, Investigation, Writing – original draft. ZF: Writing – review & editing, Formal analysis, Methodology. XZ: Funding acquisition, Supervision, Writing – review & editing. QY: Investigation, Writing – original draft. YZ: Investigation, Writing – original draft. WL: Investigation, Writing – original draft.
Funding
The author(s) declare financial support was received for the research, authorship, and/or publication of this article. This work was supported by the Special projects of research institutes in Zhejiang Province (HYS-CZ-202307).
Conflict of interest
The authors declare that the research was conducted in the absence of any commercial or financial relationships that could be construed as a potential conflict of interest.
Publisher’s note
All claims expressed in this article are solely those of the authors and do not necessarily represent those of their affiliated organizations, or those of the publisher, the editors and the reviewers. Any product that may be evaluated in this article, or claim that may be made by its manufacturer, is not guaranteed or endorsed by the publisher.
References
Borak M. D., Sarc L., Mugerli D. G., Antolic B., Brvar M. (2020). Occupational inhalation poisoning with the veterinary antibiotic tiamulin. Clin. Toxicol. (Phila) 58 (4), 287–289. doi: 10.1080/15563650.2019.1630632
Bosse M. P., Post G. (2010). Tribrissen and tiamulin for control of enteric redmouth disease. J. Fish Dis. 6 (1), 27–32. doi: 10.1111/j.1365-2761.1983.tb00048.x
Cambeiro-Pérez N., González-Gómez X., Rey-Salgueiro L., Simal-Gándara J., Martínez-Carballo E. (2020). Rapid liquid chromatographic method for the control of doxycycline and tiamulin residues and their metabolites in vivo assays with pigs: Treatment and depletion. J. Pharm. Biomed. Anal. 190, 113428. doi: 10.1016/j.jpba.2020.113428
Ciucă V. C., Rusănescu C. O., Safta V. V. (2023). Analysis of transfer of tiamulin to animal tissue after oral administration: an important factor for ensuring food safety and environmental protection. Pharmaceuticals 16 (3), 387. doi: 10.3390/ph16030387
Cromwell G. L., Stahly T. S. (1985). Efficacy of tiamulin as a growth promotant for growing swine. J. Anim. Sci. 60 (1), 14–19. doi: 10.2527/jas1985.60114x
Cui J., Wei Y., Jiang J., Xiao S., Liu X., Zhou Z., et al. (2023). Bioaccumulation, metabolism and toxicological effects of chiral insecticide malathion and its metabolites in zebrafish (Danio rerio). Chemosphere 318 137898. doi: 10.1016/j.chemosphere.2023.137898
Cuiyv C., Yongtao L., Guodong Z., Jing D., Ning X., Shun Z., et al. (2021). Temperature-dependent residue depletion regularities of tiamulin in Nile tilapia (Oreochromis niloticus) following multiple oral administrations. Front. Vet. Sci. 8. doi: 10.3389/fvets.2021.679657
Duijkeren E. V., Greko C., Pringle M., Baptiste K. E., Catry B., Jukes H., et al. (2009). Pleuromutilins: use in food-producing animals in the European Union, development of resistance and impact on human and animal health. J. Vet. Pharmacol. Ther. 32 (6), 515–533. doi: 10.1111/j.1365-2885.2009.01075.x
Fu G., Dong Y., Zhang X., Hu K. (2020). Metabolomic profiles and pathways of praziquantel in Crucian carp. Environ. Toxicol. Pharmacol. 80, 103466. doi: 10.1016/j.etap.2020.103466
Garmyn A., Vereecken M., Degussem K., Depondt W., Haesebrouck F., Martel A. (2017). Efficacy of tiamulin alone or in combination with chlortetracycline against experimental Mycoplasma gallisepticum infection in chickens. Poult. Sci. 96 (9), 3367–3374. doi: 10.3382/ps/pex105
Goethe O., Heuer A., Ma X., Wang Z., Herzon S. B. (2019). Antibacterial properties and clinical potential of pleuromutilins. Nat. Prod. Rep. 36 (1), 220–247. doi: 10.1039/c8np00042e
Hanson R. L., Matson J. A., Brzozowski D. B., Laporte T. L., Springer D. M., Patel R. N. (2002). Hydroxylation of Mutilin by Streptomyces griseus and Cunninghamella echinulata. Org. Process Res. Dev. 6 (4), 482–487. doi: 10.1021/op020028q
Irgang R., Avendaño-Herrera R. (2022). Evaluation of the in vitro susceptibility of Tenacibaculum dicentrarchi to tiamulin using minimum inhibitory concentration tests. J. Fish Dis. 45 (6), 795–799. doi: 10.1111/jfd.13604
Kavanagh F., Hervey A., Robbins W. J. (1951). Antibiotic substances from basidiomycetes: VIII. Pleurotus multilus (Fr.) Sacc. and Pleurotus passeckerianus pilat. PNAS 37 (9), 570–574. doi: 10.1073/pnas.37.9.570
Kolanczyk R. C., Serrano J. A., Tapper M. A., Schmieder P. K. (2018). A comparison of fish pesticide metabolic pathways with those of the rat and goat. Regul. Toxicol. Pharmacol. 94, 124–143. doi: 10.1016/j.yrtph.2018.01.019
Kriukova V., Kuznechova N., Gaponova V., Sabyrzyanova L. (2020). Tiamulin fumarat-new makrolide on bovine mastitis therapy in vivo and in vitro. Bio Web Conferences 17, 203. doi: 10.1051/bioconf/20201700203
Ling F., Wu Z., Jiang C., Liu L., Wang G. (2016). Antibacterial efficacy and pharmacokinetic evaluation of sanguinarine in common carp (Cyprinus carpio) following a single intraperitoneal administration. J. Fish Dis. 39 (8). doi: 10.1111/jfd.12433
Liu J., Lu G., Ding J., Zhang Z., Wang Y. (2014). Tissue distribution, bioconcentration, metabolism, and effects of erythromycin in crucian carp (Carassius auratus). Sci. Total Environ. 490, 914–920. doi: 10.1016/j.scitotenv.2014.05.055
Liu Y., Cao C., Song Y., Zhou S., Yang Y., Xu N., et al. (2021). Pharmacokinetics, bioavailability, and tissue disposal profiles of Tiamulin fumarate in Nile tilapia (Oreochromis niloticus) following oral and intravenous administrations. J. Vet. Pharmacol. Ther. 44 (4), 590–602. doi: 10.1111/jvp.12957
Peng C., Zhou S., Zhang Y., Zhang H., Zhang W., Ling S., et al. (2021). Dynamics and mechanisms of bioaccumulation and elimination of Nonylphenol in Zebrafish. Toxicology 483, 153375. doi: 10.21203/rs.3.rs-353838/v1
Schuhmacher A., Bafundo K. W., Islam K. M. S., Aupperle H., Glaser R., Schoon H. A., et al. (2006). Tiamulin and semduramicin: effects of simultaneous administration on performance and health of growing broiler chickens. Poult. Sci. 85 (3), 441–445. doi: 10.1093/ps/85.3.441
Shang R., Liang J., Liu Y., Cui Y., Wang L. (2010). Research progress on pleuromutilin antibiotics. J. Tradit. Chin. Vet. Med. 29 (3), 25–28. doi: 10.3969/j.issn.1000-6354.2010.03.008
Sun F., Yang S., Zhang H., Zhou J., Li Y., Zhang J., et al. (2017). Comprehensive analysis of tiamulin metabolites in various species of farm animals using ultra-high-performance liquid chromatography coupled to quadrupole/time-of-flight. J. Agric. Food Chem. 65 (1), 199–207. doi: 10.1021/acs.jafc.6b04377
Tan F., Lu B., Liu Z., Chen G., Liu Y., Cheng F., et al. (2020). Identification and quantification of TBBPA and its metabolites in adult zebrafish by high resolution liquid chromatography tandem mass spectrometry. Microchem. J. 154, 104566. doi: 10.1016/j.microc.2019.104566
U.S. Food and Drug Administration. (2018). Bioanalytical method validation guidance for industry. Available at: https://www.fda.gov/media/70858/download.
Wang L., Jiang S., Shi X., Wan R., Guo Y., Feng X. (2004). Tamulin: the antimicrobial susceptibility in vitro and the common toxicity in viro. Anim. Husbandry Veterinary Med. 36 (6), 3. doi: 10.3969/j.issn.0529-5130.2004.06.002
Witkamp R. F., Nijmeijer S. M., Miert A. S. V. (1996). Cytochrome P-450 complex formation in rat liver by the antibiotic tiamulin. Antimicrob. Agents Chemother. 40 (1), 50–54. doi: 10.1128/AAC.40.1.50
Xu Y., Jing X., Zhai W., Li X. (2019). The enantioselective enrichment, metabolism, and toxicity of fenoxaprop-ethyl and its metabolites in zebrafish. Chirality 32 (7), 990–997. doi: 10.1002/chir.23222
Xu C., Yan H., Zhang S. (2020). Heavy metal enrichment and health risk assessment of karst cave fish in Libo, Guizhou, China. ALEX Eng. J. 60 (1), 1885–1896. doi: 10.1016/j.aej.2020.11.036
Yang Y., Su L., Huang Y., Zhang X., Li C., Wang J., et al. (2022). Bio-uptake, tissue distribution and metabolism of a neonicotinoid insecticide clothianidin in zebrafish. Environ. Pollut. 292, 118317. doi: 10.1016/j.envpol.2021.118317
Yang F., Yang F., Kong T., Wang G., Bai D., Liu B. (2019). Pharmacokinetics of florfenicol and its metabolite florfenicol amine in crucian carp (Carassius auratus) at three temperatures after single oral administration. J. Vet. Pharmacol. Ther. 503, 446–451. doi: 10.1111/jvp.12672
Yin Z., Chai T., Mu P., Xu N., Song Y., Wang X., et al. (2016). Multi-residue determination of 210 drugs in pork by ultra-high-performance liquid chromatography–tandem mass spectrometry. J. Chromatogr. A 1463, 49–59. doi: 10.1016/j.chroma.2016.08.001
You X., Zhang G., Chen Y., Liu D., Ma D., Zhou J., et al. (2021). A novel electrochemical immunosensor for the sensitive detection of tiamulin based on staphylococcal protein A and silver nanoparticle-graphene oxide nanocomposites. Bioelectrochemistry 141, 107877. doi: 10.1016/j.bioelechem.2021.107877
Keywords: tiamulin, detection, Crucian carp, metabolites, distribution
Citation: Wang Q, Fan Z, Zhang X, Yang Q, Zhong Y and Liu W (2024) A UPLC-MS/MS method for simultaneous determination of tiamulin and its metabolites in Crucian carp (Carassius carassius): an in vivo metabolism and tissue distribution study. Front. Mar. Sci. 11:1276880. doi: 10.3389/fmars.2024.1276880
Received: 13 August 2023; Accepted: 02 January 2024;
Published: 17 January 2024.
Edited by:
Stephen J. Newman, Western Australian Fisheries and Marine Research Laboratories, AustraliaReviewed by:
Adnan H. Gora, Central Marine Fisheries Research Institute (ICAR), IndiaIrma Martinez, Centro de Investigación en Alimentación y Desarrollo A.C., Mexico
Copyright © 2024 Wang, Fan, Zhang, Yang, Zhong and Liu. This is an open-access article distributed under the terms of the Creative Commons Attribution License (CC BY). The use, distribution or reproduction in other forums is permitted, provided the original author(s) and the copyright owner(s) are credited and that the original publication in this journal is cited, in accordance with accepted academic practice. No use, distribution or reproduction is permitted which does not comply with these terms.
*Correspondence: Xiaojun Zhang, zhangxj@zjou.edu.cn