- 1Reef Biology Research Group, Department of Marine Science, Faculty of Science, Chulalongkorn University, Bangkok, Thailand
- 2Aquatic Resources Research Institute, Chulalongkorn University, Bangkok, Thailand
- 3Atmosphere and Ocean Research Institute, The University of Tokyo, Kashiwa-shi, Japan
- 4Center of Excellence for Marine Biotechnology, Department of Marine Science, Faculty of Science, Chulalongkorn University, Bangkok, Thailand
Coral reefs have been declining worldwide due to multiple threats. Various approaches for coral restoration have been employed to increase coral populations. Sexual propagation is a crucial technique that can enhance the genetic diversity of coral offspring, thereby increasing coral reef resilience in the face of a changing environment. However, the effectiveness of using sexually reared corals for transplantation depends on many factors, including the biological traits of the coral and the physical environment. This study investigated the relationship between Acropora humilis and Symbiodiniaceae during the ontogenetic stages of the coral in ex-situ conditions and after transplantation to a natural reef. Coral sexual propagation techniques were conducted to produce experimental coral colonies. The fertilization rates of coral gametes averaged 98.48 ± 0.34%. However, the survival rate of juvenile corals gradually declined to 45.96 ± 1.31% at 18 months old under hatchery conditions and to 20% after transplantation to a natural reef. Acropora humilis associated with Symbiodiniaceae lineages belonging to three genera: Symbiodinium, Cladocopium, and Durusdinium. Reared juvenile corals developed a more specific and stable association with particular Symbiodiniaceae under hatchery conditions, whereas after transplantation, corals tended to contain a higher diversity of Symbiodiniaceae lineages. These results indicate that a shift in Symbiodiniaceae composition can provide long-term benefits to corals during their ontogenetic cycle and when the environment changes.
Introduction
The establishment of a symbiotic relationship between Scleractinia corals and single-cell algae, known as zooxanthellae in the family Symbiodiniaceae, is essential for the development and survival of coral reefs (Decelle et al., 2018; LaJeunesse et al., 2018). Reef-building corals provide shelter and inorganic nutrients (e.g., ammonia, phosphate, CO2) for the Symbiodiniaceae, which, in return, assimilate and export oxygen and photosynthetic products (such as sugars and amino acids). Within this association, a large proportion of photosynthates (64%–95%) are translocated to meet the coral’s energy requirements (Muscatine, 1990; Iluz & Dubinsky, 2015; López-Londoño et al., 2022). There were 11 genera of Symbiodiniaceae that have been described based on morphological, physiological, ecological, and molecular evidence (Yorifuji et al., 2021; LaJeunesse et al., 2022). Four of these genera are commonly associated with Scleractinia corals, namely, Breviolum (clade B), Cladocopium (clade C), Durusdinium (clade D), and Symbiodinium (clade A) (Wham et al., 2017; LaJeunesse et al., 2018). Moreover, differences in Symbiodiniaceae genotypes may reflect host-specific responses to ecological or environmental gradients, as they can influence coral gene expression and present distinct ecophysiological characteristics (Berkelmans & Van Oppen, 2006; Levin et al., 2017; De Souza et al., 2022). Generally, host species in the Indo-Pacific are mostly associated with the genera Cladocopium and Durusdinium, which are found in various reef habitats (Baker, 2003; Lajeunesse, 2005). Cladocopium and Durusdinium are two well-known genera that exhibit differences in ecophysiology, which can impact their interaction with coral hosts (Hoadley et al., 2019; Abbott et al., 2021). In some reefs, for example, corals of the genus Acropora that host Cladocopium are more susceptible to bleaching, whereas those harboring Durusdinium can withstand higher temperatures (Sampayo et al., 2008; Thinesh et al., 2019). Durusdinium is considered a stress-tolerant Symbiodiniaceae; most corals that are frequently exposed to high temperature, irradiance, and turbidity were found to be associated with this genus (Rowan, 2004; Claar et al., 2020). However, there is evidence suggesting that reef-building host heat-evolved Symbiodiniaceae (e.g., Durusdinium) undergo poorer growth in non-stressful environments, highlighting trade-offs (Baker et al., 2013; Cunning et al., 2015). This may ultimately result in future declines in coral reefs owing to reduced calcification rates (Jones & Berkelmans, 2010).
The diversity of Symbiodiniaceae assemblages, when flexible in coral hosts, might provide them with the ability to rapidly cope with environmental conditions (Cunning et al., 2015; Goulet et al., 2017). Rapid acclimatization and/or adaptive bleaching of corals under a changing climate can occur through “switching” (uptake of exogenous Symbiodiniaceae from the environment) and “shuffling” (changes in the endogenous Symbiodiniaceae community composition) (Buddemeier and Fautin, 1993). These mechanisms represent an opportunity for corals to turn over their community of Symbiodiniaceae from currently susceptible to more stress-resistant genera/strains (Baker et al., 2013; Boulotte et al., 2016; Quigley et al., 2016). Coral–Symbiodiniaceae shuffling is a phenomenon more common than complete switching mechanisms. Additionally, switching requires sensitive techniques and temporal monitoring on the same coral (Goulet, 2006; Rouzé et al., 2019). Most corals can optimize their photosynthetic and metabolic performance in response to prevailing environmental conditions by changes in their Symbiodiniaceae community compositions (Cunning et al., 2018; Wang et al., 2022). For instance, the proportion of Durusdinium in Orbicella faveolata increased differently in response to short- and long-term heat stress exposure, depending on the severity of the disturbance (Cunning et al., 2015). Similarly, several studies have revealed that a large-scale spread of heat-stress tolerant symbionts is correlated with corals living in warmer oceans (Pettaya et al., 2015; Jain et al., 2021); this rapid shift in Symbiodiniaceae can potentially enhance the corals’ survival in the face of unstable environmental conditions. Nevertheless, this mechanism may not be sufficient to ensure the survival of all corals under the predicted conditions of future climate change, which can vary depending on the severity of stress, geographic locations, coral taxa, etc. (Berkelmans & Van Oppen, 2006; Cunning et al., 2018).
Several variables influence the structure of the Symbiodiniaceae assemblage in coral, including biological traits (e.g., age, life-history strategies) and environmental factors (e.g., temperature, light) (LaJeunesse et al., 2010; Stat et al., 2013). The distinct modes of Symbiodiniaceae cell acquisition (vertical and horizontal) can significantly impact the Symbiodiniaceae community in coral offspring (Ali et al., 2019; Swain et al., 2021). For example, the early developmental stages of horizontal symbiont transmission is often correlated with increased diversity of the Symbiodiniaceae communities within corals (Yuyama et al., 2012b; Yamashita et al., 2014). Conversely, vertical symbiont transmission is associated with lower Symbiodiniaceae diversity, but greater stability and co-evolution of Symbiodiniaceae with their parental colony (Chamberland et al., 2017; Quigley et al., 2017b). Offspring of broadcast spawner corals (aposymbiotic larvae and/or primary polyps) acquire symbiont cells from the surrounding environment, leading to the establishment of distinct Symbiodiniaceae community assemblages (Yamashita et al., 2014; Nitschke et al., 2016). Most newly recruited Acropora corals are associated predominantly with Durusdinium and Symbiodinium in both ex-situ and in-situ environments, whereas the parent colony is significantly associated with Cladocopium and/or Symbiodinium (Gómez-Cabrera et al., 2008; Yamashita et al., 2013). Consequently, the Symbiodiniaceae community can shift during the ontogeny in Acropora spp., potentially allowing them to acquire suitable Symbiodiniaceae to cope with environmental stress (Abrego et al., 2012; Rouzé et al., 2016; Yuyama et al., 2016).
Ongoing climate change significantly impacts coral reefs around the world (Hoegh-Guldberg et al., 2017; Thirukanthan et al., 2023). Mass bleaching events in Thai waters have become frequent, primarily due to elevated seawater temperatures and river flooding, which results in hyposalinity (Chavanich et al., 2009; True, 2012). Acropora corals are known to be a predominant species around Sattahip Bay in the upper Gulf of Thailand (Viyakarn et al., 2009; Chankong & Manthachitra, 2013). However, nearly all Acropora spp. perished after a mass bleaching event in 2010, especially at Samae San Island. Acropora humilis is particularly susceptible to environmental stressors. All populations of A. humilis have failed to recover after bleaching events, even when temperatures have returned to normal (unpublished data). As such, stock enhancement may serve as a means to mitigate coral reef degradation and foster recovery (Omori, 2019; Koch et al., 2022). Both asexual reproduction (coral fragmentation) and sexual reproduction (sexually propagated corals) are recognized as sources for coral transplantation (Omori et al., 2016; Randall et al., 2020). Our research group is focused on preserving the remaining populations of A. humilis using sexual propagation techniques, as they generate genetically diverse offspring that could enhance coral reef resilience (Randall et al., 2020; Shaver et al., 2022). To evaluate the resilience potential of corals, it is essential to investigate the relationship between the coral and their symbionts, especially at a young stage when they are more susceptible to shifts in their Symbiodiniaceae in response to environmental changes.
In this context, we aimed to examine the Symbiodiniaceae community within A. humilis from the early developmental to juvenile stages under hatchery conditions and after transplantation to the reef site. An experimental coral was prepared using a sexual propagation technique, employing a method initiated by the Reef Biology Research Group in collaboration with the Akajima Marine Science Laboratory, Japan. Investigating the composition and variability of Symbiodiniaceae communities provides insight into how coral symbioses interact throughout the ontogenetic cycle and post-transplantation. This knowledge can aid in developing restoration strategies tailored to specific reef ecosystems and environmental conditions, thereby increasing the likelihood of successful coral reef restoration and conservation.
Materials and methods
Coral species and study sites
Acropora humilis is an important reef-building coral, noted for its rapid growth and high calcification rates, which are approximately 5 mm/year–15 mm/year (Shaaban et al., 2015; Mohammed & Dar, 2017) (Figure 1). This species is prevalent in shallow reefs and reef slope habitats in Thai waters, encompassing both the Gulf of Thailand and the Andaman Sea. However, A. humilis is highly sensitive to environmental changes. Most colonies around Samae San reefs have been unable to recover following mass bleaching events. This study was conducted at three different sites: Sattahip, Chon Buri in the upper Gulf of Thailand; the Tao Mo Island (TMI: 12° 38′ 33.78'' N 100° 51′ 40.13'' E); Samae San Island, which functions as a coral hatchery (SSI: 12° 35′ 05.51'' N 100° 57′ 14.50'' E); and Had Na Baan (HNB: 12° 35′ 12'' N 100° 57′ 12'' E) (Figure 2A). Each site was selected for its accessibility and the specific type of experiment conducted.
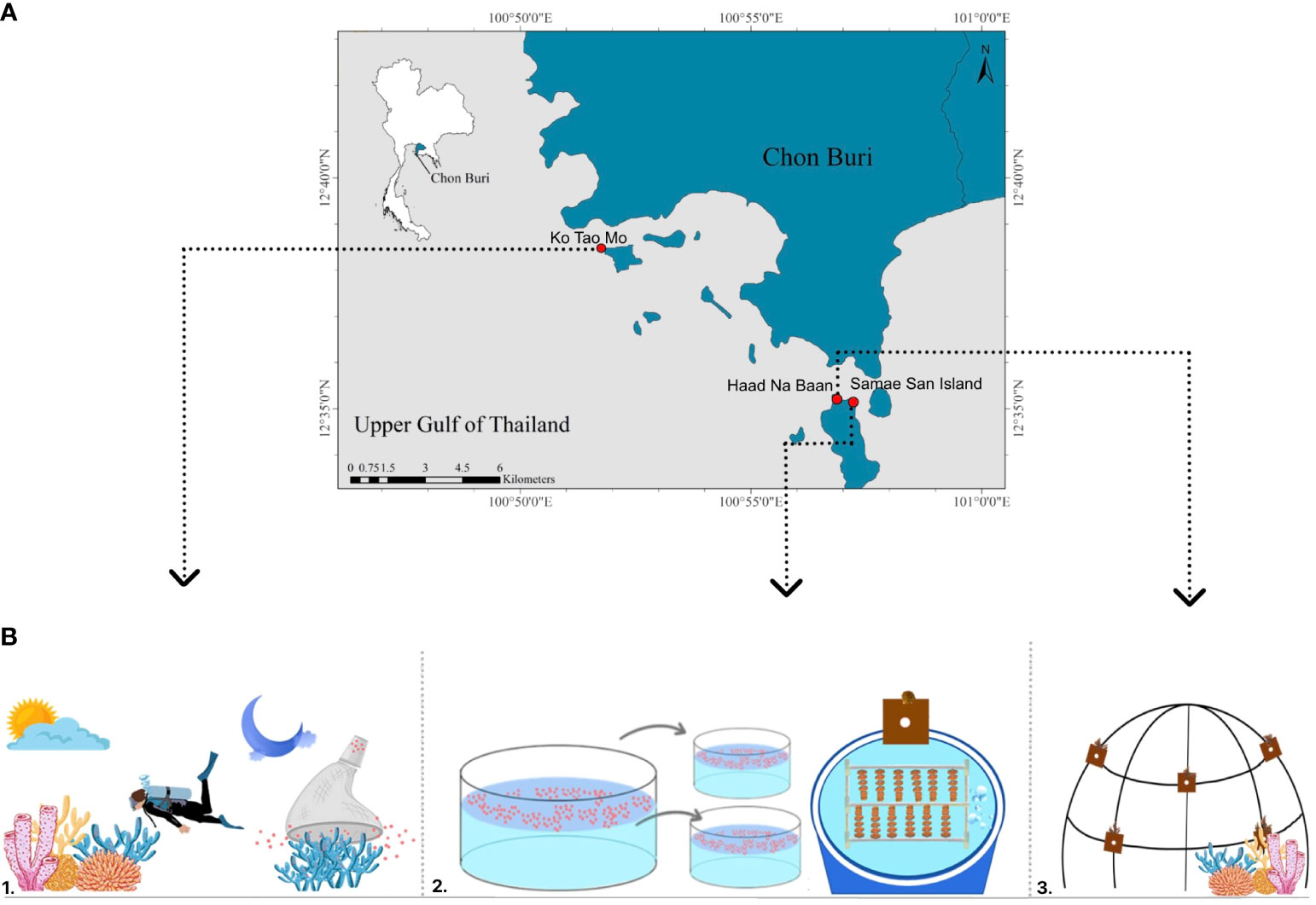
Figure 2 Location map of study areas and experimental design, Tao Mo Island (TMI), Samae San Island (SSI), and Had Na Baan (HNB) (A). Experimental design schematic: monitoring coral gamete development in in-situ (1: TMI), cultivating coral using sexual propagation techniques in-extu condition (2: SSI or coral hatchery), and transplanting reared juvenile coral to natural reef (3: HNB) (B).
The Tao Mo Island is situated within the military harbor of the Royal Thai Navy. A burgeoning reef community has established itself on the hard substrate with depths of approximately 5 m–10 m along an 800-m stretch of artificial breakwater adjacent to the island. A high density of mature A. humilis colonies, ranging from 10 cm to 120 cm in diameter, was observed at depths of 5 m–7 m.
The Samae San Island (SSI) holds particular significance as the largest (4 km²) of nine islands under the protection of the Royal Thai Navy, contributing to the Plant Genetic Conservation Project initiated by Her Royal Highness Princess Maha Chakri Sirindhorn. The coral hatcheries on SSI have been developed for cultivating and conducting experiments on corals.
Had Na Baan (HNB) features a shallow reef community, extending from approximately 3 m–7 m in depth on SSI. In this area, artificial dome-shaped structures constructed from stainless steel have been installed for the purpose of an out-planting experiment (Figure 2B).
Coral cultivation and sample collection
We sampled 10 coral fragments and gametes from 10 parent colonies at TMI during the spawning event in February from 20:00 to 20:30 h, in 2016. A gamete collector—a net with a plastic cup receptor at the end tip—was placed over the coral colony at the initial sign of spawning. Coral gametes, being positively buoyant, floated toward the surface, allowing collection into the plastic cup. Once spawning concluded, a lid sealed each cup before it was moved to the surface. All cups containing coral gametes were then stored in containers filled with seawater and transported directly to the coral hatchery on SSI for ex-situ fertilization. The gametes from each cup were gently poured into a container with 40 L of filtered seawater to facilitate fertilization. Fertilized oocytes underwent three washes with filtered seawater to remove excess sperm cells. The embryos were then distributed into five culture tanks, each containing 400 L of filtered seawater. To assess fertilization success, we counted the number of fertilized (embryos exhibiting cell division) and unfertilized (no cell division or abnormal cell development) embryos in each culture tank after 8 h, using a microscope, in five replicates (Suzuki et al., 2013; Omori, 2019). Seawater was replaced daily by 50% for 3 days to diminish impurities in the culture tanks. Terracotta tiles (5 × 5 × 1 cm), preconditioned for 2 months on the reef to promote crustose coralline algae (CCA) growth and subsequently cleaned of biofouling, were introduced to the tanks to encourage coral larva attachment. During the initial 24 h of settlement, we avoided aeration and water flow in the tanks to minimize disturbance. Continuous aeration and seawater flow were resumed after coral larvae settlement until the experiment concluded. We did not control water temperature and light intensity in the culture tanks. The settled coral was grown in flow-through systems, with each tank receiving a continuous filtered water flow at an average rate of 0.38 L/min. Sand filtration was employed to enhance water quality before entering the culture tanks. There were 10 individual colonies of settled corals randomly sampled from each tank at ages of 3, 6, 12, and 18 months. For 1-month-old corals, 15 coral spats were sampled in 10 replicates. All samples were preserved in 95% absolute ethanol for Symbiodiniaceae community analysis. The survival of post-settlement coral was recorded every 3 months up to 18 months, with survival rates based on the initial number of settled spats from the five culture tanks. Algae and other biofouling on the coral colonies were regularly removed.
Coral cultivation and maintenance protocols were adapted from Omori (2005) and Omori and Iwao (2014). At 18 months, 10 plates with reared coral colonies were transferred to HNB and affixed to artificial dome-shaped structures. We monitored the transplanted coral health bimonthly and cleared biofouling as necessary. Additionally, 10 replicates of 9-month-old (from the same batch as this experiment) and five replicates of 2-, 3-, 4-, and 5-year-old post-transplantation corals (from different hatchery batches) were collected for Symbiodiniaceae community analysis. Physical factors, such as light intensity and temperature, were recorded at SSI (2016-2017), TMI (2016), and HNB (2018) using HOBO data loggers.
DNA extraction and next-generation sequencing
Coral samples, including parent colonies and reared corals at 1, 3, 6, 12, and 18 months of age, as well as post-transplant colonies (after 9 months and 2, 3, 4, and 5 years of transplantation), were utilized for DNA extraction, respectively. A small coral fragment was air-dried to remove any excess ethanol and then pulverized using a mortar and pestle prior to proceeding with further analysis. Total genomic DNA from each sample was extracted utilizing the DNeasy Plant Mini Kit, following the manufacturer’s instructions with some modifications. The quality and concentration of the extracted DNA were assessed and adjusted using gel electrophoresis and NanoDrop 2000c (Thermo Fisher Scientific™).
To characterize the Symbiodiniaceae internal transcribed regions, both ITS-1 and ITS-2 regions were amplified employing the primer pair r18Sf(5′-CGCTCTTCCGATCTCTGGAAAGTTTCATGAACCTTAT3-′) and Sym28Sr-1st (5′-TGCTCTTCCGATCTGACCTTGTRTGACTTCATGCTA-3′). The first and second polymerase chain reactions (PCRs) were conducted in accordance with Jandang et al. (2022). The amplicon library, encompassing the second round of PCR products, was quantified with a Qubit 2.0 Fluorometer and the Agilent Bioanalyzer 2100 system. The final concentration of the DNA libraries was diluted to 8 pM and subsequently sequenced on an Illumina MiSeq platform.
Data processing and bioinformatics analysis
The quality control of the Illumina MiSeq platform output data was conducted in accordance with Shinzato et al. (2018). Quality trimming and adapter removal, as well as chimera filtering, were performed using Cutadapt and UCHIME2, respectively. Low-quality bases (Phred quality score <20) were trimmed using Cutadapt version 3.5 (Marcel, 2011), and high-quality sequences longer than 100 bp were retained for further analysis. ITS-2 gene sequences adjacent to the Sym28Sr primer were subjected to chimera filtering against the Symbiodiniaceae ITS-2 database (Shinzato et al., 2018) using the UCHIME2 algorithm (Edgar, 2016). For operational taxonomic unit (OTU) analysis, we used the Symbiodiniaceae ITS-2 database to prepare OTUs clustered at 97% sequence identity. Sequences that were trimmed and free from chimeras were aligned to the 97% identity clustering ITS-2 database to identify OTUs (Shinzato et al., 2018). The most abundant OTU sequence was selected for alignment using BLASTn. Reads that did not match Symbiodiniaceae and those of low quality were removed (Camacho et al., 2009) applying an e-value cutoff of 1e−100 and an alignment length cutoff of >100 bp. Subsequently, the number of sequences mapped to each OTU was counted. The raw sequence data were submitted to the BioProject archive under accession number PRJNA806638.
Statistical analysis
One-way ANOVA with post-hoc Tukey’s test was utilized to assess differences in physical parameters (temperature and light intensity) among the study sites. Survival data of reared corals in each culture tank were analyzed using the non-parametric test (chi-square). Pairwise comparisons were performed using the log-rank test to compare the mean survival rates across different coral ages. The Shannon and Simpson indices were employed to describe and compare alpha diversity across samples from different groups (Simpson, 1949). Significant differences in the diversity index values between samples were evaluated using the Kruskal–Wallis test, followed by post-hoc Tukey HSD analysis. To assess beta diversity, post-hoc pairwise permutation multivariate analysis of variance (PERMANOVA) was conducted using pairwiseAdonis v.0.4. This analysis focused on the relative proportions of Symbiodiniaceae types in all samples. Symbiodiniaceae communities were compared using Bray–Curtis dissimilarity among samples from relative abundance data. These dissimilarity matrices were then employed to generate non-metric multidimensional scaling (NMDS) biplots and to test for differences between samples from each group using PERMANOVA with the phyloseq v. 1.36.0 and VEGAN packages v. 2.5-7.
Results
Physical factors from three study sites
The annual average sea surface temperature (SST) at TMI (2016), HNB (2018), and SSI (2016–2017) were 30.39 ± 0.28°C, 29.94 ± 0.33°C, and 28.66 ± 0.33°C, respectively. The average temperature at the coral hatchery (SSI) was significantly lower than that of the natural reefs (TMI and HNB) (p < 0.05). However, during the summer, the temperature at all sites was approximately 0.92°C higher than the annual average. Although the monthly average SST at the study sites was within the ecological limits for coral reefs, coral bleaching still occurred in some colonies during the summer across all sites. The Samae San Island (coral hatchery) experienced the lowest seawater temperature (29.62°C) during the summer compared with the other sites. Conversely, SSI’s maximum daily average temperature reached 33.13°C in June 2016 (Figure 3). Large fluctuations in daily temperature and light intensity were also observed at SSI, with the maximum daily amplitude being 5.41 ± 0.29°C and 45.77 ± 1.75 μmol m−2 s−1, respectively. Consequently, corals reared in the hatchery were subjected to high variability in environmental conditions, which sometimes exceeded the bleaching threshold, potentially leading to a low survival rate, especially during the summer.
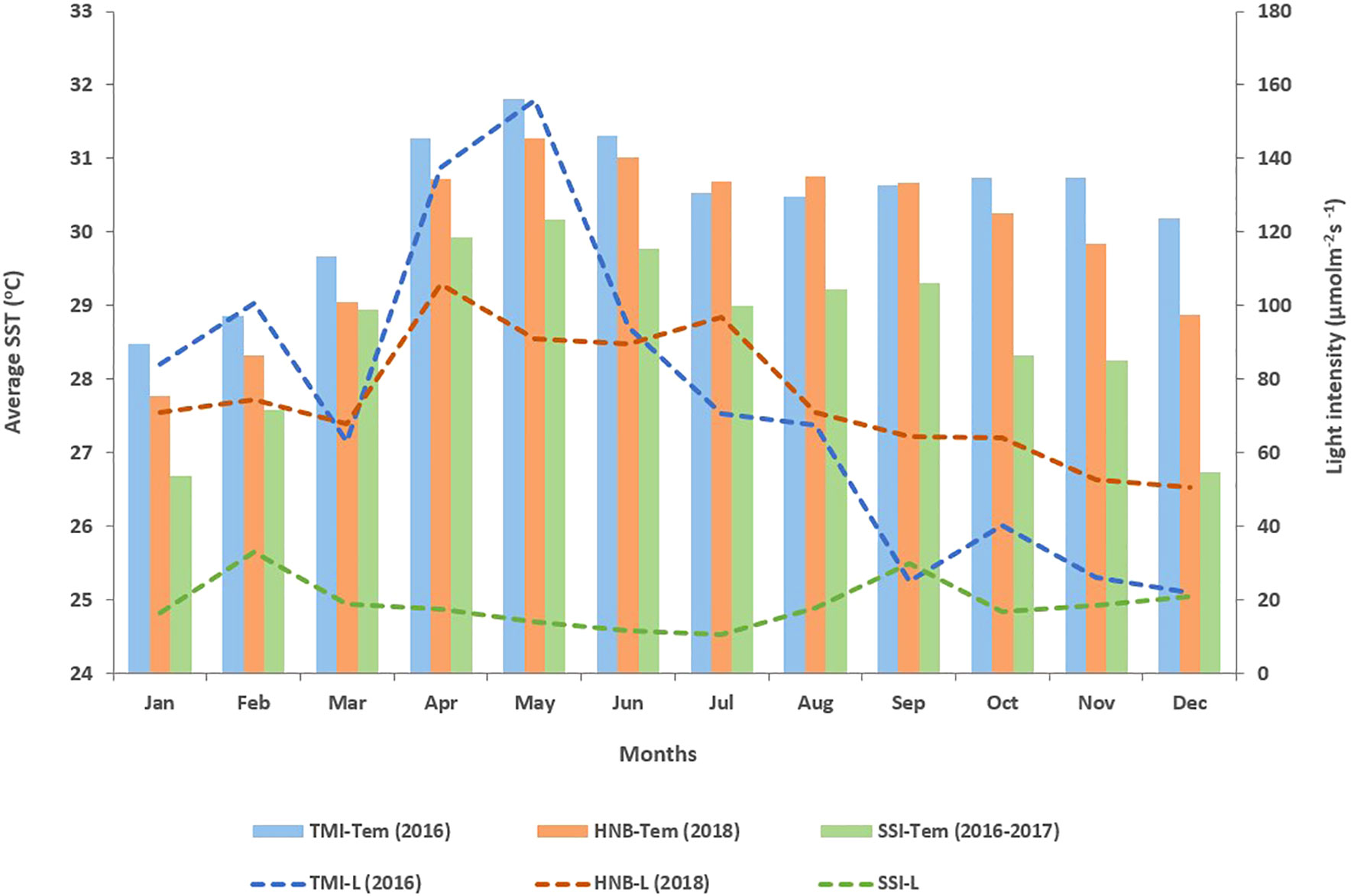
Figure 3 Average temperature and light intensity in TMI, HNB, and SSI. The bar graph illustrates the average temperature, while the dashed line depicts the light intensity at each study site (p < 0.05).
The average light intensity differed significantly between the coral hatchery (SSI) and natural environments (TMI and HNB) (p < 0.05). An unusually low light intensity was recorded at SSI, 18.76 ± 1.92 μmol m−2 s−1, whereas the ambient light intensity in the natural reef was around 50 μmol m−2 s−1 in the Gulf of Thailand. Given that the coral hatchery operates as an indoor system, a shade structure was constructed to protect against sunlight, resulting in irregularly low light levels in the coral culture tanks. On the contrary, the average light intensities at TMI and HNB were 78.85 ± 12.5 μmol m−2 s−1 and 74.85 ± 5.01 μmol m−2 s−1, respectively, which were higher than the annual average light intensity typically found in coral reefs in the upper Gulf of Thailand.
Fertilization and survivorship
The mean fertilization rate of A. humilis was 98.48 ± 0.34%. The fertilized embryos began cell division 1 h after fertilization and assumed a flat shape akin to a red blood cell after 8 h. Embryos that were abnormally shaped or round were considered unfertilized. The coral embryos then progressed to larvae (swimming stage) and settled on the artificial substrate within 4–5 days. These newly settled corals developed into primary polyps (no zooxanthellae cells were observable to the naked eye). The primary polyps budded and encrusted on the artificial substrate.
Survival rates were monitored every 3 months, revealing a gradual decline in the number of settled corals during the first 3 months, averaging around 9.46%. Notably, coral mortality was significantly higher at the 0–3-month-old stage (24.12%, p < 0.001) compared with other stages (Figure 4, Supplementary 1), especially during summer when temperatures reached 30.16°C. Overall, approximately 45% of juvenile corals across all culture tanks survived under hatchery conditions at ages 6–18 months.
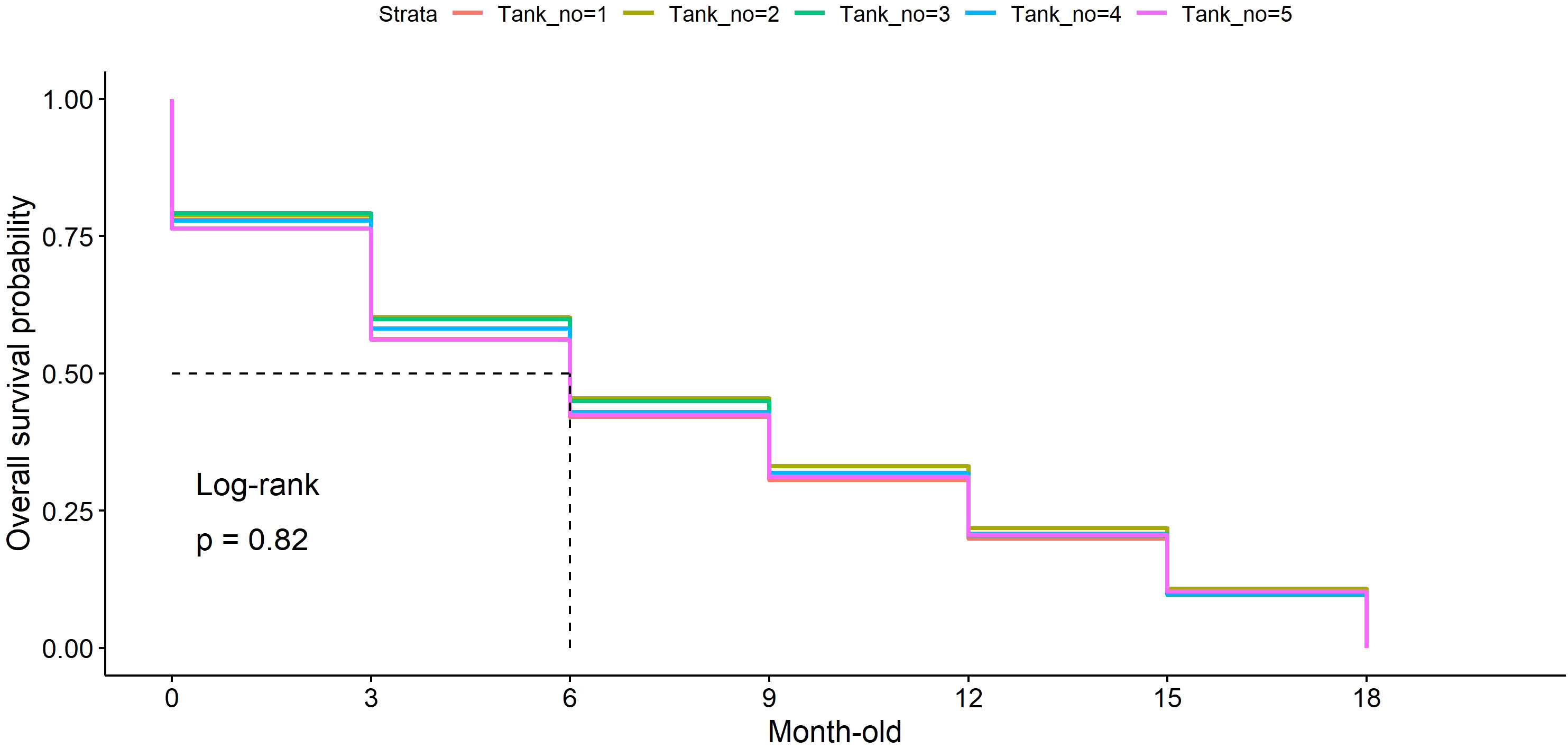
Figure 4 The survival rate of Acropora humilis under hatchery conditions. Each line color represents the survival rate observed in a different culture tank: orange (tank1, n = 174), olive green (tank2, n = 122), green (tank3, n = 215), blue (tank4, n = 112), and purple (tank5, n = 156). Values on the y-axis denote the overall survival probability of reared coral from the early stage to 18 months old, whereas the x-axis represents coral raising time.
For transplanted corals, a high mortality rate was observed within the first few months post-transplantation. At the conclusion of the 9-month experimental period at HNB, the overall survival rate of 1.5-year-old transplanted corals was 20%.
Symbiodiniaceae diversity and community
A total of 2,115,916 high-quality sequences were obtained from 72 samples (approximately 29,387 reads per sample) after filtering and trimming. These sequencing data included wild parent, reared, and transplanted colonies of A. humilis (Supplementary 2). In total, 54 lineages were detected. These OTUs included three Symbiodiniaceae genera, namely, Symbiodinium (A3), Cladocopium (predominantly of Cspc_C3, C3w, C93type1, Cspf), and Durusdinium (predominantly of D1). The dominant Symbiodiniaceae in wild parent colonies were Cladocopium (Csps_C3 and C3W) and a minority of Durusdinium (D1), whereas juvenile coral under hatchery conditions vary depending on coral age. The 1-month-old corals showed similar Symbiodiniaceae diversity to their parent, but a higher proportion of Durusdinium was detected. Approximately 44.03% of 1-month-old colonies were associated with Durusdinium D1, followed by Cladocopium Csps_C3 (33.31%), C3w (16.55%), and 10 of minority strains. The Symbiodinium A3 was found in a particular colony of 3-month-old reared coral whereas most colonies were associated predominantly with Durusdinium D1 (80.18%). Surprisingly, reared corals were shown to be harbored with almost 100% of Durusdinium D1 at 3 months of age and maintain this stable proportion until the end of the experiment (18 months of age). On the contrary, the transplanted colonies of 18-month-old reared coral were shown to change their Durusdinium proportion and gradual uptake new Cladocopium strains after 9 months (Figure 5A). Overall, the Symbiodiniaceae communities in parent, reared, and transplanted colonies were found to be significantly different. The results showed that the Symbiodiniaceae genus Cladocopium was the most common in parent colonies (98.54%), whereas Durusdinium were in reared corals (95.13%) Additionally, the transplanted corals exhibited a comparable proportion of Durusdinium (59.51%) and Cladocopium (40.48%), respectively (Figure 5B).
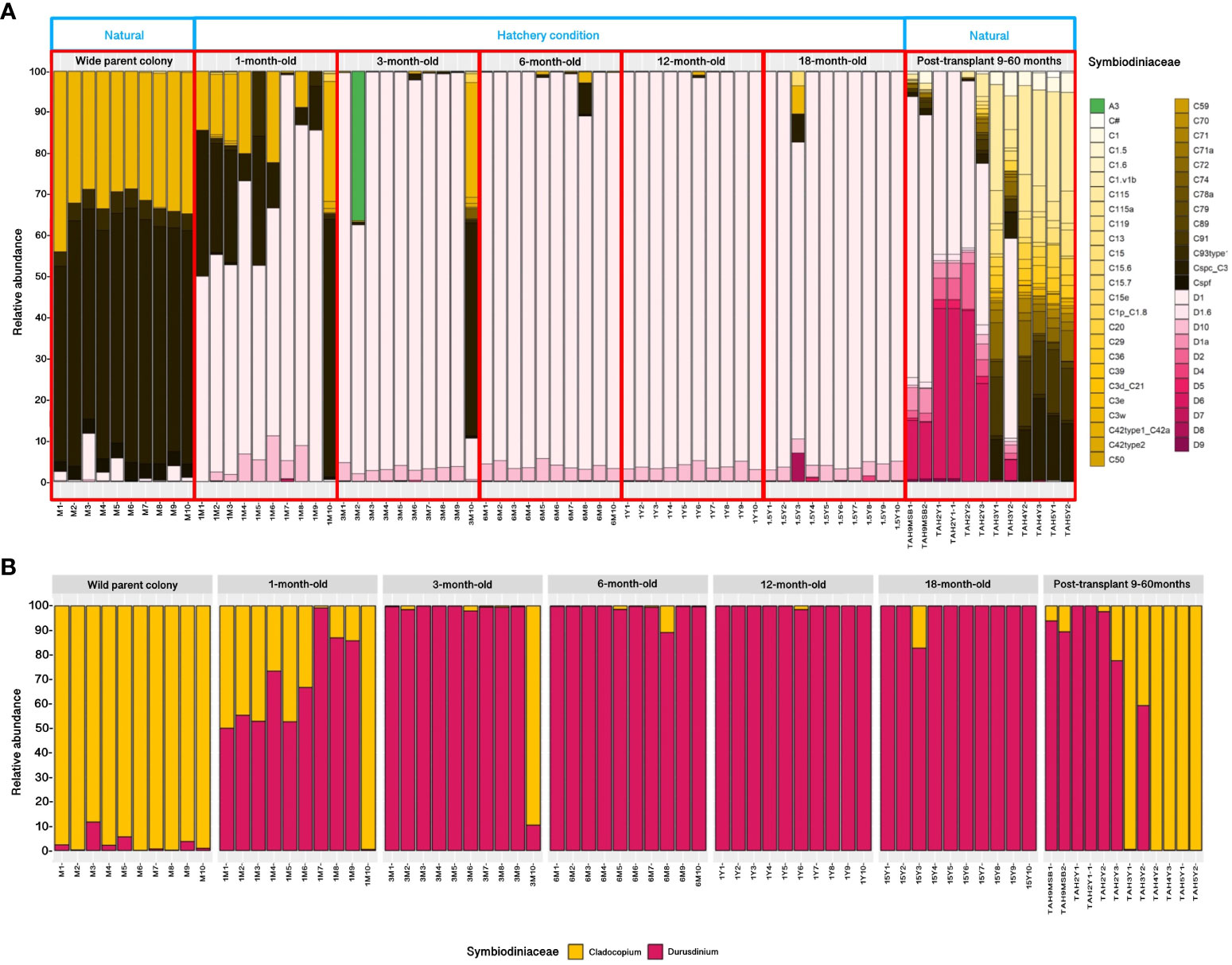
Figure 5 Relative abundance of Symbiodiniaceae communities in Acropora humilis from the wild parent, reared (different stages), and post-transplantation corals (A). An overall Symbiodiniaceae proportion (Cladocopium and Durusdinium) from three distinct coral sources (B).
The Shannon and Simpson diversity indices for Symbiodiniaceae suggested that transplanted corals possessed relatively high diversity, in contrast to the low diversity observed in reared corals (Kruskal–Wallis test, p < 0.0001). This finding indicates that transplanted corals tend to harbor Symbiodiniaceae communities with higher diversity than those in wild parent and reared coral colonies. In transplanted corals, multiple Cladocopium assemblages were present, differing from the wild parent colony, which predominantly hosted two strains (Cspc_C3 and C3W) (Figure 6). Non-metric multidimensional scaling also depicted the dissimilarity of Symbiodiniaceae communities, revealing a distinct pattern that varied significantly between communities associated with wild parent, reared, and transplanted corals (PERMANOVA, p-value = 0.001) (Figure 7).
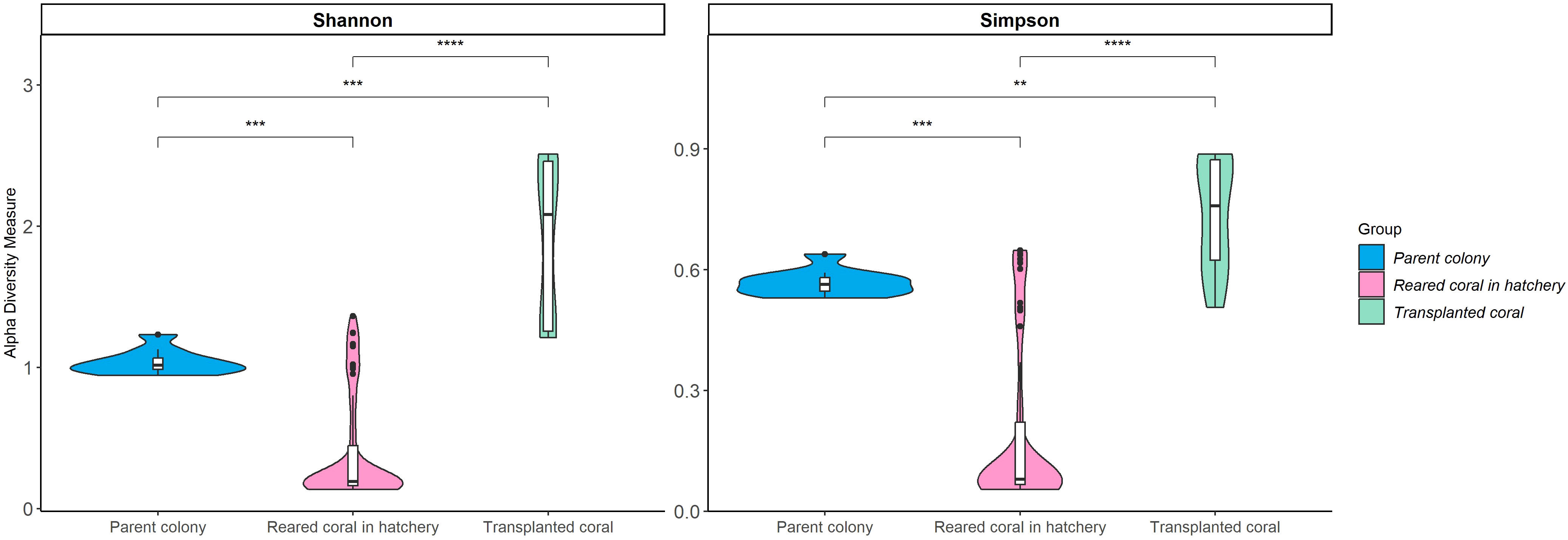
Figure 6 Alpha diversity indices (Shannon and Simpson) of Symbiodiniaceae community among Acropora humilis in different conditions. ** p < 0.01, *** p < 0.001 and **** p < 0.0001.
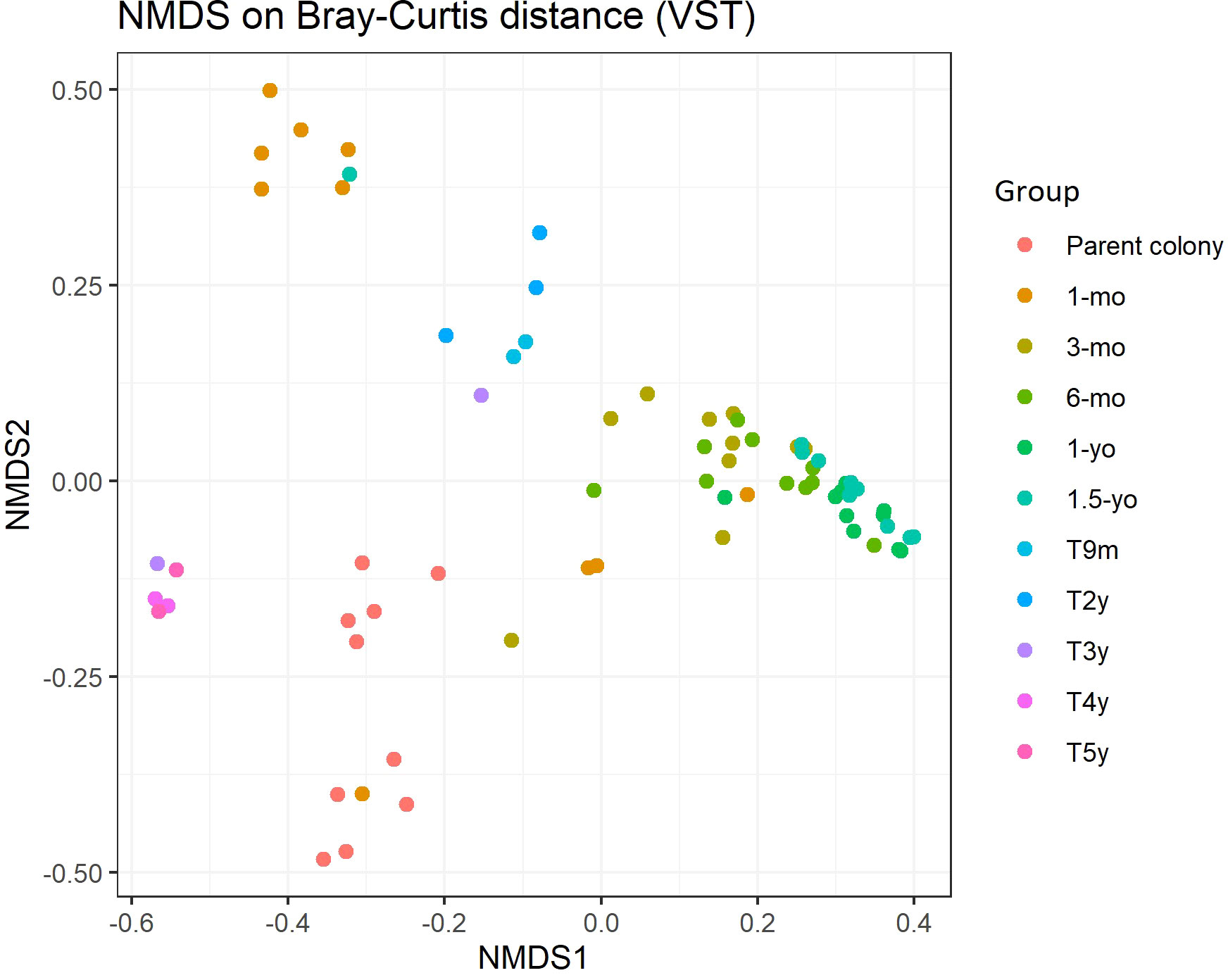
Figure 7 Non-metric multidimensional scaling (nMDS) plotting of the Symbiodiniaceae strain composition of Acropora humilis from different conditions and/or age based on Bray–Curtis dissimilarity indices.
Discussion
In this study, we observed a significant shift in the Symbiodiniaceae community composition from the early developmental to juvenile stages of Acropora humilis under hatchery conditions and following the transplantation of reared corals to a natural reef. Most early-stage reared corals (1-month-old) displayed Symbiodiniaceae genotypes similar to those of their wild parent colony, but with increased Durusdinium predominance. Furthermore, reared corals maintained a strong association with almost 100% Durusdinium from 3 to 18 months. Early life stages of Acropora spp. have been reported to harbor a broader range of Symbiodiniaceae, especially Cladocopium, Durusdinium, and Symbiodinium, under ambient conditions (Abrego et al., 2009; Yamashita et al., 2014; Quigley et al., 2017a; Yorifuji et al., 2017). However, the community of Symbiodiniaceae may vary regardless of the physical conditions, like with an increase in temperature (LaJeunesse et al., 2010; Gouezo et al., 2020; Naugle et al., 2021; Palacio-Castro et al., 2023). Juvenile Acropora corals exhibited a higher number of heat-tolerant symbionts under elevated temperatures (Yuyama et al., 2016). This study recorded the highest average temperature in June 2016 when reared corals were approximately 3 months old. Notably, coral survivorship significantly declined following the hottest month, whereas the surviving corals were predominantly associated with Durusdinium. Elevations in temperature may contribute to the preferential association of corals with Durusdinium, which has been demonstrated to confer its thermo-tolerance capacity to its host (Baker et al., 2004; Palacio-Castro et al., 2023). Hence, this may reflect the sustained exposure to higher temperatures in the culture tank, which could have aided the remaining juvenile corals in withstanding warmer conditions (Cárdenas-Alvarado et al., 2021; Palacio-Castro et al., 2023). In addition, condition of rearing in tanks with a limited volume (400 L/tank) and circulation rate of seawater in the culture tanks led to rapid temperature fluctuations, adversely affecting the survival rate of the corals. Moreover, light limitation in the coral hatchery emerged as another stressor that could disrupt the photosynthetic efficiency and metabolic interactions between coral and its symbionts (Matthews et al., 2017; Brunner et al., 2022). Environments with low light can increase cellular pigment concentration and alter coral performance; the corals may adapt by shifting their Symbiodiniaceae community composition (Ros et al., 2021; Russnak et al., 2021). Consequently, juvenile corals need to maintain a predominantly Durusdinium population, which may confer advantages in hostile environments (Abrego et al., 2009; Yuyama et al., 2012a).
While the dominance of thermo-tolerant symbionts such as Durusdinium can make corals more resilient to stressors, it might also lead to reduced calcification and growth rates compared with corals hosting the thermo-sensitive Cladocopium (Cunning et al., 2015; A. Jones & Berkelmans, 2010). Due to the lower transfer of photosynthate to the host, corals associated with Durusdinium exhibited a slower growth rate, particularly in environments limited by various factors such as light, food availability, water circulation, and biotic competition (Nakamura et al., 2011; Stat & Gates, 2011; Omori & Iwao, 2014; Gleason et al., 2018). Our reared corals had an average growth rate of 1.57 ± 0.14 year−1 (from ages 1–5 years) (unpublished data), whereas wild colonies of Acropora spp. can grow approximately three to four times faster. This discrepancy may correlate with the differences in Symbiodiniaceae types (Anderson et al., 2017; Ware et al., 2020; Weil et al., 2020).
Several studies have confirmed that juvenile Acropora exhibit greater flexibility than adult colonies due to higher Symbiodiniaceae assemblages (Little et al., 2004; Yamashita et al., 2014). Within the first 3 months of reared coral development, three genera of Symbiodiniaceae were observed, namely, Cladocopium, Durusdinium, and Symbiodinium. We also report the first presence of Symbiodinium tridacnidorum (A3) in Thai waters. Generally, Acropora larvae harboring Symbiodinium is common in many regions (Abrego et al., 2009; Yamashita et al., 2014; Quigley et al., 2017a). Most horizontally transmitting corals rely on free-living Symbiodiniaceae from surrounding environments, including seawater, some benthic organisms, and sediment (Hirose & Hidaka, 2006; Hirose et al., 2008; Sweet, 2014). Symbiodinium is recognized as an opportunistic symbiont as it has been found in corals that exhibit physiological vulnerabilities (e.g., coral disease, early developmental stages), whereas certain types can provide a degree of protection to the coral from high light stress (Suggett, 2008; LaJeunesse et al., 2010; LaJeunesse et al., 2015; Rouzé et al., 2016). Furthermore, the acquisition of Symbiodiniaceae cells in an azooxanthellate larva is exclusive with certain genera and exhibits an increasing level of selectivity later (Bay et al., 2011; Yamashita et al., 2014; Silverstein et al., 2015). Acropora larvae have demonstrated an active preference for Cladocopium, Durusdinium, and Symbiodinium, even when exposed to multiple types of Symbiodiniaceae during experiments (Adams et al., 2009; Mieog et al., 2009). Thus, during this stage, corals uniquely accommodate a particular group of Symbiodiniaceae to meet their energy needs (Gordon & Leggat, 2010; Suzuki et al., 2013; Hillyer et al., 2017) or, as Acropora spp. in the Gulf of Thailand (GoT) spawn in early summer, the hosting of multiple Symbiodiniaceae genera/types in new recruits may be advantageous for coping with the rising temperatures of the upcoming summer (Yuyama et al., 2016). Juvenile Acropora humilis (18 months old under ex-situ conditions) in this study did not exhibit the same Symbiodiniaceae proportions as that of their parent colony, aligning with previous reports by Abrego et al. (2009) and Mieog et al. (2009). These juvenile corals maintained a stable association with Durusdinium from 6 to 18 months old under hatchery conditions and even after 3 years post-transplantation to a natural reef.
Utilizing juveniles from sexually propagated corals is a viable approach for active coral restoration because they can provide greater genetic diversity compared with asexual fragmentation, thereby offering potentially higher genetic resilience in response to environmental threats (Baums, 2008; Omori & Iwao, 2014; van Oppen et al., 2017; Baums et al., 2019). The success of coral transplantation is contingent upon physical and ecological factors (Baums et al., 2019; Ladd et al., 2019). Generally, reared corals aged 1–2 years are deemed suitable for transplantation due to their higher survival rates (Omori et al., 2008). A wide range of survival rates after coral transplantation has been observed, approximately 3%–89%, depending on the size, species, and location (Omori et al., 2008; Villanueva et al., 2012; Chamberland et al., 2015; Ligson et al., 2020). When we transplanted 1.5-year-old reared corals to a natural reef, only 20% survived after 9 months. Smaller colonies or younger corals may not survive under such conditions due to disturbances such as increased sedimentation, light intensity, and temperature fluctuations (Raymundo & Maypa, 2004; Tuttle & Donahue, 2022). Therefore, exploring the heightened flexibility demonstrated during early stages is recommended to increase the success of acclimation under unfamiliar conditions at out-planting sites. Identically, examining the tipping point of flexibility in Acropora throughout its ontogenic cycle would be useful for specifically identifying the oldest and most robust stages at which the corals retain their flexible Symbiodiniaceae-conferred traits.
Additionally, the upper Gulf of Thailand is influenced by major and minor rivers, resulting in significant water and sediment discharge into the area (Zhang et al., 2022). High levels of suspended sediment can reduce photosynthesis and respiration in corals, leading to high mortality rates (Erftemeijer et al., 2012; Tuttle & Donahue, 2022). Furthermore, recent studies have suggested that reared juvenile corals tend not to shift their Symbiodiniaceae communities during the first 2 years after transplantation to a natural reef. This resistance to change may be linked to the high mortality rate of corals, as the interaction between corals and their symbiont communities might impact the corals’ resistance/resilience abilities when environmental conditions alter (Bairos-Novak et al., 2021; De Souza et al., 2022).
The juvenile Acropora corals harbored a different Symbiodiniaceae community compared with parental colonies (Abrego et al., 2009; Yorifuji et al., 2017). However, juvenile Acropora corals hosted predominantly Durusdinium starting at 3 months and began to shift their symbiont to Cladocopium at approximately 3.5 years of age in this study, aligning with a previous report by Abrego et al. (2009). The timing of the change in relative abundance of Symbiodiniaceae in juvenile Acropora to resemble that of the local adult colony is dependent on coral species and environmental conditions (Gómez-Cabrera et al., 2008; Abrego et al., 2009). Although our corals were entirely associated with several Cladocopium assemblages after 4 years of transplantation, none of the reared corals exhibited a Symbiodiniaceae structure similar to the parent colony, which mainly harbored types Cspc_C3 and C3W. Several possible explanations exist for juvenile corals hosting different types of Symbiodiniaceae compared with those found in field surveys of A. humilis: (1) reared corals may experience prolonged stress under ex-situ conditions, and even after transplantation to a new environment, they may require more time to acclimatize (Sampayo et al., 2016; DeCarlo, 2020); (2) harboring a greater diversity of Cladocopium than the parent colony could be advantageous for juveniles to cope with local stressors; (Abrego et al., 2012; Yorifuji et al., 2017); and (3) the locally available Symbiodiniaceae communities at the out-planting sites might be distinct from those at the parental field location. Such outcomes enable corals to acquire novel Symbiodiniaceae types from their surrounding environment (Boulotte et al., 2016; Scharfenstein et al., 2022).
Wild adult colonies of Acropora humilis are strongly associated with Cladocopium (Cspc_C3 and C3W in this study). Cladocopium is considered a generalist symbiont, commonly found in Indo-Pacific corals, with several lineages detected (Gong et al., 2018; Chen et al., 2019; Jandang et al., 2022). Corals hosting Cladocopium have often shown faster growth, potentially indicating a greater transfer of photosynthetically fixed carbon to their host (Cantin et al., 2009; Cunning et al., 2015). Coral sexual maturity is size-dependent; some faster-growing staghorn corals can reach maturity at around 2–4 years of age (Ligson & Cabaitan, 2021; Suzuki, 2021). Therefore, the time it takes for corals harboring Cladocopium to reach full mature size and produce offspring may be shorter, allowing them to increase their population faster than some massive corals (Van Oppen & Medina, 2020). Although stable Symbiodiniaceae lineages are present in most adult corals under ambient conditions, some corals may rapidly change their symbiont population when exposed to high seawater temperatures (Silverstein et al., 2015; Palacio-Castro et al., 2023). Such a situation is referred to as the adaptive bleaching hypothesis, occurring when corals experience bleaching events to increase heat tolerance and survive in warmer oceans (Jones et al., 2008; Howells et al., 2013; Baird et al., 2021). In this context, corals may grow slower than in non-stressful conditions due to the increased predominance of heat-evolved symbionts (Cunning et al., 2015). However, after acclimatization (shuffling or switching), corals have been shown to transition back to their pre-bleaching symbiont communities if the environment returns to optimal conditions (Coffroth et al., 2010; Kao et al., 2018).
To the best of our knowledge, this study is the first to examine the coral–Symbiodiniaceae association in A. humilis from wild and cultured colonies. In summary, we documented the distinct Symbiodiniaceae communities among wild parents, reared, and post-transplanted corals. Our reared juvenile corals exhibited the flexibility of their Symbiodiniaceae partners when changing environments. However, not all corals successfully survive varying environmental conditions; some do not acclimate and eventually perish. Despite the ability of corals to shift their Symbiodiniaceae community under stress conditions, coral survival during reef restoration in this area also depends on the initial juvenile size and the physical conditions of the transplantation site. Furthermore, extensive collections of coral species and free-living Symbiodiniaceae from the surrounding environment can enhance our understanding of coral and Symbiodiniaceae associations, as well as the ability of corals to acclimate to climate change.
Data availability statement
The datasets presented in this study can be found in online repositories. The names of the repository/repositories and accession number(s) can be found below: BioProject, PRJNA806638.
Ethics statement
The manuscript presents research on animals that do not require ethical approval for their study.
Author contributions
SJ and SC conceived the study and drafted the manuscript. SJ, YY, and CS carried out the molecular biology experiment and statistical analysis. JS and VV executed the experiments and collected samples. VV and SC coordinated the study and were involved in manuscript revising. All authors gave the final approval for publication.
Funding
The author(s) declare financial support was received for the research, authorship, and/or publication of this article. This research was supported by the Royal Golden Jubilee Ph.D. program (Grant No. PHD/0126/2558), Thailand Science Research and Innovation Fund Chulalongkorn University (DIS66230006), NRCT-JSPS Core to Core Program, CREPSUM JPJSCCB20200009, National Research Council of Thailand and Chulalongkorn University (N42A650257), Thailand Science Research and Innovation Fund Chulalongkorn University (DIS66230010), and Valeura Energy. The funders had no role in study design, data collection and analysis, decision to publish, or preparation of the manuscript.
Acknowledgments
We would like to thank the Plant Genetic Conservation Project under the Royal Initiative of Her Royal Highness Princess Maha Chakri Sirindhorn, the Naval Special Warfare Command, Royal Thai Fleet, Royal Thai Navy, and UNESCO-IOC/WESTPAC for their assistance in the fieldwork. Furthermore, we extend our appreciation to the members of the molecular marine biology section, Atmosphere and Ocean Research Institute, The University of Tokyo for their support in laboratory work. Finally, we would like to thank the members of the Reef Biology Research Group for their support during sample collection and coral cultivation.
Conflict of interest
The authors declare that the research was conducted in the absence of any commercial or financial relationships that could be construed as a potential conflict of interest.
Publisher’s note
All claims expressed in this article are solely those of the authors and do not necessarily represent those of their affiliated organizations, or those of the publisher, the editors and the reviewers. Any product that may be evaluated in this article, or claim that may be made by its manufacturer, is not guaranteed or endorsed by the publisher.
Supplementary material
The Supplementary Material for this article can be found online at: https://www.frontiersin.org/articles/10.3389/fmars.2024.1138021/full#supplementary-material
References
Abbott E., Dixon G., Matz M. (2021). Shuffling between Cladocopium and Durusdinium extensively modifies the physiology of each symbiont without stressing the coral host. Mol. Ecol. 30 (24), 6585–6595. doi: 10.1111/mec.16190
Abrego D., Van Oppen M. J. H., Willis B. L. (2009). Highly infectious symbiont dominates initial uptake in coral juveniles. Mol. Ecol. 18 (16), 3518–3531. doi: 10.1111/j.1365-294X.2009.04275.x
Abrego D., Willis B. L., van Oppen M. J. H. (2012). Impact of light and temperature on the uptake of algal symbionts by coral juveniles. PloS One 7 (11), 1–9. doi: 10.1371/journal.pone.0050311
Adams L. M., Cumbo V. R., Takabayashi M. (2009). Exposure to sediment enhances primary acquisition of Symbiodinium by asymbiotic coral larvae. Mar. Ecol. Prog. Ser. 377 (February), 149–156. doi: 10.3354/meps07834
Ali A., Kriefall N. G., Emery L. E., Kenkel C. D., Matz M. V., Davies S. W. (2019). Recruit symbiosis establishment and Symbiodiniaceae composition influenced by adult corals and reef sediment. Coral Reefs 38 (3), 405–415. doi: 10.1007/s00338-019-01790-z
Anderson K. D., Cantin N. E., Heron S. F., Pisapia C., Pratchett M. S. (2017). Variation in growth rates of branching corals along Australia’s Great Barrier Reef. Sci. Rep. 7 (1), 1–13. doi: 10.1038/s41598-017-03085-1
Baird A. H., Guest J. R., Edwards A. J., Bauman A. G., Bouwmeester J., Mera H., et al. (2021). An Indo-Pacific coral spawning database. Sci. Data 8 (1), 1–9. doi: 10.1038/s41597-020-00793-8
Bairos-Novak K. R., Hoogenboom M. O., van Oppen M. J. H., Connolly S. R. (2021). Coral adaptation to climate change: Meta-analysis reveals high heritability across multiple traits. Global Change Biol. 27 (22), 5694–5710. doi: 10.1111/gcb.15829
Baker A. C. (2003). Flexibility and specificity in coral-algal symbiosis: Diversity, ecology, and biogeography of Symbiodinium. Annu. Rev. Ecol. Evol. Systematics 34, 661–689. doi: 10.1146/annurev.ecolsys.34.011802.132417
Baker D. M., Andras J. P., Jordán-Garza A. G., Fogel M. L. (2013). Nitrate competition in a coral symbiosis varies with temperature among Symbiodinium clades. ISME J. 7 (6), 1248–1251. doi: 10.1038/ismej.2013.12
Baker A., Starger C., McClanahan T., Glynn P. W. (2004). Corals’ adaptive response to climate change. Nature 430, 741. doi: 10.1038/430741a
Baums I. B. (2008). A restoration genetics guide for coral reef conservation. Mol. Ecol. 17 (12), 2796–2811. doi: 10.1111/j.1365-294X.2008.03787.x
Baums I. B., Baker A. C., Davies S. W., Grottoli A. G., Kenkel C. D., Kitchen S. A., et al. (2019). Considerations for maximizing the adaptive potential of restored coral populations in the western Atlantic. Ecol. Appl. 29 (8), 1–23. doi: 10.1002/eap.1978
Bay L. K., Cumbo V. R., Abrego D., Kool J. T., Ainsworth T. D., Willis B. L. (2011). Infection dynamics vary between symbiodinium types and cell surface treatments during establishment of endosymbiosis with coral larvae. Diversity 3, 356–374. doi: 10.3390/d3030356
Berkelmans R., Van Oppen M. J. H. (2006). The role of zooxanthellae in the thermal tolerance of corals: A “nugget of hope” for coral reefs in an era of climate change. Proc. R. Soc. B: Biol. Sci. 273 (1599), 2305–2312. doi: 10.1098/rspb.2006.3567
Boulotte N. M., Dalton S. J., Carroll A. G., Harrison P. L., Putnam H. M., Peplow L. M., et al. (2016). Exploring the Symbiodinium rare biosphere provides evidence for symbiont switching in reef-building corals. ISME J. 10 (11), 2693–2701. doi: 10.1038/ismej.2016.54
Brunner C. A., Ricardo G. F., Uthicke S., Negri A. P., Hoogenboom M. O. (2022). Effects of climate change and light limitation on coral recruits. Mar. Ecol. Prog. Ser. 690, 65–82. doi: 10.3354/meps14055
Buddemeier R., Fautin D. (1993). Coral bleaching as an adaptive mechanism. Bioscience 43, 320–326. doi: 10.2307/1312064
Camacho C., Coulouris G., Avagyan V., Ma N., Papadopoulos J., Bealer K., et al. (2009). BLAST+: architecture and applications. BMC Bioinf. 10, 1–9. doi: 10.1186/1471-2105-10-421
Cantin N. E., Van Oppen M. J. H., Willis B. L., Mieog J. C., Negri A. P. (2009). Juvenile corals can acquire more carbon from high-performance algal symbionts. Coral Reefs 28 (2), 405–414. doi: 10.1007/s00338-009-0478-8
Cárdenas-Alvarado M. A., Nava H., González-Rodríguez A., Maldonado-López Y., Rodríguez-Lanetty M. (2021). Higher population genetic diversity within the algal symbiont Durusdinium in Pocillopora verrucosa from Mexican Pacific reefs correlates with higher resistance to bleaching after the El Niño 2015–16 event. Mar. Ecol. 42 (4), 1–13. doi: 10.1111/maec.12667
Chamberland V. F., Petersen D., Guest J. R., Petersen U., Brittsan M., Vermeij M. J. A. (2017). New seeding approach reduces costs and time to outplant sexually propagated corals for reef restoration. Sci. Rep. 7 (1), 1–12. doi: 10.1038/s41598-017-17555-z
Chamberland V. F., Vermeij M. J. A., Brittsan M., Carl M., Schick M., Snowden S., et al. (2015). Restoration of critically endangered elkhorn coral (Acropora palmata) populations using larvae reared from wild-caught gametes. Global Ecol. Conserv. 4, 526–537. doi: 10.1016/j.gecco.2015.10.005
Chankong A., Manthachitra V. (2013). Community structure of Acropora corals in the Gulf of Thailand. Galaxea J. Coral Reef Stud. 15 (Supplement), 92–100. doi: 10.3755/galaxea.15.92
Chavanich S., Viyakarn V., Loyjiw T., Pattaratamrong P., Chankong A. (2009). Mass bleaching of soft coral, Sarcophyton spp. in Thailand and the role of temperature and salinity stress. ICES J. Mar. Sci. 66 (7), 1515–1519. doi: 10.1093/icesjms/fsp048
Chen B., Yu K., Liang J., Huang W., Wang G., Su H., et al. (2019). Latitudinal variation in the molecular diversity and community composition of symbiodiniaceae in coral from the South China Sea. Front. Microbiol. 10 (JUN). doi: 10.3389/fmicb.2019.01278
Claar D. C., McDevitt-Irwin J. M., Garren M., Vega Thurber R., Gates R. D., Baum J. K. (2020). Increased diversity and concordant shifts in community structure of coral-associated Symbiodiniaceae and bacteria subjected to chronic human disturbance. Mol. Ecol. 29 (13), 2477–2491. doi: 10.1111/mec.15494
Coffroth M. A., Poland D. M., Petrou E. L., Brazeau D. A., Holmberg J. C. (2010). Environmental symbiont acquisition may not be the solution to warming seas for reef-building corals. PloS One 5 (10), e13258. doi: 10.1371/journal.pone.0013258
Cunning R., Silverstein R. N., Baker A. C. (2015). Investigating the causes and consequences of symbiont shuffling in a multi-partner reef coral symbiosis under environmental change. Proc. R. Soc. B: Biol. Sci. 282 (1809), 20141725. doi: 10.1098/rspb.2014.1725
Cunning R., Silverstein R. N., Baker A. C. (2018). Symbiont shuffling linked to differential photochemical dynamics of Symbiodinium in three Caribbean reef corals. Coral Reefs 37 (1), 145–152. doi: 10.1007/s00338-017-1640-3
DeCarlo T. M. (2020). Treating coral bleaching as weather: A framework to validate and optimize prediction skill. PeerJ 2020 (7), 1–16. doi: 10.7717/peerj.9449
Decelle J., Carradec Q., Pochon X., Henry N., Romac S., Mahé F., et al. (2018). Worldwide occurrence and activity of the reef-building coral symbiont Symbiodinium in the Open Ocean. Curr. Biol. 28 (22), 3625–3633.e3. doi: 10.1016/j.cub.2018.09.024
De Souza M. R., Caruso C., Ruiz-Jones L., Drury C., Gates R., Toonen R. J. (2022). Community composition of coral-associated Symbiodiniaceae differs across fine-scale environmental gradients in Kāne’ohe Bay. R. Soc. Open Sci. 9 (9), 212042. doi: 10.1098/rsos.212042
Edgar R. C. (2016). UCHIME2: improved chimera prediction for amplicon sequencing. BioRxiv, 074252. doi: 10.1101/074252v1%0Ahttps://www.biorxiv.org/content/10.1101/074252v1.abstract
Erftemeijer P. L. A., Riegl B., Hoeksema B. W., Todd P. A. (2012). Environmental impacts of dredging and other sediment disturbances on corals: A review. Mar. pollut. Bull. 64 (9), 1737–1765. doi: 10.1016/j.marpolbul.2012.05.008
Gleason D. F., Harbin L. R., Divine L. M., Matterson K. O. (2018). The role of larval supply and competition in controlling recruitment of the temperate coral Oculina arbuscula. J. Exp. Mar. Biol. Ecol. 506 (May), 107–114. doi: 10.1016/j.jembe.2018.06.006
Gómez-Cabrera M. C., Ortiz J. C., Loh W. K. W., Ward S., Hoegh-Guldberg O. (2008). Acquisition of symbiotic dinoflagellates (Symbiodinium) by juveniles of the coral Acropora longicyathus. Coral Reefs 27 (1), 219–226. doi: 10.1007/s00338-007-0315-x
Gong S., Chai G. J., Xiao Y., Xu L., Yu K., Li J., et al. (2018). Flexible symbiotic associations of Symbiodinium with five typical coral species in tropical and subtropical reef regions of the northern South China Sea. Front. Microbiol. 9 (NOV). doi: 10.3389/fmicb.2018.02485
Gordon B. R., Leggat W. (2010). Symbiodinium - Invertebrate symbioses and the role of metabolomics. Mar. Drugs 8 (10), 2546–2568. doi: 10.3390/md8102546
Gouezo M., Doropoulos C., Fabricius K., Olsudong D., Nestor V., Kurihara H., et al. (2020). Multispecific coral spawning events and extended breeding periods on an equatorial reef. Coral Reefs 39 (4), 1107–1123. doi: 10.1007/s00338-020-01941-7
Goulet T. L. (2006). Most corals may not change their symbionts. Mar. Ecol. Prog. Ser. 321 (January), 1–7. doi: 10.3354/meps321001
Goulet T. L., Shirur K. P., Ramsby B. D., Iglesias-Prieto R. (2017). The effects of elevated seawater temperatures on Caribbean gorgonian corals and their algal symbionts, Symbiodinium spp. PloS One 12 (2), 1–21. doi: 10.1371/journal.pone.0171032
Hillyer K. E., Dias D. A., Lutz A., Wilkinson S. P., Roessner U., Davy S. K. (2017). Metabolite profiling of symbiont and host during thermal stress and bleaching in the coral Acropora aspera. Coral Reefs 36 (1), 105–118. doi: 10.1007/s00338-016-1508-y
Hirose M., Hidaka M. (2006). Early development of zooxanthella-containing eggs of the corals Porites cylindrica and Montipora digitata: The endodermal localization of zooxanthellae. Zoological Sci. 23 (10), 873–881. doi: 10.2108/zsj.23.873
Hirose M., Yamamoto H., Nonaka M. (2008). Metamorphosis and acquisition of symbiotic algae in planula larvae and primary polyps of Acropora spp. Coral Reefs 27 (2), 247–254. doi: 10.1007/s00338-007-0330-y
Hoadley K. D., Lewis A. M., Wham D. C., Pettay D. T., Grasso C., Smith R., et al. (2019). Host–symbiont combinations dictate the photo-physiological response of reef-building corals to thermal stress. Scienctific Rep. 9, 9985. doi: 10.1038/s41598-019-46412-4
Hoegh-Guldberg O., Poloczanska E. S., Skirving W., Dove S. (2017). Coral reef ecosystems under climate change and ocean acidification. Frontier Mar. Sci. 4. doi: 10.3389/fmars.2017.00158
Howells E. J., Berkelmans R., Oppen M. J. H., Willis B. L., Ecology S., May N., et al. (2013). Historical thermal regimes define limits to coral acclimatization. Ecology 94 (5), 1078–1088. doi: 10.1890/12-1257.1
Iluz D., Dubinsky Z. (2015). Coral photobiology: new light on old views. Zoology 118 (2), 71–78. doi: 10.1016/J.ZOOL.2014.08.003
Jain S. S., Afiq-Rosli L., Feldman B., Kunning I., Levy O., Mana R. R., et al. (2021). Endosymbiont communities in pachyseris speciosa highlight geographical and methodological variations. Front. Mar. Sci. 8 (December). doi: 10.3389/fmars.2021.759744
Jandang S., Viyakarn V., Yoshioka Y., Shinzato C., Chavanich S. (2022). The seasonal investigation of Symbiodiniaceae in broadcast spawning, Acropora humilis and brooding, Pocillopora cf. damicornis corals. PeerJ 10, e13114. doi: 10.7717/peerj.13114
Jones A., Berkelmans R. (2010). Potential costs of acclimatization to a warmer climate: Growth of a reef coral with heat tolerant vs. sensitive symbiont types. PloS One 5 (5), e10437. doi: 10.1371/journal.pone.0010437
Jones A. M., Berkelmans R., Van Oppen M. J. H., Mieog J. C., Sinclair W. (2008). A community change in the algal endosymbionts of a scleractinian coral following a natural bleaching event: Field evidence of acclimatization. Proc. R. Soc. B: Biol. Sci. 275 (1641), 1359–1365. doi: 10.1098/rspb.2008.0069
Kao K. W., Keshavmurthy S., Tsao C. H., Wang J. T., Chen C. A. (2018). Repeated and prolonged temperature anomalies negate symbiodiniaceae genera shuffling in the coral Platygyra verweyi (Scleractinia; merulinidae). Zoological Stud. 57 (December), e55. doi: 10.6620/ZS.2018.57-55
Koch H. R., Matthews B., Leto C., Engelsma C., Bartels E. (2022). Assisted sexual reproduction of Acropora cervicornis for active restoration on Florida’s Coral Reef. Front. Mar. Sci. 9 (December). doi: 10.3389/fmars.2022.959520
Ladd M. C., Burkepile D. E., Shantz A. A. (2019). Near-term impacts of coral restoration on target species, coral reef community structure, and ecological processes. Restor. Ecol. 27 (5), 1166–1176. doi: 10.1111/rec.12939
Lajeunesse T. C. (2005). ‘Species’ radiations of symbiotic dinoflagellates in the Atlantic and Indo-Pacific since the Miocene-Pliocen transition. Mol. Biol. Evolution. 22, 570–581. doi: 10.1093/molbev/msi042
LaJeunesse T. C., Lee S. Y., Gil-Agudelo D. L., Knowlton N., Jeong H. J. (2015). Symbiodinium necroappetens sp. nov. (Dinophyceae): an opportunist ‘zooxanthella’ found in bleached and diseased tissues of Caribbean reef corals. Eur. J. Phycology 50 (2), 223–238. doi: 10.1080/09670262.2015.1025857
LaJeunesse T. C., Parkinson J. E., Gabrielson P. W., Jeong H. J., Reimer J. D., Voolstra C. R., et al. (2018). Systematic revision of symbiodiniaceae highlights the antiquity and diversity of coral endosymbionts. Curr. Biol. 28 (16), 2570–2580.e6. doi: 10.1016/j.cub.2018.07.008
LaJeunesse T. C., Pettay D. T., Sampayo E. M., Phongsuwan N., Brown B., Obura D. O., et al. (2010). Long-standing environmental conditions, geographic isolation and host-symbiont specificity influence the relative ecological dominance and genetic diversification of coral endosymbionts in the genus Symbiodinium. J. Biogeography 37 (5), 785–800. doi: 10.1111/j.1365-2699.2010.02273.x
LaJeunesse T. C., Wiedenmann J., Casado-Amezúa P., D’Ambra I., Turnham K. E., Nitschke M. R., et al. (2022). Revival of Philozoon Geddes for host-specialized dinoflagellates, ‘zooxanthellae’, in animals from coastal temperate zones of northern and southern hemispheres. Eur. J. Phycology 57 (2), 166–180. doi: 10.1080/09670262.2021.1914863
Levin R. A., Voolstra C. R., Agrawal S., Steinberg P. D., Suggett D. J., van Oppen M. J. H. (2017). Engineering strategies to decode and enhance the genomes of coral symbionts. Front. Microbiol. 8 (JUN). doi: 10.3389/fmicb.2017.01220
Ligson C. A., Cabaitan P. C. (2021). Survival and sexual maturity of sexually propagated Acropora verweyi corals 4 years after outplantation. Restor. Ecol. 29 (5), 1–5. doi: 10.1111/rec.13363
Ligson C. A., Tabalanza T. D., Villanueva R. D., Cabaitan P. C. (2020). Feasibility of early outplanting of sexually propagated Acropora verweyi for coral reef restoration demonstrated in the Philippines. Restor. Ecol. 28 (1), 244–251. doi: 10.1111/rec.13054
Little A. F., Van Oppen M. J. H., Willis B. L. (2004). Flexibility in algal endosymbioses shapes growth in reef corals. Science 304 (5676), 1492–1494. doi: 10.1126/science.1095733
López-Londoño T., Gómez-Campo K., Hernández-Pech X., Enríquez S., Iglesias-Prieto R. (2022). Photosynthetic usable energy explains vertical patterns of biodiversity in zooxanthellate corals. Sci. Rep. 12, 1–10. doi: 10.1038/s41598-022-25094-5
Marcel M. (2011). Cutadapt removes adapter sequences from high-throughput sequencing reads. EMBnetJ 17 (1), 10–12. doi: 10.14806/ej.17.1.200
Matthews J. L., Crowder C. M., Oakley C. A., Lutz A., Roessner U., Meyer E., et al. (2017). Optimal nutrient exchange and immune responses operate in partner specificity in the cnidarian-dinoflagellate symbiosis. Proc. Natl. Acad. Sci. U. S. A. 114, 13194–13199. doi: 10.1073/pnas.1710733114
Mieog J. C., Olsen J. L., Berkelmans R., Bleuler-Martinez S. A., Willis B. L., van Oppen M. J. H. (2009). The roles and interactions of symbiont, host and environment in defining coral fitness. PloS One 4 (7), e6364. doi: 10.1371/journal.pone.0006364
Mohammed T. A. A., Dar M. A. (2017). Coral growth and skeletal density relationships in some branching corals of the Red Sea, Egypt. J. Environ. Earth Sci. 7 (11), 66–79.
Muscatine L. (1990). “The role of symbiotic algae in carbon and energy flux in coral reefs,” in Ecosystems of the world, (Amsterdam: Elsevier) vol. 25, 75–87. Available at: http://jackckoch.us/assets/muscatine—1990—elsevier-science-publishing-company—the-role-of-symbiotic-algae-in-carbon-a.pdf.
Nakamura R., Ando W., Yamamoto H., Kitano M., Sato A., Nakamur M., et al. (2011). Corals mass-cultured from eggs and transplanted as juveniles to their native, remote coral reef. Mar. Ecol. Prog. Ser. 436, 161–168. doi: 10.3354/meps09257
Naugle M. S., Oliver T. A., Barshis D. J., Gates R. D., Logan C. A. (2021). Variation in coral thermotolerance across a pollution gradient erodes as coral symbionts shift to more heat-tolerant genera. Front. Mar. Sci. 8 (November). doi: 10.3389/fmars.2021.760891
Nitschke M. R., Davy S. K., Ward S. (2016). Horizontal transmission of Symbiodinium cells between adult and juvenile corals is aided by benthic sediment. Coral Reefs 35 (1), 335–344. doi: 10.1007/s00338-015-1349-0
Omori M. (2005). Success of mass culture of Acropora corals from egg to colony in open water. Coral Reefs 24 (4), 563. doi: 10.1007/s00338-005-0030-4
Omori M. (2019). Coral restoration research and technical developments: what we have learned so far. Mar. Biol. Res. 15 (7), 377–409. doi: 10.1080/17451000.2019.1662050
Omori M., Higa Y., Shinzato C., Zayasu Y., Nagata T., Nakamura R., et al. (2016). “Development of active restoration methodologies for coral reefs using asexual reproduction in Okinawa, Japan,” in Proceedings of the 13th International CoralReef Symposium. 369–387, (Honolulu: International Society for Reef Studies).
Omori M., Iwao K. (2014). Methods of farming sexually propagated corals and outplanting for coral reef rehabilitation; with list of references for coral reef rehabilitation through active restoration measure. Akajima Mar. Sci. Laboratory Okinawa Japan, 1–22.
Omori M., Iwao K., Tamura M. (2008). Growth of transplanted Acropora tenuis 2 years after egg culture. Coral Reefs 27 (1), 165. doi: 10.1007/s00338-007-0312-0
Palacio-Castro A. M., Smith T. B., Brandtnerise V., Snyder G. A., van Hooidonk R., Maté J. L., et al. (2023). Increased dominance of heat-tolerant symbionts creates resilient coral reefs in near-term ocean warming. Proc. Natl. Acad. Sci. 120 (8), 1–11. doi: 10.1073/pnas
Pettaya D., Whama D. C., Smith R. T., Iglesias-Prietoc R., LaJeunessea T. C. (2015). Microbial invasion of the Caribbean by an Indo-Pacific coral zooxanthella. Proc. Natl. Acad. Sci. United States America 112 (24), 7513–7518. doi: 10.1073/pnas.1502283112
Quigley K. M., Bay L. K., Willis B. L. (2017a). Temperature and water quality-related patterns in sediment-associated Symbiodinium communities impact symbiont uptake and fitness of juveniles in the genus Acropora. Front. Mar. Sci. 4 (DEC). doi: 10.3389/fmars.2017.00401
Quigley K. M., Willis B. L., Bay L. K. (2016). Maternal effects and Symbiodinium community composition drive differential patterns in juvenile survival in the coral Acropora tenuis. R. Soc. Open Sci. 3 (10), 160471. doi: 10.1098/rsos.160471
Quigley K. M., Willis B. L., Bay L. K. (2017b). Heritability of the Symbiodinium community in vertically-and horizontally-transmitting broadcast spawning corals. Sci. Rep. 7 (1), 1–14. doi: 10.1038/s41598-017-08179-4
Randall C. J., Negri A. P., Quigley K. M., Foster T., Ricardo G. F., Webster N. S., et al. (2020). Sexual production of corals for reef restoration in the Anthropocene. Mar. Ecol. Prog. Ser. 635 (February 2020), 203–232. doi: 10.3354/MEPS13206
Raymundo L. J., Maypa A. P. (2004). Getting bigger faster: Mediation of size-specific mortality via fusion in juvenile coral transplants. Ecol. Appl. 14 (1), 281–295. doi: 10.1890/02-5373
Ros M., Suggett D. J., Edmondson J., Haydon T., Hughes D. J., Kim M., et al. (2021). Symbiont shuffling across environmental gradients aligns with changes in carbon uptake and translocation in the reef-building coral Pocillopora acuta. Coral Reefs 40 (2), 595–607. doi: 10.1007/s00338-021-02066-1
Rouzé H., Lecellier G., Pochon X., Torda G., Berteaux-Lecellier V. (2019). Unique quantitative Symbiodiniaceae signature of coral colonies revealed through spatio-temporal survey in Moorea. Sci. Rep. 9 (1), 1–11. doi: 10.1038/s41598-019-44017-5
Rouzé H., Lecellier G., Saulnier D., Berteaux-Lecellier V. (2016). Symbiodinium clades A and D differentially predispose Acropora cytherea to disease and Vibrio spp. colonization. Ecol. Evol. 6 (2), 560–572. doi: 10.1002/ece3.1895
Rowan R. (2004). Thermal adaptation in reef coral symbionts. Nature 430 (7001), 742–742. doi: 10.1038/430742a
Russnak V., Rodriguez-Lanetty M., Karsten U. (2021). Photophysiological tolerance and thermal plasticity of genetically different symbiodiniaceae endosymbiont species of cnidaria. Front. Mar. Science. 8. doi: 10.3389/fmars.2021.657348
Sampayo E. M., Ridgway T., Bongaerts P., Hoegh-Guldberg O. (2008). Bleaching susceptibility and mortality of corals are determined by fine-scale differences in symbiont type. Proc. Natl. Acad. Sci. United States America 105 (30), 10444–10449. doi: 10.1073/pnas.0708049105
Sampayo E. M., Ridgway T., Franceschinis L., Roff G., Hoegh-Guldberg O., Dove S. (2016). Coral symbioses under prolonged environmental change: Living near tolerance range limits. Sci. Rep. 6 (November), 1–12. doi: 10.1038/srep36271
Scharfenstein H. J., Chan W. Y., Buerger P., Humphrey C., van Oppen M. J. H. (2022). Evidence for de novo acquisition of microalgal symbionts by bleached adult corals. ISME J. 16 (6), 1676–1679. doi: 10.1038/s41396-022-01203-0
Shaaban A. M., Tim R. M., Brian E. H., Isabelle M. C., Lance L. (2015). Growth and corallite characteristics of Kenyan scleractinian corals under the influence of sediment discharge. Int. J. Biodiversity Conserv. 7 (8), 364–377. doi: 10.5897/ijbc2015.0814
Shaver E. C., McLeod E., Hein M. Y., Palumbi S. R., Quigley K., Vardi T., et al. (2022). A roadmap to integrating resilience into the practice of coral reef restoration. Global Change Biol. 28 (16), 4751–4764. doi: 10.1111/gcb.16212
Shinzato C., Zayasu Y., Kanda M., Kawamitsu M., Satoh N., Yamashita H., et al. (2018). Using seawater to document coral-zoothanthella diversity: A new approach to coral reef monitoring using environmental DNA. Front. Mar. Sci. 5 (FEB). doi: 10.3389/fmars.2018.00028
Silverstein R. N., Cunning R., Baker A. C. (2015). Change in algal symbiont communities after bleaching, not prior heat exposure, increases heat tolerance of reef corals. Global Change Biol. 21 (1), 236–249. doi: 10.1111/gcb.12706
Stat M., Gates R. D. (2011). Clade D Symbiodinium in scleractinian corals: A “Nugget” of Hope, a selfish opportunist, an ominous sign, or all of the above? J. Mar. Biol. 2011, 1–9. doi: 10.1155/2011/730715
Stat M., Pochon X., Franklin E. C., Bruno J. F., Casey K. S., Selig E. R., et al. (2013). The distribution of the thermally tolerant symbiont lineage (Symbiodinium clade D) in corals from Hawaii: Correlations with host and the history of ocean thermal stress. Ecol. Evol. 3 (5), 1317–1329. doi: 10.1002/ece3.556
Suggett D. (2008). Photosynthesis and production of hydrogen peroxide by Symbiodinium (Pyrrhophyta) Phylotypes with different thermal tolerances. J. Phycology 44, 948–956. doi: 10.1111/j.1529-8817.2008.00537.x
Suzuki G. (2021). Sustainable large-scale coral restoration by establishing “artificial spawning hotspots”. Bulletine Japanese Fisheries Res. Educ. Agency 50 (50), 91–100.
Suzuki G., Yamashita H., Kai S., Hayashibara T., Suzuki K., Iehisa Y., et al. (2013). Early uptake of specific symbionts enhances the post-settlement survival of Acropora corals. Mar. Ecol. Prog. Ser. 494 (October), 149–158. doi: 10.3354/meps10548
Swain T. D., Lax S., Gilbert J., Backman V., Marcelino L. A. (2021). A phylogeny-informed analysis of the global coral-Symbiodiniaceae interaction network reveals that traits correlated with thermal bleaching are specific to symbiont transmission mode. MSystems 6 (3), e00266-21. doi: 10.1128/msystems.00266-21
Sweet M. J. (2014). Symbiodinium diversity within Acropora muricata and the surrounding environment. Mar. Ecol. 35 (3), 343–353. doi: 10.1111/maec.12092
Thinesh T., Meenatchi R., Jose P. A., Kiran G. S., Selvin J. (2019). Differential bleaching and recovery pattern of southeast Indian coral reef to 2016 global mass bleaching event: Occurrence of stress-tolerant symbiont Durusdinium (Clade D) in corals of Palk Bay. Mar. pollut. Bull. 145 (May), 287–294. doi: 10.1016/j.marpolbul.2019.05.033
Thirukanthan S. C., Azra M. N., Lananan F., Sara G., Grinfelde I., Rudovica V., et al. (2023). The evolution of coral reef under changing climate: A scientometric review. Animal. 13 (5), 949. doi: 10.3390/ani13050949
True J. D. (2012). “Salinity as a structuring force for near shore coral communities,” in Proceedings of the 12th International Coral Reef Symposium, Cairns, Australia, James Cook University, 9-13 July 2012.
Tuttle L. J., Donahue M. J. (2022). Effects of sediment exposure on corals: a systematic review of experimental studies. Environ. Evidence 11 (1), 1–33. doi: 10.1186/s13750-022-00256-0
van Oppen M. J. H., Gates R. D., Blackall L. L., Cantin N., Chakravarti L. J., Chan W. Y., et al. (2017). Shifting paradigms in restoration of the world’s coral reefs. Global Change Biol. 23 (9), 3437–3448. doi: 10.1111/gcb.13647
Van Oppen M. J. H., Medina M. (2020). Coral evolutionary responses to microbial symbioses. Philos. Trans. R. Soc. B: Biol. Sci. 375 (1808), 20190591. doi: 10.1098/rstb.2019.0591
Villanueva R. D., Baria M. V. B., dela Cruz D. W. (2012). Growth and survivorship of juvenile corals outplanted to degraded reef areas in Bolinao-Anda Reef Complex, Philippines. Mar. Biol. Res. 8 (9), 877–884. doi: 10.1080/17451000.2012.682582
Viyakarn V., Chavanich S., Raksasab C., Loyjiw T. (2009). New coral community on a breakwater in Thailand. Coral Reefs 28 (2), 427. doi: 10.1007/s00338-008-0453-9
Wang C., Zheng X., Li Y., Sun D., Huang W., Shi T. (2022). Symbiont shuffling dynamics associated with photodamage during temperature stress in coral symbiosis. Ecol. Indic. 145, 109706. doi: 10.1016/j.ecolind.2022.109706
Ware M., Garfield E. N., Nedimyer K., Levy J., Kaufman L., Precht W., et al. (2020). Survivorship and growth in staghorn coral (Acropora cervicornis) outplanting projects in the Florida Keys National Marine Sanctuary. PloS One 15 (5), 1–27. doi: 10.1371/journal.pone.0231817
Weil E., Hammerman N. M., Becicka R. L., Cruz-Motta J. J. (2020). Growth dynamics in Acropora cervicornis and A. prolifera in southwest Puerto Rico. PeerJ 2020 (2), 1–23. doi: 10.7717/peerj.8435
Wham D. C., Ning G., LaJeunesse T. C. (2017). Symbiodinium glynnii sp. nov., a species of stress-tolerant symbiotic dinoflagellates from pocilloporid and montiporid corals in the Pacific Ocean. Phycologia 56, 396–409. doi: 10.2216/16-86.1
Yamashita H., Suzuki G., Hayashibara T., Koike K. (2013). Acropora recruits harbor “rare” Symbiodinium in the environmental pool. Coral Reefs 32 (2), 355–366. doi: 10.1007/s00338-012-0980-2
Yamashita H., Suzuki G., Kai S., Hayashibara T., Koike K. (2014). Establishment of coral-algal symbiosis requires attraction and selection. PloS One 9 (5), e97003. doi: 10.1371/journal.pone.0097003
Yorifuji M., Harii S., Nakamura R., Fudo M. (2017). Shift of symbiont communities in Acropora tenuis juveniles under heat stress. PeerJ 2017 (12), 1–22. doi: 10.7717/peerj.4055
Yorifuji M., Yamashita H., Suzuki G., Kawasaki T., Tsukamoto T., Okada W., et al. (2021). Unique environmental Symbiodiniaceae diversity at an isolated island in the northwestern Pacific. Mol. Phylogenet. Evol. 161, 107158. doi: 10.1016/j.ympev.2021.107158
Yuyama I., Harii S., Hidaka M. (2012a). Algal symbiont type affects gene expression in juveniles of the coral Acropora tenuis exposed to thermal stress. Mar. Environ. Res. 76, 41–47. doi: 10.1016/j.marenvres.2011.09.004
Yuyama I., Ito Y., Watanabe T., Hidaka M., Suzuki Y., Nishida M. (2012b). Differential gene expression in juvenile polyps of the coral Acropora tenuis exposed to thermal and chemical stresses. J. Exp. Mar. Biol. Ecol. 430–431, 17–24. doi: 10.1016/j.jembe.2012.06.020
Yuyama I., Nakamura T., Higuchi T., Hidaka M. (2016). Different stress tolerances of juveniles of the coral Acropora tenuis associated with clades C1 and D Symbiodinium. Zoological Stud. 55, 1–9. doi: 10.6620/ZS.2016.55-19
Keywords: coral, endosymbiont, gamete, juveniles, coral culture, sexual propagation, ontogeny, resilience
Citation: Jandang S, Viyakarn V, Yoshioka Y, Shinzato C and Chavanich S (2024) Ontogenetic shifts in Symbiodiniaceae assemblages within cultured Acropora humilis across hatchery rearing and post-transplantation phases. Front. Mar. Sci. 11:1138021. doi: 10.3389/fmars.2024.1138021
Received: 05 January 2023; Accepted: 08 January 2024;
Published: 26 January 2024.
Edited by:
Hajime Kayanne, The University of Tokyo, JapanReviewed by:
Héloïse Louise Rouzé, University of Guam, GuamShashank Keshavmurthy, Academia Sinica, Taiwan
Copyright © 2024 Jandang, Viyakarn, Yoshioka, Shinzato and Chavanich. This is an open-access article distributed under the terms of the Creative Commons Attribution License (CC BY). The use, distribution or reproduction in other forums is permitted, provided the original author(s) and the copyright owner(s) are credited and that the original publication in this journal is cited, in accordance with accepted academic practice. No use, distribution or reproduction is permitted which does not comply with these terms.
*Correspondence: Suppakarn Jandang, U3VwcGFrYXJuLmpAcmlhbS5reXVzaHUtdS5hYy5qcA==; Suchana Chavanich, U3VjaGFuYS5jQGNodWxhLmFjLnRo
†Present address: Yuki Yoshioka, Marine Genomics Unit, Okinawa Institute of Science and Technology Graduate University, Okinawa, Japan
Suppakarn Jandang, Center for Ocean Plastic Studies, Research Institute for Applied Mechanics, Kyushu University, Kasuga-shi, Japan