- 1Environmental Biochemistry Group, Institute for Chemistry and Biology of the Marine Environment (ICBM), Carl von Ossietzky University Oldenburg, Wilhelmshaven, Germany
- 2Alfred Wegener Institute, Helmholtz Centre for Polar and Marine Research, Bremerhaven, Germany
- 3Helmholtz Institute for Functional Marine Biodiversity at the University of Oldenburg (HIFMB), Oldenburg, Germany
Sexually produced juvenile scleractinian corals play a key role in the adaptation process of coral reefs, as they are considered to possess an innate plasticity and thus can adjust to changing environmental parameters within a certain range. In this study we investigated in detail the early life stages of the brooding species Leptastrea purpurea to identify, categorize and visualize the critical steps of the complex transformation process from a swimming coral larva to a sessile coral recruit and later to a coral colony. For that, we performed settlement experiments using previously known cues: cycloprodigiosin (CYPRO) and crustose coralline algae (CCA) as well as novel cues: crude extracts of Pseudoalteromonas espejiana and P. piscicida to identify a general, cue-independent settlement pathway. We monitored the development of L. purpurea over 12 months using bright field and fluorescence microscopy. Also we identified the fluorescence signals of L. purpurea with confocal microscopy at four crucial development steps: (A) swimming larva, (B) metamorphosing larva, (C) coral recruit and (D) adult coral. Our methodological approach allowed us to observe an ontogenetic shift of fluorescence signals which provokes the hypothesis that certain fluorescence patterns might be connected to distinct sequential functions in the early life cycle of scleractinian corals. Our observations showed great similarities to the early development of other brooding and spawning corals, making L. purpurea a prospective candidate to be used as a model organism for coral research. Furthermore, our in-depth picture series provides a robust monitoring reference for coral nurseries or field applications and demonstrates the potential of fluorescence as an indicator to instantly determine the growth stage of a developing coral recruit.
1 Introduction
The adaptive processes of reef-building scleractinian corals in a rapidly changing environment, and thus the survival of coral reef communities, depends on sexually produced recruits (Edmunds, 2004; Negri et al., 2007; Hoegh-Guldberg, 2014; Randall et al., 2020). Juvenile scleractinian corals have greater plasticity than adult colonies to take up symbiotic partners that are adapted to the prevailing environmental conditions (Berkelmans and Van Oppen, 2006; Abrego et al., 2009; Byler et al., 2013; Yamashita et al., 2014). The resulting newly formed symbiosis between the juvenile coral and its symbiotic algae might be better prepared to deal with more routinely occurring heat waves (Cumbo et al., 2018). Up to this day, most studies investigate the impacts of anthropogenic stressors on adult scleractinians, however, the key for their survival may likely lie at the beginning of their life cycle (Bauman et al., 2015).
Mature planula larvae are usually elongated along the oral-aboral axis and swim actively with their ciliated body orienting their aboral end in front (Harrison and Wallace, 1990). During this stage, they have developed an ectoderm, endoderm, mesogleal layer and a pharynx as shown in Figures 1A, A’ (i.e., Fadlallah, 1983; Heltzel and Babcock, 2002; Hirose et al., 2008). Planula larvae reach competence when they gain the ability to respond to external cues initiating their settlement process (Gleason and Hofmann, 2011). Competent swimming larvae generally orient towards the ground to search for a suitable settlement substrate (Szmant and Meadows, 2006; Vermeij et al., 2009). Coral larval settlement is commonly defined as the combination of the two succeeding steps (1) attachment to a suitable substrate and (2) the metamorphosis and irreversible transformation into a coral recruit (Ritson-Williams et al., 2009). Swimming coral larvae contact the desired substratum with their aboral end and fuse with the settlement ground due to a calcification reaction that is triggered within the calicoblastic ectoderm (Hayward et al., 2011). The metamorphosis starts with a contraction of the larvae along the oral-aboral axis into a disc shape that is radially divided through six (in hexacorallia) primary mesenteries, which developed from gastrodermal and mesoglea cells within the larvae (Figures 1B, B’; Harrison and Wallace, 1990; Hirose et al., 2008). With further growth, the metamorphosed larvae continues to form a calcified basal plate, which is subsequently divided into segments by the septa of the primary circle (Neves and Da Silveira, 2003; Hayward et al., 2011). The oral pore of the metamorphosed larvae develops by invagination of the outer epidermal layer (Hirose et al., 2008). Within a circular shape around the oral pore, the first six (in hexacorallia) tentacle buds develop which later continue into functional tentacles (Harrison and Wallace, 1990). Furthermore, a protective theca is formed to stabilize the polyp upwards from the basal plate (Figures 1C, C’; Neves and Da Silveira, 2003). The recruit development is continued by the formation of additional circles of tentacles as well as mesenteries and septae between the primary structures (Harrison and Wallace, 1990; Neves and Da Silveira, 2003). These juvenile corals start to form a new colony by budding. Extra-tentacular budding defines the development of daughter polyps outside of the parental corallite, while inter-tentacular budding means the development inside the corallite (Matthai, 1926).
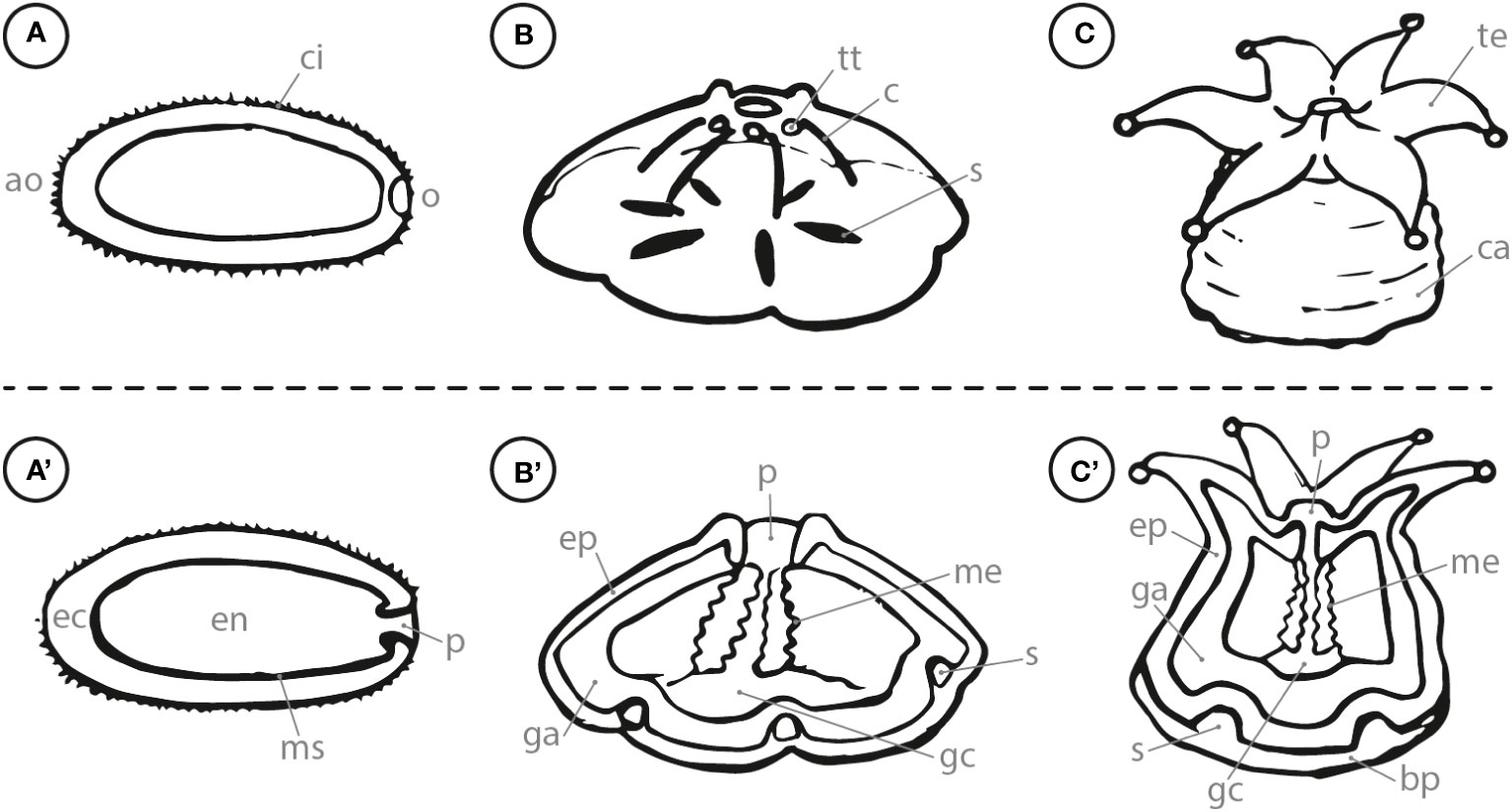
Figure 1 Side view (letter) and cross section (letter with apostrophe) of (A) swimming planula coral larva; (B) attached, metamorphosing larva; (C) coral recruit. ao, aboral; o, oral; ci, cilia; ec, ectoderm; en, endoderm; ms, mesogleal layer; p, pharynx; tt, tentacle tip; c, constriction; ep, epiderm; ga, gastroderm; me, mesenteries; s, septa; gc, gastrovascular cavity; te, tentacle; ca, calyx; bp, basal plate.
The fluorescent nature of scleractinian corals via their fluorescence proteins (FPs), has been described in prior studies (Salih et al., 2000; Dove et al., 2001; Alieva et al., 2008; Gruber et al., 2008). The most common FPs in scleractinian corals are green FPs (GFPs). However, some scleractinians contain red FPs (RFPs) and cyan FPs (CFPs) as well (reviewed by Roth, 2014). While the appearance of FPs is obvious, their role in scleractinian corals is still heavily debated. Some studies assume that the fluorescence could act as photoprotection, especially from high intensity UV radiation in shallow reef zones (Salih et al., 2000; Dove et al., 2001; Vermeij et al., 2002; Bou-Abdallah et al., 2006; Palmer et al., 2009). However, the high diversity of FPs as well as their specific localization within the coral may be related to specific functions (i.e., Gruber et al., 2008; Roth, 2014). Although the functions of FPs remain vague, fluorescence in general can act as optical indicator for corals. For example, deviations of the normal fluorescence can be used to determine the health state of corals (D’Angelo et al., 2012; Caldwell et al., 2017). In earlier life stages, such as the larval or the recruit phase, the fluorescence properties helped in identifying larvae from other floating organic particles (Nietzer et al., 2018), as well as facilitated the detection of newly settled recruits in field applications (Piniak et al., 2005; Baird et al., 2006). To this day, only a few studies investigated patterns of fluorescence distribution, development and changes during the transition of larval towards recruit and juvenile life-stages (Strader et al., 2016; Strader et al., 2018; Haryanti and Hidaka, 2019). These studies described a shift in fluorescence patterns during early development stages in different scleractinian species.
Investigating the early settlement behavior patterns of scleractinian corals is often limited by the difficult access to sexually reproduced coral larvae. Furthermore, using broadcast spawned coral larvae won’t allow continuous experimental series over longer time periods, since the planulae are only storable for a limited time and with high maintenance efforts. Here, we used the brooding coral Leptastrea purpurea (Dana, 1846), capable of releasing larvae on a daily basis and with similar settlement behavior as other widespread scleractinian coral species (Nietzer et al., 2018). Thus, the constant supply of larvae makes L. purpurea an ideal research organism to gain a wholistic view of the behavioral patterns as well as the underlying mechanism of larval settlement in scleractinian corals (Nietzer et al., 2018; Petersen et al., 2023). In this study, we followed in detail the settlement behavior and early development of L. purpurea larvae/recruits for not only 48 hours (i.e., Heyward and Negri, 1999; Kitamura et al., 2007; Tebben et al., 2011; Sneed et al., 2014; Tebben et al., 2015) but over a period of more than one year, covering all critical stages of the early post-settlement survival bottleneck (i.e., Ritson-Williams et al., 2010; Randall et al., 2020). This long-term monitoring approach was accompanied by intensive observations using light- and fluorescence-microscopic as well as confocal imaging to capture all key stages of early coral recruit development. Ultimately, this study aims to provide a reference framework for early development of scleractinian corals in general.
2 Material and methods
2.1 Collection of coral larvae
All collected coral larvae were obtained from 27 L. purpurea brood stock colonies located in the in-house aquarium system of the ICBM-Terramare in Wilhelmshaven, Germany. These colonies were originally obtained from parental colonies of the Luminao reef in Guam, USA (for more detail see Nietzer et al., 2018). The coral larvae collection was performed using the same protocol as described by Petersen et al. (2021b). In brief, the parental colonies were placed into a 1.5 L plastic container at a constant water temperature of 27°C. To guarantee an adequate oxygenation of the water body, a 6 mm thick tube with a constant air flow was attached. The next day, the adult colonies were placed back into the aquarium system, while the emitted coral larvae remained in the container. To aid the collection process, a blue flashlight (NIGHTSEA-bluestar, USA), emitting wavelength of 430-460 nm in combination with yellow barrier filter foil (#100 - Spring Yellow, Lee Filters, UK) was used to stimulate the GFPs expressed by the L. purpurea larvae (Nietzer et al., 2018; cf. Figure S1). The larvae were collected using a pipette and placed in a glass beaker filled with filtered artificial sea water (FASW, Tropic Marin® Pro-Reef salt, Prof. Dr. Biener GmbH, Wartenberg, Germany; 0.2 μm nylon syringe filter from Rotilabo, Germany) from the aquarium system and kept at 27°C.
2.2 Coral settlement experiments
All settlement experiments were performed with L. purpurea planula larvae that were not older than 7 days. However, exchanging the FASW on a weekly basis was performed to maintained sufficient water quality. Under these conditions, larvae kept swimming for longer than one month. Different settlement cues including cycloprodigiosin (CYPRO) and crude extracts derived from several Pseudoalteromonas strains were tested. A summary of settlement cues that caused morphological changes of coral larvae is presented in Table 1. For more details of the settlement experimental set-up see Petersen et al. (2021b). In brief, each tested settlement cue solved in methanol (MeOH) or pure MeOH as negative control was applied the following way: (1) target compounds were distributed evenly over the bottom of each well using a 20 µL pipette (Eppendorf, Germany) (2) the organic solvent was evaporated completely before (3) 1 mL of FASW was added to each well and (4) three coral larvae were added using a pipette (cf. Table 1) and finally (5), the well was filled with FASW to a final volume of 5 mL. To prevent the premature degradation of the respective settlement cues, assay preparation was performed under reduced light conditions. With each experiment, a negative solvent control, containing just MeOH and no extract, was applied as well. All experiments were conducted in an incubator (Certomat BS-1, Satorius, Switzerland) to maintain a constant temperature of 27°C. Furthermore, the incubator was set to alternating 12-hour dark and light conditions to mimic the natural night and day cycle. Note that all settlement experiments started with a 12 h dark cycle.
2.3 Microbial growth conditions, biofilm harvest and performed extraction
In general, the target Pseudoalteromonas colonies (cf. Table 1) were inoculated from cryo-conserved stocks onto agar plates (8.5 cm in diameter, BRAND, Germany). After 48 hours the bacterial colonies were picked and transferred into a liquid marine broth media (MB, Roth, Germany). 24 h later the liquid stock cultures were used to inoculate agar plates of the respective Pseudoalteromonas bacterial strain and incubated in complete darkness at room temperature (cf. Table 1). After 24 h, the bacterial lawn was scraped off the plate and the resulting cell paste was transferred to a 20 mL scintillation vial (BRAND, Germany) pre-filled with 10 mL of MeOH. The solution was placed in an ultra-sonic bath for five minutes and after that, the cell paste was homogenized at 12,000 rpm for 30 s using a T25 Ultra Turrax (IKA Werke GmbH & Co. KG, Germany). Finally, the homogenized crude extract was filtered through a 3 hw cellulose filter paper (Sartorius AG, Germany) and dried using a speed-vac system (Martin Christ Gefriertrocknungsanlagen GmbH, Germany). The dried, cell-free crude extract was dissolved in MeOH and brought to a concentration of 10 mg L-1. Only for the crude extract of Pseudoalteromonas rubra a purification process towards a single potent coral settlement compound was obtained (i.e., CYPRO, Petersen et al., 2021b). In brief, the isolation of the red pigment CYPRO was performed by a combined approach of liquid-liquid partitioning (LLP), a C18-based solid-phase extraction (SPE), and finally high-performance liquid chromatography using a semi-preparative C18-column and a diode array detector (HPLC-DAD). Note that CYPRO was not present in the crude extracts of isolates #2165 and #1641 (see Figure S2). All chemical extracts and compounds were stored in complete darkness at -20°C.
2.4 Monitoring of coral larvae and their developing recruits
All experiments were monitored using at least one of the four types of microscopes listed in Supplementary Table 1. Pictures of the developmental stages were mostly taken with a two mega pixel USB endoscope camera (Teslong, Shenzhen, China) with 200x magnification and six white LED lights. To monitor the changing fluorescence in coral larvae and recruits within different life stages, the combination of blue light, emitting wavelengths between 430-460 nm, and a yellow filter foil was used to visualize the autofluorescence of the corals (cf. Figure S1). For the illustration of the symbiont distribution in early development phases (Figure S3), pictures were taken with a 12MP G7 (Motorola, China) phone camera coupled with a DMIL LED inverse microscope (Leica, Germany).
For confocal measurements, individuals of each development step (i.e., swimming larvae, metamorphosing larvae, coral recruit and adult coral) that displayed the common fluorescence patterns (cf. Figure 2) were chosen. For confocal microscopy we used the laser scanning microscope TCSSP5 (Leica, Germany). The excitation wavelengths were set to 405 nm, 458 nm, 476 nm, 488 nm, 514 nm and 561 nm with the measured emission ranging up to 790 nm, whereas the starting point was dependent on the excitation wavelength. Data points were taken in approximately 3 nm intervals.
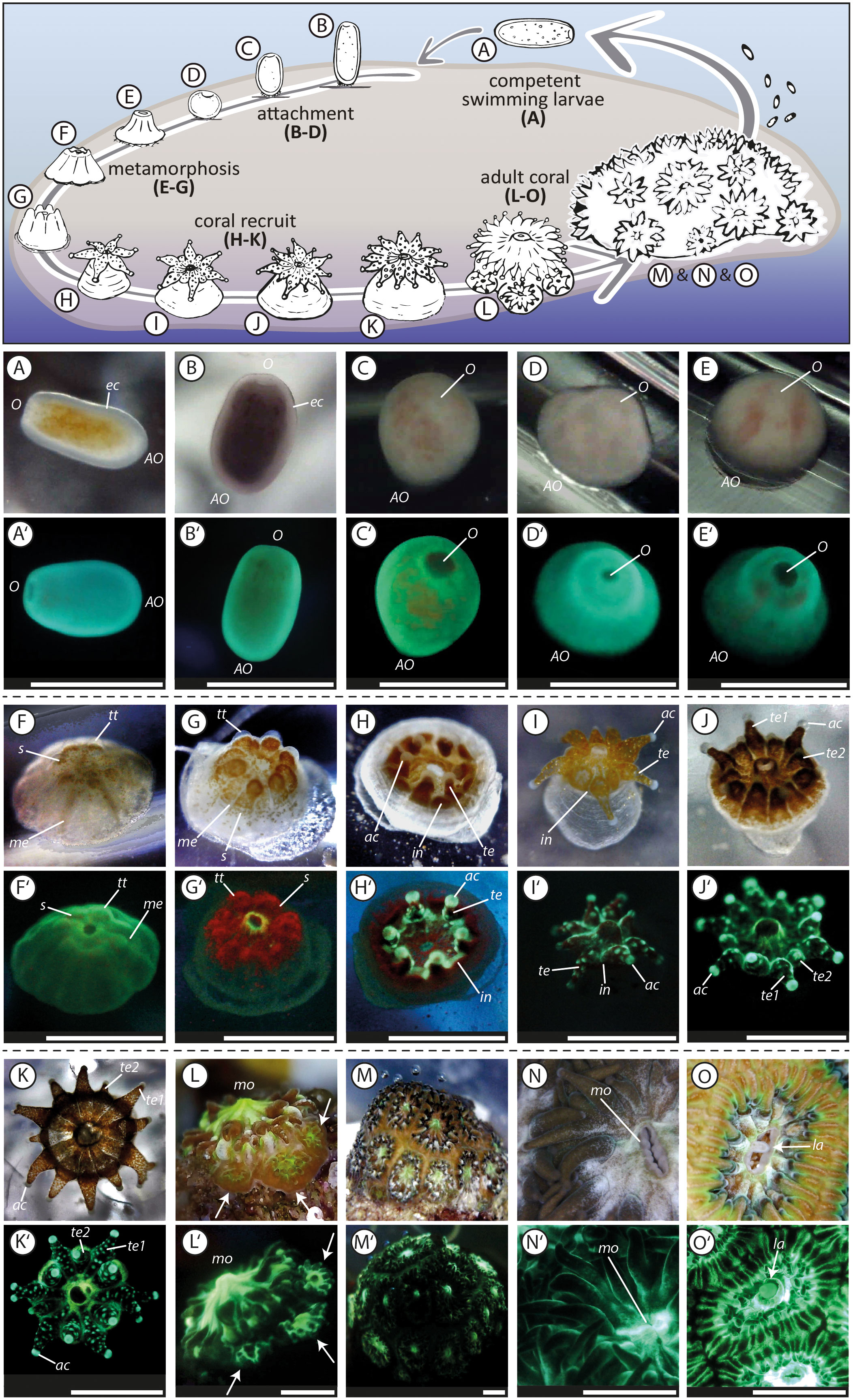
Figure 2 Life cycle of the brooding scleractinian coral Leptastrea purpurea (A, A’–O, O’). The first pictures (A, A’) were taken directly after larvae collection, the pictures (K, K’) of a juvenile polyp with 12 tentacles were taken 50 days after the initiation of the settlement experiment, the pictures (L, L’) of an juvenile coral, that started to form a colony by extra-tentacular budding, were taken after 130 days, pictures (M, M’) show the whole coral colony that has grown for one year, pictures (N, N’) show polyps of parental colonies and (O, O’) captured the release of brooded coral larvae trough the mouth of the adult polyp. The two pictures with the same letter indicate the same settlement stage but taken with a different light set-up. The picture with the plane letter was taken using white LED light, whereas the picture containing letter and apostrophe was taken using solely blue light (wavelength: 430-460 nm) and the emitted light was captured through a yellow barrier filter (see Figure S1). O, oral region; AO, aboral region; ec, ectoderm; s, symbionts; tt, tentacle tips; me, mesenteries; te, completely developed tentacle (te1, te2, primary and secondary tentacle); ac, acrospheres; in, interspaces; mo, mouth; la, larvae. Scale bar: 1mm.
3 Results and discussion
To expand current knowledge of the complex transformation of free-swimming planula larvae into sessile benthic juvenile corals, we examined in detail the early lifecycle of approximately 200 L. purpurea coral larvae. The settlement behavior of the larvae and the further morphological development of the recruits were observed over a period of 12 month and recorded in more than 5,000 images and 50 videos. These resulted in an unprecedentedly precise analysis of the different life stages in the L. purpurea settlement cascade which has been summarized in Figure 2. Our goal is to document the most important developmental steps, such as a planula coral larva (Figures 2A, A’) transforming into a coral recruit (Figures 2K, K’ recruit after 50 days) and finally forming a colony by extra-tentacular budding (Figures 2L, L’ colony after 130 days). The following four aspects that have caught our attention during our long-term monitoring approach are covered:
● First continuous, detailed observation of early coral development exceeding one year
● Different tested settlement cues reveal a unitary coral development pathway
● Fluorescence as tool to determine development and health state of coral larvae/recruits
● Species independent communalities in coral development - Leptastrea purpurea an ideal candidate for coral research
3.1 First continuous, detailed observation of early coral development exceeding one year
Among the latter tested settlement inducing cues, we analyzed in detail the most critical steps of the complex transformation process from a swimming coral larva to a sessile coral colony. This common settlement pathway of L. purpurea larvae is presented in Figure 2. We divided the settlement cascade of L. purpurea into: (1) swimming and searching behavior (Supplementary Movie 1), (2) attachment, (3) metamorphosis (Supplementary Movie 2), (4) tentacle development and (5) formation of a new colony by the beginning of extra-tentacular budding. A definition and description of each development phase (1 - 5) is described extensively in the supplemental material. To some degrees, these development patterns were already observed and identified by previous studies, using also other scleractinian species. However, no continuous detailed long-term monitoring approaches has been conducted. For example, previous studies described competent swimming coral larvae as “pear-shaped”, “barrel-shaped”, “elongated” or “rod-like” with the ability to alter their form and shape (e.g., Fadlallah, 1983; Heltzel and Babcock, 2002; Hirose et al., 2008; cf., Figure 2A). Furthermore, “ball-shaped” Fungia scutaria larvae started to attach on known settlement substrates (Schwarz et al., 1999), similar to our observations (i.e., attached larvae appeared in a round, ball-shaped form, Figures 2C, D). However, we also observed that freshly attached larvae maintained an elongated form (cf. Figure 2B), indicating that the change in shape likely represents the first step in the complex metamorphosis reaction. During the ongoing metamorphosis process, constrictions appear radially, originating from the oral pore, and give the larvae a “flower-”, or “pumpkin-shape”, as already described for L. purpurea and Acropora spp. larvae (Hirose et al., 2008; Nietzer et al., 2018; Moeller et al., 2019) and highlighted here in Figure 2F. Just before their tentacle started to develop, Fungia scutaria larvae were depicted as “volcano-shaped” (Schwarz et al., 1999) and larvae of Acropora millepora at a similar stage as “crown-shaped” (Hayward et al., 2011). These latter two steps in the metamorphosis pathway were also observed in this study, each representing however, sequential steps in the development of L. purpurea (i.e., volcano-shape, Figure 2E followed by crown-shaped, Figure 2G) before their development continued by emerging tentacles (Figure 2H). Furthermore, L. purpurea recruits continued to mature by developing secondary and third rows of septa and tentacles (cf. Figures 2I–K; Supplementary Movies 3, 4) similar to previously described Siderastrea stellata polyps by Neves and Da Silveira (2003). Next, the juvenile coral polyps continued their development with extra-tentacular budding comparably as observed and photographed in Oulastrea crispate polyps by Lam (2000). However, to the best of our knowledge, no continuous, high quality time series (including both, light and fluorescence microscopy) of the entire early development phase towards a coral colony exists up to this date. Therefore, we are convinced that our photographic record of the early life steps of L. purpurea could be of great value to compare and evaluate the development of corals during their most vulnerable early phase of life.
3.2 Different settlement cues reveal an unitary coral development pathway
In our study, settlement was induced using different settlement cues such as CYPRO (derived from P. rubra) and living CCA pieces as well as two novel crude extracts of #2165 (P. espejiana), #1641 (P. piscicida) (cf. Table 1). Most studies, focusing on the settlement behavior of coral larvae, evaluate the settlement success within the first 48 hours of the experiment (i.e., Heyward and Negri, 1999; Kitamura et al., 2007; Tebben et al., 2011; Sneed et al., 2014; Tebben et al., 2015). However, detailed long-term observations are often lacking. In this study, we extended the observation period up to 12 months to document the further development of coral larvae after successful settlement and to evaluate the long-term survival and growth of coral recruits that settled using cues specified on Table 1. So far CYPRO had only been tested for 48 hours (Petersen et al., 2021b; Petersen et al., 2023) while the two bacterial crude extracts are presented for the first time in this study. Our observations indicate that there are no detrimental effects for coral larvae settled with the here tested cues, compared to the regular used CCA fragments. While settlement initiation may vary among the used cues (cf. Table 1), the subsequent development patterns were comparable among all successful treatments. After successful settlement, all larvae developed as described in Figure 2 and Section 3.1.
3.3 Fluorescence as tool to determine development and health state of coral larvae/recruits
In our experiment, all collected L. purpurea larvae were green fluorescent (cf. Figure 2) with a fluorescence peak at 507 nm (cf. Figure 3) and settled shortly after exposure to the used settlement cue (cf. Table 1). For swimming planula larvae of several coral species, fluorescence seems to be an optical indicator for their settlement competence (e.g. Puisay et al., 2021). For example, Strader et al. (2016) indicated a diapause-like state in red fluorescing Acropora millepora coral larvae, which might be responsible for their longer dispersal. Later, Strader et al. (2018) showed a correlation of decreasing red and increasing green fluorescence in A. millepora coral larvae as possible indicators for larval settlement competence, which would also agree with our observations. Kenkel et al. (2011) investigated the fluorescence patterns of adult A. millepora colonies of different phenotypes as indicator for the settlement success of their larvae. The results showed that larvae from red parental phenotypes are less successful in settlement, compared to larvae from green phenotypes. Moreover, Puisay et al. (2021) showed that red fluorescing Pocillopora acuta larvae were dispersed over larger distances, compared to green fluorescing larvae, that settled shortly after their release. Our data, together with the results of the previous mentioned studies, strengthen the hypothesis that the fluorescing character of coral larvae may be used to predict their short-term settlement success.
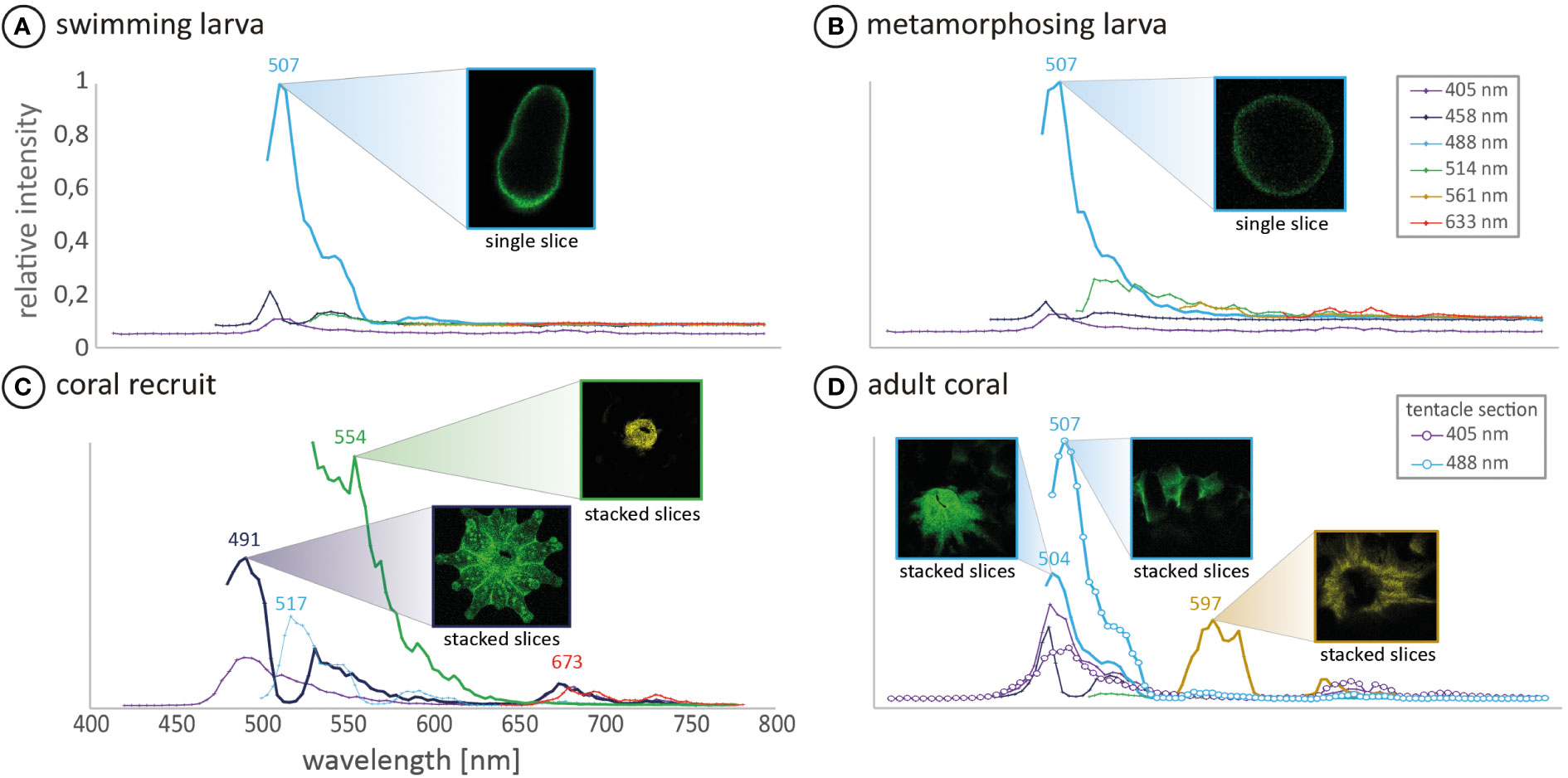
Figure 3 In vivo fluorescence spectra of (A) a Leptastrea purpurea coral larva and (B) a 48h-old metamorphosed coral larva (C) coral recruit (D) focuses on the mouth and tentacle section of a single coral polyp from an adult colony. The measured individuals of each development step displayed the common development patterns and were therefore chosen for confocal analysis. All confocal runs were excited with four different laser wavelengths of 405, 458, 488 and 514 nm. Additionally, (A, B, D) were excited with 561 nm and stage (A–C) with 633 nm. The corresponding fluorescence was recorded over a wavelength range of 420-800 nm. The fluorescence images correspond to the highest measured fluorescence of the respective emission wavelength. Note that mean intensities are not comparable between the different samples but display the fluorescence composition of the respective sample.
In later development stages, L. purpurea coral recruits show different fluorescence patterns compared to just settled larvae (Figure 2G’ compared to 2K’ and Figure 3B compared to 3C). Comparatively, Roth and colleagues (2013) and Haryanti and Hidaka (2019) observed an ontogenetic shift of fluorescence during the development of Seriatopora hystrix, Acropora tenuis, Pocillopora damincornis and Isopora palifera larvae into recruits. These authors hypothesized that the fluorescence likely fulfills different functions and therefore changes within the early life stages of larvae/recruits. In this study, we also observed clear changes in the fluorescence wavelength as well as in its spatial distribution during the transformation reaction of swimming larvae into benthic coral recruits of L. purpurea (cf. Figures 2, 3, detailed description in the supplementary material). These distinct patterns of changing fluorescence in particular anatomic structures lead to the hypothesis, that the fluorescence could have distinct multiple functions during the coral development.
Besides indicating the stage of development, the here pictured sequential fluorescence patterns could be useful to identify the tiny recruits in the field or within aquaculture applications (Piniak et al., 2005; Baird et al., 2006). Also, fluorescence-based observation could be an easy and relatively quick method for checking presence and absence as well as localizing phototrophic symbionts in the swimming or metamorphosing larvae (cf. Figure S3). Furthermore, the use of fluorescence patterns might be a simple but powerful method to assess the health state of juvenile corals, since fluorescence has shown to be an pre-indicator for bleaching stress (Haryanti and Hidaka, 2019). However, it is not clear if the patterns presented here in fluorescence distribution are representative for other coral species nor all species within the genus Leptastrea. That is, more research is needed to investigate the function of fluorescence in corals and in particular the sequential changes during the early larvae to recruit transformation.
3.4 Species independent communalities in coral development - Leptastrea purpurea an ideal candidate for coral research
The similarities in the development of L. purpurea to the latter scleractinian brooding and spawning species (as described in Section 3.1) provides further evidence for the use of this species as model organism in stony coral biology (cf., Nietzer et al., 2018). Daily larval supply over the whole year provides the opportunity for testing coral larvae and/or recruits, including settlement assays (Moeller et al., 2017; Nietzer et al., 2018; Moeller et al., 2019) and ecotoxicological tests (cf., Miller et al., 2022). Further research focusing in detail on the early development of other scleractinian species is necessary to confirm these similarities, as so far only some photographic records of settled or metamorphosed coral larvae are documented (e.g. Negri et al., 2001; Neves and Da Silveira, 2003; Kitamura et al., 2007; Hirose et al., 2008; Hayward et al., 2011; Tebben et al., 2015; Petersen et al., 2021b).
4 Conclusion
In summary, our study provides an unprecedented, detailed description of the first year after larval settlement of the brooding coral Leptastrea purpurea observed via light/fluorescence microscopy and confocal imaging. Regardless of the here used biochemical settlement cues, L. purpurea recruits followed a general developmental pathway that closely reassembles literature data from other brooding as well as spawning scleractinian coral species. This provides further evidence for the use of this species as a benchmark for coral research. Finally, we would like to encourage coral researchers to include fluorescence in future monitoring studies, as it is a simple tool and based on our observation a crucial parameter in coral development.
Data availability statement
The original contributions presented in the study are included in the article/Supplementary Material. Further inquiries can be directed to the corresponding authors.
Author contributions
LF, MK, L-EP, and PS designed the project. LF and MK did literature review. LF and MK did initial writing of the manuscript. LF and SN conducted the larval acquisition. LF, MK, and L-EP performed settlement experiments and long-term monitoring using light and fluorescence microscopy. LF, MS, MK, and UB performed confocal microscopy experiments. SN maintained the aquarium facilities. SN, L-EP, MS, UB, and PS reviewed and edited the manuscript. All authors contributed to the article and approved the submitted version.
Funding
This study was partly supported by the forschen@studium program by the Carl von Ossietzky Universität Oldenburg. PS also acknowledges funding by the Deutsche Forschungsgemeinschaft (DFG) for the last instrument grant INST 184/147-1FUGG for the high-resolution mass spectrometer Waters Synapt G2-Si.
Acknowledgments
We thank Petra Schwarz and Birgit Brinkmann for assisting with various technical issues in the laboratory and Dzikrina Nur Fatima for the identification of unknown bacteria.
Conflict of interest
The authors declare that the research was conducted in the absence of any commercial or financial relationships that could be construed as a potential conflict of interest.
Publisher’s note
All claims expressed in this article are solely those of the authors and do not necessarily represent those of their affiliated organizations, or those of the publisher, the editors and the reviewers. Any product that may be evaluated in this article, or claim that may be made by its manufacturer, is not guaranteed or endorsed by the publisher.
Supplementary material
The Supplementary Material for this article can be found online at: https://www.frontiersin.org/articles/10.3389/fmars.2023.984656/full#supplementary-material
References
Abrego D., Van Oppen M. J. H., Willis B. L. (2009). Onset of algal endosymbiont specificity varies among closely related species of acropora corals during early ontogeny. Mol. Ecol. 18, 3532–3543. doi: 10.1111/j.1365-294X.2009.04276.x
Alieva N. O., Konzen K. A., Field S. F., Meleshkevitch E. A., Hunt M. E., Beltran-Ramirez V., et al. (2008). Diversity and evolution of coral fluorescent proteins. PloS One 3, 1–12. doi: 10.1371/journal.pone.0002680
Baird A. H., Salih A., Trevor-Jones A. (2006). Fluorescence census techniques for the early detection of coral recruits. Coral Reefs 25, 73–76. doi: 10.1007/s00338-005-0072-7
Bauman A. G., Guest J. R., Dunshea G., Low J., Todd P. A., Steinberg P. D. (2015). Coral settlement on a highly disturbed equatorial reef system. PloS One 10 (5). doi: 10.1371/journal.pone.0127874
Berkelmans R., Van Oppen M. J. H. (2006). The role of zooxanthellae in the thermal tolerance of corals: A “nugget of hope” for coral reefs in an era of climate change. Proc. R. Soc B Biol. Sci. 273, 2305–2312. doi: 10.1098/rspb.2006.3567
Bou-Abdallah F., Chasteen N. D., Lesser M. P. (2006). Quenching of superoxide radicals by green fluorescent protein. Biochim. Biophys. Acta - Gen. Subj. 1760, 1690–1695. doi: 10.1016/j.bbagen.2006.08.014
Byler K. A., Carmi-Veal M., Fine M., Goulet T. L. (2013). Multiple symbiont acquisition strategies as an adaptive mechanism in the coral stylophora pistillata. PloS One 8 (3). doi: 10.1371/journal.pone.0059596
Caldwell J. M., Ushijima B., Couch C. S., Gates R. D. (2017). Intra-colony disease progression induces fragmentation of coral fluorescent pigments. Sci. Rep. 7 (1), 1–9. doi: 10.1038/s41598-017-15084-3
Cumbo V. R., Van Oppen M. J. H., Baird A. H. (2018). Temperature and symbiodinium physiology affect the establishment and development of symbiosis in corals. Mar. Ecol. Prog. Ser. 587, 117–127. doi: 10.3354/meps12441
Dana J. D. (1846). United States Exploring Expedition During the Years 1838–1842, 7 Philadelphia: Lea and Blanchard, 1–740.
D’Angelo C., Smith E. G., Oswald F., Burt J., Tchernov D., Wiedenmann J. (2012). Locally accelerated growth is part of the innate immune response and repair mechanisms in reef-building corals as detected by green fluorescent protein (GFP)-like pigments. Coral Reefs 31, 1045–1056. doi: 10.1007/s00338-012-0926-8
Dove S. G., Hoegh-Guldberg O., Ranganathan S. (2001). Major colour patterns of reef-building corals are due to a family of GFP-like proteins. Coral Reefs 19, 197–204. doi: 10.1007/PL00006956
Edmunds P. J. (2004). Juvenile coral population dynamics.pdf. Mar. Ecol. Prog. Ser. 269, 111–119. doi: 10.3354/meps269111
Fadlallah Y. H. (1983). Sexual reproduction, development and larval biology in scleractinian corals - a review. Coral Reefs 2, 129–150. doi: 10.1007/BF00336720
Gleason D. F., Hofmann D. K. (2011). Coral larvae: From gametes to recruits. J. Exp. Mar. Bio. Ecol. 408, 42–57. doi: 10.1016/j.jembe.2011.07.025
Gruber D. F., Kao H. T., Janoschka S., Tsai J., Pieribone V. A. (2008). Patterns of fluorescent protein expression in scleractinian corals. Biol. Bull. 215, 143–154. doi: 10.2307/25470695
Harrison P. L., Wallace C. (1990). Reproduction, dispersal and recruitment of scleractinian corals ecosystems of the world. 25: Coral reefs. Ecosyst. World 25 Coral Reefs (Elsevier) 25, 133–207.
Haryanti D., Hidaka M. (2019). Developmental changes in the intensity and distribution pattern of green fluorescence in coral larvae and juveniles. Galaxea J. Coral Reef Stud. 21, 13–25. doi: 10.3755/galaxea.21.1_13
Hayward D. C., Hetherington S., Behm C. A., Grasso L. C., Forêt S., Miller D. J., et al. (2011). Differential gene expression at coral settlement and metamorphosis - a subtractive hybridization study. PloS One 6 (10). doi: 10.1371/journal.pone.0026411
Heltzel P. S., Babcock R. C. (2002). Sexual reproduction, larval development and benthic planulae of the solitary coral monomyces rubrum (Scleractinia: Anthozoa). Mar. Biol. 140, 659–667. doi: 10.1007/s00227-001-0745-x
Heyward A. J., Negri A. P. (1999). Natural inducers for coral larval metamorphosis. Coral Reefs 18, 273–279. doi: 10.1007/s003380050193
Hirose M., Yamamoto H., Nonaka M. (2008). Metamorphosis and acquisition of symbiotic algae in planula larvae and primary polyps of acropora spp. Coral Reefs 27, 247–254. doi: 10.1007/s00338-007-0330-y
Hoegh-Guldberg O. (2014). Coral reef sustainability through adaptation: Glimmer of hope or persistent mirage? Curr. Opin. Environ. Sustain. 7, 127–133. doi: 10.1016/j.cosust.2014.01.005
Kenkel C. D., Traylor M. R., Wiedenmann J., Salih A., Matz M. V. (2011). Fluorescence of coral larvae predicts their settlement response to crustose coralline algae and reflects stress. Proc. R. Soc B Biol. Sci. 278, 2691–2697. doi: 10.1098/rspb.2010.2344
Kitamura M., Koyama T., Nakano Y., Uemura D. (2007). Characterization of a natural inducer of coral larval metamorphosis. Exp. Mar. Biol. Ecol. 340, 96–102. doi: 10.1016/j.jembe.2006.08.012
Lam K. K. Y. (2000). Early growth of a pioneer recruited coral oulastrea crispata (Scleractinia, faviidae) on PFA-concrete blocks in a marine park in Hong Kong, China. Mar. Ecol. Prog. Ser. 205, 113–121. doi: 10.3354/meps205113
Matthai G. (1926). Colony-formation in astraeid corals. Philos. Trans. R. Soc London 214, 313–367. doi: 10.1098/rstb.1926.0008
Miller I. B., Moeller M., Kellermann M. Y., Nietzer S., Di Mauro V., Kamyab E., et al. (2022). Towards the development of standardized bioassays for corals: Acute toxicity of the UV filter benzophenone-3 to scleractinian coral larvae. Toxics 10(5), 244. doi: 10.3390/toxics10050244
Moeller M., Nietzer S., Schils T., Schupp P. J. (2017). Low sediment loads affect survival of coral recruits: The first weeks are crucial. Coral Reefs 36, 39–49. doi: 10.1007/s00338-016-1513-1
Moeller M., Nietzer S., Schupp P. J. (2019). Neuroactive compounds induce larval settlement in the scleractinian coral leptastrea purpurea. Sci. Rep. 9, 1–9. doi: 10.1038/s41598-019-38794-2
Negri A. P., Marshall P. A., Heyward A. J. (2007). Differing effects of thermal stress on coral fertilization and early embryogenesis in four indo pacific species. Coral Reefs 26, 759–763. doi: 10.1007/s00338-007-0258-2
Negri A. P., Webster N. S., Hill R. T., Heyward A. J. (2001). Metamorphosis of broadcast spawning corals in response to bacteria isolated from crustose algae. Mar. Ecol. Prog. Ser. 223, 121–131. doi: 10.3354/meps223121
Neves E. G., Da Silveira F. L. (2003). Release of planula larvae, settlement and development of siderastrea stellata verrill 1868 (Anthozoa, scleractinia). Hydrobiologia 501, 139–147. doi: 10.1023/A:1026235832253
Nietzer S., Moeller M., Kitamura M., Schupp P. J. (2018). Coral larvae every day: Leptastrea purpurea, a brooding species that could accelerate coral research. Front. Mar. Sci. 5. doi: 10.3389/fmars.2018.00466
Palmer C. V., Modi C. K., Mydlarz L. D. (2009). Coral fluorescent proteins as antioxidants. PloS One 4 (10). doi: 10.1371/journal.pone.0007298
Petersen L.-E., Kellermann M. Y., Fiegel L. J., Nietzer S., Nietzer U., Abele D. (2023).Photodegradation of a bacterial pigment and resulting hydrogen peroxide release enable coral settlement . Sci. Rep., 1–10. doi: 10.1038/s41598-023-30470-w
Petersen L.-E., Kellermann M. Y., Nietzer S., Schupp P. J. (2021b). Photosensitivity of the bacterial pigment cycloprodigiosin enables settlement in coral larvae–light as an understudied environmental factor. Front. Mar. Sci. 8. doi: 10.3389/fmars.2021.749070
Petersen L.-E., Moeller M., Versluis D., Nietzer S., Kellermann M. Y., Schupp P. J. (2021a). Mono-and multispecies biofilms from a crustose coralline alga induce settlement in the scleractinian coral leptastrea purpurea. Coral Reefs 40 (2), 381–394. doi: 10.1007/s00338-021-02062-5
Piniak G. A., Fogarty N. D., Addison C. M., Kenworthy W. J. (2005). Fluorescence census techniques for coral recruits. Coral Reefs 24, 496–500. doi: 10.1007/s00338-005-0495-1
Puisay A., Elleaume N., Fouqueau L., Lacube Y., Goiran C., Sidobre C., et al. (2021). Parental bleaching susceptibility leads to differences in larval fluorescence and dispersal potential in pocillopora acuta corals. Mar. Environ. Res. 163. doi: 10.1016/j.marenvres.2020.105200
Randall C. J., Negri A. P., Quigley K. M., Foster T., Ricardo G. F., Webster N. S., et al. (2020). Sexual production of corals for reef restoration in the anthropocene. Mar. Ecol. Prog. Ser. 635, 203–232. doi: 10.3354/MEPS13206
Ritson-Williams R., Arnold S., Fogarty N., Steneck R. S., Vermeij M., Paul V. J. (2009). New perspectives on ecological mechanisms affecting coral recruitment on reefs. Smithson. Contrib. Mar. Sci. 37, 437–457. doi: 10.5479/si.01960768.38.437
Ritson-Williams R., Paul V. J., Arnold S. N., Steneck R. S. (2010). Larval settlement preferences and post-settlement survival of the threatened Caribbean corals. Acropora palmata A. cervicornis. Coral Reefs 29 (1), 71–81. doi:
Roth M. S., Fan T. Y., Deheyn D. D. (2013). Life history changes in coral fluorescence and the effects of light intensity on larval physiology and settlement in Seriatopora hystrix. PLoS One 8(3), e59476. doi: 10.1371/journal.pone.0059476
Roth M. S. (2014). The engine of the reef: Photobiology of the coral-algal symbiosis. Front. Microbiol. 5. doi: 10.3389/fmicb.2014.00422
Salih A., Larkum A., Cox G., Kühl M., Hoegh-Guldberg O. (2000). Fluorescent pigments in corals are photoprotective. Nature 408, 850–853. doi: 10.1038/35048564
Schwarz J. A., Krupp D. A., Weis V. M., Schwarz J. A., Krupp D. A., Weis V. M. (1999). Late larval development and onset of symbiosis in the scleractinian coral fungia scutaria. Biol. Bull. 196, 70–79. doi: 10.2307/1543169
Sneed J. M., Sharp K. H., Ritchie K. B., Paul V. J. (2014). The chemical cue tetrabromopyrrole from a biofilm bacterium induces settlement of multiple Caribbean corals. Proc. R. Soc B Biol. Sci. 281 (1786). doi: 10.1098/rspb.2013.3086
Strader M. E., Aglyamova G. V., Matz M. V. (2016). Red fluorescence in coral larvae is associated with a diapause-like state. Mol. Ecol. 25, 559–569. doi: 10.1111/mec.13488
Strader M. E., Aglyamova G. V., Matz M. V. (2018). Molecular characterization of larval development from fertilization to metamorphosis in a reef-building coral. BMC Genomics 19, 1–17. doi: 10.1186/s12864-017-4392-0
Szmant A. M., Meadows M. G. (2006). Developmental changes in coral larval buoyancy and vertical swimming behavior: Implications for dispersal and connectivity. Proc. 10th Int. Coral Reef Symp. 1, 431–437.
Tebben J., Motti C. A., Siboni N., Tapiolas D. M., Negri A. P., Schupp P. J., et al. (2015). Chemical mediation of coral larval settlement by crustose coralline algae. Sci. Rep. 5, 1–11. doi: 10.1038/srep10803
Tebben J., Tapiolas D. M., Motti C. A., Abrego D., Negri A. P., Blackall L. L., et al. (2011). Induction of larval metamorphosis of the coral acropora millepora by tetrabromopyrrole isolated from a pseudoalteromonas bacterium. PloS One 6, 1–8. doi: 10.1371/journal.pone.0019082
Vermeij M. J. A., Delvoye L., Nieuwland G., Bak R. P. M. (2002). Patterns in fluorescence over a Caribbean reef slope: The coral genus madracis. Photosynthetica. 40, 423–429. doi: 10.1023/A:1022635327172
Vermeij M. J. A., Smith J. E., Smith C. M., Vega Thurber R., Sandin S. A. (2009). Survival and settlement success of coral planulae: Independent and synergistic effects of macroalgae and microbes. Oecologia 159, 325–336. doi: 10.1007/s00442-008-1223-7
Keywords: coral larvae settlement, recruit development, scleractinian corals, confocal microscopy, fluorescence patterns
Citation: Fiegel LJ, Kellermann MY, Nietzer S, Petersen L-E, Smykala M, Bickmeyer U and Schupp PJ (2023) Detailed visualization of settlement and early development in Leptastrea purpurea reveals distinct bio-optical features . Front. Mar. Sci. 10:984656. doi: 10.3389/fmars.2023.984656
Received: 02 July 2022; Accepted: 14 February 2023;
Published: 07 March 2023.
Edited by:
Daniel Wangpraseurt, University of California, San Diego, United StatesReviewed by:
Esti Kramarsky-Winter, Ben-Gurion University of the Negev, IsraelFrancoise Cavada-Blanco, Zoological Society of London, United Kingdom
Copyright © 2023 Fiegel, Kellermann, Nietzer, Petersen, Smykala, Bickmeyer and Schupp. This is an open-access article distributed under the terms of the Creative Commons Attribution License (CC BY). The use, distribution or reproduction in other forums is permitted, provided the original author(s) and the copyright owner(s) are credited and that the original publication in this journal is cited, in accordance with accepted academic practice. No use, distribution or reproduction is permitted which does not comply with these terms.
*Correspondence: Matthias Y. Kellermann, bWF0dGhpYXMua2VsbGVybWFubkB1bmktb2xkZW5idXJnLmRl; Samuel Nietzer, c2FtdWVsLm5pZXR6ZXJAdW5pLW9sZGVuYnVyZy5kZQ==; Peter J. Schupp, cGV0ZXIuc2NodXBwQHVuaS1vbGRlbmJ1cmcuZGU=
†Present address: Lars-Erik Petersen, Department of Plant and Environmental Sciences, Weizmann Institute of Science, Rehovot, Israel
‡These authors have contributed equally to this work