- 1Institute of Marine Science and Technology, Shandong University, Qingdao, China
- 2Center Tech Tianjin Chemical Research and Design Institute, China National Offshore Oil Corporation, Tianjin, China
- 3Yellow Sea Fisheries Research Institute, Chinese Academy of Fishery Sciences, Qingdao, China
- 4Key Laboratory of Benthic Fisheries Aquaculture and Enhancement, Marine Biology Institute of Shandong Province, Qingdao, China
Marine primary production and terrestrial input are the main sources of buried carbon in sediments of marginal seas. Only marine-source carbon buried in sediments, fixed and stored by marine ecosystems, belongs to “blue carbon” and reflects marine ecosystems’ carbon sink function. The pattern of buried blue carbon in sediments, its flux, and its relationship with environmental changes remain unclear. The study aimed to investigate the composition of blue carbon in the sediments of Sanggou Bay, a special type of marginal sea. The analysis of sediment carbon sources was conducted through the C/N ratio and microscopic examination. The study also examined the long-term changes in the blue carbon burial fluxes. Results showed Blue carbon, which is sea-sourced carbon, accounted for about 23% of the total carbon content and its concentration ranged from 0.17% to 0.51%, with an average of about 0.25% ± 0.10%. The content of organic blue carbon in this sea area ranges from 0.09% to 0.26%, with an average of around 0.18% ± 0.04%. It constitutes approximately 72% of the buried blue carbon in the sediment, making it the primary component of buried blue carbon. Meanwhile, the content of inorganic blue carbon ranges from 0.01% to 0.32%. Over the past 70 years, the burial fluxes of sedimentary blue carbon, organic blue carbon and inorganic blue carbon in the Sanggou Bay are about 0.54 ± 0.22 mmol/(cm2a), 0.38 ± 0.07 mmol/(cm2a) and 0.17 ± 0.22 mmol/(cm2a), respectively; their long-term changes have been significantly affected by human aquaculture activities. Large-scale raft-rack aquaculture activities have caused a reduction in water flow velocity and an increase in the deposition of particulate organic matter, which in turn has led to the burial of organic blue carbon in the sediment. Additionally, the competition between aquaculture products and small calcareous organisms, such as mussels, foraminifera, may have inhibited the growth of small calcareous organisms. We suggest this has resulted in reduced burial fluxes of inorganic blue carbon and a decrease in its proportion among total blue carbon in the sea area. Our findings imply that aquaculture activities in Sanggou Bay had a negative impact on the burial of blue carbon in the sediments.
1 Introduction
The burial of carbon in marine sediments is an important pathway for carbon storage in the ocean. Compared to other methods of carbon storage, carbon stored in sediment is less likely to be released into the atmosphere in the short term. The IPCC has suggested that carbon buried in marine sediments is an effective way to remove CO2 from the atmosphere (Nellemann et al., 2009). About 90% of organic carbon in global ocean sediments is concentrated in marginal seas, while for inorganic carbon the percentage is over 50% (Berner, 1982; Hedges and Keil, 1995; Falkowski et al., 2000; Tesi et al., 2007; Song, 2011). Such a large quantity of buried carbon suggests that marginal sea sediments play a crucial role in the carbon cycle of marine ecosystems. Studies have shown that, due to the proximity of the marginal sea to land, the carbon buried in its sediments includes not only the carbon fixed by marine organisms, but also the carbon imported from terrestrial sources (Pocklington and Leonard, 1979; Prahl et al., 1994; Goñi et al., 1997; Bianchi et al., 2002; Burdige, 2005; Blair and Aller, 2012; Zhang et al., 2022; Tao et al., 2023). The burial of marine-derived carbon in sediment, also known as “blue carbon” (BC), reflects the sequestration of atmospheric CO2 by marine ecosystems through biological pumps (Honjo et al., 2008; Nellemann et al., 2009; Ma et al., 2014; Macreadie et al., 2019). Relatively, terrestrial carbon in sediment is fixed by land organisms, and its burial in marine sediments reflects the migration of terrestrial carbon pools to oceanic pools, rather than the direct carbon sink of CO2 by marine ecosystems. We believe that these different sourced carbons indicate distinct roles in the carbon sink function. Therefore, understanding the burial patterns of BC and non-BC in sediments can provide a more accurate understanding of the carbon sink function of marginal sea sediments and marine ecosystems (Belicka and Harvey, 2009; Bianchi, 2011).
There have been many studies focusing on the carbon sink function of sediments, and the carbon burial flux and its long-term changes in marine sediments have been studied (Thunell et al., 1992; St-Onge and Hillaire-Marcel, 2001; Justić et al., 2002; Ruiz-Fernández et al., 2007; Hayes et al., 2021; Zhao et al., 2021). The sources of organic carbon have also been discussed by isotopes or C/N, etc. (Ruttenberg and Goñi, 1997; Andrews et al., 1998; Goñi et al., 1998; Huang et al., 2001; Bouchez et al., 2014; Xing et al., 2014; Sanderman et al., 2015; Geraldi et al., 2019; Zinkann et al., 2022). However, from a BC burial perspective, the burial behavior of BC, including patterns, burial flux, and its relationship with environmental changes, remains unclear.
Nearshore aquaculture sea is a unique type of marginal sea characterized by high biological activity and a significantly higher carbon sink efficiency compared to the open ocean (Boyd et al., 2010). Besides, human aquaculture significantly disturbs the carbon cycling process in this sea (Carroll et al., 2003; Kutti et al., 2008; Ren et al., 2010; Liu et al., 2014; Xu et al., 2023). Additionally, there is potential for carbon sink enhancement in aquaculture seas. Therefore, we consider this type of sea an ideal location to identify the carbon sink function of marginal sea sediments and discuss the response of this function to increasing anthropogenic pressures. In this paper, sediment core sampling is conducted in Sangou Bay, one of China’s largest aquaculture bases. Based on an analysis of the sources of organic and inorganic carbon in sediments, we first identify the presence of burial BC in the sediments. We then explore how human aquaculture activities have affected the existing pattern and burial fluxes of BC over the past 70 years. This study attempts to identify the carbon sink function of marginal sea sediments more precisely.
2 Materials and methods
Sanggou Bay, located in Shandong province, China, is a significant aquaculture center. Since the late 1960s, macroalgae culture has a history of over 50 years. The predominant aquaculture species in Sanggou Bay are kelp, scallop, and oyster, and raft-rack culture is the primary mode of marine aquaculture (Guo et al., 1999). Currently, the aquaculture area has covered the whole Bay and extended beyond the bay (Li et al., 2023).
2.1 Sediment sampling
The sediment core, with a length of 1.78m, was collected by a gravity sediment core sampler at station RS-5 (Figure 1) in July 2014. Station RS-5 is situated at the junction of shellfish and kelp culture areas in the central part of Sanggou Bay. After being collected, the sample was kept refrigerated until it was divided. The sediment core was split into 1cm intervals for the upper 15cm and 2cm intervals for the remaining. The sub-samples were frozen and stored for future analysis.
2.2 Determination of sediment chronology
The chronology of sediment cores was rebuilt based on excess 210Pb activities (Golberg, 1963). The age of the sediment core was determined based on sample collection date and sedimentary rate. The activities of 210Pb in the sediment core were measured using a germanium detector (AMETEK Company).
2.3 Analysis of carbon and nitrogen in sediment
The sediment samples were first dried and then ground. Some ground sediments were directly detected for contents of total carbon (TC) and total nitrogen (TN) by an Elemental Analyzer Vario EL cube (Elementar Analysensysteme GmbH, German). Then, concentrated hydrochloric acid was used to fumigate ground samples for 24 hours to eliminate inorganic carbons. Subsequently, total organic carbon (TOC) was measured using the same Elemental Analyzer. The total inorganic carbon (TIC) levels were determined by subtracting TC and TOC.
2.4 Identification of organic blue carbon
The term “organic blue carbon” (OBC) in this study refers to marine-source organic carbon that is buried in sediment. And C/N ratio was used to identify the source of organic matter (Ruttenberg and Goñi, 1997; Andrews et al., 1998; Goñi et al., 1998; Kaushal and Binford, 1999; Venkatesh, 2020). The end-member values of C/N for marine and terrestrial organic matter were set at 5 and 20, respectively. The sources of TOC in Sanggou Bay sediments were analyzed using a two-end mixing model (Qian et al., 1997).
2.5 Identification of inorganic blue carbon
The term “inorganic blue carbon” (IBC) in this study refers to marine-sourced inorganic carbon that is buried in sediments, including calcareous remains of marine organisms and marine secondary carbonates. Based on current data, which indicates low levels of secondary carbonates in marine sediments globally (Sun and Turchyn, 2014), this study will only focus on inorganic carbon of marine origin. This includes calcareous remnants of various marine organisms such as shellfish, echinoderms, foraminifera, ostracoda, and coccolithophores. Secondary carbonates will not be considered in this study.
In this study, the source of inorganic carbon was distinguished by the morphological differences between terrestrial inorganic carbon minerals and marine-source inorganic carbon fractions. We first selected all calcareous remains of marine organisms in the sediments based on their morphological characteristics and then determined their total inorganic carbon. The total inorganic carbon content of these marine-source calcareous remains is the inorganic blue carbon content. For the brief operation process, we examined cleaned and sieved sediments under a microscope to identify calcareous remains of larger organisms including shellfish, echinoderms, foraminifera, ostracoda, etc. The inorganic carbon content of these remains was determined using an elemental analyzer. Carbon in the coccolithophore fossil also belongs to the marine source inorganic carbon. However, our early study found that the fossilized coccolith, examined by the polarizing microscope, was rare in Sanggou Bay sediment, so the inorganic carbon produced by coccolithophores was ignored in this study. Theoretically, the remains of freshwater mussels and other organisms may be transported into the sea by runoff, potentially interfering with the determination of marine-source inorganic carbon by the above method. However, considering the absence of large-scale runoff injection in the surrounding area of Sanggou Bay, we suggest that the impact of inorganic carbon from terrestrial organisms on determining marine inorganic carbon can be disregarded.
2.6 Calculation of carbon burial fluxes
The burial fluxes of all types of carbon in sediments were calculated using the method introduced by Dai et al. (2007).
3 Results
The vertical distribution of 210Pb in sediments in station RS-5 shows a typical attenuation trend (Figure 2), indicating that the depositional environment in this sea area is relatively stable. The sediment rate in this sea area is estimated to be 2.08 cm/a (Bai et al., 2022). The sediment core used in this study reflects the deposition of the past 70 years.
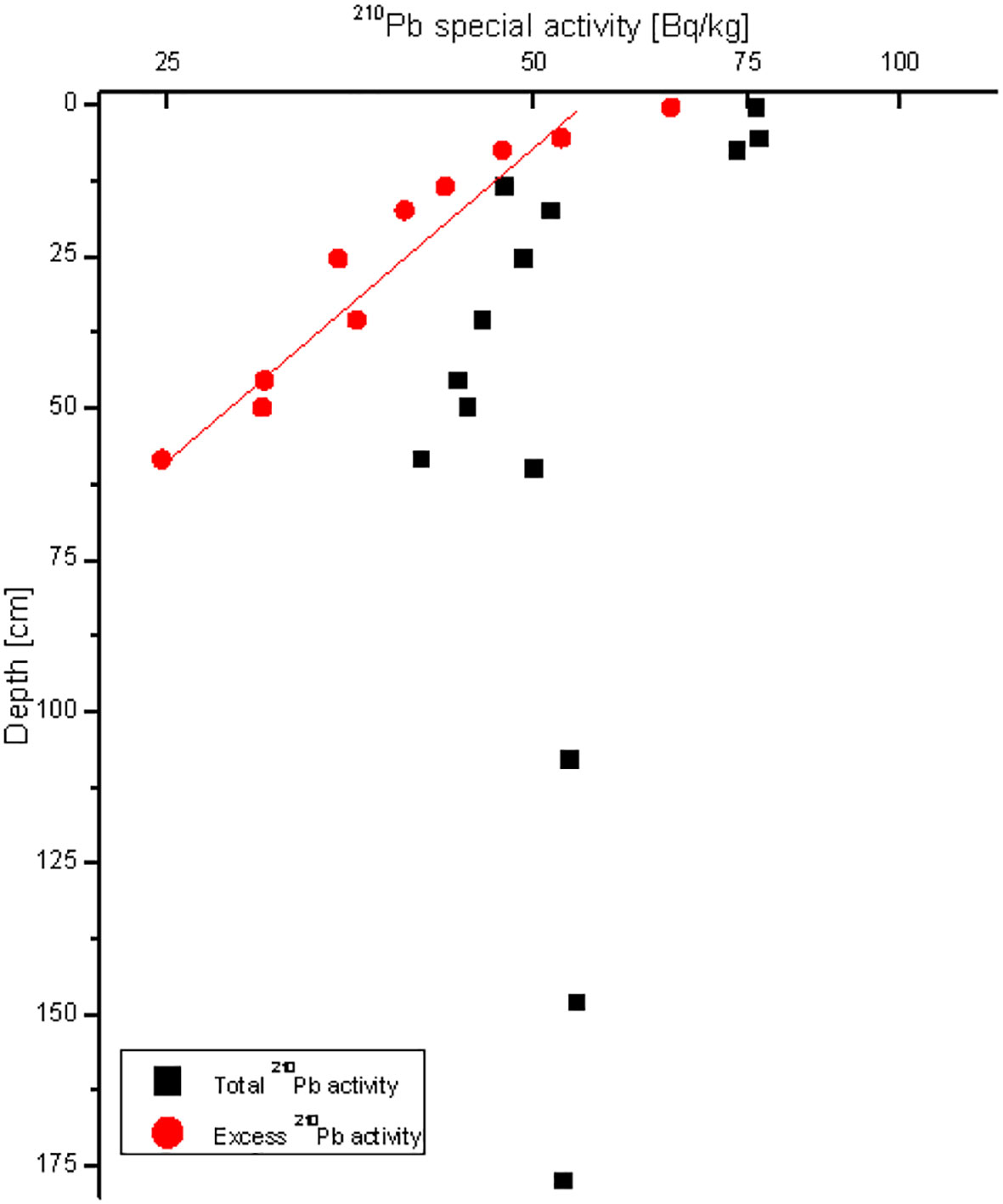
Figure 2 Vertical distributions of 210Pb activities in stations RS-5. Quadrates indicate total 210Pb activities and circles indicate excess 210Pb activities.
The total carbon content in RS-5 sediments was 0.87%~1.40% in the last 70 years (Bai et al., 2022) (Figure 3). It was slightly higher during the 1960s~1990s and then decreased significantly. The total organic carbon content fluctuates but has no overall trend, except for a significant increase from the 1970s to the 1980s, with an average of 0.37% ± 0.06%. The average content of total inorganic carbon was 0.77% ± 0.09%. Its time series resembled that of total carbon, being generally higher from the 1960s to 1990s and lower in the 1950s and 2000s. C/N ratios ranged from 7.1 to 9.4. The highest values occurred mainly before 1975, while the content was generally low after that.
The marine-source organic carbon content, estimated by C/N, averaged at about 0.18% ± 0.04%. The highest values were observed in 1965-1995, followed by the 2000s, and the lowest values occurred in 1950-1965. The average inorganic carbon from marine sources was approximately 0.08% ± 0.09%. The highest levels were observed prior to the 1980s, with a peak in the 1950s, after which the values decreased. BC refers to the carbon sequestered by marine organisms, which includes both organic and inorganic carbon from marine sources. Over the past 70 years, the concentration of BC in the sediments of Sanggou Bay has been found to range from 0.17% to 0.51%, with an average of 0.25% ± 0.10%. The highest levels of BC were recorded during the period of 1950-1965, after which there was a general decrease in concentration. Since the 1980s, the content of BC has remained at a consistently low level.
The burial fluxes of TC, TOC, TIC, OBC, IBC, and BC, in the aquaculture waters of Sanggou Bay, were estimated to be about 2.42 ± 0.22 mmol/(cm2a), 0.79 ± 0.09 mmol/(cm2a), 1.63 ± 0.19 mmol/(cm2a), 0.38 ± 0.07 mmol/(cm2a), 0.17 ± 0.22 mmol/(cm2a), and 0.54 ± 0.22 mmol/(cm2a), respectively (Figure 4). The long-term trends of burial fluxes for the various types of carbon were generally similar to the time series of their contents in the sediments. The burial fluxes of TC, TOC and TIC in Sanggou Bay were much higher than those in the global deep sea (Hayes et al., 2021), and the buried flux of TOC in Sanggou Bay was higher than those in the most part of East China Sea and Alian Bay aquaculture area (Deng et al., 2006; Pan et al., 2021), but lower than that in Yangtze River estuary (Deng et al., 2006). However, for OBC, i.e., marine organic carbon, the buried flux in Sanggou Bay was significantly higher than that in Yangtze River estuary (Deng et al., 2006), and was similar to that in Alian Bay aquaculture area (Pan et al., 2021).
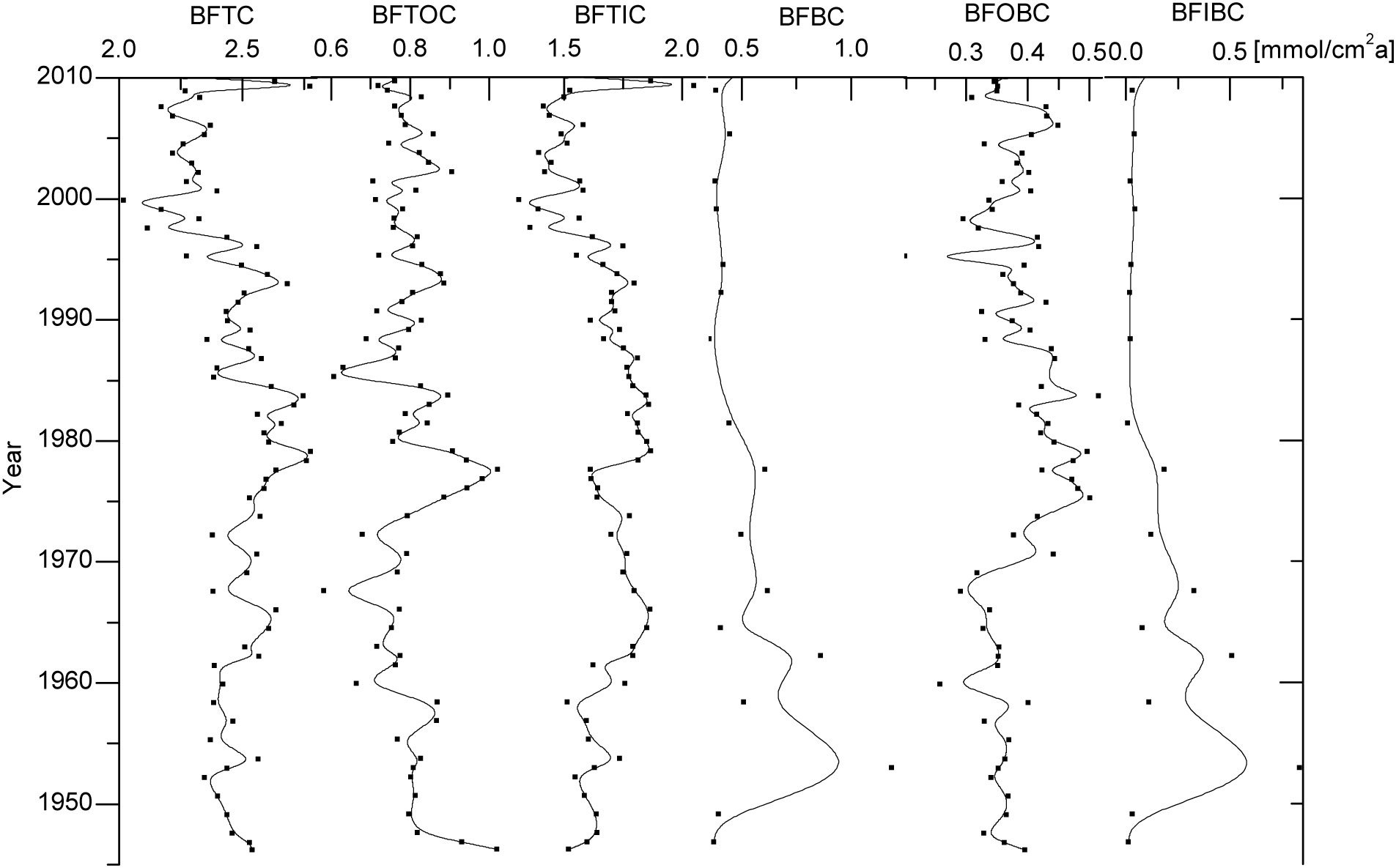
Figure 4 Long-term trends of burial fluxes of TC, TOC, TIC, BC, OBC, IBC in station RS-5. BFTC, BFTOC, BFTIC, BFBC, BFOBC and BFIBC indicate the burial flux of TC, TOC, TIC, BC, OBC and IBC respectively.
4 Discussion
4.1 Structure of the sedimentary carbon pool in Sanggou Bay
Over the past 70 years, the carbon buried in the sediments of station RS-5 is primarily inorganic carbon, with organic carbon accounting for less than 33% on average. This pattern is similar to that found in the mouth of Sanggou Bay, indicating a high proportion of inorganic carbon burial in the sediments of the bay (Liu et al., 2014).
In the sediments located in the mouth of Sanggou Bay, the organic carbon is primarily of terrestrial origin (Liu et al., 2014). However, at station RS-5, the amount of marine organic carbon in the total organic carbon is nearly equal to that from terrestrial sources, with marine organic carbon accounting for approximately 48% on average and terrestrial sources accounting for around 52% on average. On one hand, this may suggest that central Sanggou Bay has higher primary productivity compared to the mouth of the bay (Wu et al., 2016). As a result, there is more deposition and burial of marine organic matter in the area. Otherwise, it has been observed that the speed of water flow in the mouth of the bay is higher as compared to the central bay (Zhao et al., 1996; Grant and Bacher, 2001). As a result of such high-speed water flow, the deposition of marine organic carbon, which has a smaller density and particle size, is hindered in the sediment of the mouth of Sanggou Bay. While in the central bay, where the hydrodynamics is weak, marine organic matter can deposit more effectively in the sediment environment. The sediments at station RS-5 have smaller particle sizes compared to those at the mouth of the bay, which supports this point (unpublished data).
The sediments of station RS-5 contain only about 10% ± 13% of marine inorganic carbon with the main source being terrestrial input. And this is obviously different in contrast to that in the mouth of Sanggou Bay, where inorganic carbon was mainly composed of marine inorganic carbon, and with a proportion of more than 70%. This distribution trend is noticeably different from the higher proportion of marine organic carbon in sediments at station RS5 compared to the Bay mouth. It is worth noting that the lower marine inorganic carbon proportion observed at station RS-5 is in the background that we considered both shell debris and calcareous zooplankton fossils during the marine inorganic carbon discrimination in sediments at station RS-5, whereas only shell debris carbon was examined in the mouth area of Sanggou Bay. In the sediment core from the Bay mouth, a significant amount of small shellfish remains and a visible sediment layer of small shellfish remains have been discovered, which are believed to be the primary reasons for the high proportion of marine inorganic carbon. On the other hand, there is no apparent sediment layer of small shellfish remains in the sediment from station RS-5, and small shellfish remains are sporadically found in the sediment.
Marine organic and inorganic carbon comprise BC in sediments. TC buried in the RS-5 sediments has inorganic carbon as the dominant existing form, while BC is dominant in the organic form, accounting for over 70% on average. Over the past 70 years, the pattern of BC in sediments has noticeably changed. Although OBC has been the dominant form of BC throughout the entire period, the proportion of IBC was relatively higher before the 1960s, with an average of nearly 30%. However, with the development of marine aquaculture in Sanggou Bay from the end of the 1960s, the proportion of IBC in BC decreased significantly. And the average proportion decreased to less than 10% after the 1980s.
4.2 Response of composition and burial flux of sedimental BC to human aquaculture activities in Sanggou Bay
Marine aquaculture activities in Sanggou Bay began in the late 1960s and early 1970s. Correspondingly, our data show that after that time, the burial fluxes of BC and IBC in the sediments of Sanggou Bay tended to decrease compared to the previous period, while the burial flux of OBC was significantly higher than that in the previous period, and the proportion of BC in TC and the proportion of IBC in BC also decreased compared to the previous period. We suggest this phenomenon indicates the influence of human aquaculture activity on the burial of blue carbon in sediments.
With the development of macroalgae culture since the 1960s, the sedimentation of biological detritus during the growth of macroalgae can result in a significant amount of extra organic matter being buried in the sediments (Vetter and Dayton, 1999; Carroll et al., 2003; Yang et al., 2022). Additionally, the large-scale culture of macroalgae can reduce the velocity of water flow (Zhao et al., 1996; Grant and Bacher, 2001). This can enhance the deposition and burial of suspended particulate matter into the sediments, leading to an increase in the amount of marine organic matter buried in the sediments. Correspondingly, the burial flux of OBC in RS-5 sediments significantly increased since the 1960s (Figure 5). It’s worth noting that cultured macroalgae and phytoplankton in the water column have a competitive relationship. During growth, macroalgae can absorb a significant amount of nutrients from the water column. For example, kelp has excellent mechanisms for storing nutrients in vivo (Stewart et al., 2009). As a result, the availability of nutrients for phytoplankton growth is reduced, limiting their growth. Additionally, transport of organic matter from phytoplankton to sediments may decrease. However, the deposition of large amounts of organic matter from macroalgae culture has led to an increase in the buried flux of OBC in the sediments of this area, as well as an increase in the proportion of OBC in the total organic carbon, rather than a decreasing trend.
The trend of long-term change in the burial flux of IBC in the sediments of Sanggou Bay is significantly different from that of OBC. Before the start of mariculture activities, the abundance of phytoplankton provided a sufficient food source for small shellfish and calcareous zooplankton to grow vigorously. And the burial flux of IBC in sediments was high, as was its proportion among total inorganic carbon. After the late 1960s, the large-scale cultivation of kelp and other macroalgae began to restrict the growth of phytoplankton due to competition between different species. This limited the availability of food sources, which may restrict the growth of small calcareous organisms such as small shellfish (Xiao et al., 2022). Since the late 1970s and early 1980s, the aquaculture of large shellfish, such as scallops, has expanded and led to direct competition between farmed shellfish and small shellfish. This competition may have limited the growth of small shellfish and other small calcareous organisms (Galimany et al., 2017; Ferreira-Rodriguez et al., 2018; Xiao et al., 2022). Field survey results also support the view that the growth of zooplankton and benthic shellfish has been significantly affected by aquaculture activities: with the development of the aquaculture in Sanggou Bay, the zooplankton biomass decreased significantly; the abundance and diversity of benthic organisms were much lower than that before and the proportion of polychaetes increased dramatically, while the species of mollusc reduced substantially (Ji et al., 1998; Li et al., 2023). The same phenomenon was also observed in the aquaculture area in West Coast (USA) estuaries (Dumbauld et al., 2009). Large-scale farming of shellfish has made it difficult for the shells of these shellfish to be buried abundantly in sediment due to human harvesting. As a result, the decrease in the biomass of small shellfish and calcareous zooplankton may have led to a reduction in the burial rate of IBC in sediment, as well as a decrease in the proportion of IBC in the total inorganic carbon.
In Sanggou Bay, prior to aquaculture, IBC made up a significant portion of the burial flux of BC in sediments. During that period, the growth of small shellfish and calcareous zooplankton was promoted due to the abundant food provided by phytoplankton. As a result, the remains of these calcareous organisms were buried in large quantities in the sediments, leading to a relatively high flux of BC burial. After the development of aquaculture activities, the burial rate of OBC has increased. However, the burial flux of IBC decreased from about 0.28 mmol/(cm2a) before the development of aquaculture activities to about 0.08 mmol/(cm2a) in the 1980s and then continued to decline to about 0.03 mmol/(cm2a). Furthermore, the proportion of IBC in BC has reduced from 26% before aquaculture to less than 4% after the 1980s. Meanwhile, the burial of BC has been declining. We believe that the decrease in burial flux of IBC and its proportion after the appearance of aquaculture were caused by the interspecific interaction of native calcareous organisms with aquaculture organisms. Therefore, the development of aquaculture activities in Sanggou Bay has reduced the burial of BC in sediments. Aquaculture activities in this study resulted in a decrease in the burial rate of BC in the sediments. However, it is important to note that a significant amount of carbon was fixed in the aquaculture products, which also falls under the category of BC (Tang and Lui, 2016). Overall, aquaculture activities have a positive impact on the carbon sink function of the surrounding waters.
4.3 The carbon sink function of marine sediment
Marginal seas are adjacent to land, and the carbon buried in their sediments includes not only the carbon fixed by marine organisms but also terrestrial-source carbon which is transported by runoff and the atmosphere. The burial of marine carbon and terrestrial carbon in the sediments are both the carbon storage of marine sediment. However, they play different roles in the carbon cycle. Marine source carbon in sediments originates from CO2 fixed by marine organisms, which belongs to the category of BC (Nellemann et al., 2009; Mcleod et al., 2011; Macreadie et al., 2019). The burial of carbon from marine sources in sediments reflects the storage of fixed carbon in marine ecosystems. This process is an important aspect of the marine carbon sink. In contrast, terrestrial carbon originates from CO2 absorbed by green plants on land, which is classified as green carbon and represents the carbon sink function of terrestrial ecosystems (Regnier et al., 2013). The burial of terrestrial carbon in marine sediment is a form of carbon storage. However, this carbon fixation occurs in terrestrial ecosystems and does not contribute to the carbon sink function of the oceans. Generally, the process of burying BC in marine sediments indicates the carbon sink function of marine ecosystems. On the other hand, the process of burying terrestrial carbon reflects the transfer function from the carbon pool of terrestrial ecosystems to that of marine ecosystems. Of course, the burial of terrestrial carbon into marine sediments is conducive to the long-term sequestration of this part of the carbon. In other words, the burial of BC in the sediment reflects the carbon removal function of the marine ecosystem, while the burial of terrestrial carbon in the sediment reflects the carbon sequestration assistance of the marine ecosystem. It is worth noting that the sequestration of terrestrial carbon in marine ecosystems does not significantly contribute to carbon fixation. However, it can impact the carbon cycling process in marine ecosystems by adsorption, resolution, and degradation during the transportation and burial of terrestrial carbon.
In the Sanggou Bay aquaculture sea, BC accounted for only 23% of TC burial flux in the sediment. Although the amount of carbon buried in the sediments of Sanggou Bay is 1-2 orders of magnitude higher than that in the adjacent central Yellow Sea (Yang et al., 2015), the carbon sink function of the sediments of Sanggou Bay has not increased to the same extent. This is because the carbon buried in the sediments of Sanggou Bay mostly comes from terrestrial sources. Of course, with the increasing distance away from shore, the influence of terrestrial ecosystems on the marine environment gradually decreases, and the burial flux of TC in the sediments increasingly reflects the actual carbon sink function of the marine ecosystem due to the decrease of the terrestrial carbon input.
Since the beginning of the JGOFS program, carbon burial fluxes have been estimated in sediments of many marginal seas. (Thunell et al., 1992; Sayles et al., 2001; Brunskill et al., 2002; Hayes et al., 2021; Zhao et al., 2021). However, the results of this study suggested that, due to the burial of terrestrial carbon in marginal sea sediments, the achieved results on burial fluxes of TC, total organic carbon and total inorganic carbon in marginal sea sediments include the migration process of carbon pools in terrestrial ecosystems in addition to the carbon fixation and removal function in marine ecosystems. It suggests that the carbon sink function of the marginal sea sediments might have been overestimated in previous results. Exploring the division of BC fraction in sediment and its burial behavior is necessary to accurately evaluate the carbon sink function of marginal sea sediments.
Various methods have been established to estimate organic carbon in sediments, including isotopes, C/N ratios, and biomarkers (Ruttenberg and Goñi, 1997; Andrews et al., 1998; Goñi et al., 1998; Huang et al., 2001; Bouchez et al., 2014; Xing et al., 2014; Sanderman et al., 2015; Geraldi et al., 2019; Zinkann et al., 2022); however, there are still challenges in identifying the origins of inorganic carbon in sediment samples. In this study, the source resolution of inorganic carbon was initially determined based on the morphological characteristics of inorganic carbon from various sources. We are afraid that some fragments of calcareous debris were not completely picked out due to their small size, leading to lower accuracy and precision of the experimental results. And due to the heavy workload, this method was rarely used for analyzing a large number of samples. Therefore, a fast and accurate method for analyzing the source of inorganic carbon in sediments needs to be developed. This will be a key issue in studying the function of BC sinks in sediments.
5 Conclusion
The carbon buried in the sediment of marginal seas originates from both marine production and terrestrial input. Only marine carbon, buried in the sediments, is the carbon fixed and stored by the marine ecosystem, which belongs to the category of BC and reflects the carbon sink function of the marine ecosystem. In order to deeply investigate the carbon sink function of marginal sea sediments, this study attempted to identify the buried BC in sediments in Sanggou Bay aquaculture sea area based on carbon source resolution and discussed the long-term change trend of the burial flux of BC over the past 70 years. The average BC content in the sediments of Sanggou Bay aquaculture area was 0.25% ± 0.10%, accounting for 23% of TC. OBC was the main component of BC, accounting for a proportion of about 72%. The burial flux of BC in the sediment of this sea area is about 0.54 ± 0.22 mmol/(cm2a) for the past 70 years. The burial flux of sedimentary BC is influenced by human aquaculture. We suggest that with the development of aquaculture in Sanggou Bay, there has been a noticeable increase in the burial flux of OBC due to the sedimentation of more particulate organic matters resulting from aquaculture activities; however, due to the interspecies competition between aquaculture species and small calcareous organisms, the burial flux of IBC in sediments has significantly decreased. Overall, human aquaculture activities in Sanggou Bay not only reduced the burial flux of BC in the sediments but also diminished the IBC proportion among BC.
Data availability statement
The raw data supporting the conclusions of this article will be made available by the authors, without undue reservation.
Author contributions
SY: Investigation, Methodology, Writing – original draft, Writing – review & editing. QY: Investigation, Writing – review & editing. XS: Writing – review & editing. WZ: Writing – review & editing. JL: Writing – review & editing. XQ: Methodology, Writing – original draft. JC: Writing – original draft. JH: Writing – original draft. BL: Writing – review & editing. YS: Writing – review & editing.
Funding
The author(s) declare financial support was received for the research, authorship, and/or publication of this article. This work was financially supported by the National Natural Science Foundation of China (NSFC) (grant numbers: U1906216, 41606130, U1406403), the Key Research and Development Program of Shandong Province (grant numbers: 2020ZLYS04) and the Key Basic Research Program of China (grant numbers: 2015CB453303).
Acknowledgments
We thank Dr. Xin Zhou, Institute of Polar Environment, University of Science and Technology of China, Hefei, for his help in analysis of 210Pb activities. We also want to thank Mrs Hongxia Qiu, and Miss Sai Liu, Yellow Sea Fisheries Research Institute, Chinese Fisheries Science Academy, Qingdao, for her help with sample collection and sorting.
Conflict of interest
Authors SY, WZ, and BL were employed by the company Center Tech Tianjin Chemical Research and Design Institute Co. Ltd.
The remaining authors declare that the research was conducted in the absence of any commercial or financial relationships that could be construed as a potential conflict of interest.
Publisher’s note
All claims expressed in this article are solely those of the authors and do not necessarily represent those of their affiliated organizations, or those of the publisher, the editors and the reviewers. Any product that may be evaluated in this article, or claim that may be made by its manufacturer, is not guaranteed or endorsed by the publisher.
References
Andrews J. E., Greenaway A. M., Dennis P. F. (1998). Combined carbon isotope and C/N ratios as indicators of source and fate of organic matter in a poorly flushed, tropical estuary: Hunts Bay, Kingston Harbour, Jamaica. Estuarine Coast. Shelf Sci. 46 (5), 743–756. doi: 10.1006/ecss.1997.0305
Bai H. Y., Liu S., Yang Q., Huang L. F., Sun Y. (2022). High-resolution records of the rate of carbon accumulation in the shellfish aquaculture area in Sanggou Bay and its response to human aquaculture activities. Prog. Fishery Sci. 43 (5), 98–105. doi: 10.19663/j.issn2095-9869.20210419002
Belicka L. L., Harvey H. R. (2009). The sequestration of terrestrial organic carbon in Arctic Ocean sediments: A comparison of methods and implications for regional carbon budgets. Geochimica Cosmochimica Acta 73 (20), 6231–6248. doi: 10.1016/j.gca.2009.07.020
Berner R. A. (1982). Burial of organic carbon and pyrite sulfur in the modern ocean: its geochemical and environmental significance. Am. J. Sci. 282 (4), 451–473. doi: 10.2475/ajs.282.4.451
Bianchi T. S. (2011). The role of terrestrially derived organic carbon in the coastal ocean: A changing paradigm and the priming effect. Proc. Natl. Acad. Sci. 108 (49), 19473–19481. doi: 10.1073/pnas.1017982108
Bianchi T. S., Mitra S., McKee B. A. (2002). Sources of terrestrially-derived organic carbon in lower Mississippi River and Louisiana shelf sediments: implications for differential sedimentation and transport at the coastal margin. Mar. Chem. 77 (2-3), 211–223. doi: 10.1016/S0304-4203(01)00088-3
Blair N. E., Aller R. C. (2012). The fate of terrestrial organic carbon in the marine environment. Annu. Rev. Mar. Sci. 4, 401–423. doi: 10.1146/annurev-marine-120709-142717
Bouchez J., Galy V., Hilton R. G., Gaillardet J., Moreira-Turcq P., Pérez M. A., et al. (2014). Source, transport and fluxes of Amazon River particulate organic carbon: Insights from river sediment depth-profiles. Geochimica Cosmochimica Acta 133, 280–298. doi: 10.1016/j.gca.2014.02.032
Boyd C. E., Wood C. W., Chaney P. L., Queiroz J. F. (2010). Role of aquaculture pond sediments in sequestration of annual global carbon emissions. Environ. pollut. 158 (8), 2537–2540. doi: 10.1016/j.envpol.2010.04.025
Brunskill G. J., Zagorskis I., Pfitzner J. (2002). Carbon burial rates in sediments and a carbon mass balance for the Herbert River region of the Great Barrier Reef continental shelf, North Queensland, Australia. Estuarine Coast. Shelf Sci. 54 (4), 677–700. doi: 10.1006/ecss.2001.0852
Burdige D. J. (2005). Burial of terrestrial organic matter in marine sediments: A re-assessment. Global Biogeochemical Cycles 19 (4), GB4011. doi: 10.1029/2004GB002368
Carroll M. L., Cochrane S., Fieler R., Velvin R., White P. (2003). Organic enrichment of sediments from salmon farming in Norway: environmental factors, management practices, and monitoring techniques. Aquaculture 226 (1-4), 165–180. doi: 10.1016/S0044-8486(03)00475-7
Dai J., Song J., Li X., Zheng G., Yuan H., Li N. (2007). Geochemical characteristics of nitrogen and their environmental significance in Jiaozhou Bay sediment. Quaternary Sci. 27 (3), 347–356.
Deng B., Zhang J., Wu Y. (2006). Recent sediment accumulation and carbon burial in the East China Sea. Global Biogeochemical Cycles 20 (3), GB3014. doi: 10.1029/2005GB002559
Dumbauld B. R., Ruesink J. L., Rumrill S. S. (2009). The ecological role of bivalve shellfish aquaculture in the estuarine environment: a review with application to oyster and clam culture in West Coast (USA) estuaries. Aquaculture 290 (3-4), 196–223. doi: 10.1016/j.aquaculture.2009.02.033
Falkowski P., Scholes R. J., Boyle E. E. A., Canadell J., Canfield D., Elser J., et al. (2000). The global carbon cycle: a test of our knowledge of earth as a system. Science 290 (5490), 291–296. doi: 10.1126/science.290.5490.29
Ferreira-Rodriguez N., Sousa R., Pardo I. (2018). Negative effects of Corbicula fluminea over native freshwater mussels. Hydrobiologia 810, 85–95. doi: 10.1007/s10750-016-3059-1
Galimany E., Freeman C. J., Lunt J., Domingos A., Sacks P., Walters L. (2017). Feeding competition between the native oyster Crassostrea virginica and the invasive mussel Mytella charruana. Mar. Ecol. Prog. Ser. 564, 57–66. doi: 10.3354/meps11976
Geraldi N. R., Ortega A., Serrano O., Macreadie P. I., Lovelock C. E., Krause-Jensen D., et al. (2019). Fingerprinting blue carbon: rationale and tools to determine the source of organic carbon in marine depositional environments. Front. Mar. Sci. 263. doi: 10.3389/fmars.2019.00263
Goñi M. A., Ruttenberg K. C., Eglinton T. I. (1997). Sources and contribution of terrigenous organic carbon to surface sediments in the Gulf of Mexico. Nature 389 (6648), 275–278. doi: 10.1038/38477
Goñi M. A., Ruttenberg K. C., Eglinton T. I. (1998). A reassessment of the sources and importance of land-derived organic matter in surface sediments from the Gulf of Mexico. Geochimica Cosmochimica Acta 62 (18), 3055–3075. doi: 10.1016/S0016-7037(98)00217-8
Grant J., Bacher C. (2001). A numerical model of flow modification induced by suspended aquaculture in a Chinese bay. Can. J. Fisheries Aquat. Sci. 58 (5), 1003–1011. doi: 10.1139/f01-027
Guo X., Ford S. E., Zhang F. (1999). Molluscan aquaculture in China. J. Shellfish Res. 18 (1), 19–31.
Hayes C. T., Costa K. M., Anderson R. F., Calvo E., Chase Z., Demina L. L., et al. (2021). Global ocean sediment composition and burial flux in the deep sea. Global biogeochemical cycles 35 (4), e2020GB006769. doi: 10.1029/2020GB006769
Hedges J. I., Keil R. G. (1995). Sedimentary organic matter preservation: an assessment and speculative synthesis. Mar. Chem. 49 (2-3), 81–115. doi: 10.1016/0304-4203(95)00008-F
Honjo S., Manganini S. J., Krishfield R. A., Francois R. (2008). Particulate organic carbon fluxes to the ocean interior and factors controlling the biological pump: A synthesis of global sediment trap programs since 1983. Prog. Oceanography 76 (3), 217–285. doi: 10.1016/j.pocean.2007.11.003
Huang Y., Street-Perrott F. A., Metcalfe S. E., Brenner M., Moreland M., Freeman K. H. (2001). Climate change as the dominant control on glacial-interglacial variations in C3 and C4 plant abundance. Science 293 (5535), 1647–1651. doi: 10.1126/science.1060143
Ji R., Mao X., Zhu M. (1998). Impacts of coastal shellfish aquaculture on bay ecosystem. J. Oceanography Huanghai Bohai Seas 16 (1), 21–27.
Justić D., Rabalais N. N., Turner R. E. (2002). Modeling the impacts of decadal changes in riverine nutrient fluxes on coastal eutrophication near the Mississippi River Delta. Ecol. Model. 152 (1), 33–46. doi: 10.1016/S0304-3800(01)00472-0
Kaushal S., Binford M. W. (1999). Relationship between C:N ratios of lake sediments, organic matter sources, and historical deforestation in Lake Pleasant, Massachusetts, USA. J. Paleolimnology 22, 439–442. doi: 10.1023/A:1008027028029
Kutti T., Ervik A., Høisæter T. (2008). Effects of organic effluents from a salmon farm on a fjord system. III. Linking deposition rates of organic matter and benthic productivity. Aquaculture 282 (1-4), 47–53. doi: 10.1016/j.aquaculture.2008.06.032
Li W., Yu X., Jiang Z., Du M., Jia Y., et al. (2023). Assessment of benthic ecological quality of Sanggou Bay through AMBI and M-AMBI approaches (Periodical of Ocean University of China). Available at: https://link.cnki.net/urlid/37.1414.P.20231139,1341.001.
Liu S., Yang Q., Yang S., Sun Y., Yang G. (2014). The long-term records of carbon burial fluxes in sediment cores of culture zones from Sanggou Bay. Acta Oceanol Sin. 36, 30–38. doi: 10.3969/j
Ma Z., Gray E., Thomas E., Murphy B., Zachos J., Paytan A. (2014). Carbon sequestration during the Palaeocene–Eocene Thermal Maximum by an efficient biological pump. Nat. Geosci. 7 (5), 382–388. doi: 10.1038/ngeo2139
Macreadie P. I., Anton A., Raven J. A., Beaumont N., Connolly R. M., Friess D. A., et al. (2019). The future of Blue Carbon science. Nat. Commun. 10 (1), 3998. doi: 10.1038/s41467-019-11693-w
Mcleod E., Chmura G. L., Bouillon S., Salm R., Björk M., Duarte C. M., et al. (2011). A blueprint for blue carbon: toward an improved understanding of the role of vegetated coastal habitats in sequestering CO2. Front. Ecol. Environ. 9 (10), 552–560. doi: 10.1890/110004
Nellemann C., Corcoran E., Duarte C. M., et al. (2009). Blue carbon. A rapid response assessment (Norway: Birkeland Trykkeri), 1–80.
Pan Z., Tan Y., Gao Q., Dong S., Fang X., Yan J. (2021). A 120-year record of burial fluxes and source apportionment of sedimentary organic carbon in Alian Bay, China: Implication for the influence of mariculture activities, and regional environment changes. Aquaculture 535, 736421. doi: 10.1016/j.aquaculture.2021.736421
Pocklington R., Leonard J. D. (1979). Terrigenous organic matter in sediments of the St. Lawrence Estuary and the Saguenay Fjord. J. Fisheries Board Canada 36 (10), 1250–1255. doi: 10.1139/f79-179
Prahl F. G., Ertel J. R., Goñi M. A., Sparrow M. A., Eversmeyer B. (1994). Terrestrial organic carbon contributions to sediments on the Washington margin. Geochimica Cosmochimica Acta 58 (14), 3035–3048. doi: 10.1016/0016-7037(94)90177-5
Qian J., Wang S., Xue B., Chen R., Ke S. (1997). A new approach to quantitatively estimate terrestrial organic carbon in lake sediments. Chin. Sci. Bull. 42, 1655–1658. doi: 10.1007/BF02882652
Regnier P., Friedlingstein P., Ciais P., Mackenzie F. T., Gruber N., Janssens I. A., et al. (2013). Anthropogenic perturbation of the carbon fluxes from land to ocean. Nat. Geosci. 6 (8), 597–607. doi: 10.1038/ngeo1830
Ren Y., Dong S., Wang F., Gao Q., Tian X., Liu F. (2010). Sedimentation and sediment characteristics in sea cucumber Apostichopus japonicus (Selenka) culture ponds. Aquaculture Res. 42 (1), 14–21. doi: 10.1111/j.1365-2109.2010.02483.x
Ruiz-Fernández A. C., Frignani M., Tesi T., Bojórquez-Leyva H., Bellucci L. G., Páez-Osuna F. (2007). Recent sedimentary history of organic matter and nutrient accumulation in the Ohuira Lagoon, Northwestern Mexico. Arch. Environ. Contamination Toxicol. 53, 159–167. doi: 10.1007/s00244-006-0122-3
Ruttenberg K. C., Goñi M. A. (1997). Phosphorus distribution, C: N: P ratios, and δ13Coc in arctic, temperate, and tropical coastal sediments: tools for characterizing bulk sedimentary organic matter. Mar. Geology 139 (1-4), 123–145. doi: 10.1016/S0025-3227(96)00107-7
Sanderman J., Krull E., Kuhn T., Hancock G., McGowan J., Maddern T., et al. (2015). Deciphering sedimentary organic matter sources: Insights from radiocarbon measurements and NMR spectroscopy. Limnology Oceanography 60 (3), 739–753. doi: 10.1002/lno.10064
Sayles F. L., Martin W. R., Chase Z., Anderson R. F. (2001). Benthic remineralization and burial of biogenic SiO2, CaCO3, organic carbon, and detrital material in the Southern Ocean along a transect at 170 West. Deep Sea Res. Part II: Topical Stud. Oceanography 48 (19-20), 4323–4383. doi: 10.1016/S0967-0645(01)00091-1
Song J. (2011). Carbon cycling processes and carbon fixed by organisms in China marginal seas. J. Fishery Sci. China/Zhongguo Shuichan Kexue 18 (3), 703–711. doi: 10.3724/SP.J.1118.2011.00703
Stewart H. L., Fram J. P., Reed D. C., Williams S. L., Brzezinski M. A., MacIntyre S., et al. (2009). Differences in growth, morphology and tissue carbon and nitrogen of Macrocystis pyrifera within and at the outer edge of a giant kelp forest in California, USA. Mar. Ecol. Prog. Ser. 375, 101–112. doi: 10.3354/meps07752
St-Onge G., Hillaire-Marcel C. (2001). Isotopic constraints of sedimentary inputs and organic carbon burial rates in the Saguenay Fjord, Quebec. Mar. Geology 176 (1-4), 1–22. doi: 10.1016/S0025-3227(01)00150-5
Sun X., Turchyn A. V. (2014). Significant contribution of authigenic carbonate to marine carbon burial. Nat. Geosci. 7 (3), 201–204. doi: 10.1038/ngeo2070
Tang Q., Lui H. (2016). Strategy for carbon sink and its amplification in marine fisheries. Strategic Study Chin. Acad. Eng. 18 (3), 68–73.
Tao S., Wang A., Liu J. T., Ye X., Blattmann T. M., Ran C., et al. (2023). Characteristics of sedimentary organic carbon burial in the shallow conduit portion of source-to-sink sedimentary systems in marginal seas. Geochimica Cosmochimica Acta 353, 92–111. doi: 10.1016/j.gca.2023.05.006
Tesi T., Miserocchi S., Goñi M. E. A., Langone L., Boldrin A., Turchetto M. (2007). Organic matter origin and distribution in suspended particulate materials and surficial sediments from the western Adriatic Sea (Italy). Estuarine Coast. Shelf Sci. 73 (3-4), 431–446. doi: 10.1016/j.ecss.2007.02.008
Thunell R. C., Qingmin M., Calvert S. E., Pedersen T. F. (1992). Glacial-Holocene biogenic sedimentation patterns in the South China Sea: Productivity variations and surface water pCO2. Paleoceanography 7 (2), 143–162. doi: 10.1029/92PA00278
Venkatesh M. (2020). Appraisal of the carbon to nitrogen (C/N) ratio in the bed sediment of the Betwa River, Peninsular India. Int. J. Sediment Res. 35 (1), 69–78. doi: 10.1016/j.ijsrc.2019.07.003
Vetter E. W., Dayton P. K. (1999). Organic enrichment by macrophyte detritus, and abundance patterns of megafaunal populations in submarine canyons. Mar. Ecol. Prog. Ser. 186, 137–148. doi: 10.3354/meps186137
Wu W. G., Zhang J. H., Wang W., Li J. Q., Fang J. H., Liu Y., et al. (2016). Distribution of chlorophyll-a concentration and its control factors in spring in Sungo Bay. Shengtai Xuebao/Acta Ecologica Sin. 36 (15), 4855–4863. doi: 10.5846/stxb201501130102
Xiao X., Liu Y., Niu P., Chen X., Feng Z., Wang X., et al. (2022). Effects of culture of Patinopecten yessoensis and Gracilaria lemaneiformis on phytoplankton community structure based on an enclosure experiment. Process Fishery Sci. 43 (1), 66–76. doi: 10.19663/j.issn2095-9869.20200921003
Xing L., Zhao M., Gao W., Wang F., Zhang H., Li L., et al. (2014). Multiple proxy estimates of source and spatial variation in organic matter in surface sediments from the southern Yellow Sea. Organic Geochemistry 76, 72–81. doi: 10.1016/j.orggeochem.2014.07.005
Xu M., Sun C., Du Z., Zhu X. (2023). Impacts of aquaculture on the area and soil carbon stocks of mangrove: A machine learning study in China. Sci. Total Environ. 859, 160173. doi: 10.1016/j.scitotenv.2022.160173
Yang P., Tang K. W., Yang H., Tong C., Yang N., Lai D. Y., et al. (2022). Insights into the farming-season carbon budget of coastal earthen aquaculture ponds in southeastern China. Agriculture Ecosyst. Environ. 335, 107995. doi: 10.1016/j.agee.2022.107995
Yang S., Yang Q., Liu S., Cai D., Qu K., Sun Y. (2015). Burial fluxes and sources of organic carbon in sediments of the central Yellow Sea mud area over the past 200 years. Acta Oceanologica Sin. 34, 13–22. doi: 10.1007/s13131-015-0723-7
Zhang Y., Galy V., Yu M., Zhang H., Zhao M. (2022). Terrestrial organic carbon age and reactivity in the Yellow River fueling efficient preservation in marine sediments. Earth Planetary Sci. Lett. 585, 117515. doi: 10.1016/j.epsl.2022.117515
Zhao B., Yao P., Bianchi T. S., Yu Z. (2021). Controls on organic carbon burial in the Eastern China marginal seas: A regional synthesis. Global Biogeochemical Cycles 35 (4), e2020GB006608. doi: 10.1029/2020GB006608
Zhao J., Zhou S. L., Sun Y., Fang J. (1996). Research on Sanggou Bay aquaculture hydro-environment. Mar. Fishery Res. 17 (2), 68–79.
Keywords: blue carbon, sediment, burial flux, aquaculture, marginal sea, Sanggou Bay, human activity, long-term change
Citation: Yang S, Yang Q, Song X, Zhou W, Liu J, Qi X, Chen J, Huang J, Li B and Sun Y (2024) Blue carbon in sediment from Sanggou Bay: composition, burial flux and its response to human activities. Front. Mar. Sci. 10:1342750. doi: 10.3389/fmars.2023.1342750
Received: 22 November 2023; Accepted: 08 December 2023;
Published: 11 January 2024.
Edited by:
Junfu Dong, Shandong University, ChinaCopyright © 2024 Yang, Yang, Song, Zhou, Liu, Qi, Chen, Huang, Li and Sun. This is an open-access article distributed under the terms of the Creative Commons Attribution License (CC BY). The use, distribution or reproduction in other forums is permitted, provided the original author(s) and the copyright owner(s) are credited and that the original publication in this journal is cited, in accordance with accepted academic practice. No use, distribution or reproduction is permitted which does not comply with these terms.
*Correspondence: Yao Sun, sunyao@ysfri.ac.cn