- Biology Department, Faculty of Science, Taibah University, Yanbu, Saudi Arabia
This study highlights the potential of the brown algae Cystoseira myrica, collected from raw beach seaweed wastes, as a reliable source of bioactive fucoidan. Fucoidans are natural bioactive sulfated polysaccharides that are gaining popularity worldwide due to their diverse biochemical composition, attractive physical properties, and functional pharmacological activities. The aim of this work is to valorize the beach-Accumulated brown algae C. myrica by fucoidan extraction and to investigate its anticoagulant and antioxidant activity. Fucoidan was extracted using several steps of papain digestion followed by cetyltrimethylammonium bromide (CTAB) precipitation and calcium chloride treatment to avoid the coextraction of other polysaccharides. Structural features of the extracted fucoidan were investigated by Fourier transform infrared spectroscopy (FT-IR), solid-state nuclear magnetic resonance (NMR) and high-resolution mass spectroscopy. Agarose gel electrophoresis was used to confirm the purity of the isolated fucoidan from C. myrica. Anticoagulant properties were studied in vitro by activated partial thromboplastin time (aPTT) and prothrombin time (PT) assays. Furthermore, the antioxidant activity was investigated by 2,2’-azino-bis (3-ethylbenzothiazoline-6-sulfonic acid (ABTS) and Fe chelating ability. Our results indicate that the approach used was effective in extracting fucoidan with a yield of 3.07%, a high amount of sulfate (27.79%), and fucose was found to be the major monosaccharide component. The extracted fucoidan showed an interesting anticoagulant activity. It prolonged aPTT significantly at a concentration of 10 µg/ml and prolonged PT at high doses. This demonstrated that fucoidan from C. myrica may affect intrinsic pathways while having little impact on the extrinsic mechanism of coagulation. However, the extracted fucoidan significantly exhibited an interesting antioxidant capacity as shown by the higher ABTS radical scavenging activity and Ferrous ion-chelating effect. The current findings suggest that fucoidan isolated from C. myrica has unique structural, antioxidant and anticoagulant properties and offers innovative therapeutic possibilities.
1 Introduction
Marine biomass is a valuable biotechnological resource that exhibits a wide range of composition and functional capabilities, owing to the presence of diverse bioactive components such as polyphenols, peptides, and polysaccharides (Rudovica et al., 2021). Algae have garnered significant attention in the discipline of biotechnology due to their economic potential, as they are abundantly produced in nature and often discarded as waste material on beaches. The composition of algal biomass makes it a viable option for a growing array of applications, which continues to expand in scope (González Fernández et al., 2023). The presence of algae in coastal environments can be turned from an unpleasant and potentially hazardous phenomenon into a source of pharmaceutical candidates. Seaweeds are becoming more fascinating due to their potential as a renewable reservoir of bioactive chemicals (Ashour et al., 2022). The expanding range of uses for seaweeds in the food, feed (Abdelrhman et al., 2022), cosmetic, and medicine sector highlights the need to investigate their chemical composition (El-Shenody et al., 2019). A notable amount of attention is being paid to pharmaceutical candidates from new natural marine resources that have increased health-promoting qualities (Shinde et al., 2019; Jin et al., 2023). Marine species are rich in sources of beneficial metabolites. Marine polysaccharides, among the marine-based valued bioactive substances, are known as unique and attractive resources for the food and pharmaceutical industries (Mousavian et al., 2022). Great amounts of polysaccharides with different chemical properties and several bioactivities are found in seaweeds (Yu et al., 2021). Among these, fucoidan has shown promise as a therapeutic biomolecule. Fucoidans are sulfated heteropolysaccharides discovered in 1913, found in marine invertebrates and brown seaweed (Jin et al., 2013). They are predominantly made of fucose residues and sulfate groups. Fucoidan has a complex structure and is commonly composed of (1 → 3)- L-fucopyranose monomers that have a sulfate group at C-4. However, further C-2 sulfation and 2-O-a-L-fucopyranosyl branching were reported (Yu et al., 2021; Zayed et al., 2023).They also include minor amounts of protein, galactose, mannose, xylose, glucose, and uronic acids (Mak et al., 2014). They have wide ranging biological and therapeutic activities, including anti-inflammatory, antioxidant, anti-coagulant, anti-viral, anti-tumor, anti-allergic, anti-hepatopathy and immunomodulatory agents. The Food and Drug Administration (FDA) has authorized fucoidan for use in the generally recognized as safe (GRAS) category (Citkowska et al., 2019). Particularly in the recent years, there has been a significant increase in the number of published articles about fucoidan and their therapeutic effects (Wang et al., 2019; Wen et al., 2021). It has been widely reported that the chemical and pharmacological properties of fucoidan vary significantly depending on the algal species, location, harvest season, technique of extraction, and other factors (Lim and Aida, 2017; Qu et al., 2019). Cardiovascular diseases are amongst the most prevalent worldwide and their fatality rate is increasing year after year (Michel et al., 2020). Cardiovascular diseases are treated with anticoagulants, antioxidants, and anti-inflammatories (Manggau et al., 2022). Anticoagulant and antithrombotic compounds work by reducing platelet aggregation and thrombus formation, blocking the coagulation system, and interfering with future plaque extension. Fucoidans have shown promise as a preventive and alternative treatment for thrombus-induced cardiac and cerebrovascular problems. In addition to their potential applications as heparin-like effect compounds, they have also increasingly been studied for their biodegradability and non-toxicity. The body’s production of reactive nitrogen species and reactive oxygen species (ROS) encourages oxidative stress, which has several physiological effects, including risk of thrombus formation and platelet hyperactivity. Previous work has revealed that reactive oxygen species can influence both thrombus development and resolution (Gutmann et al., 2020). In addition, it is well established that platelet activation is reduced in the presence of antioxidants such as vitamin C and polyphenols (Olas, 2022), as well as being increased in the presence of ROS donors, showing the significance of ROS-mediated effects on platelet activation (Gutmann et al., 2020).
The unique ecosystems and adaptations of the Red Sea in Saudi Arabia have made it a potentially valuable resource for bioactive chemicals with remarkable biological activity. Marine macroalgae are receiving more recognition in the biotechnology sector due to their economic prospects. This is mostly due to their abundance in the environment and the fact that they are often thrown as waste material on beaches. Additionally, they are rich in distinctive bioactive chemicals. In this study, the brown algae C. myrica, a beach-cast macroalgae (Figure 1), was evaluated to isolate fucoidan as an additional, value-added product. To the best of our knowledge, no prior work has extracted fucoidan from the above-mentioned algae and studied its bioactivity. As a result, the objective of this study was to extract fucoidan by combining papain extraction, CTAB and CaCl2 precipitation from the macroalgae biomass (C. myrica) collected from the coast of Yanbu, Saudi Arabia in order to avoid the coextraction of other polysaccharides. The chemical composition and proprieties of fucoidan were assessed by colorimetric assays, Fourier-transform infrared spectroscopy (FTIR), NMR and high-resolution mass spectroscopy. Its antioxidant and anticoagulant capacities were also tested with several in vitro techniques.
2 Materials and methods
2.1 Fucoidan extraction from the biomass macroalgae
C. myrica algae were collected from coastlines in Yanbu, Saudi Arabia (Figure 1). Seaweed was immediately rinsed in seawater upon collecting and then washed several times with clean water to remove co-collected impurities. The algae were dried at low temperatures before being processed to improve the surface-to-volume ratio. Before fucoidan extraction, algae powder was treated with ethanol twice to remove low molecular weight components, pigments and liposoluble compounds and then filtered, dried, and stored at -80°C. Fucoidan, laminarin and alginate are the three principal polysaccharides found in brown algae (Li et al., 2021). Firstly, sulfated polysaccharides were extracted by enzymatic digestion with papain (100 mg) for 24 hours at 60°C (Dhahri et al., 2020). Then, fucoidan was precipitated with 0.4% (w/v) of the cationic detergent cetyltrimethylammonium bromide (CTAB) (Dhahri et al., 2020). This step is added to avoid the coextraction of the Laminarin, a polysaccharide naturally found in brown algae. Laminarin is a neutral polysaccharide and so will not react with CTAB and remain water soluble (Dobrinčić et al., 2020). Then, to eliminate alginate, polysaccharide solution was precipitated by CaCl2 2%, and the solution was kept at 4°C overnight to precipitate alginate. The supernatant was recuperated after centrifugation at 14000 rpm for 10 min. Fucoidan was precipitated by ethanol and the precipitate was recuperated by centrifugation at 10000 rpm for 20 min. To eliminate protein, fucoidan was treated by the Sevag method (Li et al., 2019). The extracted fucoidan was dialyzed with a cut-off value of 3500 Da and finally lyophilized to obtain the crude fucoidan FCm.
2.2 Physicochemical characterization
2.2.1 Chemical composition analysis
The Dische method (Dische and Shettles, 1948) and Carbazole (Cesaretti et al., 2003) method were used to determine the amount of fucose and uronic acid. Fucose and uronic acid content were estimated by using the slopes of standards. The amount of sulfate was determined by sulfate assay kit (MAK132) from sigma Aldrich. The results are represented as weight % of the dry sample. Elemental analysis was used as described by Dhahri et al. to estimate the molar ratio of carbon (C), hydrogen (H), sulfur (S), and nitrogen (N) (Dhahri et al., 2020).
2.2.2 Polysaccharide hydrolysis, derivatization, and high-resolution mass spectroscopy
According to Dhahri et al, FCm was hydrolyzed with TFA (4 M) and then derivatized using 1-phenyl-3-methyl-5-pyrazolone (PMP) as well as standards (Dhahri et al., 2023). We used an Orbitrap IDX spectrometer which is characterized by a dependable mass accuracy (5 ppm mass error) and high resolution (> 120,000) to evaluate the m/z of the extracted polysaccharides. Samples were analyzed in positive mode electrospray ionization. The derivatized samples were chromatographed using an Acquity UPLC HSS C18 column (2.1x100mm, 1.8µ). For separation, the mobile phase A was water + 0.1% formic acid + 25 mM ammonium formate. The mobile phase B was acetonitrile + 0.1% formic acid. Monosaccharide standards (fucose, galactose, glucose, xylose, arabinose mannose and rhamnose) were used for the detection and quantitation of monosaccharides in extracted fucoidan, FCm.
2.2.3 Spectroscopic analysis
The structural properties of FCm were studied by FT-IR analysis and solid-state13C NMR and compared to fucoidan standard purchased from Molekula. Briefly, samples including FCm and standard fucoidan were mixed with KBr, pressed, and examined using an FT-IR spectrometer (Nicolet FTIR iS10, Thermo Scientific, USA) with a scanning range of 400 to 4000 cm-1. The solid-state 13C NMR spectrum was analyzed using the WB Bruker 400 AVANCE III spectrometer (Bruker BioSpin, Rheinstetten, Germany) (Alkordi et al., 2015; Chisca et al., 2015; Dhahri et al., 2020).
2.2.4 Agarose gel electrophoresis
The purity of the extracted fucoidan was studied by agarose gel electrophoresis, performed as described by Stelling et al. (Stelling et al., 2019). Agarose gel (0.5%, w/v) in 0.05 M 1,3 diamino propane/acetate buffer (pH 9.0) was used to separate 10 µg of FCm and fucoidan standard. The separated samples were fixed with 0.1% CTAB before staining with 0.1% toluidine blue in acetic acid:ethanol:water (0.1:5:5, v/v). Finally, acetic acid:ethanol (0.1:1,v/v) was used for destaining.
2.3 Anticoagulant activity
The anticoagulant activity of extracted fucoidan was evaluated by using human plasma (P9523) from sigma. Before testing, the plasma was prepared as described by Escobedo et al. (Escobedo et al., 2006). Briefly, after warming at room temperature, the plasma was reconstituted by adding the appropriate amount of deionized water and the liquid was gently swirled until a suspension is formed. Plasma was used in the following assays to study the anticoagulant activity. FCm solution at various concentrations was prepared in HEPES buffer (20 mM HEPES, 150 mM NaCl, 0.1% polyethylene glycol Mr 8000, pH 7.4) (Pratt and Monroe, 1992). The clotting agents were injected into the tested samples using a multichannel pipette. The anticoagulant assays were performed on a Synergy 2 Multi-Mode Microplate reader in kinetic mode and coagulation time was calculated from the absorbance measured at 405 nm (Pratt and Monroe, 1992; Indumathi and Mehta, 2016).
2.3.1 Activated partial thromboplastin time assay
aPTT assays were performed as described by Pratt et al. (Pratt and Monroe, 1992). Briefly, 30 µl of plasma was incubated with 30 µl of various concentrations of FCm solution and 30 µl of aPTT reagent in microplate wells. Heparin was used as a reference. The plate was incubated for 5 min at 25°C and the absorbance was recorded at 405 nm for 1200 s. Assays were performed in triplicate.
2.3.2 Prothrombin time assay
PT assay was performed by mixing 30 µl of plasma with 30 µl of FCm solution at various concentrations. After 2 min of incubation, clotting was initiated by adding 30 µl of 5% thromboplastin in 25 mM in 25 mM CaCl2. The absorbance at 405 nm was recorded for 1200 s. The rate of change of absorbance was proportional to clot formation.
2.3.3 Fluorometric assay
Factor thrombin and Xa screening kits were used according to the manufacturer’s instructions to detect the direct inhibition of FCm on thrombin and factor Xa. The factor Xa inhibitor screening kit (MAK239) and thrombin Inhibitor Screening Kit (MAK243) from sigma use the capability of Factor Xa and thrombin, respectively, to cleave a synthetic substrate, thereby releasing a fluorophore, AMC, which can be measured by a fluorescence reader. In the presence of a Factor Xa inhibitor or thrombin specific inhibitors, the amount of the cleavage reaction is decreased or fully stopped. The loss in the fluorescence intensity can be correlated to the amount of inhibitor present in the assay solution.
2.4 Antioxidant activities
2.4.1 ABTS radical scavenging assay
ABTS radical cation (ABTS•+) was produced by reacting the ABTS solution (7 mM) with potassium persulfate (2.45 mM). After incubation at room temperature in the dark for 12 to 16 hours, the ABTS•+ solution was diluted with PBS to get an absorbance at 734 nm of 0.7. Then, 10 µl of FCm or fucoidan standard purchased from Molekula was mixed with 190 µl ABTS reagent in 96 well plate (Acharya, 2017). After shaking the plate and incubating for 5 minutes in the dark, the absorbance was determined at 734 nm. The following equation was used to calculate the degree of scavenging:
Where: Abs0 is the absorbance of the control (water instead of the sample) and Abs1 is the absorbance of the sample.
2.4.2 Metal chelating assay
Ferrous ion chelating activity was investigated according to the method described by Zhang et al. (Zhang et al., 2019) and compared to fucoidan standard purchased from Molekula. In 96-well microplate, the reaction mixture including 50 μL of samples at various concentrations, 160μL of deionized water and 20μL of FeSO4 solution (0.30 mmol/L) was incubated for 5 min at room temperature. 30μL of ferrozine solution (0.80 mmol/L) was then added to each well. The absorbance was measured at 562 nm, after 15 min of incubation and the capacity of samples to chelate ferrous ion was calculated using the following equation:
Abs0 is the absorbance of the control (water instead of the sample) and Abs1 is the absorbance of the sample.
2.5 Statistical analysis
Analysis was carried out in triplicate and results are presented as mean standard deviation (SD). ANOVA was used to evaluate the data, and differences are considered statistically significant if the value of p< 0.05.
3 Results and discussion
3.1 Extraction
Sulfated polysaccharides isolated from brown algae are recognized as fucoidans (Usov et al., 2022). The process of commercial manufacture of fucoidans from brown algal biomass was first proposed in 1952 by Black et al. (Black et al., 1952) and has been adjusted recently, aiming to enhance yields and purity (Jayawardena et al., 2022). Fucus vesiculosus and Ascophyllum nodosum are the two popular sources of commercial fucoidans, which have similar chemical and biological characteristics (Usov et al., 2022). Numerous studies are currently being conducted to isolate fucoidans with interesting therapeutic effects using various extraction techniques. In this study, fucoidan was extracted from Cystoseira myrica by combining papain extraction, CTAB and CaCl2 precipitation. Fucoidan was obtained with a ratio of 3.07% and contains 3.25% of uronic acid and 35.12% of fucose (Table 1). This fucoidan yield was higher than that extracted from brown seaweed (Lakshmanasenthil et al., 2014; Fauziee et al., 2021; Tsou et al., 2022). For instance, fucoidan was extracted from brown seaweed T. ornata with a yield of 1.8% (Lakshmanasenthil et al., 2014). Fucoidan obtained by isolation with water and ethanol gradient precipitation from the brown seaweed Sargassum pallidum had a ratio of isolated concentrations ranging between 0.31% to 0.96% and had low amounts of sulfate (3.84-6.85%) (Xin et al., 2016). In addition, fucoidan was extracted from S. polycystum with a ratio of 4.51 ± 0.24% and contained 38.76 ± 0.26%, 22.35 ± 0.23%, 3.9 ± 1.8%, and 4.7 ± 0.43% of carbohydrate, sulphate, uronic acid and protein, respectively (Palanisamy et al., 2017). Extraction method, seaweed harvesting season, location, and level of maturation may all influence fucoidan yield and chemical composition (Rani et al., 2017; Baba et al., 2018). Recently, Tsou et al, has extracted fucoidan samples at two temperatures (65 and 80°C) from Sargassum ilicifolium collected from five different locations in southern Taiwan and confirmed that location and temperature of extraction can significantly influence the yield (1.47%-2,73%) and the chemical composition of fucoidan (Tsou et al., 2022). The amount of sulfate and the position of sulfation have been reported to influence the bioactivity of fucoidan as well as the amount of fucose (Jayawardena et al., 2022). The amount of sulfate in the extracted fucoidan FCm is comparable to fucoidan obtained from S. polycystum (Palanisamy et al., 2017) and higher when compared to the brown seaweed S. pallidum (Xin et al., 2016). A sulfated polysaccharide extracted from C. myrica collected from the Jazan coasts, Saudi Arabia, with an antiviral effect present with an amount of sulfate of 22.3% (Mohamed et al., 2015). As shown in Table 2, the carbon (C%), hydrogen (H%) and sulfur content (S%) were comparable to commercial fucoidan. In contrast, the isolated fucoidan had low nitrogen content (N%), differing from commercial fucoidan that lacks nitrogen, indicative of the presence of some amino-containing compounds (amino sugars or protein) (Saravana et al., 2016). Additionally, the monosaccharide composition of fucoidan isolated from C. myrica was determined by high-resolution mass spectrometry (HR-MS) and summarized in Table 1. FCm contains fucose as the major sugar component with a ratio of 86.48%. In addition, galactose (0.42%), glucose (1.26%), xylose (0.42%), and arabinose (0.42%) were also detected. The result is comparable to the monosaccharide composition of fucoidan from F. vesiculosus that shows a high content of fucose of 84.0%, in addition to the presence of galactose (7.3%), xylose (6.0%) and mannose (2.0%) (Lahrsen et al., 2018). Hence, the algal species and extraction method have a strong influence on the chemical composition of fucoidan. For instance, fucoidan isolated from U. pinnatifida contains four major monosaccharides including fucose, galactose, xylose, and glucose, which were also found in different proportions in the fucoidan standard from the brown seaweed species U. pinnatifida (Koh et al., 2019). C. myrica could be an interesting source of “good quality fucoidan” given that FCm presents a high level of fucose and sulfate (January et al., 2019).
3.2 Spectroscopic analysis
FTIR and NMR analysis are efficient techniques to elucidate structural properties and have been widely used by researchers to characterize extracted fucoidan from natural sources. The FTIR spectra shown in Figure 2 indicates that the extracted sulfate polysaccharide from Cystoseira myrica presents similar structural properties to commercial fucoidan. It is possible to see two distinct absorbance bands at 3423 cm-1 and 2940cm-1, which are the most prevalent spectral features related to CH and OH stretching of polysaccharides. The wavelength range between 550-1800 cm-1 is the fingerprint region for fucoidan in the FTIR spectra. The peaks at 2929 cm-1 and 1627 cm-1 relate to CH stretching vibration and to the presence of a carbonyl group from uronic acid (Ganapathy et al., 2019). The bands ranging from 1200 to 970 cm-1 suggest the presence of C-O-C glycosidic linkages as well as C-C and C-O-C pyranose rings (Daub et al., 2020). The IR band at 1412 cm-1 can be attributed to stretching of the neutral monosaccharide -CH2 groups and the fucosyl residue -CH3 groups (Apostolova et al., 2022). The O=S=O stretching vibrations of sulfates, a distinctive characteristic of sulfated polysaccharides like fucoidans, can be attributed to the major peak between 1220 and 1270 cm-1 and a smaller peak at 583 cm-1 (Fernando et al., 2020) (Daub et al., 2020). The peak around 820 cm-1 corresponds to the bending vibration of COS (Fernando et al., 2017).
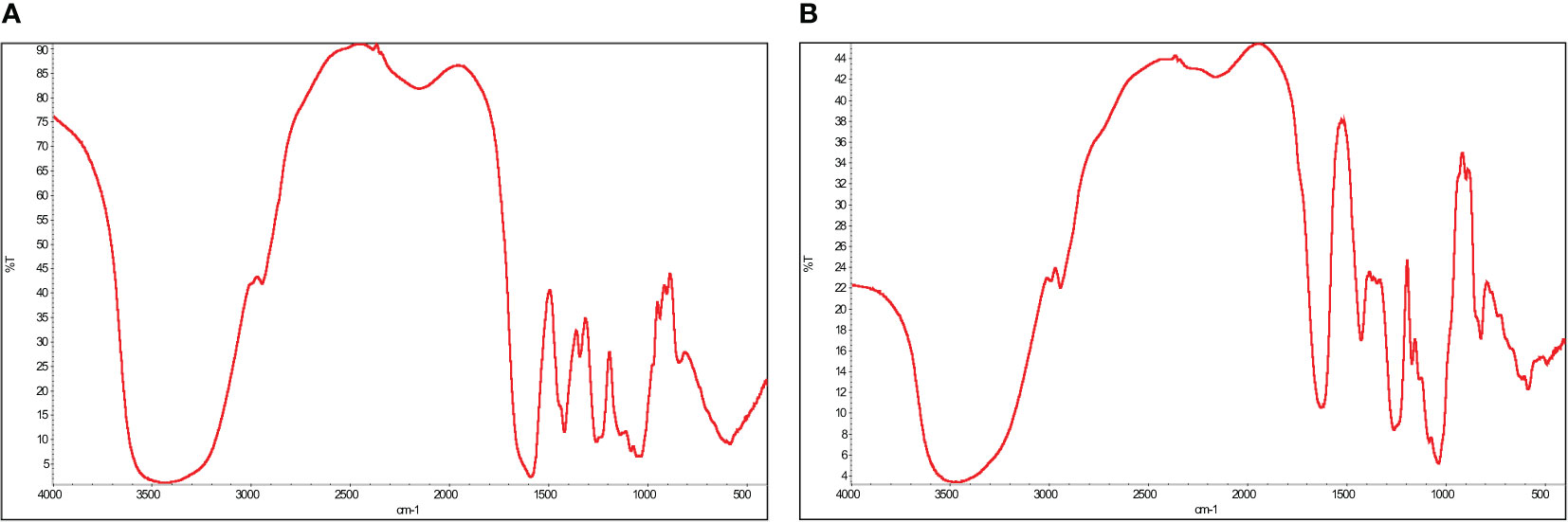
Figure 2 Fourier transform infrared analysis of the extracted fucoidan, FCm, from Cystoseira myrica (A) compared to standard fucoidan (B).
Solid-state NMR (Figure 3) showed structural similarities between extracted fucoidan and commercial fucoidan. As described in the literature, the spectra displayed strong chemical shift signals at 175.7 for fucoidan and 180.2 for FCm, that indicate the presence of the carbonyl group. In addition, an intense peak at 101.1 for fucoidan and 100.8 related to the anomeric carbon (C1)(Lim et al., 2016; Ganapathy et al., 2019). The difference between the anomeric carbons (C1) of FCm and fucoidan standard could be due to the position of sulphate groups, which influence the chemical shift of anomeric carbons, as illustrated by Daniel et al. (Daniel et al., 2001; Lim et al., 2016). The intense peaks at 17.1 ppm (FCm) and 16.96 ppm (fucoidan standard) were due to methyl groups (C6) of α-L-fucopyranose units (Synytsya et al., 2010; Lim et al., 2016). A comparable chemical shift at 17.92 ppm was obtained with α-fucose monomer. It is well recognized that location, season, and environment can affect the chemical properties of fucoidan (Lim et al., 2016).
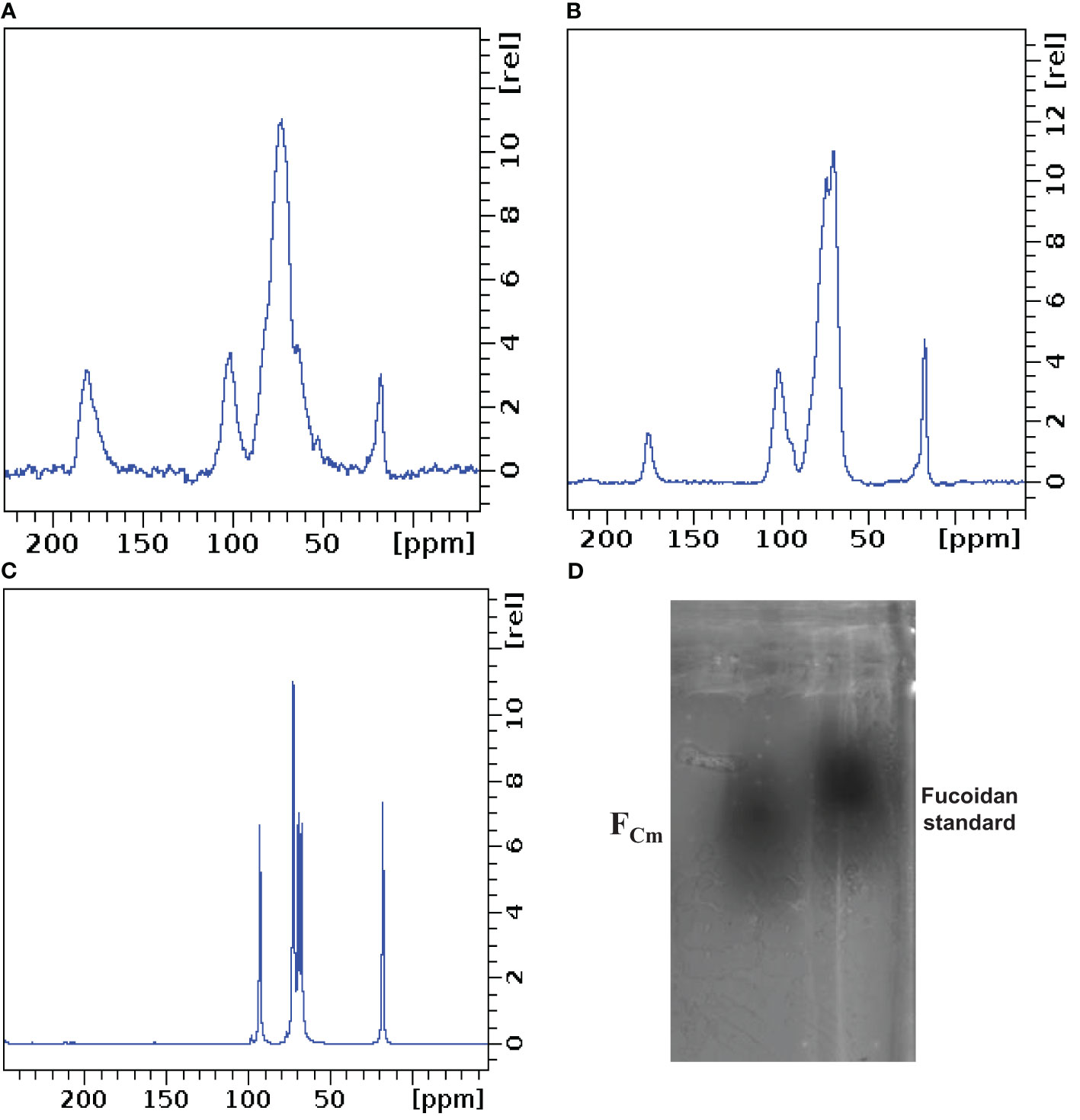
Figure 3 Solid state NMR of FCm (A), fucoidan standard (B) and fucose (C). Agarose gel electrophoresis of FCm and fucoidan (D).
3.3 Agarose gel electrophoresis
Agarose gel electrophoresis was used to confirm the purity of the isolated fucoidan from C. myrica. An agarose gel of FCm stained with toluidine blue showed a clear single spot that migrates as far as the fucoidan standard (Figure 3D), validating the purity and the presence of fucoidan in FCm. The results confirmed the effectiveness of the employed technique by combining enzymatic digestion, CTAB precipitation and calcium chloride treatment for fucoidan extraction. The small difference in the electrophoretic mobilities was caused by the complex formed between sulfated polysaccharides and diamine, which resulted in CTAB precipitation. These changes resulted from the structure of fucoidan and sulfated patterns (Ganapathy et al., 2019).
3.4 Anticoagulant activities
The anti-coagulant activity of FCm was studied in vitro by aPTT and PT assays that characterized the pathways of the cascade of coagulation. APTT assay evaluated coagulation activity via both the intrinsic and common coagulation pathways, and PT assay mostly evaluated the extrinsic pathway (Yao and Yim, 2021). These pathways converge at the common point where prothrombin is transformed to thrombin, resulting in the production of fibrin threads (Dhahri et al., 2020). In the current study, anticoagulant activity of FCm was studied by microplate reader which is highly reproducible and allows us the analysis of a large number of samples at the same time (Walkowiak et al., 1997).
As shown in Figure 4, FCm prolonged clotting time in a concentration dependent manner. Several previous studies have found that fucoidan prolongs APTT. At a concentration of 10 µg/ml, FCm prolonged aPTT significantly by 1.5-fold. FCm was more active than fucoidan isolated and purified from Ceratophyllum Submersum L. (16.38% of sulfate, 42.11% of fucose) and extended the clotting time at 500 µg/ml by approximately 2-fold compared with our fucoidan that doesn’t show any clot formation at the same concentration (Figure 4). The effect of fucoidan extracted from brown seaweed F. vesiculosus L. (27.0% of sulfate) of the Barents Sea was slightly higher than the effect of FCm that causes a prolongation of aPTT by 2.5-fold at 10 µg/ml (Pozharitskaya et al., 2020). FCm had a potential anticoagulant activity but lower than that of heparin (p<0.05). In fact, the same effect of heparin (10µg/ml) was obtained at 500 µg/ml of FCm. The effect of FCm on aPTT was more pronounced than in the PT assay as shown in Figure 5. The result of the PT assay was expressed as the ratio of the clotting time in the presence and absence of fucoidan (Figure 5). FCm doesn’t show any effect at low concentrations ranging from 10 to 100 µg/ml. However, FCm concentrations above 200 µg/ml resulted in an extension of prothrombin time. The greatest prolongation of 2.67-fold was obtained with FCm at 500 µg/ml. These findings are comparable to fucoidan isolated from F. vesiculosus L. and Tasmanian U. pinnatifida, that had no effect on PT in vitro at low concentrations, but high concentrations above 80 µg/ml and 125 µg/ml, respectively, enhanced PT (Irhimeh et al., 2009; Pozharitskaya et al., 2020). Consequently, fucoidan from C. myrica demonstrated strong anti-coagulant effects. At high doses, it prolongs PT and considerably prolongs aPTT in a concentration dependent manner. This demonstrated that FCm may affect intrinsic pathways while having little impact on the extrinsic mechanism of coagulation.
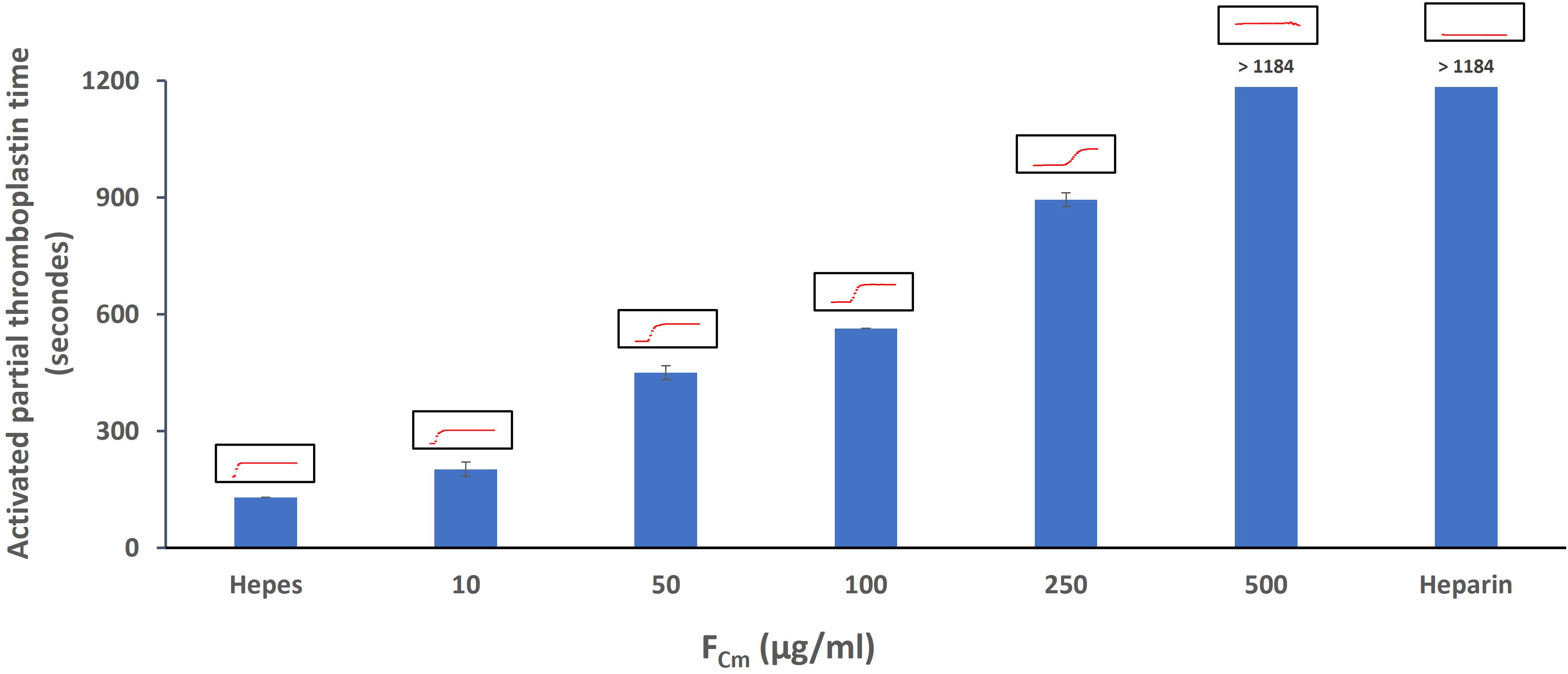
Figure 4 Activated partial thromboplastin time in the absence or presence of extracted fucoidan FCm (10 - 500 µg/mL) and standard heparin (10µg/ml). The red curves correspond to the actual instrument output of the reading on a microplate well, indicating increasing absorbance at 405 nm as a function of time.
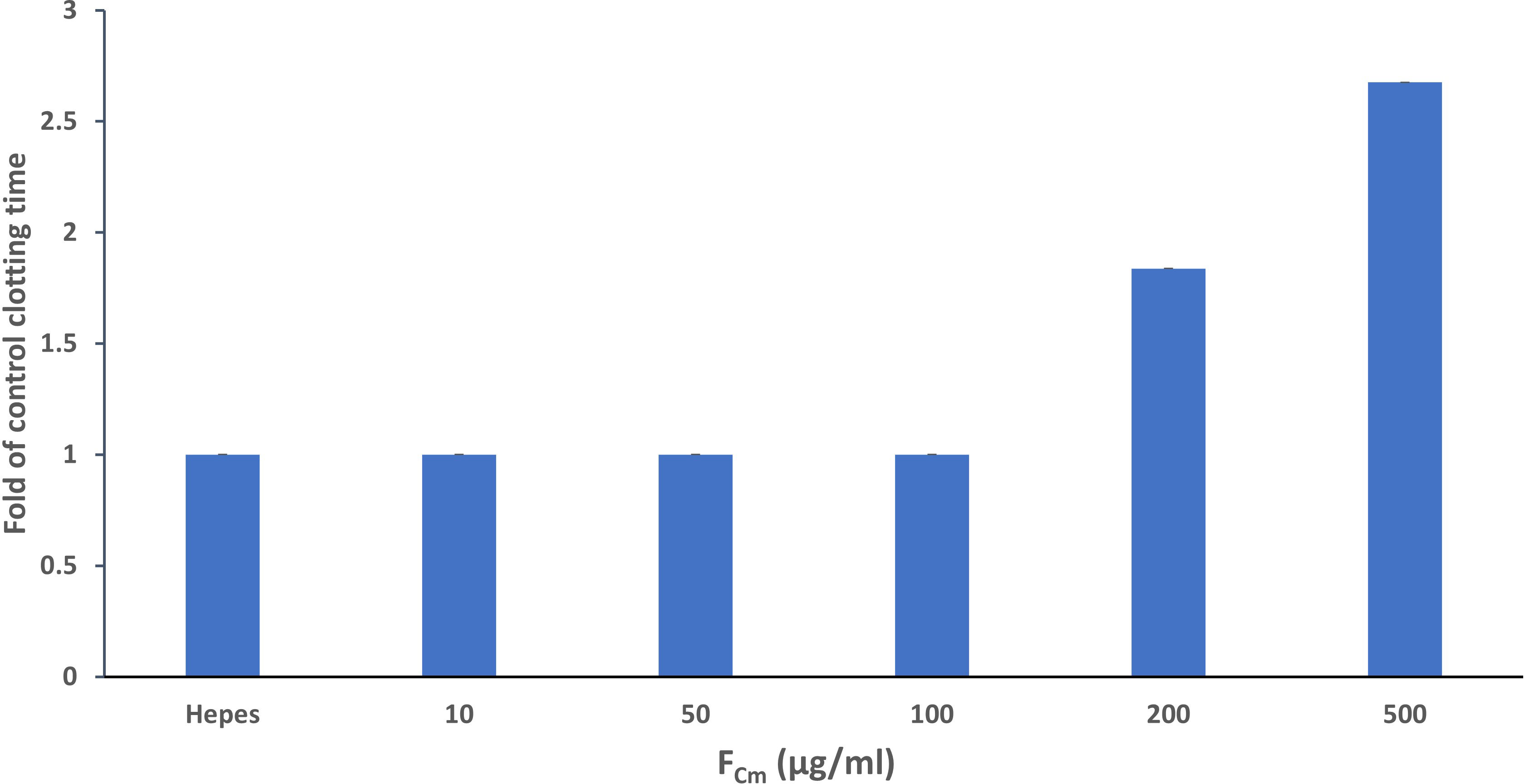
Figure 5 Prothrombin time in the absence or presence of extracted fucoidan FCm (10 –500 µg/mL). Data shown are the mean and standard deviation.
The inhibition of enzymes and cofactors of the common pathway may also cause an increase of aPTT. The common pathway begins with the activation of factor X (to factor Xa) via either the extrinsic or intrinsic pathways. It is the last step in the coagulation cascade and results in the synthesis of thrombin and fibrin (Palta et al., 2014). For this reason, fluorometric assays using thrombin and factor Xa inhibitor screening kits were employed to check the direct effect of the extracted fucoidan on thrombin or factor Xa. No inhibition was observed when thrombin or factor Xa was incubated with FCm alone over a range of concentrations (results not shown). The anticoagulant mechanism of fucoidan has been previously studied. Fucoidan, like heparin, involves antithrombin or heparin cofactor II for thrombin and factor Xa inhibition (Lapikova et al., 2008). The antithrombotic effect in vivo has also been confirmed in several studies. Ustyuzhanina et al. studied the antithrombotic activities of three fucoidans from brown seaweeds that have different structural properties including sulfate content, sulfation pattern and monosaccharide composition (Ustyuzhanina et al., 2013). Molecular weight, glycoside branching, sulfate/total sugar ratio, sulfate content and position have a significant impact on the anticoagulant activity of fucoidan (Wang et al., 2019). For instance, Jin et al. compared the anticoagulant activity of eleven fucoidans by studying the correlation between structure and anticoagulant activity. They also showed that the anticoagulant action of fucoidans was influenced by both the molar ratio of fucose to galactose and the average molecular weight (Zayed et al., 2023).
3.5 Antioxidant activities
The antioxidant activity of extracted fucoidan was studied by ABTS assay and Fe chelating test and compared to commercial fucoidan as shown in Figure 6.
3.5.1 ABTS assay
The ABTS scavenging assay depends on the decolorization of ABTS•+ when it reacts with a hydrogen-donating antioxidant (Huang et al., 2018). FCm showed ABTS radical scavenging activity dose-dependently (Figure 6A). It was also found that the antioxidant activity of FCm was 93.6% at concentrations of 5000 µg/ml and the IC50 was 1148 ± 0.02 µg/mL. The ABTS radical scavenging effect of FCm is similar to the antioxidant effect of fucoidan extracted from Kjellmaniella crassifolia (Tang et al., 2022). In contrast, commercial fucoidan shows a very low scavenging effect of 5.98 ± 0.6% at 5000 µg/ml concentration, with a comparable amount of sulfate (Table 1). Barahona et al. studied the kinetic profiles of the antioxidant capacity of seaweed polysaccharides with ABTS+ radical cations and confirmed that sulfate content and antioxidant activity were not correlated, whereas monosulfation position in the monosaccharide residues and antioxidant capacity were found to be positively correlated (Barahona et al., 2011). In addition, the link between antioxidant activity and chemical structure was studied for five fucoidans from different brown seaweeds, showing that although a sulfate group is necessary for antioxidant activity, fucoidans with high sulfate group content did not necessarily exhibit higher antioxidant activity (Ajisaka et al., 2016). The molecular mass of polysaccharides and their bioactivities are highly connected, for example Lahrse et al. have proved that pharmacological activities of depolymerized fractions obtained by hydrothermal and H2O2 treatment of fucoidan from seaweed F. vesiculosus decreased with decreasing molecular weight (Lahrsen et al., 2018).
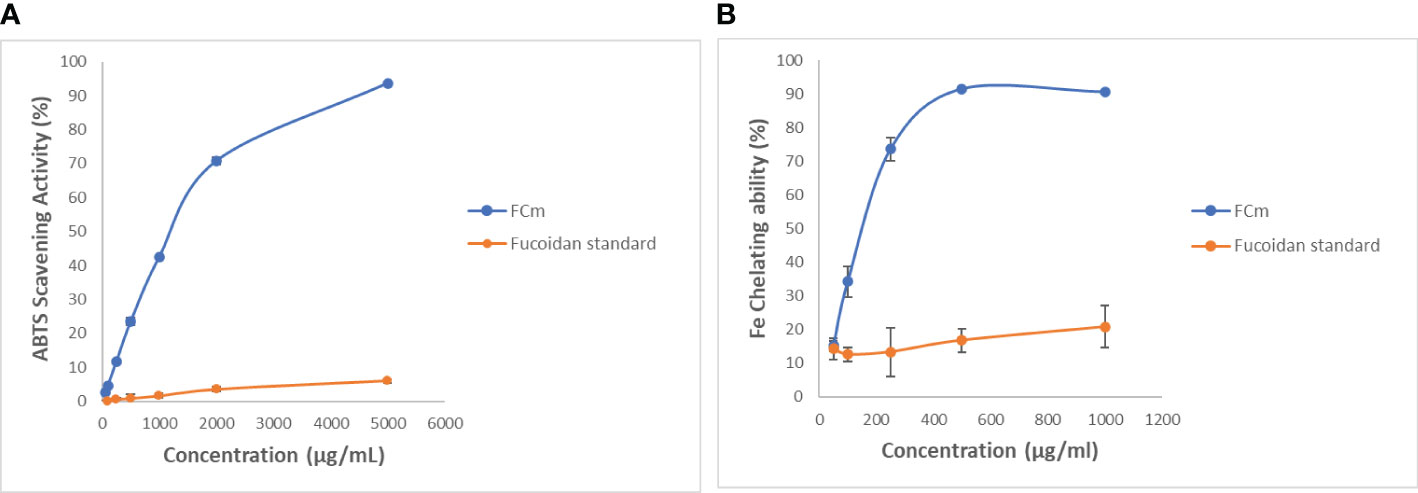
Figure 6 ABTS radical scavenging activity (A) and ferrous ion-chelating effect (B) of extracted fucoidan obtained from C. myrica and standard fucoidan at different concentrations. Data shown are the mean and standard deviation.
A low scavenging activity of 11% and 4.1% was observed for the crude fucoidan (1000 µg/ml) obtained from Fucus evanescens by hot acidic extraction and with aqueous calcium chloride solution, respectively (Imbs et al., 2015). Moreover, sulphated polysaccharides extracted from the seaweed F. vesiculosus by two different techniques (autohydrolysis and microwave-assisted extraction) showed different ABTS scavenging capacity, with a maximum of inhibition of 49.1 ± 1.1% at 1000 µg/mL (Rodriguez-Jasso et al., 2014). Fucoidan has been shown to have a variety of ABTS radical scavenging effects (Ashayerizadeh et al., 2020) that can be influenced by extraction techniques, solvents, seaweed origin, and physicochemical structures. The difference of the antioxidant activity between commercial fucoidan and extracted fucoidan could be due to coextracted secondary metabolites such as terpenoids and polyphenols (Schneider et al., 2015; Lahrsen et al., 2018), for instance. Shneider et al. have compared the antioxidant effects of four different batches of crude commercial fucoidan by DPPH test and found that they exhibited a significant batch-to-batch DPPH scavenging effect variance that could correlate with the phenolic content (Schneider et al., 2015).
3.5.2 Fe chelating ability
Ferrous ions were revealed to stimulate lipid peroxidation and have been recognized as an effective pro-oxidant in food systems (Huang et al., 2016). The ferrous ion chelating activity of FCm and commercial fucoidan at different concentrations is shown in Figure 6B. The extracted fucoidan FCm exhibited a dose-dependent ferrous ion-chelating effect. FCm was proved to have a maximal ferrous ion-chelating activity at 500 µg/ml of 91.23%. The IC50 of FCm for chelating 50% of ferrous ion was 166.9 ± 0.87 µg/ml revealing a greater metal ion-binding ability. Our results were higher than that obtained by Tsou et al. from S. ilicifolium extracted at two temperatures (65 and 80°C) with antioxidant effects of 93% and 92% at concentrations of 1000 µg/mL (Tsou et al., 2022). Furthermore, Ashayerizadeh et al. have recovered fucoidan from Sargassum tenerrimum by two water-extraction methods and their antioxidant capacity to chelate ferrous ions were 78.3% for DFM1 and 89.4% for DFM2 at 10 mg/mL concentration (Ashayerizadeh et al., 2020). The standard fucoidan (Figure 6B) revealed a weak ferrous ion-chelating effect of 20.83% at 1000 µg/ml which was comparable to the antioxidant activity of the crude fucoidan isolated from L. japonica that present a chelating effect of 24.95% at 1180 µg/ml (Wang et al., 2008). Recently, Kong et al. have isolated a fucoidan from Saccharina japonica that has the capacity of reducing cellular ROS and cell death in Vero cells stimulated by 2,2-azobis(2-amidinopropane) hydrochloride (AAPH) (Kong et al., 2022). It has previously been demonstrated that the sulfate/fucose ratio has an obvious correlation with the chelating ability (Wang et al., 2008). Also, it appears that various fucoidan processing methods and pre-treatments of seaweeds have an impact on their ion-chelating activity (Ashayerizadeh et al., 2020). According to recent research, several brown algal polysaccharides found in cell walls are related in a complicated way with polyphenols (Usov et al., 2022) that are also related to the antioxidant activity (Tsou et al., 2022). The demonstrated antioxidant activity of FCm boosts their significance as a potential new source of natural additives (Dore et al., 2013).
4 Conclusions
This study proposes an effective approach to extract fucoidan, an active polysaccharide that have therapeutic applications, from the beach-accumulated brown C. myrica algae. In the current study, the chemical properties and the purity of the isolated fucoidan were determined and confirmed using several techniques. FCm has dual anticoagulant and antioxidant properties, which may be influenced by the combination of various factors, including monosaccharide composition, the degree of sulfates, uronic acid content and others. Results indicate that fucoidan had prominent anticoagulant activity in vitro that may affect intrinsic pathways while having little impact on the extrinsic mechanism of coagulation since it significantly prolongs aPTT in a concentration dependent manner and it prolongs PT at high doses. However, FCm significantly exhibited an interesting antioxidant capacity as shown by the higher ABTS radical scavenging activity and Ferrous ion-chelating effect. According to the findings, C. myrica algae can be used as a competitive feedstock to make high-value sulfated polysaccharides like fucoidan that present beneficial effects, suggesting possible future innovative therapeutic possibilities for numerous diseases. This study provides a mechanism for scaling up marine macroalgae biomass conversion into value-added goods and particularly, the presence of the brown C. myrica algae accumulated in coastal environment can be turned from an unpleasant phenomenon into a source of pharmaceutical candidates.
Data availability statement
The original contributions presented in the study are included in the article/supplementary material. Further inquiries can be directed to the corresponding authors.
Author contributions
MD: Conceptualization, Data curation, Formal analysis, Investigation, Methodology, Project administration, Software, Writing – original draft, Writing – review & editing, Funding acquisition, Resources, Validation, Visualization.
Funding
The author(s) declare financial support was received for the research, authorship, and/or publication of this article. This research was funded by the Deputyship for Research and Innovation, Ministry of Education in Saudi Arabia through the project number 445-9-730.
Acknowledgments
The authors extend their appreciation to the Deputyship for Research and Innovation, Ministry of Education in Saudi Arabia for funding this research work through the project number 445-9-730.
Conflict of interest
The author declares that the research was conducted in the absence of any commercial or financial relationships that could be construed as a potential conflict of interest.
Publisher’s note
All claims expressed in this article are solely those of the authors and do not necessarily represent those of their affiliated organizations, or those of the publisher, the editors and the reviewers. Any product that may be evaluated in this article, or claim that may be made by its manufacturer, is not guaranteed or endorsed by the publisher.
References
Abdelrhman A. M., Ashour M., Al-Zahaby M. A., Sharawy Z. Z., Nazmi H., Zaki M. A., et al. (2022). Effect of polysaccharides derived from brown macroalgae Sargassum dentifolium on growth performance, serum biochemical, digestive histology and enzyme activity of hybrid red tilapia. Aquac. Rep. 25, 101212. doi: 10.1016/j.aqrep.2022.101212
Acharya K. (2017). Simplified methods for microtiter based analysis of in vitro antioxidant activity. Asian J. Pharm. (AJP) 11 (02).
Ajisaka K., Yokoyama T., Matsu K. (2016). Structural characteristics and antioxidant activities of fucoidans from five brown seaweeds. J. Appl. Glycosci. (1999) 63 (2), 31–37. doi: 10.5458/jag.jag.JAG-2015_024
Alkordi M. H., Haikal R. R., Hassan Y. S., Emwas A.-H., Belmabkhout Y. (2015). Poly-functional porous-organic polymers to access functionality–CO 2 sorption energetic relationships. J. Mater. Chem. A 3 (45), 22584–22590. doi: 10.1039/C5TA05297A
Apostolova E., Lukova P., Baldzhieva A., Delattre C., Molinie R., Petit E., et al. (2022). Structural characterization and in vivo anti-inflammatory activity of fucoidan from Cystoseira crinita (Desf.) Borry. Mar. Drugs 20 (11), 714. doi: 10.3390/md20110714
Ashayerizadeh O., Dastar B., Pourashouri P. (2020). Study of antioxidant and antibacterial activities of depolymerized fucoidans extracted from Sargassum tenerrimum. Int. J. Biol. Macromol. 151, 1259–1266. doi: 10.1016/j.ijbiomac.2019.10.172
Ashour M., Al-Souti A. S., Hassan S. M., Ammar G. A., Goda A. M.-S., El-Shenody R., et al. (2022). Commercial seaweed liquid extract as strawberry biostimulants and bioethanol production. Life 13 (1), 85. doi: 10.3390/life13010085
Baba B. M., Mustapha W. A. W., Joe L. S. (2018). Effect of extraction methods on the yield, fucose content and purity of fucoidan from Sargassum sp. obtained from Pulau Langkawi, Malaysia. Malaysian J. Anal. Sci. 22 (1), 87–94. doi: 10.17576/mjas-2018-2201-11
Barahona T., Chandía N. P., Encinas M. V., Matsuhiro B., Zúñiga E. A. (2011). Antioxidant capacity of sulfated polysaccharides from seaweeds. A kinetic approach. Food Hydrocoll. 25, 3, 529–535. doi: 10.1016/j.foodhyd.2010.08.004
Black W., Dewar E., Woodward F. (1952). Manufacture of algal chemicals. IV—laboratory-scale isolation of fucoidin from brown marine algae. J. Sci. Food Agric. 3, 3, 122–129. doi: 10.1002/jsfa.2740030305
Cesaretti M., Luppi E., Maccari F., Volpi N. (2003). A 96-well assay for uronic acid carbazole reaction. Carbohydr. Polymers 54 (1), 59–61. doi: 10.1016/S0144-8617(03)00144-9
Chisca S., Duong P., Emwas A.-H., Sougrat R., Nunes S. P. (2015). Crosslinked copolyazoles with a zwitterionic structure for organic solvent resistant membranes. Polymer Chem. 6 (4), 543–554. doi: 10.1039/C4PY01293C
Citkowska A., Szekalska M., Winnicka K. (2019). Possibilities of fucoidan utilization in the development of pharmaceutical dosage forms. Mar. Drugs 17 (8). doi: 10.3390/md17080458
Daniel R., Berteau O., Chevolot L., Varenne A., Gareil P., Goasdoue N. (2001). Regioselective desulfation of sulfated l-fucopyranoside by a new sulfoesterase from the marine mollusk Pecten maximus. Application to the structural study of algal fucoidan (Ascophyllum nodosum). Eur. J. Biochem. 268 (21), 5617–5626. doi: 10.1046/j.1432-1033.2001.02497.x
Daub C. D., Mabate B., Malgas S., Pletschke B. I. (2020). Fucoidan from Ecklonia maxima is a powerful inhibitor of the diabetes-related enzyme, α-glucosidase. Int. J. Biol. Macromol. 151, 412–420. doi: 10.1016/j.ijbiomac.2020.02.161
Dhahri M., Sioud S., Alsuhaymi S., Almulhim F., Haneef A., Saoudi A., et al. (2023). Extraction, characterization, and antioxidant activity of polysaccharides from Ajwa seed and flesh. Separations 10 (2), 103. doi: 10.3390/separations10020103
Dhahri M., Sioud S., Dridi R., Hassine M., Boughattas N. A., Almulhim F., et al. (2020). Extraction, characterization, and anticoagulant activity of a sulfated polysaccharide from Bursatella leachii viscera. ACS Omega 5 (24), 14786–14795. doi: 10.1021/acsomega.0c01724
Dische Z., Shettles L. B. (1948). A specific color reaction of methylpentoses and a spectrophotometric micromethod for their determination. J. Biol. Chem. 175 (2), 595–603. doi: 10.1016/S0021-9258(18)57178-7
Dobrinčić A., Balbino S., Zorić Z., Pedisić S., Bursać Kovačević D., Elez Garofulić I., et al. (2020). Advanced technologies for the extraction of marine brown algal polysaccharides. Mar. Drugs 18 (3), 168. doi: 10.3390/md18030168
Dore C. M., das C.F.A.M.G., Will L. S., Costa T. G., Sabry D. A., de Souza Rego L. A., et al. (2013). A sulfated polysaccharide, fucans, isolated from brown algae Sargassum vulgare with anticoagulant, antithrombotic, antioxidant and anti-inflammatory effects. Carbohydr. Polym. 91 (1), 467–475. doi: 10.1016/j.carbpol.2012.07.075
El-Shenody R. A., Ashour M., Ghobara M. M. E. (2019). Evaluating the chemical composition and antioxidant activity of three Egyptian seaweeds: Dictyota dichotoma, Turbinaria decurrens, and Laurencia obtusa. Braz. J. Food Technol. 22. doi: 10.1590/1981-6723.20318
Escobedo J. O., Wang W., Strongin R. M. (2006). Use of a commercially available reagent for the selective detection of homocysteine in plasma. Nat. Protoc. 1 (6), 2759–2762. doi: 10.1038/nprot.2006.399
Fauziee N. A. M., Chang L. S., Mustapha W. A. W., Nor A. R. M., Lim S. J. (2021). Functional polysaccharides of fucoidan, laminaran and alginate from Malaysian brown seaweeds (Sargassum polycystum, Turbinaria ornata and Padina boryana). Int. J. Biol. Macromol. 167, 1135–1145. doi: 10.1016/j.ijbiomac.2020.11.067
Fernando I. P. S., Dias M., Madusanka D. M. D., Han E. J., Kim M. J., Jeon Y. J., et al. (2020). Human keratinocyte UVB-protective effects of a low molecular weight fucoidan from Sargassum horneri purified by step gradient ethanol precipitation. Antioxid. (Basel) 9 (4), 340. doi: 10.3390/antiox9040340
Fernando I. P. S., Sanjeewa K. K. A., Samarakoon K. W., Lee W. W., Kim H. S., Kang N., et al. (2017). A fucoidan fraction purified from Chnoospora minima; a potential inhibitor of LPS-induced inflammatory responses. Int. J. Biol. Macromol. 104 (Pt A), 1185–1193. doi: 10.1016/j.ijbiomac.2017.07.031
Ganapathy S., Lingappa S., Naidu K., Selvaraj U., Ramachandiran S., Ponnusamy S., et al. (2019). Isolation and bioactive potential of fucoidan from marine macroalgae Turbinaria conoides. ChemistrySelect 4, 48, 14114–14119. doi: 10.1002/slct.201903548
González Fernández L. A., Castillo Ramos V., Sánchez Polo M., Medellín Castillo N. A. (2023). Fundamentals in applications of algae biomass: A review. J. Environ. Manage. 338, 117830. doi: 10.1016/j.jenvman.2023.117830
Gutmann C., Siow R., Gwozdz A. M., Saha P., Smith A. (2020). Reactive oxygen species in venous thrombosis. Int. J. Mol. Sci. 21 (6), 1918. doi: 10.3390/ijms21061918
Huang C.-Y., Kuo C.-H., Lee C.-H. (2018). Antibacterial and antioxidant capacities and attenuation of lipid accumulation in 3T3-L1 adipocytes by low-molecular-weight fucoidans prepared from compressional-puffing-pretreated Sargassum crassifolium. Mar. Drugs 16, 1, 24. doi: 10.3390/md16010024
Huang C.-Y., Wu S.-J., Yang W.-N., Kuan A.-W., Chen C.-Y. (2016). Antioxidant activities of crude extracts of fucoidan extracted from Sargassum glaucescens by a compressional-puffing-hydrothermal extraction process. Food Chem. 197, 1121–1129. doi: 10.1016/j.foodchem.2015.11.100
Imbs T. I., Skriptsova A. V., Zvyagintseva T. N. (2015). Antioxidant activity of fucose-containing sulfated polysaccharides obtained from Fucus evanescens by different extraction methods. J. Appl. Phycol. 27, 1, 545–553. doi: 10.1007/s10811-014-0293-7
Indumathi P., Mehta A. (2016). A novel anticoagulant peptide from the Nori hydrolysate. J. Funct. Foods 20, 606–617. doi: 10.1016/j.jff.2015.11.016
Irhimeh M. R., Fitton J. H., Lowenthal R. M. (2009). Pilot clinical study to evaluate the anticoagulant activity of fucoidan. Blood Coagulation Fibrinol. 20 (7), 607–610. doi: 10.1097/MBC.0b013e32833135fe
January G., Naidoo R., Kirby-McCullough B., Bauer R. (2019). Assessing methodologies for fucoidan extraction from South African brown algae. Algal Res. 40, 101517. doi: 10.1016/j.algal.2019.101517
Jayawardena T. U., Nagahawatta D., Fernando I., Kim Y.-T., Kim J.-S., Kim W.-S., et al. (2022). A review on fucoidan structure, extraction techniques, and its role as an immunomodulatory agent. Mar. Drugs 20, 12, 755. doi: 10.3390/md20120755
Jin W., Zhang Q., Wang J., Zhang W. (2013). A comparative study of the anticoagulant activities of eleven fucoidans. Carbohydr. Polymers 91 (1), 1–6. doi: 10.1016/j.carbpol.2012.07.067
Jin Y., Yu Q., Li S., Chen T., Liu D. (2023). Application of seaweed polysaccharide in bone tissue regeneration. Front. Mar. Sci. 10. doi: 10.3389/fmars.2023.1202422
Koh H. S. A., Lu J., Zhou W. (2019). Structure characterization and antioxidant activity of fucoidan isolated from Undaria pinnatifida grown in New Zealand. Carbohydr. Polym. 212, 178–185. doi: 10.1016/j.carbpol.2019.02.040
Kong Y., Wang L., Jeon Y.-J., Xu J., Gao X., Fu X. (2022). In vitro and in vivo antioxidant activities of a fucose-rich fucoidan isolated from Saccharina japonica. J. Appl. Phycol. 35, 353–364. doi: 10.1007/s10811-022-02869-x
Lahrsen E., Schoenfeld A. K., Alban S. (2018). Size-dependent pharmacological activities of differently degraded fucoidan fractions from Fucus vesiculosus. Carbohydr. Polym. 189, 162–168. doi: 10.1016/j.carbpol.2018.02.035
Lakshmanasenthil S., Vinothkumar T., Geetharamani D., Marudhupandi T., Suja G., Sindhu N. J. B., et al. (2014). Fucoidan—a novel α-amylase inhibitor from Turbinaria ornata with relevance to NIDDM therapy. Biocatal. Agric. Biotechnol. 3, 3, 66–70. doi: 10.1016/j.bcab.2014.02.003
Lapikova E., Drozd N., Tolstenkov A., Makarov V., Zvyagintseva T., Shevchenko N., et al. (2008). Inhibition of thrombin and factor Xa by Fucus evanescens fucoidan and its modified analogs. Bull. Exp. Biol. Med. 146, 328–333. doi: 10.1007/s10517-008-0267-3
Li S., Wang A., Liu L., Tian G., Xu F. (2019). Effect of deproteinization methods on the antioxidant activity of polysaccharides extracted from Lentinus edodes stipe. J. Food Measurement Characterization 13, 1382–1389. doi: 10.1007/s11694-019-00054-2
Li Y., Zheng Y., Zhang Y., Yang Y., Wang P., Imre B., et al. (2021). Brown algae carbohydrates: Structures, pharmaceutical properties, and research challenges. Mar. Drugs 19 (11), 620. doi: 10.3390/md19110620
Lim S. J., Aida W. M. W. (2017). “Extraction of sulfated polysaccharides (Fucoidan) from brown seaweed,” in Seaweed polysaccharides (Elsevier), 27–46.
Lim S. J., Aida W. M. W., Maskat M. Y., Latip J., Badri K. H., Hassan O., et al. (2016). Characterisation of fucoidan extracted from Malaysian Sargassum binderi. Food Chem. 209, 267–273. doi: 10.1016/j.foodchem.2016.04.058
Mak W., Wang S. K., Liu T., Hamid N., Li Y., Lu J., et al. (2014). Anti-proliferation potential and content of fucoidan extracted from sporophyll of New Zealand Undaria pinnatifida. Front. Nutr. 1. doi: 10.3389/fnut.2014.00009
Manggau M., Kasim S., Fitri N., Aulia N., Agustiani A., Raihan M., et al. (2022). “Antioxidant, anti-inflammatory and anticoagulant activities of sulfate polysaccharide isolate from brown alga Sargassum policystum,” in 3rd International Conference on Integrated Coastal Management & Marine Biotechnology. IOP Conference Series: Earth and Environmental Science (IOP Publishing), 012029.
Michel J., Abd Rani N. Z., Husain K. (2020). A review on the potential use of medicinal plants from Asteraceae and Lamiaceae plant family in cardiovascular diseases. Front. Pharmacol. 11, 852. doi: 10.3389/fphar.2020.00852
Mohamed S. F., Slamy T. M., Shaeiri S. Y. (2015). Characterization of sulphated polysaccharide with antiviral activity from marine brown alga Cystoseira myrica collected from Jazan coasts, KSA. Int. J. PharmTech Res. 8, 198–203.
Mousavian Z., Safavi M., Azizmohseni F., Hadizadeh M., Mirdamadi S. (2022). Characterization, antioxidant and anticoagulant properties of exopolysaccharide from marine microalgae. AMB Express 12 (1), 27. doi: 10.1186/s13568-022-01365-2
Olas B. (2022). The antioxidant, anti-platelet and anti-coagulant properties of phenolic compounds, associated with modulation of hemostasis and cardiovascular disease, and their possible effect on COVID-19. Nutrients 14 (7), 1390. doi: 10.3390/nu14071390
Palanisamy S., Vinosha M., Marudhupandi T., Rajasekar P., Prabhu N. M. (2017). Isolation of fucoidan from Sargassum polycystum brown algae: Structural characterization, in vitro antioxidant and anticancer activity. Int. J. Biol. Macromol. 102, 405–412. doi: 10.1016/j.ijbiomac.2017.03.182
Palta S., Saroa R., Palta A. (2014). Overview of the coagulation system. Indian J. Anaesthesia 58 (5), 515. doi: 10.4103/0019-5049.144643
Pozharitskaya O. N., Obluchinskaya E. D., Shikov A. N. (2020). Mechanisms of bioactivities of fucoidan from the brown seaweed Fucus vesiculosus L. of the Barents Sea. Mar. Drugs 18 (5), 275. doi: 10.3390/md18050275
Qu Y., Cao Z., Wang W., Wang N., Li X., Pan J. (2019). Monthly variations of fucoidan content and its composition in the farmed brown alga Saccharina sculpera (Laminariales, Phaeophyceae). J. Appl. Phycol. 31 (4), 2623–2628. doi: 10.1007/s10811-019-1753-x
Rani V., Shakila R., Jawahar P., Srinivasan A. (2017). Influence of species, geographic location, seasonal variation and extraction method on the fucoidan yield of the brown seaweeds of Gulf of Mannar, India. Indian J. Pharm. Sci. 79, 65–71. doi: 10.4172/pharmaceutical-sciences.1000202
Rodriguez-Jasso R. M., Mussatto S. I., Pastrana L., Aguilar C. N., Teixeira J.A.J.C.P. (2014). Chemical composition and antioxidant activity of sulphated polysaccharides extracted from Fucus vesiculosus using different hydrothermal processes. Chem. Papers 68, 2, 203–209. doi: 10.2478/s11696-013-0430-9
Rudovica V., Rotter A., Gaudêncio S. P., Novoveská L., Akgül F., Akslen-Hoel L. K., et al. (2021). Valorization of marine waste: use of industrial by-products and beach wrack towards the production of high added-value products. Front. Mar. Sci. 8, 723333. doi: 10.3389/fmars.2021.723333
Saravana P. S., Cho Y. J., Park Y. B., Woo H. C., Chun B. S. (2016). Structural, antioxidant, and emulsifying activities of fucoidan from Saccharina japonica using pressurized liquid extraction. Carbohydr. Polym. 153, 518–525. doi: 10.1016/j.carbpol.2016.08.014
Schneider T., Ehrig K., Liewert I., Alban S. J. G. (2015). Interference with the CXCL12/CXCR4 axis as potential antitumor strategy: Superiority of a sulfated galactofucan from the brown alga Saccharina latissima and fucoidan over heparins. Glycobiology 25, 8, 812–824. doi: 10.1093/glycob/cwv022
Shinde P., Banerjee P., Mandhare A. (2019). Marine natural products as source of new drugs: A patent review, (2015–2018). Expert Opin. Ther. Patents 29 (4), 283–309. doi: 10.1080/13543776.2019.1598972
Stelling M. P., de Bento A. A., Caloba P., Vilanova E., Pavão M. S. G. (2019). “Methods for isolation and characterization of sulfated glycosaminoglycans from marine invertebrates,” in The Extracellular Matrix: Methods and Protocols. Eds. Vigetti D., Theocharis A. D. (New York, NY: Springer New York), 55–70.
Synytsya A., Kim W.-J., Kim S.-M., Pohl R., Synytsya A., Kvasnička F., et al. (2010). Structure and antitumour activity of fucoidan isolated from sporophyll of Korean brown seaweed Undaria pinnatifida. Carbohydr. Polymers 81 (1), 41–48. doi: 10.1016/j.carbpol.2010.01.052
Tang S., Ma Y., Dong X., Zhou H., He Y., Ren D., et al. (2022). Enzyme-assisted extraction of fucoidan from Kjellmaniella crassifolia based on kinetic study of enzymatic hydrolysis of algal cellulose. Algal Res. 66, 102795. doi: 10.1016/j.algal.2022.102795
Tsou M. H., Lee C. C., Wu Z. Y., Lee Z. H., Lin H. M. (2022). Bioactivity of crude fucoidan extracted from Sargassum ilicifolium (Turner) C. Agardh. Sci. Rep. 12 (1), 15916. doi: 10.1038/s41598-022-19370-7
Usov A. I., Bilan M. I., Ustyuzhanina N. E., Nifantiev N.E.J.M.D. (2022). Fucoidans of Brown Algae: Comparison of Sulfated Polysaccharides from Fucus vesiculosus and Ascophyllum nodosum. Mar. Drugs 20, 10, 638. doi: 10.3390/md20100638
Ustyuzhanina N. E., Ushakova N. A., Zyuzina K. A., Bilan M. I., Elizarova A. L., Somonova O. V., et al. (2013). Influence of fucoidans on hemostatic system. Mar. Drugs 11 (7), 2444–2458. doi: 10.3390/md11072444
Walkowiak B., Kęsy A., Michalec L. (1997). Microplate reader-a convenient tool in studies of blood coagulation. Thromb. Res. 87 (1), 95–103. doi: 10.1016/S0049-3848(97)00108-4
Wang J., Zhang Q., Zhang Z., Li Z. (2008). Antioxidant activity of sulfated polysaccharide fractions extracted from Laminaria japonica. Int. J. Biol. Macromol. 42 (2), 127–132. doi: 10.1016/j.ijbiomac.2007.10.003
Wang Y., Xing M., Cao Q., Ji A., Liang H., Song S. (2019). Biological activities of fucoidan and the factors mediating its therapeutic effects: A review of recent studies. Mar. Drugs 17 (3), 183. doi: 10.3390/md17030183
Wen Y., Gao L., Zhou H., Ai C., Huang X., Wang M., et al. (2021). Opportunities and challenges of algal fucoidan for diabetes management. Trends Food Sci. Technol. 111, 628–641. doi: 10.1016/j.tifs.2021.03.028
Xin L., Bin L., Xiao-Lei W., Zhen-Liang S., Chang-Yun W. (2016). Extraction, fractionation, and chemical characterisation of fucoidans from the brown seaweed Sargassum pallidum. Czech J. Food Sci. 34, 5, 406–413. doi: 10.17221/322/2015-CJFS
Yao Y., Yim E. K. (2021). Fucoidan for cardiovascular application and the factors mediating its activities. Carbohydr. Polymers 270, 118347. doi: 10.1016/j.carbpol.2021.118347
Yu J., Li Q., Wu J., Yang X., Yang S., Zhu W., et al. (2021). Fucoidan extracted from sporophyll of Undaria pinnatifida grown in Weihai, China–chemical composition and comparison of antioxidant activity of different molecular weight fractions. Front. Nutr. 8, 636930. doi: 10.3389/fnut.2021.636930
Zayed A., Finkelmeier D., Hahn T., Rebers L., Shanmugam A., Burger-Kentischer A., et al. (2023). Characterization and cytotoxic activity of microwave-assisted extracted crude fucoidans from different brown seaweeds. Mar. Drugs 21 (1), 48. doi: 10.3390/md21010048
Keywords: marine waste, brown algae, fucoidan, sulfate, antioxidant, anticoagulant
Citation: Dhahri M (2023) Cystoseira myrica: from beach-cast seaweed to fucoidan with antioxidant and anticoagulant capacity. Front. Mar. Sci. 10:1327408. doi: 10.3389/fmars.2023.1327408
Received: 24 October 2023; Accepted: 04 December 2023;
Published: 20 December 2023.
Edited by:
El-Sayed Salama, Lanzhou University, ChinaReviewed by:
Mohamed Ashour, National Institute of Oceanography and Fisheries (NIOF), EgyptAhmed E. Alprol, National Institute of Oceanography and Fisheries (NIOF), Egypt
Copyright © 2023 Dhahri. This is an open-access article distributed under the terms of the Creative Commons Attribution License (CC BY). The use, distribution or reproduction in other forums is permitted, provided the original author(s) and the copyright owner(s) are credited and that the original publication in this journal is cited, in accordance with accepted academic practice. No use, distribution or reproduction is permitted which does not comply with these terms.
*Correspondence: Manel Dhahri, bWFuZWwuZGhhaHJpQGdtYWlsLmNvbQ==