- 1Centro de Investigaciones Biológicas del Noroeste (CIBNOR), La Paz, Baja California Sur, Mexico
- 2Université de Perpignan Via Domita, Perpignan, France
- 3School of the Environment, The University of Queensland, St. Lucia, QLD, Australia
- 4Department of Biology, The Pennsylvania State University, University Park, PA, United States
- 5Department of Biological Sciences, Louisiana State University, Baton Rouge, LA, United States
Editorial on the Research Topic
Innovative approaches to coral reef science by early career researchers
Coral reefs are facing numerous threats including climate change, ocean acidification, coastal development, pollution, overfishing, coral disease, and other disturbances (Bellwood et al., 2004; Setter et al., 2022). Improving the understanding of these ecosystems to help stem their decline requires the integration of innovative technologies. This Research Topic aims to showcase and acknowledge high-quality work from outstanding researchers in the early stages of their careers, particularly of those who obtained their Masters or PhD within the last 10 years from a wide range of fields in coral reef science. This Research Topic highlights the novel and transformative work of 14 contributions conducted by early career scientists from institutions across the globe and provides a forum for the development of the emerging technologies and the integration of these methods to address questions in coral reef ecosystems (Figure 1). The articles implement innovative approaches for reef monitoring and intervention to aid the recovery of coral reefs. They also explore new methods and models to detect and understand coral reef stressors, such as thermal and light stress, acidic water, and microplastics. In addition, different methods are used to investigate trophic strategies in mesophotic reefs, characterize sexual dimorphism, and delineate species using three-dimensional skeletal features.
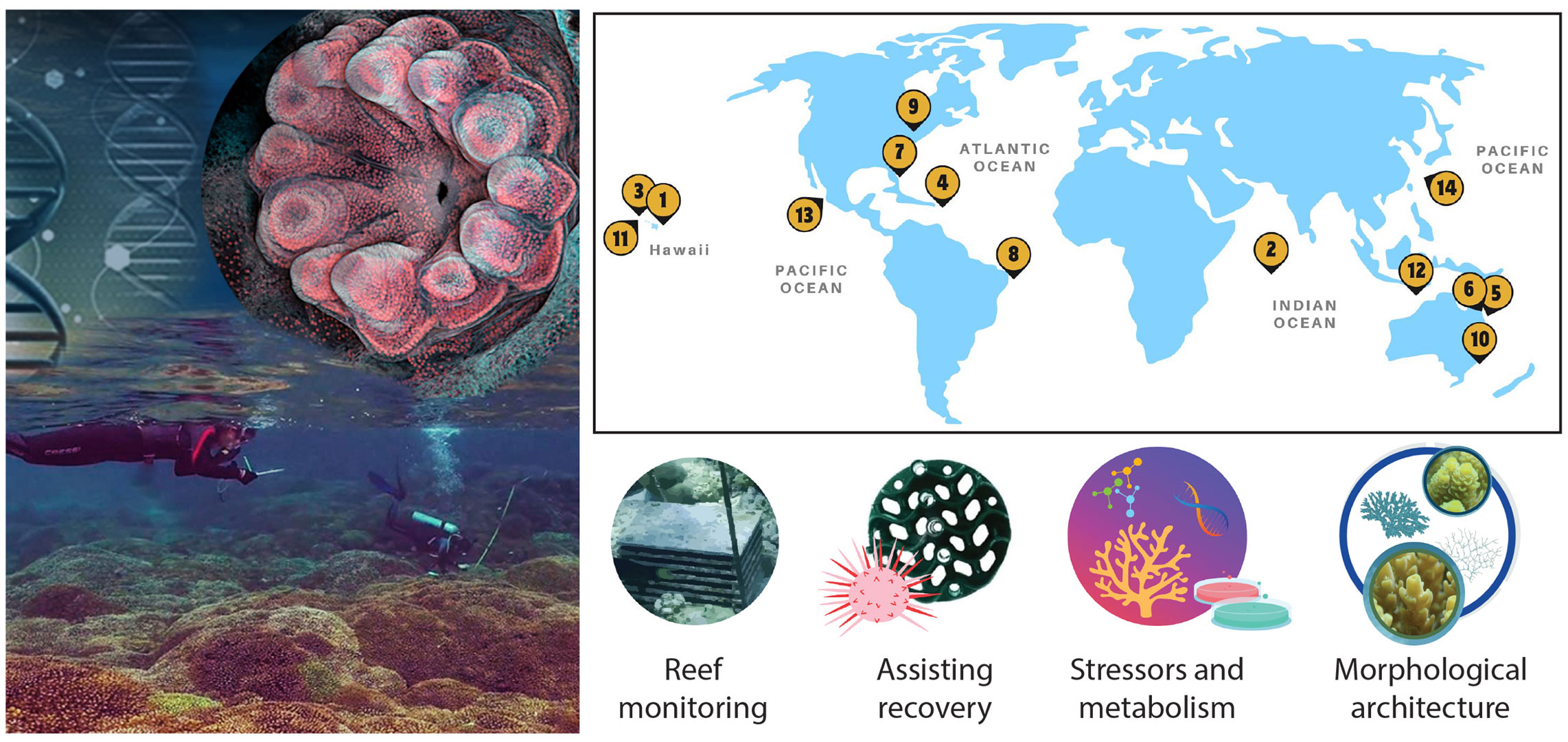
Figure 1 Geographic locations of the studies undertaken and representative topic categories of contributions to the Research Topic: Innovative Approaches to Coral Reef Science by Early Career Researchers. Reef monitoring: 1) Grady et al., 2) Steyaert et al., 3) Yadav et al.; assisting recovery: 4) Hylkema et al., 5) Röepke et al., 6) Torda & Quigley; stressors and metabolism: 7) Bove et al., 8) da Silva & Kampel, 9) Galindo-Martínez et al., 10) Buerger et al., 11) Chiles et al., 12) Carmignani et al.; morphological architecture: 13) Pedraza-Pohlenz et al., 14) Ramírez-Portilla et al. Geographic locations are based on first-author affiliations in modeling and experimental studies (6, 9, 10).
Innovative approaches in reef monitoring
Interactions between macroalgae and other benthic taxa can result in changes in community composition (Brown et al., 2018), whereby dominant taxa outcompete other species in reef ecosystems. Grady et al. present an example of how molecular genetics and scanning electron microscopy can be paired with ecological monitoring to document coral reef ecosystem change and benthic community composition reshuffling. The authors analyzed long-term ecological data (2003-2020) on the western coast of Hawaii, documenting changes in the distribution of calcifying crustose macroalgae. They also validated the presence of a newly described taxon (Ramicrusta hawaiiensis) in shallow intertidal habitats, expanding the known distribution for this taxon. This multidisciplinary study provides a baseline for monitoring ecologically important taxa and improves our understanding of algal ecology and biodiversity in Hawaii.
Cryptobenthic organisms may escape notice next to flashy teleosts and anthozoans, but they harbor high biodiversity and include members critical to coral reef function (de Goeij et al., 2013). Standardized autonomous reef monitoring structures (ARMS) have facilitated species identification and biomass estimation for these taxa (Pearman et al., 2018), but linking these data to physical reef settings has proven difficult in remote locations. Steyaert et al. employed ARMS and in situ environmental measures to assess cryptobenthic diversity and compared them to remotely sensed data. Ex situ data missed some events entirely (e.g., internal waves) and qualitatively rearranged site rankings with respect to temperature and wave height, underlying the importance of in situ data logging.
New monitoring approaches can help survey coral reefs and improve the understanding of their responses to climate change (Apprill et al., 2023). Yadav et al. used structure-from-motion photogrammetry to track spatial changes in community composition, coral bleaching, and mortality during a marine heatwave 2019 in Kāne‘ohe Bay, Hawaii. After following the fate of thousands of coral colonies during and after a bleaching event (4 months and one year after), and measuring environmental variables, the authors documented spatial heterogeneity in bleaching and mortality. Bleaching was more severe with up to 80% of corals showing signs of stress in sites dominated by heat-sensitive coral species, whereby mortality was influenced by high temperature, heat-sensitive species, and high morphology complexity of coral colonies. This study provides a novel link between heat-stress response and habitat complexity, 3D models, and a digital baseline for tracking the effects of bleaching and mortality in coral reefs.
Assisting the recovery of coral reefs
Macroalgae can become dominant in the absence of herbivory and constrain the recruitment and recovery of reef corals (Box and Mumby, 2007). The decimation of the sea urchin Diadema antillarum in the Caribbean has allowed an increase in macroalgae cover and a reduction of coral recruitment (Myhre and Acevedo-Gutiérrez, 2007). Hylkema et al. propose Assisted Natural Recovery as a new way to restore D. antillarum populations, which consists of hastening recruitment by identifying and removing barriers to natural population succession. The authors showed that lack of suitable settlement substrates and low post-settlement survival limit the recovery of D. antillarum.
Organismal behaviors can be highly sensitive indicators of environmental contaminants (Weis, 2014). Röepke et al. examined the impacts of antifouling coatings on the swimming behaviors of early-stage larvae of Acropora millepora to assess the potential uses of these non-toxic treatments in aquaculture for coral restoration. The authors show evidence for antifouling coatings effects on larval swimming, thus larval behaviors can be used as a sensitive toxicity indicator of non-lethal coral stress. By providing a guided manual on automatic image acquisition to track coral larval behaviors, the authors contribute towards developing standardized monitoring tools for cnidarian behavior with implications for improving coral health and survival in restoration efforts.
The goal of assisted evolution is to preserve and restore ecosystems by enhancing adaptative traits of species threatened by climate change (van Oppen et al., 2015). Thus, the main factors driving adaptive capacities must be quantified to determine the feasibility of success and the risks of genetic interventions in the wild. Torda & Quigley used polygenic metapopulation adaptation models to evaluate how active interventions may influence adaptation in wild populations. This study demonstrates that three factors are the main drivers of natural adaptation rates: genetic diversity, population connectivity, and additive genetic variance. Interventions are most effective in populations with low genetic diversity and during a cold-to-warm phase of an El Niño-Southern Oscillation like events. The authors also provide a global meta-analysis of the genetic diversity found in tropical reef-building corals and show how evolutionary models can be used to set thresholds and guide decision support around active genetic interventions.
Understanding coral reef stressors
Coral health is threatened by many different environmental stressors, to which they can respond in varied ways (Kaniewska et al., 2015). Studies based in laboratory experiments usually focus on single stressors and its response mechanisms, while there is limited knowledge about how the interactions among stressors affect corals (Rivera et al., 2021). Bove et al. employed a multifactorial approach in their study of the staghorn coral (Acropora cervicornis), comparing the independent and combined effects of a future ocean (warm, acidic water) and microplastics on gene expression, algal symbionts, and other members of the microbiome. Gene expression showed the strongest response, while algal and bacteria community compositions changed little. Notably, stressor interactions elicited higher expression changes in immune response genes than either single stressor. Thus, these results suggest that microplastic pollution may interact with ocean acidification and warming to trigger a more severe molecular response in this taxon, and potentially other, coral species.
Thermal stress tolerance to bleaching in corals can vary along their range of distribution (Dixon et al., 2015). Depending on the heritability of this tolerance, gene flow can potentially facilitate adaptation to warming seas (Kleypas et al., 2016). da Silva & Kampel tested for the possible impact of such connectivity using a biophysical model grounded in the life history of a common Brazilian coral (Mussismilia hispida) and decades of sea surface temperature data. While the effects of connectivity could increase or decrease the thermal stress thresholds of a given population, the positive effects were greatest at either end of the species’ range, where the challenges of warming waters should be most acute.
The loss of microalgal symbionts following heat stress can result in elevated irradiance within the coral host’s tissue leading to further photodamage and ultimately significant bleaching (Wangpraseurt et al., 2017). However, increased production of animal chromoproteins during this period can facilitate coral recovery, although the mechanisms remain obscure (Gómez-Campo et al., 2022). Galindo-Martínez et al. developed a model to evaluate the role of coral chromoproteins in setting the optical properties of coral tissues, thereby modulating their internal light environment. Their simulations suggest that up-regulation of host chromoproteins can reduce light intensity by up to 11% in hospite when present individually, and by up to 24% when present in combination. Such reductions may help prevent the full development of bleached phenotypes in stressed corals, thereby facilitating a more rapid recovery and symbiont re-colonization after bleaching.
A major driver of coral bleaching is thought to be the excess production of reactive oxygen species (ROS) within chloroplasts and mitochondria of the microalgal symbionts during heat stress (Szabó et al., 2020). However, strains of Symbiodiniaceae differ in their susceptibility to ROS production as well as their capacities for ROS scavenging (Suggett et al., 2017), contributing to differences in microalgal thermal tolerance. Buerger et al. employed flow cytometry to rapidly screen and identify thermotolerant Cladocopium strains based on intracellular stress indicators following heat exposure. Strains previously selected for their capacity to confer thermal tolerance to the coral host had the lowest ROS titers and displayed elevated ROS scavenging capacity relative to both non-selected (i.e., “wild type”) and selected, but non-thermally beneficial, strains. Their results provide further evidence for the role microalgal ROS management plays in determining holobiont thermal tolerance, and suggest that flow cytometry can be a useful tool to rapidly identify microalgal strains with enhanced thermal tolerance.
Insights into coral metabolism
Historically, metabolic analyses targeting scleractinians have primarily investigated mechanisms of holobiont carbon exchange (Furla et al., 2000). However, focus has widened to include consideration of the role other metabolic substrates, such as ammonium, may play in maintaining photosymbioses (Rädecker et al., 2015). Given the potential for rising ocean temperatures to alter rates of nitrogen uptake in reef-building corals (Rädecker et al., 2020), Chiles et al. investigated the effects of exposure to elevated temperature on host nitrogen assimilation and metabolism. Using isotopic labeling (15N) they demonstrated a compartmentalization between amino acid and dipeptide production in scleractinian corals with rapid turnover of the latter metabolite pool. This suggests that rapid microalgal dipeptide synthesis may hamper the host’s ability to regulate symbiont proliferation through nitrogen restriction and may therefore contribute to holobiont susceptibility to acute heat stress.
Little is known about how corals maintain their metabolic homeostasis in mesophotic environments, where light is scarce and sampling is challenging (Lesser et al., 2010). Carmignani et al. assessed tropic strategies of five coral species across a 40-75 m depth gradient in Western Australia, based on stable isotope analysis, photophysiological measurements, and protein concentration. Despite living at 1% of their surface irradiance, three of the studied species rely on autotrophy as the main source of metabolic energy, whereas the others appear to be more heterotrophic. This study highlights species-specific acclimation strategies and physiological limits of corals to suboptimal environments.
Approaches in morphological architecture
Reproductive modes carry different energetic costs (Hall and Hughes, 1996), which may lead to varying levels of sexual dimorphism. In modular organisms, such as corals, morphology architecture and the integration of its units (modularity) can suffer modifications in response to environmental conditions (Paz-García et al., 2015). Still, no such modularity has been investigated between sexes in corals. Pedraza-Pohlenz et al. demonstrate sexual dimorphism in the corallite dimension of two reef-building species, Porites lobata (gonochoric broadcast spawner) and Porites panamensis (gonochoric brooder). The corallite area was smaller, with higher trait integration in females than in males in P. lobata, while P. panamensis had an opposite pattern between sexes. Varying patterns of sexual dimorphism between reproductive modes may be related to the different selective pressures faced by each sex.
The study of morphological variation is crucial for understanding the role of evolution, function, and plasticity in speciation (Todd, 2008; Pereira et al., 2021). Ramírez-Portilla et al. use a three-dimensional analysis to help delineate species in the morphologically diverse and taxonomically challenging coral genus Acropora. Results revealed significant differences of the morphogroups based on 3D-variables, highlighting the potential use of this method in combination with other independent lines of evidence for the discrimination of morphologically complex organisms.
This Research Topic provides a timely compilation of innovative valuable approaches and high-quality research led by early career scientists around the world. The science presented here is spearheaded by the upcoming generation of scientific authorities. We hope this Research Topic promotes an optimistic view towards the global coral crisis. It encourages the scientific community to continue their efforts in finding effective solutions to the challenges faced by reef ecosystems in our changing world.
Author contributions
DP: Writing – original draft, Writing – review & editing. EA: Writing – original draft, Writing – review & editing. IP: Writing – original draft, Writing – review & editing. RG: Writing – original draft, Writing – review & editing. MH: Writing – original draft, Writing – review & editing.
Funding
The author(s) declare that no financial support was received for the research, authorship, and/or publication of this article.
Acknowledgments
We thank Brett Lewis for kindly providing the polyp image used in the Research Topic illustration. We appreciate the help of the reviewers and thank the authors of all articles published in this Research Topic.
Conflict of interest
The authors declare that the research was conducted in the absence of any commercial or financial relationships that could be construed as a potential conflict of interest.
Publisher’s note
All claims expressed in this article are solely those of the authors and do not necessarily represent those of their affiliated organizations, or those of the publisher, the editors and the reviewers. Any product that may be evaluated in this article, or claim that may be made by its manufacturer, is not guaranteed or endorsed by the publisher.
References
Apprill A., Girdhar Y., Mooney T. A., Hansel C. M., Long M. H., Liu Y., et al. (2023). Toward a new era of coral reef monitoring. Environ. Sci. Technol. 57, 5117–5124. doi: 10.1021/acs.est.2c05369
Bellwood D., Hughes T., Folke C., Nyström M. (2004). Confronting the coral reef crisis. Nature 429, 827–833. doi: 10.1038/nature02691
Box S. J., Mumby P. J. (2007). Effect of macroalgal competition on growth and survival of juvenile caribbean corals. Mar. Ecol. Prog. Ser. 342, 139–149. doi: 10.3354/meps342139
Brown K. T., Bender-Champ D., Kubicek A., van der Zande R., Achlatis M., Hoegh-Guldberg O., et al. (2018). The dynamics of coral-algal interactions in space and time on the southern great barrier reef. Front. Mar. Sci. 5. doi: 10.3389/fmars.2018.00181
de Goeij J. M., van Oevelen D., Vermeij M. J. A., Osinga R., Middelburg J. J., de Goeij A. F. P. M., et al. (2013). Surviving in a marine desert: the sponge loop retains resources within coral reefs. Science 342, 108–110. doi: 10.1126/science.124198
Dixon G. B., Davies S. W., Aglyamova G. V., Meyer E., Bay L. K., Matz M. V. (2015). Genomic determinants of coral heat tolerance across latitudes. Science 348, 1460–1462. doi: 10.1126/science.1261224
Furla P., Galgani I., Durand I., Allemand D. (2000). Sources and mechanisms of inorganic carbon transport for coral calcification and photosynthesis. J. Exp. Biol. 203, 3445–3457. doi: 10.1242/jeb.203.22.3445
Gómez-Campo K., Enríquez S., Iglesias-Prieto R. (2022). A road map for the development of the bleached coral phenotype. Front. Mar. Sci. 9. doi: 10.3389/fmars.2022.806491
Hall V. R., Hughes T. P. (1996). Reproductive strategies of modular organisms: comparative studies of reef-building corals. Ecology 77, 950–963. doi: 10.2307/2265514
Kaniewska P., Chan C.-K. K., Kline D., Ling E. Y. S., Rosic N., Edwards D., et al. (2015). Transcriptomic changes in coral holobionts provide insights into physiological challenges of future climate and ocean change. PloS One 10 (10), e0139223. doi: 10.1371/journal.pone.0139223
Kleypas J. A., Thompson D. M., Castruccio F. S., Curchitser E. N., Pinsky M., Watson J. R. (2016). Larval connectivity across temperature gradients and its potential effect on heat tolerance in coral populations. Global Change Biol. 22, 3539–3549. doi: 10.1111/gcb.13347
Lesser M. P., Slattery M., Stat M., Ojimi M., Gates R. D., Grottoli A. (2010). Photo accolimatization by the coral Montastraea cavernosa in the mesophotic zone: light, food, and genetics. Ecology 91, 990–1003. doi: 10.1890/09-0313.1
Myhre S., Acevedo-Gutiérrez A. (2007). Recovery of sea urchin diadema antillarum populations is correlated to increased coral and reduced macroalgal cover. Mar. Ecol. Prog. Ser. 329, 205–210. doi: 10.3354/meps329205
Paz-García D. A., Aldana-Moreno A., Cabral-Tena R. A., García-de-Léon F. J., Hellberg M. E., Balart E. F. (2015). Morphological variation and different branch modularity across contrasting flow conditions in dominant Pocillopora reef-building corals. Oecologia 178, 207–218. doi: 10.1007/s00442-014-3199-9
Pearman J. K., Leray M., Villalobos R., Machida R. J., Berumen M. L., Knowlton N., Carvalho S, et al. (2018). Cross-shelf investigation of coral reef cryptic benthic organisms reveals diversity patterns of the hidden majority. Sci. Rep. 8, 8090. doi: 10.1038/s41598-018-26332-5
Pereira C. L., Gilbert M. T. P., Araújo M. B., Matias M. G. (2021). Fine-tuning biodiversity assessments: A framework to pair eDNA metabarcoding and morphological approaches. Methods Ecol. Evol. 12, 2397–2409. doi: 10.1111/2041-210X.13718
Rädecker N., Pogoreutz C., Gegner H. M., Cardenas A., Roth F., Bougoure J., et al. (2020). Heat stress destabilizes symbiotic nutrient cycling in corals. PNAS 118, 1–11. doi: 10.1073/pnas.2022653118
Rädecker N., Pogoreutz C., Voolstra C., Wiedenmann J., Wild C. (2015). Nitrogen cycling in corals: the key to understanding holobiont functioning. Trends Microbiol. 23, 490–497. doi: 10.1016/j.tim.2015.03.008
Rivera H. E., Aichelman H. E., Fifer J. E., Kriefall N. G., Wuitchik D. M., Wuitchik S. J. S., et al. (2021). A framework for understanding gene expression plasticity and its influence on stress tolerance. Mol. Ecol. 30, 1381–1397. doi: 10.1111/mec.15820
Setter R. O., Franklin E. C., Mora C. (2022). Co-occurring anthropogenic stressors reduce the timeframe of environmental viability for the world’s coral reefs. PloS Biol. 20 (10), e3001821. doi: 10.1371/journal.pbio.3001821
Suggett D. J., Warner M. E., Leggat W. (2017). Symbiotic dinoflagellate functional diversity mediates coral survival under ecological crisis. Trends Ecol. Evol. 32, 735–745. doi: 10.1016/j.tree.2017.07.013
Szabó M., Larkum A., Vass I. (2020). A review: the role of reactive oxygen species in mass coral bleaching. Photosynthesis in Algae: Biochemical and Physiological Mechanisms (Springer: Cham), 459–488.
Todd P. A. (2008). Morphological plasticity in scleractinian corals. Biol. Rev. 83, 315–337. doi: 10.1111/j.1469-185X.2008.00045.x
van Oppen M. J.H., Oliver J. K., Putnam H. M., Gates R. D. (2015). Building coral reef resilience through assisted evolution. Proc. Natl. Acad. Sci. U.S.A. 112, 2307–2313. doi: 10.1073/pnas.1422301112
Wangpraseurt D., Holm J. B., Larkum A. W. D., Pernice M., Ralph P. J., Sugget D. J., et al. (2017). In vivo microscale measurements of light and photosynthesis during coral bleaching: Evidence for the optical feedback loop? Front. Microbiol. 8. doi: 10.3389/fmicb.2017.00059
Keywords: coral reef research, early career researchers (ECR), early career scientists, reef monitoring, assisting recovery, stressors and metabolism, morphological architecture
Citation: Paz-García DA, Armstrong EJ, Popovic I, González-Pech RA and Hellberg ME (2023) Editorial: Innovative approaches to coral reef science by early career researchers. Front. Mar. Sci. 10:1322657. doi: 10.3389/fmars.2023.1322657
Received: 16 October 2023; Accepted: 28 November 2023;
Published: 05 December 2023.
Edited by:
Christian Robert Voolstra, University of Konstanz, GermanyReviewed by:
Christine Ferrier-Pagès, Centre Scientifique de Monaco, MonacoEmma Camp, University of Technology Sydney, Australia
Copyright © 2023 Paz-García, Armstrong, Popovic, González-Pech and Hellberg. This is an open-access article distributed under the terms of the Creative Commons Attribution License (CC BY). The use, distribution or reproduction in other forums is permitted, provided the original author(s) and the copyright owner(s) are credited and that the original publication in this journal is cited, in accordance with accepted academic practice. No use, distribution or reproduction is permitted which does not comply with these terms.
*Correspondence: David A. Paz-García, ZHBhekBjaWJub3IubXg=