- 1Department of Biology and Marine Biology, University of North Carolina Wilmington, Wilmington, NC, United States
- 2Harbor Branch Oceanographic Institute, Florida Atlantic University, Fort Pierce, FL, United States
- 3Department of Marine Biology and Ecology, Rosenstiel School of Marine, Atmospheric, and Earth Science, University of Miami, Miami, FL, United States
- 4School of Chemistry and Biochemistry, Krone Engineered Biosystems Building, Georgia Institute of Technology, Atlanta, GA, United States
- 5Center for Marine and Environmental Studies, University of the Virgin Islands, St. Thomas, VI, United States
- 6Department of Oceanography and Coastal Sciences, Louisiana State University, Baton Rouge, LA, United States
- 7Center for Microbial Dynamics and Infection, Georgia Institute of Technology, Atlanta, GA, United States
Stony coral tissue loss disease (SCTLD) is destructive and poses a significant threat to Caribbean coral reef ecosystems. Characterized by the acute loss of coral tissue, SCTLD has impacted over 22 stony coral species across the Caribbean region, leading to visible declines in reef health. Based on the duration, lethality, host range, and spread of this disease, SCTLD is considered the most devastating coral disease outbreak ever recorded. Researchers are actively investigating the cause and transmission of SCTLD, but the exact mechanisms, triggers, and etiological agent(s) remain elusive. If left unchecked, SCTLD could have profound implications for the health and resilience of coral reefs worldwide. To summarize what is known about this disease and identify potential knowledge gaps, this review provides a holistic overview of SCTLD research, including species susceptibility, disease transmission, ecological impacts, etiology, diagnostic tools, host defense mechanisms, and treatments. Additionally, future research avenues are highlighted, which are also relevant for other coral diseases. As SCTLD continues to spread, collaborative efforts are necessary to develop effective strategies for mitigating its impacts on critical coral reef ecosystems. These collaborative efforts need to include researchers from diverse backgrounds and underrepresented groups to provide additional perspectives for a disease that requires creative and urgent solutions.
Introduction
Coral diseases can pose a significant threat to the health and stability of marine ecosystems, jeopardizing the biodiversity, production, and resilience of coral reefs worldwide. Unfortunately, coral reefs are also threatened by a variety of additional compounding factors including climate change, overfishing, coastal development, and pollution (e.g., Gladfelter, 1982; Hughes, 1994; Aronson and Precht, 2001; Bruno et al., 2007; Walton et al., 2018). These cumulative stressors have destroyed an estimated 50% of coral reefs worldwide since the 1950s (Eddy et al., 2021). In recent decades, it has been hypothesized that the Florida coral reef system and the Caribbean have become more susceptible to disease outbreaks due to these confounding stressors (van Woesik and Randall, 2017; Tracy et al., 2019; Vega Thurber et al., 2020; Hayes et al., 2022). Though the Caribbean contains only 8% of the global coral cover, an increase of species affected by disease and an increase is the number of diseases, over 66% of reported coral diseases in this region, make it a coral disease hotspot (Green and Bruckner, 2000; Weil, 2004).
Stony coral tissue loss disease (SCTLD) is a sub-acute to acute tissue loss disease that affects scleractinian corals and can result in complete colony mortality in a matter of months (Aeby et al., 2019; Estrada-Saldívar et al., 2020; Meiling et al., 2020; Sharp et al., 2020; Estrada-Saldívar et al., 2021). It was first observed near Miami, Florida in 2014 (Precht et al., 2016) and since this initial observation, SCTLD has spread to reefs in at least 28 countries and territories across the Caribbean (Roth, L., Kramer, P.R., Doyle, E., and O’Sullivan, C. 2020. Caribbean SCTLD Dashboard. www.agrra.org. ArcGIS Online. Accessed Oct 5, 2023). The etiological agent(s) has not yet been identified, though research suggests that is it infectious (Dobbelaere et al., 2020; Williams et al., 2021b; Truc et al., 2023) with waterborne and sediment transmission ex situ (Muller et al., 2020; Dobbelaere et al., 2022; Studivan et al., 2022a; Studivan et al., 2022b) and multiple vectors (Noonan and Childress, 2020; Rosales et al., 2020; Studivan et al., 2022a; Studivan et al., 2022b). Current hypotheses suggest that pathogenic bacteria are involved in disease progression (Aeby et al., 2019; Neely et al., 2020; Ushijima et al., 2020; Shilling et al., 2021; Walker et al., 2021; Forrester et al., 2022). The etiological agent(s) also induces transcription of genes involved pathogen detection and regeneration in the innate immune system (Traylor-Knowles et al., 2021). Since its emergence, SCTLD has caused the mortality of at least 30% of corals in the Florida coral reef system (Walton et al., 2018) and 40-60% loss in coral cover in various regions of the Caribbean (e.g. Brandt et al., 2021; Estrada-Saldívar et al., 2021; Heres et al., 2021).
Though it is possible to study disease without identifying the etiological agent (Vega Thurber et al., 2020), diagnostics, modeling, and mitigation efforts can be limited. SCTLD is one of the most impactful coral diseases ever recorded in the Caribbean, so standardization of diagnostic tools across the field along with aligned goals for future research is recommended to best navigate this outbreak. In this review, we examined host species susceptibility, disease transmission, impacts on the coral immune system, the influence of holobiont constituents, current approaches for diagnostics, intervention methods, and identify areas of future research as well as highlighting the need for multidisciplinary approaches to understand this unprecedented disease outbreak. We also aim to draw attention to the gender disparity in scientific authorship and attempt to make an effort toward equality by highlighting the need to incorporate diversity and inclusion in all aspects of scientific research.
SCTLD species susceptibility
At least 22 scleractinian coral species, approximately half the total species in the Western Atlantic and Caribbean, are known to be affected by SCTLD (Table 1; Supplementary Table 1). Notably, it is accepted that the Caribbean Acropora species do not appear to be affected; however, there is no peer reviewed data regarding this. Susceptibility among affected species varies and is typically described by the sequence of disease incidence in a species, how much of the population is affected during the epidemic phase (disease prevalence), rates of tissue loss on affected corals, and the overall impact experienced by the population. Highly susceptible species are typically affected first, exhibit high disease prevalence, fast rates of tissue loss, and show large population declines. In fact, the relative abundances of some highly susceptible species, such as Dendrogyra cylindrus, Dichocoenia stokesii, Eusmilia fastigiata, and Meandrina meandrites, declined by 90- 100% within months of local SCTLD emergence in Florida, Mexico, and USVI (Walton et al., 2018; Estrada-Saldívar et al., 2020; Brandt et al., 2021; Estrada-Saldívar et al., 2021; Neely et al., 2021a; Hayes et al., 2022). Moderate and low susceptible species are typically affected later than highly susceptible species and exhibit lower disease prevalence and tissue loss rates. However, for moderate and low susceptibility species, there can be a wide range of intra-species variability in these susceptibility characteristics, as evidenced during two ex situ transmission studies (Aeby et al., 2019; Meiling et al., 2021). These ex situ studies reported variable transmission to different assumed conspecifics under the same environmental conditions. These results may reflect differences in defense capacities among individuals of a species, including tolerance to microbial dysbiosis, as has been suggested for other coral diseases (MacKnight et al., 2022), or differences in disease signs and responses among individuals (Vega Thurber et al., 2020).
Though there is a consensus on the relative susceptibility of most species to SCTLD, there is disagreement regarding the susceptibility of Porites astreoides and Agaricia spp. In an ex situ transmission experiment, Aeby et al. (2019) reported no transmission of SCTLD from Montastraea cavernosa to P. astreoides from shared water baths. However, using a similar experimental design, Meiling et al. (2021) found lesions developed on colonies of P. astreoides after exposure to diseased Diploria labyrinthiformis. Notably, these two studies used different species of diseased source corals (M. cavernosa compared with D. labyrinthiformis) and the experiments took place in two distinct geographic regions (Florida versus the U.S. Virgin Islands) separated by four years. D. labyrinthiformis is a highly susceptible coral species that exhibits high rates of tissue loss and is often one of the first affected species in an outbreak. Compared with M. cavernosa, which is considered to be a more moderately affected coral species exhibiting lower tissue loss rates, D. labyrinthiformis may be a better “spreader” of disease. The temporal difference between the studies may also have been enough time for the pathogen(s) to evolve and become more virulent towards P. astreoides. Finally, characteristics of the exposed corals based on geographic region could also have played a role in differences observed between experiments. For example, a coral’s history of exposure to thermal and other stressors can influence its susceptibility to disease, and geography can affect the microbial communities of conspecifics, thereby affecting their tolerance to dysbiosis and disease exposure. In situ studies from Caribbean regions have observed P. astreoides with multi-focal lesions, though this occurred at low prevalence, suggesting P. astreoides is susceptible but at a low level (Alvarez-Filip et al., 2019; Brandt et al., 2021; Costa et al., 2021; Williams et al., 2021a; Hayes et al., 2022). No ex situ experiments have tested the transmission of SCTLD to Agaricia spp., however, multiple in situ studies have reported multi-focal lesions on Agaricia spp. corals at low prevalence coincident with the emergence of SCTLD on other species, therefore, Agaricia spp. could also be categorized with low susceptibility but more study is needed (Alvarez-Filip et al., 2019; Brandt et al., 2021; Combs et al., 2021; Costa et al., 2021; Heres et al., 2021; Williams et al., 2021a; Hayes et al., 2022).
While susceptibility is predominantly distinguished by incidence rates and prevalence, there are also variable lesion progression rates among species. Highly susceptible species tend to have faster lesion progression rates while moderate and low susceptible species have slower lesion progression rates (Table 1). Lesion progression rates can be further compared between age classes. Williamson et al. (2022) found faster rates of tissue loss in Colpophyllia natans recruits when compared with tissue loss rates of adults reported in other studies (Meiling et al., 2021), but slower rates in D. labyrinthiformis recruits. While there are differences in SCTLD lesion progression rates among species, even the lowest rates are magnitudes higher than other diseases causing acute tissue loss in the Caribbean and Western Atlantic, such as white plague (Clemens and Brandt, 2015) and black band (Sato et al., 2011).
Lesions presumed to be SCTLD have a range of gross morphologies that can vary among species (Figure 1). Lesions develop on any region of the coral (edge or middle) and can be focal or multifocal (Aeby et al., 2019). As the tissue is sloughed, there is a band of white denuded skeleton along the boundary of the lesion. The rate of tissue loss can be categorized into three categories defined by the width of the area of exposed skeleton: chronic (<1cm), subacute (1-5cm) and acute (>5cm) (Figure 1; Aeby et al., 2021). Some species, including Orbicella spp. and Montastraea cavernosa, have bleached margins immediately preceding the lesion boundary (Figure 2; Aeby et al., 2019). The most unique presumed SCTLD lesions are the chronic tissue loss patches that pair with dark pigmentation on Siderastrea siderea (Figure 2A; Aeby et al., 2021; Brandt et al., 2021). Although lesions present similarly among geographic regions and species, without a feasible diagnostic tool or confirmed etiological agent(s) it is not possible to definitively conclude that all corals are affected by the same disease.
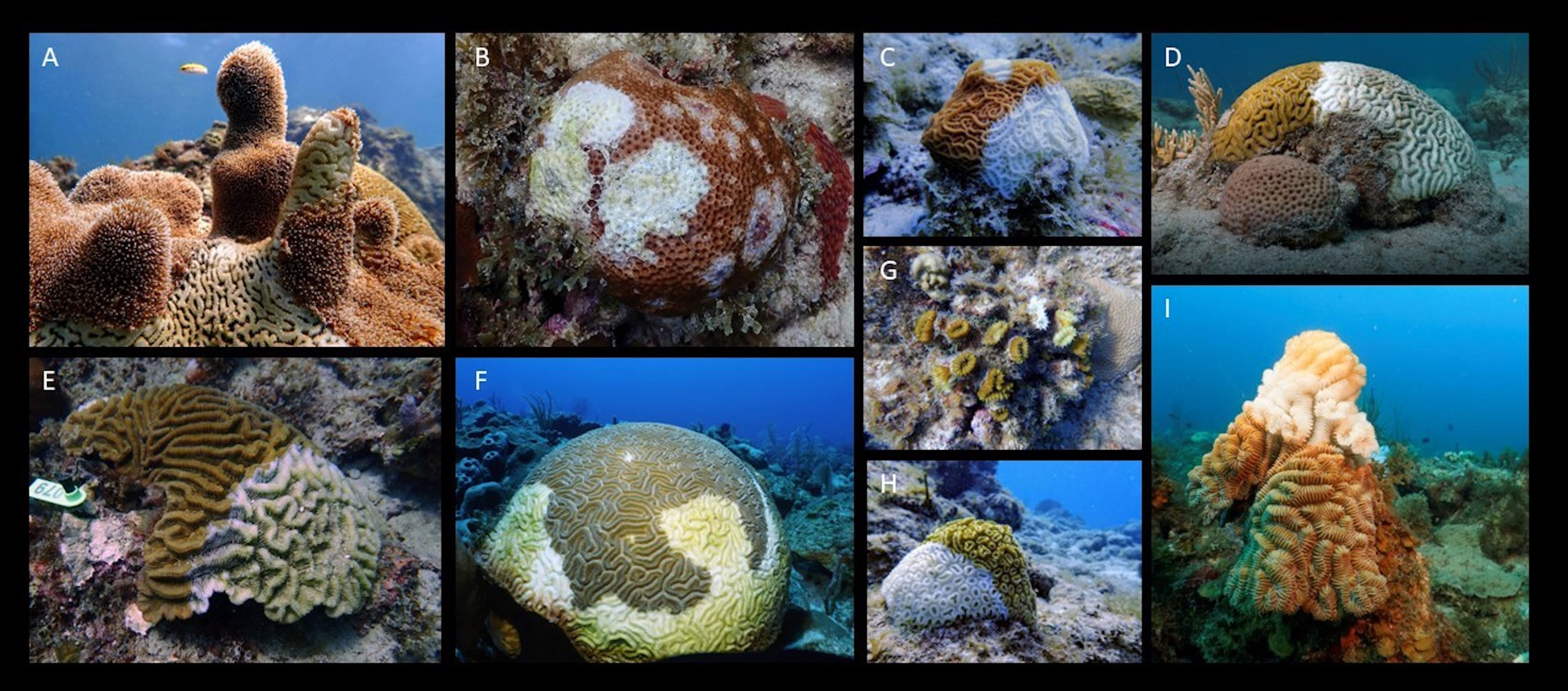
Figure 1 Photos of multiple Caribbean species of corals with visible disease lesions presumed to be stony coral tissue loss disease (SCTLD). (A) Dendrogyra cylindrus, (B) Siderastrea siderea, (C) Agaricia agaricites, (D) Pseudodiploria strigosa, (E) Colpophyllia natans, (F) Diploria labyrinthiformis, (G) Eusmilia fastigiata, (H) Dichocoenia stokesii, (I) Meandrina meandrites. Image credits: (A–C, E–I) Sonora Meiling, (D) Daniel Mele.
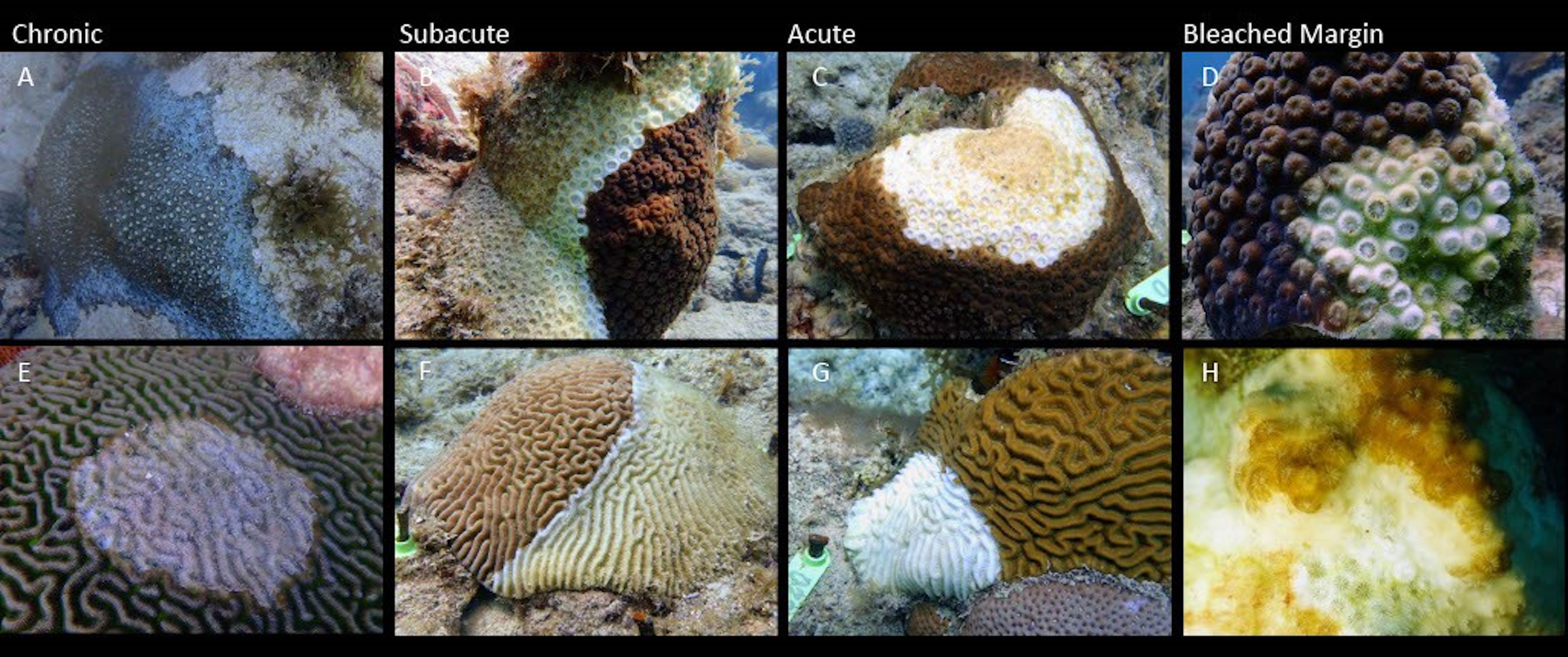
Figure 2 SCTLD gross lesion morphology. Lesions have been characterized into three categories based on the speed of lesion expansion, approximated by the linear progression of the lesion edge: chronic (<1cm, A, E), subacute (1-5cm; B, F) and acute (>5cm; C, G). Some species have bleached margins immediately preceding the lesion border (D, H). Image credit: (A–G) Sonora Meiling; (H) Rosmin Ennis.
Limitations and future directions
Species susceptibility could be influenced by a variety of factors. Susceptibility to SCTLD could potentially differ with ranges in depth in the water column, growth type, spawning type, and Symbiodiniaceae association; however, these metrics are currently unknown in relation to SCTLD, but should be recorded in future studies to aid in deciphering this complex susceptibility trend. It is also possible that secondary or coinfections (Ushijima et al., 2020) or the stage of the outbreak and how long the disease has been in the area could influence lesion progression rates. SCTLD lesion progression has been shown to stop on corals naturally (Aeby et al., 2021) which could be due to host response, pathogen evolution, environmental factors, etc.; however, the mechanisms of this are currently unknown and should be a topic of future research.
Additionally, there is considerable variability among methods for measuring lesion progression rates. For example, species susceptibility is variable for some species, such as M. cavernosa, likely because “susceptibility” is classified by different metrics among papers. Some coral disease studies have measured the advancement of the lesion edge linearly by measuring from a stationary marked point like a nail (Neely et al., 2020; Neely et al., 2021c), while others have measured linear advancement from the edge of the lesion (Elmer et al., 2021). Several studies have measured the areal expansion of each lesion through photo analysis, either 2D or 3D (Aeby et al., 2019; Meiling et al., 2020; Combs et al., 2021; Eaton et al., 2021; Meiling et al., 2021), and of these, some have reported the proportional rate of lesion expansion (Aeby et al., 2019; Meiling et al., 2020; Combs et al., 2021; Williams et al., 2021a; Williamson et al., 2022).
This variability has made it difficult to directly compare lesion progression rates among studies, yet it is an important metric for measuring the impact of disease on a colony, and when combined with mortality rates, it is an essential input for epidemiological modeling. Linear rates seem to be highly dependent on the placement of reference points as well as on the number of measurements made per lesion and per colony (Elmer et al., 2021), while proportional rates consider the impact of all lesions on a colony. We therefore recommend that the coral disease community come to a consensus on a single metric, such as the proportional rate, that can be standardized among studies.
SCTLD transmission
SCTLD was first observed near the Port of Miami in Florida in 2014 (Precht et al., 2016). This emergence coincided with the end of a bleaching event (Walton et al., 2018; Gintert et al., 2019) and a local dredging project at the port (Gintert et al., 2019; Spadafore et al., 2021). It was originally hypothesized that stress due to bleaching led to the emergence of SCTLD (Gintert et al., 2019) or bleaching in tandem with sedimentation from dredging enabled favorable conditions for this disease (Spadafore et al., 2021). Though reef sediment has been implicated as a potential SCTLD vector (Rosales et al., 2020; Studivan et al., 2022b), it was originally hypothesized that plumes and proximity to the dredging site did not correlate with coral mortality at the time of emergence (Precht, 2021) and the disease boundary radiated towards, rather than away from the dredging site (Gintert et al., 2019; Spadafore et al., 2021). However, there are contrasting views as additional research indicates that dredging in the Port of Miami caused significant impacts on the surrounding reefs due to sedimentation (Cunning et al., 2019). Since the initial observations of SCTLD near the Port of Miami, similar disease signs have been recorded along the entire Florida coral reef tract (Precht et al., 2016; Walton et al., 2018; Walton et al., 2018; Aeby et al., 2019; Muller et al., 2020; Noonan and Childress, 2020; Sharp et al., 2020; Combs et al., 2021; Williams et al., 2021a; Hayes et al., 2022) and at least 28 countries and territories in the Caribbean (Figure 3) (Kramer, P.R., Roth, L., and Lang, J. 2019. Map of Stony Coral Tissue Loss Disease Outbreak in the Caribbean. www.agrra.org. ArcGIS Online. Accessed Oct. 10, 2023) including Mexico (Alvarez-Filip et al., 2019; Estrada-Saldívar et al., 2021) the U.S. Virgin Islands (Brandt et al., 2021), Puerto Rico (Williams et al., 2021b), the Bahamas (Dahlgren et al., 2021), Belize (Lee Hing et al., 2022), Honduras (Truc et al., 2023), and Turks and Caicos (Heres et al., 2021).
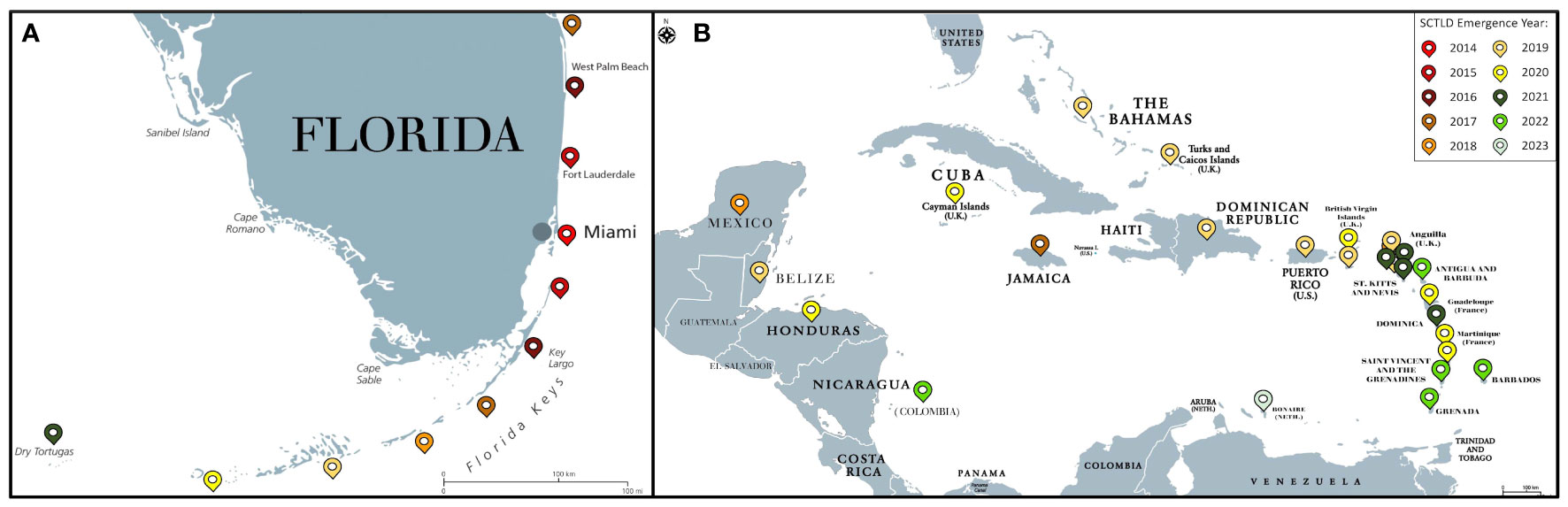
Figure 3 Map of SCTLD emergence by year for Florida (A) and the Caribbean (B) as of October 2023. Colored pins denote the year of SCTLD emergence.
There is low resistance to SCTLD among naïve coral communities; once the disease reaches a reef, only 0.05-0.1% of colonies need to be infected to trigger an outbreak (Dobbelaere et al., 2020). The incubation period ranges from four to 10 days in laboratory experimental conditions (Aeby et al., 2019; Aeby et al., 2021; Meiling et al., 2021) and six days to six months on the reef as determined from modelling (Dobbelaere et al., 2020; Guzmán-Urieta and Jordán-Dahlgren, 2021; Dobbelaere et al., 2022). It is important to note that the authors Guzmám-Urieta and Jordán-Dahlgren, 2021 used the term “white syndrome” for the outbreak described but observed both SCTLD and white plague signs. As such, we included this in our review of SCTLD studies. Most epidemiological analyses show significant clustering of diseased individuals on a reef, suggesting SCTLD is contagious among colonies (Dobbelaere et al., 2020; Williams et al., 2021b; Truc et al., 2023). These clusters, and thus the potential risk of an individual colony contracting disease from a diseased neighbor, range from 1.5 m to 140 km in studies from Florida (Muller et al., 2020; Williams et al., 2021a). However, an additional study from Florida (Sharp et al., 2020) and from Mexico (Guzmán-Urieta and Jordán-Dahlgren, 2021) found no difference in disease risk depending on the health status of neighboring corals. These findings suggest there is variability of SCTLD transmission within and among regions, or stages within an outbreak (Aeby et al., 2021). SCTLD likely spreads quickly within and among reefs due to its high virulence and generalist nature.
Although the etiological agent(s) responsible for SCTLD is (are) still unknown, various studies have suggested multiple vectors of transmission. Laboratory studies have demonstrated rapid and successful transmission via direct contact with a diseased colony (Aeby et al., 2019; Eaton et al., 2021; Titus et al., 2022), tank water shared with a diseased colony (Aeby et al., 2019; Aeby et al., 2021; Meiling et al., 2021), and indirect exposure to sediment that has contacted a diseased colony (Studivan et al., 2022b). All three modes (direct contact, waterborne, and sediment) are successful in intra- and inter-species SCTLD transmission experiments, though varying levels of susceptibility are reported as discussed above (Aeby et al., 2019; Eaton et al., 2021; Meiling et al., 2021; Studivan et al., 2022b). In addition to ex situ experiments, multiple studies have used modeling to support waterborne transmission of this disease in situ, (Dobbelaere et al., 2020; Sharp et al., 2020; Guzmán-Urieta and Jordán-Dahlgren, 2021; Dobbelaere et al., 2022) specifically, by mean barotropic currents (Dobbelaere et al., 2022) at rates up to 0.15 km/day (Muller et al., 2020; Truc et al., 2023). Some studies report higher prevalence and faster progression to areas with high exposure to wind compared to leeward areas (Alvarez-Filip et al., 2022; Truc et al., 2023). Patterns in disease observations that do not match hydrodynamic rates or connectivity, such as the disease emerging at locations like Mexico and the U.S. Virgin Islands, were up-current of known SCTLD-affected areas at the time of emergence. This suggest that other vectors, such as ballast water transport, are acting at large geographic scales (Dobbelaere et al., 2020; Dahlgren et al., 2021; Rosenau et al., 2021; Evans et al., 2022a; Studivan et al., 2022a; Studivan et al., 2022b).
Few studies have investigated the role of coral-associated organisms in SCTLD transmission, and so far, all have focused on the foureye butterflyfish, Chaetodon capistratus. Noonan and Childress (2020) found that C. capistratus associates with actively diseased colonies more than with dead or apparently healthy colonies in-situ. However, in an ex-situ experiment, Titus et al. (2022) found no strong feeding preference between diseased and apparently healthy colonies. These feeding preferences may explain the negligible effects of the butterflyfish on disease transmission rates (Titus et al., 2022). The rapid transmission of SCTLD is likely explained by other modes of transmission and may be more influenced by the large number of highly susceptible species.
Limitations and future directions
As there are contrasting results about the impact on the mortality of the surrounding reefs from the dredging project in the Port of Miami (Cunning et al., 2019; Precht, 2021), future research is trying to quantify the impacts more in depth. Additionally, it is unknown whether this dredging is connected to SCTLD but identifying the etiological agent(s) could potentially provide insight into its origin. It is also difficult to determine the rates of transmission on reefs, so it is suggested to record the stage of the outbreak, initial species impacted, and region of observation in research studies. As SCTLD continues to spread, continuous monitoring along with updating SCTLD emergence observations to an accessible domain, such as www.agrra.org/coral-disease-outbreak, is needed to continue to track this disease outbreak.
SCTLD ecological impact
Contrary to the hypothesis that higher diversity reefs have lower disease prevalence (the Diversity-Disease hypothesis; Keesing et al., 2006; Huang et al., 2016), SCTLD is more prevalent on reefs with higher diversity (Muller et al., 2020; Costa et al., 2021; Williams et al., 2021a). In many regions, disease prevalence ranged between 16-57% in the first year of disease emergence (e.g., 2016, 2018, 2019, etc.; Figure 3) (Precht et al., 2016; Alvarez-Filip et al., 2019; Estrada-Saldívar et al., 2020; Aeby et al., 2021; Brandt et al., 2021; Estrada-Saldívar et al., 2021; Williams et al., 2021a; Hayes et al., 2022), leading to losses of up to 62% coral cover (Brandt et al., 2021; Estrada-Saldívar et al., 2021; Heres et al., 2021; Kolodziej et al., 2021; Williams et al., 2021a; Hayes et al., 2022). In contrast, Dobbelaere et al. (2020) reported prevalence values of <0.4% in Florida, but this was due to an almost equal rate of full colony death and new colony infection over the investigation’s time frame. High disease prevalence is particularly impactful to coral species diversity (Alvarez-Filip et al., 2019; Estrada-Saldívar et al., 2020; Brandt et al., 2021; Heres et al., 2021; Hayes et al., 2022) because highly and moderately susceptible species are often rare, and thus can be lost quickly from a site (Costa et al., 2021; Neely et al., 2021a). However, the impact of SCTLD on integrated coral community metrics, like overall coral density and coral cover, can be low at some sites because of the rare nature and typically small size of many highly susceptible species (Costa et al., 2021). For example, the species D. stokesii and M. meandrites may be the predominant highly susceptible species at a site, but the low abundance and small sizes of these corals mean they contribute minimally to coral abundance and cover, and therefore these metrics are not as affected by SCTLD (Costa et al., 2021). Further, integrated coral community impacts are likely underestimated because in situ studies often exclude recruits and small juveniles (Williamson et al., 2022).
There are likely many interacting variables that contribute to differences in peak SCTLD prevalence and impact among reefs. For instance, in the Florida coral reef system, multiple studies have shown variable disease prevalence between nearshore and offshore reefs (Rippe et al., 2019; Muller et al., 2020; Williams et al., 2021a). Size structure of coral colonies likely also contributes to variability in SCTLD prevalence levels. Sharp et al. (2020) and Williams et al. (2021a) found that larger colonies were more likely to contract the disease, so coral communities with larger sized species and/or colonies may exhibit higher disease prevalence, which is expected. This difference in susceptibility by size may also explain why Aeby et al. (2021) found size structures dominated by small colonies at sites that had the disease longer. However, this relationship between size and disease risk may be restricted to early stages of a local outbreak, as Aeby et al. (2021); Combs et al. (2021), and Hayes et al. (2022) found no relationship between size and mortality, lesion progression rate, nor live tissue area, respectively.
The onset and shift between outbreak stages of SCTLD may be affected by seasonal temperature changes, which is common for other coral diseases, though there is considerable variability among reports. In situ observations from the U.S. Virgin Islands and from Florida have shown abatement of SCTLD incidence, lesion progression, and colony death associated with thermal stress and bleaching from elevated water temperatures (Meiling et al., 2020; Sharp et al., 2020; Williams et al., 2021a). However, other studies have found no relationship between SCTLD activity and seasonal temperature (Aeby et al., 2019; Muller et al., 2020; Hayes et al., 2022; Truc et al., 2023). SCTLD might be abated during thermal stress due to coral bleaching and the subsequent loss of the algal symbiont, which is discussed further in the Symbiodiniaceae section. The influence of seasonal temperature changes on SCTLD onset and progression remains a topic of ongoing research.
Limitations and future directions
It is important to compare the epidemiology of SCTLD among regions, as this may help in the identification of important disease characteristics and aid in the prediction of SCTLD spread and impact on new areas. However, comparisons can be difficult because SCTLD outbreaks have not been spatially or temporally distinct like other past acute tissue loss coral diseases (Cróquer et al., 2021). Tracking emergence and impacts could also be limited due to monitoring and reporting resources. SCTLD is extremely persistent (extending for nearly a decade in Florida) and becomes geographically expansive in all affected areas. The results of short-term or geographically limited studies can depend on reef characteristics, as well as the stage of the outbreak (endemic vs. epidemic). Standardizing characteristics such as the time since emergence, coral cover, community composition, and size distribution would be beneficial for future SCTLD studies.
Along with the impact SCTLD has on coral communities, the long-term impact on individual colonies affected by SCTLD that remain partially alive is still unclear. Successful spawning has been documented for some species from colonies affected by SCTLD (Quiroz et al., 2023). In an experiment that compared survival between P. strigosa recruits from crosses containing gametes collected from an SCTLD-affected colony to those from crosses containing gametes from only apparently healthy colonies, survival rates were similar (Quiroz et al., 2023). However, there is still no information on how these crosses affect disease resistance of the offspring. Future areas of research could focus on analyses to evaluate the roles of species, geographic area, stage of outbreak, environmental variables like thermal stress, and community dynamics on SCTLD epidemiology.
Coral immunity
In animals, the immune system is the fundamental defense against pathogens and non-self (Janeway, 2001; Mydlarz et al., 2006; Tran and Luallen, 2023). It can be broken into two parts: the adaptive immune system and the innate immune system (Cooper, 2010). In invertebrates, such as corals, the innate immune system (Mydlarz et al., 2006; Cooper, 2010) is the primary mechanism used to recognize foreign microorganisms and pathogens (Nyholm and Graf, 2012) (Figure 4). Innate immunity can be classified into three phases: recognition, signaling pathways, and effector responses. All three of these phases have previously been identified in corals and are activated in response to coral disease (Palmer et al., 2008; Mydlarz et al., 2010; Palmer and Traylor-Knowles, 2012). In the case of SCTLD, research concerning the role of the innate immune system has just begun.
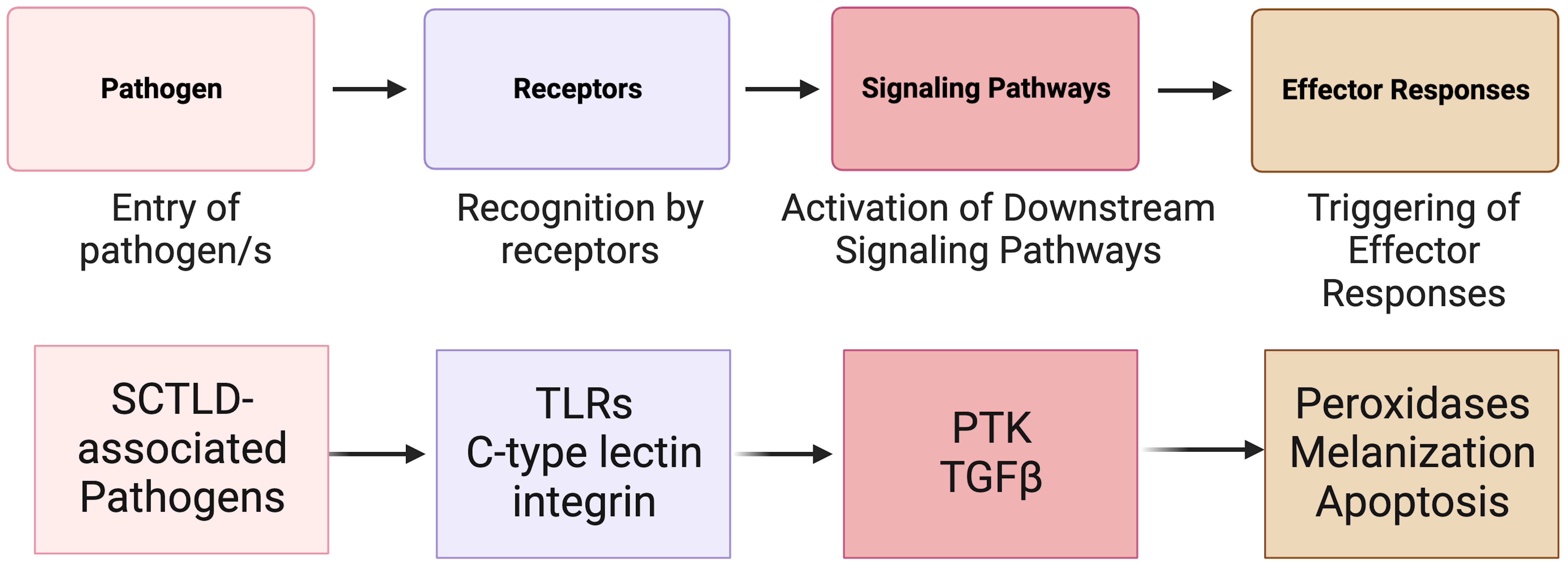
Figure 4 A simplified overview of the known invertebrate immunity components impacted by SCTLD synthesized from Traylor-Knowles et al. (2021) and Beavers et al. (2023). TLR, TOLL-like receptor; PTK, Protein Tyrosine Kinase pathway; TGFβ, Transforming Growth Factor-beta pathway.
Immunity response for SCTLD
The innate immune system in corals relies on pattern recognition receptors (PRRs) such as Toll-like receptors (TLRs), lectins, and integrins (Brower et al., 1997; Miller et al., 2007; Kvennefors et al., 2008) for the detection of non-self (Mydlarz et al., 2006) and for detection of patterns presented by microorganisms and by damaged host cells (Sato et al., 2009). After exposure to SCTLD, various coral species show significant upregulation in immune related genes associated with TLRs (Traylor-Knowles et al., 2021; Beavers et al., 2023). These include leucine-rich repeat-containing protein 1 and F-box/LRR-repeat protein 2 in M. cavernosa, leucine-rich repeat-containing protein 42-like and leucine-rich repeat-containing protein 15-like in O. faveolata, and Toll-like receptor 6 in other Caribbean species (Traylor-Knowles et al., 2021; Beavers et al., 2023). These proteins are part of a larger domain of proteins associated with pathogen response and detection (Ng and Xavier, 2011).
Integrins are another type of receptor that are important in cell migration, wound repair and immune response (Takada et al., 2007; Toledo-Hernández and Ruiz-Diaz, 2014). Recent evidence indicates that SCTLD transmission in O. faveolata induced transcription of integrin associated genes including integrin alpha-7 like, which is correlated with regeneration (Lemons and Condic, 2008). Due to their role in cell behavior and signaling, the upregulation of integrins could indicate their importance in detecting SCTLD related pathogens (Traylor-Knowles et al., 2021; Juliano et al., 2004).
Lectins are carbohydrate binding proteins that can activate signals for cell adhesion (Endo et al., 2009). After an SCTLD lesion appears, there is down regulation of genes associated with the C-type lectin domain in M. cavernosa, including galactose binding lectin domain (Traylor-Knowles et al., 2021). Galactose binding lectins play a role in the initial attachment of pathogens to host cells (Elola et al., 2007; Traylor-Knowles et al., 2021). Downregulating these lectins can reduce a foreign cell’s ability to adhere to and invade host cells. However, there is upregulation of lectin associated genes in O. faveolata, including rhamnose-binding lectin-like, which is suggested to recognize pathogenic bacteria and symbiotic Symbiodiniaceae (Zhou et al., 2017; Traylor-Knowles et al., 2021). The differential response may indicate species-specific receptor detection, but this must be further explored.
After PRRs have detected potential damage and pathogens, signals are transmitted by kinases that regulate downstream signaling pathways (Anderson et al., 2016). Several signaling pathways have been identified in corals including Protein Tyrosine Kinase (PTK) pathway and the Transforming Growth Factor-beta (TGFβ) pathway (Fuess et al., 2016; Berthelier et al., 2017). In response to SCTLD, PTK signaling triggers an immune response in O. faveolata (Traylor-Knowles et al., 2021). Additionally, after SCTLD exposure, down regulation of orthogroups linked to the (TGFβ) family suggest that this pathway is suppressed to initiate an immune response (Traylor-Knowles et al., 2021; Beavers et al., 2023). Specifically, SMAD6, which belongs to the TGF-β pathway, had significantly reduced expression in SCTLD infected corals compared to controls (Beavers et al., 2023). Suppression of this protein elevates apoptosis in human cells (Jeon et al., 2008) and there is evidence that signal transduction is decreased in species with advanced lesion progression.
Signaling pathways trigger defense mechanisms and effector responses (Palmer and Traylor-Knowles, 2012), which are specific immune mechanisms to eliminate pathogens. These mechanisms can include the production of peroxidases, melanization, and apoptosis (Palmer et al., 2008; Silva et al., 2008; Palmer et al., 2011a). SCTLD is associated with upregulation of peroxidases in O. faveolata and M. cavernosa. Peroxidases induced by pathogen exposure are often abundant in areas of tissue loss (Palmer et al., 2011b; Halliwell and Gutteridge, 2015; Abbas et al., 2019). The upregulation of peroxidases could be an antioxidant immune effector response to ROS produced by phagocytic cells, but this needs to be confirmed as peroxidases can also be induced by thermal stress (Lesser, 2006).
In both O. faveolata and M. cavernosa there is upregulation of genes associated with increased melanin deposits after an SCTLD lesion appears (Traylor-Knowles et al., 2021). Melanin deposition creates a defensive barrier that allows for the encapsulation of pathogens (Palmer et al., 2008; Mydlarz and Palmer, 2011; Parisi et al., 2020) and has been shown to increase in immunocompromised corals (Palmer et al., 2008). Increased melanin deposits in SCTLD-affected corals suggests an inflammatory-like immune response to SCTLD pathogens. In response to SCTLD transmission, corals significantly upregulate genes involved in apoptosis including programmed cell death proteins, tumor necrosis factors and apoptosis regulators (Traylor-Knowles et al., 2021; Beavers et al., 2023). The upregulation of apoptosis related genes could suggest that coordinated apoptosis is occurring to regulate wound healing and tissue loss (Traylor-Knowles et al., 2021).
Limitations and future directions
Although SCTLD transmission induces transcriptional activity at every step of the coral innate immune system (Traylor-Knowles et al., 2021), there are still many unanswered questions regarding how SCTLD impacts the immune system. For example, since SCTLD necrosis starts within the gastrodermis before moving to the epithelial layer (Aeby et al., 2019; Landsberg et al., 2020), it is crucial to sample before the presence of gross lesions to understand activating mechanisms of necrosis (Traylor-Knowles et al., 2021). Furthermore, the role of temperature variation in immune response to SCTLD needs to be deciphered as fluctuations in temperature seem to influence innate immune response parameters (Van De Water et al., 2016). Additionally, the role of peroxidases needs to be investigated to determine if increased concentrations are true immunological responses (Palmer et al., 2011b; Traylor-Knowles et al., 2021). Understanding the immune response is essential for advancing SCTLD biomarker discovery and treatment development.
The coral microbiome
The coral microbiome consists of dinoflagellates (Family Symbiodiniaceae), bacteria, archaea, fungi, and viruses. This consortium of microorgansims, along with the coral host, is referred to as the coral holobiont (Rohwer et al., 2002; Bourne et al., 2009; Blackall et al., 2015; Ainsworth et al., 2017). There are a variety of hypothesized roles for the coral microbiome (reviewed in Mohamed et al., 2023), including providing nutrition (Wild et al., 2004), preventing infection (Ritchie, 2006; Raina et al., 2009; Shnit-Orland and Kushmaro, 2009; Welsh et al., 2016; Welsh et al., 2017), and aiding coral recovery after bleaching (Peixoto et al., 2017; Santoro et al., 2021). Microbes are critical for their host’s fitness (Bourne et al., 2016), and may serve as indicators of overall coral health (Glasl et al., 2017). Understanding the interactions of these microbes can provide insight into which elements of the microbiome are critical to coral health and how they impact reef dynamics (Bythell and Wild, 2011; Ainsworth et al., 2017). In the case of SCTLD, multiple studies show the disruption and change in the coral microbiome in response to the disease (Rosales et al., 2020; Becker et al., 2021; Clark et al., 2021; Thome et al., 2021; Work et al., 2021; Huntley et al., 2022; Rosales et al., 2023).
SCTLD causes Symbiodiniaceae-host disruption
The emergence of novel coral diseases, in particular SCTLD, has emphasized the complex interactions between members of the coral holobiont and expanded research efforts beyond the coral host (Correa et al., 2009a; Thurber and Correa, 2011; Sweet et al., 2013; Soffer et al., 2014; Rosales et al., 2020; Clark et al., 2021; Schul et al., 2022; Veglia et al., 2022). Specifically, the involvement of Symbiodiniaceae in SCTLD infection and disease progression is starting to be investigated. Symbiodiniaceae populations are responsible for providing up to 90% of the coral’s nutritional demand in the form of translocated fixed carbon (Muscatine, 1990; McIlroy et al., 2022). The family is comprised of seven genera: Symbiodinium (formerly clade A), Breviolum (formerly clade B), Cladocopium (formerly clade C), Durusdinium (formerly clade D), Effrenium (formerly clade E), Fugacium (formerly clade F), and Gerakladium (formerly clade G), each comprised of species and subspecies (Baker, 2003; LaJeunesse and Thornhill, 2011; LaJeunesse et al., 2018; Davies et al., 2023), though Caribbean corals typically only associate with Symbiodinium, Breviolum, Cladocopium, and Durusdinium (Baker, 2003; Van Oppen and Burghardt, 2009). Field observations, histopathology, and laboratory-based and molecular-based studies provide strong evidence of coral-Symbiodiniaceae dysbiosis in response to SCTLD (Landsberg et al., 2020; Deutsch et al., 2021; Meiling et al., 2021; Work et al., 2021; Studivan et al., 2022b; Williamson et al., 2022; Beavers et al., 2023; Studivan et al., 2023).
SCTLD has a distinct species susceptibility hierarchy when compared to other white diseases (Table 1), which might correspond with typical Symbiodiniaceae associations. The most susceptible coral species (principally in the families Meandrinidae and Mussidae) predominantly host algal symbionts in the genus Breviolum (Baker, 2003; Lewis et al., 2018). By comparison, coral species with intermediate susceptibility (i.e., family Faviidae) to SCTLD typically have very flexible algal symbiont assemblages and can associate with multiple algal genera (Baker, 2003; Kemp et al., 2015; Silverstein et al., 2015; Rodriguez‐Casariego et al., 2022). Coral species with flexible relationships can associate with one or more Symbiodiniaceae genera at the same time or can shuffle their Symbiodiniaceae communities from one Symbiodiniaceae to another in response to an environmental change (Finney et al., 2010; Silverstein et al., 2012; Cunning and Baker, 2014; Cunning et al., 2015; Silverstein et al., 2015; Cunning et al., 2018). While it is most common to find faviid corals associating with Breviolum and Cladocopium, they have also been found to host Durusdinium and Symbiodinium (Green et al., 2014; Kemp et al., 2015; Grajales and Sanchez, 2016; Cunning et al., 2018). Finally, those which have low to no SCTLD susceptibility (such as those within the families Acroporidae and Poritidae) tend to associate exclusively with Symbiodinium (Grajales and Sanchez, 2016; Serrano et al., 2016; Palacio-Castro et al., 2021).
While evidence suggests varied Symbiodiniaceae genus level SCTLD susceptibility, species level susceptibility is still poorly understood. For example, associations with Breviolum spp. are found in both high susceptibility corals but also in Cladocora arbuscula and Oculina spp. which apparently exhibit low to no SCTLD susceptibility; however, there is no peer reviewed data regarding this. In fact, C. arbuscula and Oculina spp. associate with Breviolum psygmophilium (Thornhill et al., 2008; Leydet and Hellberg, 2016; Lewis et al., 2018), a distinct lineage of the genus Breviolum, which is different from the typical Breviolum species hosted by the most highly susceptible coral species. This suggests not all Breviolum types are equally susceptible to SCTLD. Additionally, there is physiological variation among Symbiodiniaceae species, which could confer susceptibility; however, further investigation is needed to address these intricacies.
Unlike other coral diseases, which tend to increase in prevalence in the warmer summer months (Bruno et al., 2007; Miller and Richardson, 2015; Howells et al., 2020), the prevalence of SCTLD on reefs sometimes decreases in the summer which may be attributed to the loss of algal symbionts. Notably, these observations have only been documented in Florida and the U.S. Virgin Islands where significant declines in disease prevalence, progression, and mortality have been correlated with coral bleaching (Meiling et al., 2020; Sharp et al., 2020; Williams et al., 2021a). However, there is variability among studies as natural variations in seasonal temperatures have shown no significant effects on incidence, prevalence, and/or progression (Aeby et al., 2019; Muller et al., 2020; Hayes et al., 2022). This could suggest a coral’s risk only decreases when temperatures are high enough to elicit a bleaching response, thus reducing symbiont populations and potentially holobiont diversity within the host.
In addition to the interpretation of the species susceptibility hierarchy and field observations, histopathology has shown evidence that the associated algal symbionts are affected during disease onset. Light microscopy has revealed that the algal symbionts are among the first cells to show signs of disruption which results in the rapid declines and degradation of coral tissues (Landsberg et al., 2020; Cróquer et al., 2021). Furthermore, transmission electron microscopy (TEM) has identified the presence of viral-like particles within the Symbiodiniaceae cells which are not present in the coral host. These viral-like particles resulted in various degrees of changes in the Symbiodiniaceae ultrastructure which continues to degrade over time, eventually leading to host cell death (Work et al., 2021).
Finally, various lab-based SCTLD assays and molecular-based studies show significant involvement of the Symbiodiniaceae (Deutsch et al., 2021; Meiling et al., 2021; Titus et al., 2022; Williamson et al., 2022; Beavers et al., 2023). Notably, Beavers et al. (2023) found increased expression of rab7, a known marker of Symbiodiniaceae degradation. This study also found evidence of a functional shift in the coral mucus layer, which is largely fed by translocated fixed carbon from the Symbiodiniaceae, support of a viral infection of the Symbiodiniaceae, and various degrees of disease severity driven by Symbiodiniaceae identity. These findings provide insight into the complex interactions between corals and Symbiodiniaceae during SCTLD infection.
Limitations and future directions
Given that the SCTLD causative agent(s) remain unknown, our knowledge and understanding regarding the coral microbiome is incomplete and relies on correlative evidence. Collectively, field observations, histopathology, and microscopy, along with lab- and molecular-based studies, suggest algal symbionts may play a pivotal role in SCTLD susceptibility. Differences in genera or species of Symbiodiniaceae could impact a coral’s susceptibility to SCTLD, but more research needs to be done to determine this relationship. Additionally, determining the impact of temperature and bleaching impacts in relation to SCTLD prevalence needs to be investigated further to make inferences about the role of Symbiodiniaceae in SCTLD infection.
Although coral-algal symbiosis is well-studied, there are a plethora of other microorganisms, like bacteria, viruses, fungi, and archaea, contributing to coral health which interact with other holobiont members. Even though there is a correlation between SCTLD susceptibility and Symbiodiniaceae identity, this may be part of a broader system of microbial dysbiosis. The correlation might not be driven by one specific member of microbiome, but rather a consortium of changes that occur in multiple holobiont members. Several studies have found microbial dysbiosis occurring in multiple holobiont members, triggering a cascade of effects (Boilard et al., 2020; Claar et al., 2020; Connelly et al., 2022; Bonacolta et al., 2023; Gardner et al., 2023). Under this interpretation, the relationship between SCTLD and Symbiodiniaceae identity may be the result of functional changes in the microbiome. Currently there is no conclusive evidence of this, however this has not been directly tested either and therefore further research is needed to untangle these intricacies. While research is still ongoing, understanding which member(s) of the coral holobiont are affected by SCTLD remains the utmost importance in identifying the etiological agent(s).
Bacteria are involved in SCTLD progression
The application of multiple classes of antibiotics stops or slows lesion progression (Aeby et al., 2019; Neely et al., 2020; Shilling et al., 2021; Walker et al., 2021; Forrester et al., 2022), indicating that bacteria are likely involved in SCTLD progression. Furthermore, to decipher which bacteria are involved in disease progression, next generation sequencing, which can amplify the 16S rRNA gene used for prokaryotes (Woese and Fox, 1977; Woese, 1987; Weisburg et al., 1991), and shotgun metagenomics have been used to characterize microbial community shifts in SCTLD-affected corals (Meyer et al., 2019; Rosales et al., 2020; Becker et al., 2021; Clark et al., 2021; Thome et al., 2021; Evans et al., 2022b; Huntley et al., 2022; Rosales et al., 2022; Studivan et al., 2022b). Additionally, these technologies have provided insight into potential vectors for SCTLD including reef sediments containing pathogenic microbes (Studivan et al., 2022b) and biofilm formations, assemblages of microbes that adhere to a surface, on ship hauls (Evans et al., 2022a).
The bacterial orders Flavobacteriales, Clostridiales, Rhodobacterales, Alteromonadales, Vibrionales, Rhizobiales, Campylobacterales, and Peptostreptococcales–Tissierellales are enriched at SCTLD lesions when compared to apparently healthy tissue (Meyer et al., 2019; Rosales et al., 2020; Becker et al., 2021; Clark et al., 2021; Rosales et al., 2022; Rosales et al., 2023). A meta-analysis of these studies, the largest conducted for coral disease, suggests that Rhodobacterales and Peptostreptococcales–Tissierellales are dominant in SCTLD lesion tissue (Rosales et al., 2023). These studies have also suggested multiple microbial DNA sequences that could serve as indicators for SCTLD infections (Becker et al., 2021). These SCTLD-associated microbial signatures have been found in various species of corals, across various years and geographic regions, and support the hypothesis that bacteria are involved with SCTLD pathogenesis, though might not be the initiators of the disease.
Though these technologies can potentially identify bioindicators specific to SCTLD, there are still challenges in determining disease etiology. There is a significant amount of background noise on corals due to their complex microbiome, so deciphering what is involved with disease progression can be difficult. A recent study took a different approach to studying coral disease to concentrate potentially pathogenic microorganisms and test them based on size class in infection experiments (Evans et al., 2022b). This includes utilizing tangential flow filtration in combination with sequential size fractionation to concentrate the microbial community, filter it through various filter sizes to separate different size fractions, and test the infectiousness of each size fraction. While seemingly effective at separating microbes into size classes consisting mostly of microeukaryotes, bacteria, and viral particles, an experiment using this method was deemed inconclusive (Evans et al., 2023).
Adding additional complexity to deciphering microbes associated with SCTLD, microbial composition can differ or vary across geographic regions of SCTLD zones (vulnerable, endemic, and epidemic) (Clark et al., 2021; Rosales et al., 2022; Rosales et al., 2023) and can vary based on coral species and sample area (diseased tissue vs. apparently healthy tissue) (Meyer et al., 2019; Rosales et al., 2020; Becker et al., 2021; Clark et al., 2021; Laas et al., 2021; Rosales et al., 2022; Rosales et al., 2023). These complexities can create confounding factors among sequencing data and create challenges to deciphering the microbial interactions associated with disease lesions and determining the etiological agent(s).
Additionally, it is unknown whether all SCTLD lesions stem from the same etiological agent(s). It is also possible that certain microbes are required for gross tissue loss signs or different microbes could also distinguish differences in lesion types (Aeby et al., 2019). One known microbial difference across lesion types is the co-infection of V. coralliilyticus, which was found within 20% of acute lesions on M. cavernosa and O. faveolata, but was absent in the slower progressing subacute and chronic lesions (Ushijima et al., 2020). This bacterium does not initiate disease as the primary pathogen but can exacerbate the disease if present. These intricacies create challenges in identifying the etiological agent(s) and are a topic of ongoing investigation.
Another avenue for using sequencing could be investigating the impacts of amoxicillin treatment on the coral and surrounding benthic communities before and after treatment; however, there is no published data regarding these impacts yet. In addition to determining the impacts of antibiotics on the microbial community, investigating alternative treatment options are underway. Specifically, probiotics, potentially beneficial microbes, are under consideration as a treatment for SCTLD and is discussed further in the Intervention section. The bacterium Pseudoalteromonas sp. strain McH1-7 slows SCTLD progression and, more notably, prevents transmission in the lab (Ushijima et al., 2023), providing a potential alternative to antibiotic treatment. Ongoing research is actively exploring the discovery of probiotics and the establishment of these intervention options for SCTLD and remains a valuable avenue of research.
Limitations and future directions
While microbial sequencing technologies offer valuable insights into potential bioindicators for SCTLD and provides a greater understanding of coral-associated bacteria (Hernandez-Agreda et al., 2017), it is important to acknowledge its limitations. Factors such as number of samples generated, primers selected, or sequencing region selected could introduce biases and effect analyses (Hernandez-Agreda et al., 2017). For example, the etiological agent(s) may be relatively rare within the microbiome, resulting in low detection within sequencing pipelines and subsequent loss in analyses. Additionally, bacteria may only be identified to the genus level, creating difficulty when there are significant differences among species and strains (Claesson et al., 2010; Poretsky et al., 2014; Gupta et al., 2019).
Additionally, the functionality of these microbes cannot be determined from presence or absence alone as it is possible that their presence could indicate colonizers that are associated with coral tissue breakdown (Egan and Gardiner, 2016; Gignoux-Wolfsohn et al., 2017; Meyer et al., 2019). For example, Rhodobacterales are suspected secondary pathogens or opportunistic colonizers within SCTLD lesions (Rosales et al., 2020). Sampling from more susceptible coral species and sampling during active tissue loss (Meyer et al., 2019) while recording SCTLD zone, lesion type, and sample type will aid in study comparison and provide more reliable results for microbial sequencing analyses. Standardization of sampling and methods is also vital in enabling comparisons of results across studies and further understanding of the coral microbiome and SCTLD-associated community assemblages.
Viruses
Over 60 viral families have been associated with corals (reviewed in Ambalavanan et al., 2021) with bacteriophages, viruses that infect bacteria, hypothesized to be the most dominant group (Wegley et al., 2007; Nguyen-Kim et al., 2015). Viruses can also associate with the Symbiodiniaceae and may impact coral-algal symbiosis (Correa et al., 2013). Initial observations of suspected SCTLD-affected M. cavernosa showed no discernable viral-like particles (VLPs) in Symbiodiniaceae using TEM (Landsberg et al., 2020); however, another study contradicts this observation as VLPs were observed using TEM in multiple species of corals of both apparently healthy and SCTLD-affected, including M. cavernosa (Work et al., 2021). The VLPS observed were hypothesized to be Alphaflexiviridae (Work et al., 2021). This VLP identification is supported by two draft genomes, assembled from additional samples, which found two viruses in Alphaflexiviridae across all health states (SCTLD exposed, apparently healthy, and suspected SCTLD-affected) (Veglia et al., 2022). However, the ubiquity of viruses in the ocean could create this contrasting result as latent, secondary, or coinfections are common. Additionally, these viruses might not be correlated to SCTLD because filamentous VLPs associate with a wide variety of corals and Symbiodiniaceae (Howe-Kerr et al., 2023) without having a causal relationship with SCTLD.
Limitations and future directions
Multiomics approaches are necessary to understand the coral holobiont and the roles that each member play in disease (reviewed in Mohamed et al., 2023). While the identification of VLPs in diseased coral tissue through TEM alone is not sufficient to confirm viral pathogens as causative agents, additional multiomics in tandem with TEM analysis in transmission experiments could further implicate VLPs in SCTLD if there is a significant difference between apparently healthy and diseased samples. The publicly available data generated from studies such as Veglia et al. (2022) will help resolve the functions of viruses in SCTLD spread when combined with manipulative studies. Additionally, methods such as the previously mentioned tangential flow filtration in tandem with sequencing can be used to target viruses associated with SCTLD. Finally, the use of bacteriophages to target cultured bacteria associated with SCTLD could be a potential avenue of intervention research.
SCTLD diagnostic tools
Histopathology
Histopathology is a useful tool for disease examination that involves light microscopy of thin tissue sections (Catterjee, 2014) and documentation of tissue or cellular abnormalities or changes. Historically, a lack of intentionally collected and preserved healthy and diseased coral tissues has been an impediment to coral disease detection and description (Galloway et al., 2005). In recent years, and particularly throughout the SCTLD outbreak, there has been increased effort to collect coral tissue samples for histopathological analysis to assist in the definition, identification, etiology, and ecology of emerging coral disease.
In the initial histopathological case definition of SCTLD, it was noted that the pathology first affects the basal body wall and then migrates toward the oral surface (Landsberg et al., 2020). The case definition identified gastrodermal tissue necrosis and disintegration of the mesoglea in diseased tissue and crystalline inclusion bodies in some specimens (Figures 5B, D; (Landsberg et al., 2020). In apparently healthy tissues, the gastrodermis binds tightly to the mesoglea and structures are well-defined (Figures 5A, C). However, diseased tissues have looser structure, the mesoglea and gastrodermis appear disconnected, and there is greater tissue necrosis than in healthy samples. SCTLD presents within the coral tissue as lytic necrosis, starting in the basal body wall gastrodermis and extending into the calicodermis. Lesions are more advanced in the surface body wall than the basal body wall (Landsberg et al., 2020). Additional disease characteristics include vacuolization around the symbiont, symbiont cell exocytosis, body wall breakage, gastrodermal separation, and necrosis (Landsberg et al., 2020; Meiling et al., 2021; Studivan et al., 2022b).
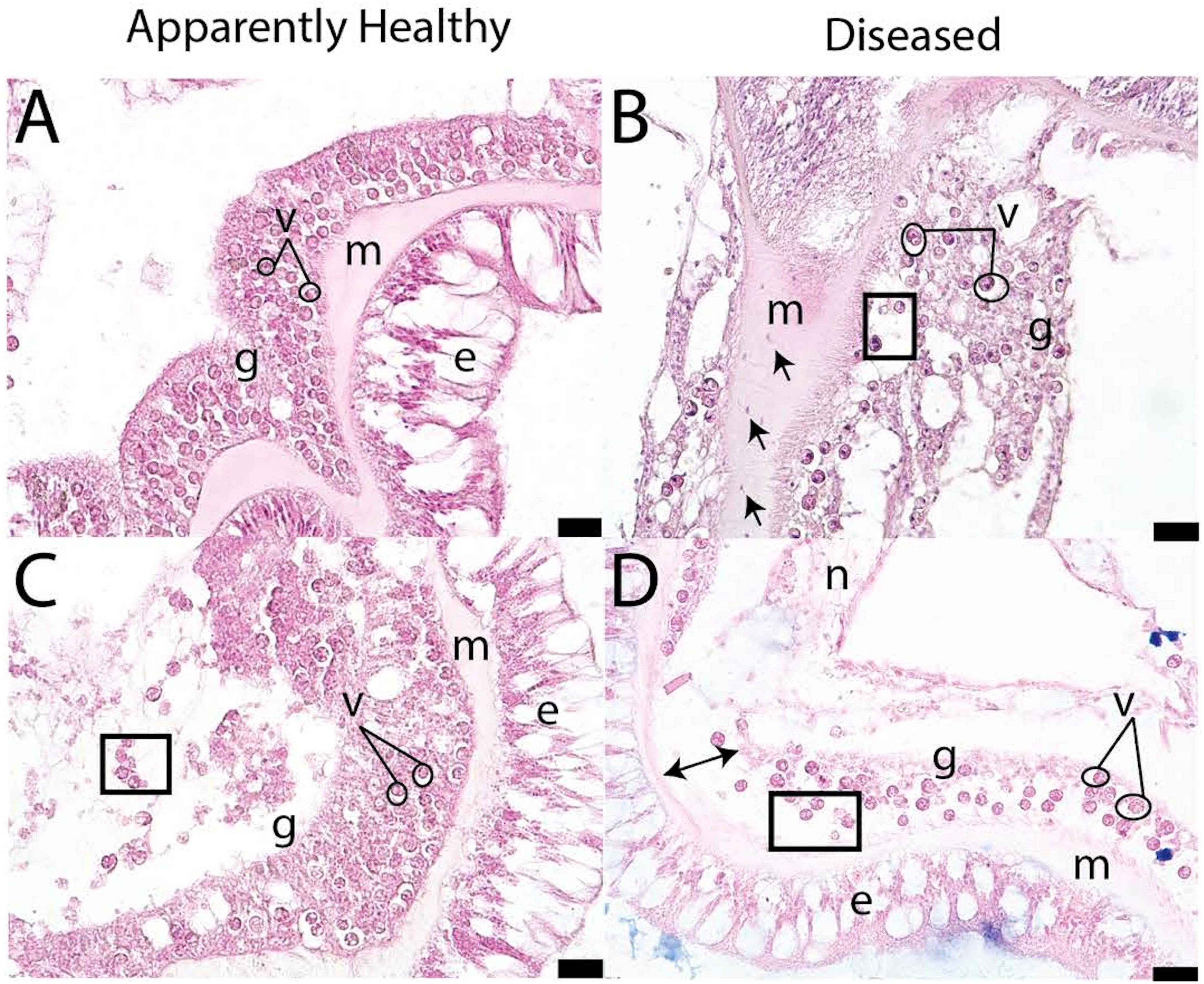
Figure 5 Pictograph of disease signs in apparently healthy (A, C) and diseased P. strigosa (B, D) (figure adapted from Meiling et al., 2021). Open circles indicate vacuolization, squares denote exocytosis, double sided arrows indicate gastrodermal separation, and single-headed arrows denote amoebocytes. Within the pictographs: m is mesoglea, e is epidermis, g is gastrodermis, and n is necrosis. Scale bar indicates 20 microns.
As histopathologists looked further into the relationship between vacuole size and disease state, an interesting pattern arose. In healthy samples, the ‘pocket’ or vacuole that surrounds the symbiont cell is closely associated. In diseased samples, the vacuole expands, which may imply that nutrient exchange from the symbiont to the coral animal is impeded (Gates et al., 1992). Analyses have shown that there is a significant increase in vacuolization around Symbiodinaceae cells in SCTLD-affected corals (Meiling et al., 2021). Vacuole size was also correlated with the gene expression of Rab7 in SCTLD affected colonies (Beavers et al., 2023). Rab7 is a known marker of Symbiodiniaceae degradation, indicating SCTLD infects the symbiont, and as the degradation continues, the vacuole expands, then limiting communication between the symbiont and the coral host. Additionally, presumed VLPs have been found in association with Symbiodinaceae cells in diseased and healthy samples using TEM (Work et al., 2021), which was discussed previously. Ciliates have also been seen in histological samples; however they could be deemed as unrelated to SCTLD (Landsberg et al., 2020) or could be associated with SCTLD tissue (Eaton et al., 2021), but more investigation needs to be done due to conflicting reports.
Evidence suggests that as SCTLD progresses, there is a disruption to the symbiont-host relationship. This appears to manifest as vacuolization of the symbiont cells and progress to symbiont cell exocytosis or in situ degradation, gastrodermal separation from the mesoglea, and tissue necrosis. This pattern of infection has been confirmed both in field collected specimens, and in specimens in which SCTLD was transmitted to apparently healthy colonies in laboratory settings.
Limitations and future directions
A complicated aspect of using histopathology to investigate coral disease is that some signs associated with infectious disease are also present in apparently healthy colonies, as a product of tank stress, sampling stress, and/or tissue processing (Landsberg et al., 2020). Distinguishing between a threshold of disease versus baseline, sampling-, or aquaria-induced stress signs is an important next step for coral histopathological studies of infectious diseases. While histopathology has proven to be a useful tool in confirming or describing coral disease patterns and pathways, it is best applied in conjunction with molecular methodologies to describe the genetic, microbial, and immunological mechanisms of coral pathologies. Additionally, TEM could be used in tandem with other methods for a more detailed view of the coral tissue and help in investigations of VLPs in relation to SCTLD. Since histopathology is time-consuming and laborious, it may be prudent to develop fast and robust histopathological diagnostics that indicate coral health status, particularly during coral disease outbreaks (Eaton et al., 2021).
Metabolomics
Metabolomics, the comprehensive study of small molecules, provides a readout of the biochemical activity and physiological state of the sampled organism (Goodacre, 2007; Viant, 2008; Patti et al., 2012; Wishart, 2019). As threats to coral reefs persist, it is vital to understand underlying chemical mechanisms that mediate symbiosis and pathogenesis between coral holobiont members during healthy and diseased states. Advancement in untargeted metabolomics-based strategies offers an opportunity to connect cellular pathways with biological mechanisms, which is critical in unravelling the biological roles of metabolites in cellular physiology. Recent studies have demonstrated the applicability of metabolomics to evaluate physiological state and identify diagnostic biomarkers in Caribbean corals (Lohr et al., 2019; Roach et al., 2020; Little et al., 2021; Roach et al., 2021).
The characterization of metabolomic profiles of M. cavernosa from a SCTLD-affected Florida reef revealed that SCTLD-affected corals displayed a higher degree of intra-metabolomic variation compared to the visually healthy corals (Deutsch et al., 2021). Inter-metabolomic variation based on health status was also observed, where a third of the top metabolites that differentiated visually healthy corals from SCTLD-affected corals were attributed to endosymbiotic Symbiodinaceae. These metabolites included diacylglyceryl-carboxyhydroxymethylcholine betaine lipids, which are also indicative of bleaching history of stony corals (Deutsch et al., 2021; Roach et al., 2021), metabolites belong to the Vitamin E pathway, and glycolipids, a key component of Symbiodiniaceae thylakoid membranes (Tchernov et al., 2004; Leblond et al., 2015; Rosset et al., 2019; Haydon et al., 2023). The varied distribution pattern of these lipids and other algal-associated features suggested the Symbiodiniaceae were affected by the onset of SCTLD (Deutsch et al., 2021). Additional metabolomics studies will enable identification and validation of biochemical pathways affected by SCTLD.
Limitations and future directions
Even though metabolic contributions of individual holobiont members are captured by profiling crude organic extracts of coral fragments using untargeted metabolomics (when present above the instrumental detection limits, see below) (Muscatine, 1990; LaJeunesse et al., 2018; Garg, 2021), it remains a challenge to assign the source of metabolites detected to the organism producing them, and to identify their chemical structures. To overcome these challenges, it is important to also characterize the metabolites produced by the coral microbiome in culture to aid in this endeavour. To do so, several culture-based methods have been developed (Sweet et al., 2011; Speare et al., 2020). While only a handful of publicly available datasets for coral microbiomes exist and the mass spectral databases generally are sparse for coral metabolomics, a repository for coral-derived bacteria (Sweet et al., 2011) and the metabolites produced has been initiated further supporting these efforts (Deutsch et al., 2022). Furthermore, methods for proper sampling of coral fragments are being developed (Greene et al., 2020). Since probiotics are a potential avenue for SCTLD intervention, metabolomic profiling will guide selection of probiotic candidates as bacteria that produce known and novel antimicrobials can be identified. The characterization of metabolomic profiles of SCTLD-associated pathogens is also vital in identifying potential mechanisms underlying host-pathogen and pathogen-microbiome interactions (Deutsch et al., 2022). The continued advancement of experimental and machine-learning based methods in metabolomics as well generation of microbial and metabolite databases will permit valuable insights into the chemical cues employed by the members of the holobiont to sense the chemical and physical environment, interact with one another, and to elucidate SCTLD impact on the host, microbiome, and endosymbionts.
Another challenge underlying the use of metabolite profiling in studying contributions of microbial members stems from difficulty in enriching the detectable diversity of low-abundance microbiome-derived bioactive molecules that remain below the detection limit of even modern mass spectrometers; however, methods that employ fractionation of extracts prior to data acquisition would overcome this limitation. Metabolite annotation, wherein several thousand detected metabolites are to be identified, is also a challenge; although the continued development of bioinformatic and machine learning-based in silico tools to aid these analyses presents exciting opportunities (Blaženović et al., 2018; Beniddir et al., 2021; Deutsch et al., 2022; Cai et al., 2023). Given that biological systems are dynamic, researchers should also focus on evaluating metabolomics profiles at discrete time points of SCTLD progression to characterize and understand the flux of metabolites. Such studies will facilitate identification of early diagnostic markers of the disease. Studies conducted on corals maintained in aquaria are also necessary to reduce the inherent variation that arises from the environment. A controlled environment will enable delineation of biochemical pathways altered by SCTLD presence from those affected by other factors present on reefs, such as dissolved reef metabolites. Such organism-specific chemical cues warrant investigation, as these molecules will readily diffuse through the water column and impact reef dynamics (Becker et al., 2023). When possible, multiple ‘omics approaches should be incorporated in a study to maximize biochemical insights into the functioning of the coral holobiont.
Intervention methods for SCTLD
General disease intervention approaches, including vaccination, selective breeding, injury/stress prevention, medications, chemotherapy, culling of infected hosts, and vector controls are common practices to reduce host susceptibility and disease transmission (McCallum et al., 2004; Smith et al., 2015; Sokolow et al., 2019). Methods primarily aimed at improving the health of individuals can also be beneficial after disease onset by decreasing disease severity and duration in a population or community (Smith et al., 2015). For SCTLD, several in situ and ex situ intervention trials have evaluated various treatments and approaches for reducing disease impacts on individuals and the coral community. These intervention approaches include amputation, culling, genetic rescue, trenching, chlorinated epoxy, antibiotics, chemotherapeutics, and probiotics. Each has been implemented on varying scales with various success.
Amputation
Amputation entails excising affected diseased coral tissue from the rest of the apparently healthy to prevent further disease progression or rescue the remaining healthy tissue (Miller et al., 2014). Removing the diseased tissue for other coral diseases has yielded varying results (Dalton et al., 2010; Beurmann et al., 2017). For example, amputation ceased tissue loss in 80% of colonies affected with Australian subtropical white syndrome (Dalton et al., 2010), but had no significant effect at arresting chronic Montipora white syndrome (Beurmann et al., 2017). For SCTLD-affected Dendrogyra cylindrus colonies, removing SCTLD-affected tissue was effective at halting the disease, but did not protect the coral from being affected with new lesions, eventually resulting in whole-colony mortality (Neely and Hower, 2019). The removal of apparently healthy pillars from diseased D. cylindrus colonies and transplantation to other areas elsewhere on the reef did not prevent future SCTLD lesions (Neely and Hower, 2019). Overall, both amputation methods resulted in poor survival rates as neither prevented eventual infection of SCTLD (Neely and Hower, 2019). However, these trials were limited to only five amputation treatments applied to one coral species, making it difficult to determine the viability of amputation as an effective intervention method if scaled-up or applied to different species.
Culling
Culling requires the removal or restriction of an infected individual from their environment to reduce possible disease spread and overall pathogen load in the system (Prentice et al., 2010). Whole coral colony culling has been discussed in various SCTLD response management plans (Johnston, 2021), however, implementation of culling as an intervention strategy on SCTLD has been limited logistically and the effectiveness of the method has not officially been reported. It is recommended, like in other disease epidemics, to implement this method at the emergence of the disease to help reduce the spread and prevalence of SCTLD (Bolzoni et al., 2014). Unfortunately, since SCTLD is already endemic on many Caribbean reefs, this is no longer a viable intervention method (Precht, 2021). This intervention strategy may also be unfeasible in many regions due to the complexity of permitting and logistics of removing massive whole coral colonies from the reef.
Genetic rescue
Aimed at preserving genetic diversity of wild populations undergoing a bottleneck event in ex situ environments, genetic rescue involves removing and relocating apparently healthy individuals or gametes for eventual use in restoration (Hagedorn and Spindler, 2014; Novak et al., 2020). Cryopreserved and thawed sperm of the highly susceptible species, Diploria labyrinthiformis, was successful at fertilizing newly collected eggs (Grosso-Becerra et al., 2021) and could be used as a method to increase the species’ genetic diversity on SCTLD impacted reefs. In response to SCTLD outbreaks, efforts have included collection and cryopreservation of coral gametes as well as collection and maintenance of susceptible coral fragments that are gene-banked and potentially spawned ex situ (Hagedorn et al., 2012; Novak et al., 2020; Grosso-Becerra et al., 2021; Neely et al., 2021b; O’Neil et al., 2021). The D. cylindrus coral population in Florida was driven to functional extinction by SCTLD outbreaks (Neely et al., 2021a). As a result, maintaining genetic diversity of the few remaining D. cylindrus was prioritized. From 2015-2019, 574 fragments representing 128 genotypes were collected from D. cylindrus colonies and then held in in situ or ex situ nurseries across five facilities in Florida and South Carolina (Neely et al., 2021b). Other rescue efforts were also prioritized in order to maximize tissue area and retain a diverse parental source for future use in sexual reproduction and restoration (Neely et al., 2021b). These rescue efforts have been removing healthy corals ahead of SCTLD emergence. The genetic rescue team has collected 2,283 coral colonies since 2016 and provide a continuous monitoring dashboard to track the number of colonies that are rescued by species (https://myfwc.maps.arcgis.com/apps/dashboards/eba7dc2cabc64f60819e6d4b084d94cd).
Trenching
Trenching creates a physical barrier or “firebreak” between diseased and apparently healthy tissue to isolate the diseased tissue from the rest of the coral colony (Aeby et al., 2015). This method has been used successfully for other coral diseases including black band and yellow band diseases (Aeby et al., 2015; Walton et al., 2018). In combination with amoxicillin treatment, 1 cm deep firebreaks were created 5 cm from SCTLD lesions with chisels and underwater angle grinders, yielding a 91% success rate on individual lesions and an 86% rate of SCTLD quiescence on colonies (Shilling et al., 2021; Walker et al., 2021). The firebreak treatment alone was less effective at halting SCTLD, ~79% success at halting SCTLD lesions and ~38% proportion of quiescence on colonies (Shilling et al., 2021). Since trenching is a very time-intensive method with no or limited beneficial effects beyond amoxicillin treatment, researchers recommend deprioritizing trenching as an intervention approach (Shilling et al., 2021).
Chlorinated epoxy
Using chlorinated epoxy as an intervention method involves mixing a ZSPAR A-788 Splash Zone two-part marine epoxy with chlorine powder (calcium hypochlorite) and applying a band of this mixture to the disease lesion (Aeby et al., 2015; Neely et al., 2021c; Shilling et al., 2021; Walker et al., 2021). Chlorinated epoxy treatments have been used with moderate success at arresting black band disease lesions (Aeby et al., 2015); however, several studies have shown that chlorinated epoxy treatment is ineffective at treating SCTLD (Lee Hing et al., 2022), as the lesion arrest rate of treated lesions was comparable to untreated lesions (Neely et al., 2021c; Shilling et al., 2021; Walker et al., 2021). This intervention method is not recommended for mitigating SCTLD due to the ineffectiveness of the method on multiple species and Florida reef locations (Neely et al., 2021c).
Antibiotic treatments
Multiple classes of antibiotics, delivery mechanisms, and concentrations have been tested for potential efficacy in halting SCTLD both ex situ and in situ. Tested antibiotic compounds include ampicillin, gentamicin, paromycin, kanamycin, chloramphenicol, sulfathiazole, nalidixic acid, and amoxicillin (Aeby et al., 2019; Miller et al., 2020). Both paromycin and gentamicin were ineffective against SCTLD, as tissue sloughing continued until the entire coral fragment reached mortality (Miller et al., 2020). Both ampicillin and nalidixic acid were also not successful (Aeby et al., 2019; Miller et al., 2020). When paired with amoxicillin, both ampicillin and kanamycin proved to be effective at times, which could be a result of the amoxicillin’s success. Overall, amoxicillin, as a standalone treatment has been the most successful SCTLD treatment, resulting in a 97% survival rate in fragments ex situ (Miller et al., 2020).
The success of the amoxicillin treatment in ex-situ trials led researchers to test it in the field. A proprietary specialized silicone-based product, termed CoralCure Ointment Base2b (CoreRx/OceanAlchemists), was developed to administer the antibiotic treatment in the field (Neely et al., 2020). Once mixed with amoxicillin trihydrate, this specialized paste releases amoxicillin over 72 hours and was developed using polymers that mimic the consistency of coral mucus (Neely et al., 2020). It adheres to the coral’s skeleton, but not the living tissue (Neely et al., 2021c). A common application method is packing the paste into 60 cc catheter-tip syringes and applying the treatment in 1 cm wide bands covering the entirety of the lesion and adhering it to both exposed skeleton and living tissue (Neely et al., 2021c; Walker et al., 2021). This amoxicillin/CoralCure Ointment Base2B paste was 88-95% effective at halting SCTLD lesions on multiple species throughout Florida including: O. faveolata, C. natans, D. labyrinthiformis, O. annularis, P. strigosa, S. siderea, M. cavernosa, P. clivosa, and D. stokesii (Neely et al., 2021c; Shilling et al., 2021; Walker et al., 2021; Lee Hing et al., 2022). Treatment experiments in the British Virgin Islands had a positive effect on the coral community compared to untreated reefs (Forrester et al., 2022), suggesting that treatment might slow the spread of SCTLD across a reef (Neely et al., 2021c; Forrester et al., 2022). Transcriptomic analysis likewise demonstrated that amoxicillin treatments resulted in the reversal of gene expression pathways to a pre-diseased state, possibly benefiting the coral immune response (Studivan et al., 2023).
Although the antibiotic paste has been successful at treating individual lesions, it does not prevent new lesions from developing and progressing; therefore, continuous monitoring, revisitation, and reapplication of antibiotics to corals every few months has been recommended (Neely et al., 2021c; Shilling et al., 2021; Walker et al., 2021; Lee Hing et al., 2022), reducing the feasibility to cover larger spatial and temporal scales. The treatment frequency needed and the overall treatment success depend on different factors including environmental conditions and the coral species’ relative susceptibility (Walker et al., 2021; Forrester et al., 2022). The amoxicillin/CoralCure Ointment Base2B treatment is currently the most successful and preferred intervention method for SCTLD outbreaks. Although effective, the amoxicillin/CoralCure Base2B method can be expensive due to the price of amoxicillin, though cheaper than full-scale restoration, and can only be purchased from a singular source, creating the need for alternative treatments.
Chemotherapeutics
Iron-chelating compounds, which sequester excess iron using organic molecules, have been suggested as a chemotherapeutic treatment and antibiotic alternative due to iron’s role in increasing disease pathogenicity. Initial ex situ trials with Chelex 100 and 2-bipyridyl (BIP) mixed with a coral dental paste did not significantly slow SCTLD progression (Miller et al., 2020). However, the trial’s limitations included a small sample size and potential interference from molecules that preferentially bound to the chelator in the artificial seawater used for water changes (Miller et al., 2020). Further research has been recommended to assess the viability of chemotherapeutic methods for treating SCTLD (Miller et al., 2020).
Probiotics
Probiotics are live microorganisms which provide health benefits to their host (Schrezenmeir and Vrese, 2001). In the context of disease intervention, probiotics operate by colonizing niches, excluding pathogens by outcompeting them for nutrients and space, and/or producing antimicrobial compounds that may degrade pathogen cell walls, inhibit pathogen growth, and disrupt cell-cell signaling (Teplitski and Ritchie, 2009). The coral probiotic hypothesis posits that bacteria play a crucial role in preventing bleaching and infectious coral diseases, and healthy coral microbiomes can contribute to resistance against multiple pathogens (Reshef et al., 2006; Brunt et al., 2007).
Antibacterial-producing microbes from apparently healthy M. cavernosa colonies resistant to SCTLD have been isolated and tested as potential probiotics (Ushijima et al., 2023). One strain isolated from disease-resistant M. cavernosa fragments, Pseudoalteromonas spp., McH1-7, was found to produce the antibacterial compounds koromicin and tetrabromopyrrole among other predicted antibacterial enzymes (Ushijima et al., 2023). During ex situ trials, M. cavernosa treated with McH1-7 had reduced mortality, slower lesion progression rates, and a 59% success rate at arresting disease progression compared to untreated colonies with active lesions (Ushijima et al., 2023). Furthermore, McH1-7 completely protected fragments against SCTLD transmission. This is the first known prophylactic intervention method, offering hope for an alternative method that might protect corals from SCTLD.
Limitations and future directions
Waterborne pathogen transmission (Aeby et al., 2019) and rapid dilution of treatment agents can make it challenging to replicate land-based intervention methods in the marine environment. Although the use of antibiotics has been successful at halting SCTLD lesions, it is important to understand the secondary impacts that the antibiotic treatments might have on the coral holobiont, surrounding organisms, and environment (Shilling et al., 2021; Walker et al., 2021). Studies have also shown antibiotics to have negative effects on corals resulting in dysbiosis in the coral microbial communities with the potential of reducing coral growth and reducing heat tolerance (Dalton et al., 2010; Zhang et al., 2018; Connelly et al., 2022). Nonetheless, given the extreme impacts of SCTLD on coral communities, the amoxicillin/CoralCure Ointment Base2B treatment remains a viable and recommended primary intervention treatment. It is also important to continue trials of different intervention treatments and to understand and reduce the risks of potential negative effects from deploying antibiotics in the environment. Furthermore, efforts are needed to optimize more preventative, potent, efficient, and cost-effective SCTLD intervention methods.
Conclusion
The complexity of SCTLD has limited our ability to identify the etiological agent(s), develop practical diagnostic tools, establish environmental drivers, understand transmission, and develop more targeted treatments. Determining the etiology of SCTLD remains a challenge due to the diversity of coral species affected and the geographic range impacted. Additionally, visual diagnosis of SCTLD in the field remains challenging due to variations in host or symbiont response (Aeby et al., 2019; Aeby et al., 2021; Meiling et al., 2021; Traylor-Knowles et al., 2021). Potential pathogen evolution and intraspecific differences in disease presentation also present complexity and inconsistency in identifying SCTLD across lesion types, species, or region. Considering these challenges, this review aimed to provide a comprehensive overview of SCTLD research with the goal of guiding future efforts toward unraveling this complex etiology and mitigating SCTLD impacts.
The diverse research on the SCTLD outbreak has highlighted another issue in the marine sciences, the contrasting lack of diversity in this same area of study. For any investigation into coral disease etiology, especially SCTLD, fostering multidisciplinary collaborations can advance the pace and scope of knowledge acquisition. While there are various issues at hand, this article comments on the disproportion of female representation in marine research. Women are less likely to be named on a scientific publication across various scientific fields at the same career stage and are less likely to be named on high-impact articles (Ross et al., 2022). These inequities are also compounded by women from underrepresented groups (Ahmadia et al., 2021). Specifically, in coral reef research, there is still a persistent gender gap in authorship that has not improved in recent decades (Ahmadia et al., 2021). As of 2018, only 33% of publications on coral reef science have contributions by authors that identified as female (Ahmadia et al., 2021). The complexity of SCTLD requires an inclusive approach to aid in research endeavors. Increasing the diversity of voices could improve research quality, provide additional perspectives, acknowledge valuable research contributions, and foster greater collaborations. A recent report from Elsevier found that female representation in research is increasing, but there is still inequality in authorship across all EU countries surveyed except Portugal (De Kleijn et al., 2020). Addressing the gender disparity in scientific authorship across all fields is an essential step towards improving research quality and enhancing inclusivity.
Additionally, these collaborative efforts should not only encompass a wide array of backgrounds and expertise from researchers, but also encompass a wide array of diagnostic methods (‘omics, TEM, histology, etc.) for both in situ and ex situ investigations. Baseline profiles of naïve healthy coral samples remain a priority to facilitate comparative analyses. Furthermore, understanding the baseline conditions of reefs prior to disease outbreaks is essential for understanding how environmental factors may influence SCTLD’s spread and impact. This comprehensive approach should involve examining timepoints before, during, and after the appearance of lesions and include exploring naïve populations to aid in diagnostics and pathogen identification. In addition to these multidisciplinary efforts, we have proposed additional future directions for SCTLD research (Figure 6). Given the increasing impact of anthropogenic and global stressors, it is likely that SCTLD will not be the last highly virulent coral disease. Therefore, the establishment of standardized methods and diverse international collaborations is essential not only for the present, but also to better prepare for disease responses in the future.
Author contributions
EP: Conceptualization, Writing – original draft, Writing – review & editing. AC: Writing – review & editing, Writing – original draft. CD: Writing – original draft, Writing – review & editing. JD: Writing – original draft, Writing – review & editing. LI: Writing – original draft, Writing – review & editing. SM: Writing – original draft, Writing – review & editing. AR: Writing – original draft, Writing – review & editing. AB: Writing – review & editing. MB: Writing – review & editing. NG: Writing – review & editing. DH: Writing – review & editing. NT: Writing – review & editing. JV: Writing – review & editing. BU: Conceptualization, Funding acquisition, Writing – review & editing.
Funding
The author(s) declare financial support was received for the research, authorship, and/or publication of this article. Funding for this review was provided by startup funds from UNCW to BU. NG and JMD were supported by the National Science Foundation (NSF) CAREER award (Award No. 2047235).
Acknowledgments
We would like to highlight this collaborative effort of seven female early career researchers on drafting different sections of this manuscript related to each one’s expertise with guidance from the other authors. As women are less likely to receive authorship on scientific publications, these authors would like to thank anyone dedicated to correcting the gender imbalance in scientific authorship. We would also like to thank the editors of the special addition along with our reviewers.
Conflict of interest
The authors declare that the research was conducted in the absence of any commercial or financial relationships that could be construed as a potential conflict of interest.
Publisher’s note
All claims expressed in this article are solely those of the authors and do not necessarily represent those of their affiliated organizations, or those of the publisher, the editors and the reviewers. Any product that may be evaluated in this article, or claim that may be made by its manufacturer, is not guaranteed or endorsed by the publisher.
Supplementary material
The Supplementary Material for this article can be found online at: https://www.frontiersin.org/articles/10.3389/fmars.2023.1321271/full#supplementary-material
References
Abbas M. N., Kausar S., Cui H. (2019). The biological role of peroxiredoxins in innate immune responses of aquatic invertebrates. Fish Shellfish Immunol. 89, 91–97. doi: 10.1016/j.fsi.2019.03.062
Aeby G., Ushijima B., Bartels E., Walter C., Kuehl J., Jones S., et al. (2021). Changing stony coral tissue loss disease dynamics through time in montastraea cavernosa. Front. Mar. Sci. 8. doi: 10.3389/fmars.2021.699075
Aeby G. S., Ushijima B., Campbell J. E., Jones S., Williams G. J., Meyer J. L., et al. (2019). Pathogenesis of a tissue loss disease affecting multiple species of corals along the Florida reef tract. Front. Mar. Sci. 6. doi: 10.3389/fmars.2019.00678
Aeby G. S., Work T. M., Runyon C. M., Shore-Maggio A., Ushijima B., Videau P., et al. (2015). First record of black band disease in the Hawaiian archipelago: Response, outbreak status, virulence, and a method of treatment. PLoS One 10, 1–17. doi: 10.1371/journal.pone.0120853
Ahmadia G. N., Cheng S. H., Andradi-Brown D. A., Baez S. K., Barnes M. D., Bennett N. J., et al. (2021). Limited progress in improving gender and geographic representation in coral reef science. Front. Mar. Sci. 8. doi: 10.3389/fmars.2021.731037
Ainsworth T. D., Fordyce A. J., Camp E. F. (2017). The other microeukaryotes of the coral reef microbiome. Trends Microbiol. 25, 980–991. doi: 10.1016/j.tim.2017.06.007
Alvarez-Filip L., Estrada-Saldívar N., Pérez-Cervantes E., Molina-Hernández A., González-Barrios F. J. (2019). A rapid spread of the stony coral tissue loss disease outbreak in the Mexican Caribbean. PeerJ 7, e8069. doi: 10.7717/peerj.8069
Alvarez-Filip L., González-Barrios F. J., Pérez-Cervantes E., Molina-Hernández A., Estrada-Saldívar N. (2022). Stony coral tissue loss disease decimated Caribbean coral populations and reshaped reef functionality. Commun. Biol. 5, 1–10. doi: 10.1038/s42003-022-03398-6
Ambalavanan L., Iehata S., Fletcher R., Stevens E. H., Zainathan S. C. (2021). A review of marine viruses in coral ecosystem. J. Mar. Sci. Eng. 9, 711. doi: 10.3390/jmse9070711
Anderson D. A., Walz M. E., Weil E., Tonellato P., Smith M. C. (2016). RNA-Seq of the Caribbean reef-building coral Orbicella faveolata (Scleractinia-Merulinidae) under bleaching and disease stress expands models of coral innate immunity. PeerJ 4, e1616. doi: 10.7717/peerj.1616
Aronson R. B., Precht W. F. (2001). Applied paleoecology and the crisis on Caribbean coral reefs. PALAIOS 16, 195–196. doi: 10.1669/0883-1351(2001)016<0195:APATCO>2.0.CO;2
Baker A. C. (2003). Flexibility and specificity in coral-algal symbiosis: diversity, ecology, and biogeography of symbiodinium. Annu. Rev. Ecology Evolution Systematics 34, 661–689. doi: 10.1146/annurev.ecolsys.34.011802.132417
Beavers K. M., Van Buren E. W., Rossin A. M., Emery M. A., Veglia A. J., Karrick C. E., et al. (2023). Stony coral tissue loss disease induces transcriptional signatures of in situ degradation of dysfunctional Symbiodiniaceae. Nat. Commun. 14, 2915. doi: 10.1038/s41467-023-38612-4
Becker C. C., Brandt M., Miller C. A., Apprill A. (2021). Microbial bioindicators of Stony Coral Tissue Loss Disease identified in corals and overlying waters using a rapid field-based sequencing approach. Environ. Microbiol 24 (3), 1166–1182. doi: 10.1111/1462-2920.15718
Becker C. C., Weber L., Zgliczynski B., Sullivan C., Sandin S., Muller E., et al. (2023). Microorganisms and dissolved metabolites distinguish Florida’s Coral Reef habitats. PNAS Nexus 2, pgad287. doi: 10.1093/pnasnexus/pgad287
Beniddir M. A., Kang K. B., Genta-Jouve G., Huber F., Rogers S., van der Hooft J. J. J. (2021). Advances in decomposing complex metabolite mixtures using substructure- and network-based computational metabolomics approaches. Nat. Prod. Rep. 38, 1967–1993. doi: 10.1039/D1NP00023C
Berthelier J., Schnitzler C. E., Wood-Charlson E. M., Poole A. Z., Weis V. M., Detournay O. (2017). Implication of the host TGFβ pathway in the onset of symbiosis between larvae of the coral Fungia scutaria and the dinoflagellate Symbiodinium sp. (clade C1f). Coral Reefs 36, 1263–1268. doi: 10.1007/s00338-017-1621-6
Beurmann S., Runyon C., Videau P., Callahan S., Aeby G. (2017). Assessment of disease lesion removal as a method to control chronic Montipora white syndrome. Dis. Aquat. Org. 123, 173–179. doi: 10.3354/dao03088
Blackall L. L., Wilson B., van Oppen M. J. H. (2015). Coral—the world’s most diverse symbiotic ecosystem. Mol. Ecol. 24, 5330–5347. doi: 10.1111/mec.13400
Blaženović I., Kind T., Ji J., Fiehn O. (2018). Software tools and approaches for compound identification of LC-MS/MS data in metabolomics. Metabolites 8, 31. doi: 10.3390/metabo8020031
Boilard A., Dubé C. E., Gruet C., Mercière A., Hernandez-Agreda A., Derome N. (2020). Defining coral bleaching as a microbial dysbiosis within the coral holobiont. Microorganisms 8, 1682. doi: 10.3390/microorganisms8111682
Bolzoni L., Tessoni V., Groppi M., De Leo G. (2014). React or wait: which optimal culling strategy to control infectious diseases in wildlife. J. Math Biol. 69, 1001–1025. doi: 10.1007/s00285-013-0726-y
Bonacolta A. M., Weiler B. A., Porta-Fitó T., Sweet M., Keeling P., Del Campo J. (2023). Beyond the Symbiodiniaceae: diversity and role of microeukaryotic coral symbionts. Coral Reefs 42, 567–577. doi: 10.1007/s00338-023-02352-0
Bourne D. G., Garren M., Work T. M., Rosenberg E., Smith G. W., Harvell C. D. (2009). Microbial disease and the coral holobiont. Trends Microbiol. 17, 554–562. doi: 10.1016/j.tim.2009.09.004
Bourne D. G., Morrow K. M., Webster N. S. (2016). Insights into the coral microbiome: underpinning the health and resilience of reef ecosystems. Annu. Rev. Microbiol. 70, 317–340. doi: 10.1146/annurev-micro-102215-095440
Brandt M. E., Ennis R. S., Meiling S. S., Townsend J., Cobleigh K., Glahn A., et al. (2021). The emergence and initial impact of stony coral tissue loss disease (SCTLD) in the United States Virgin islands. Front. Mar. Sci. 8. doi: 10.3389/fmars.2021.715329
Brower D. L., Brower S. M., Hayward D. C., Ball E. E. (1997). Molecular evolution of integrins: Genes encoding integrin β subunits from a coral and a sponge. Proc. Natl. Acad. Sci. U.S.A. 94, 9182–9187. doi: 10.1073/pnas.94.17.9182
Bruno J. F., Selig E. R., Casey K. S., Page C. A., Willis B. L., Harvell C. D., et al. (2007). Thermal stress and coral cover as drivers of coral disease outbreaks. PLoS Biol. 5, e124. doi: 10.1371/journal.pbio.0050124
Brunt J., Newaj-Fyzul A., Austin B. (2007). The development of probiotics for the control of multiple bacterial diseases of rainbow trout, Oncorhynchus mykiss (Walbaum). J. Fish Dis. 30, 573–579. doi: 10.1111/j.1365-2761.2007.00836.x
Bythell J. C., Wild C. (2011). Biology and ecology of coral mucus release. J. Exp. Mar. Biol. Ecol. 408, 88–93. doi: 10.1016/j.jembe.2011.07.028
Cai Y., Zhou Z., Zhu Z.-J. (2023). Advanced analytical and informatic strategies for metabolite annotation in untargeted metabolomics. TrAC Trends Analytical Chem. 158, 116903. doi: 10.1016/j.trac.2022.116903
Camacho-Vite C., Estrada-Saldívar N., Pérez-Cervantes E., Alvarez-Filip L. (2022). Differences in the progression rate of SCTLD in Pseudodiploria strigosa are related to colony size and morphology. Front. Mar. Sci. 9, 790818. doi: 10.3389/fmars.2022.790818
Catterjee S. (2014). Artefacts in histopathology. J. Oral. Maxillofac. Pathol. 18, S111–S116. doi: 10.4103/jomfp.JOMFP_125_15
Claar D. C., McDevitt-Irwin J. M., Garren M., Vega Thurber R., Gates R. D., Baum J. K. (2020). Increased diversity and concordant shifts in community structure of coral-associated Symbiodiniaceae and bacteria subjected to chronic human disturbance. Mol. Ecol. 29, 2477–2491. doi: 10.1111/mec.15494
Claesson M. J., Wang Q., O’Sullivan O., Greene-Diniz R., Cole J. R., Ross R. P., et al. (2010). Comparison of two next-generation sequencing technologies for resolving highly complex microbiota composition using tandem variable 16S rRNA gene regions. Nucleic Acids Res. 38, e200. doi: 10.1093/nar/gkq873
Clark A. S., Williams S. D., Maxwell K., Rosales S. M., Huebner L. K., Landsberg J. H., et al. (2021). Characterization of the microbiome of corals with stony coral tissue loss disease along Florida’s coral reef. Microorganisms 9, 2181. doi: 10.3390/microorganisms9112181
Clemens E., Brandt M. E. (2015). Multiple mechanisms of transmission of the Caribbean coral disease white plague. Coral Reefs 34, 1179–1188. doi: 10.1007/s00338-015-1327-6
Combs I. R., Studivan M. S., Eckert R. J., Voss J. D. (2021). Quantifying impacts of stony coral tissue loss disease on corals in Southeast Florida through surveys and 3D photogrammetry. PloS One 16, e0252593. doi: 10.1371/journal.pone.0252593
Connelly M. T., McRae C. J., Liu P.-J., Martin C. E., Traylor-Knowles N. (2022). Antibiotics alter pocillopora coral-symbiodiniaceae-bacteria interactions and cause microbial dysbiosis during heat stress. Front. Mar. Sci. 8. doi: 10.3389/fmars.2021.814124
Cooper E. L. (2010). Evolution of immune systems from self/not self to danger to artificial immune systems (AIS). Phys. Life Rev. 7, 55–78. doi: 10.1016/j.plrev.2009.12.001
Correa A. M. S., Brandt M. E., Smith T. B., Thornhill D. J., Baker A. C. (2009a). Symbiodinium associations with diseased and healthy scleractinian corals. Coral Reefs 28, 437–448. doi: 10.1007/s00338-008-0464-6
Correa A. M. S., Welsh R. M., Vega Thurber R. L. (2013). Unique nucleocytoplasmic dsDNA and +ssRNA viruses are associated with the dinoflagellate endosymbionts of corals. ISME J. 7, 13–27. doi: 10.1038/ismej.2012.75
Costa S. V., Hibberts S. J., Olive D. A., Budd K. A., Long A. E., Meiling S. S., et al. (2021). Diversity and disease: the effects of coral diversity on prevalence and impacts of stony coral tissue loss disease in Saint Thomas, U.S. Virgin Islands. Front. Mar. Sci. 8. doi: 10.3389/fmars.2021.682688
Cróquer A., Weil E., Rogers C. S. (2021). Similarities and differences between two deadly Caribbean coral diseases: white plague and stony coral tissue loss disease. Front. Mar. Sci. 8. doi: 10.3389/fmars.2021.709544
Cunning R., Baker A. C. (2014). Not just who, but how many: the importance of partner abundance in reef coral symbioses. Front. Microbiol. 5. doi: 10.3389/fmicb.2014.00400
Cunning R., Silverstein R. N., Baker A. C. (2015). Investigating the causes and consequences of symbiont shuffling in a multi-partner reef coral symbiosis under environmental change. Proc. R. Soc B. 282, 20141725. doi: 10.1098/rspb.2014.1725
Cunning R., Silverstein R. N., Baker A. C. (2018). Symbiont shuffling linked to differential photochemical dynamics of Symbiodinium in three Caribbean reef corals. Coral Reefs 37, 145–152. doi: 10.1007/s00338-017-1640-3
Cunning R., Silverstein R. N., Barnes B. B., Baker A. C. (2019). Extensive coral mortality and critical habitat loss following dredging and their association with remotely-sensed sediment plumes. Mar. pollut. Bull. 145, 185–199. doi: 10.1016/j.marpolbul.2019.05.027
Dahlgren C., Pizarro V., Sherman K., Greene W., Oliver J. (2021). Spatial and temporal patterns of stony coral tissue loss disease outbreaks in the Bahamas. Front. Mar. Sci. 8. doi: 10.3389/fmars.2021.682114
Dalton S. J., Godwin S., Smith S. D. A., Pereg L. (2010). Dynamics of bacterial community development in the reef coral Acropora muricata following experimental antibiotic treatment. Mar. Freshw. Res. 61, 342–350. doi: 10.1007/s00338-011-0800-0
Davies S. W., Gamache M. H., Howe-Kerr L. I., Kriefall N. G., Baker A. C., Banaszak A. T., et al. (2023). Building consensus around the assessment and interpretation of Symbiodiniaceae diversity. PeerJ 11, e15023. doi: 10.7717/peerj.15023
De Kleijn M., Jayabalasingham B., Falk-Krzesinski H.J., Collins T., Kuiper-Hoyng L., Cingolani I., Zhang , et al. (2020). The Researcher Journey Through a Gender Lens: An Examination of Research Participation, Career Progression and Perceptions Across the Globe (Elsevier). Available at: https://www.elsevier.com/gender-report
Deutsch J. M., Jaiyesimi O. A., Pitts K. A., Houk J., Ushijima B., Walker B. K., et al. (2021). Metabolomics of healthy and stony coral tissue loss disease affected montastraea cavernosa corals. Front. Mar. Sci. 8. doi: 10.3389/fmars.2021.714778
Deutsch J. M., Mandelare-Ruiz P., Yang Y., Foster G., Routhu A., Houk J., et al. (2022). Metabolomics approaches to dereplicate natural products from coral-derived bioactive bacteria. J. Nat. Prod. 85, 462–478. doi: 10.1021/acs.jnatprod.1c01110
Dobbelaere T., Holstein D. M., Muller E. M., Gramer L. J., McEachron L., Williams S. D., et al. (2022). Connecting the dots: transmission of stony coral tissue loss disease from the marquesas to the Dry Tortugas. Front. Mar. Sci. 9. doi: 10.3389/fmars.2022.778938
Dobbelaere T., Muller E. M., Gramer L. J., Holstein D. M., Hanert E. (2020). Coupled epidemio-hydrodynamic modeling to understand the spread of a deadly coral disease in Florida. Front. Mar. Sci. 7. doi: 10.3389/fmars.2020.591881
Eaton K. R., Landsberg J. H., Kiryu Y., Peters E. C., Muller E. M. (2021). Measuring Stony Coral Tissue Loss Disease Induction and Lesion Progression Within Two Intermediately Susceptible Species, Montastraea cavernosa and Orbicella faveolata. Front. Mar. Sci. 8. doi: 10.3389/fmars.2021.717265
Eddy T. D., Lam V. W. Y., Reygondeau G., Cisneros-Montemayor A. M., Greer K., Palomares M. L. D., et al. (2021). Global decline in capacity of coral reefs to provide ecosystem services. One Earth 4, 1278–1285. doi: 10.1016/j.oneear.2021.08.016
Egan S., Gardiner M. (2016). Microbial dysbiosis: rethinking disease in marine ecosystems. Front. Microbiol. 7. doi: 10.3389/fmicb.2016.00991
Elmer F., Cruz Y., Dock C., Ortega A., Wedel S., Hertler H. (2021). Determining lesion progression rate of stony coral tissue loss disease using structure-from-motion photogrammetry. GCR 32, SC12–SC17. doi: 10.18785/gcr.3201.15
Elola M. T., Wolfenstein-Todel C., Troncoso M. F., Vasta G. R., Rabinovich G. A. (2007). Galectins: matricellular glycan-binding proteins linking cell adhesion, migration, and survival. Cell. Mol. Life Sci. 64, 1679–1700. doi: 10.1007/s00018-007-7044-8
Endo Y., Nakazawa N., Iwaki D., Takahashi M., Matsushita M., Fujita T. (2009). Interactions of ficolin and mannose-binding lectin with fibrinogen/fibrin augment the lectin complement pathway. J. Innate Immun. 2, 33–42. doi: 10.1159/000227805
Estrada-Saldívar N., Molina-Hernández A., Pérez-Cervantes E., Medellín-Maldonado F., González-Barrios F. J., Alvarez-Filip L. (2020). Reef-scale impacts of the stony coral tissue loss disease outbreak. Coral Reefs 39, 861–866. doi: 10.1007/s00338-020-01949-z
Estrada-Saldívar N., Quiroga-García B. A., Pérez-Cervantes E., Rivera-Garibay O. O., Alvarez-Filip L. (2021). Effects of the stony coral tissue loss disease outbreak on coral communities and the benthic composition of Cozumel reefs. Front. Mar. Sci. 8. doi: 10.3389/fmars.2021.632777
Evans J. S., Paul V. J., Kellogg C. A. (2022a). Biofilms as potential reservoirs of stony coral tissue loss disease. Front. Mar. Sci. 9. doi: 10.3389/fmars.2022.1009407
Evans J. S., Paul V. J., Ushijima B., Kellogg C. A. (2022b). Combining tangential flow filtration and size fractionation of mesocosm water as a method for the investigation of waterborne coral diseases. Biol. Methods Protoc. 7, bpac007. doi: 10.1093/biomethods/bpac007
Evans J. S., Paul V. J., Ushijima B., Pitts K. A., Kellogg C. A. (2023). Investigating microbial size classes associated with the transmission of stony coral tissue loss disease (SCTLD). PeerJ 11, e15836. doi: 10.7717/peerj.15836
Finney J. C., Pettay D. T., Sampayo E. M., Warner M. E., Oxenford H. A., LaJeunesse T. C. (2010). The relative significance of host–habitat, depth, and geography on the ecology, endemism, and speciation of coral endosymbionts in the genus symbiodinium. Microb. Ecol. 60, 250–263. doi: 10.1007/s00248-010-9681-y
Forrester G. E., Arton L., Horton A., Nickles K., Forrester L. M. (2022). Antibiotic treatment ameliorates the impact of stony coral tissue loss disease (SCTLD) on coral communities. Front. Mar. Sci. 9. doi: 10.3389/fmars.2022.859740
Fuess L. E., Pinzόn C. ,. J. H., Weil E., Mydlarz L. D. (2016). Associations between transcriptional changes and protein phenotypes provide insights into immune regulation in corals. Dev. Comp. Immunol. 62, 17–28. doi: 10.1016/j.dci.2016.04.017
Galloway S., Woodley C. M., McLaughlin S., Work T. M., Bochsler V., Meteyer C. U., et al. (2005). “Coral disease and health workshop: Coral histopathology II, July 12-14, 2005,” in NOAA NOS NCCOS 56 and CRCP 4.
Gardner S. G., Leggat W., Ainsworth T. D. (2023). The microbiome of the endosymbiotic Symbiodiniaceae in corals exposed to thermal stress. Hydrobiologia 850, 3685–3704. doi: 10.1007/s10750-023-05221-7
Garg N. (2021). Metabolomics in functional interrogation of individual holobiont members. mSystems 6. doi: 10.1128/msystems.00841-21
Gates R. D., Baghdasarian G., Muscatine L. (1992). Temperature stress causes host cell detachment in symbiotic cnidarians: Implications for coral bleaching. Biol. Bull. 182, 324–332. doi: 10.2307/1542252
Gignoux-Wolfsohn S. A., Aronson F. M., Vollmer S. V. (2017). Complex interactions between potentially pathogenic, opportunistic, and resident bacteria emerge during infection on a reef-building coral. FEMS Microbiol. Ecol. 93. doi: 10.1093/femsec/fix080
Gintert B. E., Precht W. F., Fura R., Rogers K., Rice M., Precht L. L., et al. (2019). Regional coral disease outbreak overwhelms impacts from a local dredge project. Environ. Monit Assess. 191, 630. doi: 10.1007/s10661-019-7767-7
Gladfelter W. B. (1982). White-band disease in acropora palmata: implications for the structure and growth of shallow reefs. Bull. Mar. Sci. 32, 639–643.
Glasl B., Webster N. S., Bourne D. G. (2017). Microbial indicators as a diagnostic tool for assessing water quality and climate stress in coral reef ecosystems. Mar. Biol. 164, 91. doi: 10.1007/s00227-017-3097-x
Goodacre R. (2007). Metabolomics of a superorganism. J. Nutr. 137, 259S–266S. doi: 10.1093/jn/137.1.259S
Grajales A., Sanchez J. A. (2016). Holobiont assemblages of dominant coral species (Symbiodinium types and coral species) shape Caribbean reef community structure. Rev. la Academia Colombiana Cienc. Exactas Físicas y Naturales 40, 300–311. doi: 10.18257/raccefyn.294
Green E. A., Davies S. W., Matz M. V., Medina M. (2014). Quantifying cryptic Symbiodinium diversity within Orbicella faveolata and Orbicella franksi at the Flower Garden Banks, Gulf of Mexico. PeerJ 2, e386. doi: 10.7717/peerj.386
Green E. P., Bruckner A. W. (2000). The significance of coral disease epizootiology for coral reef conservation. Biol. Conserv. 96, 347–361. doi: 10.1016/S0006-3207(00)00073-2
Greene A., Leggat W., Donahue M. J., Raymundo L. J., Caldwell J. M., Moriarty T., et al. (2020). Complementary sampling methods for coral histology, metabolomics and microbiome. Methods Ecol. Evol. 11, 1012–1020. doi: 10.1111/2041-210X.13431
Grosso-Becerra M. V., Mendoza-Quiroz S., Maldonado E., Banaszak A. T. (2021). Cryopreservation of sperm from the brain coral Diploria labyrinthiformis as a strategy to face the loss of corals in the Caribbean. Coral Reefs 40, 937–950. doi: 10.1007/s00338-021-02098-7
Gupta S., Mortensen M. S., Schjørring S., Trivedi U., Vestergaard G., Stokholm J., et al. (2019). Amplicon sequencing provides more accurate microbiome information in healthy children compared to culturing. Commun. Biol. 2, 1–7. doi: 10.1038/s42003-019-0540-1
Guzmán-Urieta E. O., Jordán-Dahlgren E. (2021). Spatial patterns of a lethal white syndrome outbreak in pseudodiploria strigosa. Front. Mar. Sci. 8. doi: 10.3389/fmars.2021.669171
Hagedorn M., Spindler R. (2014). “The reality, use and potential for cryopreservation of coral reefs,” in Reproductive Sciences in Animal Conservation Advances in Experimental Medicine and Biology. Eds. Holt W. V., Brown J. L., Comizzoli P. (New York, NY: Springer New York), 317–329. doi: 10.1007/978-1-4939-0820-2_13
Hagedorn M., van Oppen M. J. H., Carter V., Henley M., Abrego D., Puill-Stephan E., et al. (2012). First frozen repository for the Great Barrier Reef coral created. Cryobiology 65, 157–158. doi: 10.1016/j.cryobiol.2012.05.008
Halliwell B., Gutteridge J. M. C. (2015). Free Radicals in Biology and Medicine (Oxford, United Kingdom: Oxford University Press). doi: 10.1093/acprof:oso/9780198717478.001.0001
Haydon T. D., Matthews J. L., Seymour J. R., Raina J.-B., Seymour J. E., Chartrand K., et al. (2023). Metabolomic signatures of corals thriving across extreme reef habitats reveal strategies of heat stress tolerance. Proc. R. Soc. B: Biol. Sci. 290, 20221877. doi: 10.1098/rspb.2022.1877
Hayes N. K., Walton C. J., Gilliam D. S. (2022). Tissue loss disease outbreak significantly alters the Southeast Florida stony coral assemblage. Front. Mar. Sci. 9. doi: 10.3389/fmars.2022.975894
Heres M. M., Farmer B. H., Elmer F., Hertler H. (2021). Ecological consequences of stony coral tissue loss disease in the Turks and Caicos Islands. Coral Reefs 40, 609–624. doi: 10.1007/s00338-021-02071-4
Hernandez-Agreda A., Gates R. D., Ainsworth T. D. (2017). Defining the core microbiome in corals’ Microbial soup. Trends Microbiol. 25, 125–140. doi: 10.1016/j.tim.2016.11.003
Howe-Kerr L. I., Knochel A. M., Meyer M. D., Sims J. A., Karrick C. E., Grupstra C. G. B., et al. (2023). Filamentous virus-like particles are present in coral dinoflagellates across genera and ocean basins. ISME J. 17, 1–14. doi: 10.1038/s41396-023-01526-6
Howells E. J., Vaughan G. O., Work T. M., Burt J. A., Abrego D. (2020). Annual outbreaks of coral disease coincide with extreme seasonal warming. Coral Reefs 39, 771–781. doi: 10.1007/s00338-020-01946-2
Huang Z., Langevelde F., Estrada-Peña A., Suzan G., de Boer W. F. (2016). The diversity-disease relationship: Evidence for and criticisms of the dilution effect. Parasitology 1, 1–12. doi: 10.1017/S0031182016000536
Hughes T. P. (1994). Catastrophes, phase shifts, and large-scale degradation of a Caribbean coral reef. Science 265, 1547–1551. doi: 10.1126/science.265.5178.1547
Huntley N., Brandt M. E., Becker C. C., Miller C. A., Meiling S. S., Correa A. M. S., et al. (2022). Experimental transmission of Stony Coral Tissue Loss Disease results in differential microbial responses within coral mucus and tissue. ISME Commun. 2, 46. doi: 10.1038/s43705-022-00126-3
Janeway C. A. (2001). How the immune system protects the host from infection. Microbes Infection 3, 1167–1171. doi: 10.1016/S1286-4579(01)01477-0
Jeon H.-S., Dracheva T., Yang S.-H., Meerzaman D., Fukuoka J., Shakoori A., et al. (2008). SMAD6 contributes to patient survival in non–small cell lung cancer and its knockdown reestablishes TGF-β Homeostasis in lung cancer cells. Cancer Res. 68, 9686–9692. doi: 10.1158/0008-5472.CAN-08-1083
Juliano R. L., Reddig P., Alahari S., Edin M., Howe A., Aplin A. (2004). Integrin regulation of cell signalling and motility. Biochem. Soc. Trans. 32 (3), 443–446. doi: 10.1042/bst0320443
Johnston M. A. (2021). Strategy for Stony Coral Tissue Loss Disease Prevention and Response at Flower Garden Banks National Marine Sanctuary (Galveston, TX: U.S Department of Commerce, National Oceanic and Atmospheric Administration, Flower Garden Banks National Marine Sanctuary).
Keesing F., Holt R. D., Ostfeld R. S. (2006). Effects of species diversity on disease risk. Ecol. Lett. 9, 485–498. doi: 10.1111/j.1461-0248.2006.00885.x
Kemp D. W., Thornhill D. J., Rotjan R. D., Iglesias-Prieto R., Fitt W. K., Schmidt G. W. (2015). Spatially distinct and regionally endemic Symbiodinium assemblages in the threatened Caribbean reef-building coral Orbicella faveolata. Coral Reefs 34, 535–547. doi: 10.1007/s00338-015-1277-z
Kolodziej G., Studivan M. S., Gleason A. C. R., Langdon C., Enochs I. C., Manzello D. P. (2021). Impacts of stony coral tissue loss disease (SCTLD) on coral community structure at an inshore patch reef of the upper Florida keys using photomosaics. Front. Mar. Sci. 8. doi: 10.3389/fmars.2021.682163
Kvennefors E. C. E., Leggat W., Hoegh-Guldberg O., Degnan B. M., Barnes A. C. (2008). An ancient and variable mannose-binding lectin from the coral Acropora millepora binds both pathogens and symbionts. Dev. Comp. Immunol. 32, 1582–1592. doi: 10.1016/j.dci.2008.05.010
Laas P., Ugarelli K., Absten M., Boyer B., Briceño H., Stingl U. (2021). Composition of prokaryotic and eukaryotic microbial communities in waters around the Florida reef tract. Microorganisms 9, 1120. doi: 10.3390/microorganisms9061120
LaJeunesse T. C., Parkinson J. E., Gabrielson P. W., Jeong H. J., Reimer J. D., Voolstra C. R., et al. (2018). Systematic revision of symbiodiniaceae highlights the antiquity and diversity of coral endosymbionts. Curr. Biol. 28, 2570–2580.e6. doi: 10.1016/j.cub.2018.07.008
LaJeunesse T. C., Thornhill D. J. (2011). Improved Resolution of Reef-Coral Endosymbiont (Symbiodinium) Species Diversity, Ecology, and Evolution through psbA Non-Coding Region Genotyping. PloS One 6, e29013. doi: 10.1371/journal.pone.0029013
Landsberg J. H., Kiryu Y., Peters E. C., Wilson P. W., Perry N., Waters Y., et al. (2020). Stony coral tissue loss disease in Florida is associated with disruption of host–zooxanthellae physiology. Front. Mar. Sci. 7. doi: 10.3389/fmars.2020.576013
Leblond J. D., Khadka M., Duong L., Dahmen J. L. (2015). Squishy lipids: Temperature effects on the betaine and galactolipid profiles of a C18/C18 peridinin-containing dinoflagellate, Symbiodinium microadriaticum (Dinophyceae), isolated from the mangrove jellyfish, Cassiopea xamachana. Phycological Res. 63, 219–230. doi: 10.1111/pre.12093
Lee Hing C., Guifarro Z., Dueñas D., Ochoa G., Nunez A., Forman K., et al. (2022). Management responses in Belize and Honduras, as stony coral tissue loss disease expands its prevalence in the Mesoamerican reef. Front. Mar. Sci. 9. doi: 10.3389/fmars.2022.883062
Lemons M. L., Condic M. L. (2008). Integrin signaling is integral to regeneration. Exp. Neurol. 209, 343–352. doi: 10.1016/j.expneurol.2007.05.027
Lesser M. P. (2006). OXIDATIVE STRESS IN MARINE ENVIRONMENTS: biochemistry and physiological ecology. Annu. Rev. Physiol. 68, 253–278. doi: 10.1146/annurev.physiol.68.040104.110001
Lewis A. M., Chan A. N., LaJeunesse T. C. (2018). New species of closely related endosymbiotic dinoflagellates in the greater Caribbean have niches corresponding to host coral phylogeny. J. Eukaryot. Microbiol. 66, 469–482. doi: 10.1111/jeu.12692
Leydet K. P., Hellberg M. E. (2016). Discordant coral–symbiont structuring: factors shaping geographical variation of Symbiodinium communities in a facultative zooxanthellate coral genus, Oculina. Coral Reefs 35, 583–595. doi: 10.1007/s00338-016-1409-0
Little M., George E. E., Arts M. G. I., Shivak J., Benler S., Huckeba J., et al. (2021). Three-dimensional molecular cartography of the Caribbean reef-building coral orbicella faveolata. Front. Mar. Sci. 8. doi: 10.3389/fmars.2021.627724
Lohr K. E., Khattri R. B., Guingab-Cagmat J., Camp E. F., Merritt M. E., Garrett T. J., et al. (2019). Metabolomic profiles differ among unique genotypes of a threatened Caribbean coral. Sci. Rep. 9, 6067. doi: 10.1038/s41598-019-42434-0
MacKnight N. J., Dimos B. A., Beavers K. M., Muller E. M., Brandt M. E., Mydlarz L. D. (2022). Disease resistance in coral is mediated by distinct adaptive and plastic gene expression profiles. Sci. Adv. 8, eabo6153. doi: 10.1126/sciadv.abo6153
McCallum H. I., Kuris A., Harvell C. D., Lafferty K. D., Smith G. W., Porter J. (2004). Does terrestrial epidemiology apply to marine systems? Trends Ecol. Evol. 19, 585–591. doi: 10.1016/j.tree.2004.08.009
McIlroy S. E., terHorst C. P., Teece M., Coffroth M. A. (2022). Nutrient dynamics in coral symbiosis depend on both the relative and absolute abundance of Symbiodiniaceae species. Microbiome 10, 192. doi: 10.1186/s40168-022-01382-0
Meiling S. S., Muller E. M., Lasseigne D., Rossin A., Veglia A. J., MacKnight N., et al. (2021). Variable species responses to experimental stony coral tissue loss disease (SCTLD) exposure. Front. Mar. Sci. 8. doi: 10.3389/fmars.2021.670829
Meiling S., Muller E. M., Smith T. B., Brandt M. E. (2020). 3D photogrammetry reveals dynamics of stony coral tissue loss disease (SCTLD) lesion progression across a thermal stress event. Front. Mar. Sci. 7. doi: 10.3389/fmars.2020.597643
Meyer J. L., Castellanos-Gell J., Aeby G. S., Häse C. C., Ushijima B., Paul V. J. (2019). Microbial community shifts associated with the ongoing stony coral tissue loss disease outbreak on the Florida reef tract. Front. Microbiol. 10. doi: 10.3389/fmicb.2019.02244
Miller D. J., Hemmrich G., Ball E. E., Hayward D. C., Khalturin K., Funayama N., et al. (2007). The innate immune repertoire in Cnidaria - ancestral complexity and stochastic gene loss. Genome Biol. 8, R59. doi: 10.1186/gb-2007-8-4-r59
Miller M. W., Lohr K. E., Cameron C. M., Williams D. E., Peters E. C. (2014). Disease dynamics and potential mitigation among restored and wild staghorn coral, Acropora cervicornis. PeerJ 2, e541. doi: 10.7717/peerj.541
Miller C., May L., Moffitt Z., Woodley C. (2020). “Exploratory treatments for stony coral tissue loss disease : pillar coral (Dendrogyra cylindrus),” in NOAA Technical Memorandum NOS NCCOS 245 NOAA and CRCP, Vol. 37. 78–78.
Miller A. W., Richardson L. L. (2015). Emerging coral diseases: a temperature-driven process? Mar. Ecol. 36, 278–291. doi: 10.1111/maec.12142
Mohamed A. R., Ochsenkühn M. A., Kazlak A. M., Moustafa A., Amin S. A. (2023). The coral microbiome: towards an understanding of the molecular mechanisms of coral–microbiota interactions. FEMS Microbiol. Rev. 47, fuad005. doi: 10.1093/femsre/fuad005
Muller E. M., Sartor C., Alcaraz N. I., van Woesik R. (2020). Spatial epidemiology of the stony-coral-tissue-loss disease in Florida. Front. Mar. Sci. 7. doi: 10.3389/fmars.2020.00163
Muscatine L. (1990) The role of symbiotic algae in carbon and energy flux in reef corals. Available at: https://www.semanticscholar.org/paper/The-role-of-symbiotic-algae-in-carbon-and-energy-in-Muscatine/40b336effe682ec15dbe77436da337f7d94491b0 (Accessed May 12, 2022).
Mydlarz L. D., Jones L. E., Harvell C. D. (2006). Innate immunity, environmental drivers, and disease ecology of marine and freshwater invertebrates. Source: Annu. Rev. Ecology Evolution Systematics 37, 251–288. doi: 10.2307/annurev.ecolsys.37.091305.30000011
Mydlarz L. D., McGinty E. S., Drew Harvell C. (2010). What are the physiological and immunological responses of coral to climate warming and disease? J. Exp. Biol. 213, 934–945. doi: 10.1242/jeb.037580
Mydlarz L. D., Palmer C. V. (2011). The presence of multiple phenoloxidases in Caribbean reef-building corals. Comp. Biochem. Physiol. Part A: Mol. Integr. Physiol. 159, 372–378. doi: 10.1016/j.cbpa.2011.03.029
Neely K., Hower E. (2019). FY 2018 In Situ Disease Intervention (Miami, FL: Florida Department of Environmental Protection).
Neely K. L., Lewis C. L., Lunz K. S., Kabay L. (2021a). Rapid population decline of the pillar coral dendrogyra cylindrus along the Florida reef tract. Front. Mar. Sci. 8. doi: 10.3389/fmars.2021.656515
Neely K. L., Lewis C. L., O’Neil K., Woodley C. M., Moore J., Ransom Z., et al. (2021b). Saving the last unicorns: the genetic rescue of Florida’s pillar corals. Front. Mar. Sci. 8. doi: 10.3389/fmars.2021.657429
Neely K. L., Macaulay K. A., Hower E. K., Dobler M. A. (2020). Effectiveness of topical antibiotics in treating corals affected by Stony Coral Tissue Loss Disease. PeerJ 2020. doi: 10.7717/peerj.9289
Neely K. L., Shea C. P., Macaulay K. A., Hower E. K., Dobler M. A. (2021c). Short- and long-term effectiveness of coral disease treatments. Front. Mar. Sci. 8. doi: 10.3389/fmars.2021.675349
Ng A., Xavier R. J. (2011). Leucine-rich repeat (LRR) proteins: Integrators of pattern recognition and signaling in immunity. Autophagy 7, 1082–1084. doi: 10.4161/auto.7.9.16464
Nguyen-Kim H., Bettarel Y., Bouvier T., Bouvier C., Doan-Nhu H., Nguyen-Ngoc L., et al. (2015). Coral mucus is a hot spot for viral infections. Appl. Environ. Microbiol. 81, 5773–5783. doi: 10.1128/AEM.00542-15
Noonan K. R., Childress M. J. (2020). Association of butterflyfishes and stony coral tissue loss disease in the Florida Keys. Coral Reefs 39, 1581–1590. doi: 10.1007/s00338-020-01986-8
Novak B. J., Fraser D., Maloney T. H. (2020). Transforming ocean conservation: applying the genetic rescue toolkit. Genes 11, 209. doi: 10.3390/genes11020209
Nyholm S. V., Graf J. (2012). Knowing your friends: Invertebrate innate immunity fosters beneficial bacterial symbioses. Nat. Rev. Microbiol. 10, 815–827. doi: 10.1038/nrmicro2894
O’Neil K. L., Serafin R. M., Patterson J. T., Craggs J. R. K. (2021). Repeated ex situ Spawning in Two Highly Disease Susceptible Corals in the Family Meandrinidae. Front. Mar. Sci. 8. doi: 10.3389/fmars.2021.669976
Palacio-Castro A. M., Dennison C. E., Rosales S. M., Baker A. C. (2021). Variation in susceptibility among three Caribbean coral species and their algal symbionts indicates the threatened staghorn coral, Acropora cervicornis, is particularly susceptible to elevated nutrients and heat stress. Coral Reefs 40, 1601–1613. doi: 10.1007/s00338-021-02159-x
Palmer C. V., McGinty E. S., Cummings D. J., Smith S. M., Bartels E., Mydlarz L. D. (2011a). Patterns of coral ecological immunology: variation in the responses of Caribbean corals to elevated temperature and a pathogen elicitor. J. Exp. Biol. 214, 4240–4249. doi: 10.1242/jeb.061267
Palmer C. V., Mydlarz L. D., Willis B. L. (2008). Evidence of an inflammatory-like response in non-normally pigmented tissues of two scleractinian corals. Proc. R. Soc B. 275, 2687–2693. doi: 10.1098/rspb.2008.0335
Palmer C. V., Traylor-Knowles N. (2012). Towards an integrated network of coral immune mechanisms. Proc. R. Soc. B: Biol. Sci. 279, 4106–4114. doi: 10.1098/rspb.2012.1477
Palmer C. V., Traylor-Knowles N. G., Willis B. L., Bythell J. C. (2011b). Corals use similar immune cells and wound-healing processes as those of higher organisms. PLoS One 6, e23992. doi: 10.1371/journal.pone.0023992
Parisi M. G., Parrinello D., Stabili L., Cammarata M. (2020). Cnidarian immunity and the repertoire of defense mechanisms in anthozoans. Biology 9, 283. doi: 10.3390/biology9090283
Patti G. J., Yanes O., Siuzdak G. (2012). Metabolomics: the apogee of the omics trilogy. Nat. Rev. Mol. Cell Biol. 13, 263–269. doi: 10.1038/nrm3314
Peixoto R. S., Rosado P. M., Leite D. C., de A., Rosado A. S., Bourne D. G. (2017). Beneficial microorganisms for corals (BMC): proposed mechanisms for coral health and resilience. Front. Microbiol. 8. doi: 10.3389/fmicb.2017.00341
Poretsky R., Rodriguez-R L. M., Luo C., Tsementzi D., Konstantinidis K. T. (2014). Strengths and limitations of 16S rRNA gene amplicon sequencing in revealing temporal microbial community dynamics. PLoS One 9, e93827. doi: 10.1371/journal.pone.0093827
Precht W. (2021). Failure to respond to a coral disease epizootic in Florida: Causes and consequences. Rethinking Ecol. 6, 1–47. doi: 10.3897/RETHINKINGECOLOGY.6.56285
Precht W. F., Gintert B. E., Robbart M. L., Fura R., van Woesik R. (2016). Unprecedented disease-related coral mortality in southeastern Florida. Sci. Rep. 6, 31374. doi: 10.1038/srep31374
Prentice J. C., Fox N. J., Hutchings M. R., White P. C. L., Davidson R. S., Marion (2010). When to kill a cull: factors affecting the success of culling wildlife for disease control. J. R. Soc Interface 16. doi: 10.1098/rsif.2018.0901
Quiroz S. M., Renteria R. T., Tapia G. G. R., Miller M. W., Grosso-Becerra M. V., Banaszak A. T. (2023). Coral affected by stony coral tissue loss disease can produce viable offspring. PeerJ 11, e15519. doi: 10.7717/peerj.15519
Raina J.-B., Tapiolas D., Willis B. L., Bourne D. G. (2009). Coral-associated bacteria and their role in the biogeochemical cycling of sulfur. Appl. Environ. Microbiol 75 (11). doi: 10.1128/AEM.02567-08
Reshef L., Koren O., Loya Y., Zilber-Rosenberg I., Rosenberg E. (2006). The coral probiotic hypothesis. Environ. Microbiol. 8, 2068–2073. doi: 10.1111/j.1462-2920.2006.01148.x
Rippe J. P., Kriefall N. G., Davies S. W., Castillo K. D. (2019). Differential disease incidence and mortality of inner and outer reef corals of the upper Florida Keys in association with a white syndrome outbreak. bms 95, 305–316. doi: 10.5343/bms.2018.0034
Ritchie K. B. (2006). Regulation of microbial populations by coral surface mucus and mucus-associated bacteria. Mar. Ecol. Prog. Ser. 322, 1–14. doi: 10.3354/meps322001
Roach T. N. F., Dilworth J., H. C. M., Jones A. D., Quinn R. A., Drury C. (2021). Metabolomic signatures of coral bleaching history. Nat. Ecol. Evol. 5, 495–503. doi: 10.1038/s41559-020-01388-7
Roach T. N. F., Little M., Arts M. G. I., Huckeba J., Haas A. F., George E. E., et al. (2020). A multiomic analysis of in situ coral–turf algal interactions. Proc. Natl. Acad. Sci. 117, 13588–13595. doi: 10.1073/pnas.1915455117
Rodriguez-Casariego J. A., Cunning R., Baker A. C., Eirin-Lopez J. M. (2022). Symbiont shuffling induces differential DNA methylation responses to thermal stress in the coral Montastraea cavernosa. Mol. Ecol. 31, 588–602. doi: 10.1111/mec.16246
Rohwer F., Seguritan V., Azam F., Knowlton N. (2002). Diversity and distribution of coral-associated bacteria. Mar. Ecol. Prog. Ser. 243, 1–10. doi: 10.3354/meps243001
Rosales S. M., Clark A. S., Huebner L. K., Ruzicka R. R., Muller E. M. (2020). Rhodobacterales and rhizobiales are associated with stony coral tissue loss disease and its suspected sources of transmission. Front. Microbiol. 11. doi: 10.3389/fmicb.2020.00681
Rosales S. M., Huebner L. K., Clark A. S., McMinds R., Ruzicka R. R., Muller E. M. (2022). Bacterial metabolic potential and micro-eukaryotes enriched in stony coral tissue loss disease lesions. Front. Mar. Sci. 8. doi: 10.3389/fmars.2021.776859
Rosales S. M., Huebner L. K., Evans J. S., Apprill A., Baker A. C., Becker C. C., et al. (2023). A meta-analysis of the stony coral tissue loss disease microbiome finds key bacteria in unaffected and lesion tissue in diseased colonies. ISME Commun. 3, 1–14. doi: 10.1038/s43705-023-00220-0
Rosenau N. A., Gignoux-Wolfsohn S., Everett R. A., Miller A. W., Minton M. S., Ruiz G. M. (2021). Considering commercial vessels as potential vectors of stony coral tissue loss disease. Front. Mar. Sci. 8. doi: 10.3389/fmars.2021.709764
Ross M. B., Glennon B. M., Murciano-Goroff R., Berkes E. G., Weinberg B. A., Lane J. I. (2022). Women are credited less in science than men. Nature 608, 135–145. doi: 10.1038/s41586-022-04966-w
Rosset S. L., Koster G., Brandsma J., Hunt A. N., Postle A. D., D’Angelo C. (2019). Lipidome analysis of Symbiodiniaceae reveals possible mechanisms of heat stress tolerance in reef coral symbionts. Coral Reefs 38, 1241–1253. doi: 10.1007/s00338-019-01865-x
Santoro E. P., Borges R. M., Espinoza J. L., Freire M., Messias C. S. M. A., Villela H. D. M., et al. (2021). Coral microbiome manipulation elicits metabolic and genetic restructuring to mitigate heat stress and evade mortality. Sci. Adv. 7, eabg3088. doi: 10.1126/sciadv.abg3088
Sato Y., Bourne D., Willis B. (2011). Effects of temperature and light on the progression of black band disease on the reef coral, Montiporahispida. Coral Reefs 30, 753–761. doi: 10.1007/s00338-011-0751-5
Sato S., St-Pierre C., Bhaumik P., Nieminen J. (2009). Galectins in innate immunity: dual functions of host soluble β-galactoside-binding lectins as damage-associated molecular patterns (DAMPs) and as receptors for pathogen-associated molecular patterns (PAMPs). Immunol. Rev. 230, 172–187. doi: 10.1111/j.1600-065X.2009.00790.x
Sharp W. C., Maxwell K. E. (2018). Investigating the ongoing coral disease outbreak in the Florida Keys: Collecting corals to diagnose the etiological agent(s) and establishing sentinel sites to monitor transmission rates and the spatial progression of the disease. Final Report to the Florida Department of Environmental Protection.
Schrezenmeir J., Vrese M. (2001). Probiotics, prebiotics, and synbiotics—approaching a definition 1–3. Am. J. Clin. Nutr. 73, 361s–364s. doi: 10.1093/ajcn/73.2.361s
Schul M., Mason A., Ushijima B., Sneed J. M. (2022). Microbiome and metabolome contributions to coral health and disease. Biol. Bull. 243, 76–83. doi: 10.1086/720971
Serrano X. M., Baums I. B., Smith T. B., Jones R. J., Shearer T. L., Baker A. C. (2016). Long distance dispersal and vertical gene flow in the Caribbean brooding coral Porites astreoides. Sci. Rep. 6, 21619. doi: 10.1038/srep21619
Sharp W. C., Shea C. P., Maxwell K. E., Muller E. M., Hunt J. H. (2020). Evaluating the small-scale epidemiology of the stony-coral -tissue-loss-disease in the middle Florida keys. PloS One 15, e0241871. doi: 10.1371/journal.pone.0241871
Shilling E. N., Combs I. R., Voss J. D. (2021). Assessing the effectiveness of two intervention methods for stony coral tissue loss disease on Montastraea cavernosa. Sci. Rep. 11, 1–11. doi: 10.1038/s41598-021-86926-4
Shnit-Orland M., Kushmaro A. (2009). Coral mucus-associated bacteria: a possible first line of defense. FEMS Microbiol. Ecol. 67, 371–380. doi: 10.1111/j.1574-6941.2008.00644.x
Silva M. T., Do Vale A., Dos Santos N. M. N. (2008). Secondary necrosis in multicellular animals: An outcome of apoptosis with pathogenic implications. Apoptosis 13, 463–482. doi: 10.1007/s10495-008-0187-8
Silverstein R. N., Correa A. M. S., Baker A. C. (2012). Specificity is rarely absolute in coral–algal symbiosis: implications for coral response to climate change. Proc. R. Soc B. 279, 2609–2618. doi: 10.1098/rspb.2012.0055
Silverstein R. N., Cunning R., Baker A. C. (2015). Change in algal symbiont communities after bleaching, not prior heat exposure, increases heat tolerance of reef corals. Global Change Biol. 21, 236–249. doi: 10.1111/gcb.12706
Smith P., Morrow R., Ross D. (2015). Field Trials of Health Interventions: A Toolbox. 3rd ed. Eds. Smith P., Richard M., David R. (Smith - Oxford, United Kingdom: Oxford University Press). doi: 10.1111/1753-6405.12577
Soffer N., Brandt M. E., Correa A. M. S., Smith T. B., Thurber R. V. (2014). Potential role of viruses in white plague coral disease. ISME J. 8, 271–283. doi: 10.1038/ismej.2013.137
Sokolow S. H., Nova N., Pepin K. M., Peel A. J., Pulliam J. R. C., Manlove K., et al. (2019). Ecological interventions to prevent and manage zoonotic pathogen spillover. Phil. Trans. R. Soc B 374, 20180342. doi: 10.1098/rstb.2018.0342
Spadafore R., Fura R., Precht W. F., Vollmer S. V. (2021). Multi-variate analyses of coral mortality from the 2014–2015 stony coral tissue loss disease outbreak off miami-dade county, Florida. Front. Mar. Sci. 8. doi: 10.3389/fmars.2021.723998
Speare L., Smith S., Salvato F., Kleiner M., Septer A. N. (2020). Environmental viscosity modulates interbacterial killing during habitat transition. mBio 11. doi: 10.1128/mbio.03060-19
Studivan M. S., Baptist M., Molina V., Riley S., First M., Soderberg N., et al. (2022a). Transmission of stony coral tissue loss disease (SCTLD) in simulated ballast water confirms the potential for ship-born spread. Sci. Rep. 12, 19248. doi: 10.1038/s41598-022-21868-z
Studivan M. S., Eckert R. J., Shilling E., Soderberg N., Enochs I. C., Voss J. D. (2023). Stony coral tissue loss disease intervention with amoxicillin leads to a reversal of disease-modulated gene expression pathways. Mol. Ecol 32 (19), 5294–5413. doi: 10.1111/mec.17110
Studivan M. S., Rossin A. M., Rubin E., Soderberg N., Holstein D. M., Enochs I. C. (2022b)Reef Sediments Can Act As a stony coral tissue loss disease vector. In: Frontiers in Marine Science (Accessed April 19, 2022).
Sweet M., Burn D., Croquer A., Leary P. (2013). Characterisation of the bacterial and fungal communities associated with different lesion sizes of dark spot syndrome occurring in the coral stephanocoenia intersepta. PloS One 8, e62580. doi: 10.1371/journal.pone.0062580
Sweet M. J., Croquer A., Bythell J. C. (2011). Bacterial assemblages differ between compartments within the coral holobiont. Coral Reefs 30, 39–52. doi: 10.1007/s00338-010-0695-1
Tchernov D., Gorbunov M. Y., de Vargas C., Narayan Yadav S., Milligan A. J., Häggblom M., et al. (2004). Membrane lipids of symbiotic algae are diagnostic of sensitivity to thermal bleaching in corals. Proc. Natl. Acad. Sci. 101, 13531–13535. doi: 10.1073/pnas.0402907101
Teplitski M., Ritchie K. (2009). How feasible is the biological control of coral diseases? Trends Ecol. Evol. 24, 378–385. doi: 10.1016/j.tree.2009.02.008
Thome P. E., Rivera-Ortega J., Rodríguez-Villalobos J. C., Cerqueda-García D., Guzmán-Urieta E. O., García-Maldonado J. Q., et al. (2021). Local dynamics of a white syndrome outbreak and changes in the microbial community associated with colonies of the scleractinian brain coral Pseudodiploria strigosa. PeerJ 9, e10695. doi: 10.7717/peerj.10695
Thornhill D. J., Kemp D. W., Bruns B. U., Fitt W. K., Schmidt G. W. (2008). CORRESPONDENCE BETWEEN COLD TOLERANCE AND TEMPERATE BIOGEOGRAPHY IN A WESTERN ATLANTIC SYMBIODINIUM (DINOPHYTA) LINEAGE 1. J. Phycology 44, 1126–1135. doi: 10.1111/j.1529-8817.2008.00567.x
Thurber R. L. V., Correa A. M. S. (2011). Viruses of reef-building scleractinian corals. J. Exp. Mar. Biol. Ecol. 408, 102–113. doi: 10.1016/j.jembe.2011.07.030
Titus K., O’Connell L., Matthee K., Childress M. (2022). The influence of foureye butterflyfish (Chaetodon capistratus) and symbiodiniaceae on the transmission of stony coral tissue loss disease. Front. Mar. Sci. 9. doi: 10.3389/fmars.2022.800423
Toledo-Hernández C., Ruiz-Diaz C. P. (2014). The immune responses of the coral. Inverteb. Surv. J. 11, 319–328.
Tracy A. M., Pielmeier M. L., Yoshioka R. M., Heron S. F., Harvell C. D. (2019). Increases and decreases in marine disease reports in an era of global change. Proc. R. Soc. B: Biol. Sci. 286, 20191718. doi: 10.1098/rspb.2019.1718
Tran T. D., Luallen R. J. (2023). An organismal understanding of C. elegans innate immune responses, from pathogen recognition to multigenerational resistance. Semin. Cell Dev. Biol. S1084952123000605, 77–84. doi: 10.1016/j.semcdb.2023.03.005
Traylor-Knowles N., Connelly M. T., Young B. D., Eaton K., Muller E. M., Paul V. J., et al. (2021). Gene Expression Response to Stony Coral Tissue Loss Disease Transmission in M. cavernosa and O. faveolata From Florida. Front. Mar. Sci. 8. doi: 10.3389/fmars.2021.681563
Truc M., Rivera A., Ochoa G. M., Dueñas D., Guifarro Z., Brady G., et al. (2023). Evaluating the spread of stony coral tissue loss disease in the Bay Islands, Honduras. Front. Mar. Sci. 10. doi: 10.3389/fmars.2023.1197318
Ushijima B., Gunasekera S. P., Meyer J. L., Tittl J., Pitts K. A., Thompson S., et al. (2023). Chemical and genomic characterization of a potential probiotic treatment for stony coral tissue loss disease. Commun. Biol. 6, 248. doi: 10.1038/s42003-023-04590-y
Ushijima B., Meyer J. L., Thompson S., Pitts K., Marusich M. F., Tittl J., et al. (2020). Disease diagnostics and potential coinfections by vibrio coralliilyticus during an ongoing coral disease outbreak in Florida. Front. Microbiol. 11. doi: 10.3389/fmicb.2020.569354
Van De Water J. A. J. M., Lamb J. B., Heron S. F., Van Oppen M. J. H., Willis B. L. (2016). Temporal patterns in innate immunity parameters in reef-building corals and linkages with local climatic conditions. Ecosphere 7. doi: 10.1002/ECS2.1505
Van Oppen M. J., Burghardt I. (2009). Zooxanthellae (Symbiodinium, Dinophyceae) symbioses on coral reefs. Microbiol. Aust. 30, 67. doi: 10.1071/MA09067
van Woesik R., Randall C. J. (2017). Coral disease hotspots in the Caribbean. Ecosphere 8, e01814. doi: 10.1002/ecs2.1814
Vega Thurber R. L., Mydlarz L. D., Brandt M., Harvell D., Weil E., Raymundo L., et al. (2020). Deciphering coral disease dynamics: integrating host, microbiome, and the changing environment. Front. Ecol. Evol. 8. doi: 10.3389/fevo.2020.575927
Veglia A. J., Beavers K., Van Buren E. W., Meiling S. S., Muller E. M., Smith T. B., et al. (2022). Alphaflexivirus genomes in stony coral tissue loss disease-affected, disease-exposed, and disease-unexposed coral colonies in the U.S. Virgin islands. Microbiol. Resource Announcements 11, e01199–e01121. doi: 10.1128/mra.01199-21
Viant M. R. (2008). Recent developments in environmental metabolomics. Mol. Biosyst. 4, 980–986. doi: 10.1039/b805354e
Walker B. K., Turner N. R., Noren H. K. G., Buckley S. F., Pitts K. A. (2021). Optimizing Stony Coral Tissue Loss Disease ( SCTLD ) Intervention Treatments on Montastraea cavernosa in an Endemic Zone, Vol. 8. 1–11. doi: 10.3389/fmars.2021.666224
Walton C. J., Hayes N. K., Gilliam D. S. (2018). Impacts of a regional, multi-year, multi-species coral disease outbreak in southeast Florida. Front. Mar. Sci. 5. doi: 10.3389/fmars.2018.00323
Wegley L., Edwards R., Rodriguez-Brito B., Liu H., Rohwer F. (2007). Metagenomic analysis of the microbial community associated with the coral Porites astreoides. Environ. Microbiol. 9, 2707–2719. doi: 10.1111/j.1462-2920.2007.01383.x
Weil E. (2004). “Coral reef diseases in the wider caribbean,” in Coral Health and Disease. Eds. Rosenberg E., Loya Y. (Berlin, Heidelberg: Springer), 35–68. doi: 10.1007/978-3-662-06414-6_2
Weisburg W. G., Barns S. M., Pelletier D. A., Lane D. J. (1991). 16S ribosomal DNA amplification for phylogenetic study. J. Bacteriology 173, 697–703. doi: 10.1128/jb.173.2.697-703.1991
Welsh R. M., Rosales S. M., Zaneveld J. R., Payet J. P., McMinds R., Hubbs S. L., et al. (2017). Alien vs. predator: bacterial challenge alters coral microbiomes unless controlled by Halobacteriovorax predators. PeerJ 5, e3315. doi: 10.7717/peerj.3315
Welsh R. M., Zaneveld J. R., Rosales S. M., Payet J. P., Burkepile D. E., Thurber R. V. (2016). Bacterial predation in a marine host-associated microbiome. ISME J. 10, 1540–1544. doi: 10.1038/ismej.2015.219
Wild C., Huettel M., Klueter A., Kremb S. G., Rasheed M. Y. M., Jørgensen B. B. (2004). Coral mucus functions as an energy carrier and particle trap in the reef ecosystem. Nature 428, 66–70. doi: 10.1038/nature02344
Williams S. M., García-Sais J., Sabater-Clavell J. (2021b). Prevalence of Stony Coral Tissue Loss Disease at El Seco, a Mesophotic Reef System off Vieques Island, Puerto Rico. Front. Mar. Sci. 8. doi: 10.3389/fmars.2021.668669
Williams S. D., Walter C. S., Muller E. M. (2021a). Fine scale temporal and spatial dynamics of the stony coral tissue loss disease outbreak within the lower Florida keys. Front. Mar. Sci. 8. doi: 10.3389/fmars.2021.631776
Williamson O. M., Dennison C. E., O’Neil K. L., Baker A. C. (2022). Susceptibility of Caribbean brain coral recruits to stony coral tissue loss disease (SCTLD). Front. Mar. Sci. 9. doi: 10.3389/fmars.2022.821165
Wishart D. S. (2019). Metabolomics for investigating physiological and pathophysiological processes. Physiol. Rev. 99, 1819–1875. doi: 10.1152/physrev.00035.2018
Woese C. R. (1987). Bacterial evolution. Microbiological Rev. 51, 221–271. doi: 10.1128/mr.51.2.221-271.1987
Woese C. R., Fox G. E. (1977). Phylogenetic structure of the prokaryotic domain: the primary kingdoms. Proc. Natl. Acad. Sci. U.S.A. 74, 5088–5090. doi: 10.1073/pnas.74.11.5088
Work T. M., Weatherby T. M., Landsberg J. H., Kiryu Y., Cook S. M., Peters E. C. (2021)Viral-like particles are associated with endosymbiont pathology in florida corals affected by stony coral tissue loss disease. In: Frontiers in Marine Science (Accessed February 26, 2022).
Zhang R., Zhang R., Yu K., Wang Y., Huang X., Pei J., et al. (2018). Occurrence, sources and transport of antibiotics in the surface water of coral reef regions in the South China Sea: Potential risk to coral growth. Environ. pollut. 232, 450–457. doi: 10.1016/j.envpol.2017.09.064
Keywords: tissue loss, SCTLD, coral disease, Florida reef tract, Caribbean reefs, gender disparities, inclusivity
Citation: Papke E, Carreiro A, Dennison C, Deutsch JM, Isma LM, Meiling SS, Rossin AM, Baker AC, Brandt ME, Garg N, Holstein DM, Traylor-Knowles N, Voss JD and Ushijima B (2024) Stony coral tissue loss disease: a review of emergence, impacts, etiology, diagnostics, and intervention. Front. Mar. Sci. 10:1321271. doi: 10.3389/fmars.2023.1321271
Received: 13 October 2023; Accepted: 26 December 2023;
Published: 25 January 2024.
Edited by:
Lorenzo Alvarez-Filip, National Autonomous University of Mexico, MexicoReviewed by:
Michael J. Childress, Clemson University, United StatesEsti Kramarsky-Winter, Ben-Gurion University of the Negev, Israel
Erika M. Diaz-Almeyda, New College of Florida, United States
Copyright © 2024 Papke, Carreiro, Dennison, Deutsch, Isma, Meiling, Rossin, Baker, Brandt, Garg, Holstein, Traylor-Knowles, Voss and Ushijima. This is an open-access article distributed under the terms of the Creative Commons Attribution License (CC BY). The use, distribution or reproduction in other forums is permitted, provided the original author(s) and the copyright owner(s) are credited and that the original publication in this journal is cited, in accordance with accepted academic practice. No use, distribution or reproduction is permitted which does not comply with these terms.
*Correspondence: Erin Papke, ZWdwNjMxM0B1bmN3LmVkdQ==; Blake Ushijima, dXNoaWppbWFiQHVuY3cuZWR1