- 1Division of Fish Nutrition, Biochemistry and Physiology, Indian Council of Agricultural Research-Central Institute of Fisheries Education (ICAR-CIFE), Mumbai, India
- 2Division of Fish Genetics and Biotechnology, Indian Council of Agricultural Research-Central Institute of Fisheries Education (ICAR-CIFE), Mumbai, India
- 3Department of Biology, University of Iceland, Reykjavik, Iceland
The current study reports the potential of chitosan nanoparticles for the efficient delivery of hypothalamic hormonal analogue in Labeo rohita, aiming to improve reproductive performance. Here salmon gonadotropin-releasing hormone analogue (sGnRH-a) was conjugated with chitosan nanoparticles (ChN-sGnRH-a) to evaluate its efficiency in enhancing the reproductive gene expression, hormones as well as the overall reproductive output in L. rohita. A total of 54 pairs of brooders were selected and divided into six treatments viz; C0: Negative Control (fish injected with bare chitosan nanoparticles), C: Positive Control (fish injected with Gonopro-FH®, a commercially available inducing hormone, at a dose of 0.2 ml/kg body weight of fish), T1: fish injected with ChN-sGnRH-a at a dose of 0.2 ml/kg, T2: T1 + 10 mg/ml domperidone, T3: fish injected with ChN-sGnRH-a at a dose of 0.1 ml/kg, T4: T3 + 10 mg/ml domperidone. In T2 and T4 treatments, serum hormones, Testosterone (T); Estradiol (E2); Vitellogenin (Vtg); and 17α, 20β-dihydroxyprogesterone (17α, 20β-DHP) showed the sustained elevation. The means of reproductive traits like fecundity, fertilization rate, pseudo-gonadosomatic index and spawning rate were significantly (P<0.05) higher in T2 treatment, while no significant difference was found between C and T4 treatment. The mRNA expression level of follicle-stimulating hormone (fshβ), luteinizing hormone (lhβ) and their cognate receptors was significantly better in T2 treatment, while no significant difference was found between T4 and C treatments. Further, the histological analysis of ovaries showed increased post-ovulatory follicles in C, T2 and T4 treatments. The results indicate that ChN-sGnRH-a could help in reducing the recommended dose of sGnRH-a without affecting the reproductive performance in L. rohita females. The sustained releasing mechanism of this formulation may be used as an induced breeding strategy in female L. rohita broodstock.
1 Introduction
Global fish production in 2020 reached about 178 million tons, with 49% contribution from aquaculture alone (FAO, 2022). Aquaculture is one of the most significant sources of fish supply in captive conditions. However, fish broodstock fails to reproduce spontaneously due to the absence of natural environmental conditions in captive culture systems. This failure may be due to the reproductive dysfunction in cultured fish and the disruption might start at the level of the brain-pituitary-gonad (BPG) axis (Zohar, 2021). In females, the primary reproductive dysfunction is inhibition of vitellogenesis and final oocyte maturation, while incomplete spermiation is a major issue with the males in captivity (Mylonas and Zohar, 2000; Mylonas et al., 2010), leading to inadequate production of quality seeds for the intensification of aquaculture.
Further, the simulation of natural environmental conditions at the captive conditions appears to be cumbersome due to the inadequate knowledge about the reproductive physiology of several fish species. The best alternative is to induce spawning in captivity by exploiting the application of maturation-inducing neuropeptides/hormones (Brzuska, 2011; Kucharczyk et al., 2019; Abdel-Latif et al., 2021). Several maturation-inducing hormonal preparations are commonly used in fish, viz., crude pituitary extract (CPE), gonadotropin-releasing hormones (GnRH), and, more recently, the synthetic GnRH analogues (Crim and Bettles, 1997; Zohar and Mylonas, 2001). The use of these hormone analogues is anticipated to increase the pituitary release of gonadotropic hormones (GtHs), which is crucial in gonad development, vitellogenesis and final oocyte maturation in females and spermiation in males.
The endopeptidases and exopeptidases secreted by the pituitary, kidney and liver degrade the GnRH very fast, resulting in a short half-life of GnRH, leading to insufficient ovulation and spawning (Kumar and Sharma, 2014; Hassanein et al., 2021). Multiple injections of hormones or their analogues are necessary to sustain a persistent increase in GtHs in the blood. Again, using these multiple injections increases handling stress on broodstock and may negatively affect their reproductive output (Schreck, 2010). Moreover, multiple injections are a time-consuming and labor–intensive practice, especially when dealing with a very large number of broodstocks or when broodstocks are kept in ponds or cages (Mylonas et al., 2007; Corriero et al., 2009).
A new alternative to multiple injections employed to promote a long-lasting surge of GtHs in the blood is the use of a sustained-release delivery system (Zohar, 1994). The sustained-release delivery system mainly includes a variety of GnRH analogue-delivery systems such as solid, cylindrical pellets of cholesterol implants (Ibarra et al., 2017), microspheres manufactured from copolymers of lactic acid and glycolic acid (LGA) (Mylonas et al., 1992; Zohar, 1996), biodegradable microspheres synthesized from the copolymer of fatty acid dimer and sebacic acid (Fad-Sa) (Mylonas et al., 1995), Ethylene and Vinyl Acetate (EVAc) implants (Zohar, 1996; Mylonas et al., 1998) and finally the nano-based delivery system (Rather et al., 2013; Bhat et al., 2019). The nanoconjugated delivery system extends the half-life of hormones due to its controlled and sustained delivery mechanism. In addition, the nanoparticles have been proven to offer protection against rapid degradation by peptidases and help in the targeted delivery of bioactive agents.
Although several FDA-approved molecules are being used in the preparation of micro and nanoparticle-based carriers for the efficient delivery of drugs, proteins, peptides and genes of interest. Among them, chitosan is available at cheaper rates and has received prominent attention (Wang et al., 2007). Chitosan [2-amino 2-deoxy β-D-glucan] is a deacetylated cationic polysaccharide synthesized from chitin. Due to several advantages of chitosan viz, longer shelf life, higher drug-carrying capacity (Janes et al., 2001), pH-dependent solubility (Kim et al., 2004), biocompatibility, biodegradability, low-immunogenicity and non-toxicity (Thanou et al., 2001), its nanoparticles exhibit an efficiency to replace the liposome-based delivery system.
GnRH is a decapeptide that stimulates the secretion of follicle-stimulating hormone (FSH; also called GtH1) and luteinizing hormone (LH; also called GtH2) from the adenohypophysis. It has been extensively used as a maturation-inducing agent in various forms, such as native GnRH and GnRH agonists (Mikolajczyk et al., 2002) and their sustained-delivery formulations (Zohar and Mylonas, 2001). The analogues of GnRH are derived from the functional decapeptide region of GnRH by substituting the 6th position (Gly) with D-amino acid and the 10th position with ethylamide. The modification at the sixth position contributes to the peptide’s increased half-life and stability against proteolytic digestion. Also, ethylamide (NH-CH2-CH3, abbreviated as NEt) substituted for the 10th amino acid helps to increase the binding affinity of the analogue towards its cognate receptor. GnRHa is a small, low-molecular-weight decapeptide that can be employed in controlled-release delivery systems since it is efficacious in μg doses (Mylonas and Zohar, 2000).
Several studies using nanoparticle hormonal formulations to enhance the reproductive output have been carried out in different fish species such as common carp (Rather et al., 2013), Clarias magur (Bhat et al., 2016) and goldfish (Kookaram et al., 2021). However, Rather et al. (2013) and Bhat et al. (2016) used salmon LHRH conjugated with chitosan nanoparticles as injectable formulations, while Kookaram et al. (2021) simply mixed GnRH-a with chitosan nanoparticles for oral administration in goldfish. Compared to the older studies, the current study used synthetic analogue of salmon GnRH peptide conjugated with chitosan nanoparticles in injectable form for induced breeding of Labeo rohita commonly known as rohu. The synthetic analogues of GnRH analogues are more stable and show higher efficacy than natural forms. The nanoconjugated formulation may potentially avoid multiple injections; hence, this nanotechnology intervention would be further exploited to spawn fish in captivity effectively. The present study aimed to evaluate the efficiency of chitosan nanoconjugated sGnRH analogue formulation on the induced maturation and spawning in female L. rohita broodstock.
2 Materials and methods
2.1 Chemicals
Pentasodium tripolyphosphate (TPP) and shrimp shell-derived chitosan powder, with a deacetylation degree of greater than 85% and a molecular weight of 200 kDa, were purchased from Sigma-Aldrich Corporation (St. Louis, MN). Synthetic Salmon gonadotropin-releasing hormone analogue (sGnRH-a; amino acid sequence: Glu-His-Trp-Ser-Tyr-DArg-Trp-Leu-Pro-NH-CH2-CH3) was purchased from GL Biochem (Shanghai) Ltd. All the EIA kits such as Fish 17beta-estradiol, E2 ELISA (REF: KLF0021); Fish Testosterone, T ELISA (REF: KLU0019); Fish Vitellogenin, VTG ELISA (REF: KLF0020); Fish 17α, 20β-Dihydroxyprogesterone, 17α, 20β-DHP ELISA (REF: KLF0044); Fish Glucose Transporter 4, GLUT4 ELISA (REF: KLF0150); and Cortisol, COR ELISA (REF: KLU0009) were manufactured by GENLISA™ and supplied by KRISHGEN BioSystems. All the kits were Cyprinus carpio specific, a closet relative of L. rohita as both the fishes belong to the same family, cyprinidae. Commercially available Salmon GnRH-a inducing agent, Gonopro-FH®, used in this study was manufactured by Amrit Pharmaceuticals (Aurangabad, India) and marketed by APC Nutrients, PVT. Ltd. (Telangana, India).
2.2 Synthesis of nanoparticles
With some modifications, the ionic gelation method (Calvo et al., 1997) was used to prepare chitosan nanoparticles using tripolyphosphate (TPP). Briefly, 20 mg of chitosan powder was dissolved in 100 ml of 2% acetic acid solution. The pH of the solution was maintained at 6.5. The chitosan solution was constantly stirred overnight and filtered with a nylon filter of 0.22 µm pore size. After that, negatively charged TPP solution (0.5 mg TPP per ml distilled water) was added dropwise in the ratio 5:1 (chitosan solution: TPP solution) to precipitate positively charged chitosan particles. The solution was stored overnight at 4°C before the particle size and zeta potential were analyzed.
2.3 Preparation of chitosan- nanoconjugated sGnRH-a
Chitosan-nanoconjugated sGnRH-a was prepared using a high-pressure homogenization process as described by Rather et al. (2013). A stock solution of sGnRH-a was prepared as 1 mg/ml of water. To prepare 1 ml of nanoconjugated sGnRH-a, a 20 µl of stock solution was added dropwise to chitosan nanoparticles while continuously stirring at 800 rpm for 1 hour at room temperature (Hassanein et al., 2021). The solution was incubated overnight for efficient hormonal absorption on the surface of chitosan nanoparticles.
2.4 Entrapment or loading efficiency
The percentage loading efficiency (LE%) of the chitosan-nanoconjugated sGnRH-a (ChN-sGnRH-a) was determined by UV spectrophotometry as described by Hassanein et al. (2021). Briefly, chitosan nanoparticle solution and ChN-sGnRH-a were centrifuged at 14000 rpm at 4°C for 20 minutes. The supernatant of chitosan nanoparticle solution was used as blank and the absorbance ChN-sGnRH-a supernatant was measured at 280 nm under a UV spectrophotometer. The loading efficiency was calculated as follows:
2.5 Characterization of chitosan and chitosan conjugated sGnRH-a nanoparticles
Beckman Coulter Delsa Nano C- NanoParticle Size Analyzer (Brea, CA) was used to analyze the particle size, polydispersity index (PI) and zeta potential of the individual nanoparticles. Through the use of photon correlation spectroscopy, the equipment can measure particle sizes between 0.6 nm and 7 mm. The samples were diluted with deionized water in a 1:1 ratio before loading into the cuvette. The cuvette with the sample was shaken properly and the analysis was run to determine the polydispersity index (PI) and mean particle size. Electrophoretic light scattering (ELS) technique was used to calculate the zeta potential and Fourier transform infrared spectroscopy (FTIR) was used to confirm the molecular composition and structure.
2.6 Assessment of release pattern of hormone from ChN-sGnRH-a
The approach outlined by, Mohammadpour Dounighi et al. (2012) was used with slight modification to evaluate the sGnRH-a release pattern from ChN-sGnRH-a. The assessment was conducted for 48 hours to plot the kinetic release profile of sGnRH-a from chitosan nanoparticles. Briefly, three equal aliquots of a pre-weighed sample of GnRH-ChNPs were dissolved in the phosphate buffer solution (PBS; pH 7.4). After that, the samples were kept on shaker at 600 rpm for 48 hours at 26°C. The samples were collected at regular intervals (0.5, 1, 2, 4, 6, 10, 12, 24 and 48 hours) and centrifuged for 20 minutes at 14,000 rpm and 4°C. By using a microprocessor UV-VIS double beam spectrophotometry (BioGenix system private limited) at 280 nm wavelength, the amount of free GnRH released into the supernatant was assessed. The total amount of sGnRH-a was determined using non-loaded nanoparticles as a blank, and LE% was then calculated as previously described.
2.7 Experimental setup
Fifty-four male-female pairs of L. rohita brooders were used to conduct the breeding trial. The experiment was carried out at the carp hatchery of ICAR-Central Institute of Fisheries Education, Powerkheda, Madhya Pradesh, India. The experiment was divided into six treatments and each treatment was divided into three replicates carrying three pairs of male-female L. rohita brooders in each replicate (Table 1). Standard water quality parameters viz temperature: 26.4-26.7°C, dissolved oxygen: 4.6-6.2 mg/ml, pH: 7.9-8.1, total hardness: 210–266 mg/L, ammonia: 0.17–0.26 mg/L, nitrite: 0.002–0.004 mg/L and nitrate: 0.03–0.05 mg/L were maintained for the experimental trial.
2.8 Injection dosage and blood collection
L. rohita female brooders (average body weight of 650 g) were injected sGnRH-a at a full dose of 0.2 ml/kg and half dose of 0.1 ml/kg body weight, while the Gonopro-FH® was injected at 0.2 ml/kg as recommended by Gonopro-FH® manufacturer, APC Nutrients, PVT Ltd. (Telangana, India). The commercial Gonopro-FH® formulation contains 20 µg sGnRH-a per ml solution and the same concentration of sGnRH-a was maintained per ml chitosan nanoparticle solution in ChN-sGnRH-a formulation used in the present study. So, the effective dose of sGnRH-a administered to L. rohita female brooders was 4 µg/kg body weight for C, T1 and T2 treatments, whereas for T3 and T4 treatments, it was 2 µg/kg body weight of fish. On the other hand, the L. rohita male brooders of different treatments were injected similarly but the dosage was reduced to half according to their respective female doses. The fish in all the treatments were injected on the same day. The compound was administered intramuscularly below the dorsal fin and above the lateral line with the help of a 1 mL syringe fitted with a 22-gauge needle. Initial blood samples were taken just before giving the hormone injection to the fish brooders. The fish were anesthetized with clove oil (50 µl/L) followed by blood collection from the caudal vein. Approximately 0.5 ml of blood was collected from individual fish by using a medical syringe without using an anticoagulant solution and the blood was pooled for each replicate. Three fishes were randomly selected to draw the blood before injection (marked as 0h). Subsequently, after 6h post-injection, blood samples were taken from three fish in each treatment replicate, marked with a thread, and then returned to their respective tanks. Likewise, at 12h and 18h post-injection, blood samples were collected from fish that had not been previously tagged in each replicate, marked, and subsequently returned to their respective tanks. The blood samples were centrifuged at 4000 rpm for 10 minutes to obtain serum and stored at -20°C until further analysis.
2.9 Serum hormone assay
Enzyme immunoassay (EIA) kits were used to analyze the serum hormones following the manufacturer’s instructions. Before performing the assay on L. rohita, the kits were validated using some of the samples and known standards. The assay was performed as per the protocol and the concentration of samples was calculated. The assay was repeated only for standards and the positive controls with similar concentrations (one positive control was prepared by diluting one of the standards and second and third were prepared from samples tested earlier). The similar OD values generated by the positive controls validated that the kits can be used for L. rohita samples also. To measure the reproductive hormones in serum after the treatment of nano-formulations the serum collected from different treatments were serially diluted and assay was performed, the upper limit of quantification (ULOQ) and lower limit of quantification (LLOQ) were generated and the concentrations that were in the range of assay were selected and the recovery percentage was calculated which was found to be in the range of 80-120%. On the basis of linearity, the concentration of the hormones was calculated and represented in graphical form. In the present study the coefficient of variation (CV) between the duplicates was below 15%. Here, the ELISA plates were read using BioTek Microplate Reader 800TS (Winooski, USA).
2.10 Indices of reproductive performance
All the females were examined for ripeness after 10 hours post-injection and these observations continued at 1-hour intervals by examining the genital papilla and gently pressing the abdomen with hand and squeezing towards the urogenital opening. Eggs from the ripe females were stripped and collected separately in dry and clean plastic trays. Dry method was used for egg fertilization, where the sperm from a ripe male was added directly by stripping the sperm onto the eggs. This was followed by gentle mixing for at least 30-40 seconds. The spawning rate, fecundity, fertilization rate, pseudo-gonadosomatic index (Szabó et al., 2019), hatching rate and latency period were determined using the following equations:
2.11 mRNA expression of genes
The sampling was done at the time of stripping (spawning) by taking a tissue sample from the pituitary to analyze the mRNA expression of follicle-stimulating hormone subunit beta (fshβ) and luteinizing hormone subunit beta (lhβ), and from the ovary to analyse the mRNA expression of fsh-receptor (fshr) and lh-receptor (lhr) genes. Three tissue samples from different females were taken from each treatment and preserved in RNA later (Qiagen) and stored at −80°C prior to RNA extraction.
Trizol (TRI® Reagent method, Sigma Chemical, Spain) was used to extract total RNA from the tissue samples based on the principle of acid guanidinium thiocyanate-phenol-chloroform extraction. The RNA was purified by eliminating the genomic DNA using DNase enzyme (Thermo Scientific, USA) at 37°C for 40 minutes, while Nanodrop spectrophotometer (Thermo Scientific, USA) and electrophoresis on 1% agarose gel (Supplementary Figure 1) were used to assess the quality and quantity of the isolated RNA. Further, the first strand cDNA was synthesized using 1 µg of DNase-treated RNA with the help of RevertAid H minus reverse transcriptase (Thermo Scientific, USA) according to the manufacturer’s instructions. The PCR primers of fshβ, lhβ, fshr and lhr used in this study for real-time PCR analysis were taken from the study of Pradhan et al. (2018) (Table 2). The study assessed primer efficiency to determine the optimal primer concentration for qRT-PCR. This was achieved by conducting 10-fold serial dilutions of cDNA to create a standard curve and calculate the primer efficiency (E) using the formula E (%) = (10–1/slope − 1) × 100. The resulting PCR efficiency ratios and correlation coefficient (R2) values ranged from 94 to 101% and 0.98 to 0.99, respectively, which falls within the acceptable range for performing qRT-PCR analysis. For real-time PCR, the reaction mixture in each well contained 5 µl of SYBR Green qPCR Master Mix (Thermo Scientific, USA) along with 1 µl each of forward and reverse primers, and nuclease-free water was added to make it a total volume of 9 µl. The real-time PCR program started with a pre-denaturation step at 95°C for 2 min, followed by 40 cycles (95°C for 10 s, annealing temperature of 61°C for 15 s, and 72°C for 30 s). The relative expression level of fshβ, lhβ, fshr and lhr genes was calculated according to the 2−ΔΔCT method (Livak and Schmittgen, 2001), using β-actin (actb) as a housekeeping gene. The actb was used as an internal control as it has been tested in the fish before and it remained stable compared to other housekeeping genes (Dar et al., 2020).
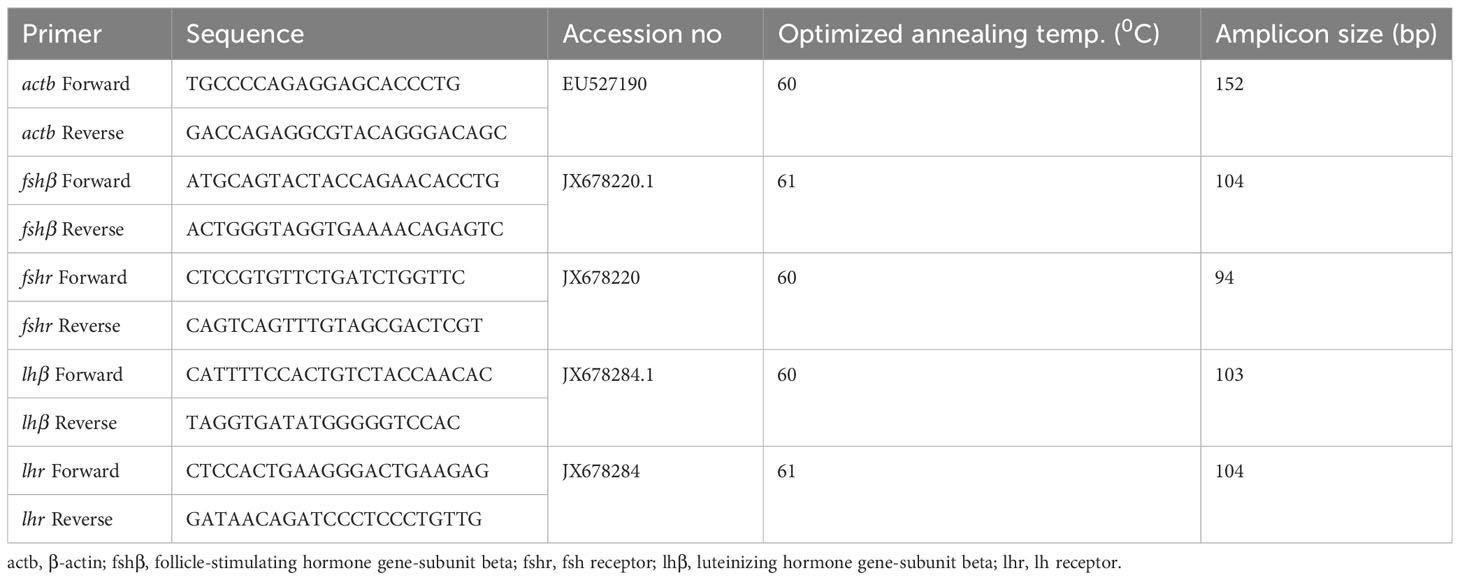
Table 2 Primers of fshβ, fshr, lhβ and lhr genes used for real-time PCR analysis in female L. rohita.
2.12 Histological analysis
Fish were anesthetized with clove oil (50 µl/l) and the ovaries were dissected and fixed in Bouin’s fluid for 24 hours. Tissues were washed in 70% alcohol and dehydrated in ascending grades of ethanol. Before embedding in paraffin wax (58–60°C congealing point), the tissues were cleaned in xylene. With the help of a rotary microtome, thin sections of 6–8 mm thickness were cut from the paraffin block. Stretched on albumenized slides, the tissue slices were then fixed at 60°C for the entire night. All of the portions were deparaffinized in xylene the following day and dehydrated in descending alcohol grades. The slides were stained with hematoxylin for 20 minutes, then differentiated with 1% alcohol and stained blue with aqueous ammonia. After washing, ready-to-use eosin was applied to the slides and then stained for 10 minutes. To capture morphological changes using microphotography, only dehydrated and well-washed sections were mounted in Dibutylphthalate Polystyrene Xylene (DPX) (Luna, 1968) and examined at a magnification of 4.2X under an Olympus microscope (FSX100).
2.13 Statistical analysis
Using the SPSS-22.0 version software, a one-way analysis of variance (ANOVA) was performed on the data following Duncan’s Multiple Range Test. The mean and standard error were used to express all data analysis. Also, ANCOVA was used to compare the fecundity depending on the body weight of each fish. Fecundity was kept as a dependable variable while the body weight as a covariate.
3 Results
3.1 In-vitro assessment of the physico-chemical properties and release patterns of ChN-sGnRH-a
The results of the physio-chemical properties and the loading efficiency of ChN-sGnRH-a are shown in Table 3. The average nanoparticle size, polydispersity index (PI) and zeta potential of ChNPs and ChN-sGnRH-a were 98.03 ± 3.91 nm vs. 175.63 ± 4.81 nm, 0.8 ± 0.01 vs. 1.18 ± 0.04, and 26.83 ± 1.42 vs. 10.2 ± 1.02 mV, respectively. The loading efficiency of the ChN-sGnRH-a was 87.75%. The FTIR spectra of ChNPs showed peaks at wavenumbers such as 3424.97 cm–1, 2928 cm–1, 1575.73 cm–1, 1417.07 cm–1, 1344 cm–1, and 1043.95 cm–1, representing the respective functional groups: hydrogen-bond (O–H); C–H bond in pyranose rings; N–H bond; C–H in CH2OH; C–N stretching vibration of type II amine; and C–O–C in glycosidic-linkages. A slight shift in the functional groups, i.e., an increase in wavenumbers, was identified in the spectra of ChNPs when sGnRH-a is added (Figure 1).

Table 3 Physico-chemical characteristics and the loading efficiency (LE) of chitosan nanoconjugated salmon GnRH-a (ChN-sGnRH-a).
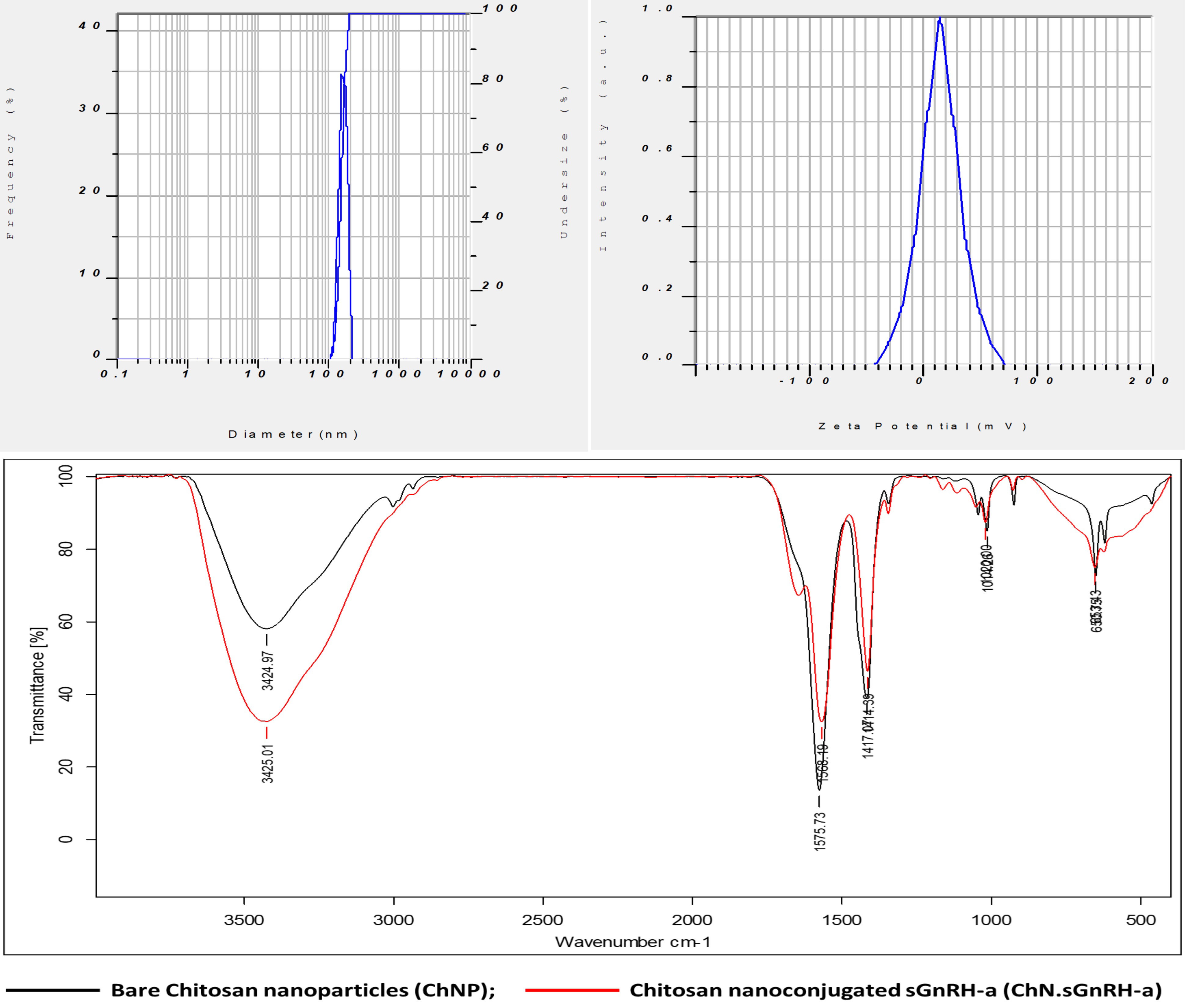
Figure 1 Particle size (nm diameter), Zeta potential (mV) and Fourier transform infrared (FTIR) spectra of chitosan nanoconjugated sGnRH-a (ChN-sGnRH-a).
About 54% of the sGnRH-a was released from the ChN-sGnRH-a within the first 12 hours of incubation at 26°C and the rest of the hormone was slowly released after the 12 hours of incubation, showing 78% released hormone during the next 24 hours. Further, the released hormone concentration at the 36th hour was observed as 95%, reaching a static value of 99% at the 48th hour (Figure 2).
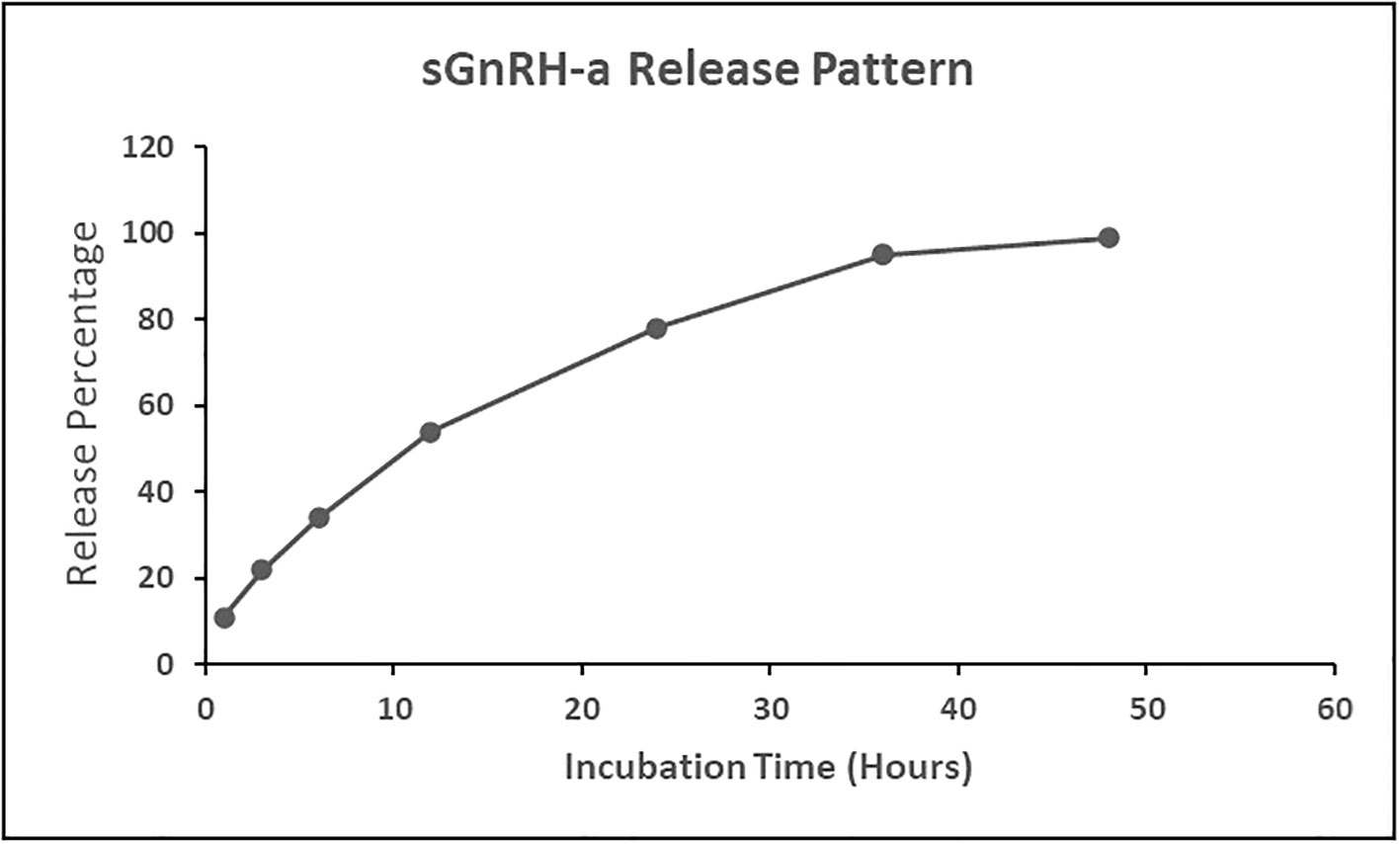
Figure 2 Release pattern of salmon gonadotropin-releasing hormone analogue (sGnRH-a) from chitosan nanoconjugated sGnRH-a (ChN-sGnRH-a).
3.2 Serum sex steroid hormones
A clear surge in the serum hormone levels was observed after using the nanoconjugated form of sGnRH-a. In the fish exposed to Ca significant (P<0.05) sudden increase in the serum hormone levels viz., testosterone, E2, Vtg and17α, 20β-DHP was observed within the first 6 hours post-injection (Figure 3). The serum hormone level of T, E2, Vtg and 17α, 20β-DHP in treatment C increased from 8.07 ± 0.07 ng/ml, 2.43 ± 0.12 ng/ml, 5.42 ± 0.17 ng/ml and 8.08 ± 0.02 ng/ml at 0 hour to a significant (P<0.05) peak of 15.65 ± 0.02 ng/ml, 11.39 ± 0.24 ng/ml, 15.35 ± 0.21 ng/ml and 21.38 ± 0.38 ng/ml at 6 hours post-injection, respectively. While in case of T2 and T4 treatments the respective peak level of Testosterone (17.56 ± 0.07 ng/ml and 16.62 ± 0.02 ng/ml), E2 (13.67 ± 0.06 ng/ml and 12.88 ± 0.07 ng/ml), Vtg (17.74 ± 0.22 ng/ml and 16.07 ± 0.11 ng/ml) and 17α, 20β-DHP (23.16 ± 0.02 ng/ml and 21.79 ± 0.05 ng/ml) were found at 12 hours post-injection. However, the fish injected with T1 and T3 formulations showed a very slow increase of these hormones and thus significantly (P<0.05) lower steroid hormone levels as compared to the rest of the treatments.
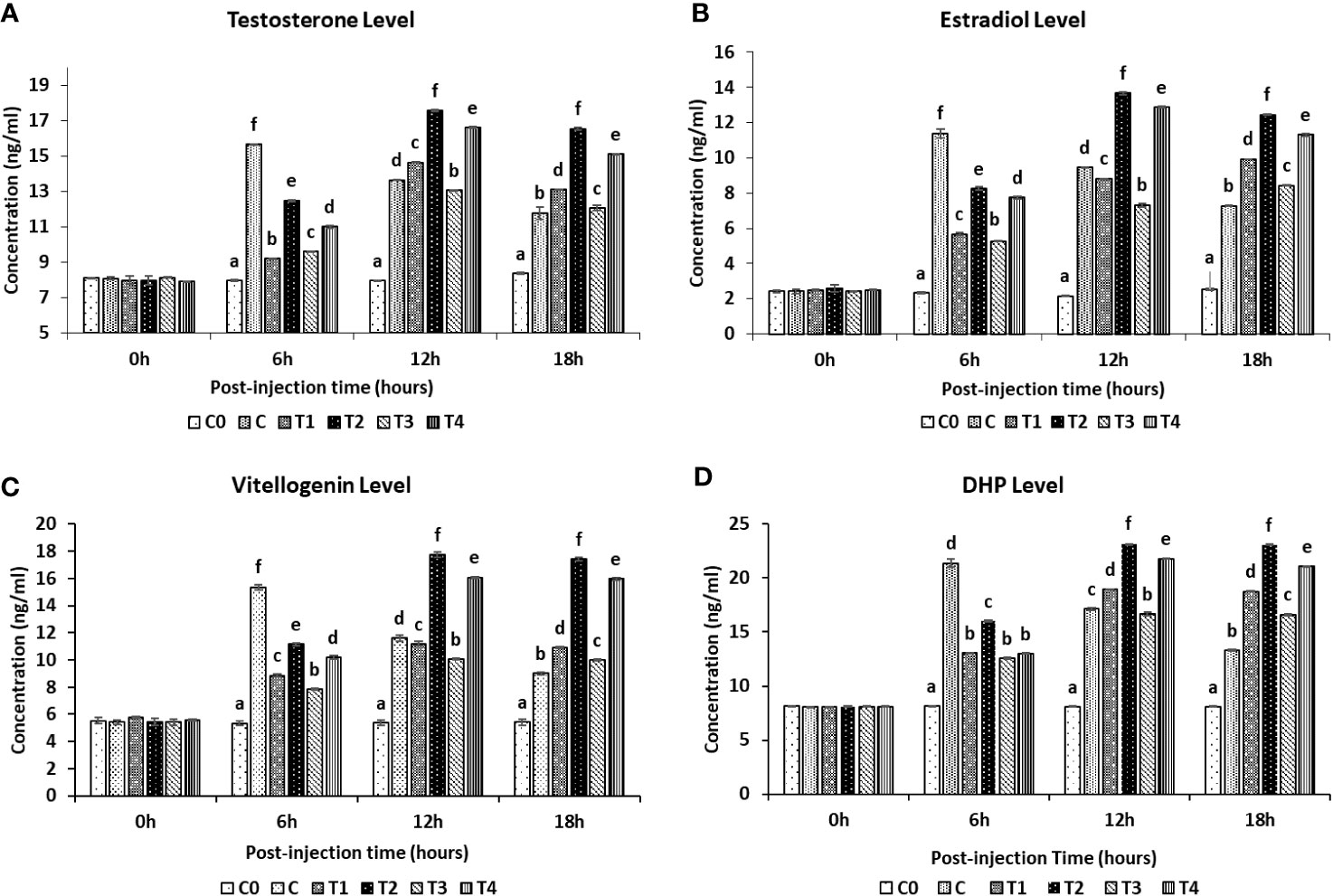
Figure 3 Reproductive serum hormones levels in female L. rohita exposed to different chitosan nanoconjugated formulations of salmon gonadotrophin-releasing hormone analogue (ChN-sGnRH-a) during the first 18 hours post-injection period. C0= Negative Control (fish injected with bare chitosan nanoparticles); C= Positive Control (Fish injected with Gonopro-FH® at 0.2 ml/kg body weight); T1= chitosan nanoconjugated salmon GnRH-a (ChN-sGnRH-a) injected at a dose of 0.2 ml/kg body weight of fish; T2= T1 + 10 mg/ml domperidone; T3= ChN-sGnRH-a injected at 0.1 ml/kg body weight; T4= T3 + 10 mg/ml domperidone. Data is calculated as mean ± SE of three observations. (A) Testosterone Level; (B) Estradiol level; (C) Vitellogenin level; (D) 17α, 20β-dihydroxy progesterone (DHP) level. Different superscript letters on bars represent significant differences between the groups (P<.05) and the groups with same superscript being not significantly different.
3.3 Serum glucose and cortisol analysis
The serum cortisol and glucose concentrations were measured at 0-, 6-, 12- and 18-hours post-injection (Figures 4, 5, respectively). The serum cortisol levels in T2 and T4 treatments were significantly (P<0.05) lower than C treatment at the 6 hours post-injection, while no significant difference was observed between these treatments at 12 hours post-injection. While the glucose level in treatment C, T1, and T3 treatments was significantly (P<0.05) higher than T2 and T4 treatments at 6 hours and 12 hours post-injection. Both cortisol and glucose levels in C0 treatment were significantly (P<0.05) lower among all the treatments throughout the post-injection intervals.
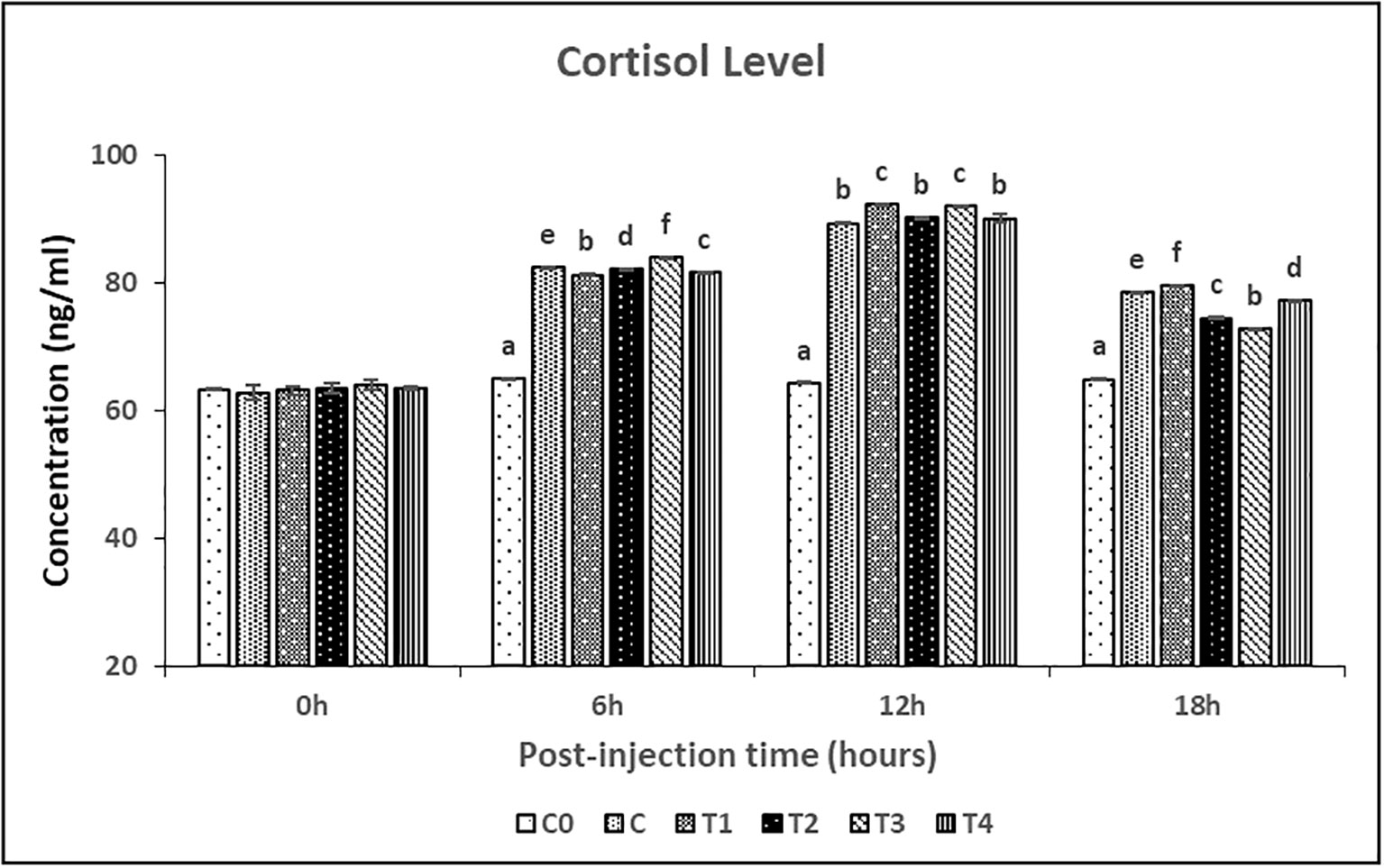
Figure 4 Cortisol levels in female L. rohita exposed to different chitosan nanoconjugated formulations of salmon gonadotrophin-releasing hormone analogue (ChN-sGnRH-a) during the first 18 hours post-injection period. C0= Negative Control (fish injected with bare chitosan nanoparticles); C= Positive Control (Fish injected with Gonopro-FH® at 0.2 ml/kg body weight); T1= chitosan nanoconjugated salmon GnRH-a (ChN-sGnRH-a) injected at a dose of 0.2 ml/kg body weight of fish; T2= T1 + 10 mg/ml domperidone; T3= ChN-sGnRH-a injected at 0.1 ml/kg body weight; T4= T3 + 10 mg/ml domperidone. Data is calculated as mean ± SE of three observations. Different superscript letters on bars represent significant differences between the groups (P<.05) and the groups with same superscript being not significantly different.
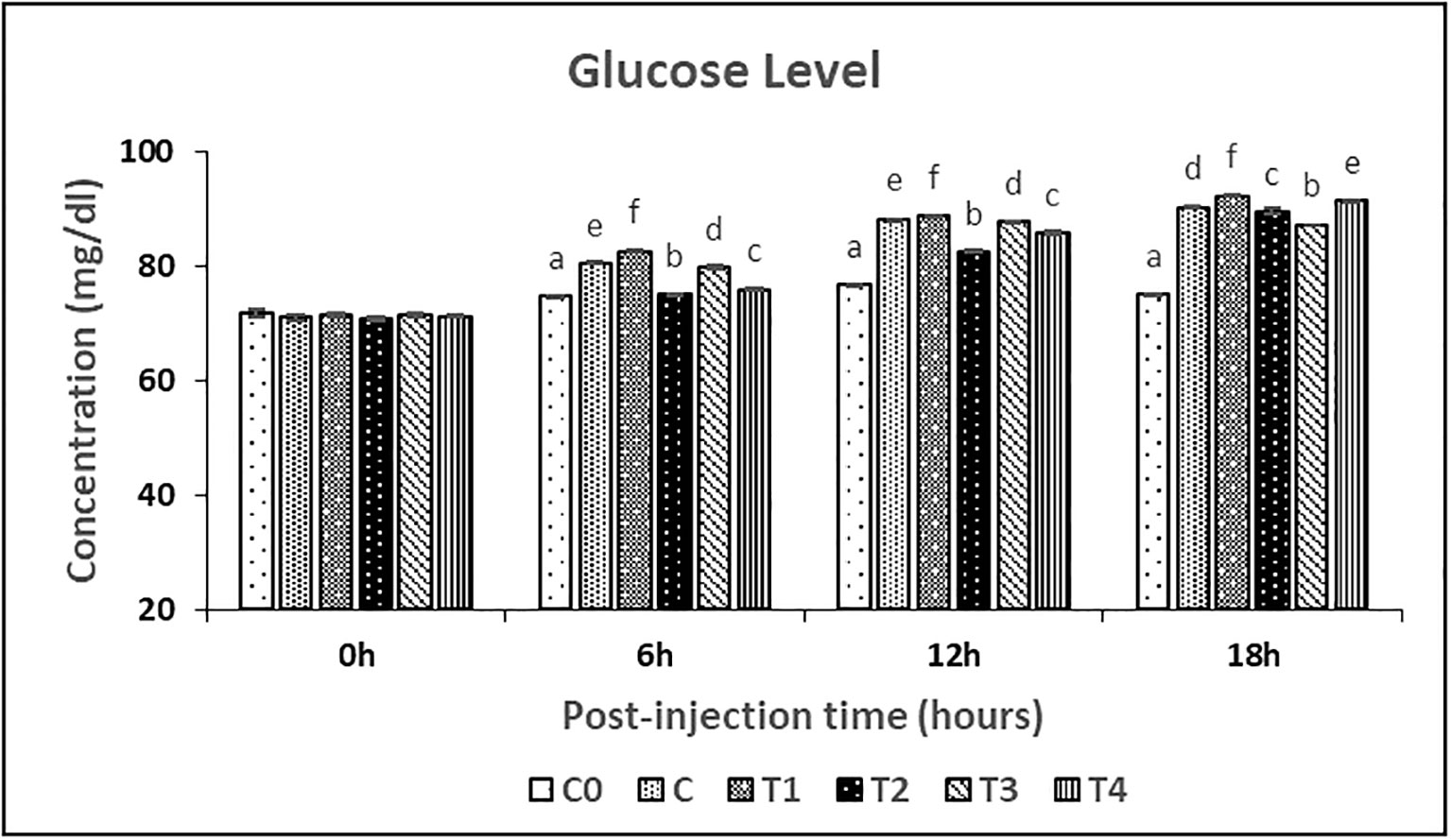
Figure 5 Glucose levels in female L. rohita exposed to different chitosan nanoconjugated formulations of salmon gonadotrophin-releasing hormone analogue (ChN-sGnRH-a) during the first 18 hours post-injection period. Different superscript letters on bars represent significant differences between the groups (P<.05) and the groups with same superscript being not significantly different.
3.4 Reproductive output
The highest fecundity, fertilization rate and spawning rate was observed in the T2 treatment (Table 4). Further, there was no significant difference (P>0.05) found in the fecundity, fertilization rate and spawning rate between C and T4 treatments. A significant lower (P<0.05) spawning rate was observed in T1 treatment. Hatching rate (%) was higher and did not show any significant difference between C, T2 and T4 treatments. Further, no spawning was found in C0 and T3 treatments. Latency period increased from 10 hours in C to 12 hours in T2 and to 14 hours in T1 and T4 treatments. Also, there was a significant difference in fecundity between the treatments [F (4,9) = 562.271, p = 0.000], whilst adjusting for body weight (Table 5). The partial Eta Squared value (0.996) indicated the effect size was large for treatments. The value showed that 99% of the variance in the fecundity is explained by the treatments when controlling for the body weight.
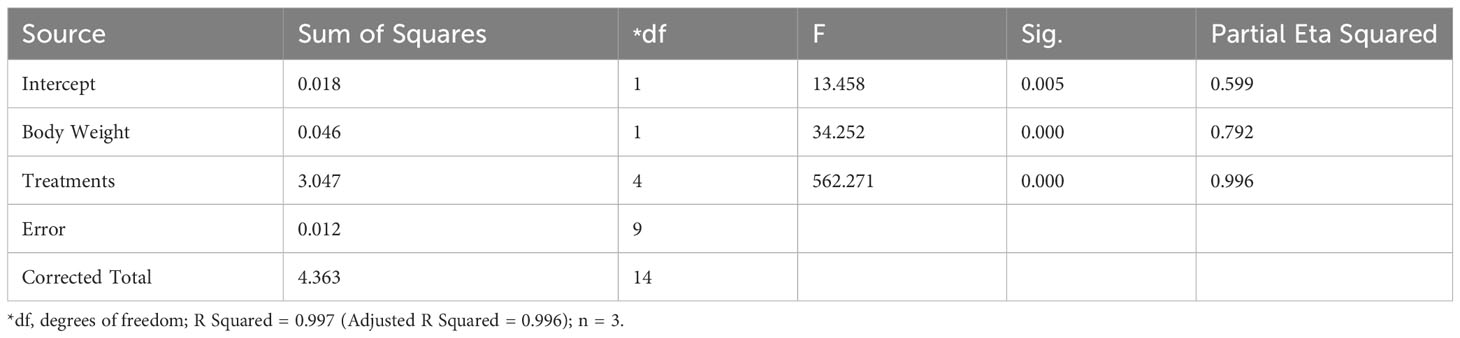
Table 5 Results of the ANCOVA for the fecundity (105 eggs) of L. rohita female injected with chitosan-nanoconjugated sGnRH-a.
3.5 mRNA expression of genes
mRNA expression studies of fshβ and lhβ genes in pituitary gland and fshr and lhr genes in ovary were performed by qRT-PCR method. The mRNAs of all genes showed a significant variation in their expression level. The mRNA expression levels of fshβ and lhβ were significantly (p< 0.05) increased in T2 followed by C and T4 treatments as compared to C0 treatment (Figure 6). Further, no significant difference was found between C and T4 treatments in terms of both fshβ and lhβ mRNA expression. However, the overall fold-change increase in gene expression on induced breeding was found to be higher for lhβ as compared to fshβ. A similar trend of increase in mRNA expression of the cognate receptors of fshand lhwas observed in female L. rohita. Significantly (p<0.05) highest fshr and lhr mRNA expression levels were found in T2 treatment (Figure 7). However, no significant difference was found between the expression levels in T4 and C treatment.
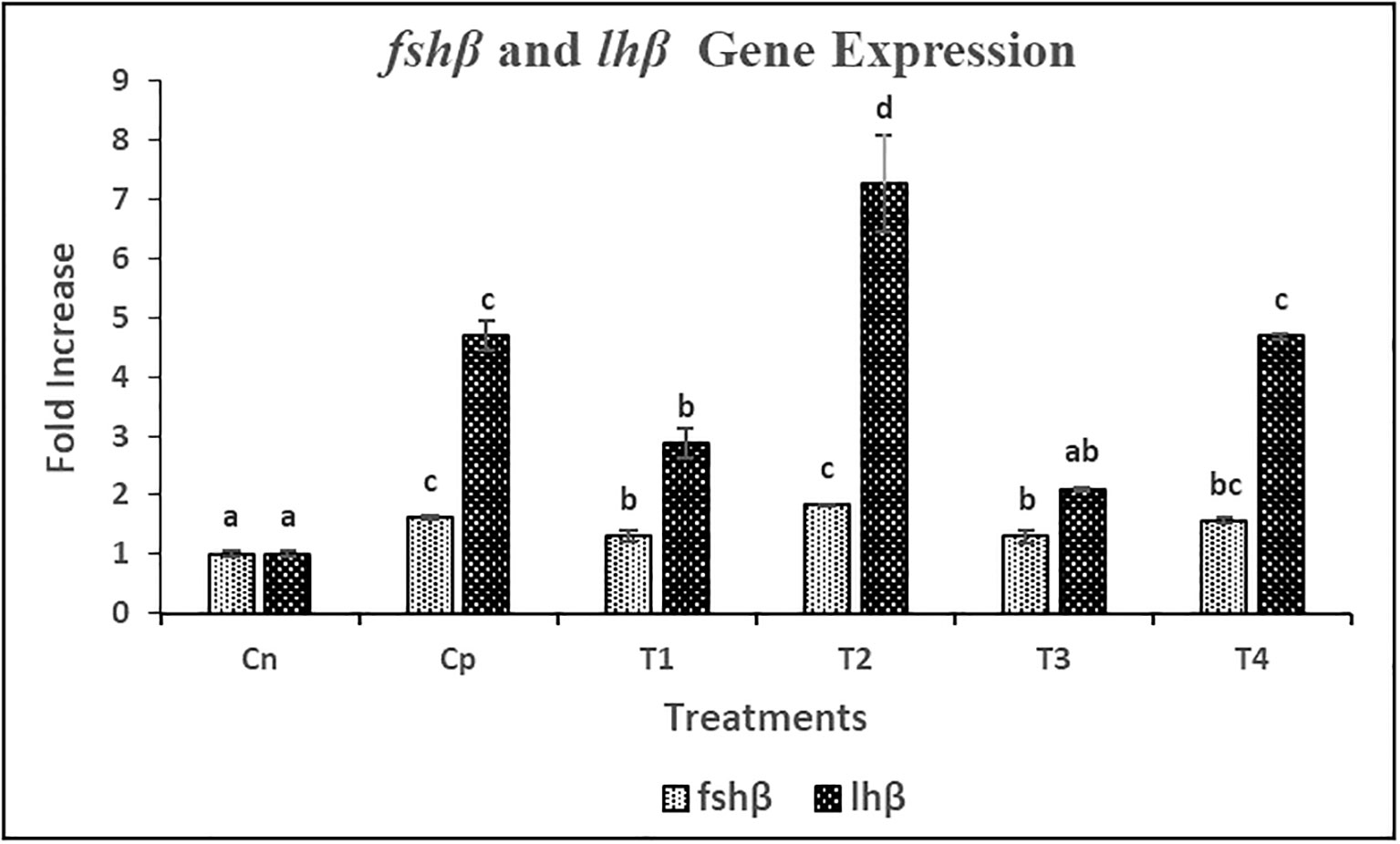
Figure 6 Relative gene expression of fshβ and lhβ against the house-keeping gene actb in female L. rohita during induced breeding with Chitosan nanoconjugated sGnRH-a. C0= Negative Control (fish injected with bare chitosan nanoparticles); C= Positive Control (Fish injected with Gonopro-FH® at 0.2 ml/kg body weight); T1= chitosan nanoconjugated salmon GnRH-a (ChN-sGnRH-a) injected at a dose of 0.2 ml/kg body weight of fish; T2= T1 + 10 mg/ml domperidone; T3= ChN-sGnRH-a injected at 0.1 ml/kg body weight; T4= T3 + 10 mg/ml domperidone. Different superscript letters on bars represent significant differences between the groups (P<.05) and the groups with same superscript being not significantly different; n = 3.
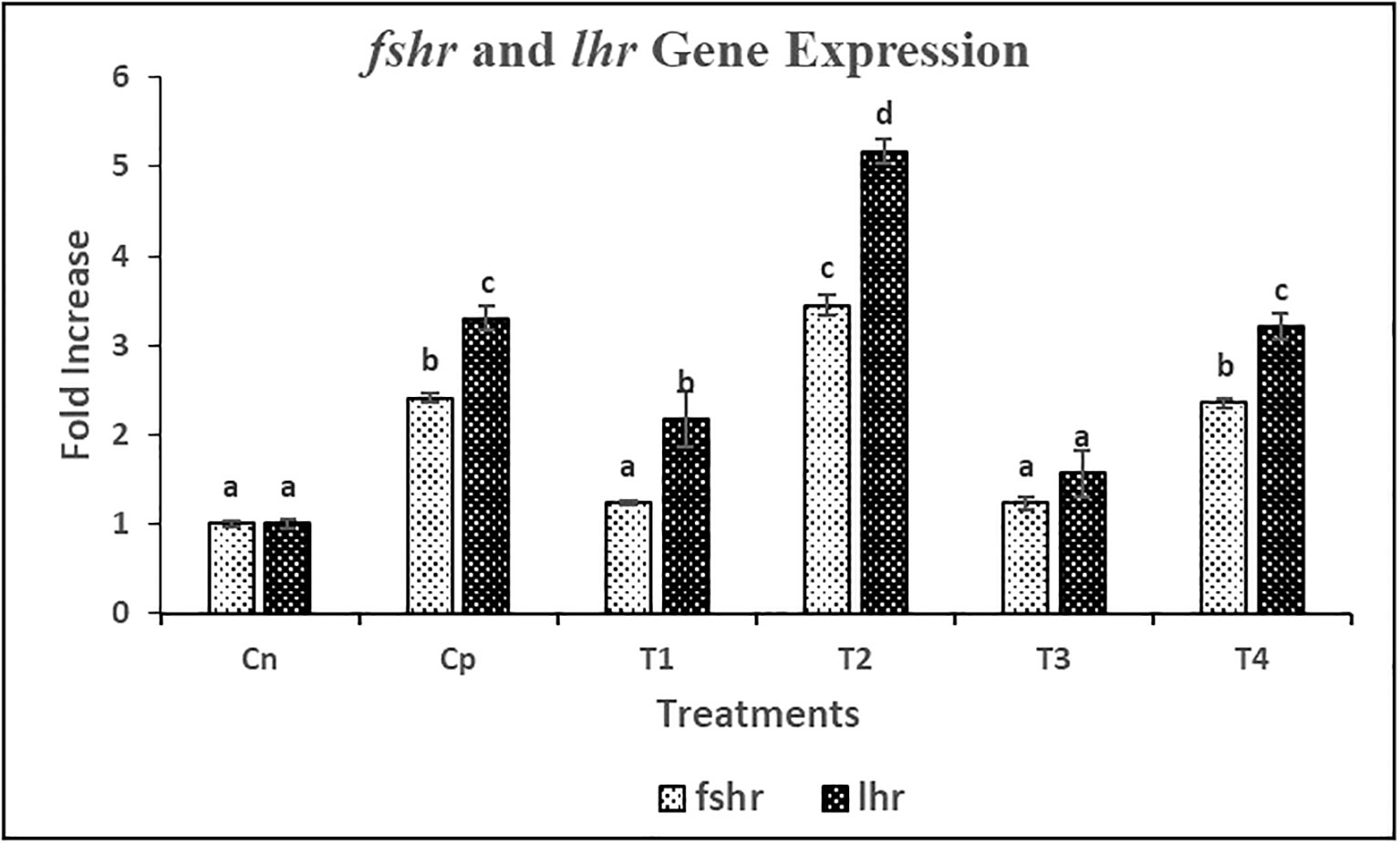
Figure 7 Relative gene expression of fshr and lhr against the house-keeping gene actb in female L. rohita during induced breeding with Chitosan nanoconjugated sGnRH-a. C0= Negative Control (fish injected with bare chitosan nanoparticles); C= Positive Control (Fish injected with Gonopro-FH® at 0.2 ml/kg body weight); T1= chitosan nanoconjugated salmon GnRH-a (ChN-sGnRH-a) injected at a dose of 0.2 ml/kg body weight of fish; T2= T1 + 10 mg/ml domperidone; T3= ChN-sGnRH-a injected at 0.1 ml/kg body weight; T4= T3 + 10 mg/ml domperidone. Different superscript letters on bars represent significant differences between the groups (P<.05) and the groups with same superscript being not significantly different; n = 3.
3.6 Histological study of gonads
The histology of the female gonads of L. rohita injected with ChN-sGnRH-a is depicted in Figure 8. The histological analysis of the ovaries in C0 group showed maturing oocytes, with vitellogenic oocytes being predominant and a few oocytes in chromatin nucleolus stage. With a predominance of vitellogenic oocytes, the ovaries in T1 and T3 respectively had partial and no spawning activity. The ovaries exposed to C, T2 and T4 groups showed abundant fully matured ova along with post-ovulatory follicles.
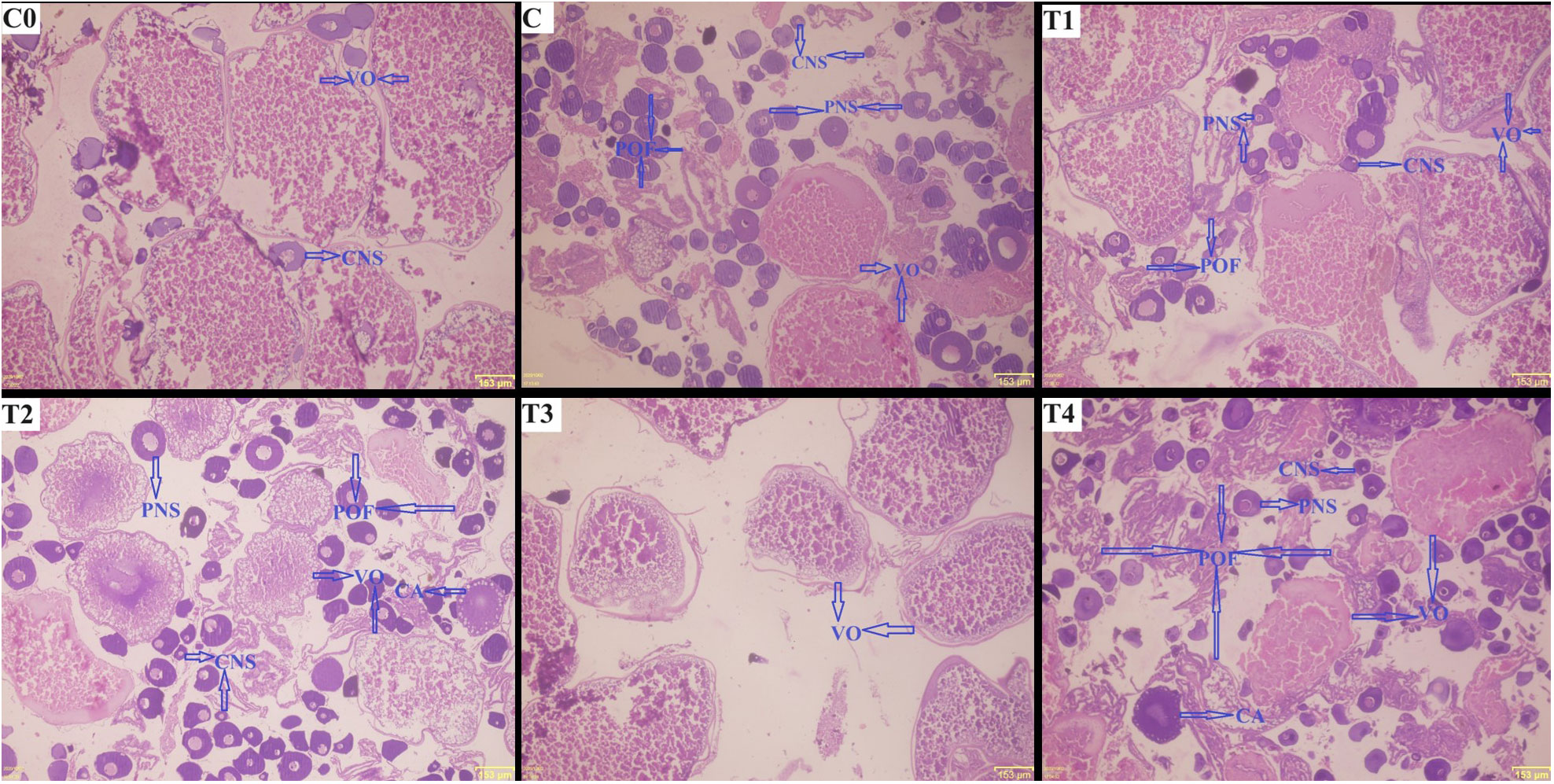
Figure 8 Histology of L. rohita ovary injected with different formulations of chitosan nanoconjugated salmon gonadotropin hormone-releasing hormone (ChN-sGnRH-a). C0= Negative Control (fish injected with bare chitosan nanoparticles); C= Positive Control (Fish injected with Gonopro-FH® at 0.2 ml/kg body weight); T1= chitosan nanoconjugated salmon GnRH-a (ChN-sGnRH-a) injected at a dose of 0.2 ml/kg body weight of fish; T2= T1 + 10 mg/ml domperidone; T3= ChN-sGnRH-a injected at 0.1 ml/kg body weight; T4= T3 + 10 mg/ml domperidone. CNS= oocytes in chromatin nucleolus stage; PNS= oocytes in perinucleolus stage; VO= vitellogenic oocyte; CA= cortical alveolus stage; POF= Post-ovulatory follicles.
4 Discussion
The present study aimed to implement a smart nano-sized delivery system strategy for GnRH-analogue to improve reproductive output in female L. rohita while reducing the required dose of injected peptide and reproductive stress. One of the critical steps in achieving this aim is the optimization of the physio-chemical properties of the nanoconjugated formulation. Among the physio-chemical properties, the size of the particles decides their ability to pass through the mucosal barriers and their intracellular uptake (Panyam and Labhasetwar, 2003). In our study, the particle size of bare chitosan nanoparticles was found to be 98.03 ± 3.91nm, while the addition of sGnRH-a increased the particle size to 175.63 ± 4.81nm (Table 3). This particle size fell within the recommended range for efficient nano-drug delivery (Gan et al., 2005; Danaei et al., 2018). Our results are supported by a previous study (Kumari et al., 2013) that concluded that trypsin addition to ChNPs increased particle size from 147 nm to roughly 220 nm. The increase in particle size after adding chitosan nanoparticles with sGnRH-a may be attributed to the aggregation between chitosan nanoparticles and sGnRH-a. This justification is confirmed by the decreased zeta potential values from 26.83 ± 1.42 mV in bare chitosan nanoparticles to 10.2 ± 1.02 mV in chitosan nanoconjugated sGnRH-a. The surface charge of a nanoparticle is determined by zeta potential, indicating particle stability. A higher Zeta potential (irrespective of the charge, + or -) indicates higher particle stability and vice-versa. On the other hand, the biological activity of a nanoconjugated particle depends mostly on the entrapment or loading efficiency of the nanocarrier. A higher loading efficiency of the nanocarrier for a peptide protects it from enzymatic degradation and consequently increases the half-life. In this study, chitosan (1 mg/ml) and TPP in a ratio of 5:1 resulted in 87.75% loading efficiency for sGnRH-a within the chitosan nanoparticle (Table 3). These results are similar to the recent studies by Hashem and Sallam (2020) and Hassanein et al. (2021), who reported a loading efficiency of 90% from Gonadorelin conjugated with chitosan-nanoparticles and 91.2% from GnRH–loaded chitosan nanoparticles, respectively. However, a lower loading efficiency of 69% for GnRH was observed by Rather et al. (2013). Thus, our results indicate that the selected physico-chemical conditions and the nanoconjugation formula used in this study are appropriate.
The FTIR spectra of ChN-sGnRH-a showed peaks at 3424.97 cm–1, 2928 cm–1, 1575.73 cm–1, 1417.07 cm–1, 1344 cm–1, and 1043.95 cm–1, representing the respective functional groups: hydrogen-bond (O–H); C–H bond in pyranose rings; N–H bond; C–H in CH2OH; C–N stretching vibration of type II amine; and C–O–C in glycosidic-linkages (Figure 1). Similar FTIR spectra of Chitosan conjugated GnRH nanoparticles were reported by Hassanein et al. (2021). A slight shift in the functional groups identified in the spectra of ChNPs was seen when sGnRH-a was added. The shift has been reported by several workers dealing with chitosan-copper oxide nanoparticles, chitosan-zinc oxide nanoparticles and chitosan–TPP (tripolyphosphate) nanoparticles (Gan et al., 2005; Loutfy et al., 2016; Badawy et al., 2018).
The in-vitro analysis of the release pattern of ChN-sGnRH-a showed 54% of the sGnRH-a released within the first 12 hours of incubation at 26°C. This higher release rate might be due to the weak bonding between the ChNP surface and the adsorbed sGnRH-a. At the same time, the rest of the conjugated hormone was slowly released from the 12–48-hours incubation period. The slow releasing pattern after 12 hours is due to the release of the entrapped or encapsulated sGnRH-a and the degradation of the bonding surface of ChN-sGnRH-a. The initial fast-releasing pattern helped to stimulate the increased surge of LH, while the slow-releasing pattern maintained the long-period GnRH concentration, thereby sustaining the surge of gonadotrophins (Mora-Huertas et al., 2011).
After analyzing the serum hormonal profile, the results revealed that the pituitary show a positive response to the ChN-sGnRh-a formulation when used along with domperidone. The fish treated with Gonopro-FH® (C) at 0.2 ml/kg, ChN-sGnRh-a at 0.2 ml/kg plus domperidone at 10 mg/ml (T2), and ChN-sGnRh-a at 0.1 ml/kg plus domperidone at 10 mg/ml (T4) showed an increased level of serum steroid hormones as compared to the treatments injected with ChN-sGnRh-a without domperidone. Furthermore, in treatment C (Gonopro-FH®), an abrupt increase in serum reproductive hormones like E2, Testosterone, Vtg and 17α, 20β-DHP was observed up to 6 hours post-injection (showing a peak at 6th hour post-injection), while the same showed an abrupt decline thereafter. A similar increase in the serum hormonal profile has been reported by Drori et al. (1994) and Rather et al. (2013) after using GnRH hormones in common carp. However, in the fish exposed to ChN-sGnRH-a along with domperidone, a sustained and controlled release pattern of these reproduction hormones was observed. The respective peaks were observed at 12th hour post-injection, indicating the resistance to endogenous enzymatic degradation of the ChN-sGnRh-a. Several studies have shown a similar trend in elevated serum E2 and testosterone levels during vitellogenesis and ovulation in different fish species (Pankhurst et al., 1986; Kobayashi et al., 1989). Moreover, Kookaram et al. (2021) reported a significant increase in serum E2 levels on oral administration of chitosan nanoparticles plus 100 μg GnRHa in goldfish, finally resulting in accelerated oocyte development and ovarian growth. Further, the serum 17α, 20β-DHP levels in the fish exposed to nanoconjugated sGnRH-a along with domperidone in treatments T2 and T4 showed a similar trend of elevated and sustained release, with a peak at 12 hours post-injection. The increased and sustained surge of these serum reproductive hormones in T2 and T4 treatments is attributed to the slow releasing mechanism of ChN-sGnRH-a and the activity of the dopamine antagonist domperidone, leading to a continuous signal to the pituitary to release gonadotropins, ultimately leading to efficient maturation and spawning. This is in agreement with the study reporting the increase of serum reproductive hormones on the administration of GnRH-loaded chitosan nanoparticles in common carp (Rather et al., 2013) and rabbit (Hassanein et al., 2021).
The overall reproductive output was analyzed in the fish injected with Gonopro-FH® and the nanoconjugated sGnRH-a with and without the dopamine antagonist domperidone. The fecundity, fertilization percentage, pseudo-gonadosomatic index (PGSI) and spawning rate in the fish injected with chitosan nanoconjugated sGnRH-a at a dose of 0.2 ml/kg along with domperidone were higher [1.47 ± 0.03 (105 eggs), 96.68 ± 0.4%, 12.76 ± 0.26 and 100%] compared to the group received Gonopro-FH® [1.23c ± 0.02 (105 eggs), 83.35 ± 2.36%, 9.02 ± 0.68 and 100%] at the same dose. Furthermore, there was no significant difference in the fecundity, fertilization percentage, pseudo-gonadosomatic index (PGSI) and spawning rate between the fish injected with nanoconjugated sGnRH-a at half dose (0.1 ml/kg) along with domperidone [1.18c ± 0.03 (105 eggs), 86.97 ± 1.14%, 8.85 ± 0.32 and 100%] and the fish injected with Gonopro-FH®. Although, the hatching rate was higher in T2 treatment (nanoconjugated sGnRH-a at a dose of 0.2 ml/kg along with domperidone), no significant difference was found between T2, C (Gonopro-FH®) and T4 (nanoconjugated sGnRH-a at a dose of 0.1 ml/kg along with domperidone) treatments. However, the fish injected without domperidone showed either incomplete spawning (in the T1 group) or a lack of spawning response (in the T3 group). Thus, it is clear from this study that dopamine antagonist plays a significant role in the reproduction of L. rohita brooders. A superior reproductive output was shown by the brooders injected with chitosan nanoconjugated sGnRH-a along with dopamine antagonist domperidone. The enhanced fertilization by1.7 times and a 2.4-fold increase in hatching rate have also been reported by Larsson et al. (1997) after using sustained delivery systems of GnRH-a in female yellowtail flounder (Pleuronectes ferruginous). Further, reproductive traits such as fecundity is often correlated with the body weight of fish. Therefore, to account for the variation in fecundity that could be attributed to the variation in body weight of fish, ANCOVA was used to compare the fecundity. The ANCOVA analysis of this study clearly indicated that the enhanced reproductive performance was more significantly contributed by the treatments as compared to the variation in the body weight of the fish. This supports the statement that ChN-sGnRH-a showed a better performance for induced breeding of L. rohita females. The latency period calculated in C was lower than the other treatments injected with ChN-sGnRH-a. This increase in latency period may be attributed to the sustained releasing mechanism of ChN-sGnRH-a, consequently leading to a continuous and controlled increase in the reproductive hormone production responsible for spawning that ultimately resulted in a better reproductive performance. On the other hand, the results of the cortisol and glucose levels in this study indicated no adverse effect of the chitosan nanoparticles on the reproduction of L. rohita females.
Apart from the environmental factors, gonadal development in fish is largely regulated by genes, especially those responsible for the production of gonadotropic hormones (GtH). Fsh and lh are among the major components of GtH that regulate gonadal development (Chen et al., 2022). The gonadotropic hormones affect reproductive tissues through related receptors, fsh receptors (fshr) and lh receptors (lhr). Both fsh and lh receptors belong to the family of glycoprotein hormone receptors (Pradhan et al., 2018).
To our knowledge, this is the first study in L. rohita showing the gonadotropins (fshβ and lhβ) and their cognate receptors (fshr and lhr) mRNA gene expression on induced breeding using synthetic sGnRH-a in female fish. The FSH mRNA in this study showed increased expression levels in female L. rohita injected with sGnRh-a compared to the fish injected with the bare nano-particles. This increased fshβ expression in pituitary was accompanied by an increased mRNA expression levels of fshr in the fish ovary. The higher fshβ and fshr mRNA expression was also reported by Pradhan et al. (2018) in female L. rohita during the spawning phase. On the other hand, the mRNA expression level of lhβ in pituitary and its receptors (lhr) in ovary was highly enhanced in the fish injected with sGnRH-a in nanoconjugated form at a dose of 0.2 ml/kg body weight (4 µg/kg sGnRH-a) along with Domperidone. This gives a clear indication that domperidone acts as dopamine antagonist to assist in uninterrupted gonadotropin secretions from the pituitary of female L. rohita. The lhr expression level in fish injected with nanoconjugated sGnRH-a at half dose of 0.1 ml/kg body weight (2 µg/kg sGnRH-a) along with domperidone (T4) was similar to that of the fish injected with Gonopro-FH® (C: Positive control). The increased lhr expression even at half dose of sGnRH-a in T4 treatment might be attributed to the sustained delivery mechanism of chitosan nanoparticles. Pradhan et al. (2018) has reported an increased lhr levels in female L. rohita at spawning phase. The higher fshβ and lhβ expression level in the pituitary of female L. rohita has led to the respective higher fshr and lhr gene expression in the ovary. The increased lhβ gene expression has been reported in greater amberjack (Nyuji et al., 2016) and salmonids (Yaron et al., 2009). Also, the highest lhr transcript level in fish can be correlated with the fully grown oocyte stage during the spawning phase in female L. rohita (Pradhan et al., 2018). Similarly, several studies have reported increased lhβ transcripts in zebrafish (Tse and Ge, 2010), bamboo leaf wrasse (Kitano et al., 2011) and Japanese eel (Kazeto et al., 2012). In addition, the increased fshr and lhr expression has also been reported in common carp when injected with carp pituitary extract (Hollander-Cohen et al., 2019). Although, the exact reason for the higher fshβ and fshr transcripts during spawning phase is not well known, studies in fish indicated that it helps in recruiting new follicles for the next reproductive cycle (Pradhan et al., 2018). All these results indicate that fshβ, lhβ and their cognate receptors play a vital role in steroidogenesis, final maturation of gonads and spawning of fish.
The results of the histology of gonads, in the current study, are consistent with the trend shown by the reproductive hormonal profile and the gene expression of gonadotropins and their receptors. The increased number of 512post-ovulatory follicles in fish gonads exposed to ChN-sGnRH-a at full and half doses (along with domperidone) indicates a better reproductive response. The biocompatible chitosan has no adverse effects (Eslahi et al., 2021) and is currently being used on a high scale in the pharmacological industries for efficient drug delivery systems (Sadoughi et al., 2020; Liu et al., 2022). The most common characteristic features of chitosan in drug delivery systems are the high drug loading capacity and prolonged drug release (Bhattarai et al., 2006). Chitosan shows better permeability due to its solubility in the aquatic medium; thus, the chitosan nanoparticles act as a promising drug carrier (Chen et al., 2008). Even at a half-dose of sGnRH-a in nanoconjugated form, sGnRH-a maintained an elevated and sustained surge of reproductive hormones because the chitosan nanoparticles used in this study aided in the prolonged and regulated release of the peptide. This half-dose injection resulted in increased maturation of follicles and subsequent ovulation, resulting in high reproductive performance in terms of fecundity, fertilization percentage and spawning rate. This is in view of the fact that nanoconjugated sGnRH-a penetrates deep into tissues by easily crossing the epithelial barriers to reach the target so efficiently (Vinogradov et al., 2002). While the nano-formulation employed in the present study led to improved reproductive outcomes in female L. rohita broodstock, it is essential to evaluate its effectiveness for other commercially important fish species in the aquaculture industry. Additionally, there is a need for an extensive investigation into the mechanism of nano-based peptide delivery systems to gain a deeper understanding of how the nanoconjugation influences the gradual release of these peptides.
5 Conclusion
The current study concludes that the formulation of chitosan nanoconjugated synthetic gonadotropin-releasing hormone analogue (ChN-sGnRH-a) could help to reduce the commercial dose of sGnRH-a to half by maintaining an elevated surge of steroid hormone levels in L. rohita. Considering the enhanced surge in serum reproductive hormones, higher gonadotropin gene expression and better reproductive performance, the sustained releasing mechanism of this formulation may be used as an induced breeding strategy in female L. rohita broodstock.
Data availability statement
The original contributions presented in the study are included in the article/Supplementary Material. Further inquiries can be directed to the corresponding author.
Ethics statement
The animal study was approved by Animal Care of ICAR- Central Institute of Fisheries Education, Mumbai, India-400061. The study was conducted in accordance with the local legislation and institutional requirements.
Author contributions
MM: Conceptualization, Data curation, Formal Analysis, Methodology, Software, Validation, Writing – original draft, Writing – review & editing. SG: Conceptualization, Data curation, Investigation, Project administration, Supervision, Validation, Visualization, Writing – review & editing. TV: Investigation, Methodology, Resources, Supervision, Validation, Writing – review & editing. SJ: Data curation, Investigation, Methodology, Software, Supervision, Validation, Visualization, Writing – review & editing. SN: Investigation, Supervision, Validation, Writing – review & editing. DR: Data curation, Investigation, Supervision, Writing – review & editing. IB: Data curation, Investigation, Validation, Writing – review & editing. CM: Data curation, Methodology, Validation, Writing – review & editing. NC: Data curation, Formal Analysis, Methodology, Validation, Writing – review & editing. SD: Data curation, Investigation, Validation, Writing – review & editing. AP: Data curation, Writing – review & editing.
Funding
The author(s) declare that no financial support was received for the research, authorship, and/or publication of this article.
Acknowledgments
The authors are thankful to the Director, ICAR-Central Institute of Fisheries Education, Mumbai for providing the laboratory facilities and the financial assistance to conduct this research.
Conflict of interest
The authors declare that the research was conducted in the absence of any commercial or financial relationships that could be construed as a potential conflict of interest.
Publisher’s note
All claims expressed in this article are solely those of the authors and do not necessarily represent those of their affiliated organizations, or those of the publisher, the editors and the reviewers. Any product that may be evaluated in this article, or claim that may be made by its manufacturer, is not guaranteed or endorsed by the publisher.
Supplementary material
The Supplementary Material for this article can be found online at: https://www.frontiersin.org/articles/10.3389/fmars.2023.1311158/full#supplementary-material
References
Abdel-Latif H. M. R., Shukry M., Saad M. F., Mohamed N. A., Nowosad J., Kucharczyk D. (2021). Effects of GnRHa and hCG with or without dopamine receptor antagonists on the spawning efficiency of African catfish (Clarias gariepinus) reared in hatchery conditions. Anim. Reprod. Sci. 231, 106798. doi: 10.1016/j.anireprosci.2021.106798
Badawy M. E. I., El-Nouby M. A. M., Marei A. E.-S. M. (2018). Development of a solid-phase extraction (SPE) cartridge based on chitosan-metal oxide nanoparticles (Ch-MO NPs) for extraction of pesticides from water and determination by HPLC. Int. J. Analytical Chem. 2018, 1–16. doi: 10.1155/2018/3640691
Bhat I. A., Ahmad I., Mir I. N., Yousf D. J., Ganie P. A., Bhat R. A. H., et al. (2019). Evaluation of the in vivo effect of chitosan conjugated eurycomanone nanoparticles on the reproductive response in female fish model. Aquaculture 510, 392–399. doi: 10.1016/j.aquaculture.2019.06.002
Bhat I. A., Rather M. A., Saha R., Pathakota G.-B., Pavan-Kumar A., Sharma R. (2016). Expression analysis of Sox9 genes during annual reproductive cycles in gonads and after nanodelivery of LHRH in Clarias batrachus. Res. Veterinary Sci. 106, 100–106. doi: 10.1016/j.rvsc.2016.03.022
Bhattarai N., Ramay H. R., Chou S.-H., Zhang M. (2006). Chitosan and lactic acid-grafted chitosan nanoparticles as carriers for prolonged drug delivery. Int. J. nanomedicine 1, 181. doi: 10.2147/nano.2006.1.2.181
Brzuska E. (2011). Artificial propagation of African catfish (Clarias gariepinus): the application of a single dose of pellets containing D-Ala6,Pro9NEt-mGnRH and dopamine inhibitor metoclopramide. Czech J. Anim. Sci. 49, 289–296. doi: 10.17221/4312-CJAS
Calvo P., Remuñán-López C., Vila-Jato J. L., Alonso M. J. (1997). Novel hydrophilic chitosan-polyethylene oxide nanoparticles as protein carriers. J. Appl. Polymer Sci. 63, 125–132. doi: 10.1002/(SICI)1097-4628(19970103)63:1<125::AID-APP13>3.0.CO;2-4
Chen H., Hu Q., Kong L., Rong H., Bi B. (2022). Effects of Temperature on the Growth Performance, Biochemical Indexes and Growth and Development-Related Genes Expression of Juvenile Hybrid Sturgeon (Acipenser baerii♀ × Acipenser schrenckii♂). Water 14, 2368. doi: 10.3390/w14152368
Chen Y., Siddalingappa B., Chan P. H., Benson H. A. (2008). Development of a chitosan-based nanoparticle formulation for delivery of a hydrophilic hexapeptide, dalargin. Pept. Sci. 90, 663–670. doi: 10.1002/bip.21055
Corriero A., Medina A., Mylonas C. C., Bridges C. R., Santamaria N., Deflorio M., et al. (2009). Proliferation and apoptosis of male germ cells in captive Atlantic bluefin tuna (Thunnus thynnus L.) treated with gonadotropin-releasing hormone agonist (GnRHa). Anim. Reprod. Sci. 116, 346–357. doi: 10.1016/j.anireprosci.2009.02.013
Crim L. W., Bettles S. (1997). Use of GnRH analogues in fish culture. Recent Adv. Mar. Biotechnol. 1, 369–382.
Danaei M., Dehghankhold M., Ataei S., Hasanzadeh Davarani F., Javanmard R., Dokhani A., et al. (2018). Impact of particle size and polydispersity index on the clinical applications of lipidic nanocarrier systems. Pharmaceutics 10, 57. doi: 10.3390/pharmaceutics10020057
Dar S. A., Chatterjee A., Rather M. A., Chetia D., Srivastava P. P., Gupta S. (2020). Identification, functional characterization and expression profiling of cytochrome p450 1A (CYP1A) gene in Labeo rohita against emamectin benzoate. Int. J. Biol. Macromolecules 158, 1268–1278. doi: 10.1016/j.ijbiomac.2020.04.215
Drori S., Ofir M., Levavi-Sivan B., Yaron Z. (1994). Spawning induction in common carp (Cyprinus carpio) using pituitary extract or GnRH superactive analogue combined with metoclopramide: analysis of hormone profile, progress of oocyte maturation and dependence on temperature. Aquaculture 119, 393–407. doi: 10.1016/0044-8486(94)90303-4
Eslahi M., Sadoughi F., Asemi Z., Yousefi B., Mansournia M. A., Hallajzadeh J. (2021). Chitosan and wnt/β-catenin signaling pathways in different cancers. Combinatorial Chem. High Throughput Screening 24, 1323–1331.
Gan Q., Wang T., Cochrane C., McCarron P. (2005). Modulation of surface charge, particle size and morphological properties of chitosan–TPP nanoparticles intended for gene delivery. Colloids Surfaces B: Biointerfaces 44, 65–73. doi: 10.1016/j.colsurfb.2005.06.001
Hashem N. M., Sallam S. M. (2020). Reproductive performance of goats treated with free gonadorelin or nanoconjugated gonadorelin at estrus. Domest. Anim. Endocrinol. 71, 106390. doi: 10.1016/j.domaniend.2019.106390
Hassanein E. M., Hashem N. M., El-Azrak K. E.-D. M., Gonzalez-Bulnes A., Hassan G. A., Salem M. H. (2021). Efficiency of gnRH–loaded chitosan nanoparticles for inducing LH secretion and fertile ovulations in protocols for artificial insemination in rabbit does. Animals 11, 440. doi: 10.3390/ani11020440
Hollander-Cohen L., Böhm B., Hausken K., Levavi-Sivan B. (2019). Ontogeny of the specificity of gonadotropin receptors and gene expression in carp. Endocr. Connect 8, 1433–1446. doi: 10.1530/EC-19-0389
Ibarra L., Navarro Flores J., Sánchez-Téllez J., Martínez Brown J., Ochoa-Bojórquez L., Rojo A. (2017). Hatchery production of pacific white snook at CIAD-unity Mazatlan, Mexico. World Aquac. Magazine 48, 25–29.
Janes K. A., Calvo P., Alonso M. J. (2001). Polysaccharide colloidal particles as delivery systems for macromolecules. Adv. Drug Deliv. Rev. 47, 83–97. doi: 10.1016/S0169-409X(00)00123-X
Kazeto Y., Kohara M., Tosaka R., Gen K., Yokoyama M., Miura C., et al. (2012). Molecular characterization and gene expression of Japanese eel (Anguilla japonica) gonadotropin receptors. jzoo 29, 204–211. doi: 10.2108/zsj.29.204
Kim T. H., Park I. K., Nah J. W., Choi Y. J., Cho C. S. (2004). Galactosylated chitosan/DNA nanoparticles prepared using water-soluble chitosan as a gene carrier. Biomaterials 25, 3783–3792. doi: 10.1016/j.biomaterials.2003.10.063
Kitano H., Irie S., Ohta K., Hirai T., Yamaguchi A., Matsuyama M. (2011). Molecular cloning of two gonadotropin receptors and their distinct mRNA expression profiles in daily oogenesis of the wrasse Pseudolabrus sieboldi. Gen. Comp. Endocrinol. 172, 268–276. doi: 10.1016/j.ygcen.2011.03.012
Kobayashi M., Aida K., Hanyu I. (1989). Involvement of steroid hormones in the preovulatory gonadotropin surge in female goldfish. Fish Physiol. Biochem. 7, 141–146. doi: 10.1007/BF00004700
Kookaram K., Mojazi Amiri B., Dorkoosh F. A., Nematollahi M. A., Mortazavian E., Abed Elmdoust A. (2021). Effect of oral administration of GnRHa+nanoparticles of chitosan in oogenesis acceleration of goldfish Carassius auratus. Fish Physiol. Biochem. 47, 477–486. doi: 10.1007/s10695-021-00926-9
Kucharczyk D., Nowosad J., Kucharczyk D. J., Kupren K., Targońska K., Wyszomirska E., et al. (2019). Out-of-season artificial reproduction of common dace (Leuciscus leuciscus L.) under controlled conditions. Anim. Reprod. Sci. 202, 21–25. doi: 10.1016/j.anireprosci.2019.01.003
Kumar P., Sharma A. (2014). Gonadotropin-releasing hormone analogs: Understanding advantages and limitations. J. Hum. Reprod. Sci. 7, 170–174. doi: 10.4103/0974-1208.142476
Kumari R., Gupta S., Singh A. R., Ferosekhan S., Kothari D. C., Pal A. K., et al. (2013). Chitosan nanoencapsulated exogenous trypsin biomimics zymogen-like enzyme in fish gastrointestinal tract. PloS One 8, e74743. doi: 10.1371/journal.pone.0074743
Larsson D. J., Mylonas C. C., Zohar Y., Crim L. W. (1997). Gonadotropin-releasing hormone analogue (GnRH-A) induces multiple ovulations of high-quality eggs in a cold-water, batch-spawning teleost, the yellowtail flounder (Pleuronectes ferrugineus). Can. J. Fisheries Aquat. Sci. 54, 1957–1964. doi: 10.1139/f97-102
Liu Z., Wang K., Peng X., Zhang L. (2022). Chitosan-based drug delivery systems: Current strategic design and potential application in human hard tissue repair. Eur. Polymer J. 166, 110979. doi: 10.1016/j.eurpolymj.2021.110979
Livak K. J., Schmittgen T. D. (2001). Analysis of relative gene expression data using real-time quantitative PCR and the 2–ΔΔCT method. Methods 25, 402–408. doi: 10.1006/meth.2001.1262
Loutfy S. A., Alam El-Din H. M., Elberry M. H., Allam N. G., Hasanin M. T. M., Abdellah A. M. (2016). Synthesis, characterization and cytotoxic evaluation of chitosan nanoparticles: in vitro liver cancer model. Adv. Nat. Sci: Nanosci. Nanotechnol. 7, 35008. doi: 10.1088/2043-6262/7/3/035008
Luna L. G. (1968) Manual of histologic staining methods of the Armed Forces Institute of Pathology (Blakiston Division, McGraw-Hill). Available at: https://scholar.google.com/scholar_lookup?title=Manual+of+histologic+staining+methods+of+the+Armed+Forces+Institute+of+Pathology&author=Luna%2C+Lee+G.&publication_year=1968 (Accessed 26, 2022).
Mikolajczyk T., Roelants I., Epler P., Ollevier F., Chyb J., Breton B. (2002). Modified absorption of sGNRH-a following rectal and oral delivery to common carp, Cyprinus carpio L. Aquaculture 203, 375–388. doi: 10.1016/S0044-8486(01)00637-8
Mohammadpour Dounighi N., Eskandari R., Avadi M. R., Zolfagharian H., Mir Mohammad Sadeghi A., Rezayat M. (2012). Preparation and in vitro characterization of chitosan nanoparticles containing Mesobuthus eupeus scorpion venom as an antigen delivery system. J. Venomous Anim. Toxins Including Trop. Dis. 18, 44–52. doi: 10.1590/S1678-91992012000100006
Mora-Huertas C. E., Fessi H., Elaissari A. (2011). Influence of process and formulation parameters on the formation of submicron particles by solvent displacement and emulsification–diffusion methods: Critical comparison. Adv. Colloid Interface Sci. 163, 90–122. doi: 10.1016/j.cis.2011.02.005
Mylonas C. C., Bridges C., Gordin H., Ríos A. B., García A., de la Gándara F., et al. (2007). Preparation and Administration of Gonadotropin-Releasing Hormone Agonist (GnRHa) Implants for the Artificial Control of Reproductive Maturation in Captive-Reared Atlantic Bluefin Tuna (Thunnus thynnus thynnus). Rev. Fisheries Sci. 15, 183–210. doi: 10.1080/10641260701484572
Mylonas C. C., Fostier A., Zanuy S. (2010). Broodstock management and hormonal manipulations of fish reproduction. Gen. Comp. Endocrinol. 165, 516–534. doi: 10.1016/j.ygcen.2009.03.007
Mylonas C. C., Hinshaw J. M., Sullivan C. V. (1992). GnRHa-induced ovulation of brown trout (Salmo trutta) and its effects on egg quality. Aquaculture 106, 379–392. doi: 10.1016/0044-8486(92)90268-P
Mylonas C. C., Tabata Y., Langer R., Zohar Y. (1995). Preparation and evaluation of polyanhydride microspheres containing gonadotropin-releasing hormone (GnRH), for inducing ovulation and spermiation in fish. J. Controlled Release 35, 23–34. doi: 10.1016/0168-3659(95)00009-W
Mylonas C. C., Woods L. C. III, Thomas P., Zohar Y. (1998). Endocrine Profiles of Female Striped Bass (Morone saxatilis) in Captivity, during Postvitellogenesis and Induction of Final Oocyte Maturation via Controlled-Release GnRHa-Delivery Systems. Gen. Comp. Endocrinol. 110, 276–289. doi: 10.1006/gcen.1998.7073
Mylonas C. C., Zohar Y. (2000). Use of GnRHa-delivery systems for the control of reproduction in fish. Rev. Fish Biol. Fish. 10, 463–491. doi: 10.1023/A:1012279814708
Nyuji M., Kazeto Y., Izumida D., Tani K., Suzuki H., Hamada K., et al. (2016). Greater amberjack Fsh, Lh, and their receptors: Plasma and mRNA profiles during ovarian development. Gen. Comp. Endocrinol. 225, 224–234. doi: 10.1016/j.ygcen.2015.10.008
Pankhurst N., Kraak G., Peter R. E. (1986). Effects of human chorionic gonadotropin, DES-GLY10 (D-ALA6) LHRH-ethylamide and pimozide on oocyte final maturation, ovulation and levels of plasma sex steroids in the walleye (Stizostedion vitreum). Fish Physiol. Biochem. 1, 45–54.
Panyam J., Labhasetwar V. (2003). Biodegradable nanoparticles for drug and gene delivery to cells and tissue. Adv. Drug Deliv. Rev. 55, 329–347. doi: 10.1016/S0169-409X(02)00228-4
Pradhan A., Nayak M., Samanta M., Panda R. P., Rath S. C., Giri S. S., et al. (2018). Gonadotropin receptors of Labeo rohita: Cloning and characterization of full-length cDNAs and their expression analysis during annual reproductive cycle. Gen. Comp. Endocrinol. 263, 21–31. doi: 10.1016/j.ygcen.2018.04.014
Rather M. A., Sharma R., Gupta S., Ferosekhan S., Ramya V. L., Jadhao S. B. (2013). Chitosan-nanoconjugated hormone nanoparticles for sustained surge of gonadotropins and enhanced reproductive output in female fish. PloS One 8, e57094. doi: 10.1371/journal.pone.0057094
Sadoughi F., Mansournia M. A., Mirhashemi S. M. (2020). The potential role of chitosan-based nanoparticles as drug delivery systems in pancreatic cancer. IUBMB Life 72, 872–883. doi: 10.1002/iub.2252
Schreck C. B. (2010). Stress and fish reproduction: The roles of allostasis and hormesis. Gen. Comp. Endocrinol. 165, 549–556. doi: 10.1016/j.ygcen.2009.07.004
Szabó T., Urbányi B., Müller T., Szabó R., Horváth L. (2019). Assessment of induced breeding of major Chinese carps at a large-scale hatchery in Hungary. Aquaculture Rep. 14, 100193. doi: 10.1016/j.aqrep.2019.100193
Thanou M., Verhoef J. C., Junginger H. E. (2001). Chitosan and its derivatives as intestinal absorption enhancers. Advanced Drug Delivery Rev. 50, S91–S101. doi: 10.1016/S0169-409X(01)00180-6
Tse A. C.-K., Ge W. (2010). Spatial localization of EGF family ligands and receptors in the zebrafish ovarian follicle and their expression profiles during folliculogenesis. Gen. Comp. Endocrinol. 167, 397–407. doi: 10.1016/j.ygcen.2009.09.012
Vinogradov S. V., Bronich T. K., Kabanov A. V. (2002). Nanosized cationic hydrogels for drug delivery: preparation, properties and interactions with cells. Advanced Drug delivery Rev. 54, 135–147. doi: 10.17615/EDB8-CT53
Wang C., Fu X., Yang L. (2007). Water-soluble chitosan nanoparticles as a novel carrier system for protein delivery. Chin. Sci. Bull. 52, 883–889. doi: 10.1007/s11434-007-0127-y
Yaron Z., Bogomoinaya A., Drori S., Biton I., Aizen J., Kulikovsky Z., et al. (2009) Spawning Induction in the Carp: Past Experience and Future Prospects - A Review. Available at: http://evols.library.manoa.hawaii.edu/handle/10524/19275 (Accessed 27, 2022).
Zohar J. (1994) Manipulation of ovulation and spawning in fish. Available at: https://patents.google.com/patent/US5288705A/en (Accessed 27, 2022).
Zohar Y. (1996). New approaches for the manipulation of ovulation and spawning in farmed fish. Bull. Natl. Res. Inst Aquacult 2, 43–48.
Zohar Y. (2021). Fish reproductive biology – Reflecting on five decades of fundamental and translational research. Gen. Comp. Endocrinol. 300, 113544. doi: 10.1016/j.ygcen.2020.113544
Keywords: nanoconjugation, sustained-release, steroids, fecundity, gene expression, histology
Citation: Malik MA, Gupta S, Varghese T, Jahageerdar S, Nayak SK, Reang D, Bhat IA, Mahadevaswamy CG, Chuphal N, Dar SA and Prabhakaran A (2023) Chitosan- hypothalamic hormonal analogue nanoconjugates enhanced the reproductive performance in Indian major carp, Labeo rohita. Front. Mar. Sci. 10:1311158. doi: 10.3389/fmars.2023.1311158
Received: 09 October 2023; Accepted: 06 November 2023;
Published: 23 November 2023.
Edited by:
Md Saydur Rahman, The University of Texas Rio Grande Valley, United StatesReviewed by:
Adnan H. Gora, Central Marine Fisheries Research Institute (ICAR), IndiaRania Fahmy Ismail, National Institute of Oceanography and Fisheries (NIOF), Egypt
Copyright © 2023 Malik, Gupta, Varghese, Jahageerdar, Nayak, Reang, Bhat, Mahadevaswamy, Chuphal, Dar and Prabhakaran. This is an open-access article distributed under the terms of the Creative Commons Attribution License (CC BY). The use, distribution or reproduction in other forums is permitted, provided the original author(s) and the copyright owner(s) are credited and that the original publication in this journal is cited, in accordance with accepted academic practice. No use, distribution or reproduction is permitted which does not comply with these terms.
*Correspondence: Subodh Gupta, sgupta@cife.edu.in