- 1National Institute for Research and Development on Marine Geology and Geoecology – GeoEcoMar, Bucharest, Romania
- 2Chemical and Biochemical Engineering Department, National University of Science and Technology POLITEHNICA Bucharest, Bucharest, Romania
It is essential to determine the concentrations of metals/metalloids in marine sediments and their contamination status to develop appropriate pollution control strategies and/or improve existing ones. Spatial distribution of aluminum (Al) and some trace elements, i.e., arsenic (As), chromium (Cr), copper (Cu), mercury (Hg), nickel (Ni), lead (Pb), and zinc (Zn), accumulated in surface sediments of the north-western (NW) Black Sea (Romanian zone) was evaluated. Sediment samples were collected in 2019 from 32 stations located at water depths in the range of 12.7–149 m. The mean values ± standard deviations (SD) of element concentrations were as follows: 3.9 ± 1.6% for Al, 11.2 ± 10.2 mg/kg for As, 64.8 ± 27.0 mg/kg for Cr, 32.7 ± 15.0 mg/kg for Cu, 0.10 ± 0.09 mg/kg for Hg, 45.3 ± 23.8 mg/kg for Ni, 24.6 ± 9.6 mg/kg for Pb, and 68.0 ± 28.0 mg/kg for Zn. These values are not significantly different (p > 0.05) from those found in a previous study on surface sediments collected in 2018 from 22 stations located in the same area, but at lower water depths (13.5–67 m). Contamination factor (CF) was used to assess the contamination status of surface sediments. The mean values of CF (0.26–1.23) reveal low to moderate contamination with Pb and low contamination with the other elements. Principal component analysis (PCA) and correlation analysis indicated that As, Cu, Ni, Hg, Pb, and Zn were associated with clay, silt, and organic matter, suggesting that these elements mainly came from the Danube discharges and also from local anthropogenic sources, whereas Al, Cr, and partly Hg, Pb, and Zn originated from rock/soil weathering and erosion. The concentration levels of As, Cu, Hg, Ni, Pb, Zn, TOC, silt, and clay were higher in sediments collected from stations generally located at higher water depths (up to 118 m), suggesting that the TEs associated with the finer carriers were transported offshore by currents and waves, whereas the concentration levels of Al, Cr, Hg, and Zn were higher in sediments with lower levels of CaCO3 content (10.1–24.3%), collected from shallower stations (water depths of 12.7–42.0 m).
1 Introduction
Trace elements (TEs) found in the coastal and marine ecosystems have become a serious concern towards ecological and human health risks due to their multiple sources, non-biodegradability, toxicity, bioaccumulation, and biomagnification along the aquatic food chain (Zhao et al., 2017; Wu et al., 2022; Zhou et al., 2022). They come from either natural sources (e.g., river discharges, rainwater runoff, volcanic eruptions, wildfires, sand/dust storms, rock/soil weathering and erosion) or anthropogenic ones (including port activities, coastal tourism, discharges of domestic, municipal, agricultural, and industrial effluents into water bodies, burning of fossil fuels, waste incineration, construction, mining, and quarrying activities, crude oil refining and other industrial processes, offshore drilling for crude oil/gas and related transport) (Sánchez-García et al., 2010; Romano et al., 2022). Moreover, TEs can be produced in situ by marine organisms (Rubio et al., 2000).
TEs enter the sea mostly through the river discharges, either adsorbed onto inorganic and organic suspended particles or in dissolved form (Remeikaitė-Nikienė et al., 2018; Sun et al., 2020). Dissolved TEs rapidly come into the particulate phase, e.g., via adsorption onto suspended particles, precipitation, flocculation (Karbassi et al., 2014; Karavoltsos et al., 2022). TEs associated with inorganic and organic suspended matter settle and accumulate in sediments, but they can be remobilized and released into the water under specific conditions, being more available to the biota, which could have significant detrimental effects on the ecosystem and human health (Omar et al., 2015; Oros, 2019). Accordingly, the surface sediments, which are both sinks and secondary sources of TEs, are important indicators of marine environment quality (Boran and Altınok, 2010; Perina et al., 2018).
Although fluvial inputs are relevant, direct discharges of wastewater/groundwater/rainwater/polluting products, coastal erosion, atmospheric deposition of particulate matter derived from natural and anthropogenic processes should not be neglected (Mülayim and Balkıs, 2015; Kay et al., 2022; Romano et al., 2022). Accumulation of TEs in marine sediments depends on various factors, e.g., water depth, water physicochemical properties (pH, salinity, temperature, dissolved oxygen and organic matter content), sediment composition and texture, intensity/direction of winds, waves, and currents, fluvial, atmospheric, and other natural or anthropogenic inputs (Oros, 2019; Constantinescu et al., 2023). Multivariate analysis, especially PCA, is widely used to identify the main factors affecting the concentration of TEs in sediments and possible pollution sources/transport pathways (Martins et al., 2012; Kahal et al., 2018; Remeikaitė-Nikienė et al., 2018; El-Sorogy et al., 2020; Perumal et al., 2021; Vilhena et al., 2021; Wang et al., 2021; Kubra et al., 2022; Zhou et al., 2022).
The Romanian Black Sea waters are subject to multiple natural and anthropogenic pressures, determined by the Danube River discharges, rock/soil weathering and erosion, port and construction activities, coastal tourism, petrochemical industry, untreated/poorly treated wastewater discharges, offshore drilling for crude oil and gas, etc. (Catianis et al., 2016; Oros, 2019; Bucşe et al., 2020). Due to its very large drainage basin (801463 km2), the Danube River, the second longest river in Europe, is a major pollution source in the NW Black Sea (Schmedtje et al., 2005; Sarı et al., 2018). Most of the studies on TE distribution in the Romanian Black Sea have focused especially on the coastal zone (Coatu et al., 2013; Catianis et al., 2016; Oros et al., 2016; Bucşe et al., 2020). Only a few researches, mainly conducted within different European projects (e.g., MISIS, ANEMONE, BRIDGE), have investigated the distribution of TEs in deeper water areas.
The present study has aimed at: (i) assessing the spatial distribution of Al and some TEs, i.e., As, Cr, Cu, Hg, Ni, Pb, and Zn, in the surface sediments from the coastal and marine environment (water depths of 12.7–149 m) of the NW Black Sea (Romanian area); (ii) evaluating their contamination status in the study area; (iii) establishing the effects of main environmental factors (water depth, sediment texture, organic matter and CaCO3 content) on element accumulation and identifying possible contamination sources by applying statistical analysis. Accordingly, the paper brings new information on the current concentration levels of some elements in the surface sediments located at water depths of up to 150 m in the Romanian Black Sea. The Romanian monitoring program carried out according to the Marine Strategy Framework Directive (MSFD) requirements is mainly focused on the coastal waters, the contamination level in the deeper waters being less monitored and studied. The data brought by this paper cover the entire Romanian shelf and could be used in the revision of the Programme of Measures for Romania, but also in other national and/or regional management plans/strategies. Knowing the values of TE concentration in marine sediments, assessing their contamination status, identifying the factors that control the element distribution in sediments and possible contamination sources are essential to develop appropriate pollution control strategies and/or to strengthen existing strategies in support of the development of relevant blue economy sectors (e.g., fisheries, aquaculture, coastal tourism) and, consequently, of increasing the well-being of coastal communities. Limiting/preventing the introduction of TEs into the marine ecosystem is a major challenge faced by scientists and managers.
2 Materials and methods
2.1 Study area
The research area, located along the Romanian coast of the Black Sea, consists of two sectors separated by Cape Midia (Figure 1).
The northern sector, which encompasses the maritime part of the Danube Delta Biosphere Reserve (DDBR), the largest biosphere reserve in the European Union (with an area of about 5800 km2 and three main distributaries, i.e., Chilia, Sulina, and Sf. Gheorghe), is strongly affected by the Danube discharges. The Danube is the most important supplier of pollutants to the Black Sea, its water and sediment discharges being of about 200 km3/year (cca. 200000 Mt/year) and 25–35 Mt/year, respectively (Panin and Jipa, 2002; Bondar and Iordache, 2016). In addition to the Danube discharges, the sea-based activities, including maritime transport, oil and gas exploitation, are potential contamination sources in this sector.
The southern sector, extended from Cape Midia to the border with Bulgaria, is subject both to the influence of the Danube and to anthropogenic pressures resulted from port and construction activities, coastal tourism, petrochemical industry, agriculture, untreated/poorly treated wastewater discharges, etc. The port of Constanta, the largest port on the Black Sea, and the satellite ports of Mangalia and Midia are located in this area. The port of Constanta has specialized terminals that operate various products, including crude oil, diesel, gas, iron ore, bauxite, coal, coke, timber, chemicals, cement, construction materials (Bucşe et al., 2022). The oil terminal located in the port of Midia, which belongs to the city of Navodari, supplies crude oil for the nearby Petromidia Refinery, the largest Romanian oil refinery. In addition to the cities of Constanta, Mangalia, and Navodari, which are important tourist centers, the southern region of the study area also includes two other localities where tourism is quite developed, i.e., the city of Eforie and commune of Tuzla. Wastewater treatment plants of Constanta, Eforie, and Mangalia are significant sources of organic matter and TEs, including Cd, Cr, Cu, Fe, Ni, Pb, and Zn (Lazăr et al., 2013). Moreover, offshore there are drilling platforms for oil and natural gas (Secrieru and Secrieru, 2002; Bucşe et al., 2020; Bucşe et al., 2022).
In the study area, the influence of the Danube freshwater extends to the south, towards Mangalia, due to the longshore current oriented from the Danube Delta to south, and also to the deep-sea zone (Panin and Jipa, 2002; Catianis et al., 2016; Constantinescu et al., 2023). Moreover, the Danube sandy sediments are remobilized from north to south, due to the longitudinal marine coastal current (Catianis et al., 2016). The jetties of the ports of Sulina, Midia, Constanta, and Mangalia are major obstacles for the transport of sediments, which are either deposited near the ports or redirected offshore (Ungureanu and Stanica, 2000). The sediment deficit from the seashore has caused a significant coastal erosion, especially in the southern sector (Ungureanu and Stanica, 2000; Anton et al., 2019).
2.2 Sediment sampling and characterization
Sediment samples were collected from 32 sampling stations (Figure 1), covering the Romanian shelf waters, in August–September 2019, on board the RV Mare Nigrum. The sampling stations were positioned as follows: 28 were along 5 transects, i.e., Sulina–E (SU01, SU03, and SU04), Sf. Gheorghe–SE (SG01, SG03, SG04, SG06, SG08, SG09, and SG14), Portita–SE (PO01, PO02, and PO04–06), Constanta–SE (CT01–03, CT05, and CT06), and Mangalia–E (MA02–08, and MA11), one was between Sulina–E and Sf. Gheorghe–SE transects (EuxRO01), and other 3 were in the Midia, Eforie, and Tuzla areas (MID02, EF02, and TZ18). Water depths (h = 12.7–149 m) and coordinates of sampling stations are specified in Table 1.
Sediment samples were collected using a Van Veen grab sampler with an opening area of 0.12 m2, and only their upper part (0–2 cm depth) was analyzed. The grain size of sediment samples was measured with a Mastersizer 2000E (ver. 5.20) laser diffraction granulometer (Malvern Instruments Ltd., Malvern, Worcestershire, UK) and Shepard’s ternary diagram was used for their lithological classification.
Total organic carbon content (TOC) was determined using a titration method involving the oxidation of carbon with excess potassium dichromate (K2Cr2O7), in the presence of concentrated sulfuric acid (H2SO4), and the back titration of excess K2Cr2O7 with Mohr’s salt, using diphenylamine as an indicator (Gaudette et al., 1974). Calcium carbonate content (CaCO3) was calculated based on a volumetric analysis method consisting in treating the sample with a hydrochloric acid (HCl) solution (0.5 N) and determining the excess acid by back titration with a sodium hydroxide (NaOH) solution (0.5 N), in the presence of phenolphthalein (Black, 1965).
The concentrations of aluminum (Al), arsenic (As), chromium (Cr), copper (Cu), nickel (Ni), lead (Pb), and zinc (Zn) were measured by X-ray fluorescence spectrometry using an ED-XRF SPECTRO XEPOS spectrometer (SPECTRO Analytical Instruments GmbH, Kleve, Germany). The concentration of mercury (Hg) was determined using a Milestone DMA-80 direct mercury analyzer (Milestone Srl, Sorisole, Bergamo, Italy). Samples were incinerated at 650°C to release Hg vapor that was trapped in a gold amalgamator and then desorbed for detection (at 260 nm) by atomic absorption spectroscopy (U.S. EPA, 2007).
The accuracy of element/compound analyses was evaluated using a certified reference material (CRM) from NCS Testing Technology Co., Ltd. (Beijing, China), i.e., NCS DC 73022. The measured and certified values of element/compound concentration in NCS DC 73022, given as mean ± standard deviation (SD) in Table 2, indicate a high accuracy of analytical methods (the percent recovery values in the range of 95.7–104.8%). The precision of element/compound analyses was checked based on the relative standard deviation (RSD) of measured concentration values (triplicate measurements). The data summarized in Table 2 indicate a high precision of analytical methods (0.23% ≤ RSD ≤ 3.05%).
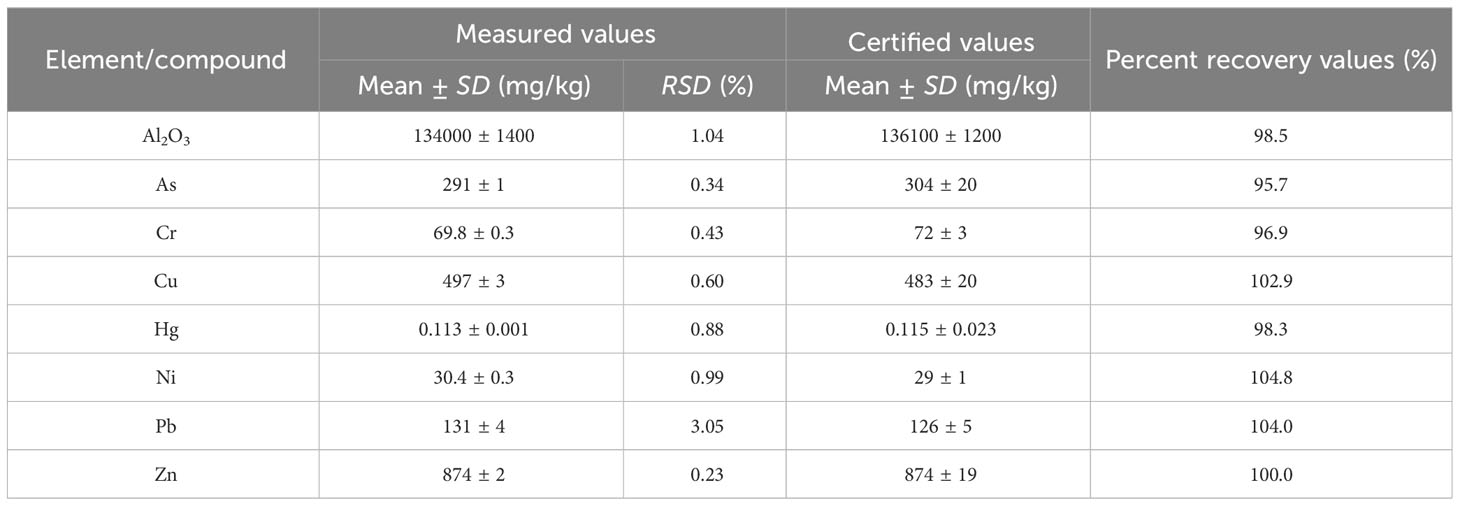
Table 2 Mean ± SD of measured and certified values of element/compound concentration in certified reference material (NCS DC 73022), relative standard deviation (RSD) of measured concentration values (triplicate measurements), and percent recovery values.
2.3 Contamination assessment
The contamination status of surface sediments was evaluated based on the contamination factor (CF), which was determined using Equation 1, where E is the element (Al, As, Cr, Cu, Hg, Ni, Pb, and Zn) concentration in the sample and Eb the background element concentration (Håkanson, 1980). Mean values of shale element concentrations reported by Turekian and Wedepohl (1961) (Alb = 8% = 80000 mg/kg, Asb =13 mg/kg, Crb = 90 mg/kg, Cub = 45 mg/kg, Hgb = 0.40 mg/kg, Nib = 68 mg/kg, Pbb = 20 mg/kg, and Znb = 95 mg/kg) were used as background values, according to other studies on the element distribution in the Black Sea (Gedik and Boran, 2013; Alkan et al., 2021; Özşeker et al., 2022). The contamination status of sediments based on CF values is as follows: low contamination for CF < 1, moderate contamination for 1 ≤ CF < 3, considerable contamination for 3 ≤ CF < 6, and very high contamination for CF ≥ 6 (Håkanson, 1980).
2.4 Data processing
Statistical analysis was performed using XLSTAT ver. 2019.1 (Addinsoft, New York, NY, USA). The Pearson correlation coefficient (r) was used to evaluate the strength of the linear correlations between different parameters. One-way analysis of variance (ANOVA) was applied to determine whether the mean values of variables in different groups were significantly different (p < 0.05) or not. Characteristic parameters of sediments were processed using PCA, a multivariate technique that is widely used to reduce the dimensionality of large data sets (Vaalgamaa and Korhola, 2004; Belivermis et al., 2016; Bucşe et al., 2021; Kapranov et al., 2021; Calcan et al., 2022; Egri et al., 2022; Moloşag et al., 2023). Spatial distributions of TOC, CaCO3, element concentrations, and contamination factors were visualized using the Ocean Data View (ODV) software, ver. 5.6.5 (Schlitzer, 2023).
3 Results and discussion
3.1 Contents of sand, silt, clay, TOC, and CaCO3 in surface sediments
Depending on the percentages of sand (Sand), silt (Silt), and clay (Clay), which are summarized in Table 3, the types of surface sediments collected from the sampling stations were mostly clayey silt and sandy silt.
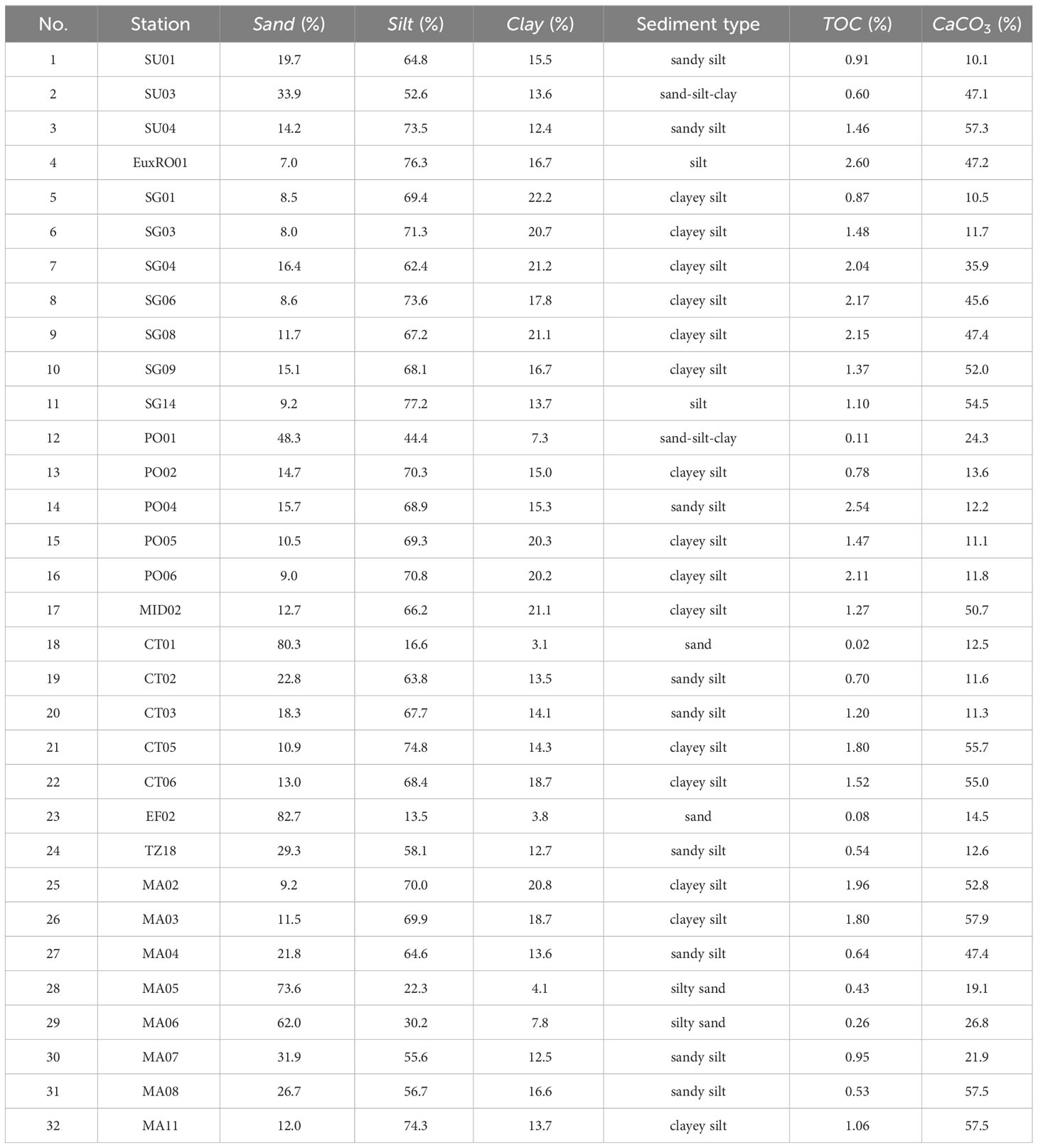
Table 3 Contents of sand (Sand), silt (Silt), clay (Clay), total organic carbon (TOC), and calcium carbonate (CaCO3) in surface sediments and sediment types in the study area.
Total organic carbon content (TOC) and calcium carbonate content (CaCO3) in surface sediments are also summarized in Table 3, and spatial distributions of TOC and CaCO3 are shown in Figure 2. The mean values ± SD of TOC (1.20 ± 0.73%) and CaCO3 (33.0 ± 19.4%) were in a good agreement with those obtained by Secrieru and Secrieru (2002) for surface sediments (0–2 cm depth) collected from Ukrainian, Romanian, and Bulgarian coasts of the Black Sea (1.53 ± 0.84% for TOC and 36.5 ± 24.4% for CaCO3). According to the data presented in Table 1, Table 3, and Figure 2, higher levels of Sand (48.3–82.7%) as well as lower levels of Clay (3.1–7.8%) and TOC (0.02–0.43%) were found in sediments from the shallowest stations (water depths of 16–27 m) located south of the Danube mouth areas, i.e., PO01, CT01, EF02, MA05, and MA06 (near Portita, Constanta, Eforie, and Mangalia). The sediments collected from the rest of the stations had the following percentages: Sand = 7.0–33.9%, Silt = 52.6–77.2%, Clay = 12.4–22.2%, and TOC = 0.53–2.60%. Higher values of TOC (> 2%) were determined in surface sediments from stations EuxRO01 (2.60%), PO04 (2.54%), PO06 (2.11%), SG04, SG06, and SG08 (2.04–2.17%), located at higher water depths (42–119 m). A particularly important component of marine sediments is CaCO3. Its concentration varied between 10.1% and 57.9%, showing an increasing trend towards the sea (Figure 2). The slightly calcareous sediments (CaCO3 in the range of 10–30%) and the calcareous ones (CaCO3 in the range of 30–50%) were found at 16 stations and 6 stations, respectively. The lowest levels of CaCO3 (10.1% and 10.5%) were identified in sediments from the stations near the mouths of Sulina (SU01) and Sf. Gheorghe (SG01) arms (water depths of 16.6 m and 20.6 m, respectively). Values of CaCO3 above 50% were found in sediments from 10 stations located at water depths in the range of 43.5–149 m, i.e., 3 stations in the eastern part of the study area (SU04, SG09, and SG14), 6 in the south (CT05, CT06, MA02, MA03, MA08, and MA11), and one in the west (MID02), the highest levels of CaCO3 (57.5–57.9%) being determined along the Mangalia-E transect (stations MA03, MA08, and MA11).
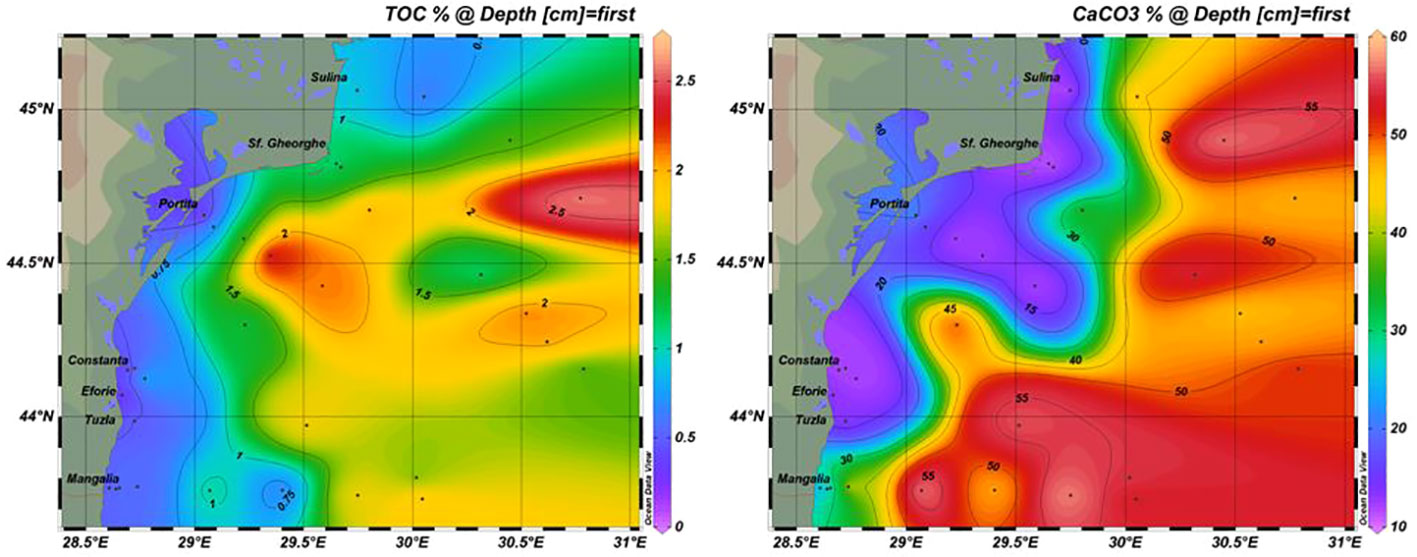
Figure 2 Spatial distributions of total organic carbon content (TOC) and calcium carbonate content (CaCO3) in surface sediments in the study area.
3.2 Element concentrations in surface sediments and potential contamination sources
Concentrations of aluminum (Al), arsenic (As), chromium (Cr), copper (Cu), mercury (Hg), nickel (Ni), lead (Pb), and zinc (Zn) in surface sediments in the study area are specified in Supplementary Table 1, and their spatial distributions are shown in Figure 3. Higher levels of As (10.0–57.1 mg/kg), Cu (33.7–62.9 mg/kg), Ni (48.0–118.0 mg/kg), Pb (26.1–50.8 mg/kg), and Zn (68.8–135.0 mg/kg) were determined in sediments from stations PO04–06, SG01, SG03, SG06, EuxRO01, MID02, SU01, CT03, and MA02. Higher levels of Hg (0.08–0.45 mg/kg) were found in sediments from PO02, PO04–06, TZ18, SG01, SG03, SG04, SG06, EuxRO01, MID02, SU01, CT02, CT03, and MA07. Moreover, the maximum values of Cu (62.9 mg/kg), Hg (0.45 mg/kg), Pb (50.8 mg/kg), and Zn (135.0 mg/kg) were found in sediments from station PO4. Higher levels of Al (5.2–7.0%) and Cr (59.8–103.0 mg/kg) were determined in sediments from PO01, PO02, EuxRO01, SG01, CT02, CT03, TZ18, SU01, and PO04. Surface sediments collected from stations MA05–07, SG03, PO05, PO06, and MID02 had also high levels of Cr (60.8–114.0 mg/kg).
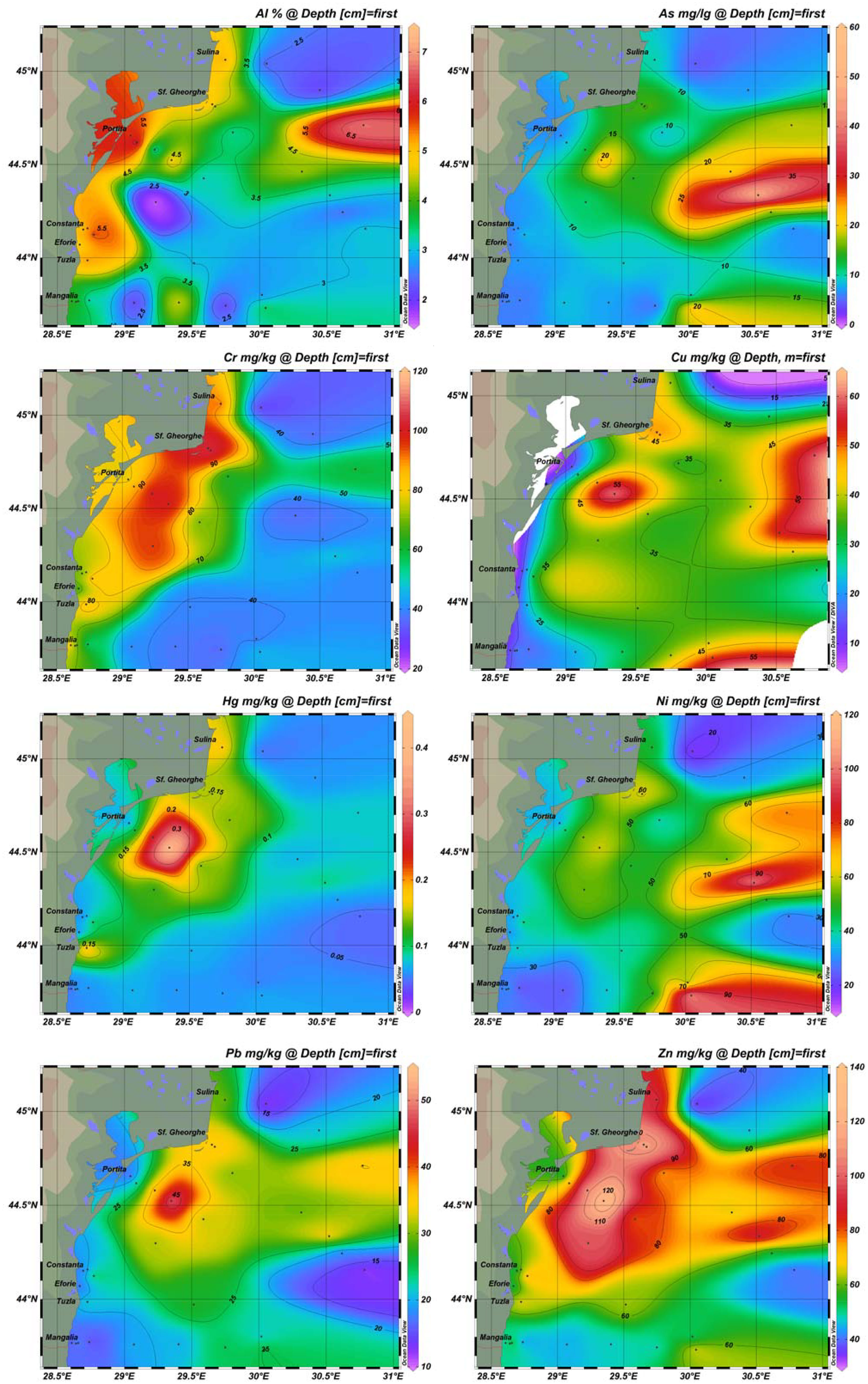
Figure 3 Spatial distributions of Al, As, Cr, Hg, Cu, Ni, Pb and Zn concentrations in surface sediments in the study area.
The study area is highly populated, especially in the summer season, and heavily anthropized, which could lead to widespread contamination with various TEs. On the one hand, the Danube River, containing various domestic, municipal, agricultural, and industrial effluents, is an important source of sediment contamination. On the other hand, there are various local anthropogenic contamination sources, including port activities (cargo and passenger transport, shipyard activities, cargo handling and storage), coastal tourism, constructions, petrochemical industry, wastewater discharges, offshore oil and gas extraction.
Fertilizers and pesticides from agricultural runoff can determine high concentrations of As, Cu, Ni, Pb, and Zn in surface sediments (Cox and Preda, 2005; Selvam et al., 2012; Remeikaitė-Nikienė et al., 2018; Vasiliu et al., 2020; Lin et al., 2021; Tang et al., 2022). As, Cr, Cu, Hg, Pb, and Zn derive from untreated/poorly treated wastewater discharges (Adamo et al., 2005; Cox and Preda, 2005; Selvam et al., 2012; Özkan, 2012; Vasiliu et al., 2020; Tang et al., 2022), whereas Ni, Pb, and Zn originate from waterborne transport (Cox and Preda, 2005; Selvam et al., 2012; Özkan, 2012; Tang et al., 2022). Anti-fouling paint used for ships is an important anthropogenic source of Cu and Zn (Cox and Preda, 2005; Lin et al., 2021). Offshore oil and gas drilling platforms (located near stations PO4 and PO5), crude oil refinery, and construction activities are potential sources of As, Cr, Cu, Hg, Ni, Pb, and Zn, which can enter the sea either through wastewater discharge or atmospheric dry/wet deposition (Adamo et al., 2005; Vasiliu et al., 2020; Lin et al., 2021). Moreover, rock/soil weathering and erosion are natural sources of TEs in surface sediments.
Potential sources of TEs and associations between selected parameters were identified by applying PCA and correlation analysis. A data matrix with 32 rows (number of samples) and 14 columns (number of variables, i.e., Al, As, Cr, Cu, Hg, Ni, Pb, Zn, h, TOC, Sand, Silt, Clay, and CaCO3) was used in PCA. The eigenvalues corresponding to PC1 (6.72), PC2 (3.74), and PC3 (1.21) were > 1. The first three principal components (PCs) explained 83.3% (48.0% + 26.7% + 8.6%) of the total variance. Only PC1 and PC2, explaining 74.7% of the total variance, were further used in PCA. The factor loadings and Pearson correlation coefficients (r) are summarized in Tables 4, 5. The results presented in Table 4 and PCA bi-plot (Figure 4) indicate that the most important variables are As, Cu, Hg, Ni, Pb, Zn, TOC, Sand, Silt, Clay, and to some extent h for PC1, and Al, Cr, h, CaCO3, and to some extent Hg, Pb, and Zn for PC2.
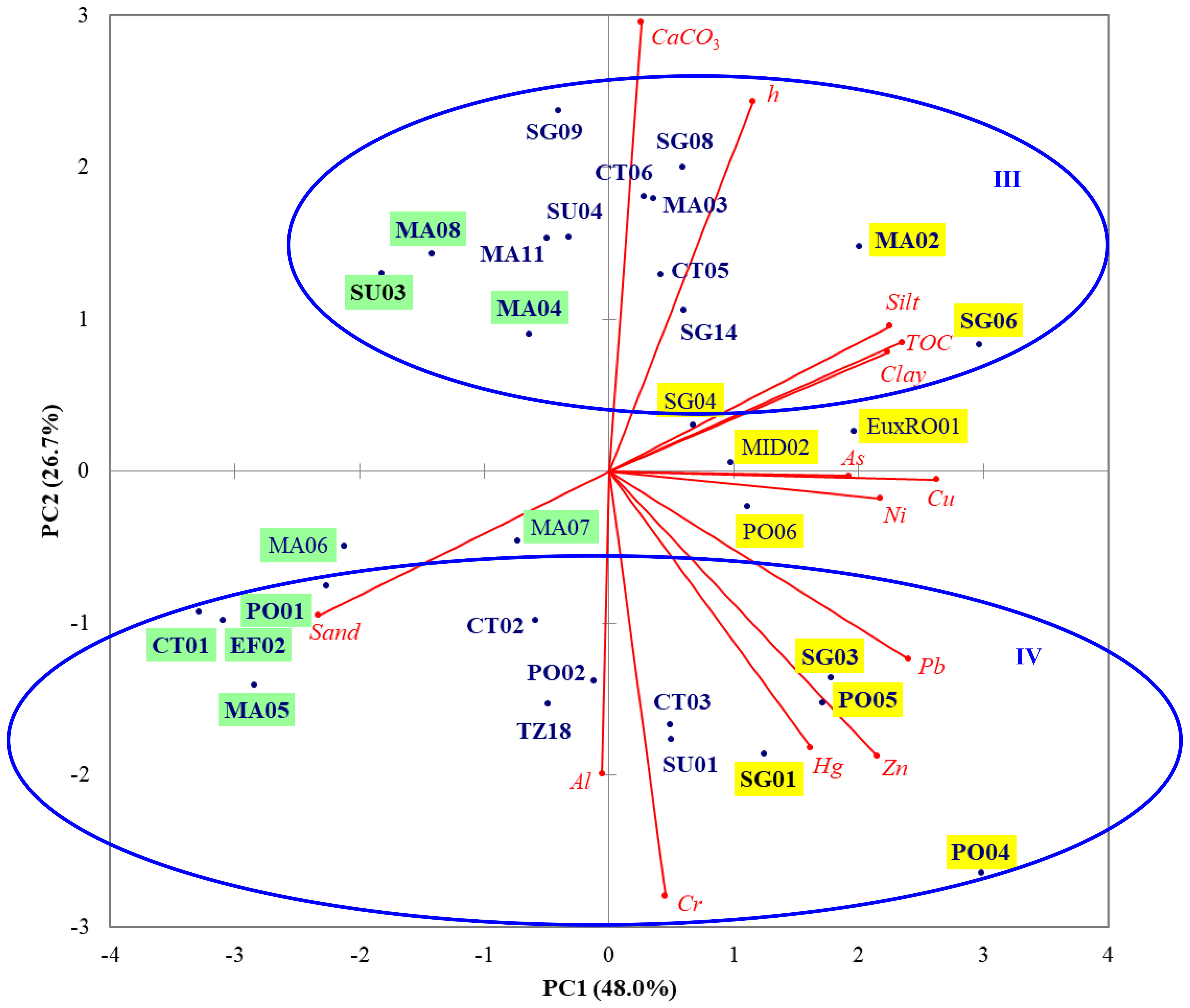
Figure 4 Projections of variables (Al, As, Cr, Cu, Hg, Ni, Pb, Zn, h, TOC, Sand, Silt, Clay, and CaCO3) and samples (collected from 32 stations) on the factor-plane PC1−PC2; group I (9 stations highlighted in green): SU03, PO01, CT01, EF02, and MA04−08; group II (10 stations highlighted in yellow): EuxRO01, SG01, SG03, SG04, SG06, PO04−06, MID02, and MA02; group III (13 stations highlighted in bold): SU03, SU04, SG06, SG08, SG09, SG14, CT05, CT06, MA02−04, MA08, and MA11; group IV (13 stations highlighted in bold): SU01, SG01, SG03, PO01, PO02, PO04, PO05, CT01−03, EF02, TZ18, and MA05.
According to the loadings for PC1 specified in Table 4, As, Cu, Hg, Ni, Pb, and Zn (loadings of 0.59–0.95) were associated with clay, silt, and organic matter particles (loadings of 0.81–0.85). Correlation analysis also indicated that As, Cu, Hg, Ni, Pb, Zn, Clay, Silt, and TOC were directly correlated with each other (0.28 ≤ r ≤ 0.93) as well as each of them was inversely correlated with Sand (-0.99 ≤ r ≤ -0.34). Consequently, the sediment grain size and TOC content had a significant influence on the distribution of As, Cu, Hg, Ni, Pb, and Zn in surface sediments. Numerous studies in the related literature reported that clay, silt, and organic matter particles are important carriers of TEs in sediments (Rubio et al., 2000; Vaalgamaa and Korhola, 2004; Selvam et al., 2012; Özkan, 2012; Dou et al., 2013; Hu et al., 2013; Xu et al., 2016; Zhao et al., 2017; Perina et al., 2018; Remeikaitė-Nikienė et al., 2018; Sun et al., 2020; Wang et al., 2021; Zhou et al., 2022). On the one hand, the finer sediment fractions (clay and silt) have a higher specific surface area than the coarser fraction (sand), giving them more binding sites for the adsorption of TEs and organic matter (Rubio et al., 2000; Xu et al., 2016; Zhou et al., 2022). On the other hand, the organic matter coatings in these fine-grained sediments retain a variety of TEs (Rubio et al., 2000). The results of PCA and correlation analysis suggest that As, Cu, Hg, Ni, Pb, and Zn were associated with fine carriers (clay, silt, and organic matter particles) and these elements originated from common sources. Although the type of each source cannot be precisely identified, it can be assumed that As, Cu, Hg, Ni, Pb, and Zn came mainly from Danube discharges, containing domestic, municipal, agricultural, and industrial effluents, but also from local anthropogenic sources (e.g., port and construction activities, coastal tourism, petrochemical industry, wastewater discharges, offshore oil and gas extraction).
The PCA bi-plot (Figure 4) indicates discrimination on the PC1 direction between the group I of stations highlighted in green (in the left quadrants) and group II of stations highlighted in yellow (in the right quadrants). For samples from group I of 9 stations, including SU03 (in the north-western part of the study area, near the mouth of the Sulina arm), PO01, CT01, and EF02 (in the west, near Portita, Constanta, and Eforie), and MA04−08 (in the south-west and south, along the Mangalia–E transect), the results of one-way ANOVA (Table 6) highlight that the mean values of h, As, Cu, Hg, Ni, Pb, Zn, TOC, Silt, and Clay are significantly lower (p < 0.05) than those for samples from group II of 10 stations, i.e., EuxRO01 (in the eastern part of the study area, between Sulina–E and Sf. Gheorghe–SE transects), SG01, SG03, SG04, and SG06 (along the Sf. Gheorghe–SE transect), PO04−06 (along the Portita–SE transect), MID02 (in the west, near the port of Midia), and MA02 (in the south-east, along the Mangalia–E transect), whereas the mean value of Sand is significantly higher for samples from group I compared to that for samples from group II. Accordingly, the levels of As, Cu, Hg, Ni, Pb, Zn, TOC, Silt, and Clay were higher in sediments collected from group II of stations generally located at higher water depths(up to 118 m), suggesting that TEs associated with these finer carriers were transported offshore by currents and waves. The spatial distribution of sampling stations in groups I and II is shown in Supplementary Figure 1.
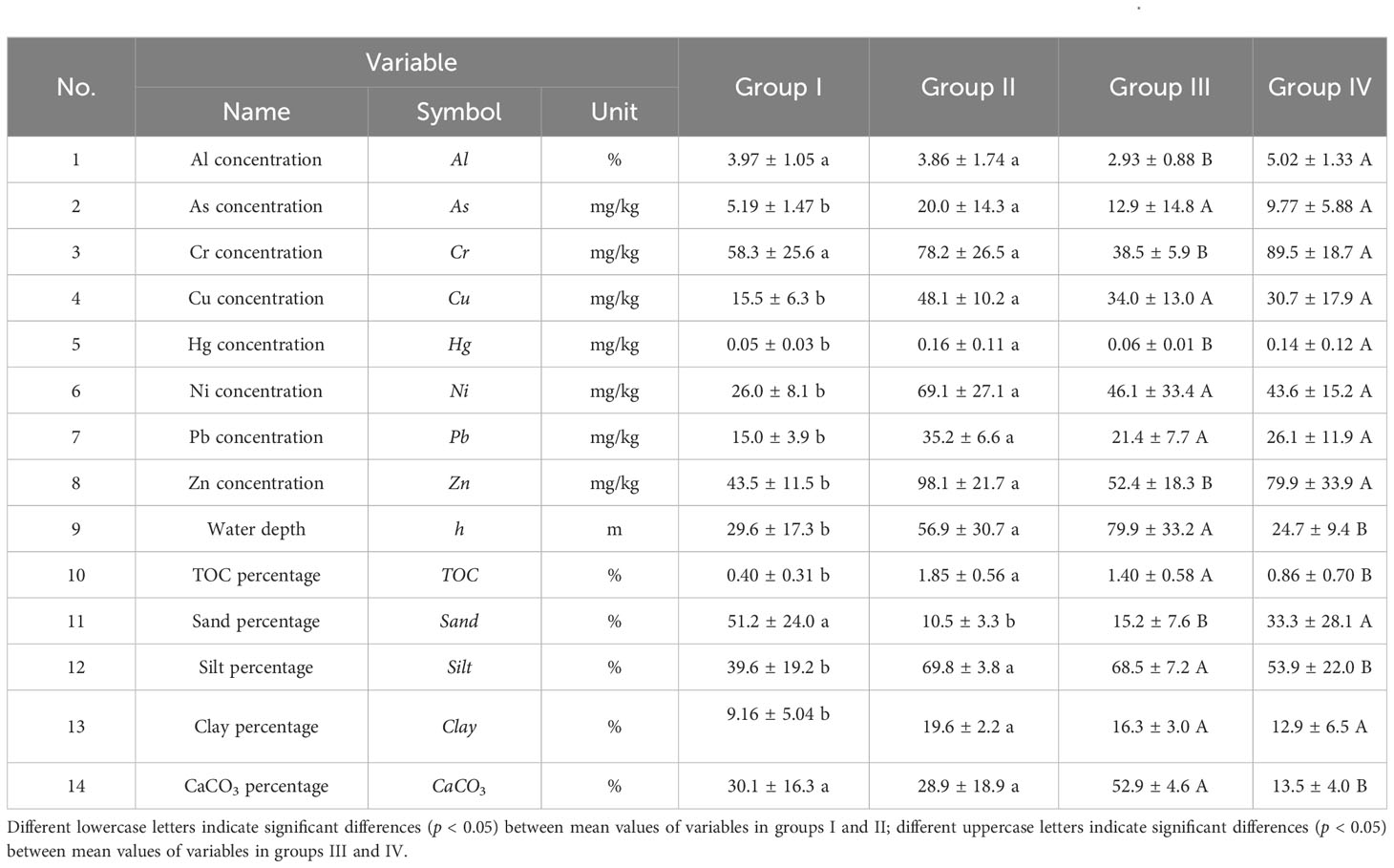
Table 6 Mean values ± SD of characteristic variables of sediment samples from the groups of stations specified in Figure 4.
The loadings of Al, Cr, Hg, Pb, and Zn on PC2 (from -0.88 to -0.39) and their correlation coefficients (0.15 ≤ r ≤ 0.93) indicate that Al, Cr, and partly Hg, Pb, and Zn could have common sources. It can be assumed that these elements originated from rock/soil weathering and erosion. Accordingly, PC2 is related to natural sources of TEs. Al, a major constituent of aluminosilicate minerals, is a typical terrigenous element. Natural sources of Cr, Hg, Pb, and Zn in sediments were reported in several studies (Cox and Preda, 2005; Ip et al., 2007; Özkan, 2012; Dou et al., 2013; Hu et al., 2013; Omar et al., 2015; Xu et al., 2016; Belhadj et al., 2017; Zhao et al., 2017; El-Sorogy et al., 2020; Sun et al., 2020; Perumal et al., 2021; Tang et al., 2022). The correlation matrix (Table 5) highlights that Al and Cr are inversely correlated with h and CaCO3 (-0.80 ≤ r ≤ -0.35). Moreover, inverse correlations were found between CaCO3 and each of Hg, Pb, and Zn (-0.46 ≤ r ≤ -0.23). Weak positive correlations between Al and each of Hg, Pb, and Zn (0.15 ≤ r ≤ 0.26) indicate that Hg, Pb, and Zn derived from both anthropogenic and natural sources. Moreover, moderate positive correlations between Cr and each of Hg, Pb, and Zn (0.43 ≤ r ≤ 0.65) suggest that Cr, Hg, Pb, and Zn could have common anthropogenic sources, e.g., untreated/poorly treated wastewater discharges, offshore oil and gas extraction.
The PCA bi-plot (Figure 4) indicates discrimination on the PC2 direction between the two groups of stations highlighted in bold and using blue ellipses, i.e., group III (in the upper quadrants) and group IV (in the lower quadrants). For samples from group III of 13 stations (located at water depths of 79.9 ± 33.2 m), including SU03 and SU04 (in the northern and north-eastern part of the study area, along the Sulina–E transect), SG06, SG08, SG09, and SG14 (in the east, along the Sf. Gheorghe–SE transect), CT05, CT06, MA02−04, MA08, and MA11 (in the south, along the Constanta–SE and Mangalia–E transects), the results of ANOVA (Table 6) reveal that the mean values of Al, Cr, Hg, and Zn are significantly lower (p < 0.05) than those for samples from group IV of 13 shallower stations (water depths of 24.7 ± 9.4 m), i.e., SU01, SG01 and SG03 (in the north-western part of the study area, near the mouths of the Sulina and Sf. Gheorghe arms), PO01, PO02, PO04, and PO05 (in the west, along the Portita–SE transect), CT01−03, EF02, TZ18, and MA05 (in the west and south-west, near Constanta, Eforie, Tuzla, and Mangalia), whereas the mean values of h and CaCO3 are significantly higher for samples from group III compared to those from group IV. The spatial distribution of sampling stations in groups III and IV is shown in Supplementary Figure 2.
The mean values ± SD and/or ranges of values of As, Cr, Cu, Hg, Ni, Pb, and Zn in surface sediments of the Black Sea, which were reported by different researchers, are summarized in Table 7. The mean values of TE concentrations in surface sediments collected in 2019 from 32 sampling stations (h = 12.7–149 m), which were obtained in this study, were not significantly different (p > 0.05) from those reported in our previous paper for surface sediments (0–2 cm depth) collected in 2018 from 22 stations located at lower water depths (h = 13.5–67 m) in the same area (Bucşe et al., 2020).
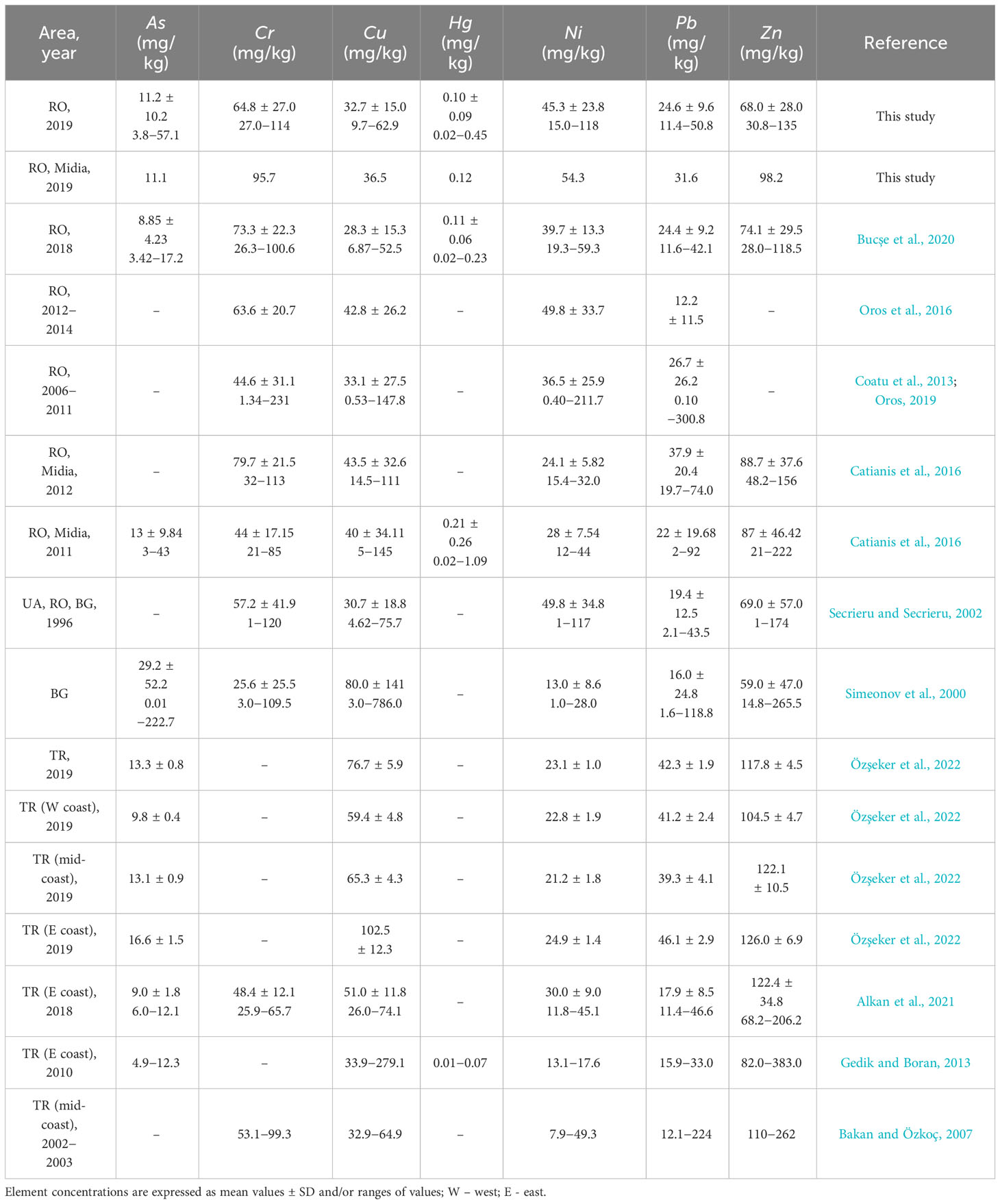
Table 7 Concentrations of arsenic (As), chromium (Cr), copper (Cu), mercury (Hg), nickel (Ni), lead (Pb), and zinc (Zn) in surface sediments of the Black Sea during 1996−2019.
The state of the marine ecosystem in Romania (2006–2014) in terms of contaminant concentrations was evaluated by Coatu et al. (2013), Oros et al. (2016), and Oros (2019). Sediment samples were taken from 40 monitoring stations located along the entire Romanian coast of the Black Sea. Compared to the data reported for the period 2006–2011 (Coatu et al., 2013; Oros, 2019), the values of contaminant concentrations in the samples collected during 2012–2014 (Oros et al., 2016) were situated in general within the same variation ranges. The values of Cr, Cu, Ni, and Pb, characterized by high dispersions, depended on the element type, sediment composition and texture, TOC, water depth, anthropogenic and natural sources of contamination. The grain size and TOC of the sediments affected significantly their contaminant contents. The values of heavy metal contents for sediments with finer texture and higher levels of TOC were higher than those obtained for coarse sediments near the shore. The Danube discharges and anthropogenic inputs in the Constanta coastal zone were the main contamination sources in the study area. The values of Cr, Cu, Ni, and Pb found in our study were generally within the limits reported by Coatu et al. (2013), Oros et al. (2016), and Oros (2019).
Catianis et al. (2016) examined the effects of potential natural and anthropogenic sources of contamination (e.g., Danube discharges, storm water runoff, waste discharges, accidental oil spills, oil refining, shipyard, and shipping activities) on contamination status of surface sediment samples collected from the port of Midia during 2011 (24 samples) and 2012 (10 samples). They found that the surface sediments were not severely polluted with TEs. The values of As, Cr, Cu, Hg, Pb, and Zn in surface sediments collected from station MID02, which were obtained in our study, were within the ranges reported by Catianis et al. (2016).
Secrieru and Secrieru (2002) analyzed sediments collected in 1996 from 26 sampling stations covering almost the entire NW part of the Black Sea (Ukrainian, Romanian, and Bulgarian areas). They assessed that oil drilling activities in Romania and Ukraine were important contamination sources in the study area. The levels of Cr, Cu, Ni, Pb and Zn reported in our study were generally within the ranges of those obtained by Secrieru and Secrieru (2002) for the top layer (0–2 cm depth) of the sediments (Table 7).
Simeonov et al. (2000) analyzed surface sediment samples from 4 different sampling sites of Bulgarian coast of the Black Sea, i.e., Gulf of Bourgas, Gulf of Varna, and 2 lakes (Beloslavsko and Varnensko) serving as a natural buffer zone between the Gulf of Varna and industrial zone. Sediment samples were taken from 39 stations located at water depths in the range of 8–50 m. PCA suggested that Cu came mainly from natural sources, whereas As, Cr, Ni, Pb, and Zn originated from anthropogenic ones, e.g., steelworks, oil refinery, glass production factory, cement and chemical plants. The mean values of As and Cu were higher than those obtained in our study, but that of Ni was lower. Generally, the levels of Ni in surface sediments along the Romanian coast of the Black Sea were higher than those reported for Bulgarian and Turkish areas (Table 7). The natural geochemical value for Ni in sediments from NW Black Sea was 5.9 mg/kg in the 1980s, but it is currently higher than 40 mg/kg (Berlinskyi and Safranov, 2016).
Özşeker et al. (2022) reported concentrations of some elements in surface sediments (0–5 cm depth) of the southern Black Sea (Turkish area). Sediment samples were collected in 2019 from 31 stations located at water depths in the range of 16–65 m. The mean values of Cu, Pb, and Zn were significantly higher than those found in our study, but that of Ni was lower. Their results revealed higher values of element concentrations for eastern Turkish coast compared to western and middle coasts (Table 7). Higher levels of Cu and Pb could be caused by landfills, international highway along the coast, and erosion of terrestrial material. Moreover, agrochemicals are important sources of Cu, Pb, and Zn.
Alkan et al. (2021) and Gedik and Boran (2013) determined element concentrations in surface sediments of the eastern Turkish coast of the Black Sea. Alkan et al. (2021) examined surface sediment samples (0–5 cm depth) collected in 2018 from 5 stations located in the drainage area of the Değirmendere Stream. Factor analysis suggested that As, Cr, Cu, and Ni came predominantly from stream discharges, whereas port activities were main sources of Pb and Zn. Gedik and Boran (2013) analyzed surface sediment samples (0–5 cm depth) taken in 2010 from 7 stations around the port of Rize. They assumed that As, Cu, Hg, Pb, and Zn originated from mining wastewater, Pb and Zn from motor vehicle emissions, Cu, Pb, and Zn from sewage discharge, industrial inputs, and surface runoff, and Ni from natural sources. The mean values of Cu and Zn reported by Gedik and Boran (2013) and Alkan et al. (2021) were significantly higher than those reported in our study, whereas the levels of Pb were similar.
Bakan and Özkoç (2007) evaluated the impact of anthropogenic activities on the accumulation of some elements in surface sediments of the middle Turkish coast. Surface sediment samples (0–5 cm depth) were collected in 2002–2003 from 5 stations around the city of Samsun. The main contamination sources in the study area were port activities and waste discharges. The mean values of Cu, Pb, and Zn were higher than those reported in our study, but that of Ni was lower.
3.3 Contamination factors
The values of contamination factors (CFE, where E = Al, As, Cr, Cu, Hg, Ni, Pb, Zn) are summarized in Supplementary Table 2, and their spatial distributions are shown in Figure 5. The results presented in Supplementary Table 2 and Figure 5 indicate the following:
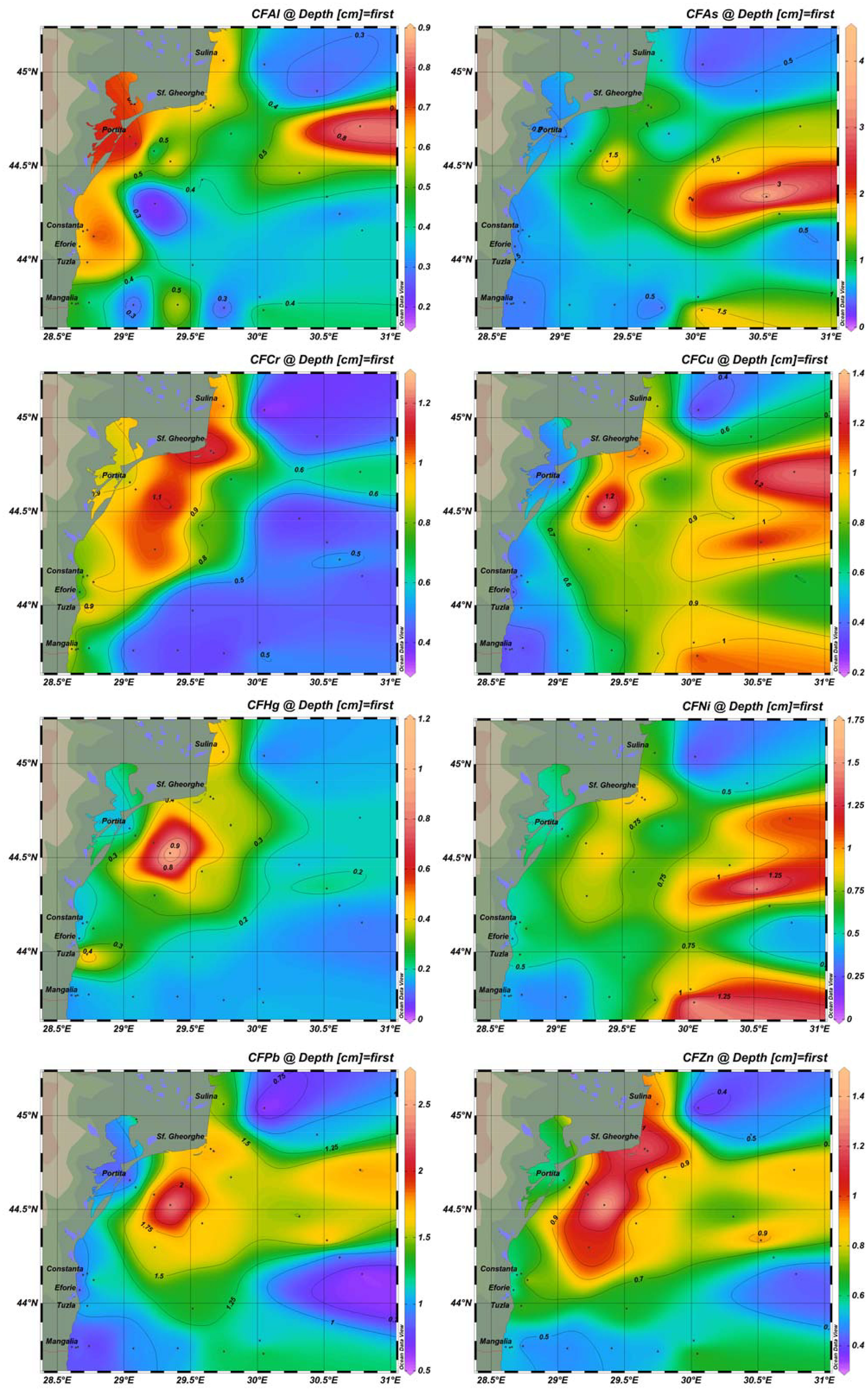
Figure 5 Spatial distributions of contamination factor (CFE, E = Al, As, Cr, Cu, Hg, Ni, Pb, Zn) in surface sediments in the study area.
- a considerable contamination with As (CFAs = 4.39) and a moderate contamination with Cu (CFCu = 1.24), Ni (CFNi = 1.71), Pb (CFPb = 1.89), and Zn (CFZn = 1.05) for sediments collected from station SG06;
- a moderate contamination (0 < CFE < 3 for all TEs and 1 ≤ CFE < 3 for at least one TE) for sediments collected from 22 stations (SU01, SU04, EuxRO01, SG01, SG03, SG04, SG14, PO02, PO04–06, MID02, CT02, CT03, CT05, CT06, TZ18, MA02–05, and MA07);
- a low contamination (0 < CFE < 1 for all TEs) for sediments collected from 9 stations (SU03, SG08, SG09, PO01, CT01, EF02, MA06, MA08, and MA11);
- the sediments from stations EuxRO01, SG06, and MA02, located at high water depths (80–118 m), had a moderate contamination with Cu, Ni, and Pb; the sediments from station SG06 (in the eastern part of the study area, along the Sf. Gheorghe–SE transect) had a considerable contamination with As and a moderate contamination with Zn, whereas those from stations EuxRO01 (in the east, between Sulina–E and Sf. Gheorghe–SE transects) and MA02 (in the south-east, along the Mangalia–E transect) had a moderate contamination with As; the TEs found in these sediments, which were associated with finer sediment fractions and organic matter, originated preponderantly from the Danube discharges, but also from local anthropogenic contamination sources;
- the sediments from station MID02, located in the area of influence of the Danube, had a moderate contamination with Cr, Pb, and Zn, mainly determined by the Danube discharges and anthropogenic inputs of pollutants from the port of Midia, oil refinery, and wastewater discharges;
- the sediments from stations SG01 and SG03 (located near the mouth of the Sf. Gheorghe arm) as well as PO04 and PO05 (located near the oil and gas drilling platforms) had a moderate contamination with Cr, Cu, Pb, and Zn; moreover, the sediments from stations SG03 and PO04 had a moderate contamination with As, whereas those from station PO04 also had a moderate contamination with Hg; the TEs found in these sediments mainly came from the Danube and wastewater discharges, but also from coastal tourism, oil and gas extraction, rock/soil weathering and erosion;
- the sediments from stations SU01 (near the port of Sulina), SU04 (along the transect Sulina–E), SG04 and SG14 (along the transect Sf. Gheorghe–SE), PO02 and PO06 (along the transect Portita–SE), CT02, CT03, CT05, and CT06 (along the transect Constanta–SE), TZ18 (near Tuzla), MA03, MA04, and MA07 (in the south and south-west, along the transect Mangalia–E) had a moderate contamination with Pb; moreover, the sediments from station SU01 had a moderate contamination with Cr and Zn, those from PO02 and CT03 a moderate contamination with Cr, and those from SG14 and PO06 a moderate contamination with As; in addition to the contamination caused by the Danube discharges, the contamination at station TZ18 could also be determined by construction activities and household waste disposal (Mureşan et al., 2018), whereas that at stations PO02 (near the holiday village of Portita), SU01 (near the port of Sulina), and CT03 (near the port of Constanta) came from different anthropogenic inputs and also from rock/soil weathering and erosion; the contamination at stations SU04, SG04, SG14, PO06, CT02, CT05, CT06, MA03, MA04, and MA07 was determined by the Danube discharges and different local anthropogenic inputs;
- the sediments from station MA05 (near the port of Mangalia) had a moderate contamination with Cr, which can be the effect of rock/soil weathering and erosion (Ungureanu and Stanica, 2000);
- the sediments collected from stations SU03 (in the north, along the transect Sulina–E), SG08 and SG09 (in the east, along the transect Sf. Gheorghe–SE, and characterized by higher water depths and CaCO3 contents), PO01 (near the holiday village of Portita), CT01 (near the port of Constanta), EF02 (near Eforie tourist resort), MA06, MA08, and MA11 (in the south-west, along the transect Mangalia–E) had a low contamination (0 < CFE < 1 for all TEs).
Based on the mean values of CFE, i.e., CFPb,m = 1.23, CFAs,m = 0.86, CFCu,m = 0.73, CFCr,m = 0.72, CFZn,m = 0.72, CFNi,m = 0.67, CFHg,m = 0.26, the sediments in the study area had a moderate contamination with Pb and a low contamination with the other TEs. However, it must be stated that the choice of background values in Equation 1 has an essential role in determining the contamination status of surface sediments.
4 Conclusions
This paper brings new information regarding the concentration levels of some elements (i.e., Al, As, Cr, Cu, Hg, Ni, Pb, and Zn) in surface sediments collected in 2019 from 32 sampling stations located in the NW Black Sea (Romanian area), at water depths in the range of 12.7–149 m. The mean values of element concentrations were as follows: 3.9% for Al, 11.2 mg/kg for As, 64.8 mg/kg for Cr, 32.7 mg/kg for Cu, 0.10 mg/kg for Hg, 45.3 mg/kg for Ni, 24.6 mg/kg for Pb, and 68.0 mg/kg for Zn.
The results of statistical analysis highlighted that As, Cu, Ni, Hg, Pb, and Zn were associated with finer carriers, i.e., clay, silt, and organic matter. Although it is difficult to identify exactly the type of each contamination source, it can be assumed that these elements mainly came from the Danube discharges and also from local anthropogenic sources, whereas Al, Cr, and partly Hg, Pb, and Zn originated from rock/soil weathering and erosion. On the one hand, the concentration levels of As, Cu, Hg, Ni, Pb, Zn, TOC, silt, and clay were higher in sediments collected from stations EuxRO01, SG01, SG03, SG04, SG06, PO04−06, MID02, and MA02, generally located at higher water depths (up to 118 m), suggesting that the TEs associated with the finer carriers were transported offshore by currents and waves. On the other hand, the concentration levels of Al, Cr, Hg, and Zn were higher in sediments collected from shallower stations (water depths of 12.7–42.0 m), i.e., SU01, SG01, SG03, PO01, PO02, PO04, PO05, CT01−03, EF02, TZ18, and MA05, having lower levels of CaCO3 content (10.1–24.3%).
The mean values of contamination factor indicated a moderate contamination with Pb (CFPb,m = 1.23) and a low contamination with the other elements (0.26 ≤ CFE,m ≤ 0.86, E = Al, As, Cr, Cu, Hg, Ni, and Zn). Except for the sediments collected from 10 stations, i.e., SU03 (in the northern part of the study area, along the transect Sulina–E), SG08 and SG09 (in the east, along the transect Sf. Gheorghe–SE, and characterized by higher water depths and CaCO3 contents), PO01 (near the holiday village of Portita), CT01 (near the port of Constanta), EF02 (near Eforie tourist resort), MA05, MA06, MA08, and MA11 (in the south-west and south, along the transect Mangalia–E), which had a low contamination with Pb (0.57 ≤ CFPb ≤ 0.99), the sediments from the other 22 stations had a moderate contamination with Pb (1.00 ≤ CFPb ≤ 2.54). Moreover, except for the sediments collected from station PO4 (located near the oil and gas drilling platforms), which had a moderate contamination with Hg (CFHg = 1.13), the sediments from the other stations had a low contamination with Hg (0.06 ≤ CFHg ≤ 0.65).
Determining TE concentration levels in marine sediments, their contamination status, the factors controlling the element distribution, and possible contamination sources are essential for improving pollution control strategies either in coastal areas or in the Danube river watershed.
Data availability statement
The original contributions presented in the study are included in the article/Supplementary Material. Further inquiries can be directed to the corresponding authors.
Author contributions
AB: Conceptualization, Formal Analysis, Methodology, Software, Writing – original draft, Investigation. OCP: Conceptualization, Formal Analysis, Methodology, Software, Writing – original draft, Supervision, Validation, Writing – review & editing. DV: Conceptualization, Methodology, Project administration, Resources, Supervision, Writing – original draft, Writing – review & editing. FR: Investigation, Methodology, Writing – original draft. NL: Investigation, Methodology, Writing – original draft. BAI: Investigation, Methodology, Writing - review & editing.
Funding
The author(s) declare financial support was received for the research, authorship, and/or publication of this article. The study was supported by the project PN 19 20 01 02 (Multidisciplinary research for the improvement of knowledge of the interaction between climate change and anthropogenic pressures and its effects on the Black Sea ecosystem).
Acknowledgments
The authors thank the crew of the RV Mare Nigrum for the excellent support during the sampling program.
Conflict of interest
The authors declare that the research was conducted in the absence of any commercial or financial relationships that could be construed as a potential conflict of interest.
Publisher’s note
All claims expressed in this article are solely those of the authors and do not necessarily represent those of their affiliated organizations, or those of the publisher, the editors and the reviewers. Any product that may be evaluated in this article, or claim that may be made by its manufacturer, is not guaranteed or endorsed by the publisher.
Supplementary material
The Supplementary Material for this article can be found online at: https://www.frontiersin.org/articles/10.3389/fmars.2023.1310164/full#supplementary-material
References
Adamo P., Arienzo M., Imperato M., Naimo D., Nardi G., Stanzione D. (2005). Distribution and partition of heavy metals in surface and sub-surface sediments of naples city port. Chemosphere 61 (6), 800–809. doi: 10.1016/j.chemosphere.2005.04.001
Alkan A., Özşeker K., Yildiz İ., Dinçer C. (2021). Determination of metal concentrations in değirmendere stream drainage area of southeastern black sea coast of Turkey. Hacettepe J. Biol. Chem. 49 (2), 117–124. doi: 10.15671/hjbc.686166
Anton C., Gasparotti C., Anton I. A., Rusu E. (2019). Analysis of the mamaia bay shoreline retreat with hard and soft orotection works. J. Mar. Sci. 1 (1), 8–18. doi: 10.30564/jmsr.v1i1.490
Bakan G., Özkoç H. B. (2007). An ecological risk assessment of the impact of heavy metals in surface sediments on biota from the mid-black sea coast of Turkey. Int. J. Environ. Stud. 64, 45–57. doi: 10.1080/00207230601125069
Belhadj H., Aubert D., Youcef N. D. (2017). Geochemistry of major and trace elements in sediments of ghazaouet bay (Western Algeria): an assessment of metal pollution. C.R.–Geosci. 349 (8), 412–421. doi: 10.1016/j.crte.2017.09.013
Belivermis M., Kılıç Ö., Çotuk Y. (2016). Assessment of metal concentrations in indigenous and caged mussels (Mytilus galloprovincialis) on entire turkish coastline. Chemosphere 144, 1980–1987. doi: 10.1016/j.chemosphere.2015.10.098
Berlinskyi N., Safranov T. (2016). Spatial and temporal variability of pollutants in the bottom sediments in the northwest part of the black sea. Environ. Probl. 1 (1), 73–76.
Black C. A. (1965). Method of soil analysis, part 2. Chemical and microbiological properties (Madison, WI: American Society of Agronomy, Inc).
Bondar C., Iordache G. (2016). Sediment transport on the Romanian section of the danube river. Geo-Eco-Mar. 22, 29–49. doi: zenodo.org/records/889809
Boran M., Altınok I. (2010). A review of heavy metals in water, sediment and living organisms in the black sea. Turkish J. Fish. Aquat. Sci. 10, 565–572. doi: 10.4194/trjfas.2010.0418
Bucşe A., Pârvulescu O. C., Vasiliu D., Lupaşcu N., Voica C. (2021). Levels of heavy metal concentration in M. galloprovincialis mollusc species from NW black sea (Romania). U.P.B. Sci. Bull. Ser. B 83 (3), 51–60.
Bucşe A., Vasiliu D., Bălan S., Pârvulescu O. C., Dobre T. (2020). Heavy metal spatial distribution and pollution assessment in the surface sediments of the north–western black sea shelf. Rev. Chim. 71 (4), 155–170. doi: 10.37358/RC.20.4.8054
Bucşe A., Pârvulescu O. C., Vasiliu D., Mureşan M. (2022). The contents of some trace elements (As, br, cu, hg, se, and zn) in mytilus galloprovincialis mussels from agigea port, Romania. Front. Mar. Sci. 9. doi: 10.3389/fmars.2022.899555
Calcan S. I., Pârvulescu O. C., Ion V. A., Răducanu C. E., Bădulescu L., Madjar R., et al. (2022). Effects of biochar on soil properties and tomato growth. Agronomy 12 (8), 1824. doi: 10.3390/agronomy12081824
Catianis I., Ungureanu C., Magagnini L., Ulazzi E., Campisi T., Stanica A. (2016). Environmental impact of the midia port-black sea (Romania) on the coastal sediment quality. Open Geosci. 8 (1), 174–194. doi: 10.1515/geo-2016-0018
Coatu V., Ţigănuş D., Oros A., Lazăr L. (2013). Analysis of hazardous substance contamination of the marine ecosystem in the Romanian black sea coast, part of the marine strategy framework directive, (2008/56/EEC) implementation. Mar. Res. J. 43 (1), 174–186.
Constantinescu A. M., Tyler A. N., Stanica A., Spyrakos E., Hunter P. D., Catianis I., et al. (2023). A century of human interventions on sediment flux variations in the danube-black sea transition zone. Front. Mar. Sci. 10. doi: 10.3389/fmars.2023.1068065
Cox M. E., Preda M. (2005). Trace metal distribution within marine and estuarine sediments of western moreton bay, queensland, Australia: relation to land use and setting. Geogr. Res. 43 (2), 173–193. doi: 10.1111/j.1745-5871.2005.00312.x
Dou Y., Li J., Zhao J., Hu B., Yang S. (2013). Distribution, enrichment and source of heavy metals in surface sediments of the eastern beibu bay, south China sea. Mar. pollut. Bull. 67 (1–2), 137–145. doi: 10.1016/j.marpolbul.2012.11.022
Egri D., Pârvulescu O. C., Ion V. A., Răducanu C. E., Calcan S. I., Bădulescu L., et al. (2022). Vine pruning-derived biochar for agronomic benefits. Agronomy 12 (11), 2730. doi: 10.3390/agronomy12112730
El-Sorogy A. S., Youssef M., Al-Kahtany K., Saleh M. M. (2020). Distribution, source, contamination, and ecological risk status of heavy metals in the red sea-gulf of aqaba coastal sediments, Saudi Arabia. Mar. pollut. Bull. 158, 1–8. doi: 10.1016/j.marpolbul.2020.111411
Gaudette H. E., Flight W. R., Toner L., Folger W. (1974). An inexpensive titration method for the determination of organic carbon in recent sediments. J. Sediment. Petrol. 44, 249–253. doi: 10.1306/74d729d7-2b21-11d7-8648000102c1865d
Gedik K., Boran M. (2013). Assessment of metal accumulation and ecological risk around rize harbor, Turkey (Southeast black sea) affected by copper ore loading operations by using different sediment indexes. Bull. Environ. Contam. Toxicol. 90, 176–181. doi: 10.1007/s00128-012-0894-2
Håkanson L. (1980). An ecological risk index for aquatic pollution control: A sediment ecological approach. Water Res. 14, 975–1001. doi: 10.1016/0043-1354(80)90143-8
Hu B., Cui R., Li J., Wei H., Zhao J., Bai F., et al. (2013). Occurrence and distribution of heavy metals in surface sediments of the changhua river estuary and adjacent shelf (Hainan island). Mar. pollut. Bull. 76 (1–2), 400–405. doi: 10.1016/j.marpolbul.2013.08.020
Ip C. C., Li X. D., Zhang G., Wai O. W., Li Y. S. (2007). Trace metal distribution in sediments of the pearl river estuary and the surrounding coastal area, south China. Environ. pollut. 147 (2), 311–323. doi: 10.1016/j.envpol.2006.06.028
Kahal A. Y., El-Sorogy A. S., Alfaifi H. J., Almadani S., Ghrefat H. A. (2018). Spatial distribution and ecological risk assessment of the coastal surface sediments from the red sea, northwest Saudi Arabia. Mar. pollut. Bull. 137, 198–208. doi: 10.1016/j.marpolbul.2018.09.053
Kapranov S. V., Karavantseva N. V., Bobko N. I., Ryabushko V. I., Kapranova L. L. (2021). Sex- and sexual maturation-related aspects of the element accumulation in soft tissues of the bivalve mytilus galloprovincialis lam. Collected off coasts of sevastopol (Southwestern crimea, black sea). Environ. Sci. pollut. Res. 28, 21553–21576. doi: 10.1007/s11356-020-12024-z
Karavoltsos S., Sakellari A., Plavšić M., Bekiaris G., Tagkouli D., Triantafyllidis A., et al. (2022). Metal complexation, FT-IR characterization, and plankton abundance in the marine surface microlayer of coastal areas in the eastern mediterranean. Front. Mar. Sci. 9. doi: 10.3389/fmars.2022.932446
Karbassi A. R., Heidari M., Vaezi A. R., Samani A. V., Fakhraee M., Heidari F. (2014). Effect of pH and Salinity on Flocculation Process of Heavy Metals during Mixing of Aras River Water with Caspian Sea Water. Environ. Earth Sci. 72, 457–465. doi: 10.1007/s12665-013-2965-z
Kay M. L., Wiklund J. A., Sun X., Savage C. A., Adams J. K., MacDonald L. A., et al. (2022). Assessment of mercury enrichment in lake sediment records from alberta oil sands development via fluvial and atmospheric pathways. Front. Environ. Sci. 10. doi: 10.3389/fenvs.2022.949339
Kubra K., Mondol A. H., Ali M. M., Palash M. A. U., Islam M. S., Ahmed A. S., et al. (2022). Pollution level of trace metals (As, pb, cr and cd) in the sediment of rupsha river, Bangladesh: assessment of ecological and human health risks. Front. Environ. Sci. 10. doi: 10.3389/fenvs.2022.778544
Lazăr L., Boicenco L., Coatu V., Oros A., Ţigănuş D., Mihailov M. E. (2013). Nutrient levels and eutrophication of the Romanian black sea waters, (2006–2011) – assessment related to the marine strategy framework directive implementation. Recherches Marines 43 (1), 162–173.
Lin H., Lan W., Feng Q., Zhu X., Li T., Zhang R., et al. (2021). Pollution and ecological risk assessment, and source identification of heavy metals in sediment from the beibu gulf, south China sea. Mar. pollut. Bull. 168, 112403. doi: 10.1016/j.marpolbul.2021.112403
Martins R., Azevedo M. R., Mamede R., Sousa B., Freitas R., Rocha F., et al. (2012). Sedimentary and geochemical characterization and provenance of the portuguese continental shelf soft-bottom sediments. J. Mar. Sys. 91 (1), 41–52. doi: 10.1016/j.jmarsys.2011.09.011
Moloşag A., Pârvulescu O. C., Ion V. A., Asănică A. C., Soane R., Moț A., et al. (2023). Effects of marine residue-derived fertilizers on strawberry growth, nutrient content, fruit yield and quality. Agronomy 13 (5), 1221. doi: 10.3390/agronomy13051221
Mülayim A., Balkıs H. (2015). Toxic metal (Pb, cd, cr, and hg) levels in rapana venosa (Valenciennes 1846), eriphia verrucosa (Forskal 1775), and sediment samples from the black sea littoral (Thrace, Turkey). Mar. pollut. Bull. 95 (1), 215–222. doi: 10.1016/j.marpolbul.2015.04.016
Mureşan M., Begun T., Voicaru C., Vasile D., Teacă A. (2018). Beach litter occurrence in sandy littoral: case study–the Romanian black sea coast. Geo-Eco-Mar. 23, 205–213. doi: zenodo.org/records/1689203
Omar M. B., Mendiguchía C., Er-Raioui H., Marhraoui M., Lafraoui G., Oulad-Abdellah M. K., et al. (2015). Distribution of heavy metals in marine sediments of tetouan coast (North of Morocco): natural and anthropogenic sources. Environ. Earth Sci. 74, 4171–4185. doi: 10.1007/s12665-015-4494-4
Oros A. (2019). Monitoring and assessment of heavy metals in the Romanian black sea ecosystem during 2006–2018, in the context of marine strategy framework directive (MSFD) 2008/56/EC implementation. Recherches Marines 49 (1), 8–33. doi: 10.55268/CM.2019.49.8
Oros A., Lazar L., Coatu V., Tiganus D. (2016). “Recent data from pollution monitoring and assessment of the Romanian black sea ecosystem, within implementation of the european marine strategy framework directive,” in Water Resour. For. Mar. Ocean Ecosyst. Conf. Proc. SGEM, Vol. 2016. 821–828.
Özkan E. Y. (2012). A new assessment of heavy metal contaminations in an eutrophicated bay (Inner Izmir Bay, Turkey). Turkish J. Fish. Aquat. Sci. 12 (1). doi: 10.4194/1303-2712-v12_1_16
Özşeker K., Erüz C., Terzi Y. (2022). Spatial distribution and ecological risk evaluation of toxic metals in the southern black sea coastal sediments. Mar. pollut. Bull. 182, 114020. doi: 10.1016/j.marpolbul.2022.114020
Panin N., Jipa D. (2002). Danube river sediment input and its interaction with the north-western black sea. Estuar. Coast. Shelf Sci. 54 (3), 551–562. doi: 10.1006/ecss.2000.0664
Perina F. C., Torres R. J., Mozeto A. A., Nascimento M. R. L., de Souza Abessa D. M. (2018). Sediment quality assessment of the tributaries of the santos-são vicente estuarine system–Brazil. Ecotoxicol. Environ. Contam. 13 (2), 25–38. doi: 10.5132/eec.2018.02.05
Perumal K., Antony J., Muthuramalingam S. (2021). Heavy metal pollutants and their spatial distribution in surface sediments from thondi coast, palk bay, south India. Environ. Sci. Eur. 33 (1), 1–20. doi: 10.1186/s12302-021-00501-2
Remeikaitė-Nikienė N., Garnaga-Budrė G., Lujanienė G., Jokšas K., Stankevičius A., Malejevas V., et al. (2018). Distribution of metals and extent of contamination in sediments from the south-eastern baltic sea (Lithuanian zone). Oceanologia 60 (2), 193–206. doi: 10.1016/j.oceano.2017.11.001
Romano E., Ausili A., Bergamin L. (2022). Coastal marine geochemical provinces and background values in sediments: A methodological approach. Front. Mar. Sci. 9. doi: 10.3389/fmars.2022.874248
Rubio B., Nombela M. A., Vilas F. J. M. P. B. (2000). Geochemistry of major and trace elements in sediments of the ria de vigo (NW Spain): an assessment of metal pollution. Mar. pollut. Bull. 40 (11), 968–980. doi: 10.1016/S0025-326X(00)00039-4
Sánchez-García L., de Andrés J. R., Martín-Rubí J. A. (2010). Geochemical signature in off-shore sediments from the gulf of cadiz inner shelf: sources and spatial variability of major and trace elements. J. Mar. Syst. 80 (3–4), 191–202. doi: 10.1016/j.jmarsys.2009.10.009
Sarı E., Çağatay M. N., Acar D., Belivermiş M., Kılıç Ö., Arslan T. N., et al. (2018). Geochronology and sources of heavy metal pollution in sediments of istanbul strait (Bosporus) outlet area, SW black sea, Turkey. Chemosphere 205, 387–395. doi: 10.1016/j.chemosphere.2018.04.096
Schmedtje U., Bachmann J., Behrendt H., Birk S., Biza P., D’Eugenio J. V., et al. (2005) The Danube River Basin District – River Basin Characteristics, Impacts of Human Activities and Economic Analysis Required under Article 5, Annex II and Annex III, and Inventory of Protected Areas Required under Article 6, Annex IV of the EU Water Framework Directive, (2000/60/EC) – part A – basin-wide overview. Available at: https://www.icpdr.org/sites/default/files/nodes/documents/danube_basin_analysis_2004.pdf.
Secrieru D., Secrieru A. (2002). Heavy metal enrichment of man-made origin of superficial sediment on the continental shelf of the north-western black sea. Estuar. Coast. Shelf Sci. 54 (3), 513–526. doi: 10.1006/ecss.2000.0671
Selvam A. P., Priya S. L., Banerjee K., Hariharan G., Purvaja R., Ramesh R. (2012). Heavy metal assessment using geochemical and statistical tools in the surface sediments of vembanad lake, southwest coast of India. Environ. Monit. Assess. 184, 5899–5915. doi: 10.1007/s10661-011-2389-8
Simeonov V., Massart D. L., Andreev G., Tsakovski S. (2000). Assessment of metal pollution based on multivariate statistical modeling of ‘Hot spot’ Sediments from the black sea. Chemosphere 41 (9), 1411–1417. doi: 10.1016/S0045-6535(99)00540-8
Sun X., Fan D., Liu M., Liao H., Zheng S., Tian Y. (2020). Budget and fate of sedimentary trace metals in the eastern China marginal seas. Water Res. 187, 116439. doi: 10.1016/j.watres.2020.116439
Tang D., Luo S., Deng S., Huang R., Chen B., Deng Z. (2022). Heavy metal pollution status and deposition history of mangrove sediments in zhanjiang bay, China. Front. Mar. Sci. 9. doi: 10.3389/fmars.2022.989584
Turekian K. K., Wedepohl K. H. (1961). Distribution of the elements in some major units of the earth’s crust. Geol. Soc Am. Bull. 72, 175–192. doi: 10.1130/0016-7606(1961)72[175:DOTEIS]2.0.CO;2
Ungureanu V. G., Stanica A. (2000). Impact of human activities on the evolution of the Romanian black sea beaches. Lakes Reserv.: Res. Manage. 5 (2), 111–115. doi: 10.1046/j.1440-1770.2000.00105.x
U.S. EPA (2007). Method 7473 (SW-846): mercury in solids and solutions by thermal decompostion, amalgamation, and atomic absorption spectrophotometry (Washington, DC).
Vaalgamaa S., Korhola A. (2004). Searching for order in chaos: a sediment stratigraphical study of a multiple-impacted bay of the baltic sea. Estuar. Coast. Shelf Sci. 59 (2), 319–332. doi: 10.1016/j.ecss.2003.09.005
Vasiliu D., Bucse A., Lupascu N., Ispas B., Gheablau C., Stanescu I. (2020). Assessment of the metal pollution in surface sediments of coastal tasaul lake (Romania). Environ. Monit. Assess. 192 (12), 749. doi: 10.1007/s10661-020-08698-0
Vilhena J. C., Amorim A., Ribeiro L., Duarte B., Pombo M. (2021). Baseline study of trace element concentrations in sediments of the intertidal zone of amazonian oceanic beaches. Front. Mar. Sci. 8. doi: 10.3389/fmars.2021.671390
Wang H., Fan Z., Kuang Z., Yuan Y., Liu H., Huang H. (2021). Heavy metals in marine surface sediments of daya bay, southern China: spatial distribution, sources apportionment, and ecological risk assessment. Front. Environ. Sci. 9. doi: 10.3389/fenvs.2021.755873
Wu J., Rong S., Wang M., Lu R., Liu J. (2022). Environmental quality and ecological risk assessment of heavy metals in the zhuhai coast, China. Front. Mar. Sci. 9. doi: 10.3389/fmars.2022.898423
Xu X., Cao Z., Zhang Z., Li R., Hu B. (2016). Spatial distribution and pollution assessment of heavy metals in the surface sediments of the bohai and yellow seas. Mar. pollut. Bull. 110 (1), 596–602. doi: 10.1016/j.marpolbul.2016.05.079
Zhao G., Ye S., Yuan H., Ding X., Wang J. (2017). Surface sediment properties and heavy metal pollution assessment in the pearl river estuary, China. Environ. Sci. pollut. Res. 24 (3), 2966–2979. doi: 10.1007/s11356-016-8003-4
Keywords: trace element, Black Sea, sediments, contamination factor, PCA
Citation: Bucşe A, Pârvulescu OC, Vasiliu D, Rădulescu F, Lupaşcu N and Ispas BA (2024) Spatial distribution of trace elements and potential contamination sources for surface sediments of the North-Western Black Sea, Romania. Front. Mar. Sci. 10:1310164. doi: 10.3389/fmars.2023.1310164
Received: 09 October 2023; Accepted: 27 December 2023;
Published: 12 January 2024.
Edited by:
Wilson Machado, Fluminense Federal University, BrazilReviewed by:
Mohamed Mohsen, Jimei University, ChinaMeng Chuan Ong, University of Malaysia Terengganu, Malaysia
Copyright © 2024 Bucşe, Pârvulescu, Vasiliu, Rădulescu, Lupaşcu and Ispas. This is an open-access article distributed under the terms of the Creative Commons Attribution License (CC BY). The use, distribution or reproduction in other forums is permitted, provided the original author(s) and the copyright owner(s) are credited and that the original publication in this journal is cited, in accordance with accepted academic practice. No use, distribution or reproduction is permitted which does not comply with these terms.
*Correspondence: Oana Cristina Pârvulescu, oana.parvulescu@yahoo.com; Dan Vasiliu, dan.vasiliu@geoecomar.ro