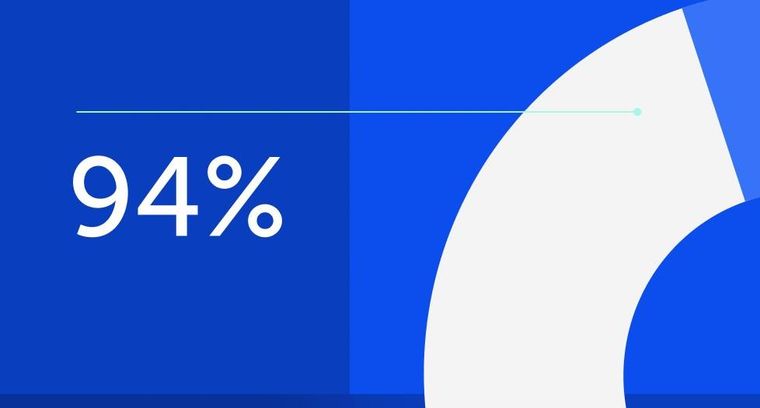
94% of researchers rate our articles as excellent or good
Learn more about the work of our research integrity team to safeguard the quality of each article we publish.
Find out more
BRIEF RESEARCH REPORT article
Front. Mar. Sci., 12 December 2023
Sec. Aquatic Physiology
Volume 10 - 2023 | https://doi.org/10.3389/fmars.2023.1306130
Reports have shown an increase in the use of disinfectants in wastewater treatment plants, prompted by the detection of residual viruses in sewage. However, the release of disinfection byproducts (DBPs) in final effluents has raised concerns about their potential adverse effects, such as endocrine disruption, on aquatic environments. Despite these concerns, few studies have examined the endocrine-disrupting effects of DBPs on fish, which may be vulnerable to DBPs. The aim of this case study was to investigate the endocrine-disrupting properties of four commonly formed DBPs: chloroiodomethane (CIM), dibromochloromethane (DBCM), bromodichloromethane (BDCM), and trichloroacetic acid (TCA) on the estrogen receptor-α in zebrafish (zERα). The results indicated that all four DBPs have high anti-estrogenic activity against zERα; with CIM, BDCM, DBCM, and TCA yielding 80.8%, 78.4%, 49.0%, and 64.1% anti-estrogenic effects on zERα, respectively. Moreover, all DBPs demonstrated negligible estrogenic effects on zERα. Our study sheds new light on the adverse effects of DBPs, particularly the endocrine-disrupting activity of CIM, which, as part of the dihalomethanes group, has received limited research attention in the past. This study shows the molecular interactions in terms of the endocrine disruption of DBP on zERα, warranting further studies to understand the overall impact of fish in affected aquatic ecosystems.
Since the emergence of the rampant Severe Acute Respiratory Syndrome Coronavirus 2 (SARS-CoV-2) pandemic, the frequent detection of coronavirus in untreated wastewater has become a widespread public concern (Bivins et al., 2020; Kataki et al., 2021) as viruses can remain infectious in faeces for an extended period of time (Casanova et al., 2009). As a result, disinfectant usage was increased to inactivate viruses in public facilities, hospitals, and wastewater treatment plants (Dewey et al., 2021). In fact, the disinfectant market grew significantly by 17.2% from 2019 to 2020 (Klemeš et al., 2021) as efforts to manage the pandemic intensified. Chlorine-based disinfectants were primarily implemented, as chlorine rapidly reacts with three chemical moieties in the viral protein, namely cysteine, tyrosine, and tryptophan amino acid residues (Noss et al., 1986; García-Ávila et al., 2020; Kály-Kullai et al., 2020; WHO, 2020). These disinfectants make up 16% of the disinfectants used, according to the United States Environmental Protection Agency (EPA) (https://cfpub.epa.gov/wizards/disinfectants/).
Apart from the desired disinfecting features, chlorine reacts with natural organic matter (NOM) or inorganic compounds, such as bromide and iodide, to form halogenated disinfection byproducts (DBPs) (Allard et al., 2015; Li et al., 2021). Halomethanes (HMs) and haloacetic acids (HAAs) are representative DBP groups derived from chlorine-based disinfectants. Trihalomethanes (THMs) and dihalomethanes (DHMs) are halogen-substituted carbon compounds with the molecular formula CHnXn, where X represents a halogen, such as fluorine, bromine, iodine, or chlorine (Waller et al., 1998). HAAs are formed by monochloramination with NOMs in water (Padhi et al., 2019). Both HMs, especially THMs and HAAs, pose carcinogenic risks and cause adverse reproductive outcomes in humans (Kujlu et al., 2020).
DBPs discharged from WWTPs directly into aquatic environments are likely to affect organisms such as fish. Many studies on endocrine disruption have focused on human reproductive defects (Ahn and Jeung, 2023; Interdonato et al., 2023). Whereas toxicological data on fish have also included histological as well as biochemical adverse effects (Lata et al., 2023). Regarding the endocrine disruption of DBPs, some studies have investigated the effects of DBPs in fish (Chaves et al., 2020; Wang et al., 2022), including endocrine disrupting effects on medaka fish estrogen receptor α (Sui et al., 2022). However, limited information exists on their effects on zebrafish estrogen receptor α (zERα) (Lee et al., 2023). Thus, this study focused on investigating the endocrine-disrupting effects of DBPs using a zERα-transfected model system. Danio rerio (zebrafish) is considered a model organism for ecotoxicological investigations as a bioindicator of environmental pollution (Dai et al., 2014). The zebrafish’s high fecundity, fast embryonic development and conserved neuroendocrine system have made it a powerful model for testing endocrine disruptors (Löhr and Hammerschmidt, 2011). Although different sensitivities for endocrine-disrupting chemicals can appear between in vivo and in vitro tests, their relative estrogenic potency is not separated by a pattern (Kolle et al., 2010). In fact, in vivo and in vitro tests have the same estrogenic activities according to the relative dose response. Furthermore, in vitro tests can more accurately demonstrate the response of a target receptor, assayed using the reporter gene assay, and provide an assessment of early targeted molecular responses caused by estrogenic and anti-estrogenic activities (Park et al., 2022). Therefore, this study adopted the in vitro reporter gene assay for the zERα-transfected model. Even though the employed reporter gene assay cannot be used to conclude on the overall impact on fish in the environment, it does show preliminary insights into the potential endocrine-disrupting effects of DBPs on a molecular level. Therefore, this study aimed to assess the in vitro potential effects of four common DBPs on zERα as an indication of endocrine disruption at a molecular level.
Chemicals were purchased from the following vendors: liquid types of chloroiodomethane (242861; Sigma-Aldrich, Germany; CIM), dibromochloromethane (206326; Sigma-Aldrich, Germany; DBCM), bromodichloromethane (139181; Sigma-Aldrich, Germany; BDCM), and crystalline type of trichloroacetic acid (T6399; Sigma-Aldrich, Germany; TCA). Based on the M-clarity program of Sigma Aldrich, CIM, DBCM, and BDCM obtained a quality level of 100, and TCA obtained a quality level of 200. Quality levels of 100 or 200 mean the ISO 9001. All the chemicals had high purity levels: CIM, DBCM, and BDCM were > 97.0% purity; TCA was American Chemical Society level purity (> 99.0%). The chemicals were dissolved in DMSO (dimethyl sulfoxide, D8418, > 99.9% purity) according to experimental methods detailed by Kim et al. (2020). Given its tight linkage with the result, the integrity of the chemical purity was ensured for both cell viability and endocrine-disrupting effects assessing experiments.
The cytotoxic effects of the DBPs were assessed with the cell counting kit-8 colourimetric assay (HY-K0301, MedChemExpress, USA; CCK-8) using HEK293 normal cells. HEK293 cell (ATCC#CRL-1573) line present in this study was purchased from the American Type Culture Collection (ATCC). This method was selected considering the advancement of alternative methods to animal testing. Additional advantages to usage include high protein production efficiency, easy transfection, rapid growth, and easier maintenance compared to other cell lines (Thomas and Smart, 2005). A competitive assay with E2 was performed for all chemicals tested in the estrogenic response reaction. Additionally, in the anti-estrogenic response experiments, 4-hydroxytamoxifen (4-HT) was used as a positive control. We conducted ligand-receptor binding competition assays by adding varying concentrations of 4-HT or DBPs depending on a 96-well plate in the presence of E2 (at a concentration where it binds 100% to the ER). To normalize the toxicity response, each well of a 96-well plate was seeded with 1 × 104 cells (n = 6, the experiment was duplicated, and three times reproducible tests were performed) and incubated in Dulbecco’s Modified Eagle Medium (DMEM) in a 37°C chamber containing 5% CO2 for 24 h. DBPs were dissolved in half-logarithmic (3.16-fold) serial dilutions in DMSO, resulting in the following exposure concentrations: 10-4.8 to 10-2.3 M for CIM, DBCM, and BDCM, and 10-8.8–10-4.5 M for TCA. DBPs were inoculated into fresh media with a final DMSO concentration of 0.5% (v/v) (n = 6). The negative control was prepared without DBPs in 0.5% DMSO. After 24 h of chemical exposure, the growth medium was aspirated, and CCK-8 was added to a 10-fold dilution with DMEM. After 2 h of incubation in 5% CO2 at 37°C, cell viability was assessed by spectrophotometric analysis at 450 nm using a TECAN microplate reader (TECAN, Männedorf, Switzerland). Cytotoxicity was expressed as a percentage of the responses, calculated using the following equation:
where V is the percentage response of cytotoxicity, OD450, s is the absorbance of the sample exposed to DBPs at 450 nm, and OD450, d is the absorbance of the sample exposed to 0.5% DMSO (negative control) at 450 nm.
Both DBP-induced estrogenic and anti-estrogenic activities were assessed using the Luciferase Reporter Assay System (E1500, Promega, Germany) in HEK293-ERE-zEsr1 cells. Cells at a concentration of 1 × 104 cells per well were seeded in a 96-well plate and incubated in DMEM with puromycin and neomycin (1 μg/mL and 0.2 μg/mL) in a 37°C chamber with 5% CO2 for 24 h. The selective estrogen receptor agonist, 17β-estradiol (E2), was used as a reference (positive control) at concentrations ranging from 10-13 to 10-9 M. DBPs in estrogenic tests were dissolved in half-logarithmic (3.16-fold) serial dilutions in DMSO, resulting in the following exposure concentrations: from 10-14 to 10-4 M for CIM; from 10-7.8 to 10-3.3 M for DBCM and BDCM; and from 10-11.8 to 10-3.3 M for TCA. Similarly, 4-hydroxytamoxifen (4-HT), a selective anti-estrogen, was used as a reference at concentrations ranging from 10-9 to 10-6 M. E2 was also used as a relative estrogen agent against 4-HT at a fixed concentration of its 100% estrogenic activity (10-10 M) to demonstrate the competitive anti-estrogenic activity of 4-HT. DBPs in anti-estrogenic tests were dissolved in half-logarithmic (3.16-fold) serial dilutions with DMSO, resulting in the final exposure concentrations: from 10-8.4 to 10-3.9 M for CIM; from 10-7.8 to 10-3.3 M for DBCM and BDCM; and from 10-7.8 to 10-3.3 M for TCA.
After 24 h of incubation with 5% CO2 at 37°C, the growth medium was aspirated, and the cells were rinsed with 1X phosphate buffer saline (pH 7.4). Passive lysis buffer (E194A, Promega, Germany) was then added and gently mixed for 10 min. The prepared lysates were added, and luciferase activities were measured using a microplate reader (TECAN, Männedorf, Switzerland) at a relative luminescence unit with 3 s integration time and 1 s settling time. The percentage induction was calculated from the responses of the estrogen receptor against E2 using the following equation:
where I is the percentage induction, OD570, s is the absorbance of the sample exposed to DBPs at 570 nm, OD570, d is the absorbance of the sample exposed to 0.5% DMSO (negative control) at 570 nm, and OD570, E2 is the maximum induced absorbance by E2.
To verify the endocrine-disrupting effects in the model cell line, a cytotoxicity test was performed before the reporter gene assay. As a result, CIM and DBCM were found to be cytotoxic to HEK293 cells (Figures 1A, B) at an LC50 of 34.1 μM (CIM) and 192.0 μM (DBCM), respectively. The response percentage exponentially decreased with increasing log CIM concentrations (Figure 1A). Specifically, 63.4 ± 7.2% of cells were nonviable at the highest inoculation concentration. Cells remained viable with no response to increasing DBCM concentrations; however, 62.4 ± 4.3% cell death was observed at the highest concentration (Figure 1B). BDCM and TCA did not show dose-dependent response percentages (Figures 1C, D).
Figure 1 Cytotoxicity of HEK 293 (human embryonic kidney 293) cells for the target DBPs, (A) CIM, (B) DBCM, (C) BDCM, and (D) TCA. Data points represent mean percentage response ± standard deviation (SD) (n = 6).
The dose-response curves of the estrogenic and anti-estrogenic responses to zERα were plotted in Figures 2A–H. The estrogenic activities of all DBPs were lower than 20% in zERα. The maximum estrogenic effects of CIM, DBCM, BDCM, and TCA were 10.4 ± 1.7%, 12.0 ± 3.1%, 8.74 ± 2.3%, and 16.9 ± 5.7%, respectively (Figures 2A–D). Conversely, all DBPs showed anti-estrogenic activities against zERα. CIM (80.8% ± 6.9% at 125 μM) and BDCM (78.4 ± 7.6% at 500 μM) showed prominent inhibition of E2 (Figures 2E, G). In contrast, DBCM (49.0 ± 4.4% at 500 μM) and TCA (64.1 ± 15.0% at 15.85 μM) showed relatively weak anti-estrogenic responses (Figures 2F, H). The EC50 of E2 (estrogen) was 0.1 nM, calculated from the 100% induction value of E2; that of 4-HT (anti-estrogen) was 0.003 nM (Figure 2).
Figure 2 Estrogenic response (%) of CIM (A), DBCM (B), BDCM (C), and TCA (D) with positive reference estrogenic activity material, black line (E2). Anti-estrogenic response (%) of CIM (E), DBCM (F), BDCM (G), and TCA (H) with reference anti-estrogenic material, black line (4-HT). Data points represent average estrogenic response percentage response ± standard deviation (SD) (n = 6).
Excessive disinfectant usage can induce DBP over-generation. However, the concentrations of DBPs detected in the environment to date have been below the cytotoxic level (Tables 1, 2). Moreover, the concentrations of DBPs in WWTPs (wastewater treatment plants) and drinking water were significantly lower than the WHO guideline values (Tables 1, 2). The guideline values of DBCM and BDCM were 32.5- and 43.3-fold lower than the lowest treatment concentrations, respectively (CIM data is absent). Although the WHO guideline value for TCA is 1,190-fold higher than the lowest treatment concentrations, a negligible cytotoxic effect from the environmental TCA exposure level can be derived from the cytotoxicity test results. No cell death was observed with exposure to BDCM and TCA (Figures 1C, D), which aligns with a previous report on the non-toxic effects of BDCM and TCA on the Sheepshead minnow, Cyprinodon variegatus (Fisher et al., 2014). Nevertheless, the cytotoxicity results suggest that attention should be paid to the endocrine-disrupting effects of DBPs in aquatic organisms. The escalating concentrations of DBPs in the ecosystem and their potential cytotoxic consequences should not be underestimated, especially considering the projected 1.7-fold increase in the demand for disinfectants (Ahuja, 2022).
Table 1 Concentrations of DBPs in WWTPs, drinking water, recommendations, and adverse effect levels.
Aside from receptor-mediated inhibition, other mechanisms of anti-estrogenic responses include protein synthesis or enzyme inhibition (Fic et al., 2014). However, the study by Kim et al. (2020) reported that the anti-estrogenic activity of DBPs was positively correlated with E2 treatment of the human estrogen receptor. Thus, the anti-estrogenic responses from the current study support estrogen receptor-mediated reactions by small molecules, such as DBPs. Estrogens interact with the ERs (estrogen receptors), while anti-estrogens (DBPs in this study) inhibit normal ER-mediated processes during ovarian development in Oryzias latipes (Kawahara and Yamashita, 2000). Specifically, these anti-estrogenic activities could adversely affect fish-sex ratios by decreasing the ratio between females and undifferentiated fish (Andersen et al., 2004). Therefore, DBP overproduction could inevitably contribute to endocrine disruption in freshwater organisms within the receiving environment.
THMs and HAAs are commonly studied in various aquatic animals, such as zebrafish, the most prominently used lower vertebrate model. THMs result in developmental aberrations, embryonic DNA damage, and mortality, whereas HAAs induce embryonal malformations (Teixidó et al., 2015). Among halogenated DBPs, iodinated forms are more toxic than chlorinated and brominated forms (Boorman, 1999). However, there are few reports of endocrine disruption by iodinated DHM (CIM in this study) in both human and animal studies because DHMs are not routinely detected in environments (Supplementary Figure 1). CIM may be as toxicologically relevant as regulated THMs and HAAs (Linge et al., 2013; Richardson and Postigo, 2015; Padhi et al., 2019). This study also found that CIM exposure resulted in the most substantial estrogenic inhibition in zERα. Therefore, environmental exposure to CIM may be more prominent, given the surge in chlorine-based disinfectants. This study therefore demonstrates the adverse consequences of DBP-induced endocrine disruptions in zERα using reporter gene responses. However, the results of this study cannot be used to predict the responses in living organisms, as it focuses solely on ligand-receptor competitive interactions between DBPs and the ligand of zERα. Therefore, further intensive in vivo tests are needed to inquire into DBPs, particularly CIM, which have less significant cytotoxicity at the current environmental concentrations but have the most concerning anti-estrogenic effects.
In the present study, the data shows that the tested DBPs, CIM, DBCM, BDCM, and TCA, had anti-estrogenic activities in zERα. Specifically, CIM, which has an 80.8% anti-estrogenic effect in zERα needs to be investigated in depth, alongside other DBPs. Although the reporter gene assay in this study may not entirely reflect the in vivo test results, it has been reported that response values induced by DBPs can indirectly represent estrogenic mimic effects (Ihara et al., 2015).
The raw data supporting the conclusions of this article will be made available by the authors, without undue reservation.
The cell lines utilized in this study were procured from the American Type Culture Collection (ATCC), designated exclusively for laboratory research purposes. They are not intended for therapeutic use in animals or humans, human or animal consumption, or diagnostic applications. Consequently, ethics approval was deemed unnecessary for this study.
SAL: Data curation, Formal analysis, Investigation, Methodology, Software, Validation, Visualization, Writing – original draft. CSR: Investigation, Writing – review & editing. CGP: Resources, Writing – review & editing. HC: Investigation, Resources, Writing – review & editing. IJ: Investigation, Writing – review & editing. CBP: Investigation, Writing – review & editing. ME: Supervision, Writing – original draft, Writing – review & editing. YJK: Conceptualization, Data curation, Funding acquisition, Project administration, Supervision, Writing – review & editing.
The author(s) declare financial support was received for the research, authorship, and/or publication of this article. This research was supported by the National Research Council of Science & Technology (NST) grant by the Strategies for Establishing Global Research Networks (NO. Global-23-004).
We gratefully thank Ian Choi (KIST Europe) for her assistance in the laboratory.
The authors declare that the research was conducted in the absence of any commercial or financial relationships that could be construed as a potential conflict of interest.
All claims expressed in this article are solely those of the authors and do not necessarily represent those of their affiliated organizations, or those of the publisher, the editors and the reviewers. Any product that may be evaluated in this article, or claim that may be made by its manufacturer, is not guaranteed or endorsed by the publisher.
The Supplementary Material for this article can be found online at: https://www.frontiersin.org/articles/10.3389/fmars.2023.1306130/full#supplementary-material
Ahn C., Jeung E. B. (2023). Endocrine-disrupting chemicals and disease endpoints. Int. J. Mol. Sci. 24 (6), 5342. doi: 10.3390/ijms24065342
Ahuja K. B. S. (2022). Surface Disinfectants Market Size - By Product (Chemical-based {Quaternary Ammonium Compounds, Hypochlorite, Alcohols, Aldehydes, Peroxides}, Bio-based), Form (Liquids, Wipes, Spray), End-user, Distribution Channel & Forecast 2021- 2027 (Delaware 19975, USA: Surface Disinfectants Market (GMI4805); Global Market Insight Inc).
Allard S., Tan J., Joll C. A., Von Gunten U. (2015). Mechanistic study on the formation of Cl-/Br-/I-trihalomethanes during chlorination/chloramination combined with a theoretical cytotoxicity evaluation. Environ. Sci. Technol. 49, 11105–11114. doi: 10.1021/acs.est.5b02624
Andersen L., Kinnberg K., Holbech H., Korsgaard B., Bjerregaard P. (2004). Evaluation of a 40-day assay for testing endocrine disrupters: effects of an anti-estrogen and an aromatase inhibitor on sex ratio and vitellogenin concentrations in juvenile zebrafish (Danio rerio). Fish Physiol. Biochem. 30, 257–266. doi: 10.1007/s10695-005-8246-3
Bivins A., Greaves J., Fischer R., Yinda K. C., Ahmed W., Kitajima M., et al. (2020). Persistence of SARS-CoV-2 in water and wastewater. Environ. Sci. Technol. Lett. 7, 937–942. doi: 10.1021/acs.estlett.0c00730
Boorman G. A. (1999). Drinking water disinfection byproducts: review and approach to toxicity evaluation. Environ. Health Perspect. 107, 207–217. doi: 10.1289/ehp.99107s1207
Casanova L., Rutala W. A., Weber D. J., Sobsey M. D. (2009). Survival of surrogate coronaviruses in water. Water Res. 43, 1893–1898. doi: 10.1016/j.watres.2009.02.002
Chaves R. S., Guerreiro C. S., Cardoso V. V., Benoliel M. J., Santos M. M. (2020). Toxicological assessment of seven unregulated drinking water Disinfection By-products (DBPs) using the zebrafish embryo bioassay. Sci. Total Environ. 742, 140522. doi: 10.1016/j.scitotenv.2020.140522
Dai Y. J., Jia Y. F., Chen N., Bian W. P., Li Q. K., Ma Y. B., et al. (2014). Zebrafish as a model system to study toxicology. Environ. Toxicol. Chem. 33, 11–17. doi: 10.1002/etc.2406
Dewey H. M., Jones J. M., Keating M. R., Budhathoki-Uprety J. (2021). Increased use of disinfectants during the COVID-19 pandemic and its potential impacts on health and safety. ACS Chem. Health Saf. 2022 29 (1), 27–38. doi: 10.1021/acs.chas.1c00026
Fic A., Žegura B., Gramec D., Mašič L. P. (2014). Estrogenic and androgenic activities of TBBA and TBMEPH, metabolites of novel brominated flame retardants, and selected bisphenols, using the XenoScreen XL YES/YAS assay. Chemosphere 112, 362–369. doi: 10.1016/j.chemosphere.2014.04.080
Fisher D., Yonkos L., Ziegler G., Friedel E., Burton D. (2014). Acute and chronic toxicity of selected disinfection byproducts to Daphnia magna, Cyprinodon variegatus, and Isochrysis galbana. Water Res. 55, 233–244. doi: 10.1016/j.watres.2014.01.056
García-Ávila F., Valdiviezo-Gonzales L., Cadme-Galabay M., Gutiérrez-Ortega H., Altamirano-Cárdenas L., Zhindón-Arévalo C., et al. (2020). Engineering, E. Considerations on water quality and the use of chlorine in times of SARS-CoV-2 (COVID-19) pandemic in the community. Case Stud. Chem. Environ. Eng. 2, 100049. doi: 10.1016/j.cscee.2020.100049
Hladik M. L., Focazio M. J., Engle M. (2014). ). Discharges of produced waters from oil and gas extraction via wastewater treatment plants are sources of disinfection by-products to receiving streams. Sci. Total Environ. 466, 1085–1093. doi: 10.1016/j.scitotenv.2013.08.008
Ihara M., Kitamura T., Kumar V., Park C.-B., Ihara M., Lee S.-J., et al. (2015). Evaluation of estrogenic activity of wastewater: comparison among in vitro ERα reporter gene assay, in vivo vitellogenin induction, and chemical analysis. Env. Sci. Technol. 49 (10), 6319–6326. doi: 10.1021/acs.est.5b01027
Interdonato L., Siracusa R., Fusco R., Cuzzocrea S., Di Paola R. (2023). Endocrine disruptor compounds in environment: Focus on women’s reproductive health and endometriosis. Int. J. Mol. Sci. 24 (6), 5682. doi: 10.3390/ijms24065682
Kály-Kullai K., Wittmann M., Noszticzius Z., Rosivall L. (2020). Can chlorine dioxide prevent the spreading of coronavirus or other viral infections? Medical hypotheses. Physiol. Int. 107, 1–11. doi: 10.1556/2060.2020.00015
Kataki S., Chatterjee S., Vairale M. G., Sharma S., Dwivedi S. K. (2021). Concerns and strategies for wastewater treatment during COVID-19 pandemic to stop plausible transmission. Resour. Conserv. Recycl. 164, 105156. doi: 10.1016/j.resconrec.2020.105156
Kawahara T., Yamashita I. (2000). ). Estrogen-independent ovary formation in the medaka fish, Oryzias latipes. Zoolog. Sci. 17, 65–68. doi: 10.2108/zsj.17.65
Kim D.-H., Park C. G., Kim Y. J. J. C. (2020). Characterizing the potential estrogenic and androgenic activities of two disinfection byproducts, mono-haloacetic acids and haloacetamides, using in vitro bioassays. Chemosphere 242, 125198. doi: 10.1016/j.chemosphere.2019.125198
Klemeš J. J., Jiang P., Van Fan Y., Bokhari A., Wang X.-C. (2021). COVID-19 pandemics Stage II–energy and environmental impacts of vaccination. Renew. Sustain. Energy Rev. 150, 111400. doi: 10.1016/j.rser.2021.111400
Kolle S., Kamp H., Huener H.-A., Knickel J., Verlohner A., Woitkowiak C., et al. (2010). ). In house validation of recombinant yeast estrogen and androgen receptor agonist and antagonist screening assays. Toxicol. In Vitro 24, 2030–2040. doi: 10.1016/j.tiv.2010.08.008
Kujlu R., Mahdavianpour M., Ghanbari F. (2020). Multi-route human health risk assessment from trihalomethanes in drinking and non-drinking water in Abadan, Iran. Environ. Sci. pollut. Res. 27, 42621–42630. doi: 10.1007/s11356-020-09990-9
Lata P., Hajam Y. A., Kumar R., Rai S. (2023). ““Endocrine-disrupting activity of xenobiotics in aquatic animals”,” in Xenobiotics in Aquatic Animals. Eds. Rather M. A., Amin A., Hajam Y. A., Jamwal A., Ahmad I. (Singapore: Springer), 67–99. doi: 10.1007/978-981-99-1214-8_2
Lee S. A., Park C. G., Esterhuizen M., Choi I., Ryu C. S., Yang J. H., et al. (2023). Investigating endocrine disrupting impacts of nine disinfection byproducts on human and zebrafish estrogen receptor alpha. Front. Biosci. 28 (3), 48. doi: 10.31083/j.fbl2803048
Li Z., Song G., Bi Y., Gao W., He A., Lu Y., et al. (2021). Occurrence and distribution of disinfection byproducts in domestic wastewater effluent, tap water, and surface water during the SARS-CoV-2 pandemic in China. Environ. Sci. Technol. 55, 4103–4114. doi: 10.1021/acs.est.0c06856
Linge K. L., Blythe J. W., Busetti F., Blair P., Rodriguez C., Heitz A. (2013). Formation of halogenated disinfection by-products during microfiltration and reverse osmosis treatment: implications for water recycling. Sep. Purif. Technol. 104, 221–228. doi: 10.1016/j.seppur.2012.11.031
Löhr H., Hammerschmidt M. (2011). Zebrafish in endocrine systems: Recent advances and implications for human disease. Annu. Rev. Physiol. 73, 183–211. doi: 10.1146/annurev-physiol-012110-142320
Noss C. I., Hauchman F. S., Olivieri V. P. (1986). Chlorine dioxide reactivity with proteins. Water Res. 20, 351–356. doi: 10.1016/0043-1354(86)90083-7
Padhi R., Subramanian S., Satpathy K. (2019). Formation, distribution, and speciation of DBPs (THMs, HAAs, ClO2–, andClO3–) during treatment of different source water with chlorine and chlorine dioxide. Chemosphere 218, 540–550. doi: 10.1016/j.chemosphere.2018.11.100
Park C. G., Singh N., Ryu C. S., Yoon J. Y., Esterhuizen M., Kim Y. J. (2022). Species differences in response to binding interactions of bisphenol A and its analogs with the modeled estrogen receptor 1 and in vitro reporter gene assay in human and zebrafish. Environ. Toxicol. Chem. 41, 2431–2443. doi: 10.1002/etc.5433
Richardson S. D., Postigo C. (2015). “Safe drinking water? Effect of wastewater inputs and source water impairment and implications for water reuse,” in Wastewater Reuse and Current Challenges (Berlin 14197, Germany: Springer), 155–182.
Richardson S. D., Thruston A. D. Jr., Krasner S. W., Weinberg H. S., Miltner R. J., Schenck K. M., et al. (2008). Integrated disinfection by-products mixtures research: comprehensive characterization of water concentrates prepared from chlorinated and ozonated/postchlorinated drinking water. J. Toxicol. Environ. Health A 71, 1165–1186. doi: 10.1080/15287390802182417
Sui S., Liu H., Yang X. (2022). Research progress of the endocrine-disrupting effects of disinfection byproducts. J. Xenobiot. 12 (3), 145–157. doi: 10.3390/jox12030013
Teixidó E., Piqué E., Gonzalez-Linares J., Llobet J. M., Gómez-Catalán J. (2015). Developmental effects and genotoxicity of 10 water disinfection by-products in zebrafish. J. Water Health 13, 54–66. doi: 10.2166/wh.2014.006
Thomas P., Smart T. G. (2005). HEK293 cell line: a vehicle for the expression of recombinant proteins. J. Pharmacol. Toxicol. Methods 51 (3), 187–200. doi: 10.1016/j.vascn.2004.08.014
Waller K., Swan S. H., DeLorenze G., Hopkins B. (1998). Trihalomethanes in drinking water and spontaneous abortion. J. Epidemiol. 9, 134–140. doi: 10.1097/00001648-199903000-00026
Wang Y., Liu H., Yang X., Wang L. (2022). Aquatic toxicity and aquatic ecological risk assessment of wastewater-derived halogenated phenolic disinfection byproducts. Sci. Total Environ. 809, 151089. doi: 10.1016/j.scitotenv.2021.151089
World Health Organization (WHO). (2000). Disinfectants and Disinfectant By-Products-Environmental Health Criteria 216. Available at: https://wedocs.unep.org/20.500.11822/29525.
World Health Organization (WHO). (2020). Water, sanitation, hygiene, and waste management for the COVID-19 virus: interim guidance, 19 March 2020 (Geneva 1211, Switzerland: World Health Organization).
Keywords: disinfection byproducts, endocrine disruption, zebrafish estrogen receptor α, chloroiodomethane (CIM), dibromochloromethane (DBCM), bromodichloromethane (BDCM), trichloroacetic acid (TCA)
Citation: Lee S-A, Ryu CS, Park CG, Cho H, Jun I, Park C-B, Esterhuizen M and Kim YJ (2023) Investigating the endocrine disruption effects of four disinfection byproducts on zebrafish estrogen receptor-α. Front. Mar. Sci. 10:1306130. doi: 10.3389/fmars.2023.1306130
Received: 09 October 2023; Accepted: 28 November 2023;
Published: 12 December 2023.
Edited by:
Carlo C. Lazado, Norwegian Institute of Food, Fisheries and Aquaculture Research (Nofima), NorwayReviewed by:
Kathryn Hassell, RMIT University, AustraliaCopyright © 2023 Lee, Ryu, Park, Cho, Jun, Park, Esterhuizen and Kim. This is an open-access article distributed under the terms of the Creative Commons Attribution License (CC BY). The use, distribution or reproduction in other forums is permitted, provided the original author(s) and the copyright owner(s) are credited and that the original publication in this journal is cited, in accordance with accepted academic practice. No use, distribution or reproduction is permitted which does not comply with these terms.
*Correspondence: Maranda Esterhuizen, bWFyYW5kYS5lc3Rlcmh1aXplbkBoZWxzaW5raS5maQ==
Disclaimer: All claims expressed in this article are solely those of the authors and do not necessarily represent those of their affiliated organizations, or those of the publisher, the editors and the reviewers. Any product that may be evaluated in this article or claim that may be made by its manufacturer is not guaranteed or endorsed by the publisher.
Research integrity at Frontiers
Learn more about the work of our research integrity team to safeguard the quality of each article we publish.