- 1Marine Science Program, Biological and Environmental Science and Engineering Division, King Abdullah University of Science and Technology (KAUST), Thuwal, Saudi Arabia
- 2KAUST Red Sea Research Center, King Abdullah University of Science and Technology, Thuwal, Saudi Arabia
- 3GEOMAR Helmholtz Centre for Ocean Research, Kiel, Germany
- 4Earth Science and Engineering Program, Physical Science and Engineering Division, King Abdullah University of Science and Technology (KAUST), Thuwal, Saudi Arabia
Octocorals (Cnidaria: Anthozoa) have a global distribution and form benthic assemblages along the depth gradient, from shallow to deep waters. They often occur below SCUBA diving limits, where they can become dominant habitat builders and aggregate different taxa. During a cruise in February 2023, one octocoral specimen was collected at 1453 m depth at Kebrit Deep, in the northern Saudi Arabian Red Sea axis, an area with extremely high temperature and salinity profiles at depth. Morphological analysis coupled with DNA barcoding using two mitochondrial markers (COI and mtMuts), revealed that the coral belongs to Acanthogorgia, a genus of azooxanthellate octocorals known to occur from 3 to 2300 m depths in cold, temperate and tropical waters. In the Red Sea, the genus was previously only known from shallower waters. Hence, we report the deepest record of the genus Acanthogorgia from the warm and saline Red Sea basin. This finding provides novel insights on deep-water octocoral diversity in the Red Sea, a still scantily explored area of the world, while emphasizing the need for further explorations at depth.
1 Introduction
Octocorals (Cnidaria: Anthozoa) occur throughout the world’s oceans and have been reported from cold, temperate and tropical waters (Fabricius and Alderslade, 2001). They are considered foundation species for marine animal forests (MAFs) (Orejas et al., 2022), supporting many marine organisms (Krieger and Wing, 2002; Cairns, 2007; Schweitzer and Stevens, 2019; Tsounis et al., 2020). Their three-dimensional colony structure provides habitat, shelter and food for a variety of other invertebrate and fish taxa (Buhl-Mortensen et al., 2010; Sànchez, 2016). In particular, given their structural complexity, they provide a substrate for habitat-specialist taxa, such as the pygmy seahorses (Lourie and Randall, 2003), and can function as a nursery for fish larvae (Baillon et al., 2012). While octocorals have been observed all along the depth gradient in different areas of the world (Daly et al., 2007), their highest diversity has been reported in mesophotic and deep-sea ecosystems (Cairns, 2007; Sánchez et al., 2021).
The octocoral genus Acanthogorgia Gray, 1857 in the family Paramuriceidae Bayer, 1956, currently comprises 57 nominal species (Kükenthal, 1908; McFadden et al., 2022). Species of Acanthogorgia form fan-shaped and densely branched colonies on slopes, walls and ridges exposed to strong currents (Fabricius and Alderslade, 2001). Colonies are azooxanthellate and often brightly colored, varied in growth form, and may reach sizes of a meter in height (Fabricius and Alderslade, 2001; McFadden et al., 2022). The genus is found in cold, temperate, and tropical areas globally. The presence of Acanthogorgia corals was reported from the Caribbean (Sánchez et al., 2019), the Mediterranean Sea (Sartoretto and Zibrowius, 2018), and the Indo-Pacific region (Rowley et al., 2019; Sánchez et al., 2019) including the Red Sea (Benayahu et al., 2019). Although previous studies indicated the occurrence of octocorals belonging to this genus from 3 to 1215 m in the Indo-Pacific region (Federated States of Micronesia; Rowley et al., 2019), the deepest global observation of Acanthogorgia specimens was reported from the US West Coast region at 2301 m (Everett et al., 2022). Similarly, the deepest known specimen in the Mediterranean basin was observed and sampled at 2180 m (Sartoretto and Zibrowius, 2018).
The Red Sea has an average depth of 450 m (Shaked and Genin, 2011) and reaches a maximum of around 2860 m at Suakin Deep in its central axis (Augustin et al., 2016). Often referred to as a young ocean basin (Purkis et al., 2012; Augustin et al., 2021), its isolation and geological history make the Red Sea an interesting setting to study patterns of marine biodiversity (Berumen et al., 2019a), especially considering that it is characterized by high water temperatures and salinities all along the depth gradient (Roder et al., 2013; Manasrah et al., 2019). Recent opportunities for Red Sea deep-water explorations along the depth and latitudinal gradients, and resulting collections of benthic organisms, have significantly enhanced our knowledge of the occurrence, composition and diversity of cnidarian-dominated animal forests (Chimienti et al., 2022; Maggioni et al., 2022; Anker et al., 2023). Octocoral diversity research from the photic to the mesophotic zone has been carried out in the north of the Gulf of Aqaba (Benayahu et al., 2017a; Benayahu et al., 2017b), but a comparable sampling effort at depth in the Saudi Arabian Red Sea is still missing and octocoral diversity remains largely overlooked at the basin scale. While isolated studies have observed deep-water corals in the region (Qurban et al., 2020), the deepest sampling of octocorals was reported by Qurban et al. (2014) at a depth of 720 m, and included the collection of organisms identified as Acanthogorgia sp. as deep as 594 m. However, such colonies were only morphologically identified, and no molecular data is available for them.
Given the challenges in the identification of octocoral specimens in situ or solely based on macro-morphological characteristics (Fabricius and Alderslade, 2001), and the often unclear description of certain taxa (McFadden et al., 2010), DNA extraction and barcoding can aid the classification of these organisms (Berntson et al., 2001). Despite limited availability of species-level molecular data for Acanthogorgia in the literature, McFadden et al. (2006) found the genus to be paraphyletic based on the analysis of mitochondrial genes and comprising two distinct, and distantly related, clades. As such, the comparison of newly generated molecular data with previously published sequences allows to assign specimens to the correspondent genetic lineage.
In this study, we report the deepest record for the genus Acanthogorgia from the Red Sea based on recent sampling efforts in the basin. Additionally, we present the phylogenetic position of the collected specimen within the family Paramuriceidae as molecularly inferred through DNA barcoding using two mitochondrial loci, and a comparison with previously published representatives of this genus and of other 19 genera in the family.
2 Materials and methods
2.1 Sample collection
Deep-sea explorations of the seafloor occurred along the central axis of the Red Sea in February 2023 aboard the R/V Aegaeo, from the Hellenic Centre for Marine Research (HCMR, Greece). Sampling was carried out using a light work class remotely operated underwater vehicle (ROV) Max Rover Mark II, equipped with color CCD video cameras and a Hydrolek electro-hydraulic 5 function manipulator arm for sampling. Videos were georeferenced with a Trackpoint II USBL positioning system. Salinities and temperatures of the seawater were measured with a CTD (Conductivity, Temperature, Depth) sensor, attached to the ROV. During an ROV dive on the 11th of February 2023, octocoral colonies were observed and one was collected at 1453 m depth in the Kebrit Deep, northern Red Sea (22.4119°N, 37.6044°E) (Figure 1A) among several sponges (Figure 1B). The specimen was processed aboard the research vessel and the apical part of one colony branch was subsampled and fixed in 99% ethanol immediately after collection for subsequent molecular analyses. The rest of the colony was air-dried for subsequent morphological identification. In the Red Sea Research Center Laboratory at KAUST, the dry colony was photographed using a Nikon D7500 camera equipped with a Nikkor 18-55mm lens (Figure 1C). Images of the polyps and skeletal elements disposition and morphology were taken using a Leica M205A stereomicroscope with a Leica DMC 5004 camera (Figure 1D). Microscopical characterization of the sclerites was achieved using a Quattro S Environmental Scanning Electron Microscope (Thermo Fisher Scientific, Wilmington, USA) (Figure 1E) at KAUST Imaging Core Lab (Thuwal, Saudi Arabia). Sclerites preparation for SEM analyses was performed by subsampling a polyp and a small part of the axis from the dry colony and dissolving them in diluted sodium hypochlorite (NaClO) after rehydration in distilled water. Sclerites were then cleaned with distilled water and ethanol (EtOH) washes before being mounted on SEM stubs and coated with a 5-nm thick iridium layer through a Q150T S turbomolecular pumped coater (Quorum Technologies, Laughton, United Kingdom). The dry colony and ethanol-preserved sample are stored at the King Abdullah University of Science and Technology (KAUST, Saudi Arabia).
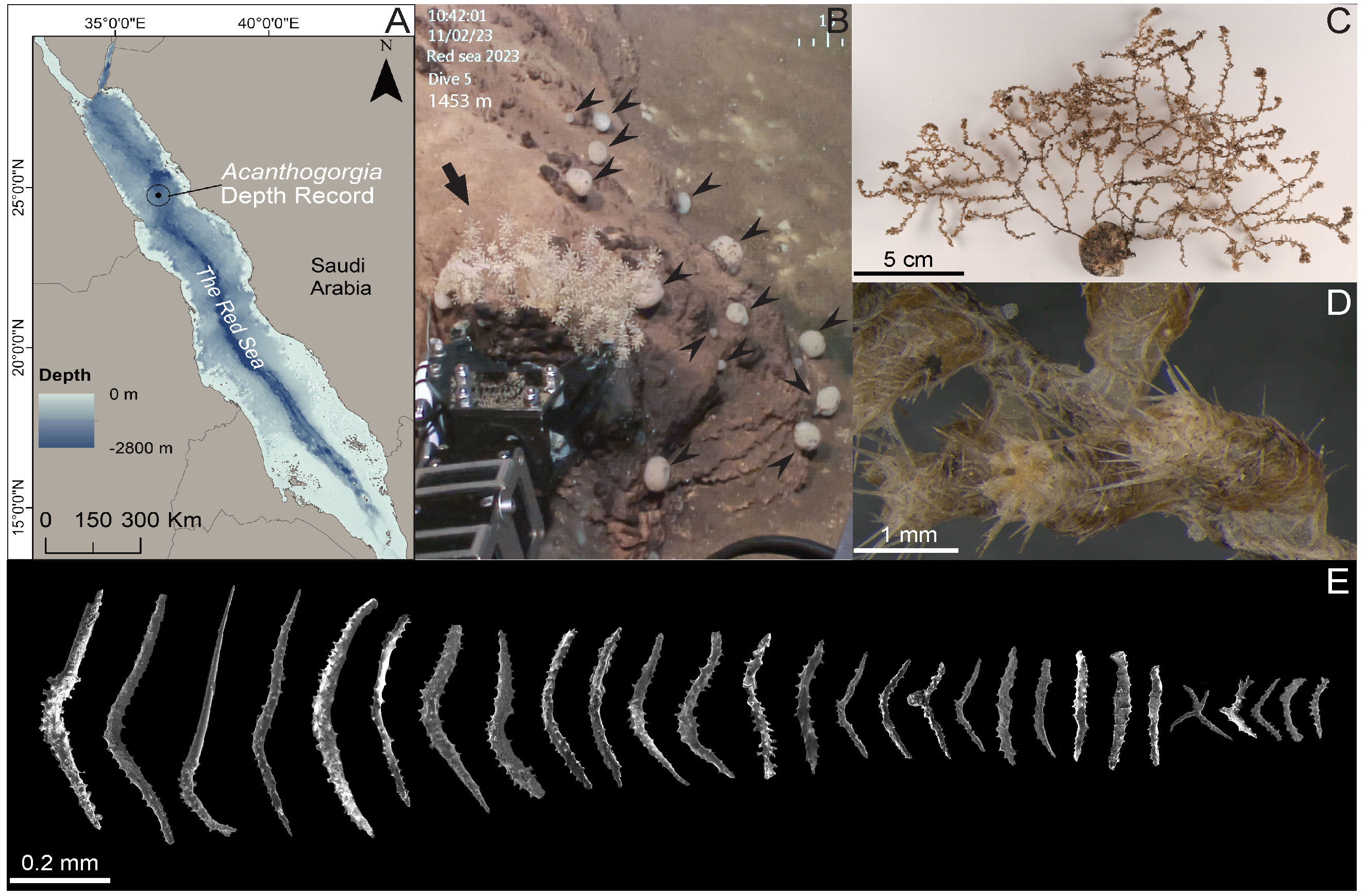
Figure 1 (A) Map of the Red Sea showing the sampling point (22.4119°N, 37.6044°E) (B) In situ picture extracted from ROV footage showing the specimen of Acanthogorgia (indicated by the arrow) at 1453 m depth surrounded by sponges (indicated by arrowheads) (C) The collected dry specimen overgrowing a sponge (D) Detail of polyp morphology and arrangement of skeletal elements and sclerites (E) SEM images showing the morphology of the sclerites.
2.2 Morphological identification
The octocoral colony was analyzed and identified to genus level at KAUST based on traditional morphological characters used in octocoral taxonomy (Gray, 1857; Kükenthal, 1908). This included the examination of macro-morphological characters, such as the colony shape and growth form (Fabricius and Alderslade, 2001), and microscopical observation of diagnostic features such as the morphology and arrangement of polyps and sclerites (Bayer et al., 1983; Grasshoff, 2000).
2.3 DNA extraction, PCR amplification, and phylogenetic analyses
Total DNA was extracted from approximately 1 cm of the ethanol-preserved specimen using a DNeasy® Blood and Tissue kit (Qiagen Inc., Hilden, Germany) and following the manufacturer’s protocol. Extracted DNA quantity and quality were assessed using a NanoDrop® 2000c spectrophotometer (Thermo Fisher Scientific, Wilmington, USA). A fragment (around 950 bp) of the Folmer region of the cytochrome oxidase I (COI) gene plus an adjacent intergenic region (igr1) was amplified using the primers COII8068F (McFadden et al., 2004) and COIOctR (France and Hoover, 2002), while around 830 bp of the mtMutS gene were amplified using the primers ND42599F (France and Hoover, 2001) and mut3458R (Sànchez et al., 2003). Polymerase chain reactions (PCRs) were performed in a final volume of 15 µL obtained with 1.2 µL of raw DNA, 1.5 µL of each primer, 3.3 µL of H2O and 7.5 µL of Multiplex PCR Master Mix (Qiagen, Hilden, Germany). The temperature profiles consisted of an initial step at 95°C for 15 min, 39 cycles of 95°C for 10 sec, 1 min at the annealing temperature (58°C for COI and 48°C for mtMutS) and 72°C for 1 min, and a final step at 72°C for 5 min. PCR products were purified with lllustra™ ExoProStar™ (Global Life Sciences Solutions Operations UK Ltd, Buckinghamshire, UK), following the manufacturer’s protocol, and directly sequenced in both forward and reverse directions using an ABI 3730xl DNA analyzer (Applied Biosystems, Massachusetts, USA) at KAUST BioSciences Core Laboratories (Thuwal, Saudi Arabia).
Forward and reverse sequences were assembled and edited using Geneious® v2023.0.1 (Biomatters Ltd., Auckland, New Zealand). Previously deposited sequences available on GenBank database (https://www.ncbi.nml.nih.gov/genbank/) and representing specimens belonging to the genus Acanthogorgia and to other 19 genera within the family Paramuriceidae were aligned to the newly produced sequences using MAFFT v7.490 (Katoh and Standley, 2013) through the E-INS-i settings. A sequence representing the species Euplexaura rhipidalis Studer, 1895 was included in the alignment and chosen as outgroup based on previously published phylogenies (McFadden et al., 2022). Alignments were manually inspected and edited using the software AliView v1.28 (Larsson, 2014). The newly produced sequences were deposited in GenBank database (https://www.ncbi.nml.nih.gov/genbank/) (Accession numbers: OR588766 and OR588767). Evolutionary models were checked through jModelTest2 (Darriba et al., 2012) on CIPRES (Miller et al., 2010), which selected TVM+I for COI and HKY+G for the mtMutS alignment. Phylogenetic trees were then inferred using both Maximum Likelihood (ML) using RAxML HPC2 (Stamatakis, 2014) and Bayesian Inference (BI) through MrBayes 3.2.6 (Ronquist et al., 2012), on the CIPRES portal, using the default parameters with 1,000 bootstrap replicates and 10,000,000 generations with 2500 burn-in values, respectively.
3 Results
3.1 Environmental conditions
The specimen was collected from the north-western Kebrit Deep, one of the depressions that characterize the northern and central Red Sea (Pautot, 1983). Kebrit Deep hosts an ambient brine pool, notably characterized by extreme conditions, including high salinities (242 PSU), anoxic waters (0 mg O2 L-1), and low pH (5.5) (Eder et al., 2001; Schmidt et al., 2015; Vestheim and Kaartvedt, 2016). The octocoral colony reported here was collected from 1453 m depth, in proximity of the brine, which has its interface at ~1465 m depth (Vestheim & Kaartvedt, 2016) and above the <5 m thick halocline that marks the transition zone to the ocean bottom water. Seawater at this location had a salinity of 41.5 PSU and temperature of 22°C. The specimen was found on the hard substrate of a sulphide chimney, surrounded by, and growing on, sponges, with a diameter smaller than 5 cm (arrowheads in Figure 1B). Several other morphologically similar colonies were also observed at similar water depths along the brine shore.
3.2 Morphological results
The examined material, consisting of one dry specimen and a fragment of the same sample preserved in 99% EtOH, presents a bushy, fan-shaped, densely branched and fragile colony (Figure 1C). Branches are thin and do not present any anastomoses, polyps arise from a dark and thin axis (Figure 1C). Polyps are tall, trumpet-shaped, contractile, but non-retractile and arranged vertically and biserially on both sides of the branches (Figures 1C, D). The coenenchyme layer is very thin and leaves the axis exposed. The surface of the polyps is covered in small spindles. Sclerites of the polyps are disposed on eight double rows on the body wall and are numerous, flat and bent or elongated. Polyps and tentacles are armed with long warty spindles which arise from the base and form a crown-shaped structure. All sclerites are colorless (Figure 1E).
3.3 Molecular results
PCRs amplifications and sequencing were successful for both COI and mtMutS regions. This allowed us to concatenate the results of the two loci sequenced in a final alignment of 1692 bp, of 98 sequences, consisting of the newly generated sequence and 97 previously published. Phylogenetic reconstructions based on both Maximum Likelihood and Bayesian Inference confirmed that the collected specimen belongs to the family Paramuriceidae and to the genus Acanthogorgia (Figure 2). The phylogenetic tree reported in Figure 2 also showed the occurrence of Acanthogorgia spp. sequences in two distinct, and not closely related, molecular lineages, highlighting the paraphyly of the genus according to analyses based on mitochondrial genes. The colony sequenced for this study appears to belong to the same lineage of previously published specimens identified as Acanthogorgia aspera Pourtalès, 1867, and phylogenetically distinct from the other lineage, which comprises species-level data for seven congeneric taxa, namely Acanthogorgia angustiflora Kükenthal & Gorzawsky, 1908, Acanthogorgia armata Verrill, 1878, Acanthogorgia breviflora Whitelegge, 1897, Acanthogorgia hedlundi Aurivillius, 1931, Acanthogorgia radians (Kükenthal & Gorzawsky, 1908), Acanthogorgia spinosa Hiles, 1899, Acanthogorgia spissa Kükenthal, 1908 (Figure 2).
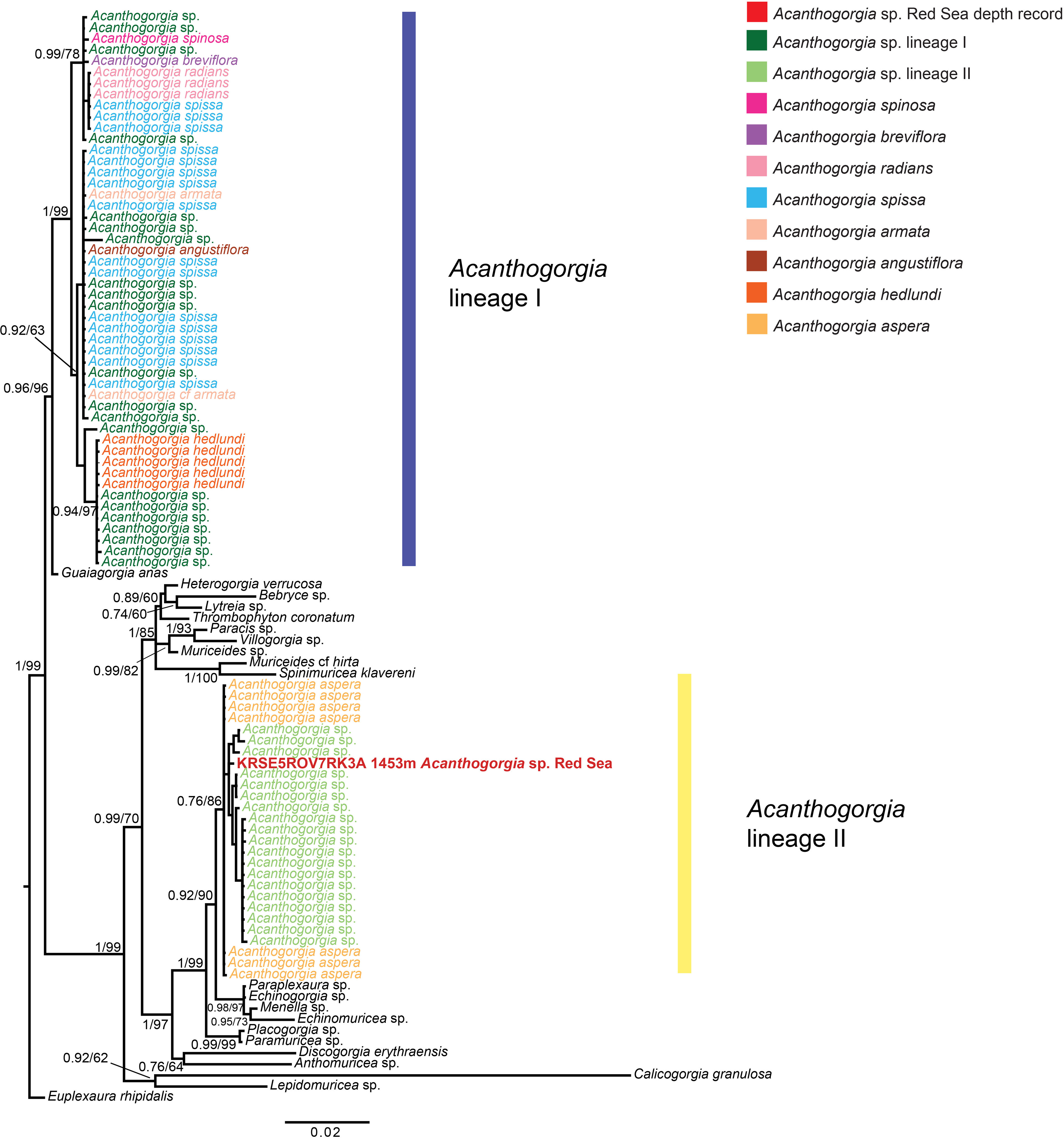
Figure 2 Bayesian Inference phylogenetic reconstruction of the octocoral family Paramuriceidae based on the concatenation of the mtMutS and COI regions, showing the phylogenetic position of the collected specimen (in red). Euplexaura rhipidalis was chosen as outgroup. Node values correspond to Bayesian posterior probability and maximum likelihood bootstrap values > 70%.
4 Discussion
According to morphological and phylogenetic analyses, the colony sampled at 1453 m depth at Kebrit Deep (Figure 1) belongs to the octocoral genus Acanthogorgia and represents the deepest record for the genus in the Red Sea and the deepest octocoral sampled in the basin.
While shallow-water coral reefs in the region have been relatively well studied over the past decade (Berumen et al., 2019b), the biodiversity of mesophotic and deep-sea coral assemblages has received less attention. Many studies focusing on octocorals from the Red Sea were based on historical material (e.g., Savigny, 1817; Kükenthal, 1913), and generally include shallow water (e.g., Thomson and Mcqueen, 1907; Verseveldt and Benayahu, 1978; Benayahu, 1990; van Ofwegen, 2016) or mesophotic specimens without clear depth indication (Benayahu et al., 2019). Although mesophotic octocorals were previously investigated in the Red Sea (Benayahu et al., 2017a; Benayahu et al., 2017b), such studies were restricted to the area of the Gulf of Aqaba, which likely reduced the levels of diversity that could be found when considering the whole basin and its depth gradient. Moreover, the majority of the octocoral specimens previously collected at depth in the Red Sea were only identified based on morphological characters (e.g., Grasshoff, 2000; Qurban et al., 2014), and molecular data matching the actual colonies are not available. Hence, the newly generated genetic data presented here contribute to a better characterization of the molecular diversity of the genus from the Red Sea basin and provide useful data for future studies addressing the depth and biogeographical distribution of the genus Acanthogorgia.
While morphological analyses allowed us to identify the collected specimen as an Acanthogorgia (Figure 1), it was not possible to reach a species-level classification based on the morphological characteristics presented in the literature (Grasshoff, 1999; McFadden et al., 2022). DNA amplification and sequencing allowed us to infer its phylogenetic position within the genus based on a comparison with previously published molecular data available in the literature and obtained from specimens sampled in other biogeographical areas (Figure 2; Supplementary Material 1). This highlighted that the collected specimen belongs to the same molecular lineage of octocorals identified as A. aspera, rather than the other lineage comprising the species A. angustiflora, A. armata, A. breviflora, A. hedlundi, A. radians, A. spinosa and A. spissa (Figure 2). Although the genus Acanthogorgia was previously reported to be paraphyletic through molecular analyses (McFadden et al., 2006), it was recently found to be monophyletic when performing phylogenomic analyses (McFadden et al., 2022). Therefore, given a lack of features that would allow a clear species distinction between the two lineages, care should be taken in the classification of Acanthogorgia specimens based on single-locus molecular markers. This mismatch between phylogenetic and phylogenomic data also highlights the need of further sampling and sequencing of this genus to better characterize and match its morphological and molecular diversity within the family Paramuriceidae (McFadden et al., 2006).
From an ecological point of view, the collection of an Acanthogorgia from an area such as the central axis of the Red Sea and at such depths emphasizes the remarkable ability of this taxon in spanning the depth gradient and withstanding a range of environmental conditions. Notably, Kebrit Deep is the only location in the Red Sea where in situ inactive hydrothermal sulphide chimneys have been found, occurring around the seawater-brine interface (Blum and Puchelt, 1991). Based on their chemistry, these chimneys are thought to have precipitated from hydrothermal fluids with a temperature of 110-130°C, however, currently the system is extinct (Blum and Puchelt, 1991). The brine pool and chimney field form a distinct habitat, with a range of microbes previously reported from the brine itself (Merlino et al., 2018), and a deep-sea community composed of bivalves, gastropods, polychaetes, and sea anemones described from the inactive chimneys (Vestheim and Kaartvedt, 2016). Nevertheless, the colony reported here is the first octocoral collected from the Kebrit Deep. Additionally, reports of hydrothermal vent fields colonization by anthozoan taxa in other deep-sea areas are scarce (Zelnio et al., 2009). This finding in such a peculiar environment, strengthens the importance of identifying similar habitats in the deep Red Sea and characterizing the benthic communities therein residing and their diversity.
Taxa known to form MAFs in other ocean basins, such as black corals and hydrozoans, have previously been described from the deep Red Sea (Chimienti et al., 2022; Maggioni et al., 2022). However, these habitats have been understudied in the deep Red Sea, and only the occurrence of deep-water coral frameworks has been reported (Qurban et al., 2020). Therefore, the presence of Acanthogorgia sp. colonies below 1000 m provides new insights into the lower limits of colonization and the vertical zonation of habitat-forming species in the basin. The genus is known to contribute to MAFs (Rossi et al., 2017) along the depth gradient and in diverse environments elsewhere in the world (e.g., Cho and Hwang, 2018; Ramiro-Sánchez et al., 2019; Sánchez et al., 2019). For instance, A. armata shows high abundances on deep-sea coral rubble in the Mediterranean (Bo et al., 2023) and in the North Atlantic Ocean (Buhl-Mortensen and Buhl-Mortensen, 2004). This is the first time Acanthogorgia is reported from an area known to host inactive sulphide chimneys and close to a brine-seawater interface. This discovery also emphasizes a need to understand how these organisms can access food and resources in extreme environments. Finally, deep coral communities and MAFs are known to be threatened by anthropogenic activities (Aguilar et al., 2017). Hence, the finding of octocoral colonies in such an unexpected area increases our knowledge on their distribution and thus provides useful spatial information for management and conservation planning, especially considering the rates of endemism of marine organisms previously reported for the Red Sea (DiBattista et al., 2016).
The Red Sea central axis exploration efforts that led to the discovery reported in this study allowed us to document for the first time the presence of octocorals deeper than 1000 m in the basin. Given the observation of other benthic organisms in the surroundings (Figure 1B), this novel finding strengthens the importance of octocoral colonies in providing habitat and substrate in the aphotic zone. While octocoral assemblages and diversity change with the bathymetric range (Sánchez et al., 2021), little is still known about their occurrence and evolution along the depth gradient of the Saudi Arabian Red Sea. Therefore, further explorations and sampling of octocoral communities at depth are needed to better characterize their diversity and conserve these ecologically important organisms.
Data availability statement
The datasets presented in this study can be found in online repositories. The names of the repository/repositories and accession number(s) can be found in the article/Supplementary Material.
Ethics statement
The manuscript presents research on animals that do not require ethical approval for their study.
Author contributions
LM: Conceptualization, Data curation, Formal analysis, Investigation, Methodology, Validation, Visualization, Writing – original draft, Writing – review & editing. MN: Investigation, Writing – review & editing, Conceptualization, Data curation, Visualization. TT: Supervision, Validation, Writing – review & editing. NO: Supervision, Writing – review & editing, Validation. NA: Supervision, Writing – review & editing, Validation, Writing – original draft. FZ: Funding acquisition, Supervision, Writing – review & editing, Resources, Validation, Writing – original draft. FB: Funding acquisition, Resources, Supervision, Writing – review & editing, Validation, Project administration, Visualization, Writing – original draft.
Funding
The author(s) declare financial support was received for the research, authorship, and/or publication of this article. This study was supported by KAUST (Ship-time on the R/V Aegaeo and ROV use by Office of the VPR; baseline research funds BAS/1/1090-01-01 awarded to FB).
Acknowledgments
The authors are grateful to the captain and crew of R/V Aegaeo for their support at sea. We further appreciate the help of the scientific parties on board, particularly the HCMR scientists and the ROV team. We are grateful to KAUST Coastal and marine core labs for organizing the research expeditions and providing technical support on board. We would like to thank Professor Catherine S McFadden for suggestions on early molecular analyses for this manuscript.
Conflict of interest
The authors declare that the research was conducted in the absence of any commercial or financial relationships that could be construed as a potential conflict of interest.
Publisher’s note
All claims expressed in this article are solely those of the authors and do not necessarily represent those of their affiliated organizations, or those of the publisher, the editors and the reviewers. Any product that may be evaluated in this article, or claim that may be made by its manufacturer, is not guaranteed or endorsed by the publisher.
Supplementary material
The Supplementary Material for this article can be found online at: https://www.frontiersin.org/articles/10.3389/fmars.2023.1305420/full#supplementary-material
References
Aguilar R., Perry A. L., López J. (2017). Conservation and Management of Vulnerable Marine Benthic Ecosystems. Eds. Rossi S., Bramanti L., Gori A., Orejas C. (Marine Animal Forests. Springer, Cham). doi: 10.1007/978-3-319-21012-4_34
Anker A., Vimercati S., Barreca F., Marchese F., Chimienti G., Terraneo T. I., et al. (2023). Mesophotic and bathyal palaemonid shrimp diversity of the red sea, with the establishment of two new genera and two new species. Diversity 15 (10), 1028. doi: 10.3390/d15101028
Augustin N., van der Zwan F. M., Devey C. W., Brandsdóttir B. (2021). 13 million years of seafloor spreading throughout the Red Sea Basin. Nat. Commun. 12 (1), e2427. doi: 10.1038/s41467-021-22586-2
Augustin N., van der Zwan F. M., Devey C. W., Ligi M., Kwasnitschka T., Feldens P., et al. (2016). Geomorphology of the central Red Sea Rift: Determining spreading processes. Geomorph 274, 162–179. doi: 10.1016/j.geomorph.2016.08.028
Baillon S., Hamel J.-F., Wareham V. E., Mercier A. (2012). Deep cold-water corals as nurseries for fish larvae. Front. Ecol. Environ. 10 (7), 351–356. doi: 10.1890/120022
Bayer F. M., Grasshoff M., Verseveldt J. (1983). Illustrated trilingual glossary of morphological and anatomical terms applied to Octocorallia. EJ Brill Leiden, 1–75. doi: 10.1163/9789004631915
Benayahu Y. (1990). Xeniidae (Cnidaria: Octocorallia) from the Red Sea with description of a new species. Zool. Meded. 64 (9), 113–120.
Benayahu Y., Bridge T. C., Colin P. L., Liberman R., McFadden C. S., Pizarro O., et al. (2019). “Octocorals of the indo-pacific,” in Mesophotic Coral Ecosystems. Coral Reefs of the World, vol. 12. Eds. Loya Y., Puglise K., Bridge T. (Springer, Cham). doi: 10.1007/978-3-319-92735-0_38
Benayahu Y., McFadden C. S., Shoham E. (2017a). Search for mesophotic octocorals (Cnidaria, Anthozoa) and they phylogeny. I. A new sclerite-free genus from Eilat, northern Red Sea. ZooKeys 680, 1–11. doi: 10.3897/zookeys.680.12727
Benayahu Y., McFadden C. S., Shoham E., van Ofwegen L. P. (2017b). Search for mesophotic octocorals (Cnidaria, Anthozoa) and they phylogeny. II. A new zooxanthellate species from Eilat, northern Red Sea. ZooKeys 676, 1–12. doi: 10.3897/zookeys.676.12751
Berntson E. A., Bayer F. M., McArthur A. G., France S. C. (2001). Phylogenetic relationships within the Octocorallia (Cnidaria: Anthozoa) based on nuclear 18S rDNA sequences. Mar. Biol. 138, 235–246. doi: 10.1007/s002270000457
Berumen M. L., Arrigoni R., Bouwmeester J., Terraneo T. I., Benzoni F. (2019b). “Corals of the Red Sea,” in Coral Reefs of the Red Sea. Eds. Voolstra C., Berumen M. L.. (Springer, Cham), 123–155. doi: 10.1007/978-3-030-05802-9_7
Berumen M. L., Voolstra C. R., Daffonchio D., Agusti S., Aranda M., Irigoien X., et al. (2019a). “The Red Sea: Environmental Gradients Shape a Natural Laboratory in a Nascent Ocean,” in Coral Reefs of the Red Sea. Eds. Voolstra C., Berumen M. L.. (Springer, Cham), 1–10. doi: 10.1007/978-3-030-05802-9_1
Blum N., Puchelt H. (1991). Sedimentary-hosted polymetallic massive sulfide deposits of the Kebrit and Shaban Deeps, Red Sea. Mineral. Deposita 26, 217–227. doi: 10.1007/BF00209261
Bo M., Enrichetti F., Betti F., Gay G., Quarta G., Calcagnile L., et al. (2023). The cold-water coral province of the eastern Ligurian Sea (NW Mediterranean Sea): historical and novel evidences. Front. Mar. Sci. 10. doi: 10.3389/fmars.2023.1114417
Buhl-Mortensen L., Vanreusel A., Gooday A. J., Levin L. A., Priede I. G., Buhl-Mortensen P., et al. (2010). Biological structures as a source of habitat heterogeneity and biodiversity on the deep ocean margins. Mar. Ecol. 31 (1), 21–50. doi: 10.1111/j.1439-0485.2010.00359.x
Buhl-Mortensen P., Buhl-Mortensen L. (2004). Distribution of deep-water gorgonian corals in relation to benthic habitat features in the Northeast Channel (Atlantic Canada). Mar. Biol. 144, 1223–1238. doi: 10.1007/s00227-003-1280-8
Cairns S. D. (2007). Deep-water corals: an overview with special reference to diversity and distribution of deep-water scleractinian corals. Bull. Mar. Sci. 81 (3), 311–322.
Chimienti G., Terraneo T. I., Vicario S., Marchese F., Purkis S. J., Eweida A. A., et al. (2022). A new species of Bathypathes (Cnidaria, Anthozoa, Antipatharia, Schizopathidae) from the Red Sea and its phylogenetic position. Zookeys 1116, 1–22. doi: 10.3897/zookeys.1116.79846
Cho I., Hwang S. (2018). A new record of Acanthogorgia species (Octocorallia: Alcyonacea: Holaxonia: Acanthogorgiidae) from Korea. J. Species Res. 7 (1), 92–96. doi: 10.12651/JSR.2018.7.1.092
Daly M., Brugler M. R., Cartwright P., Collins A. G., Dawson M. N., Fautin D. G., et al. (2007). They phylum Cnidaria: A review of phylogenetic patterns and diversity 300 years after Linnaeus. Zootaxa 1668, 127–182. doi: 10.11646/zootaxa.1668.1.11
Darriba D., Taboada G., Doallo R., Posada D. (2012). jModelTest 2: more models, new heuristics and parallel computing. Nat. Meth. 9 (8), 772–772. doi: 10.1038/nmeth.2109
DiBattista J. D., Roberts M. B., Bouwmeester J., Bowen B. W., Coker D. J., Lozano-Cortes D. F., et al. (2016). A review of contemporary patterns of endemism for shallow water reef fauna in the Red Sea. J. Biogeogr. 43 (3), 423–439. doi: 10.1111/jbi.12649
Eder W., Jahnke L. L., Schmidt M., Huber R. (2001). Microbial Diversity of the Brine-Seawater Interface of the Kebrit Deep, Red Sea, Studies via 16S rRNA Gene Sequences and Cultivation Methods. Appl. Environ. Microbiol. 67 (7), 3077–3085. doi: 10.1128/AEM.67.7.3077-3085.2001
Everett M. V., Hourigan T. F., Whitmire C. E., Clarke M. E., Cairns S. D., Horvath E. A. (2022). List of Deep-Sea Coral Taxa in the U.S. West Coast Region: Depth and Geographic Distribution (v. 2021).
Fabricius K., Alderslade P. (2001). Soft corals and sea fans: a comprehensive guide to the shallow-water genera of the Central-West Pacific, the Indian Ocean and the Red Sea (Townsville: Australian Institute of Marine Science264).
France S. C., Hoover L. L. (2001). Analysis of variation in mitochondrial DNA sequences (ND3, ND4L, MSH) among Octocorallia (= Alcyonaria) (Cnidaria: Anthozoa). Bull. Biol. Soc Wash. 10, 110–118.
France S. C., Hoover L. L. (2002). DNA sequences of the mitochondrial COI gene have low levels of divergence among deep-sea octocorals (Cnidaria: Anthozoa). Hydrobiologia 471, 149–155. doi: 10.1023/A:1016517724749
Grasshoff M. (1999). The shallow water gorgonians of New Caledonia and adjacent islands (Coelenterata: Octocorallia). Sencken. Biol. 78 (1/2), 1–121.
Grasshoff M. (2000). The Gorgonians of the Sinai coast and the strait of gubal, red sea (Coelenterata, octocorallia). Cour. Forsch. Senckenberg 224, 1–125.
Gray J. E. (1857). Description of a new genus of Gorgonidae. Proc. Zoological Soc. London 1857, 128–129. doi: 10.1111/j.1096-3642.1857.tb01216.x
Katoh K., Standley D. M. (2013). MAFFT multiple sequence alignment software version 7: improvements in performance and usability. Mol. Biol. Evol. 30, 772–780. doi: 10.1093/molbev/mst010
Krieger K. J., Wing B. L. (2002). Megafauna associations with deepwater corals (Primnoa spp.) in the Gulf of Alaska. Hydrobiologia 471, 83–90. doi: 10.1023/A:1016597119297
Kükenthal W. G. (1908). Diagnosen neuer Gorgoniden aus der Familie Plexauridae. Zool. Anz. 32 (17), 495–504.
Kükenthal W. G. (1913). Plantae chineses forrestianae. Description of new species of Cyperaceae. Notes R Bot. Garden Edinb 8, 9–10.
Larsson A. (2014). AliView: a fast and lightweight alignment viewer and editor for large data sets. Bioinformatics 30 (22), 3276–3278. doi: 10.1093/bioinformatics/btu531
Lourie S. A., Randall J. E. (2003). A new pygmy seahorse, hippocampus denise (Teleostei: syngnathidae), from the indo-pacific. Zool. Stud. 42 (2), 284–291.
Maggioni D., Terraneo T. I., Chimienti G., Marchese F., Pica D., Cairns S. D., et al. (2022). The first deep-sea stylasterid (Hydrozoa, stylasteridae) of the red sea. Diversity 14 (4), 241. doi: 10.3390/d14040241
Manasrah R., Abu-Hilal A., Rasheed M. (2019). “Physical and Chemical Properties of Seawater in the Gulf of Aqaba and Red Sea,” in Oceanographic and Biological Aspects of the Red Sea. Eds. Rasul N., Stewart I. (Springer Oceanography. Springer, Cham). doi: 10.1007/978-3-319-99417-8_3
McFadden C. S., France S. C., Sánchez J. A., Alderslade P. (2006). A molecular phylogenetic analysis of the Octocorallia (Cnidaria: Anthozoa) based on mitochondrial protein-coding sequences. Mol. Phylogenet. Evol. 41 (3), 513–527. doi: 10.1016/j.ympev.2006.06.010
McFadden C. S., Sánchez J. A., France S. C. (2010). Molecular phylogenetic insights into the evolution of octocorallia: A review. Integr. Comp. Biol. 50 (3), 389–410. doi: 10.1093/icb/icq056
McFadden C. S., Tullis I. D., Hutchinson M. B., Winner K., Sohm J. A. (2004). Variation in coding (NADH dehydrogenase subunits 2, 3, and 6) and noncoding intergenic spacer regions of the mitochondrial genome in Octocorallia (Cnidaria: Anthozoa). Mar. Biotech. 6, 516–526. doi: 10.1007/s10126-002-0102-1
McFadden C. S., van Ofwegen L., Quattrini A. (2022). Revisionary systematics of Octocorallia (Cnidaria: Anthozoa) guided by phylogenomics. Bull. Soc Syst. Biol. 1 (3). doi: 10.18061/bssb.v1i3.8735
Merlino G., Barozzi A., Michoud G., Ngugi D. K., Daffonchio D. (2018). Microbial ecology of the deep-sea hypersaline anoxic basins. FEMS Microbiol. Ecol. 94 (7), fiy085. doi: 10.1093/femsec/fiy085
Miller M. A., Pfeiffer W., Schwarts T. (2010). Creating the CIPRES Science Gateway for inference of large phylogenetic trees (New Orleans, LA: Proceedings of the Gateway Computing Environments Workshop (GCE), 1–8. doi: 10.1109/GCE.2010.5676129
Orejas C., Carreiro-Silva M., Mohn C., Reimer J. D., Samaai T., Allcock A. L., et al. (2022). Marine animal forests of the world: definition and characteristics. Res. Ideas Outcomes 8, e96274. doi: 10.3897/rio.8.e96274
Pautot G. (1983). Les fosses de la Mer Rouge: approche géomorphologique d'un stade initial d'ouverture océanique réalisée à l'aide du Seabeam. Oceanolog. Acta 6, 235–244.
Purkis S. J., Harris P. M., Ellis J. (2012). Patterns of sedimentation in the contemporary Red Sea as an analog for ancient carbonates in rift settings. J. Sediment. Res. 82 (11), 859–870. doi: 10.2110/jsr.2012.77
Qurban M. A., Krishnakumar P. K., Joydas T. V., Manikandan K. P., Ashraf T. T. M., Quadri S. I., et al. (2014). In-situ observation of deep water corals in the northern Red Sea waters of Saudi Arabia. Deep Sea Res. Part I 89, 35–43. doi: 10.1016/j.dsr.2014.04.002
Qurban M. A., Krishnakumar P. K., Joydas T. V., Manikandan K. P., Ashraf T. T. M., Sampath G., et al. (2020). Discovery of deep-water coral frameworks in the northern Red Sea waters of Saudi Arabia. Sci. Rep. 10, 15356. doi: 10.1038/s41598-020-72344-5
Ramiro-Sánchez B., González-Irusta J. M., Henry L.-A., Cleland J., Yeo I., Xavier J. R., et al. (2019). Characterization and mapping of a deep-sea sponge ground on the tropic seamount (Northeast tropical atlantic): implications for spatial management in the high seas. Front. Mar. Sci. 6. doi: 10.3389/fmars.2019.00278
Roder C., Berumen M. L., Bouwmeester J., Papathanassiou E., Al-Suwailem A., Voolstra C. R. (2013). First biological measurements of deep-sea corals from the Red Sea. Sci. Rep. 3, 2802. doi: 10.1038/srep02802
Ronquist F., Teslenko M., van der Mark P., Ayres D., Darling A., Höhna S., et al. (2012). MrBayes 3.2: efficient bayesian phylogenetic inference and model choice across a large model space. Syst. Biol. 61 (3), 539–542. doi: 10.1093/sysbio/sys029
Rossi S., Bramanti L., Gori A., Orejas C. (2017). “Marine Animal Forests,” in The Ecology of Benthic Biodiversity Hotspots (Cham: Springer). doi: 10.1007/978-3-319-21012-4
Rowley S. J., Roberts T. E., Coleman R. R., Spalding H. L., Joseph E., Dorricott M. K. L. (2019). “Pohnpei, Federated States of MICRONESIA,” in Mesophotic Coral Ecosystems. Coral Reefs of the World, vol 12. Eds. Loya Y., Puglise K., Bridge T. (Springer, Cham). doi: 10.1007/978-3-319-92735-0_17
Sánchez J. A. (2016). “Diversity and Evolution of Octocoral Animal Forests at Both Sides of Tropical America,” in Marine Animal Forests. Eds. Rossi S., Bramanti L., Gori A., Orejas Saco del Valle C. (Springer, Cham). doi: 10.1007/978-3-319-17001-5_39-1
Sánchez J. A., Dueñas L. F., Rowley S. J., Gonzalez-Zapata F. L., Vergara D. C., Montaño-Salazar S. M., et al. (2019). “Gorgonian Corals,” in Mesophotic Coral Ecosystems. Coral Reefs of the World, vol 12. Eds. Loya Y., Puglise K., Bridge T. (Springer, Cham). doi: 10.1007/978-3-319-92735-0_39
Sánchez J. A., González-Zapata F. L., Prada C., Dueñas L. F. (2021). Mesophotic Gorgonian corals evolved multiple times and faster than deep and shallow lineages. Diversity 13, 650. doi: 10.3390/d13120650
Sánchez J. A., McFadden C. S., France S. C., Lasker H. R. (2003). Molecular phylogenetic analyses of shallow-water Caribbean octocorals. Mar. Biol. 142, 975–987. doi: 10.1007/s00227-003-1018-7
Sartoretto S., Zibrowius H. (2018). Note on new records of living Scleractinia and Gorgonaria between 1700 and 2200 m depth in the western Mediterranean Sea. Mar. Biodiv. 48, 689–694. doi: 10.1007/s12526-017-0829-6
Schmidt M., Al-Farawati R., Botz R. (2015). “Geochemical classification of Brine-Filled Red Sea Deeps,” in The Red Sea. Springer Earth System Sciences. Eds. Rasul N., Stewart I. (Springer, Berlin, Heidelberg). doi: 10.1007/978-3-662-45201-1_13
Schweitzer C. C., Stevens B. G. (2019). The relationship between fish abundance and benthic community structure on artificial reefs in the Mid-Atlantic Bight, and the importance of sea whip corals Leptogorgia virgulata. PeerJ 7, e7277. doi: 10.7717/peerj.7277
Shaked Y., Genin A. (2011). “Red Sea and Gulf Of Aqaba,” in Encyclopedia of Modern Coral Reefs. Encyclopedia of Earth Sciences Series. Ed. Hopley D. (Springer, Dordrecht). doi: 10.1007/978-90-481-2639-2_129
Stamatakis A. (2014). RAxML Version 8: A tool for phylogenetic analysis and post-analysis of large phylogenesis. Bioinformatics 30, 1312–1313. doi: 10.1093/bioinformatics/btu033
Thomson J. A., Mcqueen J. M. (1907). Reports on the marine biology of the Sudanese Red Sea, VIII. Alcyonarians. Zool. J. Linn. Soc 31 (204), 48–75. doi: 10.1111/j.1096-3642.1908.tb00454.x
Tsounis G., Steele M. A., Edmunds P. J. (2020). Elevated feeding rates of fishes within octocoral canopies on Caribbean reefs. Coral Reefs 39, 1299–1311. doi: 10.1007/s00338-020-01963-1
van Ofwegen L. P. (2016). The genus litophyton forskå(Octocorallia: alcyonacea: nephtheidae) in the red sea and the western Indian ocean. Zookeys 567, 1–128. doi: 10.3897/zookeys.567.7212
Verseveldt J., Benayahu Y. (1978). Descriptions of one old and five new species of Alcyonacea (Coelenterata: Octocorallia) from the Red Sea. Zool. Meded. 53, 57–74.
Vestheim H., Kaartvedt S. (2016). A deep sea community at the Kebrit brine pool in the Red Sea. Mar. Biodiv. 46, 59–65. doi: 10.1007/s12526-015-0321-0
Keywords: Octocorallia, deep sea, gorgonians, Marine Animal Forests, depth record
Citation: Macrina L, Nolan MKB, Terraneo TI, Oury N, Augustin N, van der Zwan FM and Benzoni F (2024) The deepest record of the octocoral Acanthogorgia from the Red Sea. Front. Mar. Sci. 10:1305420. doi: 10.3389/fmars.2023.1305420
Received: 01 October 2023; Accepted: 27 December 2023;
Published: 17 January 2024.
Edited by:
Nicolas James Pilcher, Marine Research Foundation, MalaysiaReviewed by:
Maria Flavia Gravina, University of Rome Tor Vergata, ItalyLuis M. Mejía-Ortíz, Universidad de Quintana Roo, Mexico
Copyright © 2024 Macrina, Nolan, Terraneo, Oury, Augustin, van der Zwan and Benzoni. This is an open-access article distributed under the terms of the Creative Commons Attribution License (CC BY). The use, distribution or reproduction in other forums is permitted, provided the original author(s) and the copyright owner(s) are credited and that the original publication in this journal is cited, in accordance with accepted academic practice. No use, distribution or reproduction is permitted which does not comply with these terms.
*Correspondence: Laura Macrina, laura.macrina@kaust.edu.sa; Francesca Benzoni, francesca.benzoni@kaust.edu.sa