- Third Institute of Oceanography, Ministry of Natural Resources, Xiamen, China
In the context of global change, the stressors of warming and eutrophication have significant ecological implications in coastal waters. In order to examine the diversity of phytoplankton and its relationship with water quality, we conducted a survey of phytoplankton community compositions and their correlation with environmental changes over four seasons in a eutrophic bay located in the East China Sea. Through a systematic analysis, we identified diatoms and dinoflagellates as the primary dominant groups, with the species Skeletonema costatum, Skeletonema marinoi, Biddulphia sinensis, Thalassiosira eccentrica, Leptocylindrus danicus, Coscinodiscus oculus-iridis, Coscinodiscus jonesianus, and Chaetoceros knipowitschi as the most abundant species in all seasons. Significant seasonal alterations were observed in both environmental settings and phytoplankton species richness, dominance, and abundance. The phytoplankton community varied in its response to diverse aquatic environments and was principally affected by temperature, silicic acid concentrations, and suspended solids. Elevated temperatures were found to promote an increase in phytoplankton abundance. However, no clear evidence of diatom and dinoflagellate succession in relation to N:P ratio was observed across seasons. Water quality analysis illustrated that the majority of the study area exhibited a mid-eutrophic with severe organic pollution. The abundance of phytoplankton was significantly influenced by eutrophication and organic pollution. The accelerated warming process related to coastal nuclear power plants and nutrient regime alterations significantly affect the temporal shift of the phytoplankton community. These findings contribute valuable insights into the effects of eutrophic environments on the structure of phytoplankton communities in coastal aquatic systems.
1 Introduction
Coastal aquatic ecosystems possess significant global socioeconomic importance (Martínez et al., 2007), and are subject to substantial impacts from human activities and land–ocean interactions (Tagliani et al., 2003; Zhao et al., 2005). The introduction of terrestrial nutrients into coastal waters and the release of greenhouse gases have been expedited by various anthropogenic factors, including port services and shipping, aquaculture, urban domestic sewage, and industrial and agricultural wastewater, as well as agricultural nonpoint source pollution. Consequently, these human-induced activities have resulted in eutrophication and water warming (Cloern, 2001; Rice et al., 2015; Wang et al., 2018). The subsequent negative impacts, including algal blooms, organic pollution, hypoxia, and stratification, pose significant threats to the marine environment and ecosystem health (Meyer-Reil and Köster, 2000; Marcus, 2004). Within the context of global climatic change, the combined effects of rising temperatures and increased nutrient levels from eutrophication serve as prominent stressors with interconnected ecological implications in coastal waters (Miyamoto et al., 2019; Cheung et al., 2021; Wei et al., 2022), ultimately influencing the physicochemical processes of aquatic systems (Xia et al., 2016; Freeman et al., 2019; Song et al., 2022).
Phytoplankton, as the primary producer in aquatic ecosystems, play a crucial role in the microbial food web due to their ability to utilize nutrients and fix carbon (Cloern and Dufford, 2005; Zhou et al., 2008; Garmendia et al., 2011). The impact of anthropogenic nutrient inputs and global warming on marine phytoplankton has been significant (Novak et al., 2019; Wu et al., 2021). In coastal ecosystems across the globe, such as the East China Sea, the discharge of wastewater from tributaries has resulted in frequent harmful algal blooms (HABs) and subsequent fish mortality. This is primarily attributed to the increased availability of nitrogen and phosphate compared to silicon (Carstensen et al., 2007; Wang et al., 2019; Xin et al., 2019). Frequent HABs are increasingly associated with the eutrophication of coastal waters (Carstensen et al., 2007). The nutrient regime shifts have been found to impact the diversity and complexity of phytoplankton composition and biomass (Song et al., 2016; Xin et al., 2019), resulting in an increase in HAB phytoplankton species (Carstensen et al., 2007; Wells et al., 2015; Song et al., 2016) and a shift in dominant phytoplankton groups from primarily diatoms to a more balanced presence of diatoms and dinoflagellates (Wells et al., 2015; Xin et al., 2019; Song et al., 2022). Long-term studies conducted in coastal East China Sea, using extensive datasets, have revealed a decrease in diatom abundance and an increase in dinoflagellate abundance in response to higher temperatures and eutrophication. This suggests that diatoms and dinoflagellates exhibit distinct responses to warming and eutrophication (Chen et al., 2017; Xiao et al., 2018; Gao et al., 2022).
Located in the heart of China’s coastal region, Sanmen Bay serves as the primary access point to eastern Zhejiang. The region’s copious marine resources have played a pivotal role in fostering regional economic and social progress. However, the excessive exploitation of these resources, coupled with the haphazard allocation of marine territories and the extensive reclamation of land, have exerted significant strain on the natural environment and delicate ecosystems. Existing scholarly research on Sanmen Bay has primarily examined its structural density and the biodiversity of macrobenthos and fishery resources, along with their temporal and spatial distribution patterns (Yan et al., 2020; Qiu et al., 2022). Additional investigations have focused on environmental quality, interannual variation, and the correlation with macrobenthic communities (Liang et al., 2020; Meng et al., 2022). Furthermore, certain studies have explored the response of Sanmen Bay to alterations in water nutrients, wetland areas, and vegetation resulting from human activities, utilizing remote sensing and geographic information systems (Chen et al., 2015; Liu et al., 2021). In addition, previous research has examined phytoplankton extensively (Zhu et al., 2012; Liu et al., 2015; Xie et al., 2015; Chen et al., 2017). However, these studies have been constrained by the utilization of the net collection method, which proves inadequate in capturing a multitude of noncolonial small-celled (<76 μm) phytoplankton species. Consequently, this method leads to an underestimation of depth-averaged phytoplankton abundance by one to two orders of magnitude when compared to the water collection method (Jiang et al., 2020). Notably, certain investigations employing the water collection method have revealed significant heterogeneity in community composition across three distinct subregions within Sanmen Bay (Gao et al., 2022). Typically, Sanmen Bay is characterized as a partially enclosed harbor with an exposed entrance adjacent to the East China Sea. The bay’s ecological dynamics are primarily influenced by the confluence of riverine influxes, the Zhemin Coastal Current, and the Taiwan Warm Current (Zhu et al., 2012), potentially impacting the diversity and intricacy of phytoplankton composition (Xie et al., 2015; Chen et al., 2017; Jiang et al., 2020; Gao et al., 2022). Consequently, the phytoplankton composition in the bay consists of both freshwater or brackish species originating from tributaries, such as Pediastrum simplex, Phormidium tenesmue, and Melosira granulata v. austissima. Additionally, the presence of neritic eurytopic and low-salinity species, including Coscinodiscus jonesianus, Ditylum brightwellii, Synedra ulna and Prorocentrum minimum, can be attributed to the Zhemin Coastal Current (Jiang et al., 2012; Jiang et al., 2014; Gao et al., 2022). These species are the primary dominant organisms during the spring, autumn, and winter seasons (Jiang et al., 2012; Jiang et al., 2014; Gao et al., 2022). Furthermore, the presence of warm-water and high-salinity species, namely Cerataulina pelagica, Chaetoceros pseudocurvisetus, and Chaetoceros lorenzianus, has experienced a notable surge during the summer season, primarily attributed to the influence of the Taiwan Warm Current (Jiang et al., 2012; Chen et al., 2017; Gao et al., 2022). Recently, the degradation of the aquatic environment in Sanmen Bay has exerted significant strain on its natural resources and ecosystems. The ecological alterations resulting from eutrophication and escalating water temperature in the coastal region are believed to be the primary catalysts for the augmentation of phytoplankton abundance and shifts in dominant species composition (Xiao et al., 2018; Xin et al., 2019; Gao et al., 2022; Song et al., 2022).
Coastal waters exhibit the greatest susceptibility to the impacts of human activities. Given the intricate and diverse nature of anthropogenic pressure (Borja et al., 2015), the manifestations and magnitude of these effects can differ significantly across geographical locations, and even within the same area over time (Wu et al., 2021; Gao et al., 2022; Wei et al, 2022). Hence, this study uses a systematic analysis treating the temporal data as various groups to describe the shift in phytoplankton composition, abundance, and response to environmental changes in four continuous seasons in Sanmen Bay. Our objective was to examine the influence of physiochemical factors, specifically eutrophication and warming, on seasonal biotic assemblages in relation to the various sources of pollution.
2 Materials and methods
2.1 Study area
Sanmen Bay (SMB) is located on the coast of the East China Sea (Figure 1). It is a macro-tidal turbid estuary, with four tributaries and a maximum tidal range of approximately 2 m at the bay mouth and suspended sediment concentration of 1.192 kg/m3 in the middle of the bay (Shou and Zeng, 2015). Stratification in the bay is negligible due to the strong physical mixing and relatively modest freshwater input. Tidal current velocities in the bay exceed 30 cm/s at spring tide and 20 cm/s at neap tide. These velocities are greater than the critical velocity for sediment motion; therefore, turbidity in the bay is high, with average annual suspended solids (SS) of 367 mg/L (Editorial Committee of the Bay Chorography in China (ECBCC), 1992). With the rapid development of human activities, pollution in the bay is increasing, with ecological consequences (Shi, 2013; Chen et al., 2017).
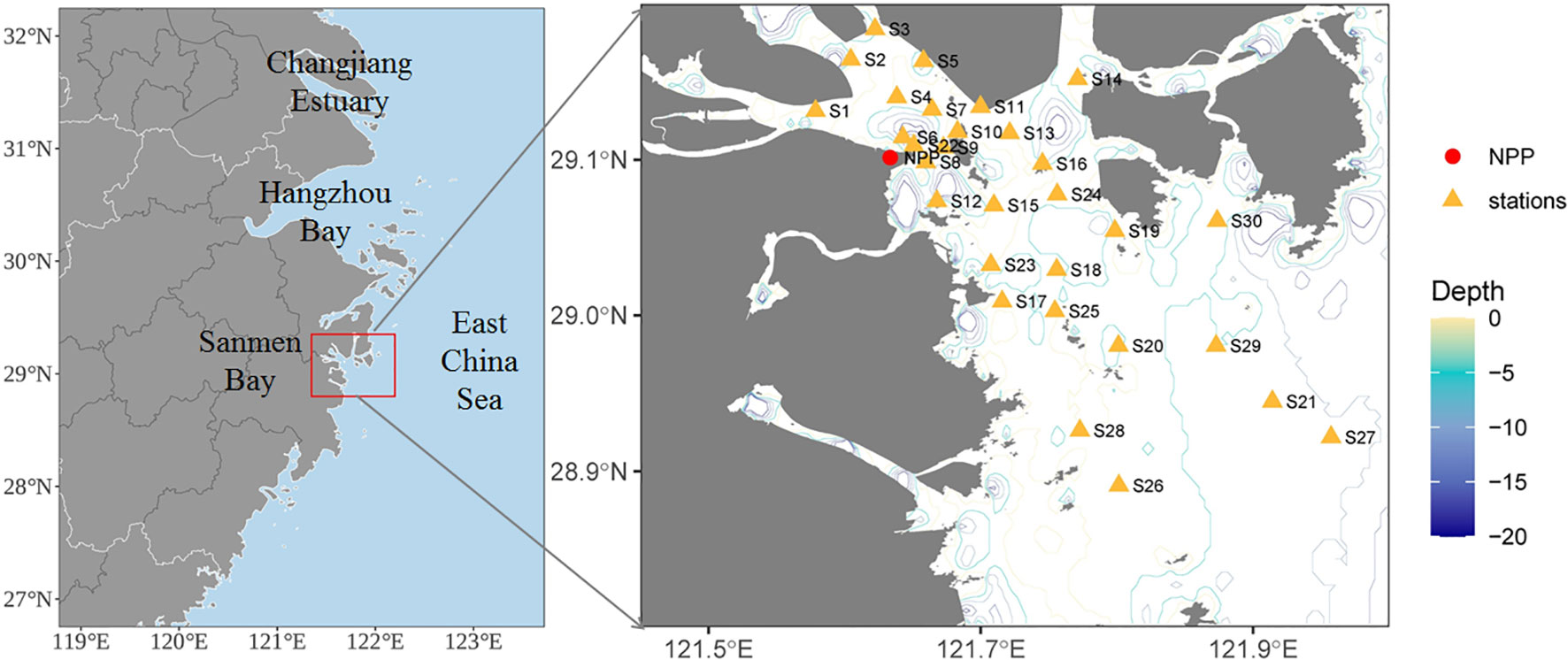
Figure 1 Location of the study area and sampling sites over four seasons in the coastal waters of Sanmen Bay, East China Sea. Red dot represents the nuclear power plant (NPP). Green rectangle represents a total of 30 stations for water sampling.
2.2 Sample collection
Four survey cruises were conducted in the SMB over the spring (April), summer (July), autumn (November), and winter (January) periods from January 2020 to November 2020, at 30 sampling stations (Figure 1). Surface seawater samples from each station were collected with 5 L Niskin bottles at 0.5 m depth. Temperature and salinity were measured in situ using a YSI salinity meter (model 30). Water samples for dissolved inorganic nutrient (nitrogen, phosphate, and silicon) analysis were filtered through a 0.45 μm cellulose acetate filter and then measured by colorimetric methods according to The Specification for Marine Monitoring (General Administration of Quality Supervision, Inspection and Quarantine (GAQSIQ, 2007a). SS samples were filtered through preweighed GF/C filters dried to a constant mass at 105°C and weighed. The dry mass of particles retained on each filter was calculated by subtracting the filter mass from the dried mass. The chemical oxygen demand (COD) was measured using alkalic potassium permanganate according to the specification of standard GB 17378.7–2007 (GAQSIQ, 2007b). The pH values of the seawater were manually measured using a pH meter (NBS scale, Mettler-Toledo, S210-k). Dissolved oxygen (DO) levels were determined via the Winkler titration method (Strickland and Parsons, 1968).
2.3 Eutrophication and organic pollution assessment
The eutrophication index (Ei) was calculated based on nutrients, using the following equation (Zhu et al., 2020).
Ei = COD × DIN × DIP × Sc, where Sc (106/4500) is the mean product of the standard concentrations of COD, dissolved inorganic nitrogen (DIN), and dissolved inorganic phosphorous (DIP). Ei < 1: no eutrophication; 1 < Ei ≤ 3: low eutrophication; 3 < Ei ≤ 9: mid-eutrophication; Ei > 9: heavy eutrophication.
The degree of organic pollution assessment was assessed using four indicators: COD, DIN, DIP, and DO (Liu et al., 2011).
Ci = CODi/CODs + DINi/DINs + DIPi/DIPs − DOi/DOs, where Ci is the organic pollution index; CODi, DINi, DIPi, and DOi are the measured values; and CODs, DINs, DIPs, and DOs are the standard type I seawater quality values of 2, 0.2, 0.015, and 6 (mg/L), respectively. For Ci, if 1 ≤ Ci ≤ 3, organic pollution is low; if Ci > 3, organic pollution is severe.
2.4 Phytoplankton identification and abundance
To determine phytoplankton composition and abundance, 500 mL of seawater was collected and preserved in formalin solution at a final concentration of 2%. In the laboratory, a 100 mL subsample was used for phytoplankton cell identification and enumeration using an inverted microscope (Zeiss Imager Z1) at 400× or 200× magnification, following previously described methods (Utermöhl, 1958). Microscopic observations were conducted according to a previous study (Fukuyo et al., 1990; Tomas, 1997; Sun and Liu, 2002). A quantitative list of dominant species was established based on the dominance index according to Tsirtsis and Karydis, 1998.
2.5 Statistical analysis
Data are given as mean values ± standard deviation (SD). One-way analysis of variance (ANOVA) and significance testing were used to compare differences among phytoplankton groups using SPSS 20.0 software (SPSS Inc., Chicago, USA). Prior to conducting the ANOVA, a normal distribution test was executed. In the event that the dataset satisfied the criteria for normal distribution (p>0.05), a T-test was employed. Conversely, if the dataset did not meet the normal distribution requirements (p<0.05), a non-parametric Wilcoxon-test was utilized for significance testing. Linear regression models and t-tests were used to explore the dynamic trends in various biological and environmental parameters. Spearman correlation analysis and least squares regression (regression variance, R2) were applied to establish relationships between groups of data, such as temperature, pH versus Ei and Ci. Canonical correspondence analysis (CCA) and aggregated boosted tree (ABT) analyses were performed to assess the relationships between environmental settings and phytoplankton communities, and to quantify the effect of key environmental settings on biological parameters. Structural equation modeling (SEM) analysis was employed to assess the relative direct and indirect impact of key explanatory variables on different phytoplankton groups. The ABT was constructed in R using the gbmplus package with 500 trees for boosting and the mgcv package with GCV smoothness estimation (Rv4.0.2). SEM results were visualized using the semPlot package (Rv4.0.2). Statistical significance was set at p < 0.05.
3 Results
3.1 Environmental settings in the SMB
Environmental settings differed significantly (p < 0.05) between seasons in most cases. The temperature in the study region had high temporal variability from 12.12 to 31.59°C in the winter and summer, respectively (Figure 2A). The variation in salinity (14.86–28.96) was classically driven by land-based influxes, with the highest in winter and a decrease from spring to autumn (Figure 2B). The average SS content reached its maximum during winter (313.74 ± 212.43) and minimum in the summer (37.09 ± 22.20), with no significant difference between spring and autumn (Figure 2C). The average DO content varied from 5.87 to 8.91 mg/L, with the highest concentration in winter (Figure 2D). The average pH varied within the range of 7.91–8.16, and the values in autumn and winter were generally higher than those in spring and summer. Nevertheless, there was no significant difference in pH between spring and summer (Figure 2E). In contrast, COD (0.31–2.94 mg/L) showed a temporally increasing trend but no significant seasonal difference from summer to winter (Figure 2F). Analogously, nutrient concentrations (i.e., DIN, DIP, and SiO3) showed explicit seasonal patterns (Figures 2G–I); especially DIN and DIP (0.20–0.85 and 0.018–0.038 mg/L, respectively), increased in the autumn.
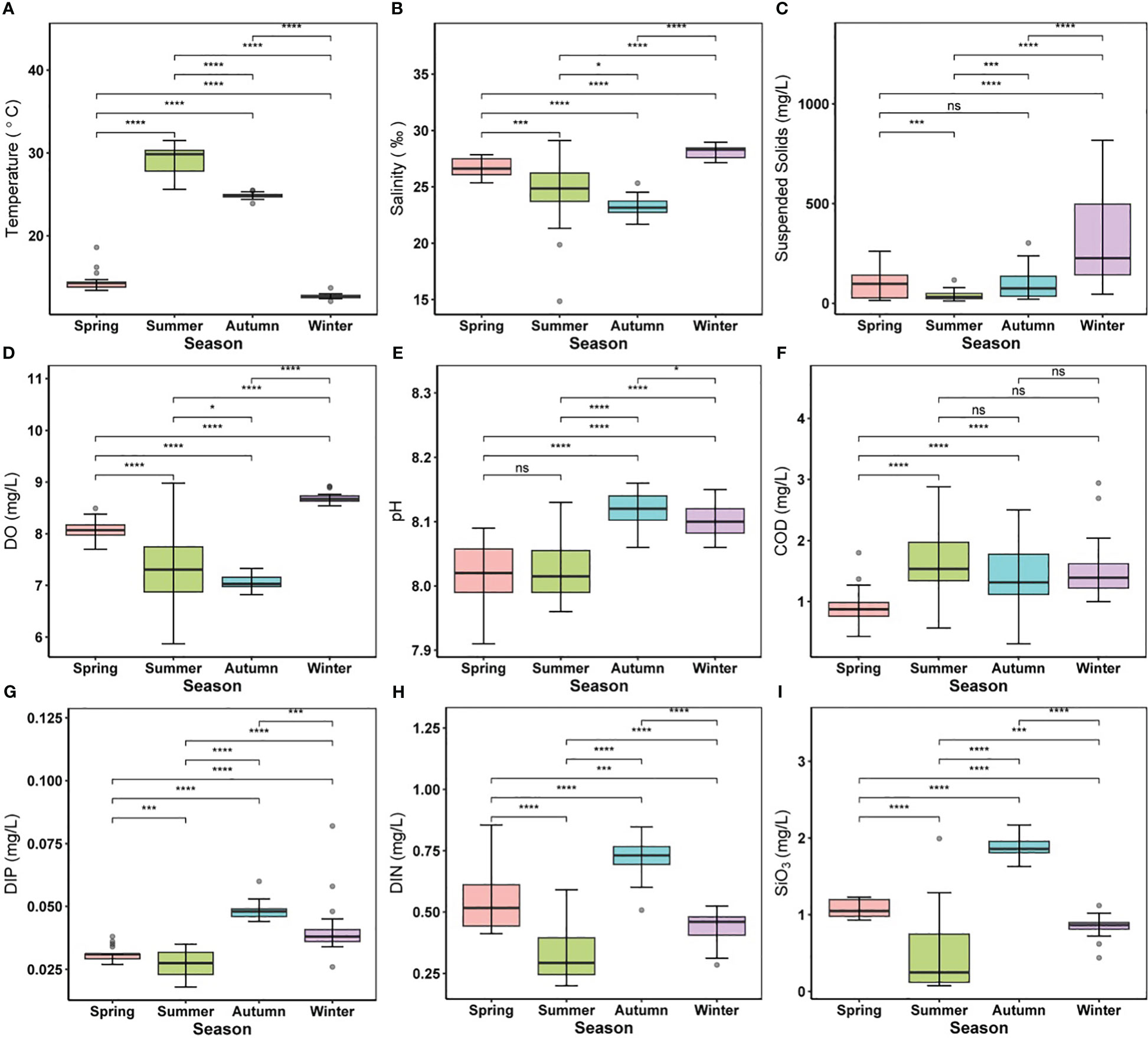
Figure 2 Alternations of environmental parameters in different seasons. (A) temperature, (B) salinity, (C) suspended solids, (D) DO, (E) pH, (F) COD, (G) DIN, (H) DIP, (I) SiO3. Different colored boxes represent four different seasons. For boxplot figures, the box border: the interquartile range; the horizontal line in the box: average value; the upper and lower vibrissae: range beyond the upper and lower quartiles, respectively; grey dots: outliers. Significance indicated by the asterisks (p-value: * <0.05, *** <0.001, **** <0.0001). ns indicates nonsignificance.
3.2 Eutrophication and organic pollution in the SMB
The average Ei index ranged between 0.90 and 21.9, with higher values during autumn and winter (Figure 3A). According to the Ei, sea areas (>20% coverage) were defined as heavily eutrophic areas year round (Ei > 9), while mid-eutrophic areas (3 < Ei ≤ 9) covered >30%, indicating a eutrophic in the entire surveyed area. In most cases, the average Ci index ranged from 2.20 to 7.43, indicating severe organic pollution. Overall, the organic pollution status in autumn was worse than in other seasons (Figure 3B). Furthermore, the temporal trends for the Ei and Ci indices were broadly similar. pH was positively correlated with both Ei and Ci indices (p < 0.001) (Figure 3C), suggesting that variation in pH has a large impact on the level of eutrophication and organic pollution. It is possible that water acidification owing to reduced pH gradually would alleviate this eutrophication and organic pollution. Both Ei and Ci indices were correlated with water temperature, but with different interactions (Figure 3D): the Ei index was positively correlated with temperature, implying that elevated temperatures (i.e., ocean warming) would intensify coastal eutrophication, in comparison, the Ci index was negatively correlated with temperature, suggesting that ocean warming would mitigate organic pollution.
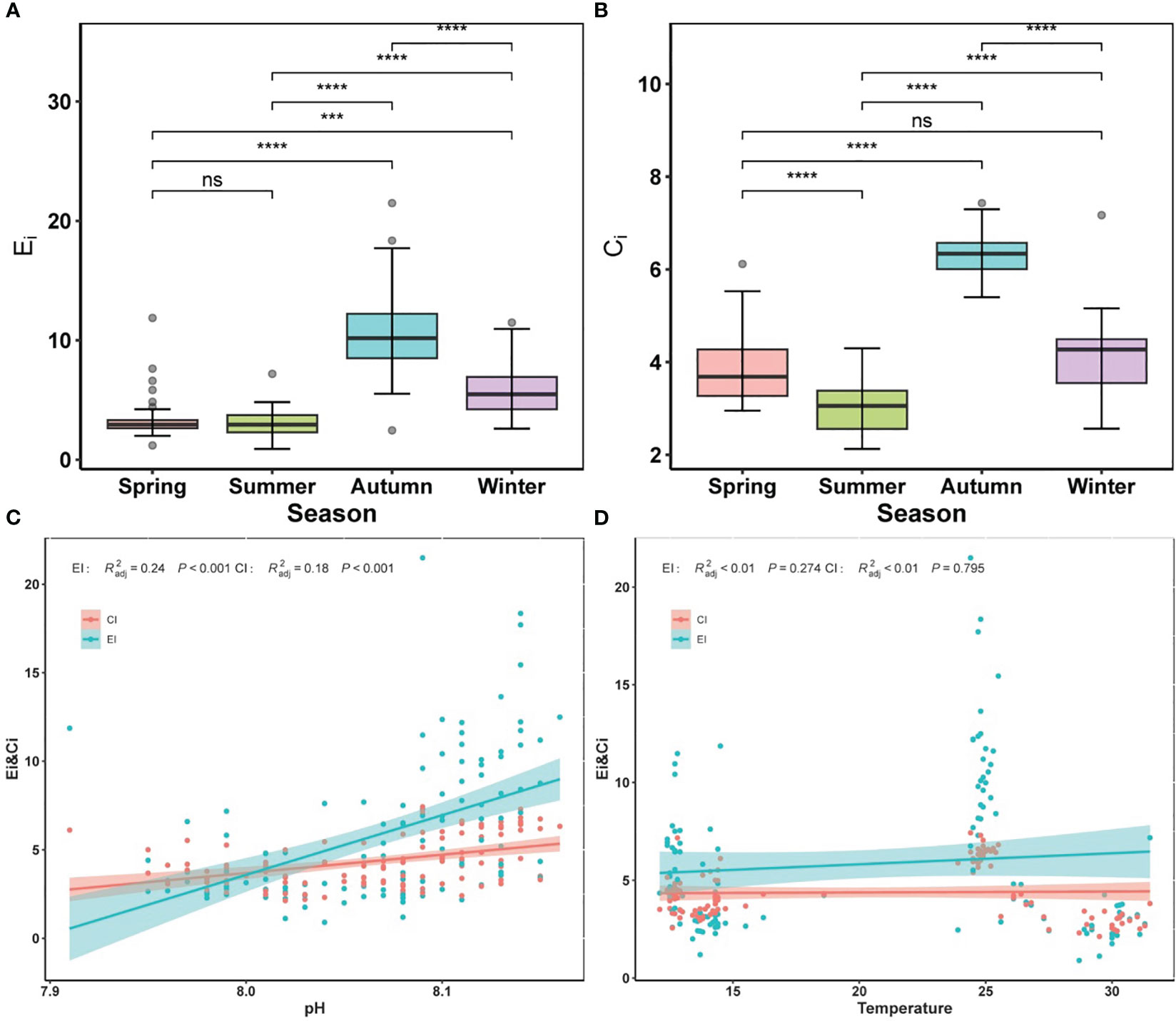
Figure 3 Alternations of eutrophication index (Ei), and organic pollution comprehensive index (Ci) in different seasons. (A) Ei, (B) Ci. (C) significant relationships between pH and Ei and Ci, (D) significant relationships between temperature and Ei and Ci. Colored lines in panels (C, D) represent the least squares regression, which shows statistically significant for both cases (R2 and p-value). Shaded areas are 95% confidence bands. Significance indicated by the asterisks (p-value: *** <0.001, **** <0.0001). ns indicates nonsignificance.
3.3 Phytoplankton community structure
A total of 118 taxa were identified over all seasons (including species, varieties, and forms), comprising 84 diatoms, 28 dinoflagellates, 1 euglenophyte, 3 cyanobacteria, and 2 chrysophytes. Diatoms were the dominant group, accounting for approximately 71.2% of the taxa and 82.6% of phytoplankton abundance on average, while dinoflagellates accounted for 23.7% of the taxa and 10.8% of phytoplankton abundance on average. There were remarkable seasonal variations in phytoplankton compositions (Figure 4), with species richness increasing from spring to summer and decreasing in cold seasons (Figure 4A). Phytoplankton abundance was also markedly higher in summer (80.04 ± 53.14 × 103 cells/L on average with a maximum of 213.8 × 103 cells/L) than in other seasons, with a nonsignificant difference between spring and autumn (Figure 4B). The abundance of the diatoms (from 4.2 to 205.8 × 103 cells/L) followed the same seasonal trend, with a nonsignificant difference between spring and autumn (Figure 4C), and the abundance of dinoflagellates in summer (3.83 ± 2.55 × 103 cells/L) was slightly greater than in other seasons, with a significant temporal difference across the four seasons (Figure 4D).
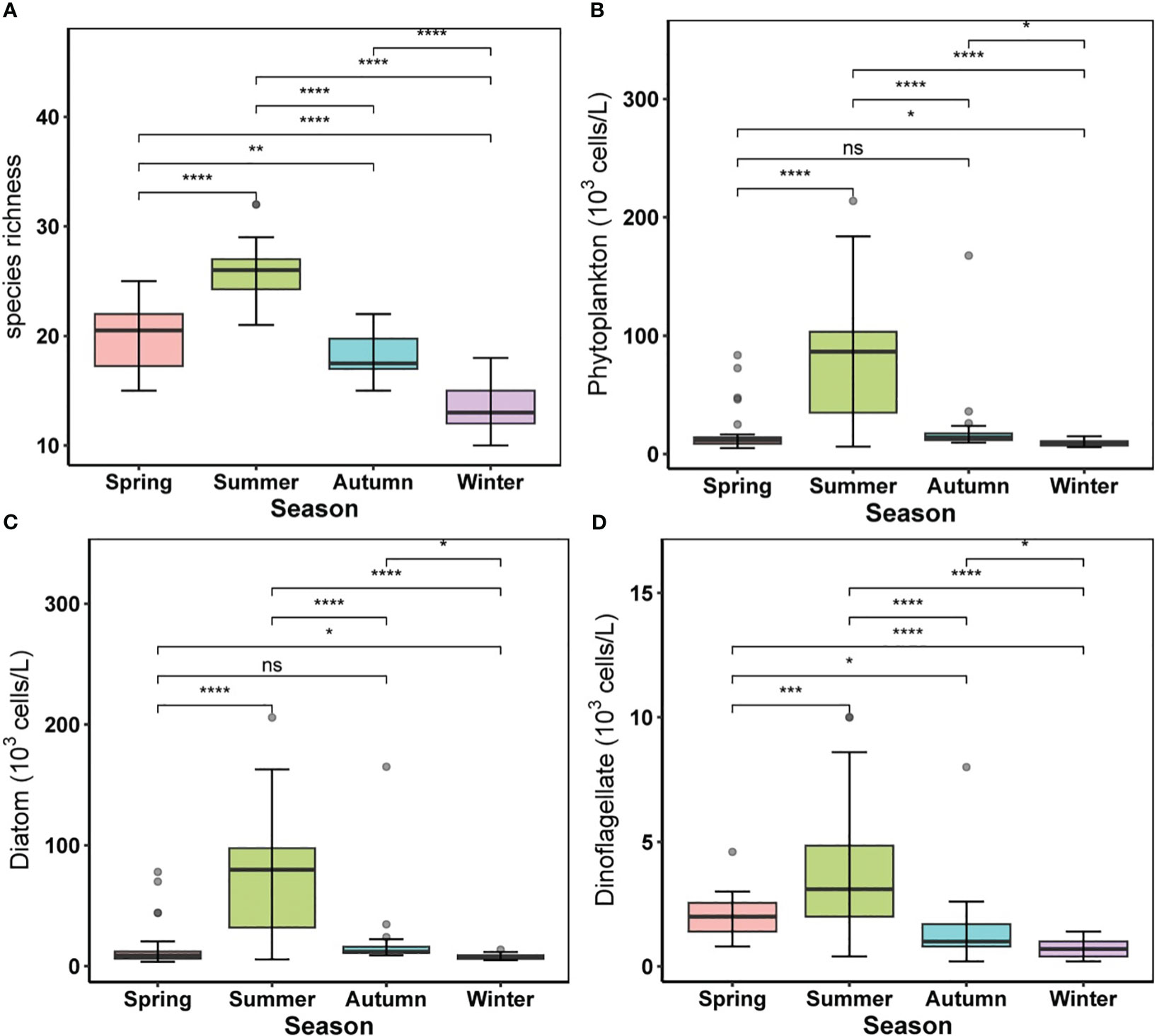
Figure 4 Alternations of phytoplankton community in different seasons. (A) species richness, (B) phytoplankton abundance, (C) diatom abundance, (D) dinoflagellate abundance. For boxplot figures, the box border: the interquartile range; the horizontal line in the box: average value; the upper and lower vibrissae: range beyond the upper and lower quartiles, respectively; grey dots: outliers. Significance indicated by the asterisks (p-value: * <0.05, ** <0.01, *** <0.001, **** <0.0001).
The dominant species were mainly colonial diatoms of either single-celled or multicellular cells (Figure 5). The chain-forming diatoms Skeletonema spp. and Paralia sulcata dominated in all seasons (Y > 0.20). The chain-forming Skeletonema. spp. was the most abundant species in all seasons (Y > 0.15) except in summer, when the dominance of Skeletonema marinoi was 0.095. The most abundant chain-forming P. sulcata and Skeletonema costatum in spring had the greatest dominances, which matched their large proportions in total phytoplankton abundance. The most dominant species in summer was small single-celled Ditylum brightwellii with a dominance of 0.218, followed by chain-forming Leptocylindrus danicus (0.196) and large single-celled Biddulphia sinensis (0.158). Some chain-forming diatoms, such as Chaetoceros lorenzianus and Chaetoceros knipowitschi, were dominant in the summer. In autumn, the predominant species were largely chain-forming Thalassionema nitzschioides, with a dominance index of up to 0.16. Colonial Thalassiosira subtilis had its highest dominance (0.083) in autumn. In winter, the maximum dominance value (0.114) was attributed to small single-celled Coscinodiscus radiates. Some large single-celled diatoms, such as Coscinodiscus oculus-iridis, Coscinodiscus jonesianus, and Coscinodiscus subtilis, were also dominant in winter. Consequently, phytoplankton community was primarily dominated by diatoms. Skeletonema marinoi, S. costatum, P. sulcata, B. sinensis, and C. oculus-iridis always dominated in this region, even though the top eight dominant species varied widely with season. These results suggest that environmental changes could affect the phytoplankton community, but that some dominant diatoms have a strong adaptability or tolerance to these changes.
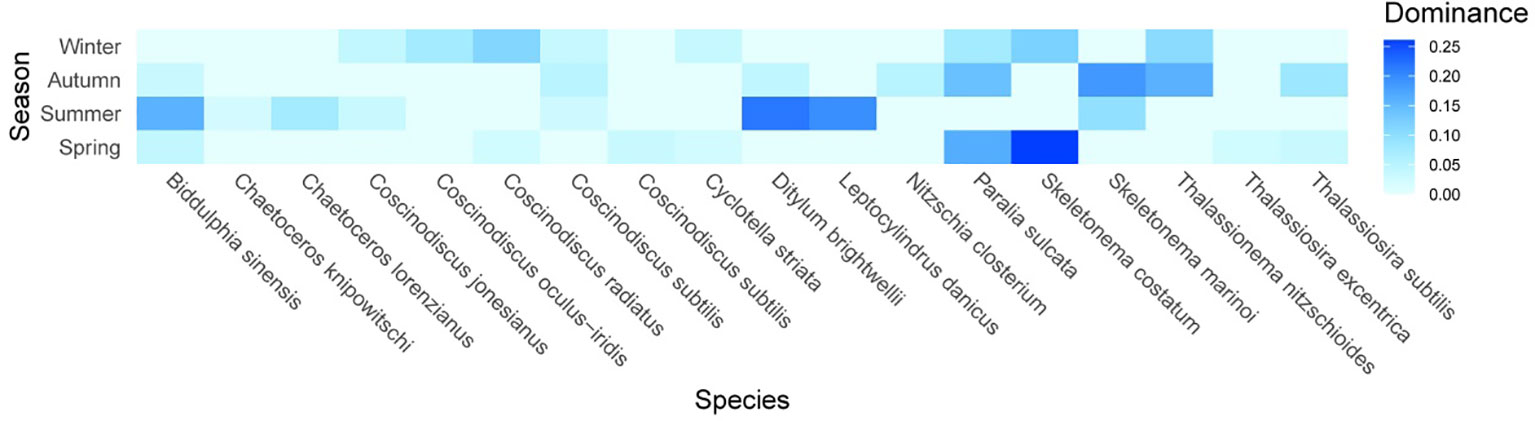
Figure 5 Alternations of phytoplankton dominant species in different seasons. The magnitude of dominance index (Y) for phytoplankton species is indicated by color intensity changing from light blue to dark blue.
3.4 Relationships between phytoplankton and water quality indicators
A CCA (Figure 6) and Spearman matrix (Figure 7A) were performed to examine the relationships between phytoplankton communities and environmental settings. Nutrients, temperature, and SS were the major variables associated with phytoplankton abundance throughout the year. Weak correlations between phytoplankton abundance and the N:P ratio were found in all seasons. The common HAB species, Skeletonema spp., was positively correlated with nutrients and the N:P ratio in spring and summer, and positively correlated with salinity and DO in autumn and winter. The chain-forming diatoms (e.g., genus Chaetoceros, P. sulcata, T. nitzschioides, and genus Thalassiosira) were highly correlated with SS and salinity. Small single-celled diatoms (e.g., Cyclotella striata, Nitzschia clostrium, and D. brightwellii) showed less correlation with environmental settings, but large single-celled diatoms (genus Coscinodiscus and B. sinensis) were correlated with high nutrients and high SS concentrations. These findings suggest that the temporal variation in dominant phytoplankton species was largely influenced by SS concentrations and N:P ratio in spring, nutrients and salinity in summer, nutrients and temperature and autumn, and nutrients in winter, respectively.
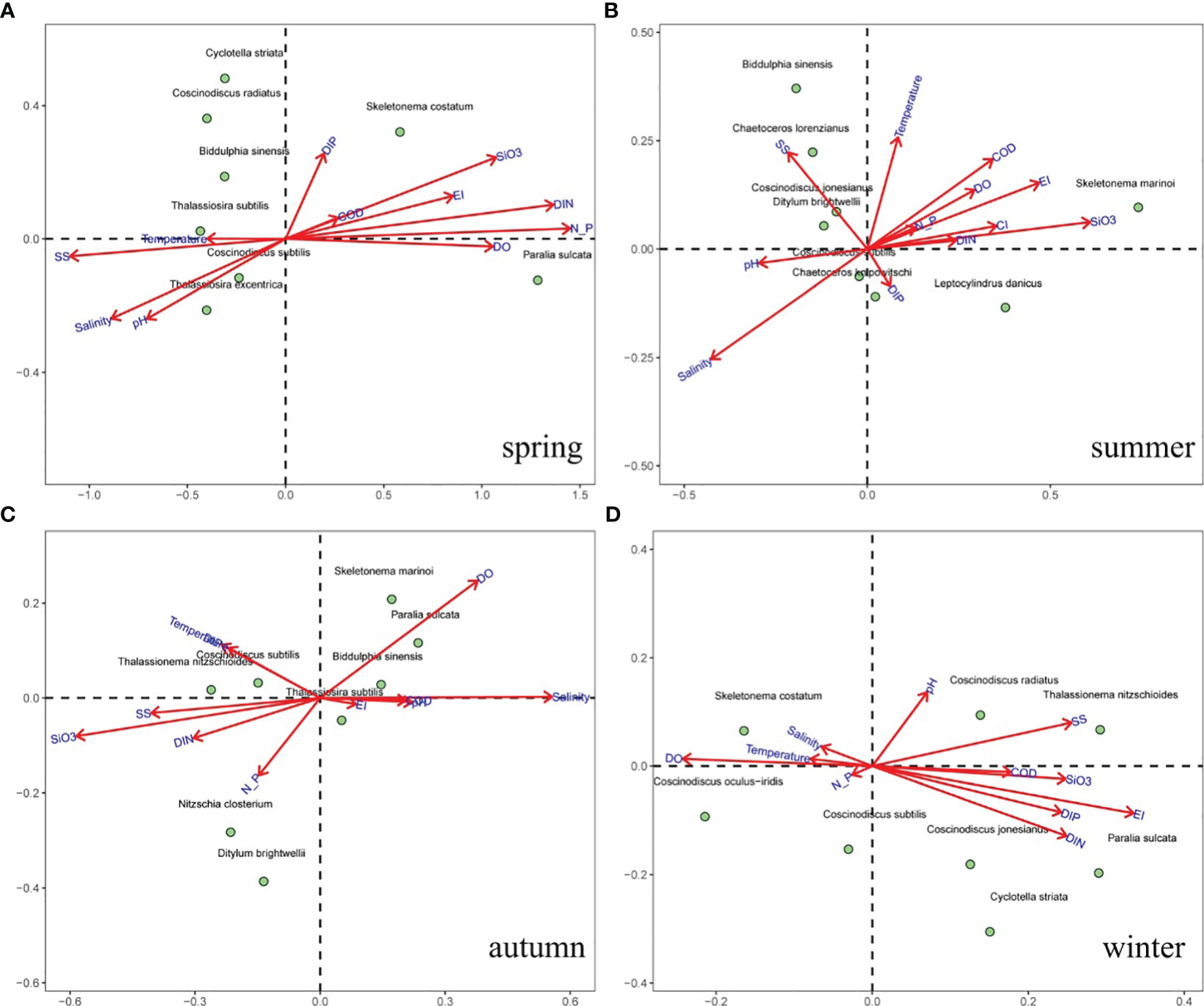
Figure 6 The CCA ordination biplots of phytoplankton abundance and environmental settings in different seasons. (A) spring, (B) summer, (C) autumn, and (D) winter.
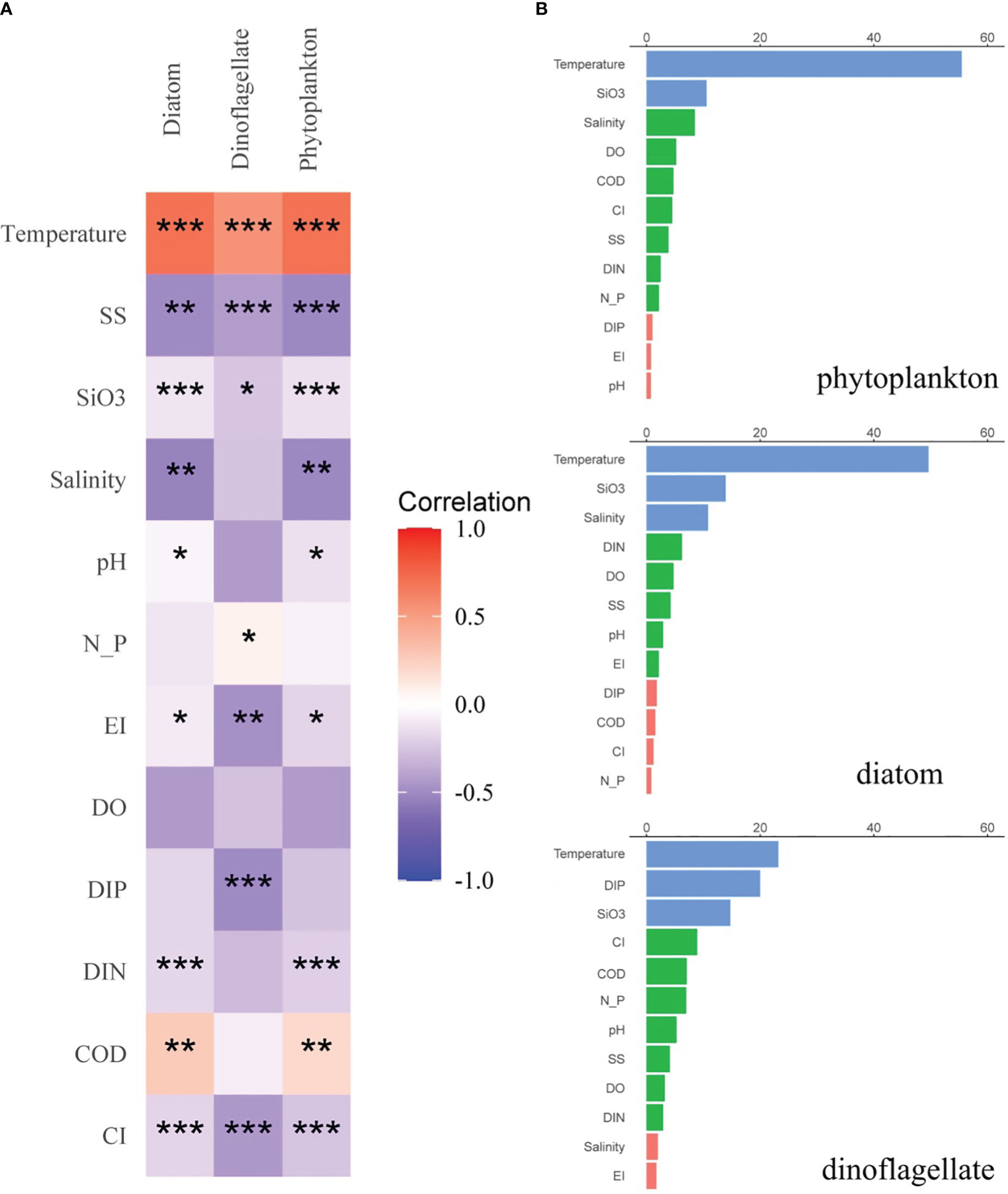
Figure 7 (A) Correlation matrix among the various environmental parameters and major phytoplankton groups over all seasons. Spearman correlation coefficients ranged from negative to positive and are indicated by color intensity changing from blue to red, respectively. Significance indicated by the asterisks (p-value: * <0.05, ** <0.01, *** <0.001). (B) ABT analysis for the relative effects of environmental settings on abundance of major phytoplankton groups over all seasons. The blue, green, and red bars represent the ranges of relative effect in >10%, 2–10%, and <2%, respectively.
An ABT analysis was ran to further quantify the effects of environmental settings on major phytoplankton populations (Figure 7B), which revealed that the major predictors were population specific. Specifically, SiO3 was the major predictor of diatom abundance. In contrast, Gymnodinium catenatum and Akashiwo sanguinea, which are dinoflagellates, experienced favorable conditions in the form of warm temperatures, low salinity, and low DIP concentrations. Thus, temperature emerged as the primary determinant of dinoflagellate abundance, and temperature, SiO3, and SS were identified as the primary predictors of overall phytoplankton, diatoms, and dinoflagellates. The relative influence of each factor was not high (<20%), except for temperature (>30%).
Consequently, the causal relationships between phytoplankton abundance and relevant environmental parameters were examined using SEM (Figure 8). We focused on interactions between temperature, salinity, N:P ratio, SiO3, Ei and Ci index (water quality assessment indices) as the importance of these variables was indicated in the above mentioned analysis (Figures 6 and 7). The model results showed that both Ei and Ci indices had a significant direct effect on phytoplankton abundance. Ei had a significant positive effect on phytoplankton abundance but a nonsignificant negative effect on diatom abundance. The Ci index had a nonsignificant negative effect on both diatom and dinoflagellate abundance. The Ei and Ci indices exhibited a positive interaction with each other. Temperature and SiO3 had positive direct effects on phytoplankton and diatom abundance, while salinity and N:P ratio had negative direct effects on phytoplankton and diatom abundance. Temperature, and N:P ration demonstrated positive direct effects on dinoflagellates, while salinity had a negative direct effect. An indirect effect of SiO3 on dinoflagellates was also indicated. Overall, temperature had the greatest total positive effect and salinity had the greatest total negative effect (Figure 8).
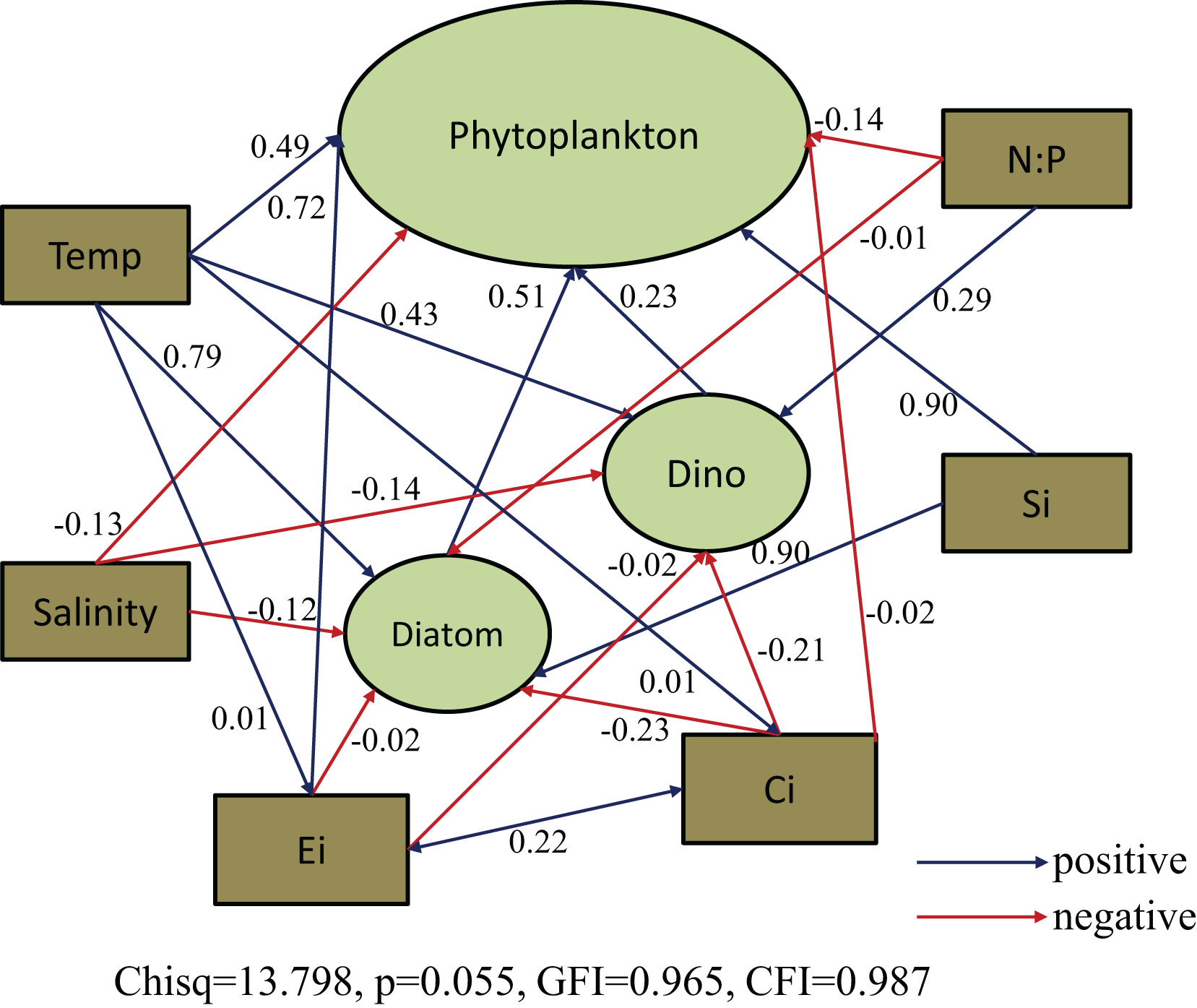
Figure 8 Structural equation model (SEM) analysis examining the effects of relevant environmental parameters (temperature, salinity, N:P ratio, SiO3) and water quality assessment indices (Ei and Ci index) on major phytoplankton groups over all seasons. Solid blue and red lines indicate significant positive and negative effects at p < 0.05, respectively, and dashed blue and red lines indicate nonsignificant positive and negative effects, respectively. Values associated with arrows represent standardized path coefficients.
4 Discussion
4.1 Nutrient regime and water temperature dynamics in the SMB
SMB has demonstrated a longstanding commitment to mariculture and related industries since the 1980s (Chen et al., 2015; Liu et al., 2021). The expansion of these activities has been extensively expanded (Jiang et al., 2012; Ye et al., 2017). The ecological integrity of the area has been persistently jeopardized by thermal discharges originating from a coastal power plant. in this area have also threatened the ecological status for a long time (Liang et al., 2021; Wang et al., 2022). Consequently, these anthropogenic activities have resulted in the excessive introduction of pollutants and nutrients, particularly nitrogen (N) and phosphorus (P), into the nearshore marine environment. This influx has not only disrupted the composition of phytoplankton communities but has also accelerated their growth. In this study, it was observed that environmental factors and water quality in the SMB exhibited noteworthy significant seasonal variation (Figures 2 and 3). Furthermore, the phytoplankton displayed distinct responses to these temporal alternations (Figures 5–8).
Eutrophication and surface warming pose significant threats to marine ecosystems, as evidenced by multiple studies (Laurent et al., 2018; Deininger and Frigstad, 2019; Freeman et al., 2019; Wei et al., 2022). The influence of nutrients on the phytoplankton community is widely acknowledged (Irwin et al., 2006; Song et al., 2016; Cloern, 2018; Lee et al., 2021). The findings from the CCA and ABT analyses provide further confirmation that seasonal variation in the phytoplankton community are primarily driven by nutrient levels (Figures 6 and 7B). Table 1 presents a comprehensive depiction of the prolonged fluctuations in averaged DIN, DIP, N/P, Ei (the eutrophication index), and dominant phytoplankton species from the 1980s to 2020. Over the course of the past five decades, there has been a discernible upward trajectory in nutrient content and N/P ratio. In comparison to the 1980s, the annual average DIN content in this investigation has experienced an approximate threefold increase, while the N/P ratio has doubled. Specifically, the N:P ratio has increased from 13.3 to 28.6 in the SMB (Gao et al., 2022), and showed an increasing trend from the 1980s to 2015 (Table 1). It was generally higher than the Redfield ratio of 16 (Redfield, 1958), with an average N:P ratio as high as 22 in spring in this study. The strong negative association between most of the dominant phytoplankton species and N:P ratio, and the positive relationship with SS, supports the inference that phytoplankton distribution in spring was principally controlled by lower SS and a higher N:P ratio (Figure 6). In addition, the SMB has experienced prolonged eutrophication as a result of its limited water exchange capacity and excessive input from tributaries. Consequently, the level of eutrophication (Ei) has significantly escalated (Table 1), leading to an increase in HAB occurrences and alterations in species composition caused by human activities (Jiang et al., 2012; Chen et al., 2017; Gao et al., 2022).
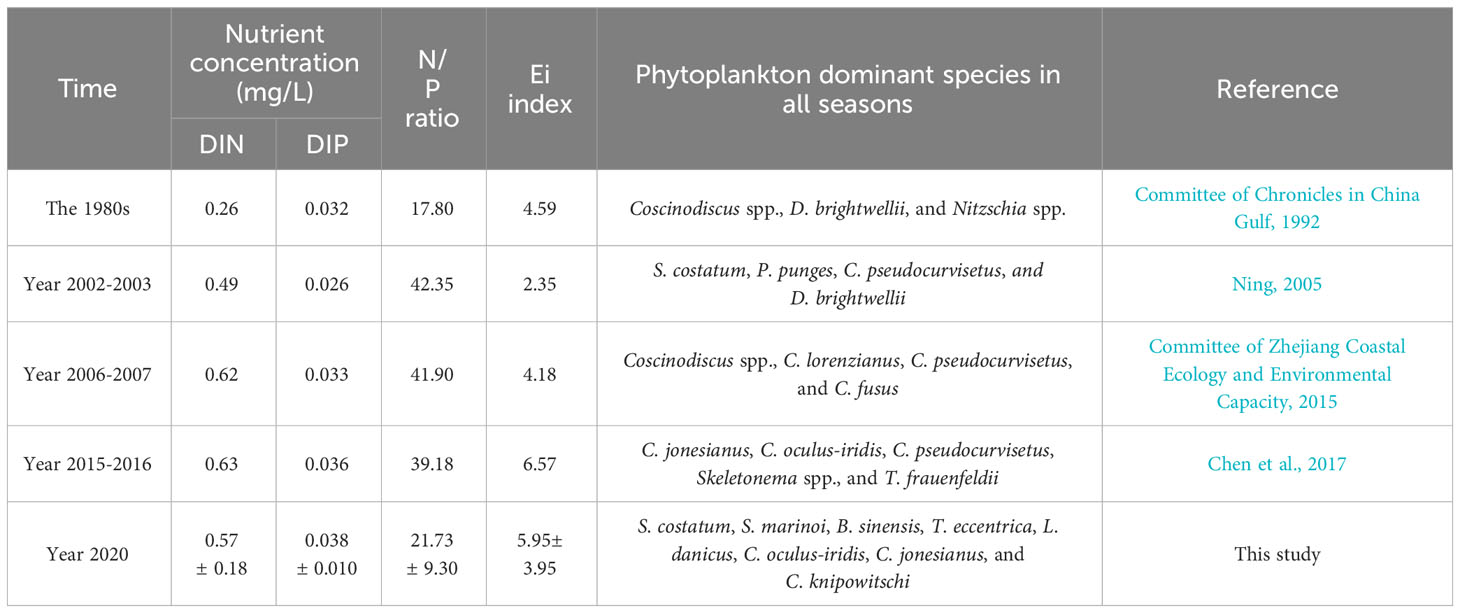
Table 1 Long-term variation in SMB in averaged nutrient concentration, N/P ratio, eutrophication index and dominant species of phytoplankton in all seasons.
In the past 50 years, the composition of dominant species in the SMB has been influenced by the presence of chain-forming diatoms, which have partially constrained the dominance of large single-celled diatoms (Table 1). The proliferation of chain-forming diatoms has been observed to occur at a faster rate under conditions of high nutrient content, as supported by studies conducted by Finkel et al. (2010); Wang et al. (2019), and Song et al. (2016). Furthermore, there has been a significant increase in the N/P ratio in the SMB over time. This increase has created a favorable environment for the growth of small-celled chain-forming diatoms, which have a lower demand for DIP compared to large single-celled diatoms and dinoflagellates. (Glibert and Burkholder, 2011; Xiao et al., 2018; Xin et al., 2019). Hence, the increasing prevalence of chain-forming diatoms surpassing large-cell dinoflagellates, such as genera Ceratium and Prorocentrum, may have contributed to the disappearance of dinoflagellates as the dominant species in this study (Figure 5). Throughout the entire duration of the study, the succession of diatoms and dinoflagellates in relation to N:P ratios did not exhibit seasonal variation (Figures 5 and 6). Correlation and ABT analysis (Figure 7) indicated that dinoflagellates possessed a stronger competitive advantage over diatoms in environments with limited biogenic factors. Furthermore, the presence of silicates exerted a substantial impact on diatoms, in addition to their utilization of nitrogen.
Phytoplankton experiencing nutrient limitations are compelled to adapt to the warming of seawater. The increase in temperatures has the potential to expedite the absorption of nutrients by phytoplankton, as evidenced by studies conducted by Berges et al., 2002; Wu et al., 2021 and Wei et al., 2022. The presence of the coastal nuclear power plant (NPP) in SMB plays a significant role in this phenomenon, as NPPs produce substantial quantities of warm water effluent (Hu, 2004), thereby substantially raising the temperature of the surrounding aquatic environment (Langford, 1990; Krishnakumar et al., 1991; Zhang et al., 2023). The thermal discharge from NPPs has the potential to affect phytoplankton survival, growth, and reproduction (Langford, 1990; Lo et al., 2004; Poornima et al., 2006; Zhang et al., 2023), resulting in changes to the upper trophic levels and modification of the ecosystem. The study observed a clear seasonal fluctuation in temperature, which, in conjunction with salinity, had a significant impact on the temporal distribution of phytoplankton (Figure 6) (e.g., Jiang et al., 2014; Song et al., 2016; Wei et al., 2022). Our findings suggest that temperature was significantly positively correlated with phytoplankton abundance, and thus ocean warming would favor high phytoplankton populations (Figures 7 and 8). Further, elevated temperatures would intensify the progress of coastal eutrophication (Figure 3D). Numerous studies have demonstrated that the concurrent effects of warming and eutrophication have the potential to amplify the growth and metabolic rates of phytoplankton, consequently leading to an increase in both the magnitude and occurrence of phytoplankton blooms, including HABs (Winder and Sommer, 2012; Xiao et al., 2018; Lee et al., 2019). Specifically, the occurrence and duration of algal blooms in subtropical eutrophic waters are frequently linked to thermal discharge from power plants (Yu et al., 2007; Jiang et al., 2019a; Jiang et al., 2019b). Nevertheless, this study did not observe any blooms throughout the four seasons (Figures 4 and 5). Previous research has shown that under eutrophic conditions, an increase in temperature resulting from thermal discharge has led to an augmentation in phytoplankton abundance in the SMB (Chen et al., 2017; Gao et al., 2022). The sequence of prevailing species in the local area was significantly influenced by the thermal discharge originating from the NPP in SMB. This discharge facilitated the growth and reproductive activities of C. jonesianus (Yang et al., 2013), ultimately resulting in the prevalence of Coscinodiscus during the winter and spring seasons, as depicted in Figures 5 and 6 of this study.
4.2 Phytoplankton community shift in the SMB
Phytoplankton, as a primary producer in aquatic ecosystems, experiences alterations in its community structure due to nutrient load and hydrologic regimes (Hart et al., 2015; Song et al., 2022). The present study reveals noteworthy seasonal fluctuations in the phytoplankton community (Figures 4–6). Additionally, the hydrologic conditions exhibited temporal variability, thereby inducing dynamic fluctuations in the phytoplankton community (Figures 4–6). In particular in spring, the low concentration of suspended solids and high transparency of the water facilitated the growth of phytoplankton, leading to an increase in both abundance and species richness (Cloern and Dufford, 2005; Jiang et al., 2019b) as depicted in Figures 2C and 4. During the summer, the Taiwan Warm Current intensified, causing a significant influx of neritic warm-water species into the bay (Zhu et al., 2012; Jiang et al., 2014). Concurrently, the intrusion of oligotrophic and highly transparent seawater resulted in reduced levels of suspended solids and nutrients (Figure 2). However, the highest abundance and species richness were found in summer accordingly (Figure 4). A previous study in the Atlantic coast of the Brazil, which had lower nutrient loads but greater light availability, also suggested a stimulated phytoplankton abundance (Cavalcanti et al., 2020). In autumn, the Zhemin Coast Current, characterized by low salinity and high nutrient content, exerted control over the system (Zhu et al., 2012; Jiang et al., 2014). This resulted in an increase in suspended solids concentration and a decrease in transparency, which disrupted the water environment (Figure 2C). Consequently, the growth of phytoplankton species was constrained, leading to a significant decline in abundance and species richness compared to the summer season (Figure 4) (Chen et al., 2017; Gao et al., 2022). However, due to the persistence of warmer water temperatures, a certain abundance of warm-water species managed to survive (Shou and Zeng, 2015; Gao et al., 2022). During the winter season, the bay experienced elevated levels of suspended solids primarily attributed to the intensified hydrodynamic forces. Additionally, the suboptimal temperature conditions hindered the growth of phytoplankton, resulting in diminished abundance and species diversity. Furthermore, phytoplankton species richness index exhibited a value of 19 across the four seasons, signifying a notable seasonal change in species composition. This occurrence has been previously recorded, as a study by Tang et al. (2018) reported a total of 12 shared species annually in the vicinity of Zhoushan Island in the East China Sea. The presence of a relatively diverse local community plays a crucial role in stabilizing the ecosystem by preserving the proportional abundance of certain species (Carr et al., 2002). Generally, coastal eutrophication had profound effects on the diversity and complexity of phytoplankton composition and biomass. Specifically, it enhanced the frequency of HAB events accompanied by severe Si limitation (Lin et al., 2001; Carstensen et al., 2007; Wang et al., 2019; Xin et al., 2019). The ABT revealed that Si was a major predictor of total phytoplankton, diatoms, and dinoflagellates (Figure 7B), indicating that the Si concentration had a significant impact on the relative proportion of dinoflagellates to diatoms. The significant impact of Si on dinoflagellates in SMB may be attributed to the presence of a widely distributed harmful algal bloom specie, A. sanguinea, which showed substantial abundance during the summer and autumn in this study. Prior research has indicated that A. sanguinea may have a preference for nitrate uptake over silicon, as evidenced by the low nitrate concentration observed (Du et al., 2011). This finding aligns with the observed negative correlation between dinoflagellates and DIN concentration throughout the present study (Figure 7A). The prevalence of A. sanguinea was observed to be prominent along the coast of Korea, resulting in blooms in autumn (Kim et al., 2019). Conversely, during the spring and summer seasons, while interspecific interactions, such asallelopathy exhibited by centric diatoms, were found to hinder the formation of these blooms (Figure 5) (e.g. Matsubara et al., 2008). This dominance of A. sanguinea aligns with the P–Si limitation observed in recent decades (Wang et al., 2019; Xin et al., 2019; Song et al., 2022).
Diatoms and dinoflagellates are of significant concern in coastal waters due to their pivotal role in aquatic ecosystems (Agustí et al., 2015). These groups exacerbate the impacts of eutrophication and ocean warming. Chen et al. (2017) demonstrated, through net collection of phytoplankton, that warm-water species such as Chaetoceros pseudocurvisetus and Palmeria hardmaniana, which became dominant in SMB in winter, facilitated the dominance of abundances. This increased dominance of genus Chaetoceros and P. hardmaniana indicates that warming influences the phytoplankton composition in SMB. Gao et al. (2022) posited that the community structure of phytoplankton in SMB was undergoing a transition towards cell miniaturization, resulting in a shift in the net collection of phytoplankton dominant species as warm-water species became more prevalent. However, our findings based on phytoplankton collected from water samples indicated that the dominance of genus Chaetoceros only increased in summer in SMB, and this genus was not dominant in other seasons (Figure 5). The dominance of small-celled chain-forming diatoms (particularly genera Skeletonema and Thalassiosira) increased from spring to autumn, while the dominance of large single-celled diatoms (e.g., genus Coscinodiscus and species D. brightwellii) increased in winter, suggesting that the shifting of phytoplankton composition toward cell miniaturization was not evident across seasons (Figures 5 and 6). This is in contradiction to reports from Hangzhou Bay in East China Sea (Zhang et al., 2015) and Daya Bay in south China Sea (Wu et al., 2017). Large diatoms such as genus Coscinodiscus and species D. brightwellii exhibit a notable ability to absorb and assimilate nutrients, enabling them to sustain a rapid growth rate (Philippart et al., 2000). This heightened nutrient consumption results in diminished nutrient levels. Consistent with the presence of the diatom genus Coscinodiscus, the winter season witnessed the second lowest nutrient concentrations in this study (Figures 2 and 6).
Dinoflagellates have been observed to dominate in conditions of lower DIP and are less sensitive to temperature compared to diatoms (Glibert et al., 2010; Wells et al., 2015). Previous studies have indicated a decrease in the proportion of diatom species in waters as the abundance of dinoflagellate species increases (Chen et al., 2017; Gao et al., 2022). This shift has been attributed to the competitive advantage of dinoflagellates over diatoms in environments characterized by higher N:P ratios and elevated temperatures (Xiao et al., 2018; Zhang et al., 2021; Wei et al., 2022). The proportions of dinoflagellates to phytoplankton abundance in the SMB were constrained by the lower temperature, higher DIP, and lower light availability (high SS) across the four seasons (Figures 7 and 8). Nitrophilous diatoms, particularly the overwhelmingly dominant genera Skeletonema, Thalassiosira and Thalassionema, were present throughout the year due to their greater tolerance to environmental variability, including low salinity and abundant nutrients (Figures 2 and 6). This finding is consistent with previous studies by Philippart et al., 2000; Shou and Zeng, 2015; Chen et al., 2017; Jiang et al., 2019a; Cavalcanti et al., 2020; and Song et al., 2022. The most dominant eurythermal species, Skeletonema spp., shows a high level of dominance in the coastal waters of the East China Sea, particularly in areas such as the Changjiang estuary (Jiang et al., 2014) and Hangzhou Bay (Zhang et al., 2015). This dominance is attributed to its ability to exploit community competition within eutrophic, low-salinity, and turbid waters. The restructuring of diatom and dinoflagellate communities in coastal ecosystems is observed to be influenced by eutrophication and warming (Glibert et al., 2010; Wells et al., 2015; Song et al., 2022). This restructuring is attributed to changes in nutrient ratios, leading to a transition from siliceous phytoplankton to nonsiliceous phytoplankton and a reduction in cell size (Liu et al., 2009; Finkel et al., 2010; Gao et al., 2022). However, further investigation is needed to fully understand the impact of warming and nutrient ratio dynamics on the succession of diatoms and dinoflagellates in the SMB, necessitating the collection of long-term datasets.
5 Conclusions
The main reason for the deterioration of the coastal environment is that a large number of pollutants produced on land are discharged into it, including inorganic nitrogen, active phosphate, and heavy metals, etc. This study conducted a systematical analysis of phytoplankton diversity in a eutrophic area of the coastal East China Sea, integrating environmental changes and water quality. This study employed a high temporal resolution. Highly significant seasonal variations in the abundance and community structure of water-collected phytoplankton and environmental settings were identified in the SMB. The results revealed significant seasonal differences in the phytoplankton community, primarily driven by variations in temperature, Si concentrations, and SS. The succession of diatoms and dinoflagellates related to N:P ratio dynamics was not evident across seasons, requiring more long-term datasets. In addition, eutrophication and organic pollution had significant direct effects on phytoplankton abundance. The significance of NPPs should not be disregarded or undervalued, particularly in relation to their impact on phytoplankton responses to eutrophication under thermal stress. As the construction of NPPs persists along China’s coastline, it is imperative to allocate additional resources towards the investigation of these responses. Additionally, unreasonable development and layout of the marine space, as well as high-intensity land reclamation, have put intense pressure on natural resources and ecosystems in SMB. These all need to bring to the attention and worth pondering with the discussion. A preliminary understanding of the current state of phytoplankton community structures and their regulation in SMB will facilitate forthcoming investigations in evaluating changes across broader spatial and extended temporal dimensions.
Data availability statement
The original contributions presented in the study are included in the article/supplementary materials, further inquiries can be directed to the corresponding author/s.
Author contributions
YW: Data curation, Investigation, Methodology, Resources, Visualization, Writing – original draft, Writing – review & editing. WW: Conceptualization, Data curation, Formal analysis, Investigation, Resources, Visualization, Writing – review & editing. YH: Data curation, Formal analysis, Investigation, Resources, Visualization, Writing – review & editing. LC: Conceptualization, Data curation, Investigation, Methodology, Writing – review & editing. XT: Conceptualization, Data curation, Investigation, Methodology, Writing – review & editing. XH: Data curation, Formal analysis, Funding acquisition, Project administration, Supervision, Validation, Writing – review & editing. LH: Data curation, Formal analysis, Funding acquisition, Project administration, Supervision, Validation, Writing – review & editing.
Funding
The author(s) declare financial support was received for the research, authorship, and/or publication of this article. This research was funded by the National Key Research and Development Program of China (contract Nos. 2022YFC2804003 and 2022YFC3102401), the National Natural Science Foundation of China (contract Nos. 42006127, 41506136 and 41306115) and the Project of Ministry of Science and Technology (contract No. GASI-01-02-04).
Acknowledgments
The authors acknowledge Dr. Senming Tang from Hongkong University for fruitful discussion and for helping to organize the manuscript. Thanks to the staff workers from Second Institute of Oceanography for their logistical support. We would also like to thank Dr. Xiuwu Sun from Third Institute of Oceanography for assistance with the determination of environmental parameters. We would like to thank MogoEdit (https://www.mogoedit.com) for its English editing during the preparation of this manuscript. We sincerely appreciate the constructive comments and suggestions of the anonymous reviewers.
Conflict of interest
The authors declare that the research was conducted in the absence of any commercial or financial relationships that could be construed as a potential conflict of interest.
Publisher’s note
All claims expressed in this article are solely those of the authors and do not necessarily represent those of their affiliated organizations, or those of the publisher, the editors and the reviewers. Any product that may be evaluated in this article, or claim that may be made by its manufacturer, is not guaranteed or endorsed by the publisher.
References
Agustí S., González-Gordillo J. I., Vaqúe D., Estrada M., Cerezo M. I., Salazar G., et al. (2015). Ubiquitous healthy diatoms in the deep sea confirm deep carbon injection by the biological pump. Nat. Commun. 6, 1–8. doi: 10.1038/ncomms8608
Berges J. A., Varela D. E., Harrison P. J. (2002). Effects of temperature on growth rate, cell composition and nitrogen metabolism in the marine diatom Thalassiosira pseudonana (Bacillariophyceae). Mar. Ecol. Prog. Ser. 225, 139–146. doi: 10.3354/meps225139
Borja A., Marin L., Muxika I., Pino L., Rodriz J. G. (2015). Is there a possibility of ranking benthic quality assessment indices to select the most responsive to different human pressure? Mar. pollut. Bull. 97 (1–2), 85–94. doi: 10.1016/j.marpolbul.2015.06.030
Carr M. H., Anderson T. W., Hixon M. A. (2002). Biodiversity, population regulation, and the stability of coral-reef fish communities. Proc. Natl. Acad. Sci. U. S. A. 99 (17), 11241–11245. doi: 10.1073/pnas.162653499
Carstensen J., Henriksen P., Heiskanen A. S. (2007). Summer algal blooms in shallow estuaries: definition, mechanisms, and link to eutrophication. Limnol. Oceanogr. 52, 370–384. doi: 10.4319/lo.2007.52.1.0370
Cavalcanti L. F., Cutrim M. V. J., Lourenço C. B., Karoline A., Sá D. D., Oliveira A. L. L., et al. (2020). Patterns of phytoplankton structure in response to environmental gradients in a macrotidal estuary of the Equatorial Margin (Atlantic coast, Brazil). Estuarine Coast. Shelf Sci. 245, 106969. doi: 10.1016/j.ecss.2020.106969
Chen X., Zhang J., Ma Y., Cui T. (2015). Monitoring and analysis of coastline changes of the Sanmen Bay with remote sensing during the past 40 years. Mar. Sci. 39 (2), 43–49. doi: 10.11759/hykx20141011004
Chen Y., Liu J. J., Gao Y. X., Shou L., Liao Y. B., Huang W., et al. (2017). Seasonal variation and the factors on net-phytoplankton in Sanmen bay. Oceanologia Limnologia Sin. 48 (1), 101–112. doi: 10.11693/hyhz20160700153
Cheung Y. Y., Cheung S., Mak J., Liu K., Xia X., Zhang X., et al. (2021). Distinct interaction effects of warming and anthropogenic input on diatoms and dinoflagellates in an urbanized estuarine ecosystem. Glob. Change Biol. 27, 3463–3473. doi: 10.1111/gcb.15667
Cloern J. E. (2001). Our evolving conceptual model of the coastal eutrophication problem. Mar. Ecol. Prog. Ser. 210, 223–253. doi: 10.3354/meps210223
Cloern J. E. (2018). Why large cells dominate estuarine phytoplankton? Limnology Oceanography 63 (S1), S392–S409. doi: 10.1002/lno.10749
Cloern J. E., Dufford R. (2005). Phytoplankton community ecology: Principles applied in san Francisco bay. Mar. Ecol. Prog. Ser. 285, 11–28. doi: 10.3354/meps285011
Committee of Chronicles in China Gulf (1992). “Sanmen Bay,” in Committee of chronicles in China Gulf Ed. Volume 5 of China gulf chronicles (Shanghai and Northern Gulf of Zhejiang Province) (Beijing: Ocean Press), 234–308.
Committee of Zhejiang Coastal Ecology and Environmental Capacity (2015). “Comprehensive evaluation of the trend of changes in marine environmental quality in Sanmen Bay,” in Zhejiang coastal ecology and environmental capacity. Eds. Sou L., Zeng J. N. (Beijing: Ocean Press), 136–228.
Deininger A., Frigstad H. (2019). Reevaluating the role of organic matter sources for coastal eutrophication, oligotrophication, and ecosystem health. Front. Mar. Sci. 6, 210. doi: 10.3389/fmars.2019.00210
Du X., Peterson W., Mcculloch A., Liu G. (2011). An unusual bloom of the dinoflagellate akashiwo sanGuinea off the Central Oregon, USA, coast in autumn 2009. Harmful Algae 10, 784–793. doi: 10.1016/j.hal.2011.06.011
Editorial Committee of the Bay Chorography in China (ECBCC) (1992). The bay chorography in China: part 5 (Beijing: China Ocean Press), 166–233.
Finkel Z. V., Beardall J., Flynn K. J., Quiqq A., Rees T. A., Raven J. A. (2010). Phytoplankton in a changing world: Cells size and elemental stoichiometry. J. Plankton Res. 32 (1), 119–137. doi: 10.1093/plankt/fbp098
Freeman L. A., Corbett D. R., Fitzgerald A. M., Lemley D. A., Quigg A., Steppe C. N. (2019). Impacts of urbanization and development on estuarine ecosystems and water quality. Estuar. Coasts 42 (7), 1821–1838. doi: 10.1007/s12237-019-00597-z
Fukuyo Y., Hideaki T., Chihara M., Matsuoka K. (1990). Red Tide Organisms in Japan, All Illustrated Taxonomic Guide. Uchida Rokakuho Publishing, Tokyo, 1–407.
GAQSIQ (2007b). The specification for marine monitoring–Part 7: Ecological survey for offshore pollution and biological monitoring (GB 17378.7–2007) (Beijing: China Standard Press).
Gao Y., Jiang Z., Chen Y., Liu J., Zhu Y., Liu X., et al. (2022). Spatial variability of phytoplankton and environmental drivers in the turbid Sanmen bay (East China Sea). Estuaries and Coasts 45, 2519–2533. doi: 10.1007/s12237-022-01104-7
Garmendia M., Revilla M. I., Bald J., Franco J., Martinez J. L., Orive E., et al. (2011). Phytoplankton communities and biomass size structure (fractionated chlorophylla), along trophic gradients of the Basque coast (northern Spain). Biogeochemistry 106 (2), 243–263. doi: 10.1007/s10533-010-9445-2
General Administration of Quality Supervision, Inspection and Quarantine (GAQSIQ) (2007a). The specification for marine monitoring–Part 4: Seawater analysis (GB 17378.4–2007) (Beijing: China Standard Press).
Glibert P. M., Allen J. I., Bouwman A. F., Brown C. W., Flynn K. J., Lewitus A. J., et al. (2010). Modeling of HABs and eutrophication: status, advances, challenges. J. Mar. Syst. 83, 262–275. doi: 10.1016/j.jmarsys.2010.05.004
Glibert P. M., Burkholder J. A. M. (2011). Harmful algal blooms and eutrophication: “strategies” for nutrient uptake and growth outside the redfield comfort zone. Chin. J. Oceanology Limnology 29 (4), 724–738. doi: 10.1007/s00343-011-0502-z
Hart J. A., Phlips E. J., Badylak S., Dix N., Petrinec K., Mathews A. L., et al. (2015). Phytoplankton biomass and composition in a well-flushed, sub-tropical estuary: the contrasting effects of hydrology, nutrient loads and allochthonous influences. Mar. Environ. Res. 112, 9–20. doi: 10.1016/j.marenvres.2015.08.010
Hu J. H. (2004). The coastal currents offshore the nuclear power plants at northern Taiwan. J. Mar. Sci. Technol. 12 (5), 355–363. doi: 10.51400/2709-6998.2256
Irwin A. J., Finkel Z. V., Schofield O. M. E., Falkowski P. G. (2006). Scaling-up from nutrient physiology to the size-structure of phytoplankton communities. J. Plankton Res. 28 (5), 459–471. doi: 10.1093/plankt/fbi148
Jiang Z., Chen Q., Zeng J., Liao Y., Liu J. (2012). Phytoplankton community distribution in relation to environmental parameters in three aquaculture systems in a Chinese subtropical eutrophic bay. Mar. Ecol. Prog. Ser. 446, 73–89. doi: 10.3354/meps09499
Jiang Z., Du P., Liu J., Chen Y., Zhu Y., Shou L., et al. (2019a). Phytoplankton biomass and size structure in Xiangshan Bay, China: current state and historical comparison under accelerated eutrophication and warming. Mar. Pollut. Bull. 142, 119–128. doi: 10.1016/j.marpolbul.2019.03.013
Jiang Z., Gao Y., Chen Y., Du P., Zhu X., Liao Y., et al. (2019b). Spatial heterogeneity of phytoplankton community shaped by a combination of anthropogenic and natural forcings in a long narrow bay in the East China Sea. Estuar. Coast. Shelf Sci. 217, 250–261. doi: 10.1016/j.ecss.2018.11.028
Jiang Z. B., Liu J. J., Chen J. F., Chen Q. Z., Yan X. J., Xuan J. L., et al. (2014). Responses of summer phytoplankton community to drastic environmental changes in the Changjiang (Yangtze River) estuary during the past 50 years. Water Res. 54, 1–11. doi: 10.1016/j.watres.2014.01.032
Jiang Z. B., Liu J. J., Zhu X. Y., Chen Y., Chen Q. Z., Chen J. F. (2020). Quantitative comparison of phytoplankton community sampled using net and water collection methods in the southern Yellow Sea. Regional Stud. Mar. Sci. 35, 2352–4855. doi: 10.1016/j.rsma.2020.101250
Kim H., Kang D., Jung S. W., Kim M. (2019). High-frequency acoustic backscattering characteristics for acoustic detection of the red tide species akashiwo sanGuinea and Alexandrium affine. J. Oceanol. Limnol. 37, 1268–1276. doi: 10.1007/s00343-019-8113-1
Krishnakumar V., Sastry J., Swamy G. N. (1991). Implication of thermal discharges into the sea-a review. Indian J. Environ. Protect. 11, 525–527. doi: AIX-24-026508
Langford T. (1990). Ecological effects of thermal discharges (London: Elsevier Applied Science Publishers), 28–103.
Laurent A., Fennel K., Ko D. S., Lehrter J. (2018). Climate change projected to exacerbate impacts of coastal eutrophication in the northern Gulf of Mexico. J. Geophys. Res. Oceans 123 (5), 3408–3426. doi: 10.1002/2017JC013583
Lee K. H., Jeong H. J., Lee K., Franks P. J., Seong K. A., Lee S. Y., et al. (2019). Effects of warming and eutrophication on coastal phytoplankton production. Harmful Algae 81, 106–118. doi: 10.1016/j.hal.2018.11.017
Lee M., Ro H., Kim Y. B., Park C. H., Baek S. H. (2021). Relationship of spatial phytoplankton variability during spring with eutrophic inshore and oligotrophic offshore waters in the East Sea, including Dokdo, Korea. J. Mar. Sci. Eng. 9 (12), 1455. doi: 10.3390/jmse9121455
Liang J., Zhou Y., Wang Z. (2021). Environmental quality assessment of the Sanmen Bay and its annual changes. J. Zhejiang Ocean. Univ. (Nat. Sci.) 40, 121–127. doi: 10.3969/j.issn.1008-830X.2021.02.004
Liang J. X., Zhou Y. D., Wang Z. M., Zhang Y. Z., Jin H. W., Xu K. D. (2020). Community structure of macrozoobenthos and its relationship with environmental factors in Sanmen Bay, Zhejiang, China. Chin. J. Appl. Ecol. 31 (9), 3187–3193. doi: 10.13287/j.1001-9332.202009.037
Lin C., Su J., Xu B., Tang Q. (2001). Long-term variations of temperature and salinity of the Bohai Sea and their influence on its ecosystem. Prog. Oceanogr. 49, 7–19. doi: 10.1016/S0079-6611(01)00013-1
Liu X., Duan X., Tian Y., Gao K., Gao F., Yin P., et al. (2021). Variation of nutrients in Sanmen Bay and its response to human activities. Mar. Geology Front. 37 (5), 46–56. doi: 10.16028/j.1009-2722.2020.052
Liu J. J., Jiang Z. B., Chen Y., Zeng J. N., Huang W., Peng L., et al. (2015). Community characteristics of net-phytoplankton in spring in Sanmen Bay, China. J. Mar. Sci. 33 (1), 74–80. doi: 10.11693/hyhz20160700153
Liu S., Lou S., Kuang C., Huang W., Chen W., Zhang J., et al. (2011). Water quality assessment by pollution-index method in the coastal waters of Hebei Province in western Bohai Sea, China. Mar. Pollut. Bull. 62 (10), 2220–2229. doi: 10.1016/j.marpolbul.2011.06.021
Liu S. M., Hong G. H., Zhang J., Ye X. W., Jiang X. L. (2009). Nutrient budgets for large Chinese estuaries. Biogeosciences 6, 2245–2263. doi: 10.5194/BG-6-2245-2009
Lo W. T., Hwang J. J., Hsu P. K., Hsieh H. Y., Tu Y. Y., Fang T. H., et al. (2004). Seasonal and spatial distribution of phytoplankton in the waters off nuclear power plants, north of Taiwan. J. Mar. Sci. Technol. 12 (5), 372–379. doi: 10.51400/2709-6998.2258
Marcus N. (2004). An overview of the impacts of eutrophication and chemical pollutants on copepods of the coastal zone. Zool. Stud. 43, 211–217. Available at: https://www.sinica.edu.tw/zool/zoolstud/43.2/211.
Martínez M. L., Intralawan A., Vázquez G., Pérez-Maqueo O., Sutton P., Landgrave R. (2007). The coasts of our world: ecological, economic and social importance. Ecol. Econ. 63, 254–272. doi: 10.1016/j.ecolecon.2006.10.022
Matsubara T., Nagasoe S., Yamasaki Y., Shikata T., Shimasaki Y., Oshima Y., et al. (2008). Inhibitory effects of centric diatoms on the growth of the dinoflagellate akashiwo sanGuinea. Nippon Suisan Gakkai Shi 74, 598–606. doi: 10.2331/suisan.74.598
Meng Z., Wei Y. J., Wang X. B., Han X. Q. (2022). Niche and interspecies association of major epifauna in Sanmen Bay of Zhangjiang Province. Oceanologia Et Limnologia Sin. 53 (5), 1269–1278. doi: 10.31497/zrzyxb.20220414
Meyer-Reil L. A., Köster M. (2000). Eutrophication of marine waters: effects on benthic microbial communities. Mar. Pollut. Bull. 41, 255–263. doi: 10.1016/S0025-326X(00)00114-4
Miyamoto Y., Nakano T., Yamada K., Hatakeyama K., Hamaguchi M. (2019). Combined effects of drift macroalgal bloom and warming on occurrence and intensity of diel-cycling hypoxia in a eutrophic coastal lagoon. Estuar. Coasts 42, 494–503. doi: 10.1007/s12237-018-0484-6
Ning X. R. (2005). “Environmental quality assessment of the Sanmen Bay and its annual changes,” in Research and evaluation on aquaculture ecology and aquaculture capacity in Leqing Bay and Sanmen Bay. Ed. Ning X. R. (Beijing: Ocean Press), 120–160.
Novak T., Godrijan J., Pfannkuchen D. M., Djakovac T., Medic N., Ivancic I., et al. (2019). Global warming and oligotrophication lead to increased lipid production in marine phytoplankton. Sci. Total Environ. 668, 171–183. doi: 10.1016/j.scitotenv.2019.02.372
Philippart C. J. M., Cadee G. C., van Raaphorst W., Riegman R. (2000). Long-term phytoplankton-nutrient interactions in a shallow coastal sea: algal community structure, nutrient budgets, and denitrification potential. Limnology Oceanography 45 (1), 131–144. doi: 10.4319/lo.2000.45.1.0131
Poornima E. H., Rajadurai M., Rao V. N. R., Narasimhan S. V., Venugopalan V. P. (2006). Use of coastal waters as condenser coolant in electric power plants: impact on phytoplankton and primary productivity. J. Therm. Biol. 31 (7), 556–564. doi: 10.1016/j.jtherbio.2006.05.009
Qiu K. C., Zhang R. L., Chao M., Cheng F. P., Zeng J. N., Peng X., et al. (2022). Spatiotemporal variations in cluster structure of fish eggs, larvae, and juveniles in the Sanmen Bay, Zheejiang, East China Sea. Oceanologia Et Limnologia Sin. 53, 684–696. doi: 10.11693/hyhz20211100285
Redfield A. C. (1958). The biological control of chemical factors in the environment. Am. Scientist 46, 205–221. doi: 10.2307/27827150
Rice E., Dam H. G., Stewart G. (2015). Impact of climate change on estuarine zooplankton: surface water warming in long island sound is associated with changes in copepod size and community structure. Estuar. Coasts 38, 13–23. doi: 10.1007/s12237-014-9770-0
Shi X. (2013). Distribution characters of nitrogen, phosphorus and other factors in surface water of Sanmen Bay in spring and autumn. J. Appl. Oceanography 32 (4), 461–467. doi: 10.3969/J.ISSN.2095-4972.2013.04.003
Shou L., Zeng J. (2015). Coastal ecological environment and environmental capacity of bays in Zhejiang Province (Beijing: Ocean Press), 154–209.
Song Y., Guo Y., Liu H., Zhang G., Zhang X., Thangaraj S., et al. (2022). Water quality shifts the dominant phytoplankton group from diatoms to dinoflagellates in the coastal ecosystem of the Bohai Bay. Mar. pollut. Bull. 183, 114078. doi: 10.1016/j.marpolbul.2022.114078
Song N. Q., Wang N., Lu Y., Zhang J. R. (2016). Temporal and spatial characteristics of harmful algal blooms in the Bohai Sea during 1952–2014. Cont. Shelf Res. 122, 77–84. doi: 10.1016/j.csr.2016.04.006
Strickland J. D. H., Parsons T. R. (1968). Determination of dissolved oxygen. In A Practical Handbook of Seawater Analysis. Fisheries Res. Board Canada Bull. 167, 71–75. doi: 10.1002/iroh.19700550118
Sun J., Liu D. (2002). The preliminary notion on nomenclature of common phytoplankton in China Sea waters. Oceanologia Limnol. Sin. 33, 271–286. doi: 10.1088/1009-1963/11/5/313
Tagliani P. R. A., Landazuri H., Reis E. G., Tagliani C. R., Asmus M. L., Sanchez-Arcilla A. (2003). Integrated coastal zone management in the Patos Lagoon estuary: Perspectives in context of developing country. Ocean Coast. Manage. 46 (9), 807–822. doi: 10.1016/S0964-5691(03)00063-2
Tang Y., Liu Q., Cheng C., Yang M., Meng Q., Zhao S. (2018). Characteristics of phytoplankton community structure in dongji sea area of zhoushan. Southwest China J. Agric. Sci. 31, 2715–2722. doi: 10.16213/j.cnki.scjas.2018.12.042
Tsirtsis G., Karydis M. (1998). Evaluation of phytoplankton community indices for detecting eutrophic trends in the marine environment. Environ. Monit. Assess. 50 (3), 255–269. doi: 10.1023/A:1005883015373
Utermöhl H. (1958). Zur vervollkommung der quantitativen phytoplankton-methodik. Limnology 9, 263–272. doi: 10.1080/05384680.1958.11904091
Wang Q., Li H., Zhang Y., Wang X., Zhang C., Xiao K., et al. (2019). Evaluations of submarine groundwater discharge and associated heavy metal fluxes in Bohai Bay, China. Sci. Total Environ. 695, 133873. doi: 10.1016/j.scitotenv.2019.133873
Wang W., Lin C., Jiang R., Liu Y., Sun X., Lin. H., et al. (2022). Distribution, source identification and environmental risk assessment of potentially toxic elements (PTEs) in the surface sediment of Sanmen Bay, Zhejiang Province, China. Mar. Pollut. Bull. 174, 113237. doi: 10.1016/j.marpolbul.2021.113237
Wang B., Xin M., Wei Q., Xie L. (2018). A historical overview of coastal eutrophication in the China seas. Mar. Pollut. Bull. 136, 394–400. doi: 10.1016/j.marpolbul.2018.09.044
Wei Y., Ding D., Gu T., Jiang T., Qu K., Sun J., et al. (2022). Different responses of phytoplankton and zooplankton communities to current changing coastal environments. Environ. Res. 215, 114426. doi: 10.1016/j.envres.2022.114426
Wells M. L., Trainer V. L., Smayda T. J., Karlson B. S. O., Trick C. G., Kudela R. M., et al. (2015). Harmful algal blooms and climate change: learning from the past and present to forecast the future. Harmful Algae 49, 68–93. doi: 10.1016/j.hal.2015.07.009
Winder M., Sommer U. (2012). Phytoplankton response to a changing climate. Hydrobiologia 698 (1), 5–16. doi: 10.1007/s10750-012-1149-2
Wu X., Liu H., Ru Z., Tu G., Ding Y. (2021). Meta-analysis of the response of marine phytoplankton to nutrient addition and seawater warming. Mar. Environ. Res. 168, 105294. doi: 10.1016/j.marenvres.2021.105294
Wu M., Wang Y., Wang Y., Yin J., Dong J., Jiang Z., et al. (2017). Scenarios of nutrient alterations and responses of phytoplankton in a changing Daya Bay, South China Sea. J. Mar. Syst. 165, 1–12. doi: 10.1016/j.jmarsys.2016.09.004
Xia R., Zhang Y., Critto A., Wu J., Fan J., Zheng Z., et al. (2016). The potential impacts of climate change factors on freshwater eutrophication: implications for research and countermeasures of water management in China. Sustainability 8, 229. doi: 10.3390/su8030229
Xiao W., Liu X., Irwin A. J., Laws E. A., Wang L., Chen B., et al. (2018). Warming and eutrophication combine to restructure diatoms and dinoflagellates. Water Res. 128, 206–216. doi: 10.1016/j.watres.2017.10.051
Xie C. Q., Ai W. M., Peng X., Chen S. B., Xie Q. L. (2015). Diversity and seasonal variation of phytoplankton in the sea near Sanmen Nuclear Power Station. Bull. Sci. Technol. 31 (7), 222–228. doi: 10.13774/j.cnki.kjtb.2015.07.055
Xin M., Wang B., Xie L., Sun X., Wei Q., Liang S., et al. (2019). Long-term changes in nutrient regimes and their ecological effects in the Bohai Sea, China. Mar. Pollut. Bull. 146, 562–573. doi: 10.1016/j.marpolbul.2019.07.011
Yan R. X., Han Q. X., Wang X. B. (2020). Community composition and diversity of macrobenthos trawled in Hangzxhou Bay and Sanmen Bay. Oceanologia Et Limnologia Sin. 51, 484–493. doi: 10.11693/hyhz20191200272
Yang H., Zhao Y., Zhang H. R., Dai G. X., Ding J., Chen J. (2013). Study on the impact of water environmental factors on dominant species of phytoplankton around the power plant in xiangshan bay. Advanced Materials Res. 610-613, 3371–3374. doi: 10.4028/www.scientific.net/AMR.610-613.3371
Ye R., Liu L., Wang Q., Ye X., Cao W., He Q., et al. (2017). Identification of coastal water quality by multivariate statistical techniques in two typical bays of northern Zhejiang Province, East China Sea. Acta Oceanologica Sin. 36 (2), 1–10. doi: 10.1007/s13131-017-0981-7
Yu J., Tang D. L., Oh I. S., Yao L. J. (2007). Response of harmful algal blooms to environmental changes in Daya Bay, China. Terr. Atmos. Ocean Sci. 18 (5), 1011–1027. doi: 10.3319/TAO.2007.18.5.1011(Oc)
Zhang J., Wang Y., Ottmann D., Cao P., Yang J., Yu J., et al. (2023). Seasonal variability of phytoplankton community response to thermal discharge from nuclear power plant in temperate coastal area. Environ. pollut. 318, 120898. doi: 10.1016/j.envpol.2022.120898
Zhang L., Xiong L., Li J., Huang X. (2021). Long-term changes of nutrients and biocenoses indicating the anthropogenic influences on ecosystem in Jiaozhou Bay and Daya Bay. China. Mar. Pollut. Bull. 168, 112406. doi: 10.1016/j.marpolbul.2021.112406
Zhang Y. X., Yu J., Jiang Z. B., Wang Q., Wang H. (2015). Variations of summer phytoplankton community related to environmental factors in a macro-tidal estuarine embayment, Hangzhou Bay, China. J. Ocean Univ. China 14 (6), 1025–1033. doi: 10.1007/s11802-015-2483-6
Zhao S. J., Jiao N. Z., Wu C. W., Liang B., Zhang S. Y. (2005). Evolution of nutrient structure and phytoplankton composition in the Jiaozhou Bay ecosystem. Chin. J. Environ. Sci. 17 (1), 95–102. doi: 10.3321/j.issn:1001-0742.2005.01.019
Zhou M. J., Shen Z. L., Yu R. C. (2008). Responses of a coastal phytoplankton community to increased nutrient input from the Changjiang (Yangtze) River. Continental Shelf Res. 28 (12), 1483–1489. doi: 10.1016/j.csr.2007.02.009
Zhu G., Noman M. A., Narale D. D., Feng W., Pujari L., Sun J. (2020). Evaluation of ecosystem health and potential human health hazards in the Hangzhou Bay and Qiantang Estuary region through multiple assessment approaches. Environ. Pollut. 264, 114791. doi: 10.1016/j.envpol.2020.114791
Keywords: phytoplankton shift, seasonal variation, Sanmen Bay, water quality assessment, elevated temperature, nutrient regime alterations
Citation: Wang Y, Wang W, Huang Y, Chang L, Tang X, He X and Lin H (2023) Scenarios of temporal environmental alterations and phytoplankton diversity in a changing bay in the East China Sea. Front. Mar. Sci. 10:1303497. doi: 10.3389/fmars.2023.1303497
Received: 28 September 2023; Accepted: 15 November 2023;
Published: 04 December 2023.
Edited by:
Meilin Wu, Chinese Academy of Sciences (CAS), ChinaReviewed by:
Kaizhi Li, Chinese Academy of Sciences (CAS), ChinaPeng Zhang, Guangdong Ocean University, China
Copyright © 2023 Wang, Wang, Huang, Chang, Tang, He and Lin. This is an open-access article distributed under the terms of the Creative Commons Attribution License (CC BY). The use, distribution or reproduction in other forums is permitted, provided the original author(s) and the copyright owner(s) are credited and that the original publication in this journal is cited, in accordance with accepted academic practice. No use, distribution or reproduction is permitted which does not comply with these terms.
*Correspondence: Xuebao He, aGV4dWViYW9AdGlvLm9yZy5jbg==; Hui Lin, bGluaHVpQHRpby5vcmcuY24=
†These authors share first authorship