- 1Shenzhen Key Laboratory of Marine Bioresource & Eco-Environmental Science, Shenzhen Engineering Laboratory for Marine Algal Biotechnology, College of Life Sciences and Oceanography, Shenzhen University, Shenzhen, China
- 2College of Physics and Optoelectronic Engineering, Shenzhen University, Shenzhen, China
- 3Center for Plant Environmental Sensing, College of Life Sciences and Oceanography, Shenzhen University, Shenzhen, China
- 4Hubei Academy of Forestry, Wuhan, Hubei, China
This study investigates the potential of natural products derived from two mangrove soil bacteria, GP521A and GP3R5, in anthracnose management in oil tea. Based on 16s rDNA sequencing, GP521A and GP3R5 were identified as Bacillus velezensis and Pseudoalteromonas caenipelagi, respectively. Both bacteria showed strong antagonistic effects towards multiple fungal pathogens on dual cultural plates. The ethyl acetate extracts of fermentation cultures of GP521A (FEGP5) and GP3R5 (FEGP3) significantly inhibited mycelial growth of both Colletotrichum camelliae MC171, C. fructicola CF-1 and Pyricularia oryzae P131 at 100 and 200 μg/mL, respectively. Furthermore, FEGP5 and FEGP3 significantly inhibited conidiation, conidial germination and appressorial formation in C. camelliae MC171 at 50 μg/mL, with stronger inhibitory effects observed in the latter. On detached oil tea leaves, 100 μg/mL of FEGP5 and FEGP3 resulted in ~ 98.0% and 97.5% reduction in diseased areas, respectively, when applied before MC171 inoculation, whereas the reduction was approximately 55.0% and 77.6%, respectively, in the curative treatments. In fresh fruits, both extracts also significantly inhibited disease development, either in preventative or curative treatments. Moreover, FEGP5 and FEGP3 demonstrated significantly lower aquatic toxicity than prochloraz at the same concentrations towards a commonly used bioindicator, Artemia salina. In conclusion, this study demonstrates the potential of marine microbes derived natural products in development of environmentally compatible biopesticides to control anthracnose in oil tea.
1 Introduction
Oil tea (Camellia. oleifera) is a Chinese most important woody oil tree species with high economic value owning to the rich oil content in seeds and high nutritional value. It is one of the four major sources of edible oil in the world (Chen, 2008; Quan et al., 2022). The history of extracting oil from oil tea in China can be traced back to over 2, 000 years ago (Zhuang, 2008). Owing to its function in preventing hypertension and arteriosclerosis, as well as other nutritional values such as antioxidant and anti-inflammation, tea oil is considered as a high-quality and healthy vegetable oil (Feas et al., 2013; Di et al., 2018), with a fatty acid composition comparable to olive oil. In 2021, the cultivation area of oil tea in China reached up to 4.6 million hectares, generating a yield of ~ 889,384 tons, and the economic value was estimated to be ~192 billion Yuan (China forestry and grassland statistics yearbook 2021). Despite the increasing market demand for tea oil, its production is severely limited by many plant diseases occurred annually, and fungal infection has been recognized as the No.1 threat.
Anthracnose of oil tea, caused by Colletotrichum spp., is one of the major fungal diseases that limiting the production of tea oil. Up till now, Colletotrichum spp. that can infect the leaves and fruits of C. oleifera mainly include: C. camelliae, C. fructicola, C. gloeosporioides, C. horii, C. siamense, C. boninense C. kahawae, C. karstii, C. fioriniae, C. aenigma and C. aeschynomenes (Li and Li, 2020; Wang et al., 2020; Li and Li, 2022; Peng et al., 2023). This anthracnose disease complex typically results in approximately 10%~ 30% reduction of tea oil each year, and the severely affected areas often experience more than 50% of economic loss. Therefore, how to effectively control the occurrence of disease has attracted more and more attention.
Current control methods for anthracnose of oil tea mainly include breeding and planting resistant plants (Savchenko, 2017), good cultivation management, and use of chemical fungicide. Among these, fungicide application is used most frequently. However, the abuse of chemical pesticides has led to a series of environmental and health issues. More importantly, repeated application of pesticides may quickly induce pathogen resistance. Due to these reasons, many governments in the world have announced policies to restrict synthetic fungicide application. As a result, biological control as a more eco-friendly alternative has become a hot spot in recent years, and there have been growing efforts in exploration of effective biological control agents in against anthracnose of oil tea. To date, a number of plant endophytic Bacillus isolates, such as B. subtilis 1-L-29 (Xu et al., 2020) and YL13 (Xia et al., 2023), B. pumilus HR10 (Dai et al., 2021), and B. tequilensis YYC155 (Zhou et al., 2022), showed strong inhibitory effects on Colletotrichum sp. growth in vitro, and some of them also demonstrated promising disease inhibition efficacy in ex vivo or pot assays. Furthermore, a few endophytic fungal isolates from C. oleifera were also proved to be effective in pathogen growth inhibition (Yu et al., 2018). However, research of biological control studies on management of oil tea anthracnose is still at the very beginning stage compared to many other diseases, and to the best of our knowledge, there is no commercial product developed yet. Therefore, studies on further exploration of novel, effective biocontrol agents and characterization of their functional mechanisms are still warranted.
In the past decade, marine microbes have gained increasing interests due to their fascinating capabilities in producing diversified bioactive compounds of various structures and bioactivities (Imhoff et al., 2011). Therefore, these microbes are emerging as an important source for novel biopesticide mining for plant disease management. In previous work, our lab has established a library of bacterial strains collected from mangrove plant rhizosphere, soil and different marine invertebrates (unpublished data). In this study, we screened this library for isolates with antifungal bioactivities in against Colletotrichum sp., and identified two isolates, GP521A and GP3R5, with broad spectrum antifungal bioactivities. These isolates were identified to be Bacillus velezensis and Pseudoalteromonas caenipelagi, respectively. To investigate the potential of these isolates in anthracnose inhibition in C. oleifera and characterize the antagonistic mechanisms, crude ethyl aetate extracts of the secondary metabolites produced in the fermentation cultures of GP521A and GP3R5 were obtained and used in subsequent assays. The effects of GP521A and GP3R5 extracts on mycelium growth, conidiation, conidia germination, appressorium formation and full virulence of C. camelliae MC171 were determined. The toxicity of these extracts towards plants and an environmental indicator organism was also determined.
2 Materials and methods
2.1 Strains and culture conditions
The bacterial strains, GP521A and GP3R5, were isolated from the mangrove soil, in Baguang Natural Reserve, Shenzhen (114.50° E, 22.64° N). The wild-type (WT) strain, Colletotrichum camelliae MC171, was isolated from a tea-oil field and kept in the Forest Protection Research Institute of Hubei Academy of Forestry. Another WT strain, Colletotrichum fructicola CF-1, was kindly provided by Dr. Guozhen Zhang (China Agricultural University, Beijing 100193, China). The rice blast causal agent, Pyricularia oryzae P131, was provided by Dr. Youliang Peng (China Agricultural University, Beijing 100193, China). GP521A and GP3R5 were cultured on Marine Agar 2216 plate (MA, BD Difco™) and fermented in SGTYP medium (5 g of starch, 5 g of glucose, 1 g of peptone, 1 g of tryptone, 1 g of yeast extract, and 17 g artificial sea salt is dissolved in 1 L double-distilled water, pH 7.4–7.6) at 28°C, and the fungal strains were cultured on Potato Dextrose Agar plate (PDA, Coolaber) in an illuminating incubator (photoperiod of 12-h light/12-h dark) at 28°C.
2.2 Dual culture assay
Fungal disks (3 mm in diameter), taken from the edge of ~7-d old colonies, were individually placed on one side of a PDA plate of 90 mm in diameter. A 10-μL droplet of GP521A or GP3R5 suspensions of OD600 = 0.9 ~1.0, which were individually grown for ~ 24 h in SGTYP medium, was inoculated to a round filter paper (~6 mm diameter), and placed to the opposite side of the fungal disks, respectively. SGTYP medium only without bacterial cells was used as the negative control. The plates were incubated at 28°C and the inhibition zone were photographed at 5 -7 days postinoculation (dpi). Three PDA plates were included in each treatment, and the experiment was repeated three times.
2.3 Bacterial identification
Bacterial DNA was extracted with the FASTDNA Spin Kit for Soil (MP Biomedicals) following the manufacturer’s instructions. The 16s rDNA region was amplified via PCR, using the forward and reverse primers, 1492R and 27F, as previously described (Song et al., 2021). The purified PCR product was sequenced at Beijing Genomics Institute. Species identification was conducted using the blastn program (http://www.ncbi.nlm.nih.gov/BLAST/), based on alignment of the sequencing results with existing 16s rDNA sequences in the ‘Nucleotide collection (nt/nt)’ database using Mega 7.0. The first ranked strain with full genus and species names and >99% similarity to the query was selected as the closest match. Sequences of the 16s rDNA region of GP3R5 and GP521A had been deposited to the GenBank database (https://www.ncbi.nlm.nih.gov/genbank/) with the accession No. OR574457 and No. OR574458, respectively.
2.4 Fermentation and extraction of secondary metabolites
GP521A and GP3R5 were cultured on MA plates for ~24 h, respectively, and colonies were picked and suspended in 100 ml SGTYP medium in a 250 ml erlenmeyer flask, and cultured at 28 °C, 220 rpm for 4 days. Then, the fermentation supernatant was collected by centrifugation (4000 rpm, 10 min), and was extracted using ethyl acetate (supernatant/ethyl acetate =1/3 (V/V)) three times. Finally, the extracted products were dissolved in dimethylsulfoxide (DMSO) to 50 mg/mL and stored in 4 °C for further assays.
2.5 Inhibition of mycelial growth in vitro
Mycelial plugs of 3-mm-diameter of P. oryzae P131, C. camelliae MC171 and C. fructicola CF-1 from 7-day-old colonies grown on PDA plates were subcultured onto fresh PDA plates, supplemented with the crude extracts of GP521A or GP3R5 to a final concentration of 100 μg/mL or 200 μg/mL, respectively. PDA plates supplemented with an equal concentration of DMSO, same as those in the crude extract treatments, were used as control. The colony diameters were measured at 5 dpi (11 dpi for C. camelliae MC171). Three PDA plates were included in each treatment, and the experiment was repeated three times.
2.6 Effects of crude extracts of GP521A and GP3R5 on conidiation, conidial germination and appressorial formation of C. camelliae MC171 in vitro
For conidiation, C. camelliae MC171 strains were grown on PDA plates supplemented with 50 μg/mL of crude extracts of GP521A and GP3R5 in dark for 5 days, followed by 3 days under continuous light at 28°C. Conidia were harvested by adding sterile distilled water and scraping the colonies with an inoculating loop. To determine conidiation of C. camelliae, the conidia were quantified by a hemocytometer under a light microscope (Olympus BX51) under 100×. For conidial germination and appressorial formation, droplets of conidial suspension of 2 × 105 spores/mL, supplemented with 50 μg/mL crude extracts of GP521A and GP3R5, and 0.1% DMSO, respectively, were inoculated onto a hydrophobic cover glass (VWR, 48366-067), and incubated at 28°C under darkness. The rates of conidial germination and appressorium formation were examined at 2 h and 24 h, respectively. One hundred spores were included in each treatment, and the experiment was repeated three times.
2.7 Virulence test
Fresh, young healthy leaves and immature healthy fruits of C. oleifera were used for virulence test. Inoculation was conducted by stab injury, and piercing wounds of leaves or fruits were made by a sterilized needle. For protective activity test, the leaves or fruits were sprayed with 100 μg/mL of crude extracts of GP521A and GP3R5, respectively, let stand for 10 minutes, and dried naturally. After 24h, mycelium blocks of C. camelliae MC171were individually inoculated to the center of each plant tissues. Leaves or fruits soaked in sterilized water supplemented with 0.2% DMSO were included as negative controls. For curative activity test, mycelial blocks of C. camelliae MC171 were inoculated onto the wounded tea-oil tree leaves or fruits directly. At 24 hpi., 100 μg/mL of crude extracts of GP521A and GP3R5 was sprayed evenly onto the surface of the leaves or fruits pre-inoculated with pathogens. Those sprayed with 0.2% DMSO were included as controls. All leaves or fruits were incubated in an illumination incubator with 90% relative humidity, maintaining full darkness for the first 24 h, and then followed by a photoperiod of 12-h light/12-h dark for 6 days. Photographs were taken at 7 dpi. Ten leaves or fruits were included in each treatment, and the experiment was repeated three times.
2.8 Toxicity assay
Artemia salina, a model organism for toxicological tests was used to determine the toxicity of crude extracts of GP521A and GP3R5 as previously described (Banti and Hadjikakou, 2021). Briefly, 1 g cysts of Artemia salina were initially soaked in 500 ml artificial seawater (17-g sea salt dissolved in distilled water) for 1 h in a cone-shaped container. Then, the container was facilitated with good aeration at 25°C and under continuous illumination. After 48 h hatching, the nauplii released from the egg shells were collected at the bright side of the cone (near the light source) by using a 20-μL micropipette (Tip was removed). An aliquot (0.3 mL) containing about 10 to 15 nauplii was introduced to each well of a 24-well plate and crude extracts of GP521A or GP3R5 and prochloraz (commercial fungicide for diseases caused by Colletotrichum sp.) were added into each well. The final volume of each well containing artificial seawater was 1 mL. The brine shrimps were determined after incubated at 25°C for 24 h by using a stereoscope. Larvae were considered dead if they did not exhibit any internal or external movement in 10 s of observation. Each experiment was repeated three times.
2.9 Statistical analysis
Three independent experiments were performed for each assay. All the statistical figures in this paper were prepared by GraphPad Prism 8 software and Adobe Illustrator 2020. Students’ t test was used to compare differences between the mean values of each compound treatment and control group (DMSO) of for all the assays. Differences were considered statistically significant at a p < 0.05, and were indicated using different letters (ns, *, **).
3 Result
3.1 Antagonistic activity of GP521A and GP3R5 against C. fructicola CF-1 and P. oryzae P131
We initially screened a previously established bacterial library for isolates with antifungal bioactivities in against two model fungal pathogens, C. fructicola CF-1 and P. oryzae P131. Two isolates, GP521A and GP3R5 were found to have obvious antagonistic bioactivity (Figure 1). GP521A formed an obvious inhibitory zone towards the edge of the mycelium of both pathogens. In contrast, GP3R5 formed a smaller inhibitory zone. These results showed that GP521A and GP3R5 might produce certain antifungal bioactive compounds.
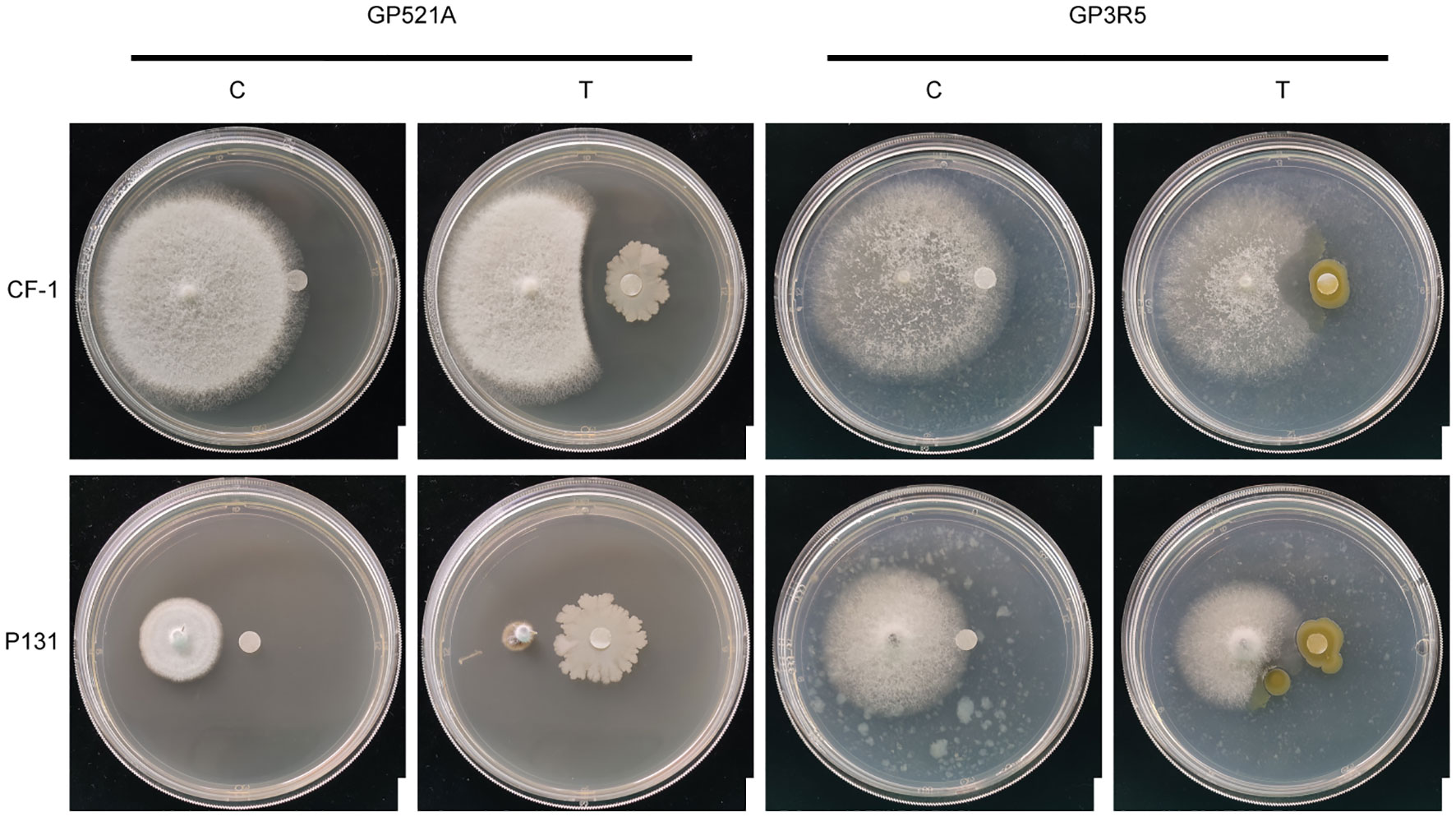
Figure 1 Observations of the antagonist activity of the two antimicrobial strains against C. fructicola CF-1 and P. oryzae P131. C: SGTYP medium without bacterial cells as the negative control. T: plates treated with the two antimicrobial strains, GP521A or GP3R5. Bar: 1 cm.
Further observation revealed that colonies of GP521A were pale white, round, wrinkled and concave in the middle, while those of GP3R5 were yellow, round and slimy on MA plates (Figure 2). Through 16S rRNA sequencing and blast analysis against known bacteria in the NCBI database, these two isolates were identified as Bacillus sp. (99.79% similarity) and Pseudoalteromonas sp. (99.86% similarity), respectively. The closest match to GP521A and GP3R5 were Bacillus velezensis strain FZB42, and Pseudoalteromonas caenipelagi strain JBTF-M23.
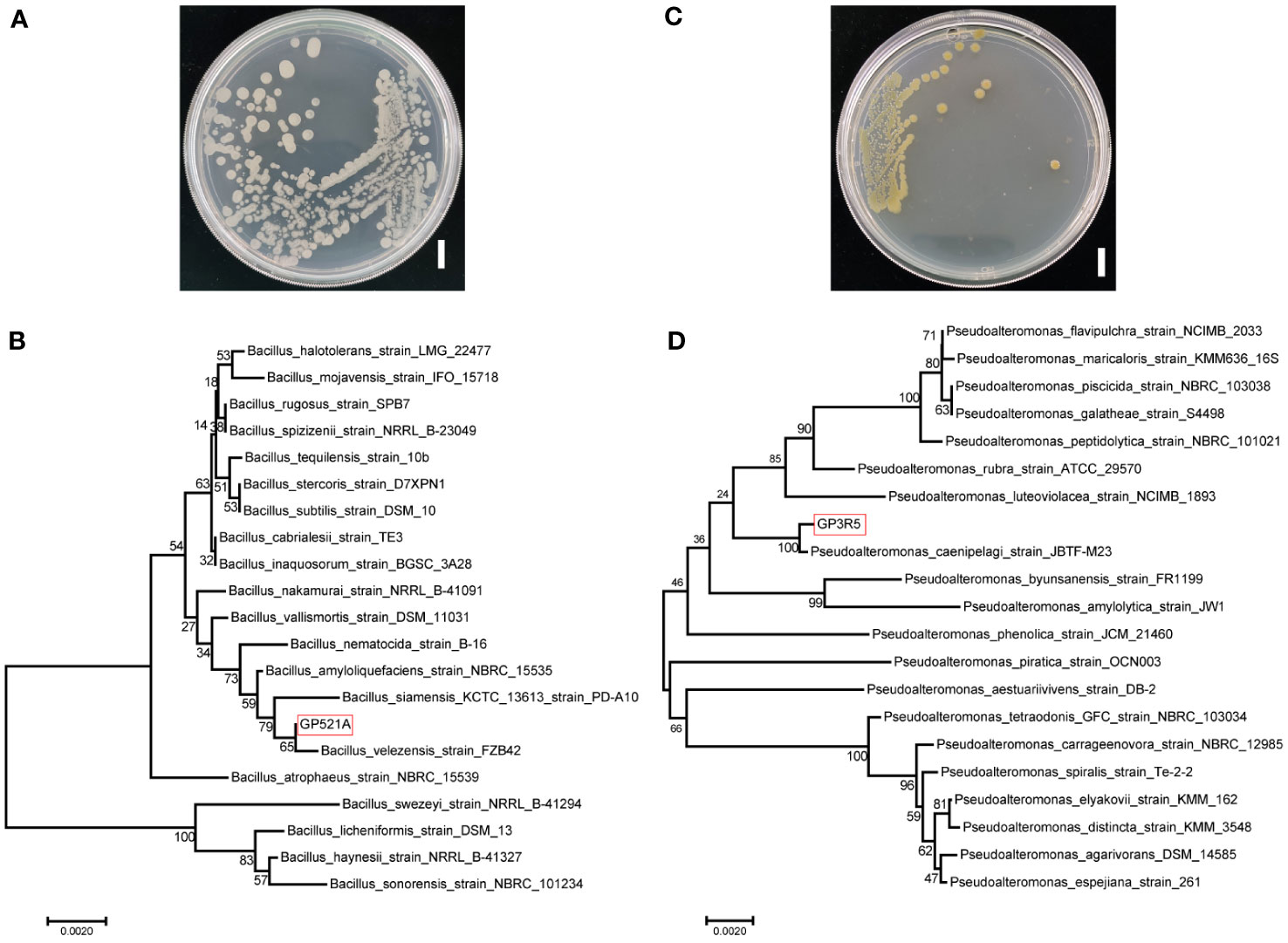
Figure 2 Identification of GP521A and GP3R5. The colony morphology of strain GP521A (A) and GP3R5 (C) Bar: 1 cm. Molecular phylogenetic analysis of GP521A (B) and GP3R5 (D) by the Neighbor-Joining method based on 16s rDNA sequences. The bootstrap values of each branch were tested by 1000 repetitions. GP521A and GP3R5 were presented by a red frame.
3.2 Fermentation extract of GP521A and GP3R5 affected the mycelia growth of C. camelliae MC171, C. fructicola CF-1 and P. oryzae P131
Given that the dual cultural plate assay suggested that GP521A and GP3R5 produce antifungal bioactive compounds, secondary metabolites of these bacteria in fermentation cultures were obtained by ethyl acetate extraction, and the effects of the crude extracts of GP521A (hereinafter called FEGP5) and GP3R5 (hereinafter called FEGP3) on pathogen growth were determined. As shown in Figure 3, mycelial growth of both anthracnose pathogens was significantly inhibited by both FEGP5 and FEGP3. When cultured on the PDA plates supplemented with DMSO for 5 or 11 days, the colony diameters of MC171 and CF-1 were 4.83 and 5.14 cm, respectively. In contrast, the diameters of MC171 and CF-1 treated with 100 μg/mL FEGP5 were 1.42 and 3.73 cm (p < 0.01for both compared to control), with an inhibition rate of 70.56 and 27.33%, respectively. Similarly, FEGP3 also significantly inhibited both MC171 and CF-1 mycelial growth when supplemented at 200 μg/mL, and the inhibition rate was 72.55% and 32.85%, respectively. To determine whether the inhibitory effects of FEGP5 and FEGP3 was specific to Colletotrichum sp., we further examined the bioactivity of these extracts to another fungal pathogen on rice, and found a similar inhibitory effect (Figure 3). Taken together, these results suggested that FEGP5 and FEGP3 may contain broad spectrum antifungal bioactive components.
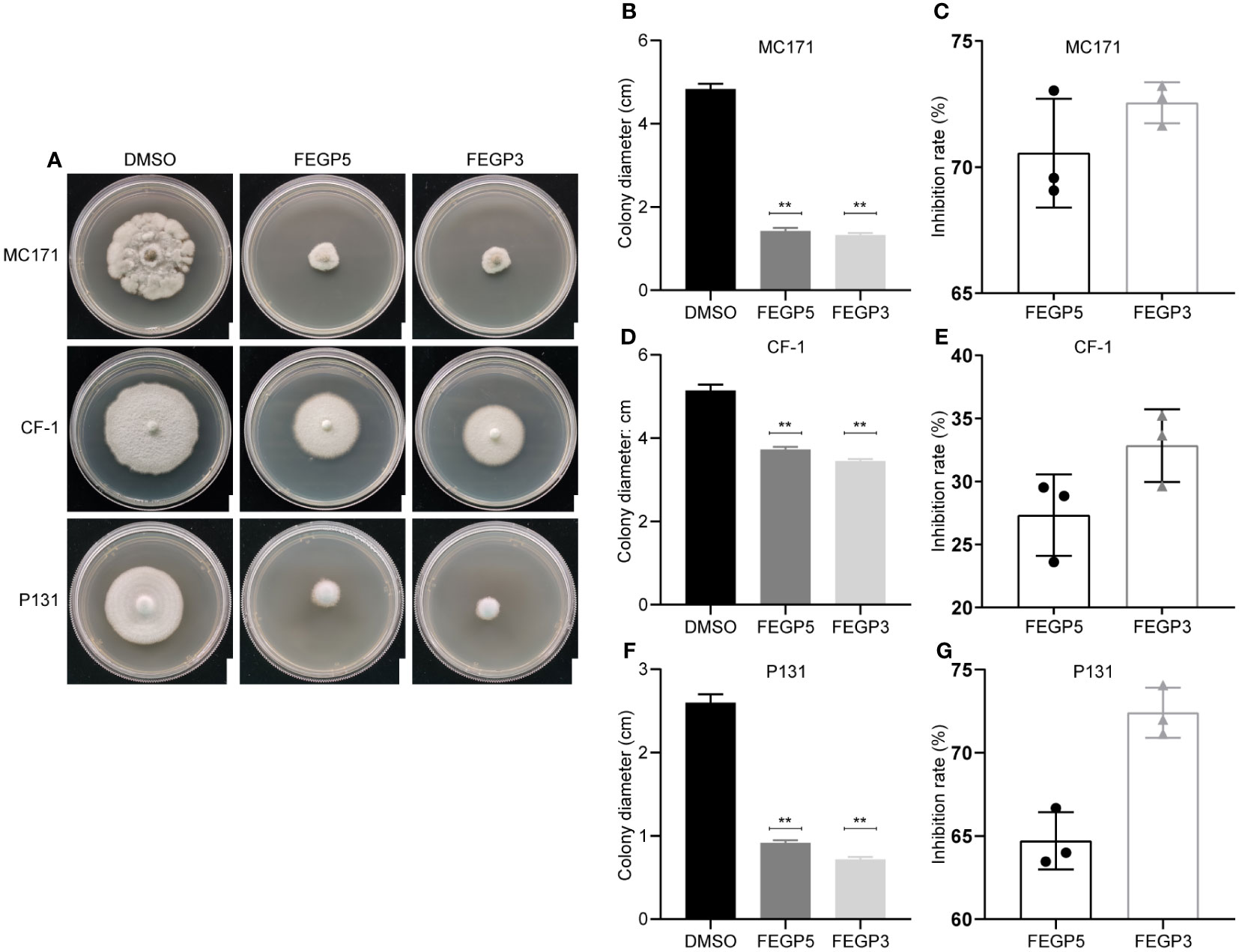
Figure 3 Effects on mycelium growth of C. camelliae MC171, C. fructicola CF-1 and P. oryzae P131 by the fermentation cultural extract of GP521A (100 μg/mL) and GP3R5 (200 μg/mL). (A) The colony morphology of MC171, CF-1 and P131 cultured on PDA plates with or without supplementation of the fermentation extract of GP521A and GP3R5. Bar: 1 cm. Statistical analysis of the colony diameter (B, D, F) and inhibition rate (C, E, G) of the indicated strains was conducted by the software GraphPad Prism 8.0 (Data are mean ± SD. one-way analysis of variance [ANOVA], **:p < 0.01). DMSO. as solvent control.
3.3 FEGP5 and FEGP3 damaged the conidiation, conidial germination and appressorium formation of C. camelliae MC171
To determine whether FEGP5 and FEGP3 has impacts on C. camelliae virulence, three pathogenic steps including conidiation, conidial germination and appressorium formation, which are all indispensable for successful infection was individually examined. As shown in Figure 4, MC171 in the control group produced almost twice as many conidia as those treated with 50 μg/mL FEGP5 and FEGP3, respectively (Figure 4A). In addition, conidial germination and appressorium formation rates of MC171 supplemented with FEGP5 was 78.49 and 73.84%, respectively, significantly lower than that of the DMSO treatment (85.59% and 90.9%, p <0.01 for both). The inhibitory effects of FEGP3 on conidial germination and appressorium formation were even stronger than FEGP5, reaching ~61.73% and 80.34% (12.39% and 18.77% for FEGP5, respectively), respectively under the same concentration (Figures 4B, C). These results suggested that FEGP5 and FEGP3 could inhibit multiple pathogenic steps and thus might be effective in control of anthracnose.
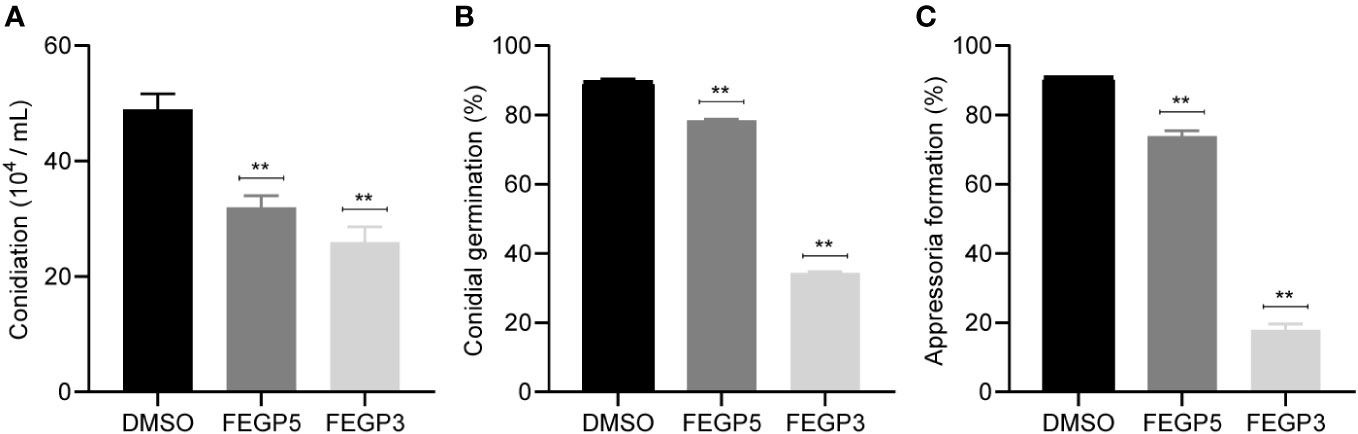
Figure 4 The fermentation extract (Final concentration was 50 μg/mL) of the strain GP521A or GP3R5 suppress conidiation (A), conidial germination (B) and appressorium formation (C) of C. camelliae MC171. (Data are mean ± SD. one-way analysis of variance [ANOVA], **:p < 0.01). DMSO: as solvent control.
3.4 FEGP5 and FEGP3 destroyed the full virulence of C. camelliae MC171
Given that FEGP5 and FEGP3 significantly inhibited the vegetative growth and virulence associated phenotypes of C. camelliae MC171, we examined the impacts of FEGP5 and FEGP3 on full virulence of C. camelliae MC171 in the young leaves and fruits of oil tea. When applied to leaves before pathogen inoculation, both FEGP5 and FEGP3 completely suppressed disease development after 7 days, while the lesion in the control group expanded to almost the whole leaf area, reaching an area of ~10.72 cm2 (Figure 5). In the curative treatments, the diseased areas in the FEGP5 and FEGP3 treatments were ~4.85 cm2 and 2.41 cm2, respectively, both significantly smaller than that of the control (10.77 cm2). Furthermore, FEGP5 and FEGP3 did not cause discoloration or any other obvious phytotoxic effects in leaves.
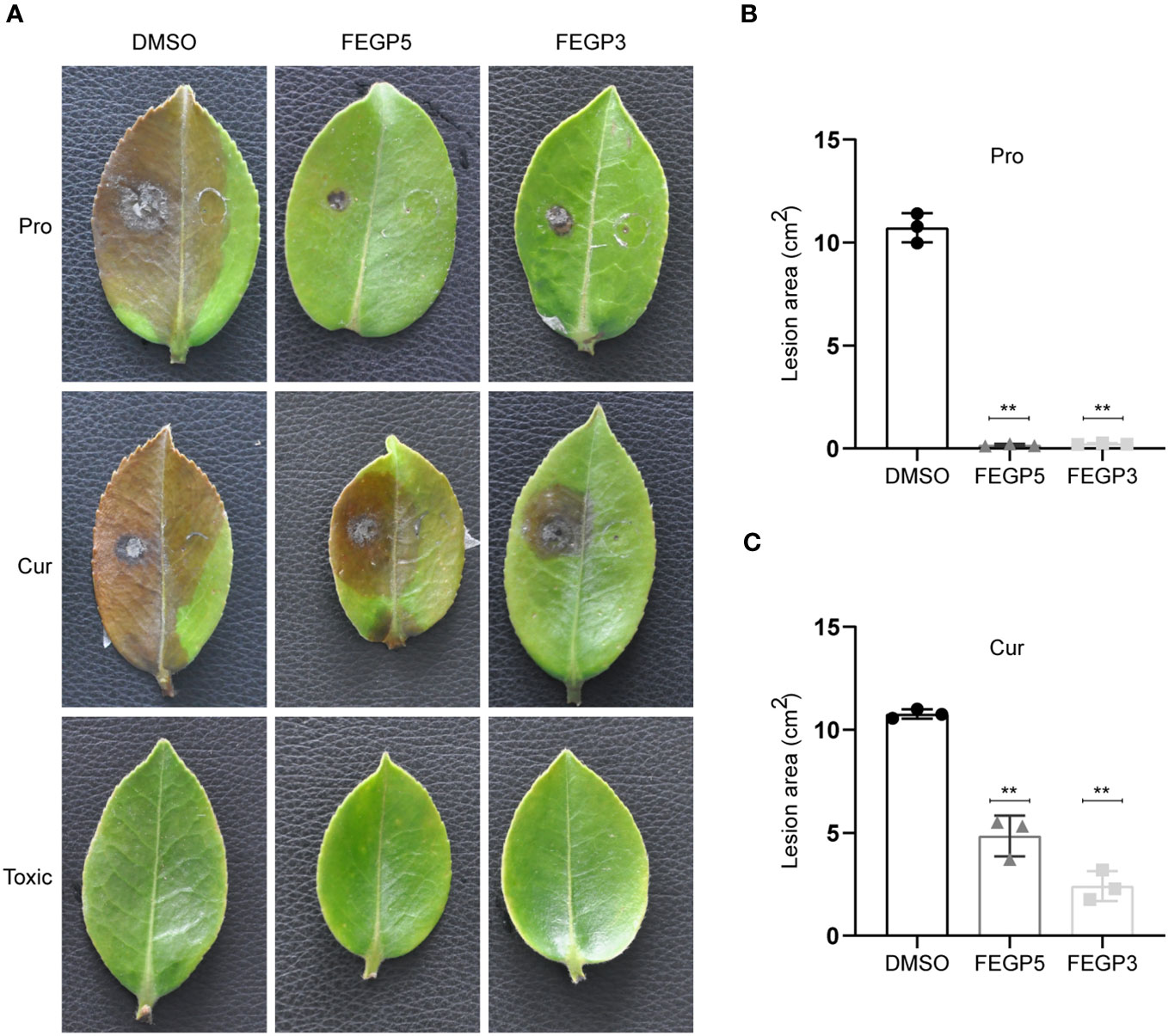
Figure 5 The fermentation extract of the strain GP521A or GP3R5 significantly inhibited the full virulence of C. camelliae MC171 in C. oleifera leaves. The image of representative samples (A), the lesion area in protective activity (B) and the lesion area in curative activity (C) of C. oleifera leaves under different treatments. (Data are mean ± SD. one-way analysis of variance [ANOVA], **:p < 0.01). Pro, protective activity; Cur, curative activity; Toxic, Toxic activity; DMSO, as solvent control.
Similar results were observed in the fruits, that both FEGP5 and FEGP3 significantly inhibited anthracnose development (Figure 6). At 7 dpi., the lesion diameter developed in the fruits pre-treated with FEGP5 and FEGP3 were ~8.04 mm and 7.59 mm, respectively, significantly smaller than that of the control (11.55 mm), and the disease inhibition rates were ~30.37% and 34.2%. In the curative activity test, the control efficacy of FEGP5 and FEGP3 reached ~62.05% and 46.48%, respectively, slightly higher than those of the preventative activity test. Taken together, these results suggested that FEGP5 and FEGP3 had strong inhibitory effects on the full virulence of C. camelliae MC171 and had great potential to be developed as novel biopesticides.
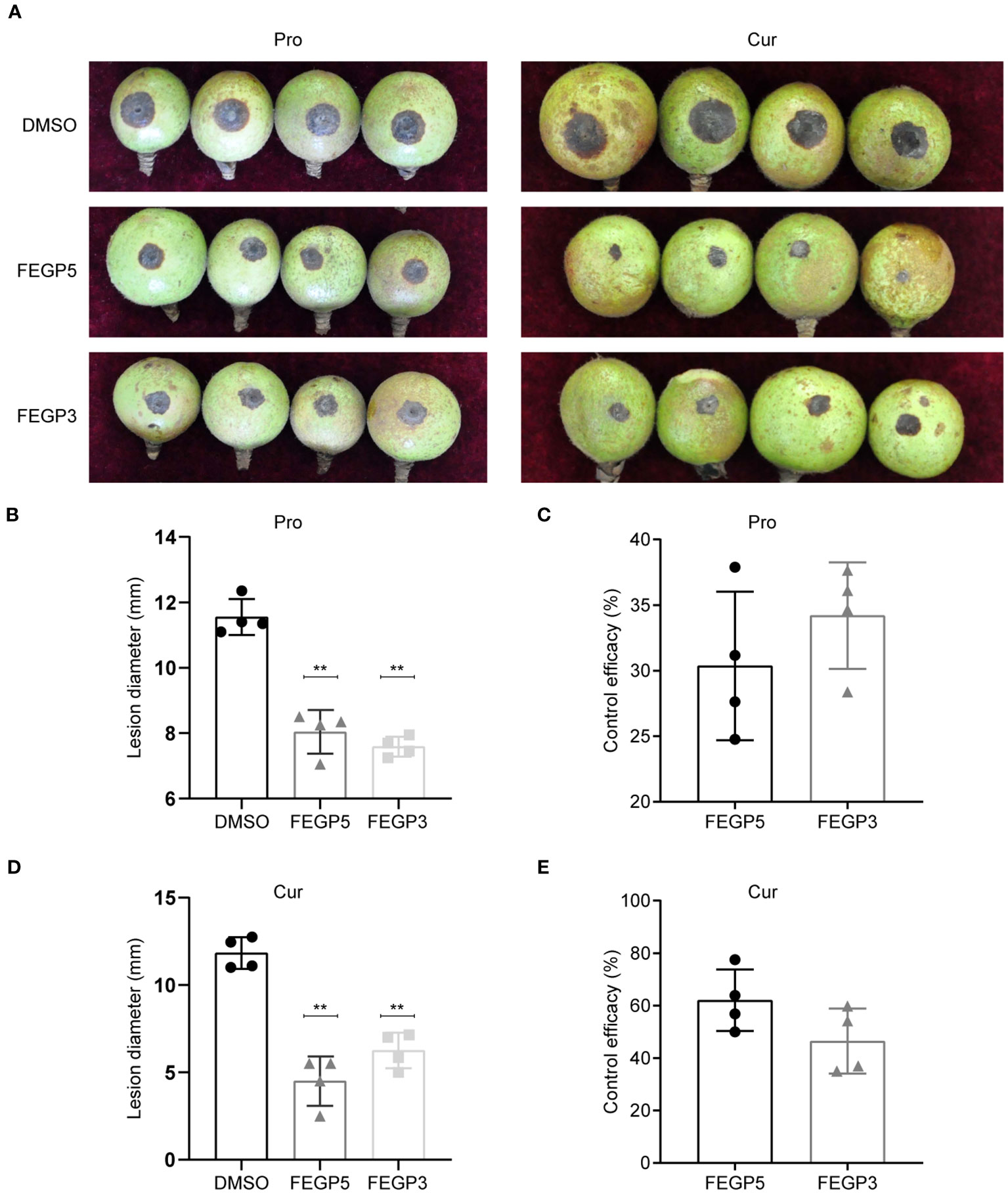
Figure 6 The fermentation extract of the strain GP521A or GP3R5significantly inhibited the full virulence of C. camelliae MC171 in C. oleifera fruits. The image of representative samples (A), Statistical analysis of the colony diameter and control efficacy of C. oleifera fruits under different treatments for protective activity (B, C) or curative activity (D, F). (Data are mean ± SD. one-way analysis of variance [ANOVA], **:p < 0.01). Pro, protective activity; Cur, curative activity; DMSO, as solvent control.
3.5 FEGP5 and FEGP3 exhibited lower environmental toxicity than commercial fungicide
Toxicity to environmental organisms is a critical consideration for any pesticide development, and thus need to be fully evaluated before a pesticide can be applied in field. As an initial step to evaluate the environmental compatibility of FEGP5 and FEGP3, we examined their impacts on the survival of Artemia salina, a common bioindicator of environmental contamination in comparison to a commercial fungicide, prochloraz. As shown in Figure 7, FEGP3 had no impacts on Artemia’s survival at 10 μg/mL, while prochloraz caused a ~30% reduction in the survival rate under the same concertation (Figure 7C). At 50 μg/mL, FEGP3 showed some toxicity, leading to a ~41% reduction in the survival rate of Artemia compared to the control (Figure 7B). However, this organism was completely killed by prochloraz under the same concentration. The toxicity of FEGP5 was also significantly lower than prochloraz, as there were only ~15.11% and 23.70% reduction in survival rates at 50 and 200 μg/mL (Figure 7A), respectively. These results showed that both FEGP5 and FEGP3 had lower environmental toxicity than one of the main fungicides for anthracnose management.
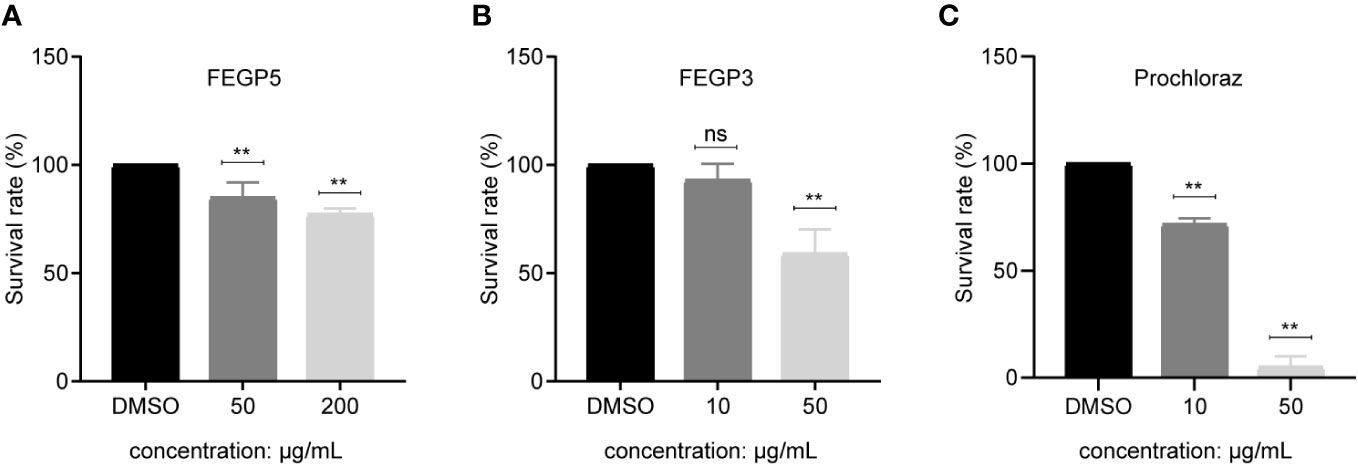
Figure 7 The effects of the fermentation extract of GP521A (A), GP3R5 (B) and prochloraz (C) on the survival rate of the Artemia salina. (Data are mean ± SD. one-way analysis of variance [ANOVA], ns, p > 0.05, **:p < 0.01). DMSO, as solvent control.
4 Discussion
The ocean occupies 70% of the earth’s surface. The extreme variation of environmental factors such as pressure, salinity, temperature and nutrient availability endows the marine environment with extraordinarily rich biodiversity and chemodiversity, which are dramatically different from those of the terrestrial environment. To date, more than 50 microbial phyla have been discovered and characterized from the marine environment, and many species are marine-exclusive. Most importantly, marine-derived natural products, especially those from marine microbes, have become a major source for innovative drug discovery. With regards to agricultural application, marine microbes are also emerging as a promising source for novel biopesticide mining. In the past decade, a large number of marine microorganisms have demonstrated promising antagonistic activities towards various plant pathogens both in vitro and in planta, suggesting great potential in plant disease management (Fudou et al., 2001; Pesic et al., 2013; Tareq et al., 2015; Du et al., 2020; Huang et al., 2020; Ma et al., 2020).
In this study, we found two isolates, GP521A and GP3R5, with strong antifungal bioactivities towards the anthracnose pathogens in oil tea. These strains were isolated from the soil of a mangrove natural reserve in China. In a previous study, bacteria of the mangrove soil ecosystem has been reported as a good source for exploration of antimicrobial compounds (Hao et al., 2019). Indeed, during the initial screening we found many isolates (>15%) from this source have antifungal bioactivities to different extent, and these two were chosen for further study due to their fast growth in plates and the best antifungal effects. GP521A was identified as Bacillus velezensis, and GP3R5 was identified as Pseudoalteromonas caenipelagi based on 16s rDNA sequencing. Pseudoalteromonas spp is a marine exclusive genus belonging to the Gammaproteobacteria class, and have been isolated from marine water and sediments worldwide (Zhou et al., 2009; Parrilli et al., 2021). Pseudoalteromonas isolates have been shown to produce a broad range of extracellular bioactive substances, such as enzymes, protease, and polysaccharides (Chen et al., 2020; Deng et al., 2022; Sun et al., 2023). Owning to these features, increasing studies have shown that these bacteria have important bioactivities, such as anticancer, antifouling and algicidal (Lovejoy et al., 1998; Soliev et al., 2015; Zeng et al., 2015; Di Guida et al., 2022). However, antifungal bioactivities have been rarely reported. To the best of our knowledge, this is the first study to prove the bioactivities of Pseudoalteromonas caenipelagi in against plant fungal diseases. Therefore, it is likely to identify novel compounds from GP3R5, and further identification of the bioactive components in its crude extract is undergoing.
Although a number of isolates from several Bacillus species have been shown to have antagonistic activities against Colletotrichum sp., these studies were all done targeting C. fructicola. We focused on C. camelliae MC171, which was repeatedly isolated from infected oil tea leaves in Macheng City, Hubei Province, China, suggesting this species might be a dominant pathovar in the local oil tea plantation (unpublished data). Therefore, this pathogen was used as a target in all subsequent assays. Bacillus spp. are known to produce various of antimicrobial compounds, and thus these bacteria are frequently used as biological control agents in plant disease management. One group of the best known antimicrobial compounds produced by Bacillus spp. is bacteriocins, such as amylolysin, subtilin, and thuricin, which shows potential antimicrobial activity and application on food preservatives, animal feeds and so on (Abriouel et al., 2011). Another is lipopeptides, such as fengycin, iturin, and surfactin, which can activate induced systemic defense in plants and play key roles in plant disease control and food safety (Ongena et al., 2007; Zhang et al., 2013; Farace et al., 2015; Meena and Kanwar, 2015). In addition, production of lytic enzymes (such as amylase and protease) and hydrogen cyanide has been shown to be responsible for the bioactivity of Bacillus cereus B8W8 in against major postharvest fungal pathogens of fruit (Khadiri et al., 2023). Different from previous reports of using Bacillus bacterial suspensions directly in against oil tea anthracnose (Xia et al., 2023; Zhou et al., 2022), we used crude extracts of the secondary metabolites produced by GP521A. Compounds obtained through ethyl acetate extraction are usually non proteinaceous, relatively small in molecular weight, and low to medium polarity. Therefore, the bioactive components of GP521A might be different from previous reports, such as YYC155, which achieved disease inhibition owning to the production of extracellular hydrolases (Zhou et al., 2022). There is a possibility that the crude extract of GP521A contains lipopeptides, which can be extracted by organic solvents. Further compound isolation and identification is needed to clarify the exact bioactive components. Although both GP521A and GP3R5 showed broad spectrum antifungal bioactivities, we observed an interesting difference between the two, that the antifungal effects of GP3R5 was not as obvious as GP521A. It could be due to a difference in either the nature or amount of bioactive compounds they produced, which needs further investigation.
Both FEGP5 and FEGP3 were capable of disrupting several pathogenic steps of MC171, from conidiation to appressorial formation, which are all indispensable to successful infection. As the crude extracts may contain more than one type of antimicrobial compound, it is highly likely that the crude extracts have multiple mode of actions, which together led to complete disease inhibition in leaves. Further experiments such as examination of the expression of pathway specific genes involved in each of these infection steps and mutant analysis will help to clarify the mechanisms involved. As the ethyl acetate extracts mostly contain small molecules, these compounds can be readily diffuse into plant cells and induce plant resistance. This might explain a better disease inhibition efficacy observed for both FEGP5 and FEGP3 in the protective treatments than in the curative treatments in leaves (Figure 5). However, an opposite trend was found in the fruits, that a better control efficacy was seen in the curative treatments rather than the protective treatments for both extracts (Figure 6). This might due to a tissue specific difference in response to compound treatment, which has been described previously by De Tender et al. (2021). Therefore, further investigation of plant responses to FEGP5 and FEGP3 treatments, such as qPCR analysis of defense related genes and examination of ROS production, will help to explain this phenomena.
A. salina is a zooplanktonic crustacean widely distributed in a variety of aquatic environments, and it is one of the main foods for many fishes and aquatic invertebrates (Zhu et al., 2018). Therefore, this organism is commonly used in toxicity tests as a bioindicator. Furthermore, A. salina has several advantages, such as ease of culturing in labs, short life cycle and high offspring production. Most importantly, A. salina is highly susceptible to environmental pollution, and the toxicity test results using this organism usually correlate well with those of rodents and humans. Therefore, A. salina is suitable for initial toxicity determination of potential pesticides. Our results demonstrated that FEGP5 and FEGP3 had much lower environmental toxicity than prochloraz, which is an imidazole fungicide widely used worldwide. This is consistent with previous reports that natural products from mangrove associated bacteria generally have much lower environmental toxicity than commercial fungicides (Hao et al., 2019). We understand that current toxicological data is not sufficient to conclude the environmental risks of FEGP5 and FEGP3, and further tests on more environmental organisms such algae and fishes are necessary in future studies. Overall, our study provides useful information for future development of environmentally compatible biopesticide using marine derived natural products in anthracnose management in oil tea.
Data availability statement
The datasets presented in this study can be found in online repositories. The names of the repository/repositories and accession number(s) can be found below: https://www.ncbi.nlm.nih.gov/genbank/, OR574457 and OR574458.
Author contributions
LF: Writing – original draft, Writing – review & editing, Data curation, Formal analysis, Methodology, Visualization. XX: Writing – original draft, Data curation, Methodology. JF: Writing – original draft. LH: Writing – original draft, Writing – review & editing.
Funding
The author(s) declare financial support was received for the research, authorship, and/or publication of this article. This work was financially supported by Scientific and Technical Innovation Council of Shenzhen (Grant No.20220811101028001).
Conflict of interest
The authors declare that the research was conducted in the absence of any commercial or financial relationships that could be construed as a potential conflict of interest.
Publisher’s note
All claims expressed in this article are solely those of the authors and do not necessarily represent those of their affiliated organizations, or those of the publisher, the editors and the reviewers. Any product that may be evaluated in this article, or claim that may be made by its manufacturer, is not guaranteed or endorsed by the publisher.
References
Abriouel H., Franz C. M., Omar N. B., Gálvez A. (2011). Diversity and applications of Bacillus bacteriocins. FEMS Microbiol. Rev. 35, 201–232. doi: 10.1111/j.1574-6976.2010.00244.x
Banti C. N., Hadjikakou S. K. (2021). Evaluation of toxicity with brine shrimp assay. Bio-Protoc. 11 (2), e3895–e3895. doi: 10.21769/BioProtoc.3895
Chen Y. Z. (2008). Oil Tea Camellia Superior Germplasm Resources (Beijing: China Forestry Publishing House).
Chen X. L., Wang Y., Wang P., Zhang Y. Z. (2020). Proteases from the marine bacteria in the genus Pseudoalteromonas: diversity, characteristics, ecological roles, and application potentials. Mar. Life Sci. Technol. 2, 309–323. doi: 10.1007/s42995-020-00058-8
Dai Y., Wu X. Q., Wang Y. H., Zhu M. L. (2021). Biocontrol potential of Bacillus pumilus HR10 against Sphaeropsis shoot blight disease of pine. Biol. Control 152, 104458. doi: 10.1016/j.biocontrol.2020.104458
Deng Y. S., Wang X. X., Xu H., Liu C., Li R., Zhang Y. Y., et al. (2022). Optimization of κ-selenocarrageenase production by Pseudoalteromonas sp. Xi13 and its immobilization. Molecules. 27, 7716. doi: 10.3390/molecules27227716
De Tender C., Vandecasteele B., Verstraeten B., Ommeslag S., Kyndt T., Debode J. (2021). Biochar-enhanced resistance to Botrytis cinerea in strawberry fruits (but not leaves) is associated with changes in the rhizosphere microbiome. Front. Plant Sci. 12. doi: 10.3389/fpls.2021.700479
Di T. M., Yang S. L., Du F. Y., Zhao L., Li X. H., Xia T., et al. (2018). Oleiferasaponin A (2), a novel saponin from Camellia oleifera Abel. seeds, inhibits lipid accumulation of HepG2 cells through regulating fatty acid metabolism. Molecules 23, 3296. doi: 10.3390/molecules23123296
Di Guida R., Casillo A., Stellavato A., Kawai S., Ogawa T., Di Meo C., et al. (2022). Capsular polysaccharide from a fish-gut bacterium induces/promotes apoptosis of colon cancer cells in vitro through Caspases’ pathway activation. Carbohydr. Polym. 278, 118908. doi: 10.1016/j.carbpol.2021.118908
Du F. Y., Ju G. L., Xiao L., Zhou Y. M., Wu X. (2020). Sesquiterpenes and cyclodepsipeptides from marine-derived fungus Trichoderma longibrachiatum and their antagonistic activities against soil-borne pathogens. Mar. Drugs 18, 165. doi: 10.3390/md18030165
Farace G., Fernandez O., Jacquens L., Coutte F., Krier F., Jacques P., et al. (2015). Cyclic lipopeptides from Bacillus subtilis activate distinct patterns of defense responses in grapevine. Mol. Plant Pathol. 16, 177–187. doi: 10.1111/mpp.12170
Feas X., Estevinho L. M., Salinero C., Vela P., Sainz M. J., Vazquez-Tato M. P., et al. (2013). Triacylglyceride, antioxidant and antimicrobial features of virgin Camellia oleifera, C reticulata and C sasanqua Oils. Molecules 18, 4573–4458. doi: 10.3390/molecules18044573
Fudou R., Iizuka T., Yamanaka S. (2001). Haliangicin, a novel antifungal metabolite produced by a marine myxobacterium. Part 1. Fermentation and biological characteristics. J. Antibiot. 54, 149–152. doi: 10.1094/PDIS-11-18-1958-RE
Hao L. Y., Wang Y., Chen X. Q., Zheng X. L., Chen S., Li S. F., et al. (2019). Exploring the potential of natural products from mangrove rhizosphere bacteria as biopesticides against plant diseases. Plant Dis. 103 (11), 2925–2932. doi: 10.1094/PDIS-11-18-1958-RE
Huang R. H., Lin W., Zhang P., Liu J. Y., Wang D., Li Y. Q., et al. (2020). Anti-phytopathogenic bacterial metabolites from the seaweed-derived fungus Aspergillus sp. D40. Front. Mar. Sci. 7, 313. doi: 10.3389/fmars.2020.00313
Imhoff J. F., Labes A., Wiese J. (2011). Bio-mining the microbial treasures of the ocean: new natural products. Biotechnol. Adv. 29 (5), 468–482. doi: 10.1016/j.bioteChadv.2011.03.001
Khadiri M., Boubaker H., Askarne L., Ezrari S., Radouane N., Farhaoui A., et al. (2023). Bacillus cereus B8W8 an effective bacterial antagonist against major postharvest fungal pathogens of fruit. Postharvest Biol. Technol. 200, 112315. doi: 10.1016/j.postharvbio.2023.112315
Li S., Li H. (2020). First report of Colletotrichum nymphaeae causing anthracnose on Camellia oleifera in China. Plant Dis. 104 (6), 1860–1860. doi: 10.1094/PDIS-09-19-2016-PDN
Li L., Li H. (2022). First report of Colletotrichum aeschynomenes causing anthracnose on Camellia oleifera in China. For. Pathol. 52 (5), e12770. doi: 10.1111/efp.12770
Lovejoy C., Bowman J. P., Hallegraeff G. M. (1998). Algicidal effects of a novel marine Pseudoalteromonas isolate (Class Proteobacteria, Gamma Subdivision) on harmful algal bloom species of the genera Chattonella, Gymnodinium, and Heterosigma. Appl. Environ. Microbiol. 64, 2806–2813. doi: 10.1128/aem.64.8.2806-2813.1998
Ma Z. W., Zhang S. Y., Sun K., Hu J. C. (2020). Identification and characterization of a cyclic lipopeptide iturin A from a marine-derived Bacillus velezensis 11-5 as a fungicidal agent to Magnaporthe oryzae in rice. J. Plant Dis. Prot. 127, 15–24. doi: 10.1007/s41348-019-00282-0
Meena K. R., Kanwar S. S. (2015). Lipopeptides as the antifungal and antibacterial agents: applications in food safety and therapeutics. Biomed. Res. Int. 2015, 473050. doi: 10.1155/2015/473050
Ongena M., Jourdan E., Adam A., Paquot M., Brans A., Joris B., et al. (2007). Surfactin and fengycin lipopeptides of Bacillus subtilis as elicitors of induced systemic resistance in plants. Environ. Microbiol. 9, 1084–1090. doi: 10.1111/j.1462-2920.2006.01202.x
Parrilli E., Tedesco P., Fondi M., Tutino M. L., Giudice A. L., de Pascale D., et al. (2021). The art of adapting to extreme environments: the model system Pseudoalteromonas. Phys. Life Rev. 36, 137–161. doi: 10.1016/j.plrev.2019.04.003
Peng X. J., Wang Q. C., Zhang S. K., Guo K., Zhou X. D. (2023). Colletotrichum species associated with Camellia anthracnose in China. Mycosphere 14 (2), 130–157. doi: 10.3897/mycokeys.99.106812
Pesic A., Baumann H. I., Kleinschmidt K., Ensle P., Wiese J., Süssmuth R. D., et al. (2013). Champacyclin, a new cyclic octapeptide from Streptomyces strain C42 isolated from the baltic Sea. Mar. Drugs 11. doi: 10.3389/fchem.2022.921246
Quan W., Wang A., Gao C., Li C. (2022). Applications of Chinese Camellia oleifera and its by-products: A review. Front. Chem. 10, 921246. doi: 10.3389/fchem.2022.921246
Savchenko I. V. (2017). Breeding new varieties and hybrids of agricultural plants. Her. Russ. Acad. Sci. 87, 104–110. doi: 10.1134/S1019331617020150
Soliev A. B., Hosokawa K., Enomoto K. (2015). Effects of prodigiosin family compounds from Pseudoalteromonas sp. 1020R on the activities of protein phosphatases and protein kinases. J. Enzy. Inhib. Med. Chem. 30, 533–538. doi: 10.3109/14756366.2014.951347
Song Z. M., Zhang J. L., Zhou K., Yue L. M., Zhang Y., Wang C. Y., et al. (2021). Anthraquinones as potential antibiofilm agents against methicillin-resistant Staphylococcus aureus. Front. Microbiol. 12. doi: 10.3389/fmicb.2021.709826
Sun X. H., Chen X. L., Wang X. F., Zhang X. R., Sun X. M., Sun M. L., et al. (2023). Cost-effective production of alginate oligosaccharides from Laminaria japonica roots by Pseudoalteromonas agarivorans A3. Microb. Cell Fact. 22, 179. doi: 10.1186/s12934-023-02170-7
Tareq F. S., Lee H. S., Lee Y. J., Lee J. S., Shin H. J. (2015). Ieodoglucomide C and ieodoglycolipid, new glycolipids from a marine-derived bacterium Bacillus licheniformis 09IDYM23. Lipids 50, 513–519. doi: 10.1007/s11745-015-4014-z
Wang Y. X., Chen J. Y., Xu X. W., Cheng J. Y., Zheng L., Huang J. B., et al. (2020). Identification and characterization of Colletotrichum species associated with anthracnose disease of Camellia oleiferain in China. Plant Dis. 104, 474–482. doi: 10.3390/f14050886
Xia Y. D., Liu J. A., Wang Z. K., He Y., Tan Q., Du Z., et al. (2023). Antagonistic activity and potential mechanisms of endophytic Bacillus subtilis YL13 in biocontrol of Camellia oleifera anthracnose. Forests 14 (5), 886. doi: 10.3390/f14050886
Xu J. X., Li Z. Y., Lv X., Yan H., Zhou G. Y., Cao L. X., et al. (2020). Isolation and characterization of Bacillus subtilis strain 1-L-29, an endophytic bacteria from Camellia oleifera with antimicrobial activity and efficient plant-root colonization. PloS One 15, e0232096. doi: 10.1371/journal.pone.0232096
Yu J., Wu Y., He Z., Li M., Zhu K., Gao B. (2018). Diversity and antifungal activity of endophytic fungi associated with Camellia oleifera. Mycobiology 46, 1–7. doi: 10.1080/12298093.2018.1454008
Zeng Z. S., Guo X. P., Li B. Y., Wang P. X., Cai X. S., Tian X. P., et al. (2015). Characterization of self-generated variants in Pseudoalteromonas lipolytica biofilm with increased antifouling activities. Appl. Microbiol. Biotechnol. 99, 10127–10139. doi: 10.1007/s00253-015-6865-x
Zhang X., Li B., Wang Y., Guo Q., Lu X., Li S., et al. (2013). Lipopeptides, a novel protein, and volatile compounds contribute to the antifungal activity of the biocontrol agent Bacillus atrophaeus CAB-1. Appl. Microbiol. Biotechnol. 97, 9525–9534. doi: 10.1007/s00253-013-5198-x
Zhou M. Y., Chen X. L., Zhao H. L., Dang H. Y., Luan X. W., Zhang X. W., et al. (2009). Diversity of both the cultivable protease-producing bacteria and their extracellular proteases in the sediments of the South China Sea. Microb. Ecol. 58, 582–590. doi: 10.1007/s00248-009-9506-z
Zhou A., Wang F., Yin J., Peng R., Deng J., Shen D., et al. (2022). Antifungal action and induction of resistance by Bacillus sp. strain YYC 155 against Colletotrichum fructicola for control of anthracnose disease in Camellia oleifera. Front. Microbiol. 13. doi: 10.3389/fmicb.2022.956642
Zhu B., Zhu S., Li J., Hui X., Wang G. X. (2018). The developmental toxicity, bioaccumulation and distribution of oxidized single walled carbon nanotubes in: Artemia salina. Toxicol. Res. (Camb). 7, 897–906. doi: 10.1039/c8tx00084k
Keywords: Camellia oleifera, anthracnose, mangrove soil bacteria, Bacillus, Pseudoalteromonas, natural product extracts, biological control
Citation: Fei L, Xu X, Feng J and Hao L (2023) Inhibition of oil tea anthracnose by natural product extracts from Bacillus and Pseudoalteromonas isolates from mangrove soil. Front. Mar. Sci. 10:1299118. doi: 10.3389/fmars.2023.1299118
Received: 22 September 2023; Accepted: 30 October 2023;
Published: 14 November 2023.
Edited by:
Dong-Lin Zhao, Chinese Academy of Agricultural Sciences, ChinaReviewed by:
Fengyu Du, Qingdao Agricultural University, ChinaXiao-Lin Chen, Huazhong Agricultural University, China
Caijuan Zheng, Hainan Normal University, China
Copyright © 2023 Fei, Xu, Feng and Hao. This is an open-access article distributed under the terms of the Creative Commons Attribution License (CC BY). The use, distribution or reproduction in other forums is permitted, provided the original author(s) and the copyright owner(s) are credited and that the original publication in this journal is cited, in accordance with accepted academic practice. No use, distribution or reproduction is permitted which does not comply with these terms.
*Correspondence: Lingyun Hao, aGFvbGluZ3l1bkBzenUuZWR1LmNu
†These authors share first authorship