- 1Key Laboratory of Plant Biotechnology of Liaoning Province, School of Life Sciences, Liaoning Normal University, Dalian, China
- 2Dalian Key Laboratory of Genetic Resources for Marine Shellfish, Liaoning Ocean and Fisheries Science Research Institute, Dalian, China
- 3Key Laboratory of Protection and Utilization of Aquatic Germplasm Resource, Ministry of Agriculture and Rural Affairs, Dalian, China
Introduction: Marine epiphytic diatoms are natural diets for multiple marine invertebrates, including sea cucumbers, sea urchins, etc. They also act as bioindicators in marine waters to assess water quality of coastal ecosystems.
Material and methods: We isolated three strains of Navicula and two strains of Nitzschia from macroalgal epiphytes. Combined concentrations of four macroelements, five trace metals, and three vitamins in f/2 medium were optimized for these diatoms using single-factor and orthogonal tests.
Results and discussion: The biomass productivities for 4-L enlarged cultures of the five isolated diatoms in their exclusively optimized f/2 media all increased by nearly 10-fold relative to f/2 medium, and that of Navicula-1 and Nitzschia-2 reached the highest, i.e., 29.52 ± 1.98 and 30.68 ± 2.84 g m-2 d-1, respectively. Moreover, the relative abundance of C20:5n3 in the exclusively optimized f/2 medium-cultured Navicula-1 notably rose by 24%, and that in Nitzschia-2 held stable at > 25%. Thus, Navicula-1 and Nitzschia-2 were screened out with outstanding growth traits and improved fatty acid compositions at high levels of combined nitrogen, phosphorus, iron, and silicon. The two diatoms have potential for becoming quality diets for marine aquaculture, and ideal candidates for effective nutrient removal.
1 Introduction
Diatoms are pivotal primary producers in aquatic ecosystems and play crucial roles in food webs as well as biogeochemical cycles. They exist in almost all aquatic habitats, including oceans, estuaries, wetlands, lakes, and even certain transient aquatic habitats (Pandey et al., 2018), and are responsible for approximately 20% of global oxygen production. The periphytic diatom cells can be established on varieties of vegetal or mineral substrates. The nature and roughness of the substrates create different species assemblages, including macroalgae and macrophytes, as well as rock, sand, and mud (Desrosiers et al., 2013). In addition, the different host macroalgae are usually adhered by various epiphytic diatoms as a portion of the periphyton assemblages (Almeida and Beltrones, 2008; Burfeid-Castellanos et al., 2021).
Periphytic diatoms have been considered effective ecological bioindicators, mainly due to their short generation times (24 h to several weeks) and their direct and sensitive responses to various biological, chemical, and physical disturbances in ecosystems (Kireta et al., 2012). These diatoms are also widely distributed in marine coastal waters. Marine periphytic diatoms have also been regarded as an emerging marine bioindicator. In mesotrophic and oligotrophic waters, nitrogen and phosphorous enrichment have altered benthic diatom species assemblage (Desrosiers et al., 2013). Their short life span and sessile traits enable them to capture ephemeral environmental variations at sampling sites. Moreover, their sensitivity to multiple environmental factors and nutrient enrichment endows characterizing species in terms of functional categories, e.g., salinity, pH, oxygen demands, nitrogen assimilation, and trophic status (Desrosiers et al., 2013; Han et al., 2023).
Municipal wastewater discharge into nearshore waters has turned into the most frequent practice of ultimate sewage disposal in coastal cities (Otim et al., 2018). However, the concentrations of nutrients, especially nitrogen and phosphorus, in multiple coastal ecosystems have increased by year, accompanied by massive increases in numerous volumes of discharge (Zhang et al., 2020). Various microalgal communities have been found to be abundant in these nearshore waters. They can considerably take in different forms of nitrogen and phosphorus (Meerssche and Pinckney, 2019). Among these pollution-resistant microalgae, the periphytic diatoms attached to macroalgae or stones are highly adaptive to the variable environment. Thus, these diatoms could be further isolated and potentially used for effective nutrient removal in municipal wastewater treatment. Meng et al. (2019) found that the periphytic diatom Navicula sp. associated with Stigeoclonium sp. and ammonia-oxidizing bacteria enhanced nitrogen and phosphorus removals in the reactors with stronger illumination. However, this study chiefly focused on the nutrient removal performance of the microalgal community, and the nutrient uptake characteristics of the mono-cultured periphytic diatom were still unclear. Currently, very limited studies on growth traits of periphytic diatoms at high levels of nutrients have been reported. They could contribute to the application of nutrient removal using potentially high-quality periphytic diatoms, especially the robust diatoms distributed around municipal wastewater discharge into nearshore waters.
In addition, the periphytic diatoms have been widely used as the essential natural diets for invertebrate marine animals because they are highly rich in polyunsaturated fatty acids (PUFAs), C20:5n3 in particular (Scholz and Liebezeit, 2013; Xie et al., 2017), and these PUFAs are closely bound up with the metamorphosis and development of larvae of invertebrates, e.g., the sea urchin Paracentrotus lividus and the red abalone Haliotis rufescens (Courtois de Viçose et al., 2012; Sánchez-Saavedra and Núñez-Zarco, 2015; Gomes et al., 2021). Hence, it is necessary to screen periphytic diatoms with high amounts of PUFAs and prominent growth properties. The screened diatoms could serve as an underlying high-quality diet for aquatic animals and be beneficial for developing aquaculture.
Despite the potential uses of the periphytic diatoms in nutrient removal or aquafeeds, only a few reports have described the impacts of culture condition changes in the periphytic diatom growth (Mercado et al., 2004; Yang et al., 2014), especially the requirements for various macro- or micronutrients. Thus, it is crucial to propose nutrient combination strategies for the significant promotion of periphytic diatom growth. In this work, in order to screen the marine epiphytic diatoms with great growth performance, we comprehensively optimized the culture medium, i.e., the general f/2 medium, for five isolated epiphytic diatoms. In addition, the variations in fatty acid profiles of the two screened diatoms with good growth traits were compared under the control f/2 and exclusively optimized f/2 media. This study aims to screen out the potentially fast-growing and nutritional marine epiphytic diatoms. These results could provide valuable insights into the underlying application of pollution-resistant epiphytic diatoms in both aquaculture and wastewater treatment industries.
2 Materials and methods
2.1 Isolation and culture of microalgal strains
Five strains of marine epiphytic diatoms, including three species of Navicula and two species of Nitzschia (Figures 1, 2), were isolated from macroalgal epiphytes in the Heishijiao intertidal zones of Dalian, China, adjacent to a sewage outlet into the sea. The epiphytes on macroalgae were first scrubbed into the sterile seawater using a banister brush. The collected epiphytes were then filtered through a 300-mesh screen to remove large particles. The filtered diatom concentrates were kept static overnight to make the diatom cells settle onto the bottom of the flasks. After the supernatants were discarded, the bottom diatom mixtures were enriched with f/2 medium. The enriched diatom cells grown exponentially were further isolated through a combination of multi-methods, including micropipettes, streaking cells across agar plates, and step dilution method. The isolated xenic epiphytic diatoms were sub-cultured in 1-L Erlenmeyer flasks and maintained in f/2 medium (Harrison and Berges, 2005) at an ambient temperature (15°C–20°C) under a natural light cycle (approximately 10 h of light duration). The diatom biofilms attached to the glass were gently collected and homogenized using a sterilized banister brush. The diatom concentrates were then transferred to a sterile 10-mL tube and kept static for 5 min to precipitate the clumps. The upper homogenous diatoms were inoculated into 400 mL of f/2 medium, and 200 mL of f/2 medium was added into the cultures every 4 days. The sub-culture lasted for a total of 12 days in 1-L flasks.
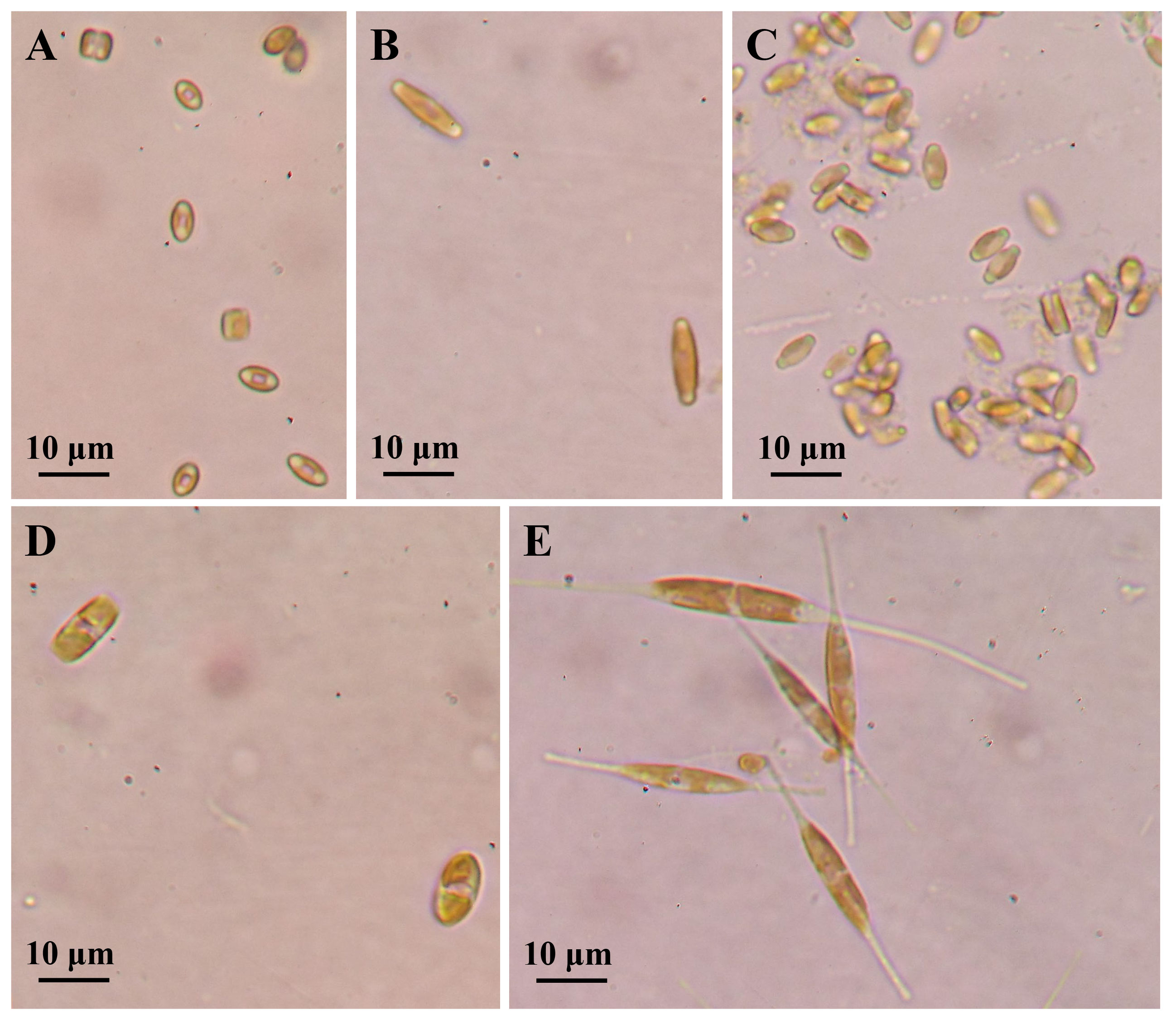
Figure 1 Cell morphology of five marine epiphytic diatoms under optical microscope. (A–C) Three species of Navicula expressed as Navicula-1, Navicula-2, and Navicula-3. (D, E) Two species of Nitzschia expressed as Nitzschia-1 and Nitzschia-2.
2.2 Experimental design and enrichment of microalgae
In this work, the optimized batch cultures (Figure 3) were conducted based on the basic f/2 medium, including the combined optimization of four macroelements (nitrogen, phosphorus, iron, and silicon) and eight microelements (five trace metal elements and three vitamins), for three species of Navicula and two species of Nitzschia (Figures 1, 2). Then, three light intensities were also detected to screen out diatoms potentially resistant to high light intensity. The subsequent enlarged cultures for Navicula spp. and Nitzschia spp. at a 4-L scale were further performed to screen the optimal diatoms with good growth traits in the exclusively optimized f/2 media. Ultimately, the fatty acid profiles of the screened diatoms in the exclusively optimized f/2 media were assessed and compared relative to those in the control (f/2 medium) (Figure 3).
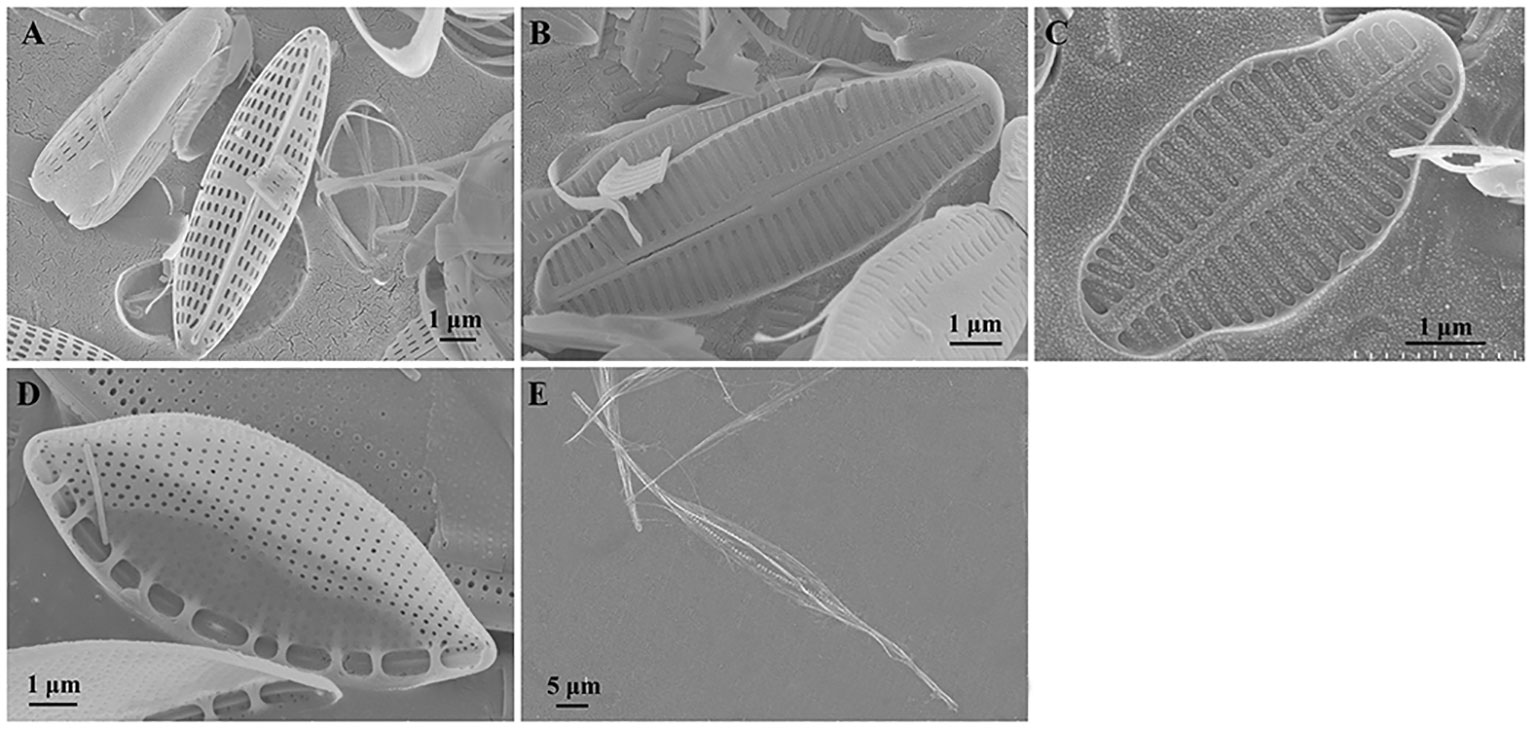
Figure 2 Cell morphology of five marine epiphytic diatoms under scanning electron microscopy. (A–C) Three species of Navicula expressed as Navicula-1, Navicula-2, and Navicula-3. (D, E) Two species of Nitzschia expressed as Nitzschia-1 and Nitzschia-2.
First, the combined concentrations of four macroelements (nitrogen, phosphorus, iron, and silicon) (Yang et al., 2014), five trace metal elements (zinc (Zn), copper (Cu), manganese (Mn), molybdenum (Mo), and cobalt (Co)), and three vitamins (vitamins thiamine (VB1), cyanocobalamin (VB12), and biotin (VH)) (Figures S1-S10, Tables S1-18) in f/2 medium were optimized for Navicula spp. and Nitzschia spp. based on the preliminary single-factor tests and orthogonal tests. Each of the four optimized f/2 media was further designed for Navicula spp. (Table 1) and Nitzschia spp. (Table 2) in terms of the growth curves and the maximum growth rates under the above-mentioned optimization conditions. The growth tests for optimization of culture media and illumination (Figure 3, Tables 1, 2) were performed in an illumination incubator (HPG-280B, Donlian Hall, China) using 10-mL glass tubes (13 × 100 mm) sealed with cotton plugs. The diatom cultures were controlled at 20°C under a 12-h light/12-h dark cycle. The light intensity was set at 100 μmol·m−2·s−1 for optimization tests of culture media.
Second, three light intensities, including 100 μmol·m−2·s−1, 200 μmol·m−2·s−1, and 350 μmol·m−2·s−1, were tested to explore the optimal illumination intensity appropriate for the isolated epiphytic diatoms grown in the exclusively optimized media (Table 3). The optical density (OD680, Multiskan GO, Thermo Fisher Scientific, Waltham, MA, USA; Wang et al., 1997) of diatom inoculum was first adjusted to 0.045 (~1 × 105 ind./mL; Courtois de Viçose et al., 2012) with sterilized seawater, and each 3-mL cell growing exponentially was transferred into the above-mentioned glass tubes following 2 days of static culture for firm attachment to glass. Then, the seawater was refreshed using 3 mL of f/2 medium, and the OD680 was monitored on Days 2, 4, 6, 8, and 10. Before measurement, a banister brush was used to completely homogenize the diatom cells attached to the bottom of the glass tube 10 times, and the homogenates were vortexed for 1 min to disperse the cells and ensure the determination accuracy. A total of 15 biological replicates of diatom cells for each treatment were prepared on Day 0. Each of the three biological replicates was taken every other day to measure the OD680, which was discarded following measurement. After the determination of OD680, the glass tube was discarded. The specific growth rate was calculated as μ = (lnOD2 − lnOD1)/(t2 − t1), where t1 and t2 are the beginning and sampling time of the culture period, respectively, and OD1 and OD2 are the corresponding optical densities at 680 nm.
Third, to further confirm the significant promotion impacts of the optimized medium on the growth of Navicula spp. and Nitzschia spp., the enlarged cultures in triplicate were conducted in 5-L flasks. Each 2 L of diatom dilutions with OD680 of 0.045 in sterilized f/2 or optimized f/2 medium was inoculated into a 5-L flask. After 4 days of initial culture, another 1 L of fresh medium was gently added into the corresponding cultures. The same volume of the above-mentioned medium was re-added following the next 4 days. The enlarged cultures of the isolated epiphytic diatoms were also conducted at an ambient temperature (15°C–20°C) under a natural light cycle, lasting for 12 days. Further, the enlarged cultures for the five diatoms at a 4-L scale were performed in their respective exclusively optimized f/2 media (Table 3). Prior to harvest, the cultures were gently agitated twice per day. Finally, the fresh diatom cells attached to the glass at the bottom of the 5-L flask were completely collected and homogenized using a long banister brush. The diatom concentrates were centrifuged (4,000 rpm, 10 min, 4°C), and the pellets were weighed to evaluate the growth performance, i.e., the biomass productivity of diatoms (Pd). The biomass productivity was calculated using the fresh weight of the xenic diatom cells, culture days, and surface area of the 5-L flask bottom, as follows:
Pd = M/(3.14 × R2 × T),
where Pd is the biomass productivity of diatoms, M is the wet weight of harvested diatom cells (g), R is the bottom radius of the 5-L conical flask for culturing diatom cells (m), and T is the culture time (days).
The collected diatom pellets were stored at −80°C until subsequent fatty acid analyses.
2.3 Cell morphology
The normal morphology of the diatom cells was observed and preliminarily characterized under a light microscope (Olympus, Tokyo, Japan) with ×400 magnification. For scanning electron microscopy (SEM) observation, 2 mL of diatom cells was first added with equal volumes of sulfuric acid, followed by boiling for 10–30 min to oxidize the organic matter. The mixture was washed with deionized water several times until a neutral level was reached. The specimens were then collected onto a polycarbonate membrane, attached to aluminum stubs, and sputter-coated with gold (JFC-1100, JEOL, Tokyo, Japan). The samples were observed, and the micrographs were taken under a JSM-6360LV SEM (JEOL, Japan) operated at 15 kV.
2.4 Fatty acid analyses
The fatty acid profiles of diatoms were determined via lipid extraction (Folch et al., 1957) and transesterification (Metcalfe and Schmitz, 1961), followed by gas chromatography (GC) analysis for fatty acid methyl esters (FAMEs), as previously reported (Yin et al., 2013). In brief, lipids were first extracted using chloroform/methanol (2:1, v/v) with ultrasonication for 15 min and then layered with potassium chloride (0.88%, m/v). The lipid extraction was repeated three times, and the merged lower organic phase was dried under gentle nitrogen flow. The extracted lipids (50–100 mg) were hydrolyzed using 0.5 M KOH–methanol at 70°C for 1 h, and the produced fatty acids were then esterified using methanol under the catalysis of boron trifluoride. FAMEs were extracted using petroleum ether and quantified using a Shimadzu GC-2010 fitted with a flame ionization detector (FID) as well as a fused silica capillary column (30 m × 0.25 mm ID × 0.3 μm film, FFAP, Dalian Institute of Chemical Physics, Chinese Academy of Sciences, China). The temperatures of the inlet and detector were 250°C and 230°C, respectively, and the split ratio was 100:1. The carrier gas was nitrogen with a purity of 99%. The column temperature was programmed from 160°C to 230°C increasing at 2°C/min and held at 230°C for 5 min. The running time was 40 min. The petroleum ether extracts were condensed under mild nitrogen flow when necessary.
2.5 Statistical analysis
The data were statistically analyzed using SPSS 19.0 and denoted as average ± standard deviation (SD, n = 3). The single-factor tests as well as the orthogonal tests (Tables S1-S4) were performed to evaluate the impacts of the four macroelements (nitrogen, phosphorus, iron, and silicon; Yang et al., 2014), five trace metals (Zn, Cu, Mn, Mo, and Co; Figures S1–S6, Tables S5-S12), and three vitamins (VB1, VB12, and VH; Figures S7-S10, Tables S13-18) in f/2 medium on the growth of diatoms. Statistical significance of the difference was determined by one-way analysis of variance and Tukey’s honestly significant difference (HSD) test with a significance level of 0.05 and Student’s t-test expressed as *p < 0.05 or **p < 0.01.
3 Results
First, the five isolated epiphytic diatoms were characterized under both bright-field and SEM (Figures 1, 2). The two lamellar plastids of Navicula spp., including Navicula-1, Navicula-2, and Navicula-3 (Figures 1A–C), were symmetrically distributed on the left and right sides of silica shell, while those of Nitzschia spp., including Nitzschia-1 and Nitzschia-2 (Figures 1D, E), were generally top- and bottom-distributed under bright-field microscopy. In addition, three species of Navicula (Figures 2A–C) were characterized by their typical raphes, and two species of Nitzschia (Figures 2D, E) had typical canal raphes under SEM.
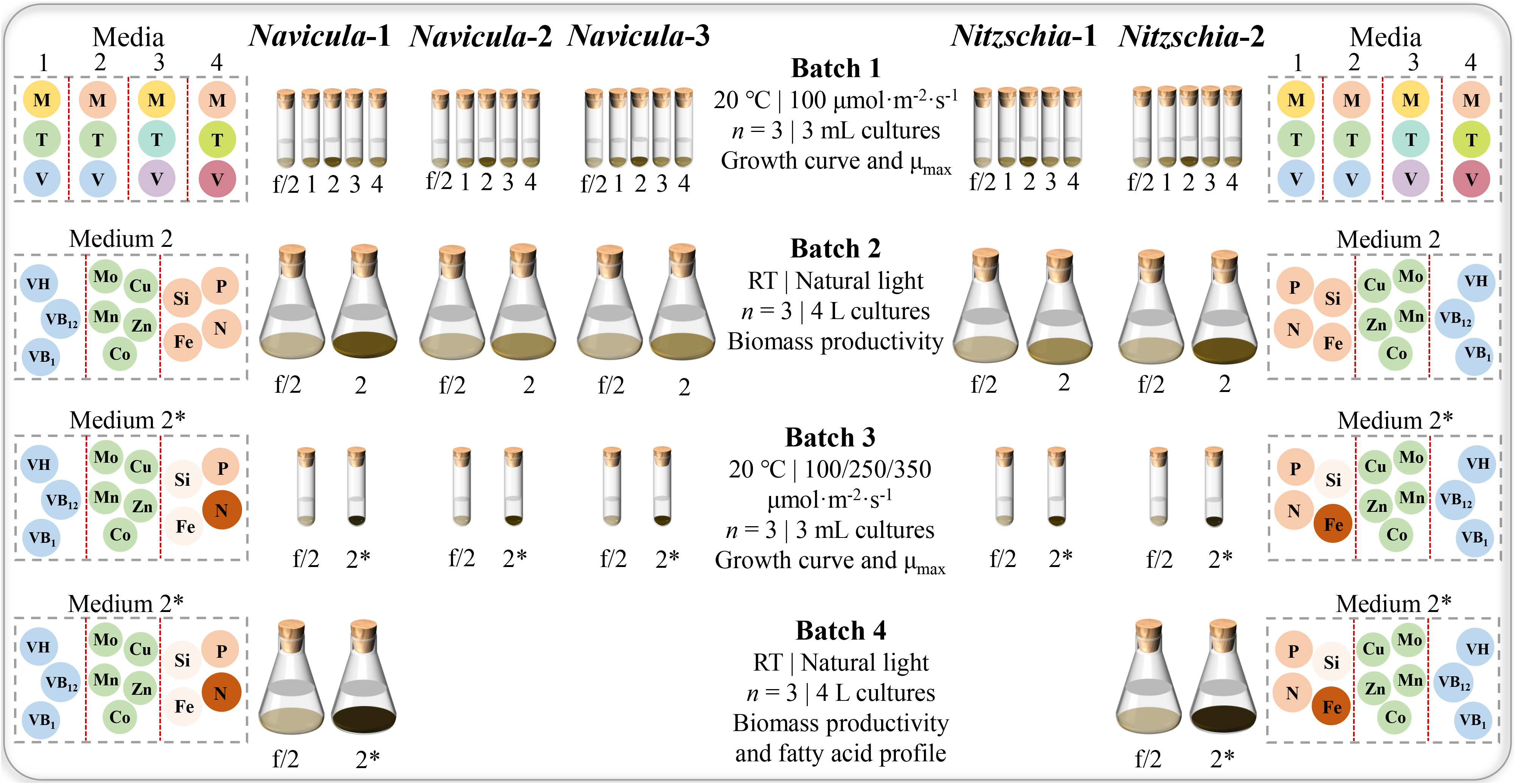
Figure 3 Experimental design for optimized cultivation and screening of five marine epiphytic diatoms. Four batches of diatom cultures in 10-mL tubes or 5-L flasks were performed in triplicate. The capital letters M, T, and V indicate macroelements (N, P, Fe, and Si), trace metals (Zn, Cu, Mn, Mo, and Co), and vitamins (VB1, VB12, and VH), respectively. The different colors of the same capital letter in Media 1, 2, 3, and 4 indicate the different concentrations, while the darker the color of the framed circle for the same element in Media 2 and 2*, the higher the concentration of the element for the same diatom. In addition, the concentration of nitrogen for Navicula was increased, and that of silicon for Navicula and Nitzschia was both reduced in Medium 2* relative to Medium 2; the concentration of iron for Navicula was reduced, and that for Nitzschia was increased. In Batch 1 of 3-mL cultures, the four optimized f/2 media for Navicula spp. and Nitzschia spp. (Media 1, 2, 3, and 4 in the figure represent optimized f/2-1, f/2-2, f/2-3, and f/2-4 media, respectively, in Tables 1, 2) were tested in terms of the growth curve, and the maximum specific growth rates, μmax, and the respective optimized f/2-2 media (Tables 1, 2) for Navicula spp. and Nitzschia spp. were screened out due to the diatoms’ good growth properties. In Batch 2 of 4-L cultures, the biomass productivities for five strains of diatoms were evaluated in their respective optimized f/2-2 media (Medium 2 in the figure). Then, the respective optimized f/2-2 media were further optimized for concentrations of nitrogen, phosphorus, and silicon and indicated as the exclusively optimized f/2 media (Medium 2* in the figure) in Table 3. In Batch 3 of 3-mL cultures, the exclusively optimized f/2 media (Medium 2* in the figure) for Navicula spp. and Nitzschia spp. were used, and three light intensities were set to assess their impacts on growth curve and μmax of five diatoms. Ultimately, Navicula-1 and Nitzschia-2 both presented more outstanding growth traits than the other diatoms, and their enlarged 4-L cultures in Batch 4 also possessed consistent biomass productivity. The corresponding fatty acid profiles of these two diatoms were then evaluated.
The results showed that the OD680 of Navicula-1, Navicula-2, Navicula-3, and Nitzschia-1 under the four optimized f/2 media were all significantly higher (p < 0.05) than that under the f/2 medium. The N:P mass ratios in the four optimized f/2 media for Navicula spp. increased from 10.8 (f/2 medium) to 23.2 or 32.7, while that for Nitzschia spp. also increased to 13.1 (Tables 1, 2). The differences for the OD680 of three species of Navicula reached up to 2.8–4.0-fold, and those for two species of Nitzschia merely reached 1.2–2.5-fold on Day 10 (Figures 4A–D). In particular, Nitzschia-2 exhibited no significantly promoted growth when cultured in the four optimized f/2 media for 10 days (Figure 4E). In addition, the maximum specific growth rates, μmax, of Navicula spp. (at Day 6 or 8) occurred later than those of Nitzschia spp. (at Day 2) (Figure 5). However, a 2- or 3-fold increase was found in μmax of three species of Navicula, and the increase in μmax in two species of Nitzschia was less than 1.5-fold under the four optimized f/2 media (Figure 5). Moreover, the μmax of Nitzschia-2 was notably higher (p < 0.05) than that of the other four epiphytic diatoms in either f/2 or the other four optimized f/2 media (Figure S11). In terms of μmax, three species of Navicula, Navicula-1, and Navicula-2 were found to be more prominent (p < 0.05) than Navicula-3 (Figure S11).
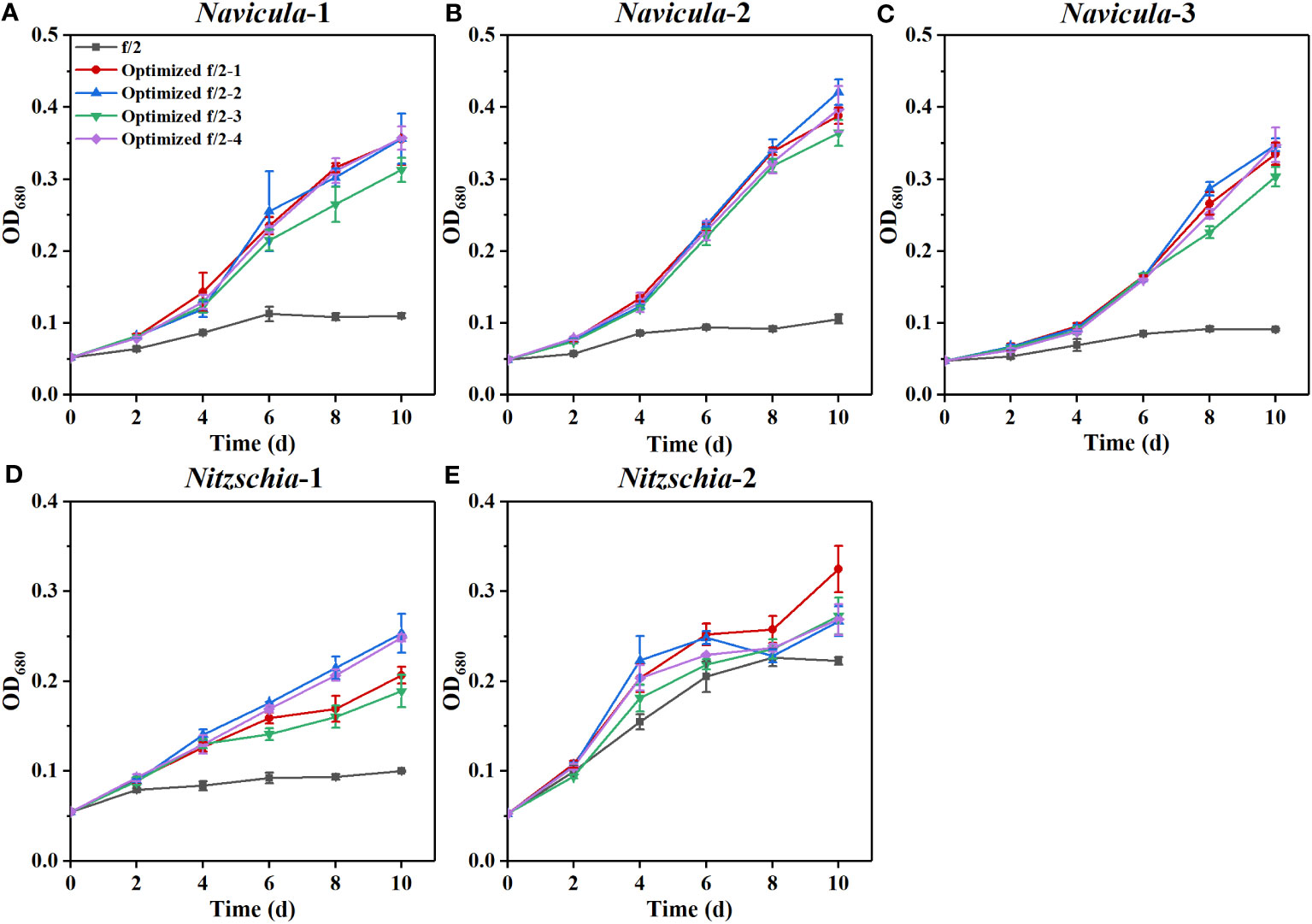
Figure 4 Effects of four optimized f/2 media on growth of five marine epiphytic diatoms. The components of the four optimized f/2 media for Navicula spp. (A–C) and Nitzschia spp. (D, E) are listed in Tables 1, 2, respectively. Values are means of three biological replicates ± SD (n = 3).
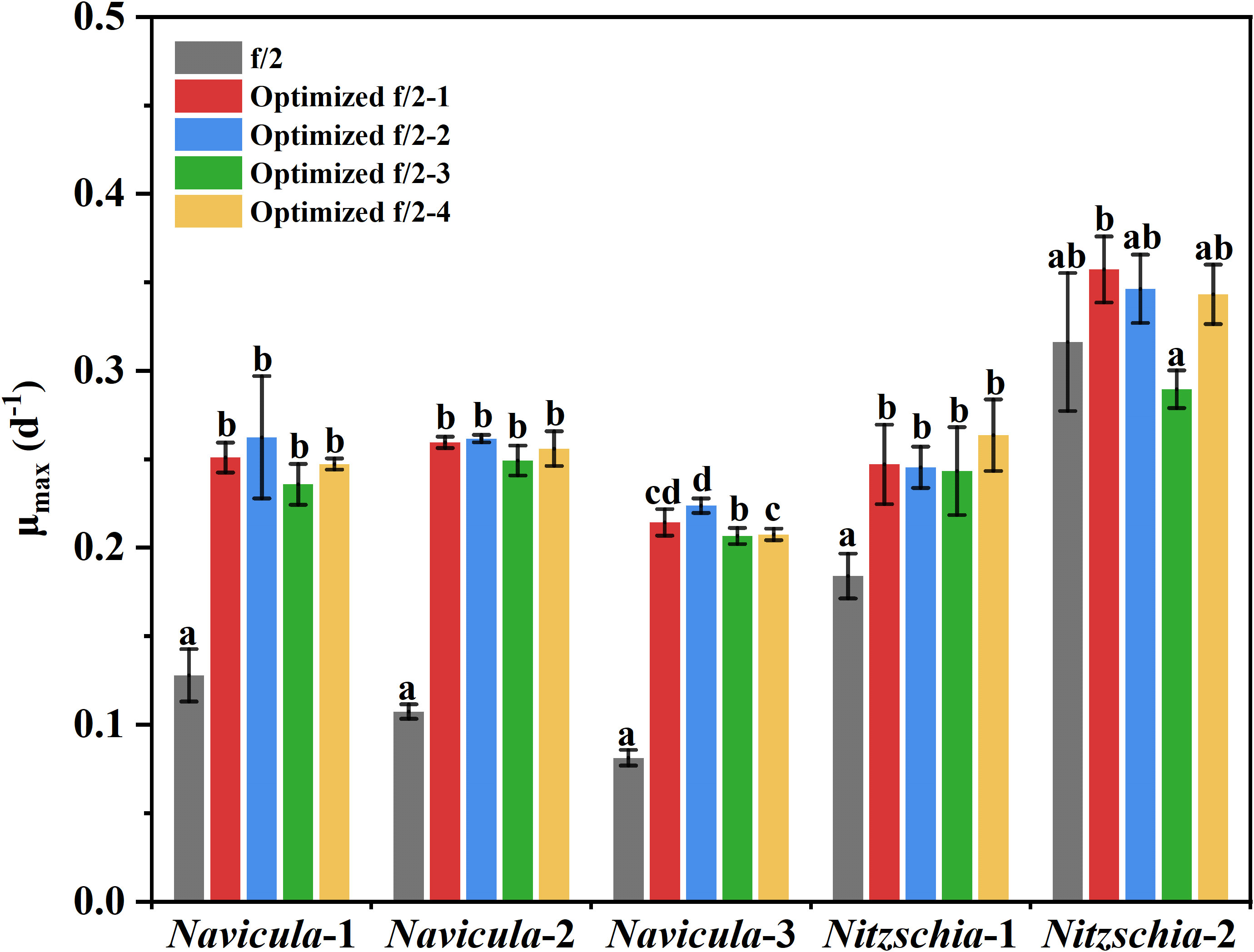
Figure 5 The maximum specific growth rates of five epiphytic diatoms in four optimized f/2 media. The μmax indicates the maximum specific growth rates of certain diatoms during certain culture days. The maximum specific growth rates of Navicula-1, Navicula-2, Navicula-3, Nitzschia-1, and Nitzschia-2 occurred on Days 6, 6, 8, 2, and 2, respectively, when they were cultured with f/2 or optimized f/2 media. The different letters labeled for the same diatom indicate the statistically significant difference by Tukey’s honestly significant difference (HSD) test. The components of the four optimized f/2 media for Navicula spp. and Nitzschia spp. are listed in Tables 1 , 2, respectively. Values are means of three biological replicates ± SD (n = 3).
Given that the optimization growth tests were conducted in 10-mL tubes, and the diatoms presented notably promoted growth rates (p < 0.05) at different levels, the optimized cultivation was then expanded to 5-L flasks for further testing the rapid growth of five diatoms. Then, the respective “optimized f/2-2” medium for Navicula spp. and Nitzschia spp. (Tables 1, 2) was chosen to assess the enlarged cultures based on the absolute growth rates of five diatoms (Figure 5). The results demonstrated that the biomass productivities of five diatoms under the optimized f/2 media were all significantly improved (p < 0.05), i.e., 6–13-fold of those under f/2 medium during 12 days of optimized cultures (Table 4). Moreover, the biomass productivities for three species of Navicula were found to be approximately 1.5-fold of those of two species of Nitzschia (Table 4).
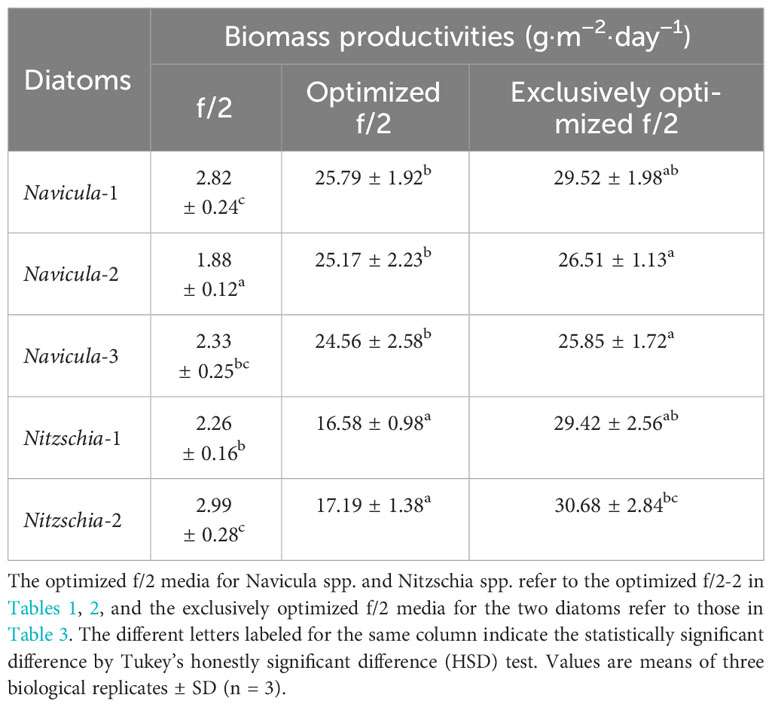
Table 4 The biomass productivities of five marine epiphytic diatoms over 12 days of enlarged cultures.
The results revealed that all the growth performances of five diatoms cultured with the exclusively optimized f/2 medium under three light intensities were much better than those cultured with the f/2 medium (Figures 6; S12). The N:P mass ratios in the exclusively optimized f/2 media for Navicula spp. and Nitzschia spp. were 2.4- and 1.2-fold of those in the f/2 medium, respectively (Table 3). Different light intensities generated no obvious impacts on the growth of the f/2 medium-cultured diatoms, except for Nitzschia-2. The growth of Navicula-2 was found to be significantly inhibited (p < 0.05) in the exclusively optimized f/2 medium when the illumination was increased to 350 μmol·m−2·s−1, while that of Navicula-1 and Navicula-3 was not prominently affected (Figures 6A–C, S12A–C, S13). Differently from Navicula spp., two species of Nitzschia both presented relatively good growth performance under the high light conditions when cultured with the exclusively optimized f/2 media (Figures 6D, E, S12D, E, S13).
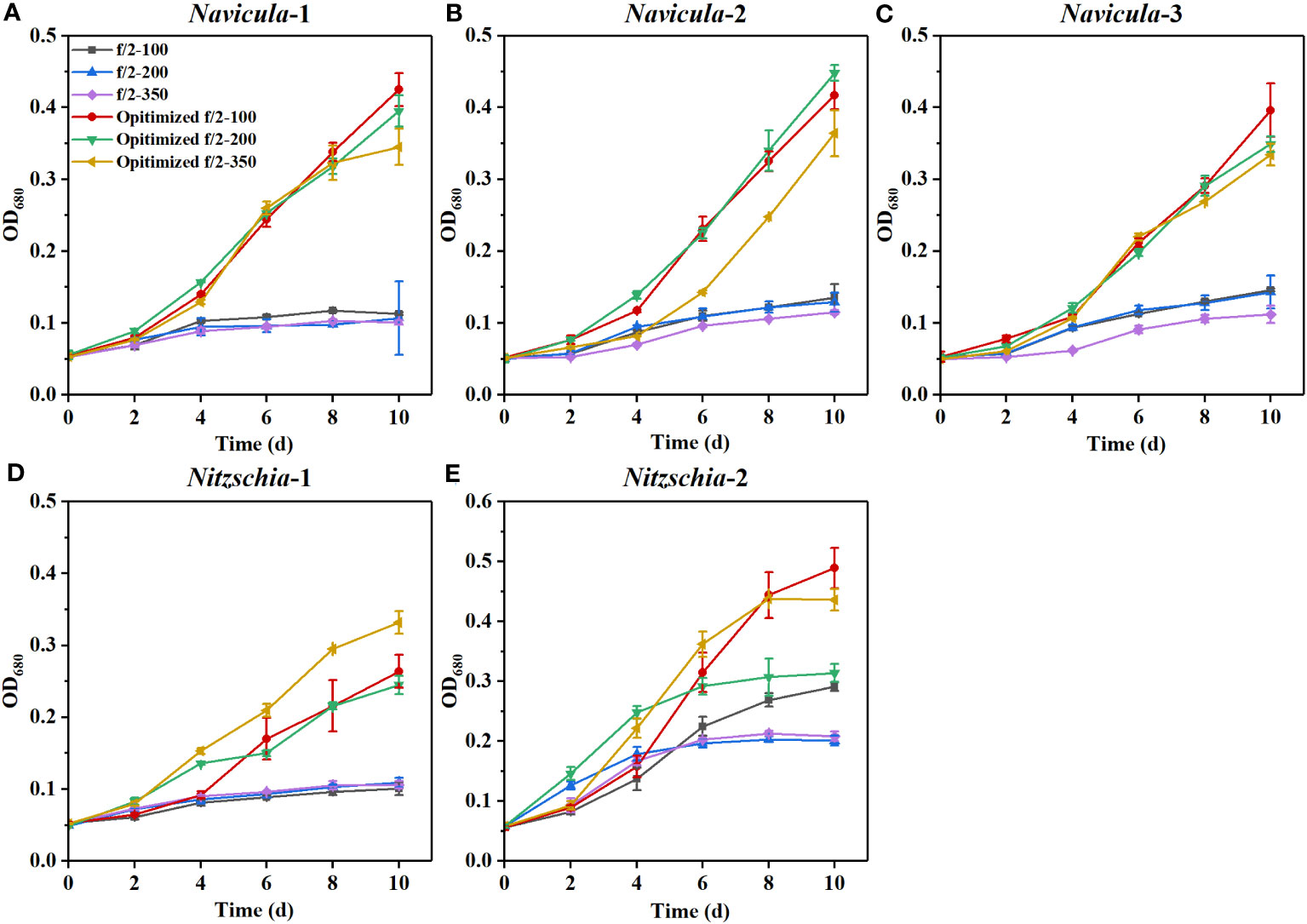
Figure 6 Effects of the exclusively optimized f/2 media on the growth of five marine epiphytic diatoms under three light intensities. The legends of “optimized f/2” denote the exclusively optimized f/2 media for Navicula spp. (A–C) and Nitzschia spp. (D, E), which are listed in Table 3. The legends of 100, 200, and 350 behind f/2 refer to the light intensities for optimization tests, i.e., 100, 200, and 350 μmol·m−2·s−1, respectively. Values are means of three biological replicates ± SD (n = 3).
From the view of the maximum specific growth rates, i.e., μmax, the most outstanding diatom turned out to be Nitzschia-2, regardless of whether it was cultured under the high light or in the exclusively optimized medium (Figure 7). The μmax of Nitzschia-2 was 1.3-2.7 times that of the other four diatoms under three light intensities, though that of the five diatoms was nearly identical in f/2 medium under light of 100 μmol·m−2·s−1. In addition, the μmax of Nitzschia spp. still occurred 2 days earlier than that of Navicula spp. However, the three species of Navicula almost could not be differentiated from each other in terms of the μmax under different culture conditions. However, once the light intensity increased to 350 μmol·m−2·s−1, the μmax of Navicula-2 and Navicula-3 remarkably decreased (p < 0.05) relative to that of Navicula-1 (Figure 7).
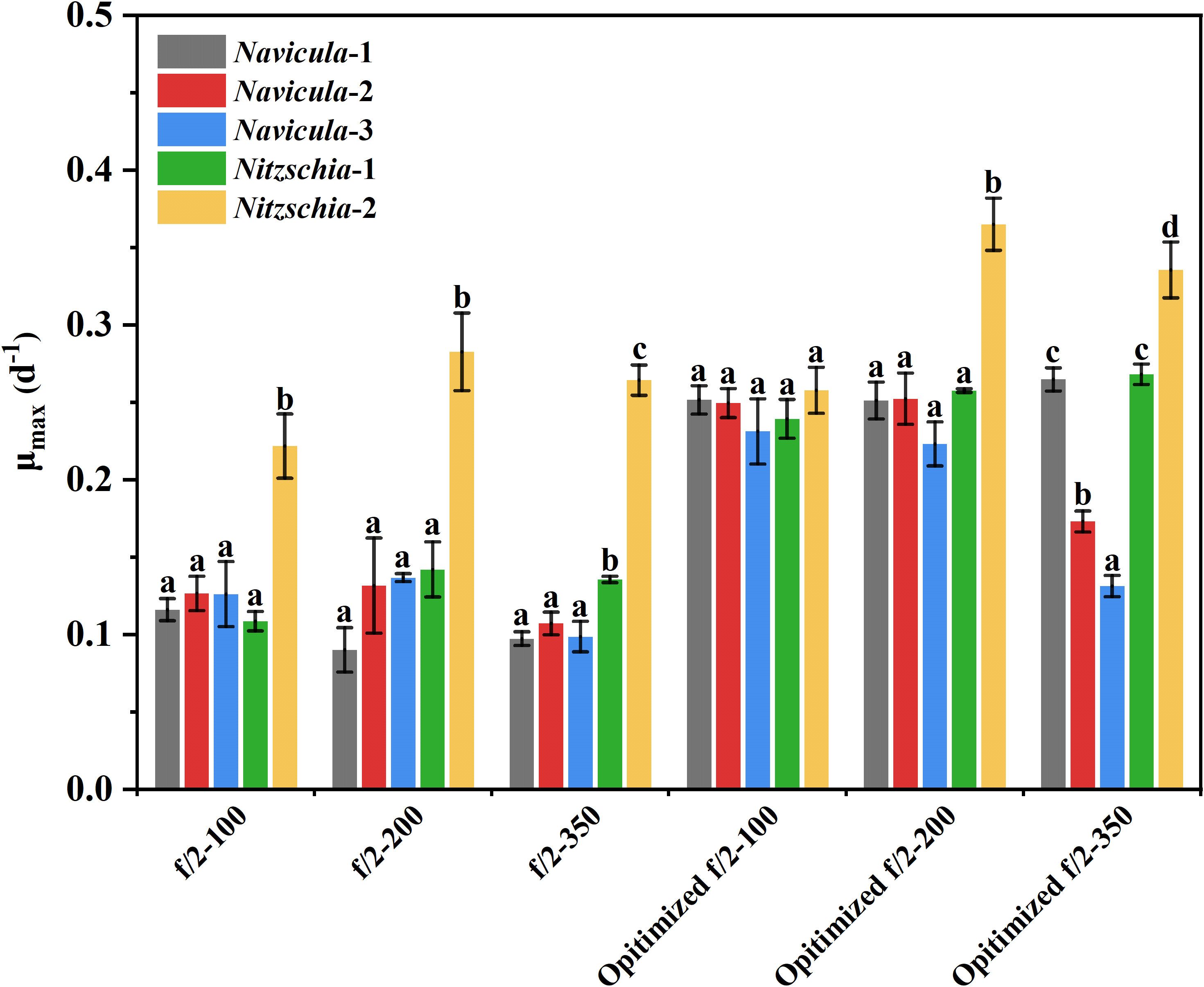
Figure 7 Comparison of the maximum specific growth rates of five epiphytic diatoms in f/2 and the exclusively optimized f/2 media under three light intensities. The μmax indicates the maximum specific growth rates of certain diatoms during certain culture days. The maximum specific growth rates of Navicula-1, Navicula-2, Navicula-3, Nitzschia-1, and Nitzschia-2 occurred on Days 6, 6, 6, 4, and 4, respectively, when they were cultured with f/2 or exclusively optimized f/2 media. The legends of “optimized f/2” denote the exclusively optimized f/2 media for Navicula spp. and Nitzschia spp., which are listed in Table 3. The different letters labeled for the same diatom indicate the statistically significant difference by Tukey’s honestly significant difference (HSD) test. The components of the exclusively optimized f/2 media for Navicula spp. and Nitzschia spp. are listed in Table 3. Values are means of three biological replicates ± SD (n = 3).
The results demonstrated that the biomass productivities of the five diatoms in the respective exclusively optimized f/2 media were all significantly higher (p < 0.05) than those in the optimized f/2-2 media listed in Tables 1, 2. In particular, Navicula-1 and Nitzschia-2 exhibited the highest biomass productivities, i.e., 29.52 ± 1.98 g·m−2·day−1 and 30.68 ± 2.84 g·m−2·day−1, respectively (Table 4). Overall, Navicula-1 and Nitzschia-2 showed greater growth performance among the five marine epiphytic diatoms.
However, the fatty acid profiles for Navicula-1 and Nitzschia-2 cultured with the exclusively optimized f/2 media at an enlarged 4-L scale were detected (Figures 8A; S14). It was interesting to note that the relative abundances of C20:5n3 in Navicula-1 cultured with the exclusively optimized f/2 medium were found to notably rise (p < 0.05) by 24%, and that of C16:1n7 significantly decreased (p < 0.05) by 28% relative to the f/2 medium (Figure 8A), though the PUFAs increased without a remarkable difference (Figure S14). In addition, the exclusively optimized f/2 medium-cultured Nitzschia-2 remained stable in the relative abundances of both PUFAs and C20:5n3, and the relative abundance of C16:1n7 was prominently reduced (p < 0.05) by 31% (Figures 8B, S14).
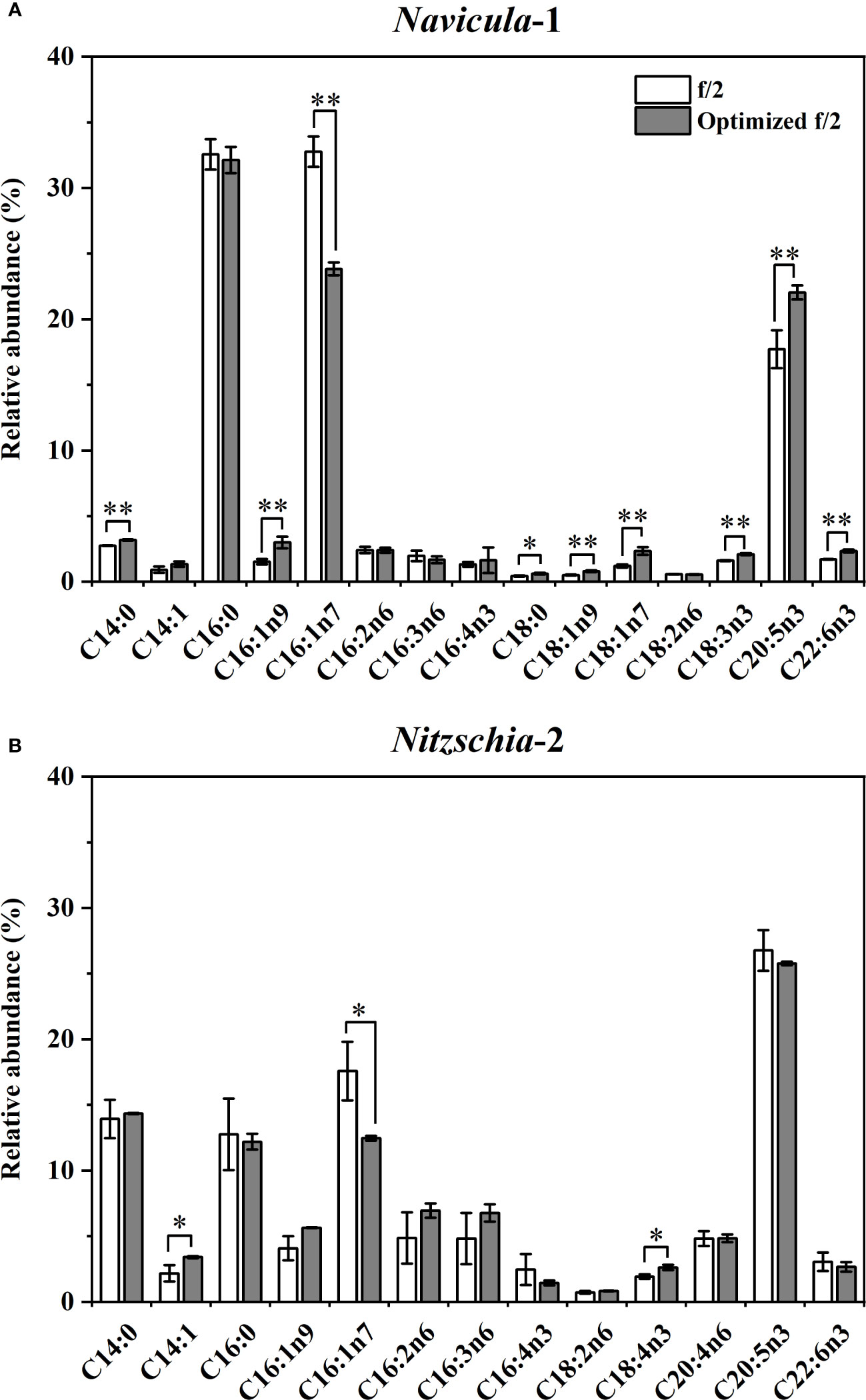
Figure 8 Effects of the exclusively optimized f/2 media on fatty acid profiles of Navicula-1 (A) and Nitzschia-2 (B). The legends of “optimized f/2” denote the exclusively optimized f/2 media for Navicula-1 and Nitzschia-2, which are listed in Table 3. Values are means of three biological replicates ± SD (n = 3). *p < 0.05; **p < 0.01.
4 Discussion
The periphytic diatoms are abundant in nutrient-rich waters and are classified as pollution bioindicators in the diversified environment. This is chiefly because these diatoms can utilize and assimilate large amounts of inorganic and organic nutrients, e.g., nitrogen and phosphorus derived from urban sewage (Kwon et al., 2013). However, periphytic diatoms have also been widely used as the key natural diets for invertebrate marine animals. It is mainly because they are highly rich in polyunsaturated fatty acids, C20:5n3 in particular, and concern the metamorphosis of the invertebrates (Chen, 2007; Sánchez-Saavedra and Núñez-Zarco, 2015; Xie et al., 2017). As such, the isolation and further screening of high-quality periphytic diatoms are necessary to potentially contribute to wastewater treatment and developing aquaculture industries.
Light drives diatom productivity, and diatoms are well-adapted to utilize flexible intensities and endure variable light exposure, dependent on seasons, latitudes, and depth. Many studies have demonstrated that the growth rates and photosynthesis in various phytoplankton species can be affected by illumination conditions (van Leeuwe et al., 2005; van de Poll et al., 2007). In this study, the enlarged cultures of the five isolated diatoms were performed in 5-L flasks under natural light cycles (approximately 10 h of light duration). The light intensity illuminated on the flasks was often up to nearly 350 μmol·m−2·s−1, especially at noon. Even so, these diatoms mostly grew vibrantly. Although the diatoms generally require not too high light intensity, the isolated epiphytic diatoms in this study might indeed be able to live under high light intensity, e.g., 350 μmol·m−2·s−1. This is probably because these isolated indigenous diatoms distributed on the intertidal macroalgae seem to be capable of enduring high light intensity. Thus, three light intensities, including 100 μmol·m−2·s−1, 200 μmol·m−2·s−1, and 350 μmol·m−2·s−1, were set to test the tolerance and adaption of the five isolated epiphytic diatoms to high irradiance. However, five strains of diatoms mostly exhibited no significantly promoted growth rates, and even presented inhibited growth rates (Figures 6, 7), when treated with high light intensities (from 100 μmol·m−2·s−1 to 350 μmol·m−2·s−1) under either f/2 or the exclusively optimized f/2 media. It has been shown that certain diatom species in adaption to high light intensities present high growth rates, high photosynthetic activity, high light saturation intensities, and competent responses to photoinhibition under different high irradiance levels (Benjamin et al., 2013; Kvernvik et al., 2020). Given that the five strains of diatoms in this work were isolated from the epiphytes on the macroalgae in the intertidal zone, the habitats that these diatoms inhabit are undergoing variable irradiations, especially in the summer high-temperature extreme days. Thus, they could likely endure high levels of irradiation and have higher biomass productivity under indoor natural light illumination.
In this study, we selected four macroelements (nitrogen, phosphorus, iron, and silicon), five trace metal elements (Zn, Cu, Mn, Mo, and Co), and three vitamins (VB1, VB12, and VH) mainly because the basal medium for culturing marine diatoms is usually f/2 medium, and the above 12 elements comprise the whole components. In addition, the pre-cultured epiphytic diatom biofilms in f/2 medium gradually turned greenish within 7 days. In this case, the diatom cells likely require higher levels of nutrients in this medium; thus, we designed single-factor as well as orthogonal tests for each element in our preliminary experiments (Yang et al., 2014, Figures S1-S10, Tables S1-18). Based on these tests, we further designed four optimized media for Navicula spp. and Nitzschia spp. (Tables 1, 2). Our results demonstrated that the isolated five strains of epiphytic diatoms were all well-adapted to high concentrations of macronutrients, including nitrogen, phosphorus, iron, and silicon. It was worth noting that the 12-day enlarged cultures for three species of Navicula in the optimized f/2 media all gradually changed from dark brown to a kind of yellow-green. It was also consistent with the change in colors of the chloroplasts under the light microscope. Hence, we assumed that the macroelement, i.e., nitrogen, was likely insufficient for Navicula spp. Additionally, when high levels of silicon stocks in optimized f/2 media were added into the sterile seawater, a few white precipitates occurred instantly, and the harvested diatom pellets were also covered with a thin layer of white precipitates. Thus, the silicon in both the optimized f/2 media for these diatoms was presumed to be surplus. Finally, the optimized f/2-2 media for Navicula spp. and Nitzschia spp. in Tables 1, 2 were further modified. The nitrogen levels for Navicula spp. were elevated, and the silicon levels for the five diatoms were all reduced (Table 3). In addition, in order to distinguish the differences between the nutrient needs of Navicula spp. and Nitzschia spp., we fine-tuned the concentrations of iron for the two genera to the same level. The two further modified media were called the exclusively optimized media for Navicula spp. and Nitzschia spp. (Table 3). Specifically, the concentrations of nitrogen, phosphorus, and iron in the exclusively optimized f/2 media for Navicula spp. and Nitzschia spp. were 2–6-fold of those in the f/2 medium. However, the concentrations of silicon for the two genera of diatoms were up to 15- and 10-fold of those in the f/2 medium (Table 3). Apart from the equivalent concentrations of phosphorus and iron for the two genera of diatoms, Navicula spp. preferred much higher levels of nitrogen (74.16 to 34.51 mg/L) and silicon (37.08 to 23.01 mg/L) relative to Nitzschia spp. in the exclusively optimized f/2 media. Hence, Navicula spp. seemed to harbor a higher capability to utilize and assimilate inorganic nitrogen and silicon than Nitzschia spp., which will be focused on and further explored in our future study. At the same time, the biomass productivities of these five diatoms in f/2 medium were found to increase by 10–14-fold in the exclusively optimized f/2 media (Table 4). Moreover, the biomass productivities of the three species of Navicula in the optimized f/2-2 medium notably rose by 5%–14% compared with those in the exclusively optimized f/2 medium, while those of the two species of Nitzschia both significantly rose by more than 77% (Table 4). These results demonstrated that the epiphytic diatoms were indeed more capable of recruiting and storing inorganic nutrients, nitrogen, and silicon in particular.
Kwon et al. (2013) investigated the uptake rates of nitrate and phosphate by four periphytic diatoms, including Achnanthes sp., Amphora sp., Navicula sp., and Nitzschia sp. However, Nitzschia sp. exhibited the highest specific uptake rates under multiple wavelengths and had been considered a useful species for phytoremediation. In this work, the selective Navicula-1 and Nitzschia-2 had outstanding growth traits and improved fatty acid profiles under high concentrations of nitrogen. They could also be potentially used for remediating nutrient-rich wastewater. Furthermore, the silicified diatoms require large amounts of silicon for growth together with nitrogen, phosphorus, and carbon, thus connecting the biogeochemical cycles of these elements. Understanding the silicon cycle is essential for deciphering the connected function of marine food webs, biogeochemical cycles, and the biological carbon pump (Tréguer et al., 2021). The fast-growing Navicula-1 and Nitzschia-2 in this work turned out to be highly adapted to silicon-abundant (34.51 mg/L and 23.01 mg/L) waters, and they were very likely to become favorable candidates for future study of the silicon cycle.
A previous study showed that silicon could become limiting to diatoms after nitrogen and phosphorus enrichment (Carrick, 1988). However, in this study, the silicon concentrations in the exclusively optimized f/2 media for Navicula spp. and Nitzschia spp. were 15- and 10-fold, respectively, of those in the control f/2 medium. In addition, nitrogen and phosphorus concentrations in the exclusively optimized f/2 media Navicula spp. and Nitzschia spp. were just 2–6-fold of those in the control f/2 medium. In general, the Redfield C:N:P ratio of 106:16:1 has been widely considered as a mean composition of the three macroelements in phytoplankton. Nutrient limitation could result in the stoichiometric ratio further away from the Redfield ratio (Spilling et al., 2015). In this case, the limiting nutrients are at the lowest level, and the non-limiting nutrients are utilized as well as over-stored. In this work, the screened optimal N:P mass ratios for Navicula spp. and Nitzschia spp. in the exclusively optimized f/2 media turned out to be 26.1:1 and 13.1:1, respectively, and they were both higher than those in the control f/2 medium (10.8). In particular, the three strains of Navicula were found to prefer more levels of nitrogen than the two strains of Nitzschia.
Vitamins and trace metals play essential roles in the growth regulation of marine microalgae. Many microalgal species require one or more vitamins as well as trace metals for growth, implying that these nutrients likely serve as limiting resources (Peperzak et al., 2000). It is necessary to make clear the vitamin and trace metal demands of microalgae greatly concerning the primary production of seas and oceans. In the f/2 medium, the above-mentioned nutrients are all included. The vitamins consist of VB1, VB12, and VH, and trace metals consist of Zn, Cu, Mn, Mo, and Co. In this study, the growth of five strains of diatoms was found to be not prominently affected by all these nutrients based on the single-factor tests as well as the orthogonal tests (Tables S1-S18, Figures S1-S10). Hence, the concentrations of these seven nutrients, i.e., 0.5–100 μg/L for vitamins and 2.54–49.44 μg/L for trace metals, in the f/2 medium appeared to be sufficient for favorable growth of the isolated diatoms in this work. Furthermore, Navicula spp. and Nitzschia spp. were verified to show outstanding growth rates and biomass productivity just in the exclusively optimized f/2 media, where the concentration of each micronutrient was the same as that in the f/2 medium. It was also indicated that the nutritional factors more important for the growth of marine epiphytic diatoms were nitrogen, phosphorus, iron, and silicon, compared with the vitamins and trace metals. Overall, in this study, the growth of the epiphytic diatoms was shown to be greatly affected by nitrogen, phosphorus, iron, and silicon, while the vitamins, trace metals, and the light intensity could just generate minimal impacts on the growth of these diatoms. Indeed, the combined higher concentrations of the above macroelements produced more than 10-fold biomass productivity for either Navicula spp. or Nitzschia spp. (Table 4), indicating the great potential of these diatoms in nutrient-rich wastewater treatment.
However, the fatty acid profiles of microalgae determine their application potential. If the algal cells are abundant in saturated or monounsaturated fatty acids, they are more suitable to become potential raw materials for biofuels (Hu et al., 2008). If they are abundant in PUFAs, they are more appropriate to serve as functional lipids that are beneficial for human health. In common, the fatty acid profiles of diatoms can alter in response to variable nutrient conditions, including nitrogen, phosphorus, iron, and silicon (Jeffryes et al., 2013; Abida et al., 2015; Yang et al., 2017; Cointet et al., 2019; Zhang et al., 2023). In particular, the saturation degree of fatty acids in nutrient-depleted or nutrient-limited algal cells significantly increased, and the lipids could also be hyper-accumulated (Zienkiewicz et al., 2016). However, Feng et al. (2011) found that Isochrysis zhangjiangensis (Chrysophyta) accumulated the highest amount of lipids (53%) when cultured in an extremely high nitrate concentration (100-fold of those in f/2 medium), though the growth of algal cells was suppressed. Nitrogen and phosphorus could affect and even take part in lipid metabolism in microalgae. In diatoms, nitrogen-containing lipids include phosphatidylcholine (PC), phosphatidylethanolamine (PE), and diaclyglycerylhydroxymethyl-N,N,N-trimethyl-beta-alanine (DGTA); all the phospholipids contain phosphorus; thus, phosphorus is closely related to lipid metabolism (Abida et al., 2015). In addition, iron has been considered one of the most essential trace mineral elements for organisms. It plays crucial roles in nitrogen utilization, chlorophyll biosynthesis, and various biochemical metabolisms in microalgae. As such, iron is a major factor affecting the growth and reproduction of algal cells (Sutak et al., 2020; Nam et al., 2021). In particular, the iron source FeCl3 added in the culture medium could increase the total lipid content and the PUFA amounts in diatoms, especially C20:5n3 and C22:6n3. Moreover, Fe3+ could also affect the expression of crucial genes involved in lipid biosynthesis in diatoms, thus altering lipid accumulation (Govindan et al., 2021). It is commonly accepted that silicon metabolism greatly concerns the frustule formation and influences the cell division in diatoms (Martin-Jezequel et al., 2000). Jeffryes et al. (2013) adopted a bioprocess strategy to enhance lipid production in the diatom Cyclotella sp. via controlled silicon addition. Thus, the fatty acid profiles and the lipid content in diatoms could be regulated by the four macroelements, including nitrogen, phosphorus, iron, and silicon. Previous studies demonstrated that nutrient limitation could dramatically alter the fatty acid composition of the diatoms, giving rise to a decline in the number of long-chain PUFAs (Breteler et al., 2005). Furthermore, the diatom feeder, e.g., the copepods, could not reach maturity (Breteler et al., 2005). In contrast, nutrient enrichment could also change the fatty acid profiles of the epiphytic diatoms, but the relative abundances of PUFAs, C20:5n3, for example, presented an increased trend in this work, which was contrary to the case for nutrient limitation (Breteler et al., 2005). Hence, either eutrophy or nutrient limitation could affect lipid production in diatoms. We explored the impacts of the optimized f/2 media on the lipid production of these indigenous diatoms. It is mainly because the epiphytic diatoms are potentially applicable as natural diets to feed the echinoderm, e.g., sea cucumbers, in the subsequent work. In addition, the fatty acid components, C20:5n3 in particular, have been largely reported to be crucial for metamorphosis development and robust growth of marine invertebrates (Chen, 2007; Sánchez-Saavedra and Núñez-Zarco, 2015; Xie et al., 2017). The fast-growing Navicula-1 in this work was rich in saturated and monounsaturated fatty acids (69%–74%), while the relative amounts of PUFAs in Nitzschia-2 accounted for approximately 50%. In spite of this, the relative amounts of the main polyunsaturated C20:5n3 for both diatoms notably improved or remained stable when these cells were cultured in the exclusively optimized f/2 media. The result was also consistent with the above-mentioned previous studies (Jeffryes et al., 2013; Abida et al., 2015; Zhang et al., 2023).
In addition, the previous study showed that Navicula sp. as a fresh diet could not only enhance growth performance but also improve digestive enzyme activity and immunity in early growth of the sea cucumber, Apostichopus japonicus (Xie et al., 2017). Moreover, another diatom, i.e., Cylindrotheca fusiformis, was also reported to be a good feed ingredient for juvenile A. japonicus (Shi et al., 2013a, Shi et al., 2013b). The appropriate addition of C. fusiformis into the feed can enhance the growth of sea cucumbers in farming ponds (Li et al., 2015). In this case, the indigenous epiphytic diatoms isolated in our study were very likely to present growth-promoting effects and essential nutrient supply; thus, they have the potential to become the natural diets for the echinoderms. Overall, the screened Navicula-1 and Nitzschia-2 presented good growth traits and optimized fatty acid compositions at high levels of not only nitrogen (3–6-fold of those in f/2 medium) but also phosphorus (2-fold of those in f/2 medium), iron (10–15-fold of those in f/2 medium), and silicon (5-fold of those in f/2 medium) (Table 3) in this study. The two diatoms are very likely to be applied for remediating nutrient-rich wastewater and further exploitation of fatty acid-based bioproducts.
5 Conclusion
In this study, three strains of Navicula and two strains of Nitzschia were isolated from macroalgal epiphytes. Navicula-1 and Nitzschia-2 were screened out due to their outstanding growth traits under the optimized media with high levels of nitrogen, phosphorus, iron, and silicon. Their biomass productivities at a 4-L enlarged scale in the exclusively optimized f/2 media both increased by 10-fold relative to the f/2 medium. Moreover, the fatty acid profiles of the two diatoms were notably improved, and their relative amounts of PUFAs, especially C20:5n3, were significantly elevated or remained stable in the exclusively optimized f/2 media. Thus, the two screened diatoms have the potential to become quality diets for marine aquaculture and are ideal candidates for effective nutrient removal.
Data availability statement
The original contributions presented in the study are included in the article/Supplementary Material. Further inquiries can be directed to the corresponding authors.
Author contributions
MY: Conceptualization, Data curation, Funding acquisition, Investigation, Methodology, Resources, Writing – original draft, Writing – review & editing. X-YX: Data curation, Investigation, Writing – original draft, Writing – review & editing. H-WH: Investigation, Writing – original draft. W-DZ: Supervision, Writing – review & editing. J-YM: Data curation, Writing – original draft. H-PL: Data curation, Writing – original draft. Q-ZW: Resources, Supervision, Writing – review & editing. XX: Conceptualization, Methodology, Resources, Writing – original draft, Writing – review & editing. ZG: Conceptualization, Funding acquisition, Supervision, Writing – review & editing.
Funding
The author(s) declare financial support was received for the research, authorship, and/or publication of this article. This work was supported by the Basic Scientific Research Project of the Education Department of Liaoning Province (LJKQZ20222365), Ph.D. Initiation Research Project of Liaoning Normal University (2022BSL009), and Petroleum Hydrocarbon Treatment Technology using Microorganisms (H2022011).
Conflict of interest
The authors declare that the research was conducted in the absence of any commercial or financial relationships that could be construed as a potential conflict of interest.
Publisher’s note
All claims expressed in this article are solely those of the authors and do not necessarily represent those of their affiliated organizations, or those of the publisher, the editors and the reviewers. Any product that may be evaluated in this article, or claim that may be made by its manufacturer, is not guaranteed or endorsed by the publisher.
Supplementary material
The Supplementary Material for this article can be found online at: https://www.frontiersin.org/articles/10.3389/fmars.2023.1292713/full#supplementary-material
References
Abida H., Dolch L. J., Mei C., Villanova V., Conte M., Block M. A., et al. (2015). Membrane glycerolipid remodeling triggered by nitrogen and phosphorus starvation in phaeodactylum tricornutum. Plant Physiol. 167, 118–136. doi: 10.1104/pp.114.252395
Almeida O. U. H., Beltrones D. A. S. (2008). Variations in the structure of epiphytic diatom assemblages in subtropical macroalgae. Hidrobiologica 18, 51–61.
Benjamin S. C., Anne J., Torsten J., Wolfram W., Maria M., Christian W. (2013). Blue light is essential for high light acclimation and photoprotection in the diatom Phaeodactylum tricornutum. J. Exp. Bot. 64, 483–493. doi: 10.1093/jxb/ers340
Breteler W. C. M. K., Schogt N., Rampen S. (2005). Effect of diatom nutrient limitation on copepod development: role of essential lipids. Mar. Ecol. Prog. Ser. 291, 125–133. doi: 10.3354/meps291125
Burfeid-Castellanos A. M., Martín-Martín R. P., Kloster M., Angulo-Preckler C., Avila C., Beszteri B. (2021). Epiphytic diatom community structure and richness is determined by macroalgal host and location in the South Shetland Islands (Antarctica). PloS One 16, e0250629. doi: 10.1371/journal.pone.0250629
Carrick H. J. (1988). and lowe, RResponse of Lake Michigan benthic algae to in situ enrichment with Si, N and P. L.Can. J. Fish. Aquat. Sci. 45, 271–279. doi: 10.1139/f88-032
Chen Y. (2007). Immobilization of twelve benthic diatom species for long-term storage and as feed for post-larval abalone Haliotis diversicolor. Aquaculture 263, 97–106. doi: 10.1016/j.aquaculture.2006.12.008
Cointet E., Wielgosz-Collin G., Bougaran G., Rabesaotra V., Gonçalves O., Méléder V. (2019). Effects of light and nitrogen availability on photosynthetic efficiency and fatty acid content of three original benthic diatom strains. PloS One 14, e0224701. doi: 10.1371/journal.pone.0224701
Courtois de Viçose G., Porta A., Viera M. P., Fernández-Palacios H. (2012). and izquierdo, M Effects of density on growth rates of four benthic diatoms and variations in biochemical composition associated with growth phase. S.J. Appl. Phycol. 24, 1427–1437. doi: 10.1007/s10811-012-9799-z
Desrosiers C., Leflaive J., Eulin A., Ten-Hage L. (2013). Bioindicators in marine waters: Benthic diatoms as a tool to assess water quality from eutrophic to oligotrophic coastal ecosystems. Ecol. Indic. 32, 25–34. doi: 10.1016/j.ecolind.2013.02.021
Feng D., Chen Z., Xue S., Zhang W. (2011). Increased lipid production of the marine oleaginous microalgae Isochrysis zhangjiangensis (Chrysophyta) by nitrogen supplement. Bioresour. Technol. 102, 6710–6716. doi: 10.1016/j.biortech.2011.04.006
Folch J., Lees M., Sloane-Stanley G. H. (1957). A simple method for the isolation and purification of total lipids from animal tissues. J. Biol. Chem. 226, 497–509. doi: 10.1016/S0021-9258(18)64849-5
Gomes A., Loureno S., Santos P. M., Raposo A., Pombo A. (2021). Effects of single and mixed-diatom diets on growth, condition, and survival of larvae of the sea urchin Paracentrotus lividus (Lamarc ). Aquacult. Int. 29, 1069–1090. doi: 10.1007/s10499-021-00676-8
Govindan N., Maniam G. P., Ab. Rahim M. H., Sulaiman A. Z., Ajit A., Chatsungnoen T. (2021). Production of renewable lipids by the diatom Amphora copulata. Fermentation 7, 37. doi: 10.3390/fermentation7010037
Han D., Sun Y., Cong J., Gao C., Wang G. (2023). Ecological distribution of modern diatom in peatlands in the northern Greater Khingan Mountains and its environmental implications. Environ. Sci. pollut. Res. 30, 36607–36618. doi: 10.1007/s11356-022-24910-9
Harrison P. J., Berges J. A. (2005). “Chapter 3 – marine culture media,” in Algal culturing techniques, 1st Edn. Ed. Andersen R. A. (Burlington: Elsevier Academic Press), 21–33.
Hu Q., Sommerfeld M., Jarvis E., Ghirardi M., Posewitz M., Seibert M., et al. (2008). Microalgal triacylglycerols as feedstocks for biofuel production: perspectives and advances. Plant J. 54, 621–639. doi: 10.1111/j.1365-313X.2008.03492.x
Jeffryes C., Rosenberger J., Rorrer G. L. (2013). Fed-batch cultivation and bioprocess modeling of Cyclotella sp. for enhanced fatty acid production by controlled silicon limitation. Algal Res. 2, 16–27. doi: 10.1016/j.algal.2012.11.002
Kireta A. R., Reavie E. D., Sgro G. V., Angradi T. R., Bolgrien D. W., Hill B. H., et al. (2012). Planktonic and periphytic diatoms as indicators of stress on great rivers of the United States: Testing water quality and disturbance models. Ecol. Indic. 13, 222–231. doi: 10.1016/j.ecolind.2011.06.006
Kvernvik A. C., Rokitta S. D., Leu E., Harms L., Gabrielsen T. M., Rost B., et al. (2020). Higher sensitivity towards light stress and ocean acidification in an Arctic sea-ice-associated diatom compared to a pelagic diatom. New Phytol. 226, 1708–1724. doi: 10.1111/nph.16501
Kwon H. K., Oh S. J., Yang H. S. (2013). Growth and uptake kinetics of nitrate and phosphate by benthic microalgae for phytoremediation of eutrophic coastal sediments. Bioresour. Technol. 129, 387–395. doi: 10.1016/j.biortech.2012.11.078
Li J., Dong S., Tian X., Shi C., Wang F., Gao Q., et al. (2015). Effects of the diatom Cylindrotheca fusiformis on the growth of the sea cucumber Apostichopus japonicus and water quality in ponds. Aquac. Int. 23, 955–965. doi: 10.1007/s10499-014-9854-7
Martin-Jezequel V., Hildebrand M., Brzezinski M. A. (2000). Silicon metabolism in diatoms: implications for growth. J. Phycol. 36, 821–840. doi: 10.1046/j.1529-8817.2000.00019.x
Meerssche E. V., Pinckney J. L. (2019). Nutrient loading impacts on estuarine phytoplankton size and community composition: Community-based indicators of eutrophication. Estuaries Coasts. 42, 504–512. doi: 10.1007/s12237-018-0470-z
Meng F., Xi L., Liu D., Huang W., Lei Z., Zhang Z., et al. (2019). Effects of light intensity on oxygen distribution, lipid production and biological community of algal-bacterial granules in photo-sequencing batch reactors. Bioresour. Technol. 272, 473–481. doi: 10.1016/j.biortech.2018.10.059
Mercado J. M., Sánchez-Saavedra. M., del P., Correa-Reyes G., Lubián L., Montero O., et al. (2004). Blue light effect on growth, light absorption characteristics and photosynthesis of five benthic diatom strains. Aquat. Bot. 78, 265–277. doi: 10.1016/j.aquabot.2003.11.004
Metcalfe L. D., Schmitz A. A. (1961). The rapid preparation of fatty acid esters for gas chromatographic analysis. Anal. Chem. 33, 363–364. doi: 10.1021/ac60171a016
Nam H. I., Shahzad Z., Dorone Y., Clowez S., Zhao K., Bouain N. (2021). Interdependent iron and phosphorus availability controls photosynthesis through retrograde signaling. Nat. Commun. 12, 7211. doi: 10.1038/s41467-021-27548-2
Otim O., Juma T., Savinelli R. (2018). The effect of a massive wastewater discharge on nearshore ocean chemistry. Environ. Monit. Assess. 190, 180. doi: 10.1007/s10661-018-6530-9
Pandey L. K., Sharma Y. C., Park J., Choi S., Han T. (2018). Evaluating features of periphytic diatom communities as biomonitoring tools in fresh, brackish and marine waters. Aquat. Toxicol. 194, 67–77. doi: 10.1016/j.aquatox.2017.11.003
Peperzak L., Gieskes W. W. C., Duin R., Colijn F. (2000). The vitamin B requirement of Phaeocystis globosa (Prymnesiophyceae). J. Plankton Res. 22, 1529–1537. doi: 10.1093/plankt/22.8.1529
Sánchez-Saavedra M. D. P., Núñez-Zarco E. (2015). Growth of red abalone, Haliotis rufescens, fed cold-stored benthic diatoms. J. World Aquacult. Soc 46, 210–218. doi: 10.1111/jwas.12182
Scholz B., Liebezeit G. (2013). Biochemical characterisation and fatty acid profiles of 25 benthic marine diatoms isolated from the Solthörn tidal flat (southern North Sea). J. Appl. Phycol. 25, 453–465. doi: 10.1007/s10811-012-9879-0
Shi C., Dong S., Pei S., Wang F., Tian X., Gao Q. (2013a). Effects of diatom concentration in prepared feeds on growth and energy budget of the sea cucumber Apostichopus japonicus (Selenka). Aquac. Res. 46, 609–617. doi: 10.1111/are.12206
Shi C., Dong S., Wang F., Gao Q., Tian X. (2013b). Effects of four fresh microalgae in diet on growth and energy budget of juvenile sea cucumber Apostichopus japonicus (Selenka). Aquaculture 416-417, 296–301. doi: 10.1016/j.aquaculture.2013.09.050
Spilling K., Ylöstalo P., Simis S., Seppälä J. (2015). Interaction effects of light, temperature and nutrient limitations (N, P and Si) on growth, stoichiometry and photosynthetic parameters of the cold-water diatom Chaetoceros wighamii. PloS One 10, e0126308. doi: 10.1371/journal.pone.0126308
Sutak R., Camadro J. M., Lesuisse E. (2020). Iron uptake mechanisms in marine phytoplankton. Front. Microbiol. 11. doi: 10.3389/fmicb.2020.566691
Tréguer P. J., Sutton J. N., Brzezinski M., Charette M. A., Devries T., Dutkiewicz S., et al. (2021). Reviews and syntheses: The biogeochemical cycle of silicon in the modern ocean. Biogeosciences 18, 1269–1289. doi: 10.5194/BG-18-1269-2021
van de Poll W. H., Visser R. J. W., Buma A. G. J. (2007). Acclimation to a dynamic irradiance regime changes excessive irradiance sensitivity of Emiliania huxleyi and Thalassiosira weissflogii. Limnol. Oceanogr. 52, 1430–1438. doi: 10.4319/lo.2007.52.4.1430
van Leeuwe M. A., van Sikkelerus B., Gieskes W. W. C., Stefels J. (2005). Taxon-specific differences in photoacclimation to fluctuating irradiance in an Antarctic diatom and a green flagellate. Mari. Ecol. Prog. Ser. 288, 9–19. doi: 10.3354/meps288009
Wang Q., Wang S., Ding M., Li M., Shi R., Cheng A. (1997). Studies on culture conditions of benthic diatoms for feeding abalone I. Effects of temperature and light intensity on growth rate. Chin. J. Oceanol. Limnol. 15, 296–303. doi: 10.1007/BF02850562
Xie X., Zhao W., Yang M., Zhao S., Wei J. (2017). Beneficial effects of benthic diatoms on growth and physiological performance in juvenile sea cucumber Apostichopus japonicus (Selenka). Aquacult. Int. 25, 287–302. doi: 10.1007/s10499-016-0028-7
Yang M., Fan Y., Wu P., Chu Y., Shen P., Xue S., et al. (2017). An extended approach to quantify triacylglycerol in microalgae by characteristic fatty acids. Front. Plant Sci. 8. doi: 10.3389/fpls.2017.01949
Yang M., Zhao W., Xie X. (2014). Effects of nitrogen, phosphorus, iron and silicon on growth of five species of marine benthic diatoms. Acta Ecologica Sinica. 34, 311–319. doi: 10.1016/j.chnaes.2014.10.003
Yin X. W., Min W. W., Lin H. J., Chen W. (2013). Population dynamics, protein content, and lipid composition of Brachionus plicatilis fed artificial macroalgal detritus and Nannochloropsis sp. diets. Aquaculture 380, 62–69. doi: 10.1016/j.aquaculture.2012.11.018
Zhang Z., Peng R., Xia X., Liu P., Chen S., Xia R., et al. (2023). Effects of iron on the growth, chlorophyll a, total lipids, and fatty acids of Chaetoceros lorenzianus. J. Appl. Phycol. 35, 639–647. doi: 10.1007/s10811-023-02912-5
Zhang X., Qi M., Chen L., Wu T., Zhang W., Wang X., et al. (2020). Recent change in nutrient discharge from municipal wastewater in China’s coastal cities and implication for nutrient balance in the nearshore waters. Estuar. Coast. Shelf S. 242, 106856. doi: 10.1016/j.ecss.2020.106856
Keywords: Navicula, Nitzschia, macroelements, biomass productivity, fatty acid profile
Citation: Yang M, Xu X-Y, Hu H-W, Zhang W-D, Ma J-Y, Lei H-P, Wang Q-Z, Xie X and Gong Z (2023) Combined application of nitrogen, phosphorus, iron, and silicon improves growth and fatty acid composition in marine epiphytic diatoms. Front. Mar. Sci. 10:1292713. doi: 10.3389/fmars.2023.1292713
Received: 12 September 2023; Accepted: 28 November 2023;
Published: 14 December 2023.
Edited by:
Melanie Hughes, CONICET Planta Piloto de Ingeniería Química (PLAPIQUI), ArgentinaReviewed by:
Patricia M Valdespino-Castillo, Berkeley Lab (DOE), United StatesRam Kumar, Central University of Bihar, India
Copyright © 2023 Yang, Xu, Hu, Zhang, Ma, Lei, Wang, Xie and Gong. This is an open-access article distributed under the terms of the Creative Commons Attribution License (CC BY). The use, distribution or reproduction in other forums is permitted, provided the original author(s) and the copyright owner(s) are credited and that the original publication in this journal is cited, in accordance with accepted academic practice. No use, distribution or reproduction is permitted which does not comply with these terms.
*Correspondence: Xi Xie, xiexim@163.com; Zheng Gong, gongzheng@lnnu.edu.cn
†These authors have contributed equally to this work