- 1Marine and Coastal Resources Institute, Faculty of Environmental Management, Songkhla, Thailand
- 2Coastal Oceanography and Climate Change Research Center, Faculty of Environmental Management, Prince of Songkla University, Songkhla, Thailand
- 3Center of Excellence in Catalysis for Bioenergy and Renewable Chemicals (CBRC), Faculty of Science, Chulalongkorn University, Bangkok, Thailand
- 4Faculty of Science and Fisheries Technology, Rajamangala University of Technology Srivijava, Trang, Thailand
- 5Faculty of Environmental Management, Prince of Songkla University, Songkhla, Thailand
- 6Health Impact Assessment Research Center, Prince of Songkla University, Songkhla, Thailand
- 7Center of Excellence on Hazardous Substance Management (HSM), Bangkok, Thailand
Heavy metal and microplastic (MP) contamination of aquatic systems is a major environmental issue that affects human health globally. Songkhla lagoon, the largest lagoon in Thailand, also faced with the environmental issues. Here, this study reported the occurrence of heavy metal and MP in 10 sites of sediment in the Songkla lagoon. From the microplastic separation, fibers were found at all stations at 68.24% (15.15 items/g) and fragments were found at 31.76% (7.05 items/g). The highest number of MP particles was found at the area near the mouth of the lagoon (5.4 items/g). The average concentrations (mg/kg) of heavy metal at all sites showed the following trend: Mg (732.54 ± 247.04) > Mn (176.74 ± 83.68) > Zn (29.36 ± 39.47) > Cu (12.31 ± 24.58) > Pb (11.07 ± 7.60) > As (5.64 ± 3.30) > Co (2.90 ± 1.38) > Cd (0.22 ± 0.17). Regarding the overall risk assessment of MPs in lagoon sediment in this study, the risk was categorized as high for the polymer hazard index. The ecological risk index found Cd and As to have high ecological risk. High EF values were observed for As and Cd, which indicated severe enrichment. Based on the Igeo calculation, the majority of sampling stations were unpolluted to moderately contaminated (Pb, Zn, Mg, and Co). Furthermore, there was no significant correlation between MPs and heavy metals, except for Zn (r = 0.697) and Cu (r = 0.61) (both p< 0.05). The results of this study might provide valuable data to develop conservation policies for coastal lagoon areas.
1 Introduction
Contamination of the ocean by plastic is a global concern due to its negative effects on marine biota. Microplastics (MPs), first defined by Thompson et al. (2004), are plastic particles smaller than 5 mm in length. MPs are widespread in the environment and in living organisms (Browne et al., 2008; Claessens et al., 2011). MPs tend to accumulate pollutants such as organic pollutants and heavy metals (Bayo et al., 2018; Liu et al., 2022), mostly on the surface of seawater because of their density compared to water. This circumstance may contribute to the interaction between these two pollutants whereby heavy metal is attached or adsorbed to the MP surface (Goh et al., 2022). Heavy metals can enter the human body through various routes of exposure including the gastrointestinal tract, inhalation, and skin contact (Alia et al., 2020). Sediments in coastal lagoon ecosystems play an important role as a major site source and sink for aquatic organisms and also for chemical substances. Sediments tend to act as reservoirs for heavy metals in marine environments and release more heavy metals into seawater when local environmental conditions change, for instance, due to changes in salinity, pH, and redox potential. Sediment therefore acts as both a sink and a source of trace metals (Rajeshkumar et al., 2018). Sediment-associated metals pose a direct risk to detrital and deposit-feeding benthic organisms and may also represent long-term sources of contamination to higher tropic levels (Mendil and Uluözlü, 2007). The distribution and accumulation of heavy metals is influenced by sediment texture, mineralogical composition, reduction/oxidation state, adsorption and desorption processes, and physical transport (Buccolieri et al., 2006). The changes in sediment oxidation/reduction state and pH influence the solubility of both metals and nutrients (Miao et al., 2006). To evaluate the potential heavy metal concentration of lagoons of the surface sediments is very important since it represents the current situation. However, sediment in lagoons or in mangrove areas also acts as a sink for MPs (Cordova et al., 2021; Pradit et al., 2022). MPs and heavy metals are typically classified as different types of pollutants, and the interconnection between them is poorly understood (Goh et al., 2022). To enhance the properties of plastics during the polymer production process, heavy metals are mainly used as additives for colorants, flame-retardants, fillers, and stabilizers. The toxic component of plastic particles increases when the metal concentrations in plastic particles are higher than those found in the water column and the bioavailability of heavy metals adsorbed to MPs is also at a high level (Holmes et al., 2012). This is likely to affect aquatic animals and finally influence human health.
Coastal lagoons are widespread along oceanic coasts globally. Many coastal lagoons are among the most productive aquatic ecosystems. Lagoons frequently have high nutrient concentrations due to both riverine fertilizer imports and efficient nutrient recycling between the sediments and the water column (Baeyens et al., 2005). Moreover, some lagoons have recently been converted to receiving reservoirs for various by-products of human activities such as urbanization, industrialization, and agriculture. These substances may affect the health of benthic and water column organisms. Both heavy metals and MPs can build up in the environment to high levels and can contaminate food chains as a result. It is therefore crucial to assess the risks posed by MPs and heavy metals to attain a comprehensive understanding of the potential dangers associated with the consumption of MPs and heavy metals by biota. Songkhla Lagoon is the biggest coastal lagoon in Thailand, with a rich biodiversity, be there have been few studies on the heavy metals and MPs in this lagoon. Therefore, the objectives of this study are as follows: 1) to determine the levels of MPs, heavy metals (As, Cd, Pb, Cu, Zn, Mg, Co, and Mn), and sediment-related variables (organic matter, grain size, and pH) in surface sediment; and 2) to assess the potential ecological risk of MPs and heavy metals in surface sediment.
2 Materials and methods
2.1 Sample collection and preparation
The study area is the lower part of Songkhla Lagoon, a shallow coastal lagoon that is Asia’s second-largest. This lagoon is located in southern Thailand between 7°08’ and 7°50’ N and 100°07’ and 100°37’ E. The lower lagoon is connected to the Gulf of Thailand by a 420 m wide and 9.5 m deep waterway (Sirinawin and Sompongchaiyakul, 2005). It is the largest natural lagoon in Thailand, located on the Malay peninsula in southern Thailand. The complex ecosystem confers Songkhla Lagoon with high biodiversity, including birds along with a rare freshwater dolphin population (Irrawaddy dolphins), while also supporting extensive fisheries. Sediment samples were collected by grab sampling at all 10 stations in the lower part of Songkhla Lagoon in December, 2022 (Figure 1). At each station, three replications were performed. Thereafter, the sediment was kept in clean plastic zip-lock bags (around 500 g of wet weight per replicate). Before the experiment, the sediment samples were separated into two parts. For the first part, samples were left to dry in an oven at 50°C for approximately 48 h. These sediments were used for analyses of MPs, heavy metals (As, Cd, Pb, Cu, Zn, Mg, Co, and Mn), and organic matter. The second part was air-dried and kept for grain size analysis.
2.2 Laboratory analysis
2.2.1 Microplastic analysis
The samples (20 g) were weighed and placed in a beaker. The sediment was then treated with 200 mL of saturated sodium chloride (NaCl) and violently agitated with a glass stirrer before being covered with aluminum foil. The NaCl approach is commonly used to remove MPs from sediments (Wang et al., 2020; Chinfak et al., 2021). The sediments in the beaker were left for 30 min. After this period, 100 ml samples from the beaker were filtered using filter net (20 µm), and 100 ml of NaCl was added into the original beaker again, before being left to stand for another 30 min. This step was repeated three times. After the filtering process, the filter net was rinsed with distilled water and transferred into another beaker. Then, 10 ml of ferrous sulfate (FeSO4) and hydrogen peroxide (H2O2) were added to the filtered samples to digest the organic matter, before covering with aluminum foil and placement on a hot plate for about 1 h for the digestion process. After this period, the filtering process was continued by filtering through GF/C filter paper (1.2 µm), and the filter paper was kept in a Petri dish. After that, all Petri dishes that contained the filter paper were oven-dried at 50°C. The MP particles were then counted and visually observed under a stereomicroscope (Olympus, model SZ61) with a light-emitting diode base. The number of MP particles was recorded, in addition to their color, shape, and size. MPs were divided into three groups: smaller than 500 µm, 500–1000 µm, and larger than 1000 µm. Polymer types of MPs were analyzed using a Fourier transform infrared spectrophotometer (FTIR, Spotlight 200i; Perkin Elmer). Wavelengths in the analysis ranged from 4000 to 400 cm–1. The obtained spectrum was compared to the reference library spectrum of each polymer type.
2.2.2 Heavy metal analysis
Analysis of heavy metals (As, Cd, Pb, Cu, Zn, Mg, Co, and Mn) was carried out by inductively Coupled Plasma Optical Emission Spectrometer (ICP-OES: Perkin Elmer Optima, model 4300 DV), followed the AOAC Official Method (AOAC, 2005). Sediment samples (0.5 g) were placed in a test tube, adding 2 ml of HNO3 (65%, Merck), and digesting in a water bath at 95°C for approximately 90 min. Then, 0.5 ml of H2O2 (30%, Merck) was added and digested for around 30 min in the temperature-controlled water bath, before being allowed to cool at room temperature and filtered through filter paper. The marine sediment-certified reference material was MESS-4. The certified value was within 90% of the analytical value. Three replicates were used to evaluate the efficiency of the analysis. All compounds used were of analytical grade and all glassware used in the study was submerged in nitric acid overnight (3% HNO3, Merck) and then cleaned three times with Milli-Q deionized water before being used.
2.2.3 Sediment characteristic analysis
2.2.3.1 Organic matter
The Walkey-Black method was used to assess the readily oxidizable organic matter content of the sediment samples (Loring and Rantala, 1992). To summarize, dried sediment samples were sieved via a 0.2 mm nonferrous sieve. The dried sediment was then transferred to a 500 mL Erlenmeyer flask. The organic carbon in the sample was then oxidized using 10 mL of 1 N potassium dichromate. To remove the chlorine, a 20 mL mixture of H2SO4 and Ag2SO4 was added. After 30 min, the mixture was diluted with 200 mL of distilled water. As a catalyst, 10 mL of 85% H3PO4 and 0.2 g of NaF were added. The diphenylamine indicator was then added.
2.2.3.2 Sediment grain size analysis
The hydrometer approach was used to analyze particle size (Gee et al., 1986). In brief, around 40 g of air-dried sediment was transferred to a 600 mL beaker and 100 to 150 mL of distilled water was added. Then, to remove organic debris, 30% hydrogen peroxide was continuously applied. The samples were heated on a hot plate at 90°C for 1 h before being incubated in a 105°C oven for 24 h. The silt was then treated with 100 mL of Calgon 5% and 50 mL of distilled water. The samples were sieved using a 63 µm sieve. The filtrate from the sieve was transferred to a 1-L sedimentation cylinder. A plunger was used to scatter the silt in the cylinder, and the density and temperature of the sample were determined using a hydrometer and a thermometer, respectively. The entire system was allowed to stand for 2 h before the density and temperature were measured again.
2.2.3.3 pH
Measurements of pH of the surface sediments were performed in the field with a combined pH meter (IQ 140 pH). At each station, the pH meter was inserted into the sediment and the value was recorded.
2.3 Data processing and statistical analysis
Statistical analysis was used to calculate the minimum, maximum, mean, and standard deviation using Microsoft Excel. The differences in the number of MP particles among the stations were analyzed by the Kruskal–Wallis method. Pearson’s correlation test was performed to determine the relationships between the number of MP particles found in sediment and heavy metal, organic matter, and grain size. A significance level of 0.05 was considered for all analyses.
2.3.1 Microplastic data analysis
2.3.1.1 Polymer hazard index
We examined both the concentration and the chemical composition of MPs in surface sediments to assess the possible hazards of MPs (Xu et al., 2018). The chemical toxicity of certain MP polymer types was taken into account in order to assess the environmental impact (Lithner et al., 2011). The following formula was used to calculate the polymer hazard of MPs (Equation 1):
where PHI is the calculated polymer hazard index caused by MP, Pn is the percentage of specific polymer types [polyethylene (PE) score 11; PET score 4] collected at each sampling location, and Sn is the hazard score of the polymer types of MPs derived from a previous study (Lithner et al., 2011).
2.3.2 Heavy metal data analysis
2.3.2.1 Enrichment factor
The sediment enrichment factor (EF) is an important index reflecting the degree of human activity regarding the accumulation of elements in the environment. It is determined by comparing the measured values with those of the control area (Han et al., 2023). Fe is commonly used as a reference element because it is a naturally abundant element (Al-Wabel et al., 2017). The enrichment factors of heavy metals were calculated using Equation (2):
where (Cn/CFe)Sediment is the ratio of the concentration of metal in the sample to the concentration of Fe and (Ci/CFe)Background is the ratio of the concentration of metal in the background to the concentration of Fe (Bayraklı et al., 2023).
The soil background values of various trace metals in the study area were not available. Thus, the geochemical background values from offshore sediments in the Gulf of Thailand from a previous study (Taylor and McLennan, 1995) were taken as reference values in this study. The background concentrations were 12,200 µg/g for Fe, 12.7 µg/g for Cu, 15 µg/g for Pb, 104 µg/g for Mn, 10 µg/g for Co, 20 µg/g for Ni, 1.5 µg/g for As, 0.098 µg/g for Cd, 71 µg/g for Zn, and 13,300 µg/g for Mg (Taylor and McLennan, 1995; Shazili et al., 1999). Ekissi et al. (2021) divided contamination into different categories based on EF values: no enrichment (EF< 1); minor enrichment (1 ≤ EF ≤ 3); moderate enrichment (3 ≤ EF ≤ 5); moderate to severe enrichment (5 ≤ EF ≤ 10); severe enrichment (10 ≤ EF ≤ 25); very severe enrichment (25 ≤ EF ≤ 50); and extreme enrichment (EF > 50) (Ekissi et al., 2021).
2.3.2.2 Geoaccumulation index (Igeo)
The degree of metal contamination or pollution in the marine environment can be determined using the geoaccumulation index (Igeo). The Igeo values were calculated as follows (Equation 3):
where Cn is the element concentration in the sediment sample and Bn is the trace metal geochemical background concentration. Due to lithogenic processes in the sediments, the background matrix correlation factor is 1.5 (Dytłow and Gorka-Kostrubiec, 2021). The geoaccumulation index divides sediment quality into seven categories: extremely contaminated (Igeo > 5); strongly to extremely contaminated (4< Igeo ≤ 5); strongly contaminated (3< Igeo ≤ 4); moderately to strongly contaminated (2< Igeo ≤ 3); moderately contaminated (1< Igeo ≤2); uncontaminated to moderately contaminated (0< Igeo< 1); and uncontaminated (Igeo< 0) (Shirani et al., 2020).
2.3.2.3 Ecological risk index (R¡)
The ecological risk index (R¡) was quantified to assess the degree of potential ecological risks of heavy metals in the surface sediment of Songkhla Lagoon. Many studies have shown that the presence of toxic heavy metals can cause different types of health problems (Tchounwou et al., 2012). The R¡ (Equation 4) was calculated according to Equation 4 as the sum of Ei (Equation 5) (Sánchez et al., 2022):
where R¡ is the sum of the possible ecological risk factors for elements in sediments, Ei is the potential ecological risk factor for the elements, and Ti is the toxic response factor for the elements. Ti values reflect the toxicity of the metals. In accordance with a report by Hakenson, the following toxic response factors were used: As = 10, Cd = 30, Pb = 5, Cu = 5, and Zn = 1 (Hakanson, 1980). Ci is the metal concentration of the sediments and Co is the metal concentration background value. According to the values obtained, R¡ was classified as follows: low (R¡< 150); moderate (150 ≤ R¡ ≤ 300); considerable (300 ≤ R¡ ≤ 600); and high (R¡ > 600).
3 Results and discussion
3.1 Abundance and occurrence of microplastics in sediment
The numbers of MP particles found at 10 stations in the lower Songkhla Lagoon are shown in Figure 2. The highest number of particles was found at station 7 (5.4 items/g), followed by sampling sties 6 (3.2 items/g), 3 (2.85 items/g), 5 (2.3 items/g), 1 (2.1 items/g), 2 (1.85 items/g), 8 (1.25 items/g), 9 (1.2 items/g), and site 4 (1.05 items/g), while the lowest number was found at site 10 (1.00 items/g). The differences in the number of MP particles among the stations were analyzed by the Kruskal–Wallis method, which found that these differences were significant (p = 0.014). Several studies of MPs in sediments in different environments in lagoons, lakes, bays, estuaries, coasts, rivers, beaches, and mangroves have found lower amounts than in the present study. Studies of MPs in the Lagoon of Tunisia found 63.8 ± 30.9 items/kg (Wakkaf et al., 2022), while in China 250.4 ± 92.0 items/kg were found (Wei et al., 2022), and in the Colombian Caribbean 0–3.1 items/kg were found (Garcés-Ordóñez et al., 2022). Elsewhere, 54–506 items/kg were found in the Lake of China (Yuan et al., 2019), while another study at the same lake found 244 ± 121 items/kg (Tang et al., 2022). In Thailand, a study on a beach found 0–33 items/kg (Jualaong et al., 2021) and another study on mangrove sediment found 106–180 items/kg (Pradit et al., 2022).
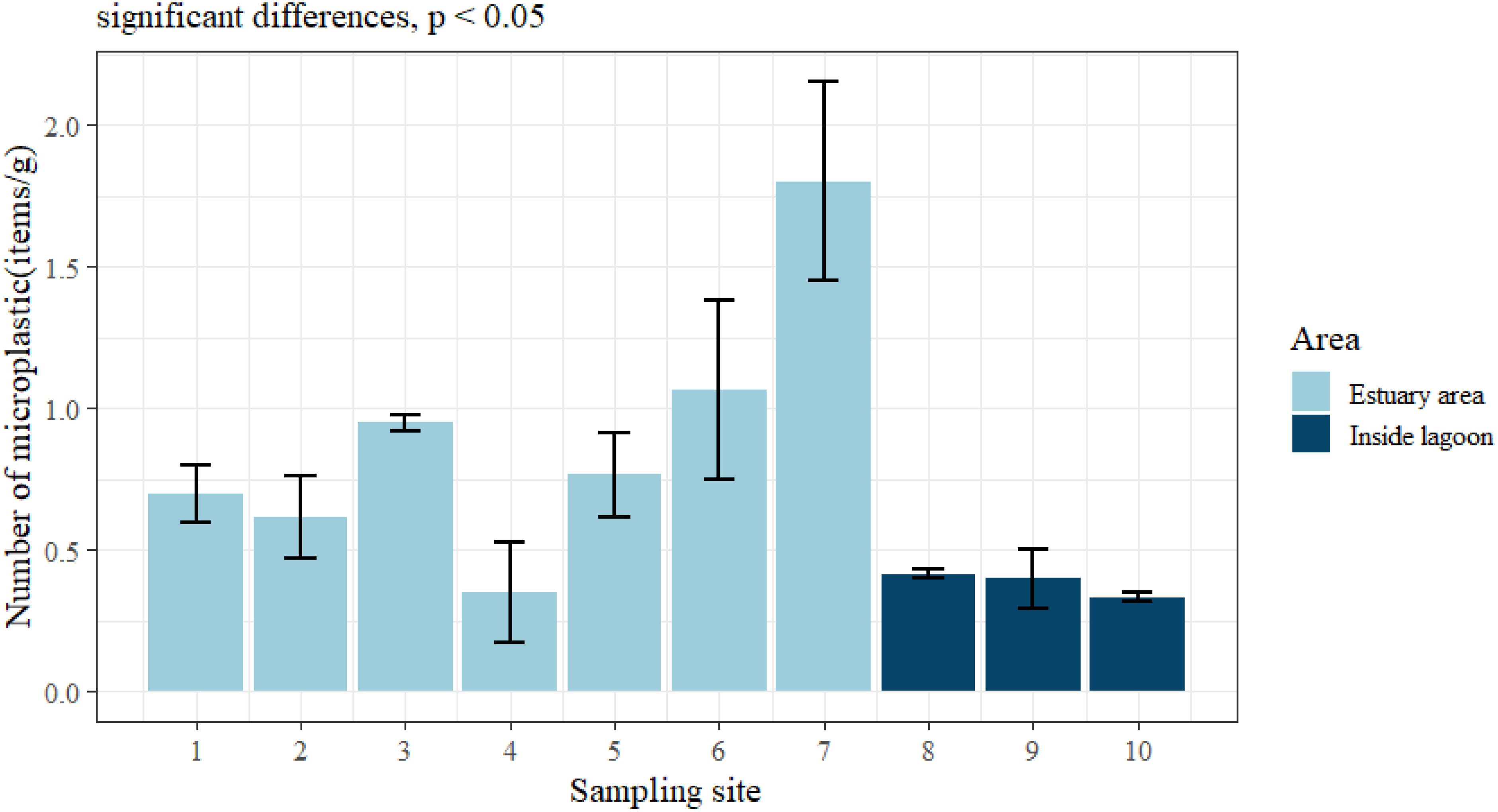
Figure 2 Microplastic distribution in the surface sediment at all sampling sites in Songkhla Lagoon.
The size and color of the MPs scattered on the surface of the sediment are shown in Figure 3. This study only found fiber and fragment shapes of MPs. Fiber was accumulated in the sediment at different stations, with statistically significant differences among them (p< 0.01). In the sediment samples, fibers were found at all sampling sites, which accounted for 68.24% (15.15 items/g) of the total MP particles, while fragments accounted for 31.76% (7.05 items/g). According to Tukey’s HSD grouping, MPs of less than 500 µm were most commonly found among all 10 stations, at a rate of 46.62% (10.35 items/g), followed by those of 500–1000 µm (31.31%, 6.95 items/g) and then those greater than 1000 µm (22.07%, 4.90 items/g). Significant differences in the sizes of MP particles in the sediment were found (p< 0.01). The colors of the MPs found on the sediment surface varied. The predominant colors in this study were black (20.50%, 4.55 items/g), blue (31.53%, 6.70 items/g), transparent (23.65%, 5.25 items/g), and other (24.32%, 5.7 items/g). Five polymers were found, namely rayon, PP, polyester, PET, and copolymer, as shown in Figure 4. In the sediment, polyester and PP were found to have the same percentage distribution, which was 33.33%, followed by rayon at 16.67%, PET at 11.11%, and copolymer at 5.56%.
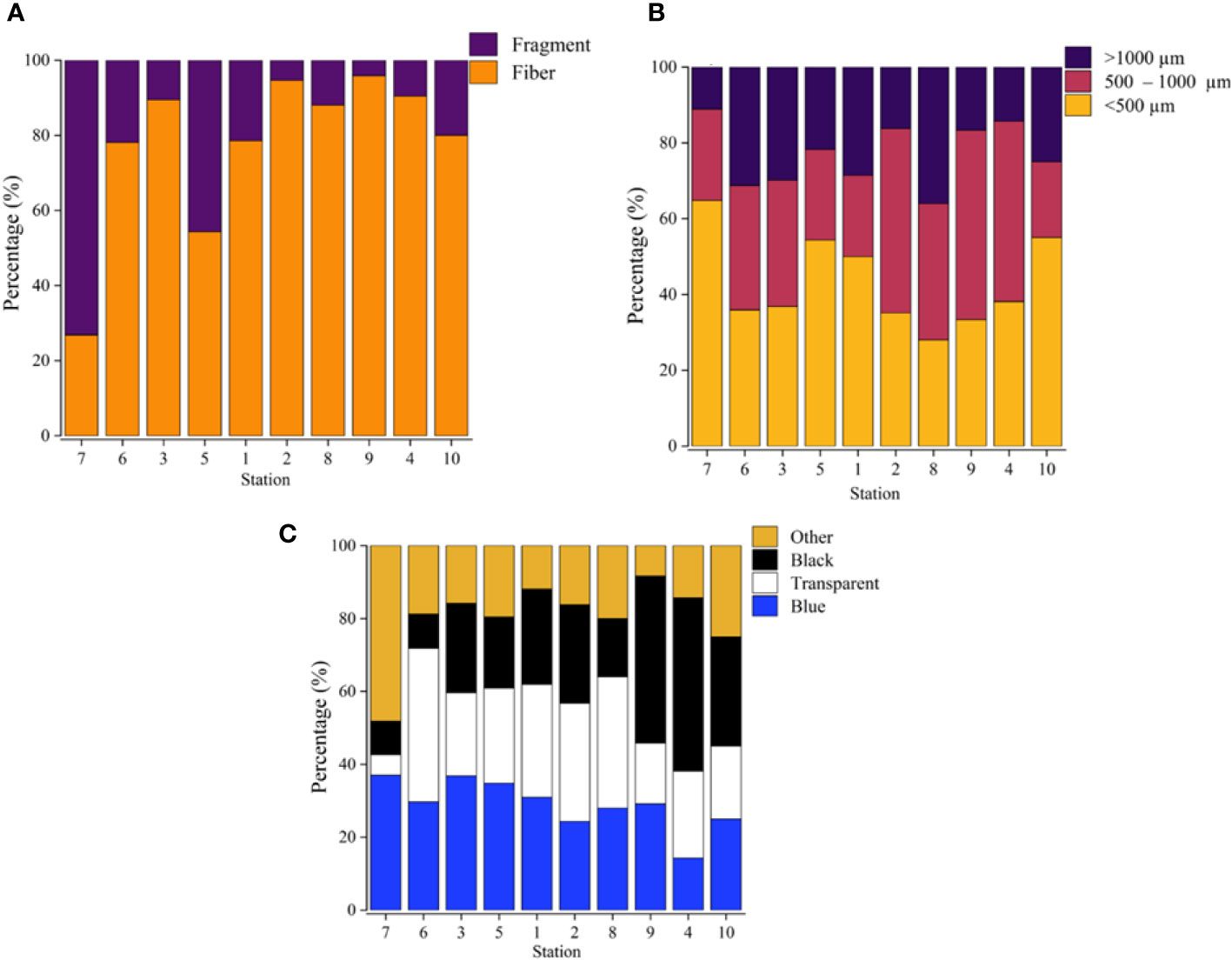
Figure 3 Distribution of microplastic characteristics in the surface sediment, classified by (A) shape, (B) size, and (C) color.
The most widely distributed polymer around the lagoon is polyester. It is common for polyester to be found in both water and sediment. This is similar to the findings in previous studies on MPs in sediment (Alam et al., 2019). Polyester, PET, and PA are important synthetic fibers used to produce synthetic fabrics such as clothing and carpets. Therefore, polyester may be derived from the deterioration of clothing when spun for washing and drying (Šaravanja et al., 2022; Wei et al., 2022). The accumulation of MPs in the environment occurs via waterways, followed by the MPs settling in the sediment over time if left undisturbed. However, in sediment, PP was found to be distributed at the same rate as polyester. The density of PP (0.89–0.92 g/cm3) was lower than that of water. In general, it was distributed more on the water surface than in the sediment. However, the longevity of this polymer causes it to accumulate and adhere to bacteria, resulting in an increase in the density and speed of sedimentation of MPs (Kershaw et al., 2011). This explains the abundance of PP in sediments, as similarly found in other studies (Yuan et al., 2019; Wakkaf et al., 2022; Wei et al., 2022). The other polymers found, rayon, PET, and copolymer, are all based on fibrous substrates, although fragments were found in smaller proportions. Rayon and PET fibers may be derived from garments and fishing equipment, which are made with rayon and PET that then deteriorate and enter the environment (Neves et al., 2015).
3.2 Relationship between microplastics, grain size, pH, and organic matter in sediment
Data on MPs found in sediment and sediment properties such as grain size (%clay, %silt, and %sand), organic matter (OM), and pH are shown in Table 1 and Figures 5, 6. The results show that clay deposits were found at stations 3, 5, 8, 9, and 10. Sand was found at sampling sites 1, 2, and 7, and silt at site 4. The pH in the sediment ranged from 7.37 to 9.37. At site 7, the highest amount of organic matter was found at 4.02%. From all 10 sediment sampling sites, it was found that site 7 had the highest organic matter value, which was related to the high MP content in the sediment there. This area also features a still water source with no tidal circulation. The sediment found was black and had a foul odor. The organic matter had completely decomposed into humus and improved the soil structure. This explanation can be linked to why MPs adhere to soil constituents since MPs can attach to mucus secreted by bacteria. In this study, the majority of MPs were less than 500 µm in size. When there is an abundance of organic matter, an abundance of MPs also accumulates in the sediment.
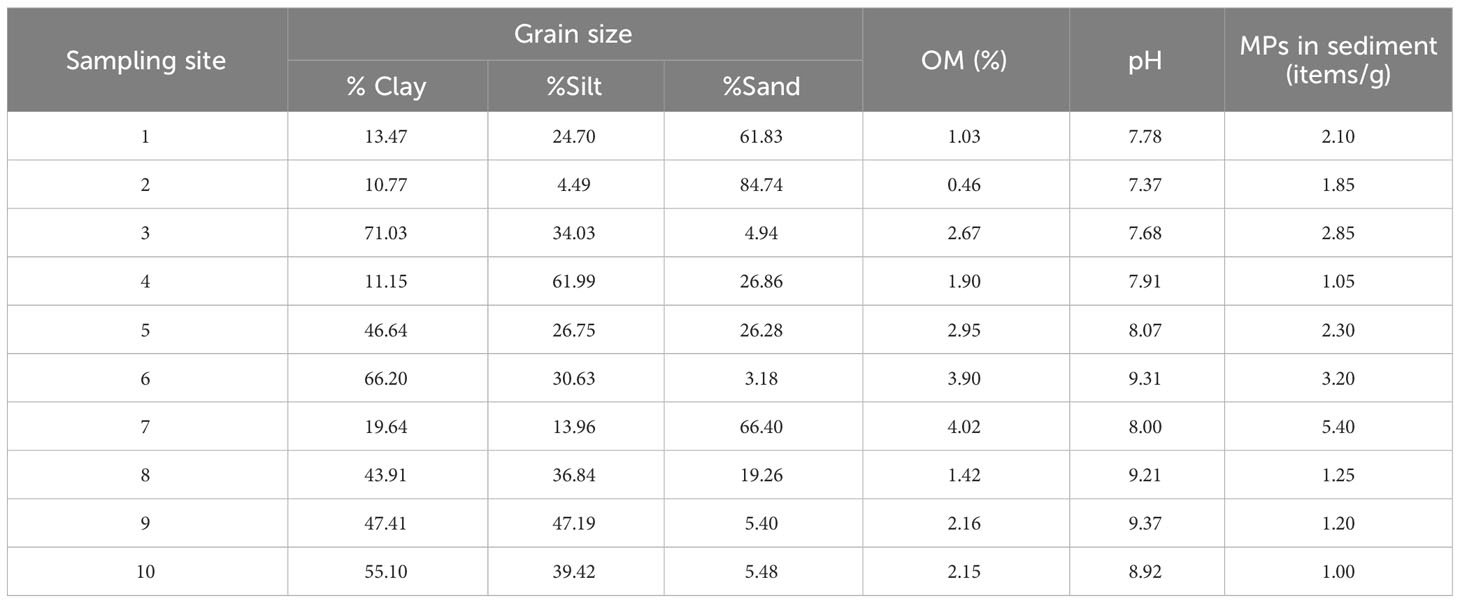
Table 1 Grain size, organic matter, pH, and the number of microplastic particles found in the sediment at each station.
In this study, there was no correlation between MP content and grain size. MP contamination in the soil resulted in a decrease in the stability of the agglomeration and enzymatic activity in the soil due to MP infiltration by reducing the absorption of nutrients into microorganisms and reducing the rate of mineralization in the soil. MPs would have no effect on the stability of soil cohesion without increased organic matter (Liang et al., 2021). The greater the MP contamination, the more difficult it is for soil particles to agglomerate, especially polyester microfibers (Lehmann et al., 2019). After analyzing the relationship between the number of MPs in sediment and sediment properties, no correlation was found between MPs, grain size, and pH. The number of MP particles contaminating the sediment was positively correlated with %OM, according to Pearson’s correlation analysis (r = 0.696, p = 0.025) (Figure 7). Surface lagoon sediment consists of minerals, organic matter, and other minor component. Due to MP and organic matter falling within the same range of density (Tian et al., 2023), MPs and OM would be deposited in the same condition. These MPs can also absorb pollutants (i.e., organic, inorganic, POPs, heavy metal) from sediment or from water on their surfaces. It can be obviously seen that the problem of MPs and marine wastes has a serious adverse impact on the environment, on living organisms, and on human food security as well (Pradit et al., 2020).
3.3 Concentrations of heavy metals in sediment
The concentrations of heavy metals (As, Cd, Pb, Cu, Zn, Mg, Co, and Mn) and their spatial distribution in the study area are summarized in Table 2. The average concentrations (mg/kg) of the trace metals are exhibited the following trend: Mg (732.54 ± 247.04) > Mn (176.74 ± 83.68) > Zn (29.36 ± 39.47) > Cu (12.31 ± 24.58) > Pb (11.07 ± 7.60) > As (5.64 ± 3.30) > Co (2.90 ± 1.38) > Cd (0.22 ± 0.17). The data show that Mg had the highest concentration in the surface sediments, while Cd was minimally accumulated. Mg is an abundant element in the Earth’s crust and in sea water, and is transferred from continents to the hydrosphere via the weathering of rock (Guo et al., 2019). The use of fertilizers from agriculture increases the concentrations of trace metals, which can be transferred to the environment and increase harmful effects on aquatic organisms through runoff from agricultural soils to water bodies (Naz et al., 2022; Nakamaru et al., 2023). Moreover, high concentrations of Mn were also found in the study area. This observation is in line with the work of Pradit et al., 2010)), who quantified the accumulation of Mn within the upper part of Songkhla Lagoon in 2010. The lagoon receives inputs from aquaculture activities such as shrimp farms and sea bass farms, along with various mining activities (Pradit et al., 2010). The mean heavy metal content in this study was compared with that in other coastal sediments around the world. The average values of As in this study are higher than the mean level reported in the Arabian Gulf, Saudi Arabia, but lower than in Chaohu Lake in China (Fang et al., 2022), Brisbane River in Australia (Duodu et al., 2016), and Badovci Lake in Kosovo (Malsiu et al., 2020). Moreover, the mean concentrations of Cd, Pb, Cu, Zn, and Mn were in the same ranges as in other coastal sediments, such as in the Arabian Gulf of Saudi Arabia, Aqaba, Saudi Arabia, and Chaohu Lake, China. Although the mean concentration of As in this study reflects strong contamination, the concentration of As is still lower than in other countries. However, it is a concern that all of the monitored heavy metals exceed the sediment quality standard, so regular monitoring of these variables should be performed.
The spatial distribution of trace metals in surface sediments is an important factor determining the level of pollution of aquatic environments, which can be affected by natural and anthropogenic factors such as weathering of parent rock, industrial wastewater, transportation, and agriculture (Dong et al., 2023). It was found that most of the trace metals had high concentrations at site 7, especially Pb, Cu, and Zn. Station 7 is connected to Samrong Canal, which receives pollutants from Songkhla City, in addition to agricultural waste from rubber plantations, the parawood industry, and the seafood processing industry (Pradit et al., 2021). It is thus possible that high concentrations of metals are derived from these human activities. This is consistent with previous reports describing how anthropogenic activities such as wastewater discharge, battery disposal, transportation, and agricultural activities can affect metal concentrations (Li et al., 2022; Moldovan et al., 2022). Cu is used as a wood preservative (Lasota et al., 2019), while the parawood industry has rapidly expanded in this area (Sompongchaiyakul and Sirinawin, 2007), indicating that it may be an important source of Cu contamination in sediment. A high concentration of Zn was found in soil at a rubbish tip. In addition, the increase in metal concentrations in outer Songkhla Lagoon may have resulted from hydrological dynamics, and water velocity in particular. However, high concentrations of Mg were found at stations 5, 6, and 7, which are connected to three important canals (U-Tapao, Phawong, and Samrong). These canals receive municipal waste from Songkhla and Hat Yai cities. It is possible that, in these highly productive agricultural areas, ammonium phosphate fertilizers are widely used (Medina et al., 2009).
3.4 Risk assessment
3.4.1 Microplastic risk assessment
MPs with a small particle size are easily consumed by marine animals such as zooplankton (Kosore et al., 2018), fish (Klangnurak and Chunniyom, 2020), and molluscs (Abidli et al., 2019), and have an impact on the health of marine organisms (Sharma and Chatterjee, 2017). A risk assessment of MPs in surface sediment based on PHI, as reported by Ranjani et al. (2021), identified five hazard levels of MP pollution (Table 3). Based on PHL value, the overall risk of MPs in lagoon sediment in this study was categorized as Hazard Level III (high risk). The PHI values calculated in this study are lower than those previously reported in India (Ranjani et al., 2021).
3.4.2 Heavy metal risk assessment
The findings on enrichment factor (EF) and geoaccumulation index (Igeo) are shown in Table 4. The EF values of trace metals varied as follows: 4.15–45.89 (mean = 19.06 ± 10.63), 3.63–48.84 (mean = 11.63 ± 9.12), 1.09–13.55 (mean = 3.80 ± 2.58), 0.99–52.04 (mean = 5.09 ± 10.31), 0.98–15.16 (mean = 2.15 ± 2.93), 0.08–0.38 (mean = 0.28 ± 0.09), 0.48–2.69 (mean = 1.46 ± 0.66), and 1.95–17.50 (mean = 8.80 ± 3.89) for As, Cd, Pb, Cu, Zn, Mg, Co, and Mn, respectively. The average EF value of Mg was lower than 1. These values indicate no enrichment at the sampling sites. The Co and Zn average concentrations were in the range of 1–3, indicating minor enrichment. For Pb, it was found in the range of 3–5, indicating moderate enrichment. Cu and Mn were in the range of 5–10, which indicates moderate to severe enrichment. High EF values were observed for As and Cd in the range of 10–25, which indicates severe enrichment. According to Zhang and Liu (2002), crustal materials or natural processes are the primary sources of metal elements with EF between 0.5 and 1.5, whereas anthropogenic activities are the primary source of metal elements with EF greater than 1.5. Based on the Igeo calculation, the majority of sampling stations were in unpolluted to moderately contaminated regions (Pb, Zn, Mg, and Co). The average Igeo values of Cu and Mn were in the range of 1–2,which showed moderate contamination. Cd showed moderate to strong contamination and As showed strong contamination. As is typically used to distinguish between natural and anthropogenic sources of heavy metals because it is a lithogenic metal that enters the soil through the weathering of rocks (Huang et al., 2020). Human activities, such as agricultural activities, mixed orchard cultivation, use of fertilizers in agriculture, and rubber plantations may contribute to heavy metal deposition, resulting in As content in surface sediment.
The ecological risk index (R¡) values of Pb, Cu, and Zn are 110, 145, and 12, respectively (Figure 8). According to the Hakenson classification, the potential ecological risk indices of Pb, Cu, and Zn are less than 150. This is classified as “low risk”, indicating that these heavy metals will not cause serious damage to the ecology of Songkhla Lagoon. Meanwhile, high R¡ values were observed for As (1128) and Cd (2047), being suggestive of high ecological risk. The main contributors to the R¡ are the most toxic elements, Cd and As. Cd and As have a long history of accumulation as pollutants and can represent very serious ecological risk factors to both ecosystems and human health.
3.5 Relationship between heavy metals and microplastics
Owing to their connection to additives used or created during the plastic production process, heavy metals, and persistent organic pollutants that exist in the environment, MPs, are frequently referred to as a “cocktail of contaminants” (Rochman, 2015). Metals such as Zn, Pb, Cr, Co, Cd, and Ti are often used as pigment-based colorants for inorganic polymers (Massos and Turner, 2017). It was observed that the areas where MPs were found most prominently also contained Pb, Cu, and Zn (Figure 9). The relationship between MPs and heavy metals in sediments from this study was calculated using Spearman’s rho correlation analysis, with the results showing that only Zn (r = 0.697) and Cu (r = 0.61) were strongly positively correlated with MP particle counts (p< 0.05). This indicates that the highly concentrated MPs in the sediment were highly contaminated with Zn and Cu, as shown in Figure 10.
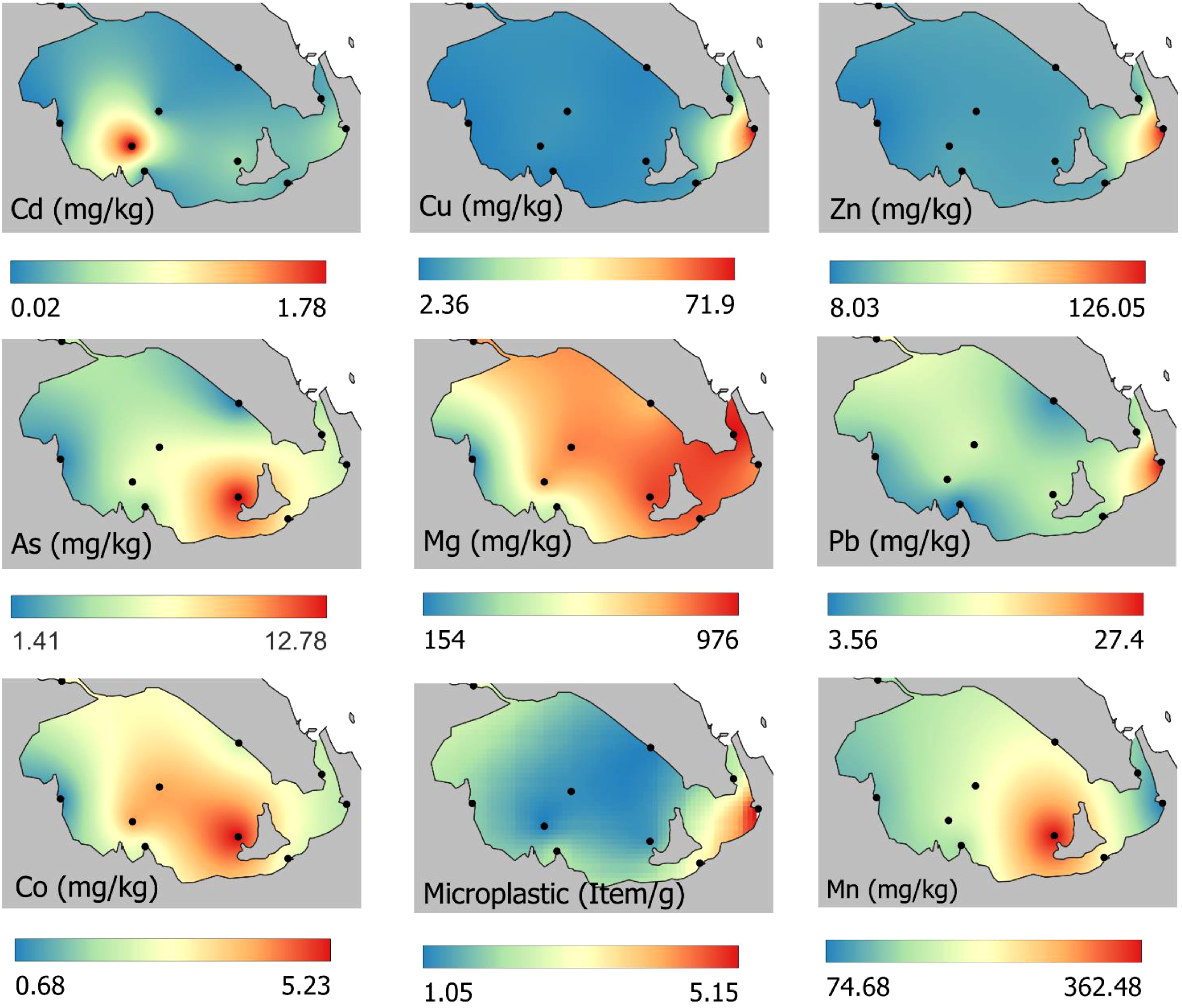
Figure 9 Heavy metal (mg/kg dry weight) and microplastic (items/g) distribution in the surface sediment of Songkhla Lagoon.
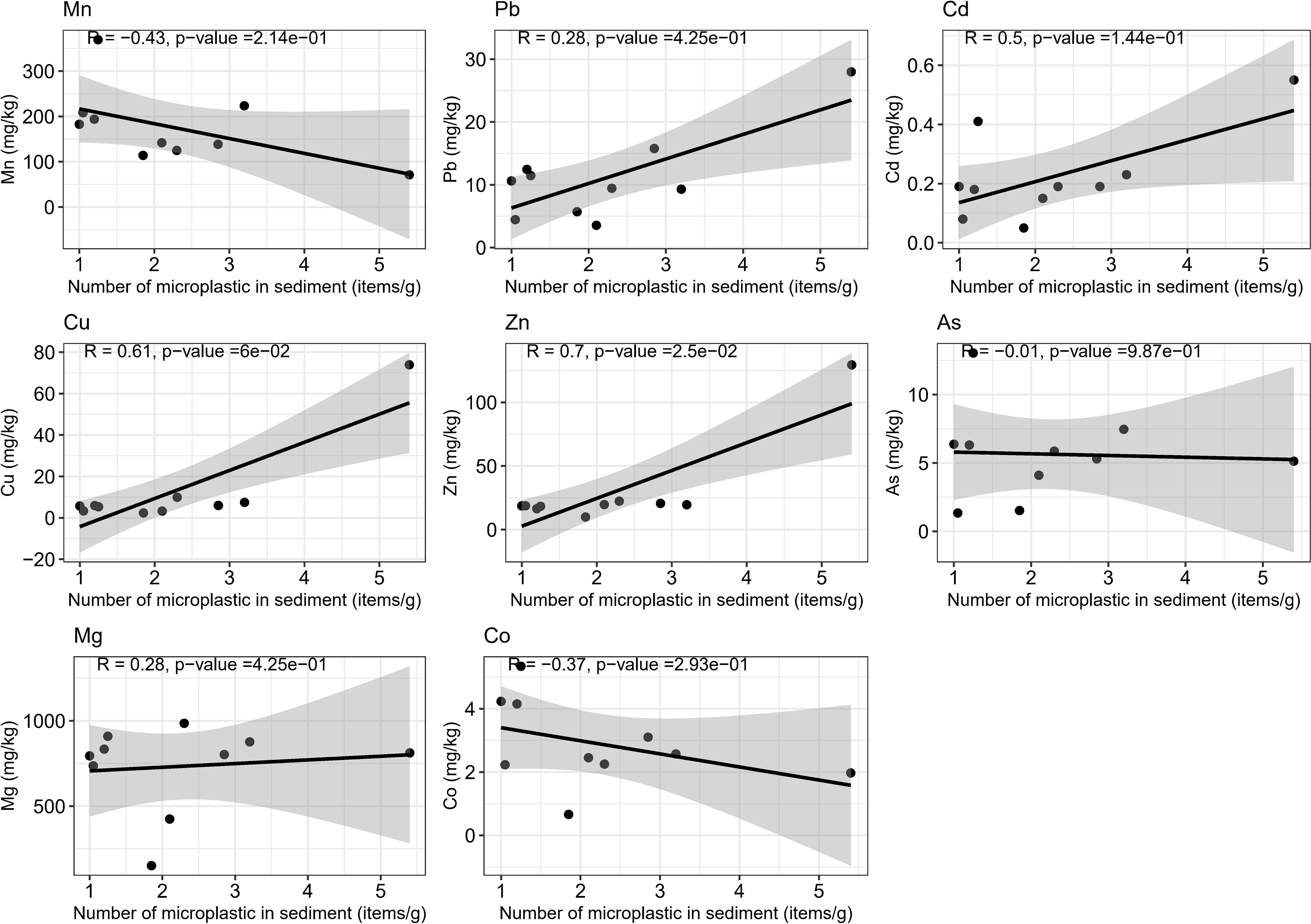
Figure 10 Relationship of heavy metals and abundance of microplastics in surface sediment in Songkhla Lagoon.
3.6 Potential sources of microplastics and heavy metals
According to the sediment data, the lagoon is considerably enriched in trace elements. The waste water is discharged into the river and lagoon. The source of MPs in the lagoon could be from waste from households, paint of fishing boats, and fishing gear (Pradit et al., 2022). These rivers collect urban waste as well as industrial and agricultural waste. The potential sources of heavy metal in the sediment of Songkhla Lagoon (the outer section) include municipal waste from the large and rapidly expanding cities of Songkhla province, as well as agricultural and industrial discharges transported via the U-Taphao canal (Sompongchaiyakul and Sirinawin, 2007; Pradit et al., 2018). As and Cu are employed in the parawood business as wood preservatives near to the lagoon (Sompongchaiyakul and Sirinawin, 2007). The high levels of As found in the soils at a rubbish tip indicate that runoff from such sites into canals, and abandoned mines near the lagoon, may also be important sources of As (Pradit et al., 2010). Notably, the pH in the sediment increased at the station near the mouth of the lagoon, which was probably due to wastewater from houses flowing into the main rivers. Water from washing clothes with detergent normally has highly alkaline components, such as OH−, CO32−, and HCO3− ions of calcium, sodium, magnesium, potassium, and ammonia. These basic conditions from laundry activities can clearly raise the pH in both water and sediment (Pradit et al., 2021). If looking at historical data on heavy metal concentrations in Songkhla Lagoon sediments from 2010 to the present (Table 5), there is a decreasing trend for As and Pb. For MPs in sediments in the lagoon there have been few studies, but the concentration of this study seems to indicate more MPs than the study in 2021. The possible explanation is that the study area in 2021 was in the canal flowing to the lagoon whereas this study area covered a section of the lagoon itself.
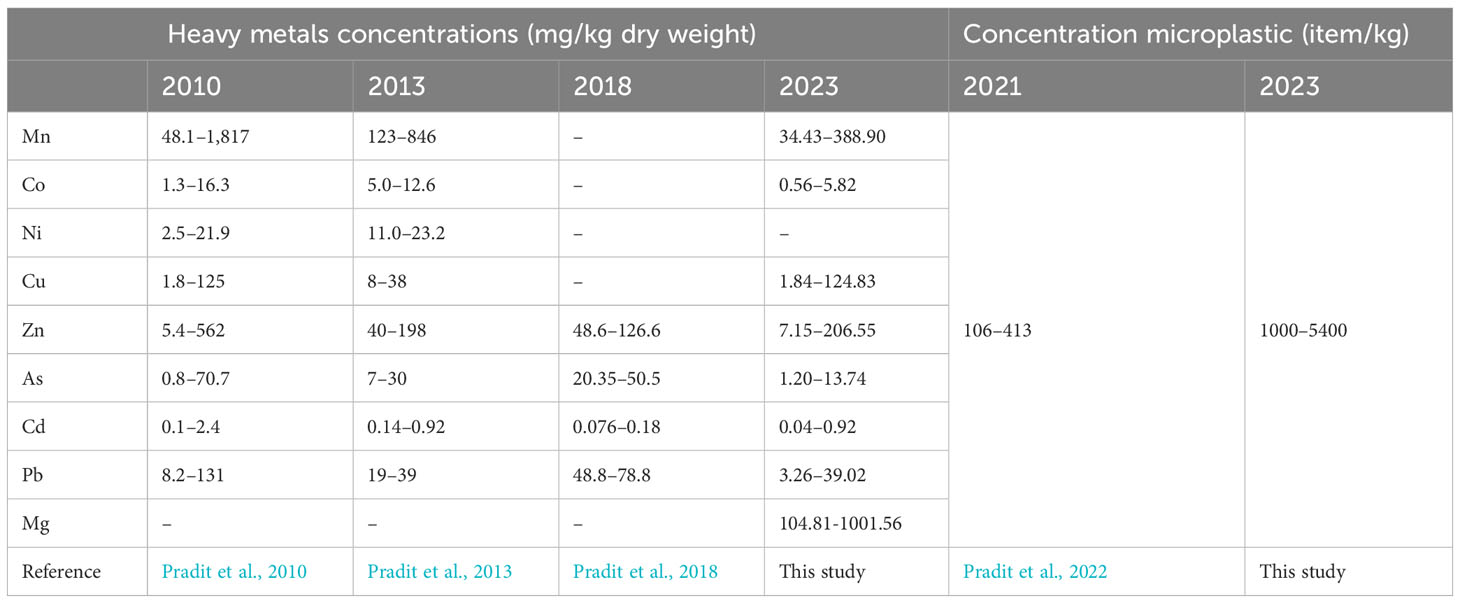
Table 5 The historical information of heavy metal and microplastic concentrations in the sediment of Songkhla Lagoon.
3.7 Conclusion
This study reports the occurrence of MPs and heavy metals in the sediment of Songkhla Lagoon, the largest lagoon in Thailand. The area where MPs particularly accumulate is near the mouth of the lagoon, possibly derived from the three main rivers flowing into the lagoon. Risk assessment of MP loading showed that MPs constitute a minor hazard, whereas the polymer hazard index indicated a high hazard. The main contributors to the R¡ are the most toxic elements, Cd and As. Cd and As have a long history of accumulation as pollutants and can represent very serious ecological risk factors to both ecosystems and human health. This study only investigated the lower Songkhla Lagoon area. The abundance and distribution of MPs and heavy metals in the lagoon sediment may lead to transfer of toxic chemicals to marine organisms and humans. The study area should be expanded to cover the middle and upper parts of the lagoon, which are the habitat of Irrawaddy dolphins.
Data availability statement
The original contributions presented in the study are included in the article/supplementary material. Further inquiries can be directed to the corresponding author.
Author contributions
SP: Conceptualization, Formal analysis, Funding acquisition, Investigation, Methodology, Project administration, Supervision, Validation, Writing – original draft, Writing – review & editing. PN: Conceptualization, Funding acquisition, Investigation, Methodology, Software, Writing – review & editing. KS: Investigation, Methodology, Writing – review & editing. PJ: Investigation, Software, Visualization, Writing – review & editing. TK: Software, Visualization, Writing – review & editing. TN: Software, Writing – review & editing. DM: Conceptualization, Funding acquisition, Investigation, Methodology, Writing – review & editing.
Funding
The author(s) declare financial support was received for the research, authorship, and/or publication of this article. This research was supported by the National Science, Research and Innovation Fund (NSRF) and Prince of Songkla University (Grant No. ENV6601203S, Ref.no. 23801).
Conflict of interest
The authors declare that the research was conducted in the absence of any commercial or financial relationships that could be construed as a potential conflict of interest.
Publisher’s note
All claims expressed in this article are solely those of the authors and do not necessarily represent those of their affiliated organizations, or those of the publisher, the editors and the reviewers. Any product that may be evaluated in this article, or claim that may be made by its manufacturer, is not guaranteed or endorsed by the publisher.
References
Abidli S., Lahbib Y., El Menif N. T. (2019). Microplastics in commercial molluscs from the lagoon of Bizerte (Northern Tunisia). Mar. Pollut. Bull. 142, 243–252. doi: 10.1016/j.marpolbul.2019.03.048
Alam F. C., Sembiring E., Muntalif B. S., Suendo V. (2019). Microplastic distribution in surface water and sediment river around slum and industrial area (Case study: ciwalengke river, majalaya district, Indonesia). Chemosphere 224, 637–645. doi: 10.1016/j.chemosphere.2019.02.188
Alia T. K. A. T. N., Hing L. S., Sim S. F., Pradit P., Ahmad A., Ong M. C. (2020). Comparative study of raw and cooked farmed sea bass (Lates calcarifer) in relation to metal content and its estimated human health risk. Mar. Pollut. Bulletin. 153, 111009. doi: 10.1016/j.marpolbul.2020.111009
Al-Wabel M. I., Sallam A. E.-A. S., Usman A. R. A., Ahmad M., El-Naggar A. H., El-Saeid M. H., et al. (2017). Trace metal levels, sources, and ecological risk assessment in a densely agricultural area from Saudi Arabia. Environ. Monit. Assess. 189, 252. doi: 10.1007/s10661-017-5919-1
AOAC (2005). "Official methods of analysis of AOAC international 18th Ed.", In: Metals and Other Elements, eds. Horwitz W., Latimer G. W.. (Maryland: Gaithersburg).pp. 46–50.
Baeyens W., Leermakers M., De Gieter M., Nguyen H. L., Parmentier K., Panutrakul S., et al. (2005). Overview of trace metal contamination in the Scheldt estuary and effect of regulatory measures. Hydrobiologia 540, 141–154. doi: 10.1007/s10750-004-7129-4
Bayo J., Guillen M., Olmos S., Jimenez P., Sanchez E., Roca M. J. (2018). Microplastics as vector for persistent organic pollutants in urban effluents: The role of polychlorinated biphenyls. Int. J. Sus. Dev. Plann. 13, 671–682. doi: 10.2495/SDP-V13-N4-671-682
Bayraklı B., Dengiz O., Özyazıcı M. A., Koç Y., Kesim E., Türkmen F. (2023). Assessment of heavy metal concentrations and behavior in cultivated soils under humid-subhumid environmental condition of the Black Sea region. Geoderma. Regional. 32, e00593. doi: 10.1016/j.geodrs.2022.e00593
Browne M., Dissanayake A., Galloway T. S., Lowe D. M., Thompson R. C. (2008). Ingested microscopic plastic translocates to the circulatory system of the mussel, Mytilus edulis (L.). Environ. Sci. Technol. 42, 5026–5031. doi: 10.1021/es800249a
Buccolieri A., Buccolieri G., Cardellicchio N., Atti A. D., Leo A. D., Maci A. (2006). Heavy metals in marine sediments of Taranto Gulf (Ionian Sea, Southern Italy). Mar. Chem. 99, 227–235. doi: 10.1016/j.marchem.2005.09.009
Chinfak N., Sompongchaiyakul P., Charoenpong C., Shi H., Yeemin T., Zhang J. (2021). Abundance, composition, and fate of microplastics in water, sediment, and shellfish in the Tapi-Phumduang river system and Bandon bay, Thailand. Sci. Total. Environ. 781, 146700. doi: 10.1016/j.scitotenv.2021.146700
Claessens M., De Meester S., Landuyt L. V., De Clerck K., Janssen C. R. (2011). Occurrence and distribution of microplastics in marine sediments along the Belgian coast. Mar. Pollut. Bull. 62, 2199–2204. doi: 10.1016/j.marpolbul.2011.06.030
Cordova M. R., Ulumuddin Y. I., Purbonegoro T., Shiomoto A. (2021). Characterization of microplastics in mangrove sediment of Muara Angke Wildlife Reserve, Indonesia. Mar. Pollut. Bull. 163, 112012. doi: 10.1016/j.marpolbul.2021.112012
Dong Q., Song C., Yang D., Zhao Y., Yan M. (2023). Contamination assessment and origin of soil heavy metals in the Danjiangkou reservoir, China. Int. J. Environ. Res. Public Health 20, 3443. doi: 10.3390/ijerph20043443
Duodu G. O., Goonetilleke A., Ayoko G. A. (2016). Comparison of pollution indices for the assessment of heavy metal in Brisbane River sediment. Environ. Pollut. 219, 1077–1091. doi: 10.1016/j.envpol.2016.09.008
Dytłow S., Gorka-Kostrubiec B. (2021). Concentration of heavy metals in street dust: an implication of using different geochemical background data in estimating the level of heavy metal pollution. Environ. Geochem. Health 43, 521–535. doi: 10.1007/s10653-020-00726-9
Ekissi D., Kinimo K. C., Kamelan T. M., Kouamelan E. P. (2021). Physicochemical characteristics and heavy metals contamination assessment in water and sediment in a tropical hydroelectric dam of sassandra river, côte d’Ivoire. JH&P 9, 27–35. doi: 10.12691/jephh-9-2-1
Fang T., Yang K., Wang H., Fang H., Liang Y., Zhao X., et al. (2022). Trace metals in sediment from Chaohu Lake in China: Bioavailability and probabilistic risk assessment. Sci. Total. Environ. 849, 157862. doi: 10.1016/j.scitotenv.2022.157862
Garcés-Ordóñez O., Saldarriaga-Vélez J. F., Espinosa-Díaz L. F., Patiño A. D., Cusba J., Canals M., et al. (2022). Microplastic pollution in water, sediments and commercial fish species from Ciénaga Grande de Santa Marta lagoon complex, Colombian Caribbean”. Sci. Total. Environ. 829, 154643. doi: 10.1016/j.scitotenv.2022.154643
Gee G. W., Bauder J. W. (1986). “Particle-size analysis” in Methods of Soil Analysis. Part 1. Physical and Mineralogical Methods, ed. Klute A. (Madison: American Society of Agronomy), 383–411. doi: 10.2136/sssabookser5.1.2ed.c15
Goh P. B., Pradit S., Towatana P., Khokkiatiwong S., Ong M. C. (2022). Laboratoty experiment on copper and lead adsorption ability of microplastics. Sains. Malaysiana. 51 (4), 993–1004. doi: 10.17576/jsm-2022-5104-04
Guo B., Zhu X., Dong A., Yan B., Shi G., Zhao Z. (2019). Mg isotopic systematics and geochemical applications: A critical review. J. Asian Earth Sci. 176, 368–385. doi: 10.1016/j.jseaes.2019.03.001
Hakanson L. (1980). An ecological risk index for aquatic pollution control: a sedimentological approach. Water Res. 14, 975–986. doi: 10.1016/0043-1354(80)90143-8
Han Y.-J., Liang R.-Z., Li H.-S., Gu Y.-G., Jiang S.-J., Man X.-T. (2023). Distribution, multi-index assessment, and sources of heavy metals in surface sediments of zhelin bay, a typical mariculture area in southern china. Toxics 11 (2), 150. doi: 10.3390/toxics11020150
Holmes L. A., Turner A., Thompson R. C. (2012). Adsorption of trace metals to plastic resin pellets in the marine environment. Environ. Pollut. 160, 42–48. doi: 10.1016/j.envpol.2011.08.052
Huang Z., Liu C., Zhao X., Dong J., Zheng B. (2020). Risk assessment of heavy metals in the surface sediment at the drinking water source of the Xiangjiang River in South China. Environ. Sci. Eur. 32, 2–9. doi: 10.1186/s12302-020-00305-w
Jualaong S., Pransilpa M., Pradit S., Towatana P. (2021). Type and distribution of microplastics in beach sediment along the coast of the Eastern Gulf of Thailand. J. Mar. Sci. Eng. 9, 1–12. doi: 10.3390/jmse9121405
Kershaw P., Katsuhiko S., Lee S., Samseth J., Woodring D., Smith J. (2011). Plastic debris in the ocean. UNEP year book.
Klangnurak W., Chunniyom S. (2020). Screening for microplastics in marine fish of Thailand: the accumulation of microplastics in the gastrointestinal tract of different foraging preferences. Environ. Sci. Pollut. Res. 27, 27161–27168. doi: 10.1007/s11356-020-09147-8
Kosore C., Ojwang L., Maghanga J., Kamau J., Kimeli A., Omukoto J., et al. (2018). Occurrence and ingestion of microplastics by zooplankton in Kenya’s marine environment: first documented evidence. Afr. J. Mar. Sci. 40, 225–234. doi: 10.2989/1814232X.2018.1492969
Lasota S., Stephan I., Horn M. A., Otto W., Noll M. (2019). Copper in wood preservatives delayed wood decomposition and shifted soil fungal but not bacterial community composition. AEM 85, e02391–e02318. doi: 10.1128/AEM.02391-18
Lehmann A., Fitschen K., Rillig M. C. (2019). Abiotic and biotic factors influencing the effect of microplastic on soil aggregation. Soil Syst. 3, 21. doi: 10.3390/soilsystems3010021
Li F., Yu X., Lv J., Wu Q., An Y. (2022). Assessment of heavy metal pollution in surface sediments of the Chishui River Basin, China. PloS One 17, e0260901. doi: 10.1371/journal.pone.0260901
Liang Y., Lehmann A., Yang G., Leifheit E. F., Rillig M. C. (2021). Effects of microplastic fibers on soil aggregation and enzyme activities are organic matter dependent. Front. Environ. Sci. 9. doi: 10.3389/fenvs.2021.650155
Lithner D., Larsson A., Dave G. (2011). Environmental and health hazard ranking and assessment of plastic polymers based on chemical composition. Sci. Total. Environ. 409, 3309–3324. doi: 10.1016/j.scitotenv.2011.04.038
Liu J., Liu H., He D., Zhang T., Qu J., Lv Y., et al. (2022). Comprehensive effects of temperature, salinity, and current velocity on the microplastic abundance in offshore area. Pol. J. Environ. Stud. 31, 1727–1736. doi: 10.15244/pjoes/142389
Loring D. H., Rantala R. T. T. (1992). Manual for the geochemical analyses of marine sediments and suspended particulate matter. Earth-Sci. Rev. 32, 235–283. doi: 10.1016/0012-8252(92)90001-A
Malsiu S., Shehu I., Stafilov T., Faiku F. (2020). Assessment of heavy metal concentrations with fractionation method in sediments and waters of the Badovci Lake (Kosovo). JEPH 2020, 14. doi: 10.1155/2020/3098594
Massos A., Turner A. (2017). Cadmium, lead and bromine in beached microplastics. Environ. Pollut. 227, 139–145. doi: 10.1016/j.envpol.2017.04.034
Medina L. C., Sartain J. B., Obreza T. A. (2009). Estimation of release properties of slow-release fertilizer materials. Horttechnology 19, 13–15. doi: 10.21273/HORTTECH.19.1.13
Mendil D., Uluözlü O. D. (2007). Determination of trace metal levels in sediment and five fish species from lakes in Tokat, Turkey. Food Chem. 101, 739–745. doi: 10.1016/j.foodchem.2006.01.050
Miao S., De Laune R. D., Jugsujinda A. (2006). Influence of sediment redox conditions on release/solubility of metals and nutrients in a Louisiana Mississippi River deltaic plain freshwater lake. Sci. Total. Environ. 371, 334–343. doi: 10.1016/j.scitotenv.2006.07.027
Moldovan A., Török A. I., Kovacs E., Cadar O., Mirea I. C., Micle V. (2022). Metal contents and pollution indices assessment of surface water, soil, and sediment from the Arieș river basin mining area, romania. Sustainability 14 (13), 8024. doi: 10.3390/su14138024
Nakamaru Y. M., Matsuda R., Sonoda T. (2023). Environmental risks of organic fertilizer with increased heavy metals (Cu and Zn) to aquatic ecosystems adjacent to farmland in the northern biosphere of Japan. Sci. Total. Environ. 884, 163861. doi: 10.1016/j.scitotenv.2023.163861
Naz S., Fazio F., Habib S. S., Nawaz G., Attaullah S., Ullah M., et al. (2022). Incidence of heavy metals in the application of fertilizers to crops (Wheat and Rice), a fish (Common carp) Pond and a human health risk assessment. Sustainability 14, 13441. doi: 10.3390/su142013441
Neves D., Sobral P., Ferreira J. L., Pereira T. (2015). Ingest of microplastic by commercial fish off the Portuguese Coast. Mar. Pollut. Bull. 101, 119–126. doi: 10.1016/j.marpolbul.2015.11.008
Pradit S., Gao Y., Faiboon A., Baeyens W., Leermakers M. (2013). Application of DET (diffusive equilibrium in thin films) and DGT (diffusive gradients in thin films) techniques in the study of the mobility of sediment-bound metals in the outer section of Songkhla Lake, Southern Thailand. Environ. Monit. Assessment. 185 (5), 4207–4220. doi: 10.1007/s10661-012-2862-z
Pradit S., Nitiratsuwan T., Towatana P., Jualaong S., Jirajarus M., Sonrnplang K., et al. (2020). Marine debris accumulation on the beach in libong, a small island in andaman sea, Thailand. Appl. Ecol. Environ. Res. 18 (4), 5461–5474. doi: 10.15666/aeer/1804_54615474
Pradit S., Noppradit P., Goh B. P., Sornplang K., Ong M. C., Towatana P. (2021). Occurrence of microplastics and trace metals in fish and shrimp from Songkhla lake, Thailand during the covid-49 pandemic. Appl. Ecol. Environ. Res. 19, 1085–1106. doi: 10.15666/aeer/1902_10851106
Pradit S., Noppradit P., Loh P.-S., Nitiratsuwan T., Le T. P. Q., Oeurng C., et al. (2022). The occurrence of microplastics in sediment cores from two mangrove areas in Southern Thailand. J. Mar. Sci. Eng. 10, 418. doi: 10.3390/jmse10030418
Pradit S., Shazili N. A. M., Pattaratumrong M. S., Chotikarn P., Kobkeatthawin T., Yucharoen M., et al. (2018). Accumulation of trace metals in mangrove plant Soneratia Caseoralis in Songkhla Lake, Thailand. Appl. Ecol. Environ. Res. 16 (4), 4081–4095. doi: 10.15666/aeer/1604_40814095
Pradit S., Wattayakorn G., Angsupanich S., Baeyens W., Leermakers M. (2010). Distribution of trace elements in sediments and biota of songkhla lake, southern Thailand. Water Air. Soil Pollut. 206, 155–174. doi: 10.1007/s11270-009-0093-x
Rajeshkumar S., Liu Y., Zhang X., Ravikumar B., Bai G., Li X. (2018). Studies on seasonal pollution of heavy metals in water, sediment, fish and oyster from the Meiliang Bay of Taihu Lake in China. Chemosphere 191, 626–638. doi: 10.1016/j.chemosphere.2017.10.078
Ranjani M., Veerasingam S., Venkatachalapathy R., Mugilarasan M., Bagaev A., Mukhanov V., et al. (2021). Assessment of potential ecological risk of microplastics in the coastal sediments of India: A meta-analysis. Mar. Pollut. Bull. 163, 111969. doi: 10.1016/j.marpolbul.2021.111969
Rochman C. M. (2015). “The complex mixture, fate and toxicity of chemicals associated with plastic debris in the marine environment” in Marine Anthropogenic Litter, eds. Bergmann M., Gutow L., Klages M. (Springer, Cham), 117–140. doi: 10.1007/978-3-319-16510-3_5
Sánchez E. R. S., Martínez J. M. E., Morales M. M., Mendoza O. T., Alberich M. V. E. (2022). Ecological and health risk assessment of potential toxic elements from a mining area (Water and Sediments): The San Juan-Taxco River System, Guerrero, Mexico. Water 14, 518. doi: 10.3390/w14040518
Šaravanja A., Pušić T., Dekanić T. (2022). Microplastics in wastewater by washing polyester fabrics. Materials 15, 2683. doi: 10.3390/ma15072683
Sharma S., Chatterjee S. (2017). Microplastic pollution, a threat to marine ecosystem and human health: a short review. Environ. Sci. Pollut. Res. 24, 21530–21547. doi: 10.1007/s11356-017-9910-8
Shazili N. A. M., Rashid M. K. A., Husain M. L., Nordin A., Ali S. (1999). “Trace metals in the surface sediments of the South China Sea, area I: Gulf of Thailand and the East Coast of Peninsular Malaysia,” in Proceedings of the first technical seminar on marine fishery resources survey in the south China sea, area I: gulf of Thailand and east coast of peninsular Malaysia, vol. 1999. (Bangkok, Thailand: Training Department, Southeast Asian Fisheries Development Center), 73–85.
Shirani M., Afzali K. N., Jahan S., Strezov V., Soleimani-Sardo M. (2020). Pollution and contamination assessment of heavy metals in the sediments of Jazmurian playa in southeast Iran. Sci. Rep. 10, 4775. doi: 10.1038/s41598-020-61838-x
Sirinawin W., Sompongchaiyakul P. (2005). Nondetrital and total metal distribution in core sediments from the U-taphao Canal, Songkhla, Thailand. Mar. Chem. 94, 5–16. doi: 10.1016/j.marchem.2004.07.007
Sompongchaiyakul P., Sirinawin W. (2007). Arsenic, Chromium and Mercury in surface sediment of Songkhla lake system, Thailand. Asian J. Water Environ. Pollut. 4, 17–24. doi: 10.1016/j.sbspro.2013.08.456
Tang N., Yu Y., Cai L., Tan X., Zhang L., Huang Y., et al. (2022). Distribution characteristics and source analysis of microplastics in urban freshwater lakes: A case study in Songkhla lake of Dongguan, China. Water 14, 1111. doi: 10.3390/w14071111
Taylor S. R., McLennan S. M. (1995). The geochemical evolution of the continental crust. Rev. Geophys 33, 241–265. doi: 10.1029/95RG00262
Tchounwou P. B., Yedjou C. G., Patlolla A. K., Sutton D. J. (2012). Heavy metals toxicity and the environment. NIH. Public Access. 101, 133–164. doi: 10.1007/978-3-7643-8340-4_6
Thompson R. C., Olsen Y., Mitchell R. P., Davis A., Rowland S. J., John A. W. G., et al. (2004). Lost at sea: where is all the plastic? Science 304, 838. doi: 10.1126/science.1094559
Tian X., Yang M., Guo Z., Chang C., Li J., Guo Z., et al. (2023). Amount and characteristics of microplastic and organic matter in wind-blown sediment at different heights within the aeolian sand saltation layer. Environ. Pollut. 327, 121615. doi: 10.1016/j.envpol.2023.121615
Wakkaf T., Zrelli R. E., Yacoubi L., Kedzierski M., Lin Y.-J., Mansour L., et al. (2022). Seasonal patterns of microplastics in surface sediments of a Mediterranean lagoon heavily impacted by human activities (Bizerte Lagoon, Northern Tunisia). Environ. Sci. Pollut. Res. 29, 76919–76936. doi: 10.1007/s11356-022-21129-6
Wang Y., Zou X., Peng C., Qiao S., Wang T., Yu W., et al. (2020). Occurrence and distribution of microplastics in surface sediments from the Gulf of Thailand. Mar. Pollut. Bull. 152, 110916. doi: 10.1016/j.marpolbul.2020.110916
Wei Y., Ma W., Xu Q., Sun C., Wang X., Gao F. (2022). Microplastic distribution and influence factor analysis of seawater and surface sediments in a typical bay with diverse functional areas: A case study in Xincun lagoon, China. Front. Environ. Sci. 10. doi: 10.3389/fenvs.2022.829942
Xu P., Peng G., Su L., Gao Y., Gao L., Li D. (2018). Microplastic risk assessment in surface waters: A case study in the Changjiang Estuary, China. Mar. Pollut. Bull. 133, 647–654. doi: 10.1016/j.marpolbul.2018.06.020
Yuan W., Liu X., Wang W., Di M., Wang J. (2019). Microplastic abundance, distribution and composition in water, sediments, and wild fish from Poyang lake, China. Ecotoxicol. Environ. Saf. 170, 180–187. doi: 10.1016/j.ecoenv.2018.11.126
Keywords: trace element, marine debris, risk assessment, coastal, organic matter
Citation: Pradit S, Noppradit P, Sornplang K, Jitkaew P, Kobketthawin T, Nitirutsuwan T and Muenhor D (2024) Microplastics and heavy metals in the sediment of Songkhla Lagoon: distribution and risk assessment. Front. Mar. Sci. 10:1292361. doi: 10.3389/fmars.2023.1292361
Received: 11 September 2023; Accepted: 18 December 2023;
Published: 09 January 2024.
Edited by:
Xuchun Qiu, Jiangsu University, ChinaReviewed by:
Gabriel-Ionut Plavan, Alexandru Ioan Cuza University, RomaniaMohamed Mohsen, Jimei University, China
Lingshi Yin, Hunan University, China
Copyright © 2024 Pradit, Noppradit, Sornplang, Jitkaew, Kobketthawin, Nitirutsuwan and Muenhor. This is an open-access article distributed under the terms of the Creative Commons Attribution License (CC BY). The use, distribution or reproduction in other forums is permitted, provided the original author(s) and the copyright owner(s) are credited and that the original publication in this journal is cited, in accordance with accepted academic practice. No use, distribution or reproduction is permitted which does not comply with these terms.
*Correspondence: Prakrit Noppradit, prakrit.n@psu.ac.th