- 1Louvain Institute of Biomolecular Science and Technology, Université catholique de Louvain, Louvain-la-Neuve, Belgium
- 2Department of Biological Sciences, University of the Pacific, Stockton, CA, United States
- 3Department of Biology, Sonoma State University, Rohnert Park, CA, United States
- 4Mass Spectrometry Laboratory, CART, MolSys Research Unit, University of Liège, Liège, Belgium
- 5Microbiology and Environmental Toxicology Department, University of California, Santa Cruz, Santa Cruz, CA, United States
Marine mammals are exposed to increasing intensities of anthropogenic stressors such as acoustic disturbance and contaminants. Correlative studies have suggested concerning shifts in behavioral and physiological status of stress-exposed individuals, which could alter the health and survival of marine mammal populations. However, functional studies of the effects of multiple stressors on marine mammals are lacking. To fill in this gap, we recently developed an ex vivo approach of precision-cut adipose tissue slices (PCATS) to study the impact of stressors on the function of an essential endocrine organ: the adipose tissue. In the present study, we investigated the impact of hormones associated with the stress response on adipose tissue from northern elephant seals (Mirounga angustirostris). Blubber biopsies were collected from 17 weaned northern elephant seal pups, separated into inner and outer layers, dissected into PCATS, and cultured for 48 hours. To mimic prolonged and short-term exposure to physiological stress, PCATS were treated with 2 µM cortisol (CORT) for the entire 48 hours or 100 nM epinephrine (EPI) for the last 12 hours of culture, respectively. Hormones were applied individually or in combination. CORT and EPI exhibited an interacting, blubber layer-dependent, effect on adipose tissue biology, as quantified by gene expression in PCATS, and release of glycerol, free fatty acids, leptin and polychlorinated biphenyls into culture media. EPI stimulated a higher rate of lipolysis than CORT in PCATS from both blubber layers. The combination of CORT and EPI upregulated the expression of adipose triglyceride lipase in inner blubber PCATS and downregulated hormone-sensitive lipase in outer blubber-derived PCATS. Expression of the leptin gene and secretion of the leptin adipokine were both decreased by EPI, while addition of CORT attenuated this effect in inner blubber PCATS only. CORT also increased the expression of the antioxidant enzyme glutathione peroxidase 3 in PCATS from both blubber layers. Polychlorinated biphenyls exhibited selective and limited mobilization from PCATS treated with stress hormones, highlighting the lipophilic properties of these toxic compounds. This study showed that physiological stress can impact several essential functions of marine mammal blubber, such as lipolysis and adipokine production.
1 Introduction
Over the past decades, marine mammals have been increasingly exposed to a variety of environmental stressors from anthropogenic sources. Studies on acoustic disturbances (e.g. boat traffic, offshore drilling, sonar), ship collisions, pollution as well as the reduction in the quantity and quality of prey suggested physiological stress-responses in marine mammals (Fair and Becker, 2000; Rolland et al., 2012; Erbe et al., 2019). Indeed, correlations between the exposure to such stressors and the levels of stress markers, such as fecal and serum glucocorticoids, were reported in numerous species of mysticetes, odontocetes, and pinnipeds (Rolland et al., 2012; Dantzer et al., 2014; Kershaw et al., 2017; Rolland et al., 2017; Champagne et al., 2018; Trumble et al., 2018; Erbe et al., 2019). In marine mammals, an exposure to environmental stressors is associated with changes in foraging, communication and resting behaviors, as well as potentially higher incidence of disease, impaired reproduction, and stranding that, together, can affect the health of individuals and more importantly, the overall survival of populations (Clark et al., 2006; Nowacek et al., 2007; Blair et al., 2016; Hing et al., 2016; Erbe et al., 2019; Mikkelsen et al., 2019). Additionally, the exposure of marine mammals to contaminants and their potential interactions with glucocorticoids are likely to alter physiological mechanisms in marine mammals (Fair and Becker, 2000; Jenssen, 2006; Peterson et al., 2023). Yet, little is known about the impact of stress hormones on the metabolism of marine mammals in vivo as studies in that field are particularly challenging for ethical and practical reasons.
In this context, investigating the effects of stress on sub-cutaneous adipose tissue, or blubber in marine mammals, is particularly relevant. In addition to being the only tissue accessible for sampling on living free-ranging individuals such as large cetaceans, this metabolically active organ plays essential roles in energy storage, insulation and the secretion of adipokines such as leptin, adiponectin and resistin (Frühbeck et al, 2001; Coelho et al., 2013; Choe et al., 2016; Kershaw et al., 2018). Leptin is a major adipokine involved in the regulation of energy homeostasis, neuroendocrine function and metabolism (Kelesidis et al., 2010). Adiponectin is involved in anti-inflammation processes, insulin sensitivity and stimulation of fatty acid oxidation, while resistin has the opposite effects (Bełtowski, 2003; McTernan et al., 2006). Stress hormones such as catecholamines (e.g. epinephrine EPI, norepinephrine) and glucocorticoids (e.g. cortisol CORT) as well as environmental pollutants are likely to affect the production of adipokines, which could impact metabolism and general health of individuals (Fried et al., 2000; Ferrante et al., 2014). Blubber is stratified in layers, with the inner blubber referring to the layer in contact with the muscles, and the outer blubber referring to the layer closest to the dermis. Both layers differ in their fatty acid composition, gene expression, and functions (Strandberg et al., 2008; Debier et al., 2012; Vanden Berghe et al., 2012; Louis et al., 2014a; Louis et al., 2015; Khudyakov et al., 2022). As a result, pollutant contents and mobilization may differ between the two layers while exposed to stress hormones.
Adipose tissue is one of the targets of stress hormones, which makes this organ highly relevant for studying the impact of stress exposure on the endocrine system and the metabolism of marine mammals in general. In cases of stress, the acute response of the sympathetic nervous system is to release catecholamines in the systemic circulation to meet the energy requirements of the body for the typical “fight or flight” response (Peckett et al., 2011). Glucocorticoids also take part in the response of the organism to stressors, with the activation of the hypothalamic-pituitary-adrenal (HPA) axis, which activates a cascade of reactions that stimulates the release of adrenocorticotropic hormone (ACTH). The binding of ACTH to its receptors in the adrenal cortex enables the synthesis of glucocorticoids that will then regulate various physiological processes such as inflammation, immunity and energy homeostasis (Peckett et al., 2011; Timmermans et al., 2019). In the case of chronic stress, the prolonged action of glucocorticoids appears to negatively impact the organism at various levels. For instance, chronic exposure to CORT is linked to neurophysiological alteration, anxiety, cardiovascular disease, inflammation and oxidative stress in humans and rodents (Elenkov et al., 1999; Espinoza et al., 2017; Zhang et al., 2021). While the individual effects of catecholamines and glucocorticoids have been well described in vitro and in vivo in biomedical systems (Xu et al., 2009; de Oliveira et al., 2011; Than et al., 2011; Stimson et al., 2017; Hua et al., 2018), there is a lack of knowledge regarding the impact of these stressors on the specific physiology of blubber in fasting-adapted diving marine mammals. Repeated exposures to stress may impair the use of lipid stores which is finely regulated and essential for energy demanding periods of fasting, lactation and breeding (Crocker et al., 2014). Stressors may also induce the mobilization of pollutants from blubber into the blood which could harm sensitive tissues and developing fetus as correlative studies have suggested (Gregoraszczuk and Ptak, 2013; Schnitzler et al., 2019). In addition, an overproduction of stress hormones may damage the endocrine system, as shown in bottlenose dolphins (Tursiops truncatus) (Clark et al., 2006). The diving ability of marine mammals may be impaired by stress hormones as well, through the induction of oxidative damages (Costantini et al., 2011; Vázquez-Medina et al., 2011).
Northern elephant seals (Mirounga angustirostris – NES) represent an ideal model to study the impact of physiological stress responses at the blubber level, because of their accessibility, capital breeding strategy, and their robustness to research handling, as shown by the absence of a stress response during blubber sampling under sedation (Champagne et al., 2012; Crocker et al., 2014). NES rely on the land for breeding, lactation, molting and for the post-natal development of pups. After a short and intense lactation, mothers leave the rookery and the pups experience a developmental fasting period on land for approximately two months (Le Boeuf et al., 2000), during which the oxidation of fat stores is increasingly stimulated (Crocker et al., 2014).
In order to draw cause-effect relationships between stress exposure and adipose tissue metabolism in marine mammals, we recently developed an ex vivo approach of precision-cut adipose tissue slices (PCATS) from NES (Debier et al., 2020). In the present study, we used this novel model to investigate the interactive effects of the two main stress hormones, CORT and EPI, on adipose tissue function and pollutant mobilization. To do so, blubber biopsies were collected from weaned NES pups and PCATS were produced separately on inner and outer blubber. Our previous study showed that PCATS generated from NES blubber remained viable for several days in culture and respond to stimulation with isoproterenol, a synthetic catecholamine (Debier et al., 2020). To mimic the response of marine mammals experiencing prolonged stress, we exposed PCATS to CORT in culture for 48 hours. EPI was added for the last 12 hours of culture. Both hormone treatments were applied individually and in combination in order to evaluate their specific and combined impacts on blubber. As a readout of tissue responses to stress hormones, we measured the lipolytic activity level of adipocytes through the release of glycerol and free fatty acids from PCATS into culture media. Lipolysis is a three-step process catalyzed by adipose triglyceride lipase (ATGL), hormone sensitive lipase (HSL) and monoglyceride lipase (MGL) that produces glycerol and free fatty acids from triglycerides (Lafontan and Langin, 2009; Coelho et al., 2013). We measured expression of genes encoding for ATGL and HSL in PCATS to determine whether they are regulated by individual and combined stress hormones in this fasting-adapted species. The impact of stressor hormones on the endocrine function of blubber was assessed by measuring the expression and secretion of the adipokine leptin as well as the expression of adiponectin and resistin. The expression of glutathione peroxidase 3 (GPx3), a key regulator of the oxidative stress in blubber and a well-known marker of corticosteroid elevation in seals in vivo, was also analyzed to measure the responsiveness of PCATS to stressors and to evaluate the combinatory effects of EPI and CORT on anti-oxidant enzymes (Crocker et al., 2016; Khudyakov et al., 2017; Deyarmin et al., 2019; Pujade Busqueta et al., 2020). Finally, we explored the mobilization of polychlorinated biphenyls (PCBs), that are persistent organic pollutants transferred to the blubber of pups through lactation (Debier et al., 2003; Debier et al., 2012), by measuring their mobilization from PCATS following stress hormone exposures. To the best of our knowledge, this is the first study that investigates the metabolic and toxic impact of the stress hormones cortisol and epinephrine on marine mammal blubber cultured ex vivo.
2 Materials and methods
2.1 Blubber collection and PCATS preparation
All methods were carried out in accordance with relevant guidelines and regulations. All capture and handling procedures were approved by the Sonoma State University Institutional Animal Care and Use Committee (IACUC #2017-56) and were performed under National Marine Fisheries Service Marine Mammal Permit #19108. Weaned NES pups were sampled at Año Nuevo State Park, CA, United States (37°06’30’’N, 122°20’10’’W), from February to April 2020. A total of 5 females (3 early and 2 late in their post-weaning fasting period, determined by percentage molt of natal pelage) and 12 males (5 early and 7 late) were sampled. Due to the limited sample size, we were not able to differentiate between early and late fasting stages in our experimental protocol. To avoid variability linked to fasting stages, sets of experiments were conducted on one fasting stage without mixing samples from early and late pups (Table 1). Anesthesia was induced by intramuscular injection of Telazol (1 mL/100 kg of estimated body mass; Telazol; Fort Dodge Animal Health, Fort Dodge, United States). Sedation was maintained through intravenous injections of ketamine as needed (Ketaset; Fort Dodge Animal Health, Fort Dodge, United States). An area on the side of the animal anterior to the lateral pelvis was cleaned with 70% ethanol and a 3-cm incision was made to cut the skin. Blubber biopsies were then collected using 6-mm biopsy punches (Uni-Punch, Premier, Plymouth, United States). Samples were rinsed in phosphate buffered saline before being transferred to pre-oxygenated sampling medium adapted from Bourez et al. (2013) and Louis et al, 2014b; Louis et al, 2014c) and warmed at 25°C. This medium contained Dulbecco’s modified eagle medium (DMEM) with 4.5 g/L glucose (Gibco, Life Technologies, Thermo Fisher Scientific, Waltham, MA, United States) supplemented with 10 mM HEPES (Life Technologies) and an antibiotic-antimycotic mixture (100 U/mL penicillin, 100 U/mL streptomycin, 250 ng/mL amphotericin (Life Technologies), 40 U/mL nystatin (Sigma-Aldrich, Saint-Louis, United States) and 1 mg/mL gentamicin (Life Technology)). After collection, blubber samples were transferred to the laboratory. Inner blubber (located closest to muscle) was separated from outer blubber (located closest to skin) by splitting biopsies in two parts, excluding the middle layer. PCATS were performed as described in Debier et al. (2020). Briefly, slices 1 mm thick and 6 mm diameter were generated using a Mouse and Rat Spinal Cord Matrices device (Ted Pella Inc., Redding, United States). Each well of 24-well plates was filled with 4 pre-weighed PCATS in 1 mL of culture medium and placed under agitation in an incubator (37°C, 5% of CO2). This culture medium was composed of DMEM with 1 g/L glucose (Gibco) supplemented with 5% fetal bovine serum (Gibco), 2% (w:v) bovine serum albumin fraction V (Research Products International, Mount Prospect, IL, United States) and an antibiotic-antimycotic mixture (100 U/mL penicillin, 100 U/mL streptomycin, 250 ng/mL amphotericin (Life Technologies), 40 U/mL nystatin (Sigma-Aldrich) and 50 μg/mL gentamicin (Life Technology)). After 2 hours, all culture media, containing cellular debris caused by slicing, were removed and replaced with fresh media containing stress hormones, as described below.
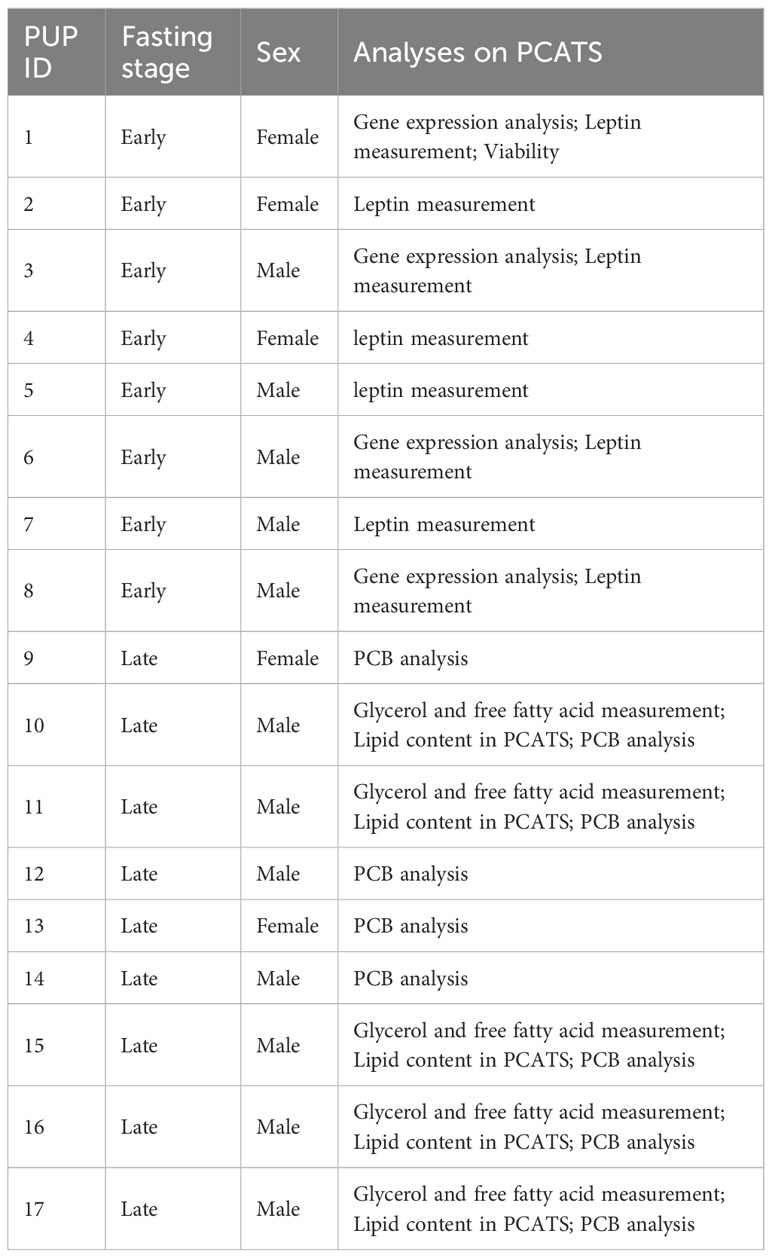
Table 1 Post-weaning fast stage and sex of northern elephant seal pups sampled for precision-cut adipose tissue slice (PCATS) experiments and downstream analyses.
2.2 Stress hormone treatments
The concentration of CORT (Sigma-Aldrich) for PCATS treatments (2 μM) was determined based on levels reported in vivo after activation of the HPA axis by exogenous ACTH administration in juvenile NES (Khudyakov et al., 2017). Based on preliminary investigation (data not shown), we determined that the minimal concentration of EPI (epinephrine bitartrate salt, Sigma-Aldrich) that was necessary to significantly induce lipolysis in NES PCATS was 100 nM, which is physiologically relevant based on levels reported in vivo in serum of NES pups and adults (Crocker et al., 2014). Preliminary experiments also showed that the maximal lipolytic response of NES PCATS to EPI happened 12 hours after EPI was added to the culture medium. CORT was dissolved in absolute ethanol and diluted in water. The final concentration of ethanol in culture media was 0.01%. EPI was dissolved in water. Based on our previous research (Debier et al., 2020), PCATS were cultured for 48 hours and culture media were collected and renewed every 12 hours (Figure 1). Three hormonal treatments were applied: i) 2 μM of CORT throughout the 48 hours of culture, ii) 100 nM of EPI added after 36 hours, for the last 12 hours of culture, and iii) 2 μM of CORT for 48 hours combined with 100 nM of EPI added after 36 hours, for the last 12 hours of culture. PCATS cultured for 48 hours in parallel without stress hormones served as a control. Experiments were conducted in triplicate for PCATS from each pup. After 48 hours, PCATS were rinsed twice in PBS and stored at -80°C for further analyses. For gene expression assays, PCATS were rinsed, flash-frozen in liquid nitrogen, and stored at -80°C.
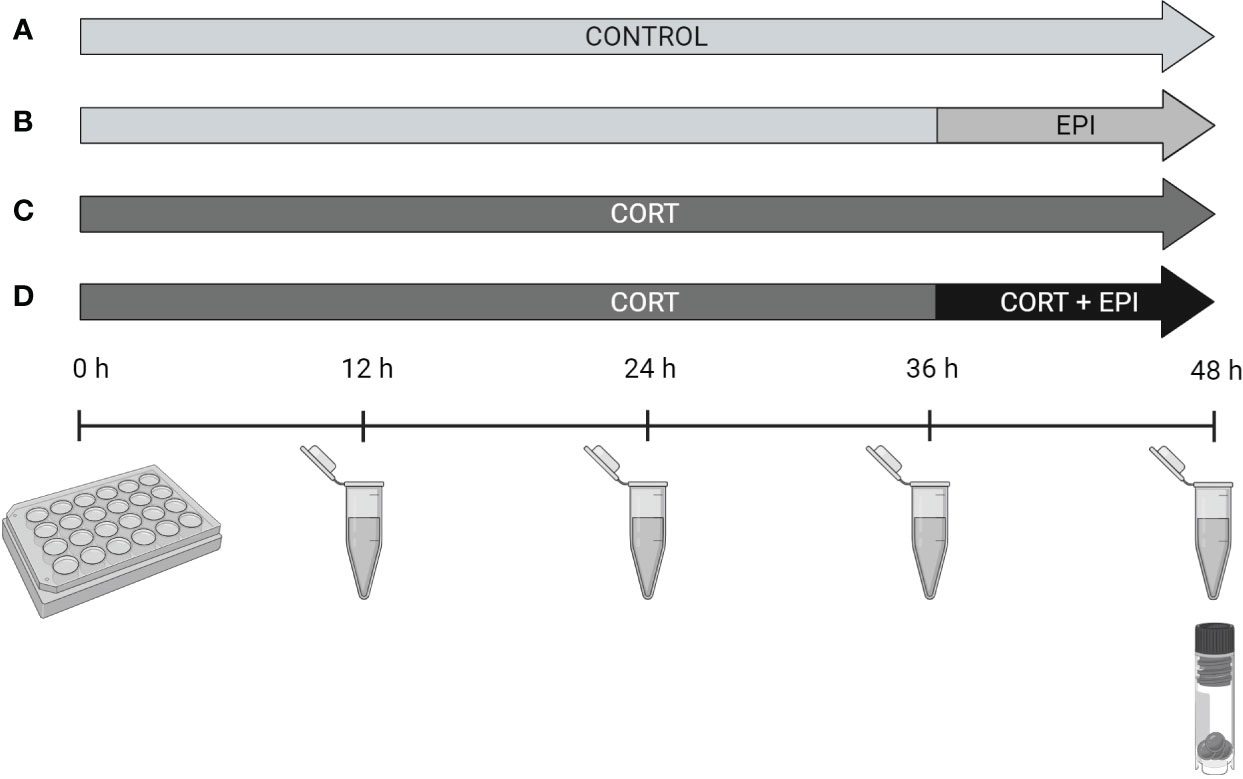
Figure 1 Illustration of the experimental design. Four conditions were applied on precision-cut adipose tissue slices from northern elephant seals during a culture of 48 hours: (A) Control consisting of culture medium only, (B) epinephrine 100 nM applied once, at 36 hours of culture (EPI), (C) cortisol 2 µM applied throughout 48 hours of culture (CORT) and (D) combination of cortisol 2 µM applied throughout 48 hours and epinephrine 100 nM added at 36 hours of culture (CORT + EPI). Culture media were collected and replaced every 12 hours. Blubber slices were flash-frozen and stored at -80°C after culture. Created with BioRender.com.
2.3 Viability
Triplicate samples from one female weaned pup sampled early in the post-weaning fast were used for this analysis (Supplementary Table 1). To assess viability of PCATS during culture treatments, we measured the lactate dehydrogenase (LDH) content in associated culture media as described in Debier et al. (2020). LDH was measured by colorimetric assay with a cytotoxicity detection kit (Cytotoxicity Detection Kit (LDH), Roche, Sigma-Aldrich) and Spectramax 190 Microplate Reader (Molecular Devices, San Jose, CA, United States) following the manufacturer’s protocol. Values were normalized by g of tissue wet weight. The LDH released into the culture medium after 48 hours was compared to the amount of LDH initially present in PCATS to calculate percentage of cell mortality (see Debier et al., 2020 for more details). Mortality remained below 10% of positive control (Supplementary Table 1).
2.4 Glycerol quantification
Samples from five male weaned pups sampled late in their post-weaning fast were used for this analysis (Table 1). Glycerol was measured in culture media collected after the last 12 hours of culture by colorimetric assay with a glycerol assay kit (MAK117, Sigma-Aldrich) and Spectramax 190 Microplate Reader (Molecular Devices) following the manufacturer’s protocol. Values were normalized by g of tissue wet weight.
2.5 Lipid quantification and profiling in culture media
Samples from five male weaned pups sampled late in their post-weaning fast were used for this analysis (Table 1). Lipids released from PCATS between 36 hours and 48 hours of culture were extracted from culture media by the Bligh and Dyer (1959) method. One of the triplicates for each condition was selected at random for each pup that was analyzed. Briefly, 800 μL of culture medium was mixed with 5 mL of ethanol/chloroform/water (2:2:1, v:v:v). An internal standard was added to quantify loss of free fatty acids during extraction (i.e. nonadecanoic acid; Larodan, Solna, Sweden). After centrifugation, the aqueous phase was used for a second extraction with 2 mL of chloroform. The chloroform phases from the two extractions were pooled and placed on a silica column (Bond Elut, Agilent Technologies, Santa Clara, CA, United States) conditioned with hexane. Free fatty acids were eluted with diethylether/acetic acid (98:2, v:v). Fatty acid methyl esters (FAMEs) formed after the methylation step with KOH/MeOH 0.1% and HCl/MeOH 1,2N (5:2, v:v) were extracted with hexane/water (2:1, v:v), and an injection standard (i.e. methyl-undecanoate; Larodan) was added to all samples. FAMEs were then separated by gas chromatography. The gas chromatograph (Trace 1310; Thermo Fisher Scientific, Milan, Italy) was equipped with an autosampler TriPlusAS (Thermo Fisher Scientific) and a Rt®-2560 capillary column (biscyanopropyl polysiloxane, 100 m length, 0.25 mm internal diameter, 0.2 μm film thickness; Restek, Bellefonte, PA, United States) with H2 as carrier gas at constant pressure (200 kPa). The oven temperature program was designed as followed: 175°C for 25 minutes, 200°C for 20 minutes, 220°C for 5 minutes and 235°C for 15 minutes. The baseline temperature was 80°C. FAMEs detection was carried out with a Flame Ionisation Detector (Thermo Fisher Scientific) maintained at 255°C and flowed by air (350 mL/minute), N2 (40 mL/minute) and H2 (35 mL/minute). Each peak in the chromatographs was identified and quantified by comparison to an external standard composed of 43 FAMEs (Larodan) with Chromeleon™ Chromatography Data System (CDS) Software 7.3 (Thermo Fisher Scientific). Results were expressed in µmol of fatty acids per g of tissue wet weight. To determine the relative abundance of fatty acid classes, fatty acids were grouped in four classes (adapted from Fowler et al., 2014 and Louis et al., 2015). Saturated fatty acids (SFAs) were composed of C6:0, C8:0, C10:0, C12:0, C13:0, C14:0, C15:0, C16:0, C18:0, C20:0, C22:0 and C24:0. Monounsaturated fatty acids (MUFAs) included C14:1 n-5, C16:1 n-7, C18:1 n-9, C18:1 n-7, C20:1 n-9, C22:1 n-9 and C24:1 n-9. Polyunsaturated fatty acids (PUFAs) were separated in ω-3 PUFAs with C18:3 n-3, C18:4 n-3, C20:3 n-3, C20:4 n-3, C20:5 n-3, C22:5 n-3, C22:6 n-3, C24:5 n-3, C24:6 n-3 and ω-6 PUFAs containing C18:2 n-6, C18:3 n-6, C20:2 n-6, C20:3 n-6, C20:4 n-6, C22:4 n-6 and C22:5 n-6. A detailed table of the level of each fatty acid measured in culture media from all the conditions is available in the Supplementary Material (Supplementary Table 2). The fatty acids C6:0, C8:0, C10:0, C13:0, C22:0, C24:0, C24:1 n-9, C24:5 n-3 and C24:6 n-3 were excluded from the analysis since their level was undetectable in all of the samples. After having summed the levels of lipids in each class, we calculated the relative abundance of the four fatty acid classes released in culture media as percentages.
2.6 Lipid content in PCATS
Samples from five male weaned pups sampled late in their post-weaning fast were used for this analysis (Table 1). PCATS from triplicates were pooled by condition for each pup (∼60 mg of PCATS). Tissues were homogenized with 800 μL water using a bead-based homogenizer (Precellys 24, Bertin Intruments, Montigny-le-Bretonneux, France). The lipid extraction protocol was similar to culture media samples (Bligh and Dyer method). After extraction, the chloroform phase was evaporated under a nitrogen flux at 30°C for 45 minutes. Lipid contents were measured by gravimetry and expressed in g of tissue wet weight.
2.7 Gene expression analyses
Samples from four weaned pups sampled early in their post-weaning fast were used for this analysis (Table 1). Ribonucleic acid (RNA) was isolated from PCATS using RNeasy Lipid Mini Kit (Qiagen, Germantown, MD, United States) according to the manufacturer’s protocol. Specifically, PCATS from replicate wells of each condition (∼60 mg) were pooled and homogenized with 1 mL of Qiazol (Qiagen) using the Bullet Blender Storm 24 (Next Advance, United States; 2 cycles of 2 minutes at 20 mHz) followed by genomic DNA disruption using a 21-gauge needle. After phase extraction with chloroform, RNA samples were purified using RNeasy columns with a 20 minutes on-column DNase I digest (Qiagen). RNA was eluted in diethylpyrocarbonate (DEPC) treated water. RNA was quantified using RNA High Sensitivity Assay on the Qubit 3.0 fluorometer (Thermo Fisher Scientific) and integrity was assessed using the RNA 6000 Pico kit on the 2100 Bioanalyzer (Agilent Technologies). RNA samples had integrity numbers (RIN) values between 8.2 and 8.9. Complementary deoxyribonucleic acid (cDNA) was synthesized using 200 ng of total RNA and SuperScript IV VILO with ezDNase kit (Thermo Fisher Scientific). The sequences for all of the primers that were used are listed in Table 2. cDNA samples were diluted 1:10. The final concentration of primers was 400 nM. PowerUp SYBR Green Master Mix (Thermo Fisher Scientific) and 2 μL of diluted cDNA were used for the quantitative polymerase chain reaction (qPCR) which was performed on the QuantStudio 5 Real-Time PCR instrument using manufacturer’s protocol (Thermo Fisher Scientific). Samples were run in triplicates. The qPCR cycle was 2 minutes at 50°C, 2 minutes at 90°C, followed by 15 seconds at 95°C and 1 minute at 60°C for 40 cycles. Two reference genes (NONO and RPL27) were selected based on their stability in expression across the samples and culture treatments (NormFinder stability values of 0.62 for NONO and 0.26 for RPL27) (Andersen et al., 2004) and that were previously validated in NES (Pujade Busqueta et al., 2020). The relative expression of target genes (delta Ct) was normalized by subtracting the geometric mean of cycle thresholds (Ct) of reference genes from the mean Ct of target genes.
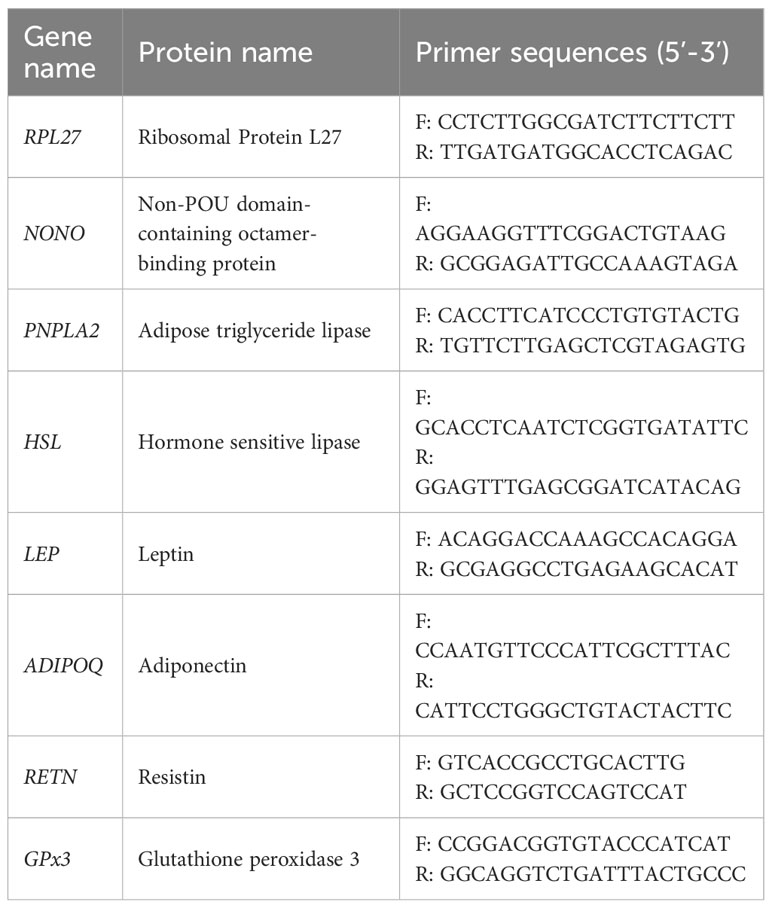
Table 2 Primer sequences for qPCR assays. Primers were designed using gene sequences from elephant seal blubber transcriptomes (from Khudyakov et al., 2017; Khudyakov et al., 2019; Pujade Busqueta et al., 2020).
2.8 Leptin quantification
Secretion of leptin into culture media was measured using a subsample of PCATS isolated from 8 pups sampled early in their post-weaning fast (Table 1). Leptin concentrations in culture media were measured using a radioimmunoassay according to the manufacturer’s protocol (Multi-Species Leptin RIA kit, Linco, St. Charles, United States) previously validated for use in NES (Ortiz et al., 2001). Two technical replicates were performed for each sample. Coefficients of variation lied between 0.16 and 5.29% and averaged 2.41%. Concentrations of leptin were normalized per g of tissue wet weight.
2.9 PCB determination in culture media and PCATS
All pups for this analysis were sampled late in their post-weaning fast (Table 1). Since levels of PCBs in culture media were very low, samples were pooled in three groups composed of media from PCATS from three pups in each group to reach a volume of 9 mL per condition per group. Corresponding PCATS samples were pooled the same way. Serum from culture media was degraded with formic acid 99% (1:1, v:v). After 5 minutes of degradation, an equivalent amount of MilliQ water (Merck Millipore, Massachusetts, USA) was added to stop the reaction. C18 SPE cartridge (Isolute, Biotage, Uppsala, Sweden) were conditioned with methanol (Biosolve, Dieuze, France), followed by MilliQ water. The samples were then transferred on the cartridge and rinsed with 10 mL of MilliQ water. Then, the column was vacuum dried for 2 hours with filtered air using activated charcoal filters. A Na2SO4 drying cartridge (Isolute, Biotage, Uppsala, Sweden) was added below the C18 prior to the elution with 15 mL of n-hexane (Biosolve, Dieuze, France). Extracts were concentrated at a volume of 2 mL, before a chromatographic purification step on an acid silica/neutral alumina column with 35 mL of n-hexane. Samples were then evaporated under nitrogen flux at 30°C to a final volume of 90 μL of n-nonane (Merck, Massachusetts, USA) and kept in fridge until injection. For PCBs measurements in blubber, PCATS were first microwaved for 1 minute and lipids were solubilized in 5 mL of n-hexane (Beltest, 2000). Samples were then sonicated for 10 minutes. After a clean-up step on the acid silica/neutral alumina column, samples were concentrated to 90 μL of n-nonane and kept in fridge until injection. Analyses were performed on an Agilent 6890 gas chromatograph (Palo Alto, CA, United States), coupled to an Autospec Ultima mass spectrometer (Waters, Manchester). Two µL of samples were injected at 275°C in splitless mode on an HT8 column (25 m x 0.22 mm x 0.25 µm; Trajan, Ringwood Victoria, Australia) at a 0.8 mL/minute He (6.0 purity; Air Liquide, Belgium) constant flow. The analysis was performed in Selected Ion Monitoring (SIM) at 10,000 mass resolution with Electronic Ionization at 35 eV. Two ions (quantifier and qualifier) were recorded for each analyte and their ion ratio was checked for interferences. The quantification of seven indicators PCBs (namely PCB-28, 52, 101, 118, 138, 153 and 180) was achieved through the isotope dilution technique using 13C12 labelled analog of every congener. Concentrations of PCBs were normalized by g of tissue wet weight. As levels of PCB-28, 52 and 101 were very low in both matrices, we focused our analysis on the four most abundant congeners (PCB-118, 138, 153 and 180) that were detected (data available in Supplementary Table 3). To evaluate the impact of stress hormones on the selective mobilization of PCBs from PCATS, we calculated the relative abundance of the four congeners stored in PCATS and released in associated culture media as percentages.
2.10 Statistical analysis
Statistical analyses were performed using JMP PRO 16.0 software. Linear mixed models were generated to determine the effect of treatments and blubber layers on glycerol, fatty acid, leptin and gene expression. For the comparison of the relative abundance of PCBs in PCATS and associated culture media, we combined levels from inner and outer-derived PCATS since no significant difference was observed between layers. For all analysis, the blubber layer from which PCATS were derived and the experimental treatment were set as fixed effects, while pup ID was used as a random effect. For analyses including males and females (i.e. leptin secretion and gene expression analysis), the sex of each pup was included as a fixed effect of the linear mixed model. The normal distribution and homoscedasticity of groups were verified. A square root transformation of glycerol, total free fatty acid and fatty acid class (SFAs, MUFAs, ω-3 PUFAs and ω-6 PUFAs) concentrations was made to meet the assumptions of the models (normal distribution and homoscedasticity of residuals). A logarithmic transformation of leptin and PCB concentrations in culture media was made to meet the assumptions of the models. Non-significant effects were excluded for post-hoc tests. Student’s t-tests were run on relevant comparisons and a Šidák correction was applied on each test. Irrelevant comparisons refer to the analysis of different treatments from different layer-derived PCATS (e.g. inner-derived PCATS in control condition vs outer-derived PCATS exposed to CORT + EPI). Non-parametric tests were performed on relative abundances of the four fatty acid classes as data distributions were not normal (Friedman rank test followed by Wilcoxon signed rank tests). The level of statistical significance was set at p < 0.05 for all analyses.
3 Results
3.1 Impact of stress hormones on lipolysis
We investigated the impact of CORT and EPI on the release of the two lipolytic products, glycerol and free fatty acids, in culture media collected after the last 12 hours of culture (from T = 36 h to T = 48 h of culture). The lipid content of the PCATS was also evaluated after 48 hours of culture. The release of both lipolytic products was impacted by stress hormones and followed a similar trend (glycerol: F3,28 = 122.09, p < 0.0001; free fatty acids: F3,28 = 222.03, p < 0.0001; Figures 2A, B). For inner blubber-derived PCATS treated with EPI and CORT + EPI, amounts of glycerol and free fatty acid release were significantly higher than control PCATS (p < 0.0001), as well as those treated with CORT alone (p < 0.0001). CORT alone also induced higher release of free fatty acids compared to control, but to a much lesser extent than EPI (p = 0.0033). In outer blubber, CORT + EPI treatment induced significantly higher release of free fatty acids than EPI alone (p < 0.0001) (Figure 2B). CORT alone also induced higher release of glycerol and free fatty acid compared to control (glycerol: p = 0.0018; free fatty acids: p = 0.0005). The amount of lipolytic products released into culture media in response to each condition did not differ between PCATS derived from inner vs those derived from outer blubber layers (glycerol: p = 0.7555; free fatty acids: p = 0.1812; Figures 2A, B).
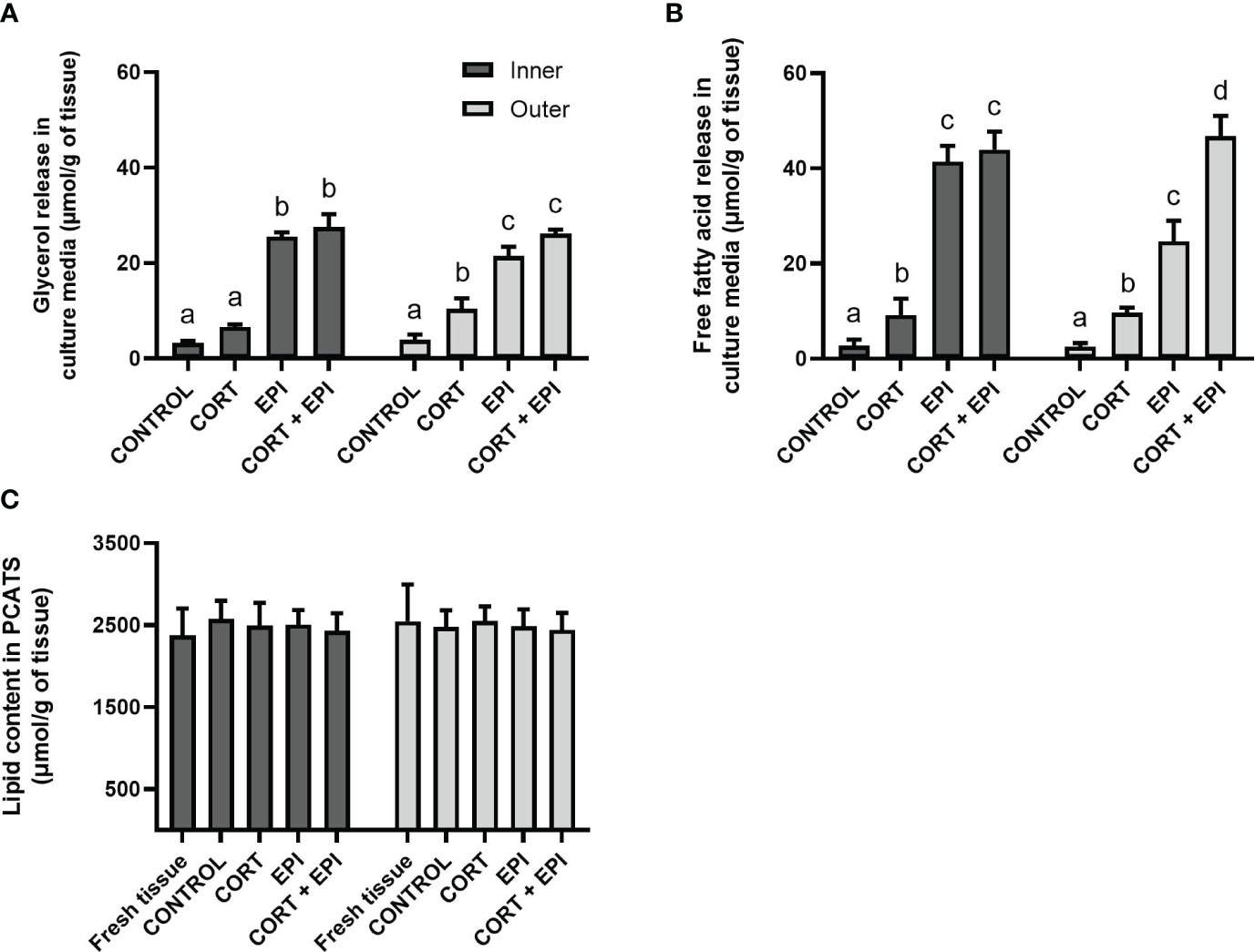
Figure 2 Analysis of lipolytic products, glycerol and free fatty acids, from precision-cut adipose tissue slices (PCATS) from northern elephant seal after ex vivo culture with stress hormones as well as lipid content of PCATS before and after exposure to stressors. PCATS were cultured for 48 hours and exposed to 2 µM cortisol for 48 hours (CORT), 100 nM EPI during the last 12 hours of culture (EPI), or to the combination of both 2 µM cortisol for 48 hours and 100 nM EPI during the last 12 hours of culture (CORT + EPI). PCATS from inner and outer blubber were cultured separately. Controls refer to culture media without added hormones. Mean ± SEM of 5 pups sampled late in their post-weaning fast are shown. (A) Glycerol released from PCATS in culture media. (B) Free fatty acids released from PCATS in culture media. (C) Lipid content of PCATS. “Fresh tissue” refers to PCATS that were analyzed directly after collection, before culture. Significant differences between treatments of PCATS within each blubber layer are represented by different letters (p < 0.05). There was no significant difference between PCATS derived from different blubber layers within each condition (p > 0.05).
The amount of lipids remaining in PCATS at the end of the culture period did not differ between treatments (p = 0.7602) or layers from which PCATS were derived (p = 0.7343; Figure 2C). Interestingly, lipid levels remained similar to those initially measured in fresh tissue before culture. Among all conditions, the amount of free fatty acids released into culture media represented between 0.5 and 2.5% of the lipids initially present in PCATS.
Both glycerol and free fatty acids resulting from the breakdown of triglycerides stored in the lipid droplet of the PCATS adipocytes should appear in the culture medium at a ratio of 1:3 (glycerol:free fatty acid). In all of the experimental conditions, the ratio was below 1:3, reflecting potential re-esterification of free fatty acids by adipocytes. By comparing the predicted level of free fatty acids based on glycerol measurement and the actual levels of fatty acids quantified in culture media, we could estimate the re-esterification of free fatty acids by adipocytes and calculate a percentage of fatty acid re-uptake (Table 3). In all conditions, statistical analysis showed a significantly lower level of fatty acid released compared to the predicted values (F1,70 = 98.91, p < 0.0001). Overall, the re-esterification rate was similar between blubber layers (p = 0.4383) even if a trend towards a higher re-esterification was observed in PCATS from outer blubber submitted to the EPI treatment. On the other hand, the re-esterification rate was significantly altered by stress hormones (F3,28 = 3.05, p = 0.0451) but post-hoc tests were not significant (p > 0.05).
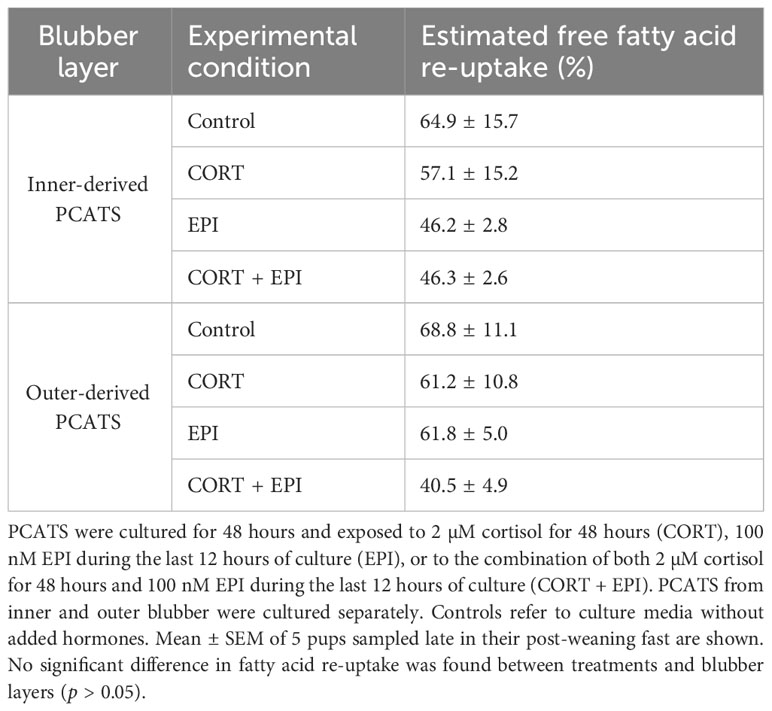
Table 3 Estimated fatty acid re-uptake from the culture media into precision-cut adipose tissue slices (PCATS) from northern elephant seals treated with stress hormones.
The relative abundance of saturated (SFAs), monounsaturated (MUFAs) and ω-3 and ω-6 polyunsaturated fatty acids (PUFAs) released from PCATS into culture media was evaluated for each condition and blubber layer (Figure 3A). We observed no distinction in the profile of fatty acid classes between treatments and blubber layers (p > 0.05).
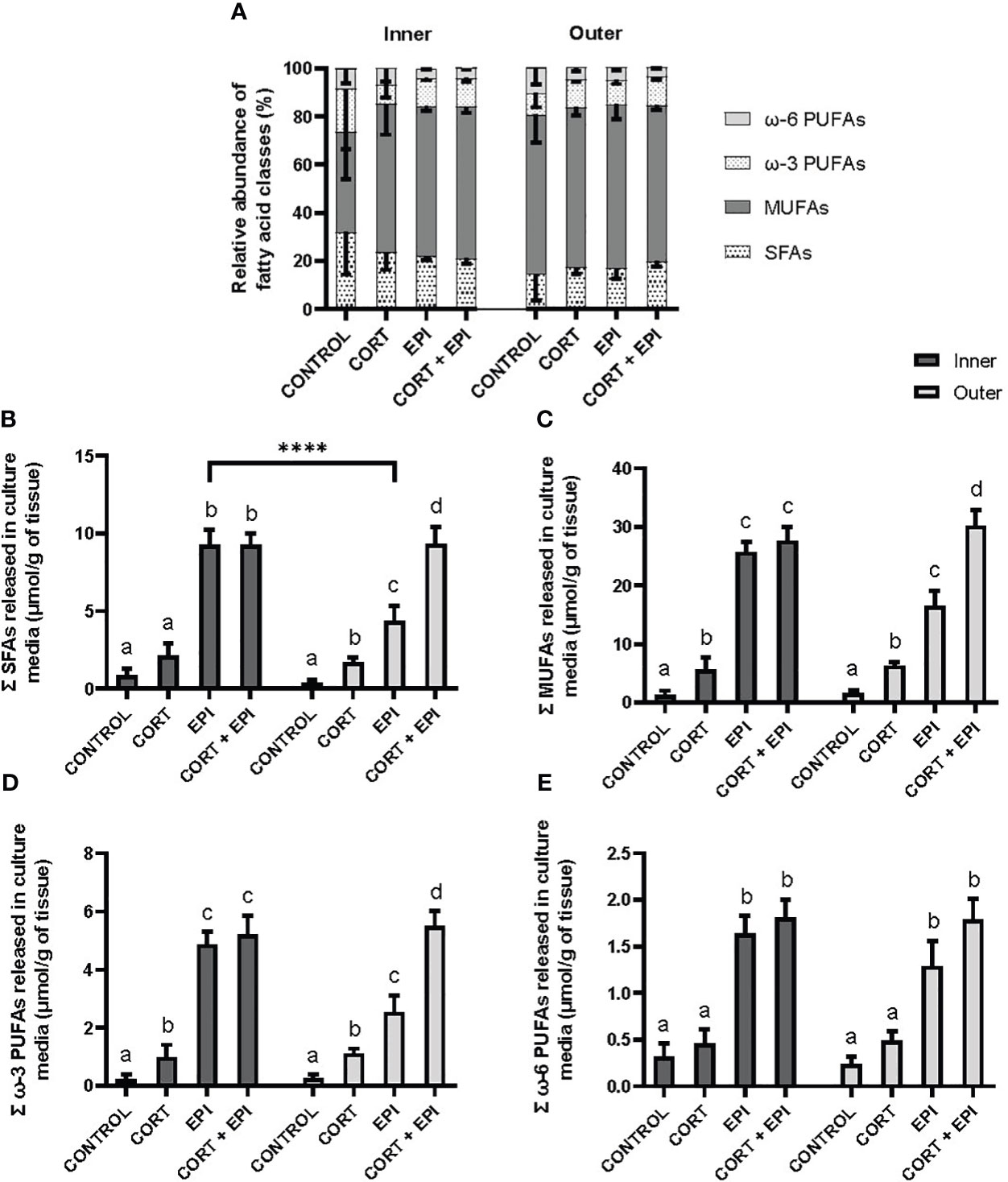
Figure 3 Analysis of the relative abundance and concentrations of four fatty acid classes released by precision-cut adipose tissue slices (PCATS) from northern elephant seals into culture media in response to stress hormones. PCATS were cultured for 48 hours and exposed to 2 µM cortisol for 48 hours (CORT), 100 nM EPI during the last 12 hours of culture (EPI), or to the combination of both 2 µM cortisol for 48 hours and 100 nM EPI during the last 12 hours of culture (CORT + EPI). PCATS from inner and outer blubber were cultured separately. Controls refer to culture media without added hormones. Mean ± SEM of 5 pups sampled late in their post-weaning fast. (A) Relative abundance of the four fatty acid classes in culture media. For each fatty acid class, no difference was observed between conditions (p > 0.05). (B) Saturated fatty acids (SFAs), (C) monounsaturated fatty acids (MUFAs) as well as (D) ω-3 and (E) ω-6 polyunsaturated fatty acids (PUFAs). Significant differences between treatments of PCATS within each blubber layer are represented by different letters (p < 0.05). Significant differences between PCATS derived from different blubber layers within each condition are represented by “****” (p < 0.0001).
Release of specific fatty acid classes (Figures 3B–E), showed a similar trend to the response of total fatty acids to stress hormones (Figure 2B). The hormone treatments had a significant impact on the concentrations of each of the four classes of lipid released from PCATS (SFAs: F3,28 = 138.78, p < 0.0001; MUFAs: F3,28 = 224.28, p < 0.0001; ω-3 PUFAs: F3,28 = 154.57, p < 0.0001; ω-6 PUFAs: F3,28 = 122.64, p < 0.0001). The layer from which PCATS were derived had a significant impact only on SFAs (F1,28 = 12.24, p = 0.0016). For inner blubber-derived PCATS, the concentration of the four classes of fatty acids in culture media was significantly higher in EPI and CORT + EPI conditions compared to control and CORT conditions (for all comparisons: p < 0.0001). The only classes of fatty acids that had higher concentrations in culture media in response to CORT treatment compared to the control were MUFAs (p = 0.0012) and ω-3 PUFAs (p = 0.0423). For outer blubber-derived PCATS, the release of all fatty acid classes increased in response to hormone treatments in the following order: Control < CORT < EPI < CORT + EPI (for all comparisons: p < 0.05) except for ω-6 PUFAs for which differences between control and CORT conditions were not significant (p = 0.0788) as well as between EPI and CORT + EPI treatments (p = 0.0798). The only significant difference between blubber layers was found in EPI-treated PCATS, where higher levels of SFAs were released from inner blubber-derived PCATS compared to those from outer blubber (p < 0.0001). The concentrations of all analyzed free fatty acids are available in the Supplementary Material (Supplementary Table 2).
The impact of stress hormones on regulation of lipolysis was also assessed at the level of gene expression, by measuring abundance of transcripts encoding two key enzymes involved in lipolysis, PNPLA2, coding for ATGL, and HSL (Figure 4). For both genes, sex of individuals had no impact on results (PNPLA2: p = 0.8230; HSL: p = 0.2301). The expression of PNPLA2 differed between the hormone treatments (F3,21 = 9.13, p = 0.0005) as well as with the blubber layers from which PCATS derived (F1,21 = 10.31, p = 0.0042). Yet, post-hoc tests exposed no difference in PNPLA2 expression between inner and outer blubber layers for each treatment (p > 0.05). In inner blubber-derived PCATS, CORT + EPI treatment significantly increased the expression of PNPLA2 compared to control (p = 0.0122) and EPI (p = 0.0247) treatments (Figure 4A). On the other hand, PNPLA2 expression in outer blubber was not altered by any of the hormone treatments (p > 0.05). The expression of HSL in PCATS was altered by hormone treatments (F3,21 = 8.29, p = 0.0008) and differed between blubber layers (F1,21 = 13.49, p = 0.0014). A significant downregulation of HSL was observed in outer blubber-derived PCATS exposed to CORT + EPI compared to control (p = 0.0008) and CORT (p = 0.0231) conditions (Figure 4B). Under CORT + EPI treatment, HSL was more expressed in inner-derived PCATS than outer-derived PCATS (p = 0.0135).
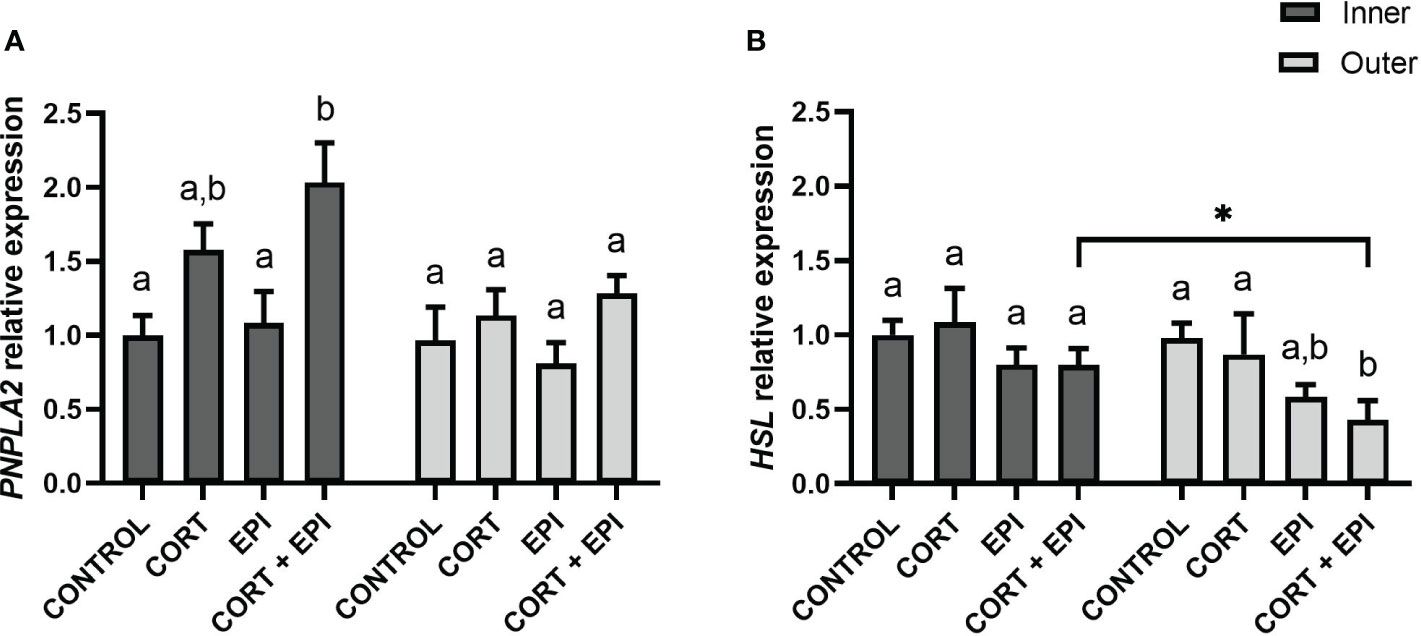
Figure 4 Relative expression of adipose triglyceride lipase (PNPLA2) and hormone-sensitive lipase (HSL) in precision-cut adipose tissue slices (PCATS) from northern elephant seals in response to stress hormones. PCATS were cultured for 48 hours and exposed to 2 µM cortisol for 48 hours (CORT), 100 nM EPI during the last 12 hours of culture (EPI), or to the combination of both 2 µM cortisol for 48 hours and 100 nM EPI during the last 12 hours of culture (CORT + EPI). PCATS from inner and outer blubber were cultured separately. Controls refer to culture media without added hormones. (A) Relative expression of PNPLA2. (B) Relative expression of HSL. Mean ± SEM of 4 pups sampled early in their post-weaning fast. Expression of PNPLA2 and HSL were normalized with reference genes (delta Ct) and expressed as relative to delta Ct values of inner blubber-derived PCATS cultured in control conditions. Significant differences between treatments of PCATS within each blubber layer are represented by different letters (p < 0.05). Significant differences between PCATS derived from different blubber layers within each condition are represented by “*” (p < 0.05).
3.2 Impact of stress hormones on adipokine gene expression and leptin secretion
We investigated the expression of LEP in PCATS (Figure 5A). Both conditions and blubber layers from which PCATS derived impacted LEP expression (conditions: F3,21 = 24.44, p < 0.0001; layers: F1,21 = 46.25, p < 0.0001). Sex of individuals had no impact on results (p = 0.1702). In PCATS derived from inner blubber, EPI downregulated LEP expression relative to control (p = 0.0329) and CORT (p = 0.0004) conditions. Interestingly, the inhibitory effect of EPI on leptin expression was offset by the presence of cortisol (CORT + EPI; p = 0.0021). In PCATS derived from outer blubber, LEP was also downregulated by EPI compared to control (p = 0.0004), CORT (p = 0.0001) and CORT + EPI conditions (p = 0.0106). The expression of LEP in inner blubber was significantly higher than in outer blubber derived-PCATS in CORT (p = 0.0478), EPI (p = 0.0161) and CORT + EPI conditions (p = 0.0032).
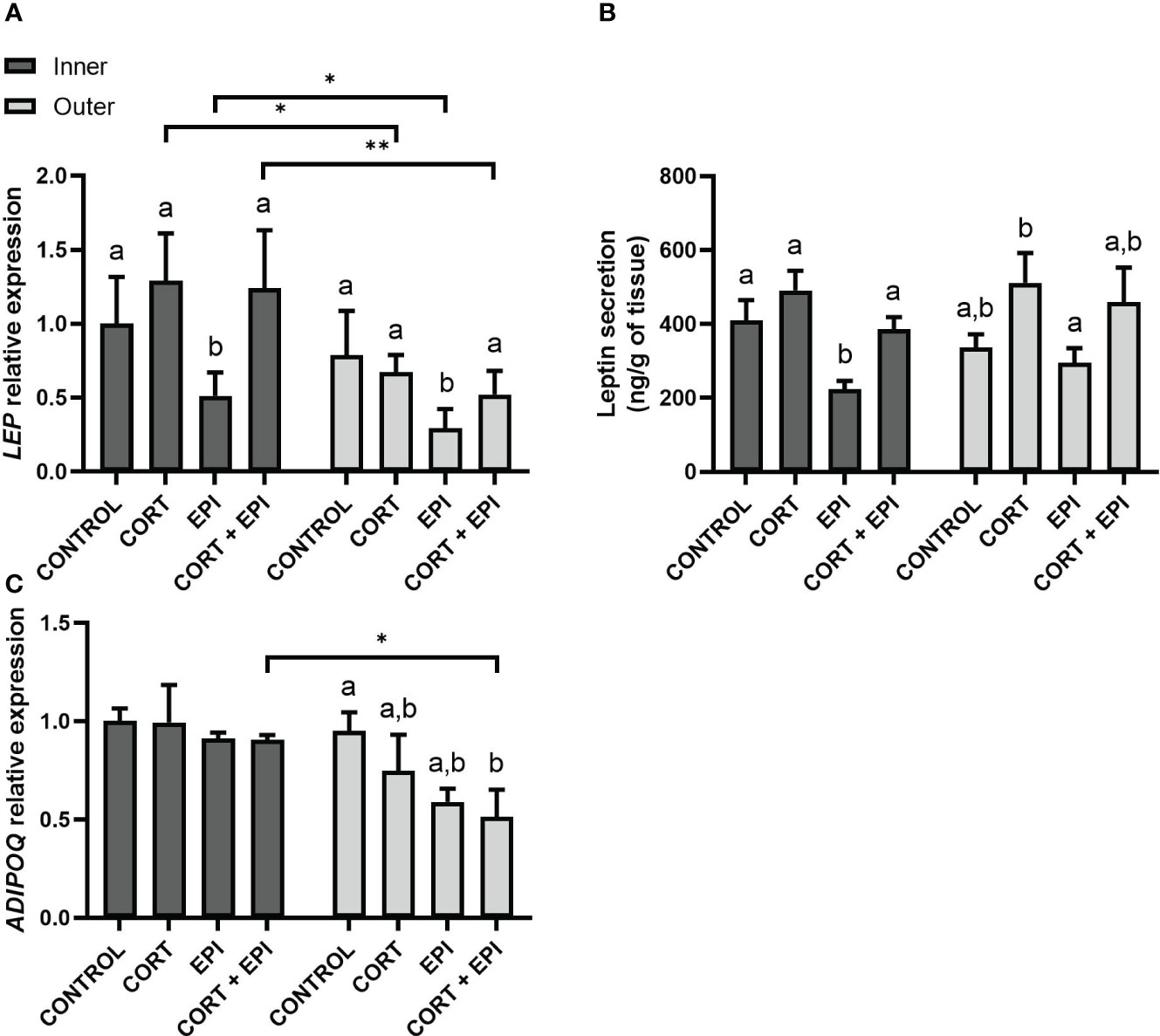
Figure 5 Secretion of leptin and relative expression of leptin (LEP) and adiponectin (ADIPOQ) in precision-cut adipose tissue slices (PCATS) from northern elephant seals in response to stress hormones. PCATS were cultured for 48 hours and exposed to 2 µM cortisol for 48 hours (CORT), 100 nM EPI during the last 12 hours of culture (EPI), or to the combination of both 2 µM cortisol for 48 hours and 100 nM EPI during the last 12 hours of culture (CORT + EPI). PCATS from inner and outer blubber were cultured separately. Controls refer to culture media without added hormones. (A) Relative expression of LEP. Mean ± SEM of 4 pups sampled early in their post-weaning fast. (B) Leptin secretion from PCATS to culture media. Mean ± SEM of 8 pups sampled early in their post-weaning fast. (C) Relative expression of ADIPOQ. Mean ± SEM of 4 pups sampled early in their post-weaning fast. Expression of LEP and ADIPOQ were normalized with reference genes (delta Ct) and expressed as relative to delta Ct values of inner blubber-derived PCATS cultured in control conditions. Significant differences between treatments of PCATS within each blubber layer are represented by different letters (p < 0.05). Significant differences between PCATS derived from different blubber layers within each condition are represented by “*” (p < 0.05) and “**” (p < 0.01).
Leptin secretion was also altered by the hormone treatments (F3,49 = 16.10, p < 0.0001) but did not vary between blubber layer from which PCATS were derived (p = 0.5871) or the sex of individuals (p = 0.4569; Figure 5B). Leptin secretion from inner blubber-derived PCATS followed a similar trend to leptin expression in PCATS (Figure 5A). Leptin secretion from inner blubber-derived PCATS was significantly lower after treatment with EPI alone compared to control (p = 0.0011) or CORT (p < 0.0001). Similar to leptin expression, the inhibitory effect of EPI on leptin secretion was counterbalanced by the presence of cortisol (CORT + EPI; p = 0.0024). Leptin secretion from outer blubber-derived PCATS was lower in response to EPI relative to CORT treatment (p = 0.0039).
The expression of genes encoding two other adipokines, adiponectin (ADIPOQ) and resistin (RETN), was also evaluated. For both genes, results did not differ between sex of individuals (ADIPOQ: p = 0.2495; RETN: p = 0.8004). Hormone treatments and the blubber layer from which PCATS derived impacted ADIPOQ (treatments: F3,21 = 3.78, p = 0.0259; layers: F1,21 = 18.71, p = 0.0003; Figure 5C). In outer blubber-derived PCATS, CORT + EPI treatment downregulated ADIPOQ compared to control (p = 0.0092), whereas no effect of treatment was observed in inner blubber. CORT + EPI-treated PCATS from inner blubber also showed higher expression of the adipokine compared to outer blubber-derived PCATS (p = 0.0148). RETN expression was not altered by the hormone treatments, and did not vary by blubber layer (treatments: p = 0.0740; layers: p = 0.0934; data not shown).
3.3 Impact of stress hormones on the expression of an antioxidant enzyme: GPx3
We explored the combinatory effect of stress hormones on antioxidant enzymes by measuring expression of the gene encoding glutathione peroxidase 3 (GPx3; Figure 6). Results did not differ between sex of individuals (p = 0.2046). Experimental conditions and blubber layers from which PCATS derived altered GPx3 expression (conditions: F3,21 = 127.84, p < 0.0001; layers: F1,21 = 6.51, p = 0.0186, post-hoc tests: p > 0.05). CORT and CORT + EPI treatments significantly upregulated GPx3 relative to control and EPI treatments in PCATS from both blubber layers (p < 0.0001). In PCATS from inner blubber, EPI treatment reduced GPx3 expression relative to control (p = 0.0356).
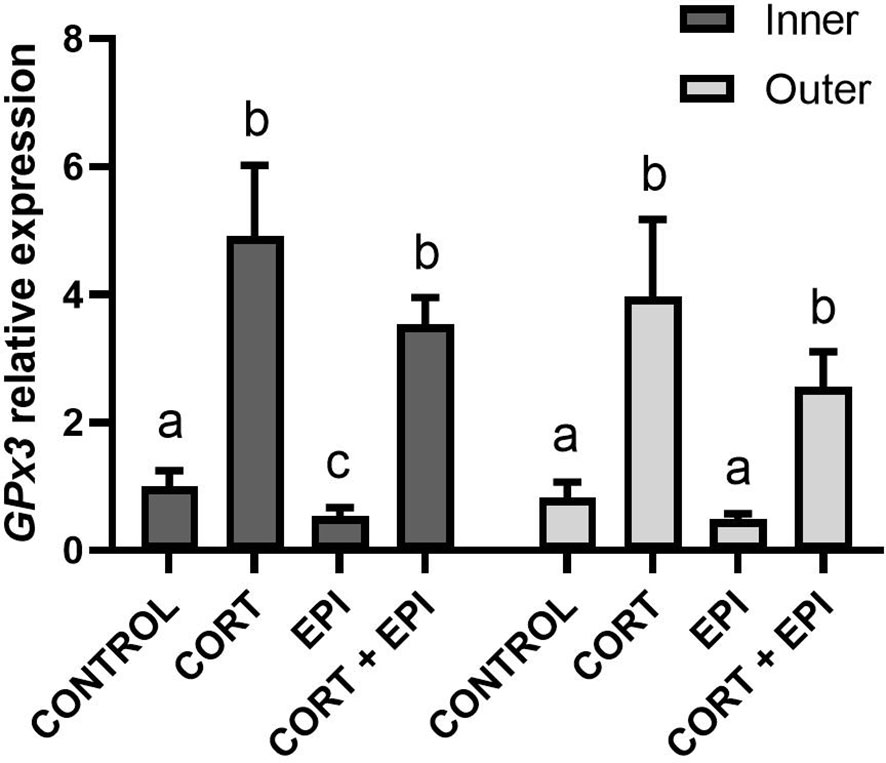
Figure 6 Relative expression of glutathione peroxidase 3 (GPx3) in precision-cut adipose tissue slices (PCATS) from northern elephant seals in response to stress hormones. PCATS were cultured for 48 hours and exposed to 2 µM cortisol for 48 hours (CORT), 100 nM EPI during the last 12 hours of culture (EPI), or to the combination of both 2 µM cortisol for 48 hours and 100 nM EPI during the last 12 hours of culture (CORT + EPI). PCATS from inner and outer blubber were cultured separately. Controls refer to culture media without added hormones. Mean ± SEM of 4 early weaned pups. Expression of GPx3 was normalized with reference genes (delta Ct) and expressed as relative to delta Ct values of inner blubber-derived PCATS cultured in control conditions. Significant differences between treatments of PCATS within each blubber layer are represented by different letters (p < 0.05). There was no significant difference between PCATS derived from different blubber layers within each condition (p > 0.05).
3.4 Impact of stressors on the mobilization of PCBs from PCATS
The sum of the four most abundant PCB congener (PCB-118, 138, 153 and 180) concentrations in PCATS did not differ over the culture period (i.e., between fresh tissue and PCATS collected at the end of the 48-hour culture period), with a low impact of the blubber layer from which PCATS were derived (F1,18 = 12.52, p = 0.0024), even though post-hoc tests showed no significant differences between layers within each treatment (p > 0.05). Hormone treatments did not affect the PCBs concentrations in the PCATS (p = 0.1640; Figure 7A). On the other hand, they had an impact on the concentration of PCBs released from PCATS (F3,3 = 15.50, p = 0.0006) regardless of the blubber layer from which PCATS were derived (p = 0.9156). Surprisingly, PCB concentrations in culture media from inner blubber PCATS decreased in response to EPI and CORT + EPI respectively, relative to control (EPI: p = 0.0022; CORT + EPI: p = 0.0047; Figure 7B). EPI treatment also induced lower concentrations than CORT (p = 0.0251). A similar trend toward a reduced level of PCB was observed in CORT + EPI relative to CORT treatment but was not significant (p = 0.0648). In outer blubber PCATS, similar differences were observed relative to control (EPI: p = 0.0284; CORT + EPI: p = 0.0014). CORT + EPI treatment induced a lower release of PCBs in culture media compared to CORT (p = 0.0078).
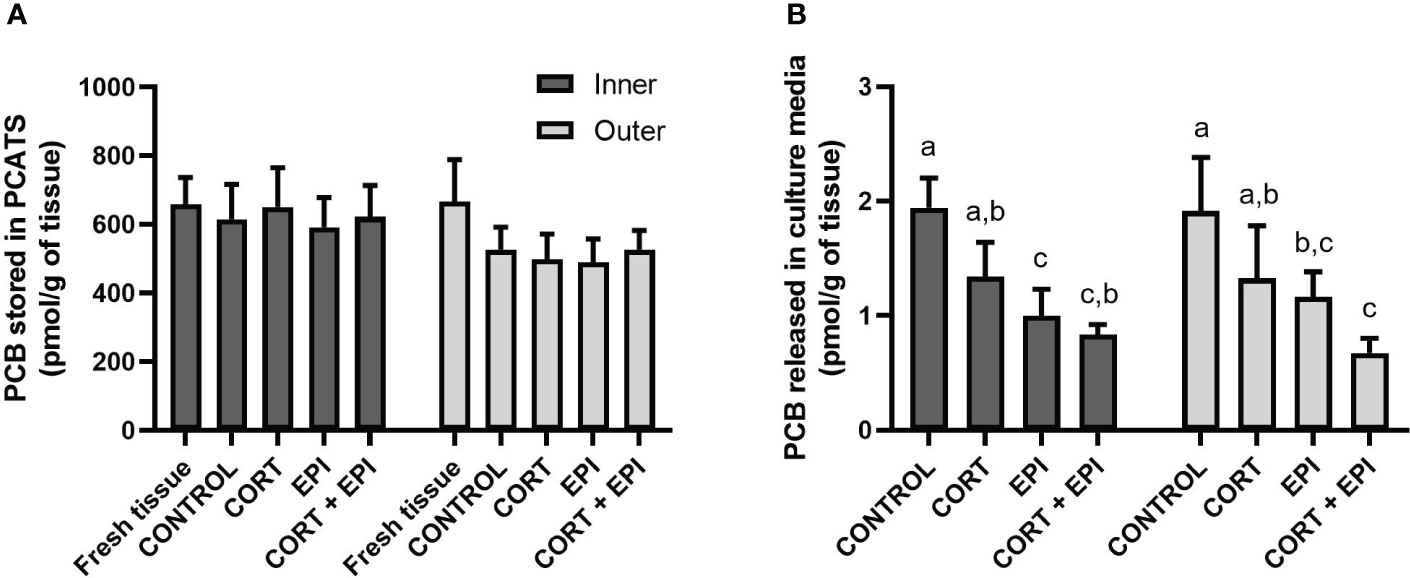
Figure 7 Levels of the most abundant PCBs (PCB-118, 138, 153 and 180) in precision-cut adipose tissue slices (PCATS) from northern elephant seals and associated culture media in response to stress hormones. PCATS were cultured for 48 hours and exposed to 2 µM cortisol for 48 hours (CORT), 100 nM EPI during the last 12 hours of culture (EPI), or to the combination of both 2 µM cortisol for 48 hours and 100 nM EPI during the last 12 hours of culture (CORT + EPI). PCATS from inner and outer blubber were cultured separately. Controls refer to culture media without added hormones. Mean of 3 groups composed of 3 pups sampled late in their post-weaning fast pooled per condition and layer ± SEM (9 pups separated in 3 groups of 3 pups). (A) Concentrations of PCBs stored within blubber. “Fresh tissue” refers to PCATS that were analyzed directly after collection, before culture. There was no significant difference within a specific condition and within a specific layer (p > 0.05). (B) Concentrations of PCBs released into culture media during the last 12 hours of culture. Significant differences between treatments of PCATS within each blubber layer are represented by different letters (p < 0.05). There was no significant difference between PCATS derived from different blubber layers within each condition (p > 0.05).
In order to assess the potential for selective mobilization of PCBs from PCATS to the surrounding culture medium, we analyzed the relative abundance of the four most abundant PCBs (PCB-118, 138, 153 and 180) in PCATS and associated culture media (Figure 8). Since the levels of PCBs were similar between PCATS derived from different blubber layers, data from inner and outer blubber PCATS were combined for these analyses. The treatment had no impact on the abundance of PCBs (p > 0.05). The relative abundance of each PCB was significantly different between PCATS and culture media except for PCB-153 (PCB-118: F1,30 = 57.21, p < 0.0001; PCB-138: F1,30 = 197.41, p < 0.0001; PCB-153: p = 0.1323; PCB-180: F1,30 = 79.12, p < 0.0001). For each condition, the relative abundance of PCB-118 was greater in culture media compared to the corresponding PCATS (p < 0.05). Conversely, the percentage of PCB-138 was lower in culture media compared to associated PCATS for each condition (PCB-138: p < 0.0001). In other words, even if levels of PCB-138 were higher than PCB-118 in the tissue, the PCATS appeared to selectively mobilize PCB-118 into the culture media. The relative abundance of PCB-180 was lower in culture media compared to PCATS in CORT, EPI and CORT + EPI treatments (p < 0.001) but not in control conditions (p = 0.0606).
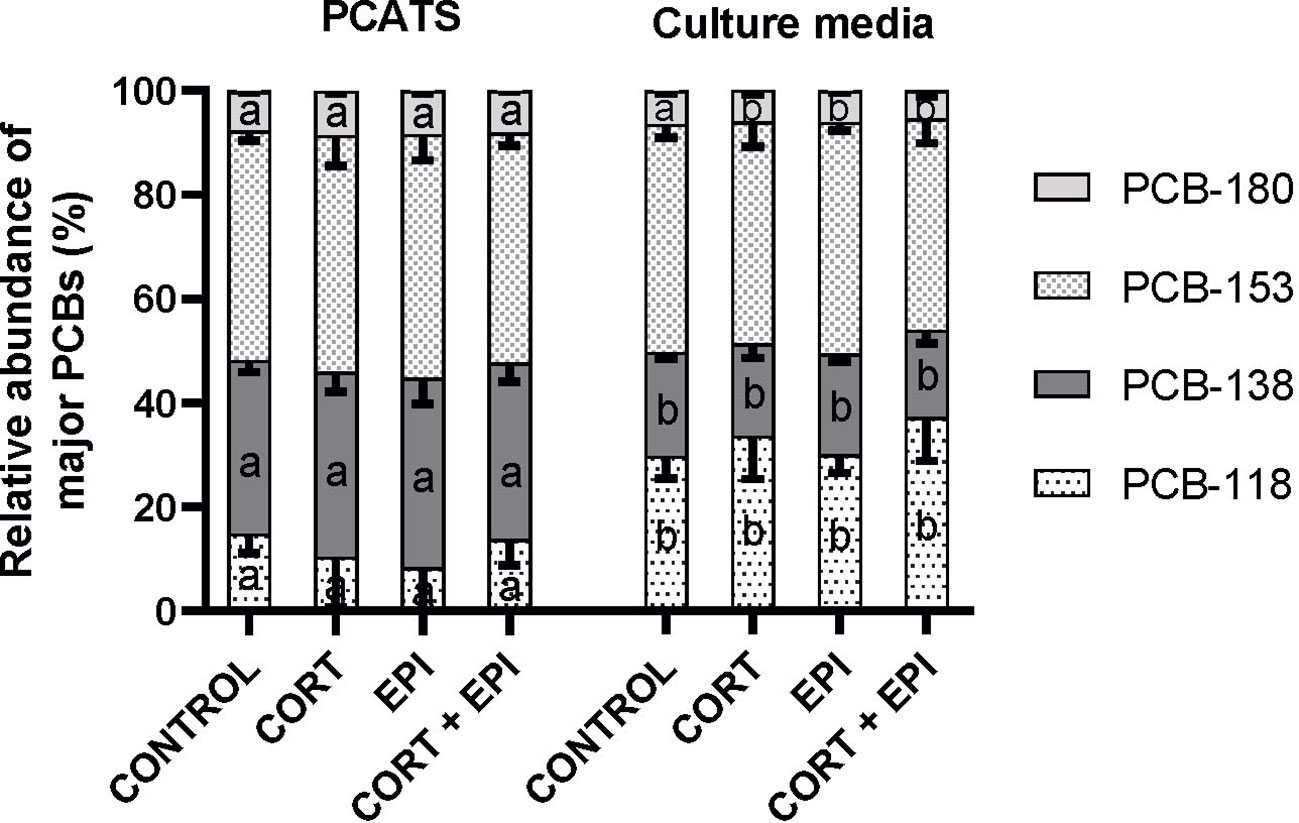
Figure 8 Relative abundance in percentages of the four most abundant PCBs (PCB-118, 138, 153 and 180) found in precision-cut adipose tissue slices (PCATS) from northern elephant seals and associated culture media. PCATS were cultured for 48 hours and exposed to 2 µM cortisol for 48 hours (CORT), 100 nM EPI during the last 12 hours of culture (EPI), or to the combination of both 2 µM cortisol for 48 hours and 100 nM EPI during the last 12 hours of culture (CORT + EPI). Inner and outer blubber samples were gathered for this analysis. Controls refer to culture media without added hormones. Mean of 3 groups composed of 3 pups sampled late in their post-weaning fast pooled per condition ± SEM (N=3, n=2). For each PCB, significant differences between groups (PCATS and culture media) within each condition are represented by different letters (p < 0.05). There was no significant difference between treatments of PCATS within each group (PCATS or culture media) (p > 0.05).
4 Discussion
We explored the metabolic responses of NES PCATS exposed to the stress hormones CORT and EPI. Our ex vivo model enabled an accurate and reproducible analysis of the effects of these two hormones on marine mammal blubber tissue. Debier et al. showed that PCATS exhibited good viability for at least 63 hours in culture with or without isoproterenol (2020). Here, cell viability remained high and was in line with levels observed in NES PCATS from previous experiments (Debier et al., 2020). The pieces of information provided by both PCATS and associated culture media offered a complementary approach to understanding the blubber response to stress hormones. The RNA extracted from PCATS after 48 hours of ex vivo culture served to highlight the major impacts of CORT and EPI on the expression of a variety of genes involved in lipolytic and endocrine processes as well as protection mechanisms against oxidative stress. We also characterized the mobilization of pollutants from PCATS in response to stress hormones.
The present study highlights the prevalence of the post-translational action of EPI on lipolysis in NES PCATS. Indeed, the release of glycerol and free fatty acids from PCATS was mainly driven by catecholamine, alone or combined with CORT, even though the expression of PNPLA2 was not increased by EPI alone. Stimulation of lipolysis by EPI in PCATS is in accordance with its known effect on the lipolytic pathway. As represented in Figure 9 and detailed in Debier et al. (2020), EPI initiates lipolysis by binding to β-adrenergic receptors located on the surface of the adipocyte membrane. This binding triggers the lipolytic cascade, involving the activation of PKA that subsequently phosphorylates HSL as well as perilipin A, a coating protein surrounding the lipid droplet. The phosphorylation of perilipins facilitates the access of lipolytic enzymes ATGL and HSL to the surface of the lipid droplet and induces the release of comparative gene identification-58 (CGI-58, also known as ABHD5 for α/β hydrolase domain-containing 5), which in turn activates ATGL. This lipase initiates the first step of lipolysis, hydrolyzing each triglyceride into one diglyceride and a free fatty acid. Once phosphorylated, activated HSL migrates to the surface of the lipid droplet where it catalyzes the break-down of diglyceride into one monoglyceride and a second free fatty acid. The last step of lipolysis takes place in the cytosol where monoglyceride lipase is mobilized to form glycerol and a third free fatty acid from a monoglyceride. Both lipolytic products are then released into the blood circulation (Duncan et al., 2007; Lafontan and Langin, 2009). Glycerol is taken up by the liver for gluconeogenesis. Released free fatty acids can either be transported to other tissues such as muscles and liver or be reabsorbed by the adipose tissue to resynthesize triglycerides (Coelho et al., 2013). In addition to its post-translational action, our parallel transcriptome study of CORT- and EPI-treated PCATS revealed that EPI also upregulated the expression of genes encoding proteins involved in the lipolytic cascade, such as AQP7, which encodes a glycerol-transporting aquaporin in adipocytes (Kashiwabara et al., 2023). Furthermore, the present study suggests that prolonged exposure to CORT alone is able to activate lipolysis in NES PCATS, although to a much lower extent than EPI. Exogeneous ACTH administration in vivo and PCATS treatment with CORT in our other studies caused upregulation of genes encoding β-adrenergic receptors in blubber (Khudyakov et al., 2017; Kashiwabara et al., 2023), including ADRB2, which encodes the sub-type of β-adrenergic receptors known to have the strongest affinity for catecholamines (Lafontan and Berlan, 1993). Decreasing levels of circulating CORT was also associated with reduced expression of forkhead box O1 (FoxO1) in in vivo ACTH administration experiments in NES (Khudyakov et al., 2017). This transcription factor has been shown to induce the expression of PNPLA2 in 3T3-L1 cell lines (Chakrabarti and Kandror, 2009). Expression of FoxO1 gene is stimulated by glucocorticoids, while its activity is stimulated by activation of the beta-adrenergic pathway and protein kinase A (PKA) in 3T3-L1 cell lines (Chakrabarti and Kandror, 2009). Therefore, glucocorticoids may indirectly stimulate lipolysis by upregulating genes related to this pathway (e.g. FoxO1), as well as increasing the sensitivity of adipocytes to EPI (e.g. ADRB2).
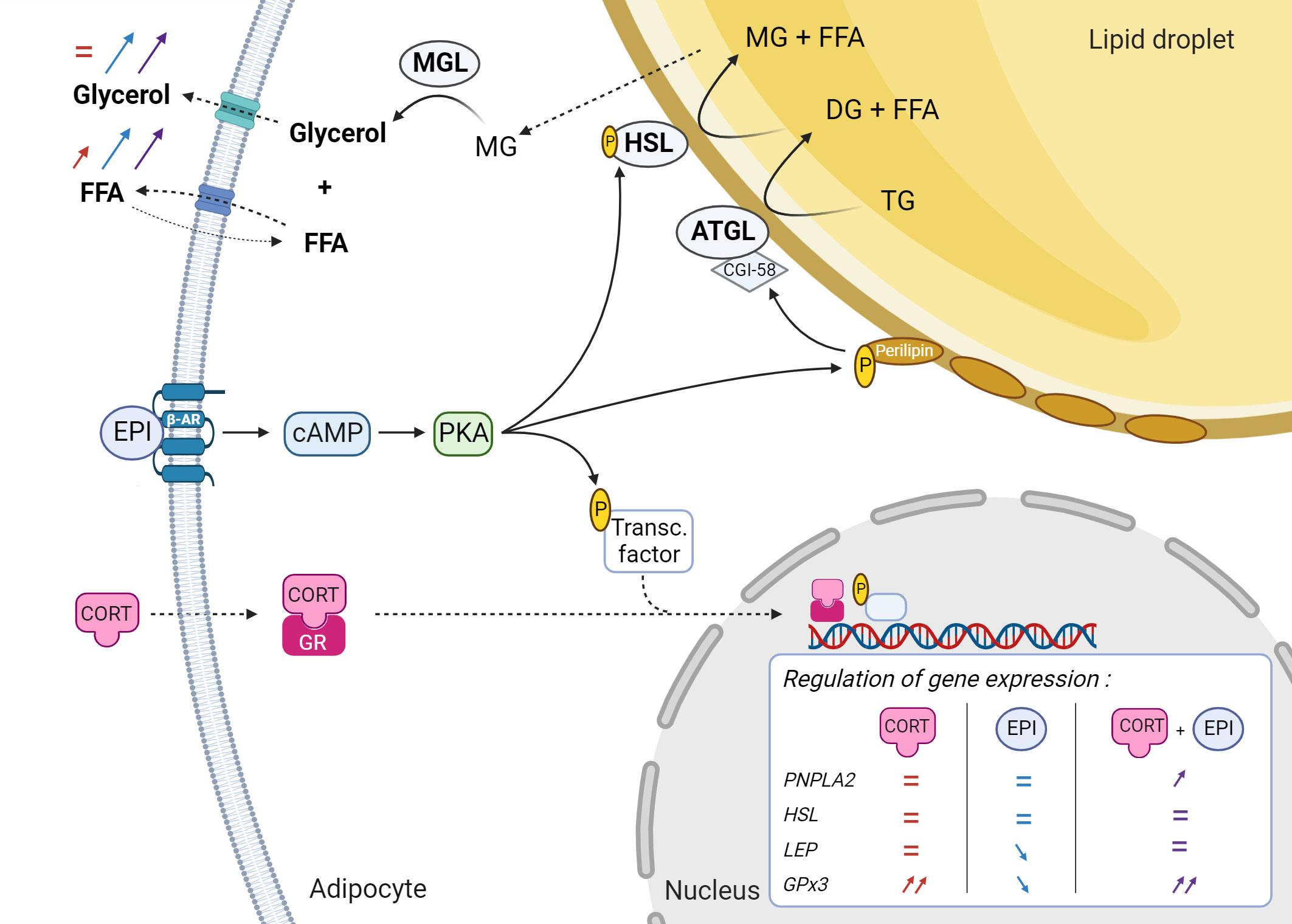
Figure 9 Proposed effects of epinephrine (EPI) and cortisol (CORT) on the function of NES adipocytes in inner blubber. Red, blue and purple arrows and equal signs refer to the magnitude of modulation relative to control after an exposure to CORT alone, EPI alone and CORT + EPI respectively. ATGL, adipose triglyceride lipase; β-AR, β-adrenergic receptor; cAMP, cyclic adenosine monophosphate; CGI-58, comparative gene identification-58; CORT, cortisol; DG, diglyceride; EPI, epinephrine; FFA, free fatty acid; GPx3, glutathione peroxidase 3; GR, glucocorticoid receptor; HSL, hormone sensitive lipase; LEP, leptin; MG, monoglyceride; MGL, monoglyceride lipase; P, phosphate; PKA, protein kinase A; PNPLA2, Patatin-like phospholipase domain-containing protein 2; TG, triglyceride. Created with BioRender.com.
The relative abundance of specific classes of fatty acid released from PCATS did not differ between treatments. Levels were similar to those previously measured in serum and blubber of early weaned NES pups, with MUFAs being the most abundant class, followed by SFAs, ω-3, and ω-6 PUFAs (Best et al., 2003; Fowler et al., 2014; Louis et al., 2015). EPI induced higher release of SFAs from inner blubber-derived PCATS compared to outer blubber, suggesting either higher lipolytic response in inner blubber or higher rate of re-uptake of SFAs in outer blubber-derived PCATS. A similar trend, although not significant, was observed for the other fatty acid classes. Yet, glycerol levels and expression of lipolytic enzymes did not vary between inner and outer blubber-derived PCATS, similar to previous studies (Debier et al., 2020), suggesting that higher re-uptake of SFAs in outer blubber is more likely. Our estimates of fatty acid re-uptake by PCATS suggested a trend towards higher rates of fatty acid re-esterification by outer blubber compared to inner blubber in response to EPI treatment (61.8 ± 5.0% in outer and 46.2 ± 2.8% in inner -derived PCATS), although the difference was not statistically significant. This difference disappeared when CORT was added to the culture medium, suggesting that this stress hormone also regulates fatty acid re-uptake during lipolysis. More investigations on the impact of CORT and EPI on fatty acid re-uptake are needed to confirm this hypothesis.
The expression of PNPLA2 in NES PCATS was only upregulated by the combination of CORT and EPI. The specific effect of CORT on PNPLA2 expression in NES is poorly known. Previous in vivo studies in juvenile NES that used exogenous ACTH administration to elevate circulating cortisol levels did not find changes in PNPLA2 expression in blubber (Khudyakov et al., 2017; Deyarmin et al., 2019). However, expression of genes encoding ATGL inhibitors (galectin-3 and early growth response protein 1) was reduced two hours after ACTH administration. During the stress recovery period (i.e. decreasing levels of circulating cortisol between 2 and 24 hours post-ACTH), the expression of forkhead box O1 (FoxO1) was downregulated, as previously mentioned (Khudyakov et al., 2017; Deyarmin et al., 2019). The impact of EPI on PNPLA2 expression is poorly referenced but may be mediated by AMP-activated protein kinase (AMPK) (Viscarra and Ortiz, 2013; Crocker, 2014). This kinase can be activated by EPI in rat adipocytes in vitro (Gaidhu et al., 2009). Activation of AMPK has also been shown to increase PNPLA2 expression and inhibit HSL activity in mouse visceral and sub-cutaneous adipocytes exposed to 100 nM EPI, the same concentration as was used in this study (Gaidhu et al., 2010). Interestingly, expression of both AMPK and PNPLA2 have been shown to increase throughout the post-weaning fast of NES pups (Viscarra et al., 2011a; Crocker et al., 2014). In the present study, only the combination of CORT and EPI significantly increased the expression of PNPLA2 compared to control in inner blubber-derived PCATS. In our transcriptome study of CORT- and EPI-treated PCATS in which inner and outer-derived samples were pooled, the combination treatment also upregulated PNPLA2 (Kashiwabara et al., 2023). This interactive effect of CORT and EPI on PNPLA2 expression could result from a combination of mechanisms, such as CORT-mediated upregulation and EPI-mediated increase in activity of key transcription factors (e.g. FoxO1) and kinases (AMPK) involved in lipolysis.
HSL expression was downregulated by the combination of stress hormones in outer blubber-derived PCATS. Expression and activity of the lipase appear to be low in NES and to decrease over fasting, concomitant with an increase in circulating cortisol levels (Viscarra et al., 2012; Crocker et al., 2014; Fowler et al., 2015; Louis et al., 2015). This may serve to regulate lipolysis throughout energetically demanding fasting periods (Crocker et al., 2014), but the underlying regulatory mechanisms remain unknown. Our results indicate that CORT might be involved in the downregulation of HSL that is observed in vivo with the progression of the fast. However, more experiments are needed to understand why the expression of HSL was suppressed only in outer blubber by hormone treatments.
The effect of stress hormones on the endocrine function of NES blubber was explored by assessing leptin gene expression in PCATS as well as leptin protein secretion from PCATS into culture media. Both leptin expression in PCATS and its secretion into culture media decreased in response to EPI. Our previous study of NES PCATS showed similar effects on leptin when lipolysis was induced by isoproterenol (Debier et al., 2020). However, the underlying mechanism by which EPI downregulates leptin is still unknown, but may be mediated by the cAMP/PKA/cAMP response element-binding protein (CREB) pathway, which has been shown to inhibit leptin secretion in human bone marrow-derived mesenchymal stem cells (hBMSCs) (Klemm et al., 2001; Kim et al., 2013; Tsubai et al., 2016; Deck et al., 2017; Caron et al., 2018). Interestingly, CORT attenuated the inhibitory effect of EPI on leptin expression and secretion in both blubber layers. Similarly, leptin expression and secretion increased in response to cortisol elevation by exogeneous ACTH administration in elephant seals and sea lions (Mashburn and Atkinson, 2008; Deyarmin et al., 2019). Our transcriptomic analysis of PCATS exposed to EPI and CORT was consistent with these results, as LEP expression was downregulated and upregulated by EPI and CORT, respectively (Kashiwabara et al., 2023). In our study, CORT counteracted the inhibiting effect of EPI, leading to secreted levels of leptin comparable to the control. These results highlight the interactive effect of catecholamines and glucocorticoids on the expression and secretion of leptin from NES PCATS.
Leptin expression was higher in inner as compared to outer blubber-derived PCATS cultured with stress hormones, similarly to the higher LEP expression in inner blubber of adult bowhead whales (Balaena mysticetus) (Ball et al., 2017). However, leptin secretion into culture media did not differ between inner- and outer blubber-derived PCATS. In our previous study using NES PCATS, leptin secretion differed between inner and outer blubber-derived PCATS obtained from weaned pups sampled during early, but not late fasting in the control condition, while the reverse was true for PCATS cultured in the presence of isoproterenol (Debier et al., 2020).
In addition to leptin, we examined the effect of stress hormones on expression of two other adipokines, adiponectin and resistin, in NES PCATS. Adiponectin expression was downregulated in response to CORT + EPI treatment relative to control in outer blubber-derived PCATS only. While the impact of EPI on adiponectin secretion is unknown, the relationship between glucocorticoids and adiponectin expression and secretion levels has been measured and varies with the life history stage of NES. Through the post-weaning fast of NES pups, circulating CORT increased while plasma adiponectin decreased, supporting our observations (Viscarra et al., 2011a and Viscarra et al., 2011b). Yet, a positive link between circulating cortisol levels and adiponectin expression in blubber was found in inner blubber from juvenile NES during haul out period (Pujade Busqueta et al., 2020). Consistent observations were made by transcriptomic analysis after repeated ACTH injections in juvenile NES during the same life history stage (Deyarmin et al., 2019). In other studies conducted on adult females during both breeding and molting seasons, decreasing circulating levels of adiponectin were associated with increased secretion of CORT (Rzucidlo et al., 2021). Conversely, a similar study related a positive correlation between circulating cortisol levels and ADIPOQ expression in late fasting female NES (Khudyakov et al., 2019). The results obtained in the present study suggest that both CORT and EPI regulate adiponectin expression in NES blubber, although their effects may vary depending on life history stage of individuals as suggested in correlative studies. In regards to resistin expression, we found that neither CORT nor EPI affected resistin expression in NES PCATS, consistent with no change in circulating resistin levels observed over fasting in adult NES (Rzucidlo et al., 2021).
Fasting, as experienced by many marine mammal species, is known to promote oxidative stress through the production of reactive oxygen species (ROS) (Crocker et al., 2016). Oxidative stress can induce cellular injuries and cell senescence, ultimately impacting growth, reproduction, and disease susceptibility (Costantini et al., 2011; Crocker et al., 2016). In this study, we measured the expression of GPx3 in PCATS following CORT and EPI exposure. The highly significant changes in GPx3 expression induced by CORT highlights the impact of this glucocorticoid on the antioxidant enzyme expression. The increase in GPx3 expression induced by CORT observed in the present ex vivo experiment is consistent with pinniped adaptations to prolonged stress periods such as fasting (Vázquez-Medina et al., 2010). Indeed, GPx3 was upregulated in blubber in response to ACTH administration in juvenile NES and was positively associated with circulating levels of CORT (Vázquez-Medina et al., 2011; Crocker et al., 2016; Pujade Busqueta et al., 2020). Similarly, transcriptomic analysis of PCATS showed that CORT treatment alone and in combination with EPI upregulated GPx3 (Kashiwabara et al., 2023). It must be noted that we did not measure GPx3 protein levels or activity or markers of oxidative damage in PCATS. Therefore, we cannot distinguish whether GPx3 upregulation is caused by stress hormones directly or indirectly, as a consequence of oxidative stress.
This study also aimed to characterize the impact of stress hormones on the mobilization of persistent organic pollutants from the blubber of weaned NES pups. As expected, blubber samples were contaminated with PCBs, which were likely transferred from mother to pup through milk (Debier et al., 2003; Debier et al., 2012; Vanden Berghe et al., 2012). PCB levels were similar to those previously reported in weaned NES pups (Louis et al., 2014a). We found that PCATS exhibited very low mobilization of PCBs in response to stress hormones. In vivo studies in grey seals (Halichoerus grypus) and NES showed that PCBs are less efficiently mobilized from blubber than are lipids during fasting and that they tend to concentrate in the remaining blubber lipids as the fast progresses (Debier et al., 2003; Vanden Berghe et al., 2010; Debier et al, 2012; Vanden Berghe et al., 2012; Louis et al., 2016). The phenomenon was also observed in our ex vivo model, since we calculated that of the 2.5% of lipids contained in PCATS that were secreted during EPI-induced lipolysis, only 0.3% of the most abundant PCBs were released, suggesting that PCBs may concentrate in the more internal, lipid-rich layers of PCATS (Figure 10).
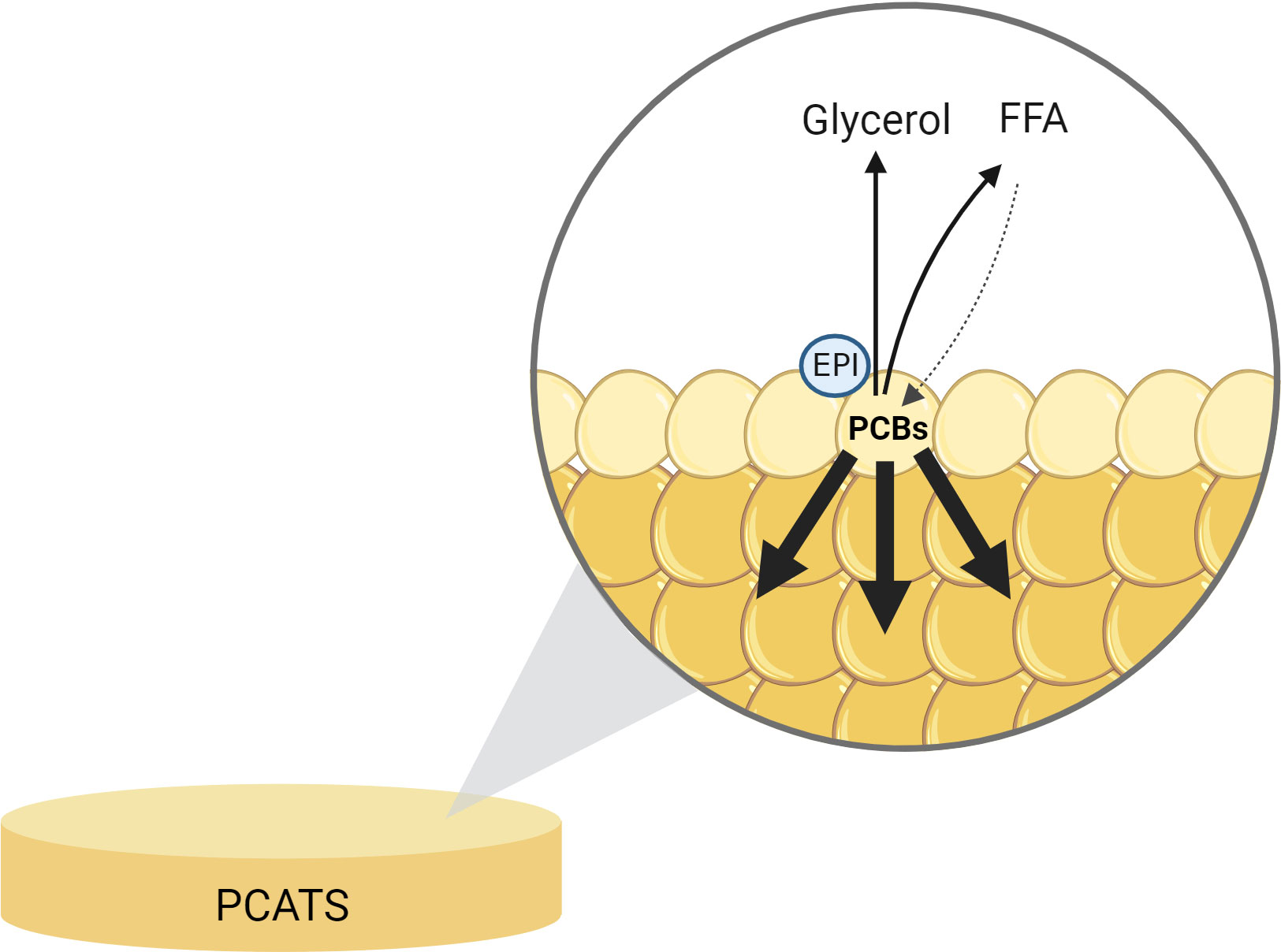
Figure 10 Illustration of the potential migration of polychlorinated biphenyls (PCBs) in deeper layers of adipocytes in a northern elephant seal precision-cut adipose tissue slice (PCATS) treated with epinephrine (EPI). PCATS were cultured for 48 hours and exposed to 100 nM EPI during the last 12 hours of culture. Glycerol and free fatty acids (FFA) were released from adipocytes. A fraction of FFA was taken up back inside adipocytes (dotted line). PCBs, already present in the blubber of NES pups before sampling, migrated in more internal layers of adipocytes from the PCATS. Created with BioRender.com.
The concentrations of PCBs did not differ between inner and outer blubber-derived PCATS. A previous in vivo study showed homogeneous distribution of PCBs between blubber layers of NES pups for up to 4 weeks after weaning (Louis et al., 2014a). On the other hand, after 7 weeks of fasting, the levels of PCBs were higher in inner blubber as compared to outer blubber, reflecting preferential mobilization of lipids from the inner layers and the subsequent concentration of PCBs inside the tissue (Louis et al., 2014a; Louis et al., 2016). In the present study, samples were collected around week 5 of the weaning fast (estimation based on the morphological characteristics of pups, such as presence of natal pelage), a period during which the differences between blubber layers might not yet be apparent.
The comparison of the relative abundances of specific PCB congeners in PCATS and associated culture media highlighted selective mobilization of PCBs according to their lipophilicity, with the exception of the most abundant PCB congener, PCB-153. PCB-118, which has a lower degree of chlorination than PCB-138 and PCB-180, and therefore, a lower lipophilicity (octanol:water partition coefficient of 6.98, 7.44 and 8.27 for PCB-118,138 and 180 respectively; Moriceau et al., 2023), was preferentially released from PCATS during lipolysis, similarly to in vivo studies of PCB levels in blubber and serum of weaned NES pups during fasting. Louis et al. described selective release of specific PCB congeners from blubber stores to the systemic circulation based on their degree of chlorination, regardless of the fasting stage (2014a, 2016). In this study, no difference between hormone treatments on mobilization of the different PCB congeners was observed. This supports the previous hypothesis that the degree of PCB lipophilicity is the primary determinant of pollutant mobilization from blubber.
The main limitations of our study are the use of both female and male pups, a limited sample size as well as individuals from different stages of the post-weaning fast (early and late) due to blubber availability and field constraints. Yet, sex of pups did not influence the present results. Our previous study on the response of NES PCATS to isoproterenol also showed no difference between sex in glycerol and leptin release from PCATS after lipolysis stimulation (Debier et al., 2020). Other studies on weaned NES pups suggest no distinction in energy expenditure and body conditions between sex during the post-weaning fast (Carlini et al., 2001; Noren et al., 2003), which is consistent with our observations. However, lipolytic rate increases through the post-weaning fast but not circulating leptin (Viscarra et al., 2011a; Crocker et al., 2016). We must thus not generalize lipolysis-related results obtained on pups sampled late in their post-weaning fast to the entire period. Yet, our findings are consistent with previous in vivo studies on the response of marine mammals to stress (Khudyakov et al., 2017; Deyarmin et al., 2019; Pujade Busqueta et al., 2020).
To summarize, the present ex vivo study using NES PCATS provides new insights into the metabolic and transcriptional response of an endocrine organ, the adipose tissue or blubber, to stress hormones in marine mammals. To the best of our knowledge, this is the first study on combinatory effects of a catecholamine and a glucocorticoid on marine mammal blubber. The concordance between this ex vivo model and previous in vivo findings highlights the relevance of the use of the PCATS method on free-ranging and protected marine mammals. We found that stress hormones stimulated lipolysis and altered expression of genes encoding lipolytic enzymes, adipokines, and a key antioxidant enzyme. We showed that CORT and EPI have opposing effects on expression and secretion of leptin, a major energy-regulating hormone secreted by blubber. We found that CORT and EPI had a combinatory effect on expression of PNPLA2, HSL, and ADIPOQ, and that CORT strongly upregulated GPx3, as shown in in vivo studies of NES. Finally, this paper also highlighted the lipophilic character of PCBs which were retained in tissues after the application of stress hormones. Overall, we showed that inner blubber exhibits higher sensitivity to stress hormones than outer blubber, consistent with the higher degree of vascularization of this blubber layer. Our results highlight the necessity of combining stressors in the context of marine ecotoxicology studies. This work contributes to our mechanistic understanding of the effects of anthropogenic stressors on marine mammal physiology and opens new avenues of investigations regarding the interactive effects of multiple stressors on marine mammal metabolism and toxicology.
Data availability statement
The raw data supporting the conclusions of this article will be made available by the authors, without undue reservation.
Ethics statement
The animal study was approved by Sonoma State University Institutional Animal Care and Use Committee (IACUC #2017-56). The study was conducted in accordance with the local legislation and institutional requirements.
Author contributions
LP: Conceptualization, Formal analysis, Investigation, Visualization, Writing – original draft, Writing – review & editing, Methodology. JK: Investigation, Resources, Writing – review & editing, Supervision. DC: Funding acquisition, Investigation, Writing – review & editing, Resources, Supervision. LV: Investigation, Writing – review & editing. GS: Investigation, Resources, Writing – review & editing. GE: Resources, Writing – review & editing, Investigation. MP: Writing – review & editing, Resources. J-FR: Conceptualization, Writing – review & editing, Resources. DS: Conceptualization, Resources, Writing – review & editing. CD: Conceptualization, Funding acquisition, Methodology, Project administration, Resources, Supervision, Writing – original draft, Writing – review & editing.
Funding
The author(s) declare financial support was received for the research, authorship, and/or publication of this article. LP is a F.R.I.A. Grant Holder of the FNRS (Fonds de la Recherche Scientifique, Belgium). The scientific mission of LP was also funded by the FNRS.
Acknowledgments
The authors are grateful to D. Costa, P. Robinson, the rangers at Año Nuevo State Park, and UC Santa Cruz’s Año Nuevo State Park for facilitating access to the animals and to G. Tinant and C. Rasse (UCLouvain, Belgium) for help with the statistical analysis of data.
Conflict of interest
The authors declare that the research was conducted in the absence of any commercial or financial relationships that could be construed as a potential conflict of interest.
Publisher’s note
All claims expressed in this article are solely those of the authors and do not necessarily represent those of their affiliated organizations, or those of the publisher, the editors and the reviewers. Any product that may be evaluated in this article, or claim that may be made by its manufacturer, is not guaranteed or endorsed by the publisher.
Supplementary material
The Supplementary Material for this article can be found online at: https://www.frontiersin.org/articles/10.3389/fmars.2023.1290472/full#supplementary-material
Abbreviations
CORT, cortisol; EPI, epinephrine; NES, northern elephant seal; PCATS, precision-cut adipose tissue slices.
References
Andersen C. L., Jensen J. L., Ørntoft T. F. (2004). Normalization of real-time quantitative reverse transcription-PCR data: a model-based variance estimation approach to identify genes suited for normalization, applied to bladder and colon cancer data sets. Cancer Res. 64 (15), 5245–5250. doi: 10.1158/0008-5472.CAN-04-0496
Ball H. C., Londraville R. L., Prokop J. W., George J. C., Suydam R. S., Vinyard C., et al. (2017). Beyond thermoregulation: metabolic function of cetacean blubber in migrating bowhead and beluga whales. J. Comp. Physiol. B: Biochem. Syst. Environ. Physiol. 187 (1), 235–252. doi: 10.1007/s00360-016-1029-6
Beltest (2000). Determination of polychlorinated biphenyls in animal feed, animal fat, egg and egg products, milk products and other foodstuffs (Beltest, Belgium: Beltest - Belac (Belgian Organisation for Accreditation)).
Bełtowski J. (2003). Adiponectin and resistin-new hormones of white adipose tissue. MedSciMonit 9 (2), 55–61.
Best N. J., Bradshaw C. J. A., Hindell M. A., Nichols P. D. (2003). Vertical stratification of fatty acids in the blubber of southern elephant seals (Mirounga leonina): Implications for diet analysis. Comp. Biochem. Physiol. B Biochem. Mol. Biol. 134 (2), 253–263. doi: 10.1016/S1096-4959(02)00252-X
Blair H. B., Merchant N. D., Friedlaender A. S., Wiley D. N., Parks S. E. (2016). Evidence for ship noise impacts on humpback whale foraging behaviour. Biol. Lett. 12 (8), 20160005. doi: 10.1098/rsbl.2016.0005
Bligh E. G., Dyer W. J. (1959). A rapid method of total lipid extraction and purification. Can. J. Biochem. Physiol. 37 (8), 911–917. doi: 10.1139/O59-099
Bourez S., Van den Daelen C., Le Lay S., Poupaert J., Larondelle Y., Thomé J. P., et al. (2013). The dynamics of accumulation of PCBs in cultured adipocytes vary with the cell lipid content and the lipophilicity of the congener. Toxicol. Lett. 216 (1), 40–46. doi: 10.1016/j.toxlet.2012.09.027
Carlini A. R., Márquez M. E. I., Ramdohr S., Bornemann H., Panarello H. O., Daneri G. A. (2001). Postweaning Duration and Body Composition Changes in Southern Elephant Seal (Mirounga leonina) Pups at King George Island. Physiol. Biochem. Zool. 74 (4), 531–540. doi: 10.1086/322168
Caron A., Lee S., Elmquist J. K., Gautron L. (2018). Leptin and brain–adipose crosstalks. Nat. Rev. Neurosci. 19 (3), 153–165. doi: 10.1038/nrn.2018.7
Chakrabarti P., Kandror K. V. (2009). FoxO1 controls insulin-dependent adipose triglyceride lipase (ATGL) expression and lipolysis in adipocytes. J. Biol. Chem. 284 (20), 13296–13300. doi: 10.1074/jbc.C800241200
Champagne C. D., Houser D. S., Costa D. P., Crocker D. E. (2012). The effects of handling and anesthetic agents on the stress response and carbohydrate metabolism in northern elephant seals. PloS One 7 (5), e38442. doi: 10.1371/JOURNAL.PONE.0038442
Champagne C. D., Kellar N. M., Trego M. L., Delehanty B., Boonstra R., Wasser S. K., et al. (2018). Comprehensive endocrine response to acute stress in the bottlenose dolphin from serum, blubber, and feces. Gen. Comp. Endocrinol. 266, 178–193. doi: 10.1016/j.ygcen.2018.05.015
Choe S. S., Huh J. Y., Hwang I. J., Kim J. I., Kim J. B. (2016). Adipose tissue remodeling: Its role in energy metabolism and metabolic disorders. Front. Endocrinol. 7 (4). doi: 10.3389/FENDO.2016.00030/BIBTEX
Clark L. S., Cowan D. F., Pfeiffer D. C. (2006). Morphological changes in the atlantic bottlenose dolphin (Tursiops truncatus) adrenal gland associated with chronic stress. J. Comp. Pathol. 135 (4), 208–216. doi: 10.1016/j.jcpa.2006.07.005
Coelho M., Oliveira T., Fernandes R. (2013). State of the art paper Biochemistry of adipose tissue: an endocrine organ. Arch. Med. Sci. 2 (2), 191–200. doi: 10.5114/aoms.2013.33181
Costantini D., Marasco V., Møller A. P. (2011). A meta-analysis of glucocorticoids as modulators of oxidative stress in vertebrates. J. Comp. Physiol. B Biochem. Syst. Environ. Physiol. 181 (4), 447–456. doi: 10.1007/S00360-011-0566-2
Crocker D. E., Champagne C. D., Fowler M. A., Houser D. S. (2014). Adiposity and fat metabolism in lactating and fasting northern elephant seals. Adv. Nutr. 5 (1), 57–64. doi: 10.3945/an.113.004663
Crocker D. E., Khudyakov J. I., Champagne C. D. (2016). Oxidative stress in northern elephant seals: Integration of omics approaches with ecological and experimental studies. Comp. Biochem. Physiol. Part A Mol. Integr. Physiol. 200, 94–103. doi: 10.1016/J.CBPA.2016.02.011
Dantzer B., Fletcher Q. E., Boonstra R., Sheriff M. J. (2014). Measures of physiological stress: A transparent or opaque window into the status, management and conservation of species? Conserv. Physiol. 2, 1. doi: 10.1093/conphys/cou023
Debier C., Crocker D. E., Houser D. S., Vanden Berghe M., Fowler M., Mignolet E., et al. (2012). Differential changes of fat-soluble vitamins and pollutants during lactation in northern elephant seal mother-pup pairs. Comp. Biochem. Physiol. Part A Mol. Integr. Physiol. 162 (4), 323–330. doi: 10.1016/J.CBPA.2012.04.001
Debier C., Pirard L., Verhaegen M., Rzucidlo C., Tinant G., Dewulf C., et al. (2020). In vitro lipolysis and leptin production of elephant seal blubber using precision-cut adipose tissue slices. Front. Physiol. 11. doi: 10.3389/fphys.2020.615784
Debier C., Pomeroy P. P., Dupont C., Joiris C., Comblin V., Le Boulengé E., et al. (2003). Quantitative dynamics of PCB transfer from mother to pup during lactation in UK grey seals Halichoerus grypus. Mar. Ecol. Prog. Ser. 247, 237–248. doi: 10.3354/meps247237
Deck C. A., Honeycutt J. L., Cheung E., Reynolds H. M., Borski R. J. (2017). Assessing the functional role of leptin in energy homeostasis and the stress response in vertebrates. Front. Endocrinol. 8. doi: 10.3389/fendo.2017.00063
de Oliveira C., Iwanaga-Carvalho C., Mota J. F., Oyama L. M., Ribeiro E. B., Oller Do Nascimento C. M. (2011). Effects of adrenal hormones on the expression of adiponectin and adiponectin receptors in adipose tissue, muscle and liver. Steroids. 76 (12), 1260–1267. doi: 10.1016/j.steroids.2011.06.004
Deyarmin J. S., McCormley M. C., Champagne C. D., Stephan A. P., Busqueta L. P., Crocker D. E., et al. (2019). Blubber transcriptome responses to repeated ACTH administration in a marine mammal. Sci. Rep. 9 (1), 2718. doi: 10.1038/s41598-019-39089-2
Duncan R. E., Ahmadian M., Jaworski K., Sarkadi-Nagy E., Sul H. S. (2007). Regulation of lipolysis in adipocytes. Annu. Rev. Nutr. 27, 79–101. doi: 10.1146/annurev.nutr.27.061406.093734
Elenkov I. J., Webster E. L., Torpy D. J., Chrousos G. P. (1999). Stress, corticotropin-releasing hormone, glucocorticoids, and the immune/inflammatory response: acute and chronic effects. Ann. N. Y. Acad. Sci. 876 (1), 1–13. doi: 10.1111/J.1749-6632.1999.TB07618.X
Erbe C., Marley S. A., Schoeman R. P., Smith J. N., Trigg L. E., Embling C. B. (2019). The effects of ship noise on marine mammals—A review. Front. Mar. Sci. 6. doi: 10.3389/fmars.2019.00606
Espinoza M. B., Aedo J. E., Zuloaga R., Valenzuela C., Molina A., Valdés J. A. (2017). Cortisol induces reactive oxygen species through a membrane glucocorticoid receptor in rainbow trout myotubes. J. Cell. Biochem. 118 (4), 718–725. doi: 10.1002/JCB.25676
Fair P. A., Becker P. R. (2000). Review of stress in marine mammals. J. Aquat. Ecosyst. Stress Recovery. 7 (4), 335–354. doi: 10.1023/A:1009968113079
Ferrante M. C., Amero P., Santoro A., Monnolo A., Simeoli R., Di Guida F., et al. (2014). Polychlorinated biphenyls (PCB 101, PCB 153 and PCB 180) alter leptin signaling and lipid metabolism in differentiated 3T3-L1 adipocytes. Toxicol. Appl. Pharmacol. 279 (3), 401–408. doi: 10.1016/j.taap.2014.06.016
Fowler M. A., Costa D. P., Crocker D. E., Shen W.-J., Kraemer F. B. (2015). Adipose triglyceride lipase, not hormone-sensitive lipase, is the primary lipolytic enzyme in fasting elephant seals (Mirounga angustirostris). Physiol. Biochem. Zool. 88 (3), 284–294. doi: 10.1086/680079
Fowler M. A., Debier C., Mignolet E., Linard C., Crocker D. E., Costa D. P. (2014). Fatty acid mobilization and comparison to milk fatty acid content in northern elephant seals. J. Comp. Physiol. Part B Biochem. Syst. Environ. Physiol. 184 (1), 125–135. doi: 10.1007/S00360-013-0787-7
Fried S. K., Ricci M. R., Russell C. D., Laferrère B. (2000). Regulation of leptin production in humans. J. Nutr. 130 (12), 3127–3131. doi: 10.1093/jn/130.12.3127s
Frühbeck G., Gómez-Ambrosi J., Muruzábal F. J., Burrell M. A. (2001). The adipocyte: A model for integration of endocrine and metabolic signaling in energy metabolism regulation. Am. J. Physiol. Endocrinol. Metab. 280, E827–E847. doi: 10.1152/ajpendo.2001.280.6.E827
Gaidhu M. P., Anthony N. M., Patel P., Hawke T. J., Ceddia R. B. (2010). Dysregulation of lipolysis and lipid metabolism in visceral and subcutaneous adipocytes by high-fat diet: role of ATGL, HSL, and AMPK. Am. J. Physiol. Cell. Physiol. 298, C961–C971. doi: 10.1152/ajpcell.00547.2009
Gaidhu M. P., Fediuc S., Anthony N. M., So M., Mirpourian M., Perry R. L. S., et al. (2009). Prolonged AICAR-induced AMP-kinase activation promotes energy dissipation in white adipocytes: novel mechanisms integrating HSL and ATGL. J. Lipid Res. 50 (4), 704. doi: 10.1194/JLR.M800480-JLR200
Gregoraszczuk E. L., Ptak A. (2013). Endocrine-disrupting chemicals: some actions of POPs on female reproduction. Int. J. Endocrinol. doi: 10.1155/2013/828532
Hing S., Narayan E. J., Thompson R. C. A., Godfrey S. S. (2016). The relationship between physiological stress and wildlife disease: consequences for health and conservation. Wildl. Res. 43, 51–60. doi: 10.1071/WR15183
Hua C., Geng Y., Niu L., Chen Q., Cai L., Tao S., et al. (2018). Stimulating lipolysis in subcutaneous adipose tissues by chronic dexamethasone administration in goats. Livest. Sci. 214, 62–67. doi: 10.1016/j.livsci.2018.05.020
Jenssen B. M. (2006). Endocrine-disrupting chemicals and climate change: A worst-case combination for arctic marine mammals and seabirds? Environ. Health Perspect. 114 (SUPPL.1), 76–80. doi: 10.1289/EHP.8057
Kashiwabara L., Pirard L., Debier C., Crocker D. E., Khudyakov J. I. (2023). Effects of cortisol, epinephrine, and bisphenol contaminants on the transcriptional landscape of marine mammal blubber. Am. J. Physiol. Regul. Integr. Comp. Physiol. 325 (5), 504–522. doi: 10.1152/ajpregu.00165.2023
Kelesidis T., Kelesidis I., Chou S., Mantzoros C. S. (2010). Narrative review: the role of leptin in human physiology: emerging clinical applications. Ann. Intern. Med. 152 (2), 93. doi: 10.1059/0003-4819-152-2-201001190-00008
Kershaw J. L., Botting C. H., Brownlow A., Hall A. J. (2018). ). Not just fat: investigating the proteome of cetacean blubber tissue. Conserv. Physiol. 6 (1). doi: 10.1093/CONPHYS/COY003
Kershaw J. L., Sherrill M., Davison N. J., Brownlow A., Hall A. J. (2017). Evaluating morphometric and metabolic markers of body condition in a small cetacean, the harbor porpoise (Phocoena phocoena). Ecol. Evol. 7 (10), 3494–3506. doi: 10.1002/ece3.2891
Khudyakov J. I., Abdollahi E., Ngo A., Sandhu G., Stephan A., Costa D. P., et al. (2019). Expression of obesity-related adipokine genes during fasting in a naturally obese marine mammal. Am. J. Physiol. Regul. Integr. Comp. Physiol. 317 (4), R521–R529. doi: 10.1152/ajpregu.00182.2019
Khudyakov J. I., Allen K. N., Crocker D. E., Trost N. S., Roberts A. H., Pirard L., et al. (2022). Comprehensive molecular and morphological resolution of blubber stratification in a deep-diving, fasting-adapted seal. Front. Physiol. 13. doi: 10.3389/FPHYS.2022.1057721/BIBTEX
Khudyakov J. I., Champagne C. D., Meneghetti L. M., Crocker D. E. (2017). Blubber transcriptome response to acute stress axis activation involves transient changes in adipogenesis and lipolysis in a fasting-adapted marine mammal. Sci. Rep. 7 (42110). doi: 10.1038/SREP42110
Kim J. M., Choi J. S., Kim Y. H., Jin S. H., Lim S., Jang H. J., et al. (2013). An activator of the cAMP/PKA/CREB pathway promotes osteogenesis from human mesenchymal stem cells. J. Cell. Physiol. 228 (3), 617–626. doi: 10.1002/JCP.24171
Klemm D. J., Leitner J. W., Watson P., Nesterova A., Reusch J. E. B., Goalstone M. L., et al. (2001). Insulin-induced Adipocyte Differentiation: activation of creb rescues adipogenesis from the arrest caused by inhibition of prenylation. J. Biol. Chem. 276 (30), 28430–28435. doi: 10.1074/JBC.M103382200
Lafontan M., Berlan M. (1993). Fat cell adrenergic receptors and the control of white and brown fat cell function. J. Lipid Res. 34, 1057–1091. doi: 10.1016/S0022-2275(20)37695-1
Lafontan M., Langin D. (2009). Lipolysis and lipid mobilization in human adipose tissue. Prog. Lipid Res. 48 (5), 275–297. doi: 10.1016/j.plipres.2009.05.001
Le Boeuf B. J., Crocker D. E., Costa D. P., Blackwell S. B., Webb P. M., Houser D. S. (2000). Foraging ecology of northern elephant seals. Ecol. Monogr. 70 (3), 353. doi: 10.2307/2657207
Louis C., Covaci A., Crocker D. E., Debier C. (2016). Lipophilicity of PCBs and fatty acids determines their mobilisation from blubber of weaned northern elephant seal pups. Sci. Total Environ. 541, 599–602. doi: 10.1016/J.SCITOTENV.2015.09.094
Louis C., Dirtu A. C., Stas M., Guiot Y., Malarvannan G., Das K., et al. (2014a). Mobilisation of lipophilic pollutants from blubber in northern elephant seal pups (Mirounga angustirostris) during the post-weaning fast. Environ. Res. 132, 438–448. doi: 10.1016/J.ENVRES.2014.04.016
Louis C., Perdaens L., Suciu S., Tavoni S. K., Crocker D. E., Debier C. (2015). Mobilisation of blubber fatty acids of northern elephant seal pups (Mirounga angustirostris) during the post-weaning fast. Comp. Biochem. Physiol. Part A: Mol. Integr. Physiol. 183, 78–86. doi: 10.1016/J.CBPA.2015.01.008
Louis C., Tinant G., Mignolet E., Thomé J. P., Debier C. (2014b). PCB-153 shows different dynamics of mobilisation from differentiated rat adipocytes during lipolysis in comparison with PCB-28 and PCB-118. PloS One 9 (9), e106495. doi: 10.1371/journal.pone.0106495
Louis C., Van den Daelen C., Tinant G., Bourez S., Thomé J. P., Donnay I., et al. (2014c). Efficient in vitro adipocyte model of long-term lipolysis: A tool to study the behavior of lipophilic compounds. In Vitro Cell. Dev. Biol. –Animal 50 (6), 507–518. doi: 10.1007/s11626-014-9733-6
Mashburn K. L., Atkinson S. (2008). Variability in leptin and adrenal response in juvenile Steller sea lions (Eumetopias jubatus) to adrenocorticotropic hormone (ACTH) in different seasons. Gen. Comp. Endocrinol. 155 (2), 352–358. doi: 10.1016/J.YGCEN.2007.05.030
McTernan P. G., Kusminski C. M., Kumar S. (2006). Resistin. Curr. Opin. Lipidol. 17 (2), 170–175. doi: 10.1097/01.MOL.0000217899.59820.9A
Mikkelsen L., Johnson M., Wisniewska D. M., van Neer A., Siebert U., Madsen P. T., et al. (2019). Long-term sound and movement recording tags to study natural behavior and reaction to ship noise of seals. Ecol. Evol. 9 (5), 2588–2601. doi: 10.1002/ece3.4923
Moriceau M. A., Cano-Sancho G., Kim M. J., Coumoul X., Emond C., Arrebola J. P., et al. (2023). Partitioning of persistent organic pollutants between adipose tissue and serum in human studies. Toxics. 11 (1), 41. doi: 10.3390/TOXICS11010041/S1
Noren D. P., Crocker D. E., Williams T. M., Costa D. P. (2003). Energy reserve utilization in northern elephant seal (Mirounga angustirostris) pups during the postweaning fast: size does matter. J. Comp. Physiol. B. Biochem. Syst. Environ. Physiol. 173 (5), 443–454. doi: 10.1007/S00360-003-0353-9
Nowacek D. P., Thorne L. H., Johnston D. W., Tyack P. L. (2007). Responses of cetaceans to anthropogenic noise. Mamm. Rev. 37 (2), 81–115. doi: 10.1111/j.1365-2907.2007.00104.x
Ortiz R. M., Wade C. E., Ortiz C. L. (2001). Effects of prolonged fasting on plasma cortisol and TH in postweaned northern elephant seal pups. Am. J. Physiol. – Regul. Integr. Comp. Physiol. 280, R790–R795. doi: 10.1152/AJPREGU.2001.280.3.R790
Peckett A. J., Wright D. C., Riddell M. C. (2011). The effects of glucocorticoids on adipose tissue lipid metabolism. Metab. Clin. Exp. 60 (11), 1500–1510. doi: 10.1016/J.METABOL.2011.06.012
Peterson S. H., Ackerman J. T., Holser R. R., McDonald B. I., Costa D. P., Crocker D. E. (2023). Mercury bioaccumulation and cortisol interact to influence endocrine and immune biomarkers in a free-ranging marine mammal. Environ. Sci. Technol. 57 (14), 5678–5692. doi: 10.1021/ACS.EST.2C08974/ASSET/IMAGES/LARGE/ES2C08974_0008.JPEG
Pujade Busqueta L., Crocker D. E., Champagne C. D., McCormley M. C., Deyarmin J. S., Houser D. S., et al. (2020). A blubber gene expression index for evaluating stress in marine mammals. Conserv. Physiol. 8 (1), coaa082. doi: 10.1093/conphys/coaa082
Rolland R. M., McLellan W. A., Moore M. J., Harms C. A., Burgess E. A., Hunt K. E. (2017). Fecal glucocorticoids and anthropogenic injury and mortality in North Atlantic right whales Eubalaena glacialis. Endanger. Species Res. 34, 417–429. doi: 10.3354/esr00866
Rolland R. M., Parks S. E., Hunt K. E., Castellote M., Corkeron P. J., Nowacek D. P., et al. (2012). Evidence that ship noise increases stress in right whales. Proc. R. Soc B: Biol. Sci. 279 (1737), 2363–2368. doi: 10.1098/rspb.2011.2429
Rzucidlo C. L., Sperou E. S., Holser R. R., Khudyakov J. I., Costa D. P., Crocker D. E. (2021). Changes in serum adipokines during natural extended fasts in female northern elephant seals. Gen. Comp. Endocrinol. 308, 113760. doi: 10.1016/J.YGCEN.2021.113760
Schnitzler J. G., Reckendorf A., Pinzone M., Autenrieth M., Tiedemann R., Covaci A., et al. (2019). Supporting evidence for PCB pollution threatening global killer whale population. Aquat. Toxicol. 206, 102–104. doi: 10.1016/j.aquatox.2018.11.008
Stimson R. H., Anderson A. J., Ramage L. E., Macfarlane D. P., de Beaux A. C., Mole D. J., et al. (2017). Acute physiological effects of glucocorticoids on fuel metabolism in humans are permissive but not direct. Diabetes Obes. Metab. 19 (6), 883–891. doi: 10.1111/dom.12899
Strandberg U., Käkelä A., Lydersen C., Kovacs K. M., Grahl-Nielsen O., Hyvärinen H., et al. (2008). Stratification, composition, and function of marine mammal blubber: The ecology of fatty acids in marine mammals. Physiol. Biochem. Zool. 81 (4), 473–485. doi: 10.1086/589108
Than A., Ye F., Xue R., Ong J. W., Poh C. L., Chen P. (2011). The crosstalks between adipokines and catecholamines. Mol. Cell. Endocrinol. 332 (1–2), 261–270. doi: 10.1016/j.mce.2010.11.002
Timmermans S., Souffriau J., Libert C. (2019). A general introduction to glucocorticoid biology. Front. Immunol. 10. doi: 10.3389/fimmu.2019.0154
Trumble S. J., Norman S. A., Crain D. D., Mansouri F., Winfield Z. C., Sabin R., et al. (2018). Baleen whale cortisol levels reveal a physiological response to 20th century whaling. Nat. Commun. 9, 4587. doi: 10.1038/s41467-018-07044-w
Tsubai T., Noda Y., Ito K., Nakao M., Seino Y., Oiso Y., et al. (2016). Insulin elevates leptin secretion and mRNA levels via cyclic AMP in 3T3-L1 adipocytes deprived of glucose. Heliyon 3, e00194. doi: 10.1016/J.HELIYON.2016.E00194
Vanden Berghe M., Mat A., Arriola A., Polain S., Stekke V., Thomé J. P., et al. (2010). Relationships between vitamin A and PCBs in grey seal mothers and pups during lactation. Environ. pollut. 158 (5), 1570–1575. doi: 10.1016/J.ENVPOL.2009.12.012
Vanden Berghe M., Weijs L., Habran S., Das K., Bugli C., Rees J. F., et al. (2012). Selective transfer of persistent organic pollutants and their metabolites in grey seals during lactation. Environ. Int. 46, 6–15. doi: 10.1016/J.ENVINT.2012.04.011
Vázquez-Medina J. P., Crocker D. E., Forman H. J., Ortiz R. M. (2010). Prolonged fasting does not increase oxidative damage or inflammation in postweaned northern elephant seal pups. J. Exp. Biol. 213, 2524–2530. doi: 10.1242/JEB.041335
Vázquez-Medina J. P., Zenteno-Savín T., Forman H. J., Crocker D. E., Ortiz R. M. (2011). Prolonged fasting increases glutathione biosynthesis in postweaned northern elephant seals. J. Exp. Biol. 214 (8), 1294. doi: 10.1242/JEB.054320
Viscarra J. A., Champagne C. D., Crocker D. E., Ortiz R. M. (2011a). 5′AMP-activated protein kinase activity is increased in adipose tissue of northern elephant seal pups during prolonged fasting-induced insulin resistance. J. Endocrinol. 209 (3), 317. doi: 10.1530/JOE-11-0017
Viscarra J. A., Ortiz R. M. (2013). Cellular mechanisms regulating fuel metabolism in mammals: role of adipose tissue and lipids during prolonged food deprivation. Metab. Clin. Exp. 62 (7), 889. doi: 10.1016/J.METABOL.2012.12.014
Viscarra J. A., Vázquez-Medina J. P., Crocker D. E., Ortiz R. M. (2011b). Glut4 is upregulated despite decreased insulin signaling during prolonged fasting in northern elephant seal pups. Am. J. Physiol. Regul. Integr. Comp. Physiol. 300 (1), 150–154. doi: 10.1152/ajpregu.00478.2010
Viscarra J. A., Vázquez-Medina J. P., Rodriguez R., Champagne C. D., Adams S. H., Crocker D. E., et al. (2012). Decreased expression of adipose CD36 and FATP1 are associated with increased plasma non-esterified fatty acids during prolonged fasting in northern elephant seal pups (Mirounga angustirostris). J. Exp. Biol. 215 (14), 2455–2464. doi: 10.1242/jeb.069070
Xu C., He J., Jiang H., Zu L., Zhai W., Pu S., et al. (2009). Direct effect of glucocorticoids on lipolysis in adipocytes. Mol. Endocrinol. 23 (8), 1161–1170. doi: 10.1210/me.2008-0464
Keywords: elephant seal, adipose tissue, precision-cut slices, cortisol, epinephrine, lipolysis, leptin, PCB mobilization
Citation: Pirard L, Khudyakov JI, Crocker DE, Van Hassel L, Scholl G, Eppe G, Page MM, Rees J-F, Smith DR and Debier C (2023) Cortisol and epinephrine alter the adipose functions and the mobilization of PCBs in adipose tissue slices from elephant seal. Front. Mar. Sci. 10:1290472. doi: 10.3389/fmars.2023.1290472
Received: 07 September 2023; Accepted: 27 October 2023;
Published: 17 November 2023.
Edited by:
MD Saydur Rahman, The University of Texas Rio Grande Valley, United StatesReviewed by:
Davina Derous, University of Aberdeen, United KingdomAubrey Converse, Northwestern University, United States
Copyright © 2023 Pirard, Khudyakov, Crocker, Van Hassel, Scholl, Eppe, Page, Rees, Smith and Debier. This is an open-access article distributed under the terms of the Creative Commons Attribution License (CC BY). The use, distribution or reproduction in other forums is permitted, provided the original author(s) and the copyright owner(s) are credited and that the original publication in this journal is cited, in accordance with accepted academic practice. No use, distribution or reproduction is permitted which does not comply with these terms.
*Correspondence: Cathy Debier, cathy.debier@uclouvain.be