- 1Laboratorio de Cultivo de Peces, Instituto del Mar del Perú (IMARPE), Esquina Gamarra y General Valle S/N - Chucuito, Callao, Peru
- 2Laboratorio de Análisis Instrumental, Instituto del Mar del Perú (IMARPE), Callao, Peru
- 3Department of Aquaculture, Centro de Investigación Científica y de Educación Superior de Ensenada (CICESE), Carretera Tijuana - Ensenada, Ensenada, Baja California, Mexico
- 4Aquaculture Programme, IRTA La Rápita, Ctra. Poble Nou, La Rápita, Tarragona, Spain
Anisotremus scapularis is a marine fish distributed along the Peruvian coast that is commercially important in local markets. IMARPE started research on this species aimed to condition breeders and control reproduction in captivity and avoid dependence on wild broodstock. Therefore, the present study characterized and compared the reproductive performance, quality of eggs and larvae between a first generation domesticated broodstock (F1B) and a wild broodstock (WB). The F1B and WB broodstocks were conditioned in recirculation systems (RAS) to spawn naturally from August 2021 to August 2022. The following parameters were recorded for each spawn, the total number of eggs, percentages of viable eggs and fertilization, and hatching, and biochemical analyses on each spawn were performed. Progeny from each spawning were assessed for survival activity index (SAI), and at 20 days’ post-hatch (DPH), the temperature tolerance index (TTI) was analyzed. WB had significantly higher (P<0.05) egg production, egg diameter, oil globule diameter, and SAI than those of F1B. However, the percentage of viable eggs and hatching showed significantly higher values (P<0.05) in F1B spawns. A low correlation (r<0.50) was observed between the evaluated reproductive parameters in A. scapularis broodstock; nevertheless, it was significant in some cases. No significant differences were observed in protein, lipid, carbohydrate, or ash content in eggs, but ash content in the 20 DPH larvae differed, with higher values in F1B. A principal component analysis (PCA), indicated a strong relationship between F1B broodstock eggs and fatty acids DHA, 16:0, 18:1n-9, and 18:2n-6; whereas fatty acids 18:0, 20:4n-3, 22:5n-3, and EPA had a high connection with WB eggs. At 20 DPH, F1B larvae had a strong link with 18:1n-9, DHA, and 18:2n-6; while WB larvae were found to have a high association with 16:1n-7, EPA, 22:5n-3, and ARA. Based on these results we concluded that F1B broodstock has better egg quality in terms of egg viability and hatching rate and similarity in most larval quality parameters compared to WB, making the F1 domesticated broodstock (F1B) an alternative to establish a broodstock under captive conditions and thus contributing to the aquaculture development of the species.
1 Introduction
The peruvian grunt Anisotremus scapularis is a marine fish distributed along the coasts of Ecuador, Peru, and Chile, from Manta (Ecuador) to Antofagasta (Chile), in rocky areas at depths of up to 25 m (Chirichigno and Velez, 1998). The Peruvian grunt is a gonochoric fish species with no sexual dimorphism and whose main spawning season spans from summer to autumn on the marine coast of Callao. It is a resource highly valued in Peru for its commercial and gastronomic qualities, known for the quality of its meat and high fillet yield (30% of total weight). Anisotremus scapularis also sustains artisanal coastal fisheries along the entire Peruvian coastline (Mendo and Wosnitza-Mendo, 2014). In relation to this, the National Aquaculture Policy to 2030 (Ministerio de la Producción, 2023) and the “Roadmap for Marine Fish Farming in Peru” [“Hoja de Ruta del Cultivo de Peces Marinos en el Perú”] (Molares, 2021) stressed the need to support and direct research towards the technological development of aquaculture based on prioritized species, such as A. scapularis. Therefore, it is important to achieve a sustained production of seed and juveniles, and for this purpose having captive broodstock and controlling reproduction are key aspects to achieve this goal.
In this respect, it is necessary to consider that the development of a sustainable aquaculture industry requires closing the culture cycle in captivity. Closing the lifecycle of any potential species and initiating a breeding selection program is essential and is best achieved by stablishing broodstock reared in captivity from juveniles (Jeréz et al., 2018). The production of domesticated broodstock batches reduces the pressure on wild populations, moreover, it serves as the underpinnings for successful farming operations in many countries, especially in Asia and the Mediterranean (Ibarra-Castro and Alvarez-Lajonchère, 2011). Additionally, the costs of feeding and maintaining broodstock are high, which is why it is important to develop methodologies to compare different stocks of broodstock in order to select individuals with high reproductive capacity (Quiñones-Arreola et al., 2015). In this regard, the quality of spawn is defined as the potential of an egg to hatch and develop into a viable larva (Kjørsvik et al., 1990; Brooks et al., 1997). In fish, the quality of eggs and larvae is highly variable and is influenced by several factors including nutritional status, stress, over-ripeness, induction of spawning, genetics and age of the broodstock, as well as water quality and other environmental factors such as photoperiod, temperature, and salinity (Ceccon et al., 2012), and the biochemical composition of eggs (Wright-Moore et al., 2019).
Therefore, developing reliable criteria to characterize egg quality and select spawns that produce highly viable offspring, is fundamental to evaluate and establish growout protocols. Extensive research on egg quality has been conducted in recent decades (Kjørsvik et al., 1990; Brooks et al., 1997; Bobe and Labbé, 2010; Migaud et al., 2013). However, the success of these efforts is still moderate in marine fish (Thorsen et al., 2003), and comparisons between studies are difficult due to the lack of standardization of methodologies and criteria used to define egg quality. Most studies include fertilization and hatching rates when evaluating egg quality, as recommended by Brooks et al. (1997).
The Instituto del Mar del Perú (IMARPE) initiated research on the conditioning and captive reproduction of A. scapularis, achieving the first spawns and, in recent years, improving the cultivation of larvae and juveniles. However, seed production relies on a batch of broodstock composed by specimens captured from the natural environment. Therefore, in order to improve the production of eggs and larvae under laboratory conditions, we propose as the main objective of this study, the conditioning and characterization of the reproductive performance and spawn quality of a first-generation broodstock born in captivity (F1), and compare egg and larval quality with spawns from wild broodstock.
2 Materials and methods
2.1 Broodstock conditioning and spawning
Broodstock fish were conditioned in seawater recirculation systems (RAS) composed of 2 sets of tanks with a total water capacity of 2.5 m3, a water pump (3/4 HP, IWAKI, Japan), a heat pump (9500 BTU, Aqua Logic, USA), a biofilter (4.5 ft3 floating bed filter, Aqua Logic, USA), and a seawater sterilizer with ultraviolet lamp (Emperor Aquatics, Aqua Logic, USA) (Carrera et al., 2018). Thirty first-generation (group F1) Peruvian grunts broodstock were housed in recirculation system 1 (RAS 1), with females weighing on average 469.89 ± 132.57 g with 27.39 ± 2.19 cm in length and males 466.23 ± 174.60 g with 27.71 ± 2.91 cm. Broodstock had been cultured for 5 years (group F1B) prior to the experiment. The wild broodstock group (WB, 30 specimens) was placed in system 2 (RAS 2) with females averaging 727.72 ± 248.59 g and 31.05 ± 4 cm and males 599.55 ± 200.75 g and 29.54 ± 3.56 cm. The sex ratio in the tanks was 1:1 in both broodstock batches. Based on the gonadosomatic index variation reported for the species in their natural environment and data that were obtained from daily satellite monitoring of oceanographic parameters in the Peruvian sea by the Remote Sensing Functional Area of IMARPE and from the National Oceanic and Atmospheric Administration (NOAA) solar calculations, fish were subjected to a natural photoperiod and thermoperiod (ranging from 11 to 13 hours of light and 17 to 20°C).
Broodstock were fed three times a week with Peruvian anchovy (Engraulis ringens) chunks supplemented with gelatin capsules containing vitamins and omega-3 fatty acids (Cota et al., 2022), at a feeding ratio between 4% and 5% of the total biomass for each culture tank. Both A. scapularis broodstock groups spawned naturally, and eggs were collected from the adjacent 100 L collection tanks attached to each culture system, which were fitted with 500 µm mesh bags. Egg production was monitored every morning from August 2021 to August 2022 for WB and from September 2021 to August 2022 for F1B for all spawns. Physicochemical parameters and nitrogen compounds of the culture systems were monitored every other day. The temperature, oxygen level, and pH were maintained at averages of 18.78 ± 0.79°C, 6.65 ± 1.21 mg L-1, and 7.30 ± 0.56, respectively. Nitrogen compounds values for total ammonia nitrogen were (0.09 ± 0.15 mg L-1), for nitrite (0.34 ± 0.24 mg L-1), and for nitrate (73.59 ± 52.50 mg L-1).
2.2 Reproductive performance and egg quality evaluation
Eggs were collected in 10 L containers from the spawn collection tanks and immediately rinsed on 500 µm mesh sieves using abundant UV sterilized seawater. The eggs were then transferred to 1 L beakers and samples were taken to count the total number of eggs per spawn.
To estimate the percent fertilization rates, three aliquots of 50 eggs each were taken and placed in Petri dishes, in each of which the number of eggs showing 2-64 blastomeres were counted using an optical microscope (Leica, Germany) and the LAS 3.4 image program.
Fertilization rate was calculated with Equation 1:
Where:
Nh = Number of eggs with 2-64 blastomeres,
Nt = Total number of eggs.
Then, the percentage of viable (floating) and non-viable (non-floating) eggs was determined. Additionally, the diameter of the eggs and the oil droplet were measured using the LAS 3.4 image program and an optical microscope (Leica, Germany).
Hatching rates (%) were calculated using 50 fertilized eggs, placed in in 500 mL beakers for incubation at 19°C, and the number of live larvae counted, approximately 40 hours after fertilization, and the following Equation 2 was used:
Where:
LV = Number of live larvae,
LM = Number of dead larvae,
HM = Number of remaining eggs.
2.3 Evaluation of larval quality
One spawn for every month from October 2021 to July 2022 (with fertilization rate > 80%) was selected from each broodstock group to assess larval quality. Larvae were cultured from day 0 DPH until 20 DPH, which corresponds to the rotifer feeding stage. The larval culture was performed using our well established protocol from the IMARPE´s Fish Culture Laboratory [Laboratorio de Cultivo de Peces] (Castro et al., 2021). Briefly, larvae were transferred to 0.5-0.7 m3 fiberglass tanks, filled with 50% of the total volume and fitted with air stones to provide gentle aeration. Additionally, the tanks were inoculated with “green water”, using a mixture of microalgae, Isochrysis galbana and Nanochloropsis oculata (ratio 1:4). Larvae were cultured at a temperature of 19°C, under static conditions (no water flow), for 3 days and then partial water changes were carried out (10%: 4-10 DPH, 20%: 11-14 DPH and 30%: 15-20 DPH). A photoperiod of 24 hours of light (HL): 0 hours of darkness (HO) was used during the first 10 DPH, followed by 12 HL: 12 HO until 20 DPH using a white fluorescent lights (1000 lux). The stocking density was 20 larvae/L.
Larval quality was assessed using larval tolerance to starvation with the Survival Activity Index (SAI) following the procedure described by Shimma and Tsujigado (1981). Briefly, three replicates of 30 newly hatched larvae were placed in 1 L beakers without feeding, and the cumulative mortality was recorded daily, using Equation 3:
Where:
N = Total number of larvae,
hi = Cumulative mortality on the i-th day,
k = Number of days elapsed until all larvae die due to starvation.
The SAI also indicates the cumulative mortality on the 3rd day after hatching. This is a potential indicator of larval quality as it reflects the quality of endogenous reserves contained in the yolk sac and the potential for larval survival (Giménez et al., 2006).
Additionally, the quality of the larvae was evaluated at the end of the experiment, on the 20 DPH, using the temperature tolerance test developed by Dhert et al. (1992), which can be considered an indicator of survival capacity in their natural environment related to the resistance inherited from the parental broodstock and the quality of the feeding during the firsts DPH (Fuentes, 2014; Santamaría-Miranda et al., 2021). The test involved subjecting the larvae to acute stress at a temperature of 32°C. For this, 500 mL beakers with seawater from the culture systems were used, which were placed inside an 80 L plastic container with the same culture water (water bath) and a 1000-watt heater to maintain a constant water temperature of 32°C. When the selected temperature was reached, 5 larvae from each tank (in duplicate) were placed in each beaker. Mortality was recorded every 5 minutes for 120 minutes. Larvae were considered dead if they appeared opaque, with a bent body, and unresponsive to any contact stimulus. The temperature tolerance index (TTI) was calculated by adding the cumulative mortalities recorded in each mentioned time interval.
During the larval culture, larval growth was monitored by measuring their length in millimeters using the LAS 3.4 image program and an optical microscope coupled with a digital camera. The larvae were anesthetized with MS-222, and their measurements were taken on the 1, 15, and 20 DPH. Survival was evaluated at the beginning and at the end of the larval culture.
2.4 Biochemical analysis
The samples for biochemical analysis were obtained from 2 spawns from each group (WB and F1B) every month during the study (October 2021 to August 2022, except for the months of March 2021 and July and August 2022, where it was analyzed from a group of broodstock), as well as from the larvae collected at the end of the larval culture (October 2021 to July 2022, except for the months of October and November 2021 and March, June, July and August 2022, where larvae were obtained from a group of broodstock or were not cultured). Approximately 10 g of viable eggs and 5 g of 20 DDE larvae per sample were collected using a 500 µm sieve and rinsed with distilled water. They were then placed in 50 mL Falcon tubes and frozen at -80°C until further analysis.
The assays were conducted by the Instrumental Analysis Laboratory [Laboratorio de Análisis Instrumental] of IMARPE. Total soluble proteins were estimated using the Hartree method (Hartree, 1972), total carbohydrates were determined using the spectrophotometric method (DuBois et al., 1956), and total lipids were assessed using a modified Folch method (Folch et al., 1957) by IMARPE, which involves lipid extraction using a Chloroform-Methanol 2:1 solvent mixture with ultrasound assistance. The fatty acids were then identified using a gas chromatograph (Varian, Netherlands) according to Ichihara and Fukubayashi (2010). For moisture analysis, 0.1 g of sample was used, which was dried in a vacuum oven at a temperature of 105°C for 16 hours and a pressure below 0.1 bar. In the case of ash analysis, 0.1 g of sample was incinerated in a muffle furnace at 550°C for 16 hours. The amino acids were hydrolyzed with 6 M HCl for 22 hours at 112°C in a block heater, then derivatized according to the Waters manufacturer’s kit, and analyzed by HPLC-FL (Hitachi, Japan).
2.5 Statistical analysis
The data were expressed as mean and standard deviation. Statistical comparisons of reproductive performance and indicators of egg and larval quality were performed between different batches of broodstock (WB and F1B) using the Student t-test and correlations were assessed using the Pearson test (Schober et al., 2018). Normality was tested using the Shapiro-Wilk statistic, and homoscedasticity of variances was tested using the Bartlett test. The non-parametric Mann-Whitney test was applied to variables that did not meet the assumptions of the Student t-test. To determine whether the fatty acid or amino acid profiles of the eggs could be used to differentiate spawns from wild and cultured broodstock (F1), a principal component analysis (PCA) and discriminant analysis were conducted. A significance level of 0.05 was used for all analyses, and the data were processed using the R statistical software (R Core Team, 2022).
3 Results
3.1 Reproductive performance and egg quality evaluation
Egg production during the study period in both F1B and WB groups is shown in Figure 1. Production from wild specimens was significantly higher (P<0.05) than the F1 generation (Figure 1). On average, wild specimens spawned a total of 90,804 ± 77,273 eggs per month, which in terms of relative fecundity (relationship between fecundity and body weight of females expressed in kg), corresponds to 206,372 eggs kg-1 female, while the F1 generation spawned 56,149 ± 33,146 eggs, that corresponds to 119,465 eggs kg-1 female. In terms of the number of spawns during the monitoring period, the F1B group produced 89 spawns, and the wild specimens produced 197 spawns (Table 1; Figure 1).
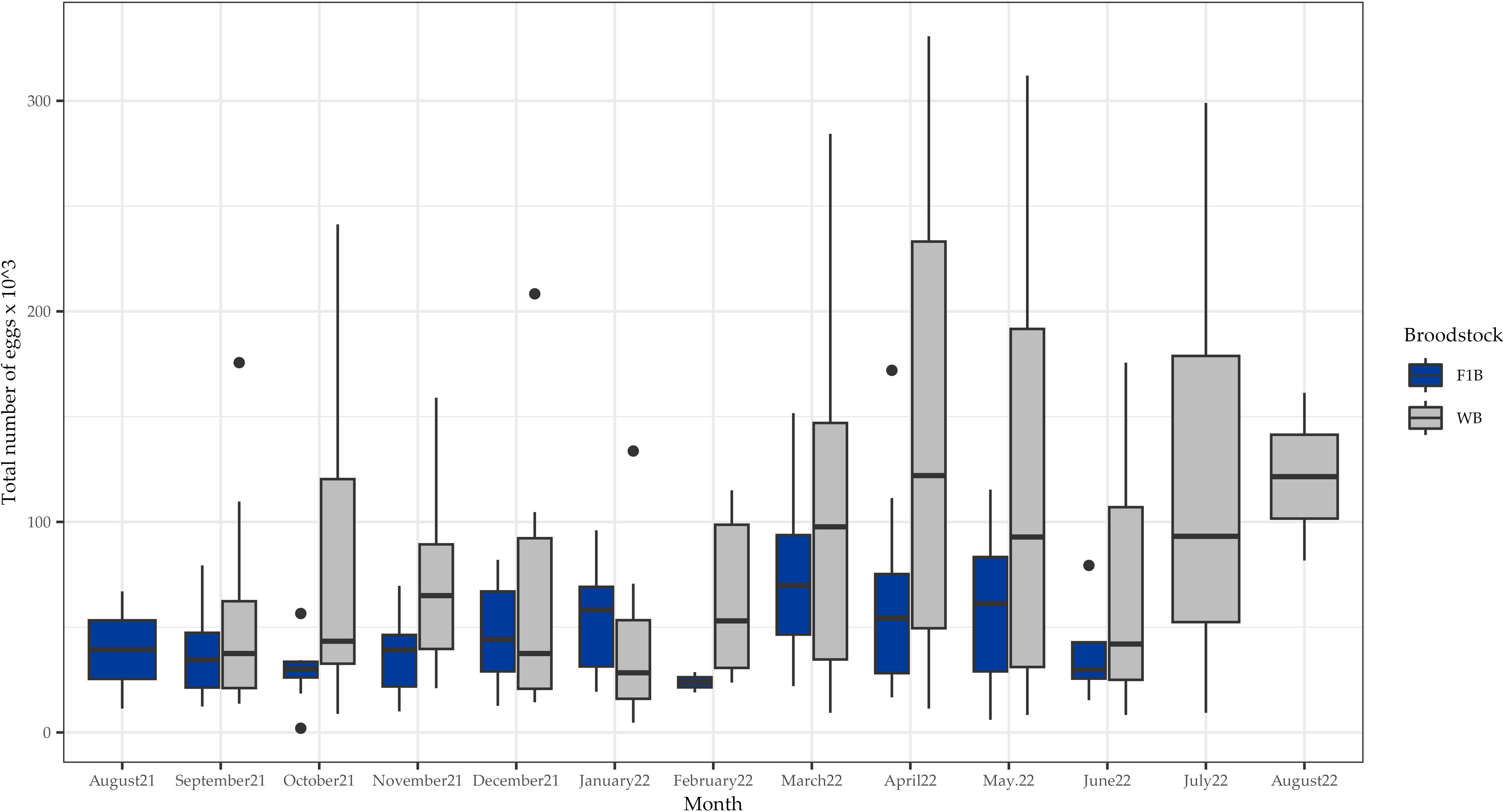
Figure 1 Total egg production (x 103) from first-generation domesticated (F1B) and wild caught (WB) Anisotremus scapularis broodstock. F1B: 89 spawns, WB: 197 spawns. A statistical significance level of 0.05 was used.
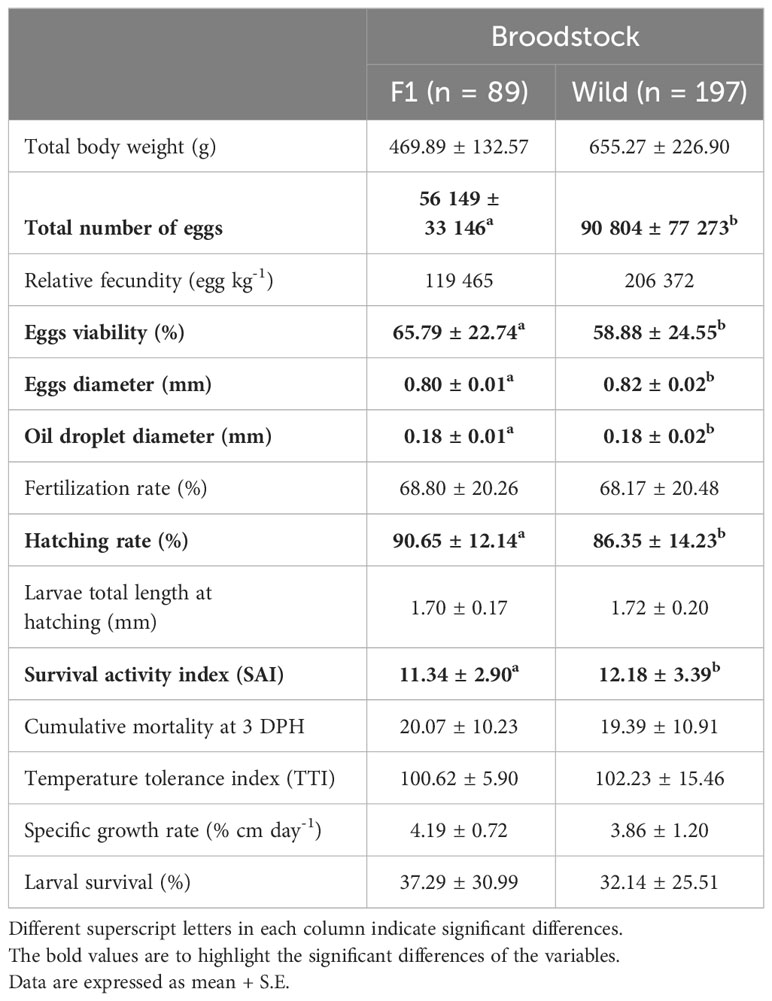
Table 1 Reproductive and larval performance of Anisotremus scapularis first-generation domesticated (F1B) and wild caught (WB) broodstock evaluated from August 2021 to August 2022.
Egg viability resulted in significant differences between groups; WB reached an average of 58.88 ± 24.55%, while F1B showed 65.79 ± 22.74%, with the latter being significantly higher. In terms of egg diameter and oil droplet size, a similar trend was observed in both cases; however, significant differences were found with larger values in wild specimens, with an average egg diameter of 0.82 ± 0.02 mm and oil droplet size of 0.18 ± 0.02 mm, compared with F1B with 0.80 ± 0.01 mm and 0.18 ± 0.01 mm, respectively. Fertilization (%) was 68.17 ± 20.48% for WB and 68.80 ± 20.26% for F1B, with no significant differences between the two groups. F1B had significantly higher hatching rates with an average of 90.65 ± 12.14%, compared with WB with an average of 86.35 ± 14.23% (Table 1).
3.2 Larval quality evaluation
There were no significant differences in the total length of hatched larvae between the two broodstock groups. With respect to the survival activity index, the larvae survived without feed up to 8 DPH, and significant differences were observed between the two broodstock groups: the SAI for WB was 12.18 ± 3.39% and 11.34 ± 2.90% for F1B (Table 1). The cumulative mortality on the 3rd day after hatching had an average of 20.07 ± 10.23% for F1B and 19.39 ± 10.91% for WB. Spawns with accumulated mortality below 10% were considered good quality spawns, while those with mortality above 35% were considered poor quality and between 10 and 35% mortality were considered intermediate quality. All spawns had intermediate quality (Figure 2).
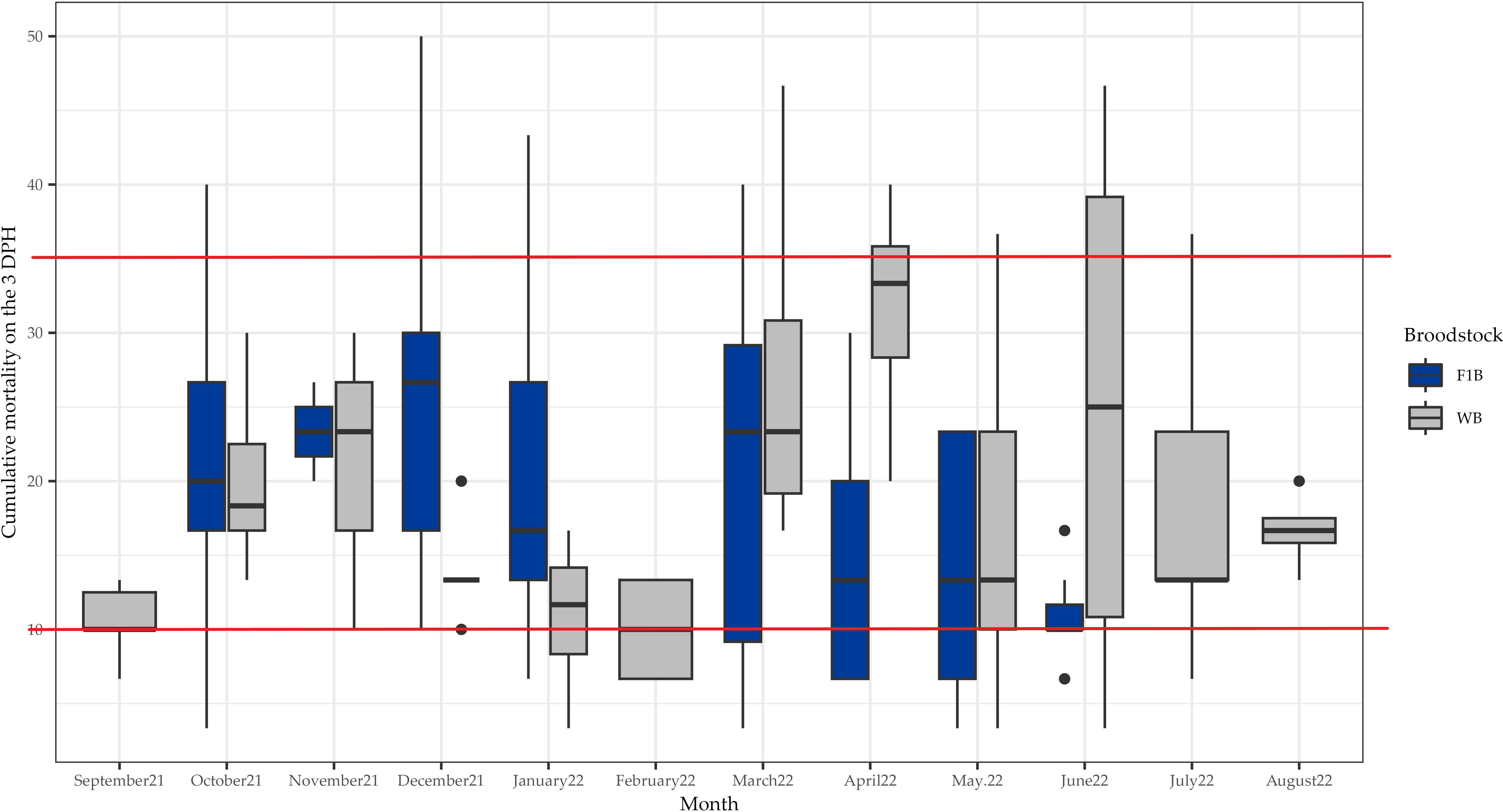
Figure 2 Box plot of accumulated mortality by day 3 (MA3) of Anisotremus scapularis first-generation domesticated (F1B) and wild caught (WB) broodstocks. F1B: 89 spawns, WB: 197 spawns. A statistical significance level of 0.05 was used.
The temperature tolerance index (TTI) of larvae from the WB batch began to show signs of low tolerance from 7.08 ± 4.69 min, and F1B from 9.29 ± 7.32 min for most of the months evaluated. The average values of the TTI from August to July for WB larvae were 102.23 ± 15.46, while for F1B larvae from August to May, it was 100.62 ± 5.90. However, no significant differences were observed between the two broodstock groups. It is also worth mentioning that the best results for F1B spawns were observed in November 2021, and March and May 2022, while for WB spawns in January, March, and July 2022 (Figure 3).
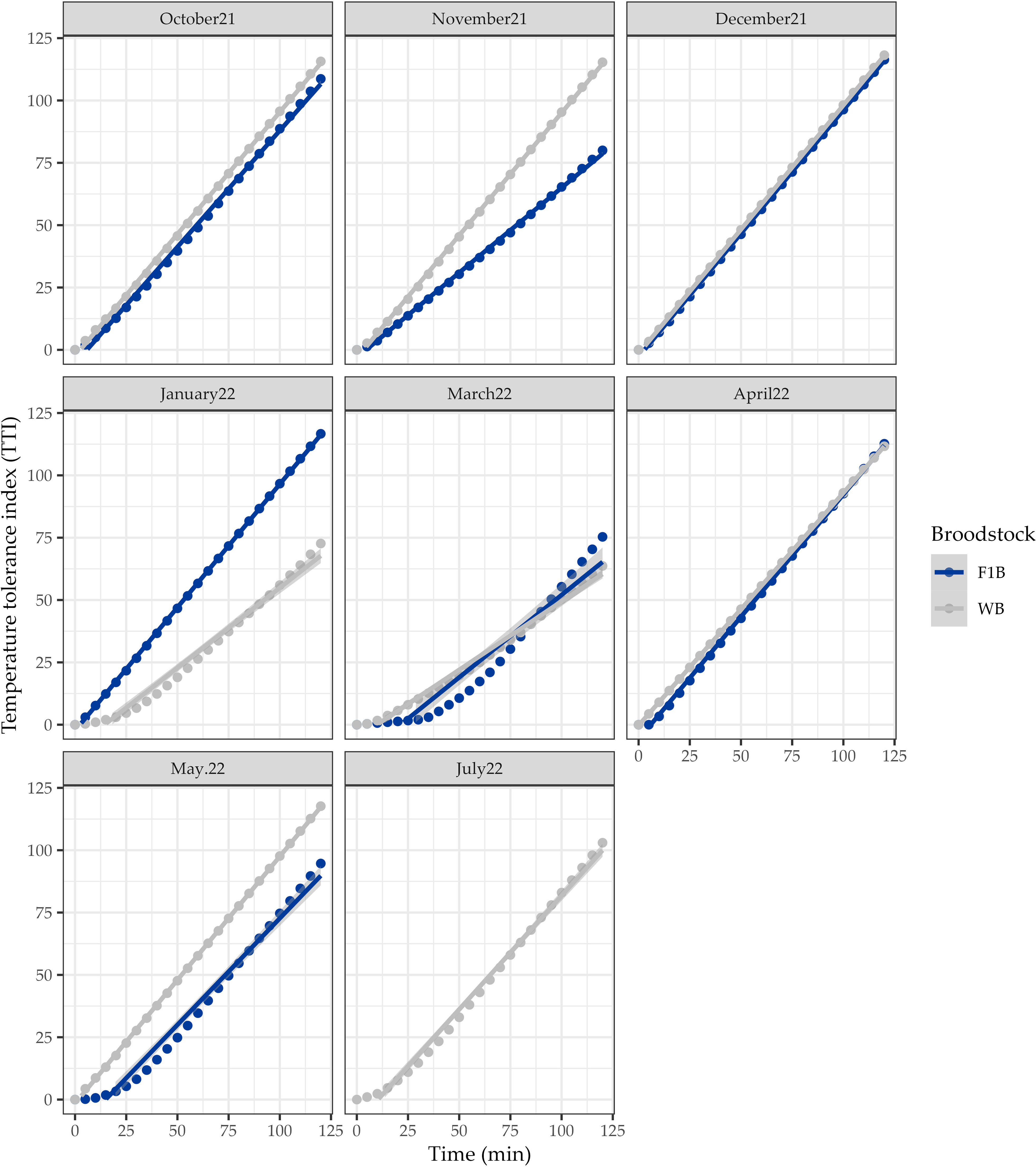
Figure 3 Temperature tolerance index (TTI) of larvae of Anisotremus scapularis first-generation domesticated (F1B) and wild caught (WB) broodstocks. F1B: 7 spawns, WB: 8 spawns. A statistical significance level of 0.05 was used.
Specific growth rate in % cm day-1 was also determined in larvae; the average for F1B was 4.19 ± 0.72% cm day-1, and 3.86 ± 1.20% cm day-1 for WB, with no significant differences between the two groups. On the other hand, the average larval survival during the study period was 32.14 ± 25.51% for WB larvae and 37.29 ± 30.99% for F1B larvae, with higher values observed for the latter group in most months evaluated. However, no significant differences were observed between groups (Table 1).
3.3 Correlation
In general, a low correlation (r<0.50) was observed between the evaluated reproductive parameters in A. scapularis broodstock. However, in some parameters significant correlations were observed (Figure 4). For example, a moderate and positive correlation was observed between the percentage of viable eggs and fertilization rate (r=0.331), egg diameter and oil droplet diameter (r=0.305), and egg diameter and larval survival (r=0.554). Moreover, a moderate and negative correlation was observed between hatching rate and larval survival (r=-0.627), and SAI and cumulative mortality at 3 DPH (r=-0.537).
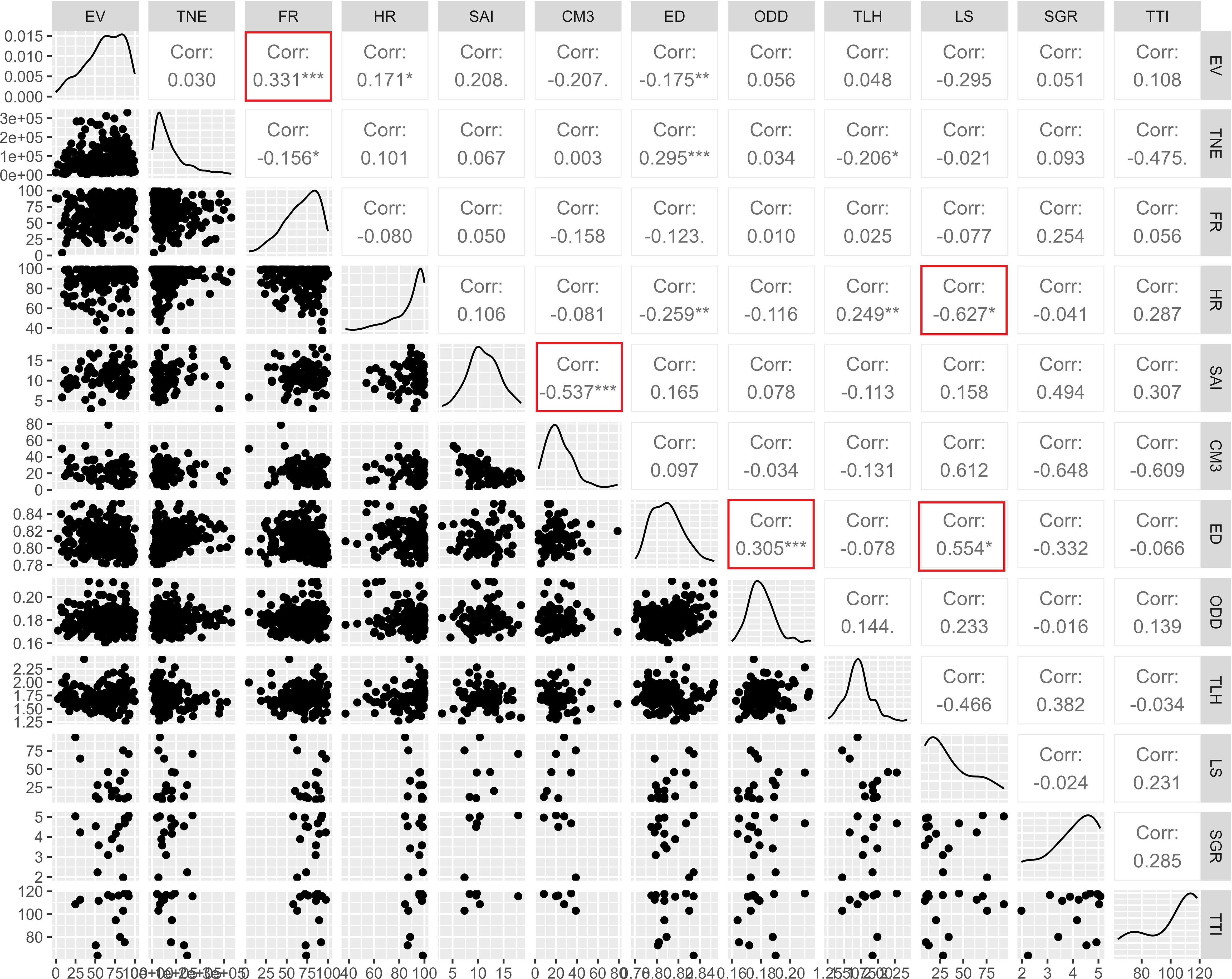
Figure 4 Pearson’s correlation coefficients between parameters measured on wild caught (WB) and first generation domesticated (F1B) spawns. Moderate and weak correlations with significant values are marked with red boxes. Symbols (*) denote the level of significance of the correlations: * p<0.05, ** p<0.01, or *** p<0.001. EV, eggs viability; TNE, total number of eggs; FR, fertilization rate; HR, hatching rate; SAI, survival activity index; CM3, cumulative mortality on the 3 DPH; ED, eggs diameter; ODD, oil droplet diameter; TLH, total length at hatching; LS, larval survival; SGR, specific growth rate; TTI, temperature tolerance index. F1B: 89 spawns, WB: 197 spawns. A statistical significance level of 0.05 was used.
3.4 Biochemical analysis of eggs and larvae
The proximal composition of eggs and 20 DPH larvae from both groups of A. scapularis broodstock is shown in Tables 2–4. No significant differences between groups were observed in protein, lipids, carbohydrates, or ash content in eggs, but significant differences were found in ash content in the 20 DPH larvae, with higher values for the F1B broodstock. Fatty acid profiles of eggs and larvae are reported in Table 3. Polyunsaturated fatty acids, in particular n-3 PUFA dominated in both groups of eggs (F1B and WB). More specifically docosahexaenoic acid (C22:6n–3) [DHA] was the most abundant fatty acid in eggs, followed by palmitic acid (16:0) and oleic acid (18:1n-9). In larvae, palmitic acid (16:0) was the highest fatty acid present in both groups (F1B and WB), followed by DHA and stearic acid (18:0). Additionally, significant differences were found in stearic acid (C18:0), eicosatetraenoic acid (20:4n-3), and docosapentaenoic acid (22:5n-3), with higher values in the WB eggs. Palmitic acid (16:0) and DHA had the highest values in the F1B eggs. In 20 DPH larvae, significant differences were observed in palmitoleic acid (16:1n-7), eicosapentaenoic acid (20:5n-3) [EPA], and arachidonic acid (20:4n-6) [ARA], with higher values in WB.
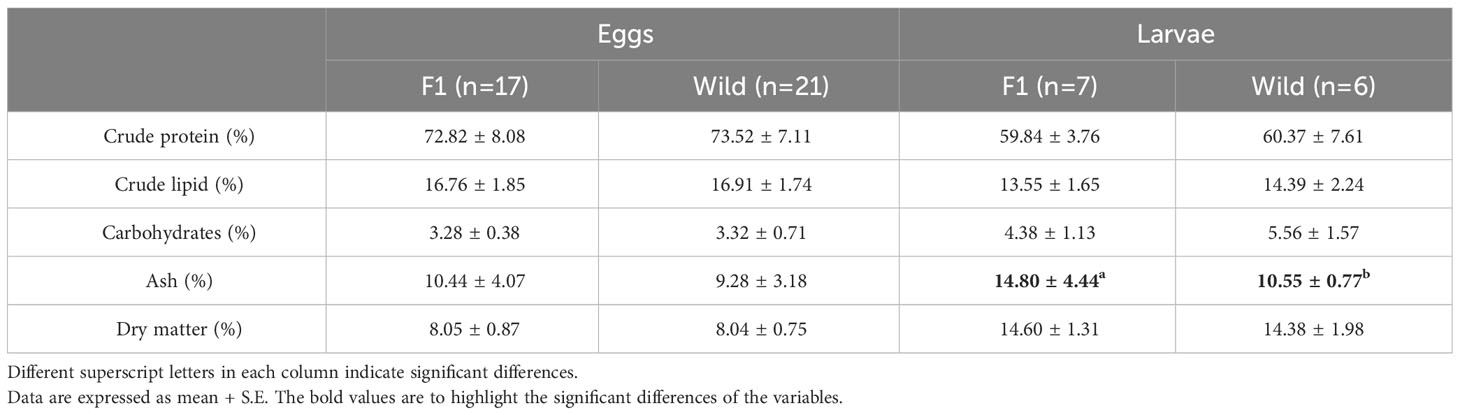
Table 2 Proximal composition of eggs and larvae from Anisotremus scapularis first-generation domesticated (F1B) and wild caught (WB) broodstocks.
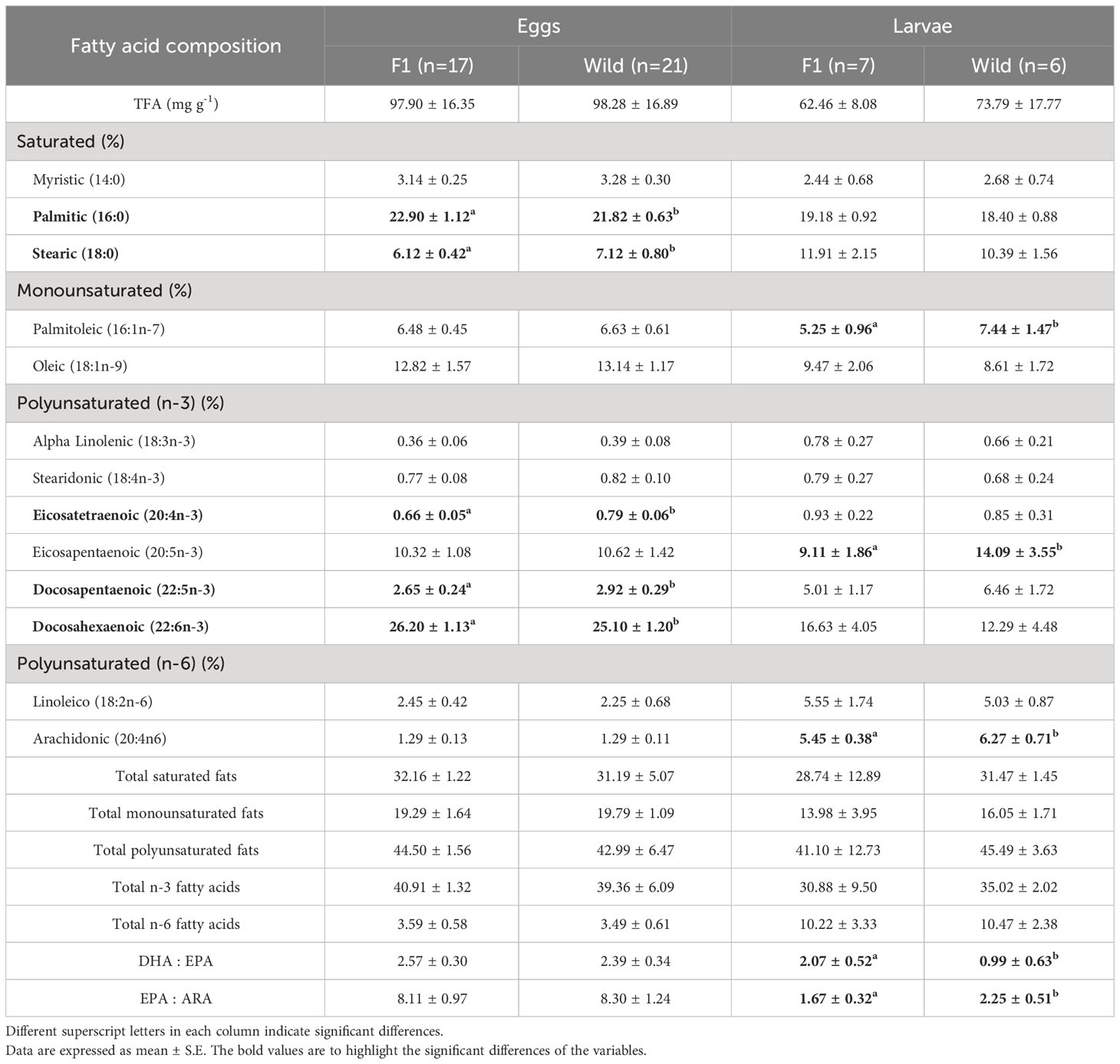
Table 3 Mean total fatty acid content (TFA, mg g-1 dry weight), and fatty acid composition (in %TFA) of eggs and larvae from Anisotremus scapularis first-generation domesticated (F1B) and wild caught (WB) broodstocks.
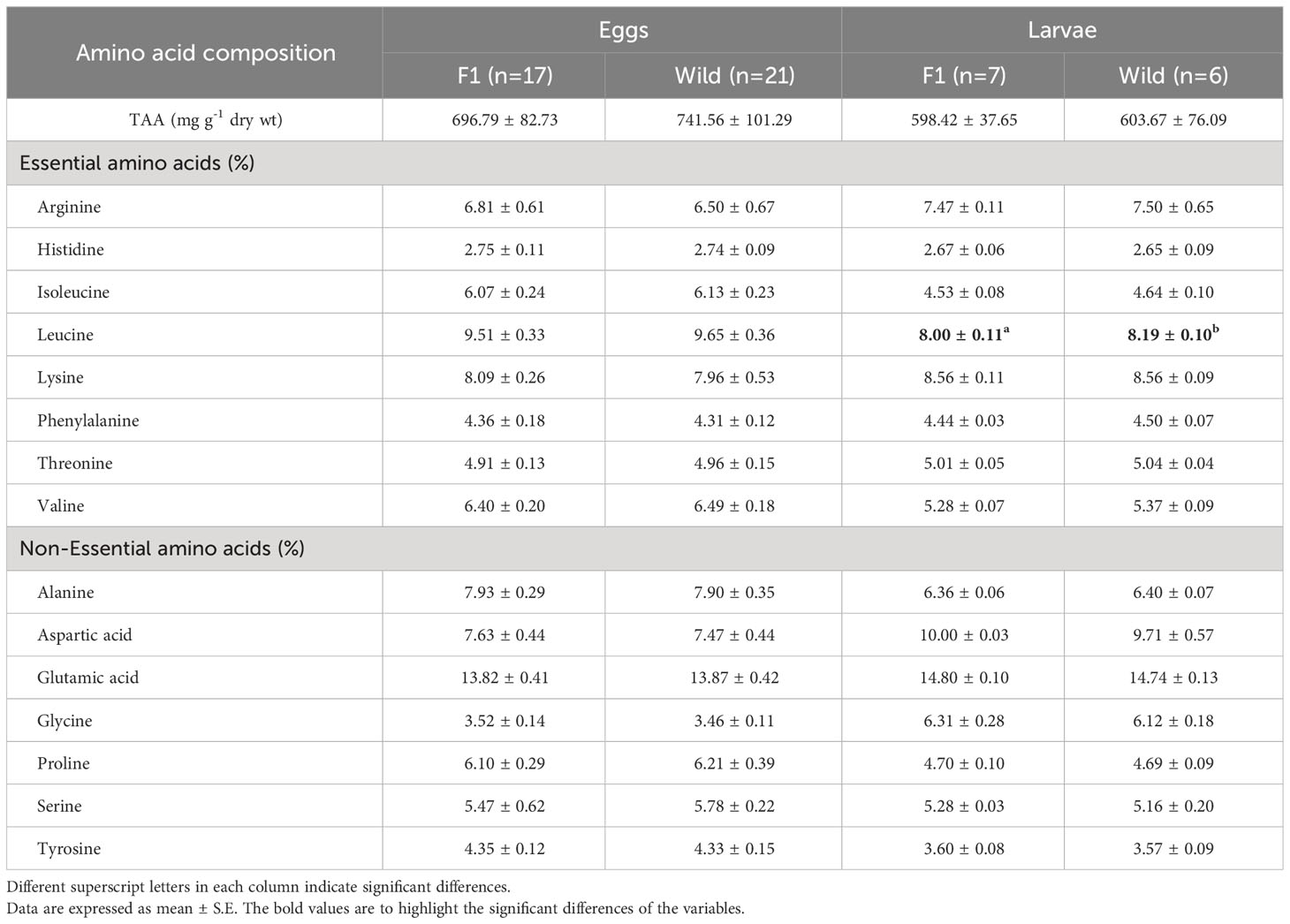
Table 4 Mean total amino acid content (TAA, mg g-1 dry weight), and amino acid composition (in %TAA) of eggs and larvae from Anisotremus scapularis first-generation (F1B) and wild caught (WB) broodstocks.
Results from the principal component analysis (PCA) of the fatty acids in eggs (Figure 5A), revealed that the first principal component explained 26.70% of the observed variability, and the second component explained 21.10%. The discriminant analysis of two components explained 47.46% of the variability and confirmed that there is no separation between the two groups of broodstock (Figure 5B). However, from the vector projections, a greater relationship between the eggs of F1B broodstock and DHA, palmitic acid (16:0), oleic acid (18:1n-9), and linoleic acid (18:2n-6); while stearic acid (18:0), eicosatetraenoic acid (20:4n-3), docosapentaenoic acid (22:5n-3), and EPA have a higher relationship with WB eggs.
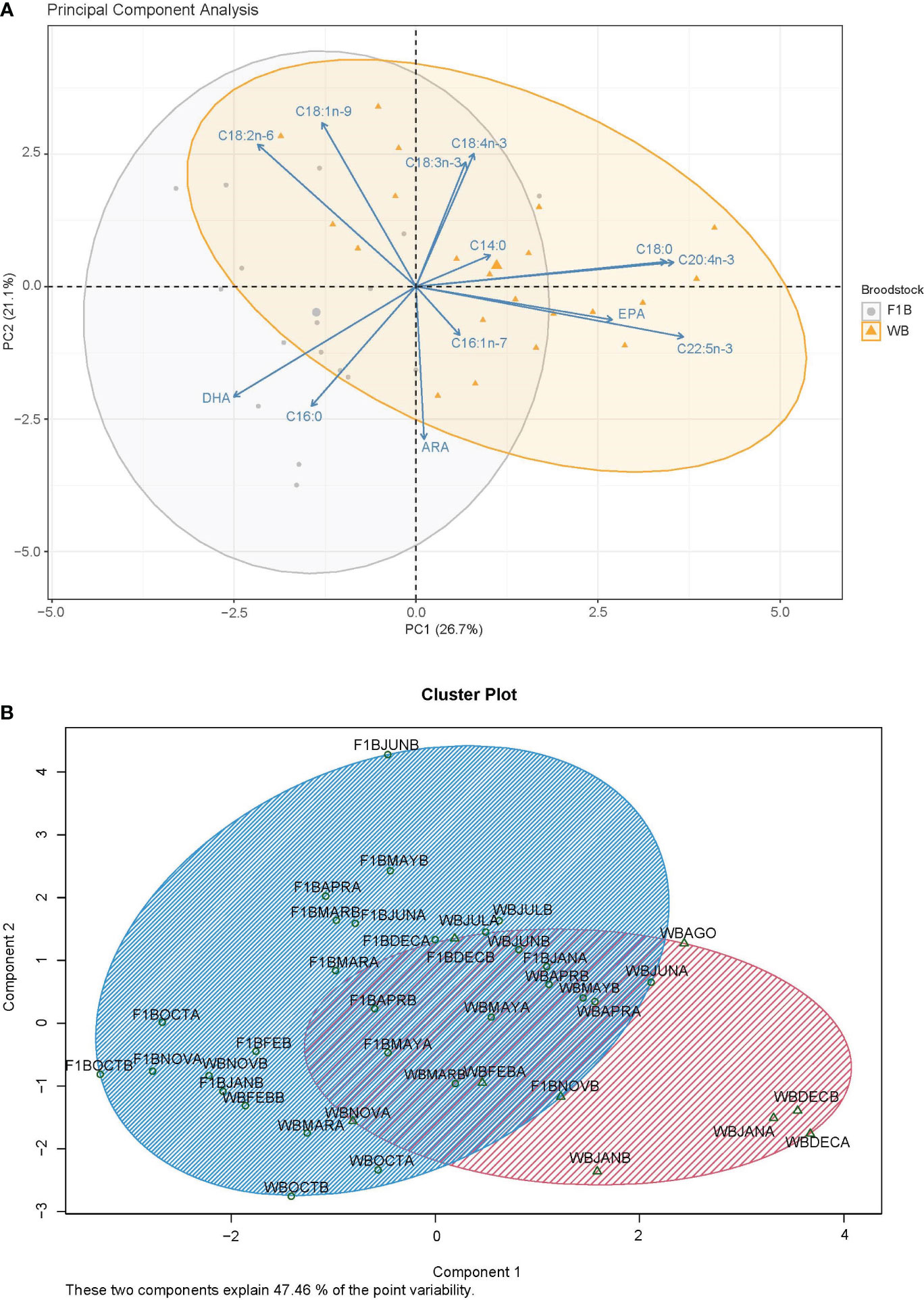
Figure 5 Principal component analysis (A) and discriminant analysis (B) of the fatty acids in eggs from Anisotremus scapularis first-generation domesticated (F1B) and wild caught (WB) broodstocks. F1: 17 spawns, WB: 21 spawns. A statistical significance level of 0.05 was used.
Results from the principal component analysis (PCA) of the larvae fatty acids (Figure 6A), showed that the first principal component explained 42.80% of the observed variability, and the second component explained 35.30%. The discriminant analysis of two components explained 74.44% of the variability, and it was observed that there is no separation between the two batches of broodstock (Figure 6B). Based on the vector projections, results show that the larvae of F1B broodstock have a higher relationship with oleic acid (18:1n-9), DHA, and linoleic acid (18:2n-6); while the larvae of WB broodstock have a higher relationship with palmitoleic acid (16:1n-7), EPA, docosapentaenoic acid (22:5n-3), and ARA.
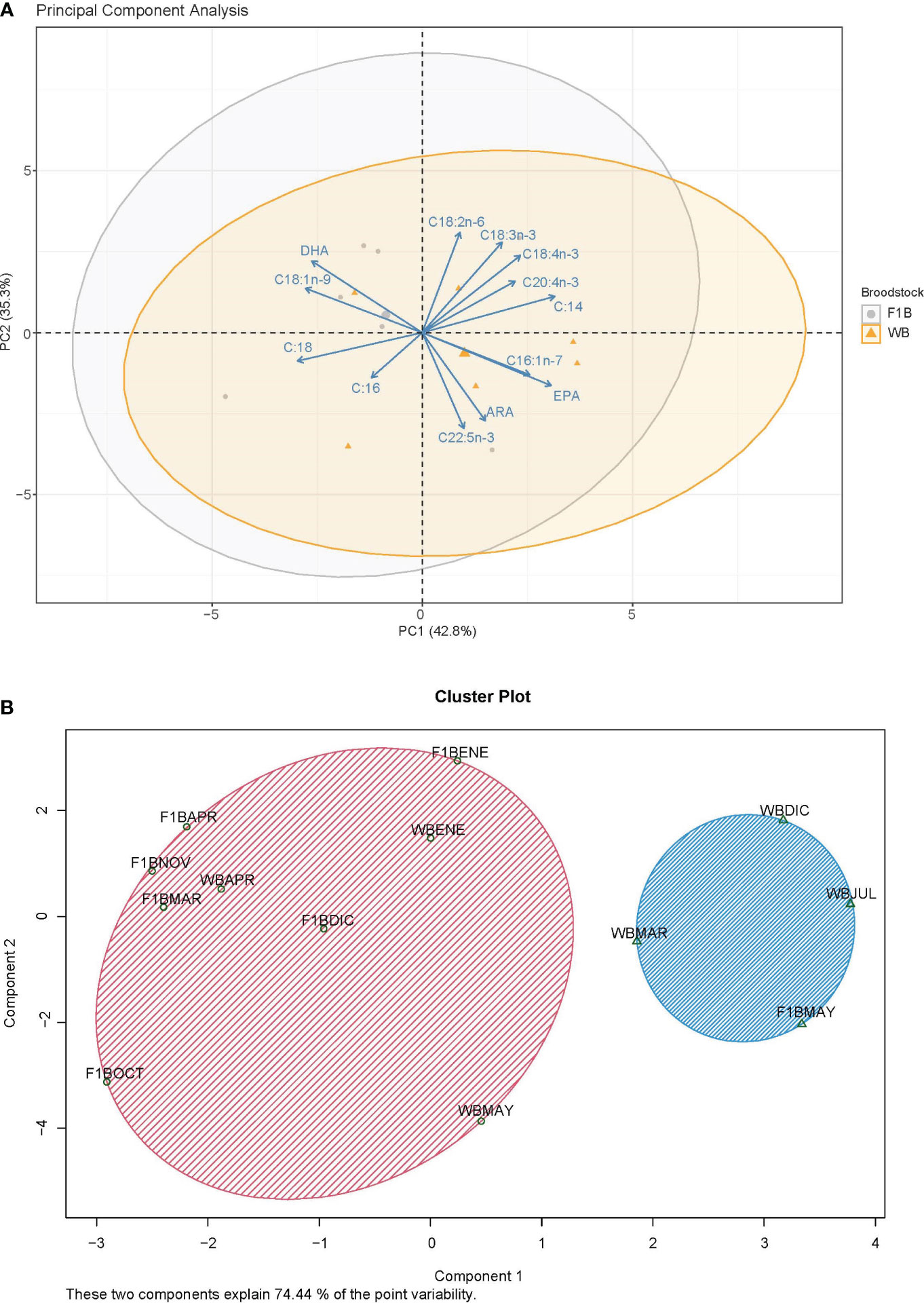
Figure 6 Principal component analysis (A) and discriminant analysis (B) of the fatty acids in larvae from Anisotremus scapularis first-generation domesticated (F1B) and wild caught (WB) broodstocks. F1: 7 spawns, WB: 6 spawns. A statistical significance level of 0.05 was used.
The total amino acid composition (Table 4) was not significantly different between the eggs of the two groups of broodstock. The amino acids found in higher proportion were glutamic acid, leucine, lysine, alanine, and aspartic acid. In 20 DPH larvae, significant differences were observed in the amino acid leucine, which was found in higher proportion in the WB batch larvae. The amino acids found in higher proportion in the larvae were glutamic acid, aspartic acid, lysine, leucine and arginine.
4 Discussion
Comparisons of egg quality and composition between wild and first-generation domesticated broodstock have been conducted in several marine fish species (Lund et al., 2008; Ceccon et al., 2012; Quiñones-Arreola et al., 2015; Soares et al., 2015; Santamaría-Miranda et al., 2021). We expected eggs from wild broodstocks would outperform F1 eggs in most quality traits evaluated, as eggs obtained from wild broodstocks are generally considered to be of higher quality (Salze et al., 2005; Ceccon et al., 2012; Santamaría-Miranda et al., 2021). However, in the F1B spawns, higher percentage of viable eggs and hatching rate were observed. Reproductive performance in fish depends on various factors such as fish size and condition, the use of hormonal treatments, nutrition, and stress management (Bobe and Labbé, 2010; Schaefer et al., 2018). In this regard, the continuous production of large quantities of high-quality gametes is essential for aquaculture (Schaefer et al., 2018). Therefore, achieving a reliable supply of high-quality eggs is a major challenge that needs to be overcome when developing aquaculture for new marine species (Mylonas et al., 2011; Bardon-Albaret and Saillant, 2017), such as Anisotremus scapularis, which is considered a prioritized new species for aquaculture in Peru (Ministerio de la Producción, 2012).
The total number of eggs, egg diameter, and oil droplets were lower in the F1B batch, which may be related to the fact that broodstock individuals were smaller (468.67 g and 27.50 cm) compared with their wild counterparts (655.27 g and 30.20 cm). For many fish species, the age or size of the female can determine the size of the eggs (Bromage et al., 1990; Kamler, 2005). On the other hand, fish that spawns for the first time often show low fecundity and low-quality spawn (Bardon-Albaret and Saillant, 2017). Therefore, the occurrence of first-time spawning females in the F1B group could explain the lower production of total eggs in this group. Similar conditions were observed in other marine fish species such as Seriola rivoliana, where wild broodstocks showed a higher spawning frequency and produced more eggs compared with the F1 generation (Quiñones-Arreola et al., 2015), which is also similar to Cyclopterus lumpus (Pountney et al., 2022). However, a different situation was observed in Lutjanus campechanus, in which wild females produced fewer eggs than captive females (Bardon-Albaret and Saillant, 2017). This result coincides with the findings reported for Gadus morhua, where the higher number of eggs produced were from the F1 group, indicating that the results vary according to the species and origin of the broodstock (Kjørsvik, 1994).
The morphological characteristics of A. scapularis eggs were described as pelagic, spherical, completely smooth, with a transparent chorion, uniform yolk, and an oil droplet (Montes et al., 2019); similar to most cultured marine fish species (e.g., Sparus aurata, Dicentrarchus labrax, Dentex dentex, Gadus morhua, Scophthalmus maximus, Solea senegales, Hippoglossus hippoglossus). These characteristics can also be used as parameters for determining egg quality (Lahnsteiner et al., 2008; Aristizabal et al., 2009; Mandal et al., 2021). Egg quality can also be defined based on the proportion of spawned and floating eggs (i.e., which are considered viable) (Giménez et al., 2006). The percentage of floating eggs is commonly used as an indicator of spawning quality in marine fish culture (Aristizabal et al., 2009), and this parameter is related to egg viability in some species (Martín et al., 2019). Flotation is achieved through the hydration process, which is a key step in successful reproduction (Carnevali et al., 2001). In the present study the quantity of viable or floating eggs, was significantly higher for F1B specimens of A. scapularis than for WB in most of the months during the bioassay, which differs from the findings reported for Lutjanus campechanus and L. guttatus, where wild females produced a greater number of viable eggs compared with captive females (Bardon-Albaret and Saillant, 2017; Chacón-Guzmán et al., 2020).
The size of fish eggs can be influenced by various factors such as the nutritional status of the broodstock, size/age of the female, spawning type (natural, induced, etc.), or the timing of spawning (beginning, middle, and end of the spawning season). In a fish species with asynchronous spawning like A. scapularis, which exhibits continuous and multiple spawns (Carrera et al., 2022), the average egg size may decrease due to the successive spawns of each individual (Lahnsteiner et al., 2008). The diameter of A. scapularis eggs has been reported to range between 0.75 to 0.77 mm for eggs from wild specimens (Montes et al., 2019), which is relatively smaller than the values observed in the present study for fish of the same origin, with an average diameter of 0.81 ± 0.07 mm. Similarly, for L. guttatus, the average egg diameter was 0.75 mm, with similar sizes for both wild and captive specimens (Chacón-Guzmán et al., 2020). In another study with the same species, Boza-Abarca et al. (2011) reported an average egg diameter of 0.72 ± 0.027 mm. In L. argentimaculatus, the reported egg diameter ranged from 0.74 to 0.81 mm (Leu et al., 2003). For Sparus aurata, the egg diameter was higher than the aforementioned species, ranging from 0.97 to 0.99 mm under captive conditions (Jerez et al., 2012). In other species such as Argyrosomus regius, F1 specimens had significantly larger egg diameters (1.04 ± 0.10 mm) compared to wild specimens (0.97 ± 0.13 mm) (Soares et al., 2015), which is different from the findings in the A. scapularis broodstock group, where F1B exhibited a smaller average diameter than that of the wild specimens (0.80 ± 0.01 mm).
Other important parameters for determining fish egg quality are the fertilization percentage and the hatching percentage (Lahnsteiner et al., 2008; Ciji et al., 2022). Percent fertilization rates for F1B specimens of A. scapularis exhibited a slightly higher average compared to those of wild origin. Similar differences were observed in L. campechanus (Bardon-Albaret and Saillant, 2017), but the opposite was found in Tor putitora, where wild specimens had higher fertilization rates than captive ones (Ciji et al., 2022). Generally, it can be said that depending on the origin of the broodstock and the rearing conditions, percent fertilization can vary. For instance, in captive specimens of Sparus aurata, the values range between 83% and 92% (Jerez et al., 2012), while in wild specimens of L. argentimaculatus, an average percent fertilization of 74.3% was obtained (Leu et al., 2003), and in wild Diplodus puntazzo, over 3 years of monitoring, values ranged from 76%, 78%, to 81% (Papadaki et al., 2008). On the other hand, in Latris lineata, the percent fertilization did not show significant differences between wild and captive specimens (Morehead et al., 2001), similar to values observed in the present study for A. scapularis. Percent hatching rates for A. scapularis, were higher for F1B group compared to the WB group. These values coincide with Argyrosomus regius, where F1 broodstock had significantly higher hatching percentages than wild broodstock (Soares et al., 2015). However, variations can be found depending on the origin of the broodstock. For example, in L. campechanus, the percent hatching for eggs of captive females was significantly lower than wild females (Bardon-Albaret and Saillant, 2017). In Sparus aurata, captive specimen percent hatching ranged from 72% to 77% (Jerez et al., 2012), and for L. argentimaculatus, percent hatching was 81.9% for eggs from wild specimens (Leu et al., 2003). In Tor putitora, wild specimens had higher hatching rates than captive specimens (Ciji et al., 2022), and in a study of wild Diplodus puntazzo broodstock, the hatching rates did not vary significantly, remaining at around 86% through all the years of study (Papadaki et al., 2008).
In a study with Lutjanus peru under laboratory conditions, the authors did not find a correlation between egg diameter and hatching or survival rate, while the egg oil droplet showed a positive correlation with larval survival (Moguel-Hernández, 2015). Santamaría-Miranda et al. (2021) found a positive correlation between the weight of L. peru females and the size of the oil droplet, but not with egg size. In L. campechanus (Bardon-Albaret and Saillant, 2017), found a low and non-significant correlation among the spawning quality parameters. In the present study, a moderate but significant positive correlation was found between larval survival and egg diameter and a negative correlation between larval survival and percent hatching. This suggests that the hatching percentage in A. scapularis could be used as a predictor of subsequent larval survival. On the other hand, SAI had negative and significant moderate correlation with cumulative mortality at 3 DPH. Other significant relationships were the number of total eggs with fertilization percentage and the egg diameter with the oil droplet diameter¸ however only a weak correlation was found.
Regarding the evaluation of the “hardiness” of the larvae using the TTI, results obtained in the present study with WB broodstock were higher than those reported by Kanazawa (1997) with 77% thermal tolerance for Pagrus major larvae and 73.90% for Lutjanus guttatus larvae from wild broodstock (Burbano et al., 2020). In larvae of the California halibut, Paralichthys californicus, an index of 1.88% was obtained at the end of the larval rearing period (Fuentes-Quesada and Lazo, 2018). Ceccon et al. (2012) compared spawns from both origins in Gadus morhua, and found that larvae from wild broodstocks were more resistant to these tolerance tests. This is contrary to what was observed in the present research, where no significant differences were found between both groups. It is worth noting that TTI values are inversely proportional to the hardiness of the larvae, where higher values represent reduced tolerance (Fuentes-Quesada and Lazo, 2018).
Values from the biochemical analysis, for protein, lipid, carbohydrate, and dry matter content were similar between the eggs and larvae from both broodstock groups. In contrast, Santamaría-Miranda et al. (2021) working with Lutjanus peru, found lower values for total proteins and carbohydrates in F1 eggs, and lipid content was lower in eggs from wild broodstock. However, Ceccon et al. (2012) in Gadus morhua, report similar findings where no temporal effects were observed for the total lipid content in both groups of eggs, and to those of Quiñones-Arreola et al. (2015) in Seriola rivoliana, where there was no difference between the two experimental groups in terms of total protein, lipid, carbohydrate or triacylglyceride content.
Total fatty acid profiles (TFA) were similar between eggs of the two broodstock groups, which can be related to the similar diet feed to both experimental groups. A similar correlation between diets and eggs was observed in gilthead seabream (Harel et al., 1994). These results were contrary to the findings of de Lund et al. (2008) in Solea solea, where the TFA content was lower in F1 broodstock eggs, although similar to the results of Ceccon et al. (2012) for Gadus morhua, where the relative total fatty acid content was similar for both cultured and wild broodstock eggs. In general, the quality of spawns can be influenced by diet and environmental conditions during oogenesis (Brooks et al., 1997; Migaud et al., 2013), which is why many aquaculturists try to manipulate fish diets with various concentrations and proportions of essential fatty acids to reach maximum egg quality (Izquierdo et al., 2005). However, for this study, the diet was similar for both groups, fed fish chunks supplemented with vitamin and fatty acid capsules (Cota et al., 2022).
In general, marine fish eggs with a high amount of DHA are considered to be of high quality, as DHA is an essential nutrient for many important biological functions including the neural development of the larvae (Sargent et al., 1999). In F1B eggs and larvae, a higher proportion of DHA was found, which could be correlated to the better growth and survival rates observed in the experimental group. Sargent et al. (1999) discussed how both the concentration and proportions of DHA, EPA, and ARA are important for the nutrition of fish larvae and suggested that appropriate proportions are in the range of 2:1 DHA : EPA and 5:1 EPA : ARA (Papanikos et al., 2008). Therefore, in addition to a DHA : EPA ratio a EPA : ARA ratio or even better a DHA : EPA:ARA ratio has been proposed as an indicator of adequate broodstock and larval diets at each stage, species, and habitat (Sargent et al., 1999; Bell and Sargent, 2003). Here, we found that the DHA : EPA ratios for the eggs were 2.57:1 and 2.27:1 for F1B and WB, respectively, and 2.07:1 and 0.99:1 in the larvae. The EPA : ARA ratio was 8.11:1 and 8.30:1 in the eggs of F1B and WB broodstock, and 1.67:1 and 2.25:1 in the larvae of each of these experimental groups. It is worth noting that eggs of the two broodstock groups did not show differences in EPA and ARA content, but the 20 DPH larvae from wild broodstock showed a higher content of these fatty acids.
In a study conducted by Lund et al. (2008) with Solea solea, using a multivariate analysis of the fatty acid profiles, it was possible to discriminate between spawns from the natural environment and cultured groups. The eggs of cultured broodstock had high levels of eicosenoic acid (20:1n-9), linoleic acid (18:2n-6), and alpha-linolenic acid (18:3n-3), while the eggs of wild fish had high levels of palmitoleic acid (16:1n-7), EPA, and ARA, indicating dietary differences between groups. Ceccon et al. (2012), reported that the total n-3 PUFA, DHA, and eicosatetraenoic acid (20:4n-3) were positively correlated and were characteristic of wild broodstock groups. On the other hand, n-6 fatty acids, such as linoleic acid (18:2n-6) and gamma-linolenic acid (18:3n-6), were negatively correlated and were characteristic of the cultured broodstock group. In the present study, a similarity in FA profiles was observed between the two broodstock groups as it was not possible to discriminate between the two of them. However, there was a high association of F1B eggs and 20 DPH larvae with palmitic acid (16:0), oleic acid (18:1n-9), DHA, and linoleic acid (18:2n-6). In the case of wild broodstock eggs and larvae, a stronger association to stearic acid (18:0), palmitoleic acid (16:1n-7), eicosatetraenoic acid (20:4n-3), docosapentaenoic acid (22:5n-3), EPA, and ARA was found.
The most prevalent amino acids measured in A. scapularis eggs from both experimental groups were glutamic acid, leucine, lysine, alanine, and aspartic acid. Essential amino acids, with the exception of tryptophan, which was not quantified, constituted 50.95% (F1B) and 50.99% (WB) of the total amino acids, with leucine, lysine, and arginine being the most abundant. In the case of 20 DPH larvae from both batches, the amino acids found in higher proportions were glutamic acid, aspartic acid, lysine, leucine, and arginine. Essential amino acids constituted 48.94% (F1B) and 49.20% (WB) of the total, with lysine, leucine, and arginine being the most prominent. Faulk and Holt (2008) observed that glutamate, leucine, alanine, proline, lysine, and aspartate are the most abundant amino acids in captive-spawned cobia (Rachycentron canadum) eggs. Ceccon et al. (2012) showed that the total amino acid content was significantly higher in eggs from wild broodstock compared to cultured Gadus morhua. In both groups, the most abundant amino acid was glutamic acid. Additionally, the authors report that leucine and threonine, were significantly higher in eggs from wild broodstock, while arginine, phenylalanine, and tryptophan were significantly higher in eggs from cultured broodstock. However, the PCA did not provide a good model to separate wild and cultured broodstock eggs. In this study, the amino acid composition was not significantly different between eggs and 20 DPH larvae from the two broodstock groups, except for leucine, which was found in higher proportions in the WB larvae. In the PCA of amino acids in eggs and 20 DPH larvae, no difference was observed in the projection of vectors by broodstock group, and there was no discrimination of amino acids by group.
5 Conclusions
Based on the results from the present study, we can conclude that the A. scapularis F1 broodstock group had better egg quality in terms of egg viability (65.79 ± 22.74%) and hatching rate (90.65 ± 12.14%). However, a lower egg production was found (119,465 eggs kg-1) and egg size (0.80 ± 0.01 mm) was smaller compared to that of wild specimens (WB). Nonetheless, F1 broodstock larvae exhibited good temperature tolerance index (100.62 ± 5.90) and similar growth rates (4.19 ± 0.72% cm day-1) and larval survival (37.29 ± 30.99%) up to 20 DPH, compared to those of the larvae from the wild spawns. Spawns showed similar total fatty acid (TFA) content between experimental groups (F1B Eggs: 97.90 ± 16.35 mg g-1, F1B 20 DPH larvae: 62.46 ± 8.08 mg g-1) and total amino acid (TAA) content (F1B Eggs: 696.79 ± 82.73 mg g-1, F1B 20 DPH larvae: 598.42 ± 37.65 mg g-1). In addition, in terms of fatty acid composition, the F1 batch exhibited a higher proportion of 16:0 and DHA in eggs and a higher DHA/EPA ratio in 20 DPH larvae. Therefore, we can recommend the use of F1 broodstock as a good alternative for the recruitment of new broodstock to produce high quality eggs and larvae under captive conditions, thereby contributing to the aquaculture development of this promising species.
Data availability statement
The original contributions presented in the study are included in the article/supplementary material. Further inquiries can be directed to the corresponding author.
Ethics statement
The animal study was approved by Ethics Committee of the Research, Innovation, and Venture Unit of the Universidad Federico Villareal - Letter N°001-2023-CEI-UIIE-FOPCA-UNFV. The study was conducted in accordance with the local legislation and institutional requirements.
Author contributions
NCM: Conceptualization, Data curation, Formal analysis, Investigation, Methodology, Project administration, Validation, Writing – original draft. LJCS: Investigation, Supervision, Writing – original draft, Conceptualization, Methodology. ACF: Investigation, Methodology, Writing – original draft, Conceptualization, Data curation. OLD: Data curation, Investigation, Methodology, Writing – original draft. LFR: Formal analysis, Investigation, Methodology, Writing – original draft. ARS: Formal analysis, Investigation, Methodology, Writing – original draft. JPL: Supervision, Visualization, Writing – review & editing. ND: Supervision, Visualization, Writing – review & editing.
Funding
The author(s) declare financial support was received for the research, authorship, and/or publication of this article. This research was supported by ProCIENCIA (Programa Nacional de Investigación Científica y Estudios Avanzados) of CONCYTEC (Consejo Nacional de Ciencia, Tecnología e Innovación Tecnológica) and IMARPE (Instituto del Mar del Perú) as part of the project “Desempeño reproductivo y calidad de huevos de la primera generación (F1) de chita Anisotremus scapularis para la producción de semilla de calidad con una proyección a escala comercial de esta especie - Contrato 005-2021-ProCIENCIA”.
Acknowledgments
We thank Instituto del Mar del Perú (IMARPE) and the laboratories of the Área Funcional de Investigaciones en Acuicultura of the Dirección General de Investigaciones en Acuicultura at IMARPE: Laboratorio de Cultivo de Peces, Laboratorio de Alimento Vivo, and Laboratorio de Análisis Instrumental, as well as M. Sc. Francisco Villamón for their help in carrying out this study.
Conflict of interest
The authors declare that the research was conducted in the absence of any commercial or financial relationships that could be construed as a potential conflict of interest.
Publisher’s note
All claims expressed in this article are solely those of the authors and do not necessarily represent those of their affiliated organizations, or those of the publisher, the editors and the reviewers. Any product that may be evaluated in this article, or claim that may be made by its manufacturer, is not guaranteed or endorsed by the publisher.
References
Aristizabal E., Suárez J., Vega A., Bargas R. (2009). Egg and larval quality assessment in the Argentinean red porgy (Pagrus pagrus). Aquaculture 287 (3-4), 329–334. doi: 10.1016/j.aquaculture.2008.10.044
Bardon-Albaret A., Saillant E. (2017). Egg quality traits and predictors of embryo and fry viability in red snapper Lutjanus campechanus. Aquaculture Rep. 7, 48–56. doi: 10.1016/j.aqrep.2017.05.004
Bell J. G., Sargent J. R. (2003). Arachidonic acid in aquaculture feeds: current status and future opportunities. Aquaculture 218, 491–499. doi: 10.1016/S0044-8486(02)00370-8
Bobe J., Labbé C. (2010). Egg and sperm quality in fish. Gen. Comp. Endocrinol. 165 (3), 535–548. doi: 10.1016/j.ygcen.2009.02.011
Boza-Abarca J., Valverde-Chavarría S., Calvo-Vargas E., Ramírez-Alvarado M., Rodríguez-Gómez E. (2011). Hormone-induced spawning of wild and captive-grown spotted rose snapper Lutjanus guttatus using carp pituitary suspension and human chorionic gonadotropin. Cienc. Marinas 37 (2), 125–139. doi: 10.7773/cm.v37i2.1802
Bromage N., Hardiman P., Jones J., Springate J., Bye V. (1990). Fecundity, egg size and total egg volume differences in 12 stocks of rainbow trout, Oncorhynchus mykiss Richardson. Aquaculture Res. 21 (3), 269–284. doi: 10.1111/j.1365-2109.1990.tb00465.x
Brooks S., Tyler C. R., Sumpter J. P. (1997). Egg quality in fish: what makes a good egg? Rev. Fish Biol. Fisheries 7, 387–416. doi: 10.1023/A:1018400130692
Burbano M. F., Torres G. A., Prieto M. J., Gamboa J. H., Chapman F. A. (2020). Increased survival of larval spotted rose snapper Lutjanus guttatus (Steindachner 1869) when fed with the copepod Cyclopina sp. and Artemia nauplii. Aquaculture 519, 734912. doi: 10.1016/j.aquaculture.2019.734912
Carnevali O., Mosconi G., Cardinali M., Meiri I., Polzonetti-Magni A. (2001). Molecular components related to egg viability in the gilthead sea bream, Sparus aurata. Mol. Reprod. Development: Incorporating Gamete Res. 58 (3), 330–335. doi: 10.1002/1098-2795(200103)58:3<330::AID-MRD11>3.0.CO;2-7
Carrera L., Cota N., Linares J., Castro A., Orihuela Toledo L., Silva E., et al. (2018). Manual para acondicionamiento y reproducción de chita Anisotremus scapularis. Informe IMARPE 45 (2), 263–276.
Carrera L. J., Fontes J. G., Santos G. R., Padilha M. C. (2022). Reproductive cycle of the female Anisotremus scapularis (Tschudi 1846) on the marine coast of Callao, basic knowledge to go towards its aquaculture. Front. Mar. Sci. 9. doi: 10.3389/fmars.2022.1033718
Castro A., Cota N., Montes M., Carrera Santos L. (2021). Protocolo del cultivo larvario de chita Anisotremus scapularis en condiciones de laboratorio. Informe Instituto del Mar. Perú 48 (1), 20–24.
Ceccon L., Bizuayehu T. T., Bolla S., Martins C., de Oliveira Fernandes J. M., Bianchini A., et al. (2012). Biochemical composition and performance of Atlantic cod (Gadus morhua L.) eggs and larvae obtained from farmed and wild broodstocks. Aquaculture 324, 267–275. doi: 10.1016/j.aquaculture.2011.10.036
Chacón-Guzmán J., Carvajal-Oses M., Toledo-Agüero P., Flores-Gatica H. (2020). Comparison of fatty acids profile of the gonads and eggs of Lutjanus guttatus (Perciformes: Lutjanidae) obtained from wild and captive broodstock. Uniciencia 34 (1), 32–59. doi: 10.15359/ru.34-1.3
Chirichigno N., Velez M. (1998). Clave para identificar los peces marinos del Perú. Instituto del Mar del Perú, 496 pp.
Ciji A., Akhtar M. S., Dubey M. K., Pandey A., Tripathi P. H., Kamalam B. S., et al. (2022). Comparative assessment of egg and larval quality traits of progeny from wild-collected and captive-matured brooders of endangered golden mahseer, Tor putitora: A prelude to quality broodstock development and seed production. Aquaculture 552, 737949. doi: 10.1016/j.aquaculture.2022.737949
Cota N., Castro A., Montes M., Carrera L. (2022). Mejoramiento de dieta de acondicionamiento de reproductores de chita Anisotremus scapularis con uso de vitaminas y ácidos grasos. Boletín IMARPE 37 (2), 2022. doi: 10.53554/boletin.v37i2.373
Dhert P., Lavens P., Sorgeloos P. (1992). Stress evaluation: a tool for quality control of hatchery-produced shrimp and fish fry. Aquaculture Europe 17 (2), 6–10.
DuBois M., Gilles K. A., Hamilton J. K., Rebers P. T., Smith F. (1956). Colorimetric method for determination of sugars and related substances. Analytical Chem. 28 (3), 350–356. doi: 10.1021/ac60111a017
Faulk C. K., Holt G. J. (2008). Biochemical composition and quality of captive-spawned cobia Rachycentron canadum eggs. Aquaculture 279 (1-4), 70–76. doi: 10.1016/j.aquaculture.2008.03.050
Folch J., Lees M., Sloane Stanley G. H. (1957). A simple method for the isolation and purification of total lipides from animal tissues. J. Biol. Chem. 226, 497–509. doi: 10.1016/S0021-9258(18)64849-5
Fuentes P. (2014). Efecto del tipo de lípido en la actividad de las lipasas digestivas durante la etapa larvaria del lenguado de California (Paralichthys californicus) (Ensenada, B.C., México: Centro de Investigación Científica y de Educación Superior de Ensenada, Baja California).
Fuentes-Quesada J. P., Lazo J. P. (2018). The effect of lipid type on lipid digestion enzymes during larval development of the California halibut, Paralichthys californicus. Aquaculture 488, 49–60. doi: 10.1016/j.aquaculture.2018.01.018
Giménez G., Estévez A., Lahnsteiner F., Zecevic B., Bell J. G., Henderson R. J., et al. (2006). Egg quality criteria in common dentex (Dentex dentex). Aquaculture 260 (1-4), 232–243. doi: 10.1016/j.aquaculture.2006.06.028
Harel M., Tandler A., Kissil G. W., Applebaum S. W. (1994). The kinetics of nutrient incorporation into body tissues of gilthead seabream (Sparus aurata) females and the subsequent effects on egg composition and egg quality. Br. J. Nutr. 72 (1), 45–58. doi: 10.1079/BJN19940008
Hartree E. F. (1972). Determination of protein: a modification of the Lowry method that gives a linear photometric response. Analytical Biochem. 48 (2), 422–427. doi: 10.1016/0003-2697(72)90094-2
Ibarra-Castro L., Alvarez-Lajonchère L. (2011). GnRHa-induced multiple spawns and volition spawning of captive spotted rose snapper, Lutjanus guttatus, at Mazatlan, Mexico. J. World Aquaculture Soc. 42 (4), 564–574. doi: 10.1111/j.1749-7345.2011.00499.x
Ichihara K. I., Fukubayashi Y. (2010). Preparation of fatty acid methyl esters for gas-liquid chromatography. J. Lipid Res. 51 (3), 635–640. doi: 10.1194/jlr.D001065
Izquierdo M. S., Montero D., Robaina L., Caballero M. J., Rosenlund G., Ginés R. (2005). Alterations in fillet fatty acid profile and flesh quality in gilthead seabream (Sparus aurata) fed vegetable oils for a long term period. Recovery of fatty acid profiles by fish oil feeding. Aquaculture 250 (1–2), 431–444. doi: 10.1016/j.aquaculture.2004.12.001
Jeréz S., Fakriadis I., Papadaki M., Martín M., Cejas J. R., Mylonas C. C. (2018). Spawning induction of first-generation (F1) greater amberjack Seriola dumerili in the Canary Islands, Spain using GnRHa delivery systems. Fishes 3 (3), 35. doi: 10.3390/fishes3030035
Jerez S., Rodríguez C., Cejas J. R., Martín M. V., Bolaños A., Lorenzo A. (2012). Influence of age of female gilthead seabream (Sparus aurata L.) broodstock on spawning quality throughout the reproductive season. Aquaculture 350, 54–62. doi: 10.1016/j.aquaculture.2012.04.018
Kamler E. (2005). Parent – egg–progeny relationships in teleost fishes: an energetics perspective. Rev. Fish Biol. Fisheries 15, 399–421. doi: 10.1007/s11160-006-0002-y
Kanazawa A. (1997). Effects of docosahexaenoic acid and phospholipids on stress tolerance of fish. Aquaculture 155 (1-4), 129–134. doi: 10.1016/S0044-8486(97)00123-3
Kjørsvik E. (1994). Egg quality in wild and broodstock cod Gadus morhua L. J. World Aquaculture Soc. 25 (1), 22–29. doi: 10.1111/j.1749-7345.1994.tb00800.x
Kjørsvik E., Mangor-Jensen A., Holmefjord I. (1990). Egg quality in fishes. Adv. Mar. Biol. 26, 71–113. doi: 10.1016/S0065-2881(08)60199-6
Lahnsteiner F., Soares F., Ribeiro L., Dinis M. T. (2008). “Egg quality determination in teleost fish,” in Methods in Reproductive Aquaculture. Eds. Cabrita E., Robles V., Herraez P. (Boca Raton, FL: CRC Press), 171–202.
Leu M., Chen I., Fang L. (2003). Natural spawning and rearing of mangrove red snapper, lutjanus agentimaculatus, larvae in captivity. Israeli J. Aquaculture - Bamidgeh 55 (1), 22–30. doi: 10.46989/001c.20332
Lund I., Steenfeldt S. J., Suhr K. I., Hansen B. W. (2008). A comparison of fatty acid composition and quality aspects of eggs and larvae from cultured and wild broodstock of common sole (Solea solea L.). Aquaculture Nutr. 14 (6), 544–555. doi: 10.1111/j.1365-2095.2007.00560.x
Mandal B., Kailasam M., Bera A., Sukumaran K., Hussain T., Biswas G., et al. (2021). Standardization of oocyte size during artificial fertilization and optimization of stocking density during indoor larval and outdoor nursery rearing of captive spotted scat (Scatophagus argus) for a viable juvenile production system. Aquaculture 534, 736262. doi: 10.1016/j.aquaculture.2020.736262
Martín I., Carazo I., Rasines I., Rodríguez C., Fernández R., Martínez P., et al. (2019). Reproductive performance of captive Senegalese sole, Solea Senegalensis, according to the origin (wild or cultured) and gender. Spanish J. Agric. Res. 17 (4), 11. doi: 10.5424/sjar/2019174-14953
Mendo J., Wosnitza-Mendo C. (2014). Reconstruction of total marine fisheries catch for Peru: 1950-2010. Fish. Centre Working Paper 201, 1–23.
Migaud H., Bell G., Cabrita E., McAndrew B., Davie A., Bobe J., et al. (2013). Gamete quality and broodstock management in temperate fish. Rev. Aquaculture 5, S194–S223. doi: 10.1111/raq.12025
Ministerio de la Producción (2012). Programa nacional de ciencia, desarrollo tecnológico e innovación en acuicultura (C+DT+i) 2013-2021 (Lima: Proyecto FAO TCP/PER/3302).
Ministerio de la Producción (2023). National Aquaculture Policy to 2030 (Lima: Decreto Supremo N° 001-2023-PRODUCE).
Moguel-Hernández I. (2015). Fisiología digestiva durante el desarrollo larval del pargo lunajero Lutjanus guttatus (La Paz, B.C.S., México: Instituto Politécnico Nacional. Centro Interdisciplinario de Ciencias Marinas).
Molares Y. (2021). Hoja de ruta para el cultivo de peces marinos en el Perú (Lima: Programa Nacional de Innovación en Pesca y Acuicultura - PNIPA).
Montes M., Castro A. M., Linares J. F., Orihuela L. I., Carrera L. J. (2019). Embryonic development of Peruvian grunt Anisotremus scapularis (Perciformes: Haemulidae). Rev. biología marina y oceanografía 54 (2), 166–173. doi: 10.22370/rbmo.2019.54.2.1881
Morehead D. T., Hart P. R., Dunstan G. A., Brown M., Pankhurst N. W. (2001). Differences in egg quality between wild striped trumpeter (Latris lineata) and captive striped trumpeter that were fed different diets. Aquaculture 192 (1), 39–53. doi: 10.1016/S0044-8486(00)00443-9
Mylonas C. C., Zohar Y., Pankhurst N., Kagawa H. (2011). “Reproduction and broodstock management” in Sparidae, Biology and Aquaculture of Gilthead Sea Bream and other Species. ed. M. A. Pavlidis and C. C. Mylonas (Hoboken, Nueva Jersey: Blackwell Publishing), 95–131. doi: 10.1002/9781444392210.ch4
Papadaki M., Papadopoulou M., Siggelaki I., Mylonas C. C. (2008). Egg and sperm production and quality of sharpsnout sea bream (Diplodus puntazzo) in captivity. Aquaculture 276 (1-4), 187–197. doi: 10.1016/j.aquaculture.2008.01.033
Papanikos N., Phelps R. P., Davis D. A., Ferry A., Maus D. (2008). Spontaneous spawning of captive red snapper, Lutjanus campechanus, and dietary lipid effect on reproductive performance. J. World Aquaculture Soc. 39 (3), 324–338. doi: 10.1111/j.1749-7345.2008.00171.x
Pountney S. M., Lein I., Selly S. L. C., Migaud H., Davie A. (2022). Comparative proximate analysis of wild and captive lumpfish (Cyclopterus lumpus) eggs show deficiencies in captive eggs and possible egg quality determinants. Aquaculture 557, 738356. doi: 10.1016/j.aquaculture.2022.738356
Quiñones-Arreola M. F., Arcos-Ortega G. F., Gracia-López V., Casillas-Hernández R., Weirich C., Morris T., et al. (2015). Reproductive broodstock performance and egg quality of wild-caught and first-generation domesticated Seriola rivoliana reared under same culture conditions. Latin Am. J. Aquat. Res. 43 (5), 953–962. doi: 10.3856/vol43-issue5-fulltext-15
R Core Team. (2022). R: A language and environment for statistical computing (Vienna, Austria: R Foundation for Statistical Computing). Available at: http://www.R-project.org/.
Salze G., Tocher D. R., Roy W. J., Robertson D. A. (2005). Egg quality determinants in cod (Gadus morhua L.): egg performance and lipids in eggs from farmed and wild broodstock. Aquaculture Res. 36, 1488–1499. doi: 10.1111/j.1365-2109.2005.01367.x
Santamaría-Miranda A., Dumas S., Pérez-Urbiola J. C., Peña R., Treviño L., Moguel-Hernández I., et al. (2021). Fatty acid composition and spawning quality in wild and captive broodstock of Pacific red snapper Lutjanus Peru. Aquaculture 538, 736577. doi: 10.1016/j.aquaculture.2021.736577
Sargent J. R., Bell J. G., McEvoy L., Tocker D., Estevez A. (1999). Recent developments in the essential fatty acid nutrition of fish. Aquaculture 177, 191–199. doi: 10.1016/S0044-8486(99)00083-6
Schaefer F. J., Overton J. L., Kloas W., Wuertz S. (2018). Length rather than year-round spawning, affects reproductive performance of RAS-reared F-generation pikeperch, Sander lucioperca (Linnaeus 1758)–insights from practice. J. Appl. Ichthyology 34 (3), 617–621. doi: 10.1111/jai.13628
Schober P., Boer C., Schwarte L. A. (2018). Correlation coefficients: appropriate use and interpretation. Anesth. analgesia 126 (5), 1763–1768. doi: 10.1213/ANE.0000000000002864
Shimma H., Tsujigado A. (1981). Some biochemical quality of bred scorpaenoid fish, Sebastiscus marmoratus, and activities of their larvae. Bull. Natl. Res. Institute Aquaculture 2, 11–20.
Soares F., Ribeiro L., Gamboa M., Duarte S., Mendes A. C., Castanho S., et al. (2015). Comparative analysis on natural spawning of F1 meagre, Argyrosomus regius, with wild broodstock spawns in Portugal. Fish Physiol. Biochem. 41 (6), 1509–1514. doi: 10.1007/s10695-015-0103-4
Thorsen A., Trippel E. A., Lambert Y. (2003). Experimental methods to monitor the production and quality of eggs of captive marine fish. J. Northwest Atlantic Fishery Sci. 33 (9), 55–70. doi: 10.2960/J.v33.a4
Wright-Moore W. D., Watanabe W. O., Bourdelais A. J., Alam M. S., Rezek T. C., Carroll P. M., et al. (2019). Spawning performance and egg quality of wild-caught and first generation southern flounder Paralichthys lethostigma broodstock induced with piscine and mammalian GnRH analogs. Aquaculture 506, 367–379. doi: 10.1016/j.aquaculture.2019.03.062
Keywords: native marine fish, captive broodstock, egg quality, larval quality, fatty acids
Citation: Cota Mamani N, Carrera Santos LJ, Castro-Fuentes A, Leon Dominguez O, Flores Ramos L, Ruiz Soto A, Lazo JP and Duncan N (2023) Reproductive performance and egg and larvae quality from first generation peruvian grunt Anisotremus scapularis (Tschudi, 1846): a comparative analysis with spawns from wild broodstock. Front. Mar. Sci. 10:1287439. doi: 10.3389/fmars.2023.1287439
Received: 01 September 2023; Accepted: 07 December 2023;
Published: 22 December 2023.
Edited by:
Salvador José Jerez Herrera, Spanish Institute of Oceanography (IEO), SpainReviewed by:
Marta Arizcun, Spanish Institute of Oceanography, SpainM. Virginia Martín, Spanish Institute of Oceanography (IEO), Spain
Copyright © 2023 Cota Mamani, Carrera Santos, Castro-Fuentes, Leon Dominguez, Flores Ramos, Ruiz Soto, Lazo and Duncan. This is an open-access article distributed under the terms of the Creative Commons Attribution License (CC BY). The use, distribution or reproduction in other forums is permitted, provided the original author(s) and the copyright owner(s) are credited and that the original publication in this journal is cited, in accordance with accepted academic practice. No use, distribution or reproduction is permitted which does not comply with these terms.
*Correspondence: Noemi Cota Mamani, bmNvdGFAaW1hcnBlLmdvYi5wZQ==