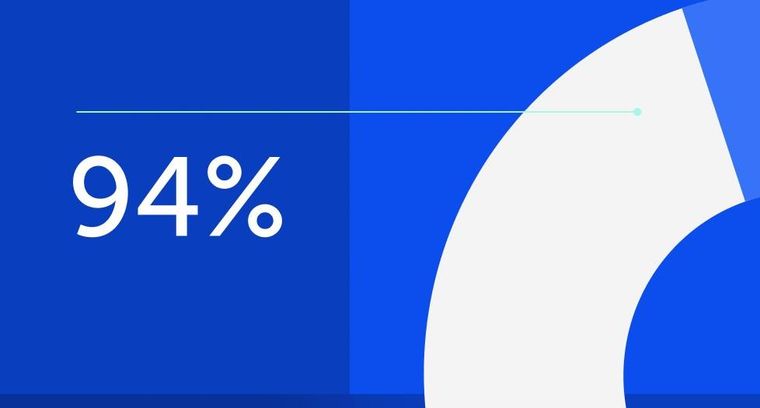
94% of researchers rate our articles as excellent or good
Learn more about the work of our research integrity team to safeguard the quality of each article we publish.
Find out more
ORIGINAL RESEARCH article
Front. Mar. Sci., 01 November 2023
Sec. Marine Pollution
Volume 10 - 2023 | https://doi.org/10.3389/fmars.2023.1283816
This article is part of the Research TopicImpacts of Emerging Contaminants and Their Ecotoxicological ConsequencesView all 10 articles
Global attention has been focused on organic UV filters due to their ubiquity and potential damage to aquatic environment, yet the effects of their life cycle exposure on fish reproduction remain unknown. In the present study, the influence of 2-phenylbenzimidazole-5-sulfonic acid (PBSA) exposure on the reproductive endocrine system of zebrafish was examined, from 6 hours post fertilization (hpf) until 150 days, at levels that near-environmentally relevant (0 to 20 μg/L). Our results showed that exposure to PBSA at 20 μg/L caused a slight decrease in the Gonadosomatic Index (GSI) of female zebrafish in the F0 generation. Furthermore, this exposure had a negatively effect on reproduction, accompanied by delayed oocyte maturation, reduced cumulative egg production and decreased fertilization rate. Additionally, offspring embryos displayed reduced egg diameter at 0.75 hpf, delayed cumulative hatching rate at 60 hpf, and increased deformities rate at 72 hpf, indicating an adverse transgenerational effect. Moreover, PBSA exposure was associated with decreased plasma levels of sex hormones of 17β-estradiol (E2) and 11-ketotestosterone (11-KT), as well as altered the transcriptional profiles of certain genes in the HPG (hypothalamic–pituitary–gonadal) and liver axis. Molecular docking (MD) simulations revealed that specific amino acid residues of PBSA interact with zebrafish estrogen receptors, confirming its xenoestrogenic properties. Therefore, exposure to PBSA during its life cycle can disturb fish reproduction through endocrine disruption, thus necessitating strict environmental regulations for the disposal of UV filters to protect ecological and public health.
Organic ultraviolet (UV) filters are a type of synthetic chemicals that are designed to protect from radiation, mainly acting as a sunscreen to prevent sunburn and skin cancer. They are also employed in personal care products, such as shampoos and cosmetics, and in industrial processes, including anti-freezing, anti-corrosion, and photo-initiator (Carve et al., 2021). Currently, there are around 55 UV filters approved for usage worldwide (Shaath, 2016), with an annually production of over 10,000 tons (Shaath and Shaath, 2005). Reports have indicated that concentrations of benzophenone-3 (BP3) and 2-ethylhexyl-4-methoxycinnamate (EHMC), two common organic UV filters, have been observed in water sources at levels of up to 3,316 µg/L and 19 µg/L, respectively (Balmer et al., 2005; Mitchelmore et al., 2019; Downs et al., 2022; Scheele et al., 2023). As a result of their widespread use, UV filters are released into the environment through wastewater treatment plants (WWTP) and recreational activities, raising growing concerns about potential environmental and human health risks.
In recent years, 2-phenylbenzimidazole-5-sulfonic acid (PBSA) has been used extensively as an organic UV filter in sunscreens and has been found in many aquatic habitats. For example, the concentration of PBSA ranged from 1.3 to 2.3 ng/L in the north-west Kerch Strain, 1.0 to 170 ng/L in the Baltic Sea, and 4490 ng/L in Australian WWTPs (Orlikowska et al., 2015; O'Malley et al., 2020). The highest concentration of PBSA in water was recorded to be over 13 μg/L in outdoor swimming pools in South Bohemia (Grabicova et al., 2013). Though most UV filters, including PBSA, are present in the environment at low concentrations, they can still have the potential biological activity by targeting certain metabolic, enzymatic or cell signaling pathways (Zhang et al., 2017; Huang et al., 2020; Wang et al., 2021). However, there is limited information available on the toxicological effects and associated risks of PBSA. Only one study showed that exposure to environmentally relevant concentration of PBSA can activate certain P450 cytochromes and alter biochemical parameters and enzyme activities in the fish plasma (Grabicova et al., 2013), indicating its potential to disrupt endocrine function. Given that UV filters are now considered as emerging endocrine disrupting chemicals (EDCs) (Ma et al., 2023; Mustieles et al., 2023; Yan et al., 2023), it is critical to evaluate the potential reproductive endocrine disruption effects of life cycle PBSA exposure and its adverse impacts on the development of offspring in aquatic species.
Zebrafish (Danio rerio) has been identified as a suitable model for studying the biological effects of EDCs (Guo et al., 2019; Qian et al., 2020). The hypothalamic-pituitary-gonadal and the liver (HPGL) axis is responsible for regulating reproduction in fish, with hormones such as follicle-stimulating hormone (FSH), luteinizing hormone (LH), 11-ketotestosterone (11-KT), estradiol (E2) and multiple molecular targets influencing gametogenesis and maturation (Mo et al., 2021; Yang et al., 2022). Environmental pollutants that interfere with these hormones in the HPGL axis can have a negative effect on fish reproductive processes, as any disruption of the endocrine system is associated with this axis. However, the consequences of PBSA exposure on fish reproduction and the growth of their offspring are still not fully understood.
Recently, structure-based molecular docking (MD) simulation has been an essential approach for uncovering the molecular mechanisms and structure-toxicity correlations of toxic substances (Shi et al., 2023; Wu et al., 2023). PBSA may activate the steroid hormone pathway via the formation of a ligand-protein complex with estrogen receptors (erα and erβ), utilizing the lock-and-key principle as a mechanism, which provides a novel insight into the mechanisms of toxicity in vivo. Therefore, the purpose of this study is to identify the amino acids of steroid hormone receptors that can interact with PBSA and generate binding sites. Additionally, it seeks to evaluate the reproductive endocrine disruption potential of PBSA through cumulative egg production, fertilization rate, gonad histopathology analysis, plasma sex hormones levels and expression levels of genes related to the HPGL axis. Furthermore, it will investigate any adverse transgenerational effects, such as the diameter of embryo, cumulative malformation rate and hatching rate in F1 generation, without continued exposure to PBSA. Finally, it will use in silico MD technique to forecast the interaction between PBSA and the estrogen receptors of zebrafish. The results of this study will provide a better understanding of the endocrine-disrupting effects of PBSA on reproduction.
Wild-type AB strain zebrafish were used in this study. Healthy adult zebrafish were raised in tanks with a temperature of 28 ± 1°C, a 14:10 light-dark cycle, and a conductivity of 500-1000 μS/cm. Zebrafish were provided with live Artemia (Jiahong Feed Co., Ltd., Tianjin, China) twice daily. The night before spawning, zebrafish were placed in tanks with a 1:1 sex ratio (6 males and 6 females per tank). In the morning, light stimulation was used to induce spawning and the embryos were collected within 30 minutes. The embryos were then rinsed in a solution of 0.8 g NaCl, 0.04 g KCl, 0.00358 g Na2HPO4, 0.006 g KH2PO4, 0.149 g CaCl2, 0.246 g MgSO4 7H2O, and 0.35 g NaHCO3, which had been dissolved in 1 liter of ultrapure water (referred to as ‘embryo medium’). Normal fertilized embryos were then selected for subsequent experimentation under a SZX12 dissecting microscope (SOPTOP, China), following the established protocol (Kimmel et al., 1995).
PBSA (2-phenylbenzimidazole-5-sulfonic acid, CAS: 27503-81-7, 96% purity) was acquired from Sigma-Aldrich. A stock solution of 200 μg/mL PBSA was prepared in dimethyl sulfoxide (DMSO), and stored in a cool dark environment (4°C) for further dilution. A working solution of PBSA was prepared by diluting the stock solution to a 10000-fold concentration prior to the experiment. Initially, 60-80 embryos per dish were cultured in a 90 mm petri dish with 30 mL of exposure medium from 6 to 120 hours post fertilization (hpf). Afterwards they were moved to 1 L plastic tanks with a density of 60 larvae/tank until 1 month old. Finally, they were kept in glass tanks (30 cm length × 20 cm width × 20 cm height) with 10-15 fish/tank in 7 L of water until 5 months old, and three parallel tanks were established for each treatment group. Throughout the entire period, the zebrafish were exposed to 0.01% DMSO (control), 0.2, 2 and 20 μg/L PBSA. All exposure solution was renewed daily to maintain a consistent PBSA concentration during the experiment, and the males and females were housed separately. For this study, the PBSA concentration of 20 μg/L was chosen based on two primary considerations. Firstly, PBSA concentrations in water have been reported to be as high as 13 μg/L in outdoor swimming pools a decade ago, which is likely to have increased due to increased attention to sun protection. Secondly, the preliminary experiment showed no gross morphological deformities at a concentration of less than 20 μg/L in an acute test in adults, and this concentration, which is close to the environmental background values, had a mortality rate of less than 5% in an acute test in embryos. Thus, 20 μg/L was decided upon as the high concentration of PBSA.
Ten males and ten females from each treatment group were randomly chosen, anesthetized with ice, and their body length, weight, and gonad weight were measured. Subsequently, the condition factor (CF = 100 × [body weight (g)/total length3 (cm)]) and gonadosomatic index (GSI = 100 × [gonad weight (g)/body weight (g)]) were calculated in order to evaluate the effect of PBSA exposure on growth in F0 zebrafish (Tao et al., 2023).
After a 5-month period of exposure, a 1:1 ratio of fish was paired and spawned in corresponding concentrations of exposure solutions to evaluate the effects of PBSA on reproductive capacity and transgenerational effects. Every morning, the eggs spawned were gathered within 30 minutes of the light being switched on and the cumulative egg number laid in the course of 7 days was recorded. Twenty fertilized eggs were randomly chosen from each group to measure the egg diameter at 0.75 hpf. The fertilization rate of the larvae at 6 hpf, the cumulative hatching rate at 60 and 72 hpf, and the cumulative malformation rate at 72 hpf were evaluated using a SOPTOP SZX12 microscope, as described in our previous article (Sun et al., 2023).
To investigate the impact of life cycle exposure to PBSA on the gonads of adult zebrafish, histopathological examination of the ovaries and testes was performed. Briefly, after anesthetizing the zebrafish on ice, intact ovaries and testis were dissected and fixed overnight in 4% paraformaldehyde (Cat. No. P1110, Solarbio, China) at 4°C. The samples were then dehydrated in graded ethanol solutions and embedded in paraffin (Cat. Q/YSQN40-91, Shanghai Huayong Paraffin Co., LTD, China), and sectioned (4 μm). Following this, the sections were stained with hematoxylin (Cat. ZLI-9610, Beijing Zhongshan Jinqiao Biotechnology Co., LTD, China) and eosin (Cat. ZLI-9613, Beijing Zhongshan Jinqiao Biotechnology Co., LTD, China) and imaged using a Shunyu EX20 biological microscope (SOPTOP, China). Subsequently, the digital images acquired were analyzed with Image J (version 1.8.0) (Schneider et al., 2012) and the proportion of different developmental stages of germ cells (Ovary: PG, primary growth; EV, early vitellogenic; MV, midvitellogenic; FG, full growth; Testis: SPG, spermatogonia; SPC, spermatocytes; SPD, spermatids) compared to the total germ cell counts were calculated, following the method described in previous studies (Chen et al., 2022).
After a 5-month period of PBSA exposure, the zebrafish were anesthetized with ice and a 3–5 μL blood sample was taken from each fish’ tail using a heparinized microcapillary tube and transferred to a 1.5 mL EP tube. This sample was then centrifuged at 12000 g for 15 minutes at 4°C and the upper layer plasma was placed in a pre-chilled EP tube and stored at −80°C for hormone analysis. To prepare the sample for analysis, 10 μL of the supernatant was diluted to 500 μL with ultrapure water (Cat. R1600, Solarbio, China), followed by the addition of 2 mL of diethyl ether used to extract the diluent twice at 2000 g for 30 minutes at 4°C. The extract was evaporated with the aid of a stream of nitrogen and the residue was then dissolved in 350 μL of ELISA buffer. Plasma from five females or males was collected in one tube with three replicates per treatment group. An enzyme-linked immunosorbent assay (ELISA) kits (Cayman Chemical, USA) was then employed to measure the plasma levels of E2 (No. 501890) and 11-KT (No. 582751) according to the manufacturer’s instructions.
Samples of brains, gonads and livers from each treatment group were collected (Supplementary Figure S1), pooled tissues (3 fish/sex per replicate with 3 replicates) were rinsed two times with ice-cold PBS and homogenized with 1 mL TRIzol reagent (Life Technology, USA) using a hand-held grinder (MY-20, Jingxin, China). Total RNA was then extracted following the manufacturer’s instructions and quantified and assess for purity using a MultiskanTMFC (Thermo, USA). The quality and integrity of the RNA were further verified by measuring the ratio of absorbance value 260:280 between 1.9-2.0 and by 1% agarose gel electrophoresis. cDNA was synthesized using Revert Aid First Stand cDNA Synthesis Kit (Thermo, USA). The PCR reaction mixtures were composed of 5 μL of TB Green® Permix Ex TaqTM II (Til RNaseH Plus) (TaKaRa, Japan), 0.4 μL of a 10 μM forward primer, 0.4 μL of a 10 μM reverse primer, 0.8 μL of cDNA and 3.4 μL of ddH2O. The PCR was then conducted using the CFX Connect Real-Time PCR Detection System (Bio-rad, USA). Primers for genes were synthesized by Sangon Biotech Co., Ltd. (Shanghai, China). Their sequences are listed in Table 1 and melting curves for all genes are available in Supplementary Figures S2–S4. The thermal cycling reaction was carried out following standard protocols, with 30 s at 95°C, followed by 40 cycles of 5 s at 95°C and 30 s at 60°C. mRNA expression levels were evaluated in three biological replicates for each treatment group. The housekeeping gene β-actin was employed as the internal reference gene to normalize the expression levels of target genes and the fold change was computed using the 2–ΔΔCT equation as described in our previous study (Livak and Schmittgen, 2001; Tao et al., 2020).
We utilized the protein models of ERα (PDB ID:6CHW) and ERβ (PDB ID:7XVY) for homologous modeling using Discovery Studio 2019 software. To identify suitable template proteins, a BLAST search was conducted in the NCBI protein database to compare the sequences of ERα and ERβ. MODELLER was then employed to construct the models based on the selected template proteins. The quality of the models was evaluated using a Ramachandran plot, with 90% of residues within the allowed region being the threshold for deeming the model satisfactory (Chen et al., 2021). Molecular docking was also used to identify the active sites of small molecules that interact with protein structures and estimate binding affinity. Cdocker, a semi-flexible docking mode based on CHARMm, was utilized in this process. The protein model obtained from homologous modeling was dehydrated and the binding pocket was then defined as the receptor. The 3D structures of BP3 (Benzophenone-3, a typical UV filter), BPA (Bisphenol A, a typical endocrine disruptor) and PBSA from the Pubchem database were used as ligands for molecular docking, which was conducted using the Cdocker module in Discovery Studio 2019. The evaluation of the result was based on Cdocker interaction energies, with higher Cdocker interaction energy indicating greater binding affinity between the protein and the ligand (Rampogu et al., 2019).
Data analysis was conducted for normality and homogeneity of variance through the Kolmogorov-Smirnov and Levene’s tests respectively, using GraphPad Prism 8.0 software (GraphPad Inc., USA). Non-paired t-test was utilized to assess the statistical differences between the control and PBSA-treated group for each sex. Two-way ANOVA followed by Sidak’s multiple comparisons test was used for all other datasets in terms of body weight, body length, CF and GSI. The results were expressed as the mean ± standard deviation (SD) and statistical significance was set at P < 0.05.
To evaluate the effects of long-term exposure to PBSA on the development of fish, we measured the growth index including body weight, body length, CF and GSI after 5 months of exposure. The results showed that there were no significant differences in body weight, body length and CF between the treatment groups and the vehicle control group (Figures 1A–C). However, a female-biased reduction in GSI value was observed in the group exposed to a concentration of 20 μg/L PBSA (Figure 1D).
Figure 1 Effects of life cycle exposure to PBSA on the growth of adult zebrafish. (A, B) Body weight (A) and body length (B) of adult zebrafish after life cycle exposure to PBSA. (C) Condition factor indices in adult zebrafish. (D) Zebrafish gonadosomatic index. Values plotted are mean ± SEM. *P < 0.05 indicate significant differences between the control and exposure groups.
The stages of adult gonadal germ cell development can be used to assess the condition of the gonads. It is possible that exposure to PBSA across the lifespan may have an impact on the normal development of the female zebrafish ovary, we then assessed the proportion of germ cells at different stages in ovaries or testes. Histological examination results indicated that PBSA had a gender-dependent effect on zebrafish germ cells. Specifically, the proportion of primary growth follicles (PG) in the ovaries was significantly increased in only 20 μg/L PBSA group, while the proportions of both mid vitellogenic follicles (MV) and full grown follicles (FG) were decreased in comparison to the control group (Figures 2A, B, other groups not shown). In contrast, PBSA had little impact on the testes (Figures 2C, D). Consequently, we selected the 20 μg/L exposure concentration for the subsequent experiments.
Figure 2 Effects of PBSA on reproduction of adult zebrafish. (A) Representative images of ovaries of adult zebrafish stained with hematoxylin-eosin (H&E) (PG, primary growth follicles; EV, early vitellogenic follicles; MV, midvitellogenic follicles; FG, full grown follicles). (B) Percentage of oocyte in ovaries at different stages. (C) Representative images of testis of adult zebrafish stained with H&E (SPG, spermatogonia; SPC, spermatocytes; SPD, spermatids). (D) Percentage of testis cells in testis at different stages. (E) Cumulative egg numbers per female over 7 days after life cycle exposure to PBSA (n = 3 × 3; 3 repeats with 3 female/3 male fish per repeat). (F) Fertilization rate (n = 3 × 3; 3 repeats with 3 female/3 male fish per repeat). Values plotted are mean ± SD. *P < 0.05, and **P < 0.01 indicate significant differences from the vehicle control.
Moreover, we evaluated the reproductive capability in fish by measuring the cumulative egg number per female over the seven-day period and the fertilization rate during spawning. Our results revealed that PBSA exposure significantly reduced the egg number and significantly impeded the fertilization rate (Figures 2E, F).
The inhibited gonadal development induced by PBSA might be related to the development of offspring embryos. To assess the influence of PBSA on offspring embryos, a study was conducted in which females exposed to PBSA across their lifespan were mated with males. Results showed that the egg diameter of the 0.75 hpf F1 embryos was visibly reduced compared to the vehicle control group (Figures 3A, B). The cumulative hatching rate was delayed at 60 hpf, but restored at 72 hpf (Figure 3C). However, the malformation rate was considerably higher at 72 hpf (Figure 3D), with deformities such as pericardial edema (PE), yolk-sac edema (YSE) and bent spine (BS) observed in F1 (Supplementary Figure S5).
Figure 3 Effects of PBSA on the development of embryos in F1 generation. (A) Representative image of F1 generation embryos at 0.75 hpf, with the red arrow denoting the egg diameter. (B) Egg diameter of embryos at 0.75 hpf (n = 3 × 20). (C) Cumulative hatching rate of F1 generation embryos at 60 and 72 hpf (n = 3 × 20). (D) Cumulative malformation rate of F1 generation embryos in 72 hpf (n = 3 × 20). Values plotted are mean ± SD. *P < 0.05, and **P < 0.01 indicate significant differences from the vehicle control.
To investigate the possibility of endocrine disruption and reproductive dysfunction caused by an imbalance in sex hormones, we tested the plasma sex hormone levels. Results from ELISA analyses revealed that the plasma levels of E2 and 11-KT were significantly decreased in the PBSA exposure group compared to the control group for both male and female zebrafish (Figures 4A, B). Notably, the decrease in E2 and 11-KT levels was more pronounced in females, indicating that PBSA had a greater effect on them.
Figure 4 Effects of PBSA on the plasma sex steroid hormone levels in adult zebrafish. E2 (A), 11-KT (B). Values plotted are mean ± SD. *P < 0.05, and **P < 0.01 indicate significant differences from the vehicle control.
We further explored the mechanisms of reproductive impairment in zebrafish caused by PBSA, by measuring the expression levels of selected genes along the HPGL axis in the brain, gonad, and liver of zebrafish after long-term exposure to PBSA (Supplementary Figure S6). In the brain, the expression levels of both erα and erβ were significantly increased in female zebrafish, whereas erα decreased and erβ remained unchanged in male zebrafish. Furthermore, gnrh2 expression was significantly increased in both female and male zebrafish, while gnrh3, lhβ and fshβ expression levels were only increased in female zebrafish, with fewer transcriptional changes observed in males (Figures 5A, B). In the ovary, the expression levels of 17βhsd, cyp19a1a and cyp11b were all significantly decreased in the PBSA exposure group compared to the control group (Figure 5C). In the testis, excluding 17βhsd, the expression levels of the cyp19a1a and cyp11b were significantly decreased in the treatment group (Figure 5D). In the liver, vtg1 and vtg2 genes were down-regulated in females but up-regulated in males (Figures 5E, F), demonstrating a gender-dependent response to PBSA exposure.
Figure 5 Effects of life cycle exposure to PBSA on the expression levels of genes in zebrafish brains (A, B), gonads (C, D), and livers (E, F). Values plotted are mean ± SD. *P < 0.05 and **P < 0.01 indicate significant differences from the vehicle control.
A BLAST comparison of protein sequences in the NCBI database was conducted, which identified sequences with 94.7% sequence identity and similarity in ERα and ERβ respectively, to be used as templates for homologous modelling. Ramachandran analysis of the protein structure revealed that the amount of ERα and ERβ residues in the allowed region was 99.5% (Supplementary Figures S7A, B), indicating that the protein structure was constructed effectively.
The results of the molecular docking and Cdocker interaction energy evaluation of three compounds (BP3, BPA, and PBSA) with both ERα and ERβ (as shown in Figures 6A, B; details displayed in Supplementary Figure S8) indicate that BP3 has an interaction energy of 32.84 kcal/mol with ERα and 17.69 kcal/mol with ERβ. BPA has an interaction energy of 39 kcal/mol with ERα and 20.43 kcal/mol with ERβ, whereas PBSA has an interaction energy of 26.89 kcal/mol with ERα and 12.96 kcal/mol with ERβ (Table 2). All three compounds appear to possess similar binding affinities to ERα and ERβ. Moreover, other significant parameters such as structure, interacting amino-acid residues number and cdocker energy are also presented in Table 2.
Figure 6 Binding modes between chemicals (BP3, BPA, and PBSA) and target proteins (ERα and ERβ). BP3-ERα, BPA-ERα, PBSA-ERα (A); BP3-ERβ, BPA-ERβ, PBSA-ERβ (B).
This study explored the effects of life cycle exposure to PBSA at near-environmentally relevant concentrations on the reproductive potential of zebrafish and the development of their offspring embryos, as well as the possible underlying mechanisms. The finding showed that PBSA inhibited oocyte maturation, decreased the levels of steroid sex hormones in the plasma, reduced fecundity, and caused malformations and growth inhibition in the F1 generation. The changes in the transcriptional profiles of genes in the HPGL axis, along with molecular docking results, provide further insight into the potential mechanisms of PBSA-induced reproductive endocrine disruption. It is suggested that PBSA exposure has a detrimental effect on female fertility and the development of their offspring, which may could lead to a decline in fish populations.
To investigate the potential impacts of life cycle exposure to PBSA on the growth and development of zebrafish, we employed a concentration range (0.2–20 μg/L) that is near-environmentally relevant. The results indicated that only the highest concentration of PBSA (20 μg/L) caused a decrease in the GSI of female fish, suggesting a potential inhibition of ovarian development and reproductive dysfunction in female zebrafish. GSI has been used as an indicator of sexual maturity and reproductive potential in fish (Huang et al., 2019; Quintaneiro et al., 2019). To further investigate this, histological analysis of the ovaries was conducted. The results showed that after exposure to 20 μg/L of PBSA, the proportion of follicles in the primary growth and early vitellogenic stages was increased, while the full grown follicle was decrease. This ovary inhibition could affect the female reproduction since the ovary is the major reproductive organ for females. Therefore, we evaluated the cumulative egg number and fertilization rate and found that they were significantly lower than the control group, indicating that PBSA is toxic to ovary development and may impede female reproduction. A similar decrease in ovary development and fecundity in fish was observed after exposure to benzophenonic UV filter BP2 and BP3 (Weisbrod et al., 2007; Tao et al., 2023). Further research is needed to uncover and explore the molecular mechanism responsible for ovarian inhibition when exposed to PBSA.
In vertebrates, gonadal development is mainly regulated by the HPG axis, which can control the levels of sex hormones. GnRH from the hypothalamus stimulates the pituitary gland to secrete gonadotropins such as LH and FSH, which then interact with FSH and LH receptors on the gonads to initiate steroidogenesis and gametogenesis (Chen et al., 2022). In our study, life cycle exposure to PBSA was found to decrease the concentration of steroid sex hormones (11-KT, E2) in the plasma, suggesting a disruption of the HPG axis. In the brain, the transcription of gnrh2, lhβ and fshβ genes was increased in females, possibly as a result of a negative feedback mechanism to enhance the pituitary’s sensitivity and the subsequent secretion of higher levels of gonadotrophins to compensate for the decreased steroid sex hormones after PBSA treatment. Further investigation is needed to gain better understanding of this. In the gonad, the transcription of 17βhsd, cyp19a1a, and cyp11b, which are enzymes responsible for the conversion of cholesterol to testosterone (T), the transformation of T to E2, and the transformation of T to 11-KT respectively (Dang et al., 2015; Yang et al., 2022), were observed to be reduced. This could be the cause of the decrease in plasma 11-KT and E2 levels observed in the PBSA treatments. Furthermore, the decrease of E2 in the treatment group could be partially attributed to the suppression of T. Taken together, the transcription of genes related to reproduction in the HPG axis was suppressed, resulting in a decrease in the levels of E2 and 11-KT. This, in turn, may have hindered gamete maturation and consequently leading to a diminished reproductive capacity of the adult zebrafish.
The elevated expression levels of steroid receptors (ERα and ERβ) in females indicated a strong xenoestrogenic behavior of PBSA. To confirm the results, an in silico molecular docking of zebrafish estrogen receptors was conducted, which revealed that PBSA had the strongest binding affinity to ERα and ERβ, similar to the typical endocrine disruptors BP3 and BPA. This was in agreement with a previous study which found that PPCPs had the strongest binding energies to ERα and Erβ (Hamid et al., 2022). Therefore, in silico MD has determined that PBSA may act as a xenoestrogen, mimicking the roles of natural ligands and thus disrupting the normal functioning of the HPGL axis.
Fish follicles secrete estradiol, prompting the liver to create the vitellogenin (vtg), a yolk protein, and initiating oocyte maturation and yolk biosynthesis. This yolk is subsequently stored and processed by the maturing oocyte to supply nourishment for the progeny, as described by Brion et al. (Brion et al., 2004; Yuan et al., 2013). The reduction of E2 concentration in the plasma after PBSA exposure may be partially accountable for the decreased expression of the vtg1 and vtg2 genes in the livers of females. Taking into account the fact female zebrafish are responsible for supplying the majority of nutrients for the F1 generation embryos (Yang et al., 2022), the inhibition of the vtg1 and vtg2 genes in females could impede the growth of the ovary and reduce the quality of eggs. As expected, we did find a reduction in egg diameter, abnormal cumulative hatching rate and an increase in malformation rate in F1 offspring. The same phenomenon was found in zebrafish after exposure to tribromophenol (TBP) for 120 days at concentrations of 0, 0.3 and 3.0 μg/L, a significant decrease in vtg1 and vtg2 expression was observed in the liver of female zebrafish, leading to an increased rate of malformation, reduced survival, and inhibited growth in their F1 larvae (Deng et al., 2010). Consequently, it can be assumed that the decreased vtg gene expression and lower plasma levels of sex hormones in female fish are likely to be responsible for the impaired fertility and inhibited growth of the F1 generation in PBSA treated fish. Further investigation is required to determine the potential molecular mechanism.
This study explored the reproductive consequences of a life cycle exposure to PBSA at near-environmentally relevant concentrations in zebrafish. Results showed that PBSA inhibited ovary development, resulting in a decrease in cumulative egg number and an increase in the proportion of primary growth follicles. Histological analysis of the gonads, sex hormones and the expression of HPGL axis related genes, particularly vtg1 and vtg2, revealed that the reproductive endocrine disruption caused by PBSA was predominantly female-biased. This reproductive endocrine disruption caused a decrease in egg diameter at 0.75 hpf, an alteration in cumulative hatching rate at 60 hpf, and an increase in malformation rate at 72 hpf in the F1 generation. Given these adverse effects on the reproductive endocrine systems, PBSA could potentially cause a decrease in fish populations due to impaired reproductive capacity.
The original contributions presented in the study are included in the article/Supplementary Material. Further inquiries can be directed to the corresponding author.
The care and use of zebrafish in this study was approved by the Institutional Animal Care and Use Committee of Guizhou Medical University. The studies were conducted in accordance with the local legislation and institutional requirements. Written informed consent was obtained from the owners for the participation of their animals in this study.
JT: Conceptualization, Funding acquisition, Supervision, Writing – review & editing. QY: Conceptualization, Data curation, Formal Analysis, Investigation, Methodology, Writing – original draft. XS: Data curation, Formal Analysis, Investigation, Writing – original draft. LT: Data curation, Methodology, Writing – original draft. YD: Formal Analysis, Methodology, Writing – original draft. YW: Formal Analysis, Methodology, Writing – original draft. WW: Formal Analysis, Methodology, Writing – original draft. XF: Formal Analysis, Methodology, Writing – original draft.
The author(s) declare financial support was received for the research, authorship, and/or publication of this article. This study was partly supported by the National Natural Science Foundation of China (No. 22166014), the Science and Technology Program of Guizhou Province (No. ZK [2023] 292), and the Guizhou Education Department Youth Science and Technology Talents Growth Project (No. KY [2021] 175).
The authors declare that the research was conducted in the absence of any commercial or financial relationships that could be construed as a potential conflict of interest.
All claims expressed in this article are solely those of the authors and do not necessarily represent those of their affiliated organizations, or those of the publisher, the editors and the reviewers. Any product that may be evaluated in this article, or claim that may be made by its manufacturer, is not guaranteed or endorsed by the publisher.
The Supplementary Material for this article can be found online at: https://www.frontiersin.org/articles/10.3389/fmars.2023.1283816/full#supplementary-material
Balmer M. E., Buser H. R., Muller M. D., Poiger T. (2005). Occurrence of some organic uv filters in wastewater, in surface waters, and in fish from swiss lakes. Environ. Sci. Technol. 39 (4), 953–962. doi: 10.1021/es040055r
Brion F., Tyler C. R., Palazzi X., Laillet B., Porcher J. M., Garric J., et al. (2004). Impacts of 17beta-estradiol, including environmentally relevant concentrations, on reproduction after exposure during embryo-larval-, juvenile- and adult-life stages in zebrafish (danio rerio). Aquat. Toxicol. 68 (3), 193–217. doi: 10.1016/j.aquatox.2004.01.022
Carve M., Allinson G., Nugegoda D., Shimeta J. (2021). Trends in environmental and toxicity research on organic ultraviolet filters: a scientometric review. Sci. Total Environ. 773, 145628. doi: 10.1016/j.scitotenv.2021.145628
Chen X., Zheng J., Zhang J., Duan M., Xu H., Zhao W., et al. (2022). Exposure to difenoconazole induces reproductive toxicity in zebrafish by interfering with gamete maturation and reproductive behavior. Sci. Total Environ. 838 (Pt 1), 155610. doi: 10.1016/j.scitotenv.2022.155610
Chen Y., Wang X., Zhai H., Zhang Y., Huang J. (2021). Identification of potential human ryanodine receptor 1 agonists and molecular mechanisms of natural small-molecule phenols as anxiolytics. ACS Omega. 6 (44), 29940–29954. doi: 10.1021/acsomega.1c04468
Dang Y., Giesy J. P., Wang J., Liu C. (2015). Dose-dependent compensation responses of the hypothalamic-pituitary-gonadal-liver axis of zebrafish exposed to the fungicide prochloraz. Aquat. Toxicol. 160, 69–75. doi: 10.1016/j.aquatox.2015.01.003
Deng J., Liu C., Yu L., Zhou B. (2010). Chronic exposure to environmental levels of tribromophenol impairs zebrafish reproduction. Toxicol. Appl. Pharmacol. 243 (1), 87–95. doi: 10.1016/j.taap.2009.11.016
Downs C. A., Bishop E., Diaz-Cruz M. S., Haghshenas S. A., Stien D., Rodrigues A., et al. (2022). Oxybenzone contamination from sunscreen pollution and its ecological threat to hanauma bay, oahu, hawaii, U.S.A. Chemosphere. 291 (Pt 2), 132880. doi: 10.1016/j.chemosphere.2021.132880
Grabicova K., Fedorova G., Burkina V., Steinbach C., Schmidt-Posthaus H., Zlabek V., et al. (2013). Presence of uv filters in surface water and the effects of phenylbenzimidazole sulfonic acid on rainbow trout (oncorhynchus mykiss) following a chronic toxicity test. Ecotox. Environ. Safe. 96, 41–47. doi: 10.1016/j.ecoenv.2013.06.022
Guo Y., Chen L., Wu J., Hua J., Yang L., Wang Q., et al. (2019). Parental co-exposure to bisphenol a and nano-tio(2) causes thyroid endocrine disruption and developmental neurotoxicity in zebrafish offspring. Sci. Total Environ. 650 (Pt 1), 557–565. doi: 10.1016/j.scitotenv.2018.09.007
Hamid N., Junaid M., Manzoor R., Duan J. J., Lv M., Xu N., et al. (2022). Tissue distribution and endocrine disruption effects of chronic exposure to pharmaceuticals and personal care products mixture at environmentally relevant concentrations in zebrafish. Aquat. Toxicol. 242, 106040. doi: 10.1016/j.aquatox.2021.106040
Huang X., Li Y., Wang T., Liu H., Shi J., Zhang X. (2020). Evaluation of the oxidative stress status in zebrafish (danio rerio) liver induced by three typical organic uv filters (bp-4, paba and pbsa). Int. J. Environ. Res. Public Health 17 (2), 651. doi: 10.3390/ijerph17020651
Huang Y., Liu J., Yu L., Liu C., Wang J. (2019). Gonadal impairment and parental transfer of tris (2-butoxyethyl) phosphate in zebrafish after long-term exposure to environmentally relevant concentrations. Chemosphere. 218, 449–457. doi: 10.1016/j.chemosphere.2018.11.139
Kimmel C. B., Ballard W. W., Kimmel S. R., Ullmann B., Schilling T. F. (1995). Stages of embryonic development of the zebrafish. Dev. Dyn. 203 (3), 253–310. doi: 10.1002/aja.1002030302
Livak K. J., Schmittgen T. D. (2001). Analysis of relative gene expression data using real-time quantitative pcr and the 2(-delta delta c(t)) method. Methods. 25 (4), 402–408. doi: 10.1006/meth.2001.1262
Ma J., Wang Z., Qin C., Wang T., Hu X., Ling W. (2023). Safety of benzophenone-type uv filters: a mini review focusing on carcinogenicity, reproductive and developmental toxicity. Chemosphere. 326, 138455. doi: 10.1016/j.chemosphere.2023.138455
Mitchelmore C. L., He K., Gonsior M., Hain E., Heyes A., Clark C., et al. (2019). Occurrence and distribution of uv-filters and other anthropogenic contaminants in coastal surface water, sediment, and coral tissue from hawaii. Sci. Total Environ. 670, 398–410. doi: 10.1016/j.scitotenv.2019.03.034
Mo A., Wang X., Yuan Y., Liu C., Wang J. (2021). Effects of waterborne exposure to environmentally relevant concentrations of selenite on reproductive function of female zebrafish: a life cycle assessment. Environ. pollut. 270, 116237. doi: 10.1016/j.envpol.2020.116237
Mustieles V., Balogh R. K., Axelstad M., Montazeri P., Marquez S., Vrijheid M., et al. (2023). Benzophenone-3: comprehensive review of the toxicological and human evidence with meta-analysis of human biomonitoring studies. Environ. Int. 173, 107739. doi: 10.1016/j.envint.2023.107739
O'Malley E., O'Brien J. W., Verhagen R., Mueller J. F. (2020). Annual release of selected uv filters via effluent from wastewater treatment plants in Australia. Chemosphere. 247, 125887. doi: 10.1016/j.chemosphere.2020.125887
Orlikowska A., Fisch K., Schulz-Bull D. E. (2015). Organic polar pollutants in surface waters of inland seas. Mar. pollut. Bull. 101 (2), 860–866. doi: 10.1016/j.marpolbul.2015.11.018
Qian L., Qi S., Zhang J., Duan M., Schlenk D., Jiang J., et al. (2020). Exposure to boscalid induces reproductive toxicity of zebrafish by gender-specific alterations in steroidogenesis. Environ. Sci. Technol. 54 (22), 14275–14287. doi: 10.1021/acs.est.0c02871
Quintaneiro C., Soares A., Costa D., Monteiro M. S. (2019). Effects of pcb-77 in adult zebrafish after exposure during early life stages. J. Environ. Sci. Health Part a-Toxic/Hazard. Subst. Environ. Eng. 54 (5), 478–483. doi: 10.1080/10934529.2019.1568793
Rampogu S., Park C., Ravinder D., Son M., Baek A., Zeb A., et al. (2019). Pharmacotherapeutics and molecular mechanism of phytochemicals in alleviating hormone-responsive breast cancer. Oxid. Med. Cell. Longev. 2019, 5189490. doi: 10.1155/2019/5189490
Scheele A., Sutter K., Karatum O., Danley-Thomson A. A., Redfern L. K. (2023). Environmental impacts of the ultraviolet filter oxybenzone. Sci. Total Environ. 863, 160966. doi: 10.1016/j.scitotenv.2022.160966
Schneider C. A., Rasband W. S., Eliceiri K. W. (2012). Nih image to imagej: 25 years of image analysis. Nat. Methods 9 (7), 671–675. doi: 10.1038/nmeth.2089
Shaath N. A. (2016). Principles and Practice of Photoprotection (Switzerland: Springer International Publishing). doi: 10.1007/978-3-319-29382-0
Shaath N. A., Shaath M. (2005). Sunscreens: Regulations and Commercial Development. 3rd ed. (Taylor & Francis).
Shi Q., Yang H., Chen Y., Zheng N., Li X., Wang X., et al. (2023). Developmental neurotoxicity of trichlorfon in zebrafish larvae. Int. J. Mol. Sci. 24 (13), 11099. doi: 10.3390/ijms241311099
Sun X., Yang Q., Jing M., Jia X., Tian L., Tao J. (2023). Environmentally relevant concentrations of organic (benzophenone-3) and inorganic (titanium dioxide nanoparticles) uv filters co-exposure induced neurodevelopmental toxicity in zebrafish. Ecotox. Environ. Safe. 249, 114343. doi: 10.1016/j.ecoenv.2022.114343
Tao J., Bai C., Chen Y., Zhou H., Liu Y., Shi Q., et al. (2020). Environmental relevant concentrations of benzophenone-3 induced developmental neurotoxicity in zebrafish. Sci. Total Environ. 721, 137686. doi: 10.1016/j.scitotenv.2020.137686
Tao J., Yang Q., Jing M., Sun X., Tian L., Huang X., et al. (2023). Embryonic benzophenone-3 exposure inhibited fertility in later-life female zebrafish and altered developmental morphology in offspring embryos. Environ. Sci. pollut. Res. 30 (17), 49226–49236. doi: 10.1007/s11356-023-25843-7
Wang J., Meng X., Feng C., Xiao J., Zhao X., Xiong B., et al. (2021). Benzophenone-3 induced abnormal development of enteric nervous system in zebrafish through mapk/erk signaling pathway. Chemosphere. 280, 130670. doi: 10.1016/j.chemosphere.2021.130670
Weisbrod C. J., Kunz P. Y., Zenker A. K., Fent K. (2007). Effects of the uv filter benzophenone-2 on reproduction in fish. Toxicol. Appl. Pharmacol. 225 (3), 255–266. doi: 10.1016/j.taap.2007.08.004
Wu L., Zeeshan M., Dang Y., Zhang Y. T., Liang L. X., Huang J. W., et al. (2023). Maternal transfer of f-53b inhibited neurobehavior in zebrafish offspring larvae and potential mechanisms: dopaminergic dysfunction, eye development defects and disrupted calcium homeostasis. Sci. Total Environ. 894, 164838. doi: 10.1016/j.scitotenv.2023.164838
Yan Y., Guo F., Liu K., Ding R., Wang Y. (2023). The effect of endocrine-disrupting chemicals on placental development. Front. Endocrinol. 14. doi: 10.3389/fendo.2023.1059854
Yang R., Wang X., Wang J., Chen P., Liu Q., Zhong W., et al. (2022). Insights into the sex-dependent reproductive toxicity of 2-ethylhexyl diphenyl phosphate on zebrafish (danio rerio). Environ. Int. 158, 106928. doi: 10.1016/j.envint.2021.106928
Yuan H. X., Xu X., Sima Y. H., Xu S. Q. (2013). Reproductive toxicity effects of 4-nonylphenol with known endocrine disrupting effects and induction of vitellogenin gene expression in silkworm, bombyx mori. Chemosphere. 93 (2), 263–268. doi: 10.1016/j.chemosphere.2013.04.075
Keywords: 2-phenylbenzimidazole-5-sulfonic acid, life cycle exposure, reproductive endocrine disruption, sex hormones, HPGL axis, transgenerational effects
Citation: Tao J, Yang Q, Sun X, Tian L, Deng Y, Wang Y, Wang W and Fan X (2023) Life cycle exposure to 2-phenylbenzimidazole-5-sulfonic acid disrupts reproductive endocrine system and induces transgenerational adverse effects in zebrafish. Front. Mar. Sci. 10:1283816. doi: 10.3389/fmars.2023.1283816
Received: 27 August 2023; Accepted: 11 October 2023;
Published: 01 November 2023.
Edited by:
Xuchun Qiu, Jiangsu University, ChinaReviewed by:
Mohamed Ahmed Ibrahim Ahmed, Assiut University, EgyptCopyright © 2023 Tao, Yang, Sun, Tian, Deng, Wang, Wang and Fan. This is an open-access article distributed under the terms of the Creative Commons Attribution License (CC BY). The use, distribution or reproduction in other forums is permitted, provided the original author(s) and the copyright owner(s) are credited and that the original publication in this journal is cited, in accordance with accepted academic practice. No use, distribution or reproduction is permitted which does not comply with these terms.
*Correspondence: Junyan Tao, anVueWFud211QDE2My5jb20=
†These authors have contributed equally to this work
Disclaimer: All claims expressed in this article are solely those of the authors and do not necessarily represent those of their affiliated organizations, or those of the publisher, the editors and the reviewers. Any product that may be evaluated in this article or claim that may be made by its manufacturer is not guaranteed or endorsed by the publisher.
Research integrity at Frontiers
Learn more about the work of our research integrity team to safeguard the quality of each article we publish.