- 1Ocean Climate Response & Ecosystem Research Department, Korea Institute of Ocean Science and Technology, Busan, Republic of Korea
- 2Department of Marine Biology, College of Fisheries Science, Pukyong National University, Busan, Republic of Korea
We investigated feeding by Calanus sinicus in the Yellow Sea over four seasons in 2019–2022 using the gut pigment method. Studying the feeding of copepods is important for understanding processes within food webs, but little is known about the feeding ecology of C. sinicus, a major consumer of primary production in the Yellow Sea. The seasonal mean ingestion rate of C. sinicus was 16.6–42.1 for copepodite stage I (CI), 24.1–41.8 for CII, and 32.7–58.5 ng chl ind.−1 d−1 for CIII, with different seasonal fluctuations in each stage. The seasonal mean ingestion rate was 45.8–114.4 for CIV, 50.2–189.3 for CV, 96.9–438.9 for females, and 69.8–132.3 ng chl ind.−1 d−1 for males, with higher values in spring and lower values in summer. The combined grazing impacts of C. sinicus from the CI to adult stages were 1.66%, 1.43%, 2.04%, and 0.65% in spring, summer, autumn, and winter, respectively. The ingestion rate of later developmental stages from CIV to adults was positively related to chlorophyll-a concentration rather than water temperature or salinity, according to a redundancy analysis. These results suggest that C. sinicus population in the Yellow Sea actively feeds on phytoplankton in spring and that most of the grazing impact is attributed to females.
Introduction
In the ocean, zooplankton transfer primary production to upper trophic levels as major consumers that regulate phytoplankton biomass and structure (Sterner, 1989; Kagami et al., 2002). Fecal pellets produced by zooplankton after ingesting prey such as phytoplankton are decomposed, providing carbon to the microbial loop (Pomeroy et al., 2007). Fecal pellets of large copepods sink to the bottom and are an important carbon source in nutrient-poor deep waters (Turner, 2015). Therefore, quantifying zooplankton feeding is essential to elucidate the carbon cycle and energy flow of marine food webs.
Laboratory culturing is a classical method for measuring the feeding rate of zooplankton. In this method, the amount of reduction in food is measured after incubating zooplankton with a measured amount of food for a certain period of time (Båmstedt et al., 1991). This technique has the advantages of a controlled environment and relatively accurate quantification, but its results inevitably differ from the ingestion rate of organisms in the actual field. Gut pigment analysis is a method of directly measuring the chlorophyll concentration associated with prey in the intestines of collected organisms. Gut pigment measurement is more realistic than artificial culturing, but ingestion rates may be underestimated because prey lacking chlorophyll or with its pigment destroyed is ignored. Nevertheless, such analysis has been used in many studies due to its convenience relative to culture-based processes (López et al., 2007; Debes et al., 2008; Yamaguchi et al., 2010; Matsuno et al., 2015), particularly in situations where feeding is investigated across a wide range of open-ocean sites, such as this study.
The copepod Calanus sinicus represents up to 44% of total zooplankton biomass in the Yellow Sea (Sun et al., 2010). Thus, it is a major contributor to the consumption of primary production and carbon cycling, and also a major food source for juvenile and small fish in this region (Huh, 1999; Jeong et al., 2016; Ko et al., 2019). Despite the importance of this species, studies on feeding by C. sinicus in offshore waters of the Yellow Sea are scarce (Li et al., 2004; Zhang et al., 2006; Huo et al., 2008). In inshore parts of the Yellow Sea, feeding by C. sinicus has been reported in Gyeonggi Bay (Shin and Choi, 1992), Taean (Song et al., 2010), and Asan Bay (Lee et al., 2012). In natural habitats, copepodites outnumber adults and have considerable biomass, but their feeding has been neglected in previous studies. Even for adults, seasonal fluctuations are still unclear. This study provides detailed and comprehensive data on the developmental and seasonal feeding of C. sinicus.
In this study, we quantified the seasonal feeding pattern of C. sinicus and its environmental drivers to elucidate its seasonality. As well as to quantify the ingestion rates of the developmental stages of C. sinicus to characterize the feeding ecology of this species in detail. This study provides basic data on the ecology of C. sinicus that will help to understand the interaction between phytoplankton and zooplankton, and energy flows and carbon cycling in the Yellow Sea food web.
Materials and methods
Sampling and environmental monitoring
In the Yellow Sea (Figure 1), we sampled copepods at nine stations in April 2019 (spring), six stations each in August 2020 (summer) and November 2021 (autumn), and seven stations in early March 2022 (winter). In summer, typhoon Bavi (950 hPa, 40 m/s) passed through the study area a few days before sampling.
A flowmeter (Model 438 115, Hydro-Bios, Kiel, Germany) attached conical net (diameter, 60 cm; 115 µm mesh) was towed vertically from the bottom to the surface. The live sample was filtered through a 115 µm net to remove seawater, frozen in liquid nitrogen, transported to the laboratory, and stored at –70°C until measurement. Because the collection time differed among stations (Table 1), no distinction was made between day and night.
Temperature and salinity were monitored at the sampling stations using a conductivity, temperature, and depth instrument (Sea-Bird 911 CTD; Sea-Bird Scientific, Bellevue, WA, USA). Temperature and salinity data from adjacent stations were included to better describe the vertical distribution. Seawater samples (500 mL) were filtered with GF/F filter paper (Whatman, UK), frozen, and transported to the laboratory. Chlorophyll a (chl-a) was extracted for 24 h in a cool, dark place (–20°C) and measured using a fluorometer (10 AU; Turner Designs, San Jose, CA, USA). Sampling was performed at four to five water depths (0, 5, 10, 20 m for stations less than 50 m and 0, 10, 20, 30, 50 for others); the resulting values were averaged and expressed as mean water column chl-a concentrations.
Gut pigment contents
Experiments were conducted on copepodite stages I–V (CI–CV) and adult (CVI) males and females of C. sinicus, without considering naupliar stages. Then, 4–100 individuals of each stage (average of 48 individuals) were selected, washed with distilled water, and subjected to chlorophyll extraction in 90% acetone for 24 h in the dark at –20°C. Fluorescence before and after acidification was measured using a fluorometer (Turner Design, Trilogy, USA) to determine gut pigment values. During the experiment, the laboratory was set to a weak light condition at 18°C, and the temperature of the sample was reduced with an ice pack to minimize pigment destruction.
The gut pigment content (ng chl ind.−1) of copepod individual (ind.) was calculated as the sum of the chl-a and phaeopigment (ng chl eq. ind.–1) concentrations, as follows (Mackas and Bohrer, 1976; Dagg and Wyman, 1983):
where f0 is the fluorescence before acidification, fa is the fluorescence after acidification, k is the machine calibration constant, and R is the acidification ratio during calibration.
Ingestion rate and grazing impact
The ingestion rate (I, ng chl ind.−1 d−1) of copepods was calculated as follows (Dagg and Wyman, 1983):
where G is the gut pigment content and E is the gut evacuation rate (min–1), which was obtained from Uye and Yamamoto (1995) and converted into a daily rate daily rate (d‒1). The population ingestion rate (mg m–2 d–1) was determined by multiplying the ingestion rate by the number of individuals (individuals m–2) for each developmental stage. Grazing impact was expressed as a percentage (%), obtained by dividing the population ingestion rate by the mean chl-a concentration of the water column.
Data analysis
The Kruskal–Wallis test with Dunn’s multiple comparison test was performed to determine the seasonal mean differences in C. sinicus gut pigment, ingestion rate, and grazing impact using GraphPad Prism v8 software (GraphPad Software, San Diego, CA, USA). To determine the relative effects of environmental factors, a redundancy analysis (RDA) was performed between the ingestion rate of the C. sinicus developmental stages and environmental factors, including surface (5 m) and bottom temperature and salinity, as well as surface (0 m) and water column mean chl-a concentrations. A preliminary RDA was performed including all environmental factors, and variables with low significance were excluded. The significance of the RDA results was checked using permutation tests. Canonical community ordination was performed using Canoco v5 (ter Braak and Smilauer, 2012).
Results
Environmental factors
In spring, surface temperatures were in the range of 8.1–12.7°C and bottom temperatures were 7.1–10.4°C (Figure 2). Salinities ranged from 31.7 to 33.3 psu in the water column. In summer, vertical mixing caused by a typhoon disturbed the isotherms in coastal areas. Surface temperatures were in the range of 20.2–26.7°C and bottom temperatures were 9.1–17.8°C. 'Salinities ranged from 30.7 to 33.1 in the water column. In autumn, surface temperatures were in the range of 17.2–19.4°C and bottom temperatures were 8.8–16.7°C. Salinities ranged from 31.1 to 32.8 in the water column. In winter, both water temperature and salinity were vertically homogeneous. surface temperatures were in the range of 4.8–8.9°C and bottom temperatures were 4.8–9.9°C. Salinities ranged from 31.9 to 33.0 psu in the water column. The surface chl-a concentrations were 0.35–3.10, 0.27–0.90, 0.28–1.63, and 0.48–8.49 µg L–1 in spring, summer, autumn, and winter, respectively, and the water column-averaged chl-a concentrations were 0.86–3.30, 0.42–0.92, 0.28–1.58, and 0.48–6.49 µg L–1, respectively (Figure 3).
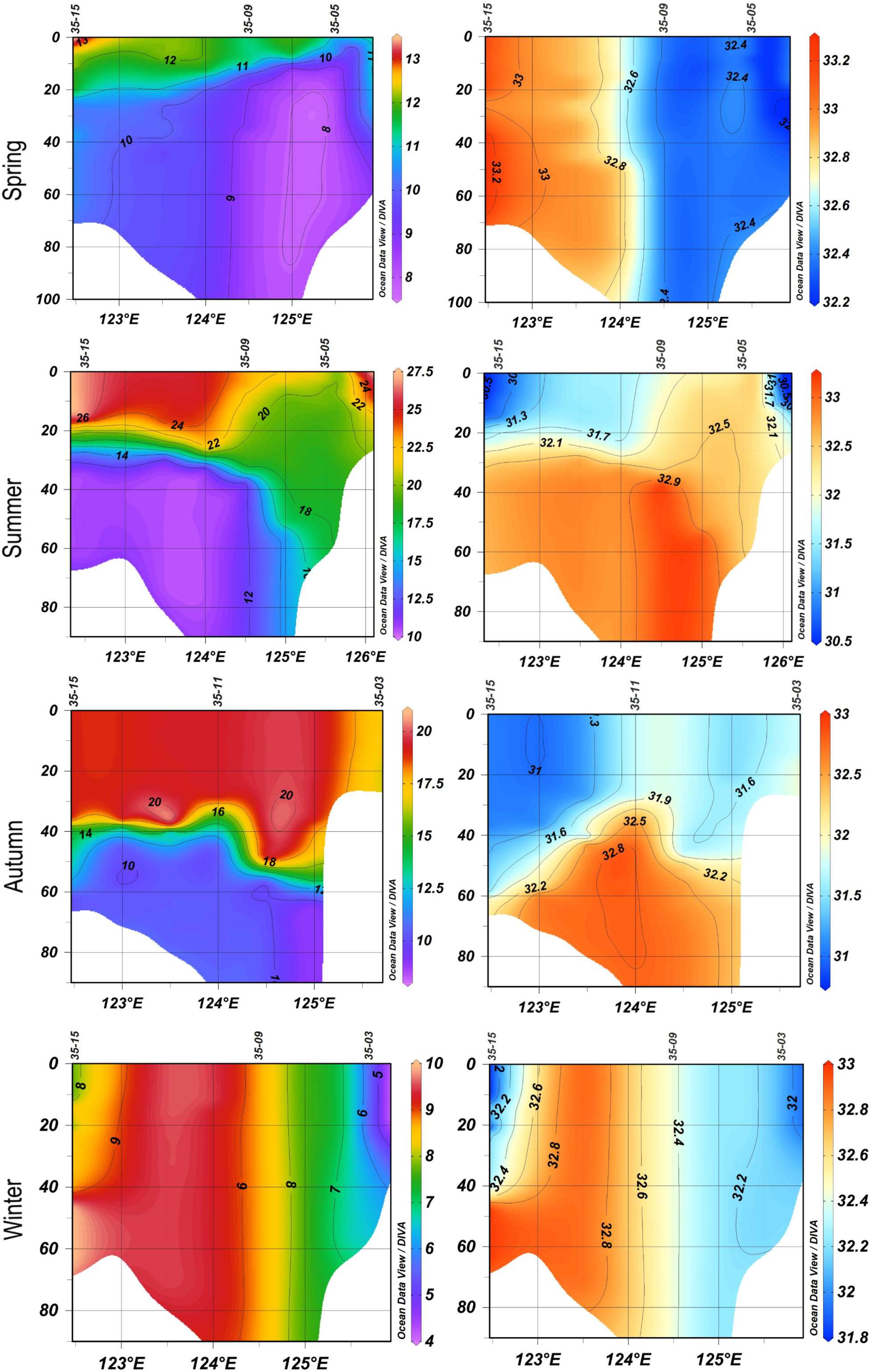
Figure 2 Vertical profiles of temperature (left, °C) and salinity (right, psu) at sampling stations located at 35°N in the four seasons.
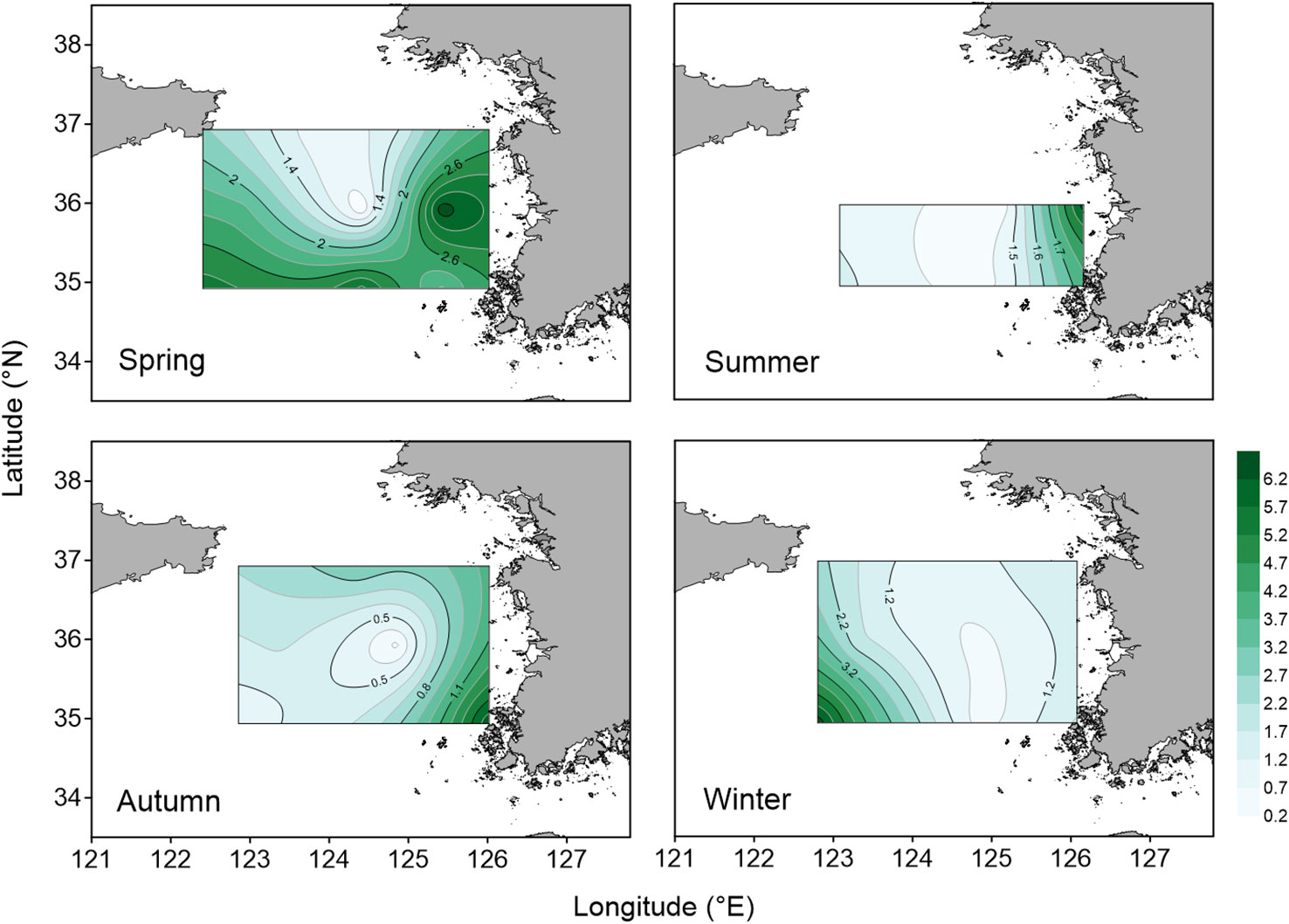
Figure 3 Seasonal variation in the spatial distribution of water column mean chlorophyll-a concentrations (µg L–1) in the four seasons.
Gut pigment contents
The seasonal mean gut pigment content of C. sinicus CI was 0.24–0.49 ng chl ind.−1, with higher values in winter and lower values in spring (Figure 4). CII was 0.39–0.46 ng chl ind.−1, with small seasonal differences. The seasonal mean gut pigment content of CIII-V was 0.45–0.81, 0.47–1.70, and 0.52–2.79 ng chl ind.−1, and that of females was 1.00–6.41 ng chl ind.−1, and that of males was 0.74–1.94 ng chl ind.−1. The values for CIII to adults were higher in spring and lower in summer. The observed seasonal differences were nonsignificant for all stages (p > 0.05).
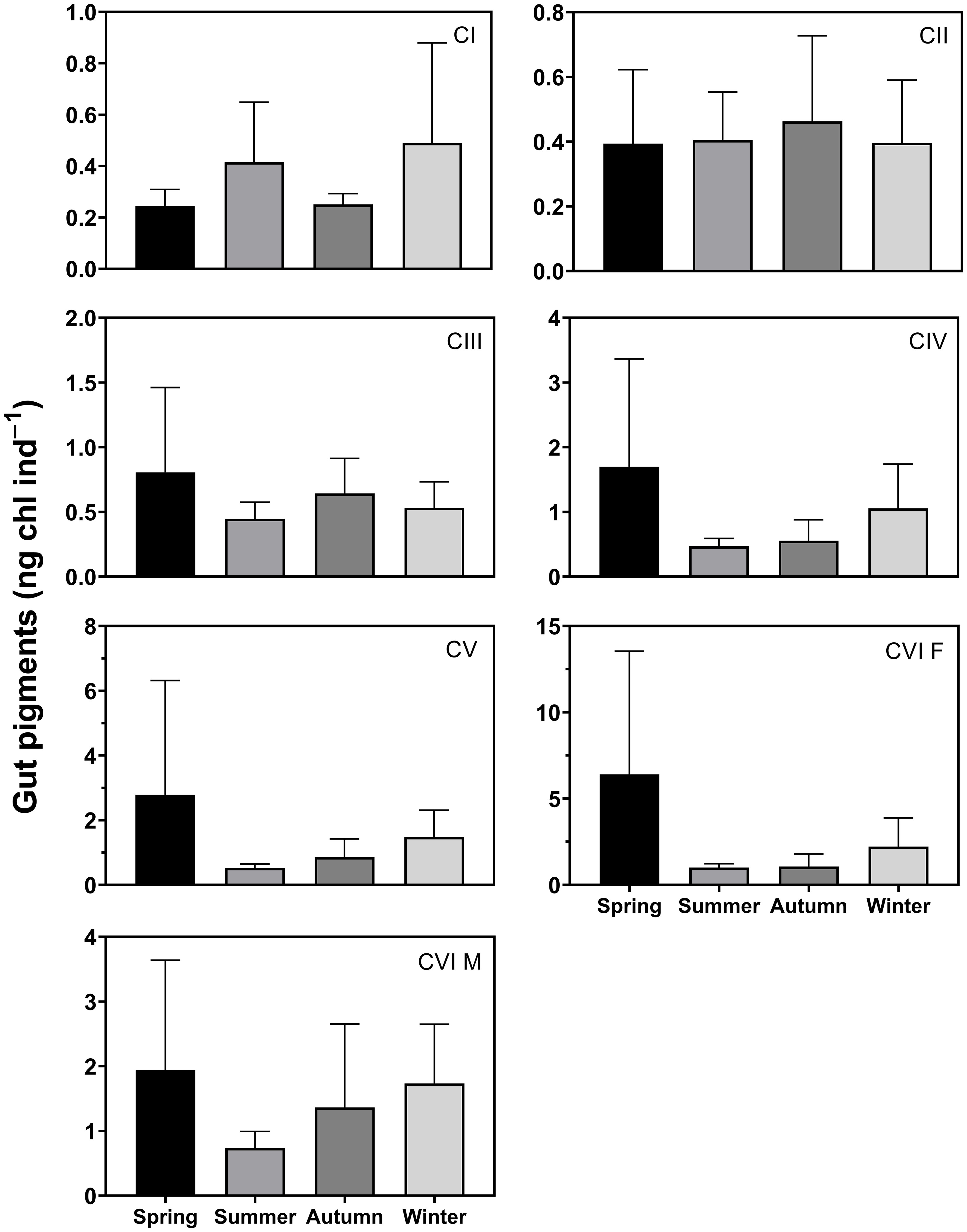
Figure 4 Seasonal variation in gut pigment content (ng chl ind.–1) of Calanus sinicus from copepodite stage I (CI) to adult female (CVI F) and male (CVI M). Error bars indicate the standard deviation.
Ingestion rate
The seasonal mean ingestion rate of C. sinicus CI was 16.6–42.1 ng chl ind.−1 d−1, with higher values in summer and lower values in spring (Figure 5). CII was 24.1–41.8 and CIII was 32.7–58.5 ng chl ind.−1 d−1, with higher values in autumn and lower values in winter. The seasonal mean ingestion rate was 45.8–114.4 for CIV, 50.2–189.3 for CV, 96.9–438.9 for females, and 69.8–132.3 ng chl ind.−1 d−1 for males. The values for CIV to adults were higher in spring and lower in summer. Seasonal differences were nonsignificant for all stages.
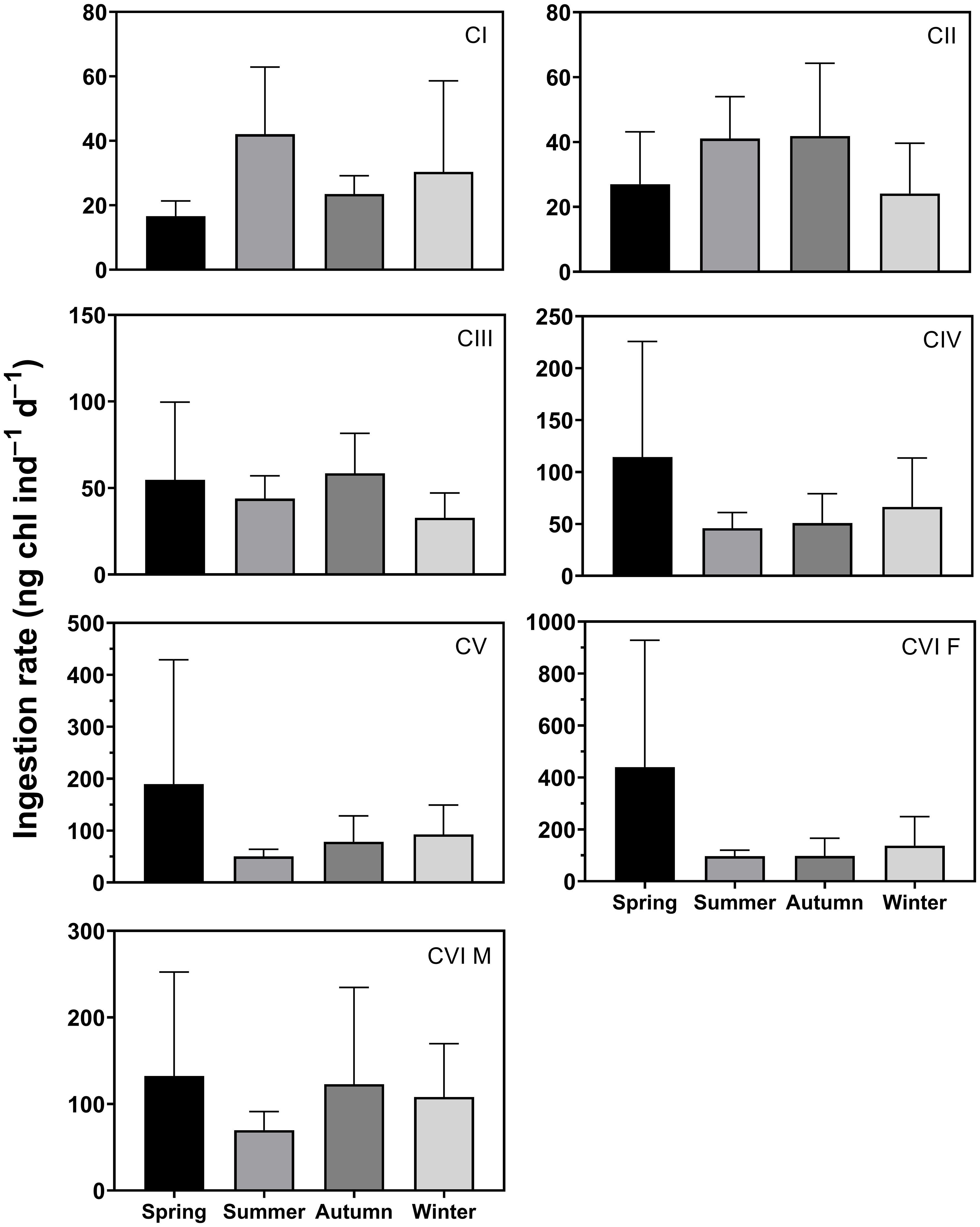
Figure 5 Seasonal variation in ingestion rate (ng chl ind.–1 d–1) of Calanus sinicus from CI to adult female (CVI F) and male (CVI M). Error bars indicate the standard deviation.
Population ingestion rate
The seasonal mean population ingestion rate of C. sinicus CI was 0.01–0.10 mg chl m–2 d–1, with higher values in spring and lower values in winter (Figure 6). The values for CII–V were 0.01–0.09, 0.01–0.15, 0.02–0.27, and 0.19–0.81 mg chl m–2 d–1; it was 0.03–0.15 in males and 0.20–1.42 mg chl m–2 d–1 in females, being higher in spring and lower in winter. The value for CIII was significantly higher in spring than summer (p = 0.043). The combined population ingestion rates of C. sinicus from the CI to adult stages were 2.98, 0.58, 0.95, 0.60 mg chl m–2 d–1 in spring, summer, autumn, and winter, respectively. That of females was the highest among stages, followed by CV, while those of the remaining stages were low (Table 2).
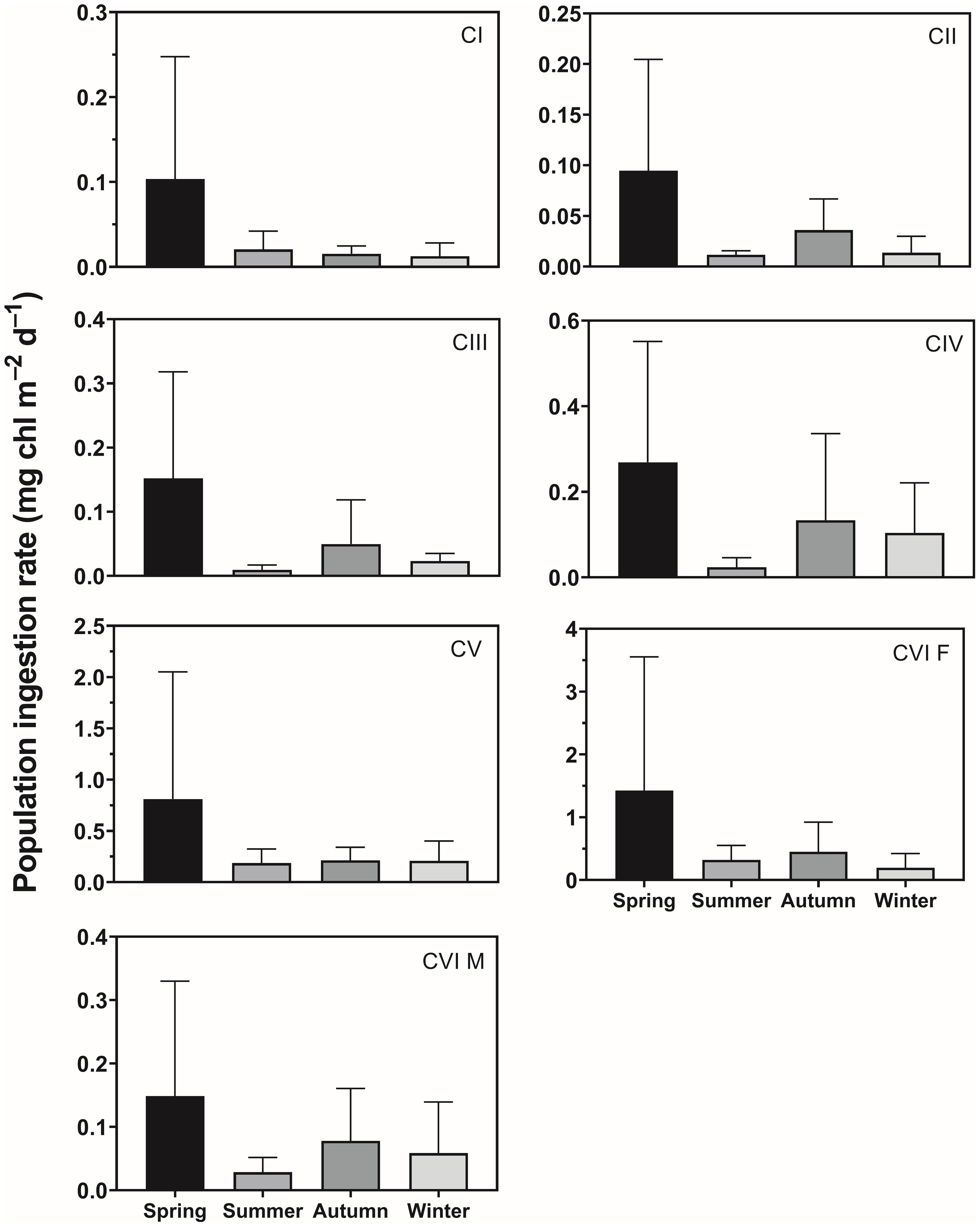
Figure 6 Seasonal variation in population ingestion rate (µg chl m–2 d–1) of Calanus sinicus from CI to adult female (CVI F) and male (CVI M). Error bars indicate the standard deviation.
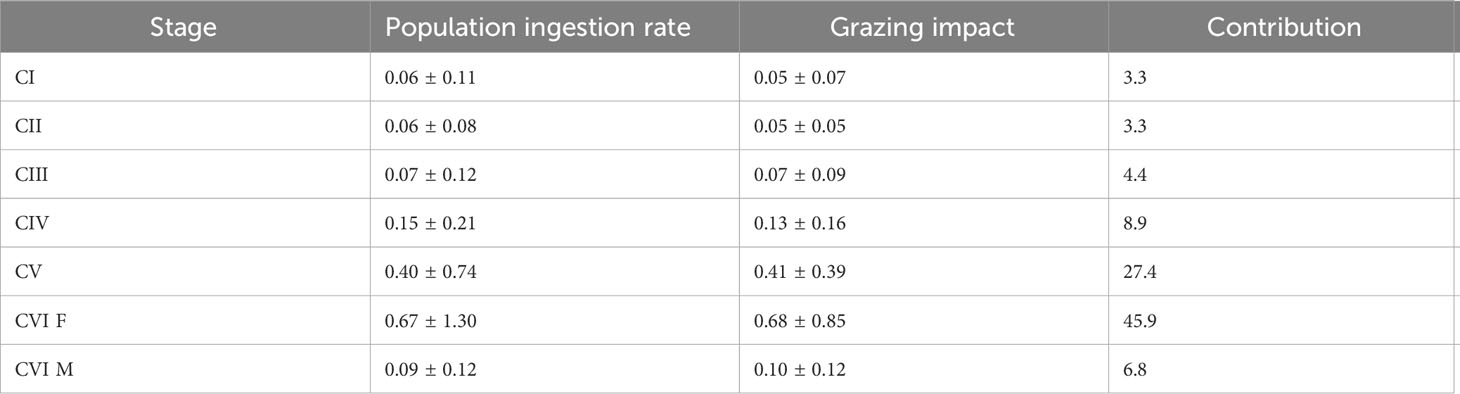
Table 2 Population ingestion rate (mg chl m‒2 d‒1), grazing impact (%), and relative grazing impact contribution (%) for each developmental stage of Calanus sinicus from copepodite stage I to adults.
Grazing impact
The seasonal mean grazing impact of C. sinicus at CI was 0.02–0.06%, with higher values in spring and lower values in winter (Figure 7). The impact of CII was 0.02–0.06%, and was highest in autumn and lowest in winter. The impact of CIII was 0.02–0.10% and that of CIV was 0.05–0.22%, with higher values in autumn and lower values in summer for both stages. The impact of CV was 0.26–0.49% and those of females and males were 0.18–1.01% and 0.07–0.16%, being higher in autumn and lower in winter. The combined grazing impacts of C. sinicus from the CI to adult stages were 1.66%, 1.43%, 2.04%, 0.65% in spring, summer, autumn, and winter, respectively. The impact of females was highest, at 45.9% of the total impact, followed by CV, at 27.4% (Table 2). Seasonal differences were nonsignificant at all stages.
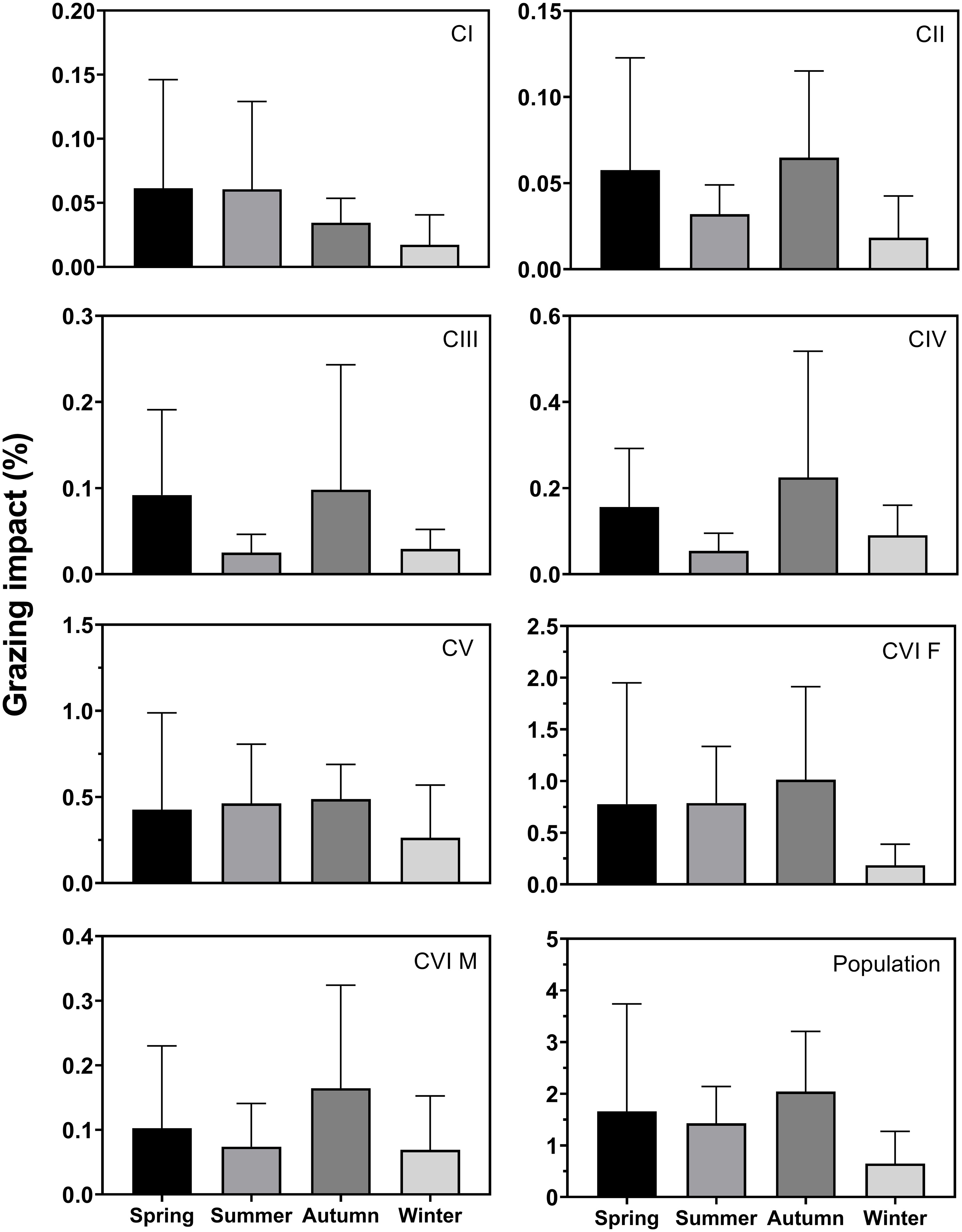
Figure 7 Seasonal variation in grazing impact (%) of Calanus sinicus from CI to adult female (CVI F) and male (CVI M) and the sum of all stages in the population. Error bars indicate the standard deviation.
Environmental influences
RDA results for the ingestion rate of C. sinicus and environmental factors in all seasons showed that CIV to adults had positive relationships with the mean chl-a concentrations in the water column (Figure 8). The early developmental stage CI-III were rather related to mean temperature in the water column. The eigenvalues of axes 1 and 2 were 0.34 and 0.06, respectively, and the cumulative percentage variance of axes 1 and 2 was 39.9% (Table 3). Environmental factors explained 97% of the variation in the ingestion rate on axes 1 and 2. The correlation coefficients for axes 1 and 2 were 0.67 and 0.73, respectively. Axis 1 and 2 were significantly related to mean chl-a concentrations (r = 0.55) and temperature (r = 0.67) in the water column, respectively.
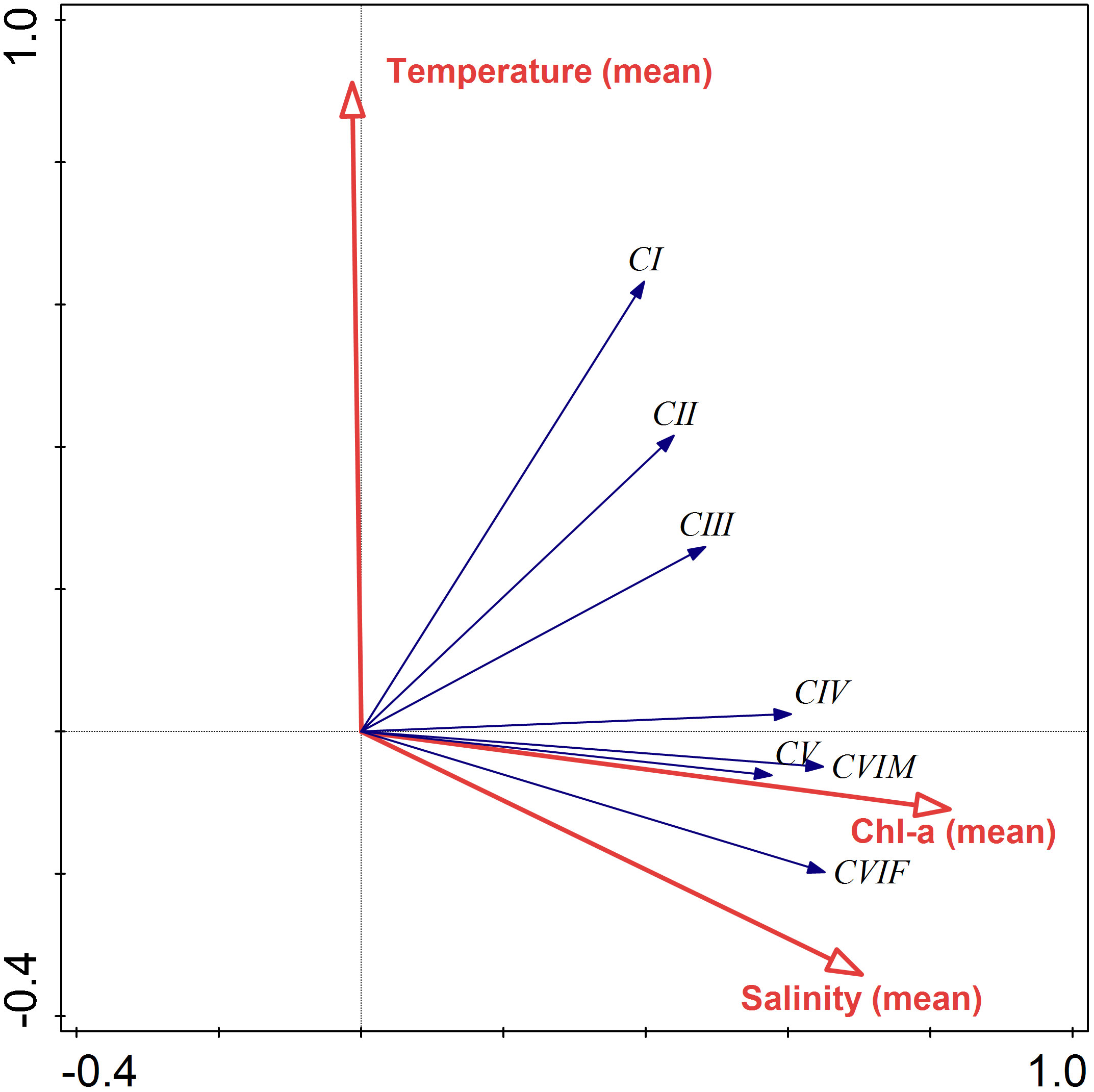
Figure 8 Redundancy analysis biplot of the year-round ingestion rate of Calanus sinicus from CI to adult female (CVI F) and male (CVI M) according to temperature (°C), salinity (psu), and chl-a concentration (µg L-1).
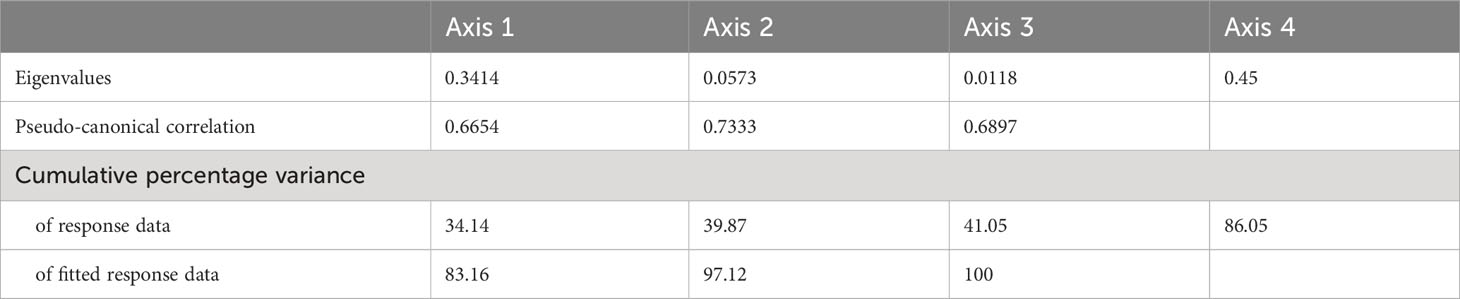
Table 3 Redundancy analysis results of year-round ingestion rate of Calanus sinicus in relation to environmental factors.
Discussion
Seasonal fluctuation
Because water temperature affects all phases of the copepod life cycle, including respiration, survival, production and development (Halsband-Lenk et al., 2002; Heine et al., 2019), it may also affect their feeding. In this study, the ingestion rate of C. sinicus on phytoplankton was particularly high in spring and decreased sharply during the summer (Figures 4–6). This is likely due to the ecological characteristics of this species, which is sensitive to water temperature. The temperature tolerance range for the survival of C. sinicus is known to be 1–27°C in the Yellow Sea (Wang et al., 2003). The optimal water temperature for C. sinicus was 20°C in the Inland Sea of Japan, and the lower and upper thermal limits for C. sinicus embryonic development were 5°C and 23°C, respectively (Uye, 1988; Uye, 2000). Therefore, high or low water temperatures in summer or winter are unfavorable to the feeding of C. sinicus. In addition to water temperature, food composition in the fields are also important factors affecting copepod feeding. C. sinicus is an omnivorous copepod that can feed on a variety of prey, including not only phytoplankton but also ciliates, flagellates, metazoan eggs and larvae, bacteria, and fungi (Huo et al., 2008; Ho et al., 2017; Yi et al., 2017; Hirai et al., 2018). In the Yellow Sea, seasonal fluctuations in phytoplankton communities have been reported, with diatom blooms occurring in spring and increased proportions of flagella and cyanobacteria in summer and autumn (Gao et al., 2013; Liu et al., 2015). Therefore, the diet of C. sinicus may vary with seasonal environmental changes in its habitat. In a previous study, herbivorous feeding supported more than 82.5% of C. sinicus egg production in spring but only 47.8% in autumn in Bohai Bay, likely driven by the prevalence of large diatoms and sufficient non-phytoplankton food sources during autumn (Zhang et al., 2006).
Developmental and sexual differences
In general, the copepod ingestion rate increases with increasing water temperature, body weight, and food concentration (Saiz and Calbet, 2011). The positive relationships between ingestion rate and body size (Figure 5) and chl-a concentration (Figure 8) were confirmed for C. sinicus in the Yellow Sea in this study. However, when developmental stage was considered, the relationship between ingestion rate in early stages (CI–CIII) and chl-a concentration were nonsignificant (Figure 8). Feeding habits and prey preferences of copepods differ with developmental stage and sex (Calbet et al., 2007; Brucet et al., 2008; Saiz et al., 2014; Meunier et al., 2016; Ismar et al., 2018). In general, the size of the ingested prey increases with increasing copepod size. Therefore, relatively large and chlorophyll-rich diatoms are more likely to be consumed by adults or late copepodites than by nauplii or early copepodites. This preference explains why chl-a concentrations were significantly correlated with C. sinicus ingestion rates only at CIV to adults in this study (Figure 8). The preferred food type may differ in association with sexual dimorphism. In laboratory experiments with three types of prey, namely, cryptophytes, haptophytes, and diatoms, females of the copepod Acartia tonsa preferred diatoms, while males preferred cryptophytes (Ismar et al., 2018). For C. sinicus, an analysis of gut contents reported that females consumed more diatoms than males (Chen et al., 2010). Methods other than chl-a measurement may produce higher ingestion rates for C. sinicus males on potential prey than are reported in this paper.
A large proportion of grazing impact is associated with CV and female individuals in the C. sinicus population (Table 2). In the Yellow Sea, spring is the main spawning season of C. sinicus (Zhang et al., 2005). As copepod egg production is related to water temperature and prey concentration expressed as chl-a concentration (Richardson and Verheye, 1998; Wang et al., 2009; Kang and Kim, 2021), copepod feeding may increase during the spawning season. On the other hand, males had ingestion rates around three times lower than females (Figure 5). Previous studies of several copepods have reported lower ingestion rates of males compared to females (van Someren Gréve et al., 2017), primarily due to the smaller size of males than females (Hirst and Kiørboe, 2014). Furthermore, during the mating season, females exhibit feeding-focused behaviors, while males engage in both mate searching and feeding behaviors (Kiørboe, 2008; Moison et al., 2013), which may increase female ingestion rates relative to male ingestion rates.
Evaluation of measured values
The gut pigment contents and ingestion rates of C. sinicus measured in this study were similar to those reported in a study of the southern Yellow Sea in summer, but higher than observations in inshore such as Taean and Asan Bay (Table 4). Li et al. (2004) noted that their results were lower than local observations in the western bay of the Yellow Sea, indicating regional differences in gut pigment contents and ingestion rates. The seasonal difference in feeding impact observed in this study was less than 1%, which is smaller than in previous studies (Table 4). This finding suggests that C. sinicus likely did not experience food limitation during any season in this study area, at least in terms of phytoplankton prey. The feeding impact of C. sinicus in this study was relatively small compared to other studies conducted in open seas (Table 4). Because the feeding impact of copepods varies greatly depending on the environment and prey conditions of the sea area, it ranges from less than 1% to 40% of phytoplankton stock (Li et al., 2003; Schnetzer and Caron, 2005; Tseng et al., 2008; Saiz and Calbet, 2011; Lee et al., 2012; Jagadeesan et al., 2017). Therefore, caution is needed when comparing the feeding impact values obtained in this study with other studies, even for the same species.
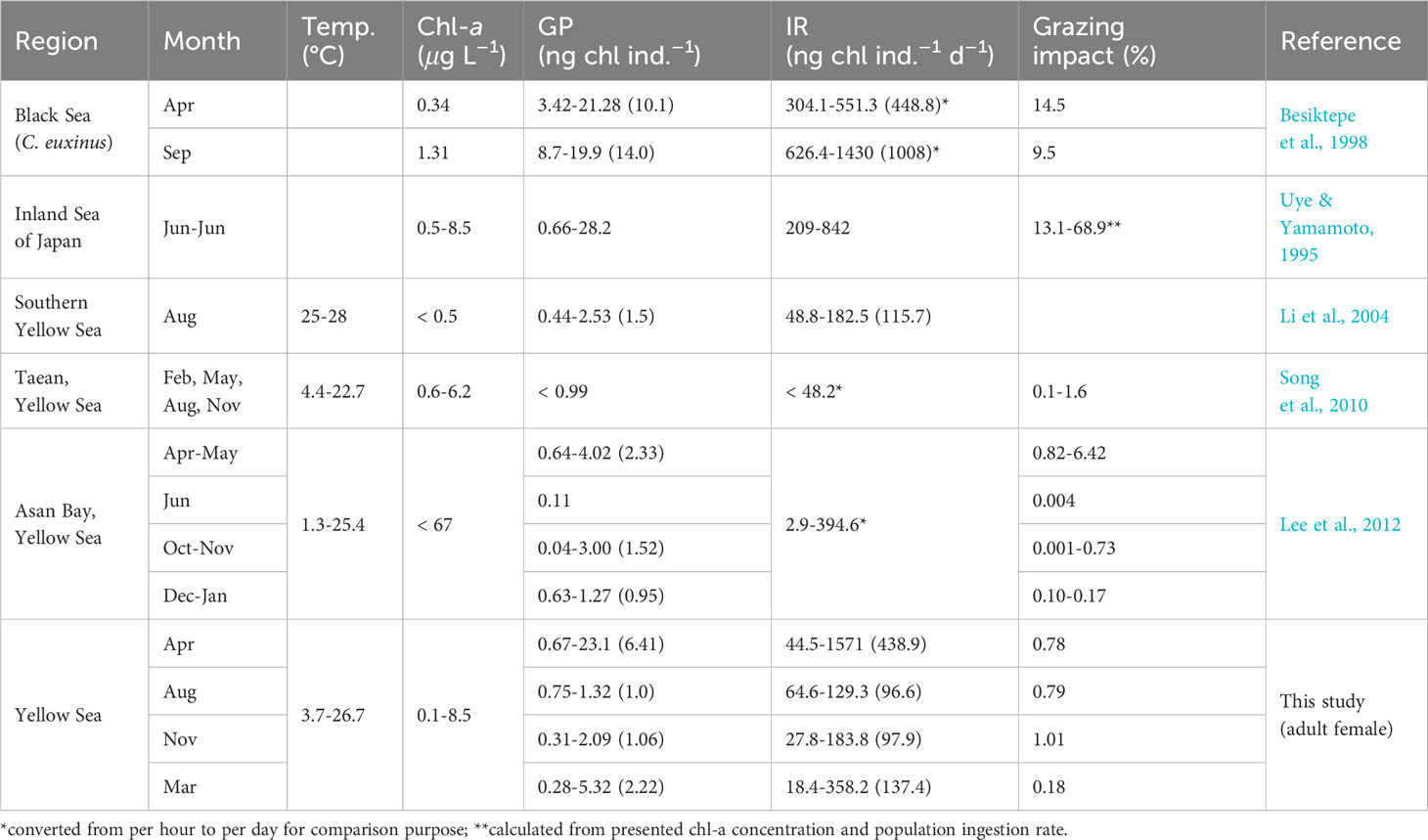
Table 4 Comparison of gut pigment, ingestion rate and grazing impact of Calanus sinicus. Parentheses indicate mean values.
Copepods have endogenous diel feeding rhythms that can be affected by external factors such as food availability, risk for predation and light intensity (Bollens and Stearns, 1992; Calbet et al., 1999; Olsen et al., 2000; Olivares et al., 2020). Diel feeding rhythm amplitudes and the time of active feeding vary among copepod species (Peterson et al., 1990; Atkinson et al., 1996; Yamaguchi et al., 2010; Lee et al., 2012). C. sinicus appears to have diel feeding rhythms of large amplitude, as the difference in gut pigment content over time was 4.20 ng chl ind–1 for adult C. sinicus in the southern Yellow Sea (Li et al., 2004). In coastal waters of Taiwan, the gut fullness of female C. sinicus was 5% greater in the morning and at midnight than in the afternoon (Chen et al., 2010). This study did not assess the impact of collection time on feeding because sampling times varied (Table 1), but it is likely that there are differences in feeding during the day and night.
Conclusion
In the Yellow Sea, feeding on phytoplankton by C. sinicus was significantly greater in spring than in other seasons, indicating that a classical food chain based on phytoplankton is operating at this time. In summer and winter, water temperature that is higher or lower than the preference of C. sinicus appears to lower its ingestion rate. In autumn, considering the relatively high-water temperature, low chl-a concentration, and low ingestion rate of C. sinicus on phytoplankton, the microbial food web appears to be dominant. The majority of the grazing impact of the C. sinicus population in the Yellow Sea was attributable to females, most likely because they require substantial energy for reproduction.
Data availability statement
The original contributions presented in the study are included in the article/supplementary material. Further inquiries can be directed to the corresponding author.
Ethics statement
The manuscript presents research on animals that do not require ethical approval for their study.
Author contributions
GK: Investigation, Writing – original draft. WP: Writing – review & editing. JN: Writing – review & editing. H-KK: Writing – review & editing.
Funding
The author(s) declare financial support was received for the research, authorship, and/or publication of this article. This work was supported by the research projects of the Korea Institute of Ocean Science and Technology (KIOST; PEA0013, PEA0111), and by the Korea Institute of Marine Science & Technology Promotion (KIMST) funded by the Ministry of Oceans and Fisheries (PM63392).
Acknowledgments
We are grateful to crew members of R/V Onnuri and R/V Isabu of KIOST.
Conflict of interest
The authors declare that the research was conducted in the absence of any commercial or financial relationships that could be construed as a potential conflict of interest.
Publisher’s note
All claims expressed in this article are solely those of the authors and do not necessarily represent those of their affiliated organizations, or those of the publisher, the editors and the reviewers. Any product that may be evaluated in this article, or claim that may be made by its manufacturer, is not guaranteed or endorsed by the publisher.
References
Atkinson A., Ward P., Murphy E. J. (1996). Diel periodicity of subantarctic copepods: relationships between vertical migration, gut fullness and gut evacuation rate. J. Plankton. Res. 18, 1387–1405. doi: 10.1093/plankt/18.8.1387
Båmstedt U., Eilertsen H. C., Tande K. S., Slagstad D., Skjoldal H. R. (1991). Copepod grazing and its potential impact on the phytoplankton development in the Barents Sea. Polar. Res. 10, 339–354. doi: 10.3402/polar.v10i2.6751
Besiktepe S., Kideys A., Unsal M. (1998). “In situ grazing pressure and diel vertical migration of female Calanus euxinus in the Black Sea,” in Eutrophication in planktonic ecosystems: food web dynamics and elemental cycling. Ed. Tamminen T. (Dordrecht: Springer), 323–332.
Bollens S. M., Stearns D. E. (1992). Predator-induced changes in the diel feeding cycle of a planktonic copepod. J. Exp. Mar. Biol. Ecol. 156, 179–186. doi: 10.1016/0022-0981(92)90244-5
Brucet S., Compte J., Boix D., López-Flores R., Quintana X. D. (2008). Feeding of nauplii, copepodites and adults of Calanipeda aquaedulcis (Calanoida) in Mediterranean salt marshes. Mar. Ecol. Prog. Ser. 355, 183–191. doi: 10.3354/meps07225
Calbet A., Carlotti F., Gaudy R. (2007). The feeding ecology of the copepod Centropages typicus (Kröyer). Prog. Oceanogr. 72, 137–150. doi: 10.1016/j.pocean.2007.01.003
Calbet A., Saiz E., Irigoien X., Alcaraz M., Trepat I. (1999). Food availability and diel feeding rhythms in the marine copepods Acartia grani and Centropages typicus. J. Plankton. Res. 21, 1009–1015. doi: 10.1093/plankt/21.5.1009
Chen M. R., Kâ S., Hwang J. S. (2010). Diet of the copepod Calanus sinicus Brodsky(Copepoda, Calanoida, Calanidae) in northern coastal waters of Taiwan during the northeast monsoon period. Crustaceana 83, 851–864. doi: 10.1163/001121610x504360
Dagg M., Wyman K. (1983). Natural ingestion rates of the copepods Neocalanus plumchrus and N. cristatus calculated from gut contents. Mar. Ecol. Prog. Ser. 13, 37–46. doi: 10.3354/meps013037
Debes H., Eliasen K., Gaard E. (2008). Seasonal variability in copepod ingestion and egg production on the Faroe shelf. Hydrobiologia 600, 247–265. doi: 10.1007/s10750-007-9238-3
Gao Y., Jiang Z., Liu J., Chen Q., Zeng J., Huang W. (2013). Seasonal variations of net-phytoplankton community structure in the southern Yellow Sea. J. Ocean. Univ. China. 12, 557–567. doi: 10.1007/s11802-013-2258-x
Halsband-Lenk C., Hirche H. J., Carlotti F. (2002). Temperature impact on reproduction and development of congener copepod populations. J. Exp. Mar. Biol. Ecol. 271, 121–153. doi: 10.1016/S0022-0981(02)00025-4
Heine K. B., Abebe A., Wilson A. E., Hood W. R. (2019). Copepod respiration increases by 7% per° C increase in temperature: A meta-analysis. Limnol. Oceanogr. Lett. 4, 53–61. doi: 10.1002/lol2.10106
Hirai J., Hamamoto Y., Honda D., Hidaka K. (2018). Possible aplanochytrid (Labyrinthulea) prey detected using 18S metagenetic diet analysis in the key copepod species Calanus sinicus in the coastal waters of the subtropical western North Pacific. Plankton. Benthos. Res. 13, 75–82. doi: 10.3800/pbr.13.75
Hirst A. G., Kiørboe T. (2014). Macroevolutionary patterns of sexual size dimorphism in copepods. Proc. R. Soc B. Biol. Sci. 281, 20140739. doi: 10.1098/rspb.2014.0739
Ho T. W., Hwang J. S., Cheung M. K., Kwan H. S., Wong C. K. (2017). DNA-based study of the diet of the marine calanoid copepod Calanus sinicus. J. Exp. Mar. Biol. Ecol. 494, 1–9. doi: 10.1016/j.jembe.2017.04.004
Huh S. H. (1999). Feeding habits of hairtail, Trichiurus lepturus. Korean. J. Ichthyol. 11, 191–197.
Huo Y. Z., Wang S. W., Sun S., Li C. L., Liu. M. T. (2008). Feeding and egg production of the planktonic copepod Calanus sinicus in spring and autumn in the Yellow Sea, China. J. Plankton. Res. 30, 723–734. doi: 10.1093/plankt/fbn034
Ismar S. M., Kottmann J. S., Sommer U. (2018). First genetic quantification of sex-and stage-specific feeding in the ubiquitous copepod Acartia tonsa. Mar. Biol. 165, 25. doi: 10.1007/s00227-017-3281-z
Jagadeesan L., Jyothibabu R., Arunpandi N., Parthasarathi S. (2017). Copepod grazing and their impact on phytoplankton standing stock and production in a tropical coastal water during the different seasons. Environ. Monit. Assess. 189, 1–21. doi: 10.1007/s10661-017-5804-y
Jeong J. M., Hwang K. S., Song S. H., Kim H. Y., Park J. H., Lee J. H. (2016). Feeding habits of juvenile and young yellow tail Seriola quinqueradiata in coastal waters of the South Sea, Korea. Korean. J. Fish. Aquat. Sci. 49, 635–641. doi: 10.5657/KFAS.2016.0635
Kagami M., Yoshida T., Gurung T., Urabe J. (2002). Direct and indirect effects of zooplankton on algal composition in in situ grazing experiments. Oecologia 133, 356–363. doi: 10.1007/s00442-002-1035-0
Kang H. K., Kim C. H. (2021). Estimation of production of the copepod Calanus sinicus during spring in the northern East China Sea. Plankton. Benthos. Res. 16, 1–10. doi: 10.3800/pbr.16.1
Kiørboe T. (2008). Optimal swimming strategies in mate-searching pelagic copepods. Oecologia 155, 179–192. doi: 10.1007/s00442-007-0893-x
Ko A., Jeong J. M., Baeck G. W. (2019). Feeding habits of the bighead croaker, Collichthys niveatus in West Sea, Korea. J. Korean. Soc Fish. Ocean. Tech. 55, 327–334. doi: 10.3796/KSFOT.2019.55.4.327
Lee D. B., Song H. Y., Park C., Choi K. H. (2012). Copepod feeding in a coastal area of active tidal mixing: diel and monthly variations of grazing impacts on phytoplankton biomass. Mar. Ecol. 33, 88–105. doi: 10.1111/j.1439-0485.2011.00453.x
Li C., Sun S., Wang R., Wang X. (2004). Feeding and respiration rates of a planktonic copepod (Calanus sinicus) oversummering in Yellow Sea Cold Bottom Waters. Mar. Biol. 145, 149–157. doi: 10.1007/s00227-004-1306-x
Li C., Wang R., Sun S. (2003). Grazing impact of copepods on phytoplankton in the Bohai Sea. Estuar. Coast. Shelf. Sci. 58, 487–498. doi: 10.1016/S0272-7714(03)00129-X
Liu X., Huang B., Huang Q., Wang L., Ni X., Tang Q., et al. (2015). Seasonal phytoplankton response to physical processes in the southern Yellow Sea. J. Sea. Res. 95, 45–55. doi: 10.1016/j.seares.2014.10.017
López E., Anadón R., Harris R. P. (2007). Functional responses of copepod nauplii using a high efficiency gut fluorescence technique. Mar. Biol. 150, 893–903. doi: 10.1007/s00227-006-0387-0
Mackas D., Bohrer R. (1976). Fluorescence analysis of zooplankton gut contents and an investigation of diel feeding patterns. J. Exp. Mar. Biol. Ecol. 25, 77–85. doi: 10.1016/0022-0981(76)90077-0
Matsuno K., Yamaguchi A., Nishino S., Inoue J., Kikuchi T. (2015). Short-term changes in the mesozooplankton community and copepod gut pigment in the Chukchi Sea in autumn: reflections of a strong wind event. Biogeosciences 12, 4005–4015. doi: 10.5194/bg-12-4005-2015
Meunier C. L., Boersma M., Wiltshire K. H., Malzahn A. M. (2016). Zooplankton eat what they need: copepod selective feeding and potential consequences for marine systems. Oikos 125, 50–58. doi: 10.1111/oik.02072
Moison M., Schmitt F. G., Souissi S. (2013). Differences in feeding activity between females and males of Temora longicornis. Ecol. Res. 28, 459–467. doi: 10.1007/s11284-013-1034-0
Olivares M., Calbet A., Saiz E. (2020). Effects of multigenerational rearing, ontogeny and predation threat on copepod feeding rhythms. Aquat. Ecol. 54, 697–709. doi: 10.1007/s10452-020-09768-8
Olsen E. M., Jørstad T., Kaartvedt S. (2000). The feeding strategies of two large marine copepods. J. Plankton. Res. 22, 1513–1528. doi: 10.1093/plankt/22.8.1513
Peterson W. T., Painting S. J., Hutchings L. (1990). Diel variations in gut pigment content, diel vertical migration and estimates of grazing impact for copepods in the southern Benguela upwelling region in October 1987. J. Plankton. Res. 12, 259–281. doi: 10.1007/BF00349702
Pomeroy L. R., leB. Williams P. J., Azam F., Hobbie J. E. (2007). The microbial loop. Oceanography 20, 28–33. doi: 10.5670/oceanog.2007.45
Richardson A. J., Verheye H. M. (1998). The relative importance of food and temperature to copepod egg production and somatic growth in the southern Benguela upwelling system. J. Plankton. Res. 20, 2379–2399. doi: 10.1093/plankt/20.12.2379
Saiz E., Calbet A. (2011). Copepod feeding in the ocean: scaling patterns, composition of their diet and the bias of estimates due to microzooplankton grazing during incubations. Hydrobiologia 666, 181–196. doi: 10.1007/s10750-010-0421-6
Saiz E., Griffell K., Calbet A., Isari S. (2014). Feeding rates and prey: predator size ratios of the nauplii and adult females of the marine cyclopoid copepod Oithona davisae. Limnol. Oceanogr. 59, 2077–2088. doi: 10.4319/lo.2014.59.6.2077
Schnetzer A., Caron D. A. (2005). Copepod grazing impact on the trophic structure of the microbial assemblage of the San Pedro Channel, California. J. Plankton. Res. 27, 959–971. doi: 10.1093/plankt/fbi049
Shin K., Choi J. (1992). The feeding behavior of the marine planktonic copepod, Calanus sinicus and Acartia clausi (A. omorii). J. Korean. Soc Oceanogr. 27, 11–18.
Song H. Y., Lee D. B., Park C. (2010). Seasonal distribution of major copepods and their feeding in the coastal area off Taean peninsula. J. Korean. Soc Oceanogr. 15, 149–157.
Sterner R. W. (1989). “The role of grazers in phytoplankton succession,” in Plankton ecology (Heidelberg: Springer), 107–170.
Sun S., Huo Y., Yang B. (2010). Zooplankton functional groups on the continental shelf of the yellow sea. Deep Sea Res. Part II Top. Stud. Oceanogr. 57, 1006–1016. doi: 10.1016/j.dsr2.2010.02.002
ter Braak C. J., Smilauer P. (2012). Canoco reference manual and user's guide: software for ordination, version 5.0. Microcomputer Power, Ithaca USA.
Tseng L. C., Kumar R., Dahms H. U., Chen Q. C., Hwang J. S. (2008). Copepod gut contents, ingestion rates and feeding impact in relation to their size structure in the southeastern Taiwan Strait. Zool. Stud. 47, 402–416.
Turner J. T. (2015). Zooplankton fecal pellets, marine snow, phytodetritus and the ocean’s biological pump. Prog. Oceanogr. 130, 205–248. doi: 10.1016/j.pocean.2014.08.005
Uye S. (1988). Temperature-dependent development and growth of Calanus sinicus (Copepoda: Calanoida) in the laboratory. Hydrobiologia 167, 285–293. doi: 10.1007/BF00026316
Uye S. (2000). Why does Calanus sinicus prosper in the shelf ecosystem of the Northwest Pacific Ocean? ICES. J. Mar. Sci. 57, 1850–1855. doi: 10.1006/jmsc.2000.0965
Uye S., Yamamoto F. (1995). In situ feeding of the planktonic copepod Calanus sinicus in the Inland Sea of Japan, examined by the gut fluorescence method. Bull. Plankton. Soc Japan. 42, 123–139.
van Someren Gréve H., Almeda R., Lindegren M., Kiørboe T. (2017). Gender-specific feeding rates in planktonic copepods with different feeding behavior. J. Plankton. Res. 39, 631–644. doi: 10.1093/plankt/fbx033
Wang S., Li C., Sun S., Ning X., Zhang W. (2009). Spring and autumn reproduction of Calanus sinicus in the Yellow Sea. Mar. Ecol. Prog. Ser. 379, 123–133. doi: 10.3354/meps07902
Wang R., Zuo T., Wang K. E. (2003). The Yellow Sea cold bottom water—an oversummering site for Calanus sinicus (Copepoda, Crustacea). J. Plankton. Res. 25, 169–183. doi: 10.1093/plankt/25.2.169
Yamaguchi A., Onishi Y., Omata A., Kawai M., Kaneda M., Ikeda T. (2010). Population structure, egg production and gut content pigment of large grazing copepods during the spring phytoplankton bloom in the Oyashio region. Deep. Sea. Res. Part II. Top. Stud. Oceanogr. 57, 1679–1690. doi: 10.1016/j.dsr2.2010.03.012
Yi X., Huang Y., Zhuang Y., Chen H., Yang F., Wang W., et al. (2017). In situ diet of the copepod Calanus sinicus in coastal waters of the South Yellow Sea and the Bohai Sea. Acta Oceanol. Sin. 36, 68–79. doi: 10.1007/s13131-017-0974-6
Zhang G. T., Li C. L., Sun S., Zhang H. Y., Sun J., Ning X. R. (2006). Feeding habits of Calanus sinicus (Crustacea: Copepoda) during spring and autumn in the Bohai Sea studied with the herbivore index. Sci. Mar. 70, 381–388. doi: 10.3989/scimar.2006.70n3381
Keywords: gut pigment, ingestion rate, grazing impact, Calanus sinicus, zooplankton
Citation: Kim G, Park W, Noh JH and Kang H–K (2023) Seasonal and developmental variation in feeding by the copepod Calanus sinicus in the Yellow Sea. Front. Mar. Sci. 10:1273734. doi: 10.3389/fmars.2023.1273734
Received: 07 August 2023; Accepted: 22 November 2023;
Published: 08 December 2023.
Edited by:
Stelios Katsanevakis, University of the Aegean, GreeceReviewed by:
Soultana Zervoudaki, Hellenic Centre for Marine Research (HCMR), GreeceS.S.S. Sarma, National Autonomous University of Mexico, Mexico
Copyright © 2023 Kim, Park, Noh and Kang. This is an open-access article distributed under the terms of the Creative Commons Attribution License (CC BY). The use, distribution or reproduction in other forums is permitted, provided the original author(s) and the copyright owner(s) are credited and that the original publication in this journal is cited, in accordance with accepted academic practice. No use, distribution or reproduction is permitted which does not comply with these terms.
*Correspondence: Hyung–Ku Kang, a2FuZ2hrQGtpb3N0LmFjLmty