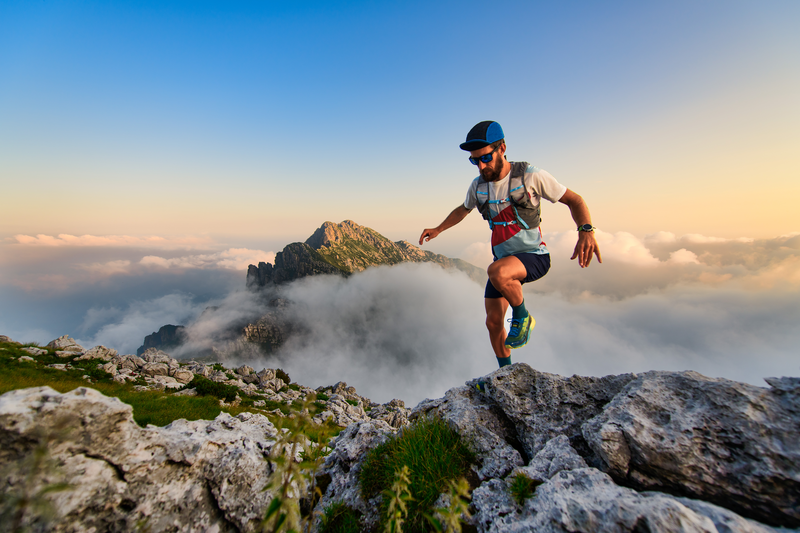
94% of researchers rate our articles as excellent or good
Learn more about the work of our research integrity team to safeguard the quality of each article we publish.
Find out more
ORIGINAL RESEARCH article
Front. Mar. Sci. , 07 November 2023
Sec. Marine Biology
Volume 10 - 2023 | https://doi.org/10.3389/fmars.2023.1272353
Temporal and geographical changes in the infections of intestinal helminths of striped dolphins, Stenella coeruleoalba were investigated in waters off the Spanish Mediterranean coast based on a sample of 233 dolphins stranded during 1982-2016. The influence of host-related factors (length and sex) and seasonality was also examined. Four helminth species had a prevalence > 5%, including adults of three cestodes, i.e, Trigonocotyle globicephalae (prevalence: 5.2%), Tetrabothrius forsteri (94.4%) and Strobilocephalus triangularis (18%); and juveniles of an acanthocephalan of the genus Bolbosoma (15.9%), identified as B. capitatum using molecular techniques. One immature specimen of Diphyllobothrium sp. (Cestoda) was also found in a single dolphin, and two juveniles of Bolbosoma balaenae in two dolphins. Trigonocotyle globicephalae seems to have low specificity for striped dolphins and was only found sporadically. Tetrabothrius forsteri and S. triangularis experienced a slight vs. a strong decrease, followed by a recovery, in infection levels throughout the study period, which are compatible with a reduction in the striped dolphin population caused by the Dolphin Morbillivirus outbreak in 1990. Infections of B. capitatum, a parasite typical from pilot whales, suddenly increased in 1990, then vanished. This rapid increase and following decrease are puzzling and can hardly be explained by a single factor. Infections of any helminth species were apparently uncorrelated to geographic origin, dolphin sex or season, but parasite load decreased with dolphin length in the case of T. forsteri and S. triangularis. To our knowledge, this study represents the first investigation of multi-decadal changes in cetacean parasites.
Long-term studies on animal populations are essential to monitor ecosystem changes, especially in the global change scenario we have been facing during the last century (Luo et al., 2011). In the marine environment, this type of studies are relatively common for free-living species, particularly those of commercial interest (e.g, Kovach et al., 2015; Pershing et al., 2015; Pang et al., 2018). In contrast, long-term surveys of marine parasite populations are very scarce. This is unfortunate because parasites represent an integral part of ecosystems that influence, inter alia, trophic web dynamics and population sizes of their hosts (Hudson et al., 1998; Lafferty et al., 2008). In particular, there is evidence suggesting that trophically-transmitted metazoan parasites (TTPs) can alter several food-web properties. TTPs can increase interaction strength through the modification of their hosts behaviour, enhancing predation on infected prey hosts (Lafferty and Morris, 1996; Moore, 2002). Similarly, they may facilitate new trophic interactions, thus increasing food-web connectivity (Lafferty et al., 2006); modulate the flow of energy along certain trophic links (Morand and Harvey, 2000; Wood et al., 2007), and decrease food-web stability (Otto et al., 2007). On the other hand, parasites can reduce the fitness of their hosts, through increased mortality, reduced fecundity, altered behaviour and/or reduced growth, among others (Kuris, 2003; Miura et al., 2006; Hasik and Siepielski, 2022). These interactions may become surprisingly complex when other environmental stressors (i.e., pollution, fisheries impacts) are also at stake (e.g., Wood et al., 2010; Sures et al., 2017). For instance, parasite diversity (and their potential associated effects) are significantly lower in fished than unfished areas (Wood and Lafferty, 2015).
There are few studies that have monitored populations of marine parasites for at least two decades, and those available deal with species of human health concern, e.g. Anisakis spp., or with host species in the life-cycle that have commercial interest or are more easily accessible for sampling, e.g. fish (Mackenzie, 1987; Fiorenza et al., 2020; Welicky et al., 2021; Diez et al., 2022; Wood et al., 2023). To our knowledge, none of these studies have focused on infections in marine top predator species.
Cetaceans are ubiquitous marine top-predators that exert significant non-linear effects on trophic webs and, therefore, represent excellent sentinels of marine ecosystem changes (Heithaus et al., 2008; Estes et al., 2016). These mammals harbour a rather diverse fauna of TTPs including digeneans, cestodes, nematodes and acanthocephalans (Fraija-Fernández et al., 2016). The life-cycle of these parasites also involves invertebrates (crustaceans, molluscs) as intermediate hosts, and a number of fish and/or cephalopods as paratenic (transport) hosts that can expand infections throughout the whole trophic web through predator-prey interactions (Raga et al., 2009). These features could make the TTPs of cetaceans particularly useful to trace trophic interactions and to monitor long-term ecosystem trends. Unfortunately, the specific identity of the intermediate and paratenic hosts of these parasites is, for the most part, unknown (Mateu et al., 2015). Therefore, this part of the cycle must currently be treated as a ‘black-box’ for interpretation purposes.
To date, surveys on TTPs of cetaceans have usually been based on small, opportunistic samples (e.g, Berón-Vera et al., 2001; Romero et al., 2014; Terracciano et al., 2020). Furthermore, with a few exceptions (Aznar et al., 1995; Mead and Potter, 1995; Herreras et al., 1997), hosts have typically been pooled for analysis, disregarding potential geographic effects on the composition and abundance of TTPs (Dailey and Perrin, 1973; Van Waerebeek et al., 1993; Dans et al., 1999; Lehnert et al., 2017). In this study, we take advantage of a well-established stranding network to investigate spatial and temporal changes (i.e. through several decades) in the infections of TTPs of a cetacean species. The striped dolphin, Stenella coeruleoalba is the most abundant cetacean species in waters off the Spanish Mediterranean coast [hereafter referred to as western Mediterranean for brevity] (Gómez de Segura et al., 2006). In 1990, this population suffered a severe epizootic outbreak caused by the Dolphin Morbillivirus (DMV), currently known as a strain of the Cetacean Morbillivirus (CeMV) (see Van Bressem et al., 2014), which presumably resulted in the death of several thousand individuals (Aguilar and Raga, 1993). Subsequent DMV outbreaks occurred in 2007 (Raga et al., 2008) and, perhaps, 2011 (Rubio-Guerri et al., 2013), but in the latter the death toll was much lower and concentrated in the Valencian Community only. On the other hand, there is evidence that this striped dolphin population has progressively shifted its diet throughout the study period, from one dominated by oceanic prey (chiefly mesopelagic squids) towards one based on neritic, demersal prey (particularly hake, Merluccius merluccius) (Gomez-Campos et al., 2011; Aznar et al., 2017).
In this study, we addressed two research questions. First, we compared the intestinal helminth fauna of striped dolphins stranded in different regions along the Spanish Mediterranean coast. The sample was composed of animals that were killed by the DMV in the summer and autumn of 1990, which minimized the confounding effects of time upon spatial variability. This geographical analysis was exploratory as we did not know whether striped dolphins in the Western Mediterranean belong to one or several population units (Gaspari et al., 2019). Second, we investigated the potential effects of mortality outbreaks in the dolphins and the dietary shift on the infection dynamics of TTPs during the last three decades. With regard to the effect of the die-offs, we expected a general decrease of infections with time because of the reduction of the striped dolphin population.
Intestinal helminths were collected from a total of 233 striped dolphins stranded along the east coast of Spain between 1982 and 2016. Permission to collect stranded dolphins was given by the wildlife services of the regional governments, which are the official institutions in charge of managing and protecting wildlife. During the 1990 epizootic, the geographic coverage included the coasts of Catalonia, Valencian Community, Murcia and eastern Andalusia; in the remaining years, sampling was restricted to the Valencian Community (Figure 1). The sample included the 52 animals examined by Mateu et al. (2014), as well as 6 of the 13 dolphins examined by Raga and Carbonell (1985), for which at least information on occurrence of all intestinal helminths was available. The remaining 175 dolphins were examined in this study, and included dolphins from every year within the time series, in order to avoid significant gaps that could affect the results. All dolphins in the final sample were > 100 cm long to exclude calves that had consumed milk only.
Figure 1 Map of the study area showing major water currents and the extent of the continental shelf in the Spanish Mediterranean. Red dashed lines indicate the four regions (numbered from 1 to 4) in which the coast was divided for the latitudinal comparison of helminth fauna of striped dolphins, Stenella coeruleoalba. Autonomous Communities are abbreviated as follows: Cat, Catalonia; VLC, Valencian Community; MU, Murcia; and AND, Andalusia. The map was created using ArcGIS Desktop version 10.8 (ESRI, 2011), and water currents were redrawn from Martínez et al. (2022).
Before necropsy, total body length was measured and sex was determined for all animals. Intestines were removed and stored at -20°C. After thawing, intestines were weighed, measured and divided into 20 sections of equal length. Each section was opened and washed over a 0.02 mm sieve using tap water. The solid content was collected in a Petri dish and examined under a stereomicroscope. The intestinal wall was also thoroughly examined for attached helminths. Acanthocephalans were left in tap water for 24 hours at 4°C to allow the proboscis to evert. All parasites were washed in saline (9 g/l), fixed and preserved in 70% ethanol, and later identified to the lowest taxonomic level possible. Cestodes were stained with iron acetocarmine (Georgiev, 1986) and identified following Delyamure (1955) and Raga, (1985). Acanthocephalans were cleared in lactophenol, drawn using a light microscope connected to a drawing tube, and identified following Petrochenko (1958); Amin and Margolis (1998) and Costa et al. (2000). All parasites were counted except in 12 dolphins stranded before 1988, for which only presence/absence data were recorded. ‘Small’ and ‘large’ plerocercoids of tetraphyllidean cestodes were not considered members of the intestinal assemblage and were not included in this study (see Aznar et al., 2007).
All individuals identified as B. vasculosum based on morphologic traits were juveniles (see the Results section), thus raising the possibility that they actually represent immature forms of other Bolbosoma species. We investigated this possibility using molecular barcoding. Two species of Bolbosoma have been recorded as adults in the Mediterranean, i.e., Bolbosoma balaenae in fin whales, Balaenoptera physalus, and Bolbosoma capitatum in long-finned pilot whales, Globicephala melas (Delyamure, 1955; Yamaguti, 1963; Raga and Balbuena, 1993; Santoro et al., 2021). We sequenced a partial (479 bp) region of the mitochondrial cytochrome c oxidase subunit I (cox1) of (i) 5 specimens of B. vasculosum from our sample (DNA extraction was attempted on a number of additional specimens, but it was unsuccessful due to poor preservation); (ii) two juvenile specimens of putative B. balaenae also collected from our sample of striped dolphins, and (iii) one adult specimen of B. capitatum from a long-finned pilot whale, Globicephala melas sampled in the study area, which was available at the collection of the Marine Zoology Department of the University of Valencia, Spain (voucher specimen ID: 13653). Available cox1 sequences from GenBank from adults of B. balaenae infecting Mediterranean fin whales, as well as from two other Bolbosoma species, were also used for comparison (Table 1).
Table 1 Data on host, locality and GenBank accession number for cox1 sequences of Bolbosoma spp. used for molecular barcoding of Bolbosoma vasculosum.
Total genomic DNA was extracted from specimens using the DNeasy Blood and Tissue Kit (Qiagen), following manufacturer’s instructions. The cox1 region was amplified using primers forward LCO1490F (5’-GGTCAACAAATCATAAAGATATTGG-3’) and reverse HC02198 (5’-TAAACTTCAGGGTGACCAAAAAATCA-3’) (Folmer et al., 1994). The concentration and quality of the extracted DNA was measured using a NanoDrop™ One (Thermo Scientific). PCR amplification reactions were performed in a total volume of 20 μL, including 1.6 μL of both forward and reverse primers (final concentration of 5 μM), 2 μL of template DNA, 4.8 μL of PCR water and 10 μL of MyFi™ DNA Polymerase (BioLine, Meridian Life Science Inc., Taunton, MA, USA). Cycling conditions were 94°C for 5 min for an initial denaturation, then 38 cycles of 94°C for 45 s, 48°C for 45 s, and 72°C for 80 s, followed by a final extension at 72°C for 7 min. Positive and negative (no template DNA) controls were used in each PCR reaction. PCR products were purified using the Nucleospin® PCR and Gel Purification Clean-up kit (Machery-Nagel, Düren, Germany). Purified amplicons were sent to Macrogen Europe (Amsterdam, The Netherlands) for sequencing with the same primers used in the PCR amplifications.
Nucleotide sequences from both strands were used to assemble consensus sequences using Geneious R7 (https://www.geneious.com). The identity of the assembled sequences was verified using the NCBI Basic Local Alignment Search Tool (BLAST). The obtained cox1 sequences, together with all cox1 sequences of Bolbosoma available in GenBank, were aligned and trimmed using Geneious R7. The accuracy of the alignment was inspected by checking the amino acid translation, using the invertebrate mitochondrial code. The total number of sequences included in the final alignment was 23. Pairwise genetic distances were calculated using the Maximum Composite Likelihood model (Tamura et al., 2004) in MEGA v.11 (Tamura et al., 2021).
Ecological terms follow Bush et al. (1997). Infection parameters were determined using the online software Quantitative Parasitology 1.0.15 (Reiczigel et al., 2019). Prevalence is the percentage of hosts that are infected by a specific helminth species, mean abundance is the average number of individuals of a helminth species per examined host, and mean intensity is the average number of individuals of a helminth species per infected host. The 95% confidence interval (CI) for prevalence was calculated with Sterne’s exact method (Reiczigel, 2003). The 95% CI for mean abundance and mean intensity of each helminth species were obtained with the bias-corrected and accelerated bootstrap method using 10000 replications (Rózsa et al., 2000).
Our dolphin sample was not a random sample of the wild striped dolphin population, but a subset of stranded animals that died from varied causes. Thus, a pertinent question is to what extent our sample could be biased regarding helminth infections if, e.g. it is enriched with heavily parasitized dolphins. We carried out a preliminary exploration of this question based on the comparison of two dolphin subsamples collected in the same period (2010-2015) for which the putative cause of death could reliably be determined based on a thorough veterinary analysis. The first one (N = 10) was composed of striped dolphins that were killed by common bottlenose dolphins, Tursiops truncatus or as a result of fisheries interactions (Crespo-Picazo et al., 2021; Izquierdo-Serrano et al., 2022). In principle, these causes of death are not related with the health condition of dolphins and should be little influenced by the infections of intestinal helminths, which were light (see the Results section). In fact, veterinary analyses indicated good body condition and no pathologies were detected. The second subsample (N = 20) included dolphins that died from diverse infectious syndromes (Unpub. data). Therefore, we hypothesized that these animals would be more prone to concomitant recruitment of TTPs due to a compromised immune response. Potential differences in abundance of each helminth species between the two subsamples were tested with Mann-Whitney tests.
The geographic analysis was carried out using 74 dolphins that died during the DMV outbreak in the summer and autumn of 1990 and stranded along the coast from Andalusia to Catalonia (Figure 1). This subsample was considered suitable for three reasons. First, it was the only sample of dolphins collected across a wide geographical range; in other years (including 2007, when the second DMV epizootic occurred), sampling was restricted to the Valencian Community; second, it was composed of animals that died from the same disease; and third, it was temporally restricted, thus reducing potential confounding seasonal or inter-annual effects.
We used two approaches to investigate latitudinal variability in helminth infections in the stranded dolphins. First, the coast was divided into four regions based on latitude, distance from coastline to continental slope, and current circulation schemes (Martínez et al., 2022) (Figure 1). The numbers of dolphins from regions 1 to 4 were 9, 33, 16 and 16, respectively (Figure 1). Differences in prevalence of each helminth species among regions were tested with exact Chi squared tests. Differences in helminth community structure were investigated using PERMANCOVA with the software PERMANOVA + for PRIMER (Anderson et al., 2008). Abundances of parasites were firstly fourth-root transformed and a Bray-Curtis similarity matrix was obtained. The full model included ‘Region’ (fixed factor) and two relevant predictors associated with individual dolphins, i.e. ‘Sex’ (fixed factor) and ‘Length’ (fixed covariate), as well as their interactions. Pseudo-F statistics under a true null hypothesis were obtained by using 20,000 permutations. Akaike Information Criterion (AIC) values were used to compare competing models with different numbers of parameters. The model with minimum AIC for small sample sizes (AICc) was considered the best model, and the rest of the models were ranked according to increasing AICc values (Johnson and Omland, 2004; Anderson et al., 2008; Aho et al., 2014). Models with values of ΔAICc ≤ 2 with respect to the best model were considered to have substantial empirical support, whereas those with ΔAICc > 4 were considered to have considerably less support (Anderson and Burnham, 2004). Akaike weights (wi) were calculated according to Anderson and Burnham (2004). It was assumed that models with wi ≤ 0.05 were unlikely to be the ‘true’ models (Anderson and Burnham, 2004).
Second, we used Generalised Additive Models (GAMs) (Zuur et al., 2009; Wood, 2017) to explore non-linear patterns in the abundance, or likelihood of occurrence, of each helminth taxon with latitude of stranding. Abundance and occurrence (presence/absence) of each parasite species were used as the response variable for each type of model, respectively, and ‘Latitude’, ‘Dolphin sex’ and ‘Dolphin length’ as the explanatory variables. For abundance data, negative binomial GAMs were fitted using a log link function; for occurrence data, binomial GAMs were fitted using a clog-log link function, which is recommended when there are considerably more zeros than ones or vice versa (Zuur et al., 2009). Model selection followed the same criteria used for the PERMANCOVA models. Collinearity between variables was checked by calculating the Variance Inflation Factor (VIF); values in between 1 and 5 were considered to indicate low collinearity (Goldstein, 1993). We further ensured the lack of dependency between covariates and factors (‘Sex’) with Mann-Whitney tests, and between the two covariates with Spearman correlation. Models were validated following Zuur et al. (2009), i.e., checking normality, homogeneity of variance, autocorrelation and over-dispersion of residuals.
GAMs were also used to investigate long-term infection changes of each helminth species. The initial models included two alternative response variables (helminth abundance or occurrence) and five predictors, i.e. ‘Group’ (with two levels, i.e., epizootic and non-epizootic)’, ‘Dolphin sex’ and ‘Season’ as fixed factors; and ‘Year’ and ‘Dolphin length’ as covariates for which smoothers were applied. Alternative models excluding dolphins affected by the DMV, or using ‘Julian day’ instead of ‘Year’, were also fitted, but the results were nearly identical to those of the models described above and are not shown. Note that ‘Latitude’ was not included in the temporal analysis because, in years other than 1990, all stranded dolphins were collected on the coasts of the Valencian Community (Figure 1).
For abundance data, negative binomial GAMs were fitted using a log link function; for occurrence data, binomial GAMs were fitted using a clog-log link function. Model selection and validation, as well as collinearity analysis between predictors, were carried out as indicated in the previous section. However, AIC was not corrected for small samples in this case because sample size (n) was large compared with the number of parameters (k), i.e. n/k > 40 (Anderson and Burnham, 2004). The lack of dependency between factors was further examined with Chi Squared tests; between covariates and factors with Kruskal-Wallis (‘Season’) or Mann-Whitney (‘Group’, ‘Sex’) tests, and between the two covariates with Spearman correlation.
All GAM models were fitted using the mgcv package (Wood, 2011) available in R version 4.2.2 (R Core Team, 2022).
Six helminth species were found in the intestine of the striped dolphins analysed. Three tetrabothriid cestodes included adult specimens, i.e. Trigonocotyle globicephalae, Tetrabothrius forsteri and Strobilocephalus triangularis, but only the two latter exhibited a prevalence > 10% and mean intensity > 10 individuals/dolphin (Table 2). A single juvenile cestode belonging to the genus Diphyllobothrium was detected in a dolphin from 1987, but identification at species level was not possible due to poor preservation (Table 2). Finally, two species of acanthocephalan were found as juvenile forms. Bolbosoma vasculosum showed an overall prevalence >15%, whereas single specimens of B. balaenae were found in two dolphins from 2011 and 2013, respectively (Table 2).
Table 2 Infection parameters (with 95% confidence intervals in parentheses and ranges in brackets) of intestinal helminths found in striped dolphins, Stenella coeruleoalba from the western Mediterranean.
The ranges of intraspecific genetic distances of Bolbosoma spp. based on cox1 sequences were as follows: 0.006 - 0.024 (B. vasculosum); 0 - 0.017 (B. balaenae); 0.004 – 0.109 (B. turbinella) and 0.004 (B. nipponicum) (Table S1). At interspecific level, specimens of B. vasculosum were indistinguishable from the specimen of B. capitatum (range: 0.006 - 0.024), showing higher distances with respect to other Bolbosoma spp. (minimum distance: 0.194) (Table S1).
Infection parameters of helminths from striped dolphins that were killed by interactions with bottlenose dolphins or fisheries were closely similar to those from dolphins that died from infectious diseases (Table 3). In fact, we did not find significant differences in abundance for any of the helminth species (Mann-Whitney tests, all p >> 0.680).
Table 3 Infection parameters (with 95% confidence intervals in parentheses and ranges in brackets) of intestinal helminths found in two samples of striped dolphins, Stenella coeruleoalba from the western Mediterranean collected during the period 2010-2015, and for which the cause of death was determined.
Of the four species found in 1990, T. forsteri, S. triangularis and B. vasculosum were detected throughout the whole study area, whereas Tri. globicephalae was apparently restricted to its southern part (Figure 2). However, we failed to detect differences in prevalence of this species between regions (exact Chi-Squared, χ2 = 2.308, 3 df, p = 0.538), nor a significant latitudinal trend (χ2 = 1.934, 1 df, p = 0.121).
Figure 2 Maps showing the localities where 74 striped dolphins, Stenella coeruleoalba were found stranded along the Mediterranean coast of Spain in 1990. Colors indicate the abundance of intestinal helminths, when present. (A) Tetrabothrius forsteri. (B) Strobilocephalus triangularis, (C) Trigonocotyle globicephalae and (D) Bolbosoma vasculosum.
There were four PERMANCOVA models that had substantial empirical support (ΔAICc ≤ 2), and two that had less support (2 < ΔAICc < 4). Among them, the simplest and with highest wi value included the intercept only (Table 4). In the rest of the models, none of the individual predictors was found to be significant (Table 4).
Table 4 Permutational Multivariate Analysis of Covariance (PERMANCOVA) accounting for the effect of region, dolphin length, dolphin sex, and their interactions, on the abundance of four intestinal helminth species found in 74 striped dolphins, Stenella coeruleoalba, stranded in the western Mediterranean in 1990.
GAMs could be fitted for T. forsteri, S. triangularis and B. vasculosum. In the case of T. forsteri, the model with lowest AICc, highest wi and lowest number of predictors included ‘Latitude’ only. There was a significant but modest positive effect of this variable on the abundance of T. forsteri (deviance explained = 13.3%, edf = 3.071, p = 0.0154) (Figure 3). Three additional negative-binomial GAMs also had substantial empirical support (ΔAICc ≤ 2), namely, those including ‘Latitude’ and ‘Dolphin length’ (ΔAICc = 0.385), ‘Latitude’ and ‘Dolphin Sex’ (ΔAICc = 1.321) or the three predictors (ΔAICc = 1.501). In all of them, the only significant variable was ‘Latitude’ (p < 0.03). In the GAMs for the abundance of S. triangularis and B. vasculosum, none of the predictors were found to be significant, and the same occurred in the models involving occurrence data (data not shown).
Figure 3 Generalized Additive Model (GAM) smoother showing the effect of latitude on the abundance of the cestode Tetrabothrius forsteri in 74 striped dolphins, Stenella coeruleoalba stranded in the western Mediterranean in 1990. The solid line is the estimated smoother and the colored bands are 95% point-wise confidence bands.
The cestodes T. forsteri, S. triangularis and Tri. globicephalae were detected throughout the whole study period, but the acanthocephalan B. vasculosum was almost exclusively found in 1990; only two additional individuals were found in 1989 and 1997, respectively (Figure 4).
Figure 4 Scatterplots representing the abundance of the four helminth species found in the intestine of striped dolphins, Stenella coeruleoalba from the western Mediterranean during the period 1987-2016. (A) Tetrabothrius forsteri (B) Strobilocephalus triangularis (C) Trigonocotyle globicephalae (D) Bolbosoma vasculosum.
Long-term trends could be investigated with GAMs in the case of T. forsteri and S. triangularis. Binomial GAMs could not meaningfully be fitted for T. forsteri due to its high prevalence (> 94%). There were four negative-binomial GAMs for abundance with substantial empirical support (ΔAIC ≤ 2) and two with less support (2 < ΔAIC < 4) (Table 5). The two simplest models included two predictors (Table 5). Among them, the one with the highest wi value explained < 10% of deviance and included the predictors ‘Year’ and ‘Dolphin length’ (Table 5). In this model, the effect of ‘Dolphin length’ was highly significant, and that of ‘Year’ just marginally so (Table 5). The abundance of T. forsteri experienced a slight decrease with a subsequent recovery throughout the study period (Figure 5A), and peaked at dolphin lengths between 130 and 150 cm, then decreased (Figure 5B). All the other competing models included ‘Length’ as a highly significant predictor; ‘Year’, ‘Group (epizootic vs. non-epizootic)’ or ‘Dolphin sex’ were included in some models, but their effects were only marginally significant, or not significant (Table 5).
Table 5 Negative binomial Generalized Additive Models (GAMs) assessing the effect of year, dolphin length, group (epizootic vs. non-epizootic), dolphin sex and season on the abundance of the cestode Tetrabothrius forsteri in striped dolphins, Stenella coeruleoalba, from the western Mediterranean during the period 1987-2016 (N = 221).
Figure 5 Generalized Additive Model (GAM) smoother showing the effect of two predictors on the infection parameters of two cestode species infecting striped dolphins, Stenella coeruleoalba in the western Mediterranean. Effects of year (A) and dolphin length (B) on the abundance of Tetrabothrius forsteri (N = 221 dolphins); effects of year (C) and dolphin length (D) on the likelihood of occurrence of Strobilocephalus triangularis (N = 233 dolphins). The solid line is the estimated smoother and the colored bands are 95% point-wise confidence bands.
In the case of Binomial GAMs accounting for the occurrence of S. triangularis in striped dolphins, there were three models with substantial empirical support (ΔAIC ≤ 2) and two with less support (2 < ΔAIC < 4) (Table 6). The model with the highest wi value included the predictors ‘Year’, ‘Dolphin length’ and ‘Dolphin sex’, while the simplest model included ‘Year’ and ‘Dolphin length’ (Table 6). The deviance explained for these models was 12.5% and 10.6%, respectively (Table 6). The effects of ‘Year’ and ‘Dolphin length’ were statistically significant in both models (Table 6). The likelihood of occurrence of S. triangularis strongly decreased from ca. 1990 (note the wide 95% CI in previous years) to 1997-1999, then increased, but without reaching the initial levels of the time series (Figure 5C). The likelihood of occurrence also decreased monotonically at increasing dolphin length (Figure 5D). In the other models; ‘Year’ and ‘Dolphin length’ remained as significant predictors; ‘Group (epizootic vs. non-epizootic)’, ‘Dolphin sex’ and ‘Season’ were included only in some models, having just marginally significant, or not significant effects (Table 6). None of the negative binomial GAMs for the abundance of S. triangularis had a suitable fit according to model validation plots, thus they are not considered further.
Table 6 Binomial Generalized Additive Models (GAMs) assessing the effect of year, dolphin length, group (epizootic vs. non-epizootic), dolphin sex and season on the likelihood of occurrence of the cestode Strobilocephalus triangularis in striped dolphins, Stenella coeruleoalba, from the western Mediterranean during the period 1982-2016 (N = 233).
The sample used in this study is composed of stranded dolphins, thus a potential concern is whether they represent an unbiased subset of animals from the wild population. First, the DMV that caused the epizootics in 1990 and 2007 is known to impair the host’s immune system (Bossart et al., 2011; Van Bressem et al., 2014). Although this effect could facilitate the establishment of parasites, thus abnormally increasing their loads (Aznar et al., 1994; Aznar et al., 2005), this is unlikely to occur in the case of TTPs. Mammals affected by other morbillivirus diseases have been reported to die between 8 hours and 2 weeks after the first clinical symptoms (Harder et al., 1990; Evermann et al., 2001; Mahy and Van Regenmortel, 2008). If the disease caused by DMV also runs its course quickly (Kennedy, 1998), the temporal window for recruitment or senescence of the helminths infecting striped dolphins would be too narrow to generate significant alterations of previous parasite loads (Mateu et al., 2014).
Second, the cause of stranding for other dolphins was not always easy to assess but included, inter alia, by-catch (Cuvertoret-Sanz et al., 2020; Izquierdo-Serrano et al., 2022), deadly interactions with bottlenose dolphins (Crespo-Picazo et al., 2021) and infectious diseases (Isidoro-Ayza et al., 2014; Cuvertoret-Sanz et al., 2020). Our results indicate that infection parameters were closely similar for ill vs. non-ill dolphins. One could wonder whether the striped dolphins belonging to the latter category exhibited non-lethal pathological conditions that made them prone to accidental catch or aggression by bottlenose dolphins; however, no such conditions were detected in any of them during the necropsies. Besides, none of the intestinal parasites found, except perhaps Diphyllobothrium sp., have pathological significance (Geraci and St. Aubin, 1987; Cuvertoret-Sanz et al., 2020), thus they were unlikely to be involved in dolphin stranding. In addition, post-mortem infection is not a possibility, since TTPs are transferred to dolphins through the consumption of infected prey. Accordingly, the load of intestinal helminths in our dolphin sample is not expected to be significantly higher than that of the wild population.
Out of the 6 helminth species found, 4, i.e., T. forsteri, Tri. globicephalae, S. triangularis and B. vasculosum, had previously been reported by Mateu et al. (2014) based on a subset of 52 striped dolphins from 1990, now included in the present study. Mateu et al. (2014) identified the juvenile specimens of Bolbosoma as B. vasculosum, as we do here, based on the most detailed descriptions of this taxon (Van Cleave, 1953; Costa et al., 2000). However, the absence of adults in a large sample of worms (n = 84, collected from 37 dolphins), which agrees with previous reports in cetaceans (Van Cleave, 1953; Fernández et al., 2004; Fraija-Fernández et al., 2016), indicates that these specimens must be juveniles of another Bolbosoma species. We cannot rule out the possibility that they belong to a new species associated with striped dolphins, but the absence of adults rather suggests that they correspond to an existing species present in other cetacean hosts. In the western Mediterranean, the only two additional species of Bolbosoma are B. balaenae and B. capitatum (Raga and Balbuena, 1993; Santoro et al., 2021). Molecular barcoding showed that our specimens of B. vasculosum were indistinguishable from B. capitatum. Nevertheless, further research using a higher number of markers and Bolbosoma specimens should be conducted to confirm our results. Since morphological similarities are also apparent between both species (Amin and Margolis, 1998; Costa et al., 2000), a taxonomic re-analysis of the concept of B. vasculosum is warranted.
Bolbosoma balaenae and Diphyllobothrium sp., were not detected by Mateu et al. (2014) and constitute exceptional records in the present survey. The former species typically infects baleen whales worldwide (Fraija-Fernández et al., 2016), with only two previous records in Stenella spp. (Dailey and Perrin, 1973). In the Mediterranean Sea, adults of B. balaenae have been reported in the only baleen whale species regularly present in this area, the fin whale Balaenoptera physalus (Santoro et al., 2021). The occurrence of juvenile specimens in striped dolphins could be interpreted as an accidental infection through shared prey.
With regard to Diphyllobothrium sp., a single immature specimen was found in our sample. The only previous record of species of Diphyllobothrium in striped dolphins corresponds to an adult of an unidentified species in the Atlantic Ocean (Jaber et al., 2006). In the study area, there are records of adults of an unidentified species of Diphyllobothrium in common bottlenose dolphins Tursiops truncatus (Raga, 1985; Quiñones et al., 2013). Since at least 7 species of this genus have been reported in odontocetes (Fraija-Fernández et al., 2016), further molecular studies are needed to determine the identity of the species occurring in our study area.
Mateu et al. (2014) did not detect a significant effect of host body length or sex on the abundance of any intestinal helminth taxa. Using now a larger sample size, we neither detected a sex effect, nor seasonal changes in infections, but we did find a significant effect of dolphin length on the abundance of T. forsteri and the prevalence of S. triangularis. Admittedly, the current sample also covers a large number of years and, therefore, inferences are more prone to confounding effects of time. However, there are also contrasting differences in the host length composition between samples that could readily explain the difference. Only 4 of 52 dolphins (7.7%) from the sample analysed by Mateu et al. (2014) could be considered sexually immature (<180 cm long, see Calzada et al., 1997), vs. 72 of 233 (30.9%) in the present study. Thus, it is likely that host length effects on parasite infections are now being detected because there is a suitable representation of small dolphins; among adult dolphins, infection parameters would vary individually regardless of host’s body length (Mateu et al., 2014).
The occurrence of S. triangularis was found to be negatively related with host length, whereas the abundance of T. forsteri peaked at ca. 140 cm, then decreased in larger dolphins. Interestingly, comparable trends appear to exist for the same parasites in other Stenella spp. Dailey and Perrin (1973) reported raw data on the abundance of T. forsteri and the occurrence of S. triangularis in a sample of pantropical spotted dolphins, S. attenuata from the eastern tropical Pacific. When we examined the relationship of both variables with host length (excluding calves), it was negative and significant, as expected (T. forsteri: Spearman correlation, rs= -0.235, n= 75, one-tailed p< 0.021; S. triangularis: logistic regression, b= -3.561, 1 df, one tailed p= 0.025).
The factor(s) accounting for such trends are difficult to ascertain. In theory, larger (older) dolphins have more chances to get infected because they are exposed to infected prey for longer and/or consume more infected prey due to their higher metabolic demands (Mateu et al., 2014; Pool et al., 2020). Since this does not occur in the striped dolphins of our study, one could argue that smaller (younger) dolphins have a weaker immune system (thus they offer more opportunities for parasite establishment) and/or feed more on specific prey that serve as intermediate hosts for T. forsteri and S. triangularis. The latter possibility opens future avenues to pinpoint relevant prey taxa for the transmission of these cestodes.
At a spatial scale of ca. 800 km (from the highest to the lowest latitude), we did not detect obvious geographical differences in the intestinal helminth assemblages of striped dolphins, except for a modest positive effect of latitude on the abundance of T. forsteri. The dolphins used for this analysis were collected in a narrow time window (summer and autumn, 1990) that minimises confounding time effects, but these animals were ill and could have performed unusual movements or suffered post-mortem transport by currents prior to stranding. However, it seems unlikely that such movements occurred at the spatial scale of the study as we compared regions ca. 200 km long on average.
Differences in helminth infection levels between localities have been observed when cetacean populations are sedentary within the spatial scale covered by the surveys (e.g., Aznar et al., 1995; Mead and Potter, 1995). In contrast, it is likely that, in the study area, striped dolphins perform large-scale movements, perhaps facilitated by the lack of obvious physical or oceanographic barriers, and this would tend to homogenise infections across the defined regions. Currently, there are no precise data on movements of striped dolphins based on satellite tagging, but evidence obtained for other Stenella spp. indicate that individuals can perform movements of > 100 km/day along the continental slope (Scott and Chivers, 2009). Mediterranean striped dolphins appear to be also highly mobile; there are reports of diel movements covering dozens of km (Gannier, 1999), and seasonal changes in abundance that would suggest migrations at the scale of hundreds of kilometres (Gómez de Segura et al., 2006; Laran and Drouot-Dulau, 2007; Panigada et al., 2011; Arcangeli et al., 2017).
Interestingly, a parasitological survey of 14 striped dolphins that were collected on the coasts of the Ligurian Sea during the 1990 epizootic reported a similar composition and comparable prevalence of helminth fauna as that of the present study (Manfredi et al., 1992). Whether or not this homogeneity was caused also by extensive dispersal of striped dolphins between these two regions is difficult to assess in this case. There is evidence of significant genetic differentiation between dolphins from the Spanish vs. Ligurian populations (Gaspari et al., 2007), thus the parasitological similarity could have resulted just from ecological convergence.
Infections of Tri. globicephalae in striped dolphins were sporadic and scattered throughout several decades. This cestode species seems to be specific to members of the subfamily Globicephalinae and, in the western Mediterranean, it has been further detected in long-finned pilot whales Globicephala melas and Risso’s dolphins Grampus griseus (Supplementary Tables S2, S3). Our findings suggest that the striped dolphin is a secondary host for this cestode, its unpredictable occurrence perhaps resulting from unusual host-parasite contacts.
On the contrary, T. forsteri and S. triangularis are typical cestode species of striped dolphins (Supplementary Tables S2, S3). In the study area, their long-term infection trends were contrasting, with infection levels decreasing slightly (T. forsteri) or strongly (S. triangularis) up to year 2000 and subsequently recovering. To understand why such decrease occurred, and why each species was affected differently, we would need detailed knowledge on the life-cycle of both parasites. Unfortunately, no such information exists, although the general life-cycle for tetrabothriid cestodes is suggested to be complex, involving zooplanktonic organisms as first intermediate hosts, and fish or cephalopods as second intermediate hosts (Hoberg, 1987; Hoberg, 1996; Hoberg and Soudachanh, 2021). Thus, a potentially vast number of both biotic and abiotic factors could influence transmission dynamics of T. forsteri and S. triangularis, as evidenced for other TTPs infecting marine mammals (Des Clers and Wootten, 1990; Des Clers and Andersen, 1995; Stobo and Fowler, 2001).
It is worth noting, however, that three aspects of the patterns found in this study are compatible with the potential effects of a sudden significant reduction of the striped dolphin population in 1990. First, the dolphin mortality decreased the number of adult worms that successfully released infective stages, which could result in lower infections in dolphins in the following years (see MacDonald and Brisson, 2022). Second, the effect should be expected to be more pronounced in S. triangularis, at least for one reason, i.e., there seems to be a smaller number of other cetacean hosts supporting this cestode population. Out of the odontocete species analysed for parasites in the study area, S. triangularis has only been further detected in bottlenose dolphins, with low prevalence, whereas T. forsteri infects three other cetacean species, some with moderate prevalence (Table S2). Finally, the increase in infection levels of T. forsteri and, particularly, S. triangularis after year 2000 would be not surprising if we consider that the striped dolphin population also experienced a fast population recovery in just a decade (Gómez de Segura et al., 2006). Note that some decoupling is expected between the recovery of the host and the parasite populations (Anderson and May, 1978). This is also congruent with the absence of a significant effect of the variable ‘Group (epizootic vs. non-epizootic)’ in our models, since the effect of the first morbillivirus outbreak seems to have extended over the next years, rather than being restricted to year 1990. In addition, this outbreak was far more severe and affected a much higher number of dolphins than the following ones in 2007 and 2011 (Raga et al., 2008; Rubio-Guerri et al., 2013), which would explain the absence of an observable effect of the other epizootic events in the abundance/prevalence of TTPs.
Long-term trends of the acanthocephalan B. capitatum (=B. vasculosum) are striking. This parasite was detected just once in striped dolphins before and after 1990, respectively, but in nearly half of those analysed in 1990 (34 of 74 dolphins), when infections were widespread across the study area. Moreover, as noted above, the striped dolphin acts as an accidental host for this parasite and likely acquired infections via prey shared with their ‘true’ cetacean hosts (see Hoberg et al., 1993; Costa et al., 2000; Gregori et al., 2012). Acanthocephalans identified as B. vasculosum have never been reported in other cetacean species from the study area, and B. capitatum only in the long-finned pilot whale, Globicephala melas, as adult (Raga and Balbuena, 1993; see also references in Table S2). The sperm whale, Physeter macrocephalus could also be a host for B. capitatum (Amin and Margolis, 1998) but has not been analysed for parasites in the study area. Therefore, these two odontocetes could be considered as the putative source of the infections observed in striped dolphins.
The reason(s) for such puzzling temporal pattern of B. capitatum (=B. vasculosum) in striped dolphin is(are) difficult to ascertain. First, we could hypothesize that, for some reason, the production of infective stages was exceptional in 1990 but, if so, the effects should last longer than a year in the trophic web. Species of Bolbosoma are relatively long-lived in paratenic hosts (Costa et al., 2000), similarly to other TTPs, for which a lifespan of several years is not uncommon (Kuhn et al., 2016). Second, we could postulate that, in 1990, striped dolphin fed on infected prey that were seldom exploited in other years. However, there is no evidence for this; although striped dolphins shifted diet from more oceanic to more neritic prey during the period 1990-2012, the change was gradual and did not entail the disappearance of any of the common fish prey taxa (Aznar et al., 2017). Third, we could argue that, in 1990, the dolphins immunocompromised by the DMV provided more favourable conditions for a longer permanence of B. capitatum in the intestine, thus significantly increasing their likelihood of detection. Yet, we did not find B. capitatum in dolphins likewise affected by the DMV in the 2007 or 2011 epizootics. Finally, we could speculate that migratory prey brought infections from elsewhere but, again, why this did occur only in 1990? In summary, the singular infection pattern of B. capitatum is hard to explain and was likely multi-causal in nature. Further monitoring of the intestinal helminth fauna of striped dolphins in the western Mediterranean could shed more light on this enigmatic parasite.
To our knowledge, this study represents the first investigation of multi-decadal changes in the parasites of a marine top-predator. It was possible to document interesting patterns than open research avenues but, as it might be expected, interpretation was difficult due to broad gaps in the basic knowledge of the parasites’ biology. Therefore, this study serves as (i) a baseline to monitor parasites of cetaceans in the western Mediterranean, (ii) a foundation for comparative studies in other geographic areas and, (iii) a plea for more integrative studies in the oceanic realm, for which parasites should be considered an integral element.
Datasets are available on request: The raw data supporting the conclusions of this article will be made available by the authors, without undue reservation. Sequence data are available in Genbank and accession numbers are provided in Table 1.
Ethical approval was not required for the study involving animals in accordance with the local legislation and institutional requirements because our study is based on dolphin carcasses that get ashore along the Spanish coast. The Spanish legal system does not require any permission from an ethics commitee in order to analyze cetacean carcasses. Nonetheless, permission and funding to collect stranded dolphins was given by the wildlife services of the regional governments, which are the official institutions in charge of managing and protecting wildlife.
AG: Conceptualization, Formal Analysis, Methodology, Writing – original draft, Data curation, Writing – review & editing. JR: Funding acquisition, Writing – review & editing. NF: Methodology, Writing – review & editing, Data curation. FA: Conceptualization, Formal Analysis, Funding acquisition, Methodology, Writing – original draft, Data curation, Supervision, Writing – review & editing.
The author(s) declare financial support was received for the research, authorship, and/or publication of this article. This work was supported by projects AICO 2021/022 of the Generalitat Valenciana and VARACOMVAL of the Biodiversity Foundation of the Spanish Ministry for the Ecological Transition and the Demographic Challenge (MITECO) under the NextGenerationEU Recovery, Transformation and Resilience Plan (PRTR). A. García-Gallego holds a Ph.D. fellowship awarded by the Spanish Ministry of Universities (FPU21/01317).
We would like to thank the veterinary staff from the Oceanogràfic Foundation, for kindly providing us with data regarding the cause of death of striped dolphins stranded in the Valencian Community during the period 2010-2015. We would also like to thank the staff from the stranding network, as well as the Wildlife Service of the Generalitat Valenciana, for their assistance with stranded dolphins and their help in obtaining biological data used in this study.
The authors declare that the research was conducted in the absence of any commercial or financial relationships that could be construed as a potential conflict of interest.
The reviewer GP declared a past collaboration with the authors NF-F, FA to the handling editor.
The author(s) declared that they were an editorial board member of Frontiers, at the time of submission. This had no impact on the peer review process and the final decision.
All claims expressed in this article are solely those of the authors and do not necessarily represent those of their affiliated organizations, or those of the publisher, the editors and the reviewers. Any product that may be evaluated in this article, or claim that may be made by its manufacturer, is not guaranteed or endorsed by the publisher.
The Supplementary Material for this article can be found online at: https://www.frontiersin.org/articles/10.3389/fmars.2023.1272353/full#supplementary-material
Aguilar A., Raga J. A. (1993). The striped dolphin epizootic in the Mediterranean sea. Ambio 22 (8), 524–528.
Aho K., Derryberry D., Peterson T. (2014). Model selection for ecologists: The worldviews of AIC and BIC. Ecology 95 (3), 631–636. doi: 10.1890/13-1452.1
Amin O. M., Margolis L. (1998). Redescription of Bolbosoma capitatum (Acanthocephala: Polymorphidae) from false killer whale off Vancouver Island, with taxonomic reconsideration of the species and synonymy of B. physeteris. J. Helminthol. Soc. Wash. 65, 179–188.
Anderson D., Burnham K. (2004). Model selection and multi-model inference. Second. NY: Springer-Verlag 63 (2020), 10.
Anderson M. J., Gorley R. N., Clarke R. K. (2008). PERMANOVA+ for PRIMER: Guide to software and statistical methods. [Computer software] (Plymouth, United Kingdom: PRIMER-E Ltd).
Anderson R. M., May R. M. (1978). Regulation and stability of host-parasite population interactions. J. Anim. Ecol. 47 (1), 219–247. doi: 10.2307/3933
Arcangeli A., Campana I., Bologna M. A. (2017). Influence of seasonality on cetacean diversity, abundance, distribution and habitat use in the western Mediterranean Sea: Implications for conservation. Aquat. Conserv. 27 (5), 995–1010. doi: 10.1002/aqc.2758
Aznar F. J., Agustí C., Littlewood D. T. J., Raga J. A., Olson P. D. (2007). Insight into the role of cetaceans in the life cycle of the tetraphyllideans (Platyhelminthes: Cestoda). Int. J. Parasitol. 37 (2), 243–255. doi: 10.1016/j.ijpara.2006.10.010
Aznar F. J., Balbuena J. A., Raga J. A. (1994). Are epizoites biological indicators of a western Mediterranean striped dolphin die-off? Dis. Aquat. Organ. 18, 159–159. doi: 10.3354/dao018159
Aznar F. J., Míguez-Lozano R., Ruiz B., de Castro A. B., Raga J. A., Blanco C. (2017). Long-term changes, (1990-2012) in the diet of striped dolphins Stenella coeruleoalba from the western Mediterranean. Mar. Ecol. Prog. Ser. 568, 231–247. doi: 10.3354/meps12063
Aznar F. J., Perdiguero D., Del Olmo A. P., Repullés A., Agustí C., Raga J. A. (2005). Changes in epizoic crustacean infestations during cetacean die-offs: The mass mortality of Mediterranean striped dolphins Stenella coeruleoalba revisited. Dis. Aquat. Organ. 67 (3), 239–247. doi: 10.3354/dao067239
Aznar F. J., Raga J. A., Corcuera J., Monzon F. (1995). Helminths as biological tags for franciscana (Pontoporia blainvillei) (Cetacea, Pontoporiidae) in Argentinian and Uruguayan waters. Mammalia 9 (43), 927. doi: 10.1515/mamm.1995.59.3.427
Berón-Vera B., Pedraza S. N., Raga J. A., Pertierra A. G., Crespo E. A., Alonso M. K., et al. (2001). Gastrointestinal helminths of Commerson1s dolphins Cephalorhynchus commersonii from central Patagonia and Tierra del Fuego. Dis. Aquat. Organ. 47 (3), 201–208. doi: 10.3354/dao047201
Bossart G. D., Romano T. A., Peden-Adams M. M., Schaefer A., McCulloch S., Goldstein J. D., et al. (2011). Clinicoimmunopathologic findings in Atlantic bottlenose dolphins Tursiops truncatus with positive cetacean morbillivirus antibody titers. Dis. Aquat. Organ. 97 (2), 103–112. doi: 10.3354/dao02410
Bush A. O., Lafferty K. D., Lotz J. M., Shostak A. W. (1997). Parasitology meets ecology on its own terms: Margolis et al. revisited. J. Parasitol. 83, 575–583. doi: 10.2307/3284227
Calzada N., Aguilar A., Grau E., Lockyer C. (1997). Patterns of growth and physical maturity in the western Mediterranean striped dolphin, Stenella coeruleoalba (Cetacea: Odontoceti). Can. J. Zool. 75 (4), 632–637. doi: 10.1139/z97-078
Costa G., Chubb J. C., Veltkamp C. J. (2000). Cystacanths of Bolbosoma vasculosum in the black scabbard fish Aphanopus carbo, oceanic horse mackerel Trachurus picturatus and common dolphin Delphinus delphis from Madeira, Portugal. J. Helminthol. 74 (2), 113–120. doi: 10.1017/S0022149X00000159
Crespo-Picazo J. L., Rubio-Guerri C., Jiménez M. A., Aznar F. J., Marco-Cabedo V., Melero M., et al. (2021). Bottlenose dolphins (Tursiops truncatus) aggressive behavior towards other cetacean species in the western Mediterranean. Sci. Rep. 11 (1), 21582. doi: 10.1038/s41598-021-00867-6
Cuvertoret-Sanz M., López-Figueroa C., Byrne A. O., Canturri A., Martí-Garcia B., Pintado E., et al. (2020). Causes of cetacean stranding and death on the Catalonian coast (western Mediterranean Sea), 2012-2019. Dis. Aquat. Organ. 142, 239–253. doi: 10.3354/dao03550
Dailey M. D., Perrin W. F. (1973). Helminth parasites of porpoises of the genus Stenella in the eastern tropical Pacific, with descriptions of two new species: Mastigonema stenellae gen. et sp. n. (Nematoda: Spiruroidea) and Zalophotrema pacificum sp. n. (Trematoda: Digenea). Fish. Bull. 71, 455–471.
Dans S. L., Reyes L. M., Pedraza S. N., Raga J. A., Crespo E. A. (1999). Gastrointestinal helminths of the dusky dolphin, Lagenorhynchus obscurus (Gray 1828), off Patagonia, in the southwestern Atlantic. Mar. Mammal Sci. 15 (3), 649–660. doi: 10.1111/j.1748-7692.1999.tb00834.x
Delyamure S. L. (1955). Helminthofauna of marine mammals (Ecology and Phylogeny) (Moscow, Russia: Akademiya Nauk SSSR).
Des Clers S. A., Andersen K. (1995). Sealworm (Pseudoterranova decipiens) transmission to fish trawled from Hvaler, Oslofjord, Norway. J. Fish Biol. 46 (1), 8–17. doi: 10.1111/j.1095-8649.1995.tb05943.x
Des Clers S. A., Wootten R. (1990). Modelling the population dynamics of the sealworm Pseudoterranova decipiens. Neth. J. Sea Res. 25 (1–2), 291–299. doi: 10.1016/0077-7579(90)90028-F
Diez G., Chust G., Andonegi E., Santurtún M., Abaroa C., Bilbao E., et al. (2022). Analysis of potential drivers of spatial and temporal changes in anisakid larvae infection levels in European hake, Merluccius merluccius (L.), from the North-East Atlantic fishing grounds. Parasitol. Res. 121 (7), 1903–1920. doi: 10.1007/s00436-022-07446-2
Estes J. A., Heithaus M., McCauley D. J., Rasher D. B., Worm B. (2016). Megafaunal impacts on structure and function of ocean ecosystems. Annu. Rev. Environ. Resour. 41, 83–116. doi: 10.1146/annurev-environ-110615-085622
Evermann J. F., Leathers C. W., Gorham J. R., McKeirnan A. J., Appel M. J. G. (2001). Pathogenesis of two strains of lion (Panthera leo) morbillivirus in ferrets (Mustela putorius furo). Vet. Pathol. 38 (3), 311–316. doi: 10.1354/vp.38-3-311
Fernández M., Aznar F. J., Montero F. E., Georgiev B. B., Raga J. A. (2004). Gastrointestinal helminths of Cuvier’s beaked whales, Ziphius cavirostris, from the western Mediterranean. J. Parasitol. 90 (2), 418–420. doi: 10.1645/GE-105R
Fiorenza E. A., Wendt C. A., Dobkowski K. A., King T. L., Pappaionou M., Rabinowitz P., et al. (2020). It’s a wormy world: Meta-analysis reveals several decades of change in the global abundance of the parasitic nematodes Anisakis spp. and Pseudoterranova spp. in marine fishes and invertebrates. Glob. Chang. Biol. 26 (5), 2854–2866. doi: 10.1111/gcb.15048
Folmer O., Black M., Hoeh W., Lutz R., Vrijenhoek R. (1994). DNA primers for amplification of mitochondrial cytochrome c oxidase subunit I from diverse metazoan invertebrates. Mol. Mar. Biol. Biotechnol. 3 (5), 294–299.
Fraija-Fernández N., Fernández M., Raga J. A., Aznar F. J. (2016). Helminth diversity of cetaceans: An update. Adv. Mar. Biol. 1, 29–100.
Gannier A. (1999). Diel variations of the striped dolphin distribution off the French Riviera (Northwestern Mediterranean Sea). Aquat. Mamm. 25 (3), 123–134.
Gaspari S., Azzellino A., Airoldi S., Hoelzel A. R. (2007). Social kin associations and genetic structuring of striped dolphin populations (Stenella coeruleoalba) in the Mediterranean Sea. Mol. Ecol. 16 (14), 2922–2933. doi: 10.1111/j.1365-294X.2007.03295.x
Gaspari S., Marsili L., Natali C., Airoldi S., Lanfredi C., Deeming C., et al. (2019). Spatio-temporal patterns of genetic diversity in the Mediterranean striped dolphin (Stenella coeruleoalba). J. Zool. Syst. Evol. Res. 57 (3), 721–734. doi: 10.1111/jzs.12265
Georgiev B. B. (1986). In toto staining method for cestodes in acetocarmine. Helminthologia 23, 279–281.
Geraci J. R., St. Aubin D. J. (1987). Effects of parasites on marine mammals. Int. J. Parasitol. 17 (2), 407–414. doi: 10.1016/0020-7519(87)90116-0
Goldstein R. (1993). Conditioning diagnostics: collinearity and weak data in regression. Technometrics 35 (1), 85–86. doi: 10.1080/00401706.1993.10484997
Gomez-Campos E., Borrell A., Cardona L., Forcada J., Aguilar A. (2011). Overfishing of small pelagic fishes increases trophic overlap between immature and mature striped dolphins in the Mediterranean Sea. PloS One 6 (9), e24554. doi: 10.1371/journal.pone.0024554
Gómez de Segura A., Crespo E. A., Pedraza S. N., Hammond P. S., Raga J. A. (2006). Abundance of small cetaceans in waters of the central Spanish Mediterranean. Mar. Biol. 150, 149–160. doi: 10.1007/s00227-006-0334-0
Gregori M., Aznar F. J., Abollo E., Roura Á., González Á.F., Pascual S. (2012). Nyctiphanes couchii as intermediate host for the acanthocephalan Bolbosoma balaenae in temperate waters of the NE Atlantic. Dis. Aquat. Organ. 99 (1), 37–47. doi: 10.3354/dao02457
Harder T., Willhaus T. H., Frey H.-R., Liess B. (1990). Morbillivirus Infections of Seals During the 1988 Epidemic in the Bay of Heligoland: III. Transmission Studies of Cell Culture-Propagated Phocine Distemper Virus in Harbour Seals (Phoca vitulina) and a Grey Seal (Halichoerus grypus): Clinical, Virological and Serological Results. J. Vet. Med. B. 37 (1–10), 641–650. doi: 10.1111/j.1439-0450.1990.tb01109.x
Hasik A. Z., Siepielski A. M. (2022). Parasitism shapes selection by drastically reducing host fitness and increasing host fitness variation. Biol. Lett. 18 (11), 20220323. doi: 10.1098/rsbl.2022.0323
Heithaus M. R., Frid A., Wirsing A. J., Worm B. (2008). Predicting ecological consequences of marine top predator declines. Trends Ecol. Evol. 23 (4), 202–210. doi: 10.1016/j.tree.2008.01.003
Herreras M. V., Kaarstad S. E., Balbuena J. A., Kinze C. C., Raga J. A. (1997). Helminth parasites of the digestive tract of the harbour porpoise Phocoena phocoena in Danish waters: A comparative geographical analysis. Dis. Aquat. Organ. 28 (3), 163–167. doi: 10.3354/dao028163
Hoberg E. P. (1987). Recognition of larvae of the Tetrabothriidae (Eucestoda): Implications for the origin of tapeworms in marine homeotherms. Can. J. Zool. 65 (4), 997–1000. doi: 10.1139/z87-158
Hoberg E. P. (1996). Faunal diversity among avian parasite assemblages: The interaction of history, ecology, and biogeography. Bull. Scand. Soc. Parasitol. 6, 65–89.
Hoberg E. P., Daoust P. Y., McBurney S. (1993). Bolbosoma capitatum and Bolbosoma sp.(Acanthocephala) from sperm whales (Physeter macrocephalus) stranded on Prince Edward Island, Canada. J. Helminthol. Soc. Wash. 60 (2), 205–210.
Hoberg E. P., Soudachanh K. M. (2021). Diversity of Tetrabothriidae (Eucestoda) among Holarctic Alcidae (Charadriiformes): Resolution of the Tetrabothrius jagerskioeldi cryptic species complex—cestodes of Alcinae—provides insights on the dynamic nature of tapeworm and marine bird faunas under the Stockholm Paradigm. MANTER: J. Parasite Biodiv. 16, 1–76. doi: 10.32873/unl.dc.manter16
Hudson P. J., Dobson A. P., Newborn D. (1998). Prevention of population cycles by parasite removal. Science 282 (5397), 2256–2258.
Isidoro-Ayza M., Ruiz-Villalobos N., Pérez L., Guzmán-Verri C., Muñoz P. M., Alegre F., et al. (2014). Brucella ceti infection in dolphins from the Western Mediterranean sea. BMC Vet. Res. 10 (1), 1–10. doi: 10.1186/s12917-014-0206-7
Izquierdo-Serrano M., Revuelta O., Miguez-Lozano R., Gozalbes P., Ruiz-Garcia D., Raga J. A., et al. (2022). Assessment of the interactions between cetaceans and fisheries at the south of the Cetacean Migration Corridor and neighboring waters (Western Mediterranean). Front. Mar. Sci. 9. doi: 10.3389/fmars.2022.981638
Jaber J. R., Pérez J., Arbelo M., Zafra R., Fernández A. (2006). Pathological and immunohistochemical study of gastrointestinal lesions in dolphins stranded in the Canary Islands. Vet. Rec. 159 (13), 410–414. doi: 10.1136/vr.159.13.410
Johnson J. B., Omland K. S. (2004). Model selection in ecology and evolution. Trends Ecol. Evol. 19 (2), 101–108. doi: 10.1016/j.tree.2003.10.013
Kennedy S. (1998). Morbillivirus infections in aquatic mammals. J. Comp. Pathol. 119 (3), 201–225. doi: 10.1016/S0021-9975(98)80045-5
Kovach R. P., Ellison S. C., Pyare S., Tallmon D. A. (2015). Temporal patterns in adult salmon migration timing across southeast Alaska. Glob. Chang. Biol. 21 (5), 1821–1833. doi: 10.1111/gcb.12829
Kuhn J. A., Frainer A., Knudsen R., Kristoffersen R., Amundsen P.-A. (2016). Effects of fish species composition on Diphyllobothrium spp. Infections in brown trout–is three-spined stickleback a key species? J. Fish Dis. 39 (11), 1313–1323. doi: 10.1111/jfd.12467
Kuris A. M. (2003). Evolutionary ecology of trophically transmitted parasites. J. Parasitol. 89, 96–100.
Lafferty K. D., Allesina S., Arim M., Briggs C. J., De Leo G., Dobson A. P., et al. (2008). Parasites in food webs: The ultimate missing links. Ecol. Lett. 11 (6), 533–546. doi: 10.1111/j.1461-0248.2008.01174.x
Lafferty K. D., Dobson A. P., Kuris A. M. (2006). Parasites dominate food web links. Proc. Natl. Acad. Sci. 103 (30), 11211–11216. doi: 10.1073/pnas.0604755103
Lafferty K. D., Morris A. K. (1996). Altered behavior of parasitized killifish increases susceptibility to predation by bird final hosts. Ecology 77 (5), 1390–1397. doi: 10.2307/2265536
Laran S., Drouot-Dulau V. (2007). Seasonal variation of striped dolphins, fin-and sperm whales’ abundance in the Ligurian Sea (Mediterranean Sea). J. Mar. Biol. Assoc. U.K. 87 (1), 345–352. doi: 10.1017/S0025315407054719
Lehnert K., Randhawa H., Poulin R. (2017). Metazoan parasites from odontocetes off New Zealand: New records. Parasitol. Res. 116 (10), 2861–2868. doi: 10.1007/s00436-017-5573-0
Luo Y., Melillo J., Niu S., Beier C., Clark J. S., Classen A. T., et al. (2011). Coordinated approaches to quantify long-term ecosystem dynamics in response to global change. Glob. Chang. Biol. 17 (2), 843–854. doi: 10.1111/j.1365-2486.2010.02265.x
MacDonald H., Brisson D. (2022). Host phenology regulates parasite–host demographic cycles and eco-evolutionary feedbacks. Ecol. Evol. 12 (3), e8658. doi: 10.1002/ece3.8658
Mackenzie K. (1987). Long-term changes in the prevalence of two helminth parasites (Cestoda: Trypanorhyncha) infecting marine fish. J. Fish Biol. 31 (1), 83–87. doi: 10.1111/j.1095-8649.1987.tb05216.x
Mahy B. W., Van Regenmortel M. H. (2008). Encyclopedia of virology (Cambridge, Massachusetts: Academic Press).
Manfredi M. T., Dini W., Ganduglia S., Podesta M., Repetto G. (1992). Parasitological findings in striped dolphins, Stenella coeruleoalba. Eur. Res. Cetac. 6, 218–219.
Martínez J., García-Ladona E., Ballabrera-Poy J., Isern-Fontanet J., González-Motos S., Allegue J. M., et al. (2022). Atlas of surface currents in the Mediterranean and Canary–Iberian–Biscay waters. J. Oper. Oceanogr. 1–23. doi: 10.1080/1755876X.2022.2102357
Mateu P., Nardi V., Fraija-Fernández N., Mattiucci S., de Sola L. G., Raga J. A., et al. (2015). The role of lantern fish (Myctophidae) in the life-cycle of cetacean parasites from western Mediterranean waters. Deep Sea Res. Part I: Oceanogr. Res. Pap. 95, 115–121. doi: 10.1016/j.dsr.2014.10.012
Mateu P., Raga J. A., Fernández M., Aznar F. J. (2014). Intestinal helminth fauna of striped dolphins (Stenella coeruleoalba) in the western Mediterranean: No effects of host body length, age and sex. Mar. Mammal Sci. 30 (3), 961–977. doi: 10.1111/mms.12096
Mead J. G., Potter C. W. (1995). Recognizing two populations of the bottlenose dolphin (Tursiops truncatus) of the Atlantic coast of North America-morphologic and ecologic considerations. IBI Rep. (International Mar. Biol. Res. Institute Kamogawa Japan) 5, 31–44.
Miura O., Kuris A. M., Torchin M. E., Hechinger R. F., Chiba S. (2006). Parasites alter host phenotype and may create a new ecological niche for snail hosts. Proc. R. Soc. B: Biol. Sci. 273 (1592), 1323–1328. doi: 10.1098/rspb.2005.3451
Morand S., Harvey P. H. (2000). Mammalian metabolism, longevity and parasite species richness. Proc. R. Soc. London. Ser. B: Biol. Sci. 267, 1999–2003. doi: 10.1098/rspb.2000.1241
Otto S. B., Rall B. C., Brose U. (2007). Allometric degree distributions facilitate food-web stability. Nature 450 (7173), 1226–1229. doi: 10.1038/nature06359
Pang Y., Tian Y., Fu C., Wang B., Li J., Ren Y., et al. (2018). Variability of coastal cephalopods in overexploited China Seas under climate change with implications on fisheries management. Fish. Res. 208, 22–33. doi: 10.1016/j.fishres.2018.07.004
Panigada S., Lauriano G., Burt L., Pierantonio N., Donovan G. (2011). Monitoring winter and summer abundance of cetaceans in the Pelagos Sanctuary (northwestern Mediterranean Sea) through aerial surveys. PLoS One 6 (7), e22878. doi: 10.1371/journal.pone.0022878
Pershing A. J., Alexander M. A., Hernandez C. M., Kerr L. A., Le Bris A., Mills K. E., et al. (2015). Slow adaptation in the face of rapid warming leads to collapse of the Gulf of Maine cod fishery. Science 350 (6262), 809–812. doi: 10.1126/science.aac9819
Petrochenko V. I. (1958). Acanthocephala of domestic and wild animals Vol. 1 (Moscow, Russia: Akademiya Nauk SSSR). Translated from Russian by Israel Program for Scientific Translations, Jerusalem, 1971.
Pool R., Chandradeva N., Gkafas G., Raga J. A., Fernández M., Aznar F. J. (2020). Transmission and predictors of burden of lungworms of the striped dolphin (Stenella coeruleoalba) in the Western Mediterranean. J. Wildl. Dis. 56 (1), 186–191. doi: 10.7589/2018-10-242
Quiñones R., Giovannini A., Raga J. A., Fernández M. (2013). Intestinal Helminth Fauna of Bottlenose Dolphin Tursiops truncatus and Common Dolphin Delphinus delphis from the Western Mediterranean. J. Parasitol. 99 (3), 576–579. doi: 10.1645/GE-3165.1
Raga J. A. (1985). Contribución al estudio del parasitismo y demás asociaciones en los cetáceos de la Península Ibérica. PhD Thesis (Universitat de València).
Raga J. A., Balbuena J. A. (1993). Parasites of the long-finned pilot whale, Globicephala melas (Traill 1809). Eur. Waters. Rep. Int. Whaling Commission Special 14, 391–406.
Raga J. A., Banyard A., Domingo M., Corteyn M., Van Bressem M.-F., Fernández M., et al. (2008). Dolphin morbillivirus epizootic resurgence, Mediterranean Sea. Emerging Infect. Dis. 14 (3), 471. doi: 10.3201/eid1403.071230
Raga J. A., Carbonell E. (1985). New dates about parasites on Stenella coeruleoalba (MEYEN 1833). Investig. Cetacea 17, 207.
Raga J. A., Fernández M., Balbuena J. A., Aznar F. J. (2009). “Parasites,” in Encyclopedia of marine mammals, 2nd ed. Eds. Perrin W. F., Thewissen J. G. M., Würsing B. (San Diego: Academic Press/Elsevier Inc.), 821–830.
R Core Team (2022). R: A language and environment for statistical computing [Computer software] (Vienna, Austria: R Foundation for Statistical Computing). Available at: https://www.R-project.org/.
Reiczigel J. (2003). Confidence intervals for the binomial parameter: Some new considerations. Stat Med. 22 (4), 611–621. doi: 10.1002/sim.1320
Reiczigel J., Marozzi M., Fábián I., Rózsa L. (2019). Biostatistics for parasitologists–a primer to quantitative parasitology. Trends Parasitol. 35 (4), 277–281. doi: 10.1016/j.pt.2019.01.003
Romero M. A., Fernández M., Dans S. L., García N. A., González R., Crespo E. A. (2014). Gastrointestinal parasites of bottlenose dolphins Tursiops truncatus from the extreme southwestern Atlantic, with notes on diet composition. Dis. Aquat. Organ. 108 (1), 61–70. doi: 10.3354/dao02700
Rózsa L., Reiczigel J., Majoros G. (2000). Quantifying parasites in samples of hosts. J. Parasitol. 86 (2), 228–232. doi: 10.1645/0022-3395(2000)086[0228:QPISOH]2.0.CO;2
Rubio-Guerri C., Melero M., Esperón F., Bellière E. N., Arbelo M., Crespo J. L., et al. (2013). Unusual striped dolphin mass mortality episode related to cetacean morbillivirus in the Spanish Mediterranean Sea. BMC Vet. Res. 9 (1), 1–6. doi: 10.1186/1746-6148-9-106
Santoro M., Palomba M., Gili C., Marcer F., Marchiori E., Mattiucci S. (2021). Molecular and morphological characterization of Bolbosoma balaenae (Acanthocephala: Polymorphidae), a neglected intestinal parasite of the fin whale Balaenoptera physalus. Parasitology 148 (11), 1293–1302. doi: 10.1017/S0031182021000925
Scott M. D., Chivers S. J. (2009). Movements and diving behavior of pelagic spotted dolphins. Mar. Mammal Sci. 25 (1), 137–160. doi: 10.1111/j.1748-7692.2008.00241.x
Stobo W. T., Fowler G. M. (2001). Sealworm (Pseudoterranova decipiens) dynamics in Sable Island grey seals (Halichoerus grypus): Seasonal fluctuations and other changes in worm infections during the 1980s. NAMMCO Sci. Publ. 3, 129–147. doi: 10.7557/3.2963
Sures B., Nachev M., Selbach C., Marcogliese D. J. (2017). Parasite responses to pollution: What we know and where we go in ‘Environmental Parasitology’. Parasit. Vectors 10 (1), 65. doi: 10.1186/s13071-017-2001-3
Tamura K., Nei M., Kumar S. (2004). Prospects for inferring very large phylogenies by using the neighbor-joining method. Proc. Natl. Acad. Sci. 101 (30), 11030–11035. doi: 10.1073/pnas.0404206101
Tamura K., Stecher G., Kumar S. (2021). MEGA11: Molecular evolutionary genetics analysis version 11. Mol. Biol. Evol. 38 (7), 3022–3027. doi: 10.1093/molbev/msab120
Terracciano G., Fichi G., Comentale A., Ricci E., Mancusi C., Perrucci S. (2020). Dolphins stranded along the Tuscan coastline (Central Italy) of the “Pelagos sanctuary”: A parasitological investigation. Pathogens 9 (8). doi: 10.3390/pathogens9080612
Van Bressem M.-F., Duignan P. J., Banyard A., Barbieri M., Colegrove K. M., De Guise S., et al. (2014). Cetacean morbillivirus: current knowledge and future directions. Viruses 6 (12), 5145–5181. doi: 10.3390/v6125145
Van Waerebeek K., Reyes J., Alfaro Shigueto J. (1993). Helminth parasites and phoronts of dusky dolphins Lagenorhynchus obscurus (Gray 1828) from Peru. Aquat. Mamm. 19, 159–169.
Welicky R. L., Preisser W. C., Leslie K. L., Mastick N., Fiorenza E., Maslenikov K. P., et al. (2021). Parasites of the past: 90 years of change in parasitism for English sole. Front. Ecol. Environ. 19 (8), 470–477. doi: 10.1002/fee.2379
Wood S. N. (2011). Fast stable restricted maximum likelihood and marginal likelihood estimation of semiparametric generalized linear models. J. R. Stat. Society: Ser. B (Statistical Methodology) 73 (1), 3–36. doi: 10.1111/j.1467-9868.2010.00749.x
Wood S. N. (2017). Generalized additive models: An introduction with R (Boca Raton, Florida: Chapman and Hall/CRC press).
Wood C. L., Byers J. E., Cottingham K. L., Altman I., Donahue M. J., Blakeslee A. M. H. (2007). Parasites alter community structure. Proc. Natl. Acad. Sci. 104 (22), 9335–9339. doi: 10.1073/pnas.0700062104
Wood C. L., Lafferty K. D. (2015). How have fisheries affected parasite communities? Parasitology 142 (1), 134–144. doi: 10.1017/S003118201400002X
Wood C. L., Lafferty K. D., Micheli F. (2010). Fishing out marine parasites? Impacts of fishing on rates of parasitism in the ocean. Ecol. Lett. 13 (6), 761–775. doi: 10.1111/j.1461-0248.2010.01467.x
Wood C. L., Welicky R. L., Preisser W. C., Leslie K. L., Mastick N., Greene C., et al. (2023). A reconstruction of parasite burden reveals one century of climate-associated parasite decline. Proc. Natl. Acad. Sci. 120 (3), e2211903120. doi: 10.1073/pnas.2211903120
Yamaguti S. (1963). Systema Helminthum. Vol. V. Acanthocephala (New York & London: Interscience Publishers, Inc).
Keywords: trophically transmitted parasites, Stenella coeruleoalba, cetaceans, long-term trends, geographical variation, western Mediterranean
Citation: García-Gallego A, Raga JA, Fraija-Fernández N and Aznar FJ (2023) Temporal and geographical changes in the intestinal helminth fauna of striped dolphins, Stenella coeruleoalba, in the western Mediterranean: a long-term analysis (1982 - 2016). Front. Mar. Sci. 10:1272353. doi: 10.3389/fmars.2023.1272353
Received: 03 August 2023; Accepted: 23 October 2023;
Published: 07 November 2023.
Edited by:
Marco Casu, University of Sassari, ItalyReviewed by:
Georgios Gkafas, University of Thessaly, GreeceCopyright © 2023 García-Gallego, Raga, Fraija-Fernández and Aznar. This is an open-access article distributed under the terms of the Creative Commons Attribution License (CC BY). The use, distribution or reproduction in other forums is permitted, provided the original author(s) and the copyright owner(s) are credited and that the original publication in this journal is cited, in accordance with accepted academic practice. No use, distribution or reproduction is permitted which does not comply with these terms.
*Correspondence: Alicia García-Gallego, YWxpY2lhLmdhcmNpYS1nYWxsZWdvQHV2LmVz
Disclaimer: All claims expressed in this article are solely those of the authors and do not necessarily represent those of their affiliated organizations, or those of the publisher, the editors and the reviewers. Any product that may be evaluated in this article or claim that may be made by its manufacturer is not guaranteed or endorsed by the publisher.
Research integrity at Frontiers
Learn more about the work of our research integrity team to safeguard the quality of each article we publish.