- 1Marine Geospatial Ecology Lab, Nicholas School for the Environment, Duke University, Durham, NC, United States
- 2Tethys Research Institute, Milano, Italy
- 3Biology Department & ECOMARE, University of Aveiro, Aveiro, Portugal
- 4EcoOcéan Institut, Montpellier, France
- 5Observatoire Pelagis UAR 3462, CNRS-La Rochelle University, La Rochelle, France
- 6Blue World Institute of Marine Research and Conservation, Veli Losinj, Croatia
- 7Croatian Natural History Museum, Zagreb, Croatia
- 8Marine Biodiversity, Habitat and Species Protection Unit, National Institute for Italian Institute for Environmental Protection and Research, Rome, Italy
- 9Association AL LARK, Cancale, France
- 10Marine Mammal Group, Oceanographic Research Center of Vigo, Marine Environment Department, Spanish Institute of Oceanography – Spanish National Research Council, Vigo, Spain
The ACCOBAMS Survey Initiative (ASI) is the first synoptic large-scale survey of the entire ACCOBAMS Area and as such it plays a key role in filling the current gaps in our biological and ecological knowledge of large vertebrate species occurring in the region. Data gathered during the ASI were analyzed in a distance sampling surface-modelling framework to assess the summer distribution, densities and patterns, as well as to investigate the correlates of these parameters, for large vertebrate species and taxa in the Mediterranean Basin. Static and dynamic explanatory variables, including water depth (m), distance to depth contours (km), distance to canyons and seabed slope (km), sea surface temperature (°C), mixed layer depth (m) and levels of chlorophyll-a (mg/l), were considered to predict density and compute its variance spatially at a resolution of 10x10 km. A strong longitudinal gradient from low densities in the east to high densities in the west is shared by most taxa. In addition, several taxa also showed a less marked latitudinal gradient varying in direction according to species, and finally, a few of them exhibited patchy distributions.
1 Introduction
In the context of the general decline of biodiversity, the need for monitoring programs at large spatial and temporal scales is widely recognized (Balmford et al., 2005; Pereira and Cooper, 2006; Tiralongo et al., 2019), where the aim is to assess changes in species occurrence to predict long-term responses to anthropogenic pressures and global changes. In this context, measuring distributions and densities of species is crucial to inform conservation and management (Grand et al., 2007).
Historically, the systematic collection of data to assess baseline species population parameters and species responses to stressors has been heterogeneous across the Mediterranean Basin (Bianchi and Morri, 2000) and this is particularly true for cetaceans (Mannocci et al., 2018a). While existing knowledge on the distribution and occurrence of megafauna is abundant during the summer and in the western and northern sectors of the Mediterranean, relatively little information exists for other seasons and for the eastern and southern portions of the basin, where knowledge is primarily anecdotal. This uneven distribution of research effort, limited in both space and time, has led to large gaps in our knowledge of marine megafauna occurrence and distribution. The need for systematic surveys across the entire Mediterranean region has been stressed by many. In response, the Agreement on the Conservation of Cetaceans of the Black Sea, Mediterranean Sea and Contiguous Atlantic Area (ACCOBAMS) organized and conducted in 2018 the ACCOBAMS Survey Initiative (ASI) project (Panigada et al., 2023) aimed at monitoring marine megafauna and anthropogenic activities with the ultimate goal of contributing to fill the current gaps in knowledge.
Large scale multi-species monitoring programs for megafauna have been established outside the Mediterranean Sea (e.g., CODA, 2009) with the primary goal to obtain the first comprehensive estimates of abundance of encountered species and to evaluate these estimates in an ecological and conservation context. To date, the continuation of these well-established programs and the planning of new ones is not only justified by their effectiveness to inform management and conservation decisions but is also a legal requirement under the European Union Habitats and Marine Strategy Framework Directives (HD and MSFD respectively) and, albeit in a less prescriptive way, by the Barcelona Convention.
The Mediterranean Sea, although in general considered an oligotrophic sea, is a hotspot of biodiversity, hosting several endemic and rare species. At the same time, it is also subject to ever increasing anthropogenic pressures, with the potential to afflict critical habitats, Important Marine Mammal Areas (Tetley et al., 2022) and the species therein, therefore posing a serious threat to their persistence.
Amongst cetaceans, eleven species, considered sub-populations of their north Atlantic counterparts, are known to occur regularly in the Mediterranean Sea (ACCOBAMS, 2021a): the fin whale Balaenoptera physalus (Linnaeus, 1758), the sperm whale Physeter macrocephalus (Linnaeus, 1758), the Cuvier’s beaked whale Ziphius cavirostris (Cuvier, 1823), the common dolphin Delphinus delphis (Linnaeus, 1758), the long-finned pilot whale Globicephala melas (Traill, 1809), the Risso’s dolphin Grampus griseus (Cuvier, 1812), the killer whale Orcinus orca (Linnaeus, 1758), the striped dolphin Stenella coeruleoalba (Meyen, 1833), the rough-toothed dolphin Steno bredanensis (Cuvier, 1823), and the common bottlenose dolphin Tursiops truncates (Montagu, 1821). For most of these populations, the Mediterranean Basin is the relevant unit for monitoring, assessment and conservation actions.
Alongside cetaceans, in the Mediterranean Sea, 73 species of sharks, rays and chimaeras have been recorded (Dulvy et al., 2016), with 31 species endangered or critically endangered. Some shark species, particularly large predatory ones, have suffered a steep decline from >96 to >99.99% in the last decades, often due to overfishing and bycatch (Ferretti et al., 2008). Similarly, of 32 species of rays that are found in the Mediterranean, half are facing extinction risk (Dulvy et al., 2016). Together with species of conservation concern, the Mediterranean is home to commercially lucrative species such as the swordfish (Xiphias gladius (Linnaeus, 1758)) and the bluefin tuna (Thunnus thynnus (Linnaeus, 1758)), whose stocks have dramatically reduced due to overexploitation and whose fishery has been subjected to strong restrictive and rebuilding measures introduced by the International Commission for the Conservation of Atlantic Tunas (ICCAT)1.
The patterns of occurrence and distribution of species are influenced by a variety of physical, biological, and environmental parameters operating at different spatial and temporal scales (e.g., Hillebrand, 2004; Whitehead et al., 2008; Tittensor et al., 2010; Torreblanca et al., 2022), as well as by the variability of these parameters due to natural fluctuations and human induced changes (e.g., MacLeod, 2009; Lu et al., 2023; Snell et al., 2023).
The presence of decreasing eastward longitudinal gradients in the Mediterranean Sea has been reported for primary productivity, as well as for the distribution and abundance of benthic and pelagic species (Danovaro et al., 1999; Quignard and Tomasini, 2000; Ben Rais Lasram et al., 2009; López-Sandoval et al., 2010; Bonnet et al., 2011; Danovaro et al., 2020). Bonnet et al. (2011) described the Western Mediterranean as oligotrophic and the Eastern Mediterranean as ultra-oligotrophic, reporting that planktonic N2 fixation has a clear decreasing trend from West to East. The authors also found that the N2 fixation in the western basin can sustain up to 35% of new primary production, while it seems negligible in the eastern basin. In the Strait of Gibraltar, there are overlapping, and inverse flows of water, whereby Atlantic waters enter at the surface and Mediterranean waters leave at depth. As a result, the surface water currents produce a general flow of Atlantic waters towards the east with many eddies and diversions of branches on its way, resulting in the formation of cyclonic circuits due to the Coriolis effect. There is no return system at the surface; the return occurs in the intermediate layers coming from the Levantine basin which has a general movement towards the west but also following large cyclonic circuits (Rodríguez Martínez, 1982; Miller and Ketchum, 1983).
The purpose of this paper is to explore if the same prevailing longitudinal distribution gradients from west to east, and other less marked or less prevalent patterns, are observed particularly in cetaceans, but also in some elasmobranchs and large fish of both conservation and commercial importance. We discuss the results alongside the significant variables that likely explain the predicted estimates and that can help to understand the observed patterns and gradients. The present work, focused on spatial patterns in densities of marine megafauna and their possible ecological significance, is paralleled by another paper dealing with basin-wide abundance estimates of cetaceans and their monitoring and conservation implications (Panigada et al., 2023).
2 Materials and methods
2.1 Study area
The entire Mediterranean expands up to 2.6 million km2 (of which we covered around 73%) with an average depth of 1,460 m, and a maximum depth of 5,267 m along the Hellenic Trench in the Ionian Sea (Rodríguez Martínez, 1982). In general, the Mediterranean Sea has a quite narrow continental shelf, with a few exceptions such as the eastern coast of Tunisia and the Adriatic Sea, and large areas of open deep sea (Coll et al., 2010) (Figure I.1 in Supplementary Materials). The morpho-bathymetry, the water circulation (Figure I.2 in Supplementary Materials) and the general oceanographic conditions of the Mediterranean Sea are complex (e.g., Bethoux, 1979; Bas, 2009; Brosolo et al., 2012). The basin is characterized by several regional and local features and strong environmental patterns (Danovaro et al., 1999) with both longitudinal and latitudinal, as well as depth gradients, affecting primary and biological productivity. It is also considered as a concentration basin, where evaporation is higher particularly in the eastern basin, increasing salinity and decreasing sea level, which in turn promotes the flow of Atlantic water into the Mediterranean (Rodríguez Martínez, 1982; Miller and Ketchum, 1983). The sea surface temperature (Sst) in the Mediterranean has two very different regimes, in winter (typically December to March) and summer (usually June to September) with spring and autumn being transition periods (Pastor et al., 2018). During summer, when this survey was carried out, the highest Sst values are found in the south-eastern Mediterranean, the Lybian coast and, in some years, the south Tyrrhenian Sea. While the coolest areas are in the Alboran Sea/Strait of Gibraltar and the Gulf of Lyon (Pastor et al., 2018).
2.2 Survey design and data collection
The Mediterranean region (excluding the waters off Lybia and Egypt) was divided in 32 blocks to best accommodate logistical needs, presence of airports, jurisdictional boundaries and robustness of the sampling design. Transects were designed systematically with equal coverage probability, with an equal-angle zig-zag configuration, using the software Distance 7.3 (Thomas et al., 2010). The survey, conducted in passing mode (Dawson et al., 2008), was planned with a ratio effort/area varying between 2.7 and 3.6% in each block. Specific details on survey design are provided in Panigada et al. (2023).
A distance-sampling approach was used for marine mammals and large fish, such as elasmobranchs. Accordingly, for these taxa, the declination angle to the sighting was collected when the animal or group of animals were abeam to the plane, and subsequently used to calculate the perpendicular distance to the transect used to account for animals/groups detectability via distance sampling methods (Buckland, 2001). On the other hand, a strip-sampling approach was used for sun fish (most probably Mola mola (Linnaeus, 1758), as it is the most common sunfish in the Mediterranean, over Mola alexandrine (Ranzani, 1839) (Sawai et al., 2018), although definitive species could not be distinguished from the airplane).
Environmental conditions potentially affecting the detectability of the animals (e.g. Beaufort sea state, glare severity and angle, turbidity, cloud coverage and an overall subjective assessment of the detection conditions) were recorded at the beginning of each transect and whenever they changed. Data collected during sightings included species (identified to the lowest possible taxonomic level), school size and declination angle (measured with a hand-held clinometer) in the case of species for which a line transect approach was used.
More specific details on data collection are provided in Panigada et al. (2023), while a description of the field procedures and protocols for data collection are described in Lambert et al. (2019) and followed well established procedures in aerial distance sampling surveys (e.g., Laran et al., 2017).
2.3 Data analysis
2.3.1 Areas and subareas
The study area has been subdivided into 5 areas for the analyses (Figure I.3 in Supplementary Materials), defined as the Atlanticl (33,720 km2), the Western Mediterranean (MedW – 499,002 km2), the Central Mediterranean (MedC – 601,262 km2), the Adriatic Sea (135,783 km2), and the Eastern Mediterranean (MedE – 632,983 km2), totaling 1,902,749 km2. Although the study area was originally divided in 32 strata, for the purpose of this study, larger sectors were used for the final analysis (referred to as “Areas” in Figure I.3 in Supplementary Materials) to better reflect existing knowledge on the ecology and distribution of the observed species.
2.3.2 Model-based analysis
Design-based analyses of the dataset arising from the ASI are presented and discussed by Panigada et al. (2023). Data on Chelonids (e.g., Caretta caretta) were integrated with existing datasets in the region and are presented and discussed by (DiMatteo et al., 2022); consequently, they are not included in this study. For the aims of this paper, density surface models within a distance sampling framework were used to predict species’ density as a function of environmental, oceanographic and physiographic covariates (the list of tested covariates is presented in Table III.2 in the Supplementary Materials). A spatial grid at a resolution of 10x10 km was overlaid to the survey area to associate explanatory covariates values to on-effort segments within each grid cell to predict density spatially. The resolution of the grid cells was chosen as the finest consistent resolution that captures all available covariates. Covariate values were then assigned to the centroid of each grid cell.
The count of groups in each segment was used as the response variable. The density of groups was modelled using a Generalized Additive Model (GAM) with a logarithmic link function, and a Tweedie error distribution, very close to a Poisson distribution but allowing for some over-dispersion. The general structure of the model was:
where the offset ai is the effective search area for the ith segment (calculated as the length of the segment multiplied by twice the esw), Θ is the intercept, fk are smoothed functions of the explanatory covariates, and zik is the value of the kth explanatory covariate in the ith segment. The esw was obtained for each species or species group from their detection function, according to the covariates included in it. The abundance is then estimated by multiplying the density by the survey area.
Abundance for each taxon in each grid cell was estimated by multiplying the abundance of groups, predicted from the best fitting model, by the mean group size estimated for each area or the modelled group sizes if spatial variation was observed. In the case of modelled group sizes, the observed group size of each sighting was taken as a response variable, no offset was used, and the distribution family was negative binomial. For fin whales, however, given the very small group sizes, the number of animals was modelled directly (instead of two steps). The same framework was used as the one used for the model of groups but using the number of individuals instead of the number of groups as the response variable.
Variance of abundance was estimated by a parametric bootstrap procedure, also called “posterior simulation” (Miller et al., 2022). The delta method was used to combine the coefficient of variation (CV) from the bootstrap with the CV from the detection function and from the model. The 95% Confidence Interval (CI) was obtained using the final CV and assuming the estimates were lognormally distributed. All modelling was carried out using the statistical software R.4.0.3 (R Core Team, 2020) using the mgcv 1.8-33 package (Wood, 2017).
For some small areas not surveyed (highlighted in Figure I.3 in Supplementary Materials), predictions were extrapolated from surrounding areas and according to their environmental covariates. However, no extrapolation was produced for the large un-surveyed area in the south-eastern portion of the Mediterranean Sea, as the environmental covariates in such a large area in the edge of the study area might be too different and outside the ranges measured in the surveyed areas.
For those species for which robust model-based analyses could not be performed due to a limited sample size, density estimates based on Conventional Distance Sampling (CDS) analyses were calculated per Area. Additionally, to highlight potential latitudinal or longitudinal gradients and to help compare the distribution of sighting location by species or functional group, a plot of staggered distribution of sightings coordinates was created. For each species or functional group, the ridges in the plot show the probability density function of the sightings’ latitude and longitude estimated via a kernel density method. Plots were prepared using the stat_density_ridges() function in the package ggridges (Wilke, 2022) for the software for statistical analyses R (R Core Team, 2023) with an automatic scaling of the ridgelines computed at 2.77.
3 General results
About 91% (55,167 km) of the planned transects (60,808 km) were monitored on effort (Figure I.3 in Supplementary Materials). Table III.1 in the Supplementary Materials summarizes sightings’ information of cetaceans, elasmobranchs and large fish; among cetaceans, striped dolphins were the most commonly observed species, followed by bottlenose dolphins and to a lesser extent, Risso’s dolphins. In 18% of sightings (n= 146) it was not possible to discriminate between striped and common dolphins. Among elasmobranchs, the spinetail devil ray (Mobula mobular (Bonnaterre, 1788)) was the most sighted species, followed by unidentified sharks. Sunfish was the most sighted large bony fish in terms of number of encounters, although tunas represented the larger number of individuals observed.
Table 1 shows the parameters and selected covariates for the density surface modelling for each species or group of species for cetaceans, and Table 2 for elasmobranch and large bony fish. Figures 1–12 present the results of model-based abundance estimates for those species with sufficient sightings to allow running models. The maps present predicted numbers of animals per 100 km2. Associated uncertainty maps (CVs) are shown in the Supplementary Materials.
All results provided here, including Table IV.1 to Table IV.13 in the Supplementary Materials, which show the abundances estimates per area (Figure I.3 in Supplementary Materials) for the species considered in this paper come from the model-based analysis. Results from the design-based analysis are given in Panigada et al. (2023). Figure 13 shows the density distribution of recorded latitude and longitude at sighting location by species or functional group.
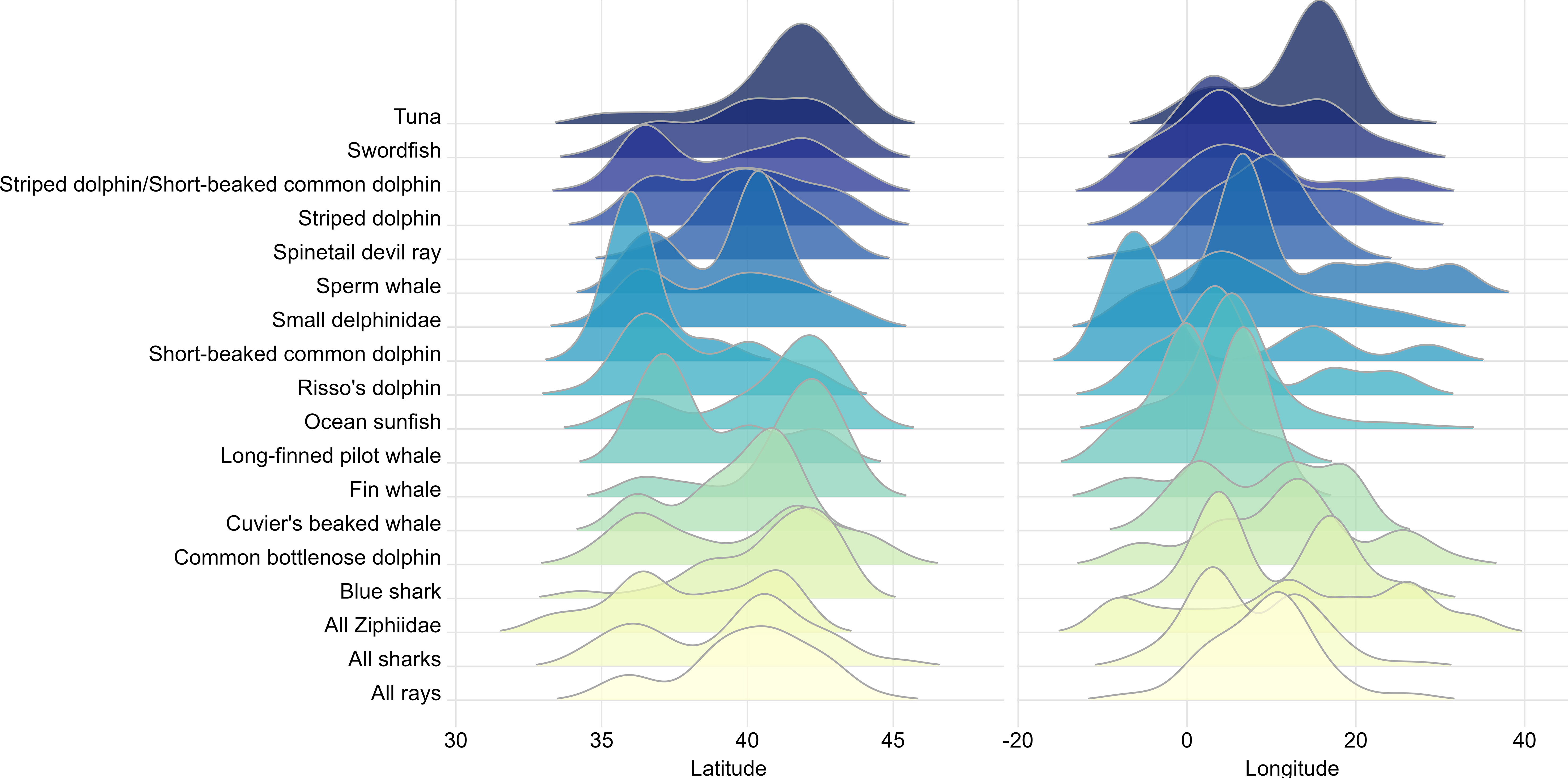
Figure 13 Staggered distribution of recorded latitude and longitude (in degrees) at sighting location by species or functional group.
4 Species account
For each taxon, the overall density and its distribution pattern derived from the present analyses are highlighted. These results are followed by taxon-specific comparisons with previous literature on density estimates and ecology. The interpretation of the broad picture about marine megafauna distribution patterns in the Mediterranean Sea is left for the general discussion. The results for the Atlantic contiguous area are not discussed in this paper. The plots of the smooth functions for all models are shown in Supplementary Materials.
4.1 Fin whale
Overall density estimate for fin whales was 0.8 10-3 ind.km-2 (CV=0.29) (Table IV.1 in the Supplementary Materials) and mean group size was 1.5 animals per group. The highest density was found in the Western Mediterranean area (2.3 10-3 ind.km-2, CV=0.29), notably in pelagic waters from the Ligurian Sea to north of the Balearic Islands (Figure 3). Model-based estimated densities were one order of magnitude lower (Adriatic Sea and Central Med) or nil (Eastern Med) in the other areas.
This general pattern of the distribution of fin whales in the Mediterranean basin is in accordance with previous papers (e.g., Forcada et al., 1995; Notarbartolo di Sciara et al., 2003; Notarbartolo di Sciara et al., 2016; Panigada et al., 2017a). The species strong preference for pelagic habitats, with several groups detected at depths of 2000 metres or more, is also in line with previous works (e.g., Cotté et al., 2009; Panigada et al., 2017b).
While long distance latitudinal movements of fin whales within the Mediterranean have been reported (Panigada et al., 2017b), these movements primarily occur at the beginning of the spring season, while during the summer the species shows a rather limited distributional range, concentrated in the waters of the Corso-Ligurian-Provençal Basin (Geijer et al., 2016; Notarbartolo di Sciara et al., 2016). The species has been reported in the eastern basin, but in a practically negligible way (Stephens et al., 2021). The same pattern was found in (Mannocci et al., 2018a).
4.2 Risso’s dolphin
The basin-wide density of Risso’s dolphins was estimated at 13.6 10-3 ind.km-2 (CV=0.25) (Table IV.2 in the Supplementary Materials), with highest values in the Western and, to a lower extent, Central Mediterranean. Modelled densities in the other two areas were only 3 10-3 ind.km-2. The mean group size was 6 animals per group (CV=0.18). Highest densities were in oceanic waters from the Alborán Sea, north of the Balearic Islands to off the central Algerian slope (Figure 4). Smaller concentrations were found off the slope of Provence and Liguria.
Our results agree with previous knowledge supporting a higher occurrence of this species in the Western part of the Mediterranean Sea (Gómez de Segura et al., 2008; Laran et al., 2017). Although the species is often considered to be associated with continental slopes, during the ASI it was sighted mainly in offshore oceanic environment.
The Risso’s dolphin in the Mediterranean basin is one of the least-known cetacean species and has been the subject of only few dedicated studies (e.g., Azzellino et al., 2016; Borrell et al., 2021; Cipriano et al., 2022; Luna et al., 2022; Minoia et al., 2023). The species is known for its strong habitat preferences, with individuals primarily encountered in relatively small groups, favoring slope areas, mainly in the north-western Basin (Bearzi et al., 2011). They are also regularly reported in the Alborán Sea, the Gulf of Vera and the Provençal basin, where their range includes deep offshore waters (Cañadas et al., 2002; Cañadas et al., 2005; Laran et al., 2017). Mannocci et al. (2018a) showed higher densities over the continental shelf across the Mediterranean Sea (only depth was included in their models), with most of their observations occurring in the Western Mediterranean and only a few in the Central Mediterranean and southern Adriatic.
4.3 Common bottlenose dolphin
The overall density of bottlenose dolphins was estimated as 28.4 10-3 ind.km-2 (CV=0.15) (Table IV.3 in the Supplementary Materials), with a mean group size of 7.4 animals (CV=0.17). Highest densities were in the Adriatic area (60 10-3 ind.km-2) and lowest densities in the Eastern Mediterranean area. More specifically, the distribution was patchy, with favorable sectors in predominantly shelf and slope habitats of the Alborán, northern Balearic Islands-to-Gulf of Lion, northern Tyrrhenian, northern Adriatic, southern Sicily-Malta-Tunisian shelf, and the Aegean subareas, separated by extended stretches of low densities (Figure 5).
The present results are in gross agreement with existing knowledge on the coastal and shelf preferences of this species (Bearzi et al., 2009; Mannocci et al., 2018a). However, the bottlenose dolphin is also present in oceanic waters, albeit at lower densities, and this seems to be more notable in winter (Laran et al., 2017).
4.4 Striped and common dolphins
The two species are considered jointly because an uncertainty often lies in distinguishing common and striped dolphins from a plane during an aerial survey. Therefore, a specific species assemblage (striped or common dolphin) was used when a clear distinction between both species was not possible. The overall estimated density of this category was 111.7 10-3 ind.km-2 (CV= 0.19) (Table IV.4 in the Supplementary Materials). Another assemblage was called “small dolphins”, which included striped, common and unidentified small dolphins (which were most probably one of these two species), with an estimated density of 390.2 10-3 ind.km-2 (CV=0.09) (Table IV.5 in the Supplementary Materials). Regarding this last assemblage, the Western Mediterranean area displayed densities of 972.9 10-3 ind.km-2, about one order of magnitude above the other three areas; lowest densities were in the Adriatic and Eastern Mediterranean areas. More specifically, the vast majority of sightings of both groupings were recorded from the Alborán Sea, the Balearic Sea, the Gulf of Lions to the Ligurian Sea (Figure 5, Figure 6, Table IV.4 and Table IV.5 in the Supplementary Materials). Almost no sightings of this guild were recorded in the northern Adriatic Sea and the Levantine Basin.
Striped dolphins could be unequivocally identified in 258 encounters. The estimated basin wide density for striped dolphin was 234.7 10-3 ind.km-2 (CV= 0.15) (Table IV.6 in the Supplementary Materials), with densities in the Western Mediterranean about 5-10 times higher than anywhere else. The highest densities were mostly found in slope and oceanic waters along the northwestern part of the Western Mediterranean area, from the Alborán Sea to the Ligurian Sea, all the way through the Balearic Islands and off the Gulf of Lion. The Tyrrhenian Sea, the Moroccan and Algerian plateau and the waters of the Ionian Sea and the southern Adriatic Sea showed slightly lower densities (Figure 4).
Overall, only 32 sightings of common dolphins, mostly encountered in the western portion of the Basin and in the Strait of Sicily, have been recorded during the aerial surveys (Table III.1 in the Supplementary Materials), mainly between latitude 33-38° North. No spatial modelling could be done for this species due to the small sample size.
The striped dolphin has previously been observed primarily in the offshore waters of the Mediterranean Sea, where the largest groups were also observed, indicating a strong preference for deep pelagic waters (e.g., Azzellino et al., 2008). Overall, the present data support the existing evidence that the western basin represents the most important striped dolphin habitat in the region (Mannocci et al., 2018a).
Regarding the common dolphin, Mannocci et al. (2018a), with a much larger dataset, showed an extreme gradient in which the density was high in the Alborán Sea, being very low or close to zero in the rest of the Mediterranean, except for a small patch in the north-eastern section of the Aegean Sea and northern Adriatic Sea. Vella et al. (2021) did a compilation of knowledge on this species in the Mediterranean, reporting similar distribution patterns. Therefore, this survey corroborates previous knowledge that common dolphins have a strong longitudinal gradient, with most of their density concentrated in the West and very little as we progress to the East. This result shows the strongest pattern in the staggered distribution plot in Figure 13.
4.5 Sperm, pilot and Cuvier’s beaked whales
Sperm whales, being deep divers, are difficult to detect from airplanes, and only 10 groups were detected (Table III.1 in the Supplementary Materials). They were encountered in both the eastern and western basin, with sightings only recorded within 35° to 40° of latitude North, mainly along the Hellenic Trench and in the offshore waters of the Sea of Sardinia (Figure 7). With such low number of observations, it was not possible to create a detection function and therefore estimate abundance from the aerial survey. A density estimate is provided, however by Boisseau et al. (submitted) from acoustic survey of the ASI vessel-based survey. Mannocci et al. (2018a), which had enough observations to model sperm whale density, found the highest densities in the Western Mediterranean and western Ionian Sea, in agreement with the abundance estimates from acoustic surveys (Lewis et al., 2018) and data from the vessel-based component of the ASI (Boisseau et al., submitted).
Long-finned pilot whales were only encountered west of 12° E of longitude, with a strong preference for deep pelagic waters. Largest groups of this species were observed in the Alborán Sea, along the coast of Morocco and in the Gulf of Lion. Relatively smaller pods were observed in the Ligurian Sea within the waters of the Pelagos Sanctuary (Figure 7). The distribution of sightings and estimated abundance confirms the almost exclusive presence of this species in the Western Mediterranean Sea (Canadas and Sagarminaga, 2000; Notarbartolo di Sciara, 2016; Verborgh et al., 2016; Mannocci et al., 2018b).
Cuvier’s beaked whales have been mostly sighted in areas rich in canyons in the Ionian Sea and the Hellenic Trench, the deep southern Adriatic Sea, the Central Tyrrhenian Sea, the Balearic and the Alborán Seas (Figure 7). The ASI results confirm existing knowledge on the basin-wide presence of the Cuvier’s beaked whale and, at the same time, confirm that the species occurs in relatively small patches at low densities. Mannocci et al., 2018a predicted higher densities in areas between 1000 and 2000 m depth across the whole basin using only static covariates (depth and slope), despite most of the observations being in the Alborán Sea. Cañadas et al., 2018 found similar patterns as the observations registered here, with the highest densities in the Alborán Sea, and other patches of high density in the Ligurian Sea, Central Thyrrenian Sea and Ionian Sea. Being a deep diving species, ship-board surveys with an acoustic array represent an important complement to study the distribution of this species (Boisseau et al., submitted).
4.6 Rays
Rays, most of them being identified as spinetail devil rays, had an overall surface density of 13.2 10-3 ind.km-2 (Cv=0.15) (Tables IV.7 and IV.8 in the Supplementary Materials) and the western and Central Mediterranean areas had the highest densities at about 20 10-3 ind.km-2. The central part of the Western Mediterranean, from Valencia and the Balearic Islands to Corsica and Sardinia, shows the highest density values (Figure 8 for spinetail devil rays).
Notarbartolo di Sciara et al. (2015) estimated a summer density of 25.7 10-3 ind.km-2 (Cv=0.13) in the northern part of the Western Mediterranean between 2009 and 2013, in the same order of magnitude as the estimates reported here for the Western Mediterranean (0.0285 individuals per km2).
4.7 Sharks
Sharks, only about 10% of which were identified as blue shark Prionace glauca (Linnaeus, 1758), had an overall surface density of 13.9 10-3 ind.km-2 (CV=0.26) (Tables IV.9 and IV.10 in the Supplementary Materials), with the highest densities found in the Adriatic Sea, followed by the western and Central Mediterranean areas. The distribution pattern for sharks was fairly patchy, with spots of high densities around the Balearic Islands and along the Tyrrhenian coast of Italy, and other patches in the northern and southern Adriatic, on the Tunisian plateau and in the Alborán Sea (Figure 9). The Eastern Mediterranean area was uniformly low in shark density.
4.8 Swordfish
The basin-wide swordfish surface density was estimated at 14 10-3 ind.km-2 (CV=0.11) (Table IV.11 in the Supplementary Materials), with highest figures found in the Western Mediterranean (24.4 10-3 ind.km-2), and values one order of magnitude lower in the Eastern Mediterranean. Hotspots of swordfish densities were from the Alborán Sea and the Algerian coast to the Gulf of Lion, and to a lesser extent in the Tyrrhenian, the western Ionian and the central Adriatic (Figure 10).
Similarly, Lauriano et al. (2017) estimated a summer density of 10 10-3 ind.km-2 in the same area between 2009 and 2010, in the same order of magnitude as the estimates from ASI for the Western Mediterranean (0.024 individuals per km2).
4.9 Sunfish
The ocean sunfish was present at the surface at an overall density of 14.9 10-3 ind.km-2 (CV= 0.36) (Table IV.12 in the Supplementary Materials), with up to 47.9 10-3 ind.km-2 in the Western Mediterranean and low to very low densities everywhere else. The hotspot of highest density was in the north of the Western Mediterranean, from the shelf of the Gulf of Lion all the way to Ligurian Sea through oceanic waters of the northern Algero-Provençal Basin (Figure 11). Patches of intermediate densities were found in either shelf or oceanic waters, in the northern Adriatic Sea, the Alborán Sea and the north-eastern Levantine Basin.
By comparison, densities of 1000 10-3 ind.km-2 were estimated in the North Western Mediterranean during the summer with hotspots in the Gulf of Lion and along the Provençal slope in the summer, and further offshore in the middle of the north-Western Mediterranean in the winter (Grémillet et al., 2017). This estimate is much higher than the present one for the same area, even when one consider that Grémillet et al.,’s estimate was corrected for availability bias by using a g(0) = 0.4 to account for time spent at the surface by ocean sunfish.
4.10 Tuna
Tunas (all species observed) were present at the surface at an overall density of 252.7 10-3 ind.km-2 (CV=0.39) (Table IV.13 in the Supplementary Materials), with up to 898.3 10-3 ind.km-2 in the Western Mediterranean and much lower densities everywhere else. Tunas were encountered mostly in the north-Western Mediterranean (Balearic Sea, Gulf of Lyon and Ligurian Sea, Figure 12), where they had the larger number of individuals per group. They were also encountered, with smaller group sizes, in the eastern Thyrrenian Sea and the Adriatic Sea, and to a lesser extent in the Aegean Sea and South of Sicily. Only one group was observed in the easternmost part of the basin.
5 General discussion
5.1 Strengths and weaknesses
This paper presents the model-based results of the first large-scale synoptic aerial survey of the entire Mediterranean region conducted in the summer of 2018, and provides the baseline density estimates and maps for several large vertebrates occurring in the basin, including cetaceans, elasmobranchs and large teleosts.
Although the values presented here are underestimates of the actual densities, as they have not been corrected for availability and perception biases, known to negatively affect estimates, we consider this limitation not to significantly affect the possibility of comparing the observed spatial patterns. With the exception of sperm whales, we can assume consistent diving behavior of each species across the study area, and therefore the availability biases are likely to be consistent over the monitored area. Male and female sperm whales segregate at different latitudes across most of their range when non breeding, with single males occurring at higher latitudes primarily engaging in feeding activities, and females occurring at lower latitudes within family units. This social structure is accompanied with striking differences in the use of habitats between the two sexes as well as different diving ad feeding behaviors (e.g., Pirotta et al., 2011; Pirotta et al., 2020a; Pirotta et al., 2020b). As a result, availability bias for males and females observed during aerial surveys might be different. During the ASI, most of sperm whale sightings were recorded at lower latitudes where family units rather than adult males are known to occur (e.g., Gannier et al., 2002) and as such the estimates presented here might be more relevant to groups or family units of this species. For this species, abundance estimated from visual aerial line transect distance sampling surveys is likely to be negatively biased because of the long dive times of these species, and passive acoustic approaches might produce more robust estimates (e.g., Barlow and Taylor, 2005; Boisseau et al., submitted; Lewis et al., 2018). The perception bias, although more variable across the region because of the differences in observers’ experience, was mitigated by a pre-survey training for all observers and teams. It should also have been minimized by the use of similar airplanes, all implementing identical standardized protocols, including the compulsory use of bubble windows and the same flight speed and altitude. In addition, it is unlikely that the possible differences in observer experiences and perception biases could have any spatial trend, potentially biasing the results presented here.
It is important to note that Mediterranean Sea basin-wide estimates for cetaceans and other mega vertebrates, with the exception of Cuvier’s beaked whales (Cañadas et al., 2018) and loggerhead turtles (DiMatteo et al., 2022), from visual surveys have never been obtained before, thus making comparisons with existing knowledge, for some species and geographic areas, rather difficult. Furthermore, the ASI is the only existing snapshot of the whole basin (all surveys in all areas done almost simultaneously over a short period of time), while the aforementioned estimates for Cuvier’s beaked whales and sea turtles merge datasets arising from surveys conducted over several years, different areas and different survey platforms. Historically, most of the effort in the region has been allocated along coastal areas and, despite research on cetaceans has been going on for well over three decades, several portions of the Mediterranean have never been, or at best very minimally, monitored in the past (Mannocci et al., 2018b). At sea monitoring has been more substantial in the Western Mediterranean Sea, in particular during the last decade, with several aerial surveys focusing on marine megafauna, with a main focus on cetaceans, and taking place in particular in its central and north-western sectors (e.g., Gomez de Segura et al., 2006; Panigada et al., 2011; Panigada et al., 2017b; Fortuna et al., 2014; Lauriano et al., 2014; Bauer et al., 2015a; Bauer et al., 2015b; Notarbartolo di Sciara et al., 2015; Laran et al., 2017; Lauriano et al., 2017; Fortuna et al., 2018). It is also noteworthy the ICCAT aerial surveys focusing on bluefin tuna conducted annually, in the last decade, in the Levatine Sea, Ionian Sea, Tyrrhenian sea and Algerian-Provençal Sea (e.g., Cañadas and Vazquez, 2020).
Moreover, some areas in the eastern and southern portions of the Basin remain unexplored to date. In fact, some portions of the easternmost and southernmost Mediterranean were not surveyed during the ASI due to logistic constraints, geopolitical issues and lack of research permits. Accordingly, in these areas it is still difficult to assess the occurrence, distribution and abundance of cetaceans and marine megafauna therein. Despite pre-existing knowledge in these areas, direct comparisons cannot be made with the present results and further data collection and analyses are necessary to eventually assess local trends.
All the species considered in this study show geographical patterns that reflect their ecological, biological and life-history traits as well as strong eastward longitudinal gradients, with density being relatively higher in the western portion of the Mediterranean Sea and decreasing towards the eastern basin. Similarly, the uncertainty on the predictions is higher in the eastern basin, and in most cases also in the southern portions of the study area where density has been estimated.
5.2 Dominant spatial patterns
The highest values of densities for most species presented here were obtained for the Western Mediterranean. For eight of the 11 taxa considered here, a longitudinal gradient with highest densities at the western end of the basin was the most prominent feature of their distributions. Other patterns were visible in bottlenose dolphins, which showed a predominantly patchy distribution generally associated to shelf habitats. Similarly, although the current data were too limited, Cuvier’s beaked whales are considered to exhibit a patchy distribution in areas of slope with extensive canyons. Sharks showed a combination of strong longitudinal gradient combined with a strong patchiness of the highest density hotspots. Finally, a latitudinal component of the distribution was also clearly visible in fin whales, rays and sunfish, and to a lesser extent in striped dolphins and sharks as well.
The main aggregations of fin whales were in the deeper waters of the Corso-Liguro-Provençal Basin and the western part of the Pelagos Sanctuary. Striped dolphins were found to have their highest densities (>1 individual per km2) in the Alborán Sea, with high densities also estimated across the Corso-Liguro-Provençal Basin. The highest densities of bottlenose dolphins were encountered west of 18°E (eastern tip of Italy). Although Risso’s dolphins were seen in both the west and east, highest densities were apparent in the Algerian, Corso-Liguro-Provençal and Balearic Basins. Sperm whales exhibited clustering throughout the survey blocks, with highest densities in the Eastern Mediterranean, which includes the known important area for this species of the Hellenic Trench in Greece.
According to the knowledge of decreasing longitudinal gradients in the Mediterranean Sea from the west to the east at the levels of primary productivity (López-Sandoval et al., 2010; Bonnet et al., 2011), distribution and abundance of benthic and pelagic species (Tecchio et al., 2011; Danovaro et al., 2020) and fish richness (Quignard and Tomasini, 2000; Ben Rais Lasram et al., 2009), it seems reasonable to think that the distribution of the cetacean, elasmobranch and large fish species described in this work is a reflection of the distribution patterns of the primary productivity and of their prey.
Data availability statement
The datasets presented in this study can be found in online repositories. The names of the repository/repositories and accession number(s) can be found below: https://accobams.org/asi-data-access-request/.
Ethics statement
Ethical approval was not required for the study involving animals in accordance with the local legislation and institutional requirements because this research did not include handling of any animal. It was just observation from airplanes.
Author contributions
AC: Conceptualization, Data curation, Formal Analysis, Investigation, Methodology, Validation, Writing – original draft, Writing – review & editing. NP: Conceptualization, Writing – review & editing. HA: Investigation, Writing – review & editing. LD: Investigation, Writing – review & editing. ND: Investigation, Writing – review & editing. GD: Investigation, Writing – review & editing. JG: Investigation, Writing – review & editing. DH: Investigation, Writing – review & editing. SL: Investigation, Writing – review & editing. GL: Investigation, Writing – review & editing. MP: Writing – review & editing, Investigation. VR: Writing – review & editing. JV: Investigation, Writing – review & editing. SP: Conceptualization, Investigation, Supervision, Writing – review & editing.
Funding
The author(s) declare financial support was received for the research, authorship, and/or publication of this article. The ACCOBAMS Survey Initiative was supported by all riparian countries and has received substantial financial support from the MAVA Foundation, the Prince Albert II of Monaco Foundation, the International Fund for Animal Welfare, the Ministry of Agriculture and Fisheries, Food and Environment of Spain, the French Agency for Biodiversity, the Italian Ministry of the Environment and Protection of Land and Sea, the Government of the Principality of Monaco and from the operational program for the implementation of the European Maritime and Fisheries Fund in the Republic of Slovenia.
Acknowledgments
Special thanks to the members of the ASI Contact Group, the ASI Steering Committee, observers and team leaders, the ACCOBAMS Secretariat and the ACCOBAMS Scientific Committee for their continuous support to the development of the ACCOBAMS Survey Initiative project since its inception. Thanks to the government organizations that provided logistical support in the field and/or the necessary authorizations, and to the aircraft companies and their pilots, without whom the ACCOBAMS Survey Initiative would not have been possible. The authors are grateful to the Italian Ministry for the Environment and Energy Security for the voluntary contribution offered to ACCOBAMS in support of the publication of this article. Special thanks also to PELAGIS Observatory (UMS 3462, CNRS-La Rochelle University) for training the observers. Parts of this manuscript has been released as a final report at https://accobams.org/wp-content/uploads/2021/11/ASI-Med-Report-updated.pdf, (ACCOBAMS, 2021b).
Conflict of interest
The authors declare that the research was conducted in the absence of any commercial or financial relationships that could be construed as a potential conflict of interest.
Publisher’s note
All claims expressed in this article are solely those of the authors and do not necessarily represent those of their affiliated organizations, or those of the publisher, the editors and the reviewers. Any product that may be evaluated in this article, or claim that may be made by its manufacturer, is not guaranteed or endorsed by the publisher.
Supplementary material
The Supplementary Material for this article can be found online at: https://www.frontiersin.org/articles/10.3389/fmars.2023.1270917/full#supplementary-material
Footnotes
References
ACCOBAMS (2021a). Conserving Whales, Dolphins and Porpoises in the Mediterranean Sea, Black Sea and adjacent areas: an ACCOBAMS status report. Eds. Notarbartolo di Sciara G., Tonay Monaco: A. M. (ACCOBAMS). Available at: https://accobams.org/wp-content/uploads/2022/03/ACCOBAMS_ConservingWDP_web_2022.pdf.
ACCOBAMS (2021b). Estimates of abundance and distribution of cetaceans, marine mega-fauna and marine litter in the Mediterranean Sea from 2018-2019 surveys. Eds. Panigada S., Boisseau O., Canadas A., Lambert C., Laran S., McLanaghan R., Moscrop A. (Monaco: ACCOBAMS - ACCOBAMS Survey Initiative Project), 177.
Azzellino A., Airoldi S., Gaspari S., Lanfredi C., Moulins A., Podestà M., et al. (2016). “Risso’s Dolphin, Grampus griseus, in the Western Ligurian Sea,” in Advances in Marine Biology (Elsevier), 205–232. doi: 10.1016/bs.amb.2016.08.003
Azzellino A., Gaspari S., Airoldi S., Nani B. (2008). Habitat use and preferences of cetaceans along the continental slope and the adjacent pelagic waters in the western Ligurian Sea. Deep Sea Res. Part I: Oceanogr. Res. Pap. 55, 296–323. doi: 10.1016/j.dsr.2007.11.006
Balmford A., Bennun L., ten Brink B., Cooper D., Côté I. M., Crane P., et al. (2005). The convention on biological diversity’s 2010 target. Science 307, 212–213. doi: 10.1126/science.1106281
Barlow J., Taylor B. L. (2005). Estimates of sperm whale abundance in the northeastern temperate pacific from a combined acoustic and visual survey. Mar. Mammal Sci. 21, 429–445. doi: 10.1111/j.1748-7692.2005.tb01242.x
Bas C. (2009). The Mediterranean: a synoptic overview. Contrib. to Sci., 25–39. doi: 10.2436/20.7010.01.57
Bauer R., Bonhommeau S., Brisset B., Fromentin J. (2015a). Aerial surveys to monitor bluefin tuna abundance and track efficiency of management measures. Mar. Ecol. Prog. Ser. 534, 221–234. doi: 10.3354/meps11392
Bauer R. K., Fromentin J.-M., Demarcq H., Brisset B., Bonhommeau S. (2015b). Co-occurrence and habitat use of fin whales, striped dolphins and atlantic bluefin tuna in the northwestern mediterranean sea. PloS One 10, e0139218. doi: 10.1371/journal.pone.0139218
Bearzi G., Fortuna C. M., Reeves R. R. (2009). Ecology and conservation of common bottlenose dolphins Tursiops truncatus in the Mediterranean Sea. Mammal Rev. 39, 92–123. doi: 10.1111/j.1365-2907.2008.00133.x
Bearzi G., Reeves R., Remonato E., Pierantonio N., Airoldi S. (2011). Risso’s dolphin Grampus griseus in the Mediterranean Sea. Mamm. Biol. - Z. für Säugetierkunde 76, 385–400. doi: 10.1016/j.mambio.2010.06.003
Ben Rais Lasram F., Guilhaumon F., Mouillot D. (2009). Fish diversity patterns in the Mediterranean Sea: deviations from a mid-domain model. Mar. Ecol. Prog. Ser. 376, 253–267. doi: 10.3354/meps07786
Bethoux J. P. (1979). Budgets of the Mediterranean Sea-Their dependance on the local climate and on the characteristics of the Atlantic waters. Oceanol. Acta 2, 157–163.
Bianchi C. N., Morri C. (2000). Marine biodiversity of the mediterranean sea: situation, problems and prospects for future research. Mar. pollut. Bull. 40, 367–376. doi: 10.1016/S0025-326X(00)00027-8
Bonnet S., Grosso O., Moutin T. (2011). Planktonic dinitrogen fixation along a longitudinal gradient across the Mediterranean Sea during the stratified period (BOUM cruise). Biogeosciences 8, 2257–2267. doi: 10.5194/bg-8-2257-2011
Borrell A., Gazo M., Aguilar A., Raga J. A., Degollada E., Gozalbes P., et al. (2021). Niche partitioning amongst northwestern Mediterranean cetaceans using stable isotopes. Prog. Oceanogr. 193, 102559. doi: 10.1016/j.pocean.2021.102559
Buckland S. T. (2001). Introduction to distance sampling: estimating abundance of biological populations. (Oxford; New York: Oxford University Press).
Brosolo L., Mascle J., Loubrieu B. (2012). Morphobathymetric Map of the Mediterranean Sea, publication CCGM/CGMW, UNESCO: Paris. Available at: https://sextant.ifremer.fr/geonetwork/srv/api/records/fcb72f2c-0033-4706-9ed7-ad36ffaf1024.
Cañadas A., Aguilar De Soto N., Aissi M., Arcangeli A., Azzolin M., B-Nagy A., et al. (2018). The challenge of habitat modelling for threatened low density species using heterogeneous data: the case of Cuvier’s beaked whales in the Mediterranean. Ecol. Indic. 85, 128–136. doi: 10.1016/j.ecolind.2017.10.021
Canadas A., Sagarminaga R. (2000). The Northeastern Alboran Sea, an important breeding and feeding ground for the long-finned pilot whale (Globicephala melas) in the Mediterranean Sea. Mar. Mammal Sci. 16, 513–529. doi: 10.1111/j.1748-7692.2000.tb00948.x
Cañadas A., Sagarminaga R., De Stephanis R., Urquiola E., Hammond P. S. (2005). Habitat preference modelling as a conservation tool: proposals for marine protected areas for cetaceans in southern Spanish waters. Aquat. Conserv.: Mar. Freshw. Ecosyst. 15, 495–521. doi: 10.1002/aqc.689
Cañadas A., Sagarminaga R., García-Tiscar S. (2002). Cetacean distribution related with depth and slope in the Mediterranean waters off southern Spain. Deep Sea Res. Part I: Oceanogr. Res. Pap. 49, 2053–2073. doi: 10.1016/S0967-0637(02)00123-1
Cañadas A., Vazquez A. (2020) 2010-2019 Aerial Survey Data Re-Analysis. SHORT-TERM CONTRACT ICCAT GBYP 07/2019 Final report. Available at: https://www.iccat.int/GBYP/Docs/Aerial_Survey_Phase_9_Data_Analysis.pdf.
Cipriano G., Carlucci R., Bellomo S., Santacesaria F. C., Fanizza C., Ricci P., et al. (2022). Behavioral pattern of Risso’s dolphin (Grampus griseus) in the gulf of taranto (Northern Ionian sea, Central-Eastern Mediterranean sea). JMSE 10, 175. doi: 10.3390/jmse10020175
CODA (2009). Cetacean Offshore Distribution and Abundance in the European Atlantic (St. Andrews, Scotland: University of Saint Andrews).
Coll M., Piroddi C., Steenbeek J., Kaschner K., Lasram F. B. R., Aguzzi J., et al. (2010). The biodiversity of the mediterranean sea: estimates, patterns, and threats. PloS One 5, e11842. doi: 10.1371/journal.pone.0011842
Cotté C., Guinet C., Taupier-Letage I., Mate B., Petiau E. (2009). Scale-dependent habitat use by a large free-ranging predator, the Mediterranean fin whale. Deep Sea Res. Part I: Oceanogr. Res. Pap. 56, 801–811. doi: 10.1016/j.dsr.2008.12.008
Danovaro R., Dinet A., Duineveld G., Tselepides A. (1999). Benthic response to particulate fluxes in different trophic environments: a comparison between the Gulf of Lions–Catalan Sea (western-Mediterranean) and the Cretan Sea (eastern-Mediterranean). Prog. Oceanogr. 44, 287–312. doi: 10.1016/S0079-6611(99)00030-0
Danovaro R., Fanelli E., Canals M., Ciuffardi T., Fabri M.-C., Taviani M., et al. (2020). Towards a marine strategy for the deep Mediterranean Sea: Analysis of current ecological status. Mar. Policy 112, 103781. doi: 10.1016/j.marpol.2019.103781
Dawson S., Wade P., Slooten E., Barlow J. (2008). Design and field methods for sighting surveys of cetaceans in coastal and riverine habitats. Mammal Rev. 38, 19–49. doi: 10.1111/j.1365-2907.2008.00119.x
DiMatteo A., Cañadas A., Roberts J., Sparks L., Panigada S., Boisseau O., et al. (2022). Basin-wide estimates of loggerhead turtle abundance in the Mediterranean Sea derived from line transect surveys. Front. Mar. Sci. 9. doi: 10.3389/fmars.2022.930412
Dulvy N., Allen D., Ralph G., Walls R. (2016). The conservation status of Sharks, Rays and Chimaeras in the Mediterranean Sea. doi: 10.13140/RG.2.2.22020.53129
Ferretti F., Myers R. A., Serena F., Lotze H. K. (2008). Loss of large predatory sharks from the mediterranean sea: large sharks in the mediterranean. Conserv. Biol. 22, 952–964. doi: 10.1111/j.1523-1739.2008.00938.x
Forcada J., Notarbartolo di Sciara G., Fabbri F. (1995). Abundance of fin whales (Balenoptera physalus) and striped dolphins (Stenella coeruloalba) summering in the Corso-Ligurian Basin. Mammalia 59, 127–140. doi: 10.1515/mamm.1995.59.1.127
Fortuna C. M., Kell L., Holcer D., Canese S., Jr E. F., Mackelworth P., et al. (2014). Summer distribution and abundance of the giant devil ray (Mobula mobular) in the Adriatic Sea: Baseline data for an iterative management framework. Scientia Marina 78, 227–237. doi: 10.3989/scimar.03920.30D
Fortuna C. M., Cañadas A., Holcer D., Brecciaroli B., Donovan G. P., Lazar B., et al. (2018). The coherence of the european union marine natura 2000 network for wide-ranging charismatic species: a mediterranean case study. Front. Mar. Sci. 5. Available at: https:///www.frontiersin.org/articles/10.3389/fmars.2018.00356
Gannier A., Drouot V., Goold J. C. (2002). Distribution and relative abundance of sperm whales in the Mediterranean Sea. Mar. Ecol. Prog. Ser. 243, 281–293. doi: 10.3354/meps243281
Geijer C. K. A., Notarbartolo di Sciara G., Panigada S. (2016). Mysticete migration revisited: are Mediterranean fin whales an anomaly? Mam Rev. 46, 284–296. doi: 10.1111/mam.12069
Gómez de Segura A., Crespo E. A., Pedraza S. N., Hammond P. S., Raga J. A. (2006). Abundance of small cetaceans in waters of the central Spanish Mediterranean. Mar. Biol. 150, 149–160. doi: 10.1007/s00227-006-0334-0
Gómez de Segura A., Hammond P. S., Raga J. A. (2008). Influence of environmental factors on small cetacean distribution in the Spanish Mediterranean. J. Mar. Biol. Assoc. United Kingdom 88, 1185–1192. doi: 10.1017/S0025315408000386
Grand J., Cummings M. P., Rebelo T. G., Ricketts T. H., Neel M. C. (2007). Biased data reduce efficiency and effectiveness of conservation reserve networks. Ecol. Lett. 10, 364–374. doi: 10.1111/j.1461-0248.2007.01025.x
Grémillet D., White C. R., Authier M., Dorémus G., Ridoux V., Pettex E. (2017). Ocean sunfish as indicators for the ‘rise of slime.’. Curr. Biol. 27, R1263–R1264. doi: 10.1016/j.cub.2017.09.027
Hillebrand H. (2004). Strength, slope and variability of marine latitudinal gradients. Mar. Ecol. Prog. Ser. 273, 251–267. doi: 10.3354/meps273251
Lambert C., Authier M., Dorémus G., Gilles A., Hammond P., Laran S., et al. (2019). The effect of a multi-target protocol on cetacean detection and abundance estimation in aerial surveys. R. Soc Open Sci. 6, 190296. doi: 10.1098/rsos.190296
Laran S., Pettex E., Authier M., Blanck A., David L., Dorémus G., et al. (2017). Seasonal distribution and abundance of cetaceans within French waters- Part I: The North-Western Mediterranean, including the Pelagos sanctuary. Deep Sea Res. Part II: Topical Stud. Oceanogr. 141, 20–30. doi: 10.1016/j.dsr2.2016.12.011
Lauriano G., Pierantonio N., Donovan G., Panigada S. (2014). Abundance and distribution of Tursiops truncatus in the Western Mediterranean Sea: An assessment towards the Marine Strategy Framework Directive requirements. Mar. Environ. Res. 100, 86–93. doi: 10.1016/j.marenvres.2014.04.001
Lauriano G., Pierantonio N., Kell L., Cañadas A., Donovan G., Panigada S. (2017). Fishery-independent surface abundance and density estimates of swordfish (Xiphias gladius) from aerial surveys in the Central Mediterranean Sea. Deep Sea Res. Part II: Topical Stud. Oceanogr 141:102–114. doi: 10.1016/j.dsr2.2017.04.019
Lewis T., Boisseau O., Danbolt M., Gillespie D., Lacey C., Leaper R., et al. (2018). Abundance estimates for sperm whales in the Mediterranean Sea from acoustic line-transect surveys. J. cetacean Res. Manage. 18, 103–117. doi: 10.47536/jcrm.v18i1.437
López-Sandoval D. C., Fernández A., Marañón E. (2010). Dissolved and particulate primary production along a longitudinal gradient in the Mediterranean Sea. Biogeosciences 8, 815–825. doi: 10.5194/bg-8-815-2011
Lu Y., Liu G., Cheung W. W. L., Xian Y., Chen W., Yu D. (2023). Anthropogenic footprints are invading global habitats of Indo-Pacific humpback dolphins. Geogr. Sustain. 4, 58–69. doi: 10.1016/j.geosus.2022.12.001
Luna A., Sánchez P., Chicote C., Gazo M. (2022). Cephalopods in the diet of Risso’s dolphin (Grampus griseus ) from the Mediterranean Sea: a review. Mar. Mammal Sci. 38, 725–741. doi: 10.1111/mms.12869
MacLeod C. (2009). Global climate change, range changes and potential implications for the conservation of marine cetaceans: a review and synthesis. Endang. Species. Res. 7, 125–136. doi: 10.3354/esr00197
Mannocci L., Roberts J. J., Halpin P. N. (2018b). Development of Exploratory Marine Species Density Models in the Mediterranean Sea (Durham, North Carolina: Duke University Marine Geospatial Ecology Lab).
Mannocci L., Roberts J. J., Halpin P. N., Authier M., Boisseau O., Bradai M. N., et al. (2018a). Assessing cetacean surveys throughout the Mediterranean Sea: a gap analysis in environmental space. Sci. Rep. 8, 3126. doi: 10.1038/s41598-018-19842-9
Miller D. L., Becker E. A., Forney K. A., Roberts J. J., Cañadas A., Schick R. S. (2022). Estimating uncertainty in density surface models. PeerJ 10, e13950. doi: 10.7717/peerj.13950
Minoia L., Consales G., Mazzariol S., Mancusi C., Terracciano G., Ceciarini I., et al. (2023). Preliminary assessment of persistent organic pollutants (POPs) in tissues of Risso’s dolphin (Grampus griseus) specimens stranded along the Italian coasts. Mar. pollut. Bull. 186, 114470. doi: 10.1016/j.marpolbul.2022.114470
Notarbartolo di Sciara G. (2016). “Chapter One - Marine Mammals in the Mediterranean Sea: An Overview,” in Advances in Marine Biology Mediterranean Marine Mammal Ecology and Conservation. Eds. Notarbartolo Di Sciara G., Podestà M., Curry B. E. (Academic Press), 1–36. doi: 10.1016/bs.amb.2016.08.005
Notarbartolo di Sciara G., Castellote M., Druon J.-N., Panigada S. (2016). “Chapter Three - Fin Whales, Balaenoptera physalus: At Home in a Changing Mediterranean Sea?,” in Advances in Marine Biology Mediterranean Marine Mammal Ecology and Conservation. Eds. Notarbartolo Di Sciara G., Podestà M., Curry B. E. (Academic Press), 75–101. doi: 10.1016/bs.amb.2016.08.002
Notarbartolo di Sciara G., Lauriano G., Pierantonio N., Cañadas A., Donovan G., Panigada S. (2015). The devil we don’t know: investigating habitat and abundance of endangered giant devil rays in the North-Western Mediterranean sea. PloS One 10, 0141189. doi: 10.1371/journal.pone.0141189
Notarbartolo di Sciara G., Zanardelli M., Jahoda M., Panigada S., Airoldi S. (2003). The fin whale Balaenoptera physalus (L. 1758) in the Mediterranean Sea: Fin whales in the Mediterranean. Mammal Rev. 33, 105–150. doi: 10.1046/j.1365-2907.2003.00005.x
Panigada S., Donovan G. P., Druon J.-N., Lauriano G., Pierantonio N., Pirotta E., et al. (2017b). Satellite tagging of Mediterranean fin whales: working towards the identification of critical habitats and the focussing of mitigation measures. Sci. Rep. 7, 3365. doi: 10.1038/s41598-017-03560-9
Panigada S., Lauriano G., Burt L., Pierantonio N., Donovan G. (2011). Monitoring winter and summer abundance of cetaceans in the pelagos sanctuary (Northwestern Mediterranean sea) through aerial surveys. PloS One 6, e22878. doi: 10.1371/journal.pone.0022878
Panigada S., Lauriano G., Donovan G., Pierantonio N., Cañadas A., Vázquez J. A., et al. (2017a). Estimating cetacean density and abundance in the Central and Western Mediterranean Sea through aerial surveys: implications for management. Deep Sea Res. Part II: Topical Stud. Oceanogr. 141, 41–58. doi: 10.1016/j.dsr2.2017.04.018
Panigada S., Pierantonio N., Araújo H., David L., Di-Méglio N., Dorémus G., et al (2023). The ACCOBAMS Survey Initiative: the first synoptic assessment of cetacean abundance in the Mediterranean Sea through aerial surveys. Front. Mar. Sci. 10, 1270513. doi: 10.3389/fmars.2023.1270513
Pastor F., Valiente J. A., Palau J. L. (2018). Sea surface temperature in the mediterranean: trends and spatial patterns, (1982–2016). Pure Appl. Geophys. 175, 4017–4029. doi: 10.1007/s00024-017-1739-z
Pereira H. M., Cooper D. H. (2006). Towards the global monitoring of biodiversity change. Trends Ecol. Evol. 21, 123–129. doi: 10.1016/j.tree.2005.10.015
Pirotta E., Brotons J. M., Cerdà M., Bakkers S., Rendell L. E. (2020a). Multi-scale analysis reveals changing distribution patterns and the influence of social structure on the habitat use of an endangered marine predator, the sperm whale Physeter macrocephalus in the Western Mediterranean Sea. Deep Sea Res. Part I: Oceanogr. Res. Pap. 155, 103169. doi: 10.1016/j.dsr.2019.103169
Pirotta E., Matthiopoulos J., MacKenzie M., Scott-Hayward L., Rendell L. (2011). Modelling sperm whale habitat preference: a novel approach combining transect and follow data. Mar. Ecol. Prog. Ser. 436, 257–272. doi: 10.3354/meps09236
Pirotta E., Vighi M., Brotons J. M., Dillane E., Cerdà M., Rendell L. (2020b). Stable isotopes suggest fine-scale sexual segregation in an isolated, endangered sperm whale population. Mar. Ecol. Prog. Ser. 654, 209–218. doi: 10.3354/meps13502
Quignard J.-P., Tomasini J. A. (2000). Mediterranean fish biodiversity. Biol. marina Mediterr. 7, 1–66.
R Core Team (2020) R: A Language and Environment for Statistical Computing. Available at: https://www.R-project.org/.
R Core Team (2023) R: A Language and Environment for Statistical Computing. Available at: https://www.R-project.org/.
Sawai E., Yamanoue Y., Nyegaard M., Sakai Y. (2018). Redescription of the bump-head sunfish Mola alexandrini (Ranzani 1839), senior synonym of Mola ramsayi (Giglioli 1883), with designation of a neotype for Mola mola (Linnaeus 1758) (Tetraodontiformes: Molidae). Ichthyol. Res. 65, 142–160. doi: 10.1007/s10228-017-0603-6
Snell M., Baillie A., Berrow S., Deaville R., Penrose R., Perkins M., et al. (2023). An investigation into the effects of climate change on baleen whale distribution in the British Isles. Mar. pollut. Bull. 187, 114565. doi: 10.1016/j.marpolbul.2022.114565
Stephens G., Akkaya Bas A., Hardy J., Araç N., Lyne P. (2021). Sightings and stranding reports of Fin whales (Balaenoptera physalus) in the Levantine Sea, with recent sightings from Turkey. jcrm 22, 55–60. doi: 10.47536/jcrm.v22i1.212
Tecchio S., Ramírez-Llodra E., Sardà F., Company J., Palomera I., Mechó A., et al. (2011). Drivers of deep Mediterranean megabenthos communities along longitudinal and bathymetric gradients. Mar. Ecol. Prog. Ser. 439, 181–192. doi: 10.3354/meps09333
Tetley M. J., Braulik G. T., Lanfredi C., Minton G., Panigada S., Politi E., et al. (2022). The important marine mammal area network: a tool for systematic spatial planning in response to the marine mammal habitat conservation crisis. Front. Mar. Sci. 9. doi: 10.3389/fmars.2022.841789
Thomas L., Buckland S. T., Rexstad E. A., Laake J. L., Strindberg S., Hedley S. L., et al. (2010). Distance software: design and analysis of distance sampling surveys for estimating population size. J. Appl. Ecol. 47, 5–14. doi: 10.1111/j.1365-2664.2009.01737.x
Tiralongo F., Lillo A. O., Tibullo D., Tondo E., Martire C. L., D’Agnese R., et al. (2019). Monitoring uncommon and non-indigenous fishes in Italian waters: One year of results for the AlienFish project. In Regional Stud. Mar. Sci. 28, 100606). doi: 10.1016/j.rsma.2019.100606
Tittensor D. P., Mora C., Jetz W., Lotze H. K., Ricard D., Berghe E. V., et al. (2010). Global patterns and predictors of marine biodiversity across taxa. Nature 466, 1098–1101. doi: 10.1038/nature09329
Torreblanca E., Báez J.-C., Real R., Macías D., García-Barcelona S., Ferri-Yañez F., et al. (2022). Factors associated with the differential distribution of cetaceans linked with deep habitats in the Western Mediterranean Sea. Sci. Rep. 12, 12918. doi: 10.1038/s41598-022-14369-6
Vella A., Murphy S., Giménez J., de Stephanis R., Mussi B., Vella J. G., et al. (2021). The conservation of the endangered Mediterranean common dolphin (Delphinus delphis): Current knowledge and research priorities. Aquat. Conserv: Mar. Freshw. Ecosyst. 31, 110–136. doi: 10.1002/aqc.3538
Verborgh P., Gauffier P., Esteban R., Giménez J., Cañadas A., Salazar-Sierra J. M., et al. (2016). Conservation status of long-finned pilot whales, globicephala melas, in the mediterranean sea. Adv. Mar. Biol. 75, 173–203. doi: 10.1016/bs.amb.2016.07.004
Whitehead H., McGill B., Worm B. (2008). Diversity of deep-water cetaceans in relation to temperature: implications for ocean warming: Cetacean diversity, temperature and global warming. Ecol. Lett. 11, 1198–1207. doi: 10.1111/j.1461-0248.2008.01234.x
Wilke C. O. (2022) ggridges: Ridgeline Plots in “ggplot2”. Available at: https://wilkelab.org/ggridges/.
Keywords: Mediterranean, density surfaces, modeling, cetacean, elasmobranch, large fish, abundance, distance sampling
Citation: Cañadas A, Pierantonio N, Araújo H, David L, Di Meglio N, Dorémus G, Gonzalvo J, Holcer D, Laran S, Lauriano G, Perri M, Ridoux V, Vázquez JA and Panigada S (2023) Distribution patterns of marine megafauna density in the Mediterranean Sea assessed through the ACCOBAMS Survey Initiative (ASI). Front. Mar. Sci. 10:1270917. doi: 10.3389/fmars.2023.1270917
Received: 01 August 2023; Accepted: 06 October 2023;
Published: 18 December 2023.
Edited by:
Holly Crystal Raudino, Department of Biodiversity, Conservation and Attractions (DBCA), AustraliaReviewed by:
Francesco Tiralongo, University of Catania, ItalyDimitrios K. Moutopoulos, University of Patras, Greece
Copyright © 2023 Cañadas, Pierantonio, Araújo, David, Di Meglio, Dorémus, Gonzalvo, Holcer, Laran, Lauriano, Perri, Ridoux, Vázquez and Panigada. This is an open-access article distributed under the terms of the Creative Commons Attribution License (CC BY). The use, distribution or reproduction in other forums is permitted, provided the original author(s) and the copyright owner(s) are credited and that the original publication in this journal is cited, in accordance with accepted academic practice. No use, distribution or reproduction is permitted which does not comply with these terms.
*Correspondence: Ana Cañadas, YW5hLmNhbmFkYXNAZHVrZS5lZHU=