- 1Centro de Investigación e Innovación para el Cambio Climático, Facultad de Ciencias, Universidad Santo Tomás, Santiago, Chile
- 2Programa de Doctorado en Conservación y Gestión de la Biodiversidad, Facultad de Ciencias, Universidad Santo Tomás, Santiago, Chile
- 3Centro de Investigación Austral Biotech, Facultad de Ciencias, Universidad Santo Tomás, Santiago, Chile
- 4Programa de Doctorado en Ciencias mención Biodiversidad y Biorecursos, Universidad Católica de la Santísima Concepción, Concepción, Chile
- 5Departamento de Ecología, Facultad de Ciencias, Universidad Católica de la Santísima Concepción, Concepción, Chile
- 6Departamento de Ecología y Biodiversidad, Facultad de Ciencias de la Vida, Universidad Andres Bello, Santiago, Chile
- 7Centro de Investigaciones marinas de Quintay, Facultad de Ciencias de la Vida, Universidad Andres Bello, Valparaíso, Chile
Parasites can alter the reproductive performance of their hosts, and to avoid or mitigate the resulting fitness loss, hosts may increase their current reproductive output to compensate for the future loss due to the parasitic infection. Fecundity compensation can be exploited by parasites for their own transmission (exploitation of host compensatory responses by parasites). However, this phenomenon has rarely been reported in second intermediate hosts of trematodes and its mechanisms and consequences largely unexplored. Along the east coast of the South Pacific, the second intermediate host, the mollusk Fissurella crassa, has been observed to display higher muscular foot, greater shell length and weight, and a higher gonadosomatic index when parasitized by metacercariaes of Proctoeces humboldti compared to non-parasitized hosts. In this study, we examined the histology, biochemistry (glucose, lipids, and proteins), and levels of sex hormones (estradiol and progesterone) in both parasitized and non-parasitized female individuals of F. crassa. Our findings revealed that the gonad of parasitized limpets had a higher density of oocytes, but these had a smaller individual area. Additionally, the gonadal tissue of parasitized limpets exhibited lower glucose content but higher lipid content. Notably, the levels of progesterone increased with parasite intensity. These results suggest that F. crassa possesses the ability to compensate for the negative effects of parasites by increasing the number of oocytes through biochemical and hormonal mechanisms. Our study contributes to the limited research on the impact of metacercariae on the reproduction of second intermediate hosts. Furthermore, we discuss how these changes in parasitized limpets could benefit parasite transmission.
1 Introduction
Several parasites induce phenotypic alterations in their hosts, which can enhance the parasites’ fitness at the expense of the hosts (see references in Fayard et al., 2020). These parasite-induced alterations can affect various traits, including physiology, reproduction, and appearance (Poulin, 1995; Moore, 2002; Thomas et al., 2005; Cézilly and Perrot-Minnot, 2010). Among the parasites in coastal ecosystems, digenean trematodes are highly abundant and often utilize mollusks as their first and second intermediate hosts (Bush et al., 1997; Poulin, 1998; Kuris et al., 2008). In first intermediate hosts, trematode infections can lead to reduced reproductive output or complete reproductive impairment (Sorensen and Minchella, 2001; Hall et al., 2007; Granovitch et al., 2009). Hosts, in response to the fitness loss, may evolve non-immunological defenses such as fecundity compensation, by adjusting their reproductive traits (Agnew et al., 2000; Hurd, 2009; Parker et al., 2011). However, the impact of trematodes on the reproduction of second intermediate hosts, as well as the existence of this type of non-immunological defenses, remains understudied (but see Fostera, 1958; Serbina, 2014; Parietti et al., 2020). The mechanisms underlying fecundity compensation are not fully understood, but previous research suggests that non-immunological defenses against parasites involve energetic, hormonal, and physiological pathways that involve a molecular dialogue between hosts and parasites (Hall et al., 2007; Pike et al., 2019; Gomez de Leon et al., 2021). In this study, we examined the histology, biochemistry, and sex hormone levels in the female gonads of Fissurella crassa, a limpet species parasitized by the digenean Proctoeces humboldti in the metacercaria stage. This host-parasite system is one of the most extensively studied systems on the eastern Pacific coast, in which previous research has demonstrated greater gonadal biomass of parasitized limpets compared to non-parasitized ones (Aldana et al., 2014; Aldana et al., 2020; García-Huidobro et al., 2020).
Mollusk-trematode interactions have been used as a model to investigate energy allocation strategies in parasitized organisms (Sandland and Minchella, 2003). When hosts are unable to resist infection through immune defense, they may compensate for the damage caused by parasites, adjusting their life history traits (Lefévre et al., 2008). Fecundity compensation and gigantism are two strategies that involve changes in life history traits and have been widely studied in mollusks serving as the first intermediate hosts for trematodes (Sorensen and Minchella, 2001; Ebert et al., 2004). Fecundity compensation involves a greater allocation of resources to reproduction or an earlier sexual maturity of adult or juvenile host individuals, respectively (Minchella and LoVerde, 1981; Thornhill et al., 1986). However, the recognition of these phenotypic alterations in second intermediate hosts is unclear, as the nature and outcome of host-parasite interactions may depend on species, life cycles, and contextual factors (Aldana et al., 2020; Liu and Gaines, 2022). From the parasite’s perspective, fecundity compensation benefits mechanisms that enhance their own fitness, such as prolonging its life within the host or increasing its transmission rates (Schmid-Hempel, 2021). Compensatory host responses to parasitism, including changes in fitness-related traits like survival, growth, fecundity, and competitiveness, can align with the transmission routes of parasites (Lefévre et al., 2008). Parasites can exploit selected compensatory responses in other ecological contexts, mimicking the causes that induce them and thus becoming the causal agents of the host’s compensatory response (Thomas et al., 2012).
In the coastal regions of Chile and Peru, the interaction between the fellodistomid trematode Protoeces humboldti George-Nascimento and Quiroga, 1983, and its second intermediate host, the keyhole limpets Fissurella spp. (Vetigastropoda), has received considerable attention (see Aldana et al., 2009; Aldana et al., 2014). The Fissurella limpets comprise a diverse group of 13 herbivorous species inhabiting intertidal and subtidal zones (McLean, 1984; Oliva and Castilla, 1992). The different species of Fissurella are dioecious and do not present evidence of sexual reversal or hermaphroditism (but see Olivares et al., 2009), they lack external sexual dimorphism and the sex is recognized by the color of the gonad, pale yellow to white in males and green in females (after dissection of the limpet) (Bretos, 1978; Bretos et al., 1983; Bretos et al., 1988; Bretos and Chihuailaf, 1990).
Proctoeces humboldti has a three-host life cycle (Oliva, 1984; Oliva and Zegers, 1988; George-Nascimento et al., 1998; Balboa et al., 2001; Oliva et al., 2018). In mussels, its first intermediate host, the parasite produces cercariae that then infect the gonad of keyhole limpets Fissurella spp., its second intermediate host, in which they develop into metacercariae. Infected limpets are subsequently ingested by the definitive host, the clingfish Syciases sanguineus, where the parasite reaches sexual maturity. In Fissurella species in general and in F. crassa in particular, the non-encysted metacercaria of P. humboldti is only found in the gonad, and is considered a progenetic metacercariae with precocious development that would facilitate the transmission of the parasite in intertidal conditions where the probability of consumption by S. sanguineus is low (Oliva and Huaquín, 2000; Balboa et al., 2001; Aldana, 2007; Oliva and Álvarez, 2011; Valdivia et al., 2014).
Previous studies on Proctoeces humboldti have suggested a positive effect of the parasite on its host Fissurella crassa (Aldana et al., 2014; Aldana et al., 2020; García-Huidobro et al., 2020). Parasitized individuals show larger shell length, muscle foot biomass, and gonadosomatic index (GSI) compared to non-parasitized individuals (Aldana et al., 2014). The increased body size of parasitized limpets is maintained across three localities in the southern Pacific of Chile, separated by approximately 1500 km (García-Huidobro et al., 2020). Moreover, particularly in areas of high biological productivity, parasitized individuals exhibited lower oxygen consumption than non-parasitized ones, suggesting lower metabolic demand in infected individuals (Aldana et al., 2020). Despite the positive impact of P. humboldti on body size and reproduction (GSI) in F. crassa, there is still a knowledge gap regarding the reproductive and biochemical aspects of parasitized limpets that allow this phenomenon to be described as the fecundity compensation. In addition, few examples exist of fecundity compensation in mollusks serving as second intermediate hosts for trematodes, and no studies have explored the underlying mechanisms of this phenomenon (but see Fostera, 1958; Serbina, 2014). In this study, we compared the histology, biochemistry, and sex hormone levels in the female gonads of F. crassa parasitized and non-parasitized by P. humboldti. Based on the positive effect of P. humboldti on the gonadosomatic index of F. crassa (Aldana et al., 2014; Aldana et al., 2020; García-Huidobro et al., 2020), our hypothesis is that the size and density of oocytes, the amount of glucose, proteins, and lipids, and the levels of estradiol and progesterone will be higher in parasitized individuals than in non-parasitized individuals, suggesting a potential mechanism of fecundity compensation in this host-parasite system.
2 Methods
2.1 Sample collection
In January 2019 (austral summer), a total of 121 individuals of Fissurella crassa with shell lengths ranging from 31 to 75 mm were collected from Quintay (33° 11′ S 71° 41′ W). In the laboratory, the limpet individuals were measured at their maximum shell length and dissected by removing their gonad. The gonad was examined under a stereomicroscope to verify the parasite presence. The gonad of 68 individuals was used for the gonadosomatic index evaluation, and the gonad of 53 female specimens of similar proportion between parasitized and non-parasitized were used for histological and biochemical analysis.
2.2 Gonadosomatic index evaluation of parasitized and non-parasitized F. crassa
The gonad tissue of each limpet was weighed (g), and sifted with water under high pressure. We examined the material retained in a 0.5-mm mesh sieve under a stereomicroscope, and counted and weighed all specimens of the P. humboldti parasite. The GSI was estimated as the proportion of gonadal mass to the body weight of the limpet (LB) and in parasitized individuals it considered the biomass of the parasite (PB) subtracted from the gonadal biomass (GB) [GSI= (GB-PB/LB)*100]. Additionally, the parasite biomass:GSI ratio for each host [PB/GSI], was used as an estimator for parasite severity or the proportion of F. crassa gonad (per unit of body size) that is occupied by P. humboldti (see Heins, 2012). The number of growth rings of each shell was also recorded in order to estimate limpet age (Bretos, 1980; Aldana et al., 2014; Aldana et al., 2020), thus correcting for age differences when comparing the GSI between parasitized and non-parasitized individuals.
2.3 Histological and biochemical analysis of parasitized and non-parasitized F. crassa
The female individuals were divided into three groups for evaluation: (1) gonadal histology (n=23), (2) measurement of glucose, lipids, and proteins in gonadal tissue (n=10), and (3) quantification of estradiol levels and progesterone in gonadal tissue (n=20).
(a) Gonadal histology
The gonad of 23 female F. crassa individuals was dissected, and in each of them, three pieces from the anterior (AS), middle (MS), and posterior (PS) sectors (see Supplementary Figure 1), were fixed in 10% saline formaldehyde. The remaining gonad was carefully examined under a stereomicroscope to confirm the presence and quantity of parasites (parasite intensity, Bush et al., 1997). The fixed gonadal sectors (14 parasitized and 9 non-parasitized) were dehydrated in ascending ethanol solutions, cleared with xylene, and embedded in paraffin. Three sections of 5 μm thickness were cut for each gonadal sector, mounted on glass slides, and stained with Harry’s hematoxylin-eosin (Supplementary Figure 1). From the three sections, 8 to 10 areas of 0.5 mm2 were randomly selected, and in each area the number of oocytes was counted (oocyte density). At the same time, between 8 and 10 photographs were taken to estimate the area (μm2) of each oocyte and its corresponding gelatinous layer (referred to as jelly coat). All photographs were taken with a Jenoptik® microscope camera mounted on a compound microscope (BX51 Olympus®). The images were analyzed using the image-processing software ProgRes® CapturePro 2.7.
(b) Glucose, lipids, and proteins content in gonads
The gonad of 10 female F. crassa individuals was dissected and carefully examined under a stereomicroscope to confirm the presence of P. humboldti specimens. Along with quantifying the number of parasites per host individual, gonadal tissue was extracted to quantify sex steroids (see below). The gonad tissue samples (~10 g wet weight, 5 parasitized and 5 non-parasitized) were frozen at -80°C and sent to the Laboratory of Hydrobiological Resources (LHR) at the Universidad Católica de la Santísima Concepción (UCSC, Concepción, Chile). At the LHR, the samples were dried using a lyophilizer (Operon, FDU-7012), ground, and stored in 15 mL Falcon tubes for the analysis of glucose, lipids, and proteins using the protocols described in Lazo-Andrade et al., 2021a, Lazo-Andrade et al., 2021b.
The total glucose content was quantified for 20 mg of dried sample (DW). The samples were homogenized with distilled water in a 1.5 mL Eppendorf tube and analyzed with the colorimetric Glucose IVD kit (Spinreact) using the quantitative determination method of the IVD Spin React kit for 20 min. Two hundred microliters of the reaction mixture from the kit were extracted, placed in a microplate (Biotek Elx808), and the absorbance was read at a wavelength of 490 nm using spectroscopy. The final glucose content was calculated according to Tietz (1995).
The total lipid content was measured using 20 mg of dry weight (DW) from each sample, weighed with a Sartorius digital analytical balance (d = 0.1 mg). The samples were mixed with 5 mL of the dichloromethane:methanol (2:1) solvent in 20 mL amber vials, following the method described by Folch et al. (1957), modified by Cequier-Sánchez et al. (2008). The samples were incubated in this solution in a 3L ultrasonic bath (MRC, AC-120H) with ultrapure water (Mili-Q) at 6°C for 10 min. Afterward, each sample was mixed with 4 mL of potassium chloride (0.88%) in centrifuge tubes, homogenized in a vortex for 15 s (Select Vortexer, model SBS100-2), and centrifuged (Boeco, model S-8) at 6°C and 1500 rpm for 5 min. The precipitate of each sample was collected in a group of amber empty vials, which were previously primed with dichloromethane-methanol (2:1), to measure their dry weights. The total amount of lipids in the gonad tissue was calculated as the difference between the dry weights of the empty amber vials and their dry weights after the collection of the lipids.
For protein content, 20 mg of dry sample (DW) was homogenized with distilled water in a 1.5 mL Eppendorf tube and analyzed with a colorimetric kit (Bio-Rad DC protein assay) following the manufacturer’s instructions. This kit is based on the methodology described by Lowry et al. (1951). Five microliters of the sample were mixed with the kit’s product, placed in a microplate (Biotek Elx808), and the absorbance was read at a wavelength of 750 nm using spectroscopy. The protein content was determined using a calibration curve based on the readings of successive dilutions of bovine albumin serum from the kit (BM-0880, BSA Winkler).
(c) Quantification of sex steroids in gonads
Variations in the reproductive development of mollusks can be evaluated by measuring the levels of steroids (e.g., estradiol and progesterone), as several studies have shown that these hormones play a role in the regulation of invertebrate reproduction processes (Wang and Croll, 2004; Gauthier-Clerc et al., 2006; Croll and Wang, 2007; Lafont and Mathieu, 2007; Chong et al., 2019). The gonad of 20 female F. crassa individuals was carefully examined under a stereomicroscope to confirm the presence and quantity of parasites. Later, the gonads (11 parasitized and 9 non-parasitized) were frozen with dry ice and preserved at -80°C. Gonad samples (160-190 mg) were individually homogenized in ultrapure water (1:5 w:w) and sonicated twice for 30 s. Four hundred microliters of 25 mM HCl were added to 500 μl of the homogenate and allowed to stand for 15 min at 40°C. Then, 1.25 ml of 0.07 M Na2HPO4 (pH 7.4) was added before the organic extraction (Siah et al., 2022). Homogenates were mixed with 10 ml of dichloromethane, stirred for 1 minute, and allowed to stand. The aqueous phase was separated and subjected to a second extraction with 12 ml of dichloromethane. The organic phases from the first and second extractions were evaporated at 30°C using ultra-pure nitrogen to prevent oxidation of the steroids. The dried extracts were resuspended in 400 μl of the EIA solution (EIA kits from Cayman Chemicals). From the gonadal extracts, the concentrations of estradiol and progesterone were estimated using an enzyme-linked immunosorbent assay (ELISA) kit following the manufacturer’s instructions (Cayman Chemical Company). The solution was quantified spectrophotometrically in a microplate reader (model Autobio Phomo mark) at an absorbance of 405 nm. The results were normalized by the weight of the gonad and expressed as pg hormone per g wet tissue. The standard curve for estradiol was constructed in a range of 6.6 to 4000 pg/ml, and for progesterone in a range of 15.6 to 1000 pg/ml.
2.4 Statistical analysis
General Linear Model (GLM) was used to compare the GSI between parasite condition (P and NP) and ages (two to four growth rings). Additionally, the relationship between parasite biomass:GSI ratio and GSI was explored using a linear regression analyses (GLM). Preliminary analyses did not show any differences in jelly coat, oocyte area, and oocyte density between gonad sectors (AS, MS, and PS). Thus, to avoid pseudoreplication problems, the jelly coat, oocyte area, and oocyte density were averaged for each individual. Histological and biochemical data (Jelly coat, Oocyte area, Oocyte density, Glucose, Lipids, Proteins, Estradiol and Progestore) were compared between parasite condition using the shell length as co-variable (GLM) with a Poisson “link” function (link=“log”). In addition, the relationship between the amount of sex steroids and the parasite intensity was determined by Pearson correlation analyses. All analyzes were performed using R (R Core Team, 2023).
3 Results
Our results indicate that 59.5% of F. crassa individuals harbored between 1 and 32 individuals of the P. humboldti parasite. The gonadosomatic index of limpets increases from two to four growth rings (Figure 1). However, independent of age and consistent with previous reports (Aldana et al., 2014; Aldana et al., 2020; García-Huidobro et al., 2020), parasitized limpets show a higher GSI than non-parasitized limpets (Figure 1, Table 1). The log-normal fit regression analysis (GLM) shows that the GSI does not vary significantly with increasing parasite:host biomass ratio (χ2(1, 23)= 0.15, P= 0.696, R2pseudo= 0.03. Figure 2).
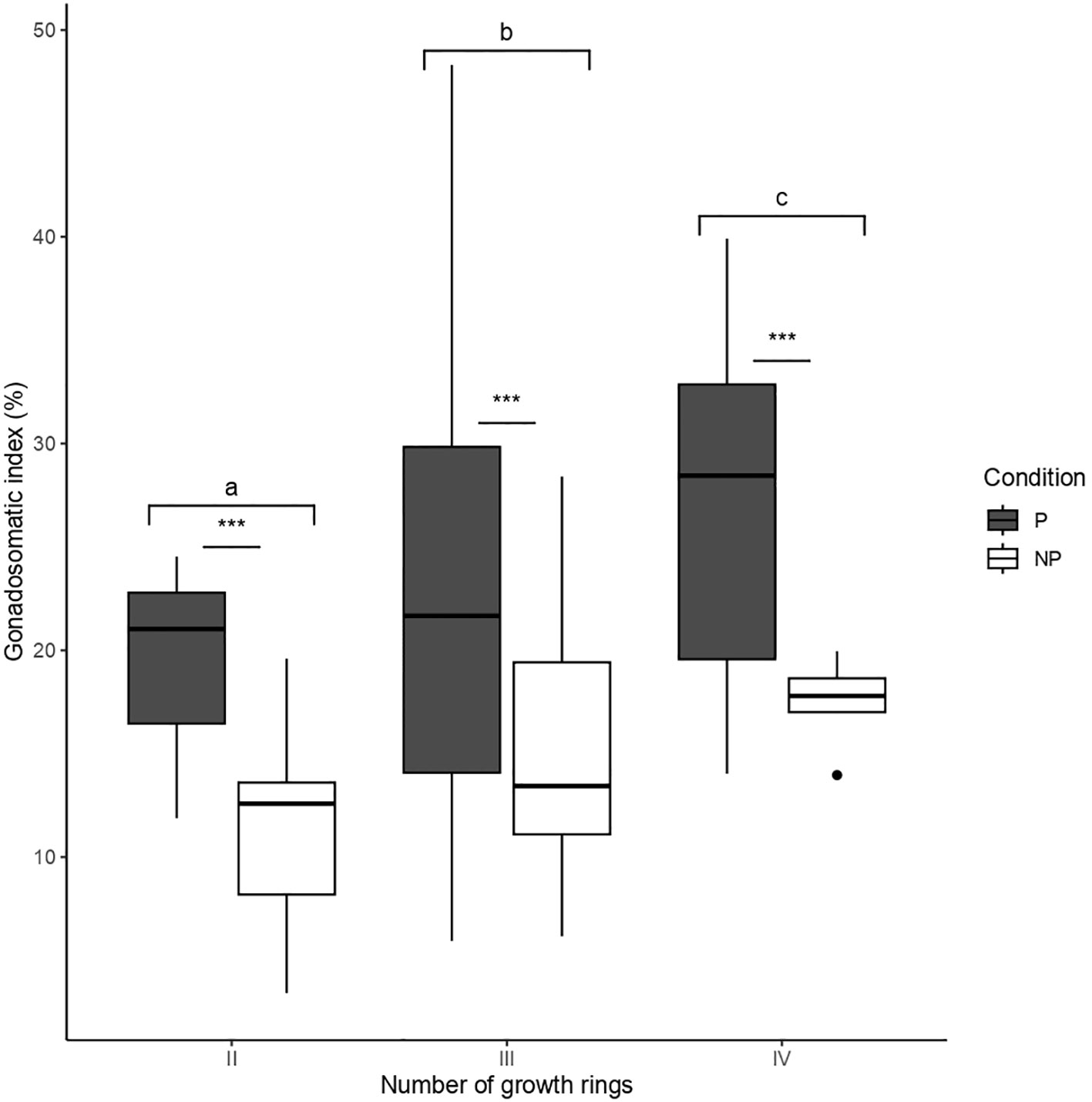
Figure 1 Average gonadosomatic index of Fissurella crassa limpets according to parasitism condition and the number with different numbers of growth rings (age). Asterisks and letters indicate significant differences between parasitized and non-parasitized, and between those with different growth rings number, respectively.
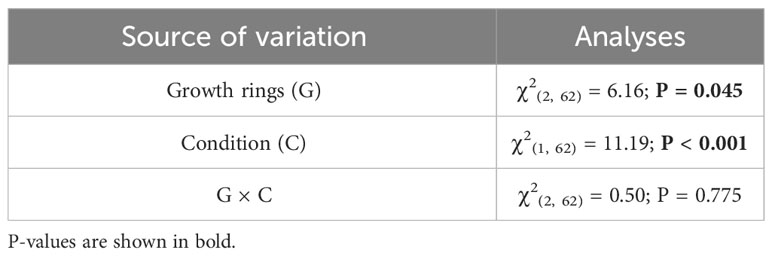
Table 1 Generalized linear model for the comparison of Gonadosomatic index between growth rings and parasitized and non-parasitized F. crassa individuals.
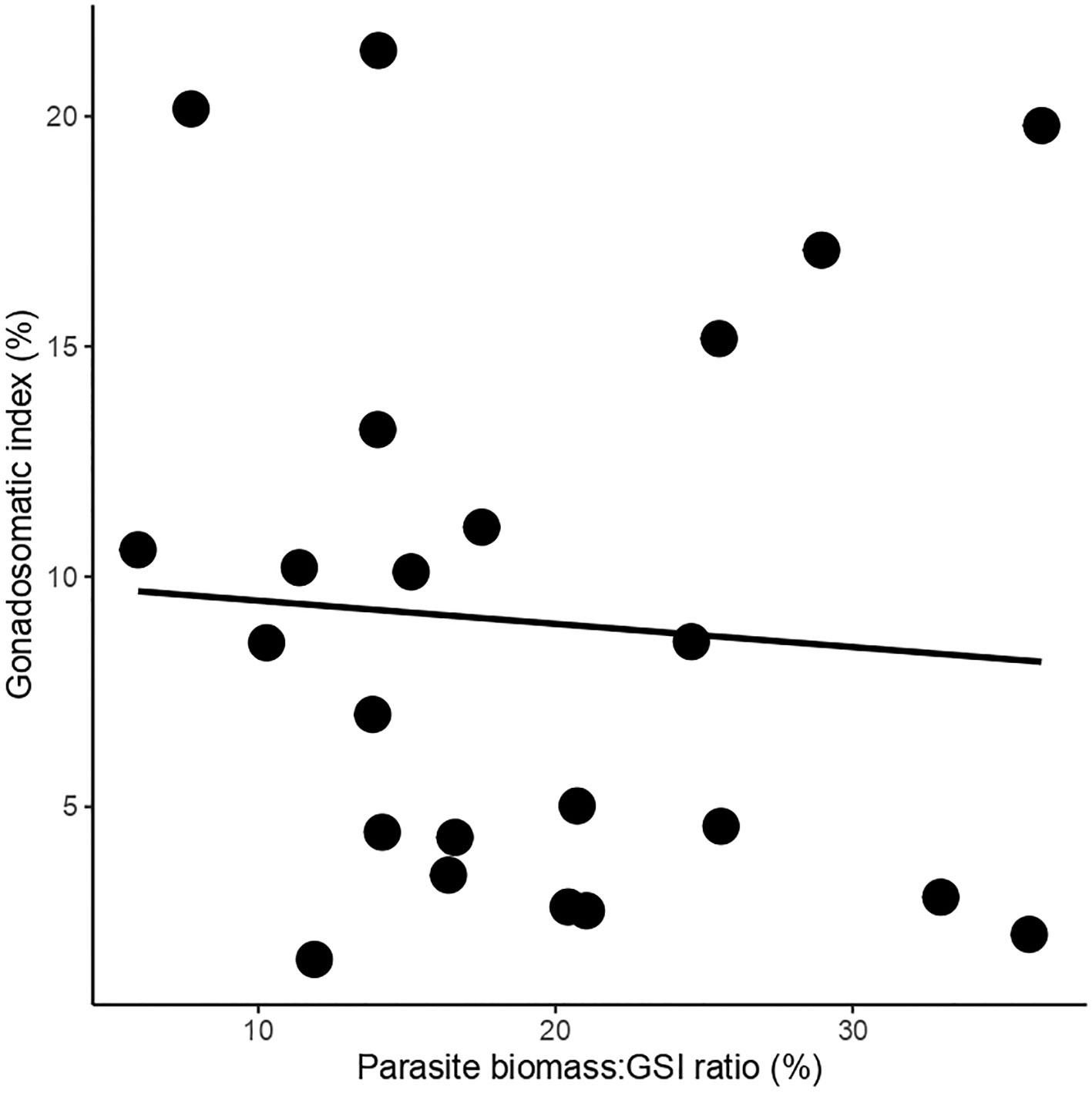
Figure 2 Relationship between the gonadosomatic index (GSI) and the parasite biomass:GSI ratio, for each Fissurella crassa host individual.
Gonadal histology of parasitized and non-parasitized F. crassa
In general, histological characterization of the limpet gonad reveals an epithelium covering the ovary with cubic cells and underlying connective tissue. A septum, composed of muscular fibers and other fibrillar elements, extends into the gonad (Figure 3A). Most oocytes display a nucleus and a gelatinous layer that covers the oocyte (Figure 3B). However, parasitized gonads, show tissue damage around the oocytes and a collagen-like fibrillar element around the parasite; while more distant from the site of parasite insertion, near the periphery of the gonad, normal tissue with epithelium and connective tissue is observed (Figures 3C, D).
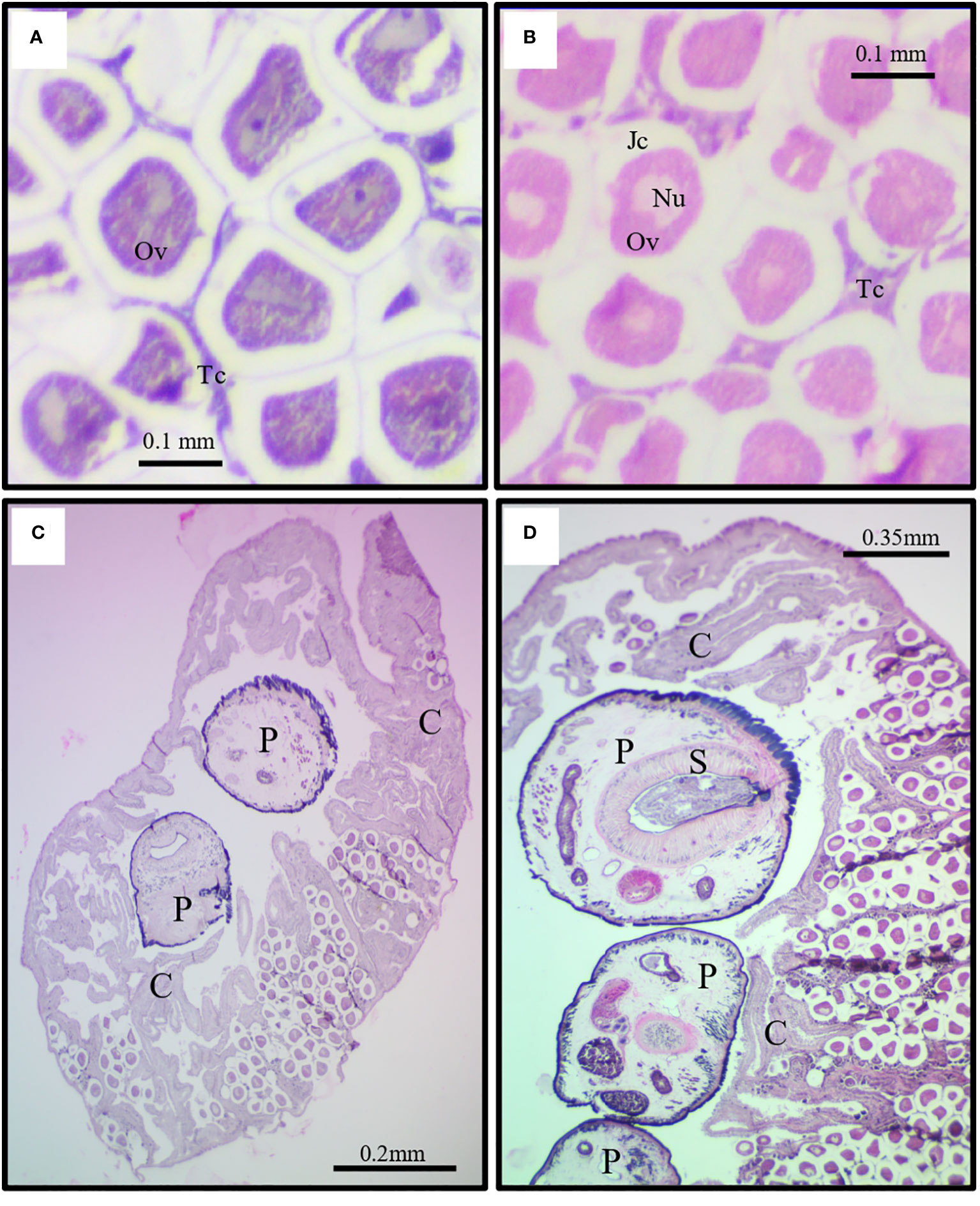
Figure 3 Histological sections of (A) non-parasitized and (B-D) parasitized female gonads of (F) crassa with the digenean P. humboldti. Tc, connective tissue; Ov, oocyte; Jc, jelly coat; Nu, oocyte nucleus; P, P. humboldti parasite; S, sucker; C, collagen-like fibrillar element.
Quantitatively, although the jelly coat area was similar between parasitized and non-parasitized gonads (Table 2, Figure 4A), the area and density of oocytes presented significant differences with opposite trends, associated with the parasitism condition. While oocyte area was greater in non-parasitized compared to parasitized gonads (Table 2, Figure 4B), oocyte density was greater in parasitized than in non-parasitized gonads (Table 2, Figure 4C). These results considered the effect of host length on the variation of each response variable (Table 2, Figure 4).
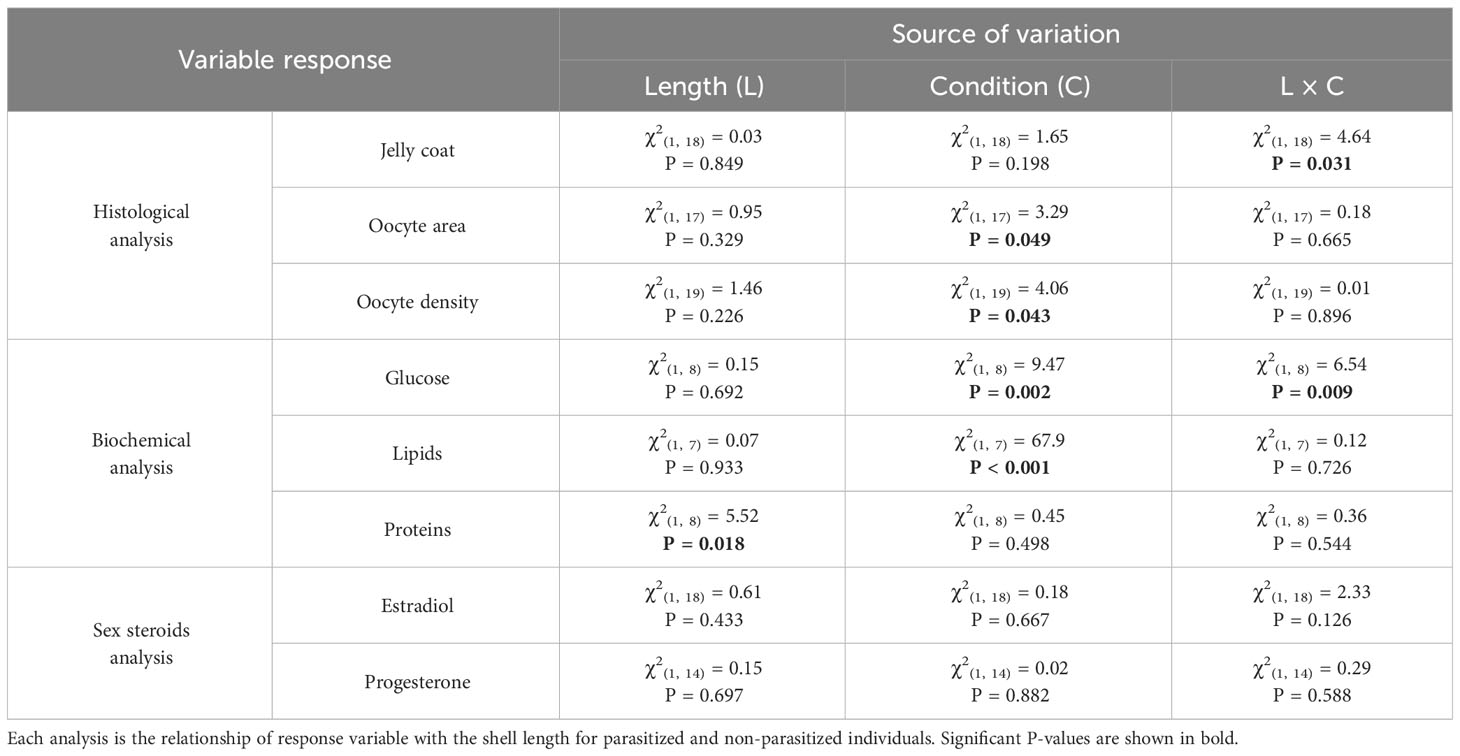
Table 2 Generalized linear model results for Histological analysis (Jelly coat, Oocyte area and Oocyte density), Biochemical analysis (Glucose, Lipids and Proteins), and Sex steroids amounts (Estradiol and Progesterone) of F. crassa gonads.
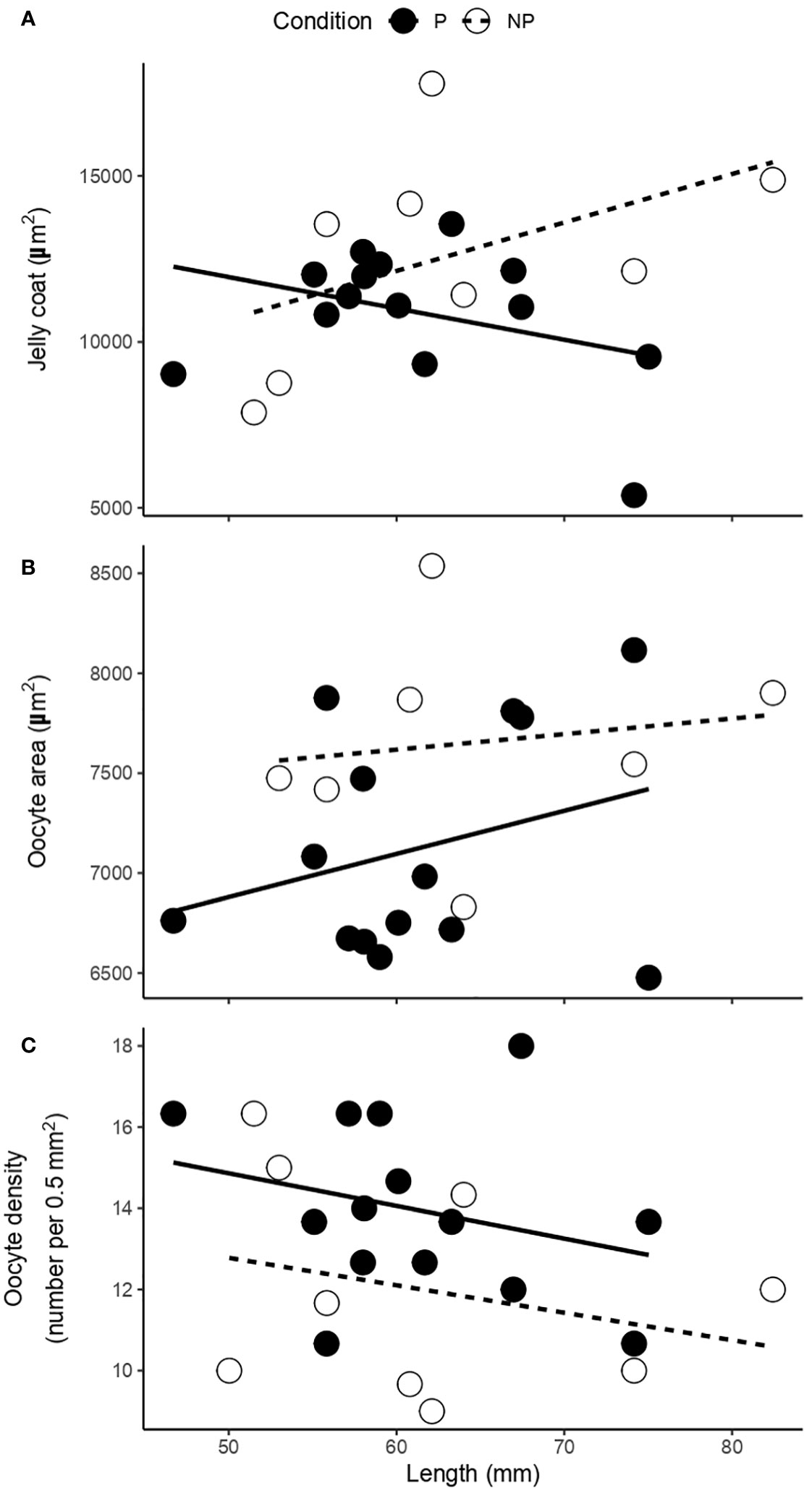
Figure 4 Relationship between shell length and (A) jelly coat, (B) oocyte area, and (C) oocyte density of female gonads of F. crassa parasitized (P) and non-parasitized (NP) by the digenean P. humboldti.
Glucose, lipids, and proteins content in gonads of parasitized and non-parasitized F. crassa
While the amount of glucose was greater in non-parasitized than in parasitized gonads (Table 2, Figure 5A), parasitized limpets presented around 44% more lipids in their gonad than non-parasitized limpets (Table 2, Figure 5B). The amount of proteins, on the other hand, was similar between gonads of different infection conditions (Table 2, Figure 5C). These results also considered the effect of host length on the variation of each response variable (Table 2, Figure 5).
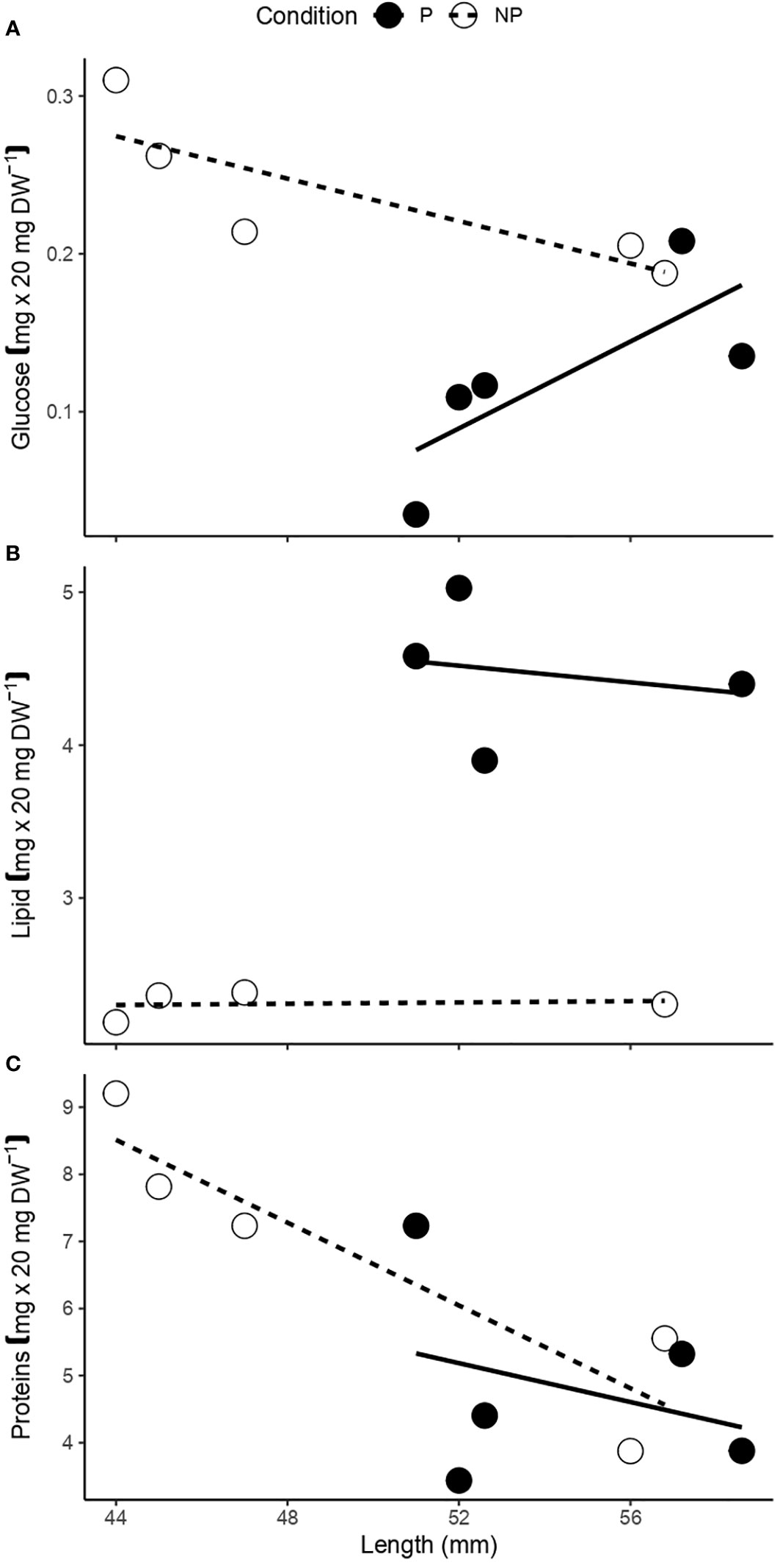
Figure 5 Relationship between shell length and the amount of (A) glucose, (B) lipids, and (C) proteins, present in the female gonad of F. crassa parasitized (P) and non-parasitized (NP) by the digenean P. humboldti.
Quantification of sex steroids in gonads of parasitized and non-parasitized F. crassa
Neither host length nor parasitism condition were significantly important on the variation in hormone levels (estradiol and progesterone, Table 2, Figures 6A, B). However, the level progesterone increased significantly with parasite intensity (Estradiol: F(1, 8) = 0.64, R2 = 0.07, P = 0.445. Progesterone: F(1, 6) = 6.74, R2 = 0.52, P = 0.040. Figures 6C, D).
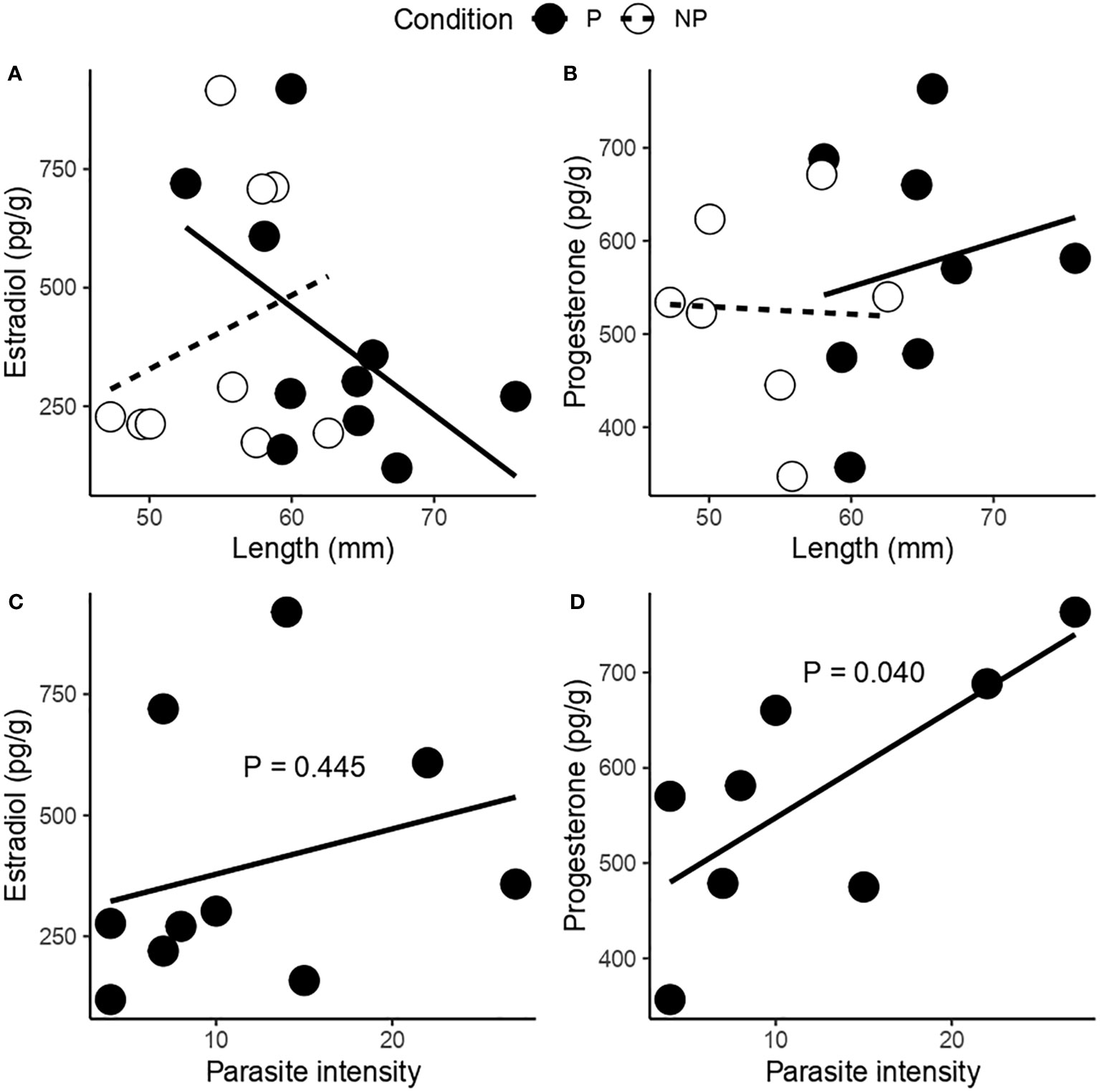
Figure 6 Relationship between shell length and the amount of (A) estradiol, and (B) progesterone present in the female gonad of F. crassa parasitized (P) and non-parasitized (NP) by the digenean P. humboldti. Relationship between parasite intensity and the amount of (C) estradiol, and (D) progesterone present in the female gonad of F. crassa parasitized by the digenean P. humboldti.
4 Discussion
The increase in the gonadosomatic index (GSI) associated with the presence of P. humboldti (Figure 1) is consistent with previous reports for F. crassa from the central zone of Chile (Aldana et al., 2014; Aldana et al., 2020; García-Huidobro et al., 2020). In the present study we also show that parasitized limpets have gonads with a higher oocyte density (with a smaller area, Figures 4B, C), lower amount of glucose (Figure 5A), and greater amount of lipids (Figure 5B) than non-parasitized limpets. Additionally, a positive association between the number of parasites and the amount of progesterone in gonadal tissue was observed (Figure 6D). A host may respond to the presence of some trematode infections by varying its investment into current reproduction, in quantity and/or quality of offspring (Duffield et al., 2017), to make up for lost future reproduction (Minchella and LoVerde, 1981; Minchella, 1985; Lafferty, 1993; Krist, 2001). This fecundity compensation could be associated with instances where the host cannot effectively prevent the establishment of an infection or immunologically suppress it (Parker et al., 2011) and has been widely reported in invertebrate first intermediate hosts of trematodes (Minchella and LoVerde, 1981; Thornhill et al., 1986; Lafferty, 1993; Krist, 2001). Consequently, infected hosts should attempt to achieve maximum reproductive success before the cumulative costs of infection become detrimental to their fitness (Agnew et al., 2000). In this sense, our results suggest that second intermediate host F. crassa can also compensate for the effect of P. humboldti metacercaria, increasing the GSI, mediated by biochemical and hormonal pathways.
Data on the effects of trematodes on the reproduction of their second mollusk intermediate hosts are scarce and isolated. The response of the snail Bithynia troscheli to the presence of trematode metacercariae constitutes, to our knowledge, the first evidence of fecundity compensation in a second intermediate host (Serbina, 2014). The increase in the percentage of reproductively active host females, at low infection levels, was considered as a mechanism that could compensate for the subsequent reduction in snail fecundity, as a result of the increase in trematode intensity (Serbina, 2014). Studies carried out on the shrimp Palaemonetes argentinus, the second intermediate host for the digenean Microphalus szidati, demonstrated that parasitized females produced more embryos, but had more egg loss throughout embryonic development than non-parasitized females (Merlo et al., 2016; Parietti et al., 2020). In this sense, female shrimp should produce a greater number of offspring at the early season to compensate for egg losses as a result of being parasitized. Similarly, the higher density (Figure 4C) and smaller area (Figure 4B) of oocytes in parasitized F. crassa, i.e. greater number of oocytes, would also be a compensatory response for the posterior reduction in fecundity due to the parasite.
In a study carried out on limpets F. crassa from Perú, Oliva (1992) reported structural and functional disorganization of the gonadal tissue in parasitized individuals by Proctoeces humboldti, preventing sexual identification of the gonad and inhibiting the gametogenesis process in advanced infections. However, the gonad of specimens collected from the northern and central-southern areas of Chile does not present evidence of damage of this type associated with P. humboldti, and even in particular conditions, parasitized limpets present more gonad with respect to the body (GSI) than those that are not parasitized (Aldana et al., 2014; Aldana et al., 2020; García-Huidobro et al., 2020). On the other hand, induced spawning in F. crassa from northern Chile showed that the fecundity of limpets, estimated as the number of eggs/mm of shell, decreased significantly with increasing parasite intensity of P. humboldti (Oliva and Vega, 1994). In this sense, the increase in the IGS and ultimately, the number of parasitized F. crassa oocytes could compensate for the subsequent reduction in the number of spawned eggs. Although it is unknown whether this decrease in spawning is a widespread phenomenon in Chile, its association with the increase in parasite intensity, which according to our results is positively related to the level of progesterone (Figure 6D), suggests a compensatory response of parasitized F. crassa against the oocyte losses during spawning. In this sense, increased fecundity would reflect a trade-off between the number and size of eggs (Einum and Fleming, 2000; Rollingson and Hutchings, 2013), with eggs from infected females having a smaller area, as has been observed in other host-parasite systems (Heins, 2012; Parietti et al., 2020).
Previous studies have shown that parasitized limpets F. crassa have more parasite intensity in sites where the GSI is also higher, suggesting that the increase in gonadal biomass could provide more space for the accumulation of metacercariae (Aldana et al., 2014; Aldana et al., 2020; García-Huidobro et al., 2020). In fact, our results show that the proportion of F. crassa gonad (per unit of body size) occupied by P. humboldti increases, although the gonadal mass/body mass ratio of the limpet (GSI) remains relatively stable (Figure 2). Shell alterations of gastropods induced by parasite trematodes have been reported in several systems as a strategy that would allow the parasites to increase the volume within the host and provide more space for the accumulation of greater numbers of individuals (McCarthy et al., 2004; Hay et al., 2005; Levri et al., 2005; Lagrue et al., 2007; Thieltges, 2009). For example, changes in the shell shape of the periwinkle Littorina saxatilis caused by the trematode Microphallus piriformes resulted in a 12% increase in the total volume inside the shell (McCarthy et al., 2004). The resulting increase in space is particularly advantageous for trematodes with abbreviated life-cycles, allowing parasite growth and reproduction without further reduction of host tissue. Consistently, it has been proposed that P. humboldti metacercariae would abbreviate their life cycle through progenesis, developing precociously into an adult due to the low probability of consumption by the definitive host (Oliva and Huaquín, 2000; Balboa et al., 2001; Aldana, 2007; Oliva and Álvarez, 2011). However, regardless of transmission opportunities, the long residence time of P. humboldti in Fissurella (George-Nascimento et al., 1998; Balboa et al., 2001; Aldana, 2007), would promote precocious maturity and egg production by the parasite in response to some internal developmental clock (Poulin & Cribb, 2002; Lagrue and Poulin, 2009).
Until now, the greater fecundity of parasitized limpets compared to non-parasitized limpets of the same age had been attributed to the increase in body size of F. crassa associated with P. humboldti (Aldana et al., 2014; Aldana et al., 2020). Here, we present evidence that the increase in reproductive performance of parasitized limpets could also be mediated by an increase in oocyte density or, equivalently, gonadal biomass per unit volume. That is, assuming that a parasitized gonad (minus the parasites) and a non-parasitized one occupy the same space/volume, the parasitized gonad will have a greater biomass. If this effect of P. humboldti on the oocyte density of F. crassa is coupled to the increase in body size and therefore the greater gonadal space/volume of limpets, an even greater reproductive performance of parasitized F. crassa is expected. From the host’s perspective, however, the reproductive performance evaluated through the GSI shows astonishing results. Firstly, it is greater in parasitized than in non-parasitized limpets; and secondly, it remains relatively stable as the number of parasites increases, instead of decreasing as would be expected given the energetic costs of parasite maintenance (Price, 1980; Poulin, 2007; Goater et al., 2014) and expression of host phenotypic alterations (Maure et al., 2013). In this context, parasitized F. crassa limpets appear to be showing greater reproductive effort than their non-parasitized counterparts. However, this capacity, as well as a lower energy demand associated with infection, occur in highly productive sites close to upwelling centers such as Quintay where this study was carried out (Aldana et al., 2020; García-Huidobro et al., 2020). The availability and quality of food in these environments would benefit the energy budget of F. crassa (Pulgar et al., 2013) and attenuate the conflict for resources with P. humboldti, promoting the welfare of the parasite-host system.
In general, elevated levels of glycogen and/or lipids have been identified in several host-helminth systems (Amat et al., 1991; Jokela et al., 1993; Plaistow et al., 2001; Ponton et al., 2005; Korkofigas et al., 2016) associated with the numerous energetic demands experienced. Likewise, the gonad of parasitized F. crassa presented a higher level of lipids, but a lower level of glucose (Figures 5A, B). Particularly, non-immunological defenses such as the compensatory responses in fecundity described for F. crassa, are costly to activate and maintain (Parker et al., 2011) and the lower glucose content in infected gonad supports this proposal. Similarly, Gammarus insensibilis amphipods that are more active when infected with metacercariae of the trematode Microphallus papillorobustus, had a lower glucose level than the uninfected ones (Ponton et al., 2005). In addition, the higher lipid concentration in parasitized limpets compared to non-parasitized ones may be related to the increase in oocyte density, as lipids are considered to be the nutritional source for gonad development (e.g., Soudant et al., 1996; Ke and Li, 2013). On the other hand, alterations in protein levels in gastropods during trematode infection suggest that their occurrence is associated with the identity of the host-parasite systems, food availability, and environmental conditions (Thompson, 1983). Our findings did not reveal any difference in protein quantity between parasitized and non-parasitized gonads (Figure 5C), suggesting that specific enzymatic processes may be functioning adequately even in parasitized individuals. Unfortunately, unlike previous studies (e.g., Jones, 1989; Santarém et al., 1994), we did not assess protein, amino acid and/or lipids composition in relation to parasitism condition. Information on the structure and composition of macromolecules could be useful when comparing parasitized and non-parasitized individuals.
Knowledge of the endocrine system’s role in the maturation of sex organs and the induction of sexual behavior is relatively limited in invertebrates. Although the information obtained from different species strongly suggests the involvement of vertebrate-type steroids in the control of mollusk reproduction (Lafont and Mathieu, 2007; Fernandes et al., 2011), its endogenic biosynthesis and its role in endocrine regulation has been questioned (Scott, 2012; Scott, 2013; Scott, 2018). Recent studies on possible pathways for sex steroid synthesis and regulation, have provided evidence for the hypothesis of endogenous production of sex steroids and gonadal maturity regulation by invertebrates (Thitiphuree et al., 2018; Zhang et al., 2020). Thus, considering that there is evidence against but also in support of the involvement of steroidal factors in the reproduction of mollusks (see review in Pandian, 2017), it is appropriate to evaluate the relationship between these factors and parasitism by P. humboldti, which influences the GSI of F. crassa. In this regard, although estradiol and progesterone levels showed a similar magnitude between parasitized and non-parasitized gonads (Figures 6A, B), progesterone levels increased with parasite intensity (Figure 6D). Fluctuations in testosterone, estradiol and progesterone levels in the hemolymph of F. cumingi limpets were related to the reproductive state, with a greater presence of estradiol and testosterone associated with periods of vitellogenesis and gonadal renewal (increase in GSI), while a greater progesterone level during spawning events or lower reproductivity activity (Honour, 2002). Similarly, the ovarian concentration of progesterone in F. crassa also presented variations around the year, with a peak prior to the period of maximum IGS and another peak after a secondary increased IGS (Del Campo et al., 2005). Thus, the increase in steroid hormones, including estradiol and progesterone, may explain the increase in the number of oocytes in infected limpets, although only progesterone increased with parasite intensity.
Parasite-mediated host phenotypic alterations have traditionally been examined as host adaptations that reduce the harmful consequences of infection, parasite adaptations that facilitate its transmission, or secondary outcomes of infection without adaptive value (Minchella, 1985; Moore, 2002; Thomas et al., 2005). However, from an evolutionary perspective, host phenotypic changes following infection could be trade-offs between host and parasite strategies, where natural selection should favor parasites that are able to initiate compensatory host responses that benefit parasite transmission and host fitness (Lefévre et al., 2008). In this scenario, such interactions could move a continuum between parasitism and mutualism where the environmental context would have a key role (Leung and Poulin, 2008; Fellous and Salvaudon, 2009). In the interaction F. crassa-P. humboldti, fecundity compensation could be a mechanism used by both the host and the parasite (Lefévre et al., 2008), which would not only mitigate the negative impact if the parasite on the host’s spawning, but also provide greater space to the progenetic metacercariae. The release of P. humboldti eggs into the environment could be synchronized with host spawning. Empirical data from different systems are required to elucidate the mechanistic processes behind these phenomena, as well as an integrated consideration from different disciplines (e.g. parasitology, physiology, biochemistry and ecology), in the performance of closely interacting species.
Data availability statement
The raw data supporting the conclusions of this article will be made available by the authors, without undue reservation.
Ethics statement
The animal study was approved by Daniel Valenzuela Universidad Santo Tomás. The study was conducted in accordance with the local legislation and institutional requirements.
Author contributions
MG-H: Conceptualization, Data curation, Formal analysis, Funding acquisition, Investigation, Methodology, Resources, Supervision, Visualization, Writing – original draft, Writing – review & editing. MR: Investigation, Methodology, Writing – original draft. NF: Investigation, Methodology, Resources, Writing – review & editing. TB: Investigation, Methodology, Resources, Writing – review & editing. FG-R: Investigation, Methodology, Writing – original draft. ÁU: Investigation, Methodology, Resources, Writing – review & editing. JP: Conceptualization, Funding acquisition, Investigation, Resources, Writing – original draft, Writing – review & editing. MA: Conceptualization, Funding acquisition, Investigation, Methodology, Resources, Writing – original draft, Writing – review & editing.
Funding
The author(s) declare financial support was received for the research, authorship, and/or publication of this article. MG-H and MA appreciate the support of FONDECYT 11220593 and 1220866 respectively. JP acknowledge FONDECYT 1200813.
Conflict of interest
The authors declare that the research was conducted in the absence of any commercial or financial relationships that could be construed as a potential conflict of interest.
Publisher’s note
All claims expressed in this article are solely those of the authors and do not necessarily represent those of their affiliated organizations, or those of the publisher, the editors and the reviewers. Any product that may be evaluated in this article, or claim that may be made by its manufacturer, is not guaranteed or endorsed by the publisher.
Supplementary material
The Supplementary Material for this article can be found online at: https://www.frontiersin.org/articles/10.3389/fmars.2023.1266405/full#supplementary-material
References
Agnew P., Koella J. C., Michalakis Y. (2000). Host life history responses to parasitism. Microbes Infect. 2, 891–896. doi: 10.1016/S1286-4579(00)00389-0
Aldana M. (2007). Variación espacial de la interacción hospedador-parásito: la importancia de la densidad poblacional de los hospedadores ( Santiago, Chile: Pontificia Universidad Católica de Chile, Santiago), 214. Ph.D. Thesis.
Aldana M., Gonzalez K., Loor G., Pulgar J., Marquet P. A. (2009). First intermediate host of the digenean trematode Proctoeces lintoni (Fellodistomidae) in Chile. J. Parasitol. 95, 1408–1414. doi: 10.1645/GE-2004.1
Aldana M., Pulgar J., Hernández B., George-Nascimento M., Lagos N. A., García-Huidobro M. R. (2020). Context-dependence in parasite effects on keyhole limpets. Mar. Environ. Res. 157, 104923. doi: 10.1016/j.marenvres.2020.104923
Aldana M., Pulgar J. M., Orellana N., Ojeda F. P., García-Huidobro M. R. (2014). Increased parasitism of limpets by a trematode metacercaria in fisheries management areas of central Chile: effects on host growth and reproduction. EcoHealth 11, 215–226. doi: 10.1007/s10393-013-0876-9
Amat F., Gozalbo A., Navarro J. C., Hontoria F., Varó I. (1991). Some aspects of Artemia biology affected by cestode parasitism. Hydrobiologia 212, 39–44. doi: 10.1007/BF00025985
Balboa L., George-Nascimento M., Ojeda F. P. (2001). Differential precocious sexual development of Proctoeces lintoni (Digenea: fellodistomidae) in three sympatric species of Keyhole limpets Fissurella spp. may affect transmission to the final host. J. Parasitol. 87, 1164–1167. doi: 10.1645/0022-3395(2001)087[1164:DPSDOP]2.0.CO;2
Bretos M. (1978). Growth in the keyhole limpet Fissurella crassa Lamarck (Mollusca: Archaeogastropoda) in northern Chile. Veliger 21, 268–273.
Bretos M. (1980). Age determination in the keyhole limpet Fissurella crassa Lamarck (Archeogastropoda: Fissurellidae), based on shell growth rings. Biol. Bull. 159, 606–612. doi: 10.2307/1540826
Bretos M., Chihuailaf R. (1990). Biometría y otros aspectos biológicos de Fissurella pulchra (Mollusca: Prosobranquia). Rev. Biol. Mar. Oceanog. 25, 1–14.
Bretos M., Gutiérrez J., Espinoza Z. (1988). Estudios biológicos para el manejo de Fissurella picta. Medio Ambiente 9, 55–62.
Bretos M., Tesoneri I., Alvarez L. (1983). The biology of Fissurella maxima Sowerby (Mollusca: Archaeogastropoda) in Northern Chile. 2. Notes on its reproduction. Biol. Bull. 165, 559–568. doi: 10.2307/1541465
Bush A. O., Lafferty K. D., Lots J., Shostak A. (1997). Parasitology meet ecology in its own terms: Margolis et al. revisited. J. Parasitol. 83, 575–583. doi: 10.2307/3284227
Cequier-Sánchez E., Rodríguez C., Ravelo A. G., Zárate R. (2008). Dichloromethane as a solvent for lipid extraction and assessment of lipid classes and fatty acids from samples of different natures. J. Agric. Food. Chem. 56, 4297–4303. doi: 10.1021/jf073471e
Cézilly F., Perrot-Minnot M. J. (2010). Interpreting multidimensionality in parasite-induced phenotypic alterations: panselectionism versus parsimony. Oikos 119, 1224–1229. doi: 10.1111/j.1600-0706.2010.18579.x
Chong F., Enríquez M., Aldana D. (2019). Cuantificación de hormonas 17β - estradiol y progesterona en el caracol rosa Lobatus gigas (Mollusca, Gastropoda). Rev. Biol. Trop. 67, 708–715. doi: 10.1551/rbt.v67i4.34793
Croll R. P., Wang C. (2007). Possible roles of sex steroids in the control of reproduction in bivalve molluscs. Aquaculture 272, 76–86. doi: 10.1016/j.aquaculture.2007.06.031
Del Campo A., Huaquín L. G., Urquieta B. (2005). Germinal line and progesterone concentration of the ovary in Fisurella crassa (Lamarck 1822). Av. Cienc. Vet. 20, 54–60.
Duffield K., Bowers E., Sakaluk S., Sadd B. (2017). A dynamic threshold model for terminal investment. Behav. Ecol. Sociobiol. 71, 1–17. doi: 10.1007/s00265-017-2416-z
Ebert D., Carius H. J., Little T., Decaestecker E. (2004). The evolution of virulence when parasites cause host castration and gigantism. Amm. Nat. 164, S19–S32. doi: 10.1086/424606
Einum S., Fleming I. A. (2000). Highly fecund mothers sacrifice offspring survival to maximize fitness. Nature 405, 565–567. doi: 10.1038/35014600
Fayard M., Dechaume-Moncharmont F. X., Wattier R., Perrot-Minnot M. J. (2020). Magnitude and direction of parasite-induced phenotypic alterations: a meta-analysis in acanthocephalans. Biol. Rev. 95, 1233–1251. doi: 10.1111/brv.12606
Fellous S., Salvaudon L. (2009). How can your parasites become your allies? Trends. Parasitol. 25, 62–66. doi: 10.1016/j.pt.2008.11.010
Fernandes D., Loi B., Porte C. (2011). Biosynthesis and metabolism of steroids in molluscs. J. Steroid. Biochem. Mol. Biol. 127, 189–195. doi: 10.1016/j.jsbmb.2010.12.009
Folch J., Lees M., Stanley G. S. (1957). A simple method for the isolation and purification of total lipids from animal tissues. J. Biol. Chem. 226, 497–509. doi: 10.1016/S0021-9258(18)64849-5
Fostera R. (1958). The effects of trematode metacercariae (Brachylaemidae) on the slugs Milax sowerbii Férussac and Agriolimax reticulatus Müller. Parasitology 48, 261–268. doi: 10.1017/s0031182000021235
García-Huidobro M. R., Aldana M., Varas O., Pulgar J., García-Herrera C., Abarca-Ortega A., et al. (2020). Geographical variability and parasitism on body size, reproduction and shell characteristics of the keyhole limpet Fissurella crassa (Mollusca: Vetigastropoda). Mar. Eviron. Res. 161, 105060. doi: 10.1016/j.marenvres.2020.105060
Gauthier-Clerc S., Pelledirn J., Amiard J. C. (2006). Estradiol-17beta and testosterone concentrations in male and female Mya arenaria (Mollusca Bivalvia) during the reproductive cycle. Gen. Comp. Endocrinol. 145, 133–139. doi: 10.1016/j.ygcen.2005.08.004
George-Nascimento M., Balboa L., Aldana M., Olmos V. (1998). Las lapas Fissurella spp. (Mollusca: archeogastropoda) y el pejesapo Sicyases sanguineus (Pisces: gobiesocidae) son huéspedes secuenciales de Proctoeces lintoni (Digenea: fellodistomidae) en Chile. Rev. Chil. Hist. Nat. 71, 169–176.
George-Nascimento M., Quiroga H. (1983). Descripción de una nueva especie de trematodo proctoeces humboldti n. sp. (Fellodistomidae) parásito de las lapas fissurella spp. brugie 1789 (Mollusca: Archeogastropoda). Parasitol. al Día 7, 100–103.
Goater T. M., Goater C. P., Esch G. W. (2014). Parasitism: the diversity and ecology of animal parasites (Cambridge: Cambridge University Press).
Gomez de Leon C. T., Ostoa-Saloma P., Segovia-Mendoza M., Del Rio-Araiza V. H., Morales-Montor J. (2021). Environmental parasitology and its impacts on the host neuroimmuneendocrine network. Front. Biosci. Landmark 26, 431–443. doi: 10.2741/4900
Granovitch A. I., Yagunova E. B., Maximovich A. N., Sokolova I. M. (2009). Elevated female fecundity as a possible compensatory mechanism in response to trematode infestation in populations of Littorina saxatilis (Olivi). Int. J. Parasitol. 39, 1011–1019. doi: 10.1016/j.ijpara.2009.02.014
Hall S. R., Becker C., Cáceres C. E. (2007). Parasitic castration: a perspective from a model of dynamic energy budgets. Integr. Comp. Biol. 47, 295–309. doi: 10.1093/icb/icm057
Hay K. B., Fredensborg B. L., Poulin R. (2005). Trematode-induced alterations in shell shape of the mud snail Zeacumantus subcarinatus (Prosobranchia: Batillariidae). J. Mar. Biol. Ass. U.K. 85, 989–992. doi: 10.1017/S0025315405012002
Heins D. C. (2012). Fecundity compensation in the three-spined stickleback Gasterosteus aculeatus infected by the diphyllobothriidean cestode Schistocephalus solidus. Biol. J. Linn. Soc 106, 807–819. doi: 10.1111/j.1095-8312.2012.01907.x
Honour J. (2002). Análisis abiótico, hormonal y patológicos de la fisiología reproductiva de Fissurella cumingi (Reeve 1849) para la definición de innovaciones tecnológicas productivas ( Santiago, Chile: Universidad Nacional Andrés Bello), 147. Lic. Thesis.
Hurd H. (2009). Evolutionary drivers of parasite-induced changes in insect life-history traits from theory to underlying mechanisms. Adv. Parasitol. 68, 85–110. doi: 10.1016/S0065-308X(08)00604-0
Jokela J., Uotila L., Taskinen J. (1993). Effect of the castrating trematode parasite Rhipidocotyle fennica on energy allocation of fresh-water clam Anodonta piscinalis. Func. Ecol 7, 332–338. doi: 10.2307/2390213
Jones D. (1989). Protein expression during parasite redirection of host (Trichoplusia ni) biochemistry. Insect. Biochem. 19, 445–455. doi: 10.1016/0020-1790(89)90026-7
Ke Q. Z., Li Q. (2013). Annual dynamics of glycogen, lipids, and proteins during the reproductive cycle of the surf clam Mactra veneriformis from the north coast of Shandong Peninsular, China. Invertebr. Reprod. Dev. 57, 49–60. doi: 10.2983/035.035.0211
Korkofigas E., Park T., Sparkes T. C. (2016). Acanthocephalan-related variation in the pattern of energy storage of a behaviorally and physiologically modified host: field data. Parasitol. Res. 115, 339–345. doi: 10.1007/s00436-015-4753-z
Krist A. C. (2001). Variation in fecundity among populations of snails is predicted by prevalence of castrating parasites. Evol. Ecol. Res. 3, 191–197.
Kuris M. A., Hechinger R. F., Shaw J. C., Whitney K. L., Aguirre-Macedo L., Boch C. A., et al. (2008). Ecosystem energetic implications of parasite and free-living biomass in three stuaries. Nature 454, 515–518. doi: 10.1038/nature06970
Lafferty K. D. (1993). Effects of parasitic castration on growth, reproduction and population dynamics of the marine snail Cerithidea californica. Mar. Ecol. Prog. Ser. 96, 229–237. doi: 10.3354/meps096229
Lafont R., Mathieu M. (2007). Steroids in aquatic invertebrates. Ecotoxicology 16, 109–130. doi: 10.2307/2265536
Lagrue C., Kaldonski N., Perrot-Minnot M., Motreul S., Bollache L. (2007). Modification of hosts’ behavior by a parasite: Field evidence for adaptive manipulation. Ecology 88, 2839–2847. doi: 10.1890/06-2105.1
Lagrue C., Poulin R. (2009). Life cycle abbreviation in trematode parasites and the developmental time hypothesis: is the clock ticking? J. Evol. Biol. 22, 1727–1738. doi: 10.1111/j.1420-9101.2009.01787.x
Lazo-Andrade J., Guzmán-Rivas F. A., Espinoza P., García-Huidobro M. R., Aldana M., Pulgar J., et al. (2021a). Temporal variations in the bioenergetic reserves of Concholepas concholepas (Gastropoda: Muricidae) in contrasting coastal environments from the Humboldt current system. J Sea. Res. 167, 101970. doi: 10.1016/j.seares.2020.101970
Lazo-Andrade J., Guzmán-Rivas F. A., Espinoza P., García-Huidobro M. R., Aldana M., Pulgar J., et al. (2021b). Spatio-temporal changes in the biochemical parameters of the fishery resource Concholepas concholepas (Gastropoda: Muricidae) in the Southeastern Pacific Ocean. Reg. Stud. Mar. Sci. 44, 101735. doi: 10.1016/j.rsma.2021.101735
Lefévre T., Roche B., Poulin R., Hurd H., Renaud F., Thomas F. (2008). Exploiting host compensatory responses: the must of manipulation? Trends. Parasitol. 24, 435–439. doi: 10.1016/j.pt.2008.06.006
Leung T. L. F., Poulin R. (2008). Parasitism, commensalism, and mutualism: Exploring the many shades of symbioses. Vie Mieliu 58, 107–115.
Levri E. P., Dillard J., Martin T. (2005). Trematode infection correlates with shell shape and defence morphology in a freshwater snail. Parasitology 130, 699–708. doi: 10.1017/S0031182005007286
Liu O. R., Gaines S. D. (2022). Environmental context dependency in species interactions. PNAS 119, e2118539119. doi: 10.1073/pnas.2118539119
Lowry O. H., Rosebrough N. J., Farr A. L., Randall R. J. (1951). Protein measurement with the folin phenol reagent. J. Biol. Chem. 193, 265–275. doi: 10.1016/S0021-9258(19)52451-6
Maure F., Brodeur J., Hughes D., Thomas F. (2013). How much energy should manipulative parasites leave to their hosts to ensure altered behaviours? J. Exp. Biol. 216, 43–46. doi: 10.1242/jeb.073163
McCarthy H. O., Fitzpatrick S. M., Irwin S. W. B. (2004). Parasite alteration of host shape: a quantitative approach to gigantism helps elucidate evolutionary advantages. Parasitology 128, 7–14. doi: 10.1017/s0031182003004190
McLean J. H. (1984). Systematics of Fissurella in the Peruvian and magellanic faunal provinces (Gastropoda: Prosobranchia). Contribut. Sci. 354, 1–70. doi: 10.5962/p.226839
Merlo M. J., Parietti M., Ituarte R. B. (2016). Influence of Microphallus szidati Martorelli 1986 (Trematoda) on the fecundity of the second intermediate host, Palaemonetes argentinus Nobili 1901 (Decapoda: Natantia). J. Crustacean Biol. 36, 94–98. doi: 10.1163/1937240X-00002394
Minchella D. J. (1985). Host-life variation in response to parasitism. Parasitology 90, 205–216. doi: 10.1017/s0031182000049143
Minchella D. J., LoVerde P. T. (1981). A cost of increased early reproductive effort in the snail Biomphalaria glabrata. Amm. Nat. 118, 876–881. doi: 10.1086/283879
Oliva M. E. (1984). Proctoeces Chilensis nueva especie (Trematoda: Strigeatoidea: Fellodistomidae), parásito en Syciases sanguineus Müller & Tronschel 1843 (Pisces: Telostei) (Chile: Boletín de la Sociedad de Biología de Concepción), 87–92.
Oliva M. E. (1992). Parasitic castration in Fissurella crassa (Archaeogastropoda) due to an adult digenea, Proctoeces lintoni (Fellodistomidae). Mem. Inst. Oswaldo Cruz 87, 37–42. doi: 10.1590/S0074-02761992000100007
Oliva M. E., Álvarez C. (2011). Is a vertebrate a better host for a parasite than an invertebrate host? Fecundity of Proctoeces cf lintoni (Digenea: fellodistomidae), a parasite of fish an gastropod in northern Chile. Parasitol. Res. 109, 1731–1734. doi: 10.1007/s00436-011-2489-y
Oliva D., Castilla J. C. (1992). Guía para el reconocimiento y morfometría de diez especies del género Fissurella (ruguíere 1789) (Mollusca: gastropoda) comunes en la pesquería y conchales indígenas de Chile central y sur. Gayana 56, 77–108.
Oliva M. E., Huaquín L. (2000). Progenesis in Proctoeces lintoni (fellodistomidae) parasite of Fissurella crassa (Mollusca: archeogastropoda) in latitudinal gradient in the pacific coast of South America. J. Parasitol. 86, 768–772. doi: 10.1645/0022-3395(2000)086[0768:PIPLFA]2.0.CO;2
Oliva M. E., Valdivia I. M., Cárdenas L., Muñoz G., Escribano R., George-Nascimento M. (2018). A new species of Proctoeces and reinstatement of Proctoeces humboldti George-Nascimento and Quiroga 1983 (Digenea: fellodistomidae) base on molecular and morphological evidence. Parasitol. Int. 67, 159–169. doi: 10.1016/j.parint.2017.10.004
Oliva M. E., Vega A. A. (1994). Effect of Proctoeces lintoni (Digenea) on the fecundity of Fissurella crassa (Archaeogastropoda). Mem. Inst. Oswaldo Cruz. 89, 225. doi: 10.1590/S0074-02761994000200020
Oliva M. E., Zegers J. (1988). Variaciones intraespecificas del adulto de Proctoeces lintoni Siddiqi & Cable 1960 (Trematoda: fellodistomidae) en hospedadores vertebrados e invertebrados. Stud. Neotrop. Fauna Environ. 23, 189–195. doi: 10.1080/01650528809360760
Olivares P. A., Jofré M. D., Álvarez M. C., Bustos-Obregón E. (2009). Hermafroditismo funcional de la gónada de Fissurella crassa (Mollusca: Fissurellidae). Int. J. Morphol. 27, 509–514. doi: 10.4067/S0717-95022009000200034
Pandian T. J. (2017). Reproduction and development in Mollusca (Kerela, India: CRC Press Taylor & Francis Group).
Parietti M., Merlo M. J., Natal M., Méndez Casariego M. A. (2020). Reproductive compensation in female Palaemonetes argentinus (Decapoda: Natantia) due to Microphallus szidati (Trematoda) infection. J. Helminthol. 94, e204, 1–5. doi: 10.1017/S0022149X20000917
Parker B. J., Brribeau S. M., Laughton A. M., de Roode J. C., Gerardo N. M. (2011). Non-immunological defense in an evolutionary framework. Trends. Ecol. Evol. 26, 242–248. doi: 10.1016/j.tree.2011.02.005
Pike V. L., Ford S. A., King K. C., Rafalu-Mohr C. (2019). Fecundity compensation is dependent on the generalized stress response in a nematode host. Ecol. Evol. 9, 11957–11961. doi: 10.1002/ece3.5704
Plaistow S. J., Troussard J.-P., Cézilly F. (2001). The effect of the acanthocephalan parasite Pomphorhynchus laevis on the lipid and glycogen content of its intermediate host Gammarus pulex. Int. J. Parasitol. 31, 346–351. doi: 10.1016/S0020-7519(01)00115-1
Ponton F., Biron D. G., Joly C., Helluy S., Duneau D., Thomas F. (2005). Ecology of parasitically modified populations: a case study from a gammarid-trematode system. Mar. Ecol. Prog. Ser. 299, 205–215. doi: 10.3354/meps299205
Poulin R. (1995). Adaptive changes in the behavior of parasitized animals: a critical review. Int. J. Parasitol. 25, 1371–1383. doi: 10.1016/0020-7519(95)00100-x
Poulin R. (1998). Evolution and phylogeny of behavioural manipulation of insect hosts by parasites. Parasitology 116, S3–S11. doi: 10.1017/s0031182000084894
Poulin R. (2007). Evolutionary Ecology of Parasites. 2nd ed. (Princeton, New Jersey: Princeton University Press).
Poulin R., Cribb T. H. (2002). Trematode life cycles: short is sweet? Trends Parasitol. 18, 176–183. doi: 10.1016/s1471-4922(02)02262-6
Price P. W. (1980). Evolutionary biology of parasites, Princeton (New Jersey, USA: Princeton University Press).
Pulgar J., Aldana M., Alvarez M., García-Huidobro M. R., Molina P., Morales J. P., et al. (2013). Upwelling effects food availability, impacting the morphological and molecular conditions of the herviborous limpet Fissurella crassa (Mollusca: archeogastropoda). J. Mar. Biol. Assoc. U.K. 93, 797–802. doi: 10.1017/S0025315412001415
R Core Team (2023). R: A language and environment for statistical computing (Vienna, Austria: R Foundation for Statistical Computing). Available at: https://www.R-project.org/.
Rollingson N., Hutchings J. A. (2013). The relationship between offspring size and fitness: Integrating theory and empiricism. Ecology 94, 315–324. doi: 10.1890/2-0552.1
Sandland G. J., Minchella D. J. (2003). Costs of immune defense: an enigma wrapped in an environmental cloak? Trends. Parasitol. 19, 571–574. doi: 10.1016/j.pt.2003.10.006
Santarém M. M., Robledo J. A. F., Figueras A. (1994). Seasonal changes in hemocytes and serum defense factors in the blue mussel Mytilus galloprovincialis. Dis. Aquat. Org. 18, 217–222. doi: 10.3354/dao018217
Schmid-Hempel P. (2021). Evolutionary parasitology: The integrated study of infections, immunology, ecology, and genetics. 2nd ed. (Oxford, United Kingdom: Oxford University Press).
Scott A. P. (2012). Do mollusks use vertebrate sex steroids as reproductive hormones? Part I. Critical appraisal of the evidence for the presence, biosynthesis and uptake of steroids. Steroids 77, 1450–1468. doi: 10.1016/j.steroids.2012.08.009
Scott A. P. (2013). Do mollusks use vertebrate sex steroids as reproductive hormones? II. Critical review of the evidence that steroids have biological effects. Steroids 78, 268–281. doi: 10.1016/j.steroids.2012.11.006
Scott A. P. (2018). Is there any value in measuring vertebrate steroids in invertebrates? Gen. Comp. Endocr. 265, 77–82. doi: 10.1016/j.ygcen.2018.04.005
Serbina E. A. (2014). The influence of trematode metacercariae on the individual fecundity of Bithyna troscheli (Gastropa: Bithyniidae). Parazitologiia 48, 3–19. doi: 10.1515/ap-2015-0006
Siah A., Pellerin J., Benosman A., Gagné J. P., Amiard J. C. (2022). Seasonal gonad progesterone pattern in the soft-shell clam Mya arenaria. Comp. Biochem. Physiol. Part. A Mol. Integr. Physiol. 132, 499–511. doi: 10.1016/s1095-6433(02)00095-8
Sorensen R. E., Minchella D. J. (2001). Snail-trematode life history interactions: past trends and future directions. Parasitology 123, S3–S18. doi: 10.1017/s0031182001007843
Soudant P., Marty Y., Moal J., Samain J. F. (1996). Fatty acids and eggs quality in great scallop. Aquacult. Int. 4, 191–200. doi: 10.1007/BF00117381
Thieltges D. W. (2009). Contribution of parasites to intra- and inter-site variation in shell morphology of a marine gastropod. J. Mar. Biol. Assoc. UK. 89, 563–568. doi: 10.1017/S0025315408002294
Thitiphuree T., Nagasawa K., Osada M. (2018). Molecular identification of steroidogenesis-related genes in scallops and their potential roles in gametogenesis. J. Steroid. Biochem. 186, 22–33. doi: 10.1016/j.jsbmb.2018.09.004
Thomas F., Adamo S., Moore J. (2005). Parasitic manipulation: where are we and where should we go? Behav. Process. 68, 185–199. doi: 10.1016/j.beproc.2004.06.010
Thomas F., Rigaud T., Brodeur J., Stearns S. C. (2012). Evolutionary routes leading to host manipulation by parasites, in Host manipulation by parasites. Eds. Hughes D., Brodeur J., Thomas F. (Oxford, United Kingdom: Oxford Academic).
Thompson S. N. (1983). Biochemical and physiological effects of metazoan endoparasites on their host species. Comp. Biochem. Physiol. 74B, 183–211. doi: 10.1016/0305-0491(83)90001-9
Thornhill J. A., Jones J., Kusel J. R. (1986). Increased oviposition and growth in immature Biomphalaria glabrata after exposure to Schistosoma mansoni. Parasitology 93, 443–450. doi: 10.1017/s0031182000081166
Valdivia I. M., Criscione C. D., Cárdenas L., Durán C. P., Oliva M. E. (2014). Does a facultative precocious life cycle predispose the marine trematode Proctoeces cf. lintoni to inbreeding and genetic differentiation among host species? Int. J. Parasitol. 44, 183–188. doi: 10.1016/j.ijpara.2013.10.008
Wang C., Croll R. P. (2004). Effects of sex steroids on gonadal development and gender determination in the sea scallop, Placopecten magellanicus. Aquaculture 238, 483–498. doi: 10.1016/j.aquaculture.2004.05.024
Keywords: Proctoeces humboldti, second intermediate host, Reproductive performance, Gonadal histology, Biochemical responses
Citation: García-Huidobro MR, Reyes M, Fuentes NC, Bruna T, Guzmán-Rivas F, Urzúa Á, Pulgar J and Aldana M (2024) Host-parasite dialogue: fecundity compensation mechanisms of Fissurella crassa. Front. Mar. Sci. 10:1266405. doi: 10.3389/fmars.2023.1266405
Received: 24 July 2023; Accepted: 26 December 2023;
Published: 17 January 2024.
Edited by:
Jeff Shimeta, RMIT University, AustraliaReviewed by:
Andrei Granovitch, Saint Petersburg State University, RussiaLiliana Rojo, Centro de Investigación Biológica del Noroeste (CIBNOR), Mexico
Copyright © 2024 García-Huidobro, Reyes, Fuentes, Bruna, Guzmán-Rivas, Urzúa, Pulgar and Aldana. This is an open-access article distributed under the terms of the Creative Commons Attribution License (CC BY). The use, distribution or reproduction in other forums is permitted, provided the original author(s) and the copyright owner(s) are credited and that the original publication in this journal is cited, in accordance with accepted academic practice. No use, distribution or reproduction is permitted which does not comply with these terms.
*Correspondence: M. Roberto García-Huidobro, mgarciahuidobro@santotomas.cl; Marcela Aldana, marcelaaldana@santotomas.cl