- 1State Key Laboratory of Freshwater Ecology and Biotechnology, Institute of Hydrobiology, Chinese Academy of Sciences, Wuhan, China
- 2University of Chinese Academy of Sciences, Beijing, China
- 3Limnology Laboratory, Department of Biological Sciences and Centre for Ecosystem Research and Implementation, Middle East Technical University, Ankara, Türkiye
- 4School of Life Sciences, Nanchang University, Nanchang, China
- 5College of Animal Science, Guizhou University, Guiyang, China
- 6Agronomy and Life Science Department, Zhaotong University, Zhaotong, China
- 7Zhaotong Yudong Reservoir Administration, Zhaotong, China
- 8Wuhan Sino-Hiking Technology Company Limited, Wuhan, China
- 9China Renewable Energy Engineering Institute, Beijing, China
- 10Department of Ecoscience, Aarhus University, Aarhus, Denmark
- 11Sino-Danish Centre for Education and Research, Beijing, China
- 12Institute of Marine Sciences, Middle East Technical University, Mersin, Türkiye
- 13Institute for Ecological Research and Pollution Control of Plateau Lakes, School of Ecology and Environmental Science, Yunnan University, Kunming, China
Stocking of filter-feeding fish (mainly Hypophthalmichthys molitrix and Aristichthys nobilis) is a common method used in lakes and reservoirs in (sub)tropical China to control phytoplankton, but the results are ambiguous and lack long-term data to support. We analysed a decade (2010-2020) of monitoring data from a subtropical plateau reservoir, southwest China, to which filter-feeding fish were stocked annually. We found that the total phytoplankton biomass, cyanobacteria biomass and average individual mass of phytoplankton decreased significantly during the study period despite absence of nutrient concentration reduction. However, the grazing pressure of zooplankton on phytoplankton also decreased markedly as judged from changes in the ratio of zooplankton biomass to phytoplankton biomass and Daphnia proportion of total zooplankton biomass. This is likely a response to increasing predation on zooplankton by the stocked fish. Our results also indicated that water temperature, total phosphorus and water level promoted phytoplankton growth. Our results revealed that filter-feeding fish contributed to the decline in the biomass of phytoplankton but that it also had a strong negative effect on the grazing pressure of zooplankton on phytoplankton, even in this deep reservoir where zooplankton may have a better chance of survival through vertical migration. The particular strong effect on zooplankton is most likely due to imbalance of stocking and harvesting of fish. In the management of eutrophic reservoirs, the reduction of external nutrient loading should have highest priority. In highland (low temperature) deep-water eutrophic reservoirs, stocking of filter-feeding fish may help to control filamentous phytoplankton provided that the fish stocking is properly managed. The optimal stocking intensity of filter-feeding fish that can help control phytoplankton in such reservoirs without excessive impact on large-bodied zooplankton is a topic for further elucidation, however.
1 Introduction
Cyanobacteria blooms caused by eutrophication are threatening the health of freshwater ecosystems (Amorim et al., 2017; Zhang et al., 2019). The ecosystem services, aquatic organisms and human beings who depend on freshwater resources are all affected by this environmental problem (Olokotum et al., 2020). Various in-lake techniques have been developed in eutrophic waters to restore water quality and prevent lake deterioration in addition to external nutrient loading reduction. One of these techniques is biomanipulation, which involves controlling phytoplankton with large-bodied zooplankton, achieved by planktivorous fish removal to reduce predation pressure on zooplankton (Shapiro et al., 1975; Jeppesen et al., 2005). Increasing the grazing pressure on phytoplankton, including cyanobacteria directly by stocking of filter-feeding fish (mainly silver carp Hypophthalmichthys molitrix and bighead carp Aristichthys nobilis) is another technique. Filter-feeding fish influence phytoplankton through trophic cascades, (Attayde and Hansson, 2001; Jeppesen et al., 2012; Lemmens et al., 2018) and have been widely used in (sub)tropical regions in China (Xie and Liu, 2001; Guo et al., 2015; Lin et al., 2020; Lin et al., 2021). However, the effectiveness of phytoplankton control by filter-feeding fish remains controversial.Some studies have suggested that filter-feeding fish is a useful method to suppress phytoplankton and enhance water quality in shallow lakes (Starling, 1993; Guo et al., 2015), while other results demonstrated that filter-feeding fish cannot control phytoplankton instead increase the biomass or density of phytoplankton, b ecause grazing pressure of zooplankton is reduced by indiscriminate filter feeding from fish (Lin et al., 2020; Mao et al., 2020; Lin et al., 2021). Others again have argued that in the cases of large individual phytoplankton as the dominant species, filter-feeding fish are able to suppress these effectively (Domaizon and Devaux, 1999; Guo et al., 2015).
There may also be variations in the outcome of filter-feeding fish stocking with lake depth. In most experiments conducted in shallow lakes and mesocosms in the subtropics, filter-feeding fish were not able to control the phytoplankton despite massive stocking (Mao et al., 2020; Shen et al., 2021). In fact, they may even stimulate the growth of nanoplankton by preying on zooplankton and by sediment disturbance, potentially bringing nutrients to the water (Jeppesen et al., 2012; Zhang et al., 2016a). Compared with shallow lakes, it may be easier for filter-feeding fish to control phytoplankton in highland reservoirs as studies have shown that zooplankton gets lower predation pressure form fish in deep lakes and reservoirs than in shallow lakes (Jeppesen et al., 1997), and cladocerans in deep reservoirs may have more opportunity to avoid predation by vertical migration (Jeppesen et al., 1997; Hart, 2011). There may also be better chances for zooplankton population recovery when fish predation is released (Lin et al., 2014). These zooplankton may contribute to the control of nanoplankton that filter-feeding fish cannot feed on. The low mean annual temperature in deep and highland subtropical lakes may also make phytoplankton control less challenging than in lowland, shallow, subtropical lakes (Jeppesen et al., 2012; Mao et al., 2020), where high temperatures stimulate growth of phytoplankton.
To reveal how the silver carp and bighead carp affect phytoplankton and zooplankton, we conducted a decade-long study (from January 2010 to December 2020) in Yudong Reservoir, a highland eutrophic reservoir. We hypothesised that the continuous filter-feeding fish stockings would alleviate the cyanobacteria blooms and reduce the biomass of phytoplankton in this deep highland (low temperature) subtropical reservoir, although it might also lead to reduction in zooplankton biomass and their grazing on phytoplankton, though this effect would be less than in shallow lakes, as zooplankton may have a better chance of survival through vertical migration (Jeppesen et al., 1997).
2 Materials and methods
2.1 Study area
The Yudong Reservoir, located at the upper stream of Hengjiang River (a tributary of the lower Jinsha River) in Zhaotong City, is one of eight large reservoirs (capacity > 108 m3) in Yunnan Province, China (Figure 1). As a comprehensive water conservancy project, Yudong Reservoir supplies water for 800,000 people and irrigates 250 km2 of farmland. It is a typical deep valley plateau reservoir with an average elevation of almost 2000 m. The maximum and average water depths are 84.4 m and about 30.3 m, respectively. The area of the Yudong Reservoir is 13.6 km2, and its total storage capacity is 3.64×108 m3, with an annual exchange coefficient of 0.8-1.0. In its 709 km2 watershed, mainly consisting of high mountains and small valley plains, agriculture is the major economic activity. The area has distinct dry and rainy seasons. The annual precipitation is 700-800 mm, and precipitation in the rainy season (May to October) is about 90% of the whole year’s rainfall.
The Yudong Reservoir was fully filled in 2000, followed by fish stocking and fishery activities. At first, the purpose of stocking was to enhance the local economic income. However, the water quality deteriorated, affected by the ecological deterioration and rural non-point source pollution in the watershed. Since 2008, cyanobacteria blooms have often occurred, causing serious threats to the safety of the drinking water supply. Therefore, the management strategy was adjusted to control phytoplankton by stocking filter-feeding fish. The quantities of stocking were determined by the local management department based on the year’s phytoplankton density and water transparency. And these stocking were completed in the late spring of each year, normally in April, before the rainy season. The average weight of the stocked silver carp was 6.2 ± 0.8g, average total length was 3.0 ± 0.5cm and the average weight of the bighead carp was 48.5 ± 5.8g, average total length was 15 ± 2.5cm. The fishing of silver carp and bighead carp was mainly carried out by net trawling, supplemented by gillnet fishing. Individuals with a body weight < 5kg were released back.
2.2 Sampling and processing
2.2.1 Water parameters and fish
The data about Yudong Reservoir water quality were obtained from the Zhaotong Yudong Reservoir Administration. Water samples were collected at 5 fixed long-term monitoring sites monthly (Figure 1) from January 2010 to December 2020 by the department’s professional monitoring team following the Environmental Quality Standards for Surface Water (China, 2002a) and the standard of the Guidance on Sampling Techniques (China, 2009). Chemistry analyses were completed according to the Standard Methods for the Examination of Water and Wastewater (China, 2002b). The water quality parameters included 10 variables, including pH, water temperature (WT, °C), conductivity (Cond, μs/cm), total phosphorus (TP, mg/L), total nitrogen (TN, mg/L), ammonia (NH4-N, mg/L), nitrate (NO3-N, mg/L), water transparency (SD, m), water level (WL, m) and chlorophyll a (Chl-a, μg/L). The monthly sampling dates were selected based on weather conditions to ensure that the water samples were not affected by rainfall, strong winds and runoff. The analytical quality control was carried out in accordance with APHA (2005), with reference to previous studies (Table S1; Wu et al., 2018; Qu et al., 2020).
Filter-feeding fish data on Yudong Reservoir from 2010 to 2020 were provided by the Zhaotong Yudong Reservoir Administration. The annual stocking and harvesting weights, as well as CPUE of filter-feeding fish, were recorded in detail.
2.2.2 Plankton community
We collected 1 L depth-integrated water from each site once a month. After treated with 10 mL Lugol’s iodine solution and sedimentation more than 48 h, the residue was collected and examined under ×100 to ×400 magnification to enumerate phytoplankton. The phytoplankton species were identified following the taxonomic classification of Hu (2006). The biomass of common phytoplankton taxa was calculated based on cell size measurements using formulae from Zhang and Huang (1991). The approximation dry weight of phytoplankton was obtained from corresponding biological volume multiplied by 0.29 (Reynolds, 1984).
We also collected zooplankton samples every month in some years (2011, 2013, 2015, 2018, 2019 and 2020). At each sampling, we filtered 10 L depth-integrated water with 64 mm net and preserved the samples in 4% formaldehyde. Crustacean zooplankton (cladocerans, copepodites and adult copepods) were counted at microscope with an ocular micrometer. Species represented by more than 100 individuals were identified according to Chiang and Du (1979). Crustacean zooplankton biomass (wet weight) was calculated by formulas from Dumont et al. (1975) and Huang (1999) based on length measurements of 30 individuals of each taxon. Rotifers were counted at ×100 magnification, and the biomass was calculated according to the standard weights obtained from several lakes in the Yangtze River (He et al., 2018).
2.3 Statistical analyses
2.3.1 Generalized additive model
We used generalized additive models (GAMs) on monthly data to uncover the temporal trend in variables using the “gam” function and cubic regression spline from the R-package “mgcv” (Wood, 2020). All parameters besides pH and water level were log-transformed [log10(X+1)] to reduce distributional skewness. Spearman rank correlation coefficient (ρ) with year was calculated, when the temporal change of parameter was not linear.
2.3.2 Piecewise structural equation model
Piecewise structural equation modelling (pSEM) was used to elucidate the influences of filter-feeding fish and environmental variables on the phytoplankton and zooplankton communities through different pathways. Different from SEM, piecewise SEM has a more flexible mathematical framework and a wider range of use (less restrictions on the original dataset). We assumed that filter-feeding fish could not only directly reduce phytoplankton biomass through grazing effects but also indirectly by changing the predation pressure of zooplankton. Environmental variables can also directly affect the phytoplankton biomass through bottom-up effects. All response variables were transformed to achieve normal distributions, as for the GAMs. During the model building process, factors that affect the degree of fit were removed. The model fit was assessed by Fisher’s C statistics (Shipley, 2013).
2.3.3 Top-down control
We calculated several indicators to assess the trophic cascade effects of filter-feeding fish stocking via predation on zooplankton. The proportion of large-bodied cladocerans (species sensitive to fish grazing) within the total zooplankton biomass, zooplankton and cladoceran body mass were used to indicate the predation pressure on the zooplankton community (Jackson et al., 2007; Jeppesen et al., 2011). In addition, the ratio of zooplankton biomass to phytoplankton biomass was calculated to reflect the grazing pressure of zooplankton on phytoplankton (Jeppesen et al., 2003; Jeppesen et al., 2011). The average individual mass of phytoplankton was calculated using total phytoplankton biomass divided by total phytoplankton abundance (Yvon-Durocher et al., 2011; Zhang et al., 2016b).
2.4 Data analysis strategy
We first used GAMs to analyse the trend of environmental variables, zooplankton, phytoplankton and top-down strength (zooplankton biomass to phytoplankton, Zoop : Phty) over time. Second, Spearman correlation analyses and redundancy analysis (RDA) were conducted to analysis the relationships between filter-feeding fish stocking, environmental variables and phytoplankton and zooplankton taxonomic groups. Hierarchical partitioning (HP) was conducted to estimate the contributions of environmental factors to the variance in the phytoplankton biomass and several indicators of top-down control (Walsh and Mac Nally, 2013). Finally, piecewise SEM analyses were performed to show the influence of filter-feeding fish on the phytoplankton community in deep reservoirs represented by the Yudong Reservoir. We used the Friedman test to check the condition of phytoplankton average individual biomass and cyanobacteria biomass. All graphs and statistical analysis were performed by the RStudio software. The results were considered to be significant when p < 0.05 or p < 0.01. As zooplankton data were partially missing, the only data from the years for which zooplankton data were available were used in the Spearman correlation analysis, the redundancy analysis, the hierarchical partitioning and the construction of the piecewise structural model.
3 Results
3.1 Dynamics of the environmental variables and fish
From 2010 to 2020, the average TP in Yudong Reservoir was 0.13 mg/L and remained high (Figure 2; Table S2). TN, NH4-N and NO3-N showed a significant upward trend (ρ = 0.27, ρ = 0.13 and ρ = 0.25, respectively). The mean concentration of TN was 1.14 mg/L. Chl-a decreased significantly (ρ = -0.68) but remained high, with an annual average value of 34 μg/L in 2020. Cond and SD increased (ρ = 0.41 and ρ = 0.13), while WT, pH and WL did not change significantly during the study period (Figures 2; S1). The contribution of each variable to principal components is displayed in Figure S2.
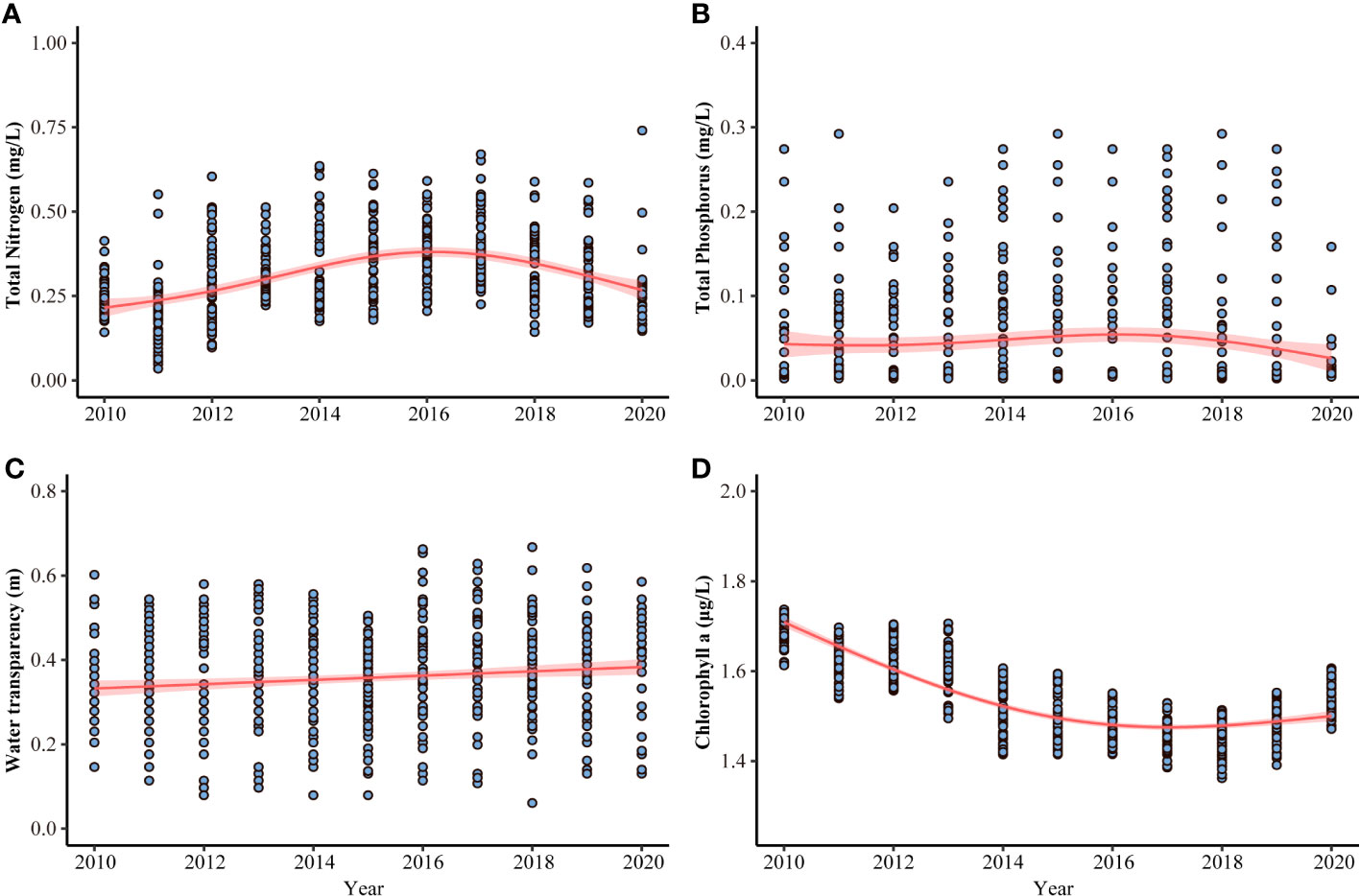
Figure 2 Time series of monthly total nitrogen (A), total phosphorus (B), water transparency (C) and chlorophyll-a (D) in Yudong reservoir. The solid line represents the regression line fitted with monthly data using generalized additive models (GAMs). The 95% confidence intervals from the GAM model are shaded.
Fishing was organised annually with the primary aim of nutrient removal. However, the annual average catches of fish were far lower than the amount stocked (Figure 3).
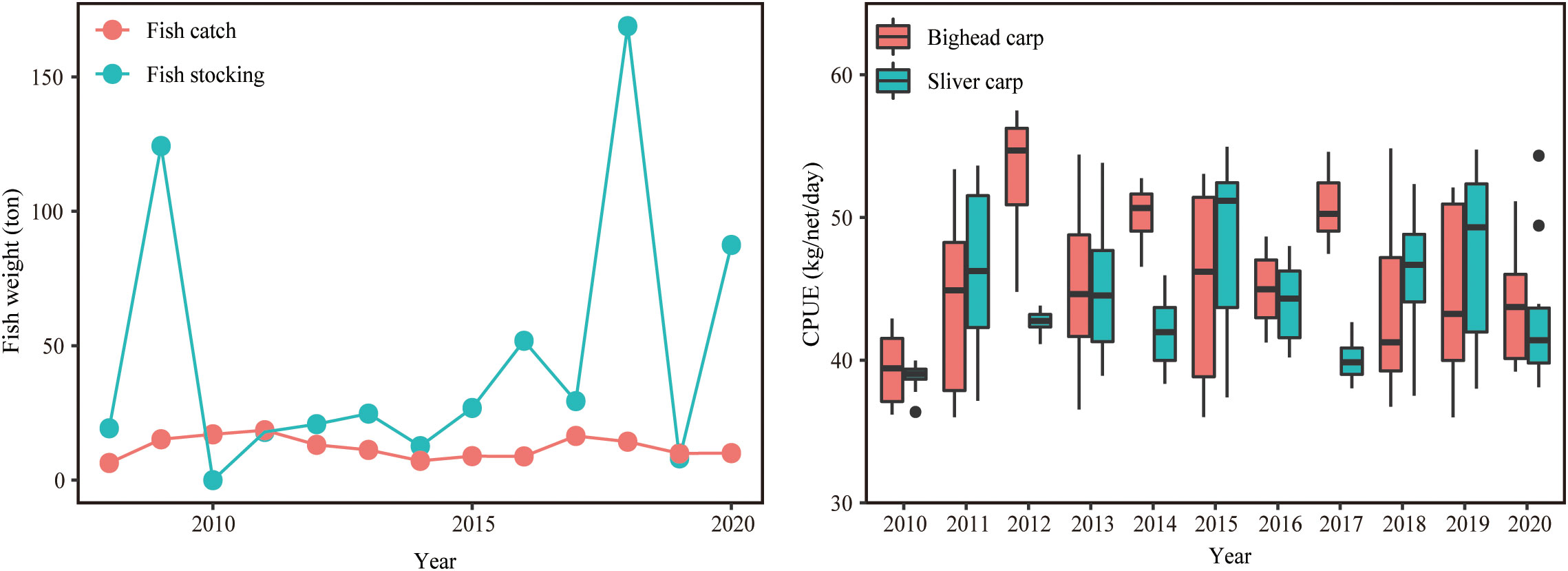
Figure 3 Time series of fish stocking, fish harvesting and CPUE obtained from the Zhaotong Yudong Reservoir Administration (2010–2020).
3.2 Dynamics of the plankton community
The biomass of phytoplankton decreased significantly (ρ = -0.206), from 14.6 mg/L in 2010 to 3.6 mg/L in 2020 (24% of that in 2010) (Figure 4A). Meanwhile, the cyanobacteria biomass decreased significantly (Friedman test, p<0.01). the average individual biomass of phytoplankton decreased, from 5.3×10-6 μg/ind to 0.3×10-6 μg/ind (Friedman test, p<0.01) (Figure S3). The zooplankton biomass (ρ = -0.938) decreased significantly to an average of 0.04 mg/L in 2020, which was 1% of the level in 2011 (Figure 4B). Coinciding with this, the ratio of zooplankton biomass: phytoplankton biomass decreased markedly from 1.79 to 0.01 (ρ = -0.865, Figure 4C). Daphnia% in terms of biomass, zooplankton body mass and cladoceran body mass showed similar decreasing trends (ρ = -0.589, ρ = -0.178 and ρ = -0.329, Figure 4).
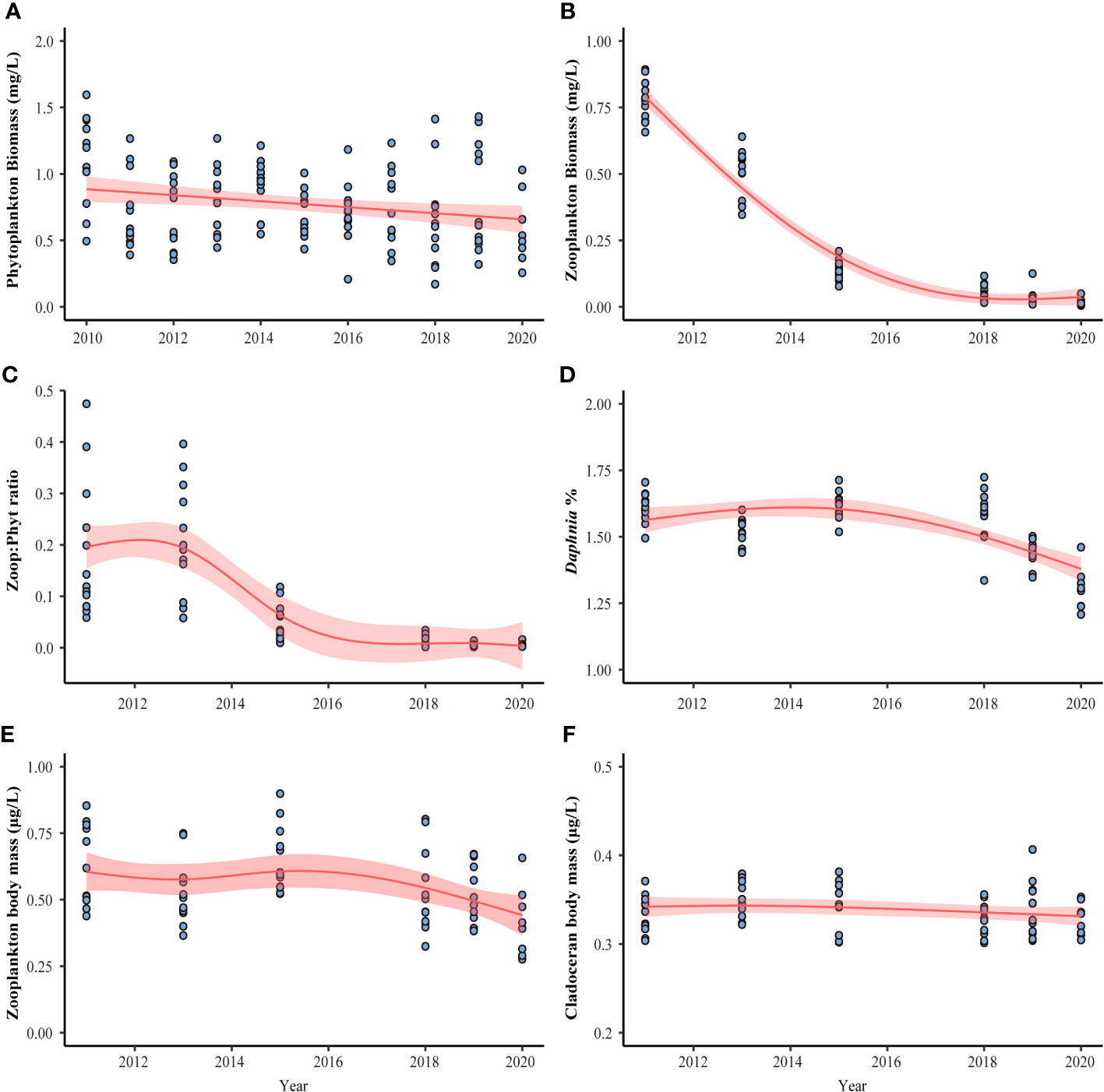
Figure 4 Time-series of phytoplankton biomass (A), zooplankton biomass (B), the ratio of zooplankton biomass to phytoplankton biomass ratio (Zoop: Phyt, (C), Daphnia biomass percentage (Daphnia %, (D), zooplankton body mass (E) and cladoceran body mass (F) in Yudong Reservoir.
3.3 Environmental factors driving plankton resource
The effects of environmental variables on phytoplankton biomass were evaluated by redundancy analysis. The first and second ordinal axes of RDA explained a total of 98.8%. The results indicated that the biomass and average individual biomass of phytoplankton were mainly affected by fish, TN, TP and WT (Figure 5A).
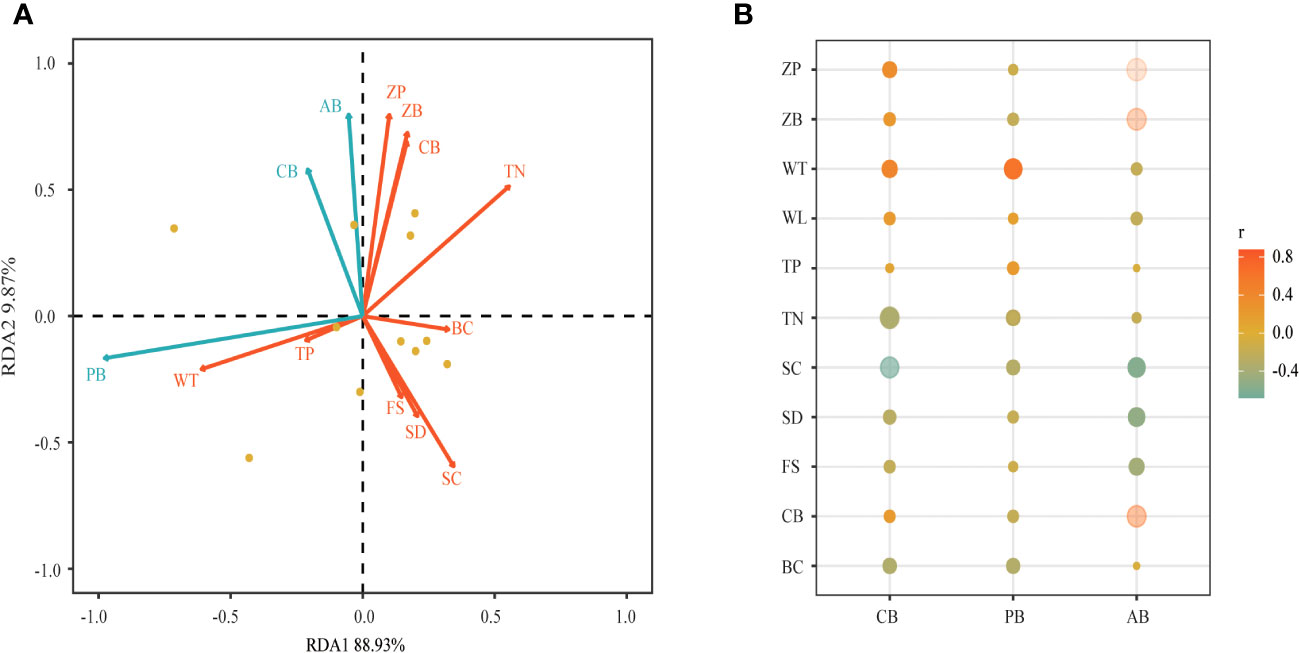
Figure 5 Redundancy analysis (A) and Spearman correlation analysis (B) of phytoplankton between several environmental indicators and top-down effect indicators. FS, fish stocking; BC, bighead carp CPUE; SC, silver carp CPUE; PB, phytoplankton biomass; AB, average individual mass of phytoplankton; PB, phytoplankton biomass; CB, cyanobacteria biomass; TP, total phosphorus; TN, total nitrogen; WT, water temperature; WL, water level; SD, water transparency; ZP, zooplankton biomass, phytoplankton biomass; ZB, zooplankton biomass.
Both silver carp CPUE and bighead carp CPUE and thus total fish stocking had significant negative correlations with the cyanobacteria biomass and the average individual biomass of phytoplankton. TP and WT promoted the phytoplankton biomass, including cyanobacteria, and the water level increasing also can promote the phytoplankton biomass. However, there was a negative correlation between TN and phytoplankton, including cyanobacteria (Figure 5B).
For all three response variables (phytoplankton biomass, cyanobacteria biomass and average individual mass of phytoplankton), hierarchical partitioning revealed WT (2.5 to 36.4%) and silver carp CPUE (2.4% to 37.2%) to be the most important influencing variables (Figure 6A), showing the largest independent effects on variance. For the zooplankton variables, phytoplankton biomass (16.3 to 19.7%), cyanobacteria biomass (12.9 to 19.3%) and silver carp CPUE (14.2 to 17.1) were the most important influencing variables (Figure 6B). The results of RDA and HP revealed that phytoplankton and cyanobacteria in the reservoir were mainly affected by silver carp CPUE and WT. Phytoplankton and cyanobacteria were affected more by silver carp than zooplankton, and zooplankton was affected by both top-down and bottom-up factors.
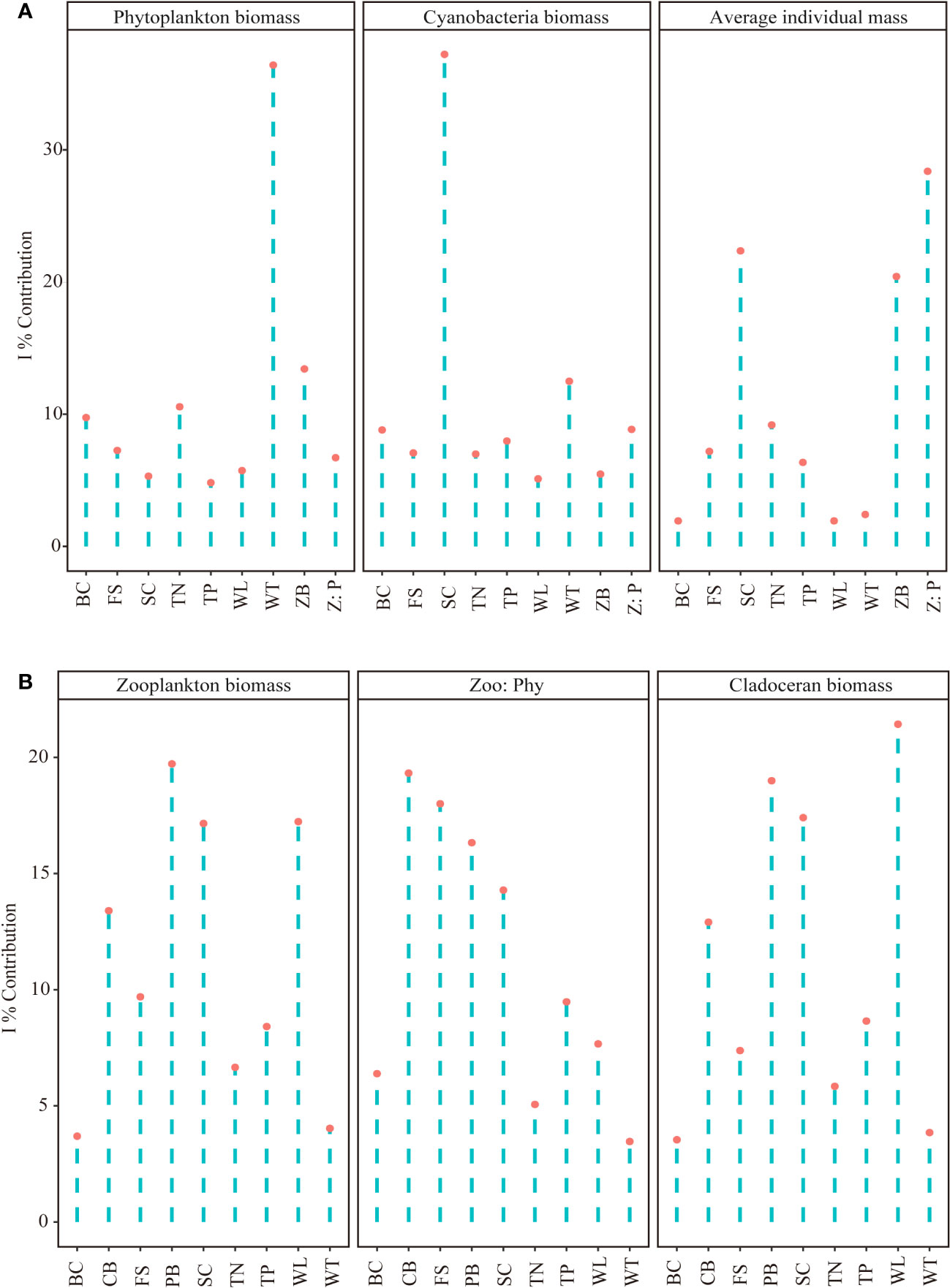
Figure 6 Comparison of predictors explaining variance of zooplankton (A) and phytoplankton (B) in Yudong Reservoir with % independent contribution by hierarchical partitioning. (% I). FS, fish stocking; BC, bighead carp CPUE; SC, silver carp CPUE; PB, phytoplankton biomass; CB, cyanobacteria biomass; TP, total phosphorus; TN, total nitrogen; WT, water temperature; WL, water level; ZP, zooplankton biomass, phytoplankton biomass; ZB, zooplankton biomass.
The results of the piecewise SEM overall concur with the other analyses. The filter-feeding effects on zooplankton and phytoplankton were significant, indicating that filter-feeding fish contributed importantly to the reduction of zooplankton and phytoplankton biomass. The grazing pressure of zooplankton was weak, and the negative correlation between zooplankton and phytoplankton was not obvious. By contrast, TP and WT promoted the growth of phytoplankton, while bighead carp CPUE and total fish stocking also had negative correlations with cyanobacteria biomass and the average individual biomass of phytoplankton. Higher phytoplankton biomass may decrease water transparency. TN also had a negative relationship with phytoplankton (Figure 7).
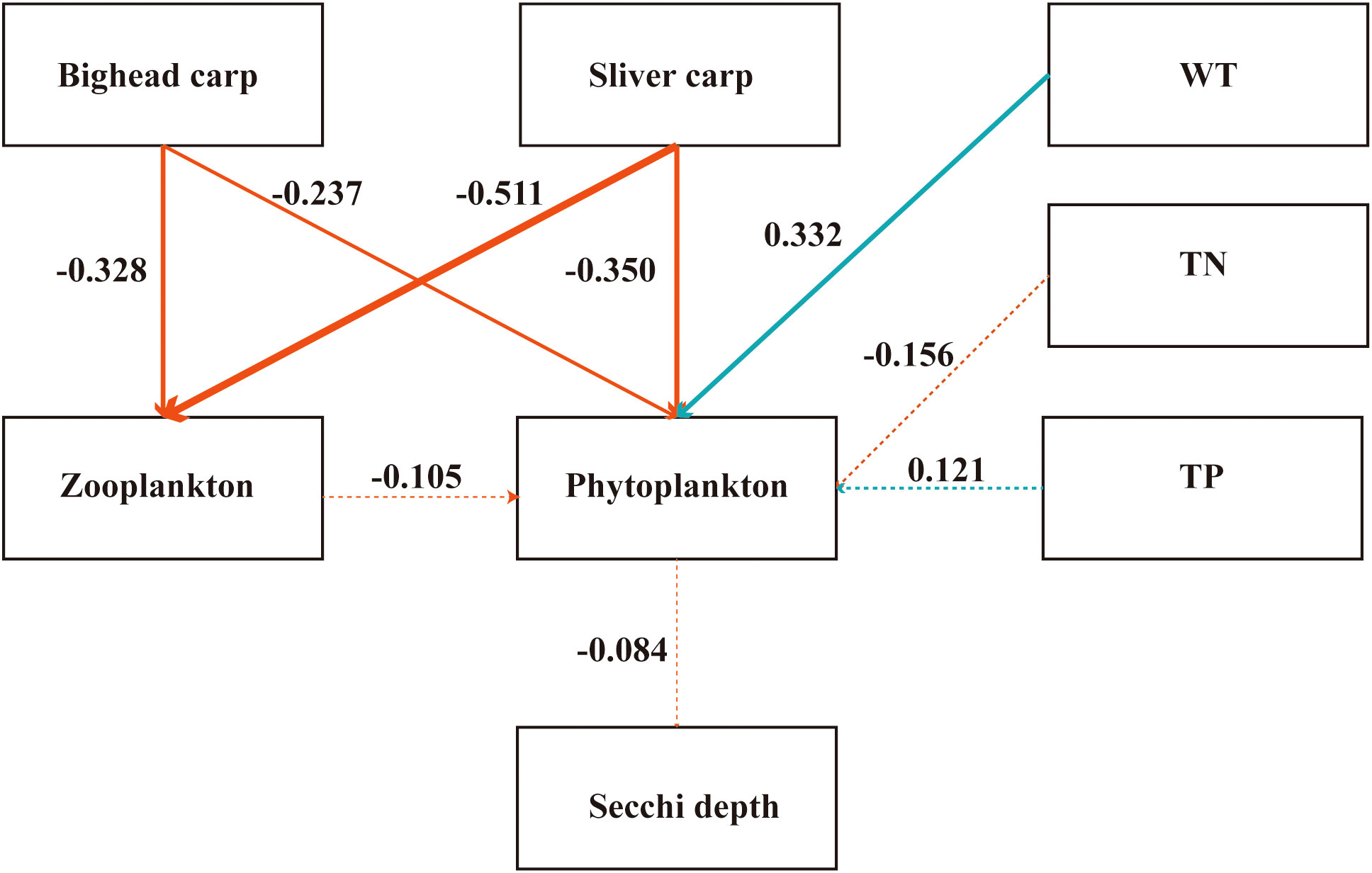
Figure 7 Piecewise structural equation model (pSEM) of the direct and indirect effects of bighead carp and silver carp stocking on phytoplankton in Yudong Reservoir. Fisher’s C = 48.739; df = 18; P = 0.185; AIC = 50.53. Orange, blue and dashed arrows represent negative, positive and non-significant paths, respectively. The thickness of paths represents the magnitude of the standardised regression coefficient or effect sizes given on the arrows.
4 Discussion
Using Yudong Reservoir as a case study, we discussed the effects of environment parameters and filter-feeding fish as well as the contribution difference of trophic cascades on phytoplankton in deep highland reservoirs. Coinciding with the continuous introduction of filter-feeding fish into Yudong Reservoir, we found that the phytoplankton biomass and average individual mass of phytoplankton decreased significantly despite no reduction in nutrient level. Moreover, the biomass of zooplankton, including cladocerans, has declined significantly in recent years. The body size of zooplankton and cladocerans decreased as well, indicating strong top-down control on zooplankton by the fish.
4.1 Effects of environmental factors on phytoplankton
Even though the Yudong Reservoir is located in a subtropical climate, its high-altitude level and its large depth leads to a lower average WT than typically found in lowland subtropical lakes (e, g., Lake Qiandao and Lake Taihu) (Mao et al., 2020; Su et al., 2021), and this may dampen the growth of cyanobacteria (Li et al., 2020; Nwankwegu et al., 2020). Supporting this view, our correlation analysis indicated that, under the current eutrophic conditions (high TN and TP), increased WT promoted phytoplankton growth (Figure 6).
The RDA results illustrated that the phytoplankton biomass was negatively correlated with TN (Figure 5). pSEM also indicated that high TN concentrations do not promote phytoplankton biomass (Figure 7). This phenomenon has also been found in other eutrophication lakes and considered due to N subsidy-stress effects (Mao et al., 2020). Filstrup et al. (2018) suggested when TN concentration is more than 2 mg/L, it might negatively influence phytoplankton (Chla) concentrations. Mao et al. (2020) demonstrated the same trend in Lake Taihu where the mean TN value was 3.4 mg/L. However, whether high TN is of importance for the decrease in phytoplankton biomass in our study or not is an open question as it coincides with the increase in the net stocking (stocking minus harvesting) of bigheaded carp (Figure 3). We also found that water level fluctuation can affect the phytoplankton biomass (Figure 5B). Rising water levels due to excessive precipitation may result in higher input of nutrients from the catchment to the reservoir, promoting phytoplankton growth. The precipitation season of Yudong reservoir is concentrated, mainly from May to October. High nutrient concentrations made Yudong Reservoir unable to meet the standards of an ideal water source indicating the original management strategy is not sufficient (Table S2; China, 2002a). To reduce pollution from catchments, local legislation like rational use of phosphorus fertilizer and establish more sewage treatment plants have been introduced to strengthen watershed management. Restricted areas have also been established to reduce nutrient runoff generated by dispersed waste disposal and to prevent soil erosion (Wu et al., 2012; Lian et al., 2018). However, the complex residential and farming conditions in the catchment of the reservoir make it challenging to obtain high water quality, and until target loadings are reached, other methods must be applied of which stocking of filter feeding fish is one possibility (Lin et al., 2014; Han et al., 2020; Xiang et al., 2021).
4.2 Effects of filter-feeding fish and zooplankton on phytoplankton
Our results from Yudong Reservoir indicate that filter-feeding fish, especially silver carp, contributed to the reduction of phytoplankton, especially the larger specimens (Figures 5, 7). Thus, an increase in CPUE of silver carp significantly reduces the biomass of phytoplankton and cyanobacteria (Figure 5), and the average individual size of the phytoplankton declined despite no reduction in nutrient concentrations (Figure S3). The results are consistent with previous research showing that silver carp can feed on large-bodied phytoplankton and decrease the average body size of phytoplankton. However, nanoplankton cannot be effectively controlled, especially if zooplankton grazing is also reduced (Zhao et al., 2013; Shen et al., 2021). Since the filtering efficiency of silver carp depends on the interval of gill rakers and on the size of phytoplankton, silver carp cannot filter phytoplankton whose size is less than 10 μm (Vörös et al., 1997; Radke and Kahl, 2002). The higher density of gill rakers makes silver carp more efficient to filter phytoplankton than bighead carp, silver carp might feed more phytoplankton even in the same scenario (Xie and Liu, 2001). Accordingly, we found that the effect of bighead carp on phytoplankton in Yudong Reservoir was weaker than that of silver carp at similar CPUEs (Figures 5B, 7).
In addition to fish, zooplankton can effectively control phytoplankton provided that they are not too heavily predated upon by fish as evidenced in numerous biomanipulation experiments involving fish removal in temperate lakes (Shapiro et al., 1975; Søndergaard et al., 2007). Filter-feeding fish, however, decreased biomass of large-bodied zooplankton (Shen et al., 2021; Mao et al., 2023) as seen in Yudong Reservoir after years of filter fish stocking (Figure 4). Thus, we found a substantial decrease in large-bodied zooplankton, a smaller cladoceran body weight and a decreased ratio of zooplankton biomass to phytoplankton biomass, all indicated a higher grazing pressure on zooplankton and weaker pressure on phytoplankton in the reservoir (Figure 4; Jeppesen et al., 2011). Such results are not unexpected given the imbalance between stocking and harvesting of filter-feeding fish in Yudong Reservoir (Figure 3), especially at the end of the research phase, where the changes in the zooplankton metrics were largest (Figure 4). A lower stocking of carp or a larger harvest would, therefore, be required to better exert zooplankton control over phytoplankton (Lin et al., 2021; Mao et al., 2023). The top-down effect of phytoplankton in the Yudong Reservoir mainly came from filter-feeding fish, and the effect from zooplankton was weak.
5 Conclusions
Our results revealed that stocking of filter feeding fish (mainly silver carp and bighead carp) most likely were the key drivers of the observed reduction of the biomass of phytoplankton and cyanobacteria and the decrease in phytoplankton average size in Yudong Reservoir. Stocking also led to a major reduction of large-bodied zooplankton, which, if abundant, are the most efficient grazers on phytoplankton. Imbalance in stocking and harvesting over the years likely contributed to the loss of effective grazers. Furthermore, the fish stocking did not solve the eutrophication problem as chlorophyll-a and phytoplankton biomass remained high. In the management of the Yudong and other eutrophic reservoirs, a reduction of external nutrient loading should, therefore, be given top priority. Our results indicate that in highland (low temperature) deep-water eutrophic reservoirs, unlike observations from lowland shallow lakes, stocking of filter-feeding fish may contribute to the control of filamentous phytoplankton provided that the fish stocking is properly managed. The optimal stocking intensity of filter-feeding fish that can help control phytoplankton without excessive impact on large-bodied zooplankton in highland reservoirs is a topic for further investigation, however.
Data availability statement
The original contributions presented in the study are included in the article/Supplementary Materials, further inquiries can be directed to the corresponding author.
Ethics statement
The animal study was approved by Research Ethics Committee of Institute of Hydrobiology, Chinese Academy of Sciences. The study was conducted in accordance with the local legislation and institutional requirements.
Author contributions
CZ: conceptualization, methodology, form analysis, investigation, data curation, validation, writing–original draft. FM: methodology, investigation, data curation, validation, writing-review and editing. BL: methodology, investigation and data curation. DZ: methodology, investigation and data curation. XC: form analysis and investigation. YX: form analysis and investigation. JB: writing-review and editing. RD: writing-review and editing. HJ: writing-review and editing. EJ: conceptualization, writing-review and editing. MD: conceptualization, funding acquisition, writing-review and editing. All authors contributed to the article and approved the submitted version.
Funding
This work was financially supported by the National Key R & D Program of China (2022YFB3206903), the National Natural Science Foundation of China (32172955), the Science and Technology Poverty Alleviation Program of the Chinese Academy of Sciences (KFJ-FP-201905 and KFJ-FP-202102), and the Guizhou Province Science and Technology Support Plan (Qiankehe Support [2022] General 129). EJ was supported by the TÜBITAK program BIDEB2232 (Project 118C250). CZ was supported by the China Scholarship Council (CSC 202204910443).
Acknowledgments
We would like to thank the staff of Zhaotong Yudong Reservoir Administration for their help. We also would like to thank Anne Mette Poulsen for linguistic assistance.
Conflict of interest
Author RD is employed by Wuhan Sino-Hiking Technology Company Limited, Wuhan, China.
The remaining authors declare that the research was conducted in the absence of any commercial or financial relationships that could be construed as a potential conflict of interest.
Publisher’s note
All claims expressed in this article are solely those of the authors and do not necessarily represent those of their affiliated organizations, or those of the publisher, the editors and the reviewers. Any product that may be evaluated in this article, or claim that may be made by its manufacturer, is not guaranteed or endorsed by the publisher.
Supplementary material
The Supplementary Material for this article can be found online at: https://www.frontiersin.org/articles/10.3389/fmars.2023.1251469/full#supplementary-material
References
Amorim C. A., Ulisses C., Moura A. N. (2017). Biometric and physiological responses of Egeria densa Planch. cultivated with toxic and non-toxic strains of Microcystis. Aquat. Toxicol. 191, 201–208. doi: 10.1016/j.aquatox.2017.08.012
APHA (2005). Standard Methods for the Examination of Water and Wastewater. 21st edition (Washington, DC, USA: American Public Health Association, American Water Works Association, Water Environment Federation).
Attayde J. L., Hansson L. A. (2001). Fish-mediated nutrient recycling and the trophic cascade in lakes. Can. J. Fish. Aquat. Sci. 58, 1924–1931. doi: 10.1139/f01-12
Chiang S. C., Du N. S. (1979). Fauna Sinica, Crustacea, Freshwater Cladocera (Beijing: Science press).
China M. (2002a) Environmental Quality Standards for Surface Water. Available at: https://english.mee.gov.cn/Resources/standards/water_environment/quality_standard/200710/t20071024_111792.shtml (Accessed June 1, 2002).
China M. (2002b). Standard Methods for the Examination of Water and Wastewater (Version 4) China (Beijing: Environmental Science Press).
China M. (2009) Water Quality Guidance on Sampling Techniques. Available at: https://www.mee.gov.cn/ywgz/fgbz/bz/bzwb/jcffbz/200910/t20091010_162158.shtml (Accessed November 1, 2009).
Dumont H. J., Van de Velde I., Dumont S. (1975). The dry weight estimate of biomass in a selection of Cladocera, Copepoda and Rotifera from the plankton, periphyton and benthos of continental waters. Oecologia 19, 75–97. doi: 10.1007/BF00377592
Domaizon I., Devaux J. (1999). Experimental study of the impacts of silver carp on plankton communities of eutrophic Villerest reservoir. Aquat. Ecol. 33, 193–204. doi: 10.1023/A:1009984713183
Filstrup C. T., Wagner T., Oliver S. K., Stow C. A., Webster K. E., Stanley E. H., et al. (2018). Evidence for regional nitrogen stress on chlorophyll a in lakes across large landscape and climate gradients. Limnol. Oceanogr. 63, 324–339. doi: 10.1002/lno.10742
Guo L., Wang Q., Xie P., Tao M., Zhang J., Niu Y., et al. (2015). A non-classical biomanipulation experiment in Gonghu Bay of Lake Taihu: control of Microcystis blooms using silver and bighead carp. Aquac. Res. 46, 2211–2224. doi: 10.1111/are.12375
Han Q., Tong R., Sun W., Zhao Y., Yu J., Wang G., et al. (2020). Anthropogenic influences on the water quality of the Baiyangdian Lake in North China over the last decade. Sci. Total Environ. 701, 134929. doi: 10.1016/j.scitotenv.2019.134929
Hart R. C. (2011). Zooplankton biomass to chlorophyll ratios in relation to trophic status within and between ten South African reservoirs: Causal inferences, and implications for biomanipulation. Water SA 37, 513–522. doi: 10.4314/wsa.v37i4.9
He H., Jin H., Jeppesen E., Li K., Liu Z., Zhang Y. (2018). Fish-mediated plankton responses to increased temperature in subtropical aquatic mesocosm ecosystems: implications for lake management. Water Res. 144, 304–311. doi: 10.1016/j.watres.2018.07.055
Hu H. J. (2006). The Freshwater Algae of China: Systematics, Taxonomy and Ecology (Beijing: Science Press).
Huang X. F. (1999). Survey Observation and Analysis of Lake Ecology (Beijing: Standards Press of China).
Jackson L. J., Lauridsen T. L., Søndergaard M., Jeppesen E. (2007). A comparison of shallow Danish and Canadian lakes and implications of climate change. Freshw. Biol. 52, 1782–1792. doi: 10.1111/j.1365-2427.2007.01809.x
Jeppesen E., Jensen J. P., Jensen C., Faafeng B., Hessen D. O., Søndergaard M., et al. (2003). The impact of nutrient state and lake depth on top-down control in the pelagic zone of lakes: a study of 466 lakes from the temperate zone to the Arctic. Ecosystems 6, 313–325. doi: 10.1007/PL00021503
Jeppesen E., Jensen J. P., Søndergaard M., Lauridsen T., Pedersen L. J., Jensen L. (1997). Top-down control in freshwater lakes: the role of nutrient state, submerged macrophytes and water depth. Hydrobiologia 342, 151–164. doi: 10.1023/A:1017046130329
Jeppesen E., Noges P., Davidson T. A., Haberman J., Noges T., Blank K., et al. (2011). Zooplankton as indicators in lakes: a scientific-based plea for including zooplankton in the ecological quality assessment of lakes according to the European Water Framework Directive (WFD). Hydrobiologia 676, 279–297. doi: 10.1007/s10750-011-0831-0
Jeppesen E., Søndergaard M., Lauridsen T. L., Davidson T. A., Liu Z., Mazzeo N., et al. (2012). Biomanipulation as a restoration tool to combat eutrophication: recent advances and future challenges. Adv. Ecol. Res. 47, 411–488. doi: 10.1016/B978-0-12-398315-2.00006-5
Jeppesen E., Søndergaard M., Mazzeo N., Meerhoff M., Branco C., Huszar V. (2005). Lake restoration and biomanipulation in temperate lakes: relevance for subtropical and tropical lakes (Enfield: Science Publisher).
Lemmens P., Declerck S. A., Tuytens K., Vanderstukken M., De Meester L. (2018). Bottom-up effects on biomass versus top-down effects on identity: a multiple-lake fish community manipulation experiment. Ecosystems 21, 166–177. doi: 10.1007/s10021-017-0144-x
Li Y., Nwankwegu A. S., Huang Y., Norgbey E., Paerl H. W., Acharya K. (2020). Evaluating the phytoplankton, nitrate, and ammonium interactions during summer bloom in tributary of a subtropical reservoir. J. Environ. Manage. 271, 110971. doi: 10.1016/j.jenvman.2020.110971
Lian Y., Li C., Ye S., Li W., Liu J., Li Z. (2018). Fish spatial distribution patterns and controlling factors in Yudong Reservoir, Yunnan Plateau. J. Lake Sci. 30 1755–1765. doi: 10.18307/2018.0626
Lin Q., Chen Q., Peng L., Xiao L., Lei L., Jeppesen E. (2020). Do bigheaded carp act as a phosphorus source for phytoplankton in (sub) tropical Chinese reservoirs? Water Res. 180, 115841. doi: 10.1016/j.watres.2020.115841
Lin Q., Jiang X., Han B. P., Jeppesen E. (2014). Does stocking of filter-feeding fish for production have a cascading effect on zooplankton and ecological state? A study of fourteen (sub) tropical Chinese reservoirs with contrasting nutrient concentrations. Hydrobiologia 736, 115–125. doi: 10.1007/s10750-014-1896-3
Lin Q., Zeng D., Guo T., Peng L. (2021). Filter-feeding fish (Hypophthalmichthys molitrix) mediated phosphorus recycling versus grazing pressure as drivers of the trophic cascade in large enclosures subsidized by allochthonous detritus. Water Res. 204, 117579. doi: 10.1016/j.watres.2021.117579
Mao Z., Cao Y., Gu X., Zeng Q., Chen H., Jeppesen E. (2023). Response of zooplankton to nutrient reduction and enhanced fish predation in a shallow eutrophic lake. Ecol. Appl. 33, e2750. doi: 10.1002/eap.2750
Mao Z., Gu X., Cao Y., Zhang M., Zeng Q., Chen H., et al. (2020). The role of top-down and bottom-up control for phytoplankton in a subtropical shallow eutrophic lake: evidence based on long-term monitoring and modeling. Ecosystems 23, 1449–1463. doi: 10.1007/s10021-020-00480-0
Nwankwegu A. S., Li Y., Huang Y., Wei J., Norgbey E., Lai Q., et al. (2020). Nutrient addition bioassay and phytoplankton community structure monitored during autumn in Xiangxi Bay of Three Gorges Reservoir, China. Chemosphere 247, 125960. doi: 10.1016/j.chemosphere.2020.125960
Olokotum M., Mitroi V., Troussellier M., Semyalo R., Bernard C., Montuelle B., et al. (2020). A review of the socioecological causes and consequences of cyanobacterial blooms in Lake Victoria. Harmful Algae 96, 101829. doi: 10.1016/j.hal.2020.101829
Qu X., Chen Y., Liu H., Xia W., Lu Y., Gang D. D., et al. (2020). A holistic assessment of water quality condition and spatiotemporal patterns in impounded lakes along the eastern route of China's South-to-North water diversion project. Water Res. 185, 116275. doi: 10.1016/j.watres.2020.116275
Radke R. J., Kahl U. (2002). Effects of a filter-feeding fish [silver carp, Hypophthalmichthys molitrix (Val.)] on phyto-and zooplankton in a mesotrophic reservoir: results from an enclosure experiment. Freshw. Biol. 47, 2337–2344. doi: 10.1046/j.1365-2427.2002.00993.x
Reynolds C. F. (1984). The Ecology of Freshwater Phytoplankton (Cambridge: Cambridge University Press).
Shapiro J., Lamarra V., Lynch M. (1975). “Biomanipulation: an ecosystem approach to lake restoration,” in Proc. Symp. on water quality management through biological control (Florida: Univ. Florida and US Environ. Prot. Agency), 85–95.
Shen R., Gu X., Chen H., Mao Z., Zeng Q., Jeppesen E. (2021). Silver carp (Hypophthalmichthys molitrix) stocking promotes phytoplankton growth by suppression of zooplankton rather than through nutrient recycling: An outdoor mesocosm study. Freshw. Biol. 66, 1074–1088. doi: 10.1111/fwb.13700
Shipley B. (2013). The AIC model selection method applied to path analytic models compared using ad-separation test. Ecology 94, 560–564. doi: 10.1890/12-0976.1
Søndergaard M., Jeppesen E., Lauridsen T. L., Skov C., Van Nes E. H., Roijackers R., et al. (2007). Lake restoration: successes, failures and long-term effects. J. Appl. Ecol. 44, 1095–1105. doi: 10.1111/j.1365-2664.2007.01363.x
Starling F. L. D. R. M. (1993). Control of eutrophication by silver carp (Hypophthalmichthys molitrix) in the tropical Paranoa Reservoir (Brasília, Brazil): a mesocosm experiment. Hydrobiologia 257, 143–152. doi: 10.1007/BF00765007
Su H., Pan J., Feng Y., Yu J., Liu J., Wang L., et al. (2021). Stocking alien carp leads to regime shifts in native fish populations: Evidence from long-term observation and ecological modeling of a Chinese reservoir. Ecol. Indic. 132, 108327. doi: 10.1016/j.ecolind.2021.108327
Vörös L., Oldal I., Présing M., Balogh K. (1997). Size-selective filtration and taxon-specific digestion of plankton algae by silver carp (Hypophthalmichthys molitrix Val.). Hydrobiologia 342, 223–228. doi: 10.1023/A:1017039423485
Walsh C., Mac Nally R. (2013). hier.part: hierarchical partitioning (Vienna: R package version 1.0-4. R Project for Statistical Computing).
Wood S. N. (2020). Package “mgcv”. Available at:https://cran.r-project.org/web/packages/mgcv/index.html.
Wu Z., Wang X., Chen Y., Cai Y., Deng J. (2018). Assessing river water quality using water quality index in Lake Taihu Basin, China. Sci. Total Environ. 612, 914–922. doi: 10.1016/j.scitotenv.2017.08.293
Wu W., Yang K., Wang Z., Li B., Liu Y. (2012). Community structure and seasonal succession of phytoplankton in Yudong Reservoir of Yungui-plateau. J. Hydroecol. 33, 69–75.
Xiang R., Wang L., Li H., Tian Z., Zheng B. (2021). Water quality variation in tributaries of the Three Gorges Reservoir from 2000 to 2015. Water Res. 195, 116993. doi: 10.1016/j.watres.2021.116993
Xie P., Liu J. (2001). Practical success of biomanipulation using filter-feeding fish to control cyanobacteria blooms: a synthesis of decades of research and application in a subtropical hypereutrophic lake. Sci. World J. 1, 337–356. doi: 10.1100/tsw.2001.67
Yvon-Durocher G., Montoya J. M., Trimmer M., Woodward G. U. Y. (2011). Warming alters the size spectrum and shifts the distribution of biomass in freshwater ecosystems. Glob. Change Biol. 17, 1681–1694. doi: 10.1111/j.1365-2486.2010.02321.x
Zhang Z., Cao Y., Jeppesen E., Li W. (2016b). The response of Vallisneria spinulosa (Hydrocharitaceae) and plankton to pulse addition of inorganic nitrogen with different loading patterns. Hydrobiologia 767, 175–184. doi: 10.1007/s10750-015-2494-8
Zhang Y., Cheng L., Li K., Zhang L., Cai Y., Wang X., et al. (2019). Nutrient enrichment homogenizes taxonomic and functional diversity of benthic macroinvertebrate assemblages in shallow lakes. Limnol. Oceanogr. 64, 1047–1058. doi: 10.1002/lno.11096
Zhang X., Liu Z., Jeppesen E., Taylor W. D., Rudstam L. G. (2016a). Effects of benthic-feeding common carp and filter-feeding silver carp on benthic-pelagic coupling: implications for shallow lake management. Ecol. Eng. 88, 256–264. doi: 10.1016/j.ecoleng.2015.12.039
Keywords: phytoplankton, filter-feeding fish, trophic cascade, highland reservoir, fish stocking
Citation: Zhang C, Mei F, Luo B, Zhang D, Cheng X, Xiong Y, Bao J, Duan R, Jiang H, Jeppesen E and Duan M (2023) Phytoplankton control by stocking of filter-feeding fish in a subtropical plateau reservoir, southwest China. Front. Mar. Sci. 10:1251469. doi: 10.3389/fmars.2023.1251469
Received: 01 July 2023; Accepted: 11 September 2023;
Published: 27 September 2023.
Edited by:
Weijie Wang, China Institute of Water Resources and Hydropower Research, ChinaCopyright © 2023 Zhang, Mei, Luo, Zhang, Cheng, Xiong, Bao, Duan, Jiang, Jeppesen and Duan. This is an open-access article distributed under the terms of the Creative Commons Attribution License (CC BY). The use, distribution or reproduction in other forums is permitted, provided the original author(s) and the copyright owner(s) are credited and that the original publication in this journal is cited, in accordance with accepted academic practice. No use, distribution or reproduction is permitted which does not comply with these terms.
*Correspondence: Ming Duan, ZHVhbm1pbmdAaWhiLmFjLmNu