- 1Integrative Science Center of Germplasm Creation in Western China (CHONGQING) Science City & Southwest University, Chongqing, China
- 2Key Laboratory of Freshwater Fish Reproduction and Development (Ministry of Education), Key Laboratory of Aquatic Science of Chongqing, School of Life Sciences, Southwest University, Chongqing, China
Vitellogenesis is the most important stage of ovarian maturation in fish, involving the synthesis and transport of essential yolk substances and their complex mechanisms and coordination process. The liver is the main tissue involved in the vitellogenesis of oviparous animals, but studies of vitellogenesis in fish rarely include the liver and ovary as a whole. The aim of this study was to explore the molecular mechanism and associated regulatory factors behind vitellogenesis in Sichuan bream (Sinibrama taeniatus). The different stages of oogenesis were first identified by successive histological observations. Then, ovary and liver tissues that developed to 115 days (stage II, previtellogenesis stage), 165 days (stage III, vitellogenesis stage) and 185 days (stage IV, late-vitellogenesis stage) were collected for transcriptomic and metabolomic analyses, and serum testosterone (T), 17β-estradiol (E2), vitellogenin (Vtg), triiodothyronine (T3), and thyroxine (T4) levels were measured at the corresponding stages. We found that energy redistribution during vitellogenesis is mainly regulated through glycolysis, fatty acid biosynthesis and the citrate cycle pathway. In the liver, energy metabolism was promoted by activating glucolipid metabolic pathways to provide sufficient ATP, but at the same time, the ovary tends to retain nutrients rather than decompose them to produce energy. In addition, we have identified several key factors involved in the metabolism of neutral lipids, polar lipids, amino acids and vitamins, which are involved in the assembly and transport of important yolk nutrients. The initiation of vitellogenesis was found to be associated with a surge in serum E2 levels, but the sustained increase in Vtg levels in the late stage may be due more to upregulation of the estrogen receptor. These results provide valuable information about the regulation of ovarian development in cultured fish.
1 Introduction
The process of vitellogenesis in teleosts is generally characterized by the synthesis and secretion of vitellogenin (Vtg) by the liver under estrogen stimulation, its transportation to the ovaries through blood circulation, uptake by growing oocytes, and subsequent processing into yolk proteins (YPs) (Kime, 1993; Lubzens et al., 2017). As the primary precursors of YPs, Vtg is the main source of polar lipids and amino acids nutrition in oocytes (Finn and Fyhn, 2010). Additionally, Vtg functions as a specialized carrier of lipid soluble vitamins, inorganic ions and hormones (Babin et al., 2007; Lubzens et al., 2017). The process of vitellogenesis in fish represents a crucial and intricate stage of oogenesis, involving morphological and structural alterations in oocytes (Tyler and Sumpter, 1996; Le Menn et al., 2007), as well as the coordination and allocation of nutrient and energy reserves within the organism (Perez et al., 2007). These factors collectively determine the nutritional status of eggs and subsequently impact offspring survival (Hiramatsu et al., 2015).
The causes of vitellogenesis are intricate and dynamic (Hiramatsu et al., 2013), necessitating a comprehensive understanding of the underlying mechanisms and coordinated processes to enhance species reproduction management and conservation. Previous studies have elucidated the prevailing vitellogenesis pattern, contingent upon the regulation of the hypothalamic-pituitary-gonadal (HPG) axis (Nagahama and Yamashita, 2008; Grier et al., 2009; Levavi-Sivan et al., 2010). The hypothalamus mediates the transmission of crucial signals from the central nervous system through the release of gonadotropin-releasing hormone (GnRH), which stimulates the pituitary gland to produce follicle stimulating hormone (FSH) and luteinizing hormone (LH), ultimately inducing estradiol (E2) secretion in the ovaries (Zohar et al., 2010). E2 forms dimers with the estrogen receptors, guiding Vtg synthesis in the liver and subsequent release into the circulation. Then, Vtg is incorporated into the developing oocyte to support its growth (McBride et al., 2015).Factors that control vitellogenesis in fish may affect the HPG axis at different levels, leading to the initiation or delay of development (Good and Davidson, 2016). The previous studies have generally concluded that vitellogenesis is directly regulated by E2 in vivo, as evidenced by the progressive increases in plasma FSH levels and follicular fshr mRNA expression, both of which enhance pituitary-to-ovarian signaling, thereby further promoting E2 synthesis (Luckenbach et al., 2008; Guzman et al., 2014). In the endocrine regulation of the ovary, StAR, Cyp1a1, Hsd3 and Cyp19a1 proteins appear to be common targets of gonadotropin-mediated upregulation of expression during the vitellogenesis stage (Ings and van der Kraak, 2006; Kagawa et al., 2003; Nakamura et al., 2003; Montserrat et al., 2004; Kazeto et al., 2006; Zhang et al., 2013). In addition to sex hormones, there is increasing evidence of a cross-talk between the reproductive and thyroid systems in teleosts due to the fact that thyroid hormones often exhibit seasonal rhythms associated with reproduction (Duarte-Guterman et al., 2014; De Vincentis et al., 2018). The administration of triiodothyronine (T3) in goldfish significantly enhanced the expression of estrogen receptor alpha (ERα) in the liver, thereby promoting an increase in Vtg levels through its interaction with E2 (Nelson and Habibi, 2016). The field of nutrigenomics believes that ensuring adequate body nutrition and lipid reserves can affect vitellogenesis via nerve center regulation of gene expression (Taranger et al., 2010; Sullivan and Yilmaz, 2018). The addition of 8% fish oil to the diet of spotted scat (Scatophagus argus) compared to 8% soybean oil can effectively promote the accumulation of yolk in the ovaries by regulating the expression of genes related to steroid hormones, glucose and lipid metabolic pathways, with an increased proportion of n-3 long chain polyunsaturated fatty acids, such as docosahexaenoic acid (DHA) and eicosapentaenoic acid (EPA), in the ovaries (Wang et al., 2022). Meanwhile, dietary supplementation with fish oil increased the serum vitellogenin levels of female spotted scat and upregulated the expression of the reproduction-related genes vtga, vtgb, and zp4, as well as fabp2 and mfsd2ab, which are related to lipid absorption and transport in the liver (Wang et al., 2021).
China is the world’s largest aquaculture producer, and traditional carp species occupy an important share of the inland aquaculture industry (Tacon and Metian, 2015; Edwards et al., 2019; Newton et al., 2021). The long time required for ovarian maturation of carp species, especially before vitellogenesis (Sehgal and Toor, 1995; Song and Wessel, 2005), has significantly limited the development of the aquaculture industry. Therefore, investigating the molecular mechanisms that initiate vitellogenesis and seeking regulation is an unavoidable issue in aquaculture production. In oviparous vertebrates, the liver plays a crucial role in gonadal development and is primarily responsible for synthesizing and providing essential nutrients during vitellogenesis to support oocyte growth (Levi et al., 2009; Xu et al., 2016; Gloux et al., 2019). However, studies on fish vitellogenesis often separate the ovaries from the liver (Osachoff et al., 2016; Xu et al., 2016; Bertolini et al., 2020; Meng et al., 2022), and only a few reports on crustacean aquatic animals have combined them to investigate the mechanisms underlying vitellogenesis (Feng et al., 2022; Li et al., 2022). Sichuan bream (Sinibrama taeniatus) is a small economic Cyprinidae fish endemic to the upper reaches of the Yangtze River (Luo et al., 2014), usually breeding once or twice a year under natural conditions, with the youngest female sexually mature individual in the field over one year old (Li et al., 2015). At present, by optimizing breeding conditions in the laboratory, we can make adults spawn once every 2 weeks, and the sexual maturation time of juveniles has been greatly reduced compared to those in the wild at the same time points. Based on its easy-to-regulate characteristics, Sichuan bream can serve as a good model for exploring key factors in the ovarian development of Cyprinidae fish. The purpose of this study was to explore the biological changes related to the ovarian maturation process, especially the vitellogenesis stage, in juvenile Sichuan bream by regularly measuring their growth performance, ovarian development status, serum steroid hormones, thyroid hormones and Vtg levels and the combination of transcriptomic and metabolomic analysis of ovary and liver. The results of this study will help us gain insight into the biological changes that occur during ovarian development and vitellogenesis to help regulate the captive breeding of economic fish.
2 Materials and methods
2.1 Ethics statement
All experimental protocols were approved by Southwest University, and the study was carried out under the protocols of the Institutional Animal Care and Use Committee of Southwest University.
2.2 Experimental fish and sample collection
The experimental fish (a total of 350 individuals) were artificially bred Sinibrama taeniatus, with the parents being 3 females and 3 males from one population collected from natural rivers of the Upper Yangtze River. Fish were maintained in indoor tanks (50 × 40 × 30 cm) with a water circulating system at 26°C under a 14 L:10 D lighting cycle and fed twice daily using commercial feed.
The experiment lasted from November 2021 to May 2022, and the sampling times were as follows: samples were collected every 2 days from 1-30 days after hatching, every 5 days from 30-60 days, and every 10 days after 60 days. Ten fish were dissected after anesthesia with tricaine methanesulfonate (MS-222) each time. Individual measurements of body length and body weight were taken. Ovaries were removed and weighed to determine the gonadosomatic index (GSI = [gonad weight/body weight] × 100) and then fixed in Bouin’s solution for further histological analysis to determine the timing of each stage of oogenesis.
At 115 (stage II), 165 (stage III), and 185 days (stage IV) after hatching, another 6 female individuals were sampled. Blood, ovary and liver samples of each individual were collected as follows: (a) blood samples were centrifuged (4°C, 4000 r/min, 10 min) to collect serum and stored at -80°C for the determination of serum sex hormones and vitellogenin levels; (b) parts of the ovary were fixed in Bouin’s solution for further histological analysis; (c) remaining ovary and liver samples were each divided into 3 portions and stored at -80°C for RNA isolation, transcriptome analysis and metabolome analysis.
2.3 Histological analysis
Fixed ovary tissues were dehydrated with serial dilutions of ethanol, embedded in paraffin, sectioned serially at 5-8 μm in thickness (Leica RM 2235), and then double stained with hematoxylin and eosin for histological analysis to distinguish the different stages of ovarian development (Lowerre-Barbieri et al., 2011; Yin et al., 2019). All micrographs were taken using a light microscope (Nikon Eclipse 80i), and oocyte diameters were recorded.
2.4 Serum biochemical analyses
Fish ELISA Kits (Jiangsu Meimian Industrial Co., Ltd., Jiangsu, China) were used to determine serum concentrations of 17β-estradiol (E2), testosterone (T), vitellogenin (Vtg), triiodothyronine (T3), and thyroxine (T4) according to the manufacturer’s instructions. Measure the absorbance (OD) value using a microplate reader (Rayto RT-6100, USA) at a wavelength of 450nm to calculate the concentration. Serum samples from six fish in each group were tested.
2.5 Transcriptomic analysis
Total RNA from stage II, stage III, and stage IV ovaries and liver tissues were extracted using Trizol reagent (TaKaRa Bio, Japan) according to the manufacturer’s protocol, and the quality of total RNA was assessed using an Agilent 2100 Bioanalyzer system (Agilent Technologies, USA). For more detailed instructions, refer to our previous work (Yuan et al., 2020). The qualified ovary and liver samples (total RNA concentration ≥ 10 μg; RNA integrity number ≥ 8) were used for sequencing library construction with a paired-end sample preparation kit (Illumina Inc., USA), and the library was sequenced on an Illumina HiSeq 2000 sequencer.
Before proceeding to the subsequent analysis step, the raw sequencing data were filtered to remove the noise reads that contained adapters, ploy-N reads, and low-quality reads by fastp (Chen et al., 2018). The resulting clean reads were subsequently mapped onto a Sinibrama taeniatus genome using the aligner HISAT2 (Kim et al., 2019). Gene expression quantification was calculated using RNA-Seq by Expectation-Maximization (RSEM), and they were converted to Fragments Per Kilobase of exon model per Million mapped fragments (FPKM), thereby generating normalized gene/transcript expression levels (Li and Dewey, 2011). The DESeq2 R package (Love et al., 2014) was used to analyze differential expression between the two groups with adjusted P values. The threshold for significant differential expression was established at adjusted P value < 0.05 and absolute log2FC ≥ 1. The clusterProfiler R package (Wu TZ. et al., 2021) was used to perform Gene Ontology (GO) and Kyoto Encyclopedia of Genes and Genomes (KEGG) enrichment analyses of differentially expressed genes (DEGs), and results were visualized using ggplot2 package.
2.6 Experimental validation by quantitative real-time PCR
Quantitative real-time PCR (qRT-PCR) was performed with TB Green ® Premix Ex TaqTM II (Takara Bio) on a QuantStudio 1 (Thermo Fisher, USA). Ten DEGs were selected in each of the ovary and liver samples. According to the genome and transcriptome data, the primers of the target gene were designed (see Supplementary Table A.1). β-actin was used as the internal reference gene, and the 2−ΔΔCT method was used to analyze the expression level of genes.
2.7 Metabolomics analysis
Ovary and liver tissues from stage II, stage III and stage IV were used for metabolomic analysis. A 25 mg sample was weighed into an EP tube, and 500 μL extract solution (methanol: acetonitrile: water = 2:2:1, with isotopically labeled internal standard mixture) was added. Then, the samples were homogenized at 35 Hz for 4 min and sonicated for 5 min in an ice-water bath. The homogenization and sonication cycle were repeated 3 times. Then, the samples were incubated for 1 h at -40°C and centrifuged at 12000 rpm (RCF = 13800 (×g), R = 8.6 cm) for 15 min at 4°C. The resulting supernatant was transferred to a fresh glass vial for analysis. The quality control (QC) sample was prepared by mixing an equal aliquot of the supernatants from all of the samples.
LC-MS/MS analyses were performed using an UHPLC system (Vanquish, Thermo Fisher Scientific) with a UPLC BEH Amide column (2.1 mm × 100 mm, 1.7 μm) coupled to an Orbitrap Exploris 120 mass spectrometer (Orbitrap MS, Thermo) (Wang et al., 2016). The mobile phase consisted of 25 mmol/L ammonium acetate and 25 mmol/L ammonium hydroxide in water (pH = 9.75) (A) and acetonitrile (B). The autosampler temperature was 4°C, and the injection volume was 2 μL. The Orbitrap Exploris 120 mass spectrometer was used for its ability to acquire MS/MS spectra in information-dependent acquisition (IDA) mode by the acquisition software (Xcalibur, Thermo). In this mode, the acquisition software continuously evaluates the full scan MS spectrum. The ESI source conditions were set as follows: sheath gas flow rate, 50 Arb; Aux gas flow rate, 15 Arb; capillary temperature, 320°C; full MS resolution, 60000; MS/MS resolution, 15000; collision energy, 10/30/60 in NCE mode; spray voltage, 3.8 kV (positive) or -3.4 kV (negative).
The raw data were converted to the mzXML format using ProteoWizard and processed with an in-house program that was developed using R and based on XCMS for peak detection, extraction, alignment, and integration (Smith et al., 2006). Then, an in-house MS2 database (BiotreeDB) was applied for metabolite annotation. The cutoff for annotation was set at 0.3. The compounds identified in positive mode (POS) and negative mode (NEG) were consolidated prior to subsequent analysis.
The resulting data were fed into the SIMCA16 software package (Umetrics, Umea, Sweden) for principal component analysis (PCA) and orthogonal projections to latent structures–discriminate analysis (OPLS–DA). In the OPLS-DA model, R2Y is used to represent the interpretation rate, and Q2 is used to represent the prediction rate of the model. Theoretically, the closer R2Y and Q2 are to 1, the better the model is. The variable importance in the projection (VIP) was sorted according to the overall contribution of each variable to the OPLS-DA model, and those metabolites with a VIP ≥ 1 and P value < 0.05 were considered significant differences. The differentially expressed metabolites (DMs) were annotated with KEGG pathway analysis using metaboanalyst software (www.metaboanalyst.ca).
2.8 Statistics
All data are presented as the mean value ± standard deviation. One-way ANOVA was used for comparisons between groups, followed by Duncan’s multiple range test, and P < 0.05 was considered significant. All statistical analyses were performed using SPSS 23 software (SPSS, Chicago, IL, USA).
3 Results
3.1 Stage of oogenesis
Several key stages of oogenesis in Sichuan bream were identified by histological observation. Within 40 days after hatching, there were only primordial germ cells in the gonads, which cannot be used to distinguish between males and females, and the germ cells subsequently pass through the following four developmental stages that eventually lead to egg maturation. Stage I: 40-60 days, the ovary is filled with clusters of oogonia undergoing vigorous mitosis (Figure 1A). Stage II: 60-150 days, oocytes enter the primary growth stage. The nucleus lacks a large central nucleolus, and multiple small nucleoli begin to appear on the inside of the nuclear membrane margins (Figure 1B). In addition, oocyte size continues to increase, and protoplasts continue to accumulate at this stage (Figure 1C). Stage III: 150-180 days, the appearance of yolk vesicles (or cortical alveoli) marks the entry of the oocyte into the secondary growth stage and the initiation of vitellogenesis (Figure 1D). Subsequently, there is a rapid accumulation of yolk material within the cell accompanied by an increase in the number of yolk vesicles (Figure 1E). Stage IV: After 180 days, entering the stage of late-vitellogenesis. Yolk granules dominate the ooplasm of the largest oocytes (Figure 1F). Following yolk accumulation, artificial induction can be employed to facilitate ovary maturation.
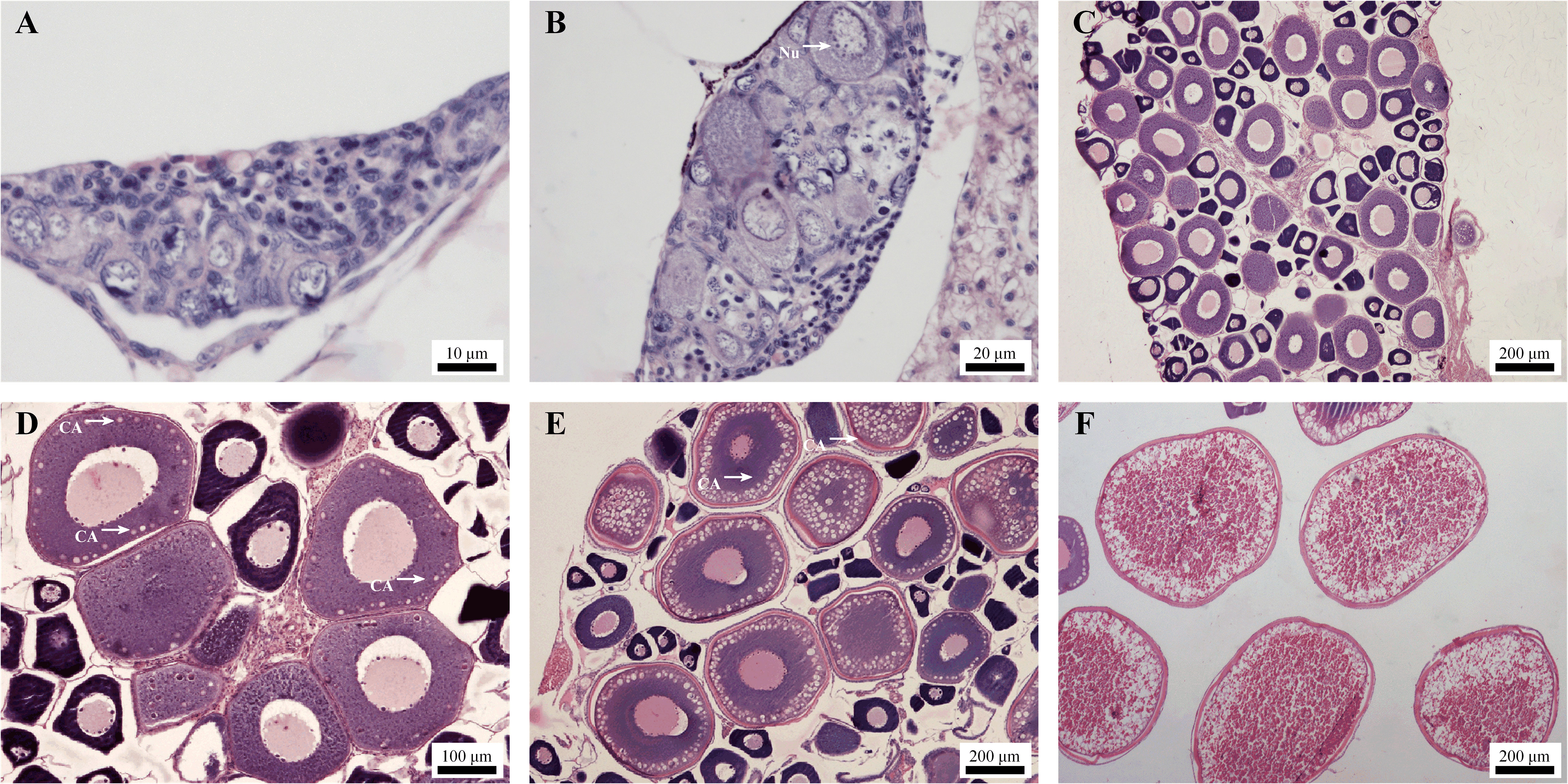
Figure 1. Micrographs of ovaries at different developmental stages. (A) On day 45, ovary in stage I; (B) On day 60, ovary in early-primary growth stage. Nu, Nucleolus; (C) On day 100, ovary in mid- primary growth stage; (D) On day 150, ovary in early-vitellogenesis stage. CA, cortical alveoli; (E) On day 165, ovary in mid-vitellogenesis stage; (F) On day 185, ovary in late-vitellogenesis stage.
3.2 Growth performance
Body length, body weight, GSI and oocyte diameter were recorded during oogenesis, and all values showed a gradual increase throughout the developmental stages. Notably, the increase in body length and body weight slowed or even “paused” upon entering stage III (Figure 2A). In contrast, ovarian development was significantly promoted in stage III compared to the long stage II, as reflected by the increase in GSI and oocyte diameter (Figure 2B), indicating that the material and energy metabolism in the body is undergoing dramatic changes at this critical stage.
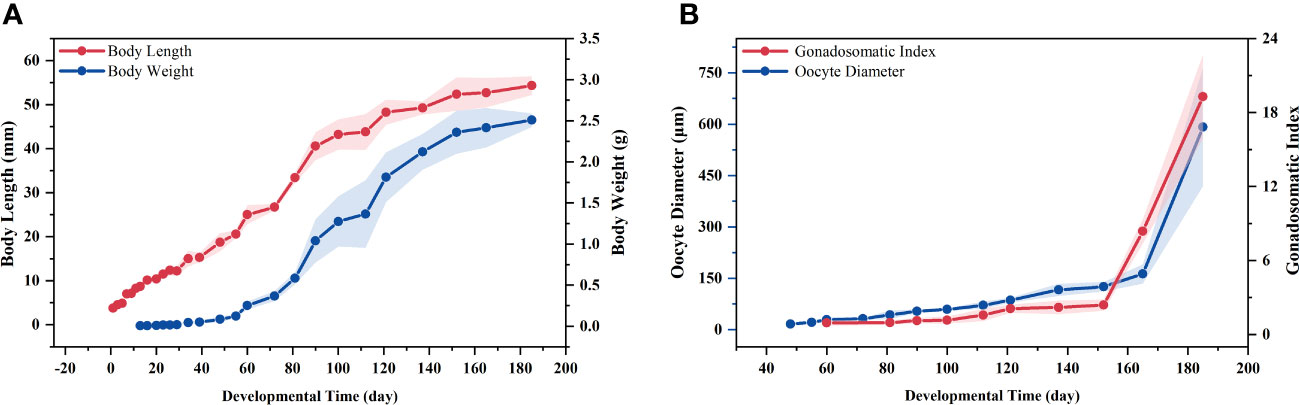
Figure 2. Changes in biometric parameters during oogenesis. (A) Body length and body weight; (B) Gonadosomatic index (GSI) and oocyte diameter.
3.3 Serum biochemistry
The levels of serum testosterone (T), 17β-estradiol (E2), vitellogenin (Vtg), triiodothyronine (T3), and thyroxine (T4) were measured during stages II, III and IV of oogenesis. Serum T levels were maintained at low levels at different stages, and there was no significant difference between the stages (P > 0.05) (Figure 3A). Compared to stage II, serum E2 levels increased significantly in stages III and IV (P < 0.05), but there was no significant difference between stages III and IV (P > 0.05) (Figure 3B). Serum Vtg levels continued to increase in stages II, III and IV, with significant differences between all groups (P < 0.05) (Figure 3C). In addition, the two serum thyroid hormones levels exhibited distinct patterns. Serum T3 levels demonstrated a high degree of similarity across the three developmental stages (P > 0.05) (Figure 3D), while T4 levels followed the same trend as Vtgs and continued to rise during vitellogenesis, with significant differences between groups (P < 0.05) (Figure 3E).
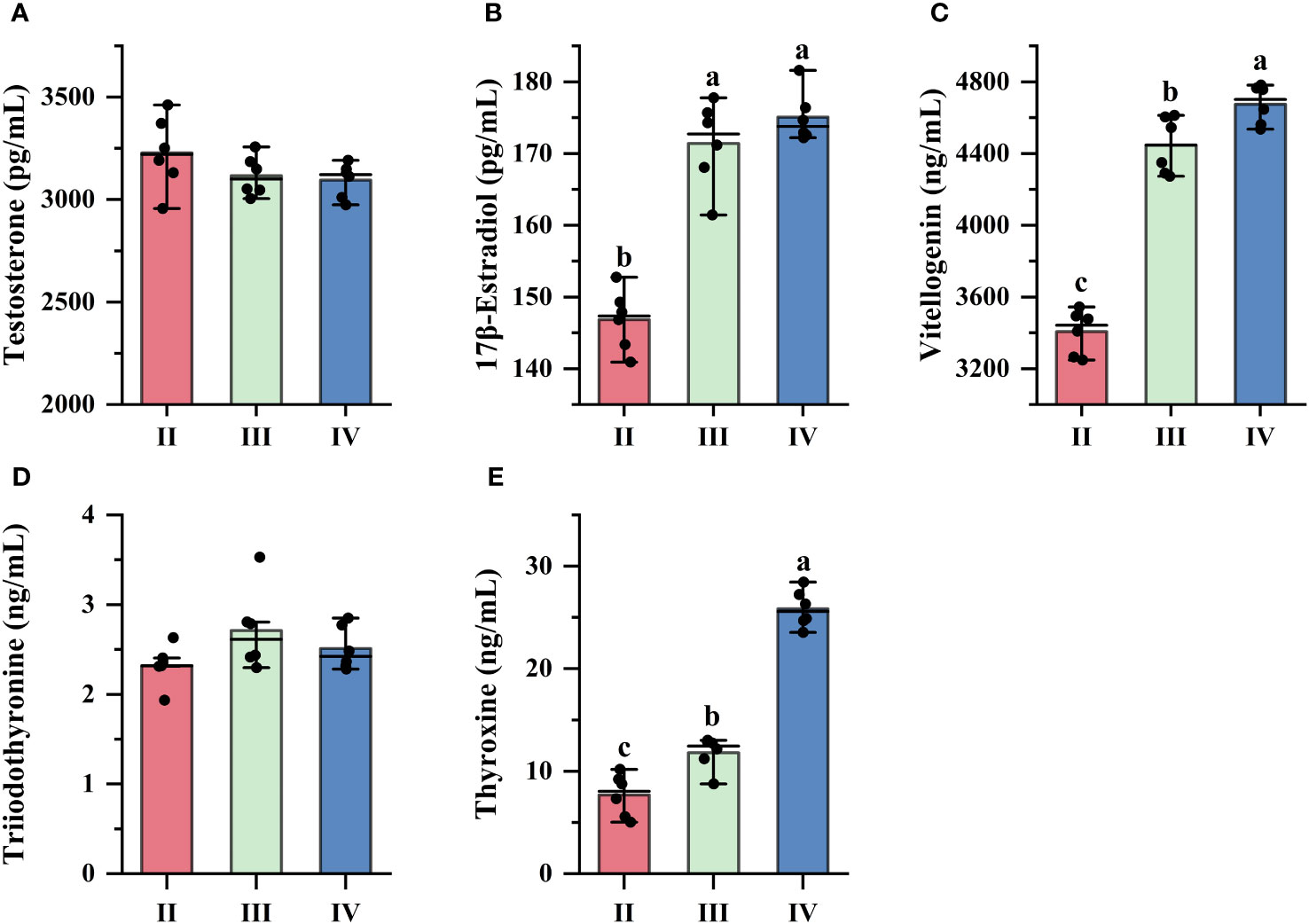
Figure 3. Changes in serum biochemical levels. (A) Testosterone; (B) 17β-estradiol; (C) Vitellogenin; (D) Triiodothyronine; (E) Thyroxine. The x-axis indicates different developmental stages of the ovary. a,b,c Mean values with unlike letters were significantly different (P < 0.05).
3.4 Transcriptomic analysis
The RNA-Seq analysis was conducted on ovarian and liver samples collected from three distinct developmental stages. After preprocessing and filtering the low-quality sequences, 144.06 M, 149.55 M and 152.75 M total clean reads were obtained for ovaries at stages II, III and IV, respectively, while 137.29 M, 130.23 M and 127.74 M total clean reads were obtained for liver samples at the corresponding stages. The Q20, Q30 and total mapping values of all samples were analyzed to show the high quality of sequencing and alignment (Supplementary Table A.2).
The results of differential expression analysis showed that the comparison of stage II and stage IV resulted in the highest number of DEGs in the ovary, with 1150 DEGs upregulated and 1054 DEGs downregulated (Figure 4A). Conversely, the lowest number of DEGs was obtained in the comparison of stage III and stage IV ovaries. Notably, 609 (581 + 29) DEGs produced in stage II versus stage III and in stage II versus stage IV are identical, maybe implying that the ovary undergoes similar regulation in the two stages after entering vitellogenesis compared to the previtellogenesis stage (Figure 4B). The number of DEGs in the liver did not exhibit significant variation across different stages, with the highest number of DEGs observed in the comparisons between stages III and IV (Figure 4C). Additionally, the quantitative relationship between the comparison groups in the liver exhibited less variability compared to that in the ovaries (Figure 4D).
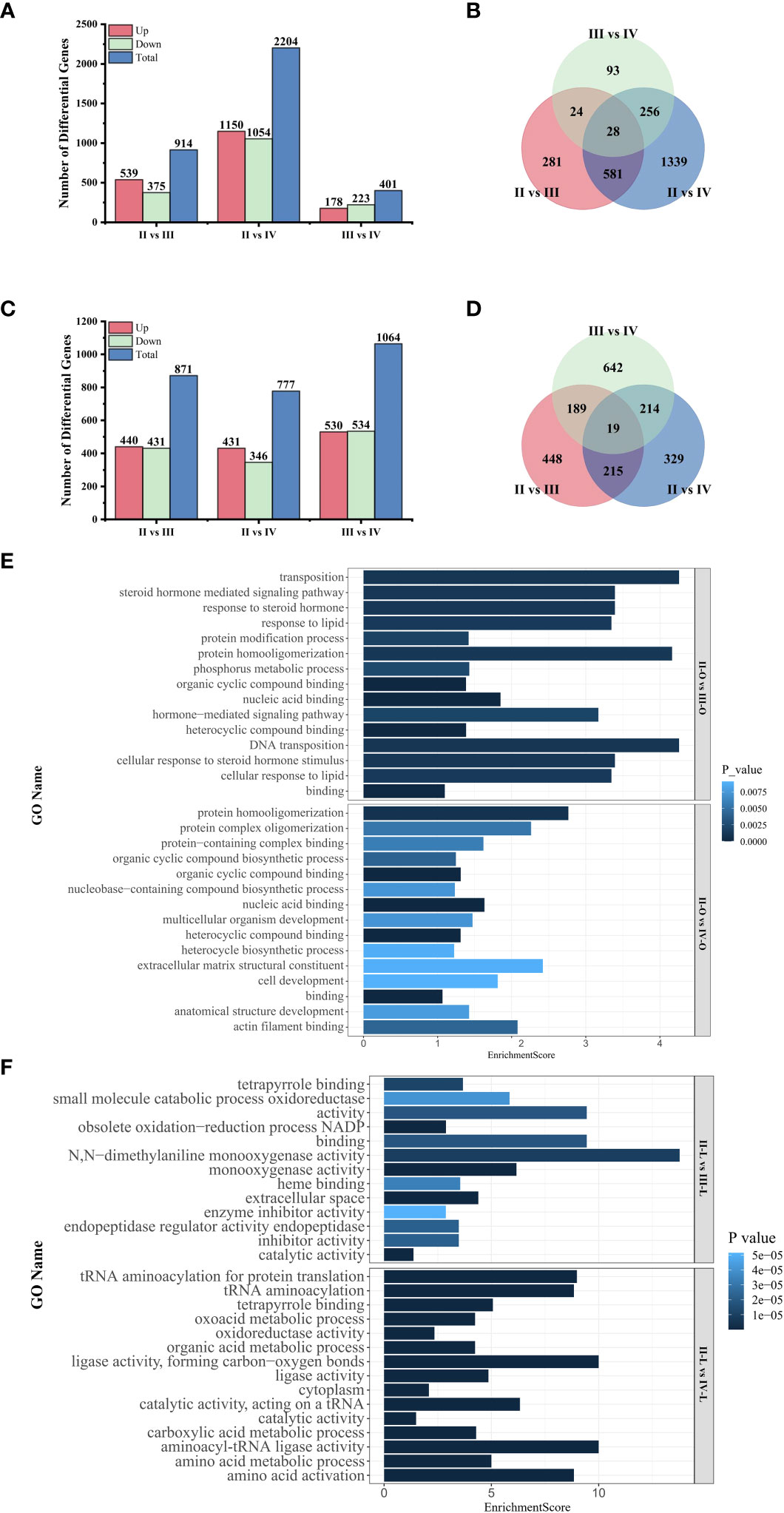
Figure 4. Transcriptome analysis among the comparison groups. (A) Number of DEGs in the ovary; (B) Venn diagram of DEGs in the ovary; (C) Number of DEGs in the liver; (D) Venn diagram of DEGs in the liver; (E) GO enrichment analysis of DEGs in the ovary; (F) GO enrichment analysis of DEGs in the liver.
In this paper, we focused on the differences between pre- and post-vitellogenesis, so we performed enrichment analysis for the DEGs generated in the comparison of stage II with stage III and stage II with stage IV to explore their functional categories. The top 15 GO terms showed that DEGs in the ovary (Figure 4E) were mainly associated with “binding”, including “nucleic acid binding” and “organic cyclic compound binding” after the start of vitellogenesis. In the comparison between stage II and stage III, DEGs also affected the “response to steroid hormone” and the “response to lipid”. DEGs in the liver (Figure 4F) were mainly related to “catalytic activity” and “oxidoreductase activity” during vitellogenesis, and DEGs in stage IV particularly affected “amino acid activation” and “amino acid metabolic processes”.
In the comparison of stage II with stages III and IV, DEGs in the ovary were significantly enriched in 24 and 34 pathways by KEGG analysis (Figure 5A), while DEGs in the liver were enriched in 89 and 80 pathways (Figure 5B), respectively. During vitellogenesis, ovarian development is controlled by a complex regulatory network, in which the functions of DEGs are mainly related to environmental information processing, and several signal transduction pathways, such as the “PI3K-Akt signaling pathway”, the “MAPK signaling pathway” and the “Rap1 signaling pathway”, are significantly enriched. In addition, three hormone-related pathways were enriched in stage IV, including “ovarian steroidogenesis”, “thyroid hormone signaling pathway” and “cortisol synthesis and secretion”. In the liver, DEGs were mainly enriched in pathways related to energy metabolism, such as “glycolysis/gluconeogenesis”, “fatty acid biosynthesis” and “citric acid cycle (TCA cycle)”. In addition, pathways related to lipid metabolism, amino acid metabolism and vitamin metabolism, such as “Glycerophospholipid metabolism”, “Alanine, aspartate and glutamate metabolism” and “Retinol metabolism”, were also significantly enriched, which indicates involvement of the synthesis or assembly processes of yolk nutrients in the liver.
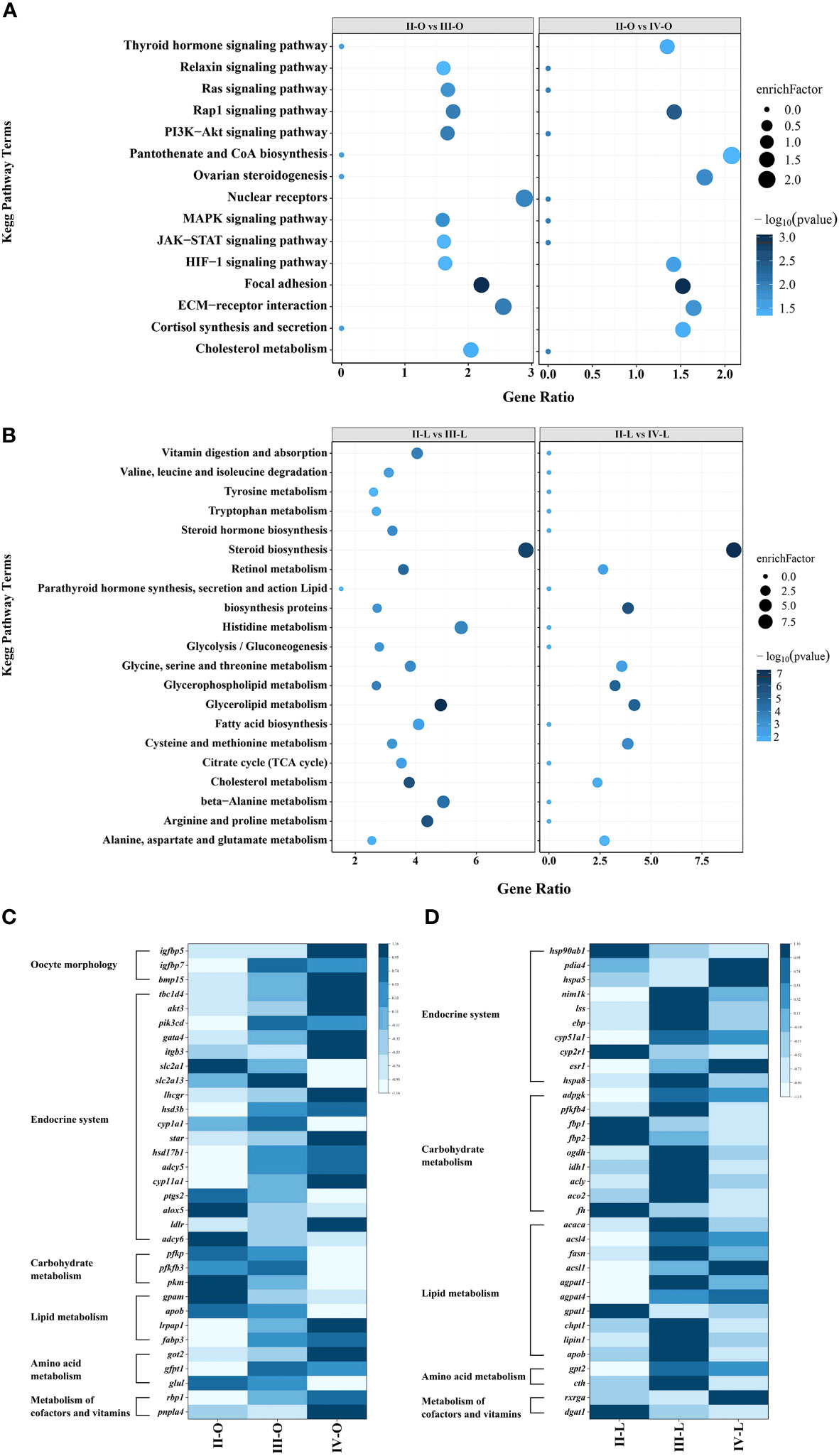
Figure 5. KEGG enrichment analysis and heat map of the DEGs. (A) Enriched KEGG pathways in the ovary; (B) Enriched KEGG pathways in the liver; (C) Heat map of DEGs involved in key pathways in the ovary; (D) Heat map of DEGs involved in key pathways in the liver.
To explore the key factors regulating vitellogenesis, we focused on DEGs in the ovary and/or liver involved in cellular morphological changes, the endocrine system, carbohydrate metabolism, lipid metabolism, amino acid metabolism, cofactors and vitamin metabolic pathways. Heat maps were constructed using Z score (Auman et al., 2007) normalized values to reflect the expression patterns of DEGs at different stages (Figures 5C, D).
3.5 Validation of RNA-seq data by qRT-PCR
The RNA-Seq data was validated by selecting 10 DEGs from the ovary (rbp1, pla2g3, got2, slc2a1, pkm, pfkp, pfkfb3, hsd17b1, cyp1a1, pnpla4) and liver (fbp1, agpat1, apob, vtg1, esr1, fbp2, adpgk, hsp90b, gpt2, lipin1) for qRT-PCR analysis, respectively. The results showed highly similar trends between the RNA-Seq and qRT-PCR results (Figures 6A, B), indicating the reliability of the RNA-Seq results.
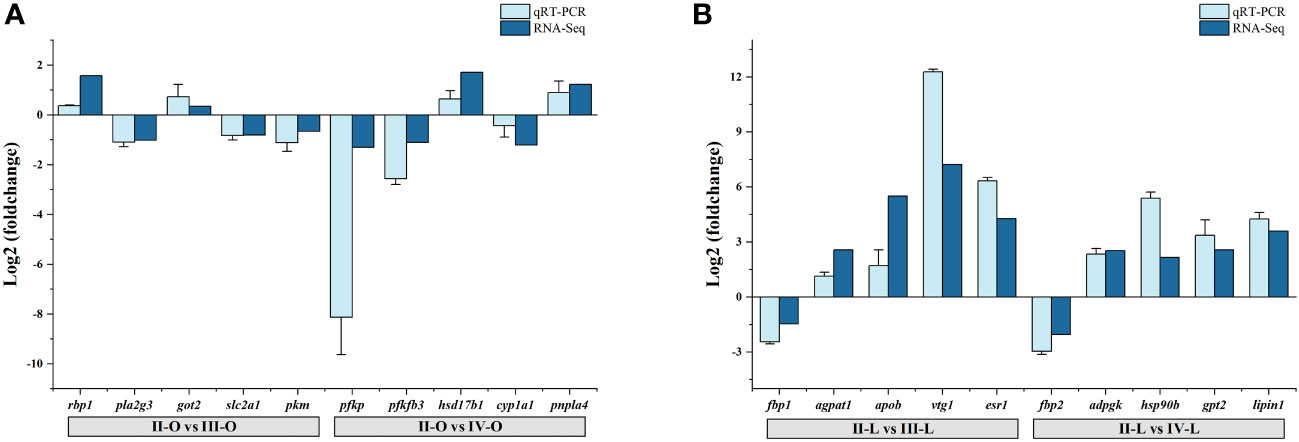
Figure 6. qRT-PCR validation of DEGs generated from the RNA-Seq results. (A) DEGs in the ovary; (B) DEGs in the liver.
3.6 Metabolomic analysis
To facilitate comparison between groups, metabolites obtained from positive and negative mode identifications were rearranged and combined for subsequent analysis. The normalized data obtained were analyzed by PCA and OPLS-DA using multivariate analysis. The PCA score plots show the trends of the data from the ovaries and liver at different stages (Figures 7A, B), which means that the ovaries and liver have distinct metabolic profiles at different stages. The OPLS-DA score plots showed significant separation between all comparison groups (Supplementary Figure A.1). The values of the classification parameters R2Y and Q2 were both stable with adequate goodness-of-fit and predictive ability, which indicates that subsequent DMs could be analyzed. DMs between groups were screened with VIP ≥ 1 and P value < 0.05 as criteria and successfully annotated by metabolite identification level 2 rather than level 1. The number of DMs in the ovary and liver when comparing stages is shown in Figures 7A, B.
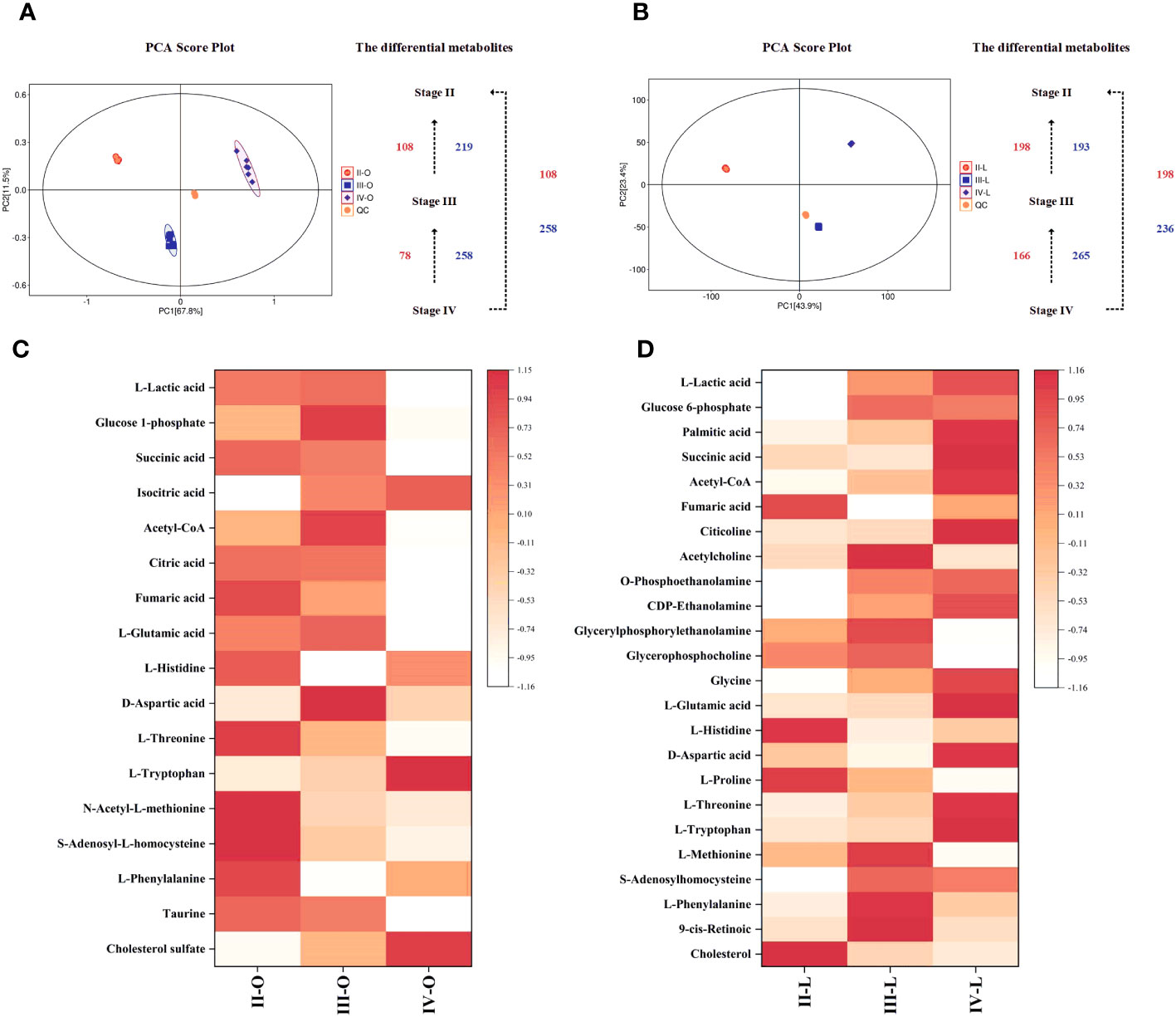
Figure 7. Metabolomic analysis among the comparison groups. (A) PCA score plot and DMs in the ovary; (B) PCA score plot and DMs in the liver; (C) Heat map of Screened DMs in the ovary; (D) Heat map of Screened DMs in the liver.
Pathway analysis of DMs was performed using MetaboAnalyst software to analyze their impact on the ovary and liver during vitellogenesis. Multiple DMs involved in carbohydrate metabolism (L-Lactic acid, Glucose 6-phosphate, Glucose 1-phosphate, Palmitic acid, Isocitric acid, Citric acid, Succinic acid, Acetyl-CoA, Fumaric acid), lipid metabolism (Citicoline, Acetylcholin, O-Phosphoethanolamine, CDP-Ethanolamine, Glyceryl-phosphorylethanolamine, Glycerophosphocholine), amino acid metabolism (Glycine, L-Glutamic acid, L-Histidine, D-Aspartic acid, L-Prolin, L-Threonin, L-Tryptophan, S-Adenosyl-L-homocysteine, N-Acetyl-L-methionine, L-Methionine, S-Adenosylhomocysteine, L-Phenylalanine), metabolism of cofactors and vitamins (9-cis-Retinoic) and steroid hormone biosynthesis (Taurin, Cholesterol sulfate, Cholesterol) were screened by correlating transcriptome KEGG enrichment results, and heatmaps were constructed to visualize their expression patterns at different stages (Figures 7C, D).
4 Discussion
In most teleosts, ovarian development is a dynamic process with both stages and continuity (Patino and Sullivan, 2002). In this paper, on the basis of existing studies (Lowerre-Barbieri et al., 2011; Yin et al., 2019), the different developmental stages of the Sichuan bream ovary were determined by the histological characterization of oocyte renewal. Female juvenile Sichuan bream can complete yolk accumulation and reach sexual maturity in 6 months under laboratory culture conditions, which is much shorter than the sexual maturation time of individuals in the field (Li et al., 2015). Perhaps due to the complexity of oocyte vitellogenesis, it requires a longer preparation time (stage II), which occupies approximately half of the length of the ovary maturation time. Therefore, this study investigated the influence of the liver and ovary on vitellogenesis during oocyte maturation mainly by combining transcriptomics and metabolomics.
4.1 Thyroid hormones regulate ovarian development
The thyroid hormones (THs), primarily thyroxine (T4) and triiodothyronine (T3), play pivotal roles in regulating physiological processes such as growth, morphogenesis, and reproduction in vertebrates (Stacey et al., 1984; Brown et al., 2014). The correlation between circulating THs and reproductive cycles in teleosts has been demonstrated by several studies. The peak plasma THs levels were observed during gametogenesis in certain species, such as rainbow trout (Osborn et al., 1978) or brook trout (White and Henderson, 1977), while climbing perch (Chakraborti and Bhattacharya, 1984) and sea lamprey (Sower et al., 1985) exhibited peak THs levels during spawning. Researchers gradually recognized the potential crosstalk mechanism between the thyroid and reproductive axes and attempted to modulate reproductive physiological processes in fish with THs, which achieved significant outcomes (Lam and Loy, 1985; Soyano et al., 1993). We found that key DEGs involved in “thyroid hormone synthesis” in the liver (hsp90b, pdia4, hspa5, nim1k) and “thyroid hormone signaling pathway” in the ovary (itgb3, pik3cd, akt3, tbc1d4, gata4) were both significantly upregulated, which would promote the synthesis and function of THs (Yi et al., 2020). The serum T3 level in Sichuan bream was found to be much lower than that of T4, which is consistent with the observed trend in other carp species such as grass carp (Ma et al., 2021) and Chinese rare minnow (Li et al., 2014). In addition, we noted that serum T4 levels, but not T3 levels, rose consistently during vitellogenesis, paralleling changes in serum Vtg levels. T4 is the primary form of thyroid hormone synthesized in organisms and it is converted into the more biologically active T3 through the iodothyronine deiodinase (Dio2) (St Germain et al., 2009; Habibi et al., 2012).
Previous studies have demonstrated that T4 exerts a stimulatory effect on metabolism and the bioactivity of sex hormones, while also facilitating the uptake of vitellogenin by tilapia oocytes (Lam, 2007). Therefore, it can be inferred that T4 could be necessary for fish reproduction. Undeniably, T3 has also been shown to be involved in the synthesis of vitellogenin in fish liver. The administration of thyroid hormones with distinct biological activities to Poecilia reticulata (Lam and Loy, 1985) and goldfish (Nelson and Habibi, 2016) can promote vitellogenesis through synergistic effects on the HPG axis. In our study, although serum T3 levels exhibited an increase during vitellogenesis, the observed change was not statistically significant. This lack of significance may be attributed to the potent biological activity of T3 (Deal and Volkoff, 2020), which mitigates any pronounced surge in demand for it. Future research should incorporate hormone treatment experiments to investigate further the underlying physiological mechanisms through which thyroid hormones regulate vitellogenesis.The growth of oocytes occurs throughout ovarian development, particularly during vitellogenesis, when their diameter significantly increases to accommodate the accumulation of material in cells due to nutrient intake (Tyler and Sumpter, 1996; Lubzens et al., 2017). It is widely recognized that THs are involved in promoting oocyte growth by stimulating the expression of insulin-like growth factors (IGFs) (Eivers et al., 2004; Molla et al., 2019). We detected increased expression of IGF-binding proteins (IGFBPs), including igfbp5 and igfbp7, in stages III and IV of the ovary. These proteins are known to interact with IGFs and play a role in IGF signaling in vivo (Artico et al., 2021; Waters et al., 2022). In addition to the TH-IGF pathway, bone morphogenetic factor 15 (bmp15) is thought to be a key factor in the regulation of primary oocyte growth and granulosa cell development in mammals and teleosts (Juengel et al., 2004; Moore and Shimasaki, 2005). Transcriptomic data showed that bmp15 is significantly upregulated in ovarian stage IV, which coincides with the stage of rapid oocyte growth. Interestingly, while the ovaries gained additional growth, the rapid growth of the body seemed to be inhibited, including the factors of body length and body weight. This is not uncommon in fish. Some scholars believe that the early stage of fish sexual maturation is accompanied by the acceleration of somatic cell growth, and in the later stage, somatic cell growth decreases because resources are directed to gonadal development (Norberg et al., 2001). For most teleosts, drastic physiological changes often occur in this critical growing stage, regulating energy metabolism through the liver and reallocating accumulated reserves for vitellogenesis (Bertolini et al., 2020).
4.2 Energy metabolism in the liver and ovary
After entering the vitellogenesis stage, liver DEGs are significantly enriched in pathways related to ATP production, which may be indispensable for the initiation of vitellogenesis. L-lactic acid and glucose 6-phosphate were found to be upregulated by metabolomic assays in both stage III and stage IV in the liver, indicating that the liver is undergoing vigorous glucose metabolism (Boles, 1998; Yao et al., 2019). Combined with the results of transcriptomic analysis, we found that the upregulation of DEGs catalyzes several key unidirectional links in the glycolytic pathway, including ADP-dependent glucokinase (adpgk) (Grudnik et al., 2018) and 6-phosphofructo-2-kinase (pfkfb4) (Yi et al., 2019). Meanwhile, fructose-1,6-bisphosphatases (fbp1 and fbp2) were downregulated in the unidirectional link of gluconeogenesis, implying that the liver supplies energy to the organism by promoting glycolysis and inhibiting the gluconeogenesis pathway during vitellogenesis. In addition, fatty acids are important substrates for energy metabolism, and we found that palmitic acid, an important intermediate in fatty acid biosynthesis (Zhang et al., 2023), was significantly upregulated in stage IV in the liver. Combined with the results of transcriptome analysis, the process of fatty acid biosynthesis in the liver was comprehensively and profoundly promoted during vitellogenesis, as reflected by the significant upregulation of acetyl-CoA carboxylase (acaca), long-chain fatty acid-CoA ligase (acsl4, acsl1), and fatty acid synthase (fasn), which catalyze almost all of the key steps in fatty acid biosynthesis (Mu et al., 2021). Carbohydrates and fatty acids are eventually metabolized to acetyl coenzyme A, which then enters the citrate cycle (TCA cycle) to produce ATP for life activities (Zheng et al., 2021). Our study showed that during vitellogenesis, several DMs (succinic acid, acetyl-CoA) and DEGs (ogdh, idh1, acly, aco2) are upregulated in the liver, contributing to the release of energy via TCA cycle pathway. Fish ovarian development is an energy-intensive process (Song et al., 2018), and our study shows that energy metabolism in the liver was promoted by upregulating glucolipid metabolic pathways to provide sufficient ATP for vitellogenesis.
The ovary undergoes completely different regulation from the liver in pathways related to energy metabolism. We detected by transcriptome analysis that 6-phosphofructo-2-kinase (pfkfb3), ATP-dependent 6-phosphofructokinase (pfkp) and pyruvate kinase (pkm) were significantly downregulated in stage IV and that they inhibited ovarian glucose catabolism. The bidirectional glucose transporter protein GLUTs, located on the cell membrane, are encoded by the SLC gene and responsible for glucose transport between the cell and the blood (Augustin, 2010; Levy et al., 2010). Herein, slc2a1 and slc2a13 were downregulated in stage IV ovaries, which inhibited glucose transport and supported our hypothesis to some extent. DMs involved in the TCA cycle, such as succinate, acetyl coenzyme A, citrate and fumarate, were also found to be downregulated during vitellogenesis, suggesting that oocytes tend to retain nutrients rather than decompose them to produce energy at this stage.
4.3 Molecular cargo biosynthetic processes
It has been suggested that the start of puberty in fish is associated with the level or rate of accumulation of lipid stores (Taranger et al., 2010). Several DEGs and DMs involved in lipid metabolism are upregulated in the liver during vitellogenesis, activating the “glycerophospholipid metabolism” and “glycerol ester metabolism” pathways, which contribute to the synthesis and transport of key lipid components. As an essential component of oocyte nutrition, neutral lipids are stored in the cells in the form of oil droplets in some fish (Patino and Sullivan, 2002). This lipid component has been shown to consist mainly of triglycerides (TAG), which are assembled in the liver with apolipoproteins (Apob) to form very low-density lipoproteins (VLDL) and are released into the bloodstream as the main lipid component secreted by the liver (Shelness and Ledford, 2005; Hiramatsu et al., 2013). A recent study reported a conserved pathway for TAG synthesis via “glycerol-3-phosphate” (Li et al., 2018). We detected significant upregulation of acylglycerophosphate acyltransferase (agpat1), Phosphatidate phosphatase (lpin1) and apob expression in the liver during vitellogenesis, which together facilitate the synthesis and assembly of neutral lipids in the liver.
Circulating VLDL enters the oocyte mainly by the following two pathways: 1) receptor (Vldlr)-mediated endocytosis; and 2) processing of VLDL to low-density lipoprotein by ovarian lipoprotein lipase (LPL), with the released free fatty acids (FFAs) that enter the oocyte to regenerate neutral oil droplets (Hiramatsu et al., 2013). Differential expression of vldr and lpl was not found in this study, but lrpap1 expression was found to be upregulated in both stage III and stage IV. Past studies have speculated that Lrpap1 might function as a chaperone protein to promote the correct folding of LPLs and the formation of intramolecular disulfide bonds (Van Leuven et al., 1998), and its upregulation may be related to the formation of lipid droplets by VLDL in the ovarian absorption circulation. In addition, expression of the fatty acid binding protein (fabp3) was upregulated in ovarian stage IV, which has been widely shown to contributed to the intracytoplasmic transport of free fatty acids after crossing the oocyte plasma membrane (Liu et al., 2007; Thirumaran and Wright, 2014). These DEGs contribute to neutral lipid synthesis and transport and support the concept of a non-endocytotic pathway of oocyte lipidation in Sichuan bream.
In addition to neutral lipids, polar lipids are synthesized in the liver and stored in the lipovitellin structural domain of Vtg, which becomes the main lipid component carried and transported by Vtg (Lubzens et al., 2017). The main component of polar lipids is phosphatidylcholine, which is barely hydrolyzed during the maturation stage of the oocyte and serves as an essential yolk nutrient store for the development of the offspring (Yilmaz et al., 2016). Past studies have reviewed the general pattern of synthesis of phosphatidylcholine using fatty acids and acetyl-CoA as substrates (Jackowski et al., 2000). We found that DEGs, including agpat4, gpat1, and chpt1, are upregulated in stage III and/or stage IV, which helps the liver synthesize essential lipid components during vitellogenesis to supply Vtg formation.
We found that many DMs and DEGs were enriched in amino acid synthesis and metabolism-related pathways in both stage III and stage IV. The levels of glutamic acid, glycine, histidine, aspartic acid, proline, threonine, tryptophan, methionine, cysteine, and phenylalanine were found to be significantly elevated in the liver during vitellogenesis by metabolomic assays, suggesting that the liver’s requirement for amino acids is associated with increased protein synthesis. Past studies have identified Vtg proteins from English Sole (Pleuronectes vetulus) by native PAGE (Roubal et al., 1997), with the highest glutamate content (12.6%) and lowest histidine abundance (2.5%). In addition, we compared the amino acid composition of Vtg in various fish (Tao et al., 1993; Fazielawanie et al., 2013; Maltais et al., 2014) and found that alanine or glutamic acid was generally higher in fish, and there were lower levels of glycine, histidine, aspartic acid, proline, threonine, methionine and phenylalanine. The increase in glutamate in organisms is critical, and high levels of glutamate may lead to the production of other amino acids, such as aspartate and alanine, via glutamate-oxaloacetate transaminase (Got) and glutamate pyruvate transaminase (Gpt), and these amino acids may eventually serve as substrates for the synthesis of Vtg or participate in other biological processes (Korsgaard, 1990). We detected significant upregulation of got2 and gpt2 in stage IV ovaries and livers, respectively, suggesting a conversion of glutamate to other amino acids; meanwhile, aspartate was an upregulated DM in stage IV ovaries, in agreement with our transcriptome results. In addition, cysteines were also found to be associated with the Vtg structure; its two small structural domains (β’-c and Ct) together contain 14 highly conserved cysteine residues (Lubzens et al., 2017).
In recent years, Vtg has received some attention as an important carrier of lipolytic vitamins such as vitamin A and vitamin E. In particular, vitamin A is transported into the egg in the form of retinol to regulate gene transcription in developing embryos (Balmer and Blomhoff, 2002) or determine egg quality (Izquierdo et al., 2001; Palace and Werner, 2006). We found that the DM 9-cis-retinoic was upregulated in stages III and IV of the liver; this metabolite is activation form of retinol (Kane, 2012). Its specific precursor 9-cis-retinal is an important isomer of retinol and coexists in the circulation (Saeed et al., 2017). Information on the transport and uptake of the vitamin by developing oocytes in fish is scarce, and we speculate that 9-cis-retinoic may be the predominant form present and transported in Sichuan bream. Additionally, 9-cis-retinoic is a high-affinity ligand for retinoid X receptors (RXRs). We found that rxrga is upregulated in liver stage IV, which mediates the activity of 9-cis-retinoic acid and agrees with our hypothesis.
Retinol-binding protein 1 (Rbp1) is an intracellular regulator of vitamin A metabolism and retinoid transport (Levi et al., 2008). We found significant upregulation of rbp1 in both stages III and IV in the ovary, which facilitates vitamin A uptake and storage by oocytes. Moreover, significant upregulation of pnpla4 was detected in ovarian stage IV, which has been reported to regulate access to retinol from retinol-ester storage depots in vertebrates (Holmes, 2012).
4.4 Vitellogenesis: response to estrogen
It has been well confirmed that the progression of vitellogenesis is usually accompanied by a rapid increase in circulating E2 to induce Vtg production in the liver (Lubzens et al., 2010). DEGs involved in “ovarian steroidogenesis” were significantly enriched in the comparison between stage II and stage IV of the ovary, where star, cyp11a1, hsd3b and hsd17b1 were significantly upregulated and mediated the process of transporting cholesterol from the cytoplasm to the mitochondrial inner membrane and converting it to E2 through a series of enzymatic reactions (Nyuji et al., 2020). This mechanism is supported by the serum E2 levels at different stages, which increased significantly (P < 0.05) after the onset of vitellogenesis compared to stage II. We found that while serum E2 levels increased significantly, T remained at a lower level throughout the vitellogenesis process. Although we detected the upregulation of taurine in stages III and IV of the ovaries, this metabolite has been shown to be closely associated with elevated T levels in fish and mammals (Yang et al., 2013; Garcia-Reyero et al., 2014), suggesting that T acts as a substrate and is actively involved in E2 synthesis rather than being released directly into the bloodstream. In this study, while serum Vtg levels were found to be significantly elevated in both stages III and IV (P < 0.05), E2 levels did not show significant differences between stages III and IV, suggesting that other key factors may be involved in the persistent elevation of Vtg levels.
Traditionally, it is widely accepted that E2 exerts its effects through binding to the intracellular nuclear estrogen receptors (ERs). E2 forms dimers with ERs and subsequently bind to the estrogen response elements in the promoter region of the Vtg gene, leading to transcription and translation through genomic effects (Gruber et al., 2004). To date, three estrogen receptor subtypes have been described for fish, including estrogen receptor 1 (esr1), estrogen receptor 2b (esr2b) and estrogen receptor 2a (esr2a) (Wang et al., 2021). Vtg synthesis in teleosts is generally thought to be mediated primarily by esr1 (Cerda et al., 2008). However, it has been reported that knockdown of ERα, ERβ1, and ERβ2 in fish all led to a significant decrease in Vtg gene expression levels (Nelson and Habibi, 2010; Griffin et al., 2013). In addition, unique but also overlapping roles in the estrogen-dependent actions of these two receptor subtypes were found in mice lacking ERα, Erβ, or both (Matthews and Gustafsson, 2003). Knockdown of ERs in goldfish liver cells (Nelson and Habibi, 2010) and zebrafish embryos (Griffin et al., 2013) revealed that stimulation with E2 increased the expression of ERβs in particular, which activated ERα and ultimately contributed to vitellogenesis. The present study found that esr1, but not esr2, was upregulated in stages III and IV of the liver, consistent with changes in Vtg levels, confirming the important role of esr1 in Vtg production. However, further investigation is needed to understand the role of ERβs.
In recent years, the role of the membrane-bound G protein-coupled estrogen receptor (GPER) in fish vitellogenesis has been well define (Wu XJ. et al., 2021). Activation of GPER by E2 leads to rapid activation of G proteins and cell membrane-associated secondary signaling such as generation of cAMP and EGFR-signaling, which may stimulate vtg transcription through rapid nongenomic effect (Filardo and Thomas, 2005; Prossnitz and Barton, 2011). Previous study have demonstrated that knockdown of the zebrafish gper gene leads to delayed ovarian development and a significant decrease in serum Vtg level (Wu XJ. et al., 2021). Furthermore, treatment with GPER agonist (G-1) or antagonist (G-15) significantly activated or inhibited the expression of zebrafish Vtg genes in the liver, suggesting that GPER plays a crucial role in regulating vitellogenesis (Chen et al., 2019). We found significant upregulation of gper expression in both stage III and stage IV of the liver (results not shown), which is similar to the expression profile of esr1 and parallel to changes in serum Vtg levels. Therefore, it is reasonable to believe that GPER, as an essential estrogen membrane receptor, contributes significantly to vitellogenesis in Sinibrama taeniatus.
5 Conclusion
This study identified the time required for ovarian maturation in juvenile Sichuan bream (Sinibrama taeniatus) under laboratory culture conditions and explored the complex process of vitellogenesis by combining growth performance, serum biochemistry, transcriptomic and metabolomic analyses. The dynamic changes in gene expression and metabolites revealed that energy reallocation during vitellogenesis is mainly regulated by carbohydrate and lipid metabolic pathways, and several key factors involved in the assembly and transport of important yolk nutrients have been detected. In addition, we found that the initiation of vitellogenesis was associated with a surge in serum E2 levels, but the sustained increase in Vtg levels at the late stage appears to be more attributable to the upregulation of estrogen receptors, which should be further confirmed in future studies. Overall, this study seems to be the first report to investigate the complex metabolic processes that occur in the liver and the ovary during vitellogenesis by transcriptomics and metabolomics in fish, providing valuable information about the regulation of ovarian development in fish.
Data availability statement
The datasets presented in this study can be found in online repositories. The names of the repository/repositories and accession number(s) can be found below: https://www.ncbi.nlm.nih.gov/, PRJNA949537.
Ethics statement
The animal study was approved by Institutional Animal Care and Use Committee of Southwest University. The study was conducted in accordance with the local legislation and institutional requirements.
Author contributions
ZW and ZZ contributed to conception and design of the study. ZZ, QZ and LW collected the samples. HW performed the bioinformatics analysis. SW and SL performed the molecular experiments. ZZ wrote the manuscript. QZ and DY reviewed and edited the manuscript. All authors contributed to the article and approved the submitted version.
Funding
This work was supported by the Chongqing Technology Innovation and Application Development Special Key Project (Grant No. CSTB2023TIAD-KPX0023) and the Ministry of Agriculture of China (Grant No. 4141900024).
Acknowledgments
Thanks to all the authors for contributing to this article. We are also grateful to all the laboratory members for their technical advice and helpful discussions.
Conflict of interest
The authors declare that the research was conducted in the absence of any commercial or financial relationships that could be construed as a potential conflict of interest.
Correction note
A correction has been made to this article. Details can be found at: 10.3389/fmars.2025.1612351.
Publisher’s note
All claims expressed in this article are solely those of the authors and do not necessarily represent those of their affiliated organizations, or those of the publisher, the editors and the reviewers. Any product that may be evaluated in this article, or claim that may be made by its manufacturer, is not guaranteed or endorsed by the publisher.
Supplementary material
The Supplementary Material for this article can be found online at: https://www.frontiersin.org/articles/10.3389/fmars.2023.1243767/full#supplementary-material
References
Artico L. L., Laranjeira A. B. A., Campos L. W., Correa J. R., Zenatti P. P., Carvalheira J. B. C., et al. (2021). Physiologic IGFBP7 levels prolong IGF1R activation in acute lymphoblastic leukemia. Blood Adv. 5 (18), 3633–3646. doi: 10.1182/bloodadvances.2020003627
Augustin R. (2010). The protein family of glucose transport facilitators: it's not only about glucose after all. IUBMB Life 62 (5), 315–333. doi: 10.1002/iub.315
Auman J. T., Boorman G. A., Wilson R. E., Travlos G. S., and Paules R. S. (2007). Heat map visualization of high-density clinical chemistry data. Physiol. Genomics 31 (2), 352–356. doi: 10.1152/physiolgenomics.00276.2006
Babin P. J., Carnevali O., Lubzens E., and Schneider W. J. (2007). Molecular aspects of oocyte vitellogenesis in fish (Dordrecht, Netherlands: Springer). Po Box 17, 3300 Aa.
Balmer J. E. and Blomhoff R. (2002). Gene expression regulation by retinoic acid. J. Lipid Res. 43 (11), 1773–1808. doi: 10.1194/jlr.R100015-JLR200
Bertolini F., Jorgensen M. G. P., Henkel C., Dufour S., and Tomkiewicz J. (2020). Unravelling the changes during induced vitellogenesis in female European eel through RNA-Seq: What happens to the liver? PloS One 15 (8), 19. doi: 10.1371/journal.pone.0236438
Boles E. (1998). The role of glucose 6-phosphate in yeast glycolysis. Trends Microbiol. 6 (12), 472–472. doi: 10.1016/s0966-842x(98)01403-6
Brown C. L., Urbinati E. C., Zhang W. M., Brown S. B., and McComb-Kobza M. (2014). Maternal thyroid and glucocorticoid hormone interactions in larval fish development, and their applications in aquaculture. Rev. Fish. Sci. Aquac. 22 (3), 207–220. doi: 10.1080/23308249.2014.918086
Cerda J., Bobe J., Babin P. J., Admon A., and Lubzens E. (2008). Functional genomics and proteomic approaches for the study of gamete formation and viability in farmed finfish. Rev. Fish. Sci. 16, 56–72. doi: 10.1080/10641260802324685
Chakraborti P. and Bhattacharya S. (1984). Plasma thyroxine levels in fresh-water perch - influence of season, gonadotropins, and gonadal-hormones. Gen. Comp. Endocrinol. 53 (2), 179–186. doi: 10.1016/0016-6480(84)90240-5
Chen S. F., Zhou Y. Q., Chen Y. R., and Gu J. (2018). fastp: an ultra-fast all-in-one FASTQ preprocessor. Bioinformatics. 34(17), 884–890. doi: 10.1093/bioinformatics/bty560
Chen Y., Tang H. P., He J. N., Wu X., Wang L., Liu X. C., et al. (2019). Interaction of nuclear ERs and GPER in vitellogenesis in zebrafish. J. Steroid Biochem. Mol. Biol. 189, 10–18. doi: 10.1016/j.jsbmb.2019.01.013
Deal C. K. and Volkoff H. (2020). The role of the thyroid axis in fish. Front. Endocrinol. 11. doi: 10.3389/fendo.2020.596585
De Vincentis S., Monzani M. L., and Brigante G. (2018). Crosstalk between gonadotropins and thyroid axis. Minerva Ginecol. 70 (5), 609–620. doi: 10.23736/s0026-4784.18.04271-5
Duarte-Guterman P., Navarro-Martin L., and Trudeau V. L. (2014). Mechanisms of crosstalk between endocrine systems: Regulation of sex steroid hormone synthesis and action by thyroid hormones. Gen. Comp. Endocrinol. 203, 69–85. doi: 10.1016/j.ygcen.2014.03.015
Edwards P., Zhang W. B., Belton B., and Little D. C. (2019). Misunderstandings, myths and mantras in aquaculture: Its contribution to world food supplies has been systematically over reported. Mar. Pol. 106, 9. doi: 10.1016/j.marpol.2019.103547
Eivers E., McCarthy K., Glynn C., Nolan C. M., and Byrnes L. (2004). Insulin-like growth factor (IGF) signalling is required for early dorso-anterior development of the zebrafish embryo. Int. J. Dev. Biol. 48 (10), 1131–1140. doi: 10.1387/ijdb.041913ee
Fazielawanie N. M. R., Siraj S. S., Harmin S. A., and Ina-Salwany M. Y. (2013). Isolation and partial characterization of Asian sea bass (Lates calcarifer) Vitellogenin. Fish Physiol. Biochem. 39 (2), 191–200. doi: 10.1007/s10695-012-9690-5
Feng Q. M., Liu M. M., Cheng Y. X., and Wu X. G. (2022). Comparative transcriptome analysis reveals the process of ovarian development and nutrition metabolism in chinese mitten crab, eriocheir sinensis. Front. Genet. 13. doi: 10.3389/fgene.2022.910682
Filardo E. J. and Thomas P. (2005). GPR30: a seven-transmembrane-spanning estrogen receptor that triggers EGF release. Trends Endocrinol. Metab. 16 (8), 362–367. doi: 10.1016/j.tem.2005.08.005
Finn R. N. and Fyhn H. J. (2010). Requirement for amino acids in ontogeny of fish. Aquac. Res. 41 (5), 684–716. doi: 10.1111/j.1365-2109.2009.02220.x
Garcia-Reyero N., Ekman D. R., Habib B. T., Villeneuve D. L., Collette T. W., Bencic D. C., et al. (2014). Integrated approach to explore the mechanisms of aromatase inhibition and recovery in fathead minnows (Pimephales promelas). Gen. Comp. Endocrinol. 203, 193–202. doi: 10.1016/j.ygcen.2014.03.022
Gloux A., Duclos M. J., Brionne A., Bourin M., Nys Y., and Rehault-Godbert S. (2019). Integrative analysis of transcriptomic data related to the liver of laying hens: from physiological basics to newly identified functions. BMC Genomics 20 (1), 16. doi: 10.1186/s12864-019-6185-0
Good C. and Davidson J. (2016). A review of factors influencing maturation of atlantic salmon, salmo salar, with focus on water recirculation aquaculture system environments. J. World Aquacult. Soc 47 (5), 605–632. doi: 10.1111/jwas.12342
Grier H. J., Uribe Aranzabal M. C., and Patino R. (2009). “The Ovary, Folliculogenesis, and Oogenesis in Teleosts,” in Reproductive Biology and Phylogeny of Fishes. Ed. Jamieson B. G. M. (6000 Broken Sound Parkway Nw, Ste 300, Boca Raton, Fl 33487-2742 USA: Crc Press-Taylor & Francis Group), 25–84.
Griffin L. B., January K. E., Ho K. W., Cotter K. A., and Callard G. V. (2013). Morpholino-Mediated Knockdown of ER alpha, ER beta a, and ER beta b mRNAs in Zebrafish (Danio rerio) Embryos Reveals Differential Regulation of Estrogen-Inducible Genes. Endocrinology 154 (11), 4158–4169. doi: 10.1210/en.2013-1446
Gruber C. J., Gruber D. M., Gruber I. M. L., Wieser F., and Huber J. C. (2004). Anatomy of the estrogen response element. Trends Endocrinol. Metab. 15 (2), 73–78. doi: 10.1016/j.tem.2004.01.008
Grudnik P., Kaminski M. M., Rembacz K. P., Kuska K., Madej M., Potempa J., et al. (2018). Structural basis for ADP-dependent glucokinase inhibition by 8-bromo-substituted adenosine nucleotide. J. Biol. Chem. 293 (28), 11088–11099. doi: 10.1074/jbc.RA117.001562
Guzman J. M., Luckenbach J. A., Yamamoto Y., and Swanson P. (2014). Expression profiles of fsh-regulated ovarian genes during oogenesis in coho salmon. PloS One 9 (12), 22. doi: 10.1371/journal.pone.0114176
Habibi H. R., Nelson E. R., and Allan E. R. O. (2012). New insights into thyroid hormone function and modulation of reproduction in goldfish. Gen. Comp. Endocrinol. 175 (1), 19–26. doi: 10.1016/j.ygcen.2011.11.003
Hiramatsu N., Luo W., Reading B. J., Sullivan C. V., Mizuta H., Ryu Y. W., et al. (2013). Multiple ovarian lipoprotein receptors in teleosts. Fish Physiol. Biochem. 39 (1), 29–32. doi: 10.1007/s10695-012-9612-6
Hiramatsu N., Todo T., Sullivan C. V., Schilling J., Reading B. J., Matsubara T., et al. (2015). Ovarian yolk formation in fishes: Molecular mechanisms underlying formation of lipid droplets and vitellogenin-derived yolk proteins. Gen. Comp. Endocrinol. 221, 9–15. doi: 10.1016/j.ygcen.2015.01.025
Holmes R. S. (2012). Vertebrate patatin-like phospholipase domain-containing protein 4 (PNPLA4) genes and proteins: a gene with a role in retinol metabolism. 3 Biotech. 2 (4), 277–286. doi: 10.1007/s13205-012-0063-7
Ings J. S. and van der Kraak G. J. (2006). Characterization of the mRNA expression of StAR and steroidogenic enzymes in zebrafish ovarian follicles. Mol. Reprod. Dev. 73 (8), 943–954. doi: 10.1002/mrd.20490
Izquierdo M. S., Fernandez-Palacios H., and Tacon A. G. J. (2001). Effect of broodstock nutrition on reproductive performance of fish. Aquaculture 197 (1-4), 25–42. doi: 10.1016/s0044-8486(01)00581-6
Jackowski S., Wang J., and Baburina I. (2000). Activity of the phosphatidylcholine biosynthetic pathway modulates the distribution of fatty acids into glycerolipids in proliferating cells. BBA-Mol. Cell. Biol. Lipids 1483 (3), 301–315. doi: 10.1016/s1388-1981(99)00203-6
Juengel J. L., Bodensteiner K. J., Heath D. A., Hudson N. L., Moeller C. L., Smith P., et al. (2004). Physiology of GDF9 and BMP15 signalling molecules. Anim. Reprod. Sci. 82-3, 447–460. doi: 10.1016/j.anireprosci.2004.04.021
Kagawa H., Gen K., Okuzawa K., and Tanaka H. (2003). Effects of luteinizing hormone and follicle-stimulating hormone and insulin-like growth factor-I on aromatase activity and P450 aromatase gene expression in the ovarian follicles of red seabream, Pagrus major. Biol. Reprod. 68 (5), 1562–1568. doi: 10.1095/biolreprod.102.008219
Kane M. A. (2012). Analysis, occurrence, and function of 9-cis-retinoic acid. BBA-Mol. Cell. Biol. Lipids 1821 (1), 10–20. doi: 10.1016/j.bbalip.2011.09.012
Kazeto Y., Ijiri S., Adachi S., and Yamauchi K. (2006). Cloning and characterization of a cDNA encoding cholesterol side-chain cleavage cytochrome P450 (CYP11A1): Tissue-distribution and changes in the transcript abundance in ovarian tissue of Japanese eel, Anguilla japonica, during artificially induced sexual development. J. Steroid Biochem. Mol. Biol. 99 (2-3), 121–128. doi: 10.1016/j.jsbmb.2005.12.004
Kim D., Paggi J. M., Park C., Bennett C., and Salzberg S. L. (2019). Graph-based genome alignment and genotyping with HISAT2 and HISAT-genotype. Nat. Biotechnol. 37 (8), 907. doi: 10.1038/s41587-019-0201-4
Kime D. E. (1993). ‘Classical’ and ‘non-classical’ reproductive steroids in fish. Rev. Fish Biol. Fish. 3 (2), 160–180. doi: 10.1007/BF00045230
Korsgaard B. (1990). Estrogen-treatment and its influence on protein-synthesis and amino-acid-metabolism in zoarces-viviparus (L) males. Fish Physiol. Biochem. 8 (2), 121–127. doi: 10.1007/bf00004439
Lam T. J. (2007). Hormones and egg/larval quality in fish. J. World Aquacult. Soc 25 (1), 2–12. doi: 10.1111/j.1749-7345.1994.tb00798.x
Lam T. J. and Loy G. L. (1985). Effect of L-thyroxine on ovarian development and gestation in the viviparous guppy, poecilia-reticulata. Gen. Comp. Endocrinol. 60 (2), 324–330. doi: 10.1016/0016-6480(85)90330-2
Le Menn F., Cerda J., and Babin P. J. (2007). Ultrastructural aspects of the ontogeny and differentiation of ray-finned fish ovarian follicles (Po Box 17, 3300 Aa Dordrecht, Netherlands: Springer).
Levavi-Sivan B., Bogerd J., Mananos E. L., Gomez A., and Lareyre J. J. (2010). Perspectives on fish gonadotropins and their receptors. Gen. Comp. Endocrinol. 165 (3), 412–437. doi: 10.1016/j.ygcen.2009.07.019
Levi L., Levavi-Sivan B., and Lubzens E. (2008). Expression of genes associated with retinoid metabolism in the trout ovarian follicle. Biol. Reprod. 79 (3), 570–577. doi: 10.1095/biolreprod.107.066548
Levi L., Pekarski I., Gutman E., Fortina P., Hyslop T., Biran J., et al. (2009). Revealing genes associated with vitellogenesis in the liver of the zebrafish (Danio rerio) by transcriptome profiling. BMC Genomics 10, 17. doi: 10.1186/1471-2164-10-141
Levy B., Wang D., Ullner P. M., Engelstad K., Yang H., Nahum O., et al. (2010). Uncovering microdeletions in patients with severe Glut-1 deficiency syndrome using SNP oligonucleotide microarray analysis. Mol. Genet. Metab. 100 (2), 129–135. doi: 10.1016/j.ymgme.2010.03.007
Li Z. H., Chen L., Wu Y. H., Li P., Li Y. F., and Ni Z. H. (2014). Effects of waterborne cadmium on thyroid hormone levels and related gene expression in Chinese rare minnow larvae. Comp. Biochem. Physiol. C-Toxicol. Pharmacol. 161, 53–57. doi: 10.1016/j.cbpc.2014.02.001
Li B. and Dewey C. N. (2011). RSEM: accurate transcript quantification from RNA-Seq data with or without a reference genome. BMC Bioinf. 12, 16. doi: 10.1186/1471-2105-12-323
Li T. Y., Song L. T., Sun Y., Li J. Y., Yi C., Lam S. M., et al. (2018). Tip60-mediated lipin 1 acetylation and ER translocation determine triacylglycerol synthesis rate. Nat. Commun. 9, 14. doi: 10.1038/s41467-018-04363
Li B., Xie C.-Y., Zhang T., and Wang Z.-J. (2015). The reproductive biology of sinibrama taeniatus from the Minjiang river (in Meishan city). Chin. J. Zoology 50 (4), 563–570. doi: 10.13859/j.cjz.201504008
Li Z., Zhou M. Y., Ruan Y., Chen X. L., Ren C. H., Yang H., et al. (2022). Transcriptomic analysis reveals yolk accumulation mechanism from the hepatopancreas to ovary in the pacific white shrimp Litopenaeus vannamei. Front. Mar. Sci. 9. doi: 10.3389/fmars.2022.948105
Liu R. Z., Saxena V., Sharma M. K., Thisse C., Thisse B., Denovan-Wright E. M., et al. (2007). The fabp4 gene of zebrafish (Danio rerio) - genomic homology with the mammalian FABP4 and divergence from the zebrafish fabp3 in developmental expression. FEBS J. 274 (6), 1621–1633. doi: 10.1111/j.1742-4658.2007.05711.x
Love M. I., Huber W., and Anders S. (2014). Moderated estimation of fold change and dispersion for RNA-seq data with DESeq2. Genome Biol. 15 (12), 38. doi: 10.1186/s13059-014-0550-8
Lowerre-Barbieri S. K., Brown-Peterson N. J., Murua H., Tomkiewicz J., Wyanski D. M., and Saborido-Rey F. (2011). Emerging issues and methodological advances in fisheries reproductive biology. Mar. Coast. Fish. 3 (1), 32–51. doi: 10.1080/19425120.2011.555725
Lubzens E., Bobe J., Young G., and Sullivan C. V. (2017). Maternal investment in fish oocytes and eggs: The molecular cargo and its contributions to fertility and early development. Aquaculture 472, 107–143. doi: 10.1016/j.aquaculture.2016.10.029
Lubzens E., Young G., Bobe J., and Cerda J. (2010). Oogenesis in teleosts: How fish eggs are formed. Gen. Comp. Endocrinol. 165 (3), 367–389. doi: 10.1016/j.ygcen.2009.05.022
Luckenbach J. A., Iliev D. B., Goetz F. W., and Swanson P. (2008). Identification of differentially expressed ovarian genes during primary and early secondary oocyte growth in coho salmon, Oncorhynchus kisutch. Reprod. Biol. Endocrinol. 6, 15. doi: 10.1186/1477-7827-6-2
Luo H. H., Yang Q. R., Qian L. I., Chong L. I., Chen B. Y., and Ping-Yu L. V. (2014). A preliminary study on fish habitat characteristics in the National Nature Reserve for the rare and endemic fishes in the upper reaches of the Yangtze River. Freshwater Fisheries. 6, 44–48. doi: 10.13721/j.cnki.dsyy.2014.06.004
Ma P., Hu Z. Y., Li L., Li D. P., and Tang R. (2021). Dietary selenium promotes the growth performance through growth hormone-insulin-like growth factor and hypothalamic-pituitary-thyroid axes in grass carp (Ctenopharyngodon idella). Fish Physiol. Biochem. 47 (4), 1313–1327. doi: 10.1007/s10695-021-00974-1
Maltais D., Dupont-Cyr B. A., Roy R. L., and Le Francois N. R. (2014). Purification and partial characterization of vitellogenin from spotted wolffish (Anarhichas minor) and development of an enzyme-linked immunosorbent assay for the determination of gender and sexual maturity. Fish Physiol. Biochem. 40 (1), 279–294. doi: 10.1007/s10695-013-9843-1
Matthews J. and Gustafsson J.-A. (2003). Estrogen signaling: a subtle balance between ER alpha and ER beta. Mol. Interventions 3 (5), 281–292. doi: 10.1124/mi.3.5.281
McBride R. S., Somarakis S., Fitzhugh G. R., Albert A., Yaragina N. A., Wuenschel M. J., et al. (2015). Energy acquisition and allocation to egg production in relation to fish reproductive strategies. Fish. Fish. 16 (1), 23–57. doi: 10.1111/faf.12043
Meng F. Y., Sun S., Xu X., Yu W. X., Gan R. P., Zhang L. H., et al. (2022). Transcriptomic analysis provides insights into the growth and maturation of ovarian follicles in the ricefield eel (Monopterus albus). Aquaculture 555, 14. doi: 10.1016/j.aquaculture.2022.738251
Molla M. H. R., Hasan M. T., Jang W. J., Diaz C. D. S., Appenteng P., Marufchoni H., et al. (2019). Thyroid hormone-induced swim bladder and eye maturation are transduced by IGF-1 in zebrafish embryos. Aquac. Res. 50 (11), 3462–3470. doi: 10.1111/are.14305
Montserrat N., Gonzalez A., Mendez E., Piferrer F., and Planas J. V. (2004). Effects of follicle stimulating hormone on estradiol-17 beta production and P-450 aromatase (CYP19) activity and mRNA expression in brown trout vitellogenic ovarian follicles in vitro. Gen. Comp. Endocrinol. 137 (2), 123–131. doi: 10.1016/j.ygcen.2004.02.011
Moore R. K. and Shimasaki S. (2005). Molecular biology and physiological role of the oocyte factor, BMP-15. Mol. Cell. Endocrinol. 234 (1-2), 67–73. doi: 10.1016/j.mce.2004.10.012
Mu T., Hu H. H., Ma Y. F., Feng X. F., Zhang J., and Gu Y. L. (2021). Regulation of key genes for milk fat synthesis in ruminants. Front. Nutr. 8. doi: 10.3389/fnut.2021.765147
Nagahama Y. and Yamashita M. (2008). Regulation of oocyte maturation in fish. Dev. Growth Diff. 50, S195–S219. doi: 10.1111/j.1440-169X.2008.01019.x
Nakamura I., Kusakabe M., and Young G. (2003). Regulation of steriodogenic enzyme mRNAs in rainbow trout (Oncorhynchus mykiss) ovarian follicles in vitro. Fish Physiol. Biochem 28 (1-4), 355–356. doi: 10.1023/B:FISH.0000030586.74085.0f
Nelson E. R. and Habibi H. R. (2010). Functional significance of nuclear estrogen receptor subtypes in the liver of goldfish. Endocrinology 151 (4), 1668–1676. doi: 10.1210/en.2009-1447
Nelson E. R. and Habibi H. R. (2016). Thyroid hormone regulates vitellogenin by inducing estrogen receptor alpha in the goldfish liver. Mol. Cell. Endocrinol. 436 (C), 259–267. doi: 10.1016/j.mce.2016.08.045
Newton R., Zhang W. B., Xian Z. X., McAdam B., and Little D. C. (2021). Intensification, regulation and diversification: The changing face of inland aquaculture in China. Ambio 50 (9), 1739–1756. doi: 10.1007/s13280-021-01503-3
Norberg B., Weltzien F. A., Karlsen O., and Holm J. C. (2001). Effects of photoperiod on sexual maturation and somatic growth in male Atlantic halibut (Hippoglossus hippoglossus L.). Comp. Biochem. Physiol. B-Biochem. Mol. Biol. 129 (2-3), 357–365. doi: 10.1016/s1096-4959(01)00320-7
Nyuji M., Hongo Y., Yoneda M., and Nakamura M. (2020). Transcriptome characterization of BPG axis and expression profiles of ovarian steroidogenesis-related genes in the Japanese sardine. BMC Genomics 21 (1), 18. doi: 10.1186/s12864-020-07080-1
Osachoff H. L., Brown L. L. Y., Tirrul L., van Aggelen G. C., Brinkman F. S. L., and Kennedy C. J. (2016). Time course of hepatic gene expression and plasma vitellogenin protein concentrations in estrone-exposed juvenile rainbow trout (Oncorhynchus mykiss). Comp. Biochem. Physiol. D-Genomics Proteomics 19, 112–119. doi: 10.1016/j.cbd.2016.02.002
Osborn R. H., Simpson T. H., and Youngson A. F. (1978). Seasonal and diurnal rhythms of thyroidal status in rainbow-trout, salmo-gairdneri richardson. J. Fish Biol. 12 (6), 531–540. doi: 10.1111/j.1095-8649.1978.tb04199.x
Palace V. P. and Werner J. (2006). Vitamins A and E in the maternal diet influence egg quality and early life stage development in fish: a review. Sci. Mar. 70, 41–57. doi: 10.3989/scimar.2006.70s241
Patino R. and Sullivan C. V. (2002). Ovarian follicle growth, maturation, and ovulation in teleost fish. Fish Physiol. Biochem. 26 (1), 57–70. doi: 10.1023/a:1023311613987
Perez M. J., Rodriguez C., Cejas J. R., Martin M. V., Jerez S., and Lorenzo A. (2007). Lipid and fatty acid content in wild white seabream (Diplodus sargus) broodstock at different stages of the reproductive cycle. Comp. Biochem. Physiol. B-Biochem. Mol. Biol. 146 (2), 187–196. doi: 10.1016/j.cbpb.2006.10.097
Prossnitz E. R. and Barton M. (2011). The G-protein-coupled estrogen receptor GPER in health and disease. Nat. Rev. Endocrinol. 7 (12), 715–726. doi: 10.1038/nrendo.2011.122
Roubal W. T., Lomax D. P., Willis M. L., and Johnson L. L. (1997). Purification and partial characterization of English sole (Pleuronectes vetulus) vitellogenin. Comp. Biochem. Physiol. B-Biochem. Mol. Biol. 118 (3), 613–622. doi: 10.1016/s0305-0491(97)00241-1
Saeed A., Hoekstra M., Hoeke M. O., Heegsma J., and Faber K. N. (2017). The interrelationship between bile acid and vitamin A homeostasis. BBA-Mol. Cell. Biol. Lipids 1862 (5), 496–512. doi: 10.1016/j.bbalip.2017.01.007
Sehgal H. S. and Toor H. S. (1995). Effect of stocking density on ovarian maturation, offspring fitness and growth of common carp. Aquaculture 129 (1-4), 113–117. doi: 10.1016/0044-8486(94)00232-d
Shelness G. S. and Ledford A. S. (2005). Evolution and mechanism of apoliprotein B-containing lipoprotein assembly. Curr. Opin. Lipidol. 16 (3), 325–332. doi: 10.1097/01.mol.0000169353.12772.eb
Smith C. A., Want E. J., O'Maille G., Abagyan R., and Siuzdak G. (2006). XCMS: Processing mass spectrometry data for metabolite profiling using Nonlinear peak alignment, matching, and identification. Anal. Chem. 78 (3), 779–787. doi: 10.1021/ac051437y
Song Y. F., Tan X. Y., Pan Y. X., Zhang L. H., and Chen Q. L. (2018). Fatty Acid beta-Oxidation Is Essential in Leptin-Mediated Oocytes Maturation of Yellow Catfish Pelteobagrus fulvidraco. Int. J. Mol. Sci. 19 (5), 24. doi: 10.3390/ijms19051457
Song J. L. and Wessel G. M. (2005). How to make an egg: transcriptional regulation in oocytes. Differentiation 73 (1), 1–17. doi: 10.1111/j.1432-0436.2005.07301005.x
Sower S. A., Plisetskaya E., and Gorbman A. (1985). Changes in plasma steroid and thyroid-hormones and insulin during final maturation and spawning of the sea lamprey, petromyzon-marinus. Gen. Comp. Endocrinol. 58 (2), 259–269. doi: 10.1016/0016-6480(85)90342-9
Soyano K., Saito T., Nagae M., and Yamauchi K. (1993). Effects of thyroid-hormone on gonadotropin-induced steroid-production in medaka, oryzias-latipes, ovarian follicles. Fish Physiol. Biochem. 11 (1-6), 265–272. doi: 10.1007/bf00004574
Stacey N. E., Mackenzie D. S., Marchant T. A., Kyle A. L., and Peter R. E. (1984). Endocrine changes during natural spawning in the white sucker, catostomus-commersoni .1. Gonadotropin, growth-hormone, and thyroid-hormones. Gen. Comp. Endocrinol. 56 (3), 333–348. doi: 10.1016/0016-6480(84)90076-5
St Germain D. L., Galton V. A., and Hernandez A. (2009). Minireview: defining the roles of the iodothyronine deiodinases: current concepts and challenges. Endocrinology 150 (3), 1097–1107. doi: 10.1210/en.2008-1588
Sullivan C. V. and Yilmaz O. (2018). “Vitellogenesis and Yolk Proteins, Fish,” in Encyclopedia of Reproduction, 2nd ed. Ed. Skinner M. K. (Oxford: Academic Press), 266–277.
Tacon A. G. J. and Metian M. (2015). Feed matters: satisfying the feed demand of aquaculture. Rev. Fish. Sci. Aquac. 23 (1), 1–10. doi: 10.1080/23308249.2014.987209
Tao Y. X., Hara A., Hodson R. G., Woods L. C., and Sullivan C. V. (1993). Purification, characterization and immunoassay of striped bass (Morone-saxatilis) vitellogenin. Fish Physiol. Biochem. 12 (1), 31–46. doi: 10.1007/bf00004320
Taranger G. L., Carrillo M., Schulz R. W., Fontaine P., Zanuy S., Felip A., et al. (2010). Control of puberty in farmed fish. Gen. Comp. Endocrinol. 165 (3), 483–515. doi: 10.1016/j.ygcen.2009.05.004
Thirumaran A. and Wright J. M. (2014). Fatty acid-binding protein (fabp) genes of spotted green pufferfish (Tetraodon nigroviridis): comparative genomics and spatial transcriptional regulation. Genome 57 (5), 289–301. doi: 10.1139/gen-2014-0059
Tyler C. R. and Sumpter J. P. (1996). Oocyte growth and development in teleosts. Rev. Fish Biol. Fish. 6 (3), 287–318. doi: 10.1007/BF00122584
Van Leuven F., Thiry E., Stas L., and Nelissen B. (1998). Analysis of the human LRPAP1 gene coding for the lipoprotein receptor-associated protein: Identification of 22 polymorphisms and one mutation. Genomics 52 (2), 145–151. doi: 10.1006/geno.1998.5413
Wang T., Jiang D. N., Shi H. J., Mustapha U. F., Deng S. P., Liu Z. L., et al. (2021). Liver transcriptomic analysis of the effects of dietary fish oil revealed a regulated expression pattern of genes in adult female spotted scat (Scatophagus argus). Front. Mar. Sci. 8. doi: 10.3389/fmars.2021.784845
Wang T., Liu Z. L., Li G. L., Mustapha U. F., Ndandala C. B., Shi H. J., et al. (2022). Ovary transcriptomic analysis reveals regulation effects of dietary fish oil on hormone, lipid, and glucose metabolism in female adult spotted scat (Scatophagus argus). Front. Mar. Sci. 9. doi: 10.3389/fmars.2022.935968
Wang J. L., Zhang T., Shen X. T., Liu J., Zhao D. L., Sun Y. W., et al. (2016). Serum metabolomics for early diagnosis of esophageal squamous cell carcinoma by UHPLC-QTOF/MS. Metabolomics 12 (7), 10. doi: 10.1007/s11306-016-1050-5
Waters J. A., Urbano I., Robinson M., and House C. D. (2022). Insulin-like growth factor binding protein 5: Diverse roles in cancer. Front. Oncol. 12. doi: 10.3389/fonc.2022.1052457
White B. A. and Henderson N. E. (1977). Annual variations in circulating levels of thyroid-hormones in brook trout, salvelinus-fontinalis, as measured by radioimmunoassay. Can. J. Zool.-Rev. Can. Zool. 55 (3), 475–481. doi: 10.1139/z77-064
Wu T. Z., Hu E. Q., Xu S. B., Chen M. J., Guo P. F., Dai Z. H., et al. (2021). clusterProfiler 4.0: A universal enrichment tool for interpreting omics data. Innovation-Amsterdam 2 (3), 11. doi: 10.1016/j.xinn.2021.100141
Wu X. J., Williams M. J., Kew K. A., Converse A., Thomas P., and Zhu Y. (2021). Reduced vitellogenesis and female fertility in gper knockout zebrafish. Front. Endocrinol. 12. doi: 10.3389/fendo.2021.637691
Xu G. C., Du F. K., Li Y., Nie Z. J., and Xu P. (2016). Integrated application of transcriptomics and metabolomics yields insights into population-asynchronous ovary development in Coilia nasus. Sci. Rep. 6, 11. doi: 10.1038/srep31835
Yang J. C., Lin S. M., Feng Y., Wu G. F., and Hu J. M. (2013). “Taurine Enhances the Sexual Response and Mating Ability in Aged Male Rats,” in Taurine 8, Vol 2: Nutrition and Metabolism, Protective Role, and Role in Reproduction, Development, and Differentiation. Eds. ElIdrissi A. and Lamoreaux W. J. (New York: Springer), 347–355.
Yao K., Zhou Q. X., Liu D. M., Chen S. M., and Yuan K. (2019). Comparative proteomics of the metabolic pathways involved in L-lactic acid production in Bacillus coagulans BCS13002 using different carbon sources. LWT-Food Sci. Technol. 116, 9. doi: 10.1016/j.lwt.2019.108445
Yi M., Ban Y. Y., Tan Y. X., Xiong W., Li G. Y., and Xiang B. (2019). 6-Phosphofructo-2-kinase/fructose-2,6-biphosphatase 3 and 4: A pair of valves for fine-tuning of glucose metabolism in human cancer. Mol. Metab. 20, 1–13. doi: 10.1016/j.molmet.2018.11.013
Yi S. K., Liu L. F., Zhou L. F., Zhao B. W., Wang W. M., and Gao Z. X. (2020). Screening of biomarkers related to ovarian maturation and spawning in blunt snout bream (Megalobrama amblycephala) based on metabolomics and transcriptomics. Mar. Biotechnol. 22 (2), 180–193. doi: 10.1007/s10126-019-09943-5
Yilmaz O., Prat F., Ibanez A. J., Koksoy S., Amano H., and Sullivan C. V. (2016). Multiple vitellogenins and product yolk proteins in European sea bass (Dicentrarchus labrax): Molecular characterization, quantification in plasma, liver and ovary, and maturational proteolysis. Comp. Biochem. Physiol. B-Biochem. Mol. Biol. 194, 71–86. doi: 10.1016/j.cbpb.2015.11.010
Yin M., Xie C., Deyong P. U., Huang J., and Wang Z. (2019). Microstructure of oogenesis in Sinibrama taeniatus. South China Fisheries Science. 15, 127–132. doi: cnki:sun:nfsc.0.2019-02-015
Yuan D. Y., Chen X. H., Gu H. R., Zou M., Zou Y., Fang J., et al. (2020). Chromosomal genome of Triplophysa bleekeri provides insights into its evolution and environmental adaptation. GigaScience 9 (11), 14. doi: 10.1093/gigascience/giaa132
Zhang J., Wang T., Yang C. J., Wu R. X., Xi L. J., and Ding W. (2023). Integrated proteomics and metabolomics analysis revealed the mechanisms underlying the effect of irradiation on the fat quality of Chinese bacon. Food Chem. 413, 10. doi: 10.1016/j.foodchem.2023.135385
Zhang Y., Zhang S., Liu Z. X., Zhang L. H., and Zhang W. M. (2013). Epigenetic modifications during sex change repress gonadotropin stimulation of cyp19a1a in a teleost ricefield eel (Monopterus albus). Endocrinology 154 (8), 2881–2890. doi: 10.1210/en.2012-2220
Zheng X. C., Jiang W. B., Zhang L., Espe M., Abasubong K. P., Zhang C. N., et al. (2021). Investigation of the regulatory effect of icariin on the hepatopancreas glycolipid metabolism and energy metabolism of female Eriocheir sinensis during exogenous vitellogenesis based on the transcriptomics and metabolomics. Aquac. Nutr. 27 (6), 2066–2083. doi: 10.1111/anu.13341
Keywords: Sinibrama taeniatus, vitellogenesis, vitellogenin, transcriptomics, metabolomics Sinibrama taeniatus, metabolomics
Citation: Zhao Z, Zhao Q, Wang H, Wei L, Wang S, Li S, Yuan D and Wang Z (2023) Integrated transcriptomic and metabolomic analyses identify key factors in the vitellogenesis of juvenile Sichuan bream (Sinibrama taeniatus). Front. Mar. Sci. 10:1243767. doi: 10.3389/fmars.2023.1243767
Received: 21 June 2023; Accepted: 27 September 2023;
Published: 10 October 2023; Corrected: 10 July 2025.
Edited by:
Dong Han, Chinese Academy of Sciences (CAS), ChinaReviewed by:
Khor Waiho, University of Malaysia Terengganu, MalaysiaZhifeng Liu, Chinese Academy of Fishery Sciences (CAFS), China
Ye Yuan, Shantou University, China
Yu Chen, Southern Marine Science and Engineering Guangdong Laboratory (Guangzhou), China
Copyright © 2023 Zhao, Zhao, Wang, Wei, Wang, Li, Yuan and Wang. This is an open-access article distributed under the terms of the Creative Commons Attribution License (CC BY). The use, distribution or reproduction in other forums is permitted, provided the original author(s) and the copyright owner(s) are credited and that the original publication in this journal is cited, in accordance with accepted academic practice. No use, distribution or reproduction is permitted which does not comply with these terms.
*Correspondence: Zhijian Wang, d2FuZ3pqMTk2OUAxMjYuY29t