- 1Experimental Zooprophylactic Institute of Lombardy and Emilia Romagna, Ferrara, Italy
- 2Department of Biological, Geological and Environmental Sciences, University of Catania, Catania, Italy
- 3Ente Fauna Marina Mediterranea, Scientific Organization for Research and Conservation of Marine Biodiversity, Avola, Italy
- 4Fondazione Cetacea Onlus, Riccione, Italy
- 5National Research Council, Institute of Biological Resources and Marine Biotechnologies, Ancona, Italy
Epibiont communities can be used as useful ecological indicators, providing information on the ecology and health conditions of their hosts. In this study, we analyzed the cirriped community from a total of 117 dead specimens of Caretta caretta (Linnaeus, 1758) collected in the north Adriatic between the years 2020 and 2022. We recorded a total of six different species distributed in five genera of cirripeds. The two most abundant species were Chelonibia testudinaria (Linnaeus, 1758) and Platylepas hexastylos (Linnaeus, 1758), located in different areas of the body; the former mainly on the carapace, while the latter mainly on the skin. We analyzed their abundance and distribution pattern on the sea turtle’s body and used the findings to deduce the health conditions and ecological aspects of stranded specimens of C. caretta, providing new data on this threatened and vulnerable species and its epibionts. A total of 11 specimens of C. caretta were affected by DTS (Debilitative Turtle Syndrome), these specimens exhibited a significant barnacle infestation on all body parts, markedly higher than the specimens of C. caretta not affected by DTS. Studies of associated barnacles in sea turtles should be encouraged among researchers as complementary tool to infer habitat use and health status of sea turtle species.
Introduction
Sea turtles are one of several examples of a broadly distributed group that has historically suffered population declines caused by fishing bycatch and hunting of adult turtles and harvesting of eggs (Dodd, 1988; Jackson et al., 2001; Ernst and Lovich, 2009). These declines have led to worldwide conservation efforts since the 1950s (Hamann et al., 2010). In the Mediterranean Sea, the only common and widely distributed species of sea turtle is Caretta caretta (Linnaeus, 1758), commonly known as the “loggerhead sea turtle” (Prato et al., 2022). The other two species recorded in the basin are Chelonia mydas (Linnaeus, 1758) and Dermochelys coriacea (Vandelli, 1761). While the former nests in some areas of the Mediterranean, the latter is an occasional visitor (Casale et al., 2018). The Adriatic Sea represents an important marine sector of the Mediterranean basin with regard to C. caretta ecology. While the southern part of the Adriatic Sea has been identified as a significant growth habitat (Casale et al., 2007), the shallow waters of the central and northern Adriatic are recognized as some of the most crucial neritic feeding grounds in the region for specific populations (Margaritoulis et al., 2003; Lazar et al., 2004; Zbinden et al., 2008).
A large variety of marine organisms have the ability to colonize surfaces and develop encrusting communities (Schärer, 2003). These organisms are called epibiotic if they live or grow attached to other living organisms (called “basibionts”), such as corals, shelled mollusks, decapod crustaceans, sea turtles and cetaceans (Wahl, 1989; Ramos-Rivera et al., 2021; Ten et al., 2022). The most common epibionts include cirripedes (Crustacea, Cirripedia), which exhibit high diversity (Bille and Spitzner, 2021) and live in a great variety of natural (and also artificial) substrates, including rocky shores, mangroves, deep-sea hydrothermal vents and cold seeps (Darwin, 1854; Anderson and Underwood, 1994; Chan et al., 2021). Cirripedes are also often the dominant component of biofouling on hard substrates (both natural and artificial), as they are perfectly adapted to sessile life. This high degree of adaptability is due to their peculiar larval stage (cypris). Indeed, Crisp (1984) stated that they are “the top of the evolution of sessile organisms.” The members of the superfamily Coronuloidea Leach, 1817 are currently divided into five families, namely, Austrobalanidae Newman & Ross, 1976, Bathylasmatidae Newman & Ross, 1971, Chelonibiidae Pilsbry, 1916, Coronulidae Leach, 1817 and Tetraclitidae Gruvel, 1903. Some of these species are obligate commensals of sea turtles and cetaceans, while other are more generalist species. The so called “turtle barnacles” indicate barnacle species that exclusively or preferentially live on carapace, plastron or skin of chelonians (Ross and Newman, 1967; Ross and Frick, 2011; Hayashi et al., 2013). It has been demonstrated that chelonibiids have been turtle-dwelling species for more than 30 million years (Collareta et al., 2023).
Sea turtles host a considerable variety of epibiont communities (including ectoparasites) of algae and invertebrates (Caine, 1986; Kitsos et al., 2005; Robinson et al., 2017; Serio et al., 2020). The qualitative and quantitative composition of epibionts can offer indications on the ecology, behavior and health status of their hosts (Killingley and Lutcavage, 1983; Flint et al., 2009; Pfaller et al., 2014; Doell et al., 2017). For example, one recent study suggested that epibionts can be an indication of the foraging regions used by C. caretta and specialized habitat use (Silver-Gorges et al., 2021). Furthermore, quali-quantitative composition of barnacle species found on the body, as well as the chemical analysis of their shells, may provide useful information on different biological and ecological aspects of sea turtles (Hayashi and Tsuji, 2008). However, the paucity of knowledge about different life aspects of barnacles, such as growth rates and age determination, limit the utilization of barnacles as ecological indicators. Some studies have demonstrated that barnacles serve as effective environmental indicators, capturing variations such as water flow strength in estuaries – where they display phenotypic plasticity in response to flow (Reustle et al., 2023) - and the prevalence of microplastics in their environment (Zhang et al., 2022). Moreover, it was demonstrated, for example, that data on spatio-temporal patterns of sea turtles can be inferred through analyses of chemical composition of the barnacle shells. This happens because the chemical composition varies according to different chemical-physical parameters, such as salinity and temperature of the different water body masses that the sea turtle has gone through during its life and the barnacles were exposed to (Killingley and Lutcavage, 1983). Sea turtles are used by barnacles as an attachment substrate that can optimize, thanks to the swimming movements of the sea turtle, the suspension and filter feeding strategies (Frick et al., 2002; Frick et al., 2004). More than 15 species of corolunoid were recorded as epibionts on sea turtles, with members of the genera Chelonibia Leach, 1817, Platylepas Gray, 1825, Chelolepas Ross & Frick, 2007, Cylindrolepas Pilsbry, 1916, Stomatolepas Pilsbry, 1910, Stephanolepas Fischer, 1886, and Calyptolepas Frick, Zardus & Lazo-Wasem, 2010. Furthermore, of all the genera mentioned above, the last five were found only on sea turtles (Carriol and Vader, 2002; Frick et al., 2010a; Frick et al., 2010b; Frick et al., 2011). A study showed that stranded turtles were in bad health conditions with epibiont loads significantly higher than those of nesting or foraging turtles (Deem et al., 2009). Previous reports have suggested an increased occurrence of Chelonibia testudinaria (Linnaeus, 1758) on turtles found in benthic habitats of the continental shelf, where turtle density is usually high (Casale et al., 2004a; Casale et al., 2004b; Collareta and Bianucci, 2021). Barnacle–turtle associations has been considered a borderline symbiotic relationship between mutualism and parasitism. This association is not considered a phoresis, where an organism (phoretic) attaches itself to another (host) for the purpose of travel (White et al., 2018). Rather, this association is usually considered a case of commensalism, which literally means “feeding at a common table” (Van Beneden, 1878). In this association, the commensal (barnacles) benefits and the other (turtle) remains unaffected. However, this relationship can assume other nuances depending on the health status of the sea turtle and of the barnacle species involved. Different factors, such as environmental, physiological and behavioral ones, determine the recruitment of barnacle species and their survival on sea turtles; while the relationship between barnacles’ density, spatial distribution and size and sea turtle health condition has only rarely been demonstrated (Nájera-Hillman et al., 2012; Rubin et al., 2016). In all cases, commensalism is rarely reported as obligate and specific (Wahl, 1989) and no benefits to sea turtles have been demonstrated by the association with barnacles, though some authors have suggested that barnacle coverage may provide a disruptive camouflage (Wahl, 1989; Kobayashi, 2000). In most cases of association, the presence of barnacles on the body of sea turtles is benign under normal conditions and chemical signaling from host to commensal may be a key element (Zardus and Hadfield, 2004). In other cases, chronically debilitated specimens of C. caretta showed a heavy barnacle’s coverage (Deem et al., 2009; Stacy et al., 2018). These specimens are characterized by lethargy and emaciation, and often show various injuries and trauma caused by hooks and other fishing gears and by collision with boats (Casal and Orós, 2009). Unlike specimens not affected by Debilitative Turtle Syndrome (DTS) the ones ill-affected have significantly lower body condition index, with altered parameters in total white blood cell (WBC) count, glucose (Glc), total protein, calcium (Ca), respiratory burst, etc. (Stacy et al., 2018).
It is still unclear if massive barnacle aggregations occurring in some debilitated or sick sea turtles are responsible for the death of their host. In this regard, some authors suggested that such specimens were already in poor health conditions due to other factors prior to the massive colonization of epibionts (Deem et al., 2009; Novarini et al., 2009). In fact, when in good health conditions, sea turtles regularly participate in symbiotic cleaning associations with shrimps, crabs and fishes (Davenport, 1994; Losey et al., 1994; Dellinger et al., 1997; Frick and Slay, 2000; Frick et al., 2004; Sazima et al., 2010) and scrape off epibionts by rubbing the body underwater against hard structures (Frick and McFall, 2007). This behavior helps to control epibiont colonization (Schofield et al., 2006). Extensive seasonal migrations may also affect the quali-quantitative composition of the epibiotic community in healthy turtles (Reich et al., 2010). Many of the factors that affect barnacle colonization on sea turtles are environmental, including water flow rate, which has a major influence on settlement variability. Furthermore, the competition and hence the distribution among barnacle species can be affected by the different hydrodynamic areas on the carapace, characterized by different flow patterns and drag (Logan and Morreale, 1994; Nájera-Hillman et al., 2012). The presence of different barnacle species in different microhabitats represented by different body parts of the sea turtles indicated their niche partitioning (Motlagh et al., 2020). A large number of epibionts were recorded on sea turtles, and especially on C. caretta. However, despite the important information that they can provide on sea turtles’ life aspects, the relationships between barnacles, and between them and their host, are still poorly known. Casale et al. (2012a) demonstrated that barnacle’s species composition on sea turtles collected at Lampedusa (central Mediterranean Sea, Italy) varies not only among different geographic areas, but also among different habitats within the same geographic area. Indeed, it was demonstrated for some barnacle’s species their presence in association with different habitats frequented by sea turtles: e.g. pelagic vs. benthic ones (Casale et al., 2004a).
With the present study on the epibiotic community of cirripeds associated with loggerhead sea turtles in the Adriatic Sea, we aim to provide important new data on the localization and abundance of barnacles that can be useful to infer on health condition and biological and ecological aspects of C. caretta specimens. Data here provide baseline information that is important for the development of conservation strategies and management of endangered sea turtles species. Indeed, epibionts can be used to better understand geographical distribution, migration routes, habitat preferences, as well as health status of sea turtles.
Material and methods
A total of 117 dead loggerhead sea turtles (Caretta caretta) found stranded in varying conditions and states of preservation were analyzed from 2020 to 2022 to assess barnacle loads. Specimens were found beached along the North Adriatic coast (along the stretch of coast that extends from the mouth of the river Po to the mouth of the Reno, for a total length of about 28 km) and transported to the Istituto Zooprofilattico Sperimentale della Lombardia e dell’Emilia Romagna (IZSLER) for routinary necropsy analyses. Before conducting the necropsy, we assessed the condition of the carcasses. Ideally, necropsies were carried out on fresher carcasses. Our study is based on carcasses categorized as code 1 (fresh carcass, less than 24 hours post mortem) or code 2 (moderate decomposition), following the standardized necropsy protocols NETCET (Poppi and Marchiori, 2013).
Sampling for epibionts was conducted by collecting barnacles from the dorsal and ventral area of each turtle, recording position on the body and the area occupied for each barnacle. Barnacles were collected by scraping the carapace and skin with a bistoury and were immediately preserved in 70% ethanol. A total of 26,498 cirripeds were collected on the 117 dead specimens of C. caretta; of this, a total of 3,464 (13.1%) specimens were randomly selected for measurement (width and length in mm) and identification to species level, following the identification key provided by Igić (2007). This percentage of barnacles is hence representative of the total number of barnacles present on sea turtles analyzed. These data were used to calculate species composition, abundance and determine position of barnacles for each sea turtle.
Photographs of barnacles on different body parts of C. caretta were analyzed before removal using ImageJ, a java-based analysis software, that allowed us to identify the specific position of each barnacle on carapace, plastron and other parts of turtle bodies, and size of each barnacle. The initial step involved establishing the measurement scale. To achieve this, an ABFO scale was added to each photograph. Using the “line” tool, a straight line was drawn between two points of known distance on the ABFO scale. The line was then selected, and the “Analyze” > “Set Scale” option was chosen. In the “Set Scale” window, the length of the line in pixels was displayed. Subsequently, the known distance and units of measurement were entered into the appropriate fields. From that point on, measurements were displayed using these settings. If the pixel-to-length relationship was previously determined through another measurement, this information could be directly inputted into the “Set Scale” window. Following this procedure for each barnacle, both length and width were measured. To take measurements, the “line” tool was selected, and a single click was made to specify the starting point on the edge of the barnacle. Then, the mouse was dragged to the opposite edge of the barnacle to determine the endpoint.
Each specimen of C. caretta was measured to the nearest 0.1 cm using a soft tape considering its curved carapace length (CCL) and assigned to a specific life stage: juveniles (CCL 15–50 cm), subadults (CCL 51–77 cm) and adults (CCL>77 cm) (Peckham et al., 2007).
Two-way ANOVA (α = 0.05) was used to compare the abundance of barnacles in relation to body size of C. caretta (CCL in cm).
One-sample Wilcoxon test (α = 0.05) was used to compare the overall barnacle’s abundance in different body parts of C. caretta: head-neck, carapace, plastron, forelimbs, hindlimbs and tail.
A two-way ANOVA (α = 0.05) was performed to compare barnacle’s abundance in different body parts of C. caretta per each species.
A paired t-test (α = 0.05) was used to compare the barnacle load of C. caretta affected by DTS (Debilitative Turtle Syndrome) versus unaffected specimens. To assess if the turtles were affected by the DTS, the recovery center, where the individuals were hospitalized, conducted a clinical examination. Animals with severely damaged skin, anemic, hyporeactive, and completely colonized by barnacles were considered to have DTS.
Results
The total number of C. caretta specimens analyzed was 117. Most of these were juveniles (55.6% of 117, equivalent to 65 turtles), followed by subadults (35.9% of 117, equivalent to 42 turtles), with only a small proportion being adults (8.5% of 117, equivalent to 10 turtles). We identified six different species and five genera of cirripeds, namely: Amphibalanus eburneus (Gould, 1841), Chelonibia caretta (Spengler, 1790), Chelonibia testudinaria (Linnaeus, 1758), Lepas anatifera Linnaeus, 1758, Platylepas hexastylos (Fabricius, 1798) and Stomatolepas elegans (Costa, 1838). Eleven out of one hundred seventeen specimens examined showed DTS (Debilitative Turtle Syndrome). All these DTS specimens were juveniles with a CCL ranging from 17.5 to 24.5 cm.
There was no correlation between the size of the sea turtles and the number of Cirripedia they carried [F (2, 4) = 3.903, p = 0.1148)] (Figure 1). On the contrary, the overall barnacle abundance was statistically different depending on the body part (W = 21.00, p = 0.0312), with most barnacles (52,9%) concentrated on the carapace (Figure 2). The majority of barnacles was specifically located on the costal (41,7%) and vertebral scutes (31,4%). Another aspect highlighted during barnacle inspection was the marked aggregation behavior of barnacles (Figure 3).
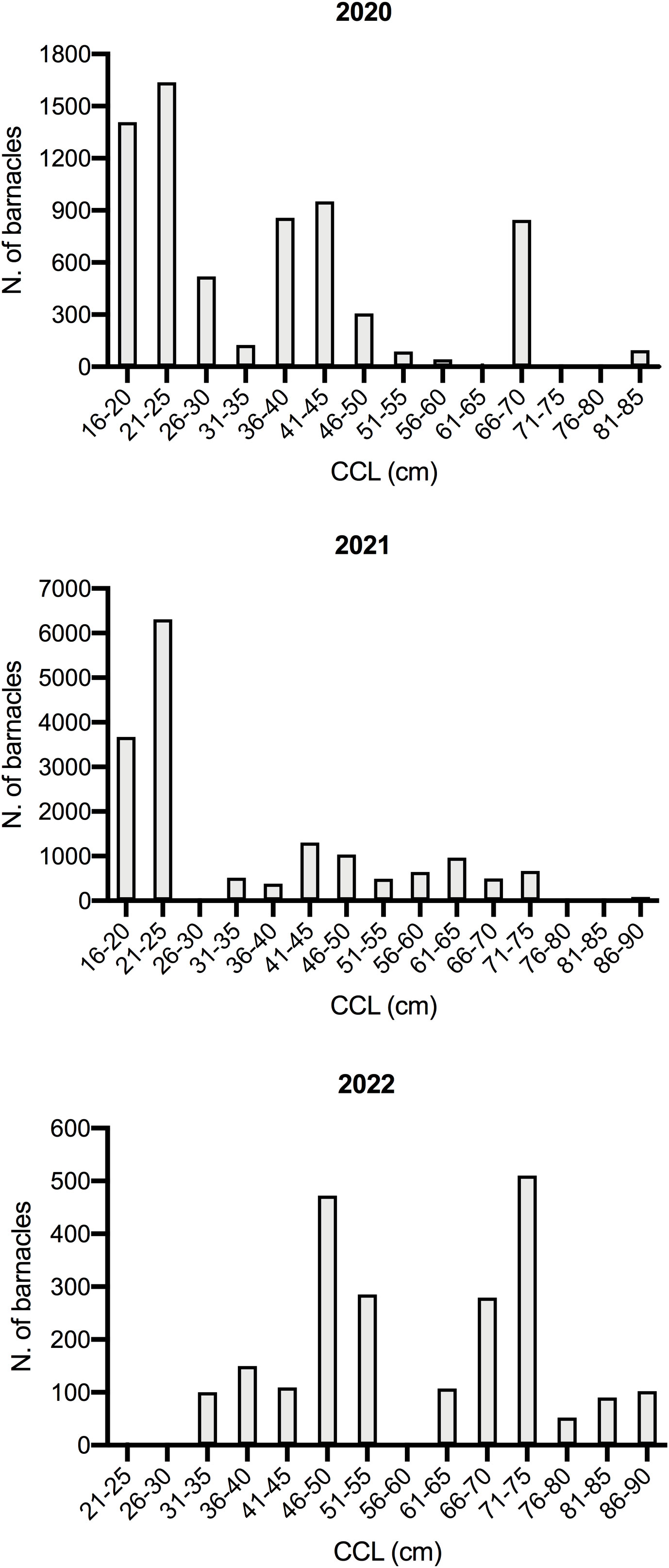
Figure 1 Distribution of barnacles found on dead loggerhead turtles stranded in the North Adriatic coast from 2020-2022, across different turtle curved carapace lengths (CCL in cm).
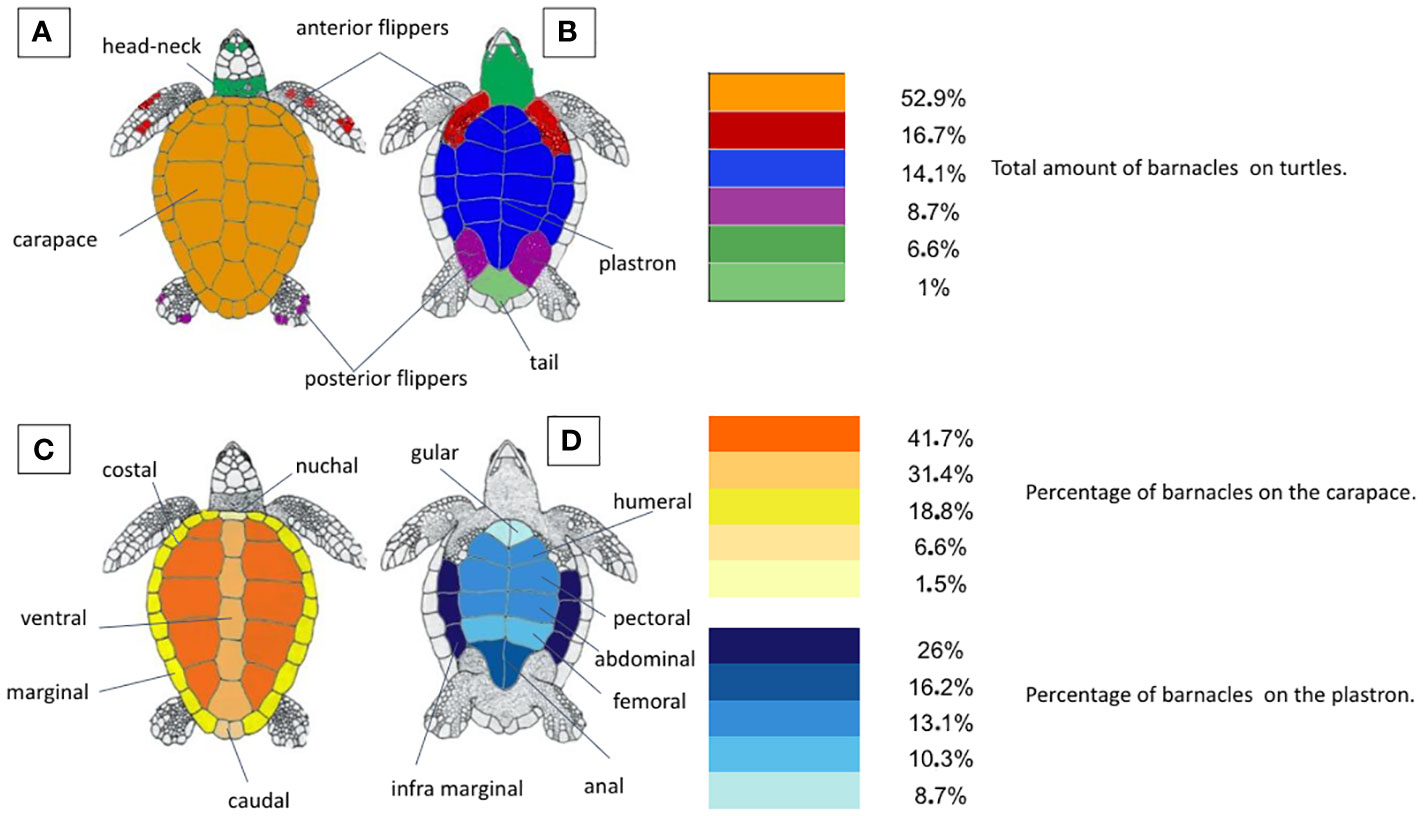
Figure 2 (A, B) Total barnacle distribution (all species) on Caretta caretta, dorsal and ventral body surface; (C, D) barnacle distribution on C. caretta carapace and plastron.
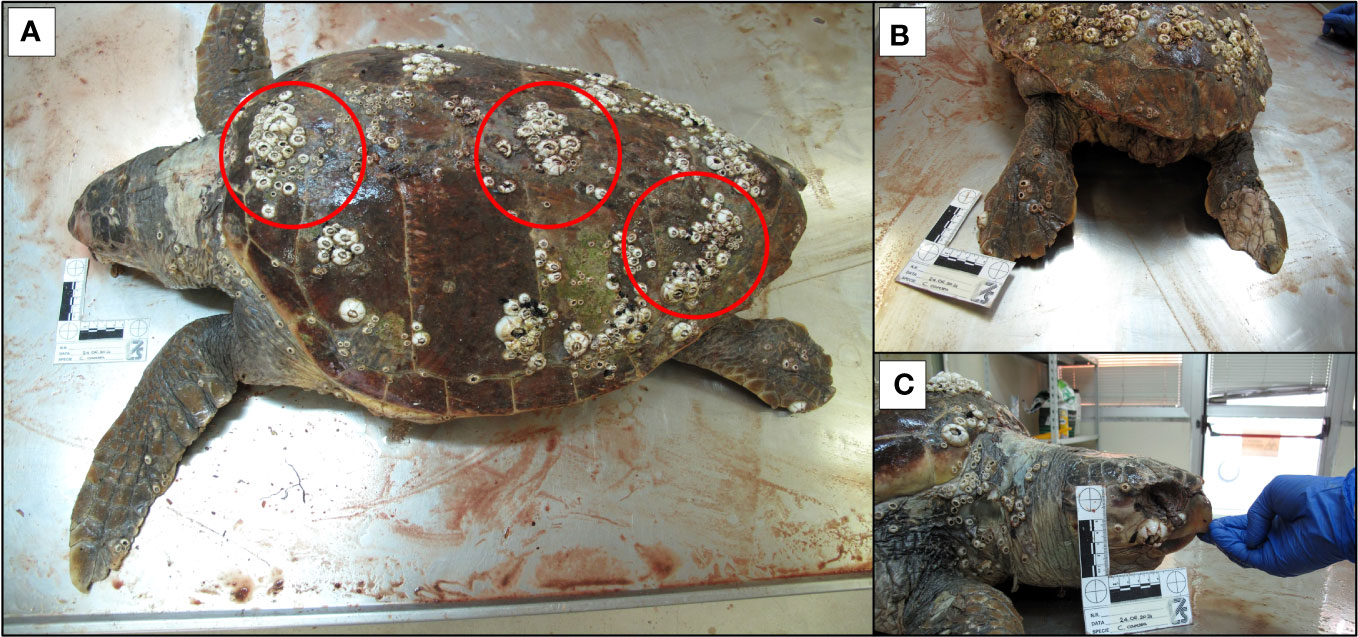
Figure 3 Barnacle aggregation (all species, with prevalence of C. testudinaria) on the carapace of C. caretta (A) detail of posterior (B) and anterior (C) part of the body.
The most abundant cirriped was C. testudinaria (Figure 4). This barnacle was present in 90.6% of specimens of sea turtle studied, and was the most abundant on the carapace (~59%), followed by the fore limbs (~15%), plastron (~13%), hind limbs (~9%), head/neck (~4%) and tail (<1%). Furthermore, some specimens of C. caretta also carried C. testudinaria complemental males (Zardus and Hadfield, 2004) (Figure 5). The second most abundant cirriped was Platylepas hexastylos (Fabricius, 1798), found in 45.3% of specimens of C. caretta analyzed. Unlike C. testudinaria, it was found to be more abundant in the soft parts of the turtle represented by the skin (e.g., head, neck, fore- and hind limbs, tail) (Figure 6) than hard parts (carapace and plastron). Similar to C. testudinaria, Chelonibia caretta was also primarily found on the carapace, albeit in lower abundance and less common compared to its congeneric species (it was found only in 13.7% of specimens studied). On the other hand, Stomatolepas elegans was found exclusively on soft parts (Figure 7), and only in 5.1% of studied specimens of C. caretta. Lepas anatifera (order Scalpellomorpha) was also identified (in 0.85% of studied specimens); this species was found on the carapace only in low abundance. Finally, similarly to L. anatifera, Amphibalanus eburneus (Gould, 1841) was recorded in low abundance and on the carapace only, in 0.85% of investigated specimens. The abundance/preference of the different barnacle species per body part was statistically significant (F (5, 25) = 3.537, p = 0.0149). Chelonibia testudinaria exhibited a well-distributed abundance across all the size classes examined, as shown in Figure 8.
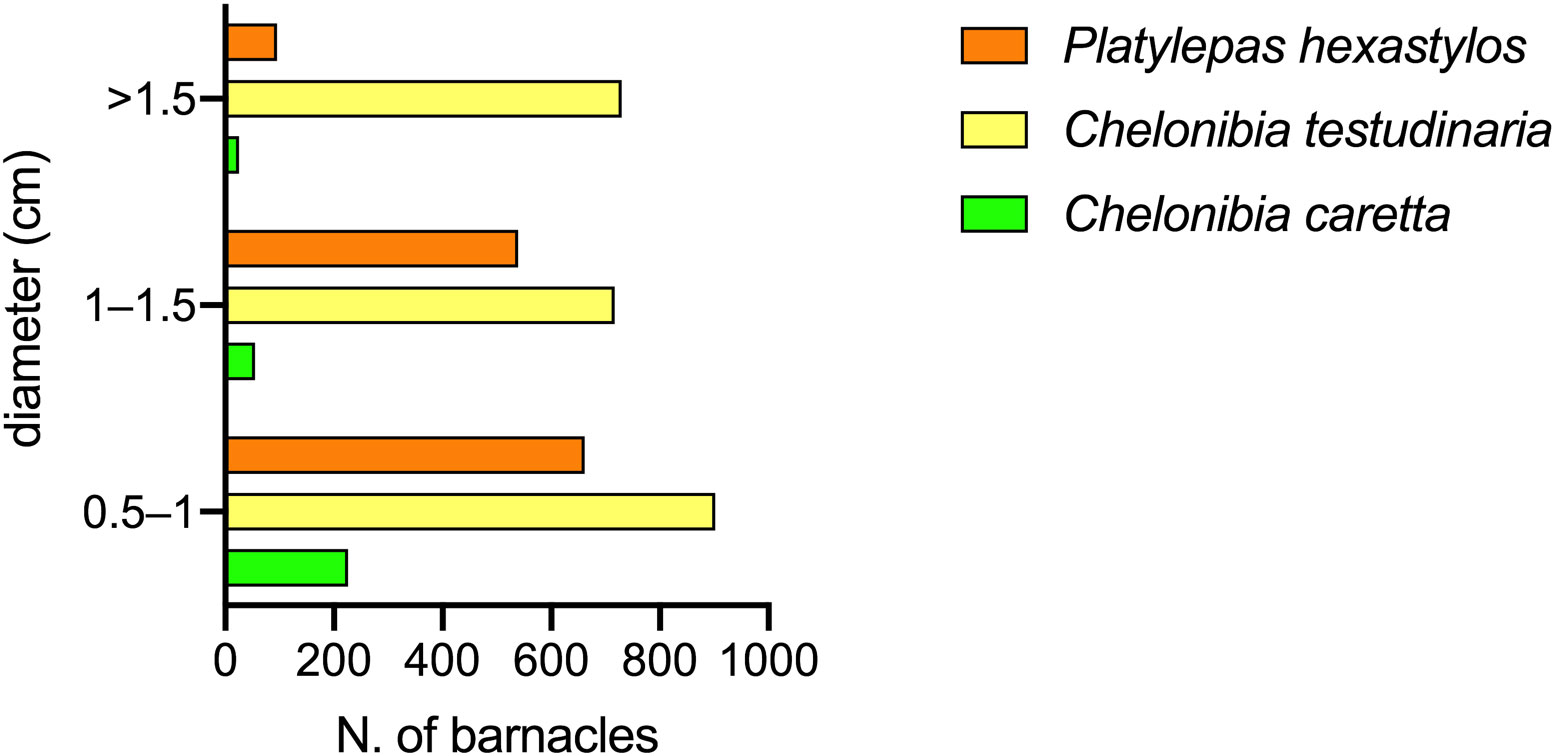
Figure 8 Barnacles size per species found on C. caretta; only the three most abundant species are showed.
Finally, evidence (t = 3.767, df = 5, p = 0.0131) does show a link between turtles affected by DTS– so small individuals – and the prevalence of epibionts (Figure 9). In terms of the size, the largest cirriped species recorded was Chelonibia testudinaria (Table 1). Indeed, specimens affected by DTS exhibited a significant barnacle infestation on all body parts, markedly higher than the specimens of C. caretta not affected by DTS (Table 2).
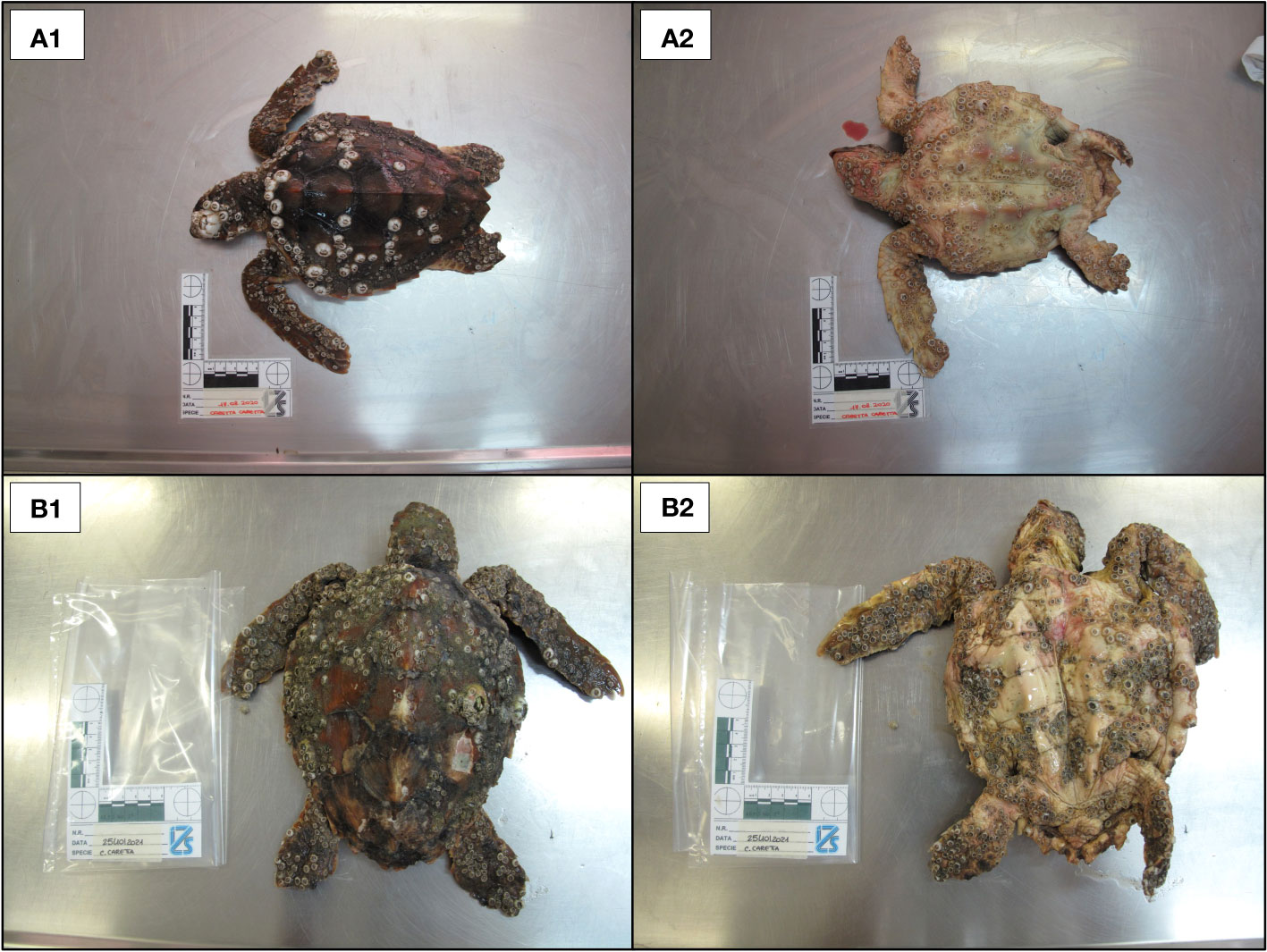
Figure 9 (A) C. caretta affected by Debilitative Turtle Syndrome (DTS), dorsal (A1) and ventral (A2) view; (B) another specimen of C. caretta affected by DTS, dorsal (B1) and ventral (B2) view.

Table 1 Sizes of barnacles collected on C. caretta specimens; AL, average length; AW, average width; LR, length range; WR, width range.
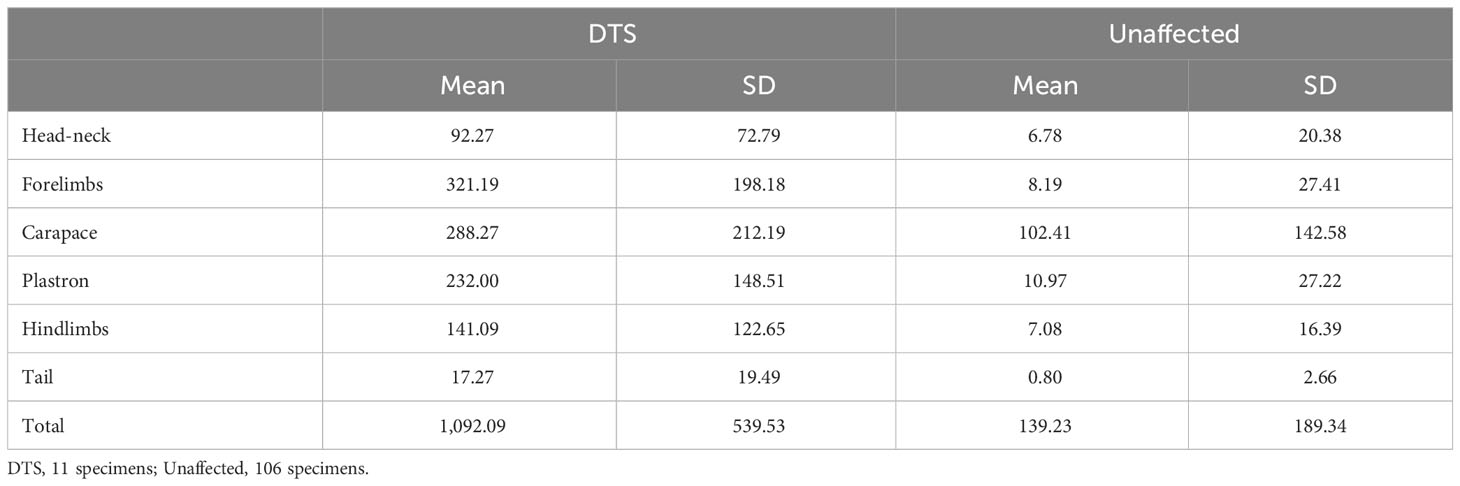
Table 2 Barnacle abundance on different body parts between C. caretta specimens affected by DTS (Debilitative Turtle Syndrome) versus unaffected specimens.
Necropsies conducted on C. caretta specimens in 2020 revealed signs of drowning, such as swallowing seawater and having a red neck, as well as the presence of foam in the windpipe (Figure 10).
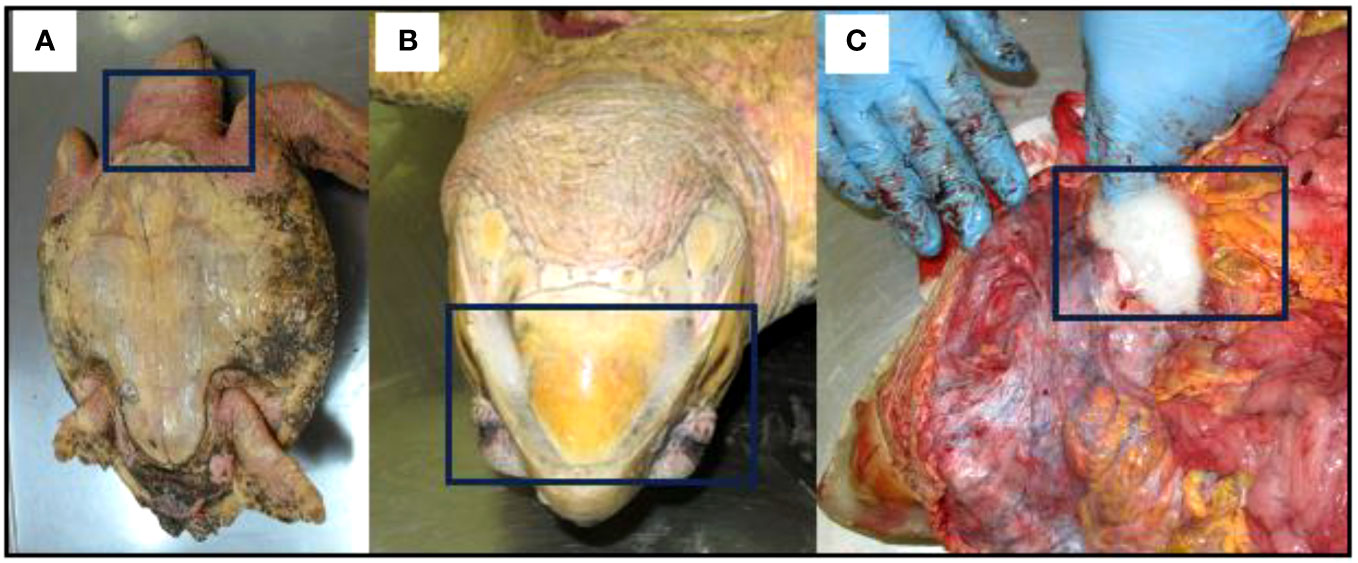
Figure 10 Caretta caretta with evidence of drowning (A) swallow and red neck (B, C) foam produced in the windpipe.
Discussion
This study represents a useful baseline assessment of barnacle species composition, abundance and distribution on loggerhead turtles that forage in the North Adriatic Sea, thereby providing valuable data on the ecology of the species. Indeed, as suggested by Casale et al. (2012b), each barnacle species showed marked preferences for hosts frequenting pelagic vs. benthic habitats. Furthermore, as we reported in this research, the different species of cirripeds showed clear preferences for the different parts of the body of the host, as these represent specific microhabitats with different conditions, such as food availability and hydrodynamics. It was demonstrated that juveniles of C. caretta undergo a deterministic ontogenetic habitat shift, with immature specimens inhabiting neritic (late stage juveniles) and oceanic (early stage juveniles) waters (Bolten et al., 2003). However, in some cases, this ontogenetic shift is not well defined among individuals, depending on food availability and specific habitat characteristics (Casale et al., 2008; Casale et al., 2012b). In our study, the prevalence of juvenile individuals and the associated composition of barnacles suggested that in the investigated area of the North Adriatic, juveniles of C. caretta prefer a benthic feeding behavior to a pelagic one. Indeed, the preference of specific species of barnacles depending on the different habitat frequented by sea turtles was directly and indirectly supported by some authors (e.g., García Gallego, 2018; Ten et al., 2019). For instance, the high abundance of C. testudinaria on turtles frequenting benthic habitats of the continental shelve was suggested by Casale et al., (2004a). In these habitats, the turtle density is usually high. In the present study, C. testudinaria has been found to be the most abundant species on Adriatic specimens of C. caretta. The pattern of distribution of C. testudinaria on the plastron of C. caretta has not been previously investigated, and scant evidence is available for green turtles (Chelonia mydas) (Lazo-Wasem et al., 2011). In this species, the scraping activity during foraging of seagrasses and algae allow sea turtles to remove (or prevent barnacles from attaching), especially the barnacles of the central area of the plastron. On the other hand, barnacles present on the marginal area of the plastron are more difficult to remove due to their peripheral position (López-Mendilaharsu et al., 2005).
Photographic analysis of our samples showed that barnacles were more abundant in the costal scutes of the carapace (52,9%). This can be explained by the fact that in this area of the body, water flow can be lower compared to other areas, and this allows for a higher potential for barnacle feeding. Likewise, other studies demonstrated that barnacles are more abundant in the vertebral zones compared to marginal ones of the carapace (Matsuura and Nakamura, 1993; Pfaller et al., 2008). Carapace hydrodynamics probably affect epibiont distributions, with differential drag and water flow patterns (Logan and Morreale, 1994). Moreover, resting turtles often place their front flippers over the marginal scutes, which may impede colonization and contribute to the lower density of barnacles there (Pfaller et al., 2006). Smaller individuals are concentrated in the marginal scutes of the carapace and the largest in the anterior part of carapace. This can happen because of a different potential in feeding due to the water flow. Indeed, barnacles located in the rear and marginal areas of the carapace are subjected to lower water flow than those located in other regions in which it is present a higher water flow (Moriarty et al., 2008). This latter work also demonstrated that C. testudinaria is capable of substantial post-settlement locomotion that generally occurs from areas of relatively low flow to higher ones. The likelihood of cyprid settlement is increased by the lower shear stress environment on the posterior region of the carapace. However, in this position barnacles found no optimal position for feeding: the significative turbulence due to the shape of the shell and to the presence of other epibionts positioned anteriorly makes feeding more difficult and therefore result in slower growth (Mullineaux and Butman, 1991). Moreover, microeddies due to the presence of adjacent epibionts would alter flow direction, thus reducing the particulate matter amount for barnacles located further to the rear than the others, as particulate matter can be consumed by other barnacles before it reaches them (Pfaller et al., 2006). Moriarty et al. (2008) discovered that barnacles change their location relative to other individuals on the same scute, thus exhibiting independent movement from one another, and this behavior occurs frequently. Hence, barnacles settled in non-optimal positions actively seek an alternative, searching for a more favorable position as they grow. This would explain at least in part the high incidence of C. testudinaria in the anterior and medial part of the body of sea turtles. This indicates us that to understand the processes that underlie the quali-quantitative distribution of the various species of barnacles (and epibionts in general) on sea turtles it is necessary to consider and study various factors (e.g., biology and ecology of barnacle species, hydrodynamics, environmental parameters, sea turtles’ behavior). Analysis of photos of dead specimen of C. caretta and species identification activity of epibionts showed that P. hexastylos and S. elegans aggregate on the skin of turtles, whilst C. testudinaria and C. caretta settle on the carapace and plastron, as demonstrated also by other authors (Casale et al., 2004a; Casale et al., 2012a). This can also be explained by different attachment methods of P. hexastylos and S. elegans, both of which use hook-like structures to anchor themselves to the soft (skin) parts of sea turtle’s body. Another important observation is the absence of any correlation between turtle size and the number of barnacles recorded. This could be due to the fact that pheromones released from conspecifics attract cyprid larvae independently of turtle size. Attractive pheromones have been found in several barnacle species (Dreanno et al., 2007). It is therefore probable that epibiont barnacles, in addition to the chemical signals useful to find their basibiont, also are attracted by aggregation pheromones produced by conspecifics (Nogata and Matsumura, 2006); more research is necessary to confirm this hypothesis. So, barnacle abundance seems not to be affected by turtle size, while their attachment position seems to be affected mainly by carapace water flow and plastron abrasion against the sea floor, according with Nájera-Hillman et al. (2012). The only confirmed relationship appears to be between the high number of barnacles and turtles affected by debilitative turtles’ syndrome (Fernández et al., 2015; Rubin et al., 2016). DTS has become a growing concern for sea turtles. Turtles with DTS are typically emaciated, anemic, hypoglycemic, and are often completely or almost completely covered by barnacles when caught or find stranded (Fernández et al., 2015). Excessive stationary floating due to debilitation may place the turtle in water conditions and concentrations of plankton ideal for abundant cyprid attachment (Sloan et al., 2014). This condition was previously shown to typically affect juveniles, a fact confirmed by the CCL measurements taken during necropsy. It has been demonstrated that in these extreme and unique cases, the epibiont load may double the volume and mass in juvenile sea turtles resulting in increased drag caused by the substantial barnacle infestation (Bjorndal et al., 2003). This could further amplify the potentially negative effects of epibiosis on sea turtles already in bad health condition impeding the ability to forage efficiently. Debilitation results in stationary floating and decreased grooming. Furthermore, debilitated turtle carapaces may in some way be more attractive to barnacle cyprids as they display atypical, multifocal beta keratin thickness and they also present keratin eating copepods on the carapace (Badillo et al., 2006). On the other hand, sea turtles in good health conditions keep the abundance of epibionts in check exhibiting self-grooming patterns. Indeed, these healthy specimens are usually observed actively rubbing against hard submerged structures to remove epibionts (Heithaus et al., 2002; Schofield et al., 2006; Frick and McFall, 2007). Furthermore, it was suggested that the expenditure of self-grooming behaviors may differ between sick and healthy sea turtles (Rubin et al., 2016). Another interesting finding is that the communities of barnacles seem to be aggregated. This suggests that when a cypris larva attach on a sea turtle that already carries another individual of the same species, the settlement area is not selected simply by chance but is determined by the previous presence of the epibiont. In other words, the presence of conspecifics on sea turtle acts as an “attraction point” to other. Like most crustacean species, the reproduction of barnacles is characterized by copulation, although they have the peculiar feature of being sessile organisms when adults. Although barnacles are hermaphroditic species and this increases the opportunities of copulation, in all cases at least two mature individuals of the same species are necessary to be on the same sea turtle at a short distance (Kelly and Sanford, 2010; Casale et al., 2012a). Moreover, it is known that C. testudinaria needs receptive neighbors for cross-fertilization during breeding (Zardus and Hadfield, 2004); thus, it is not surprising that barnacles were found to aggregate (Nájera-Hillman et al., 2012). Chelonibia testudinaria have been found to have complemental males on their edges, confirming this reproduction strategy and that the conditions for these epibionts were good.
This research underlines the importance of analysis on barnacles attached to sea turtles, as they can provide a variety of information about different ecological and biological aspects of their host, such as migration, health conditions and feeding behavior, helping the scientific community to monitor C. caretta populations, and sea turtle populations in general. Although the data here reported comes from specimens sampled in a relatively small area and found dead (although most of them were freshly dead), some studies offer strong quantitative evidence that certain barnacles species have the potential to serve as ecological indicators of habitat (Casale et al., 2004a; Casale et al., 2012b; Ten et al., 2019). The study of the qualitative and quantitative composition and distribution of barnacles on C. caretta can also be used to infer the habitat of incidental captures of sea turtles. In the Adriatic Sea a high number of sea turtles are incidentally captured by different fishing gears (Lazar and Tvrtkovic, 1995; Affronte and Scaravelli, 2001; Casale et al., 2004b). Every year, in this area, more than 11,000 sea turtles are captured as bycatch, with the majority being captured by bottom trawlers (Casale, 2011). Lepadomorph (Lepas anatifera) and balanomorph (Platylepas spp.) barnacles are more commonly associated with turtles frequenting epipelagic habitats (or floating at sea) and then caught by pelagic longlines or drift nets (Almón et al., 2019; Ten et al., 2019). On the other hand, the other balanomorph barnacles, namely Chelonibia spp. and Stomatolepas elegans, are more commonly associated with turtles frequenting benthic habitats and then caught by bottom and demersal neritic fishing gears (Casale et al., 2004b; Ten et al., 2019). Indeed, the observation that stranded sea turtles had also come into contact with fishing gear is realistic, as interactions with fisheries are the most common cause of mortality among Mediterranean specimens of C. caretta (Carreras et al., 2004). The main cause of mortality due to trawling is forced apnea during trawling activities. This aspect was also demonstrated by our analysis, during which we found several specimens with signs of drowning typically resulting from contact with fishing gear, such as swallowing seawater and having a red neck, as well as the presence of foam in the windpipe.
Previous research on the different communities of barnacles found on sea turtles have recommended that basibiont individuals should be included in epibiont studies only if they can be regarded as representative of normal conditions (Casale et al., 2012a; Ten et al., 2019). Indeed, in dead specimens of sea turtles washed ashore for a long period of time, the epibiont community may have been post-mortem changed. In our case, most specimens of C. caretta were freshly dead, hence the barnacle’s communities found on sea turtles’ body can be considered as representative of “normal conditions”.
Conclusions
Sea turtles are essential to marine ecosystems: as they maintain habitat health, provide nutrients to other species, control jellyfish populations, and host epibiont communities. Several factors determine the presence, distribution and species composition of barnacles, and epibionts in general, on sea turtles. The process that underlines the dynamics of colonization, localization and reproduction of barnacles are still little known, and have we only begun to understand them quite recently. These biological and ecological processes (e.g., settling, feeding, growth, reproduction) of epibiotic barnacles are strictly linked to behavioral and ecological aspects of sea turtles. Hence, the study of epibiotic fauna of sea turtles, and of other marine organisms, can be considered a cost-effective tool to investigate habitat use and habits of sea turtles, helping to track the habitat shift and migration of sea turtles in the Mediterranean. Using barnacles as ecological indicators is a cost-effective method to aid in sea turtle conservation efforts, providing insights into their ecological aspects like distribution, migration routes, habitat preferences, and health status.
Data availability statement
The original contributions presented in the study are included in the article/supplementary material. Further inquiries can be directed to the corresponding author.
Ethics statement
Ethical review and approval was not required for the study on animals in accordance with the local legislation and institutional requirements.
Author contributions
FT: conceptualization; statistical analysis; write the original draft and revised it; scientific supervision; barnacles identification. MB: conceptualization; image analysis; write the original draft and revised it; barnacles identification; data collection. SR: conceptualization; revised the draft; funds; data collection. SI: revised the original draft; images curation. VA: data collection; revised the draft. All authors contributed to the article and approved the submitted version.
Acknowledgments
We are grateful to the ENPA (national animal protection association) in Lagosanto (Ferrara), especially to Marco Pozzi and Viviana Prati; the Fondazione Cetacea in Riccione (Rimini), especially Sauro Pari and the Carabinieri Biodiversità in Punta Marina (Ravenna), in particular Ten. Col. Giovanni Nobili and M.llo Capo Emanuele Battani for their active and collaborative role. We are grateful too to the two reviewers for their valuable suggestions that helped us in improving our manuscript.
Conflict of interest
The authors declare that the research was conducted in the absence of any commercial or financial relationships that could be construed as a potential conflict of interest.
Publisher’s note
All claims expressed in this article are solely those of the authors and do not necessarily represent those of their affiliated organizations, or those of the publisher, the editors and the reviewers. Any product that may be evaluated in this article, or claim that may be made by its manufacturer, is not guaranteed or endorsed by the publisher.
References
Affronte M., Scaravelli D. (2001). Analysis of stranded sea turtles in the north-western Adriatic Sea. Zoology Middle East. 24, 101–108. doi: 10.1080/09397140.2001.10637888
Almón B., Ariza P., Pérez-Dieste J. (2019). First record of the epibiont barnacle Platylepas hexastylos (Crustacea: Cirripedia: Platylepadidae) from Galician coast Front. Mar. Sci. Conference Abstract: XX Iberian Symposium on Marine Biology Studies (SIEBM XX). doi: 10.3389/conf.fmars
Anderson M. J., Underwood A. J. (1994). Effects of substratum on the recruitment and development of an intertidal estuarine fouling assemblage. J. Exp. Mar. Biol. Ecology. 184, 217–236. doi: 10.1016/0022-0981(94)90006-X
Badillo F. J., Puig L., Montero F. E., Raga J. A., Aznar F. J. (2006). Diet of Balaenophilus spp. (Copepoda: Harpacticoida): feeding on keratin at sea? Mar. Biol., 151:751–758. doi: 10.1007/s00227-006-0521-z
Bille J., Spitzner F. A. (2021). A morphological comparative approach of different Barnacle species in Gullmarfjord with special focus on the cirripeds. Environment. Sci. 1, 5–19. doi: 10.18452/23141
Bjorndal K. A., Bolten A. B., Dellinger T., Delgado C., Martins H. R. (2003). Compensatory growth in oceanic loggerhead sea turtles: response to a stochastic environment. Ecology 84, 1237–1249. doi: 10.1890/0012-9658(2003)084[1237:CGIOLS]2.0.CO;2
Bolten A. B., Lutz P. L., Musick J. A., Wyneken J. (2003). Variation in sea turtle life history patterns: neritic vs. oceanic developmental stages. Biol. sea turtles. 2, 243–257.
Caine E. A. (1986). Carapace epibionts of nesting loggerhead sea turtles: Atlantic coast of USA. J. Exp. Mar. Biol. Ecology. 95, 15–26. doi: 10.1016/0022-0981(86)90084-5
Carreras C., Cardona L., Aguillar A. (2004). Incidental catch of the loggerhead turtle Caretta caretta off the Balearic Islands (western Mediterranean). Biol. Conserv. 117, 321–329. doi: 10.1016/j.biocon.2003.12.010
Carriol R.-P., Vader W. (2002). Occurrence of Stomatolepas elegans (Cirripedia: Balanomorpha) on a leatherback turtle from Finnmark, northern Norway. J. Mar. Biol. Assoc. United Kingdom 82, 1033–1034. Cambridge University Press. doi: 10.1017/S0025315402006604
Casal A. B., Orós J. (2009). Plasma biochemistry and hematology values in juvenile loggerhead sea turtles undergoing rehabilitation. Veterinary Rec. 164, 663–665. doi: 10.1136/vr.164.21.663
Casale P. (2011). Sea turtle by-catch in the Mediterranean. Fish Fisheries. 12, 299–316. doi: 10.1111/j.1467-2979.2010.00394
Casale P., Abbate G., Freggi D., Conte N., Oliverio M., Argano R. (2008). Foraging ecology of loggerhead sea turtles Caretta caretta in the central Mediterranean Sea: evidence for a relaxed life history model. Mar. Ecol. Prog. Series. 372, 265–276. doi: 10.3354/meps07702
Casale P., Broderick A. C., Camiñas J. A., Cardona L., Carreras C., Demetropoulos A., et al. (2018). Mediterranean Sea turtles: current knowledge and priorities for conservation and research. Endangered Species Res. 36, 229–267. doi: 10.3354/esr00901
Casale P., Broderick A. C., Freggi D., Mencacci R., Fuller W. J., Godley B. J., et al. (2012b). Long-term residence of juvenile loggerhead turtles to foraging grounds: a potential conservation hotspot in the Mediterranean. Aquat. Conservation: Mar. Freshw. Ecosystems. 22, 144–154. doi: 10.1002/aqc.2222
Casale P., D’Addario M., Freggi D., Argano R. (2012a). Barnacles (Cirripedia, Thoracica) and associated epibionts from sea turtles in the central Mediterranean. Crustaceana. 85, 533–549. doi: 10.1163/156854012x634393
Casale P., Freggi D., Basso R., Argano R. (2004a). Epibiotic barnacles and crabs as indicators of Caretta caretta distribution and movements in the Mediterranean Sea. J. Mar. Biol. Assoc. United Kingdom. 84, 1005–1006. doi: 10.1017/S0025315404010318h
Casale P., Freggi D., Basso R., Vallini C., Argano R. (2007). A model of area fidelity, nomadism, and distribution patterns of loggerhead sea turtles (Caretta caretta) in the Mediterranean Sea. Mar. Biol. 152, 1039–1049. doi: 10.1007/s00227-007-0752-7
Casale P., Laurent L., De Metrio G. (2004b). Incidental capture of marine turtles by the Italian trawl fishery in the north Adriatic Sea. Biol. Conserv. 119, 287–295. doi: 10.1016/j.biocon.2003.11.013
Chan B. K., Dreyer N., Gale A. S., Glenner H., Ewers-Saucedo C. E., Pérez-Losada M., et al. (2021). The evolutionary diversity of barnacles, with an updated classification of fossil and living forms. Zoological J. Linn. Society. 193, 789–846. doi: 10.1093/zoolinnean/zlaa160
Collareta A., Bianucci G. (2021). The occurrence of the coronuloid barnacle Chelonibia Leach 1817 as an encruster on mammalian bone in the central Mediterranean Sea. Acta Adriatica 62, 83–92. doi: 10.1007/s12542-022-00641-7
Collareta A., Rasser M. W., Frey E., Harzhauser M. (2023). Turtle barnacles have been turtle riders for more than 30 million years. PalZ. 97, 353–363. doi: 10.1007/s12542-022-00641-7
Crisp D. J. (1984). “Overview of research in marine invertebrate larvae 1940–80,” in Marine biodeterioration: an interdispciplinary study. Ed. Coslow J. D., Tipper R. C. (Annapolis, Maryland: Naval Institute Press), 103–126.
Darwin C. R. (1854). A monograph on the sub-class Cirripedia, with figures of all the species. The Balanidæ, (or sessile cirripedes) Vol. 2 (Verrucidæ. London: The Ray Society).
Davenport J. A. (1994). Cleaning association between the oceanic crab Planes minutus and the loggerhead sea turtle Caretta caretta. J. Mar. Biol. Assoc. United Kingdom. 74, 735–737. doi: 10.1017/S0025315400047780
Deem S. L., Norton T. M., Mitchell M., Segars A. L., Alleman A. R., Cray C., et al. (2009). Comparison of blood values in foraging, nesting, and stranded loggerhead turtles (Caretta caretta) along the coast of Georgia, USA. J. Wildlife Diseases. 45, 41–56. doi: 10.7589/0090-3558-45.1.41
Dellinger T., Davenport J., Wirtz P. (1997). Comparisons of social structure of Columbus crabs living on loggerhead sea turtles and inanimate flotsam. J. Mar. Biol. Assoc. United Kingdom. 77.1, 185–194. doi: 10.1017/S0025315400033865
Dodd C. K. (1988). Synopsis of the biological data on the loggerhead sea turtle Caretta caretta (Linnaeus 1758). Biol. Rep. 88, 1–110.
Doell S. A., Connolly R. M., Limpus C. J., Pearson R. M., Van de Merwe J. P. (2017). Using growth rates to estimate age of the sea turtle barnacle Chelonibia testudinaria. Mar. Biol. 164, 1–7. doi: 10.1007/s00227-017-3251-5
Dreanno C., Kirby R. R., Clare A. S. (2007). Involvement of the barnacle settlement-inducing protein complex (SIPC) in species recognition at settlement. J. Exp. Mar. Biol. ecology. 351, 276–282. doi: 10.1016/j.jembe.2007.07.003
Ernst C. H., Lovich J. E. (2009). Turtles of the United States and Canada. 2nd ed (Baltimore: Johns Hopkins University Press).
Fernández I., Retamal M. A., Mansilla M., Yáñez F., Campos V., Smith C., et al. (2015). Analysis of epibiont data in relation with the Debilitated Turtle Syndrome of sea turtles in Chelonia mydas and Lepidochelys olivacea from Concepción coast, Chile. Latin Am. J. Aquat. Res. 43, 1024–1029. doi: 10.3856/vol43-issue5-fulltext-23
Flint M., Patterson-Kane J. C., Limpus C. J., Work T. M., Blair D., Mills P. C. (2009). Postmortem diagnostic investigation of disease in free-ranging marine turtle populations: a review of common pathologic findings and protocols. J. Veterinary Diagn. Invest. 21, 733–759. doi: 10.1177/104063870902100601
Frick M. G., McFall G. (2007). Self-grooming by loggerhead turtles in Georgia, USA. Mar. Turtle Newsletter. 118, 15.
Frick M. G., Slay C. K. (2000). Caretta caretta (loggerhead sea turtle) epizoans 31 (United States:Herpetological Review). 31, 102–103.
Frick M. G., Williams K. L., Markesteyn E. J., Pfaller J. B., Frick R. E. (2004). New records and observations of epibionts from loggerhead sea turtles. Southeast Nat. 3, 613–620. doi: 10.1656/1528-7092(2004)003[0613:NRAOOE]2.0.CO;2
Frick M. G., Williams K. L., Veljacic D. C. (2002). New records of epibionts from loggerhead sea turtles Caretta caretta (L.). Bull. Mar. Science. 70, 953–956.
Frick M. G., Zardus J. D., Lazo-Wasem E. A. (2010a). A new Stomatolepas barnacle species (Cirripedia: Balanomorpha: Coronuloidea) from leatherback sea turtles. Bull. Peabody Museum Natural History. 51, 123–136. doi: 10.3374/014.051.0102
Frick M. G., Zardus J. D., Lazo-Wasem E. A. (2010b). A new coronuloid barnacle subfamily, genus and species from cheloniid sea turtles. Bull. Peabody Museum Natural History 51 (2), 169–177. doi: 10.3374/014.051.0203
Frick M. G., Zardus J. D., Ross A., Senko J., Montano-Valdez D., Bucio-Pacheco M., et al. (2011). Novel records and observations of the barnacle Stephanolepas muricata (Cirripedia: Balanomorpha: Coronuloidea); including a case for chemical mediation in turtle and whale barnacles. J. Natural History 45 (11-12), 629–640. doi: 10.1080/00222933.2010.534563
García Gallego I. (2018). Barnacles’ classification of sea turtles in baja California Sur (México: Gran Canaria Bachelor’s thesis). Available at: https://accedacris.ulpgc.es/bitstream/10553/75048/2/0749069_00000_0000.pdf.
Hamann M., Godfrey M. H., Seminoff J. A., Arthur K., Barata P. C. R., Bjorndal K. A., et al. (2010). Global research priorities for sea turtles: informing management and conservation in the 21st century. Endangered species Res. 11, 245–269. doi: 10.3354/esr00279
Hayashi R., Chan B. K., Simon-Blecher N., Watanabe H., Guy-Haim T., Yonezawa T., et al. (2013). Phylogenetic position and evolutionary history of the turtle and whale barnacles (Cirripedia: Balanomorpha: Coronuloidea). Mol. Phylogenet. Evolution. 67, 9–14. doi: 10.1016/j.ympev.2012.12.018
Hayashi R., Tsuji K. (2008). Spatial distribution of turtle barnacles on the green sea turtle, Chelonia mydas. Ecol. Res. 23, 121–125. doi: 10.1007/s11284-007-0349-0
Heithaus M., Dill L., Marshall G., Buhleier B. (2002). Habitat use and foraging behavior of tiger sharks (Galeocerdo cuvier) in a seagrass ecosystem. Mar. Biol. 140, 237–248. doi: 10.1007/s00227-001-0711-7
Jackson J. B. C., Kirby M. X., Berger W. H., Bjorndahl K. A., Botsford L. W., Bourque B. J. (2001). Historical overfishing and the recent collapse of coastal ecosystems. Science. 293, 629–638. doi: 10.1126/science.1059199
Kelly M. W., Sanford E. (2010). The evolution of mating systems in barnacles. J. Exp. Mar. Biol. Ecology. 392, 37–45. doi: 10.1016/j.jembe.2010.04.009
Killingley J. S., Lutcavage M. (1983). Loggerhead turtle movements reconstructed from 18O and 13C profiles from commensal barnacle shells. Estuarine Coast. Shelf Science. 16, 345–349. doi: 10.1016/0272-7714(83)90152-X
Kitsos M. S., Christodoulou M., Arvanitidis C., Mavidis M., Kirmitzoglou I., Koukouras A. (2005). Composition of the organismic assemblage associated with Caretta caretta. J. Mar. Biol. Assoc. United Kingdom. 85, 257–261. doi: 10.1017/S0025315405011136h
Kobayashi M. (2000). An analysis of the growth based on the size and age distributions of the hawksbill sea turtle inhabiting Cuban waters. Japanese J. Veterinary Res. 48.2, 129–135.
Lazar B., Margaritoulis D., Tvrtković N. (2004). Tag recoveries of the loggerhead sea turtle Caretta caretta in the eastern Adriatic Sea: implications for conservation. J. Mar. Biol. Assoc. United Kingdom. 84, 475–480. doi: 10.1017/S0025315404009488h
Lazar B., Tvrtkovic N. (1995). Marine turtles in the eastern part of the Adriatic Sea: preliminary research. Oceanographic Literature Review. 12, 1106.
Lazo-Wasem E. A., Pinou T., de Niz A. P., Feuerstein A. (2011). Epibionts associated with the nesting marine turtles Lepidochelys olivacea and Chelonia mydas in Jalisco, Mexico: a review and field guide. Bull. Peabody Museum Natural History. 52, 221–240. doi: 10.3374/014.052.0203
Logan P., Morreale S. J. (1994). “Hydrodynamic drag characteristics of juvenile L. kempii, C. mydas and C. caretta,” in Proceedings of the 13th Annual Symposium on Sea Turtle Biology and Conservation. (Jekyll Island, Georgia: University of California Libraries). pp 205–208.
López-Mendilaharsu M., Gardner S. C., Seminoff J. A., Riosmena-Rodriguez R. (2005). Identifying critical foraging habitats of the green turtle (Chelonia mydas) along the Pacific coast of the Baja California peninsula, Mexico. Aquat. conservation: Mar. Freshw. ecosystems. 15, 259–269. doi: 10.1002/aqc.676
Losey G. S., George H., Balazs L. A. (1994). Cleaning symbiosis between the wrasse, Thalassoma duperry, and the green turtle, Chelonia mydas (Copeia) 3, 684–690.
Margaritoulis D., Argano R., Baran I., Bentivegna F., Bradai M. N., Camiñas J. A., et al. (2003). “Loggerhead turtles in the Mediterranean: present knowledge and conservation perspectives,” in Loggerhead sea turtles. Eds. Bolten A. B., Witherington B. E. (Washington: Smithsonian Institution Press), 175–198.
Matsuura I., Nakamura K. (1993). Attachment pattern of the turtle barnacle Chelonibia testudinaria on carapace of nesting loggerhead turtle Caretta caretta. Nippon Suisan Gakkaishi. 1993, 59(10). doi: 10.2331/suisan.59.1803
Moriarty J. E., Sachs J. A., Jones K. (2008). Directional locomotion in a turtle barnacle, Chelonibia testudinaria, on green turtles, Chelonia mydas. Mar. turtle newsletter. 119, 1–4.
Motlagh S. H., Nasrolahi A., Rezaie-Atagholipour M. (2020). Species assemblage and distribution of turtle barnacles (Cirripedia: Coronuloidea) on foraging green sea turtles (Chelonia mydas) in the Persian Gulf. J. Natural History. 54, 2113–2123. doi: 10.1080/00222933.2020.1837276
Mullineaux L. S., Butman C. A. (1991). Initial contact, exploration and attachment of barnacle (Balanus amphitrite) cyprids settling in flow. Mar. Biol. 110.1, 93–103. doi: 10.1007/BF01313096
Nájera-Hillman E., Bass J. B., Buckham S. (2012). Distribution patterns of the barnacle, Chelonibia testudinaria, on juvenile green turtles (Chelonia mydas) in Bahia Magdalena, Mexico. Rev. mexicana biodiversidad 83 (4), 1171–1179. doi: 10.7550/rmb.27444
Nogata Y., Matsumura K. (2006). Larval development and settlement of a whale barnacle. Biol. Letters. 2, 92–93. doi: 10.1098/rsbl.2005.0409
Novarini N., Mizzan L., Verza E., Vianello C. (2009). Segnalazioni di tartarughe marine in Laguna di Venezia e lungo le coste venete–Anno 2009 (Reptilia, Testudines). Boll. Mus. Civ. St. Nat. Venezia. 59, 109–115.
Peckham S. H., Díaz D. M., Walli A., Ruiz G., Crowder L. B., Nichols W. J. (2007). Small-scale fisheries bycatch jeopardizes endangered Pacific loggerhead turtles. PloS One 2, 10. doi: 10.1371/journal.pone.0001041
Pfaller J. B., Alfaro-Shigueto J., Balazs G. H., Ishihara T., Kopitsky K., Mangel J. C., et al. (2014). Hitchhikers reveal cryptic host behavior: new insights from the association between Planes major and sea turtles in the Pacific Ocean. Mar. Biol. 161, 2167–2178. doi: 10.1007/s00227-014-2498-3
Pfaller J. B., Bjorndal K. A., Reich K. J., Williams K. L., Frick M. G. (2006). Distribution patterns of epibionts on the carapace of loggerhead turtles, Caretta caretta. Mar. Biodiversity Records 1.5381, 1–4. doi: 10.1017/S1755267206003812
Pfaller J. B., Frick M. G., Reich K. J., Williams K. L., Bjorndal K. A. (2008). Carapace epibionts of loggerhead turtles (Caretta caretta) nesting at Canaveral National Seashore, Florida. J. Nat. Hist. 42, 1095–1102. doi: 10.1080/00222930701877565
Poppi L., Marchiori E. (2013). “Standard protocol for post-mortem examination on sea turtles,” in Network for the conservation of cetaceans and sea turtles in the adriatic (Venice), 35.
Prato O. O., Paduano V., Baldi G., Bonsignore S., Callea G., Camera C., et al. (2022). Minor sea turtle nesting areas may remain unnoticed without specific monitoring: The case of the largest Mediterranean Island (Sicily, Italy). Animals 12, 1221. doi: 10.3390/ani12091221
Ramos-Rivera B. S., Castro-Mondragon H., Kuk-Dzul J. G., Flores-Rodriguez P., Flores-Garza R. (2021). Diversity of epibionts associated with Lepidochelys olivacea (Eschscholtz 1829) sea turtle nesting in the Mexican South Pacific. Animals. 11, 1734. doi: 10.3390/ani11061734
Reich K. J., Bjorndal K. A., Frick M. G., Witherington B. E., Johnson C., Bolten A. B. (2010). Polymodal foraging in adult female loggerheads (Caretta caretta). Mar. Biol. 157, 113–121. doi: 10.1007/s00227-009-1300-4
Reustle J. W. N. L., Belgrad B. A., MkKee A., Smee D. L. (2023). Barnacles as biological flow indicators. PeerJ. 11, e15018. doi: 10.7717/peerj.15018
Robinson N. J., Lazo-Wasem E. A., Paladino F. V., Zardus J. D., Pinou T. (2017). Assortative epibiosis of leatherback, olive ridley and green sea turtles in the Eastern Tropical Pacific. J. Mar. Biol. Assoc. United Kingdom. 97, 1233–1240. doi: 10.1017/S0025315416000734
Ross A., Frick M. F. (2011). Nomenclatural emendations of the family-group names Cylindrolepadinae, Stomatolepadinae, Chelolepadinae, Cryptolepadinae, and Tubicinellinae of Ross & Frick 2007—including current definitions of family-groups within the Coronuloidea (Cirripedia: Balanomorpha). Zootaxa. 3106, 60–66. doi: 10.11646/zootaxa.3106.1.4
Ross A., Newman W. A. (1967). Eocene Balanidae of Florida, including a new genus and species with a unique plan of “ turtle-barnacle” organization. Am. Museum novitates 2288, 1-21.
Rubin A., Hamman E., Frick M., Mott C., Frazer T. (2016). The relationship between barnacles and green turtle health. J. Undergraduate Res. 17, 1–5.
Sazima C., Grossman A., Sazima I. (2010). Turtle cleaners: reef fishes foraging on epibionts of sea turtles in the tropical Southwestern Atlantic, with a summary of this association type. Neotrop. Ichthyol. 8 (1), 187–192. doi: 10.1590/S1679-62252010005000003
Schärer M. T. (2003). A survey of the epibiota of Eretmochelys imbricata (Testudines: Cheloniidae) of Mona Island, Puerto Rico. Rev. Biol. tropical. 51, 87–90.
Schofield G., Katselidis K. A., Dimopoulos P., Pantis J. D., Hays G. C. (2006). Behaviour analysis of the loggerhead sea turtle Caretta caretta from direct in-water observation. Endangered Species Res. 2, 71–79. doi: 10.3354/esr002071
Serio D., Furnari G., Moro I., Sciuto K. (2020). Molecular and morphological characterization of Melanothannus testudinis sp. nov. (Rhodophyta, Rhodomelaceae) and its distinction from Polysiphonia carettia. Phycologia 4, 281–291. doi: 10.1080/00318884.2020.1752531
Silver-Gorges I., Ingels J., Dos Santos G. A. P., Valdes Y., Pontes L. P., Silva A. C., et al. (2021). Epibionts reflect spatial and foraging ecology of Gulf of Mexico loggerhead turtles (Caretta caretta). Front. Ecol. Evolution. 9. doi: 10.3389/fevo.2021.696412
Sloan K., Zardus J. D., Jones M. L. (2014). Substratum fidelity and early growth in Chelonibia testudinaria, a turtle barnacle especially common on debilitated loggerhead (Caretta caretta) sea turtles. Bull. Mar. Science. 90, 581–597. doi: 10.5343/bms.2013.1033
Stacy N. I., Lynch J. M., Arendt M. D., Avens L., McNeill J. B., Cray C., et al. (2018). Chronic debilitation in stranded loggerhead sea turtles (Caretta caretta) in the southeastern United States: Morphometrics and clinicopathological findings. PloS One 13, e0200355. doi: 10.1371/journal.pone.0200355
Ten S., Pascual L., Pérez-Gabaldon M. I., Tomas J., Doménech F., Aznar F. J., et al. (2019). Epibiotic barnacles of sea turtles as indicators of habitat use and fishery interactions: an analysis of juvenile loggerhead sea turtles, Caretta caretta, in the western Mediterranean. Ecol. Indic. 107, 105672. doi: 10.1016/j.ecolind.2019.105672
Ten S., Raga J. A., Aznar F. J. (2022). Epibiotic fauna on cetaceans worldwide: A systematic review of records and indicator potential. Front. Mar. Science. 9. doi: 10.3389/fmars.2022.846558
Wahl M. (1989). Marine epibiosis. Fouling and antifouling: some basic aspects. Mar. Ecol. Prog. series. 58, 175–189. doi: 10.3354/meps058175
White E. M., Clark S., Manire C. A., Crawford B., Wang S., Locklin J., et al. (2018). Ingested micronizing plastic particle compositions and size distributions within stranded post-hatchling sea turtles. Environ. Sci. technology. 52, 10307–10316. doi: 10.1021/acs.est.8b02776
Zardus J. D., Hadfield M. G. (2004). Larval development and complemental males in Chelonibia testudinaria, a barnacle commensal with sea turtles. J. Crustacean Biol. 24, 409–421. doi: 10.1651/C-2476
Zbinden J. A., Aebischer A., Margaritoulis D., Arlettaz R. (2008). Important areas at sea for adult loggerhead sea turtles in the Mediterranean Sea: satellite tracking corroborates findings from potentially biased sources. Mar. Biol. 153, 899–906. doi: 10.1007/s00227-007-0862-2
Keywords: loggerhead sea turtle, cirripeds, barnacles, Mediterranean Sea, threatened species, fishery
Citation: Baruffaldi M, Rubini S, Ignoto S, Angelini V and Tiralongo F (2023) Learning from Caretta caretta (Linnaeus, 1758) epibionts: a study from the Adriatic Sea. Front. Mar. Sci. 10:1243153. doi: 10.3389/fmars.2023.1243153
Received: 20 June 2023; Accepted: 03 October 2023;
Published: 19 October 2023.
Edited by:
Suncica Bosak, University of Zagreb, CroatiaReviewed by:
Carlos Jimenez, Enalia Physis Environmental Research Centre (ENALIA), CyprusAna Rita Patrício, MARE-ISPA, Portugal
Copyright © 2023 Baruffaldi, Rubini, Ignoto, Angelini and Tiralongo. This is an open-access article distributed under the terms of the Creative Commons Attribution License (CC BY). The use, distribution or reproduction in other forums is permitted, provided the original author(s) and the copyright owner(s) are credited and that the original publication in this journal is cited, in accordance with accepted academic practice. No use, distribution or reproduction is permitted which does not comply with these terms.
*Correspondence: Francesco Tiralongo, ZnJhbmNlc2NvLnRpcmFsb25nb0B1bmljdC5pdA==