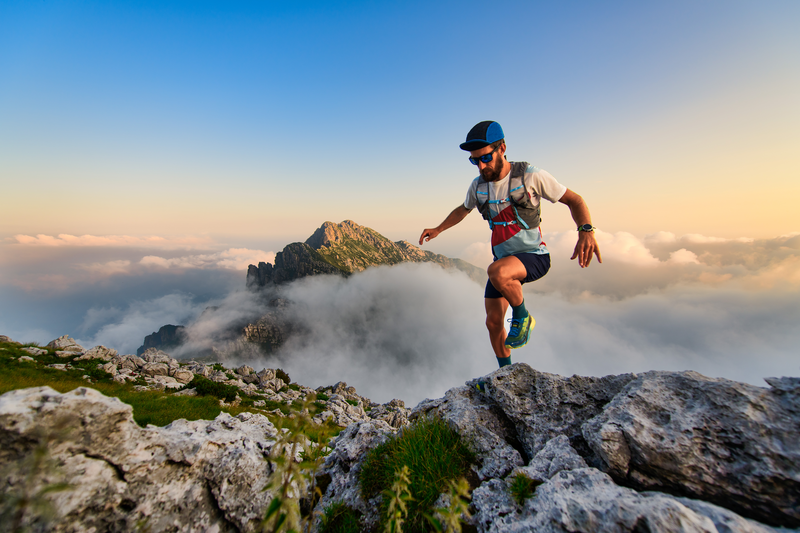
95% of researchers rate our articles as excellent or good
Learn more about the work of our research integrity team to safeguard the quality of each article we publish.
Find out more
SYSTEMATIC REVIEW article
Front. Mar. Sci. , 27 September 2023
Sec. Marine Pollution
Volume 10 - 2023 | https://doi.org/10.3389/fmars.2023.1240969
Over the past few decades, the scientific community has extensively investigated microplastics (MPs) and their interactions with marine organisms. MPs ingestion has been one of the most commonly examined topics across marine fauna. In this study, we present a systematic review conducted to gather information on the MPs ingestion by different taxonomic groups, collect MPs abundances and characteristics, and explore the relative literature evolution. Additionally, through meta-analysis, we focused on marine commercial species, aiming to estimate the mean MPs ingestion quantities in Pisces, Mollusca, Crustacea, and Echinodermata. A total of at least 822 marine species were reported to have ingested MPs for the period of 1972–2021. Pisces (class: Teleostei) was the most frequently examined group including 513 species reported to have ingested at least one MP and exhibited the widest range of mean MPs ingestion quantities [0.07–16.33 MPs per individual (MPs/ind) GIT] among the different families. The studies that analyzed soft tissue focused mainly on Gastropoda, Bivalvia, Malacostraca, and Echinodermata (8.85 MPs/ind, 3.64 MPs/ind, and 1.03 MPs/ind, 1.56 MP/g respectively). In total, MPs’ most frequently found characteristics were fibers, measuring less than 1 mm, black in color, and composed of polyethylene. The wide range of MPs descriptions concerning their characteristics and the notable high heterogeneity of the estimated values of MPs ingestion among and within most families highlights the importance of adopting harmonized methodological protocols to more effectively address the MPs issue.
Since the 1950s, the growing production of plastics has arisen concerns over their impact on the natural environment. Plastics’ properties, such as durability, resistance to degradation, lightweight, and low cost are the main reasons that plastics became extensively used in numerous domestic and industrial applications (UNEP, 2016). Plastic production transitioned from multiple- to single-use plastics, commonly intended for packaging and consumer products (UNEP, 2018). In 2016, up to 23 million Mt of plastics entered the aquatic environments, according to estimations based on the total plastic waste generated globally (Borrelle et al., 2020).
Microplastics (MPs) are plastics less than 5 mm in size, formed by the fragmentation of larger plastic items in the marine environment (secondary MPs) or produced in that size for industrial and commercial purposes (primary MPs) (Arthur et al., 2009). Secondary MPs are the most frequent MPs in the marine environment with fibers being the most found type (Browne, 2015; Gago et al., 2018). MPs are present in all marine habitats, from the sediments collected along coastlines to the seawater of mid-ocean gyres (Cole et al., 2011) and hadal bottom waters (Peng et al., 2018). MPs are potentially accessible by marine fauna (Andrady, 2015; UNEP, 2016), as their small size favors being ingested by a wide range of marine organisms at all trophic levels (Wright et al., 2013). MPs’ characteristics, mainly size and color, affect the incident of ingestion as they often resemble the natural, preferable prey of the organisms and, thus, it is more likely to be ingested (Shaw and Day, 1994; Carson et al., 2013; Wright et al., 2013; Ory et al., 2017).
Ingested MPs can physically impact marine organisms by the blockage or the injury of the internal tissues that may even be fatal (e.g., Oliveira et al., 2013; Wright et al., 2013; Rochman, 2015; Lusher et al., 2017; Egbeocha et al., 2018). In ingestion incidents, MPs also have an adverse effect on various biological functions of the marine organisms, such as alterations of the development and reproduction patterns, decrease of the survival and fecundity rates, increase of the mortality rates, promotion of inflammatory responses, and disruption of the endocrine system (Lee et al., 2013; Avio et al., 2015; Capolupo et al., 2018; Green et al., 2019; Piccardo et al., 2020; Provenza et al., 2020; Teng et al., 2021). MPs ingestion effect on marine organisms has been commonly examined under laboratory-controlled conditions; however, frequently, the selected concentration of MPs was usually higher than those occurring in the natural environments (Bucci et al., 2020). The substances added to the plastics throughout the production process have also been found capable of provoking a toxic effect on marine organisms (Andrady and Rajapakse, 2019). Plastic additives, such as plasticizers, stabilizers, flame retardants, and colorants are not covalently linked to the plastic and, thus, are potentially released to the environment and/or the tissues of the organism when ingested (ECHA, 2020).
Humans consume a variety of aquatic and marine species, primarily marine finfish (33%), mollusks (14%), and crustaceans (11%) as their primary sources of protein (FAO, 2022). Human exposure to MPs through diet has been assessed globally, and the MPs intake varied significantly depending on the different taxonomic groups of commercial marine fauna, the methodological approaches, and the reported measurement units of MPs content (Garrido Gamarro and Costanzo, 2022). At regional level, knowledge about MPs occurrence in edible tissue and the potential effect on human health through their consumption is still lacking (Barboza et al., 2018; Walkinshaw et al., 2020).
In the context of this study, a global systematic review was conducted aiming to (a) map and assess the existing knowledge and the temporal evolution of MPs ingestion by marine biota and specifically investigate differences among the various taxonomic groups and (b) quantify the MPs ingestion by commercial marine organisms.
The Preferred Reporting Items for Systematic Reviews and Meta-Analyses (PRISMA) guidelines and protocol were applied for this systematic review (Moher et al., 2009), and the SciVerse Scopus Engine was used to collect articles (http://www.info.sciverse.com/). The engine search included articles up to 31/12/2021 and consisted of the terms: (“microplastic” OR “microfiber” OR “plastics”) AND (“sea” OR “ocean” OR “marine” OR “coastal” OR “pelagic”) AND (“organisms” OR “biota” OR “species” OR “specimen”) AND (“ingestion” OR “content”). Known peer-reviewed papers not retrieved through the Scopus search were added manually into the dataset.
The systematic review was structured in four stages: identification, screening, eligibility, and inclusion in the analysis (Figure 1). All unique publications were included in the first stage. The articles were screened, and information related to each study was retrieved (Title, Year of publication, DOI). Non-English, non-peer-reviewed, and non-related to MPs ingestion by marine organisms’ publications were excluded. Aiming to target the ingestion that occurred under natural conditions in the marine environment, studies corresponding to MPs ingestion from survey-based methodological approaches (samples collected through research expeditions or marketplace surveys) were included. Thus, studies based on manipulative experiments, field setting experiments, and simulation studies were also excluded. Throughout the eligibility stage, a full-text review was conducted, and the same exclusion criteria were applied. The collected information at this stage included: (a) the study area (type of ecosystem, sampling area classified in marine realms, and provinces based on Spalding et al, 2007, 2012 or the origin country in case of samples collected from marketplaces), (b) the research (research topic, study design/methodological approach, and examined tissue), (c) the organism [taxonomic groups, species, sample size, and verification of ingestion (YES/NO), number of individuals with MPs], and (d) the MPs [number of total ingested MPs, MPs per individual or per mass unit (g) accompanied with a measure of variability, percentage, and description of the most frequently ingested type, size, color, and polymer material]. The selection of MPs descriptors and the precautions of preventing MPs contamination (e.g., use of appropriate clothing and materials and examination of blank samples) were based on the European Commission Joint Research Center guidelines on the collection, extraction, and reporting of MPs from marine organisms (Hanke et al., 2013). If the above-described information was grouped with findings of plastics greater than 5 mm in size and could not be distinguished, it was excluded from the analysis.
Figure 1 Flow diagram of the articles examined in the four stages of PRISMA. The numbers in brackets correspond to the number of articles included at each step. Source of PRISMA Flow Diagram: Moher et al. (2009).
Online databases were used for the further collection of data related to the taxonomy (class and family) and the feeding behavior of the examined organism: FishBase for Pisces (Froese and Pauly, 2022), Avibase (Lepage, 2014) for Aves, and SeaLifeBase (Palomares and Pauly, 2022) for the rest of the groups of the organisms. Additionally, the World Register of Marine Species (WoRMS) database was used to double-check the taxonomy of each species (Ahyong et al, 2022). The commercial status of the species was classified into five categories: (a) “highly commercial,” (b) “commercial,” (c) “minor commercial,” (d) “subsistence fisheries,” and (e) “of potential interest” based on the FAO statistics on fisheries retrieved from Froese and Pauly (2022) and Palomares and Pauly (2022).
The collected data were organized into spreadsheets, and R Studio was used for further processing and visualization (R Core Team, 2021). QGIS (QGIS Development Team, 2022) was used for mapping, as well as the combined geospatial vector of coastal and pelagic ecoregions provided by Nature Conservancy (2012).
To examine the ingestion of MPs among marine fauna, the retrieved records were analyzed to produce quantitative and qualitative results (Figure 1). Meta-analysis was conducted to aggregate and compare the ingestion among different studies and species. The meta-analysis focused on Pisces, Mollusca, Crustacea, and Echinodermata as these taxonomic groups included commercially important species (Supplementary Material, List of marine species that have ingested MPs.xlsx). MPs ingestion was registered for each species within each study as the number of MP items per individual or per unit mass (g). The standard deviation (SD) or standard error (SE) was also retrieved from the analyzed studies, and the latter was converted to SD [ ] (Higgins and Green, 2011). The analyzed tissue was also taken into consideration for grouping the data. The total number of records exceeded the final number of studies, as multiple species and/or multiple incidents of MPs ingestion were reported within studies. For each study, the mean values of MPs ingestion per individual or per unit mass (g) and their corresponding SD were weighted and grouped at the family level (Supplementary Section S1.1).
The “metafor” package (Viechtbauer, 2010) was used in R Studio (R Core Team, 2021) to estimate the ingestion of the different groups and families, employing a multilevel analysis approach with a random effect linear model and the DerSimonian-Laird t2 estimator (DerSimonian and Laird, 1986). Cochran’s Q test was used to assess heterogeneity across the examined variables (study and taxonomic group) (Viechtbauer, 2010; Harrer et al., 2022). The weighting of the outcomes was estimated by the inverse summarized matrix of the covariances of the variables and the potential outliers were detected by the examination of the residuals and the influential studies (Viechtbauer, 2010). For families with records originating from a single study (e.g., multiple species from the same family), the findings were grouped and organized into tables (Supplementary Table S1). In cases where only one incident of MPs ingestion was reported by a study, further analysis could not be conducted and, once again, the reported values of MPs ingestion and the examined species organized into tables for providing a better understanding of MPs ingestion range (Supplementary Table S1).
The primary search (identification stage) included 3,378 articles from 1957 to 2021, which mainly originated from the literature search on the Scopus database and a few additional publications that were imported manually (Figure 1). Through the screening process and the application of the inclusion criteria, 2,363 articles proceed to the full-text assessment stage. The inclusion stage consisted of 345 articles producing 1,967 individual records that were analyzed to assess MPs ingestion among the different taxonomic groups (Figure 1). A subset of 281 articles (1,667 records) was referred to species with a commercial status and was further analyzed. Specifically, the MPs ingestion mean values grouped at the family level within each study (as described above) concluded to 336 grouped records (k) intended for meta-analysis purposes.
Although the term “microplastic(s),” as commonly used today, was introduced at a later stage (Authur et al., 2009), the interaction of marine organisms with plastics present in the marine environment is dated back to the 1950s (Snoke, 1957). MPs were described as pellets, granules, or plastic fragments on beaches (Merrell, 1980; Shiber, 1982; Gregory, 1983) and in seawater (Carpenter and Smith, 1972; Carpenter et al., 1972). Ingestion of polystyrene by fish species—in the shape of spherules, sizing on average below 5 mm and intended for manufacturers’ uses—was first published in 1972 (Carpenter et al., 1972). Incidents of plastic ingestion continued to be highlighted through the following decades, although the size of the plastic particles was not always described. In recent years, an increasing number of published studies focusing on MPs ingestion by marine fauna was recorded; similar is the trend in records (Figure 2).
Figure 2 Temporal evolution of publications related to MPs ingestion by marine organisms for the period of 1972–2021. Left: The number of published articles. Right: The number of records within the articles. Consecutive years with no values are represented in the figures with the gray x-axis breakage.
From 1972 to 2021, species of 13 marine biota groups were analyzed under the scope of MPs ingestion, including 29 classes and 332 families. At least 1,043 marine species were examined, and MPs ingestion was confirmed for 822 different marine species. The most frequently examined groups were Pisces (685 species), Aves (102 species), Mollusca (97 species), and Crustacea (73 species). Echinodermata (22 species), Mammalia (18 species), Polychaeta (13 species), and Cnidaria (12 species) had a lower number of examined species, while each of the rest of the groups (Reptilia, Porifera, Ctenophora, Tunicata, and Chaetognatha) included up to seven species. Overall, 43.9% of the total studies focused their research on MPs ingestion by Pisces, followed by Mollusca (21%), Crustacea (11%), Aves (5.6%), Echinodermata (4.1%), and Mammalia (3.7%) (Figure 3).
Figure 3 Percentage of the total articles examined for the incidence of MPs ingestion across marine fauna per biota group. The black slice aggregates all biota groups with contributions equal or less than 1% of the total articles (Porifera, 1%; Tunicata, 1%; Ctenophora, 0.5%; Chaetognatha, 0.2%).
As an indicator for exploring the research intensity within studies, the total number of examined species per article for each biota group was calculated. Pisces and Aves presented the highest mean number of examined species per article, 10.2 and 8.5 species per article, respectively (Figure 4).
Figure 4 Box plot of the examined number of marine species per article for each biota group. The mean value of the examined number of species per article for each biota group is presented with the x symbol and the median with the horizontal line within each box. In case of no visual display of the median within boxes, the median is equal to the minimum value. The vertical whiskers in each box present the maximum value of the biota group.
The percentage of the species found to have ingested MPs was 78.8% of the total species examined (Figure 5). The species that were reported to ingest at least one MP corresponded to 29 classes and 300 families. MPs ingestion occurred for 513 species of Pisces, 94 species of Mollusca, 68 species of Crustacea, 65 species of Aves, 21 species of Echinodermata, 18 species of Mammalia, 13 species of Polychaeta, 11 species of Cnidaria, and seven species of Reptilia, while the rest of the groups included fewer species (Supplementary Material, List of marine species that have ingested MPs.xlsx).
Figure 5 Percentage of the species having ingested MPs among the total examined species for each biota group. The total number of species that ingested MPs among the total examined species is presented with the black bar (%).
Pisces, Mollusca, Crustacea, and Echinodermata included species of commercial interest. In summary, most of the species were categorized as “Commercial” (53%), followed by “Highly Commercial” (27%) and “Minor Commercial” (19%), while species “of potential interest to fisheries” and species of “subsistence fisheries” reached 1% in total. Pisces had the greatest number of species examined, with 20 species accumulating more than 20% of the records of MPs ingestion of the group. The most frequently examined species for Crustacea was Nephrops norvegicus (Linnaeus, 1758, Norway lobster) (17% of the total records of Crustacea), followed by Aristeus antennatus (Risso, 1816, blue and red shrimp) (9%) and Chionoecetes opilio (Fabricius, 1788, snow crab) (9%). Most of the research on MPs ingestion by Mollusca and Echinodermata focused on fewer species, as only three species of Mollusca (Mytilus galloprovincialis (Lamarck, 1819), Crassostrea gigas (Thunberg, 1793), and Mytilus edulis (Linnaeus, 1758)) and one species of Echinodermata [Paracentrotus lividus (Lamarck, 1816)] covered at least half of the total records of each group.
The total number of the MPs retrieved from the tissues of the examined specimens ranged from 0 to almost 6 million particles. The latter was estimated for an analyzed group of 15 individuals of Pleoticus muelleri (Bate, 1888) (Crustacea) purchased from the marketplace of Singapore and originated from Atlantic Ocean (Curren et al., 2020). For studies focusing on Mollusca, 1,945 MPs were collected from Magallana gigas (Thunberg, 1793) (class: Bivalvia) from bays in Mexico (Lozano-Hernández et al., 2021). More than 2,000 MPs were found in 200 specimens of Apostichopus japonicus (Selenka, 1867) that belonged to Echinodermata (class: Holothuroidea) and were sampled in China (Mohsen et al., 2019), while 4,566 MPs were isolated from Solea solea (Linnaeus, 1758) (Pisces, class: Teleostei) (N = 533) in the Adriatic Sea (Pellini et al., 2018).
Data on MPs characteristics (type, size, color, and polymer material) were extracted from the groups that had commercial species, thus, Pisces, Mollusca, Crustacea, and Echinodermata. In Table 1, the description and percentage of the most abundant type, size, color, and polymer material of the found MPs within each study for each biota group were summarized. Overall, fibers (71% of the total records) were the most dominant type of MPs found in the examined specimens (N = 494 records). Fibers were the most frequently found type among each group, Pisces (67.6%), Mollusca (77.6%), Crustacea (61.1%), and Echinodermata (75%) (Table 1). “Fragments and particles”‘ followed fibers, while “pellets and films” were reported as the most abundant type in a few studies. Various values and size classes were used to describe the most abundant size of MPs, while “< 1 mm” was in total the most frequently reported size class (67.6% of the total records, N = 251 records), and for each commercial group (Table 1). MPs color had the widest range of descriptions, as 20 different colors and mixed descriptions of colors were reported in the reviewed studies as the most frequent. The black color was the most frequently reported color among Pisces, Mollusca, and Crustacea, followed by blue and transparent, while blue was the most frequent for Echinodermata (N = 282 records). Twenty different polymer materials have been identified by different studies as the most abundant materials of the found MPs; this information was provided by only 84 articles. In general, polyethylene (PE) was the most frequently detected polymer material, while in Pisces both PE and semi-cellulosic fibers were equally reported as the most frequently found materials (Table 1).
Table 1 Summary table of MPs characteristics in total and for each biota group of commercial interest.
Pisces, Mollusca, Crustacea, and Echinodermata were selected for the meta-analysis of MPs ingestion based on their commercial characterization, concluding to a subset of 281 studies with 1,667 observations that were further examined based on the data provided and 336 grouped records of MPs ingestion mean values included in the analysis (see Materials and Methods). The analysis was conducted for each biota group primarily in the level of class and if the sample size was considered appropriate, then the analysis was conducted at the level of family.
Most of the commercial species analyzed were sampled around Northern European Seas and Mediterranean Sea, followed by Cold and Warm Temperate Northwest Pacific, South China Sea, and Sunda Shelf, mostly in coastal provinces rather than pelagic (Figure 6).
Figure 6 Global distribution of marine commercial species (Pisces, Mollusca, Crustacea, and Echinodermata) being reported to have ingested at least one MP. The sampling of the species occurred through scientific expeditions. Most species were sampled in the coastal and pelagic marine provinces of Europe and Southeast/East Asia (Northern European Seas, Mediterranean Sea, Cold and Warm Temperate Northwest Pacific, South China Sea and Sunda Shelf). Marine provinces are based on Spalding et al. (2007, 2012). Source of GIS layers used to produce the map: The Nature Conservancy (2012).
Pisces analysis consisted of two classes, Elasmobranchii and Teleostei with the vast majority of the species been commercially important (Supplementary Material, List of marine species that have ingested MPs.xlsx). Elasmobranchii (five species) included species characterized as “commercial” and “minor commercial”. The estimated mean value of MPs ingestion for Elasmobranchii was 0.92 (± 0.46 SE) MPs per individual (MPs/ind), with high heterogeneity (Q test, p< 0.0001, k = 6, and CI = 95%), and the examined tissue was the gastrointestinal tract (GIT) (Figure 7). Overall, Elasmobranchii had a small sample size of only six records, corresponding to four families. In the level of family, MPs/ind was estimated for two families, with Scyliorhinidae (cat sharks), having the smallest mean value among the families, and Rajidae (skates), a moderate one (0.14 ± 0.09 MPs/ind and 1.17 ± 0.58 MPs/ind, respectively). The rest of the two families were examined by only one study, each targeting the species Hypanus guttatus (Bloch & Schneider, 1801, family: Dasyatidae) and Galeus melastomus (Rafinesque, 1810, family: Pentanchidae) (Supplementary Table S1). Thus, among the families of Elasmobranchii, the mean estimated and reported values of MPs ingestion (GIT tissue analyzed) ranged from 0.14 MPs/ind to 2.4 MPs/ind.
Figure 7 Forest plot of the estimation of the mean MPs/ind for the Elasmobranchii. The black box presents the mean value of MPs/ind accompanied by a horizontal line of the standard error. The studies (left part of the forest plot) are the studies used in the analysis. In the right part, the mean value is presented with the CI = 95% and the weight for each record. The blue polygon presents the estimation of the mean value in CI = 95%.
Teleostei (at least 217 species and group records) was the most frequently examined class across marine fauna, producing an overall mean value of 2.87 (± 0.57) ingested MPs/ind for findings referring to GIT examination (Q test, p< 0.0001, k =222, and CI = 95%). At the level of family, Teleostei consisted of data records of MPs ingestion from 76 families. Half of the families had been examined only by one study and concerned one species or several species of the same Family (Supplementary Table S1). For the rest of the families the mean values of MPs ingestion were estimated and presented in Table 2. Considering both estimated mean values in the context of this study and reported mean values by the original studies, the range of MPs ingestion per individual examined, among the different families, ranged from 0.07 MPs/ind to 16.33 MPs/ind. The most frequently examined species belonged to families of Clupeidae (herrings, shads, sardines, and menhadens), Engraulidae (anchovies), Sparidae (porgies), Carangidae (jacks and pompanos), Scombridae (mackerels, tunas, and bonitos), and Mugilidae (mullets) (Table 2).
Table 2 Summary table of estimated mean values of ingested MPs for the families of Teleostei (Pisces).
Mollusca analysis was based on the data retrieved from 26 articles (19 species) and expressed in MPs mean numbers per individual and the soft tissue analyzed (Supplementary Material, List of marine species that have ingested MPs.xlsx). Three classes were included in the analysis: Bivalvia (82.1% of the total records), Gastropoda (15.5%), and only one record of Cephalopoda (Supplementary Table S1). For Bivalvia and Gastropoda, the mean values of MPs ingestion were estimated and Bivalvia had a mean value of 3.64 ± 0.50 ingested MPs/ind (Q test, k = 35, p< 0.0001, and CI = 95%) for the soft tissue analyzed. The heterogeneity was considered high, and further analysis was conducted aiming to detect potential outliers. After the application of the exclusion criteria, a new mean value of 1.47 ingested MPs/ind ± 0.02 was obtained (Q test, k = 12, p = 0.1180, and CI = 95%). To further investigate the heterogeneity, a further analysis was conducted at the level of family. Bivalvia included nine families with four of them having only one record each (Supplementary Table S1). Most of the families included species of “commercial” or “highly commercial” interest (Supplementary Material, List of marine species that have ingested MPs.xlsx). For the rest five families, the mean value of MPs ingestion was estimated and Veneridae (venus clams) had the highest mean values (7.32 ± 2.72 MPs/ind, Q test, k = 7, p< 0.0001, and CI = 95%), followed by Pharidae (4.96 ± 0.49 MPs/ind, Q test, k = 2, p = 0.09, and CI = 95%), Mytilidae (3.23 ± 0.86 MPs/ind, Q test, k = 13, p< 0.0001, CI = 95%), Ostreidae (3.03 ± 0.94 MPs/ind, Q test, k = 5, p< 0.0001, and CI = 95%), and Pectinidae (2.31 ± 0.95 MPs/ind, Q test, k = 3, p< 0.0001, and CI = 95%) (Table 3). The exclusion criteria were applied for Mytilidae and Ostreidae, and the new mean values of MPs ingestion are presented in Table 3. Considering MPs ingestion for all nine families (Table 3 and Supplementary Table S1), their mean values ranged from 1.2 MPs/ind to 7.32 MPs/ind (soft tissue analyzed). Gastropoda had a mean estimated value of 8.85 ± 1.22 MPs/ind for the soft tissue of the specimens examined, with low heterogeneity (Q test, k = 5, p = 0.1251, and CI = 95%).
Only one study examined Dosidicus gigas (D’Orbigny, 1835) (Cephalopoda, family: Ommastrephidae), but it cannot be directly compared with the other classes as different tissues were analyzed (Supplementary Table S1).
Crustacea corresponded to two classes: Malacostraca and Thecostraca (10 species) (Supplementary Material, List of marine species that have ingested MPs.xlsx). Half of the examined species had a “commercial” or “minor commercial” characterization, while for the rest of the species, this information was lacking. The most frequently examined species belonged to Malacostraca, while Thecostraca had only one study examining one species of the class with a mean value of 0.33 MPs/g (Supplementary Table S1).
Malacostraca were grouped based on the common measurement unit (MPs per individual and MPs per gram) and tissue analyzed (soft tissue and GIT). Malacostraca had an estimated mean value of 3.02 MPs/g, although it could not be compared with Thecostraca as results originated from different tissues, and an estimated mean value of 1.03 MPs/ind for the soft tissue analyzed (Table 4). For the records referring to the investigation of MPs in the GIT of Malacostraca, the estimated mean value was 4.77 ± 2.56 (Q test, k = 7, p< 0.0001, CI = 95%), four times higher than the respective estimated mean value produced by the records of the soft tissue. Although heterogeneity of the class was high for all the subgroups analyzed, the further analysis in the level of the families could not be applied.
Table 4 Summary table of estimated mean values of ingested MPs/ind or MPs/g (column: Unit of measurement) for Malacostraca class of the Crustacea.
Echinodermata had the fewest records among the analyzed groups, with only five records expressed in the same unit of measurement of MPs content (MPs/g) and analysis of the same tissue of the specimen (soft tissue). The findings referred to three classes, where each class was represented by one family, Pterasteridae (class: Asteroidea, starfish), Ophiosphalmidae (class: Ophiuridea, brittle star), and Echinidae (class: Echinidea, sea urchins); thus, in this case, the level of class and family coincided. Echinidae had species that were characterized as “commercial,” while the species of the rest of the families had no commercial interest (Supplementary Material, List of marine species that have ingested MPs.xlsx). Paracentrotus lividus (Lamarck, 1816) (Echinidae) was the only commercial species and had findings expressed as MPs/ind and MPs/g (as the other classes/families of different groups); thus, they were selected to be presented in Supplementary Table S1.
Based on the data, the overall estimated mean value of Echinodermata was estimated for the soft tissue analyzed and was 1.56 ± 0.10 MPs/g (Q test, k = 5, p< 0.001, CI = 95%) (Figure 8). No further analysis was conducted as the sample size of the group was considered small.
Figure 8 Forest plot of the estimation of the mean MPs/g for the Echinodermata. The black box presents the mean value of MPs/g accompanied by a horizontal line of the standard error. The studies (left part of the forest plot) are the studies used in the analysis. In the right part, the mean value is presented with the CI = 95% and the weight for each record. The grey polygon presents the estimation of the mean value in CI = 95%.
Summarizing the findings of the analysis (Table 5), Malacostraca had the highest mean value of MPs/ind, followed by Teleostei and Elasmobranchii. When soft tissue was analyzed, Gastropoda had the highest mean values of MPs/ind, followed by Bivalvia and Malacostraca. Echinodermata had the fewest records among the examined groups with the overall mean value of the group 1.56 MPs/g (soft tissue analyzed). Concerning the mean range of MPs ingestion among the families for each class (when applicable), Teleostei had the widest range expressed in MPs/ind (GIT).
Table 5 Summary table of the findings produced by the meta-analysis applied for Pisces, Mollusca, Crustacea, and Echinodermata.
Under this review, the occurrence of MPs ingestion by marine organisms covered a period of 49 years. The research interest began to grow substantially starting in 2016 when the number of published articles focusing on MPs ingestion by marine fauna starting from a few studies in 2015 was doubled and continued to increase almost exponentially through 2021 (Figure 2 left); similar is the trend in the incidents of ingestion assessed within the articles (Figure 2 right).
Similar findings for MPs-related literature have been previously published (Barboza and Gimenez, 2015; Qin et al., 2020). The overall growth of the research literature could be linked with the policy landmarks related to plastic pollution. In 2015, the UN General Assembly launched the Sustainable Development Goals (SDGs) (UN RES/70/1, 2015). SDG 14 targeted the reduction of marine pollution, including an indicator [14.1.1(b)] devoted to reducing plastics and MPs density in marine habitats. At the European level, the Marine Strategy Framework Directive (Directive 2008/65/EC) included Descriptor 10, which is devoted to marine litter, while the related criterion D10C3, as defined in a European Commission Decision in 2017, depicts the urgency of highlighting microliter ingestion by marine organisms and maintaining it at a level that does not negatively impact them (Commission Decision 2017/848/EC and European Commission, 2017). In 2015, (Directive 2015/56/EC, European Commission, 2015) for the mitigation of plastic bags was introduced, which although not directly related to MPs, it considered the production of smaller plastic particles through degradation (“oxo-degradable plastic carrier bags shall mean plastic carrier bags made of plastic materials that include additives, which catalyze the fragmentation of the plastic material into micro-fragments). Thus, recent policies combined with the overall effort of the research community highlighted the urgency of quantifying and monitoring MPs ingestion by marine fauna.
More than 1,000 marine species were examined for MPs ingestion, while at least 822 marine species have been reported to have ingested MPs. In a few studies, MPs ingestion was described for a group of different species, while in other cases, only the common name was referred; thus, those records were excluded for identifying the number of total species that examined and had ingested MPs. Previous literature reviews referred to 208 marine species of verified MPs ingestion (Gall and Thompson, 2015), followed by 494 examined species with 67% to have ingested MPs (Markic et al., 2020), and, most recently, 555 fish species (Savoca et al., 2021) and 568 species including both marine and freshwater ones (Hossain and Olden, 2022) were documented as having ingested plastic litter
The overall increase of marine species found to have ingested MPs could be expected as derived from the raise of published articles and records of examined species within articles.
The occurrence of MPs ingestion was investigated in marine species from 29 classes and 332 families, while the most frequently examined class was Teleostei (Pisces) with a mean value of 10.2 species examined per article. The latter could be expected, as Teleostei are the largest group within vertebrates consisting of approximately 70 orders and 500 families (Ravi and Venkatesh, 2018). Additionally, several species of the Teleostei class have been proposed for MPs biomonitoring at a regional level or have been commonly used as bioindicator species for other pollutants (such as metals and additives) (Bray et al., 2019; Zhang et al., 2020; De Witte et al., 2022). Similarly, Mollusca and especially Bivalvia have been widely examined for MPs ingestion. Mussels have been globally accepted and proposed as small-scale bioindicators, as they are considered appropriate species for estimating the level of contamination (Fossi et al., 2018; Li et al., 2019; Cho et al., 2021; Ding et al., 2021). Crustacea studies followed in the aspect of total numbers examining MPs ingestion. On the contrary, Aves had fewer studies than Crustacea and Mollusca but produced a higher number of species examined. Aves have been already used as a bioindicator for monitoring plastic ingestion with Fulmarus glacialis (Linnaeus, 1761) being a well-known example of regional biomonitoring in the North Sea (Galgani et al., 2010).
The total percentage of species that had ingested MPs (Figure 5) was considered high, as almost 80% of the total examined species had ingested at least one MP item. It is also important to mention that studies with no significant findings, where MPs ingestion was not detected or occurred in very low levels, might have been underrepresented in the literature (publication bias) and may have affected the estimations (Müller, 2021; Salerno et al., 2021).
The total number of MPs and MPs characteristics (type, size, color, and polymer material) of the most frequently found MPs were retrieved. The sample size of the analyzed specimens could explain the high number of found MPs, although the conditions occurring in the species’ habitats may also be a contributing factor (Mohsen et al., 2019; Lozano-Hernández et al, 2021). High abundances of total retrieved MPs came from species sampled from coastal areas and semi-enclosed basins that are highly urbanized areas and constitute reservoirs of intense MPs presence (Llorca et al., 2020; Wang et al., 2020; Martellini et al., 2018; Song et al., 2018). Fibers were the most dominant type of found MPs across all groups examined, in line with previous studies. Fibers, due to their high abundance and small size, may have wide effects on many organisms (Bajt, 2021; Rebelein et al., 2021). MPs most frequent size class was “< 1 mm,” mostly referring to fibers, a result previously highlighted by other studies (Lim et al., 2022). As the “smallest” size classes of MPs potentially may be present among all sizes of organisms (Wright et al., 2013), the MPs size affects the occurrence of MPs ingestion and could be a valuable measurement for various research scopes, such as selective feeding-focused studies or research on the ingestion or transfer of plastic additives (Ašmonaitė et al., 2018; Lehtiniemi et al., 2018).
MPs color had a wide range of descriptions and appeared as the most subjective MPs descriptor. The difficulty occurred when more than one color was used to characterize the shade of a MP item (e.g. transparent/white and blue/green) or the color was characterized based on its brightness (e.g. light, dark), thus, summarizing those findings was challenging. Under this review, black was reported as the most frequently reported color, followed by blue and transparent. However, this result obtained with the precondition that all other descriptions not clearly defining the color were excluded. A recent review by Lim et al. (2022) focusing on ingested MPs characteristics by fish concluded that blue is the most frequently found color, followed by black and transparent. The differences between the findings of this study and the previously published could possibly be explained by the exclusion approach that was applied for the description of the color (Lim et al., 2022). The weathering of MPs before ingestion is expected to affect the overall appearance of MPs, including color (Liu et al., 2020; Alimi et al., 2022). The importance of the color of MPs has previously been related to the feeding selectivity where MPs resemble prey items (Andrady, 2015; Zhao et al., 2022). As color is a description based on visual observation, the use of a standard color scale could contribute to the improvement of comparability among different reports (Andrady, 2015).
PE was the most abundant polymer material ingested by marine fauna, followed by PA and semi-cellulose. PE [as both high density (HDPE) and low density (LDPE)] dominates the plastics market and as its density is lower (ρ = 0.91 g/cm3–0.95 g/cm3) than the average seawater density is mainly floating on the sea surface (GESAMP, 2019). Higher density MPs, such as PA (ρ = 1.02 g/cm3–1.15 g/cm3) are more likely to sink in the water column and end up on the benthic habitats (GESAMP, 2019); thus, their presence in Echinodermata could be explained as they are benthic organisms. It should be mentioned that MPs less dense than water as PE could also be present in seafloor as biofouling increases their density or when included in fecal pellets after ingestion and marine snow (Erni-Cassola et al., 2019). MPs composition was a descriptor provided for a small number of studies. This could be explained by the fact that polymer analysis was usually applied to a subset of the total number of MPs found, and in the case of studies with several species, the correspondence between the MPs material and the specific species may be lacking. Another reason could be that the chemical characterization of MPs requires specialized equipment and protocols, while their type, size, and color can commonly be identified through visual observation using stereo- (or dissecting) microscopy, which is considered more accessible and potentially cost-effective (Primpke et al., 2020a; Primpke et al., 2020b).
The retrieved data used in the meta-analysis came from studies conducted in coastal water rather than in pelagic habitats (Figure 6). Previous findings focusing on plastics and MPs ingestion by fishes also suggested that, commonly, the coastal habitats were more representative than the pelagic and spatial differences of MPs ingestion have been reported (Savoca et al., 2021; Zazouli et al., 2022). Thus, regional action plans and national strategic measures on monitoring MPs pollution incorporating grounds of important commercially species could also act as an important tool for identifying and tackling the potential MPs intake by marine organisms (UNEP, 2018).
In a primary effort to examine MPs ingestion among the different groups and based on their taxonomic level, the MPs ingestion records were grouped by the measurement units and tissue examined. Several classes or families had findings from only one study; thus, they could not be analyzed further (Supplementary Table S1). When the gastrointestinal tract was analyzed, Malacostraca had the highest values, followed by Teleostei and Elasmobranchii. Unfortunately, not comparable records were available for Bivalvia that constitute a class with commercially important representative species. For the records that soft tissue was analyzed, Gastropoda had the highest mean values of MPs/ind, followed by Bivalvia and Malacostraca (Table 5). Once again, this finding could not be directly compared with Teleostei that was the most frequently examined class.
In general, based on the data retrieved from the publications and the analysis conducted, it is evident that standardized reporting could contribute to the quantification of MPs ingestion and, thus, potentially the strengthening of understanding the issue of MPs ingestion. Especially, through the process of retrieving data for meta-analysis purposes a significant number of studies were excluded as the necessary information was not reported and could not be estimated by the provided data. More detailed available information could benefit future approaches focusing on examining the origin of the heterogeneity among the groups, highlighting possible differences between the taxonomic levels as previously reported (Sánchez-Hernández, 2020).
Under this review, species of Pisces (Teleostei) was the most frequently examined and recorded with MPs. The mean value of Teleostei was 2.87 MPs/ind, while similar findings (2.76 MPs/GIT) have been reported from Zazouli et al. (2022), including species of open- and closed-water bodies.
The consumption of food commodities contaminated with MPs have raised concerns, as they could pose a threat to human health (Garrido Gamarro and Costanzo, 2022); however, studies examining MPs abundances in edible tissue of fishes were scarce (Akhbarizadeh et al., 2019). Several groups such as sardines, anchovies, herrings, and sprats belong to fishes that in cases could be further processed and consumed as a whole (Karami et al., 2017) had their estimated mean values ranging from 2.77 MPs/ind to 4.26 MPs/ind (Table 2). Recently, Danopoulos et al. (2020) meta-analyzed the ingested MPs by several groups such as anchovies, sardines, lance, and so forth, focusing on their potential consumption by humans and produced summary results expressed in MPs/g, thus without further transformations no comparison could take place with the results of this study. For the species of Bivalvia, the digestive tract is not usually removed and, thus, is eaten (Renzi et al., 2018) and have been recognized as the main source of MPs intake through seafood consumption (Garrido Gamarro et al., 2020; Garrido Gamarro and Costanzo, 2022). The estimated mean value of the class was 3.64 MPs/ind with high heterogeneity (Table 3). For mussels and oysters that the sample size was appropriate, the exclusion criteria (see Material and Methods) were applied, and the new estimated values of mean MPs/ind were in both cases smaller than the primary estimates (Table 3). The diversity among laboratory procedures and the selected sample size have already been mentioned that could add an overestimation to the findings of the meta-analysis (Hanke et al., 2013; Bai et al., 2023).
Malacostraca had both records for soft tissue and GIT of the examined specimens, and the latter was estimated three times higher than the mean estimated values for the soft tissue (MPs/ind). Previous findings have highlighted the higher abundances of MPs in inedible tissues (such as GIT) (Daniel et al., 2020). Among the examined groups, Echinodermata had the fewest records for the meta-analysis purposes expressed in MPs/g, highlighting the difficulty of comparing the findings among the groups as the same measurement unit and analyzed tissue was only applied in a class of Crustacea.
The lack of information about specific classes and families also made difficult the investigation of factors that could affect MPs consumption at a lower taxonomic level. Although the number of the scientific publications related to MPs ingestion is increasing and the gaps of knowledge about MPs ingestion by marine fauna have been partially covered throughout the last years, the need for harmonized protocols that has been previously highlighted, as well as the improvement in the accuracy of the findings is still remaining (Bessa et al., 2019; Dehaut et al., 2019; GESAMP, 2019; Lusher et al., 2020; Primpke et al., 2020a; Provencher et al., 2020). Future efforts of the scientific community, addressing or taking into consideration those challenges, could contribute on improving the understanding about MPs ingestion.
Within the reviewed studies, the MPs were examined in more than 1,000 marine species and MPs ingestion was confirmed in at least 822 species. The most researched class was Teleostei. The most abundant MPs were fibers, less than 1 mm in size, of black color, and composed of PE. The estimated mean of MPs/ind ingestion quantities varied among the different taxonomic groups, and high heterogeneity was presented between and within different classes.
Based on the current focus of the scientific community on the MPs ingestion, the harmonization of the protocols is important to be empowered and implemented, as it could not only mitigate the heterogeneity among the findings but could also provide a better understanding of the issue. Various marine organisms are important seafood worldwide, and the potential exposure of humans through consumption has been verified. Thereof, the monitoring of the MPs ingestion by marine organisms is crucial to mitigate the negative effect on marine species but also humans. Finally, building analytic approaches considering the different variables that affect the ingestion of MPs by marine organisms (such as feeding grounds, feeding strategy, age of the organisms, and the spatial distribution of MPs in the marine environment) could also be a valuable approach.
The original contributions presented in the study are included in the article/Supplementary Material. Further inquiries can be directed to the corresponding author.
DM, SK, and EK contributed to the conception and design of this study. DM collected the data and performed the analysis. SK contributed analysis tools. EK and M-VB contributed with supervising the study. DM, FT, and SI contributed in writing the original manuscript. All authors contributed to the article and approved the submitted version.
The research work for Dimitra Marmara was supported by the Hellenic Foundation for Research and Innovation (HFRI) under the HFRI PhD Fellowship grant (Fellowship Number: 1331). Additionally, this research for University of Catania was funded by “fondi di ateneo 2020–2022, Università di Catania, linea OpenAccess".
Authors wish to thank the reviewers for their comments that improved the manuscript.
The authors declare that the research was conducted in the absence of any commercial or financial relationships that could be construed as a potential conflict of interest.
All claims expressed in this article are solely those of the authors and do not necessarily represent those of their affiliated organizations, or those of the publisher, the editors and the reviewers. Any product that may be evaluated in this article, or claim that may be made by its manufacturer, is not guaranteed or endorsed by the publisher.
The Supplementary Material for this article can be found online at: https://www.frontiersin.org/articles/10.3389/fmars.2023.1240969/full#supplementary-material
Ahyong S., Boyko C. B., Bailly N., Zullini A. (2022) World register of marine species. Available at: https://www.marinespecies.org.
Akhbarizadeh R., Moore F., Keshavarzi B. (2019). Investigating microplastics bioaccumulation and biomagnification in seafood from the Persian Gulf: a threat to human health? Food Addit. Contam. Part A. Chem. Anal. Control. Expo. Risk Assess. 36 (11), 1696–1708. doi: 10.1080/19440049.2019.1649473
Alimi O. S., Claveau-Mallet D., Kurusu R. S., Lapointe M., Bayen S., Tufenkji N. (2022). Weathering pathways and protocols for environmentally relevant microplastics and nanoplastics: What are we missing? J. Hazardous. Mater. 423, 126955. doi: 10.1016/j.jhazmat.2021.126955
Andrady A. L. (2015). “Persistence of plastic litter in the oceans,” in Marine anthropogenic litter (Cham; Heidelberg; New York; Dordrecht; London: Springer), 57–72, ISBN: ISBN 978-3-319-16510-3.
Arthur C., Baker J. E., Bamford H. A. (2009). Proceedings of the international research workshop on the occurrence, effects, and fate of microplastic marine debris (University of Washington Tacoma, Tacoma, WA, USA). Available at: https://marinedebris.noaa.gov/proceedings-second-research-workshop-microplastic-marine-debris.
Ašmonaitė G., Larsson K., Undeland I., Sturve J., Carney Almroth B. (2018). Size matters: ingestion of relatively large microplastics contaminated with environmental pollutants posed little risk for fish health and fillet quality. Environ. Sci. Technol. 52 (24), 14381–14391. doi: 10.1021/acs.est.8b04849
Avio C. G., Gorbi S., Milan M., Benedetti M., Fattorini D., d’Errico G., et al. (2015). Pollutants bioavailability and toxicological risk from microplastics to marine mussels. Environ. pollut. 198, 211–222. doi: 10.1016/j.envpol.2014.12.021
Bai R., Fan R., Xie C., Liu Q., Liu Q., Yan C., et al. (2023). Microplastics are overestimated due to poor quality control of reagents. J. Hazardous. Mater. 459, 132068. doi: 10.1016/j.jhazmat.2023.132068
Bajt O. (2021). From plastics to microplastics and organisms. FEBS Open Bio 11 (4), 954–966. doi: 10.1002/2211-5463.13120
Barboza L. G. A., Gimenez B. C. G. (2015). Microplastics in the marine environment: current trends and future perspectives. Mar. pollut. Bull. 97 (1-2), 5–12. doi: 10.1016/j.marpolbul.2015.06.008
Barboza L. G. A., Vethaak A. D., Lavorante B. R., Lundebye A. K., Guilhermino L. (2018). Marine microplastic debris: An emerging issue for food security, food safety and human health. Mar. pollut. Bull. 133, 336–348. doi: 10.1016/j.marpolbul.2018.05.047
Bessa F., Frias J., Kögel T., Lusher A., Andrade J. M., Antunes J., et al. (2019). Harmonized protocol for monitoring microplastics in biota (Belgium: JPI-Oceans BASEMAN project). Available at: https://www.jpi-oceans.eu/en/publications?id=507.
Borrelle S. B., Ringma J., Law K. L., Monnahan C. C., Lebreton L., McGivern A., et al. (2020). Predicted growth in plastic waste exceeds efforts to mitigate plastic pollution. Science 369 (6510), 1515–1518. doi: 10.1126/science.aba3656
Bray L., Digka N., Tsangaris C., Camedda A., Gambaiani D., de Lucia G. A., et al. (2019). Determining suitable fish to monitor plastic ingestion trends in the Mediterranean Sea. Environ. pollut. 247, 1071–1077. doi: 10.1016/j.envpol.2019.01.100
Browne M. A. (2015). Sources and pathways of microplastics to habitats (Springer Cham Heidelberg New York Dordrecht London: Marine anthropogenic litter), 229–244, ISBN: ISBN 978-3-319-16510-3.
Bucci K., Tulio M., Rochman C. M. (2020). What is known and unknown about the effects of plastic pollution: A meta-analysis and systematic review. Ecol. Appl. 30 (2), e02044. doi: 10.1002/eap.2044
Capolupo M., Franzellitti S., Valbonesi P., Lanzas C. S., Fabbri E. (2018). Uptake and transcriptional effects of polystyrene microplastics in larval stages of the Mediterranean mussel Mytilus galloprovincialis. Environ. pollut. 241, 1038–1047. doi: 10.1016/j.envpol.2018.06.035
Carpenter E. J., Anderson S. J., Harvey G. R., Miklas H. P., Peck B. B. (1972). Polystyrene spherules in coastal waters. Science 178 (4062), 749–750. doi: 10.1126/science.178.4062.749
Carpenter E. J., Smith J. K.L. (1972). Plastics on the sargasso sea surface. Science 175 (4027), 1240–1241. doi: 10.1126/science.175.4027.1240
Carson H. S., Nerheim M. S., Carroll K. A., Eriksen M. (2013). The plastic-associated microorganisms of the North Pacific Gyre. Mar. pollut. Bull. 75 (1-2), 126–132. doi: 10.1016/j.marpolbul.2013.07.054
Cho Y., Shim W. J., Jang M., Han G. M., Hong S. H. (2021). Nationwide monitoring of microplastics in bivalves from the coastal environment of Korea. Environ. pollut. 270, 116175. doi: 10.1016/j.envpol.2020.116175
Cole M., Lindeque P., Halsband C., Galloway T. S. (2011). Microplastics as contaminants in the marine environment: a review. Mar. pollut. Bull. 62 (12), 2588–2597. doi: 10.1016/j.marpolbul.2011.09.025
Commission Decision 2017/848/EC, European Commission. (2017). Commission decision (EU) 2017/848 of laying down criteria and methodological standards on good environmental status of marine waters and specifications and standardised methods for monitoring and assessment, and repealing decision 2010/477/EU. Off. J. Eur. Union. L125/43.
Curren E., Leaw C. P., Lim P. T., Leong S. C. Y. (2020). Evidence of marine microplastics in commercially harvested seafood. Front. Bioeng. Biotechnol. 8. doi: 10.3389/fbioe.2020.562760
Daniel D. B., Ashraf P. M., Thomas S. N. (2020). Microplastics in the edible and inedible tissues of pelagic fishes sold for human consumption in Kerala, India. Environ. pollut. 266, 115365. doi: 10.1016/j.envpol.2020.115365
Danopoulos E., Jenner L. C., Twiddy M., Rotchell J. M. (2020). Microplastic contamination of seafood intended for human consumption: a systematic review and meta-analysis. Environ. Health Perspect. 128 (12), 126002. doi: 10.1289/EHP7171
Dehaut A., Hermabessiere L., Duflos G. (2019). Current frontiers and recommendations for the study of microplastics in seafood. Trends Analytical. Chem. 116, 346–359. doi: 10.1016/j.trac.2018.11.011
DerSimonian R., Laird N. (1986). Meta-analysis in clinical trials. Control. Clin. Trials. 7, 177–188. doi: 10.1016/0197-2456(86)90046-2
De Witte B., Catarino A. I., Vandecasteele L., Dekimpe M., Meyers N., Deloof D., et al. (2022). Feasibility study on biomonitoring of microplastics in fish gastrointestinal tracts. Front. Mar. Sci. 8. doi: 10.3389/fmars.2021.794636
Ding J., Sun C., He C., Li J., Ju P., Li F. (2021). Microplastics in four bivalve species and basis for using bivalves as bioindicators of microplastic pollution. Sci. Total. Environ. 782, 146830. doi: 10.1016/j.scitotenv.2021.146830
Directive 2008/65/EC, European Commission. (2008). Directive 2008/56/EC of the european parliament and of the council of 17 june 2008 establishing a framework for community action in the field of marine environmental policy (Marine strategy framework directive). Off. J. Eur. Union. Available at: https://eur-lex.europa.eu/legalcontent/EN/TXT/?uri=celex%3A32008L0056.
Directive 2015/56/EC, European Commission. (2015). Directive 2015/720 of the european parliament and of the council of 29 april 2015 amending directive 94/62/EC as regards reducing the consumption of lightweight plastic carrier bags. Off. J. Eur. Union. Available at: https://eur-lex.europa.eu/legal-content/EN/TXT/?uri=celex%3A32015L0720.
ECHA (2020). Opinion on an Annex XV Dossier Proposing Restrictions on Intentionally-Added Microplastics. Committee for Risk Assessment (RAC), Committee for Socio-economic Analysis (SEAC). ECHA/RAC/RES-O-0000006790-71-01/F, ECHA/SEAC/RES-O-0000006901-74-01/F.
Egbeocha C. O., Malek S., Emenike C. U., Milow P. (2018). Feasting on microplastics: ingestion by and effects on marine organisms. Aquat. Biol. 27, 93–106. doi: 10.3354/ab00701
Erni-Cassola G., Zadjelovic V., Gibson M. I., Christie-Oleza J. A. (2019). Distribution of plastic polymer types in the marine environment; A meta-analysis. J. Hazardous. Mater. 369, 691–698. doi: 10.1016/j.jhazmat.2019.02.067
FAO (2022). The State of World Fisheries and Aquaculture 2022. Towards Blue Transformation. Rome, FAO. doi: 10.4060/cc0461en.
Fossi M. C., Pedà C., Compa M., Tsangaris C., Alomar C., Claro F., et al. (2018). Bioindicators for monitoring marine litter ingestion and its impacts on Mediterranean biodiversity. Environ. pollut. 237, 1023–1040. doi: 10.1016/j.envpol.2017.11.019
Gago J., Carretero O., Filgueiras A. V., Viñas L. (2018). Synthetic microfibers in the marine environment: A review on their occurrence in seawater and sediments. Mar. pollut. Bull. 127, 365–376. doi: 10.1016/j.marpolbul.2017.11.070
Galgani F., Fleet D., Van Franeker J., Katsanevakis S., Maes T., Mouat J., et al. (2010). Marine strategy framework directive - task group 10 report marine litter. Ed. Zampoukas N. (Luxembourg (Luxembourg): Publications Office of the European Union). Available at: https://publications.jrc.ec.europa.eu/repository/handle/JRC58104, ISBN: ISBN 978-92-79-15653-3.
Gall S. C., Thompson R. C. (2015). The impact of debris on marine life. Mar. pollut. Bull. 92 (1-2), 170–179. doi: 10.1016/j.marpolbul.2014.12.041
Garrido Gamarro E., Costanzo V. (2022). Microplastics in food commodities – A food safety review on human exposure through dietary sources. Food Safety and Quality Series No. 18 (Rome: FAO). doi: 10.4060/cc2392en
Garrido Gamarro E., Ryder J., Elvevoll E. O., Olsen R. L. (2020). Microplastics in fish and shellfish–a threat to seafood safety? J. Aquat. Food Product. Technol. 29 (4), 417–425. doi: 10.1080/10498850.2020.1739793
GESAMP (2019). Guidelines or the monitoring and assessment of plastic litter and microplastics in the ocean. Eds. Kershaw P. J., Turra A., Galgani F., 130, IMO/FAO/UNESCO-IOC/UNIDO/WMO/IAEA/UN/UNEP/UNDP/ISA Joint Group of Experts on the Scientific Aspects of Marine Environmental Protection). Rep. Stud. GESAMP No. 99. (United Nations Office Nairobi: United Nations Environment Programme (UNEP)).
Green D. S., Colgan T. J., Thompson R. C., Carolan J. C. (2019). Exposure to microplastics reduces attachment strength and alters the haemolymph proteome of blue mussels (Mytilus edulis). Environ. pollut. 246, 423–434. doi: 10.1016/j.envpol.2018.12.017
Gregory M. R. (1983). Virgin plastic granules on some beaches of eastern Canada and Bermuda. Mar. Environ. Res. 10 (2), 73–92. doi: 10.1016/0141-1136(83)90011-9
Hanke G., Galgani F., Werner S., Oosterbaan L., Nilsson P., Fleet D., et al. (2013). Guidance on Monitoring of Marine Litter in European Seas: a guidance document within the Common Implementation Strategy for the Marine Strategy Framework Directive. (Luxembourg: Publications Office of the European Union). doi: 10.2788/99475
Harrer M., Pim C., Toshi A. F., David D. E. (2022). “Doing meta-analysis with R,” in A hands-on guide, 1st ed. (Boca Raton: CRC Press), ISBN: ISBN: 9781003107347.
Higgins J. P. T., Green S. (2011). Cochrane handbook for systematic reviews of interventions (The Cochrane Collaboration). Available at: http://handbook-5-1.cochrane.org.
Hossain M. A., Olden J. D. (2022). Global meta-analysis reveals diverse effects of microplastics on freshwater and marine fishes. Fish. Fisheries. 23 (6), 1439–1454. doi: 10.1111/faf.12701
Karami A., Golieskardi A., Ho Y. B., Larat V., Salamatinia B. (2017). Microplastics in eviscerated flesh and excised organs of dried fish. Sci. Rep. 7 (1), 5473. doi: 10.1038/s41598-017-05828-6
Lee K. W., Shim W. J., Kwon O. Y., Kang J. H. (2013). Size-dependent effects of micro polystyrene particles in the marine copepod Tigriopus japonicus. Environ. Sci. Technol. 47 (19), 11278–11283. doi: 10.1021/es401932b
Lehtiniemi M., Hartikainen S., Näkki P., Engström-Öst J., Koistinen A., Setälä O. (2018). Size matters more than shape: Ingestion of primary and secondary microplastics by small predators. Food webs. 17, e00097. doi: 10.1016/j.fooweb.2018.e00097
Lepage D. (2014) Avibase, the world bird database. Available at: http://avibase.bsc-eoc.org/.
Li J., Lusher A. L., Rotchell J. M., Deudero S., Turra A., Bråte I. L. N., et al. (2019). Using mussel as a global bioindicator of coastal microplastic pollution. Environ. pollut. 244, 522–533. doi: 10.1016/j.envpol.2018.10.032
Lim K. P., Lim P. E., Yusoff S., Sun C., Ding J., Loh K. H. (2022). A meta-analysis of the characterisations of plastic ingested by fish globally. Toxics 10 (4), 186. doi: 10.3390/toxics10040186
Liu P., Zhan X., Wu X., Li J., Wang H., Gao S. (2020). Effect of weathering on environmental behavior of microplastics: Properties, sorption and potential risks. Chemosphere 242, 125193. doi: 10.1016/j.chemosphere.2019.125193
Llorca M., Álvarez-Muñoz D., Ábalos M., Rodríguez-Mozaz S., Santos L. H., León V. M., et al. (2020). Microplastics in Mediterranean coastal area: Toxicity and impact for the environment and human health. Trends Environ. Analytical. Chem. 27, e00090. doi: 10.1016/j.teac.2020.e00090
Lozano-Hernández E. A., Ramírez-Álvarez N., Mendoza L. M. R., Macías-Zamora J. V., Sánchez-Osorio J. L., Hernández-Guzmán F. A. (2021). Microplastic concentrations in cultured oysters in two seasons from two bays of Baja California, Mexico. Environ. pollut. 290, 118031. doi: 10.1016/j.envpol.2021.118031
Lusher A., Hollman P., Mendoza-Hill J. (2017). Microplastics in fisheries and aquaculture: status of knowledge on their occurrence and implications for aquatic organisms and food safety (Rome: FAO), ISBN: ISBN 978-92-5-109882-0.
Lusher A., Munno K., Hermabessiere L., Carr S. (2020). Isolation and extraction of microplastics from environmental samples: an evaluation of practical approaches and recommendations for further harmonization. Appl. Spectrosc. 74, 1049–1065. doi: 10.1177/0003702820938993
Markic A., Gaertner J. C., Gaertner-Mazouni N., Koelmans A. A. (2020). Plastic ingestion by marine fish in the wild. Crit. Rev. Environ. Sci. Technol. 50 (7), 657–697. doi: 10.1080/10643389.2019.1631990
Martellini T., Guerranti C., Scopetani C., Ugolini A., Chelazzi D., Cincinelli A. (2018). A snapshot of microplastics in the coastal areas of the Mediterranean Sea. TrAC. Trends Analytical. Chem. 109, 173–179. doi: 10.1016/j.trac.2018.09.028
Merrell J. T.R. (1980). Accumulation of plastic litter on beaches of Amchitka Island, Alaska. Mar. Environ. Res. 3 (3), 171–184. doi: 10.1016/0141-1136(80)90025-2
Moher D., Liberati A., Tetzlaff J., Altman D. G., PRISMA Group (2009). Preferred reporting items for systematic reviews and meta-analyses: the PRISMA statement. Ann. Internal Med. 151 (4), 264–269. doi: 10.7326/0003-4819-151-4-200908180-00135
Mohsen M., Wang Q., Zhang L., Sun L., Lin C., Yang H. (2019). Microplastic ingestion by the farmed sea cucumber Apostichopus japonicus in China. Environ. pollut. 245, 1071–1078. doi: 10.1016/j.envpol.2018.11.083
Müller C. (2021). Not as bad as it seems? A literature review on the case of microplastic uptake in fish. Front. Mar. Sci. 8. doi: 10.3389/fmars.2021.672768
Nature Conservancy (2012). Marine ecoregions and pelagic provinces of the world (Cambridge (UK): The Nature Conservancy). GIS layers developed by The Nature Conservancy with multiple partners, combined from Spalding et al. (2007) and Spalding et al. (2012). doi: 10.1016/j.ocecoaman.2011.12.016
Oliveira M., Ribeiro A., Hylland K., Guilhermino L. (2013). Single and combined effects of microplastics and pyrene on juveniles (0+ group) of the common goby Pomatoschistus microps (Teleostei, Gobiidae). Ecol. Indic. 34, 641–647. doi: 10.1016/j.ecolind.2013.06.019
Ory N. C., Sobral P., Ferreira J. L., Thiel M. (2017). Amberstripe scad Decapterus muroadsi (Carangidae) fish ingest blue microplastics resembling their copepod prey along the coast of Rapa Nui (Easter Island) in the South Pacific subtropical gyre. Sci. Total. Environ. 586, 430–437. doi: 10.1016/j.scitotenv.2017.01.175
Palomares M. L. D., Pauly D. (2022). SeaLifeBase (World Wide Web electronic publication). Available at: www.sealifebase.org.
Pellini G., Gomiero A., Fortibuoni T., Ferrà C., Grati F., Tassetti A. N., et al. (2018). Characterization of microplastic litter in the gastrointestinal tract of Solea solea from the Adriatic Sea. Environ. pollut. 234, 943–952. doi: 10.1016/j.envpol.2017.12.038
Peng X., Chen M., Chen S., Dasgupta S., Xu H., Ta K., et al. (2018). Microplastics contaminate the deepest part of the world’s ocean. Geochem. Perspect. Lett. 9 (1), 1–5. doi: 10.7185/geochemlet.1829
Piccardo M., Provenza F., Grazioli E., Cavallo A., Terlizzi A., Renzi M. (2020). PET microplastics toxicity on marine key species is influenced by pH, particle size and food variations. Sci. Total. Environ. 715, 136947. doi: 10.1016/j.scitotenv.2020.136947
Primpke S., Christiansen S. H., Cowger W., De Frond H., Deshpande A., Fischer M., et al. (2020a). Critical assessment of analytical methods for the harmonized and cost-efficient analysis of microplastics. Appl. Spectrosc. 74 (9), 1012–1047. doi: 10.1177/0003702820921465
Primpke S., Fischer M., Lorenz C., Gerdts G., Scholz-Böttcher B. M. (2020b). Comparison of pyrolysis gas chromatography/mass spectrometry and hyperspectral FTIR imaging spectroscopy for the analysis of microplastics. Analytical. Bioanal. Chem. 412, 8283–8298. doi: 10.1007/s00216-020-02979-w
Provencher J. F., Covernton G. A., Moore R. C., Horn D. A., Conkle J. L., Lusher A. L. (2020). Proceed with caution: The need to raise the publication bar for microplastics research. Sci. Total. Environ. 748, 141426. doi: 10.1016/j.scitotenv.2020.141426
Provenza F., Piccardo M., Terlizzi A., Renzi M. (2020). Exposure to pet-made microplastics: particle size and pH effects on biomolecular responses in mussels. Mar. Pollut. Bull. 156, 111228. doi: 10.1016/j.marpolbul.2020.111228
QGIS Development Team (2022). QGIS geographic information system (Open Source Geospatial Foundation Project). Available at: http://qgis.osgeo.org.
Qin F., Du J., Gao J., Liu G., Song Y., Yang A., et al. (2020). Bibliometric profile of global microplastics research from 2004 to 2019. Int. J. Environ. Res. Public Health 17 (16), 5639. doi: 10.3390/ijerph17165639
Ravi V., Venkatesh B. (2018). The divergent genomes of teleosts. Annu. Rev. Anim. Biosci. 6, 47–68. doi: 10.1146/annurev-animal-030117-014821
R Core Team (2021). R: A language and environment for statistical computing (Vienna, Austria: R Foundation for Statistical Computing). Available at: https://www.R-project.org.
Rebelein A., Int-Veen I., Kammann U., Scharsack J. P. (2021). Microplastic fibers—underestimated threat to aquatic organisms? Sci. Total. Environ. 777, 146045. doi: 10.1016/j.scitotenv.2021.146045
Renzi M., Blašković A., Bernardi G., Russo G. F. (2018). Plastic litter transfer from sediments towards marine trophic webs: a case study on holothurians. Mar. Pollut. Bull. 135, 376–385. doi: 10.1016/j.marpolbul.2018.07.038
Rochman C. M. (2015). “The complex mixture, fate and toxicity of chemicals associated with plastic debris in the marine environment,” in Marine anthropogenic litter (Cham; Heidelberg; New York; Dordrecht; London: Springer), 117–140, ISBN: ISBN 978-3-319-16510-3.
Salerno M., Berlino M., Mangano M. C., Sarà G. (2021). Microplastics and the functional traits of fishes: A global meta-analysis. Global Change Biol. 27 (12), 2645–2655. doi: 10.1111/gcb.15570
Sánchez-Hernández J. (2020). Taxonomy-based differences in feeding guilds of fish. Curr. Zool. 66 (1), 51–56. doi: 10.1093/cz/zoz015
Savoca M. S., McInturf A. G., Hazen E. L. (2021). Plastic ingestion by marine fish is widespread and increasing. Global Change Biol. 27 (10), 2188–2199. doi: 10.1111/gcb.15533
SciVerse Scopus Engine. Available at: http://www.info.sciverse.com.
Shaw D. G., Day R. H. (1994). Colour-and form-dependent loss of plastic micro-debris from the North Pacific Ocean. Mar. pollut. Bull. 28 (1), 39–43. doi: 10.1016/0025-326X(94)90184-8
Shiber J. G. (1982). Plastic pellets on Spain's ‘Costa del Sol’beaches. Mar. pollut. Bull. 13 (12), 409–412. doi: 10.1016/0025-326X(82)90014-5
Snoke L. R. (1957). Resistance of organic materials and cable structures to marine biological attack. Bell. System. Tech. J. 36 (5), 1095–1127. doi: 10.1002/j.1538-7305.1957.tb01504.x
Song Y. K., Hong S. H., Eo S., Jang M., Han G. M., Isobe A., et al. (2018). Horizontal and vertical distribution of microplastics in Korean coastal waters. Environ. Sci. Technol. 52 (21), 12188–12197. doi: 10.1021/acs.est.8b04032
Spalding M. D., Agostini V. N., Rice J., Grant S. M. (2012). Pelagic provinces of the world): a biogeographic classification of the world’s surface pelagic waters. Ocean. Coast. Manage. 60, 19–30. doi: 10.1016/j.ocecoaman.2011.12.016
Spalding M. D., Fox H. E., Allen G. R., Davidson N., Ferdaña Z. A., Finlayson M., et al. (2007). Marine Ecoregions of the World: a bioregionalization of coast and shelf areas. BioScience 57, 573–583. doi: 10.1641/B570707
Teng J., Zhao J., Zhu X., Shan E., Zhang C., Zhang W., et al. (2021). Toxic effects of exposure to microplastics with environmentally relevant shapes and concentrations: Accumulation, energy metabolism and tissue damage in oyster Crassostrea gigas. Environ. pollut. 269, 116169. doi: 10.1016/j.envpol.2020.116169
UN RES/70/1. (2015). UN General Assembly, Transforming our world: the 2030 Agenda for Sustainable Development, 21 October. Available at: https://www.refworld.org/docid/57b6e3e44.htm.
UNEP (2016). EA.2/Res.11 Marine plastic litter and microplastics (Nairobi, Kenya: United Nations Environment Programme).
UNEP (2018). Addressing marine plastics: A systemic approach - Stocktaking report (Nairobi, Kenya: Notten, United Nations Environment Programme).
Viechtbauer W. (2010). Conducting meta-analyses in R with the metafor package. J. Stat. Software. 36 (3), 1–48. doi: 10.18637/jss.v036.i03
Walkinshaw C., Lindeque P. K., Thompson R., Tolhurst T., Cole M. (2020). Microplastics and seafood: lower trophic organisms at highest risk of contamination. Ecotoxicol. Environ. Saf. 190, 110066. doi: 10.1016/j.ecoenv.2019.110066
Wang T., Hu M., Song L., Yu J., Liu R., Wang S., et al. (2020). Coastal zone use influences the spatial distribution of microplastics in Hangzhou Bay, China. Environ. pollut. 266, 115137. doi: 10.1016/j.envpol.2020.115137
Wright S. L., Thompson R. C., Galloway T. S. (2013). The physical impacts of microplastics on marine organisms: a review. Environ. pollut. 178, 483–492. doi: 10.1016/j.envpol.2013.02.031
Zazouli M., Nejati H., Hashempour Y., Dehbandi R., Fakhri Y. (2022) Occurrence of microplastics (MPs) in the gastrointestinal tract of fishes: A global systematic review and meta-analysis and meta-regression. Sci. Total. Environ. 815, 152743. doi: 10.1016/j.scitotenv.2021.152743
Zhang C., Wang S., Sun D., Pan Z., Zhou A., Xie S., et al. (2020). Microplastic pollution in surface water from east coastal areas of Guangdong, South China and preliminary study on microplastics biomonitoring using two marine fish. Chemosphere 256, 127202. doi: 10.1016/j.chemosphere.2020.127202
Keywords: plastic, pollution, marine litter, marine environment, marine organisms, accumulation, stomachic content, meta-analysis
Citation: Marmara D, Katsanevakis S, Brundo M-V, Tiralongo F, Ignoto S and Krasakopoulou E (2023) Microplastics ingestion by marine fauna with a particular focus on commercial species: a systematic review. Front. Mar. Sci. 10:1240969. doi: 10.3389/fmars.2023.1240969
Received: 15 June 2023; Accepted: 28 August 2023;
Published: 27 September 2023.
Edited by:
Chiara Gambardella, National Research Council (CNR), ItalyReviewed by:
Silvia Morgana, National Research Council (CNR), ItalyCopyright © 2023 Marmara, Katsanevakis, Brundo, Tiralongo, Ignoto and Krasakopoulou. This is an open-access article distributed under the terms of the Creative Commons Attribution License (CC BY). The use, distribution or reproduction in other forums is permitted, provided the original author(s) and the copyright owner(s) are credited and that the original publication in this journal is cited, in accordance with accepted academic practice. No use, distribution or reproduction is permitted which does not comply with these terms.
*Correspondence: Dimitra Marmara, ZG1hcm1hcmFAbWFyaW5lLmFlZ2Vhbi5ncg==
Disclaimer: All claims expressed in this article are solely those of the authors and do not necessarily represent those of their affiliated organizations, or those of the publisher, the editors and the reviewers. Any product that may be evaluated in this article or claim that may be made by its manufacturer is not guaranteed or endorsed by the publisher.
Research integrity at Frontiers
Learn more about the work of our research integrity team to safeguard the quality of each article we publish.