- 1Xiamen Key Laboratory for Feed Quality Testing and Safety Evaluation, Fisheries College of Jimei University, Xiamen, China
- 2Fujian Engineering Research Center of Aquatic Breeding and Healthy Aquaculture, Fisheries College of Jimei University, Xiamen, China
β-conglycinin is one of the major soy antigen proteins in soybean meal (SBM) and exhibits growth inhibition and intestinal health damage in grouper, but the underlying mechanisms are still poorly understood. In this study, orange-spotted grouper (Epinephelus coioides) was used to investigate whether the poor growth performance induced by dietary β-conglycinin levels is associated with intestinal structural integrity disruption, the intestinal apoptosis and intestinal microbiota. The basal diet (FM diet) was formulated to contained 48% protein and 12% fat without SBM supplementation. Fish meal protein in the FM diet was replaced by SBM to prepare a high SBM diet (SBM diet). β-conglycinin at 3% and 7% were added into FM diets to prepare two diets (B-3 and B-7). Triplicate groups of fish (20 fish/tank) were fed one of the experimental diets twice daily in a feeding period of 8 weeks. Compared with FM diet, fish fed diets SBM and B-7 had decreased the growth rate, hepatosomatic index, whole-body lipid and ash contents, and increased whole-body moisture content. However, the maximum growth was observed for diet B-3 and was not different from that of FM diet. The liver total antioxidant capacity and glutathione peroxidase activity, the muscle layer thickness of middle and distal intestine, and the mucosal fold length of distal intestine were lower, while liver malondialdehyde content, intestinal diamine oxidase activity, d-lactic acid and endotoxin contents, and the number of intestinal apoptosis were higher in SBM and B-7 groups than that in FM and/or B-3 groups. SBM and B-7 diets down-regulated the intestinal expression of tight junction genes (occludin, claudin-3 and ZO-1), apoptosis genes (bcl-2 and bcl-xL) and anti-inflammatory factor genes (IκBα, TGF-β1 and IL-10), but up-regulated the intestinal expression of apoptosis genes (caspase-3, caspase-8 and caspase-9) and pro-inflammatory factor genes (NF-κB1, RelA, TAK1, IKK, MyD88, TNF-α, IL-1β, and IL-8) vs FM and/or B-3 diets. The richness and diversity indexes of OTUs, Chao1, ACE, Shannon and Simpson were not affected by dietary treatments. The relative abundances of intestinal bacteria (phylum Proteobacteria and genus Vibrio) were generally higher, and relative abundance of phylum Tenericutes was lower in SBM and/or B-7 groups than that in B-3 group. The above results indicate that high dietary β-conglycinin level, rather than intermediate level could decrease liver antioxidant capacity, reshape the intestinal microbiota, and impair the intestinal normal morphology through disrupting the intestinal tight junction structure, increasing intestinal mucosal permeability, and promoting intestinal apoptosis, which in turn triggers intestinal inflammatory responses and the occurrence of enteritis, and ultimately leads to poor growth performance in fish.
1 Introduction
Aquaculture is an increasingly important contributor of protein source to meet the demand of the steady growth of the world population and the improvement of people’s living standards for high-quality animal protein products (Han et al., 2018; Boyd et al., 2022). As the main types of fish farming to produce food fish, semi-intensive and intensive aquaculture relies heavily on artificial feeds (Han et al., 2018). However, farmed marine fish require more high quality feed protein than terrestrial animals (Zhao et al., 2021). Fish meal (FM), a processed product of marine catches, is the priority of protein ingredients for intensification and shift to farmed marine fish due to its high nutritional value (Tacon, 2020). Over the past decades, however, the limited marine fishery catches resources have caused the stagnant production of FM (Han et al., 2018). Moreover, the rapidly growing global aquaculture industry has exacerbated the tight supply of FM (Han et al., 2018; FAO, 2020). Therefore, there is a consensus in the industry to find suitable feed proteins to replace FM (Hardy, 2010).
Soybean meal (SBM) is widely used in aquafeeds as the major plant protein source, and is the most potential substitute for FM due to its relatively high protein quality, massive production, and availability over other plant proteins (Wang et al., 2017; Zhao et al., 2021). However, most fishes, especially carnivorous fishes have SBM intolerance when they receive a diet with a high level of SBM (Baeverfjord and Krogdahl, 1996; Booman et al., 2018; Zhu et al., 2021). It is well-known that many anti-nutritional factors (ANFs) present in SBM provoke the response of intolerance, usually accompanied with a reduction of fish ability to digest nutrients (Chen et al., 2019), the so-called SBM-induced enteritis (SBMIE) (Carmona, 2008; Bai et al., 2017). Among the ANFs, soy antigen proteins are believed to be the main ANFs that induces SBMIE both in mammals (He et al., 2015; Wu et al., 2016a; Wu et al., 2016b) and fish (Ringø et al., 2006). The proteins have high thermal stability (Soumeh et al., 2019) and strong immunogenicity (Hu et al., 2021), and are difficult to deactivate to use conventional methods (Jiang et al., 2015).
Soy antigen proteins belong to soy globulins and can be subdivided into four types: 2S (α-conglycinin), 7S (β-conglycinin and γ-conglycinin), 11S (glycinin) and 15S (polymers of glycinin) according to their sedimentation rates, of which, glycinin and β-conglycinin account for about 40% and 30% of total soy globulin respectively (Renkema, 2001; Zhu et al., 2011). The two types of antigen protein, therefore, have become the major concern for studying sensitization on animals and its underlying mechanisms (He et al., 2015). Recent results showed that soy antigen proteins have high antigenic activity (Li et al., 2017; Hu et al., 2021; Shan et al., 2021), promote intestinal permeability (Han et al., 2019; Scott et al., 2019; Zhang Y. L. et al., 2021), induce specific antigen-antibody reactions and T lymphoid cell-mediated hypersensitivity delay by stimulating the immune system (Gu et al., 2016a; Li et al., 2020; Yin et al., 2021a; He et al., 2022), trigger apoptosis of intestinal epitheliums (Peng et al., 2018; Duan et al., 2019a; Peng et al., 2019; Wang et al., 2022; Yi et al., 2022) through the production of reactive oxygen species (Li et al., 2019; Li et al., 2020; Zhang et al., 2020; Yi et al., 2022), disrupt intestinal microbiota homeostasis (Han et al., 2019; He et al., 2022) and develop into pathological reactions with allergies and enteritis as the main symptoms (Zhang et al., 2013; Booman et al., 2018; Zheng et al., 2020; Zhu et al., 2021), eventually leading to poor growth (Zhao et al., 2021; Yi et al., 2022). Great progress has been made in this regard in recent years, but the research on the negative impact of soy antigen proteins on fish is limited, and the underlying mechanisms involving enteritis and allergy induced by soy antigen proteins in fish are still unclear.
Grouper is an important marine fish that has been farmed along Southeast Asia coasts including the southeast coast of China (Shapawi et al., 2019). The annual production of grouper in China reached 204,119 tons in 2021, ranking third in the production of marine fish farming in China (Bureau of Fisheries and Ministry of Agriculture, 2022). Like other carnivorous fish, grouper also exhibit high sensitivity and low tolerance to soybean by-products (Wang et al., 2017; He et al., 2020; Zhao et al., 2021). As one of the main soy antigen proteins, β-conglycinin has strong immunogenicity and can cause enteritis in fish (Gu et al., 2016a; Li et al., 2017; Peng et al., 2018; Duan et al., 2019a; Li et al., 2020; Hu et al., 2021). Recently, the effects of dietary soy antigen proteins on intestinal immune function and intestinal microbiota of hybrid grouper (Epinephelus fuscoguttatus ♀× Epinephelus lanceolatus ♂) were investigated (He et al., 2022). However, the effects of soy antigen proteins on the apoptosis of intestinal epitheliums and intestinal permeability of grouper have not been reported yet. In the present study, orange-spotted grouper (Epinephelus coioides), another important grouper species, was used as target fish was selected to investigate the effects of dietary β-conglycinin inclusion levels on the growth, apoptosis of intestinal epitheliums intestinal permeability and intestinal microbiota of grouper to illustrate the relationship between intestinal health and β-conglycinin and its mechanism of action, which provides a reference for the rational development and utilization of soybean by-products in feed of marine fish.
2 Materials and methods
2.1 Experimental diets and feeding trial
A basal diet (FM diet) was formulated to contain 48% protein and 12% lipid using FM, casein and gelatin as the protein source, fish oil, soy oil and soy lecithin as the main lipid source, and corn starch as the filler. On the basis of the FM diet, 60% FM protein was replaced by SBM in the FM diet to make a high-SBM diet (SBM diet). To determine the effects of antigen protein in SBM, the β-conglycinin was included at two inclusion levels (3% and 7%) in the FM diet to prepare two experimental diets (B-3 diet and B-7 diet). The dietary 3% and 7% β-conglycinin were calculated according to the substitution of SBM for 30% and 60% FM protein, respectively. The β-conglycinin inclusion levels were at the expense of the decrease of casein and gelatin to ensure B-3 and B-7 diets contain protein and lipid levels identical to FM and SBM diets. The β-conglycinin product (purity 90.10%) was provided by Professor Guo Shuntang at China Agricultural University. All feed ingredients were ground in a hammer mill (GH-20B, Jiangyin Kejia Machinery Manufacturing Co., Ltd., Jiangyin, Jiangsu, China) and passed through a 60-mesh sieve, then weighed and uniformly mixed. The liquid ingredients (marine fish oil and soy oil) were added to the dry feed ingredients, and then freshwater was added to make a dough. The dough was then pelleted through a 3 mm die using cold press extrusion (CD4XITS, South China University of Technology, Guangzhou, Guangdong, China). The pellets were dried in a ventilated oven at 55°C for 24 h, then sealed in plastic bags and stored in a refrigerator at -20°C. The feed ingredients and proximate composition of experimental diets are shown in Table 1.
This experiment was conducted in a circulating water rearing system with a temperature control device at Haikang Base of Fujian Dabeinong Technology Company (Zhangzhou, Fujian, China). Before the experiment, juvenile groupers were fed commercial feed for 4-weeks of accommodation. A total of 240 fish with similar size were randomly divided into 4 groups, each with triplicate tanks and at a stocking density of 20 fish per tank. The fish in each group were fed one of the diets twice daily (7:30 and 17:30) for a feeding period of 56 days. Uneaten feed was collected by siphoning 30 min after each feeding, then feces were removed. The collected uneaten feed was dried for 24 h at 65°C and weighed for the calculation of feed intake. After cleaning, the water body consumed by sewage cleaning was refilled with sand-filtered fresh seawater until the reservoir water returned to the original level. Dissolved oxygen was measured daily and nitrite-N was monitored twice a week using a multi-parameter photome (HI83200; Hanna Instruments, Woonsocket, Rhode Island). During the experimental period, the dissolved oxygen level was > 5.7 mg/L, the water temperature was 28.5°C, and ammonia nitrogen content was < 0.23 mg/L.
2.2 Sample collection
At the end of the 56-day feeding trial, the fish in each tank were caught and anesthetized with a dose of 100 mg/L solution of MS-222 (tricaine methane sulfonate, Sigma-Aldrich Shanghai Trading Co. Ltd., Shanghai) and then the fish number and bulk weight were recorded to determine weight gain rate (WGR), specific growth rate (SGR), feed efficiency (FE), and feeding rate (FR). Ten fish per tank were randomly caught and anesthetized with MS-222 (100 mg/L), followed by individually weighing to determine the condition factor (CF). Blood was then collected using a 2-mL heparinized syringe from the caudal vein, followed by centrifugation (1027 g, 4°C and 10 min). Plasma samples were separated, pooled by tank, and stored in a refrigerator at -80°C for the determination of diamine oxidase (DAO) activity and the contents of d-lactic acid (D-Lac) and endotoxin (ET). After hemostasis, the livers and intestines were aseptically removed. The livers were weighed for the calculation of hepatosomatic index (HSI). The intestine was washed with ice-cold sterile PBS to remove the intestinal digesta. Then the liver and intestinal samples were pooled in a tank and quickly frozen with liquid nitrogen and stored at -80°C for the subsequent biochemical analysis and the assay for intestinal microbiota. For the intestinal histology observation and the terminal deoxynucleotidyl transferase-mediated dUTP nick end labeling (TUNEL) detection, two fish were caught from each tank and dissected to obtain the whole intestinal tract, followed by the removal of digesta. The intestinal tract was then divided into the proximal, middle, and distal segments (i.e. PI, MI, and DI, respectively), according to the method described by Anguiano et al. (2013). Another four fish per tank were randomly captured, put in plastic bags, and stored at − 20°C until determination of whole-body proximate composition analysis.
2.3 Proximate composition analysis
The proximate composition of ingredients, diets, and whole-body fish samples were determined according to standard methods of AOAC (1995).. Dry matter was determined by drying the samples at 105°C to a constant weight. The crude protein was determined by the Dumas combustion method (Elementar® Rapid N, Alpha Resources LLC, Michigan, USA). The crude lipid content was measured by the Soxtec extraction method by using Soxtec Avanti 2050 (Foss Tecator AB). Ash was measured in the residues of samples burned in a muffle furnace at 550°C for 8 h.
2.4 Liver and plasma component analysis
The glutathione peroxidase (GSH-Px), superoxide dismutase (SOD), catalase (CAT), total antioxidant capacity (T-AOC), and malondialdehyde (MDA) in the liver samples were determined using commercial kits (Nanjing Jiancheng Bioengineering Institute, Nanjing, Jiangsu, China) according to the manufacturer’s instructions. The diamine oxidase (DAO) activity and contents of d-lactic acid (D-Lac), endotoxin (ET), and endothelin-1 (ET-1) in plasma were determined using enzyme-linked immunosorbent assay kits according to the protocols of the same manufacturer.
2.5 Histological analysis
After formalin fixation, all the intestine segments were removed and washed with normal saline, then fixed in 4% Bouin’s solution for 24 h, rinsed with 70% ethanol solution, and finally immersed in 70% ethanol solution until histological processing was performed (Niu et al., 2021). The fixed intestine segments were embedded in paraffin and 5-μm sections were cut by using a rotary microtome (KD-2258S, China). The serial histological sections were then mounted on glass slides, and stained with hematoxylin and eosin for morphometric analysis. Pictures were examined under a light microscope (Leica DM5500B, Germany), and digital images were acquired and processed with a digital camera (Leica DFC450) equipped with the image program LAS AF (Version 4.3.0 Leica). Five slides were prepared for each intestine segment sample and 30 measurements were made to determine the mucosal fold number (nMF), muscle layer thickness (tML), and mucosal fold length (lMF).
For the detection of apoptotic cells, the TUNEL staining was carried out using a TUNEL Apoptosis Detection Kit (Vazyme Biotech, Nanjing, China) according to the manufacturer’s instruction. Briefly, sections mounted on a slide were deparaffinized with xylene and washed twice with deionized water. The rehydrated sections were digested with proteinase K for 4 min at 37°C. The samples were then covered with TdT Reaction Buffer for 10 min at 37°C, followed by incubation in a TUNEL reaction mix containing EdUTP and TdT enzyme for 1 h at 37°C. After incubation, the samples were washed twice with PBS for 5 min and subsequently incubated with streptavidin-HRP and DAB working solution for 30 min at 37°C in the dark. Finally, the samples were washed with 3% BSA in PBS (pH 7.4) for 5 min and counterstained with DAPI. The slides were viewed under a fluorescence microscope with green fluorescence set at 520 nm. The cells stained red indicated apoptotic cells.
2.6 Intestinal microbiota assay
The total DNA of the intestinal samples was extracted using a DNA extraction kit (Omega Bio-teK, Norcross, GA, USA) according to the manufacturer’s instructions. The integrity and quality, purity and quantity of DNA samples were assessed by electrophoresis on a 1% (w/v) agarose gel and the spectrophotometer method (NanoDrop 2000, Wilmington, DE, U.S. 260nm/280nm optical density ratio), respectively. The V3-V4 region of the 16S rDNA of intestinal bacteria was amplified by polymerase chain reaction (PCR) using the forward primer 338F (5’-ACTCCTACGGGAGGCAGCAG-3’) and the reverse primer 806R (5’-GGACTACNNGGGTATCTAAT-3’). The PCR amplification system solution included 30 ng DNA sample (X), 1 μL forward primer (5 µM), 1 µL reverse primer (5 µM), 3 µL BSA (2 ng/µL), 12.5 µL 2xTaq Plus Master Mix and 7.5-X µL ddH2O. The PCR amplification program consisted of pre-denaturation at 95°C for 5 min; denaturation at 95°C for 45 s, annealing at 55°C for 50 s, and extension at 72°C for 45 s, 32 cycles; extension at 72°C for 10 min. Subsequently, high-throughput sequencing was performed using Illumina Miseq PE300 at Beijing Allwegene technology Co., Ltd (Beijing, China). A library of small fragments was constructed using paired-end for sequencing, and the data was passed through QIIME (v1.8.0) for the removal of low-quality sequences and chimeras. Based on 97% sequence similarity, similar sequences were assigned to the same operational taxonomic units (OTU). Species classification information corresponding to each OTU was obtained by comparing with the silva database, and alpha diversity analysis (Shannon, ACE, and Chao1) was performed using Mothur software (version 1.31.2). Based on the weighted uniface distance, the heat map of the R (v3.1.1) software package was used for clustering analysis. After the UniFrac algorithm, the information on system evolution was used to compare the difference of species communities among samples, and beta diversity analysis was performed
2.7 RNA extraction and expression analysis
The total RNA was extracted from the intestinal samples using TRIzol® reagent (Invitrogen, Carlsbad, CA, USA) according to the manufacturer’s instructions. Isolated RNA was quantified using the NanoDrop ND-2000 Spectrophotometer, and its integrity was confirmed by agarose gel electrophoresis. The cDNA was generated from 1 μg DNase-treated RNA and synthesized by a PrimeScriptTM RT Reagent Kit with gDNA Eraser (TaKaRa, Dalian, China). Real-time PCR was employed to determine mRNA levels based on the TB GreenTM Premix Ex TaqTM II (Tli RNaseH Plus) (TaKaRa, Dalian, China) using a QuantStudioTM Real-Time PCR System (ABI) quantitative thermal cycler. The fluorescent quantitative PCR solution consisted of 10 μL TB Green Premix Ex TaqTM II (Tli RNaseH Plus) (2×), 0.8 μL PCR forward primer (10 μM), 0.8 μL PCR reverse primer (10 μM), 2.0 μL RT reaction (cDNA solution), and 6 μL dH2O. The thermal program included 30 s at 95°C, 40 cycles at 95°C for 5 s, 60°C for 30 s, and a melting curve step. The sequences of primers are shown in Table 2. All amplicons were initially separated by agarose gel electrophoresis to ensure that they were of the correct size. β-actin served as the internal reference gene to normalize cDNA loading. The gene expression levels of the target genes were analyzed by the 2-ΔΔCt method (Schmittgen and Livak, 2008) after verifying that the primers were amplified with an efficiency of approximately 100% (Hanaki et al., 2014), and the data for all treatment groups were compared with the data for the control group.
2.8 Statistical analysis
All data were presented as the mean and standard error of the mean (SEM). The data were analyzed using a one-way analysis of variance (ANOVA) to test for differences between treatments and then the Student-Neuman-Keuls multiple comparison test was performed after confirming the normality and homogeneity of variance using the Kolmogorov-Smirnov test and Levene’s test in SPSS Statistics 20.0 (SPSS, Michigan Avenue, Chicago, IL, USA). The data expressed as percentages or ratios were subjected to the data conversion before statistical analysis. P-values < 0.05 were considered statistically significant.
3 Results
3.1 Growth performance and proximate composition
The results of growth performance are presented in Table 3. The SBM group had the lowest WG, SGR, and CF among all treatments (P < 0.05). Group B-3 exhibited the highest WG and SGR, and the values were higher (P < 0.05) than that of B-7 group, but were not different (P > 0.05) from that of the FM group. The CF in the SBM group was lower (P < 0.05) than that of other groups, and there was no significant (P > 0.05) difference among other groups. There was no difference (P > 0.05) in HSI between groups SBM, B-3 and B-7, but they were higher (P < 0.05) than that in group FM. The FR of the SBM group was lower (P < 0.05) than that of the B-3 group, but not different (P > 0.05) from that in groups FM and B-7. The FE and survival were not affected (P > 0.05) by dietary treatments.
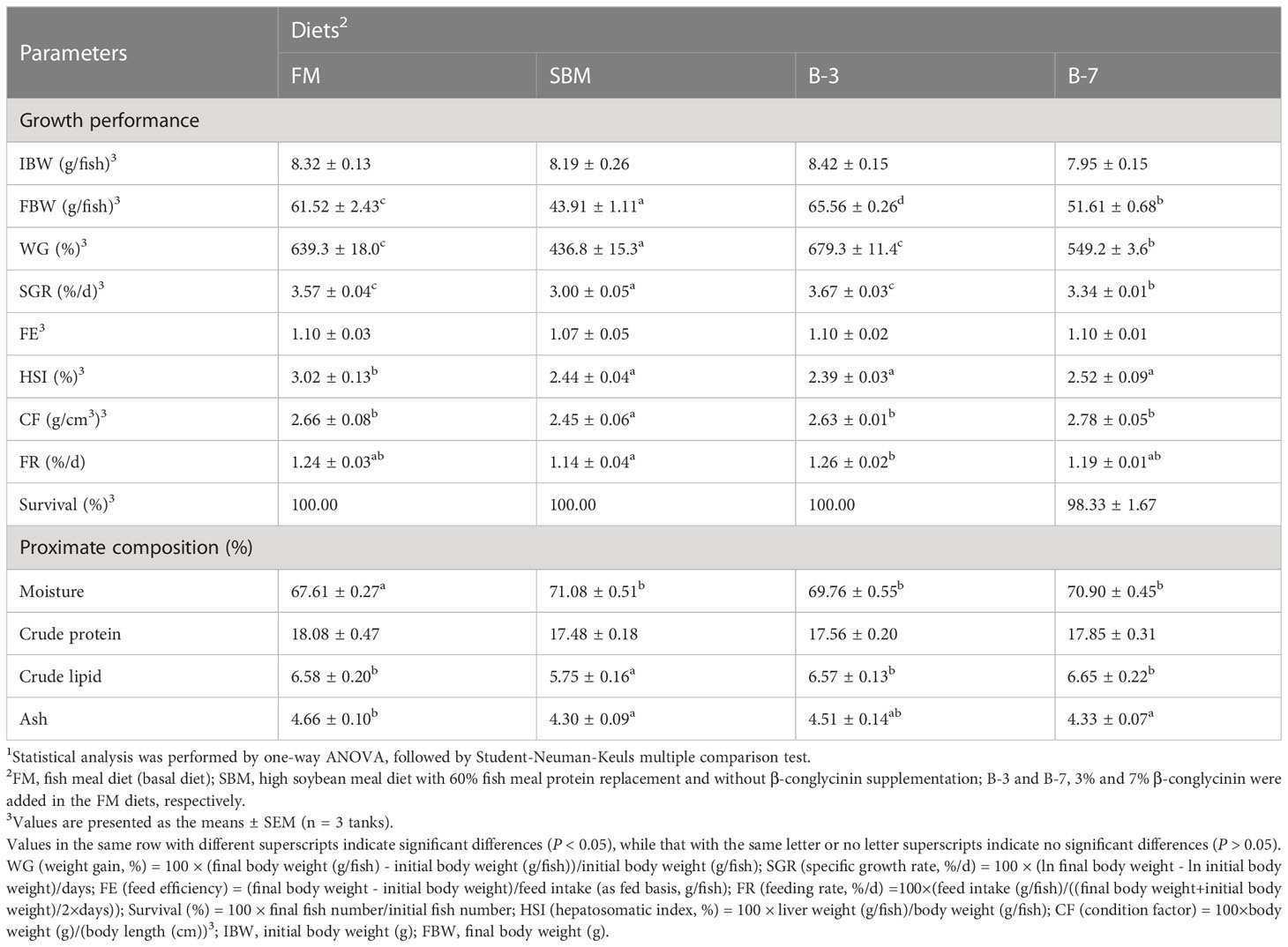
Table 3 Effects of β-conglycinin addition in diets on growth performance of orange-spotted groupers in a 56-day feeding period.
Groups SBM, B-3 and B-7 had higher (P < 0.05) whole-body moisture contents compared with the FM group, but no variation was observed among the three groups. Groups FM, B-3 and B-7 had higher (P < 0.05) whole-body lipid contents compared with group SBM, but there was no variation (P > 0.05) among the three groups. Whole-body ash content followed a pattern similar to whole-body lipid content, in response to dietary treatments. The whole-body protein content did not differ (P > 0.05) across dietary treatments.
3.2 Liver antioxidant capacity
The liver T-AOC, GSH-Px activities in FM and B-3 groups were generally higher (P < 0.05) than that in SBM and B-7 groups, except that GSH-Px activity in the B-7 group was similar (P > 0.05) to that of FM and B-3 groups (Table 4). The changes of liver MDA values were opposite to that of T-AOC, in response to dietary treatments. Dietary treatments did not affect liver activities of SOD and CAT (P > 0.05). There were no significant differences (P > 0.05) in liver T-AOC and MDA values, liver SOD and GSH-Px activities between the B-3 group and FM group, and the same was true for the values between the B-7 group and the SBM group.
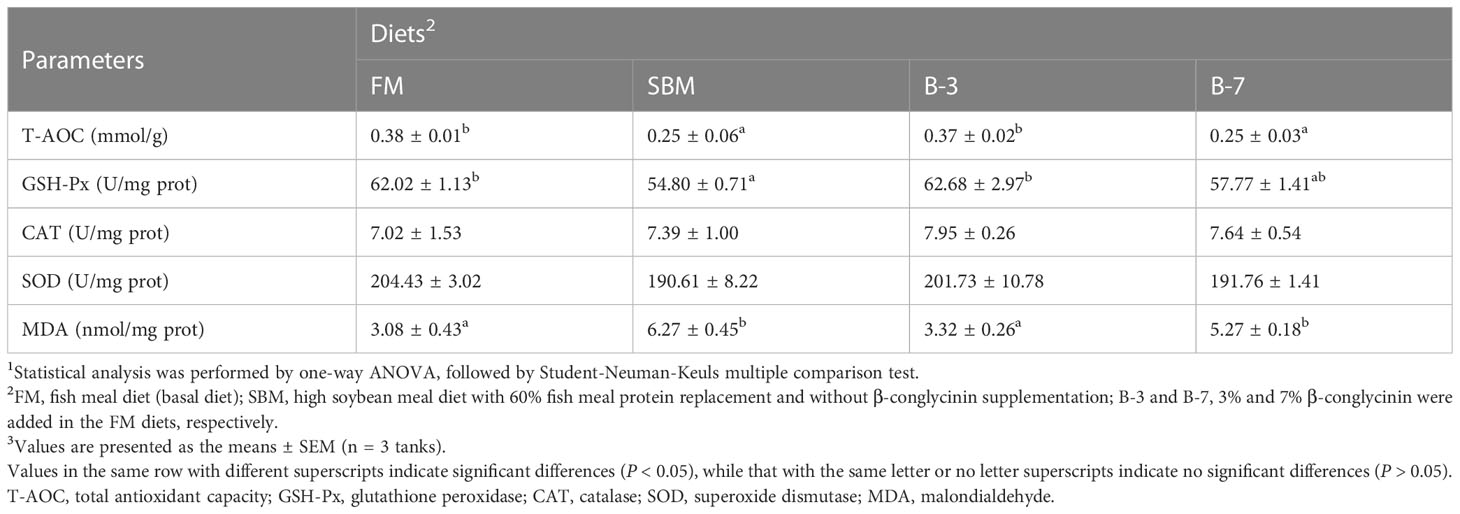
Table 4 Effects of β-conglycinin addition in diets on liver antioxidant indices of orange-spotted groupers in a 56-day feeding period.
3.3 Gut morphological analysis
As shown in Figure 1, the single-layer columnar epithelial cells were tightly connected and a large number of goblet cells in the intestinal mucosa were observed in the FM group. Compared with the FM group, the intestinal structure of MI and DI of the SBM group was damaged, and there was an obvious irregular arrangement of single-layer columnar epithelial cells, a significant reduction in the number of goblet cells in the intestinal mucosa, and many intestinal epithelial cells separated from lamina propria. Both B-3 and B-7 groups exhibited slight damage to intestinal structure compared with the FM group and the B-7 group had greater intestinal structure damage in comparison with the B-3 group.
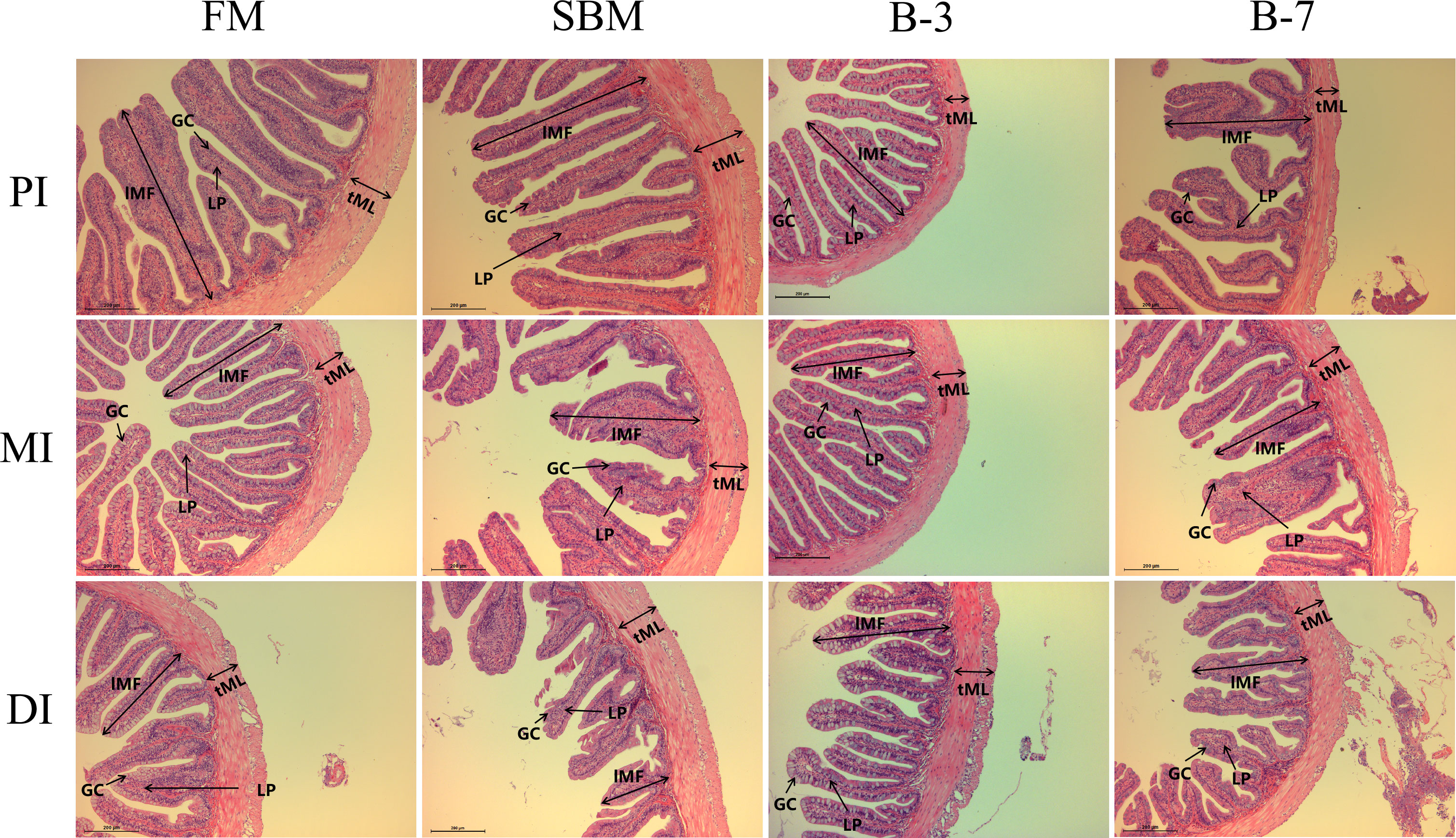
Figure 1 Histological examinations of the proximal intestine (PI), mid intestine (MI), and distal intestine (DI) in the 4 groups of fish fed the diets in a 56-day feeding period (magnification 100). FM, basal diet (control); SBM, high-soybean meal diet; B-3, 3% β-conglycinin addition in FM diet; B-7, 7% β-conglycinin addition in FM diet; LP, lamina propria; GC, goblet cells.
The SBM group had the thinnest tML value for MI among treatments, and the value was lower (P < 0.05) than that of the FM group, but not different (P > 0.05) from that of other groups (Figure 2). The tML and lMF values for DI of SBM and B-7 groups were lower compared with FM and B-3 groups. There were no differences (P > 0.05) in tML and lMF values for DI between the B-3 group and FM group, and the same was true for DI between the B-7 group and SBM group. The tML for PI, lMF for PI and MI, and nMF for PI, MI, and DI were not affected (P > 0.05) by dietary treatments.
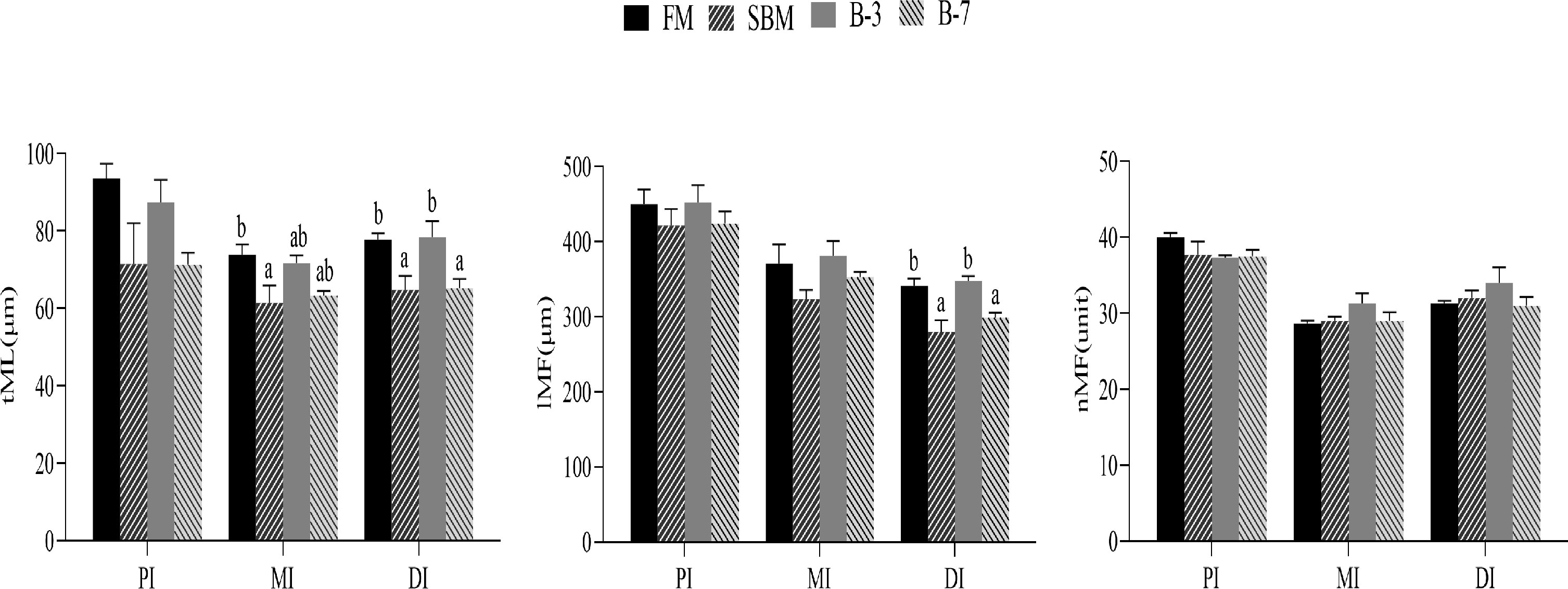
Figure 2 Histological examinations of the proximal intestine (PI), middle intestine (MI), and distal intestine (DI) in the 4 groups of fish fed the diets in a 56-day feeding period (magnification 100). Data are represented as means ± SEM (n = 3 tanks) and analyzed by one-way ANOVA. Values in the same row with different superscripts indicate significant differences (P < 0.05), while that with the same letter or no letter superscripts indicate no significant differences (P > 0.05). FM, basal diet (control); SBM, high-soybean meal diet; B-3, 3% β-conglycinin addition in FM diet; B-7, 7% β-conglycinin addition in FM diet; tML, muscle layer thickness; lMF, mucosal fold length; nMF, mucosal fold number.
As shown in Figure 3, the mRNA levels of genes (occludin, claudin-3 and ZO-1) related to intestinal tight junction structure in SBM and B-7 groups were down-regulated (P < 0.05) compared with FM and B-3 groups. There were no significant differences (P > 0.05) in mRNA levels for the three genes between the B-3 group and FM group, and the same was true for the three genes between the B-7 group and SBM group.
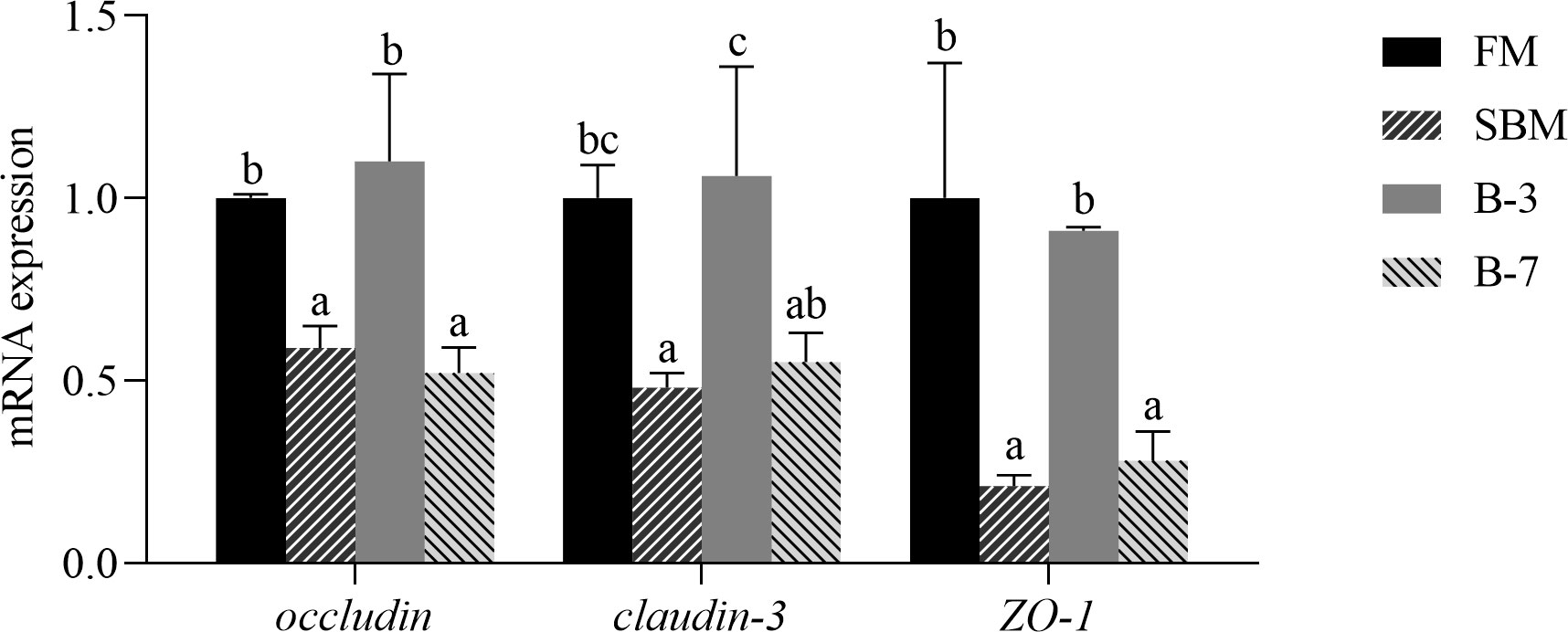
Figure 3 The expression of genes related to intestinal tight junction functions in the 4 groups of fish fed the diets in a 56-day feeding period. Data are represented as means ± SEM (n = 3 tanks) and analyzed by one-way ANOVA. Bars for each gene with different superscripts indicate significant differences (P < 0.05), while that with the same letter or no letter superscripts indicate no significant differences (P > 0.05). FM, basal diet (control); SBM, high-soybean meal diet; B-3, 3% β-conglycinin addition in FM diet; B-7, 7% β-conglycinin addition in FM diet.
3.4 Intestinal mucosal permeability
Table 5 showed that plasma DAO activity, and plasma D-lac and ET contents in SBM and B-7 groups were higher (P < 0.05) than that in FM and B-3 groups. There were no differences (P > 0.05) in intestinal DAO activity, D-lac and ET contents between the B-3 group and FM group, and the same was true for the values between the B-7 group and SBM group.
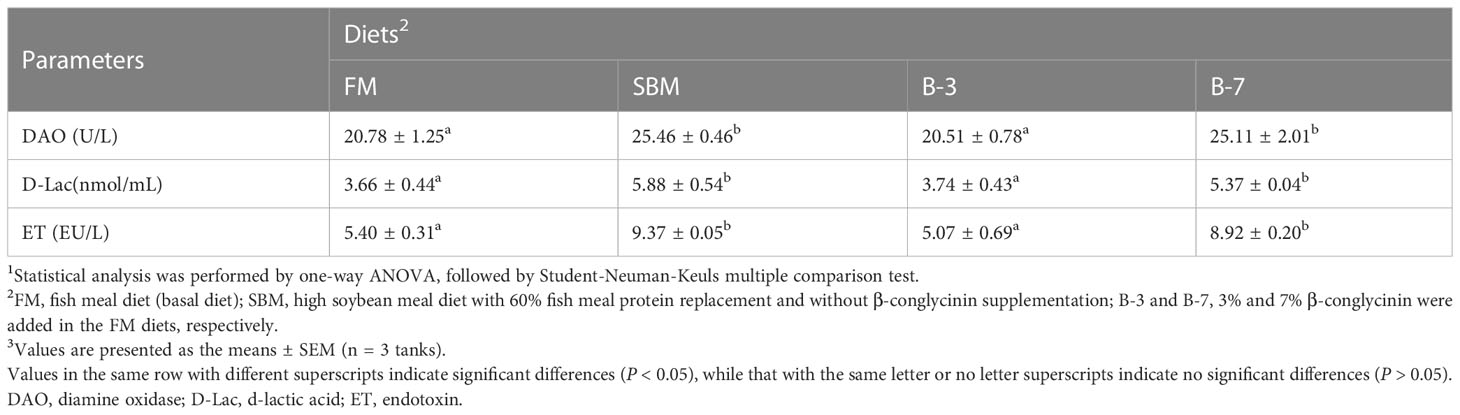
Table 5 Effects of β-conglycinin addition in diets on the plasma components in regard to intestinal mucosal permeability of orange-spotted groupers in a 56-day feeding period.
3.5 Distal intestinal cell apoptosis
As shown in Figure 4, the number of intestinal cell apoptosis of DI in groups SBM and B-7 showed obvious positive signaling compared to that of FM and B-3 groups. The SBM group had the highest number of intestinal cell apoptosis among treatments, followed by the B-7 group, and the values of the SBM and B-7 groups were higher than that in FM and B-3 groups.
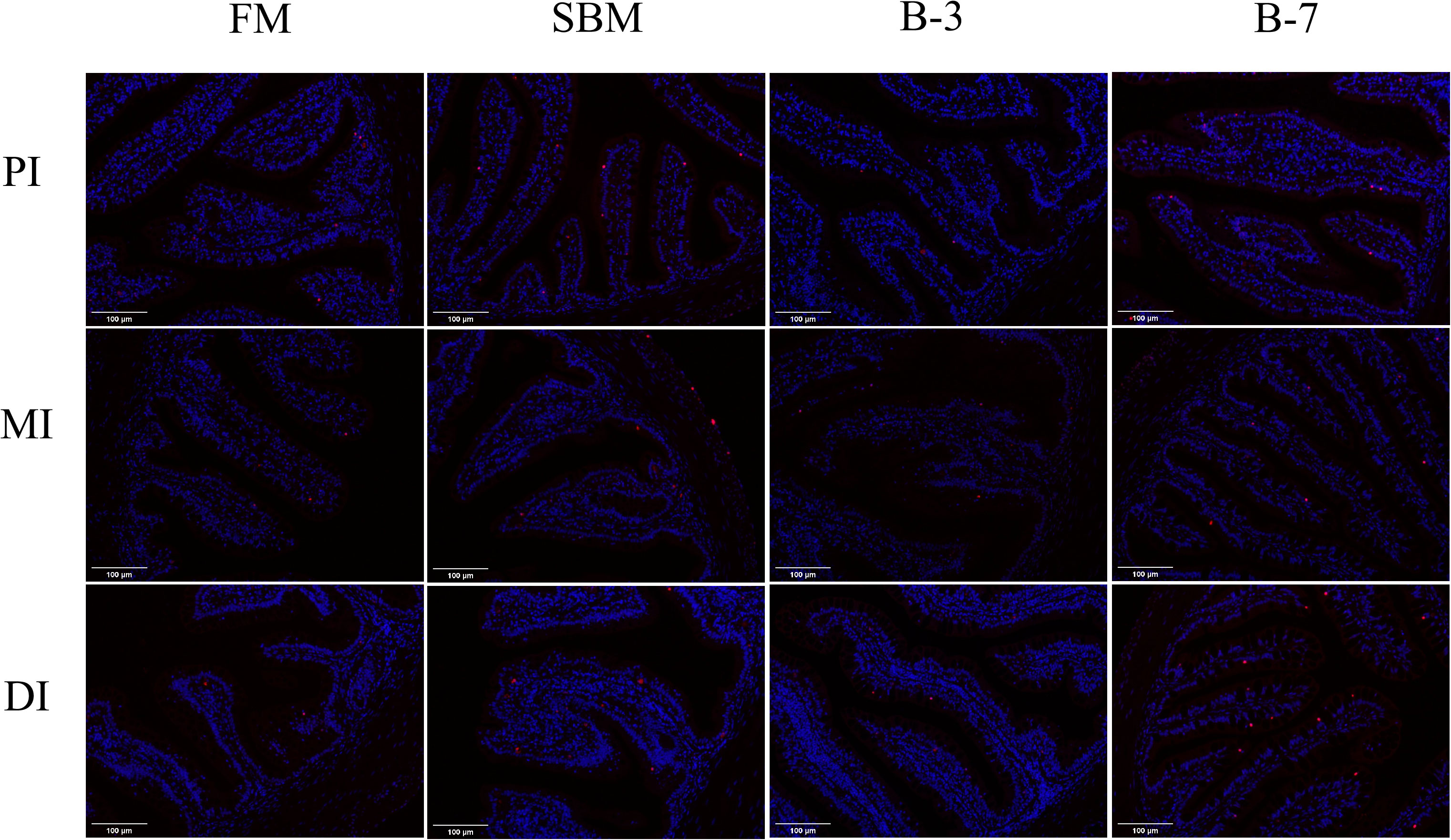
Figure 4 Changes of intestinal cell apoptosis in the 4 groups of fish fed the diets in a 56-day feeding period (magnification 200). PI, MI and DI represent the proximal, mid and distal intestines respectively; red particles refer to apoptotic cells. FM, basal diet (control); SBM, high-soybean meal diet; B-3, 3% β-conglycinin addition in FM diet; B-7, 7% β-conglycinin addition in FM diet.
The mRNA levels of intestinal caspase-3, caspase-8 and caspase-9 genes were higher (P < 0.05) and the bcl-2 and bcl-xL genes were lower (P < 0.05) in SBM and B-7 groups than in FM and B-3 groups (Figure 5). No differences (P > 0.05) in the values for the five genes between the B-3 group and FM group were observed, and the same was true for the five genes between SBM and B-7 groups.
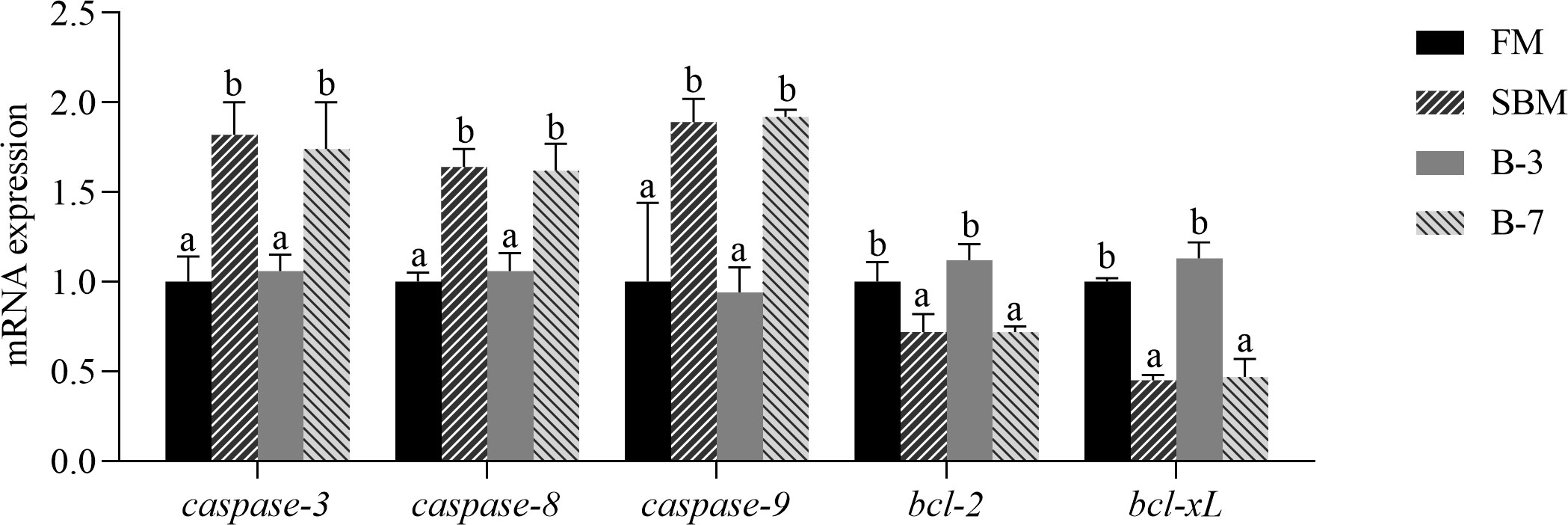
Figure 5 The expression of intestinal cell apoptosis genes in the 4 groups of fish fed the diets in a 56-day feeding period. Data are represented as means ± SEM (n = 3 tanks) and analyzed by one-way ANOVA. Bars for each gene with different superscripts indicate significant differences (P < 0.05), while that with the same letter or no letter superscripts indicate no significant differences (P > 0.05). FM, basal diet (control); SBM, high-soybean meal diet; B-3, 3% β-conglycinin addition in FM diet; B-7, 7% β-conglycinin addition in FM diet; bcl-2, B cell lymphoma 2; bcl-xL, B cell lymphoma xL.
3.6 Gut microbiota
As shown in Table 6, the richness and the diversity of bacterial community in the intestine was not affected (P > 0.05) by dietary treatments.
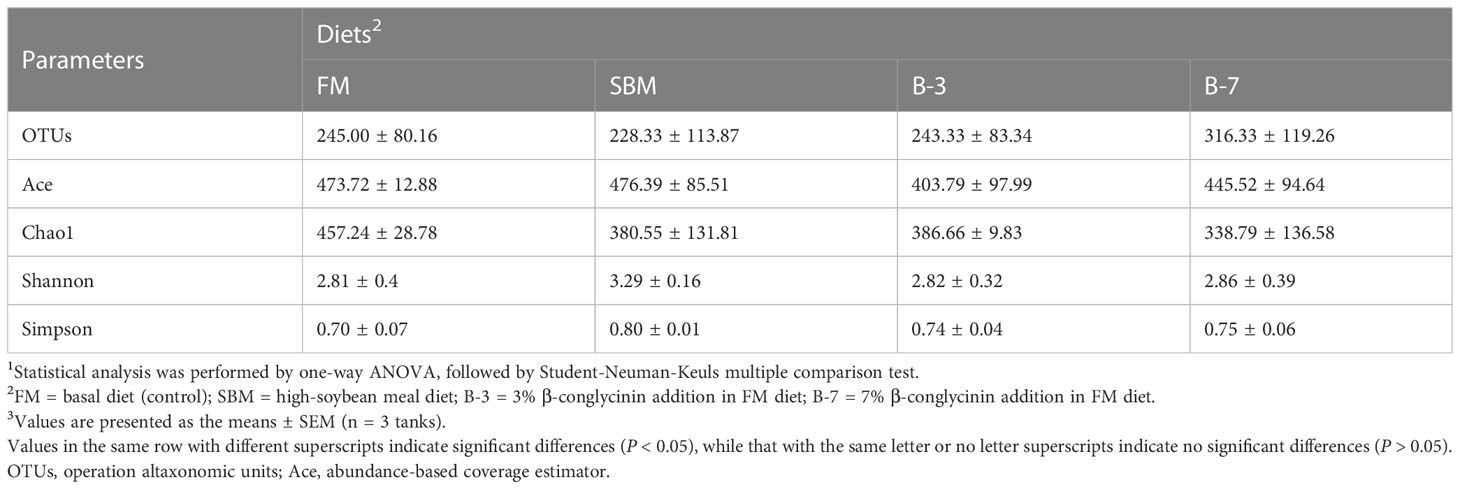
Table 6 Richness and diversity index of bacterial community in intestinal samples of orange-spotted groupers in a 56-day feeding period.
As shown in Figure 6A, the Proteobacteria (relative abundance 62.62%), Tenericutes (19.34%), Actinobacteria (9.92%), Firmicutes (3.69%), Cyanobacteria (1.42%) and Bacteroidetes (1.12%) are the dominant intestinal bacteria at the phylum level. The relative abundances of the first four dominant phyla were listed in Figure 6B. Compared with the FM group, the relative abundance of Proteobacteria in the SBM group was higher (P < 0.05) than that in the B-3 group, but was not different (P > 0.05) from those of other groups. However, the relative abundance of Tenericutes in SBM and B-7 groups were lower (P < 0.05) than that in FM and B-3 groups. There was no difference in relative abundance of Actinobacteria among treatments (P > 0.05). The relative abundance of Firmicutes in the SBM group was lower (P < 0.05) than that in the FM group, and was not different (P > 0.05) from B-3 and B-7 groups.
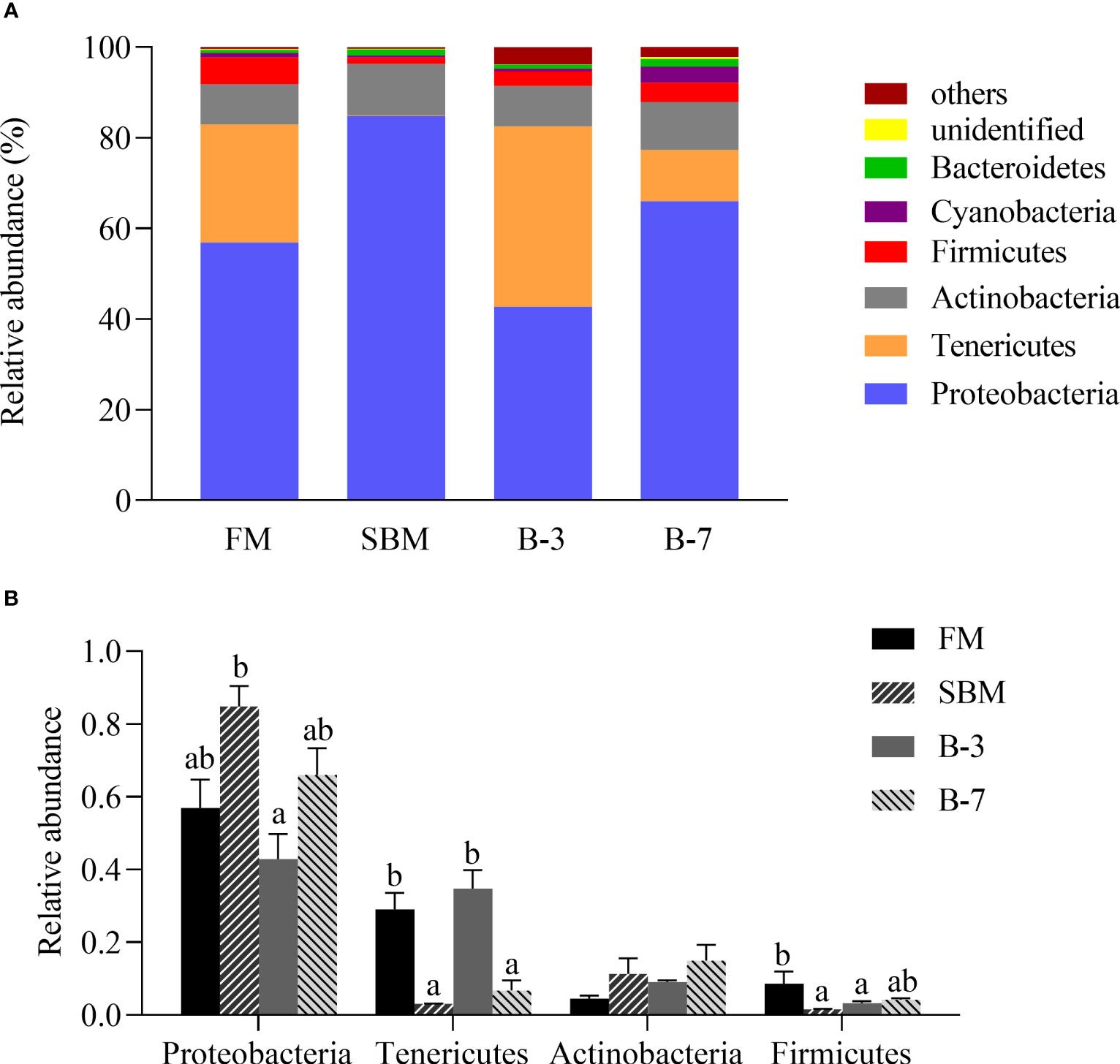
Figure 6 The distal intestine microbiota at phylum levels in the four groups of fish fed the experimental diets in a 56-day feeding period. (A) Relative abundances of the intestine microbiota and (B) Relative abundances for the top-four phylum level. Data are represented as means ± SEM (n = 3 tanks) and analyzed using ANOVA. Bars for each bacterium with different superscripts indicate significant differences (P < 0.05), while that with the same letter or no letter superscripts indicate no significant differences (P > 0.05). FM, basal diet (control); SBM, high-soybean meal diet; B-3, 3% β-conglycinin addition in FM diet; B-7, 7% β-conglycinin addition in FM diet.
As shown in Figure 7A, the dominant bacterial genera in the intestine of all four treatments were Achromobacter (relative abundance 31.35%), Arthrobacter (9.64%), Vibrio (4.98%), Bacillus (2.31%), Hyphomicrobium (1.50%), Sediminibacterium (0.64%), Chroococcidiopsis (0.73%) and Enterovibrio (0.19%). The relative abundances of the four most dominant genera were listed in Figure 7B. The relative abundance of Vibrio in the SBM group was higher (P < 0.05) than that in other groups, and there was no difference among other groups. However, the abundances of the Achromobacter, Arthrobacter and Bacillus were not affected (P > 0.05) by dietary treatments.
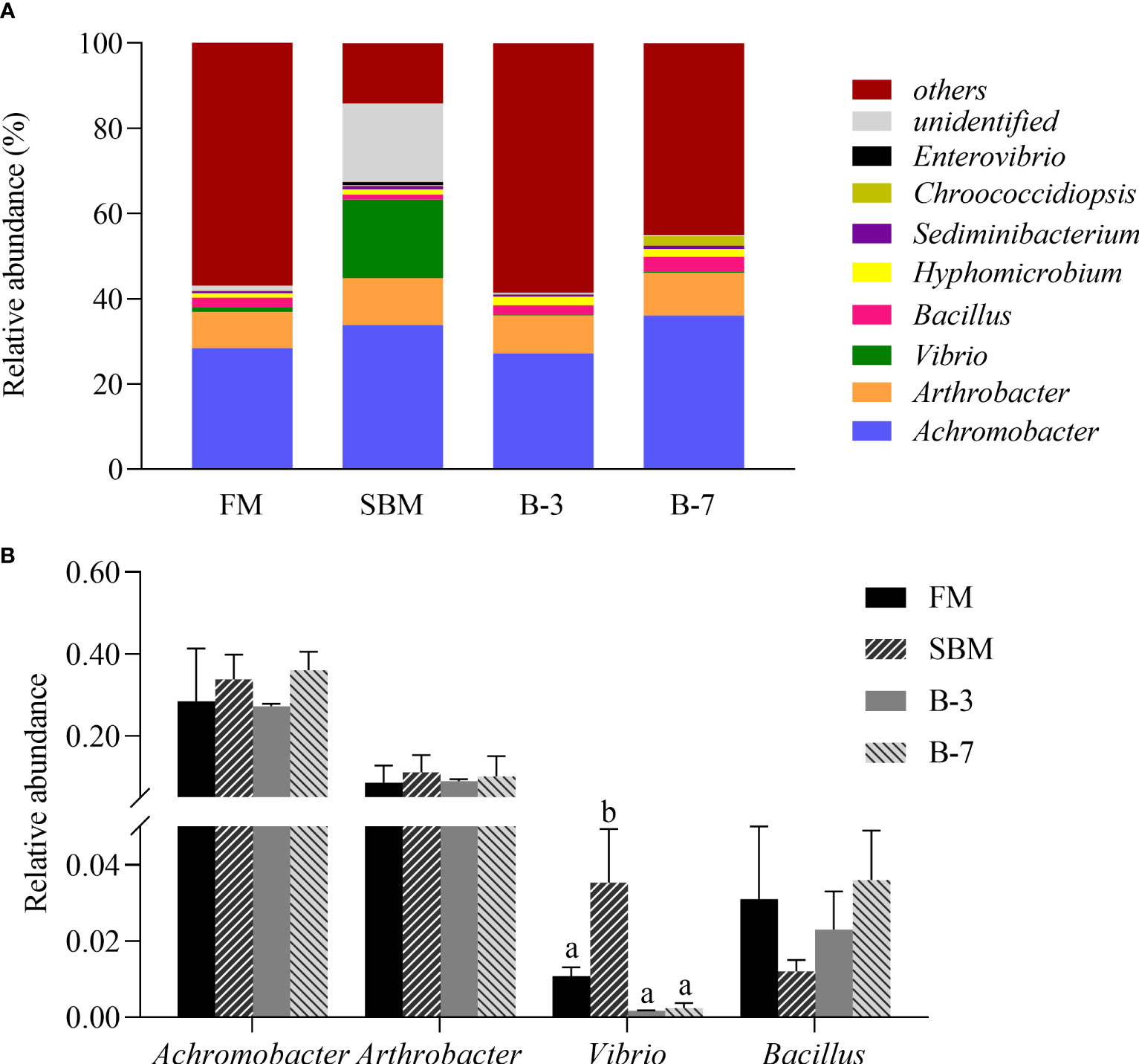
Figure 7 The relative abundance of intestine microbiota at genus levels in the 4 groups of fish fed the experimental diets in a 56-day feeding period. (A) Relative abundances of the intestine microbiota and (B) Relative abundances for the top-four genus level. Data are represented as means ± SEM (n = 3 tanks) and analyzed by one-way ANOVA. Bars for each bacterium with different superscripts indicate significant differences (P < 0.05), while that with the same letter or no letter superscripts indicate no significant differences (P > 0.05). FM, basal diet (control); SBM, high-soybean meal diet; B-3, 3% β-conglycinin addition in FM diet; B-7, 7% β-conglycinin addition in FM diet.
3.7 mRNA expressions of intestinal inflammatory factor genes
As shown in Figure 8, SBM and B-7 groups showed an upregulated (P < 0.05) mRNA level for NF-κB1, RelA, TAK1, IKK, MyD88, TNF-α, IL-1β, and IL-8 genes in comparison with FM and B-3 groups. In contrast, SBM and B-7 groups showed a downregulated (P < 0.05) mRNA level for IκBα, TGF-β1 and IL-10 genes. No differences (P > 0.05) of the 11 genes between the B-3 group and FM group was observed, and the same was true for the 11 genes between SBM and B-7 groups.
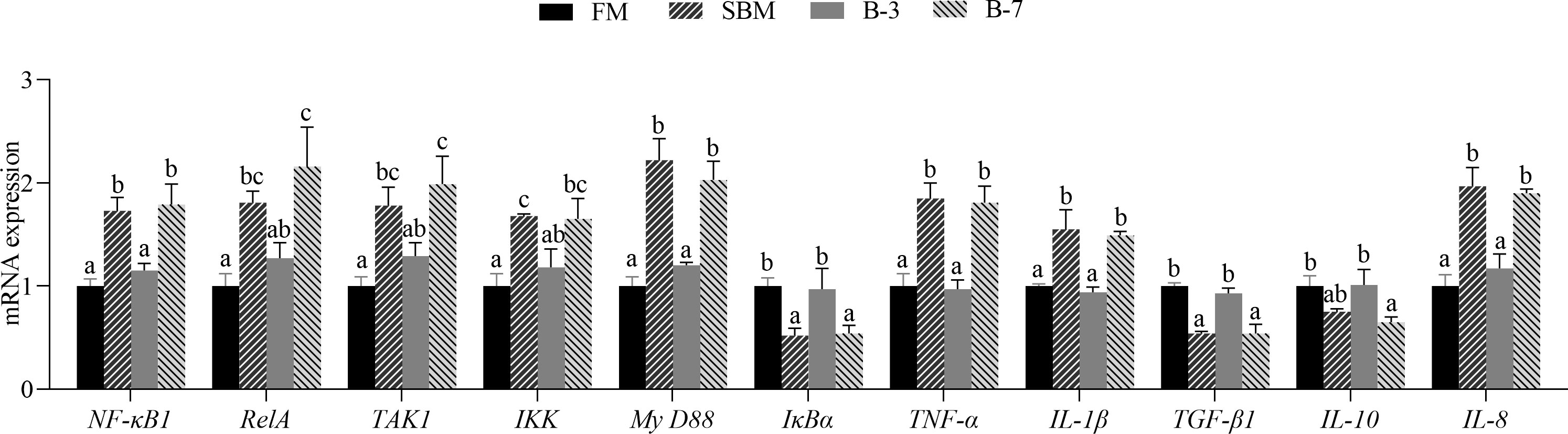
Figure 8 The expression of intestinal inflammatory factor genes in the 4 groups of fish fed the diets in a 56-day feeding period. Data are represented as means ± SEM (n = 3 tanks) and analyzed by one-way ANOVA. Bars for each gene with different superscripts indicate significant differences (P < 0.05), while that with the same letter or no letter superscripts indicate no significant differences (P > 0.05). FM, basal diet (control); SBM, high-soybean meal diet; B-3, 3% β-conglycinin addition in FM diet; B-7, 7% β-conglycinin addition in FM diet; NF-κB1, nuclear factor kappa-B1; RelA, transcription factor p65; TAK1, transforming growth factor activated kinase-1; IKK, inhibitor of kappa B kinase; MyD88, myeloid differentiation factor 88; IκBα, inhibitor of κB alpha; TNF-α, tumor necrosis factor-alpha; IL-1β, interleukin-1 beta; TGF-β1, transforming growth factor-beta 1.
4 Discussion
4.1 Growth performance and proximate composition
β-conglycinin is recognized as one of the main anti-nutritional factors in SBM due to its strong immunogenicity (Hu et al., 2021). In this study, two inclusion levels (3% and 7%) of β-conglycinin identical to the SBM substitution for 30% and 60% FM protein respectively were used to investigate its impacts on the growth performance of grouper. Unexpectedly, 3% β-conglycinin improved growth and feed utilization in grouper than the FM diet. As expected,7% β-conglycinin addition lowered the growth rate of grouper compared with those fed the FM diet, the practice achieved better growth and feed utilization in comparison with SBM diets. Similarly, several studies have also reported a negative effect of dietary high levels of β-conglycinin on the growth rate and feed utilization of other fish species (Zhang et al., 2013; Li et al., 2017; Duan et al., 2019a; Yin et al., 2021a; He et al., 2022). However, in this study, fish fed diets 3% and 7% β-conglycinin had the HSI similar to the SBM group but exhibited reduced HSI vs the control. The similar effect of dietary β-conglycinin addition was observed in a study with Jian carp (Cyprinus carpiovar Jian) (Zhang et al., 2013). Furthermore, both SBM and β-conglycinin diets promoted whole-body moisture contents and reduced whole-body ash contents in this study and previous studies with turbot (Scophthalmus maximus) (Gu et al., 2016b) and barramundi (Lates calcarifer) (Ma et al., 2018), which was generally paralleled with the HSI. This may indicate the lower nutritional status of fish caused by the feeding of high β-conglycinin diets, as observed in fish fed high SBM diets. Consistent with the study with golden crucian carp (Cyprinus carpio × Carassius auratus) (Li et al., 2019), whole-body lipid contents of grouper fed β-conglycinin diets were not different from those fed the FM diet, but higher than those fed the SBM diet.
4.2 Liver antioxidant capacity
Like the SBM diet, fish fed 7% β-conglycinin diet exhibited lower T-AOC value and GSH-Px activity, and increased MDA content in the liver compared with those fed the FM diet. This result indicates high β-conglycinin addition can cause an enhancement in hepatic lipid peroxidation, a reflection of hepatic oxidative damage to some extent. The enhancement of hepatic lipid peroxidation by high β-conglycinin was paralleled with the elevated MDA contents, and declined T-AOC value and GSH-Px activity in our current study and previous studies with Jian carp (Zhang et al., 2013) and golden crucian carp (Li et al., 2019). However, this negative effect was not rendered in fish fed 3% β-conglycinin diet in the present study, and the same result was observed in golden crucian carp fed 2% β-conglycinin diets (Li et al., 2019). Therefore, the intermediate β-conglycinin level is relatively safe for hepatic antioxidant damage in fish.
4.3 Intestinal structural integrity
The intestinal structure integrity plays an important role in the maintenance of normal digestion and absorption status and intestinal disease resistance as wall as a crucial barrier against exogenous pathogens in fish (Song et al., 2022). For histology and morphology, intestinal tML, lMF and nMF are usually used as indicators to measure its digestion and absorption function (Niu et al., 2021). In this study, 7% β-conglycinin addition decreased tML and lMF of MI and DI, which agreed with the observations in other fish species (Gu et al., 2016b; Duan et al., 2019a; Li et al., 2021; Luo et al., 2023). Unlike 7% β-conglycinin diet, 3% β-conglycinin diet did not show significant alterations in the intestinal morphology vs FM diet. This indicates the dietary intermediate inclusion level of β-conglycinin has no damage to intestinal structure of fish (Li et al., 2021).
TLR2 gene deficient mice develop severe enteritis and bleeding, accompanied by intestinal inflammation and cell apoptosis, as well as intestinal mucosal barrier dysfunction mediated by intestinal junction proteins, ZO-1, and claudin-3 (Gibson et al., 2008). In order to better understand the effects of dietary β-conglycinin on the intestinal structure integrity, the intestinal tight junction function of fish at the mRNA level was determined in the present study. Like high SBM diets, 7% β-conglycinin diets down regulated the mRNA levels of claudin-3, occludin and ZO-1 of intestine vs FM diet. Our current results were supported by previous studies with other fish when fed high β-conglycinin diets (Zheng et al., 2020; Yin et al., 2021a). However, the mRNA levels of the three genes in fish fed 3% β-conglycinin diets did not differ from that fed the FM diet. Interestingly, an up-regulation of intestinal mRNA levels of claudin-3 was reported in hybrid grouper fed 1.5% β-conglycinin diet (Yin et al., 2021a). It can be seen that dietary high β-conglycinin level rather than intermediate β-conglycinin level disrupts the intestinal structure integrity by disrupting the function of intestinal tight junction proteins of fish.
The intestinal mucosa provides an important barrier against external bacterial invasion. When the intestinal mucosa barrier is impaired, bacterial-derived substances such as ET and D-Lac are able to invade deeper intestinal mucosal layers to enter the blood circulation (Han et al., 2020). The increase of plasma DAO activity, ET and D-Lac content caused by SBM addition is associated with the decrease in the integrity of the intestinal structure or the increase of intestinal mucosal permeability (Zhao et al., 2021). In the present study, like SBM diets, fish fed 7% β-conglycinin diets had higher plasma DAO activity, D-Lac and ET contents vs FM diets. This was consistent with the previous study with turbot fed 8% β-conglycinin diet (Zheng et al., 2020). However, the adverse effect was not triggered by 3% β-conglycinin diet. These results indicate that high β-conglycinin could impair intestinal mucosa barrier through increasing the intestinal permeability of fish.
4.4 Intestinal cell apoptosis
Intestinal cell apoptosis caused by dietary high β-conglycinin inclusion is mediated by caspase family (Duan et al., 2019a; He et al., 2022), as observed in other studies, that is, feeding high SBM diets led to the up-regulation of intestinal apoptosis gene expression (caspase-3, caspase-8 and caspase-9) (Zhang B. L. et al., 2022; Zhao et al., 2023). In this study, the intestinal TUNEL fluorescence staining and the intestinal caspase-3, caspase-8 and caspase-9 mRNA expression levels were used to further determine the effects of dietary β-conglycinin on intestinal apoptosis of grouper. The results showed that both SBM and 7% β-conglycinin diets enhanced the number of intestinal apoptotic cells and the expression levels of caspase-3, caspase-8 and caspase-9 genes compared with fish fed the FM diet, but this effect did not occur in fish fed 3% β-conglycinin diet. These indicate that intestinal cell apoptosis of fish could be triggered by dietary high β-conglycinin through promoting the activation of intestinal caspases.
Besides the caspase-mediated intestinal apoptosis, the NF-κB signaling pathway regulating intestinal apoptosis was determined in this study. The activation of NF-κB can induce an up-regulation of mRNA levels of bcl-2 and bcl-xL genes, as a result of a reduction in mitochondrial membrane permeability and a block of cytochrome C release to inhibit apoptosis (Leber et al., 2007). The cytokine TNF-α acts as an activator of NF-κB signaling pathway, whose up-regulation will stimulate NF-κB1/RelA to enter the nucleus and activates genes with DNA binding sites for NF-κB, leading to the occurrence of apoptosis, which in turn induces the down-regulation of mRNA expression of downstream bcl-2 and bcl-xL genes (Heissmeyer et al., 2001). In this study, down-regulated mRNA levels of bcl-2 and bcl-xL genes and up-regulated mRNA levels of NF-κB and TNF-α genes were observed in fish fed the SBM and 7% β-conglycinin diets, which agreed with the results in a study with grass carp fed high β-conglycinin diets (Duan et al., 2019a). Although there are few research reports on the effect of dietary β-conglycinin levels on the intestinal cell apoptosis of fish, glycinin, another major antigen protein, has also been found to render this effect in other fish, that is, high glycinin diets down-regulated intestinal bcl-2 mRNA expression and up-regulated intestinal TNF-α mRNA expression (Zhang Y. L. et al., 2021; Yi et al., 2022). Therefore, dietary high β-conglycinin could enhance intestinal cell apoptosis by down-regulating anti-apoptosis bcl-2 and up-regulating expression of pro-apoptosis TNF-α through NF-κB signaling pathway.
4.5 Intestinal microbiota
Numerous and complex intestinal microbiota are interdependent with the host, forming a relatively independent intestinal microbiota homeostasis, which is inseparable from nutrition, immunity and metabolism (McKenney and Pamer, 2015). The intestinal microbiota of fish is affected by the environment, temperature, and especially by the source, composition and type of feed (Bereded et al., 2022). For instance, high SBM diets decreased intestinal abundance of Firmicutes and Actinobacteria and increased intestinal abundance of Cyanobacteria and Tenericutes at the phylum level, as well as lowered the abundances of Fictibacillus and Lactobacillus and increased Mycoplasma at the genus level in largemouth bass (Micropterus salmoides) (Chen et al., 2021). In the present study, the predominant bacterial phyla in the intestine of grouper were Proteobacteria, Tenericutes, Actinobacteria and Firmicutes. Interestingly, the abundances of Proteobacteria were generally higher in fish fed the SBM and 7% β-conglycinin diets than those fed the 3% β-conglycinin and FM diets. The increased abundance of Proteobacteria was also observed in largemouth bass fed SBM diets (Ren et al., 2022) and hybrid grouper fed 6% β-conglycinin diets (Yin et al., 2021b). Proteobacteria includes many pathogenic bacteria that produce a large amount of lipopolysaccharides (Lin et al., 2020), and which exacerbate stroke by activating NF-κB to produce large amounts of pro-inflammatory cytokines (Hakoupian et al., 2021). In contrast, the abundances of Tenericutes were higher in fish fed FM and 3% β-conglycinin diets than those fed SBM and 7% β-conglycinin diets. It is still unclear whether Tenericutes belongs to one of beneficial bacterial phyla in fish, which needs further study (Wang et al., 2021). The intestinal enrichment of Proteobacteria and reduction of Tenericutes may reflect an imbalanced profile of intestinal microbiota (Shin et al., 2015). In addition, our present study showed that SBM and β-conglycinin diets reduced abundance of Firmicutes, which was consistent with the results in hybrid grouper fed 6% β-conglycinin diet (Yin et al., 2021b).
The genus Vibrio such as Vibrio anguallanim is harmful to many fish species (Frans et al., 2011). In this study, the genus Vibrio is one of the dominant bacterial genera in the intestine of fish fed SBM diet (Figure 7A), which was consistent with the results in a previous study with turbot fed SBM diet (Zhang W. et al., 2022). This might partly explain the phenomenon that dietary high SBM can induce enteritis in fish by providing the conditions for the colonization of opportunistic pathogens in the intestine. However, dietary β-conglycinin addition did not render the similar responses to SBM diets, the changing tendency of intestinal bacterial composition of high β-conglycinin diets is generally similar to that of FM diets, which may be related to other anti-nutritional factors.
4.6 Gene expression of intestinal inflammatory factors
The intestinal tract of fish is not only a place for digestion and absorption of nutrient, but also an important organ for immune defense. In addition to acting as a physical barrier, its epithelial cells can also secrete cytokines and chemokines to regulate immune cells and resist the invasion of pathogens (Ferguson et al., 2010). As an important nuclear transcription factor, NF-κB is also involved in the regulation of inflammation through inducing the expression of various pro-inflammatory genes including TNF-α, IL-1β, IL-6, IL-12 (Liu et al., 2022). IL-1β receptor-associated kinase is mobilized through Myd88 to activate IRAKs when cells are stimulated by IL-1β. Subsequently, a downstream molecular transformation growth factor TAK1 is triggered. Then activates IκB kinase, resulting in degradation of the IκB protein and released of NF-κB dimer. NF-κB1 and NF-κB p65 (RelA) then entered the nucleus and bind to target genes in the nucleus, promoting the release of pro-inflammatory factors (IL-8, IL-1β, TNF-α) and inhibiting anti-inflammatory factors (IL-10, TGF-β1) (Trung and Lee, 2020). In addition, TNF-α activates mitogen-activated protein kinase kinase kinases (MAKKKs) by binding to their receptors, and subsequently activates IκB protein, which in turn activates NF-κB-related pathways and their downstream inflammatory responses (Garg and Aggarwal, 2002).
Previous studies showed that the intestinal mRNA levels of RelA, TNF-α, IL-1β, IL-8, Myd88, and/or IKK genes of fish were up-regulated by high SBM diets (Gu et al., 2016b; Wang et al., 2017; Zhang W. et al., 2021; Liu et al., 2022). Similarly, in this study, SBM diets exhibited up-regulated intestinal mRNA levels of TNF-α, IL-1β, Myd88, IKK-α, TAK1, RelA, NF-κB1, and IL-8 genes similar to 7% β-conglycinin diets did, the down-regulated intestinal mRNA levels of TGF-β1, IL-10, and IκBα genes were observed in fish fed both SBM and 7% β-conglycinin diets. This finding was supported by the results of previous studies on other fish fed 7% or 8% β-conglycinin diets with an intestinal up-regulation of RelA, IKKα, IKKβ, IKKγ, IL-1β, IL-8, and/or Myd88 and a down-regulation of TGF-β1, IL-10, and/or IκBα genes (Duan et al., 2019b; Liu et al., 2019; He et al., 2022). The above results showed that high β-conglycinin addition could cause inflammation in the intestine through NF-κB pro-inflammatory signaling pathway and related anti-inflammatory pathways.
5 Conclusions
The growth performance of fish could be restricted by dietary high β-conglycinin, which was accompanied with the enhancement of hepatic lipid peroxidation. Meanwhile, dietary high β-conglycinin could increase the intestinal mucosal permeability and damage the intestinal structural integrity by disrupting intestinal tight junction structure and inducting intestinal apoptosis through promoting the activation of intestinal caspases. Furthermore, dietary high β-conglycinin could alter intestinal bacterial profile and provide the conditions for the colonization of opportunistic pathogens in the intestine, triggering intestinal inflammatory responses, thereby promoting the occurrence of enteritis of the fish species.
Data availability statement
The datasets presented in this study can be found in online repositories. This data can be found here: NCBI accession number: PRJNA980955.
Ethics statement
The animal study was reviewed and approved by Jimei University, Xiamen.
Author contributions
LY: Methodology, Data curation, Writing-original draft. XZ: Investigation, Data curation. YY: Investigation, Visualization. KW: Validation, Investigation. YS: Data curation, Formal analysis. JY: Conceptualization, Supervision, Writing- review & editing, Funding acquisition. All authors contributed to the article and approved the submitted version.
Funding
This work was supported by the funding from the National Natural Science Foundation of China (Grant No. 32072990), and the Science and Technology Major/Special Project of Fujian Province (No. 2021NZ029022).
Conflict of interest
The authors declare that the research was conducted in the absence of any commercial or financial relationships that could be construed as a potential conflict of interest.
Publisher’s note
All claims expressed in this article are solely those of the authors and do not necessarily represent those of their affiliated organizations, or those of the publisher, the editors and the reviewers. Any product that may be evaluated in this article, or claim that may be made by its manufacturer, is not guaranteed or endorsed by the publisher.
References
Anguiano M., Pohlenz C., Buentello A., Gatlin D. M. (2013). The effects of prebiotics on the digestive enzymes and gut histomorphology of red drum (Sciaenops ocellatus) and hybrid striped bass (Morone chrysops×M. saxatilis). Brit. J. Nutr. 109, 623–629. doi: 10.1017/s0007114512001754
AOAC (1995). Official methods of analysis of AOAC international. 16th Edn (Arlington. VA, USA: Association of Official Analytical Chemists).
Baeverfjord G., Krogdahl A. (1996). Development and regression of soybean meal induced enteritis in Atlantic salmon, Salmo salar L., distal intestine: a comparison with the intestines of fasted fish. J. Fish Dis. 19 (5), 375–387. doi: 10.1046/j.1365-2761.1996.d01-92.x
Bai N., Gu M., Xu X., Xu B., Krogdahl Å. (2017). Protective effects of mannan oligosaccharides on turbot Scophthalmus maximus suffering from soy enteropathy. Aquaculture 476, 141–151. doi: 10.1016/j.aquaculture.2017.04.005
Bereded N. K., Abebe G. B., Fanta S. W., Curto M., Waidbacher H., Meimberg H., et al. (2022). The gut bacterial microbiome of Nile tilapia (Oreochromis niloticus) from lakes across an altitudinal gradient. Bmc. Microbiol. 22 (1), 87. doi: 10.1186/s12866-022-02496-z
Booman M., Forster I., Vederas J. C., Groman D. B., Jones S. R. M. (2018). Soybean meal-induced enteritis in Atlantic salmon (Salmo salar) and Chinook salmon (Oncorhynchus tshawytscha) but not in pink salmon (O. gorbuscha). Aquaculture 483, 238–243. doi: 10.1016/j.aquaculture.2017.10.025
Boyd C. E., McNevin A. A., Davis R. P. (2022). The contribution of fisheries and aquaculture to the global protein supply. Food Security 14 (3), 805–827. doi: 10.1007/s12571-021-01246-9
Bureau of Fisheries, Ministry of Agriculture (2022). China Fishery statistics yearbook (Beijing, China: China Agriculture Press).
Carmona P. A. U. (2008). Etiology of soybean-induced enteritis in fish (the Netherlands: Wageningen University and Research).
Chen W. J., Chang K., Chen J. L., Zhao X. Y., Gao S. Y. (2021). Dietary sodium butyrate supplementation attenuates intestinal inflammatory response and improves gut microbiota composition in largemouth bass (Micropterus salmoides) fed with a high soybean meal diet. Fish Physiol. Biochem. 47, 1805–1819. doi: 10.1007/s10695-021-01004-w
Chen Y., Ma J., Huang H., Zhong H. (2019). Effects of the replacement of fishmeal by soy protein concentrate on growth performance, apparent digestibility, and retention of protein and amino acid in juvenile pearl gentian grouper. Plos. One 14 (12), e0222780. doi: 10.1371/journal.pone.0222780
Duan X. D., Feng L., Jiang W. D., Wu P., Liu Y., Kuang S. Y., et al. (2019a). Dietary soybean β-conglycinin suppresses growth performance and inconsistently triggers apoptosis in the intestine of juvenile grass carp (Ctenopharyngodon idella) in association with ROS-mediated MAPK signalling. Aquacult. Nutr. 25 (4), 770–782. doi: 10.1111/anu.12895
Duan X. D., Jiang W. D., Wu P., Liu Y., Jiang J., Tan B. P., et al. (2019b). Soybean β-conglycinin caused intestinal inflammation and oxidative damage in association with NF-κB, TOR and Nrf2 in juvenile grass carp (Ctenopharyngodon idella): varying among different intestinal segments. Fish Shellfish Immun. 95, 105–116. doi: 10.1016/j.fsi.2019.10.021
FAO (2020). “The state of world fisheries and aquaculture 2020,” in Sustainability in action [Rome: Food Agric. Organ. United Nations (FAO)]. doi: 10.4060/ca9229en
Ferguson R. M. W., Merrifield D. L., Harper G. M., Rawling M. D., Mustafa S., Picchietti S., et al. (2010). The effect of Pediococcus acidilactici on the gut microbiota and immune status of on-growing red tilapia (Oreochromis niloticus). J. Appl. Microbiol. 109 (3), 851–862. doi: 10.1111/j.1365-2672.2010.04713.x
Frans I., Michiels C. W., Bossier P., Willems K. A., Lievens B., Rediers H. (2011). Vibrio anguillarum as a fish pathogen: virulence factors, diagnosis and prevention. J. Fish Dis. 34 (9), 643–661. doi: 10.1111/j.1365-2761.2011.01279.x
Garg A. K., Aggarwal B. B. (2002). Reactive oxygen intermediates in TNF signaling. Mol. Immunol. 39 (9), 509–517. doi: 10.1016/s0161-5890(02)00207-9
Gibson D. L., Ma C., Rosenberger C. M., Bergstrom K. S., Valdez Y., Huang J. T., et al. (2008). Toll-like receptor 2 plays a critical role in maintaining mucosal integrity during citrobacter rodentium-induced colitis. Cell Microbiol. 10 (2), 388–403. doi: 10.1111/j.1462-5822.2007.01052.x
Gu M., Bai N., Xu W., Zhou H., Zhang W., Mai K. (2016a). Effects of dietary β-conglycinin and glycinin on digestive enzymes activities, intestinal histology and immune responses of juvenile turbot Scophthalmus maximus. Aquac. Res. 47 (3), 1001–1008. doi: 10.1016/j.aquaculture.2017.05.008
Gu M., Bai N., Zhang Y. Q., Krogdahl Å. (2016b). Soybean meal induces enteritis in turbot Scophthalmus maximus at high supplementation levels. Aquaculture 464, 286–295. doi: 10.1016/j.aquaculture.2016.06.035
Hakoupian M., Ferino E., Jickling G. C., Amini H., Stamova B., Ander B. P., et al. (2021). Bacterial lipopolysaccharide is associated with stroke. Sci. Rep. 11 (1), 6570. doi: 10.1038/s41598-021-86083-8
Han D., Shan X. J., Zhang W. B., Chen Y. S., Wang Q. Y., Li Z. J., et al. (2018). A revisit to fishmeal usage and associated consequences in Chinese aquaculture. Rev. Aquacult. 10 (2), 493–507. doi: 10.1111/raq.12183
Han F. L., Wang X. D., Guo J. L., Qi C. L., Xu C., Luo Y., et al. (2019). Effects of glycinin and β-conglycinin on growth performance and intestinal health in juvenile Chinese mitten crabs (Eriocheir sinensis). Fish Shellfish Immun. 84, 269–279. doi: 10.1016/j.fsi.2018.10.013
Han R., Wang L., Zhao Z. G., You L. J., Pedisić S., Kulikouskaya V., et al. (2020). Polysaccharide from Gracilaria Lemaneiformis prevents colitis in Balb/c mice via enhancing intestinal barrier function and attenuating intestinal inflammation. Food Hydrocolloid. 109, 106048. doi: 10.1016/j.foodhyd.2020.106048
Hanaki K. I., Ike F., Kajita A., Yasuno W., Yanagiba M., Goto M., et al. (2014). A broadly reactive one-step SYBR Green I real-time RT-PCR assay for rapid detection of murine norovirus. PloS One 9 (5), e98108. doi: 10.1371/journal.pone.0098108
Hardy R. W. (2010). Utilization of plant proteins in fish diets: effects of global demand and supplies of fishmeal. Aquac. Res. 41, 770–776. doi: 10.1111/j.1365-2109.2009.02349.x
He L., Han M., Qiao S., He P., Li D., Li N., et al. (2015). Soybean antigen proteins and their intestinal sensitization activities. Curr. Protein Pept. Sc. 16 (7), 613–621. doi: 10.2174/1389203716666150630134602
He Y. F., Liang J. F., Dong X. H., Liu H. Y., Yang Q. H., Zhang S., et al. (2022). Soybean β-conglycinin and glycinin reduced growth performance and the intestinal immune defense and altered microbiome in juvenile pearl gentian groupers Epinephelus fuscoguttatus♀ × Epinephelus lanceolatus♂. Anim. Nutr. 9 (2), 193–203. doi: 10.1016/j.aninu.2021.11.001
He Y. F., Ye G. L., Chi S. Y., Tan B. P., Dong X. H., Yang Q. H., et al. (2020). Integrative transcriptomic and small RNA sequencing reveals immune-related miRNA-mRNA regulation network for soybean meal-induced enteritis in hybrid grouper, Epinephelus fuscoguttatus♀× Epinephelus lanceolatus♂. Front. Immunol. 11. doi: 10.3389/fimmu.2020.01502
Heissmeyer V., Krappmann D., Hatada E. N., Scheidereit C. (2001). Shared pathways of IkB kinase-induced SCF(βTrCP)-mediated ubiquitination and degradation for the NF-κB precursor p105 and IκBa. Mol. Cell Biol. 21 (4), 1024–1035. doi: 10.1128/mcb.21.4.1024-1035.2001
Hu J. Q., Yuan L. Y., An G. J., Zhang J. S., Zhao X. W., Liu Y., et al. (2021). Antigenic activity and epitope analysis of β-conglycinin hydrolyzed by pepsin. J. Sci. Food Agr. 101 (4), 1396–1402. doi: 10.1002/jsfa.10752
Jiang W. D., Hu K., Zhang J. X., Liu Y., Jiang J., Wu P., et al. (2015). Soyabean glycinin depresses intestinal growth and function in juvenile jian carp (Cyprinus carpiovar jian): protective effects of glutamine. Brit. J. Nutr. 114 (10), 1569–1583. doi: 10.1017/s0007114515003219
Leber B., Lin J. L., Andrews D. W. (2007). Embedded together: the life and death consequences of interaction of the Bcl-2 family with membranes. Apoptosis 12, 897–911. doi: 10.1007/s10495-007-0746-4
Li Y. X., Hu H. B., Liu J. T., Yang P., Zhang Y. J., Ai Q. H., et al. (2017). Dietary soya allergen β-conglycinin induces intestinal inflammatory reactions, serum-specific antibody response and growth reduction in a carnivorous fish species, turbot Scophthalmus maximus L. Aquac. Res. 48 (8), 4022–4037. doi: 10.1111/are.13224
Li L., Li M., Zhu R., Yu Z., Wang J. Y., Duan J., et al. (2019). Effects of β-conglycinin on growth performance, antioxidant capacity and intestinal health in juvenile golden crucian carp, Carassius auratus. Aquac. Res. 50 (11), 3231–3241. doi: 10.1111/are.14278
Li L., Zhu R., Li M., Yu Z., Wang H. H., Quan Y. A., et al. (2020). Effects of β-conglycinin on growth performance, antioxidant capacity and immunity in Rhynchocypris lagowski dybowski. Aquacult. Nutr. 26 (6), 2059–2073. doi: 10.1111/anu.13147
Li L., Zhu R., Yu Z., Liu J., Qu Z. H., Wu L. F. (2021). Effects of β-conglycinin on intestinal structure and intestinal permeability in Rhynchocypris lagowski Dybowski. Anim. Nutr. 27 (6), 1946–1958. doi: 10.1111/anu.13331
Lin T. L., Shu C. C., Chen Y. M., Lu J. J., Wu T. S., Lai W. F., et al. (2020). Like cures like: pharmacological activity of anti-inflammatory lipopolysaccharides from gut microbiome. Front. Pharmacol. 11. doi: 10.3389/fphar.2020.00554
Liu Y., Chen Z. C., Dai J. H., Yang P., Xu W. Q., Ai Q. H., et al. (2019). Sodium butyrate supplementation in high-soybean meal diets for turbot (Scophthalmus maximus L.): effects on inflammatory status, mucosal barriers and microbiota in the intestine. Fish Shellfish Immun. 88, 65–75. doi: 10.1016/j.fsi.2019.02.064
Liu P. Q., Li Y., Wang W. L., Bai Y. Z., Jia H. M., Yuan Z. W., et al. (2022). Role and mechanisms of the NF-kB signaling pathway in various developmental processes. Biomed. Pharmacother. 153, 113513. doi: 10.1016/j.biopha.2022.113513
Luo Q. H., Qian R. D., Qiu Z. S., Yamamoto F. Y., Du Y. Y., Lin X. W., et al. (2023). Dietary α-ketoglutarate alleviates glycinin and β-conglycinin induced damage in the intestine of mirror carp (Cyprinus carpio). Front. Immunol. 14. doi: 10.3389/fimmu.2023.1140012
Ma Z. H., Hassan M. M., Allais L., He T., Leterme S., Ellis A. V., et al. (2018). Replacement of fishmeal with commercial soybean meal and EnzoMeal in juvenile barramundi Lates calcarifer. Aquac. Res. 49 (10), 3258–3269. doi: 10.1111/are.13790
McKenney P. T., Pamer E. G. (2015). From hype to hope: the gut microbiota in enteric infectious disease. Cell 163 (6), 1326–1332. doi: 10.1016/j.cell.2015.11.032
Niu X. J., Qian X. L., Feng H. M., Yi K., Li D., Chen W. J., et al. (2021). Growth and metabolic responses of grouper juveniles (Epinephelus coioides) fed diets containing varying levels of leucine. Aquaculture 534, 736281. doi: 10.1016/j.aquaculture.2020.736281
Peng C. L., Cao C. M., He M. C., Shu Y. S., Tang X. B., Wang Y. H., et al. (2018). Soybean glycinin-and β-conglycinin-induced intestinal damage in piglets via the p38/JNK/NF-κB signaling pathway. J. Agric. Food Chem. 66 (36), 9534–9541. doi: 10.1021/acs.jafc.8b03641
Peng C. L., Ding X. D., Zhu L., He M. C., Shu Y. S., Zhang Y., et al. (2019). β-conglycinin-induced intestinal porcine epithelial cell damage via the nuclear factor κB/mitogen-activated protein kinase signaling pathway. J. Agric. Food Chem. 67 (32), 9009–9021. doi: 10.1021/acs.jafc.9b02784
Qin Y. M., He L. Y., Wang Y. F., Li D., Chen W. J., Ye J. D. (2022). Growth performance, fatty acid composition, and lipid metabolism are altered in groupers (Epinephelus coioides) by dietary fish oil replacement with palm oil. Anim. Nutr. 8 (1), 102–113. doi: 10.1016/j.aninu.2021.04.007
Ren X., Ma H. J., Liu X. X., Wu Y. B. (2022). Effects of taurine supplementation on growth, feed utilization, antioxidant capacity, and intestinal microflora of largemouth bass fed a low fish meal diet. N. Am. J. Aquacult. 84 (3), 285–294. doi: 10.1002/naaq.10239
Renkema J. M. S. (2001). Formation, structure and rheological properties of soy protein gels (The Netherlands: Wageningen University).
Ringø E., Sperstad S., Myklebust R., Refstie S., Krogdahl Å. (2006). Characterisation of the microbiota associated with intestine of Atlantic cod (Gadus morhua L.): the effect of fish meal, standard soybean meal and a bioprocessed soybean meal. Aquaculture 261 (3), 829–841. doi: 10.1016/j.aquaculture.2006.06.030
Schmittgen T. D., Livak K. J. (2008). Analyzing real-time PCR data by the comparative C-T method. Nat. Protoc. 3, 1101–1108. doi: 10.1038/nprot.2008.73
Scott R. J., Brito L. F., Lavanya R., Schmidt M., Herman E. M., Schinckel A. P. (2019). A swine model of soy protein-induced food allergenicity: implications in human and swine nutrition. Anim. Front. 9 (3), 52–59. doi: 10.1093/af/vfz025
Shan D. D., Yu H. S., Lyu B., Fu H. L. (2021). Soybean β-conglycinin: structure characteristic, allergenicity, plasma lipid-controlling, prevention of obesity and non-alcoholic fatty liver disease. Curr. Protein Pept. Sc. 22 (12), 831–847. doi: 10.2174/1389203722666211202151557
Shapawi R., Ching F. F., Senoo S., Mustafa S. (2019). Nutrition, growth and resilience of tiger grouper (Epinephelus fuscoguttatus) × giant grouper (Epinephelus lanceolatus) hybrid-a review. Rev. Aquacult. 11, 1285–1296. doi: 10.1111/raq.12292
Shin N. R., Whon T. W., Bae J. W. (2015). Proteobacteria: microbial signature of dysbiosis in gut microbiota. Trends Biotechnol. 33 (9), 496–503. doi: 10.1016/j.tibtech.2015.06.011
Song T., Qin Y. M., Ke L. E., Wang X. X., Wang K., Sun Y. Z., et al. (2022). Dietary lactoferrin supplementation improves growth performance and intestinal health of juvenile orange-spotted groupers (Epinephelus coioides). Metabolites 12 (10), 915. doi: 10.3390/metabo12100915
Soumeh E. A., Mohebodini H., Toghyani M., Shabani A., Ashayerizadeh A., Jazi V. (2019). Synergistic effects of fermented soybean meal and mannan-oligosaccharide on growth performance, digestive functions, and hepatic gene expression in broiler chickens. Poult. Sci. 98 (12), 6797–6807. doi: 10.3382/ps/pez409
Tacon A. G. J. (2020). Trends in global aquaculture and aquafeed production: 2000–2017. Rev. Fish Sci. Aquac. 28 (1), 43–56. doi: 10.1080/23308249.2019.1649634
Trung N. B., Lee P. T. (2020). Functional characterization of myeloid differentiation factor 88 in Nile tilapia (Oreochromis niloticus). Comp. Biochem. Phys. B 250, 110485. doi: 10.1016/j.cbpb.2020.110485
Wang W. Z., Huang J. S., Zhang J. D., Wang Z. L., Li H. Z., Amenyogbe E., et al. (2021). Effects of hypoxia stress on the intestinal microflora of juvenile of cobia (Rachycentron canadum). Aquaculture 536, 736419. doi: 10.1016/j.aquaculture.2021.736419
Wang L., Sun Z. F., Xie W. N., Peng C. L., Ding H. Y., Li Y., et al. (2022). 11S glycinin up-regulated NLRP-3-induced pyroptosis by triggering reactive oxygen species in porcine intestinal epithelial cells. Front. Vet. Sci. 9. doi: 10.3389/fvets.2022.890978
Wang Y. R., Wang L., Zhang C. X., Song K. (2017). Effects of substituting fishmeal with soybean meal on growth performance and intestinal morphology in orange-spotted grouper (Epinephelus coioides). Aquacult. Rep. 5, 52–57. doi: 10.1016/j.aqrep.2016.12.005
Wu J. J., Cao C. M., Ren D. D., Zhang Y., Kou Y. N., Ma L. Y., et al. (2016a). Effects of soybean antigen proteins on intestinal permeability, 5-hydroxytryptamine levels and secretory IgA distribution in the intestine of weaned piglets. Ital. J. Anim. Sci. 15 (1), 174–180. doi: 10.1080/1828051x.2016.1148559
Wu J. J., Zhang Y., Dong J. H., Cao C. M., Li B., Feng S. B., et al. (2016b). Allergens and intestinal damage induced by soybean antigen proteins in weaned piglets. Ital. J. Anim. Sci. 15 (3), 437–445. doi: 10.1080/1828051x.2016.1200441
Yi L. Y., Liu J. W., Yang H. J., Mo A. J., Zhai Y. X., Wang S., et al. (2022). Effects of dietary glycinin on oxidative damage, apoptosis and tight junction in the intestine of juvenile hybrid yellow catfish, Pelteobagrus fulvidraco female symbol × Pelteobaggrus vachelli male symbol. Int. J. Mol. Sci. 23 (19), 11198. doi: 10.3390/ijms231911198
Yin B., Liu H. Y., Tan B. P., Dong X. H., Chi S. Y., Yang Q. H., et al. (2021a). MHC II-PI3K/Akt/mTOR signaling pathway regulates intestinal immune response induced by soy glycinin in hybrid grouper: protective effects of sodium butyrate. Front. Immunol. 11. doi: 10.3389/fimmu.2020.615980
Yin B., Liu H. Y., Tan B. P., Dong X. H., Chi S. Y., Yang Q. H., et al. (2021b). Dynamic alterations of the distal intestinal microbiota, transcriptome, and metabolome of hybrid grouper by β-conglycinin with reconciliations by sodium butyrate in feed. Front. Mar. Sci. 8. doi: 10.3389/fmars.2021.705332
Yin B., Liu H. Y., Tan B. P., Dong X. H., Chi S. Y., Yang Q. H., et al. (2021a). Dietary supplementation of β-conglycinin, with or without sodium butyrate on the growth, immune response and intestinal health of hybrid grouper. Sci. Rep. Uk. 11 (1), 17298. doi: 10.1038/s41598-021-96693-x
Zhang Y. L., Duan X. D., Feng L., Jiang W. D., Wu P., Liu Y., et al. (2021). Soybean glycinin disrupted intestinal structural integrity related to aggravation of apoptosis and downregulated transcription of tight junction proteins in the intestine of juvenile grass carp (Ctenopharyngodon idella). Aquaculture 531, 735909. doi: 10.1016/j.aquaculture.2020.735909
Zhang J. X., Guo L. Y., Feng L., Jiang W. D., Kuang S. Y., Liu Y., et al. (2013). Soybean β-conglycinin induces inflammation and oxidation and causes dysfunction of intestinal digestion and absorption in fish. Plos. One 8 (3), e58115. doi: 10.1371/journal.pone.0058115
Zhang Y. L., Jiang W. D., Duan X. D., Feng L., Wu P., Liu Y., et al. (2020). Soybean glycinin caused NADPH-oxidase-regulated ROS overproduction and decreased ROS elimination capacity in the mid and distal intestine of juvenile grass carp (Ctenopharyngodon idella). Aquaculture 516, 734651. doi: 10.1016/j.aquaculture.2019.734651
Zhang W., Tan B. P., Deng J. M., Dong X. H., Yang Q. H., Chi S. Y., et al. (2021). The single-molecule long-read sequencing of intestine after soy meal-induced enteritis in juvenile pearl gentian grouper, Epinephelus fuscoguttatus♀× Epinephelus lanceolatus♂. Front. Mar. Sci. 8. doi: 10.3389/fmars.2021.688601
Zhang W., Tan B. P., Deng J. M., Yang Q. H., Chi S. Y., Pang A. B., et al. (2022). PRR-mediated immune response and intestinal flora profile in soybean meal-induced enteritis of pearl gentian groupers, Epinephelus fuscoguttatus female symbol × Epinephelus lanceolatus male symbol. Front. Immunol. 13. doi: 10.3389/fimmu.2022.814479
Zhang B. L., Zhang Y., Cui M. C., Zhang M. Y., Xu J. N., Zhang Z., et al. (2022). Comparison of the performance of raw and Lactobacillus paracasei fermented soybean meal in diets for turbot (Scophthalmus maximus L.): growth, intestinal morphology, apoptosis, tight junction, and microbiota. Aquac. Rep. 24, 101184. doi: 10.1016/j.aqrep.2022.101184
Zhao X. Q., Wang Y. F., Wang X. X., Ye J. D. (2021). Growth performance, plasma components, and intestinal barrier in grouper (Epinephelus coioides) are altered by dietary fish meal replacement with extruded soybean meal. Aquacult. Rep. 21, 100863. doi: 10.1016/j.aqrep.2021.100863
Zhao J. H., Yang X., Qiu Z. S., Zhang R. F., Xu H., Wang T. (2023). Effects of tributyrin and alanyl-glutamine dipeptide on intestinal health of largemouth bass (Micropterus salmoides) fed with high soybean meal diet. Front. Immunol. 14. doi: 10.3389/fimmu.2023.1140678
Zheng J., Yang P., Dai J. H., Yu G. J., Ou W. H., Xu W. Q., et al. (2020). Dynamics of intestinal inflammatory cytokines and tight junction proteins of turbot (Scophthalmus maximus l.) during the development and recovery of enteritis induced by dietary β-conglycinin. Front. Mar. Sci. 7. doi: 10.3389/fmars.2020.00198
Zhu C., Jiang Z. Y., Zheng C. T., Wang S. K., Wang L. (2011). Soybean antigenic protein: composition and allergy mechanisms. Chin. J. Anim. Nutr. 23 (12), 2053–2063. doi: 10.3969/j.issn.1006-267x.2011.12.003
Zhu W. Q., Yuan X. Q., Luo H. J., Shao J. C., Chen X. H. (2021). High percentage of dietary soybean meal inhibited growth, impaired intestine healthy and induced inflammation by TLR-MAPK/NF-κB signaling pathway in large yellow croaker (Larimichthys crocea). Aquacult. Rep. 20, 100735. doi: 10.1016/j.aqrep.2021.100735
Keywords: β-conglycinin, inflammatory response, intestinal microbiota, apoptosis, orange-spotted grouper
Citation: Yang L, Zhao X, Yin Y, Wang K, Sun Y and Ye J (2023) Dietary high β-conglycinin reduces the growth through enhancing hepatic lipid peroxidation and impairing intestinal barrier function of orange-spotted grouper (Epinephelus coioides). Front. Mar. Sci. 10:1237387. doi: 10.3389/fmars.2023.1237387
Received: 09 June 2023; Accepted: 29 June 2023;
Published: 18 July 2023.
Edited by:
Lei Chen, Guangdong Ocean University, ChinaReviewed by:
Yanan Zhao, Guangdong Ocean University, ChinaXiaohui Dong, Guangdong Ocean University, China
Jianchun Shao, Fujian Agriculture and Forestry University, China
Copyright © 2023 Yang, Zhao, Yin, Wang, Sun and Ye. This is an open-access article distributed under the terms of the Creative Commons Attribution License (CC BY). The use, distribution or reproduction in other forums is permitted, provided the original author(s) and the copyright owner(s) are credited and that the original publication in this journal is cited, in accordance with accepted academic practice. No use, distribution or reproduction is permitted which does not comply with these terms.
*Correspondence: Jidan Ye, eWp5amRAam11LmVkdS5jbg==