- 1College of Science and Engineering, Flinders University, Adelaide, SA, Australia
- 2South Australian Research and Development Institute, Aquatic Science Centre, Adelaide, SA, Australia
- 3College of Animal Science and Veterinary Medicine, Shenyang Agricultural University, Shenyang, China
- 4Fisheries College, Ocean University of China, Qingdao, China
Cryopreservation is a technique to maintain biological materials’ physiological and genetic stability at an ultralow temperature. For commercially important livestock or aquatic species, gamete and embryo cryopreservation could play a significant role in breeding programs and commercial production. For example, it could help overcome key problems such as asynchronous maturation and an unbalanced sex ratio. However, the physiochemical stresses imposed by cryopreservation can negatively affect gametes and embryos, leading to a poor survival rate. Recent studies on cryoinjury have demonstrated that the cryosensitivity of lipids is one of the key causes of cryodamage in mammalians, as lipid compositions in membranes of gametes and embryos are closely related to their cryoresistance. In addition, the cryotolerance of gametes and embryos in some mammalian species has been improved by lipid modification. However, studies on the role of lipids in the cryopreservation of gametes, embryos, and larvae are rare in fish and shellfish. Therefore, this review focuses on recent methodological advances to improve cryotolerance by lipid modification, including lipid application or manipulation in human and livestock sperm, oocytes, and embryos, and how these novel approaches could improve cryopreservation techniques in aquatic species, especially for oocytes and embryos.
1 Introduction
Cryopreservation is a technique to store living materials (such as gametes, embryos, larvae, cells, and tissues) at an ultralow temperature to maintain their long-term physiological and genetic stability (Kopeika et al., 2015; Dhali et al., 2019). Cryopreservation of gametes, embryos and larvae (fish and shellfish) could play a significant role in reproductive and genetic improvement programs for commercially important livestock or aquatic species. It could also reduce the costs of transporting live animals for breeding and manage issues related to asynchronous maturation and unbalanced sex ratios (Huang et al., 2019; Díaz et al., 2021). The development of gamete and embryo cryopreservation protocols has been an integral step in advancing assisted reproductive technology (ART). From the first human infant derived from frozen sperm in 1953 (Bunge and Sherman, 1953) to the first human live birth after embryo cryopreservation in 1984 (Zeilmaker et al., 1984), ART in humans has become a mature technique. Cryopreservation techniques have also been greatly improved in livestock and pet animals (Galiguis et al., 2014; Mandal et al., 2014; Nagashima et al., 2015). In addition, the technical details of the governing factors and mechanisms contributing to cryoinjury have been gradually revealed (Kopeika et al., 2015; Dhali et al., 2019).
Several physical and chemical factors causing severe cryoinjuries have been reported in previous reviews in mammalian species (Amstislavsky et al., 2019; Dhali et al., 2019). Among them, intracellular ice crystal formation, osmotic shock, free radicals such as reactive oxygen and nitrogen species (ROS and RNS), and lipid phase transition (LPT; a transition from a liquid phase to a crystalline-gel phase) are the primary factors causing cryoinjuries (Figueroa et al., 2019). Additionally, many adverse impacts of cryopreservation have been identified. For example, frozen-thawed sperm are characterized by lower motility, acrosome integrity, and mitochondrial membrane potential, resulting in low fertilization capacity (O’Connell et al., 2002; Ozkavukcu et al., 2008; Ugur et al., 2019). Fracture and membrane damage, mitochondrial dysfunction, and rupture and disruption of the cytoskeleton structure can negatively impact the further development of cryopreserved oocytes and embryos (Saunders and Parks, 1999; Kasai, 2002; Iussig et al., 2019; Gualtieri et al., 2021). At the molecular level, genomic DNA lesions and mitochondrial DNA damage have been detected in cryopreserved mammalian gametes and embryos (Lin and Tsai, 2012; Valcarce et al., 2013; Liu et al., 2016). Additionally, epigenetic and transcriptomic profiles are also susceptive to the stress caused by cryopreservation (Chatterjee et al., 2017; Barberet et al., 2020).
Lipids are hydrophobic or amphiphilic molecules, including fatty acids (FAs), sterols, phospholipids (PLs) and triglycerides, and function as energy, signaling transduction, and cell membrane components (Fahy et al., 2009; Subramaniam et al., 2011). Lipid droplets (LDs) are also a significant component of oocytes, embryos and early-stage larvae in aquatic species. In gamete and embryo cryopreservation in mammalian species, among all chemical constituents, lipids such as the PLs - the main component of the plasma membrane, are the most susceptible to freezing damage (Quinn, 1985; Sieme et al., 2015). During the freezing process, LPT occurs, which is accompanied by the alteration in PLs structure and composition (Jung et al., 2014; Fang et al., 2016; Jung et al., 2021). The membrane then loses its high elasticity and becomes rigid, resulting in poor membrane permeability and hydrophobic molecule diffusion (Amstislavsky et al., 2019). Ultimately, this process leads to the loss of cellular functionality (Hinkovska-Galcheva et al., 1989; Schuffner et al., 2001; Fang et al., 2016). Therefore, LPT at the nonphysiological temperature is considered one of the major causes of freezing damage. In general, mammalian gametes or embryos with lower LPT temperatures are likely to have better cryotolerance (Drobnis et al., 1993; Ghetler et al., 2005). Given that the LPT temperature is greatly dependent on lipid composition, features of the lipid profile are related to the cryoresistance of gametes and embryos. For example, the ratio between cholesterol and PL, and unsaturation rates are related to cryotolerance of spermatozoa (Waterhouse et al., 2006; Oldenhof et al., 2012), and lipid-rich oocytes or embryos usually exhibit high cryosensitivity. Therefore, lipid modification has become one of the key approaches to further improve post-thaw performances in mammalian gamete and embryo cryopreservation. For example, cryotolerance has been enhanced significantly by delipidation in lipid-rich oocytes and embryos (Amstislavsky et al., 2019) and membrane lipid replacement (MLR) in sperm (Vireque et al., 2016).
In aquatic animals, cryopreservation of oocytes, embryos and larvae is more challenging than sperm. Apart from their larger size and complex structure, the higher lipid content is another key factor that contributes to the chanlleges. For example, the egg lipid content is 32.4% on a wet weight basis in the whitefish Coregonus albula (V’uorela et al., 1979) and 38% on a dry weight basis in the Pacific oyster Magallana gigas (Massapina, 1999). These lipids serve as a vital energy reservoir for the early development of aquatic species as their digestive system has not fully evolved. Since lipid content and composition can affect cryotolerance, it is anticipated that lipid modification could play a similar role as in mammalians and improve the cryopreservation techniques in aquatic animals. Indeed, a few studies have demonstrated the potential of lipid modification in cryopreservation of gametes and larvae in aquatic animals. For instance, embryos produced from broodstock fed with diet supplemented with a fish oil showed a better permeability to cryoprotectants in Prochilodus lineatus (Costa et al., 2018). The supplement of exogenous lipids in cryoprotectants resulted in the improved performances in post-thaw fish sperm, and coral and oyster larvae (Cirino et al., 2021; Díaz et al., 2021; Zhu et al., 2023).
This review aims to update the recent development in lipid manipulation in the cryopreservation of sperm (both mammalian and aquatic species), oocytes (mammalian species), embryos (mammalian species), and larvae (aquatic species) and explore their applications to overcome some key challenges in aquatic species. In this review, we first discuss the effect and modification of lipids in the sperm, oocyte and embryo cryopreservation in mammalian species. We then present the recent development of lipid manipulation in cryopreservation of aquatic species. Finally we draw conclusions and propose future studies in aquatic species.
2 The effect of lipids on sperm cryopreservation in mammalian species
2.1 Current status of sperm cryopreservation
The cryopreservation of sperm is a reliable technique and has been extensively used in human ART and genetic germplasm conservation in livestock, and pet animals (Mandal et al., 2014).
This technique, however, has also showed several adverse effects on post-thaw spermatozoa, such as a decrease in motility and viability, the increase of single-strand DNA lesions, and the elevation of abnormal morphological characteristics (O’Connell et al., 2002; Ozkavukcu et al., 2008; Figueroa et al., 2019). It has been found that fluidity and permeability of the membrane serve a significant part in sperm cryoresistance and are related to the nature and percentage of PLs, polyunsaturated fatty acids (PUFAs), and cholesterol (Waterhouse et al., 2006; Oldenhof et al., 2012).
2.2 Plasma membrane lipid composition and sperm quality
Membrane lipid composition is diverse among organisms, cell type, organelle, membrane, bilayer-leaflet, and membrane subdomain levels (Harayama and Riezman, 2018). Compared with other tissues, spermatozoa membrane lipid is characterized by high PUFAs, especially dipolyunsaturated fatty acid (Bell et al., 1997; Fang et al., 2016). Given the low LPT temperature of PUFAs and the kinks of double bonds in PUFAs hindering the acyl chains from packing, PUFAs increase overall membrane fluidity (Israelachvili et al., 1980; Sieme et al., 2015). In addition to the small size of spermatozoa, the high quantity of PUFAs also contributes to better cryoresistance in spermatozoa than in oocytes and embryos.
Spermatozoa membrane lipid composition varies between individuals, ages, and seasons (Kelso et al., 1996; Cerolini et al., 1997; Argov-Argaman et al., 2013a Argov-Argaman et al., 2013b). These variations contribute to the difference in fresh sperm quality including motility and viability. In boar semen, for example, several lipid parameters such as the total lipid content, cholesterol, PL, n-3 PUFAs and Docosahexaenoic acid (DHA) are positively associated with sperm quality. On the other hand, saturated fatty acids (SFAs) and the ratio of n-6 to n-3 PUFAs are negatively correlated with sperm quality (Am-in et al., 2011). Similarly, in humans, PUFAs (especially DHA) play a significant role in normal sperm motility, concentration, and morphology, whereas monounsaturated fatty acids (MUFAs) adversely influence the quality parameters (Aksoy et al., 2006; Andersen et al., 2016).
2.3 Effect of cryopreservation on sperm lipid composition
The alteration of lipid composition in sperm can result in irreversible damage to the cellular membrane and the shift of cell homeostasis after cryopreservation (Schiller et al., 2000; Maldjian et al., 2005). In general, the changes can be summarized as follows: First, PUFAs and SFAs are commonly decreased and increased respectively as the result of lipid peroxidation after sperm cryopreservation in humans and domestic mammalians (Alvarez and Storey, 1992; Schiller et al., 2000; Maldjian et al., 2005). Second, cryopreservation can reduce cholesterol levels (Cerolini et al., 2001). Cholesterol plays a vital role in the structure and function of cell membranes, including the stability, permeability and fluidity of the cell membrane and the microenvironment of membrane proteins (Crockett, 1998; Partyka et al., 2016; Zhang et al., 2019). The loss of cholesterol can trigger the degeneration of the plasma membrane and apoptosis (Aitken, 2011). Third, the alteration of PLs composition and the translocation of membrane PLs have been found in frozen-thawed sperm (Schuffner et al., 2001; Fang et al., 2016). Normally, the distribution of PLs on the bilayer of the cellular membrane is asymmetric. For example, phosphatidylserine (PS), phosphatidylinositol (PI), and phosphatidylethanolamine (PE) are primarily distributed on the cytoplasmic leaflet, while Phosphatidylcholine (PC) and sphingomyelin (SM) are mainly located on the outer leaflet (Quinn, 2004; Fadeel and Xue, 2009).
The asymmetric distribution of PLs plays an important role in maintaining the physiological function of cells. Some specific proteins (e.g. protein kinase C, annexin, membrane skeletal proteins) are distributed on the cytoplasmic side by binding with PS (Manno et al., 2002). In the frozen-thawed sperm of ram, a significant reduction of PLs (PS, PI, PE, PG), an increase of diphosphatidylglycerol (DPG), and translocation of PLs between the cytoplasmic and outer layers were observed (Fang et al., 2016). Additionally, externalizations of PS and DPG were detected in cryopreserved ram sperm (Hinkovska-Galcheva et al., 1989; Schuffner et al., 2001). Hinkovska-Galcheva et al. (1989) suggested that the externalization of DPG inhibited the subsequent acrosome reaction and eventually impaired the fertilization capacity of sperm.
2.4 Lipid manipulation and sperm cryopreservation
Maintaining the lipid composition (PUFAs, cholesterol, PLs) and the physiological function of the membrane has been one of the key methods to improve the success rate of spermatozoa cryopreservation in many investigations (Ferreira et al., 2018). Egg yolk has become a common ingredient in the sperm extender in many mammalian species due to its protective influence (Anzar et al., 2019), which was first identified in bull semen (Phillips and Lardy, 1940). Despite the complex composition of egg yolks, low-density lipoprotein (LDL) plays a significant role in protecting spermatozoa in cryopreservation (Prapaiwan et al., 2015). In addition to egg yolk and LDL, other lipids, such as FAs and cholesterol, can also protect sperm during cryopreservation (Purdy and Graham, 2004; Moore et al., 2005; Hossain et al., 2007).
Three main strategies are currently used to modify the lipid compositions to counteract the cryopreservation stress in mammalian sperm. The first is the supplementation of PUFAs in diet, which has enhanced the performance of post-thaw sperm in a few species, such as water buffalo, and goat (Souza et al., 2019; Silva et al., 2020). The second is the in vitro sperm incubation with exogenous lipids to improve sperm cryotolerance in humans, bovine, and swine (He et al., 2001; Röpke et al., 2011; Ferreira et al., 2018). The third and most common strategy is the supplementation of exogenous lipids in the cryopreservation extender, which can provide almost instantaneous protection from cold shock and freezing to increase the sperm cryoresistance (Quinn et al., 1980; He et al., 2001; Vireque et al., 2016).
While the mechanism of freezing protection of sperm by exogenous lipids is not fully understood, some theories have been proposed. First, a “loose interaction” between PL and membrane bilayers was inferred by Quinn et al. (1980) and Simpson et al. (1987) in ram and boar sperm, where the protection of PC against the cold shock was instantaneous and could be readily disrupted by a gentle wash. Second, the adhesion of PL micelles to and possible formation of PL protective film on the membrane surface have been suggested for the improvement of sperm cryotolerance in many studies (Ricker et al., 2006; Zhang et al., 2009; Vireque et al., 2016). Third, the monomeric transfer and the fusion between the liposome and sperm membrane bilayers were detected by Gadella et al. (1999) using 6-(7-nitrobenz-2-oxa-1,3-diazol-4-yl) amino-caproyl (C6NBD) labeled PLs in boar. The incorporation of exogenous lipids into the sperm has also been confirmed by 14C-labeled FAs and octadecyl rhodamine B (Neill and Masters, 1972; Vasquez and Roldan, 1997). Therefore, the properties of the sperm membrane can be modified by manipulating exogenous lipids, which could improve sperm cryotolerance.
It is worth noting that the application of antioxidants in combination with exogenous lipids could enhance sperm cryoresistance by neutralizing the ROS produced during cryopreservation as lipids (especially PUFAs) are highly susceptible to this chemical (Ortega Ferrusola et al., 2009; Towhidi and Parks, 2012; Towhidi et al., 2013).
3 The impact of lipids on oocyte and embryo cryopreservation in mammalian species
3.1 Current status of oocyte and embryo cryopreservation
Aside from maternal genetic material, oocytes provide essential nutrients, energy, and mitochondria for subsequent development after fertilization (Kopeika et al., 2015). On the other hand, the embryo contain genetic material from both maternal and paternal sides. Compared to sperm, the larger size of oocytes and embryos reduces their cryotolerance (Pai et al., 2021). As oocytes and embryos share similarities in freezing sensitivity, the methods to modify their lipid compositions are discussed together.
After decades of effort, the viability of post-thaw oocytes and embryos in mammalians has improved substantially (Tharasanit and Thuwanut, 2021). In human, for example, the live birth rates of cryopreserved embryos and oocytes reach 41% and 32%, respectively (Fraison et al., 2023). In bovine, this rate has been improved to the fresh control level of 53% (Gómez et al., 2020). Compared with sperm, the substantially lower surface-to-volume ratio and higher cytoplasmic lipid content in oocytes and embryos also contribute to their higher cryosensitivity in mammalians (Amstislavsky et al., 2019). In oocytes and embryors, lipids also aggregate into LDs, which are often structurally bound to key cellular organelles, such as mitochondrion and endoplasmic reticulum (ER), cytoskeleton (microfilaments and microtubules), and cellular membrane (Guo et al., 2009; Zhou and Li, 2009). While this structural association has yet to be fully understood, the cluster of LDs, ER, and mitochondrion facilitate lipid metabolism (Guo et al., 2009). LDs normally contain triglycerides, PLs, sterols, and FAs (Dunning et al., 2014) and serve as an energy resource during the development of oocytes and embryos (Romek et al., 2011; Amstislavsky et al., 2019). The phase separation and further consolidation of LDs during cryopreservation could reduce the chance of successful cryopreservation of oocytes and embryos (Amstislavsky et al., 2019).
3.2 Lipid composition and cryopreservation of oocytes and embryos
Similar to sperm, the occurrence of LTP during cryopreservation is the primary source of cryodamage of oocytes and embryos (Arav et al., 1996; Amstislavsky et al., 2019), which can change the properties of cellular membrane and disrupt its function (Quinn, 1985; Van Meer et al., 2008). Changes in lipid composition have also been observed in post-thaw oocytes and embryos. For instance, in comparison with the fresh controls, the levels of certain PLs were significantly lower in post-thaw bovine embryos (three lysophosphatidylcholines; Janati Idrissi et al., 2021) and mouse oocytes [phosphatidic acid (PA), lysophosphatidic acid (LPA), lysophosphatidylglycerol (LPG); Jung et al., 2021].
Lipid content and composition in oocytes and embryos are species-specific and important for assessing their quality and potential cryotolerance (Pereira and Marques, 2008). Empirically, those species with lipid-rich oocytes and embryos (e.g. pig and domestic cat) have poor cryoresistance (Nagashima et al., 1999; Pereira and Marques, 2008; Galiguis et al., 2014). However, a higher proportion of unsaturated lipids can also produce a better cryosurvival rate due to lower LPT temperatures (Amstislavsky et al., 2019). For example, in comparison with bovine or ovine, the higher survival rate of cryopreserved embryos of domestic cats is attributed to being richer in unsaturated lipids (Pope, 2014; Amstislavsky et al., 2019). Lipid compositions also vary among breeding strains, individuals and seasons (Zeron et al., 2001; Sudano et al., 2012). For example, by using the MALDI-MS/MS laser-induced fragmentation technique (LIFT), Sudano et al. (2012) revealed that embryos of Simmental subspecies showed a better cryosurvival rate than Nellore subspecies due to significant differences in particular PCs [e.g. PC(32:0), PC (34:1), PC (34:2) and PC (36:5)], and suggested these PCs be used as biomarkers to predict the outcome of cryopreservation.
3.3 Lipid modification and oocyte and embryo cryopreservation
The modification of lipid composition is an important strategy to further improve the cryopreservation technique in mammalian oocytes and embryos in recent years. The main strategies include: 1) nutritional management; 2) delipidation using mechanical methods; 3) delipidation using chemical methods; and 4) cholesterol level modification. They are summerized in Table 1.
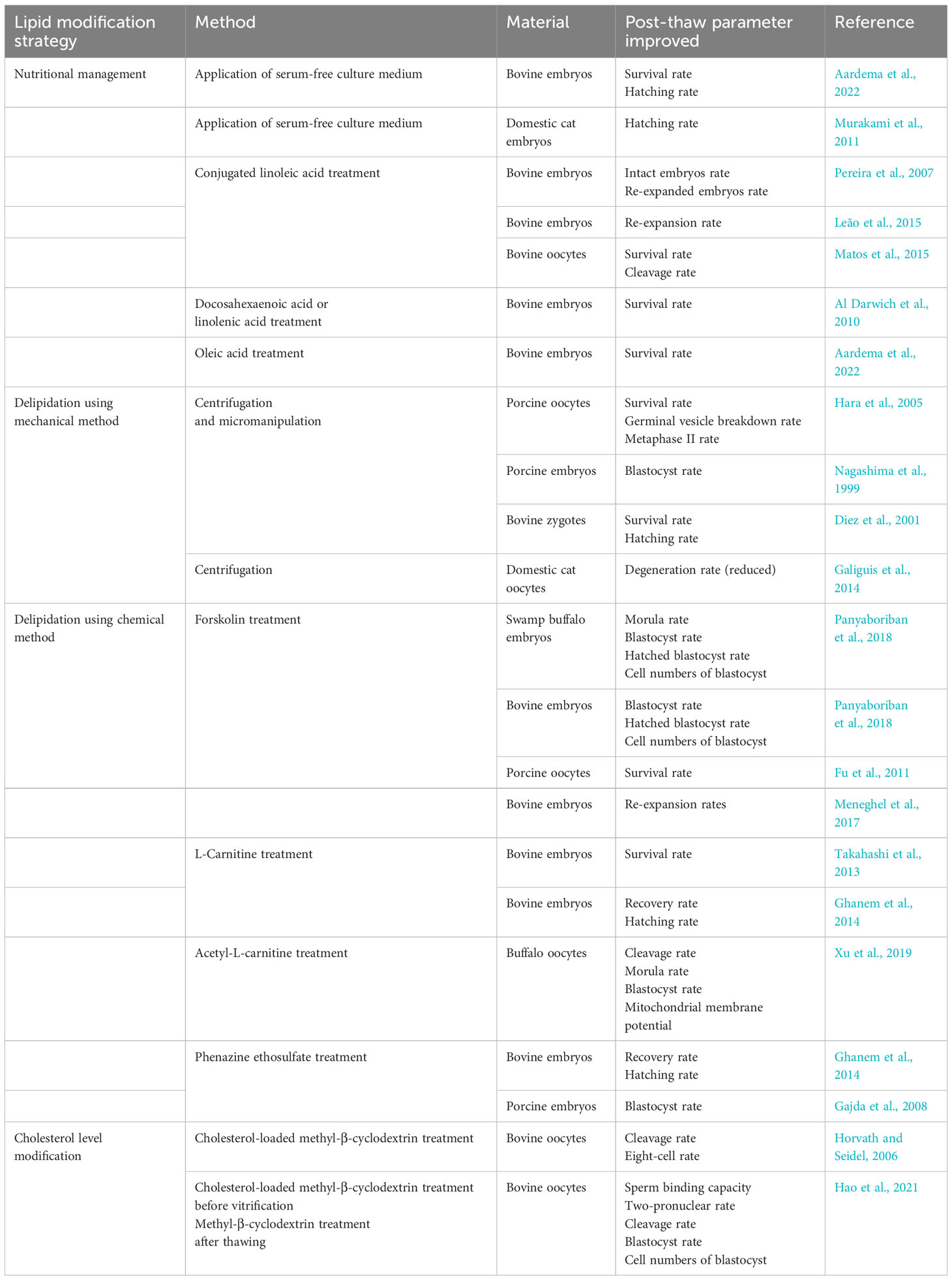
Table 1 Lipid modification for improving the cryopreservation of oocytes and embryos in mammalian species.
3.3.1 Nutritional management
The lipid composition of a female diet can affect the quality of oocytes and embryos (Childs et al., 2008a; Childs et al., 2008b; Wonnacott et al., 2010), which can affect their resistance to freezing. For instance, a diet with the addition of PUFAs can increase the quality of oocytes in ewes (Zeron et al., 2002). The increase of long-chain PUFA content in the follicle component could lower the midpoint of the LPT temperature of sheep oocytes and result in a better membrane integrity rate after chilling (Zeron et al., 2002).
As lipid is the primary energy resource for oocytes and embryos, the lipid content can be reduced if the nutrient restriction is applied in vitro (Abe et al., 2002). Sudano et al. (2011) also found that when a high fetal calf serum (FCS) concentration was used in the culture medium, LDs accumulated in both fresh and post-thaw bovine embryos, leading to a lower re-expansion rate after vitrification. Compared to the whole sheep serum, delipidated sheep serum in the culture medium could reduce the LDs content of ovine cumulus-oocyte complexes (Barrera et al., 2018). The application of serum-free culture medium has improved the survival rates of post-thaw bovine and domestic cat embryos (Abe et al., 2002; Murakami et al., 2011).
Some FAs have also been supplemented in the in vitro culture medium to improve the cryosurvival of mammalian oocytes and embryos by altering their lipid compositions (Al Darwich et al., 2010; Leão et al., 2015; Aardema et al., 2022). For example, conjugated linoleic acid (CLA) could reduce the accumulation of lipids to large- and medium-size LDs by inhibiting the expression and activities of stearoyl–CoA desaturase and lipoprotein lipase (Pariza et al., 2001). CLA could also improve membrane fluidity by incorporation between CLA fatty acyl residues and SM or PC (Leão et al., 2015), and it has a better free radical scavenging property than linoleic acid or methyl linoleate (Fagali and Catalá, 2008). Due to these three functions, CLA-treated bovine embryos showed a higher survival rate after cryopreservation than the untreated control (Pereira et al., 2007; Leão et al., 2015). In addition to CLA, the supplementation of linolenic acid, docosahexaenoic acid (DHA), and oleic acid in the in vitro medium could also improve the cryosurvival of bovine embryos (Al Darwich et al., 2010; Karaşahin, 2019; Aardema et al., 2022).
3.3.2 Delipidation using mechanical methods
The mechanical approach is an option to remove lipids in lipid-rich oocytes and embryos (Nagashima et al., 1994; Nagashima et al., 1999). LDs can be extruded by centrifugation under a hypertonic condition or removed by micromanipulation after polarization by centrifugation. Both methods have significantly improved the post-thaw survival rate and further developmental capacity of porcine oocytes, embryos, and domestic cat zygotes (Hara et al., 2005; Karja et al., 2006; Nagashima et al., 1994). Furthermore, when the lipid polarization method was used in domestic cat oocytes, the cryosurvival rate was improved in partially polarized cat oocytes (Galiguis et al., 2014). In contrast, the developmental competence was compromised in fully polarized oocytes, probably due to the adverse effect of lipid redistribution (Galiguis et al., 2014).
3.3.3 Delipidation using chemical methods
In comparison with mechanical delipidation, the in vitro chemical treatment is a more prevalent lipid modification method in cryopreservation of oocytes and embryos. Forsklin, L-carnitine, and Phenazine ethosulfate are the main chemicals used in the culture medium to enhance lipolysis (Borges and Vireque, 2019).
Forskolin
Forskolin triggers adenylate cyclase, which can raise the cyclic adenosine monophosphate (cAMP) level and induce lipolysis (Paschoal et al., 2016). When 10 μM forskolin was applied in the medium for in vitro maturation, the development competence of porcine oocytes was not impaired and showed higher resistance against cryopreservation (Fu et al., 2011). Likewise, after incubation with 5.0 μM forskolin for 24 hours, bovine embryos had less lipid and greater cryotolerance (Meneghel et al., 2017). Besides diminishing lipid content, forskolin could also attenuate cytoskeleton actin filament damages caused by vitrification in bovine oocytes (Meneghel et al., 2017).
L-carnitine
In animal cells, the primary role of L-carnitine is to transfer long-chain FAs across the inner mitochondrial membrane for the subsequent β-oxidation. It is, therefore, an enhancer of lipid metabolism (Longo et al., 2016; Borges and Vireque, 2019). L-carnitine supplementation could increase the rate of zygote development to the blastocyst stage and improve the survival rate after cryopreservation in bovine (Takahashi et al., 2013). L-carnitine could also reduce ROS formation during vitrification (Sprícigo et al., 2017). Thus, the supplementation of L-carnitine could not only lower the density of LDs and modify the PL composition, but it could also enhance the physiological function of mitochondria (Somfai et al., 2011; Xu et al., 2019).
Phenazine ethosulfate
Phenazine ethosulfate (PES) can oxidize nicotinamide adenine dinucleotide phosphate hydrogen to nicotinamide adenine dinucleotide phosphate and arouse the pentose-phosphate pathway to generate more Adenosine triphosphate (ATP) through glucose metabolism (De La Torre-Sanchez et al., 2006; Barceló-Fimbres and Seidel, 2007). The lipid accumulation in bovine and porcine embryos could be inhibited when incubated with PES (De La Torre-Sanchez et al., 2006; Gajda et al., 2011). Ghanem et al. (2014) found that PES-treated bovine embryos showed enhanced cryotolerance.
3.3.4 Cholesterol level modification
A high ratio between cholesterol and PL in the cytoplasm membrane is usually associated with higher membrane fluidity, especially at low temperatures, meaning that cholesterol content in the membrane bilayer can affect the cryotolerance of oocytes (Horvath and Seidel, 2006). Methyl-β-cyclodextrin (MBC) is a water-soluble cyclic heptasaccharide that can be used to deliver hydrophobic substances, such as cholesterol or FAs, through its hydrophobic cylindrical cavity (Brewster and Loftsson, 2007). Horvath and Seidel (2006) showed that Cholesterol-loaded Methyl-β-cyclodextrin (CLC) treated oocytes had a better cleavage and 8-cells rate compared to untreated oocytes in bovine vitrification, although the advantage was not apparent in subsequent development. Conversely, Arcarons et al. (2017) found that CLC treatment did not significantly affect the cleavage and blastocyst rate in the same species. Nevertheless, the expression of some development-related genes (e.g. DNMT3A and BAX) indicates that oocytes treated by CLC could have a better quality, especially when they are vitrified at the germinal vesicle stage (Arcarons et al., 2017).
4 Lipid manipulation in cryopreservation of aquatic species
4.1 Current status of cryopreservation of gametes, embryos and larvae
The first successful fish sperm cryopreservation in aquatic species was reported in 1953 (Blaxter, 1953), almost at the same time as that of domestic mammalians. However, despite sperm cryopreservation having become a lucrative global industry in the livestock sector, it has not been widely applied to commercially important aquatic species (Tiersch et al., 2007; Migaud et al., 2013). The development of cryopreservation technique in oocytes, embryos, and larvae in aquatic species is still in its early stages, and has focused on the selection of cryoprotectant, optimization of freezing rate, and assessment of developmental stages suitable for freezing (Martínez-Páramo et al., 2017). The potential application of cryopreservation in the aquaculture industry is significant, as it could resolve key issues during seed production, such as the unbalanced sex ratio of broodstock, asynchronous sexual maturation, long-distance broodstock transportation, and seasonal constraints. This technique could also play an essential role in germplasm resource protection of rare breeding varieties and endangered species, especially in the face of natural disasters, environmental pollution, and disease outbreak (Liu et al., 2020a; Díaz et al., 2021).
To date, hundreds of fish sperm cryopreservation protocols have been reported and comprehensively reviewed by Cabrita et al. (2010), Tsai and Lin (2012), and Martínez-Páramo et al. (2017). Sperm cryopreservation techniques have also been developed for most farmed and some ecologically important aquatic invertebrates (Diwan et al., 2020). The freezing of oocytes, embryos, and larvae in aquatic organisms is more challenging than that of spermatozoa. In comparison with sperm, the additional challenges are their poor membrane permeability, large size, high lipid content, and high sensitivity to temperature shock (Martínez-Páramo et al., 2017; Diwan et al., 2020). Although studies on cryopreservation of early-stage oocytes (Tsai et al., 2009), ovarian tissue fragments (Anil et al., 2011), and primordial germ cells or a genital ridge (Kobayashi et al., 2007; Higaki et al., 2010; Inoue et al., 2012) have occurred in some fish species, these methods are subject to the success of subsequent in vitro maturation or transplantation. Compared with fish, the cryopreservation of oocytes, embryos and larvae of aquatic invertebrates is more promising due to their holoblastic cleavage, relatively less egg lipid content, and smaller size (Martínez-Páramo et al., 2017). To date, studies on larval cryopreservation have been reported in crustaceans (Subramoniam and Newton, 1993; Huang et al., 2017; Diwan et al., 2020) and echinoderms (Paredes, 2016; Dupré and Carvajal, 2019), and successful cryopreservation of oocytes, embryos and/or larvae has been published in mollusks (Tervit et al., 2005; Liu and Li, 2015; Liu et al., 2020b; Heres et al., 2021) and corals (Daly et al., 2018), although the post-thaw oocyte survival rates were low (Tervit et al., 2005; Liu and Li, 2015). Progresses of molluscan larval cryopreservation have been summarized recently by Yang and Huo (2022).
4.2 Lipid modification and cryopreservation
As with livestock, the relationship between gamete or embryo quality and lipid composition has been established in many aquatic species, especially those of commercial importance (Mansour et al., 2011; Beirão et al., 2012; Glandon et al., 2016; Díaz et al., 2018). However, studies on lipid composition and cryopreservation are limited in aquatic species. Based on mammalian studies, it can be assumed that membrane fluidity, permeability, and lipid composition can play a similar role in the cryosurvival of gametes, embryos and larvae in aquatic animals, which has been demonstrated recently in Pacific oysters (Zhu et al., 2023). Therefore, lipid manipulations could enhance cryotolerance in aquatic species.
4.2.1 Lipid modification through nutritional management
To improve gamete quality, gamete lipid modification through dietary manipulation during broodstock conditioning is common in aquatic species (Helm et al., 1973; Fernández-Palacios et al., 1997; Asturiano et al., 2001; Ehteshami et al., 2011; Diogo et al., 2015; Valdebenito et al., 2015). Promising results have been reported in a few studies related to sperm or oocyte cryotolerance. For instance, a fish oil-supplement diet for broodstocks of Prochilodus lineatus led to a higher amount of total PUFAs, n-6 PUFAs, and long-chain PUFAs, and a lower amount of total MUFAs in the embryos. Those embryos also presented better permeability to cryoprotectants (1,2-Propanediol; Costa et al., 2018). Bivalves usually do not have sufficient capability to elongate and desaturate short-chain saturated FAs to long-chain PUFAs (de Moreno et al., 1976; de Moreno et al., 1977; Helm et al., 1991). Hence dietary lipid profiles can significantly affect the composition of FAs in bivalves (Langdon and Waldock, 1981; Dudognon et al., 2014). In Pacific oysters, when the broodstock was fed with microalgae containing a high fraction of PUFAs during cold preconditioning at 9 °C, the fertilization rate of post-thaw oocytes was significantly increased in comparison with the control (Adams et al., 2013). These authors have proposed that the absolute content of PUFAs, rather than the ratios between PUFAs and MUFAs or SFAs, plays a vital role in cryotolerance of post-thaw oocytes.
4.2.2 Lipid modification and sperm cryopreservation
In addition to lipid modification through diet, there have been a few examples of improving sperm tolerance by supplementary FAs, cholesterol, and LDLs in fish sperm extenders (Table 2). According to Lahnsteiner et al. (2009), when FAs (including palmitic acid, arachidonic acid, linoleic acid, and arachidic acid) were added to the rainbow trout (Oncorhynchus mykiss) sperm motility-inhibiting extenders, the motility rate and the average path velocity were improved after 72 h storage at 4°C. However, their cryotolerance was not enhanced in the same study. On the contrary, when the arachidonic acids were used in the freezing medium of Atlantic salmon (Salmo salar), the membrane integrity and fertility rate of post-thaw sperm were significantly increased (Díaz et al., 2021). It is worth mentioning that the protective effect of egg yolk in the extender in the study by Lahnsteiner et al. (2009) was likely to be veiled by its LDL component (Pérez-Cerezales et al., 2010). The impact of cholesterol in sperm cryopreservation is species-specific. Its addition had no positive effect on the viability of cryopreserved sperm in S. Salar (Díaz et al., 2021), whereas showed significant improvement in cryoresistence in the common carp (Cyprinus carpio) when was used at a dose of 1.5 mg cholesterol per 120 × 106 spermatozoa (Yildiz et al., 2015).
4.2.3 Lipid modification and oocyte and larval cryopreservation
Total lipid extracts from aquatic invertebrates present cryoprotective abilities when they were added into the medium to cryopreserve molluscan primary larval cells (Odintsova et al., 2001; Odintsova et al., 2006; Kostetsky et al., 2008). For example, the post-thaw survival rate of Mytilus trossulus trochophore larval cells was increased from 5% to 13% by the addition of lipid extract of Crenomytilus grayanus, which was further improved to 35% when antioxidants (vitamin C and vitamin E) were supplemented (Kostetsky et al., 2008). The lipid profile analysis revealed that the addition of lipid extracts and antioxidants effectively increased percentages of MUFAs, PUFAs, n-3 PUFAs, n-6 PUFAs, and the unsaturation index and reduced the percentage of SFAs in the post-thaw larval cells (Kostetsky et al., 2008).
Attempts have also been made to alter the lipid composition of oocytes and larvae to improve cryoresistance. For example, Salinas-Flores et al. (2008) cultured the oocytes of M. gigas with CLC and MBC to increase and decrease the cholesterol level in the oocyte, respectively. Although the incorporation of cholesterol in oocytes was confirmed by fluorescence assessment, the treated and untreated oocytes showed similar post-thaw fertilization rates. In corals, Cirino et al. (2021) reported a methodology to improve the cryoresistance of coral larvae by adding exogenous lipids and gold nanoparticles to the vitrification solution. The vitality rate of vitrified Seriatopora caliendrum larvae was increased by erucic acid liposomes, whereas the settlement rate of vitrified Pocillopora verrucosa larvae was enhanced by PE liposomes. In addition, the survival rate of post-thaw M. gigas larvae was significantly improved by including 1-palmitoyl-2-oleoyl-sn-glycero-3-phosphocholine (POPC) and α-tocopherol in the cryopreservation medium (Zhu et al., 2023).
5 Conclusions and future research in aquatic species
The development of oocyte, embryo and larvae cryopreservation techniques is still in its early stages in aquatic organisms, although progresses have been made in some species, especially in bivalves. In comparison with mammalians, aquatic species possess even higher intracellular lipid content in oocytes, embryos and early-stage larvae (prior to feeding), which means that the cryopreservation of these materials might be more challenging. Therefore, the introduction of lipid modification might be a cornerstone in the development of cryopreservation techniques in aquatic species. The following will be the primary aspects the future studies should focus on.
While the relationship between lipid composition and gamete quality and/or development capability has been investigated in some species, most of these studies have not been focused on cryopreservation. Therefore, investigations on the relationship between specific lipid composition and cryotolerance would be a key research priority, which could be achieved by manipulating nutritional ingredients. Theoretically, when broodstock are fed with a diet rich in PUFAs during gametogenesis, they are likely to yield gametes with lower LPT, thus enhancing the cryoresistance of both gametes produced and the resulting embryos and larvae.
Given its effectiveness in larvae cryopreservation in some bivalve and coral species, the application of exogenous lipids (e.g. PLs, and lipid extract from hydrobiontes) in the cryoprotectant is likely to offer a new strategy to optimize existing or develop new larvae cryopreservation techniques. Due to the hydrophobic nature of exogenous lipids, they usually present in the form of liposomes in extenders or cryoprotectants. Their particle size and other properties could affect their interaction with plasma membrane and subsequently the cryopreservation outcomes. For example, sonicating the extender containing egg yolk has resulted in smaller liposomes and better post-thaw motility in donkey sperm compared to that without the treatment (Zhang et al., 2018). In the study by Cirino et al. (2021), the application of gold nanoparticles played a significant role in the success of coral larval cryopreservation because gold nanoparticles can change the biophysic features of liposomes such as zeta potential, temperature of LPT (Mady et al., 2012). Therefore, understanding the biophysical characteristics of liposomes and their effects on the cryopreserved materials will be beneficial for the further improvement of cryopreservation techniques.
Furthermore, partial removal of yolk through micromanipulation would be worth trying to improve the cryosurvival of oocytes, embryos, and larvae in aquatic animals when their cryopreservation has been investigated by optimizing other parameters. This method may not only hold significance academically but could also be critical to establish cryopreservation techniques, although the application of this technique might be limited to specific requirements such as gene banking and difficult to meet the quantity demands for commercial hatchery production. With the development of extended in vitro oocyte culture techniques in aquatic species, chemical delipidation and in virto lipid modulation could become practicable. This method would have broader applications than micromanipulation, since it could manipulate a larger quantity of materials.
As the vitrification method has been routinely used in the cryopreservation of oocyts and embryos in some mammalian species, including lipid-rich materials (Amstislavsky et al., 2019; Du et al., 2021; Tharasanit and Thuwanut, 2021), and has also been successfully applied in a couple of coral species (Daly et al., 2018; Narida et al., 2023), the integratation of lipid modification and vitrification is likely to become a novel technological pathway for the cryopreservation of oocytes, embryos, and larvae in aquatic organisms.
Author contributions
XZ conceived and developed the idea and prepared the draft of the manuscript. YZ, YL, and YT helped for the collection of references and provided comments and suggestions to improve the manuscript. PM-E, JQ, and XL have critically gone through the draft and finalized the manuscript. All authors contributed to the article and approved the submitted version.
Acknowledgments
Mr. XZ is grateful for the financial support of the Australian Government Research Training Program Scholarship (AGRTPS) for his PhD study at Flinders University. Dr. YZ acknowledges the financial support provided by the Overseas Training Program for Colleges and Universities of Liaoning Province (2020GJWYB017).
Conflict of interest
The authors declare that the research was conducted in the absence of any commercial or financial relationships that could be construed as a potential conflict of interest.
Publisher’s note
All claims expressed in this article are solely those of the authors and do not necessarily represent those of their affiliated organizations, or those of the publisher, the editors and the reviewers. Any product that may be evaluated in this article, or claim that may be made by its manufacturer, is not guaranteed or endorsed by the publisher.
References
Aardema H., Bertijn I., Van Tol H., Rijneveld A., Vernooij J., Gadella B. M., et al. (2022). Fatty acid supplementation during in vitro embryo production determines cryosurvival characteristics of bovine blastocysts. Front. Cell Dev. Biol. 10. doi: 10.3389/fcell.2022.837405
Abe H., Yamashita S., Satoh T., Hoshi H. (2002). Accumulation of cytoplasmic lipid droplets in bovine embryos and cryotolerance of embryos developed in different culture systems using serum-free or serum-containing media. Mol. Reprod. Dev. 61, 57–66. doi: 10.1002/mrd.1131
Adams S. L., Salinas-Flores L., Lim M. H. (2013). Diet conditioning of Pacific oyster, Crassostrea gigas , broodstock to improve oocyte cryopreservation success. J. Shellfish Res. 32, 391–399. doi: 10.2983/035.032.0219
Aitken R. J. (2011). The capacitation-apoptosis highway: oxysterols and mamMalian sperm function. Biol. Reprod. 85, 9–12. doi: 10.1095/biolreprod.111.092528
Aksoy Y., Aksoy H., Altınkaynak K., Aydın H. R., Özkan A. (2006). Sperm fatty acid composition in subfertile men. Prostaglandins Leukot. Essent. Fatty Acids 75, 75–79. doi: 10.1016/j.plefa.2006.06.002
Al Darwich A., Perreau C., Petit M. H., Papillier P., Dupont J., Guillaume D., et al. (2010). Effect of PUFA on embryo cryoresistance, gene expression and AMPKα phosphorylation in IVF-derived bovine embryos. Prostaglandins Other Lipid Mediators 93, 30–36. doi: 10.1016/j.prostaglandins.2010.06.002
Alvarez J. G., Storey B. T. (1992). Evidence for increased lipid peroxidative damage and loss of superoxide dismutase activity as a mode of sublethal cryodamage to human sperm during cryopreservation. J. Androl. 13, 232–241.
Am-in N., Kirkwood R. N., Techakumphu M., Tantasuparuk W. (2011). Lipid profiles of sperm and seminal plasma from boars having normal or low sperm motility. Theriogenology 75, 897–903. doi: 10.1016/j.theriogenology.2010.10.032
Amstislavsky S., Mokrousova V., Brusentsev E., Okotrub K., Comizzoli P. (2019). Influence of cellular lipids on cryopreservation of mamMalian oocytes and preimplantation embryos: a review. Biopreserv. Biobank. 17, 76–83. doi: 10.1089/bio.2018.0039
Andersen J. M., Rønning P. O., Herning H., Bekken S. D., Haugen T. B., Witczak O. (2016). Fatty acid composition of spermatozoa is associated with BMI and with semen quality. Andrology 4, 857–865. doi: 10.1111/andr.12227
Anil S., Zampolla T., Zhang T. (2011). Development of in vitro culture method for zebrafish ovarian tissue fragment. Cryobiology 63, 311–312. doi: 10.1016/j.cryobiol.2011.09.025
Anzar M., Rajapaksha K., Boswall L. (2019). Egg yolk-free cryopreservation of bull semen. PLoS One 14, e0223977. doi: 10.1371/journal.pone.0223977
Arav A., Zeron Y., Leslie S. B., Behboodi E., Anderson G. B., Crowe J. H. (1996). Phase transition temperature and chilling sensitivity of bovine oocytes. Cryobiology 33, 589–599. doi: 10.1006/cryo.1996.0062
Arcarons N., Morató R., Vendrell M., Yeste M., López-Bejar M., Rajapaksha K., et al. (2017). Cholesterol added prior to vitrification on the cryotolerance of immature and in vitro matured bovine oocytes. PLoS One 12, e0184714. doi: 10.1371/journal.pone.0184714
Argov-Argaman N., Mahgrefthe K., Zeron Y., Roth Z. (2013a). Season-induced variation in lipid composition is associated with semen quality in Holstein bulls. Reproduction 145, 479–489. doi: 10.1530/REP-12-0498
Argov-Argaman N., Mahgrefthe K., Zeron Y., Roth Z. (2013b). Variation in lipid profiles within semen compartments—the bovine model of aging. Theriogenology 80, 712–721. doi: 10.1016/j.theriogenology.2013.05.024
Asturiano J. F., Sorbera L. A., Carrillo M., Zanuy S., Ramos J., Navarro J. C., et al. (2001). Reproductive performance in male European sea bass (Dicentrarchus labrax, L.) fed two PUFA-enriched experimental diets: a comparison with males fed a wet diet. Aquaculture 194, 173–190. doi: 10.1016/S0044-8486(00)00515-9
Barberet J., Barry F., Choux C., Guilleman M., Karoui S., Simonot R., et al. (2020). What impact does oocyte vitrification have on epigenetics and gene expression? Clin. Epigenet. 12, 121. doi: 10.1186/s13148-020-00911-8
Barceló-Fimbres M., Seidel G. E. (2007). Effects of either glucose or fructose and metabolic regulators on bovine embryo development and lipid accumulation in vitro. Mol. Reprod. Dev. 74, 1406–1418. doi: 10.1002/mrd.20700
Barrera N., dos Santos Neto P. C., Cuadro F., Bosolasco D., Mulet A. P., Crispo M., et al. (2018). Impact of delipidated estrous sheep serum supplementation on in vitro maturation, cryotolerance and endoplasmic reticulum stress gene expression of sheep oocytes. PLoS One 13, e0198742. doi: 10.1371/journal.pone.0198742
Beirão J., Zilli L., Vilella S., Cabrita E., Fernández-Díez C., Schiavone R., et al. (2012). Fatty acid composition of the head membrane and flagella affects Sparus aurata sperm quality. J. Appl. Ichthyol. 28, 1017–1019. doi: 10.1111/jai.12085
Bell M. V., Dick J. R., Buda Cs. (1997). Molecular speciation of fish sperm phospholipids: Large amounts of dipolyunsaturated phosphatidylserine. Lipids 32, 1085–1091. doi: 10.1007/s11745-997-0140-y
Blaxter J. H. S. (1953). Sperm storage and cross-fertilization of spring and autumn spawning herring. Nature 172, 1189–1190. doi: 10.1038/1721189b0
Borges E. D., Vireque A. A. (2019). Updating the impact of lipid metabolism modulation and lipidomic profiling on oocyte cryopreservation. Euro. Med. J. 4, 79–87. doi: 10.33590/emj/10310074
Brewster M. E., Loftsson T. (2007). Cyclodextrins as pharmaceutical solubilizers. Adv. Drug Deliv. Rev. 59, 645–666. doi: 10.1016/j.addr.2007.05.012
Bunge R. G., Sherman J. K. (1953). Fertilizing capacity of frozen human spermatozoa. Nature 172, 767–768. doi: 10.1038/172767b0
Cabrita E., Sarasquete C., Martínez-Páramo S., Robles V., Beirão J., Pérez-Cerezales S., et al. (2010). Cryopreservation of fish sperm: applications and perspectives: Cryopreservation of fish sperm. J. Appl. Ichthyol. 26, 623–635. doi: 10.1111/j.1439-0426.2010.01556.x
Cerolini S., Kelso K. A., Noble R. C., Speake B. K., Pizzi F., Cavalchini L. G. (1997). Relationship between spermatozoan lipid composition and fertility during aging of chickens. Biol. Reprod. 57, 976–980. doi: 10.1095/biolreprod57.5.976
Cerolini S., Maldjian A., Pizzi F., Gliozzi T. (2001). Changes in sperm quality and lipid composition during cryopreservation of boar semen. Reproduction 121, 395–401. doi: 10.1530/rep.0.1210395
Chatterjee A., Saha D., Niemann H., Gryshkov O., Glasmacher B., Hofmann N. (2017). Effects of cryopreservation on the epigenetic profile of cells. Cryobiology 74, 1–7. doi: 10.1016/j.cryobiol.2016.12.002
Childs S., Carter F., Lynch C. O., Sreenan J. M., Lonergan P., Hennessy A. A., et al. (2008a). Embryo yield and quality following dietary supplementation of beef heifers with n-3 polyunsaturated fatty acids (PUFA). Theriogenology 70, 992–1003. doi: 10.1016/j.theriogenology.2008.06.008
Childs S., Hennessy A. A., Sreenan J. M., Wathes D. C., Cheng Z., Stanton C., et al. (2008b). Effect of level of dietary n-3 polyunsaturated fatty acid supplementation on systemic and tissue fatty acid concentrations and on selected reproductive variables in cattle. Theriogenology 70, 595–611. doi: 10.1016/j.theriogenology.2008.04.002
Cirino L., Tsai S., Wang L.-H., Chen C.-S., Hsieh W.-C., Huang C.-L., et al. (2021). Supplementation of exogenous lipids via liposomes improves coral larvae settlement post-cryopreservation and nano-laser warming. Cryobiology 98, 80–86. doi: 10.1016/j.cryobiol.2020.12.004
Costa R., da S., Souza F. M. S., Senhorini J. A., Bashiyo-Silva C., Verissímo-Silveira R., et al. (2018). Fatty acid influence on Prochilodus lineatus (Characiformes, Prochilodontidae) embryo cryopreservation parameters. Aquac. Res. 49, 2714–2722. doi: 10.1111/are.13732
Crockett E. L. (1998). Cholesterol function in plasma membranes from ectotherms: membrane-specific roles in adaptation to temperature. Am. Zool. 38, 291–304. doi: 10.1093/icb/38.2.291
Daly J., Zuchowicz N., Nuñez Lendo C. I., Khosla K., Lager C., Henley E. M., et al. (2018). Successful cryopreservation of coral larvae using vitrification and laser warming. Sci. Rep. 8, 15714. doi: 10.1038/s41598-018-34035-0
De La Torre-Sanchez J. F., Gardner D. K., Preis K., Gibbons J., Seidel G. E. (2006). Metabolic regulation of in vitro-produced bovine embryos. II. Effects of phenazine ethosulfate, sodium azide and 2,4-dinitrophenol during post-compaction development on glucose metabolism and lipid accumulation. Reprod. Fertil. Dev. 18, 597. doi: 10.1071/RD05064
de Moreno J. E. A., Moreno V. J., Brenner R. R. (1976). Lipid metabolism of the yellow clam, Mesodesma mactroides: 2-Polyunsaturated fatty acid metabolism. Lipids 11, 561–566. doi: 10.1007/BF02532902
de Moreno J. E. A., Moreno V. J., Brenner R. R. (1977). Lipid metabolism of the yellow clam, Mesodesma mactroides: 3-Saturated fatty acids and acetate metabolism. Lipids 12, 804–808. doi: 10.1007/BF02533268
Dhali A. P., Kolte A., Mishra A. C., Roy S., Bhatta R. (2019). “Cryopreservation of oocytes and embryos: current status and opportunities,” in Infertility, Assisted Reproductive Technologies and Hormone Assays. Ed. Sheriff D.S. (London, United Kingdom: IntechOpen). doi: 10.5772/intechopen.81653
Díaz R., Lee-Estévez M., Figueroa E., Ulloa-Rodríguez P., Sepúlveda N., Farias J. G. (2018). Study of the membrane lipid composition of Atlantic salmon ( Salmo salar ) spermatozoa and its relation with semen quality. Aquac. Res. 49, 2603–2607. doi: 10.1111/are.13713
Díaz R., Quiñones J., Short S., Contreras P., Ulloa-Rodríguez P., Cancino-Baier D., et al. (2021). Effect of exogenous lipids on cryotolerance of Atlantic salmon (Salmo salar) spermatozoa. Cryobiology 98, 25–32. doi: 10.1016/j.cryobiol.2021.01.004
Diez C., Heyman Y., Le Bourhis D., Guyader-Joly C., Degrouard J., Renard J. P. (2001). Delipidating in vitro-produced bovine zygotes: Effect on further development and consequences for freezability. Theriogenology 55, 923–936. doi: 10.1016/S0093-691X(01)00454-X
Diogo P., Martins G., Gavaia P., Pinto W., Dias J., Cancela L., et al. (2015). Assessment of nutritional supplementation in phospholipids on the reproductive performance of zebrafish, Danio rerio (Hamilton 1822). J. Appl. Ichthyol. 31, 3–9. doi: 10.1111/jai.12733
Diwan A. D., Harke S. N., Gopalkrishna, Panche A. N. (2020). Cryobanking of fish and shellfish egg, embryos and larvae: an overview. Front. Mar. Sci. 7. doi: 10.3389/fmars.2020.00251
Drobnis E. Z., Crowe L. M., Berger T., Anchordoguy T. J., Overstreet J. W., Crowe J. H. (1993). Cold shock damage is due to lipid phase transitions in cell membranes: A demonstration using sperm as a model. J. Exp. Zool. 265, 432–437. doi: 10.1002/jez.1402650413
Du X., Zhuan Q., Cheng K., Luo Y., Hou Y., Zhu S., et al. (2021). Cryopreservation of porcine embryos: Recent updates and progress. Biopreserv. Biobank. 19, 210–218. doi: 10.1089/bio.2020.0074
Dudognon T., Lambert C., Quere C., Auffret M., Soudant P., Kraffe E. (2014). Mitochondrial activity, hemocyte parameters and lipid composition modulation by dietary conditioning in the Pacific oyster Crassostrea gigas. J. Comp. Physiol. B 184, 303–317. doi: 10.1007/s00360-013-0800-1
Dunning K. R., Russell D. L., Robker R. L. (2014). Lipids and oocyte developmental competence: the role of fatty acids and β-oxidation. Reproduction 148, R15–R27. doi: 10.1530/REP-13-0251
Dupré E., Carvajal J. (2019). Cryopreservation of embryos and larvae of the edible sea urchin loxechinus albus (Molina 1782). Cryobiology 86, 84–88. doi: 10.1016/j.cryobiol.2018.11.005
Ehteshami F., Christianus A., Rameshi H., Harmin S. A., Saad C. R. (2011). The effects of dietary supplements of polyunsaturated fatty acid on pearl oyster, Pinctada margaritifera L., gonad composition and reproductive output: The effects of dietary supplements of PUFA on pearl oyster. Aquac. Res. 42, 613–622. doi: 10.1111/j.1365-2109.2010.02658.x
Fadeel B., Xue D. (2009). The ins and outs of phospholipid asymmetry in the plasma membrane: roles in health and disease. Crit. Rev. Biochem. Mol. Biol. 44, 264–277. doi: 10.1080/10409230903193307
Fagali N., Catalá A. (2008). Antioxidant activity of conjugated linoleic acid isomers, linoleic acid and its methyl ester determined by photoemission and DPPH techniques. Biophys. Chem. 137, 56–62. doi: 10.1016/j.bpc.2008.07.001
Fahy E., Subramaniam S., Murphy R. C., Nishijima M., Raetz C. R. H., Shimizu T., et al. (2009). Update of the LIPID MAPS comprehensive classification system for lipids. J. Lipid Res. 50, S9–S14. doi: 10.1194/jlr.R800095-JLR200
Fang Y., Blair H., Zhong R., Sun H., Zhou D. (2016). Optimizing the freezing rate for ovine semen cryopreservation: phospholipid profiles and functions of the plasma membrane and quality and fertilization of spermatozoa. Small Rumin. Res. 139, 46–51. doi: 10.1016/j.smallrumres.2016.04.012
Fernández-Palacios H., Izquierdo M., Robaina L., Valencia A., Salhi M., Montero D. (1997). The effect of dietary protein and lipid from squid and fish meals on egg quality of broodstock for gilthead seabream (Sparus aurata). Aquaculture 148, 233–246. doi: 10.1016/S0044-8486(96)01312-9
Ferreira G., Costa C., Bassaizteguy V., Santos M., Cardozo R., Montes J., et al. (2018). InCubation of human sperm with micelles made from glycerophospholipid mixtures increases sperm motility and resistance to oxidative stress. PLoS One 13, e0197897. doi: 10.1371/journal.pone.0197897
Figueroa E., Lee-Estevez M., Valdebenito I., Watanabe I., Oliveira R. P. S., Romero J., et al. (2019). Effects of cryopreservation on mitochondrial function and sperm quality in fish. Aquaculture 511, 634190. doi: 10.1016/j.aquaculture.2019.06.004
Fraison E., Huberlant S., Labrune E., Cavalieri M., Montagut M., Brugnon F., et al. (2023). Live birth rate after female fertility preservation for cancer or haematopoietic stem cell transplantation: a systematic review and meta-analysis of the three main techniques; embryo, oocyte and ovarian tissue cryopreservation. Hum. Reprod. 38, 489–502. doi: 10.1093/humrep/deac249
Fu X.-W., Wu G.-Q., Li J.-J., Hou Y.-P., Zhou G.-B., Lun-Suo, et al. (2011). Positive effects of Forskolin (stimulator of lipolysis) treatment on cryosurvival of in vitro matured porcine oocytes. Theriogenology 75, 268–275. doi: 10.1016/j.theriogenology.2010.08.013
Gadella B. M., Miller N. G. A., Colenbrander B., Van Golde L. M. G., Harrison R. A. P. (1999). Flow cytometric detection of transbilayer movement of fluorescent phospholipid analogues across the boar sperm plasma membrane: Elimination of labeling artifacts. Mol. Reprod. Dev. 53, 108–125. doi: 10.1002/(SICI)1098-2795(199905)53:1<108::AID-MRD13>3.0.CO;2-K
Gajda B., Romek M., Grad I., Krzysztofowicz E., Bryla M., Smorag Z. (2011). Lipid content and cryotolerance of porcine embryos cultured with phenazine ethosulfate. Cryo Lett. 32, 349–357.
Gajda B., Smorag Z., Bryla M. (2008). Effect of phenazine ethosulfate on porcine blastocyst development, apoptosis, and cryotolerance after open pulled straw vitrification. Reprod. Fertil. Dev. 20, 118. doi: 10.1071/RDv20n1Ab75
Galiguis J., Gómez M. C., Leibo S. P., Pope C. E. (2014). Birth of a domestic cat kitten produced by vitrification of lipid polarized in vitro matured oocytes. Cryobiology 68, 459–466. doi: 10.1016/j.cryobiol.2014.02.012
Ghanem N., Ha A.-N., Fakruzzaman Md., Bang J.-I., Lee S.-C., Kong I.-K. (2014). Differential expression of selected candidate genes in bovine embryos produced in vitro and cultured with chemicals modulating lipid metabolism. Theriogenology 82, 238–250. doi: 10.1016/j.theriogenology.2014.03.024
Ghetler Y., Yavin S., Shalgi R., Arav A. (2005). The effect of chilling on membrane lipid phase transition in human oocytes and zygotes. Hum. Reprod. 20, 3385–3389. doi: 10.1093/humrep/dei236
Glandon H. L., Michaelis A. K., Politano V. A., Alexander S. T., Vlahovich E. A., Reece K. S., et al. (2016). Impact of environment and ontogeny on relative fecundity and egg quality of female oysters (Crassostrea virginica) from four sites in northern chesapeake bay. Biol. Bull. 231, 185–198. doi: 10.1086/691066
Gómez E., Carrocera S., Martín D., Pérez-Jánez J. J., Prendes J., Prendes J. M., et al. (2020). Efficient one-step direct transfer to recipients of thawed bovine embryos cultured in vitro and frozen in chemically defined medium. Theriogenology 146, 39–47. doi: 10.1016/j.theriogenology.2020.01.056
Gualtieri R., Kalthur G., Barbato V., Di Nardo M., Adiga S. K., Talevi R. (2021). Mitochondrial dysfunction and oxidative stress caused by cryopreservation in reproductive cells. Antioxidants 10, 337. doi: 10.3390/antiox10030337
Guo Y., Cordes K. R., Farese R. V., Walther T. C. (2009). Lipid droplets at a glance. J. Cell Sci. 122, 749–752. doi: 10.1242/jcs.037630
Hao T., Zhang P., Hao H., Du W., Pang Y., Zhao S., et al. (2021). The combination treatment of cholesterol-loaded methyl-β-cyclodextrin and methyl-β-cyclodextrin significantly improves the fertilization capacity of vitrified bovine oocytes by protecting fertilization protein JUNO. Reprod. Dom. Anim. 56, 519–530. doi: 10.1111/rda.13890
Hara K., Abe Y., Kumada N., Aono N., Kobayashi J., Matsumoto H., et al. (2005). Extrusion and removal of lipid from the cytoplasm of porcine oocytes at the germinal vesicle stage: Centrifugation under hypertonic conditions influences vitrification. Cryobiology 50, 216–222. doi: 10.1016/j.cryobiol.2005.01.003
Harayama T., Riezman H. (2018). Understanding the diversity of membrane lipid composition. Nat. Rev. Mol. Cell Biol. 19, 281–296. doi: 10.1038/nrm.2017.138
He L., Bailey J. L., Buhr M. M. (2001). Incorporating lipids into boar sperm decreases chilling sensitivity but not capacitation potential. Biol. Reprod. 64, 69–79. doi: 10.1095/biolreprod64.1.69
Helm M. M., Holland D. L., Stephenson R. R. (1973). The effect of supplementary algal feeding of a hatchery breeding stock of ostrea edulis l. on larval vigour. J. Mar. Biol. Assoc. U. K. 53, 673–684. doi: 10.1017/S0025315400058872
Helm M. M., Holland D. L., Utting S. D., East J. (1991). Fatty acid composition of early non-feeding larvae of the European flat oyster, Ostrea edulis. J. Mar. Biol. Assoc. U. K. 71, 691–705. doi: 10.1017/S0025315400053248
Heres P., Troncoso J., Paredes E. (2021). Larval cryopreservation as new management tool for threatened clam fisheries. Sci. Rep. 11, 15428. doi: 10.1038/s41598-021-94197-2
Higaki S., Eto Y., Kawakami Y., Yamaha E., Kagawa N., Kuwayama M., et al. (2010). Production of fertile zebrafish (Danio rerio) possessing germ cells (gametes) originated from primordial germ cells recovered from vitrified embryos. REPRODUCTION 139, 733–740. doi: 10.1530/REP-09-0549
Hinkovska-Galcheva V., Petkova D., Koumanov K. (1989). Changes in the phospholipid composition and phospholipid asymmetry of ram sperm plasma membranes after cryopreservation. Cryobiology 26, 70–75. doi: 10.1016/0011-2240(89)90034-5
Horvath G., Seidel G. E. (2006). Vitrification of bovine oocytes after treatment with cholesterol-loaded methyl-β-cyclodextrin. Theriogenology 66, 1026–1033. doi: 10.1016/j.theriogenology.2006.03.004
Hossain M. S., Tareq K. M. A., Hammano K.-I., Tsujii H. (2007). Effect of fatty acids on boar sperm motility, viability and acrosome reaction: Fatty acids induce acrosome reaction. Reprod. Med. Biol. 6, 235–239. doi: 10.1111/j.1447-0578.2007.00191.x
Huang Z., Gao L., Hou Y., Zhu S., Fu X. (2019). Cryopreservation of farm animal gametes and embryos: recent updates and progress. Front. Agr. Sci. Eng. 6, 42. doi: 10.15302/J-FASE-2018231
Huang X., Zhuang P., Feng G., Zhao F., Liu J., Zhang T., et al. (2017). Cryopreservation of Chinese mitten crab, Eriocheir sinensis H. Milne Edwards 1853 (Decapoda, Brachyura), embryos by vitrification. Crustaceana 90, 1765–1777. doi: 10.1163/15685403-00003730
Inoue D., Fujimoto T., Kawakami Y., Yasui G. S., Yamaha E., Arai K. (2012). Vitrification of primordial germ cells using whole embryos for gene-banking in loach, Misgurnus anguillicaudatus. J. Appl. Ichthyol. 28, 919–924. doi: 10.1111/jai.12058
Israelachvili J. N., Marčelja S., Horn R. G. (1980). Physical principles of membrane organization. Quart. Rev. Biophys. 13, 121–200. doi: 10.1017/S0033583500001645
Iussig B., Maggiulli R., Fabozzi G., Bertelle S., Vaiarelli A., Cimadomo D., et al. (2019). A brief history of oocyte cryopreservation: Arguments and facts. Acta Obstet. Gynecol. Scand. 98, 550–558. doi: 10.1111/aogs.13569
Janati Idrissi S., Le Bourhis D., Lefevre A., Emond P., Le Berre L., Desnoës O., et al. (2021). Lipid profile of bovine grade-1 blastocysts produced either in vivo or in vitro before and after slow freezing process. Sci. Rep. 11, 11618. doi: 10.1038/s41598-021-90870-8
Jung G. T., Lee J. H., Park D., Ahn J. M., Um D.-E., Shin H., et al. (2021). Lipidomic changes in mouse oocytes vitrified in PEG 8000-supplemented vitrification solutions. Cryobiology 99, 140–148. doi: 10.1016/j.cryobiol.2020.11.004
Jung J., Shin H., Bang S., Mok H. J., Suh C. S., Kim K. P., et al. (2014). Analysis of the phospholipid profile of metaphase II mouse oocytes undergoing vitrification. PLoS One 9, e102620. doi: 10.1371/journal.pone.0102620
Karaşahin T. (2019). The effect of oleic and linoleic acid addition to the culture media on bovine embryonic development following vitrification. Pol. J. Vet. Sci. 22, 661–666. doi: 10.24425/pjvs.2019.129978
Karja N. W. K., Otoi T., Wongsrikeao P., Murakami M., Agung B., Fahrudin M., et al. (2006). In vitro development and post-thaw survival of blastocysts derived from delipidated zygotes from domestic cats. Theriogenology 65, 415–423. doi: 10.1016/j.theriogenology.2005.04.029.
Kasai M. (2002). Advances in the cryopreservation of mamMalian oocytes and embryos: Development of ultrarapid vitrification: Ultrarapid vitrification of oocytes/embryos. Reprod. Med. Biol. 1, 1–9. doi: 10.1046/j.1445-5781.2002.00004.x
Kelso K. A., Cerolini S., Noble R. C., Sparks N. H. C., Speake B. K. (1996). Lipid and antioxidant changes in semen of broiler fowl from 25 to 60 weeks of age. Reproduction 106, 201–206. doi: 10.1530/jrf.0.1060201
Kobayashi T., Takeuchi Y., Takeuchi T., Yoshizaki G. (2007). Generation of viable fish from cryopreserved primordial germ cells. Mol. Reprod. Dev. 74, 207–213. doi: 10.1002/mrd.20577
Kopeika J., Thornhill A., Khalaf Y. (2015). The effect of cryopreservation on the genome of gametes and embryos: principles of cryobiology and critical appraisal of the evidence. Hum. Reprod. Update 21, 209–227. doi: 10.1093/humupd/dmu063
Kostetsky E. Y., Boroda A. V., Odintsova N. A. (2008). Changes in the lipid composition of mussel (Mytilus trossulus) embryo cells during cryopreservation. Biophysics 53, 299–303. doi: 10.1134/S000635090804012X
Lahnsteiner F., Mansour N., McNiven M. A., Richardson G. F. (2009). Fatty acids of rainbow trout (Oncorhynchus mykiss) semen: Composition and effects on sperm functionality. Aquaculture 298, 118–124. doi: 10.1016/j.aquaculture.2009.08.034
Langdon C. J., Waldock M. J. (1981). The effect of algal and artificial diets on the growth and fatty acid composition of Crassostrea gigas Spat. J. Mar. Biol. Assoc. U. K. 61, 431–448. doi: 10.1017/S0025315400047056
Leão B. C. S., Rocha-Frigoni N. A. S., Cabral E. C., Coelho M. B., Ferreira C. R., Eberlin M. N., et al. (2015). Improved embryonic cryosurvival observed after in vitro supplementation with conjugated linoleic acid is related to changes in the membrane lipid profile. Theriogenology 84, 127–136. doi: 10.1016/j.theriogenology.2015.02.023
Lin C., Tsai S. (2012). The effect of cryopreservation on DNA damage, gene expression and protein abundance in vertebrate. Ital. J. Anim. Sci. 11, e21. doi: 10.4081/ijas.2012.e21
Liu T., Gao J., Zhou N., Mo M., Wang X., Zhang X., et al. (2016). The effect of two cryopreservation methods on human sperm DNA damage. Cryobiology 72, 210–215. doi: 10.1016/j.cryobiol.2016.04.004
Liu Y., Gluis M., Miller-Ezzy P., Han J., Qin J., Zhan X., et al. (2020a). Development of a programmable freezing technique on larval cryopreservation in the Pacific oyster Crassostrea gigas. Aquaculture 523, 735199. doi: 10.1016/j.aquaculture.2020.735199
Liu Y., Gluis M., Miller-Ezzy P., Qin J., Han J., Zhan X., et al. (2020b). Development of a programmable freezing technique on larval cryopreservation in Mytilus galloprovincialis. Aquaculture 516, 734554. doi: 10.1016/j.aquaculture.2019.734554
Liu Y., Li X. (2015). Successful oocyte cryopreservation in the blue mussel Mytilus galloprovincialis. Aquaculture 438, 55–58. doi: 10.1016/j.aquaculture.2015.01.002
Longo N., Frigeni M., Pasquali M. (2016). Carnitine transport and fatty acid oxidation. Biochim. Biophys. Acta Mol. Cell Res. 1863, 2422–2435. doi: 10.1016/j.bbamcr.2016.01.023
Mady M. M., Fathy M. M., Youssef T., Khalil W. M. (2012). Biophysical characterization of gold nanoparticles-loaded liposomes. Phys. Med. 28, 288–295. doi: 10.1016/j.ejmp.2011.10.001
Maldjian A., Pizzi F., Gliozzi T., Cerolini S., Penny P., Noble R. (2005). Changes in sperm quality and lipid composition during cryopreservation of boar semen. Theriogenology 63, 411–421. doi: 10.1016/j.theriogenology.2004.09.021
Mandal R., Badyakar D., Chakrabarty J. (2014). Role of membrane lipid fatty acids in sperm cryopreservation. Arch. Androl. 2014, 1–9. doi: 10.1155/2014/190542
Manno S., Takakuwa Y., Mohandas N. (2002). Identification of a functional role for lipid asymmetry in biological membranes: Phosphatidylserine-skeletal protein interactions modulate membrane stability. Proc. Natl. Acad. Sci. U.S.A. 99, 1943–1948. doi: 10.1073/pnas.042688399
Mansour N., Lahnsteiner F., McNiven M. A., Richardson G. F., Pelletier C. S. (2011). Relationship between fertility and fatty acid profile of sperm and eggs in Arctic char, Salvelinus alpinus. Aquaculture 318, 371–378. doi: 10.1016/j.aquaculture.2011.05.023
Martínez-Páramo S., Horváth Á., Labbé C., Zhang T., Robles V., Herráez P., et al. (2017). Cryobanking of aquatic species. Aquaculture 472, 156–177. doi: 10.1016/j.aquaculture.2016.05.042
Massapina C. (1999). Oocyte and embryo quality in Crassostrea gigas (Portuguese strain) during a spawning period in Algarve, South Portugal. Aquat. Living Resour. 12, 327–333. doi: 10.1016/S0990-7440(99)00115-1
Matos J. E., Marques C. C., Moura T. F., Baptista M. C., Horta A. E. M., Soveral G., et al. (2015). Conjugated linoleic acid improves oocyte cryosurvival through modulation of the cryoprotectants influx rate. Reprod. Biol. Endocrinol. 13, 60. doi: 10.1186/s12958-015-0059-3
Meneghel M., Dall’Acqua P. C., Ambrogi M., Leão B. C. S., Rocha-Frigoni N. A. S., Mingoti G. Z. (2017). Lipid content and cryotolerance of in vitro-produced bovine embryos treated with forskolin before vitrification. Pesq. Vet. Bras. 37, 395–400. doi: 10.1590/s0100-736x2017000400015
Migaud H., Bell G., Cabrita E., McAndrew B., Davie A., Bobe J., et al. (2013). Gamete quality and broodstock management in temperate fish. Rev. Aquac. 5, S194–S223. doi: 10.1111/raq.12025
Moore A. I., Squires E. L., Graham J. K. (2005). Adding cholesterol to the stallion sperm plasma membrane improves cryosurvival. Cryobiology 51, 241–249. doi: 10.1016/j.cryobiol.2005.07.004
Muchlisin Z. A., Sarah P. I., Aldila D. F., Eriani K., Hasri I., Batubara A. S., et al. (2020). Effect of Dimethyl sulfoxide (DMSO) and egg yolk on sperm motility, fertility and hatching rates of depik Rasbora tawarensis (Pisces: Cyprinidae) eggs after short-term cryopreservation. Aquac. Res. 51, 1700–1705. doi: 10.1111/are.14516
Murakami M., Dong Y. J., Suzuki T., Taniguchi M., Kaedei Y., Sato Y., et al. (2011). Development and subsequent cryotolerance of domestic cat embryos cultured in serum-free and serum-containing media. Cryobiology 63, 170–174. doi: 10.1016/j.cryobiol.2011.06.002
Nagashima H., D.A. Cameron R., Kuwayama M., Young M., Beebe L., W. Blackshaw A., et al. (1999). Survival of porcine delipated oocytes and embryos after cryopreservation by freezing or vitrification. J. Reprod. Dev. 45, 167–176. doi: 10.1262/jrd.45.167
Nagashima H., Kashiwazaki N., Ashman R. J., Grupen C. G., Seamark R. F., Nottle M. B. (1994). Removal of cytoplasmic lipid enhances the tolerance of porcine embryos to chilling. Biol. Reprod. 51, 618–622. doi: 10.1095/biolreprod51.4.618
Nagashima J. B., Sylvester S. R., Nelson J. L., Cheong S. H., Mukai C., Lambo C., et al. (2015). Live births from domestic dog (Canis familiaris) embryos produced by in vitro fertilization. PLoS One 10, e0143930. doi: 10.1371/journal.pone.0143930
Narida A., Tsai S., Hsieh W.-C., Wen Z.-H., Wang L.-H., Huang C.-L., et al. (2023). First successful production of adult corals derived from cryopreserved larvae. Front. Mar. Sci. 10. doi: 10.3389/fmars.2023.1172102
Neill A. R., Masters C. J. (1972). Metabolism of fatty acids by bovine spermatozoa. Biochem. J. 127, 375–385. doi: 10.1042/bj1270375
O’Connell M., McClure N., Lewis S. E. M. (2002). The effects of cryopreservation on sperm morphology, motility and mitochondrial function. Hum. Reprod. 17, 704–709. doi: 10.1093/humrep/17.3.704
Odintsova N. A., Ageenko N. V., Kiselev K. V., Sanina N. M., Kostetsky E. Y. (2006). Analysis of marine hydrobiont lipid extracts as possible cryoprotective agents. Int. J. Refrig. 29, 387–395. doi: 10.1016/j.ijrefrig.2005.07.010
Odintsova N. A., Boroda A. V., Velansky P. V., Kostetsky E. (2009). The fatty acid profile changes in marine invertebrate larval cells during cryopreservation. Cryobiology 59, 335–343. doi: 10.1016/j.cryobiol.2009.09.006
Odintsova N., Kiselev K., Sanina N., Kostetsky E. (2001). Cryopreservation of primary cell cultures of marine invertebrates. Cryo Lett. 22, 299–310.
Oldenhof H., Friedel K., Akhoondi M., Gojowsky M., Wolkers W. F., Sieme H. (2012). Membrane phase behavior during cooling of stallion sperm and its correlation with freezability. Mol. Membr. Biol. 29, 95–106. doi: 10.3109/09687688.2012.674161
Ortega Ferrusola C., González Fernández L., Morrell J. M., Salazar Sandoval C., Macías García B., Rodríguez-Martinez H., et al. (2009). Lipid peroxidation, assessed with BODIPY-C11, increases after cryopreservation of stallion spermatozoa, is stallion-dependent and is related to apoptotic-like changes. Reproduction 138, 55–63. doi: 10.1530/REP-08-0484
Ozkavukcu S., Erdemli E., Isik A., Oztuna D., Karahuseyinoglu S. (2008). Effects of cryopreservation on sperm parameters and ultrastructural morphology of human spermatozoa. J. Assist. Reprod. Genet. 25, 403–411. doi: 10.1007/s10815-008-9232-3
Pai H., Baid R., Palshetkar N., Pai A., Pai R., Palshetkar R. (2021). Oocyte cryopreservation - current scenario and future perspectives: A narrative review. J. Hum. Reprod. Sci. 14, 340. doi: 10.4103/jhrs.jhrs_173_21
Panyaboriban S., Tharasanit T., Chankitisakul V., Swangchan-Uthai T., Techakumphu M. (2018). Treatment with chemical delipidation forskolin prior to cryopreservation improves the survival rates of swamp buffalo (Bubalus bubalis) and bovine (Bos indicus) in vitro produced embryos. Cryobiology 84, 46–51. doi: 10.1016/j.cryobiol.2018.08.003
Paredes E. (2016). Biobanking of a marine invertebrate model organism: the sea urchin. J. Mar. Sci. Eng. 4, 7. doi: 10.3390/jmse4010007
Pariza M. W., Park Y., Cook M. E. (2001). The biologically active isomers of conjugated linoleic acid. Prog. Lipid Res. 40, 283–298. doi: 10.1016/S0163-7827(01)00008-X
Partyka A., Bonarska-Kujawa D., Sporniak M., Strojecki M., Niżański W. (2016). Modification of membrane cholesterol and its impact on frozen–thawed chicken sperm characteristics. Zygote 24, 714–723. doi: 10.1017/S0967199416000022
Paschoal D., Sudano M., Maziero R., Guastali M., Magalhães L., Landim-Alvarenga F., et al. (2016). Cryopreservation of in vitro produced bovine embryos after lipid decrease with forskolin. Reprod. Fertil. Dev. 28, 212. doi: 10.1071/RDv28n2Ab165
Pereira R. M., Baptista M. C., Vasques M. I., Horta A. E. M., Portugal P. V., Bessa R. J. B., et al. (2007). Cryosurvival of bovine blastocysts is enhanced by culture with trans-10 cis-12 conjugated linoleic acid (10t,12c CLA). Anim. Reprod. Sci. 98, 293–301. doi: 10.1016/j.anireprosci.2006.03.015
Pereira R. M., Marques C. C. (2008). Animal oocyte and embryo cryopreservation. Cell Tissue Bank. 9, 267–277. doi: 10.1007/s10561-008-9075-2
Pérez-Cerezales S., Martínez-Páramo S., Beirão J., Herráez M. P. (2010). Evaluation of DNA damage as a quality marker for rainbow trout sperm cryopreservation and use of LDL as cryoprotectant. Theriogenology 74, 282–289. doi: 10.1016/j.theriogenology.2010.02.012
Phillips P. H., Lardy H. A. (1940). A yolk-buffer pabulum for the preservation of bull semen. J. Dairy Sci. 23, 399–404. doi: 10.3168/jds.S0022-0302(40)95541-2
Pope C. E. (2014). Aspects of in vivo oocyte production, blastocyst development, and embryo transfer in the cat. Theriogenology 81, 126–137. doi: 10.1016/j.theriogenology.2013.09.006
Prapaiwan N., Tharasanit T., Punjachaipornpol S., Yamtang D., Roongsitthichai A., Moonarmart W., et al. (2015). Low-density lipoprotein improves motility and plasma membrane integrity of cryopreserved canine epididymal spermatozoa. Asian Australas. J. Anim. Sci. 29, 646–651. doi: 10.5713/ajas.15.0572
Purdy P. H., Graham J. K. (2004). Effect of adding cholesterol to bull sperm membranes on sperm capacitation, the acrosome reaction, and fertility. Biol. Reprod. 71, 522–527. doi: 10.1095/biolreprod.103.025577
Quinn P. J. (1985). A lipid-phase separation model of low-temperature damage to biological membranes. Cryobiology 22, 128–146. doi: 10.1016/0011-2240(85)90167-1
Quinn P. J. (2004). “Plasma membrane phospholipid asymmetry,” in Phospholipid Metabolism in Apoptosis Subcellular Biochemistry. Eds. Quinn P. J., Kagan V. E. (Boston: Kluwer Academic Publishers), 39–60. doi: 10.1007/0-306-47931-1_3
Quinn P. J., Chow P. Y. W., White I. G. (1980). Evidence that phospholipid protects ram spermatozoa from cold shock at a plasma membrane site. Reproduction 60, 403–407. doi: 10.1530/jrf.0.0600403
Ricker J. V., Linfor J. J., Delfino W. J., Kysar P., Scholtz E. L., Tablin F., et al. (2006). Equine sperm membrane phase behavior: the effects of lipid-based cryoprotectants. Biol. Reprod. 74, 359–365. doi: 10.1095/biolreprod.105.046185
Romek M., Gajda B., Krzysztofowicz E., Kepczynski M., Smorag Z. (2011). New technique to quantify the lipid composition of lipid droplets in porcine oocytes and pre-implantation embryos using Nile Red fluorescent probe. Theriogenology 75, 42–54. doi: 10.1016/j.theriogenology.2010.06.040
Röpke T., Oldenhof H., Leiding C., Sieme H., Bollwein H., Wolkers W. F. (2011). Liposomes for cryopreservation of bovine sperm. Theriogenology 76, 1465–1472. doi: 10.1016/j.theriogenology.2011.06.015
Salinas-Flores L., Adams S. L., Lim M. H. (2008). Cholesterol addition and removal in pacific oyster oocytes does not improve cryopreservation success. CryoLetters 29, 391–398.
Saunders K. M., Parks J. E. (1999). Effects of cryopreservation procedures on the cytology and fertilization rate of in vitro-matured bovine oocytes. Biol. Reprod. 61, 178–187. doi: 10.1095/biolreprod61.1.178
Schiller J., Arnhold J., Glander H.-J., Arnold K. (2000). Lipid analysis of human spermatozoa and seminal plasma by MALDI-TOF mass spectrometry and NMR spectroscopy — effects of freezing and thawing. Chem. Phys. Lipids. 106, 145–156. doi: 10.1016/S0009-3084(00)00148-1
Schuffner A., Morshedi M., Oehninger S. (2001). Cryopreservation of fractionated, highly motile human spermatozoa: effect on membrane phosphatidylserine externalization and lipid peroxidation. Hum. Reprod. 16, 2148–2153. doi: 10.1093/humrep/16.10.2148
Sieme H., Oldenhof H., Wolkers W. (2015). Sperm membrane behaviour during cooling and cryopreservation. Reprod. Domest. Anim. 50, 20–26. doi: 10.1111/rda.12594
Silva L. K. X., Lourenço J. B., da Silva A. O. A., de Sousa J. S., Silva A. G. M. E., Dos Reis A. N., et al. (2020). Increased quality of in natura and cryopreserved semen of water buffaloes supplemented with saturated and unsaturated fatty acids from the palm oil industry. Anim. Reprod. 17, e20200522. doi: 10.1590/1984-3143-ar2020-0522
Simpson A. M., Swan M. A., White I. G. (1987). Susceptibility of epididymal boar sperm to cold shock and protective action of phosphatidylcholine. Gamete Res. 17, 355–373. doi: 10.1002/mrd.1120170408
Somfai T., Kaneda M., Akagi S., Watanabe S., Haraguchi S., Mizutani E., et al. (2011). Enhancement of lipid metabolism with L-carnitine during in vitro maturation improves nuclear maturation and cleavage ability of follicular porcine oocytes. Reprod. Fertil. Dev. 23, 912. doi: 10.1071/RD10339
Souza R. S., Barbosa L. P., Aguiar C. S., Vieira R. L. A., Ribeiro M. O., Araújo R.C.d. S., et al. (2019). Cryopreservation of semen from goats fed a diet supplemented with flaxseed. Rev. Bras. saúde Prod. anim. 20, e0112020. doi: 10.1590/s1519-9940210112020
Sprícigo J. F., Morató R., Arcarons N., Yeste M., Dode M. A., López-Bejar M., et al. (2017). Assessment of the effect of adding L-carnitine and/or resveratrol to maturation medium before vitrification on in vitro-matured calf oocytes. Theriogenology 89, 47–57. doi: 10.1016/j.theriogenology.2016.09.035
Subramaniam S., Fahy E., Gupta S., Sud M., Byrnes R. W., Cotter D., et al. (2011). Bioinformatics and systems biology of the lipidome. Chem. Rev. 111, 6452–6490. doi: 10.1021/cr200295k
Subramoniam T., Newton S. S. (1993). Cryopreservation of penaeid prawn embryos. Curr. Sci. 65, 176–178.
Sudano M. J., Paschoal D. M., da Silva Rascado T., Magalhães L. C. O., Crocomo L. F., de Lima-Neto J. F., et al. (2011). Lipid content and apoptosis of in vitro-produced bovine embryos as determinants of susceptibility to vitrification. Theriogenology 75, 1211–1220. doi: 10.1016/j.theriogenology.2010.11.033
Sudano M. J., Santos V. G., Tata A., Ferreira C. R., Paschoal D. M., MaChado R., et al. (2012). Phosphatidylcholine and sphingomyelin profiles vary in Bos taurus indicus and Bos taurus taurus in vitro- and in vivo-produced blastocysts. Biol. Reprod. 87, 1–11. doi: 10.1095/biolreprod.112.102897
Takahashi T., Inaba Y., Somfai T., Kaneda M., Geshi M., Nagai T., et al. (2013). Supplementation of culture medium with L-carnitine improves development and cryotolerance of bovine embryos produced in vitro. Reprod. Fertil. Dev. 25, 589. doi: 10.1071/RD11262
Tervit H. R., Adams S. L., Roberts R. D., McGowan L. T., Pugh P. A., Smith J. F., et al. (2005). Successful cryopreservation of Pacific oyster (Crassostrea gigas) oocytes. Cryobiology 51, 142–151. doi: 10.1016/j.cryobiol.2005.06.001
Tharasanit T., Thuwanut P. (2021). Oocyte cryopreservation in domestic animals and humans: principles, techniques and updated outcomes. Animals 11, 2949. doi: 10.3390/ani11102949
Tiersch T. R., Yang H., Jenkins J. A., Dong Q. (2007). Sperm cryopreservation in fish and shellfish. Soc Reprod. Fertil. Suppl. 65, 493–508.
Torres T. M., Almeida-Monteiro P. S. D., Nascimento R. V. D., Pereira V. A., Ferreira Y. M., Lobato J. S., et al. (2022). Sperm cryopreservation of Prochilodus brevis using different concentrations of non-permeable cryoprotectants. Anim. Reprod. 19, e20210083. doi: 10.1590/1984-3143-ar2021-0083
Towhidi A., Parks J. E. (2012). Effect of n-3 fatty acids and α-tocopherol on post-thaw parameters and fatty acid composition of bovine sperm. J. Assist. Reprod. Genet. 29, 1051–1056. doi: 10.1007/s10815-012-9834-7
Towhidi A., Zeinoaldini S., Ardebili R., Dadashpour Davachi N., Nasiri A. H. (2013). Combined n-3 fatty acids and α-tocopherol supplementation improved the ovine sperm cryosurvival. Iran. J. Biotechnol. 11, 238–243. doi: 10.5812/ijb.14469
Tsai S., Lin C. (2012). Advantages and applications of cryopreservation in fisheries science. Braz. Arch. Biol. Technol. 55, 425–434. doi: 10.1590/S1516-89132012000300014
Tsai S., Rawson D. M., Zhang T. (2009). Studies on chilling sensitivity of early stage zebrafish (Danio rerio) ovarian follicles. Cryobiology 58, 279–286. doi: 10.1016/j.cryobiol.2009.02.002
Ugur M. R., Saber Abdelrahman A., Evans H. C., Gilmore A. A., Hitit M., Arifiantini R. I., et al. (2019). Advances in cryopreservation of bull sperm. Front. Vet. Sci. 6. doi: 10.3389/fvets.2019.00268
Valcarce D. G., Cartón-García F., Riesco M. F., Herráez M. P., Robles V. (2013). Analysis of DNA damage after human sperm cryopreservation in genes crucial for fertilization and early embryo development. Andrology 1, 723–730. doi: 10.1111/j.2047-2927.2013.00116.x
Valdebenito I. I., Gallegos P. C., Effer B. R. (2015). Gamete quality in fish: evaluation parameters and determining factors. Zygote 23, 177–197. doi: 10.1017/S0967199413000506
Van Meer G., Voelker D. R., Feigenson G. W. (2008). Membrane lipids: where they are and how they behave. Nat. Rev. Mol. Cell Biol. 9, 112–124. doi: 10.1038/nrm2330
Vasquez J. M., Roldan E. R. S. (1997). Phospholipid metabolism in boar spermatozoa and role of diacylglycerol species in the De Novo formation of phosphatidylcholine. Mol. Reprod. Dev. 47, 105–112. doi: 10.1002/(SICI)1098-2795(199705)47:1<105::AID-MRD14>3.0.CO;2-0
Vireque A. A., Tata A., Silva O. F. L. L. O., LoTurco E. G., Azzolini A., Ferreira C. R., et al. (2016). Effects of n-6 and n-3 polyunsaturated acid-rich soybean phosphatidylcholine on membrane lipid profile and cryotolerance of human sperm. Fertil. Steril. 106, 273–283.e6. doi: 10.1016/j.fertnstert.2016.03.044
V’uorela R., Kaitaranta J., Linko R. R. (1979). Proximate composition of fish roe in relation to maturity. Food Res. Int. 12, 186–188. doi: 10.1016/S0315-5463(79)73133-6
Waterhouse K. E., Hofmo P. O., Tverdal A., Miller R. R. (2006). Within and between breed differences in freezing tolerance and plasma membrane fatty acid composition of boar sperm. Reproduction 131, 887–894. doi: 10.1530/rep.1.01049
Wonnacott K. E., Kwong W. Y., Hughes J., Salter A. M., Lea R. G., Garnsworthy P. C., et al. (2010). Dietary omega-3 and -6 polyunsaturated fatty acids affect the composition and development of sheep granulosa cells, oocytes and embryos. Reproduction 139, 57–69. doi: 10.1530/REP-09-0219
Xu H.-Y., Geng S.-S., Li T.-T., Fu Q., Lu S.-S., Liang X.-W., et al. (2019). Maturation of buffalo oocytes in vitro with acetyl-L-carnitine improves cryotolerance due to changes in mitochondrial function and the membrane lipid profile. Reprod. Fertil. Dev. 31, 386. doi: 10.1071/RD18102
Yang H., Huo Y. (2022). Review of molluscan larval cryopreservation and application to germplasm cryobanking and commercial seed production. Aquaculture 547, 737491. doi: 10.1016/j.aquaculture.2021.737491
Yildiz C., Yavas I., Bozkurt Y., Aksoy M. (2015). Effect of cholesterol-loaded cyclodextrin on cryosurvival and fertility of cryopreserved carp (Cyprinus carpio) sperm. Cryobiology 70, 190–194. doi: 10.1016/j.cryobiol.2015.01.009
Zeilmaker G. H., Alberda A. T., van Gent I., Rijkmans C. M. P. M., Drogendijk A. C. (1984). Two pregnancies following transfer of intact frozen-thawed embryos. Fertil. Steril. 42, 293–296. doi: 10.1016/S0015-0282(16)48029-5
Zeron Y., Ocheretny A., Kedar O., Borochov A., Sklan D., Arav A. (2001). Seasonal changes in bovine fertility: relation to developmental competence of oocytes, membrane properties and fatty acid composition of follicles. Reproduction 121, 447–454. doi: 10.1530/rep.0.1210447
Zeron Y., Sklan D., Arav A. (2002). Effect of polyunsaturated fatty acid supplementation on biophysical parameters and chilling sensitivity of ewe oocytes. Mol. Reprod. Dev. 61, 271–278. doi: 10.1002/mrd.1156
Zhang S. S., Hu J. H., Li Q. W., Jiang Z. L., Zhang X. Y. (2009). The cryoprotective effects of soybean lecithin on boar spermatozoa quality. Afr. J. Biotechnol. 8, 6476–6480. doi: 10.5897/AJB09.1070
Zhang J., Li Q., Wu Y., Wang D., Xu L., Zhang Y., et al. (2019). Cholesterol content in cell membrane maintains surface levels of ErbB2 and confers a therapeutic vulnerability in ErbB2-positive breast cancer. Cell Commun. Signal. 17, 15. doi: 10.1186/s12964-019-0328-4
Zhang H., Ye H., Shao Y., Wu S., Yu J., Ji C., et al. (2018). The effects of egg yolk concentration and particle size on donkey semen preservation. J. Equine. Vet. Sci. 65, 19–24. doi: 10.1016/j.jevs.2018.03.002
Zhou G., Li N. (2009). Cryopreservation of porcine oocytes: recent advances. Mol. Hum. Reprod. 15, 279–285. doi: 10.1093/molehr/gap016
Keywords: cryopreservation, lipid application, sperm, oocyte, embryo, larva, aquaculture
Citation: Zhu X, Miller-Ezzy P, Zhao Y, Qin J, Tang Y, Liu Y and Li X (2023) Lipid modification to improve cryotolerance of gametes, embryos and larvae and its potential application in aquaculture species: a review. Front. Mar. Sci. 10:1235958. doi: 10.3389/fmars.2023.1235958
Received: 07 June 2023; Accepted: 28 August 2023;
Published: 11 September 2023.
Edited by:
Khor Waiho, University of Malaysia Terengganu, MalaysiaReviewed by:
Estefania Paredes, University of Vigo, SpainMaocang Yan, Zhejiang Mariculture Research Institute, China
Copyright © 2023 Zhu, Miller-Ezzy, Zhao, Qin, Tang, Liu and Li. This is an open-access article distributed under the terms of the Creative Commons Attribution License (CC BY). The use, distribution or reproduction in other forums is permitted, provided the original author(s) and the copyright owner(s) are credited and that the original publication in this journal is cited, in accordance with accepted academic practice. No use, distribution or reproduction is permitted which does not comply with these terms.
*Correspondence: Xiaoxu Li, xiaoxu.li@sa.gov.au; Yibing Liu, liuyibing@ouc.edu.cn