- 1Mokarran Protection Society, BP 245 Avatoru, Rangiroa, French Polynesia
- 2Andromède Océanologie, Mauguio, France
- 3Southern Shark Ecology Group, College of Science and Engineering, Flinders University, Adelaide, SA, Australia
- 4Florida International University, Institute of Environment, Department of Biological Sciences, Miami, FL, United States
- 5MARBEC, Univ Montpellier, CNRS, IFREMER, IRD, Sète, France
- 6Department of Marine Science, University of Otago, Dunedin, New Zealand
- 7Direction des Ressources Marines, Papeete, French Polynesia
- 8New Caledonian Agronomic Institute, Campus Anse Vata IRD, Nouméa, New Caledonia
The occurrence and seasonality of the Critically Endangered great hammerhead shark, Sphyrna mokarran, is data deficient in the Central Pacific region. Using photo-identification and laser-photogrammetry, we describe the seasonal population of great hammerhead sharks in the Tiputa pass (Rangiroa atoll) and Tuheiava pass (Tikehau atoll) in the Tuamotu archipelago of French Polynesia. During the austral summer of 2020 and 2021, we recorded a female-biased aggregation of at least 55 individuals (54 females; 1 unknown sex), representing an unprecedented number of S. mokarran in one study. All measured sharks were likely mature with pre-caudal lengths ranging from 147 cm to 297 cm (n = 35). Videos from citizen scientists recorded over a 15-year period enable us to identify 30 additional individuals between 2006 and 2019, with strong evidence of sexual segregation during the year. Our findings revealed seasonal residency (n = 32) of up to 6 days/month and for up to 5 months at the study site during the austral summer. We also demonstrated site fidelity with 32 individuals returning to the same atoll for up to 12 years between the first and last sighting, and with limited evidence of connectivity between the two sites. Our analysis also provides preliminary insight into the environmental factors driving S. mokarran aggregation in the Tiputa pass, suggesting a marked influence of the lunar cycle and of ocellated eagle rays (Aetobatus ocellatus) abundance. This study, conducted in situ with non-invasive methods offers a unique opportunity to study a great hammerhead shark population in a near-pristine ecosystem and provides important life-history elements for the Central Pacific region.
1 Introduction
The great hammerhead shark, Sphyrna mokarran (Rüppell, 1837), is an upper-trophic level predator (Cliff, 1995; Mourier et al., 2013), occurring in tropical and temperate latitudes (Compagno, 1984; Macbeth et al., 2009). It is a highly mobile species, found both in coastal and pelagic waters (Hammerschlag et al., 2011; Graham et al., 2016; Guttridge et al., 2017), that engage in large-scale pelagic migrations of up to 3,000 km (Hammerschlag et al., 2011; Graham et al., 2016; Guttridge et al., 2017). Great hammerhead sharks are particularly vulnerable to anthropogenic pressures such as commercial (targeted and bycatch) and recreational fisheries due to their k-selected life-history characteristics, i.e., late maturity (8 – 20 years of age) (Cortés, 2000; Harry et al., 2011), low fecundity (litter size ranging 6 – 42 pups) (Compagno, 1984; Stevens and Lyle, 1989), slow growth (k = 0·079 – 0·11) (Piercy et al., 2010; Harry et al., 2011), and long gestation periods (10 – 11 months) (Stevens and Lyle, 1989; Harry et al., 2011) which occurs on a biennial reproductive cycle (Stevens and Lyle, 1989). Great hammerhead sharks also suffer from high at-vessel and post-release mortality due to an elevated stress response to capture (Morgan and Burgess, 2007; Gallagher et al., 2014). High likelihood of post-release mortality and slow life-history traits combined with increasing anthropogenic pressures has led to population decline across the species range and to great hammerhead sharks being globally listed as Critically Endangered on the IUCN (International Union for Conservation of Nature) Red List of Threatened Species (Rigby et al., 2019) on the Convention on Migratory Species (CMS, 2019) and on the Convention on International Trade of Endangered Species (CITES, 2019) Appendix II.
Most studies on great hammerhead sharks have been conducted in the northwest Atlantic Ocean (Piercy et al., 2010; O’Connell and Leurs, 2015; Guttridge et al., 2017; Doan and Kajiura, 2020; Heim et al., 2021; Macdonald et al., 2021), southwest Pacific Ocean (Stevens and Lyle, 1989; Harry et al., 2011; Raoult et al., 2019), and to a lesser extent in the Western Indian Ocean (Cliff, 1995) and in the Arabian Gulf (Hsu et al., 2020). Extensive industrial fishing in those regions over the past half-century has resulted in an unprecedented increase in the risk of extinction of sharks and in particular of pelagic sharks (Pacoureau et al., 2021; Walls and Dulvy, 2021). The Central Pacific Ocean, however, provides a unique opportunity to investigate the ecology and population dynamics of shark species in a relatively pristine environment where targeted fishing pressure has been minimal to non-existent (Ward-Paige and Worm, 2017). Several nations within the Central Pacific Ocean have protected shark populations, notably via fishing bans. In French Polynesia, for example, all shark species have been protected within its exclusive economic zone (EEZ) by the environmental code (Art. A 121-10-1) since 2012, with limited targeted shark fishing occurring prior (Stahl, 2006). As a result, French Polynesia has the highest probability of shark sightings, with one of the highest shark abundance and diversity in the world (MacNeil et al., 2020). Yet, limited information about great hammerhead shark populations from the Central Pacific Ocean or French Polynesia is currently available. First records of great hammerhead sharks in the region originate from the Tuamotu archipelago (Figure 1) by the Pa’umotu (people from the Tuamotu), who mentioned sightings of the Tamataroa (great hammerhead shark in the local dialect) from the beginning of the 20th century (Hervé, 1929). Nowadays, great hammerhead sharks are of particular economic value for the local diving industry that has developed in the Western Tuamotu archipelago of French Polynesia (Figure 1). Since 2011, the Polynesian Shark Observatory (ORP) has collected elasmobranch sightings recorded by dive instructors throughout French Polynesia (Séguigne et al., 2023). These citizen science surveys showed that great hammerhead sharks’ occurrence seasonally increases during the austral summer (i.e., from December to March) in the Western Tuamotu archipelago, specifically in Rangiroa and Tikehau, two atolls 15 km apart (with both passes 78 km apart) (Figure 1).
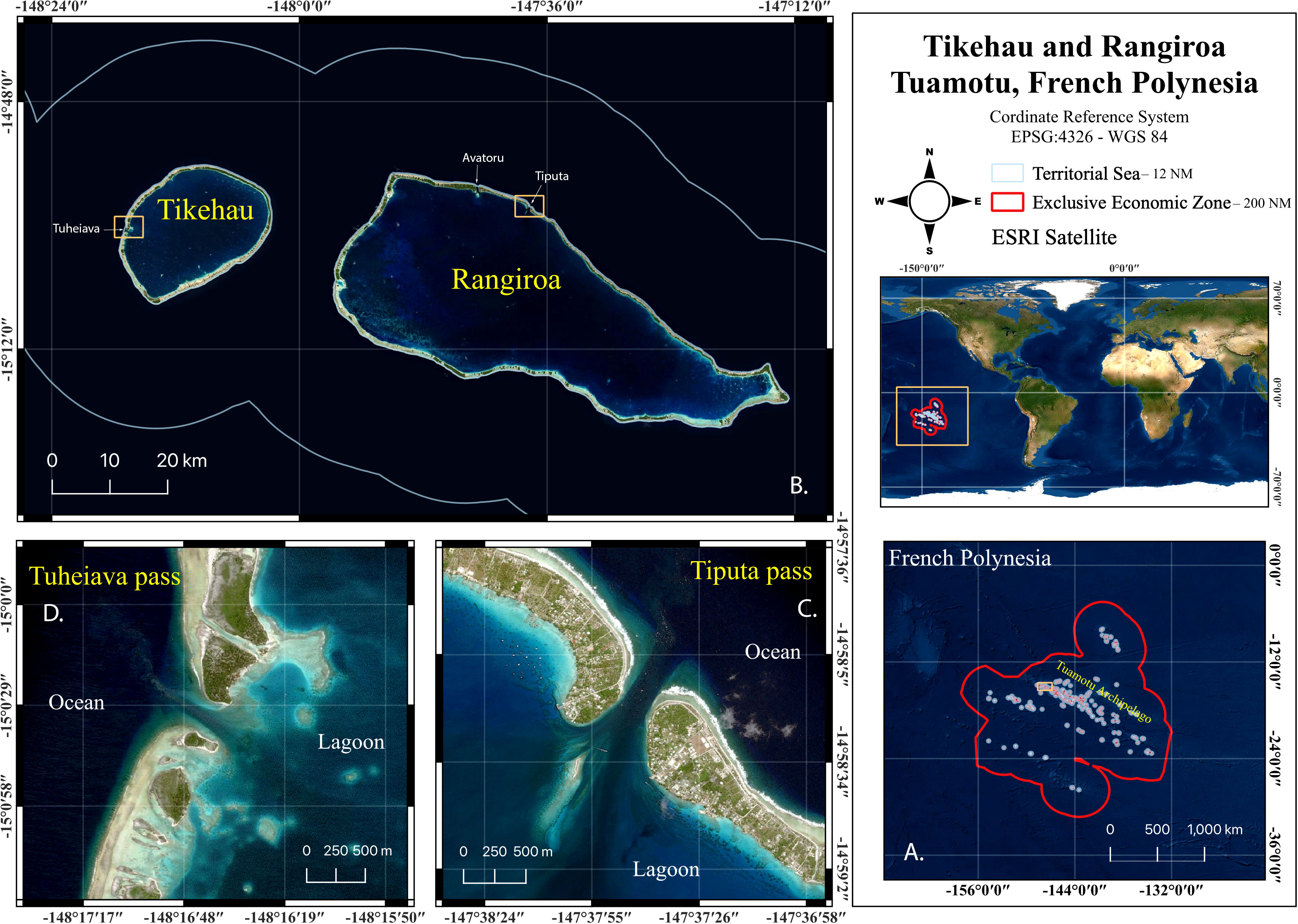
Figure 1 (A) Tuamotu Archipelago location in French Polynesia. (B) Tikehau and Rangiroa Atoll. (C) Tiputa pass, Location A. (D) Tuheiava pass, Location B. (Satellite imagery ©ESRI).
Based on the frequent sightings and vulnerability of the species, the non-governmental organisation Mokarran Protection Society (MPS) was created in 2019 in Rangiroa atoll to bridge local ecological knowledge and science-based conservation in collecting data on S. mokarran to inform conservation and management of the species. Understanding the population dynamic and structure of the great hammerhead shark in this understudied region is key to establishing abundance baselines and monitoring of the population to address shark sanctuary regulations (Ward-Paige and Worm, 2017). Our study used underwater visual census (UVC) survey data conducted by the MPS over two austral summers (2019/2020 and 2020/2021) to characterise the great hammerhead shark population in the Tiputa Pass (Rangiroa) (Figure 1) and Tuheiava Pass (Tikehau) (Figure 1). We used photo-identification (photo-ID) and laser-photogrammetry given their easy implementation, cost-effectiveness, and non-invasiveness, reducing the impact on the shark’s natural behaviour, compared to more invasive techniques (e.g., use of bait to attract individuals, Heim et al., 2021). Specifically, we assessed the number of individuals, sex ratios, seasonal residency patterns at both sites, and investigated potential drivers of occurrence and habitat use in the Tiputa pass, the only site surveyed throughout the whole sampling period for logistic reasons. We coupled the seasonal scientific surveys undertaken by the MPS with citizen science data collected over a 15-year period (i.e., all year long) to investigate long-term site fidelity.
2 Materials and methods
2.1 Study sites and sampling method
Our study focused on two main sites: The Tiputa pass, mentioned hereafter as location A, in Rangiroa atoll, the second largest lagoon in the world (Stoddart, 1969) (14°58’22.1 “S; 147°37’45.5 “W; Figure 1) and the Tuheiava pass, mentioned hereafter as location B, in Tikehau atoll (15°00’29.8 “S; 148°16’40.1 “W; Figure 1). Location A is a 240 x 950 m opening on the atoll barrier reef and ranges from 14 – 60 m depth. A second pass in Rangiroa atoll, the Avatoru pass (14°56’28.1 “S; 147°42’44.6 “W), mentioned hereafter as location A.bis, is located 10 km west (Figure 1) of location A. Historically, it was the first dive site on Rangiroa atoll and was gradually abandoned in the early 2010s in favour of location A, due to the absence of great hammerhead shark sightings. Nowadays, all dives are exclusively taking place in location A. Due to our logistical dependence to local dive centers, the location A.bis is only considered here for exploratory purposes, with only 1% of the dives in Rangiroa conducted there. Location A was gridded into 0.01 km² to facilitate the spatial distribution of the observations (Supplementary Figure 1). The “Hammerhead plateau” (Supplementary Figure 1, E1 area) is 45 – 60 m deep on the north-western part of the location A and is known by local divers as the best location to observe great hammerhead sharks. The location B is the only pass of Tikehau atoll and is located 78 km west of the location A (Intes, 1984). It is a smaller and shallower pass, i.e., 150 x 500 m and 4 – 40 m deep. Tikehau atoll circumference has few land ridges, such that oceanic water overflows during high tides through shallow (< 1 m deep) channels (Harmelin, 1985), named hoa by Polynesians, that connect the ocean to the lagoon. As a result, the current in location B is less powerful than in location A.
Between January to February 2020 and December 2020 to March 2021, 221 dives (n = 123 days) were conducted in location A, 23 dives (n = 15 days) were conducted in location B (in January and February 2021) and 2 dives (n = 2 days) were conducted in location A.bis. Dives were conducted using closed-circuit rebreathers, open-circuits scuba, or free-diving, during incoming currents as the outgoing tides have dangerously strong currents with poor visibility, making the pass inoperable for divers. Each diving team consisted of two to six divers equipped with a camera and lasers to enable photo-ID and laser photogrammetry. In location A, during each dive, teams made a mandatory passage through the Hammerhead plateau (coming from the west, north or east side) before drifting into the pass with a random trajectory.
After each dive, the number of different great hammerhead sharks sighted was established based on identifiable individuals, number of sharks sighted at the same time on the Hammerhead plateau and then number of sharks sighted when drifting into the pass (i.e., the ones sighted into the pass could not be the same as the ones sighted earlier on the Hammerhead plateau, as current was incoming and sharks in the pass were seen going out of the pass against the current). Using this method, shark numbers were possibly underestimated, but never overestimated.
2.2 Structure and characteristics of the population
2.2.1 Photo-identification
We used four cameras’ types to take photographs and videos of great hammerhead sharks: a GoPro® 4 (1080p) in early 2020, and a GoPro® 8 (2.7K), a SONY A7 II (24.3 MP), and a CANON D5 (30.4 MP) in austral summer 2020 – 2021. Photographs of great hammerhead sharks were taken to catalog all sharks sighted on the day. Individual great hammerhead sharks were identified using natural markings (e.g., spots, and dark patterns on the body and fin-tips), fins morphology (e.g., notches, outgrowth, shortened), scars (e.g., wounds, scratches, depigmentation marks), and ventral pigmentation (Domeier and Nasby-Lucas, 2007; O’Connell and Leurs, 2015; Guttridge et al., 2017) (Figure 2; Table 1). Given the fast-healing capacity of elasmobranchs (Chin et al., 2015), we only used scars for intra-annual identification (within a season). Photo-identification surveys of other sharks using the same method as this study have been extensively used (Domeier and Nasby-Lucas, 2007; Buray et al., 2009; Gubili et al., 2009; Towner et al., 2013; Bègue, 2017). We assigned each distinctive feature to one of 13 body regions: right flank, left flank, cephalofoil, first dorsal fin, posterior edge of the first dorsal fin, right and left pectoral fin, right and left pelvic fin, second dorsal fin, anal fin, caudal fin, and ventral surface. Sex was determined based on clasper presence. We used a minimum of two distinctive marks to identify individuals, unless one feature was sufficiently explicit (e.g., absent second dorsal fin). Each photo was analysed by the same two observers to validate identification and matching between two individuals. A first round of identification and matching was conducted after each sampling period by a first observer. A second observer then went through the database again. Sightings with only one matching trait, with poor-quality pictures that prevented identification, or for which both observers disagreed, were considered to have a low confidence index. Only observations with a high confidence index are considered here. Seasonal residency (i.e., as a form of temporal attachment to a limited area, Chapman et al., 2015; in our case the study site and its surrounding lagoon or open ocean area) was determined when an individual was sighted within at least one lunar cycle (~ one month) between the first and last sighting or with more than one sighting within a month of the austral summer (extending from November to May). The MPS created a network of dive instructors who shared photographs and videos of great hammerhead sharks from December 2019 to May 2021. A total of 29 sightings out of 275 reported were used to assess their visitation and residency patterns outside of the MPS surveys. In addition, the MPS randomly obtained 1058 photographs and videos of great hammerhead sharks from recreational scuba-divers in location A, A.bis and B from February 2006 to December 2019 (prior to the first MPS sampling campaign). A total of 109 out of 388 sightings enabled identification of great hammerhead sharks, based on the same criterias as described above. These historic and additional data, combined with our focused survey, enabled us to assess the residency and long-term site fidelity (i.e., repeated use of a particular area throughout a shark’s life, Speed et al., 2010) of great hammerhead sharks over a 15-year period, i.e., from February 2006 to May 2021, and to identify new individuals regardless of the season. We considered a great hammerhead shark to have site fidelity to a pass when sighted over at least two austral summers.
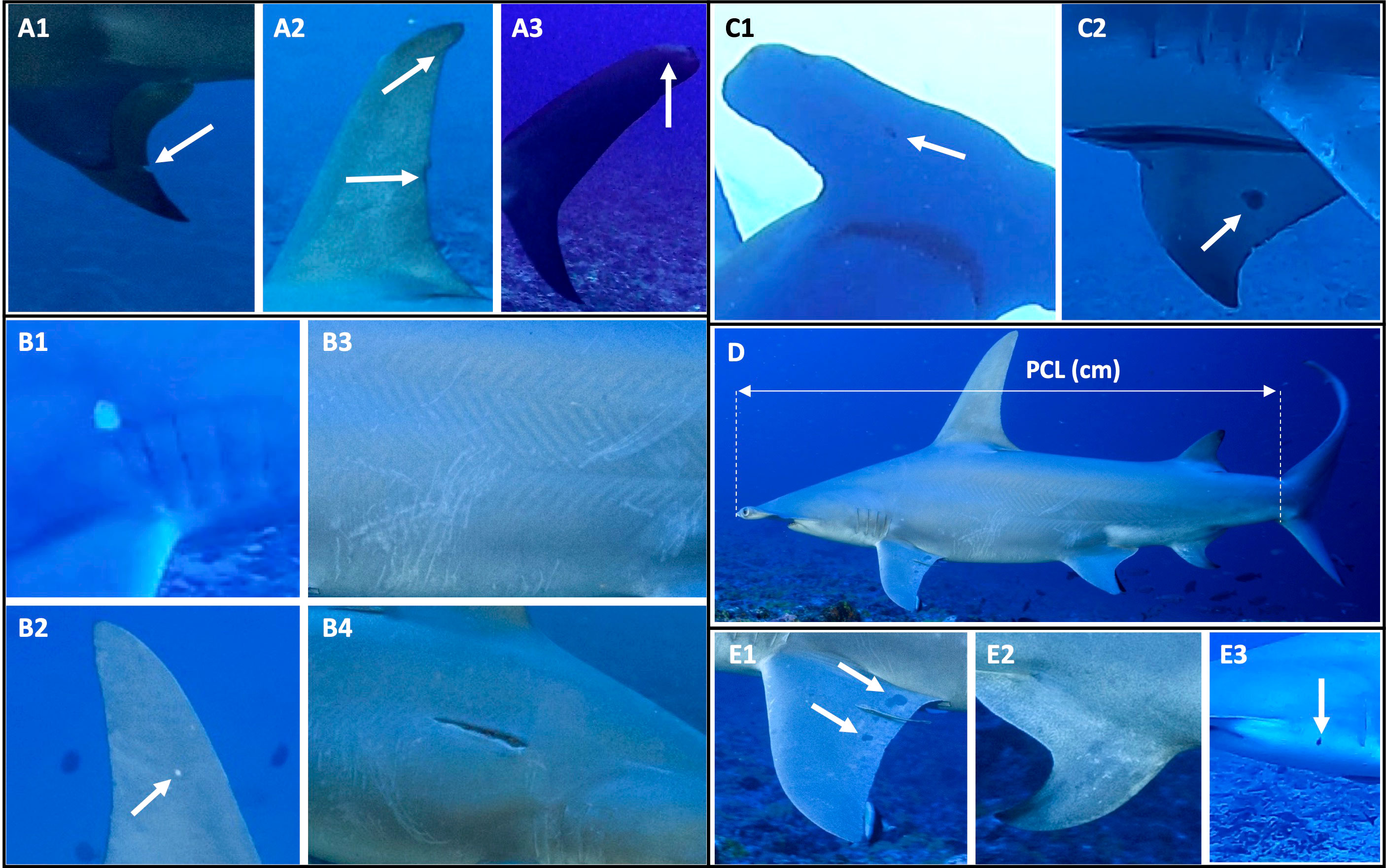
Figure 2 Distinctive traits for S. mokarran identification (A) fin morphology: 1) notches, 2) outgrowths, 3) truncated or shortened fins. (B) distinctive for one season only: 1) white patches (around the head), 2) blisters, 3) scratches, 4) open wound or scars. (C) ventral features: 1) black dots, 2) dark patches. (D) Pre-Caudal length. (E) Natural marks on fin-tips or body: 1) dark patches, 2) dark pattern of fin-tips, 3) black dots (Pictures from ©MPS and ©Thomas Pavy).
2.2.2 Laser-photogrammetry
On 225 dives over 123 days of diving, a laser-photogrammetry system, as described in Deakos (2010) and Rohner et al. (2011), was used in situ with a GoPro® 4 (1080p; 48 frames per second; narrow field of view) in 2019 – 2020 and a GoPro® 8 (2.7K; 60 frames per second; narrow field of view) in season 2020 – 2021, with two parallel laser pointers (BALP-LG05-B150 < 5mM) separated by 30 or 50 cm (Supplementary Figure 2). Lasers were projected onto the flank of the shark and used as a reference length. We used the resulting images to measure: pre-caudal length (PCL), fork length (FL), total length (TL), and height of the 1st dorsal fin (DH) from the side, and cephalofoil width (HW) with footage from above (Supplementary Figure 3). Pre-caudal length was used as the reference size for identification as it excludes the caudal fin, which tends to flex during swimming, thus shortening total length (Cliff, 1995; Rohner et al., 2015).
Three sources of inaccuracies can occur when using laser photogrammetry (Deakos, 2010): (1) image distortion – due to the airspace between the GoPro® lens and the waterproof housing refracting the incoming light and distorting the captured image, (2) non-parallel alignment of the lasers and (3) the parallax error leading to shark size being underestimated due to the relative spacing between pointers increasing as shark body position veers away from being perpendicular to the lasers. To quantify distortion, an in-situ experiment was carried out and consisted of filming a grid underwater in a parallel layout. The distortions were adjusted using the Photoshop® software (CS5 version) (correction of -12 out of +100/-100 range) to restore the straight angles from the grid (Supplementary Figure 4). This coefficient was applied to all the extracted photographs. The parallel alignment and spacing of the lasers were calibrated before each dive using a metal plate marked with 30 and 50-cm distances and placed 5 and 10 m away. The parallax error was assessed by measuring an underwater object of known length (84 cm) at different angles: 10°, 20°, 30° and 40° at a 5 m distance to estimate a reasonable margin of error (Supplementary Figure 5). The percentage errors were 2.4%, 7.1%, 14.3%, and 27.4%, respectively. Once underwater, divers had no precise tools to estimate the angle of observation and thus correct a potential horizontal parallax error in situ. As a result, we excluded all images that were taken with an angle of incidence greater than 10°. Therefore, all our measurements were estimated to have an error not greater than 2.4%. Perpendicularity (+/- 10°) was established according to the alignment of the anterior part of the cephalofoil, the two pectoral fins, the two pelvic fins and, if applicable, the maximum development of the caudal fin to measure a TL in the horizontal plane; and the disappearance of dorsal fins and caudal fin in the vertical plane (from above). Size measurements from laser photogrammetry pictures were carried out using ad hoc software on the model of ImageJ (Rasband, 1997-2015). Due to geographical connectivity, we used Australian-referenced measurements to assess the maturity of the individuals sighted. The stretched total length (LST) at maturity ranges from 210 to 228 cm (LST 50%) on Australian coastlines (Stevens and Lyle, 1989; Harry et al., 2011). Using the equation derived from Harry et al. (2011) and being conservative, we converted LST into PCL and considered individuals as potentially mature from 146 cm PCL.
2.3 Influence of the biotic and abiotic drivers on the presence of S. mokarran in location A
2.3.1 Biotic and abiotic drivers
To assess the influence of water temperature on the seasonal occurrence of great hammerhead sharks, we positioned a temperature logger (HOBO® Pro v2 data logger; accuracy ± 0.2°C) at the Hammerhead plateau (57 m depth). Water temperature was recorded every 30 min during the austral summer 2020 – 2021 only (i.e., 6 January to 28 February 2021).
Daily lunar age was obtained from the equation derived from Stephen R. Schmitt's algorithm: age of the moon = integer ([(age of the moon on March 1st) + (number of days since March 1st)] modulo 29.53) during the austral summer of 2019 – 2020 and 2020 – 2021 to assess its influence on the occurrence of great hammerhead sharks in the pass. Lunar data was expressed in days (Day 0 – 29), with the new moon on day 0, the first quarter on day 7, full moon on day 15, and the last quarter on day 22.
Great hammerhead shark diet includes different species of rays (Strong et al., 1990; Chapman and Gruber, 2002; Raoult et al., 2019). The ocellated eagle ray (Aetobatus ocellatus, Khul, 1823) is thus potential prey for the great hammerhead shark in the Central Pacific region. The presence and absence of A. ocellatus was recorded during each dive from 19 December 2020 to 4 March 2021 to evaluate the effect of potential prey presence and abundance on great hammerhead shark’s occurrence in the location A. We used a coded binary: absence = 0 and presence = 1. Number of rays were counted until 7, after which we used abundance classes: [7 – 10], [11 – 15], [16 – 30], [31 – 50], [51 – 100].
2.3.2 Statistical analysis
We analysed the impact of biotic and abiotic factors on the occurrence of great hammerhead sharks in location A using the statistical environment software R Studio (Version 4.0.4; R Core Team, 2021). First, we tested the influence of biotic and abiotic drivers on the occurrence of great hammerhead sharks using a generalized linear model (GLM) following Venables and Ripley (2002) and Dobson and Barnett (2018) for each season (2019 – 2020 and 2020 – 2021) with a binomial family. Abiotic factors include site (i.e., location A/B (to check the site effect); categorical variable, fixed), current (i.e., inflow/outflow/slack water; categorical variable, fixed), water temperature at 57 m (continuous variable, fixed), moon age (continuous variable, fixed), day phase (i.e., morning and afternoon; categorical variable, fixed), and hour of dives (continuous variable, fixed). Sex (male/female; categorical variable fixed) was the only biotic factor included in the models. The presence of potential prey, which is also a biotic factor (e.g., A. ocellatus), was not included in the model because it was recorded only from 19 December 2020 to 4 March 2021. Instead of a GLM, the Kruskal-Wallis Chi-squared test was employed to assess differences in occurrence between the two datasets (S. mokarran vs. A. ocellatus) during the overlapping period (19 December 2020 to 4 March 2021), with a limit of significance of <0.01. Also, for the 2020 – 2021 season, the water temperature was not included in the model because it was not recorded throughout the Austral summer period. Instead of a GLM, the water temperature was analysed by univariate analysis with the Wilcoxon test with the stats R package (R core team). All other variables were analysed through the GLM for each dataset, and then, univariate analysis was performed to decipher the observed variations for each significant variable in the previous GLM with Bonferroni p adjusted < 0.01.
For the moon age analysis, the distribution of presence/absence sightings was tested with a Wilcoxon test and Chi-squared test (with a limit of significance of <0.01) with the stats R package (R core team). All graphics were produced with the ggplot2 R package (Wickham, 2011). All scripts and data used for these analyses are freely available at https://github.com/PLStenger/Mokarran_II allowing the automatization, tracing, and reproduction of the analyses.
3 Results
3.1 Characteristics of the identified great hammerhead sharks in location A and B
3.1.1 Number of individuals, sex ratio and sexual maturity
In locations A and A. bis, during January to February 2020 and December 2020 to March 2021, 50 great hammerhead sharks (49 in location A and one in location A.bis) were identified across 369 sightings, comprising 49 females and one animal of unknown sex (sex ratio of 0/49) (Table 2). Great hammerhead sharks were recorded on 144 out of 225 dives (64.0%) over 123 days of diving. Between one to 11 individuals were sighted per dive on the Hammerhead plateau and across the pass, with a maximum of six great hammerhead sharks in the same field of view on the Hammerhead plateau. The number of great hammerhead shark identified during austral summer 2020 – 2021 (n = 27) was slightly higher than during season 2019 – 2020 (n = 23), likely due to a longer sampling period and to a larger amount of great hammerhead sharks’ photographs shared by dive instructors, which increased identification likelihood (Supplementary Materials on identification). In location B, five great hammerhead sharks, all females (sex ratio of 0/5), were identified across 23 sightings, on 11 out of 23 dives (47.8%) over 15 days. Between one to four solitary individuals were sighted randomly per dives across the pass. All three datasets (MPS data, additional data from dive instructors and historic data) record a total of 1075 sightings of great hammerhead sharks in locations A, A.bis and B combined, between February 2006 and May 2021. Among these 1075 sightings, a total of 166 (89 from the MPS data, 27 from instructors’ data and 49 from historic data) match the 55 identified great hammerhead sharks (Supplementary Table 1). In locations A, the PCL ranged between 147 and 297 cm (209.1 ± 29.7 cm, mean ± S.D cm; n = 105 sightings) over the two seasons combined, indicating that all measured individuals might be mature (Table 3). In location B, size ranged between 225 and 264 cm PCL (248.3 ± 15.9 cm; n = 4 sightings) over one season (Table 4), also indicating likely maturity of the female great hammerhead sharks.
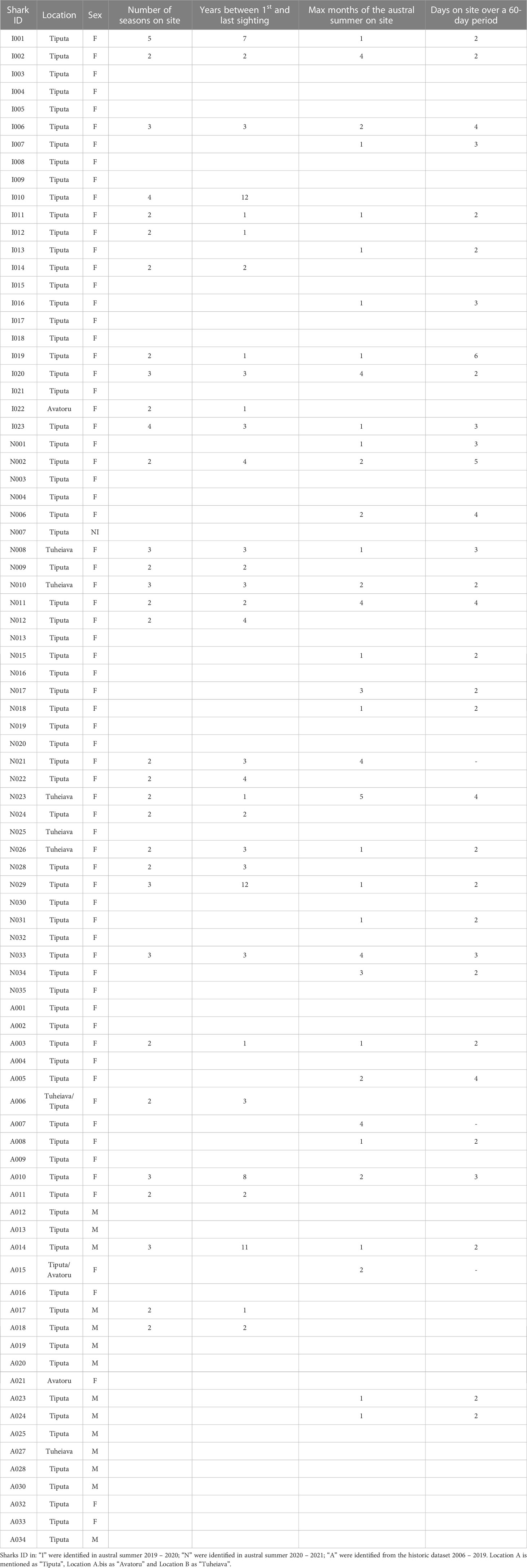
Table 2 Overview of the seasonal residency and site fidelity characteristics of the identified individuals.
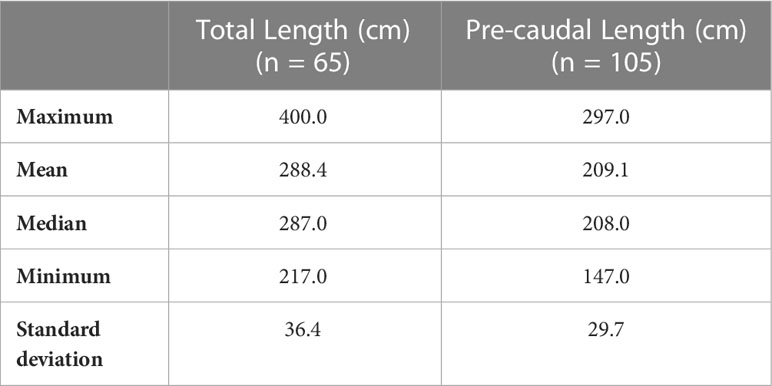
Table 3 Maximum, minimum, median, mean and standard deviation of PCL and TL (cm) measured during the austral summer from January 2020 to March 2021 in location A.
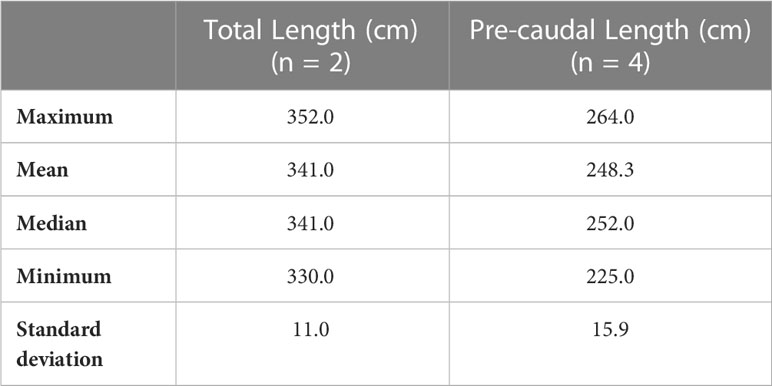
Table 4 Maximum, minimum, median, mean and standard deviation of PCL and TL (cm) measured during the austral summer from January 10th 2020 to February 9th 2021 in location B.
The analysis of the historic database enabled us to identify 30 additional individuals among 54 sightings (48 in location A, two in location A.bis, and four in location B) through February 2006 and December 2019, with a sex ratio of 14/16 (n = 13 males in location A; n = 1 male in location B) (Table 2; Supplementary Table 1). Males were primarily sighted from August to October and females during the austral summer months from November to May, which is consistent with what we observed during the main survey and suggests distinct temporal habitat use and segregation between males and females on the two atolls. Maturity of these 30 additional individuals cannot be confirmed as no laser photogrammetry was conducted during these historic dives. Identified individuals are presented with a shark ID code in Table 2.
3.1.2 Residency pattern and site fidelity
Based on the sharks identified during the main survey (January 2020 – March 2021) on the two atolls combined, 25 (45.5%; 21 in location A and A.bis combined, four in location B) out of the 55 individuals identified demonstrated residency behaviour around the passes, where diving activities and sightings occur. Great hammerhead sharks were sighted for a period of up to five months (e.g., shark N23; between December 2020 and April 2021), although two months was the mean time of observation during the austral summer. Re-sighting ranged between one to six occurrences over one to five months and up to six days/per month (Table 2). One seasonal resident (I19) re-sighted on six occasions during a 37-day timeframe, demonstrated the fast- healing capabilities of a 17 cm horizontal open wound (Supplementary Figure 6). Out of the 30 individuals identified from the historic database, seven (n = 23.3%; four females, three males) showcased residency behaviour around the sites, with one (A15) sighted on both location A.bis and location A (10 km apart) one month apart. Males (n = 3; 21.4%) were only sighted over one month, up to twice per month. Among the 70 females identified from the three datasets (MPS, instructors and historic images), 29 of them (41.4%; 25 in location A and A.bis combined, four in location B) demonstrated seasonal residency (Table 2). In the location A, sightings occurred in 72.8% of the time on the “Hammerhead plateau” (outer part of the pass), with sharks roving around in the area, not providing evidence of origin nor destination (lagoon or open ocean/outer reef).
From the 55 great hammerheads sharks identified during the main surveys on both atolls, 25 (45.5%; 21 sharks in locations A and A.bis combined and four in location B) were re-sighted across two to five different austral summer seasons, not necessarily consecutive. Two great hammerhead sharks (N29 and I10) were sighted over a period of up to 12 years, with three years between first and last sighting being the average time of site fidelity. Most sightings occurred between December and February but extended from November to May in both atolls. No specific pattern of fidelity stands out, with individuals being sighted every season, every two season or every three + season, sometimes all three patterns combined for the same individual. A total of 34 (61.8%) great hammerhead sharks out of the 55 identified demonstrated either seasonal residency (n = 25), site fidelity (n = 25) or both behaviours (n = 15). Out of the 30 additional individuals, seven (23.3%; three males, four females) demonstrated site fidelity, with one male (A14) being sighted three different years (during August and September) across an 11-year period. Overall, out of the 70 females identified from the three datasets, 29 of them (n = 41.4%; 24 in location A and A.bis combined, five in location B) demonstrated site fidelity (Table 2), of which 17 also showed seasonal residency.
No great hammerhead sharks identified in 2019 – 2021 were sighted at both sites (locations A and B). However, one female-identified from the historic dataset (A06) demonstrated geographical connectivity between location A and B, during the austral summers of 2014 – 2015 and 2017 – 2018. The first sighting was reported on January 2015 in location B and a second observation was made over the same month (unknown exact day) in location A. This female was re-sighted two seasons later in location B (on December 2017 and April 2018) with the last observation on May 2018 in location A. It is the only evidence of geographical connectivity between the two atolls.
3.2 Influence of the biotic and abiotic drivers on the presence of S. mokarran in location A
Temperature data was only recorded during the 2020 – 2021 season, and not included in the GLM analysis. Great hammerhead sharks were sighted between 26.6°C - 28.9°C (median value at 27.7°C), which is 74.2% of the recorded temperature range during deployment (26.4°– 29.5°C). The Wilcoxon test showed differences in presence/absence (1/0) of great hammerhead sharks in 57- meters depth with P value = 0.032 (Figure 3).
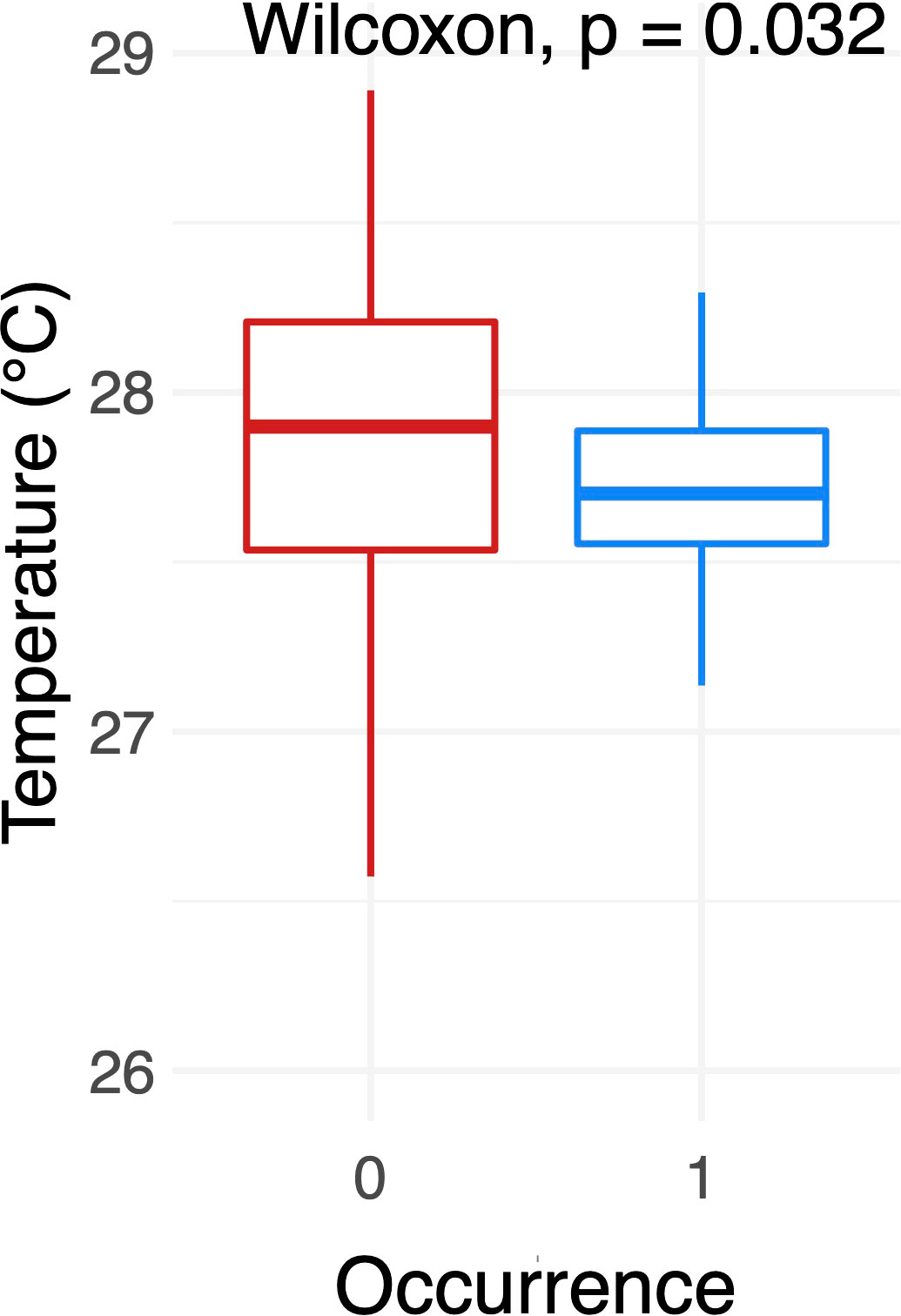
Figure 3 Difference in water temperature according to presence (1) / absence (0) of Sphyrna mokarran at location A (Tiputa Pass).
Across both seasons (2019 – 2020 and 2020 – 2021) moon age was the only factor that significantly affected shark sightings (adjusted P value < 0.001 for both seasons). Sightings increased during days close to the full moon, with a peak two days before the full moon (13th day of the lunar cycle) (Figure 4). The distribution of presence/absence observations were significantly different (Wilcoxon test P value < 0.001), and only present observations were significantly affected by moon age (Chi-squared test P value < 0.001).
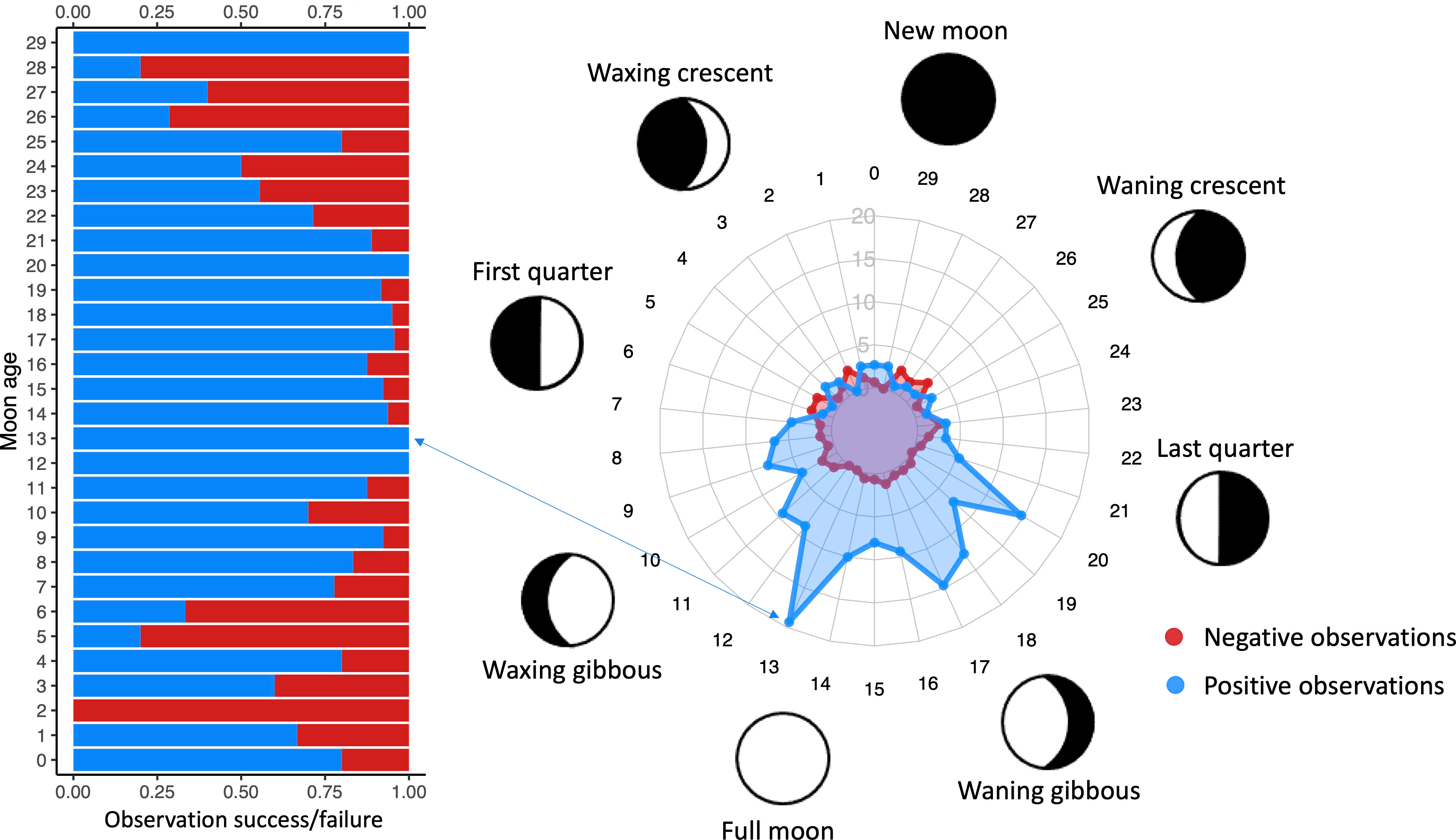
Figure 4 Radar plot of presence and absence of S. mokarran relative to the moon age, and bar plot in fraction numbers (from 0 to 1) of these observations, location A (Tiputa Pass).
During the period from 19 December 2020 to 4 March 2021, ocellated eagle rays were sighted 73.1% of times in location A (95 out of the 130 dives) and 81.5% of the observations took place around the “Hammerhead plateau” (E1 area, see Supplementary Figure 1). Although the Kruskal-Wallis Chi-squared demonstrated differences in occurrence between the two datasets (S. mokarran vs A. ocellatus; P-value <0.001), the two distributions were similar, with a peak, with a maximum peak at the end of January and beginning of February, and a second smaller peak at the end of February (Figure 5). On two occasions around the full moon period (29 January/day 15 of the moon cycle and 22 – 23 of February/day 10 and 11 of the moon cycle), we observed a steep decline in A. ocellatus presence, which also matched a decrease of great hammerhead shark sightings. On 14 April 2021, predation of a great hammerhead shark on an ocellated eagle ray was observed (Figure 6) on the east outer reef of location A (F2 area, see Supplementary Figure 1).
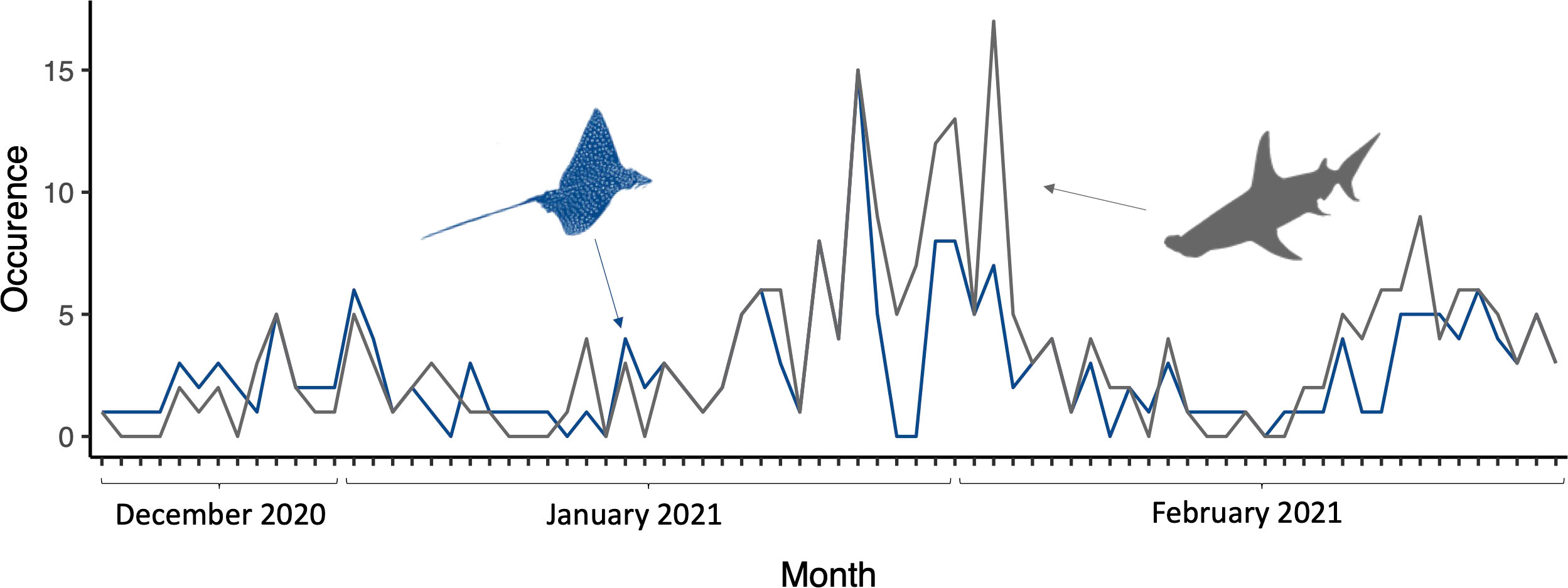
Figure 5 Temporal distribution of both S. mokarran (in grey) and A. ocellatus (in blue) occurrence from December 2020 to February 2021, location A (Tiputa Pass).
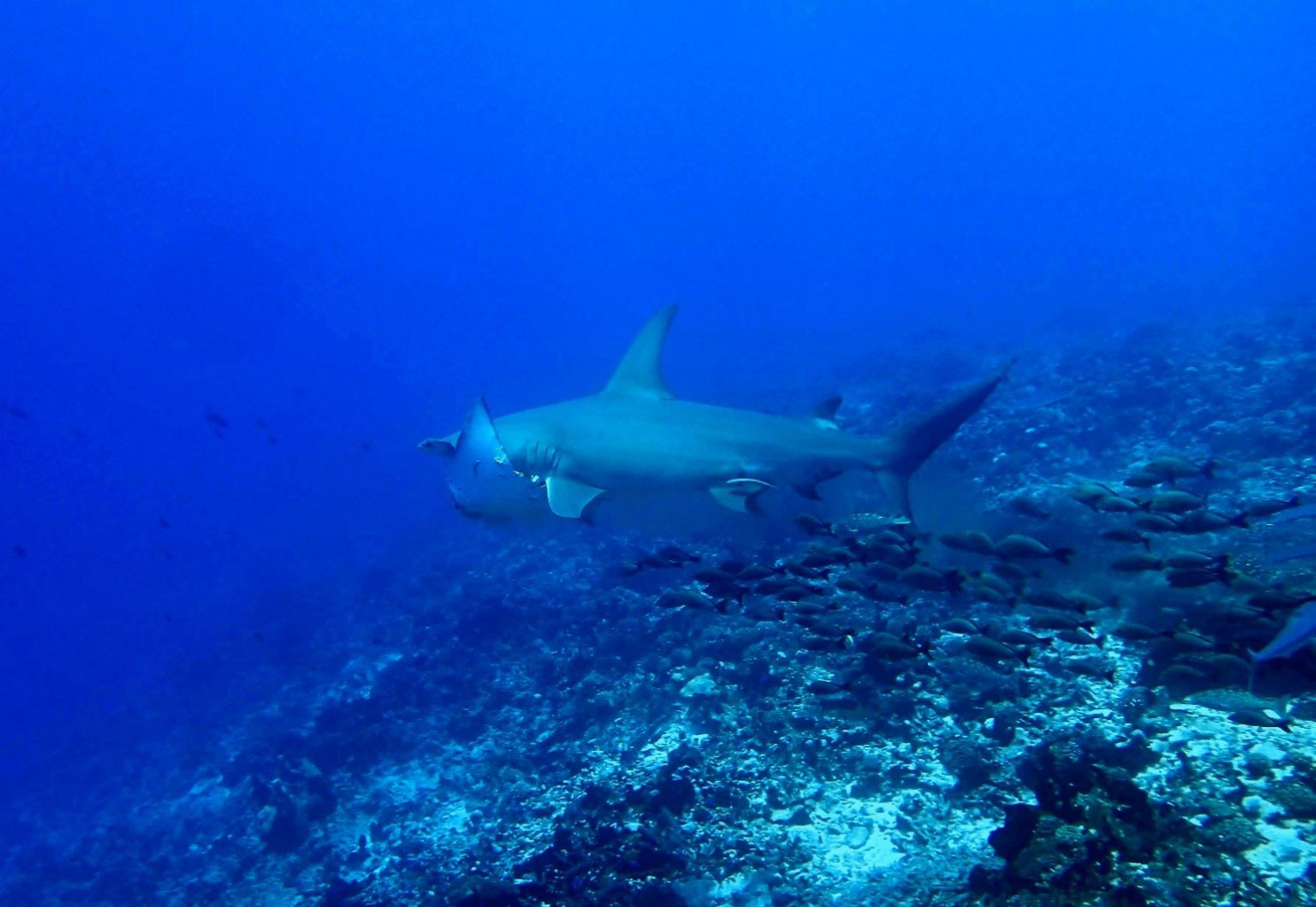
Figure 6 Predation by a great hammerhead shark S. mokarran on A. ocellatus (April 14th, 2021) in the Tiputa pass, location A. Picture by ©Damien Cortet from Y AKA Plongée, Rangiroa.
4 Discussion
Our study shows that the Western Tuamotu archipelago and Rangiroa atoll are seasonally important for great hammerhead sharks, with a total of 55 individuals identified among 167 sightings obtained across three locations. Results provided evidence of philopatric behaviour for great hammerhead sharks, with more than half of the sharks demonstrating seasonal residency, long-term site fidelity or both. All individuals measured were likely mature and a strong female bias in the sex ratio stands out during the austral summer. Thirty additional great hammerhead sharks were identified from the historic dataset, with further evidence of sexual segregation between males and females and insights on the philopatric behaviours and geographical connectivity between the two sites. In location A, the moon age was the only significant factor affecting shark sightings during both seasons, with observation rate increasing shortly before and after the full moon. Temperature data recorded only during the 2020 – 2021 season showed that great hammerhead sharks were sighted between 26.6°C and 28.9°C and the abundance of ocellated eagle rays and great hammerhead sharks sighted was synchronous throughout the season.
4.1 Identification of great hammerhead sharks
We used photo-ID, with a combination of fins morphology and natural body markings, to identify 55 individual great hammerhead sharks, proving the effectiveness of photo-ID without the need to attract sharks using bait or other food-based attractant, as has been the case in previous studies for great hammerhead and other species (e.g., Buray et al., 2009; Bègue, 2017; Guttridge et al., 2017; Heim et al., 2021). Few published data exists on population size estimates of great hammerhead sharks around the world, with the only sources coming from fishery activities in the northwest Atlantic, Gulf of Mexico and Indian Ocean (Rigby et al., 2019). The only other region with an estimate of great hammerhead sharks’ abundance is in Bimini, Bahamas. According to Guttridge et al. (2017) and Heim et al. (2021) , between 26 to 28 great hammerhead sharks were identified within a 4-month period, which is consistent with our findings (e.g., n = 27 individuals in 3 months of surveys in 2020 – 2021; n = 23 in 2 months of surveys 2019 – 2020). To our knowledge, the number of sharks identified in Bimini is not reported, but is expected to be at least 70+ individuals between 2000 and 2017 based on the evidence provided in Guttridge et al. (2017) and Heim et al. (2021) (e.g., number of identified sharks and period of sampling). In our study, individual sharks could not be identified in 283 sightings due to a lack of distinctive markings on their body or too distant an approach. Considering the number of great hammerhead sharks identifiable over the two seasons of study (n = 55) and over 15 years of randomly collected diver photos (n = 30), the population of great hammerhead sharks in the Western Tuamotu is of at least 85 individuals and is likely to be larger. However, a mark-recapture study would be necessary to estimate population size accurately, but was outside the scope of this study. These results highlight the importance of continued monitoring efforts for this species in the archipelago.
4.2 Sexual segregation and maturity
All sharks sighted during the main surveys (but one could not be sexed) were identified as females. They were mainly sighted roving around the bottom of the plateau, independently from one another. Although described as a solitary species (Rigby et al., 2019), the number of female great hammerhead sharks naturally sighted at the same time, in the same location, suggests the presence of an aggregation site, as described in Morrow, 1948 (i.e., a chance grouping of individuals brought into a given locality by some external factor or factors not concerned with relationships between individuals), which differs from school formation, known in scalloped hammerheads sharks (Gallagher and Klimley, 2018). The results from the historic dataset suggest that males are essentially observed between August and October, which supports our lack of males sighted during the austral summers of 2019 – 2020 and 2020 – 2021. Sexual segregation in great hammerhead sharks has not been reported in fishery-based studies (Stevens and Lyle, 1989; Cliff, 1995; Piercy et al., 2010; O’Connell and Leurs, 2015; Raoult et al., 2019; Hsu et al., 2020), unlike other hammerhead species, such as the scalloped hammerhead sharks (Sphyrna lewini, Griffith and Smith, 1834) (Klimley, 1987; Stevens and Lyle, 1989; Harry et al., 2011; Noriega et al., 2011); or other marine species (Sims, 2005; Wearmouth and Sims, 2008). Yet, all three studies conducted on the great hammerhead sharks in Bimini, Bahamas, show a female-dominated population (Guttridge et al., 2017; Heim et al., 2021; Guttridge et al., 2022). In contrast to our results, the few males identified in Bimini are observed during the same period as the females (i.e., December to May), which could be explained by the provisioning occurring in Bimini, attracting all sharks within range of the olfactory stimulus and could suggest that male great hammerhead sharks in Rangiroa and Tikehau atolls remain at a distance from the passes during the austral summers (e.g., off-shore or within the lagoon).
Based on our estimated sizes, all female sharks sighted in this study are large enough to be mature. Interestingly, the austral summer matches the identified parturition period of the great hammerhead sharks in the southern hemisphere, e.g., off the coast of northern (December – January) (Stevens and Lyle, 1989) and eastern (October – November) (Harry et al., 2011) Australia. This temporal separation in habitat use by male and female great hammerhead sharks could thus results from pre or post-breeding strategies (Sims, 2005; Wearmouth and Sims, 2008; Noriega et al., 2011). There is no mention of juvenile or neonate great hammerhead sharks in both passes, but fishers report the presence of adult and small hammerheads inside the lagoon, although species identification is uncertain (Barker et al., 2017) as S. lewini is also present in the region. Lagoons and their protected warm-shallow-coastal waters are known to serve as nursery areas for various shark species. These environmental characteristics are also considered beneficial for embryonic development and post-partum recovery (Guttridge et al., 2022). This raises the question of the possible existence of a gestation/nursery area in the vicinity. The first ever described nursery for the species has likely been identified off the south-eastern coast of Florida (Macdonald et al., 2021), providing evidence that such functional areas may exist for the species. A large citizen survey with local fishers who operate daily throughout the lagoon is currently being conducted in Rangiroa and Tikehau atolls to further investigate this hypothesis.
4.3 Seasonal residency, regional connectivity and site fidelity
Seasonal (e.g., extending from November to May) residency was reported in 41.4% of the 70 females identified from 2006 to 2021 and in 46.3% of the 54 females identified from the austral summer months of 2019 – 2020 and 2020 – 2021, with individual variation in frequency of sightings and length of stay (i.e., between first and last observation of the season) and no apparent site variation. However, considering that one female (A06) moved between the two sites (location A and B) in 2015 and 2018 within the same month, the question of scale of the residency arises. Other species in the Sphyrnidae family, such as the bonnethead shark (Sphyrna tiburo, FishBase et al. (2023); Heupel et al., 2006) or the scalloped hammerhead shark (S. lewini; Gallagher and Klimley, 2018) are known to exhibit residency. In Bimini, Bahamas, Guttridge et al. (2017) used a combination of acoustic tracking and photo-ID to report seasonal and regional residency and movements of the great hammerheads shark at the Florida-Bahamas scale. Residency was assessed using a residency index (RI; e.g., total number of days a shark is detected divided by the number of days it could potentially be detected), ranging from 0.01 to 0.68 depending on the location and time of year (Guttridge et al., 2017). The RI was higher during winter months (i.e., January – March) in Bimini, which matches our results in the Tuamotu archipelago, although occurring in the south hemisphere. The seasonal and regional residency at Bimini was correlated with the long-standing provisioning activities taking place in the area and the decrease in temperature (Guttridge et al., 2017). This contrasts with our study sites where the occurrence of the great hammerhead sharks is timed with an increase in water temperature (See section 4.4.1). Also, wildlife provisioning has been prohibited in French Polynesia since 2017 (environmental code; annex to the law of the land n° 2017-25, Art. LP. 2200-1), so no touristic activities in the area can influence the residency behaviour of the species. Great hammerhead sharks were mainly sighted on the “Hammerhead plateau” with no indication of provenance, but when sighted within location A (i.e., by divers drifting with the incoming current) they were mainly observed coming out of the lagoon (T. Boube, pres. obs.). Great hammerhead sharks could therefore remain within the lagoon and move out into the pass at variable frequency during the incoming current (i.e., implying that sharks would mainly enter the lagoon during outgoing current) in response to a prey aggregation pulse (Chapman et al., 2015; Guttridge et al., 2017; see section 4.4.3). In location B, great hammerhead sharks were sighted randomly within the whole length of the pass, with no apparent indication of origin (T. Boube, pres. obs.). The geographical connectivity established between the two sites indicate that they can head out to other nearby sites. The seasonal residency could then be considered at the Western Tuamotu Archipelago scale. However, the fact that only one individual out of the 85 identified showed connectivity between these two atolls could also suggest the occurrence of two distinct population with few overlaps in the sites frequented. The female (A06) that was observed at the two atolls was a large individual. It is therefore possible that female great hammerhead sharks extend their home range once they reach a certain size. Genetic analyses would provide valuable insights into gene flow patterns and population connectivity. The use of photo-ID may have limited our ability to detect the full extent of the seasonal residency (e.g., representative calculation of a RI) and movements between locations A and B, which could be assessed using tracking technology (e.g., satellite or acoustic tracking) (Rohner et al., 2022).
Site fidelity was reported in 41.4% of the 70 females identified from 2006 to 2021 and in 46.3% of the 54 females identified from the austral summer months of 2019 – 2020 and 2020 – 2021, with a regular return ranging between a one to three-year period in location B and between a one to 12-year period in location A. This represents the longest site fidelity reported for the species. Long-term site fidelity behaviour has previously been documented for the great hammerhead sharks of both sexes in Bimini, Bahamas (Guttridge et al., 2017; Heim et al., 2021), with a maximum fidelity established across a 7-year period and a return use of up to four consecutive winter seasons. It is suggested to be linked to foraging behaviour as the region provides an abundance of prey (e.g., small elasmobranchs and teleost) (Strong et al., 1990; Chapman and Gruber, 2002; Roemer et al., 2016; Guttridge et al., 2017). The long-term and seasonal site fidelity documented in our study could also be linked to the increase in prey availability (see section 4.4.3), resulting in a feeding site fidelity (Speed et al., 2010; Chapman et al., 2015) as is suggested in the Bahamas. But this explanation appears partial when considering the occurrence of a mature female-biased population during this time of year, raising the question of a possible pupping/parturition site fidelity behaviour (Speed et al., 2010; Chapman et al., 2015), see section 4.3). Chapman et al. (2015) notes that site fidelity can be motivated by different activities such as feeding and pupping at the same time. Indeed, in the middle of the oligotrophic Pacific Ocean, the geomorphology of atolls generates very productive systems (i.e., lagoon and passes) (Michel et al., 1971), providing abundant seasonal food source and a diversity of potential key habitats for great hammerhead sharks. Thus, an abundance of resources may explain the long-term site fidelity and seasonal residency behaviour of 25.7% (n=18) of all the females identified (percentage likely underestimated by the method used). The philopatric behaviour of male great hammerhead shark is beyond the scope of this study as the main surveys focused on the austral summer season (e.g., female biased), however, results from the historic database show preliminary evidence of site fidelity to location A.
4.4 Impact of biotic and abiotic drivers on the great hammerhead shark presence in location A
4.4.1 Temperature
The influence of temperature on the great hammerhead shark’s range (vertical and horizontal) has been relatively well studied (Calich, 2016; Queiroz et al., 2016; Guttridge et al., 2022) and is presumed to play a major role in the timing of migration (Guttridge et al., 2017), as is often the case in ectothermic species (Huey and Stevenson, 1979). The thermocline in the oceanic Tuamotu waters is characterized as stable (e.g., between 25° – 29°C all year long) and deep (e.g., around 150 – 200 m depth), with lagoon waters being warmer (+ 1°C on average) and influencing water temperature only in the immediate vicinity of the passes (Rancher and Rougerie, 1995). Our recording site (i.e., the “Hammerhead plateau”) is located on the outer part of the pass and is thus exposed to both lagoon and oceanic water influences. Great hammerhead sharks were recorded between 26.6°C – 28.9°C, in 74.2% of the temperature range over two months. This is a slightly warmer and narrower temperature range than presented by Calich (2016) (i.e., 25°– 29.9°C) and Guttridge et al. (2022) (i.e., 23°– 28°C), but it is consistent with the surface layer temperature described in Rancher and Rougerie (1995), indicating that vertical distribution of the species in the 0 – 150 m layer in the region should be independent from temperature variation. However, our results are limited as they only include two summer months of recording. Interestingly and in contrast to these preliminary data, in Guttridge et al. (2017), great hammerheads appeared to leave their residential waters when temperature exceeded a 26°C threshold. Since many studies highlight the role of temperature in regulating sexual segregation (Robbins, 2007) or seasonal movements and migrations of large coastal and highly mobile shark species (Kessel et al., 2014; Queiroz et al., 2016; Guttridge et al., 2017), future research should include temperature monitoring throughout the year to explore drivers of arrival time in location A.
4.4.2 Moon cycle governing great hammerhead shark seasonal presence
The lunar cycle influences biological processes (e.g., reproduction cycle), movements (e.g., horizontal and vertical migration), and foraging behaviour of marine life (e.g., prey-predator interactions) (Vianna et al., 2013; Ikegami et al., 2014; Rivas et al., 2015; Fallows et al., 2016; Mourier et al., 2019). The direct or indirect effect of this cycle on the occurrence and movements of great hammerhead sharks to location A is difficult to establish, as physical factors and the biological responses are likely interconnecting. Variation of moonlight induced by different lunar phases is an obvious physical effect, especially at nighttime. As night illumination increases with full moon, the sensory abilities of predators and their ability to detect preys could increase as well. Although this theory has been supported in terrestrial environments (Penteriani et al., 2011; Prugh and Golden, 2014), no study has demonstrated its direct effect on marine predators. The deployment of motion sensing dataloggers would be useful to assess change in activity during the full moon and better understand the causal factors. Another interesting potential physical factor is presented by Nishimura and Fukushima (2009), which suggests that changes in geomagnetic fields during the full moon are perceived by animals, in response to an increase in their magnetoreception sensitivity during that same moon phase. Sharks are able to sense and respond to changes in geomagnetic stimuli (Meyer et al., 2005), using it for orientation and migration purposes (Keller et al., 2021). Tide is also another environmental variable linked to the lunar cycle but its influence varies according to parameters, such as the location (e.g., distance from the moon and location of the sun), the local sea bottom topography and the currents (Melchior, 1983). Given the atypical geomorphology of the study site (e.g., the top of a 1300 m coral barrier exposed to alternating in and outgoing currents), the influence of tide in relation to the full moon is difficult to assess (French et al., 2021).
4.4.3 Ocellated eagle rays’ and other preys’ abundance as a potential driver of great hammerhead shark seasonal occurrence
Seasonal movements and aggregations in relation to high prey abundance have been reported in many shark species (Heyman et al., 2001; Heithaus et al., 2002; Torres et al., 2006; Mourier et al., 2016; Logan et al., 2020). The great hammerhead shark is known to migrate according to food availability in the northwest Atlantic Ocean (Guttridge et al., 2017). Our results show a clear relationship between the number of great hammerhead sharks and ocellated eagle rays in location A across the austral summer period (Figure 5), which is complementary with Séguigne et al. (2023) that showed a synchrony of observations of the two species in location A on a multi-year scale. The gathering of A. ocellatus is thus a predictable event that sharks could try to intercept, indicating that predation is a likely driving factor of the seasonal presence of great hammerhead sharks. Numerous visual observations of hunting (Strong et al., 1990; Chapman and Gruber, 2002; this study Figure 6), stomach content analyses (Stevens and Lyle, 1989; Cliff, 1995), and stable isotope analyses (Raoult et al., 2019) have shown that rays are significant prey for great hammerhead sharks. Few studies have been conducted on A. ocellatus in French Polynesia (Berthe et al., 2018), however social gathering of schools of rays is a behaviour known to be linked to reproduction phases in the genus Aetobatus (Bassos-Hull et al., 2014; Bacchet et al., 2017). Local observations of mating behaviour of pairs of ocellated eagle rays in sub-surface waters within Rangiroa lagoon during the austral summer, supports the hypothesis that ocellated eagle rays gather in location A for pre-reproduction strategies (i.e., courtship) and could enter the lagoon for mating (Tricas, 1980; Pratt and Carrier, 2005). It would thus explain the variation in numbers of ocellated eagle rays in the pass and consequently the number of predators, as mating pairs would be easier prey for great hammerhead sharks inside the lagoon (Carrier et al., 2004). However, this correlation between the number of great hammerhead sharks and ocellated eagle rays should be interpreted with caution as environmental and other biotic factors could drive increase and variation in the abundance of both species.
Reef sharks (e.g., grey reef shark, Carcharhinus amblyrhynchos, Bleeker, 1856 and blacktip reef sharks, Carcharhinus limbatus, Müller and Henle, 1839) are common prey of great hammerhead sharks (Raoult et al., 2019; Doan and Kajiura, 2020) and predation on grey reef sharks has also been described in French Polynesia (Mourier et al., 2013). Looking at dive instructors’ observation data from Séguigne et al. (2023), grey reef sharks’ abundance in the pass tends to decrease between January/May. However, based on anecdotal observations, the overall abundance of grey reefs does not vary during the year, only the location of the groups within the pass during this period, with grey reef sharks moving to another side of the pass, less frequented by divers and closer to the Hammerhead plateau. The continuous annual presence of grey reef sharks in location A does not, therefore, seem to explain the seasonal peak in great hammerhead sharks’ presence on its own, but could be an additional driver in the seasonal presence of A. ocellatus. Stable isotope analyses should help better understand the relative importance of prey abundance and predatory opportunities to the occurrence of great hammerhead sharks in location A. In the Atlantic, great hammerhead sharks are also known to synchronise their horizontal movements with predictable spawning aggregations of preys such as Atlantic tarpon Megalops atlanticus (Valenciennes, 1847) and permit Trachinotus falcatus (Linnaeus, 1758) off the Florida Keys and Bahamas (Lowerre-Barbieri et al., 2021; Griffin et al., 2022; Guttridge et al., 2022). Similar spawning aggregation events occur in the Tuamotu passes during the austral spring and summer for the longnose emperors (Lethrinus olivaceus, Valenciennes, 1830) (Filous et al., 2021) and the bigeye scads (Selar crumenophthalmus, Bloch, 1793), which could possibly play a role in the horizontal movements of great hammerheads shark locally.
5 Conclusion
Traditionally in the Polynesian culture, the austral summer is called Matarii i ni’a (from mid-November to mid-May) and represents a season of abundance (Henry, 1951) during which the reproduction of many reef and lagoon fishes occurs (Sissons, 2014). In contrast, Matarii I raro (from mid-May to mid-November), represents a season of scarcity (Henry, 1951), often leading to fishing restrictions. Like the Polynesians, the great hammerhead shark seems to take advantage of Matarii i ni’a for needs inherent to its life cycle. This study provides the first life-history characteristics of the great hammerhead shark in the Central Pacific Ocean with an unprecedented in-situ sampling effort conducted without provisioning and capture. The use of non-invasive techniques reveals specific features of its seasonal population. At least 54 different females were present in two atolls of the Western Tuamotu archipelago over two years during the austral summer period. The combination of sexual segregation and site-specific philopatric behavior during the summer months suggests an occurrence in the area related to critical life-history needs. This timeframe matches an increase in prey concentration and diversity for the great hammerhead sharks and an increase in water temperature after the winter months. Rangiroa and Tikehau atolls could include various essential habitats (foraging, parturition, resting) for the species, from deep outer reefs to rich shallow lagoon waters. The results of this study highlight the importance of these atolls for the conservation of great hammerhead sharks, where it still occurs in high numbers. Out of the 80+ atolls that compose the Tuamotu archipelago, at least 18 of them report great hammerhead shark sightings by fishers, inside or outside lagoons. Future studies on the species regional and fine-scale movements could provide all year-round clues on essential habitats location inside the lagoons and migration patterns within the archipelago, to inform long-term species-specific conservation planning at local and regional scales.
Data availability statement
The datasets presented in this study can be found in online repositories. The names of the repository/repositories and accession number(s) can be found in the article/Supplementary Material.
Ethics statement
The study was approved by the DIREN (Environmental Department of French Polynesia). Non-invasive scientific techniques were applied, no provisioning or capture were conducted for this study. An ethical approach guide was established for all divers involved; they received training.
Author contributions
TB, AG, NF, FB and J-MJ, were responsible for project conception funding acquisition and administration. TB, AG, C-SA, NF, EL, AB, TT, YF, FB, TP, VJ, AM, MB, JB, BR, LR, AL, FC, MH, JMJ and PLS conducted fieldwork. TB, AG, C-SA, NF, EL, YF, FB, J-MJ and P-LS contributed to conception and design of the study. TB, AG, C-SA, AK, AB and P-LS conducted data analysis. TB, P-LS, CSA, CH, YP, JM and JT wrote the first draft of the manuscript. TB, AG, and J-MJ helped with fieldwork logistics and permits. All authors have validated the manuscript and given constructive comments. All authors contributed to the article and approved the submitted version.
Funding
This work was supported by our financial partners “Blancpain” and the “Banque de Tahiti”.
Acknowledgments
This study could not have been successfully completed without the Direction de l’Environnement (DIREN) of French Polynesia and our financial partners Blancpain and “Banque de Tahiti”. We thank the municipality of Rangiroa and Tikehau; Andromède Océanologie; The Polynesian Shark Observatory (ORP) with Nicolas Buray; Thomas Perceval and Christophe Réquillart; Christian Lumbreras; our dedicated volunteers Jean-Marc Belin and Sterenn Gallou; our network of contributing photographers (alphabetical order): Francis Abbott, Sauveur Adamo, Vincent Albertini, Christian Allanic, Nicolas Amiel, Arnaud Avare, Sophie Babel, Bernard Beaussier, Michel Bègue, Xavier Béziers, Christiane Borg Barthet, Jimmy Caire, Mathias Castello, David Chandeleur, Damien Cortet, Angélique De Stéphano, Bertrand Feuillet, Kori Garza, Jean-Marie Gayard, Etienne Guitton, Volker Heitmann, Christian Heylen, Alain Hurpeau, Anne Hurpeau, Christophe Kraether, Mathieu Junker, Baptiste Le Bouil, Eva Lefebvre, Manuel Lefevre, Erick Maillet, Charles Marque, Gerard Martigny, Ugo Mazzavillani, Rémi Millot, Marc Mouton, Jaime Paumier, Francisque Protche, Chloé Rauchs, Serge Renault, Marine Pacchiano, Peter Schneider, Albert Sholz, Didier Théron, Yoshino Katuhilo and Sophie Vanruymbeke; our network of contributing divers (alphabetical order): Laurent Dureau, Raphael Ferreira, Bélen Ferro, Jean-Louis Jacquelle, Sébastien Hubert, Yves Lefèvre, Mao Manuarii, Martin Marquez, Alizée Même, Claudio Pena, Gaël Rossi, Yukari Sugimoto, and Yannick Wagner; the local dive operators “Rangiroa Plongée”, “YAKA Plongée”, “CCR Rangiroa”, “Top Dive Rangiroa”, “Rangiroa Diving Center”, “Raie Manta Club Rangiroa” “Raie Manta Club Tikehau”, “Tikehau Plongée”, “Coco Dive”, “Hititemanava Tikehau Excursion”.
Conflict of interest
The authors declare that the research was conducted in the absence of any commercial or financial relationships that could be construed as a potential conflict of interest.
Publisher’s note
All claims expressed in this article are solely those of the authors and do not necessarily represent those of their affiliated organizations, or those of the publisher, the editors and the reviewers. Any product that may be evaluated in this article, or claim that may be made by its manufacturer, is not guaranteed or endorsed by the publisher.
Supplementary material
The Supplementary Material for this article can be found online at: https://www.frontiersin.org/articles/10.3389/fmars.2023.1234059/full#supplementary-material
References
Bacchet P., Lefevre Y., Zysman T. (2017). Guide des poissons de Tahiti et de ses iles. Cinquième édition. Au vent Des. îles collect. Nat. environ. d’Océanie. 84p.
Barker A. M., Frazier B. S., Bethea D. M., Gold J. R., Portnoy D. S. (2017). Identification of young-of-the-year great hammerhead shark Sphyrna mokarran in northern Florida and South Carolina. J. Fish Biol. 91 (2), 664–668. doi: 10.1111/jfb.13356
Bassos-Hull K., Wilkinson K.A, Hull P.T., Dougherty D.A., Omori K.L., Ailloud L.E., et al. (2014). Life history and seasonal occurrence of the spotted eagle ray, Aetobatus narinari, in the eastern Gulf of Mexico. Environ. Biol. fishes 97 (9), 1039–1056. doi: 10.1007/s10641-014-0294-z
Bègue M. (2017). Photo-identification et évaluation de la fidélité du requin tigre (Galeocerdo cuvier) à un site de nourrissage artificiel à Tahiti (Polynésie française). Biodiversité Ecol.
Berthe C., Waqalevu V., Latry L., Besson M., Lerouvreur F., Siu, et al. (2018). Distribution patterns of ocellated eagle rays, Aetobatus ocellatus, along two sites in Moorea Island, French Polynesia. Cybium 42 (4), 313–320.
Bleeker (1856). FishBase Carcharhinus amblyrhynchos (Bleeke) (World Register of Marine Species). Available at: https://www.marinespecies.org/aphia.php?p=taxdetails&id=217337 (Accessed 2023-08-12).
Bloch. (1793). FishBase Selar crumenophthalmus (Bloc) (World Register of Marine Species). Available at: https://www.marinespecies.org/aphia.php?p=taxdetails&id=159646 (Accessed 2023-08-12).
Buray N., Mourier J., Planes S., Clua E. (2009). Underwater photo-identification of sicklefin lemon shark, Negaprion acutidens, at Moorea (French Polynesia). Cybium 33, 21e27.
Calich H. (2016). Identifying suitable habitat for three highly migratory sharks (Great hammerhead, tiger, and bull) and assessing their spatial vulnerability to commercial longline fishing in the southwest atlantic ocean and gulf of Mexico University of Miami. Open Access Theses.
Carrier J. C., Musick J., Heithau M. (2004). Biology of Sharks and their Relatives. Chapter 17: Predator-Prey Interactions (Boca Raton: CRC Press). 616 pp.
Chapman D. D., Feldheim K. A., Papastamatiou Y. P., Hueter R. E. (2015). There and back again: a review of residency and return migrations in sharks, with implications for population structure and management. Annu. Rev. Mar. Sci. 7, 547–570. doi: 10.1146/annurev-marine-010814-015730
Chapman D. D., Gruber S. H. (2002). A further observation of the prey-handling behavior of the great hammerhead shark, Sphyrna mokarran: Predation upon the spotted eagle ray, Aetobatus narinari. Bull. Mar. Sci. 70 (3), 947–952.
CITES. (2019). Appendices I, II and III of the Convention on International Trade in Endangered Species of Wild Fauna and Flora (CITES) (CITES).
Chin A., Mourier J., Rummer J. L. (2015). Blacktip reef sharks (Carcharhinus melanopterus) show high capacity for wound healing and recovery following injury. Conserv. Physiol. 3 (1), cov062. doi: 10.1093/conphys/cov062
Cliff G. (1995). Sharks caught in the protective gill nets off KwaZulu-Natal, South Africa. 8. The great hammerhead Sphyrna mokarran (Ruppell,1835). S Afr J. Mar. Sci. 15, 105–114. doi: 10.2989/025776195784156331
CMS. (2019). Appendices I and II of the Convention on the Conservation of Migratory Species of Wild Animals (CMS), as amended by the Conference of the Parties in 1985, 1988, 1991. 2002, 2005, 2008. and 2020 (CMS).
Compagno L. J. V. (1984). FAO species catalogue, Vol. 4, Sharks of the world. An annotated and illustrated catalogue of shark species known to date. Part 2. Carcharhiniforms. FAO Fish. Synop. 4 (Pt 2), 251–655.
Cortés E. (2000). Life history patterns and correlations in sharks. Rev. Fish. Sci. 8, 299–344. doi: 10.1080/10408340308951115
Deakos M. (2010). Paired-laser photogrammetry as a simple and accurate system for measuring the body size of free-ranging manta rays Manta alfredi. Aquat. Biol. 10, 1–10. doi: 10.3354/ab00258
Doan M. D., Kajiura S. M. (2020). Adult blacktip sharks (Carcharhinus limbatus) use shallow water as a refuge from great hammerheads (Sphyrna mokarran). J. Fish Biol. 96 (6), 1530–1533. doi: 10.1111/jfb.14342
Dobson A. J., Barnett A. G. (2018). An Introduction to Generalized Linear Models. 4th ed (Boca Raton, Florida, USA: Chapman and Hall/CRC). doi: 10.1201/9781315182780
Domeier M. L., Nasby-Lucas N. (2007). Annual re-sightings of photographically identified white sharks (Carcharodon carcharias) at an eastern Pacific aggregation site (Guadalupe Island, Mexico). Mar. Biol. 150, 977–984. doi: 10.1007/s00227-006-0380-7
Fallows C., Fallows M., Hammerschlag N. (2016). Effects of lunar phase on predator-prey interactions between white shark (Carcharodon carcharias) and Cape fur seals (Arctocephalus pusillus pusillus). Environ. Biol. Fishes 99 (11), 805–812. doi: 10.1007/s10641-016-0515-8
Filous A., Daxboeck C., Beguet T., Cook C. (2021). The life history of longnose emperors (Lethrinus olivaceus) and a data-limited assessment of their stock to support fisheries management at Rangiroa Atoll, French Polynesia. J. Fish Biol. 100 (3), 1–13. doi: 10.1111/jfb.1497
FishBaseFroese R., Pauly D. Editors. (2023). Sphyrna tiburo (Linnaeus, 1758). Accessed through: World Register of Marine Species at: https://www.marinespecies.org/aphia.php?p=taxdetails&id=158517on2023-08-2
French L. A., Midway S. R., Evans D. H., Burgess G. H. (2021). Shark side of the moon: are shark attacks related to lunar phase? Front. Mar. Sci. 8. doi: 10.3389/fmars.2021.745221
Gallagher A. J., Klimley A. P. (2018). The biology and conservation status of the large hammerhead shark complex: The great, scalloped, and smooth hammerheads. Rev. Fish Biol. Fish. 28, 777–794. doi: 10.1007/s11160-018-9530-5
Gallagher A., Serafy J., Cooke S., Hammerschlag N. (2014). Physiological stress response, reflex impairment, and survival of five sympatric shark species following experimental capture and release. Mar. Ecol. Prog. Ser. 496, 207–218. doi: 10.3354/meps10490
Graham F., Rynne P., Estevanez M., Luo J., Ault J. S., Hammerschlag N. (2016). Use of marine protected areas and exclusive economic zones in the subtropical western North Atlantic Ocean by large highly mobile sharks. Divers. Distrib 22, 534–546. doi: 10.1111/ddi.12425
Griffith & Smith. (1834). FishBase Sphyrna lewini (Griffith & Smit) (World Register of Marine Species). Available at: https://www.marinespecies.org/aphia.php?p=taxdetails&id=105816 (Accessed 2023-08-12).
Griffin L. P., Casselberry G. A., Lowerre-Barbieri S. K., Acosta A., Adams A. J., Cooke S. J., et al. (2022). Predator-prey landscapes of large sharks and game fishes in the Florida Keys. Ecol. Appl. 32 (5), e2584. doi: 10.1002/eap.2584
Gubili C., Johnson R., Gennari E., Oosthuizen W.H., Kotze D., Meÿer M., et al. (2009). Concordance of genetic and fin photo identification in the great white shark, Carcharodon carcharias, off Mossel Bay, South Africa. Mar. Biol. 156, 2199–2207. doi: 10.1007/s00227-009-1233-y
Guttridge T. L., Müller L., Keller A. B., Bond M. E., Grubbs R. D., Winram W., et al. (2022). Vertical space use and thermal range of the great hammerhead (Sphyrna mokarran), (Rüppell 1837) in the western North Atlantic. J. Fish Biol 4, pp. 1–3. doi: 10.1111/jfb.15185
Guttridge T. L., Van Zinnicq Bergmann M. P. M., Bolte C., Howey L. A., Finger J. S., Kessel S. T., et al. (2017). Philopatry and regional connectivity of the great hammerhead shark, sphyrna mokarran in the U.S. and Bahamas. Front. Mar. Sci. 4. doi: 10.3389/fmars.2017.00003
Hammerschlag N., Gallagher A. J., Lazarre D. M., Slonim C. (2011). Range extension of the Endangered great hammerhead shark Sphyrna mokarran in the Northwest Atlantic: preliminary data and significance for conservation. Endanger Species Res. 13, 111–116. doi: 10.3354/esr00332
Harmelin V. (1985). “Contribution à l’étude de l’atoll de Tikehau – Présentation générale de l’atoll. ORSTOM Polynésie française,” in OCEANOGRAPHIE (French Polynesia: ORSTOM). ISSN _0755 _3412Notes et documents n° 24.
Harry A. V., Macbeth W. G., Guttridge A. N., Simpfendorfer C. A. (2011). The life histories of endangered hammerhead sharks (Carcharhiniformes, Sphyrnidae) from the east coast of Australia. J. Fish Biol. 78, 2026–2051. doi: 10.1111/j.1095-8649.2011.02992.x
Heim V., Dhellemmes F., Smukall M. J., Gruber S. H., Guttridge T. L. (2021). Effects of food provisioning on the daily ration and dive site use of great hammerhead sharks, sphyrna mokarran. Front. Mar. Sci. 8. doi: 10.3389/fmars.2021.628469
Heithaus M. R., Dill L. M., Marshall G. J., Buhleier B. (2002). Habitat use and foraging behaviour of tiger sharks (Galeocerdo cuvier) in a seagrass ecosystem. Mar. Biol. 140, 237–248. doi: 10.1007/s00227-001-0711-7
Henry M. T. (1951). Terminologie et système de calcul » in Tahiti aux temps anciens, ed. Publication de la SdO 1 (Paris, France: Société des Océanistes). 330–342.
Hervé M. F. (1929). Noms des requins des Tuamotu. Bulletin de la Société d’études océaniennes. Polynésie Orientale, Tomme III (Papeete – Polynésie française: Société des Études océaniennes), (N°10). N°32 - Aout 1929.
Heupel M. R., Simpfendorfer C. A., Collins A. B., Tyminski J.P.. (2006). Residency and movement patterns of bonnethead sharks, Sphyrna tiburo, in a large Florida estuary. Environ. Biol. Fish 76, 47–67. doi: 10.1007/s10641-006-9007-6
Heyman W. D., Graham R. T., Kjerfve B., Johannes R. E. (2001). Whale sharks Rhincodon typus aggregate to feed on fish spawn in Belize. Mar. Ecol. Prog. Ser. 215, 275–282. doi: 10.3354/meps215275
Hsu H. H., Nazeer Z. M., Lin Y. J., Panickan P., Al-Abdulkader K., Loughland R., et al. (2020). Biological aspects of juvenile great hammerhead sharks Sphyrna mokarran from the Arabian Gulf. Mar. Freshw. Res. 72, 110–117. doi: 10.1071/mf19368
Huey R. B., Stevenson R. D. (1979). Integrating thermal physiology and ecology of ectotherms: a discussion of approaches. Am. Zool 19, 357–366. doi: 10.1093/icb/19.1.357
Ikegami T., Takeuchi Y., Hur S.-P., Takemura A. (2014). Impacts of moonlight on fish reproduction. Mar. Genomics 14, 59–66. doi: 10.1016/j.margen.2013.11.007
Intes A. (1984). Présentation générale de l'atoll - in "L'atoll de Tikehau (archipel des Tuamotu, Polynésie Française) premiers résultats" (French Polynesia: ORSTOM), O.R.S.T.O.M. Tahiti~ Notes et Doc.
Keller B. A., Putman N. F., Grubbs R. D., Portnoy D. S., Murphy T. P. (2021). Map-like use of Earth’s magnetic field in sharks. Curr. Biol. 13, 2881–2886. doi: 10.1016/j.cub.2021.03.103
Kessel S. T., Chapman D. D., Franks B. R., Gedamke T., Gruber S. H., Newman J. M., et al. (2014). Predictable temperature-regulated residency, movement and migration in a large, highly mobile marine predator (Negaprion brevirostris). Mar. Ecol. Prog. Ser. 514, 175–190. doi: 10.3354/meps10966
Khul. (1823). FishBase Aetobatus ocellatus (Kuh (World Register of Marine Species). Available at: https://www.marinespecies.org/aphia.php?p=taxdetails&id=278243 (Accessed 2023-08-12).
Klimley A. P. (1987). The determinants of sexual segregation in the scalloped hammerhead, Sphyrna lewini. Environ. Biol. Fish 18, 27–40. doi: 10.1007/BF00002325
Linnaeus (1758). Systema naturae per regna tria naturae, secundum classes, ordines,genera, species, cum characteribus, differentiis, synonymis, locis 824.
Logan R. K., Vaudo J. J., Sousa L. L., Sampson M., Wetherbee B. M., Shivji M. S. (2020). Seasonal movements and habitat use of juvenile smooth hammerhead sharks in the Western North Atlantic Ocean and significance for management. Front. Mar. Sci. 7. doi: 10.3389/fmars.2020.566364
Lowerre-Barbieri S. K., Friess C., Griffin L. P., Morley D., Skomal G. B., Bickford J. W., et al. (2021). Movescapes and eco-evolutionary movement strategies in marine fish: Assessing a connectivity hotspot. Fish Fish. 22 (6), 1321–1344. doi: 10.1111/faf.12589
Macbeth W. G., Geraghty P. T., Peddemors V. M., Gray C. A. (2009). Observer-based study of targeted commercial fishing for large shark species in waters off northern New South Wales (New South Wales, Australia: Industry & Investment NSW, Sydney). Final Report Series No. 114.
Macdonald C., Jerome J., Pankow C., Perni N., Black K., Shiffman D., et al. (2021). First identification of probable nursery habitat for critically endangered great hammerhead Sphyrna mokarran on the Atlantic Coast of the United States. Conserv. Sci. Practice. 3, e418. doi: 10.1111/csp2.418
MacNeil M. A., Chapman D. D., Heupel M., Simpfendorfer C.A., Heithaus M., Meekan M., et al. (2020). Global status and conservation potential of reef sharks. Nature 583, 801–806. doi: 10.1038/s41586-020-2519-y
Meyer C. G., Holland K. N., Papastamatiou Y. P. (2005). Sharks can detect changes in the geomagnetic field. J. R. Soc Interface 2, 129–130. doi: 10.1098/rsif.2004.0021
Michel A., Colin C., Desrosières R., Oudot C. (1971). Observations sur l'hydrologie et le plancton des abords et de la zone des passes de l'atoll de Rangiroa, Cah. ORSTOM, sér. Océanogr 3, 375–402.
Morgan A., Burgess G. H. (2007). At-vessel fishing mortality for six species of sharks caught in the Northwest Atlantic and Gulf of Mexico. Gulf Caribb Res. 19, 123. doi: 10.18785/gcr.1902.15
Mourier J., Ballesta L., Clua E., Planes S. (2019). Visitation patterns of camouflage groupers Epinephelus polyphekadion at a spawning aggregation in Fakarava inferred by acoustic telemetry. Coral Reefs 38, 909–916. doi: 10.1007/s00338-019-01814-8
Mourier J., Maynard J., Parravicini V., Ballesta L., Clua E., Domeier M. L., et al. (2016). Extreme inverted trophic pyramid of reef sharks supported by spawning groupers, current biology. Current Biology 26, 15, 2011–2016. doi: 10.1016/j.cub.2016.05.058
Mourier J., Planes S., Buray N. (2013). Trophic interactions at the top of the coral reef food chain. Coral Reefs 1, 285. doi: 10.1007/s00338-012-0976-y
Müller & Henle. (1839). FishBase Carcharhinus limbatus (Müller & Henl) (World Register of Marine Species). Available at: https://www.marinespecies.org/aphia.php?p=taxdetails&id=105793 (Accessed 2023-08-12).
Nishimura T., Fukushima M. (2009). Why animals respond to the full moon: magnetic hypothesis. Biosci. Hypotheses 2, 399–401. doi: 10.1016/j.bihy.2009.06.006
Noriega R., Werry J. M., Sumpton W., Mayer D., Lee S. Y. (2011). Trends in annual CPUE and evidence of sex and size segregation of Sphyrna lewini: management implications in coastal waters of north eastern Australia. Fish Res. 110, 472–477. doi: 10.1016/j.fishres.2011.06.005
O’Connell C. P., Leurs G. (2015). A minimally invasive technique to assess several life-history characteristics of the endangered great hammerhead shark Sphyrna mokarran (2016). J. Fish Biol. 88, 1257–1264. doi: 10.1111/jfb.12900
Pacoureau N., Rigby C. L., Kyne P. M., Sherley R.B, Winker H., Carlson J.K., et al. (2021). Half a century of global decline in oceanic sharks and rays. Nature 589, 567–571. doi: 10.1038/s41586-020-03173-9
Penteriani V., Kuparinen A., del Mar Delgado M., Lourenço R., Campioni L. (2011). Individual status, foraging effort and need for conspicuousness shape behavioural responses of a predator to moon phases. Anim. Behav. 82 (2), 413–420. doi: 10.1016/j.anbehav.2011.05.027
Piercy A. N., Carlson J. K., Passerotti M. S. (2010). Age and growth of the great hammerhead shark, Sphyrna mokarran, in the north-western Atlantic Ocean and Gulf of Mexico. Mar. Freshw. Res. 61, 992–998. doi: 10.1071/MF09227
Pratt H. L., Carrier J. C. (2005). “Elasmobranch courtship and mating behavior,” in Reproductive Biology and Phylogeny of Chondrichthyes. Ed. Hamlett W. C. (Boca Raton: William C. Hamlett), 129–170.
Prugh R., Golden C. (2014). Does moonlight increase predation risk? Meta-analysis reveals divergent responses of nocturnal mammals to lunar cycles. J. Anim. Ecol. 83, 504–514. doi: 10.1111/1365-2656.12148
Queiroz N., Humphries N. E., Mucientes G., Hammerschlag N., Lima F. P., Scales K. L., et al. (2016). Ocean-wide tracking of pelagic sharks reveals extent of overlap with longline fishing hotspots. Proc. Natl. Acad. Sci. U.S.A. 113, 1582–1587. doi: 10.1111/1365-2656.12148
R Core Team. (2021). R: A language and environment for statistical computing (Vienna, Austria: R Foundation for Statistical Computing). Available at: https://www.R-project.org/.
Rancher J., Rougerie F. (1995). The oceanic environment of the Tuamotu archipelago (French Polynesia), Oceanol. Acta 18, 43–60.
Raoult V., Broadhurst M. K., Peddemors V. M., Williamson J. E., Gaston T. F. (2019). Resource use of great hammerhead sharks (Sphyrna mokarran) off eastern Australia. J. Fish Biol. 95, 1430–1440. doi: 10.1111/jfb.14160
Rigby C. L., Barreto R., Carlson J., Fernando D., Fordham S., Francis M. P., et al. (2019). Sphyrna mokarran. The IUCN Red List of Threatened Species (Gland, Switzerland: International Union for Conservation of Nature). e.T39386A2920499.
Rivas M. L., Santidrián Tomillo P., Uribeondo J. D., Marco A. (2015). Leatherback hatchling sea-finding in response to artificial lighting: interaction between wavelength and moonlight. J. Exp. Mar. Biol. Ecol. 463, 143–149. doi: 10.1016/j.jembe.2014.12.001
Robbins R. L. (2007). Environmental variables affecting the sexual segregation of great white sharks Carcharodon carcharias at the Neptune Islands South Australia. J. fish Biol. 70 (5), 1350–1364. doi: 10.1111/j.1095-8649.2007.01414.x
Roemer P. R., Gallagher A. J., Hammerschlag N. (2016). Shallow water tidal flat use and associated specialized foraging behavior of the great hammerhead shark (Sphyrna mokarran). Mar. Freshw. Behav. Physiol 49 (4), 235–249. doi: 10.1080/10236244.2016.1168089
Rohner C. A., Richardson A. J., Marshall A. D., Weeks S. J., Pierce S. J. (2011). How large is the world’s largest fish? Measuring whale sharks Rhincodon typus with laser photogrammetry. J. Fish Biol. 78, 378–385. doi: 10.1111/j.1095-8649.2010.02861.x
Rohner C. A., Richardson A. J., Prebble C. E. M., Marshall A. D., Bennett M. B., Weeks S. J., et al. (2015). Laser photogrammetry improves the size and demographic estimates for whale sharks. PeerJ 3, e886. doi: 10.7717/peerj.886
Rohner C. A., Venables S. K., Cochran J. E., Prebble C. E., Kuguru B. L., Berumen M. L., et al. (2022). The need for long-term population monitoring of the world’s largest fish. Endangered Species Res. 47, 231–248. doi: 10.3354/esr01177
Rüppell. (1837). FishBase Sphyrna mokarran (Rüppel) (World Register of Marine Species). Available at: https://www.marinespecies.org/aphia.php?p=taxdetails&id=105817 (Accessed 2023-08-12).
Séguigne C., Mourier J., Clua É., Buray N., Planes S. (2023). Citizen science provides valuable data to evaluate elasmobranch diversity and trends throughout the French Polynesia’s shark sanctuary. PloS One 18 (3), e0282837. doi: 10.1371/journal.pone.0282837
Sims D. W. (2005). “Differences in habitat selection and reproductive strategies of male and female sharks,” in Sexual segregation in vertebrates: ecology of the two sexes (Cambridge, UK: Cambridge University Press), 127–147.
Sissons J. (2014). The Polynesian iconoclasm. Religious revolution and the seasonality of power Vol. 160 (New York: Berghahn). p. ill., index.
Speed C. W., Field I. C., Meekan M. G., Bradshaw C. J. A. (2010). Complexities of coastal shark movements and their implications for management. Mar. Ecol. Prog. Ser. 408, 275–293. doi: 10.3354/meps08581
Stahl L. (2006). Le Code de l'environnement de la Polynésie française, Revue juridique de l'Environnement (Cachan, France: Lavoisier), Vol. 1. 5–19.
Stevens J. D., Lyle J. M. (1989). Biology of three hammerhead sharks (Eusphyra blochii, Sphyrna mokarran and S. lewini) from northern Australia. Mar. Freshw. Res. 40, 129–146. doi: 10.1071/MF9890129
Stoddart D. R. (1969). Reconnaissance geomorphology of Rangiroa atoll, Tuamotu Archipelago. Atoll Res. Bull. 125, 1–31. doi: 10.5479/si.00775630.125.1
Strong W. R., Snelson F. F., Gruber S. H. (1990). Hammerhead shark predation on stingrays – an observation of prey handling by Sphyrna mokarran. Copeia 3, 836–840. doi: 10.2307/1446449
Torres L. G., Heithaus M. R., Delius B. (2006). Influence of teleost abundance on the distribution and abundance of sharks in Florida Bay, USA. Hydrobiologia 569 (1), 449–455. doi: 10.1007/s10750-006-0148-6
Towner A. V., Wcisel M. A., Reisinger R. R., Edwards D., Jewell O. J. D. (2013). Correction: gauging the threat: the first population estimate for white sharks in South Africa using photo identification and automated software. PloS One 8 (6), 1–7. doi: 10.1371/annotation/2ac91258-bfcc-40f4-adee-3f855b1a4be7
Tricas T. C. (1980). Courtship and mating-related behaviors in myliobatid rays. Copeia 1980, 673 553–556. doi: 10.2307/1444540
Valenciennes. (1830). FishBase Lethrinus olivaceus (Valencienne) (World Register of Marine Species). Available at: https://www.marinespecies.org/aphia.php?p=taxdetails&id=212069 (Accessed 2023-08-12).
Valenciennes. (1847). FishBase Megalops atlanticus (Valencienne) (World Register of Marine Species). Available at: https://www.marinespecies.org/aphia.php?p=taxdetails&id=126430 (Accessed 2023-08-12).
Venables W. N., Ripley B. D. (2002). “Random and Mixed Effects,” in Modern Applied Statistics with S. Statistics and Computing (New York, NY: Springer). doi: 10.1007/978-0-387-21706-2_10
Vianna G., Meekan M., Meeuwig J., Speed C. (2013). Environmental Influences on Patterns of Vertical Movement and Site Fidelity of Grey Reef Sharks (Carcharhinus amblyrhynchos) at Aggregation Sites. PloS One 8 (4), 1–13. doi: 10.1371/journal.pone.0060331
Walls R. H. L., Dulvy N. K. (2021). Tracking the rising extinction risk of sharks and rays in the Northeast Atlantic Ocean and Mediterranean Sea. Sci. Rep. 11, 15397. doi: 10.1038/s41598-021-94632-4
Ward-Paige C. A., Worm B. (2017). Global evaluation of shark sanctuaries, Global Environmental Change (Amsterdam: Elsevier), Vol. 47. 174–189, ISSN 0959-3780.
Wearmouth V. J., Sims D. W. (2008). Sexual segregation of marine fish, reptiles, birds and mammals: behaviour patterns, mechanisms and conservation implications. Adv. Mar. Biol. 54, 107–170. doi: 10.1016/S0065-2881(08)00002-3
Keywords: Central Pacific, photo-identification, laser-photogrammetry, sexual segregation, seasonal residency, site fidelity, environmental drivers
Citation: Boube T, Azam C-S, Guilbert A, Huveneers C, Papastamatiou YP, Mourier J, Trujillo JE, Femmami N, Kunovsky A, Bersani F, Laurent E, Bousseyroux A, Thellier T, Follin Y, Pavy T, Jeandel V, Mataarere A, Burlot M, Bouyeure J, Rigoreau B, Rigoreau L, Lenormand A, Chalabi F, Hayek M, Jeandel J-M and Stenger P-L (2023) First insights into the population characteristics and seasonal occurrence of the great hammerhead shark, Sphyrna mokarran (Rüppell, 1837) in the Western Tuamotu archipelago, French Polynesia. Front. Mar. Sci. 10:1234059. doi: 10.3389/fmars.2023.1234059
Received: 03 June 2023; Accepted: 04 August 2023;
Published: 29 August 2023.
Edited by:
David M. P. Jacoby, Lancaster University, United KingdomReviewed by:
Austin Gallagher, Beneath the Waves, Inc., United StatesNatascha Wosnick, Federal University of Paraná, Brazil
Copyright © 2023 Boube, Azam, Guilbert, Huveneers, Papastamatiou, Mourier, Trujillo, Femmami, Kunovsky, Bersani, Laurent, Bousseyroux, Thellier, Follin, Pavy, Jeandel, Mataarere, Burlot, Bouyeure, Rigoreau, Rigoreau, Lenormand, Chalabi, Hayek, Jeandel and Stenger. This is an open-access article distributed under the terms of the Creative Commons Attribution License (CC BY). The use, distribution or reproduction in other forums is permitted, provided the original author(s) and the copyright owner(s) are credited and that the original publication in this journal is cited, in accordance with accepted academic practice. No use, distribution or reproduction is permitted which does not comply with these terms.
*Correspondence: Tatiana Boube, tatiana.boube@gmail.com; Pierre-Louis Stenger, pierrelouis.stenger@gmail.com