- 1Centro Oceanográfico de Murcia, Instituto Español de Oceanografía (IEO-CSIC), San Pedro del Pinatar, Murcia, Spain
- 2Instituto de Investigación en Medio Ambiente y Ciencia Marina (IMEDMAR−UCV), Universidad Católica de Valencia SVM, Calpe, Alicante, Spain
- 3Centro Oceanográfico de Vigo, Instituto Español de Oceanografía (IEO-CSIC), Vigo, Spain
The Mar Menor lagoon (SE Spain) suffers a severe eutrophication process aggravated since the collapse of the lagoon in 2016. Together with it, the populations of the flat oyster Ostrea edulis have been decimated in the lagoon, but also in the European seas, where the species and the habitats provided by it have mainly disappeared. The Mar Menor Oyster Initiative’s main objectives are gaining knowledge on the nutrient extraction capability of the Mar Menor flat oyster and developing the required tools for restoration and bioextraction actions. The project RemediOS, within the framework of the Mar Menor Oyster Initiative, aims to obtain flat oyster seed using the decimated local broodstock to provide the initiative with an oyster population to work with. The present work describes the results of the first attempt of Mar Menor local broodstock reproduction in captivity. Larvae produced in the hatchery accounted for 57.5 million, of which 11.8 million were cultured and 0.68 million survived to settlement and metamorphosis.
1 Introduction
During the last 50 years, eutrophication has been identified as one of the main problems affecting aquatic ecosystems (Karydis and Kitsiou, 2012). This nutrient excess has provoked massive microalgae blooms that have severe consequences in the ecosystem [hypoxia, decrease of water clarity, fisheries decline, loss of biodiversity, etc (Smolders et al., 2006)]. In marine environments, coastal waters have been more susceptible to it due to their proximity to heavily populated areas, especially in marine lagoons and other paralic environments where large water renewal time makes them more vulnerable to nutrient enrichment (Kennish and Paerl, 2010).
The Mar Menor (MM) lagoon, with its 135 km2 and 645 hm3, is the largest coastal hyperhaline lagoon in the Mediterranean basin. A constant rise of chlorophyll a level has been observed in the lagoon since the 1980s (Ruiz et al., 2020; IEO-CSIC, 2023), with the threat of eutrophication beginning in the early 1990s with the nutrient increase noted when high peaks of chlorophyll a were reported from the area close to the Albujon ravine, which provides the main freshwater supply of the lagoon (Pérez-Ruzafa et al., 2005; Ruiz et al., 2020). The lagoon suffered for decades from the constant increase of nutrients coming in, together with other pollutants, until its recent ecological collapse in 2015, when a massive phytoplankton bloom deteriorated strongly the environmental conditions and erased most of the macrophytes and benthic fauna (Ruiz et al., 2020). The eradication of the natural ecological community destabilized the lagoon, reducing its resilience and hence making it more vulnerable against future perturbations, as has been observed in several ecological crises after 2015, including anoxic episodes (Álvarez-Rogel et al., 2020; Ruiz et al., 2020; IEO-CSIC, 2023).
Bivalves and the ecosystem services they provide have been used to mitigate the effects of eutrophication and recover the ecosystem functionality as a nature-based solution (Rose et al., 2014; Galimany et al., 2020). Bivalves are filter feeders that fed on suspended particulate matter such as phytoplankton, detritus, micro-zooplankton, bacteria and dissolved organic matter (Gosling, 2015), thus improving water clarity and quality by extracting nutrients and other pollutants (Ismail et al., 2014; Ayvazian et al., 2021). Moreover, bivalves such as mussels and oysters are ecosystem engineers, creating biogenic structures or reefs, which help to improve biodiversity by acting as a natural hatchery and habitat for many species and providing coastal protection (Smaal et al., 2019; zu Ermgassen et al., 2020b).
In this context, The Mar Menor Oyster Initiative (MMOI, https://noraeurope.eu/spain-the-mar-menor-oyster-initiative/) was launched in 2020 at the Native Oyster Restoration Alliance (Pogoda et al., 2019) 3rd Conference. The MMOI aims to gain knowledge on the nutrient extraction capability of the MM flat oyster (Ostrea edulis) and develop the necessary tools for restoration and bioextraction actions. The selection of the flat oyster as the bioremediation species was based on the actual presence of the species in the lagoon and the existence in the 1980s–1990s of a population over 130 million oysters (Rosíque, 1994). This population vanished through the years because of the degradation of the environmental conditions, the negative effect of the sponge Cliothosa hancocki, and the massive proliferation of the invasive macroalgae Caulerpa prolifera (Taxon Estudios Ambientales SL, 2006).
The last assessment of the state of the MM oyster beds was carried out in 2006 (Taxon Estudios Ambientales SL, 2006), and no sampling has been carried out since then. This study estimated from a partial sampling that the population had decreased by more than 10-fold compared to the 1990s. Currently and according to the observations of divers, the population has practically disappeared, so we considered that it would be very difficult for it to be able to recover on its own.
Thus, the main challenge faced by MMOI was the limited availability of individuals to study and to use in restoration/bioextraction actions, which implied the need to produce hatchery seed using aquaculture techniques. In terms of biosecurity, the best recommendation for seed production is to use local broodstock and water (Fitzsimons et al., 2020; zu Ermgassen et al., 2020c). With the purpose of using local breeders, Albentosa et al., (2023) collected some adult oyster specimens from the lagoon in order to determine their potential nutrient extraction capacity, species identity, and genetic diversity and their proximity to other flat oyster populations in Europe. This study concluded that the species was O. edulis, which exhibited a genetic diversity similar to those described for other European oyster beds and were genetically related to the Mediterranean oysters near the MM (Albentosa et al., 2023). After this initial study (Albentosa et al., 2023), the MMOI focused on building an experimental oyster hatchery for seed production using water from the lagoon itself. The use of seawater from the MM to supply the hatchery may have represented an additional challenge to the inherent difficulties of flat osyter seed production (Colsoul et al., 2021). The studies focused on flat oyster production are limited (Colsoul et al., 2021) and challenging because of several factors such as mortalities associated to larval metamorphosis, unexplained larval mass mortality events, and the presence of pathogens which can provoke large mortalities, like Bonamia ostreae (zu Ermgassen et al., 2020a; Gray et al., 2022; zu Ermgassen et al., 2023).
This study presents the results of the RemediOS project, which constitutes a proof of concept for the production of flat oyster seed from broodstock collected from the MM using water from the lagoon itself.
2 Methods
2.1 Hatchery setup and microalgae culture
The RemediOS hatchery was designed as a small-scale experimental hatchery for the proof of concept and as a place for the dissemination of nutrient bioextraction and oyster restoration concepts. The hatchery consisted of a 60 m2 empty space divided in two equal rooms of 30 m2. The rooms were enclosed and divided by a sailcloth for temperature isolation. One of the rooms was used for microalgae production, half of it to scale up the culture using different volumes (250 mL, 2 L, and 6 L) and the other half for production in 40-L plastic bags. For the microalgae culture, water was filtered using activated carbon filter and to 0.5 µm together with a skimmer. Previous to inoculation in all the different volumes, chlorine was used for water disinfection (0.5 mL L-1 of a 40-g L-1 solution), and thiosulfate (0.5 mL L-1 of a 100-g L-1 solution) was used for chlorine neutralization.
Strains of the four species of microalgae cultured were obtained from Toralla Marine Science Station culture collection: Tisochrysis lutea (ECC038), Diacronema lutheri (ECC015), Tetraselmis suecica (ECC036), and Phaeodactylum tricornutum (ECC028), with an organic weight per million cells of 0.043, 0.021, 0.225, and 0.015 mg, respectively, for each microalgae. Phytoplankton was cultured with 24 h of 6500K light period and a temperature of 19–22°C. The culture of phytoplankton was adapted following the recommendations in Helm et al. (Helm et al., 2004).
The other room was used for flat oyster culture in the different phases described below (broodstock, larvae, and seed). For oyster culture, water intake was pumped from MM lagoon, and it was filtered up to 0.5 µm through a series of cartridge filters including activated carbon and sterilized by UV.
2.2 Broodstock
The breeding season studied was from the end of February to mid-June, when the broodstock was returned to the sea. For that, a total of 36 individuals were collected between February 24 and March 11, 2022 through scuba diving from the surrounding areas of the Baron Island (37° 41′ 46″ N 0° 45′ 13″ W) located in the MM lagoon (SE Spain) and transported to laboratory in coolers with MM water. At the time of collection, the field water temperature was 15°C. All individuals were cleaned using a metal brush and then immersed for 20 min in a bath of freshwater with a 5-mL L-1 dilution of bleach to eliminate epiphytes and to disinfect the shells externally. Biometric data was measured for each oyster (length, height, and width), according to Bayne (2017), together with the wet weight. The adults collected had a mean length of 93.1 ± 12.1 mm, height of 106.1 ± 14.3 mm, and width of 41.4 ± 8.1 mm. The mean wet weight (WW) was 230.2 ± 74.4 g, with a mean dry weight (DW) of 1.6 ± 0.4 g [the DW was estimated through length–DW relationships estimated from individuals dissected in the study of Albentosa et al., (2023)].
The individuals were maintained in a flow-through system during the entire reproductive season from March to late June. The system consisted of three 60-L tanks where the individuals were distributed (n = 12 for each tank). Temperature and salinity were measured daily and maintained at natural conditions in the range of 14–27°C and ≃42 PSU. The water flow rate for each tank was 120 L h-1 (10 L per individual h-1), and the microalgae concentration was ≃2.50 mm3 L-1 in cell volume and ≃ 45,000 cells mL-1 in cell number. The diet composition was based on a mixture of the four microalgae cultured in a proportion of 25% ± 10% in volume depending on the culture conditions. The conditions used for broodstock were an adaptation of those of Helm et al. (2004). The ingestion in adults was monitored two times a week during the whole period. For this, the initial concentration of the diet, the concentration at the outflow of the tanks, and the flow rate were measured. The clearance rate (CR) was calculated according to Hildreth and Crip, (1976), and the ingestion as the product of CR and the microalgae’s organic weight.
Three times per week, the individuals and their tanks were cleaned using pressurized freshwater, including tank disinfection using bleach once a week. Furthermore, once a month, the individuals were subjected to a 20-min bath in freshwater with a 5-mL-L-1 dilution of bleach (37 g L-1 of chlorine).
2.3 Larvae
A 100-µm mesh sieve was disposed at the water outflow of each broodstock tank to retain larvae released at swarming events. Sieves were checked and cleaned every morning and frequently controlled during the workday. The larvae collected in the sieves were transferred to a 1-L test tube. Then, they were gently mixed, and two samples of 1 mL were collected to be counted in a Sedgewick Rafter chamber.
Afterwards, the larvae were transferred to 70–350-L cylindroconical tanks in a batch system at a concentration of 5.5 ± 0.9 larvae mL-1, with the exception of three tanks which held higher larval densities of 13.4 ± 1.0 larvae mL-1 in March. The larval tanks were maintained at 20°C–22°C, and the salinity was at actual MM levels of ≃42 PSU. Every day, the larvae were fed with a concentration of 2.5–3 mm3 L-1 in cell volume, 40,000–50,000 cells mL-1 in cell number, using the same diet composition as that for adults. The conditions used for the larvae were an adaptation of those of Helm et al. (2004). Ingestion was specifically controlled in four of the larvae batches to obtain ingestion data. For the ingestion calculation, the food concentration of the tank was measured 24 h after feeding during the whole batch period. This ingestion was expressed in function of the number of larvae. Those four batches came from two different swarming events: two from a swarming on the May 1 and the other two on May 5. The larval density was between 5.0 and 7.0 larvae mL-1. Those batches exhibited a larval survival before settlement at 74%, 68%, 97%, and 43%.
Three times a week, the larvae tanks were drained and cleaned, and the water therein was renewed. During this process, the larvae were inspected weekly under a microscope to estimate the growth (maximum length) and mortality and to check their mobility and the presence of microalgae in the stomach being indicative of good health.
2.4 Seed
Once the eye-spot and/or active foot was observed in at least 50% of the larvae, they were transferred to a 30-cm-diameter container equipped with a 200-µm mesh. The larval density at this moment was ≃1,000 larvae cm-2, with the exception of the first two batches in March, which were held at a density of ≃500 larvae cm-2 due to a higher availability of space in the hatchery. The bottom of the sieve was previously covered with 30 mL of 200–500-µm oyster shell powder as settlement substrate. The same feeding protocol used for larvae feeding was applied during 1 week. The conditions used for larvae settlement were an adaptation of those of Helm et al. (2004).
Once settlement was completed and the seed was sampled (see the paragraph below), it was fed daily with a dose of 1.2%–0.4% of the seed wet weight depending on the seed size distributed in doses throughout the day using a peristaltic pump and with the same diet composition as the one provided to adults. After reaching a size of >1 mm, the seed was transferred to perforated plastic trays of 40 cm × 30 cm and grouped in size classes with a 500-µm to 1-mm mesh sieve to retain the seed. The trays were disposed in 500-L square tanks with a density of 0.09–0.17 g of live weight cm-2 depending on the seed size. Water temperature was maintained at 20°C–22°C and salinity at actual MM levels of ≃ 42 PSU. The conditions used for the flat oyster seed were an adaptation of those of Helm et al. (2004).
Once a month, the seed was sampled to estimate growth, mortality, number of individuals, and to distribute the seed into groups depending on the size class. The individuals’ biometry was measured as previously described for broodstock. Each size group was weighed, and length was measured to determine seed growth. Afterwards, all groups were redistributed into new groups. Each new group was weighed again, and a small sample between 3 and 15 g, depending on the size class, was weighed, and the number of individuals was counted to estimate the total number of individuals in each size group.
2.5 Statistical analysis
The Pearson’s correlation coefficient (Benesty et al., 2009) between larval density and survival was calculated.
3 Results
The broodstock cleared 38.9% of the diet, therefore giving the diet ingested as 6.43% of the DW of the individuals. Five adults died during the experiment period.
A total of 29 swarming events were observed. A swarming event was considered when each batch of larvae obtained from a tank in a single day could be related to one or several oysters. The first release of larvae was observed on March 21 from two adults collected 10 days before. Swarming events were recorded from March to mid-June when broodstock was returned to the sea. The water temperature during this time ranged between 15°C and 25°C (Figure 1). Five of the swarming events were not counted due to management circumstances. From the other 24 events, 57.5 million larvae were obtained (1.6 million larvae per adult) (Table 1). A total of 11.8 million larvae (20.5%) were cultured in the hatchery, while the rest of them were discarded due to the lack of space in the facilities. Considering the total of larvae cultured, 5.1 million larvae (43.6%) reached competence or pediveliger stage, when eye-spot and/or active foot was observed, and 0.68 million (5.8%) survived through metamorphosis, reaching post-larvae stage or spat and attaching effectively to the substrate (Table 1). The mean settlement rate was 13.3%.
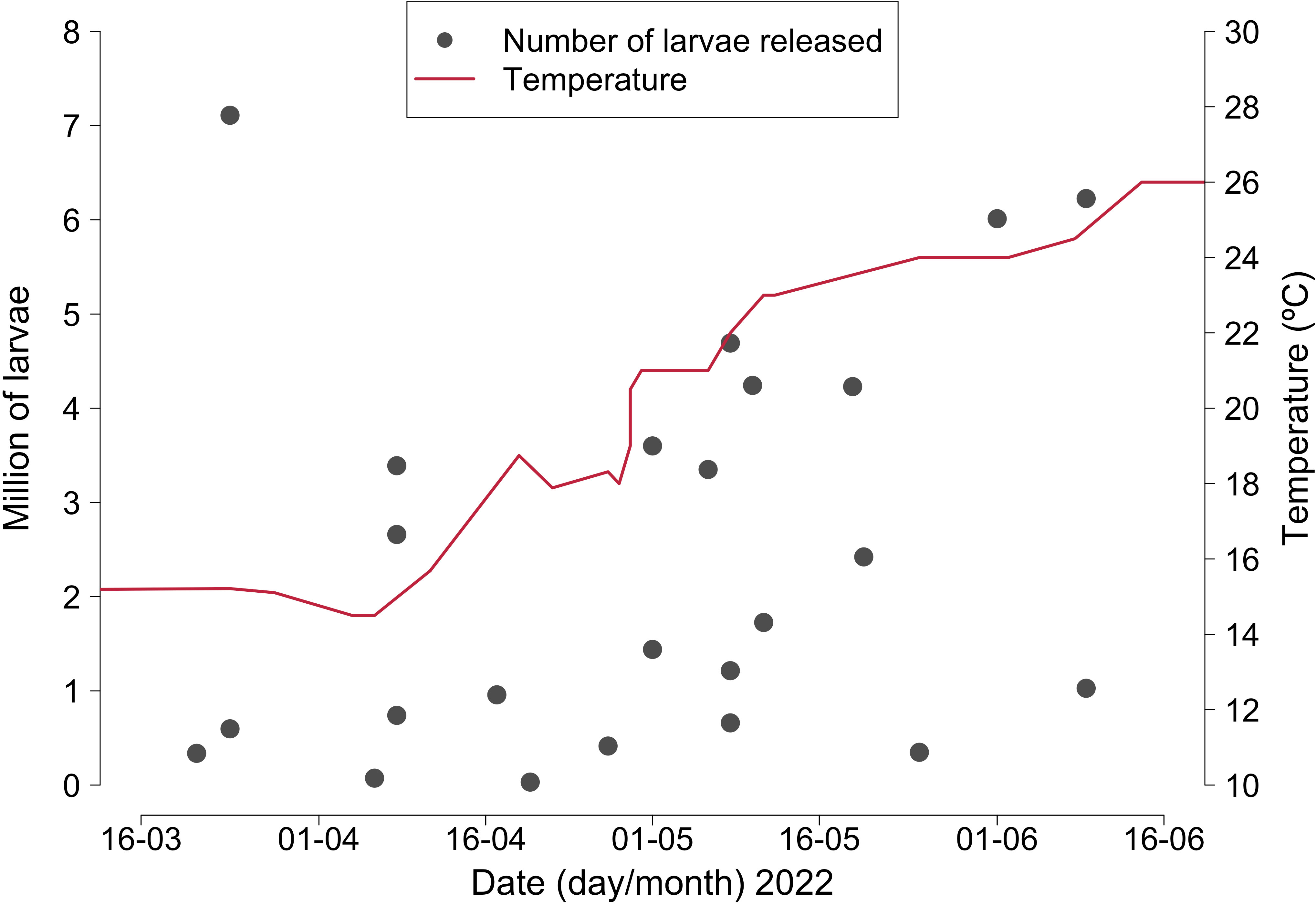
Figure 1 Million of larvae released by broodstock and temperature of broodstock maintenance during the reproductive season in 2022.
The three batches cultured at high larvae density in March (13.4 ± 1.0 larvae mL-1) showed a lower survival of 23% compared to the larvae cultured at a lower density (5.5 ± 0.9 larvae mL-1), which showed a survival of 73%. A negative significant correlation of -0.79 was observed between larval density and survival (df = 12, p < 0.001).
Larvae at release exhibited a size of ≃170 µm and reached maximum length after 16–20 days with ≃290 µm (growth rate = 5.54 µm day-1) (Figure 2). In total, 50% of eye-spot and/or active foot was usually observed between 17 and 20 days, with a minimum of 15 days and a maximum of 22 days. During the culture period, the ingestion rate increased together with larval size at up to a maximum of 0.5 µg of organic matter (OM) larvae-1 day-1 on day 17, when a decline was observed (data related to four batches) (Figure 2).
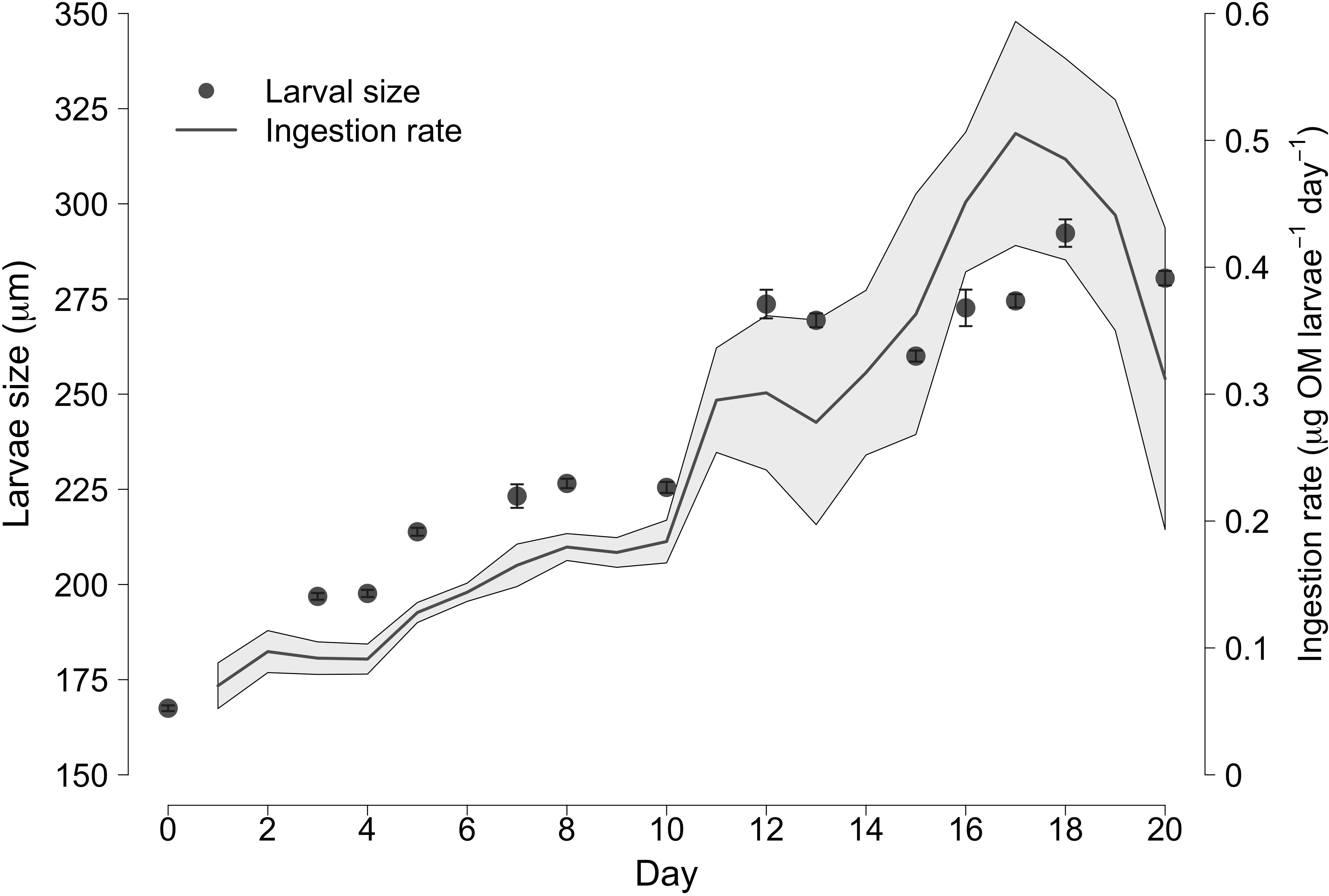
Figure 2 Mean larval size and ingestion (in µg) of organic matter (OM) per larvae and day in four of the cultured batches from release to settlement.
In June 2022, the hatchery accounted a total of 398,317 seed individuals with a size range between 0.5 and 6 mm (Figure 3). The total number of seeds decreased drastically over the following months with different mortalities related always to the individual in the lower size class. The 30,000 seeds maintained in September did not show any additional mortality during the following months (Figure 3). By November 2022, the seed size ranged from 4 to 15 mm.
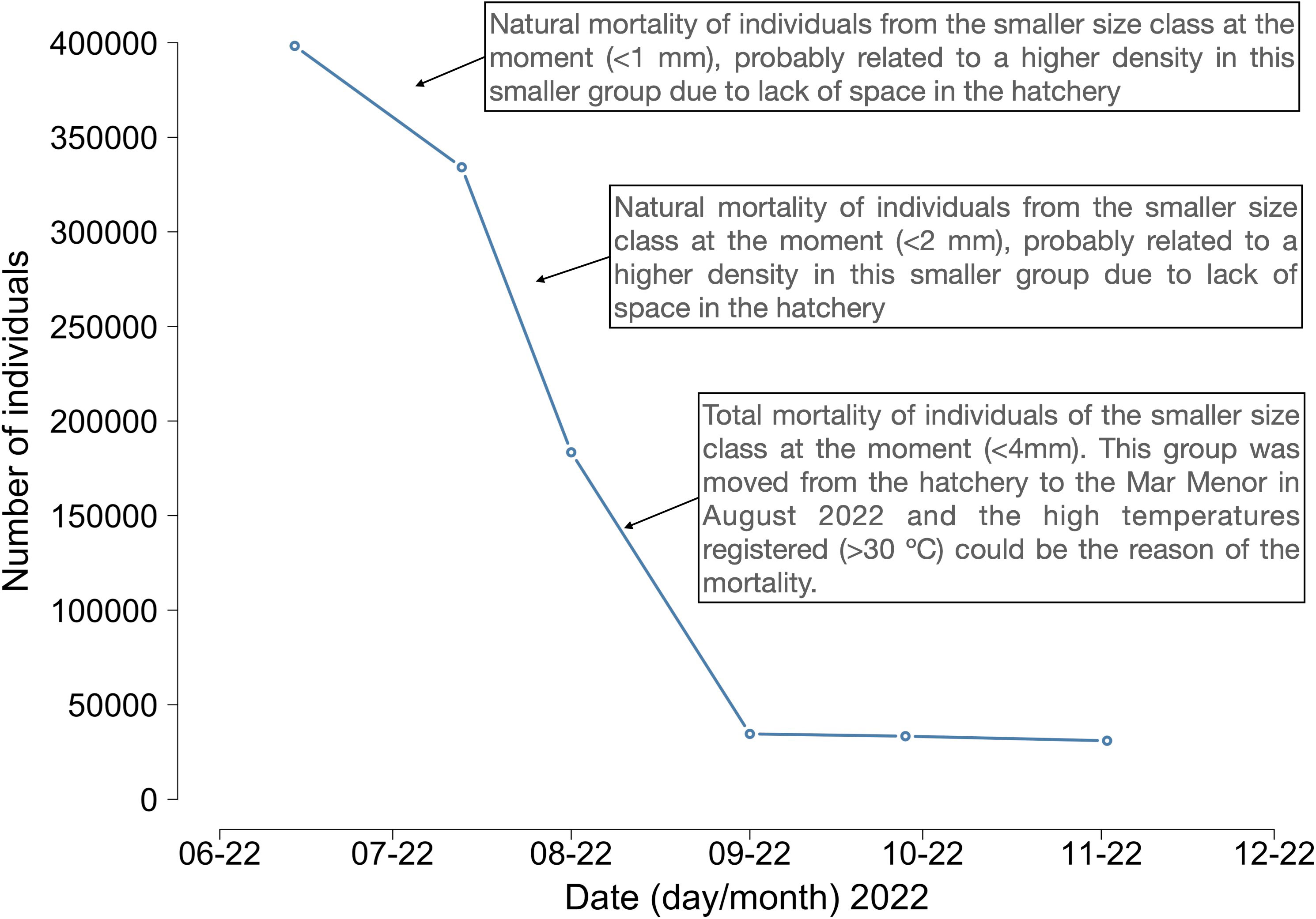
Figure 3 Number of seed individuals obtained in the hatchery with an explanation of the three main mortalities observed.
4 Discussion
The present work showed the viability of producing seed using the population of flat oyster inhabiting the MM. In the Spanish Mediterranean Sea, the flat oyster can start spawning at temperatures of 12°C to 13°C (Bromley et al., 2016), which agrees with the first releases of larvae observed in March with a water temperature of 15°C. In fact, Cano et al. (1997) observed the first larvae in the MM plankton at 14°C. Larval release stopped at a temperature of 25°C at the beginning of June, and by the end of June, adults were returned to the Mar Menor. Similarly, Abellán Martinez et al. (1989) observed the first larvae in April, a little later than during the present work, and detected larvae until August. Therefore, it would be possible that more larvae could be obtained during the summer if the broodstock would have been maintained. The fertility observed for the MM broodstock, with 1.5 million of larvae per oyster, coincides with the observations reviewed by Colsoul et al. (2021) for an oyster size of 90 mm.
The larvae survival rates obtained during April (97%) are comparable to the survival rates of 99% obtained by Robert et al. (2017) at 25°C and using a diet with Chaetoceros neogracile and T. lutea. In March, a lower survival rate (28%, Table 1) was observed compared to those of other months (>70%, Table 1), which could be related to the higher larval density in some of the tanks (5.5 vs. 13.4 larvae mL-1). During this month, the tanks with a lower larval density had a survival rate of 65%, while the tanks with a higher density had a survival rate of 23%. The presence of contaminants such as heavy metals and detergents can provoke higher mortalities in larvae Colsoul et al. (2021). However, a carbon filter was used, which helps to remove certain contaminants, and different survival rates were observed for batches cultured at the same time. It seems unlikely to think that the concentration of those contaminants could significantly change in seawater in a short period of time. Another explanation could be the presence of bacteria such as Vibrio sp., which can also cause large mortalities in oyster cultures (Helm et al., 2004). These bacteria could originate in non-treated broodstock, microalgae production, and the larval culture (Colsoul et al., 2021) and be responsible of the different survival rates observed in the different batches.
Larval growth rate (5.54 µm day-1) was in the middle of the usual growth rates reported for the species in other hatcheries, with a range observed to be between 1.4 and 11 µm day-1 for different conditions (González-Araya and Robert, 2018; Jacobs et al., 2020; da Costa et al., 2023). Despite the fact that the growth rate was similar to those reported in some of the cited works, the pelagic larval period was much longer (a total of 17–20 days) when compared to those of other hatcheries, where periods below 14 days were observed (Gonzalez Araya et al., 2012; Mesías-Gansbiller et al., 2013; González-Araya and Robert, 2018). However, longer periods have been reported as up to 38 days (Jacobs et al., 2020).
The settlement rates were low compared to the 68% obtained by Robert et al., (2017), with a higher rate during March at 21.4%. In the following months, the survival rate was even lower, with 6.5% and 7.1% obtained for April and May, respectively. This difference could be related to different factors. One explanation could be that the first larvae obtained in March came from field fertilization, where the food available may have contributed to a higher quality of these spawns. In fact, the higher survival rate during settlement was only observed for the first two larval batches (34% of survival vs. <5% during the rest of March). On the other hand, the lower density of larvae during the two initial batches (≃500 larvae cm-2) compared to the rest of them (≃1,000 larvae cm-2) could lead to a higher survival rate.
The seed obtained in the hatchery suffered a great mortality during the initial months of life, with a decrease of almost 400,000 individuals to 30,000 individuals in 3 months. The mortality was always observed in the smaller size classes, as have been observed for other bivalves such as Mytilus edulis, which could be associated to inherent higher metabolic rates (Rodhouse et al., 1984). Moreover, space constraints in the hatchery forced us to prioritize the well-being of larger individuals, which resulted in rearing smaller size classes at high densities, and thus it may have impacted negatively on spat survival (Lauzon-Guay et al., 2005). The presence of pathogenic Vibrios could have also played a role in these mortalities, affecting the smaller and weaker individuals (Dubert et al., 2017).
For both larval and seed production, specific diets could be used in order to improve the growth and survival. During the present work, phytoplankton production was affected by the implementations of new protocols in the recently built hatchery. Therefore, diets were given according to the availability of the different microalgae instead of prioritizing a specific mix of species—for example, a specific diet of C. neogracile and T. lutea is recommended to increase the larval survival rate and the settlement rate (Robert et al., 2017; da Costa et al., 2023).
5 Conclusions
The objective of obtaining seed using MM broodstock was achieved at the first trial. This new stock of oyster seed will be used in future actions of the MMOI to evaluate the bioremediation capacity of the oysters and to carry out a restoration pilot. However, further advances could be done to improve the survival and development of oyster larvae, specially to increase the settlement success, such as improving the diet quality using a more controlled combination of microalgae and the use of lower densities during culture.
Data availability statement
The raw data supporting the conclusions of this article will be made available by the authors, without undue reservation.
Ethics statement
Ethical review and approval was not required for the study on animals in accordance with the local legislation and institutional requirements.
Author contributions
SH wrote the first draft of the manuscript, organized the database, and conducted data analysis. FC and MA elaborated the conception and design of the study and provided support with data interpretation. MA provided financial support. SH, AH, and MA performed the experiment. All authors contributed to the article and approved the submitted version.
Funding
Author SH was supported by the Generalitat Valenciana and the European Social Fund under postdoctoral Grant APOSTD/2021/177.
Acknowledgments
We appreciate the work done by Francisco Gomez in the maintenance and feeding of the oyster. This research has been performed in the scope of the RemediOS Project, developed with the collaboration of the Biodiversity Foundation (Spanish Ministry for Ecological Transition and the Demographic Challenge), through the Pleamar Program, and co-financed by the European Maritime and Fisheries Fund (EMFF).
Conflict of interest
The authors declare that the research was conducted in the absence of any commercial or financial relationships that could be construed as a potential conflict of interest.
Publisher’s note
All claims expressed in this article are solely those of the authors and do not necessarily represent those of their affiliated organizations, or those of the publisher, the editors and the reviewers. Any product that may be evaluated in this article, or claim that may be made by its manufacturer, is not guaranteed or endorsed by the publisher.
References
Abellán Martinez E., García Alcázar A., Pérez Camacho A., García García B. (1989). Preliminary study on the reproductive cycle of oysters (Ostrea edulis L.) in Mar Menor (SE Spain). Aquacult.: Biotechnol. progress 1. Bredene: Eur. Aquacult. Society. 1, 279–286.
Albentosa M., Akinyemi M. I., Vera M., Ibarrola I., Filgueira R., Galimany E., et al. (2023). Recovery of eutrophized marine ecosystems using the European flat oyster, Ostrea edulis. Aquat. Conservation: Mar. Freshw. Ecosyst. 33 (7), 645–660. doi: 10.1002/aqc.3926
Álvarez-Rogel J., Barberá G. G., Maxwell B., Guerrero-Brotons M., Díaz-García C., Martínez-Sánchez J. J., et al. (2020). The case of Mar Menor eutrophication: State of the art and description of tested Nature-Based Solutions. Ecol. Eng. 158, 106086. doi: 10.1016/j.ecoleng.2020.106086
Ayvazian S., Mulvaney K., Zarnoch C., Palta M., Reichert-Nguyen J., McNally S., et al. (2021). Beyond bioextraction: the role of oyster-mediated denitrification in nutrient management. Environ. Sci. Technol. 55 (21), 14457–14465. doi: 10.1021/acs.est.1c01901
Bayne B. L. (2017). “Biology of Oysters,” in Developments in Aquaculture and Fisherires Science, vol. 41 . Ed. Bayne B. (London: Elsevier), 209–329.
Benesty J., Chen J., Huang Y., Cohen I. (2009). “Pearson correlation coefficient,” in Noise reduction in speech processing (Heidelberg, Germany: Springer), 1–4.
Bromley C., McGonigle C., Ashton E. C., Roberts D. (2016). Bad moves: Pros and cons of moving oysters – A case study of global translocations of Ostrea edulis Linnaeus, 1758 (Mollusca: Bivalvia). Ocean Coast. Management 122, 103–115. doi: 10.1016/j.ocecoaman.2015.12.012
Cano J., Rosique M. J., Rocamora J. (1997). Influence of environmental parameters on reproduction of european flat oyster (Ostrea edulis L.) in a coastal lagoon (Mar Menor, Southeastern Spain). J. Molluscan Stud. 63 (2), 187–196. doi: 10.1093/mollus/63.2.187
Colsoul B., Boudry P., Pérez-Parallé M. L., Bratoš Cetinić A., Hugh-Jones T., Arzul I., et al. (2021). Sustainable large-scale production of European flat oyster (Ostrea edulis) seed for ecological restoration and aquaculture: a review. Rev. Aquacult. 13 (3), 1423–1468. doi: 10.1111/raq.12529
da Costa F., González-Araya R., Robert R. (2023). Using combinations of microalgae to condition European flat oyster (Ostrea edulis) broodstock and feed the larvae: Effects on reproduction, larval production and development. Aquaculture 568, 739302. doi: 10.1016/j.aquaculture.2023.739302
Dubert J., Barja J. L., Romalde J. L. (2017). New insights into pathogenic vibrios affecting bivalves in hatcheries: present and future prospects. Front. Microbiol. 8. doi: 10.3389/fmicb.2017.00762
Fitzsimons J. A., Branigan S., Gillies C. L., Brumbaugh R. D., Cheng J., DeAngelis B. M., et al. (2020). Restoring shellfish reefs: Global guidelines for practitioners and scientists. Conserv. Sci. Practice 2 (6), e198. doi: 10.1111/csp2.198
Galimany E., Lunt J., Freeman C. J., Houk J., Sauvage T., Santos L., et al. (2020). Bivalve feeding responses to microalgal bloom species in the Indian river lagoon: the potential for top-down control. Estuaries Coasts 43 (6), 1519–1532. doi: 10.1007/s12237-020-00746-9
Gonzalez Araya R., Mingant C., Petton B., Robert R. (2012). Influence of diet assemblage on Ostrea edulis broodstock conditioning and subsequent larval development. Aquaculture 364-365, 272–280. doi: 10.1016/j.aquaculture.2012.08.036
González-Araya R., Robert R. (2018). Larval development and fatty acid composition of Ostrea edulis (L.) fed four different single diets from conditioning to pre-settlement. Aquacult. Res. 49 (5), 1768–1781. doi: 10.1111/are.13631
Gray M. W., Alexander S. T., Beal B. F., Bliss T., Burge C. A., Cram J. A., et al. (2022). Hatchery crashes among shellfish research hatcheries along the Atlantic coast of the United States: A case study of production analysis at Horn Point Laboratory. Aquaculture 546, 737259. doi: 10.1016/j.aquaculture.2021.737259
Helm M. M., Lovatelli A., Bourne N. (2004). Hatchery culture of bivalves: a practical manual (Rome: FAO Fisheries).
Hildreth D. I., Crip D. J. (1976). A corrected formula for calculation of filtration rate of bivalve molluscs in an experimental flowing system. J. Mar. Biol. Assoc. United Kingdom 56 (1), 111–120. doi: 10.1017/S0025315400020476
IEO-CSIC. (2023). Informe de actualización de resultados del programa de monitorización y estudio del Mar Menor de febrero de 2023 (IEO-CSIC). Available at: http://www.ieo.es/es_ES/web/ieo/mar-menor.
Ismail N. S., Müller C. E., Morgan R. R., Luthy R. G. (2014). Uptake of Contaminants of Emerging Concern by the Bivalves Anodonta californiensis and Corbicula fluminea. Environ. Sci. Technol. 48 (16), 9211–9219. doi: 10.1021/es5011576
Jacobs P., Greeve Y., Sikkema M., Dubbeldam M., Philippart C. J. M. (2020). Successful rearing of Ostrea edulis from parents originating from the Wadden Sea, the Netherlands. Aquacult. Rep. 18, 100537. doi: 10.1016/j.aqrep.2020.100537
Karydis M., Kitsiou D. (2012). Eutrophication and environmental policy in the Mediterranean Sea: a review. Environ. Monit. Assessment 184 (8), 4931–4984. doi: 10.1007/s10661-011-2313-2
Kennish M. J., Paerl H. W. (2010). Coastal lagoons: critical habitats of environmental change (London: CRC Press).
Lauzon-Guay J.-S., Dionne M., Barbeau M. A., Hamilton D. J. (2005). Effects of seed size and density on growth, tissue-to-shell ratio and survival of cultivated mussels (Mytilus edulis) in Prince Edward Island, Canada. Aquaculture 250 (3), 652–665. doi: 10.1016/j.aquaculture.2005.03.049
Mesías-Gansbiller C., Silva A., Maneiro V., Pazos A., Sánchez J. L., Pérez-Parallé M. L. (2013). Effects of chemical cues on larval settlement of the flat oyster (Ostrea edulis L.): A hatchery approach. Aquaculture 376-379, 85–89. doi: 10.1016/j.aquaculture.2012.11.022
Pérez-Ruzafa A., Marcos C., Gilabert J. (2005). “The ecology of the Mar Menor coastal lagoon: a fast-changing ecosystem under human pressure,” in Coastal Lagoons: Ecosystem Processes and Modeling for Sustainable Use and Development (Boca Ratón, Florida: CRC Press), 392–422.
Pogoda B., Brown J., Hancock B., Preston J., Pouvreau S., Kamermans P., et al. (2019). The Native Oyster Restoration Alliance (NORA) and the Berlin Oyster Recommendation: bringing back a key ecosystem engineer by developing and supporting best practice in Europe. Aquat Living Resour. 32, 13. doi: 10.1051/alr/2019012
Robert R., Vignier J., Petton B. (2017). Influence of feeding regime and temperature on development and settlement of oyster Ostrea edulis (Linnaeus, 1758) larvae. Aquacult. Res. 48 (9), 4756–4773. doi: 10.1111/are.13297
Rodhouse P. G., Roden C. M., Hensey M. P., Ryan T. H. (1984). Resource allocation in Mytilus edulis on the shore and in suspended culture. Mar. Biol. 84 (1), 27–34. doi: 10.1007/BF00394523
Rose J. M., Bricker S. B., Tedesco M. A., Wikfors G. H. (2014). A role for shellfish aquaculture in coastal nitrogen management. Environ. Sci. Technol. 48 (5), 2519–2525. doi: 10.1021/es4041336
Rosíque M. J. (1994). Estudio del banco de ostra plana (Ostrea edulis L.) del Mar Menor: posibilidades de explotación (Universidad de Murcia). PhD Thesis. University of Murcia. Murcia, Spain.
Ruiz J. M., Albentosa M., Aldeguer B., Álvarez-Rogel J., Antón J., Belando M. D., et al. (2020). Informe de evolución y estado actual del Mar Menor en relación al proceso de eutrofización y sus causas (Informe de asesoramiento técnico del Instituto Español de Oceanografía (IEO).
Smaal A. C., Ferreira J. G., Grant J., Petersen J. K., Strand Ø. (2019). Goods and services of marine bivalves (Cham, Switzerland: Springer Nature).
Smolders A. J. P., Lamers L. P. M., Lucassen ECHET, van der Velde G., Roelofs J. G. M. (2006). Internal eutrophication: How it works and what to do about it—a review. Chem. Ecol. 22 (2), 93–111. doi: 10.1080/02757540600579730
Taxon Estudios Ambientales SL (2006). Evaluación del banco natural de ostra plana del Mar Menor. DT06063. Consejería de agricultura y agua. Servicio de Pesca y Acuicultura. Región de Murcia. Murcia, Spain.
zu Ermgassen P. S. E., Bonačić K., Boudry P., BromLey C. A., Cameron T. C., Colsoul B., et al. (2020a). Forty questions of importance to the policy and practice of native oyster reef restoration in Europe. Aquat. Conservation: Mar. Freshw. Ecosyst. 30 (11), 2038–2049. doi: 10.1002/aqc.3462
zu Ermgassen P., Colsoul B., Debney A., Fabra M., Hancock B., Helmer L., et al. (2020c). “Biosecurity in native oyster restoration,” in European Native Oyster Habitat Restoration Handbook (UK & Ireland: The Zoological Society of London, London, UK).
zu Ermgassen P. S. E., Strand Å, Bakker N., Blanco A., Bonačić K., Boudry P., et al. (2023). Overcoming Ostrea edulis seed production limitations to meet ecosystem restoration demands in the UN decade on restoration. Aquat Living Resour. 36, 16. doi: 10.1051/alr/2023012
Keywords: restoration, aquaculture, hatchery, coastal lagoon, Ostrea edulis, larvae
Citation: Hernandis S, da Costa F, Hernández-Contreras Á and Albentosa M (2023) Hatchery seed production of flat oysters from the Mar Menor lagoon. Front. Mar. Sci. 10:1231686. doi: 10.3389/fmars.2023.1231686
Received: 30 May 2023; Accepted: 01 September 2023;
Published: 29 September 2023.
Edited by:
Bernadette Pogoda, Alfred Wegener Institute Helmholtz Centre for Polar and Marine Research (AWI), GermanyReviewed by:
Domitilia Coutinha Matias, Instituto Português do Mar e Atmosfera, PortugalJosê Lino Vieira De Oliveira Costa, University of Lisbon, Portugal
Bérenger Colsoul, Alfred Wegener Institute Helmholtz Centre for Polar and Marine Research (AWI), Germany
Copyright © 2023 Hernandis, da Costa, Hernández-Contreras and Albentosa. This is an open-access article distributed under the terms of the Creative Commons Attribution License (CC BY). The use, distribution or reproduction in other forums is permitted, provided the original author(s) and the copyright owner(s) are credited and that the original publication in this journal is cited, in accordance with accepted academic practice. No use, distribution or reproduction is permitted which does not comply with these terms.
*Correspondence: Marina Albentosa, bWFyaW5hLmFsYmVudG9zYUBpZW8uY3NpYy5lcw==