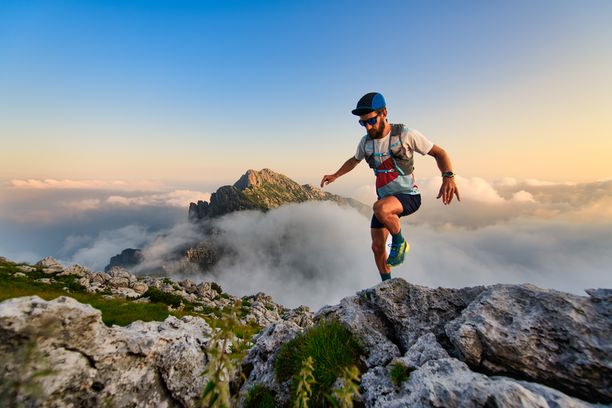
95% of researchers rate our articles as excellent or good
Learn more about the work of our research integrity team to safeguard the quality of each article we publish.
Find out more
REVIEW article
Front. Mar. Sci., 19 September 2023
Sec. Deep-Sea Environments and Ecology
Volume 10 - 2023 | https://doi.org/10.3389/fmars.2023.1225114
This article is part of the Research TopicSubmarine Canyons: Human Connections to the Deep SeaView all 12 articles
Litter is ubiquitous in the ocean, interacting with fauna and causing impacts that are unquantified at present. Mainly sourced from land, marine litter is very persistent, and undergoes slow degradation upon settling on the ocean floor. Submarine canyons contain more litter than other oceanographic features due to hydrological processes, but study of litter in canyons is made difficult by logistical requirements. Monitoring and quantification of marine litter often do not consider interactions between fauna and litter, meaning impacts are largely unconsidered and unknown. Among publications that have reported litter-fauna (L-F) interactions in canyons, the large majority occur in the Mediterranean Sea, and the most reported interaction is of corals entangled in fishing gear. When it occurs, the reporting of L-F interactions is unstandardised, resulting in a lack of global comparison and trend analysis. A standardised, comprehensive framework for the reporting of L-F interactions has been created and includes 6 major categories: entanglement, ingestion, smothering, habitat provision, adaptive behaviour, and encountering (entanglement and smothering occur on abiotic features as well). Use of the framework will aid in research collaboration and creation of a global dataset of L-F interactions. Impacts resulting from interactions are plentiful, most coming from entanglement and smothering.
Marine litter is becoming increasingly prevalent, causing effects often unknown and unquantified (Canals et al., 2021). Defined by the United Nations Environment Programme (UNEP), marine litter is “any persistent, manufactured or processed solid material discarded, disposed of or abandoned in the marine and coastal environment” (United Nations Environment Programme [UNEP], 2009). The focus of this review is marine litter larger than micro-litter, which is sized at over 5 mm in diameter and includes meso-, macro-, and mega-litter (Joint Group of Experts on the Scientific Aspects of Marine Environmental Protection [GESAMP], 2019); these categories will be collectively referred to as ‘marine litter’. Most marine litter comes from land-based sources such as coastal landfills, industrial pollution and storm water discharge (Katsanevakis, 2008); but some, especially fishing gear, originates from the ocean via boats, offshore platforms, and aquaculture (United Nations Environment Programme [UNEP], 2009; Galgani et al., 2015). Once in the ocean, litter is transported by currents before sinking to the seabed and accumulating (Watters et al., 2010), sometimes thousands of kilometres from its point of origin (Ryan and Moloney, 1993). The degradation of marine litter is slow, especially in the deep sea, where there is a lack of ultraviolet light, temperatures are cold, and oxygen is limited (Andrady, 2015). Plastic, which makes up the majority of marine litter (Derraik, 2002; Katsanevakis, 2008; Galgani et al., 2015) is essentially non-degradable in the cool, dark deep sea (Ryan and Moloney, 1993; Gregory, 2009; Nevill, 2011). Abandoned, lost or otherwise discarded fishing gear (ALDFG) is a type of marine plastic litter that is very prevalent, especially due to increases in fishing activity and gear durability over the last 50 years (Macfadyen et al., 2009; Watson, 2012). Approximately 1% of fishing gear per ship is lost each year (Gilman et al., 2016), being transported through currents before snagging or sinking (Katsanevakis, 2008). Rocks and coralline structures provide habitat for diverse species and thus are often heavily fished, so fishing gear is found to concentrate on such rugose features (Savini et al., 2014; Tubau et al., 2015; Melli et al., 2017; van den Beld et al., 2017; Consoli et al., 2018a; Angiolillo et al., 2022). Even if inputs decrease, marine litter already in the ocean will remain prevalent, snagged on features and resting on the seafloor (Keller et al., 2010). As such, the persistence of marine litter means transport can occur over long time periods, causing impacts through its movement and settlement (Vieira et al., 2014).
Marine litter may interact with fauna while travelling via ocean currents and after descending to the seafloor. For purposes of research, when an organism comes into contact with litter, it is termed a litter-fauna (L-F) interaction. The organism may or may not be affected by such interactions. Litter-fauna interactions began to be reported in the 1960s, mainly as entanglement and ingestion, which are the easiest to observe and quantify (Ryan, 2015; Bajaj et al., 2021). Reports in the 1970s described litter providing habitat through colonisation by algae and bryozoans (Carpenter and Smith, 1972; Venrick et al., 1973; Holmström, 1975). By the 1980s, more interactions had been observed, and the impacts of marine litter presence became a concern, so solutions started to be explored (Ryan, 2015; Bajaj et al., 2021). Since then, further research and quantification has occurred, with new focus on microplastics and toxin transfer from plastics to seawater and into the food chain (Ryan, 2015). In 1997, there were 267 species reported to be either entangled by or to have ingested marine litter (Laist, 1987); by 2015, Kühn et al. (2015) tallied 557 interacting species, while Gall and Thompson (2015) found 693. Gall and Thompson (2015) further reported that plastic resulted in the majority of interactions: synthetic rope and netting caused entanglement, and plastic fragments were ingested. Additionally, 17% of interactions reported had occurred to near-threatened, vulnerable, endangered, or critically endangered species, according to the IUCN Red List (Gall and Thompson, 2015).
Research on L-F interactions is limited at present and is often reported peripherally in studies with other purposes. In order to quantify and compare global occurrence of L-F interactions, a standardised reporting framework is needed. Currently, research papers reporting interactions vary in level of detail; often, the observed litter, interaction, and species type are not quantified. Some researchers have created their own categories for data categorisation purposes, but non-uniformity means global comparison between datasets is an arduous task. Table 1 displays the L-F interactions categories that have so far been used in reporting. While not standardised, themes exist; for example, entanglement, coverage, and colonisation or habitat provision are often reported (Gall and Thompson, 2015; Kühn et al., 2015).
Table 1 Reporting categories utilised in litter-fauna interactions reporting by individual research teams, ordered chronologically.
While the prevalence of L-F interactions has been noted, research on such interactions is still limited at present (Ramirez-Llodra, 2020), and may continue to be so while a standardised framework is not widely utilised. Through conduction of a critical literature review, a litter-fauna interactions framework is proposed with the goal of promoting standardised reporting and, thus, creation of a dataset of L-F interactions that are occurring in all ocean zones. The described quantification of the number and type of species interacting with litter will help create sensitivity and vulnerability assessments to understand the true impact of litter on benthic marine ecosystems.
Litter-fauna interactions result in impacts that harm species, but ‘harm’ is so far unquantified, and due to non-standardised measurement methods, impacts are not fully monitored or even considered (Galgani et al., 2013; Sherrington et al., 2016). For example, between the two most commonly reported interactions, entanglement and ingestion, assessment inabilities result in discrepancies in impact count. It is reported that 79% of entanglement interactions, but only 4% of ingestion interactions, result in direct harm or death, possibly due to difficulties in observing ingestion and quantifying its sublethal effects (Gall and Thompson, 2015). Even the most plainly visible L-F interactions are undercounted; it is estimated that 80% of seals that are entangled die and do not wash up on shore (Fowler, 1982). Due to difficulties in observation and reporting, sporadic individual interactions do not represent population-level effects (Gall and Thompson, 2015; Kühn et al., 2015; Galgani et al., 2018). On the floor of the deep sea especially, the lack of litter data means that effects on deep-sea fauna are unknown and largely unstudied (Canals et al., 2021). However, while widespread impacts are not well understood, that does not mean they do not exist (Koelmans et al., 2013). Endangered or threatened species can be easily impacted by interactions (Macfadyen et al., 2009) whose effects may result in biodiversity loss (Galgani et al., 2013).
Legislation has been enacted to reduce the input of marine litter, largely targeting ocean-based sources. For example, Annex V of the International Convention for the Prevention of Pollution from Ships, (International Maritime Organization [IMO], 1997), regulates dumping of litter from ships, completely banning the disposal of plastics at sea. The London Protocol, adopted in 1996 and entered into force in 2006, to which 53 countries adhere, bans most ship-based dumping (International Maritime Organization [IMO], 2019). The accumulation of litter along open-ocean shipping routes (Gjerde, 2006) means the targeting of ocean-based litter sources can be beneficial. However, since the initial writing of the two described pieces of legislation, it has been discovered that up to 80% of marine litter comes from land (North Pacific Fishery Management Council [NPFMC], 2011). Land-sourced litter legislation occurs on a country-by-country or even city-by-city basis. Successful methods to reduce land-sourced marine litter include educational campaigns, increased waste receptacles, improved waste management plans, and beach clean-up activities (Matthews and Doyle, 2012).
While policy increasingly regulates general marine litter, legislation to decrease L-F interactions specifically is lacking. The Marine Strategy Framework Directive (MSFD), which was created to protect European marine ecosystems, calls its member states to achieve Good Environmental Status (GES) in their waters. There are 11 descriptors to be met for achievement of GES, and marine litter is one. As such, achievement of GES requires there to be minimal marine litter presence. To assess GES based on marine litter, impacts due to interactions are monitored, namely impacts of ingestion (EC, 2010) and of entanglement (EC, 2022). As described, L-F interactions are beginning to be considered important benchmarks for marine litter presence and environmental health, but further analysis and policy creation will be necessary to better regulate L-F interactions.
Submarine canyons contain significantly more litter than any other oceanographic feature (Pham et al., 2014; Tubau et al., 2015). Described as keystone structures for their provision of habitat heterogeneity and facilitation of nutrient upwelling, submarine canyons are V-shaped valleys with steep walls incised into continental shelves and margins (Vetter et al., 2010; Fernandez-Arcaya et al., 2017). Submarine canyons are formed by mass slumping, landslides, and turbidity currents, and they act as conduits for the transport of sediment, debris, and anthropogenic litter from canyon heads to their deep-sea floors (Fernandez-Arcaya et al., 2017; Ramirez-Llodra, 2020). Linear litter items such as ropes, fishing lines, and nets are commonly found snagged on rugose canyon walls, while rounded objects such as plastic fragments, bottles, and cans are transported to canyon floors (Lastras et al., 2016). Canyons closer to shore and populated cities contain the highest litter densities (Galgani et al., 2000; Pham et al., 2014; Pierdomenico et al., 2019); however, marine litter is still found in offshore canyons, even those in protected, no-fishing zones (Marin and Aguilar, 2012).
The abundance of marine litter in submarine canyons and the general occurrence of marine L-F interactions suggests that interactions and impacts are occurring within submarine canyons. However, financial, logistical, and technical implications make research of submarine canyons and the deep sea challenging compared to that of shallow-water areas (Barnes et al., 2009; Galgani et al., 2015; Tekman et al., 2017; Canals et al., 2021). Such challenges leave the deep sea, which makes up half of the surface of the earth, grossly understudied (Barnes et al., 2009; Ramirez-Llodra et al., 2011; Galgani et al., 2015); over 95% of the deep sea is unexplored (van den Beld, 2017). As such, there is a need for increased research into submarine canyon L-F interactions.
The current research aims to fill gaps in knowledge in two ways. Firstly, a standardised L-F interactions framework is proposed, sourced from interaction types reported ocean-wide, and will help increase the ability for global interactions comparisons found in all oceanic zones. Additionally, the current documentation of L-F interactions occurring in submarine canyons will be presented, categorised using the standardised framework, providing both L-F interactions knowledge at present and a baseline dataset to be augmented upon further L-F interactions observations within canyons.
A critical literature review was undertaken following the PRISMA methodology (Page et al., 2021) to amalgamate L-F interactions and impacts reported to occur in all ocean zones, from which the L-F interactions framework was derived. Additionally, papers reporting marine L-F interactions within submarine canyons were used to complete a synthesis review of the current state of research. The body of papers read was derived from three separate groupings, the first two resulting from keywords searches using Google Scholar, and the third resulting from relevant citations found within papers read. The first, general keywords search was conducted by entering the keywords ‘marine AND debris* AND/OR litter* AND/OR interact* AND/OR seafloor* AND/OR seabed* AND fauna AND -micro’ into Google Scholar. In order to find additional papers focusing on canyons, a second, specific keywords search was undertaken by using the terms from the first search plus AND canyon* AND “deep sea*” AND/OR “deep-sea”. The final search was performed on 7th May, 2023. See Figure 1 for a decision tree depicting the creation of the body of papers to be read, starting with all papers found in the general and specific keywords search, and selecting for papers with relevant data. Peer-reviewed publications with data on L-F interactions with micro-litter or in simulated laboratory experiments were excluded from the primary data categories. Relevant citations within the publications from the keywords searches were recorded and read. The literature review was deemed exhaustive when all relevant citations in each paper read had already been included in the review.
Figure 1 Criteria for inclusion or exclusion in the systematic review of papers resulting from the keywords searches and data gathered from each evidence source.
All L-F interactions mentioned or reported were categorised by type. Synonyms describing the same type of interaction were recorded using the most common term, and similar interactions were amalgamated into groups. Overarching categories of interactions were finalised into groupings of the main types of marine L-F interactions that occur, creating a framework. Each description of an impact resulting from an interaction was likewise categorised by type. Impact types were combined to be written as one term if synonymic, and similar types of impacts were grouped together; primary, secondary, and tertiary impacts were recorded as such. Primary impacts occur as direct results of interactions, secondary impacts occur as direct results of primary impacts, and tertiary impacts occur as direct results of secondary impacts.
From papers reporting primary data on L-F interactions and impacts within submarine canyons, the following information was recorded for comparison and analysis:
• taxa (categorised by phylum, the most specific level possible)/features affected,
• type of litter,
• type of interaction,
• global canyon location,
• location of interaction within the canyon,
• maximum depth range of study, and
• initial intention of data collection.
As canyons are complex systems with transport and mixing throughout, all locations within canyons were considered in data extraction.
The critical literature review produced a body of scientific articles and grey literature that provides background on the current state of L-F interactions, with an emphasis on submarine canyons. A total of 4,086 papers resulted from the two keywords searches, of which 222 mentioned L-F interactions and were retained for the next stage of screening (Figure 2). An additional 137 papers which mentioned L-F interactions were found through in-text citations while reviewing the literature from the two keyword searches, which were used to build the L-F interactions framework. Almost two-thirds (n=221) of papers mentioning or reporting L-F interactions have been published in the past 10 years, starting in 2013 (Figure 3). Out of 359 papers found that mentioned L-F interactions, 23 contained primary data of such interactions occurring in submarine canyons (Figure 2). All 23 of those papers have been published in the past 14 years, since 2009 (Figure 3).
Figure 2 Screening process for papers to be included in the critical literature review and number of papers included at each step, following the PRISMA methodology (Page et al., 2021).
Figure 3 Publication year of papers that were included in the critical literature review, delineated by type of paper. Light blue: number of papers that mention Litter-fauna (L-F) interactions but have no primary interaction data. Medium blue: number of papers with primary data on L-F interactions, not including micro-litter ingestion or simulated experiments. Dark blue: number of papers with primary data on L-F interactions in or along submarine canyons, not including micro-litter ingestion.
A comprehensive L-F interactions framework has been devised, based on categorisation of L-F interactions in all ocean zones mentioned in the literature review. The six litter-fauna interaction categories are as follows: entanglement, ingestion, smothering, habitat provision, adaptive behaviour, and encountering. While biotic interactions are most often mentioned, a smaller framework for interactions between litter and abiotic features consists of entanglement and smothering. Abiotic features are those that are not living and are not derived from organisms that were once living. If no biotic or abiotic L-F interaction is observed, the piece of litter should be reported as such. See Table 2 for L-F interactions framework, including synonyms and common examples.
Entanglement in marine litter is defined as fauna becoming ensnared by a piece of litter (Figures 4A–C). An entangled individual may remain mobile or become immobilised. Also reported as tangling and entrapment, entanglement occurs largely due to fishing gear and packing bands (United Nations Environment Programme [UNEP] and National Oceanic and Atmospheric Administration [NOAA], 2012; Morris and Seasholes, 2014). Impacts are mainly mechanical, and include reduced feeding and difficulty breathing, as suggested in Rhizoprionodon lalandii sharks in southeast Brazil found emaciated with plastic debris collars (Sazima et al., 2002), increased metabolic rate, seen in captive Callorhinus ursinus fur seals from St. Paul Island, Alaska (Feldkamp et al., 1988), wounds or abrasions, as seen in Asia-Pacific coral reefs (Lamb et al., 2018), and even drowning and death, which are difficult to observe (Fowler, 1982). Abiotic entanglement occurs when litter snags on ocean features, degrading, altering, or obstructing habitat (Gilman et al., 2021).
Figure 4 Litter-fauna entanglement, smothering, habitat provision, and encountering examples. Entanglement: (A) Plastic bag snagged on a deep-sea gorgonian (source: Schlining et al., 2013 © MBARI 2006). (B) Echinoid entangled (mobile entanglement) in rope (© JNCC/Cefas, 2017). (C) Ghost trapping of red crabs and banded shrimp (source: Quattrini et al., 2015). Smothering: (D) Abiotic smothering: plastic bags smothering the seafloor (source: Taviani et al., 2019). (E) Abiotic smothering: mattress smothering the seafloor (source: Taviani et al., 2019). Habitat provision: (F) Tire serving as substrate for an anemone and sea cucumber, shelter for a rockfish (source: Schlining et al., 2013 © MBARI 2009). (G) Shoe serving as shelter for a rockfish (source: Schlining et al., 2013 © MBARI 2010). Encountering: (H) Fish Lepidion eques within 2 body lengths of monofilament fishing line (© JNCC/Cefas, 2017). (I) Anemone Actinauge richardi within 2 body lengths of rope (© JNCC/Cefas, 2017). (J) Two individuals of A richardi and one Munida (small lobster opposite rope from the right-most anemone) touching rope (© JNCC/Cefas, 2017).
Ingestion of marine litter is defined as fauna consuming marine litter (Table 3). The main source of ingestion is degraded micro-litter (United Nations Environment Programme [UNEP] and National Oceanic and Atmospheric Administration [NOAA], 2012); it can, therefore, be difficult to observe ingestion as it occurs. Ingestion may occur purposefully or inadvertently, and impacts include digestion blockages, as seen in Chelonia mydas sea turtles in southeastern Brazil (Di Beneditto and Awabdi, 2014), internal injuries, as suggested by Gramentz (1988) in species of loggerhead sea turtles, false senses of satiation, as seen in albatross chicks on Midway Atoll (Auman et al., 1997), and transport of chemical pollutants adsorbed to ingested litter, as suggested by Rochman et al. (2013) in Oryzias latipes fish.
Smothering by marine litter is defined as litter settling on top of fauna and blocking water flow (Figures 4D, E). Also reported as ‘covering,’ smothering is mainly caused by wide pieces of litter, such as bags, fabric, and sheets of various materials (United Nations Environment Programme [UNEP] and National Oceanic and Atmospheric Administration [NOAA], 2012). Smothering can also occur on abiotic features where no fauna is discernible. Impacts include preventing filter feeders from feeding, as suggested in sponges by Bergmann and Klages (2012), and creation of anoxic sediment, as exemplified by plastic bags placed in intertidal waters off the coast of Dublin, Ireland (Green et al., 2015).
Habitat provision by marine litter is defined as litter acting as either substrate or shelter for fauna (Figures 4F, G). Substrate provision occurs when sessile and encrusting species grow on pieces of litter, as seen in the settlement of sea anemones, hydroids, and crinoids on litter in the Arctic deep sea (Bergmann and Klages, 2012), and in the settlement of species on a shipping container not typically found in the soft sediment of the area (Taylor et al., 2014). Shelter provision occurs when species take cover in the cavities of litter, either temporarily or permanently, such as Munida crustaceans making burrows of plastic and cloth in the Bay of Biscay (van den Beld et al., 2017). In the use of litter as substrate or shelter, species may settle on mobile materials that are transported by currents, potentially causing the spread of invasive species; in the review by Gall and Thompson (2015), there were 259 species reported to use litter as rafts, and 6 of those were invasives.
Adaptive behaviour to marine litter is defined as the adaptation of a species to the presence of litter, using it in ways other than habitat provision. Examples include use as egg laying substrates, as seen in Sepia officinalis cuttlefish and Loligo vilgaris squid in the Northern Adriatic Sea (Moschino et al., 2019), aggregation sites, exemplified by Cidaris cidaris and Pleisionika species being often found around litter accumulations in submarine canyons south of Italy (Pierdomenico et al., 2018), and carrying litter, as seen by Paromola cuvieri off the southern coast of Sardinia (Figure 9, Image E: Taviani et al., 2017). Impacts include alteration of natural systems and behaviours, with so far unquantified effects. Adaptive behaviour is the least understood of all the L-F interactions (Barros et al., 2020).
Encountering marine litter is defined as a species coming across litter but not interacting with it in any of the 5 ways listed above (Figures 4H–J). Examples of encountering include touching, collision, or general disturbance from litter, and impacts may include altered behaviour or wounds. While the interaction ‘encountering’ is not often reported as such, Tekman et al. (2017) reported contact between litter and fauna to occur in 80% of its 60 observed interactions on the Arctic seafloor, largely with suspension feeders. When any part of a species is within two of its body lengths of a piece of litter, it is considered to have encountered the litter in a non-contact manner. Impacts of non-touching encountering include proximity to toxin leaching from the litter and increased likelihood of one of the other types of interactions.
Interactions do not occur in isolation but cause ensuing impacts on fauna. For examples, see Katsanevakis (2008); Barreiros and Raykov (2014); Lamb et al. (2018) and Angiolillo and Fortibuoni (2020). Connections between interactions and their primary, secondary, and tertiary impacts, as discovered in the literature review, have been amalgamated using common standardised terminology. Entanglement and smothering resulted in the largest number of possible primary impacts, with 6 each in biotic interactions, and 2 and 5, respectively, in abiotic interactions. See Figure 5 for chord diagrams of the relationship between biotic and abiotic interactions and their respective resulting primary effects on marine ecosystems.
Figure 5 Left: Litter-fauna interactions (bold) and their respective primary impacts on fauna. Number of primary impacts from each interaction: Entanglement-6; Ingestion-5; Smothering-6; Habitat Provision-4; Adaptive Behaviour-2; Encountering-3 (not including the potential for occurrence of the other interaction types). Right: Abiotic litter interactions (bold) and their respective impacts on fauna and ocean features. Number of primary impacts from each interaction: Entanglement-2; Smothering-5. Diagrams created using datasmith.org.
Information extracted from the 23 papers reporting primary data on L-F interactions within submarine canyons is displayed in Figure 6. See Table 4 for further details on country, phylum, litter category, and interaction type from the 23 papers. Papers included in the matrix are referred to in Appendix A.
Figure 6 Trends in canyon litter-fauna interactions reporting, categorised by country of research, with each category organised from L to R. Maximum survey depth categorised by depth (metres); location within canyon is organised by most commonly observed location of interaction in each publication, followed by ‘multiple locations’ and ‘not specified’; survey type is organised by most commonly used method; data collection is organised by category; phylum affected is organised by most commonly affected phylum; interaction observed is categorised by most commonly observed interaction; litter type is organised according to the framework used by the MSFD (Source: Hanke et al., 2013).
Table 4 Primary reports of litter-fauna interactions in submarine canyons detailed by country, phylum with Cnidaria order (Cnidaria is the most commonly observed interacting phylum), litter category with plastic type [categories from the MSFD framework (Hanke et al., 2013)], and interaction type.
The categories of survey method and data collection method were each dominated by one type (Figure 6). ROVs were used in 19 of the 23 papers, making them the most commonly used survey tool to encounter L-F interactions in submarine canyons. Of the 23 papers, 15 collected data indirectly, meaning L-F interactions were observed incidentally during a survey that had other aims. Of those papers, 13 occurred at the time of surveying, and 2 occurred retrospectively, upon reviewing the footage for another purpose. Of the 8 reports that directly intended to search for litter-fauna interactions, 5 were retrospective and 3 had the initial goal of quantifying litter-fauna interactions.
Among all taxa, entanglement and habitat provision were most commonly observed, each reported at least once by 19 papers (Figure 6). Smothering and adaptive behaviour were each reported 3 times, 4 papers observed encountering, and ingestion was never reported. The most commonly observed interactions took place between plastic and Cnidarians; 21 papers reported at least one instance of plastic interacting with fauna, and 19 papers reported at least one instance of the phylum Cnidaria interacting with litter. In total, 15 of the 23 papers reported corals entangled by fishing nets or lines, making coral entanglement with plastic fishing gear the most commonly reported L-F interaction in submarine canyons.
Globally, the majority of litter-fauna interactions occurring in canyons were reported from Europe, mainly in the Mediterranean Sea (Figure 7). At 7 papers, Italy has produced the largest number of such publications by a single country. Within the canyons, maximum survey depth and location of interactions vary. Of the 23 papers analysed, 19 presented results from deep sea surveys, while 2 occurred largely outside of the zone of the deep sea, with maximum depths under 250 m. All papers reported on benthic interactions except Santín et al. (2020), which found sponges colonising plastic entangled in ROV rope as it was pulled up from a canyon. Among benthic surveys, the most common locations of L-F interactions were canyon flanks and heads, each with 6 reports.
Figure 7 Global locations of the 23 reports of litter-fauna interactions occurring in submarine Canyons. Legend indicates the dot colour based on the number of scientific publications. Inset depicts European locations of litter-fauna interactions occurring in submarine canyons, making up 18 out of 23 total global reports. Each bubble indicates one scientific publication, unless otherwise labelled.
Submarine canyons provide habitat and harbour biodiversity, especially for vulnerable slow-growing species like cold-water corals (CWCs), meaning the pressure of litter can cause long-lasting physical damage (Giusti et al., 2019; Lartaud et al., 2020). At present, research on L-F interactions in submarine canyons is extremely limited, with reports coming from 23 scientific publications, only 8 of which whose primary aim was to investigate L-F interactions. The amount of deep-sea litter research is disproportionate to the number of canyons that exist. Globally, at least 9,477 submarine canyons are found in the ocean, making up approximately 11% of the area of continental slopes (Harris et al., 2014). The prevalence of submarine canyons, combined with their provision of habitat and transport of materials such as litter to the deep sea, means that the minimal data on L-F interactions likely does not signify a lack of interactions, but a lack of research.
Submarine canyon research is made difficult by constraints such as equipment, financing, and logistics (Barnes et al., 2009; Galgani et al., 2015; Tekman et al., 2017; Canals et al., 2021). Trawl surveys are commonly used in benthic litter analyses due to low costs and litter retrieval ability, but L-F interactions are disturbed during the trawling process, thus minimising the advantages of this method (Angiolillo and Fortibuoni, 2020). The benefits of manoeuvrability and access to the deep sea make ROVs the best option for submarine canyon L-F interactions research, but their cost and variation in image resolution can be limiting factors (Canals et al., 2021). Among the 23 papers with L-F interactions in canyons, inconsistencies in taxon level reported required fauna to be compared at the phylum level, making for poor taxonomic resolution. In order to quantify and classify marine litter and L-F interactions, a combination of visual and trawling surveys may provide the most comprehensive results (Tubau et al., 2015). With increasingly descriptive data, even if total global reports stay low, impacts of L-F interactions on fauna and ecosystems may begin to be hypothesised and assessed. In order to better understand the existence and impacts of litter in submarine canyons, standardised reporting of litter in general (Hernandez et al., 2022), and of L-F interactions specifically, will help to start compiling standardised datasets and allow for global comparability and elucidation of trends and true ecological impact. For example, currently under negotiation is the Global Plastics Treaty, which aims to address plastic pollution worldwide (Plastics Europe, 2023); the binding legislature proposed by this treaty could benefit from a standardised framework to help in reporting and monitoring.
Litter finds its way to the ocean due to unsustainable, linear production and consumption processes (Thompson et al., 2011). Producers are not concerned with the end of a product’s life, proper recycling centres are few and far between, and there is a disconnect between product use and far away disposal, promoting irresponsible waste practices (Thompson et al., 2011). When litter originates at sea, such as ALDFG, dumping may occur due to costliness of repairing damaged nets (Macfadyen et al., 2009), or boats not even being equipped to bring deep sea fishing nets back to shore (Brown et al., 2005). Both small- and large-scale policy and action will be required to reduce the presence of, and therefore faunal interactions with, marine litter. Mitigation strategies generally follow one of two paths: remove what is already present or stop the input of new litter, the latter of which is widely considered to be more advantageous, being more cost-effective and sustainable (United Nations Environment Programme [UNEP], 2009; Consoli et al., 2019; Hohn et al., 2020). While it will take further research to globally quantify marine litter impacts, it is advantageous to take action now to mitigate marine litter and prevent further harm than is already occurring (National Research Council [NRC], 2009; Kühn et al., 2015). A comprehensive strategy is needed that combines public-facing advocacy with shifts to circular production and consumption cycles, global litter prevention policies, and improved recycling technology, all while ensuring that each effort is conducted in a sustainable, long-lasting manner (Borrelle et al., 2020).
Several limitations of this research must be considered to increase transparency. The literature review, though deemed exhaustive using the keywords search method, potentially missed reports of L-F interactions within submarine canyons if such reports had misaligned keywords or a lack of keywords entirely. Future literature reviews of the same topic may prevent the issue by utilising more than one search method. Of the papers read, all but one reported L-F interactions within canyons in the Northern Hemisphere, providing potential bias in trend analyses. The limited location of studies was corroborated by the results found by Hernandez et al. (2022), so a potential solution is seemingly to collate ongoing research on litter in submarine canyons and grow the dataset.
At present, any marine L-F interactions reported globally are sporadic in timescale and in quantity of data presented. The overwhelming majority of primary data on L-F interactions in submarine canyons comes from the Mediterranean Sea. The global locations of general marine litter surveys at depths greater than 50 m presented by Hernandez et al. (2022) corroborate this finding: most sites were in the Mediterranean Sea, and research sites specifically in submarine canyons solely existed in the Northern Hemisphere. The first assessment of anthropogenic impact in Australian canyons was reported in 2022 (Taviani et al., 2023). In creating a standardised L-F interactions framework, the ease of reporting will be increased, thus encouraging L-F interactions observed to be studied and recorded. With reports from additional locations and even depths, a global dataset can begin to be compiled, helping track trends and make comparisons worldwide. Further, L-F interaction data will be critical in the creation of sensitivity and vulnerability assessments for marine flora and fauna, as the ubiquitous nature of litter in the ocean suggests impacts on biota that are not yet quantified.
The L-F interactions framework proposed and tested within the present research has the potential to be applied globally, increasing the breadth of marine litter data while encouraging standardised reporting approaches and, thus, connectivity between researchers. The body of evidence that contributed to the design of the framework reported on L-F interactions occurring in all ocean zones, from the surface to the benthos and from the shore to the open ocean. As such, the framework is applicable for use in all aquatic research, not just those studying submarine canyons. The framework is intentionally broad, providing 6 main categories of interactions, 2 of which also occur with abiotic features. Each interaction category was designed through comprehensive review of reported interactions and categories used previously. Additionally, impacts were considered in the delineation of categories; specific types of interactions within each of the 6 groupings cause similar impacts to the fauna or features involved.
Within each broad category, there is potential for subdivision based on interactions observed, which will provide higher data resolution. For example, in a location with a high incidence of encountering, such interactions may be split into the categories of contact and non-contact. Within the wide auspice of entanglement, ghost fishing or coral snagging may be separated; within the umbrella term of habitat provision, shelter vs. substrate provision may be specified. The L-F interactions framework may be added to or receive alterations as it is utilised by researchers, strengthening it for increased global applicability. Overall, we propose a ‘call to action’ to the submarine canyon and deep-sea researcher community-at-large with the goal being implementation of the litter-fauna interaction framework to kickstart connectivity and comparability between researchers observing L-F interactions all over the world. Without this standardised reporting method and analysis of global trends, the types and scale of the long-term ecological impacts of marine litter cannot truly be assessed.
AB: research, framework creation, manuscript draft JD: conception and design of the study, manuscript editing AD: conception and design of the study, manuscript editing. All authors contributed to the article and approved the submitted version.
The authors declare that the research was conducted in the absence of any commercial or financial relationships that could be construed as a potential conflict of interest.
All claims expressed in this article are solely those of the authors and do not necessarily represent those of their affiliated organizations, or those of the publisher, the editors and the reviewers. Any product that may be evaluated in this article, or claim that may be made by its manufacturer, is not guaranteed or endorsed by the publisher.
Andrady A. L. (2015). “Persistence of plastic litter in the oceans,” in Marine anthropogenic litter. Eds. Bergmann M., Gutow L., Klages M. (Cham: Springer), 57–72. doi: 10.1007/978-3-319-16510-3_3
Angiolillo M., Fortibuoni T. (2020). Impacts of marine litter on mediterranean reef systems: From shallow to deep waters. Front. Mar. Sci. 7. doi: 10.3389/fmars.2020.581966
Angiolillo M., Giusti M., Rossi L., Tunesi L. (2022). A dendrophyllia ramea population in the ionian sea (Central mediterranean sea) threatened by anthropogenic impacts. Front. Mar. Sci. 9. doi: 10.3389/fmars.2022.838274
Angiolillo M., La Mesa G., Giusti M., Salvati E., Di Lorenzo B., Rossi L., et al. (2021a). New records of scleractinian cold-water coral (CWC) assemblages in the southern Tyrrhenian Sea (western Mediterranean Sea): Human impacts and conservation prospects. Prog. Oceanography 197, 1–21. doi: 10.1016/j.pocean.2021.102656
Auman H. J., Ludwig J. P., Giesy J. P., Colborn T. (1997). ‘Plastic ingestion by Laysan Albatross chicks on Sand Island, Midway Atoll, in 1994 and 1995’. Albatross Biol. Conserv., 239–244.
Bajaj R., Kulkarni N., Raut R., Garg S. (2021). Sea debris: A review of marine litter detection techniques. Int. J. Innovative Res. Technol. 7 (8), 119–125.
Barnes D. K. A., Galgani F., Thompson R. C., Barlaz M. (2009). Accumulation and fragmentation of plastic debris in global environments. Philos. Trans. R. Soc. B 364, 1985–1998. doi: 10.1098/rstb.2008.0205
Barreiros J. P., Raykov V. S. (2014). Lethal lesions and amputation caused by plastic debris and fishing gear on the loggerhead turtle Caretta caretta (Linnaeus, 1758). Three case reports from Terceira Island, Azores (NE Atlantic). Mar. pollut. Bull. 86, 518–522. doi: 10.1016/j.marpolbul.2014.07.020
Barros F., Santos D., Reis A., Martins A., Dodonov P., Nunes J. A. C. C. (2020). Choosing trash instead of nature: Sea urchin covering behavior. Mar. pollut. Bull. 155, 1–4. doi: 10.1016/j.marpolbul.2020.111188
Bergmann M., Klages M. (2012). Increase of litter at the Arctic deep-sea observatory HAUSGARTEN. Mar. pollut. Bull. 64, 2734–2741. doi: 10.1016/j.marpolbul.2012.09.018
Borrelle S. B., Ringma J., Law K. L., Monnahan C. C., Lebreton L., McGivern A., et al. (2020). Predicted growth in plastic waste exceeds efforts to mitigate plastic pollution. Science 369, 1515–1518. doi: 10.1126/science.aba3656
Brown J., Macfadyen G., Huntington T., Magnus J., Tumilty J. (2005). Ghost fishing by lost fishing gear, institute for european environmental policy. Available at: https://citeseerx.ist.psu.edu/viewdoc/download?doi=10.1.1.114.8547&rep=rep1&type=pdf.
Canals M., Pham C. K., Bergmann M., Gutow L., Hanke G., van Sebille E., et al. (2021). The quest for seafloor macrolitter: a critical review of background knowledge, current methods and future prospects. Environ. Res. Lett. 16 (2), 1–29. doi: 10.1088/1748-9326/abc6d4
Carpenter E. J., Smith K. L. Jr. (1972). Plastics on the sargasso sea surface. Science 175, 1240–1241. doi: 10.1126/science.175.4027.1240
Consoli P., Andaloro F., Altobelli C., Battaglia P., Campagnuolo S., Canese S., et al. (2018a). Marine litter in an EBSA (Ecologically or Biologically Significant Area) of the central Mediterranean Sea: Abundance, composition, impact on benthic species and basis for monitoring entanglement. Environ. pollut. 236, 405–415. doi: 10.1016/j.envpol.2018.01.097
Consoli P., Falautano M., Sinopoli M., Perzia P., Canese S., Esposito V., et al. (2018b). ‘Composition and abundance of benthic marine litter in a coastal area of the central Mediterranean Sea’. Mar. pollut. Bull. 136, 243–247. doi: 10.1016/j.marpolbul.2018.09.033
Consoli P., Romeo T., Angiolillo M., Canese S., Esposito V., Salvati E., et al. (2019). Marine litter from fishery activities in the Western Mediterranean Sea: The impact of entanglement on marine animal forests. Environ. pollut. 249, 472–481. doi: 10.1016/j.envpol.2019.03.072
Consoli P., Sinopoli M., Deidun A., Canese S., Berti C., Andaloro F., et al. (2020). The impact of marine litter from fish aggregation devices on vulnerable marine benthic habitats of the central Mediterranean Sea. Mar. pollut. Bull. 152, 1–8. doi: 10.1016/j.marpolbul.2020.110928
Derraik J. G. B. (2002). The pollution of the marine environment by plastic debris: a review. Mar. pollut. Bull. 44, 842–852. doi: 10.1016/S0025-326X(02)00220-5
Di Beneditto A. P. M., Awabdi D. R. (2014). Marine debris, megafauna, fish, turtle, dolphins, Brazilian coastal area. Mar. pollut. Bull. 88 (1-2), 86–90. doi: 10.1016/j.marpolbul.2014.09.020
European Commission (2010). EU Commission Decision of 1 September 2010 on criteria and methodological standards on good environmental status of marine waters. (2010/477/EU) Off. J. Eur. Union 232, 1424.
European Commission (2022) D10 Marine litter. Available at: https://mcc.jrc.ec.europa.eu/main/dev.py?N=28&O=223&titre_chap=D10+Marine+litter.
Feldkamp S. D., Costa D. P., DeKrey G. K. (1988). Energetic and behavioral effects of net entanglement on juvenile northern fur seals, callorhinus ursinus. Fishery Bull. 87, 85–94.
Fernandez-Arcaya L., Ramirez-Llodra E., Aguzzi J., Allcock A. L., Davies J. S., Dissanayake A., et al. (2017). Ecological role of submarine canyons and need for canyon conservation: A review. Front. Mar. Sci. 4. doi: 10.3389/fmars.2017.00005
Fowler C. W. (1982). “Interactions of Northern Fur seals and commercial fisheries’, in Kenneth Sabol (ed.) Transactions of the forty-third North American Wildlife and Natural Resources Conference. Washington, DC: United States Department of Commerce. pp. 278-292.
Galgani F., Hanke G., Maes T. (2015). “Global distribution, composition and abundance of marine litter,” in Marine anthropogenic litter. Eds. Bergmann M., Gutow L., Klages M. (Springer Open), 29–56. doi: 10.1007/978-3-319-16510-3_2
Galgani F., Hanke G., Werner S., De Vrees L. (2013). Marine litter within the European Marine Strategy Framework Directive. ICES J. Mar. Sci. 70 (6), 1055–1064. doi: 10.1093/icesjms/fst122
Galgani F., Leaute P., Moguedet P., Souplet A., Verin Y., Carpentier A., et al. (2000). Litter on the sea floor along European coasts. Mar. pollut. Bull. 40 (6), 516–527. doi: 10.1016/S0025-326X(99)00234-9
Galgani F., Pham C. K., Claro F., Consoli P. (2018). Marine animal forests as useful indicators of entanglement by marine litter. Mar. pollut. Bull. 135, 735–738. doi: 10.1016/j.marpolbul.2018.08.004
Gall S. C., Thompson R. C. (2015). The impact of debris on marine life. Mar. pollut. Bull. 92, 170–179. doi: 10.1016/j.marpolbul.2014.12.041
Gilman E., Chopin F., Suuronen P., Kuemlangan B. (2016). Abandoned, lost and discarded gillnets and trammel nets: Methods to estimate ghost fishing mortality, and the status of regional monitoring and management (Rome, Italy: Food and Agriculture Organization of the United Nations).
Gilman E., Musyl M., Suuronen P., Chaloupka M., Gorgin S., Wilson J., et al. (2021). Highest risk abandoned, lost and discarded fishing gear. Sci. Rep. 11, 1–11. doi: 10.1038/s41598-021-86123-3
Gjerde K. M. (2006). “Ecosystems and biodiversity in deep waters and high seas,” in UNEP regional seas report and studies (Switzerland: UNEP/IUCN).
Gramentz D. (1988). Involvement of loggerhead turtle with the plastic, metal, and hydrocarbon pollution in the central mediterranean. Mar. pollut. Bull. 19 (1), 11–13. doi: 10.1016/0025-326X(88)90746-1
Green D. S., Boots B., Blockley D. J., Rocha C., Thompson R. (2015). Impacts of discarded plastic bags on marine assemblages and ecosystem functioning. Environ. Sci. Technol. 49 (9), 1–37. doi: 10.1021/acs.est.5b00277
Gregory R. M. (2009). Environmental implications of plastic debris in marine settings –entanglement, ingestion, smothering, hangers-on, hitch-hiking and alien invasions. Philos. Trans. R. Soc. B 364, 2013–2025. doi: 10.1098/rstb.2008.0265
Hanke G., Galgani F., Werner S., Oosterbaan L., Nilsson P., Fleet D., et al. (2013). Guidance on Monitoring of Marine Litter in European Seas. EUR 26113 Luxembourg (Luxembourg): Publications Office of the European Union. JRC83985 doi: 10.2788/99475
Harris P. T., Macmillan-Lawler M., Rupp J., Baker E. K. (2014). ‘Geomorphology of the oceans’. Mar. Geology 352, 4–24. doi: 10.1016/j.margeo.2014.01.011
Hernandez I., Davies J. S., Huvenne V. A. I., Dissanayake A. (2022). Marine litter in submarine canyons: A systematic review and critical synthesis. Front. Mar. Sci. 9. doi: 10.3389/fmars.2022.965612
Hohn S., Acevedo-Trejos E., Abrams J. F., de Moura J. F., Spranz R., Merico A. (2020). The long-term legacy of plastic mass production. Sci. Total Environ. 746, 1–8. doi: 10.1016/j.scitotenv.2020.141115
Holmström A. (1975). Plastic films on the bottom of the Skagerack. Nature 255, 622–623. doi: 10.1038/255622a0
International Maritime Organization [IMO] (1997). MARPOL 73/78: consolidated edition 1997 (London: International Maritime Organization), 349–355. Available at: https://vdoc.pub/download/marpol-73-78-33i5ha4808d0.
International Maritime Organization [IMO] (2019) Convention on the prevention of marine pollution by dumping of wastes and other matter. Available at: https://www.imo.org/en/OurWork/Environment/Pages/London-Convention-Protocol.aspx.
Joint Group of Experts on the Scientific Aspects of Marine Environmental Protection [GESAMP] (2019). Guidelines for the monitoring and assessment of plastic litter in the ocean (UNEP).
Katsanevakis S. (2008). “Marine debris, a growing problem: sources, distribution, composition, and impacts,” in Marine pollution: new research. Ed. Hofer T. N. (New York, NY: Nova Science Publishers, Inc.), 53–100.
Keller A. A., Fruh E. L., Johnson M. M., Simon V., McGourty C. (2010). Distribution and abundance of anthropogenic marine debris along the shelf and slope of the US West Coast. Mar. pollut. Bull. 60, 692–700. doi: 10.1016/j.marpolbul.2009.12.006
Koelmans A. A., Gouin T., Thompson R., Wallace N., Arthur C. (2013). Plastics in the marine environment. Environ. Toxicol. Chem. 33 (1), 5–10. doi: 10.1002/etc.2426
Kühn S., Bravo Rebolledo E. L., van Franeker J. A. (2015). “Deleterious effects of litter on marine life,” in Marine anthropogenic litter. Eds. Bergmann M., Gutow L., Klages M. (Springer), 75–116.
Laist D. W. (1987). Overview of the biological effects of lost and discarded plastic debris in the marine environment. Mar. pollut. Bull. 18, 319–326. doi: 10.1016/S0025-326X(87)80019-X
Lamb J. B., Willis B. L., Fiorenza E. A., Couch C. S., Howard R., Rader D. N., et al. (2018). Plastic waste associated with disease on coral reefs. Science 359 (6374), 460–462. doi: 10.1126/science.aar3320
Lartaud F., Meistertzheim A. L., Reichert J., Ziegler M., Peru E., Ghiglione J. F. (2020). “Plastics: an additional threat for coral ecosystems,” in Perspectives on the marine animal forests of the world. Eds. Rossi S., Bramanti L. (Switzerland: Springer), 469–485. doi: 10.1007/978-3-030-57054-5_14
Macfadyen G., Huntington T., Cappell R. (2009). Abandoned, lost or otherwise discarded fishing gear (Rome: United Nations Environment Programme (UNEP) and Food and Agriculture Organization of the United Nations (FAO). Available at: https://wedocs.unep.org/bitstream/handle/20.500.11822/13603/rsrs185r.pdf?sequence=1&%3BisAllowed.
Marin P., Aguilar R. (2012). “Mediterranean submarine canyons 2012: pending protection,” in Mediterranean submarine canyons. Ed. Würtz M. (Gland, Switzerland and Málaga, Spain: IUCN), 191–205.
Matthews T. R., Doyle E. (2012). Marine litter in the caribbean: Five case studies. Proc. 64th Gulf Caribbean Fisheries Institute, 64, 172–179.
Melli V., Angiolillo M., Ronchi F., Canese S., Giovanardi O., Querin S., et al. (2017). The first assessment of marine debris in a Site of Community Importance in the north-western Adriatic Sea (Mediterranean Sea). Mar. pollut. Bull. 114 (2), 821–830. doi: 10.1016/j.marpolbul.2016.11.012
Morris J., Seasholes B. (2014). How green is that grocery bag ban? An assessment of the environmental and economic effects of grocery bag bans and taxes (Reason Foundation). Available at: https://reason.org/wp-content/uploads/2014/06/how_green_bag_ban.pdf.
Moschino V., Riccato F., Fiorin R., Nesto N., Picone M., Boldrin A., et al. (2019). Is derelict fishing gear impacting the biodiversity of the Northern Adriatic Sea? An answer from unique biogenic reefs. Sci. Total Environ. 663, 387–399. doi: 10.1016/j.scitotenv.2019.01.363
National Research Council [NRC] (2009). Tackling marine debris in the 21st century (Washington, D.C: The National Academies Press).
Nevill J. (2011). Submission to the select committee on marine parks in south Australia (Victoria, Australia: OnlyOnePlanet Consulting). Available at: http://www.onlyoneplanet.com/marineSAsubmissionMPAs_2011.pdf.
North Pacific Fishery Management Council [NPFMC] (2011). Appendix C: non-fishing effects on EFH in the arctic (Arctic FMP). Available at: https://media.fisheries.noaa.gov/dam-migration/arctic1-fmp-akr.pdf.
Page M. J., McKenzie J. E., Bossuyt P. M., Boutron I., Hoffmann T. C., Mulrow C. D., et al. (2021). The PRISMA 2020 statement: an updated guideline for reporting systematic reviews. BMJ 372 (71). doi: 10.1136/bmj.n71
Pham C. K., Ramirez-Llodra E., Alt C. H. S., Amaro T., Bergmann M., Canals M., et al. (2014). Marine litter distribution and density in European seas, from the shelves to deep basins. PloS One 9 (4), 1–13. doi: 10.1371/journal.pone.0095839
Pierdomenico M., Casalbore D., Chiocci F. L. (2019). Massive benthic litter funnelled to deep sea by flash-flood generated hyperpycnal flows. Sci. Rep. 9, 1–10. doi: 10.1038/s41598-019-41816-8
Plastics Europe (2023) The global plastics treaty. Available at: https://plasticseurope.org/changingplasticsforgood/global-plastics-treaty/.
Ramirez-Llodra E. (2020). “Deep-sea ecosystems: biodiversity and anthropogenic impacts,” in The law of the seabed. Ed. Banet C. (Netherlands: Brill-Nijhoff), 36–60. doi: 10.1163/9789004391567_004
Ramirez-Llodra E., Tyler P. A., Baker M. C., Bergstad O. A., Clark M. R., Escobar E., et al. (2011). ‘Man and the last great wilderness: Human impact on the deep sea’. PloS One 6 (7), 1–25. doi: 10.1371/journal.pone.0022588
Rochman C. M., Browne M. A., Halpern B. S., Hentschel B. T., Hoh E., Karapanagioti H. K., et al. (2013). Classify plastic waste as hazardous. Nature 494, 169–171. doi: 10.1021/es303700s
Ryan P. G. (2015). “A brief history of marine litter research,” in Marine anthropogenic litter. Eds. Bergmann M., Gutow L., Klages M. (Cham: Springer), 1–25. doi: 10.1007/978-3-319-16510-3_1
Ryan P. G., Moloney C. L. (1993). Marine litter keeps increasing. Nature 361, 23. doi: 10.1038/361023a0
Savini A., Vertino A., Marchese F., Beuck L., Freiwald A. (2014). Mapping Cold-Water Coral Habitats at Different Scales within the Northern Ionian Sea (Central Mediterranean): An Assessment of Coral Coverage and Associated Vulnerability. PloS One 9. doi: 10.1371/journal.pone.0087108
Sazima I., Gadig O. B. F., Namora R. C., Motta F. S. (2002). Plastic debris collars on juvenile carcharhinid sharks (Rhizoprionodon lalandii) in southwest Atlantic. Mar. pollut. Buletin 44, 1147–1149. doi: 10.1016/S0025-326X(02)00141-8
Sherrington C., Darrah C., Hann S., Cole G., Corbin M. (2016). “Study to support the development of measures to combat a range of marine litter sources,” in Report for european commission DG environment. Available at: https://www.kosmopedia.org/wp-content/uploads/2020/09/MSFD-Measures-to-Combat-Marine-Litter.pdf.
Tekman M. B., Krumpen T., Bergmann M. (2017). Marine litter on deep Arctic seafloor continues to increase and spreads to the North at the HAUSGARTEN observatory. Deep-Sea Res. I 120, 88–99. doi: 10.1016/j.dsr.2016.12.011
Thompson R. C., La Belle B. E., Bouwman H. (2011). “Marine debris: defining a global environmental challenge,” in A STAP advisory document (Nairobi: UNEP), 1–28. Available at: https://www.thegef.org/sites/default/files/council-meeting-documents/C.40.Inf_.14_Marine_Debris.pdf.
Tubau X., Canals M., Lastras G., Rayo X., Rivera J., Amblas D. (2015). Marine litter on the floor of deep submarine canyons of the Northwestern Mediterranean Sea: The role of hydrodynamic processes. Prog. Oceanography 134, 379–403. doi: 10.1016/j.pocean.2015.03.013
Unger B., Herr H., Benke H., Böhmert M., Burkhardt-Holm P., Dähne M., et al. (2017). Marine debris in harbour porpoises and seals from German waters. Mar. Environ. Res. 130, 77–84. doi: 10.1016/j.marenvres.2017.07.009
United Nations Environment Programme [UNEP] (2009). Marine litter: A global challenge (Nairobi: UNEP), 1–234.
United Nations Environment Programme [UNEP], National Oceanic and Atmospheric Administration [NOAA] (2012) The Honolulu Strategy: A global framework for prevention and management of marine debris. Available at: https://wedocs.unep.org/20.500.11822/10670.
van den Beld I. (2017). Coral habitats in submarine canyons of the Bay of Biscay: distribution, ecology and vulnerability (Université de Bretagne Occidentale).
Venrick E. L., Backman T. W., Bartram W. C., Platt C. J., Thornhill M. S., Yates R. E. (1973). Man-made objects on the surface of the central North Pacific ocean. Nature 241, 271. doi: 10.1038/241271a0
Vetter E. W., Smith C. R., De Leo F. C. (2010). Hawaiian hotspots: enhanced megafaunal diversity in submarine canyons on the oceanic islands of Hawaii. Mar. Ecol. 31, 183–199. doi: 10.1111/j.1439-0485.2009.00351.x
Vieira R. P., Raposo I. P., Sobral P., Gonçalves J. M. S., Bell K. L. C., Cunha M. R. (2014). Lost fishing gear and litter at Gorringe Bank (NE Atlantic). J. Sea Res. 100, 91–98. doi: 10.1016/j.seares.2014.10.005
Watson M. (2012). Marine debris along the florida keys reef tract- mapping, analysis and perception study (University of Miami).
Woodall L. C., Robinson L. F., Rogers A. D., Narayanaswamy B. E., Paterson G. L. J. (2015). Deep-sea litter: a comparison of seamounts, banks and a ridge in the Atlantic and Indian Oceans reveals both environmental and anthropogenic factors impact accumulation and composition. Front. Mar. Sci. 2 (3). doi: 10.3389/fmars.2015.00003
Angiolillo M., di Lorenzo B., Farcomeni A., Bo M., Bavestrello G., Santangelo G., et al. (2015). Distribution and assessment of marine debris in the deep Tyrrhenian Sea (NW Mediterranean Sea, Italy). Mar. pollut. Bull. 92, 149–159. doi: 10.1016/j.marpolbul.2014.12.044
Angiolillo M., Gérigny O., Valente T., Fabri M.-C., Tambute E., Rouanet E., et al. (2021b). Distribution of seafloor litter and its interaction with benthic organisms in deep waters of the Ligurian Sea (Northwestern Mediterranean). Sci. Total Environ. 788, 1–20. doi: 10.1016/j.scitotenv.2021.147745
Cau A., Alvito A., Moccia D., Canese S., Pusceddu A., Rita C., et al. (2017). Submarine canyons along the upper Sardinian slope (Central Western Mediterranean) as repositories for derelict fishing gears. Mar. pollut. Bull. 123, 357–364. doi: 10.1016/j.marpolbul.2017.09.010
Cerrillo-Escoriza J., Lobo F. J., Puga-Bernabéu Á., Rueda J. L., Bárcenas P., Sánchez-Guillamón O., et al. (2023). Origin and driving mechanisms of marine litter in the shelf-incised Motril, Carchuna, and Calahonda canyons (northern Alboran Sea). Front. Mar. Sci. doi: 10.3389/fmars.2023.1098927
D’Onghia G., Calculli C., Capezzuto F., Carlucci R., Carluccio A., Grehan A., et al. (2017). Anthropogenic impact in the Santa Maria di Leuca cold-water coral province (Mediterranean Sea): Observation and conservation straits. Deep Sea Res. Part II: Topical Stud. Oceanography 145, 87–101. doi: 10.1016/j.dsr2.2016.02.012
Enrichetti F., Dominguez-Carrió C., Toma M., Bavestrello G., Canese S., Bo M. (2020). Assessment and distribution of seafloor litter on the deep Ligurian continental shelf and shelf break (NW Mediterranean Sea). Mar. pollut. Bull. 151, 1–14. doi: 10.1016/j.marpolbul.2019.110872
Fabri M.-C., Pedal L., Beuck L., Galgani F., Hebbeln D., Freiwald A. (2014). Megafauna of vulnerable marine ecosystems in French mediterranean submarine canyons: Spatial distribution and anthropogenic impacts. Deep Sea Res. Part II: Topical Stud. Oceanography 104, 184–207. doi: 10.1016/j.dsr2.2013.06.016
Giusti M., Canese S., Fourt M., Bo M., Innocenti C., Goujard A., et al. (2019). Coral forests and Derelict Fishing Gears in submarine canyon systems of the Ligurian Sea. Prog. Oceanography 178, 1–16. doi: 10.1016/j.pocean.2019.102186
Lastras G., Canals M., Ballesteros E., Gili J.-M., Sanchez-Vidal A. (2016). Cold-water corals and anthropogenic impacts in la fonera submarine canyon head, Northwestern mediterranean sea. PloS One 11 (5), 1–36. doi: 10.1371/journal.pone.0155729
Moccia D., Cau A., Bramanti L., Carugati L., Canese S., Follesa M. C., et al. (2021). Spatial distribution and habitat characterization of marine animal forest assemblages along nine submarine canyons of Eastern Sardinia (central Mediterranean Sea). Deep Sea Res. Part I: Oceanographic Res. Papers 167. doi: 10.1016/j.dsr.2020.103422
Mordecai G., Tyler P. A., Masson D. G., Huvenne V. E. I. (2011). Litter in submarine canyons off the west coast of Portugal. Deep-Sea Res. II 58, 2489–2496. doi: 10.1016/j.dsr2.2011.08.009
Orejas C., Gori A., Lo Iacono C., Puig P., Gili J.-M., Dale M. R. T. (2009). Cold-water corals in the Cap de Creus canyon, northwestern Mediterranean: spatial distribution, density and anthropogenic impact. Mar. Ecol. Prog. Ser. 397, 37–51. doi: 10.3354/meps08314
Pierdomenico M., Cardone F., Carluccio A., Casalbore D., Chiocci F., Maiorano P., et al. (2018). Megafauna distribution along active submarine canyons of the central Mediterranean: relationships with environmental variables. Prog. Oceanography 171, 49–69. doi: 10.1016/j.pocean.2018.12.015
Quattrini A. M., Nizinski M. S., Chaytor J. D., Demopoulos A. W. J., Roark E. B., France S. C., et al. (2015). Exploration of the canyon-incised continental margin of the Northeastern United States reveals dynamic habitats and diverse communities. PloS One 10 (10), 1–32. doi: 10.1371/journal.pone.0139904
Ramirez-Llodra E., De Mol B., Company J. B., Coll M., Sardà F. (2013). Effects of natural and anthropogenic processes in the distribution of marine litter in the deep Mediterranean Sea. Prog. Oceanography 118, 273–287. doi: 10.1016/j.pocean.2013.07.027
Santín A., Grinyó J., Bilan M., Ambroso S., Puig P. (2020). First report of the carnivorous sponge Lycopodina hypogea (Cladorhizidae) associated with marine debris, and its possible implications on deep-sea connectivity. Mar. pollut. Bull. 159, 1–7. doi: 10.1016/j.marpolbul.2020.111501
Schlining K., von Thun S., Kuhnz L., Schlining B., Lundssten L., Stout N. J., et al. (2013). Debris in the deep: Using a 22-year video annotation database to survey marine litter in the Monterey Canyon, central California, USA. Deep-Sea Res. I 79, 96–105. doi: 10.1016/j.dsr.2013.05.006
Taviani M., Angeletti L., Canese S., Cannas R., Cardone F., Cau A., et al. (2017). The “Sardinian cold-water coral province” in the context of the Mediterranean coral ecosystems. Deep-Sea Res. II 145, 61–78. doi: 10.1016/j.dsr2.2015.12.008
Taviani M., Angeletti L., Cardone F., Montagna P., Danovaro R. (2019). A unique and threatened deep water coral-bivalve biotope new to the Mediterranean Sea offshore Naples megalopolis. Sci. Rep. 9 (1), 1–12. doi: 10.1038/s41598-019-39655-8
Taviani M., Foglini F., Castellan G., Montagna P., McCulloch M. T., Trotter J. A. (2023). First assessment of anthropogenic impacts in submarine canyon systems off southwestern Australia. Sci. Total Environ. 857 (1), 1–12. doi: 10.1016/j.scitotenv.2022.159243
Taylor J. R., DeVogelaere A. P., Burton E. J., Frey O., Lundsten L., Kuhnz L. A., et al. (2014). Deep-sea faunal communities associated with a lost intermodal shipping container in the Monterey Bay National Marine Sanctuary, CA. Mar. pollut. Bull. 83 (1), 92–106. doi: 10.1016/j.marpolbul.2014.04.014
van den Beld I. M. J., Guillaumont B., Menot L., Bayle C., Arnaud-Haond S., Bourillet J.-F. (2017). Marine litter in submarine canyons of the Bay of Biscay. Deep-Sea Res. II 145, 142–152. doi: 10.1016/j.dsr2.2016.04.013
Keywords: marine litter, benthic fauna, litter-fauna interaction, submarine canyon, seafloor
Citation: Bruemmer AL, Dissanayake A and Davies JS (2023) Marine litter-fauna interactions: a standardised reporting framework and critical review of the current state of research with a focus on submarine canyons. Front. Mar. Sci. 10:1225114. doi: 10.3389/fmars.2023.1225114
Received: 18 May 2023; Accepted: 03 August 2023;
Published: 19 September 2023.
Edited by:
Cinzia Corinaldesi, Marche Polytechnic University, ItalyReviewed by:
Lucy Cheryl Woodall, University of Oxford, United KingdomCopyright © 2023 Bruemmer, Dissanayake and Davies. This is an open-access article distributed under the terms of the Creative Commons Attribution License (CC BY). The use, distribution or reproduction in other forums is permitted, provided the original author(s) and the copyright owner(s) are credited and that the original publication in this journal is cited, in accordance with accepted academic practice. No use, distribution or reproduction is permitted which does not comply with these terms.
*Correspondence: Jaime Selina Davies, SmFpbWUuRGF2aWVzQHVuaWdpYi5lZHUuZ2k=
Disclaimer: All claims expressed in this article are solely those of the authors and do not necessarily represent those of their affiliated organizations, or those of the publisher, the editors and the reviewers. Any product that may be evaluated in this article or claim that may be made by its manufacturer is not guaranteed or endorsed by the publisher.
Research integrity at Frontiers
Learn more about the work of our research integrity team to safeguard the quality of each article we publish.