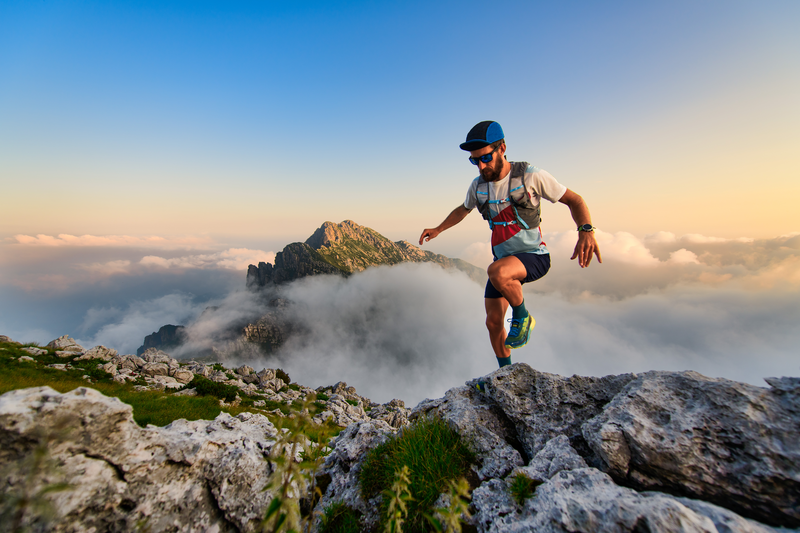
95% of researchers rate our articles as excellent or good
Learn more about the work of our research integrity team to safeguard the quality of each article we publish.
Find out more
REVIEW article
Front. Mar. Sci. , 09 October 2023
Sec. Marine Biology
Volume 10 - 2023 | https://doi.org/10.3389/fmars.2023.1225067
This article is part of the Research Topic The Biology and Conservation of Elasmobranchs and Chimaeras View all 12 articles
In this review, we summarize the state of knowledge of the influence of light on the activity and physiology of elasmobranchs (sharks, skates, rays, and sawfish). These are a diverse group with great economic and ecological importance. The long-term success of a species is largely determined by its ability to respond to changes in its environment. Light plays an important role for many marine species in signaling rhythmic environmental changes which are part of daily and annual cycles. Behavioral and physiological changes by organisms in response to these signals have evolved enabling them to maximize survival and reproductive success. In an environment with increased levels of artificial light at night (ALAN), deleterious changes in activity and physiology can occur. By summarizing what is known about the influence of light on elasmobranch activity, it can be concluded that ALAN is likely to have a negative impact on elasmobranchs at the individual and population level. We also discuss the example of intentional nocturnal light pooling by the tourism industry to attract whale sharks (Rhincodon typus) and manta rays (Mobula spp.) and recommend regulation of this activity.
Light is a fundamental signal for living organisms to organize processes ranging from the molecular scale to coordinated behaviors across entire populations (Mishra and Kumar, 2017; Lincoln, 2019; Yan et al., 2020). The role of rhythmic variation in natural light sources, primarily the sun, in regulating activity such as feeding and breeding is well established for many clades (Guh et al., 2019). It is likely, if currently under researched, that such rhythmic variation plays a similar role for elasmobranchs (sharks, skates, rays, and sawfish). This clade contains a diverse range of species fulfilling important ecological roles as top predators, prey, and scavengers (Myers et al., 2007; Ferretti et al., 2010; Dulvy et al., 2017). Many elasmobranchs also have a direct value for humans as a food source (Dulvy et al., 2017; Glaus et al., 2019) and, increasingly, through ecotourism (Gallagher and Hammerschlag, 2011; Cisneros-Montemayor et al., 2013; Huveneers et al., 2017; Mieras et al., 2017). Due in part to their long generation time and low fecundity, many elasmobranch species are currently at risk of population decline or extinction (García et al., 2008; Lucifora et al., 2011; Dulvy et al., 2014).
Organisms have evolved with light being a reliable predictor of environmental cues (Hut and Beersma, 2011; Ashton et al., 2022). Disruption of such cues impacts daily and seasonal biology (Falcón et al., 2020; Fishbein et al, 2021).The introduction of artificial light at night (ALAN) in elasmobranch habitats therefore has the potential to compound other threats to elasmobranch populations as daily and seasonal rhythms are disrupted. On land, the influence of ALAN has been characterized for many clades (Gaston et al., 2017; Falcón et al., 2020; Sanders et al., 2020; Burt et al., 2023). In the ocean, however, its influence is less well known. In 2017, 22% of coastal areas were exposed to ALAN. This has induced changes in the physiology of marine organisms (Davies et al., 2014; Marangoni et al., 2022). The area exposed to ALAN is known to be increasing by more than 2% each year (Kyba et al., 2017). Understanding the likely influence of both intentionally and unintentionally introduced ALAN on elasmobranchs requires an understanding of the role of light in establishing rhythms in physiological and behavioral activity.
Much ALAN is the unintentional outcome of human activities (Levin et al., 2020), however there is increasing occurrence of ‘light pooling.’ Here, multiple bright lights, exceeding 4000 Watts, are shone on the ocean surface leading to an increase in biological activity and the attraction of both micro and macrofauna, including sharks and rays, with larger species feeding on smaller species (Jauharee, 2014; Zareer, 2022). Light pooling is conducted to attract whale sharks (Rhincodon typus) and manta rays including (Mobula birostris) by the tourism industry in Hawaii, Palau, and the Maldives (Shaahunaz, 2017; Passoni and Saponari, 2019; Passoni, 2021). Whale shark tourism is an important source of income in many countries, including the Maldives, where it was valued at 9.4 million USD to the Maldivian economy in 2014 (Cagua et al., 2014). The global value of manta ray tourism was estimated at 140 million USD in 2013 (O’Malley et al., 2023). Despite the conservation benefits of macrofauna focused ecotourism, such activities can also be damaging when unregulated (Harvey-Carroll et al., 2021; Gayford et al., 2023). The impacts of newly introduced activities such as light pooling, should thus be investigated.
Biological rhythms are highly conserved across taxa (Kumar and Sharma, 2018). Rhythmic activity can broadly be split into diurnal or diel (daily) and seasonal (annual) activity (Lincoln, 2019). Although many studies refer to diel, diurnal, and circadian rhythms interchangeable, there is an important distinction to be made. Where both diel and diurnal rhythms refer to activity which follows a 24-hour cycle, circadian rhythms refer to activity which is endogenous and can be demonstrated to follow an internal control (Vitaterna et al., 2001; Vetter, 2018). Thanks in part to the influence of external cues, circadian rhythms generally also follow a 24-hour cycle. They are, however ‘free running’ meaning they persist when cues are removed, and lose synchrony with the external environment (Golombek and Rosenstein, 2010; Cox and Takahashi, 2019). Circadian rhythms are the endogenous ‘pacemaker’ controlling diel activity while circannual rhythms control seasonal behavior. For the purposes of this review, we use the term ‘diel rhythm’ to refer to any activity with a 24-hour cycle to avoid confusion with the term diurnal behavior, which refers to activity which peaks during daylight hours.
To make sensible decisions about priority areas of ALAN mitigation and minimize negative anthropogenic impacts on elasmobranch species, it is first necessary to understand how light controls behavior and physiology in the subclass. In this review, we synthesis the current state of knowledge about how light influences elasmobranch behavioral and physiological activity. Through a systematic review, we investigate the known occurrences of diel and circadian rhythms in elasmobranchs, and how light affects them. We discuss the case of light pooling by the tourism industry as a case study for an emerging source of ALAN and recommend that this practice be regulated.
A systematic review of primary literature on the influence of light on elasmobranch activity was carried out using the Web of Science Database (Clarivate, 2022) and Google Scholar (Google, 2022). The terms Elasmobranch AND (“photoperiod” OR “entrainment” OR “zeitgeber” OR “circadian organization” OR “clock gene*” OR “extra-ocular photoreceptor*” OR “deep brain photoreceptor*” OR “artificial light at night” OR “ALAN” OR “pineal gland*” OR “light pollution” OR “circadian oscillator” OR “light exposure” OR “light pulse” OR “circadian rhythm*” OR “circadian organization” OR circadian) were searched and all resulting peer reviewed literature evaluated for relevance. Within reviews, cited literature was searched and evaluated for relevance.
The initial review of primary literature identified 54 unique studies from Google scholar and the Web of Science Database which reported an impact of either light intensity or photoperiod on elasmobranch activity or physiology. A further five relevant studies were identified by searching cited literature in reviews (Table 1).
Table 1 Summary of findings of the systematic review into the impact of light on elasmobranch behavioural and physiological activity.
In four studies (Nelson and Johnson, 1970; Finstad and Nelson, 1975; Gleiss et al., 2017; Kelly et al., 2020), a definitive influence of light on elasmobranch behavior in isolation of other factors was reported. Under controlled laboratory conditions an individual nocturnal horn shark (Heterodontus francisci) (n = 1) was shown by Nelson and Johnson (1970) to exhibit diel rhythms in locomotor activity influenced by light exposure. This activity became arrhythmic in the absence of light or under constant light and was re-established under a 12-12 light-dark (LD) cycle. Under constant light, locomotive behavior was diminished, whereas under constant darkness, near continuous locomotion occurred. When subjected to both one- and seven-hour phase shifts, locomotion activity patterns were immediately changed to match the corresponding light levels. The entrainment speed and lack of rhythmicity during constant photoperiods indicated no circadian endogeneity.
This study also provides proof of endogenous circadian rhythms cued by light in an elasmobranch; the nocturnal swell shark (Cephaloscyllium ventriosum). An individual swell shark (n = 1) shifted to constant darkness maintained a 24-hour cycle in locomotor activity, however this began to drift with peak activity shifting by 0.6-hours each day resulting in a nine-hour phase shift following 15 days of constant darkness. The reintroduction of 12-12-hour LD cycle resulted in the slow reestablishment of the 24-hour cycle, taking three days for locomotion to be synchronized with light periods. Following one week on a 12-12-hour photoperiod, the shark was held under constant light conditions for 18 days. This resulted in a shift in activity, with a seven-hour shift in peak activity by day 18, characteristic of true endogenous circadian behavior. Unlike synchronization following exposure to constant darkness, synchronization to an LD cycle following continuous light conditions was immediate. A one-hour shift in the LD cycle resulted in a corresponding shift in peak activity, which generally anticipated the dark phase. Furthermore, the sharks were able to track a seven-hour light shift.
The re-establishment of rhythmicity in behavior matching that of the photoperiod was evident for both the horned and swell shark when they were returned to a 12-12-hour LD conditions. This is clear evidence for an influence of light on shark activity although the study was limited in sample number and consideration of long-term effects. Importantly, in this study no food was given to isolate the effect of light as a zeitgeber (a stimulus capable of entraining biological rhythms).
Finstad and Nelson (1975) found that wild horn shark movement activity (leaving cave count) peaked 60-90 minutes after sunset; corresponding to 0.03 lux environmental levels. Under laboratory conditions, with a 12-12-hour LD cycle (light = 8 lux) horn sharks (n = 2) displayed cyclic activity, (passing sensors, binary) with anticipation of dark periods. When moved to constant darkness, all rhythmicity was immediately lost, and activity became irregular.
Three sharks were then held under constant lighting conditions: 0.2 lux for days 3-18. 0.13 lux for days 19-25 and finally during days 26-30, sharks were held in complete darkness. Marked differences were identified between individuals, as has been observed in other taxa (Guyomarc’h et al., 1998). When held under constant light conditions of 0.2 lux, behavioral rhythmicity of all sharks was found to drift, demonstrating a phase advance of activity. This would be expected of an endogenous circadian clock. Continual exposure to 0.13 lux resulted in individual differences. A loss of rhythmicity occurred for 2/3 sharks. Interestingly, one of the two sharks with initial complete loss of rhythmicity began to establish slight rhythmicity of behavior during the final days of constant 0.13 lux conditions. The final shark maintained complete rhythmicity, which appeared to drift by one hour earlier each day. When the three sharks were moved to complete darkness an immediate and complete loss of rhythmicity occurred (Finstad and Nelson, 1975). This experiment demonstrated the importance of light in controlling behavior in elasmobranchs, regardless of the presence of endogenous circadian rhythms.
In freshwater, Gleiss et al. (2017) showed that the crepuscular and night-time movement activity of sawfish (Pristis pristis) tagged with accelerometers (n = 13) is driven by light. Sawfish activity was shown to be elevated prior to twilight. In addition, they investigated the influence of water temperatures on diel vertical migrations (measured by using Time Depth Recording (TDR) devices). These were found to respond to alterations in water temperatures independently of circadian accelerometer activity. This is noteworthy as the only study in this review to show a decoupling between two aspects of elasmobranch behavior and the influence of photoperiod and water temperature.
Kelly et al. (2020), studied swimming (distance and time) of two shark species; the Port Jackson (Heterodontus portusjacksoni) (n = 8) and draughtsboard (Cephaloscyllium isabellum) (n = 8) shark under a 12-12-hour LD cycle, 6-6-hour LD cycle, constant light, and constant darkness. Under the 12-12-hour LD cycle, swimming activity in both species peaked during the dark phase. Under a ‘force desynchrony’ paradigm of a 6-6-hour LD cycle, peak in swimming activity of the Port Jackson shark closely followed dark phases, however, a 12-12-hour circadian pattern in activity was still detected during the first and third day under these conditions. Regardless of underlying circadian rhythms, sharks were found to swim more during dark phases. This indicates that activity is entrained by external light, however elasmobranchs may have a reduced capacity to follow light cycles shorter than 24-hours. Similarly, under a 6-6-hour LD cycle, draughtsboard sharks displayed higher swimming activity during the dark phase with no increase during the light phase reported. The swimming rhythmicity of Port Jackson sharks was disrupted after 48-hours in either constant light or darkness. Port Jackson sharks retained an attenuated circadian activity rhythm (activity levels dramatically decreased) for the first 24-hours of constant conditions. Draughtsboard sharks appeared to maintain rhythmic behavior under constant conditions. During these experiments, animals were fed every 72-hours, with the timing of feeding coinciding with the second day of each lighting regime. Feeding has the potential to act as a strong entrainment factor (Shibata et al., 2010; Carneiro and Araujo, 2012; Trzeciak and Steele, 2022), potentially influencing results. The short time frame of this study (72-hours for each lighting regime) also limits a full assessment of the longer-term effects of light on elasmobranch activity.
20 other studies implicated light as a cue for diel or seasonal rhythms across 22 species of elasmobranch but did not isolate light from other environmental cues such as sea surface temperature, seafloor water temperature, wind speed, or tides (Table 1). Diel rhythms in movement have been associated with daily photoperiods include based on accelerometer (Kneebone et al., 2018; Kadar et al., 2019; Byrnes et al., 2021) and TDR (Nelson et al., 1997; Andrews et al., 2009; Gallant et al., 2016; Kneebone et al., 2018; Byrnes et al., 2021) tagging studies. An influence of photoperiods on movement has also been inferred based on broad scale trends in shark abundances, such as daily aggregations (Brunnschweiler and Barnett, 2013; Nosal et al., 2014), rate of movement (Cartamil et al., 2003), and bycatch rates (Niella et al., 2021b). Seasonal behaviors associated with solar and lunar photoperiods include aggregation (Grubbs et al., 2007; Nosal et al., 2014; Kajiura and Tellman, 2016; Ayres et al., 2021; Niella et al., 2021a), migration (Kessel et al., 2014; Bangley et al., 2021), site fidelity (Vaudo and Lowe, 2006; Grubbs et al., 2007; Kneebone et al., 2012; Dudgeon et al., 2013; Nosal et al., 2014), residency (Kneebone et al., 2012; Kessel et al., 2014), and diving (Andrews et al., 2009).
Demski (1990) proposed that gametogenesis and reproductive behavior in elasmobranchs is controlled via photic input to the retina and pineal gland, which is analogous to other vertebrates (Bertolucci and Foà, 2004; Golombek and Rosenstein, 2010; Cassone, 2014). They collated data on elasmobranch photic neural projections and endocrine systems and documented the overlap of projections from both the retina and pineal gland to areas of the brain involved in sex steroid production, including gonadotropin-releasing hormone (GnRH). They proposed that this effects gonad physiology and indicated strong evidence for the role of the pineal gland in the production of pituitary gonadotrophins (GTHs). GnRH is the major neuropeptide modulating reproduction in vertebrates (Gorbman and Sower, 2003; Chen and Fernald, 2008; Roch et al., 2011) including elasmobranchs (Awruch, 2013). Extensive projections of pineal neurons throughout the brain of skate (Raja montagui) and dogfish (Scyliorhinus canicula) have since been mapped by (Mandado et al., 2001). Projections were found to be wide reaching, and largely conserved between teleosts, amphibians, and elasmobranchs. Pineal projections were identified in the only area of the dogfish brain producing GnRH. The authors concluded that the midbrain sGnRH immunoreactive nucleus is a core part of pineal pathways and heavily involved in photic induced control of brain function of the pineal gland (Figure 1) (Mandado et al., 2001).
Figure 1 An updated version of the pathway for light dependent control of reproductive behavior proposed by (Demski, 1990). Support for this pathway has been presented in (Mandado et al., 2001; Awruch, 2013). Light is detected by photoreceptors in the retina and pineal gland. Photic projections signal gonadotropin-releasing hormone (GnRH)-producing regions of the brain, stimulating the production and release of GnRH, which in turn stimulates production and release of gonadotrophins (GTHs). Within reproductive organs (testes and ovaries), GTHs stimulates the production/release of reproductive hormones, such as progestins, androgens and estrogen, which in turn lead to gametogenesis and reproductive behaviors.
A direct influence of light on aspects of elasmobranch physiology have been reported for three species (Mull et al., 2008; Mull et al., 2010; Waltrick et al., 2014), although this influence is difficult to disentangle from other environmental conditions (e.g. water temperature). Waltrick et al. (2014) reported concentrations of the reproductive hormone, 17β-estradiol, and ovarian follicle size to be positively correlated with day length and water temperature in the Australian sharpnose shark (Rhizoprionodon taylori). Mull et al., 2010 found that progesterone concentrations in female round stingrays (Urobatis halleri) were significantly positively correlated with day length and water temperature. In males of the same species, Mull et al., 2008 reported gonadosomatic index (GSI) and plasma 11-ketotestosterone levels to be significantly negatively correlated with photoperiod, with an additional influence of an undefined change in day length on 11-ketotestosterone. Plasma testosterone levels were negatively correlated with both photoperiod and temperature, with photoperiod demonstrating a stronger influence. These three studies present findings that are consistent with photoperiodic regulation of seasonal behavior in other taxa, such as birds and mammals (Dawson et al., 2001; Hazlerigg and Wagner, 2006).
More broadly, seasonal rhythms in shark physiology have been observed in five species (Table 1). Concentrations of the reproductive hormone, T4, were found to follow seasonal rhythms in whitetip reef sharks (Triaenodon obesus) by (Crow et al., 1999). Blood cholesterol levels were found to follow seasonal rhythms in small-spotted catshark (Scyliorhinus canicula) by (Valls et al., 2016). Seasonal changes in sevengill shark (Notorynchus cepedianus) immune function indicators (lymphocyte and heterophil counts along with granulocyte to lymphocyte ratio) have also been documented (Sueiro et al., 2019). The highest testosterone and sperm motility has been reported in captive sand tiger sharks (Carcharias taurus) when environmental conditions mimic natural seasonal photoperiods and temperatures (Wyffels et al., 2020). Finally, Sumpter and Dodd (1979) report that pituitary gonadotropin (involved in photic control of reproduction in non-mammalian vertebrates (Pérez, 2022)) concentrations in mature female lesser spotted dogfish (Scyliorhinus canicula) are up to 100 times higher between February and April than other months, coinciding with peak egg-laying and highest levels of GSI.
The rhythmicity of animal behavior is largely influenced by light, alongside temperature and food availability (Häfker and Tessmar-Raible, 2020). In wild non-model organisms, it is often a challenge to disentangle the influence of different environmental cues. This can be compounded by the fact that studies of captive sharks often neglect to record water temperatures (e.g., Casterlin and Reynolds, 1979). Some inference must therefore take place when considering the influence of light on elasmobranch activity. It is likely that the prevalence of diel rhythms in sharks should be considered an initial indication of a light cued activity, although other factors such as water temperature or prey activity are likely involved. The diurnal and nocturnal activity of sharks has been reviewed by (Hammerschlag et al., 2017).
In literature recovered during this review, 33 elasmobranch species were reported to display some form of diel rhythm (Table S1). There were no reported instances in which diel rhythms were absent. 15 species of elasmobranch were reported to be nocturnal; three species were reported to be diurnal, and crepuscular activity was reported in seven species. Rhythmicity in either depth or presence at a location was reported for seven species (Table S1).
Diel behavior can be directly inferred from the observation of behavior or physiological markers or indirectly through, for example, bycatch reports. Diel rhythms in diving activity have been reported for 12 species and in swimming speed for two species of elasmobranch; the blue (Prionace glauca) and common thresher (Alopias vulpinus) sharks (Sciarrotta and Nelson, 1977; Cartamil et al., 2012). Elasmobranch physiology was also reported to follow diel rhythms by four studies with diel rhythms in metabolic rates reported for three species (Table S1). One bycatch study demonstrated that the blue shark (Prionace glauca) and shortfin mako (Isurus oxyrinchus) are largely caught during the night and between 00:00 and 04:00 respectively (Rodrigues et al., 2022).
Vertebrates use external light cues to modulate diel and seasonal rhythms (Figure 2, Tosini et al., 2001; Cowan et al., 2017; Mishra and Kumar, 2017; Liddle et al., 2022). The mechanisms of detection of external light varies across taxa, but the result (rhythmic hormone production) is highly conserved. In mammals, light stimulation is restrained to the retina, whereas in birds, teleosts, amphibians, and reptiles, light input is transduced by both ocular and non-ocular photoreceptors (Aschoff et al., 1982; Katherine Tamai et al., 2003; Nishiwaki-Ohkawa and Yoshimura, 2016). The mechanism for modulation of light cued rhythms in elasmobranchs is not fully established. Only the elephant shark (Callorhinchus milii) and the lantern shark (Etmopterus spinax) have been screened for, and found to possess, extra-ocular photoreceptors (Davies et al., 2012; Delroisse et al., 2018). The presence and responsiveness of non-ocular photoreceptors suggests that non-ocular control of rhythmic activity can occur.
Figure 2 Elasmobranchs can detect light through a variety of ocular and extra-ocular receptors in tissues such as the pineal and skin.
In non-mammalian vertebrates proteins, called opsins, have been linked to photic control of the endocrine system, such as breeding, circadian behavior and locomotion (Pérez et al., 2019; Dekens et al., 2022). Es-encephalopsin, a non-visual ciliary opsin has been identified in the in the ventral skin of the velvet belly lantern shark (Etmopterus spinax, (Delroisse et al., 2018). Melanopsins are a class of extensively studied non-visual opsin. They exist in two main classes: opn4m (mammalian-like) and the opn4x isoform (xenopus like). In mammalian vertebrates, opn4ms are found exclusively within the eye and are implicated with circadian rhythm regulation and melatonin production. In non-mammalian vertebrates, opn4x and opn4ms are present in the retina, pineal gland, skin, and deep brain regions (Davies et al., 2012). Davies et al. (2012) reported three melanopsin genes in the elephant shark (Callorhinchus milii). Two of these belonged to opn4m class (opn4m1 and opn4m2) and the third was the opn4x class. All melanopsins were found to be expressed in elephant shark eyes. Opn4m2 was found to be expressed in the fin, gills, hypothalamus, liver, skin, and testes. Opn4x was found throughout the brain, fin, gills, hypothalamus, kidney, liver, snout, skin, and testes. It has been proposed that melanopsins are involved in photoentrainment of circadian behavior, displaying different spectral sensitivity for deep-sea bioluminescence and bright-light environments (Davies et al., 2012). The wide expression of opsins in elasmobranchs is analogous to that seen in teleosts, which are capable of photoentrainment (Frøland Steindal and Whitmore, 2019; Steindal and Whitmore, 2020).
(Hamasaki and Streck, 1971) demonstrated light sensitivity of the pineal gland in dogfish (Squalus acanthias). Following exposure to as little as 4.3x10-4 lumens for 1 second, distinctive neuronal activity was detected through electrophysiology. This gland has extensive neuronal connections throughout the brain and humoral outputs, indicating the importance of photic influenced brain function. The pineal projections seen in elasmobranchs are largely similar to those found in teleosts, who are thought to display photic controlled breeding (Mandado et al., 2001).
Elasmobranchs are diverse and many aspects of their behavior, physiology, and role in ecosystems are understudied. This presents challenges for drawing firm general conclusions about their activity and conservation needs. It is clear, however, that light is a strong driver in establishing and regulating diel rhythms across elasmobranch taxa, which in turn likely controls seasonal behavior. Given the importance of light for modulating activity, it is of critical importance that the impact of anthropogenic alterations to natural light cycles be assessed. Among the literature reviewed, there is a clear absence of this research. Consequently, recommendations for minimizing the impact of light pollution on elasmobranchs must be based on inference from known aspects of elasmobranch ecology and other taxa.
Exposure to light outside of natural cycles is highly likely to disrupt rhythmic physiological and behavioral activity of individual elasmobranchs. The metanalysis conducted by (Sanders et al., 2020) demonstrated wide ranging effects of ALAN on organisms’ life history traits, physiology, population structure, and activity patterns resulting from as little as 1 lux in both terrestrial and marine ecosystems. Extensive studies have demonstrated that ALAN can have catastrophic effects on physiology and behavior as it disrupts the immune and endocrine system leading to impairments in reproduction and health (Sanders et al., 2020; Bumgarner and Nelson, 2021; Liu et al., 2022). For example, it has been demonstrated that, when subjected to ALAN, 0% of clownfish (Amphiprion ocellaris) eggs hatch (Fobert et al., 2019). A 36% decrease in survival, 51% weight reduction, and significant changes to metabolism have been identified in damselfish (Chromis viridis) and juvenile orange-fin anemonefish (Amphiprion chrysopterus) exposed to ALAN (Hillyer et al., 2021; Schligler et al., 2021). Similarly, multiple reviews have demonstrated unpredictable light regimes can disrupt circadian rhythms, negatively impacting health by disrupting multiple physiological systems, leading to disease and lowering offspring survival rates (Vetter, 2018; Chellappa et al., 2019; Maury, 2019; Rijo-Ferreira and Takahashi, 2019; Hou et al., 2020; Fishbein et al., 2021; Fatima et al., 2022; Lane et al., 2022). Cumulatively, disruption of life history in many individuals leads to population level effects such as reduced population growth rate and resilience to exploitation, hampering conservation efforts (Longcore and Rich, 2004; Davies et al., 2014; Gaston et al., 2017).
The case of light pooling being used to attract whale sharks and manta rays by the tourism industry should be considered as an emerging source of ALAN. Light pooling is reported in Hawaii, Palau and the Maldives (Shaahunaz, 2017; Passoni and Saponari, 2019; Passoni, 2021). It may also occur in other locations. During 2023, light pooling excursions were run by multiple operators for several hours at a time in the South Ari Atoll Marine Protected Area (SAMPA) in the Maldives throughout the night (7pm-8am). The frequency and duration of light pooling events has yet to be quantified.
Whale sharks and manta rays exhibit strong diel rhythms (Graham et al., 2005; Wilson et al., 2006; Brunnschweiler and Sims, 2011; Robinson et al., 2017; Lassauce et al., 2020; Andrzejaczek et al., 2021). Manta rays are thought to dive deeper at night than during the day (Lassauce et al., 2020; Andrzejaczek et al., 2021). In shallow waters whale sharks have been found to dive deeper during the day and shallower at night. There is evidence that this pattern may be reversed in deeper waters however this is not always the case (Tyminski et al., 2015). Furthermore accelerometer readings have demonstrated strong crepuscular activity in whale sharks (Gleiss et al., 2013). A single pulse of light is known to be sufficient to disrupt circadian rhythm in a plethora of organisms (Leloup and Goldbeter, 2001) while white light exposure during the night can lead to decreases in melatonin and gonadotrophin levels in European perch (Perca fluviatilis) (Brüning et al., 2016). There have been suggestions that whale shark diving behavior is determined by prey availability rather than abiotic environmental cues (Gleiss et al., 2013). Prey availability is likely to be strongly influenced by ALAN. Demersal zooplankton, which form a large component of whale shark and manta ray diets, are known to exhibit skototaxis (movement towards darkness) (Rohner et al., 2013; Couturier et al., 2013). Light pooling is therefore likely to alter the diet of target species. It is likely then that light pooling leads to physiological stress in whale sharks and manta rays as a result of the mismatch between internal physiology and the environment caused by alterations to two major zeitgebers; light and food (Figure 3).
Figure 3 (A) In the context of wildlife tourism, light pooling involves the intentional shining of bright lights (> 4000 watts) into the ocean at night (Photo source: Marloes Otten, photographer/videographer). This practice is currently unregulated and the impact on elasmobranch behavior and physiology are unknown. (B) Based on a review of available literature, we predict artificial light at night (ALAN) to have multiple negative impacts on elasmobranch individuals and populations.
Outside of direct impacts to individuals and cumulative impacts to populations, light pooling may alter the ability of elasmobranchs to regulate populations on lower trophic levels. There are also likely to be impacts on numerous nontarget species, such as Indo-Pacific bottlenose dolphins (Tursiops aduncus) and other elasmobranchs, such as nurse sharks (Ginglymostoma cirratum) which have all been documented during light pooling excursions.
Traditionally, emerging human activities harmful to wildlife have been permitted to occur until enough proof can be gathered of negative impacts to influence policy (Wilson et al., 2011). The precautionary principal in conservation counters this harmful dynamic by promoting the regulation of new practices before they have an opportunity to become harmful, based on what data is available (Meyers, 1993; Fisher et al., 2006; Wilson et al., 2011; Cooney and Dickson, 2012). As an endangered species and source of over 9.4 million USD to the Maldivian economy in 2014 (Cagua et al., 2014; Pierce and Norman, 2016), conservation of whale sharks should be a priority when designing and regulating tourism activities. It is not currently possible to predict how light pooling activities can be conducted in a manner that will allow individuals sufficient time between encounters to recover. We therefore recommend a precautionary approach to safeguard the species. In this way the issue of harmful new practices outpacing regulation can be avoided (Cooney and Dickson, 2012).
We recommend that the frequency and duration of light pooling events by the tourism industry be quantified and that targeted research should be conducted into the impact of light pooling at different intensities and pulse durations on elasmobranch health and physiology. This should be done opportunistically, making use of currently unregulated light pooling activities. The endorsement of light pooling by conservation and research bodies should also be avoided until further evidence can be gathered. The output of such research could be used to conduct a risk assessment for various management strategies allowing recommendations for best practice to be made and minimizing the negative impacts of light pooling on elasmobranch health.
Until such research has been conducted, we recommend that incidents of light pooling be reduced and tightly regulated to avoid negative impacts for individuals and/or the population. We recommend that i) both the frequency and duration of light pooling encounters be limited to the hours immediately proceeding sunset and preceding sunrise, leaving animals with some level of natural darkness each night, ii) light pooling be prohibited in marine protected areas (MPAs), iii) tour operators offering light pooling be required to hold a licence which demonstrates they have been informed of the potential negative impact of the practice on wildlife, and iv) white lights be switched off and the encounter proceed under red lights when sharks or manta rays are present. Light of longer wavelengths (red, above 639 nm) has been shown to have lesser impacts on the circadian system when compared to higher wavelengths (blue light, under 465 nm) (Brainard et al., 2008; Rahman et al., 2008; Park et al., 2013; Di Rosa et al., 2015; Sánchez-Vázquez et al., 2019). It is important to note that red light likely still induces some circadian disruption (Dauchy et al., 2015; Bonmati-Carrion et al., 2017).
Light is a clear driver of behavior and physiology across the elasmobranch subclass. There are a great deal of questions remaining regarding the exact mechanisms of this control, how this varies between taxa, and the complex interactions between light and other environmental factors. In combination with well-established research into how the disruption of natural light rhythms effects all other taxa, we predict that artificial light at night (ALAN) is likely to have multiple disruptive and negative impacts on elasmobranch behavior and physiology. Taxa specific research should be conducted to confirm this in cases where economically important species, such as the whale shark and manta rays, are experiencing increasing levels of ALAN. The emergence of light pooling has the potential to confound current elasmobranch conservation efforts. We recommend that a precautionary approach be taken and light pooling by the tourism industry be regulated.
Both authors contributed equally. JH-C conceived the study which was further developed in collaboration with DC. JH-C prepared Table 1 and illustrations. DC prepared Figures 1–3. JH-C and DC wrote the manuscript and contributed to revisions. All authors contributed to the article and approved the submitted version.
JH-C was funded by MWSRP.
The authors would like to thank Irthisham Zareer and Clara Canovas Perez for their valuable advice regarding light pooling. They would also like to thank Prof. Karin Harding for her assistance getting this manuscript to publication.
The authors declare that the research was conducted in the absence of any commercial or financial relationships that could be construed as a potential conflict of interest.
All claims expressed in this article are solely those of the authors and do not necessarily represent those of their affiliated organizations, or those of the publisher, the editors and the reviewers. Any product that may be evaluated in this article, or claim that may be made by its manufacturer, is not guaranteed or endorsed by the publisher.
The Supplementary Material for this article can be found online at: https://www.frontiersin.org/articles/10.3389/fmars.2023.1225067/full#supplementary-material
Andrews K. S., Williams G. D., Farrer D., Tolimieri N., Harvey C. J., Bargmann G., et al. (2009). Diel activity patterns of sixgill sharks, Hexanchus griseus: the ups and downs of an apex predator. Anim. Behav. 78 (2), 525–536. doi: 10.1016/J.ANBEHAV.2009.05.027
Andrzejaczek S., Schallert R. J., Forsberg K., Arnoldi N. S., Cabanillas-Torpoco M., Purizaca W., et al. (2021). Reverse diel vertical movements of oceanic manta rays off the northern coast of Peru and implications for conservation. Ecol. Solutions Evidence 2 (1), e12051. doi: 10.1002/2688-8319.12051
Aschoff J., Daan S., Groos G. A. (1982). Vertebrate circadian systems : structure and physiology (Berlin Heidelbeg New york: Springer Berlin Heidelberg). Available at: https://books.google.co.uk/books?hl=en&lr=&id=icLuCAAAQBAJ&oi=fnd&pg=PA1&dq=vertebrate+circadian+systems&ots=7Xq9AM81iy&sig=ag6rR8NTZSrXiFaePN8qLu9nmN0#v=onepage&q=amphibians&f=false.
Ashton A., Foster R. G., Jagannath A. (2022). Photic entrainment of the circadian system. Int. J. Mol. Sci. 23 (2), 729. doi: 10.3390/IJMS23020729
Awruch C. A. (2013). Reproductive endocrinology in chondrichthyans: The present and the future. Gen. Comp. Endocrinol. 192, 60–70. doi: 10.1016/J.YGCEN.2013.05.021
Ayres K. A., Ketchum J. T., Gonzalez-Armas R., Galvan-Magana F., Hearn A., Elorriaga-Verplancken F. R., et al. (2021). Seasonal aggregations of blacktip sharks Carcharhinus limbatus at a marine protected area in the Gulf of California, assessed by unoccupied aerial vehicle surveys. Mar. Ecol. Prog. Ser. 678, 95–107. doi: 10.3354/MEPS13897
Bangley C. W., Edwards M. L., Mueller C., Fisher R. A., Aguilar R., Heggie K., et al. (2021). Environmental associations of cownose ray (Rhinoptera bonasus) seasonal presence along the U.S. Atlantic Coast. Ecosphere 12 (9), e03743. doi: 10.1002/ECS2.3743
Barnett A., Payne N. L., Semmens J. M., Fitzpatrick R. (2016). Ecotourism increases the field metabolic rate of whitetip reef sharks. Biol. Conserv. 199, 132–136. doi: 10.1016/j.biocon.2016.05.009
Bass N. C., Day J., Guttridge T. L., Knott N. A., Brown C., Bass N. C., et al. (2021). Intraspecific variation in diel patterns of rocky reef use suggests temporal partitioning in Port Jackson sharks. Mar. Freshw. Res. 72 (10), 1445–1456. doi: 10.1071/MF20204
Bertolucci C., Foà A. (2004). Extraocular photoreception and circadian entrainment in nonmammalian vertebrates. Chronobiol. Int. 21 (4-5), 501–519. doi: 10.1081/CBI-120039813
Bonmati-Carrion M. A., Baño-Otalora B., Madrid J. A., Rol M. A. (2017). Light color importance for circadian entrainment in a diurnal (Octodon degus) and a nocturnal (Rattus norvegicus) rodent. Sci. Rep. 7 (1), 8846. doi: 10.1038/s41598-017-08691-7
Bouyoucos I. A., Montgomery D. W., Brownscombe J. W., Cooke S. J., Suski C. D., Mandelman J. W., et al. (2017). Swimming speeds and metabolic rates of semi-captive juvenile lemon sharks (Negaprion brevirostris, Poey) estimated with acceleration biologgers. J. Exp. Mar. Biol. Ecol. 486, 245–254. doi: 10.1016/J.JEMBE.2016.10.019
Brainard G. C., Sliney D., Hanifin J. P., Glickman G., Byrne B., Greeson J. M., et al. (2008). Sensitivity of the human circadian system to short-wavelength (420-nm) light. J. Biol. rhythms 23 (5), 379–386. doi: 10.1177/0748730408323089
Brewster L. R., Dale J. J., Guttridge T. L., Gruber S. H., Hansell A. C., Elliott M., et al. (2018). Development and application of a machine learning algorithm for classification of elasmobranch behaviour from accelerometry data. Mar. Biol. 165 (4), 1–19. doi: 10.1007/S00227-018-3318-Y/METRICS
Brüning A., Hölker F., Franke S., Kleiner W., Kloas W. (2016). Impact of different colours of artificial light at night on melatonin rhythm and gene expression of gonadotropins in European perch. Sci. Total Environ. 543, 214–222. doi: 10.1016/J.SCITOTENV.2015.11.023
Brunnschweiler J. M., Barnett A. (2013). Opportunistic visitors: long-term behavioural response of bull sharks to food provisioning in Fiji. PloS One 8 (3), e58522. doi: 10.1371/JOURNAL.PONE.0058522
Brunnschweiler J. M., Sims D. W. (2011). “Diel oscillations in whale shark vertical movements associated with meso-and bathypelagic diving,” in American fisheries society symposium (American Fisheries Society), vol. 76, 1–14.
Bumgarner J. R., Nelson R. J. (2021). Light at night and disrupted circadian rhythms alter physiology and behavior. Integr. Comp. Biol. 61 (3), 1160–1169. doi: 10.1093/ICB/ICAB017
Burt C. S., Kelly J. F., Trankina G. E., Silva C. L., Khalighifar A., Jenkins-Smith H. C., et al. (2023). The effects of light pollution on migratory animal behavior. Trends Ecol. Evol. doi: 10.1016/J.TREE.2022.12.006
Byrnes E. E., Daly R., Leos-Barajas V., Langrock R., Gleiss A. C. (2021). Evaluating the constraints governing activity patterns of a coastal marine top predator. Mar. Biol. 168 (1), 1–15. doi: 10.1007/S00227-020-03803-W/FIGURES/4
Cagua E. F., Collins N., Hancock J., Rees R. (2014). Whale shark economics: A valuation of wildlife tourism in South Ari Atoll, Maldives. PeerJ 2014 (1), e515. doi: 10.7717/peerj.515
Carneiro B. T., Araujo J. F. (2012). Food entrainment: major and recent findings. Front. Behav. Neurosci. 6, 83. doi: 10.3389/fnbeh.2012.00083
Cartamil D. P., Vaudo J. J., Lowe C. G., Wetherbee B. M., Holland K. N. (2003). Diel movement patterns of the Hawaiian stingray, Dasyatis lata: Implications for ecological interactions between sympatric elasmobranch species. Mar. Biol. 142 (5), 841–847. doi: 10.1007/S00227-003-1014-Y/FIGURES/5
Cartamil D., Wegner N. C., Aalbers S., Sepulveda C. A., Baquero A., Graham J. B. (2012). Diel movement patterns and habitat preferences of the common thresher shark (Alopias vulpinus) in the Southern California Bight. Mar. Freshw. Res. 61, 596–604. doi: 10.1071/MF09153
Cassone V. M. (2014). Avian circadian organization: A chorus of clocks. Front. Neuroendocrinol. 35 (1), 76–88. doi: 10.1016/J.YFRNE.2013.10.002
Casterlin M. E., Reynolds W. W. (1979). Diel activity patterns of the smooth dogfish shark, Mustelus canis. Bull. Mar. Sci. 29 (3), 440–442.
Chellappa S. L., Vujovic N., Williams J. S., Scheer F. A. J. L. (2019). Impact of circadian disruption on cardiovascular function and disease. Trends Endocrinol. Metab. 30 (10), 767–779. doi: 10.1016/J.TEM.2019.07.008
Chen C. C., Fernald R. D. (2008). GnRH and GnRH receptors: distribution, function and evolution. J. Fish Biol. 73 (5), 1099–1120. doi: 10.1111/J.1095-8649.2008.01936.X
Cisneros-Montemayor A. M., Barnes-Mauthe M., Al-Abdulrazzak D., Navarro-Holm E., Sumaila U. R. (2013). Global economic value of shark ecotourism: implications for conservation. Oryx 47 (3), 381–388. doi: 10.1017/S0030605312001718
Coffey D. M., Royer M. A., Meyer C. G., Holland K. N. (2020). Diel patterns in swimming behavior of a vertically migrating deepwater shark, the bluntnose sixgill (Hexanchus griseus). PloS One 15 (1), e0228253. doi: 10.1371/JOURNAL.PONE.0228253
Cooney R., Dickson B. (2012). Biodiversity and the precautionary principle: risk, uncertainty and practice in conservation and sustainable use (New York: Routledge).
Couturier L. I., Rohner C. A., Richardson A. J., Marshall A. D., Jaine F. R., Bennett M. B., et al. (2013). Stable isotope and signature fatty acid analyses suggest reef manta rays feed on demersal zooplankton. PloS One 8 (10), e77152. doi: 10.1371/journal.pone.0077152
Cowan M., Azpeleta C., López-Olmeda J. F. (2017). Rhythms in the endocrine system of fish: a review. J. Comp. Physiol. B 187, 8. doi: 10.1007/S00360-017-1094-5
Cox K. H., Takahashi J. S. (2019). Circadian clock genes and the transcriptional architecture of the clock mechanism. J. Mol. Endocrinol. 63 (4), R93–R102. doi: 10.1530/JME-19-0153
Crow G. L., Ron B., Atkinson S., Rasmussen L. (1999). Serum T4 and serum T3 concentrations in immature captive whitetip reef sharks, triaenodon obesus. J. Exp. zoology 284, 500–504. doi: 10.1002/(SICI)1097-010X(19991001)284:5
Dauchy R. T., Wren M. A., Dauchy E. M., Hoffman A. E., Hanifin J. P., Warfield B., et al. (2015). The influence of red light exposure at night on circadian metabolism and physiology in Sprague–Dawley rats. J. Am. Assoc. Lab. Anim. Sci. 54 (1), 40–50.
Davies T. W., Duffy J. P., Bennie J., Gaston K. J. (2014). The nature, extent, and ecological implications of marine light pollution. Front. Ecol. Environ. 12 (6), 347–355. doi: 10.1890/130281
Davies W. I. L., Tay B. H., Zheng L., Danks J. A., Brenner S., Foster R. G., et al. (2012). Evolution and functional characterisation of melanopsins in a deep-sea chimaera (Elephant shark, callorhinchus milii). PloS One 7 (12), e51276. doi: 10.1371/JOURNAL.PONE.0051276
Dawson A., King V. M., Bentley G. E., Ball G. F. (2001). Photoperiodic control of seasonality in birds. J. Biol. Rhythms 16 (4), 365–380. doi: 10.1177/074873001129002079
Dekens M. P. S., Fontinha B. M., Gallach M., Pfl€ Ugler S., Tessmar-Raible K. (2022). Melanopsin elevates locomotor activity during the wake state of the diurnal zebrafish. EMBO Rep. 23 (5), e51528. doi: 10.15252/EMBR.202051528
Delroisse J., Duchatelet L., Flammang P., Mallefet J. (2018). De novo transcriptome analyses provide insights into opsin-based photoreception in the lanternshark Etmopterus spinax. PloS One 13 (12), e0209767. doi: 10.1371/JOURNAL.PONE.0209767
Demski L. S. (1990). Neural substrates for photic control of elasmobranch sexual development and behavior. J. Exp. Zool. 256 (5 S), 121–129. doi: 10.1002/JEZ.1402560517
Di Rosa V., Frigato E., López-Olmeda J. F., Sánchez-Vázquez F. J., Bertolucci C. (2015). The light wavelength affects the ontogeny of clock gene expression and activity rhythms in zebrafish larvae. PloS One 10 (7), e0132235. doi: 10.1371/journal.pone.0132235
Dudgeon C. L., Lanyon J. M., Semmens J. M. (2013). Seasonality and site fidelity of the zebra shark, Stegostoma fasciatum, in southeast Queensland, Australia. Anim. Behav. 85 (2), 471–481. doi: 10.1016/J.ANBEHAV.2012.12.013
Dulvy N. K., Fowler S. L., Musick J. A., Cavanagh R. D., Kyne P. M., Harrison L. R., et al. (2014). Extinction risk and conservation of the world’s sharks and rays. ELife 2014 (3), e00590. doi: 10.7554/eLife.00590.001
Dulvy N. K., Simpfendorfer C. A., Davidson L. N. K., Fordham S. V., Bräutigam A., Sant G., et al. (2017). Challenges and priorities in shark and ray conservation. Curr. Biol. 27 (11), R565–R572. doi: 10.1016/J.CUB.2017.04.038
Falcón J., Torriglia A., Attia D., Viénot F., Gronfier C., Behar-Cohen F., et al. (2020). Exposure to artificial light at night and the consequences for flora, fauna, and ecosystems. Front. Neurosci. 14. doi: 10.3389/FNINS.2020.602796
Fatima N., Sonkar G. K., Singh S. (2022). Circadian mechanism disruption is associated with dysregulation of inflammatory and immune responses: a systematic review. Beni-Suef University. J. Basic Appl. Sci. 11 (1), 1–12. doi: 10.1186/S43088-022-00290-4
Ferretti F., Worm B., Britten G. L., Heithaus M. R., Lotze H. K. (2010). Patterns and ecosystem consequences of shark declines in the ocean. Ecol. Lett. 13 (8), 1055–1071. doi: 10.1111/J.1461-0248.2010.01489.X
Finstad W. O., Nelson D. R. (1975). Circadian activity rhythm in the horn shark, heterodontus francisci: effect of light intensity. Bull. South. California Acad. Sci. 74 (1), 20–26. doi: 10.3160/0038-3872-74.1.20
Fishbein A. B., Knutson K. L., Zee P. C. (2021). Circadian disruption and human health. J. Clin. Invest. 131 (19), e148286. doi: 10.1172/JCI148286
Fisher E. C., Jones J. S., von Schomberg R. (2006). Implementing the precautionary principle: perspectives and prospects. (Great Britain: Edward Elgar Publishing Limited).
Fitzpatrick R., Abrantes K. G., Seymour J., Barnett A. (2011). Variation in depth of whitetip reef sharks: does provisioning ecotourism change their behaviour? Coral Reefs 30, 569–577. doi: 10.1007/s00338-011-0769-8
Fobert E. K., Da Silva K. B., Swearer S. E. (2019). Artificial light at night causes reproductive failure in clownfish. Biol. Lett. 15 (7), 20190272. doi: 10.1098/RSBL.2019.0272
Frøland Steindal I. A., Whitmore D. (2019). Circadian clocks in fish—what have we learned so far? Biology 8 (1), 17. doi: 10.3390/biology8010017
Gallagher A. J., Hammerschlag N. (2011). Global shark currency: the distribution, frequency, and economic value of shark ecotourism. Curr. Issues Tourism 14 (8), 797–812. doi: 10.1080/13683500.2011.585227
Gallant J. J., Rodríguez M. A., Stokesbury M. J. W., Harvey-Clark C. (2016). Influence of environmental variables on the diel movements of the Greenland shark (Somniosus microcephalus) in the st. Lawrence estuary. Can. Field-Naturalist 130 (1), 1–14. doi: 10.22621/CFN.V130I1.1784
García V. B., Lucifora L. O., Myers R. A. (2008). The importance of habitat and life history to extinction risk in sharks, skates, rays and chimaeras. Proc. R. Soc. B: Biol. Sci. 275 (1630), 83–89. doi: 10.1098/rspb.2007.1295
Gaston K. J., Davies T. W., Nedelec S. L., Holt L. A. (2017). Impacts of artificial light at night on biological timings. Annu. Rev. Ecology Evolution Systemat. 48 (1), 49–68. doi: 10.1146/annurev-ecolsys-110316-022745
Gayford J. H., Pearse W. D., de la Parra Venegas R., Whitehead D. A. (2023). Quantifying the behavioural consequences of shark ecotourism. Sci. Rep. 13 (1), 12938. doi: 10.1038/s41598-023-39560-1
Glaus K. B. J., Adrian-Kalchhauser I., Piovano S., Appleyard S. A., Brunnschweiler J. M., Rico C. (2019). Fishing for profit or food? Socio-economic drivers and fishers’ attitudes towards sharks in Fiji. Mar. Policy 100, 249–257. doi: 10.1016/J.MARPOL.2018.11.037
Gleiss A. C., Morgan D. L., Whitty J. M., Keleher J. J., Fossette S., Hays G. C. (2017). Are vertical migrations driven by circadian behaviour? Decoupling of activity and depth use in a large riverine elasmobranch, the freshwater sawfish (Pristis pristis). Hydrobiologia 787 (1), 181–191. doi: 10.1007/S10750-016-2957-6/TABLES/3
Gleiss A. C., Wright S., Liebsch N., Wilson R. P., Norman B. (2013). Contrasting diel patterns in vertical movement and locomotor activity of whale sharks at Ningaloo Reef. Mar. Biol. 160 (11), 2981–2992. doi: 10.1007/S00227-013-2288-3-3/FIGURES/6
Golombek D. A., Rosenstein R. E. (2010). Physiology of circadian entrainment. Physiol. Rev. 90 (3), 1063–1102. doi: 10.1152/physrev.00009.2009
Gorbman A., Sower S. A. (2003). Evolution of the role of GnRH in animal (Metazoan) biology. Gen. Comp. Endocrinol. 134 (3), 207–213. doi: 10.1016/J.YGCEN.2003.09.018
Gordon I. (1993). Pre-copulatory behaviour of captive sandtiger sharks, Carcharias taurus. Environ. Biol. Fishes 38 (1–3), 159–164. doi: 10.1007/BF00842912/METRICS
Graham R. T., Roberts C. M., Smart J. C. R. (2005). Diving behaviour of whale sharks in relation to a predictable food pulse. J. R. Soc. Interface 3 (6), 109–116. doi: 10.1098/RSIF.2005.0082
Grubbs R. D., Musick J. A., Conrath C. L., Romine J. G. (2007). Long-term movements, migration, and temporal delineation of a summer nursery for juvenile sandbar sharks in the chesapeake bay region. Am. Fisheries Soc. Symposium 50, 87–107.
Guh Y. J., Tamai T. K., Yoshimura T. (2019). The underlying mechanisms of vertebrate seasonal reproduction. Proc. Japan Academy Ser. B 95 (7), 343–357. doi: 10.2183/PJAB.95.025
Guyomarc’h C., Lumineau S., Richard J. P. (1998). Circadian rhythm of activity in Japanese quail in constant darkness: variability of clarity and possibility of selection. Chronobiol. Int. 15 (3), 219–230. doi: 10.3109/07420529808998685
Häfker N. S., Tessmar-Raible K. (2020). Rhythms of behavior: are the times changin’? Curr. Opin. Neurobiol. 60, 55–66. doi: 10.1016/J.CONB.2019.10.005
Hamasaki D. I., Streck P. (1971). Properties of the epiphysis cerebri of the small-spotted dogfish shark, Scyliorhinus caniculus L. Vision Res. 11 (3), 189–IN3. doi: 10.1016/0042-6989(71)90184-2
Hammerschlag N., Skubel R., Calich H., Nelson E., Shiffman D., Wester J., et al. (2017). Nocturnal and crepuscular behavior in elasmobranchs: a review of movement, habitat use, foraging, and reproduction in the dark. Bull. Mar. Sci. 93 (2), 355–374. doi: 10.5343/bms.2016.1046
Harvey-Carroll J., Stewart J. D., Carroll D., Mohamed B., Shameel I., Zareer I. H., et al. (2021). The impact of injury on apparent survival of whale sharks (Rhincodon typus) in South Ari Atoll Marine Protected Area, Maldives. Sci. Rep. 11 (1), 937. doi: 10.1038/s41598-020-79101-8
Hazlerigg D. G., Wagner G. C. (2006). Seasonal photoperiodism in vertebrates: from coincidence to amplitude. Trends Endocrinol. Metab. 17 (3), 83–91. doi: 10.1016/J.TEM.2006.02.004
Heupel M. R. (2007). Exiting terra ceia bay: an examination of cues stimulating migration from a summer nursery area. Am. Fisheries Soc. Symposium 50, 265–280.
Hillyer K. E., Beale D. J., Shima J. S. (2021). Artificial light at night interacts with predatory threat to alter reef fish metabolite profiles. Sci. Total Environ. 769, 144482. doi: 10.1016/J.SCITOTENV.2020.144482
Hou Y., Liu L., Chen X., Li Q., Li J. (2020). Association between circadian disruption and diseases: A narrative review. Life Sci. 262, 118512. doi: 10.1016/J.LFS.2020.118512
Hut R. A., Beersma D. G. M. (2011). Evolution of time-keeping mechanisms: early emergence and adaptation to photoperiod. Philos. Trans. R. Soc. B: Biol. Sci. 366 (1574), 2141–2154. doi: 10.1098/RSTB.2010.0409
Huveneers C., Meekan M. G., Apps K., Ferreira L. C., Pannell D., Vianna G. M. S. (2017). The economic value of shark-diving tourism in Australia. Rev. Fish Biol. Fisheries 27 (3), 665–680. doi: 10.1007/S11160-017-9486
Jauharee A. R. (2014). Livebait fishing- night time (Maldives: Maldives Marine Research Centre, Ministry of Fisheries, Marine Resources and Agriculture).
Kadar J., Ladds M., Mourier J., Day J., Brown C. (2019). Acoustic accelerometry reveals diel activity patterns in premigratory Port Jackson sharks. Ecol. Evol. 9 (16), 8933–8944. doi: 10.1002/ECE3.5323
Kajiura S. M., Tellman S. L. (2016). Quantification of massive seasonal aggregations of blacktip sharks (Carcharhinus limbatus) in southeast florida. PloS One 11 (3), e0150911. doi: 10.1371/JOURNAL.PONE.0150911
Katherine Tamai T., Vardhanabhuti V., Arthur S., Foulkes N. S., Whitmore D. (2003). Flies and fish: birds of a feather. J. Neuroendocrinol. 15 (4), 344–349. doi: 10.1046/j.1365-2826.2003.00974.x
Kelly M. L., Murray E. R. P., Kerr C. C., Radford C. A., Collin S. P., Lesku J. A., et al. (2020). Diverse activity rhythms in sharks (Elasmobranchii). J. Biol. Rhythms 35 (5), 476–488. doi: 10.1177/0748730420932066
Kessel S. T., Chapman D. D., Franks B. R., Gedamke T., Gruber S. H., Newman J. M., et al. (2014). Predictable temperature-regulated residency, movement and migration in a large, highly mobile marine predator (Negaprion brevirostris). Mar. Ecol. Prog. Ser. 514, 175–190. doi: 10.3354/MEPS10966
Kneebone J., Chisholm J., Skomal G. B. (2012). Seasonal residency, habitat use, and site fidelity of juvenile sand tiger sharks Carcharias taurus in a Massachusetts estuary. Mar. Ecol. Prog. Ser. 471, 165–181. doi: 10.3354/meps09989
Kneebone J., Winton M., Danylchuk A., Chisholm J., Skomal G. B. (2018). An assessment of juvenile sand tiger (Carcharias taurus) activity patterns in a seasonal nursery using accelerometer transmitters. Environ. Biol. Fishes 101 (12), 1739–1756. doi: 10.1007/S10641-018-0821-4/FIGURES/8
Kumar V., Sharma A. (2018). Common features of circadian timekeeping in diverse organisms. Curr. Opin. Physiol. 5, 58–67. doi: 10.1016/J.COPHYS.2018.07.004
Kyba C. C. M., Kuester T., De Miguel A. S., Baugh K., Jechow A., Hölker F., et al. (2017). Artificially lit surface of Earth at night increasing in radiance and extent. Sci. Adv. 3 (11), e1701528. doi: 10.1126/SCIADV.1701528/SUPPL_FILE/1701528_SM.PDF
Lane J. M., Qian J., Mignot E., Redline S., Scheer F. A. J. L., Saxena R. (2022). Genetics of circadian rhythms and sleep in human health and disease. Nat. Rev. Genet. 24 (1), 4–20. doi: 10.1038/s41576-022-00519-z
Lassauce H., Chateau O., Erdmann M. V., Wantiez L. (2020). Diving behavior of the reef manta ray (Mobula alfredi) in New Caledonia: More frequent and deeper night-time diving to 672 meters. PloS One 15 (3), e0228815. doi: 10.1371/journal.pone.0228815
Lavender E., Aleynik D., Dodd J., Illian J., James M., Wright P. J., et al. (2021). Environmental cycles and individual variation in the vertical movements of a benthic elasmobranch. Mar. Biol. 168 (11), 1–18. doi: 10.1007/S00227-021-03973-1/METRICS
Lear K. O., Whitney N. M., Morris J. J., Gleiss A. C. (2021). Temporal niche partitioning as a novel mechanism promoting co-existence of sympatric predators in marine systems. Proc. R. Soc. B 288 (1954), 20210816. doi: 10.1098/RSPB.2021.0816
Legare B., Skomal G., DeAngelis B. (2018). Diel movements of the blacktip shark (Carcharhinus limbatus) in a Caribbean nursery. Environ. Biol. Fishes 101, 1011–1023. doi: 10.1007/s10641-018-0755-x
Leloup J. C., Goldbeter A. (2001). A molecular explanation for the long-term suppression of circadian rhythms by a single light pulse. Am. J. Physiol. - Regul. Integr. Comp. Physiol. 280 (4 49-4), 1206–1212. doi: 10.1152/AJPREGU.2001.280.4.R1206/ASSET/IMAGES/LARGE/H60410490007.JPEG
Levin N., Kyba C. C., Zhang Q., de Miguel A. S., Román M. O., Li X., et al. (2020). Remote sensing of night lights: A review and an outlook for the future. Remote Sens. Environ. 237, 111443. doi: 10.1016/J.RSE.2019.111443
Liddle T. A., Stevenson T. J., Majumdar G. (2022). Photoperiodic regulation of avian physiology: From external coincidence to seasonal reproduction. J. Exp. Zool. Part A: Ecol. Integr. Physiol. 337 (9–10), 890–901. doi: 10.1002/JEZ.2604
Lincoln G. (2019). A brief history of circannual time. J. Neuroendocrinol. 31 (3), e12694. doi: 10.1111/JNE.12694
Liu J. A., Meléndez-Fernández O. H., Bumgarner J. R., Nelson R. J. (2022). Effects of light pollution on photoperiod-driven seasonality. Hormones Behav. 141, 105150. doi: 10.1016/J.YHBEH.2022.105150
Longcore T., Rich C. (2004). Ecological light pollution. Front. Ecol. Environ. 2 (4), 191–198. doi: 10.1890/1540-9295
Lucifora L. O., García V. B., Worm B. (2011). Global diversity hotspots and conservation priorities for sharks. PloS One 6 (5), e19356. doi: 10.1371/JOURNAL.PONE.0019356
Mandado M., Molist P., Anadón R., Yáñez J. (2001). A DiI-tracing study of the neural connections of the pineal organ in two elasmobranchs (Scyliorhinus canicula and Raja montagui) suggests a pineal projection to the midbrain GnRH-immunoreactive nucleus. Cell Tissue Res. 303 (3), 391–401. doi: 10.1007/S004410000328/METRICS
Marangoni L. F. B., Davies T., Smyth T., Rodríguez A., Hamann M., Duarte C., et al. (2022). Impacts of artificial light at night in marine ecosystems—A review. Global Change Biol. 28 (18), 5346–5367. doi: 10.1111/GCB.16264
Maury E. (2019). Off the clock: from circadian disruption to metabolic disease. Int. J. Mol. Sci. 20 (7), 1597. doi: 10.3390/IJMS20071597
Meese E. N., Lowe C. G. (2020). Active acoustic telemetry tracking and tri-axial accelerometers reveal fine-scale movement strategies of a non-obligate ram ventilator. Movement Ecol. 8 (1), 1–17. doi: 10.1186/S40462-020-0191-3/TABLES/3
Mieras P. A., Harvey-Clark C., Bear M., Hodgin G., Hodgin B. (2017). The economy of shark conservation in the northeast pacific: the role of ecotourism and citizen science. Adv. Mar. Biol. 78, 121–153. doi: 10.1016/BS.AMB.2017.08.003
Mishra I., Kumar V. (2017). Circadian basis of seasonal timing in higher vertebrates. Biol. Rhythm. Res. 48 (5), 723–738. doi: 10.1080/09291016.2017.1345447
Mull C. G., Lowe C. G., Young K. A. (2008). Photoperiod and water temperature regulation of seasonal reproduction in male round stingrays (Urobatis halleri). Comp. Biochem. Physiol. Part A: Mol. Integr. Physiol. 151 (4), 717–725. doi: 10.1016/J.CBPA.2008.08.029
Mull C. G., Lowe C. G., Young K. A. (2010). Seasonal reproduction of female round stingrays (Urobatis halleri): Steroid hormone profiles and assessing reproductive state. Gen. Comp. Endocrinol. 166 (2), 379–387. doi: 10.1016/J.YGCEN.2009.12.009
Myers R. A., Baum J. K., Shepherd T. D., Powers S. P., Peterson C. H. (2007). Cascading effects of the loss of apex predatory sharks from a coastal ocean. Science 315 (5820), 1846–1850. doi: 10.1126/SCIENCE.1138657/SUPPL_FILE/MYERS.SOM.PDF
Nelson D. R., Johnson R. H. (1970). Diel Activity Rhythms in the Nocturnal, Bottom-Dwelling Sharks, Heterodontus francisci and Cephaloscyllium ventriosum. Copeia 1970 (4), 732. doi: 10.2307/1442315
Nelson D. R., McKibben J. N., Strong W. R., Lowe C. G., Sisneros J. A., Schroeder D. M., et al. (1997). An acoustic tracking of a megamouth shark, Megachasma pelagios: A crepuscular vertical migrator. Environ. Biol. Fishes 49 (4), 389–399. doi: 10.1023/A:1007369619576/METRICS
Niella Y., Smoothey A. F., Taylor M. D., Peddemors V. M., Harcourt R. (2021a). Environmental drivers of fine-scale predator and prey spatial dynamics in sydney harbour, Australia, and adjacent coastal waters. Estuaries Coasts 45, 1465–1479. doi: 10.1007/s12237-021-01020-2
Niella Y., Wiefels A., Almeida U., Jaquemet S., Lagabrielle E., Harcourt R., et al. (2021b). Dynamics of marine predators off an oceanic island and implications for management of a preventative shark fishing program. Mar. Biol. 168 (4), 1–18. doi: 10.1007/S00227-021-03852-9/FIGURES/10
Nishiwaki-Ohkawa T., Yoshimura T. (2016). Molecular basis for regulating seasonal reproduction in vertebrates. J. Endocrinol. 229 (3), R117–R127. doi: 10.1530/JOE-16-0066
Nixon A. J., Gruber S. H. (1988). Diel metabolic and activity patterns of the lemon shark (Negaprion brevirostris). J. Exp. Zool. 248 (1), 1–6. doi: 10.1002/JEZ.1402480102
Nosal A. P., Caillat A., Kisfaludy E. K., Royer M. A., Wegner N. C. (2014). Aggregation behavior and seasonal philopatry in male and female leopard sharks Triakis semifasciata along the open coast of southern California, USA. Mar. Ecol. Prog. Ser. 499, 157–175. doi: 10.3354/MEPS10632
O’Malley M. P., Lee-Brooks K., Medd H. B. (2023). The global economic impact of manta ray watching tourism. PloS One 8 (5), e65051. doi: 10.1371/journal.pone.0065051
Park M. S., Shin H. S., Kim N. N., Lee J., Kil G. S., Choi C. Y. (2013). Effects of LED spectral sensitivity on circadian rhythm-related genes in the yellowtail clownfish, Amphiprion clarkii. Anim. Cells Syst. 17 (2), 99–105. doi: 10.1080/19768354.2013.779935
Passoni M. (2021) Diving with Whale Sharks - Everything You Should Know, blog.padi.com. Available at: https://blog.padi.com/diving-with-whale-sharks-everything-you-should-know/ (Accessed 11 September 2023).
Passoni M., Saponari S. (2019) Manta Ray Diving - The Top 12 Best Places on Earth, blog.padi.com. Available at: https://blog.padi.com/best-places-to-dive-with-manta-rays/ (Accessed 11 September 2023).
Pérez J. H. (2022). Light receptors in the avian brain and seasonal reproduction. J. Exp. Zool. Part A: Ecol. Integr. Physiol. 337 (9–10), 985–993. doi: 10.1002/JEZ.2652
Pérez J. H., Tolla E., Dunn I. C., Meddle S. L., Stevenson T. J. (2019). A comparative perspective on extra-retinal photoreception. Trends Endocrinol. Metab. 30 (1), 39–53. doi: 10.1016/J.TEM.2018.10.005
Rahman S. A., Kollara A., Brown T. J., Casper R. F. (2008). Selectively filtering short wavelengths attenuates the disruptive effects of nocturnal light on endocrine and molecular circadian phase markers in rats. Endocrinology 149 (12), 6125–6135. doi: 10.1210/en.2007-1742
Rijo-Ferreira F., Takahashi J. S. (2019). Genomics of circadian rhythms in health and disease. Genome Med. 11, 1. doi: 10.1186/S13073-019-0704-0
Robinson D. P., Jaidah M. Y., Bach S. S., Rohner C. A., Jabado R. W., Ormond R., et al. (2017). Some like it hot: Repeat migration and residency of whale sharks within an extreme natural environment. PloS One 12 (9), e0185360. doi: 10.1371/journal.pone.0185360
Roch G. J., Busby E. R., Sherwood N. M. (2011). Evolution of gnRH: diving deeper. Gen. Comp. Endocrinol. 171 (1), 1–16. doi: 10.1016/J.YGCEN.2010.12.014
Rodrigues L., dos S., Kinas P. G., Cardoso L. G. (2022). Optimal setting time and season increase the target and reduce the incidental catch in longline fisheries: a Bayesian beta mixed regression approach. ICES J. Mar. Sci. 79 (4), 1245–1258. doi: 10.1093/ICESJMS/FSAC049
Rohner C. A., Couturier L. I. E., Richardson A. J., Pierce S. J., Prebble C. E. M., Gibbons M. J., et al. (2013). Diet of whale sharks Rhincodon typus inferred from stomach content and signature fatty acid analyses. Mar. Ecol. Prog. Ser. 493, 219–235. doi: 10.3354/MEPS10500
Sánchez-Vázquez F. J., López-Olmeda J. F., Vera L. M., Migaud H., López-Patiño M. A., Míguez J. M. (2019). Environmental cycles, melatonin, and circadian control of stress response in fish. Front. Endocrinol. 10, 279. doi: 10.3389/fendo.2019.00279
Sanders D., Frago E., Kehoe R., Patterson C., Gaston K. J. (2020). A meta-analysis of biological impacts of artificial light at night. Nat. Ecol. Evol. 5 (1), 74–81. doi: 10.1038/s41559-020-01322-x
Schligler J., Cortese D., Beldade R., Swearer S. E., Mills S. C. (2021). Long-term exposure to artificial light at night in the wild decreases survival and growth of a coral reef fish. Proc. R. Soc. B 288 (1952), 20210454. doi: 10.1098/RSPB.2021.0454
Sciarrotta T. C., Nelson D. R. (1977). Diel behavior of the blue shark, Prionace glauca, near Santa Catalina Island, California. Fisheries Bull. 75:3, 519–528.
Shaahunaz F. (2017) Fear soars as whale sharks go off radar in South Ari atoll (The Edition Maldives). Available at: https://edition.mv/whale_shark/2495 (Accessed 01 May 2023).
Shibata S., Hirao A., Tahara Y. (2010). Restricted feeding-induced entrainment of activity rhythm and peripheral clock rhythm. Sleep Biol. Rhythms 8 (1), 18–27. doi: 10.1111/J.1479-8425.2009.00417.X
Spaet J. L. Y., Butcher P. A., Manica A., Lam C. H. (2022). Spatial dynamics and fine-scale vertical behaviour of immature eastern australasian white sharks (Carcharodon carcharias). Biology 11 (12), 1689. doi: 10.3390/BIOLOGY11121689/S1
Steindal I. A. F., Whitmore D. (2020). Zebrafish circadian clock entrainment and the importance of broad spectral light sensitivity. Front. Physiol. 11. doi: 10.3389/FPHYS.2020.01002/BIBTEX
Sueiro M. C., Awruch C. A., Irigoyen A. J., Argemi F., Palacios M. G. (2019). Seasonality of immunological and health-state parameters of wild broadnose sevengill shark, Notorynchus cepedianus. Physiol. Biochem. Zool. 92 (1), 24–36. doi: 10.1086/700573/ASSET/IMAGES/LARGE/FG2.JPEG
Sumpter J. P., Dodd J. M. (1979). The annual reproductive cycle of the female lesser spotted dogfish, Scyliorhinus canicula L., and its endocrine control. J. Fish Biol. 15 (6), 687–695. doi: 10.1111/J.1095-8649.1979.TB03678.X
Tosini G., Bertolucci C., Foà A. (2001). The circadian system of reptiles: a multioscillatory and multiphotoreceptive system. Physiol. Behav. 72 (4), 461–471. doi: 10.1016/S0031-9384(00)00423-6
Trzeciak J. R., Steele A. D. (2022). Studying food entrainment: Models, methods, and musings. Front. Nutr. 9. doi: 10.3389/FNUT.2022.998331
Tyminski J. P., de la Parra-Venegas R., Cano J. G., Hueter R. E. (2015). Vertical movements and patterns in diving behavior of whale sharks as revealed by pop-up satellite tags in the eastern gulf of Mexico. PloS One 10 (11), e0142156. doi: 10.1371/JOURNAL.PONE.0142156
Valls E., Navarro J., Barría C., Coll M., Fernández-Borràs J., Rotllant G. (2016). Seasonal, ontogenetic and sexual changes in lipid metabolism of the small-spotted catshark (Scyliorhinus canicula) in deep-sea free-living conditions. J. Exp. Mar. Biol. Ecol. 483, 59–63. doi: 10.1016/J.JEMBE.2016.07.001
Vaudo J. J., Lowe C. G. (2006). Movement patterns of the round stingray Urobatis halleri(Cooper) near a thermal outfall. J. Fish Biol. 68 (6), 1756–1766. doi: 10.1111/J.0022-1112.2006.01054.X
Vedor M., Mucientes G., Hernández-Chan S., Rosa R., Humphries N., Sims D. W., et al. (2021). Oceanic diel vertical movement patterns of blue sharks vary with water temperature and productivity to change vulnerability to fishing. Front. Mar. Sci. 8. doi: 10.3389/FMARS.2021.688076/BIBTEX
Vetter C. (2018). Circadian disruption: What do we actually mean? Eur. J. Neurosci. 51 (1), 531–550. doi: 10.1111/EJN.14255
Vitaterna M. H., Takahashi J. S., Turek F. W. (2001). Overview of circadian rhythms. Alcohol Res. Health 25 (2), 85.
Waltrick D., Jones S. M., Simpfendorfer C. A., Awruch C. A. (2014). Endocrine control of embryonic diapause in the Australian sharpnose shark rhizoprionodon taylori. PloS One 9 (7), e101234. doi: 10.1371/JOURNAL.PONE.0101234
Wheeler C. R., Kneebone J., Heinrich D., Strugnell J. M., Mandelman J. W., Rummer J. L. (2022). Diel rhythm and thermal independence of metabolic rate in a benthic shark. J. Biol. Rhythms 37 (5), 484–497. doi: 10.1177/07487304221107843
Whitney N. M., Papastamatiou Y. P., Holland K. N., Lowe C. G. (2007). Use of an acceleration data logger to measure diel activity patterns in captive whitetip reef sharks, Triaenodon obesus. Aquat. Living Resour. 20 (4), 299–305. doi: 10.1051/ALR:2008006
Wilson H. B., Joseph L. N., Moore A. L., Possingham H. P. (2011). When should we save the most endangered species? Ecol. Lett. 14, 886–890. doi: 10.1111/j.1461-0248.2011.01652.x
Wilson S. G., Polovina J. J., Stewart B. S., Meekan M. G. (2006). Movements of whale sharks (Rhincodon typus) tagged at Ningaloo Reef, Western Australia. Mar. Biol. 148 (5), 1157–1166. doi: 10.1007/s00227-005-0153-8
Wyffels J. T., George R., Adams L., Adams C., Clauss T., Newton A., et al. (2020). Testosterone and semen seasonality for the sand tiger shark Carcharias taurus. Biol. Reprod. 102 (4), 876–887. doi: 10.1093/BIOLRE/IOZ221
Yan L., Smale L., Nunez A. A. (2020). Circadian and photic modulation of daily rhythms in diurnal mammals. Eur. J. Neurosci. 51 (1), 551–566. doi: 10.1111/EJN.14172
Keywords: elasmobranch, shark, artificial light at night, ALAN, ecotourism, elasmobranch conservation, elasmobranch physiology, light pollution
Citation: Carroll D and Harvey-Carroll J (2023) The influence of light on elasmobranch behavior and physiology: a review. Front. Mar. Sci. 10:1225067. doi: 10.3389/fmars.2023.1225067
Received: 18 May 2023; Accepted: 21 September 2023;
Published: 09 October 2023.
Edited by:
Elizabeth Grace Tunka Bengil, University of Kyrenia, CyprusReviewed by:
Daniel M. Coffey, Texas A&M University Corpus Christi, United StatesCopyright © 2023 Carroll and Harvey-Carroll. This is an open-access article distributed under the terms of the Creative Commons Attribution License (CC BY). The use, distribution or reproduction in other forums is permitted, provided the original author(s) and the copyright owner(s) are credited and that the original publication in this journal is cited, in accordance with accepted academic practice. No use, distribution or reproduction is permitted which does not comply with these terms.
*Correspondence: Jessica Harvey-Carroll, amVzc2ljYS5jYXJyb2xsQGJpb2Vudi5ndS5zZQ==; Daire Carroll, ZGFpcmUuY2Fycm9sbEBiaW9lbnYuZ3Uuc2U=
†These authors have contributed equally to this work and share first authorship
Disclaimer: All claims expressed in this article are solely those of the authors and do not necessarily represent those of their affiliated organizations, or those of the publisher, the editors and the reviewers. Any product that may be evaluated in this article or claim that may be made by its manufacturer is not guaranteed or endorsed by the publisher.
Research integrity at Frontiers
Learn more about the work of our research integrity team to safeguard the quality of each article we publish.