- 1Center of Excellence for Science and Technology-Integration of Mediterranean Region (STIM), Faculty of Science, University of Split, Split, Croatia
- 2Department of Applied Science, Institute for Adriatic Crops and Karst Reclamation, Split, Croatia
- 3University Department of Marine Studies, University of Split, Split, Croatia
The cosmopolitan centric diatom Thalassiosira rotula produces compounds in its natural habitat that can inhibit copepod reproduction. Moreover, it has been reported to possess compounds with therapeutic effects beneficial for health care. In this experiment, the extraction yield, total phenolic content (TPC), antioxidant activity by 2,2-diphenyl-1-picrylhydrazyl (DPPH) radical scavenging ability, ferric reducing/antioxidant power (FRAP) and oxygen radical absorbance capacity (ORAC), and chemical profile by gas chromatography-mass spectrometry (GC-MS) analyses of T. rotula were investigated. Extractions were performed with 50% and 70% ethanol. A higher extraction yield [0.21 ± 0.01 g extract/g dry weight (DW) diatom] was observed for 70% ethanol. In addition, higher TPC (5.80 ± 0.32 mg gallic acid equivalents (GAE)/g DW diatom) and antioxidant activity [DPPH inhibition of 17.53% ± 0.56%, FRAP of 766.67 ± 34.69 µM Trolox equivalents (TE), and ORAC of 58.87 ± 2.03 µM TE] were observed for this extract. Myristic acid, palmitelaidic acid, palmitic acid, eicosapentaenoic acid, 24-methylenecholesterol, and docosapentaenoic acid were identified as dominant compounds in both extracts, while extraction in 70% ethanol yielded a higher content of fatty acids such as myristic acid, eicosapentaenoic acid, docosapentaenoic acid, and sterol 24-methylenecholesterol. Thus, it can be concluded that extraction of T. rotula with 70% ethanol improves antioxidant activity and provides a higher yield of compounds such as polyunsaturated fatty acids and sterols. Therefore, the species T. rotula could be considered a sustainable source of essential fatty acids and other bioactive compounds for further applications.
1 Introduction
The number of microalgae species is estimated to be about 300,000, making them a remarkable natural resource for investigations with possible use of the results in various biotechnological applications (Rumin et al., 2020; Duran et al., 2021). The use of marine microalgae to produce valuable compounds is recognized as a renewable and environmentally friendly production system. In general, microalgae are capable of producing nutraceuticals such as pigments (carotenoids), polyunsaturated fatty acids (PUFAs), sterols, phenolic compounds, terpenes, and sulfated polysaccharides (Menaa et al., 2021; Silva et al., 2022). Microalgae and their metabolites have been studied for applications in agriculture, food, pharmaceutical, and cosmetic industries (Borowitzka, 2018; Michalak et al., 2020; Alvarez et al., 2021; Lafarga & Acién, 2022). In the marine environment, diatoms are a predominant group of microalgae responsible for over 40% of the total primary production of the oceans (Field et al., 1998).
With the improvement of microscopy, metabarcoding, analytical analysis, and genetic analysis, research related to the use of diatoms for industrial applications has expanded considerably (Sharma et al., 2021). One of the genera that has been studied for the production of bioactive compounds is Thalassiosira (Sabia et al., 2018; Bhattacharjya et al., 2020; Marella & Tiwari, 2020; Baldisserotto et al., 2021). Thalassiosira sp. are known as marine centric diatoms with lipid content greater than 50% (Bhattacharjya et al., 2020). Lipids are among the major components of the intracellular content of diatom cells and account for nearly 25% of the dry weight (DW) (Yi et al., 2017). Diatoms contain a wide range of fatty acids, from C14:0 to C22:6, and many strains are considered excellent sources of n-3 fatty acids. Eicosapentaenoic acid (EPA), docosahexaenoic acid (DHA), and docosapentaenoic acid (DPA) are important n-3 fatty acids found in microalgae (Li et al., 2019; Bárcenas-Pérez et al., 2021). The use of microalgal oil could reduce the pressure of intensive fishing on stocks and the risks associated with contamination with heavy metals, organic pollutants, and seasonal variations present in fish oil (Alves Martins et al., 2013; Remize et al., 2021). In contrast to the extraction of microalgae oil, the extraction and purification of fish oil (EPA and DHA) are difficult due to the removal of fishy odor and instability caused by the high content of PUFA (Li et al., 2019). However, consumption of these fatty acids provides health benefits in preventing and reducing the risk of various diseases such as coronary heart disease, diabetes, hypertension, inflammatory and autoimmune diseases, and neurodegenerative diseases such as Parkinson’s disease, Alzheimer’s disease, and depression (Calder, 2017). In addition to PUFA, diatoms are also known to be a good source of sterols, essential triterpenoids found in all eukaryotes (Jaramillo-Madrid et al., 2020). Sterols are compounds with numerous biological activities and classified as safe compounds for food use by the consensus panel of the European Atherosclerosis Society (Aldini et al., 2014; Ras et al., 2014; Wang & Seibert, 2017; Cutignano et al., 2022). Diatoms are capable of synthesizing animal, plant, and algal sterols (Gallo et al., 2020). One of the most abundant sterols in centric diatoms is 24-methylenecholesterol, an important intermediate in the biosynthesis of phytosterols (Rampen et al., 2010; Gallo et al., 2020; Frleta et al., 2022). Phytosterols have gained popularity in recent decades due to their health-promoting properties (Luo et al., 2015). They have shown antioxidant, anti-inflammatory, and antidiabetic activity, as well as cholesterol-lowering, hepatoprotective, and neuroprotective ability (Sañé et al., 2021; Jie et al., 2022). The extraction protocol for all mentioned compounds and the choice of solvent play a crucial role and directly affect the extract yield as well as the biological activity and chemical profile of the extracts. Hydroalcoholic mixtures are considered a good choice for extraction because they allow the extraction of compounds with a wide range of polarities (Jacotet-Navarro et al., 2018). In addition, the use of “green” solvents such as ethanol for extraction is known to be environmentally friendly, and such extracts are acceptable for use in the food industry. Currently, research efforts are focused on maximizing the extraction of the desired compounds using ultrasound, the less time-consuming, environmentally friendly, and convenient method. A previous study reported higher biological activity of extracts prepared with a hydroalcoholic mixture compared to extracts prepared with water or ethanol alone (Silva et al., 2022). In the literature, the highest yield of bioactive compounds for the hydroalcoholic mixtures is reported in the range of 50%–80% (Jacotet-Navarro et al., 2018; Monteiro et al., 2020; Čagalj et al., 2021). The aim of this study was to 1) compare the differences in extraction yield and antioxidant capacity of the extracts when different hydroalcoholic mixtures were used for the extractions, 2) identify and compare the dominant compounds in Thalassiosira rotula extracts with potential for biotechnological applications.
2 Materials and methods
2.1 Cultivation and harvesting
The diatom T. rotula (CIM861) shown in Figure 1 was isolated from fresh seawater sample from the northern Adriatic Sea by the Center for Marine Research of the Ruđer Bošković Institute (Rovinj, Croatia). A standardized purification protocol was used to establish a culture, and the strain was additionally confirmed by molecular identification. The diatom was cultured in six Erlenmeyer flasks in a volume of 5 L. Each flask contained 1.5 L of F/2 medium and was inoculated with 150 mL of the same inoculum (105 cells/mL). The F/2 culture medium was prepared according to the previously described recipe (Andersen, 2010). T. rotula was cultured at 17°C and exposed to a 16:8 light:dark cycle with LED light (Led GNC Minu Deep AM140, Sicce, Pozzoleone, Italy) of 2,500 lux intensity. Diatom biomass was harvested at the stationary phase by filtration with glass microfiber filters (Grade GF/F Whatman). Biomass collected on the filter was transferred to falcon tubes using a cell scraper. Prior to extraction, samples were freeze-dried (FreeZone 2.5, Labconco, Kansas City, MO, USA).
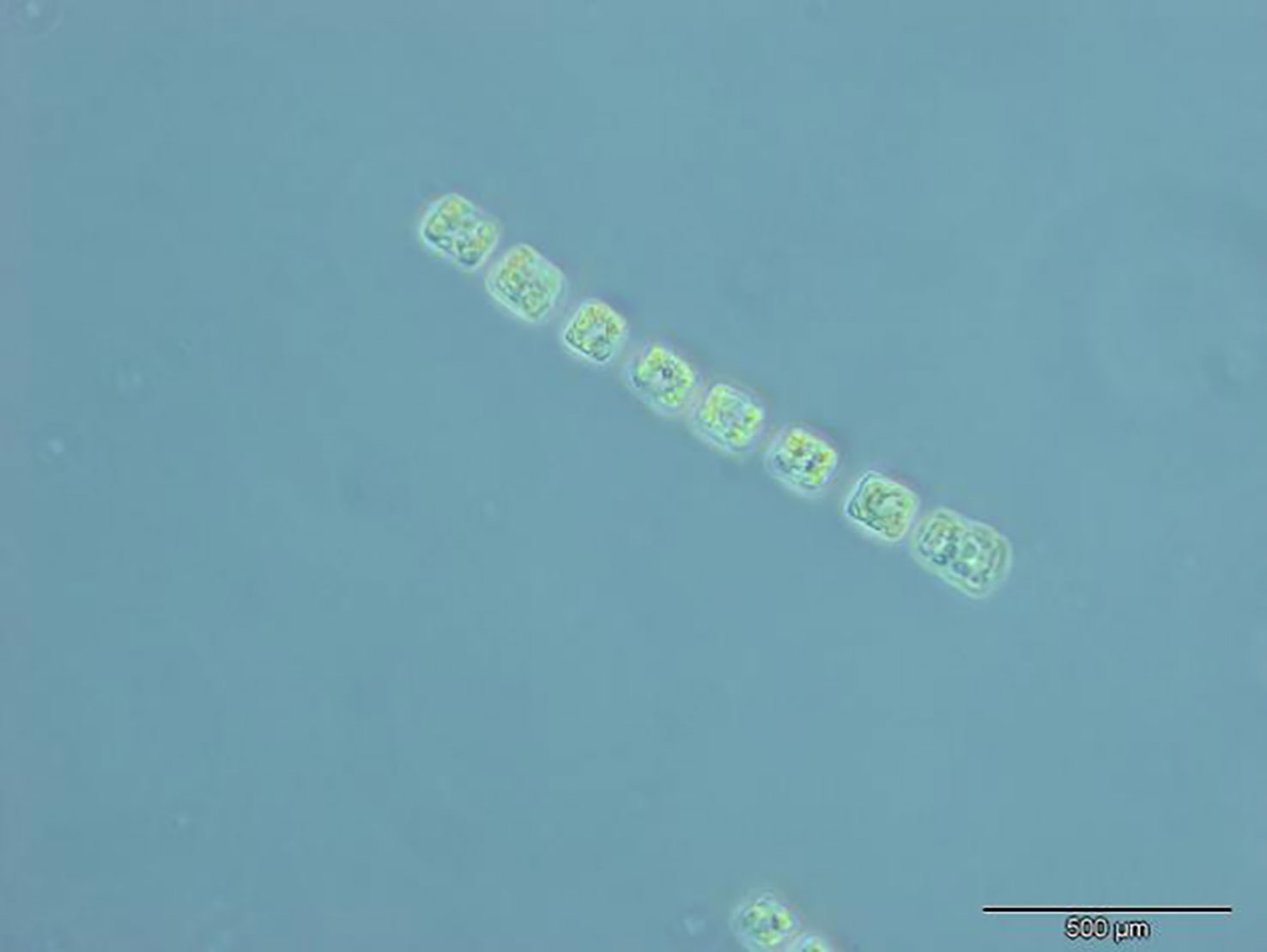
Figure 1 Diatom Thalassiosira rotula under the light microscope (BX51, Olympus, Japan) at 400× magnification.
2.2 Extraction of diatom biomass
Freeze-dried diatom biomass was extracted in 50% and 70% ethanol by ultrasound-assisted extraction (UAE) using an ultrasonic bath (Transsonic Tp 310H, Elma Schmidbauer GmbH, Singen, Germany) at a frequency of 40 kHz and 40°C for 1 h. After extraction, the samples were centrifuged (Centric 150, Tehtnica, Slovenia) for 10 min at room temperature and 2,515 × g. The supernatants obtained were filtered through a 0.45-µm mixed cellulose ester filter (LGG, Meckenheim, Germany) and concentrated using a centrifugal evaporator (RC10-22, Jouan, Herblain, France).
2.3 Determination of total phenolic content and antioxidation activity assays
The determination of total phenolic content (TPC) in diatom extracts was performed by the Folin–Ciocalteu method (Amerine and Ough, 1980). Briefly, 1.5 mL of distilled water and 25 µL of T. rotula extracts were mixed with 25 µL of Folin–Ciocalteu reagent. After adding the reagent, the mixtures were stirred and left for 1 min. Then, 475 µL of distilled water and 375 µL of 20% sodium carbonate solution were added. Samples were left for 2 h at room temperature in the dark, and absorbance was measured at 765 nm using a spectrophotometer (SPECORD 200 Plus, Edition 2010, Analytik Jena AG, Jena, Germany). Results were expressed as milligrams of gallic acid equivalents (GAE) per gram of dry weight (mg GAE/g DW diatom).
The antioxidant potential of T. rotula extracts was evaluated using ferric reducing/antioxidant power (FRAP), ability to scavenge 2,2-diphenyl-1-picrylhydrazyl (DPPH) radicals, and oxygen radical absorbance capacity (ORAC).
Samples were diluted 1:10 for the FRAP assay. FRAP was used to measure reducing activity (Benzie & Strain, 1996). Briefly, 300 µL of the FRAP reagent solution was added to the microplate wells, and absorbance was measured at 592 nm using a plate reader (Synergy HTX Multi-Mode Reader, BioTek Instruments, Inc., Winooski, VT, USA). The change in absorbance was recorded after 4 min following the addition of 10 µL of sample in FRAP reagent. The difference in absorbance between the FRAP reagent before addition of the sample and 4 min after addition was compared to a value determined for the Trolox standard solution.
The ability of diatom extracts to scavenge DPPH radicals was also evaluated in 96-well microplates (Čagalj et al., 2022). A volume of 290 µL of DPPH radical solution with an initial absorbance of 1.2 nm was pipetted into the microplate wells, and measurements were performed at 517 nm. One hour after addition of 10 µL of the T. rotula extracts to the wells, the decrease in absorbance was measured using a plate reader (Synergy HTX Multi-Mode Reader, BioTek Instruments, Inc., Winooski, VT, USA). The antioxidant activity of diatom extracts was expressed as the percentage of inhibition of DPPH radicals (% inhibition).
The ORAC technique was performed according to previously described procedures (Prior et al., 2003; Burčul et al., 2018). After a 1:10 dilution, 25 µL of the samples were added to wells of a microtiter plate that contained 150 µL of 4.2 mM fluorescein (3’,6’-dihydroxyspiro[isobenzofuran-1(3H),9’-[9H] xanthan]-3-one). The plates were thermostatted at 37°C for 30 min, and then 25 µL of 2,2'-Azobis (2-amidinopropane) dihydrochloride, AAPH was added. Measurements were performed at excitation and emission wavelengths of 485 and 520 nm every minute for 80 min. Results were expressed in µM Trolox equivalents (µM TE). All assays were performed in triplicate.
2.4 Chemical analyses
Compounds were identified according to Frleta et al. (2022). Briefly, 50 µL of derivatizing agent (NO-Bis (trimethylsilyl)trifluoroacetamide-BSFA) was added to dry T. rotula extracts. Gas chromatography (GC; Nexis GC-2030, Shimadzu, Kyoto, Japan) equipped with mass spectrometry (MS) detector (Shimadzu QP2020 NX) and split/splitless injection port was used to identify the compounds. Analyses were performed using ultrapure helium as the carrier gas (flow rate = 1 mL/min) and a fused silica capillary column (length 30 m × inside diameter 0.25 mm i.d., film thickness 0.25 m) (SH-5MS, Shimazu, Japan). The mass spectrometer was calibrated using perfluorotributylamine at an electron impact ionization energy of 70 eV. Analyses were performed using a full MS scan (35–750 m/z). The column temperature program included an equilibration time of 3 min, the initial temperature of 120°C was held for 3 min and then increased to 292°C at a rate of 5°C/min, followed by a final increase to 320°C at a rate of 30°C/min and isothermal holding for 17 min. The derivatized compounds were identified by comparing their mass spectra and retention times of trimethylsilyl (TMS) derivatives with commercial databases NIST 2020 and Wiley (Oberacher, 2012; NIST, 2022). All samples were injected and analyzed in duplicate.
2.5 Statistical analyses
Analyses of variance (one-way ANOVA, followed by Fisher’s least significant difference test) were used to express the statistical difference between samples in terms of extract yield, TPC, FRAP, DPPH, and ORAC assays. Analyses were performed using Statgraphics Centurion-Ver.16.1.11 (StatPoint Technologies, Inc., Warrenton, VA, USA).
3 Results and discussion
3.1 Extraction yield
The extraction was performed with hydroalcoholic mixtures to extract compounds with a wide range of polarities. Moreover, these solvents were chosen to obtain higher yields of bioactive compounds and to preserve their activity. The extract yields obtained from T. rotula are shown in Figure 2. Extraction with 70% ethanol resulted in a higher yield (0.21 ± 0.01 g extract/g DW diatom) compared to 50% ethanol (0.17 ± 0.01 g extract/g DW diatom).
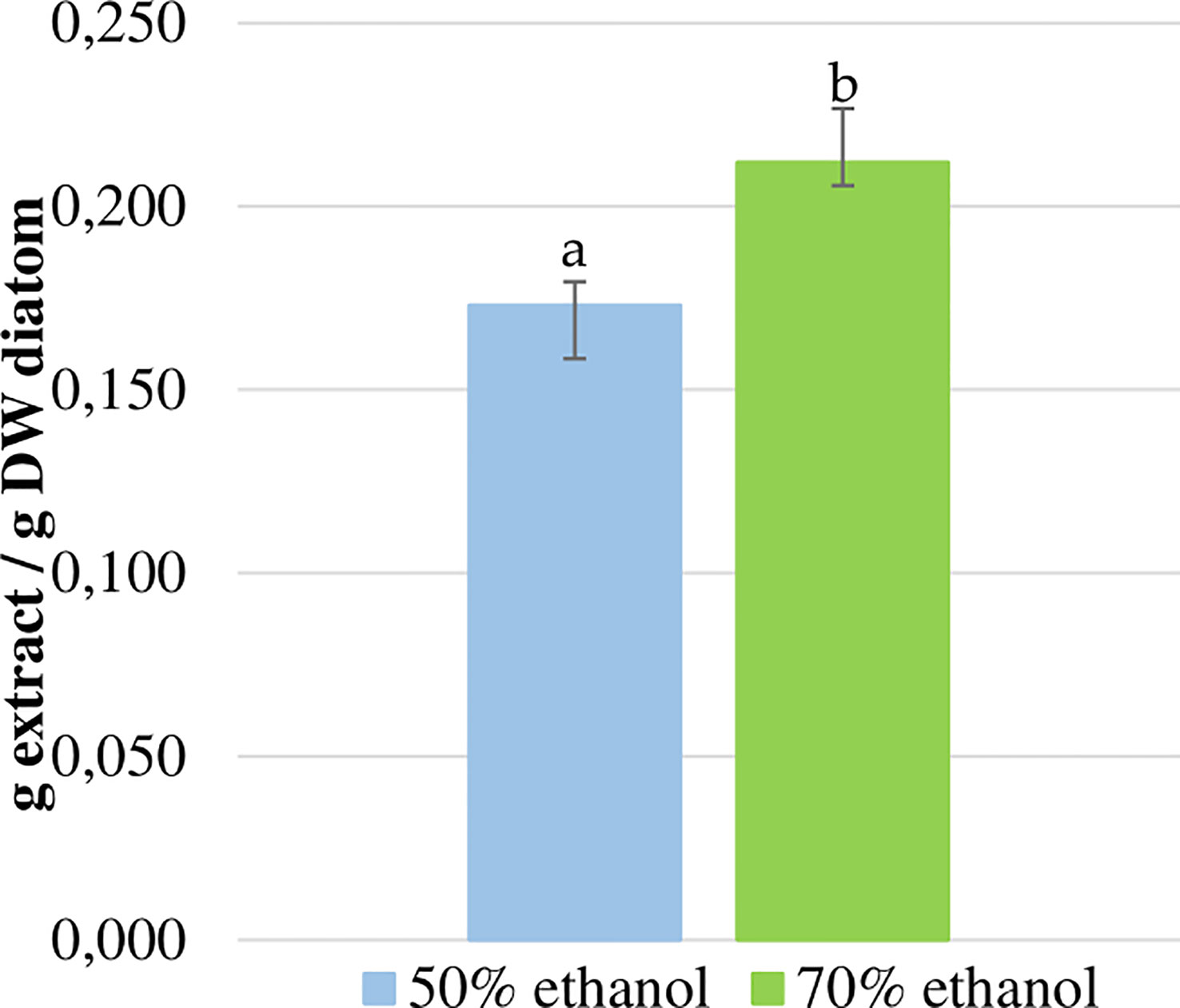
Figure 2 The average mass of extract obtained by extraction with 50% and 70% ethanol/g of dry weight of diatom Thalassiosira rotula. Different letters indicate statistically significant differences (p < 0.05).
One of the biggest challenges in reducing production costs is maximizing extraction yields from microalgal biomass (Svenning et al., 2020). In diatom extraction, the choice of solvent and extraction method plays a critical role in solubilizing compounds from diatom frustules (Suroy et al., 2014). Svenning et al. (2020) reported sonication as an effective method for the disruption of diatom cells, while the extraction yield of lipids was highly dependent on the polarity of the solvent. Mixtures of ethanol and water have a synergistic effect in which the water acts as a swelling agent while the ethanol breaks the bonds between the matrix and solutes, which is also evident in this study where a higher extraction yield is reported for the 70% ethanol extraction (Şahin & Şamlı, 2013). In the extraction of two microalgal species, Nannochloropsis gaditana and Chlorella sp., ethanol-water mixtures resulted in higher extraction yields compared to methanol-water mixtures (Monteiro et al., 2020). In addition, higher extraction yields were observed for both species in UAE with 80% ethanol compared to 50% ethanol.
3.2 GC-MS compound identification
The identified compounds in derivatized form in ethanolic extracts of the diatom T. rotula are listed in Table 1 with their retention index (RI), similarity, proportion, molecular weight, and mass spectra [relative intensity (m/z)]. The amount of compound in the sample was calculated based on the areas obtained and refers only to the proportion in the injected sample, since the method is not quantitative. All compounds were identified based on matched RI and similarity spectra ≥90% and confirmed according to previous studies (Zulu et al., 2018; Bhattacharjya et al., 2020; Gallo et al., 2020; Frleta et al., 2022).
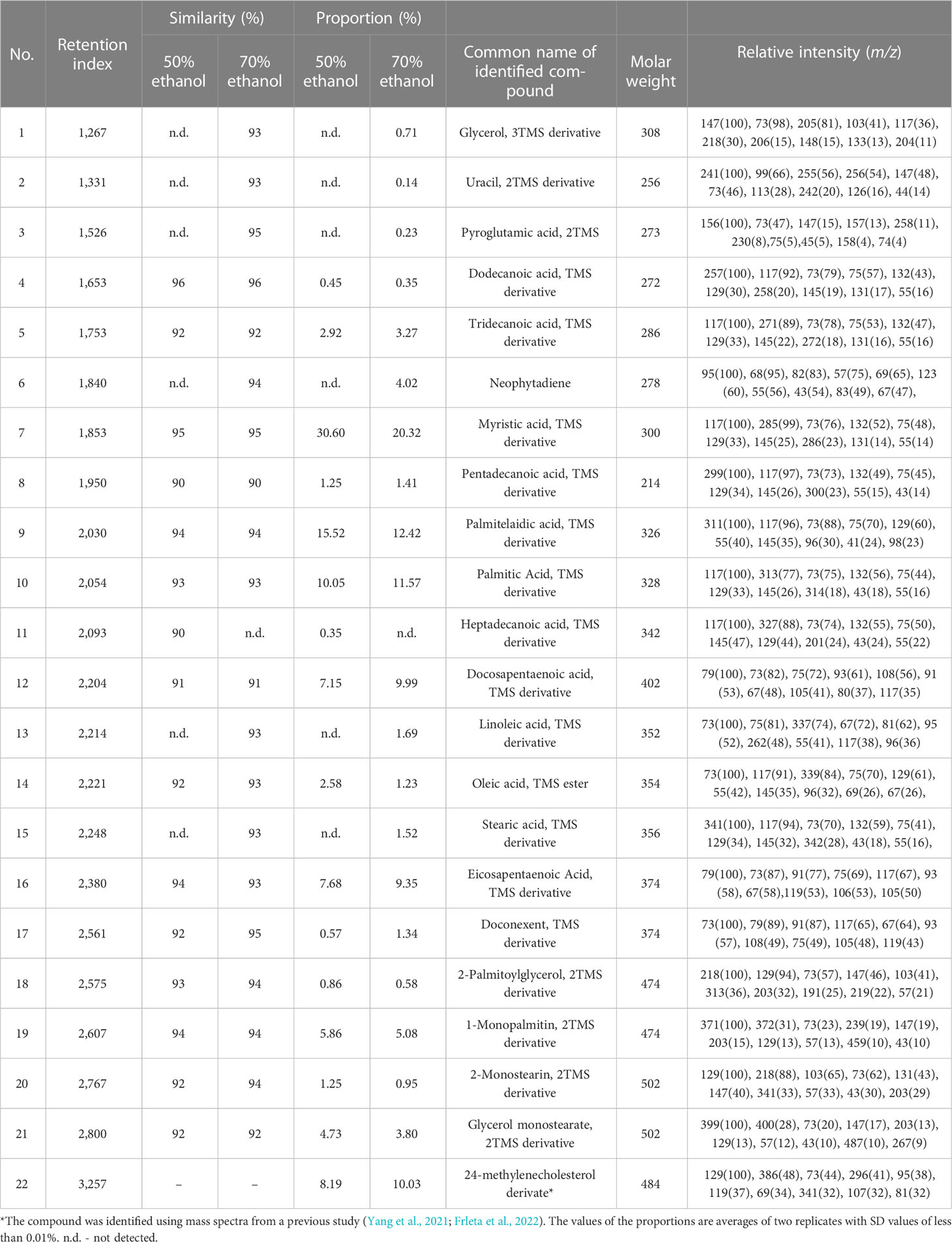
Table 1 List of compounds identified in diatom Thalassiosira rotula extracted by 50% and 70% ethanol.
A total of 16 compounds were identified by GC-MS in the 50% ethanolic extracts, while 19 compounds were identified in the 70% ethanolic extracts. In the 50% ethanolic extract, dominant compounds with a proportion greater than 10% were fatty acids, namely, myristic acid (30.6%), palmitelaidic acid (15.52%), and palmitic acid (10.05%). Compared to the 50% ethanolic extract, the content of myristic acid and palmitelaidic acid in the 70% ethanolic extract was lower by 34% and 8%, respectively, while the content of palmitic acid was higher by 15%. The predominant compounds were the same in both extracts, except for 24-methylenecholesterol, the proportion of which exceeded 10% only in the 70% ethanolic extract. Its amount was almost 20% higher in the 70% ethanolic extract. High levels of two PUFAs, DPA and EPA, were found in both extracts. However, higher efficiency in the extraction of PUFA is obtained by extraction with the higher ethanol content in the solvent. Nearly 10% of DPA and EPA were detected in a 70% ethanol extract, while a 50% ethanol extract contained nearly 30% and 18% less of these PUFAs, respectively. In addition to neophytadiene, which constituted 4.02% in the 70% ethanol extract, linoleic and stearic acids, glycerol, pyroglutamic acid, and uracil were identified, but in lower amounts, while they were not detected in the 50% ethanol extract. On the other hand, no heptadecanoic acid was identified in the 70% extract, which was present in the 50% ethanolic extract in an amount of 0.35%.
In conclusion, six compounds were found to be dominant in both extracts. These are listed in Table 2 along with their chemical formula, subclass in chemical classification, and reported biological activities.
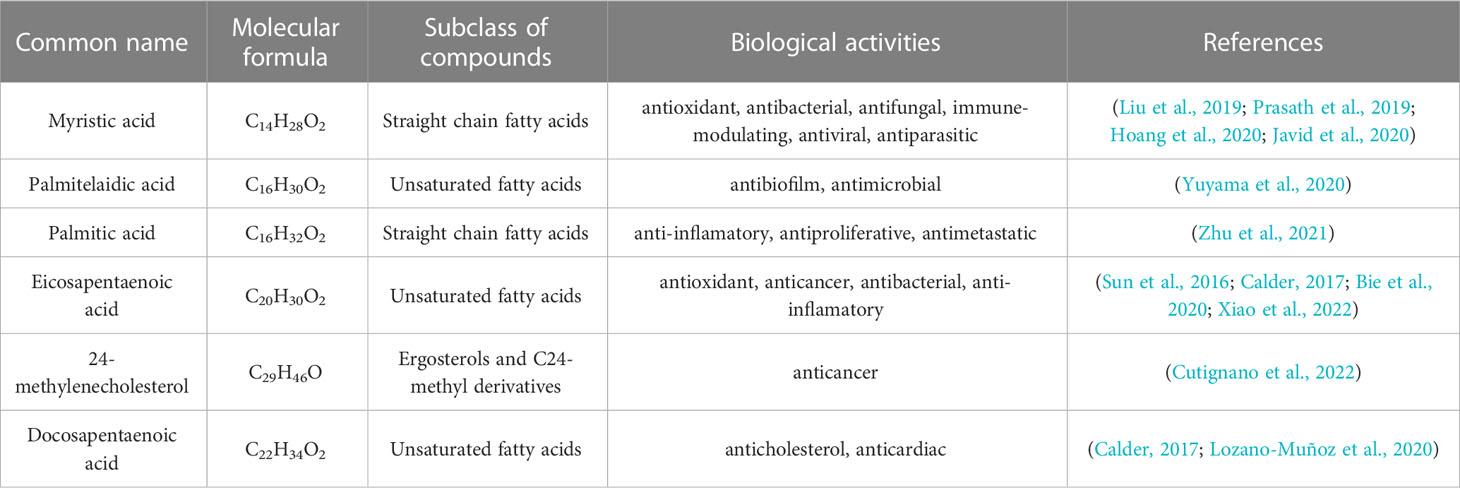
Table 2 The main compounds identified in 50% and 70% ethanol extracts of the diatom Thalassiosira rotula.
The predominant fatty acids in diatoms are myristic, palmitic, palmitelaidic, and EPA, which is also confirmed in this study (Yi et al., 2017; Zulu et al., 2018). Sabia et al. (2018) reported the predominance of myristic acid, palmitic acid, and palmitoleic acid in Thalassiosira pseudonana. Myristic acid, also known as C14:0, is present in all living organisms. This natural bioactive compound could be used as a potential agent against drug-resistant Candida species, while Javid et al. (2020) highlighted the antiparasitic, antiviral, antifungal, and anticancer properties as the main activities of myristic acid (Prasath et al., 2019; Javid et al., 2020). On the other hand, palmitic acid (C16:0) is an important compound from which the biosynthetic pathway of two classical fatty acid families starts: n-3 (such as DPA) and n-6 (such as EPA) (Nieri et al., 2023). Zhu et al. (2021) reported antiproliferative and antimetastatic activities of palmitic acid against prostate cancer. Palmitelaidic acid is the trans isomer of palmitoleic acid. In a study on the control of biofilm formation of Gram-negative and Gram-positive bacteria by palmitelaidic acid, 25% inhibition of Escherichia coli at a concentration of 256 µg mL-1 and 21% inhibition of Staphylococcus aureus at a concentration of 16 µg mL-1 were observed by this unsaturated fatty acid (Yuyama et al., 2020).
Diatoms also contain large amounts of PUFA n-3 fatty acids (Maadane et al., 2015). In this study, nearly 20% of EPA and DPA were extracted from T. rotula with 70% ethanol. DPA is an intermediate compound between EPA and DHA and has many health benefits, such as lowering the risk of coronary heart disease and plasma cholesterol levels, while EPA is important for neurological development, has an anti-inflammatory effect, and lowers the risk of hypertension, Crohn’s disease, and coronary heart disease (Calder, 2017; Lozano-Muñoz et al., 2020). Bie et al. (2020) reported the antitumor activity of EPA on ovarian cells by enhancing the immunomodulatory activity. In oral infections, it showed antibacterial activities against periodontal diseases (Sun et al., 2016).
As a good source of sterols, centric diatoms of the genus Thalassiosira are characterized by a dominance of the phytosterol 24-methylenecholesterol (>75%), also known as 24-methycholesta-5,24(28)-dien-3β-ol (Rampen et al., 2010; Gallo et al., 2020; Nieri et al., 2023). In accordance with our results, Jaramillo-Madrid et al. (2020) reported the presence of 24-methylenecholesterol in three Thalassiosira species, including T. rotula. Cutignano et al. (2022) were the first to report the cytotoxic and antiproliferative activity of 24-methylenecholesterol extracted from T. rotula on MCF7 breast cancer and A549 lung cancer cell lines in their study. These results suggest that extraction with 70% ethanol may be suitable for the preparation of an extract with a higher content of this sterol for further biomedical applications.
3.3 Total phenolic content and antioxidation capacity
The TPC of diatom extracts is shown in Figure 3. Due to their different polarities, hydroalcoholic mixtures should be the best choice for the extraction of phenolic compounds (Medina-Torres et al., 2017). T. rotula extracted with 70% ethanol had an almost 2-fold higher phenolic content (5.80 ± 0.32 mg GAE/g DW biomass) compared to the extract obtained with 50% ethanol (3.17 ± 0.29 mg GAE/g DW biomass).
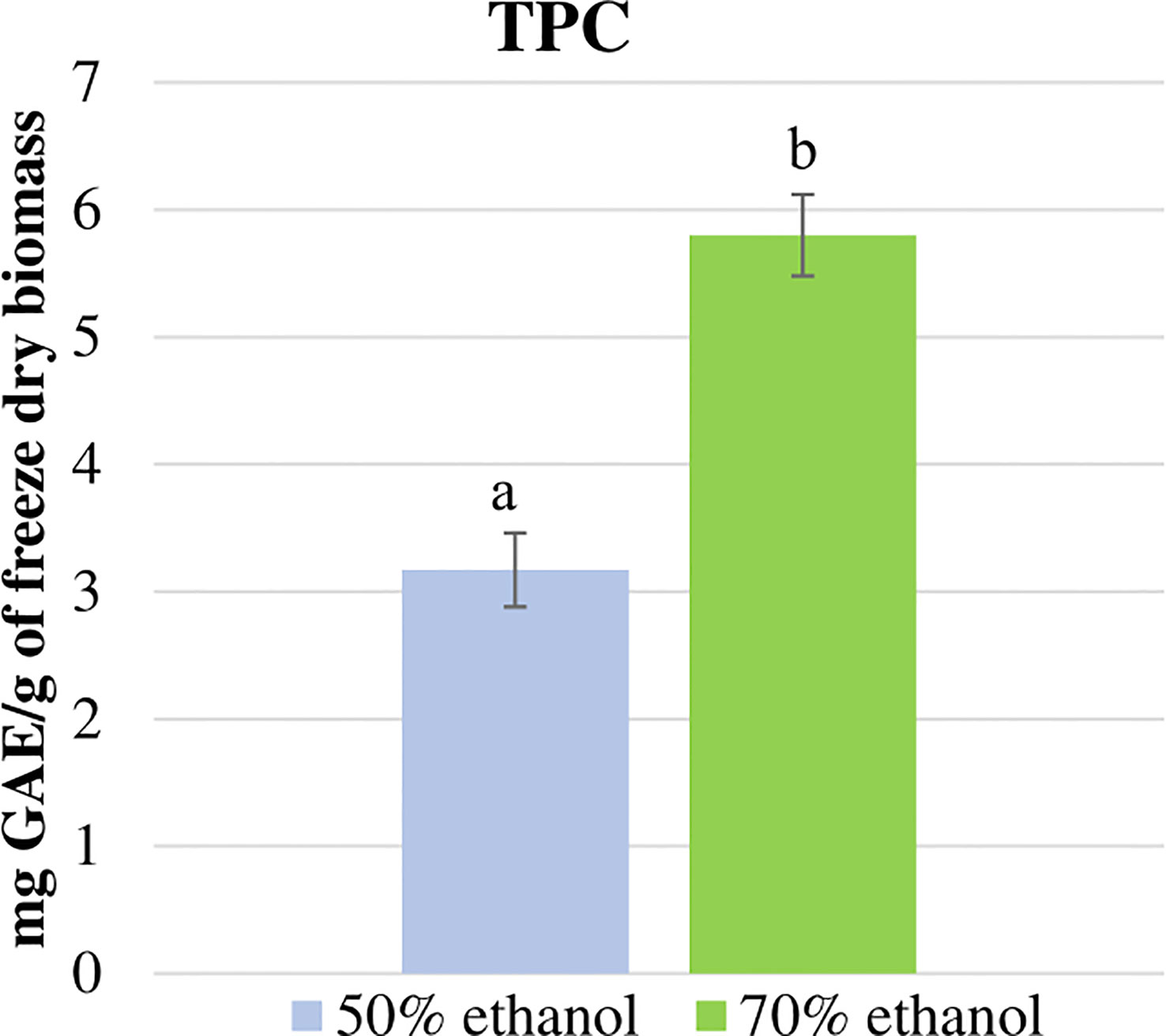
Figure 3 Total phenolic content (TPC) in the extracts obtained by extraction with 50% and 70% ethanol/g of freeze-dried diatom Thalassiosira rotula. Different letters indicate statistically significant differences (p < 0.05).
When comparing our results with other Thalassiosira strains, Syed Ali et al. (Asha Shalini et al., 2019) found a higher amount of TPC in the methanolic extract of T. weissflogii (11.34 mg GAE g-1 DW). On the other hand, Hemalatha et al. (2015) found more than 10-fold lower TPC for T. subtilis methanolic extract compared to our strain. Moreover, authors tested different extracts, methanol, acetone, and hexane, from which methanolic extract showed the highest phenolic content. It is evident that the phenolic content varies greatly between species and is also influenced by the choice of solvent.
The full antioxidant potential of T. rotula extracts was measured using FRAP, DPPH, and ORAC assays, which are based on different mechanisms of action. DPPH and ORAC are based on hydrogen atom transfer, while FRAP is based on electron transfer (Granato et al., 2018). The results are shown in Figure 4. T. rotula extracted at 70% showed higher antioxidant activity in this study, which was measured by three different methods. Using the FRAP assay, almost 3-fold higher reducing activity was observed for the 70% ethanolic extract (766.67 ± 34.69 µM TE). The percentage of inhibition of DPPH radicals was 12-fold lower for the 50% ethanol extract, while the ability of the 70% ethanol extract to scavenge peroxyl radicals was more than 47% higher than that of the 50% ethanol extract.
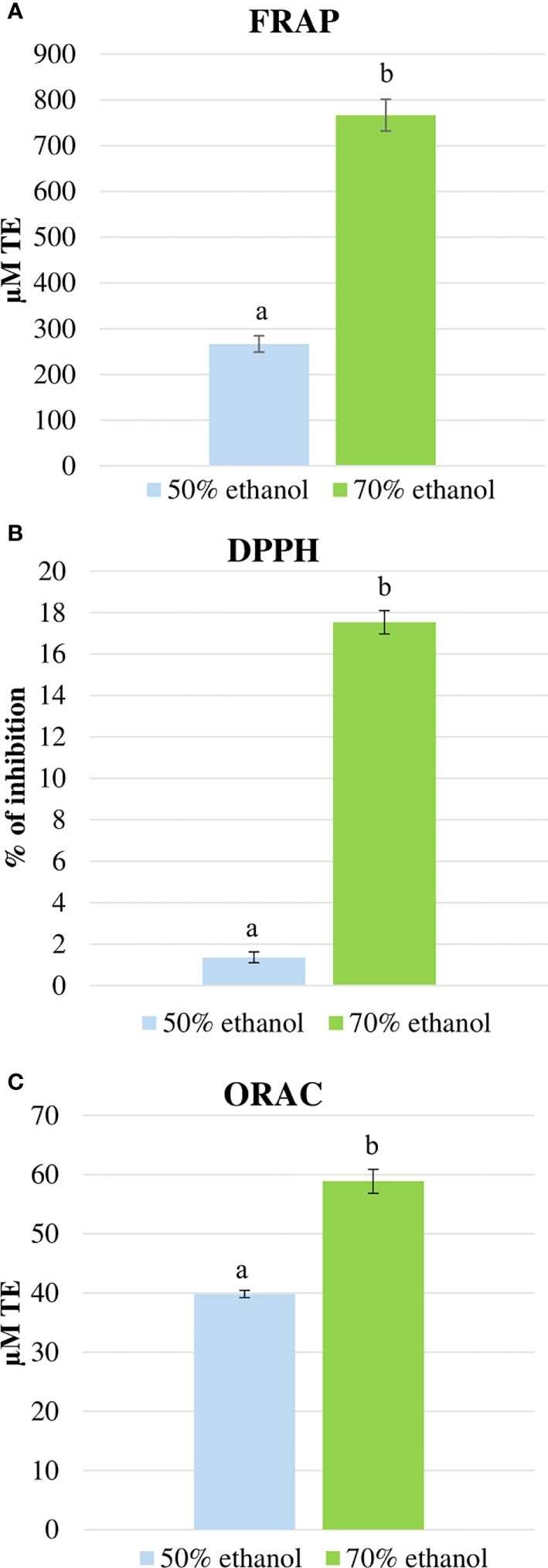
Figure 4 Ferric reducing/antioxidant power (FRAP) (A), 2,2-diphenyl-1-picrylhydrazyl radical scavenging ability (DPPH) (B), and the oxygen radical absorbance capacity (ORAC) (C) results for the 50% and 70% ethanolic extract of Thalassiosira rotula. Different letters denote statistically significant differences (p < 0.05).
The choice of extraction solvent had a significant effect on the variation of DPPH inhibition (Henriques et al., 2007; Hemalatha et al., 2015). Compared to the acetone and hexane extract, Hemalatha et al. (2015) showed the highest DPPH inhibition with the methanol extract of T. subtilis (12.51%). The methanol extract showed 46% lower inhibition in their study compared to our results for the 70% ethanol extract of T. rotula. Silva et al. (2022) reported almost three times higher percent DPPH inhibition by the ethanolic extract of the diatom Skeletonema marinoi compared to the water extract. This is similar to our study, where an increase in antioxidant activity was observed for the extract with a higher percentage of ethanol in the solvent mixture in all assays used.
4 Conclusions
The results confirmed that extraction in 70% ethanol provided a higher extraction yield of diatom T. rotula. In addition, the 70% ethanol extract showed higher TPC and antioxidant capacity by three methods. Myristic acid, palmitelaidic acid, palmitic acid, EPA, and DPA were identified as dominant compounds in both extracts. In addition, 24-methylenecholesterol was identified in 70% ethanolic extract in amounts greater than 10%. All of the above compounds have been shown to be biologically active in previous studies, and some compounds such as EPA are already being used commercially as therapeutics and food supplements. Higher yields of commercially important compounds (e.g., EPA), as well as compounds that could be used in the pharmaceutical industry in the future (e.g., 24-methylenecholesterol), were extracted in 70% ethanol. The results confirm that T. rotula is a diatom with a wide range of compounds that have exceptional potential. Therefore, future work should focus on studying the biological activities of the identified compounds in vivo, clinical trials, and methods to increase diatom production for commercial use.
Data availability statement
The raw data supporting the conclusions of this article will be made available by the authors without undue reservation.
Author contributions
RF: conceptualization, methodology, formal analysis, investigation, data curation, writing—original draft preparation, and visualization. MP: methodology, validation, formal analysis, data curation, and writing—review and editing. MČ: methodology, formal analysis, data curation, and writing—review and editing. VŠ: conceptualization, methodology, validation, investigation, writing—review and editing, visualization, and supervision. All authors contributed to the article and approved the submitted version.
Funding
This research was partially supported under the project STIM–REI, Contract Number: KK.01.1.1.01.0003, a project funded by the EU through the ERDF–the Operational Programme Competitiveness and Cohesion 2014‐2020 (KK.01.1.1.01).
Acknowledgments
The authors would like to thank Dr. Daniela Marić Pfannkuchen from the Laboratory for Evolutionary Ecology of the Center for Marine Research of the Ruđer Bošković Institute (Rovinj, Croatia) for the donation of the Thalassiosira rotula strain from the institute culture collection. The authors would also like to thank Elda Vitanović from the Institute for Adriatic Crops and Karst Reclamation for providing equipment purchased within KK.01.1.1.04.0002 for chemical identification of T. rotula strain.
Conflict of interest
The authors declare that the research was conducted in the absence of any commercial or financial relationships that could be construed as a potential conflict of interest.
Publisher’s note
All claims expressed in this article are solely those of the authors and do not necessarily represent those of their affiliated organizations, or those of the publisher, the editors and the reviewers. Any product that may be evaluated in this article, or claim that may be made by its manufacturer, is not guaranteed or endorsed by the publisher.
Supplementary material
The Supplementary Material for this article can be found online at: https://www.frontiersin.org/articles/10.3389/fmars.2023.1221417/full#supplementary-material
References
Aldini R., Micucci M., Cevenini M., Fato R., Bergamini C., Nanni C., et al. (2014). Antiinflammatory effect of phytosterols in experimental murine colitis model: prevention, induction, remission study. PLoS One 9 (9), e108112. doi: 10.1371/journal.pone.0108112
Alvarez A. L., Weyers S. L., Goemann H. M., Peyton B. M., Gardner R. D. (2021). Microalgae, soil and plants: A critical review of microalgae as renewable resources for agriculture. Algal Res. 54, 102200. doi: 10.1016/j.algal.2021.102200
Alves Martins D., Rocha F., Castanheira F., Mendes A., Pousão-Ferreira P., Bandarra N., et al. (2013). Effects of dietary arachidonic acid on cortisol production and gene expression in stress response in Senegalese sole (Solea senegalensis) post-larvae. Fish Physiol. Biochem. 39 (5), 1223–1238. doi: 10.1007/s10695-013-9778-6
Asha Shalini A., Syed Ali M., Anuradha V., Yogananth N., Bhuvana P. (2019). GCMS analysis and invitro antibacterial and anti-inflammatory study on methanolic extract of Thalassiosira weissflogii. Biocatal. Agric. Biotechnol. 8 (7), 1463–1481. doi: 10.1016/j.bcab.2019.101148
Baldisserotto C., Sabia A., Guerrini A., Demaria S., Maglie M., Ferroni L., et al. (2021). Mixotrophic cultivation of Thalassiosira pseudonana with pure and crude glycerol: Impact on lipid profile. Algal Res. 54, 102194. doi: 10.1016/j.algal.2021.102194
Bárcenas-Pérez D., Lukeš M., Hrouzek P., Kubáč D., Kopecký J., Kaštánek P., et al. (2021). A biorefinery approach to obtain docosahexaenoic acid and docosapentaenoic acid n-6 from Schizochytrium using high performance countercurrent chromatography. Algal Res. 55, 102241. doi: 10.1016/j.algal.2021.102241
Benzie I. F. F., Strain J. J. (1996). The Ferric Reducing Ability of Plasma (FRAP) as a measure of “Antioxidant power”: the FRAP assay. Anal. Biochem. 239 (1), 70–76. doi: 10.1006/abio.1996.0292
Bhattacharjya R., Marella T. K., Tiwari A., Saxena A., Singh P. K., Mishra B. (2020). Bioprospecting of marine diatoms Thalassiosira, Skeletonema and Chaetoceros for lipids and other value-added products. Mar. Drugs 10 (5), 1–10. doi: 10.1016/j.biortech.2020.124073
Bie N., Han L., Meng M., Zhang Y., Guo M., Wang C. (2020). Anti-tumor mechanism of eicosapentaenoic acid (EPA) on ovarian tumor model by improving the immunomodulatory activity in F344 rats. J. Funct. Foods 65, 103739. doi: 10.1016/j.jff.2019.103739
Borowitzka M. A. (2018). “Microalgae in Medicine and Human Health: A Historical Perspective.” in Microalgae in Health and Disease Prevention Levine I. A., Fleurence J. ed. (Kidlington, United Kingdom: Elsevier), 195–210.
Burčul F., Generalić Mekinić I., Radan M., Rollin P., Blažević I. (2018). Isothiocyanates: cholinesterase inhibiting, antioxidant, and anti-inflammatory activity. J. Enzyme Inhib. Med. Chem. 33 (1), 577–582. doi: 10.1080/14756366.2018.1442832
Čagalj M., Skroza D., Razola-Díaz M. D. C., Verardo V., Bassi D., Frleta R., et al. (2022). Variations in the Composition, Antioxidant and Antimicrobial Activities of Cystoseira compressa during Seasonal Growth. Mar. Drugs 20 (64), 1–14. doi: 10.3390/md20010064
Čagalj M., Skroza D., Tabanelli G., Özogul F., Šimat V. (2021). Maximizing the antioxidant capacity of Padina pavonica by choosing the right drying and extraction methods. Processes 9 (4), 587. doi: 10.3390/pr9040587
Calder P. C. (2017). Omega-3 fatty acids and inflammatory processes: From molecules to man. Biochem. Soc Trans. 45 (5), 1105–1115. doi: 10.1042/BST20160474
Cutignano A., Conte M., Tirino V., Del Vecchio V., De Angelis R., Nebbioso A., et al. (2022). Cytotoxic Potential of the Marine Diatom Thalassiosira rotula: Insights into Bioactivity of 24-Methylene Cholesterol. Mar. Drugs 20 (10), 595. doi: 10.3390/md20100595
Duran S. K., Kumar P., Sandhu S. S. (2021). A review on microalgae strains, cultivation, harvesting, biodiesel conversion and engine implementation. Biofuels 12 (1), 91–102. doi: 10.1080/17597269.2018.1457314
Field B.C., Behremdeld M. J., Randerson J. T., Falkowski P. (1998). Primary production of the biosphere: integrating terrestrial and oceanic components. Science 281, 237–240: 5374. doi: 10.1126/science.281.5374.237
Frleta R., Popović M., Smital T., Šimat V. (2022). Comparison of growth and chemical profile of diatom Skeletonema grevillei in bioreactor and incubation-shaking cabinet in two growth phases. Mar. Drugs 20 (11), 697. doi: 10.3390/md20110697
Gallo C., Landi S., d’Ippolito G., Nuzzo G., Manzo E., Sardo A., et al. (2020). Diatoms synthesize sterols by inclusion of animal and fungal genes in the plant pathway. Sci.Rep 10 (1), 1–13. doi: 10.1038/s41598-020-60993-5
Granato D., Shahidi F., Wrolstad R., Kilmartin P., Melton L. D., Hidalgo F. J., et al. (2018). Antioxidant activity, total phenolics and flavonoids contents: Should we ban in vitro screening methods? Food Chem. 264, 471–475. doi: 10.1016/j.foodchem.2018.04.012
Hemalatha A., Parthiban C., Saranya C., Girija K., Anantharaman P. (2015). Evaluation of antioxidant activities and total phenolic contents of different solvent extracts of selected marine diatoms. Indian J. Mar. Sci. 44 (10), 1630–1636.
Henriques M., Silva A., Rocha J. (2007). “Extraction and quantification of pigments from a marine microalga: a simple and reproducible method,” in Communicating current research and educational topics and trends in applied microbiology (FORMATEX), 586–593.
Hoang L., Beneš F., Fenclová M., Kronusová O., Švarcová V., Řehořová K., et al. (2020). Phytochemical composition and in vitro biological activity of Iris spp. (iridaceae): A new source of bioactive constituents for the inhibition of oral bacterial biofilms. Antibiotics 9 (7), 1–24. doi: 10.3390/antibiotics9070403
Jacotet-Navarro M., Laguerre M., Fabiano-Tixier A. S., Tenon M., Feuillère N., Bily A., et al. (2018). What is the best ethanol-water ratio for the extraction of antioxidants from rosemary? Impact of the solvent on yield, composition, and activity of the extracts. Electrophoresis 39 (15), 1946–1956. doi: 10.1002/elps.201700397
Jaramillo-Madrid A. C., Ashworth J., Ralph P. J. (2020). Levels of diatom minor sterols respond to changes in temperature and salinity. J. Mar. Sci. Eng. 8 (2), 85. doi: 10.3390/JMSE8020085
Javid S., Purohit M. N., Yogish Kumar H., Ramya K., Mithuna N. F. A., Salahuddin M. D., et al. (2020). Semisynthesis of myristic acid derivatives and their biological activities: A critical insight. J. Biol. Act. Prod. Nat. 10 (6), 455–472. doi: 10.1080/22311866.2020.1865836
Jie F., Yang X., Wu L., Wang M., Lu B. (2022). Linking phytosterols and oxyphytosterols from food to brain health: origins, effects, and underlying mechanisms. Food Sci. Nutr. 62 (13), 3613–3630. doi: 10.1080/10408398.2020.1867819
Lafarga T., Acién G. (2022). Microalgae for the food industry: from biomass production to the development of functional foods. Foods 11 (5), 765. doi: 10.3390/foods11050765
Levine I. A., Fleurence J. (2018). “Microalgae in medicine and human health: A historical perspective,” in Microalgae in Health and Disease Prevention (Academic Press), 195–210. doi: 10.1016/B978-0-12-811405-6.00009-8
Li X., Liu J., Chen G., Zhang J., Wang C., Liu B. (2019). Extraction and purification of eicosapentaenoic acid and docosahexaenoic acid from microalgae: A critical review. Algal Res. 43, 101619. doi: 10.1016/j.algal.2019.101619
Liu C., Yuan C., Ramaswamy H. S., Ren Y., Ren X. (2019). Antioxidant capacity and hepatoprotective activity of myristic acid acylated derivative of phloridzin. Heliyon 5 (5), e01761. doi: 10.1016/j.heliyon.2019.e01761
Lozano-Muñoz I., Muñoz S., Díaz N. F., Medina A., Bazaes J., Riquelme C. (2020). Nutritional enhancement of farmed salmon meat via non-GMO Nannochloropsis gaditana: Eicosapentaenoic acid (EPA, 20:5 n-3), docosapentaenoic acid (DPA, 22:5 n-3) and vitamin D3 for human health. Molecules 25 (20), 4615. doi: 10.3390/molecules25204615
Luo X., Su P., Zhang W. (2015). Advances in microalgae-derived phytosterols for functional food and pharmaceutical applications. Mar. Drugs 13 (7), 4231–4254. doi: 10.3390/md13074231
Maadane A., Merghoub N., Ainane T., El Arroussi H., Benhima R., Amzazi S., et al. (2015). Antioxidant activity of some Moroccan marine microalgae: Pufa profiles, carotenoids and phenolic content. J. Biotechnol. 215, 13–19. doi: 10.1016/j.jbiotec.2015.06.400
Marella T. K., Tiwari A. (2020). Marine diatom Thalassiosira weissflogii based biorefinery for co-production of eicosapentaenoic acid and fucoxanthin. Bioresour. Technol. 307, 123245. doi: 10.1016/j.biortech.2020.123245
Medina-Torres N., Ayora-Talavera T., Espinosa-Andrews H., Sánchez-Contreras A., Pacheco N. (2017). Ultrasound assisted extraction for the recovery of phenolic compounds from vegetable sources. Agronomy 7 (3), 47. doi: 10.3390/agronomy7030047
Menaa F., Wijesinghe U., Thiripuranathar G., Althobaiti N. A., Albalawi A. E., Khan B. A., et al. (2021). Marine algae-derived bioactive compounds: A new wave of nanodrugs? Mar. Drugs 19 (9), 1–36. doi: 10.3390/md19090484
Michalak I., Dmytryk A., Chojnacka K. (2020). “Algae Cosmetics.” in Encyclopedia of Marine Biotechnology Se-Kwon K. ed. (Pondicherry, India: Wiley), 65–85.
Monteiro M., Santos R. A., Iglesias P., Couto A., Serra C. R., Gouvinhas I., et al. (2020). Effect of extraction method and solvent system on the phenolic content and antioxidant activity of selected macro- and microalgae extracts. J. Appl. Phycol. 32 (1), 349–362. doi: 10.1007/s10811-019-01927-1
Nieri P., Carpi S., Esposito R., Costantini M., Zupo V. (2023). Bioactive molecules from marine diatoms and their value for the nutraceutical industry. Nutrients 15 (2), 1–23. doi: 10.3390/nu15020464
NIST (2022)NIST chemistry webBook. In: NIST Standard Reference Database Number 69. Available at: https://www.nist.gov/ (Accessed 1 May 2023).
Prasath K. G., Sethupathy S., Pandian S. K. (2019). Proteomic analysis uncovers the modulation of ergosterol, sphingolipid and oxidative stress pathway by myristic acid impeding biofilm and virulence in Candida albicans. J. Proteomics. 208, 103503. doi: 10.1016/j.jprot.2019.103503
Prior R. L., Hoang H., Gu L., Wu X., Bacchiocca M., Howard L., et al. (2003). Assays for hydrophilic and lipophilic antioxidant capacity (oxygen radical absorbance capacity (ORACFL)) of plasma and other biological and food samples. J. Agric. Food Chem. 51 (11), 3273–3279. doi: 10.1021/jf0262256
Rampen S. W., Abbas B. A., Schouten S., Damsté J. S. S. (2010). A comprehensive study of sterols in marine diatoms (Bacillariophyta): Implications for their use as tracers for diatom productivity. Limnol. Oceanogr. 55 (1), 91–105. doi: 10.4319/lo.2010.55.1.0091
Ras R. T., Geleijnse J. M., Trautwein E. A. (2014). LDL-cholesterol-lowering effect of plant sterols and stanols across different dose ranges: A meta-analysis of randomised controlled studies. Br. J. Nutr. 112 (2), 214–219. doi: 10.1017/S0007114514000750
Remize M., Brunel Y., Silva J. L., Berthon J. Y., Filaire E. (2021). Microalgae n-3 PUFAs production and use in food and feed industries. Mar. Drugs 19 (2), 1–29. doi: 10.3390/MD19020113
Rumin J., Nicolau E., de Oliveira R. G., Fuentes-Grünewald C., Picot L. (2020). Analysis of scientific research driving microalgae market opportunities in Europe. Mar. Drugs 18 (5), 264. doi: 10.3390/md18050264
Şahin S., Şamlı R. (2013). Optimization of olive leaf extract obtained by ultrasound-assisted extraction with response surface methodology. Ultrason. Sonochem. 20 (1), 595–602. doi: 10.1016/j.ultsonch.2012.07.029
Sabia A., Clavero E., Pancaldi S., Salvadó Rovira J. (2018). Effect of different CO2 concentrations on biomass, pigment content, and lipid production of the marine diatom Thalassiosira pseudonana. Appl. Microbiol. Biotechnol. 102 (4), 1945–1954. doi: 10.1007/s00253-017-8728-0
Sañé E., Del Mondo A., Ambrosino L., Smerilli A., Sansone C., Brunet C. (2021). The recent advanced in microalgal phytosterols: bioactive ingredients along with human-health driven potential applications. Food Rev. Int. 39 (4), 1859–1878. doi: 10.1080/87559129.2021.1938115
Sharma N., Simon D. P., Diaz-Garza A. M., Fantino E., Messaabi A., Meddeb-Mouelhi F., et al. (2021). Diatoms biotechnology: various industrial applications for a greener tomorrow. Front. Mar. Sci. 8. doi: 10.3389/fmars.2021.636613
Silva M., Kamberovic F., Uota S. T., Kovan I. M., Viegas C. S. B., Simes D. C., et al. (2022). Microalgae as potential sources of bioactive compounds for functional foods and pharmaceuticals. Appl. Sci. 12 (12), 5877. doi: 10.3390/app12125877
Sun M., Zhou Z., Dong J., Zhang J., Xia Y., Shu R. (2016). Antibacterial and antibiofilm activities of docosahexaenoic acid (DHA) and eicosapentaenoic acid (EPA) against periodontopathic bacteria. Microb. Pathog. 99, 196–203. doi: 10.1016/j.micpath.2016.08.025
Suroy M., Moriceau B., Boutorh J., Goutx M. (2014). Fatty acids associated with the frustules of diatoms and their fate during degradation-A case study in Thalassiosira weissflogii. Deep Sea Res. 86, 21–31. doi: 10.1016/j.dsr.2014.01.001
Svenning J. B., Dalheim L., Vasskog T., Matricon L., Vang B., Olsen R. L. (2020). Lipid yield from the diatom Porosira glacialis is determined by solvent choice and number of extractions, independent of cell disruption. Sci. Rep. 10 (1), 1–10. doi: 10.1038/s41598-020-79269-z
Wang J. K., Seibert M. (2017). Prospects for commercial production of diatoms. Biotechnol. Biofuels 10 (1), 1–13. doi: 10.1186/s13068-017-0699-y
Xiao B., Li Y., Lin Y., Lin J., Zhang L., Wu D., et al. (2022). Eicosapentaenoic acid (EPA) exhibits antioxidant activity via mitochondrial modulation. Food Chem. 373, 131389. doi: 10.1016/j.foodchem.2021.131389
Yang J., Li C., Zhang Y. (2021). Engineering of Saccharomyces cerevisiae for 24-methylene-cholesterol production. Biomolecules 11 (11), 1–13. doi: 10.3390/biom11111710
Yi Z., Xu M., Di X., Brynjolfsson S., Fu W. (2017). Exploring valuable lipids in diatoms. Front. Mar. Sci. 4. doi: 10.3389/fmars.2017.00017
Yuyama K. T., Rohde M., Molinari G., Stadler M., Abraham W. R. (2020). Unsaturated fatty acids control biofilm formation of Staphylococcus aureus and other gram-positive bacteria. Antibiotics 9 (11), 1–11. doi: 10.3390/antibiotics9110788
Zhu S., Jiao W., Xu Y., Hou L., Li H., Shao J., et al. (2021). Palmitic acid inhibits prostate cancer cell proliferation and metastasis by suppressing the PI3K/Akt pathway. Life Sci. 286, 120046. doi: 10.1016/j.lfs.2021.120046
Keywords: diatom, Thalassiosira rotula, antioxidant activity, chemical profile, myristic acid, EPA, DPA, 24-methylenecholesterol
Citation: Frleta Matas R, Popović M, Čagalj M and Šimat V (2023) The marine diatom Thalassiosira rotula: chemical profile and antioxidant activity of hydroalcoholic extracts. Front. Mar. Sci. 10:1221417. doi: 10.3389/fmars.2023.1221417
Received: 12 May 2023; Accepted: 17 July 2023;
Published: 09 August 2023.
Edited by:
Nitin Trivedi, Institute of Chemical Technology, IndiaReviewed by:
Suncica Bosak, University of Zagreb, CroatiaNirmal Mazumder, Manipal Academy of Higher Education, India
Copyright © 2023 Frleta Matas, Popović, Čagalj and Šimat. This is an open-access article distributed under the terms of the Creative Commons Attribution License (CC BY). The use, distribution or reproduction in other forums is permitted, provided the original author(s) and the copyright owner(s) are credited and that the original publication in this journal is cited, in accordance with accepted academic practice. No use, distribution or reproduction is permitted which does not comply with these terms.
*Correspondence: Vida Šimat, vida@unist.hr