- 1Red Sea Research Center (RSRC) and Computational Biology Research Center (CBRC), Biological and Environmental Sciences and Engineering Division (BESE), King Abdullah University of Science and Technology (KAUST), Thuwal, Saudi Arabia
- 2Department of Biology, University of Konstanz, Konstanz, Germany
Climate change is turning formerly pristine ecosystems into ever-changing states, causing major disturbance and biodiversity loss. Such impacted marine ecosystems and organisms exhibit clear microbiome shifts that alter their function. Microbiome-targeted interventions appear as feasible tools to support organismal and ecosystem resilience and recovery by restoring symbiotic interactions and thwarting dysbiotic processes. However, microbiome restoration and rehabilitation are perceived as drastic measures, since they alter ‘natural relationships’. What is missing from this notion is that microbiomes already drastically differ from any pre-anthropogenic state. As such, our perception and definition of even ‘pristine states’ may in fact represent an already disturbed/derived condition. Following this, we argue that restoring and rehabilitating marine microbiomes are essential tools to mitigate ecosystem and organismal decline.
Background
Microorganisms continue to shape our planet (Cavicchioli et al., 2019; Lopez et al., 2019) and the biology of most living organisms (Bosch and McFall-Ngai, 2011; McFall-Ngai et al., 2013; Wilkins et al., 2019; Santoro et al., 2021). Consequently, microbiology thus far has focused on exploring microbial diversity, distribution, and response to environmental change (Lozupone and Knight, 2007; Rachid et al., 2012; Gilbert et al., 2014; Fonseca et al., 2018). In addition, current research explores to utilize microbial-based capacities for restoration (de Jesus et al., 2015; Berg et al., 2021; Peixoto et al., 2021), biotechnological application (Cross et al., 2019; Modolon et al., 2020; Vilela et al., 2021), and promotion of organismal health and resilience (Sanders et al., 2019; Woodhams et al., 2020; Berg et al., 2021; Peixoto et al., 2021), predominantly from a anthropocentric point of view (Cavicchioli et al., 2019).
Probiotics and other microbial therapies (Daliri et al., 2018; Wegh et al., 2019; Peixoto et al., 2022), for example, have been administered to a variety of organisms, including humans (Liu et al., 2021), plants (do Carmo et al., 2011; Berg et al., 2020; Bziuk et al., 2022), and wildlife (D’Alvise et al., 2012; Küng et al., 2014; Hoyt et al., 2019; Rosado et al., 2019; Daisley et al., 2020), representing a growing industry currently worth ~USD15 billion per year (van den Nieuwboer et al., 2016).
Despite the favorable prospect of microbiome interventions, from an ecological rather than an economic perspective, we must consider that microbes themselves are threatened by global change. In particular host-associated beneficial microbial symbionts - the very agents to counter ecological decline (Peixoto et al., 2022) - may be as sensitive to environmental impact as animals and plants (Cavicchioli et al., 2019; Averill et al., 2022). Recent surveys have emphasized that Earth’s microbial biodiversity is under threat and highlighted the urgency of conserving and restoring soil microbiomes (Averill et al., 2022), although these concepts are still poorly explored for marine conservation.
Notably, global change-related impacts, such as warmer temperatures, droughts, and weather extremes, have been causing the emergence and spread of new pathogens (Robert et al., 2015; Nnadi and Carter, 2021; Tiedje et al., 2022) as well as an overall increase of pathogens in ecosystems such as coral reefs (Haas et al., 2016). Decreased host-specificity and the increase of pathogens, hypermutators, and antimicrobial resistance genes were defined as signatures of declines of plant microbiomes in the Anthropocene (Berg and Cernava, 2022). Such microbiomes are termed ‘pathobiomes’ in contrast to ‘beneficiomes’, a term used to signify microbes that are members indicative of a healthy microbiome, where ‘healthy’ is defined as the absence of signs of disease and/or a dysbiotic process (Figure 1).
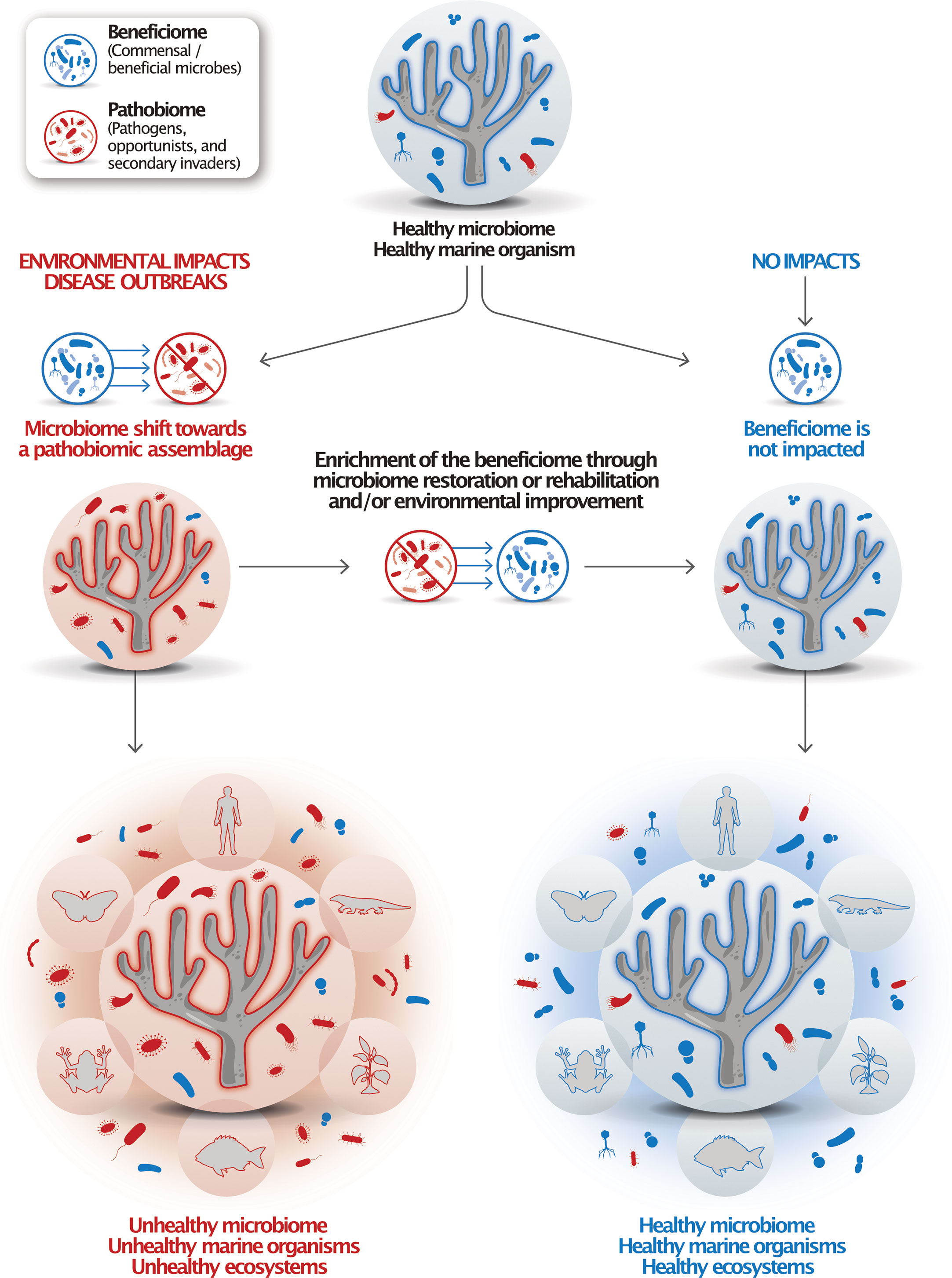
Figure 1 The ‘pathobiome’ and ‘beneficiome’ as types of microbial assemblages to indicate deteriorated and healthy marine organisms. Healthy marine organisms harbor and rely on healthy microbiomes. When impacted by stressors, these microbiomes tend to shift towards a pathobiotic assemblage, which can cause disease and, ultimately, death. Microbiome rehabilitation is focused on through the use of retaining or restoring a healthy microbiome, the beneficiome, which could be achieved through the application of probiotics, microbiome transplants, or other microbial therapies. Healthy organisms interact with other marine organisms and contribute to ecosystem health. Under the One Health concept, marine organismal and ecosystem health are connected to terrestrial organism and ecosystem health.
Marine ecosystems have been heavily impacted by human activities (Jackson et al., 2001), and even the most remote deep-sea areas can be heavily contaminated with pollutants (Vilela et al., 2022). The impact can be at the organismal or at the ecosystem level. Most notably, coral reefs have been decimated by ongoing environmental impact over decades (Eddy et al., 2021), compounded by forecasts of massive future losses in the coming decades driven by global climate change and local anthropogenic impact (Knowlton et al., 2021) that threaten their very existence (Kleypas et al., 2021). Likewise, this has impacted and will further affect marine microbiomes (associated with marine organisms or ecosystems), including the possible extinction of an unknown diversity of obligate microbial symbionts that are yet to be discovered (Weinbauer and Rassoulzadegan, 2007). Microbiomes are characteristic microbial communities that shape or compose specific ecological niches, establishing dynamic interactions with species, populations, and ecosystems at large, being crucial for their functioning and health (Berg et al., 2020).
‘The shifting baseline trap’ - pristine-like marine ecosystems and microbiomes no more
Dysbiotic processes, i.e., the breakdown of symbiotic interactions between a host and its associated microbiome (Petersen and Round, 2014), are frequently triggered by environmental disturbance (Egan and Gardiner, 2016). In such cases, beneficial or commensal microbes that are sensitive to shifts in environmental conditions are replaced by pathogens or opportunistic microbes (Sweet and Bulling, 2017). Microbiome restructuring towards a more pathobiomic assemblage is hypothesized to be the main cause of coral disease and is also apparent in coral bleaching caused by thermal stress (Boilard et al., 2020). The notion that microbiome deterioration underlies the deterioration of organismal and, by extension, ecosystem health, is prevalent to the point where diseases traditionally considered as non-communicable (non-transmittable), such as cardiovascular diseases, cancer, or certain lung diseases in humans, are now discussed to harbor a microbiome component, e.g. with regard to disease transmission or even the underlying cause of the disease (Finlay et al., 2020). Besides corals, other marine organisms such as kelps, seagrasses, fishes, and mangrove plants have also been highly affected by anthropogenically-driven dysbiotic processes (Harvell et al., 1999; Filbee-Dexter et al., 2016; Minich et al., 2018; Conte et al., 2021; Tye et al., 2022).
Mass mortality of marine organisms can cause severe shifts in ecosystem structure, prominent examples being the widespread lethal stony coral tissue loss disease (SCTLD) (Precht, 2021), the devastating (and anthropogenic impact-associated) sea star wasting disease (SSWD) decimating populations of asteroids (Echinodermata) (Hewson et al., 2019; Jaffe et al., 2019), or the climate change-associated spread of oyster diseases (Cook et al., 1998; Soniat et al., 2009; Epstein, 2010). All of these examples of marine die-offs are associated with microbiome changes (Aquino et al., 2020; Clark et al., 2021).
These marine organisms are critical for their respective marine ecosystems and “planetary homeostasis” (Dyke and Weaver, 2013). Consequently, altered microbiome states of these organisms are at the core of alarming losses of marine biodiversity (Peixoto et al., 2022), and microbiomes will continue to shift towards more pathogenic assemblages in pace with ongoing global change. Importantly, increasing rates of environmental change induced by human activity have been apparent for the better part of the last century for many anthropogenic actions considered (e.g., fertilizer consumption, transport, tourism, etc.) (Steffen et al., 2011). As such, we fall victim to the ‘shifting baseline trap’, i.e. the perception that the already altered state experienced by the previous generation is/was the pristine state, even though in reality it already represented a disturbed/derived condition (Soga and Gaston, 2018).
Microbiome stewardship to attain rehabilitation
Even if current conditions are reversed immediately, in many cases, it is too late for “nature to heal itself” (Duarte et al., 2020; Knowlton et al., 2021), and significant losses are expected over the coming years before things (may) get better (Kleypas et al., 2021). Although any solution needs to incorporate reducing CO2 emissions and mitigating local stressors eventually, active restoration efforts are equally important to buffer the gap and buy time (Knowlton et al., 2021; Voolstra et al., 2021). Our objective and obligation must ensure that sufficient foundational species and genetic diversity survive to assist in the long-term recovery of marine ecosystems once carbon neutrality is reached.
Marine probiotics have recently been introduced as a means to support conservation and restoration of marine wildlife (Peixoto et al., 2017; Peixoto et al., 2021; Voolstra et al., 2021; Peixoto et al., 2022). Such ‘enhanced restoration’ or rehabilitation (Voolstra et al., 2021, 2023) extends and clarifies the concept of restoration because i) it is unclear whether restoration to past (microbial) configurations under current environmental conditions is an actual remedy for stress susceptibility and also due to ii) uncertainties associated with the nature of past “pristine” microbial configurations (given our shifting baseline perception and ignorance what “pristine” actually means or signifies; see below). Although marine microbiome manipulation is still in its infancy (Blackall et al., 2020), integrated actions and global networks have been built to accelerate such research and development in the face of alarming marine biodiversity losses (Allard et al., 2020). The high toll of inaction must be weighed against the potential caveats associated with microbial manipulation. A roadmap for the risk assessment and safe development of probiotics to protect wildlife has been recently proposed (Peixoto et al., 2022).
Here we extend such reasoning and propose that marine conservation should generally consider microbiomes as one of its key components. Such efforts should not be defined as microbial manipulation since the eventual aim is the reversal of deteriorated states of organisms and ecosystems to more pristine states by means of the restoration/rehabilitation of associated/underlying microbiomes (Figure 2). It is crucial to highlight that already degraded ecosystems are unlikely to harbor “pristine-like” microbiomes (Leite et al., 2018) and that there is no certainty of what such pristine microbiomes would look like. Consequentially, an altered (in a sense manipulated) microbiome is already present in many marine ecosystems, often enriched with potential pathogens. We are witnessing a continuous alteration of microbiomes, in line with the pace and magnitude of environmental change. Accordingly, microbiome restoration/rehabilitation is a way to minimize impacts caused by ongoing changes, rather than it being the cause of these changes. If we accept microbiome change as a consequence of continuous environmental change, our obligation should ensure such changes are towards a less pathogenic, neutral or, ideally, beneficial assemblage. The correct use of these terms and concepts is key for the development and public acceptance of the nascent field of microbiome stewardship (Peixoto et al., 2022) with parallels to terrestrial restoration/rehabilitation (e.g., reforestation) (Nave et al., 2018; Bziuk et al., 2022) and medical approaches (e.g., fecal transplants) (Daliri et al., 2018) (Figure 2). Microbes are a key factor connecting and supporting humans, animals, and ecosystem functioning under the ‘One Health’ concept (Trinh et al., 2018), representing a docile target for restoration/rehabilitation (Peixoto et al., 2022).
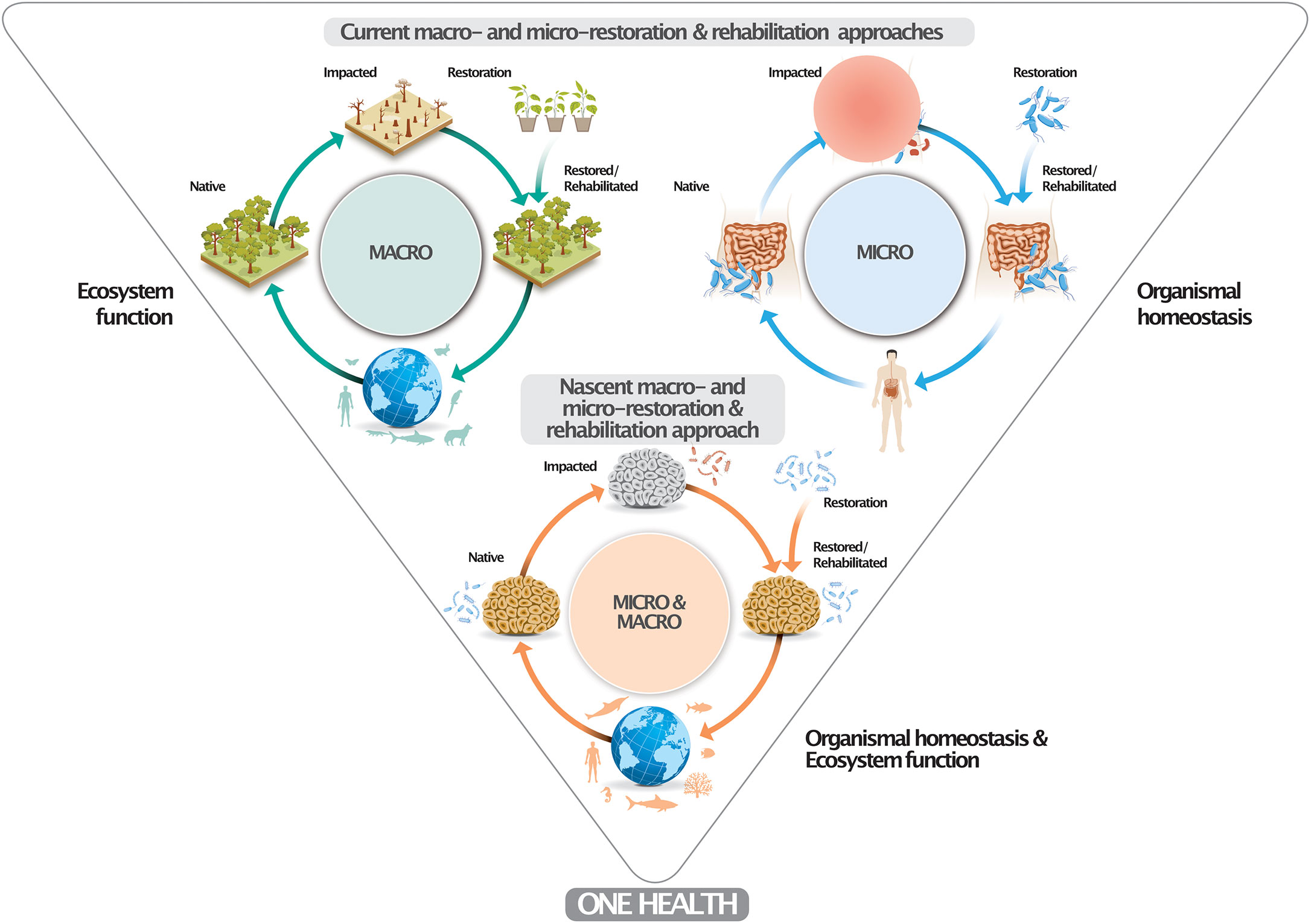
Figure 2 Conceptual framework of a marine micro-macro-restoration/-rehabilitation approach. The upper part depicts current macro- and micro-restoration/-rehabilitation approaches at the ecosystem- (i.e., reforestration) and organismal-level (i.e., human microbiome fecal transplants) to ascertain ecosystem function and organismal homeostasis. Marine microbiomes have been altered due to global change and local impact. The proposed marine microbiome restoration/rehabilitation approach (e.g., by means of probiotic provisioning) restores organismal homeostasis and ecosystem health (function). Similar to degraded forests and dysbiotic human gut microbiomes, impacted marine microbiomes can be restored or rehabilitated to minimize or prevent the establishment of pathobiomes, which also contributes to human well-being. The One Health concept signifies that healthy organisms in healthy ecosystems make for healthy humans.
In a nutshell
Innovative efforts to conserve, restore, and rehabilitate marine microbiomes can contribute to the protection of marine ecosystems and increase the adaptive capacity of marine organisms and ecosystems to survive climate change (Voolstra et al., 2021; Peixoto et al., 2022). Impacted microbiomes, on the other hand, offer a clear path for opportunistic pathogens to thrive and spread, which may lead to pandemics and wildlife die-offs (Averill et al., 2022; Peixoto et al., 2022; Tiedje et al., 2022). Associated management approaches to rehabilitate degraded/impacted marine microbiomes range from improvement of environmental conditions to probiotic or other microbial-based interventions (Peixoto et al., 2019). Despite the promise of microbiome manipulation, it is often perceived as unnatural and overly intervening. Here we lay out the need to consider (i) how the ‘shifting baseline trap’, the notion that the previous ecosystem state was pristine, may obscure our sight of long-term ongoing deterioration; (ii) the need to adopt a terminology of ‘microbiome restoration’, or more accurately ‘microbiome rehabilitation’, as opposed to the term ‘microbiome manipulation’; (iii) the notion that most marine microbiomes are already altered (and potentially damaged). The ongoing debate on whether microbial therapies will cause damage should be replaced by a discussion on how (and whether it is possible) to safely restore them. This notion is still very incipient (or null) for marine ecosystems and not even fully scrutinized for terrestrial systems.
Data availability statement
The original contributions presented in the study are included in the article/supplementary material. Further inquiries can be directed to the corresponding authors.
Author contributions
Both authors contributed equally to this work.
Acknowledgments
RSP acknowledges funding from King Abdullah University of Science and Technology (KAUST) (grants FCC/1/1973-51-01 and BAS/1/1095-01-01). CRV acknowledges acknowledges support by the Deutsche Forschungsgemeinschaft (DFG, German Research Foundation) project number 15951622. CRV and RSP acknowledge funding from KAUST (OSR-2021-NTGC-4984).
Conflict of interest
The authors declare that the research was conducted in the absence of any commercial or financial relationships that could be construed as a potential conflict of interest.
Publisher’s note
All claims expressed in this article are solely those of the authors and do not necessarily represent those of their affiliated organizations, or those of the publisher, the editors and the reviewers. Any product that may be evaluated in this article, or claim that may be made by its manufacturer, is not guaranteed or endorsed by the publisher.
References
Allard S. M., Costa M. T., Bulseco A. N., Helfer V., Wilkins L. G. E., Hassenrück C., et al. (2020). Introducing the mangrove microbiome initiative: identifying microbial research priorities and approaches to better understand, protect, and rehabilitate mangrove ecosystems. mSystems 5, e00658–20. doi: 10.1128/mSystems.00658-20
Aquino C. A., Besemer R. M., DeRito C. M., Kocian J., Porter I. R., Raimondi P. T., et al. (2020). Evidence that microorganisms at the animal-water interface drive Sea star wasting disease. Front. Microbiol. 11, 610009. doi: 10.3389/fmicb.2020.610009
Averill C., Anthony M. A., Baldrian P., Finkbeiner F., van den Hoogen J., Kiers T., et al. (2022). Defending earth’s terrestrial microbiome. Nat. Microbiol. 7, 1717–1725. doi: 10.1038/s41564-022-01228-3
Berg G., Cernava T. (2022). The plant microbiota signature of the Anthropocene as a challenge for microbiome research. Microbiome 10, 54. doi: 10.1186/s40168-021-01224-5
Berg G., Kusstatscher P., Abdelfattah A., Cernava T., Smalla K. (2021). Microbiome modulation-toward a better understanding of plant microbiome response to microbial inoculants. Front. Microbiol. 12, 650610. doi: 10.3389/fmicb.2021.650610
Berg G., Rybakova D., Fischer D., Cernava T., Vergès M.-C. C., Charles T., et al. (2020). Microbiome definition re-visited: old concepts and new challenges. Microbiome 8, 103.
Blackall L. L., Dungan A. M., Hartman L. M., van Oppen M. J. H. (2020). Probiotics for corals. Microbiol. Aust. 41, 100–104. doi: 10.1071/MA20025
Boilard A., Dubé C. E., Gruet C., Mercière A., Hernandez-Agreda A., Derome N. (2020). Defining coral bleaching as a microbial dysbiosis within the coral holobiont. Microorganisms 8. doi: 10.3390/microorganisms8111682
Bosch T. C. G., McFall-Ngai M. J. (2011). Metaorganisms as the new frontier. Zoology 114, 185–190. doi: 10.1016/j.zool.2011.04.001
Bziuk N., Maccario L., Sørensen S. J., Schikora A., Smalla K. (2022). Barley rhizosphere microbiome transplantation–a strategy to decrease susceptibility of barley grown in soils with low microbial diversity to powdery mildew. Front. Microbiol. 1748. doi: 10.3389/fmicb.2022.830905
Cavicchioli R., Ripple W. J., Timmis K. N., Azam F., Bakken L. R., Baylis M., et al. (2019). Scientists’ warning to humanity: microorganisms and climate change. Nat. Rev. Microbiol. 17, 569–586. doi: 10.1038/s41579-019-0222-5
Clark A. S., Williams S. D., Maxwell K., Rosales S. M., Huebner L. K., Landsberg J. H., et al. (2021). Characterization of the microbiome of corals with stony coral tissue loss disease along florida’s coral reef. Microorganisms 9. doi: 10.3390/microorganisms9112181
Conte C., Rotini A., Manfra L., D’Andrea M. M., Winters G., Migliore L. (2021). The seagrass holobiont: what we know and what we still need to disclose for its possible use as an ecological indicator. Water 13, 406. doi: 10.3390/w13040406
Cook T., Folli M., Klinck J., Ford S., Miller J. (1998). The relationship between increasing Sea-surface temperature and the northward spread ofPerkinsus marinus(Dermo) disease epizootics in oysters. Estuar. Coast. Shelf Sci. 46, 587–597. doi: 10.1006/ecss.1997.0283
Cross K. L., Campbell J. H., Balachandran M., Campbell A. G., Cooper C. J., Griffen A., et al. (2019). Targeted isolation and cultivation of uncultivated bacteria by reverse genomics. Nat. Biotechnol. 37, 1314–1321. doi: 10.1038/s41587-019-0260-6
Daisley B. A., Pitek A. P., Chmiel J. A., Al K. F., Chernyshova A. M., Faragalla K. M., et al. (2020). Novel probiotic approach to counter paenibacillus larvae infection in honey bees. ISME J. 14, 476–491. doi: 10.1038/s41396-019-0541-6
Daliri E. B.-M., Tango C. N., Lee B. H., Oh D.-H. (2018). Human microbiome restoration and safety. Int. J. Med. Microbiol. 308, 487–497. doi: 10.1016/j.ijmm.2018.05.002
D’Alvise P. W., Lillebø S., Prol-Garcia M. J., Wergeland H. I., Nielsen K. F., Bergh Ø., et al. (2012). Phaeobacter gallaeciensis reduces vibrio anguillarum in cultures of microalgae and rotifers, and prevents vibriosis in cod larvae. PloS One 7, e43996. doi: 10.1371/journal.pone.0043996
de Jesus H. E., Peixoto R. S., Cury J. C., van Elsas J. D., Rosado A. S. (2015). Evaluation of soil bioremediation techniques in an aged diesel spill at the Antarctic peninsula. Appl. Microbiol. Biotechnol. 99, 10815–10827. doi: 10.1007/s00253-015-6919-0
do Carmo F. L., dos Santos H. F., Martins E. F., van Elsas J. D., Rosado A. S., Peixoto R. S. (2011). Bacterial structure and characterization of plant growth promoting and oil degrading bacteria from the rhizospheres of mangrove plants. J. Microbiol. 49, 535–543. doi: 10.1007/s12275-011-0528-0
Duarte G. A. S., Villela H. D. M., Deocleciano M., Silva D., Barno A., Cardoso P. M., et al (2020). Heat waves are a major threat to turbid coral reefs in Brazil. Front. Mar. 7, 179. doi: 10.3389/fmars.2020.00179
Dyke J. G., Weaver I. S. (2013). The emergence of environmental homeostasis in complex ecosystems. PloS Comput. Biol. 9, e1003050. doi: 10.1371/journal.pcbi.1003050
Eddy T. D., Lam V. W. Y., Reygondeau G., Cisneros-Montemayor A. M., Greer K., Palomares M. L. D., et al. (2021). Global decline in capacity of coral reefs to provide ecosystem services. One Earth 4, 1278–1285. doi: 10.1016/j.oneear.2021.08.016
Egan S., Gardiner M. (2016). Microbial dysbiosis: rethinking disease in marine ecosystems. Front. Microbiol. 7, . doi: 10.3389/fmicb.2016.00991
Epstein P. (2010). The ecology of climate change and infectious diseases: comment. Ecology 91, 925–928. doi: 10.1890/09-0761.1
Filbee-Dexter K., Feehan C. J., Scheibling R. E. (2016). Large-Scale degradation of a kelp ecosystem in an ocean warming hotspot. Mar. Ecol. Prog. Ser. 543, 141–152. doi: 10.3354/meps11554
Finlay B. B., CIFAR Humans, Microbiome (2020). Are noncommunicable diseases communicable? Science 367, 250–251. doi: 10.1126/science.aaz3834
Fonseca E. D. S., Peixoto R. S., Rosado A. S., Balieiro F. D. C., Tiedje J. M., Rachid C. T. C. D. C. (2018). The microbiome of eucalyptus roots under different management conditions and its potential for biological nitrogen fixation. Microb. Ecol. 75, 183–191. doi: 10.1007/s00248-017-1014-y
Gilbert J. A., Jansson J. K., Knight R. (2014). The earth microbiome project: successes and aspirations. BMC Biol. 12, 69. doi: 10.1186/s12915-014-0069-1
Haas A. F., Fairoz M. F. M., Kelly L. W., Nelson C. E., Dinsdale E. A., Edwards R. A., et al. (2016). Global microbialization of coral reefs. Nat. Microbiol. 1, 16042. doi: 10.1038/nmicrobiol.2016.42
Harvell C. D., Kim K., Burkholder J. M., Colwell R. R., Epstein P. R., Grimes D. J., et al. (1999). Emerging marine diseases–climate links and anthropogenic factors. Science 285, 1505–1510. doi: 10.1126/science.285.5433.1505
Hewson I., Sullivan B., Jackson E. W., Xu Q., Long H., Lin C., et al. (2019). Perspective: something old, something new? review of wasting and other mortality in asteroidea (Echinodermata). Front. Mar. Sci. 6. doi: 10.3389/fmars.2019.00406
Hoyt J. R., Langwig K. E., White J. P., Kaarakka H. M., Redell J. A., Parise K. L., et al. (2019). Field trial of a probiotic bacteria to protect bats from white-nose syndrome. Sci. Rep. 9, 9158. doi: 10.1038/s41598-019-45453-z
Jackson J. B., Kirby M. X., Berger W. H., Bjorndal K. A., Botsford L. W., Bourque B. J., et al. (2001). Historical overfishing and the recent collapse of coastal ecosystems. Science 293, 629–637. doi: 10.1126/science.1059199
Jaffe N., Eberl R., Bucholz J., Cohen C. S. (2019). Sea Star wasting disease demography and etiology in the brooding sea star leptasterias spp. PloS One 14, e0225248. doi: 10.1371/journal.pone.0225248
Kleypas J., Allemand D., Anthony K., Baker A. C., Beck M. W., Hale L. Z., et al. (2021). Designing a blueprint for coral reef survival. Biol. Conserv. 257, 109107. doi: 10.1016/j.biocon.2021.109107
Knowlton N., Corcoran E., Felis T., de Goeij J., Grottoli A., Harding S., et al. (2021). ““Rebuilding coral reefs: a decadal grand challenge,”,” in Rebuilding coral reefs: a decadal grand challenge, vol. 56. (Bremen: International Coral Reef Society and Future Earth Coasts).
Küng D., Bigler L., Davis L. R., Gratwicke B., Griffith E., Woodhams D. C. (2014). Stability of microbiota facilitated by host immune regulation: informing probiotic strategies to manage amphibian disease. PloS One 9, e87101. doi: 10.1371/journal.pone.0087101
Leite D. C. A., Salles J. F., Calderon E. N., Castro C. B., Bianchini A., Marques J. A., et al. (2018). Coral bacterial-core abundance and network complexity as proxies for anthropogenic pollution. Front. Microbiol. 9, 833. doi: 10.3389/fmicb.2018.00833
Liu A., Ma T., Xu N., Jin H., Zhao F., Kwok L.-Y., et al. (2021). Adjunctive probiotics alleviates asthmatic symptoms via modulating the gut microbiome and serum metabolome. Microbiol. Spectr. 9, e0085921. doi: 10.1128/Spectrum.00859-21
Lopez J. V., Peixoto R. S., Rosado A. S. (2019). Inevitable future: space colonization beyond earth with microbes first. FEMS Microbiol. Ecol. 95. doi: 10.1093/femsec/fiz127
Lozupone C. A., Knight R. (2007). Global patterns in bacterial diversity. Proc. Natl. Acad. Sci. U.S.A. 104, 11436–11440. doi: 10.1073/pnas.0611525104
McFall-Ngai M., Hadfield M. G., Bosch T. C. G., Carey H. V., Domazet-Lošo T., Douglas A. E., et al. (2013). Animals in a bacterial world, a new imperative for the life sciences. Proc. Natl. Acad. Sci. U.S.A. 110, 3229–3236. doi: 10.1073/pnas.1218525110
Minich J. J., Morris M. M., Brown M., Doane M., Edwards M. S., Michael T. P., et al. (2018). Elevated temperature drives kelp microbiome dysbiosis, while elevated carbon dioxide induces water microbiome disruption. PloS One 13, e0192772. doi: 10.1371/journal.pone.0192772
Modolon F., Barno A. R., Villela H. D. M., Peixoto R. S. (2020). Ecological and biotechnological importance of secondary metabolites produced by coral-associated bacteria. J. Appl. Microbiol. 129, 1441–1457. doi: 10.1111/jam.14766
Nave L. E., Domke G. M., Hofmeister K. L., Mishra U., Perry C. H., Walters B. F., et al. (2018). Reforestation can sequester two petagrams of carbon in US topsoils in a century. Proc. Natl. Acad. Sci. U. S. A. 115, 2776–2781. doi: 10.1073/pnas.1719685115
Nnadi N. E., Carter D. A. (2021). Climate change and the emergence of fungal pathogens. PloS Pathog. 17, e1009503. doi: 10.1371/journal.ppat.1009503
Peixoto R. S., Rosado P. M., Leite D. C., de A., Rosado A. S., Bourne D. G. (2017). Beneficial microorganisms for corals (BMC): proposed mechanisms for coral health and resilience. Front. Microbiol. 8, 341. doi: 10.3389/fmicb.2017.00341
Peixoto R. S., Sweet M., Bourne D. G. (2019). Customized medicine for corals. Front. Mar. Sci. 6, 686. doi: 10.3389/fmars.2019.00686/full
Peixoto R. S., Sweet M., Villela H. D. M., Cardoso P., Thomas T., Voolstra C. R., et al. (2021). Coral probiotics: premise, promise, prospects. Annu. Rev. Anim. Biosci. 9, 265–288. doi: 10.1146/annurev-animal-090120-115444
Peixoto R. S., Voolstra C. R., Sweet M., Duarte C. M., Carvalho S., Villela H., et al. (2022). Harnessing the microbiome to prevent global biodiversity loss. Nat. Microbiol. 7, 1726–1735. doi: 10.1038/s41564-022-01173-1
Petersen C., Round J. L. (2014). Defining dysbiosis and its influence on host immunity and disease. Cell. Microbiol. 16, 1024–1033. doi: 10.1111/cmi.12308
Precht W. (2021). Failure to respond to a coral disease epizootic in Florida: causes and consequences. Rethink. Ecol. 6, 1–47. doi: 10.3897/rethinkingecology.6.56285
Rachid C. T. C. C., Piccolo M. C., Leite D. C. A., Balieiro F. C., Coutinho H. L. C., van Elsas J. D., et al. (2012). Physical-chemical and microbiological changes in cerrado soil under differing sugarcane harvest management systems. BMC Microbiol. 12, 170. doi: 10.1186/1471-2180-12-170
Robert V., Cardinali G., Casadevall A. (2015). Distribution and impact of yeast thermal tolerance permissive for mammalian infection. BMC Biol. 13, 18. doi: 10.1186/s12915-015-0127-3
Rosado P. M., Leite D. C. A., Duarte G. A. S., Chaloub R. M., Jospin G., Nunes da Rocha U., et al. (2019). Marine probiotics: increasing coral resistance to bleaching through microbiome manipulation. ISME J. 13, 921–936. doi: 10.1038/s41396-018-0323-6
Sanders M. E., Merenstein D. J., Reid G., Gibson G. R., Rastall R. A. (2019). Probiotics and prebiotics in intestinal health and disease: from biology to the clinic. Nat. Rev. Gastroenterol. Hepatol. 16, 605–616. doi: 10.1038/s41575-019-0173-3
Santoro E. P., Borges R. M., Espinoza J. L., Freire M., Messias C. S. M. A., Villela H. D. M., et al. (2021). Coral microbiome manipulation elicits metabolic and genetic restructuring to mitigate heat stress and evade mortality. Sci. Adv. 7, eabg3088. doi: 10.1126/sciadv.abg3088
Soga M., Gaston K. J. (2018). Shifting baseline syndrome: causes, consequences, and implications. Front. Ecol. Environ. 16, 222–230. doi: 10.1002/fee.1794
Soniat T. M., Hofmann E. E., Klinck J. M., Powell E. N. (2009). Differential modulation of eastern oyster (Crassostrea virginica) disease parasites by the El-Niño-Southern oscillation and the north Atlantic oscillation. Int. J. Earth Sci. 98, 99–114. doi: 10.1007/s00531-008-0364-6
Steffen W., Persson A., Deutsch L., Zalasiewicz J., Williams M., Richardson K., et al. (2011). The anthropocene: from global change to planetary stewardship. Ambio 40, 739–761. doi: 10.1007/s13280-011-0185-x
Sweet M. J., Bulling M. T. (2017). On the importance of the microbiome and pathobiome in coral health and disease. Front. Mar. Sci. 4. doi: 10.3389/fmars.2017.00009
Tiedje J. M., Bruns M. A., Casadevall A., Criddle C. S., Eloe-Fadrosh E., Karl D. M., et al. (2022). Microbes and climate change: a research prospectus for the future. MBio 13, e0080022. doi: 10.1128/mbio.00800-22
Trinh P., Zaneveld J. R., Safranek S., Rabinowitz P. M. (2018). One health relationships between human, animal, and environmental microbiomes: a mini-review. Front. Public Health 6, 235. doi: 10.3389/fpubh.2018.00235
Tye S. P., Siepielski A. M., Bray A., Rypel A. L., Phelps N. B. D., Fey S. B. (2022). Climate warming amplifies the frequency of fish mass mortality events across north temperate lakes. Limnol. Oceanogr. Lett. 7, 510–519. doi: 10.1002/lol2.10274
van den Nieuwboer M., van de Burgwal L. H. M., Claassen E. (2016). A quantitative key-opinion-leader analysis of innovation barriers in probiotic research and development: valorisation and improving the tech transfer cycle. PharmaNutrition 4, 9–18. doi: 10.1016/j.phanu.2015.09.003
Vilela C. L. S., Damasceno T. L., Thomas T., Peixoto R. S. (2022). Global qualitative and quantitative distribution of micropollutants in the deep sea. Environ. Pollut. 307, 119414. doi: 10.1016/j.envpol.2022.119414
Vilela C. L. S., Villela H. D. M., Rachid C. T. C. D. C., do Carmo F. L., Vermelho A. B., Peixoto R. S. (2021). Exploring the diversity and biotechnological potential of cultured and uncultured coral-associated bacteria. Microorganisms 9. doi: 10.3390/microorganisms9112235
Voolstra C. R., Peixoto R. S., Ferrier-Pagès C. (2023). Mitigating the ecological collapse of coral reef ecosystems: effective strategies to preserve coral reef ecosystems: effective strategies to preserve coral reef ecosystems. EMBO Rep. 24, e56826. doi: 10.15252/embr.202356826
Voolstra C. R., Suggett D. J., Peixoto R. S., Parkinson J. E., Quigley K. M., Silveira C. B., et al. (2021). Extending the natural adaptive capacity of coral holobionts. Nat. Rev. Earth Environ. 2, 747–762. doi: 10.1038/s43017-021-00214-3
Wegh C. A. M., Geerlings S. Y., Knol J., Roeselers G., Belzer C. (2019). Postbiotics and their potential applications in early life nutrition and beyond. Int. J. Mol. Sci. 20. doi: 10.3390/ijms20194673
Weinbauer M. G., Rassoulzadegan F. (2007). REVIEW: extinction of microbes: evidence and potential consequences. Endanger. Species Res. 3, 205–215. doi: 10.3354/esr003205
Wilkins L. G. E., Leray M., O’Dea A., Yuen B., Peixoto R. S., Pereira T. J., et al. (2019). Host-associated microbiomes drive structure and function of marine ecosystems. PloS Biol. 17, e3000533. doi: 10.1371/journal.pbio.3000533
Keywords: microbiome restoration, rehabilitation, probiotics, microbial therapy, climate change, anthropogenic impacts, biodiversity loss
Citation: Peixoto RS and Voolstra CR (2023) The baseline is already shifted: marine microbiome restoration and rehabilitation as essential tools to mitigate ecosystem decline. Front. Mar. Sci. 10:1218531. doi: 10.3389/fmars.2023.1218531
Received: 07 May 2023; Accepted: 16 June 2023;
Published: 30 June 2023.
Edited by:
Daniel Wangpraseurt, University of California, San Diego, United StatesReviewed by:
Marco Fusi, Edinburgh Napier University, United KingdomCopyright © 2023 Peixoto and Voolstra. This is an open-access article distributed under the terms of the Creative Commons Attribution License (CC BY). The use, distribution or reproduction in other forums is permitted, provided the original author(s) and the copyright owner(s) are credited and that the original publication in this journal is cited, in accordance with accepted academic practice. No use, distribution or reproduction is permitted which does not comply with these terms.
*Correspondence: Raquel S. Peixoto, cmFxdWVsLnBlaXhvdG9Aa2F1c3QuZWR1LnNh; Christian R. Voolstra, Y2hyaXN0aWFuLnZvb2xzdHJhQHVuaS1rb25zdGFuei5kZQ==