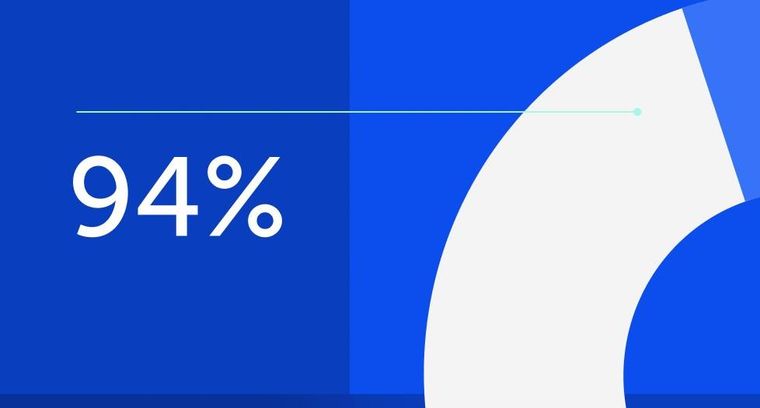
94% of researchers rate our articles as excellent or good
Learn more about the work of our research integrity team to safeguard the quality of each article we publish.
Find out more
ORIGINAL RESEARCH article
Front. Mar. Sci., 22 August 2023
Sec. Marine Biology
Volume 10 - 2023 | https://doi.org/10.3389/fmars.2023.1217779
This article is part of the Research TopicCurrent Research on Fish Otoliths and their ApplicationsView all 10 articles
Fish diversity and ecology in the ocean’s mesopelagic zone are understudied compared to other marine regions despite growing interest in harvesting these potential resources. Otoliths can provide a wealth of taxonomic and life history information about fish, which can help fill these knowledge gaps; however, there has been relatively little research to date on the otoliths of mesopelagic species. Here, a species-specific image library was assembled of sagittal otoliths from 70 mesopelagic fishes belonging to 29 families collected in the western North Atlantic Ocean. Images of adult sagittal otoliths from 12 species were documented and photographed for the first time. The fish were identified to species with a combination of morphological characters and DNA barcoding. Regressions between otolith size and fish length are presented for the six species with the largest sample sizes in this study. This otolith image library, coupled with otolith-length and width to fish-length relationships, can be used for prey identification and back-calculation of fish size, making it a valuable tool for studies relating to food webs in the important yet poorly understood mesopelagic zone. In addition, the 44 fish barcodes generated in this study highlight the benefit of using an integrative taxonomic approach to studies of this nature, as well as add to existing public databases that enable cryptic species and metabarcoding analyses of mesopelagic species.
Mesopelagic fishes are the most abundant fishes in the global ocean (Gjøsaeter and Kawaguchi, 1980; Irigoien et al., 2014). As such, they play a critical role in carbon and energy transfer and contribute to trophic connectivity, especially due to the vertical migration behavior performed by many species (Davison et al., 2013; St. John et al., 2016; Saba et al., 2021). In spite of their abundance, mesopelagic fish species are understudied, and gaps exist in our knowledge of many species’ life history strategies (Caiger et al., 2021). Pelagic predators such as white sharks (Carcharodon carcharias), Atlantic bluefin tuna (Thunnus thynnus), and swordfish (Xiphias gladius) have been found to dive down to feed on mesopelagic fishes, highlighting the importance of the mesopelagic zone to surface systems and fisheries (Karakulak et al., 2009; Battaglia et al., 2013; Gaube et al., 2018; Braun et al., 2019; Arostegui et al., 2022). In one study, mesopelagic and benthopelagic fishes were found to be a large percentage of Atlantic bluefin tuna’s diet (Battaglia et al., 2013). For analysis of digested prey, Battaglia et al. (2013) drew on taxonomic keys, including otolith atlases (Tuset et al., 2008; Battaglia et al., 2010). Mesopelagic otolith identification keys, therefore, are essential for accurate reconstruction of prey for many commercially important species, and for the study of important food web dynamics that include mesopelagic fishes.
Otoliths, or ear stones, are calcareous structures located in the otic capsule in the inner ear of teleost fishes. There are three pairs of otoliths found in most teleosts: sagittae, lapilli, and asterisci, with the sagittae being the largest structure in most fishes, with the exception of Ostariophysi (Campana and Neilson, 1985; Assis, 2003; Volpedo and Fuchs, 2010). Otolith size and shape can be used for taxonomic identification due to their high interspecific variation and intraspecific consistency (Campana, 2004; Yedier and Bostanci, 2022). Gut content analyses commonly use otoliths found in stomachs to identify prey items (Jackson et al., 1998; Brophy et al., 2009; Karakulak et al., 2009; Battaglia et al., 2013). Thus, comprehensive documentation of otoliths for species is essential to increase confidence in identification and facilitate our understanding of mesopelagic food webs.
Several otolith atlases have been constructed as taxonomic identification guides for specific geographic regions (Smale et al., 1995; Rivaton and Bourret, 1999; Campana, 2004; Tuset et al., 2008; Battaglia et al., 2010; Jones and Morales, 2014; Conversani et al., 2017; Volpedo et al., 2017). However, the otoliths for many mesopelagic species are still either undocumented or poorly documented. Furthermore, atlases often only present images from one individual per species. Additional visual documentation is therefore needed to assess not only interspecific differences in otolith morphology, but also intraspecific variation that might occur due to geographical separation and/or ontogenetic changes.
Otoliths can also provide useful ecological insights, as otolith size is correlated with fish size. Especially in gut content analyses, otoliths, along with other calcified structures such as scales, can be used to estimate the size and weight of prey consumed (Jobling and Breiby, 1986; Jackson et al., 1998; Harvey et al., 2000; Brophy et al., 2009). A simple linear regression of otolith size and fish size has been commonly used to describe these relationships for many fish species, though environmental conditions may affect the relationships between calcified structures and fish size (Harvey et al., 2000; Smoliński and Berg, 2022). There are few studies detailing the otolith size and fish size relationships of mesopelagic species, and limited work on these relationships in the western North Atlantic Ocean (Battaglia et al., 2010).
Our study focuses on documenting otoliths from mesopelagic fish species in the western North Atlantic Ocean. Our objectives were to compile species-specific otolith images of mesopelagic fishes and to describe the relationships between otolith length and width and fish standard length. Mesopelagic fishes are often very difficult to identify, due to subtle morphological differences between species, damage during the collection process, or cryptic species traits (Kenchington et al., 2017). As such, the fish specimens used in this study were identified to species with a combination of morphological analyses and DNA barcoding of the mitochondrial cytochrome c oxidase subunit I (COI) gene. The resulting species-specific otolith library and associated collection metadata and barcodes deepen our understanding of variation in the shape and size of otoliths from mesopelagic fishes, documents otoliths of species not previously examined, and facilitate future integrative taxonomic research (Dayrat, 2005).
Fishes were collected on six cruises between 2014 and 2019, five of which were in the Slope Sea off the northeast United States in the western North Atlantic Ocean (waters between the continental shelf and the Gulf Stream; (Csanady and Hamilton, 1988; Richardson et al., 2016), and a sixth cruise in Bahamian waters (Figure 1; see Table S1 for details on the location, and collection depths for each specimen). Three specimens were included from a cruise in Bahamian waters, and these specimens belong to species found throughout the Atlantic Ocean and overlap in distribution with the main study area in the Slope Sea. Sampling was performed with a variety of gear types, including a midwater trawl (as described in De Robertis et al., 2017, but without recapture nets, and we used a 0.64 cm (1/4”) knotless cod-end liner and 3.5 m2 NETS midwater-trawl doors), a 1m2 and 10m2 Multiple Opening Closing Net and Environmental Sensing System (MOCNESS, Wiebe et al., 1976), and a single 10m2 MOCNESS net configured like a Tucker trawl. Fish were flash-frozen in liquid nitrogen or a -80°C freezer upon collection.
Figure 1 Map of fish specimen collection locations. Open circles indicate the locations of each tow used to collect specimens.
Fish specimens were visually identified to the species level when unequivocal (and when morphological characters and/or meristic counts were reliably intact) with the aid of fish identification keys and other sources (e.g. Bigelow, 1965; Nafpaktitus et al., 1977; Carpenter, 2002). Individuals previously flash-frozen were photographed, and standard length (SL) and weight measurements were recorded before dissection. The length was measured to the nearest millimeter using a ruler, and weight was measured to the nearest 0.0001 g using a Mettler Toledo AB204-S scale. Muscle tissue was excised from fish specimens and stored at -80°C until DNA extraction. Heads were removed and frozen at -20°C for future otolith extraction.
DNA barcoding, utilizing the COI marker gene, was conducted for fish that were difficult to identify by morphological criteria alone. Genomic DNA was extracted from the tissue samples with DNEasy Extraction Kits (Qiagen, Germantown, MD, USA) according to the manufacturer’s protocol. The COI gene was amplified with PCR with primers from either Folmer et al. (1994) or Ward et al. (2005) (Fish F1/R1 or Fish F2/R2) (Table S2). The PCR program consisted of 95°C for 3 minutes, 35 cycles of 95°C for 30 seconds, 48°C for 30 seconds, 72°C for 1 minute, and 72°C for 5 minutes. PCR products were visualized on an agarose gel with GelRed (Biotium, Hayward, CA, USA). Amplicons were purified with Qiaquick PCR purification kits (Qiagen, Germantown, MD, USA), quantified using a Nanodrop ND-1000 spectrophotometer (Thermo Fisher Scientific, Waltham, MA, USA), and sent for sequencing to Eurofins Genomics (https://www.eurofinsgenomics.com). Chromatograms were aligned and consensus sequences were generated with Geneious version 9.0.5 (Biomatters, Inc). Sequences were compared to the GenBank database to identify taxonomic matches. All sequences were deposited in GenBank (accession numbers OP114527 – OP114570).
Sagittal otoliths from 171 adult fish were extracted from the otic capsule and placed in 95% ethanol and cleaned of any extra membranous material using forceps. Dry otoliths were placed in plastic vials and labeled by fish sample and left or right side when known. Sagittal otoliths of all samples were photographed with a Canon EOS Rebel T5i camera mounted to a Leica 10447436 1.6x camera tube on a Leica MZ16A stereomicroscope. Otoliths that were chipped or damaged badly were excluded from further analysis. Photographs of sagittal otoliths were taken with reflected light against a black background for the otolith library. The microscope magnification was recorded for each photograph. Photographs were taken at each microscope magnification with a ruler placed in the field of view to convert pixels to millimeters.
Images were analyzed and measured for otolith length (OL) and otolith width (OW). Measurements were taken by calibrating the magnification of each otolith photograph to millimeters and using the computer image analysis system ImageJ (https://imagej.nih.gov/ij/). Otolith length was measured by recording the “greatest distance from the anterior rostrum to the posterior edge, parallel to the sulcus” (sensu Harvey et al., 2000). Otolith width was measured as the distance between the dorsal and ventral edges, perpendicular to the sulcus (Battaglia et al., 2010). For more information on otolith terminology and morphology, see Tuset et al. (2008).
Adobe Photoshop was used for image processing, for instance to enhance visual contrast where necessary. All species from the study were included in the visual identification library. Due to the nature of otolith morphological change through ontogeny, only otoliths from adult specimens were chosen to provide the most representative visualization of unique otolith morphological features that develop over the course of the fish’s life. Additional photographs of all individuals are publicly available through the WHOI repository (https://hdl.handle.net/1912/66723, DOI: 10.26025/1912/66723).
Simple linear regressions were fitted to OL vs. fish SL, and OW vs. fish SL for six species. These relationships were examined for species that had seven or more individuals. Species that had fewer samples were not included in this analysis due to there being too few individuals to create an informative regression. Numerous studies have shown statistically insignificant differences between left and right otoliths for the purpose of identification and linear regressions (Harvey et al., 2000; Bilge, 2013; Jones and Morales, 2014; Battaglia et al., 2015; Dehghani et al., 2016; Wang et al., 2019), so the otolith side was chosen at random or one side was picked over the other if one was damaged or chipped.
A total of 70 fish species, from 29 families, were identified. Forty-four specimens were DNA barcoded (Table S2). All but three of the barcode sequences were 99-100% identical to reference sequences on GenBank. Typically, within-species variation in COI sequences is within 1% (Ward et al., 2005; Ward et al., 2009), and 97-98% identity is used as a species-level threshold in COI metabarcoding taxonomic assignment for fish (e.g. Hatzenbuhler et al., 2017; Kimmerling et al., 2018; Duke and Burton, 2020). For nearly all specimens, our results were indicative of species identification. A few fish showed possible species-level matches to multiple species, and for these, the species assignments were based on the visual assessment. These included two specimens that were >99% identical to both Scopelogadus beanii and Scopelogadus mizolepis reference sequences, consistent with previous studies that have noted indistinguishable COI sequences from these nominally different species (Ward et al., 2009; Kenchington et al., 2017). The other specimens that had a high percent identity to multiple species were Borostomias mononema (similar to Neonesthes capensis), and Notoscopelus kroyeri (similar to Notoscopelus elongatus). The three specimens that did not show ≥99% similarity to any reference sequences were identified visually as follows: Ariomma lurida (closest GenBank match was Ariomma lurida with 97.5% identity), Paracaristius maderensis (closest GenBank match was Caristius macropus with 94.7% identity), and Astronesthes sp. (closest GenBank match was Astronesthes formosana with 94.9% identity) (Table S2).
Our otolith catalog features photos from all 70 species, grouped by family (Figures 2–5). Twelve of the 70 otoliths are from species whose adult otoliths have not previously been documented with photographs. These species are: Ariomma lurida, Aristostomias tittmanni, Bathylagichthys greyae, Bonapartia pedaliota, Dolicholagus longirostris, Dolopichthys karsteni, Howella brodiei, Melanostomias bartonbeani, Nansenia longicauda, Paracaristius maderensis, Photostomias goodyeari, and Pollichthys mauli. Among these 12 species, two had sketches drawn of these otoliths but no photographs (Bonapartia pedaliota and Dolicholagus longirostris), and the remaining 10 species have not previously had their otoliths documented in any capacity (Table S3).
Figure 2 Sagittal otoliths from the family Myctophidae. Metadata in caption below for each otolith includes: species, fish SL (mm); photographed from distal (D), proximal (P) or unknown (U) view; orientation to top of figure either anterior (A), posterior (P) or unknown (U); left (L), right (R) or unknown (U) otolith. (A) Benthosema glaciale, 50, D, A, R; (B) Bolinichthys photothorax, 64, D, A, U; (C) Ceratoscopelus maderensis, 56, D, A, L; (D) Ceratoscopelus warmingii, 61, D, A, L; (E) Diaphus perspicillatus, 42, P, A, L; (F) Hygophum hygomii, 48, D, A, L; (G) Lampadena speculigera, 88, P, A, L; (H) Lepidophanes guentheri, 62, D, A, U; (I) Lobianchia gemellarii, 90, D, A, L; (J) Myctophum affine, 42, P, A, U; (K) Myctophum obtusirostre, 30, P, A, U; (L) Myctophum punctatum, 32, D, A, U; (M) Nannobrachium atrum, 108, P, U, U; (N) Nannobrachium lineatum, 100, P, U, L; (O) Notoscopelus caudispinosus, 71, P, A, U; (P) Notoscopelus kroyeri, 38, D, A, U; (Q) Notoscopelus resplendens, 67, P, A, R; (R) Symbolophorus veranyi, 52, P, A, L; (S) Taaningichthys bathyphilus, 60, P, U, R. Scale bar below each otolith is 0.5 mm.
Figure 3 Sagittal otoliths from Stomiiform fishes. Metadata in caption below for each otolith includes: species, fish SL (mm); photographed from distal (D), proximal (P) or unknown (U) view; orientation to top of figure either anterior (A), posterior (P) or unknown (U); left (L), right (R) or unknown (U) otolith. (A) Bonapartia pedaliota 48, D, A, L; (B) Cyclothone microdon, 49, U, U, R; (C) Sigmops elongatus, 176, D, A, U; (D) Pollichthys mauli, 42, U, U, U; (E) Vinciguerria nimbaria, 40, U, U, U; (F) Argyropelecus aculeatus, 57, D, U, L; (G) Argyropelecus hemigymnus, 29, D, A, L; (H) Sternoptyx diaphana, 26, D, A, R; (I) Trigonolampa miriceps, 240, U, A, L; (J) Aristostomias tittmanni, 94, U, U, L; (K) Astronesthes sp., 50, U, U, U; (L) Borostomias mononema, 130, U, A, U; (M) Chauliodus sloani, 208, U, U, R; (N) Idiacanthus fasciola, 215, D, U, L; (O) Malacosteus niger, 218, P, U, R; (P) Melanostomias bartonbeani, 241, U, A, L; (Q) Photostomias goodyeari, 107 U, U, R; (R) Photostomias guernei, 93, U, U, L; (S) Stomias boa, 149, U, U, R; (T) Stomias longibarbatus, 233, U, U, U. Scale bar below each otolith is 0.5 mm.
Figure 4 Sagittal otoliths from mesopelagic fishes. Metadata in caption below for each otolith includes: species, fish SL (mm); photographed from distal (D), proximal (P) or unknown (U) view; orientation to top of figure either anterior (A), posterior (P) or unknown (U); left (L), right (R) or unknown (U) otolith. (A) Alepisaurus ferox, U, U, U, U; (B) Bathylagichthys greyae, 133, U, A, R; (C) Bathylagus euryops, 120, U, A, U; (D) Dolicholagus longirostris, 119, D, A, L; (E) Chlorophthalmus agassizi, 46, P, A, U; (F) Derichthys serpentinus, 265, U, U, U; (G) Coccorella atlantica, 141, P, A, L; (H) Xenolepidichthys dalgleishi, 31, U, U, U; (I) Melanonus zugmayeri, 131, U, U, L; (J) Nansenia longicauda, 116, P, P, L; (K) Physiculus fulvus, 43, D, A, U; (L) Nemichthys scolopaceus, 485, U, A, L; (M) Arctozenus risso, 161, P, A, L; (N) Serrivomer beanii, 535, D, A, R; (O) Serrivomer lanceolatoides, 270, D, U, L. Scale bar below each otolith is 0.5 mm.
Figure 5 Sagittal otoliths from mesopelagic fishes. Metadata in caption below for each otolith includes: species, fish SL (mm); photographed from distal (D), proximal (P) or unknown (U) view; orientation to top of figure either anterior (A), posterior (P) or unknown (U); left (L), right (R) or unknown (U) otolith. (A) Anoplogaster cornuta, 108, U, U, L; (B) Ariomma lurida, 40, D, A, U; (C) Antigonia capros, 22, D, U, U; (D) Paracaristius maderensis, 80, U, U, U; (E) Dolopichthys karsteni, 46, U, A, U; (F) Chiasmodon niger, 106, P, U, L; (G) Diplospinus multistriatus, U, P, A, L; (H) Nealotus tripes, 146, D, A, L; (I) Howella brodiei, 97, P, U, R; (J) Poromitra capito, 39, D, A, L; (K) Poromitra megalops, 65, U, U, L; (L) Scopleogadus beanii, 112, U, U, L; (M) Scopelogadus mizolepis, 109, U, U, L; (N) Cubiceps pauciradiatus, 88, D, A, U; (O) Scombrolabrax heterolepis, 95, D, A, U; (P) Benthodesmus simonyi, 229, P, U, U. Scale bar below each otolith is 0.5 mm.
Otolith length and width are positively correlated with fish SL (Figures 6, 7; Table 1). All species we examined show a significant relationship between OL and SL, and OW and SL (p-values less than 0.006). The correlation coefficients ranged from 0.52 to 0.99. Regressions using otolith length had higher r2 values than regressions using otolith width for eight of the twelve regressions.
Table 1 Relationships of fish standard length (SL, mm) to otolith length (OL, mm) and otolith width (OW, mm).
Our photographic library, like other similar atlases (eg. Campana, 2004; Tuset et al., 2008; Battaglia et al., 2010; Jones and Morales, 2014), provides informative documentation of sagittal otolith shapes and sizes of mesopelagic fishes. The fish specimens in our library were identified with a combination of morphological criteria and DNA barcoding.
This extensive otolith atlas serves as a taxonomic guide with applications in biological, paleontological, and archaeological studies, particularly those exploring food web analyses. Otoliths recovered from gut contents can be a valuable ecological tool in reconstructing food web dynamics. Furthermore, mesopelagic fishes are understudied, and the role that mesopelagic species play in the biological carbon pump is recognized to be significant; however, major gaps in our knowledge of mesopelagic ecology exist, making it a priority for research (St. John et al., 2016; Martin et al., 2020; Saba et al., 2021)
In addition to the otolith library, the DNA barcodes generated in this study add to existing barcode databases which are foundational for DNA metabarcoding (e.g. Bucklin et al., 2016). Metabarcoding is increasingly used in environmental DNA (eDNA), zooplankton, and gut content studies (e.g. Casey et al., 2019; Ruppert et al., 2019; Blanco-Bercial, 2020; Govindarajan et al., 2021). Most of our barcodes were matched to barcodes already in GenBank, but our specimens add to knowledge of intraspecific variation, which is critical for detecting cryptic diversity and biogeographical patterns (Gaither et al., 2016; Kenchington et al., 2017; Christiansen et al., 2018). Furthermore, the association of our barcodes with our otolith data will facilitate future integrative taxonomic work (i.e., that incorporates multiple lines of evidence, including both genetic and morphological) (Dayrat, 2005). Of the 12 otoliths presented here that have not been previously described and photographed, seven were identified to the species level with DNA barcoding, highlighting the importance of integrative taxonomic work. Additional documentation and analyses of both otoliths and DNA barcodes of mesopelagic fishes should be conducted in order to aid in our understanding of the ecology, trophic dynamics, and importance of these species.
Numerous considerations are important when using otoliths as a means of identifying species. Otoliths resist degradation and are stable when dry; though, calcium carbonate is soluble in acid, meaning that if they remain in the stomach for too long, otoliths, especially small ones, can dissolve and lose their species-specific morphology (Jobling and Breiby, 1986; Campana, 2004). Ontogenetic morphological change in otoliths is also important to consider, as shape and features vary throughout the course of development (de Carvalho et al., 2015; Jawad et al., 2018). Otoliths collected from young fishes may not yet have formed a species-specific shape, rendering fish identification via otolith morphology impossible (Campana, 2004; Deng et al., 2013). To account for this, we only extracted otoliths from adult specimens, based on the representative sizes of adults in the literature for each species. However, we did not determine ages, and many species had small sample sizes, so it is possible that a photograph presented may not be completely characteristic of the adult form of a species. Due to a lack of samples representing all age classes, we were unable to characterize ontogenetic changes in otolith morphology.
This library does not include otolith morphological descriptions; however, there are studies that focus on otolith morphometrics that can be used in conjunction with this library for more precision in identifying closely related species (Tuset et al., 2003; Škeljo and Ferri, 2012; Jawad et al., 2018). Additionally, abnormalities in otolith shape have been found in species, due to genetic or environmental factors (Yedier et al., 2023). In one study examining otoliths of the benthopelagic fish, Atherinella brasiliensis, four of 45 sagittal otoliths were determined to be anomalous in shape (de Carvalho Lapuch et al., 2022). As some of the species presented here had small samples sizes, there is a possibility that the otolith presented is not characteristic of that species, but rather anomalous. Future work should focus on describing the surface morphology and characteristics of the otoliths presented here to aid in identifying species and detecting anomalous otoliths, especially for the otoliths that are documented here for the first time.
Our study found that otolith size (length and width) and fish standard length are significantly correlated in the species we examined. Both otolith length and otolith width led to informative, statistically significant regressions. However, the SL regressions had higher adjusted r2 values when using the OL compared to OW for a majority of the regressions examined. Otolith length commonly shows a more robust correlation to fish length than otolith width (Dehghani et al., 2016). For species that have otoliths that are easily chipped like Sigmops elongatus, OW can still provide an informative regression and may be more practical in certain circumstances. This tactic could likely be employed in species such as Bathylagichthys greyae and Dolicholagus longirostris, as their pyriform otolith shape is similar to that of Sigmops elongatus.
While these regressions can be useful, otolith-size to fish-length relationships can vary in different populations of the same species or with varying growth rates (Campana, 2004). Otolith degradation from an extended period of time in the predator’s stomach has been documented, which could make estimates of fish size inaccurate (Jobling and Breiby, 1986). Otolith-size to fish-size relationships have also been found to be less clear for the juvenile stages of fishes (Hare and Cowen, 1995). Additional samples per species would enable a better understanding of the otolith-size to fish-size relationships, especially for the species with small sample sizes for which we do not present regressions. A greater number of specimens could also help improve the accuracy of the regressions by broadening the range of otolith sizes used to fit otolith-size to fish-size regressions. Many other similar studies benefit from larger sample sizes (eg. Bilge, 2013; Battaglia et al., 2015). We also were unable to statistically analyze differences between left and right-side otoliths due to damage on some otoliths, so we included the left or the right otolith from each individual in our regressions. Future work should test for differences between otolith side for the species presented here.
Sagittal otoliths of a large diversity of ecologically important mesopelagic fishes are documented here in this library. Furthermore, an integrative taxonomic process including visual and genetic identification proved to be highly effective for this work, enabling the identification of species with otoliths not previously documented. Given the relative paucity of research on mesopelagic fishes, and otolith documentation of these species in particular, this work effectively builds on that limited knowledge base, deepening our understanding of the ecology and trophodynamics of fishes in the mesopelagic. However, there is still much to understand, and more detailed analyses of otoliths, including the lapilli and asterisci, morphological descriptions, as well as additional documentation of DNA barcodes of mesopelagic fishes would continue to further this knowledge.
The datasets presented in this study can be found in online repositories. The names of the repository/repositories and accession number(s) can be found in the article/Supplementary Material.
The animal study was approved by WHOI Animal Care and Use Committee. The study was conducted in accordance with the local legislation and institutional requirements.
JL, PC, and LQ designed the study. AL, HS, JJ, JL, HM, and PC collected samples. LQ, HM, PC, JL and AG performed analyses. LQ wrote the manuscript with input from all authors. All authors contributed to the article and approved the submitted version
This work was part of the Woods Hole Oceanographic Institution’s Ocean Twilight Zone Project, funded as part of the Audacious Project housed at TED. Sample collection on Northeast U.S. Shelf Long-Term Ecological Research (NES-LTER) cruises was enabled by funding from the National Science Foundation [grant OCE-1655686 to HS and JL].
We thank Sarah Glancy, Thomas Heine, and Betsy Broughton for assistance with sample collection, and Rene Francolini, Katie Pell, and Erin Frates for assistance with DNA barcoding.
The authors declare that the research was conducted in the absence of any commercial or financial relationships that could be construed as a potential conflict of interest.
All claims expressed in this article are solely those of the authors and do not necessarily represent those of their affiliated organizations, or those of the publisher, the editors and the reviewers. Any product that may be evaluated in this article, or claim that may be made by its manufacturer, is not guaranteed or endorsed by the publisher.
The Supplementary Material for this article can be found online at: https://www.frontiersin.org/articles/10.3389/fmars.2023.1217779/full#supplementary-material
Arostegui M. C., Gaube P., Woodworth-Jefcoats P. A., Kobayashi D. R., Braun C. D. (2022). Anticyclonic eddies aggregate pelagic predators in a subtropical gyre. Nature 609 (7927), 535–540. doi: 10.1038/s41586-022-05162-6
Assis C. A. (2003). The lagenar otoliths of teleosts: their morphology and its application in species identification, phylogeny and systematics. J. Fish Biol. 62 (6), 1268–1295. doi: 10.1046/j.1095-8649.2003.00106.x
Battaglia P., Andaloro F., Consoli P., Esposito V., Malara D., Musolino S., et al. (2013). Feeding habits of the Atlantic bluefin tuna, Thunnus thynnus (L. 1758), in the central Mediterranean Sea (Strait of Messina). Helgoland Mar. Res. 67 (1), 97–107. doi: 10.1007/s10152-012-0307-2
Battaglia P., Malara D., Ammendolia G., Romeo T., Andaloro F. (2015). Relationships between otolith size and fish length in some mesopelagic teleosts (Myctophidae, Paralepididae, Phosichthyidae and Stomiidae). J. Fish Biol. 87 (3), 774–782. doi: 10.1111/jfb.12744
Battaglia P., Malara D., Romeo T., Andaloro F. (2010). Relationships between otolith size and fish size in some mesopelagic and bathypelagic species from the Mediterranean Sea (Strait of Messina, Italy). Scientia Marina 74 (3), 605–612. doi: 10.3989/scimar.2010.74n3605
Bigelow H. B. (1965). Fishes of the Western North Atlantic, Part four. Order isospondyli (part), suborder argentinoidea, suborder stomiatoidea, suborder esocoidea, suborder bathylaconoidea, order giganturoidei. Sears Foundation Mar. Res. 1, 599.
Bilge G. (2013). Otolith size—fish size relations in the jewel lanternfish, Lampanyctus crocodilus (Actinopterygii: Myctophiformes: Myctophidae), from deepwater environment of the southern Aegean Sea. Acta Ichthyologica Et Piscatoria 43 (4), 293–296. doi: 10.3750/AIP2013.43.4.05
Blanco-Bercial L. (2020). Metabarcoding analyses and seasonality of the zooplankton community at BATS. Front. Mar. Sci. 7. doi: 10.3389/FMARS.2020.00173/BIBTEX
Braun C. D., Gaube P., Afonso P., Fontes J., Skomal G. B., Thorrold S. R. (2019). Assimilating electronic tagging, oceanographic modelling, and fisheries data to estimate movements and connectivity of swordfish in the North Atlantic. ICES J. Mar. Sci. 76 (7), 2305–2317. doi: 10.1093/icesjms/fsz106
Brophy J. T., Murphy S., Rogan E. (2009). The diet and feeding ecology of short-beaked common dolphin (Delphinus delphis) in the northeast Atlantic. Paper SC/61/SM 14. Int. Whaling Commission, 1–18.
Bucklin A., Lindeque P. K., Rodriguez-Ezpeleta N., Albaina A., Lehtiniemi M. (2016). Metabarcoding of marine zooplankton: prospects, progress and pitfalls. J. Plankton Res. 38 (3), 393–400. doi: 10.1093/PLANKT/FBW023
Caiger P. E., Lefebve L. S., Llopiz J. K. (2021). Growth and reproduction in mesopelagic fishes: a literature synthesis. ICES J. Mar. Sci. 78 (3), 765–781. doi: 10.1093/ICESJMS/FSAA247
Campana S. E. (2004). Photographic atlas of fish otoliths of the Northwest Atlantic Ocean. Ottawa, Ontario: NRC Research Press. 284. doi: 10.1139/9780660191089
Campana S. E., Neilson J. D. (1985). Microstructure of fish otoliths. Can. J. Fisheries Aquat. Sci. 42 (5), 1014–1032. doi: 10.1139/f85-127
Carpenter K. E. (Ed.) (2002). “The living marine resources of the Western Central Atlantic. Volume 2: Bony fishes part 1 (Acipenseridae to Grammatidae),” in FAO species identification guide for fishery purposes & American society of ichthyologists and herpetologists special publication, vol. 5 (Rome: FAO), 601–1374.
Casey J. M., Meyer C. P., Morat F., Brandl S. J., Planes S., Parravicini V. (2019). Reconstructing hyperdiverse food webs: Gut content metabarcoding as a tool to disentangle trophic interactions on coral reefs. Methods Ecol. Evol. 10 (8), 1157–1170. doi: 10.1111/2041-210X.13206
Christiansen H., Dettai A., Heindler F. M., Collins M. A., Duhamel G., Hautecoeur M., et al. (2018). Diversity of mesopelagic fishes in the Southern Ocean - a phylogeographic perspective using DNA barcoding. Front. Ecol. Evol. 6 (SEP). doi: 10.3389/FEVO.2018.00120/BIBTEX
Conversani V. R. M., Brenha-Nunes M. R., Santificetur C., Giaretta M. B., Siliprandi C. C., Rossi-Wongtschowski C. L. D. B. (2017). Atlas of marine bony fish otoliths (sagittae) of Southeastern-Southern Brazil Part VII: Atheriniformes, Beloniformes, Beryciformes, Zeiformes, Syngnathiformes, Scorpaeniformes and Tetraodontiformes. Braz. J. Oceanography 65 (3), 400–447. doi: 10.1590/s1679-87592017134306503
Csanady G. T., Hamilton P. (1988). Circulation of slopewater. Continental Shelf Res. 8 (5–7), 565–624. doi: 10.1016/0278-4343(88)90068-4
Davison P. C., Checkley D. M., Koslow J. A., Barlow J. (2013). Carbon export mediated by mesopelagic fishes in the northeast Pacific Ocean. Prog. Oceanography 116, 14–30. doi: 10.1016/j.pocean.2013.05.013
Dayrat B. (2005). Towards integrative taxonomy. Biol. J. Linn. Soc. 85 (3), 407–415. doi: 10.1111/j.1095-8312.2005.00503.x
de Carvalho B. M., Vaz-dos-Santos A. M., Spach H. L., Volpedo A. V. (2015). Ontogenetic development of the sagittal otolith of the anchovy, Anchoa tricolor, in a subtropical estuary. Scientia Marina 79 (4), 409–418. doi: 10.3989/scimar.04218.31A
de Carvalho Lapuch I., Carvalho B. M. D., Baptista Metri C. (2022). First record of anomalous otoliths in Atherinella brasiliensis. J. Appl. Ichthyology 38, 109–113. doi: 10.1111/jai.14255
Dehghani M., Kamrani E., Salarpouri A., Sharifian S. (2016). Otolith dimensions (length, width), otolith weight and fish length of Sardinella sindensis (Day 1878), as index for environmental studies, Persian Gulf, Iran. Mar. Biodiver. Rec. 9, 44. doi: 10.1186/s41200-016-0039-0
Deng X., Wagner H.-J., Popper A. N. (2013). Interspecific variations of inner ear structure in the deep-sea fish family Melamphaidae. Anatomical Rec. 296 (7), 1064–1082. doi: 10.1002/ar.22703
De Robertis A., Taylor K. C., Williams K., Wilson C. (2017). Species and size selectivity of two midwater trawls used in an acoustic survey of the Alaska Arctic. Deep-Sea Res. II 135, 40–50. doi: 10.1016/j.dsr2.2015.11.014
Duke E. M., Burton R. S. (2020). Efficacy of metabarcoding for identification of fish eggs evaluated with mock communities. Ecol. Evol. 10 (7), 3463–3476. doi: 10.1002/ECE3.6144
Folmer O., Black M., Hoeh W., Lutz R., Vrijenhoek R. (1994). DNA primers for amplification of mitochondrial cytochrome c oxidase subunit I from diverse metazoan invertebrates. Mol. Mar. Biol. Biotechnol. 3 (5), 294–299.
Gaither M. R., Bowen B. W., Rocha L. A., Briggs J. C. (2016). Fishes that rule the world: circumtropical distributions revisited. Fish Fisheries 17 (3), 664–679. doi: 10.1111/FAF.12136
Gaube P., Braun C. D., Lawson G. L., McGillicuddy D. J., Penna A., Skomal G. B., et al. (2018). Mesoscale eddies influence the movements of mature female white sharks in the Gulf Stream and Sargasso Sea. Sci. Rep. 8 (1), 7363. doi: 10.1038/s41598-018-25565-8
Gjøsaeter J., Kawaguchi K. (1980). A review of the world resources of mesopelagic fish. FAO Fisheries Tech. Paper 193, 1–151.
Govindarajan A. F., Francolini R. D., Jech J. M., Lavery A. C., Llopiz J. K., Wiebe P. H., et al. (2021). Exploring the use of environmental DNA (eDNA) to detect animal taxa in the mesopelagic zone. Front. Ecol. Evol. 9. doi: 10.3389/FEVO.2021.574877/BIBTEX
Hare J. A., Cowen R. K. (1995). Effect of age, growth rate, and ontogeny on the otolith size – fish size relationship in bluefish, Pomatomus saltatrix, and the implications for back-calculation of size in fish early life history stages. Can. J. Fisheries Aquat. Sci. 52 (9), 1909–1922. doi: 10.1139/f95-783
Harvey J. T., Loughlin T. R., Perez M., Oxman D. S. (2000). Relationship between fish size and otolith length for 63 species of fishes from the Eastern North Pacific Ocean. NOAA Tech. Rep. NMFS 150, 1–36.
Hatzenbuhler C., Kelly J. R., Martinson J., Okum S., Pilgrim E. (2017). Sensitivity and accuracy of high-throughput metabarcoding methods for early detection of invasive fish species. Sci. Rep. 7 (1), 1–10. doi: 10.1038/srep46393
Irigoien X., Klevjer T. A., Røstad A., Martinez U., Boyra G., Acuña J. L., et al. (2014). Large mesopelagic fishes biomass and trophic efficiency in the open ocean. Nat. Commun. 5 (1), 3271. doi: 10.1038/ncomms4271
Jackson G. D., McKinnon J. F., Lalas C., Ardern R., Buxton N. G. (1998). Food spectrum of the deepwater squid Moroteuthis ingens (Cephalopoda: Onychoteuthidae) in New Zealand waters. Polar Biol. 20 (1), 56–65. doi: 10.1007/s003000050276
Jawad L. A., Sabatino G., Ibáñez A. L., Andaloro F., Battaglia P. (2018). Morphology and ontogenetic changes in otoliths of the mesopelagic fishes Ceratoscopelus maderensis (Myctophidae), Vinciguerria attenuata and V. poweriae (Phosichthyidae) from the Strait of Messina (Mediterranean Sea). Acta Zoologica 99 (2), 126–142. doi: 10.1111/azo.12197
Jobling M., Breiby A. (1986). The use and abuse of fish otoliths in studies of feeding habits of marine piscivores. Sarsia 71 (3–4), 265–274. doi: 10.1080/00364827.1986.10419696
Jones W. A., Morales M. M. (2014). Catalog of otoliths of select fishes from the California Current System (UC San Diego: Scripps Institution of Oceanography). Available at: http://escholarship.org/uc/item/5m69146s.
Karakulak F. S., Salman A., Oray I. K. (2009). Diet composition of bluefin tuna (Thunnus thynnus L. 1758) in the Eastern Mediterranean Sea, Turkey. J. Appl. Ichthyology 25 (6), 757–761. doi: 10.1111/J.1439-0426.2009.01298.X
Kenchington E. L., Baillie S. M., Kenchington T. J., Bentzen P. (2017). Barcoding Atlantic Canada’s mesopelagic and upper bathypelagic marine fishes. PloS One 12 (9), e0185173. doi: 10.1371/JOURNAL.PONE.0185173
Kimmerling N., Zuqert O., Amitai G., Gurevich T., Armoza-Zvuloni R., Kolesnikov I., et al. (2018). Quantitative species-level ecology of reef fish larvae via metabarcoding. Nat. Ecol. Evol. 2 (2), 306–316. doi: 10.1038/S41559-017-0413-2
Martin A., Boyd P., Buesseler K., Cetinic I., Claustre H., Giering S., et al. (2020). The oceans’ twilight zone must be studied now, before it is too late. Nature 580 (7801), 26–28. doi: 10.1038/d41586-020-00915-7
Nafpaktitus B., Backus R., Craddock J., Haedrich R., Robison B., Karnella C. (1977). Fishes of the Western North Atlantic, part 7. Order iniomi (Myctophiformes): neoscopelids, lanternfishes, and atlantic mesopelagic zoogeographyes of the Western North Atlantic. Sears Foundation Mar. Res. 1, 299.
Richardson D. E., Marancik K. E., Guyon J. R., Lutcavage M. E., Galuardi B., Lam C. H., et al. (2016). Discovery of a spawning ground reveals diverse migration strategies in Atlantic bluefin tuna (Thunnus thynnus). Proc. Natl. Acad. Sci. United States America 113 (12), 3299–3304. doi: 10.1073/PNAS.1525636113
Rivaton J., Bourret P. (1999). Les otolithes des poissons de l’Indo-Pacifique. IRD. Doc. Sci. Tech. II 2, 378.
Ruppert K. M., Kline R. J., Rahman M. S. (2019). Past, present, and future perspectives of environmental DNA (eDNA) metabarcoding: A systematic review in methods, monitoring, and applications of global eDNA. Global Ecol. Conserv. 17, e00547. doi: 10.1016/J.GECCO.2019.E00547
Saba G. K., Burd A. B., Dunne J. P., Hernández-León S., Martin A. H., Rose K. A., et al. (2021). Toward a better understanding of fish-based contribution to ocean carbon flux. Limnology Oceanography 66 (5), 1639–1664. doi: 10.1002/lno.11709
Škeljo F., Ferri J. (2012). The use of otolith shape and morphometry for identification and size-estimation of five wrasse species in predator-prey studies. J. Appl. Ichthyology 28 (4), 524–530. doi: 10.1111/j.1439-0426.2011.01925.x
Smale M. J., Watson G. W., Hecht T. (1995). Otolith atlas of Southern African marine fishes. Ichthyological Monographs, 1. Grahamstown, South Africa: J.L.B. Smith Institute of Ichthyology. xiv, 253. doi: 10.5962/bhl.title.141860
Smoliński S., Berg F. (2022). Varying relationships between fish length and scale size under changing environmental conditions–multidecadal perspective in Atlantic herring. Ecol. Indic. 134, 108494. doi: 10.1016/j.ecolind.2021.108494
St. John M. A., Borja A., Chust G., Heath M., Grigorov I., Mariani P., et al. (2016). A dark hole in our understanding of marine ecosystems and their services: Perspectives from the mesopelagic community. Front. Mar. Sci. 3 (MAR). doi: 10.3389/FMARS.2016.00031/BIBTEX
Tuset V. M., Lombarte A., Assis C. A. (2008). Otolitos de peces del mediterráneo occidental y del atlántico central y nororiental. Scientia Marina, 72 (S1), 7–198. doi: 10.3989/scimar.2008.72s17
Tuset V. M., Lombarte A., González J. A., Pertusa J. F., Lorente M. (2003). Comparative morphology of the sagittal otolith in Serranus spp. J. Fish Biol. 63 (6), 1491–1504. doi: 10.1111/j.1095-8649.2003.00262.x
Volpedo A. V., Fuchs D. V. (2010). Ecomorphological patterns of the lapilli of Paranoplatense Siluriforms (South America). Fisheries Res. 102 (1-2), 160–165. doi: 10.1016/j.fishres.2009.11.007
Volpedo A. V., Thompson G. A., Avigliano E. (2017). Atlas de otolitos de peces de Argentina (Buenos Aires: CAFP-BACAPES).
Wang Y., Zhang J., Chen Z., Jiang Y., Xu S., Li Z., et al. (2019). Age and growth of Myctophum asPerum in the South China Sea based on otolith microstructure analysis. Deep Sea Res. Part II: Topical Stud. Oceanography 167, 121–127. doi: 10.1016/j.dsr2.2018.07.004
Ward R. D., Hanner R., Hebert P. D. N. (2009). The campaign to DNA barcode all fishes, FISH-BOL. J. Fish Biol. 74 (2), 329–356. doi: 10.1111/j.1095-8649.2008.02080.x
Ward R. D., Zemlak T. S., Innes B. H., Last P. R., Hebert P. D. N. (2005). DNA barcoding Australia’s fish species. Philos. Trans. R. Soc. B: Biol. Sci. 360 (1462), 1847–1857. doi: 10.1098/RSTB.2005.1716
Wiebe P. H., Burt K. H., Boyd S. H., Morton A. W. (1976). A multiple opening/closing net and environmental sensing system for sampling zooplankton. J. Mar. Res. 34 (3), 313–326.
Yedier S., Bostanci D. (2022). Molecular and otolith shape analyses of Scorpaena spp. in the Turkish seas. Turkish J. Zoology 46 (1), 78–92. doi: 10.3906/zoo-2105-26
Keywords: sagitta, midwater fish, DNA barcoding, otolith, integrative taxonomy
Citation: Quigley LA, Caiger PE, Govindarajan AF, McMonagle H, Jech JM, Lavery AC, Sosik HM and Llopiz JK (2023) Otolith characterization and integrative species identification of adult mesopelagic fishes from the western North Atlantic Ocean. Front. Mar. Sci. 10:1217779. doi: 10.3389/fmars.2023.1217779
Received: 05 May 2023; Accepted: 07 August 2023;
Published: 22 August 2023.
Edited by:
Josipa Ferri, University of Split, CroatiaReviewed by:
Alejandra Vanina Volpedo, University of Buenos Aires, ArgentinaCopyright © 2023 Quigley, Caiger, Govindarajan, McMonagle, Jech, Lavery, Sosik and Llopiz. This is an open-access article distributed under the terms of the Creative Commons Attribution License (CC BY). The use, distribution or reproduction in other forums is permitted, provided the original author(s) and the copyright owner(s) are credited and that the original publication in this journal is cited, in accordance with accepted academic practice. No use, distribution or reproduction is permitted which does not comply with these terms.
*Correspondence: Lucinda A. Quigley, bHF1aWdsZXlAdWNzZC5lZHU=; Joel K. Llopiz, amxsb3BpekB3aG9pLmVkdQ==
Disclaimer: All claims expressed in this article are solely those of the authors and do not necessarily represent those of their affiliated organizations, or those of the publisher, the editors and the reviewers. Any product that may be evaluated in this article or claim that may be made by its manufacturer is not guaranteed or endorsed by the publisher.
Research integrity at Frontiers
Learn more about the work of our research integrity team to safeguard the quality of each article we publish.