- 1Seafood and Nutrition, Norwegian Institute of Marine Research (IMR), Bergen, Norway
- 2AZTI, Food Research, Basque Research and Technology Alliance (BRTA), Derio, Bizkaia, Spain
- 3AZTI, Marine Research, Basque Research and Technology Alliance (BRTA), Pasaia, Gipuzkoa, Spain
- 4Department of Clinical Medicine, University of Bergen, Bergen, Norway
Introduction: We are having pressing issues of global food insecurity and malnutrition. Mesopelagic communities in the North Atlantic have been estimated to have high biomasses of organisms. Some of these low-trophic organisms are known to be nutrient-dense and may thus contribute to food security and nutrition. Here, we aim to understand the variation in nutrient and undesirable substance concentrations in a common mesopelagic species, Maurolicus muelleri in the North Atlantic Ocean.
Methods: We sampled the M. muelleri from the Bay of Biscay (BB), Norwegian fjords (NF), and the North Sea (NS). The concentrations of micro- and macronutrients, undesirable metals, and persistent organic pollutants (POPs) were measured in composite whole fish samples.
Results: We found no difference across the sampling areas in the selected micronutrients except that the NF and NS samples had higher vitamin A1 concentrations than the BB samples. The NF samples had higher concentrations of fat, fatty acids, and POPs but lower concentrations of cadmium than the BB and NS samples; the differences in fat and fatty acids were only marginal in the NF-BB pair. The BB samples had lower arsenic concentrations than the NS samples, and lower concentrations of erucic acid and mercury than the NF and NS samples. Comparing the measured values against existing EU regulation values for nutrients and undesirable substances for human consumption, we found that the samples from NS and BB may cause food safety concerns due to their high cadmium concentrations, while the M. muelleri from all the sampling areas are qualified as good sources of iron, selenium, vitamin A1, and ω-3 fatty acids.
Discussion: This study confirms that M. muelleri from the North Atlantic Ocean may play an important role in food security and nutrition. However, potential variations in nutrient and undesirable substance concentrations related to seasonality, fish body size, and maturity level shall be taken into consideration prior to exploiting such a marine resource. Further understanding of trophic ecology, life cycles, and productivity of M. muelleri is essential to investigate the drivers behind the observed variation in nutrient and undesirable substance concentrations.
Introduction
Global food security remains under challenge to date (FAO, 2022; Abay et al., 2023; Alabi and Ngwenyama, 2023), and malnutrition is one of the most pressing issues (FAO, 2022; Stevens et al., 2022), particularly in sub-Saharan Africa, southern Asia and the Caribbean (Zurayk, 2020; FAO, 2022). Approximately one in two children under five years of age suffer from deficiencies in vitamins and other essential nutrients globally (UNICEF, 2019). Deficiencies in certain essential nutrients may cause certain diseases. Iron deficiency-induced anaemia (Camaschella, 2015; Stevens et al., 2022), iodine deficiency disorders (Pearce and Zimmermann, 2023), and vitamin A deficiency-induced eye diseases (Rice et al., 2004; Zhao et al., 2022) are still prevalent globally or regionally.
Aquatic foods are recognised as one of the key solutions to combat global hunger and malnutrition (Smith et al., 2010; Tacon and Metian, 2013; Hicks et al., 2019; Costello et al., 2020; Golden et al., 2021; Cai and Leung, 2022). However, current aquatic food systems are challenged by multiple global and regional threats, including policies (e.g. trade policies, Chan et al., 2019), trade (Smith et al., 2010), climate change (Tanentzap et al., 2020; Tigchelaar et al., 2021), pollution (Hallgren et al., 2014), overfishing (Jackson et al., 2001; Scheffer et al., 2005) and post-harvest loss (Ahmed, 2008; Aulakh et al., 2013). Generating knowledge of aquatic foods may help address some of these challenges. Nevertheless, new marine resources of high quality and quantity can contribute to combating global hunger and malnutrition.
Mesopelagic communities are one of the least studied marine systems compared to others with commercial interests. However, it is suggested that they may play an important role in food security and nutrition (FSN) (Standal and Grimaldo, 2020; Kourantidou and Jin, 2022; Fjeld et al., 2023). Mesopelagic fishes are often low in trophic level and diverse (Eduardo et al., 2022) and are estimated to contain more than ten billion tons of biomass globally (Irigoien et al., 2014; Standal and Grimaldo, 2021). In addition, some mesopelagic species have been found to be dense in essential nutrients such as iodine, selenium, vitamin A1 and ω-3 fatty acids (e.g. Alvheim et al., 2020; Grimaldo et al., 2020; Voronin et al., 2023). Aquatic foods are a better source of a range of micronutrients than other common animal-based foods, and an increase in the intake of aquatic foods has been shown to have a major impact on public health (Golden et al., 2021). This evidently points to the potential of utilising mesopelagic species as feed and for human consumption (Fjeld et al., 2023). However, nutrient concentrations in wild-caught seafood often vary greatly geographically (Hicks et al., 2019), and relevant data for mesopelagic species are lacking. This may have implications for potential utilisation of mesopelagic species.
Undesirable substances in aquatic food are yet a concern (e.g. García et al., 2000; Bank et al., 2020) because they can cause human health issues (Qin et al., 2010; WHO, 2010; Ruzzin, 2012; Shi et al., 2019). These undesirable substances include microplastic (Lusher et al., 2016; Barboza et al., 2018), metals and metalloids (e.g. mercury, arsenic, and lead), and persistent organic pollutants (POPs) such as polychlorinated biphenyls (PCBs), polybrominated flame-retardants (PBDEs), dioxins and furans (Corsolini et al., 2005; Panseri et al., 2019; Wiech et al., 2020). Some mesopelagic species have been shown to have relatively low undesirable substance concentrations (Wiech et al., 2020; Berntssen et al., 2021). Yet, potential differences in undesirable substance concentrations have been observed among some mesopelagic species (e.g. Wiech et al., 2020). This may intrinsically result from the differences in the basal undesirable substance concentrations (Islam and Tanaka, 2004; Beiras, 2018). Thus, further investigation to infer the risks and benefits of exploiting mesopelagic species for FSN is required.
The North Atlantic Ocean provides valuable fishing grounds for many countries to nourish regional and global populations (Pauly and Maclean, 2003). While most of the local fisheries focus on the traditional and/or commercial species (Rose, 2007), mesopelagic species remain as an opportunity to increase the productivity of aquatic food (Grimaldo et al., 2020). Maurolicus muelleri is one of the most abundant mesopelagic species in the North Atlantic Ocean (Salvanes and Stockley, 1996; Salvanes and Kristoffersen, 2001; Rees et al., 2020). Its biomass estimation in Southern Norway and West of the British Isles was conducted in 1971–1976 with both echosounders and trawls, which resulted in a stock size of between 20,000 and 1,600,000 tons (Gjøsæter, 1986). Recently, several trial fisheries also suggested the high biomass of M. muelleri in Iceland (46,000 and 18,000 tons in 2009 and 2010, respectively; Standal and Grimaldo, 2021), the North Sea (1,500 tons of mostly M. muelleri; Bjordal and Thorvaldsen, 2020), and the Bay of Biscay (70,000 to 160,000 tons in 2014-2017; Sobradillo et al., 2019). To assess the potential of fisheries of M. muelleri to contribute to FSN, it is essential to understand their quality (nutrients against undesirable substances) and how this may vary within the North Atlantic Ocean.
In this study, we aimed to investigate the concentrations of nutrients and undesirable substances in M. muelleri from different sampling areas in the North Atlantic Ocean to examine potential geographic variations. Specifically, we analysed the concentrations of key nutrients and undesirable substances in the M. muelleri from three sampling areas (Bay of Biscay, Norwegian fjords, and North Sea); compared these concentrations with EU regulation values for nutrients and undesirable substances in food; and detected any differences in these values across the sampling areas.
Materials and methods
Sample collection
The Institute of Marine Research Norway (IMR) conducted mesopelagic trawls in Norwegian fjords (NF, Figure 1; including Bjørnafjorden, Boknafjorden, and Osterfjorden) during December 2018 (data published in Alvheim et al., 2020, and Wiech et al., 2020), March 2020 and May 2020, and in the North Sea (NS, Figure 1) during March 2020. Two pelagic otter trawls with equal-sized mesh, one 35 m2 and one 350 m2 openings were deployed (Table 1). AZTI conducted mesopelagic trawls in the Bay of Biscay (BB, Figure 1) during September 2019 and September 2020 using a Gloria HOD 352 pelagic trawl (Table 1). After landing, M. muelleri individuals were identified, and a subsample of the catch (approximately 100 individuals in BB and at least 50 in NF and NS) was used to estimate the size distribution of the catch by measuring the standard length. All samples were then frozen on board at -18 °C and kept in the dark to avoid potential degradation of light-sensitive compounds (e.g. vitamins).
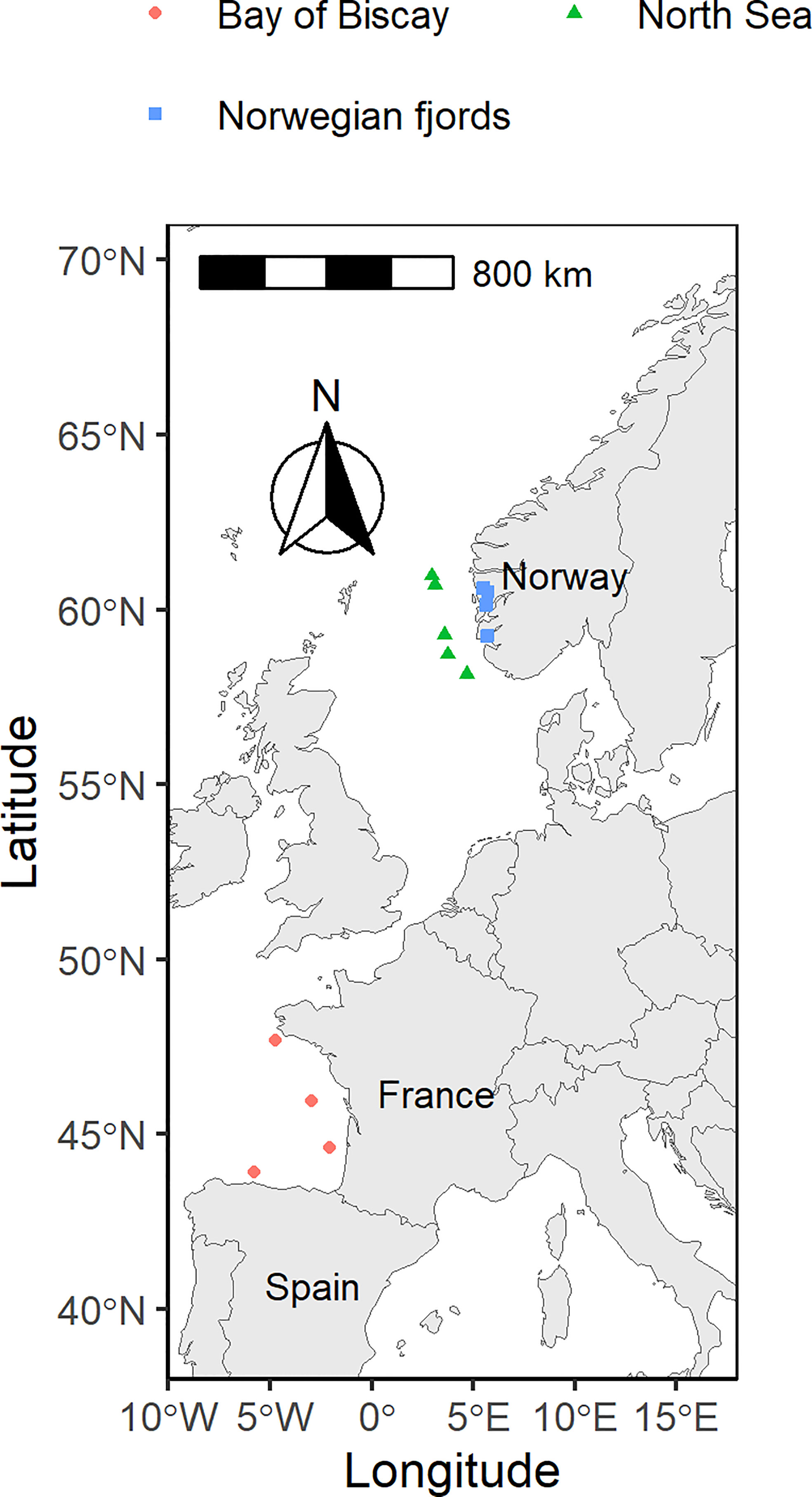
Figure 1 Map of sampling sites for each sampling area in the Bay of Biscay, Norwegian fjords, and the North Sea. Each dot represents the centre of the trawl transect.
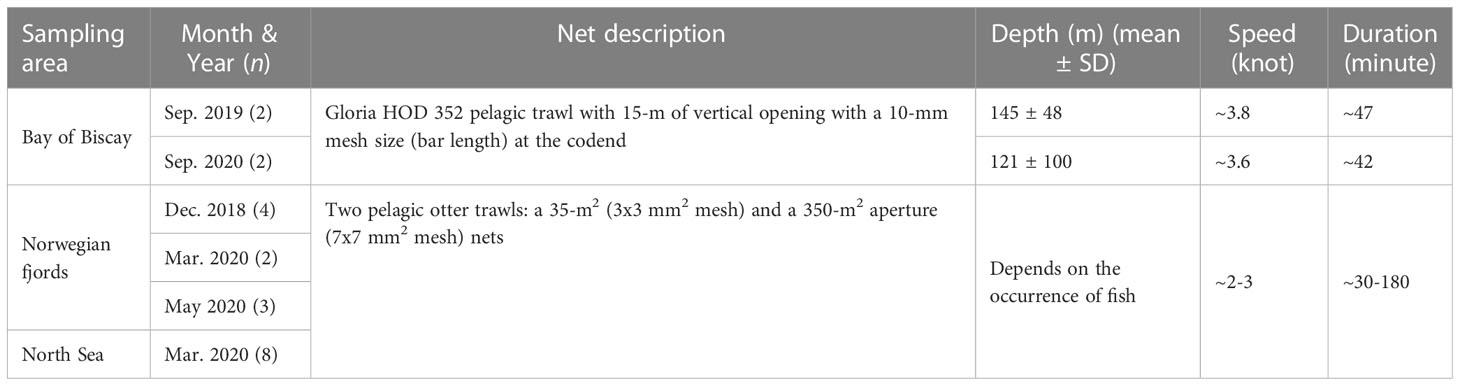
Table 1 Mesopelagic sampling information of materials collected in this study, including sampling area, sampling period (month & year), the number of trawls for each period (n), net description, the depth, speed and duration of trawls.
Substance analyses
From each trawl, we made one or two composite sample(s) by selecting and homogenising at least 25 whole M. muelleri individuals. We analysed important substances (including both nutrients and undesirable substances) based on existing relevant studies (i.e. Alvheim et al., 2020; Wiech et al., 2020) and EU regulations on nutrients and undesirable substances (for all analysed substances, see Appendix 1) with analytical methods (Table 2) which are accredited according to NS-EN ISO/IEC 17025 (2017) or are holding the status of National Reference Laboratory (NRL).
Data analysis
We first screened the data. For nutrients with less than 25% of measured values below the limit of quantification (hereafter<LOQ) for all the sampling areas, we chose the upper bound (UB) values for the data analysis, i.e. the LOQ value. Otherwise, we excluded the substance with more than 25% of values<LOQ for at least one sampling area (e.g. vitamin A2 and vitamin D3). For all undesirable substances with measured values<LOQ, we used the UB values. The data collected in NF were from three different months (Table 1), they were pooled together due to no statistically significant difference found across the sampling months except for arsenic (Appendix 2).
To decide whether the measured nutrient concentrations could be defined as a source of a given nutrient, we compared them with existing EU regulation values (i.e. thresholds). For minerals and vitamins, we adopted EU Regulation 1169/2011 (https://eur-lex.europa.eu/LexUriServ/LexUriServ.do?uri=OJ:L:2011:304:0018:0063:en:PDF). The threshold for a significant source of a given micronutrient refers to whether the product contains 15% or more of the nutrient reference values (NRVs) per 100 g. Here, we calculated these thresholds to concentrations by . For macronutrients, which only included fatty acids (FAs) in this study, we compared the total ω-3 FAs (expressed as the sum of eicosapentaenoic acid [EPA] and docosahexaenoic acid [DHA]) with the EU Nutrition Claim in EU Regulation 1047/2012 (https://food.ec.europa.eu/safety/labelling-and-nutrition/nutrition-and-health-claims/nutrition-claims_en), which included a threshold for a source of ω-3 FAs, and a threshold that the food item contains high ω-3 FAs.
To decide whether the measured concentration of an undesirable substance was low/high risk to human consumers for a given undesirable substance, we compared them with the maximal levels (MLs) for food established in EU Regulation 1881/2006 (https://faolex.fao.org/docs/pdf/eur68134.pdf).
To categorise the measured substance values from each sampling area, we compared the 25th and 75th quantiles with the thresholds and interpreted the categorisation accordingly (Table 3). Because the sample size of substance values for each sampling area was relatively low, we did not assume a normal distribution of the substance values. Thus, to detect differences across sampling areas for each substance, we compared the substance values using the non-parametric Wilcoxon test in Rstudio 1.4.1106 (Team, 2009). The difference between a pair of sampling areas was considered significant if p-value was below 0.05; and such a difference was considered marginal if p-value was not below 0.05 and the median value of one sampling area was outside of the 25th or 75th quantile of another sampling area (i.e. the box in the boxplot, Figure 2).
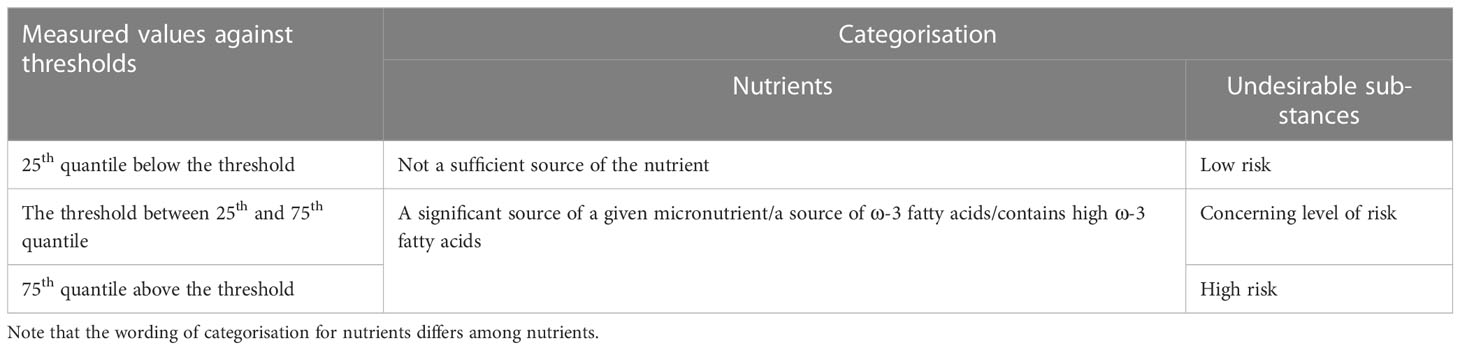
Table 3 Categorisation based on substance values against established relevant EU regulation values (i.e. threshold).
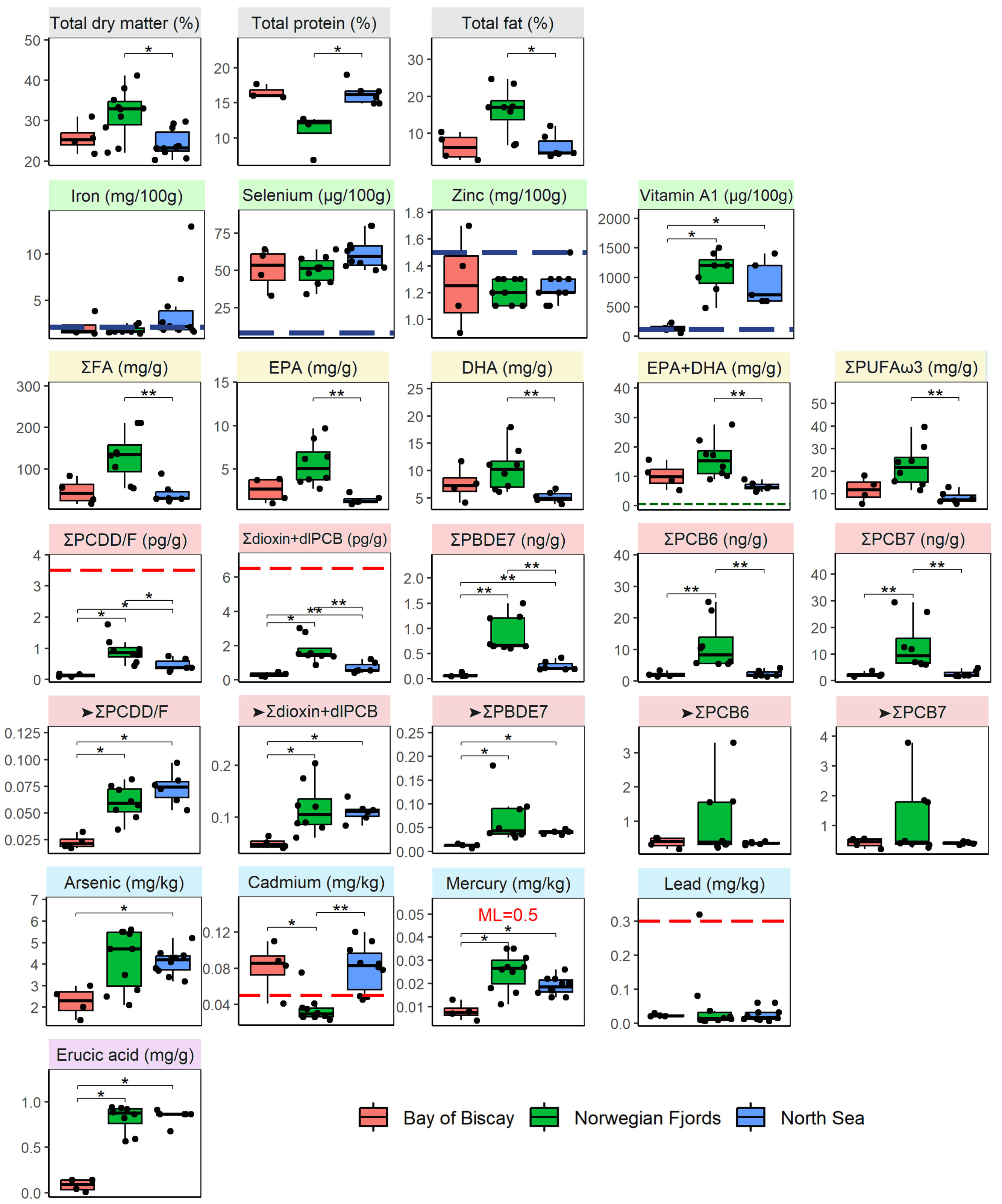
Figure 2 Non-parametric comparison of gross proximate ▬, nutrient (micro- ▬ and macro- ▬) and undesirable substance concentrations (persistent organic pollutants ▬, metals & metalloids ▬, and others ▬) of the Maurolicus muelleri in wet weight from the Bay of Biscay, Norwegian fjords, and the North Sea. Blue long-dashed line indicates the concentration for a significant source of a micronutrient; green dashed line indicates the concentration for a source of omega-3 fatty acids (ω-3 FAs; the lower line) or it contains high ω-3 FAs (the higher line); red long-dashed line indicates the maximum level (ML) for an undesirable substance; for mercury, the ML is annotated. ➤: the fat-corrected values. Statistical significance (non-significance not shown) using Wilcox test: padjusted*< 0.05 and **< 0.01. EPA, eicosapentaenoic acid; DHA, docosahexaenoic acid; PUFA, polyunsaturated FA; PCDD/F, total dioxin including PCDD (polychlorinated dibenzodioxin) and PCDF (polychlorinated dibenzofuran); PBDE, polybrominated diphenyl ether; PCB, polychlorinated biphenyl; and dl-PCB, dioxin-like PCB.
Results
We conducted four mesopelagic trawls in the Bay of Biscay (BB), nine in Norwegian fjords (NF), and eight in the North Sea (NS) (Table 1). In total, we collected four composite samples from BB, and ten from NF and NS (nBay of Biscay = 4, nNorwegian fjord = 10, and nNorth Sea = 10). The M. muelleri collected in BB had standard lengths (SLs) ranging from 25.19 ± 4.87 to 32.37 ± 3.56 mm; those collected in NF had SLs ranging from 23.57 ± 2.63 to 51.17 ± 11.47 mm; and those collected in NS had SLs ranging from 28.40 ± 7.90 to 50.60 ± 7.30 mm.
In total, we analysed three gross proximate, ten essential elements, three vitamins, 37 individual FAs, six hazardous metals and metalloids, 43 POPs, and the sum of all fatty alcohols (Appendix 1). We selected 22 substances while excluding two important nutrients, vitamin A2 and vitamin D3, from the analyses due to high percentages of values<LOQ (Table 4, Figure 2, Appendix 1).
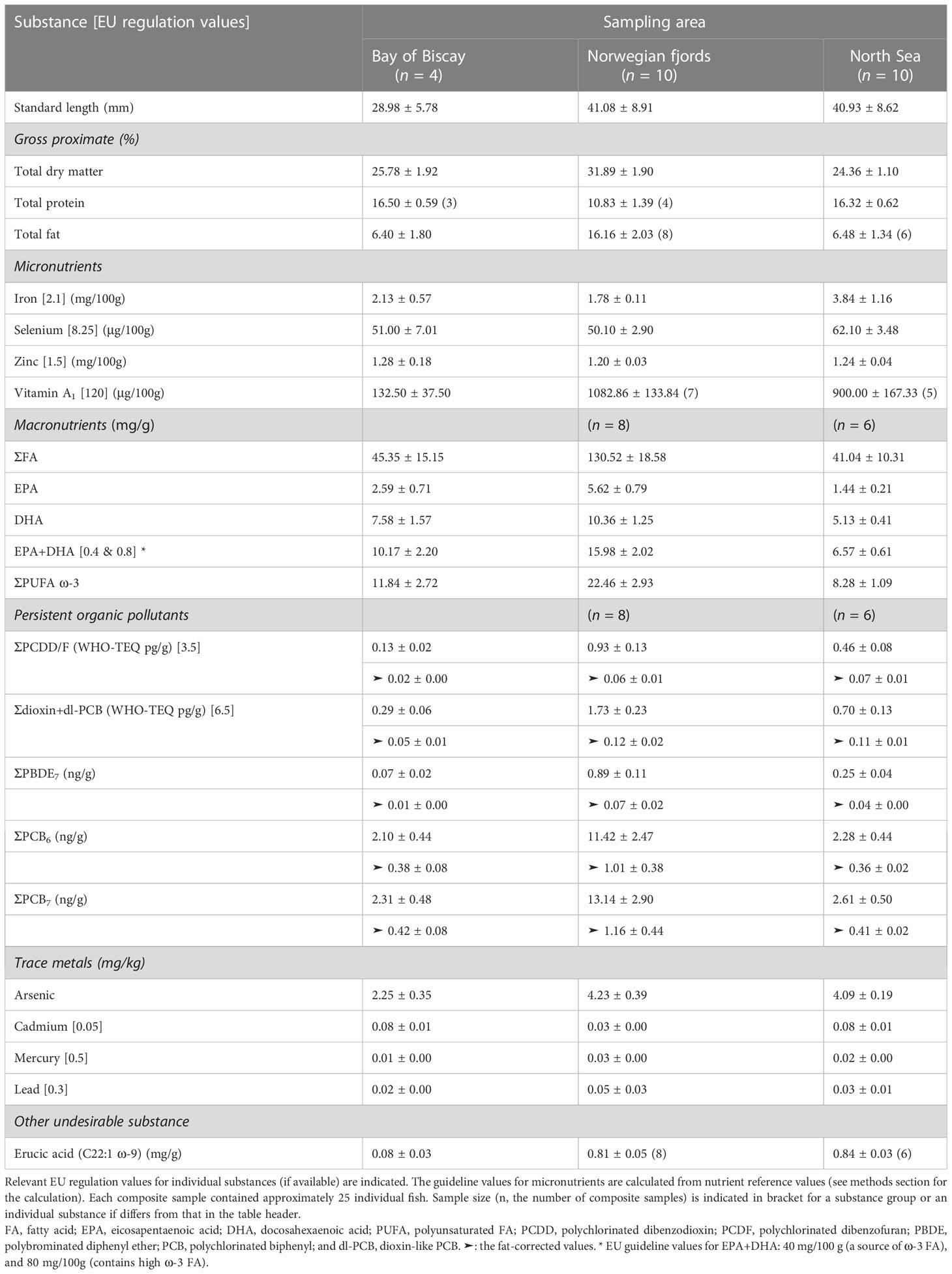
Table 4 Summary of standard length and important substance values (mean ± S.E.; in wet weight) measured for Maurolicus muelleri composite samples of whole fish individuals collected from the Bay of Biscay, Norwegian fjords (Bjørnafjorden, Boknafjorden, and Osterfjorden) and the North Sea.
In terms of gross proximate, the M. muelleri from NF had a dry matter content (31.89 ± 1.90%) statistically significantly higher than that from NS (24.36 ± 1.10%); and that from NS was similar to that from BB (25.78 ± 1.92%; Table 4, Figure 2). Similar trends were found in fat content (NF, NS, and BB: 16.16 ± 2.03%, 6.48 ± 1.34%, and 6.40 ± 1.82%, respectively; Table 4, Figure 2), while completely opposite trends were found in protein concentration (10.83 ± 1.39%, 16.32 ± 0.62%, and 16.50 ± 0.59%, respectively; Table 4, Figure 2).
Micronutrients
There were statistically significant differences in the vitamin A1 concentrations. The NF and NS samples had higher vitamin A1 concentrations (1082.86 ± 133.84, and 900.00 ± 167.33 μg/100g [NF, and NS, respectively]; Table 4, Figure 2) than the BB samples (132.50 ± 37.50 μg/100g; Table 4, Figure 2), while no difference was observed in the other micronutrients across the sample areas (Table 4, Figure 2). Compared with relevant EU regulation values, the M. muelleri from all the sampling areas were shown to be significant sources of selenium/Se (51.00 ± 7.01, 50.10 ± 2.90, 62.10 ± 3.48, and 8.25 μg/100g [BB, NF, NS, and the regulation value, respectively]) and vitamin A1 (132.50 ± 37.50, 1082.86 ± 133.84, 900.00 ± 167.33, and 120 μg/100g [BB, NF, NS, and the regulation value, respectively]); and the M. muelleri from BB and NS was a significant source of iron/Fe (2.13 ± 0.57, 3.84 ± 1.16, and 2.1 mg/100g [BB, NS, and the regulation value, respectively]; Table 4, Figure 2).
Macronutrients
The M. muelleri from NF had higher FA concentrations (e.g. EPA+DHA = 15.98 ± 2.02 mg/g; Table 4, Figure 2) than those from NS (statistically significant; e.g. EPA+DHA = 6.57 ± 0.61 mg/g) and BB (marginal; e.g. EPA+DHA = 10.17 ± 2.20 mg/g). The M. muelleri from all the sampling areas had EPA+DHA concentrations surpassing the relevant EU regulation value for ‘source of ω-3 FAs’ (0.8 mg/g; Table 4, Figure 2).
Undesirable substances
In wet weight, the M. muelleri from NF had statistically significantly higher concentrations of all selected POPs (e.g. PCDD/F = 0.93 ± 0.13 WHO-TEQ pg/g; Table 4, Figure 2) but lower concentrations of cadmium/Cd (0.03 ± 0.00 mg/kg) than those from BB (e.g. PCDD/F = 0.13 ± 0.02 WHO-TEQ pg/g, and Cd = 0.08 ± 0.01 mg/kg) and NS (e.g. PCDD/F = 0.46 ± 0.08 WHO-TEQ pg/g, and Cd = 0.08 ± 0.01 mg/kg). The samples from NS had higher concentrations of PCDD/F, dioxin+dl-PCB (0.70 ± 0.13 WHO-TEQ pg/g; Table 4, Figure 2), PBDE7 (0.25 ± 0.04 ng/g), and arsenic/As (4.09 ± 0.19 mg/kg) than those from BB (dioxin+dl-PCB = 0.29 ± 0.06 WHO-TEQ pg/g, PBDE7 = 0.07 ± 0.02 ng/g, As = 2.25 ± 0.35 mg/kg). The M. muelleri from NF and NS had higher mercury/Hg (0.03 ± 0.00 mg/kg, and 0.02 ± 0.00, respectively; Table 4, Figure 2) and erucic acid concentrations (0.81 ± 0.05 mg/g, and 0.84 ± 0.03 mg/g, respectively) than those from BB (Hg = 0.01 ± 0.00 mg/kg, erucic acid = 0.08 ± 0.03 mg/g). After fat correction, the PCDD/F, dioxin+dl-PCB, and PBDE7 levels were statistically significantly higher in the samples from NF (e.g. ➤PCDD/F = 0.06 ± 0.01 WHO-TEQ pg/g; Table 4, Figure 2) and NS (e.g. ➤PCDD/F = 0.07 ± 0.01 WHO-TEQ pg/g) than those from BB (e.g. ➤PCDD/F = 0.02 ± 0.00 WHO-TEQ pg/g), while no difference was detected in PCB6 (1.01 ± 0.38, 0.36 ± 0.02, and 0.38 ± 0.08 ng/g [NF, NS, and BB, respectively]) and PCB7 levels (1.16 ± 0.44, 0.41 ± 0.02, and 0.42 ± 0.08 ng/g) across the sampling areas (Table 4, Figure 2).
Considering the established maximum levels (MLs), the M. muelleri from all the sampling areas had PCDD/F, dioxin+dl-PCB (0.29 ± 0.06, 1.73 ± 0.23, and 0.70 ± 0.13 WHO-TEQ pg/g [BB, NF, and NS, respectively]; Table 4, Figure 2), Hg, and lead/Pb concentrations (0.02 ± 0.00, 0.05 ± 0.03, and 0.03 ± 0.01 mg/kg) below the MLs for human consumption (Table 4, Figure 2). However, the Cd concentrations measured in the BB and NS samples exceeded the ML for human consumption (0.05 mg/kg; Table 4, Figure 2), assuming that the M. muelleri will be consumed whole due to its small size as a convention similar to other small fishes (Roos et al., 2007; Longley et al., 2014; Kolding et al., 2019).
Discussion
Our study confirmed the potential roles of Maurolicus muelleri from the North Atlantic Ocean for food security and nutrition by proving a novel nutrient-dense and low-risk aquatic food. However, there were statistically significant differences in the concentrations of several nutrients and undesirable substances across the sampling areas, which may influence the utilisation of this new marine resource.
Roles in nutrition provision
The M. muelleri from all the sampling areas in the North Atlantic Ocean were found to have several benefits. According to relevant EU regulations, they were qualified as significant sources of Se and vitamin A1 and contained high ω-3 FAs; and those from BB and NS were qualified as a significant source of Fe.
Compared with other mesopelagic species in BB (Chouvelon et al., 2022) and assuming the dry matter content of M. muelleri is 25% (units are in dm, otherwise in ww), the M. muelleri from BB were rather low in the concentrations of Fe (FeBB = 85.20, FeOthers = 47.9-333.3 mg/kg dm), Se (SeBB = 2.04, SeOthers = 1.68-2.91 mg/kg dm), and Zn (ZnBB = 51.20, ZnOthers = 24.5-103.7 mg/kg dm). This is the case for the M. muelleri from NF and NS, except that the Se concentration in the NS samples was rather high (SeNS = 2.48 mg/kg dm).
Compared with other animal food products, the M. muelleri from all the sampling areas had higher concentrations of vitamin A1 and DHA than whole Sardina pilchardus (vitamin A1 = 115 ± 32.7 μg/100g and DHA = 0.87 ± 0.15 g/100g; Aakre et al., 2020), a higher concentration of Se than whole Engraulis encrasicolus (Se = 38.2 ± 2.1 μg/100g; Aakre et al., 2020), filet of commercial fishes (e.g. SeSalmo salar = 17 and SeGadus morhua = 25 μg/100g; Alvheim et al., 2020) and other animal products (e.g. Sepork/beef = 6 and Sechicken = 12 μg/100g; Alvheim et al., 2020). These comparisons may involve multiple tissue types, and data from whole fish samples may contain greater variability than that from a single tissue type. Such variability is likely due to different accumulation mechanisms among substances in tissues/organs (Landrier et al., 2012; Shukla et al., 2018), and possibly gut contents of individual whole fish. While this variability may have made the comparisons across tissue types less robust, it nevertheless highlights the possible range in the substance yields from the consumer’s perspective.
Vitamin D3 is another important micronutrient (Holick, 2007; Amrein et al., 2020) which can be obtained from seafood (Lund, 2013). In our study, most of the measured vitamin D3 concentrations were<LOQ (LOQ = 0.01 mg/kg) except in two instances (0.01 [from BB] and 0.02 mg/kg [from NS]; Appendix 1). According to the EU Regulation No 1169/2011, a food item that provides 15% of 5 μg/100 g vitamin D3 (or 0.0075 mg/kg in concentration) can be qualified as a significant source of vitamin D3. Because the LOQ of our method was higher than the regulation value, we cannot confirm whether the M. muelleri collected in this study is a significant source of vitamin D3.
Malnutrition might be exacerbated due to the pandemic, food shortage (Workie et al., 2020; Zurayk, 2020), and climate change (Gomez-Zavaglia et al., 2020), while the global population is projected to increase. Thus, alternative productive and nutritious foods such as M. muelleri are important to help combat malnutrition, for example, reducing the risk of iron deficiency-induced anaemia (Aikawa et al., 2006; Beck et al., 2014) which is prevalent globally (Stevens et al., 2022). Its high Se concentration may help provide a protective effect against cardiovascular diseases (Mozaffarian, 2009) and Hg poisoning (Gochfeld and Burger, 2021).
Food safety
The M. muelleri from all the sampling areas were found to have low risk. All the examined undesirable substances had low concentrations except for the high Cd concentrations in the BB and NS samples when compared the measured concentrations against the MLs in relevant EU regulations. We further compared the BB and NS Cd concentrations with the EU regulation on undesirable substances in animal feed under EU Directive 2002/32/EC (https://eur-lex.europa.eu/legal-content/EN/TXT/PDF/?uri=CELEX:02002L0032-20191128&from=EN) and found that they were below the ML (assuming an 88% dry weight). This suggests their potential usage as a feed ingredient, similar to some earlier findings (Berntssen et al., 2021).
The M. muelleri from our sampling areas had lower Cd concentrations than those reported from the Mid Atlantic Ridge (Grimaldo et al., 2020), and lower Hg concentrations than those from the Azores (Monteiro et al., 1996).
Compared with other mesopelagic species from BB (Chouvelon et al., 2022) and assuming the dry matter content of M. muelleri is 25%, the M. muelleri from BB were higher in As concentration than these mesopelagic fishes (AsBB = 90.00, AsOthers = 3.83-53.60 mg/kg dm); this is also the case for Cd (CdBB = 3.20, CdOthers = 0.03-2.43 mg/kg dm), and Pb (PbBB = 0.80, PbOthers = 0.03-0.18 mg/kg dm). While the Hg concentrations in the M. muelleri from BB were similar to these mesopelagic species (HgBB = 0.40, HgOthers = 0.090-1.365 mg/kg dm). These differences persist when comparing the M. muelleri from NF and NS with these mesopelagic species except that the Cd concentration in the NF samples was rather low (CdNF = 1.2 mg/kg dm), and the Hg concentration in the NF samples was rather high (HgNF = 1.2 mg/kg dm).
Compared with other mesopelagic species from NF (Wiech et al., 2020), the M. muelleri from all the sampling areas had lower concentrations of As, and Pb than Meganyctiphanes norvegica (As = 28 ± 19, Pb = 0.086 ± 0.075 mg/kg), As, and Hg than Pasiphaea sp. (As = 22 ± 19, Hg = 0.038 ± 0.02 mg/kg), As, and Pb than Eusergestes arcticus(As = 9.5 ± 4.2, Pb = 0.01 ± 0.006 mg/kg), and Pb than Benthosema glaciale (Pb = 0.016 ± 0.017 mg/kg). The M. muelleri from NF and NS had higher concentrations of total fat and erucic acid than the aforementioned mesopelagic species from NF; while those from BB and NS had lower concentrations of total PCDD/F, PBDE7, PCB6, and PCB7 than these mesopelagic species from NF.
There are other undesirable substances that were not included in the present study. For example, wax esters may pose some health hazards (e.g. keriorrhea) when consumed in large amounts (Schots et al., 2020) and are commonly found among mesopelagic species (Voronin et al., 2022, 2023). However, the wax ester concentrations in the NF and NS samples were low (in both wet weight and % of FA; Appendix 1), which is similar to some of the previous findings (Wiech et al., 2020; Voronin et al., 2023).
Observed differences and possible drivers
Some of the analysed substances had great variation across the sampling areas, and there are multiple possible drivers behind them. The differences in the gross proximate across the sampling areas indicate differential accumulation mechanisms for the M. muelleri. Evidently, fishes from northern regions and/or collected during colder seasons tended to have larger lipid reserves (Schultz and Conover, 1997) and unsaturated FAs concentrations (Hazel, 1984) than those from the south or collected during warmer seasons. This matches with our data that the samples from NF (colder) had higher fat content than the samples from NS & BB samples (warmer). Additionally, smaller-body sized fishes often have lower lipid reserves than larger-sized ones (Schultz and Conover, 1997; Toppe et al., 2007); this has been shown among M. muelleri (Olsen et al., 2020). In our study, such a body size-fat correlation was apparent between the BB and NF samples but not between the NF and NS samples (Table 4). The body sizes of the NF and NS samples were similar, yet the fat content of the NF samples was statistically significantly higher than that of the NS samples (Table 4). This was likely caused by the sampling during different months/seasons (NF: March, May, and December; NS: March) and high food availability in the fjords due to the seasonal algal blooming (Cembella et al., 2005; Marquardt et al., 2016). Moreover, biological activities such as spawning can lead to transferring essential nutrients to the eggs and metabolising the stored fat for reproduction, thus resulting in the loss of some substances in a short period (Boran and Karaçam, 2011; Fuiman and Faulk, 2013; Shadyeva et al., 2019). Although M. muelleri has a protracted spawning season (Gjøsæter, 1981; d’Elbée et al., 2009), the samples collected in BB were practically outside of the spawning season (spawning season: at least between March and September; Alvarez et al., 2023), while those in NF and NS partially or entirely included spawning individuals (spawning season: March to September; Gjøsæter, 1981). Thus, we could not examine the effect of spawning here. Lastly, M. muelleri can have complex population structures (Ikeda, 1994; Rasmussen et al., 2009) with individuals of different life stages occurring in the same location, potentially resulting in variability in the substance concentrations.
For the macronutrients, the trend in fat content (i.e. NF > NS [statistically significant] and NF > BB [marginal]) was also observed for total FAs and individual FAs/FA groups, possibly for similar reasons such as the lower temperature and higher food availability due to the seasonal algal blooming in NF than in BB and NS (e.g. Donnelly et al., 1990; Zlatanos and Laskaridis, 2007; Boran and Karaçam, 2011). Among the micronutrients, the only observed difference across the sampling areas was the lower concentration of vitamin A1 in the BB samples than in the NF and NS samples. This was likely due to the seasonal variation in vitamin A1 which has been observed among a few other fish species (Bridges, 1965; Temple et al., 2006). Since our knowledge of vitamin A1 is rather limited, we cannot disentangle the temporal effects from the spatial effects based on our data.
The POP concentrations (in wet weight) showed similar variation patterns across the sampling areas to those of fat/FAs that higher POP concentrations were found in the NF samples than in the BB and NS samples. Such a co-occurrence of fat/FAs and POPs has been observed in other aquatic systems including the Mediterranean pelagic fishes (Cembella et al., 2005; Romanić et al., 2021) and the commercial fishes from the North East Atlantic Ocean (Ho et al., 2021). This co-occurrence likely results from the interaction between POPs and lipids (Elskus et al., 2005) that POPs often accumulate in fatty tissues and their bioavailability may be affected by the lipid composition. In contrast, the fat-corrected POP levels showed different patterns across the sampling areas compared with those from the uncorrected concentrations. The statistically significant differences in POP concentrations between the NF and NS samples were attenuated, and no statistically significant difference across the sampling areas remained in PCB6 and PCB7 concentrations. Because the samples were collected during different seasons, the anticipated variation in fat content across the sampling areas may drive the variation in POP concentrations to some extent. In addition, POP concentrations can be different at the base of the food web geographically across the large sampling areas (Batool et al., 2016), resulting from the grasshopper effect of POPs (Fernández and Grimalt, 2003). This may have led to higher basal POP in NF and NS than in BB. Besides, the samples collected from one of the selected fjords, Osterfjorden (OF), had higher PBDE7, PCB6, and PCB7 concentrations and the pertinent fat-corrected levels than those from the other two fjords; this has also been observed among a few other fish species (Frantzen and Måge, 2016). Because the fat content of the OF samples were lower than those from the other two fjords (Appendix 3), the POP concentrations in OF may not be fat-driven. We suspect that such an observation may result from the high basal PBDE7, PCB6, and PCB7 concentrations in OF. Overall, all the measured POP concentrations were statistically significantly lower than the MLs, thus, the sampled M. muelleri had low risks of POPs to human health.
The samples from both NF and NS had higher Hg concentrations than those from BB. However, this trend is opposite from what has been observed in different fish species from the south to the north in the North East Atlantic Ocean (Azad et al., 2019). Because Hg concentration correlates positively with body size (Monteiro et al., 1996), our contradictive result suggests that body size-dependent bioaccumulation may play a predominant role here as the NF and NS samples had greater body size than the BB samples (Table 4). On the other hand, the NS samples had Hg concentrations marginally lower than the NF samples while both had similar body sizes (Figure 2). This is in accordance with earlier findings in several other fish species collected from NS and NF (Azad et al., 2019).
The As concentrations, however, were lower in BB than in NF and NS. It may likely result from different temperatures, which has been observed in As concentrations globally (higher in polar regions than in tropical regions; Fattorini et al., 2006), as well as the body size-dependent bioaccumulation of As (Zhang et al., 2022). Additionally, the higher As concentrations in the NF samples than the NS samples may result from different temperatures or fat contents during the sampling seasons (i.e. March, May, and December vs. May, respectively; Appendix 2). There is no authorised ML for arsenic in European legislation. To assess the potential risk from As, the toxic forms must be considered. Earlier work has shown that only a small fraction of the total arsenic in M. Muelleri was present in the most toxic inorganic form (Wiech et al., 2020). And a recent study performing As speciation found large proportions of potentially toxic arseno-lipids (Tibon et al., 2022). But as the toxicity of arseno-lipids depends on the present As species, a complete analytical characterisation of present compounds is needed to evaluate the risk connected to arseno-lipids.
The higher Cd concentrations in the M. muelleri from BB and NS than those from NF are consistent with earlier findings between NF and offshore systems (Wiech et al., 2020) and with another common mesopelagic species B. glaciale (Bjordal and Thorvaldsen, 2020; Wiech et al., 2020). One possible driver may be the Cd distribution in seawater. Cadmium is known to behave similarly to phosphate (de Baar et al., 1994), that it is depleted close to the surface (where primary production takes place) and enriched in deeper waters (where organic matter is decomposed). Thus, higher concentrations of Cd are expected in BB and NS which receive water from oceanic deep-water systems than in NF which receive a mixture of oceanic water and fresh water.
Most of the examined undesirable substances had concentrations lower than the relevant MLs, yet some had great variation across the sampling areas. Also, POPs may have a cumulative adverse effect on human health (Bank et al., 2020) and other undesirable substances like Hg may mask the positive effects of essential nutrients on human health (Kris-Etherton et al., 2002). Thus, it is crucial to monitor not only the mean values but also the variation in concentrations to further influence their usage (food or feed). One possible way to further reduce the POP concentrations is to process the fish for fish oil and fish meals through the established methods (Berntssen et al., 2021).
Other drivers
Both biological and environmental drivers are important in determining the substance concentrations of the M. muelleri in the present study. However, many drivers from these two dimensions are entangled and may synergistically affect the substance concentrations in fish. Apart from the discussed drivers, there are others that may incorporate both dimensions. For example, temporospatial variation in food availability (e.g. algal blooming in NF) can affect the substance concentrations in fish (Boran and Karaçam, 2011) as well as their feeding strategies. Since much of the substances in fish come from their diet (Grimaldo et al., 2020), apart from their geographic distribution (Batool et al., 2016), mesopelagic food webs may be supported by multiple food sources (Ianiri and McCarthy, 2023) with different basal substance compositions (e.g. upwelling, offshore and nearshore phytoplankton, and terrestrial runoff). While the prey specialisation is relatively low among mesopelagic fishes (Bernal et al., 2015), the substance concentrations in fish are thus partially determined by the contributions of prey from different basal food sources. Thus, it is important to understand the nutrient flow in mesopelagic species, which may nevertheless provide insights into the possible environmental impacts and sustainability of potential fisheries. For mesopelagic systems, these are particularly vital (Hidalgo and Browman, 2019; Grimaldo et al., 2020; Standal and Grimaldo, 2021; Fjeld et al., 2023) due to their trophic functions in nutrient cycling, carbon fixation (Li et al., 2022; Schadeberg et al., 2023), and mediating ocean health (Bank et al., 2020).
Limitations and future work
In this study, we chose nutrients and undesirable substances based on relevant studies and EU regulations. However, there is no EU regulation on a few important undesirable substances including As, wax esters, and PBDE. As they may pose threats to human health, it is important to develop regulations on them to further ensure food safety of current and emerging aquatic foods.
We mentioned the importance of body size on the concentrations of some nutrients and undesirable substances. However, in the present study, only the body size of a group of individuals for each catch was measured, which reduced the resolution in variability to understand the effect of body size on the substance concentrations. It is ideal to analyse the substance concentrations at the individual level. Unfortunately, the current methods require a higher mass of materials than one individual of a small fish such as M. muelleri to analyse the full suite of nutrients and undesirable substances. Thus, future development of analytical methods is essential to enable high-resolution individual-based substance analyses of small mesopelagic fishes.
Nevertheless, we suggested that the season of collection and spawning activities may have caused some uncertainty in the analyses. The sampling of mesopelagic species at IMR and AZTI takes into consideration of the cruise planning and financial feasibility. Targeted sampling of mesopelagic species is challenging, and cruise activity was limited. This led to sampling at different seasons in different areas and varying size distributions. As M. muelleri is known to spawn in batches over a protracted spawning period and shows mixed schooling behaviours, it was not possible to foresee their maturity levels prior to sampling. These factors may have an influence on the substance concentrations. However, our datasets nevertheless have important implications on the geographic differences in the substance concentrations of the M. muelleri in the North Atlantic Ocean, while pointing to important future work to better inform the sampling design and improve the resolution of such analyses by disentangling these potentially confounding factors.
Conclusion
We confirmed the potential roles of Maurolicus muelleri in food security and nutrition. There are statistically significant differences in the concentrations of several substances across the sampling areas, including vitamin A1, dioxin, cadmium, and mercury. These differences were probably related to differential basal substance concentrations, body sizes/maturity levels of samples, and sampling periods. One general trend we found is that the M. muelleri from Norwegian fjords tended to contain higher concentrations of fat, fatty acids, and lipophilic undesirable substances than those from the Bay of Biscay and the North Sea, however, the drivers behind are unclear. Overall, the M. muelleri from all the sampling areas are good sources of several essential nutrients (e.g. Se and vitamin A1) with low concentrations of undesirable substances (e.g. mercury) according to available EU regulations for food. However, the variability in some of these substances (e.g. cadmium) should be closely monitored to provide important indications on the usages of this marine resource (i.e. for food or feed).
Data availability statement
The original contributions presented in the study are included in the article/Supplementary Material. Further inquiries can be directed to the corresponding author.
Ethics statement
Ethical review and approval was not required for the animal study because We do not deal with live animals in this study. All the animal data we used here was from previous studies/projects.
Author contributions
YZ, AA, LM, and MW contributed to the conception and design of the work. YZ, MK, CB, BI, PA, GB, MB, and MW contributed to the sample collection and data analysis. YZ and MW contributed to the drafting the work. All authors contributed to the revising the work and approved for publication of the content. All authors agreed to be accountable for all aspects of the work in ensuring that questions related to the accuracy or integrity of any part of the work are appropriately investigated and resolved.
Funding
The funding was provided by multiple sources including: SIS: contribution to the sub-project ‘New marine resources as food and feed (Ocean to Oven)’ within the umbrella of ‘Strategic priorities for Institute of Marine Research’ (299554/F40) funded by the Norwegian Research Council. MEESO: EU H2020 research and innovation programme, Grant Agreement No 817669. Fish in Food Systems (15586), Institute of Marine Research, Norway. Total Effect of Seafood (15779), Institute of Marine Research, Norway. The funding contributed to the collection and analyses of samples, data analysis, writing of the manuscript, relevant publication and working hours.
Acknowledgments
We are grateful for the funders who have contributed to this study. They are: SIS: contribution to the sub-project ‘New marine resources as food and feed (Ocean to Oven)’ within the umbrella of ‘Strategic priorities for Institute of Marine Research’ (299554/F40) funded by the Norwegian Research Council; MEESO: EU H2020 research and innovation programme, Grant Agreement No 817669; Fish in Food Systems (15586), Institute of Marine Research, Norway; and Total Effect of Seafood (15779), Institute of Marine Research, Norway. We are grateful for the cruise members involved in the sample collection, and the laboratory staff for analysing the samples.
Conflict of interest
The authors declare that the research was conducted in the absence of any commercial or financial relationships that could be construed as a potential conflict of interest.
Publisher’s note
All claims expressed in this article are solely those of the authors and do not necessarily represent those of their affiliated organizations, or those of the publisher, the editors and the reviewers. Any product that may be evaluated in this article, or claim that may be made by its manufacturer, is not guaranteed or endorsed by the publisher.
Supplementary material
The Supplementary Material for this article can be found online at: https://www.frontiersin.org/articles/10.3389/fmars.2023.1213612/full#supplementary-material
References
Aakre I., Bøkevoll A., Chaira J., Bouthir F. Z., Frantzen S., Kausland A., et al. (2020). Variation in nutrient composition of seafood from North West Africa: implications for food and nutrition security. Foods 9, 1516. doi: 10.3390/foods9101516
Abay K. A., Breisinger C., Glauber J., Kurdi S., Laborde D., Siddig K. (2023). The Russia-Ukraine war: implications for global and regional food security and potential policy responses. Global Food Secur. 36, 100675. doi: 10.1016/j.gfs.2023.100675
Ahmed A. A. (2008). Post-harvest losses of fish in developing countries. Nutr. Health 19, 273–287. doi: 10.1177/026010600801900403
Aikawa R., Khan N. C., Sasaki S., Binns C. W. (2006). Risk factors for iron-deficiency anaemia among pregnant women living in rural Vietnam. Public Health Nutr. 9, 443–448. doi: 10.1079/PHN2005851
Alabi M. O., Ngwenyama O. (2023). Food security and disruptions of the global food supply chains during COVID-19: building smarter food supply chains for post COVID-19 era. Br. Food J. 125, 167–185. doi: 10.1108/BFJ-03-2021-0333
Alvarez P., Korta M., Garcia D., Boyra G. (2023). Life history strategy of Maurolicus muelleri (Gmenlin 1789) in the Bay of Biscay. Hydrobiology 2, 289–313. doi: 10.3390/hydrobiology2020019
Alvheim A. R., Kjellevold M., Strand E., Sanden M., Wiech M. (2020). Mesopelagic species and their potential contribution to food and feed security–a case study from Norway. Foods 9, 344. doi: 10.3390/foods9030344
Amrein K., Scherkl M., Hoffmann M., Neuwersch-Sommeregger S., Köstenberger M., Tmava Berisha A., et al. (2020). Vitamin D deficiency 2.0: an update on the current status worldwide. Eur. J. Clin. Nutr. 74, 1498–1513. doi: 10.1038/s41430-020-0558-y
Aulakh J., Regmi A., Fulton J. R., Alexander C. E. (2013). Estimating post-harvest food losses: developing a consistent global estimation framework. In Proceedings of the Agricultural & Applied Economics Association’s 2013 AAEA & CAES Joint Annual Meeting, Washington, DC, USA, 4–6 August 2013.
Azad A. M., Frantzen S., Bank M. S., Nilsen B. M., Duinker A., Madsen L., et al. (2019). Effects of geography and species variation on selenium and mercury molar ratios in Northeast Atlantic marine fish communities. Sci. Total Environ. 652, 1482–1496. doi: 10.1016/j.scitotenv.2018.10.405
Bank M. S., Metian M., Swarzenski P. W. (2020). Defining seafood safety in the anthropocene. Environ. Sci. Technol. 54, 8506–8508. doi: 10.1021/acs.est.0c03505
Barboza L. G. A., Vethaak A. D., Lavorante B. R., Lundebye A.-K., Guilhermino L. (2018). Marine microplastic debris: an emerging issue for food security, food safety and human health. Mar. pollut. Bull. 133, 336–348. doi: 10.1016/j.marpolbul.2018.05.047
Batool S., Ab Rashid S., Maah M. J., Sarfraz M., Ashraf M. A. (2016). Geographical distribution of persistent organic pollutants in the environment: a review. J. Environ. Biol. 37, 1125.
Beck K. L., Conlon C. A., Kruger R., Coad J. (2014). Dietary determinants of and possible solutions to iron deficiency for young women living in industrialized countries: a review. Nutrients 6, 3747–3776. doi: 10.3390/nu6093747
Beiras R. (2018). Marine pollution: sources, fate and effects of pollutants in coastal ecosystems (Amsterdam: Elsevier).
Bernal A., Olivar M. P., Maynou F., de Puelles M. L. F. (2015). Diet and feeding strategies of mesopelagic fishes in the western Mediterranean. Prog. Oceanogr. 135, 1–17. doi: 10.1016/j.pocean.2015.03.005
Berntssen M. H., Thoresen L., Albrektsen S., Grimaldo E., Grimsmo L., Whitaker R. D., et al. (2021). Processing mixed mesopelagic biomass from the North-East Atlantic into aquafeed resources; implication for food safety. Foods 10, 1265. doi: 10.3390/foods10061265
Biancarosa I., Espe M., Bruckner C., Heesch S., Liland N., Waagbø R., et al. (2017). Amino acid composition, protein content, and nitrogen-to-protein conversion factors of 21 seaweed species from Norwegian waters. J. Appl. Phycol. 29, 1001–1009. doi: 10.1007/s10811-016-0984-3
Bjordal Å., Thorvaldsen K. G. (2020). Forsøksfiske etter mesopelagiske arter 2019-Oppsummerende rapport. (Norway: Rapport fra havforskningen).
Boran G., Karaçam H. (2011). Seasonal changes in proximate composition of some fish species from the Black Sea. Turkish J. Fish. Aquat. Sci. 11, 330–340. doi: 10.4194/trjfas.2011.0101
Bridges C. (1965). Variability and relationships of fish visual pigments. Vision Res. 5, 239–251. doi: 10.1016/0042-6989(65)90002-7
Cai J., Leung P. (2022). Unlocking the potential of aquatic foods in global food security and nutrition: a missing piece under the lens of seafood liking index. Global Food Secur. 33, 100641. doi: 10.1016/j.gfs.2022.100641
Camaschella C. (2015). Iron-deficiency anemia. New Engl. J. Med. 372, 1832–1843. doi: 10.1056/NEJMra1401038
Cembella A. D., Ibarra D. A., Diogene J., Dahl E. (2005). Harmful algal blooms assessment in fjordsand. Oceanography 18, 158. doi: 10.5670/oceanog.2005.51
CEN (1999). Foodstuffs–Determination of vitamin A by high performance liquid chromatography-Part 1: Measurement of all-trans-retinol and 13-cis-retinol (Comitè Europèen de Normalisation prEN), 12823-1, 1–13.
Chan C. Y., Tran N., Pethiyagoda S., Crissman C. C., Sulser T. B., Phillips M. J. (2019). Prospects and challenges of fish for food security in Africa. Global Food Secur. 20, 17–25. doi: 10.1016/j.gfs.2018.12.002
Chouvelon T., Munschy C., Bruzac S., Caurant F., Churlaud C., Crochet S., et al. (2022). High inter-species variability in elemental composition of the twilight zone fauna varies implications for predators and exploitation by humans. Environ. Res. 204, 112379. doi: 10.1016/j.envres.2021.112379
Corsolini S., Ademollo N., Romeo T., Greco S., Focardi S. (2005). Persistent organic pollutants in edible fish: a human and environmental health problem. Microchem. J. 79, 115–123. doi: 10.1016/j.microc.2004.10.006
Costello C., Cao L., Gelcich S., Cisneros-Mata M. Á., Free C. M., Froehlich H. E., et al. (2020). The future of food from the sea. Nature 588, 95–100. doi: 10.1038/s41586-020-2616-y
de Baar H. J., Saager P. M., Nolting R. F., van der Meer J. (1994). Cadmium versus phosphate in the world ocean. Mar. Chem. 46, 261–281. doi: 10.1016/0304-4203(94)90082-5
d’Elbée J., Castège I., Hémery G., Lalanne Y., Mouchès C., Pautrizel F., et al. (2009). Variation and temporal patterns in the composition of the surface ichthyoplankton in the southern Bay of Biscay (W. Atlantic). Continental Shelf Res. 29, 1136–1144. doi: 10.1016/j.csr.2008.12.023
de Normalisation C. E. (2009). Foodstuffs–determination of vitamin D by high performance liquid chromatography-measurement of cholecalciferol (D3) or ergocalciferol (D2) (Brussels, Belgium: Comité Européen de Normalisation).
Donnelly J., Torres J., Hopkins T., Lancraft T. (1990). Proximate composition of Antarctic mesopelagic fishes. Mar. Biol. 106, 13–23. doi: 10.1007/BF02114670
Eduardo L. N., Bertrand A., Lucena-Fredou F., Villarins B. T., Martins J. R., Afonso G. V. F., et al. (2022). Rich and underreported: first integrated assessment of the diversity of mesopelagic fishes in the Southwestern Tropical Atlantic. Front. Mar. Sci. 9. doi: 10.3389/fmars.2022.937154
Elskus A. A., Collier T. K., Monosson E. (2005). “Interactions between lipids and persistent organic pollutants in fish,” in Biochemistry and Molecular Biology of Fishes, vol. 6 . Eds. Mommsen T. P., Moon T. W. (St. Luis: Elsevier), 119–152.
FAO, I, UNICEF, WFP, WHO (2022). The state of food security and nutrition in the world 2022. Rome: FAO.
Fattorini D., Notti A., Regoli F. (2006). Characterization of arsenic content in marine organisms from temperate, tropical, and polar environments. Chem. Ecol. 22, 405–414. doi: 10.1080/02757540600917328
Fernández P., Grimalt J. O. (2003). On the global distribution of persistent organic pollutants. Chimia 57, 514–514. doi: 10.2533/000942903777679000
Fjeld K., Tiller R., Grimaldo E., Grimsmo L., Standal I.-B. (2023). Mesopelagics–new gold rush or castle in the sky? Mar. Policy 147, 105359. doi: 10.1016/j.marpol.2022.105359
Frantzen S., Måge A. (2016). “Fremmedstoffer i villfisk med vekt på kyst-nære farvann. Brosme, lange og bifangstarter,” in Gjelder tall for prøver samlet inn i 2013-2015 (Bergen: NIFES).
Fuiman L. A., Faulk C. K. (2013). Batch spawning facilitates transfer of an essential nutrient from diet to eggs in a marine fish. Biol. Lett. 9, 20130593. doi: 10.1098/rsbl.2013.0593
García L. M., Porte C., Albaigés J. (2000). Organochlorinated pollutants and xenobiotic metabolizing enzymes in W. Mediterranean mesopelagic fish. Mar. pollut. Bull. 40, 764–768. doi: 10.1016/S0025-326X(00)00023-0
Gjøsæter J. (1981). Life history and ecology of Maurolicus muelleri (Gonostomatidae) in Norwegian waters. FiskDir. Skr. Ser. Havunders. 17, 109–131.
Gjøsæter J. (1986). Estimates of the abundance of mesopelagic fish off southern Norway and west of the British isles 1971-1976. Flodevigen Rapp 1, 1–22.
Gochfeld M., Burger J. (2021). Mercury interactions with selenium and sulfur and the relevance of the Se: Hg molar ratio to fish consumption advice. Environ. Sci. pollut. Res. 28, 18407–18420. doi: 10.1007/s11356-021-12361-7
Golden C. D., Koehn J. Z., Shepon A., Passarelli S., Free C. M., Viana D. F., et al. (2021). Aquatic foods to nourish nations. Nature 598, 315–320. doi: 10.1038/s41586-021-03917-1
Gomez-Zavaglia A., Mejuto J. C., Simal-Gandara J. (2020). Mitigation of emerging implications of climate change on food production systems. Food Res. Int. 134, 109256. doi: 10.1016/j.foodres.2020.109256
Grimaldo E., Grimsmo L., Alvarez P., Herrmann B., Møen Tveit G., Tiller R., et al. (2020). Investigating the potential for a commercial fishery in the northeast Atlantic utilizing mesopelagic species. ICES J. Mar. Sci. 77, 2541–2556. doi: 10.1093/icesjms/fsaa114
Hallgren P., Nicolle A., Hansson L. A., Brönmark C., Nikoleris L., Hyder M., et al. (2014). Synthetic estrogen directly affects fish biomass and may indirectly disrupt aquatic food webs. Environ. Toxicol. Chem. 33, 930–936. doi: 10.1002/etc.2528
Hazel J. R. (1984). Effects of temperature on the structure and metabolism of cell membranes in fish. Am. J. Physiol.-Regulatory Integr. Comp. Physiol. 246, R460–R470. doi: 10.1152/ajpregu.1984.246.4.R460
Hicks C. C., Cohen P. J., Graham N. A., Nash K. L., Allison E. H., D’Lima C., et al. (2019). Harnessing global fisheries to tackle micronutrient deficiencies. Nature 574, 95–98. doi: 10.1038/s41586-019-1592-6
Hidalgo M., Browman H. I. (2019). Developing the knowledge base needed to sustainably manage mesopelagic resources. ICES J. Mar. Sci. 76, 609–615. doi: 10.1093/icesjms/fsz067
Ho Q. T., Bank M. S., Azad A. M., Nilsen B. M., Frantzen S., Boitsov S., et al. (2021). Co-Occurrence of contaminants in marine fish from the North East Atlantic Ocean: implications for human risk assessment. Environ. Int. 157, 106858. doi: 10.1016/j.envint.2021.106858
Holick M. F. (2007). Vitamin D deficiency. New Engl. J. Med. 357, 266–281. doi: 10.1056/NEJMra070553
Ianiri H. L., McCarthy M. D. (2023). Compound specific δ15N analysis of amino acids reveals unique sources and differential cycling of high and low molecular weight marine dissolved organic nitrogen. Geochim. Cosmochim. Acta 344, 24–39. doi: 10.1016/j.gca.2023.01.008
Ikeda T. (1994). Growth and life cycle of the mesopelagic fish Maurolicus muelleri (Sternoptychidae) in Toyama Bay, southern Japan Sea. Bull. Plankton Soc. Japan (Japan) 40, 127–138.
Irigoien X., Klevjer T. A., Røstad A., Martinez U., Boyra G., Acuña J. L., et al. (2014). Large Mesopelagic fishes biomass and trophic efficiency in the open ocean. Nat. Commun. 5, 1–10. doi: 10.1038/ncomms4271
Islam M. S., Tanaka M. (2004). Impacts of pollution on coastal and marine ecosystems including coastal and marine fisheries and approach for management: a review and synthesis. Mar. pollut. Bull. 48, 624–649. doi: 10.1016/j.marpolbul.2003.12.004
Jackson J. B., Kirby M. X., Berger W. H., Bjorndal K. A., Botsford L. W., Bourque B. J., et al. (2001). Historical overfishing and the recent collapse of coastal ecosystems. Science 293, 629–637. doi: 10.1126/science.1059199
Julshamn K., Maage A., Norli H. S., Grobecker K. H., Jorhem L., Fecher P., et al. (2007). Determination of arsenic, cadmium, mercury, and lead by inductively coupled plasma/mass spectrometry in foods after pressure digestion: NMKL interlaboratory study. J. AOAC Int. 90, 844–856. doi: 10.1093/jaoac/90.3.844
Kjeldahl J. (1883). Neue methode zur bestimmung des stickstoffs in organischen körpern. Z. für analytische Chemie 22, 366–382. doi: 10.1007/BF01338151
Kolding J., van Zwieten P. A., Marttin F., Funge-Smith S., Poulain F. (2019). Freshwater small pelagic fish and fisheries in the main African great lakes and reservoirs in relation to food security and nutrition. Fisheries and Aquaculture Technical Paper No. 642 (Rome: FAO), 124 pp.
Kourantidou M., Jin D. (2022). Mesopelagic–epipelagic fish nexus in viability and feasibility of commercial-scale mesopelagic fisheries. Natural Res. Model. 35, e12350. doi: 10.1111/nrm.12350
Kris-Etherton P. M., Harris W. S., Appel L. J. (2002). Fish consumption, fish oil, omega-3 fatty acids, and cardiovascular disease. Circulation 106, 2747–2757. doi: 10.1161/01.CIR.0000038493.65177.94
Landrier J.-F., Marcotorchino J., Tourniaire F. (2012). Lipophilic micronutrients and adipose tissue biology. Nutrients 4, 1622–1649. doi: 10.3390/nu4111622
Li J., Liu Z., Lin B., Zhao Y., Cao J., Zhang X., et al. (2022). Zooplankton fecal pellet characteristics and contribution to the deep-sea carbon export in the southern South China Sea. J. Geophys. Res.: Oceans 127, e2022JC019412. doi: 10.1029/2022JC019412
Longley C., Thilsted S. H., Beveridge M., Cole S., Nyirenda D. B., Heck S., et al. (2014). The role of fish in the first 1,000 days in Zambia (Brighton: Institute of Development Studies), 27–35. Special Collection.
Lund E. K. (2013). Health benefits of seafood; is it just the fatty acids? Food Chem. 140, 413–420. doi: 10.1016/j.foodchem.2013.01.034
Lusher A. L., O'Donnell C., Officer R., O'Connor I. (2016). Microplastic interactions with North Atlantic mesopelagic fish. ICES J. Mar. Sci. 73, 1214–1225. doi: 10.1093/icesjms/fsv241
Marquardt M., Vader A., Stübner E. I., Reigstad M., Gabrielsen T. M. (2016). Strong seasonality of marine microbial eukaryotes in a high-Arctic fjord (Isfjorden, in West Spitsbergen, Norway). Appl. Environ. Microbiol. 82, 1868–1880. doi: 10.1128/AEM.03208-15
Meier S., Mjøs S. A., Joensen H., Grahl-Nielsen O. (2006). Validation of a one-step extraction/methylation method for determination of fatty acids and cholesterol in marine tissues. J. Chromatogr. A 1104, 291–298. doi: 10.1016/j.chroma.2005.11.045
Monteiro L., Costa V., Furness R., Santos R. (1996). Mercury concentrations in prey fish indicate enhanced bioaccumulation in mesopelagic environments. Mar. Ecol. Prog. Ser. 141, 21–25. doi: 10.3354/meps141021
Mozaffarian D. (2009). Fish, mercury, selenium and cardiovascular risk: current evidence and unanswered questions. Int. J. Environ. Res. Public Health 6, 1894–1916. doi: 10.3390/ijerph6061894
Olsen R. E., Strand E., Melle W., Nørstebø J. T., Lall S. P., Ringø E., et al. (2020). Can mesopelagic mixed layers be used as feed sources for salmon aquaculture? Deep Sea Res. Part II: Topical Stud. Oceanogr. 180, 104722. doi: 10.1016/j.dsr2.2019.104722
Panseri S., Chiesa L., Ghisleni G., Marano G., Boracchi P., Ranghieri V., et al. (2019). Persistent organic pollutants in fish: biomonitoring and cocktail effect with implications for food safety. Food Additives Contaminants: Part A 36, 601–611. doi: 10.1080/19440049.2019.1579926
Pauly D., Maclean J. (2003). In a perfect ocean: the state of fisheries and ecosystems in the North Atlantic Ocean (Washington: Island Press).
Pearce E. N., Zimmermann M. B. (2023). The prevention of iodine deficiency: a history. Thyroid 33, 143–149. doi: 10.1089/thy.2022.0454
Qin Y. Y., Leung C. K. M., Leung A. O. W., Wu S. C., Zheng J. S., Wong M. H. (2010). Persistent organic pollutants and heavy metals in adipose tissues of patients with uterine leiomyomas and the association of these pollutants with seafood diet, BMI, and age. Environ. Sci. pollut. Res. 17, 229–240. doi: 10.1007/s11356-009-0251-0
Rasmussen L. B., Hollenbach B., Laurberg P., Carlé A., Hög A., Jørgensen T., et al. (2009). Serum selenium and selenoprotein P status in adult Danes - 8-year followup. J. Trace Elem. Med. Biol. 23, 265–271. doi: 10.1016/j.jtemb.2009.03.009
Rees D. J., Poulsen J. Y., Sutton T. T., Costa P. A., Landaeta M. F. (2020). Global phylogeography suggests extensive eucosmopolitanism in mesopelagic fishes (Maurolicus: sternoptychidae). Sci. Rep. 10, 1–12. doi: 10.1038/s41598-020-77528-7
Reksten A. M., Bøkevoll A., Frantzen S., Lundebye A.-K., Kögel T., Kolås K., et al. (2020). Sampling protocol for the determination of nutrients and contaminants in fish and other seafood–the EAF-Nansen Programme. MethodsX 7, 101063. doi: 10.1016/j.mex.2020.10106
Rice A. L., West K. P. Jr., Black R. E. (2004). “Vitamin A deficiency,” in Comparative quantification of health risks: global and regional burden of disease attributable to selected major risk factors. (Geneva: WHO). vol. 1. , 0211–0256.
Romanić S. H., Jovanović G., Mustać B., Stojanović-Đinović J., Stojić A., Čadež T., et al. (2021). Fatty acids, persistent organic pollutants, and trace elements in small pelagic fish from the eastern Mediterranean Sea. Mar. pollut. Bull. 170, 112654. doi: 10.1016/j.marpolbul.2021.112654
Roos N., Wahab M. A., Chamnan C., Thilsted S. H. (2007). The role of fish in food-based strategies to combat vitamin A and mineral deficiencies in developing countries. J. Nutr. 137, 1106–1109. doi: 10.1093/jn/137.4.1106
Rose G. A. (2007). Cod: the ecological history of the North Atlantic fisheries (St. John’s: Breakwater books).
Ruzzin J. (2012). Public health concern behind the exposure to persistent organic pollutants and the risk of metabolic diseases. BMC Public Health 12, 1–8. doi: 10.1186/1471-2458-12-298
Salvanes A., Stockley B. (1996). Spatial variation of growth and gonadal developments of Maurolicus muelleri in the Norwegian Sea and in a Norwegian fjord. Mar. Biol. 126, 321–332. doi: 10.1007/BF00347456
Schadeberg A., Kraan M., Groeneveld R., Trilling D., Bush S. (2023). Science governs the future of the mesopelagic zone. NPJ Ocean Sustainability 2, 2. doi: 10.1038/s44183-023-00008-8
Scheffer M., Carpenter S., de Young B. (2005). Cascading effects of overfishing marine systems. Trends Ecol. Evol. 20, 579–581. doi: 10.1016/j.tree.2005.08.018
Schots P. C., Pedersen A. M., Eilertsen K.-E., Olsen R. L., Larsen T. S. (2020). Possible health effects of a wax ester rich marine oil. Front. Pharmacol. 11, 961. doi: 10.3389/fphar.2020.00961
Schultz E. T., Conover D. O. (1997). Latitudinal differences in somatic energy storage: adaptive responses to seasonality in an estuarine fish (Atherinidae: Menidia menidia). Oecologia 109, 516–529. doi: 10.1007/s004420050112
Shadyeva L., Romanova E., Romanov V., Spirina E., Lyubomirova V., Shlenkina T., et al. (2019). “Forecast of the nutritional value of catfish (Clarias gariepinus) in the spawning period,” in IOP Conference Series: Earth and Environmental Science. 012218.
Shi L., Brunius C., Bergdahl I. A., Johansson I., Rolandsson O., Donat Vargas C., et al. (2019). Joint analysis of metabolite markers of fish intake and persistent organic pollutants in relation to type 2 diabetes risk in Swedish adults. J. Nutr. 149, 1413–1423. doi: 10.1093/jn/nxz068
Shukla A. K., Behera S. K., Pakhre A., Chaudhari S. (2018). Micronutrients in soils, plants, animals and humans. Indian J. Fertil. 14, 30–54.
Smith M. D., Roheim C. A., Crowder L. B., Halpern B. S., Turnipseed M., Anderson J. L., et al. (2010). Sustainability and global seafood. Science 327, 784–786. doi: 10.1126/science.1185345
Sobradillo B., Boyra G., Martinez U., Carrera P., Peña M., Irigoien X. (2019). Target strength and swimbladder morphology of Mueller’s pearlside (Maurolicus muelleri). Sci. Rep. 9, 17311. doi: 10.1038/s41598-019-53819-6
Standal D., Grimaldo E. (2020). Institutional nuts and bolts for a mesopelagic fishery in Norway. Mar. Policy 119, 104043. doi: 10.1016/j.marpol.2020.104043
Standal D., Grimaldo E. (2021). Lost in translation? practical-and scientific input to the mesopelagic fisheries discourse. Mar. Policy 134, 104785. doi: 10.1016/j.marpol.2021.104785
Stevens G. A., Beal T., Mbuya M. N., Luo H., Neufeld L. M., Addo O. Y., et al. (2022). Micronutrient deficiencies among preschool-aged children and women of reproductive age worldwide: a pooled analysis of individual-level data from population-representative surveys. Lancet Global Health 10, e1590–e1599. doi: 10.1016/S2214-109X(22)00367-9
Tacon A. G., Metian M. (2013). Fish matters: importance of aquatic foods in human nutrition and global food supply. Rev. fish. Sci. 21, 22–38. doi: 10.1080/10641262.2012.753405
Tanentzap A. J., Morabito G., Volta P., Rogora M., Yan N. D., Manca M. (2020). Climate warming restructures an aquatic food web over 28 years. Global Change Biol. 26, 6852–6866. doi: 10.1111/gcb.15347
Team, R. D. C (2009) A language and environment for statistical computing. Available at: http://www.R-project.org.
Temple S., Plate E., Ramsden S., Haimberger T., Roth W.-M., Hawryshyn C. (2006). Seasonal cycle in vitamin A1/A2-based visual pigment composition during the life history of coho salmon (Oncorhynchus kisutch). J. Comp. Physiol. A 192, 301–313. doi: 10.1007/s00359-005-0068-3
Tibon J., Amlund H., Gomez-Delgado A. I., Berntssen M. H., Silva M. S., Wiech M., et al. (2022). Arsenic species in mesopelagic organisms and their fate during aquafeed processing. Chemosphere 302, 134906. doi: 10.1016/j.chemosphere.2022.134906
Tigchelaar M., Cheung W. W., Mohammed E. Y., Phillips M. J., Payne H. J., Selig E. R., et al. (2021). Compound climate risks threaten aquatic food system benefits. Nat. Food 2, 673–682. doi: 10.1038/s43016-021-00368-9
Toppe J., Albrektsen S., Hope B., Aksnes A. (2007). Chemical composition, mineral content and amino acid and lipid profiles in bones from various fish species. Comp. Biochem. Physiol. Part B: Biochem. Mol. Biol. 146, 395–401. doi: 10.1016/j.cbpb.2006.11.02
UNICEF (2019). The state of the world’s children 2019: children, food and nutrition: growing well in a changing world (New York: UNICEF).
Voronin V. P., Artemenkov D. V., Orlov A. M., Murzina S. A. (2022). Lipids and fatty acids in some mesopelagic fish species: general characteristics and peculiarities of adaptive response to deep-water habitat. J. Mar. Sci. Eng. 10, 949. doi: 10.3390/jmse10070949
Voronin V. P., Artemenkov D. V., Orlov A. M., Murzina S. A. (2023). Fatty acid spectra in mesopelagic fishes of the Myctophidae and Stomiidae families collected in the North East Atlantic. Diversity 15, 166. doi: 10.3390/d15020166
Wiech M., Silva M., Meier S., Tibon J., Berntssen M. H., Duinker A., et al. (2020). Undesirables in mesopelagic species and implications for food and feed safety–insights from Norwegian fjords. Foods 9, 1162. doi: 10.3390/foods9091162
Workie E., Mackolil J., Nyika J., Ramadas S. (2020). Deciphering the impact of COVID-19 pandemic on food security, agriculture, and livelihoods: a review of the evidence from developing countries. Curr. Res. Environ. Sustainability 2, 100014. doi: 10.1016/j.crsust.2020.100014
Zhang W., Miao A.-J., Wang N.-X., Li C., Sha J., Jia J., et al. (2022). Arsenic bioaccumulation and biotransformation in aquatic organisms. Environ. Int. 163, 107221. doi: 10.1016/j.envint.2022.107221
Zhao T., Liu S., Zhang R., Zhao Z., Yu H., Pu L., et al. (2022). Global burden of vitamin A deficiency in 204 countries and territories from 1990–2019. Nutrients 14, 950. doi: 10.3390/nu14050950
Zlatanos S., Laskaridis K. (2007). Seasonal variation in the fatty acid composition of three Mediterranean fish–sardine (Sardina pilchardus), anchovy (Engraulis encrasicholus) and picarel (Spicara smaris). Food Chem. 103, 725–728. doi: 10.1016/j.foodchem.2006.09.013
Keywords: mesopelagic, food security, food safety and quality, nutrient, contaminant, marine resource
Citation: Zhu Y, Azad AM, Kjellevold M, Bald C, Iñarra B, Alvarez P, Boyra G, Berntssen M, Madsen L and Wiech M (2023) Differences in nutrient and undesirable substance concentrations in Maurolicus muelleri across the Bay of Biscay, Norwegian fjords, and the North Sea. Front. Mar. Sci. 10:1213612. doi: 10.3389/fmars.2023.1213612
Received: 28 April 2023; Accepted: 29 June 2023;
Published: 20 July 2023.
Edited by:
Snorre Bakke, NTNU Ålesund, NorwayReviewed by:
Francesca Falco, National Research Council (CNR), ItalyEnrique Lozano, University of Las Palmas de Gran Canaria, Spain
Copyright © 2023 Zhu, Azad, Kjellevold, Bald, Iñarra, Alvarez, Boyra, Berntssen, Madsen and Wiech. This is an open-access article distributed under the terms of the Creative Commons Attribution License (CC BY). The use, distribution or reproduction in other forums is permitted, provided the original author(s) and the copyright owner(s) are credited and that the original publication in this journal is cited, in accordance with accepted academic practice. No use, distribution or reproduction is permitted which does not comply with these terms.
*Correspondence: Yiou Zhu, yiou.mike.zhu@hi.no