- 1Dipartimento di Scienze della Vita e dell’Ambiente, Università Politecnica delle Marche, Ancona, Italy
- 2National Biodiversity Future Centre, Palermo, Italy
- 3Dipartimento di Scienze e Ingegneria della Materia, dell’Ambiente ed Urbanistica, Università Politecnica delle Marche, Ancona, Italy
Introduction: Passive and active restoration approaches have been often considered as different alternatives to achieve the ecological restoration of a degraded site. This false dichotomy has been overcome in terrestrial ecosystems, for which a range of restoration approaches have often been applied within the same restoration project, creating a continuum of interventions. In the marine environment, the combined approach based on passive and active restoration is less studied.
Methods: Here, we tested the possibility of actively restoring a macroalgal population in a historically polluted industrial site, subjected to decadal passive restoration (i.e., unassisted, or spontaneous recovery following cessation of industrial activity), in the Mediterranean Sea. Recently, it has been demonstrated that in the site under scrutiny, there is no longer any sign of the historical contamination or impact on benthic fauna therefore the environmental conditions are indistinguishable from surrounding non-impacted areas. We used artificial breakwater barriers already present in the site to restore a population of the brown algae Gongolaria barbata. The intervention was conducted by applying an in situ recruitment approach and a complementary action using cages to exclude the herbivores. The G. barbata recruits were transplanted on the artificial reefs and after they reached the maximum growth inside the cages, one cage was opened and another one was completely removed to also test the grazing pressure. The associated benthic assemblages were also analysed in terms of meio- and macrofaunal abundance, the richness of taxa, and taxonomic composition.
Results and discussion: Our results indicate that, at least over a relatively short period (i.e., 6 months), the use of the cages represents a useful tool to let the macroalgae grow enough to counterbalance the herbivory pressure. The associated fauna below the macroalgae was characterized by a different taxonomic composition when compared to controls (i.e., artificial reefs without the macroalgae), particularly when considered the rare taxa. In conclusion, the combination of passive and active restoration can be considered a useful approach to restore marine sites degraded by historical industrial activities.
1 Introduction
Facing unprecedented rates of loss and change at all levels of biological diversity, the increasing efforts to conserve marine coastal ecosystems appear still insufficient, since the natural recovery of ecosystems can be difficult in a reasonable time frame (Lotze et al., 2006; Cardinale et al., 2012). Indeed, even when the source of impact is removed, the ecosystem recovery to pristine conditions can last from decades to centuries (Lotze et al., 2011). Thus, in the frame of ecological restoration, passive or unassisted restoration (mainly considered as a conservation measure rather than genuine restoration, mostly aimed at mitigating human threats) can be insufficient to halt or reverse trajectories of change (Jones et al., 2018; Lindegren et al., 2018). To this end, active restoration (i.e., the process of actively assisting the recovery of an ecosystem that has been degraded, damaged, or destroyed) is considered one of the most promising strategies to foster ecosystems recovery (Aronson and Alexander, 2013; Fraschetti et al., 2021).
The distinction between active and passive restoration persists in much research and policy today (Chazdon et al., 2021). However, in the framework of the UN Decade on Ecosystem Restoration, it has recently suggested replacing this dichotomy with a continuum-based process framework (2021-2030, UNGA Resolution 73/284; Chazdon et al., 2021). The main distinction between “passive” and “active” restoration lies primarily in the timing and extent of human interventions, and cannot be considered as opposite aspects, but rather as complementary. The actions required to eliminate human pressures are essential for enabling ecosystem recovery processes, regardless of the kind of restoration interventions applied (Chazdon et al., 2021).
Previous investigations in marine ecosystems suggested that to reduce and reverse biodiversity loss, the “passive” restoration (e.g., obtained through the institution of marine protected areas, MPAs) should be complemented by “active” restoration (e.g., transplants, translocations; van Tatenhove et al., 2021). However, also “Other Effective Area-based Conservation Measures” (OECMs: locally managed no-take areas, fishing restricted areas, ecological corridors, and trawling bans established through long-term management plans; Diz et al., 2018) or areas interdicted to human activities for decades due to historical pollution can be optimal candidates as restoration sites, particularly those that no longer present signals of environmental damage and have characteristics indistinguishable from the surrounding non-interdicted areas (Corinaldesi et al., 2022; Fanelli et al., 2023).
In this regard, historically and chronically polluted sites, are under the attention of policy makers and environmental managers in the USA (by the U.S. Environmental Protection Agency - EPA) and Canada (by the Canadian Council of Ministry of the Environment - CCME) (Ausili et al., 2020). The European Union (EU) identified soil contamination as a priority for the collection of policy-relevant soil data at the European scale (COM(2006)231). Regarding the marine realm, the EU recognized the need to review current legislation, such as the Directive on Sewage Sludge and the Directive on Integrated Pollution Prevention and Control (IPPC) and to assess whether there are any synergies between the current legislation and the Water (2000/60/EC), the Marine Strategy (2008/56/EC) Framework Directives, as well as with the Barcelona (1976), OSPAR (1992), and HELCOM (1992) Conventions (Panagos et al., 2013; Ausili et al., 2020). In Italy, polluted sites defined as “Sites of National Interest” (SNI), require priority actions of environmental remediation by governmental laws due to the high levels of environmental and human risk (Ausili et al., 2020). In the SNI under scrutiny here, previous studies suggested a natural recovery of the marine area over 2 decades of restrictions on human activities, including fishing and shipping (Corinaldesi et al, 2022). Following the concept of restoration as a continuum of interventions, these sites are excellent candidates for active restoration implementation.
Macroalgal forests are among the most studied rocky-bottom habitats for their potential success in restoration interventions (Fabbrizzi et al., 2023). In the Mediterranean Sea, macroalgal forests are dominated by Cystoseira sensu lato (Fucales brown algae) species and form the most productive and diversified ecosystems, thus providing a plethora of ecosystem services. At the same time, these forests are among the habitats most threatened by human impacts. Although macroalgal forests are regressing in the whole Mediterranean basin, important steps forward in their restoration in terms of approaches, techniques, and interventions have been made in the last years, so that nowadays guidelines are available for several species (Cebrian et al., 2021). In 2009, an amendment of the Mediterranean Action Plan (Annex IV, SPA/BD Protocol – United Nations Environment Programme) adopted within the framework of the Barcelona Convention (1976), identified the conservation of all but one (Cystoseira compressa) Mediterranean Cystoseira s.l. species as a priority. Despite the robust legislative framework, specific conservation measures for the protection of these habitat-forming species have never been implemented (Fraschetti et al., 2011). Indeed, these species are not listed in the Habitat Directive annexes (Directive 92/43 EEC), but only the habitat they colonize (1170 “Reefs”).
These macroalgal forests play an important role in sustaining high levels of biodiversity, due to their role as primary producers and the organic matter availability which they provide (Bianchelli et al., 2016a; Bianchelli et al., 2016b; Bianchelli and Danovaro, 2020). For this reason, restoration interventions are necessary, especially for those populations that are not able to recover naturally (Gianni et al., 2013; Fraschetti et al., 2021). However, by definition, “ecological restoration” aims to recreate, initiate, or accelerate the recovery of an ecosystem that has been disturbed, so that the success should be measured by monitoring not only the survival of the restored populations but also the biodiversity recovery of the associated biodiversity.
Meio- and macrobenthic communities are characterized by high structural and trophic-functional diversity and have key roles in benthic food webs and in maintaining their functionality, thus providing information on the ecosystem’ health status (Bianchelli and Danovaro, 2020; Fanelli et al., 2023). Therefore, their abundance, biomass, and diversity can be investigated in restoration interventions of different macroalgae species and used as indicators of success (Semprucci et al., 2013; Bianchelli et al., 2016a; Bianchelli et al., 2016b; Corinaldesi et al., 2022; Bianchelli et al., submitted).
In this study, we carried out a restoration intervention of a Gongolaria barbata population on the breakwater barriers in the Site of National Interest (SNI) of Falconara Marittima in the Central-Western Adriatic Sea (Italy, Mediterranean Sea). The SNI is a historically polluted and degraded environment, enclosed among the “problem areas” of the European seas (Andersen et al., 2022). The interdiction of the marine area to any human use for about 20 years has been reported to have positive effects on the benthic ecosystems, which currently show no sign of alteration (Corinaldesi et al., 2022; Fanelli et al., 2023). Therefore, this area putatively represents an optimal site for restoration. Studies on the use of artificial reefs, which are already present on site, as substrates for the active restoration of lost natural populations of Cystoseira s.l. are limited, but these could be very useful to foster the rehabilitation of degraded and artificial habitats (Susini et al., 2007; Perkol-Finkel et al., 2012; Ferrario et al., 2015).
Breakwater barriers, like many other artificial marine substrates, contribute to the alteration of coastal habitats, threatening biodiversity and impairing ecosystem functioning (Perkol-Finkel et al., 2012; Gianni et al., 2013). Artificial structures are typically characterized by lower levels of biodiversity than natural ones and are often dominated by opportunistic and invasive species (Bulleri and Chapman, 2010, Ferrario et al., 2015). Even if they can be exploited as a substrate for macroalgae restoration interventions and to increase local ecological value, the growth of canopy algae could be impaired by possible less favourable abiotic conditions and by higher biotic disturbance from both consumptive and non-consumptive interactions on the artificial structures compared to the natural reef (Ferrario et al., 2015).
Literature information about the historical presence of G. barbata in the area under scrutiny is lacking although the presence of Cystoseira spp was reported along the Adriatic coasts, including the western-central side of the basin (Perkol-Finkel and Airoldi, 2010). Along this coast attempts of promoting the growth of threatened species of Cystoseira s.l. on coastal infrastructures were also carried out to rehabilitate these habitats (i.e., restore or improve some aspects of an ecosystem but not necessarily fully restore all its components to their original, undisturbed state; Roni et al., 2005; Perkol-Finkel et al., 2012).
In the present study, to increase the restoration success, the active intervention was complemented with the protection of the G. barbata transplants from macro- and mesograzers through cages. Previous studies, indeed, showed a high grazing pressure in the same investigated site due to the Pachygrapsus marmoratus crab (Bianchelli et al., submitted), which has been specifically reported to be a grazer of Cystoseira s.l. on artificial reefs (Gianni et al., 2018). The success of restoration interventions was measured in terms of algal growth but also of associated benthic meio- and macrofaunal abundance and biodiversity. An additional laboratory experiment was also performed to better understand the mechanisms and the rates of herbivory of P. marmoratus on G. barbata, and to provide a first calibration of the grazing management in future restoration actions.
Overall, the objective of the present investigation is assessing the transplanting success of the macroalgal population of G. barbata in a historically polluted industrial site, combining active and passive restoration. More specifically, we tested the null hypothesis that using cages to control the grazing pressure, the transplanted G. barbata do not grow over time and that the associated meio- and macrofaunal assemblages (considered here as a proxy of biodiversity) did not change in terms of abundance, richness of taxa and taxonomic composition.
2 Materials and methods
2.1 Study area and selection of species for restoration intervention
The study area is the Site of National Interest (SNI) of Falconara Marittima (43°38’54’’N 13°21°35’’E), established under Law No. 179 of July 31, 2002, by Decree of the Minister of Environment and Territorial Protection of February 26th, 2003 (Corinaldesi et al., 2022; Figure 1). The SNI covers a marine-coastal area of about 1’200 ha and a territory of about 108 ha; it includes industrial settlements with high environmental impact, such as the API Raffineria S.p.A. and the disused area of the industrial plant “ex Montedison”, which produced phosphatic fertilizers from pyrite and phosphorite.
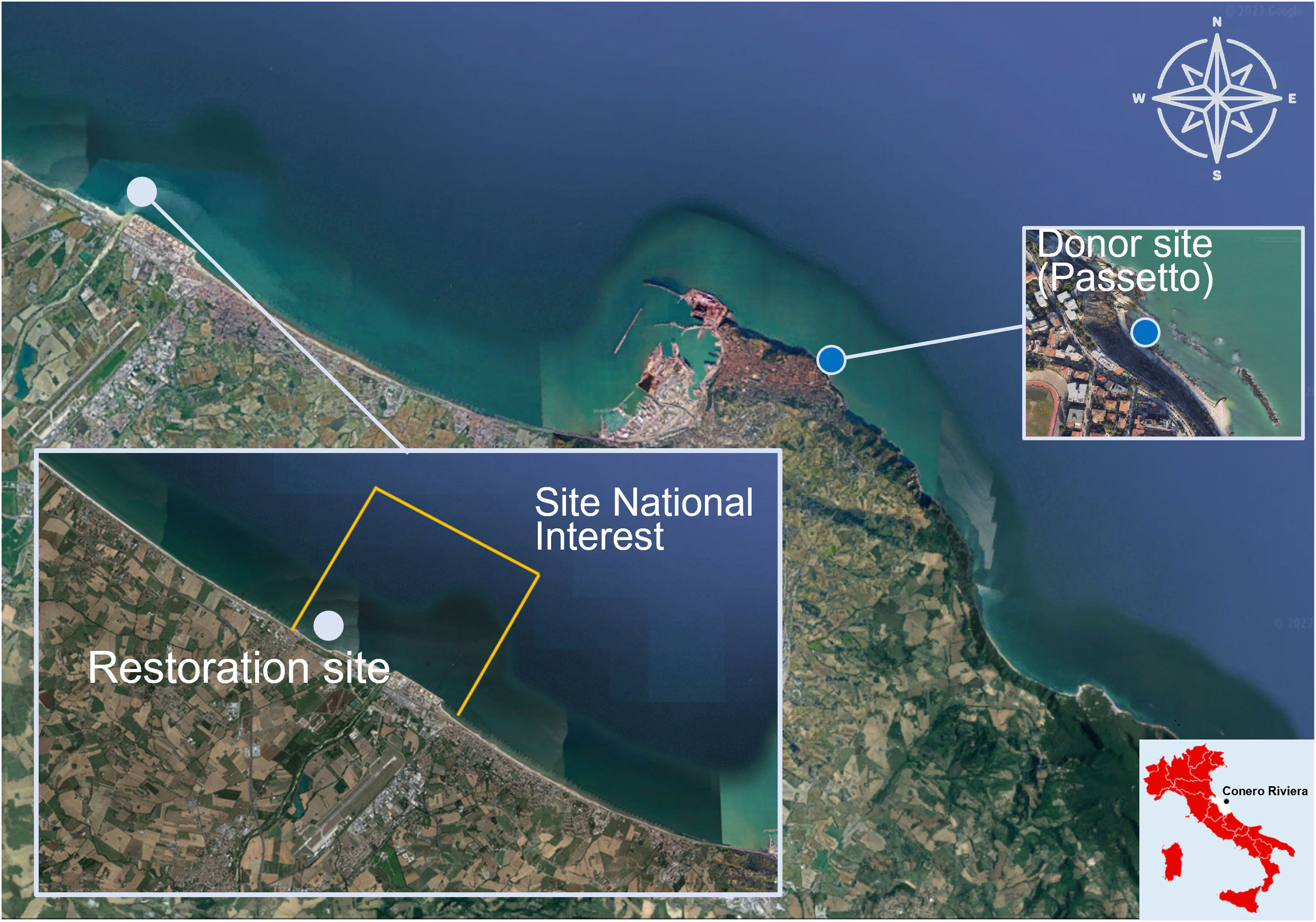
Figure 1 Study area. Orange lines: boundaries of SNI area, light blue dot: restoration site inside SNI (Lat: 43°38’54’’N; Long: 13°21°35’’E), dark blue dot: donor site. The map was generated using Map data ©2019 Google.
Although the SNI is included in the Area with High Risk of Environmental Crisis Falconara and Lower Esino Valley (AERCA) (ARPAM, https://www.arpa.marche.it/siti-di-interesse-nazionale), the anthropogenic impact is no longer detectable in the marine area and the marine sediment could be classified as “meso-eutrophic” (Pusceddu et al., 2009), a common condition in many areas of the Adriatic Sea (Bianchelli et al., 2016a; Bianchelli et al., 2018). However, in the terrestrial environment, soil and groundwater show contaminant levels above the thresholds (Legambiente, 2021). In the coastal area facing the SNI, there are 11 breakwater barriers, extending from the “ex Montedison” industrial plant to the mouth of the Esino River.
G. barbata (Fucales) lives in shallow and sheltered rocky environments and is considered a threatened species by the Barcelona Convention. This species is quite rare along the coasts of the Mediterranean Sea, 107 populations have been recorded in recent years along Italian coasts across the Central and Southern Tyrrhenian, Sardinian, Adriatic and Northern Ionian Seas (Tamburello et al., 2022). Local extinctions have been documented between 1979 and 2013–2016, whereas few populations naturally recovered by 2000–2008 (Tamburello et al., 2022).
2.2 Restoration intervention
The G. barbata specimens used for transplantation in the SNI were obtained from in-situ recruitment carried out at Passetto (Ancona), where during February 2021 3 artificial structures were positioned under reproductive adults of G. barbata (the donor population: Bianchelli et al., submitted). The structures were equipped with clay tiles characterized by rough surfaces to let the zygotes root and grow (Verdura et al., 2018).
In July 2021, the 3 structures with the G. barbata juveniles were moved from Passetto to the SNI of Falconara Marittima. The most distant breakwater barrier from the Esino River mouth was selected as receiving site since it corresponds to the lowest level of transport of sediment, freshwater, and nutrients.
A complementary action based on the installation of cages to exclude the herbivores due to the high grazing pressure in the area (Bianchelli et al., submitted) was also carried out. As a result, each experimental unit was composed of an artificial structure with G. barbata juveniles and the cage to exclude grazers. The cages were positioned at about 1-meter depth in the landward part of the barrier and were built with a galvanized iron mesh with 12 mm mesh and were 40 cm in length, 25 cm in width, and 20 cm in height and had a removable lid (Figure 2). The experimental units were fixed to the artificial reef with the drill and stainless-steel screws.
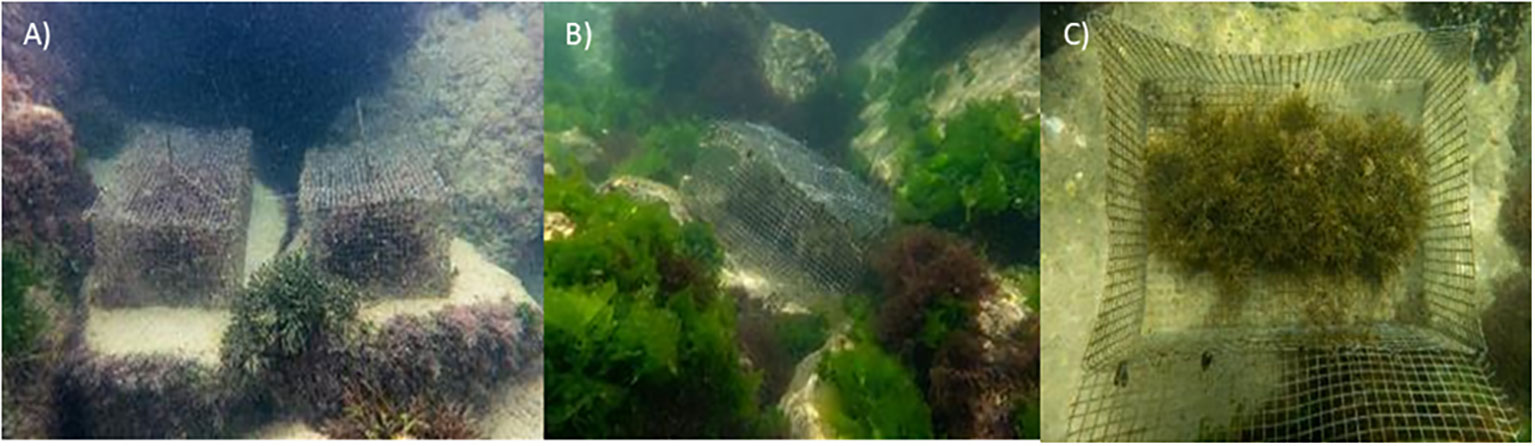
Figure 2 Three experimental units (A, B) composed each of the artificial structure with G. barbata juveniles and cage, and G. barbata juveniles inside one of the cages (C).
On 21st October 2021, following a strong storm, the cage of the third structure (structure 3) was uprooted and one of the five clay tails was lost. Since we observed that in the other two structures the algae’ heights reached the lid of the cages, the same day, the lid of the first cage (structure 1) was removed to let the algae grow and test the resistance of G. barbata grown against grazers.
2.3 Indicators of success: algal growth and associated biodiversity
The response variables chosen (G. barbata individuals’ height and canopy coverage, meio- and macrofaunal abundance and diversity) allowed us to monitor the growth of G. barbata over time and changes in the associated biodiversity. The height and the canopy coverage of the G. barbata were used to monitor its growth. The height was measured in 10 individuals for each experimental unit (abundance 5-15 cm-2 individuals), whereas the canopy coverage was assessed as % over a standardized surface (corresponding to the area of the cage).
Meiofauna and macrofauna (investigated in terms of total abundance, richness of taxa, and taxonomic composition) were used as a proxy for the associated communities. Sampling for meio- and macrofauna analyses was carried out comparing assemblages under the structures with a substrate with no transplanted macroalgae (used as control), approximately every 2 months, from transplantation to SNI in July until January 2022. A modified manual corer with an inner diameter of 9 cm was used to take 3 replicated samples from the hard substrate nearby cages (1 replicate for each experimental unit) and 3 replicates far from them (as a reference). Samples were then collected in plastic bags (Danovaro and Fraschetti, 2002). The samples were then frozen at -20°C until the analyses at lab.
For the extraction of meio- and macrofauna, the samples were subjected to ultrasonic treatment (3 x 1 minutes) and filtered on 20 and 500 µm meshes, for separating meio- and macrofauna. For meiofauna, an extraction based on centrifugation in a density gradient, with Ludox HS40 gel solution was used (Heip et al., 1985; Danovaro, 2010). The samples were then placed in 50 ml falcon with 70% ethanol and Rose Bengal and kept refrigerated for 2-3 days to allow the staining of the sample. The abundance of organisms was determined by using the stereomicroscope with magnifications from 16 to 40X.
2.4 Grazing experiment
During the sampling survey in October 2021, individuals of P. marmoratus were photographed while eating G. barbata settled on the third structure, deprived of the cage after the sea storm. After 18 days, on the same structure, grazers completely removed G. barbata individuals. Since no herbivorous fish were observed along the breakwaters during the entire period, an experiment was conducted in the aquarium to investigate P. marmoratus grazing on the algae. To do this, in December 2021, 4 specimens of P. marmoratus, together with a small stone covered by numerous individuals of G. barbata less than 15 cm high, were taken from the donor site Passetto (Figure 3).
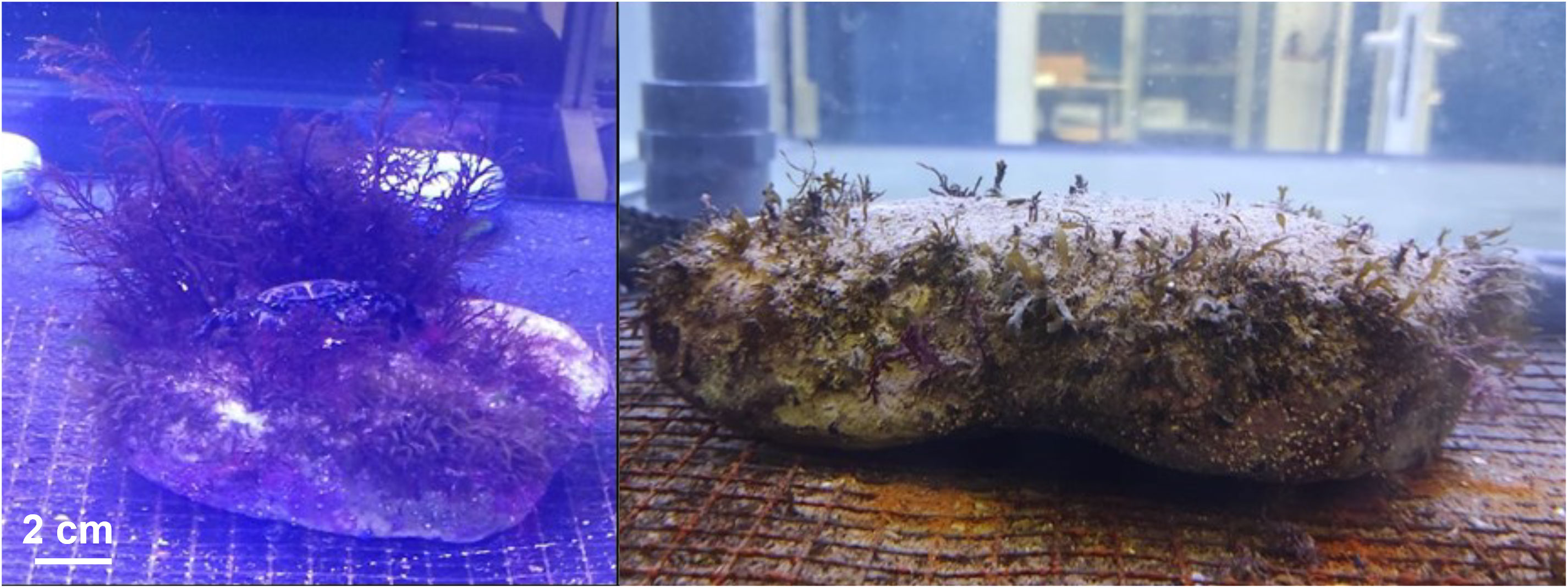
Figure 3 P. marmoratus grazing on G. barbata at the beginning of the experiment (left panel) and the stone completely grazed at the end of the experiment (right panel).
The crabs and the rock with the algae were transferred inside a tank of 200 L, inside which was placed a rubber mat as a substrate to facilitate the movement of animals, which was not given any type of additional food. The photoperiod of the tank was set at seven hours of light and 17 h of dark, and the water temperature was 13°C.
The number of G. barbata individuals and their heights were measured across 20 days, every 4-5 days, for a total of 6 sampling times. Through an underwater camera, videos were also made taking photos at an interval of 30 seconds one from each other, to shoot crabs while eating G. barbata.
2.5 Data processing and statistical analyses
For algal growth, the experimental design considered 2 factors as source of variability: Time, fixed with 17 levels (corresponding to the sampling times), and Structure, fixed with 3 levels. The analysis considered 9-10 replicated measures each time in each structure. For the analysis of the associated biodiversity, the experimental design considered 2 factors as source of variability: Site (fixed, 2 levels: cages and control) and Time (fixed, 4 levels, corresponding to the sampling times), with 3 replicates. For algal growth and abundance in the experiment on grazing, the experimental design considered only Time as source of variance (fixed factor, with 6 levels).
The experimental designs were applied to permutational analyses of variance (PERMANOVA), in uni- (for algal growth, total meio- and macrofaunal abundance) and multivariate context (for taxonomic composition of the meio- and macrofaunal assemblages) and based on Euclidean distance and Bray-Curtis similarity matrices, respectively. Analyses on meio- and macrofaunal taxonomic composition were repeated considering the whole assemblages and considering only the rare taxa. When significant differences were observed, pair wise tests were performed to establish between which levels significant differences were present. To visualize differences in the taxonomic composition, bi-plot were also produced following CAP (Canonical Analysis of Principal coordinates) analysis.
All statistical analyses were done with the PRIMER7 software package.
3 Results
3.1 Gongolaria barbata growth
During the recruitment phase (from March ‘21 to July ‘21) at Piscinetta del Passetto, the heights of G. barbata individuals significantly grew over time, with no difference among the structures (Figure 4A). After the transplant at SNI carried out in July ‘21, algae significantly grew until September ‘21, both in terms of height and canopy coverage (heights range 13.7-15.0 cm and canopy coverage 60.4-81.8%, Figures 4A, B). In October ‘21 a storm destroyed one of the cages (Structure 3), resulting in the complete disappearance of the algae from the structure in one month (Figure 4A, dark green dots). On that occasion, the cage lid was removed from Structure 1. In Structures 1 and 2, algal heights and canopy coverage did not significantly change over the following 3 months. At the beginning of January ‘23, another storm destroyed the remaining two cages, however, on that occasion the algae were not detached and their eight and canopy coverage did not change until the end of the experiment (end of January ‘22). At the end of the experiment, the algae heights, and their canopy coverage in the Structure 1 (without the lid) were higher than in the cage with the closed lid (Structure 2).
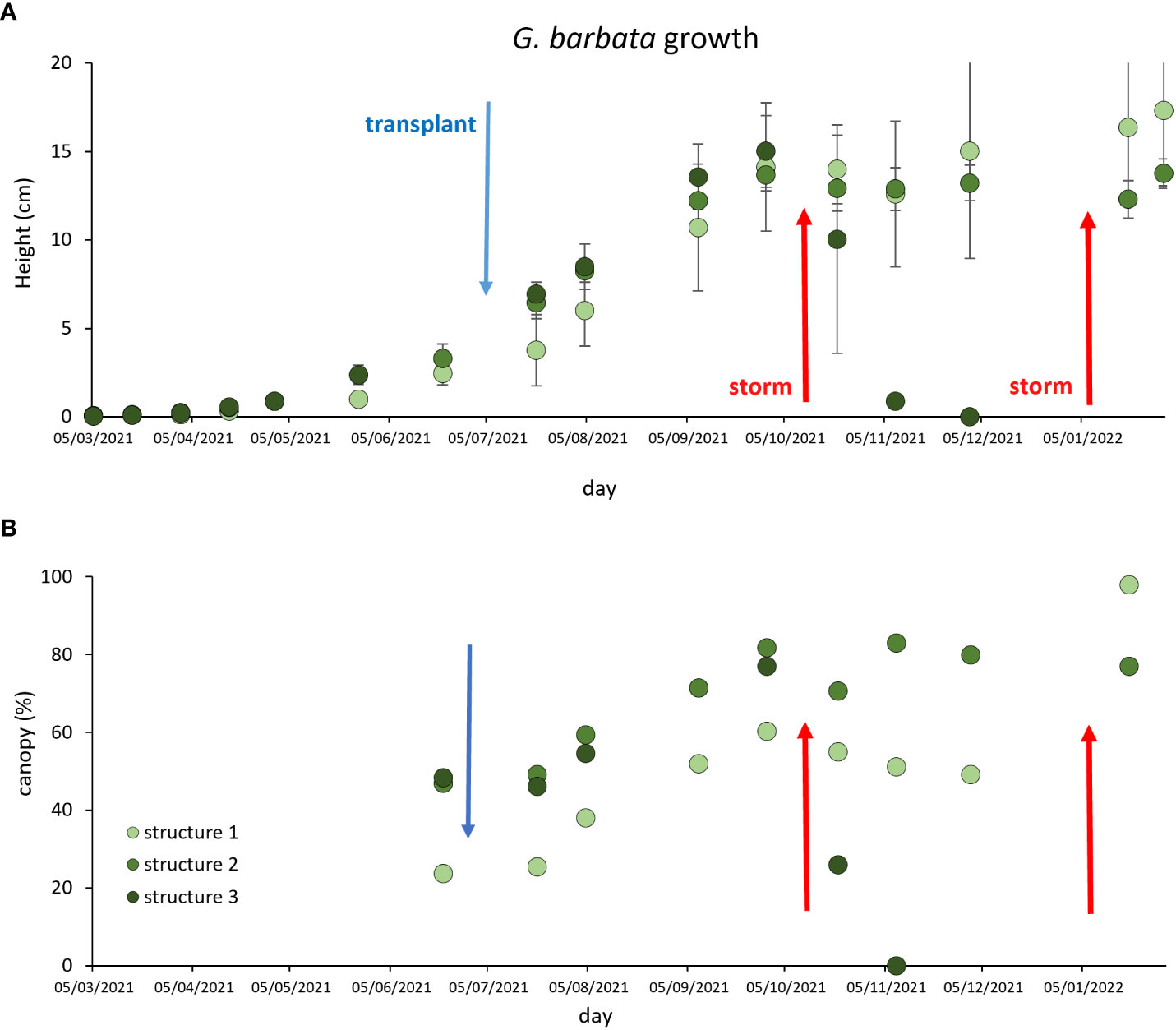
Figure 4 G. barbata growth during the recruitment phase at Passetto and after the transplant within the SNI (blue arrow), in term of algal height (A) and canopy coverage (B). Red arrows indicate storms, the first of which destroyed one of the cages (in October ‘21), the second one destroyed the two remaining cages (in January ‘22).
3.2 Associated meio- and macrofaunal diversity
Meiofaunal abundance showed significant differences over time but not between cages and the control site (Table 1A, Figure 5A). The richness of meiofaunal taxa was higher in the control site in July ‘21 and underneath the cages in the last sampling time (January ‘22) (Figure 5B). The overall richness of taxa (i.e., cumulatively in control and cages sites) increased over time until November ‘21 and corresponded to the richness of taxa underneath the cages in the last sampling time (Figure 5B).
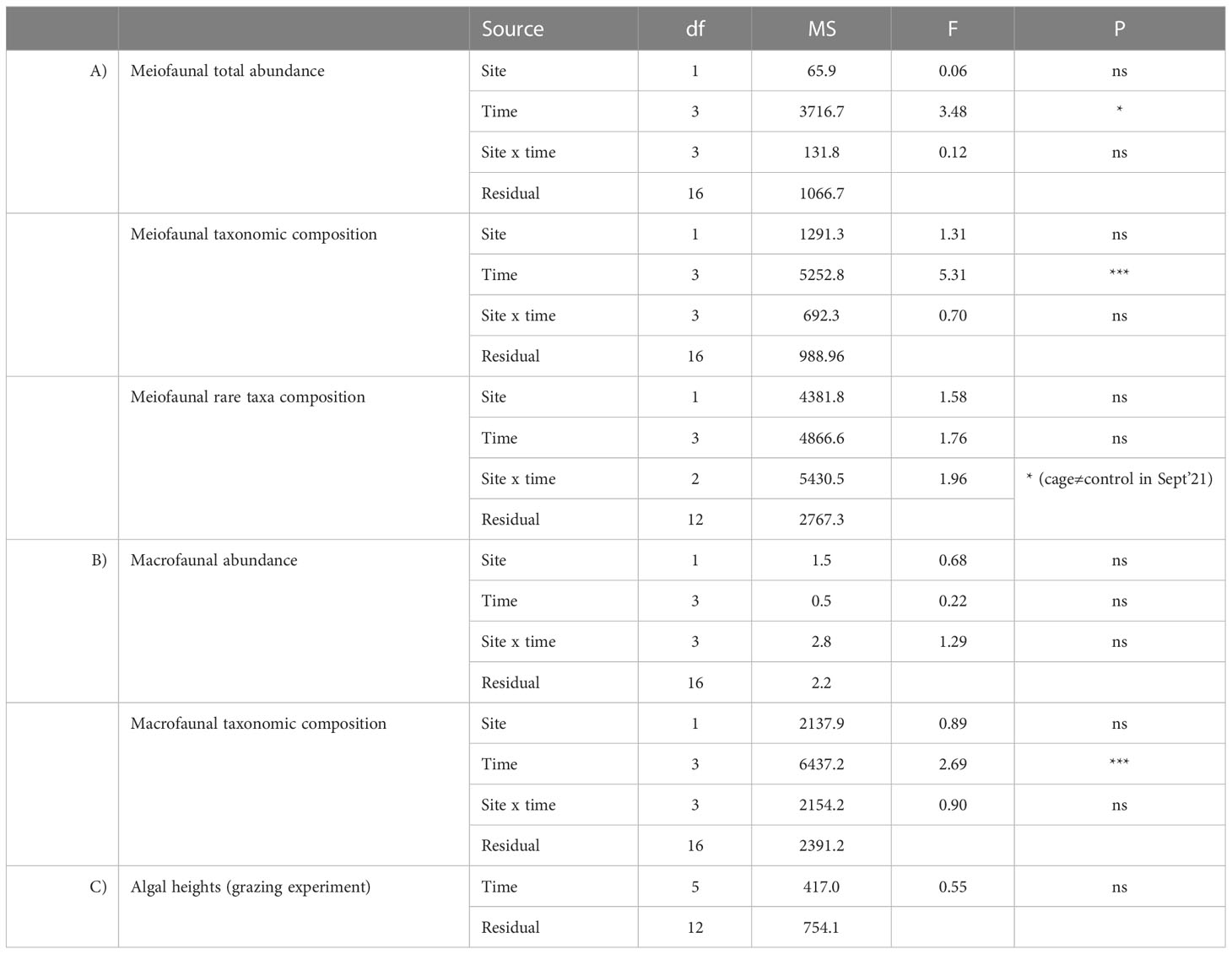
Table 1 Output of PERMANOVA analyses testing for the effect of factors Site and Time on meiofaunal abundance, taxonomic and rare taxa composition (A), macrofaunal abundance and taxonomic composition (B), and the effect of time on the algal heights during the grazing experiment (ns = not significant, * = p < 0.05; *** = p < 0.001).
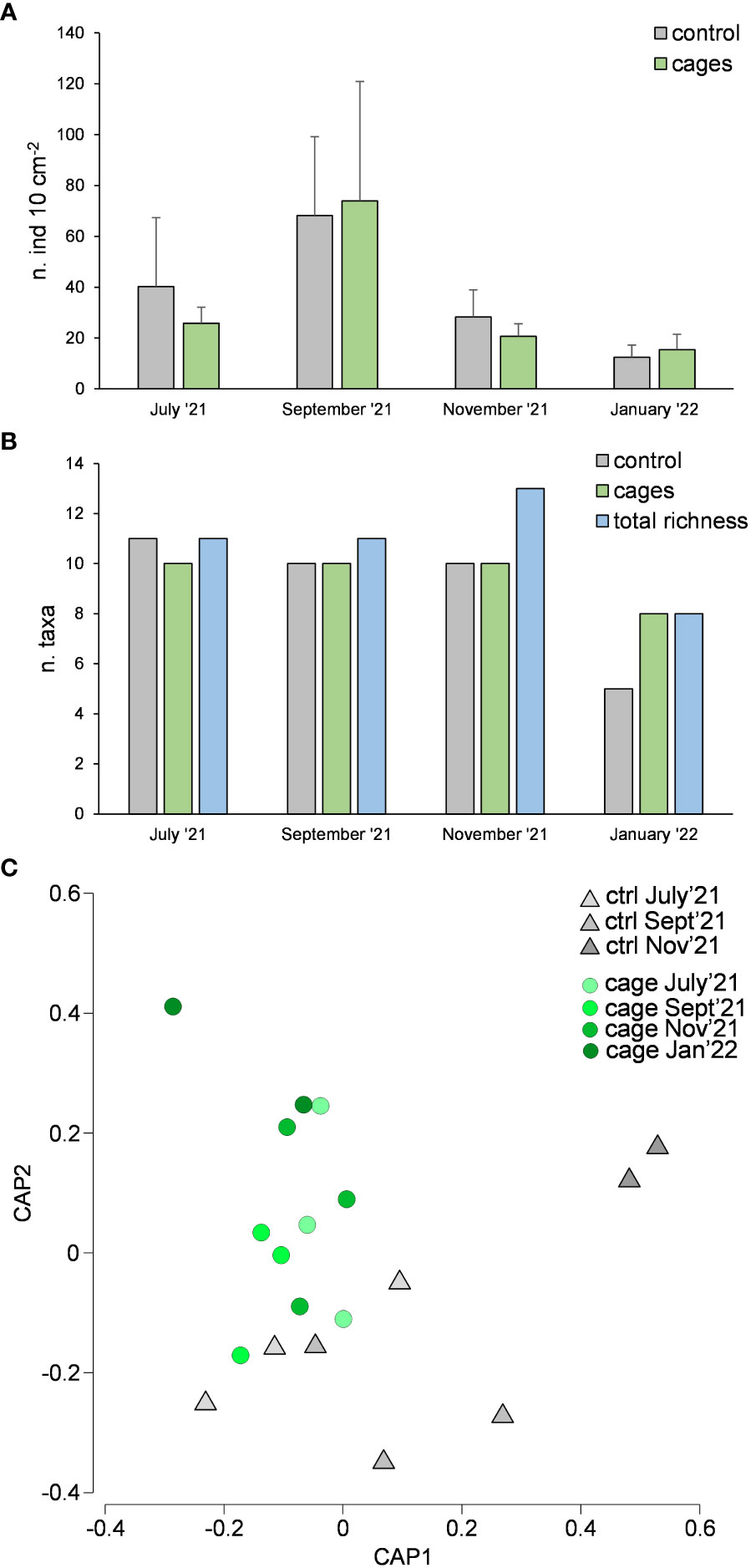
Figure 5 Meiofaunal abundance (A), richness of taxa (B) and taxonomic composition considering rare taxa (C) underneath the cages with G. barbata and in the control site (without G. barbata and cages).
The assemblages were dominated by Nematoda (52-57% and 36-67% in control site and beneath the cages, respectively), Copepoda (11-37% and 9-30%), Polychaeta (2-5% and 3-10%), Bivalvia (2-32% and 2-44%) and Kinorhyncha (2% and 0-2%). The rare taxa (i.e., representing each <1% of the assemblages) observed were: Oligochaeta, Amphipoda, Ostracoda, Isopoda, Cumacea, Gasteropoda, Acarina, Tanaidacea and Hydrozoa in the control site; and Oligochaeta, Cladocera, Amphipoda, Ostracoda, Isopoda, Cumacea, Gasteropoda, Acarina, Tanaidacea, Hydrozoa and Picnogonida underneath the cages. Considering only the rare taxa, significant differences were observed between cages and control site (Table 1A, Figure 5C). In the last sampling time (January 2022) rare taxa were observed only underneath the cages with G. barbata.
Macrofaunal abundance did not show significant differences over time nor between cages and the control site (Table 1B, Figure 6A). The richness of macrofaunal taxa was higher in the control site only in July ‘21, where the values were higher underneath the cages in September and November 2021 (Figure 6B). The overall richness of taxa was higher than that observed in the control or cages (Table 1B, Figure 6B).
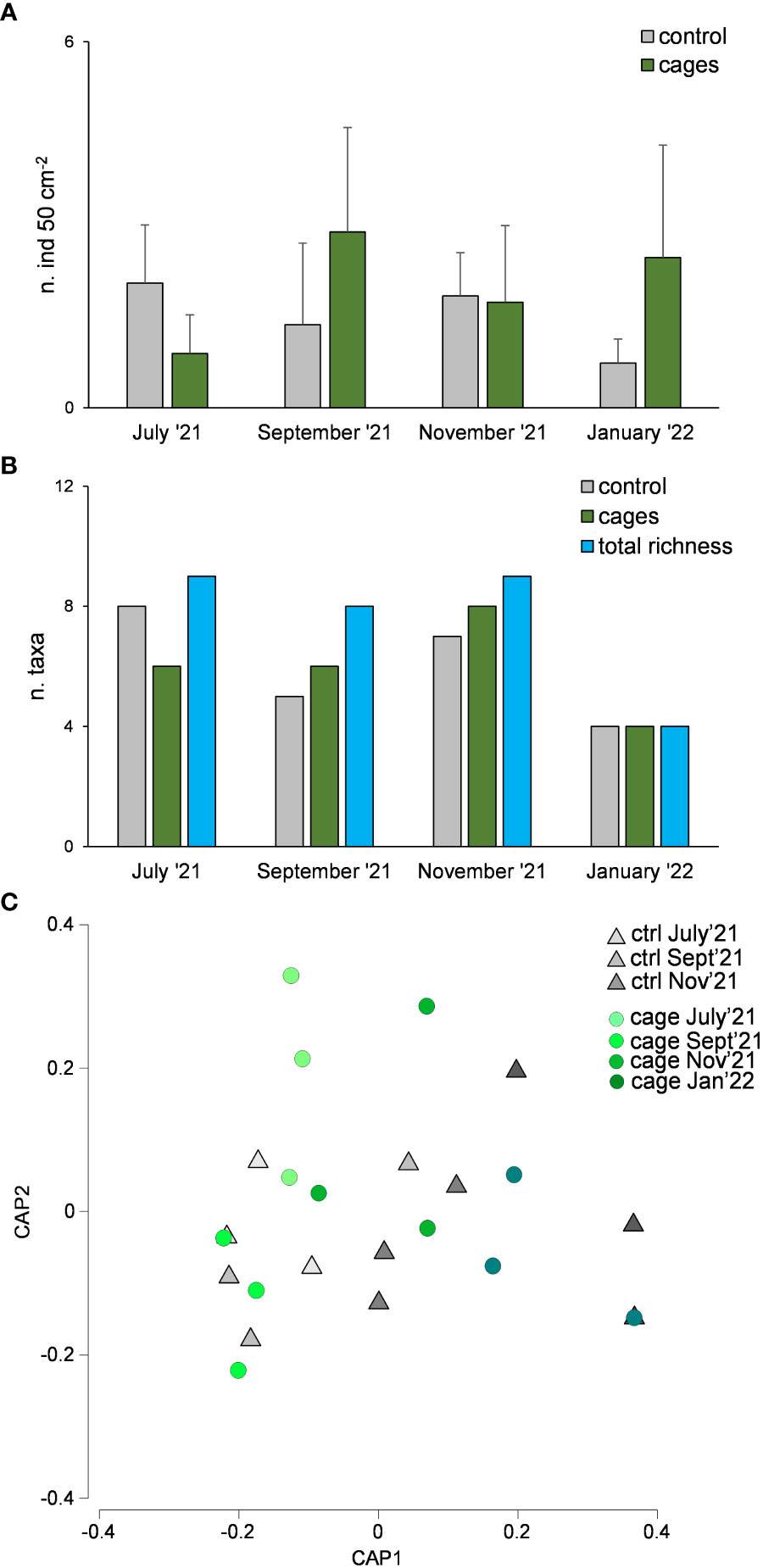
Figure 6 Macrofaunal abundance (A), richness of taxa (B) and taxonomic composition (C) underneath the cages with G. barbata and in the control site (without G. barbata and cages).
The assemblages were dominated by Polychaeta (21-49% and 27-64% in the control site and beneath the cages, respectively), Nematoda (7-41% and 4-53%), Bivalvia (3-21% and 0-13%) and Hydrozoa (0-50% and 0-55%). Significant differences were observed only among sampling times but not between cages and control site (Table 1B, Figure 6C).
3.3 Grazing rates and mechanisms
The number of the algae individuals on the stone was 27 at the beginning of the experiment, decreased by ca. 50% after 12 days and the individuals were completely grazed after 30 days (Figure 7A). PERMANOVA analyses did not reveal any significant differences in the algal heights across time (Table 1C, Figure 7B). The recorded videos confirmed that P. marmoratus grazes on G. barbata detaching the individuals from the basal disc of the algae, irrespective of their height.
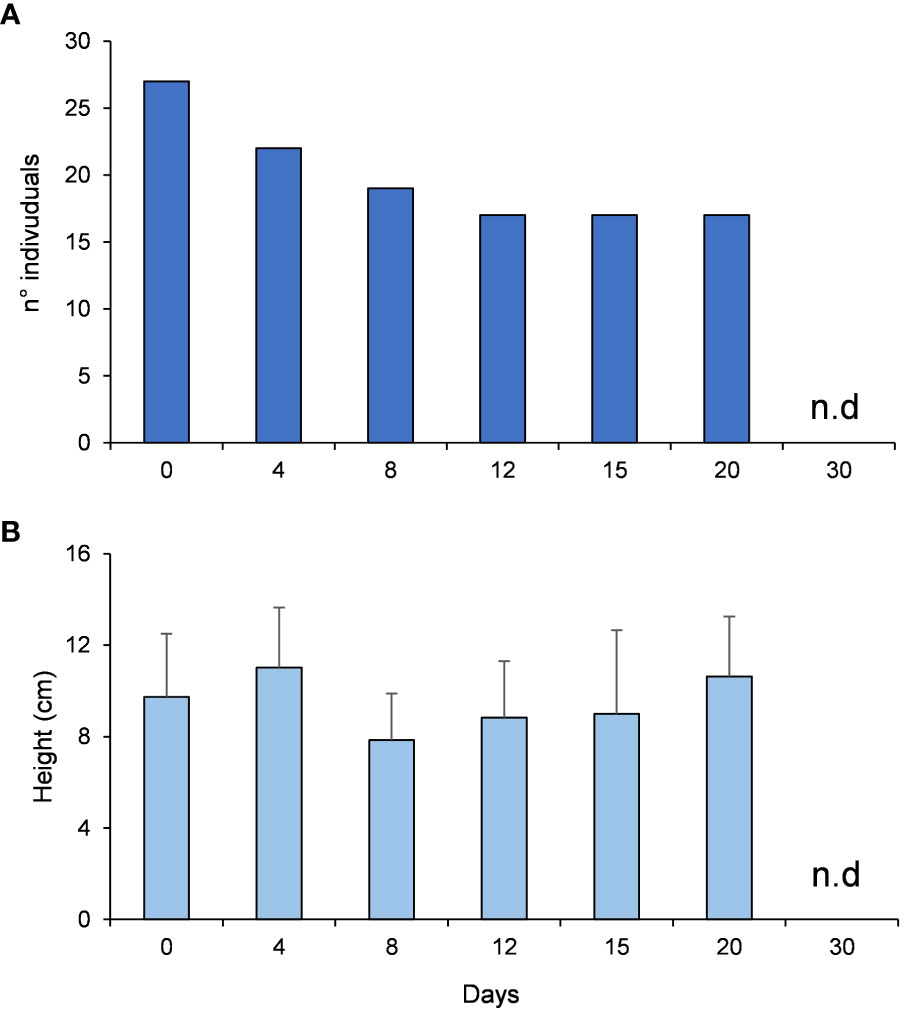
Figure 7 G. barbata number of individuals (A) and heights (B) during the experiment on grazing rates and mechanism.
4 Discussion
4.1 Integrating “passive” and “active” restoration
In this study, the SNI of Falconara Marittima, was selected for testing the possibility of rehabilitating the marine ecosystem through a transplant experiment of a population of G. barbata. Fucales forests can indeed be transplanted into degraded habitats where they were historically present, but also in areas where they had never been recorded in order to rehabilitate the habitat and increase its ecological value (Gianni et al., 2013).
Being interdicted to human activities for decades due to historical pollution and presenting nowadays characteristics indistinguishable from the surrounding non-interdicted areas, this SNI represents an optimal candidate as restoration site to implement combined passive and active restoration interventions (Corinaldesi et al., 2022; Fanelli et al., 2023).
Coastal infrastructures, such as breakwater barriers, ports, and offshore installations, are an ideal harsh substrate for the conservation of threatened marine species and can represent suitable substrates for “active restoration” interventions (Perkol-Finkel et al., 2012). In this regard, very few attempts were carried out in the Northern Adriatic Sea, where breakwater barriers represent a hard substrate different from the surrounding sandy habitats, using G. barbata on coastal defence structures, with encouraging results (Perkol-Finkel et al., 2012). However, in contrast to previous findings reporting a very low survival percentage (on average 20-30%, Perkol-Finkel et al., 2012), we obtained high survival rates, high levels of growth and canopy coverage. Differences between our and previous results may rely on the fact that we applied an approach integrating “passive” and “active” restoration, as an intervention continuum and not a mere action of transplant. In particular, the area in which we transplanted G. barbata was evaluated a priori as suitable for restoration as it no longer showed signs of pollution (Corinaldesi et al., 2022; Fanelli et al., 2023). We argue that this is a critical step to evaluate the success of “passive” restoration before implementing a transplant action on coastal infrastructures as breakwater barriers.
Moreover, we successfully managed the herbivory pressure using cages (see Paragraph 4.3), as also reported in previous studies (Perkol-Finkel et al., 2012; Orlando-Bonaca et al., 2021). The only factor threatening the success of the transplant was the autumnal storm that occurred in October, which destroyed one of the cages and allowed us to test the grazing pressure over G. barbata individuals of 15 cm height. This allows us to confirm that restoration must be considered a continuous process needing maintenance, in this case, the possible replacement of the lost cage. Also, the outcomes of previous studies highlighted that unpredictable climatic events are one of the major threats impairing the restoration process of G. barbata (Savonitto et al., 2021). The ongoing climate change, provoking temperature raising or stochastic and more and more frequent strong events (e.g., frequent storms, heatwaves), represents the biggest challenge to face when putting in force restoration interventions at the local level (Garrabou et al., 2022; Orlando-Bonaca et al., 2022). Climate change events can affect the survival and the reproduction of the target species but also impair the transplants themselves, destroying the infrastructures used to implement the reintroduction of the target populations (Savonitto et al., 2021; present study). Moreover, population vulnerability to warming would have to be considered before designing and investing in a long-term and successful restoration plan and climatic refugia should be considered as priority areas for habitat-forming species restoration (Verdura et al., 2021).
4.2 Restoration success and effects on biodiversity
In this restoration experiment the total abundance, richness of taxa, and the taxonomic composition of meio- and macrofaunal assemblages were assessed underneath the structures with macroalgae and in the control (artificial reef’ substrate with no transplanted macroalgae), to assess potential biodiversity changes led by G. barbata. Meio- and macrofauna abundance did not show significant differences between the control and the restoration site but only changes over time. This is probably due to seasonal fluctuations and the effect of the sea storms occurring during the experiment (Danovaro and Fraschetti, 2002). However, in the last sampling period, the richness of meiofaunal taxa was higher underneath the structures with macroalgae than in the control site, whereas the macrofaunal richness of taxa was the same underneath the structures and in the control. Similarly, meio- and macrofauna taxonomic composition significantly changed over time and the only difference between structures with macroalgae and control sites was observed in the composition of meiofaunal rare taxa. Fucales forests typically attract and sustain high levels of benthic biodiversity, particularly related to meiofaunal and macrofaunal herbivores which feed on the macroalgae, but also other consumers, thus provoking an increase in the richness of taxa (Bianchelli et al., 2016b). Moreover, macroalgae form three-dimensional habitats, hosting a lot of associated species (as epiphytes), due to the high number of ecological niches available (Veiga et al., 2014). Indeed, our data indicate that the overall richness of taxa (i.e., cumulatively in control and underneath cages) was higher than those observed in each site, reflecting that G. barbata host taxa were otherwise absent.
Overall, our results let us hypothesize a positive response by meio- and macrofaunal assemblages, which could have a positive effect on the entire food web, due to their ecological role. However, a longer monitoring period is required to ascertain a consistent and durable response from the benthic community after the restoration intervention in terms of biodiversity enhancement.
4.3 Complementary actions in restoration: herbivory management
Herbivores can drastically reduce algal and seagrass canopies, thus Sarpa salpa, Paracentrotus lividus and Arbacia lixula have been defined as important ecosystem modifiers (Tamburello et al., 2022 and citations therein). Since fluctuations in their populations cannot be easily controlled, grazers are considered one of the main constraints in macroalgal restoration actions (Orlando-Bonaca et al., 2021), and devices to prevent access by grazers (fish and sea urchins) and herbivore removal/culling should be considered (Gianni et al., 2018; Cebrian et al., 2021). Moreover, the knowledge of the critical threshold density of herbivores affecting recruitment success in macroalgae is still to be ascertained and will vary depending on environmental conditions (Cebrian et al., 2021). However, in this study, the main herbivores were represented by benthic meso- or macro-grazers (i.e., Pachigrapsus marmoratus), so the use of cages to protect the small juveniles of G. barbata was an effective complementary action to obtain macroalgal adults even in presence of high grazing pressure (Cebrian et al., 2021; Monserrat et al., 2023; Bianchelli et al., submitted).
In addition, we could estimate that when the algae reached 10-15 cm height (in January ‘22, Figure 4), they were grown enough to counteract the herbivory pressure. The use of cages to exclude grazers has indeed allowed the algae to grow and survive six months after the transplant in the SNI (except for structure 3, damaged by the sea storm after 3 months after the transplant). Especially in the early stages of the experiment, the presence of cages is essential to avoid grazing, and this has also been proven by other studies conducted on artificial reefs. In this regard, previous investigations (Perkol-Finkel et al., 2012) demonstrated that the use of cages with 1x1 mm mesh for the G. barbata restoration in artificial barriers allows the algae to survive and to have a growth comparable to natural populations, but with very low survival percentage. In the present study, we observed different factors possibly impairing the transplant success: i) the storm destroying one of the cages and ii) the lid of the cages, limiting the growth in height of individuals. Overall, our field results indicate that the G. barbata needs to reach at least a minimum height of ca. 10-15 cm and 80% in canopy coverage before removing the cages. Moreover, the laboratory experiment of grazing demonstrated that P. marmoratus (apparently the main grazer, Gianni et al., 2018) detach the algae from the basal disc. This means that, in our case, cages can be built without lids to avoid grazing and at the same time allow for algae growth. This could also impair the growth of epiphytes on the cages’ mesh; indeed, these species are in competition for resources with G. barbata and they could modify the irradiance and the water exchange conditions (Cebrian et al., 2021). Moreover, in future restoration interventions, it would be necessary to transplant a very large number of algae individuals to counteract the grazing pressure by P. marmoratus.
Despite the difficulties encountered mainly due to stochastic events (bad weather and sea storms) G. barbata survived and grew over time; this demonstrates that the cages play a fundamental role in the success of restoration interventions in artificial reefs, at least for the survival of juvenile algae and until they become resistant to grazing pressure.
5 Conclusions and perspectives
This study demonstrates that the complementary approach based on passive and active restoration is successful also in marine degraded ecosystems. In this regard, the SNIs, even when passively recovered (depending on their contamination levels and environmental characteristics) are optimal sites to implement restoration interventions and rehabilitate the habitats they host. The use of cages for grazing management in restoration interventions along breakwater structures is efficient and permits the survival and growth of macroalgae over time. However, finding thresholds in terms of algal growth to counteract the herbivory pressure is a key aspect to succeed in restoring real macroalgae forests, particularly when stochastic events compromise the use of cages or other devices typically used in restoration interventions (i.e., artificial substrates, grazers deterrents).
The present investigation also reveals that benthic biodiversity in terms of richness of taxa can recover and favor the presence of rare taxa (for meiofauna), also over a short time (6 months). Overall, our results let us suggest that restoration interventions may have a positive role in enhancing biodiversity, however, to corroborate our data, it would be necessary to lengthen the monitoring period by at least one year to cover all seasons also to assess the self-sustaining potential of the transplanted population.
Also, technical aspects can improve the success of the intervention: as an example, a high number of macroalgal individuals, together with the design of specific cages (e.g., without lids), should be considered in a restoration intervention on artificial substrates, since they help to counterbalance the grazing pressure.
Overall, we can conclude that restoration interventions on artificial substrates in OECMs or areas interdicted to human activities for decades due to historical pollution such as the SNIs offer a great possibility to achieve the targets of the UN Decade on Ecosystem Restoration.
Data availability statement
The raw data supporting the conclusions of this article will be made available by the authors, without undue reservation.
Author contributions
SB, RD and CC conceived the work. SB and CC wrote the manuscript. FM and MM performed the experiment and the laboratory analyses. All authors revised the manuscript and agreed to be accountable for the content of the work. All authors contributed to the article and approved the submitted version.
Funding
This study was conducted in the framework of the EASME–EMFF (Sustainable Blue Economy) Project AFRIMED (http://afrimed-project.eu/, grant agreement N. 789059), supported by the European Community, and the project BIOBLUTECH “Blue Biotechnologies for restoring marine ecosystems of the contaminated Site of National Interest (SIN) ex Montedison (Falconara M.ma), ID 9204 supported by Cariverona Foundation. This study was also supported by the National Recovery and Resilience Plan (NRRP), Mission 4 Component 2 Investment 1.4 - Call for tender No. 3138 of 16 December 2021, rectified by Decree n.3175 of 18 December 2021 of Italian Ministry of University and Research funded by the European Union – NextGenerationEU; Award Number: Project code CN_00000033, Concession Decree No. 1034 of 17 June 2022 adopted by the Italian Ministry of University and Research, Project title “National Biodiversity Future Center - NBFC”.
Acknowledgments
Virginia Rossi and Giulia Morello are also acknowledged for their help during the field and lab activities.
Conflict of interest
The authors declare that the research was conducted in the absence of any commercial or financial relationships that could be construed as a potential conflict of interest.
Publisher’s note
All claims expressed in this article are solely those of the authors and do not necessarily represent those of their affiliated organizations, or those of the publisher, the editors and the reviewers. Any product that may be evaluated in this article, or claim that may be made by its manufacturer, is not guaranteed or endorsed by the publisher.
References
Aronson J., Alexander S. (2013). Ecosystem restoration is now a global priority: time to roll up our sleeves. Restor. Ecol. 21, 293–296. doi: 10.1111/rec.12011
Andersen J. H., Caetano M., Grouhel-Pellouin A., Harvey E. T., Reker J., Murray C. J. (2022). Mapping of contamination problem areas in Europe’s seas using a multi-metric indicator-based assessment tool. Front. Mar. Sci. 9, 1037914. doi: 10.3389/fmars.2022.1037914
Ausili A., Bergamin L., ROmano E. (2020). Environmental status of Italian coastal marine areas affected by long history of contamination. Front. Environ. Sci. 8. doi: 10.3389/FENVS.2020.00034
Bianchelli S., Buschi E., Danovaro R., Pusceddu A. (2016b). Biodiversity loss and turnover in alternative states in the Mediterranean Sea: a case study on meiofauna. Sci. Rep. 6, 34544. doi: 10.1038/srep34544
Bianchelli S., Buschi E., Danovaro R., Pusceddu A. (2018). Nematode biodiversity and benthic trophic state are simple tools for the assessment of the environmental quality in coastal marine ecosystems. Ecol. Indic. 95, 270–287. doi: 10.1016/j.ecolind.2018.07.032
Bianchelli S., Danovaro R. (2020). Impairment of microbial and meiofaunal ecosystem functions linked to algal forest loss. Sci. Rep. 10 (1), 19970. doi: 10.1038/s41598-020-76817-5
Bianchelli S., Fraschetti S., Martini F., Lo Martire M., Nepote E., Ippoliti D., et al. Macroalgal forest restoration: the effect of the foundation species. Submitted to Frontiers in Marine Science, this issue.
Bianchelli S., Pusceddu A., Buschi E., Danovaro R. (2016a). Trophic status and meiofauna biodiversity in the Northern Adriatic Sea: insights for the assessment of good environmental status. Mar. Environ. Res. 113, 18–30. doi: 10.1016/j.marenvres.2015.10.010
Bulleri F., Chapman M. G. (2010). The introduction of coastal infrastructure as a driver of change in marine environments. J. Appl. Ecol. 47 (1), 26–35. doi: 10.1111/j.1365-2664.2009.01751.x
Cardinale B. J., Duffy J. E., Gonzales A., Hooper D. U., Perrings C., Venail P., et al. (2012). Biodiversity loss and its impact on humanity. Nature 486, 59–67. doi: 10.1038/nature11148
Cebrian E., Tamburello L., Verdura J., Guarnieri G., Medrano A., Linares C., et al. (2021). A roadmap for the restoration of Mediterranean macroalgal forests. Front. Mar. Sci. 8. doi: 10.3389/fmars.2021.709219
Chazdon R. L., Falk D. A., Banin L. S., Wagner M., Wilson S. J., Grabowski R. C., et al. (2021). The intervention continuum in restoration ecology: rethinking the active–passive dichotomy. Restor. Ecol., e13535. doi: 10.1111/rec.13535
Corinaldesi C., Bianchelli S., Rastelli E., Varrella S., Canensi S., Gambi C., et al. (2022). The paradox of an unpolluted coastal site facing a chronically contaminated industrial area. Front. Mar. Sci. 8. doi: 10.3389/fmars.2021.813887
Danovaro R. (2010). Methods for the study of deep-sea sediments, their functioning and biodiversity (Taylor & Francis Group, Boca Raton: CRC Press).
Danovaro R., Fraschetti S. (2002). Meiofaunal vertical zonation on hard-bottoms: comparison with soft-bottom meiofauna. Mar. Ecol. Prog. Ser. 230, 159–169. doi: 10.3354/meps230159
Diz D., Johnson D., Riddell M., Rees S., Battle J., Gjerde K., et al. (2018). Mainstreaming marine biodiversity into the SDGs: The role of other effective area-based conservation measures (SDG 14.5). Mar. Policy 93, 251–261. doi: 10.1016/j.marpol.2017.08.019
Fabbrizzi E., Giakoumi S., De Leo F., Tamburello L., Chiarore A., Colletti A., et al. (2023). The challenge of setting restoration targets for macroalgal forests under climate changes. J. Environ. Manage. 326, 116834. doi: 10.1016/j.jenvman.2022.116834
Fanelli E., Dell'Anno A., Nepote E., Lo Martire M., Musco L., Bianchelli S., et al. (2023). Positive effects of two decades of passive ecological restoration in a historically polluted marine site. Front. Mar. Sci. 10, 1199654. doi: 10.3389/fmars.2023.1199654
Ferrario F., Ivesa L., Jaklin A., Perkol-Finkel S., Airoldi L. (2015). The overlooked role of biotic factors in controlling the ecological performance of artificial marine habitats. J. Appl. Ecol. 53 (1), 16–24. doi: 10.1111/1365-2664.12533
Fraschetti S., Guarnieri G., Bevilacqua S., Boero F. (2011). Conservation of Mediterranean habitats and biodiversity countdowns: what information do we really need? Aquat. Conserv.: Mar. Freshw. Ecosyst. 21, 299–306. doi: 10.1002/aqc.1185
Fraschetti S., Mc Owen C., Papa L., Papadopoulou N., Bilan M., Boström C., et al. (2021). Where is more important than how in coastal and marine ecosystems restoration. Front. Mar. Sci. 8. doi: 10.3389/fmars.2021.626843
Garrabou J., Gómez-Gras D., Medrano A., Cerrano C., Ponti M., Schlegel R., et al. (2022). Marine heatwaves drive recurrent mass mortalities in the Mediterranean Sea. Global Change Biol. 28, 5708–5725. doi: 10.1111/gcb.16301
Gianni F., Bartolini F., Airoldi L., Ballesteros E., Francour P., Guidetti P., et al. (2013). Conservation and restoration of marine forests in the Mediterranean Sea and the potential role of Marine Protected Areas. Adv. Oceanogr. Limnol. 4 (2), 83–101. doi: 10.1080/19475721.2013.845604
Gianni F., Bartolini F., Airoldi L., Mangialajo L. (2018). Reduction of herbivorous fish pressure can facilitate focal algal species forestation on artificial structures. Mar. Environ. Res. 138, 102–109. doi: 10.1016/j.marenvres.2018.04.007
Heip C., Vincx M., Vranken G. (1985). The ecology of marine nematodes. Oceanogr. Mar. Biol.: Annu. Rev. 23, 399–489.
Jones H. P., Jones P. C., Barbier E. B., Blackburn R. C., Benayas J. M. R., Holl K. D., et al. (2018). Restoration and repair of Earth’s damaged ecosystems. Proc. R. Soc. B 285, 20172577. doi: 10.1098/rspb.2017.2577
Legambiente. (2021). Liberi dai veleni, per il risanamento ambientale e il diritto alla salute nei territori dell’Italia inquinata. Focus/ La bonifica del Sito di Interesse Nazionale “Falconara marittima”, Agosto 2021. Available at: https://www.legambiente.it/wp-content/uploads/2021/08/SIN-Falconara-Marittima.pdf.
Lindegren M., Holt B. G., MacKenzie B. R., Rahbek C. (2018). A global mismatch in the protection of multiple marine biodiversity components and ecosystem services. Sci. Rep. 8, 4099. doi: 10.1038/s41598-018-22419-1
Lotze H. K., Coll M., Magera A. M., Ward-Paige C., Airoldi L. (2011). Recovery of marine animal populations and ecosystems. Trends Ecol. Evol. 26, 595–605. doi: 10.1016/j.tree.2011.07.008
Lotze H. K., Lenihan H. S., Bourque B. J., Bradbury R. H., Cooke R. G., Kay M. C., et al. (2006). Depletion, degradation, and recovery potential of estuaries and coastal seas. Science 312, 1806–1809. doi: 10.1126/science.1128035
Monserrat M., Verdura J., Comeau S., Cottalorda J.-M., Priouzeau F., Romero G., et al. (2023). The role of grazers in early-life stages of Cystoseira sensu lato can be crucial in the restoration of marine forests. Front. Mar. Sci. 10. doi: 10.3389/fmars.2023.1176780
Orlando-Bonaca M., Pitacco V., Slavinec P., Šiško M., Makovec T., Falace A. (2021). First restoration experiment for Gongolaria barbata in Slovenian coastal waters. What can go wrong? Plants 10 (2), 239. doi: 10.3390/plants10020239
Orlando-Bonaca M., Savonitto G., Asnaghi V., Trkov D., Pitacco V., Šiško M., et al. (2022). Where and how - new insight for brown algal forest restoration in the Adriatic. Front. Mar. Sci. 9. doi: 10.3389/fmars.2022.988584
Panagos P., Van Liederkranz M., Yigini Y., Montanarella L. (2013). Contaminated Sites in Europe: review of the current situation based on data collected through a European Network. J. Environ. Public Health 158764, 11 pages. doi: 10.1155/2013/158764
Perkol-Finkel S., Airoldi L. (2010). Loss and recovery potential of marine habitats: an experimental study of factors maintaining resilience in subtidal algal forests at the Adriatic Sea. PLoS ONE 5 (5), e10791. doi: 10.1371/journal.pone.0010791
Perkol-Finkel S., Ferrario F., Nicotera V., Airoldi L. (2012). Conservation challenges in urban seascapes: Promoting the growth of threatened species on coastal infrastructures. J. Appl. Ecol. 49 (6), 1457–1466. doi: 10.1111/j.1365-2664.2012.02204.x
Pusceddu A., Dell’Anno A., Fabiano M., Danovaro R. (2009). Quantity and bioavailability of sediment organic matter as signatures of benthic trophic status. Mar. Ecol. Prog. Ser. 375, 41–52. doi: 10.3354/meps07735
Roni P., Hanson K., Beechie T., Pess G., Pollock M., Bartley D. M. (2005). Habitat rehabilitation for inland fisheries. Global review of effectiveness and guidance for rehabilitation of freshwater ecosystems Vol. 2005 (Rome: Food and Agriculture Organization of The United Nations).
Savonitto G., de la Fuente G., Tordoni E., Ciriaco S., Srijemsi M., Bacaro G., et al. (2021). Addressing reproductive stochasticity and grazing impacts in the restoration of a canopy-forming brown alga by implementing mitigation solutions. Aquat. Conserv.: Mar. Freshw. Ecosyst. 31 (7), 1611–1623. doi: 10.1002/aqc.3555
Semprucci F., Frontalini F., Covazzi-Harriague A., Coccioni R., Balsamo M. (2013). Meio-and macrofauna in the marine area of the Monte St. Bartolo natural Park (Central Adriatic Sea, Italy). Scientia Marina 77 (1), 189–199. doi: 10.3989/scimar.03647.26A
Susini M. L., Mangialajo L., Thibaut T., Meinesz A. (2007). Development of a transplantation technique of Cystoseira amentacea var. stricta and Cystoseira compressa. Hydrobiologia 580, 241–244. doi: 10.1007/s10750-006-0449-9
Tamburello L., Chiarore A., Fabbrizzi E., Colletti A., Franzitta G., Grech D., et al. (2022). Can we preserve and restore overlooked macroalgal forests? Sci. Total Environ. 806, 150855. doi: 10.1016/j.scitotenv.2021.150855
van Tatenhove J. P. M., Ramírez-Monsalve P., Carballo-Cárdenas E., Papadopoulou N., Smith C. J., Alferink L., et al. (2021). The governance of marine restoration: insights from three cases in two European seas. Restor. Ecol. 29, e13288. doi: 10.1111/rec.13288
Veiga P., Rubal M., Sousa-Pinto I. (2014). Structural complexity of macroalgae influences epifaunal assemblages associated with native and invasive species. Mar. Environ. Res. 101, 115–123. doi: 10.1016/j.marenvres.2014.09.007
Verdura J., Sales M., Ballesteros E., Cefalì M., Cebrian M. (2018). Restoration of a canopy-forming alga based on recruitment enhancement: methods and long-term success assessment. Front. Plant Sci. 9. doi: 10.3389/fpls.2018.01832
Keywords: passive and active restoration, Gongolaria barbata, artificial barriers, indicators of success, associated biodiversity, meiofauna, macrofauna, grazing management
Citation: Bianchelli S, Martini F, Lo Martire M, Danovaro R and Corinaldesi C (2023) Combining passive and active restoration to rehabilitate a historically polluted marine site. Front. Mar. Sci. 10:1213118. doi: 10.3389/fmars.2023.1213118
Received: 27 April 2023; Accepted: 31 July 2023;
Published: 21 August 2023.
Edited by:
Bernardo Antonio Perez Da Gama, Fluminense Federal University, BrazilReviewed by:
Martina Orlando-Bonaca, National Institute of Biology, SloveniaKelly Cristina Tonello, Federal University of São Carlos, Brazil
Copyright © 2023 Bianchelli, Martini, Lo Martire, Danovaro and Corinaldesi. This is an open-access article distributed under the terms of the Creative Commons Attribution License (CC BY). The use, distribution or reproduction in other forums is permitted, provided the original author(s) and the copyright owner(s) are credited and that the original publication in this journal is cited, in accordance with accepted academic practice. No use, distribution or reproduction is permitted which does not comply with these terms.
*Correspondence: Silvia Bianchelli, c2lsdmlhLmJpYW5jaGVsbGlAdW5pdnBtLml0