- Research Department, Norwegian Polar Institute, Tromsø, Norway
The Svalbard Archipelago, Norway, is warming rapidly, resulting in environmental change that is likely already affecting the underwater soundscape, a critical habitat feature for marine mammals. Baseline information about current sound levels is needed to monitor future changes. Fixed-location recorders were used in this study to characterize the soundscape and study the occurrence and phenology of marine mammals at two locations within Svalbard. Kongsfjorden (2017-2018), on the west coast, has already undergone vast environmental change, whereas M2 (2019-2020), on the east coast, remains more Arctic. The results of the study reveal that the soundscapes and species assemblages differed markedly west vs east. In the west, Arctic species were detected from winter to summer and migrant marine mammal species were detected occasionally through the autumn. In the east, Arctic species were detected constantly on an almost year-round basis, and a few migrant species were detected during summer. Vessels were detected more often in the west than in the east, in accordance with AIS traffic data that indicated more boat traffic in Kongsfjorden in the west than in the east of the archipelago. In terms of soundscapes, geophonies (wind, ice) were the main factors determining the sound energy at both locations. Kongsfjorden’s soundscape was characterized by glacier-melt sounds with moderate levels of anthropogenic noise and some biophonies (marine mammals). At M2, sea-ice noises shaped the soundscape, which was otherwise dominated by biophonies from Arctic marine mammals. At the east coast site there was little impact from anthropophonies (vessels). This study provides information on Svalbard’s current underwater soundscape, which is likely to be transformed in the future due to ongoing climate change, with the west coast reflecting future conditions in many Arctic regions if ship-traffic is not regulated.
Introduction
The Arctic has experienced dramatic environmental change in recent decades, with increasing temperatures, reduced sea-ice cover, increased primary production and borealization of the biota throughout all trophic levels (Cottier et al., 2007; Fossheim et al., 2015; Frey et al., 2017; Polyakov et al., 2017; Storrie et al., 2018; Stroeve and Notz, 2018; Ershova et al., 2021; Bengtsson et al., 2022). Many of these changes are leading to increased pressure to explore and exploit resources in this region, which has previously been sheltered from many types of anthropogenic activities due to its remoteness and logistical challenges associated with extensive ice cover. Reduced sea ice has already resulted in increased interest in tourism, fishing, oil and gas exploration, marine mining, trans-oceanic shipping, and other activities (PAME, 2019; PAME, 2021). Encroachment of these activities into the Arctic is changing the underwater soundscape of the region, which has, at least seasonally, been considered to have one of lowest sound levels in the world (Insley et al., 2017; PAME, 2019; Halliday, 2021).
Increasing ocean noise levels are a concern for marine organisms (e.g., Au and Hastings, 2008). Arctic marine mammals have received a considerable amount of attention in the context of climate change because these animals provide valuable ecosystem services and are of public interest. Currently, Arctic marine mammal populations are facing multiple climate change related stressors simultaneously, including loss of habitat, competition from temperate species shifting their distributions northward, as well as increased levels of exposure to diseases and parasites and increased levels of noise (Jensen et al., 2010; Kovacs et al., 2011; Laidre et al., 2015; Van Wormer et al., 2019; Huntington et al., 2020). Noise disturbance is an important factor in the context of marine mammal long-distance communication, finding food and also navigating (PAME, 2019). Some Arctic marine mammal species have been shown to be sensitive to disturbance from oil and gas exploration and ship traffic more generally, which currently dominates the non-natural soundscape in the Arctic (Stafford, 2021). Demonstrated effects of such disturbance varies from decreased calling rates and avoidance behavior to interruption of feeding (Heide-Jørgensen et al., 2013; PAME, 2019; Halliday et al., 2020; Martin et al., 2022).
In the context of rapid change, it is important to characterize the different elements that make-up the current underwater soundscapes across regions in the Arctic, both in areas that have already experienced considerable change and in those that are modestly pristine (Merchant et al., 2016). Several sound types must be evaluated because a soundscape is composed of geophysical sounds (e.g., sea-ice, wind), anthropogenic sounds (e.g., shipping, oil and gas exploration) as well as biological sounds (e.g., marine mammals, fish and crustaceans) (Hildebrand, 2009; Pijanowski et al., 2011; Stafford, 2021). Providing baseline knowledge about the relative contributions of these different elements to the overall soundscape is important to monitor future changes, identify potential overlaps of anthropogenic activities with vulnerable species and reduce the impact that noise pollution might have through area-based management (Dekeling et al., 2014; Reeves et al., 2014). Studying the biological components of the soundscape provides relevant information about the distribution, timing of migration and mating of species that are often very challenging to study in conventional ways (Stafford et al., 2012; Ahonen et al., 2019).
Despite the acoustics work that has been done in the Arctic, many spatial and temporal knowledge gaps still exist regarding regional soundscapes (PAME, 2019). For example, in the Northeast Atlantic High Arctic, only one study reporting ambient sound levels exists for areas east of Svalbard and into the Barents Sea Region (De Vreese et al., 2018), and only a handful of studies of soundscapes have been done in coastal area in western Svalbard, despite this region being an important hotspot for marine mammals (Sanjana et al., 2018; Ashokan et al., 2020; Madan et al., 2020; Hamilton et al., 2021). Some studies have been focused on geophysical sounds (iceberg bubbling, iceberg calving; Glowacki et al., 2018; Mahanty et al., 2020) or species-specific biological sounds (e.g. Van Parijs et al., 2001; de Vincenzi et al., 2019; Llobet et al., 2021), but increasingly there is a need to document noise from anthropogenic sources in the context of the biological community (Ahonen et al., 2017; Ashokan et al., 2020; Madan et al., 2020; Sanjana et al., 2021; Stafford, 2021; Richard et al., 2023).
In this study, we examine the ambient sound levels recorded at two locations: Kongsfjorden, in the west part of Svalbard and M2, in the northeast of the archipelago. We also studied the presence of vocal marine mammal species, and analyzed the potential influence of sea-ice and vessel traffic (using noise metrics, presence of vessel noise (signals) per hour and AIS data). Our study sites, despite their proximity, are experiencing climate change at very different rates; the east coast retains largely Arctic conditions, while west coast fjords in Svalbard, including Kongsfjorden, have experienced Atlantification with dramatic losses of sea ice and increased temperatures (e.g., Ivanov et al., 2012; Muckenhuber et al., 2016; Hop and Wiencke, 2019; Tverberg et al., 2019). Both sites were previously heavily ice-covered and home to rich Arctic endemic marine mammal communities. In the past, the deep cold water generated in winter in Kongsfjorden, in combination with the sea ice formation, prevented Atlantic Water from flooding the fjord. However, during the winter of 2006, a change in wind patterns lead to an unusual flooding of the West Spitsbergen shelf with Atlantic Water, leading to open water conditions that have occurred during many more winters since (Tverberg et al., 2019). Sea-ice extent, duration, and thickness, as well as snow cover on fast-ice have experienced dramatic declines in Kongsfjorden over the last 20 years, affecting pelagic and benthic production that have in turn affected higher trophic levels (Pavlova et al., 2019; Polyakov et al., 2020; Descamps and Strøm, 2021). M2 is located south of Kvitøya, in the northeastern side of the archipelago, north of the Polar Front on the Barents Sea shelf. This environment is dominated by cold and stratified Polar Water (PW) and the area remains covered in drifting sea ice most of the fall, winter, and early spring, although there is strong interannual variation in ice conditions (Renner et al., 2018; Lundesgaard et al., 2021; Lundesgaard et al., 2022). The whole Barents Sea Region is expected to become one of the first Arctic areas to experience ice-free winter conditions (Barton et al., 2018; Årthun et al., 2021; Isaksen et al., 2022), so our current west coast site is expected to become the norm for the whole Archipelago in the decades ahead. The changes in the environment are likely to simultaneously affect the soundscapes and the marine mammal communities. The study can be regarded as a current baseline for modern-day Svalbard, but the west likely serves as a precursor for the east.
Materials and methods
The acoustics records used herein were obtained using two Autonomous Underwater Recorders for Acoustic Listening (AURAL M2, Multi-Électronique Inc.; HTI-96-MIN hydrophone with receiving sensitivity of -164 ± 1 dB re1 V/μPa with a 16 dB system gain and frequency response from 5 Hz to 32.8 kHz) deployed on stationary oceanographic moorings located around the High Arctic Svalbard Archipelago (Figure 1). The western recorder was placed in Kongsfjorden, on the northwest side of the Svalbard Archipelago. The eastern location was situated 50 km south of Kvitøya, in the northeastern corner of the archipelago; this site will henceforth be referred to as M2.
The sampling rate for both recorders was 32768 Hz, providing an effective frequency range of 10-16384 Hz. The recorders’ duty cycle was 15 min every hour for Kongsfjorden, and 12 min every hour for M2. We used the most recent, available, recording period for Kongsfjorden (2017-2018) and the only available period for M2 (2019-2020). Details about the AURAL locations, depths and recording periods are presented in Table 1.
Marine mammal and anthropogenic sounds
Spectrograms of each acoustic file (one for each hour) were generated using the bioacoustics software Ishmael (version 3.0) to manually screen, listen and register marine mammal vocalizations and anthropogenic sounds such as vessel noise and airgun blasts (10-8192 Hz, FFT 2048/4096, 75% overlap, Hanning window). Presence was scored when at least one clear signal of each sound source was found in an hourly file. The number of hours with acoustic presence per day (0-24) was the resulting data obtained for all the species and anthropogenic noise sources. The acoustic presence of species was determined based on well-documented, species-specific sounds of marine mammals (e.g., Stirling et al., 1987; Terhune, 1994; Stafford et al., 2012; Oliveira et al., 2013; Parisi et al., 2017; Ahonen et al., 2019; Nieukirk et al., 2020; Romagosa et al., 2020). Some biological sounds were not identified to the species level. For example, unknown odontocete sounds were grouped in this study, because many tonal sounds are common to both white whales (Delphinapterus leucas) and narwhals (Monodon monoceros). However, low-frequency clicks were considered evidence of narwhal presence (Blackwell et al., 2018; Ahonen et al., 2019). Additionally, some low frequency sounds (non-song vocalizations) produced by bowhead whales (Balaena mysticetus) and/or and humpback whales (Megaptera novaeanlgiae) were too similar to separate; these were not assigned as an indicator for either species (see Videsen et al., 2021). Bowhead whale signals were broken into simple calls and sequences versus songs (Stafford et al., 2012). From the second week of July, when open water suddenly dominated the east coast site, simple calls could not be assigned to either bowhead whales or humpbacks whales, except for the growls and whups of humpback whales as described by Fournet et al. (2015).
For fin (Balaenoptera physalus) and blue whales (Balaenoptera musculus), the spectrogram-correlation-modality program in Ishmael (Mellinger and Clark, 2000) was used to detect the main periods when these species were present. The recordings were down-sampled to 500 Hz, and then a low-pass filter (below 100 Hz) and a spectrogram equalization (30 s) were applied to reduce noise in the low frequency band from vessels and strumming. The spectrogram correlation parameters for the blue whale consisted of a 10 s long down-sweep from 18 to 16 Hz, with a detection contour width of 3 Hz and a 1 s FFT with 75% overlap. For the fin whale, the spectrogram correlation parameter was a 2s down-sweep from 30 to 19 Hz with a contour width of 7 Hz (Ahonen et al., 2021). Due to the presence of low frequency noises from vessels and strumming noise, this automated detection method did not provide enough information for a final acoustic presence determination for these species. Thus, the data from these auto-detectors were corrected manually, adding the false negatives, and removing the false positives from the dataset. An example of each species-specific sound found in the dataset is shown in spectrograms (Supplementary Material Figure 1). Daily presence was reported as the number of hours per day (0−24) with at least one clear fin or blue whale call per file.
Sea ice and wind
Sea ice data obtained from the Norwegian Meteorological Institute1 were used to calculate daily sea ice cover for each study site. Previous studies have reported that some pinnipeds, like bearded seals, can be heard as far away as 20 km (Cleator et al., 1989; MacIntyre et al., 2013). Other species targeted in the present study have an even larger detection range, such as the baleen whales (Tervo et al., 2012). Thus, a radius of 30 km around each recorder was chosen in the present study to extract the ice information, with the aim of characterizing the ice habitat on a scale large enough to be relevant for the species occurring in an area, yet small enough to still have a locally accurate depiction of conditions.
One thousand points were randomly generated (Gaussian distribution) within a radius of 30 km from the recorder at each location. Land points were excluded from the analyses. Points falling in adjacent fjords or outside of the line-of-sight of the recorder were also eliminated. The rest of the points were used to extract the ice information from a shape file corresponding to the ice report for each day over the study period. The function “over” from the R package “sp 1.3-1” was used for the analyses (Bivand et al., 2008). When ice information was missing for a particular day, the information was retrieved from the shape file available for the closest day. Ice cover is presented as the proportion of the study area (radius 30 km around the recorder) covered by a given ice class (0-10%, >10-39%, >40-69%, >70-89%, >90-99%, Fast-Ice).
Wind data was obtained from two different sources. For Kongsfjorden, wind speed observations were available from the meteorological station in Ny-Alesund2. Daily mean wind speed observations were obtained to study the wind speed trend during the study period in Kongsfjorden. No on-site observations were available for M2. For this location, the daily mean surface wind speed predictions were obtained from the NOAA’s climate diagnostic center3 (NCEP/NCAR reanalysis 1; 2.5 degrees x 2.5 degrees spatial resolution). Simple linear regression models were performed to get an understanding of the relationship between the dependent variable broadband noise (sound pressure levels at 50-1000 Hz) and wind speed (m/s).
Vessel traffic data
Data from the Automatic Identification System (AIS) for vessels was obtained from The Norwegian Coastal Administration4. Three different distance buffers (10, 25 and 50 km) were plotted around the two AURAL locations, together with an additional buffer of 100 km for M2, where the propagation of distant sounds is likely less restricted by landmasses compared to Kongsfjorden. AIS location points contain information such as the Maritime Mobile Service Identity (MMSI, conveying the ship ID), position and time. These were combined with the distance buffers, and the points falling on land, related to buoys or not relatable to vessels, were eliminated (QGIS 3.16 Hannover). Then, csv files from the resulting interceptions were exported and analyzed in R (R version 4.0.5) to calculate the number of vessels per day in each area. In Kongsfjorden, the area for which the number of vessels was calculated was corrected for the assumed directionality of the sound. Thus, boats within the distance classes, but out of the line of sight of the recorder, were not included in the analysis. The number of vessels per day were plotted within the distance classes for each location against the number of hours per day with acoustic presence of vessels, to visualize how the latter variable represents vessel traffic in an area. The same study period was not available for both locations. At M2, the only data available are likely biased downward somewhat from normal traffic density as a consequence of the global pandemic. For that reason, a multiyear trend with the number of vessels per month by vessel type from 2016 to 2021 was generated for both locations, in order to understand if the pandemic restrictions affected our results or the differences observed were part of an ongoing trend. The vessel categories were harmonized into ten categories: authorities, cargo, fishing, industrial, passenger, passenger/cruise, recreational, research, other and unknown. The vessel types from the IHS Fairplay level 5 classification included in each simplified category can be found in Supplementary Material Table 1.
Soundscape analysis
Power Spectral Densities (PSD) and empirical probability densities are an effective method for comparing soundscapes among locations and throughout time because they provide statistically more robust results of sound energy across the frequency spectrum when compared to other conventional ways of reporting sound levels (Madsen, 2005; PAME, 2019). The PSD analyses performed in this study focused on the 10-4000 Hz frequency band (1 Hz resolution, 60 s spectral averages, 50% overlap, Hanning window) for both sites using the PAMGuide package in MATLAB (Merchant et al., 2015). Long-term spectrograms (LTS) were generated to visualize the temporal extent of different sound sources, their contribution to the sound energy and their segmentation across the selected frequency band. Additionally, empirical probability densities were computed with the annual PSD statistical plots to examine the distribution probabilities of sound energy across the frequency band including percentile levels (1, 5, 50, 95, 99) and root mean squared - RMS - (which is a measure of average loudness - Merchant et al., 2013) using MATLAB (version R2022a, Mathworks, Natick, Massachusetts, USA). Subsequently, monthly median PSDs were plotted for each location to compare the seasonal variation in sound energy across the frequency band in detail, so different contributions from seasonal sound sources could be ascertained.
In a third analysis, the RMS Sound Pressure Levels (SPL) were calculated for the one-Third Octave Level (TOL) bands centered at 63 Hz, 125 Hz and 250 Hz using the PAMGuide package and custom-made MATLAB code. One RMS SPL value per file was obtained, averaging 15 and 12 min of recording in Kongsfjorden and M2 respectively. The 63 Hz and 125 Hz TOL are of particular interest to assess the influence of shipping noise on the soundscape, as defined by the European Union Marine Strategy Framework Directive (2008/56/EC; Dekeling et al., 2014). The 250 Hz TOL was also explored, as it has been associated with broadband shipping noise (Merchant et al., 2014). These TOLs were generated for every month at each location, to compare the sound levels around these frequencies between seasons. An extra broadband, expanding from 50 to 1000 Hz, was used to calculate the broadband SPL. This frequency band encompasses geophonic soundscape processes like the sea-ice stress, periods of high winds and glacier melt in glacial fjords (Hildebrand, 2009; Petit et al., 2012).
Results
Marine mammal and anthropogenic sounds
The AURALs recorded the acoustic presence of 11 marine mammal species, including five resident Arctic endemic species; bowhead whales, narwhals, white whales, bearded seals (Erignathus barbatus), and walruses (Odobenus rosmarus) as well as harp seals (Pagophilus groenlandicus), which are a subarctic pack-ice breeding (referred to henceforth as a regionally migrant) species and five seasonal migrant cetacean species, sei whale (Balaenoptera borealis), fin whale, blue whale, humpback whale and sperm whale (Physeter macrocephalus). The presence of these species varied seasonally and by location. Three out of eight species recorded in Kongsfjorden were Arctic species, the other five were seasonal migrants (Figure 2A). Long periods of vocal presence by the Arctic resident species were recorded in the eastern location; four out of eight species at M2 were Arctic residents (Figure 2B).
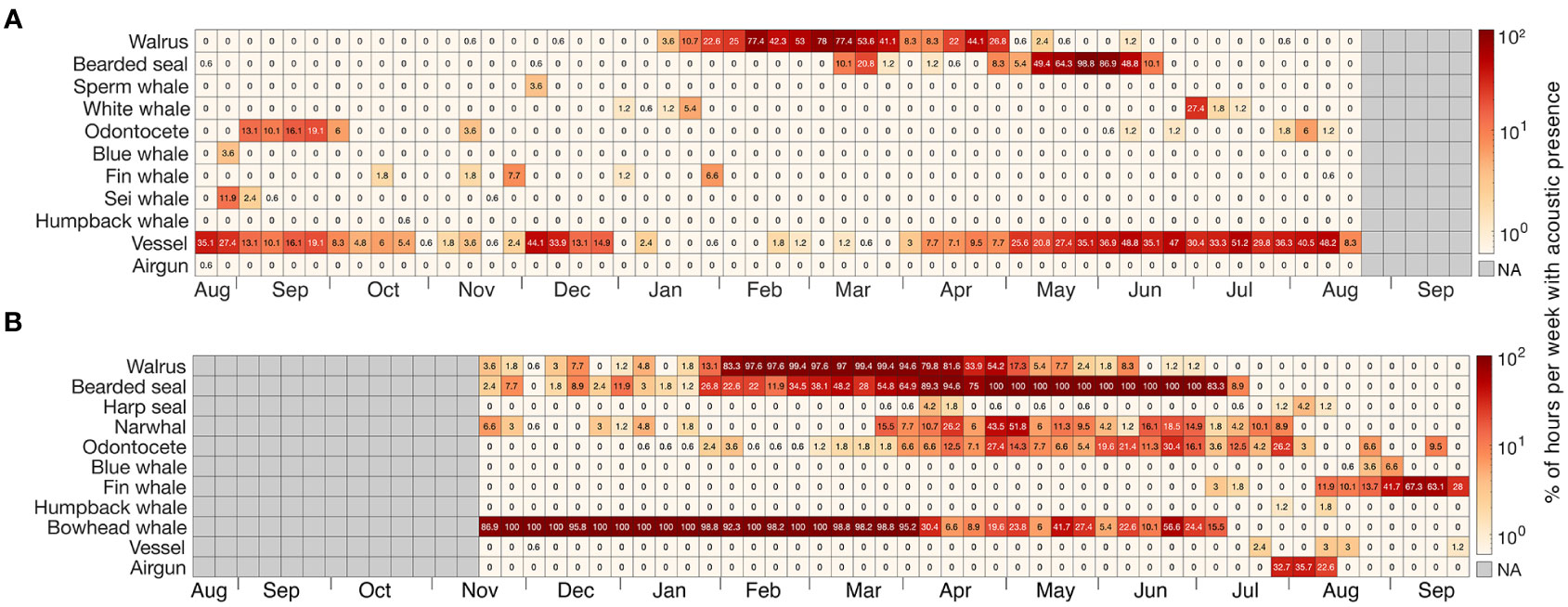
Figure 2 Heatmap representing the percentage of hours per week with acoustic detections from the different marine mammal species and anthropogenic sound sources manually detected in Kongsfjorden (A) and M2 (B). The grey areas represent periods with no data.
In Kongsfjorden, walruses were vocally active between mid-January and mid-May, with a peak from mid-February to mid-March (Figure 2A). Bearded seal vocalized from mid-March to mid-June, with a constant presence through May and June. White whales could only be identified in two discrete periods of the year, January, and July, although other odontocete vocalizations detected in Kongsfjorden were likely also produced by white whales. The seasonally migrant species (blue, fin, sei, sperm and humpback whales) were detected sporadically during the summer through to the onset of winter. These species were acoustically absent from February to June. Vessel noises were detected throughout most of the year, except during some few weeks mid-winter (between January-March). Vessels were most common in the summer (June, July, and August). Air guns were detected only during the first week of August (2017) in Kongsfjorden.
At M2, walruses were detected almost every week from November to June, with a peak in vocal activity occurring in February and March, when they were detected almost every hour (Figure 2B). Bearded seals were similarly detected nearly every week from November until July, with a peak in vocal activity occurring from April until June, and they were not detected after mid-July. Harp seals were detected intermittently between March and August. Narwhals were detected occasionally between November and January, and every week between mid-March and late-July. During this period, unidentified odontocete vocalizations were also detected that were most likely also from narwhals. Bowhead whales were detected every week for nine months from November to mid-July, vocalizing continuously between November and late-March. Singing behavior was detected between November and late-March (Supplementary Material Figure 2). Seasonal baleen whales (blue, fin and humpback whales) were occasionally detected from July to September; with a distinct peak in September when 60% of sampling periods had signals from fin whales. Vessels were detected only rarely, in July, August, September and December. Air guns were detected over a three-week period starting in late-July through to mid-August (2020).
Sea ice data
The AURAL in Kongsfjorden had much less ice cover around it compared to M2 in eastern Svalbard, with the area around the recorder (30 km radius) having a maximum of 20% ice cover, although some drift-ice was present most months of the year (Figure 3A). From February to May the fjord had its most extensive sea-ice. In March and April high concentrations of ice covered approximately half of the fjord’s area. At M2, very close drift-ice covered the entire sampling area from November 2019 until June 2020 (Figure 3B), with some few, short, lighter ice periods in late-March and early April, when a large open lead connected Kvitøya (near M2) with Nordaustlandet (Figure 1) and east-Spitsbergen. Open water conditions prevailed around M2 by mid-July.
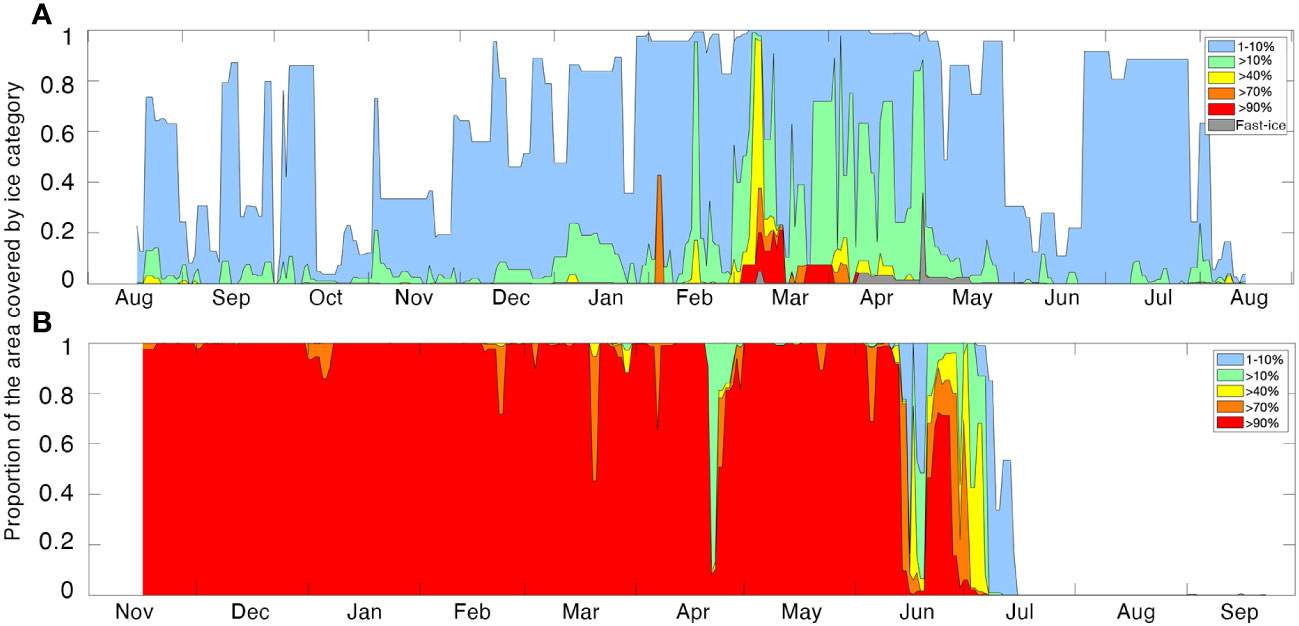
Figure 3 Daily sea-ice concentrations at Kongsfjorden (2017-2018, (A), and at M2 (2019-2020, (B) during the study period. Sea-ice cover is expressed as the proportion of the study area (radius= 30 km around the recorder) covered by a given ice class as indicated in the legend. Periods with no color indicate ice free periods.
Vessel traffic data
Figure 4 presents the number of vessels per distance category each day according to AIS data (Figure 4A), together with the number of hours with acoustic vessel detections per day. Kongsfjorden had by far the heaviest traffic levels, with more than 10 vessels per day from May through September within 50 km of the recorder, and 20+ boats daily were common in July and August (Figure 4BI). Acoustic detections and AIS data matched well through much of the year, except in December, when a few vessels (2 within 25 km) resulted in many acoustic detections (up to 20 h per day).
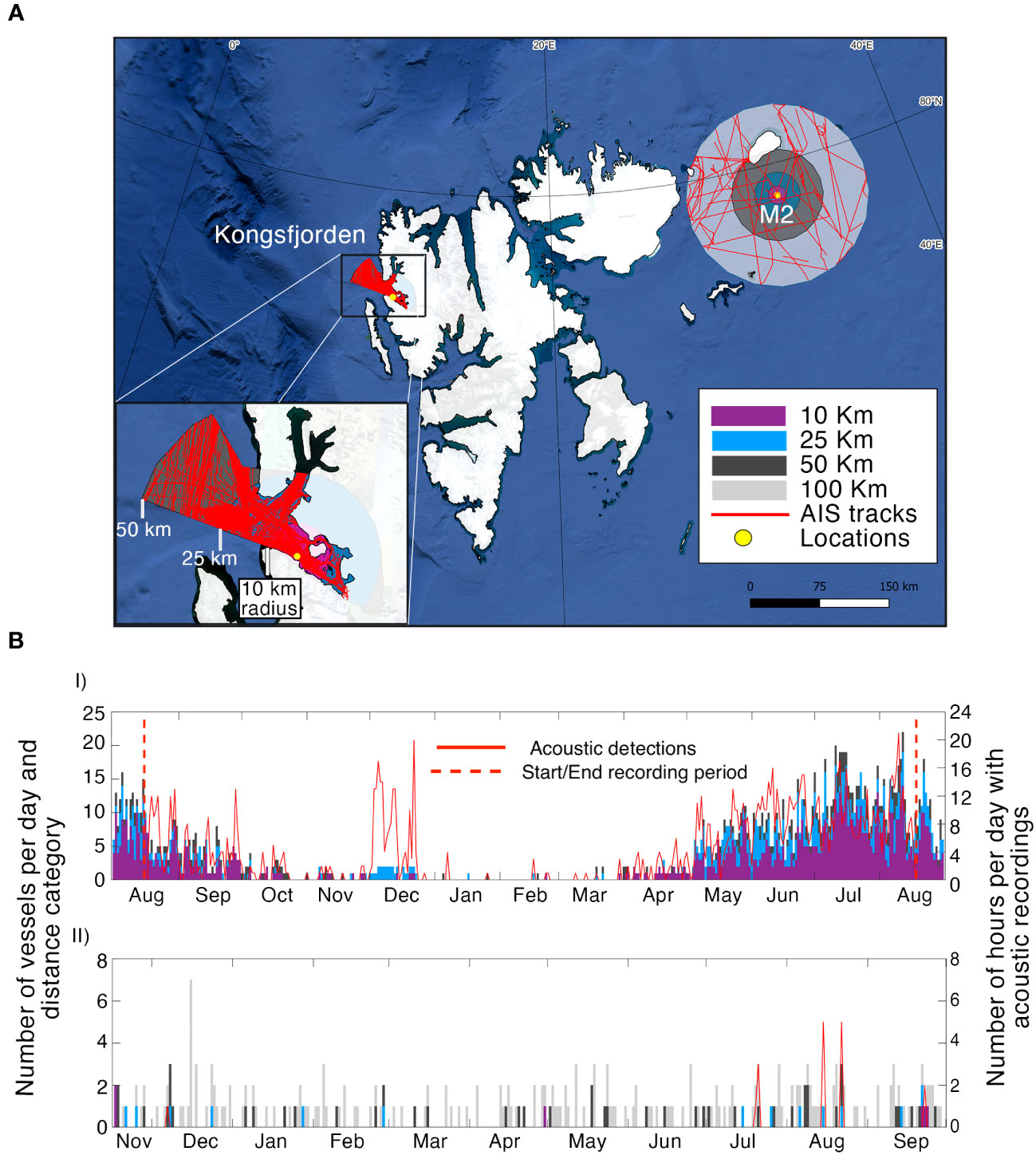
Figure 4 Year-round AIS traffic data within different distance classes plotted in a map, spanning the study period at each location around the recorders as indicated by the legend (A). The number of vessels per day and distance category indicated by the legend, plotted versus the number hours with acoustic presence from vessels at Kongsfjorden (2017-2018, (BI) and M2 (2019-2021, (BII). The vertical red dashed lines indicate the beginning and end of the recording period at Kongsfjorden, whereas the recording period fits the temporal span of the figure at M2.
M2 had little traffic, but there were vessels every month in the 100 and 50 km distance categories, and vessels within 25 km over a period of several months. There was a maximum of 7 vessels within 100 km in the busiest period in December, and for most of the year, the number of vessels was below 4 (Figure 4BII). The acoustic detections matched well with the AIS data at short distances (within 25 km) but sounds from vessels were not detected at longer distances at M2 (50-100 km).
The multiyear traffic trend showed remarkable differences in monthly numbers of boats within 50 km of the recorders in Kongsfjorden and M2. The number of vessels per month increased year by year in Kongsfjorden, from a maximum of 65 vessels per month in July 2016 to a maximum of 112 vessels per month in July 2019 (Supplementary Material Figure 3A). The trend collapsed in 2020 when the global pandemic changed traffic patterns worldwide (Ryan et al., 2021). The maximum number of vessels per month remained relatively constant over the 5-year period at M2 (Supplementary Material Figure 3B). Additionally, the types of vessels found at the two locations also differed. In Kongsfjorden, a high number of vessels belonging to “Passenger”, “Passenger/Cruise” and “Recreational” category were present, especially during the summer months, related to tourism activities. At M2, “Fishing” and “Passenger/Cruise” vessels were the most common until 2018, after which a higher proportion of “Cargo” vessels were present in the area. M2 traffic seemed to be highly influenced by sea-ice cover (Supplementary Material Figure 3B top panel).
Soundscape analysis
The LTS from Kongsfjorden was dominated by acoustic signatures from glacier melt, vessel traffic and strumming noise generated by the mooring (Figure 5A). Three intermittent noise bands were present year-round at about 15 Hz, 50 Hz and 90 Hz, related to the cable strumming from the mooring itself. No clear signatures from biological sounds could be identified in the LTS. Vessel noise extended from just above 10 Hz up to a few 100 Hz and was seen in almost every month, decreasing during winter, and becoming ubiquitous during the summer (Figure 5A). A silent period coincided with the maximum ice cover in the fjord during the month of March, observed as a deep blue color at all frequencies. The median PSD monthly plot for Kongsfjorden showed little seasonal variation and was skewed towards a peak of energy centered at 800 Hz year-round (Figure 5B).
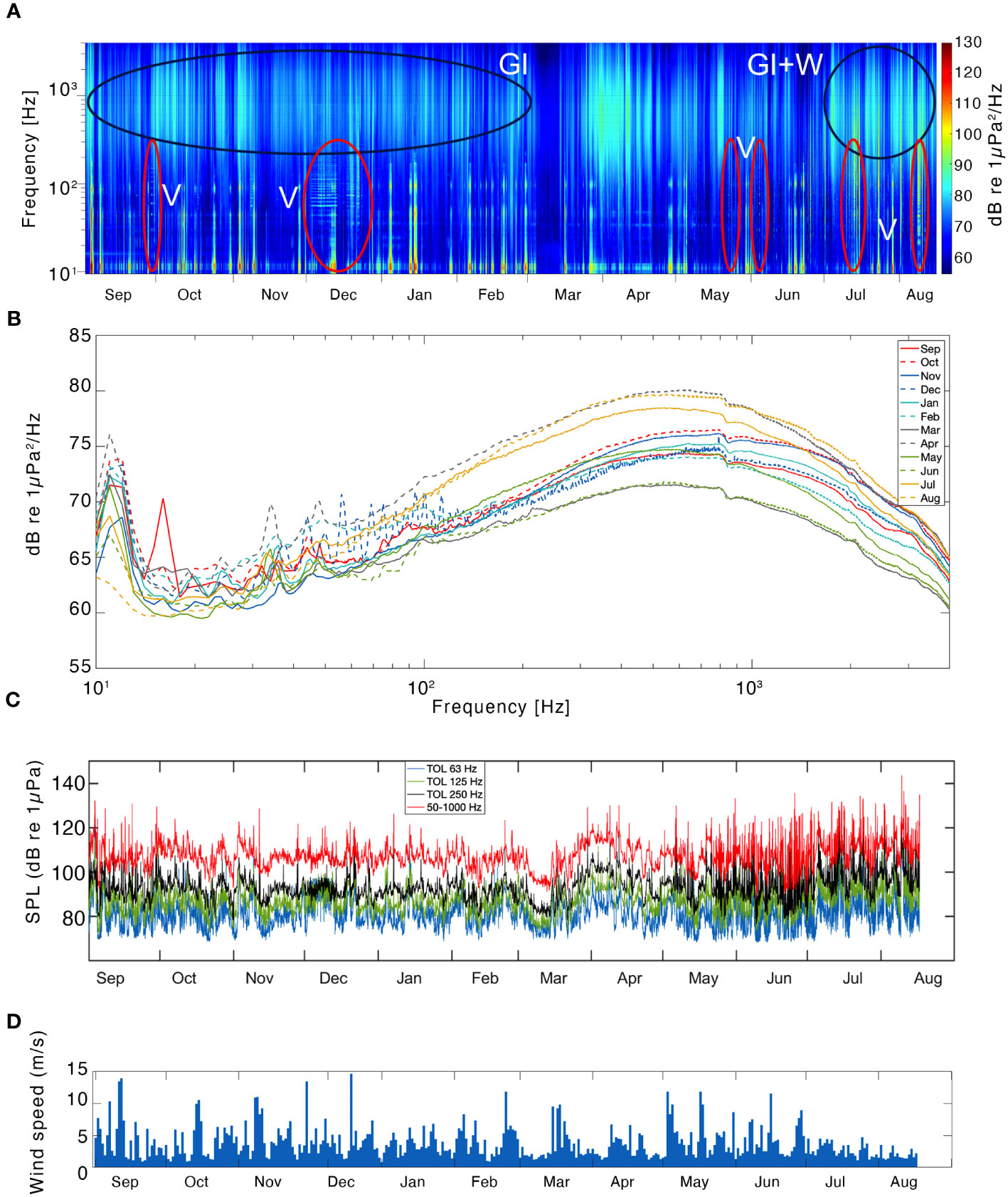
Figure 5 Results from the soundscape analysis in Kongsfjorden. A long-term spectrogram (LTS) indicating the frequency band (primary y-axis, logarithmic scale) and the power spectral density (PSD) of the sound in a color scale- from low intensity in blue, to high intensity in red (secondary y-axis; re 1 μPa2/Hz), illustrating the concentration of sound energy along the frequency band 10-4000 Hz and the study period (2017-2018) in Kongsfjorden (A). Black ellipses indicate sound energy from geophonies: glacier ice (GI) and wind (W). Red ellipses indicate sound energy from anthropophonies: vessels (V). The median PSD per month, as indicated in the legend, along the frequency band 10-4000 Hz (logarithmic scale) at Kongsfjorden (B). The mean SPL in the 1/3 octave bands centered at 63 Hz (blue), 125 Hz (green), 250 Hz (black) and the broadband 50-1000 Hz (red) plotted for Kongsfjorden (C). The daily mean wind-speed through the recording period at Kongsfjorden (D).
For most of the year in Kongfjorden, the SPL showed a trend with TOL centered at 63 Hz being the lowest, followed by TOL centered at 125 Hz, 250 Hz and the 50-1000 Hz broadband levels being the highest year-round. This trend was disrupted in December and occasionally during September, late-June through August, when low frequency TOL levels approached the higher broadband levels (Figure 5C). The highest SPLs were reached during the summer months, when they passed the120 dB (re 1μPa) on few occasions. The wind speed data shows that the winds were higher during late summer and autumn (September-December, Figure 5D), but no evident increase in broadband SPL could be observed during that period due to wind, as can be observed in the very low linear fit of SPL versus wind speed (R-squared= 0.0027; Supplementary Material Figure 4A).
The LTS for M2 was dominated by broadband noise from sea-ice, marine mammal biophonies and flow and strumming noise from the mooring below 50 Hz (Figure 6A). From November to mid-April, physical stresses within the sea ice increased the broadband PSD greatly. Sea-ice break-up events were detected as overall high PSDs in late-April, mid-June, and July 2020, whereas a silent period with high sea-ice concentration occurred in May. Biophonies were present year-round in the LTS at M2. Bowhead whale vocalizations increased PSD levels at 0.2-1 kHz from November to April, reflecting a shift from songs (higher frequency) to call sequences through the winter; singing behavior occurred in December, January, and March 2020 (Figure 6A). Bearded seals were detected at 0.5-0.6 kHz from May to July (Figure 6A). A signature from fin whale chorusing, centered at 20 Hz and 125 Hz, started in August 2020. Airguns increased the PSDs in the 80-300 Hz range during late-July and early-August. Only one signature from vessels was observed in late August, as a bracketed signal at 20-80 Hz (Figure 6A).
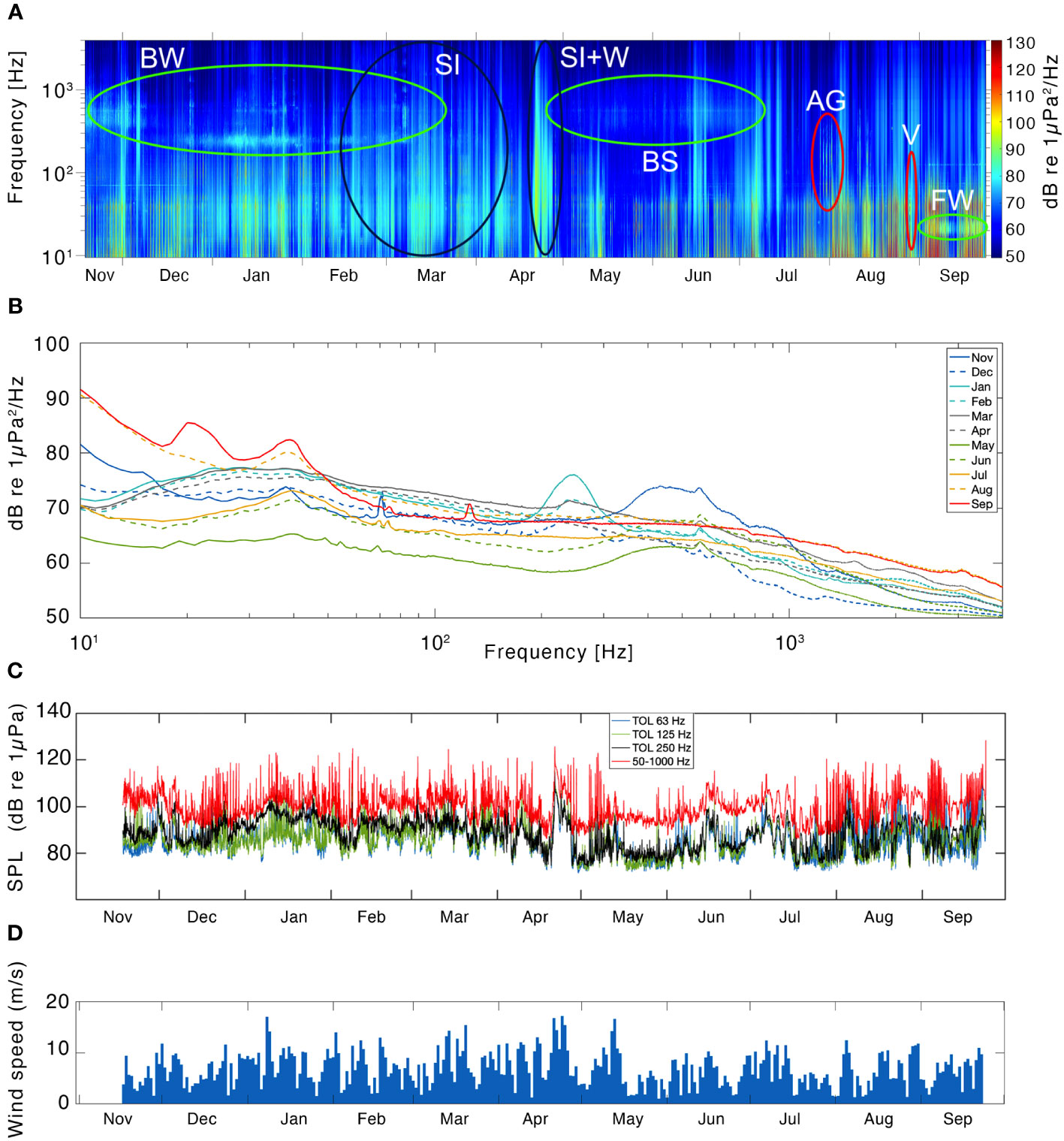
Figure 6 Results from the soundscape analysis at M2. A long-term spectrogram (LTS) indicating the frequency band (primary y-axis, logarithmic scale) and the power spectral density (PSD) of the sound in a color scale- from low intensity in blue, to high intensity in red (secondary y-axis; re 1 μPa2/Hz), illustrating the concentration of sound energy along the frequency band 10-4000 Hz and the study period 2019-2020 (A). Black ellipses indicate sound energy from geophonies: Sea ice (SI). Green ellipses indicate sound energy from marine mammal vocalizations: bowhead whale (BW), bearded seal (BS) and fin whales (FW). Red ellipses indicate sound energy from anthropophonies: airguns (AG) and vessels (V). The median PSD per month, as indicated in the legend, across the frequency band 10-4000 Hz (logarithmic scale) during the study period 2019-2020 (B). The mean SPL in the 1/3 octave bands centered at 63 Hz (blue), 125 Hz (green), 250 Hz (black) and the broadband 50-1000 Hz (red) plotted for the study period (2019-2020, at M2 (C). The daily mean wind-speed through the recording period at M2 (D).
The monthly median PSD for M2 showed a variety of signals throughout the year. August and September 2020 showed the highest median PSDs above 1 kHz, probably due to the absence of sea-ice allowing for higher frequency noise from wind and waves. May had the lowest PSDs of the season (Figure 6B). Bowhead whales caused an increase in median PSD of roughly 6 dB (re 1 μPa2/Hz) at 0.5-0.6 kHz during the month of November when they sang constantly, and again raised the sound levels by roughly 9 dB at 0.2-0.3 kHz when they shifted to simple calls and sequences in January 2020. From April onward, a sustained peak in PSD centered at 0.5-0.6 kHz was caused by bearded seals, with an increase in 6 dB above the background noise. A clear signal from fin whale 135 Hz vocalizations generated an increase of 3 dB in the median PSD during September 2020, while their 20 Hz pulses were masked by mooring noise.
At M2, SPL in TOL centered at low frequencies remained lower than the broadband levels during periods of stable ice cover, with a constant gap of roughly 10 dB (January-July 2020). An increase in SPL in TOLs centered at low frequencies, but also in broadband levels, occurred during periods without sea-ice, due to the effect of wind on the mooring and consequently on the sound recorded (strumming noise; April, July to September, Figure 6C). Sharper increases in the 250 Hz TOL band compared to the other frequency bands reflected the constant bowhead whale vocal sequencies (January 2020). At M2, the wind speed was generally higher than in Kongsfjorden, often exceeding the 15 m/s during winter and spring (Figure 6D) and wind noise was reflected in higher broadband SPL levels compared to Kongsfjorden, especially in late April, when the break-up of the sea-ice coincided with higher wind speeds and higher broadband SPL. A linear relationship between broadband SPL and wind speed was observed at M2, with higher R-Squared when the sea-ice cover at the location was below 40% (R-squared= 0.3792) than when the sea-ice cover was above 40% (R-squared= 0.2239; Supplementary Material Figure 4B).
The year-round power spectral density plots showed that the Kongsfjorden soundscape is strongly skewed towards high median PSD levels centered at higher frequencies (Figure 7A, Table 2). In contrast, the median PSD levels under 100 Hz were below 70 dB. The RMS levels showed the influence of transient high amplitude sounds from vessels, which elevated the PSD levels to >90 dB below 100 Hz; strumming noise might also have contributed to this source of noise. At M2 (Figure 7B), the highest median PSD levels were centered at frequencies <100 Hz, and usually did not rise above 75 dB. The much higher but stable RMS levels found at these frequencies are due to noise generated by the mooring itself during periods with high winds in summer and autumn when there was open-water, and some current and sea-ice derived mooring noise elevated the spectral density in the lower frequencies as well.
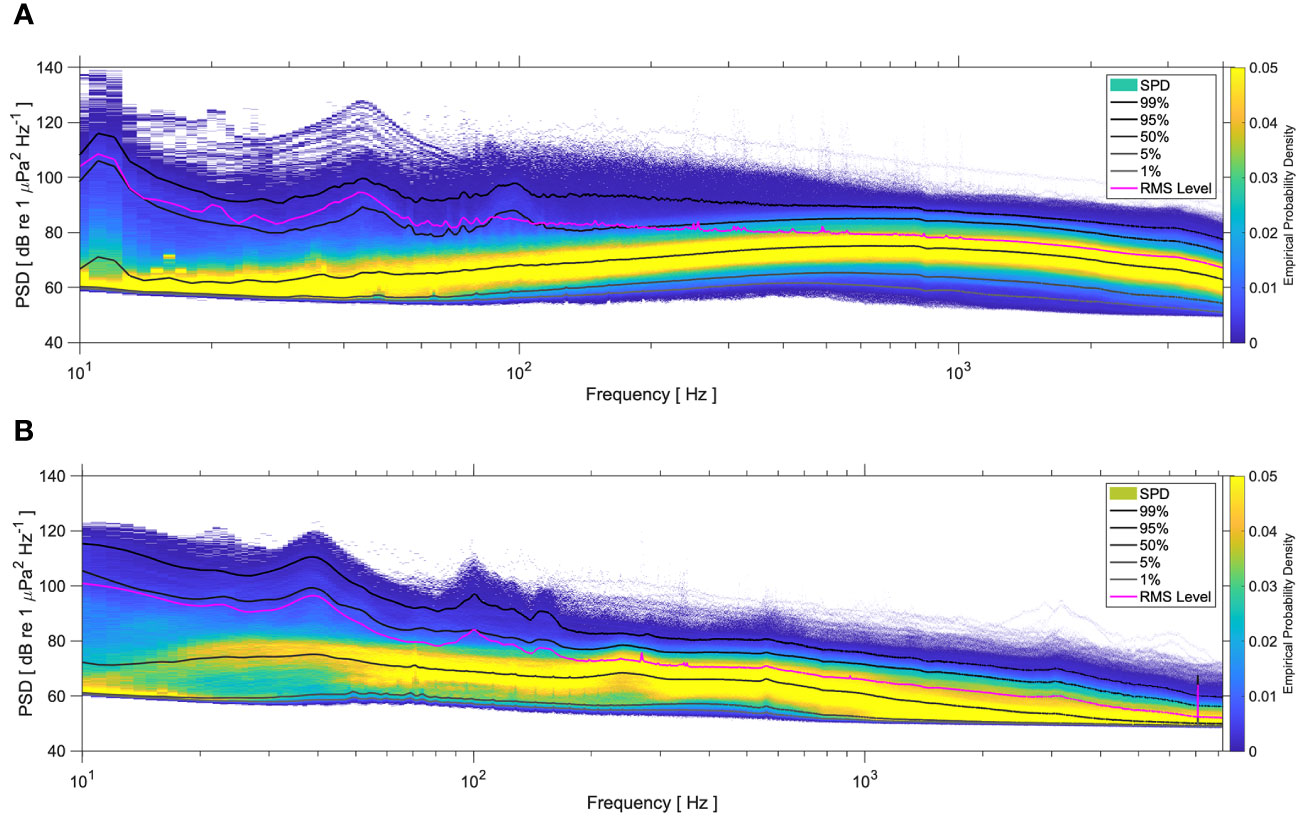
Figure 7 Percentiles (1%, 5%, 50%, 95%, 99%), root mean square (RMS) and empirical probability densities (color scale) showing the distribution of power spectral density (PSD, re 1 μPa2/Hz) in the 10-4000 Hz frequency band (logarithmic scale) year-round at Kongsfjorden (2017-2018, (A) and M2 (2019-2020, (B).

Table 2 Median and RMS (in brackets) power spectral densities (PSD) centered at 10, 50, 100, 500 and 1000 Hz at the AURAL sites in this study.
Discussion
This study compares the underwater soundscapes from two locations in the Svalbard Archipelago, one on the west coast (Kongsfjorden), where Atlantification is advanced, and one on the east coast (M2), which retains more Arctic climatic conditions. It provides baseline data that can serve as a reference point for soundscape studies in the decades to come and the west coast might serve as a precursor for what is likely to be the future situation throughout the archipelago, and more broadly across the Arctic. Anthropogenic influences are clearly more pronounced in the soundscape on the west side of the archipelago, where ship traffic is a common component of the soundscape. The eastern site remains more pristine, although seismic blasting was detected over a longer period at the eastern site. The manual detections performed for one complete season at each location provide insight into the marine mammal community, including identifying the timing of migration for several seasonally resident species as well as documenting a surprisingly high presence of narwhal and bowhead whales on the east side of the archipelago throughout much of the year. The study site in eastern Svalbard was more heavily used by resident, endemic marine mammals in general compared with the site on the west of the archipelago. In contrast, migratory whales were somewhat more common in the west, where Atlantification of the ecosystem is more advanced.
Arctic endemic marine mammals
Walrus vocalizations were detected on both sides of the archipelago, with 3 months of presence in Kongsfjorden, and for a period roughly twice as long - half a year at M2. A peak in vocal detections occurred in February and March on both the west and the east sides of Svalbard. M2 is located in an area where tracking studies have previously shown that male walruses spend time during the breeding periods in early winter and spring (Jan-Feb-Mar; Stirling et al., 1987; Freitas et al., 2009; Lowther et al., 2015). In accordance with Madan et al. (2020), walruses were detected throughout the breeding period in Kongsfjorden. With the current expansion of the number of walruses in the archipelago, more areas are likely to come into use across broader seasonal time frames (Lydersen et al., 2008; Kovacs et al., 2014), though summer observations of walruses in Kongsfjorden are still rare (Bengtsson et al., 2021).
Bearded seal sounds were detected intermittently for four months in Kongsfjorden, while this species was nearly continuously vocally present for almost nine months at M2 (commencing in November). Male bearded seals perform trilling vocalizations to advertise their breeding condition, so the presence of their trill call types is a good proxy for the mating season at a given location (Van Parijs et al., 2001; Llobet et al., 2021). The short vocal season in Kongsfjorden in this study, compared to studies in the 1990s and early 2000’s, suggests reduced mating activity in the fjord. The mismatch between ice-cover and the mating season in 2018 is consistent with results from the 2015 season recently published by Llobet et al. (2021). The seasonal pattern of bearded seal vocalizations reported in this study in eastern Svalbard is similar to two northerly locations on the east side of Svalbard (Llobet et al., 2021), with several months of high levels of vocal activity that suddenly drop off when the drift ice disappears periodically. Two vocalization types that had not been previously reported in Svalbard (Risch et al., 2007; Parisi et al., 2017) were recorded in the current study; these resemble type-5 calls reported by Agafonov and Chernetsky (2018) for the White Sea population. “boomerang trill” (Supplementary Material Figure 1BI) and “drop sweep” (Supplementary Material Figure 1BII) are proposed as names for these call types, following a nomenclature that resembles the one used by Parisi et al. (2017).
Narwhals were detected only in eastern Svalbard. At M2, signals from narwhals were detected sporadically from November to January, and between March and late July this species was present every week. It is likely that all the “odontocete” acoustic signals documented in this location are in fact from narwhals, but this could not be confirmed without the presence of the low frequency clicks that are a good diagnostic feature for identification of this species (Ahonen et al., 2019). Few observations of narwhals are available from Svalbard shelf waters (Storrie et al., 2018), although the tracking study by Lydersen et al. (2007) suggests that they likely spend time around Noraustlandet in summer. A survey conducted in August 2015 to count ice whales in the marginal ice zone north of Svalbard reported and estimated 837 narwhals, mainly located deep into the polar pack-ice (Vacquié-Garcia et al., 2017). Tracking studies from Greenland suggest that narwhals target a narrow sea-temperature niche and prefer high ice concentrations (Heide-Jørgensen et al., 2020). This species is sensitive to ocean noise and anthropogenic disturbances (Heide-Jørgensen et al., 2021; Tervo et al., 2021; Williams et al., 2022). Narwhal are structured in highly subdivided metapopulations and show little genetic flow between groups (Hobbs et al., 2019; Louis et al., 2020). Narwhal from Svalbard waters are closely related to those from Northeast Greenland, and they are markedly different from those in Southeast Greenland (Louis et al., 2020). Ahonen et al. (2019) have demonstrated a year-round presence of narwhals in the Fram Strait, between Northeast-Greenland and Svalbard. The current study reports the presence of this species in eastern Svalbard shelf waters for several months of the year for the first time, as animals from this population have otherwise been detected only occasionally east of Svalbard, most of them around Franz-Josef Land (Figure 1, Belikov and Boltunov, 2002). During the present study, they were detected most commonly when heavy ice-conditions prevailed, although they were also vocally active during the second half of July in open water. They were detected in 8.9% of the available files during the last week of July 2020, overlapping with seismic air guns, posing a direct risk of anthropogenic disturbance to this population at this site (Heide-Jørgensen et al., 2021; Tervo et al., 2021; Williams et al., 2022).
At the M2 location there were weekly detections of bowhead whales from November until July, with singing behavior peaking in November and shifting towards sequence-based vocal behavior with some singing in late-December (Supplementary Material Figure 2), January and March. Complex singing behavior is indicative of mating activity in bowhead whales (Stafford, 2022). There are relatively few observations of bowhead whales close to the coast in Svalbard but trends over recent decades suggest a northward shift during summer (Storrie et al., 2018; Bengtsson et al., 2022). Offshore, in the Fram Strait west of Svalbard there is an extended vocal presence of bowhead whales throughout the year (Stafford et al., 2012; Ahonen et al., 2017; Stafford et al., 2018). A survey conducted in August 2015 estimated 343 bowhead whales in an area of 53000 km2 in the pack-ice north of Svalbard (Vacquié-Garcia et al., 2017), and a genetic study recently confirmed that these animals belong to a unique stock, with its own genetic legacy (Bachmann et al., 2021). Tracking data from animals marked in Fram Strait showed that they dispersed throughout much of the historical range of the species, from the coast of east Greenland via Svalbard and over to Russian waters and were strongly affiliated with sea-ice (Kovacs et al., 2020). The present study confirms that bowhead whales overwinter on the northeastern shelf of Svalbard. They vocalized nearly constantly over a 5-month period. They also show a wide repertoire of complex songs, similar to what has been reported from western Fram Strait (Stafford et al., 2018), rather than the modest singing behavior reported in eastern Fram Strait (Tomisch et al., 2022), suggesting that this area is another mating area for the Spitsbergen population.
White whales were detected during a few weeks in January and during June-July (2018) in Kongsfjorden. Further vocal activity from odontocetes was detected during the study, but only in these two periods is it certain to have been white whales. The restricted sampling frequency of the AURALs in Svalbard does not cover the frequencies of echolocation clicks from white whales. However, it is likely that the odontocete vocalizations recorded in Kongsfjorden are all from white whales, as low-frequency clicks (typical of narwhals - Blackwell et al., 2018; Ahonen et al., 2019; Zahn et al., 2021) were never detected in Kongsfjorden, and white whales are often seen in this fjord, especially during the summer and autumn (Vacquié-Garcia et al., 2020; Bengtsson et al., 2022).
Seasonal marine mammals
Six seasonally migrant marine mammals were identified during this study. Two of these, sperm whales and sei whales, were detected only in the west. The sperm whale recordings comprised series of slow clicks. This type of vocalization has been hypothesized to have a communication function among mature males (Jaquet et al., 2001). The presence of sperm whales on the shelf west of Svalbard has been previously documented through visual observations (Storrie et al., 2018; Bengtsson et al., 2022). However, the late season sounds recorded in December were surprising, as it has previously been thought that most sperm whales leave the archipelago in the autumn to migrate to southern areas to breed. The detection of sei whales in Kongsfjorden between late-August and mid-September was more in line with the normal season for migrant cetaceans. This species has only been sighted rarely in shelf areas in western Svalbard; it is believed that they prefer waters further offshore along the continental slope (Prieto et al., 2014; Storrie et al., 2018). However, visual misidentification of sei whale by non-experts is likely an issue in shelf areas, where they can be confused easily with the more abundant fin whales (Prieto et al., 2012; Storrie et al., 2018). This makes acoustic detection of species-specific vocalizations a particularly valuable tool for identifying sei whale presence.
Fin whales were detected both on the west and east coasts of Svalbard. This species was recorded intermittently from late-October to late-January and then was heard again in August (2018) in Kongsfjorden. The late autumn and early winter calls recorded from fin whales in this study support recent articles that have demonstrated that some fin whales reside in the High Arctic year-round (Ahonen et al., 2021; Papale et al., 2023). However, most fin whales are thought to migrate to breeding or more southerly feeding areas during the winter; first shifting from inshore areas to deeper waters and then leaving the High Arctic in the autumn/early winter (Lydersen et al., 2020). In eastern Svalbard, fin whales were detected frequently in September. Similar to the other migratory whales, fin whales have been observed at progressively higher latitudes over the last 15 years in Svalbard (Bengtsson et al., 2022). Blue whale acoustic signals were also detected on both coasts; blue whale 16-18 Hz calls were detected in late August in Kongsfjorden, and in late-August through until early-September at M2. Similarly, humpback whales were also detected on both coasts. They were recorded occasionally in October (2017) in Kongsfjorden, and in July and August (2020) at M2. In the open water period in summer, many mysticete vocalizations were detected, especially at M2, but it was not possible to separate humpbacks from bowheads definitively, and it is possible that both species were on the east side at this time. However, it is most likely that most of the summer vocalizations were produced by humpback whales. Humpback whales occur in significant numbers east of Svalbard during summer (Storrie et al., 2018; Bengtsson et al., 2022); this species makes up 11.3% of all cetacean observations in recent years around Svalbard (Bengtsson et al., 2022). More detailed studies are needed to investigate and differentiate summer vocalizations between these two species.
Minke whales were not acoustically detected in this study, though they are common in shelf waters on both sides of Svalbard (Storrie et al., 2018; Bengtsson et al., 2022). This is attributable to their quiet nature in this region, which is likely linked to the fact that the animals in Svalbard are mostly females (Andersen et al., 2003). Further increases in the detections of balaenopterid whales are expected in the future around Svalbard given the Atlantification of the region detected at other trophic levels (Berge et al., 2015; Fossheim et al., 2015; Ershova et al., 2021) but monitoring of quiet species such as minke whales will have to be done via visually surveys. Harp seals were detected only on the recorders in eastern Svalbard. Species-specific calls (Terhune, 1994) were heard occasionally between April and August 2020. This timing coincides with their seasonal movements into the northern Barents Sea and Svalbard region, where animals from both the Greenland Sea and the White Sea breeding stocks feed during summer (Haug et al., 1994; Folkow et al., 2004; Nordøy et al., 2008).
Anthropogenic activities
Two sources of anthropogenic noise were detected in the present study – air guns from seismic surveys and ship traffic. Air guns were detected only briefly in Kongsfjorden, in August, while air-gun noises were detected at M2 over a period of three weeks in July and August. Vessels were detected in all weeks from August (2017) to January (2018), and from April (2018) until the end of the study period in Kongsfjorden. The highest rate of acoustic detections of ships in the area occurred in June, July and August at this location. A high rate of vessel noise was also detected during December 2017 in Kongsfjorden. However, AIS analysis showed that only a few vessels were present in the area at that time. This situation was further investigated because these vessels caused a significant increase in the sound levels and a persistent noise signal in the spectrograms. A time-lapse of the monthly traffic was plotted in the Norwegian Coastal Administration web service5 for Kongsfjorden and the MMSI numbers were used to categorize the type of vessels in the area during that month6. Only 8 vessels were found in the entire month, 7 out of 8 were fishing vessels, and 4 of these were trawlers that targeted northern shrimp7 (Pandalus borealis) for many days in a row. This activity seems to have created the persistent noise signal detected in December 2017 (Supplementary Material Figure 5). In marked contrast to the situation in Kongsfjorden, only a few vessels were acoustically detected at M2, in part because ice cover was extensive for most of the study period; ice in this region is highly variable interannually and undoubtedly impacts anthropogenic noise levels. The covid pandemic could also have played a role in the extreme quiet period during the summer of 2020 as underwater noise worldwide was greatly reduced at this time because of the low level of economic activity including shipping and tourism (Ryan et al., 2021). However, results from the AIS data indicate that a pronounced change in the number and type of vessels present in M2 occurred prior to the pandemic – in the 2018/2019 season. Therefore, changes in traffic observed in this location might be related to other socioeconomic factors. These results also support the idea that a further reduction in sea-ice cover in the region will contribute to the increase in vessel traffic derived from different economic activities. The reduction in traffic due to the pandemic was evident in Kongsfjorden, where the number of boats involved in tourism activities dropped in 2020, following many years with an upward trend in this activity.
Ship traffic noise can be a problem for marine mammals. Several studies in the Arctic have demonstrated negative impacts from ship traffic, including reduced vocal rates in response to vessels (white whales, Halliday et al., 2019; Martin et al., 2022). Bearded seals in Alaska increase the amplitude of their calls when ambient sound levels increase, up to a threshold at which they ceased signaling (Fournet et al., 2021). In this study, vessels were detected 50% of the time in the period when bearded seal vocal activity peaked in Kongsfjorden.
Marine mammals are also sensitive to seismic blasts (Reeves et al., 2014; Nowacek et al., 2015). Bowhead whales react to air gun pulses by increasing their calling rates until the amplitude of air-gun pulses reaches a threshold at which they stop vocalizing (Blackwell et al., 2015). Narwhals react to air-gun pulses even when they are embedded in background noise (Tervo et al., 2021). In this study, vocal detections of narwhals at M2 ceased the same week that air guns were detected for the first time. This source of anthropogenic noise also overlapped with the presence of harp seals, humpback, and fin whales in this study.
One of the limitations of this study was that information about noise propagation in our locations was limited (Sanjana et al., 2018; Sanjana et al., 2021; Richard et al., 2023). The implementation of such data in the study would allow us to assess the active acoustic space from which our recorders receive ship noise at any given time. Without such data, the presence or absence of acoustic signals from boats could be biased. On the other hand, combining this information with AIS data split into distance buffers and PSD plots, increased our ability to access relative levels of potential contribution of ships to the soundscape and assess disturbance to marine mammals from these sound sources. Using AIS analysis areas shaped by the line of sight was chosen with the intention of leaving out potential ship traffic information from adjacent fjords or areas that might not reach our recorders, although we are aware that some of the noise received at our recorders could come from locations out of sight. In Kongsfjorden a few boats increased the PSD and TOL levels in some seasons noticeably and given high rates of daily acoustic detections, highlights the utility of our approach in emphasizing the different issues that noise can pose for marine mammals depending on its character. Constant versus transient noise, the season of occurrence and the type of vessel that produces such noise are all factors to be considered when assessing the impact of traffic noise on marine mammals. Noise propagation models and more experimental studies are necessary to assess this impact in Svalbard, but our study provides a first overview showing that Kongsfjorden’s soundscape is sometimes noisy despite the high latitude of this location and that it is certainly currently more affected by vessel noise than M2. It also highlights the vulnerability of M2 to an increase of anthropogenic noise in the near future; the sea-ice is the main characteristic of the soundscape of the M2 site currently, but it is also the main safeguard against the increase of anthropogenic noise in this region. M2 biophonies are shaped by species that depend on sea-ice cover (bowhead whales, bearded seals, walruses and narwhals).
Soundscape analysis
The soundscape results in Kongsfjorden were typical for fjords with glaciers at their termini in Svalbard (Glowacki et al., 2018; Ashokan et al., 2020), where the higher PSD are centered at frequencies near 1 kHz (Tęgowski et al., 2011; Petit et al., 2012). This pattern was consistent year-round, with little variation in contributions to the soundscape as inferred from the monthly PSD plots, due to the constant melting signal at high frequencies, the presence of vessel traffic most of the year and the lack of biophonies at a soundscape scale. The lowest sound levels were found in March, due to the higher sea-ice extents in the fjord at this time (Geyer et al., 2016). The highest levels of sound occurred during April (due to break-up of the ice) and during summer. Median PSD values showed peaks related to ship traffic in the low frequency range during June, July, August and September in the SPL plots, with TOLs centered at 63, 125 and 250 Hz showing a greater increase in SPL. The maximum values exceeded 120 decibels many times during this period, which is defined by NOAA as a disturbance threshold for all marine mammals (Southall et al., 2008; Halliday et al., 2017). Therefore, vessel traffic in Kongsfjorden can already be considered a relevant stressor. A few shrimp trawlers that were continuously active within 25 km of the recording device during December 2017, caused a sustained increase in SPL in the 63 and 125 Hz TOLs, generating a persistent signal on the LTS and increasing the monthly median PSD in those frequencies as well. This finding highlights the importance of investigating the type of activity and its acoustic impact rather than targeting just the number of vessels as an impact indicator.
At M2, periods with extensive ice cover (late-May) were generally very quiet, although some periods when the ice cover was extensive but probably more dynamic showed high broadband energy, as was the case from January to February. Sea ice was the main factor shaping the geophysical soundscape and the sound levels overall at M2. The absence of sea ice led to high sound levels from wind at this site. Biophonies were detected at a seasonal scale at the eastern location and contributed to increased sound levels at M2. The prolonged period in which endemic Arctic species were vocally present in eastern Svalbard, together with the increasing presence of seasonal species, contributed to a more diverse soundscape throughout the season in eastern Svalbard compared to the west. M2’s soundscape was richer in endemic Arctic marine mammal signals, and even some behavioral and ecological processes became evident at the soundscape scale. Bearded seals and bowhead whales left a remarkably similar signature in the increase of monthly median PSD centered at 0.5-0.6 kHz, frequency that both species seemed to exploit. A segregation in different acoustic niches (niche hypothesis, acoustic niche hypothesis; Hutchinson, 1957; Krause, 1993) seems to exist in the different dimensions of the soundscape. When bowhead whales emit broadband songs in November, walruses and bearded seals vocalized only occasionally. As bowhead whales shifted from songs to vocal sequences, walruses reached the peak of their vocal activity centered at 2 kHz. Bearded seals, whose sounds overlap with bowhead whale frequencies, seem to take over and reach maximum vocal activity levels from April-May, when bowhead whales and walruses drop their vocal activity. This has been proposed to be a property of soundscapes emerging from minimally disturbed ecosystems where species have coevolved to efficiently exploit the soundscape in part by minimizing interference (Sueur and Farina, 2015).
In conclusion, this study provides a useful baseline for the current acoustic soundscape and marine mammal community composition in an interesting High Arctic site – the Svalbard Archipelago – which is one of the fastest changing regions in the world, including the little-studied east coast. It was surprising that ship noise at the west coast site already came close to maximum recommended levels for ensuring the safety of marine mammals from an ocean noise perspective. It is uncertain whether the current noise levels are in keeping with the intentions of the EUs MSFD guidelines that suggest the noise should not harm or disturb marine mammals (both of which are challenging to demonstrate unless levels are extreme). Studying two different areas that have been differentially impacted by climate change also provides insight into the change that is likely to occur in the coming decades in sites that are currently still retaining an Arctic character. In future, studies should include 1) validated acoustic propagation models to avoid bias and better understand how vessel noise propagates in both fjord and open water locations with different bathymetries, 2) more fine-scale oceanographic data should be studied to explore drivers of biotic change and 3) multiyear acoustic data should be analyzed to track trends through time.
Data availability statement
The datasets presented in this study can be found in online repositories. The names of the repository/repositories and accession number(s) can be found below: https://data.npolar.no/dataset/?filter-created_by=samuel.7.arg@gmail.com&sort=-created-Repository: Norwegian Polar Datacentre by the Norwegian Polar Institute.
Author contributions
Data collection was facilitated by KK, CL and HA. SL and HA led the analyses. All authors participated in study design and the writing of the manuscript. All authors contributed to the article and approved the submitted version.
Funding
This study was funded by the Svalbard Environmental Fund (grant no. 19/8), the Norwegian Research Council (NRC-ARK project no. 313678) and the Norwegian Polar Institute. Mooring services on the east side of Svalbard were provided under the NRC project Arven etter Nansen project infrastructure and the Kongsfjorden mooring was serviced by SIOS.
Acknowledgments
We thank Kristin Fossan (NPI) and Daniel Vogedes (UiT – Jørgen Berge’s Lab), for servicing the instruments and downloading the data from the AURALS. We want to thank Kate M. Stafford and Michelle Fournet for their valuable advice during the analysis of bowhead and humpback whale sounds, Isabel Charrier and Heloise Frouin-Mouy for their help with some pinniped sounds, thanks to Jack Terhune for his time spent helping us with the harp seal underwater sounds.
Conflict of interest
The authors declare that the research was conducted in the absence of any commercial or financial relationships that could be construed as a potential conflict of interest.
Publisher’s note
All claims expressed in this article are solely those of the authors and do not necessarily represent those of their affiliated organizations, or those of the publisher, the editors and the reviewers. Any product that may be evaluated in this article, or claim that may be made by its manufacturer, is not guaranteed or endorsed by the publisher.
Supplementary material
The Supplementary Material for this article can be found online at: https://www.frontiersin.org/articles/10.3389/fmars.2023.1208049/full#supplementary-material
Footnotes
- ^ https://cryo.met.no/
- ^ https://seklima.met.no/
- ^ https://psl.noaa.gov/data/gridded/data.ncep.reanalysis.html
- ^ https://www.kystverket.no/
- ^ https://kystdatahuset.no/tallogstatistikk/sporing
- ^ https://www.marinetraffic.com/
- ^ https://www.fiskeridir.no/Yrkesfiske
References
Agafonov A. V., Chernetsky A. D. (2018). Underwater acoustic signaling of bearded seals (Erignatus barbatus) in the White Sea. Oceanology (Wash DC) 58, 847–855. doi: 10.1134/S0001437018070019
Ahonen H., Stafford K. M., de Steur L., Lydersen C., Wiig Ø., Kovacs K. M. (2017). The underwater soundscape in western Fram Strait: Breeding ground of Spitsbergen’s endangered bowhead whales. Mar. pollut. Bull. 123, 97–112. doi: 10.1016/j.marpolbul.2017.09.019
Ahonen H., Stafford K. M., Lydersen C., Berchok C. L., Moore S. E., Kovacs K. M. (2021). Interannual variability in acoustic detection of blue and fin whale calls in the Northeast Atlantic High Arctic between 2008 and 2018. Endang. Species Res. 45, 209–224. doi: 10.1016/j.marpolbul.2017.09.019
Ahonen H., Stafford K. M., Lydersen C., de Steur L., Kovacs K. M. (2019). A multi-year study of narwhal occurrence in the western Fram Strait—detected via passive acoustic monitoring. Polar Res. 38. doi: 10.33265/polar.v38.3468
Andersen L. W., Born E. W., Dietz R., Haug T., Øien N., Bendixen C. (2003). Genetic population structure of minke whales Balaenoptera acutorostrata from Greenland, the North East Atlantic and the North Sea probably reflects different ecological regions. Mar. Ecol. Prog. Ser. 247, 267–280. doi: 10.3354/meps247263
Årthun M., Onarheim I. H., Dörr J., Eldevik T. (2021). The seasonal and regional transition to an ice-free Arctic. Geophys. Res. Lett. 48 (1), e2020GL090825. doi: 10.1029/2020GL090825
Ashokan M., Latha G., Thirunavukkarasu A. (2020). Underwater ambient noise in kongsfjorden, spitsbergen, during the summers of 2015 and 2016. Arctic 73, 386–392. doi: 10.14430/arctic70499
Au W. L. W., Hastings M. C. (2008). “Hearing in marine animals,” in Principles of marine bioacoustics. Eds. Au W. W. L., Hastings M. C. (New York, NY: Springer US), 337–400.
Bachmann L., Cabrera A. A., Heide-Jørgensen M. P., Shpak O. V., Lydersen C., Wiig Ø., et al. (2021). Mitogenomics and the genetic differentiation of contemporary Balaena mysticetus (Cetacea) from Svalbard. Zool. J. Linn. Soc 191, 1192–1203. doi: 10.1093/zoolinnean/zlaa082
Barton B. I., Lenn Y. D., Lique C. (2018). Observed Atlantification of the Barents Sea causes the Polar Front to limit the expansion of winter sea ice. J. Phys. Oceanogr. 48 (8), 1849–1866. doi: 10.1175/JPO-D-18-0003.1
Belikov S. E., Boltunov A. N. (2002). Distribution and migrations of cetaceans in the Russian Arctic according to observations from aerial ice reconnaissance. NAMMCO Sci. Publ 4, 69–86. doi: 10.7557/3.2838
Bengtsson O., Hamilton C. D., Lydersen C., Andersen M., Kovacs K. M. (2021). Distribution and habitat characteristics of pinnipeds and polar bears in the Svalbard Archipelago 2005–2018. Polar. Res. 40. doi: 10.33265/polar.v40.5326
Bengtsson O., Lydersen C., Kovacs K. M. (2022). Cetacean spatial trends from 2005 to 2019 in Svalbard, Norway. Polar. Res. 41. doi: 10.33265/polar.v41.7773
Berge J., Heggland K., Lønne O. J., Cottier F., Hop H., Gabrielsen G. W., et al. (2015). First records of atlantic mackerel (Scomber scombrus) from the svalbard archipelago, Norway, with possible explanations for the extensions of its distribution. Arctic 68, 54–61. doi: 10.14430/arctic4455
Bivand R. S., Pebesma E. J., Gómez-Rubio V., Pebesma E. J. (2008). Applied spatial data analysis with R (New York: Springer).
Blackwell S. B., Nations C. S., McDonald T. L., Thode A. M., Mathias D., Kim K. H., et al. (2015). Effects of airgun sounds on bowhead whale calling rates: evidence for two behavioral thresholds. PloS One 10, e0125720. doi: 10.1371/journal.pone.0125720
Blackwell S. B., Tervo O. M., Conrad A. S., Sinding M. H. S., Hansen R. G., Ditlevsen S., et al. (2018). Spatial and temporal patterns of sound production in East Greenland narwhals. PloS One 13, e0198295. doi: 10.1371/journal.pone.0198295
Cleator H. J., Stirling I., Smith T. G. (1989). Underwater vocalizations of the bearded seal (Erignathus barbatus). Can. J. Zool. 67, 1900–1910. doi: 10.1139/z89-272
Cottier F. R., Nilsen F., Inall M. E., Gerland S., Tverberg V., Svendsen H. (2007). Wintertime warming of an Arctic shelf in response to large-scale atmospheric circulation. Geophys. Res. Lett. 34 (10). doi: 10.1029/2007GL029948
Dekeling R. P. A., Tasker M. L., van der Graaf A. J., Ainslie M. A., Andersson M. H., André M., et al. (2014). Monitoring Guidance for Underwater Noise in European Seas, Part II: Monitoring Guidance Specifications. A guidance document within the Common Implementation Strategy for the Marine Strategy Framework Directive by MSFD Technical Subgroup on Underwater Noise. Luxembourg: Publications Office of the European. Available at: https://repository.oceanbestpractices.org/handle/11329/1237
Descamps S., Strøm H. (2021). As the Arctic becomes boreal: Ongoing shifts in a high-Arctic seabird community. Ecology 102 (11), e03485. doi: 10.1002/ecy.3485
de Vincenzi G., Parisi I., Torri M., Papale E., Mazzola S., Nuth C., et al. (2019). Influence of environmental parameters on the use and spatiotemporal distribution of the vocalizations of bearded seals (Erignathus barbatus) in Kongsfjorden, Spitsbergen. Polar Biol. 42, 1241–1254. doi: 10.1007/s00300-019-02514-3
De Vreese S., van der Schaar M., Weissenberg J., Erbs F., Kosecka M., Solé M., et al. (2018). Marine mammal acoustic detections in the Greenland and Barents Sea 2013-2014 seasons. Sci. Rep. 8, 1–14. doi: 10.1038/s41598-018-34624-z
Ershova E. A., Kosobokova K. N., Banas N. S., Ellingsen I., Niehoff B., Hildebrandt N., et al. (2021). Sea ice decline drives biogeographical shifts of key Calanus species in the central Arctic Ocean. Glob. Change Biol. 27, 2128–2143. doi: 10.1111/gcb.15562
Folkow L. P., Nordøy E. S., Blix A. S. (2004). Distribution and diving behaviour of harp seals (Pagophilus groenlandicus) from the Greenland Sea stock. Polar Biol. 27, 281–298. doi: 10.1007/s00300-004-0591-7
Fossheim M., Primicerio R., Johannesen E., Ingvaldsen R. B., Aschan M. M., Dolgov A. V. (2015). Recent warming leads to a rapid borealization of fish communities in the Arctic. Nat. Clim. Change 5, 673–677. doi: 10.1038/nclimate2647
Fournet M. E. H., Silvestri M., Clark C. W., Klinck H., Rice A. N. (2021). Limited vocal compensation for elevated ambient noise in bearded seals: implications for an industrializing Arctic Ocean. Proc. R. Soc B 288, 20202712. doi: 10.1098/rspb.2020.2712
Fournet M. E., Szabo A., Mellinger D. K. (2015). Repertoire and classification of non-song calls in Southeast Alaskan humpback whales (Megaptera novaeangliae). J. Acoust. Soc Am. 137, 1–10. doi: 10.1121/1.4904504
Freitas C., Kovacs K. M., Ims R. A., Fedak M. A., Lydersen C. (2009). Deep into the ice: over-wintering and habitat selection in male Atlantic walruses. Mar. Ecol. Prog. Ser. 375, 247–261. doi: 10.3354/meps07725
Frey K., Comiso J. C., Cooper L. W., Gradinger R. R., Grebmeyer J. M., Tremblay J. E., et al. (2017). Arctic ocean primary productivity. Arctic report card update 2016 (Silver Spring: NOAA).
Geyer F., Sagen H., Hope G., Babiker M., Worcester P. F. (2016). Identification and quantification of soundscape components in the Marginal Ice Zone. J. Acoust. Soc Am. 139, 1873–1885. doi: 10.1121/1.4945989
Glowacki O., Deane G. B., Moskalik M. (2018). The intensity, directionality, and statistics of underwater noise from melting icebergs. Geophys. Res. Lett. 45, 4105–4113. doi: 10.1029/2018GL077632
Halliday W. D. (2021). Underwater sound levels in the Arctic: Filling knowledge gaps. Geophys. Res. Lett. 48 (15), e2021GL094607. doi: 10.1029/2021GL094607
Halliday W. D., Insley S. J., Hilliard R. C., de Jong T., Pine M. K. (2017). Potential impacts of shipping noise on marine mammals in the western Canadian Arctic. Mar. pollut. Bull. 123, 73–82. doi: 10.1016/j.marpolbul.2017.09.027
Halliday W. D., Pine M. K., Insley S. J. (2020). Underwater noise and Arctic marine mammals: Review and policy recommendations. Environ. Res. 28, 438–448. doi: 10.1139/er-2019-0033
Halliday W. D., Scharffenberg K., MacPhee S., Hilliard R. C., Mouy X., Whalen D., et al. (2019). Beluga vocalizations decrease in response to vessel traffic in the Mackenzie River Estuary. Arctic 72, 337–346. doi: 10.14430/arctic69294
Hamilton C. D., Lydersen C., Aars J., Biuw M., Boltunov A. N., Born E. W., et al. (2021). Marine mammal hotspots in the Greenland and Barents Seas. Mar. Ecol. Prog. Ser. 659, 3–28. doi: 10.3354/meps13584
Haug T., Nilssen K. T., Øien N., Potelov V. (1994). Seasonal distribution of harp seals (Phoca groenlandica) in the Barents Sea. Polar Res. 13, 163–172. doi: 10.1111/j.1751-8369.1994.tb00446.x
Heide-Jørgensen M. P., Blackwell S. B., Tervo O. M., Samson A. L., Garde E., Hansen R. G., et al. (2021). Behavioral response study on seismic airgun and vessel exposures in narwhals. Front. Mar. Sci. 665. doi: 10.3389/fmars.2021.658173
Heide-Jørgensen M. P., Blackwell S. B., Williams T. M., Sinding M. H. S., Skovrind M., Tervo O. M., et al. (2020). Some like it cold: Temperature-dependent habitat selection by narwhals. Ecol. Evol. 10, 8073–8090. doi: 10.1002/ece3.6464
Heide-Jørgensen M. P., Hansen R. G., Westdal K., Reeves R. R., Mosbech A. (2013). Narwhals and seismic exploration: Is seismic noise increasing the risk of ice entrapments? Biol. Conserv. 158, 50–54. doi: 10.1016/j.biocon.2012.08.00
Hildebrand J. A. (2009). Anthropogenic and natural sources of ambient noise in the ocean. Mar. Ecol. Prog. Ser. 395, 5–20. doi: 10.3354/meps08353
Hobbs R. C., Reeves R. R., Prewitt J. S., Desportes G., Breton-Honeyman K., Christensen T., et al. (2019). Global review of the conservation status of monodontid stocks. Mar. Fish. Rev. 81 (3-4), 1–62. doi: 10.7755/MFR.81.3–4.1
Hop H., Wiencke C. (2019). “The ecosystem of kongsfjorden, svalbard,” in The ecosystem of kongsfjorden, svalbard. Eds. Hop H., Wiencke C. (Cham: Springer International Publishing), 1–20.
Huntington H. P., Danielson S. L., Wiese F. K., Baker M., Boveng P., Citta J. J., et al. (2020). Evidence suggests potential transformation of the Pacific Arctic ecosystem is underway. Nat. Clim. Change 10, 342–348. doi: 10.1038/s41558-020-0695-2
Hutchinson C. E. (1957). Concluding remarks, coldspring harbor symposium. Quant. Biol. 22, 415–427. doi: 10.1101/SQB.1957.022.01.039
Insley S. J., Halliday W. D., de Jong T. (2017). Seasonal patterns in ocean ambient noise near sachs harbour, northwest territories. Arctic 70, 239–248. doi: 10.14430/arctic4662
Isaksen K., Nordli Ø., Ivanov B., Køltzow M. A., Aaboe S., Gjelten H. M., et al. (2022). Exceptional warming over the Barents area. Sci. Rep. 12 (1), 9371. doi: 10.1038/s41598-022-13568-5
Ivanov V. V., Alexeev V. A., Repina I., Koldunov N. V., Smirnov A. (2012). Tracing atlantic water signature in the arctic sea ice cover east of svalbard. Adv. Meteorol., 2012. doi: 10.1155/2012/201818
Jaquet N., Dawson S., Douglas L. (2001). Vocal behavior of male sperm whales: Why do they click? J. Acoust. Soc Am. 109, 2254–2259. doi: 10.1121/1.1360718
Jensen S. K., Aars J., Lydersen C., Kovacs K. M., Åsbakk K. (2010). The prevalence of Toxoplasma gondii in polar bears and their marine mammal prey: evidence for a marine transmission pathway? Polar Biol. 33, 599–606. doi: 10.1007/s00300-009-0735-x
Kovacs K. M., Aars J., Lydersen C. (2014). Walruses recovering after 60+ years of protection in Svalbard, Norway. Polar Res. 33, 26034. doi: 10.3402/polar.v33.26034
Kovacs K. M., Lydersen C., Overland J. E., Moore S. E. (2011). Impacts of changing sea-ice conditions on Arctic marine mammals. Mar. Biodiv. 41, 181–194. doi: 10.1007/s12526-010-0061-0
Kovacs K. M., Lydersen C., Vacquiè-Garcia J., Shpak O., Glazov D., Heide-Jørgensen M. P. (2020). The endangered Spitsbergen bowhead whales’ secrets revealed after hundreds of years in hiding. Biol. Lett. 16, 20200148. doi: 10.1098/rsbl.2020.0148
Krause B. L. (1993). The niche hypothesis: a virtual symphony of animal sounds, the origins of musical expression and the health of habitats. Soundscape Newslett. 6, 6–10.
Laidre K. L., Stern H., Kovacs K. M., Lowry L., Moore S. E., Regehr E. V., et al. (2015). Arctic marine mammal population status, sea ice habitat loss, and conservation recommendations for the 21st century. Conserv. Biol. 29, 724–737. doi: 10.1111/cobi.12474
Llobet S. M., Ahonen H., Lydersen C., Berge J., Ims R., Kovacs K. M. (2021). Bearded seal (Erignathus barbatus) vocalizations across seasons and habitat types in Svalbard, Norway. Polar Biol. 44, 1273–1287. doi: 10.1111/cobi.12474
Louis M., Skovrind M., Samaniego Castruita J. A., Garilao C., Kaschner K., Gopalakrishnan S., et al. (2020). Influence of past climate change on phylogeography and demographic history of narwhals, Monodon monoceros. Proc. R. Soc B 287, 20192964. doi: 10.1098/rspb.2019.2964
Lowther A. D., Kovacs K. M., Griffiths D., Lydersen C. (2015). Identification of motivational state in adult male Atlantic walruses inferred from changes in movement and diving behavior. Mar. Mamm. Sci. 31, 1291–1313. doi: 10.1111/mms.12224
Lundesgaard Ø., Sundfjord A., Lind S., Nilsen F., Renner A. H. (2022). Import of Atlantic Water and sea ice controls the ocean environment in the northern Barents Sea. Ocean Sci. 18 (5), 1389–1418. doi: 10.21334/npolar.2022.1a68b156
Lundesgaard Ø., Sundfjord A., Renner A. H. (2021). Drivers of interannual sea ice concentration variability in the Atlantic water inflow region north of Svalbard. J. Geophys. Res.: Oceans 126 (4). doi: 10.1029/2020JC016522
Lydersen C., Aars J., Kovacs K. M. (2008). Estimating the number of walruses in Svalbard from aerial surveys and behavioural bata from satellite telemetry. Arctic 61, 119–128.
Lydersen C., Martin A. R., Gjertz I., Kovacs K. M. (2007). Satellite tracking and diving behaviour of sub-adult narwhals (Monodon monoceros) in Svalbard, Norway. Polar Biol. 30, 437–442. doi: 10.1007/s00300-006-0200-z
Lydersen C., Vacquié-Garcia J., Heide-Jørgensen M. P., Øien N., Guinet C., Kovacs K. M. (2020). Autumn movements of fin whales (Balaenoptera physalus) from Svalbard, Norway, revealed by satellite tracking. Sci. Rep. 10, 1–13. doi: 10.1038/s41598-020-73996-z
MacIntyre K. Q., Stafford K. M., Berchok C. L., Boveng P. L. (2013). Year-round acoustic detection of bearded seals (Erignathus barbatus) in the Beaufort Sea relative to changing environmental conditions 2008-2010. Polar Biol. 36, 1161–1173. doi: 10.1007/s00300-013-1337-1
Madan M. M., Latha G., Ashokan M., Raguraman G., Thirunavukkarasu A. (2020). Identification of soundscape components and their temporal patterns in Kongsfjorden, Svalbard Archipelago. Polar Sci. 26, 100604. doi: 10.1016/j.polar.2020.100604
Madsen P. T. (2005). Marine mammals and noise: Problems with root mean square sound pressure levels for transients. J. Acoust. Soc Am. 117, 3952–3957. doi: 10.1121/1.1921508
Mahanty M. M., Latha G., Venkatesan R., Ravichandran M., Atmanand M. A., Thirunavukarasu A., et al. (2020). Underwater sound to probe sea ice melting in the Arctic during winter. Sci. Rep. 10, 1–7. doi: 10.1038/s41598-020-72917-4
Martin M. J., Halliday W. D., Storrie L., Citta J. J., Dawson J., Hussey N. E., et al. (2022). Exposure and behavioral responses of tagged beluga whales (Delphinapterus leucas) to ships in the Pacific Arctic. Mar. Mamm. Sci. 39 (2), 387–421. doi: 10.1111/mms.12978
Mellinger D. K., Clark C. W. (2000). Recognizing transient low-frequency whale sounds by spectrogram correlation. J. Acoust. Soc Am. 107, 3518–3529. doi: 10.1121/1.429434
Merchant N. D., Barton T. R., Thompson P. M., Pirotta E., Dakin D. T., Dorocicz J. (2013). Spectral probability density as a tool for ambient noise analysis. J. Acoust. Soc Am. 133, EL262–EL267. doi: 10.1121/1.4794934
Merchant N. D., Brookes K. L., Faulkner R. C., Bicknell A. W. J., Godley B. J., Witt M. J. (2016). Underwater noise levels in UK waters. Sci. Rep. 6, 36942. doi: 10.1038/srep36942
Merchant N. D., Fristrup K. M., Johnson M. P., Tyack P. L., Witt M. J., Blondel P., et al. (2015). Measuring acoustic habitats. Meth. Ecol. Evol. 6, 257–265. doi: 10.1111/2041-210X.12330
Merchant N. D., Pirotta E., Barton T. R., Thompson P. M. (2014). Monitoring ship noise to assess the impact of coastal developments on marine mammals. Mar. pollut. Bull. 78, 85–95. doi: 10.1016/j.marpolbul.2013.10.058
Muckenhuber S., Nilsen F., Korosov A., Sandven S. (2016). Sea ice cover in Isfjorden and Hornsund, Svalbard, (2000–2014) from remote sensing data. Cryosphere 10, 149–158. doi: 10.5194/tc-10-149-2016
Nieukirk S. L., Mellinger D. K., Dziak R. P., Matsumoto H., Klinck H. (2020). Multi-year occurrence of sei whale calls in North Atlantic polar waters. J. Acoust. Soc Am. 147 (3), 1842–1850. doi: 10.1121/10.0000931
Nordøy E. S., Folkow L. P., Potelov V., Prischemikhin V., Blix A. S. (2008). Seasonal distribution and dive behaviour of harp seals (Pagophilus groenlandicus) of the White Sea–Barents Sea stock. Polar Biol. 31 1119–1135. doi: 10.1007/s00300-008-0453-9
Nowacek D. P., Clark C. W., Mann D., Miller P. J., Ronsenbaum H. C., Golden J. S., et al. (2015). Marine seismic surveys and ocean noise: time for coordinated and prudent planning. Front. Ecol. Environ. 13, 378–386. doi: 10.1890/130286
Oliveira C., Wahlberg M., Johnson M., Miller P. J., Madsen P. T. (2013). The function of male sperm whale slow clicks in a high latitude habitat: Communication, echolocation, or prey debilitation? J. Acoust. Soc Am. 133 (5), 3135–3144. doi: 10.1121/1.4795798
PAME (2019). Underwater noise in the Arctic: A state of knowledge report, Rovaniemi, May 2019 (Akureyri: Protection of the Arctic Marine Environment (PAME) Secretariat).
PAME (2021). Underwater noise pollution from shipping in the arctic, may 2021 (Akureyri: Protection of the Arctic Marine Environment (PAME) Secretariat).
Papale E., Pelagetti M., Pedrazzi G., Buscaino G. (2023). Occurrence and patterns of fin whale songs reveal alternative migration strategies in Svalbard Islands, Norway. Sci. Rep. 13, 4436. doi: 10.1038/s41598-023-31665-x
Parisi I., de Vincenzi G., Torri M., Papale E., Mazzola S., Bonanno A., et al. (2017). Underwater vocal complexity of Arctic seal Erignathus barbatus in Kongsfjorden (Svalbard). J. Acoust. Soc Am. 142, 3104–3115. doi: 10.1121/1.5010887
Pavlova O., Gerland S., Hop H. (2019). “Changes in sea-ice extent and thickness in Kongsfjorden, Svalbard, (2003–2016),” in The ecosystem of Kongsfjorden, Svalbard. Eds. Hop H., Wiencke C. (Springer, Cham), vol. 2, 105–136. doi: 10.1007/978-3-319-46425-1_4
Petit C., Nystuen J., O’Neel S. (2012). Listening to glaciers: Passive hydroacoustics near marine-terminating glaciers. Oceanography (Wash D C) 25, 104–105. doi: 10.5670/oceanog.2012.81
Pijanowski B. C., Villanueva-Rivera L. J., Dumyahn S. L., Farina A., Krause B. L., Napoletano B. M., et al. (2011). Soundscape ecology: The science of sound in the landscape. Bioscience 61, 203–216. doi: 10.1525/bio.2011.61.3.6
Polyakov I. V., Alkire M. B., Bluhm B. A., Brown K. A., Carmack E. C., Chierici M., et al. (2020). Borealization of the Arctic Ocean in response to anomalous advection from sub-Arctic seas. Front. Mar. Sci. 7. doi: 10.3389/fmars.2020.00491
Polyakov I. V., Pnyushkov A. V., Alkire M. B., Ashik I. M., Baumann T. M., Carmack E. C., et al. (2017). Greater role for Atlantic inflows on sea-ice loss in the Eurasian Basin of the Arctic Ocean. Science 356, 285–291. doi: 10.1111/j.1365-2907.2011.00195.x
Prieto R., Janiger D., Silva M. A., Waring G. T., Gonçalves J. M. (2012). The forgotten whale: a bibliometric analysis and literature review of the North Atlantic sei whale Balaenoptera borealis. Mammal Rev. 42 (3), 235. doi: 10.1111/j.1365-2907.2011.00195.x
Prieto R., Silva M. A., Waring G. T., Gonçalves J. M. A. (2014). Sei whale movements and behaviour in the North Atlantic inferred from satellite telemetry. Endanger. Species Res. 26, 103–113. doi: 10.3354/esr00630
Reeves R. R., Ewins P. J., Agbayani S., Heide-Jørgensen M. P., Kovacs K. M., Lydersen C., et al. (2014). Distribution of endemic cetaceans in relation to hydrocarbon development and commercial shipping in a warming Arctic. Mar. Policy 44, 375–389. doi: 10.1016/j.marpol.2013.10.005
Renner A. H. H., Sundfjord A., Janout M. A., Ingvaldsen R. B., Beszczynska-Möller A., Pickart R. S., et al. (2018). Variability and redistribution of heat in the Atlantic Water boundary current north of Svalbard. J. Geophys. Res.: Oceans 123 (9), 6373–6391. doi: 10.1029/2018JC013814
Richard G., Mathias D., Collin J., Chauvaud L., Bonnel J. (2023). Three-dimensional anthropogenic underwater noise modeling in an Arctic fjord for acoustic risk assessment. Mar. pollut. Bull. 187, 114487. doi: 10.1016/j.marpolbul.2022.114487
Risch D., Clark C. W., Corkeron P. J., Elepfandt A., Kovacs K. M., Lydersen C., et al. (2007). Vocalizations of male bearded seals, Erignathus barbatus: classification and geographical variation. Anim. Behav. 73, 747–762. doi: 10.1016/j.anbehav.2006.06.012
Romagosa M., Baumgartner M., Cascão I., Lammers M. O., Marques T. A., Santos R. S., et al. (2020). Baleen whale acoustic presence and behaviour at a Mid-Atlantic migratory habitat, the Azores Archipelago. Sci. Rep. 10 (1), 4766. doi: 10.1038/s41598-020-61849-8
Ryan J. P., Joseph J. E., Margolina T., Hatch L. T., Azzara A., Reyes A., et al. (2021). Reduction of low-frequency vessel noise in monterey bay national marine sanctuary during the COVID-19 pandemic. Front. Mar. Sci. 8. doi: 10.3389/fmars.2021.656566
Sanjana M. C., Latha G., Raguraman G. (2021). Anthropogenic sound field and noise mapping in an Arctic fjord during summer. Mar. pollut. Bull. 173, 113035. doi: 10.1016/j.marpolbul.2021.113035
Sanjana M. C., Latha G., Thirunavukkarasu A., Venkatesan R. (2018). Ambient noise field and propagation in an Arctic fjord Kongsfjorden, Svalbard. Polar Sci. 17, 40–19. doi: 10.1080/09524622.2008.9753846
Southall B. L., Ellison W. T., Finneran J. J., Gentry R. L., Greene Jr. C. R., et al. (2008). Marine mammal noise-exposure criteria: initial scientific recommendations. Bioacoustics 17 (1–3), 273–275. doi: 10.1080/09524622.2008.9753846
Stafford K. M. (2021). The changing arctic marine soundscape. Arctic report card: update for 2021 (United states: National Oceanic and Atmospheric Administration. Office of oceanic and atmospheric research. Global ocean monitoring and observing (GOMO) program). doi: 10.25923/jagc-4a84
Stafford K. M. (2022). “Singing behavior in the bowhead whale,” in ethology and behavioral ecology of marine mammals book series (EBEMM) Ed. Clark C. W., Garland E. C. (Springer, Cham), 277–295.
Stafford K. M., Lydersen C., Wiig Ø., Kovacs K. M. (2018). Extreme diversity in the songs of Spitsbergen’s bowhead whales. Biol. Lett. 14, 20180056. doi: 10.1098/rsbl.2018.0056
Stafford K. M., Moore S. E., Berchok C. L., Wiig Ø., Lydersen C., Hansen E., et al. (2012). Spitsbergen’s endangered bowhead whales sing through the polar night. Endang. Species Res. 18, 95–103. doi: 10.3354/esr00444
Stirling I., Calvert W., Spencer C. (1987). Evidence of stereotyped underwater vocalizations of male Atlantic walruses (Odobenus rosmarus rosmarus). Can. J. Zool. 65, 2311–2321. doi: 10.1139/z87-348
Storrie L., Lydersen C., Andersen M., Wynn R. B., Kovacs K. M. (2018). Determining the species assemblage and habitat use of cetaceans in the Sva lbard Archipelago, based on observations from 2002 to 2014. Polar Res. 37, 1463065. doi: 10.1080/17518369.2018.1463065
Stroeve J., Notz D. (2018). Changing state of Arctic sea ice across all seasons. Environ. Res. Lett. 13, 103001. doi: 10.1088/1748-9326/aade56
Sueur J., Farina A. (2015). Ecoacoustics: the ecological investigation and interpretation of environmental sound. Biosemiotics 8, 493–502. doi: 10.1007/s12304-015-9248-x
Tęgowski J., Deane G., Lisimenka A., Blondel P. (2011). “Detecting and analyzing underwater ambient noise of glaciers on Svalbard as indicator of dynamic processes in the Arctic,” in Proceedings of the 4th UAM Conference, Kos, Greece. Eds. Papadakis J. S., Jørnø L. B. 1149–1154.
Terhune J. M. (1994). Geographical variation of harp seal underwater vocalizations. Can. J. Zool. 72, 892–897. doi: 10.1139/z94-121
Tervo O. M., Blackwell S. B., Ditlevsen S., Conrad A. S., Samson A. L., Garde E., et al. (2021). Narwhals react to ship noise and airgun pulses embedded in background noise. Biol. Lett. 17, 20210220. doi: 10.1098/rsbl.2021.0220
Tervo O. M., Christoffersen M. F., Simon M., Miller L. A., Jensen F. H., Parks S. E., et al. (2012). High source levels and small active space of high-pitched song in bowhead whales (Balaena mysticetus). PloS One 7, e52072. doi: 10.1371/journal.pone.0052072
Tomisch K., Hiemer K., Boebel O., Burkhardt E., Spiesecke S., van Opzeeland I. (2022). Acoustic presence and vocal repertoire of bowhead whales (Balaena mysticetus) in eastern and central Fram Strait. Front. Remote Sens. 83. doi: 10.3389/frsen.2022.907105
Tverberg V., Skogseth R., Cottier F., Sundfjord A., Walczowski W., Inall M. E., et al. (2019). “The Kongsfjorden transect: seasonal and inter-annual variability in hydrography,” in The ecosystem of kongsfjorden (Svalbard: Springer), 49–104.
Vacquié-Garcia J., Lydersen C., Marques T. A., Aars J., Ahonen H., Skern-Mauritzen M., et al. (2017). Late summer distribution and abundance of ice-associated whales in the Norwegian High Arctic. Endang. Species Res. 32, 59–70. doi: 10.3354/esr00791
Vacquié-Garcia J., Lydersen C., Marques T. A., Andersen M., Kovacs K. M. (2020). First abundance estimate for white whales Delphinapterus leucas in Svalbard, Norway. Endang. Species Res. 41, 253–263. doi: 10.3354/esr01016
Van Parijs S. M., Kovacs K. M., Lydersen C. (2001). Spatial and temporal distribution of vocalising male bearded seals: implications for male mating strategies. Behaviour 138, 905–922. doi: 10.1163/156853901753172719
Van Wormer E., Mazet J. A. K., Hall A., Gill V. A., Boveng P. L., London J. M., et al. (2019). Viral emergence in marine mammals in the North Pacific may be linked to Arctic sea ice reduction. Sci. Rep. 9, 1–11. doi: 10.1038/s41598-019-51699
Videsen S. K. A., Simon M., Johnson M., Madsen P. T., Christiansen F. (2021). Cryptic vocal behavior of foraging humpback whales on feeding grounds in West Greenland. J. Acoust. Soc Am. 150, 2879–2887. doi: 10.1121/10.0006735
Williams T. M., Blackwell S. B., Tervo O., Garde E., Sinding M. H. S., Ritcher B., et al. (2022). Physiological responses of narwhals to anthropogenic noise: A case study with seismic airguns and vessel traffic in the Arctic. Funct. Ecol. 36, 2251–2266. doi: 10.1111/1365-2435.14119
Keywords: antropophonies, arctic endemic species, atlantic arctic, biophonies, borealization, geophonies, ocean noise, ship traffic
Citation: Llobet SM, Ahonen H, Lydersen C and Kovacs KM (2023) The Arctic and the future Arctic? Soundscapes and marine mammal communities on the east and west sides of Svalbard characterized through acoustic data. Front. Mar. Sci. 10:1208049. doi: 10.3389/fmars.2023.1208049
Received: 18 April 2023; Accepted: 13 October 2023;
Published: 26 October 2023.
Edited by:
Philippe Blondel, University of Bath, United KingdomReviewed by:
Jaroslaw Tegowski, University of Gdansk, PolandIlse Catharina Van Opzeeland, Alfred Wegener Institute Helmholtz Centre for Polar and Marine Research (AWI), Germany
Copyright © 2023 Llobet, Ahonen, Lydersen and Kovacs. This is an open-access article distributed under the terms of the Creative Commons Attribution License (CC BY). The use, distribution or reproduction in other forums is permitted, provided the original author(s) and the copyright owner(s) are credited and that the original publication in this journal is cited, in accordance with accepted academic practice. No use, distribution or reproduction is permitted which does not comply with these terms.
*Correspondence: Heidi Ahonen, Heidi.Ahonen@npolar.no