- 1Programa de Pós-graduação em Ecologia e Conservação da Biodiversidade, Universidade Estadual de Santa Cruz (UESC), Ilhéus, Bahia, Brazil
- 2Laboratório de Etnoconservação e Áreas Protegidas (LECAP), Universidade Estadual de Santa Cruz (UESC), Ilhéus, Bahia, Brazil
- 3Laboratório de Aves Aquáticas e Tartarugas Marinhas, Instituto de Ciências Biológicas, Universidade Federal do Rio Grande (FURG), Rio Grande, Rio Grande do Sul, Brazil
- 4Instituto de Ciências Biológicas, Universidade Federal do Rio Grande (FURG), Rio Grande, Rio Grande do Sul, Brazil
- 5Programa de Pós-Graduação em Sistemas Aquáticos Tropicais, Universidade Estadual de Santa Cruz (UESC), Ilhéus, Bahia, Brazil
- 6Departamento de Ciências Agrárias e Ambientais, Universidade Estadual de Santa Cruz (UESC), Ilhéus, Bahia, Brazil
- 7Investigador Associado CESIMAR/CENPAT, Puerto Madryn, Chubut, Argentina
Stable isotope analysis is an approach that allows inferring feeding ecology and trophic structure of populations, especially for species that are difficult to observe in natural habitats, such as marine turtles. This study investigated the niche breadth of adult and juvenile green sea turtles (Chelonia mydas) through δ15N and δ13C values in carapace scutes. During 2017 and 2018, tissues were collected from juveniles in a feeding area in southeastern Brazil (n = 60) and from adults in a nesting area in northeastern Brazil, at Fernando de Noronha (n = 21). Our results revealed no temporal variations in the isotopic values of adult green turtles and the mean δ15N values are compatible with the isotopic values of primarily herbivorous animals. In southeastern Brazil, the δ13C values and isotopic niche breadth differences observed in juvenile green turtles suggest that variations in habitat (i.e., channel between the municipalities of Ilhabela and São Sebastião - “SSC” and region comprising the other two municipalities of Ubatuba and Caraguatatuba - “CAU”, as defined in this study based on oceanographic current dynamics) can influence their isotopic niche. The isotopic values observed in juvenile green turtles demonstrated a high degree of feeding behavior plasticity and individual variability, which were possibly influenced by the dynamic of oceanographic currents and seasonal nutrient inputs. These findings help to elucidate the feeding behavior of green turtles at different life stages and the potential influence of oceanographic current dynamics in key feeding sites for juvenile green turtles.
Introduction
Understanding the feeding ecology of species is critical for their conservation since food acquisition is directly related to survival, growth rate, and reproductive success (Bjorndal, 1985; Balazs, 1995). The life history of the green sea turtle (Chelonia mydas) is an iconic example of ontogenetic change in diet and habitat use, as these animals exhibit an oceanic-neritic developmental pattern (Bolten, 2003). Green turtles have an omnivorous diet (oceanic phase) during the first few years after hatching, switching to a mainly herbivorous diet when they are 3–6 years old to finally be recruited into neritic habitats (Bjorndal, 1985; Bjorndal, 1997; Seminoff et al., 2002). However, recent studies have revealed regional differences in the duration of this process (Cardona et al., 2010; Vélez-Rubio et al., 2016), with high levels of omnivory after recruitment (Cardona et al., 2009; González-Carman et al., 2013; Vélez-Rubio et al., 2016), including a persistent carnivorous diet in some individuals and populations during the adult phase (e.g. Hatase et al., 2006). Furthermore, Esteban et al. (2020) and Quiñones et al. (2022) identified that sea surface temperature may also have a high potential to influence omnivorous behavior.
Stomach content and stable isotope analyses are commonly used to evaluate the diet of animals. Stable isotope analysis, in animals sampled over a period of time or through the analysis of tissues that allow a temporal analysis, provides valuable insights into the isotopic niche of a species and its potential variations, since individuals from the same population do not necessarily have consistent or homogenous foraging strategies over time, changing the consumed food resources (Bearhop et al., 2004; Martínez del Rio et al., 2009). The isotopic niche has been represented by data plotted in multivariate space (δ15N versus δ13C), which is comparable to the “n-dimensional” space that contains what ecologists refer to as the niche, because an animal’s chemical composition is directly influenced by what it consumes (bionomic axes) as well as the habitat in which it lives (scenopoetic axes) (Newsome et al., 2007).
Nitrogen stable isotope values (δ15N) change during metabolism, as 15N is preferentially retained (in detriment of 14N) in animal body during excretion, causing the δ15N values in animal tissues generally being 3 to 5‰ higher in comparison to its food source, which makes this isotope useful to determine trophic level (DeNiro and Epstein, 1981; Minagawa and Wada, 1984). Carbon isotopic ratios (expressed as δ13C) are enriched in coastal benthic habitats, compared to oceanic pelagic environments (DeNiro and Epstein, 1978), thus, they provide an indication of the animal’s habitat. The δ13C values are not as useful as δ15N values to assess the trophic level, as it is typically only ~1‰ higher in comparison to food sources. These stable isotopes have been used to examine the diet of sea turtles through different life stages (Reich et al., 2007) as well as the use of feeding areas in several populations worldwide (e.g. Hatase et al., 2002; Hatase et al., 2006; Zbinden et al., 2011; Ceriani et al., 2012; Eder et al., 2012; Pajuelo et al., 2012; Seminoff et al., 2012; Tucker et al., 2014; Vander-Zanden et al., 2014).
However, it is also necessary to consider that different environments may present isotopically distinct baseline organisms (Michener and Kaufman, 2007). If very different values of δ15N are found in different regions for primary producers, consumers will also reflect these different values between these regions, since they are enriched in 15N in relation to their diet (Minagawa and Wada, 1984). Furthermore, sources of nitrogen reaching the sea can alter the δ15N baseline values of marine biota, due to land runoff, atmospheric deposition, NO3- and N2 (Minagawa and Wada, 1984; Sánchez et al., 2020). Variations in δ13C values are caused by temperature, carbon dioxide concentration in surface water and the differences in plankton synthesis or metabolism (Rubenstein and Hobson, 2004). The δ13C values of primary producers vary between ocean basins, higher offshore and decreasing towards the coast (Clementz and Koch, 2001). In addition, pelagic environments, which have phytoplankton as the base of the food chain, are more 13C depleted than neritic regions (Ceriani et al., 2012).
Troina et al. (2020) characterized the stable carbon and nitrogen isotope composition patterns at the shelf break and continental slope in the subtropical western South Atlantic Ocean. In the southeast region of Brazil, they observed an increase in δ13C values from the shelf break towards the offshore areas, which was attributed to the influence of continental water input and upwelling events along the shelf break. Conversely, δ15N values exhibited an opposite trend, with higher values observed along the shelf break due to the intrusion of upwelled deep-water nutrients. Additionally, the study revealed a latitudinal decrease in δ13C values in the offshore region from north to south, while an increase in δ15N values was observed towards the south latitudes.
In Brazil, herbivory had been described as the main dietary pattern of the nesting female green turtles in coastal-benthic environments in Atol das Rocas (03°51’S; 33°49’W; Agostinho et al., 2021), in the tropical northeastern area. Also in Atol das Rocas, Filippos et al. (2021) found high plasticity among individuals, both in the use of habitat and food resources. Campos and Cardona (2019) found that juvenile neritic green turtles from Praia do Forte (12°38’S; 38°05’W - Bahia state, Brazil) generally had herbivorous diets showing few temporal changes in diet or habitat switch, while most juvenile green turtles sampled in Ubatuba (23°26’S; 45°05’W - São Paulo state, Brazil) showed omnivorous diets with high levels of individual and temporal variability.
Here we investigated the niche breadth of juvenile and adult green turtles in feeding and nesting areas in the western South Atlantic Ocean. Fernando de Noronha, the nesting area, is an archipelago and covers a total area of 26 km2, 70% of which is encompassed by the Marine National Park of Fernando de Noronha – PARNAMAR. The remaining area comprises the Environmental Protection Area (APA - Fernando de Noronha – Atol das Rocas - São Pedro e São Paulo) (Serafini et al., 2010). The archipelago is one of the main nesting areas of the green turtle in Brazil (Bellini, 1996). No studies specifically describing the feeding behavior of adult green turtles in the region were found. However, Gitirana and Souza (2012) reported a higher occurrence of subadult green turtles exhibiting feeding behavior on a beach in the region. This particular beach is characterized by high abundance of marine flora, including various species of algae and extensive seagrass beds. The north coast of the state of São Paulo, the feeding area, is described as an important feeding area for the juvenile green turtles (e.g., Gallo et al., 2006; Romanini, 2014; Fernandes et al., 2017; Silva et al., 2017), and the most frequently consumed items are primarily marine algae from the Phyla Rodophyta and Chlorophyta. They also consume animal matter, with a significant contribution from bryozoans, jellyfish, and filament structures of bivalves (byssus) (Romanini, 2014). Mixed-stock analyzes of the southwestern Atlantic juvenile green turtle population identified their origin as being represented in large percentage by the Ascension Island, with lesser contributions from Surinam/Aves and Trindade (Naro-Maciel et al., 2007; Coelho et al., 2018). Because this area is located at the tropic–temperate convergence zone (25°S), it is considered a strategic geographical position for acclimating recruited C. mydas (at between 2 and 8 years old) (Coelho et al., 2018).
In sea turtles, the carapace scute tissues are continuously produced; hence, as the turtle grows and the bony shell increases in area, scute accumulates and becomes thicker over the older areas, while areas of recent growth expansion are covered only by thin, young scute tissue. Once produced, scute is inert (keratin) and, although it is susceptible to wear, retains a history of diet and habitat (Reich et al., 2007). According to previous studies (Reich et al., 2007; Cardona et al., 2010; Vander-Zanden et al., 2013b), each 30 µm thick layer integrates approximately 54 days, although the associated variance is unknown and hence should be considered as a coarse estimate only (Campos and Cardona, 2019). Thus, the whole carapace tissue profile could integrates from 0.8 years in juveniles to 6.5 years in adults (Vander-Zanden et al., 2013b), with anterior portions of each scute representing a more recent assimilation time window, while posterior areas are oldest (Reich et al., 2007).
Changes in foraging strategies caused by local and regional processes can increase anthropogenic threats to turtles according to habitat use (Santos et al., 2015). This research investigated the niche breadth of juvenile and adult green turtles in feeding and nesting areas in the western South Atlantic Ocean, through stable isotope analysis of carbon and nitrogen. For the adult turtles we further assessed temporal feeding patterns in isotopic niches, by analyzing two regions of the same scute. Likewise, we investigated the potential influence of two distinct habitat types, characterized by dynamics of oceanographic currents, on the isotopic niche breadth of juvenile green turtles. We hypothesize that the primary productivity generated due to the dynamics of the water masses contributes to the availability of different trophic resources for juvenile green turtles feeding in the region.
Materials and methods
Study sites
Fernando de Noronha (03°50’S; 32°4’W) (Figure 1) is a small volcanic archipelago composed by 21 islands and islets in the equatorial South Atlantic Ocean, located in the state of Pernambuco, in northeast Brazil, and is situated 345 km from the nearest continental coast (Cabo de São Roque, Rio Grande do Norte state) (Serafini et al., 2010).
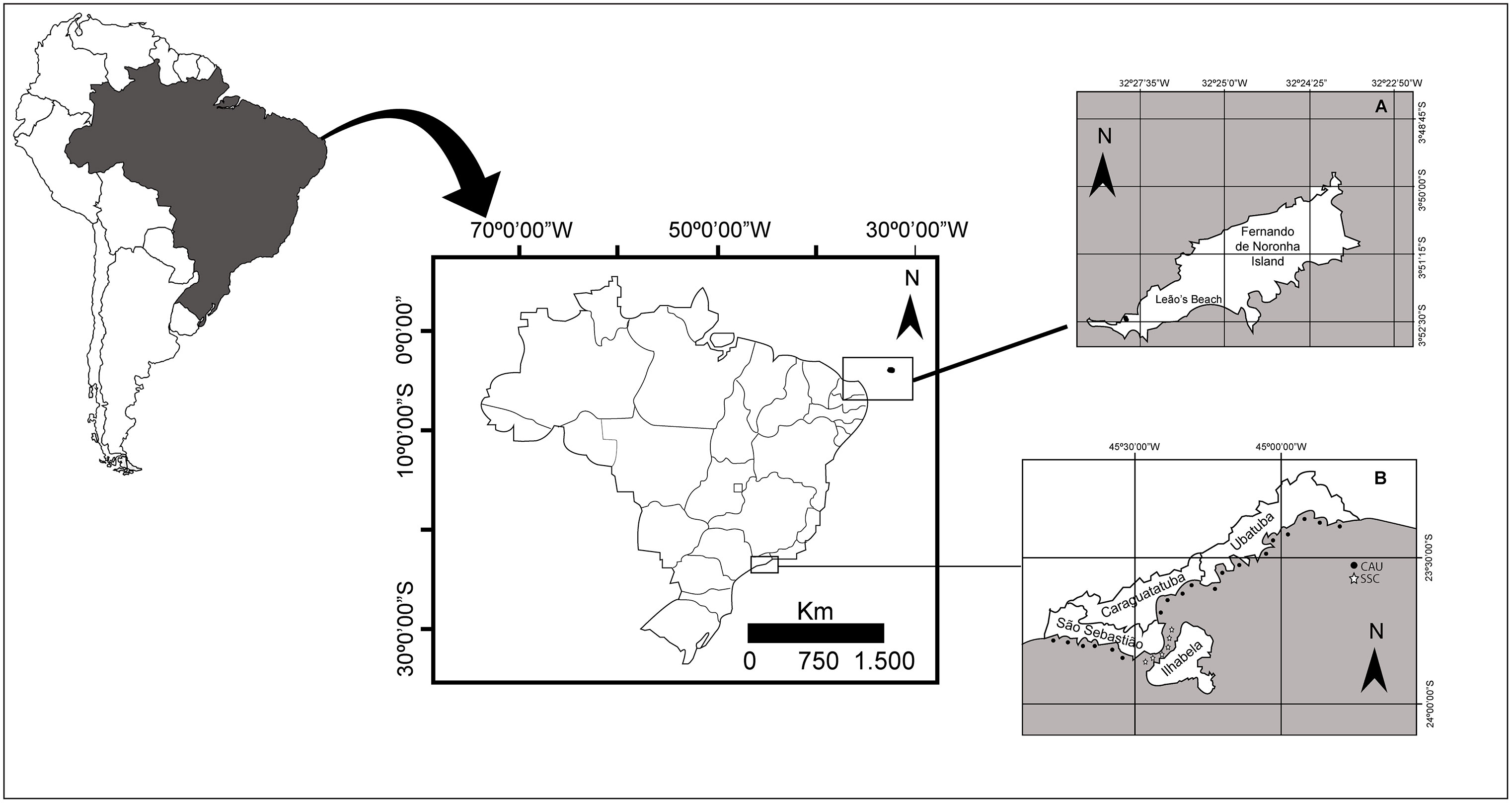
Figure 1 Map of the study regions. (A) Fernando de Noronha island, in northeast Brazil; and (B) North coast of the state of São Paulo, in southeast Brazil.
The north coast of the state of São Paulo (23°45’S; 45°24’W) (Figure 1), is situated in the central region of the southeastern Brazilian continental shelf. It encompasses three main water bodies: Coastal Water (CW), South Atlantic Central Water (SACW), and Tropical Water (TW) (Supplementary Table S1). CW and TW are surface waters characterized by oligotrophic conditions (Aidar and Mesquita, 1993). These waters receive seasonal enrichment from SACW, which brings nutrients from the ocean floor, resulting in increased phytoplankton biomass and primary production rates within the mixed layer (Castro-Filho et al., 1987). During the summer, nutrient-rich SACW moves closer to the coast, typically occupying the central and outer regions of the continental shelf (20–100 m), while CW is found in a narrow coastal strip. In winter, when SACW is restricted outside the continental shelf, thermal gradients become reduced and stratification diminishes (Castro-Filho, 1990).
The São Sebastião Channel (SSC; 23°41’–23°53.5’S, 45°19’–45°30’W), which runs parallel to the coast, separates the mainland from the island of São Sebastião (the main island of the Ilhabela archipelago). It acts as a barrier to the adjacent coast of Caraguatatuba and Ubatuba, hereafter referred to as ‘CAU’, and functions as a wind tunnel, affecting marine currents, and sedimentation patterns (Castro-Filho, 1990). The SSC has a length of 24 km and is 5.8 km wide at its north entrance and 6.4 km wide at its south entrance. It covers a total area of 150 km2 and has an average depth of 28 m at the north entrance and 20 m at the south entrance (Furtado, 1995). The sea currents in the SSC mainly flow towards the northeast, except during the summer when a two-layer flow in the opposite direction settles, with deep flow moving towards the northeast. The bottom current is influenced by the intrusion of SACW, while CW predominates for the rest of the year (Castro-Filho et al., 1987). These water movements lead to vertical stratification, characterized by a strong thermocline at a intermediate depth (Castro-Filho et al., 1987). Additionally, these dynamics contribute to the individual biodiversity of each water body, adding complexity to the overall food chain in the SSC (Oliveira and Marques, 2007). We hypothesize that these factors potentially contribute to the availability of different food resources for turtles in these two habitats, both spatially and seasonally.
Due to these oceanographic characteristics, the study region of São Paulo coast was divided into the following two large areas: the ‘SSC’ region, between the municipalities of Ilhabela and São Sebastião, and the ‘CAU’ region, comprising the other two municipalities of Ubatuba and Caraguatatuba. This division was necessary to distinguish oceanographic characteristics of these two “ecosystems”.
Turtle sampling
Carapace samples were collected at different locations and life stages: juveniles in the north coast of the state of São Paulo, southeastern Brazil, and adults were sampled at Leão’s beach, the main nesting site for green turtles in the archipelago of Fernando de Noronha.
In partnership with Projeto TAMAR (Tartarugas Marinhas - National Marine Turtle Conservation Program), between April 2017 and March 2018, 21 adult female green turtle carapace samples were collected at Fernando de Noronha. To evaluate possible temporal differences in isotopic niche occupation for adult turtles, a 6 mm diameter sample was collected from the posterior (oldest) carapace scute and the anterior (youngest) portion of the second lateral scute (Supplementary Figure S1; adapted from Reich et al., 2007). We used sterile 6-mm biopsy punches to remove carapace samples, encompassing the full depth of the scute from the surface (oldest scute) to the origin (newest scute), and thus integrate a variable period of several years (Reich et al., 2007; Vander-Zanden et al., 2013b). The samples and biometric data were collected during the females’ nesting period, after the first eggs were laid in the nest. Due to logistical difficulties, to preserve the research material it was stored in tubes with NaCl, and subsequently refrigerated (-20°C) until analysis.
For juvenile green sea turtles from southeastern Brazil, the curvilinear carapace length (CCL) was measured with flexible tape and the second lateral carapace scute of dead individuals was collected, stored in a dry place, and subsequently brushed and frozen (-20°C). In total, 60 juvenile green turtle samples were obtained, 30 from each one of the areas – ‘SSC’ and ‘CAU’ (Table 1). Sample size of juvenile green sea turtles was defined a priory, to make the project feasible and large enough to provide sufficient power for the proposed analyses and the study validity. The different preservation methods used do not alter the isotopic composition (Barrow et al., 2008).
Additionally, to analyze the possible effects of water bodies on juvenile green turtles isotopic niches, in partnership with Instituto Argonauta para Conservação Costeira e Marinha (Argonauta Institute for Coastal and Marine Conservation), sampling of green turtles took place during 2017 austral summer and autumn, corresponding to warm months (January to May) and in the austral winter and early spring (June to October), corresponding to cold months.
Laboratory methodology
Turtle carapace samples were washed with distilled water, dried in an oven at 60°C for 24 h, powdered, weighed (0.5 to 0.7 mg), and placed in sterile tin capsules. Samples were analyzed in an isotopic ratio mass spectrometer attached to an elementary analyzer, at the University of New Mexico, Center for Stable Isotopes (UNM-CSI). Internal standards with known isotope values of soy, casein, whey protein, and tuna were analyzed for every 12 samples. The repeatability of standard values based on the standard deviation was 0.08‰ and 0.03‰, for δ13C and δ15N, respectively. The international reference standards for carbon and nitrogen were Vienna Pee Dee Belemnite (VPDB) and atmospheric air, respectively. Values are expressed in δ notation, in parts per thousand (‰), according to Bond and Hobson (2012) equation:
where Rsample and Rstandard are the corresponding ratios of 13C/12C or 15N/14N.
The C:N ratio used as a quality control measure for stable carbon and nitrogen isotope measurements in keratin was considered as values <3.5. This threshold is based on the understanding that lipid concentrations are uniformly low within this range, and therefore, extraction or normalization is unnecessary to prevent any effect on the δ13C values (Post et al., 2007).
Statistical analysis
All data were tested for normality and homoscedasticity using the Shapiro-Wilk and Levene’s tests. Assumptions of normality and homoscedasticity were not violated, so the mean δ15N and δ13C values between juvenile turtle of SSC and the CAU, and between the posterior (oldest) carapace scute and the anterior (youngest) portion of the second lateral scute of adult turtles, were compared using the independent t-test and paired t-test, respectively. The results were considered significant when p< 0.05.
To investigate the isotopic niche breadth of the different groups analyzed in this study, the standard ellipse area corrected for small sample sizes (SEAc) was calculated using the Stable Isotope Bayesian Ellipses (SIBER) package in R (Jackson et al., 2011). The default ellipse was plotted for each group. The overlaps between niches were calculated using the SEAc value (Jackson et al., 2011).
We used generalized linear models (GLM; McCullagh and Nelder, 1989; Zeleis et al., 2008) to test the influence of variables on the δ15N and δ13C values in tissues of juvenile turtles. The general model was composed of the response variable (δ15N and δ13C values in separate models) the predictor variables (curvilinear carapace length - CCL; environmental areas - CAU and SSC, and season of the year - summer and winter), the last two of which are categorical variables; the interaction terms were also included in the model. We used the package ‘MuMIn’ (Barton, 2019) to compare models and the best model was chosen by the lowest AIC value (Akaike, 1974). The best probability distribution family of the data was tested using the package ‘fitdistrplus’ (Delignette-Muller and Dutang, 2015) and was chosen through the lowest AIC value. The gamma distribution was used for the model with the response variable δ15N values and the Gaussian distribution was used for the model with the response variable δ13C values.
All the statistical analyses were performed in R language (R Core Team, 2020).
Results
The C:N ratio for keratin stable carbon and nitrogen isotope measurements in adult and juvenile green turtles was <3.5 for all samples.
Adult green turtles
The CCL varied from 98.4 to 125.2 cm, with mean ± 1 standard deviation of 111.6 ± 3.3 cm (n = 21). The isotope values for adult turtle carapaces showed mean ± standard deviation of -18.02 ± 2.10‰ for δ13C (-21.7 to -13.1‰) and 7.73 ± 1.53‰ for δ15N (5.4 to 11.5‰) for samples representing the oldest portion of the carapace and -18.00 ± 2.06‰ for δ13C (-21.6 to -13.3‰) and 7.80 ± 1.56‰ for δ15N (4.7 to 11.8‰) for the samples representing the youngest portion of the carapace (Figure 2; Supplementary Table S2). The carbon and nitrogen isotope values of the anterior and posterior portion of the carapace did not show significant difference (t = 0.19, df = 20, p = 0.85 and t = 0.40, df = 20, p = 0.69, respectively).
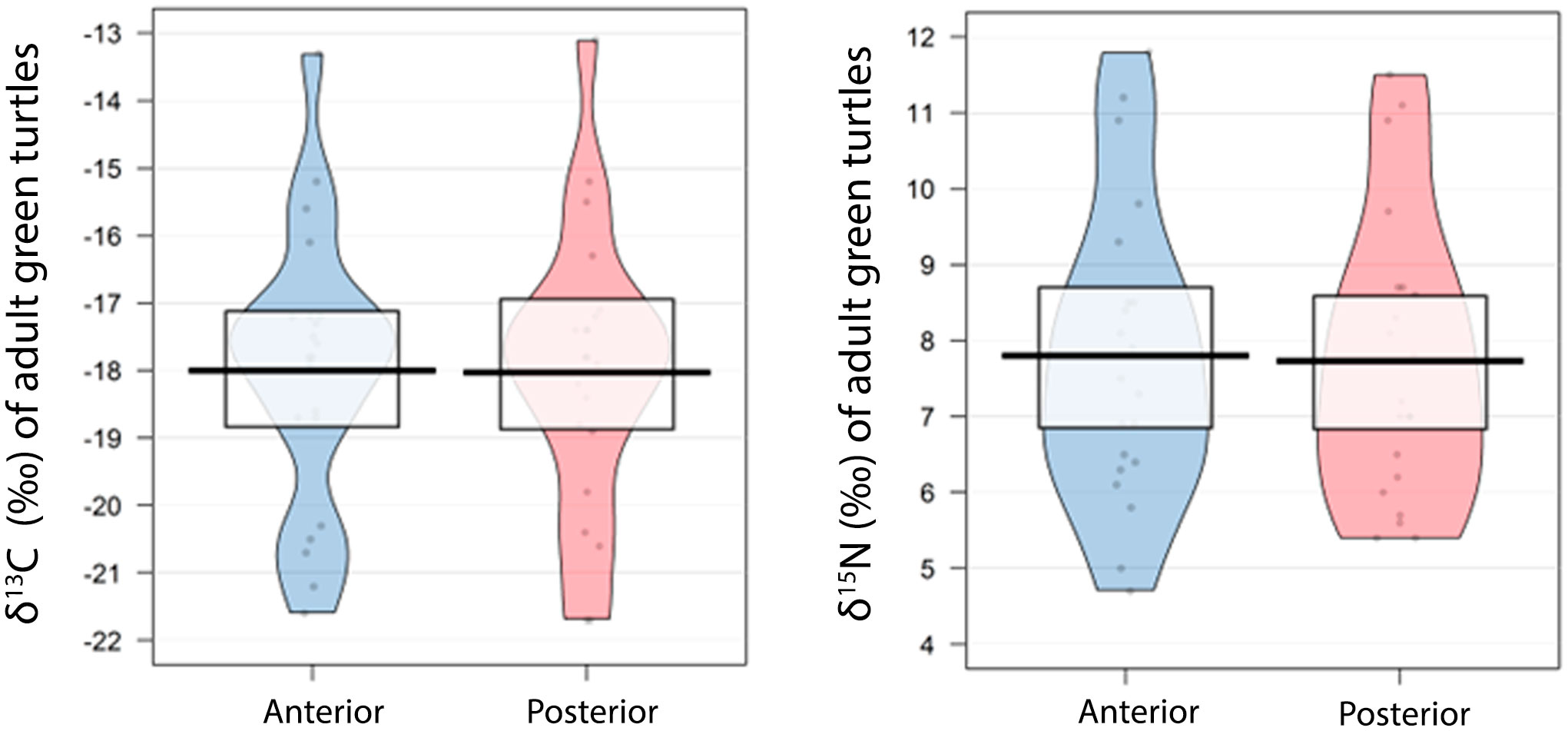
Figure 2 Plots of mean δ15N and δ13C values for samples taken from posterior (oldest) and anterior (youngest) portions of the second lateral scute of adult green turtles (n = 21). Points are the raw data, black line represents the mean, bean is the confidence interval.
In adult green turtles, the area of ellipse overlap was 96% of the isotopic niche of newest portion of the carapace of turtles with the isotopic niche of oldest portion, and 95.6% of the isotopic niche of oldest portion of the carapace of turtles with the isotopic niche of newest portion (Figure 3A).
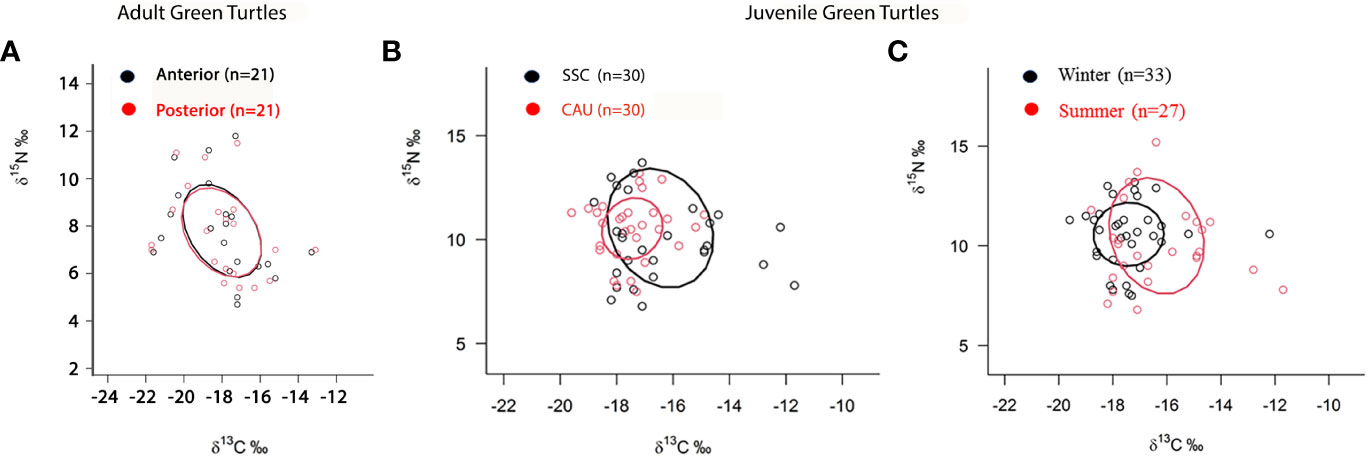
Figure 3 Area of ellipse overlap of (A) isotopic niche of samples of the posterior (oldest) and of anterior (youngest) carapace scute of adult green sea turtles, Chelonia mydas, in southeastern Brazil, (B) comparison of isotopic niches of juvenile green turtles between the São Sebastião Channel (SSC) and coast of Caraguatatuba and Ubatuba (CAU) and (C) for winter and summer, in southeastern Brazil.
Juvenile green turtles
The CCL varied from 28.0 to 55.0 cm with mean 40.4 ± 5.8 cm (n = 60). The isotopic values in carapace scutes of juvenile turtles had mean ± standard deviation of -16.93 ± 1.19‰ for δ13C (-19.6 to -11.7‰) and 10.36 ± 1.43‰ for δ15N (6.8 to 15.2‰) (Figure 4). Considering only juvenile green turtles from the SSC, the mean was -16.45 ± 1.51‰ for δ13C and 10.53 ± 2.03‰ for δ15N. Juvenile green turtles of CAU had a mean of -17.45 ± 0.82‰ for δ13C and 10.53 ± 1.13‰ for δ15N (Supplementary Table S2), and thus, SSC and CAU areas differed by δ13C values (t = 2.35, df = 29, p = 0.02), but not δ15N values (t = 0.03, df = 29, p = 0.97). Besides that, SSC turtles had a greater isotopic niche breadth compared to the CAU turtles.
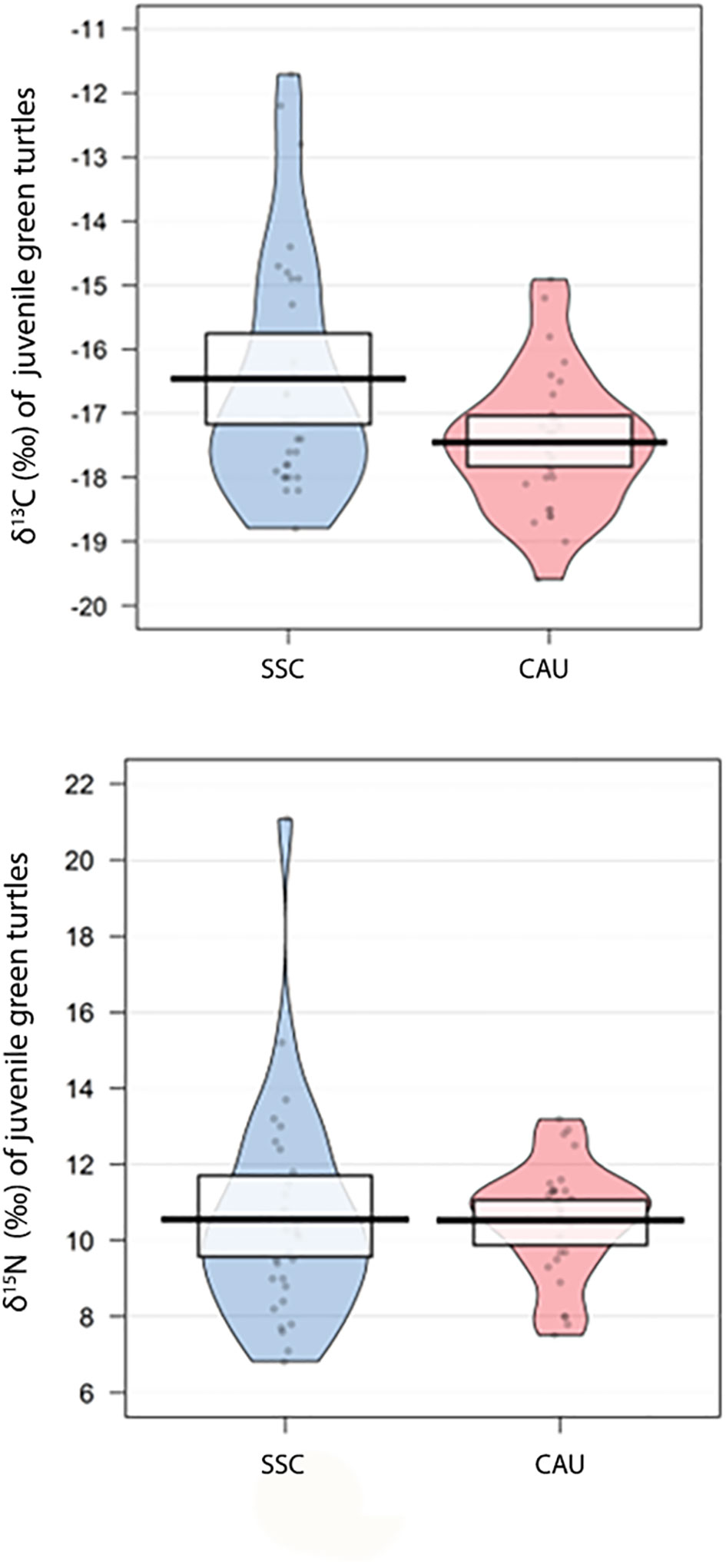
Figure 4 Plots of mean δ15N and δ13C values for juvenile green turtles from São Sebastião channel (SSC; n = 30) and the coast of Caraguatatuba and Ubatuba (CAU; n = 30). Points are the raw data, black line represents the mean, bean is the confidence interval.
The area of ellipse overlap was 39.1% of the SSC turtles with the isotopic niche of CAU turtles and 91.9% of the isotopic niche of the CAU turtles with the isotopic niche of SSC turtles (Figure 3B). Regarding seasons, a significant difference was observed between juvenile turtles sampled in winter and summer when compared mean isotope values of δ13C (t = 2.69, df = 26, p = 0.01), but not δ15N values (t = 0.035, df = 26, p = 0.97). Turtles sampled in winter had δ13C values of -17.41 ± 0.86‰ and δ15N values of 10.57 ± 1.21‰ and presented a 72.4% overlap of the isotopic niche with the niche of the turtles stranded in summer, which had δ13C values of -16.38 ± 1.45‰ and δ15N values of 10.50 ± 2.0‰ and presented a 41.3% overlap of the isotopic niche with that of the turtles stranded in winter (Figure 3C).
The GLMs indicated that the only assessed variable that was significantly correlated with δ15N values was CCL, but a clear trend was not observed (Figure 5). This variable did not have a significant effect on δ13C, but for δ13C values the model with the best fit indicated that only season of the year had a significant influence on the observed values (Table 2).
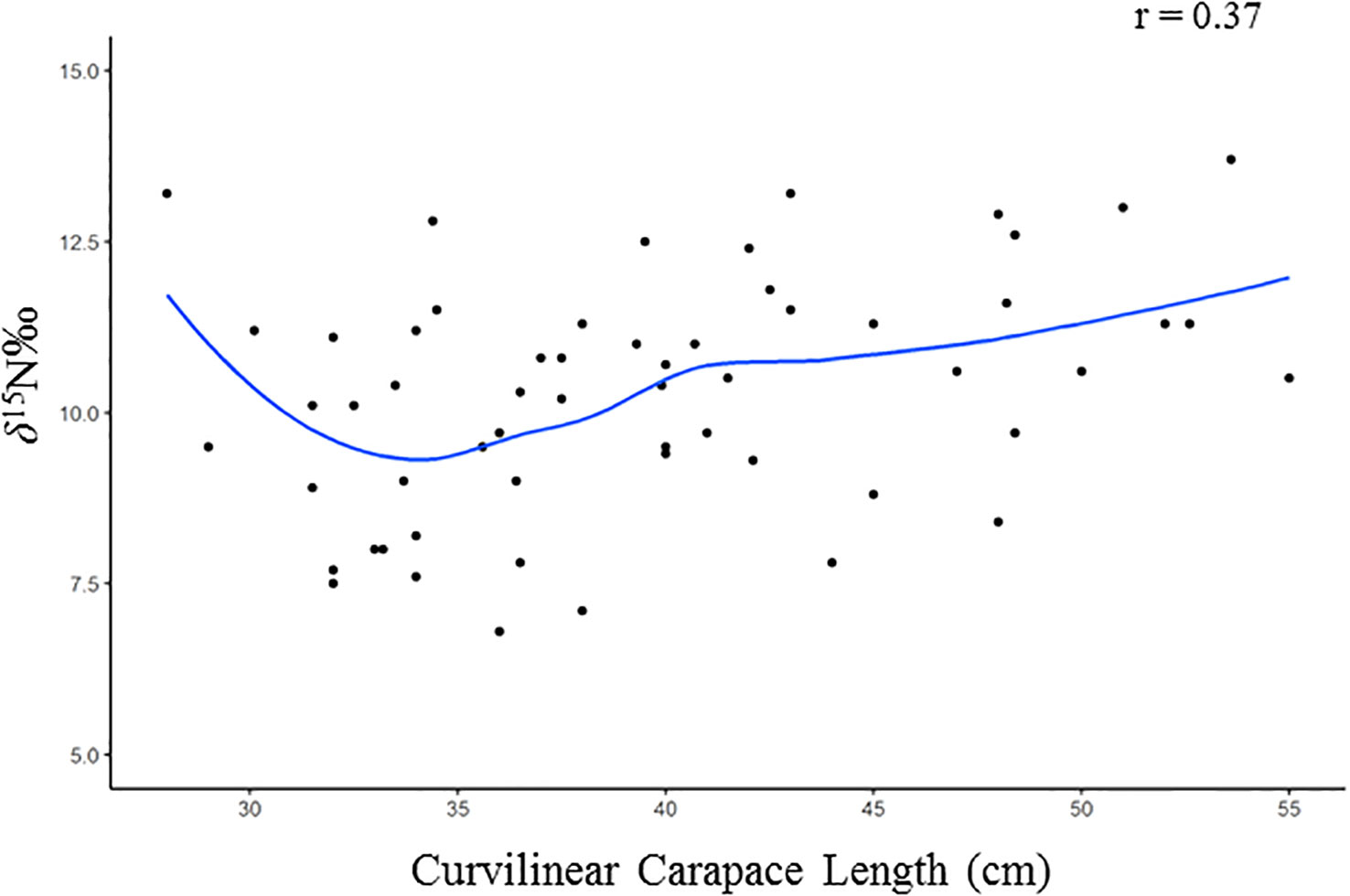
Figure 5 Correlation between δ15N values and Curvilinear Carapace Length (CCL, in cm) of juvenile green turtles, Chelonia mydas in southeastern Brazil. The blue lines indicate the trend lines.
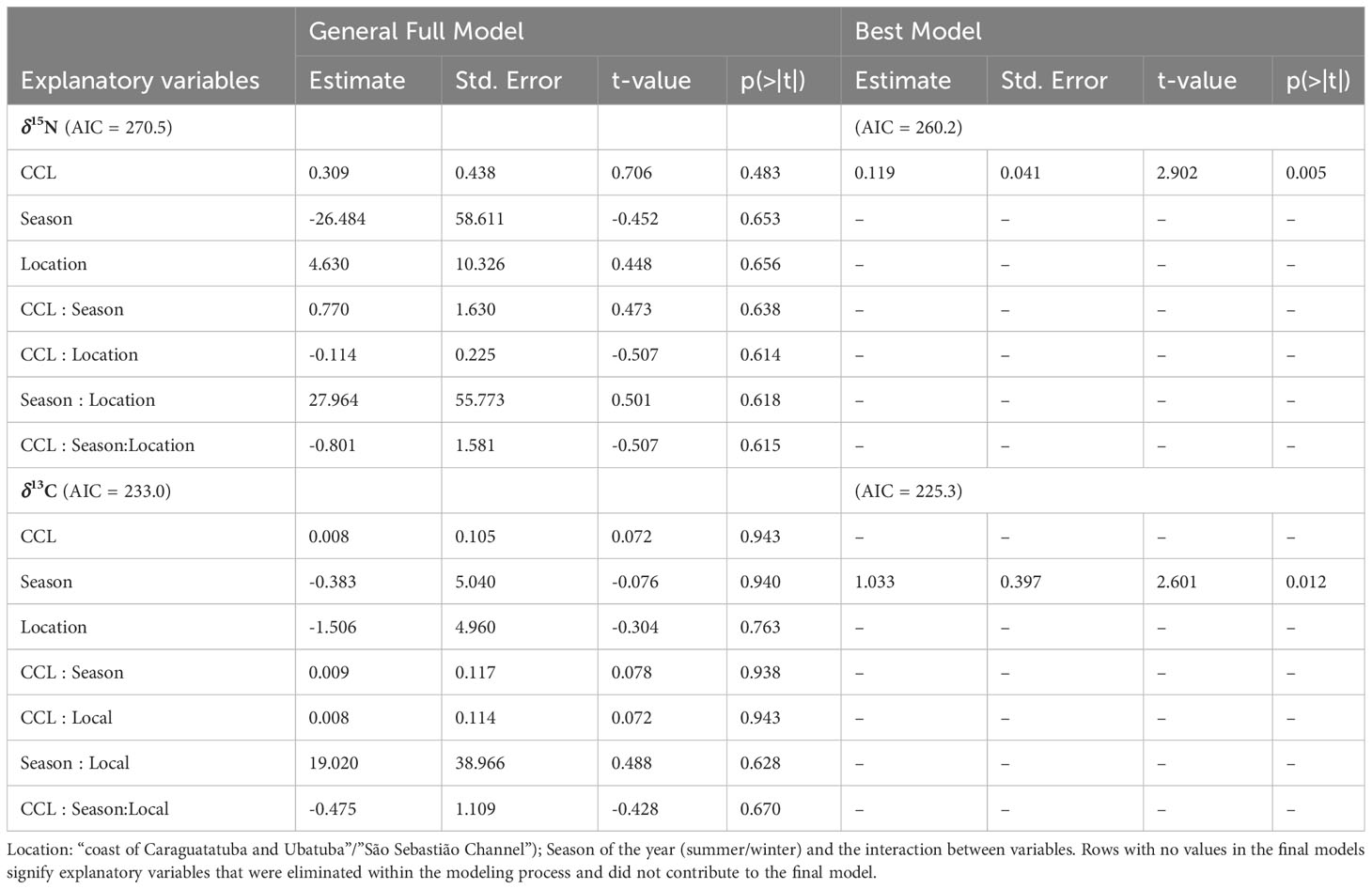
Table 2 Results of generalized linear models (GLMs) composed by the response variables (isotope values of δ15N and δ13C) and the predictor variables (curvilinear carapace length-CCL).
Discussion
We present the carbon and nitrogen isotopic values of juvenile and adult green turtles in feeding and nesting areas in Brazil, southwestern Atlantic Ocean, to assess the isotopic niche breadth of these animals and the potential variability and plasticity in their feeding behavior. We considered temporal analysis for adult green turtles and different habitats based on oceanographic dynamics and seasonal fluctuations for juvenile green turtles. Our results revealed no temporal variations in the isotopic values of adult green turtles and the mean δ15N values are compatible with the isotopic values of primarily herbivorous animals. The isotopic values observed in juvenile green turtles demonstrated a high degree of feeding behavior plasticity and individual variability, which were possibly influenced by the dynamic of oceanographic currents and seasonal nutrient inputs.
Adult green turtles
The comparison between the isotope values of samples representing the oldest and the youngest portions of the carapace of adult green sea turtles from Fernando de Noronha indicated that the same niche is occupied between these temporal windows (mean δ15N of 7.73‰ and 7.80‰, mean δ13C of -18.02‰ and -18.00‰, respectively). Considering that samples from the posterior part of the carapace scute retain a history of diet and habitat (Reich et al., 2007), the results contradict the prediction that such samples reflect a higher trophic level, due to the diet with a greater tendency to omnivory presented by these animals in their juvenile stage. A possible explanation is that the collected tissues reflected a period in which the individuals had already made the transition from an omnivorous diet to a predominantly herbivorous diet, but it is also necessary to consider the possible differences in isotopic values due to different baselines in feeding areas that the adult green turtles of Fernando de Noronha occupied in the period of up to 6.5 years, which is the time that samples of whole carapace tissue profile of an adult individual can integrate (Vander-Zanden et al., 2013b). Although no published studies on the trophic ecology of adult green turtles in Fernando de Noronha were found, Gitirana and Souza (2012) documented a high occurrence of subadult green turtles (60 – 90 cm CCL) showing feeding behavior on a beach characterized by the presence of various algae species and seagrass in Fernando de Noronha. This information may help clarify the result we found regarding the absence of a temporal difference in the feeding behavior observed in adult green turtles.
Regarding the mean δ13C values, -18.0‰ is also similar to those reported by Agostinho et al. (2021) in the carapace of adult green turtles from the nearby Atol das Rocas (-17.9‰, ranging from -12.4 to -21.14‰). It is known that δ13C high values are associated with coastal-benthic environments (Fry, 2008). Although the δ13C values found for the adult green turtle from Fernando de Noronha were more negative and associated with more pelagic environments, the mean δ15N values are compatible with the isotopic values of primarily herbivorous animals (Vander-Zanden et al., 2013a; Vander-Zanden et al., 2013b). These values may be influenced by the location of Fernando de Noronha, an archipelago located 345 km from the nearest continental coast, but where adult green turtles can have herbivorous feeding habits in shallow waters.
Juvenile green turtles
Juvenile green turtles settle into neritic habitats when their CCL reaches 25 – 50 cm (Williams et al., 2014; Howell et al., 2016; Williard et al., 2017). Sea turtles in the present study had little variation in CCL (28 – 55 cm) for the range described for green turtles recruited to the neritic habitat and the GLM result indicated that CCL was the only variable explaining significantly δ15N values, although these variables presented a weak relationship (Figure 5).
In a recent study, Campos and Cardona (2019), when studying the individual variability in the settlement of juvenile green turtles, describe that in Ubatuba there is a high individual variability in the diet of turtles and that this is probably related to a lower and highly seasonal availability of food, when compared to tropical areas in Brazil, and also due to individual differences in their growth rate (higher in tropics, e.g. Lima et al., 2021). This shed light to the weak relationship found in our study between the size of individuals and δ15N values, due to the possible individual variability in the diet of turtles. Indeed, if there were a strong relationship between these variables could indicate that newly recruited turtles (smaller in size) have differences in feeding compared to resident turtles (larger in size).
Individuals from the northern coast of the state of São Paulo also seem adjust foraging according to food availability and benefit from the large supply of nutrients from the SACW. Romanini (2014) describes the feeding ecology of juvenile green turtles conducted in the same study region, where there is a contribution of animal matter in the diet of turtles, as well as a variety of food items among individuals, despite their preference for marine algae. Other studies have reported high levels of omnivory in resident green turtles elsewhere (e.g., Cardona et al., 2009; Burkholder et al., 2011; Lemons et al., 2011; Russell et al., 2011; González-Carman et al., 2013). This intraspecific variability can be expected, as this species occupies a wide diversity of habitats in tropical and warm-temperate waters around the world (Wallace et al., 2010). Furthermore, their trophic plasticity ensures survival in a wide range of local conditions (Santos et al., 2015).
Troina et al. (2020) analyzed δ13C and δ15N in zooplankton samples collected along the south and southeast Brazilian oceanic waters, providing novel information regarding the patterns of these isotopic values in the southwestern Atlantic Ocean. Considering the average δ15N value we found (10.36 ± 1.43‰) and the baseline described for the southeastern Brazilian oceanic waters, where δ15N values in animal tissues are generally 3 to 5‰ higher compared to their food source (DeNiro and Epstein, 1981; Minagawa and Wada, 1984), it is possible to suggest that the juvenile green turtles sampled in this study occupy at least one trophic level higher than a solely herbivorous diet. However, the values found also resemble those described by Vélez-Rubio et al. (2018) for macroalgae in the Uruguayan region that demonstrates the seasonal movement of juvenile turtles in the southwestern Atlantic Ocean between Uruguayan and south Brazilian waters, which contributes to the variation in δ15N values.
It is also important to note that the current study analyzed juvenile green turtles that were stranded on the beach. Consequently, it was not possible to investigate a wide size-range, which could have interfered in the detection of a possible change in foraging niche among turtles, as found by Madeira et al. (2022) for green turtles sampled over a wide size-range in a feeding area in the Bijagós archipelago, in Guinea Bissau, for example.
The δ13C values found for juvenile green turtles are lower than expected for coastal sites and are close to the range of values found for turtles in the oceanic life stage described by Vander-Zanden et al. (2013b) (-18.8 to -12.7‰) in the Bahamas, for example. The range of δ13C values found by Campos and Cardona (2019) for juvenile green turtles also at Ubatuba, is similar to values we found (-20.5 to -14.9‰). These authors described that the range of isotopic values found for these turtles is compatible with an omnivorous diet or a plant-based diet from Uruguay (Vélez-Rubio et al., 2018) that would thus represent a recent arrival to the sampling area, also presenting high levels of individual and temporal variability, suggesting a complex life history with frequent changes in diet and habitats.
When we analyzed individuals in two groups, the SSC and CAU turtle groups shown ellipses of isotopic niches which occupy the same position in the isospace, albeit at different breadths, as the SSC turtles had a significantly larger niche breadth than those of the CAU, indicating they are more generalist and/or individual variability. The mean values of δ13C between the two areas also differed. As higher δ13C values are generally associated with coastal-benthic environments (Fry, 2008), it is also possible that turtles sampled in the SSC are more coastal-benthic consumers than the group of turtles sampled in the CAU.
In a study that compared the trophic model of the SSC with the internal continental shelf adjacent to the island of São Sebastião, Rocha et al. (2007) found higher values of total biomass, capacity for development, and total transfer in the SSC, which further confirms the SSC as a nutrient rich area. According to Pires-Vanin et al. (2014) despite being a relatively small area, the SSC has a high number of macrofauna invertebrate species compared to that over the adjacent continental shelf. These differences in resource availability between areas may explain the greater niche breadth found in juvenile green turtles of the SSC.
The isotopic niche breadths of juvenile green turtles also differed seasonally, with isotopic niche of individuals sampled in summer with a greater breadth than winter turtles. In summer, the intrusion of cold, saline, nutrient-rich water occurs close to the bottom layer in the study region (Castro-Filho and Miranda, 1998) which promotes eutrophication pulses at medium depths. In the winter, SACW retreats to the continental shelf and the inner shelf is filled with the warm, low saline CW (Castro-Filho and Miranda, 1998). Thus, the oceanographic characteristics of the region should also strongly influence the results found for the different niche breadths between seasons, as in the summer, due to the large intake of nutrients brought by SACW, when turtles have greater variability of food items. Similarly, Quiñones et al. (2022) also found differences in the feeding patterns of juvenile and subadult green turtles in Peru, influenced by seasonal water temperatures.
In Argentina, green turtles sampled in summer feed mainly on gelatinous plankton (González-Carman et al., 2013) and one of the hypotheses for the basically carnivorous diet is the high intraspecific competition, where macroalgae beds are available. Because intraspecific competition could decrease the growth rate in juvenile green turtles (Bjorndal et al., 2000) authors suggest that some individuals migrate seasonally to temperate regions and feed on the resources available in the region. In southeastern Brazil, where the present study was carried out, there is a high supply of nutrients brought by SACW in the summer, thus we hypothesized that green turtles expand the variability of food items ingested and become more generalist, thus decreasing intraspecific competition. The intraspecific competition possibly occurs predominantly in winter, a period in which food resources are scarcer due to the prevalence of CW, a nutrient-poor body of water that prevails in the region during this period of the year, and turtles from colder southward regions such as Argentina, Uruguay and southern Brazil move to the area (Silva et al., 2017).
The season of the year was indicated by the GLM analysis as a significant variable explaining variation in δ13C values, with turtles sampled in summer with higher mean value than those sampled in winter, suggesting that they are more coastal-benthic consumers. These values often decrease from coastal environment to oceanic environment and from shallow to deeper waters (Hill et al., 2006; Michener and Kaufman, 2007; Nerot et al., 2012), which may indicate that, in summer, these animals were feeding closer to the coast and in shallower waters. This difference may also occur due to the influence of the different water masses that act seasonally in the region, where the upwelling caused by SACW in the summer increases growth and availability of phyto and zooplankton biomass (Lopes et al., 2006), thus providing nutrients in shallower and coastal waters for foraging turtles.
In conclusion, the results of this study suggest that oceanographic characteristics and their influence on food resource availability can influence the trophic niche of juvenile green turtles in feeding areas of the southwestern Atlantic Ocean. These characteristics and the dynamics of water bodies play a crucial role in determining the availability of nutrients in the environment, which in turn affects the feeding behavior and sites of juvenile turtles. Stable isotope analysis of carbon and nitrogen in sea turtle tissues revealed that juvenile turtles from the north coast of the state of São Paulo exhibit high plasticity in their feeding behavior, adapting their diet based on the availability and quality of food resources. The weak relationship found in our study between the size of juvenile turtles and δ15N values, which may be related to the possible individual variability in the diet of turtles. Furthermore, adult green sea turtles from Fernando de Noronha were identified as primarily herbivorous consumers, feeding in shallow waters despite having more negative δ13C values compared to adult turtles from other places. This variation in isotopic values can be attributed to the offshore location of Fernando de Noronha. Places such as the SSC, which have their own characteristics due to the dynamics of oceanographic currents, can be key sites for feeding juvenile turtles. The identification of these sites and their contributions to the development of sea turtles is of great importance for the conservation of these threatened animals.
Data availability statement
The raw data supporting the conclusions of this article will be made available by the authors, without undue reservation.
Ethics statement
The animal study was approved by Licença Sistema de Autorização e Informação em Biodiversidade SISBIO 58049-1. The study was conducted in accordance with the local legislation and institutional requirements.
Author contributions
Conceptualization: AF, LB, AS, AB, CZ Data Curation: AF Formal Analysis: AF Laboratory analysis: AB, LB Investigation: AF Methodology: AF, AS Project Administration: AF, AS Supervision: AS, LB Validation: AF, LB, AS Writing – Original Draft Preparation: AF, LB, AS Writing – Review & Editing AF, LB, AS, LB, AB, CZ. All authors contributed to the article and approved the submitted version.
Funding
We acknowledge the Programa de Monitoramento de Praias - Bacia de Santos - PMP-BS, an activity developed to attend the requirements of the federal environmental licensing of Petrobras’ activities in the production and flow of oil and natural gas in the Santos Basin, conducted by IBAMA. The objective of this project is to assess the possible impacts of oil production and flow activities on birds, turtles and marine mammals, through monitoring of beaches and veterinary care for live animals and necropsy of animals found dead. The project is carried out from Laguna/SC to Saquarema/RJ, being divided into 15 sections. The ARGONAUTA institute monitors the Section 10, between São Sebastião and Ubatuba.
Acknowledgments
We would like to thank Instituto Argonauta para Conservação Costeira e Marinha, PMP-BS and Fundação Projeto Tamar, for its support during data and sample collection and to everyone who collaborated during the research; Fernando Azevedo Faria and Gustavo Menezes for their support and help with statistical analysis; Manuella Dultra de Jesus, Andréia Martucci Esteves, Jéssica Maldonado and Pedro Marques for support during sample collection; CAPES for the doctoral scholarship for the first author, CNPq for the productivity grants to professors Alexandre Schiavetti, Leandro Bugoni, and Adalto Bianchini and to UESC for the postdoctoral fellowship for Cleverson Zapelini. We also thank the reviewers for suggestions to improve earlier versions of the manuscript.
Conflict of interest
The authors declare that the research was conducted in the absence of any commercial or financial relationships that could be construed as a potential conflict of interest.
Publisher’s note
All claims expressed in this article are solely those of the authors and do not necessarily represent those of their affiliated organizations, or those of the publisher, the editors and the reviewers. Any product that may be evaluated in this article, or claim that may be made by its manufacturer, is not guaranteed or endorsed by the publisher.
Supplementary material
The Supplementary Material for this article can be found online at: https://www.frontiersin.org/articles/10.3389/fmars.2023.1203900/full#supplementary-material
References
Agostinho K. F. F., Monteiro L. R., Carvalho C. E. V., Beneditto A. P. M. (2021). Stable isotopes as measure of niche breadth of nesting green turtles (Chelonia mydas) on Rocas Atoll, Brazil. Rev. Iberoam. Cienc. Ambient. 12, 82–89. doi: 10.6008/CBPC2179-6858.2021.003.0008
Aidar E., Mesquita H. S. L. (1993). “Oceanografia biológica: fitobioensaios,” in Levantamento Oceanográfico da Área Diretamente Afetada por Efluentes dos Emissários Submarinhos de Esgotos da SABESP, Entre os Municípios de São Sebastião e Mongaguá, Estado de São Paulo. Eds. Ikeda Y., Giannini. R. (FUNDESPA/IOUSP), 194–216.
Akaike H. (1974). A new look at the statistical model identification. IEEE Trans. Automat. Contr. 19, 716–723. doi: 10.1109/TAC.1974.1100705
Balazs G. H. (1995). “Status of sea turtles in the Central Pacific Ocean,” in Biology and Conservation of Sea Turtles. Ed. Bjorndal K. A. (Washington, DC.: Smithsonian Institution Press), 243–252.
Barrow L. M., Bjorndal K. A., Reich K. J. (2008). Effects of preservation method on stable carbon and nitrogen isotope values. Physiol. Biochem. Zool. 81, 688–693. doi: 10.1086/588172
Barton K. (2019) MuMIn: multi-model inference. R package version 1.43.15. Available at: http://cran.r-project.org/package=MuMIn.
Bearhop S., Adams C. E., Waldron S., Fuller R. A., Macleod H. (2004). Determining trophic niche width: a novel approach using stable isotope analysis. J. Anim. Ecol. 73, 1007–1012. doi: 10.1111/j.0021-8790.2004.00861.x
Bellini C. (1996). Reproduction and feeding of marine turtles in the Fernando de Noronha archipelago, Brazil. Mar. Turtle Newsl. 74, 12–13.
Bjorndal K. A. (1985). Nutritional ecology of sea turtles. Copeia 1985, 736–751. doi: 10.2307/1444767
Bjorndal K. A. (1997). “Foraging ecology and nutrition of sea turtles,” in The Biology of Sea Turtles. Eds. Lutz P. L., Musick J. A. (Boca Raton: CRC Press), 199–231.
Bjorndal K. A., Bolten A. B., Chaloupka M. Y. (2000). Green turtle somatic growth model: evidence for density dependence. Ecol. Appl. 10, 269–282. doi: 10.1890/1051-0761(2000)010[0269:GTSGME]2.0.CO;2
Bolten A. B. (2003). “Variation in sea turtle life history patterns: neritic vs. oceanic developmental stages,” in The Biology of Sea Turtles. Eds. Lutz P. L., Musick J. A. (Boca Raton: CRC Press), 243–257.
Bond A. L., Hobson K. A. (2012). Reporting stable-isotope ratios in ecology: recommended terminology, guidelines and best practices. Waterbirds 35, 324–331. doi: 10.1675/063.035.0213
Burkholder D. A., Heithaus M., Thomson J. A., Fourqurean J. W. (2011). Diversity in trophic interactions of green sea turtles Chelonia mydas on a relatively pristine coastal foraging ground. Mar. Ecol. Prog. Ser. 439, 277–293. doi: 10.3354/meps09313
Campos P., Cardona L. (2019). Individual variability in the settlement of juvenile green turtles in the western South Atlantic Ocean: relevance of currents and somatic growth rate. Mar. Ecol. Prog. Ser. 614, 173–182. doi: 10.3354/meps12909
Cardona L., Aguilar A., Pazos L. (2009). Delayed ontogenic dietary shift and high levels of omnivory in green turtles (Chelonia mydas) from the NW coast of Africa. Mar. Biol. 156, 1487–1495. doi: 10.1007/s00227-009-1188-z
Cardona L., Campos P., Levy Y., Demetropoulos A., Margaritoulis D. (2010). Asynchrony between dietary and nutritional shifts during the ontogeny of green turtles (Chelonia mydas) in the Mediterranean. J. Exp. Mar. Biol. Ecol. 393, 83–89. doi: 10.1016/j.jembe.2010.07.004
Castro-Filho B. M. (1990). Wind driven currents in the channel of São Sebastião: winter. Bol. Inst. Oceanogr. São Paulo 38, 111–132. doi: 10.1590/S0373-55241990000200002
Castro-Filho B. M., Miranda L. B. (1998). “Physical oceanography of the western Atlantic continental shelf located between 4°N and 34°S coastal segment,” in The Sea. Eds. Robinson A. R., Brink. K. H. (New York: John Wiley Publishers), 209–251.
Castro-Filho B. M., Miranda L. B., Miyao S. Y. (1987). Condições hidrográficas na plataforma continental ao largo de Ubatuba: variações sazonais e em média escala. Bol. Inst. Oceanogr. São Paulo 35, 135–151. doi: 10.1590/S0373-55241987000200004
Ceriani S. A., Roth J. D., Evans D. R., Weishampel J. F., Ehrhart L. M. (2012). Inferring foraging areas of nesting loggerhead turtles using satellite telemetry and stable isotopes. PLoS One 7, e0045335. doi: 10.1371/journal.pone.0045335
Clementz M. T., Koch P. L. (2001). Differentiating aquatic mammal habitat and foraging ecology with stable isotopes in tooth enamel. Oecologia 129, 461–472. doi: 10.1007/s004420100745
Coelho V. F., Domit C., Broadhurst M. K., Nishizawa H., Prosdocimi L., Almeida F. S. (2018). Intra-specific variation in skull morphology of juvenile Chelonia mydas in the southwestern Atlantic Ocean. Mar. Biol. 165, 174. doi: 10.1007/s00227-018-3429-5
Delignette-Muller M. L., Dutang C. (2015). fitdistrplus: an R package for fitting distributions. Stat. Software 64, 1–34. doi: 10.18637/jss.v064.i04
DeNiro M. J., Epstein S. (1978). Influence of the diet on the distribution of carbon isotopes in animals. Geochim. Cosmochim. Acta 42, 495–506. doi: 10.1016/0016-7037(78)90199-0
DeNiro M. J., Epstein S. (1981). Influence of diet on the distribution of nitrogen isotopes in animals. Geochim. Cosmochim. Acta 45, 341–351. doi: 10.1016/0016-7037(81)90244-1
Eder E., Ceballos A., Martins S., Pérez-Garcia H., Marín I., Marco A., et al. (2012). Foraging dichotomy in loggerhead sea turtles Caretta caretta off northwestern Africa. Mar. Ecol. Prog. Ser. 470, 113–122. doi: 10.3354/meps10018
Esteban N., Mortimer J. A., Stokes H. J., Laloë J.-O., Unsworth R. K. F., Hays G. C. (2020). A global review of green turtle diet: sea surface temperature as a potential driver of omnivory levels. Mar. Biol. 167, 183. doi: 10.1007/s00227-020-03786-8
Fernandes A., Bondioli A. C. V., Solé M., Schiavetti A. (2017). Seasonal variation in the behavior of sea turtles at a Brazilian foraging area. Chelonian Conserv. Biol. 16, 93–102. doi: 10.2744/CCB-1200.1
Filippos L. S., Taniguchi S., Baldassin P., Pires T., Montone R. C. (2021). Persistent organic pollutants in plasma and stable isotopes in red blood cells of Caretta caretta, Chelonia mydas and Lepidochelys olivacea sea turtles that nest in Brazil. Mar. Pollut. Bull. 167, 112283. doi: 10.1016/j.marpolbul.2021.112283
Furtado V. (1995). Sedimentação quaternária no Canal de São Sebastião. Bol. Inst. Oceanogr. São Paulo 11, 27–35.
Gallo B. M. G., Macedo S., Giffoni B. B., Becker J. H., Barata P. C. R. (2006). Sea turtle conservation in Ubatuba, southeastern Brazil, a feeding area with incidental capture in coastal fisheries. Chelonian Conserv. Biol. 5, 93–101. doi: 10.2744/1071-8443(2006)5[93:STCIUS]2.0.CO;2
Gitirana H. M., Souza A. T. (2012). Notes on the spatial distribution and foraging behavior of green turtles at the Fernando de Noronha archipelago, northeastern Brazil. Mar. Turtle Newsl. 132, 9–12.
González-Carman V., Botto F., Gaitán E., Albareda D., Campagna C., Mianzan H. (2013). A jellyfish diet for the herbivorous green turtle Chelonia mydas in the temperate SW Atlantic. Mar. Biol. 161, 339–349. doi: 10.1007/s00227-013-2339-9
Hatase H., Sato K., Yamaguchi M., Takahashi K., Tsukamoto K. (2006). Individual variation in feeding habitat use by adult female green sea turtles (Chelonia mydas): are they obligately neritic herbivores? Oecologia 149, 52–64. doi: 10.1007/s00442-006-0431-2
Hatase H., Takai N., Matsuzawa Y., Sakamoto W., Omuta K., Goto K., et al. (2002). Size-related differences in feeding habitat use of adult female loggerhead turtles Caretta caretta around Japan determined by stable isotope analyses and satellite telemetry. Mar. Ecol. Prog. Ser. 233, 273–281. doi: 10.3354/meps233273
Hill J. M., McQuaid C. D., Kaehler S. (2006). Biogeographic and nearshore-offshore trends in isotope ratios of intertidal mussels and their food sources around the coast of southern Africa. Mar. Ecol. Prog. Ser. 318, 63–73. doi: 10.3354/meps318063
Howell L. N., Reich K. J., Shaver D. J., Landry-Jr. A. M., Gorga C. C. (2016). Ontogenetic shifts in diet and habitat of juvenile green sea turtles in the north western Gulf of Mexico. Mar. Ecol. Prog. Ser. 559, 217–229. doi: 10.3354/meps11897
Jackson A. L., Richard I., Parnell A. C., Bearhop S. (2011). Comparing isotopic niche widths among and within communities: SIBER – Stable Isotope Bayesian Ellipses in R. J. Anim. Ecol. 80, 595–602. doi: 10.1111/j.1365-2656.2011.01806.x
Lemons G., Lewison R., Komoroske L., Gaos A., Lai C. T., Dutton P., et al. (2011). Trophic ecology of green sea turtles in a highly urbanized bay: insights from stable isotopes and mixing models. J. Exp. Mar. Biol. Ecol. 405, 25–32. doi: 10.1016/j.jembe.2011.05.012
Lima E. H. S. M., Awabdi D. R., Melo M. T. D., Giffoni B., Bugoni L. (2021). Nonlethal capture of green sea turtles (Chelonia mydas) in fishing weirs as an opportunity for population studies and conservation. Mar. Environ. Res. 170, 105437. doi: 10.1016/j.marenvres.2021.105437
Lopes R. M., Katsuragawa M., Dias J. F., Montú M. A., Muelbert J. H., Gorri C., et al. (2006). Zooplankton and ichthyoplankton distribution on the southern Brazilian shelf: an overview. Sci. Mar. 70, 189–202. doi: 10.3989/scimar.2006.70n2189
Madeira F. M., Rebelo R., Catry P., Neiva J., Barbosa C., Regalla A., et al. (2022). Fine-scale foraging segregation in a green turtle (Chelonia mydas) feeding ground in the Bijago’s archipelago, Guinea Bissau. Front. Mar. Sci. 9, 984219. doi: 10.3389/fmars.2022.984219
Martínez del Rio C., Wolf N., Carleton S. A., Gannes L. Z. (2009). Isotopic ecology ten years after a call for more laboratory experiments. Biol. Rev. 84, 91–111. doi: 10.1111/j.1469-185X.2008.00064
Michener R. H., Kaufman L. (2007). “Stable isotope ratios as tracers in marine food webs: an update,” in Stable Isotopes in Ecology and Environmental Science. Eds. Michener R., Lajtha K. (Malden: Blackwell Publishing Ltd), 238–282.
Minagawa M., Wada E. (1984). Stepwise enrichment of δ15N along food chains: further evidence and the relation between δ15N and animal age. Geochim. Cosmochim. Acta 48, 1135–1140. doi: 10.1016/0016-7037(84)90204-7
Naro-Maciel E., Becker J. H., Lima E. H. S. M., Marcovaldi M. A., DeSalle R. (2007). Testing dispersal hypotheses in foraging green sea turtles (Chelonia mydas) of Brazil. J. Hered. 98, 29–39. doi: 10.1093/jhered/esl050
Nerot C., Lorrain A., Grall J., Gillikin D. P., Munaron J. M., Le Bris H., et al. (2012). Stable isotope variations in benthic filter feeders across a large depth gradient on the continental shelf. Estuar. Coast. Shelf Sci. 96, 228–235. doi: 10.1016/j.ecss.2011.11.004
Newsome S. D., Martínez del Rio C., Bearhop S., Phillips D. L. (2007). A niche for isotopic ecology. Front. Ecol. Environ. 5, 429–436. doi: 10.1890/060150.01
Oliveira O. M. P., Marques A. C. (2007). “Dinâmica sazonal de massas de água no Canal de São Sebastião (SE Brasil) de Março de 2005 a Maio de 2006,” in XII Congresso Latino-Americano de Ciências do Mar, Florianópolis (AOCEANO -Associação Brasileira de Oceanografia).
Pajuelo M., Bjorndal K. A., Reich K. J., Vander-Zander H. B., Hawkes L. A., Bolten A. B. (2012). Assignment of nesting loggerhead turtles to their foraging areas in the northwest Atlantic using stable isotopes. Ecosphere 3, 89. doi: 10.1890/ES12-00220.1
Pires-Vanin A. M. S., Muniz P., Bromberg S. (2014). Inventory of the marine soft bottom macrofauna of São Sebastião channel, southeastern Brazilian continental shelf. Check List 10, 795–807. doi: 10.15560/10.4.795
Post D. M., Layman C. A., Arrington D. A., Takimoto G., Quattrochi J., Montaña C. G. (2007). Getting to the fat of the matter: models, methods and assumptions for dealing with lipids in stable isotope analyses. Oecologia 152, 179–189. doi: 10.1007/s00442-006-0630-x
Quiñones J., Paredes-Coral E., Seminoff J. A. (2022). Foraging ecology of green turtles (Chelonia mydas) in Peru: relationships with ontogeny and environmental variability. Mar. Biol. 169, 139. doi: 10.1007/s00227-022-04126-8
R Core Team (2020). R: A Language and Environment for Statistical Computing (Vienna, Austria: R Foundation for Statistical Computing).
Reich K. J., Bjorndal K. A., Bolten A. B. (2007). The ‘lost years’ of green turtles: using stable isotopes to study cryptic life stages. Biol. Lett. 3, 712–714. doi: 10.1098/rsbl.2007.0394
Rocha G. R. A., Rossi-Wongtschowski C. L. D. B., Pires-Vanin A. M. S., Soares L. S. H. (2007). Trophic models of São Sebastião channel and continental shelf systems, SE Brazil. Pan-Am. J. Aquat. Sci. 2, 149–162.
Romanini E. (2014). “Ecologia alimentar de tartarugas-verdes, Chelonia mydas (Linnaeus 1758), em Ilhabela e Ubatuba – litoral norte de São Paulo, Brasil,” in Monography (São Paulo: Universidade de São Paulo).
Rubenstein D. R., Hobson K. A. (2004). From birds to butterflies: animal movement patterns and stable isotope. Trends Ecol. Evol. 19, 256–263. doi: 10.1016/j.tree.2004.03.017
Russell D. J., Hargrove S., Balazs G. H. (2011). Marine sponges, other animal food, and nonfood items found in digestive tracts of the herbivorous marine turtle Chelonia mydas in Hawaii. Pac. Sci. 65, 375–381. doi: 10.2984/65.3.375
Sánchez A., Anguas-Cabrera D., Camacho-Cruz K., Ortiz-Hernández M. C., Aguíñiga-García S. (2020). Spatial and temporal variation of the δ15N in Thalassia testudinum in the Mexican Caribbean, (2009–2017). Mar. Freshw. Res. 71, 905–912. doi: 10.1071/MF19105
Santos R. G., Silva A. M., Batista M. B., Horta P. A. (2015). Regional and local factors determining green turtle Chelonia mydas foraging relationships with the environment. Mar. Ecol. Prog. Ser. 529, 265–277. doi: 10.3354/meps11276
Seminoff J. A., Benson S. R., Arthur K. E., Eguchi T., Dutton P. H., Tapilatu R. F., et al. (2012). Stable isotope tracking of endangered sea turtles: validation with satellite telemetry and δ15N analysis of amino acids. PLoS One 7, e0037403. doi: 10.1371/journal.pone.0037403
Seminoff J. A., Resendiz A., Nichols W. J. (2002). Diet of east Pacific green turtles (Chelonia mydas) in the central Gulf of California, Mexico. J. Herpetol. 36, 447–453. doi: 10.2307/1566189
Serafini T. Z., França G. B., Andriguetto-Filho J. M. (2010). Brazilian oceanic islands: known biodiversity and its relation to the history of human use and occupation. J. Integr. Coast. Zone Manage. 10, 281–301.
Silva B. M. G., Bugoni L., Almeida B. A. D. L., Giffoni B. B., Alvarenga F. S., Brondizio L. S., et al. (2017). Long-term trends in abundance of green sea turtles (Chelonia mydas) assessed by non-lethal capture rates in a coastal fishery. Ecol. Indic. 79, 254–264. doi: 10.1016/j.ecolind.2017.04.008
Troina G. C., Dehairs F., Botta S., Couto J., Di Tullio J. C., Elskens M., et al. (2020). Zooplankton-based δ13C and δ15N isoscapes from the outer continental shelf and slope in the subtropical western South Atlantic. Deep Sea Res. Part I 159, 103235. doi: 10.1016/j.dsr.2020.103235
Tucker A. D., MacDonald B. D., Seminoff J. A. (2014). Foraging site fidelity and stable isotope values of loggerhead turtles tracked in the Gulf of Mexico and northwest Caribbean. Mar. Ecol. Prog. Ser. 502, 267–279. doi: 10.3354/meps10655
Vander-Zanden H. B., Arthur K. E., Bolten A. B., Popp B. N., Lagueux C. J., Harrison E., et al. (2013a). Trophic ecology of a green turtle breeding population. Mar. Ecol. Prog. Ser. 476, 237–249. doi: 10.3354/meps10185
Vander-Zanden H. B., Bjorndal K. A., Bolten A. B. (2013b). Temporal consistency and individual specialization in resource use by green turtles in successive life stages. Oecologia 173, 767–777. doi: 10.1007/s00442013-2655-2
Vander-Zanden H. B., Pfaller J. B., Reich K. J., Pajuelo M., Bolten A. B., Willians K. L. (2014). Foraging areas differentially affect reproductive output and interpretation of trends in abundance of loggerhead turtles. Mar. Biol. 161, 585–598. doi: 10.1007/s00227-013-2361-y
Vélez-Rubio G. M., Cardona L., López-Mendilaharsu M., Martinez-Souza G., Carranza A., Campos P., et al. (2018). Pre and post-settlement movements of juvenile green turtles in the southwestern Atlantic Ocean. J. Exp. Mar. Biol. Ecol. 501, 36–45. doi: 10.1016/j.jembe.2018.01.001
Vélez-Rubio G. M., Cardona L., López-Mendilaharsu M., Martínez-Souza G., Carranza A., González-Paredes D., et al. (2016). Ontogenetic dietary changes of green turtles (Chelonia mydas) in the temperate southwestern Atlantic. Mar. Biol. 163, 57. doi: 10.1007/s00227-016-2827-9
Wallace B. P., DiMatteo A. D., Hurley B. J., Finkbeiner E. M., Bolten A. B., Chaloupka M. Y., et al. (2010). Regional Management Units for marine turtles: a novel framework for prioritizing conservation and research across multiple scales. PLoS One 5, e0015465. doi: 10.1371/jornal.pone.0015465
Williams N. C., Bjorndal K. A., Lamont M. M., Carthy R. R. (2014). Winter diets of immature green turtles (Chelonia mydas) on a northern feeding ground: integrating stomach contents and stable isotope analyses. Estuar. Coasts 37, 986–994. doi: 10.1007/s12237-013-9741-x
Williard A. S., Hall A. G., Fujisaki I., McNeill J. B. (2017). Oceanic overwintering in juvenile green turtles Chelonia mydas from a temperate latitude foraging ground. Mar. Ecol. Prog. Ser. 564, 235–240. doi: 10.3354/meps12013
Zbinden J. A., Bearhop S., Bradshaw P., Gill B., Margaritoulis D., Newton J., et al. (2011). Migratory dichotomy and associated phenotypic variation in marine turtles revealed by satellite tracking and stable isotope analysis. Mar. Ecol. Prog. Ser. 421, 291–302. doi: 10.3354/meps08871
Keywords: isotopic niche, ontogenetic changes, South Atlantic Ocean, stable isotopes, turtle size
Citation: Fernandes A, Bugoni L, Bianchini A, Zapelini C and Schiavetti A (2023) Isotopic niches of juvenile and adult green turtles (Chelonia mydas) in feeding and nesting areas in Brazil, southwestern Atlantic Ocean. Front. Mar. Sci. 10:1203900. doi: 10.3389/fmars.2023.1203900
Received: 11 April 2023; Accepted: 24 August 2023;
Published: 18 September 2023.
Edited by:
Jose Carlos Báez, Spanish Institute of Oceanography (IEO), SpainReviewed by:
Ana Rita Patrício, MARE-ISPA, PortugalVictor Hugo Valiati, University of the Rio dos Sinos Valley, Brazil
Damián Gustavo Vales, CONICET Centro de Estudios de Sistemas Marinos (CESIMAR), Argentina
Copyright © 2023 Fernandes, Bugoni, Bianchini, Zapelini and Schiavetti. This is an open-access article distributed under the terms of the Creative Commons Attribution License (CC BY). The use, distribution or reproduction in other forums is permitted, provided the original author(s) and the copyright owner(s) are credited and that the original publication in this journal is cited, in accordance with accepted academic practice. No use, distribution or reproduction is permitted which does not comply with these terms.
*Correspondence: Amanda Fernandes, mandifernandes@gmail.com