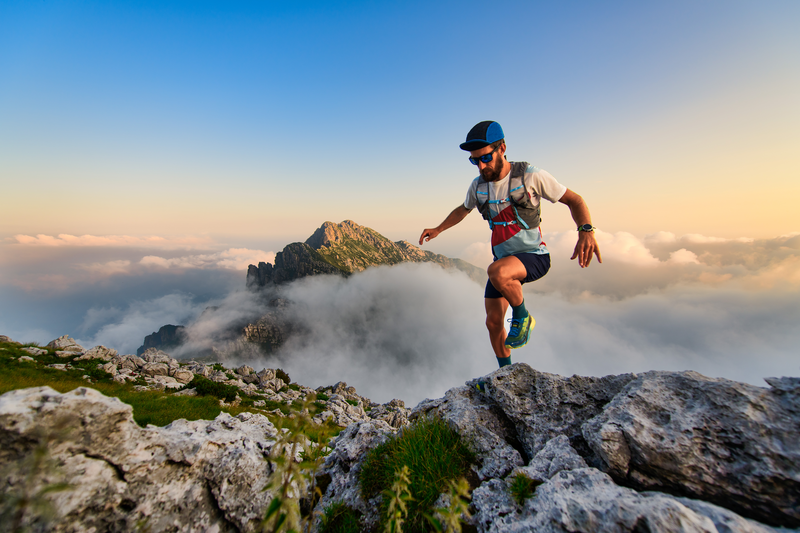
94% of researchers rate our articles as excellent or good
Learn more about the work of our research integrity team to safeguard the quality of each article we publish.
Find out more
ORIGINAL RESEARCH article
Front. Mar. Sci. , 24 July 2023
Sec. Marine Ecosystem Ecology
Volume 10 - 2023 | https://doi.org/10.3389/fmars.2023.1199654
This article is part of the Research Topic Restoration of Coastal Marine Ecosystems View all 22 articles
The Mediterranean Sea is one of the most exploited regions of the world’s oceans. Here industrial activities have determined either acute or long-term impacts on coastal marine ecosystems. In this study, we investigated macrofauna distribution and diversity, and food-web functioning in a coastal area of the Mediterranean Sea facing an industrial chemical plant abandoned in the ‘90s to assess benthic ecosystem health. This area has been identified as a Site of National Interest (SNI) since 2002 and has been closed to any human activity awaiting to be remediated according to national laws. Our results indicate that, two decades after the SNI declaration (a decade after the plant decommissioning), there is no longer any sign of the impact of historical contaminations on macrofauna and benthic food web functioning. Overall, all the thirty-six sites showed high/good ecological quality according to the score assigned by AMBI and M-AMBI indexes, reflecting the absence of chronic impacts. Our findings reveal, for the first time, the positive effects of passive restoration (i.e., unassisted, or spontaneous recovery following cessation of anthropogenic impacts) on historically impacted coastal ecosystems since their health conditions, in terms of both abiotic (environmental variables and contaminant concentration) and biotic (macrofauna diversity and community composition, and benthic food-web structure) factors, were indistinguishable from surrounding non-impacted areas. These findings also suggest that other effective area-based conservation measures (OECMs) could be useful not only for biodiversity conservation of vulnerable and priority habitats in larger ocean sectors but also to promote the passive recovery of historically contaminated ecosystems.
Coastal environments include essential marine habitats (e.g., seagrass meadows, rocky reefs, estuaries, soft-sediment habitats) that support marine biodiversity and food webs and provide key ecosystem goods and services for human well-being (Coll et al., 2010; Barbier, 2017). At the same time, coastal areas have a long-lasting legacy of environmental impacts due to increasing urbanization, industrialization, and the consequent wastewater discharge of contaminants, and resource exploitation, which have led to the progressive loss of marine biodiversity and habitats (Cardinale et al., 2012; Pereira et al., 2012; Halpern et al., 2015; Hay Mele et al., 2020; Soto-Navarro et al., 2021). Anthropogenic impacts impairing coastal environments also act in synergy with other stressors such as extreme climate events (i.e., heat waves), thus determining a progressive alteration of the trophic food webs and ecosystem functioning (Micheli et al., 2013; He and Silliman, 2019; Danovaro et al., 2018).
Along the Mediterranean coasts, there are more than 200 active, dismissed, or decommissioned refineries, petrochemical, and chemical plants that, over time, have determined persistent and chronic impacts on the ecosystems due to the release of inorganic and organic contaminants, including fertilizers, hydrocarbons, PCBs, and heavy metals (Gambi et al., 2020). Several industrial-contaminated sites have a relevant impact on coastal ecosystems even after decades of industrial activity cessation (Loures, 2015; Bertocci et al., 2019; Ausili et al., 2020; Morroni et al., 2020; Gambi et al., 2022). In particular, high concentrations of polycyclic aromatic hydrocarbons (PAHs) and heavy metals have been widely reported to cause adverse effects on benthic communities at all levels, from prokaryotes to metazoans, and alter the biodiversity, food-web structure, and ecosystem functioning (Louati et al., 2013; Aylagas et al., 2017; Gambi et al., 2020; Hay Mele et al., 2020; Mayer-Pinto et al., 2020; Morroni et al., 2020; Tangherlini et al., 2020; Raymond et al., 2021).
The Italian legislation has assigned the status of “Sites of National Interest” (SNIs) to the most polluted coastal and terrestrial sites affected by industrial activity, thus requiring specific recovery plans to reduce the risk for human and ecosystem health (Ausili et al., 2020; Corinaldesi et al., 2022). The SNIs are indeed characterized by persistent contamination levels even several decades after the cessation of industrial activities (Romano et al., 2004; Gambi et al., 2020; Morroni et al., 2020; Naidu et al., 2021). The coastal area of Falconara Marittima (Northwest Adriatic Sea, Mediterranean Sea), which is also included among the European “problem areas” based on the contamination levels in water, sediments and biological components (Andersen et al., 2019), has been declared a SNI since 2002 and has been interdicted to any anthropogenic activities (e.g., shipping, fishery, and bathing). Such levels of contaminants have been reported to be harmful to macrobenthic communities (Josefson et al., 2008). A recent investigation conducted on the benthic ecosystems of this SNI, in front of a chemical industry abandoned more than three decades ago, revealed that heavy metals (As, Hg, and Al), C > 12 aliphatic hydrocarbons and total PAHs are currently low and without significant impact on meiofauna assemblages (Corinaldesi et al., 2022). However, the observed biological response could be due to the specific tolerance and fast resilience of the meiofaunal component (Whomersley et al., 2009; Schratzberger and Somerfield, 2020) while other benthic components, i.e., larger animals such as macro-megafauna, could better reflect long-lasting negative effects on biodiversity and ecosystem functioning (Bessa et al., 2014; Kim et al., 2020; Raymond et al., 2021; Gammal et al., 2023; Hilgendag et al., 2022).
Macrofaunal assemblages respond to environmental changes depending on their species-specific sensitivity/tolerance (Han et al., 2021), providing information on the different degrees of impact on biodiversity and ecosystem functioning (Gray et al., 1988; Hewitt et al., 2016; Ellis et al., 2017; Momota and Hosokawa, 2021). Nonetheless, macrofaunal assemblages in coastal benthic ecosystems play crucial functional roles by i) channelling the matter and energy flow to higher trophic levels, ii) influencing nutrient cycling and secondary production; and iii) contributing to the burial, diagenesis, and transfer of pollutants along food webs (Constable, 1999; Jędruch et al., 2019). For all these reasons, macrofauna has been proven to be one of the most effective biological indicators (Borja et al., 2000; Nepote et al., 2022; Magni et al., 2023).
Food-web functioning is crucial for the good health of marine ecosystems and provides important indications of the impacts of anthropogenic activities on benthic ecosystems (Castro-Jiménez et al., 2021; Alp and Cucherousset, 2022). Stable isotope analysis (hereafter defined as SIA) is a useful tool for investigating the trophic interactions within marine assemblages (Boecklen et al., 2011; Fanelli et al., 2015) and the isotopic analysis of the niche size (quantified as convex hull, standard ellipse area, or kernel utilization density; Jackson et al., 2011; Eckrich et al., 2020; Bada et al., 2022) has been also applied to assess food web response to different stressors, including chemical pollution (Hogsden and Harding, 2012; Alp and Cucherousset, 2022).
In the present study, we assessed the effects of passive restoration (i.e., unassisted, or spontaneous recovery following cessation of anthropogenic impacts; Perrow and Davy, 2002; Jones et al., 2018; Fraschetti et al., 2021) on benthic biodiversity and food-web functioning in the SNI of Falconara Marittima (Northwest Adriatic Sea, Mediterranean Sea), by comparing taxa richness, biomass and diversity of macrofauna and their food-web structure within and outside the SNI. Results of this study expand the knowledge on the current health status and resilience of benthic ecosystems after the cessation of industrial activities.
The study area is located on the western coast of the central Adriatic Sea (Mediterranean Sea, Figure 1), in Falconara Marittima (Italy).
Figure 1 Study area and location of Control (C1 and C2= transects to the north of the SNI area, and C3= transect to the south of the SNI area) and impact (M1, M2, and M3) transects.
The marine area of the SNI of Falconara Marittima has an extension of 1200 ha and is characterized by the presence of the abandoned “Montedison SPA” and the still-active “API SPA” Oil Refinery. In addition, the SNI includes the mouth of the Esino River. Our investigation has been carried out in 2019 in front of the former Montedison industrial plant (Figure 1). This company started its production of superphosphate in 1919, then in 1944 the plant was acquired by the Montecatini Company and used as a warehouse by the British Royal Army Service Corps, and finally, from 1966 to 1990 (decommissioning year) the chemical pole has produced fertilizers using pyrite and phosphorous, which have been reported to contaminate soil and groundwater of the surrounding area, together with heavy metals, fluorides, hydrocarbons and PAHs (Regione Marche, 2009). The SNI of Falconara Marittima was declared in 2002 and the coastal area was confined and included in the high-risk area in 2003.
Three transects (defined as M1, M2, and M3) were investigated in the SNI area in front of the Montedison plant, and three transects (C1, C2, and C3) were selected outside the SNI, two transects to the north (C1 and C2) of the SNI area, and one to the south (C3). Each transect, perpendicular to the coastline, included 6 stations located at 3, 4, 5, 6, 10, and 12 m depth (see SOM Table S1). In each station, three independent replicates of sediment samples were collected onboard the R/V Actea with a Van Veen grab of 40 x 20 cm (surface of 0.08 m2). Sediment samples were sieved on board with a 500 µm mesh-size sieve to retain the macrofauna organisms. Each sample was fixed in 70% ethanol solution and stored at 4°C.
At all sampling stations, water temperature and salinity were measured using a CTD probe (SBE19 equipped with additional sensors for O2, fluorescence, turbidity, and pH911-plus sensor). Grain size and contaminants concentration (C > 12 and C< 12 aliphatic hydrocarbons, PAHs, PCBs, heavy metals, and metalloids) as well as phytopigments and biochemical components of the sedimentary organic matter contextually analyzed with macrofauna samples have been previously reported (Corinaldesi et al., 2022) and summarized in Supplemental Online Material.
To analyze the main current regime, a 1MHz Nortek ASC AWAC Acoustic Profiler, deployed at 1.8 km far from the coast (13.36E, 43.66N, close to the M3 transect in the SNI area) from February 13 to April 14, 2020, was used. The current meter was placed at 0.5 m from the bottom and the currents were monitored for 10 minutes at intervals of 30 minutes over 4 depth cells with a 2 m vertical spacing. To avoid the side lobe echo near the sea surface the topmost cell was removed. To avoid the side lobe interference, the closest cell to the sea surface was removed. The Integrate Marine Observing System toolbox (IMOS toolbox by Australian National Mooring Network and Australian Ocean Data Network) was used for data parsing including quality control to remove spikes and outliers. Data processing was performed using the software Storm (Nortek) and hourly currents were computed from the 30 min data by averaging three sequential half-hour observations in the time range ±30 min around each hour. The data are represented in the Supplementary Material (Figures S1, S2).
Samples of macrofauna were sorted under a stereomicroscope (x10 and x40), and organisms were identified at the lowest taxonomic level possible and counted. Specimens were then oven-dried at 60°C for 24 hours and weighted for dry biomass estimates. Both abundance (ind/m2) and biomass (g DW/m2) were obtained by standardizing abundance and biomass data to the grab surface. Polychetes and molluscs (many of them represented by juveniles) were mostly identified to the genus and family levels, while Crustacea and Echinodermata were identified to the species level. Therefore, since part of the samples was identified at the family or genus level (see the complete list of all taxa identified in Table S1), the level of family was used for the calculations of the various metrics. It is commonly acknowledged that family-level identification is sufficient to assess the impacts on marine soft bottom assemblages in routine monitoring programs (e.g., Somerfield and Clarke, 1995; Lampadariou et al., 2005; Chessman et al., 2009; Dauvin et al., 2016; Romano et al., 2016; Pitacco et al., 2019). Some authors even concluded that environmental quality assessments conducted at the family and genus levels are even more accurate than those at conducted the species level (Checon and Amaral, 2017). Families are also likely to be a good predictor of species-level variation related to natural environmental gradients (De Biasi et al., 2003; Dethier and Schoch, 2006). This is because there is a considerable degree of redundancy in species-level data (Clarke and Warwick, 1998; Olsgard et al., 1998) and thus, the analysis at higher taxonomic levels might act to reduce the confounding effects of species-level responses to natural variation and allow the effects of anthropogenic disturbance to be more clearly detected (Dauvin et al., 2003). Taxa richness was estimated by the DIVERSE routine in PRIMER7 (Clarke and Gorley, 2006). As species and higher taxa richness strongly correlate with each other (Volvenko et al., 2023), species richness can be assessed using genus, family, or order richness and here taxa richness was calculated using the level of family, as in other studies (Cooper and Barry, 2017; De Smet et al., 2017; Bevilacqua et al., 2018). The AZTI Marine Biotic Index (AMBI, Borja et al., 2000) and Multivariate AMBI indices (M-AMBI, Carvalho et al., 2006; Dauvin and Ruellet, 2007; Cai et al., 2014; Tweedley et al., 2015) were calculated to assess the environmental status of the different communities according to condition, transects, and depths. Both indices were estimated through the AMBI software, freely available at https://ambi.azti.es/download/. They were both robust to aggregation of macrofaunal data to the family level (Forde et al., 2013).
The most abundant taxa identified in the investigated area were used for stable isotope analyses. Selected taxa were oven-dried for 24 hours at 60°C. Dried samples were converted into a fine powder with a mortar and pestle. For each taxon, two or three replicates (when possible) were weighed (ca 0.3 - 1.3 mg) and placed into tin capsules. Since inorganic carbon could lead to an increase of δ13C, because it is isotopically heavier than most carbon of organic origin and could reflect the isotopic signature of environmental carbon (Schlacher and Connolly, 2014), samples containing carbonates (i.e., spicules or exoskeleton) were acidified with HCl 1M, by adding it drop by drop to the sample until bubble cessation. Samples for the analysis of N were not acidified, as several studies demonstrated that the acidification procedure can alter the N signal (Kolasinski et al., 2008). After that, samples were oven-dried again for 24 hours at 60°C. Samples were analyzed through an elemental analyser (Thermo Flash EA 1112) for the determination of total carbon and nitrogen, coupled through a continuous flux to an isotope-ratio mass spectrometer (IRMS, Thermo Delta Plus XP) for δ13C and δ15N at the Laboratory of Stable Isotopes Ecology of the University of Palermo (Italy). A stable isotope ratio was expressed, in relation to international standards (atmospheric N2 and Vienna PeeDee Belemnite for δ15N and δ13C, respectively), as:
where R = 13C/12C or 15N/14N. Analytical precision based on standard deviations of internal standards (International Atomic Energy Agency IAEA-CH-6; IAEA-NO-3; IAEA-N-2) ranged from 0.10 to 0.19‰ for δ13C and 0.02 to 0.08‰ for δ15N.
To test for differences among all variables investigated between SNI and Control transects and among water depths, uni- and multivariate distance-based permutational analyses of variance were applied (PERMANOVA; Anderson et al., 2008). All the statistical analyses were carried out considering 3 factors as sources of variance: Condition (fixed, 2 levels: SNI vs. Control); Transect (random and nested in Condition, 3 levels for each Condition: northern Control C1, northern Control C2, southern Control C3, SNI M1, SNI M2, and SNI M3) and Depth (fixed and crossed, 6 levels: 3, 4, 5, 6, 10, 12 m). PERMANOVA was run on the Euclidean distance matrix for univariate data (abundance, biomass and taxa richness) and on the Bray-Curtis resemblance matrix of 4th root-transformed abundance data for multivariate data (taxa composition), with 9999 permutations and with “permutation of residuals under a reduced model” as permutation method, significant p-values were set at p<0.05. A CAP analysis (Canonical Analysis of Principal Coordinates, Anderson and Willis, 2003) was run on the factor(s) found to be significant according to PERMANOVA results to visualize the pattern found. A SIMPER analysis was then performed to identify the species/taxa that most contributed to the similarity/dissimilarity in each condition and transect, and that was responsible for the dissimilarities. SIMPER test was run using Bray-Curtis similarity on the 4th root-transformed abundance square matrix with a cut-off percentage for low contribution at 60%.
Multivariate multiple regression analysis (Distance-based linear models, DistLM) was performed to determine whether macrofaunal taxonomic composition (used as the response variable) was potentially influenced by environmental characteristics (grain size, depth, temperature, and salinity), presence of different contaminants (PAHs, C > 12 and C< 12 aliphatic hydrocarbons, PCB, heavy metals, and metalloids such as As, Cu, Hg, Ni, Cr) and/or trophic resources (phytopigments, biopolymeric C concentrations -BPC) (all abiotic data were tested for differences by univariate PERMANOVA using the same design described above). Before running the subsequent analyses, the mean-effects range medium-quotient (m-ERM-q quotient) was calculated for both heavy metals and PAHs based on the available ERM values for these contaminants (Kowalska et al., 2018, see also Corinaldesi et al., 2022). Then variables were tested for collinearity, excluding values >0.6 according to the number of samples (Dormann et al., 2013). The DistLM was run using the “step-wise” selection procedure and the AIC (Akaike Information Criterion) model selection criterion (Anderson et al., 2008). In the model, only the concentration of contaminants present in the first 10 cm depth of the sediments was used since most of the macrofaunal organisms usually inhabit the shallowest layer of sediment with abundance decreasing with depth (Janas et al., 2019).
For the Stable Isotope Analysis (SIA), since the biomass obtained was not sufficient to allow the analysis of each taxon/species per transect and depth (the minimum required biomass for SIA is 1 mg of dry weight per replicate), for each taxon/species specimens, from transects within each condition, were merged and depths were unified as follows: depth 1 comprises samples from 3 m, depth 2 from 4-6 m and depth 3 from 10-12 m. Additionally, each taxon was assigned to a trophic guild (TG), based on literature evidence for that specific species/taxon or a similar taxon (i.e. co-generic/same family, etc.), as follows: Ca (carnivore), OS (opportunistic scavenger), DF (deposit feeder) and SF (suspension feeder). Then, uni- and multivariate PERMANOVA tests were conducted on a modified experimental design from the one used for community analysis, with three crossed factors, Condition (fixed, 2 levels: SNI and Control), Depth (fixed, 3 levels: 1, 2 and 3) and TG (fixed, 4 levels: Ca, OS, DF, and SF).
To reveal key aspects of trophic structure and to detect any effect of contamination across the food web, niche-based metrics (traditional convex Hull - TA, standard ellipse area - SEA, containing ca. 40% of the data and representing the core isotopic niche, and standard ellipse areas corrected for small sample size – SEAc, Layman, et al., 2007; Jackson et al., 2011) were also estimated through the R routine SIBER (Stable Isotope Bayesian Ellipses in R; Jackson et al., 2011).
Finally, to provide an overview of the trophic structure of the macrofauna assemblage of the area, stable isotope data were merged at the taxon level and a cluster analysis and a nMDS were run to visualize the separation of species/taxon according to the TG. The trophic level (TL) of each taxon was calculated according to Post (2002) as TL=[(δ 15Ni- δ15Nbase)/TEF]+λ, where δ15Ni is the mean δ15N value of the i-species, δ15Nbase is the δ15N of the species used as a baseline, here Tellina spp., a primary consumer, TEF is the trophic enrichment factor here set at 2.56 (Vanderklift and Ponsard, 2003) and Λ is the trophic level of the species used as a baseline, that is 2, being a primary consumer. Uni- and multivariate PERMANOVA, pairwise tests, CAP, DistLM, Draftsman plot, and SIMPER tests were carried out using the routines included in the software PRIMER 7+ (Clarke and Gorley, 2006).
Results of the environmental variables, chemical contaminants, and biochemical components of the sediment organic matter in the investigated sediments have been reported by Corinaldesi et al. (2022) and are briefly presented in Table S2. To summarize, the sedimentary grain size was generally characterized by a high percentage of sand (from 39 to 99%) within a 10-m depth. An increase of the silt-clay fraction (15–45%) was observed between 10 and 12-m depth, being such differences significant (Table S3). However, the gravel fraction was generally very low in all the stations investigated (<5%). The temperature of the bottom water of the Control transects ranged from 19.62 to 21.27°C, whereas in the SNI transects from 19.93 to 20.97°C. Salinity in the Control and SNI transects ranged from 37.59 to 37.92 and from 37.38 to 37.75, respectively, with slightly, though significant, higher values at 10-12 m (Table S3). Steady currents showed a mean speed of 13 cms-1 flowing southeasts, notwithstanding the proximity to the coast (see Figures S1-2).
Heavy metal and metalloid concentrations varied among the different transects investigated without a clear pattern. However, the highest concentrations of Hg, Cu, Cd, and As were observed in the sediment of the station at 12 m depth in the M3 transect, being such differences almost significant only for Cu (Table S3). The highest concentrations of aliphatic hydrocarbons C > 12 were observed in some stations of the SNI transects, especially at 12 m depth in the M1 transect (4,000 mg kg–1) as well as the total PAH concentrations at 3 m (51.98 μg kg–1). The concentrations of C< 12 and C > 12 hydrocarbons in surface sediments (0-10 cm) were similar or higher than in the sub-superficial layers (10–20 cm). PCB concentrations showed values much lower than thresholds established by the national (D. M. 152/2006, 173/2016) and international laws (e.g., Canadian Council of Ministers of the Environment, 2002). However, all the observed differences related to metals and metalloids, PAHs and hydrocarbons were not significant (Table S3).
The biochemical components of the sedimentary organic matter significantly changed with water-column depth both in the SNI and Control transects. In addition, the concentrations of phytopigments were significantly higher in the C3 Control transect (4.76 ± 0.83 μg g–1) than in the other transects, where values were very similar. Protein concentrations were on average lower in M1 and M2 transects (0.44 ± 0.09 – 0.52 ± 0.6 mg g–1) than in the other transects, while carbohydrate and lipid concentrations were significantly higher in the C3 transects. Overall, the highest average values of BPC concentrations were observed in the Control transects, especially in C3 (0.82 ± 0.06 mgC g–1) compared to those observed within the SNI area. In both SNI and Control transects BPC concentrations were on average 0.6 mgC g−1, while the contribution of autotrophic C to BPC was ca. 23.5%.
Overall, a total of 69 taxa, 53 families (22 polychaetes, 13 molluscans, 16 crustaceans and 2 echinoderms), and 18 species were identified (see Table S1). Juvenile bivalves were the most abundant group at all transects, especially at 3-4 m depths (Figure S3). Spionid polychaetes and venerid bivalves were dominant in the whole area (Figure S3). Macrofaunal abundances along the SNI transects ranged, on average, from 1649.4 ± 337.4 to 2016.7 ± 296.2 ind. m–2, in M2 and M1, respectively, and along the Control transects from 1799.4 ± 338.5 to 2278.6 ± 465.1 ind. m–2, C1 and in C3, respectively (Figure 2A). In general, macrofaunal biomass was higher at 4-6-m depth than at 3 m and 10-12 m depth (Figure 2B), and in the Control transects varied from 37.7 ± 7.2 to 58.4± 2.6 g m–2 (in C2 and C1, respectively) and in the SNI transects from 44.7 ± 7.9 to 56.9 ± 7.3 g m–2 (in M3 and M2 transects, respectively; Figure 2B). Taxa richness (indicated as SR) was similar in the Control (SR=17.42 ± 1) and SNI (SR=16.68 ± 0.92) transects (Figure 2C), being on average higher at 5-m depth in the Control than in the SNI transects (57.7 ± 1.5 in the Control vs. 50.3 ± 2.3 in the SNI transects) (Figure 2C).
Figure 2 Bathymetric distribution (from 3 m to 10 m depths) of macrofaunal abundance, biomass, richness of taxa, and community structure in the sediments of Control (C1, C2, and C3) and SNI (M1, M2, and M3) transects (mean ± ES for abundance and biomass). (A) Macrofauna abundances in the different transects and depths, (B) biomasses in the different transects and depths; (C) richness of taxa in the different stations and transects, and (D) community structure in the different transects and depths. The group “Others” contains Echinodermata, Phoronida, and Nemertea. The PERMANOVA analyses showed no significant effect of the factor “Condition” on macrofaunal abundance, biomass, and richness of taxa (Table S4A). A significant effect of the interaction “Transect (Condition) × Depth” was observed on macrofaunal abundance and biomass, indicating differences among transects and depths within each area (Control and SNI). Biomass significantly varied between 3 m and 4 m depths (Table S4B), while analyzing changes between pairs of adjacent depths (i.e., 3 vs. 4 m, 4 vs. 5 m and so on) within the Control or SNI condition, significant differences were highlighted (Table S4C). Comparing biomass values among transects within the Control or SNI conditions, significant differences were observed within pairs of Control transects (C1 vs. C2 and C2 vs. C3; Table S3), and between pairs of SNI transects. Taxa richness significantly varied with the factor depth (p< 0.001). In particular, the pairwise tests revealed significant differences among the two extremes of the depth range explored (i.e., 3 m vs. 12 m), whereas no significant differences were present when comparing adjacent depths (Table S4B).
Macrofaunal assemblages were typically dominated by polychaetes, crustaceans, and molluscs (Figure 2D). PERMANOVA did not show any significant difference for the factor “Condition” (i.e., between assemblages at SNI and Control transects; Table S5A), while significant differences were observed among all Control transects, and M1 vs. M3 transects of the SNI. In addition, differences were found across bathymetric gradients (3 m vs. 4-m depths and 6 m vs. 10-m depths; Tables S5A–D). Although the PERMANOVA output indicated the significant interaction “condition x depth” (Table S5A), the related pairwise test did not provide significant differences between Control and SNI transects at any depths (Table S5C).
The CAP plot carried out on the factor “Depth” highlighted a clear horse-shoe distribution of the samples as a function of the depth (Figure 3, left), while CAP run on the factor “Transect” separated partially the SNI from the Control transects (Figure 3, right). A clear segregation was evident for some samples of the C1 and C3 transects along the x-axis.
Figure 3 CAP plot representing the separation of samples collected in the SNI and Control transects, as a function of depth (left) and transect (right).
SIMPER analyses showed similar average dissimilarities among the composition of macrofaunal assemblages at the different depths of Control (on average, 38.40%, range 32.86 to 47.32%) or SNI (on average 36.73, range 30.53 to 43.83%) transects (Table S6), separately, with the highest dissimilarity observed at 12-m depth in both cases. A higher average dissimilarity was found comparing the Control and SNI transects in macrofaunal assemblage compositions, ranging from 52.2 to 64.0% (at 12 and 5-m depth, respectively).
The AMBI and M-AMBI indices revealed a generally good environmental status for the whole area, with all the stations classified as undisturbed or slightly disturbed, according to the AMBI index (Figure S4), or defined in a high-good ecological status, according to the M-AMBI index (Figure S5).
The DISTLM model was carried out by excluding depth and temperature from the dataset due to their significant correlation with other variables. DISTLM results for the whole dataset (e.g., SNI and Control transects together) indicated that the percentage of sand was the main explanatory variable accounting for 26% of the total variance (AIC=102.66): the model run only considering Control transects provided similar results, with salinity and % sand as the main drivers contributing together for 74% of the total variance. In the SNI transects the main driver was salinity, which explains alone 48% of the total variance (AIC=46.97) (Table S7).
The most abundant taxa, according to the previous results and occurring at both SNI and Control areas, selected for the SIA were four taxa of bivalves (Chamelea gallina, Tellina spp., Donax spp., and Spisula subtruncata), two taxa of gastropods (Tritia neritea and Tritia spp.), two crustacean taxa (the amphipod Ampelisca sp. and the tanaid Apseudopsis latreillii), one species and three families of polychaetes (Owenia fusiformis and Nephthyidae, Flabelligeridae, Spionidae). Additionally, other two non-indigenous bivalves were found and analyzed for SIA, Ruditapes philippinarumn and Anadara transversa.
Overall, the highest and the lowest δ13C values were recorded for the two species of bivalves, i.e., Tellina spp. (14.02‰ ± 0.45) and Chamelea gallina (-19.32‰ ± 0.18), respectively, both from the SNI area. The highest δ15N values were detected in nephtyid polychaetes (10.74‰ ± 0.36) from the SNI and the lowest in Tellina spp. (4.29‰ ± 0.12) from the Control area, respectively (Table S8). The PERMANOVA analysis performed on both δ13C and δ15N, evidenced significant differences for factor “depth” and “trophic group (TG)” (Table S9A), with communities located at intermediate depths (4-6 m) showing greater δ15N values (Table S9B and Figure 4A) than those inhabiting deeper bottoms (10-12 m), but no differences occurred for the factor “Condition”. The pairwise comparisons for trophic groups highlighted a significant separation between carnivores and suspension feeders, deposit feeders and suspension feeders, and suspension feeders and opportunistic scavengers for all the combinations tested (i.e., δ13C-δ15N and δ15N and δ13C separately) (Table S9B). As far as δ15N values are concerned, significant differences were observed also for the factor “Condition”, specifically at the trophic group level between Control and SNI for suspension feeders and opportunistic scavengers (Table S9, Figure 4B), being the δ15N values of suspension feeders lower and that of opportunistic scavengers higher in the SNI than in the Control transects. Conversely, δ13C values of the different TG were similar in the SNI and Control areas (Figure 4C).
Figure 4 Box plots of the (A) mean λ15N values of the macrofauna most representative species in the whole area according to depth, (B) mean λ15N, and (C) λ13C values of the different trophic groups in Control (C) and SNI area. Depth: 1 = 1-3 m; 2 = 4-6 m, 3 = 10-12 m; TG: Ca, carnivore; OS, opportunistic scavenger; DF, deposit feeder; SF, suspension feeder.
Standard ellipses showed that the two macrofauna communities (at the Control and SNI area) have similar δ13C and δ15N variability (Table 1), with almost complete overlap of standard ellipses (SEAc) (Figure 5).
Table 1 Standard ellipse area (SEA), standard ellipse area corrected for sample size (SEAc), and Total Convex Hull for Control and SNI macrofauna collected in the study area.
Figure 5 δ13C - δ15N scatterplot with standard ellipses corrected for small sample size population (SEAC) of the two macrofauna communities (p interval=0.4) in the study area.
Both nMDS and cluster analyses, carried out on δ13C and δ15N values merged by taxon, irrespective of depth and condition showed a food web organized into three trophic levels, with carnivore polychaetes (Nephthyidae) and two taxa of scavenger gastropods (Tritia neritea and Tritia spp.) occupying the highest trophic level (TL=4) and all suspension and deposit feeders distributed between TL 2 and 3, and clustered together (Figure 6A). A further separation within TL 2 occurred between DF which generally showed higher δ13C and δ15N values and SF (Figure 6B).
Figure 6 Output of cluster (A) and nMDS (B) analyses carried out on the average values of δ15N and δ13C of taxa collected in the whole area for the factor trophic group (TG). Ca, carnivore; OS, opportunistic scavenger; DF, deposit feeder; SF, suspension feeder.
Recent insights obtained at the SNI of Falconara Marittima (Corinaldesi et al., 2022) showed that meiofaunal abundance, biomass and community structure changed among stations regardless of the distance from the abandoned chemical industrial plant, which was dismissed three decades before the investigation, while all human activities ceased with the SNI establishment in 2002. The concentrations of heavy-metals and organic contaminants in the sediments of the investigated area were, indeed, generally lower than those expected to induce harmful biological effects (Long et al., 1995). However, studies on macrobenthic communities reported that the full recovery of coastal marine ecosystems from inorganic and organic pollution may be slow, and can take more than a decade (Josefson et al., 2008; Schwing et al., 2020) or even more for attainment of the composition and diversity of original biotic components (especially considering short-lived and high-turnover biological component; Borja et al., 2010). The present study in the SNI of Falconara Marittima expands information on the effect of historical industrial contaminations on macrobenthic communities and their food-web structure. Since the investigated area of the SNI was interdicted to any anthropogenic activities since 2002, this study provides insights into the responses of benthic ecosystems to passive restoration.
We found that macrofaunal abundance and biomass within the SNI were similar to the values found in the Control areas. Also, the cumulative richness of taxa was similar in the SNI and the Control areas. Further confirmation of the lack of negative impacts from the industrial activities on macrofaunal assemblages was obtained by the analysis of their taxonomic composition, which showed similar bathymetric gradients in the area facing the decommissioned industrial plant and the Control sediments. Therefore, our results indicate that the entire area, including both the SNI and the Control sites, was characterized by a homogeneous composition of the macrofaunal assemblage, mostly represented by opportunistic and tolerant taxa which are typically dominant in the coastal area of the North and Central Adriatic Sea (Simonini et al., 2009; Spagnolo et al., 2019). In particular, we found that all the investigated area was dominated by venerid bivalves (mostly Chamelea gallina), characteristic of the well-sorted fine sands, and widely reported in the Adriatic Sea from ca. 4-5 m down to 10-12 m depth (Romanelli et al., 2009). Among polychaetes the most abundant taxa were represented by spionids and the species Owenia fusiformis, which are indicators of impacted environments often present near river mouths (Putro, 2007; Pinto et al., 2009; Sivadas et al., 2010) and commonly found in the North and Central Adriatic Sea (Semprucci et al., 2010; Frontalini et al., 2011; Nasi et al., 2020). Since these two taxa were equally distributed within the Control and SNI transects, we can argue that the whole area was characterized by a similar benthic macrofauna diversity.
The most common crustacean family found was Apseudidae, represented exclusively by the species Apseudopsis latreillii (Milne-Edwards) (Table S1), a sensitive species to sewage discharge (de-la-Ossa-Carretero et al., 2010). These results suggest the lack of impacts due to anthropogenic contamination at present. Accordingly, the AMBI and M-AMBI indices indicated a homogeneous environmental status within and outside the former industrialized area with all the investigated stations showing a good/high ecological status. These results are consistent with those previously reported in the same area based on m-ERM-q (Effects-Range-Median; Long et al., 1995) values for heavy metals and PAHs, which indicated low environmental risk levels, with the only exception of a station in the SNI site very close to a stream outfall, classified as at high risk (Corinaldesi et al., 2022). As further support of the lack of differences between the SNI and the Control area’s communities, the analysis of drivers revealed that the macrofauna composition in the whole area was mostly influenced by sedimentary characteristics (the % of sand in the sediments) and thermohaline conditions (salinity and temperature, which were highly-correlated), while neither hydrocarbons nor heavy metals were found to be the main controlling factors of macrofauna communities at the Control or SNI transects. Previous studies revealed that changes in salinity and temperature may influence benthic communities (Semprucci et al., 2010). Consistently, in the present study, the changes in the thermohaline conditions observed in both the Control and SNI areas (variation between the maximum and minimum salinity and temperature values, 0.34 PSU and 1.58°C and 0.37 PSU and 1.04°C; respectively), which were probably attributed to the presence of fresh water inputs (Esino river and the Rubiano torrent), could explain the significant role of these factors in shaping the composition of macrofauna assemblages.
From the analysis of the benthic food web, we observed the typical structure of benthic macrofauna inhabiting the Mediterranean soft bottoms (Romano et al., 2016), which is organized into three main trophic levels, from suspension feeders (located at trophic level-TL2) to carnivores and opportunistic scavengers (TL 4). The similar isotopic values (both δ15N and δ13C together and separately) found in the common macrofaunal taxa in the SNI and Control transects further support the finding of the absence of a specific impact on the macrofaunal assemblages due to the chemical plant of the Falconara SNI. The last industrial activity of the chemical plant was the production of fertilizers, which are known to provoke an increase in δ15N values in marine sediments and in turn in the benthic fauna living therein (Alonso-Hernández et al., 2017 and references cited), while the fossil fuel emission necessary for the industrial plant’s functioning has been reported to determine a λ13C decrease (Rumolo et al., 2011 and reference cited). However, we did not find 13C-depletion and 15N-enrichment in the analyzed taxa of the SNI and Control areas. The significant differences observed in the δ13C and δ15N values between specimens inhabiting intermediate depths (4-6 m) and deeper bottoms (10-12 m) can be attributed to the gradual decrease of terrigenous inputs offshore, as observed in other coastal areas of the Mediterranean influenced by river runoff (Darnaude et al., 2004). When only δ15N values were considered, some significant differences for suspension feeders and, to a lesser extent, for scavengers between SNI and Control transects were observed. Since the majority of the investigated samples from the Control sites were collected south of the mouth of the Esino River (C3 transect), changes in δ15N values could be attributed to local environmental variability of the nitrogen baseline related to river inputs (Galloway et al., 2004). River outflows usually increase the nitrogen load in the coastal environment (Boyer et al., 2006), which could have caused in turn an enrichment in the δ15N levels in the species living close to the river mouth (Fanelli et al., 2013). However, since the food web structure of the macrofauna assemblages at the two investigated sites (Control vs. SNI) was similar, all the differences concerning the benthic food web of macrofauna assemblages observed in the whole area are mainly due to natural variability. A similar trophic structure without any clear signs of the effects of contamination was also revealed by stable isotope-based community metrics, with Standard Ellipse Areas (as a proxy of isotopic niche width) showing similar values in SNI and Control benthic communities, and almost complete overlapping, suggesting the lack of anthropogenic impacts on the food web attributes (Alp and Cucherousset, 2022).
Overall, our findings indicate that although few stations in the SNI showed a certain degree of organic contamination, no stations can be defined as having a low-quality ecological status. We argue that the dispersion of contaminants due to the West Adriatic current, which flows along the Italian coast southward (Wang and Pinardi, 2002; Bignami et al., 2007), which can be intensified by Bora wind events (Paklar et al., 2001; Cushman-Roisin et al., 2013), can have contributed to the passive restoration of the area. The current measurement collected during the sampling period confirmed the expected pattern (Figures S1, S2) of a flowing southward current. These high-energy hydrodynamic conditions allow the biocoenosis of Fine Well-Sorted Sands or FWSS (SFBC, Sables Fins Bien Calibres in French, Pérès and Picard, 1964) to take place in the coastal area of the whole Region (Semprucci et al., 2010). The FWSS biocoenosis is recognized to be resistant and resilient under an array of human pressures (Dauvin et al., 2017) and the highly- hydrodynamic conditions likely prevent from accumulating contaminants, also when they are associated with organic matter.
Other factors besides the hydrodynamic regime could have concurred to the natural recovery such as microbial degradation (Röling and Van Verseveld, 2002; Acosta-González and Marqués, 2016; Dell’Anno et al., 2021) and bioturbation of opportunistic or tolerant burrowing macrofauna (including for example Spionidae, Owenidae and Paraonidae among polychaetes, and Photidae among crustaceans; Dauvin et al., 2017) that can adapt to human-impacted conditions (Fabi et al., 2009; Simonini et al., 2009). Bioturbation may represent a nature-based remediation strategy as it can improve sediment oxygenation, thus promoting resilience to anthropogenic impacts (Lam-Gordillo et al., 2022).
As further evidence of the good health status of the SNI area, we also discovered habitats with high ecological value, such as sabellarid bioconstructions (Franzitta et al., 2022 and Figure S6). These habitats are known to provide important ecosystem services such as sediment stabilization and mitigation of coastal erosion (Desroy et al., 2011; Lisco et al., 2017; Jones et al., 2020) and supporting coastal biodiversity (Bertocci et al., 2017; Gravina et al., 2018; Ingrosso et al., 2018; Bonifazi et al., 2019) and ecosystem functioning (Jones et al., 2020; Muller et al., 2021). Sabellaria spinulosa reefs are also included in the Habitat “Reefs” (code 1170) and listed under Annex I of the Habitats Directive 92/42/EEC.
Overall, this study expands knowledge on the health of the coastal benthic ecosystems affected by historical contaminations such as the SNI of Falconara Marittima, where the lack of a significant impact on macrofauna assemblages and food-web functioning, corroborates information obtained from the previous study on meiofauna (Corinaldesi et al., 2022). This two-pronged approach was used for the first time in a historically polluted marine site, confirming that the joint assessment of meio- and macrofauna are a robust tool to assess the environmental quality of marine ecosystems, as previously reported for others coastal environments (Fanelli et al., 2022; Magni et al., 2022) subjected to natural or anthropogenic impacts.
The present investigation reveals that after decades from the decommissioning of the industrial chemical plant located in the Falconara Marittima SNI, the coastal ecosystem recovery was achieved. It is likely that healthy conditions recovered even earlier but, due to the lack of previous studies in the area, this is difficult to ascertain. Our findings support the evidence that historically impacted marine coastal ecosystems and identified as “problem areas” under the law, can passively recover if characterized by highly performing self-purification conditions (i.e., hydrodynamic features) and that OECMs, by limiting marine resource exploitation and human pressures on ecosystems, can be useful not only to conserve marine biodiversity but also to favor the resilience of impacted ecosystems. At the same time, it is urgently necessary to better understand the passive ecological restoration times of historically polluted coastal areas in order to adopt management plans tailored to their specific characteristics, and eventually consider the implementation of an active restoration if these times are too long.
The original contributions presented in the study are included in the article/Supplementary Material. Further inquiries can be directed to the corresponding author.
CC, AD’A, and RD conceived the study. CC, ML, LM, and AD’A defined sampling strategy. ML, MM, and AC participated in the sampling activity. MM and ML performed laboratory analyses. EF, SB, and CG supervised laboratory activities. EF, CC, EN, CG, SB, SV, AD’A, PF, FM, and LM contributed to data elaboration and interpretation. EF, SV, and EN contributed to data visualization. EF, EN, and CC drafted the first version of the manuscript. All authors contributed to the article and approved the submitted version.
This study has been supported by the project BIOBLUTECH “Blue Biotechnologies for restoring marine ecosystems of the contaminated Site of National Interest (SIN) ex Montedison (Falconara M.ma), ID 9204 supported by Cariverona Foundation. This work was also supported by the H2020 project MERCES (GA N. 689518) and by the European Union’s EMFF programme project AFRIMED (GA EASME/EMFF/2017/1.2.1.12/S4/01/SI2.789059).
We thank Dr. Lisa Pola for her support in the analyses of macrofauna, and Dr. Giulia Lucia for her participation in the sampling and laboratory activities. The authors also thank three reviewers whose comments helped to improve the first version of the paper.
The authors declare that the research was conducted in the absence of any commercial or financial relationships that could be construed as a potential conflict of interest.
All claims expressed in this article are solely those of the authors and do not necessarily represent those of their affiliated organizations, or those of the publisher, the editors and the reviewers. Any product that may be evaluated in this article, or claim that may be made by its manufacturer, is not guaranteed or endorsed by the publisher.
The Supplementary Material for this article can be found online at: https://www.frontiersin.org/articles/10.3389/fmars.2023.1199654/full#supplementary-material
Acosta-González A., Marqués S. (2016). Bacterial diversity in oil-polluted marine coastal sediments. Curr. Opin. Biotechnol. 38, 24–32. doi: 10.1016/j.copbio.2015.12.010
Alonso-Hernández C. M., Garcia-Moya A., Tolosa I., Diaz-Asencio M., Corcho-Alvarado J. A., Morera-Gomez Y., et al. (2017). Tracing organic matter sources in a tropical lagoon of the Caribbean Sea. Continental Shelf Research 148, 53–63. doi: 10.1016/j.csr.2017.08.001
Alp M., Cucherousset J. (2022). Food webs speak of human impact: Using stable isotope-based tools to measure ecological consequences of environmental change. Food Webs 30, e00218. doi: 10.1016/j.fooweb.2021.e00218
Andersen J. H., Harvey T., Murray C., Green N., Reker J. (2019). Contaminants in Europe’s Seas. Moving Towards a Clean, Non-Toxic Marine Environment (Copenhagen: European Environment Agency).
Anderson M. J., Gorley R. N., Clarke K. R. (2008). PERMANOVA + for PRIMER: Guide to Software and Statistical Methods. Primer-E (Plymouth, England).
Anderson M. J., Willis T. J. (2003). Canonical analysis of principal coordinates: A useful method of constrained ordination for ecology. Ecology 84, 511–525. doi: 10.1890/0012-9658(2003)084[0511:CAOPCA]2.0.CO;2
Ausili A., Bergamin L., Romano E. (2020). Environmental status of Italian coastal marine areas affected by long history of contamination. Front. Environ. Sci. 8. doi: 10.3389/fenvs.2020.00034
Aylagas E., Borja Á., Tangherlini M., Dell’Anno A., Corinaldesi C., Michell C. T., et al. (2017). A bacterial community-based index to assess the ecological status of estuarine and coastal environments. Mar. pollut. Bull. 114, 679–688. doi: 10.1016/j.marpolbul.2016.10.050
Bada N., Da Ros Z., Rindi F., Busi S., Azzurro E., Derbal F., et al. (2022). Seasonal trophic ecology of the invasive crab Percnon gibbesi (Brachyura, Plagusiidae) in the southwestern Mediterranean: Insights from stomach contents and stable isotope analyses. Mar. Environ. Res. 173, 105513. doi: 10.1016/j.marenvres.2021.105513
Barbier E. B. (2017). Marine ecosystem services. Curr. Biol. 27, R507–R510. doi: 10.1016/j.cub.2017.03.020
Bertocci I., Badalamenti F., Lo Brutto S., Mikac B., Pipitone C., Schimmenti E., et al. (2017). Reducing the data-deficiency of threatened European habitats: Spatial variation of sabellariid worm reefs and associated fauna in the Sicily Channel, Mediterranean Sea. Mar. Environ. Res. 130, 325–337. doi: 10.1016/j.marenvres.2017.08.008
Bertocci I., Dell’Anno A., Musco L., Gambi C., Saggiomo V., Cannavacciuolo M., et al. (2019). Multiple human pressures in coastal habitats: variation of meiofaunal assemblages associated with sewage discharge in a post-industrial area. Sci. Total Environ. 655, 1218–1231. doi: 10.1016/j.scitotenv.2018.11.121
Bessa F., Gonçalves S. C., Franco J. N., André J. N., Cunha P. P., Marques J. C. (2014). Temporal changes in macrofauna as response indicator to potential human pressures on sandy beaches. Ecol. Indic. 41, 49–57. doi: 10.1016/j.ecolind.2014.01.023
Bevilacqua S., Ugland K. I., Plicanti A., Scuderi D., Terlizzi A. (2018). An approach based on the total-species accumulation curve and higher taxon richness to estimate realistic upper limits in regional species richness. Ecol. Evol. 8 (1), 405–415. doi: 10.1002/ece3.3570
Bignami F., Sciarra R., Carniel S., Santoleri R. (2007). Variability of Adriatic Sea coastal turbid waters from SeaWiFS imagery. J. Geophys. Res. Ocean. 112(C3). doi: 10.1029/2006JC003518
Boecklen W. J., Yarnes C. T., Cook B. A., James A. C. (2011). On the use of stable isotopes in trophic ecology. Annu. Rev. Ecol. Evol. Syst. 42, 411–440. doi: 10.1146/annurev-ecolsys-102209-144726
Bonifazi A., Lezzi M., Ventura D., Lisco S., Cardone F., Gravina M. F. (2019). Macrofaunal biodiversity associated with different developmental phases of a threatened Mediterranean Sabellaria alveolata (Linnaeus 1767) reef. Marine Environ. Res. 145, 97–111. doi: 10.1016/j.marenvres.2019.02.009
Borja Á., Dauer D. M., Elliott M., Simenstad C. A. (2010). Medium- and long-term recovery of estuarine and coastal ecosystems: patterns, rates and restoration effectiveness. Estuaries Coasts 33 (6), 1249–1260. doi: 10.1007/s12237-010-9347-5
Borja A., Franco J., Pérez V. (2000). A marine Biotic Index to establish the ecological quality of soft-bottom benthos within European estuarine and coastal environments. Mar. pollut. Bull. 40, 1100–1114. doi: 10.1016/S0025-326X(00)00061-8
Boyer E. W., Howarth R. W., Galloway J. N., Dentener F. J., Green P. A., Vörösmarty C. J. (2006). Riverine nitrogen export from the continents to the coasts. Global Biogeochemical Cycles 20(1). doi: 10.1029/2005GB002537
Cai W., Borja Á., Liu L., Meng W., Muxika I., Rodríguez J. G. (2014). Assessing benthic health under multiple human pressures in Bohai Bay (China), using density and biomass in calculating AMBI and M-AMBI. Mar. Ecol. 35, 180–192. doi: 10.1111/maec.12067
Canadian Council of Ministers of the Environment. (2002). Canadian environmental quality guidelines (Vol. 2). Canadian Council of Ministers of the Environment.
Cardinale B. J., Duffy J. E., Gonzalez A., Hooper D. U., Perrings C., Venail P., et al. (2012). Biodiversity loss and its impact on humanity. Nature 486, 59–67. doi: 10.1038/nature11148
Carvalho S., Gaspar M. B., Moura A., Vale C., Antunes P., Gil O., et al. (2006). The use of the marine biotic index AMBI in the assessment of the ecological status of the Óbidos lagoon (Portugal). Mar. pollut. Bull. 52, 1414–1424. doi: 10.1016/j.marpolbul.2006.04.004
Castro-Jiménez J., Bǎnaru D., Chen C. T., Jiménez B., Muñoz-Arnanz J., Deviller G., et al. (2021). Persistent organic pollutants burden, trophic magnification and risk in a pelagic food web from coastal NW mediterranean sea. Environ. Sci. Technol. 55, 9557–9568. doi: 10.1021/acs.est.1c00904
Checon H. H., Amaral A. C. Z. (2017). Taxonomic sufficiency and the influence of rare species on variation partitioning analysis of a polychaete community. Mar. Ecol. 38, e12384. doi: 10.1111/maec.12384
Chessman B., Williams S., Besley C. (2009). Bioassessment of streams with macroinvertebrates: effect of sampled habitat and taxonomic resolution. J. North Am. Benthol. Soc 26, 546–565. doi: 10.1899/06-074.1
Clarke K. R., Warwick R. M. (1998). Quantifying structural redundancy in ecological communities. Oecologia 113, 278–289.
Coll M., Piroddi C., Steenbeek J., Kaschner K., Lasram F. B. R., Aguzzi J., et al. (2010). The biodiversity of the Mediterranean Sea: Estimates, patterns, and threats. PloS One 5, e11842. doi: 10.1371/journal.pone.0011842
Constable A. J. (1999). Ecology of benthic macro-invertebrates in soft-sediment environments: A review of progress towards quantitative models and predictions. Austral Ecol. 24, 452–476. doi: 10.1046/j.1442-9993.1999.00977.x
Cooper K. M., Barry J. (2017). A big data approach to macrofaunal baseline assessment, monitoring and sustainable exploitation of the seabed. Sci. Rep. 7 (1), 12431. doi: 10.1038/s41598-017-11377-9
Corinaldesi C., Bianchelli S., Rastelli E., Varrella S., Canensi S., Gambi C., et al. (2022). The paradox of an unpolluted coastal site facing a chronically contaminated industrial area. Front. Mar. Sci. 8. doi: 10.3389/fmars.2021.813887
Cushman-Roisin B., Gacic M., Poulain P. M., Artegiani A. (2013). Physical oceanography of the Adriatic Sea: past, present and future (Dordrecht, Netherlands: Springer Science & Business Media).
Danovaro R., Nepote E., Martire M., Ciotti C., De Grandis G., Corinaldesi C., et al. (2018). Limited impact of beach nourishment on macrofaunal recruitment/settlement in a site of community interest in coastal area of the Adriatic Sea (Mediterranean Sea). Mar. pollut. Bull. 128, 259–266. doi: 10.1016/j.marpolbul.2018.01.033
Darnaude A. M., Salen-Picard C., Polunin N. V. C., Harmelin-Vivien M. L. (2004). Trophodynamic linkage between river runoff and coastal fishery yield elucidated by stable isotope data in the Gulf of Lions (NW Mediterranean). Oecologia 138, 325–332. doi: 10.1007/s00442-003-1457-3
Dauvin J. C., Andrade H., de-la-Ossa-Carretero J. A., Del-Pilar-Ruso Y., Riera R. (2016). Polychaete/amphipod ratios: An approach to validating simple benthic indicators. Ecol. Indic. 63, 89–99. doi: 10.1016/j.ecolind.2015.11.055
Dauvin J. C., Bakalem A., Baffreau A., Delecrin C., Bellan G., Lardicci C., et al. (2017). The well sorted fine sand community from the western Mediterranean Sea: A resistant and resilient marine habitat under diverse human pressures. Environ. pollut. 224, 336–351. doi: 10.1016/j.envpol.2017.02.013
Dauvin J. C., Gomez Gesterira J. L., Salvande Fraga M. (2003). Taxonomic sufficiency: an overview of its use in the monitoring of sublittoral benthic communities after oil spills. Mar. pollut. Bull. 46, 252–255. doi: 10.1016/S0025-326X(03)00033-X
Dauvin J. C., Ruellet T. (2007). Polychaete/amphipod ratio revisited. Mar. pollut. Bull. 55, 215–224. doi: 10.1016/j.marpolbul.2006.08.045
De Biasi A. M., Gai F., Pacciardi L. (2003). Benthic assemblage in a «Catino» of the Meloria Shoals (Southern Ligurian Sea). Atti Soc. tosc. Sci. nat., Mem., Serie B, 110.
de-la-Ossa-Carretero J. A., Del-Pilar-Ruso Y., Giménez-Casalduero F., Sánchez-Lizaso J. L. (2010). Sensitivity of tanaid Apseudes latreillei (Milne-Edwards) populations to sewage pollution. Mar. Environ. Res. 69, 309–317. doi: 10.1016/j.marenvres.2009.12.005
Dell’Anno F., Rastelli E., Tangherlini M., Corinaldesi C., Sansone C., Brunet C., et al. (2021). Highly contaminated marine sediments can host rare bacterial taxa potentially useful for bioremediation. Front. Microbiol. 12. doi: 10.3389/fmicb.2021.584850
De Smet B., Pape E., Riehl T., Bonifácio P., Colson L., Vanreusel A. (2017). The community structure of deep-sea macrofauna associated with polymetallic nodules in the eastern part of the Clarion-Clipperton Fracture Zone. Front. Mar. Sci. 4, 103. doi: 10.3389/fmars.2017.00103
Desroy N., Dubois S. F., Fournier J., Ricquiers L., Le Mao P., Guerin L., et al. (2011). The conservation status of Sabellaria alveolata (L.) (Polychaeta: Sabellariidae) reefs in the bay of mont-saint-michel. Aquat. Conserv. Mar. Freshw. Ecosyst. 21, 462–471. doi: 10.1002/aqc.1206
Dethier M. N., Schoch G. C. (2006). Taxonomic sufficiency in distinguishing natural spatial patterns on an estuarine shoreline. Marine Eco. Progress Series 306, 41–49. doi: 10.3354/meps306041
Dormann C. F., Elith J., Bacher S., Buchmann C., Carl G., Carré G., et al. (2013). Collinearity: A review of methods to deal with it and a simulation study evaluating their performance. Ecography (Cop.). 36, 27–46. doi: 10.1111/j.1600-0587.2012.07348.x
Eckrich C. A., Albeke S. E., Flaherty E. A., Bowyer R. T., Ben-David M. (2020). rKIN: Kernel-based method for estimating isotopic niche size and overlap. J. Anim. Ecol. 89, 757–771. doi: 10.1111/1365-2656.13159
Ellis J. I., Clark D., Atalah J., Jiang W., Taiapa C., Patterson M., et al. (2017). Multiple stressor effects on marine infauna: Responses of estuarine taxa and functional traits to sedimentation, nutrient and metal loading. Sci. Rep. 7, 12013. doi: 10.1038/s41598-017-12323-5
Fabi G., Manoukian S., Spagnolo A. (2009). Impact of an open-sea suspended mussel culture on macrobenthic community (Western Adriatic Sea). Aquaculture 289, 54–63. doi: 10.1016/j.aquaculture.2008.12.026
Fanelli E., Azzurro E., Bariche M., Cartes J. E., Maynou F. (2015). Depicting the novel Eastern Mediterranean food web: a stable isotopes study following Lessepsian fish invasion. Biol. Invasions 17, 2163–2178. doi: 10.1007/s10530-015-0868-5
Fanelli E., Di Giacomo S., Gambi C., Bianchelli S., Da Ros Z., Tangherlini M., et al. (2022). Effects of local acidification on benthic communities at shallow hydrothermal vents of the Aeolian Islands (Southern Tyrrhenian, Mediterranean Sea). Biology 11, 321. doi: 10.3390/biology11020321
Fanelli E., Papiol V., Cartes J. E., Rumolo P., López-Pérez C. (2013). Trophic webs of deep-sea megafauna on mainland and insular slopes of the NW Mediterranean: A comparison by stable isotope analysis. Mar. Ecol. Prog. Ser. 490, 199–221. doi: 10.3354/meps10430
Forde J., Shin P. K., Somerfield P. J., Kennedy R. M. (2013). M-AMBI derived from taxonomic levels higher than species allows Ecological Status assessments of benthic habitats in new geographical areas. Ecol. Indic. 34, 411–419. doi: 10.1016/j.ecolind.2013.05.014
Franzitta G., Colletti A., Savinelli B., Lo Martire M., Corinaldesi C., Musco L. (2022). Feasibility of the sabellarid reef habitat restoration. Front. Mar. Sci. 9. doi: 10.3389/fmars.2022.854986
Fraschetti S., McOwen C., Papa L., Papadopoulou N., Bilan M., Boström C., et al. (2021). Where is more important than how in coastal and marine ecosystems restoration. Front. Mar. Sci. 8. doi: 10.3389/fmars.2021.626843
Frontalini F., Semprucci F., Coccioni R., Balsamo M., Bittoni P., Covazzi-Harriague A. (2011). On the quantitative distribution and community structure of the meio and macrofaunal communities in the coastal area of the Central Adriatic Sea (Italy). Environ. Monit. Assess. 180, 325–344. doi: 10.1007/s10661-010-1791-y
Galloway J. N., Dentener F. J., Capone D. G., Boyer E. W., Howarth R. W., Seitzinger S. P., et al. (2004). Nitrogen cycles: past, present, and future. Biogeochemistry 70, 153–226. doi: 10.3389/fmars.2017.00103
Gambi C., Canals M., Corinaldesi C., Dell’Anno A., Manea E., Pusceddu A., et al. (2022). Impact of resuspended mine tailings on benthic biodiversity and ecosystem processes: The case study of Portmán Bay, Western Mediterranean Sea, Spain. Environ. pollut. 301, 119021. doi: 10.1016/j.envpol.2022.119021
Gambi C., Dell’Anno A., Corinaldesi C., Lo Martire M., Musco L., Da Ros Z., et al. (2020). Impact of historical contamination on meiofaunal assemblages: The case study of the Bagnoli-Coroglio Bay (southern Tyrrhenian Sea). Mar. Environ. Res. 156, 104907. doi: 10.1016/j.marenvres.2020.104907
Gammal J., Hewitt J., Gladstone-Gallagher R., Thrush S., Douglas E., Lohrer A., et al. (2023). Stressors increase the impacts of coastal macrofauna biodiversity loss on ecosystem multifunctionality. Ecosystems 26(3), 539–552. doi: 10.1007/s10021-022-00775-4
Gravina M. F., Cardone F., Bonifazi A., Bertrandino M. S., Chimienti G., Longo C., et al. (2018). Sabellaria spinulosa (Polychaeta, Annelida) reefs in the Mediterranean Sea: Habitat mapping, dynamics and associated fauna for conservation management. Est. Coast. Shelf Sci. 200, 248–257. doi: 10.1016/j.ecss.2017.11.017
Gray J. S., Aschan M., Carr M. R., Clarke K. R., Green R. H., Pearson T. H., et al. (1988). Analysis of community attributes of the benthic macrofauna of Frierfjord/Langesundfjord and in a mesocosm experiment. Mar. Ecol. Prog. Ser., 151–165. doi: 10.3354/meps046167
Halpern B. S., Frazier M., Potapenko J., Casey K. S., Koenig K., Longo C., et al. (2015). Spatial and temporal changes in cumulative human impacts on the world’s ocean. Nat. Commun. 6, 1–7. doi: 10.1038/ncomms8615
Han C., Xu Z., Liu X. (2021). Characteristics of macrofaunal assemblages and their relationships with environmental factors in a semi-enclosed bay. Mar. pollut. Bull. 167, 112348. doi: 10.1016/j.marpolbul.2021.112348
Hay Mele B., Russo L., Crocetta F., Gambi C., Dell’Anno A., Danovaro R., et al. (2020). Ecological assessment of anthropogenic impact in marine ecosystems: The case of Bagnoli Bay. Mar. Environ. Res. 158, 104953. doi: 10.1016/j.marenvres.2020.104953
He Q., Silliman B. R. (2019). Climate change, human impacts, and coastal ecosystems in the anthropocene. Curr. Biol. 29, R1021–R1035. doi: 10.1016/j.cub.2019.08.042
Hewitt J. E., Ellis J. I., Thrush S. F. (2016). Multiple stressors, nonlinear effects and the implications of climate change impacts on marine coastal ecosystems. Glob. Change Biol. 22, 2665–2675. doi: 10.1111/gcb.13176
Hilgendag I. R., Swanson H. K., Lewis C. W., Ehrman A. D., Power M. (2022). Mercury biomagnification in benthic, pelagic, and benthopelagic food webs in an Arctic marine ecosystem. Sci. Total Environ. 841, 156424. doi: 10.1016/j.scitotenv.2022.156424
Hogsden K. L., Harding J. S. (2012). Anthropogenic and natural sources of acidity and metals and their influence on the structure of stream food webs. Environ. pollut. 162, 466–474. doi: 10.1016/j.envpol.2011.10.024
Ingrosso G., Abbiati M., Badalamenti F., Bavestrello G., Belmonte G., Cannas R., et al. (2018). Mediterranean bioconstructions along the italian coast. Adv. Mar. Biol. 79, 61–136. doi: 10.1016/bs.amb.2018.05.001
Jędruch A., Bełdowska M., Ziółkowska M. (2019). The role of benthic macrofauna in the trophic transfer of mercury in a low-diversity temperate coastal ecosystem (Puck Lagoon, southern Baltic Sea). Environ. Monit. Assess. 191, 1–25. doi: 10.1007/s10661-019-7257-y
Jackson A. L., Inger R., Parnell A. C., Bearhop S. (2011). Comparing isotopic niche widths among and within communities: SIBER - Stable Isotope Bayesian Ellipses in R. J. Anim. Ecol. 80, 595–602. doi: 10.1111/j.1365-2656.2011.01806.x
Janas U., Burska D., Kendzierska H., Pryputniewicz-Flis D., Łukawska-Matuszewska K. (2019). Importance of benthic macrofauna and coastal biotopes for ecosystem functioning–Oxygen and nutrient fluxes in the coastal zone. Estuar. Coast. Shelf Sci. 225, 106238. doi: 10.1016/j.ecss.2019.05.020
Jones A. G., Denis L., Fournier J., Desroy N., Duong G., Dubois S. F. (2020). Linking multiple facets of biodiversity and ecosystem functions in a coastal reef habitat. Mar. Environ. Res. 162, 105092. doi: 10.1016/j.marenvres.2020.105092
Jones H. P., Jones P. C., Barbier E. B., Blackburn R. C., Rey Benayas J. M., Holl K. D., et al. (2018). Restoration and repair of Earth’s damaged ecosystems. Proc. R. Soc B Biol. Sci. 285, 20172577. doi: 10.1098/rspb.2017.2577
Josefson A. B., Hansen J. L. S., Asmund G., Johansen P. (2008). Threshold response of benthic macrofauna integrity to metal contamination in West Greenland. Mar. Poll. Bull. 56 (7), 1265–1274. doi: 10.1016/j.marpolbul.2008.04.028
Kim H. G., Song S. J., Bae H., Noh J., Lee C., Kwon B. O., et al. (2020). Natural and anthropogenic impacts on long-term meiobenthic communities in two contrasting nearshore habitats. Environ. Int. 134, 105200. doi: 10.1016/j.envint.2019.105200
Kolasinski J., Rogers K., Frouin P. (2008). Effects of acidification on carbon and nitrogen stable isotopes of benthic macrofauna from a tropical coral reef. Rapid Commun. Mass Spectrom. 22, 2955–2960. doi: 10.1002/rcm.3694
Kowalska J. B., Mazurek R., Gąsiorek M., Zaleski T. (2018). Pollution indices as useful tools for the comprehensive evaluation of the degree of soil contamination–A review. Environ. Geochem. Health 40, 2395–2420. doi: 10.1007/s10653-018-0106-z
Lam-Gordillo O., Huang J., Barceló A., Kent J., Mosley L. M., Welsh D. T., et al. (2022). Restoration of benthic macrofauna promotes biogeochemical remediation of hostile sediments; An in situ transplantation experiment in a eutrophic estuarine-hypersaline lagoon system. Sci. Total Env. 833, 155201. doi: 10.1016/j.scitotenv.2022.155201
Lampadariou N., Karakassis I., Pearson T. H. (2005). Cost/benefit analysis of a benthic monitoring programme of organic benthic enrichment using different sampling and analysis methods. Mar. pollut. Bull. 50, 1606–1618. doi: 10.1016/j.marpolbul.2005.06.030
Layman C. A., Arrington D. A., Montaña C. G., Post D. M. (2007). Can stable isotope ratios provide for community‐wide measures of trophic structure? Ecology 88(1), 42–48. doi: 10.1890/0012-9658(2007)88[42:CSIRPF]2.0.CO;2
Lisco S., Moretti M., Moretti V., Cardone F., Corriero G., Longo C. (2017). Sedimentological features of Sabellaria spinulosa biocontructions. Mar. Petrol. Geology 87, 203–212. doi: 10.1016/j.marpetgeo.2017.06.013
Long E. R., Macdonald D. D., Smith S. L., Calder F. D. (1995). Incidence of adverse biological effects within ranges of chemical concentrations in marine and estuarine sediments. Environ. Manage. 19, 81–97. doi: 10.1007/BF02472006
Louati H., Ben Said O., Soltani A., Got P., Mahmoudi E., Cravo-Laureau C., et al. (2013). The roles of biological interactions and pollutant contamination in shaping microbial benthic community structure. Chemosphere 93, 2535–2546. doi: 10.1016/j.chemosphere.2013.09.069
Loures L. (2015). Post-industrial landscapes as drivers for urban redevelopment: Public versus expert perspectives towards the benefits and barriers of the reuse of post-industrial sites in urban areas. Habitat Int. 45, 72–81. doi: 10.1016/j.habitatint.2014.06.028
Magni P., Semprucci F., Gravina M. F. (2022). Joint analysis of macrofaunal and meiofaunal assemblages improves the assessment of lagoonal environmental heterogeneity. Est. Coas. Shelf Sci. 266, 107740. doi: 10.1016/j.ecss.2021.107740
Magni P., Vesal S. E., Giampaoletti J., Como S., Gravina M. F. (2023). Joint use of biological traits, diversity and biotic indices to assess the ecological quality status of a Mediterranean transitional system. Ecol. Indic. 147, 109939. doi: 10.1016/j.ecolind.2023.109939
Mayer-Pinto M., Ledet J., Crowe T. P., Johnston E. L. (2020). Sublethal effects of contaminants on marine habitat-forming species: a review and meta-analysis. Biol. Rev. 95, 1554–1573. doi: 10.1111/brv.12630
Micheli F., Halpern B. S., Walbridge S., Ciriaco S., Ferretti F., Fraschetti S., et al. (2013). Cumulative human impacts on Mediterranean and Black Sea marine ecosystems: Assessing current pressures and opportunities. PloS One 8, e79889. doi: 10.1371/journal.pone.0079889
Momota K., Hosokawa S. (2021). Potential impacts of marine urbanization on benthic macrofaunal diversity. Sci. Rep. 11, 4028. doi: 10.1038/s41598-021-83597-z
Morroni L., d’Errico G., Sacchi M., Molisso F., Armiento G., Chiavarini S., et al. (2020). Integrated characterization and risk management of marine sediments: The case study of the industrialized Bagnoli area (Naples, Italy). Mar. Environ. Res. 160, 104984. doi: 10.1016/j.marenvres.2020.104984
Muller A., Poitrimol C., Nunes F. L. D., Boyé A., Curd A., Desroy N., et al. (2021). Musical chairs on temperate reefs: species turnover and replacement within functional groups explain regional diversity variation in assemblages associated with honeycomb worms. Front. Mar. Sci. 8. doi: 10.3389/fmars.2021.654141
Naidu R., Biswas B., Willett I. R., Cribb J., Kumar Singh B., Paul Nathanail C., et al. (2021). Chemical pollution: A growing peril and potential catastrophic risk to humanity. Environ. Int. 156, 106616. doi: 10.1016/j.envint.2021.106616
Nasi F., Auriemma R., Relitti F., Bazzaro M., Cassin D., Cibic T. (2020). Structural and functional response of coastal macrofaunal community to terrigenous input from the Po River (northern Adriatic Sea). Estuar. Coast. Shelf Sci. 235, 106548. doi: 10.1016/j.ecss.2019.106548
Nepote E., Corinaldesi C., Dell’Anno A., Greco S., Lo Martire M., Danovaro R. (2022). Positive effects of the decrease of hydraulic dredging due to the SARS-CoV-2 pandemic on benthic fauna and clam harvesting in the northern Adriatic (Mediterranean Sea). Aquat. Sci. Manage. 10, 8. doi: 10.35800/jasm.v10i1.37485
Olsgard F., Somerfield P. J., Carr M. R. (1998). Relationships between taxonomic resolution, macrobenthic community patterns and disturbance. Mar. Ecol. Prog. Ser. 172, 25–36. doi: 10.3354/meps172025
Paklar G. B., Isakov V., Koračin D., Kourafalou V., Orlić M. (2001). A case study of bora-driven flow and density changes on the Adriatic shelf (January 1987). Cont. Shelf Res. 21, 1751–1783. doi: 10.1016/S0278-4343(01)00029-2
Pereira H. M., Navarro L. M., Martins I. S. (2012). Global biodiversity change: The Bad, the good, and the unknown. Annu. Rev. Environ. Resour. 37, 25–50. doi: 10.1146/annurev-environ-042911-093511
Pérès J. M., Picard J. (1964). Nouveau manuel de bionomie benthique de la Mer Méditerranée (Marseille; France: Station Marine d’Endoume).
Perrow M., Davy A. (2002). Handbook of Ecological Restoration (Cambridge: Cambridge University Press). doi: 10.1017/CBO9780511549984
Pinto R., Patrício J., Baeta A., Fath B. D., Neto J. M., Marques J. C. (2009). Review and evaluation of estuarine biotic indices to assess benthic condition. Ecol. Indic. 9, 1–25. doi: 10.1016/j.ecolind.2008.01.005
Pitacco V., Mistri M., Aleffi I. F., Lardicci C., Prato S., Tagliapietra D., et al. (2019). The efficiency of taxonomic sufficiency for identification of spatial patterns at different scales in transitional waters. Mar. Environ. Res. 144, 84–91. doi: 10.1016/j.marenvres.2019.01.001
Post D. M. (2002). Using stable isotopes to estimate trophic position: models, methods, and assumptions. Ecology 83(3), 703–718. doi: 10.1890/0012-9658(2002)083[0703:USITET]2.0.CO;2
Putro S. P. (2007). Spatial and temporal patterns of the macrobenthic assemblages in relation to environmental variables. J. Coast. Dev. 10, 153–169.
Raymond C., Gorokhova E., Karlson A. M. L. (2021). Polycyclic aromatic hydrocarbons have adverse effects on benthic communities in the baltic sea: implications for environmental status assessment. Front. Environ. Sci. 9. doi: 10.3389/fenvs.2021.624658
Regione Marche (2009) Regione Marche. Available at: http://www.ambiente.regione.marche.it/Portals/0/Ambiente/Sitiinquinati/2009pianobonifiche_relazionegenerale.pdf (Accessed October 5, 2022).
Röling W. F. M., Van Verseveld H. W. (2002). Natural attenuation: What does the subsurface have in store? Biodegradation 13, 53–64. doi: 10.1023/A:1016310519957
Romano E., Ausili A., Zharova N., Celia Magno M., Pavoni B., Gabellini M. (2004). Marine sediment contamination of an industrial site at Port of Bagnoli, Gulf of Naples, Southern Italy. Mar. pollut. Bull. 49, 487–495. doi: 10.1016/j.marpolbul.2004.03.014
Romano C., Fanelli E., D’Anna G., Pipitone C., Vizzini S., Mazzola A., et al. (2016). Spatial variability of soft-bottom macrobenthic communities in northern Sicily (Western Mediterranean): Contrasting trawled vs. untrawled areas. Mar. Environ. Res. 122, 113–125. doi: 10.1016/j.marenvres.2016.10.002
Romanelli M., Cordisco C. A., Giovanardi O. (2009). The long-term decline of the Chamelea gallina L. (Bivalvia: Veneridae) clam fishery in the Adriatic Sea: is a synthesis possible? Acta Adriatica 50(2), 171–205.
Rumolo P., Barra M., Gherardi S., Marsella E., Sprovieri M. (2011). Stable isotopes and C/N ratios in marine sediments as a tool for discriminating anthropogenic impact. J. Environ. Monit. 13, 3399–3408. doi: 10.1039/c1em10568j
Schlacher T. A., Connolly R. M. (2014). Effects of acid treatment on carbon and nitrogen stable isotope ratios in ecological samples: A review and synthesis. Methods Ecol. Evol. 5, 541–550. doi: 10.1111/2041-210X.12183
Schratzberger M., Somerfield P. J. (2020). Effects of widespread human disturbances in the marine environment suggest a new agenda for meiofauna research is needed. Sci. Total Environ. 728, 138435. doi: 10.1016/j.scitotenv.2020.138435
Schwing P. T., Montagna P. A., Joye S. B., Paris C. B., Cordes E. E., McClain C. R., et al. (2020). A synthesis of deep benthic faunal impacts and resilience following the deepwater horizon oil spill. Front. Mar. Sci. 7. doi: 10.3389/fmars.2020.560012
Semprucci F., Boi P., Manti A., Covazzi Harriague A., Rocchi M., Colantoni P., et al. (2010). Benthic communities along a littoral of the Central Adriatic Sea (Italy). Helgol. Mar. Res. 64, 101–115. doi: 10.1007/s10152-009-0171-x
Simonini R., Grandi V., Massamba-N’Siala G., Iotti M., Montanari G., Prevedelli D. (2009). Assessing the ecological status of the North-western Adriatic Sea within the European Water Framework Directive: A comparison of Bentix, AMBI and M-AMBI methods. Mar. Ecol. 30, 241–254. doi: 10.1111/j.1439-0485.2009.00281.x
Sivadas S., Ingole B., Nanajkar M. (2010). Benthic polychaetes as good indicators of anthropogenic impact. Indian J. Mar. Sci. 39, 201–211.
Somerfield P. J., Clarke K. R. (1995). Taxonomic levels, in marine community studies, revisited. Mar. Ecol. Prog. Ser. 127, 113–119. doi: 10.3354/meps127113
Soto-Navarro J., Jordá G., Compa M., Alomar C., Fossi M. C., Deudero S. (2021). Impact of the marine litter pollution on the Mediterranean biodiversity: A risk assessment study with focus on the marine protected areas. Mar. pollut. Bull. 165, 112169. doi: 10.1016/j.marpolbul.2021.112169
Spagnolo A., Cuicchi C., De Biasi A. M., Ferrà C., Montagnini L., Punzo E., et al. (2019). Effects of the installation of offshore pipelines on macrozoobenthic communities (northern and central Adriatic Sea). Mar. pollut. Bull. 138, 534–544. doi: 10.1016/j.marpolbul.2018.12.003
Tangherlini M., Corinaldesi C., Rastelli E., Musco L., Armiento G., Danovaro R., et al. (2020). Chemical contamination can promote turnover diversity of benthic prokaryotic assemblages: The case study of the Bagnoli-Coroglio bay (southern Tyrrhenian Sea). Mar. Environ. Res. 160, 105040. doi: 10.1016/j.marenvres.2020.105040
Tweedley J. R., Warwick R. M., Potter I. C. (2015). Can biotic indicators distinguish between natural and anthropogenic environmental stress in estuaries? J. Sea Res. 102, 10–21. doi: 10.1016/j.seares.2015.04.001
Vanderklift M. A., Ponsard S. (2003). Sources of variation in consumer-diet delta 15N enrichment: a meta-analysis. Oecologia 136, 169–182. doi: 10.1007/s00442-003-1270-z
Volvenko I. V., Gebruk A. V., Katugin O. N., Vinogradov G. M., Orlov A. M. (2023). What can supraspecies richness tell us? Geographical Res., 1–9. doi: 10.1111/1745-5871.12594
Wang X. H., Pinardi N. (2002). Modeling the dynamics of sediment transport and resuspension in the northern Adriatic sea. J. Geophys. Res. Ocean. 107, 18–11. doi: 10.1029/2001jc001303
Keywords: passive restoration, benthic biodiversity, food webs, industrial contamination, environmental quality, Mediterranean Sea
Citation: Fanelli E, Dell’Anno A, Nepote E, Martire ML, Musco L, Bianchelli S, Gambi C, Falco P, Memmola F, Coluccelli A, Meola M, Varrella S, Danovaro R and Corinaldesi C (2023) Positive effects of two decades of passive ecological restoration in a historically polluted marine site. Front. Mar. Sci. 10:1199654. doi: 10.3389/fmars.2023.1199654
Received: 03 April 2023; Accepted: 05 July 2023;
Published: 24 July 2023.
Edited by:
Susana Carvalho, King Abdullah University of Science and Technology, Saudi ArabiaReviewed by:
Gustavo Fonseca, Federal University of São Paulo, BrazilCopyright © 2023 Fanelli, Dell’Anno, Nepote, Martire, Musco, Bianchelli, Gambi, Falco, Memmola, Coluccelli, Meola, Varrella, Danovaro and Corinaldesi. This is an open-access article distributed under the terms of the Creative Commons Attribution License (CC BY). The use, distribution or reproduction in other forums is permitted, provided the original author(s) and the copyright owner(s) are credited and that the original publication in this journal is cited, in accordance with accepted academic practice. No use, distribution or reproduction is permitted which does not comply with these terms.
*Correspondence: Cinzia Corinaldesi, Yy5jb3JpbmFsZGVzaUBzdGFmZi51bml2cG0uaXQ=
Disclaimer: All claims expressed in this article are solely those of the authors and do not necessarily represent those of their affiliated organizations, or those of the publisher, the editors and the reviewers. Any product that may be evaluated in this article or claim that may be made by its manufacturer is not guaranteed or endorsed by the publisher.
Research integrity at Frontiers
Learn more about the work of our research integrity team to safeguard the quality of each article we publish.