- 1College of River and Ocean Engineering, Chongqing Jiaotong University, Chongqing, China
- 2Chongqing Research Academy of Ecology and Environmental Sciences, Chongqing, China
- 3Chongqing Yi Da Environmental Protection Engineering Co., Ltd., Chongqing, China
Microplastics are prevalent in the environment and have a strong affinity to pollutants owing to their large specific surface area and hydrophobicity. Once ingested, microplastics transport pollutants into organisms. This study investigated bisphenol A (BPA) desorption behavior from three microplastic materials, namely, polystyrene (PS), polypropylene (PP), and polyamide (PA), under simulated biological gastrointestinal conditions. The results showed that BPA can rapidly desorb from microplastic carriers under simulated gastrointestinal conditions, with different BPA desorption percentages in the order of PP > PS > PA. This was related to the amorphous structure and functional groups of the polymers. The BPA desorption behavior of microplastics in gastric juices was not significantly affected by pH; however, within the pH range of intestinal juices, the BPA desorption percentage increased significantly as the pH increased. The increase in Na+ concentration in the gastrointestinal tract exhibited a certain inhibitory effect on BPA desorption from microplastics owing to the salting-out effect. The temperature of digestive juices positively affected BPA desorption, suggesting that endothermic organisms are more susceptible to it. Our findings help elucidate the potential health risks of exposure to microplastics and their sorbed pollutants in the environment.
1 Introduction
Plastic products are widely used in human life owing to their versatility. However, because of their strong stability, discarded plastics can remain in the environment for a long time. These plastics are gradually decomposed into smaller fragments through a series of physicochemical and biological processes (Habib et al., 2020). Plastic particles smaller than 5 mm are called microplastics, and their widespread prevalence in the environment enables their accidental ingestion by various organisms (Desforges et al., 2015). Microplastics have been found in a variety of animals, including those consumed by humans (Windsor et al., 2019; Zheng et al., 2019). Owing to their persistence in the environment, microplastics inevitably encounter a series of other pollutants, which can have a strong affinity for microplastic surfaces owing to their large specific surface area and strong hydrophobicity (Wang et al., 2020). Furthermore, during the manufacture of plastics, organic additives are often used to modify the properties of polymers (Cheng et al., 2020), and the resulting microplastics can function as a pollutant source in the environment.
Bisphenol A (BPA) is an endocrine disruptor widely present in the environment and is an essential additive in plastic production (Corrales et al., 2015). BPA can damage biomes by disrupting the endocrine system of organisms (Simonelli et al., 2017). The BPA concentrations associated with the presence of microplastics in the environment usually range from ng·g−1 to mg·g−1, and the concentration of BPA in edible fish is higher than that of other bisphenols (e.g., BPB, BPF, and BPS) (Vandenberg et al., 2019; Barboza et al., 2020). When BPA-containing microplastics are ingested by fish, BPA is likely to be desorbed in the fish digestive tract. Furthermore, some microplastics can translocate from the gut into the circulatory system (Browne et al., 2008), thereby increasing the distribution of these contaminants in different tissues and posing health risks to organisms.
This study aimed to explore the desorption behavior of BPA-contaminated microplastics under different conditions of the digestive system, which have not been investigated in detail in previous studies. We hypothesized that the desorption of BPA from microplastics would be enhanced under the gastrointestinal conditions of organisms compared to that under freshwater conditions. To verify this hypothesis, three common microplastics were selected to adsorb BPA to saturation, and they were exposed to fresh water. Then, in vitro digestion models under different conditions were used to evaluate their desorption properties.
2 Materials and methods
2.1 Raw materials
We selected polystyrene (PS), polypropylene (PP), and polyamide (PA) microplastics for this study. These three types of microplastics are widely detected in water worldwide (Jiang et al., 2019). Considering the biological effects of microplastic particle size (the smaller the particle size, the more likely it is to be inadvertently ingested) (Zu et al., 2023), we chose 50 μm-sized (average particle size) microplastics. PS, PP, and PA microplastics (50 μm) were purchased from Dongguan Shunjie Plastic Technology Co., Ltd., and BPA was sourced from Beijing Bailingwei Technology Co., Ltd. Sodium taurocholate, bovine serum albumin, and pepsin were purchased from Hefei Qiansheng Biotechnology, Co. Ltd.; sodium chloride was purchased from Tianjin Zhiyuan Chemical Reagent Co., Ltd.; and acetonitrile and methanol were purchased from Sichuan Xilong Chemical Co., Ltd.
2.2 Preparation of microplastics and digestive juice
The simulated digestive juice was configured according to the relevant components found in the digestive system of aquatic organisms (Wagstaff and Petrie, 2022). The gastric juice was composed of pepsin and NaCl at concentrations of 3.2 g·L−1 and 100 mmol·L−1, respectively, and the pH was adjusted to 2.0 using HCl to simulate an acidic gastric environment. The intestinal fluid was composed of bovine serum albumin, sodium taurocholate, and NaCl at concentrations of 5 g·L−1, 10 mmol·L−1, and 100 mmol·L−1, respectively. The pH was adjusted to 9.0 using NaOH to simulate the weakly alkaline intestinal environment.
To explore the general pattern of BPA desorption by microplastics in these digestive fluids, 1.0 g each of microplastics (PS, PP, and PA) were placed in BPA solutions at concentrations of 0.01, 0.05, 0.10, 0.50, and 1.00 mg·L−1 before the experiment. The solution was shaken at 25 ± 1°C and 200 rpm for 96 h. Preliminary experiment (kinetic adsorption) results showed that 96 h was sufficient to achieve equilibrium adsorption of BPA on PP, PS and PA microplastics.
Liquid chromatography–mass spectrometry (ACQUITY, TQD, Waters Corp, USA) was used to measure the BPA content in the liquid phase, and the adsorbed amount was calculated based on the difference in concentration before and after adsorption. At different initial concentrations of BPA (0.01, 0.05, 0.10, 0.50, and 1.00 mg·L−1), the BPA adsorption capacity of PP was 0.006, 0.018, 0.043, 0.067, and 0.103 mg·g−1; that of PS was 0.009, 0.024, 0.053, 0.083, and 0.111 mg·g−1; and that of PA was 0.014, 0.036, 0.063, 0.092, and 0.131 mg·g−1, respectively.
2.3 Batch experiments
1) Desorption kinetics: A total of 100 mg of PS, PP, and PA microplastics (at an adsorption equilibrium of 1.00 mg·L−1 BPA) were placed in 30 mL of ultrapure water (18°C), gastric juice (18°C and 37°C), and intestinal fluid (18°C and 37°C). The different temperatures represent the different gastrointestinal environments of ectothermic and endothermic organisms. The samples were shaken at 100 rpm for 72 h. Samples were drawn at 0.5, 1, 3, 5, 7, 9, 12, 24, 36, 48, 60, and 72 h, and their BPA concentrations were assessed after filtration and separation.
2) Desorption thermodynamics: A total of 100 mg of PS, PP, and PA microplastics (at an adsorption equilibrium of 0.01–1.00 mg·L−1 BPA) was used for the desorption thermodynamic experiments. The desorption duration was 72 h, and the remaining steps and parameters were similar to those in the desorption kinetics experiment.
3) The effects of different environmental factors in the simulated gastrointestinal tract were investigated. A total of 100 mg of PS microplastics (at an adsorption equilibrium of 1.00 mg·L−1 BPA) were subjected to desorption experiments in gastric and intestinal juices under different conditions, with a desorption duration of 72 h. Control conditions included pH (2, 4, 6, 8, and 10), Na+ concentration (10, 50, 100, and 1000 mmol·L−1), and temperature (18, 24, 30, and 37°C). The remaining steps and parameters were similar to those in the desorption kinetics experiment.
The desorption experiment was performed three times, and blank groups were prepared to eliminate any error caused by adsorption to the container surface. Considering the limited amount of digestive fluid in the digestive tract of real organisms, the concentration of microplastics in the digestive fluid after the microplastics are ingested by organisms is likely to be quite high. Therefore, a high concentration of microplastics was set in the experiment (much higher than that of microplastics in the real environment), which was also similar to the concentration of microplastics used in previous studies (Coffin et al., 2019b). Before conducting the batch experiments, controlled experiments were conducted, and they confirmed that BPA was not detected in the microplastic-only system.
The desorption kinetics of BPA from microplastics can be described by a first-order kinetic model, as follows (Johnson et al., 2001):
where is the BPA load in microplastics at time t, mg·g−1; is the initial load of BPA in the microplastics, mg·g−1; and are the fast and slow desorption rates, respectively, h−1; and and are the BPA fractions in the fast and slow adsorption stages, respectively, for which + = 1.
The desorption thermodynamics of BPA from microplastics can be described by the Langmuir (Ju et al., 2021) and Freundlich (Chen and Zhang, 2014) models, as follows:
where is the BPA concentration in the solid phase at equilibrium, mg·g−1; is the saturated load of BPA in the solid phase, mg·g−1; is the equilibrium concentration of the solute, mg·L−1; and are Langmuir and Freundlich constants, respectively; and n is the surface heterogeneity constant.
The degree of reversibility of the reaction was assessed using the hysteresis index (HI) (Berhane et al., 2016):
where and denote the concentration of the adsorbate on the adsorbent during the desorption and adsorption process, respectively; and T and denote the temperature and equilibrium concentration in the liquid phase, respectively.
2.4 Statistical analysis
SPSS (version 21, IBM, USA) was used for data analysis. The difference between two groups of data was analyzed using t-tests. One-way analysis of variance (ANOVA) and post-hoc Tukey multiple comparisons were used to determine the significance of differences between the experimental groups. Pearson coefficient was used for the correlation analysis. The average of three independent experiments was used as the result of each experiment, and values are presented as mean ± standard deviation.
3 Results and discussion
3.1 Desorption kinetics in different matrices
The desorption kinetics of BPA from PP, PS, and PA in water and the gastric and intestinal juices of ectothermic and endothermic organisms were investigated. BPA was rapidly desorbed from the three microplastics, and the desorbed amount exceeded 80% of equilibrium desorption within 12 h (Figure 1). Subsequently, the increase in BPA concentration in the desorption solution decelerated until it reached equilibrium. Most exposed microplastics have a limited residence time (from hours to days) in the gastrointestinal tract of animals, thus the rapid desorption of BPA from microplastic carriers is of great significance. Liu et al. (2020) reported that atorvastatin (ATV) and amlodipine (AML) can be rapidly desorbed from PS microplastics. Bakir et al. (2014) demonstrated that PVC and PE sorbed with dichlorodiphenyltrichloroethane, phenanthrene, and perfluorooctanoic acid can reach desorption equilibrium within a short time. These results suggest that upon ingestion, these contaminants can be rapidly transferred from the microplastic to the digestive juices and can subsequently enter the circulatory system through the intestinal walls.
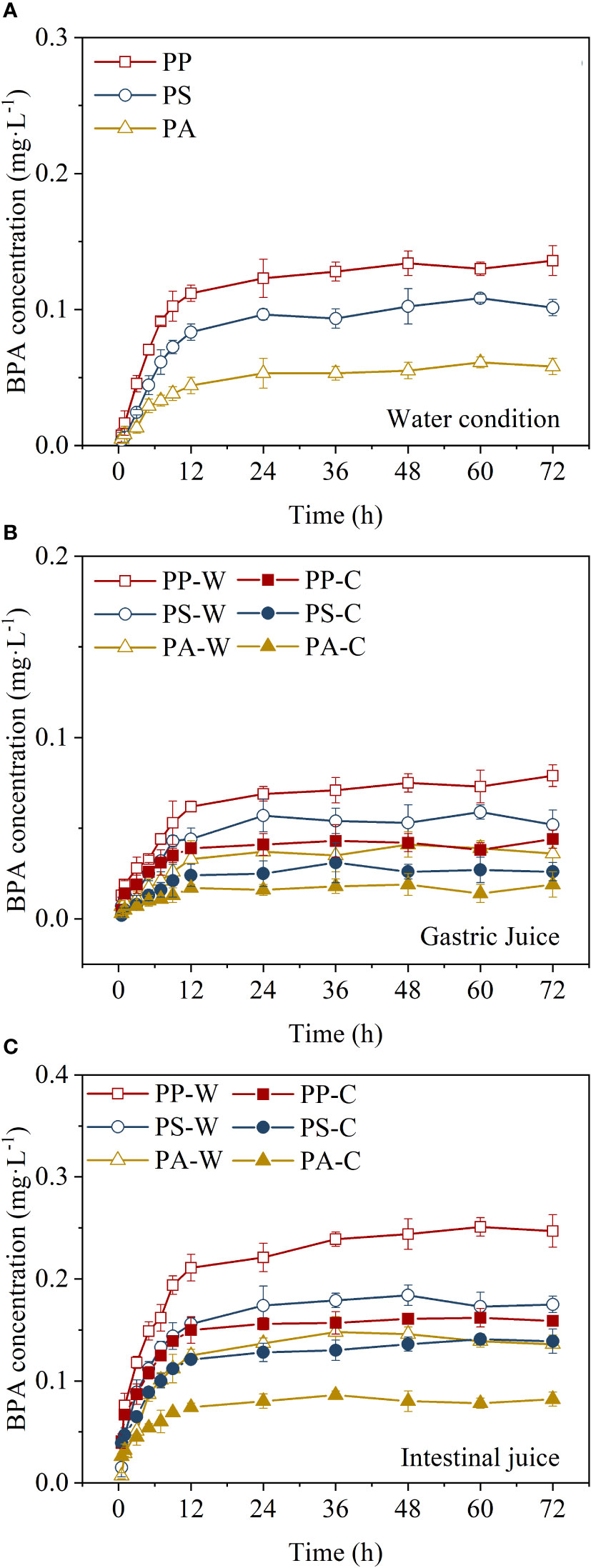
Figure 1 Bisphenol A (BPA) concentrations in matrices containing polystyrene (PS), polypropylene (PP), and polyamide (PA) microplastics: (A) water, (B) gastric juice, and (C) intestinal juice. C and W represent the simulated digestive juices of ectothermic (18°C) and endothermic organisms (37°C), respectively.
A first-order kinetic model was used to fit the BPA desorption from microplastics data (Table 1). The desorption fractions (Ffast) of PP, PS, and PA microplastics in the fast desorption stage were higher in the digestive juices of endothermic organisms than in those of ectothermic organisms. This phenomenon indicates that endothermic animals are more susceptible to BPA-carrying microplastics, which is likely related to the enhanced desorption of BPA from microplastics in warmer environments. For the same species (ectothermic or endothermic organisms), the BPA released in intestinal juices was substantially higher than that in gastric juice, and the release in gastric juice was lower than that in water (Figure 1). The desorption fraction in the fast desorption stage showed the following trend: intestinal juice > water > gastric juice.
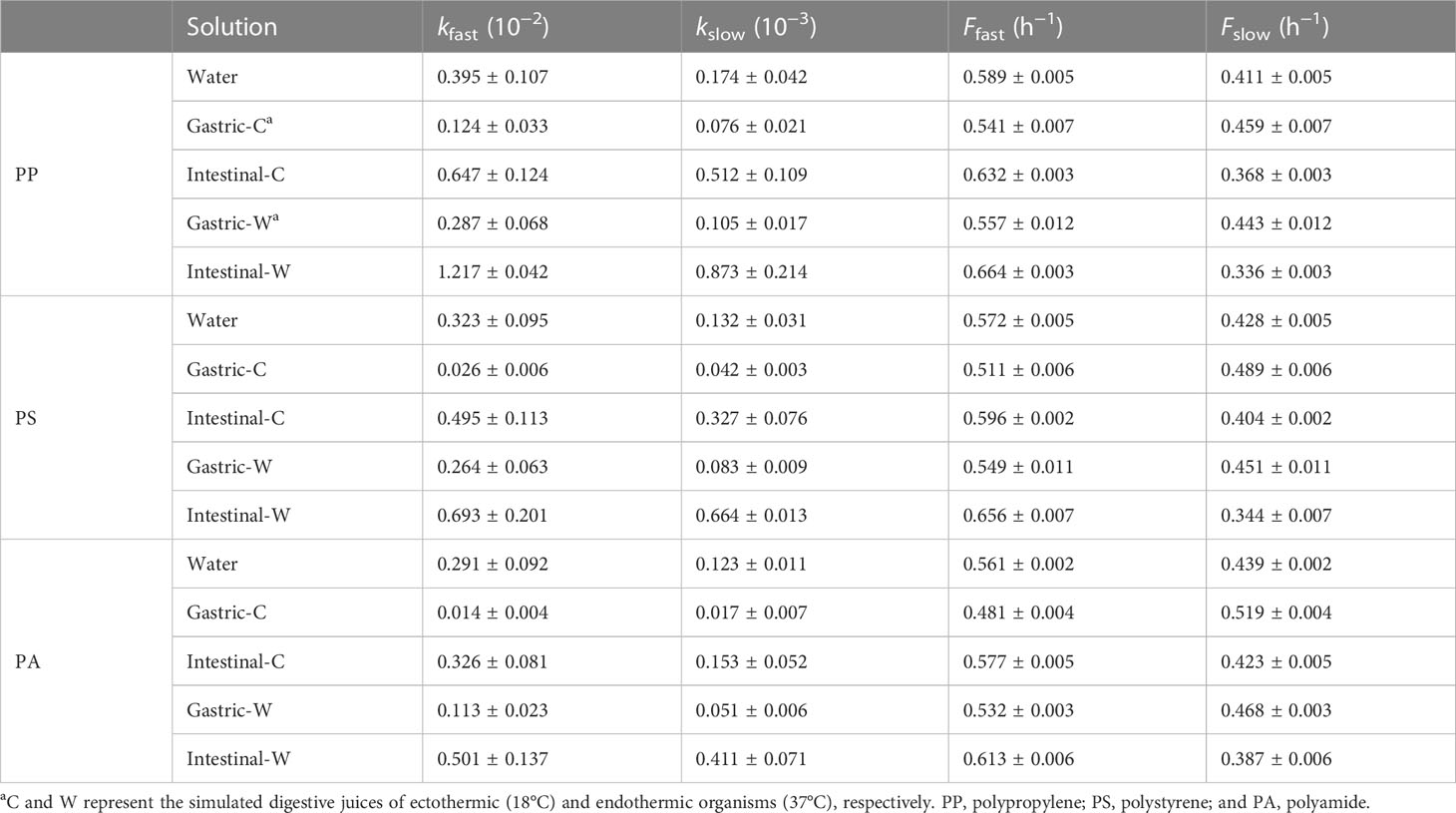
Table 1 Results of fitting first-order kinetic model for bisphenol A (BPA) desorption from microplastics in water and simulated gastric and intestinal juices.
The fast desorption rate constant (kfast) indicates that BPA can be rapidly desorbed from microplastics in a short period in intestinal juices. The ratio between the fast desorption rate constants of gastric juice and water was 0.05–0.82, whereas that between intestinal juice and water was 1.12–3.08. These differences can be attributed to the different components of the digestive juices (Liu et al., 2020). In addition, different pH conditions in gastric and intestinal juices may cause BPA to exist in the matrix in different forms (Bis(OH)2, Bis(OH)O−, and BisO22−), thereby leading to differences in BPA desorption rates in different environments (Han et al., 2012). This is further discussed in Section 3.3.
In general, different types of polymers have different adsorption capacities for BPA; in other words, the amount of BPA that can be loaded onto different microplastics varies (Li et al., 2021). The differences in the amount of BPA carried and BPA concentrations in solid and aqueous phases can lead to differences in the desorption amount of different microplastics. Therefore, the desorption percentage, rather than desorption capacity, was used to further evaluate the desorption of BPA from different microplastics in the digestive juices.
As shown in Figure 2, within the investigated concentration range, the desorption percentages of the three microplastics to BPA in different matrices were substantially different. The desorption percentage of BPA from PP ranged from 13.6–79.1%, whereas that of PS and PA ranged from 6.4–46.5% and 5.1–34.6%, respectively. Evidently, regardless of water, gastric juice, or intestinal juice, the desorption percentage of BPA from the rubbery PP polymer was substantially higher than that of from glassy PS and PA polymers. This might be related to the greater fluidity and free volume of molecular chains in rubbery polymers. Rubbery domains are flexible and highly accessible for hydrophobic organic pollutants, which might accelerate the movement of the latter (Guo et al., 2012). By contrast, the strong cohesion and cross-linking of glassy polymers may hinder the diffusion of pollutants in non-crystalline regions (Fu et al., 2021).
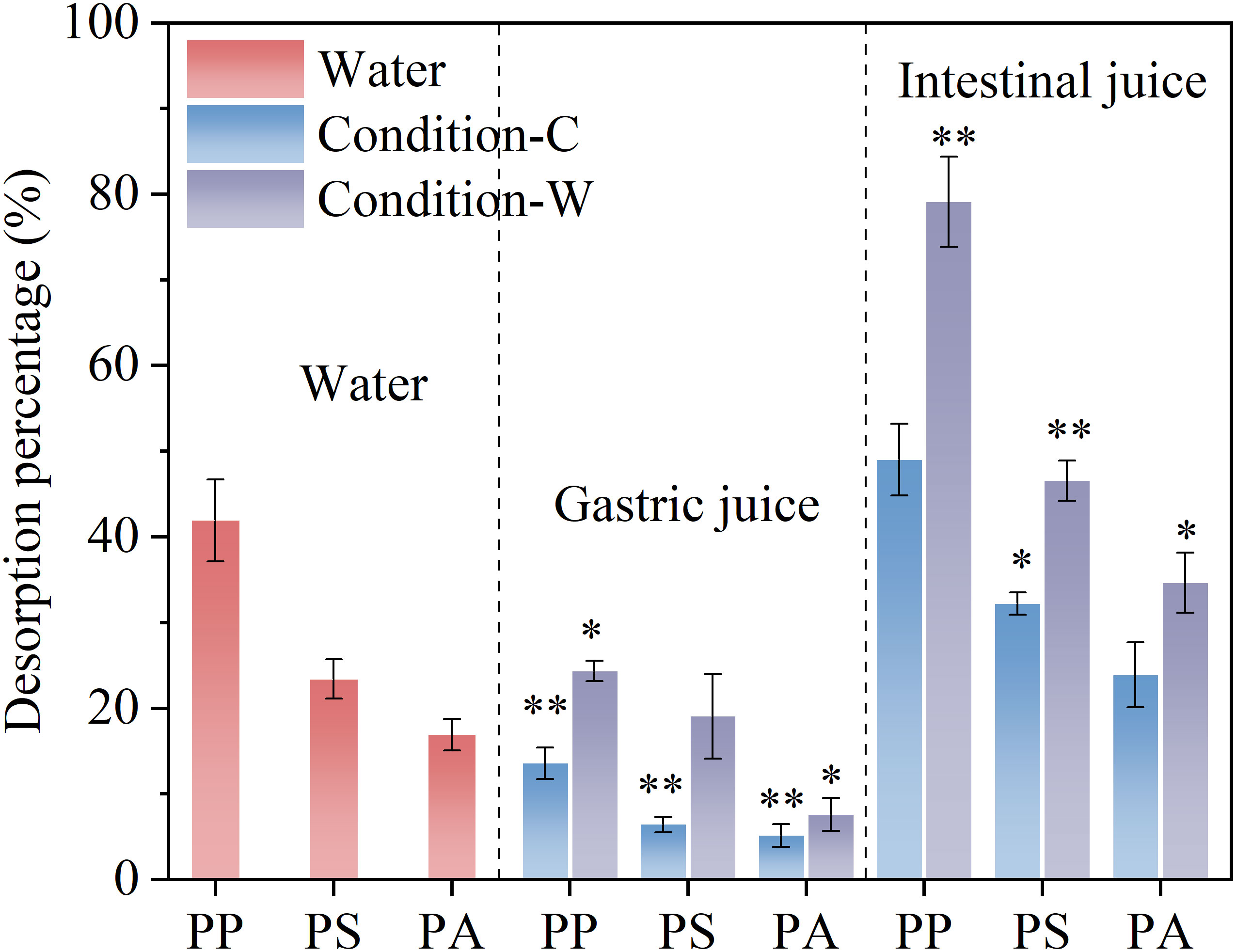
Figure 2 Bisphenol A (BPA) desorption percentage from three microplastic materials (polypropylene [PP], polystyrene [PS], and polyamide [PA]) in different matrices. C and W represent the simulated digestive juices of ectothermic (18°C) and endothermic organisms (37°C), respectively. (*significant difference compared with water; **extremely significant difference).
Among the three microplastics, PA presented the weakest BPA desorption, which was attributed to the irreversible adsorption of BPA on PA resulting from hydrogen bonds. The amide bond (-CO-NH-) in the PA molecular chain can be used as a hydrogen bond acceptor, while the hydroxyl group (-OH) in BPA can be used as a hydrogen bond donor, which results in the interaction of BPA and PA via hydrogen bonding (Liu et al., 2019b). The strong hydrogen bond energy (2–40 KJ/mol) contributes to more stable binding sites, hindering BPA desorption from microplastics.
Compared with water, gastric juices significantly inhibited BPA desorption from microplastics, whereas the intestinal juices of endothermic animals significantly promoted BPA desorption, which is consistent with the previous conclusions obtained by fitting the first-order kinetic model. These results suggest that when BPA-contaminated microplastics are ingested by organisms, BPA can be released in large quantities from certain polymers into the organism’s gut and that endothermic animals are more susceptible to such forms of complex pollution.
3.2 Desorption thermodynamics in different matrices
Figure 3 shows the desorption isotherms of BPA in water, gastric, and intestinal juices using the Langmuir and Freundlich models to describe the relationship between the BPA concentration in the solid phase (qe) and in solution upon desorption equilibrium (Ce) (Table 2) (Wu et al., 2020). Among the five matrices, the correlation coefficient (r2) obtained by fitting the Freundlich model ranged from 0.941–0.996, which was higher than the values of the Langmuir model (0.883–0.977). The better fitting effect of the Freundlich model indicate that multilayer desorption was crucial for BPA desorption from PP, PS, and PA microplastics (Putra et al., 2009). The surface heterogeneity constant n obtained by fitting the Freundlich model reflects the desorption trends, and the 1/n values obtained by fitting the BPA desorption in the five matrices were all less than 1, indicating that the desorption process was nonlinear and that the desorbed amount increased as the initial BPA adsorption on each microplastic increased (Wu et al., 2020). In addition, the Freundlich adsorption constant KF is related to the spontaneity of the reaction. In water and gastric juices, the KF values of each microplastic were higher than those in intestinal juices, suggesting that the degree of BPA desorption from microplastics in water and gastric juices was low.
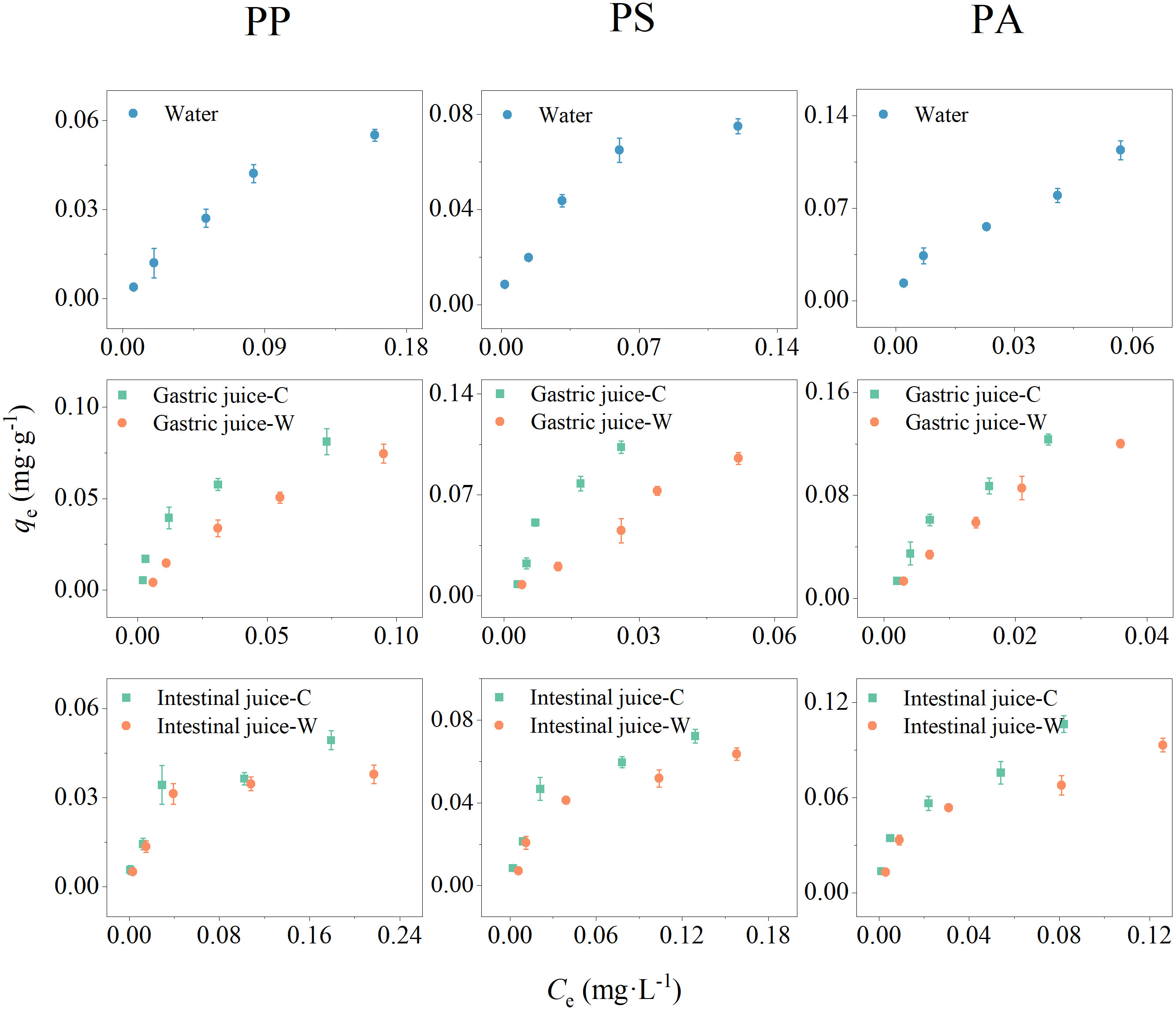
Figure 3 Desorption isotherms of bisphenol A (BPA) from three microplastic materials (polypropylene [PP], polystyrene [PS], and polyamide [PA]) in water, gastric juices, and intestinal juices. C and W represent the simulated digestive juices of ectothermic (18°C) and endothermic organisms (37°C), respectively.
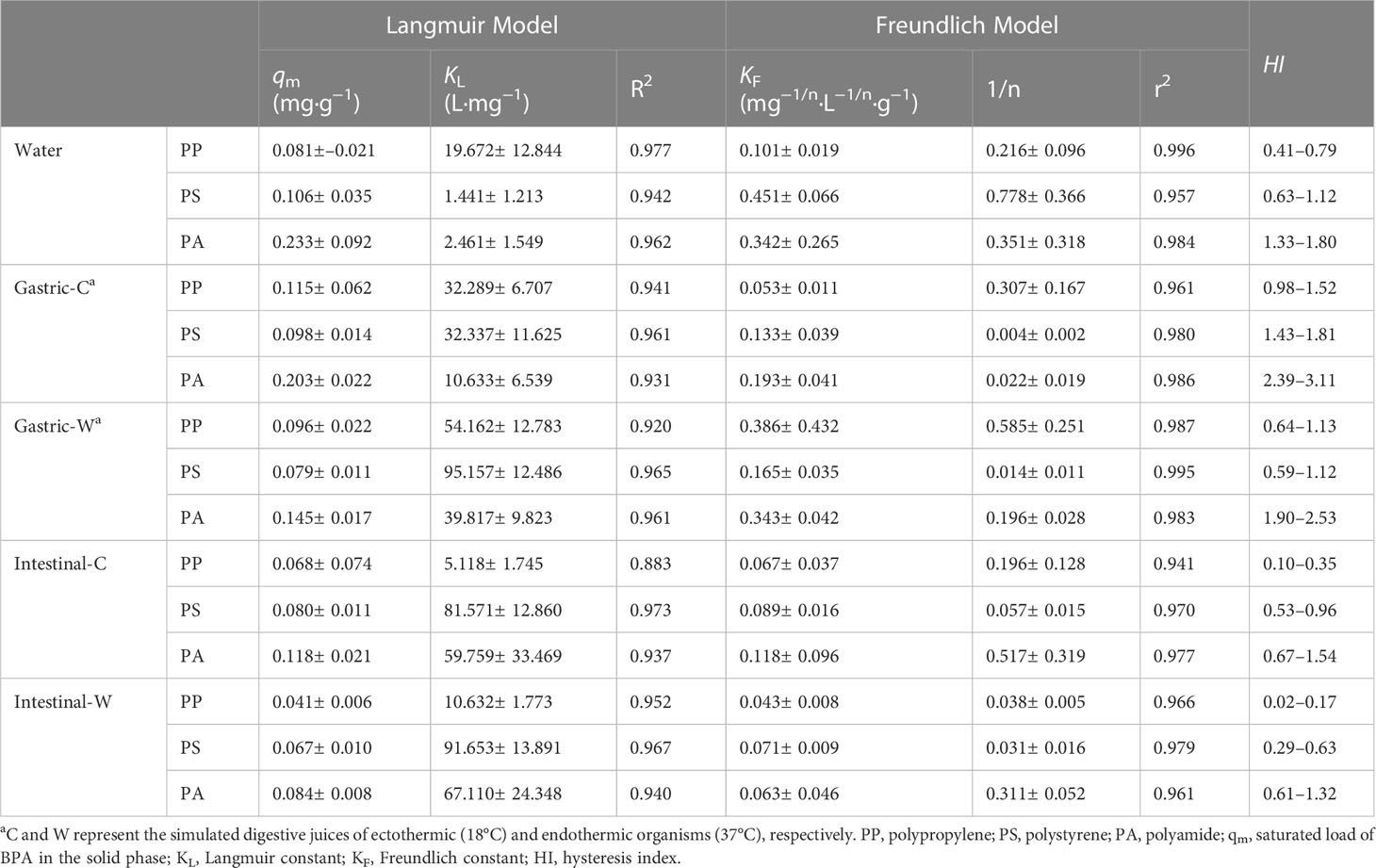
Table 2 Results of fitting Langmuir and Freundlich models for bisphenol A (BPA) desorption from microplastics in water and simulated gastric and intestinal juices.
Based on the desorption thermodynamic data, the desorption hysteresis coefficient HI of BPA from microplastics in different matrices was calculated (Table 2). BPA desorption from microplastics in all five matrices experienced hysteresis at varying degrees depending on the type of matrix and microplastic. For the same polymer, the BPA desorption hysteresis was the largest in gastric juices and the smallest in intestinal juices. However, for the same matrix, the degree of desorption hysteresis was in this order: PA > PS > PP.
The main causes of desorption hysteresis are generally the loss of adsorbate (caused by degradation, volatilization, or improper operation), irreversible binding of adsorbate at specific adsorption sites, and capture of adsorbate by the internal structure of the adsorbent (Monson, 2012). Because the results exhibited strong regularity, the loss of adsorbate is unlikely the dominant reason for desorption hysteresis. Compared to rubbery plastics (i.e., PP), hydrophobic organic pollutants diffuse more easily into the interior of glassy plastics (PS and PP), which may be detrimental to their desorption (Wu et al., 2020). In addition, the benzene rings in PS can form π-π conjugation with benzene compounds (BPA), thereby enhancing the adsorption between the two and increasing its irreversibility (Shen et al., 2018). Similarly, hydrogen bonds strengthen the binding interactions between PA and BPA, which increases hysteresis during desorption (Zhou et al., 2014). However, the reason for the different BPA desorption hysteresis in different matrices might be related to other environmental factors, which are further discussed in Section 3.3.
3.3 Influence of gastrointestinal environmental factors on desorption
As mentioned in the previous section, the amount of BPA desorbed by the same type of microplastics exhibited vast differences in digestion matrices. On the one hand, this may be related to the presence of different components in the digestive juices, including pepsin, sodium taurocholate, and bovine serum albumin (Liu et al., 2020). On the other hand, it might also be related to the different gastrointestinal environments (Han et al., 2012). In fact, significant differences in the amount of microplastic-mediated contamination in the gastrointestinal tract of different organisms in the same exposed environment have been reported (Coffin et al., 2019a). Although these differences cannot be directly attributed to the different environmental conditions of the gastrointestinal tract, they are undeniably affected by the environmental factors of the gastrointestinal tract.
As shown in Figure 4, there was a large difference between the BPA desorption percentage in gastric and intestinal juices as the pH increased. Within the pH range of gastric juices, BPA desorption was not significantly affected by pH (p > 0.05). However, within the pH range of intestinal juices, the BPA desorption percentage increased significantly as the pH increased. Although BPA is a neutral molecule with a dissociation constant (pKa) of 9.56, its two polar hydroxyl groups allow it to exist as three different forms in different pH solutions (Han et al., 2012). When the pH of the digestive juices is relatively low, BPA mainly exists as an uncharged molecule; however, it gradually dissociates into monovalent and divalent anions (Bis(OH)O− and BisO22−) as the pH increases. As the surfaces of PS microplastics are negatively charged under weakly acidic or alkaline conditions, the electrical repulsion between bisphenol anions and the negatively charged surfaces of PS microplastics may be the main reason for the increased desorption percentage of BPA in intestinal juices.
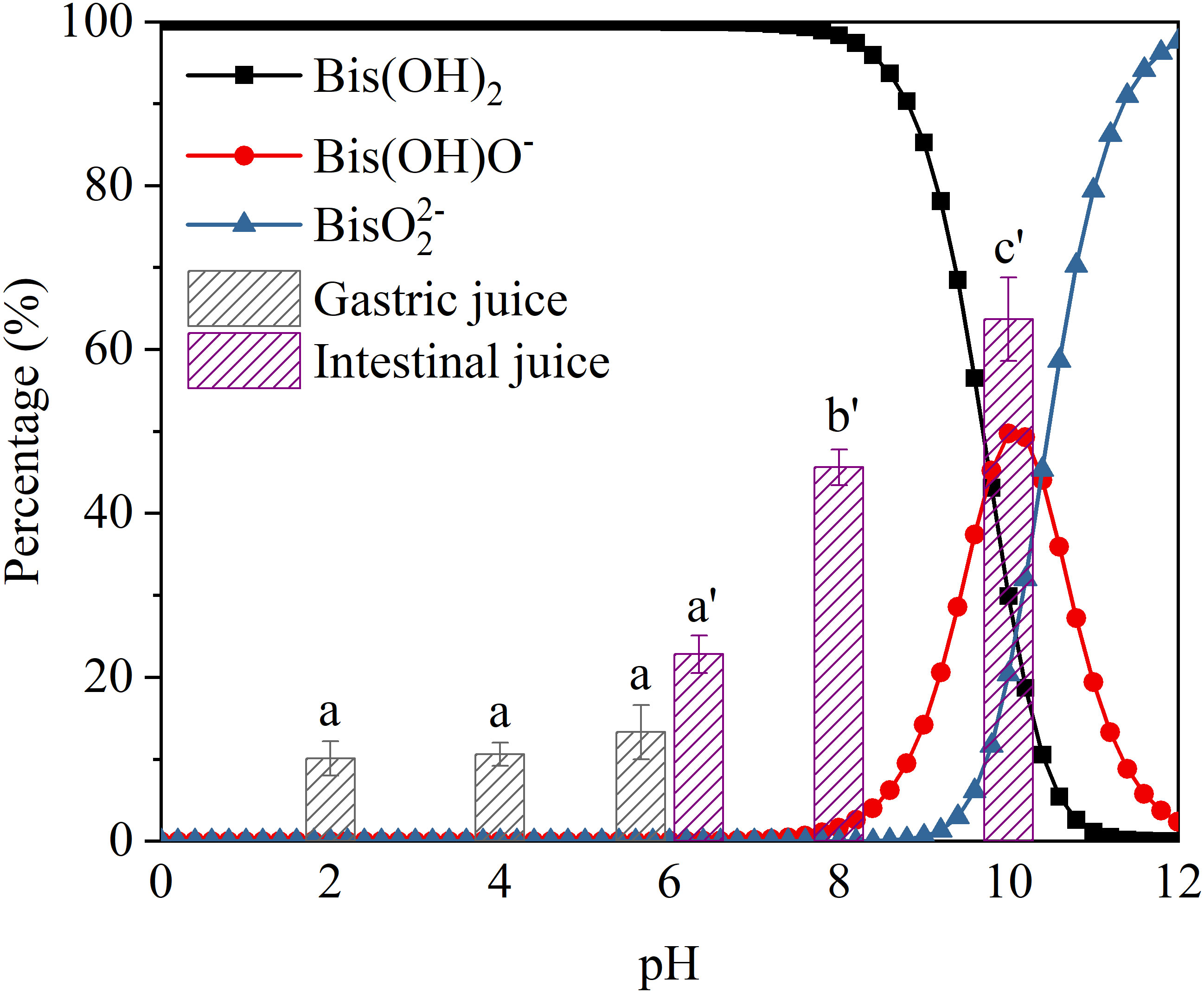
Figure 4 Bisphenol A (BPA) forms and the desorption percentage of BPA in simulated gastric and intestinal juices at different pH values. Significant differences between desorption rates at different pH values in each digestive juice are denoted using letters.
In addition, the BPA deprotonation in alkaline solution enhances BPA solubility (Gong et al., 2009), which can alter the partitioning of BPA between solid and aqueous phases, thereby enhancing BPA desorption from microplastics. The pH of digestive juices can significantly affect the desorption of fluoxetine, propranolol, atorvastatin, ketamine, and carbamazepine sorbed onto microplastics in the gastrointestinal tract (McDougall et al., 2022). Wu et al. (2022) explored the desorption potential of 17β-estradiol and 17α-ethinylestradiol on microplastics and similarly reported that pH changes can modify the distribution of substances in both solid and aqueous phases by altering their hydrophobic and electrostatic interactions. These findings suggest that the pH conditions of the gastrointestinal tract of organisms can significantly affect the desorption behavior of pollutants from microplastics. For microplastics sorbed with BPA, although BPA is not desorbed in large quantities in the stomach, organisms with a higher pH in the intestinal environment may be more susceptible to desorption. Furthermore, because the pH of the gastrointestinal tracts of organisms varies between fasting and eating, BPA biotoxicity mediated by microplastics may differ substantially before and after eating.
The desorption percentages of BPA from microplastics in gastric and intestinal juices with different ion concentrations (Na+) are shown in Figure 5. In the concentration range of 10–1000 mmol·L−1, the salt ions significantly inhibited BPA desorption from the microplastics. On the one hand, high concentrations of salt ions can induce the salting-out of organic compounds (Liu et al., 2019a). The hydrophobic surfaces of microplastics provide a good attachment point for BPA, thus weakening their distribution in the liquid phase. On the other hand, the biophysical properties of proteins in digestive juices may change under the stress of high ionic concentrations, thus affecting the hydrophobic binding sites on the surface of the microplastics. This then disrupts the BPA equilibrium in the solid and aqueous phases, subsequently hindering its further desorption (Mahapatra and Roy, 2019).
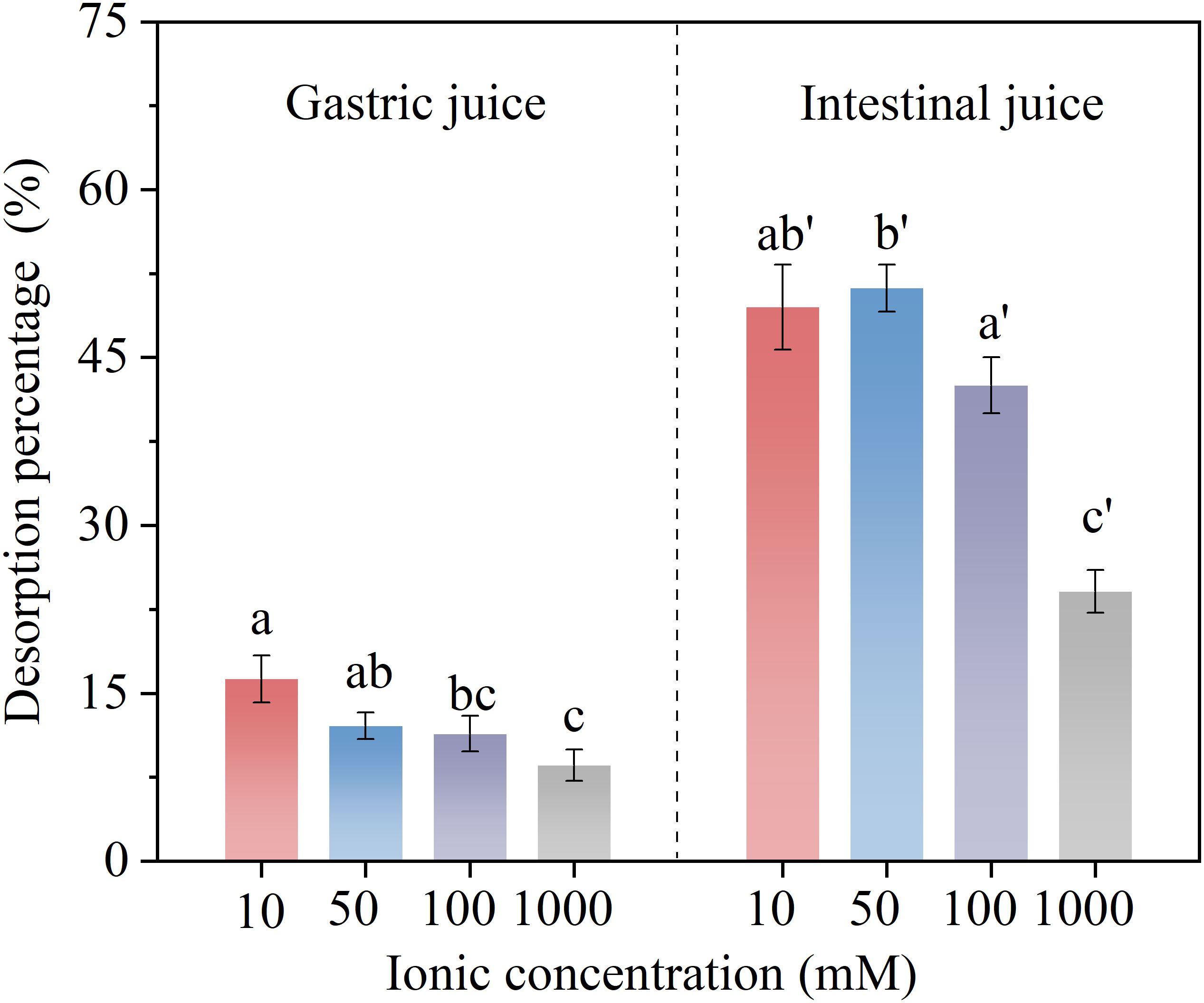
Figure 5 Desorption percentage of bisphenol A (BPA) in simulated gastric and intestinal juices at different ionic (Na+) concentrations. Significant differences between desorption rates at different ionic concentrations in each digestive juice are denoted using letters.
In addition, homogeneous aggregation of microplastics plays an important role in highly concentrated salt solutions (Lu et al., 2018). Aggregates with denser structures can further hinder BPA desorption from their surfaces. As mentioned above, electrical repulsion is the main reason for the higher desorption percentage of BPA in intestinal juices. Upon addition of ions, the ionic diffusion and electrostatic attraction between oppositely charged ions lead to the adsorption of Na+ onto the surface of microplastics, which neutralizes part of the negative charge on the microplastic surface (Alimi et al., 2018). This then weakens the electrical repulsion between microplastics and Bis(OH)O− or BisO22−, thereby decreasing the desorption percentage.
The BPA desorption percentage in digestive juices at different temperatures (18, 24, 30, and 37°C) is shown in Figure 6. The increase in temperature significantly enhanced the desorption of BPA in the gastric and intestinal juices from 7.0 ± 0.8% and 30.1 ± 1.7% to 20.3 ± 1.7% and 41.9 ± 2.6%, respectively. Temperature changes can alter the van der Waals forces between molecules, surface tension between substances, and solubility of organic pollutants, which may affect the interaction between organic pollutants and microplastics (Alsarraf et al., 2021). As the temperature increases, the mobility and solubility of the molecules also increase, which, to some extent, facilitates BPA desorption from microplastics (Zhan et al., 2016). In addition, under certain temperature conditions, chemical bonds may be broken, and the surface tension of microplastic particles may be reduced, thereby adversely affecting the binding between microplastics and organic compounds, which shifts the equilibrium towards desorption (Chen et al., 2019). Although Liu et al. (2020) observed that excessive temperature inhibited the desorption of ATV and AML in digestive juices to a certain extent, the significant positive correlation between the fast desorption percentage constant Kfast and temperature confirmed the positive effect of temperature on desorption. In summary, in the gastrointestinal temperature range, an increase in temperature significantly improved BPA desorption from microplastics. Therefore, we speculate that the risk of ingesting BPA-contaminated microplastics is greater for organisms with higher body temperatures than in those with lower body temperatures. Because ectothermic organisms have no mechanism to regulate body temperature, the risk of pollutant release caused by changes in body temperature when the ambient temperature rises cannot thus be ignored.
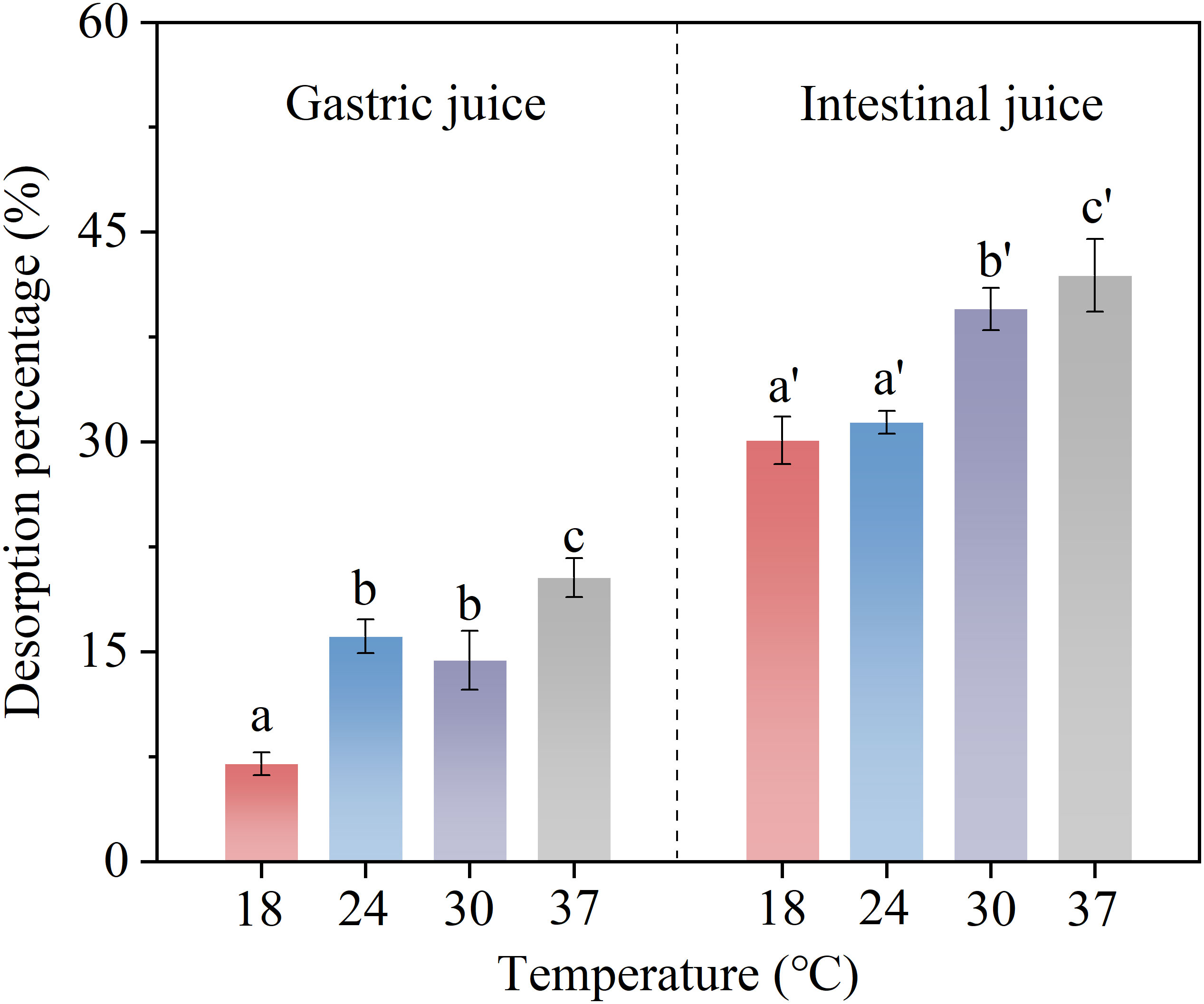
Figure 6 Desorption percentage of bisphenol A (BPA) in simulated gastric and intestinal juices at different temperatures. Significant differences between desorption rates at different temperatures in each digestive juice are denoted using letters.
4 Conclusions
Our results showed that BPA was rapidly desorbed from microplastic carriers under simulated gastrointestinal conditions and that multilayer desorption was crucial for the desorption process. The desorption of BPA from different polymers can vary because of the different crystalline structures and functional groups associated with the polymers. Under specific pH, ion concentration, or temperature conditions, the desorption of BPA from microplastics in the simulated digestive tract of organisms was enhanced. The main interactions that influence desorption are electrostatic interaction, hydrophobic interaction, and van der Waals forces. Microplastics exhibited desorption hysteresis in the simulated gastrointestinal environment, and the degree of BPA desorption from microplastics was substantially higher in the intestinal environment than in the gastric environment. In addition, microplastics are desorbed at a higher percentage in the gastrointestinal environment of endothermic organisms than in that of ectothermic organisms. These results suggest that when BPA-contaminated microplastics are ingested by organisms, the specific gastrointestinal environment can increase the bioaccessibility of these pollutants, leading to health hazards. In addition, to explore the general pattern of BPA desorption from microplastics in digestive fluids, the initial saturation of the microplastics must be carried out in a higher concentration of BPA solution. However, it is more likely that microplastics would be exposed to a lower level of BPA in real environments where both contaminants co-occur. In this case, the mass of adsorbed BPA at equilibrium would likely be much lower. Therefore, additional studies that better simulate real environmental conditions are needed to further assess the impact of microplastic intake on the health of organisms.
Data availability statement
The raw data supporting the conclusions of this article will be made available by the authors, without undue reservation.
Author contributions
WL: Conceptualization, Writing - Original Draft; BZ: Conceptualization, Supervision; LL and JianL: Review; JiawL and XM: Editing. All authors contributed to the article and approved thesubmitted version.
Funding
This study was financially supported by the Natural Science Foundation of Chongqing, China (grant number cstc2020jcyj-msxmX0763).
Conflict of interest
Author XM is employed by the company Chongqing Yi Da Environmental Protection Engineering Co., Ltd.
The remaining authors declare that the research was conducted in the absence of any commercial or financial relationships that could be construed as a potential conflict of interest.
Publisher’s note
All claims expressed in this article are solely those of the authors and do not necessarily represent those of their affiliated organizations, or those of the publisher, the editors and the reviewers. Any product that may be evaluated in this article, or claim that may be made by its manufacturer, is not guaranteed or endorsed by the publisher.
References
Alimi O. S., Farner Budarz J. F., Hernandez L. M., Tufenkji N. (2018). Microplastics and nanoplastics in aquatic environments: aggregation, deposition, and enhanced contaminant transport. Environ. Sci. Technol. 52, 1704–1724. doi: 10.1021/acs.est.7b05559
Alsarraf J., Al-Rashed A. A. A. A., Alnaqi A. A., Goldanlou A. S. (2021). Dominance of cohesion of EG-water molecules over van der waals force between SiO2-ZnO nanoparticles in the liquid interface. Powder Technol. 379, 537–546. doi: 10.1016/j.powtec.2020.10.079
Bakir A., Rowland S. J., Thompson R. C. (2014). Enhanced desorption of persistent organic pollutants from microplastics under simulated physiological conditions. Environ. Pollut. 185, 16–23. doi: 10.1016/j.envpol.2013.10.007
Barboza L. G. A., Cunha S. C., Monteiro C., Fernandes J. O., Guilhermino L. (2020). Bisphenol a and its analogs in muscle and liver of fish from the north East Atlantic ocean in relation to microplastic contamination. exposure and risk to human consumers. J. Hazard. Mater. 393, 122419. doi: 10.1016/j.jhazmat.2020.122419
Berhane T. M., Levy J., Krekeler M. P. S., Danielson N. D. (2016). Adsorption of bisphenol a and ciprofloxacin by palygorskite-montmorillonite: effect of granule size, solution chemistry and temperature. Appl. Clay Sci. 132-133, 518–527. doi: 10.1016/j.clay.2016.07.023
Browne M. A., Dissanayake A., Galloway T. S., Lowe D. M., Thompson R. C. (2008). Ingested microscopic plastic translocates to the circulatory system of the mussel, Mytilus edulis (L.). Environ. Sci. Technol. 42, 5026–5031. doi: 10.1021/es800249a
Chen S., Tan Z., Qi Y., Ouyang C. (2019). Sorption of tri-n-butyl phosphate and tris(2-chloroethyl) phosphate on polyethylene and polyvinyl chloride microplastics in seawater. Mar. pollut. Bull. 149, 110490. doi: 10.1016/j.marpolbul.2019.110490
Chen Y., Zhang D. (2014). Adsorption kinetics, isotherm and thermodynamics studies of flavones from Vaccinium bracteatum thunb leaves on NKA-2 resin. Chem. Eng. J. 254, 579–585. doi: 10.1016/j.cej.2014.05.120
Cheng H., Luo H., Hu Y., Tao S. (2020). Release kinetics as a key linkage between the occurrence of flame retardants in microplastics and their risk to the environment and ecosystem: a critical review. Water Res. 185, 116253. doi: 10.1016/j.watres.2020.116253
Coffin S., Huang G. Y., Lee I., Schlenk D. (2019a). Fish and seabird gut conditions enhance desorption of estrogenic chemicals from commonly ingested plastic items. Environ. Sci. Technol. 53, 4588–4599. doi: 10.1021/acs.est.8b07140
Coffin S., Lee I., Gan J., Schlenk D. (2019b). Simulated digestion of polystyrene foam enhances desorption of diethylhexyl phthalate (DEHP) and in vitro estrogenic activity in a size-dependent manner. Environ. Pollut. 246, 452–462. doi: 10.1016/j.envpol.2018.12.011
Corrales J., Kristofco L. A., Steele W. B., Yates B. S., Breed C. S., Williams E. S., et al. (2015). Global assessment of bisphenol a in the environment: review and analysis of its occurrence and bioaccumulation. Dose-Response 13, 1559325815598308. doi: 10.1177/1559325815598308
Desforges J. P., Galbraith M., Ross P. S. (2015). Ingestion of microplastics by zooplankton in the northeast pacific ocean. Arch. Environ. Contam. Toxicol. 69, 320–330. doi: 10.1007/s00244-015-0172-5
Fu L., Li J., Wang G., Luan Y., Dai W. (2021). Adsorption behavior of organic pollutants on microplastics. Ecotoxicol. Environ. Saf. 217, 112207. doi: 10.1016/j.ecoenv.2021.112207
Gong R., Liang J., Chen J., Huang F. (2009). Removal of bisphenol a from aqueous solution by hydrophobic sorption of hemimicelles. Int. J. Environ. Sci. Technol. 6, 539–544. doi: 10.1007/BF03326093
Guo X., Wang X., Zhou X., Kong X., Tao S., Xing B. (2012). Sorption of four hydrophobic organic compounds by three chemically distinct polymers: role of chemical and physical composition. Environ. Sci. Technol. 46, 7252–7259. doi: 10.1021/es301386z
Habib R. Z., Thiemann T., Al Kendi R. A. (2020). Microplastics and wastewater treatment plants–a review. J. Water Resour. Prot. 12, 1–35. doi: 10.4236/jwarp.2020.121001
Han W., Luo L., Zhang S. (2012). Adsorption of bisphenol a on lignin: effects of solution chemistry. Int. J. Environ. Sci. Technol. 9, 543–548. doi: 10.1007/s13762-012-0067-1
Jiang C., Yin L., Li Z., Wen X., Luo X., Hu S., et al. (2019). Microplastic pollution in the rivers of the Tibet plateau. Environ. pollut. 249, 91–98. doi: 10.1016/j.envpol.2019.03.022
Johnson M. D., Keinath T. M., Weber W. J. (2001). A distributed reactivity model for sorption by soils and sediments. 14. characterization and modeling of phenanthrene desorption rates. Environ. Sci. Technol. 35, 1688–1695. doi: 10.1021/es001391k
Ju W. J., An J., Jho E. H. (2021). Adsorption characteristics of cd and Pb on microplastic films generated in agricultural environment. J. Korean Soc Environ. Eng. 43, 32–42. doi: 10.4491/KSEE.2021.43.1.32
Li S., Ma R., Zhu X., Liu C., Li L., Yu Z., et al. (2021). Sorption of tetrabromobisphenol a onto microplastics: behavior, mechanisms, and the effects of sorbent and environmental factors. Ecotoxicol. Environ. Saf. 210, 111842. doi: 10.1016/j.ecoenv.2020.111842
Liu F. F., Liu G. Z., Zhu Z. L., Wang S. C., Zhao F. F. (2019a). Interactions between microplastics and phthalate esters as affected by microplastics characteristics and solution chemistry. Chemosphere 214, 688–694. doi: 10.1016/j.chemosphere.2018.09.174
Liu X., Shi H., Xie B., Dionysiou D. D., Zhao Y. (2019b). Microplastics as both a sink and a source of bisphenol a in the marine environment. Environ. Sci. Technol. 53, 10188–10196. doi: 10.1021/acs.est.9b02834
Liu P., Wu X., Liu H., Wang H., Lu K., Gao S. (2020). Desorption of pharmaceuticals from pristine and aged polystyrene microplastics under simulated gastrointestinal conditions. J. Hazard. Mater. 392, 122346. doi: 10.1016/j.jhazmat.2020.122346
Lu S., Zhu K., Song W., Song G., Chen D., Hayat T., et al. (2018). Impact of water chemistry on surface charge and aggregation of polystyrene microspheres suspensions. Sci. Total Environ. 630, 951–959. doi: 10.1016/j.scitotenv.2018.02.296
Mahapatra K., Roy S. (2019). An insight into the folding and stability of Arabidopsis thaliana SOG1 transcription factor under salinity stress in vitro. Biochem. Biophys. Res. Commun. 515, 531–537. doi: 10.1016/j.bbrc.2019.05.183
McDougall L., Thomson L., Brand S., Wagstaff A., Lawton L. A., Petrie B. (2022). Adsorption of a diverse range of pharmaceuticals to polyethylene microplastics in wastewater and their desorption in environmental matrices. Sci. Total Environ. 808, 152071. doi: 10.1016/j.scitotenv.2021.152071
Monson P. A. (2012). Understanding adsorption/desorption hysteresis for fluids in mesoporous materials using simple molecular models and classical density functional theory. Micropor. Mesopor. Mater. 160, 47–66. doi: 10.1016/j.micromeso.2012.04.043
Putra E. K., Pranowo R., Sunarso J., Indraswati N., Ismadji S. (2009). Performance of activated carbon and bentonite for adsorption of amoxicillin from wastewater: mechanisms, isotherms and kinetics. Water Res. 43, 2419–2430. doi: 10.1016/j.watres.2009.02.039
Shen T., Gao M., Ding F., Zeng H., Yu M. (2018). Organo-vermiculites with biphenyl and dipyridyl gemini surfactants for adsorption of bisphenol a: structure, mechanism and regeneration. Chemosphere 207, 489–496. doi: 10.1016/j.chemosphere.2018.05.054
Simonelli A., Guadagni R., De Franciscis P., Colacurci N., Pieri M., Basilicata P., et al. (2017). Environmental and occupational exposure to bisphenol a and endometriosis: urinary and peritoneal fluid concentration levels. Int. Arch. Occup. Environ. Health 90, 49–61. doi: 10.1007/s00420-016-1171-1
Vandenberg L. N., Hunt P. A., Gore A. C. (2019). Endocrine disruptors and the future of toxicology testing–lessons from CLARITY–BPA. nat. rev. Endocrinology 15, 366–374. doi: 10.1038/s41574-019-0173-y
Wagstaff A., Petrie B. (2022). Enhanced desorption of fluoxetine from polyethylene terephthalate microplastics in gastric fluid and sea water. Environ. Chem. Lett. 20, 975–982. doi: 10.1007/s10311-022-01405-0
Wang J., Coffin S., Schlenk D., Gan J. (2020). Accumulation of HOCs via precontaminated microplastics by earthworm eisenia fetida in soil. Environ. Sci. Technol. 54, 11220–11229. doi: 10.1021/acs.est.0c02922
Windsor F. M., Tilley R. M., Tyler C. R., Ormerod S. J. (2019). Microplastic ingestion by riverine macroinvertebrates. Sci. Total Environ. 646, 68–74. doi: 10.1016/j.scitotenv.2018.07.271
Wu J., Lu J., Wu J. (2022). Effect of gastric fluid on adsorption and desorption of endocrine disrupting chemicals on microplastics. Front. Environ. Sci. Eng. 16, 1–13. doi: 10.1007/s11783-022-1525-8
Wu P., Tang Y., Jin H., Song Y., Liu Y., Cai Z. (2020). Consequential fate of bisphenol-attached PVC microplastics in water and simulated intestinal fluids. Environ. Sci. Ecotechnol. 2, 100027. doi: 10.1016/j.ese.2020.100027
Zhan Z., Wang J., Peng J., Xie Q., Huang Y., Gao Y. (2016). Sorption of 3,3’,4,4’-tetrachlorobiphenyl by microplastics: a case study of polypropylene. Mar. pollut. Bull. 110, 559–563. doi: 10.1016/j.marpolbul.2016.05.036
Zheng K., Fan Y., Zhu Z., Chen G., Tang C., Peng X. (2019). Occurrence and species-specific distribution of plastic debris in wild freshwater fish from the pearl river catchment, China. Environ. Toxicol. Chem. 38, 1504–1513. doi: 10.1002/etc.4437
Zhou X., Wei J., Liu K., Liu N., Zhou B. (2014). Adsorption of bisphenol a based on synergy between hydrogen bonding and hydrophobic interaction. Langmuir 30, 13861–13868. doi: 10.1021/la502816m
Keywords: microplastic, bisphenol A, desorption, gastrointestinal tract, bioaccessibility, risk assessment
Citation: Li W, Zu B, Li L, Li J, Li J and Mei X (2023) Desorption of bisphenol A from microplastics under simulated gastrointestinal conditions. Front. Mar. Sci. 10:1195964. doi: 10.3389/fmars.2023.1195964
Received: 29 March 2023; Accepted: 03 May 2023;
Published: 12 May 2023.
Edited by:
Jinping Cheng, The Education University of Hong Kong, Hong Kong SAR, ChinaReviewed by:
Liyuan Qiang, Shihezi University, ChinaDefu He, East China Normal University, China
Qiqing Chen, East China Normal University, China
Copyright © 2023 Li, Zu, Li, Li, Li and Mei. This is an open-access article distributed under the terms of the Creative Commons Attribution License (CC BY). The use, distribution or reproduction in other forums is permitted, provided the original author(s) and the copyright owner(s) are credited and that the original publication in this journal is cited, in accordance with accepted academic practice. No use, distribution or reproduction is permitted which does not comply with these terms.
*Correspondence: Bo Zu, enVib0BjcWp0dS5lZHUuY24=