- 1Key Laboratory of Integrated Regulation and Resources Development on Shallow Lakes of Ministry of Education, Hohai University, Nanjing, China
- 2College of Environment, Hohai University, Nanjing, China
The widespread distribution of nanoplastics and nanomaterials in aquatic environments is of great concern. Nanoplastics have been found to modulate the toxicity of other environmental pollutants in organisms, while few studies have focused on their influences on nanomaterials. Thus, this study evaluated the influences of polystyrene (PS) nanoplastics on the toxicity of silver nanoparticles (AgNPs) to zebrafish (Danio rerio) embryos, including acute toxicity, oxidative stress, apoptosis, immunotoxicity, and metabolic capability. The results showed that the presence of PS nanoplastics could act as a carrier of the co-existing AgNPs in waters. The release ratio of Ag+ from AgNPs was up to 4.23%. The lethal effects of AgNPs on zebrafish embryos were not significantly changed by the co-added PS nanoplastics. Whereas, the alterations in gene expression related to antioxidant and metabolic capability in zebrafish (sod1, cat, mt2, mtf-1, and cox1) caused by AgNPs were significantly enhanced by the presence of PS nanoplastics, which simultaneously lowered the apoptosis and immunotoxicity (caspase9, nfkβ, cebp, and il-1β) induced by AgNPs. It suggests the presence of PS nanoplastics suppressed the AgNPs-induced genotoxicity in zebrafish. The released Ag+ from AgNPs may be responsible for the toxicity of AgNPs in zebrafish, while the subsequent absorption and agglomeration of AgNPs and the released Ag+ on PS nanoplastics may alleviate the toxicity.
1 Introduction
Plastic pollution is one of the biggest challenges in the environment worldwide due to the huge production and extremely low recovery over the whole life cycle. It is estimated that approximately 12,000 Mt of plastic waste will be released into the natural environment by 2050 if current trends continue (Geyer et al., 2017). Under various natural and anthropogenic forces, plastic waste is further fragmented into small particle sizes, generating microplastics with a size of less than 5 mm. Recently, microplastics have been ubiquitously detected in different waters, including seawater, fresh water, drinking water, and even bottled water (Tekman et al., 2020; Kumar et al., 2021; Sridharan et al., 2021). Research into the environmental impacts of microplastics in aquatic environments is thereby sharply increasing.
In the aquatic environment, microplastics are easily ingested by aquatic organisms due to their small size, thereby inhibiting growth, disrupting predation behavior, and causing histopathological damage (Davies et al., 2021; Wei et al., 2021; Hoseini et al., 2022). The ingested microplastics may further accumulate and even transfer up the food chain, finally entering the human body (Huang et al., 2021). Currently, microplastics have been continuously detected in the human lung (Jenner et al., 2022), breastmilk (Ragusa et al., 2022), blood (Leslie et al., 2022), and even placenta (Ragusa et al., 2021). Hence, a greater understanding of the biological effects and ecological risks of microplastics in water is highlighted.
In addition to the common damage caused by microplastic itself, its carrier effects with the co-existing pollutants are of particular concern. Due to its small size and large specific surface area, microplastic can easily interact with and adsorb various pollutants from the surrounding environment, such as endocrine-disrupting compounds, heavy metals, and pharmaceuticals, playing a vital role in the transportation and toxicity of the co-existing pollutants (Thiagarajan et al., 2021; Hu et al., 2022). However, the outcomes of such interactions for aquatic biota are often unforeseeable, and the results are often confusing (Bhagat et al., 2021). For instance, Zhang et al. (2022) suggested that the carrier effects of microplastics enhanced the accumulation and toxic effects of roxithromycin on fish, showing a sensitization effect. On the other hand, microplastics alleviated the biological stress caused by arsenic in the earthworm Metaphire californica, playing a dilution and protective effect (Wang et al., 2019). In some cases, the sensitization and protective effects of microplastics may appear alternately, depending on the concentration variations of microplastics and co-existing pollutants. As a result, it highlights the need for a deep understanding of the carrier effects of microplastics on the co-existing pollutants in aquatic environments once they encounter each other. To date, the knowledge regarding the carrier effects of microplastics is mainly focused on persistent organic pollutants, heavy metals, and pharmaceuticals. Study on the interactions between microplastics and engineered nanomaterials is still limited, though both of them are constantly released into the aquatic environment through similar pathways, including wastewater, facility agriculture, and landfill (Gautam and Tiwari, 2020; Zhang et al., 2020).
Research on the combined effects of microplastics and nanomaterials on aquatic systems is of particular interest since the presence of these pollutants in waters is of great concern with respect to food safety and human health. In addition, nanomaterials show a similar sorption potential as microplastics (Scott-Fordsmand et al., 2017), which further prompts scientists and the public to pursue their interactions. Among the nanomaterials, silver nanoparticles (AgNPs) have the highest annual output of 800 tons and have been applied in more than 400 consumer products (Wang et al., 2019; Tortella et al., 2020; Li et al., 2022). In addition to the benefits from the application, AgNPs also inevitably entered the aquatic environment during their use and disposal, with an estimated concentration of up to 2.2 μg/L in surface water (Sun et al., 2016). The occurrence of AgNPs in waters has received widespread attention due to their potential risks to the biota. Lekamge et al. (2019) found that AgNPs changed the feeding behavior of Daphnia magna and further transferred up the food chain. During zebrafish embryonic development, AgNPs were able to cause apoptosis, inhibit erythropoiesis, and interfere with the formation of skeletal and cardiac muscle fibrosis (Xin et al., 2015; Cui et al., 2016; Xu et al., 2018). Given that microplastics and AgNPs appear to share similar transportation and accumulation pathways from sources to natural environments, this provides more opportunities for microplastics and AgNPs to capture and interact with each other in waters (Li et al., 2022). However, only a limited number of reports have studied the toxic impacts of microplastics and nanomaterials on aquatic biota and did not include AgNPs. Considering the micro-sized plastics in aquatic environments could degrade subsequently into nano-sizes (Mattsson et al., 2015), which may have a different impact on the toxicity of nanomaterials in aquatic organisms than the larger ones due to their small size, high surface curvature, and large surface area. Hence, the combined effects of nanoplastics and AgNPs on aquatic organisms need to be assessed critically.
Among these plastics, polystyrene (PS) is one of the most important materials from the modern plastic industry and has been used all over the world due to its excellent physical properties and low cost (Yousif and Haddad, 2013). In addition, PS is also one of the components of plastic debris commonly found in aquatic environments (Lu et al., 2016). Given that the presence of nanoplastics and AgNPs in waters could capture and interact with each other, herein it is hypothesized that the presence of nanoplastics would further alter the toxicity of AgNPs in aquatic organisms. Zebrafish (Danio rerio), a model aquatic organism, has been wildly used for acute and chronic tests. Considering embryos are more ethical, economical, and sensitive than those on adult fish treated with different pollutants (Yang et al., 2016), the zebrafish embryo was used as the target organism in the present study. Therefore, the current study investigates the interactive effects of plain PS nanoplastics and nano-sized AgNPs on zebrafish at early life stages. The responses of acute toxicity, oxidative stress, apoptosis, innate immune, and metabolic function of zebrafish embryos were integrally evaluated. In addition, a silver ions treatment was also introduced as a positive reference for AgNPs to study the interaction mechanism between nanoplastics and AgNPs in fish. The results will broaden our understanding of the interactions of microplastics and nanomaterials in aquatic environments.
2 Materials and methods
2.1 Materials
Plain polystyrene (PS) nanoplastics were purchased from Big Goose Scientific (Tianjin, China). All the PS nanoplastics were dispersed in deionized water with a mass concentration of 10 mg/mL and a size of 0.1 μm (density: 1.05 g/cm3). The fresh stock solution of AgNPs was obtained from XFNANO Materials Tech (Nanjing, China). AgNPs were prepared in ultrapure water with a mass concentration of 0.1mg/mL and a size of 15 ± 5 nm. All the stocks of PS-MPs and AgNPs were stored at 4 °C in the dark. Other chemicals used in this study, such as AgNO3, were purchased from Sinopharm Group (Beijing, China). Ultrapure water was prepared by a Millipore Milli-Q integral water purification system (Millipore, Milford, USA).
22 Animal cultures
Adult wild-type zebrafish (TU strain) were kindly provided by the Model Animal Research Center of Nanjing University, and then maintained in the laboratory for at least two weeks before exposure, according to previous procedures (Yan et al., 2019). All fish were cultured in a recirculation system with a temperature of 28 ± 1°C and a photoperiod of 14:10 h light: dark. The fish were fed with fresh brine shrimp two times per day.
The spawning of fish was carried out in a spawning aquarium for 24 h acclimatization and then stimulated by light. All fertilized eggs were collected via collection chambers and inspected using a stereomicroscope (SZX16, Olympus, Japan). The healthy fertilized eggs at 4 h post-fertilization (hpf) were used for further exposure.
2.3 Preparation and characterization of PS nanoplastics and AgNPs
All exposure solutions were freshly prepared in ultrapure water with ultrasonic treatment for 20 min to ensure uniform dispersion. The number concentrations of the PS nanoplastics prepared in test solutions with 10 mg/L were approximately 1.80 × 1010 particles/mL.
The structural morphology of PS nanoplastics, AgNPs, and their mixture in exposure solution was characterized by High-Resolution Transmission Electron Microscopy (TEM) (JEM-2100F, JEOL, Japan). The zeta potentials and z-average hydrodynamic diameters of the AgNPs alone and in combination with PS microplastics in the suspension solution during the exposure periods were also examined using a Zetasizer Nano ZS90 (Malvern, UK), as described by Calisi et al. (2022).
2.4 Ionic release from AgNPs
To evaluate the released Ag+ from different concentrations of AgNPs particles in different solutions before the exposure experiment as in previous studies (Osborne et al., 2013; van Aerle et al., 2013), 5 mL of exposure solutions were collected from each treatment and then passed through a 10 KDa ultrafiltration centrifuge tube (13600 g, 10 min). Since the pore size of the centrifuge tube is close to 1 nm, it can easily trap AgNPs and release Ag+ (Huang et al., 2019). The concentrations of the released Ag+ were determined by an inductively coupled plasma mass spectrometry (ICP-MS) (Thermon Fisher Scientific, USA).
2.5 Experimental design
All fertilized eggs were individually placed in a 24-well plate containing 2 mL of exposure solution. All exposure experiments were semi-static and the solutions were replaced daily with a replacement rate of 90% v/v to maintain stable exposure concentrations. All test solutions were maintained at pH 7.0 ± 0.1 during the exposure periods. The whole experiments were kept in illumination incubators at the temperature of 28 ± 1°C with a photoperiod of 14/10 h light/dark. During the exposure periods, the dead zebrafish embryos or larvae were removed promptly to prevent negative effects on other healthy individuals. Each exposure experiment was run in three independent replicates.
2.5.1 Acute toxicity test
The acute toxicity test was conducted in accordance with the OECD guidelines for fish embryo acute toxicity test (Test No. 236), which is intended to determine the acute or lethal toxicity of chemicals in the embryonic stages of zebrafish. Briefly, newly fertilized zebrafish embryos were exposed to different concentrations of AgNPs alone (0, 0.5, 1, 2, 4, and 8 mg/L), AgNPs + PS nanoplastics (the same concentrations as AgNPs alone plus 10 mg/L of PS), and Ag+ + PS nanoplastics (0, 10, 20, 40, 60, and 160 μg/L of Ag+ plus 10 mg/L of PS) for a period of 96 h, respectively. In each treatment, twenty embryos were included. Each test was conducted with five replicates. The dead or immobility embryos were checked in time during the 96 h exposure periods using an Olympus SZX16 stereomicroscope. Every 24 h, four apical observations are recorded as indicators of lethality: (i) coagulation of fertilized eggs, (ii) lack of somite formation, (iii) lack of detachment of the tail-bud from the yolk sac, and (iv) lack of heartbeat. No food was provided during the acute exposure periods. At the end of the exposure period, acute toxicity was determined based on a positive outcome in any of the four apical observations recorded, and the LC50 was calculated. And then, the toxic unit (TU) approach was used to assess the toxicity of AgNPs and PS nanoplastics mixtures. The toxicity of the mixture was termed additive when TU was equal to 1 at 50% effect, while it was called synergistic or antagonistic when TU was less than or greater than 1 (Meyer et al., 2015).
2.5.2 Subacute exposure experiment
Based on the results of the acute toxicity test of AgNPs in this study and previous reports (van Aerle et al., 2013; Cambier et al., 2018), the exposure concentration of AgNPs in the subacute exposure experiment was selected as 50 μg/L, which was about 1/10 of the 96 h LC50 in this study. Zebrafish embryos at 4 hpf were exposed to control treatment, AgNPs alone treatment (50 μg/L), and a mixture treatment containing AgNPs and PS (50 μg/L + 10 mg/L), respectively. Meanwhile, an additional treatment containing Ag+ and PS (2.5 μg/L + 10 mg/L) was conducted to serve as the positive treatment to explain the toxic effect of AgNPs on zebrafish caused by the released Ag+. Given that the released amounts of Ag+ from AgNPs were generally less than 5% in the present study and previous studies (Osborne et al., 2013; van Aerle et al., 2013), the 5% of the release ratio from AgNPs was selected as the Ag+ concentration (2.5 μg/L). Each experiment was conducted in three independent replicates containing 60 embryos per replicate. All fish were checked daily and the dead one was removed in time. The concentrations of AgNPs and Ag+ in testing medium were checked every day by ICP-MS.
2.6 Biological responses analyses
After exposure for 120 h, all zebrafish larvae were collected and stored in liquid nitrogen in time. The total RNA of each sample was isolated using a MiniBEST universal RNA extraction kit (TaKaRa, Japan). The quality of the extracted RNA was verified by gel electrophoresis and the ratio of absorbance at the wavelength of 260/280 nm. Then, the RNA was reversely transcribed to cDNA using the iScript cDNA synthesis kit (Bio-Rad, USA) according to the manufacturer’s instructions.
The expression of several important genes involved in oxidative stress (sod1, cat, and hsp70), apoptosis (bax, caspase9, and caspase3), innate immune (nfkβ, cebp, and il-1β), and metabolic function (mt2, mtf-1, cox1, and abcb4) in zebrafish were determined using qRT-PCR according to previous studies, and β-Actin was selected as the reference gene (Khan et al., 2019; Lu et al., 2022). The primer pairs were designed and synthesized by Shanghai Shenggong (Shanghai, China). The primer sequences of each target gene are listed in Table 1. Quantitative real-time polymerase chain reaction (qRT-PCR) was then conducted using a Bio-Rad CFX96 Touch qRT-PCR determination system (Bio-Rad, USA). The PCR amplification program was set as pre-denaturation for 10 min at 95°C, denaturation for 15 s at 95°C, extension for 1 min at 60°C, and 40 cycles in total. The data were obtained as threshold cycle (Ct) values.
2.7 Data analyses
The relative expression of the target gene was quantified by the 2-ΔΔCt method, where the calculation formula of ΔΔCt was as follows:
Data analyses were conducted with SPSS 20 statistical software (IBM, USA). All results were expressed in the form of mean ± standard deviation. The median lethal concentration (LC50) value was calculated using Probit analysis at 48 and 96 h. The normality and homogeneity of the data were determined by Shapiro-Wilk and Levene tests, respectively. The Tukey post-test method after a one-way analysis of variance (ANOVA) was then used to determine the significance between different treatments. The difference was considered statistically significant with a p-value below 0.05.
3 Results and discussion
3.1 Characterization of AgNPs and PS nanoplastics
As shown in Figures 1A, B, the AgNPs and PS nanoplastics were spherical particles and dispersible well in different exposure solutions without significant agglomeration. However, in the mixture treatment (Figure 1C), the AgNPs were mostly adsorbed on the PS nanoplastics. A similar result has been also reported by Li et al. (2020), who found that the presence of AgNPs in water solutions could be significantly captured on the surface of PS nanoplastics through a monolayer adsorption process.
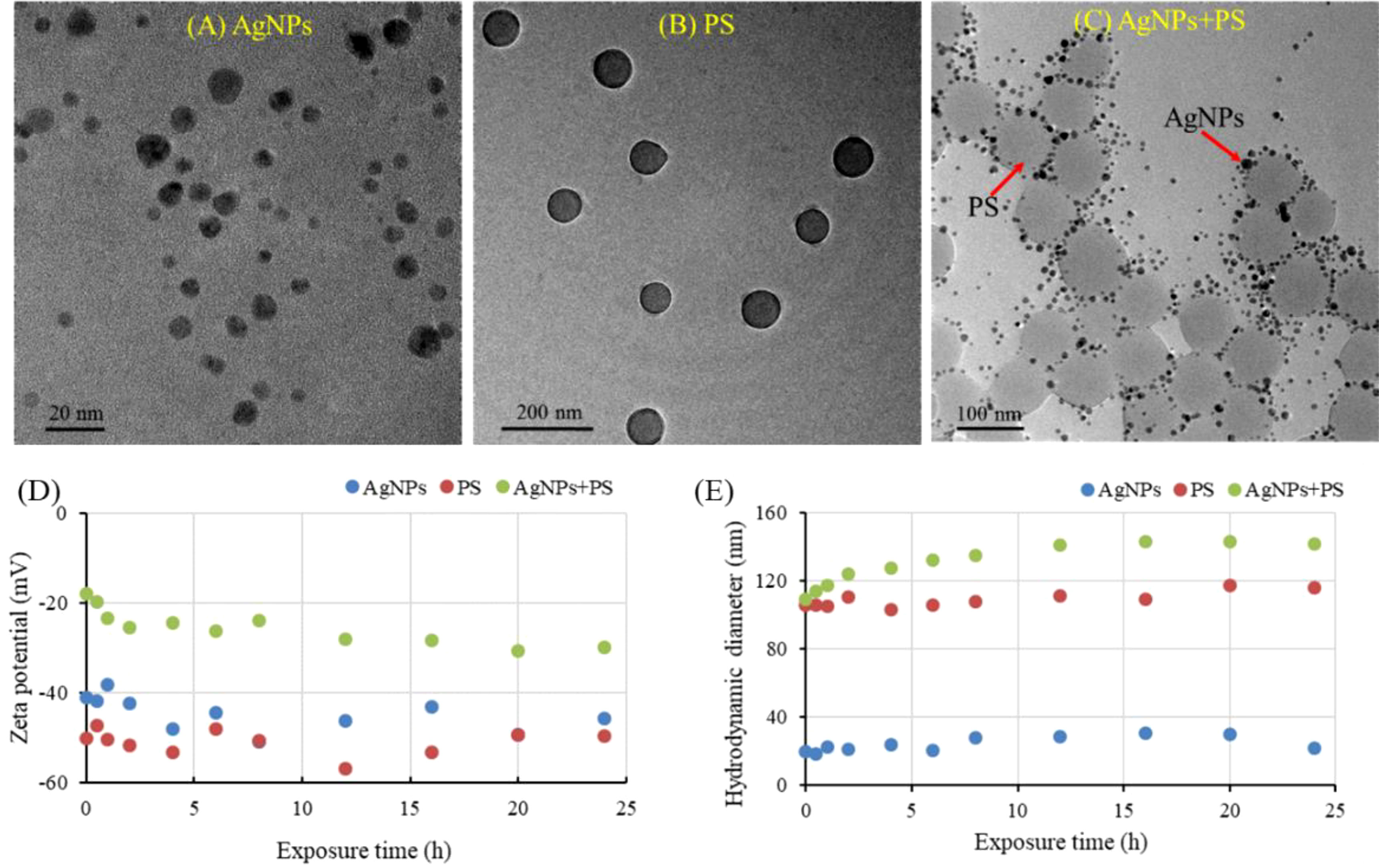
Figure 1 TEM characterization (A–C), zeta potential (D) and hydrodynamic sizes (E) of AgNPs, PS nanoplastics, and their mixture in exposure solutions.
To further verify the carrier effect of PS nanoplastics on the AgNPs, the zeta potentials and hydrodynamic sizes of AgNPs alone and in combination with PS nanoplastics during the exposure periods are summarized in Figures 1D, E. Both the AgNPs and PS nanoplastics were negatively charged, indicating the stability, polydispersity, and the existence of electric repulsion between them. The zeta potentials of the mixtures were sharply raised to -17.9 eV and then slowly reduced to -29.9 eV, which were also much higher than that of AgNPs and PS nanoplastics alone. The elevated zeta potentials may further reduce the electrostatic repulsion between particles, aggravating the agglomeration of AgNPs and PS nanoplastics. In addition, the hydrodynamic sizes of AgNPs and PS nanoplastics alone showed a stable behavior during the exposure periods, with an average size of 21.8 nm and 116.2 nm, respectively. In the mixtures, AgNPs and PS nanoplastics aggregated rapidly, with a hydrodynamic size high to 143.4 nm. It confirms that the presence of PS nanoplastics in aquatic environments could act as a carrier of AgNPs, which may further alter the behaviors and effects of AgNPs in aquatic environments.
3.2 Ionic release of AgNPs
The release of dissolved Ag+ from AgNPs at different concentrations in the presence of PS nanoplastics (10 mg/L) is shown in Table 2. The limit of quantification of dissolved Ag+ in water was 0.04 μg/L. The release ratios of soluble Ag+ from AgNPs ranged from 0.6% to 4.23%, which increased with the increase of AgNPs concentrations. Similarly, van Aerle et al. (2013) reported that the highest dissolution of Ag+ for the AgNPs in different adopted exposure conditions was below 5%. A variable dissolution of AgNP was also demonstrated by Osborne et al. (2013), who found the release ratio of Ag+ from AgNPs in zebrafish embryo culture water varied between 0.1 and 2%. Hence, exposure to silver nitrate at 2.5 μg/L in the presence of PS nanoplastics was conducted to provide a likely worst-case scenario for the highest dissolution of Ag+ from the AgNPs in different exposure conditions.
3.3 Acute toxicity
The 48-h and 96-h acute toxicities of AgNPs, the mixture of AgNPs and PS nanoplastics, and the mixture of Ag+ and PS nanoplastics are listed in Table 3. The results showed that the lethal concentration of 50% (LC50) of AgNPs was 1.03 and 0.62 mg/L after exposure to 48 h and 96 h, respectively. This value was consistent with the reported acute toxicity of AgNPs with 14 different properties (Cunningham et al., 2013). After co-exposure to PS nanoplastics, the acute toxicity caused by AgNPs in zebrafish was not significantly altered. In addition, the values of TU for the AgNPs and PS nanoplastics mixtures were close to 1 (0.88-1.12), suggesting that the mixture followed a trend of additive effects. In previous studies, both the reduction and enhancement of PS nanoplastics on the toxic effects caused by nanomaterials on aquatic biota have been reported (Gunasekaran et al., 2020). These different alterations in acute toxicity may be attributed to the changes in the aggregative potentials between microplastics and nanomaterials, as well as the released ions from the adsorbed nanomaterials on microplastics. In this study, the 48 h and 96-h LC50 of zebrafish treated with Ag+ and PS were ten-fold lower than that of AgNPs + PS nanoplastics, respectively. It suggests that the released Ag+ from the adsorbed AgNPs on PS nanoplastics may be supposed to enhance the acute toxicity of AgNPs in zebrafish, but the adsorption of AgNPs on PS nanoplastics may significantly alleviate the acute toxicity caused by the released Ag+. Similar results have been found by Lai et al. (2020), who found that co-exposure to nanomaterials markedly alleviated the acute toxicity induced by Ag+ in vivo. Therefore, it is necessary to study the influences of PS nanoplastics on the toxicity of AgNPs.

Table 3 The acute toxicity of AgNPs alone and in combination with PS nanoplastics in zebrafish embryos.
3.4 Oxidative stress
Oxidative stress is an imbalance between the production and removal of reactive oxygen species in organisms (Yan et al., 2018). In previous studies, the presence of both PS nanoplastics and AgNPs has been reported to induce the antioxidant defense in zebrafish (Choi et al., 2010). In this study, the expression of the antioxidase superoxide dismutase (sod1) gene in zebrafish was significantly reduced by AgNPs (Figure 2). The inhibitive effect on the antioxidase caused by AgNPs suggests that the exposed fish may lose the capacity to scavenge oxidative damage through SOD, showing a compensatory inhibition. For the catalase (cat) gene, exposure to AgNPs did not alter its expression in fish, which may be also excessive consumption of CAT to scavenge oxidative damage. Generally, the SOD and CAT systems have been regarded as the first defense line to scavenge the reactive oxygen species (ROS) in biota. The induced ROS can be rapidly metabolized by SOD into H2O2, and then decomposed by CAT (Wen et al., 2018). However, the high large production of ROS may further suppress the antioxidase. Hence, the low expression of the antioxidase genes caused by AgNPs may associate with the high oxidative damage in fish. This result was then confirmed by the high expression of heat shock protein hsp70 in zebrafish (Figure 2C), which was 3.64-fold higher than that in the control.
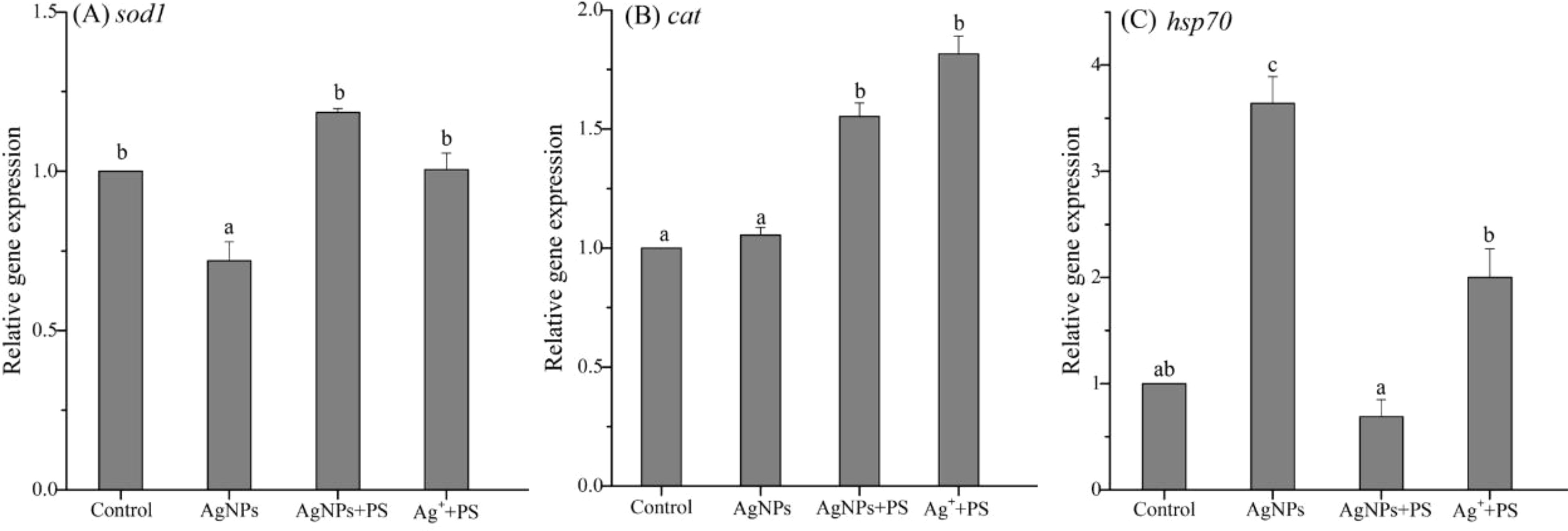
Figure 2 Expression of genes related to oxidative stress in zebrafish embryos treated with AgNPs alone, the mixture of AgNPs and PS nanoplastics, and the mixture of Ag+ and PS nanoplastics (A) sod1, (B) cat, and (C) hsp70. Values sharing different lowercase letters (a–c) in the same gene are significantly different between different treatments.
After co-exposure to PS nanoplastics, the gene expression of the antioxidase (sod1 and cat) in zebrafish was significantly enhanced, with a 1.65 and 1.47-fold increase than that caused by AgNPs alone. Meanwhile, the presence of PS nanoplastics significantly reduced the transcription level of hsp70 gene stimulated by AgNPs, making it back to the control line. These results indicate that the presence of PS nanoplastics could weaken the oxidative stress caused by AgNPs on zebrafish through enhancing the antioxidase and inhibiting oxidative damage, showing a protective role. Similarly, Gunasekaran et al. (2020) found a dose-dependent increment in the ROS generation and lipid peroxidation in marine microalgae Dunaliella salina treated with ZnO particles, which were considerably reduced by the presence of plain PS nanoplastics. Given that PS nanoplastics could also stimulate oxidative stress on aquatic organisms (Ding et al., 2018), the oxidative damage of AgNPs on zebrafish was reduced by PS microplastics may be attributed to the formation of agglomeration. In addition, combined exposure to Ag+ and PS nanoplastics also resulted in similarly inhibitive effects on the oxidative stress on zebrafish when compared to that treated with AgNPs alone. It suggests that the released Ag+ from the adsorbed AgNPs on PS nanoplastics may be partially responsible for the oxidative stress on zebrafish stimulated by AgNPs alone, but the weak adsorption of Ag+ on PS nanoplastics may further alleviate the toxicity.
3.5 Apoptosis
The expression of genes related to apoptosis (bax, caspase9, and caspase3) in zebrafish larvae are shown in Figure 3. The results showed that the gene expression of bax in zebrafish was significantly up-regulated by AgNPs when compared to the control, as well as the gene expression of caspase9. When the PS nanoplastics were added, the gene expression of caspase9 was significantly decreased by more than 30%, while the bax was not significantly altered. When zebrafish were co-exposed to Ag+ and PS nanoplastics, the gene expression of bax and caspase9 were slightly up-regulated when compared to the control, but the expression of caspase3 gene was significantly enhanced.
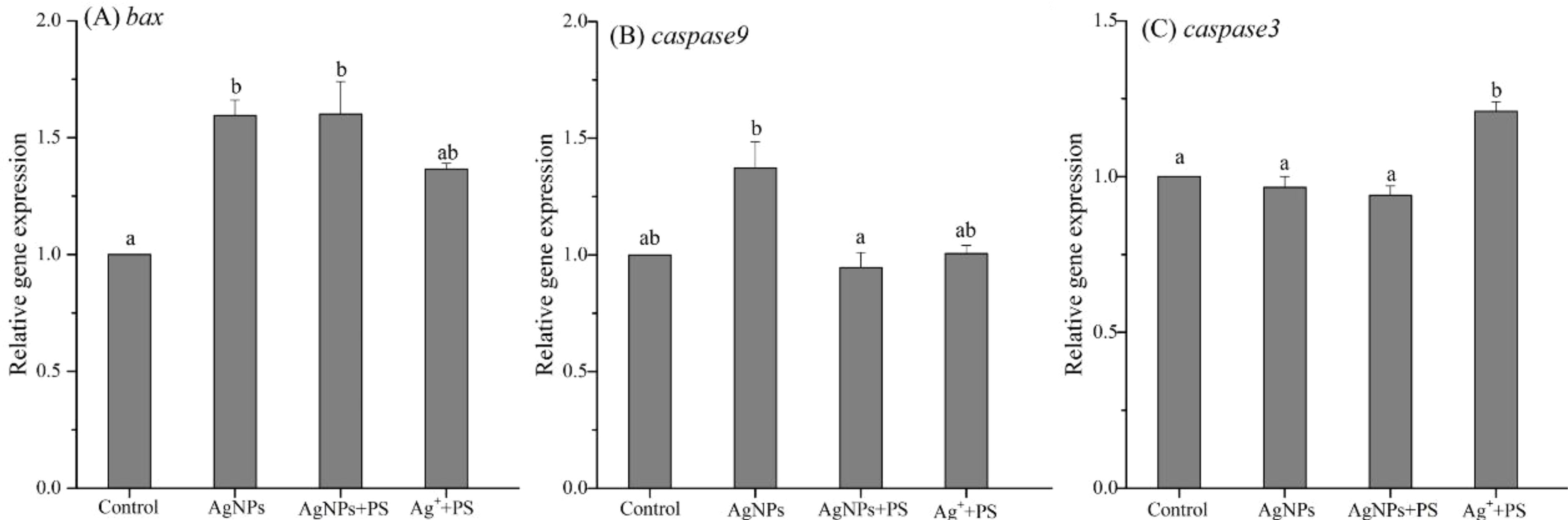
Figure 3 Expression of genes related to apoptosis in zebrafish embryos treated with AgNPs alone, the mixture of AgNPs and PS nanoplastics, and the mixture of Ag+ and PS nanoplastics (A) bax, (B) caspase9, and (C) caspase3. Values sharing different lowercase letters (a–b) in the same gene are significantly different between different treatments.
Bax is a member of the Bcl-2 family mainly located in the cytoplasm (Choi et al., 2010; Ma et al., 2018). After early apoptosis, bax could transfer from the cytoplasm to mitochondria and assist with cytochrome C to open the pro-apoptotic pathway. The released cytochrome C may further activate caspase9 located upstream of the apoptosis pathway. In the downstream, Caspase3 could receive signals and eventually lead to cell apoptosis. Obviously, in this study, exposure to AgNPs and AgNPs + PS nanoplastics mainly stimulated the early apoptosis of zebrafish cells, causing damage to cell structure and function. However, the presence of PS nanoplastics inhibited the overexpression of caspase9 gene caused by AgNPs, which may be attributed to the hindrance on the release of cytochrome C in the mitochondrial-mediated apoptosis pathway. Hence, the presence of PS nanoplastics plays a protective role in the mitochondria apoptosis pathway caused by AgNPs. In addition, the positive treatment with the mixture of Ag+ and PS nanoplastics resulted in the activation of the whole apoptosis pathways, especially the downstream of caspase3, which implies the outcomes of apoptosis in zebrafish cells. The difference between the two mixture treatments once again confirmed that the presence of PS nanoplastics alleviated the apoptosis caused by AgNPs in zebrafish, which may result from the formation of aggregation between AgNPs and PS nanoplastics.
3.6 Innate immune response
The innate immune system is the first line of defense against different pollutants and is also a prerequisite for enhancing the adaptive immune response (Xu et al., 2013). The oxidative damage caused by pollutants has been suggested as a pathway to induce immunotoxicity in organisms. Hence, it is not surprising to the AgNPs-induced immunotoxicity in zebrafish because of an increase in oxidative stress. The expression of genes related to innate immune in zebrafish embryos was investigated, including nfkβ, cebp, and il-1β, and the results are shown in Figure 4. After exposure to AgNPs, all innate immune genes in zebrafish were significantly up-regulated by 1.7-fold, 1.57-fold, and 1.62-fold compared to the control group, respectively. Among these innate immune responses, the activated NFKβ could induce the production of pro-inflammatory cytokines and then induce inflammation in organisms. The cebp gene is involved in cell growth, proliferation, differentiation, cell cycle arrest, and apoptosis (Ramachandran et al., 2018). The il-1β gene is responsible for the accumulation of phagocytes in the infected area of pollutants in organisms, and subsequently activates macrophages and neutrophils to defend against damage (Brun et al., 2018; Aksakal and Ciltas, 2019). The increase in the expression of these genes in zebrafish indicated that the innate immune system of zebrafish larvae was under the stress of AgNPs. When the PS nanoplastics were co-added, the increased expression of these genes was all inhibited by 18%-23%, which was consistent with the enhanced gene expression of the antioxidase in zebrafish. Hence, the presence of PS nanoplastics may further weaken the AgNPs-induced immunotoxicity in zebrafish via inhibiting oxidative damage.
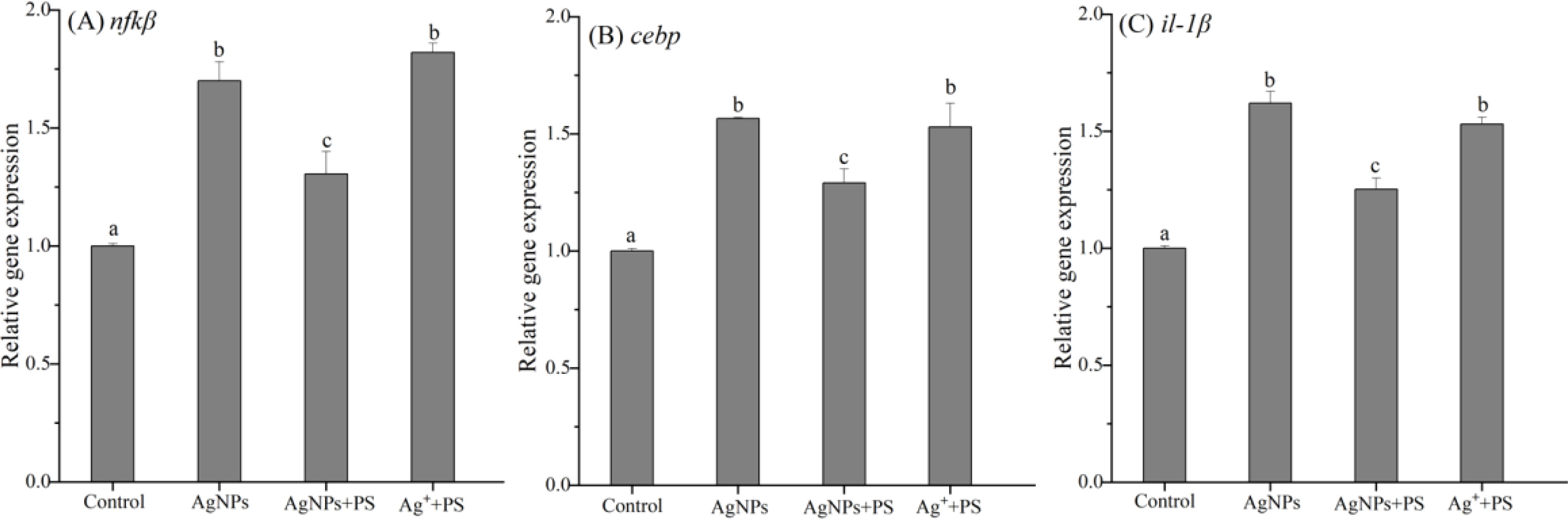
Figure 4 Expression of genes related to innate immune in zebrafish embryos treated with AgNPs alone, the mixture of AgNPs and PS nanoplastics, and the mixture of Ag+ and PS nanoplastics (A) nfkb, (B) cebp, and (C) il-1b. Values sharing different lowercase letters (a–c) in the same gene are significantly different between different treatments.
In the positive treatment, the relative expression of nfkβ, cebp, and il-1β gene in zebrafish was significantly up-regulated by 1.53 to 1.82 times of the control, which was also much higher than that in the mixture of AgNPs and PS nanoplastics. This finding indicates that the combined exposure to Ag+ and PS exerted greater pressure on the zebrafish immune system than that in the AgNPs + PS treatment. Previous studies have found that the presence of PS nanoplastics in waters could aggravate the toxicity of gold and copper ions and then trigger a significant immune response in zebrafish (Lee et al., 2019; Santos et al., 2022). However, the formation of hetero-aggregates between nanoplastics and AgNPs may further decrease the immunotoxicity in zebrafish caused by Ag+. The different sorption profiles of metallic ions and nanomaterials on nanoplastics may be responsible for the distinguishing combined effects on zebrafish.
3.7 Metabolic function
The gene expression of metabolic function in zebrafish embryos treated with AgNPs alone or in combination with PS nanoplastics was studied, including mt2, mtf-1, cox1, and abcb4. As shown in Figure 5A, exposure to AgNPs stimulated the mt2 gene in zebrafish by 1.42-fold when compared with the control, which was significantly enhanced by the presence of PS nanoplastics with a 1.69 times increase. In addition, a 1.81-fold increase in the mt2 gene expression was also observed in zebrafish treated with Ag+ + PS nanoplastics compared to the control. In general, exposure to metal could induce the production of metallothionein in organisms and help to scavenge metal pollutants from the body through chelating with metal ions. The enhancement of PS nanoplastics on the production of metallothionein may imply a greater efficiency in the scavenging of Ag+ from zebrafish. This result has been also confirmed in discus fish (Symphysodon aequifasciatus) exposed to PS nanoplastics and metal cadmium (Wen et al., 2018). Similar to the expression of mt2 gene, the mtf-1 gene was also up-regulated in the three treatments, and the two mixture treatments exhibited a significant up-regulation trend (Figure 5B). Since the mtf-1 gene plays an important role in regulating the metal detoxification processes and is responsible for transcriptional activation of the mt gene (Ramachandran et al., 2018), the higher activation in mtf-1 gene caused by PS nanoplastics suggests a stronger scavenger of metal from zebrafish. The presence of PS nanoplastics may significantly trigger the metal detoxification function in zebrafish larvae.
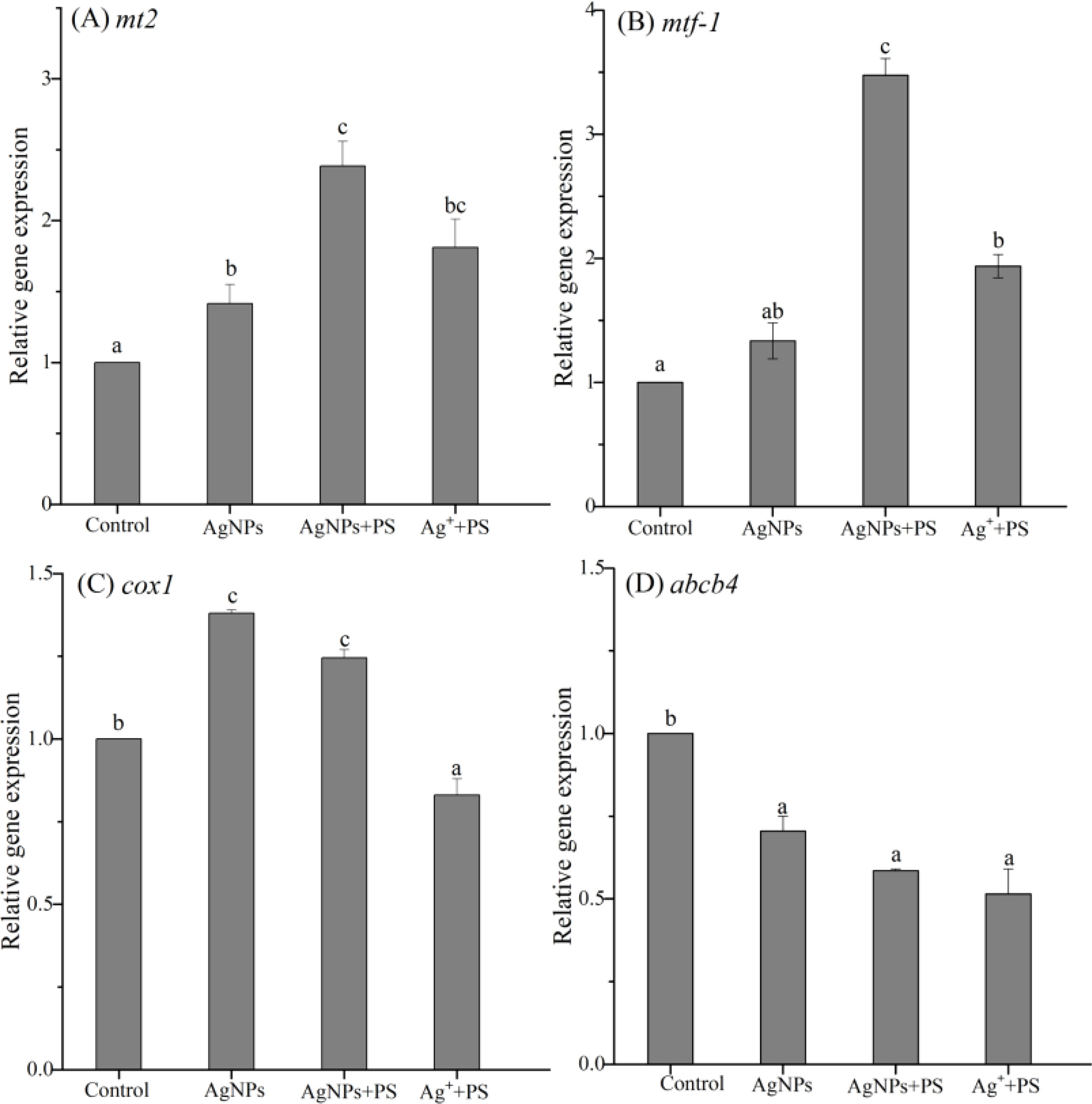
Figure 5 Expression of genes related to metabolic function in zebrafish embryos treated with AgNPs alone, the mixture of AgNPs and PS nanoplastics, and the mixture of Ag+ and PS nanoplastics (A) mt2, (B) mtf-1, (C) cox1, and (D) abcb4. Values sharing different lowercase letters (a–c) in the same gene are significantly different between different treatments.
Cytochrome c oxidase (cox) is mainly involved in the binding of metal ions and the electron transfer of mitochondria in the energy metabolism in organisms (Hu et al., 2016). The expression of cox1 gene in zebrafish is shown in Figure 5C. Compared with the control, exposure to AgNPs alone or in combination with PS nanoplastics induced a significant increase in cox1 gene by 38% and 25%, respectively. Similarly, Shen et al. (2020) found that the cox1 gene in fungi Fusarium solani was 41-fold up-regulated by AgNPs after 24 h of exposure. The energy metabolism processes related to cox1 gene in zebrafish mitochondria were also stimulated by AgNPs with different particle sizes and surface coatings (Liu et al., 2019). The activation of cox1 gene in zebrafish suggests a high stress on the mitochondrial metabolism caused by AgNPs, which was further declined by PS nanoplastics although the distinction was not obvious. On the contrary, the mixture of Ag+ and PS nanoplastics resulted in an inhibitive effect on the relative gene expression by 0.83-fold. This obvious distinction between AgNPs and Ag+ implies that the cox1 gene in zebrafish has a specific response to AgNPs but not the related ion. Dedeh et al. (2015) illustrated that the gene involved in the brain mitochondrial respiration (cox1) of zebrafish displayed a 3-fold increased expression in response to gold NPs compared to ionic gold. Hence, the expression of cox1 gene could be used as a specific biomarker to assess AgNPs stress.
ATP binding cassette (ABC) transporter in organisms plays a critical role in excreting toxic substances from cells and the efflux activity of exogenous pollutants in zebrafish cells is related to the gene of ABCB4 (Yan et al., 2018; Ma et al., 2020). In this study, the expression of the abcb4 gene in all treatments was significantly suppressed when compared to the control, while the differences between the three treatments were not significant (Figure 5D). The inhibition in the abcb4 gene suggests that the efflux capacity of zebrafish embryos was severely weakened in the presence of AgNPs and Ag+. Meanwhile, the co-existence of PS nanoplastics did not alter the efflux potential in zebrafish, which may further enhance the accumulation of exogenous pollutants in the body, leading to a serious risk to zebrafish.
In general, exposure to AgNPs induced an increase in oxidative damage, apoptosis, immunotoxicity, and metabolic capability in zebrafish embryos in most cases. The release of dissolved Ag+ from AgNPs may be partially responsible for the toxicity in zebrafish caused by AgNPs. The co-existence of PS nanoplastics in aquatic environments further suppressed these biological effects, playing a protective role in most cases. The variation in toxicity caused by PS nanoplastics may be partially attributed to the absorption of the released Ag+ from AgNPs, which could reduce the toxicity. In addition, the formation of agglomeration with AgNPs and PS nanoplastics would also alleviate the toxicity caused by AgNPs in zebrafish.
4 Conclusions
This study confirmed that the presence of PS nanoplastics could interact with AgNPs in aquatic environments, and further altered the toxicity of AgNPs in zebrafish embryos. The results reveal that PS nanoplastics could act as a carrier of AgNPs in aquatic environments. The presence of PS nanoplastics did not alter the acute toxicity of AgNPs in zebrafish embryos. Exposure to AgNPs significantly stimulated the gene expression of oxidative stress, apoptosis, immune response, and metabolic function. However, the co-added PS nanoplastics alleviated these negative effects by up-regulating the gene expression of antioxidase and metabolic functions in zebrafish, reflecting an inhibitory effect on the AgNPs-induced genotoxicity. The absorption and agglomeration of AgNPs and the released Ag+ with PS nanoplastics may be partly contributed to the inhibitory effects caused by PS nanoplastics. This study confirmed that the presence of PS nanoplastics could interact with AgNPs in aquatic environments, and further altered the toxicity of AgNPs in zebrafish embryos.
Data availability statement
The original contributions presented in the study are included in the article/supplementary material. Further inquiries can be directed to the corresponding author.
Ethics statement
The animal study was reviewed and approved by the Animal Care and Use Committee of Hohai University.
Author contributions
ZY performed the conceptualization, writing, supervision, and funding acquisition. YZ and PZ contributed to the methodology, formal analysis, and writing. XB and PS performed methodology and writing. All authors contributed to the article and approved the submitted version.
Funding
This study was financially supported by the National Natural Science Foundation of China (51979080), and the risk assessment and control project of emerging pollutants of the Geological Survey of Jiangsu Province (819073116).
Conflict of interest
The authors declare that the research was conducted in the absence of any commercial or financial relationships that could be construed as a potential conflict of interest.
Publisher’s note
All claims expressed in this article are solely those of the authors and do not necessarily represent those of their affiliated organizations, or those of the publisher, the editors and the reviewers. Any product that may be evaluated in this article, or claim that may be made by its manufacturer, is not guaranteed or endorsed by the publisher.
References
Aksakal F. I., Ciltas A. (2019). Impact of copper oxide nanoparticles (CuO NPs) exposure on embryo development and expression of genes related to the innate immune system of zebrafish (Danio rerio). Comp. Biochem. Physiol. C: Comp. Pharmacol. 223, 78–87. doi: 10.1016/j.cbpc.2019.05.016
Bhagat J., Nishimura N., Shimada Y. (2021). Toxicological interactions of microplastics/nanoplastics and environmental contaminants: current knowledge and future perspectives. J. Hazard. Mater. 405, 123913. doi: 10.1016/j.jhazmat.2020.123913
Brun N. R., Koch B. E. V., Varela M., Peijnenburg W. J. G. M., Spaink H. P., Vijver M. G. (2018). Nanoparticles induce dermal and intestinal innate immune system responses in zebrafish embryos. Environ. Sci. Nano 5, 904–916. doi: 10.1039/c8en00002f
Calisi A., Lorusso C., Gallego-Urrea J.-A., Hassellöv M., Dondero F. (2022). Ecotoxicological effects of silver nanoparticles in marine mussels. Sci. Total Environ. 851, 158113. doi: 10.1016/j.scitotenv.2022.158113
Cambier S., Rogeberg M., Georgantzopoulou A., Serchi T., Karlsson C., Verhaegen S., et al. (2018). Fate and effects of silver nanoparticles on early life-stage development of zebrafish (Danio rerio) in comparison to silver nitrate. Sci. Total Environ. 610, 972–982. doi: 10.1016/j.scitotenv.2017.08.115
Choi J. E., Kim S., Ahn J. H., Youn P., Kang J. S., Park K., et al. (2010). Induction of oxidative stress and apoptosis by silver nanoparticles in the liver of adult zebrafish. Aquat. Toxicol. 100, 151–159. doi: 10.1016/j.aquatox.2009.12.012
Cui B., Ren L., Xu Q. H., Yin L. Y., Zhou X. Y., Liu J. X. (2016). Silver_ nanoparticles inhibited erythrogenesis during zebrafish embryogenesis. Aquat. Toxicol. 177, 295–305. doi: 10.1016/j.aquatox.2016.06.005
Cunningham S., Brennan-Fournet M. E., Ledwith D., Byrnes L., Joshi L. (2013). Effect of nanoparticle stabilization and physicochemical properties on exposure outcome: acute toxicity of silver nanoparticle preparations in zebrafish (Danio rerio). Environ. Sci. Technol. 47, 3883–3892. doi: 10.1021/es303695f
Davies H. L., Robb H., Cox K. D., Covernton G. A., Eastham T. M., Alexander H. J., et al. (2021). A preliminary analysis of ingestion and egestion of microplastic fibres in the acorn barnacle Balanus glandula. J. Exp. Mar. Biol. Ecol. 542, 151589. doi: 10.1016/j.jembe.2021.151589
Dedeh A., Ciutat A., Treguer-Delapierre M., Bourdineaud J. P. (2015). Impact of gold nanoparticles on zebrafish exposed to a spiked sediment. Nanotoxicology 9, 71–80. doi: 10.3109/17435390.2014.889238
Ding J., Zhang S., Razanajatovo R. M., Zou H., Zhu W. (2018). Accumulation, tissue distribution, and biochemical effects of polystyrene microplastics in the freshwater fish red tilapia (Oreochromis niloticus). Environ. pollut. 238, 1–9. doi: 10.1016/j.envpol.2018.03.001
Gautam R. K., Tiwari I. (2020). Humic acid functionalized magnetic nanomaterials for remediation of dye wastewater under ultrasonication: application in real water samples, recycling and reuse of nanosorbents. Chemosphere 245, 125553. doi: 10.1016/j.chemosphere.2019.125553
Geyer R., Jambeck J. R., Law K. L. (2017). Production, use, and fate of all plastics ever made. Sci. Adv. 3, 1700782. doi: 10.1126/sciadv.1700782
Gunasekaran D., Chandrasekaran N., Jenkins D., Mukherjee A. (2020). Plain polystyrene microplastics reduce the toxic effects of ZnO particles on marine microalgae Dunaliella salina. J. Environ. Chem. Eng. 8, 104250. doi: 10.1016/j.jece.2020.104250
Hoseini S. M., Khosraviani K., Delavar F. H., Arghideh M., Zavvar F., Hoseinifar S. H., et al. (2022). Hepatic transcriptomic and histopathological responses of common carp, Cyprinus carpio, to copper and microplastic exposure. Mar. pollut. Bull. 175, 113401. doi: 10.1016/j.marpolbul.2022.113401
Hu Y.-D., Pang H.-Z., Li D.-S., Ling S.-S., Lan D., Wang Y., et al. (2016). Analysis of the cytochrome c oxidase subunit 1 (COX1) gene reveals the unique evolution of the giant panda. Gene 592, 303–307. doi: 10.1016/j.gene.2016.07.029
Hu L., Zhao Y., Xu H. (2022). Trojan Horse in the intestine: a review on the biotoxicity of microplastics combined environmental contaminants. J. Hazard. Mater.s 439, 129652. doi: 10.1016/j.jhazmat.2022.129652
Huang W., Song B., Liang J., Niu Q., Zeng G., Shen M., et al. (2021). Microplastics and associated contaminants in the aquatic environment: a review on their ecotoxicological effects, trophic transfer, and potential impacts to human health. J. Hazard. Mater. 405, 124187. doi: 10.1016/j.jhazmat.2020.124187
Huang B., Wei Z. B., Yang L. Y., Pan K., Miao A. J. (2019). Combined toxicity of silver nanoparticles with hematite or plastic nanoparticles toward two freshwater algae. Environ. Sci. Technol. 53, 3871–3879. doi: 10.1021/acs.est.8b07001
Jenner L. C., Rotchell J. M., Bennett R. T., Cowen M., Tentzeris V., Sadofsky L. R. (2022). Detection of microplastics in human lung tissue using mu FTIR spectroscopy. Sci. Total Environ. 831, 154907. doi: 10.1016/j.scitotenv.2022.154907
Khan I., Bahuguna A., Krishnan M., Shukla S., Lee H., Mine S.-H., et al. (2019). The effect of biogenic manufactured silver nanoparticles on human endothelial cells and zebrafish model. Sci. Total Environ. 679, 365–377. doi: 10.1016/j.scitotenv.2019.05.045
Kumar R., Sharma P., Manna C., Jain M. (2021). Abundance, interaction, ingestion, ecological concerns, and mitigation policies of microplastic pollution in riverine ecosystem: a review. Sci. Total Environ. 782, 146695. doi: 10.1016/j.scitotenv.2021.146695
Lai Y., Dong L., Zhou H., Yan B., Chen Y., Cai Y., et al. (2020). Coexposed nanoparticulate Ag alleviates the acute toxicity induced by ionic ag+in vivo. Sci. Total Environ. 723, 138050. doi: 10.1016/j.scitotenv.2020.138050
Lee W. S., Cho H. J., Kim E., Huh Y. H., Kim H. J., Kim B., et al. (2019). Bioaccumulation of polystyrene nanoplastics and their effect on the toxicity of au ions in zebrafish embryos. Nanoscale 11, 3173–3185. doi: 10.1039/c8nr09321k
Lekamge S., Miranda A. F., Ball A. S., Shukla R., Nugegoda D. (2019). The toxicity of coated silver nanoparticles to Daphnia carinata and trophic transfer from alga Raphidocelis subcapitata. PloS One 14, 214398. doi: 10.1371/journal.pone.0214398
Leslie H. A., van Velzen M. J. M., Brandsma S. H., Vethaak A. D., Garcia-Vallejo J. J., Lamoree M. H. (2022). Discovery and quantification of plastic particle pollution in human blood. Environ. Int. 163, 107199. doi: 10.1016/j.envint.2022.107199
Li P., Liu J., Zhang H. (2022). Insights into the interaction of microplastic with silver nanoparticles in natural surface water. Sci. Total Environ. 805, 150315. doi: 10.1016/j.scitotenv.2021.150315
Li P., Zou X., Wang X., Su M., Chen C., Sun X., et al. (2020). A preliminary study of the interactions between microplastics and citrate-coated silver nanoparticles in aquatic environments. J. Hazard. Mater. 385, 121601. doi: 10.1016/j.jhazmat.2019.121601
Liu H., Wang X., Wu Y., Hou J., Zhang S., Zhou N., et al. (2019). Toxicity responses of different organs of zebrafish (Danio rerio) to silver nanoparticles with different particle sizes and surface coatings. Environ. pollut. 246, 414–422. doi: 10.1016/j.envpol.2018.12.034
Lu C., Lv Y., Kou G., Liu Y., Liu Y., Chen Y., et al. (2022). Silver nanoparticles induce developmental toxicity via oxidative stress and mitochondrial dysfunction in zebrafish (Danio rerio). Ecotoxicol. Environ. Saf. 243, 113993. doi: 10.1016/j.ecoenv.2022.113993
Lu Y., Zhang Y., Deng Y., Jiang W., Zhao Y., Geng J., et al. (2016). Uptake and accumulation of polystyrene microplastics in zebrafish (Danio rerio) and toxic effects in liver. Environ. Sci. Technol. 50, 4054–4060. doi: 10.1021/acs.est.6b00183
Ma Y.-B., Lu C.-J., Junaid M., Jia P.-P., Yang L., Zhang J.-H., et al. (2018). Potential adverse outcome pathway (AOP) of silver nanoparticles mediated reproductive toxicity in zebrafish. Chemosphere 207, 320–328. doi: 10.1016/j.chemosphere.2018.05.019
Ma Y.-P., Sun L.-H., Li S.-Y., Ni Y.-X., Cao Z.-Y., Chen M.-X., et al. (2020). Modulation of steroid metabolism and xenobiotic biotransformation responses in zebrafish (Danio rerio) exposed to triadimefon. Environ. pollut. 262, 114145. doi: 10.1016/j.envpol.2020.114145
Mattsson K., Hansson L.-A., Cedervall T. (2015). Nano-plastics in the aquatic environment. Environ. Sci. Processes Impacts 17, 1712–1721. doi: 10.1039/C5EM00227C
Meyer J.-S., Ranville J.-F., Pontasch M., Gorsuch J.-W., Adams W.-J. (2015). Acute toxicity of binary and ternary mixtures of cd, Cu, and zn to Daphnia magna. Environ. Toxicol. Chem. 34, 799–808. doi: 10.1002/etc.2787
Osborne O. J., Johnston B. D., Moger J., Baalousha M., Lead J. R., Kudoh T., et al. (2013). Effects of particle size and coating on nanoscale Ag and TiO2 exposure in zebrafish (Danio rerio) embryos. Nanotoxicology 7, 1315–1324. doi: 10.3109/17435390.2012.737484
Ragusa A., Notarstefano V., Svelato A., Belloni A., Gioacchini G., Blondeel C., et al. (2022). Raman microspectroscopy detection and characterisation of microplastics in human breastmilk. Polymers 14, 14132700. doi: 10.3390/polym14132700
Ragusa A., Svelato A., Santacroce C., Catalano P., Notarstefano V., Carnevali O., et al. (2021). Plasticenta: first evidence of microplastics in human placenta. Environ. Int. 146, 106274. doi: 10.1016/j.envint.2020.106274
Ramachandran R., Krishnaraj C., Kumar V. K. A., Harper S. L., Kalaichelvan T. P., Yun S.-I. (2018). In vivo toxicity evaluation of biologically synthesized silver nanoparticles and gold nanoparticles on adult zebrafish: a comparative study. 3 Biotech. 8, 441. doi: 10.1007/s13205-018-1457-y
Santos D., Luzio A., Felix L., Bellas J., Monteiro S. M. (2022). Oxidative stress, apoptosis and serotonergic system changes in zebrafish (Danio rerio) gills after long-term exposure to microplastics and copper. Comp. Biochem. Physiol. C: Comp. Pharmacol. 258, 109363. doi: 10.1016/j.cbpc.2022.109363
Scott-Fordsmand J. J., Navas J. M., Hund-Rinke K., Nowack B., Amorim M. J. B. (2017). Nanomaterials to microplastics: swings and roundabouts. Nano Today 17, 7–10. doi: 10.1016/j.nantod.2017.09.002
Shen T., Wang Q., Li C., Zhou B., Li Y., Liu Y. (2020). Transcriptome sequencing analysis reveals silver nanoparticles antifungal molecular mechanism of the soil fungi Fusarium solani species complex. J. Hazard. Mater. 388, 122063. doi: 10.1016/j.jhazmat.2020.122063
Sridharan S., Kumar M., Singh L., Bolan N. S., Saha M. (2021). Microplastics as an emerging source of particulate air pollution: a critical review. J. Hazard. Mater. 418, 126245. doi: 10.1016/j.jhazmat.2021.126245
Sun T. Y., Bornhoft N. A., Hungerbuhler K., Nowack B. (2016). Dynamic probabilistic modeling of environmental emissions of engineered nanomaterials. Environ. Sci. Technol. 50, 4701–4711. doi: 10.1021/acs.est.5b05828
Tekman M. B., Wekerle C., Lorenz C., Primpke S., Hasemann C., Gerdts G., et al. (2020). Tying up loose ends of microplastic pollution in the Arctic: distribution from the sea surface through the water column to deep-sea sediments at the hausgarten observatory. Environ. Sci. Technol. 54, 4079–4090. doi: 10.1021/acs.est.9b06981
Thiagarajan V., Alex S. A., Seenivasan R., Chandrasekaran N., Mukherjee A. (2021). Interactive effects of micro/nanoplastics and nanomaterials/pharmaceuticals: their ecotoxicological consequences in the aquatic systems. Aquat. Toxicol. 232, 105747. doi: 10.1016/j.aquatox.2021.105747
Tortella G. R., Rubilar O., Duran N., Diez M. C., Martinez M., Parada J., et al. (2020). Silver nanoparticles: toxicity in model organisms as an overview of its hazard for human health and the environment. J. Hazard. Mater. 390, 121974. doi: 10.1016/j.jhazmat.2019.121974
van Aerle R., Lange A., Moorhouse A., Paszkiewicz K., Ball K., Johnston B. D., et al. (2013). Molecular mechanisms of toxicity of silver nanoparticles in zebrafish embryos. Environ. Sci. Technol. 47, 8005–8014. doi: 10.1021/es401758d
Wang H.-T., Ding J., Xiong C., Zhu D., Li G., Jia X.-Y., et al. (2019). Exposure to microplastics lowers arsenic accumulation and alters gut bacterial communities of earthworm Metaphire californica. Environ. Pollut. 251, 110–116. doi: 10.1016/j.envpol.2019.04.054
Wei Q., Hu C.-Y., Zhang R.-R., Gu Y.-Y., Sun A.-L., Zhang Z.-M., et al. (2021). Comparative evaluation of high-density polyethylene and polystyrene microplastics pollutants: uptake, elimination and effects in mussel. Mar. Environ. Res. 169, 105329. doi: 10.1016/j.marenvres.2021.105329
Wen B., Jin S.-R., Chen Z.-Z., Gao J.-Z., Liu Y.-N., Liu J.-H., et al. (2018). Single and combined effects of microplastics and cadmium on the cadmium accumulation, antioxidant defence and innate immunity of the discus fish (Symphysodon aequifasciatus). Environ. pollut. 243, 462–471. doi: 10.1016/j.envpol.2018.09.029
Xin Q., Zhang Q., Cheng J. (2015). Effects of silver nanoparticles and silver ions on the early development of zebrafish embryos and toxicity mechanisms. Asian J. Ecotoxicol. 10, 55–64.
Xu Q.-H., Guan P., Zhang T., Lu C., Li G., Liu J.-X. (2018). Silver nanoparticles impair zebrafish skeletal and cardiac myofibrillogenesis and sarcomere formation. Aquat. Toxicol. 200, 102–113. doi: 10.1016/j.aquatox.2018.04.018
Xu H., Yang M., Qiu W., Pan C., Wu M. (2013). The impact of endocrine-disrupting chemicals on oxidative stress and innate immune response in zebrafish embryos. Environ. Toxicol. Chem. 32, 1793–1799. doi: 10.1002/etc.2245
Yan Z., Lu G., Sun H., Bao X., Jiang R., Liu J., et al. (2019). Comparison of the accumulation and metabolite of fluoxetine in zebrafish larva under different environmental conditions with or without carbon nanotubes. Ecotoxicol. Environ. Saf. 172, 240–245. doi: 10.1016/j.ecoenv.2019.01.089
Yan Z., Sun H., Jiang R., Dong H., Yang H., Liu J., et al. (2018). Accumulation, metabolite and active defence system responses of fluoxetine in zebrafish embryos: influence of multiwalled carbon nanotubes with different functional groups. Aquat. Toxicol. 205, 204–212. doi: 10.1016/j.aquatox.2018.10.018
Yang Y., Liu W., Mu X., Qi S., Fu B., Wang C. (2016). Biological response of zebrafish embryos after short-term exposure to thifluzamide. Sci. Rep. 6, 38485. doi: 10.1038/srep38485
Yousif E., Haddad R. (2013). Photodegradation and photostabilization of polymers, especially polystyrene: review. Springerplus 2, 398. doi: 10.1186/2193-1801-2-398
Zhang P., Lu G., Sun Y., Zhang J., Liu J., Yan Z. (2022). Aged microplastics change the toxicological mechanism of roxithromycin on Carassius auratus: size-dependent interaction and potential long-term effects. Environ. Int. 169, 107540. doi: 10.1016/j.envint.2022.107540
Keywords: PS nanoplastics, silver nanoparticles, oxidative stress, immunotoxicity, metabolic function
Citation: Yan Z, Zhou Y, Zhu P, Bao X and Su P (2023) Polystyrene nanoplastics mediated the toxicity of silver nanoparticles in zebrafish embryos. Front. Mar. Sci. 10:1195125. doi: 10.3389/fmars.2023.1195125
Received: 28 March 2023; Accepted: 18 May 2023;
Published: 05 June 2023.
Edited by:
Wilson Machado, Fluminense Federal University, BrazilReviewed by:
Diego Nogueira, Federal University of Santa Catarina, BrazilJulian Alberto Gallego Urrea, Horiba Europe, Sweden
Copyright © 2023 Yan, Zhou, Zhu, Bao and Su. This is an open-access article distributed under the terms of the Creative Commons Attribution License (CC BY). The use, distribution or reproduction in other forums is permitted, provided the original author(s) and the copyright owner(s) are credited and that the original publication in this journal is cited, in accordance with accepted academic practice. No use, distribution or reproduction is permitted which does not comply with these terms.
*Correspondence: Zhenhua Yan, hwahuer@hhu.edu.cn