- 1College of Food Science and Technology, Modern Biochemistry Experimental Center, Guangdong Ocean University, Zhanjiang, China
- 2Shenzhen Institute of Guangdong Ocean University, Shenzhen, China
In recent years, microplastics (MPs) have received extensive attention due to their bioaccumulation and toxic effects on organisms. However, there are few studies on MPs in coastal waters ecosystem and the relationship between MPs and microorganisms. Thus, to understand the concentration and characteristics of MPs and explore the impact of MPs on microbial community, we collected sediment samples from the coastal waters of Shenzhen, a developed city in southern China, and then analyzed them. The results showed that the concentration of MPs in the sediments of eastern Shenzhen was 119 ± 18 items/kg, while the concentration of MPs in the sediments of western Shenzhen, in which coastal waters were heavily polluted, was as high as 664 ± 197 items/kg. micro-FTIR analysis showed that the copolymers of polystyrene (PS) (39.64%) and polyethylene (PE) (16.26%) were the main types of MPs in Shenzhen coastal waters. According to optical microscopy, MPs (51.52%) with a size range of 0.1-0.5 mm dominated sediments in Shenzhen coastal watershed. Fibrous black plastics and film white plastics were the main forms of MPs in sediment samples studied in eastern and western Shenzhen. Metagenome and metabonomics analysis showed that MPs had an impact on microbial diversity in sediments, the structure and composition of microbial community. What’s more, through a functional prediction analysis, MPs may have different effects on the functions of various microbial communities. The study on the effects of MPs on microorganisms in the sediments of coastal waters ecosystem can provides a basis for further study on the interaction between MPs and microorganisms in similar habitats.
1 Introduction
Plastic products are widely used in human life because of their light weight, corrosion resistance and low price (Thompson et al., 2004; Baldwin et al., 2016). Microplastics (MPs) are defined as plastic particles less than 5 mm in diameter, mainly produced by dissociation of larger items, commodities and industrial additives (Horton et al., 2017; Kitahara and Nakata, 2020; Zhu et al., 2022a). MPs can exist almost anywhere in the environment, such as seawater, fresh water, beaches, sediments, soil and air (Ding et al., 2019; Zhang et al., 2020; Yan et al., 2022; Zhu et al., 2022a). Since MPs can be transported in global oceans under the influence of wind and surface current, they also can adsorb persistent organic pollutants or heavy metals from surrounding seawater, and can be eaten by marine organisms with different trophic levels (Wang et al., 2018a; Wayman and Niemann, 2021). As a carrier, MPs transport pollutants into the food web, which is considered as one of the major health risks of marine organisms (Li et al., 2020; Covernton et al., 2022; Jiang et al., 2023). Therefore, it is of great ecological significance to understand the concentration, distribution and characteristics of MPs in the environment.
In coastal waters ecosystems, sediments are not only the sink of land-based MPs, but also the source of MPs in seawater (Qian et al., 2018; Wu et al., 2022a). Studies have shown that the abundance of MPs in sediments is often higher than that in water bodies (Wu et al., 2020; Ding et al., 2022). Although some plastics usually have a smaller density than water, due to the impact of wind, hydrodynamic conditions, bioenrichment and the shape of MPs proper, etc., MPs in water bodies will settle and accumulate in sediments (Talbot and Chang, 2022). Sediments are also a special ecological environment that integrates chemicals and microorganisms into one (Seeley et al., 2020; Li et al., 2022a; Li et al., 2022b). Marine sediments are the most complex microbial habitats on the earth, and microorganisms in sediments often have morphological and physiological specificity to adapt to their special environment (Seeley et al., 2020; Hu et al., 2021; Zhu et al., 2022b). Studies have found that MPs in sediments have two characteristics, one is that they can serve as particulate pollutants, and the other is that they can adsorb other pollutants or microorganisms due to the surface structure and thermodynamic properties of MPs (Khalid et al., 2021; Qi et al., 2021; Liu et al., 2022b). Some MPs from sediments enter the ocean, while others are remained in polluted areas permanently or semi-permanently and accumulate year by year, especially in some enclosed or semi-enclosed bays and wharves (Boskovic et al., 2021; Yang et al., 2021). When MPs are reserved in sediments, they can only be degraded by organisms and remain for a relatively long time, because the MPs in sediments cannot be photolyzed by UV light (Wu et al., 2022b). This will significantly raise the pressure of local environment. Therefore, there is a close relationship between MPs and microorganisms. MPs contain toxic pollutants and release phthalate or other additives during degradation, all of these will affect the diversity and function of microorganisms (Chen et al., 2021; Zhu et al., 2022a). In coastal marine ecosystems, especially in coastal waters largely disturbed by human beings, studies on the relationship between MPs and the microbial community in sediments are still limited.
Shenzhen, a coastal city, has developed rapidly in the past 40 years and now become an international metropolis (Duan et al., 2021). However, little is known about the concentration, distribution and characteristics of MPs, microbial diversity and ecological strategies of communities in sediments from Shenzhen coasts. In addition, the metabolic potential of microorganisms in offshore sediments hasn’t been well characterized. Recent developments in omics-based techniques and their integration have contributed towards advances in the fields of environmental biology. The focus of this study is the presence of MPs and microorganisms in sediments and their relevant influence. The objectives of this study are as follows: (1) to investigate the abundance, distribution and characteristics of MPs in sediments from Shenzhen coastal waters; (2) to explore the effects of MPs on the structure and function of microbial community in sediments, and lay a foundation for the study of the interaction between MPs and microorganisms. This comprehensive study has important implications for understanding the regional or global distribution and potential impact of MPs pollution on microorganisms, and may be a guide for governments in developing effective plastic waste control programs.
2 Materials and methods
2.1 Sampling and environmental information
In this study, Shenzhen coastal waters were selected as the research area, and its location is shown in Figure 1. Shenzhen was an economically developed coastal city in southeastern China. By the end of 2021, Shenzhen had a permanent resident population of 17.5601 million, with 1.5532 million in Futian District, 214,200 in Yantian District, 1.7958 million in Nanshan District, 4.4766 million in Bao’an District, and 156,200 in Dapeng New Area (Xu et al., 2022). Sediment samples were collected from 10 sites, including 4 sites (E10, E21, E41, and E71) in eastern Shenzhen and 6 sites (W11, W12, W14, W15, W17, and W20) in western Shenzhen. At each site, three aliquots as replicates, and each one is 10 m apart. In addition, 25 L of seawater was collected at each site using a water harvester. The samples were put into an encoded aluminum foil sealing bag and stored at 4°C to analyze the physicochemical properties of sediments and extraction of MPs. Environmental properties (e.g. salinity, total nitrogen (TN), and pH) were detected by KLL-Q-2 portable water level and water quality measuring instrument (Shanghai Haiheng Electromechanical Instrumentation Co., China) with half of samples from different sampling sites. Determination of Vibrio abundance using cell culture (Zhang et al., 2021). At the same time, the samples used for microbiological analysis were stored in -20°C.
2.2 Extraction of MPs
In the laboratory, all glassware was washed with distilled water, so that there would be no MPs pollution during the experiment. After freeze-drying, 300 g was weighed from each sample and placed in a glass beaker. After that, 250 mL saturated NaCl solution was added and stirred for 10 min, so that the MPs can be suspended. After precipitation for 12 h, the supernatant was vacuum suction filtered using a 3 μm stainless steel sieve and NaCl was removed by washing with distilled water repeatedly. The material on the membrane was transferred to a glass beaker, and 30% H2O2 was added to remove the organic matter, and the material was suspended in saturated NaCl solution. The supernatant was filtered with a 3 μm steel sieve and NaCl was removed by rinsing with distilled water for at least 5 times. The MPs were transferred from the steel sieve to a petri dish for optical microscopy.
2.3 Observation and identification
The morphological data of MPs were statisticized with an optical microscope (Olympus BX-51, Japan). According to the identification rules of previous studies, the MPs were divided into five size grades: <0.1 mm, 0.1-0.5 mm, 0.5-1 mm, 1-2 mm and 2-5 mm, the shapes were divided into fibrous, film and granular (Li et al., 2020). The colors were divided into black, blue, red, white and transparent, etc. A microscopic Fourier transform infrared spectroscope (micro-FTIR; Thermo Fisher, USA) was used. An acquisition time of 3 sec and 16 confocal scans were used, and the micro-FTIR spectrum of each plastic item was recorded with a resolution of 8 cm-1 between 4,000 and 650 cm-1. Each spectrum was compared with the OMNIC Standard Spectral Library. When the matching degree was higher than 70%, the suspicious particles can be identified as MPs. The actual quantity of MPs was calculated based on micro-infrared spectral analysis.
2.4 Metagenomic DNA extraction, library preparation and sequencing of microorganisms in sediments
According to the manufacturer’s instructions, each genomic DNA was extracted with a commercial kit (QIAmp) and the bacteria were eluted from the microfiltration membrane. The integrity and purity of DNA were detected by 1% agarose gel. For DNA samples, high-throughput sequencing was performed on V4-V5 region of 16S rRNA gene using universal primer sets 515F and 907R. PCR primers were synthesized by Invitrogen Corp. (Invitrogen, Carlsbad, CA, USA). PCR reaction was performed with 25 μl 2x Premix Taq (Takara Biotechnology, Dalian Co. Ltd, China). The primer was 1 μl (10 mM) each, the template was 3 μl (20 ng/μl), and the volume was 50 μl. Thermal cycle amplification reaction: initialized at 94°C for 5 min; denatured at 94°C for 30 s; annealed at 52°C for 30 s and stretched at 72°C for 30 s, with a total of 30 cycles. At last, the final extension was performed at 72°C for 10min. The PCR instrument was BioRad S1000 (Bio-Rad Laboratory, CA, USA). The length and concentration of PCR product were detected by 1% agarose gel electrophoresis, and then purified by EZNA gel Extraction Kit (Omega, USA). The quality of sequencing library was evaluated on Qubit@ 2.0 fluorometer and Agilent 2100 Bioanalyzer System. Sequencing was performed using Illumina Mi Seq Double-terminal (250 bp×2) Sequencing Platform (Illumina, San Diego, CA, USA).
2.5 Analysis of microbial metabolites of sediments
After freeze-drying, the samples were weighed into an EP tube, and 1,000μL extract (acetonitrile: methanol: water=2:2:1) with 1g/mL internal standard was added. After 30s of whirlpool, the sample was homogenized at 35 Hz for 4 min, and then sonicated on ice for 5 min. Homogenization and ultrasound were performed for 5 cycles. And then the samples were incubated at -20°C for 1h and centrifuged at 11,000 rpm at 4°C for 15 min. The resulting supernatant was transferred to a fresh glass bottle for analysis. The quality control (QC) samples were prepared by mixing equal amounts of supernatant from all samples. The resulting supernatant was transferred to a fresh glass bottle for analysis. After the original data was converted to mzXML format by ProteoWizard software, a self-written R package (whose kernel was XCMS) was used for peak identification, peak extraction, peak alignment, integration and other treatments, and then matched with BiotreeDB (V2.1) self-built secondary mass spectral database for material annotation, and the cutoff value of algorithm score was set to 0.3.
2.6 Omics data processing
Analysis steps of species composition and diversity of samples: Kraken2 (whose parameter was confidence 0.2) was used to annotate and classify all valid sequences of all samples. After Kraken was used, the metagenomic sequencing data were classified. We continued to use Bracken (default parameter) to classify the classification results obtained by Kraken2, and then adopted Bayesian Reestimation of Abundance to estimate the species level or generic abundance of metagenomic samples.
Basic steps of metagenomic functional analysis: HUMAnN2 software was used to compare the sequences after quality control and de-hosting using protein database (UniRef90) (based on DIAMOND); reads that failed the comparison were filtered out (HUMAnN2 default comparison parameters: translated query coverage threshold=90.0, prescreen threshold=0.01, evalue threshold=1.0, translated subject coverage threshold=50.0); the relative abundance of each protein of UniRef90 (RPKM, reads per kilobase per million, the abundance after the number of reads (mapped reads) succeeded in comparison and gene length of samples were corrected) was counted. According to the correspondence between the ID of UniRef90 and the ID of each functional database (mainly from LinkDB), the relative abundance of the corresponding function of each functional database was counted. From the relative abundance table of the function of each database, the relative abundance histogram display, Circos chart display, abundance clustering heat map display, LEfSe analysis of inter-group functional difference, pair-wise multiple comparison, DunnTest analysis of inter-group functional difference, histogram analysis of the origin of species with significantly different functions, coloring of KEGG pathway map, and correlation analysis between function and environmental factors (or other omic data) were carried out.
Basic steps of resistance gene annotation: FMAP software was used to compare the sequences after quality control and de-hosting of each sample with CARD database (based on DIAMOND), and sequences that failed the comparison were filtered out; parameter: -e0.00001 (evalue threshold). According to the comparison results, the number of reads of each sample compared to each ARO reference sequence was counted, so as to calculate the relative abundance (RPKM, reads per kilobase per million, the abundance after the number of reads (mapped reads) succeeded in comparison and gene length of samples were corrected. Finally, starting from the abundance of ARO, abundance histogram display and abundance clustering heat map display were conducted.
Correlation analysis: Redundancy analysis (RDA) mainly relied on R language VEGAN package and visualized using ggplot2.
2.7 Quality assessment and quality control
Throughout the sample collection, sample separation and sample analysis, we took precautions to avoid contamination from external plastics. To put it simply, all glass containers were soaked in dilute nitric acid overnight and then rinsed with deionized water for three times. All chemical reagents were filtered via a 0.45 μm glass film before use. Microscopy was done in a laboratory with the windows being closed, so as to reduce air pollution. During the field sampling and analysis, cotton-padded clothes, gloves and masks should be worn to prevent contamination from external synthetic fiber. The solution preparation and extraction of MPs had been carried out in a laminar flow cabinet. The program blank (ultrapure water) was run in the same program to make sure that there was no plastic contamination during analysis.
3 Results and discussion
3.1 The abundance, distribution and characteristics of MPs
3.1.1 Occurrence and distribution of MPs
Coastal waters were also identified as areas vulnerable to MPs pollution. MPs pollution in sediment system was an urgent problem. As shown in Figures 2 and S1, MPs were detected in marine sediments at 10 sites in Shenzhen coastal waters. The concentration of MPs in marine sediments was 101–963 items/kg, with an average concentration of 446 ± 318 items/kg. The concentration of MPs in the sediments of western Shenzhen was measured at 664 ± 197 items/kg, while in the sediments of eastern Shenzhen, it was found to be 119 ± 18 items/kg. In 2021, Shenzhen’s gross domestic product (GDP) reached 3,066.485 billion yuan, with a per capita GDP of 70,800 yuan, reflecting its development comparable to that of developed countries (Zhong, 2022). Notably, western Shenzhen displayed robust economic performance with a GDP of 2,855.268 billion yuan and a population of 16.5726 million, while eastern Shenzhen showed relatively lower economic figures with a GDP of 204.144 billion yuan and a smaller population of 921,700 (Zhong, 2022). And the industrial and human activities in the western part of Shenzhen are much higher than those in the eastern part. The abundance of MPs in the offshore of Shenzhen is not much affected by fishing activities, because the fishing industry in Shenzhen is dominated by pelagic fishing. We can clearly observe that the concentration of MPs in marine sediments of western Shenzhen was much higher than that in eastern Shenzhen, 9.5 times at most. The development of all kinds of industrial and human activities can lead to the occurrence of plastic waste and other pollutants in coastal waters. W15 was a mangrove area, since mangroves can intercept MPs, W15 became a place with the highest MPs pollution in Shenzhen (Liu et al., 2022a). As one of the largest transit centers in China, W17 was considered as an important distribution center and transit port in southern China. Due to its geographical location and the introduction of modern machinery, a large number of items and products are distributed from here and thus probably lead to significant MPs contamination. W14 was located downstream of the estuary, near the most densely populated area, and had the second highest MPs abundance in Shenzhen. This was probably because the physical processes involved in the flow of MPs in coastal areas were complex and influenced by a variety of environmental factors. Injecting MPs into upstream water can greatly increase the MPs abundance of sediments in downstream water. Eastern Shenzhen has developed tourism vigorously and the coastal waters are clean, so the pollution level of MPs is low. In this study, relatively high abundances of MPs were observed in the coastal sediments of the Shenzhen, with a mean of 446 ± 318 items/kg, which was much higher than those in the marine sediments of the Chilean fjords, Oslofjord, the Belgian coast, Canadian Arctic, Tarragona coast, Zhuhai and East China Sea.
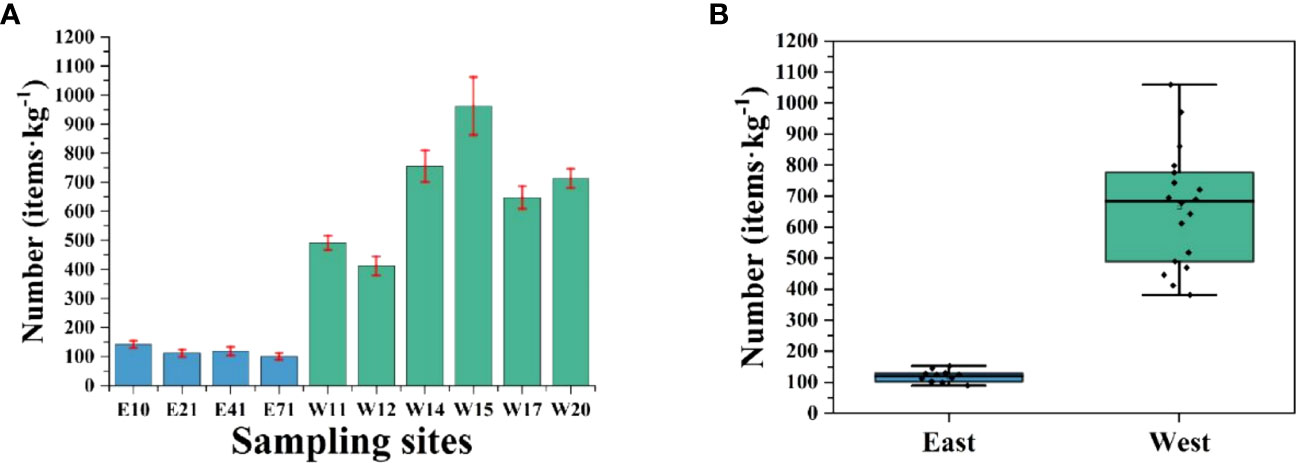
Figure 2 Concentration of MPs in different sampling sites across Shenzhen. (A) MPs concentration (items·kg−1) of 4 sampling sites (E10, E21, E41, and E71) in the eastern part and 6 sampling sites (W11, W12, W14, W15, W17, and W20) in the western part of Shenzhen; (B) MPs concentration (items·kg−1) comparison between the eastern and western regions of Shenzhen.
3.1.2 Surface morphology of MPs
The shape, size and color of MPs may change the exposure pathway of MPs to human body, and affect the potential ecological risk of MPs in sediments. In this study, the analysis of MPs can be divided into three major shapes, that is, fibrous, film and fragmented, as shown in previous studies. Specifically, fragmented MPs were bulky MPs with a small piece or part of plastic. MPs films were more likely secondary MPs packages formed by the degradation of large flexible plastics, and a very thin plastic layer can be observed. Fibrous was the most common form of MPs, and these types of MPs had a long and thin appearance. Different shapes of MPs in the sediments from eastern and western Shenzhen had different proportions, as shown in Figure 3. Fibrous was the predominant form of MPs in the sediment samples of interest from eastern and western Shenzhen, accounting for 63.77% of the total MPs (Figures 3A, B), and the results were similar to the detection results of MPs in waters along the Black Sea of Bulgaria (Pojar et al., 2021). These fibers may derive from fishing tools, which were often damaged during fishing activities, or transported to the aquatic system through sewage discharge. The proportion of MPs film in western Shenzhen was obviously higher than that in eastern Shenzhen, which was probably caused by the dense residential areas and industrial areas in western Shenzhen. Based on previous studies, it has been observed that plastic fragments tend to accumulate along the shoreline and undergo physical abrasion with sand, resulting in the generation of MPs fragments (Carvalho et al., 2021). Consequently, the proportions of MPs fragments were relatively bigger in beach sites E10 and E71, as depicted in Figure 3B.
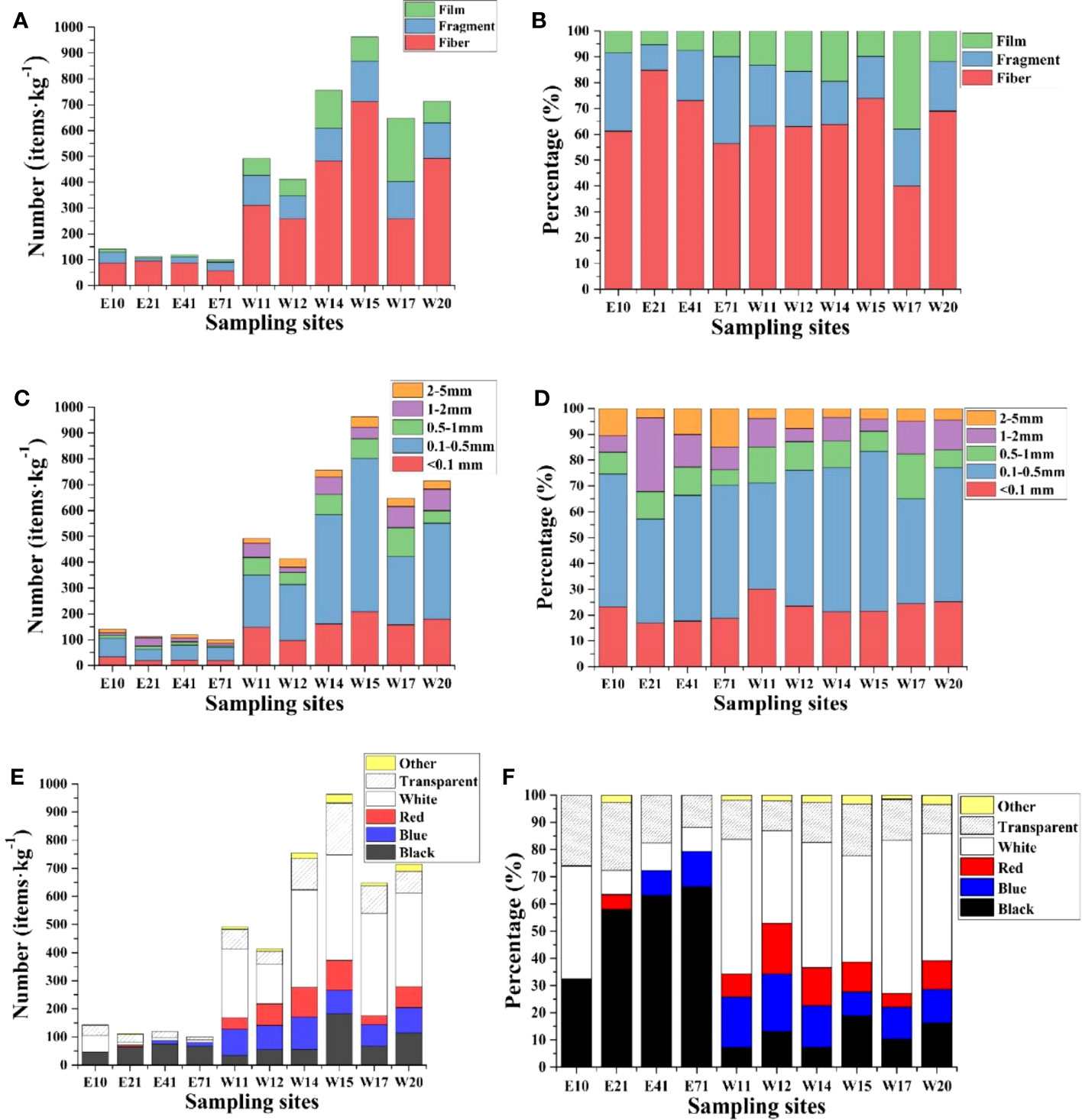
Figure 3 Number and percentage of MPs in different sampling sites, according to shape (A, B), size (C, D), and color (E, F).
MPs can be divided into five groups according to size grades (<0.1mm, 0.1-0.5mm, 0.5-1mm, 1-2mm and 2-5mm). According to the size distribution summarized in Figure 3C and d, MPs with a size range of 0.1-0.5mm dominated sediments in Shenzhen coastal watershed, accounting for 51.52% of the total number of detected debris. Among the detected MPs, 23.41% were found to be MPs with a size of less than 0.1 mm, 10.61% with a diameter of 0.5 to 1mm, 9.37% with a diameter of 1 to 2mm and 5.09% with a diameter of 2 to 5mm. The size distribution ratios of MPs in sediments were similar (Figure 3D).
This was consistent with the particle size of MPs observed in the sediments of coastal cities. The high proportion of 0.1-0.5 mm MPs was also proved by previous studies. For example, the main size of MPs detected in the Saigon River was <0.25 mm (Lahens et al., 2018), while after investigating 29 Great Lakes in the world, Baldwin et al. (2016) discovered that 72.00% of MPs were within the range of 0.35-0.99 mm. Turbulence induced by tides may strongly affect the exchange of MPs between the Maozhou River (W14) and Pearl River Estuary. The small-sized MPs in sediments were more susceptible to interference and resuspended in water, thereby entering the aquifer through the gap of bedrock and leading to a higher risk of human exposure (Panno et al., 2019).
Colors were also considered as a fundamental issue affecting the intake of MPs by organisms (Abayomi et al., 2017). For this reason, the colors of MPs were recorded in this study (Figures 3E, F), including black, blue, red, white, transparent and others. The results showed that black MPs accounted for the largest proportion in the waters of eastern Shenzhen, up to 53.38%, and these black fibers may be produced by fishing nets used for fishing activities. Transparent MPs were the second largest category MPs (20.68%). White MPs accounted for the largest proportion in the waters of western Shenzhen, up to 45.33%. Transparent MPs were the second largest category MPs (14.70%). The high proportions of white and transparent MPs in high industrial and residential areas were consistent with many previous studies (Wang et al., 2019; Marti et al., 2020; Cai et al., 2022). Reportedly, this was caused by the spill of plastics production from surrounding industries and fading during strong weathering processes, for example, tides and waves (Cho et al., 2019).
3.1.3 Identification of polymers in MPs
Micro-FTIR analysis showed that there were mainly five types of polymers in the sediments of coastal waters of Shenzhen (Figures 4A, B): polystyrene (PS), polyethylene (PE), polyethylene terephthalate (PET), polypropylene (PP) and polyamide (PA). The polymer composition in MPs varied with eastern and western Shenzhen. The copolymer of PE and PS was the main type of MPs, which accounted for 16.26% and 39.64% of the total polymers in samples from waters around Shenzhen, respectively. In sediment samples from eastern Shenzhen (Figure 4C), the proportion of PS (42%) was the largest among the detected plastic polymers, followed by PA (23.42%), PP (16.88%). PE was usually widely used in the packaging and manufacturing of disposable tableware. In sediment samples from western Shenzhen (Figure 4C), the proportion of PE (44.28%) was the largest among the detected plastic polymers. PE was a frequently detected type of MPs polymer and had a wide range of applications, mainly derived from fragments of rigid plastics and packages. PP was used to make hard plastic tools and furniture, such as textile floor coverings, carpets and fishing nets. Likewise, the diversity of polymer types detected was smaller than that of MPs collected from Nordic coastal waters (Kooi et al., 2017; Bronzo et al., 2021). Even if the density of PE and PP in water was lower than that of water, they can settle and accumulate in sediments, which was affected by wind, hydrodynamic conditions, bioenrichment and the shape of MPs proper, etc (Kooi et al., 2017). The main types of PP and PE based on environmental samples are relatively less risky than PVC. Due to the change of molecular structure, PVC is one of the most widely used plastics with unique chemical properties. After entering the environment, it will release carcinogenic monomers and inherent plasticizers (Bronzo et al., 2021). Generally speaking, the diversity of MPs was attributed to its relationship with different sources of incoming plastic waste and relatively strong coast types.
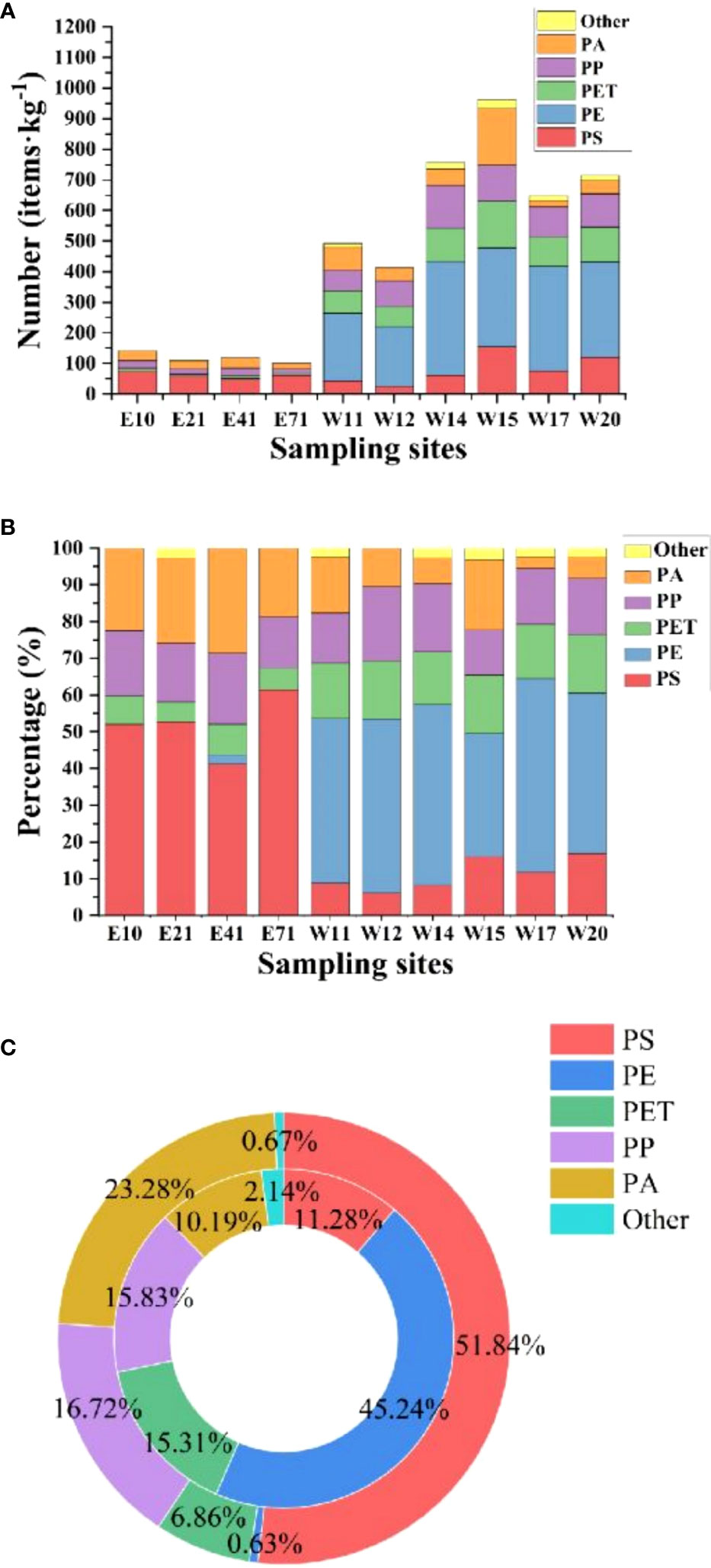
Figure 4 Polymer types of MPs in sediments of the seas around Shenzhen. (A, B) Number and percentage of MPs. (C) Comparison of MPs abundance in eastern and western Shenzhen. The outer circle represents the eastern sample, and the inner circle represents the western sample.
3.2 Relationship between MPs and microbial community
Microorganisms drove biogeochemical cycles, which connected abiological and biological processes in the environment and were intricately linked with plastic fragments (Harrison et al., 2014; McCormick et al., 2014). As MPs settled to the seabed, they were buried and fused into aerobic sediment-water interface, biodisturbance region and anoxic sediments. Coastal marine ecosystems directly received MPs from the land runoff, undesirable waste management, storm drains, sewer overflow, and sewage treatment plant discharge. It was particularly important to explore the effects of MPs on microbial community of coastal marine sediments. As a unique microbial habitat, MPs can not only change the community structure, but also influence the microbial function, and potentially affect the ecological function of microbial community in ecosystem (Kong et al., 2018; Wang et al., 2018b).
3.2.1 Effect of MPs on the diversity of microbial community
Figure 5A indicates a significant correlation between MPs and microbial community characteristics. The similarity of microbial composition in all samples was analyzed by means of PCoA, and the results showed that samples from eastern sites were obviously separated from those from western sites (Figures 5B, S1). Three duplicate samples from the same site were clustered together, indicating that there was good consistency within this group.
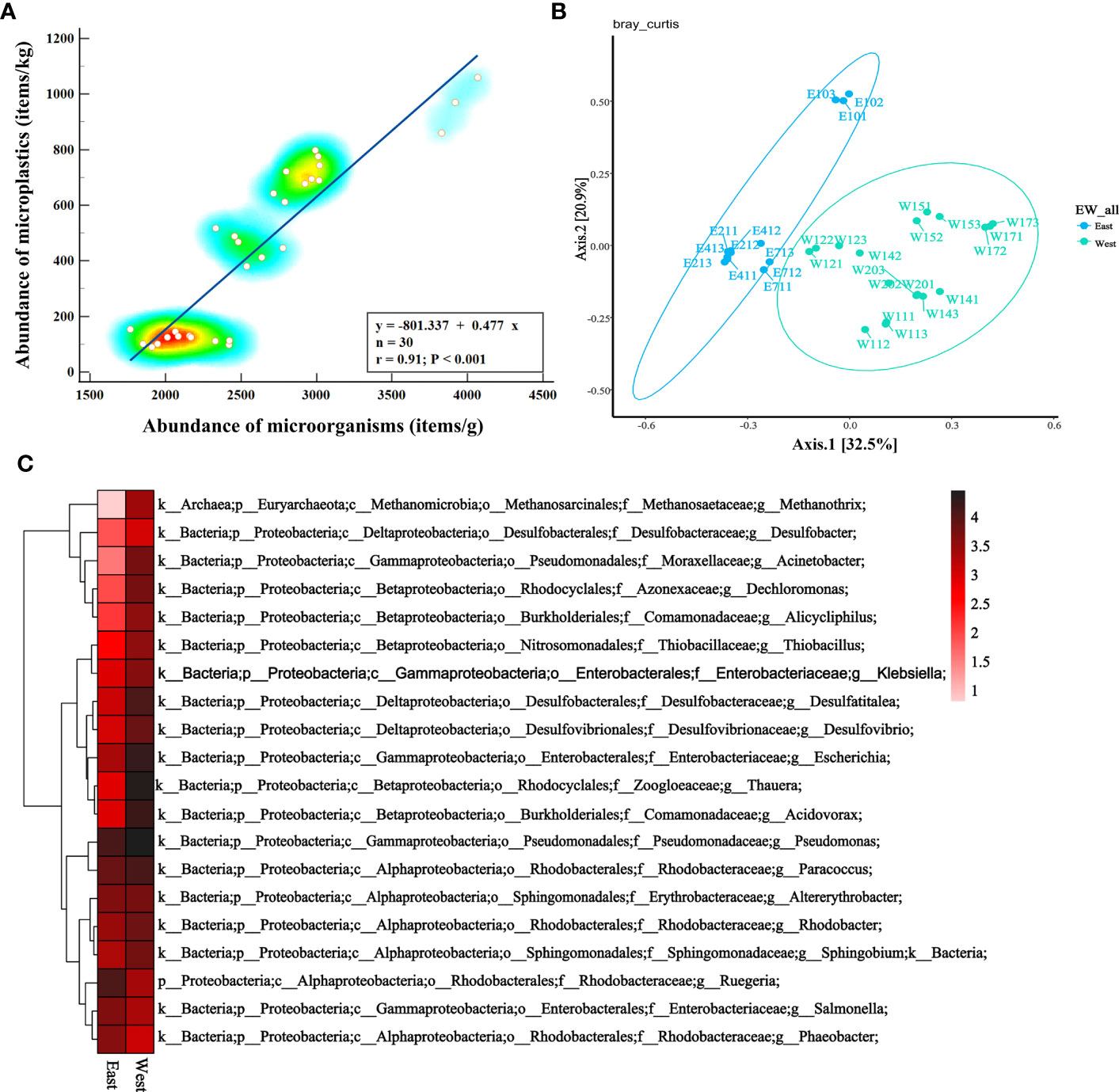
Figure 5 (A) Spearman correlation analysis to analyze the relationship between MPs concentration and microbial community characteristics in the genus level. (B) PCoA based on Bray Curtis distance matrix. (C) Relative distribution of eastern and western sample at the genus level (top 20 relative abundance).
The results of microbial composition indicated that the bacteria in sediment samples were dominated by Proteus, Bacteroides, Planus, Chloromyces, Acidophilic Bacteria, Actinomycetes, and Cyanobacteria, accounting for 76.70% of all microorganisms on average (Figures S2A, S3A). As shown in Figures 5C and S2B, the eastern samples exhibited higher levels of Aspergillus (87.63%), with the highest level observed in sample E41 (90.61%) at the phylum level. Conversely, the western samples showed higher levels of Aspergillus (83.70%). Sample E9s had the highest abundance of Bacteroidetes (94.93%) among all samples. Euryarchaeota (2.12%) had relatively higher abundance in the western samples, about 10 times higher than in the eastern samples. At the genus level (Figure 5C), compared to the eastern region, western samples had higher levels of Thauera (5.41%), Pseudomonas (4.67%), Escherichia (3.61%), Desulfatitalea (3.64%), and Acidovorax (2.97%). Other microorganisms like Salmonella (6.54%), Ruegeria (6.30%), Altererythrobacter (3.68%), and others were enriched in eastern sediment samples.
At the species level, abundant types were Salmonella enterica, Escherichia coli, Desulfatitalea tepidiphila, Pseudomonas aeruginosa, Altererythrobacter ishigakiensis, and Thauera aminoaromatica (Figure S3B). Escherichia coli, Desulfatitalea tepidiphila, Thauera aminoaromatica, Thauera sp. MZ1T, and Methanothrix soehngenii were more prevalent in western samples, while microorganisms like Altererythrobacter ishigakiensis, Ruegeria lacuscaerulensis, Phaeobacter gallaeciensis, Ruegeria sp. AD91A, and Tropicimonas sediminicola were enriched in eastern sediment samples. Altererythrobacter ishigakiensis removed polycyclic aromatic hydrocarbon with high molecular weight from petroleum contaminated environment (Park et al., 2019). Ruegeria lacuscaerulensis, Ruegeria sp. AD91A played an important role in the carbon and sulfur cycle of marine ecosystem. Phaeobacter gallaeciensis can produce the potent antibacterial compound tropodithietic acid, which inhibited both fish and human pathogenic bacteria, as well as many non-pathogenic bacteria (Arahal et al., 2018; Shao et al., 2019). Desulfatitalea tepidiphila and Thiobacillus denitrificans can oxidize reduced sulfides and were present in various sulfur-rich environments (Kubo et al., 2014). Methanothrix soehngenii was a class of archaebacteria that can ferment inorganic or organic compounds anaerobically into methane and carbon dioxide (Ohtsubo et al., 1992). These phenomena are attributed to the higher concentration of microplastics in western Shenzhen. The presence of microplastics can indeed alter the microorganisms’ living environment by providing a novel bio-attachment surface, potentially leading to changes in their growth and distribution patterns.
In addition, we used metagenomic sequencing data to predict the KEGG pathway of microbial community at each sampling point (Figures 6A, S4). The top five pathways in our samples were about amino acid metabolism, carbohydrate metabolism, energy metabolism, and metabolism of cofactors. The KEGG models of functional differences of these important pathways were not highly similar, which suggested that there were potential similar functions between these eastern sediments and western sediments as a whole. However, there were also some obvious differences in the relative abundance of functions, for example, the western prediction pathways (e.g. Carbohydrate metabolism, Global and overview maps, Cellmotility, Chemical structure transformation maps, and Cellularcommunity prokaryotes) were more abundant than eastern ones.
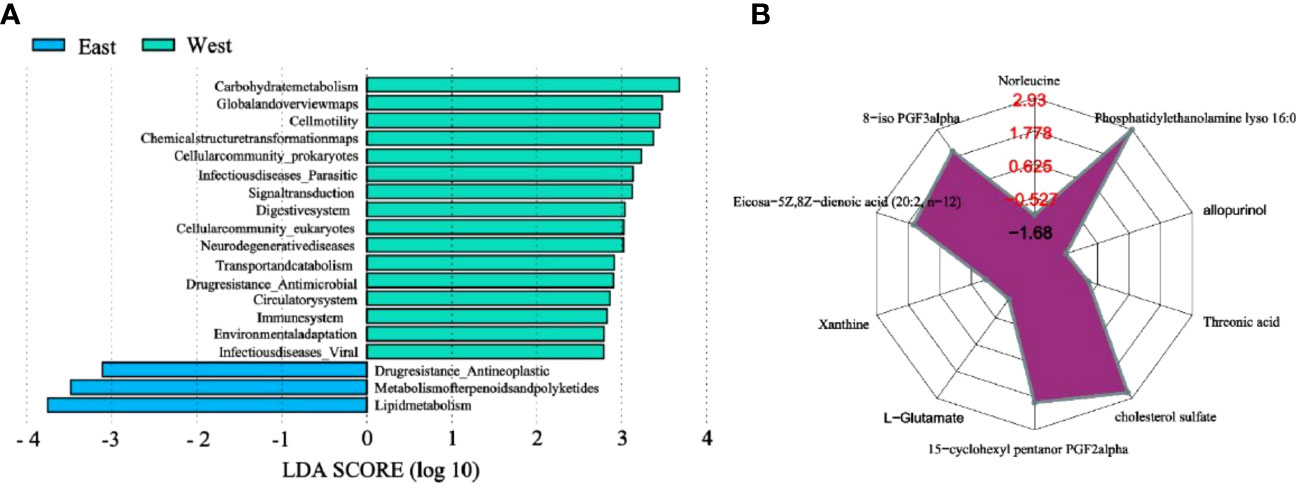
Figure 6 (A) LEfSe Analysis of basic Metabolic Pathway of KEGG. (B) Radar map analysis of differential metabolites. For each group of comparisons, we calculate the corresponding ratio for the quantitative values of differential metabolites, and take 2 as the base logarithmic conversion, which is expressed in red font, and each grid line represents a multiple of the difference. Purple shadows are made up of multiple lines of difference for each substance.
We calculated Euclidean distance matrix using the quantitative value of differential metabolites, clustered the differential metabolites by complete linkage, and displayed them with thermodynamic chart (Figure S5). The results are shown in Figures 6B and S5, and there were obvious differences in microbial metabolites between eastern and western Shenzhen. Phosphatidylethanolamine lyso 16:0, cholesterol sulfate, 15-cyclohexyl pentanor PGF2alpha, Eicosa-5Z,8Z-dienoic acid (20:2, n-12), 8-iso PGF3alpha in the west and Norleucine, allopurinol, Threonic acid, L-Glutamate, Xanthine in the east. However, different microorganisms may have similar functions. For this reason, microbial composition may change significantly in response to external pressure, while the main functional structure of microbial community remained relatively stable (Qian et al., 2018). The presence of microplastics may affect the microbial habitat by providing new biological attachment surfaces or by releasing organic material that can lead to changes in microbial metabolic pathways (Li et al., 2020).
3.2.2 Correlation analysis between MPs and other environmental factors and microorganisms
In order to explore the correlation analysis between MPs and other environmental factors and microorganisms, we analyzed environmental factors of each sample in western Shenzhen and eastern Shenzhen (Figure 7; Table S1). Dissolved oxygen (DO), TN, total phosphorus (TP), chemical oxygen demand (COD), harmful marine vibrio (HMV), transparence and MPs were also significantly correlated with microbial composition (p=0.001). In general, the water quality in the west coast was worse and may affect the microbial community around the coastline (Li et al., 2018a).
RDA analysis showed that at the phylum level (Figure 7A), the most abundant Proteobacteria in samples from eastern sites was significantly negatively correlated with the abundance of MPs, while Euryarchaeota found in western samples, which was about 10 times higher than the relative abundance of eastern samples, was significantly positively correlated with the abundance of MPs. Euryarchaeota, as an important and ubiquitous archaebacteria, made great contributions to the global energy cycle (Ma et al., 2020). It was universally known that Euryarchaeota got involved in the production of greenhouse gas, and methane was the final product of anaerobic respiration. This biological process accounted for about 74% of atmospheric methane emitted on the earth. In addition to the production of methane, Euryarchaeota was also found to oxidize methane, fix nitrogen, reduce nitrate and metabolize sulfur and iron (Evans et al., 2019). This also suggested that the pollution degree of coastal waters in western Shenzhen was far greater than that of eastern Shenzhen.
At the genus level (Figure 7B), the abundant bacterial colonies, Salmonella (6.54%), Ruegeria (6.30%), Altererythrobacter (3.68%), Phaeobacter (2.90%), Paracoccus (2.15%) and Klebsiella (1.34%) at eastern sites, the abundant bacterial colony Pseudomonas (4.67%) at western sites were significantly negatively correlated with MPs abundance. The dominant bacteria of Salmonella appeared in eastern Shenzhen, and Salmonellosis was one of the zoonotic diseases of great significance to public health (Eng et al., 2015). After infection, humans and animals can present as asymptomatic carriers, or show fatal disease with clinical symptoms, which may aggravate morbidity or mortality, or lower the reproductive productivity of animals (Eng et al., 2015). This may be attributed to the discharge of waste water from numerous breeding areas in the east. Altererythrobacter had the ability to degrade polycyclic aromatic hydrocarbon with high molecular weight. Ruegeria played an important role in the carbon and sulfur cycle of marine ecosystem (Arahal et al., 2018). Phaeobacter can produce an effective antibacterial compound, thiodithionic acid, which can inhibit both pathogenic bacteria in fish and humans and many non-pathogenic bacteria (Breider et al., 2017). While Thauera (5.41%), Escherichia (3.61%), Desulfatitalea (3.64%) and Acidovorax (2.97%) found in western samples, which had higher relative abundance than eastern samples, were significantly positively correlated with MPs abundance. Thauera, a typical genus of denitrifying bacteria, is widely found and dominant in a variety of biological treatment processes of wastewater (Mao et al., 2014). Thauera have a wide range of carbon and nitrogen metabolic pathways, which enable them to remove carbon, nitrogen and phosphorus from wastewater (Mao et al., 2014). Desulfatitalea and Thiobacillus exist in all kinds of sulfur-rich environments and can oxidize reduced sulfides (Han et al., 2021; He et al., 2021). Methanothrix is a class of archaebacteria that can ferment inorganic or organic compounds anaerobically into methane and carbon dioxide (Li et al., 2018b). MPs, as well as polycyclic aromatic hydrocarbon and other organic compounds adsorbed by plastics, offer nutrition to some bacteria with hydrocarbon degradation function. MPs are not only the source of carbon, but also can absorb organic carbon and offer nutrition to some bacteria with carbon metabolism function. This may lead to the increase of genes with carbon metabolism function in microbial communities in western Shenzhen. The existence of MPs changes the composition of microbial community and the cycling processes of carbon, nitrogen and sulfur in sediments.
In the CCA/RDA ordination chart, environmental factors are indicated by arrows, and the length of the arrow line represents the degree of correlation between an environmental factor and community distribution and species distribution (explain the size of variance). The longer the arrowhead is, the greater the correlation is, and vice versa. The angle between the arrow line and the sorting axis represents the correlation between an environmental factor and the sorting axis, the smaller the angle, the higher the correlation, and vice versa. When the angle between environmental factors is acute, it means that there is a positive correlation between the two environmental factors, and a negative correlation when the angle is obtuse. Each point represents a species, the larger the point, the higher the corresponding species abundance, and the gray dots represent the species with lower abundance.
4 Conclusion
MPs are detected in marine sediments at 10 sites of Shenzhen coastal waters. The concentration of MPs in marine sediments ranges from 101 to 963 items/kg, and is 446 ± 318 items/kg on average. The concentration of MPs in marine sediments of western Shenzhen is much higher than that in eastern Shenzhen, 9.5 times at most. Micro-FTIR analysis shows that the copolymers of PS (39.64%) and PE (16.26%) in Shenzhen coastal waters are the main types of MPs. These potential disparities can be attributed to differences in economic development and population density between the eastern and western parts of Shenzhen. It was obtained through Spearman correlation analysis that the presence of MPs has a great impact on microbial diversity, which further affects the functioning of the microbial community. In conclusion, this study presents a comprehensive investigation of MPs pollution in Shenzhen Coastal Waters, shedding light on the interaction between MPs and microorganisms in the coastal marine environment, providing a valuable basis for future research in this field.
Data availability statement
The sequences obtained in this study have been deposited in the NCBI Sequence Read Archive (SRA) under BioProject numbers PRJNA1010718.
Author contributions
RZ: Supervision; Validation; Project administration; Funding acquisition. YL: Experiments, Formal analysis, Data curation, Conceptualization, Writing - review &editing. All authors contributed to the article and approved the submitted version.
Funding
This research was supported by The Future Industrial Development Fund of Shenzhen (JCYJ20170413111950426).
Conflict of interest
The authors declare that the research was conducted in the absence of any commercial or financial relationships that could be construed as a potential conflict of interest.
Publisher’s note
All claims expressed in this article are solely those of the authors and do not necessarily represent those of their affiliated organizations, or those of the publisher, the editors and the reviewers. Any product that may be evaluated in this article, or claim that may be made by its manufacturer, is not guaranteed or endorsed by the publisher.
Supplementary material
The Supplementary Material for this article can be found online at: https://www.frontiersin.org/articles/10.3389/fmars.2023.1189876/full#supplementary-material
References
Abayomi O. A., Range P., Al-Ghouti M. A., Obbard J. P., Almeer S. H., Ben-Hamadou R. (2017). Microplastics in coastal environments of the Arabian Gulf. Mar. pollut. Bull. 124 (1), 181–188. doi: 10.1016/j.marpolbul.2017.07.011
Arahal D. R., Lucena T., Rodrigo-Torres L., Pujalte M. J. (2018). Ruegeria denitrificans sp nov., a marine bacterium in the family Rhodobacteraceae with the potential ability for cyanophycin synthesis. Int. J. Systematic Evolutionary Microbiol. 68 (8), 2515–2522. doi: 10.1099/ijsem.0.002867
Baldwin A. K., Corsi S. R., Mason S. A. (2016). Plastic debris in 29 great lakes tributaries: relations to watershed attributes and hydrology. Environ. Sci. Technol. 50 (19), 10377–10385. doi: 10.1021/acs.est.6b02917
Boskovic N., Joksimovic D., Pekovic M., Perosevic-Bajceta A., Bajt O. (2021). Microplastics in surface sediments along the montenegrin coast, adriatic sea: types, occurrence, and distribution. J. Mar. Sci. Eng. 9 (8), 13. doi: 10.3390/jmse9080841
Breider S., Freese H. M., Sproer C., Simon M., Overmann J., Brinkhoff T. (2017). Phaeobacter porticola sp nov., an antibiotic-producing bacterium isolated from a sea harbour. Int. J. Systematic Evolutionary Microbiol. 67 (7), 2153–2159. doi: 10.1099/ijsem.0.001879
Bronzo L., Lusher A. L., Schoyen M., Morigi C. (2021). Accumulation and distribution of microplastics in coastal sediments from the inner Oslofjord, Norway. Mar. pollut. Bull. 173, 11. doi: 10.1016/j.marpolbul.2021.113076
Cai M. G., Liu M. Y., Qy H. Y., Cui Y. Z., Zhang M. Y., Huang P., et al. (2022). Transport of microplastics in the South China Sea: A review. Gondwana Research 108, 45–59. doi: 10.1016/j.atmosenv.2021.118389
Carvalho J. P. S., Silva T. S., Costa M. F. (2021). Distribution, characteristics and short-term variability of microplastics in beach sediment of Fernando de Noronha Archipelago, Brazil. Mar. pollut. Bull. 166, 10. doi: 10.1016/j.marpolbul.2021.112212
Chen Y. G., Zhang Y., Zhang Z. Z. (2021). Occurrence, effects, and biodegradation of plastic additives in sludge anaerobic digestion: A review. Environ. pollut. 287, 11. doi: 10.1016/j.envpol.2021.117568
Cho Y., Shim W. J., Jang M., Han G. M., Hong S. H. (2019). Abundance and characteristics of microplastics in market bivalves from South Korea. Environ. pollut. 245, 1107–1116. doi: 10.1016/j.envpol.2018.11.091
Covernton G. A., Cox K. D., Fleming W. L., Buirs B. M., Davies H. L., Juanes F., et al. (2022). Large size (>100-mu m) microplastics are not biomagnifying in coastal marine food webs of British Columbia, Canada. Ecol. Appl. 32 (7), 24. doi: 10.1002/eap.2654
Ding J. F., Jiang F. H., Li J. X., Wang Z. X., Sun C. J., Wang Z. Y., et al. (2019). Microplastics in the coral reef systems from xisha islands of south China sea. Environ. Sci. Technol. 53 (14), 8036–8046. doi: 10.1021/acs.est.9b01452
Ding R., Ouyang F., Peng D., You J., Ding L., Ouyang Z. Z., et al. (2022). A case study of distribution and characteristics of microplastics in surface water and sediments of the seas around Shenzhen, southern coastal area of China. Sci. Total Environ. 838, 9. doi: 10.1016/j.scitotenv.2022.156063
Duan J. H., Han J., Cheung S. G., Chong R. K. Y., Lo C. M., Lee F. W. F., et al. (2021). How mangrove plants affect microplastic distribution in sediments of coastal wetlands: Case study in Shenzhen Bay, South China. Sci. Total Environ. 767, 10. doi: 10.1016/j.scitotenv.2020.144695
Eng S. K., Pusparajah P., Ab Mutalib N. S., Ser H. L., Chan K. G., Lee L. H. (2015). Salmonella: A review on pathogenesis, epidemiology and antibiotic resistance. Front. Life Sci. 8 (3), 284–293. doi: 10.1080/21553769.2015.1051243
Evans P. N., Boyd J. A., Leu A. O., Woodcroft B., Parks D. H., Hugenholtz P., et al. (2019). An evolving view of methane metabolism in the Archaea. Nat. Rev. Microbiol. 17 (4), 219–232. doi: 10.1038/s41579-018-0136-7
Han F., Li X., Zhang M. R., Liu Z., Han Y. F., Li Q., et al. (2021). Solid-phase denitrification in high salinity and low-temperature wastewater treatment. Bioresource Technol. 341, 9. doi: 10.1016/j.biortech.2021.125801
Harrison J. P., Schratzberger M., Sapp M., Osborn A. M. (2014). Rapid bacterial colonization of low-density polyethylene microplastics in coastal sediment microcosms. BMC Microbiol. 14, 15. doi: 10.1186/s12866-014-0232-4
He C., Zhang B. G., Lu J. P., Qiu R. (2021). A newly discovered function of nitrate reductase in chemoautotrophic vanadate transformation by natural mackinawite in aquifer. Water Res. 189, 9. doi: 10.1016/j.watres.2020.116664
Horton A. A., Walton A., Spurgeon D. J., Lahive E., Svendsen C. (2017). Microplastics in freshwater and terrestrial environments: Evaluating the current understanding to identify the knowledge gaps and future research priorities. Sci. Total Environ. 586, 127–141. doi: 10.1016/j.scitotenv.2017.01.190
Hu H., Jin D. F., Yang Y. Y., Zhang J., Ma C. P., Qiu Z. M. (2021). Distinct profile of bacterial community and antibiotic resistance genes on microplastics in Ganjiang River at the watershed level. Environ. Res. 200, 10. doi: 10.1016/j.envres.2021.111363
Jiang S., Wang J., Wu F., Xu S., Liu J., Chen J. (2023). Extensive abundances and characteristics of microplastic pollution in the karst hyporheic zones of urban rivers. Sci. total Environ. 857 (Pt 3), 159616. doi: 10.1016/j.scitotenv.2022.159616
Khalid N., Aqeel M., NOman A., Khan S. M., Akhter N. (2021). Interactions and effects of microplastics with heavy metals in aquatic and terrestrial environments. Environ. pollut. 290, 13. doi: 10.1016/j.envpol.2021.118104
Kitahara K. I., Nakata H. (2020). Plastic additives as tracers of microplastic sources in Japanese road dusts. Sci. Total Environ. 736, 8. doi: 10.1016/j.scitotenv.2020.139694
Kong X., Jin D. C., Jin S. L., Wang Z. G., Yin H. Q., Xu M. Y., et al. (2018). Responses of bacterial community to dibutyl phthalate pollution in a soil- vegetable ecosystem. J. Hazardous Materials 353, 142–150. doi: 10.1016/j.jhazmat.2018.04.015
Kooi M., van Nes E. H., Scheffer M., Koelmans A. A. (2017). Ups and downs in the ocean: effects of biofouling on vertical transport of microplastics. Environ. Sci. Technol. 51 (14), 7963–7971. doi: 10.1021/acs.est.6b04702
Kubo K., Kojima H., Fukui M. (2014). Vertical distribution of major sulfate-reducing bacteria in a shallow eutrophic meromictic lake. Systematic Appl. Microbiol. 37 (7), 510–519. doi: 10.1016/j.syapm.2014.05.008
Lahens L., Strady E., Kieu-Le T. C., Dris R., Boukerma K., Rinnert E., et al. (2018). Macroplastic and microplastic contamination assessment of a tropical river (Saigon River, Vietnam) transversed by a developing megacity. Environ. pollut. 236, 661–671. doi: 10.1016/j.envpol.2018.02.005
Li C. C., Gan Y. D., Dong J. Y., Fang J. H., Chen H., Quan Q., et al. (2020). Impact of microplastics on microbial community in sediments of the Huangjinxia Reservoir-water source of a water diversion project in western China. Chemosphere 253, 12. doi: 10.1016/j.chemosphere.2020.126740
Li W. W., Khalid H., Zhu Z., Zhang R. H., Liu G. Q., Chen C., et al. (2018b). Methane production through anaerobic digestion: Participation and digestion characteristics of cellulose, hemicellulose and lignin. Appl. Energy 226, 1219–1228. doi: 10.1016/j.apenergy.2018.05.055
Li L., Li F. B., Deng M., Wu C. X., Zhao X. L., Song K., et al. (2022a). Microplastics distribution characteristics in typical inflow rivers of Taihu lake: Linking to nitrous oxide emission and microbial analysis. Water Res. 225, 11. doi: 10.1016/j.watres.2022.119117
Li W. L., Wang Z. C., Li W. P., Li Z. (2022b). Impacts of microplastics addition on sediment environmental properties, enzymatic activities and bacterial diversity. Chemosphere 307, 12. doi: 10.1016/j.chemosphere.2022.135836
Li M., Zhao L., Ma J. J., Zhao N., Luo J., Wang C. M., et al. (2018a). Vibrio vulnificus in aquariums is a novel threat to marine mammals and public health. Transboundary Emerging Dis. 65 (6), 1863–1871. doi: 10.1111/tbed.12967
Liu Y., Jiang W. Y., Liao Y. Y., Sun R. K., Hu J. L., Lu Z. F., et al. (2022b). Separation of false-positive microplastics and analysis of microplastics via a two-phase system combined with confocal Raman spectroscopy. J. Hazardous Materials 440, 11. doi: 10.1016/j.jhazmat.2022.129803
Liu X. Y., Liu H. T., Chen L., Wang X. H. (2022a). Ecological interception effect of mangroves on microplastics. J. Hazardous Materials 423, 8. doi: 10.1016/j.jhazmat.2021.127231
Ma C. L., Coffinet S., Lipp J. S., Hinrichs K. U., Zhang C. L. (2020). Marine group II euryarchaeota contribute to the archaeal lipid pool in northwestern pacific ocean surface waters. Front. Microbiol. 11. doi: 10.3389/fmicb.2020.01034
Mao Y. P., Xia Y., Wang Z. P., Zhang T. (2014). Reconstructing a Thauera genome from a hydrogenotrophic-denitrifying consortium using metagenomic sequence data. Appl. Microbiol. Biotechnol. 98 (15), 6885–6895. doi: 10.1007/s00253-014-5756-x
Marti E., Martin C., Galli M., Echevarria F., Duarte C. M., Cozar A. (2020). The Colors of the Ocean Plastics. Environmental Science & Technology 54 (11), 6594–6601. doi: 10.1021/acs.est.9b06400
McCormick A., Hoellein T. J., Mason S. A., Schluep J., Kelly J. J. (2014). Microplastic is an abundant and distinct microbial habitat in an urban river. Environ. Sci. Technol. 48 (20), 11863–11871. doi: 10.1021/es503610r
Ohtsubo S., Demizu K., Kohno S., Miura I., Ogawa T., Fukuda H. (1992). Comparison of acetate utilization among strains of an aceticlastic methanogen, Methanothrix soehngenii. Appl. Environ. Microbiol. 58 (2), 703–705. doi: 10.1128/aem.58.2.703-705.1992
Panno S. V., Kelly W. R., Scott J., Zheng W., McNeish R. E., Holm N., et al. (2019). Microplastic contamination in karst groundwater systems. Groundwater 57 (2), 189–196. doi: 10.1111/gwat.12862
Park S., Park J. M., Oh T. K., Yoon J. H. (2019). Altererythrobacter insulae sp. nov., a lipolytic bacterium isolated from a tidal flat. Int. J. Systematic Evolutionary Microbiol. 69 (4), 1009–1015. doi: 10.1099/ijsem.0.003260
Panno S. V., Kelly W. R., Scott J., Zheng W., McNeish R. E., Holm N., et al. (2019). Microplastic contamination in karst groundwater systems. Groundwater 57 (2), 189–196. doi: 10.1111/gwat.12862
Pojar I., Kochleus C., Dierkes G., Ehlers S. M., Reifferscheid G., Stock F., et al. (2021). Quantitative and qualitative evaluation of plastic particles in surface waters of the Western Black Sea. Environ Pollut 268 (10), 115724. doi: 10.1016/j.envpol.2020.115724
Qi K., Lu N., Zhang S. Q., Wang W. W., Wang Z. R., Guan J. N. (2021). Uptake of Pb(II) onto microplastic-associated biofilms in freshwater: Adsorption and combined toxicity in comparison to natural solid substrates. J. Hazardous Materials 411, 12. doi: 10.1016/j.jhazmat.2021.125115
Qian H. F., Zhang M., Liu G. F., Lu T., Qu Q., Du B. B., et al. (2018). Effects of soil residual plastic film on soil microbial community structure and fertility. Water Air Soil pollut. 229 (8), 11. doi: 10.1007/s11270-018-3916-9
Seeley M. E., Song B., Passie R., Hale R. C. (2020). Microplastics affect sedimentary microbial communities and nitrogen cycling. Nat. Commun. 11 (1), 10. doi: 10.1038/s41467-020-16235-3
Shao X., Cao H. Y., Zhao F., Peng M., Wang P., Li C. Y., et al. (2019). Mechanistic insight into 3-methylmercaptopropionate metabolism and kinetical regulation of demethylation pathway in marine dimethylsulfoniopropionate-catabolizing bacteria. Mol. Microbiol. 111 (4), 1057–1073. doi: 10.1111/mmi.14211
Talbot R., Chang H. J. (2022). Microplastics in freshwater: A global review of factors affecting spatial and temporal variations*. Environ. pollut. 292, 11. doi: 10.1016/j.envpol.2021.118393
Thompson R. C., Olsen Y., Mitchell R. P., Davis A., Rowland S. J., John A. W. G., et al. (2004). Lost at sea: Where is all the plastic? Science 304 (5672), 838–838. doi: 10.1126/science.1094559
Wang F., Wong C. S., Chen D., Lu X. W., Wang F., Zeng E. Y. (2018a). Interaction of toxic chemicals with microplastics: A critical review. Water Res. 139, 208–219. doi: 10.1016/j.watres.2018.04.003
Wang J., Wang M. X., Ru S. G., Liu X. S., et al. (2019). High levels of microplastic pollution in the sediments and benthic organisms of the South Yellow Sea, China. Science of the Total Environment 651, 1661–1669. doi: 10.1016/j.scitotenv.2018.10.007
Wang Z. G., You Y. M., Xu W. H., Chen W. J., Zeng J., Zhao X. S., et al. (2018b). Dimethyl phthalate altered the microbial metabolic pathways in a Mollisol. Eur. J. Soil Sci. 69 (3), 439–449. doi: 10.1111/ejss.12545
Wayman C., Niemann H. (2021). The fate of plastic in the ocean environment - a minireview. Environ. Science-Processes Impacts 23 (2), 16. doi: 10.1039/d0em00446d
Wu X. W., Liu P., Zhao X. L., Wang J. Y., Teng M. M., Gao S. X. (2022b). Critical effect of biodegradation on long-term microplastic weathering in sediment environments: A systematic review. J. Hazardous Materials 437, 13. doi: 10.1016/j.jhazmat.2022.129287
Wu C. C., Ma Y. J., Wang D., Shan Y. P., Song X. P., Hu H. Y., et al. (2022a). Integrated microbiology and metabolomics analysis reveal plastic mulch film residue affects soil microorganisms and their metabolic functions. J. Hazardous Materials 423, 12. doi: 10.1016/j.jhazmat.2021.127258
Wu P. F., Tang Y. Y., Dang M., Wang S. Q., Jin H. B., Liu Y. S., et al. (2020). Spatial-temporal distribution of microplastics in surface water and sediments of Maozhou River within Guangdong-Hong Kong-Macao Greater Bay Area. Sci. Total Environ. 717, 11. doi: 10.1016/j.scitotenv.2019.135187
Xu Y., Luo F., Chen S., He B. (2022). Study on the coordination between distribution of public service facilities and population characteristics in shenzhen metropolitan area. Urban Plann. Journal No. 273 (S1), 70–76. doi: 10.16361/j.upf.202207010
Yan M. Q., Yang J. T., Sun H. W., Liu C. G., Wang L. (2022). Occurrence and distribution of microplastics in sediments of a man-made lake receiving reclaimed water. Sci. Total Environ. 813, 9. doi: 10.1016/j.scitotenv.2021.152430
Yang L., Zhang Y. L., Kang S. C., Wang Z. Q., Wu C. X. (2021). Microplastics in freshwater sediment: A review on methods, occurrence, and sources. Sci. Total Environ. 754, 20. doi: 10.1016/j.scitotenv.2020.141948
Zhang R., Liu Y., Zhao X. F., Zhao Z. H., Zhang H. L., Huang X. P., et al. (2021). High-throughput sequencing reveals the spatial distribution variability of microbial community in coastal waters in Shenzhen. Ecotoxicology 30 (7), 1429–1436. doi: 10.1007/s10646-021-02391-9
Zhang Q., Xu E. G., Li J. N., Chen Q. Q., Ma L. P., Zeng E. Y., et al. (2020). A review of microplastics in table salt, drinking water, and air: direct human exposure. Environ. Sci. Technol. 54 (7), 3740–3751. doi: 10.1021/acs.est.9b04535
Zhong Z. (2022). Exploring the Path to Achieve Common Prosperity in Shenzhen. Master’s thesis (Communist Party of China Jilin Provincial Party School (Jilin Administrative Institute). doi: 10.27474/d.cnki.gzjld.2022.000037
Zhu F. X., Yan Y. Y., Doyle E., Zhu C. Y., Jin X., Chen Z. H., et al. (2022a). Microplastics altered soil microbiome and nitrogen cycling: The role of phthalate plasticizer. J. Hazardous Materials 427, 10. doi: 10.1016/j.jhazmat.2021.127944
Keywords: microplastics pollution, coastal waters, microbial community, function prediction, Shenzhen
Citation: Zhang R and Liu Y (2023) Multi-omics-based approach reveals the effects of microplastics on microbial abundance and function of sediments in Shenzhen coastal waters. Front. Mar. Sci. 10:1189876. doi: 10.3389/fmars.2023.1189876
Received: 20 March 2023; Accepted: 11 August 2023;
Published: 04 September 2023.
Edited by:
Xiaoshou Liu, Ocean University of China, ChinaReviewed by:
Xiao-Hua Zhang, Ocean University of China, ChinaWenzhe Xu, Tianjin University of Science and Technology, China
Copyright © 2023 Zhang and Liu. This is an open-access article distributed under the terms of the Creative Commons Attribution License (CC BY). The use, distribution or reproduction in other forums is permitted, provided the original author(s) and the copyright owner(s) are credited and that the original publication in this journal is cited, in accordance with accepted academic practice. No use, distribution or reproduction is permitted which does not comply with these terms.
*Correspondence: Yu Liu, 13692423536@163.com