- Department of Biology, Faculty of Marine Sciences, Instituto Universitario de Investigación en Estudios Ambientales y Recursos Naturales i-UNAT, Universidad de Las Palmas de Gran Canaria, Las Palmas, Spain
In this study, we monitored changes in cell wall sulfated galactans in the red seaweed Grateloupia imbricata after the rapid (48 h) induction of cystocarp maturation and carpospore development by the volatile hormone methyl jasmonate (MEJA). Synthesis of sulfated galactans, carrageenans, which requires sulfated UDP galactose, was followed by expression analysis of genes encoding phosphoglucomutase (PGM), galactose-1-phosphate uridyltransferase (GALT), and those responsible for sulfate assimilation (S-transporter and sulfate adenylyltransferase, SAT). In addition, the expression of carbohydrate sulfotransferase and galactose-6-sulfurylase responsible for the addition and removal of sulfate groups to the galactans backbone were evaluated. Structural changes, such as thallus softening, were analyzed using Fourier-transformed infrared spectroscopy (FTIR) spectra, and ploidy was assessed using flow cytometry. The results showed downregulation of most of the genes encoding precursors or those in charge of transformation into sulfated hexoses for cell wall synthesis. Furthermore, alterations in the FTIR spectrum of carrageenan from MEJA-induced fertile thalli, such as the disappearance and flattening of absorption bands, were observed. The promptness of these events and the ploidy of thalli and spores seem to confirm the induction of mitotic production of haploid spores by MEJA.
1 Introduction
The regulation and control of the life cycle of seaweeds have received considerable attention, particularly in cultivars of economic interest, which are affected by seasonality and abiotic factors such as light and temperature (Avila et al., 1999). Moreover, spore production, recruitment, and the population structure result from the fact that all the cycle phases are occasionally encountered in the field in the expected proportion or may even be absent (Santos and Duarte, 1996), have also been valued. This assessment involves the occurrence of heterosporous (with meiospores and mitospores) or even parthenogenetic (without male fecundation) specimens (Pereira and Ribeiro-Claro, 2016), although the conditions that induce their formation remain unknown.
Red seaweeds, such as the carrageenophytic red alga Grateloupia imbricata, deploy a three-phase life cycle (polysiphonia-type) with alternating sporophytic (2n) and gametophytic (n) phases and the carposporophytic (2n) phase, which is associated with the female gametophyte (Figure 1). The carposporophyte comprises carpospores clustered inside a structure with cover and exit pores, a set known as the cystocarp, which is formed after fecundation and the profound structural transformation of the female gametophyte at its spot localization over the thallus surface (Delivopoulos, 2003). In the case of G. imbricata, the diploid sporophyte is very rare in nature, and male gametophytes (or spermatangia in potential monoicous specimens) are absent or extremely ephemeral to sustain several dominant female populations that develop cystocarps regularly in several populations.
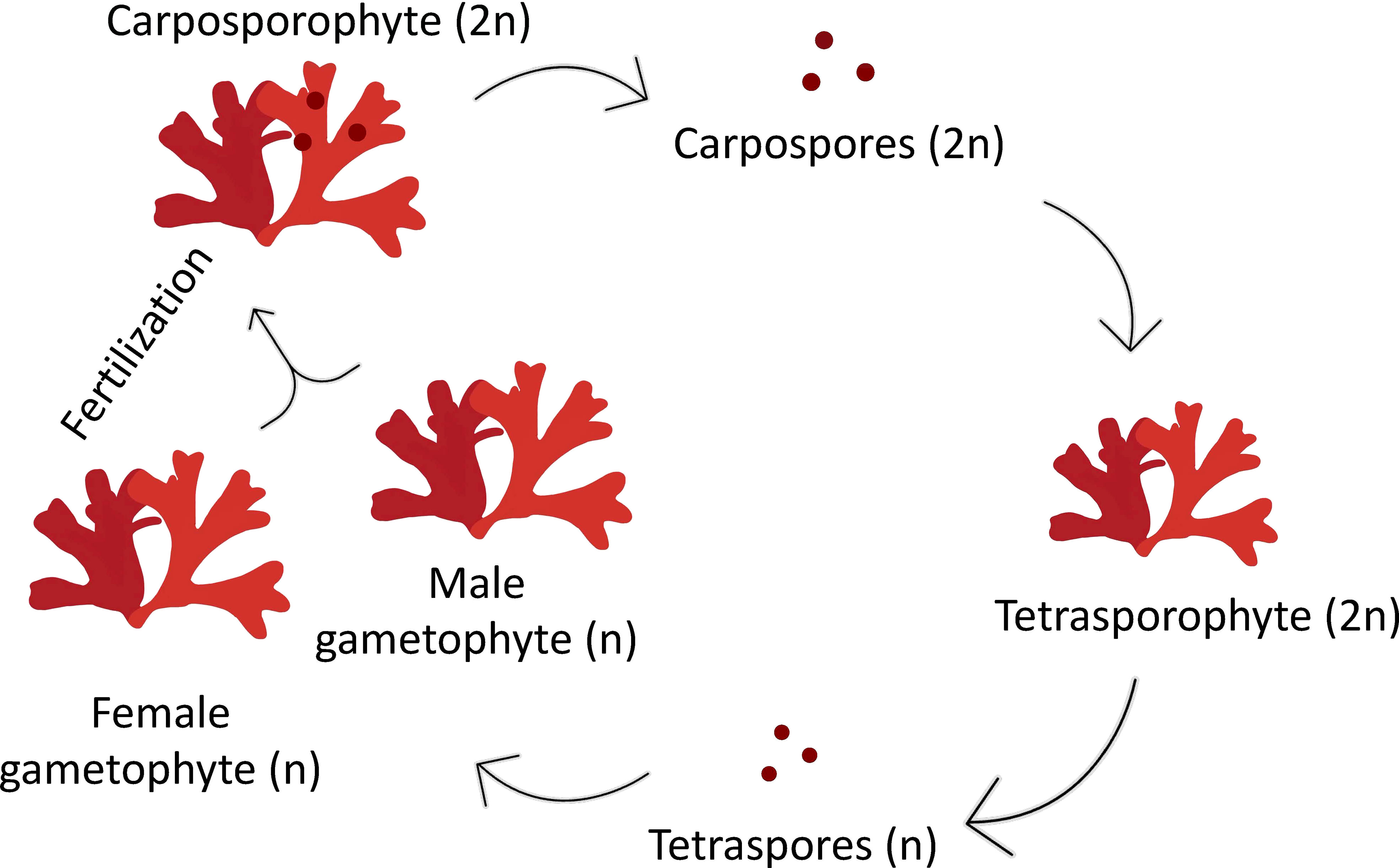
Figure 1 Diagram of a triphasic life cycle in the red alga Grateloupia imbricata comprising gametophytes (haploids, male and female), the carposporophyte, that develops on the female gametophyte after fertilization, and the sporophyte (diploid). Note that sporophyte, when mature produce haploid tetraspores, after meiosis, that are released, settled and grow into male or female gametophytes.
Together with life cycle stages and thalli development, several plant regulatory substances have been reported to be associated with carposporogenesis in red seaweeds. For example, the polyamine spermine and the corresponding enzymatic and genetic machinery induce the maturation of the cystocarp in the red seaweeds G. imbricata and Gracilaria cornea (Guzmán-Urióstegui et al., 2002; Sacramento et al., 2004; Sacramento et al., 2007; Garcia-Jimenez et al., 2016; Stirk and van Staden, 2014).
Two volatiles, ethylene and methyl jasmonate (MEJA), significantly caused drastic changes in the reproductive status of the red algae Pterocladiella capillacea and G. imbricata in the time periods of 7 d for ethylene and 2 d for MEJA. Furthermore, evidence has shown that ethylene induces the maturation of tetrasporangia in the sporophyte phase (2n) of P. capillacea (Garcia-Jimenez and Robaina, 2012) and of cystocarps of G. imbricata (Garcia-Jimenez and Robaina, 2017), which undoubtedly affect thallus softening and location of reproductive structures, and hence the synthesis of sulfated galactans.
Sulfated galactans show different degrees of sulfation and molecular conformation, rendering three main types of carrageenans (κ-, ι-, and λ types). These types of carrageenans are recognized through the analysis of FTIR spectra, whose absorption bands are characteristic of the different functional groups of the galactan backbone (Garcia-Tasende et al., 2013). Carrageenans from Grateloupia sp. have been identified with a preference for κ- carrageenan and ι- to a lesser extent. As previously reported in naturally collected samples, the expression of genes that encode the addition (sulfotransferases) and removal (sulfurylases) of sulfate groups to the galactan backbone has been associated with alterations in the thallus development of G. imbricata such as thallus softening and subsequent reconstitution of the cell wall after disclosure and maturation of cystocarps, and even early release of spores (Garcia-Jimenez et al., 2020). Likewise, other gene expressions for carrageenan biosynthesis are also related to changes in development stages of G. imbricata (Garcia-Jimenez et al., 2020), and to the life cycle phases of the red seaweed Chondrus crispus (tetrasporophyte and gametophyte stages; Lipinska et al., 2020).
The synthesis of cell wall sulfated galactans requires S-transporters, activation of the S-source (SAT), and available precursors. The main precursor, sulfated UDP-galactose, comes from the phosphate-hexose pool. UDP-galactose is obtained from galactose-1-phosphate using galactose-1-phosphate uridyltransferase (GALT). UDP glucose is derived from glucose-1-phosphate, which is converted from glucose-6-phosphate by phosphoglucomutase (PGM) (Oesterhelt et al., 1996; Ciancia et al., 2020). Moreover, sulfation of hexoses may occur through different transporters and forms of inorganic (oxidized form such as SO4 2-) and organic (reduced form such as methionine) sulfur, as these S-forms are also products of the assimilation pathways (del Rosario-Santana et al., 2022). Additionally, S-adenosylmethionine (SAM) is a pivotal compound for ethylene synthesis besides a precursor of the sulfur-containing amino acid methionine and a donor of methyl groups for methyl jasmonate synthesis from acid jasmonic (McQueney et al., 2000). Gene expressions related to carrageenan synthesis in ethylene-induced fertile thalli of G. imbricata were affected by transport machinery and assimilation of different S-sources as the synthesis of cell-wall sulfated galactans proceeds through sulfated-UDP galactose units (del Rosario-Santana et al., 2022). MEJA provokes rapid (48 h) thallus softening, a reduction in the maturation period, and the dehiscence of cystocarps (Garcia-Jimenez et al., 2016; Garcia-Jimenez et al., 2017, Garcia-Jimenez and Robaina, 2019, Montero-Fernández et al., 2016); however, its interaction with the synthesis of sulfated galactans is unknown.
This study aimed to 1) determine the expression of key genes encoding S-transport (S-transport), S-activation (SAT), and galactan precursor synthesis (PGM and GALT). The addition of sulfate groups through two sequences of two sulfotransferases (ST1 and ST2) and removal using two sequences of two gene sulfurylases (SYI and SYII) were also followed; 2) establish if reduced and oxidized S-forms affect galactan synthesis; 3) analyze spectroscopic traits of sulfated galactans to infer structural changes associated with MEJA that induce a rather rapid (48 h) cystocarp maturation; and 4) finally connect with the ploidy of the carpospores, which contributes to discerning whether maturation eventually occurs in a short time for spore release.
2 Materials and methods
2.1 Plant material and culture conditions
Infertile thalli from the carrageenophytic G. imbricata were collected along the northeastern coast of Gran Canaria on the Canary Islands. Thalli were placed in 500 mL vessels (3 g per vessel) and cultivated separately with a reduced source of S, such as methionine (10 mM), and others oxidized as magnesium sulfate (1.6 mM) for 3 d. Cystocarp development was elicited by spraying three times with a solution of 100 μM MEJA (Sigma Co., St. Louis, MO, USA) in 0.01% (v/v) ethanol in autoclaved seawater solution (Garcia-Jimenez et al., 2016) and then kept for 1 h under a light source of 50 μmol photons μm-2 s-1. Thalli were cultivated for 48 h (MEJA-induced fertile thalli) (Figure 2). Thalli were maintained at 20 ± 2°C under an 18 h light (50 μmol photons m-2 s-1): 6 h dark photoperiod in a growth chamber. Thallus development from infertile (i.e. not showing cystocarps) to induced-fertile thalli (i.e. with light red dots indicative of cystocarps) was verified through the expression of ornithine decarboxylase (ODC) as a reproduction marker gene in red seaweeds (Garcia-Jimenez et al., 2017; Garcia-Jimenez et al., 2018, Montero-Fernández et al., 2016). The ODC gene expression was always downregulated in fertile thalli (2.45 ± 0.15 × 10-2 copies μL-1 in infertile thalli and 0.99 ± 0.05 × 10-2 copies μL-1 in fertile thalli).
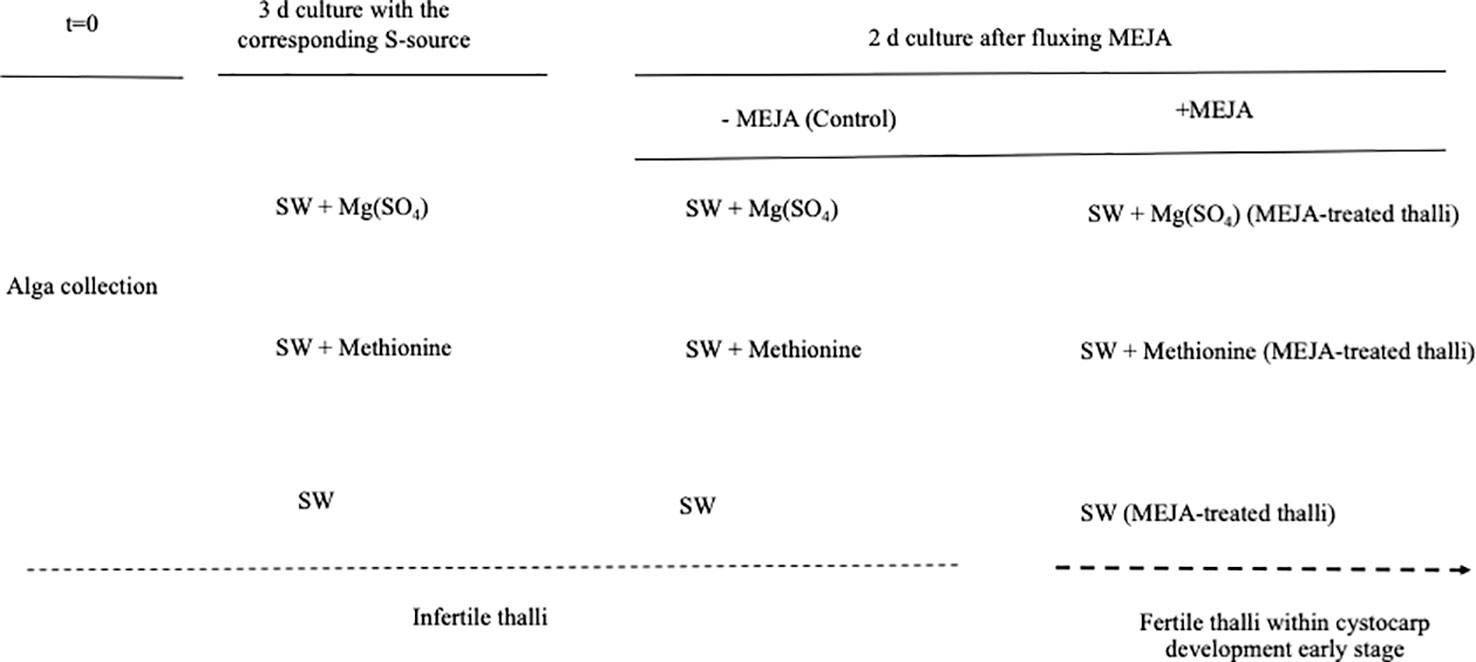
Figure 2 Scheme showing timeline for determination of gene expression in Grateloupia imbricata. Infertile thalli (not showing cystocarps) were cultured in presence of methionine and MgSO4 for 3 days, and thalli treated with methyljasmonate (MEJA) elicited early stages of cystocarp development (fertile thalli). Dotted line indicates transition from infertile thalli (without cystocarps) to fertile thalli (within cystocarps). Note that end time was 5 days and different controls are indicated.
2.2 Effect of S-source and MEJA on the synthesis of cell-wall sulfated galactans in G. imbricata
The synthesis of cell-wall-sulfated galactans was analyzed in infertile and MEJA-induced fertile thalli cultivated separately in the presence of one of the S sources detailed above. Gene expression of the S-transporter (S-transport) and S-activation (sulfate adenylyltransferase; SAT) was determined (Figure 3). In addition, genes encoding phosphoglucomutase (PGM) and galactose-1-phosphate uridyltransferase (GALT) were also considered precursors of galactan synthesis. Similarly, two contigs of carbohydrate sulfotransferase (ST1 and ST2), two contigs annotated as galactose-6-sulfurylase type I (SYI.1 and SYI.2), and two contigs of galactose- 6-sulfurylase type II (SYII.1 and SYII. 2) were examined (Figure 3).
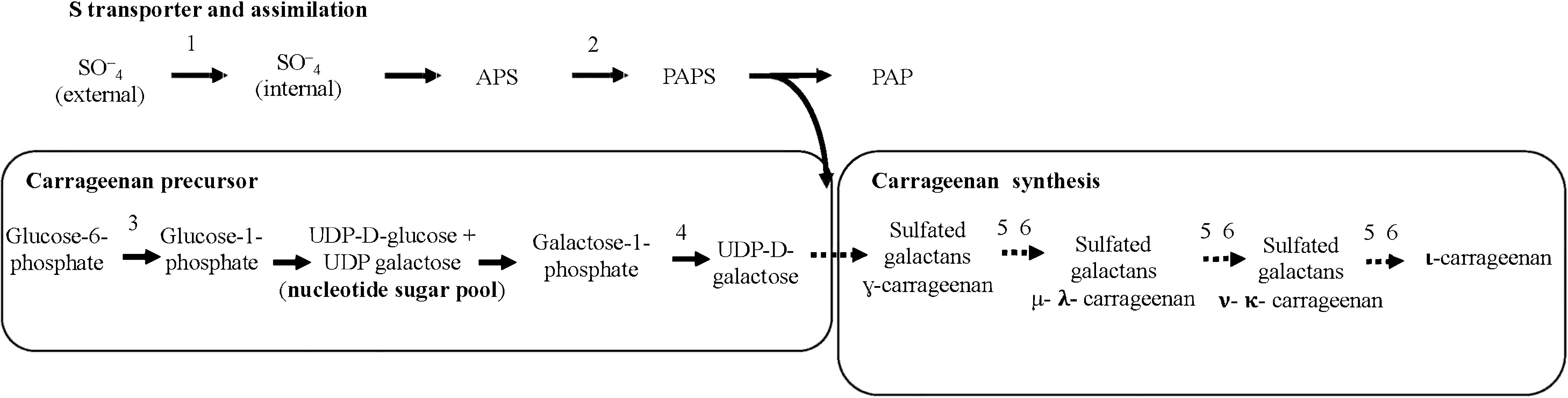
Figure 3 Schematic biosynthetic pathway for sulfate assimilation and synthesis of carrageenan. PAPS, 3′phosphoadenosine-5′phosphosulfate; APS, adenosine 5´-phosphosulfate; S transporter (S-transport); 2, sulfate adenylyltransferase (SAT); 3, phosphoglucomutase (PGM); 4, galactose 1 phosphate uridyltransferase (GALT); 5, carbohydrate sulfotransferase (ST); 6, galactose-6-sulfurylase (SY), I and II.
Untreated (control) samples were cultured and processed in parallel. Field controls were analyzed at 0, 3, and 5 d. All samples were assayed in triplicate with two independent replicates for each experiment. At the end of this period, samples of the thalli were frozen at 80°C until RNA was isolated.
2.3 RNA extraction
Total RNA was separately extracted from the upper half (100 mg) of the thalli using 1 mL TRIzol reagent (Sigma, St. Louis, MO, USA) according to the manufacturer’s instructions. The isolated RNA samples were individually suspended in 20 μL of 1 M Tris-HCl (pH 8), 0.5 M EDTA, and treated with DNase (1 Umg-1, Promega, Madison, WI, USA) to destroy contaminating DNA. Total RNA was quantified using a TrayCell cuvette and Beckman Coulter DU 530 spectrophotometer. Next, the RNA extracted from each sample (approximately 1 μg) was reverse transcribed in the presence of oligo (dT) and primers with randomly generated sequences from an iScript cDNA synthesis kit (Bio-Rad, Hercules, CA, USA). The reverse transcription procedure was performed at 25°C for 5 min, 42°C for 30 min, and 85°C for 5 min. The integrity of cDNA was validated using a NanoDrop spectrophotometer (Thermo Fisher Scientific, Waltham, MA, USA). The products were stored at 4°C until used.
2.4 Droplet digital PCR primers and protocol implementation
For the quantification of each target transcript by ddPCR, QX200 ddPCR EvaGreen Supermix (Bio-Rad) was used according to the manufacturer’s instructions. Briefly, for each sample, a PCR mix (final volume, 20 μL) was prepared to contain 1.5 μL of cDNA, 10 μL of QX200 ddPCR Eva Green Supermix, and 0.22 μL of each primer (10 μM), and then loaded into a cartridge. An oil droplet (70 μL) was loaded into each cartridge and covered with a gasket. Each cartridge was introduced individually into the droplet generator, and approximately 40 μL were transferred to the amplification plate. For each gene, three replicates were analyzed for each MEJA-treated and non-treated sample (control). Primers for ddPCR were designed based on the cDNA sequences of the G. imbricata transcriptome (Table 1). PCR amplification was performed with a C1000 Touch Thermal Cycler (Bio-Rad) using the following conditions: an initial step at 95°C for 5 min, followed by 40 cycles of 95°C for 30 s, an experimentally determined annealing temperature for each gene for 1 min, 72°C for 45 s; a single step at 4°C for 5 min; and a temperature ramping from 4°C to 90°C at a rate of 2°C s-1 for 5 min. After amplification, each sample was quantified using the QuantaSoft v1.7.4 software (Bio-Rad). Data from the merged wells (corresponding to each group of replicates) were retrieved, and the concentration of each group was calculated as the average number of transcript copies per μL.
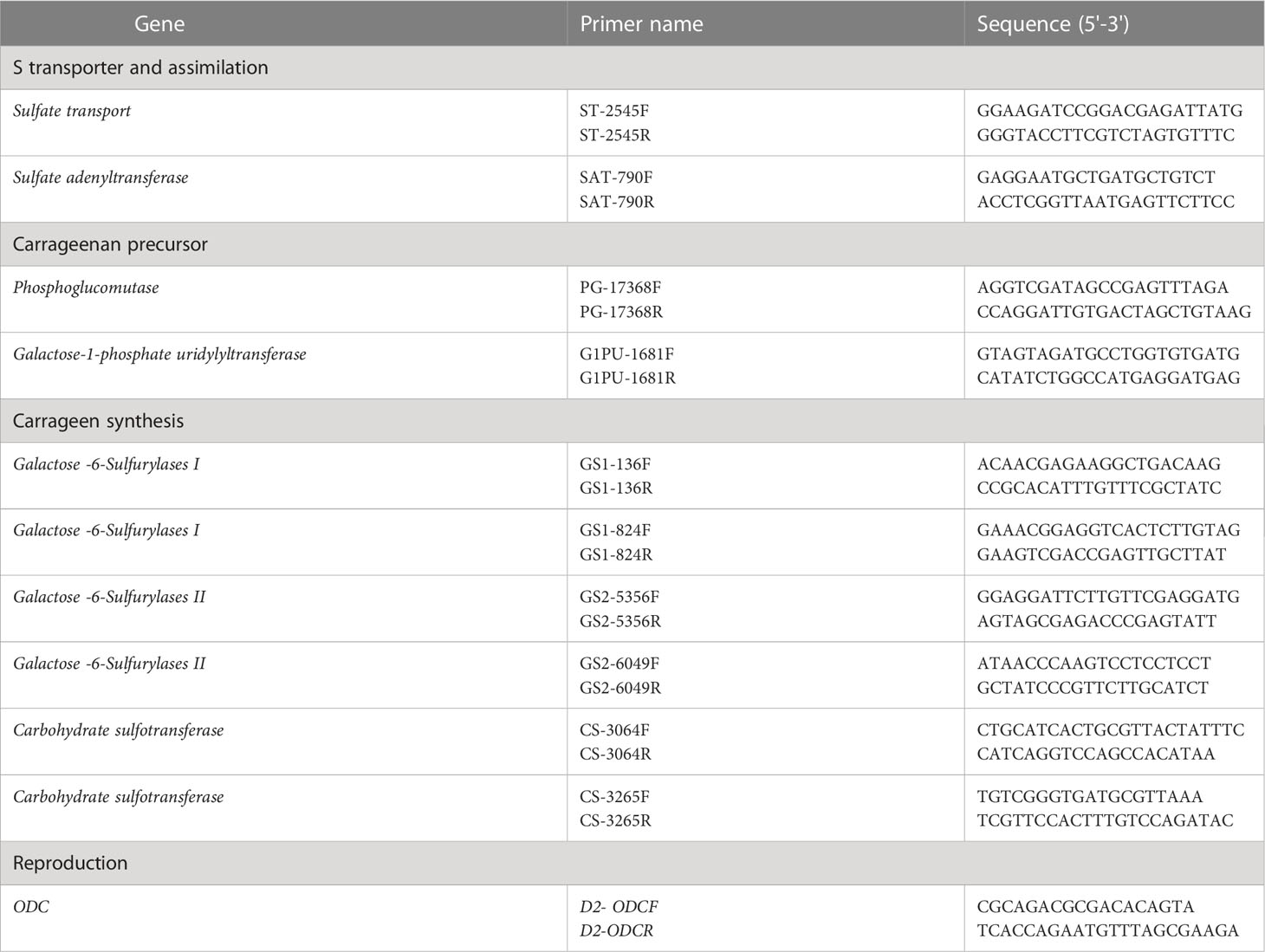
Table 1 Sequences of the forward (F) and reverse (R) primers for each gene involved in S-transport and assimilation (sulfate transport, sulfate adenyltransferase),as carrageenan precursor (phosphoglucomutase, galactose-1-phosphate uridylyltransferase), in carrageenan synthesis (Galactose-6-Sulfurylases I and II, Carbohydrate sulfotransferase), and marker gene of reproduction in red seaweeds (ODC).
2.5 Evaluation of sulfated galactans (carrageenans) of G. imbricata through the Fourier-transformed infrared spectroscopy
To extract sulfated galactans, a modified procedure from Imjongjairak et al. (2016) was used. Briefly, milled seaweed (3.0 g) was successively extracted with distilled water (50 mL) at 53°C for 16 h under constant mechanical stirring. Centrifugation was performed at 3300 × g for 10 min. Then, the supernatant was collected and pelleted by adding three volumes of 99.5% ethanol (v/v). The pellet was stored at 4°C overnight, and then, the precipitate was re-dissolved in distilled water.
To analyze the galactans qualitatively, 10 mg of the precipitate was mixed well with 150 mg of potassium bromide powder (Sigma). A force of approximately 3 ton cm-2 was then applied for several minutes. The measurements were performed immediately using an RS/1 FTIR spectrometer (UNICAM). Spectra were taken in intervals from 2000 to 400 cm-1 with a resolution of 2 cm-1.
Sulfated galactans from infertile, fertile, and MEJA-induced fertile thalli from G. imbricata were assayed. As a reference, samples from ethylene-induced fertile thalli of G. imbricata and commercial carrageenan (Sigma Commercial Grade, Type 1) were also evaluated. All samples were assayed in duplicate for each type.
2.6 Ploidy in collected gametophytic thalli, in vitro released spores and spores from MEJA-induced fertile thalli
Ploidy was analyzed in naturally collected gametophytic thalli, in spontaneously in vitro released spores from carposporophyte thalli, and spores released from MEJA-treated thalli. MEJA treatment occurred as previously described. However, unlike the spontaneously released spores that took 1 month, MEJA spores were released after 48 h. Ploidy determination was performed using flow cytometry (MACSQuant® Analyzer 10). For the extraction of the nuclei, samples were crushed, and 500 µL of the LB01 buffer (15 mM Tris, 2 mM Na2EDTA, 0.5 mM spermine tetrahydro chloride, 80 mM KCl, 20 mM NaCl, and 0.1% (v/v) Triton X-100) was added at pH 7.5 adjusted with 1 M NaOH. Then, the slurry was filtered through a 0.22 µm nylon mesh, and 4′,6-diamidine-2′-phenylindole dihydrochloride (DAPI, Sigma) was added at 0.01 µg µL-1 final concentration. Flow cytometry data were acquired and analyzed according to the manufacturer’s instructions.
2.7 Data analysis
Gene expression (transcript copies × μL) is reported as mean ± standard deviation (SD). Statistical comparisons of concentrations were performed using R software (https://www.r-project.org; accessed on May 20, 2022). A one-way ANOVA followed by the post hoc tests Tukey HSD and Dunnett T3 was used to detect significant differences (p ≤ 0.01) between genes, each gene and their respective controls, and the same gene between the treatment of methionine and SO4.
3 Results
3.1 Effect of S-source and MEJA on the synthesis of cell-wall sulfated galactans in G. imbricata
Two general observations were made regarding S-sources and MEJA as a plant volatile growth regulator, together with sulfated galactan synthesis, carrageenan. The first S-source altered the expression of genes responsible for S transport, assimilation, and sulfated-galactan synthesis precursors. Genes encoding S-transport and SAT were upregulated in the presence of a reduced source of S (methionine), whereas in the presence of SO4, the expression was downregulated compared to methionine. However, non-significant differences were observed compared to the control (Figure 4A). Furthermore, PGM and GALT expression seemed to be mainly affected by methionine, as the transcript numbers were significantly different from those in the sulfate and control groups (Figure 4B).
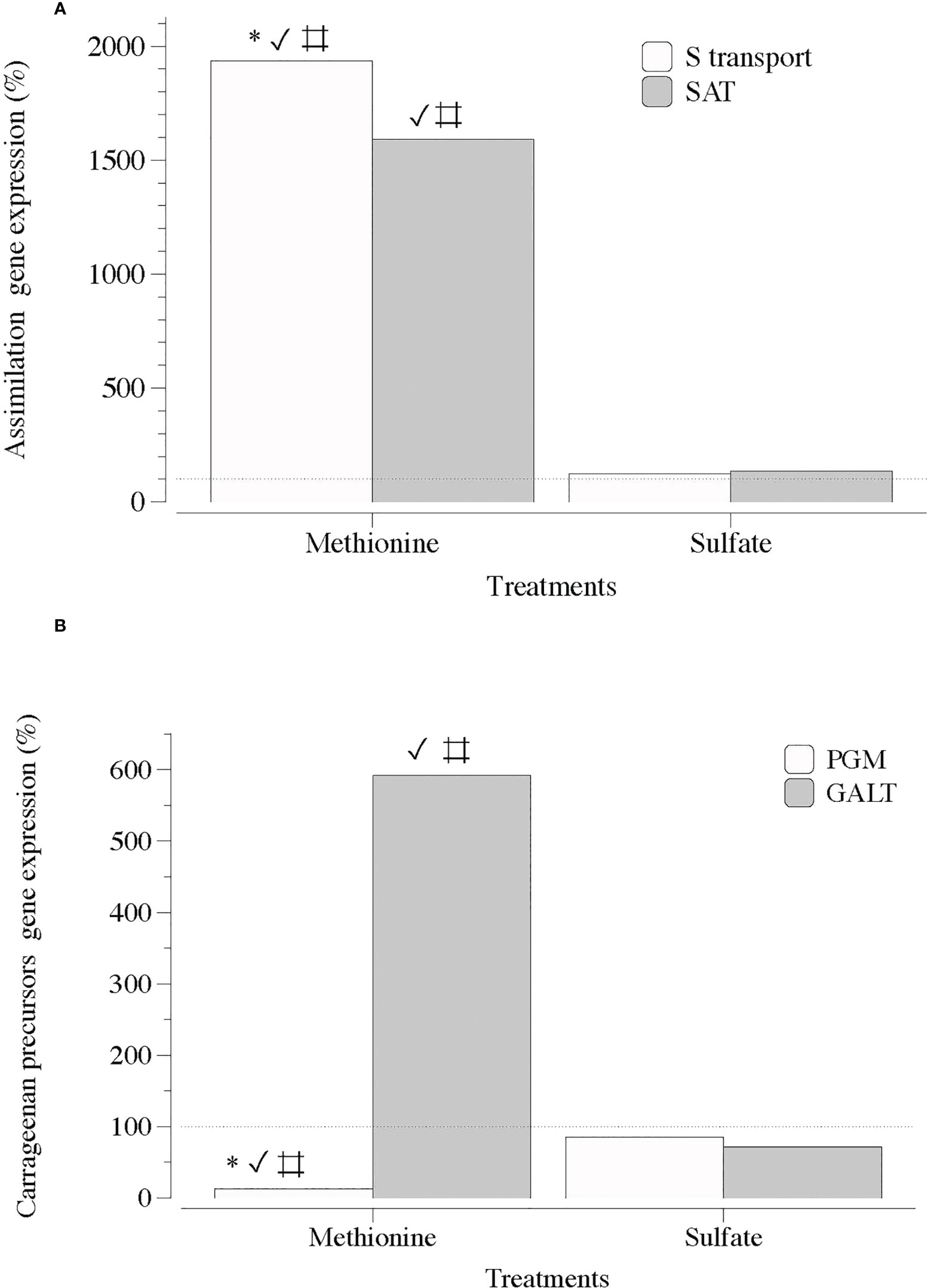
Figure 4 Expression of genes that encode S-transporter and activation (S-transport and SAT) and precursor of carrageenan synthesis (PGM and GALT) in thalli of Grateloupia imbricata cultured separately in exogenous methionine and in MgSO4 for 3 days. (A) Genes encoding a sulfate transporter (S-transport) and sulfate adenylyltransferase (SAT) (B) Genes encoding phosphoglucomutase (PGM) and galactose 1 phosphate uridyltransferase (GALT). Expressions (copies μL-1) are shown as a percentage relative to expression in untreated thalli at day 3 (100%, dashed horizontal line). Untreated thalli gene expression (i.e. 100%) were S-transport = 88 ± 10-3, SAT = 66 ± 3.10 × 10-3, PGM = 130 ± 4 × 10-3 and GALT = 112 ± 2.50 × 10-3. * means significant difference (p < 0.01) between S transport and SAT and between PGM and GALT; # is difference between each gene with its control (without treatment), and ✓ shows differences for the same gene between treatment of methionine and SO4.
In contrast, two sequences of genes encoding carbohydrate sulfotransferases (ST1 and ST2) responsible for the addition of sulfate groups exhibited a significant transcript number in the presence of SO4 (Figure 5). Transcripts of galactose-6-sulfurylase (SY), which are responsible for the removal of sulfate groups, showed a non-defined pattern regardless of the sequence of contigs (1 and 2) and type of SY (SYI and SYII; Figures 6A, B).
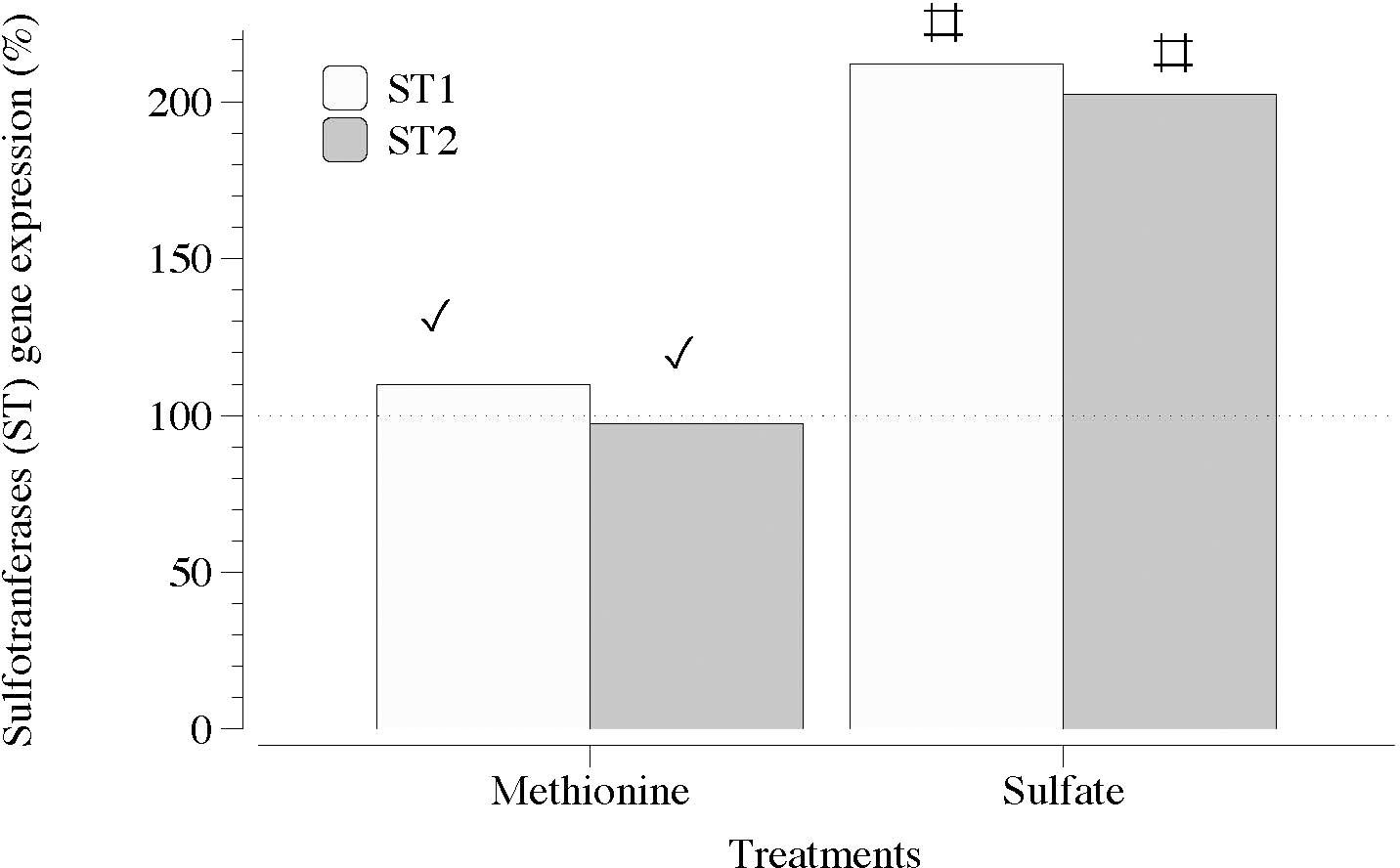
Figure 5 Expression of genes that encode carbohydrate sulfotransferase (ST1 and ST2) in thalli of Grateloupia imbricata cultured separately in exogenous methionine and in MgSO4 for 3 days. Expressions (copies μL-1) are shown as a percentage relative to expression in untreated thalli at day 3 (100%, dashed horizontal line). Untreated thalli gene expression (i.e., 100%) were ST1 = 50 ± 2.7 × 10-3 and ST2 = 78 ± 0.50 × 10-3. # means significant difference (p < 0.01) between each gene and its control (without treatment), and ✓ shows differences for the same gene between treatment of methionine and SO4.
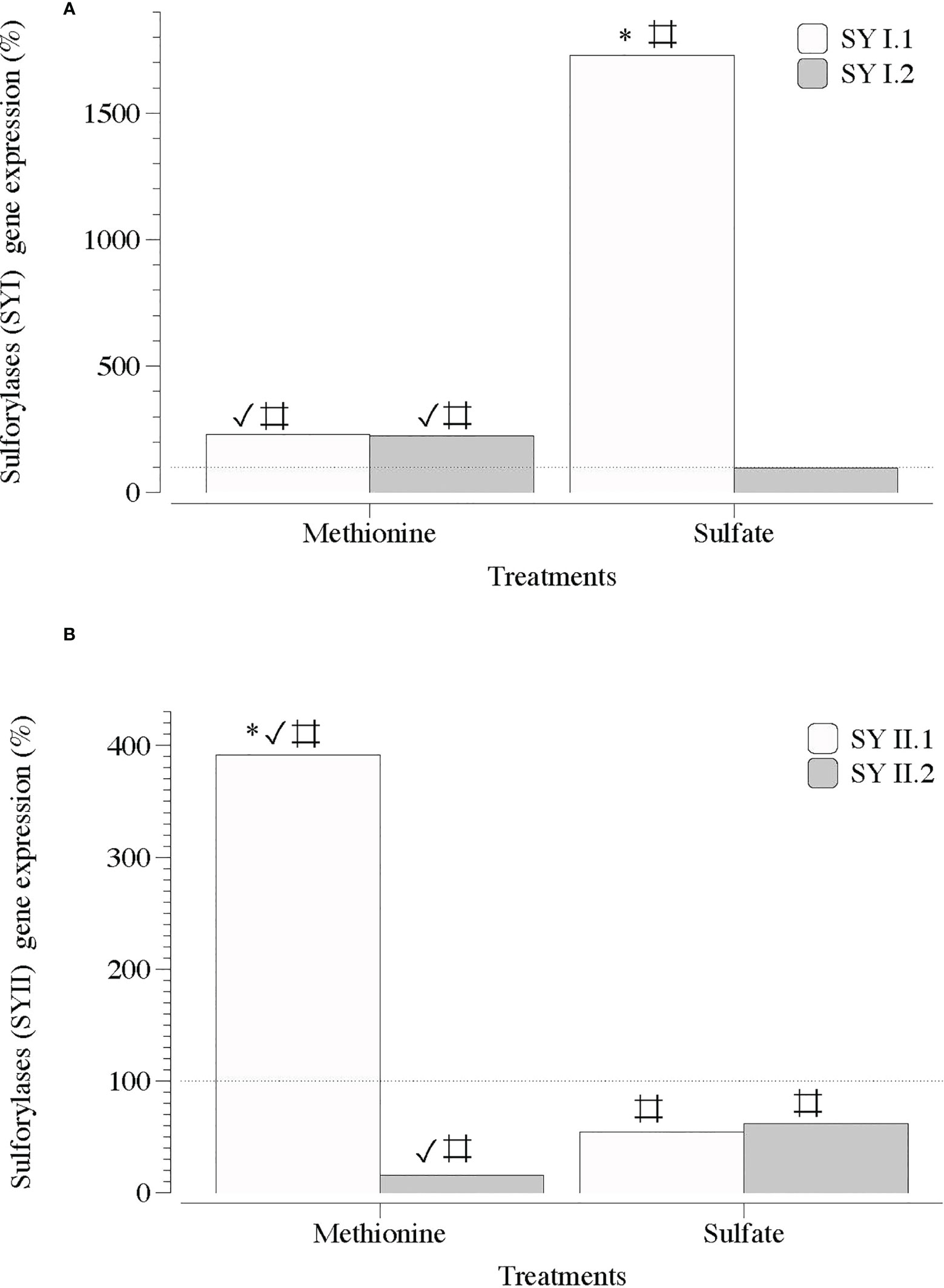
Figure 6 Expression of genes that encode galactose 6 sulfurylase (SYI and SYII) in infertile thalli of Grateloupia imbricata cultured separately in exogenous methionine and in MgSO4 for 3days. Expressions (copies μL-1) are shown as a percentage relative to expression in untreated thalli at day 3 (100%, dashed horizontal line). Untreated thalli gene expression (i.e., 100%) were for SYI.1 and SYI.2 = 231.5 ± 3.2 × 10-3 and for SYII.1 and SYII.2 = 225 ± 2.8 × 10-3. * means significant difference (p < 0.01) between SY1.1 and SYI.2 and between SYI1.1 and SYII.2. # is difference between each gene and its control (without treatment), and ✓ shows differences for the same gene between treatment of methionine and SO4.
Second, MEJA downregulated the expression of genes encoding S-transport, S-assimilation, precursors, and galactan synthesis. Thus, the expression of genes involved in the assimilation processes of S, such as S-transport and SAT, was completely downregulated in the presence of MEJA, regardless of the S source, that is, methionine or SO4 (Figure 7A). In addition, the expression of genes encoding precursors for the synthesis of cell-wall galactans was differentially altered if methionine and SO4 were present (Figure 7B).
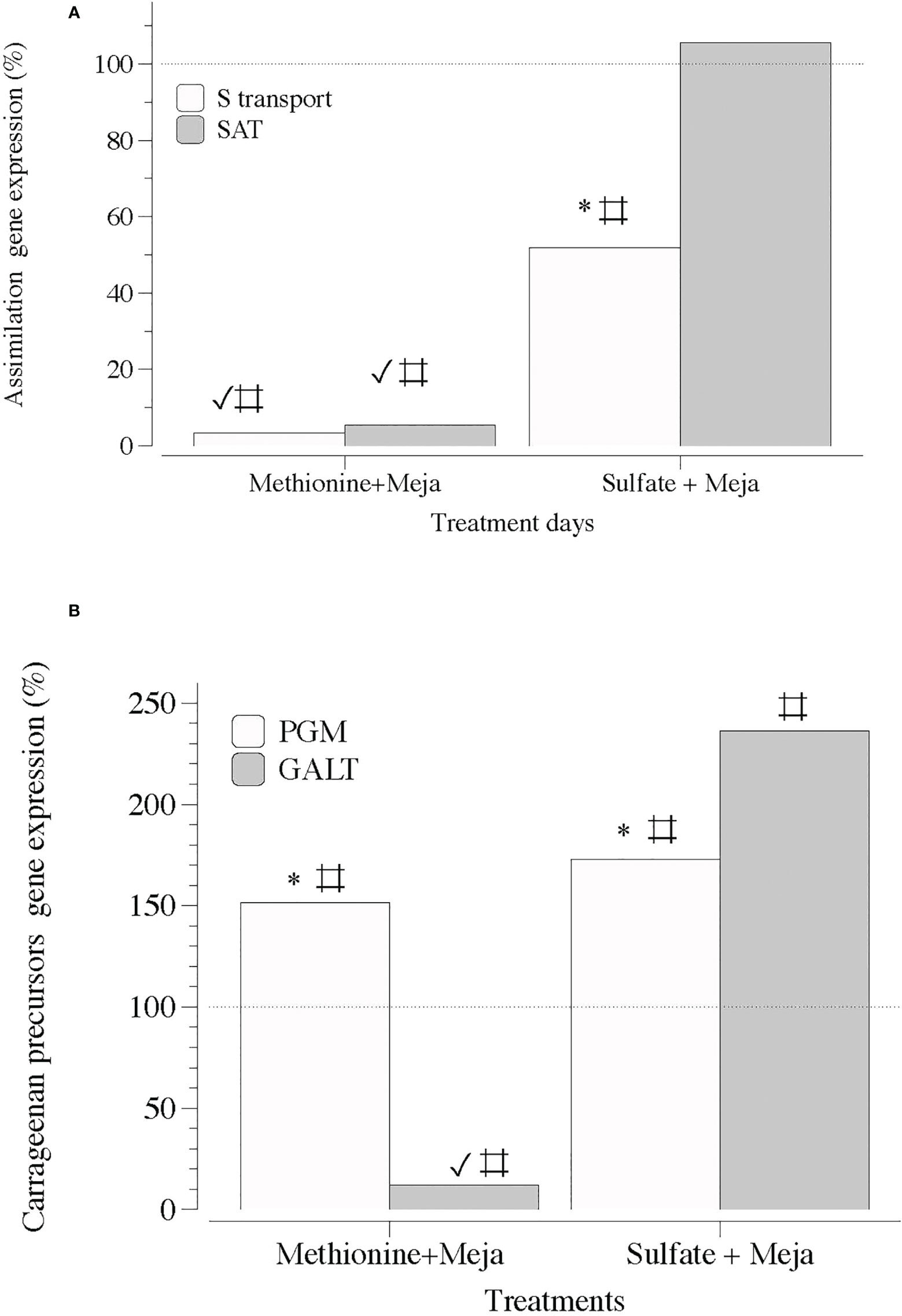
Figure 7 Expression of genes that encode S-transporter and activation (S-transport and SAT) and precursor synthesis of sulfated polysaccharides (PGM and GALT) at day 5 in thalli of Grateloupia imbricata cultured separately in exogenous methionine and in MgSO4 for 3 days plus 2 days in methyljasmonate (S-source plus MEJA). (A) Genes encoding a sulfate transporter (S-transport) and sulfate adenylyltransferase (SAT) (B) Genes encoding phosphoglucomutase (PGM) and galactose 1 phosphate uridyltransferase (GALT). Expressions (copies μL-1) are shown as a percentage relative to expression in treated thalli at day 3 for methionine and MgSO4 respectively (100%, dashed horizontal line). For methionine, thalli gene expression (i.e., 100%), S-transport = 1704 ± 0.33; SAT = 1050 ± 0.63; PGM = 16.5 ± 0.53 and GALT= 663 ± 8. 7 × 10-2. For MgSO4, S-transport = 110 ± 10-3; SAT = 89 ± 9.5 × 10-4; PGM = 111 ± 3.1 × 10-3 and GALT = 80 ± 12 × 10-3. * means significant difference (p < 0.01) between S-transport and SAT and between PGM and GALT at day 5. # is difference between each gene and its control (thalli without treatment). ✓ shows differences for the same gene between treatment of MEJA + methionine and MEJA + SO4.
Notably, the transcript numbers for the two sequences of genes encoding the addition of sulfate groups to the galactan backbone, ST1 and ST2, were considerably lower in the presence of MEJA (Figure 8). MEJA also altered the expression of the two contigs of each type of sulfurylase (SYI and SYII) without a consistent pattern (Figures 9A, B).
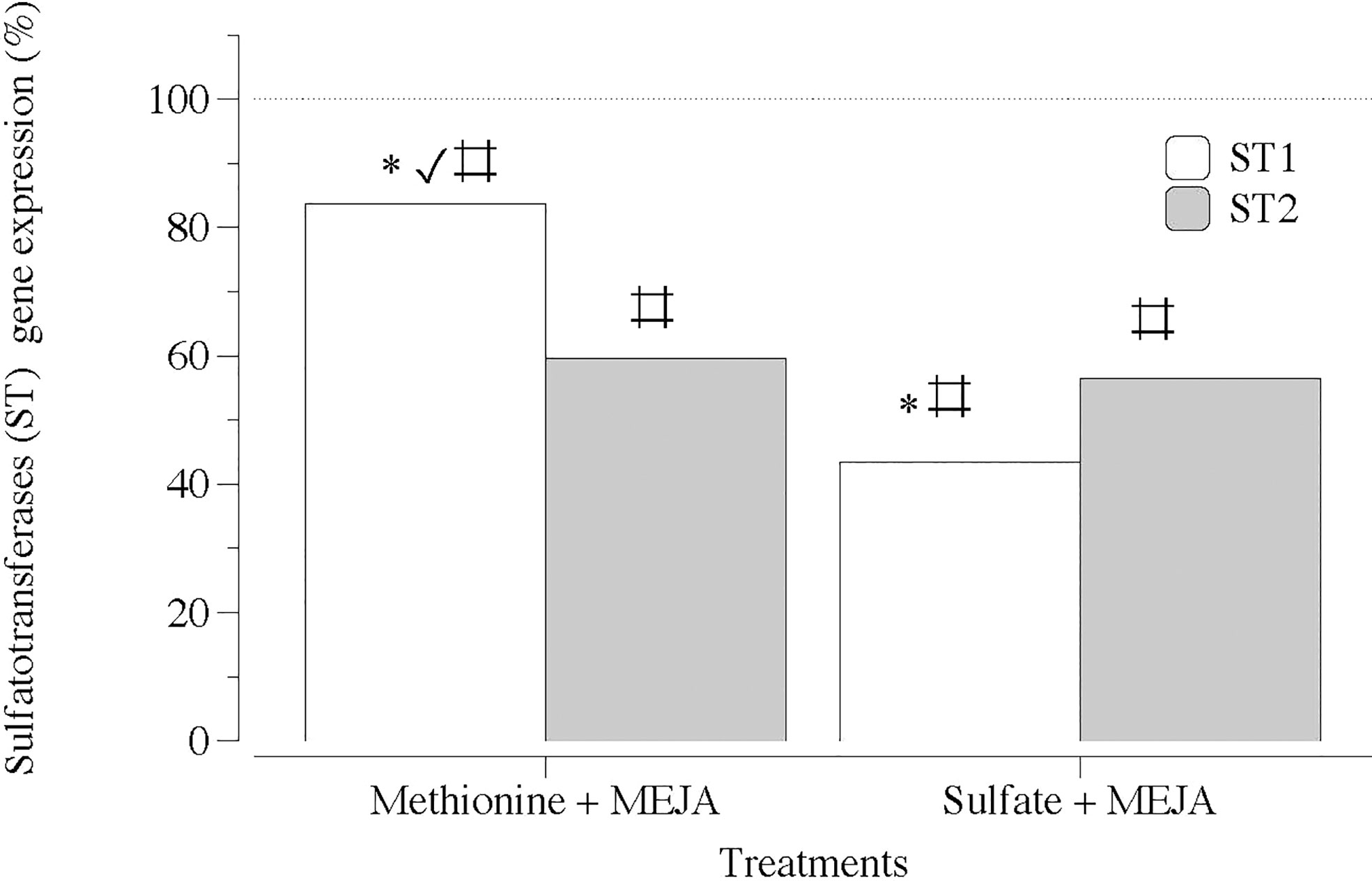
Figure 8 Expression of genes that encode carbohydrate sulfotransferase (ST1 and ST2) at day 5 in thalli of Grateloupia imbricata cultured separately in exogenous methionine and in MgSO4 for 3 days plus 2 days in methyljasmonate (S-source plus MEJA). Expressions (copies μL-1) are shown as a percentage relative to expression in treated thalli at day 3 for methionine and for MgSO4, respectively (100%, dashed horizontal line). For methionine, thalli gene expression (i.e. 100%), ST1 = 55 ± 0.43 and ST2 = 75.9 ± 11.2 × 10-2. For MgSO4, ST1 = 106 ± 2.94 × 10-3 and ST2 = 158 ± 3.2 × 10-3 * means significant difference (p < 0.01) between ST1 and ST2 at day 5. # is difference between each gene and its control (thalli without treatment). ✓ shows differences for the same gene between treatment of MEJA + methionine and MEJA + SO4.
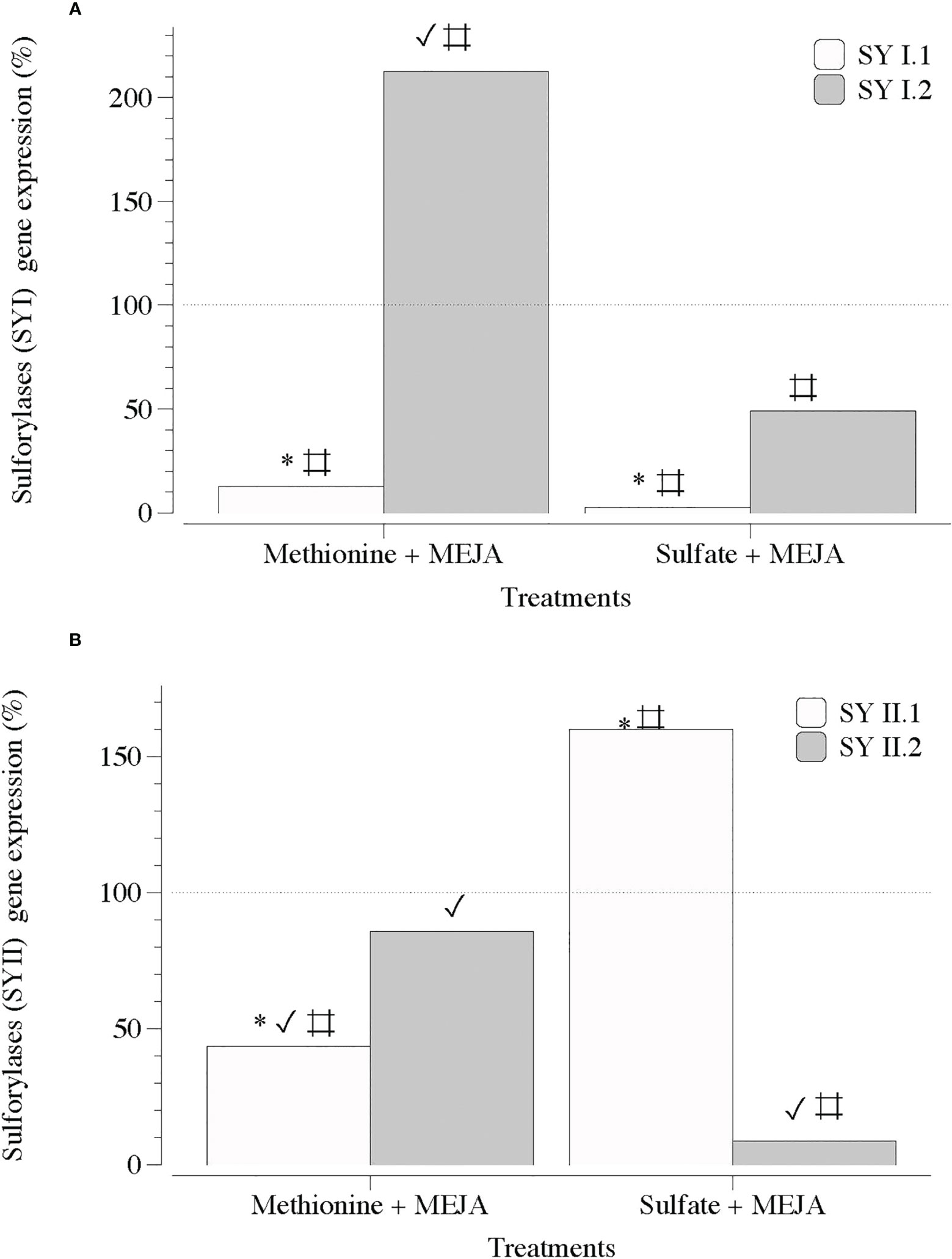
Figure 9 Expression of genes that encode galactose 6 sulfurylase at day 5 in thalli of Grateloupia imbricata cultured separately in exogenous methionine and in MgSO4 for 3 days plus 2 days in methyljasmonate (S-source plus MEJA). (A) Genes encoding galactose 6 sulfurylase (SYI.1 and SYI.2). (B). Genes encoding galactose 6 sulfurylase (SYII.1 and SYII.2). Expressions (copies μL-1) are shown as a percentage relative to expression in treated thalli at day 3 for methionine and for MgSO4, respectively (100%, dashed horizontal line). For methionine, thalli gene expression (i.e., 100%), SYI.1 = 531.99 ± 4.6 × 10-3; SYI.2 = 520.56 ± 9.6 × 10-2; SYII.1 = 880.43 ± 6.1 × 10-4 and SYII.2 = 33.99 ± 11.6 × 10-5. For MgSO4, SYI.1 = 4000 ± 18 × 10-2; SYI.2 = 227.75 ± 3.2 × 10-2; SYII.1 = 122.28 ± 2.8 × 10-3 and SYII.2 = 139.2 ± 3.7 × 10-3 * means significant difference (p < 0.01) between SYI.1 and SYI.2 and between SYII.1 and SYII.2 at day 5. # is difference between each gene and its control (thalli without treatment). ✓ shows differences for the same gene between treatment of MEJA + methionine and MEJA + SO4.
3.2 Evaluation of sulfated galactans of G. imbricata through the Fourier-transformed infrared spectroscopy
Spectral regions from 740 to 1742 cm-1 were assumed to be the fingerprint regions for determining sulfated galactans, particularly carrageenan (Table 2 and references). Spectra of commercial carrageenan were superimposed on spectra corresponding to carrageenan extracted from infertile and fertile thalli and fertile thalli induced by MEJA. Commercial carrageenan was introduced as a control as well as carrageenan from ethylene-induced fertile thalli of G. imbricata (Figure 10). As shown in Figure 10 and Table 2, the carrageenan spectra of the MEJA-induced fertile thalli showed a considerable decrease in the vibration bands. Moreover, to evaluate these changes in spectra, area counts were used as a measure of the degree of alteration of the carrageenan profile; thus, we could infer the effect of MEJA on the synthesis of sulfated galactans. Thus, the spectrum of sulfated galactans from thalli cultured in the presence of MEJA was assumed to be altered by the diminution of vibration bands in terms of relative area counts in the FTIR spectra through a qualitative comparison between the spectra of commercial carrageenan and those from the control, infertile, and fertile thalli. Flattening in spectra of sulfated galactans from fertile thalli induced by MEJA was associated with a diminution of all functional groups in area counts, approximately 70–80% and 20–40% in regions between 1010–1030 and 1070–1085 cm-1 compared to that of commercial carrageenan (Table 3).
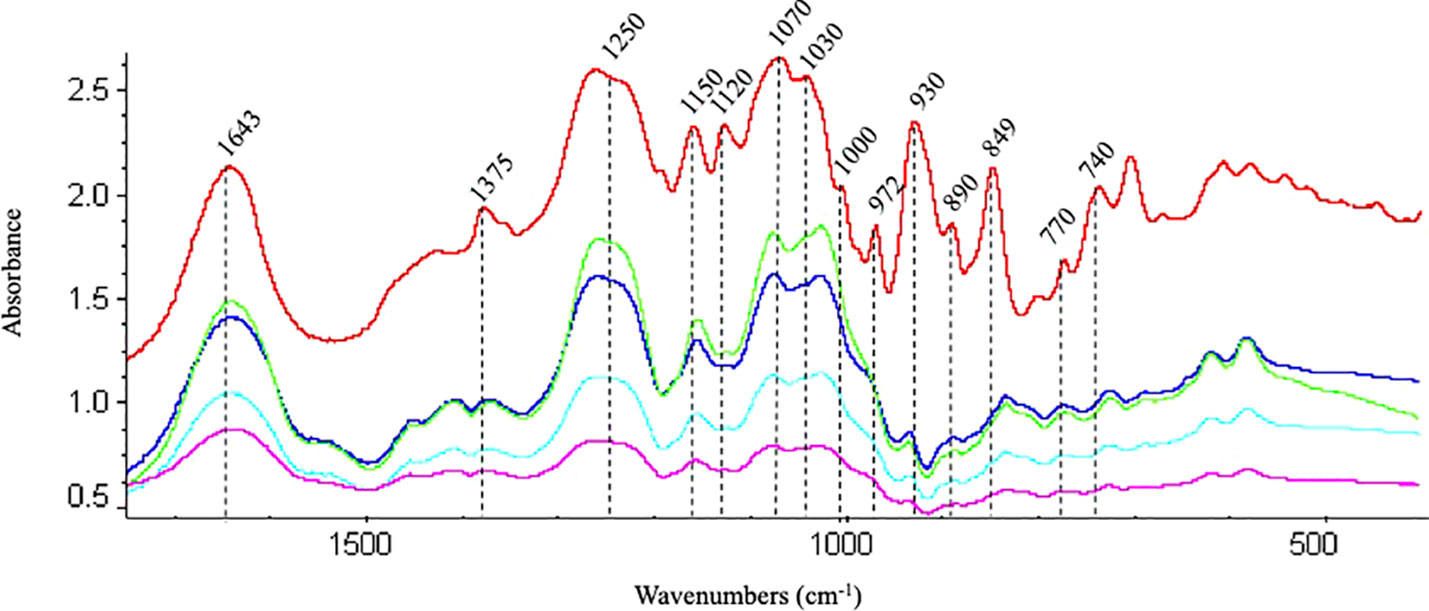
Figure 10 Fingerprint region of the FTIR spectra of commercial carrageenan (red line); carrageenan extracted from infertile (blue), fertile (green) thalli, methyljasmonate-induced fertile thalli (pink) and ethylene-induced fertile thalli (light blue) of Grateloupia imbricata.
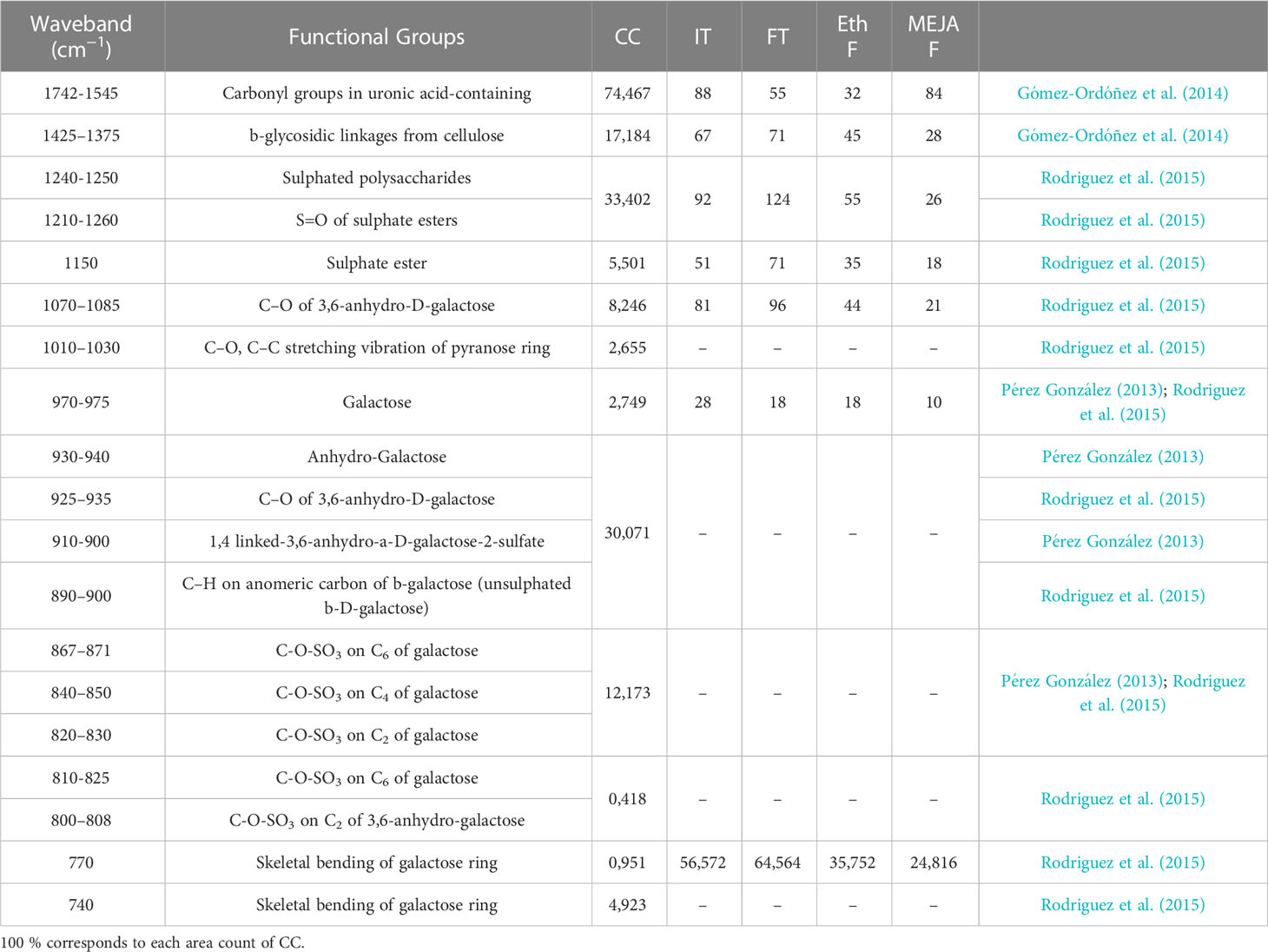
Table 3 Area counts extracted of FTIR spectra for each functional groups detailed in commercial carrageenan (CC; as in Table 2), and percentages of area (%) of each functional group reported for carrageenan extracted from infertile (not showing cystocarps; IT) and fertile (with light red dots indicative of cystocarps; FT) thalli, and from induced-fertile thalli by methyljasmonate (MEJA F) and by ethylene (Eth F).
3.3 Ploidy in collected gametophytic thalli, in vitro-released spores, and spores from MEJA -induced fertile thalli
The assessment of ploidy through flow cytometry cannot be assured as no differences between the ploidy patterns of haploid gametophytic thalli and in vitro-released spores and MEJA-induced spores were encountered (Figure 11).
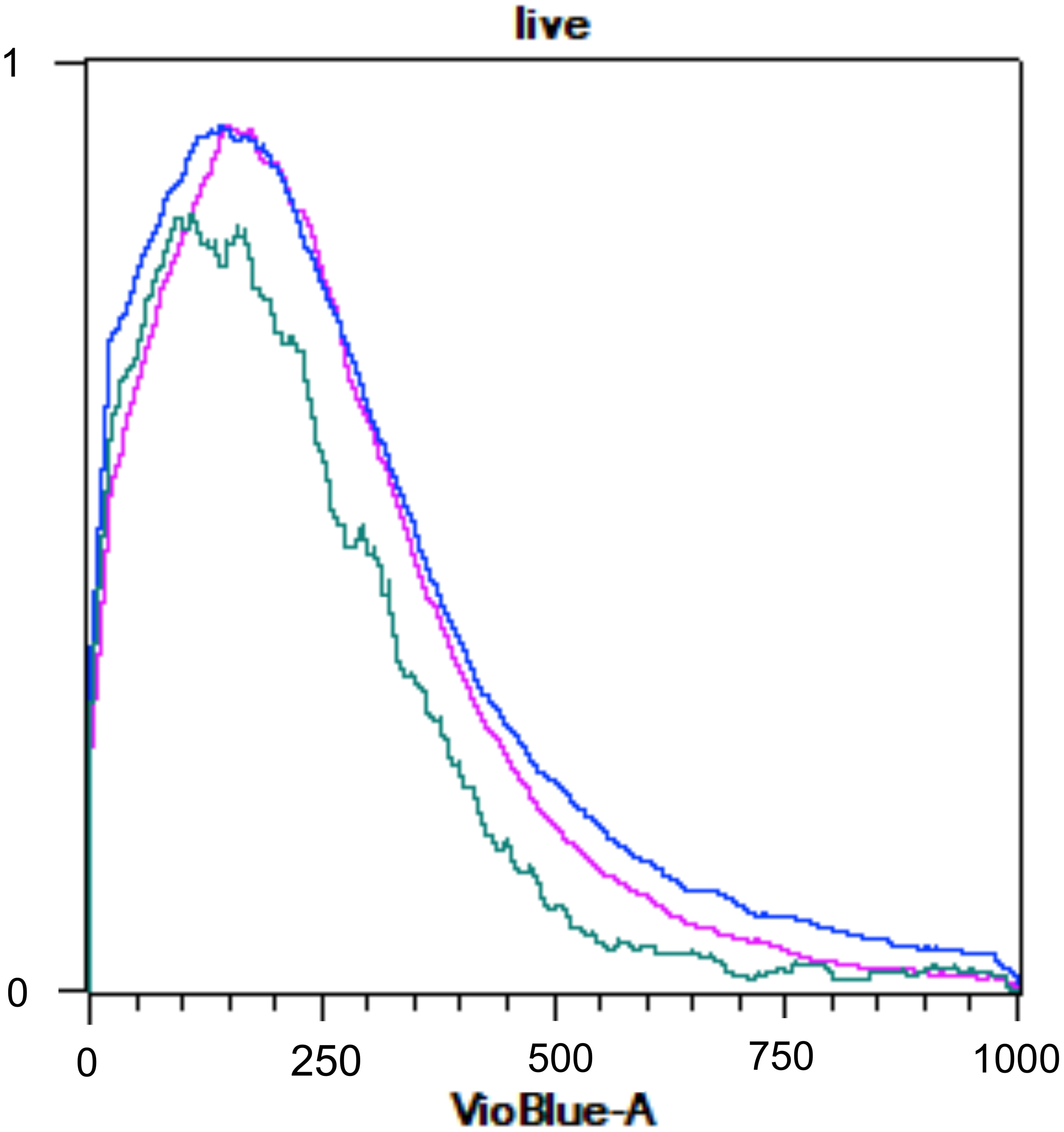
Figure 11 Flow cytometry histogram of Grateloupia imbricata showing naturally released-spores (pink), spores from methyljasmonate (MEJA) treatment (dark blue) and infertile thalli (without cystocarps; light blue). The x-represents fluorescence of 4′,6-diamidine-2′-phenylindole dihydrochloride (DAPI; VioBlueA channel) and y-axis represents relative DNA content.
4 Discussion
The study of the effect of the sulfur source (oxidized or reduced) on carrageenan synthesis, as the effects of hormones on sporulation are produced, is entirely relevant, as differences in growth and changes in cell wall sulfated galactans, carrageenan, have been reported owing to different life cycle stages and environmental conditions in red algae. Sulfur also plays a central role in the synthesis of hormones, such as ethylene or jasmonates (via S-adenosyl methionine, SAM), which affect algal reproduction. Particularly, the effect of ethylene on carrageenan synthesis during carposporogenesis in G. imbricata has recently been detailed (del Rosario-Santana et al., 2022). Ethylene downregulated the expression of sulfated-galactan synthesis precursors in the fertile stage of thalli of G. imbricata. The expression of these genes is associated with the source of S-sources. Thus, it is understood that gene expressions for galactan synthesis were modified in thalli cultured in the presence of a reduced source of S, such as methionine, compared to an oxidized source, such as magnesium sulfate (del Rosario-Santana et al., 2022).
MEJA also has immediate effects on algal reproduction, with changes in the expression of genes related to cystocarp maturation and spore release occurring in a short time, such as 48 h against 30 d for spontaneously released spores (Garcia-Jimenez et al., 2016; Garcia-Jimenez et al., 2017). In addition to reducing time, MEJA boosts cystocarp maturation and dehiscence, that is, the opening of cystocarps and carpospore release (Garcia-Jimenez et al., 2016). Furthermore, MEJA synthesis relies on SAM because jasmonic acid is methylated through the cleavage of a methyl group from SAM to produce MEJA (Yang and Hoffmann, 1984).
Accordingly, the subsequent attempts in this complex condition of seaweed reproduction and metabolism should lead to deciphering the interactions between sulfated galactan synthesis of red algal cell wall components by studying different S-sources and MEJA as an inducer of algal reproduction. Consequently, two observations were made concerning the S source and MEJA.
First, methionine upregulated expressions of genes involved in the transport and assimilation of S, that is, S-transport and SAT transcript numbers were increased compared to those of sulfate and for thalli without treatment (Figure 4A), similarly as previously reported in ethylene-induced fertile thalli for 7 d (del Rosario-Santana et al., 2022). Furthermore, when sulfur is assimilated, methionine affects the UDP pool, downregulating the conversion of glucose 6P to 1P by PGM and transforming it to UDP galactose by GALT (Figure 4B). These results are consistent with those previously reported, where gene expression may be regulated by a pool of sulfur organic compounds in algae, in contrast to what occurs in the presence of sulfate, which is non-limiting to seawater. In contrast, galactan synthesis requires sulfate to be added to the backbone of UDP-galactose through sulfotransferases, as UDP-galactose is a precursor of sulfate-galactan synthesis. Thus, the expression of two gene sequences encoding two sulfotransferases (ST1 and ST2) was upregulated as SO4 was available (Figure 5; Supplementary Figure S1).
Secondly, MEJA downregulated the gene expression of S-transport and S-assimilation in the presence of methionine (Figure 7A), and an imbalance in the gene expression of sulfated galactan precursors in PGM and GALT occurred (Figure 7B). This imbalance seems to disrupt the pool of UDP-glucose and UDP, as a severe reduction in transcripts was observed in the presence of methionine and MEJA (Figures 7A, B). PGM and GALT have been described as keys for the development of spores in higher plants, as PGM contributes to polysaccharide synthesis for spore protection, and GALT controls the synthesis to accommodate the differential compounds of the cell wall (Egli et al., 2010). Similar considerations have been reported in red seaweed when infertile thalli of G. imbricata transitioned to ethylene-induced fertile thalli (del Rosario-Santana et al., 2022). Furthermore, MEJA provoked a downregulation of the ST gene machinery together with softening of the thalli and coincided with the maturation and dehiscence of cystocarps in a short time of 48 h (Figure 8). As transpired with ethylene, ST1 and ST2 gene expression trends could reveal different roles, such as ST1 promoting neutral polysaccharides and mucilage resembling those of fertile thalli from the field and those induced by ethylene (Garcia-Jimenez et al., 2020; del Rosario-Santana et al., 2022). Meanwhile, a drastic ST2 downregulated expression would be constrained to the presence of MEJA and associated with the development of reproductive structures (cystocarps; Figure 8 vs. Figure 5), as also reported for ethylene-treated thalli (del Rosario-Santana et al., 2022; Shao and Duan, 2022). Thus, it can be inferred that if ST gene expression is sustained or downregulated, then changes in the expression of two sequences for each sulfurylase gene (SYI and SYII) could mirror those ST expressions or simply provide an unrestrained boost in sulfated group removal on the galactan backbone to promote the softening of thalli and the location of reproductive structures (Figures 9A, B). Nonetheless, although more insight is required, these ST and SY gene expression patterns indicate the existence of residual transcripts after MEJA signaling. This implies that MEJA suppresses carrageenan synthesis by disrupting the genes involved in carrageenan precursor synthesis and those in charge of addition (mainly ST2), and the removal of sulfate groups by acting on the two gene sequences of each SY (Supplementary Figure S1). This suppression coincides with the elicitation and maturation of cystocarps and softening of thalli (Garcia-Jimenez and Robaina, 2017; Garcia-Jimenez and Robaina, 2019; Garcia-Jimenez et al., 2020).
As revealed by FTIR spectra, the dysregulation of galactan synthesis was illustrated with carrageenan vanishing in MEJA-treated fertile thalli. Notably, the presence of MEJA disrupted the functional groups of carrageenan (Table 2) and flattened the spectral peaks, with a reduction in area counts (Table 3, Figure 10). This result also supports that carrageenan synthesis was suppressed, as non-flattening was observed in the spectra of carrageenan extracted from ethylene-induced fertile thalli, similar to the commercial carrageenan spectra and those of fertile and infertile thalli (Figure 10).
Furthermore, MEJA, which induces the maturation and dehiscence of cystocarps and spore release in an extremely short period, may induce mitosis to form n spores, affecting ploidy. As theoretically assumed, the life cycle of G. imbricata comprises three phases: gametophytes (n), free-living and isomorphic sporophytes (2n), and carposporophytes (2n), a reproductive structure (cystocarp) that develops in the female gametophyte, more than a living being in itself. Therefore, our results, obtained by monitoring ploidy using flow cytometry, indicate that carpospores induced by MEJA could be haploids (n). G. imbricata is a species introduced to the Canary Islands (Garcia-Jimenez et al., 2008), and it could be plausible that the introduced specimens display an incomplete life cycle based on the propagation of the female gametophyte (n) through the production of spores, or mitospores (n), without the need for fertilization. To date, G. imbricata (formerly G. doryphora) male thalli have never been reported, nor have tetrasporophytic thalli naturally collected along the coasts of Gran Canaria. However, the multiplicity and variants of the life cycle with alterations of the theoretical model are well known, particularly in algae and red algae (Vieira et al., 2018; Krueger-Hadfield, 2020; Heesch et al., 2021). This would reveal new perspectives for the investigation of the volatile substances in the cell cycle rather than in the life cycle, particularly MEJA, owing to its rapid and direct action.
Data availability statement
The datasets presented in this study can be found in online repositories. The names of the repository/repositories and accession number(s) can be found below: https://www.ncbi.nlm.nih.gov/genbank/, PRJNA309128.
Author contributions
PG-J conceived, designed, and wrote the manuscript. DR-S conducted the experiments. PG-J and RR discussed the manuscript. Authors read and approved the manuscript. All authors contributed to the article and approved the submitted version.
Funding
General funding from Universidad de Las Palmas de Gran Canaria, and Project TED2021-129249B-I00 funded by Ministerio de Ciencia e Innovación (Spain). Publication fees have been charged by the project ULPGC Excellence, funded by the Consejería de Economía, Conocimiento y Empleo del Gobierno de Canarias.
Acknowledgments
A fellowship from the Universidad de Las Palmas de Gran Canaria to DdRS is acknowledged. Authors also wish to thank Dr. Leandro Fernández Pérez of the University of Las Palmas de Gran Canaria for their skillful assistance with flow cytometer.
Conflict of interest
The authors declare that the research was conducted in the absence of any commercial or financial relationships that could be construed as a potential conflict of interest.
Publisher’s note
All claims expressed in this article are solely those of the authors and do not necessarily represent those of their affiliated organizations, or those of the publisher, the editors and the reviewers. Any product that may be evaluated in this article, or claim that may be made by its manufacturer, is not guaranteed or endorsed by the publisher.
Supplementary material
The Supplementary Material for this article can be found online at: https://www.frontiersin.org/articles/10.3389/fmars.2023.1188493/full#supplementary-material
Supplementary Figure 1 | Heatmap for expressions of genes encoding transport and assimilation of S-source (S transport and SAT), precursors of carrageenan (PGM and GALT) and the addition (ST1 and ST2) and removal (SYI and SYII) of sulfate groups to the galactans backbone in Grateloupia imbricata. Thalli of G. imbricata were treated with methionine (METH) and sulfate (SO4) for 3 d, and with a S-source plus methyljasmonate (METH.MEJA and SO4. MEJA), as a plant volatile growth regulator, for 1 h. Expression of genes in presence of a S source is shown normalized to seawater and, in presence of MEJA to those corresponding to treatments with METH and SO4 respectively. Dark red indicating highly expressed genes, light blue and red indicating intermediately expressed genes, and dark blue representing low expression values.
References
Avila M., Candia A., Núñez M., Romo H. (1999). Reproductive biology of Gigartina skottsbergii (Gigartinaceae, Rhodophyta) from Chile. Hydrobiologia 398–399, 149–157. doi: 10.1007/978-94-011-4449-0_17
Ciancia M., Matulewicz M. C., Tuvikene R. (2020). Structural diversity in galactans from red seaweeds and its influence on rheological properties. Front. Plant Sci. 11. doi: 10.3389/fpls.2020.559986
Delivopoulos S. G. (2003). Ultrastructure of auxiliary and gonimoblast cells during carposporophyte development in the red alga Cryptopleura ruprechtiana (Delesseriaceae, Ceramiales, Rhodophyta). Biol. Cell 95 (6), 383–392. doi: 10.1016/S0248-4900(03)00085-6
del Rosario-Santana D., Robaina R. R., Garcia-Jimenez P. (2022). S-Assimilation influences in Carrageenan Biosynthesis Genes during Ethylene-Induced Carposporogenesis in Red Seaweed Grateloupia imbricata. Mar. Drugs 20 (7), 436. doi: 10.3390/md20070436
Egli B., Kölling K., Köhler C., Zeeman S. C., Streb S. (2010). Loss of cytosolic phosphoglucomutase compromises gametophyte development in arabidopsis. Plant Physiol. 154 (4), 1659–1671. doi: 10.1104/pp.110.165027
Garcia-Jimenez P., Brito-ROmano O., Robaina R. R. (2016). Occurrence of jasmonates during cystocarp development in the red alga Grateloupia imbricata. J. Phycology 52 (6), 1085–1093. doi: 10.1111/jpy.12467
Garcia-Jimenez P., Geraldino P. J. L., Boo S. M., Robaina R. R. (2008). Red alga Grateloupia imbricata (Halymeniaceae), a species introduced into the Canary Islands. Phycological Res. 56 (3), 166–171. doi: 10.1111/j.1440-1835.2008.00498.x
Garcia-Jimenez P., Mantesa S. R., Robaina R. R. (2020). Expression of genes related to carrageenan synthesis during carposporogenesis of the red seaweed Grateloupia imbricata. Mar. Drugs 18 (9), 432. doi: 10.3390/MD18090432
Garcia-Jimenez P., Montero-Fernández M., Robaina R. R. (2017). Molecular mechanisms underlying Grateloupia imbricata (Rhodophyta) carposporogenesis induced by methyl jasmonate. J. Phycology 53 (6), 1340–1344. doi: 10.1111/jpy.12594
Garcia-Jimenez P., Montero-Fernández M., Robaina R. R. (2018). Analysis of ethylene-induced gene regulation during carposporogenesis in the red seaweed Grateloupia imbricata (Rhodophyta). J. Phycology 54 (5), 681–689. doi: 10.1111/jpy.12762
Garcia-Jimenez P., Robaina R. R. (2012). Effects of ethylene on tetrasporogenesis in Pterocladiella capillacea (Rhodophyta). J. Phycology 48 (3), 710–715. doi: 10.1111/j.1529-8817.2012.01156.x
Garcia-Jimenez P., Robaina R. R. (2017). Volatiles in the aquatic marine ecosystem: Ethylene and related plant hormones and sporulation in red seaweeds. Syst. Biol. Mar. Ecosyst, 99–116. doi: 10.1007/978-3-319-62094-7_5
Garcia-Jimenez P., Robaina R. R. (2019). “Insight into the Mechanism of Red Alga Reproduction. What Else Is Beyond Cystocarps Development?,” in Systems Biology (USA: IntechOpen). doi: 10.5772/intechopen.83353
Garcia-Tasende M., Cid M., Fraga M. I. (2013). Qualitative and quantitative analysis of carrageenan content in gametophytes of Mastocarpus stellatus (Stackhouse) Guiry along Galician coast (NW Spain). J. Appl. Phycology 25 (2), 587–596. doi: 10.1007/s10811-012-9893-2
Gómez-Ordóñez E., Jiménez-Escrig A., Rupérez P. (2014). Bioactivity of sulfated polysaccharides from the edible red seaweed Mastocarpus stellatus. Bioactive Carbohydrates Dietary Fibre. 3, 29–40. doi: 10.1016/j.bcdf.2014.01.002
Guzmán-Urióstegui A., Garcia-Jimenez P., Marián F., Robledo D., Robaina R. (2002). Polyamines influence maturation in reproductive structures of Gracilaria cornea (Gracilariales, Rhodophyta). J. Phycology 38 (6), 1169–1175. doi: 10.1046/j.1529-8817.2002.01202.x
Heesch S., Serrano-Serrano M., Barrera-Redondo J., Luthringer R., Peters A. F., Destombe C., et al. (2021). Evolution of life cycles and reproductive traits: Insights from the brown algae. J. Evolutionary Biol. 34 (7), 992–1009. doi: 10.1111/jeb.13880
Imjongjairak S., Ratanakhanokchai K., Laohakunjit N., Tachaapaikoon C., Pason P., Waeonukul R. (2016). Biochemical characteristics and antioxidant activity of crude and purified sulfated polysaccharides from Gracilaria fisheri. Bioscience Biotechnology Biochem. 80 (3), 524–532. doi: 10.1080/09168451.2015.1101334
Krueger-Hadfield S. A. (2020). What’s ploidy got to do with it? Understanding the evolutionary ecology of macroalgal invasions necessitates incorporating life cycle complexity. Evolutionary Appl. 13 (3), 486–499. doi: 10.1111/eva.12843
Lipinska A. P., Collén J., Krueger-Hadfield S. A., Mora T., Ficko-Blean E. (2020). To gel or not to gel: differential expression of carrageenan-related genes between the gametophyte and tetasporophyte life cycle stages of the red alga Chondrus crispus. Sci. Rep. 10 (1), 11498. doi: 10.1038/s41598-020-67728-6
McQueney M. S., Anderson K. S., Markham G. D. (2000). Energetics of S-adenosylmethionine synthetase catalysis. Biochemistry 39 (15), 4443–4454. doi: 10.1021/bi992876s
Montero-Fernández M., Robaina R. R., Garcia-Jimenez P. (2016). In silico characterization of DNA motifs associated with the differential expression of the ornithine decarboxylase gene during in vitro cystocarp development in the red seaweed Grateloupia imbricata. J. Plant Physiol. 195, 31–38. doi: 10.1016/j.jplph.2016.03.004
Oesterhelt C., Schnarrenberger C., Gross W. (1996). PhosphOmannomutase and phosphoglucomutase in the red alga Galdieria sulphuraria. Plant Sci. 121, 19–27. doi: 10.1016/S0168-9452(96)04475-5
Pereira L., Ribeiro-Claro P. (2016). “Morphological, cytological and chemical analysis of the heterosporous Gigartina Pistillata,” in Carrageenans: Sources and Extraction Methods, Molecular Structure, Bioactive Properties and Health Effects, 147–159. doi: 10.1007/978-3-030-92174-3_6
Pérez González C. M. (2013). Caracterización biológica y química de Kappaphycus alvarezii de Panamá (Universidad de Las Palmas de Gran Canaria).
Rodriguez D., Freitas A. C., Pereira L., Rocha-Santos T. A. P., Vasconcelos M. W., Roriz M., et al. (2015). Chemical composition of red, brown and green macroalgae from Buarcos bay in Central West Coast of Portugal. Food Chem. 183, 197–207. doi: 10.1016/j.foodchem.2015.03.057
Sacramento A. T., Garcia-Jimenez P., Alcázar R., Tiburcio A. F., Robaina R. R. (2004). Influence of polyamines on the sporulation of Grateloupia (Halymeniaceae, Rhodophyta). J. Phycology 40 (5). doi: 10.1111/j.1529-8817.2004.03183.x
Sacramento A. T., Garcia-Jimenez P., Robaina R. R. (2007). The polyamine spermine induces cystocarp development in the seaweed Grateloupia (Rhodophyta). Plant Growth Regul. 53 (3), 887–894. doi: 10.1007/s10725-007-9212-0
Santos R., Duarte P. (1996). Fecundity, spore recruitment and size in Gelidium sesquipedale (Gelidiales, Rhodophyta). Hydrobiologia 326–327, 223–228. doi: 10.1007/BF00047811
Shao Z., Duan D. (2022). “The cell wall polysaccharides biosynthesis in seaweeds: a molecular perspective. Front. Plant Sci. 13, 902823. doi: 10.3389/fpls.2022.902823
Stirk W. A., van Staden J. (2014). “Plant growth regulators in seaweeds: Occurrence, regulation and functions,” in Advances in Botanical Research, vol. 71. (Academic Press Inc), 125–159. doi: 10.1016/B978-0-12-408062-1.00005-6
Vieira V. M., Engelen A. H., Huanel O. R., Guillemin M. L. (2018). Differentiation of haploid and diploid fertilities in Gracilaria Chilensis affect ploidy ratio. BMC Evolutionary Biol. 18 (1), 1–11. doi: 10.1186/s12862-018-1287-x
Keywords: carbohydrate sulfotransferase, carrageenan, galactose-1-phosphate uridyltransferase, galactose-6-sulfurylase, jasmonates, phosphoglucomutase, red seaweed
Citation: Del Rosario-Santana D, Robaina R and Garcia-Jimenez P (2023) Jasmonates disrupt carrageenan synthesis during carposporogenesis in the red seaweed Grateloupia imbricata. Front. Mar. Sci. 10:1188493. doi: 10.3389/fmars.2023.1188493
Received: 17 March 2023; Accepted: 25 July 2023;
Published: 11 August 2023.
Edited by:
Yang Jin, Johns Hopkins University, United StatesReviewed by:
Manoj Kumar, University of Technology Sydney, AustraliaDaniel Robledo, National Polytechnic Institute of Mexico (CINVESTAV), Mexico
Copyright © 2023 Del Rosario-Santana, Robaina and Garcia-Jimenez. This is an open-access article distributed under the terms of the Creative Commons Attribution License (CC BY). The use, distribution or reproduction in other forums is permitted, provided the original author(s) and the copyright owner(s) are credited and that the original publication in this journal is cited, in accordance with accepted academic practice. No use, distribution or reproduction is permitted which does not comply with these terms.
*Correspondence: Pilar Garcia-Jimenez, cGlsYXIuZ2FyY2lhQHVscGdjLmVz