- 1Sanya Institute of South China Sea Geology, Guangzhou Marine Geological Survey & National Engineering Research Center for Gas Hydrate Exploration and Development, Sanya, China
- 2Key Laboratory of Marine Mineral Resoures, Ministry of Natural Resources, Guangzhou Marine Geological Survey, China Geological Survey Guangzhou, Guangzhou, China
- 3Research Institute of Petroleum Exploration and Development, PetroChina, Beijing, China
- 4School of Marine Sciences, Sun Yat-sen University & Southern Marine Science and Engineering Guangdong Laboratory (Zhuhai), Zhuhai, China
Most of the natural gas hydrate sources in the world are biogenic gas, but there are still many unsolved problems in the formation process of biogenic gas. The gas source of gas hydrates in the northern South China Sea is also controversial, and the evaluation of biogas source rock in hydrate development areas lacks experimental basis and quantitative data. In order to solve these problems, this paper used the sediments obtained from the GMGS2 hydrate drilling voyage to simulate the biological gas production. Through the culture experiment, the relationship between sedimentary gas production, temperature and sediment characteristics in the hydrate development area of Dongsha Sea area was comparatively studied, and the results were compared with typical biological gas fields. The results showed that CH4 and CO2 were the main gas products, and a small amount of H2 was formed. Methane yield is closely related to temperature, and the optimal temperature range in the study area is 20°C~ 40°C. At the same time, it is found that organic carbon content (TOC) has no significant correlation with methane yield, and it is not suitable to be used as an index for the evaluation of biogas source rock. Comprehensive analysis shows that the methane production rate in the study area is relatively high and the hydrocarbon generation potential is great, which meets the gas source conditions for the formation of gas hydrate ore body. The main factors affecting biomethane yield include available organic matter, bacterial community and environmental factors.
1 Introduction
Natural gas hydrate is known as the high efficiency and clean strategic replacement energy in the 21st century, with the advantage of ample resources and wide distribution (Wan et al., 2022b). In recent years, a lot of achievements have been obtained in the exploration of gas hydrate in the northern South China Sea (Zhang et al., 2020a), while there is still some controversy on the gas source (Wu et al., 2003; Wu et al., 2007; Su et al., 2010; Fu et al., 2011; He et al., 2013; Su et al., 2013; Liang et al., 2016; Zhang et al., 2017; Hu et al., 2018 ; Zhang et al., 2018). Geochemical data show that the main gas source is biogenic gas (Chen et al., 2022). However it is generally believed that the amount of biogenic gas in terrestrial basins is very small, and only a little of them can accumulate to form gas fields. Therefore, it’s still controversial whether the amount of biogenic gas generated from sediments in the northern South China Sea is sufficient to meet the gas hydrate accumulation (Pandey et al., 2016; Wan et al., 2022a). Most studies on gas source conditions only have presented merely qualitative analyses, and lacked experimental basis and quantitative data for the evaluation of biogas source rocks (Gao et al., 2011).
It has been suggested that most hydrates in the world originate from biogenic gas, however there are still many unsolved problems in the formation process of biogenic gas, for example, the influencing factors of biogas production, the effect of temperature on the gas production process, the relationship between TOC parameters representing organic matter abundance and gas production. These problems are the basis for establishing and improving biogas formation theory and biogas resource evaluation technology (Liu et al., 2015), while biological gas production simulation experiment is an important way to solve these problems. However, due to high requirements of such time-consuming experiments, there have been few studies in this field. So far, there is no report on the biological gas production simulation experiment using shallow sediments in the South China Sea.
Based on this, this paper takes Dongsha area as an example to conduct biogas production simulation experiment with sediments obtained from GMGS2 hydrate drilling voyage, to study the production characteristics and rules and influencing factors of biogas, in order to provide experimental basis for hydrate gas source analysis and biogas source rock evaluation.
1.1 Geological background and samples
The Dongsha area is located in the continental slope zone of the northeast South China Sea and the southwest of Taiwan Island, with a water depth of 200~3000 m. The Dongsha Sea area consists of the eastern Pearl River Mouth Basin, South Taiwan Basin and parts of the South Bijia Basin in structure. It is an important gas hydrate distribution area in the northern South China Sea, with good accumulation conditions and great resource potential (Gong et al., 2008).The Dongsha Sea area is in a unique tectonic location and has experienced geological events such as basin expansion, early faults, and regional thermal deposition. This area has the temperature and pressure conditions of natural gas hydrate, as well developed source rock systems and high sedimentation rates. In 2013, Guangzhou Marine Geological Survey conducted the GMGS2 hydrate drilling voyage in Dongsha Sea area (Figure 1). Core-taking operations were carried out at five stations, and gas hydrate samples were obtained for the first time in the South China Sea. The gas hydrates found in the drilling are distributed in multiple layers, mainly in the range of 8~94 meter below sea floor (mbsf), and the thickness of single layer is between 15~35 m. At the same time, a variety of hydrates in massive, vein-like, tuberous and dispersed state are developed, with high saturation between 45% and 100%. The content of methane in hydrate decomposition gas is more than 99%, and isotopic evidence shows that it is biogenic methane (Zhang et al., 2015; Kong et al., 2018).
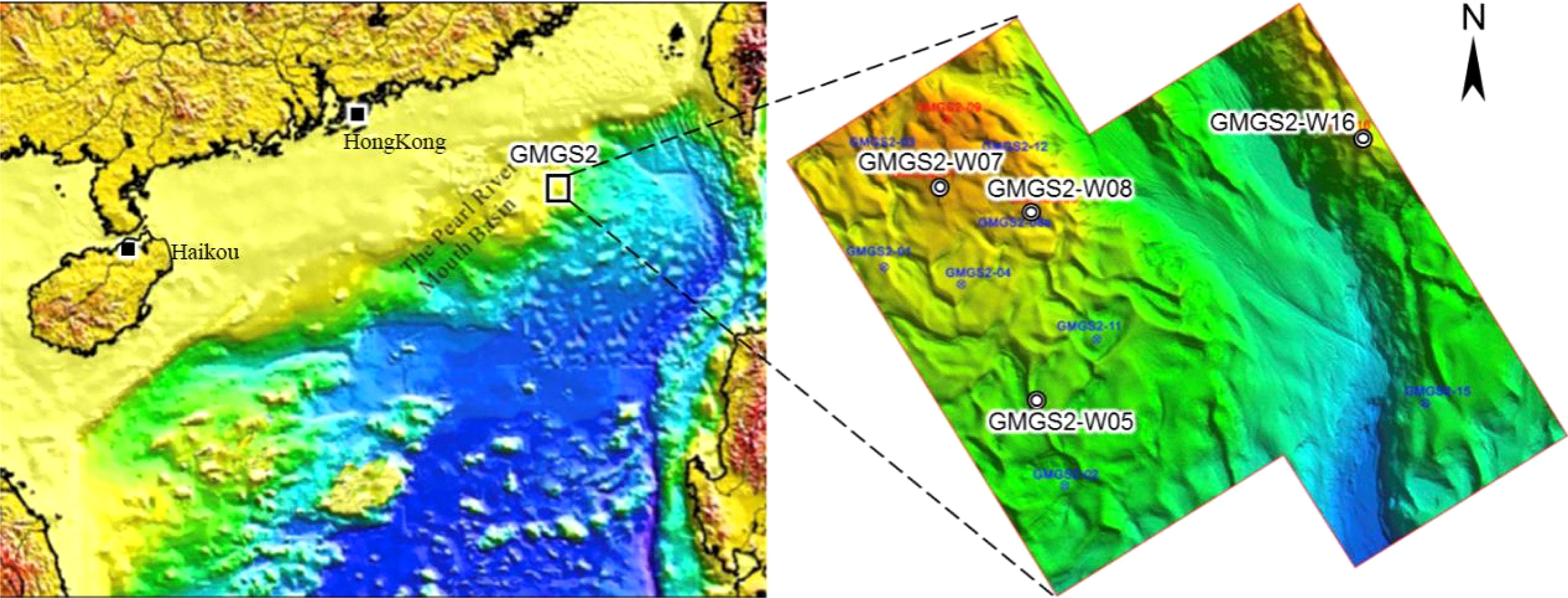
Figure 1 Seabed geomorphology and sampling location in the study area (base map modified according to Zhang et al., 2015).
In this paper, 20 samples were collected from the cores of GMGS2 in the hydrate development zone of the Dongsha Sea, and 20 samples were selected from the cores of GMGS2-W05, GMGS2-W07, GMGS2-W08 and GMGS2-W16 stations (hereinafter referred to as W05, W07, W08 and W16) where natural gas hydrate samples were drilled as sediments for biogas simulation experiments (Figure 1, Table 1). The lithology of the sample was gray-green silty clay. The background data showed that the mudstone content and organic matter abundance in the study area were high; The average total organic carbon content of samples from multiple sites was 0.6-0.7% and the maturity level was low. This makes the area more susceptible to biogenic methane formation. Samples are kept in the low-temperature core library of the Guangzhou Marine Geological Survey during the period from the seafloor to laboratory simulations.
2 Methods and experimental procedures
2.1 Sample preparation
The selected twenty sediment samples were air-dried at room temperature and ground into powder. Each sample was divided into five parts and there are a hundred parts in total. The total organic carbon content (TOC) was tested: add 3~5 g samples to a culture bottle, label and record (Table 1).
2.2 Strains domestication and enrichment
Domestication of simulated experimental strains, The primary bacterial culture and biogas digester in source rock of biogas producing area were selected for enrichment. The mixture of highly active sludge culture from distiller’s lees was used as the starting strain of simulation experiment. Enriching and culturing at 38°C for multiple times, until the activity of the strains was stable, some strains were taken and then heated to further culture at 38°C, 45°C and 55°C respectively.
2.3 Inoculation and culture
Five samples were selected from each sample for simulation experiments at room temperature (18-22°C)、38°C、45°C、55°C and 65°C, and 100 groups in total. During inoculation, simulated samples were inoculated with strains at 38°C at room temperature, and strains corresponding to their respective temperature points were inoculated at 38°C, 45°C and 55°C respectively. Considering that it is difficult to obtain strains with good activity in a short time at 65°C, the simulated samples at 65°C were inoculated with strains at 55°C, and then cultured at 65°C after the activity of the strains was stable. The inoculation process was carried out in a sealed anaerobic operating chamber. The configuration of culture medium and selection of culture bottle are same with Jianming Gong et al (Gong et al., 2009). The simulated culture lasted for 195 days.
2.4 Product detection
Three samples with a larger sample size were selected as process monitoring samples, and the CH4 content in these samples was regularly monitored by gas chromatography to determine the production of methane from products at different temperature points. After the experiment finishes, the composition and gas production of gas products are detected. Gas production was obtained by internal standard quantitative method of gas group, gas product components were obtained by gas chromatography, and gas production rate could be calculated according to gas production and sample weight.
3 Results and Discussion
3.1 Composition characteristics of gas products
In one hundred experiments, a certain amount of gas products were generated, mainly CH4 and CO2, and a small amount of H2 was generated in some experiments (Figure 2). CO2 and CH4 do not show good correlation over time under the same temperature conditions, so the monitoring results will not be presented. The simulation results of samples at different depths at the same station and the simulation results of the same sample at different temperatures were respectively compared. The results show that the content of gas components is closely related to the experimental temperature, but has no obvious correlation with the sediment depth. At room temperature and 38°C, the content of CH4 was the highest and the content of CO2 was the least. At 45°C, the content of CH4 in the products decreased significantly, the average proportion was 11%, and the lowest less than 1%; more than 80%of the gas product was CO2.
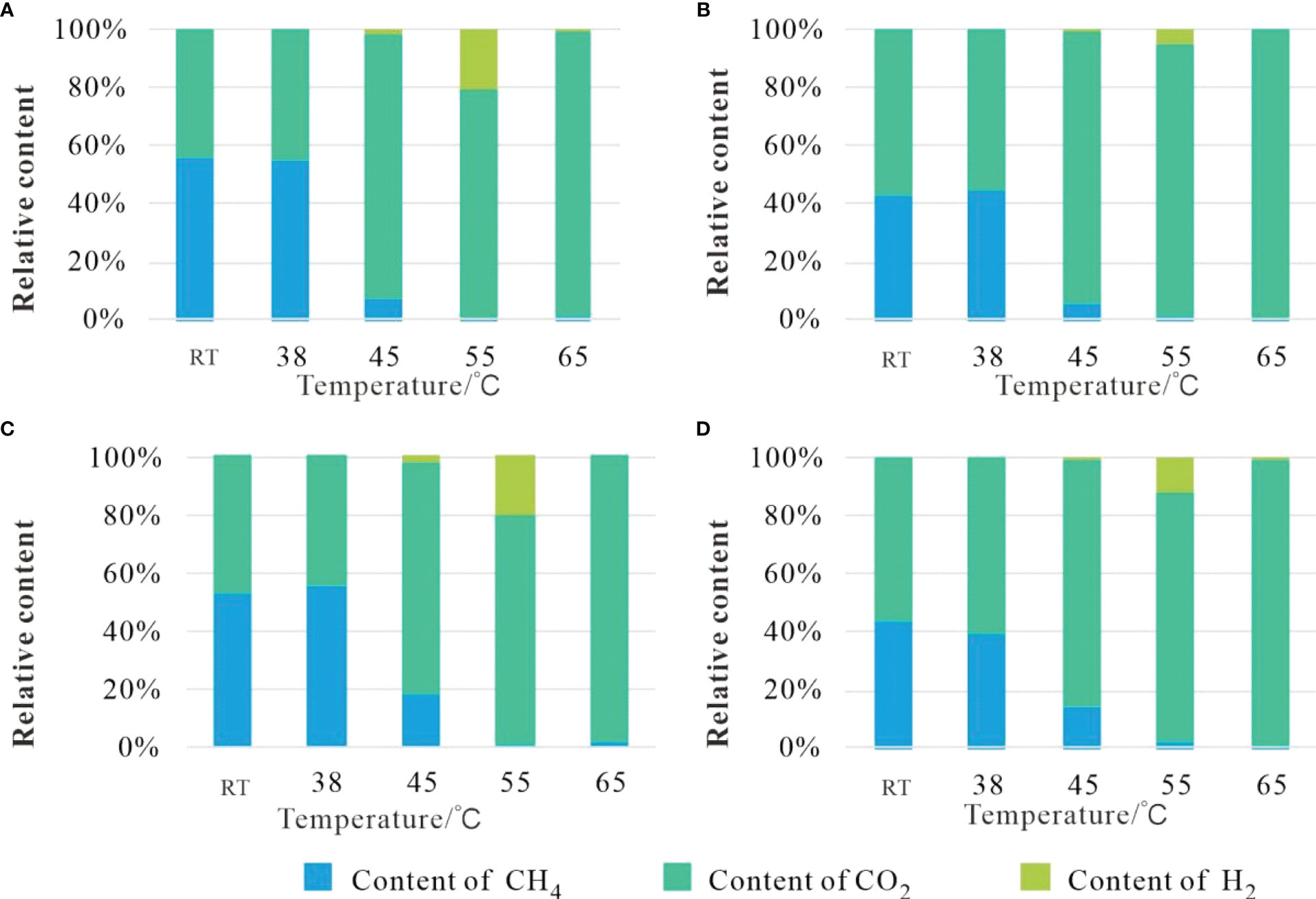
Figure 2 Composition of gas products from samples at different stations (A) W05; (B) W07; (C) W08; (D) W16.
The proportion of H2 in the products is very low on the whole, but the proportion of H2 in five groups of experiments (GZ06-55, GZ11-55, GZ12-55, GZ16-55, GZ17-55) is relatively high, the highest is 1.1%, the average of these five groups is 0.9%, and they are all products at 55°C, and H2 generated at other temperatures are all below 0.1%. The five samples were distributed at W05, W08 and W16, respectively, with relatively scattered distribution on the plane and no obvious pattern. The higher H2 content should be related to the ambient temperature, indicating that at this temperature, the hydrogen-consuming methanogens in the sediment samples began to become active and dominant, and CO2 reduction may become the main route of methanogenesis.
3.2 Yield characteristics and rules
3.2.1 Characteristics of methane production at different temperatures
In this paper, the unit of methane production was ml/g sediment. It should be noted that ml/gTOC is used as the unit of methane production in some literature. Since there is no significant positive correlation between TOC and methane production (more hereof later), this paper suggests that it is unreasonable to use this unit to measure methane production efficiency.
The variation of methane production rates (calculated by methane production per unit sediment) of the three samples at the station W05 under different temperatures is basically the same (Figure 3). The yield curves of GZ06 and GZ16 samples almost overlap, and both of them have the highest yields at room temperature, which are 0.82 and 0.78ml/g sediment, respectively, which decrease slightly at 38°C and below 0.1 ml/g sediment at 45°C. The methane yield of GZ01 samples was 0.35 ml/g at room temperature and 38°C, and decreased sharply to less than 0.1 ml/g at 45°C. Among the 4 samples in W07, two samples reached the highest and the second highest yield at room temperature and 38°C, respectively, while the other two samples showed the opposite trend, and their yield decreased sharply above 45°C. The samples in the W08 and W16 are basically consistent with the two states in the above two sites.
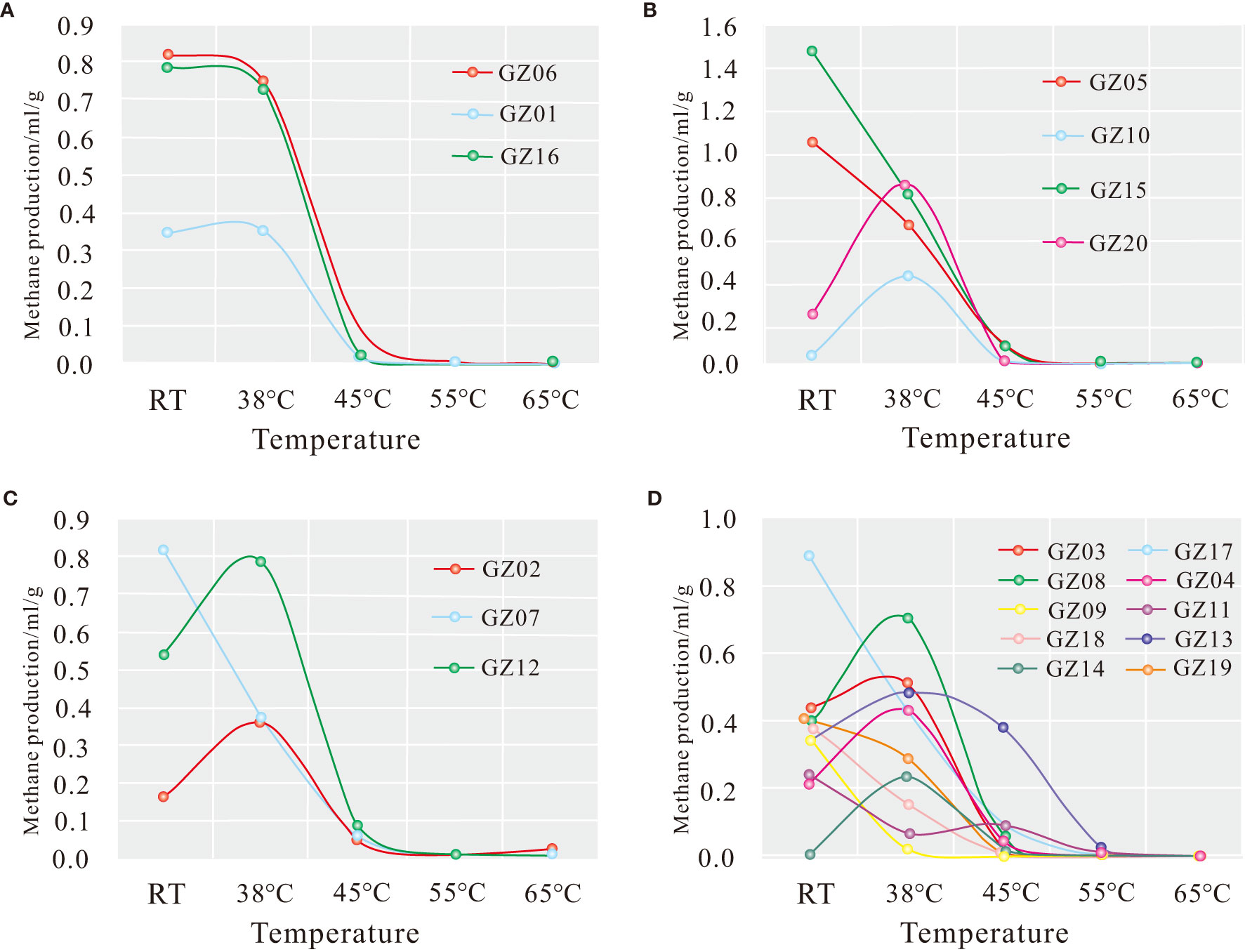
Figure 3 Relationship between methane production and temperature of samples at different sites (A) W05; (B) W07; (C) W08; (D) W16.
In conclusion, temperature is the most important environmental factor to control the activity of methanogens (Liu et al., 2020), and 20~40°C is the optimal temperature for the formation of biomethane in the study area, with the highest methane production rate. Combined with the geothermal gradient, based on the experimental results of the same sample at different temperatures, the gas production state of sediments at different depths in geological environment can be predicted. According to the results of this experiment, 55°C can be used as the upper limit of biogenic gas temperature in the study area, and the main gas production interval is between 20 and 40°.C Combined with the geothermal gradient of 34°C/km in the study area, it is estimated that the shallow sediments at 1400m are still in the biogenic gas stage, while the deep sediments at 1400 mbsf are less likely to yield biogenic gas (Wei et al., 2018). The yield is negligible and the main gas range should be between 400 and 1100m.
Methane production at the optimal temperature in the study area is concentrated in the range of 0.3~0.5 and 0.7~0.9ml/g sediments, with a maximum of 1.47ml/g sediments and an average of 0.48 mL/g sediments (Figure 4). Sebei Gas field in Qaidam Basin is a typical Quaternary biogenic gas field. A gas yield obtained in previous simulation gas production experiments with the deposits of this gas field was 0.35mL/g sediment. The simulation results of Yinggehai Basin, Qiongdongnan Basin and Baoshan Basin in Yunnan Province are also mainly lower than 1 mL/g sediment, with some higher values; and the produced gas in these areas has accumulated to form natural gas pools (Table 2). On the whole, compared with the results of biogas simulation experiments in other areas, the methane production rate at the optimal temperature in the study area is relatively high, showing that the shallow sediments in the study area have great hydrocarbon generation potential, and the area has a good gas source basis for the formation of gas hydrate ore bodies. In continental lacustrine basins, biogas is easily dispersed and difficult to accumulate after formation, so the preservation conditions of biogas in marine environment may be different from that in continental lacustrine basins. Therefore, according to the results of this experiment, it is suggested that when evaluating whether biogas produced by sediments in Dongsha Sea area can form hydrate, Gas production is sufficient and it is important to investigate whether biogas generated by sediments have been dissipated before the accumulation and formation of hydrate.
Due to different temperatures, the change of biomethane yield presents many common characteristics, and the following basic understandings can be obtained: (1) there is a good correlation between gas production and temperature under the action of microorganisms, which indicates that ambient temperature is an important factor affecting the activity of methanogens; (2) there is an optimal temperature range in the gas generation process (i.e., the temperature when the methane production rate is the maximum), which is mainly concentrated between 20°C and 40°C, which is speculated to be the temperature range where the metabolism of methanogens is the most active, which is basically consistent with previous reports. (3) when the ambient temperature reaches 45°C, the yield is very small; and when it reaches above 55°C, it is basically close to 0. 55°C may be the upper limit of methanogenic microbial activity temperature range. This temperature may also be a possible temperature range for CO2 reduction to produce methane, as higher levels of H2 are formed at this temperature. (4) under the optimal temperature, the methane yield is relatively high and the hydrocarbon generation potential is large, which has the gas source basis for the formation of hydrate ore body, biogenic methane may play an important role.
3.2.2 Characteristics of methane yield at different depths
In this experiment, samples at different depths can produce gas. Under room temperature and 38°C, the variation characteristics of methane production with depth are consistent or relatively similar, showing a certain rule (Figure 5). With the increase of depth, the methane yield generally shows a V-shaped or wavy change. The methane yield of shallow samples is generally large, and a minimum value will appear with the increase of depth. When the depth increases again, the yield will increase or rise again and again.
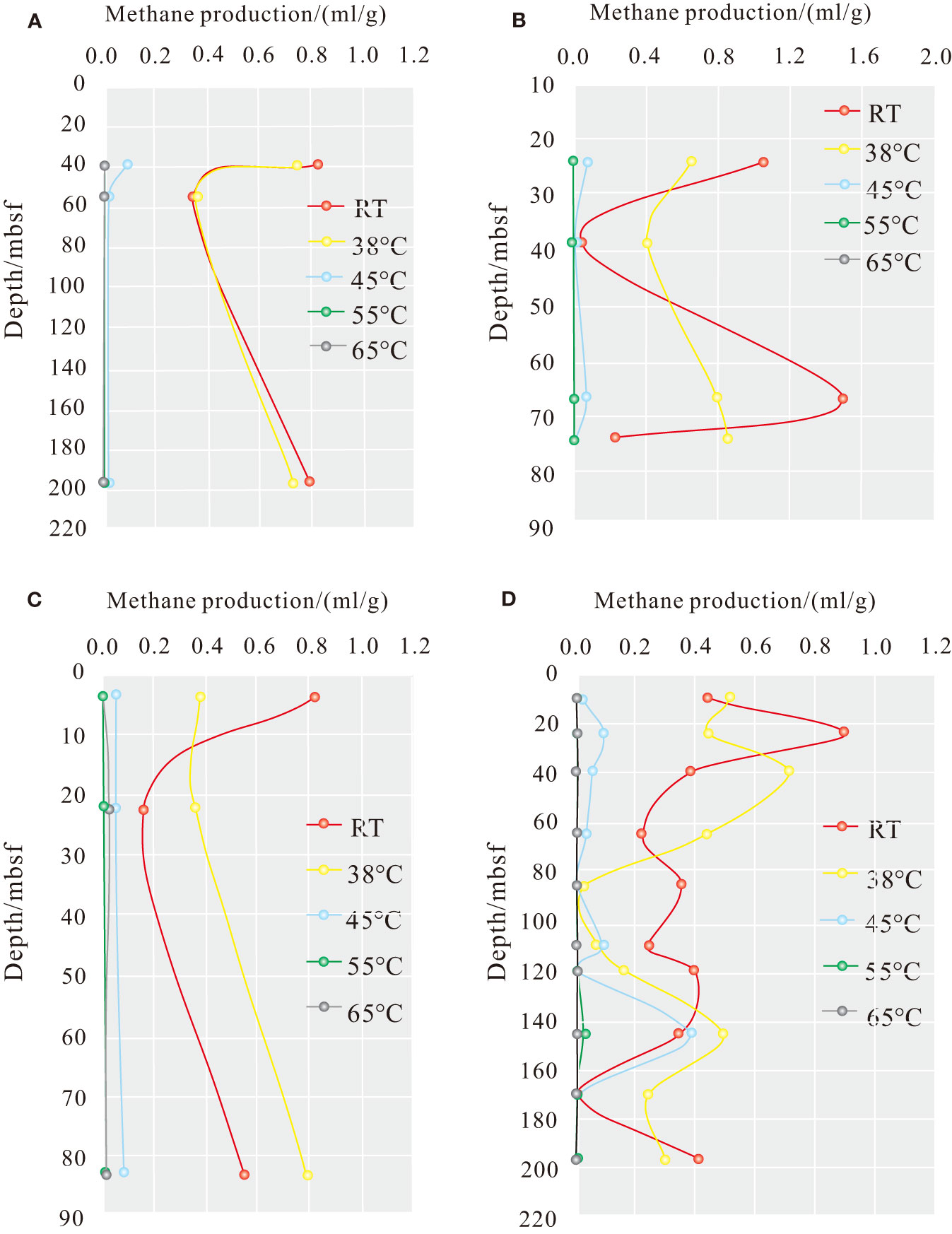
Figure 5 Relationship between methane yield and depth of samples at different sites (RT, room temperature) (A) W05; (B) W07; (C) W08; (D) W16.
At the same site, samples at different depths have different organic matter compositions favorable for microorganisms. Secondly, the characteristics and biomass of methanogens in the samples are different, which may be the main reason for the difference in gas yield of samples at different depths at the same temperature in the experiment.
The low point of methane production in shallow samples may be caused by the active sulfate-reducing bacteria in the sediments (Fan et al., 2015), which inhibits the activity of methanogens (Guan et al., 2016). The competition for H2 and acetate between sulfate-reducing bacteria and methanogens affects the production of methane (Whiticar et al., 1986; Guan et al., 2018; Zhang et al., 2022). With the increase of depth, the activity of sulfate-reducing bacteria was gradually limited, and the methane yield began to increase. When the temperature is above 45°C, the activity of methanogenic bacteria is obviously weakened, and the methane yield is small, and there is no obvious change with the depth.
3.3 Discussion on influencing factors of methane production
To explore the influencing factors of biomethane yield is the premise of establishing evaluation index of biogas source rock. The essence of biogas is natural gas formed by the biochemical interaction between organic matter and microorganism (Min et al., 2014). The generation of biogas can be achieved through acetic acid fermentation and CO2 reduction. Carbon dioxide reduction reaction is the main source of microbial genesis gas, most of the methane producing bacteria can be through carbon dioxide reduction to produce methane, fermentation is mainly acetate deacidification to form methyl, and then converted into methane. Any factors that strengthen or weaken these two processes will have an impact on gas production. Based on the analysis of the results of this experiment, it is concluded that there are three key factors affecting the formation of biomethane in sediments: the first is the richness of organic matter; the second is the biomass and activity of methanogens; the third is environmental factors.
3.3.1 Available organic matter
Analysis of the relationship between methane yield and organic carbon (TOC) of sediment samples at the optimal temperature of 20 samples showed that there was no significant correlation between the yield and TOC (Figure 6). Under greenhouse conditions, the methane yield of sediments with the same TOC is also unstable, varying in a wide range. For example, the methane yield of GZ04 and GZ06 samples is between 42.7 and 161.2 ml/g TOC, and that of all samples is between 0.64 and 248 ml/g TOC. Similarly, Rashid and Vilks (1977) studied biogenic methane in Holocene sediments of a Canadian basin and also showed that there was no direct correlation between methane production and organic carbon (Rashid and Vilks, 1977). Gong et al (2009) conducted a similar biological gas production simulation experiment on the sediment samples of the “hydrate ridge” in the west coast of the United States (Gong et al., 2009; Wei et al., 2018), and the data also showed similar results.
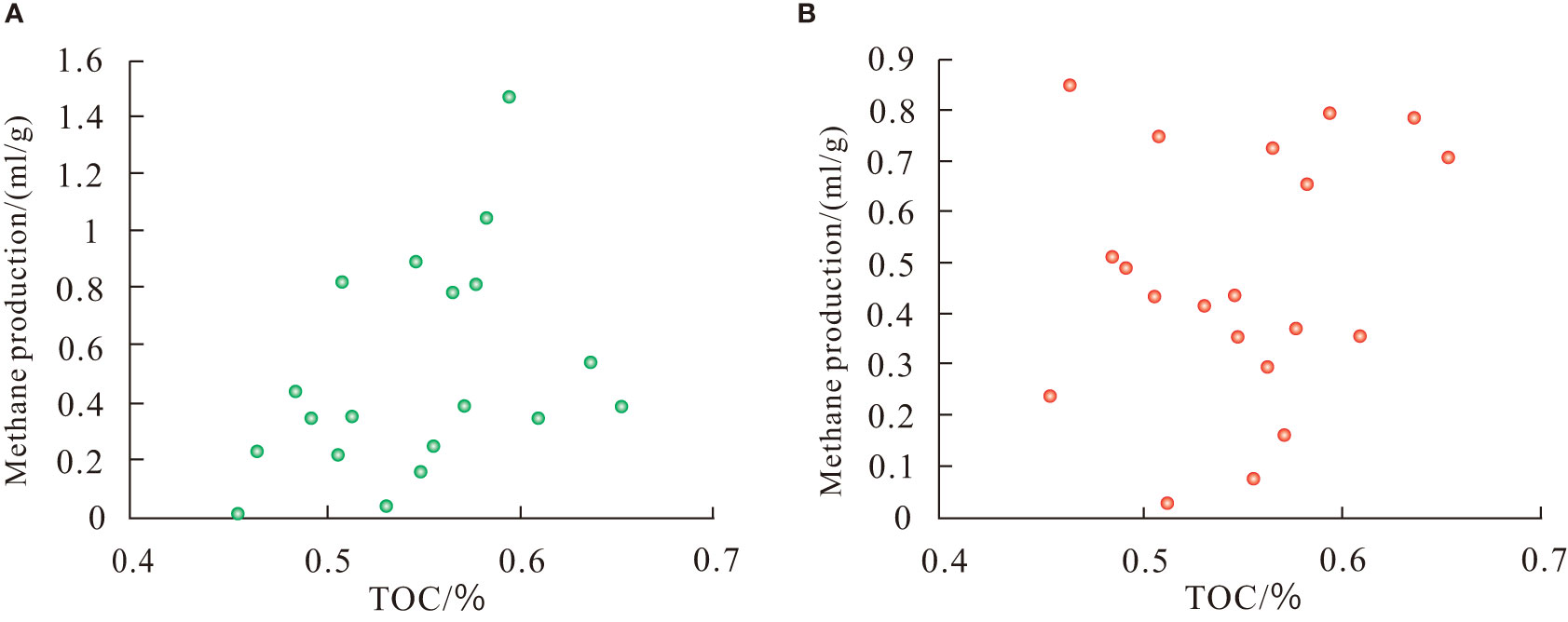
Figure 6 Intersection diagram of sediment organic carbon content (TOC) and methane production at optimal temperature (A) Room temperature; (B) 38°C.
According to the available experimental data,it can be seen that there is no significant correlation between TOC and methane yield. This indicates that TOC cannot be simply used to replace the index of organic matter abundance that affects gas production. TOC is one of the indicators of hydrocarbon generation potential because it can be used to characterize the abundance of organic matter, which is based on the theory of hydrocarbon generation by pyrolysis. The generation of biogas is a biochemical reaction in a strictly anaerobic environment, and its process is completely different from that of hydrocarbon generation by pyrolysis. TOC cannot accurately reflect the amount of effective organic matter available to microorganisms in organic matter. Two samples with the same TOC may not have the same content of organic matter available to microorganisms. What really affects methane production is the amount of metabolizable organic carbon contained in the sample, which is not currently available for accurate values. This is why the TOC of some samples is basically the same, and the methane production rates differ greatly when the ambient temperature and experimental time are the same.
Studies have shown that in the biogenic stage of sediments, methanogens and other microorganisms consume mainly cellulose, acetic acid and amino acids in organic matter, while some kerogen and other components cannot be directly utilized by bacteria and become invalid organic matter. The degree of difficulty for the carbon in different organic matters to be converted into gaseous carbon (CH4 and CO2) varies greatly, and the conversion ratio varies greatly, with the highest conversion rate reaching 69% and the lowest 2% (Zhang et al., 2008).
It can be seen that the types and characteristics of organic matter are important factors affecting the methane yield, which mainly depends on the amount of effective components that can be directly utilized by microorganisms. The quantity of these active components may be used as the index of organic matter abundance to evaluate the quality of biogas source rocks, which is called effective organic matter abundance in this paper. It should be a comprehensive index, which should contain the abundance information of specific components, and how to characterize, it still need a lot of detailed work based on geochemical process studies, laboratory simulation experiments and geological facts.
3.3.2 Bacterial community
The formation of biomethane is an anaerobic fermentation process, which can be divided into four stages. Each stage requires a different microbial community. The microorganisms involved in the whole process consist of many strains, including hydrolytic bacteria, fermentative bacteria, hydrogen and acetic acid producing bacteria, methanogens and other microbial groups (Brandon et al., 2012; Fan et al., 2017).
Methanogens are the last and most important part of this complex gas production process. They are a class of bacteria capable of anaerobic digestion of inorganic or organic compounds into methane gas. It relies on other microorganisms to degrade organic matter into simple compounds for reuse, and finally metabolizes methane through biochemical pathways under the action of other anaerobic bacteria and biological enzymes (Taiki et al., 2016). The two ways to form biomethane involve different bacterial communities, and methanogens are also the general term of one kind of bacteria rather than single kind of bacteria. The differences in the characteristics of bacterial communities in sediments directly determine which kinds of gas production routes occur and the intensity of gas production.
Therefore, the composition, biomass and activity of bacterial communities in sediments directly affect the production and yield of biomethane. In this process, many specific mechanisms are still unclear, but it has been repeatedly confirmed as one of the main factors affecting the methane yield.
3.3.3 Environmental factors
The results show that the biomethane yield is closely related to temperature, and temperature is the most important environmental factor affecting the methane yield. At present, most known methanogens are mesophilic. If the temperature is too low or too high, its activity will be affected and the biochemical action will be weakened or ceased. Under the optimal temperature, the microorganisms are the most active and the gas production rate is the highest.
The effect of depth on methane yield is mainly indirectly influenced by the difference of temperature and organic matter at different depths. Environmental pH value and other factors affect the yield of biomethane by influencing microbial activity (Giovana et al., 2018). In detail, different pH values affect the growth rate and product synthesis of bacteria by affecting the activity of related enzymes, cell membrane permeability, metabolic processes, and internal group decomposition, and may eventually change the gas production. In this experiment, the chemical composition of the pore water is still retained during the natural air drying of the sample. Redox reactions and their products have a certain effect on the formation of methane. The magnitude of the effect and how to eliminate the effect of pore water still require further experiments. In addition, a relatively arid climate, a saline environment with certain salinity and a relatively fast deposition rate are considered to be conducive to the preservation of organic matter, and thus play a positive role in the formation of biomethane (Qi et al., 1997).
4 Conclusion
(1) The biological gas production simulation experiment was conducted in the sediments in the natural gas hydrate development area of Dongsha Sea. The gas products were mainly composed of CH4 and CO2, and a small amount of H2 was generated in some experiments.
(2) In the biochemical gas production stage, the methane production rate is closely related to temperature. The most methane production occurred between 20°C and 40°C in the study area, and stopped about > 55°C. It is speculated that the shallow sediments in the seabed of the study area at 1400m are still in the biochemical gas production stage.
(3) The maximum methane yield in the study area can reach 1.47ml/g sediment, and the average is 0.48 mL/g sediment. Compared with other biological gas fields, the methane yield under the optimal temperature in the study area is relatively high, and the hydrocarbon generation potential is large, in favor of the formation of natural gas hydrate ore body (Zhang et al., 2019; Zhang et al., 2020b). The preservation and accumulation conditions of biogas may be the key to the formation of hydrate ore bodies.
(4) There is no significant correlation between organic carbon content (TOC) and methane yield. Using TOC as an evaluation index of resource potential of biological gas source rocks is not suitable here. This paper proposes effective organic matter instead of TOC, and further work is needed to establish this index.
(5) The factors affecting methane production include available organic matter, bacterial community and environmental factors. In detail, this is: the metabolizable organic carbon in organic matter, the control of the activity and quantity of different types of methanogens, the possible influence of pore water and pH.
Data availability statement
The original contributions presented in the study are included in the article/supplementary material. Further inquiries can be directed to the corresponding author.
Author contributions
PS: Writing—original draft, Methodology, Data analysis. WW: Methodology, Data analysis. CS: Writing—original draft, Data analysis. JLi: Methodology, Data analysis. WH: Methodology, Data analysis. ZX: Writing—original draft, Data analysis. JLia: Supervision. FW: Methodology, Project administration. ZW: Main idea, Methodology, Writing—review and editing. All authors contributed to the article and approved the submitted version.
Funding
This work was supported by the Guangdong Province Marine Economic Development (Six Major Marine Industries) Special Fund Project (No. [2021] No. 58), the Fourteenth Five-Year Plan” Prospective Basic Major Scientific and Technological Projects of CNPC (2021DJ4901), Hainan Research Institute of China Engineering Science and Technology Development Strategy (No. 21-HN-ZD-02-3);Guangzhou Science and Technology Planning Project (202201011434).
Conflict of interest
The authors declare that the research was conducted in the absence of any commercial or financial relationships that could be construed as a potential conflict of interest.
Publisher’s note
All claims expressed in this article are solely those of the authors and do not necessarily represent those of their affiliated organizations, or those of the publisher, the editors and the reviewers. Any product that may be evaluated in this article, or claim that may be made by its manufacturer, is not guaranteed or endorsed by the publisher.
References
Brandon R. B., Fumio I., Yuki M., Taiki F., Carme H., Antoni R., et al. (2012). Bacterial dominance in subseafloor sediments characterized by methane hydrates. FEMS Microbiolo Eco 81 (1), 88–98. doi: 10.1111/j.1574-6941.2012.01311.x
Chen C. M., Zhong L. F., Wan Z. F., Cheng C. Y., Zhou W., Xu X. (2022). Geochemical characteristics of cold-seep carbonates in shenhu area, south China Sea. J. Oceanology Limnology. 40 (3), 969–985. doi: 10.1007/s00343-021-1112-z
Fan X. L., Guo R. B., Yuan X. Z., Qiu Y. L., Yang M., Wang F., et al. (2015). Biogas production from macrocystis pyrifera biomass in seawater system. Bioresource Technolo. 197, 339–347. doi: 10.1016/j.biortech.2015.08.128
Fan X. B., Liang Q. Y., Liu M. Y., Yu T. T., Wang Y., Wang F. P. (2017). The diversity and richness of archaea in the northern continental slope of south China Sea. Microbiol. China. 44 (7), 1589–1601. doi: 10.13344/j.microbiol.china.170159
Fu N., Lin Q., Liu Y. L. (2011). Analysis on potential gas source of gas hydrate from the original characteristics of shallow gas in the north of the south China Sea. Geoscience 25 (2), 332–339. doi: 10.3969/j.issn.1000-8527.2011.02.017
Gao Y., Jin Q., Shuai Y. H., Wang H. (2011). Genetic types and accumulation conditions of biogas in bohaiwan basin. Natural Gas Geoscience. 22 (03), 407–414. doi: 10.1002/9781444350302.wbhe1011
Gao L., Song J. (1998). Modeling lab and resource evaluation of biogenic gas in baoshan basin, yunnan province. J. Chengdu Univ. Technology. 25 (4), 487–494. doi: 10.1088/0256-307X/16/9/027
Giovana O. F., Paulo I. H., Juline M. W., Tatiana V. V., Piter D. F. G., Caio B. L., et al. (2018). Rapid isolation of culturable microalgae from a tropical shallow lake system. J. Appl. Phycol. 30 (3), 1807–1819. doi: 10.1080/09593330.2015.1069900
Gong J. M., Cao Z. M., Chen J. W., Zhang M., Li J., Yang G. F. (2009). Simulation experiment on gas generation of sediments in area discovered gas hydrate. Sci. China. 039 (002), 204–211. doi: 10.1016/S1874-8651(10)60080-4
Gong J. L., Sun X. M., Li Xu., Lu H. F. (2017). Contribution of thermogenic organic matter to the formation of biogenic gas hydrate: evidence from geochemical and microbial characteristics of hydrate-containing sediments in the taixinan basin, south China Sea. Mar. Petrol Geol. 80, 432–449. doi: 10.1016/j.marpetgeo.2016.12.019
Gong Y. H., Wu S. G., Zhang G. X., Wang H. B., Liang J. Q., Guo Y. Q., et al. (2008). Relation between gas hydrate and geologic structures in dongsha islands Sea area of south China Sea. Mar. Geology Quaternary Geology. 28 (1), 99–104. doi: 10.16562/j.cnki.0256-1492.2008.01.013
Guan H. X., Daniel B., Jorn P., Liang Q. Y., Feng D., Yang S. X., et al. (2018). Lipid biomarker patterns of authigenic carbonates reveal fluid composition and seepage intensity at haima cold seeps, south China Sea. J. Asian Earth Sci. 168, 163–172. doi: 10.1016/j.jseaes.2018.04.035
Guan H. X., Feng D., Wu N. Y., Chen D. f. (2016). Methane seepage intensities traced by biomarker patterns in authigenic carbonates from the south China Sea. Org Geochem. 91, 109–119. doi: 10.1016/j.orggeochem.2015.11.007
He J. X., Yan W., Zhu Y. H., Zhang W., Gong F. X., Liu F. L., et al. (2013). Bio genetic and sub biogenetic gas resource potential and genetic types of natural gas hydrates in the northern marginal basins of south China Sea. Natural Gas Industry 033 (006), 121–134. doi: 10.3787/j.issn.1000-0976.2013.06.023
Hu Y., Luo M., Chen L. Y., Liang Q. Y., Feng D., Tao J., et al. (2018). Methane source linked to gas hydrate system at hydrate drilling areas of the south China Sea: porewater geochemistry and numerical model constraints. J. Asian Earth Sci. 168, 87–95. doi: 10.1016/j.jseaes.2018.04.028
Kong Y., Lei H. Y., Xu J., Wang B., Pan F. L., Zhang J., et al. (2018). The Coupling Between Microorganisms and Natural Gas Hydrates in the Northern South China Sea. Journal of xiamen university (Natural science). 57(06):768-777 doi: 10.6043/j.issn.0438-0479.201805029
Liang J. Q., Zhang G. X., Lu J. A., Su P. B., Sha Z. B., Gong Y. H., et al. (2016). Accumulation characteristics and genetic models of natural gas hydrate reservoirs in the NE slope of the south China Sea. Natural Gas Industry. 36 (010), 157–162. doi: 10.3787/j.issn.1000-0976.2016.10.020
Liu Y., Li Q., Lin X. Y., Kong Y., Xiao Q. L. (2020). Evolution characteristics of biogas in seabed sediments and their influencing factors on gas sources. Mar. Geology Quaternary Geology. 40 (06), 169–177. doi: 10.16562/j.cnki.0256-1492.2020081303
Liu C., Wang J., Ji X. Y., Qian H. L., Huang L. L., Lu X. H. (2015). The biomethane producing potential in China: a theoretical and practical estimation. Chin. J. Chem. Eng. 24 (7), 920–928. doi: 10.1016/j.cjche.2015.12.025
Min W., Yu Z. A., Zheng J., Zhang H. X. (2014). Microbial diversity and biogenic methane potential of a thermogenic-gas coal mine. Int. J. Coal Geol. 134-135, 96–107. doi: 10.1016/j.coal.2014.09.008
Pandey R., Haroalani S., Feng R. M., Zhang J., Liang Y. N. (2016). Changes in gas storage and transport properties of coal as a result of enhanced microbial methane generation. Fuel. 179, 114–123. doi: 10.1016/j.fuel.2016.03.065
Qi H. F., Guan D. S., Qian Y. B., Zhang X., Huang B. J., Yan J. F, et al. (1997). Biogenic gas accumulation conditions in China. Mar. Origin Petroleum Geology. 03), 64. doi: CNKI:SUN:HXYQ.0.1997-03-017
Rashid M. A., Vilks G. (1977). Environmental controls of methane production in Holocene basin in eastern. Org Geochem. 1, 53–59. doi: 10.1016/0146-6380(77)90008-0
Song N. N. (2010). Biogenic gas generation model and source rock evaluation in sanhu area of qaidam basin (Yangtze: Yangtze University).
Su P. B., Lei H. Y., Liang J. Q., Sha Z. B., Fu S. Y., Gong Y. H. (2010). Characteristics of gas source in the waters of shenhu and their significance to gas hydrate accumulation. Natural Gas Industry. 30 (10), 103–108. doi: 10.3787/j.issn.1000-0976.2010.10.025
Su P. B., Liang J. Q., Sha Z. B., Fu S. Y. (2013). Gas sources condition of gas hydrate formation in shenhu deep water zone. J. Southwest Petroleum University. 36 (2), 1–8. doi: 10.11885/j.issn.1674-5086.2013.10.16.01
Taiki K., Hideyoshi Y., Hiroshi A. T., Miki A., Tetsuya F., Susumu S. (2016). Changes in microbial communities associated with gas hydrates in subseafloor sediments from the nankai trough. FEMS Microbiolo Eco 92 (8), fiw093. doi: 10.1093/femsec/fiw093
Wan Z. F., Luo J. S., Yang X. L., Zhang W., Liang J. Q., Zuo L. H., et al. (2022a). The thermal effect of submarine mud volcano fluid and its influence on the occurrence of gas hydrates. J. Mar. Sci. Eng. 10 (6), 832. doi: 10.3390/jmse10060832
Wan Z. F., Zhang W., Ma C., Liang J. Q., Li A., Meng D. J., et al. (2022b). Dissociation of gas hydrates by hydrocarbon migration and accumulation-derived slope failures: an example from the south china sea. Geosci. Frontiers. 13 (2), 101345. doi: 10.1016/j.gsf.2021.101345
Wei J. G., Yang S. X., Liang J. Q., Lu J. A., Liu S. X., Zhang W. (2018). Impact of seafloor drilling on methane seepage — enlightenments from natural gas hydrate drilling site GMGS2-16,northern south China Sea. Mar. Geology Quaternary Geology. 038 (005), 63–70. doi: 10.16562/j.cnki.0256-1492.2018.05.006
Whiticar M. J., Faber E., S M. (1986). Biogenic methane formation in marine and freshwater environments: CO2 reduction vs. acetate fermentation–isotope evidence. Geochim Cosmochim Ac. 50 (5), 693–709. doi: 10.1016/0016-7037(86)90346-7
Wu N. Y., Zhang H. Q., Yang S. X., Liang J. Q., Wang H. B. (2007). Preliminary discussion on natural gas hydrate (NGH) reservoir system of shenhu area, north slope of south China Sea. Natural Gas Industry. 27 (9), 1–6. doi: 10.3321/j.issn:1000-0976.2007.09.001
Wu B. H., Zhang G. X., Zhu Y. H., Lu Z. Q., Chen B. Y. (2003). Progress of gas hydrate investigation in China offshore. Earth Sci. Frontiers. 10 (1), 177–189. doi: 10.3321/j.issn:1005-2321.2003.01.021
Zhang G. X., Chen F., Sha Z. B., Liang J. Q., Su X., Lu H. F. (2017). The geological evolution process of natural gas hydrate reservoirs in the northeastern south China Sea. Earth Sci. Frontiers. 24 (4), 015–023. doi: 10.13745/j.esf.yx.2016-12-27
Zhang W., Chen C. M., Su P. B., Wan Z. F., Huang W., Shang J. J., et al. (2022). Formation and implication of cold-seep carbonates in the southern south China Sea. J. Asian Earth Sci. 241, 105485. doi: 10.1016/j.jseaes.2022.105485
Zhang G. X., Liang J. Q., Lu J. A., Yang S. X., Zhang M., Holland M., et al. (2015). Geological features, controlling factors and potential prospects of the gas hydrate occurrence in the east part of the pearl river mouth basin, south China Sea. Mar. Petrol Geol. 67, 356–367. doi: 10.1016/j.marpetgeo.2015.05.021
Zhang W., Liang J. Q., Su P. B., Wei J. G., Sha Z. B., Lin L., et al. (2018). Migrating pathways of hydrocarbons and their controlling effects associated with high saturation gas hydrate in shenhu area, northern south China Sea. Geology China. 045 (001), 1–14. doi: 10.12029/gc20180101
Zhang W., Liang J. Q., Wan Z. F., Su P. B., Huang W., Wang L. F., et al. (2020a). Dynamic accumulation of gas hydrates associated with the channel-levee system in the shenhu area, northern south China Sea. Mar. Petrol Geol. 117, 104354. doi: 10.1016/j.marpetgeo.2020.104354
Zhang W., Liang J. Q., Wei J. G., Lu J. A., Su P. B., Lin L., et al. (2019). Geological and geophysical features of and controls on occurrence and accumulation of gas hydrates in the first offshore gas-hydrate production test region in the shenhu area, northern south China Sea. Mar. Petrol Geol. 114, 104191. doi: 10.1016/j.marpetgeo.2019.104191
Zhang W., Liang J. Q., Yang X. L., Su P. B., Wan Z. F. (2020b). The formation mechanism of mud diapirs and gas chimneys and their relationship with natural gas hydrates: insights from the deep-water area of qiongdongnan basin, northern south China Sea. Int. Geology Review. 62 (7-8), 789–810. doi: 10.1080/00206814.2018.1491014
Keywords: Dongsha area, simulation experiment, biogas, optimal temperature, gas production, GMGS2
Citation: Su P, Wei W, Shi C, Li J, Han W, Xiao Z, Liang J, Wang F and Wan Z (2023) Gas production characteristics of biogenic gas simulated in hydrate-developing area of Dongsha area, South China Sea. Front. Mar. Sci. 10:1184641. doi: 10.3389/fmars.2023.1184641
Received: 13 March 2023; Accepted: 19 April 2023;
Published: 05 June 2023.
Edited by:
Dong Feng, Shanghai Ocean University, ChinaReviewed by:
Jinan Guan, Guangzhou Institute of Energy Conversion (CAS), ChinaJiliang Wang, Institute of Deep-Sea Science and Engineering (CAS), China
Copyright © 2023 Su, Wei, Shi, Li, Han, Xiao, Liang, Wang and Wan. This is an open-access article distributed under the terms of the Creative Commons Attribution License (CC BY). The use, distribution or reproduction in other forums is permitted, provided the original author(s) and the copyright owner(s) are credited and that the original publication in this journal is cited, in accordance with accepted academic practice. No use, distribution or reproduction is permitted which does not comply with these terms.
*Correspondence: Feifei Wang, Feifeiwang2017@163.com; Zhifeng Wan, wanzhif@mail.sysu.edu.cn