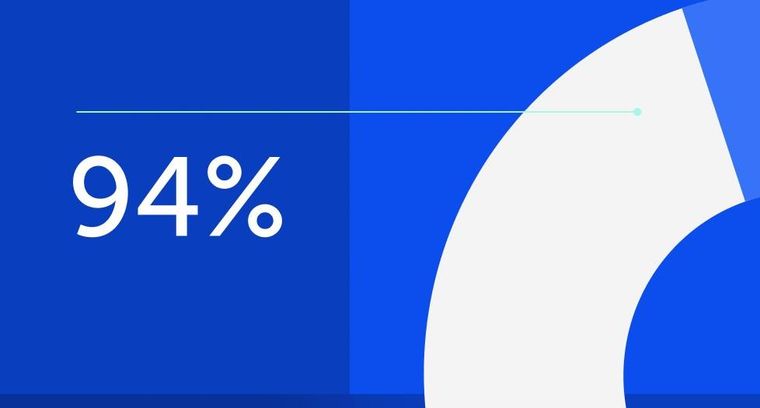
94% of researchers rate our articles as excellent or good
Learn more about the work of our research integrity team to safeguard the quality of each article we publish.
Find out more
ORIGINAL RESEARCH article
Front. Mar. Sci., 04 August 2023
Sec. Marine Pollution
Volume 10 - 2023 | https://doi.org/10.3389/fmars.2023.1182294
This article is part of the Research TopicModel Organisms in Marine PollutionView all 4 articles
Introduction: Estuarine ecosystems are under high anthropogenic pressure and receive a wide Q7 variety of contaminants, including metals. They can remain trapped in estuarine sediments at high concentrations for long periods but eventually they can be released to the overlying water and become toxic for the resident benthic biota. As a consequence, biomonitoring programs surveying the health status of estuaries and coastal areas count with benthic species for the assessment of health disturbances in these specific ecosystems. The present investigation aims at recognizing toxicopathic effects elicited in estuarine life stages of the benthic fish Solea senegalensis on exposure to waterborne Cd, a model compound for metal contamination.
Methods: Biological responses to metal exposure were assessed based on the application of a “biomarker + histopathology” approach. Two-year old sole juveniles were exposed to various concentrations of waterborne Cd for 3 and 7 days (Control; Low Cd: 1 µg/l; Mid Cd: 10 µg/l and High Cd: 1000 µg/l). Liver samples were collected for chemical analysis at day 7. Biological samples were collected at days 3 and 7 for biochemical (brain and liver), histochemical (liver) and histopathological analysis (liver, gills and gonad).
Results: Brain acetyl cholinesterase was inhibited after 7 days exposure, indicating neurotoxic effects on exposure to 1000 µg Cd/l. Hepatic catalase and glutathione-Stransferase were induced at day 3 and inhibited at day 7, which suggests a bell-shaped response. A dose-dependent lysosomal membrane destabilization in hepatocytes was recorded at day 7. In parallel, histopathological lesions in gills, liver and gonad were more frequent at day 7 in soles exposed to high Cd concentrations.
Discussion: Overall, the “biomarker + histopathology” approach revealed that waterborne Cd causes toxicopathic effects in sole juveniles upon exposure to environmentally relevant concentrations (10 µg Cd/l). The effects were clearly time dependent, and thus it is conceivable that more severe biological effects would be elicited by these low concentrations of Cd at longer exposure periods. Therefore, biomarkers and histopathology in combination provide early-warning indications of altered health status after waterborne Cd exposure in sole juveniles, a prospective sentinel for biological effects assessment of metal contamination in pollution monitoring programs in estuaries and coastal areas.
Estuarine ecosystems under anthropogenic pressure endure a wide variety of contaminants, including metals, which occur dissolved in water or accumulated in the sediment. Globally, the concentration of metals in marine sediments has increased by 5-10 times in the last century (Sharifuzzaman et al., 2016); these can remain trapped in the sediment for long periods but they can also be released to the overlying water and become toxic for the resident benthic biota (Eggleton and Thomas, 2004);. Thus, because they live in close contact with the sediment and are consequently under the influence of both waterborne and sediment contamination, there is a growing interest in benthic species to be used as potential sentinels in pollution monitoring programs in estuaries.
Sole (Solea spp.), a benthic fish, is an emerging sentinel suitable for coastal pollution monitoring (S. senegalensis: Fonseca et al., 2011; Gonçalves et al., 2013; S. solea: Jebali et al., 2013; Constenla et al., 2021; Solea spp.: Siscar et al., 2013; Solé et al., 2013; Briaudeau et al., 2019). Larvae inhabit upstream areas, young juveniles extend throughout the estuary and old juveniles or young adults concentrate in the outer estuary and coastal areas, whilst sexually mature adults migrate offshore to spawning grounds (Primo et al., 2013). Thus, old juveniles and young adults may serve as pollution sentinels in estuaries (S. senegalensis: Oliva et al., 2010; Gonçalves et al., 2013; S. solea: Amara et al., 2007; Cuevas et al., 2015a; Constenla et al., 2021: Solea spp.: Briaudeau et al., 2019) whilst adults from offshore fishing grounds are used to determine pollutant tissue levels in seafood safety programs (Solea spp: Siscar et al., 2013; Solé et al., 2013). The interpretation of these field data relays on knowledge acquired in laboratory studies of controlled exposure of test organisms to model chemicals.
Laboratory experiments have shown that the response of flatfish to pollutant insult varies along its life cycle (e.g. larvae vs. juveniles; Cao et al., 2010); however, exposure experiments with sole are mainly restricted to early life stages that are not representative of estuaries and coastal areas regarding biological effects assessment in pollution monitoring programs (Costa et al., 2009; Oliva et al., 2009; Kalman et al., 2010; Camacho et al., 2020; Ortiz-Delgado et al., 2021; Pavlaki et al., 2021). Therefore, these studies have a limited value to transfer laboratory results to the field realm. Fortunately, although more research is still needed, sole adults, and juveniles to a lesser extent, are being increasingly used as test organisms in laboratory experiments (S. senegalensis: Solé et al., 2008; Ghribi et al., 2019; Briaudeau et al., 2020; Briaudeau et al., 2021). In addition, it is worth mentioning that although both species of sole are present and used in monitoring programs, most ecotoxicological studies made in laboratory conditions use S. senegalensis for experimentation, as it is easily available from farming production.
Together with the life stage, the route of exposure is also crucial regarding the interpretation of data on metal accumulation and biological effects in fish (Guo et al., 2019; Pavlaki et al., 2021). Available data often refer to laboratory experiments based on intraperitoneal injection (Kalman et al., 2010; Solé et al., 2014), dietary exposure (Pavlaki et al., 2021) or whole-sediment exposure (Salamanca et al., 2008; Costa et al., 2010; Briaudeau et al., 2020). Certainly, dietary intake of metals seems to be a major route of exposure in teleosts (Mathews and Fisher, 2009); yet, waterborne exposure may also be relevant, as shown in sole in response to a variety of pollutants (Solé et al., 2008; Oliva et al., 2009; Briaudeau et al., 2021).
Cadmium is a highly toxic metal commonly used as model contaminant in toxicological experimentation (Nordberg, 2009; Levit, 2010). It accumulates in fish tissues, as shown in adults in field studies and in adults or early life stages after peritoneal injection or dietary exposure to the metal (Roméo et al., 2000; Atli et al., 2006; Bouraoui et al., 2008, Carriquiriborde and Ronco, 2008). Likewise, Cd accumulation has been reported in fish after waterborne exposure in adults (van Dyk et al., 2007; Pereira et al., 2016), fries (Hansen et al., 2002) and young juveniles (Alvarado et al., 2005; Kumar et al., 2008), mainly in freshwater species, but never in sole to our knowledge. Exceptionally, Cd accumulation was recently investigated in young juvenile S. senegalensis via a combined waterborne and dietary exposure (Pavlaki et al., 2021).
Exposure to Cd provokes responses in cells of marine organisms, including some recommended as endpoints for biological effect assessment in pollution monitoring programs (UNEP/RAMOGE, 1999; Davies and Vethaak, 2012; OSPAR Commission, 2013). Cadmium and other neurotoxic pollutants inhibit acetylcholinesterase (AChE) in sole and other fish species (Solé et al., 2012; Siscar et al., 2013; Burgeot et al., 2017; Ortiz-Delgado et al., 2021). As other metals do, Cd also produces oxidative stress (Regoli et al., 2011; Alijani et al., 2017), which is reflected by enhanced production of reactive oxygen species (ROS), disturbance of antioxidant defences and alterations in xenobiotic metabolism (Livingstone, 2001; Regoli and Giuliani, 2014). The presence of Cd has been related with changes in superoxide dismutase (SOD) and catalase (CAT) activities in fish liver, both in metal contaminated sites and upon exposure in the laboratory (Atli et al., 2006; Oliva et al., 2012a; Souid et al., 2013). Exposure to metals and other pollutants in mixtures enhances hepatic glutathione-S-transferase (GST) activity in sole (Fonseca et al., 2011; Ghribi et al., 2019). Lysosomal alterations are effect biomarkers used in fish as general responses to a variety of environmental stressors (Köhler et al., 2002; JAMP, 2003; Baršienė et al., 2006; ICES, 2006). Particularly, the lysosomal membrane stability is a core biomarker used as a guide parameter for biological effect assessment in marine pollution monitoring (UNEP/RAMOGE, 1999; Law et al., 2010; OSPAR Commission, 2010; HELCOM, 2012; UNEP/MAP, 2014; ICES, 2015). Hepatocytes of fish collected from polluted sites typically present lysosomal enlargement, membrane destabilisation and changes in lysosomal content (Broeg et al., 2002; Baršienė et al., 2006; Zorita et al., 2008; Burgeot et al., 2017). Alike, Cd exposure under controlled laboratory conditions causes comparable lysosomal alterations in mollusc digestive cells and flatfish hepatocytes (Lekube et al., 2000; Alvarado et al., 2005; Marigómez et al., 2005; Izagirre et al., 2014). Further on, histopathological lesions in gills and liver are a powerful signal of the effects caused by exposure to pollutants in flatfish, including sole (Bernet et al., 1999; Stentiford et al., 2003; Feist et al., 2004). Multi-organ histopathological approaches have been applied in sole in field and laboratory studies (Costa et al., 2009; Oliva et al., 2013; Cuevas et al., 2015a; Cuevas et al., 2015b; Briaudeau et al., 2020; Briaudeau et al., 2021). The use of different target organs for histopathological assays is essential to identify alterations in vital functions such as metabolic processes (e.g. in liver), respiratory functions (e.g. gills) and reproduction (e.g. gonads). Histopathology has provided indication of Cd toxicity in fish (van Dyk et al., 2007) and in particular in sole (Costa et al., 2013); as shown in 4-month old juveniles, in which ecologically relevant concentrations (10 µg Cd/l) provoked complex histopathological alterations linked to organ-specific responses (Costa et al., 2013).
In this context, the present investigation aimed at identifying toxicopathic effects in waterborne Cd exposed 2-years old Solea senegalensis, which are potential sentinels for pollution monitoring in estuaries and coastal areas. For this purpose, the “biomarker + histopathology” approach was applied in order to identify dose-response relationships integrating responses elicited at different levels of biological complexity.
Solea senegalensis 2-year old juveniles (24.5 ± 1.8 cm length; 165.0 ± 37.1 g total wet-weight) were acquired from a fish farm (Ferme Marine de l’Adour, Stolt Sea Farm, Anglet, France) and transported to the Research Centre for Experimental Marine Biology and Biotechnology “Plentziako Itsas Estazioa” (PiE-UPV/EHU; Plentzia Marine Station) prior experimentation. Fish were exposed for 7 d to different concentrations of Cd; a control level refereeing to the Cd concentration of seawater with no added Cd; two environmentally relevant levels as Low Cd = 1 µg/l and Mid Cd = 10 µg/l; and one high concentration selected to induce short-term biological responses as High Cd = 1000 µg/l). Stocking density was 4-6 kg/m2. Each experimental group (n=26) was placed in a closed-system in a 500 l capacity tank with continuous aeration. Water changes were performed every second day to ensure optimal water conditions: pH=8, salinity=31-33 PSU, temperature=13-14°C, dissolved O2 = 6-8 mg/l and total ammonia=0 mg/l. Photoperiod throughout the experiment was set at 12:12 h light:dark. Fish were fed with commercial food from BioMar Iberia S.A. (Dueñas, Spain): 0.3g/fish/day. Samples of liver, gills, brain and gonad were collected from each experimental group (n = 13) after 3 and 7 d of exposure to Cd.
For each experimental group, water samples were collected 3, 24 and 48 h after contaminant load. Analyses of Cd content in water (1:10, v/v) were performed by inductively coupled plasma with mass detector (7700×, Agilent Technologies, Palo Alto, USA) using a MicroMist micro-uptake glass concentric nebulizer (Glass Expansion, West Melbourne, Victoria, Australia). In order to reduce MO+ formation in the plasma, the spray chamber was Peltier cooled at 2°C. Finally, standard nickel cones (sample and skimmer) were generally used. The acquisition masses and integration times provided more than sufficient sensitivity to meet all certified values. The optimization of the ICP-MS conditions was achieved by adjusting the torch position and tuning for reduced oxide and doubly charged ion formation with a standard tuning solution containing 1.0 μg/l of 7Li, 24Mg, 59Co, 89Y, 140Ce and 205Tl in 1.0% HNO3. This equipment includes a collision cell (He gas, ORS3 system, Agilent Technologies©) for discriminate spectral interferences with high performance for all the trace metals considered in here. In addition, EPA 6020 recommendations were followed for interference overcoming such as correction equations for cadmium.
Biometric data (wet-weight; length; liver wet-weight; gonad wet-weight) were recorded throughout the experiment to calculate the condition factor K, the hepatosomatic index HSI and the gonadosomatic index GSI, as calculated in Briaudeau et al. (2021).
Liver samples were collected at day 7 for chemical analysis (n=6 per experimental group) and were pooled to obtain a minimum of 1 g dry weight per group. After lyophilisation, tissue samples were digested in an acid mixture (2HCl:1HNO3) at 180°C for 15min, using a microwave system (MARS 5 Xpress CEM Corporation Instrument). Cadmium content was determined by Inductively Coupled Plasma with mass detector (7700x, Agilent Technologies, Palo Alto, USA) using a MicroMist micro-uptake glass concentric nebulizer (Glass Expansion, West Melbourne, Victoria, Australia).
Liver and brain samples (n=6 per experimental group) were collected at days 3 and 7 for biochemical analysis. After sample homogenisation (0.1 M potassium phosphate buffer, pH 7.4; 1:4 for liver and 1:5 for brain) and centrifugation (30 min, 12000 g, 4°C), the liver post-mitochondrial supernatant (PMS) was used to determine catalase (CAT), superoxide dismutase (SOD) and glutathione-S-transferase (GST) enzyme activities and the brain PMS was used for acetylcholinesterase (AChE) activity using a BioTek Eon microplate spectrophotometer. Enzyme activities were expressed as a function of the protein concentration in the samples, which was determined in triplicate at 595 nm according to the Bradford’s method adapted to microplate and using bovine serum albumin as standard (Guilhermino et al., 1996). All enzyme assays were performed at 25°C.
AChE activity was determined according to the Ellman’s colorimetric method adapted to microplate (Guilhermino et al., 1996) at 412 nm. AChE activity was expressed as specific activity (nmol DTNB/min/mg prot). GST activity was determined by the Habig’s method adapted to microplate and using bovine serum albumin as standard (Guilhermino et al., 1996). Enzyme activity was measured at 340 nm for 6 min and expressed as nmol/min/mg prot. CAT activity was determined by the method of Claiborne (1985) as absorbance decrease at 240 nm. Results were expressed as μmol H2O2/min/mg prot. SOD activity was determined by a colorimetric method using a SIGMA kit (SOD Determination kit; ref: SIGMA 19160) at 450 nm and SOD activity (inhibition rate %) was calculated following manufacturer’s protocol and expressed as nmol/min/mg prot.
Liver samples (n=12 per experimental group) were collected at days 3 and 7 for histochemical analysis. Lysosomal membrane stability (LMS) was assessed after histochemical demonstration of the acid phosphatase activity in hepatocyte lysosomes (UNEP/RAMOGE, 1999), as detailed by Briaudeau et al. (2020); Briaudeau et al. (2021). Lysosomal structural changes were determined by image analysis after histochemical demonstration of β-glucuronidase activity in fish hepatocytes (Alvarado et al., 2005), as detailed by Briaudeau et al. (2020); Briaudeau et al. (2021). Intracellular accumulation of neutral lipids was assessed according to Marigómez and Baybay-Villacorta (2003), after Oil Red O (ORO) staining.
Gill, liver and gonad samples (n=12 per experimental group) were collected at days 3 and 7 for histopathological examination. Gills were fixed in Bouin’s solution (24 h, 4°C) and rinsed in formic acid (8% v/v, 24 h). Liver and gonad samples were fixed in 4% neutral buffered formaldehyde (24 h, 4°C). Fixed samples were dehydrated in a graded series of ethanol, cleared and embedded in paraffin (Leica ASP 300S). Sections (5 μm) were obtained using a rotary microtome (Leica RM 2125RTS) and stained with haematoxylin-eosin (H&E).
Gonad histological sections were examined at a light microscope to determine individuals’ gender and gamete developmental stages. Male gamete developmental stages were classified in five stages as described by García-López et al. (2006). Female gamete developmental stages were classified in four stages as described by Murua and Motos (2006).
The histopathological examination of gill, liver and gonad samples was based on previous publications (BEQUALM, 2001; Costa and Costa, 2008; Zorita and Cuevas, 2014; Briaudeau et al., 2019). For each histopathological lesion identified, the percentage occurrence of the alteration within an experimental group was calculated in gills and liver and for each gender in gonads. This parameter is referred to as lesion prevalence. Only lesions with a persistent occurrence were included in the calculation of histopathological indices.
For each organ, each histopathological lesions identified was given a weight w (1: minimal; 2: moderate; 3: severe) and a score value a (from 0 to 4 based on the tissue area affected and the degree of cellular change observed). The weighted histopathological index (I) were calculated for each individual and each organ as: where “wj” is the weight of the jth histopathological alteration and “aj” the score given to the jth alteration for the individual h.
The IBR index (Beliaeff and Burgeot, 2002; Broeg and Lehtonen, 2006; Devin et al., 2014) was calculated according to Marigómez et al. (2013) based on the integration of biomarkers (AChE, GST, LP) and histopathological indices (Igills, Iliver), as previously applied in sole (Briaudeau et al., 2020; Briaudeau et al., 2021). Parameters were selected to illustrate responses recorded at different levels of biological complexity.
Statistical analyses were carried out using IBM SPSS Statistics Base 22.0. Normality of data (Shapiro’s test) and homogeneity of variance (Levene’s test) were tested before statistical analysis. Two-way ANOVAs were performed to analyse the effects of the waterborne concentration of Cd ([Cd]), exposure time (T) and their combination ([Cd]×T) on all parameters. In the case of non-parametric variables, a logarithmic transformation was applied (CAT, NvL and VvNL). For normal data, differences between experimental groups were tested using the parametric one-way ANOVA test with a Duncan’s post-hoc test. Differences throughout exposure time were tested with the Student’s t-test. For non-normal data set, the non-parametric Kruskal-Wallis’ test with the Dunn’s post-hoc test was used to analyse differences in biological data between experimental groups and the Mann-Whithney’s U-test was used to detect differences throughout exposure time. The Z-score test and the Pearson’s Chi2-test were used to determine significant differences in histopathological lesion prevalence between experimental groups and throughout exposure time. Significant differences in chemical data were tested with the Z-score test. The parametric Pearson’s correlation test was used to assess associations between concentrations of Cd in water and in liver samples. Level of significance for all analyses was p =0.05.
The concentrations of Cd measured in seawater in the different experimental groups were slightly lower than the corresponding nominal ones but in accordance with the gradient of Cd concentrations selected for the experimental setup (Table 1). The Cd concentration in liver after 7-day exposure was below detection limits except in High Cd exposed sole, in which the metal was clearly accumulated above its concentration in seawater (Table 1).
Table 1 Cd concentrations (µg/l) determined in water samples after 3, 24, and 48 hr of contaminant load.
Overall, the length (24.5 ± 1.8 cm) and wet-weight (165.0 ± 37.1 g) were constant throughout the experiment. Likewise, the indices K (1.1 ± 0.1), HSI (1.0 ± 0.3), GSImale (0.08 ± 0.10) and GSIfemale (0.94 ± 0.19) did not differ between exposure groups.
Brain AChE activity was significantly affected by the interaction between Cd concentration and exposure time ([Cd]×T; 2-way ANOVA; Table 2), varying from 88.50 ± 4.12 nmol/min/mg prot in Low Cd exposed soles at day 7 to 66.19 ± 2.85 nmol/min/mg prot in High Cd exposed soles at day 7 (Figure 1A). Thus, AChE activities tended to increase in Control and Low Cd exposed soles at day 7 whilst activities from High Cd exposed soles decreased at day 7 and were lower than levels detected in other exposure groups. Hepatic CAT activity was significantly affected by Cd concentration and the [Cd]×T interaction (2-way ANOVA; Table 2). The highest CAT activity, detected in High Cd exposed soles at day 3, decreased significantly at day 7, being lower than levels recorded in the other exposure groups (Figure 1B). Hepatic GST activity was affected by exposure time and the [Cd]×T interaction (2-way ANOVA; Table 2). Thus, levels of GST activity detected at day 3 were higher in Cd exposed soles than in Control soles and decreased in all experimental groups at day 7 (Figure 1C). In contrast, hepatic SOD activity only varied with time (2-way ANOVA; Table 2), being higher at day 7 than at day 3 for all the exposure groups (Figure 1D).
Table 2 Summary of the 2-way ANOVAs performed to analyse the effects of [Cd] (d.f.: 3), time of exposure (d.f.: 1) and their combination (“[Cd] × T”, d.f.: 3) on biomarkers and histopathology (lesion stages and indices) in S. senegalensis exposed to different concentrations of [Cd] for 3 and 7 days.
Figure 1 Brain acetylcholinesterase (A) and hepatic catalase (B), glutathione-S-transferase (C) and superoxide dismutase (D) enzyme activities measured in S. senegalensis exposed to different concentrations of Cd for 3 and 7 days. Different letters indicate significant differences among experimental groups of the same sampling time; asterisks indicate significant differences between exposure times for each treatment (p<0.05); AChE, acetylcholinesterase; CAT, catalase; GST, glutathione-S-transferase; SOD, superoxide dismutase.
Lysosomal LP was significantly affected by Cd concentration, exposure time and the [Cd]×T interaction (2-way ANOVA; Table 2); ranging from 30.48 ± 1.64 min in Control soles at day 7 to 7.48 ± 1.00 min in High Cd exposed soles at day 7 (Figure 2A). LP increased at day 7 in Control soles whilst it decreased in all Cd exposed groups, in particular in the case of Mid Cd and High Cd groups (Figure 2A). Lysosomal VvL and NvL were significantly affected by Cd concentration and the [Cd]×T interaction (2-way ANOVA; Table 2). At day 3, the lowest VvL was recorded in High Cd exposed soles whilst the values of other lysosomal biomarkers were constant between exposure groups (Figures 2B-D). At day 7, the highest VvL and the lowest S/VL were detected in Mid Cd exposed soles (Figures 2B, C) and NvL only decreased in Low Cd exposed soles (Figure 2D). VvNL was significantly affected by Cd concentration (2-way ANOVA; Table 2); ranging from 0.046 ± 0.013 µm3/µm3 (High Cd soles, at day 7) to 0.252 ± 0.106 µm3/µm3 (Low Cd soles, at day 3; Figure 2E). Thus, VvNL raised transiently in Low Cd exposed soles at day 3 and then returned to levels similar to those of the other experimental groups at day 7.
Figure 2 Lysosomal membrane stability (A), lysosomal structural changes (B-D) and intracellular neutral lipid volume density (E) assessed in liver of S. senegalensis exposed to different concentrations of Cd for 3 and 7 days. Different letters indicate significant differences among experimental groups of a same sampling time; asterisks indicate significant differences between exposure times for each treatment (p<0.05). LP, labilisation period; VvL, lysosomal volume density; S/VL, lysosomal surface to volume ratio; NvL, lysosomal numerical density; VvNL, volume density of neutral lipids.
Gill histopathological lesions included lamellar capillary aneurysm (Figure 3A), epithelial lifting (Figure 3B), hypertrophy of squamous epithelium (Figure 3C) and chloride cell hyperplasia (Figure 3D). The high prevalence of lamellar capillary aneurysm and epithelial lifting did not vary between experimental groups (Table 3). In contrast, hypertrophy of squamous epithelium was more frequently recorded upon Cd exposure, in particular at day 7. At day 3, chloride cell hyperplasia was recorded at mild prevalence in all groups, except in Mid Cd exposed soles where prevalence was high. At day 7, the prevalence of this lesion increased with Cd concentration (Table 3). Yet, the prevalence of hypertrophy of squamous epithelium and chloride cell hyperplasia in the Control group was lower at day 7 than day 3. No tumours were detected in gills.
Figure 3 Histological sections (5 µm) of S. senegalensis exposed to different concentrations of Cd for 3 and 7 days, stained with hematoxylin-eosin. (A) Gill tissue showing capillary aneurysm (arrows); (B) severe case of gill epithelial lifting (arrows); (C) high degree of hypertrophy of squamous epithelium; (D) gill section showing a case of chloride cell hyperplasia (segment) with slight epithelial lifting (arrow); (E) hepatic tissue containing melanomacrophage centres (dotted circle) and fat vacuolation (arrow); (F) hepatic hyperaemia with accumulation of erythrocytes in blood vessels; h: hyperaemia; s: sinusoid; (G) presence of a large lymphocytic infiltration in liver (arrow); (H) severe case of hepatic necrosis. Black scale bar: 50 µm; white scale bar: 100 µm.
Table 3 Prevalence (%) for gill, liver and female gonad histopathological lesions recorded in S. senegalensis (n=13 per experimental group; except for gonad#) at days 3 and 7.
Hepatocytes often appeared extensively occupied by fat vacuoles in all experimental groups (98.1%; Figure 3E). Haemorrhage, hyperaemia (Figure 3F) and hydropic vacuolation of epithelial cells of bile ducts were recorded at mild prevalence (<40%), with higher values detected in Cd exposure groups at day 7 (Table 3). Similarly, lymphocytic infiltration (Figure 3G) was observed at mild prevalence in all experimental groups (<25%), except in soles exposed to High Cd for 7 d where this lesion was moderately frequent (61.5%). Necrosis (Figure 3H) was recorded at moderate to high prevalence, with highest values detected in soles exposed to High Cd (days 3 and 7) and to Mid Cd (day 7). The prevalence of MMCs (Figure 3E) and concentric periductal fibrosis of bile ducts increased in all experimental groups from day 3 to day 7 and did not show a clear relation with Cd concentration (Table 3).
Upon microscopic examination of gonad tissue, most soles were at an early stage of gamete development. Males mostly presented immature testis (42.6%) and early spermatogenesis stage (53.2%); and although one case of early vitellogenesis (Stage II) was identified, most females (98.2%) presented primary growth oocytes (Stage I). The only histopathological lesion identified in testis was the necrosis and was recorded in soles exposed to Mid Cd for 3 d (14.3%) and High Cd for 7 d (28.6%). Hyperaemia, MMCs and pyknotic oocytes were only occasionally observed (Table 3). Lymphocytic infiltration was rarely recorded, except in soles exposed to High Cd for 3 d (50%). Similarly, a mild prevalence of necrosis was recorded in most experimental groups but in High Cd exposed soles it was high at day 7 (Table 3). Highest prevalence of atresia was recorded in High Cd soles at day 3 (75.0%) and day 7 (66.7%). The prevalence of lipids in oocytes varied with Cd concentration at day 7, reaching 100% in soles exposed to High Cd (Table 3).
Total index (Itot) and liver index (Iliver) were significantly affected by Cd concentration and exposure time; whilst the gill index (Igills) and female gonad index (Igonad) were only affected by Cd concentration (2-way ANOVA; Table 2). Thus, Itot and Iliver increased in Cd exposed soles at day 7, with higher values at increasing Cd concentration (Figures 4A, C). In contrast, no significant increase was detected from day 3 to day 7 in Igills. Yet, higher Igills values were recorded in Mid Cd and High Cd exposed soles than in Control soles at day 7 (Figure 4B). In the case of Igonad, the lowest index values were recorded in Control soles and the highest values in soles exposed to High Cd (Figure 4D).
Figure 4 Total histopathological index (Itot), gill index (Igills), liver index (Iliver) and female gonad index (Igonad) of S. senegalensis exposed to different concentrations of Cd for 3 and 7 days. Different letters indicate significant differences among experimental groups of the same sampling time; asterisks indicate significant differences between exposure times for each treatment (p<0.05).
The IBR/n index ranged from 0.07 in Control soles at day 7 to 3.47 in soles exposed to High Cd for 7 d (Figure 5). Overall, higher IBR/n index levels were recorded in Cd exposed soles than in Control soles, in a dose dependent manner at day 7 (Control<Low Cd<Mid Cd<High Cd; Figure 5).
Figure 5 Radar plots constructed using five selected biological parameters (AChE, GST, LP, Igills and Iliver) for each experimental group of juvenile S. senegalensis exposed to different concentrations of Cd for 3 and 7 days; and IBR/n index calculated on the basis of these radar plots.
The lowest concentration of Cd measured in water samples was recorded for the control group and resembled the annual average quality standard described for transitional and coastal waters by the Water Framework Directive (2000/60/EC). The low concentration of Cd selected for the present study is representative of the maximum allowable concentration described for surface waters by the Directive 2008/105/EC and is similar to levels detected in contaminated estuaries (Waeles et al., 2004). Although much higher, the mid Cd concentration used herein is still considered environmentally relevant as it is comparable to levels detected in highly contaminated estuaries (Neff, 2002). In contrast, the highest concentration of Cd was used to induce rapid and acute biological effects of Cd in an attempt to identify response mechanisms. Chemical analysis of water samples indicated that levels of contaminant applied throughout the experiment were lower than the original nominal concentrations. Indeed, Cd behavior in seawater is affected by different mechanisms such as adsorption and precipitation, among others (Sigel et al., 2013). Nevertheless, present results also indicated that the gradient of concentrations was maintained between experimental groups and that exposure was constant throughout time. After 7 days of experimentation, levels of Cd detected in liver of fish exposed to low and mid concentrations of Cd did not differ from the Control group, indicating that no bioaccumulation of the metal was recorded for these groups. In contrast, Cd was accumulated in liver of fish exposed to 1000 µg Cd/l for 7 days, reaching a concentration comparable to that reported in wild sole from a metal polluted estuary (Vicente-Martorell et al., 2009). Fish are known to accumulate Cd in liver, at least after injection, dietary exposure or when exposed to high waterborne concentrations of the metal (Kim et al., 2004; Jayakumar and Paul, 2006; Kumar et al., 2008), which has been linked to oxidative stress, oxidative damage, neurotoxicity and multi-organ histopathology (Bouraoui et al., 2008; Costa et al., 2013; Souid et al., 2013; Pereira et al., 2016). As a result, toxicopathic effects are likely to occur in juvenile S. senegalensis exposed to High Cd in the present study. Conversely, hepatic Cd was below detection limits at environmental realistic concentrations of the metal in seawater (10 µg Cd/l or below). Seemingly, Cd accumulation in liver is less relevant after light waterborne exposure than after dietary or strong waterborne exposure (Guo et al., 2019). Nevertheless, multi-organ toxicopathic effects have been reported in juvenile S. senegalensis upon exposure to Cd concentration in the 0.5-10 µg Cd/l range, especially in liver (Costa et al., 2013).
Cadmium was reported to inhibit AChE activity in fish brain (van der Oost et al., 2003; Davies and Vethaak, 2012). Presently, AChE activity recorded in control soles resembles the values of the normal range elsewhere reported in sole. Thus, although they are higher than those recorded in farmed fry and adult S. senegalensis (Solé et al., 2012), they are absolutely comparable to those measured in wild juvenile S. solea from a reference site (Jebali et al., 2013). However, AChE activity was reduced to around a 30-40% in individuals exposed to 1000 µg Cd/l for 7 days and to a lesser extent in those exposed to 10 µg Cd/l. It is accepted that a 20% reduction in AChE in fish indicates exposure to neurotoxic compounds (Davies and Vethaak, 2012). This is in agreement with previous studies demonstrating fish AChE inhibition upon exposure to Cd and other metals (Oliva et al., 2012b; Jebali et al., 2013; Ghribi et al., 2019). In comparison, exposure to environmentally relevant concentrations of 1-10 µg Cd/l does not seem to cause AChE enzyme inhibition in the short term (7 days) in the present experimental conditions; however, some signs of response on exposure to 10 µg Cd/l suggest that effects might occur at longer exposure periods. Indeed, Cd toxicity in fish brain has been reported to be time and dose-dependent (Green and Planchart, 2018) where a short-term exposure (<24 hr) may induce expression of some apoptotic and detoxifying genes (Gonzalez et al., 2006) and a longer-term exposure may lead to tissue damage (e.g. disruption of nerve function; light sensitivity; increased ROS production). In the present study, the short-term inhibition of the AChE enzyme indicates the blockage of acetylcholine hydrolysis. The consequent acetylcholine accumulation in cholinergic synapses may lead to nervous malfunctions upon longer exposure (Jebali et al., 2006).
Cadmium accumulation in fish liver stimulates ROS production, activating antioxidant defences (e.g., CAT, GST and SOD induction; Bouraoui et al., 2008; Souid et al., 2013). Accordingly, CAT activity was affected by exposure to Cd with values ranging 10-90 µmol/min/mg prot. Presently, CAT activity in control soles (≈25-30 µmol/min/mg prot) was comparable to that registered in control juvenile S. senegalensis in other experimental setups (Solé et al., 2008) and in wild Solea spp. from reference sites (Oliva et al., 2012a; Jebali et al., 2013). The transient increase of CAT activity recorded at day 3 in Cd exposed soles indicates an activation of the antioxidant defence system, particularly in soles exposed to 1000 µg Cd/l (51.1 ± 15.9 µmol/min/mg prot). This is in agreement with observations made by Souid et al. (2013), where CAT activity was quickly induced (24 hr) in S. aurata exposed to waterborne Cd at a concentration of 0.5 mg Cd/l. However, 7 days exposure to 1000 µg Cd/l led to a decline in CAT activity to low levels (9.6 ± 3.7 µmol/min/mg prot), comparable to those recorded upon laboratory exposure to naturally contaminated sediments (Briaudeau et al., 2020) and to waterborne B[a]P (Briaudeau et al., 2021). CAT inhibition was also reported in wild juvenile S. senegalensis from polluted sites (Oliva et al., 2012a). Indeed, CAT inhibition may occur in response to ROS overproduction exceeding the antioxidant protection capacity of the cell (Roméo et al., 2000; Kalman et al., 2010). Certainly, the pattern of CAT activity in response to pollutants in fish liver may be disparate (induction or inhibition) depending on the exposure time and the concentration of chemicals (Regoli et al., 2002). GST enzyme induction has been recorded in fish liver upon exposure to a variety of pollutants both through waterborne and sediment exposure (Regoli et al., 2002; Salamanca et al., 2008). Accordingly, a brief increase of GST activity was recorded herein in response to Cd exposure at day 3 (60-70 nmol/min/mg prot), reaching levels similar to those recorded in juvenile S. senegalensis after laboratory exposure to naturally contaminated sediments (Briaudeau et al., 2020) and waterborne B[a]P (Briaudeau et al., 2021). Thus, it seems that, in all the Cd exposed soles, GST enzyme activity is at least transiently induced at day 3, indicating an activation of the antioxidant defence system (Regoli et al., 2002; van der Oost et al., 2003). However, a longer exposure to Cd for 7 days led to a decline in GST activity (30-40 nmol/min/mg prot). Interestingly, GST inhibition was described in wild S. senegalensis juveniles from highly polluted areas (Fonseca et al., 2011). Overall, the response pattern of GST activity may be intricate depending on the chemical and on the exposure conditions (van der Oost et al., 2003; Fonseca et al., 2011). For instance, in Cd exposed catfish, hepatic GST activity was induced after 24-72 h Cd exposure but inhibited after 96 h (Mani et al., 2014). SOD activity is enhanced upon exposure to pollutants (Regoli et al., 2002; Dos Santos Carvalho et al., 2012); however, it seems to be a fast transient response. For instance, SOD activity was quickly induced in S. aurata after 4 h exposure to waterborne Cd (0.5 mg/l) and further on inhibited (35% decrease) within 24 h (Souid et al., 2013). Presently hepatic SOD activity did not change with Cd exposure but was higher at day 7 (≈155 mmol/min/mg prot) than at day 3 (≈125-130 mmol/min/mg prot) in all the experimental groups, including Control individuals. It is thus conceivable that SOD response time to Cd exposure was shorter that 3 d and that changes in enzyme activity recorded from day 3 to day 7 would appear to be effects of experimentation, as all experimental groups were affected. Overall, the progression of antioxidant defense and oxidative damage seems to be intricate in fish. Thus, for instance, in juvenile Japanese flounder, Paralichthys olivaceus, exposed to waterborne Cd (48 μg Cd/L) for 80 days SOD and LPO increased but GST decreased as Cd concentration increased, whilst CAT and GSH did not change (Cao et al., 2010). Nevertheless, in agreement with our present results, Cd accumulation in fish stimulates ROS production, resulting in lipid peroxidation and alterations in antioxidant defenses such as SOD, CAT, GSH and GST (Roméo et al., 2000; Livingstone, 2001; Firat et al., 2009; Cao et al., 2010).
Changes in lysosomal structure and membrane integrity are core biomarkers for biological effects assessment and they have been applied in laboratory experiments to demonstrate sub-cellular effects of Cd exposure in molluscs (Marigómez et al., 2005; Izagirre et al., 2014) and fish species (Roméo et al., 2000; Alvarado et al., 2005). Presently, LP values recorded in hepatocytes of Control soles (≈20-30 min) are similar to those previously recorded in healthy fish (Broeg et al., 2002; Viarengo et al., 2007; Zorita et al., 2008). Conversely, in Cd exposed soles LP decreased from around 20 min at day 3 to 7-12min at day 7, especially in soles exposed to 1000 µg Cd/l sw. Lysosomal membrane destabilisation may occur when autophagy is enhanced to maintain cellular health status (Moore et al., 2013), once the antioxidant defence system is overloaded, as recorded herein. The reduced LP values observed after Cd exposure resemble those reported in S. senegalensis and other fish species exposed to pollutants (Baršienė et al., 2006; Zorita et al., 2008; Burgeot et al., 2017; Briaudeau et al., 2021). LP values recorded in control and Cd exposed fish from the present study are essential reference data to support the use of lysosomal biomarkers for the demonstration of metal toxicity in juvenile soles. Lysosomal membrane impairment may generate an outflow of hydrolases thus affecting cellular functions and potentially leading to histopathological alterations (Köhler et al., 2002; Viarengo et al., 2007). For instance, lysosomal membrane destabilisation in hepatocytes of dab, Limanda limanda, was seemingly related to high oxyradical production and prevalence of histopathological lesions along a pollution gradient in the German Bight (Köhler et al., 1992). Likewise, lysosomal membrane destabilisation may be accompanied with lysosomal enlargement evidenced by changes in size and numbers of the lysosomes (Alvarado et al., 2005; Izagirre and Marigómez, 2009). However, in the present study, we have not obtained clear evidence of lysosomal enlargement. In most of the experimental groups, lysosomes observed were overall scarce and small (e.g. in comparison with control and reference individuals of other fish species; Alvarado et al., 2005; Izagirre, 2007). Similarly, although lipid accumulation in fish liver may be considered an early indicator of liver injury (Köhler et al., 2002), the present results on intracellular accumulation of neutral lipids are not conclusive.
Histopathological lesions are typically described as medium-term consequences to contaminant exposure (Bernet et al., 1999), and herein they were already recorded in gills, liver and gonads after 7 days exposure to Cd. However, overall they were recorded at low lesion stages, suggesting a low impact of Cd at tissue-level. Gill histopathology is useful for assessing early biological responses to Cd in S. senegalensis (Costa et al., 2013) used to detect tissue-level effects of recent stress conditions. Gill lesions identified in the present study were reported previously in wild fish from contaminated sites (Stentiford et al., 2003; Oliva et al., 2013; Briaudeau et al., 2019) and after laboratory exposure to metals (Oliva et al., 2009). Hypertrophy of squamous epithelium and chloride cell hyperplasia in gills were more frequently observed in Cd exposed sole than in control, especially at day 7. Alike, haemorrhage, hyperaemia, hydropic vacuolation of epithelial cells of bile ducts, lymphocytic infiltration and necrosis were observed at higher prevalence in the liver of soles exposed to 10-1000 µg Cd/l for 7 days than in controls. These lesions were previously reported in fish liver upon exposure to a variety of pollutants including e.g. Cd, pesticides and B[a]P (Oliveira Ribeiro et al., 2005; van Dyk et al., 2007; Briaudeau et al., 2021). Particularly, exposure to concentrations as low as 0.5 µg Cd/l for 28 days elicited comparable histopathological lesions in the liver of sole fry (Costa et al., 2013). In contrast, several lesions previously described in sole in response to pollutants such as MMCs and concentric periductal fibrosis of bile ducts (Costa et al., 2011; Zorita and Cuevas, 2014; Briaudeau et al., 2019; Briaudeau et al., 2020) were not related to the concentration of Cd in the present study. In female gonad, lymphocytic infiltration, oocyte atresia and necrosis, and lipidosis were recorded at mild lesion stages and were more frequently found in Cd exposed soles, especially upon 1000 µg Cd/l, than in control ones. These lesions were previously reported in fish subject to environmental stress (Cuevas et al., 2015b). The use of histopathological indices integrating pathological importance and degree of severity of each lesion in different organs permitted to observe a clear general tissue-level effect of Cd. This was more remarkable in the case of the weighted histopathological indices (Bernet et al., 1999; Costa et al., 2009). Values of Iliver (≈10) and Igonad (0.5-2) calculated for the control soles were comparable with those measured in wild fish from non-severely contaminated areas (Cuevas et al., 2015a; Cuevas et al., 2015b). Overall, the highest indices (Iliver=16.3 ± 1.3; Igonad=10.7 ± 2.2) were detected in Cd exposed soles, particularly in those exposed to 1000 µg Cd/l at day 7. Similarly, comparably high Iliver and Igonad were recorded in sole juveniles exposed to contaminated sediments and to waterborne B[a]P exposure (Briaudeau et al., 2020; Briaudeau et al., 2021).
The use of the IBR/n index contributed to an integrated evaluation of biological responses and minimized the potential uncertainty of individual biomarkers (Marigómez et al., 2013; Baudou et al., 2019). Thus, IBR/n values were related with Cd concentration and exposure time (IBR/nHighCd>IBR/nMidCd>IBR/nLowCd>IBR/nControl). Alike, successive biological responses elicited by Cd were depicted by radar plot profiles: neurotoxicity and altered antioxidant enzyme activities and lysosomal system anticipated histopathological lesions. Besides, at day 3 the main contributors to IBR/n were biochemical responses related to neurotoxicity (AChE) and antioxidant defence (GST) along with gill histopathological lesions; whereas at day 7 all the biological responses in the star plots contributed to IBR/n. Likewise, a dose dependent effect was only clearly envisaged at day 7.
In conclusion, the assessment of biological responses at different levels of biological complexity in Solea senegalensis juveniles exposed to waterborne Cd for 7 days indicated an early dose-dependent decline in health condition. Thus, a 3-day exposure to Cd caused dose-dependent oxidative stress (CAT and GST induction/inhibition) and lysosomal membrane destabilisation, whereas neurotoxicity (AChE inhibition) and gill, liver and gonad histopathological lesions were mainly recorded at day 7. These effects were very remarkable upon exposure to a non-environmentally relevant Cd concentration (1000 µg Cd/l) but were also, to some extent, elicited at environmentally relevant concentrations of the metal (10 µg Cd/l). Thus, as the effects are clearly time dependent, it is conceivable that more severe biological effects would be elicited by these low concentrations of the metal at longer exposure periods, which describes an environmental scenario. Therefore, the present study evidences the toxicopathic effects of waterborne Cd in sole juveniles and supports the use of biomarkers and histopathology approaches as early-warning indicators of altered health status in sole juveniles, prospective sentinels for pollution monitoring in estuaries and coastal areas. It also suggests the need for future experimental studies using juvenile S. senegalensis exposed to environmental concentrations of Cd for longer period, in order to further study dose and time-dependency identified here.
The original contributions presented in the study are included in the article/supplementary material, further inquiries can be directed to the corresponding author/s.
The animal study was reviewed and approved by Comité de Ética de Experimentación Animal (CEEA, UPV/EHU).
TB: Methodology, Investigation, Visualization, Writing original draft, Editing. GG-L: Investigation, Visualization. IZ: Methodology, Formal analysis, Writing, Review. IM: Conceptualization, Funding acquisition, Writing, Supervision. UI: Conceptualization, Investigation, Supervision, Writing, Review. All authors contributed to the article and approved the submitted version.
This work was funded by Spanish MICINN (CTM2017-87766-R), by the University of the Basque Country UPV/EHU (UFI 11/37) and by the Basque Government through Consolidated Research Groups Grant (IT810-B and IT1302-19). TB profited from a PhD Scholarship provided by the Spanish MINECO.
The authors wish to thank the MSc students Edurne Huerga and Luis Alejandro Alves Dos Santos and the PiE-UPV/EHU lab technician Irune Valenciano for their invaluable support during experimentation and sample analysis. The authors also wish to thank for technical and human support provided by SGIker (UPV/EHU/ERDF, EU).
The authors declare that the research was conducted in the absence of any commercial or financial relationships that could be construed as a potential conflict of interest.
All claims expressed in this article are solely those of the authors and do not necessarily represent those of their affiliated organizations, or those of the publisher, the editors and the reviewers. Any product that may be evaluated in this article, or claim that may be made by its manufacturer, is not guaranteed or endorsed by the publisher.
Alijani R. A., Movahedinia A., Rastgar S. (2017). Fish liver biomarkers for heavy metal pollution: a review article. Amer. J. Toxicol. 2, 1–8.
Alvarado N. E., Buxens A., Mazón L. I., Marigómez I., Soto M. (2005). Cellular biomarkers of exposure and biological effect in hepatocytes of turbot (Scophthalmus maximus) exposed to Cd, Cu and Zn and after depuration. Aquat. Toxicol. 74, 110–125. doi: 10.1016/j.aquatox.2005.03.024
Amara R., Meziane T., Gilliers C., Hermel G., Laffargue P. (2007). Growth and condition indices in juvenile sole (Solea solea) measured to assess the quality of essential fish habitat. Mar. Ecol. Prog. Ser. 351, 201–208. doi: 10.3354/meps07154
Atli G., Alptekin. O., Tükel. S., Canli. M. (2006). Response of catalase activity to Ag+, Cd2+, Cd6+, Cu2+ and Zn2+ in five tissues of freshwater fish Oreochromis niloticus. comp. Bioch. Physiol. 143C, 218–224. doi: 10.1016/j.cbpc.2006.02.003
Baršienė J., Lethonen K., Koehler A., Broeg K., Vuorinen P. J., Lang T., et al. (2006). Biomarker responses in flounder (Platichthys flesus) and mussel (Mytilus edulis) in the Klaipeda-Buyinge area (Baltic Sea). Mar. pollut. Bull. 53, 422–436. doi: 10.1016/j.marpolbul.2006.03.009
Baudou F. G., Ossana N. A., Castañé P. M., Mastrángelo M. M., González Núñez A. A., Palacio M. J., et al. (2019). Use of integrated biomarker indexes for assessing the impact of receiving waters on a native neotropical teleost fish. Sci. Tot. Environ. 650, 1779–1786. doi: 10.1016/j.scitotenv.2018.09.342
Beliaeff B., Burgeot T. (2002). Integrated biomarker response: a useful tool for ecological risk assessment. Environ. Toxicol. Chem. 21, 1316–1322. doi: doi: 10.1002/etc.5620210629
BEQUALM (2001) Biological effects quality assurance in monitoring programmes. Available at: http://www.bequalm.org/about.htm
Bernet D., Schmidt H., Meier W., Burkhardt-Holm P., Wahli T. (1999). Histopathology in fish: a proposal for a protocol to assess aquatic pollution. J. Fish Dis. 22, 25–35. doi: doi: 10.1046/j.1365-2761.1999.00134.x
Bouraoui Z., Banni M., Ghedira J., Clerandeau C., Guerbej H., Narbonne J. F., et al. (2008). Acute effects of cadmium on liver phase I and phase II enzymes and metallothionein accumulation on sea bream Sparus aurata. Fish Physiol. Biochem. 34, 201–207. doi: 10.1007/s10695-007-9177-y
Briaudeau T., Alves Dos Santos L. A., Zorita I., Izagirre U., Marigómez I. (2021). Biological responses and toxicopathic effects elicited in Solea senegalensis juveniles by waterborne exposure to benzo[a]pyrene. Mar. Environ. Res. 170, 105351. doi: 10.1016/j.marenvres.2021.105351
Briaudeau T., Zorita I., Cuevas N., Franco J., Marigómez I., Izagirre U. (2019). Multi-annual survey of health status disturbance in the Bilbao estuary (Bay of Biscay) based on sediment chemistry and juvenile sole (Solea spp.) histopathology. Mar. pollut. Bull. 145, 126–137. doi: 10.1016/j.marpolbul.2019.05.034
Briaudeau T., Zorita I., Izagirre U., Marigómez I. (2020). Biological responses and toxicopathic effects elicited in Solea senegalensis juveniles on exposure to contaminated sediments under laboratory conditions. Sci. Tot. Environ. 731, 138849. doi: 10.1016/j.scitotenv.2020.138849
Broeg K., Koehler A., Von Westernhagen H. (2002). Disorder and recovery of environmental health monitored by means of lysosomal stability in liver of European flounder (Platichthys flesus L.). Mar. Environ. Res. 54, 569–573. doi: 10.1016/s0141-1136(02)00174-5
Broeg K., Lehtonen K. K. (2006). Indices for the assessment of environmental pollution of the Baltic Sea coasts: integrated assessment of a multi-biomarker approach. Mar. pollut. Bull. 53, 508–522. doi: 10.1016/j.marpolbul.2006.02.004
Burgeot T., Akcha F., Ménard D., Robinson C., Loizeau V., Brach-Papa C., et al. (2017). Integrated monitoring of chemicals and their effects on four sentinel species, Limanda limanda, Platichthys flesus, Nucella lapillus and Mytilus sp., in Seine Bay: a key step towards applying biological effects to monitoring. Mar. Environ. Res. 124, 92–105. doi: 10.3390/app10061993
Camacho C., Maulvault A. L., Santos M. S., Barbosa V., Fogaça F. H. S., et al (2010). Mercury in Juvenile Solea senegalensis: Linking Bioaccumulation, Seafood Safety, and Neuro-Oxidative Responses under Climate Change-Related Stressors. Appl. Sci. 10, 193. doi: 10.3390/app10061993
Camacho C., Maulvault A. L., Santos M. S., Barbosa V., Fogaça F. H. S., Pousão-Ferreira P., et al (2020). Mercury in Juvenile Solea senegalensis: Linking Bioaccumulation, Seafood Safety, and Neuro-Oxidative Responses under Climate Change-Related Stressors. Appl. Sci. 10, 1993. doi: 10.3390/app10061993
Cao L., Huang W., Liu J., Yin X., Dou S. (2010). Accumulation and oxidative stress biomarkers in Japanese flounder larvae and juveniles under chronic cadmium exposure. Comp. Biochem. Physiol. 151C, 386–392. doi: 10.1016/j.cbpc.2010.01.004
Carriquiriborde P., Ronco A. E. (2008). Distinctive accumulation patterns of Cd(II), Cu(II), and Cr(VI) in tissue of the South American teleost, pejerrey (Odontesthes bonariensis). Aquat. Toxicol. 86, 313–322. doi: 10.1016/j.aquatox.2007.11.011
Claiborne A. (1985). “Catalase activity,” Eds. Greenwald R. A. in CRC handbook of methods in oxygen radical research (Boca Raton, Florida: CRC Press), 283–284. doi: 10.1201/9781351072922
Constenla M., Soler-Membrives A., Besada V., Carrassón M. (2021). Impact assessment of a large river on the sediments and fish from its continental shelf: using Solea solea as sentinel in the ebro river mouth (NW Mediterranean, Spain). Environ. Sci. pollut. Res. Int. 29 (11), 15713–15728. doi: 10.1007/s11356-021-16408-7
Costa P. M., Caeiro S., Costa M. H. (2013). Multi-organ histological observations on juvenile Senegalese soles exposed to low concentrations of waterborne cadmium. Fish Physiol. Biochem. 39, 143–158. doi: 10.1007/s10695-012-9686-1
Costa P. M., Caeiro S., Diniz M. S., Lobo J., Martins M., Ferreira A. M., et al. (2010). A description of chloride cell and kidney tubule alterations in the flatfish Solea senegalensis exposed to moderately contaminated sediments from the Sado estuary (Portugal). J. Sea Res. 64, 465–472. doi: 10.1016/j.seares.2010.01.005
Costa P. M., Caeiro S., Lobo J., Martins M., Ferreira A. M., Caetano M., et al. (2011). Estuarine ecological risk based on hepatic histopathological indices from laboratory and in situ tested fish. Mar. pollut. Bull. 62, 55–65. doi: 10.1016/j.marpolbul.2010.09.009
Costa P. M., Costa M. H. (2008). Biochemical and histopathological endpoints of in vivo cadmium toxicity in Sparus aurata. Cienc. Mar. 34, 349–361. doi: 10.7773/cm.v34i3.1394
Costa P. M., Diniz M. S., Caeiro S., Lobo J., Martins M., Ferreira A. M., et al. (2009). Histological biomarkers in liver and gills of juvenile Solea senegalensis exposed to contaminated estuarine sediments: a weighted indices approach. Aquat. Toxicol. 92, 202–212. doi: 10.1016/j.aquatox.2008.12.009
Cuevas N., Zorita I., Costa P. M., Larreta J., Franco J. (2015b). Histopathological baseline levels and confounding factors in common sole (Solea solea) for marine environmental risk assessment. Mar. Environ. Res. 110, 162–173. doi: 10.1016/j.marenvres.2015.09.002
Cuevas N., Zorita I., Costa P. M., Quincoces I., Larreta J., Franco J. (2015a). Histopathological indices in sole (Solea solea) and hake (Merluccius merluccius) for implementation of the European Marine Strategy Framework Directive along the Basque continental shelf (SE bay of Biscay). Mar. pollut. Bull. 94, 185–198. doi: 10.1016/j.marpolbul.2015.02.030
Davies I. M., Vethaak A. D. (2012). Integrated marine environmental monitoring of chemicals and their effects (ICES Cooperative Research Report No. 315 Copenhagen, Denmark: ICES), 277. doi: 10.17895/ices.pub.5403
Devin S., Burgeot T., Giambérini L., Minguez L., Pain-Devin S. (2014). The integrated biomarker response revisited: optimization to avoid misuse. Environ. Sci. pollut. Res. 21, 2448–2454. doi: 10.1007/s11356-013-2169-9
Dos Santos Carvalho C., Bernusso V. A., De Araújo H. S. S., Espíndola E. L. G., Fernandes M. N. (2012). Biomarker responses as indication of contaminant effects in Oreochromis niloticus. Chemosphere 89, 60–69. doi: 10.1016/j.chemosphere.2012.04.013
Eggleton J., Thomas K. V. (2004). A review of factors affecting the release and bioavailability of contaminants during sediment disturbance events. Environ. Internat. 30, 973–980. doi: 10.1016/j.envint.2004.03.001
Feist S. W., Lang T., Stentiford G. D., Köhler A. (2004). Biological effects of contaminants: use of liver pathology of the European flatfish dab (Limanda limanda l.) and flounder (Platichthys flesus L.) for monitoring. ICES Techniques Mar. Environ. Sci. No. 38 ICES Copenhagen 38, 42. doi: 10.25607/OBP-226
Firat O., Cogun H. Y., Aslanyavrusu S., Kargin F. (2009). Antioxidant responses and metal accumulation in tissues of Nile tilapia Oreochromis niloticus under Zn, Cd and Zn+Cd exposures. J. Appl. Toxicol. 29, 295–301. doi: 10.1002/jat.1406
Fonseca V. F., França S., Serafim A., Company R., Lopez B., Bebianno M. J., et al (2011). Multi-biomarker responses to estuarine habitat contamination in three fish species: Dicentratus labrax, Solea senegalensis and Pomatoschistus microps. Aquat Toxicol 102, 216–227. doi: 10.1016/j.aquatox.2011.01.018
García-López A., Fernández-Pasquier V., Couto E., Canario A. V. M., Sarasquete C., Martínez-Rodríguez G. (2006). Testicular development and plasma sex steroid levels in cultured male Senegalese sole Solea senegalensis kaup. Gen. Comp. Endocrinol. 147, 343–351. doi: 10.1016/j.ygcen.2006.02.003
Ghribi R., Correia A. T., Elleuch B., Nunes B. (2019). Testing the impact of contaminated sediments from the Southeast marine coast of Tunisia on biota: a multibiomarker approach using the flatfish Solea senegalensis. Environ. Sci., 1–18. doi: 10.1007/s11356-019-05872-x
Gonçalves C., Martins M., Costa M. H., Caeiro S., Costa P. M. (2013). Ecological risk assessment of impacted estuarine areas: integrating histological and biochemical endpoints in wild Senegalese sole. Ecotoxicol. Environ. 95, 202–211. doi: 10.1016/j.ecoenv.2013.06.004
Gonzalez P., Baudrimont M., Boudou A., Bourdineaud J.-P. (2006). Comparative effects of direct cadmium contamination on gene expression in gills, liver, skeletal muscles and brain of the zebrafish (Danio rerio). BioMetals 19, 225–235. doi: 10.1007/s10534-005-5670-x
Green A. J., Planchart A. (2018). The neurological toxicity of heavy metals: a fish perspective. Comp. Biochem. Physiol. 208, 12–19. doi: 10.1016/j.cbpc.2017.11.008
Guilhermino L., Lopes M. C., Carvalho A. P., Soares A. M. V. M. (1996). Acetylcholinesterase activity in juveniles of Daphnia magna Straus. Bull. Environ. Contam. Toxicol. 57, 979–985. doi: 10.1007/s001289900286
Guo Z., Ni Z., Ye H., Xiao J., Chen L., Green I., et al. (2019). Simultaneous uptake of cd from sediment, water and diet in a demersal marine goby Mugilogobius chulae. J. Hazard. Mater. 364, 143–150. doi: 10.1016/j.jhazmat.2018.09.045
Hansen J. A., Welsh P. G., Lipton J., Suedkamp M. J. (2002). The effects of long-term cadmium exposure on the growth and survival of juvenile bull trout (Salvelinus confluentus). Aquat. Toxicol. 58, 165–174. doi: 10.1016/s0166-445x(01)00233-8
HELCOM (2012). Development of a set of core indicators: Interim report of the HELCOM CORESET project. PART A. Description of the selection process. Balt. Sea Environ. Proc. No. 129.ICES, 2006. Report of the ICES/BSRP Sea-going Workshop on Fish Disease Monitoring in the Baltic Sea (WKFDM), 5-12 December 2005. ICES CM 2006/BCC:02. 89 pp.
ICES (2015). “Lysosomal membrane stability in mussels,” in ICES techniques in marine environmental sciences no. 56. Eds. Martínez-Gómez C., Bignell J., Lowe D. (Copenhagen: ICES), 41. doi: 10.17895/ices.pub.5084
ICES (2006). “Report of the ICES/BSRP Sea-going Workshop on Fish Disease Monitoring in the Baltic Sea (WKFDM), ICES, 89.
Izagirre U. (2007). Contribution to the interpretation of lysosomal biomarkers in marine organisms based on the mechanistic understanding of the lysosomal responses to pollutants (Bilbao, Spain: University of the Basque Country).
Izagirre U., Errasti A., Bilbao E., Múgica M., Marigómez I. (2014). Combined effects of thermal stress and Cd on lysosomal biomarkers and transcription of genes encoding lysosomal enzymes and HSP70 in mussels, Mytilus galloprovincialis. Aquat Toxicol 149, 145–156. doi: 10.1016/j.aquatox.2014.01.013
Izagirre U., Marigómez I. (2009). Lysosomal enlargement and lysosomal membrane destabilisation in mussel digestive cells measured by an integrative index. Environ. pollut. 157, 1544–1553. doi: 10.1016/j.envpol.2009.01.011
JAMP (Joint Assessment and Monitoring Program) (2003). JAMP guidelines for contaminant-specific biological effects monitoring (Oslo and Paris Commissions).
Jayakumar P., Paul V. I. (2006). Patterns of cadmium accumulation of the catfish Clarias batrachus (Linn.) exposed to sublethal concentration of cadmium chloride. Vet. Arh. 76, 167–177.
Jebali J., Banni M., Guerbej H., Almeida E. A., Banaoui A., Boussetta H. (2006). Effects of malathion and cadmium on acetylcholinesterase activity and metallothionein levels in the fish Seriola dumerilli. Fish Physiol. Biochem. 32, 93–98. doi: 10.1007/s10695-006-0041-2
Jebali J., Sabbagh M., Banni M., Kamel N., Ben-Kheder S., M’hmadi N., et al. (2013). Multiple biomarkers of pollution effects in Solea solea fish on the Tunisia coastline. Environ. Sci. pollut. Res. 20, 3812–3821. doi: 10.1007/s11356-012-1321-2
Kalman J., Riba I., Del Valls A., Blasco J. (2010). Comparative toxicity of cadmium in the commercial fish species Sparus aurata and Solea senegalensis.. Ecotoxicol. Environ. Saf. 73, 306–311. doi: 10.1016/j.ecoenv.2009.10.013
Kim S.-G., Jee J.-H., Kang J.-C. (2004). Cadmium accumulation and elimination in tissues of juvenile olive flounder, Paralichthys olivaceus after sub-chronic cadmium exposure. Environ. pollut., 127, 117–123. doi: 10.1016/s0269-7491(03)00254-9
Köhler A., Deisemann H., Lauritzen B. (1992). Histological and cytochemical indices of toxic injury in the liver of dab Limanda limanda. Mar. Ecol. Prog. Ser. 91, 141–153. doi: 10.3354/MEPS091141
Köhler A., Wahl E., Söffker K. (2002). Functional and morphological changes of lysosomes as prognostic biomarkers of toxic liver injury in a marine flatfish (Platichthys flesus (L)). Environ. Toxicol. Chem. 21, 2434–2444. doi: 10.1002/etc.5620211124
Kumar P., Prasad Y., Ranjan R., Swarup D., Pattanaik A. K., Patra R. C. (2008). Accumulation pattern of cadmium in tissues of Indian catfish Clarias batrachus. Anim. Nutr. Feed. Technol. 8, 115–119.
Law R., Hanke G., Angelidis M., Batty J., Bignert A., Dachs J., et al. (2010). Marine strategy framework directive - task group 8 report contaminants and pollution effects. EUR 24335 EN e Joint Research Centre Scientific and Technical Reports. In: Scientific and Technical Research SeriesOffice for Official Publications of the European Communities, Luxembourg, 161. doi: 10.2788/85887
Lekube X., Cajaraville M. P., Marigómez I. (2000). Use of polyclonal antibodies for the detection of changes induced by cadmium in lysosomes of aquatic organisms. Sci. Total Environ. 247, 201–212. doi: 10.1016/s0048-9697(99)00491-x
Levit S. M. (2010). A literature review of effects of cadmium on fish (Bozeman, Montana: Centre for Science in Public Participation).
Livingstone D. R. (2001). Contaminant-stimulated reactive oxygen species production and oxidative damage in aquatic organisms. Mar. Poll. Bull. 42, 656–666. doi: 10.1016/s0025-326x(01)00060-1
Mani R., Meena K., Valivittan K., Suresh A. (2014). Glutathione-S-Transferase and catalase activity in different tissues of marine catfish Arius arius on exposure to cadmium. Int. J. Pharm. Pharm. Sci. 6, 326–332.
Marigómez I., Baybay-Villacorta L. (2003). Pollutant-specific and general lysosomal responses in digestive cells of mussels exposed to model organic chemicals. Aquat. Toxicol. 64, 235–257. doi: 10.1016/s0166-445x(03)00056-0
Marigómez I., Garmendia L., Soto M., Orbea A., Izagirre U., Cajaraville M. P. (2013). Marine ecosystem health status assessment through integrative biomarker indices: a comparative study after the prestige oil spill “Mussel watch”. Ecotoxicology 22, 486–505. doi: 10.1007/s10646-013-1042-4
Marigómez I., Izagirre U., Lekube X. (2005). Lysosomal enlargement in digestive cells of mussels exposed to cadmium, benzo[a]pyrene and their combination. Comp. Biochem. Physiol. 141, 188–193. doi: 10.1016/j.cca.2005.06.005
Mathews T., Fisher N. S. (2009). Dominance of dietary intake of metals in marine elasmobranch and teleost fish. Sci. Total Environ. 407, 5156–5161. doi: 10.1016/j.scitotenv.2009.06.003
Moore M. N., Viarengo A. G., Somerfield P. J., Sforzini S. (2013). Linking lysosomal biomarkers and ecotoxicological effects at higher biological levels. Ecol. Biomarkers 5, 107–130. doi: 10.1201/b13036-9
Murua H., Motos L. (2006). Reproductive strategy and spawning activity of the European hake Merluccius merluccius (L.) in the bay of Biscay. J. Fish Biol. 69, 1288–1303. doi: 10.1111/j.1095-8649.2006.01169.x
Neff J. M. (2009). Bioaccumulation in Marine Organisms: Effect of Contaminants from Oil Well Produced Water. Elsevier Science Publishers, Amsterdam 452. doi: 10.1016/B978-008043716-3/50006-3
Neff J. M. (2002). Bioaccumulation in Marine Organisms: Effect of Contaminants from Oil Well Produced Water. Elsevier Science Publishers, Amsterdam 452. doi: 10.1016/B978-008043716-3/50006-3
Nordberg G. F. (2009). Historical perspectives on cadmium toxicology. Toxicol. Appl. Pharmacol. 238, 192–200. doi: 10.1016/j.taap.2009.03.015
Oliva M., Garrido M. C., Sales Márquez D., González de Canales M. L. (2009). Sublethal and lethal toxicity in juvenile Senegal sole (Solea senegalensis) exposed to copper: a preliminary toxicity range-finding test. Exp. Toxicol. Pathol. 61, 113–121. doi: 10.1016/j.etp.2008.06.001
Oliva M., González de Canales M. L., Gravato C., Guilhermino L., Perales J. A. (2010). Biochemical effects and polycyclic aromatic hydrocarbons (PAHs) in senegal sole (Solea senegalensis) from a huelva estuary (SW Spain). Ecotoxicol. Environ. Saf. 73, 1842–1851. doi: 10.1016/j.ecoenv.2010.08.035
Oliva M., Perales J. A., Gravato C., Guilhermino L., Galindo-Riano M. D. (2012a). Biomarkers responses in muscle of Senegal sole (Solea senegalensis) from a heavy metals and PAHs polluted estuary. Mar. Poll. Bull. 64, 2097–2108. doi: 10.1016/j.marpolbul.2012.07.017
Oliva M., Vicente J. J., Gravato C., Guilhermino L., Galindo-Riano M. D. (2012b). Oxidative stress biomarkers in Senegal sole, Solea senegalensis, to assess the impact of heavy metal pollution in a huelva estuary (SW spain): seasonal and spatial variation. Ecotoxicol. Environ. Saf. 75, 151–162. doi: 10.1016/j.ecoenv.2011.08.017
Oliva M., Vicente-Martorell J. J., Galindo-Riaño M. D., Perales J. A. (2013). Histopathological alterations in Senegal sole, Solea senegalensis, from a polluted huelva estuary (SW, Spain). Fish Physiol. Biochem. 39, 523–545. doi: 10.1007/s10695-012-9717-y
Oliveira Ribeiro C. A., Vollaire Y., Sanchez-Chardi A., Roche H. (2005). Bioaccumulation and the effects of organochlorine pesticides, PAH and heavy metals in the eel (Anguilla anguilla) at the camargue nature reserve, France. Aquat. Toxicol. 74, 53–69. doi: 10.1016/j.aquatox.2005.04.008
Ortiz-Delgado J. B., Funes V., Albendín G., Scala E., Sarasquete C. (2021). Environmental toxicity of malathion during Senegalese sole, Solea senegalensis larval development and metamorphosis: histopathological disorders and effects on type b esterases and CYP1A enzymatic systems. Toxicology 36, 1894–1910. doi: 10.1002/tox.23310
OSPAR Commission (2010). “Hazardous substances,” in Quality status report 2010 (London: OSPAR Commission). 37–52.
OSPAR Commission (2013). Background document and technical annexes for biological effects monitoring, update 2013 (London, UK: OSPAR Commission) 37–52.
Pavlaki M. D., Morgado R. G., Ferreira V., Rocha R. J. M., Soares A. M. V. M., Calado R., et al. (2021). Cadmium accumulation and kinetics in Solea senegalensis tissues under dietary and water exposure and the link to human health. Water 13, 522. doi: 10.3390/w13040522
Pereira L. S., Ribas J. L. C., Vicari T., Silva S. B., Stival J., Baldan A. P., et al. (2016). Effects of ecologically relevant concentrations of cadmium in a freshwater fish. Ecotoxicol. Environ. Saf. 130, 29–36. doi: 10.1016/j.ecoenv.2016.03.046
Primo A. L., Azeiteiro U. M., Marques S., Martinho F., Baptista J., Pardal M. A. (2013). Colonization and nursery habitat use patterns of larval and juvenile flatfish species in a small temperate estuary. J. Sea Res. 76, 126–134. doi: 10.1016/j.seares.2012.08.002
Regoli F., Giuliani M. E. (2014). Oxidative pathways of chemical toxicity and oxidative stress biomarkers in marine organisms. Mar. Environ. Res. 93, 106–117. doi: 10.1016/j.marenvres.2013.07.006
Regoli F., Giuliani M. E., Benedetti M., Arukwe A. (2011). Molecular and biochemical biomarkers in environmental monitoring: a comparison of biotransformation and antioxidant defense systems in multiple tissues. Aquat. Toxicol. 1055, 56–66. doi: 10.1016/j.aquatox.2011.06.014
Regoli F., Gorbi S., Frenzilli G., Nigro M., Corsi I., Focardi S., et al. (2002). Oxidative stress in ecotoxicology: from the analysis of individual antioxidants to a more integrated approach. Mar. Environ. Res. 54, 419–423. doi: 10.1016/s0141-1136(02)00146-0
Roméo M., Bennani N., Gnassia-Barelli M., Lafaurie M., Girard J. P. (2000). Cadmium and copper display different responses towards oxidative stress in the kidney of the sea bass Dicentrarchus labrax. Aquat. Toxicol. 48, 185–194. doi: 10.1016/s0166-445x(99)00039-9
Salamanca M. J., Jiménez-Tenorio N., González de Canales M. L., Del Valls T. A. (2008). Evaluation of the toxicity of an oil spill conducted through bioassays using the fish Solea senegalensis Cienc. Mar. 34, 339–348. doi: 10.7773/cm.v34i3.1403
Sharifuzzaman S. M., Rahman H., Ashekuzzaman S. M., Islam M. M., Chowdhury S. R., Hossain M. S. (2016). “Heavy metals accumulation in coastal sediments,” in Environmental remediation technologies for metal-contaminated soils. Eds. Hasegawa H., Rahman I. M. M., Rahman M. A. (Japan: Springer), 21–42. doi: 10.1007/978-4-431-55759-3_2
Sigel A., Sigel H., Sigel R. K. (2013). Cadmium: from toxicity to essentiality; metal ions in life sciences (The Netherlands: Springer: Dordrecht). doi: 10.1007/978-94-007-5179-8
Siscar R., Torreblanca A., Palanques A., Solé M. (2013). Metal concentrations and detoxification mechanisms in Solea solea and Solea senegalensis from NW Mediterranean fishing grounds. Mar. Poll. Bull. 77, 90–99. doi: 10.1016/j.marpolbul.2013.10.026
Solé M., Fortuny A., Mananos E. (2014). Effects of selected xenobiotics on hepatic and plasmatic biomarkers in juveniles of Solea senegalensis. Environ. Res. 135, 227–235. doi: 10.1016/j.envres.2014.09.024
Solé M., Lima D., Reis-Henriques M. A., Santos M. M. (2008). Stress biomarkers in juvenile Senegal sole, Solea senegalensis, exposed to the water-accommodated fraction of the “Prestige” fuel oil. bull. Environ. Contam. Toxicol. 80, 19–23. doi: 10.1007/s00128-007-9289-1
Solé M., Manzanera M., Bartolomé A., Tort L., Caixach J. (2013). Persistent organic pollutants (POPs) in sediments from fishing grounds in the NW Mediterranean: ecotoxicological implications for the benthic fish solea sp. Mar. Poll. Bull. 67, 158–165. doi: 10.1016/j.marpolbul.2012.11.018
Solé M., Vega S., Varó I. (2012). Characterization of type “B” esterases and hepatic CYP450 isoenzymes in Senegalese sole for their further application in monitoring studies. Ecotoxicol. Environ. Saf. 78, 72–79. doi: 10.1016/j.ecoenv.2011.11.013
Souid G., Souayed N., Yaktiti F., Maaroufi K. (2013). Effect of acute cadmium exposure on metal accumulation and oxidative stress biomarker of Sparus aurata. ecotoxicol. Environ. Saf. 89, 1–7. doi: 10.1016/j.ecoenv.2012.12.015
Stentiford G. D., Longshaw M., Lyons B. P., Jones G., Green M., Feist S. W. (2003). Histopathological biomarkers in estuarine fish species for the assessment of biological effects of contaminants. Mar. Environ. Res. 55, 137–159. doi: 10.1016/s0141-1136(02)00212-x
UNEP/MAP (2014). Report of the correspondence group on monitoring, pollution and litter (CORMON). monitoring guidance on ecological objective 9: contaminants (Athens (Greece: UNEP(DEPI)/MED WG.394/5 and 394/7), 8–9.
UNEP/RAMOGE (1999). Manual on the biomarkers recommended for the MED POL biomonitoring programme (Athens, Greece: UNEP), 40.
van der Oost R., Beyer J., Vermeulen N. P. E. (2003). Fish bioaccumulation and biomarkers in environmental risk assessment: a review. Environ. Toxicol. Pharmacol. 13, 57–149. doi: 10.1016/s1382-6689(02)00126-6
van Dyk J. C., Pieterse G. M., Van Vuren J. H. J. (2007). Histological changes in the liver of Oreochromis mossambicus (Cichlidae) after the exposure to cadmium and zinc. Ecotoxicol. Environ. Saf. 66, 432–440. doi: 10.1016/j.ecoenv.2005.10.012
Viarengo A., Lowe D., Bolognesi C., Fabbri E., Koehler A. (2007). The use of biomarkers in biomonitoring: a 2-tier approach assessing the level of pollutant-induced stress syndrome in sentinel organisms. Comp. Biochem. Physiol. 146C, 281–300. doi: 10.1016/j.cbpc.2007.04.011
Vicente-Martorell J. J., Galindo-Riaño M. D., García-Vargas M., Castro-Granado D. (2009). Bioavailability of heavy metals monitoring water, sediments and fish species from a polluted estuary. J. Hazard. Mater. 162, 823–836. doi: 10.1016/j.jhazmat.2008.05.106
Waeles M., Riso R. D., Maguer J.-F., Le Corre P. (2004). Distribution and chemical speciation of dissolved cadmium and copper in the Loire estuary and North Biscay continental shelf, France. Estuar. Coast. Shelf Sci. 59, 49–57. doi: 10.1016/j.ecss.2003.07.009
Zorita I., Cuevas N. (2014). Protocol for fish disease assessment in marine environmental monitoring using common sole (Solea solea, Linnaeus 1758) as sentinel organism: identification of externally visible diseases and liver histopathology Vol. 21 (AZTI-Tecnalia: Revista de Investigación Marina), 1.
Keywords: estuaries, sentinel species, sole, cadmium, biological effects, biomarkers, lysosomes, histopathology
Citation: Briaudeau T, Guerrero-Limón G, Zorita I, Marigómez I and Izagirre U (2023) Toxicopathic effects of waterborne Cd in sole juveniles, a prospective pollution monitoring sentinel for estuarine ecosystems. Front. Mar. Sci. 10:1182294. doi: 10.3389/fmars.2023.1182294
Received: 08 March 2023; Accepted: 29 June 2023;
Published: 04 August 2023.
Edited by:
Lucía Viñas Diéguez, Spanish Institute of Oceanography (IEO), SpainReviewed by:
Ketil Hylland, University of Oslo, NorwayCopyright © 2023 Briaudeau, Guerrero-Limón, Zorita, Marigómez and Izagirre. This is an open-access article distributed under the terms of the Creative Commons Attribution License (CC BY). The use, distribution or reproduction in other forums is permitted, provided the original author(s) and the copyright owner(s) are credited and that the original publication in this journal is cited, in accordance with accepted academic practice. No use, distribution or reproduction is permitted which does not comply with these terms.
*Correspondence: Tifanie Briaudeau, dGlmYW5pZS5icmlhdWRlYXVAZWh1LmV1cw==
Disclaimer: All claims expressed in this article are solely those of the authors and do not necessarily represent those of their affiliated organizations, or those of the publisher, the editors and the reviewers. Any product that may be evaluated in this article or claim that may be made by its manufacturer is not guaranteed or endorsed by the publisher.
Research integrity at Frontiers
Learn more about the work of our research integrity team to safeguard the quality of each article we publish.